- 1Blue Forest, Sacramento, CA, United States
- 2School of Informatics, Computing, and Cybersystems Northern Arizona University, Flagstaff, AZ, United States
- 3Vibrant Planet, Truckee, CA, United States
- 4University of California, Berkeley, Berkeley, CA, United States
- 5University of Pennsylvania, Philadelphia, PA, United States
- 6Verra, Washington, DC, United States
- 7American Forest Foundation, Washington, DC, United States
- 8National Forest Foundation, Missoula, MT, United States
Restoring a resilient forest structure in California’s American River watershed in the Sierra Nevada mountains can generate up to $6,100 per acre in carbon revenue from increased forest carbon and market-ready biomass utilization pathways, potentially fully funding forest management. Employing a dynamic performance benchmark (DPB) framework, this study models the impacts of restoring resilience to high-risk forests via forest thinning followed by prescribed fire. These practices show an initial carbon cost, but ultimately reduce carbon emissions from wildfire and increase carbon storage compared to a no-treatment counterfactual scenario by 35 tCO2e per acre on average, with market-ready biomass utilization pathways adding another 6–23 tCO2e average benefit per acre. Treatments enhance carbon stability by shifting carbon storage from dense, overcrowded small trees to more dispersed, fire-resilient large trees and reduces fire severity (flame length) by 78% five years post-treatment. Compared to pretreatment levels, treatment decreases the number of trees on the landscape by 74% while increasing carbon storage by 6% at the end of the 25-year simulation. To reduce investor risk into nature-based solutions focused on increasing carbon stability in fire adapted forests and generate carbon revenue from fuel treatments, accurate predictive tools are needed. To maximize certainty of carbon benefits, landscape level treatments, DPBs, and ex-post carbon crediting will be critical. This study shows that carbon revenue from traditional markets or novel carbon contribution programs can help close the funding gap for forest restoration in California while underscoring the need for innovative conservation finance mechanisms to support ecosystem resilience and climate mitigation goals.
1 Introduction
Forests are vital for carbon storage (Pan et al., 2011; Harris et al., 2021), yet disturbances from fire, drought, climate change, and human activities such as logging and deforestation lead to fluxes in carbon levels and complicate measurements and predictions of carbon stocks (Duffy et al., 2021; Hurteau et al., 2008). In California, continued forest carbon storage plays a critical role in the development of pathways to achieve the state’s goal of Carbon Neutrality by 2045 (2024). However, California’s forests are overstocked due to past logging practices and fire suppression policies which have significantly decreased average tree size while increasing fuel load and continuity, stand density, and canopy cover (Scholl and Taylor, 2010; Collins et al., 2011; Knapp et al., 2013). These trends are exacerbated by global warming and increased aridity which have led to an eightfold increase in summer fire extent since 1972 (Williams et al., 2019). These fires pose a significant threat to the durability of forest carbon storage (Tyukavina et al., 2022) and have led to average emissions of 19 million tCO2e annually between 2000 and 2020 (California Air Resources Board, 2021).
California’s forest ecosystems co-evolved with periodic fires, which helped to maintain ecosystem integrity (DellaSala et al., 2017; Perry et al., 2011; Hessburg et al., 2016). Currently these fire adapted forests are out of equilibrium with climate and are experiencing high mortality from severe drought and wildfire (Hill et al., 2023) which threatens the forest’s carbon carrying capacity (Goodwin et al., 2020; Hurteau et al., 2019). Accomplishing the State’s goal of treating one million acres a year via forest thinning and prescribed burning can mitigate the risk of severe wildfires (Foster et al., 2020) and increase the resiliency of forests to wildfire and other disturbance (Kennedy and Johnson, 2014; Stephens et al., 2020). Nonetheless, forest management aimed at enhancing carbon stability initially lowers carbon stocks via biomass removal, which presents a challenge to achieve near-term objectives (North et al., 2012; Liang et al., 2018).
Historically, low and mixed-severity fires have played a crucial role in stabilizing carbon stocks in forests by maintaining carbon in fewer, larger trees, which accumulate carbon at higher rates (Hurteau et al., 2016; Stephenson et al., 2014). North et al. (2022) provides a historical benchmark for a resilient forest structure – those able to withstand disturbances such as fire while maintaining their core functions and structure. However, forest management involves balancing multiple objectives, including carbon storage, timber production, watershed protection, recreation, wildlife habitat, and cultural values alongside resilience (Clawson, 1977). Navigating these goals, particularly when wildfire resilience and carbon benefits may not immediately align, presents a complex challenge characterized by social, political, financial, and logistical dynamics (Bowes and Krutilla, 1985).
Forest management in the U.S. has traditionally been funded by federal appropriations and timber sales revenue (Quesnel Seipp et al., 2023). To successfully treat one million acres a year in California, over two billion dollars would be needed annually, assuming per acre costs between $2000–2,500 per acre once preparation and planning, thinning, and pile burning is accounted for (Chang, 2021; Hartsough et al., 2008). At these rates, California alone would exceed the total, non-recurring allocations for national forest treatments in under three years (Visualizing Federal Funding for Wildfire Management and Response, 2023), highlighting the need for novel sources of revenue. Conservation finance leveraging voluntary or compliance carbon markets offer a potential additional funding source. But nature based carbon market credibility has increasingly come under scrutiny due to lack of transparency (Delacote, 2024), critiques of over crediting due to scientifically inaccurate protocols (Badgley et al., 2022), unfounded assumptions for leakage rates (Haya et al., 2020), challenges of permanence (Kaarakka et al., 2023), and over-simplified carbon accounting practices (Haya et al., 2023).
These critiques of carbon markets often focus on issues associated with baseline scenarios - the theoretical “business as usual” or counterfactual conditions needed to measure the impact of carbon finance projects on carbon removal or emission reductions, which then determine the generation of carbon credits. The critiques of forestry offsets in California’s Cap and Trade system highlight both inaccurate fixed assumptions and static baselines (Badgley et al., 2022) as well as fundamental issues with design of the policy. The majority of California’s Cap and Trade offsets are traditional Improved Forest Management (IFM) projects (So et al., 2023) which incentivize lengthened harvest rotations to increase forest biomass and carbon levels relative to a standard harvest rotation baseline. These projects largely increase competition stress among trees and elevate fire risks in already overstocked forests, putting California’s compliance carbon markets in direct conflict with state wildfire prevention goals (Herbert et al., 2022). These critiques of carbon market forestry projects in California and elsewhere have highlighted that (1) accurate baselines are critical for effective forest carbon offsets (2) traditional static baseline assumptions about ecological and economic factors used to construct counterfactual scenarios tend to be inaccurate and overly simplistic, leading to overgeneration of carbon credits and (3) extended rotation age IFM protocols in California may increase the risk of high-severity fires.
The movement of carbon accounting protocols toward Dynamic Performance Benchmarks (DPB) (e.g., Verra’s IFM methodology VM0045 and ARR methodology VM0047) offer a potential way to enhance the accuracy of counterfactual estimates of carbon stocks, increase carbon credit quality, and adapt to fire-prone ecosystems (Haya et al., 2023; Fick et al., 2021), although there is risk and uncertainty with all forms of MRV. DPBs are generated for counterfactual scenarios by comparing observed changes in a project area to changes in a similar reference region over time, allowing for the generation of carbon credits ex-post, based on actual observed outcomes. In contrast, traditional static baselines for counterfactual scenarios are typically established using fixed assumptions about ecological and economic factors, sometimes leading to the generation of carbon credits ex-ante, based on predicted outcomes and assumptions about the counterfactual scenario (Michaelowa et al., 2021). This mismatch between assumed and observed carbon levels in the counterfactual scenario is at the heart of current market criticisms. Although DPBs show promise to increase the quality of carbon markets, projects will only be viable with accurate predictions of the timing and quantity of carbon benefits. Funders and investors need assurance that the risk associated with the future carbon credit repayment is manageable. This paper uses methods grounded in DPB principles to predict the timing and quantity of carbon benefits from forest treatment in fire-prone forests. Predictive analyses will be critical to the development of ex-post and DPB methodologies and crediting.
While DPBs improve the certainty and accuracy of carbon benefits and boost buyer confidence, they create significant financing challenges and uncertainty for project developers. DPBs enhance the precision of carbon benefit measurement but reduce certainty around the timing and amount of carbon revenue. Forest restoration projects require substantial upfront capital for management costs, including thinning, prescribed fire, project setup, and administrative oversight. Currently, static baselines help secure early funding by providing estimated credits for validation and registration. These assumed counterfactuals simplify credit prediction, reduce investor risk, and support financial mechanisms like advanced market commitments that provide upfront funding for project implementation.
In contrast, DPBs complicate prediction of carbon benefit and revenue generation because the counterfactual scenario is based on a reference region which is constantly observed in real time and used to measure the carbon impact at the treatment site. This methodological shift is more accurate but introduces greater uncertainty in both the timing and quantity of carbon revenue, due to factors outside of a project developers’ control, such as wildfire, climate change, and drought which are captured by a DPB. Financial tools like Environmental Impact Bonds, designed to generate social and environmental outcomes along with financial returns, can help attract funding in earlier stages and address funding challenges (Brand et al., 2021). However, attracting impact-oriented finance for projects using ex-post DPBs will require highly accurate projections of the timing and quantity of ex-post carbon credits to ensure uptake by the market, which is made challenging by the inherently shifting nature of DPBs. Projections can also enable planning for repeated treatments and recurring management to enhance the permanence and durability of carbon benefits – key challenges that DPBs do not inherently solve.
This analysis couples forest growth models with the cumulative probability of fire to explore the carbon impacts of thinning and prescribed fire treatments aimed at restoring a fire-resilient forest structure. We use predictive tools aligned with the framework of a DPB protocol (Duffy, 2023) and current methods (Yackulic et al., 2024) to explore the timing and quantity of future carbon credits that could be generated. We examine the potential and temporal dynamics of stacking carbon income from ex-post increased forest carbon and biomass utilization pathways to increase funding for forest resilience treatments. Although there have been initial grey literature assessments of dynamic basely protocols (BeZero Carbon, 2023) and explorations of the potential of DPBs to increase certainty and quality of carbon credits, this is the first analysis to model carbon benefits in fire prone forests through a carbon finance lens. The American River watershed (see Figure 1) is at high risk for wildfire and critical for municipal and irrigation water, hydropower production, carbon storage, wildlife, recreation and thus proves as a useful case study. Ultimately, we assess whether integrating carbon finance with other novel funding sources leveraging the multiple benefits of forest restoration (Quesnel Seipp et al., 2023) such as water security (Guo et al., 2023) could contribute to closing the multi-billion-dollar funding gap for forest restoration in California.
