- 1Department of Forest and Conservation Sciences, Faculty of Forestry, University of British Columbia, Vancouver, BC, Canada
- 2Ts'msyen Nation, Prince Rupert, BC, Canada
- 3Department of Forest Ecosystems and Society, Oregon State University, Corvallis, OR, United States
Introduction
Common mycorrhizal networks (CMNs) are networks of mycorrhizal fungal hyphae held in common by at least two plants (Horton, 2015; Rillig et al., 2024) and were first discovered in the laboratory by Reid and Woods (1969) and later supported by Finlay and Read (1986) and Perry et al. (1989). Simard et al. (1997c) contributed to this knowledge by investigating underground transmission of carbon between ectomycorrhizal paper birch and Douglas-fir as well as arbuscular mycorrhizal western redcedar trees in the mixed temperate rainforests of western Canada. This body of pioneering work was followed by decades of creative peer-reviewed research by many scientists investigating the structure and function of CMNs in various forests around the world (see reviews including Newman, 1988; Simard et al., 2012; Horton, 2015; Tedersoo et al., 2020; Klein et al., 2023, and others). Research on the role of CMNs in the regenerative nature of forest ecosystems has recently come under targeted criticism by Karst et al. (2023), Henriksson et al. (2023), and Robinson et al. (2023). These criticism articles question the veracity and interpretations of the peer reviewed research. They were triggered by the memoir of Simard (2021), who told of how her experiences shaped her research, and what she thinks her findings about CMNs mean for forests in Canada. An important aspect of our roles as scientists is to ensure clarity of our research to support future informed thought and investigations. In the following paragraphs, we address some of the questions and perceptions about CMNs raised by these authors.
Context
A brief review of the four main questions scientifically investigated about CMNs in forests provide context for our response to the criticisms. Notably, the three criticism articles generally dismiss evidence for all four questions. The first question, whether CMNs exist in forest ecosystems, has been investigated over the past five decades using increasingly sophisticated tools, from microscopy to DNA sequencing, microsatellites, and isotopic tracing. These studies, in our view, have revealed that CMNs can connect the roots of trees with other trees (see Figure 1; Beiler et al., 2010), as well as with compatible seedlings, shrubs, or mycoheterotrophic herbs (Selosse et al., 2006; Tedersoo et al., 2020; Authier et al., 2022; Merckx et al., 2024). The second question investigated has been whether CMNs facilitate nutrient, carbon, water or infochemical transfer among trees. This has also been demonstrated, and most studies show multiple belowground pathways functioning simultaneously, including CMNs, mycorrhizal roots, and soil (Simard et al., 1997a,c; Song et al., 2015; Horton, 2015; Klein et al., 2016). The third question, how resource transfer between trees varies in forests, has been investigated using field and associated greenhouse and lab studies. These studies have demonstrated that transfer is affected by a range of factors in forests, including the light, water, nutrient and health status of donor and recipient trees (Simard et al., 1997c; Teste et al., 2010; Song et al., 2015; Klein et al., 2016), and the characteristics of the fungal species in the CMN (Teste et al., 2009; Merckx et al., 2024). A fourth question, whether membership in the CMN affects performance of trees, has also been investigated and results reveal this is also context dependent, as would be expected in complex systems like forests (Levin, 2005). Nevertheless, evidence exists that linking into CMNs in forests can affect establishment of seedlings (Booth and Hoeksema, 2010; Teste et al., 2009; Bingham and Simard, 2011), growth or carbon status of mature trees (Klein et al., 2016; Birch et al., 2021), and performance of mycoheterotrophic plants (Selosse et al., 2006).
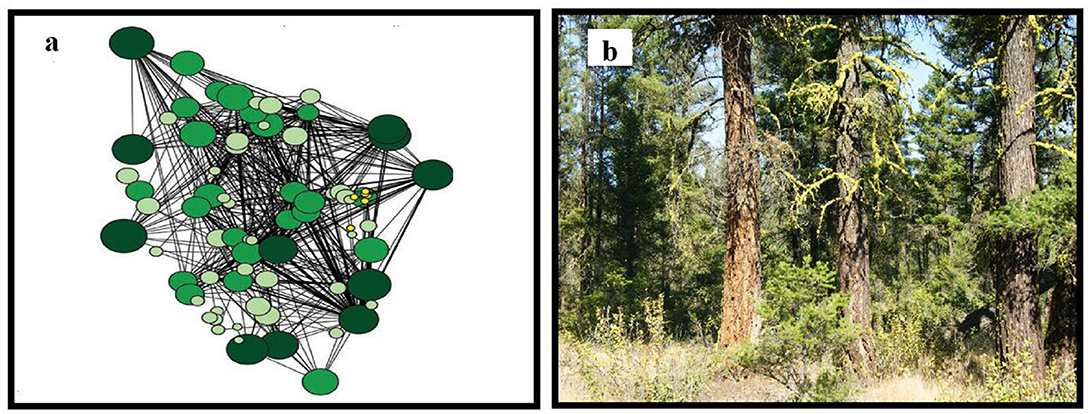
Figure 1. Temperate interior Douglas-fir forest in British Columbia with mutigenerational age and multilayered size structure. The panel includes: (a) map of CMN typology from Beiler et al. (2010), where interior Douglas-fir trees are connected through shared colonization of Rhizopogon spp. genets in a 30 m × 30 m plot (green circles = Douglas-fir, sized relative to stem diameter; black lines = genet linkages). (b) Interior Douglas-fir forest typical of where CMN mapping was conducted (photo credit: W. J. Roach).
