- 1Department of Ecology, Swedish University of Agricultural Sciences, Uppsala, Sweden
- 2Department of Geosciences and Natural Resource Management, University of Copenhagen, Frederiksberg, Denmark
Introduction: Bark and longhorn beetles have become of increasing concern as invasive pests as they are transported across the globe. The larch bark beetle Ips cembrae and the larch longhorn beetle Tetropium gabrieli are two species that have recently established in Scandinavia. We asked the following questions for both I. cembrae and T. gabrieli: (i) How does habitat availability affect the relative population density? (ii) How is the colonization probability related to the relative population density? (iii) What is the relationship between relative population densities of the beetles and enemy pressure?
Methods: The relative population density of the two beetle species were assessed with two pheromone-baited traps for each beetle species in 36 pure larch stands distributed in southern Sweden and Denmark. In addition, colonization success was recorded on five cut larch trees in 30 of the 36 larch stands.
Results: Ips cembrae was trapped in 19 stands and had colonized cut trees in two stands, whereas Tetropium gabrieli was trapped in 18 stands and had colonized cut trees in 12 stands. Both species showed a positive relationship between the relative population density and the probability of colonization of the cut trees. For I. cembrae a higher population size was required for colonization than for T. gabrieli. The relative population density of both species was positively related to the amount of habitat.
Discussion: The difference in the population size required for colonization between the two studied species might be explained by their different reproductive strategies. The results are discussed in terms of host location and mate finding, and predation pressure.
1 Introduction
Establishments of non-native species in new areas are a major challenge for forest ecosystems around the globe (Vilà et al., 2010; Simberloff et al., 2013), and their number have increased in the last decades (Brockerhoff and Liebhold, 2017). Increasing global trade volumes are a major factor for the increase in the unintended introductions of non-native species (Everett, 2000; Haack, 2006; Meurisse et al., 2019). Although species might be unwittingly transported, only a small percentage of incursions result in establishment (Liebhold and Tobin, 2008). Often incursions fail due to lack of suitable habitat (Brockerhoff and Liebhold, 2017), or too low numbers of arriving individuals (Brockerhoff et al., 2014). The number of arriving individuals is thought to be one of the most important driving forces behind the success of non-native species establishment (Lockwood et al., 2005; Drake and Lodge, 2006; Simberloff, 2009; Chase et al., 2023). After initial establishment in a new region, the spread, and subsequent colonization of new localities in the region, can be expected to be influenced by both population pressure and species-specific traits. In this study, we compare the success of colonization of new localities of two bark- and wood-boring beetle species that have recently established in Denmark and southern Sweden, and that differ regarding their life history traits.
Establishment success and spread of non-native species commonly follows the principles of the Allee effect (Taylor and Hastings, 2005), which describes the relationship between population density and individual fitness (Lewis and Kareiva, 1993; Berec et al., 2007) in relation to population growth. The Allee threshold defines the population density below which population growth will be negative and population establishment will fail. To distinguish species-specific factors contributing to the Allee effect, the Allee effect is divided into component and demographic Allee effects (Stephens et al., 1999). Component Allee effects use life history traits, like mate finding, foraging efficiency and enemy pressure, to understand their impact on the realized population growth rate (Courchamp and Macdonald, 2001; Grünbaum and Veit, 2003; Gascoigne et al., 2009; Tobin et al., 2011; Fauvergue, 2013; Bürgi et al., 2015).
Species life history traits are key behavioral predictors when it comes to success of establishment and spread because they determine how the component Allee effects contribute to the Allee threshold (Ducatez and Shine, 2019; Davies and Robert Britton, 2021). Previous research has shown that dispersal ability, location of suitable habitat (South and Kenward, 2001) and the duration of the flight period (Kolar and Lodge, 2001), are important determinants in population expansions. In addition, the reproduction strategies and the number of produced offspring influence probability of colonization and also establishment and spread (Caswell et al., 2003; Rhainds, 2010; Sekar, 2012; Allen et al., 2017; Demidko et al., 2021). Other factors, such as reduced impact from natural enemies (Roy et al., 2011) and competition (Berec and Mrkvička, 2013), may also affect the probability of establishment and spread (Roy et al., 2011).
Besides differences in life history traits of species, habitat fragmentation is another important factor affecting the establishment and spread of non-native species by influencing dispersal, mortality, foraging efficiency and mate finding (Drake and Lodge, 2006; Dewhirst and Lutscher, 2009; Maciel and Lutscher, 2015; Musgrave et al., 2015; Walter et al., 2016). Different species may be affected at different spatial scales (Holland et al., 2004, 2005; Martin and Fahrig, 2012) or at different phases in the establishment and spread process (Johnson et al., 1992).