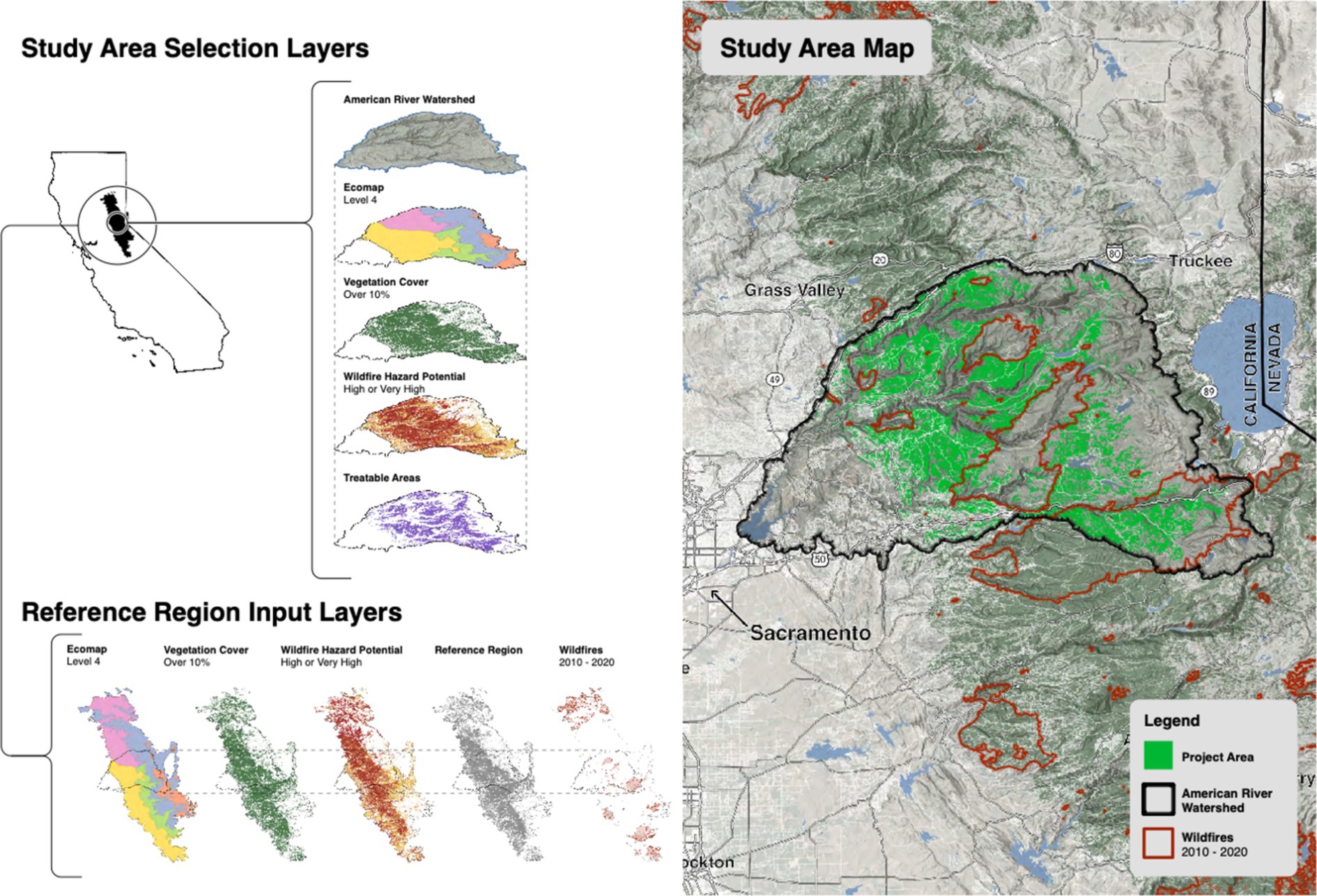
Figure 1. Map of the study area and reference region. The 287,021-acre study area includes all forested, high fire risk, and treatable areas within the American River watershed. A 2,860,418-acre reference region, based on ecoregions within the study area, was established to gather accurate fire extent and severity data for the model.
In this manuscript we aim to answer the following key questions:
1. What are the carbon dynamics associated with restoring resilience to the American River watershed?
2. What is the economic value and certainty of different sources of carbon benefits?
2 Materials and methods
2.1 Methodological overview
This study aims to determine whether restoring forest resilience can enhance carbon storage and generate carbon revenue by comparing the outcomes of two distinct scenarios: one where forests are treated to reduce fire risk and a counterfactual where no action is taken. The assumption in traditional forest management is that removing biomass leads to decreased carbon stocking levels, but this overlooks the long-term benefits of reducing fire severity and protecting carbon stocks over the long term. Our analysis interrogates this assumption by quantifying the carbon implications of forest management in high fire-risk landscapes over a 25-year period, focusing on the American River watershed in California’s central Sierra Nevada mountains.
We simulate two scenarios—treatment and no-treatment—using the Forest Vegetation Simulator (FVS), statistical modeling, and the cumulative probability of fire to model the carbon dynamics associated with forest treatment (see Figure 2). FVS, developed by the U.S. Forest Service, is used to model forest stand dynamics and treatment outcomes using inventory data. Calibrated for U.S. regions, it is widely applied to simulate fire behavior and management impacts (FVS, 2024). Grounding our analysis in real-world data, we apply remote sensing techniques to determine historical fire extent and severity, using publicly accessible Google Earth Engine code (Parks et al., 2014), and simulate future fire scenarios using FVS. A range of future fire extent scenarios are explored using Monte Carlo simulations, allowing us to estimate aboveground live carbon fluxes in the treatment and no treatment scenarios given a range of fire extents and to model the potential carbon revenue generated from (1) monetizing the increased forest carbon stock created from reduced wildfire emissions (2) biomass utilization.
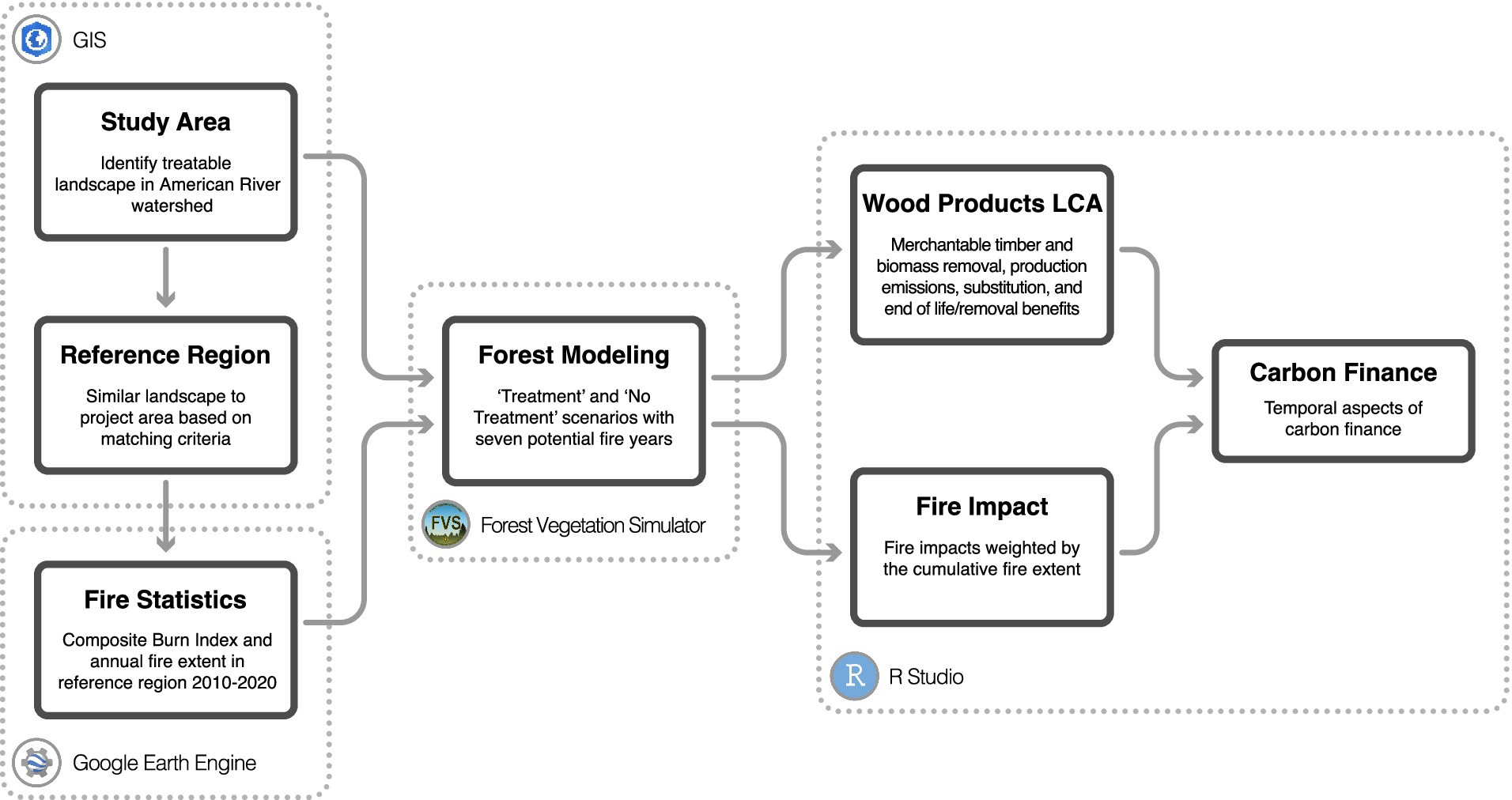
Figure 2. Workflow illustrating the study’s methods for analyzing treatment and no-treatment forest scenarios. The process starts by identifying treatable landscapes, followed by forest modeling using fire statistics from the reference region to simulate seven potential fire years. Carbon impacts from biomass utilization pathways and timber products are assessed through a life cycle assessment (LCA), while fire impacts are weighted by cumulative fire extent. The results are then used to inform carbon finance calculations.
2.2 Developing the counterfactual: treatment vs. no treatment
To assess the carbon benefits of treatment, we compare two scenarios: a treatment scenario that represents active forest management and a no-treatment scenario that reflects the status quo of unmanaged forests. This counterfactual is necessary for measuring the impact of interventions over time, as it provides a baseline against which to evaluate carbon outcomes.
In the treatment scenario, we model forest thinning and prescribed fire over a 25-year period using data from Treemap (Riley et al., 2021) and the Forest Vegetation Simulator (FVS), targeting a Stand Density Index (SDI) of 175 through forest thinning, which is approximately 30% of the maximum SDI for the region and represents an empirically resilient forest structure (North et al., 2022). Thinning is limited to high fire risk areas (Dillon and Gilbertson-Day, 2020) which are treatable (Kelsey et al., 2017; North et al., 2015) and to trees with a diameter at breast height (DBH) of less than 30 inches, which is aligned with current forest practices. After thinning, prescribed fires are applied to reduce surface fuels, covering 70% of each stand.