This body of research investigating the structure and function of CMNs has fundamentally shifted how scientists understand forests (Perry et al., 2008), not only as collections of individual trees and plants competing for resources (Nyland, 2016), but as connected systems of multiple complex interactions (Simard et al., 2012; Beiler et al., 2015). Whenever there is such a fundamental shift in knowledge, however, there is resistance (Rowell, 2017), and the three critiques by Karst et al. (2023), Henriksson et al. (2023), and Robinson et al. (2023) generally conclude this body of research is inadequate to inform our understanding of forests. In the following paragraphs, we discuss the main points raised by these authors, with more detailed responses to select statements in Table 1.
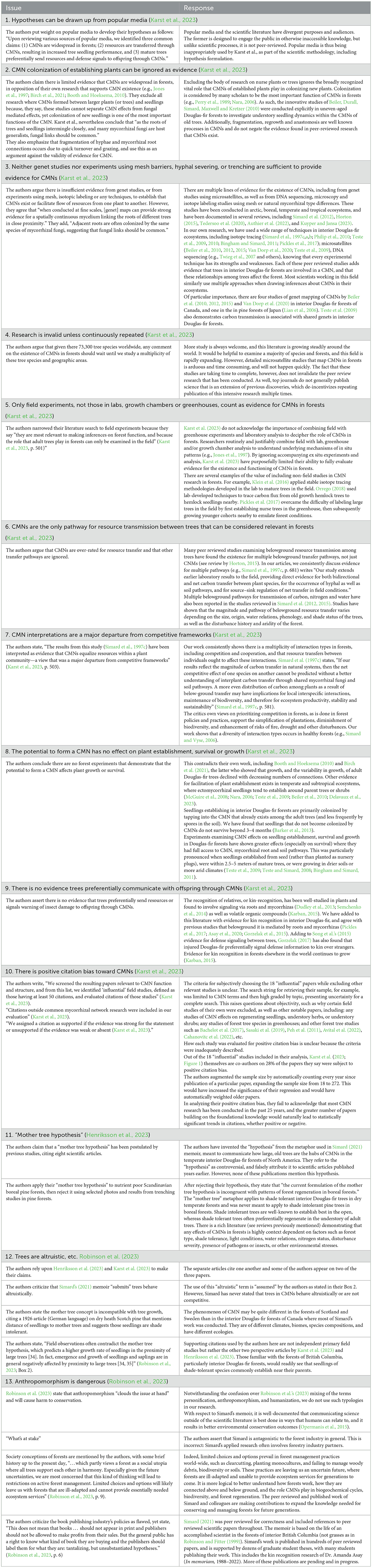
Table 1. Issues raised in Karst et al. (2023), Henriksson et al. (2023), and Robinson et al. (2023).
Discussion
In the first paper, Karst et al. (2023) provide negative commentary against public media interpretations of the memoir of Simard (2021) to open their case that there is a positive citation bias regarding the role of CMNs in forests. They argue there is insufficient evidence that CMNs contribute to regeneration processes in forests. There are several weaknesses in their analysis as follows. First, their search of CMN research in the primary literature (Reporting Summary, https://www.nature.com/articles/s41559-023-01986-1#additional-information) is narrow and incomplete. As detailed in Table 1, they rely on subjective evaluations to determine which 18 studies to choose for their analysis, and then use unclear criteria to determine whether these papers have a positive bias. For example, they choose to exclude all studies investigating nurse tree facilitation of seedling establishment because they argue CMN effects cannot be disentangled from mycorrhizal colonization benefits (Table 1). However, scholars have argued facilitation of seedling establishment is likely the most important function of CMNs in natural ecosystems (Perry et al., 1989; Nara, 2006; Horton, 2015; Rillig et al., 2024). For each of the 18 selected papers, Karst et al. (2023) automatically counted the number of years in the paper's publication record, which augmented their sample size to 273 and increased the power of their tests. The rationale for this enhancement of sample size was vague and lacked evidence that each year was represented by a citation bias in the literature. In their analysis, they also failed to adjust their methods to account for the inherent growth of sample size over time as researchers increase numbers of investigations in an emergent field. Regardless, whether there is any citation bias in the literature, this does not override the fact that studies show all tree species are mycorrhizal dependent and CMN-dependent facilitation and transfer have been demonstrated (Klein et al., 2023; Rillig et al., 2024). It is unfortunate these key points were overlooked in the review process.
Karst et al. (2023) further imply that CMN-related field studies discount the role of alternative belowground resource transfer pathways to CMNs, such as through the soil or disconnected networks. Karst's criticism is echoed by Robinson et al. (2023) and Henriksson et al. (2023). However, as shown repeatedly by Simard et al. (1997a,c), Simard et al. (2012), and verified by others as detailed above, belowground transfer among trees occurs through multiple belowground transfer pathways simultaneously. All of these studies discussed alternative explanations to CMNs, contrary to the criticisms they did not. Regardless of this, Simard et al. (1997c) found that a fraction (18%) of carbon isotope was transmitted to arbuscular mycorrhizal western redcedar compared with that between ectomycorrhizal paper birch and Douglas-fir, providing experimental evidence for carbon transfer through the CMN and simultaneously and to a lesser degree through the soil. Here, all three species had comparably vigorous and overlapping mycorrhizal roots, as naturally occurred in the surrounding forest (Simard et al., 1997c; Twieg et al., 2007), rendering results that were realistic of the natural system. If, as Karst et al. (2023) imply, transfer patterns in Simard et al. (1997c) could be accounted for by differing root densities, and hence the gathering power of the different species, logic dictates that arbuscular mycorrhizal cedar growing among the two ectomycorrhizal trees would gather only one-fifth of the soil resources going to the ectomycorrhizal trees. Given the comparable health and vigor of the cedar roots and shoots to the other species of seedlings in the study, however, this is highly unlikely. Moreover, Karst's implication that the existence of one pathway rules out another, when they claim that transfer via mycorrhizal fungi had not been shown here in the field, misses the complexity of forest ecosystems.
