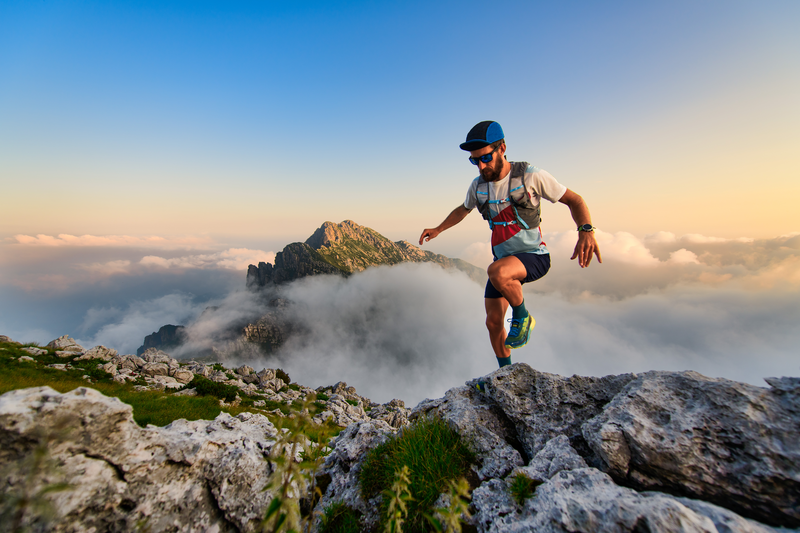
94% of researchers rate our articles as excellent or good
Learn more about the work of our research integrity team to safeguard the quality of each article we publish.
Find out more
ORIGINAL RESEARCH article
Front. For. Glob. Change
Sec. Forest Management
Volume 8 - 2025 | doi: 10.3389/ffgc.2025.1346224
The final, formatted version of the article will be published soon.
You have multiple emails registered with Frontiers:
Please enter your email address:
If you already have an account, please login
You don't have a Frontiers account ? You can register here
In southern China, four species of the Taxus genus are distributed, and identifying their suitable habitats plays an important role in species conservation. This study used the MaxEnt model to simulate and predict the potential suitable habitat distribution for these four species. The prediction model utilized 257 occurrence points from the four yew species, along with historical climate and topographic data, and incorporated the distribution of nature reserves in China. The model’s accuracy was validated using the Area Under the Receiver Operating Characteristic Curve (AUC) to compare the effect of different function models on prediction accuracy. The results showed that the AUC values for all four yew species exceeded 0.9, indicating a high level of reliability in the model predictions. The study results showed that the high-suitability area for Taxus wallichiana var. chinensis is 13,943.62 km², with nature reserves covering 7.49% of this area; Taxus wallichiana var. mairei has a high-suitability area of 11,950.97 km², with a coverage rate of 2.44%; Taxus wallichiana has a high-suitability area of 9,784.43 km², with a coverage rate of 10.52%; and Taxus yunnanensis has a high-suitability area of 7,226.57 km², with a coverage rate of 6.47%. The high-suitability areas for T. wallichiana var. chinensis are concentrated in the Qinling, Wuyi, and Hengduan mountain ranges, while T. wallichiana var. mairei has a broader distribution, primarily in the Wuyi, Qinling, Hengduan, and southeastern Himalayas. The distributions of T. wallichiana and T. yunnanensis are concentrated in the Hengduan Mountains and southeastern Himalayas.The study suggests expanding the network of protected areas, constructing ecological corridors, and introducing cultivation efforts to enhance population stability within high-suitability zones. For T. wallichiana var. mairei and T. wallichiana var. chinensis, it is recommended to attempt more reintroduction efforts, while for T. wallichiana and T. yunnanensis, habitat protection should be prioritized. The establishment of additional natural protected areas and the construction of ecological corridors linking these protected areas are important ways to strengthen the conservation of yew species.
Keywords: Taxus species, MAXENT model, Habitat suitability simulation, Nature reserve, receiver operating characteristic curve
Received: 29 Nov 2023; Accepted: 04 Feb 2025.
Copyright: © 2025 Zhou, Liu, Hu, Xie and Shao. This is an open-access article distributed under the terms of the Creative Commons Attribution License (CC BY). The use, distribution or reproduction in other forums is permitted, provided the original author(s) or licensor are credited and that the original publication in this journal is cited, in accordance with accepted academic practice. No use, distribution or reproduction is permitted which does not comply with these terms.
* Correspondence:
Mu Liu, College of Forestry, Jiangxi Agricultural University, Nanchang, Jiangxi Province, China
Juhua Hu, School of Computer and Information Engineering, Jiangxi Agricultural University, Nanchang, Jiangxi Province, China
Guanhong Xie, College of Forestry, Jiangxi Agricultural University, Nanchang, Jiangxi Province, China
Yakui Shao, Jiangsu Normal University, Xuzhou, 221116, Jiangsu Province, China
Disclaimer: All claims expressed in this article are solely those of the authors and do not necessarily represent those of their affiliated organizations, or those of the publisher, the editors and the reviewers. Any product that may be evaluated in this article or claim that may be made by its manufacturer is not guaranteed or endorsed by the publisher.
Research integrity at Frontiers
Learn more about the work of our research integrity team to safeguard the quality of each article we publish.