In the no-treatment scenario, no active management is applied. These unmanaged forests, characterized by high tree density and fuel loads, are more vulnerable to severe wildfires.
2.3 Modeling fire dynamics and cumulative carbon impact
To ensure our simulations accurately reflect fire dynamics, we base our analysis on historical data from a reference region ecologically similar to the treatment area. Between 2010 and 2020, this region experienced 174 fires, with an annual mean of 5.12% of the landscape burning, ranging from 0.05 to 14.86%. Of the burned acres, 32% were classified as high-severity, with a Composite Burn Index (CBI) above 2.25 (Miller et al., 2009). This high-severity proportion is used to parametrize the no-treatment fire scenarios in FVS, with fire data sourced through Google Earth Engine and following the methods of Parks et al. (2014).
To simulate the impacts of treatment versus no-treatment scenarios, we model seven fire years for each scenario: no fire and fires occurring in years 1, 5, 10, 15, 20, and 25. These simulations provide outputs which can then be weighted by the assumed cumulative extent of fire to create an integrated prediction of carbon levels over time.
To model the long-term carbon implications of wildfire by weighting the model outputs, we calculate the running annual, cumulative extent of fire across the landscape over the 25-year simulation period. Using historical fire data, we develop a cumulative probability density function to represent the average cumulative fire extent for any given year. For each year in the simulation, the cumulative extent of fire is calculated by multiplying the observed annual fire extent (5.12% on average) by a factor representing that simulation year. This value indicates the proportion of the landscape expected to have burned by that point in time. The cumulative extent is then used to weight and integrate the modeled fire and no-fire scenarios for both treatment and no-treatment conditions. For instance, if the cumulative extent after five years is 25% (5 years * 5% annual extent), the final carbon outputs are adjusted so that 25% of the final outputs reflect the results from the model run with wildfire, while 75% reflects the model run without wildfire.
Cumulative Wildfire Extent
Where:
AE = observed annual extent of fire.
n = number of years.
Net Carbon (tCO2e per acre)
Where:
represents carbon under fire scenario j at time t.
represents carbon under no fire scenario at time t.
j represents fire occurrence timestep 1, 5, 10, 15, 20, 25.
CWE represents the cumulative probability that wildfire has occurred.
This cumulative extent approach allows us to model the progression of fire risk over time, illustrating how different fire extents can influence carbon storage across the landscape. It is a key tool for understanding the long-term impact of wildfire on carbon stocks under different forest management and fire scenarios.
To account for the inherent variability in wildfire extent, we use a Monte Carlo simulation. For each iteration, we randomly sample the annual fire extent from a normal distribution based on observed data, which ranges from 0.05 to 14.86%, with a mean value of 5.12%. This random sampling introduces variability into the fire modeling for both the treatment and no-treatment scenarios. By running 100 iterations, we generate a wide range of possible fire and carbon outcomes, allowing us to evaluate the variability in carbon dynamics driven by differences in fire extents and inherently performing a sensitivity analysis on assumptions of annual fire extent.
2.4 Carbon dynamics of biomass utilization
We evaluate the potential carbon benefits of utilizing the biomass removed during forest thinning, dividing biomass into two categories: merchantable timber and low-value biomass. Merchantable timber consists of species like Douglas fir and ponderosa pine, while low-value biomass includes small trees, bushes, and logging slash. Low-value biomass typically has no market value, represents a large disposal cost to land managers, and release stored carbon into the atmosphere when it disposed of via pile burning.
To assess the carbon benefits of different low-value biomass utilization options, we model five pathways: biopower production, where biomass is converted into electricity through combustion; biochar production, where biomass is pyrolyzed into stable carbon for soil or other applications; fuels, which convert biomass into biofuels; biomass burial, where biomass is stored in underground conditions to prevent decomposition; and fuels with Carbon Capture and Sequestration (CCS), which captures and stores carbon emissions from biofuels production. Each biomass utilization pathway offers distinct carbon benefits, depending on the durability of the carbon sequestered in wood products, the potential for carbon storage with CCS, and the emission reductions achieved by replacing high-carbon fossil fuel feedstocks with lower-carbon biomass feedstocks.
To quantify the carbon benefits, we calculate the amount of low-value biomass removed per acre (average 14.3 bone dry tonnes (BDT) per acre) and apply carbon benefit multipliers for each utilization pathway based on existing life cycle analyses (Elias et al., 2023; Cabiyo et al., 2021; Github, 2022). These carbon benefits are expressed in terms of tCO2e per acre to harmonize the different pools of carbon benefits. Full cost modeling is beyond the scope of this paper, which focuses explicitly on revenue estimates rather than a complete cost–benefit analysis. Fuels with CCS generate the highest carbon benefits, followed by biomass burial, while biochar and biopower provide pathways with lower but immediate carbon sequestration potential.
2.5 Economic valuation of carbon benefits
The final step in our analysis is to evaluate the economic viability of carbon finance to support large-scale forest restoration efforts by monetizing the carbon benefits associated with both increased forest carbon and biomass utilization pathways. The goal is to quantify how revenue from carbon credits can offset the costs of forest treatments over the 25-year period.
To estimate potential carbon revenue from increased forest carbon, we compare the aboveground live carbon stocks in the treatment and no-treatment scenarios. The increase in carbon stock represents the emissions avoided due to reduced fire severity in the treatment scenario. By utilizing current carbon market prices for similar types of carbon credits such as reforestation and other nature-based carbon removal credits (ranging from $35 to $75 per tCO2e), we estimate the revenue generated from avoiding carbon emissions under different fire scenarios.
Biomass utilization offers a second source of carbon revenue by diverting low-value biomass from pile burning or decomposition, which would otherwise release all its carbon into the atmosphere. By converting this biomass into biochar, fuels, or burying it, carbon can be sequestered, generating revenue through carbon-beneficial pathways. Revenue sources for these products include the Voluntary Carbon Market (VCM), the Low Carbon Fuel Standard (LCFS), the Renewable Fuel Standard (RFS), and 45Q tax incentives, with prices ranging from $50 to $150 per tCO2e depending on the specific market.
We combine the revenue from increased forest carbon with the revenue from biomass utilization to estimate the total financial benefit of forest restoration under the treatment scenario and ultimately, demonstrate the potential of carbon finance to contribute to forest restoration.
3 Results
3.1 Forest modeling: sawtimber, biomass, and carbon removed in the treatment scenario
Forest thinning in year five and prescribed fire in year nine result in a significant reduction of aboveground live carbon in the treatment scenario, covering 287,021 modeled acres in the American River watershed. However, thinning fails to restore 15% of stands to a resilient Stand Density Index (SDI) of 175, as the treatment is restricted to trees with a diameter at breast height (DBH) of less than 30 inches, which is common practice. Many trees in these stands exceed this threshold, underscoring the challenge of achieving forest resilience under current management limitations. On average, the thinning removes 10.2 thousand board feet (MBF) per acre of merchantable timber – containing 2.2 tCO2e – and 14.3 bone dry tonnes (BDT) per acre of low-value biomass, containing 26.4 tCO2e (see Table 1).

Table 1. Summary of statistics for low-value biomass removed, including bone dry tonnes (BDT), thousand board feet (MBF) of merchantable timber, and carbon content (tCO2e) for each biomass category.
3.2 Carbon dynamics in the treatment vs. no treatment scenario
After completing the thinning and prescribed fire treatments, the treatment scenario begins to accumulate carbon and demonstrates greater resilience to wildfire than the no-treatment scenario, measured by the gradual increase in carbon stock in the presence of fire. Initially, the no-treatment scenario continues to accumulate carbon, but its average per-acre carbon stock starts to decrease significantly within 10 years of the simulation due to the increased cumulative probability of fire. Nine years after the treatments are complete, the carbon levels in the treatment scenario have rebounded and begin accumulating between 3 and 6 tCO2e per acre per year, totaling 35 tCO2e per acre on average by the end of the simulation, compared to the no-treatment scenario. In an increased forest carbon DPB crediting scenario, these carbon benefits could be monetized. Other greenhouse gasses could also be accounted for but are not considered in this study.
Notably, at the end of the simulation, mean aboveground live carbon levels per acre in the treatment scenario are 6% higher than levels at the beginning of the simulation, despite a decrease of 74% in the number of trees per acre. While treatment initially removes carbon from the landscape, the resilience provided by the new forest structure results in carbon benefits at the end of the simulation compared to the no-treatment counterfactual (see Figure 3).
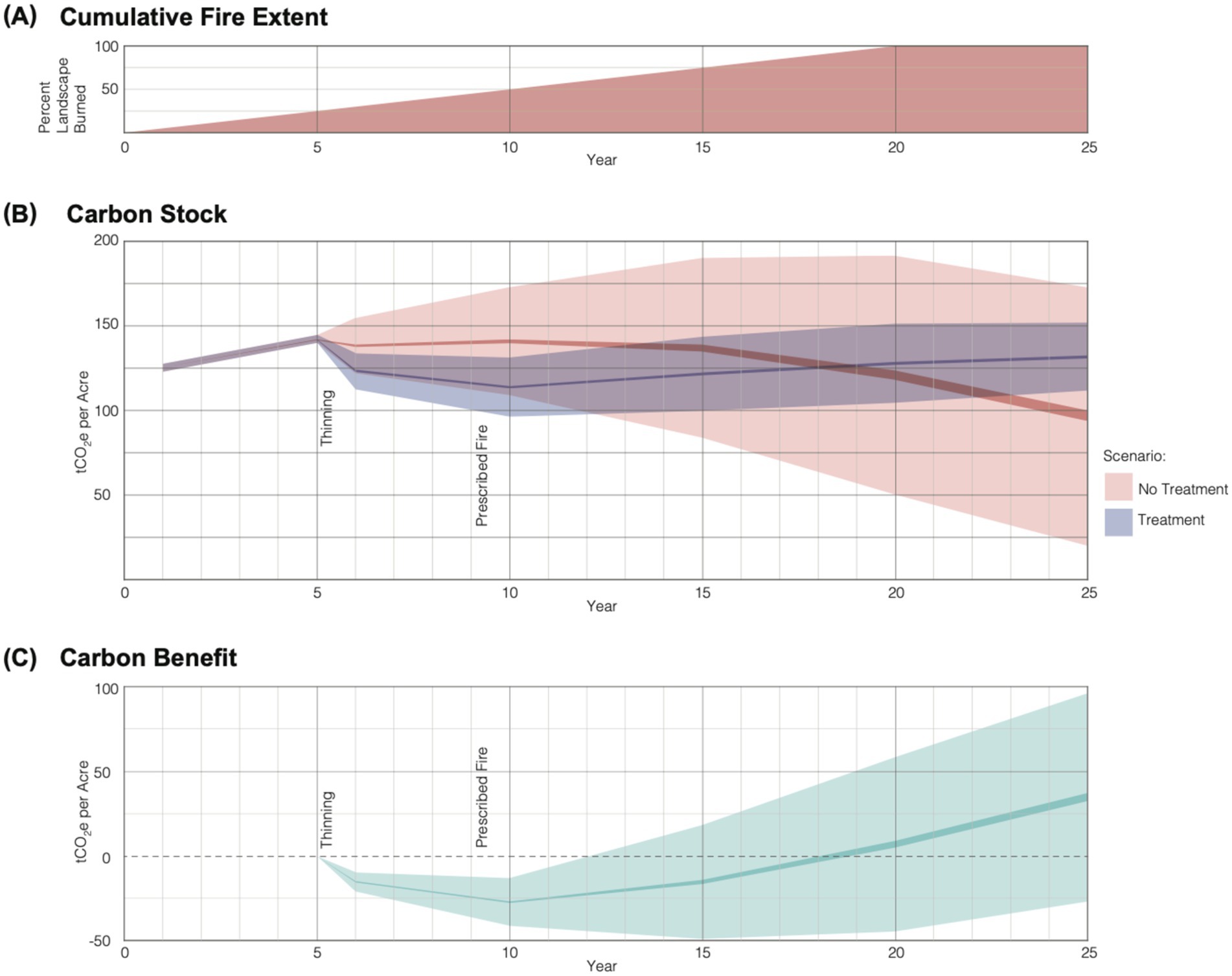
Figure 3. Panel (A) shows the cumulative probability of fire extent over time for treated and untreated scenarios, indicating the increasing likelihood of landscape burning as time progresses. The cumulative probability reflects the proportion of the landscape expected to burn by any given year, based on recent fire extents. Panel (B) illustrates changes in aboveground live carbon (tCO2e per acre) over 25 years for treated and untreated scenarios, with thinning and prescribed burning marked. Variability in carbon outcomes is based on Monte Carlo simulations using fire data from 2010 to 2020 across the 2,860,418-acre reference region, with fire extents ranging from 0.05 to 14.86% and an average of 5.12% annually. Lighter shaded regions represent one standard deviation from the mean (per-acre variability), while darker shaded regions represent the standard error around the mean (landscape-level variability). Panel (C) displays the carbon benefit (tCO2e in living biomass per acre) from treatment over time, showing the difference in carbon storage between treated and untreated scenarios. Lighter shading indicates variability in individual acre benefits, while darker shading represents the average landscape-scale benefit. After 25 years, the mean per-acre carbon storage in the treatment scenario is 35 tCO2e higher than in the no-treatment scenario.