The three criticism articles imply that the carbon transfer measured would be insignificant to plants because they say isotopes in these studies did not enter recipient shoots (Karst et al., 2023; Henriksson et al., 2023; Robinson et al., 2023). However, our studies have repeatedly demonstrated that significant amounts of carbon are transferred into both shoots and roots of recipient seedlings through CMNs and other pathways (e.g., Simard et al., 1997a,b,c; Teste et al., 2009; Philip et al., 2010; Song et al., 2015). In keeping with these findings, Klein et al. (2016) and Cahanovitc et al. (2022) have also found transfer into shoots of trees. This type of transfer has been associated with establishment of seedlings and mycoheterotrophic plants (see above; Van Der Heijden and Horton, 2009; Rillig et al., 2024; Merckx et al., 2024). Moreover, the requirement of transfer into shoots assumes that carbon supply is the limiting factor in the photosynthetic process, and dismisses the likelihood that carbon subsidies to roots and associated mycorrhizae enhance the gathering of soil nutrients essential for photosynthesis. Perry and Oetter (2024) found that growth of Douglas-fir in low light was limited by magnesium, not carbon supply, indicating the essential role of mycorrhizas in productivity. As with other issues, the critics are not looking at the bigger picture.
Karst et al. (2023) further argued that our research ignores the important role of competition in forest dynamics, possibly because CMN research in general has generated interpretations regarding cooperative relationships from trading of resources or infochemicals. However, none of our articles negate the process of competition in forests. Instead, we repeatedly discuss that multiple types of species interactions occur simultaneously, including competition, and that understanding forest dynamics must account for this complexity (Perry et al., 1989; Simard et al., 1997c, 2012; Simard and Vyse, 2006). This complexity is readily evident in the mature, multicohort, multi-storied temperate forests where these experiments were conducted, where seedlings readily establish in the understory of old trees following small scale natural disturbances (Figure 1). In younger plantation forests absent of old trees and where competition may be less intense, CMNs may play a lesser role in forest dynamics. Nevertheless, many of our experiments tracing resource transfer between trees through belowground pathways have been conducted in recently planted forests (e.g., Simard et al., 1997c; Teste et al., 2009), and we have also successfully mapped the architecture of CMNs in young Douglas-fir forests (Van Dorp et al., 2020), suggesting CMNs also exist in young temperate forests.
Our discovery of kin recognition in trees has also been discounted by Karst et al. (2023) because the precise belowground mechanism by which trees recognize their genetic relatives remains elusive (Gorzelak et al., 2015; Pickles et al., 2017; Asay et al., 2020). This dismissal is short-sighted because the novel discovery could, for example, lead to a greater diversity of reforestation practices in western Canada, which currently relies heavily on clearcutting with artificial regeneration (Simard and Vyse, 2006). By providing support for natural regenerative processes as an alternative or supplement to even-aged plantations, which are vulnerable to climate-related failures and wildfires (Clason et al., 2022), this finding could help in a transition toward more resilient regeneration methods, such as use of overstory retention (Franklin et al., 2018; Simard et al., 2020). In a large-scale experiment crossing a 900-km climate gradient in interior Douglas-fir forests (Simard et al., 2020; Roach et al., 2021), for example, Simard et al. (2021) and Harris et al. (2025) have found that increasing levels of overstory retention facilitate regeneration success in increasingly arid regional climates.
Henriksson et al. (2023) mistakenly compare our work in interior Douglas-fir forests of Canada to boreal pine forests in Europe, which have not been part of our research. The individual papers we have published provide results applicable to the temperate forests where the studies occurred (e.g., Simard et al., 1997c; Teste et al., 2009; Beiler et al., 2010; Bingham and Simard, 2011). It is well-known that different species in specific ecosystems behave differently, and studies in the boreal pine forests of Scandinavia (Henriksson et al., 2023) may thus not find that CMNs play the same role as shade tolerant Douglas-firs in British Columbia (Beiler et al., 2015). Shade-intolerant pines in boreal forests, for example, require open conditions, whereas shade-tolerant Douglas-firs in dry temperate forests of Canada naturally regenerate in the shade and CMNs of parent trees. Our results are place-based and context dependent, and the absence or difference of a phenomenon in one forest does not negate its validity elsewhere (Zahra et al., 2021).
In the same vein as Henriksson et al. (2023), Karst et al. (2023), and Robinson et al. (2023) suggest our peer reviewed articles on belowground carbon transfer in temperate Douglas-fir forests of Canada inappropriately generalize to forests elsewhere in the world. This is incorrect; we have not made such statements in the literature. The evidence is clear, however, that mycorrhizas play a strong role in the nature of interactions among trees. For example, large analyses of global temperate and tropical forest biomes suggest there is strong positive feedback among conspecifics in ectomycorrhizal forests, but negative feedback in arbuscular mycorrhizal forests (Bennett et al., 2017; Pither et al., 2018; Delavaux et al., 2023). These authors suggest that mycorrhizal colonization by conspecifics (Bennett et al., 2017; Pither et al., 2018) or CMNs (Zahra et al., 2021; Delavaux et al., 2023) may play a role in structuring these disparate forest types. Further hypothesis testing is needed to clarify the role of CMNs in different forest biomes, disturbance histories, and stand structures. It is important to move forward in understanding these phenomena, which could be important in developing nature-based solutions to climate change (Drever et al., 2021).
Robinson et al. (2023) erroneously refer to “the mother tree hypothesis,” and claim that it is harmful to forests of Europe. However, they have misused the metaphor of the mother tree in Simard's (2021) memoir meant for conveying scientific meaning to the public about the regenerative nature of interior Douglas-fir forests in Canada. Robinson et al. (2023) also refer to “recent reviews” to support their claims, but only cite those of Henriksson et al. (2023) and Karst et al. (2023). Robinson et al. (2023) further conflate two different types of mycorrhizas—arbuscular- and ecto-mycorrhizas—in comparing CMN carbon and nutrient transfer mechanisms to our work, which is specifically on ectomycorrhiza in Douglas-fir forests of Canada. These two types of mycorrhizal fungi are evolutionarily divergent with distinct hosts and ecological functions (Bennett et al., 2017; Haq et al., 2024).
Karst et al. (2023) suggest a strict set of requirements that must be met before any research on CMNs be considered valid in forests, but these are unrealistic to satisfy in the field given the relational, variable and dynamic nature of forests (Klein et al., 2023; Rillig et al., 2024). While it is not uncommon that lab tests or greenhouse experiments need reconciling with ex-situ methodologies, this is true for any scientific study of complex natural systems, and does not mean discarding valid investigation. Indeed, any researcher examining relationships in complex systems such as forests must confront the limitations of their experimentation and invoke a version of Occam's Razor to interpret their findings. It is the responsibility of anyone criticizing research to offer alternative explanations that have a probability greater than those interpreted from the published experiments. However, Karst et al. (2023) do not, nor do they provide new information that warrants discounting peer reviewed research. A more recent investigation proposes expanding terminology to identify mycorrhizal-dependent direct and indirect effects, but this does not diminish research investigating the role of CMNs in forest ecosystems (Rillig et al., 2024).
Finally, “Finding the Mother Tree” (Simard, 2021) was written in laypeople's terms so the public can critically assess whether industrial forest management, focused on timber extraction (Nyland, 2016; Ashton and Kelty, 2018), is serving the interests of our collective wellbeing (Mazzocchi, 2012). Forests are best understood through the multitude of relationships, connections, interdependencies and feedbacks that shape them, and CMNs are part of these processes (Perry et al., 1989; Simard et al., 2012; Beiler et al., 2015; Bennett et al., 2017). The intent of Simard (2021) was to engage people in how CMNs help shape temperate forests, and whether the unraveling of these connections and relationships through industrial forest management is worth the risks of biodiversity loss and climate change (Moomaw et al., 2019; IPCC, 2023; Ripple et al., 2024; Betts et al., 2024), or whether more holistic approaches are needed (Mazzocchi, 2012; Filotas et al., 2014; Sands et al., 2023; Robinson et al., 2021; Drever et al., 2021; Mina et al., 2022). Metaphors such as “mother tree” were used to help make these concepts more relatable to the public. Indeed, tree metaphors have been deeply understood in cultures worldwide for millennia (Blicharska and Mikusiński, 2014; Arnold, 2021), as well as in western scientific literature [e.g., the use of “family” in taxonomy; the translation, “mother,” for the scientific genus name of hemlock, Tsuga (Pojar and MacKinnon, 1994; Farjon, 2010)]. Moreover, many Indigenous communities have place-based cultural practices that depict their ancient understanding of relationships in forests, including of elder trees or mother trees (Baumflek et al., 2021; Ryan, 2014; Turner, 2008; Turner et al., 2000; Wickham et al., 2022), and these could inform culturally revitalized land stewardship practices as climate changes (Charnley et al., 2007).
In summary, we argue that our peer-reviewed literature on CMNs stands up against the targeted criticisms of Karst et al. (2023), Henriksson et al. (2023), and Robinson et al. (2023), which appear to distort then undermine peer-reviewed findings. Their claims that our scientific articles (i) discount the role of alternative transfer pathways to CMNs, (ii) negate the importance of competition in forests, (iii) did not find carbon transfer to shoots, or (iv) that we extended our findings to all forests, are simply not true. These criticism articles fail to provide any new experimental evidence that would discount the peer reviewed literature. In our view, our research has contributed to a meaningful paradigm shift in how we view forests and this may be important for developing more holistic approaches for protecting, restoring and managing forests in our changing climate.
Author contributions
SS: Writing – original draft. TR: Writing – review & editing. DP: Writing – review & editing.
Funding
The author(s) declare that no financial support was received for the research, authorship, and/or publication of this article.
Conflict of interest
The authors declare that the research was conducted in the absence of any commercial or financial relationships that could be construed as a potential conflict of interest.
The reviewer MH declared a shared affiliation with the author(s) SS and TR to the handling editor at the time of review.
Generative AI statement
The author(s) declare that no Gen AI was used in the creation of this manuscript.
Publisher's note
All claims expressed in this article are solely those of the authors and do not necessarily represent those of their affiliated organizations, or those of the publisher, the editors and the reviewers. Any product that may be evaluated in this article, or claim that may be made by its manufacturer, is not guaranteed or endorsed by the publisher.
References
Arnold, C. (2021). Reciprocal relationships with trees: rekindling indigenous wellbeing and identity through the Yuin ontology of oneness. Aust. Geog. 52, 131–147. doi: 10.1080/00049182.2021.1910111
Asay, A. K., Simard, S. W., and Dudley, S. A. (2020). Altering neighborhood relatedness and species composition affects interior Douglas-Fir size and morphological traits with context-dependent responses. Front. Ecol. Evol. 8:578524. doi: 10.3389/fevo.2020.578524
Ashton, M. S., and Kelty, M. J. (2018). The Practice of Silviculture: Applied Forest Ecology. Hoboken, NJ: John Wiley and Sons.