While the carbon benefits of forest treatment vary on a per-acre basis, this variability decreases significantly at larger scales. As shown in Figure 3, the standard error and standard deviation around the mean for per-acre benefits of aboveground live carbon suggest that, while individual acre outcomes are uncertain, the carbon benefits of landscape-scale treatment are much more certain. This increased certainty at larger scales arises because landscape-scale projects average out variability in factors such as treatment effectiveness, site conditions, and fire behavior, reducing the influence of outliers and providing more reliable estimates of carbon.
Based on the Monte Carlo simulation, which assume future fire extents follow the pattern of observed data from 2010 to 2020 (with a minimum of 0.05%, a maximum of 14.86%, and a mean of 5.12%), the treatment scenario is expected to surpass the no-treatment scenario in terms of average per-acre aboveground live carbon approximately nine years after treatment completion. However, this assumes that fire extent and severity will remain constant over the 25-year modeling period, which is a conservative assumption and likely underestimates future fire risks. Given projections that fire activity in the Sierra Nevada could increase by over 50% by 2040, with fire extent expanding by more than 55% (Gutierrez et al., 2021), our conservative approach was chosen to avoid overestimating the potential carbon impacts of restoration projects that may generate carbon credits.
Altering the assumed annual fire extent significantly affects the timeline for predicted carbon benefits. In simulations, we assumed an annual fire extent of 5.12%. If the fire extent increases to 7%, carbon benefits emerge after seven years instead of nine (as shown in Figure 3). Conversely, a 3% annual fire extent delays carbon benefits to 14 years. This underscores the sensitivity of carbon outcomes to fire extent assumptions and the importance of accurate fire projections when modeling carbon benefits and the need for ex-post DPBs to accurately account for carbon benefits of forest treatment.
3.3 Timber, low-value biomass, and carbon benefits of biomass utilization
Biomass pile burning, which we assume releases all the carbon stored in the biomass to the atmosphere, produces the worst carbon outcomes in this analysis. When compared to pile burning, all other modeled biomass utilization pathways demonstrate clear carbon benefits (see Figure 4). Fuels with CCS provide the highest relative carbon benefits, primarily because they capture and permanently store emissions. Biomass burial ranks second in terms of carbon benefits, largely due to minimal process emissions associated with burying or storing biomass in conditions that prevent decomposition.
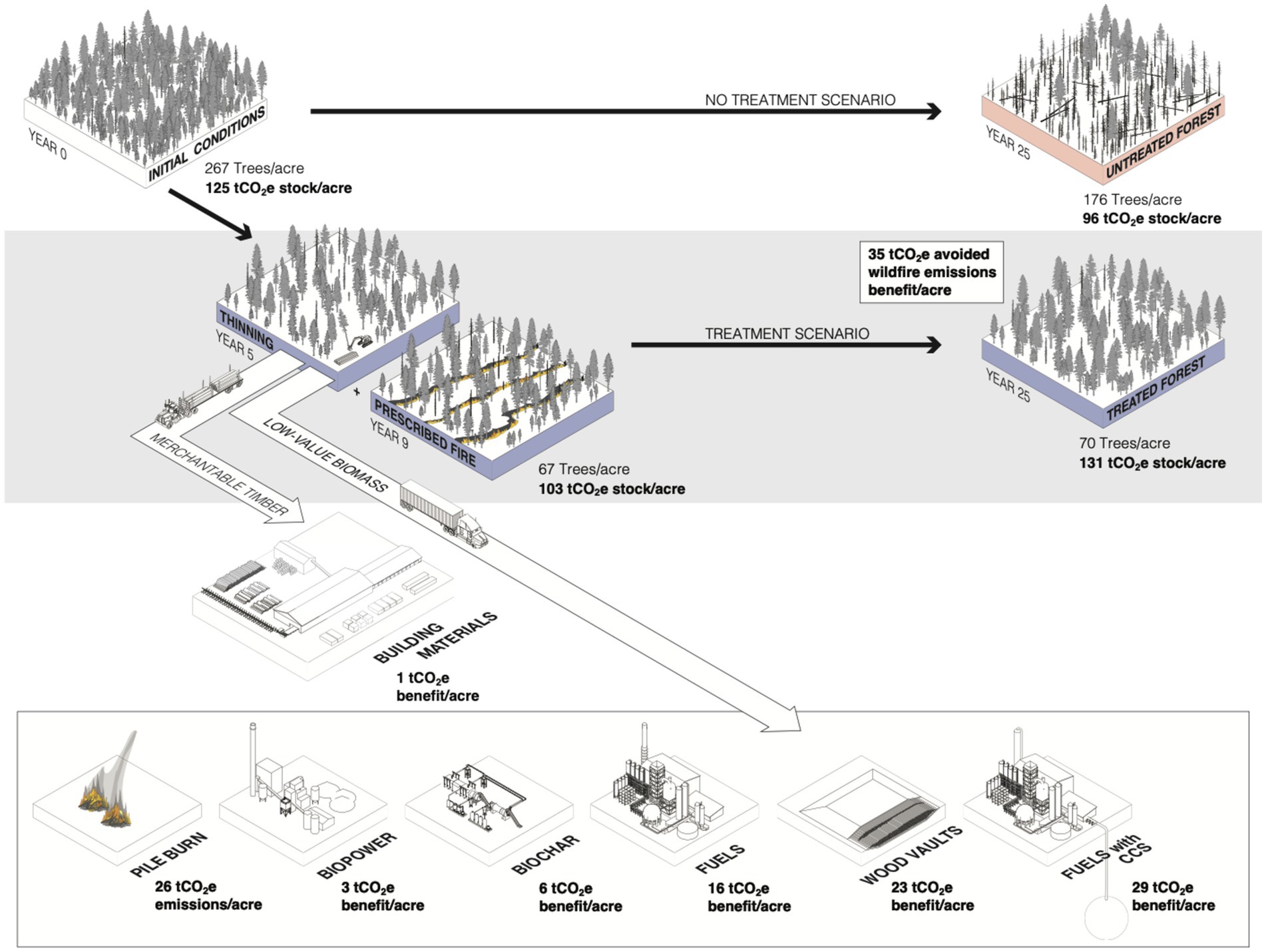
Figure 4. Comparison of trees per acre and aboveground carbon stocks for treatment and no-treatment scenarios. The treatment scenario, which includes thinning in year 5 and prescribed fire in year 9, results in 35 tCO2e higher carbon storage by the end of the simulation. Carbon benefits from various biomass utilization pathways—including merchantable timber, pile burning, biopower, biochar, biomass burial, fuels, and fuels with CCS—are shown for an average acre, which yields 14.3 BDT of low-value biomass and 10.2 MBF of merchantable timber.
Biopower offers the lowest carbon benefits among the utilization pathways, primarily because the process involves converting biomass into electricity, which releases a significant portion of the carbon content during combustion. Meanwhile, traditional building materials sequester about 59% of their carbon content once deposited in landfills (Skog, 2008).
Biochar presents lower carbon benefits than fuels with CCS but provides immediate opportunities for monetization. The market readiness of biochar technology makes it an attractive option for project developers aiming to generate short-term revenue from biomass utilization or reduce disposal costs. Although its carbon benefits are not as substantial as other pathways, biochar’s practical and immediate application enhances its appeal for reducing emissions in the short term (Elias et al., 2024).
3.4 Economic valuation of carbon benefits
The potential revenue from carbon benefits associated with restoring forest resilience is substantial, particularly through voluntary and compliance carbon markets. Our analysis focuses on tCO2e, aligning with market standards, and assumes recent market prices. Over the first five years of the project, low-value biomass can generate between $2,900 and $4,300 per acre in the fuels with CCS scenario, providing 29 tCO2e benefit per acre. The fuels scenario offers between $800 and $1,600 per acre, while biochar generates between $600 and $900 per acre. Biomass burial shows significant potential, generating between $2,300 and $3,500 per acre with 23 tCO2e benefit per acre through the VCM. Although fuels with CCS offer the highest carbon benefits, this pathway is currently limited by a lack of biomass supply chains at scale and large capital expenditure needs. The 35 tCO2e benefit per acre from increased forest carbon and increased forest carbon in the treatment scenario could yield $1,200 to $2,600 per acre, assuming carbon prices ranging from $35 to $75 per tCO2e.
Notably, the most technologically mature options, biochar and biomass burial, provide significant revenue sources when combined with increased forest carbon. Pairing biochar with increased forest carbon could generate between $1,800 and $3,500 per acre, while the combination of low-value biomass burial and increased forest carbon could yield between $3,500 and $6,100 (see Table 2).