Authier, L., Violle, C., and Richard, F. (2022). Ectomycorrhizal networks in the anthropocene: from natural ecosystems to urban planning. Front. Plant Sci. 13:900231. doi: 10.3389/fpls.2022.900231
Avital, S., Rog, I., Livne-Luzon, S., Cahanovitc, R., and Klein, T. (2022). Asymmetric belowground carbon transfer in a diverse tree community. Mol. Ecol. 31, 3481–3495. doi: 10.1111/mec.16477
Bachelot, B., Uriarte, M., McGuire, K. L., Thompson, J., and Zimmerman, J. (2017). Arbuscular mycorrhizal fungal diversity and natural enemies promote coexistence of tropical tree species. Ecology 98, 712–720. doi: 10.1002/ecy.1683
Barker, J. S., Simard, S. W., Jones, M. D., and Durall, D. M. (2013). Ectomycorrhizal community fungal assembly on regenerating Douglas-fir after wildfire and clearcut harvesting. Oecologia 172, 1179–1189. doi: 10.1007/s00442-012-2562-y
Baumflek, M., Kassam, K. A., Ginger, C., and Emery, M. R. (2021). Incorporating biocultural approaches in forest management: insights from a case study of Indigenous plant stewardship in Maine, USA and New Brunswick, Canada. Soc. Nat. Res. 34, 1155–1173. doi: 10.1080/08941920.2021.1944411
Beiler, K. J., Durall, D. M., Simard, S. W., Maxwell, S. A., and Kretzer, A. M. (2010). Architecture of the wood-wide web: Rhizopogon spp. genets link multiple Douglas-fir cohorts. New Phyt. 185, 543–553. doi: 10.1111/j.1469-8137.2009.03069.x
Beiler, K. J., Simard, S. W., and Durall, D. M. (2015). Topology of Rhizopogon spp. mycorrhizal meta- networks in xeric and mesic old-growth interior Douglas-fir forests. J. Ecol. 103, 616–628. doi: 10.1111/1365-2745.12387
Beiler, K. J., Simard, S. W., Lemay, V., and Durall, D. M. (2012). Vertical partitioning between sister species of Rhizopogon fungi on mesic and xeric sites in an interior Douglas-fir forest. Mol. Ecol. 21, 6163–6174. doi: 10.1111/mec.12076
Bennett, J. A., Maherali, H., Reinhart, K. O., Lekberg, Y., Hart, M. M., Klironomos, J., et al. (2017). Plant-soil feedbacks and mycorrhizal type influence temperate forest population dynamics. Science 355, 181–184. doi: 10.1126/science.aai8212
Betts, M. G., Yang, Z., Gunn, J. S., and Healey, S. P. (2024). Congruent long-term declines in carbon and biodiversity are a signature of forest degradation. Glob. Change Biol. 30:e17541. doi: 10.1111/gcb.17541
Bingham, M. A., and Simard, S. W. (2011). Do mycorrhizal network benefits to survival and growth of interior Douglas-fir seedlings increase with soil moisture stress? Ecol. Evol. 1, 306–316. doi: 10.1002/ece3.24
Birch, J. D., Simard, S. W., Beiler, K. J., and Karst, J. (2021). Beyond seedlings: ectomycorrhizal fungal networks and growth of mature Pseudotsuga menziesii. J. Ecol. 109, 806–818. doi: 10.1111/1365-2745.13507
Blicharska, M., and Mikusiński, G. (2014). Incorporating social and cultural significance of large old trees in conservation policy. Cons. Biol. 28, 1558–1567. doi: 10.1111/cobi.12341
Booth, M. G., and Hoeksema, J. D. (2010). Mycorrhizal networks counteract competitive effects of canopy trees on seedling survival. Ecology 91, 2294–2302. doi: 10.1890/09-1139.1
Cahanovitc, R., Livne-Luzon, S., Angel, R., and Klein, T. (2022). Ectomycorrhizal fungi mediate belowground carbon transfer between pines and oaks. ISME J. 16:5. doi: 10.1038/s41396-022-01193-z
Charnley, S., Fischer, A. P., and Jones, E. T. (2007). Integrating traditional and local ecological knowledge into forest biodiversity conservation in the Pacific Northwest. For. Ecol. Manage. 246, 14–28. doi: 10.1016/j.foreco.2007.03.047
Clason, A. J., Farnell, I., and Lilles, E. B. (2022). Carbon 5–60 years after fire: planting trees does not compensate for losses in dead wood stores. Front. For. Glob. Change 5:868024. doi: 10.3389/ffgc.2022.868024
Delavaux, C. S., LaManna, J. A., Myers, J. A., Phillips, R. P., Aguilar, S., Allen, D., et al. (2023). Mycorrhizal feedbacks influence global forest structure and diversity. Comm. Biol. 6, 1–11. doi: 10.1038/s42003-023-05410-z
Drever, C. R., Cook-Patton, S. C., Akhter, F., Badiou, P. H., Chmura, G. L., Davidson, S. J., et al. (2021). Natural climate solutions for Canada. Sci. Adv. 7:eabd6034. doi: 10.1126/sciadv.abd6034
Dudley, S. A., Murphy, G. P., and File, A. L. (2013). Kin recognition and competition in plants. Funct. Ecol. 27, 898–906. doi: 10.1111/1365-2435.12121
Filotas, E., Parrott, L., Burton, P. J., Chazdon, R. L., Coates, K. D., Coll, L., et al. (2014). Viewing forests through the lens of complex systems science. Ecosphere 5, 1–23. doi: 10.1890/ES13-00182.1
Finlay, R., and Read, D. J. (1986). The structure and function of the vegetative mycelium of ectomycorrhizal plants. I. Translocation of 14C-labeled carbon between plants interconnected by a common mycelium. New Phytol. 103, 143–156. doi: 10.1111/j.1469-8137.1986.tb00603.x
Franklin, J. F., Johnson, K. N., and Johnson, D. L. (2018). Ecological Forest Management. Longrove, IL: Waveland Press.