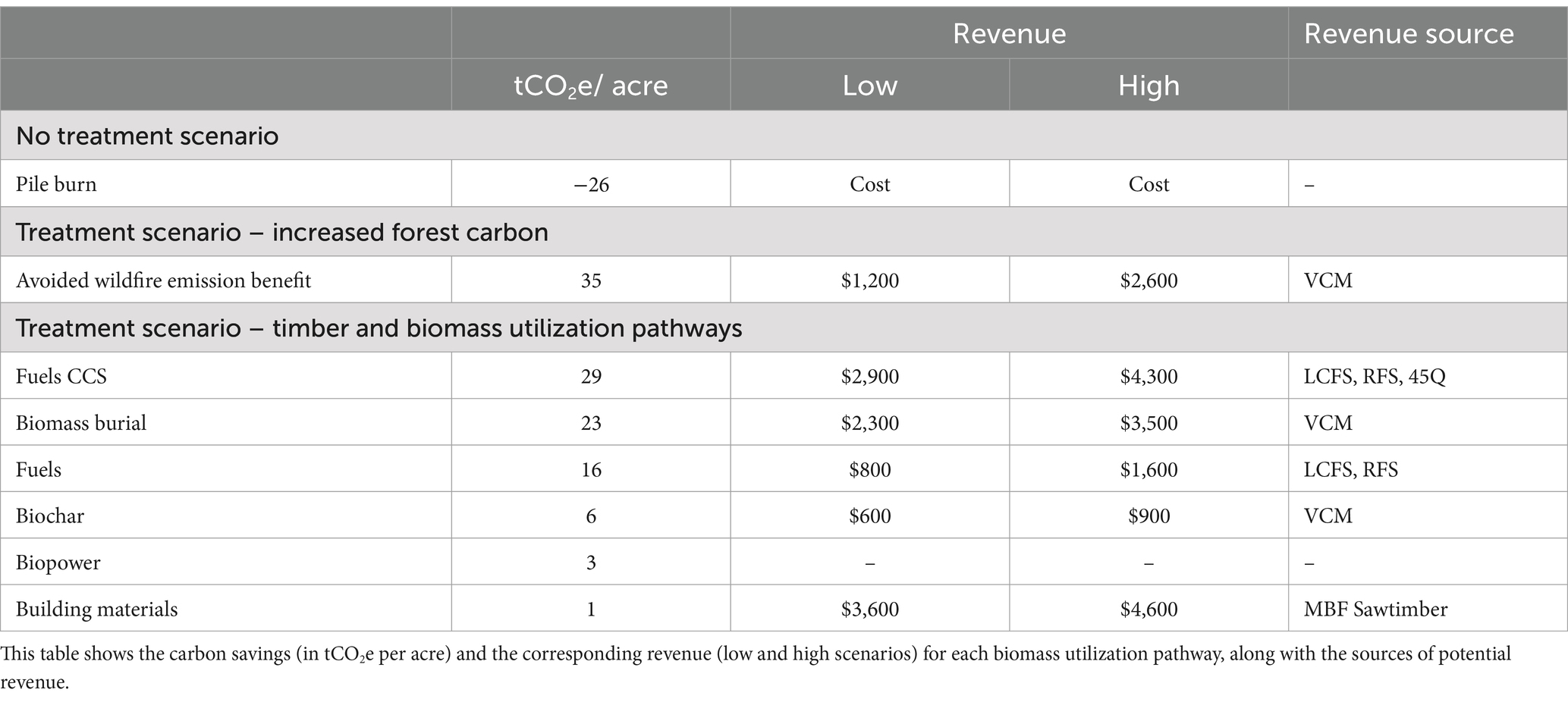
Table 2. Comparison of carbon benefits and potential revenue from different biomass utilization pathways and increased forest carbon from avoided wildfire emissions.
4 Discussion
4.1 Carbon finance and forest restoration benefits
Restoring resilience to fire-adapted forests in the central Sierra Nevada enhances carbon storage durability and provides monetizable carbon benefits over a 25-year project lifespan. Our analysis shows that carbon finance can generate up to $6,100 per acre—with $3,500 per acre from Carbon Dioxide Removal (CDR) credits via low-value biomass burial and up to $2,600 per acre from increased forest carbon via avoided wildfire emissions. Given current management costs of $2,000 to $2,500 per acre, federal and state governments need to secure $2 to $2.5 billion annually to treat one million acres per year, which represents up to half of current federal allocations (Visualizing Federal Funding for Wildfire Management and Response, 2023). Carbon revenues from $3,500 to $6,100 per acre may be able to offset biomass disposal costs while generating revenue from increased carbon stocks and biomass utilization, although disposal costs were not examined here.
Carbon finance for fire-adapted forest management can also support California’s Sustainable Insurance Strategy by funding costly hazardous fuel treatments that reduce wildfire risk in vulnerable communities. The Sustainable Insurance Strategy aims to address the growing challenge of insuring properties in high-risk wildfire areas by promoting proactive risk reduction measures and making insurance more accessible and affordable. From a climate governance perspective, this strategy aligns with broader efforts to integrate climate risk management into policy frameworks, incentivizing resilience-building activities that reduce long-term vulnerabilities to climate-related disasters. For many non-industrial private landowners who rely on the FAIR Plan – a last-resort insurance option that provides basic fire coverage for homeowners and businesses unable to obtain insurance through traditional markets – carbon finance offers a means to fund fuel reduction treatments that would otherwise be prohibitively expensive. By mitigating fire risk, carbon finance not only delivers climate benefits and protects ecosystems and local economies, but also helps stabilize insurance markets, improving access to affordable coverage in fire-prone areas.
This is one of the few studies to explore potential carbon revenue from fuel treatments in fire-prone ecosystems (Alcasena et al., 2021; Huang and Sorensen, 2011). To our knowledge, it is the first to simultaneously assess the carbon dynamics of restored forest resilience (North et al., 2022), the potential for carbon revenue from increased forest carbon associated with fuel treatments, and the carbon revenue from biomass utilization.
4.2 Carbon dynamics, treatment impacts, and fire severity
Restoring forest resilience is crucial for maintaining carbon storage and ensuring the long-term health and benefits of forests. Over a 25-year modeling period, restoration resulted in an average increase of 35 tCO₂e per acre in live biomass carbon compared to no treatment, with benefits accruing within 10 years after treatment completion. Yackulic et al. (2024) used recent remote sensing data and found that carbon benefits from recent fuel reduction projects in California can accrue in under five years. Our study intentionally incorporates management designed to restore resilience (North et al., 2022) and maximize carbon stability, which requires removing more biomass than typical fuel reduction practices and takes longer for benefits to accrue. This likely explains why recent fuel treatment projects have shown carbon benefits manifest more quickly than our simulations (Yackulic et al., 2024). Moreover, wildfire severity has generally increased since the 2010–2020 period used to parameterize this study and higher fire severity on average leads to faster carbon benefit accrual. Alongside the carbon benefits of treatment, we found that burying all low-value biomass or converting it into biochar—identified as the most market-ready products in our analysis—could yield an additional 23 tCO₂e and 6 tCO₂e per acre, respectively. Fuels with CCS provide the highest carbon benefits but have not yet been able to attract large-scale investment or established the long-term supply contracts necessary to support capital-intensive production.
Restoring resilience reduced fire severity by 78% five years post-treatment in this study, aligning with previous research showing that combining thinning with prescribed fire reduces flame length, overall fire intensity, and results in less severe fire behavior (Piqué and Domènech, 2018; Butler et al., 2012; Agee and Lolley, 2006; Agee and Skinner, 2005). These results are contingent on treatment frequency, intensity, and duration, which should be further refined to understand potential outcomes. Additionally, external factors such as climate variability, pest outbreaks, and land-use changes may influence the long-term effectiveness of these approaches and merit additional research. However, this is the first study to specifically explore treatments designed to restore empirically grounded forest resilience (North et al., 2022).
Interestingly, the treatment scenario exhibited a slight increase in carbon levels at the end of the simulation compared to the beginning. Treatment for a resilient forest structure, which led to an 78% reduction in trees per acre at year 25, not only enhanced fire resilience but also marginally increased carbon stores relative to levels at the beginning of the simulation. This increase primarily resulted from the redistribution of carbon from many densely packed, small trees to dispersed, large trees. Previous analyses have indicated that current carbon stocks are disproportionately concentrated in small trees within homogenous forests, elevating the risk of losses due to wildfire, drought, and other disturbances foster (Foster et al., 2020; Hurteau et al., 2011). These findings underscore the importance of aligning treatment prescriptions with resilience to maximize magnitude and durability of carbon storage.
This analysis deliberately employs historical observations of fire extent and severity from 2010 to 2020, thereby minimizing the potential impacts of climate change, the anticipated annual increase in fire extent, and the trend of escalating fire severity. These estimates are conservative when assessing the impacts of treatment relative to the no-treatment counterfactual scenario Gutierrez et al. (2021) forecasted an increase in the number of fires in the Sierra by over 50% and an increase in fire extent by over 55% by 2040 which is in line with both historical observations and other predictions of future fire extent and severity (Miller et al., 2009; Kane et al., 2015; Schwartz et al., 2015; Miller and Safford, 2012; Yue et al., 2013). However, the implications of utilizing historical estimates for predicting carbon stocking relative to contemporary levels remain uncertain, underscoring the significance of establishing accurate baselines. Put simply, conservative assumptions regarding the escalation of fire extent and severity likely understate the benefits of treatment compared to the no-treatment scenario. Conversely, maintaining constant the effects of climate change, such as drought and temperature rises, may lead to an overestimation of the treatment’s impact compared to current carbon levels. Nonetheless, the relative enhancement in carbon stability resulting from the restoration of resilience to forests is evident, as forest carbon shifts toward fewer, larger trees that are more capable of withstanding wildfires. The integration of forest carbon into policy decisions necessitates clear baseline assumptions for carbon stocks (be it current levels or future levels in the absence of management) and refined projections for forest carbon stocking amidst climate change.
4.3 Biomass utilization and carbon dioxide removal (CDR) strategies
Although the carbon benefits of increased forest carbon require nuanced assumptions about baselines, the carbon benefits of low-value biomass burial, biochar, and fuels are clear. While using biomass for fuels like hydrogen combined with carbon capture and sequestration offers significant carbon benefits, the required capital is enormous (Elias et al., 2024), and consistent, contracted feedstock supply is critical to attract investors (CLERE, 2020). Given the current ad-hoc nature of supply chains for low-value biomass from forest restoration projects, less capital-intensive strategies like biomass burial and biochar are more promising in the near term. Moreover, biomass burial offers higher carbon removal efficiency compared to fuels, transferring more of the embedded carbon to long term carbon storage.
However, concerns about the durability and permanence of these CDR strategies persist, raising important questions about the environmental safeguards needed to prevent replicating widespread credibility problems in the VCM. The long-term sustainability of these pathways depends on factors such as site conditions, transportation emissions, and lifecycle analyses of carbon outcomes. Further research should assess how these strategies perform under varying ecological conditions and management scenarios to better understand their implications for long-term carbon storage. Nevertheless, there are several options for managing carbon outcomes in overstocked forests, especially when restoration projects have flexibility in deciding where wood products and wood waste are directed. With growing interest in technologies like biochar, wood burial, and biofuels, forest restoration now provides more opportunities for achieving low-carbon outcomes.
4.4 Challenges in carbon finance for forest resilience and future directions
Carbon finance in forest ecosystems has been fraught with challenges due to inaccurate methodologies and baselines, leading to carbon credits that do not represent actual emission reductions. DPBs and ex-post revenue offer a potential solution by shifting carbon markets toward an observable, results-based approach using scalable methods based on remote sensing. However, this increased certainty does not inherently solve issues of permanence and durability and introduces funding challenges, as revenue becomes more uncertain and is generated in the future under current carbon market structure. In this analysis, approximately half of the carbon income would be generated more than ten years after project completion, assuming benefits are monetized after they are observed, not before.
Permanence and leakage are both critical principles in carbon finance, ensuring that credited carbon benefits endure over time and carbon emissions are not simply increased elsewhere (Yin, 2024). Achieving permanence is particularly challenging in fire-adapted forests, where dynamic disturbances such as wildfire, drought, and climate change can jeopardize long-term carbon storage. Regular repeated treatments, either thinning or prescribed fire, are needed to ensure long term carbon benefits. While ex-post, dynamic monitoring does not fully resolve these challenges, it provides high-certainty methods to track permanence over time and incentivize recurring treatments to maintain carbon levels and wildfire resilience. Leakage is not a significant concern for fire treatments, as these treatments create a shadow effect—where one acre treated reduces fire severity and emissions on surrounding untreated acres, amplifying the benefits beyond the treated area.
Scaling up to landscape or jurisdictional levels can address many of the challenges inherent in project-level carbon finance, such as accurately identifying baselines and determining additionality. Large-scale projects reduce variability and risk by averaging outcomes across broader spatial extents, providing greater confidence in carbon benefits while potentially lowering per-unit costs. Jurisdictional approaches, in particular, assess carbon outcomes across entire administrative regions, allowing for dynamic, system-wide changes to be accounted for in carbon accounting. This aggregate perspective improves the reliability of carbon assessments, enhances investor confidence, and better aligns with regional climate goals.
Corporate investors have increasingly been motivated by market competitiveness and company values have increasingly led to a willingness to invest in higher-cost carbon finance projects with higher certainty of benefits as well as significant local co-benefits (Lou et al., 2023). Ecosystem Marketplace research has found that projects offering “beyond carbon” environmental and social co-benefits – such as preserving biodiversity, ensuring water security, or supporting local economies – are more attractive to investors (Procton, 2024). DPBs and remote sensing measurement, reporting, and verification (MRV) approaches, such as those discussed here, may be able to provide a high-integrity product for companies interested in carbon finance while, hopefully, displace the supply of low-quality offsets that can undermine market integrity (Trencher et al., 2024). Importantly, predictions like those used in this paper should only be used for investor planning and to inform recurring treatments that enhance the permanence and durability of carbon benefits, rather than to generate credits.
Emerging finance structures such as milestone-based Advance Market Commitments (AMC) and Environmental Impact Bonds (EIB) could unlock significant funding by tying up-front financing to the success of carbon benefit generation. AMCs function by guaranteeing future purchases of carbon benefits at a pre-agreed price, providing investors with the certainty needed to de-risk initial capital expenditures. Similarly, EIBs offer a pay-for-success model, where investors provide upfront funding for restoration projects and receive returns based on verified environmental outcomes. This approach was recently illustrated by the World Bank’s Amazon Reforestation Outcome Bond, which linked up-front financing to project milestones and the value of the carbon credits generated.
By aligning financial incentives with measurable outcomes, these mechanisms not only reduce investor risk but also create accountability for project implementers. For example, tying funding to specific milestones, such as verified carbon sequestration or successful establishment of fire-resilient landscapes, ensures that resources are allocated efficiently and that projects deliver tangible results. Additionally, these structures encourage collaboration among stakeholders, including governments, private investors, and local communities, to achieve shared climate goals.
By reducing risk and offering financial incentives for achieving measurable outcomes, these mechanisms provide powerful tools for expanding carbon finance in fire-prone forests. In fire-adapted landscapes, where upfront costs for forest treatments are high and long-term carbon benefits are often uncertain, these innovative structures can help bridge the gap between project funding needs and investor requirements for returns, fostering greater scalability and impact.
Although this analysis primarily addresses traditional carbon markets, the methodologies employed are compatible with novel carbon contribution initiatives that do not necessarily aim to offset corporate emissions or generate carbon credit, per se. A carbon contribution model assigns carbon benefits to specific observed actions or projects and can be funded through voluntary contributions, corporate sponsorship, government grants and subsidies, impact investors, or public-private partnerships. This approach seeks to offer a more transparent and precise accounting of carbon benefits, concentrating on the tangible outcomes of conservation or restoration efforts rather than focusing on equivalence between carbon emissions and carbon offsets. Carbon contributions initiatives can encourage stringent monitoring and verification to ensure the authenticity and verifiability of the attributed carbon benefits. By focusing on the direct benefits of management, these programs potentially foster more effective and accountable climate action while avoiding the complexities of traditional carbon offset markets. This approach also reduces financial speculation, as fungible credits are generally not generated, thereby potentially increasing the portion of each ‘carbon dollar’ that reaches land managers (Blanchard et al., 2024). Carbon contribution initiatives may be particularly well-suited to DPBs methodologies, enabling accurate impact tracking of initial project contributions without the creation of conventional carbon credits.
Large, landscape-scale projects decrease risk by increasing certainty in benefits, supporting the rationale for novel financing. The uncertainty of carbon benefits and revenue tied to incremental increases in carbon benefits is high for any individual acre but decreases as projects scale. The carbon benefits in aboveground live biomass when treatment is done at scale are roughly 32 to 38 tCO2e per acre - but between −27 to 97 tCO2e per acre for treatment of a single acre. This highlights the importance of landscape level restoration to increase carbon benefit certainty. However, this analysis is temporally limited and further research is needed to determine the longevity of single, landscape scale treatments which restore resilience. To ensure the permanence of carbon benefits from restoring resilience, reintroducing regular, low-severity fire at scale will undoubtedly be necessary (Odland et al., 2021; Molina et al., 2018; Rabin et al., 2022). This analysis shows that extensive forest treatments to restore a resilient forest structure has more durable carbon benefits than less extensive treatment. Extensive treatments are more expensive, but the increased initial expense extends the longevity of treatment impacts on wildfire resilience and carbon stocks (Collins et al., 2014). Cheaper, less extensive forest management practices will require more regular reentry and likely increased management costs over time. In essence, restoring resilience has a higher return on investment even though upfront treatment costs may be higher. From a project finance standpoint, coupling carbon revenue from increased forest carbon with carbon revenue from biomass utilization revenue can alleviate up-front project costs by generating revenue in the project’s initial years and further enabling durable forest treatments.
To enable conservation finance through carbon benefits for fire adapted forests, several key areas require attention, underscoring the need to rethink the role of carbon markets. First, the DPB approach must be validated, refined, and incorporated into a methodology. This will help ensure carbon credits are based on high-quality, durable carbon benefits and that emerging carbon contribution programs use the highest quality MRV available to account for the carbon impacts of their investments. Second, durability of carbon benefits from fire resilience treatments needs to be ensured through recurring future treatments, which is the consensus in the literature (Aponte et al., 2014; Stephens et al., 2012), although this study only examines the effects of thinning followed by one prescribed fire. Third, CDR credits like those from biochar and biomass burial need coupling with rigorous environmental safeguards to prevent reversals. Fourth, predictive carbon and financial models, such as the one used in this paper, need refining alongside investors to ensure confidence and maintain academic rigor. In essence, the successful harnessing of carbon markets for the restoration and resilience of fire-prone forests hinges on creating accurate baselines and reimagining carbon project finance.
Data availability statement
The raw data supporting the conclusions of this article will be made available by the authors, without undue reservation.
Author contributions
ME: Conceptualization, Data curation, Formal analysis, Investigation, Methodology, Writing – original draft, Writing – review & editing. EY: Data curation, Formal analysis, Methodology, Writing – original draft. KD: Conceptualization, Investigation, Methodology, Supervision, Writing – original draft. PS: Investigation, Methodology, Supervision, Writing – original draft. DS: Conceptualization, Methodology, Supervision, Writing – original draft. NP: Conceptualization, Formal analysis, Visualization, Writing – original draft. SP: Conceptualization, Supervision, Writing – original draft. JD: Conceptualization, Writing – original draft. CB: Conceptualization, Writing – original draft.
Funding
The author(s) declare that financial support was received for the research, authorship, and/or publication of this article. This work was partially funded by the National Forest Foundation, as a part of a joint effort with the USFS Southwest Pacific Research Station Agreement #21-JV-11272131-043 Expanding Forest Management and Promoting Ecosystem Services through access to Environmental Markets: Modeling Carbon Markets.
Acknowledgments
We are grateful to Dr. Andrew Jones, Dr. Lara Kueppers, and Dr. Matthew Potts for their invaluable feedback and insightful comments, which greatly helped refine and improve this manuscript. Their expertise in forest ecology, climate resilience, and forest management provided critical perspectives that strengthened the analysis and presentation of our findings. We also acknowledge the use of ChatGPT, a generative AI language model developed by OpenAI (version October 2024), to assist in editing the manuscript. The content edited using this generative AI was thoroughly reviewed for factual accuracy and plagiarism by the authors.
Conflict of interest
The authors declare that the research was conducted in the absence of any commercial or financial relationships that could be construed as a potential conflict of interest.
Generative AI statement
The authors declare that Generative AI was used in the creation of this manuscript. AI was used to assist in editing this manuscript by identifying areas of redundancy, reviewing for clarity, and recommending improvements for sections that were unclear or ambiguously worded. Specifically, ChatGPT, a generative AI model, was used to provide feedback based on line numbers throughout the manuscript, ensuring each section was refined for coherence and conciseness. The AI highlighted specific line numbers where improvements could be made, including simplifying complex sentences, improving narrative flow, and ensuring consistent terminology.
Publisher’s note
All claims expressed in this article are solely those of the authors and do not necessarily represent those of their affiliated organizations, or those of the publisher, the editors and the reviewers. Any product that may be evaluated in this article, or claim that may be made by its manufacturer, is not guaranteed or endorsed by the publisher.