Gorzelak, M. A. (2017). Kin-selected signal transfer through mycorrhizal networks in Douglas-fir (Doctoral dissertation). University of British Columbia.
Gorzelak, M. A., Asay, A. K., Pickles, B. J., and Simard, S. W. (2015). Inter-plant communication through mycorrhizal networks mediates complex adaptive behaviour in plant communities. AoB Plants 7:plv050. doi: 10.1093/aobpla/plv050
Haq, H., Hauer, A., Singavarapu, B., Christel, H., Cesarz, S., Eisenhauer, N., et al. (2024). The interactive effect of tree mycorrhizal type, mycorrhizal type mixture and tree diversity shapes rooting zone soil fungal communities in temperate forest ecosystems. Funct. Ecol. 1–14. doi: 10.1111/1365-2435.14651
Harris, T. C., Roach, J. W., Miller, E. M., and Simard, S. W. (2025). The interactive role of climatic transfer distance and overstory retention on Douglas-fir seedling survival and height growth in interior British Columbia. Glob. Change Biol. doi: 10.1111/gcb.70027
Henriksson, N., Marshall, J., Högberg, M. N., Högberg, P., Polle, A., Franklin, O., et al. (2023). Re-examining the evidence for the mother tree hypothesis – resource sharing among trees via ectomycorrhizal networks. New Phyt. 239, 19–28. doi: 10.1111/nph.18935
IPCC (2023). Climate Change 2023: Synthesis Report. Geneva: Sixth Assessment Report of the Intergovernmental Panel on Climate Change.
Jones, M. D., Durall, D. M., Harniman, S. M. K., Classen, D. C., Simard, S. W., et al. (1997). Ectomycorrhizal diversity on Betula papyrifera and Pseudotsuga menziesii seedlings grown in the greenhouse or outplanted in single-species and mixed plots in southern BC. Can. J. For. Res. 27, 1872–1889. doi: 10.1139/x97-160
Karst, J., Jones, M. D., and Hoeksema, J. D. (2023). Positive citation bias and overinterpreted results lead to misinformation on common mycorrhizal networks in forests. Nat. Ecol. Evol. 7:4. doi: 10.1038/s41559-023-01986-1
Klein, T., Rog, I., Livne-Luzon, S., van der Heijden, M. G. A., and Körner, C. (2023). Belowground carbon transfer across mycorrhizal networks among trees: Facts, not fantasy. Open Res. Europe 3:168doi: 10.12688/openreseurope.16594.1
Klein, T., Siegwolf, R. T. W., and Körner, C. (2016). Belowground carbon trade among tall trees in a temperate forest. Science 352, 342–344. doi: 10.1126/science.aad6188
Kuyper, T., and Jansa, J. (2023). Arbuscular mycorrhiza: advances and retreats in our understanding of the ecological functioning of the mother of all root symbioses. Plant Soil 489, 1–48. doi: 10.1007/s11104-023-06045-z
Levin, S. (2005). Self-organization and the emergence of complexity in ecological systems. Bioscience 55, 1075–1079. doi: 10.1641/0006-3568(2005)055[1075:SATEOC]2.0.CO;2
Lian, C., Narimatsu, M., Nara, K., and Hogetsu, T. (2006). Tricholoma matsutake in a natural Pinus densiflora forest. New Phyt. 171, 825–836. doi: 10.1111/j.1469-8137.2006.01801.x
Mazzocchi, F. (2012). Complexity and the reductionism–holism debate in systems biology. WIREs Syst. Biol. Med. 4, 413–427. doi: 10.1002/wsbm.1181
McGuire, K. L., Henkel, T. W., Cerda, I. G. D., Villa, G., Edmund, F., and Andrew, C. (2008). Dual mycorrhizal colonization of forest-dominating tropical trees and the mycorrhizal status of non-dominant tree and liana species. Mycorrhiza 18, 217–222. doi: 10.1007/s00572-008-0170-9
Merckx, V. S. F. T., Gomes, S. I. F., Wang, D., Verbeek, C., Jacquemyn, H., Zahn, F. E., et al. (2024). Mycoheterotrophy in the wood-wide web. Nat. Plants 10, 710–718. doi: 10.1038/s41477-024-01677-0
Mina, M., Messier, C., Duveneck, M. J., Fortin, M.-J., and Aquilué, N. (2022). Managing for the unexpected: building resilient forest landscapes to cope with global change. Glob. Change Biol. 28, 4323–4341. doi: 10.1111/gcb.16197
Moomaw, W. R., Masino, S. A., and Faison, E. K. (2019). Intact forests in the US: Proforestation mitigates climate change and serves the greatest good. Front. For. Glob. Change 2:27. doi: 10.3389/ffgc.2019.00027
Nara, K. (2006). Ectomycorrhizal networks and seedling establishment during early primary succession. New Phyt. 169, 169–178. doi: 10.1111/j.1469-8137.2005.01545.x
Newman, E. I. (1988). Mycorrhizal links between plants: their functioning and ecological significance. Adv. Ecol. Res. 18, 243–270. doi: 10.1016/S0065-2504(08)60182-8
Nyland, R. D. (2016). Silviculture Concepts and Applications, 3rd Edn. Long Grove, IL: Waveland Press, Inc.
Opermanis, O., Kalnins, S. N., and Aunins, A. (2015). Merging science and arts to communicate nature conservation. J. Nat. Cons. 28, 67–77. doi: 10.1016/j.jnc.2015.09.005
Orrego, G. (2018). Western Hemlock Regeneration on Coarse Woody Debris Is Facilitated by Linkage Into a Mycorrhizal Network in an Old-Growth Forest (M.Sc. thesis). UBC, Vancouver, BC.