Supplementary material
The Supplementary material for this article can be found online at: https://www.frontiersin.org/articles/10.3389/ffgc.2024.1507554/full#supplementary-material
References
Agee, J., and Lolley, M. (2006). Thinning and prescribed fire effects on fuels and potential fire behavior in an eastern cascades Forest, Washington, USA. Fire Ecol. 2, 3–19. doi: 10.4996/fireecology.0202003
Agee, J. K., and Skinner, C. N. (2005). Basic principles of forest fuel reduction treatments. For. Ecol. Manag. 211, 83–96. doi: 10.1016/j.foreco.2005.01.034
Alcasena, F. J., Rodrigues, M., Gelabert, P., Ager, A., Salis, M., Améztegui, A., et al. (2021). Fostering carbon credits to finance wildfire risk reduction Forest Management in Mediterranean Landscapes. Land 10, 10–13. doi: 10.3390/land10101104
Aponte, C., Tolhurst, K. G., and Bennett, L. T. (2014). Repeated prescribed fires decrease stocks and change attributes of coarse woody debris in a temperate eucalypt forest. Ecol. Appl. 24, 976–989. doi: 10.1890/13-1426.1
Badgley, G., Freeman, J., Hamman, J. J., Haya, B., Trugman, A. T., Anderegg, W. R. L., et al. (2022). Systematic over-crediting in California’s forest carbon offsets program. Global Change Biol. 28, 1433–1445. doi: 10.1111/gcb.15943
BeZero Carbon. (2023). Assessment of Verra’s new VM0047 ARR methodology. Available at: https://bezerocarbon.com/insights/assessment-of-verra-s-new-vm0047-arr-methodology?utm_source=chatgpt.com (Accessed December 5, 2024).
Blanchard, L, Anderegg, WRL, and Haya, B. Instead of carbon offsets, we need ‘contributions’ to forests (SSIR). (2024). Available at: https://ssir.org/articles/entry/forest-contributions-carbon-offsets (Accessed March 17, 2024).
Bowes, M. D., and Krutilla, J. V. (1985). “Chapter 12 multiple use management of public forestlands” in Handbook of natural resource and energy economics Eds. Allen V. Kneese and James L. Sweeney (Amsterdam, Netherlands: The publisher, North-Holland Elsevier), 531–569.
Brand, M. W., Seipp, K. Q., Saksa, P., Ulibarri, N., Bomblies, A., Mandle, L., et al. (2021). Environmental impact bonds: a common framework and looking ahead. Environ Res 1:023001. doi: 10.1088/2634-4505/ac0b2c
Butler, B., Ottmar, R., Rupp, T., Jandt, R., Miller, E., Howard, K., et al. (2012). Quantifying the effect of fuel reduction treatments on fire behavior in boreal forests. Can. J. For. Res. 43, 97–102. doi: 10.1139/cjfr-2012-0234
Cabiyo, B., Fried, J. S., Collins, B. M., Stewart, W., Wong, J., and Sanchez, D. L. (2021). Innovative wood use can enable carbon-beneficial forest management in California. Proc. Natl. Acad. Sci. USA 118:e2019073118. doi: 10.1073/pnas.2019073118
California Air Resources Board. Public comment draft California’s historical fire activity before modern fire suppression. (2021).
Carbon Neutrality by 2045. (2024). Office of Planning and Research. Available at: https://opr.ca.gov/climate/carbon-neutrality.html (Accessed March 17, 2024).
Chang, H. (2021). The cost of Forest thinning operations in the United States west: A systematic literature review and model development : Northern Arizona University.
CLERE. The lack of long-term feedstock supply impedes capital investment in California’s wood utilization opportunities. (2020). Available at: https://www.clereinc.com/_files/ugd/21f5c9_a8402d7ae16e472e85d0b6295804fa02.pdf (Accessed March 17, 2024).
Collins, B. M., Das, A. J., Battles, J. J., Fry, D. L., Krasnow, K. D., and Stephens, S. L. (2014). Beyond reducing fire hazard: fuel treatment impacts on overstory tree survival. Ecol. Appl. 24, 1879–1886. doi: 10.1890/14-0971.1
Collins, B. M., Everett, R. G., and Stephens, S. L. (2011). Impacts of fire exclusion and recent managed fire on forest structure in old growth Sierra Nevada mixed-conifer forests. Ecosphere 2:51. doi: 10.1890/ES11-00026.1
Delacote, P. (2024). Strong transparency required for carbon credit mechanisms. Nature Sustainabil. 7, 706–713. doi: 10.1038/s41893-024-01310-0
DellaSala, D. A., Hutto, R. L., Hanson, C. T., Bond, M. L., Ingalsbee, T., Odion, D., et al. (2017). Accommodating mixed-severity fire to restore and maintain ecosystem integrity with a focus on the Sierra Nevada of California, USA. Fire Ecol. 13, 148–171. doi: 10.4996/fireecology.130248173
Dillon, GK, and Gilbertson-Day, JW. (2020). Wildfire Hazard potential for the United States (270-m), version 2020. Available at: https://www.fs.usda.gov/rds/archive/Catalog/RDS-2015-0047-3 (Accessed February 9, 2023).
Duffy, K. A., Schwalm, C. R., Arcus, V. L., Koch, G. W., Liang, L. L., and Schipper, L. A. (2021). How close are we to the temperature tipping point of the terrestrial biosphere? Sci. Adv. 7:3. doi: 10.1126/sciadv.aay1052
Elias, M., Dees, J., Cabiyo, B., Saksa, P., and Sanchez, D. L. (2023). Financial analysis of innovative wood products and carbon finance to support Forest restoration in California. For. Prod. J. 73, 31–42. doi: 10.13073/FPJ-D-22-00049
Elias, M., Sanchez, D. L., Saksa, P., Hunt, J., and Remucal, J. (2024). Market analysis of coupled biochar and carbon credit production from wildfire fuel reduction projects in the western USA. Biofuels Bioproducts Biorefining 18, 1226–1237. doi: 10.1002/bbb.2614
Fick, S. E., Nauman, T. W., Brungard, C. C., and Duniway, M. C. (2021). Evaluating natural experiments in ecology: using synthetic controls in assessments of remotely sensed land treatments. Ecol. Appl. 31:e02264. doi: 10.1002/eap.2264
Foster, D. E., Battles, J. J., Collins, B. M., York, R. A., and Stephens, S. L. (2020). Potential wildfire and carbon stability in frequent-fire forests in the Sierra Nevada: trade-offs from a long-term study. Ecosphere 11:e03198. doi: 10.1002/ecs2.3198
FVS. (2024). What is FVS? Available at: https://www.fs.usda.gov/fvs/whatis/index.shtml?utm_source=chatgpt.com (Accessed December 5, 2024).
Github. (2022). Carbon-removal-source-materials/Project Applications at main Frontierclimate/carbon-removal-source-materials. Available at: https://github.com/frontierclimate/carbon-removal-source-materials/tree/main/Project%20Applications (Accessed March 17, 2024).
Goodwin, M. J., North, M. P., Zald, H. S. J., and Hurteau, M. D. (2020). Changing climate reallocates the carbon debt of frequent-fire forests. Glob. Chang. Biol. 26, 6180–6189. doi: 10.1111/gcb.15318
Guo, H., Goulden, M., Chung, M. G., Nyelele, C., Egoh, B., Keske, C., et al. (2023). Valuing the benefits of forest restoration on enhancing hydropower and water supply in California’s Sierra Nevada. Sci. Total Environ. 876:162836. doi: 10.1016/j.scitotenv.2023.162836
Gutierrez, A. A., Hantson, S., Langenbrunner, B., Chen, B., Jin, Y., Goulden, M. L., et al. (2021). Wildfire response to changing daily temperature extremes in California’s Sierra Nevada. Science. Advances 7:1. doi: 10.1126/sciadv.abe6417
Harris, N., Gibbs, D., Baccini, A., Birdsey, R., de Bruin, S., Farina, M., et al. (2021). Global maps of twenty-first century forest carbon fluxes. Nat. Clim. Chang. 11, 234–240. doi: 10.1038/s41558-020-00976-6
Hartsough, B. R., Abrams, S., Barbour, R. J., Drews, E. S., McIver, J. D., Moghaddas, J. J., et al. (2008). The economics of alternative fuel reduction treatments in western United States dry forests: financial and policy implications from the National Fire and fire surrogate study. Forest Policy Econ. 10, 344–354. doi: 10.1016/j.forpol.2008.02.001
Haya, B., Cullenward, D., Strong, A. L., Grubert, E., Heilmayr, R., Sivas, D. A., et al. (2020). Managing uncertainty in carbon offsets: insights from California’s standardized approach. Clim. Pol. 20, 1112–1126. doi: 10.1080/14693062.2020.1781035
Haya, B., Evans, S., Brown, L., Bukoski, J., Butsic, V., Cabiyo, B., et al. (2023). Comprehensive review of carbon quantification by improved forest management offset protocols. Front For Glob Change 21:6. doi: 10.3389/ffgc.2023.958879
Herbert, C., Haya, B. K., Stephens, S. L., and Butsic, V. (2022). Managing nature-based solutions in fire-prone ecosystems: competing management objectives in California forests evaluated at a landscape scale. Front For Glob Change 5:181. doi: 10.3389/ffgc.2022.957189
Hessburg, P. F., Spies, T. A., Perry, D. A., Skinner, C. N., Taylor, A. H., Brown, P. M., et al. (2016). Tamm review: management of mixed-severity fire regime forests in Oregon, Washington, and northern California. For. Ecol. Manag. 366, 221–250. doi: 10.1016/j.foreco.2016.01.034
Hill, A. P., Nolan, C. J., Hemes, K. S., Cambron, T. W., and Field, C. B. (2023). Low-elevation conifers in California’s Sierra Nevada are out of equilibrium with climate. PNAS Nexus. 2:1. doi: 10.1093/pnasnexus/pgad004
Huang, C. H., and Sorensen, C. (2011). The economic value of selling carbon credits from restored forests: a case study from the Navajo Nation’s tribal forests. West. J. Appl. For. 26, 37–45. doi: 10.1093/wjaf/26.1.37
Hurteau, M. D., Koch, G. W., and Hungate, B. A. (2008). Carbon protection and fire risk reduction: toward a full accounting of forest carbon offsets. Front. Ecol. Environ. 6, 493–498. doi: 10.1890/070187
Hurteau, M. D., Liang, S., Martin, K. L., North, M. P., Koch, G. W., and Hungate, B. A. (2016). Restoring forest structure and process stabilizes forest carbon in wildfire-prone southwestern ponderosa pine forests. Ecol. Appl. 26, 382–391. doi: 10.1890/15-0337
Hurteau, M. D., North, M. P., Koch, G. W., and Hungate, B. A. (2019). Managing for disturbance stabilizes forest carbon. Proc. Natl. Acad. Sci. 116, 10193–10195. doi: 10.1073/pnas.1905146116
Hurteau, M. D., Stoddard, M. T., and Fulé, P. Z. (2011). The carbon costs of mitigating high-severity wildfire in southwestern ponderosa pine. Glob. Chang. Biol. 17, 1516–1521. doi: 10.1111/j.1365-2486.2010.02295.x
Kaarakka, L., Rothey, J., and Dee, L. E. (2023). Managing forests for carbon–status of the forest carbon offset markets in the United States. PLOS Climate 2:e0000158. doi: 10.1371/journal.pclm.0000158
Kane, V. R., Cansler, C. A., Povak, N. A., Kane, J. T., McGaughey, R. J., Lutz, J. A., et al. (2015). Mixed severity fire effects within the rim fire: relative importance of local climate, fire weather, topography, and forest structure. For. Ecol. Manag. 358, 62–79. doi: 10.1016/j.foreco.2015.09.001
Kelsey, R, Smith, E, Biswas, T, McColl, C, Wilson, K, and Wilson, D. Regional prioritization of Forest restoration across California’s Sierra Nevada. (2017). 16. Available at: https://www.scienceforconservation.org/assets/downloads/tnc_Prioritizing_Forest_Restoration_hr.pdf (Accessed July 12, 2023).