Peh, K., Lewis, S. L., and Lloyd, J. (2011). Mechanisms of monodominance in diverse tropical tree-dominated systems. J. Ecol. 99, 891–898. doi: 10.1111/j.1365-2745.2011.01827.x
Perry, D. A., Amaranthus, M. P., Borchers, J. G., Borchers, S. L., and Brainerd, R. E. (1989). Bootstrapping in ecosystems: Internal interactions largely determine productivity and stability in biological systems with strong positive feedback. Bioscience 39, 230–237. doi: 10.2307/1311159
Perry, D. A., and Oetter, D. R. (2024). Thirty years of forest productivity in a mountainous landscape: The Yin and Yang of topography. Ecosphere 15:e4865. doi: 10.1002/ecs2.4865
Perry, D. A., Oren, R., and Hart, S. C. (2008). Forest Ecosystems. Baltimore, MD: The Johns Hopkins University Press.
Philip, L., Simard, S., and Jones, M. D. (2010). Pathways for below-ground carbon transfer between paper birch and Douglas-fir seedlings. Plant Ecol. Divers. 3, 221–233. doi: 10.1080/17550874.2010.502564
Pickles, B. J., Wilhelm, R., Asay, A. K., Hahn, A. S., Simard, S. W., and Mohn, W. W. (2017). Transfer of 13C between paired Douglas-fir seedlings reveals plant kinship effects and uptake of exudates by ectomycorrhizas. New Phyt. 214, 400–411. doi: 10.1111/nph.14325
Pither, J., Pickles, B. J., Simard, S. W., Ordonez, A., and Williams, J. W. (2018). Below-ground biotic interactions moderated the postglacial range dynamics of trees. New Phytol. 220, 1148–1160. doi: 10.1111/nph.15203
Pojar, J., and MacKinnon, A. (1994). Plants of Coastal British Columbia. Vancouver, BC: Lone Pine Publishing.
Reid, C. P. P., and Woods, F. W. (1969). Translocation of 14C-labeled compounds in mycorrhizae and its implications in interplant nutrient cycling. Ecology 50, 179–187. doi: 10.2307/1934844
Rillig, M. C., Lehmann, A., Lanfranco, L., Caruso, T., and Johnson, D. (2024). Clarifying the definition of common mycorrhizal networks. Funct. Ecol. 2024, 1–7. doi: 10.1111/1365-2435.14545
Ripple, W. J., Wolf, C., van Vuuren, D. P., Gregg, J. W., and Lenzen, M. (2024). An environmental and socially just climate mitigation pathway for a planet in peril. Env. Res. Lett. 19:021001. doi: 10.1088/1748-9326/ad059e
Roach, W. J., Simardm, S. W., Defrenne, C. E., Pickles, B. J., Lavkulich, L. M., and Ryan, T. L. (2021). Tree diversity, site index, and carbon storage decrease with aridity in Douglas-fir forests in western Canada. Front. For. Glob. Change 4:682076. doi: 10.3389/ffgc.2021.682076
Robinson, D. G., Ammer, C., Polle, A., Bauhus, J., Aloni, R., Annighöfer, P., et al. (2023). Mother trees, altruistic fungi, and the perils of plant personification. Trends Plant Sci. 29, 20–31. doi: 10.1016/j.tplants.2023.08.010
Robinson, D. G., and Fitter, A. (1999). The magnitude and control of carbon transfer between plants linked by a common mycorrhizal network. J. Exp. Bot. 50, 9–13. doi: 10.1093/jxb/50.330.9
Robinson, J. M., Gellie, N., MacCarthy, D., Mills, J. G., O'Donnell, K., and Redvers, N. (2021). Traditional ecological knowledge in restoration ecology: a call to listen deeply, to engage with, and respect Indigenous voices. Rest. Ecol. 29:e13381. doi: 10.1111/rec.13381
Rowell, A. (2017). Green Backlash: Global Subversion of the Environmental Movement. New York, NY: Routledge.
Ryan, T. L. (2014). Territorial Jurisdiction: The Cultural and Economic Significance of Eulachon Thaleichthys pacificus in the North-Central Coast Region of British Columbia (PhD Diss.). UBC, Vancouver, BC.
Sands, B., Machado, M. R., White, A., Zent, E., and Gould, R. (2023). Moving towards an anti-colonial definition for regenerative agriculture. Agric. Hum. Values. 40, 1697–1716. doi: 10.1007/s10460-023-10429-3
Sasaki, T., Konno, M., Hasegawa, Y., Imaji, A., Terabaru, M., Nakamura, R., et al. (2019). Role of mycorrhizal associations in tree spatial distribution patterns based on size class in an old-growth forest. Oecologia 189, 971–980. doi: 10.1007/s00442-019-04376-2
Selosse, M.-A., Richard, F., He, X., and Simard, S. W. (2006). Mycorrhizal networks: Des liaisons dangereuses? Trends Ecol. Evol. 21, 621–628. doi: 10.1016/j.tree.2006.07.003
Semchenko, M., Saar, S., and Lepik, A. (2014). Plant root exudates mediate neighbour recognition and trigger complex behavioural changes. New Phyt. 204, 631–637. doi: 10.1111/nph.12930
Simard, S., and Vyse, A. (2006). Trade-offs between competition and facilitation: a case study of vegetation management in the interior cedar–hemlock forests of southern British Columbia. Can. J. For. Res. 36, 2486–2496. doi: 10.1139/x06-150
Simard, S. W., Asay, A. K., Beiler, K. J., Bingham, M. A., Deslippe, J. R., He, X., et al. (2015). “Resource transfer between plants through ectomycorrhizal networks. Ecol. Stud. 224, 133–176. doi: 10.1007/978-94-017-7395-9_5
Simard, S. W., Beiler, K. J., Bingham, M. A., Deslippe, J. R., Philip, L. J., and Teste, F. P. (2012). Mycorrhizal networks: mechanisms, ecology and modelling. Fung. Biol. Rev. 26, 39–60. doi: 10.1016/j.fbr.2012.01.001
Simard, S. W., Durall, D. M., and Jones, M. D. (1997b). Carbon allocation and carbon transfer between Betula papyrifera and Pseudotsuga menziesii seedlings using a 13C pulse-labeling method. Plant Soil. 191, 41–55.