Kennedy, M. C., and Johnson, M. C. (2014). Fuel treatment prescriptions alter spatial patterns of fire severity around the wildland–urban interface during the wallow fire, Arizona, USA. For. Ecol. Manag. 318, 122–132. doi: 10.1016/j.foreco.2014.01.014
Knapp, E. E., Skinner, C. N., North, M. P., and Estes, B. L. (2013). Long-term overstory and understory change following logging and fire exclusion in a Sierra Nevada mixed-conifer forest. For. Ecol. Manag. 310, 903–914. doi: 10.1016/j.foreco.2013.09.041
Liang, S., Hurteau, M. D., and Westerling, A. L. (2018). Large-scale restoration increases carbon stability under projected climate and wildfire regimes. Front. Ecol. Environ. 16, 207–212. doi: 10.1002/fee.1791
Lou, J., Hultman, N., Patwardhan, A., and Mintzer, I. (2023). Corporate motivations and co-benefit valuation in private climate finance investments through voluntary carbon markets. NPJ Clim Action 2, 3–5. doi: 10.1038/s44168-023-00063-4
Michaelowa, A, Michaelowa, K, and Hermwille, L, Espelage a. towards net zero: dynamic baselines for internationalmarketmechanisms. CIS working paper. (2021). Available at: https://ethz.ch/content/dam/ethz/special-interest/gess/cis/cis-dam/CIS_2021/CIS%20WP%20107.pdf (Accessed April 14, 2024).
Miller, J. D., and Safford, H. (2012). Trends in wildfire severity: 1984 to 2010 in the Sierra Nevada, Modoc plateau, and southern cascades, California, USA. Fire Ecol. 8, 41–57. doi: 10.4996/fireecology.0803041
Miller, J. D., Safford, H. D., Crimmins, M., and Thode, A. E. (2009). Quantitative evidence for increasing Forest fire severity in the Sierra Nevada and southern Cascade Mountains, California and Nevada, USA. Ecosystems 12, 16–32. doi: 10.1007/s10021-008-9201-9
Molina, J. R., García, J. P., Fernández, J. J., and Rodríguez y Silva, F. (2018). Prescribed fire experiences on crop residue removal for biomass exploitations. Application to the maritime pine forests in the Mediterranean Basin. Sci. Total Environ. 612, 63–70. doi: 10.1016/j.scitotenv.2017.08.182
North, M., Brough, A., Long, J., Collins, B., Bowden, P., Yasuda, D., et al. (2015). Constraints on mechanized treatment significantly limit mechanical fuels reduction extent in the Sierra Nevada. J. For. 113, 40–48. doi: 10.5849/jof.14-058
North, M., Collins, B. M., and Stephens, S. (2012). Using fire to increase the scale, benefits, and future maintenance of fuels treatments. J. For. 110, 392–401. doi: 10.5849/jof.12-021
North, M. P., Tompkins, R. E., Bernal, A. A., Collins, B. M., Stephens, S. L., and York, R. A. (2022). Operational resilience in western US frequent-fire forests. For. Ecol. Manag. 507:120004. doi: 10.1016/j.foreco.2021.120004
Odland, M. C., Goodwin, M. J., Smithers, B. V., Hurteau, M. D., and North, M. P. (2021). Plant community response to thinning and repeated fire in Sierra Nevada mixed-conifer forest understories. For. Ecol. Manag. 495:119361. doi: 10.1016/j.foreco.2021.119361
Pan, Y., Birdsey, R. A., Fang, J., Houghton, R., Kauppi, P. E., Kurz, W. A., et al. (2011). A large and persistent carbon sink in the World’s forests. Science 333, 988–993. doi: 10.1126/science.1201609
Parks, S. A., Dillon, G. K., and Miller, C. (2014). A new metric for quantifying burn severity: the relativized burn ratio. Remote Sens. 6, 1827–1844. doi: 10.3390/rs6031827
Perry, D. A., Hessburg, P. F., Skinner, C. N., Spies, T. A., Stephens, S. L., Taylor, A. H., et al. (2011). The ecology of mixed severity fire regimes in Washington, Oregon, and northern California. For. Ecol. Manag. 262, 703–717. doi: 10.1016/j.foreco.2011.05.004
Piqué, M., and Domènech, R. (2018). Effectiveness of mechanical thinning and prescribed burning on fire behavior in Pinus nigra forests in NE Spain. Sci. Total Environ. 618, 1539–1546. doi: 10.1016/j.scitotenv.2017.09.316
Procton, A. State of the voluntary carbon market: on the path to maturity. Ecosystem Marketplace. (2024). Available at: https://www.ecosystemmarketplace.com/publications/2024-state-of-the-voluntary-carbon-markets-sovcm/ (Accessed march 17, 2024).
Quesnel Seipp, K., Maurer, T., Elias, M., Saksa, P., Keske, C., Oleson, K., et al. (2023). A multi-benefit framework for funding forest management in fire-driven ecosystems across the Western U.S. J. Environ. Manag. 344:118270. doi: 10.1016/j.jenvman.2023.118270
Rabin, S. S., Gérard, F. N., and Arneth, A. (2022). The influence of thinning and prescribed burning on future forest fires in fire-prone regions of Europe. Environ. Res. Lett. 17:055010. doi: 10.1088/1748-9326/ac6312
Riley, K. L., Grenfell, I. C., Finney, M. A., and Wiener, J. M. (2021). TreeMap, a tree-level model of conterminous US forests circa 2014 produced by imputation of FIA plot data. Sci. Data 8:11. doi: 10.1038/s41597-020-00782-x
Scholl, A. E., and Taylor, A. H. (2010). Fire regimes, forest change, and self-organization in an old-growth mixed-conifer forest, Yosemite National Park, USA. Ecol. Appl. 20, 362–380. doi: 10.1890/08-2324.1
Schwartz, M., Butt, N., Dolanc, C. R., Holguin, A., Moritz, M., North, M., et al. (2015). Increasing elevation of fire in the Sierra Nevada and implications for forest change. Ecosphere 6, 1–10. doi: 10.1890/ES15-00003.1
Skog, K. E. (2008). Sequestration of carbon in harvested wood products for the United States. For. Prod. J. 58, 56–72.
So, Ivy, Haya, Barbara, and Elias, Micah. Berkeley carbon trading project voluntary registry offset database. (2023). Available at: https://gspp.berkeley.edu/research-and-impact/centers/cepp/projects/berkeley-carbon-trading-project/offsets-database (Accessed March 17, 2024).
Stephens, S. L., Battaglia, M. A., Churchill, D. J., Collins, B. M., Coppoletta, M., Hoffman, C. M., et al. (2020). Forest restoration and fuels reduction: convergent or divergent? Bioscience :134, 85–101. doi: 10.1093/biosci/biaa134
Stephens, S. L., Collins, B. M., and Roller, G. (2012). Fuel treatment longevity in a Sierra Nevada mixed conifer forest. For. Ecol. Manag. 285, 204–212. doi: 10.1016/j.foreco.2012.08.030
Stephenson, N. L., Das, A. J., Condit, R., Russo, S. E., Baker, P. J., Beckman, N. G., et al. (2014). Rate of tree carbon accumulation increases continuously with tree size. Nature 507, 90–93. doi: 10.1038/nature12914
Trencher, G., Nick, S., Carlson, J., and Johnson, M. (2024). Demand for low-quality offsets by major companies undermines climate integrity of the voluntary carbon market. Nat. Commun. 15:6863. doi: 10.1038/s41467-024-51151-w
Tyukavina, A., Potapov, P., Hansen, M. C., Pickens, A. H., Stehman, S. V., Turubanova, S., et al. (2022). Global trends of Forest loss due to fire from 2001 to 2019. Front. Remote Sens. 3:3. doi: 10.3389/frsen.2022.825190
Visualizing Federal Funding for Wildfire Management and Response. (2023). Federation of American Scientists. Available at: https://fas.tghp.co.uk/publication/visualizing-federal-funding-for-wildfire-management-and-response/ (Accessed December 21, 2023).
Williams, A. P., Abatzoglou, J., Gershunov, A., Guzman-Morales, J., Bishop, D. A., Balch, J., et al. (2019). Observed impacts of anthropogenic climate change on wildfire in California. Earth’s Future 7, 892–910. doi: 10.1029/2019EF001210
Yackulic, E., Elias, M., Duffy, K., Plumb, S., Shannon, J., Gilbert, S., et al. (2024). Rising from the ashes: Quantifying treatment effectiveness in mixd conifer forests of the central sierras (in review). Front For Glob Change.
Yin, R. (2024). Global forest carbon: Policy, economics and finance. Abingdon, Oxon: Routledge, Taylor & Francis Group.
Keywords: carbon finance, carbon markets, nature-based solutions, forest resilience, forest management, wildfire mitigation, biomass utilization, dynamic performance benchmark
Citation: Elias M, Yackulic E, Duffy K, Saksa P, Sanchez DL, Pevzner N, Plumb S, Dyszynski J and Bracer C (2025) Carbon finance for forest resilience in California. Front. For. Glob. Change. 7:1507554. doi: 10.3389/ffgc.2024.1507554
Edited by:
Samuel Evans, California Department of Forestry and Fire Protection, United StatesReviewed by:
Gui Jin, China University of Geosciences Wuhan, ChinaRunsheng Yin, Michigan State University, United States
Copyright © 2025 Elias, Yackulic, Duffy, Saksa, Sanchez, Pevzner, Plumb, Dyszynski and Bracer. This is an open-access article distributed under the terms of the Creative Commons Attribution License (CC BY). The use, distribution or reproduction in other forums is permitted, provided the original author(s) and the copyright owner(s) are credited and that the original publication in this journal is cited, in accordance with accepted academic practice. No use, distribution or reproduction is permitted which does not comply with these terms.
*Correspondence: Micah Elias, bWljYWhAYmx1ZWZvcmVzdC5vcmc=