Simard, S. W., Jones, M. D., Durall, D. M., Perry, D. A., Myrold, D. D., and Molina, R. (1997a). Reciprocal transfer of carbon isotopes between ectomycorrhizal Betula papyrifera and Pseudotsuga menziesii. New Phytol. 137, 529–542. doi: 10.1046/j.1469-8137.1997.00834.x
Simard, S. W., Perry, D. A., Jones, M. D., Myrold, D. D., Durall, D. M., and Molina, R. (1997c). Net transfer of carbon between ectomycorrhizal tree species in the field. Nature 388, 579–582. doi: 10.1038/41557
Simard, S. W., Roach, W. J., Beauregard, J., Burkart, J., Cook, D., Law, D., et al. (2021). Partial retention of legacy trees protects mycorrhizal inoculum potential, biodiversity, and soil resources while promoting natural regeneration of interior Douglas-fir. Front. For. Glob. Change 3:620436. doi: 10.3389/ffgc.2020.620436
Simard, S. W., Roach, W. J., Defrenne, C. E., Pickles, B. J., Snyder, E. N., Robinson, A., et al. (2020). Harvest intensity effects on carbon stocks and biodiversity are dependent on regional climate in Douglas-fir forests of British Columbia. Front. For. Glob. Change 3:88. doi: 10.3389/ffgc.2020.00088
Song, Y. Y., Simard, S. W., Carroll, A., Mohn, W. W., and Zeng, R. S. (2015). Defoliation of interior Douglas-fir elicits carbon transfer and stress signaling to ponderosa pine neighbors through ectomycorrhizal networks. Sci. Rep. 5:8495. doi: 10.1038/srep08495
Tedersoo, L., Bahram, M., and Zobel, M. (2020). How mycorrhizal associations drive plant population and community biology. Science 367:eaba1223. doi: 10.1126/science.aba1223
Teste, F. P., and Simard, S. W. (2008). Mycorrhizal networks and distance from mature trees alter patterns of competition and facilitation in dry Douglas-fir forests. Oecologia 158, 193–203. doi: 10.1016/j.funeco.2008.11.003
Teste, F. P., Simard, S. W., Durall, D. M., Guy, R. D., and Berch, S. M. (2010). Net carbon transfer occurs under soil disturbance between Pseudotsuga menziesii var. glauca seedlings in the field. J. Ecol. 98, 429–439. doi: 10.1111/j.1365-2745.2009.01624.x
Teste, F. P., Simard, S. W., Durall, D. M., Guy, R. D., Jones, M. D., and Schoonmaker, A. L. (2009). Access to mycorrhizal networks and roots of trees: importance for seedling survival and resource transfer. Ecology 90, 2808–2822. doi: 10.1890/08-1884.1
Turner, N. J. (2008). The Earth's Blanket: Traditional Teachings for Sustainable Living. Madeira Park, BC: D&M Publishers.
Turner, N. J., Ignace, M. B., and Ignace, R. (2000). Traditional ecological knowledge and wisdom of Aboriginal peoples in British Columbia. Ecol. Appl. 10, 1275–1287. doi: 10.1890/1051-0761(2000)010[1275:TEKAWO]2.0.CO;2
Twieg, B. D., Durall, D. M., and Simard, S. W. (2007). Ectomycorrhizal fungal succession in mixed temperate forests. New Phytol. 176, 437–447. doi: 10.1111/j.1469-8137.2007.02173.x
Van Der Heijden, M. G., and Horton, T. R. (2009). Socialism in soil? The importance of mycorrhizal fungal networks for facilitation in natural ecosystems. J. Ecol. 97, 1139–11150. doi: 10.1111/j.1365-2745.2009.01570.x
Van Dorp, C. H., Simard, S. W., and Durall, D. M. (2020). Resilience of Rhizopogon-Douglas-fir mycorrhizal networks 25 years after selective logging. Mycorrhiza 30, 467–474. doi: 10.1007/s00572-020-00968-6
Wickham, S. B., Augustine, S., Forney, A., Mathews, D. L., Shackelford, N., Trant, A. J., et al. (2022). Incorporating place-based values into ecological restoration. Ecol. Soc. 27:32. doi: 10.5751/ES-13370-270332
Keywords: common mycorrhizal network, carbon transfer, regeneration, complex adaptive systems, temperate forests
Citation: Simard SW, Ryan TSL and Perry DA (2025) Opinion: Response to questions about common mycorrhizal networks. Front. For. Glob. Change 7:1512518. doi: 10.3389/ffgc.2024.1512518
Received: 16 October 2024; Accepted: 24 December 2024;
Published: 21 January 2025.
Edited by:
Verena C. Griess, ETH Zürich, SwitzerlandReviewed by:
Miranda Hart, University of British Columbia, CanadaCopyright © 2025 Simard, Ryan and Perry. This is an open-access article distributed under the terms of the Creative Commons Attribution License (CC BY). The use, distribution or reproduction in other forums is permitted, provided the original author(s) and the copyright owner(s) are credited and that the original publication in this journal is cited, in accordance with accepted academic practice. No use, distribution or reproduction is permitted which does not comply with these terms.
*Correspondence: Suzanne W. Simard, c3V6YW5uZS5zaW1hcmRAdWJjLmNh