In this study, we investigate how relative population densities of the European larch bark beetle, Ips cembrae (Heer) (Curculionidae), and the larch longhorn beetle, Tetropium gabrieli (Weise) (Cerambycidae), influence their colonization success. Both species are non-native in the study area and breed in the non-native larch trees (genus Larix), which occur in highly fragmented forest patches. Bark beetles and longhorn beetles, have become of increasing concern as invasive species as they are easily transported in wooden products and wood packing material used in shipping of goods (Brockerhoff et al., 2006; Haack, 2006; Vilardo et al., 2022).
We ask the following questions for I. cembrae and T. gabrieli: (i) How does habitat availability (amount of larch in the surrounding landscape), affect the relative population density? (ii) How is the colonization probability related to the relative population density? (iii) What is the relationship between local population densities of beetles and enemy pressure? Our hypotheses are that: (1) the relative population density is influenced by the availability of larch stands in the surrounding landscape, hence we expect higher populations in areas with larger total areas of larch stands; (2) a minimum population size is required for colonization in new localities and this threshold value is influenced by species reproductive strategies; (3) there are no relationships between the relative population densities of the two beetles and their enemies because the enemies are generalist species.
2 Materials and methods
2.1 Study system
Both I. cembrae and T. gabrieli are native to the Alps in Central Europe where European larch (Larix decidua, Mill.) is the host tree. Although Siberian larch (Larix sibirica, Ledeb.) occurred naturally in Scandinavia shortly after the last glacial period (Kullman, 1998), it disappeared from the Scandinavian peninsula probably due to changes in the climatic conditions (Larsson-Stern, 2003). In Sweden, the first recorded plantation of European larch dates from 1763 (Schotte, 1917 in Larsson-Stern, 2003). Today, Larix x eurolepis, (A Henry), a hybrid between the European and the Japanese larch, (L. kaempferi, Lamb. Carr), is the most planted larch variety in Sweden (Johansson, 2012; Larsson-Stern, 2003). In southern Sweden (Götaland), larch constitutes 0.2% of the total growing stock including all tree species (Swedish University of Agricultural Sciences, 2022) while in Denmark larch constitutes 3.4% of total growing stock (Nord-Larsen et al., 2020). The increased use of larch in forest plantations is thought to have enabled I. cembrae and T. gabrieli to spread and establish in new areas in Europe (Ravn, 2012; Lindelöw et al., 2015; Lynikiene et al., 2021; Grégoire et al., 2024).
The current known distribution of I. cembrae outside its native area ranges from UK and the Netherlands in the west (Luitjes, 1974; Alexander, 2007), to Denmark and Sweden in the north (Ravn, 2012; Lindelöw et al., 2015; Grégoire et al., 2024). Ips cembrae generally colonizes weakened trees. In the native range outbreaks of I. cembrae have occurred after storm feelings and snow-breakages (Grégoire and Evans, 2004; Grodzki, 2008). The flight period in central Europe occurs between the end of April to the middle of September (Grucmanová et al., 2014; Holuša et al., 2014). It is not known if I. cembrae is attracted to host volatiles although it is known that I. cembrae is not attracted to α-pinene (Stoakley et al., 1978). The male initiates colonization of breeding substrate by boring into the bark. After successful colonization, an aggregation pheromone is released by the males that is strongly attractive to both males and females. The males create a mating chamber and is joined by three to five females, which create maternal galleries where they oviposit. After producing a first brood the parent beetles may emerge to establish a second brood (sister brood) in another tree. The species can have one to two generations per year, depending on environmental conditions.
The current distribution of T. gabrieli outside its native area ranges from the UK in the west to Denmark and southern Sweden, and recently Lithuania, towards the northeast (Crawshay, 1907; Ericson, 2010; Kahanpää, 2017; Lynikiene et al., 2021). Like I. cembrae, T. gabrieli is considered a secondary pest, but when larch trees are weakened due to drought it may cause considerable tree mortality (Evans et al., 2004). The flight period starts in May and extends to early August in southern Sweden (Schroeder et al., 2020). Mating may take place right after the emergence of the new generation, possibly even before dispersal, as well as later in the summer (Hanks, 1999). Thus, in contrast to I. cembrae, mated females can colonize (i.e., lay their eggs) host trees independent of males, and aggregation with conspecifics to overwhelm tree defence is not required. It is not known if T. gabrieli uses host tree volatiles to locate breeding substrate, but it is shown that the species was not attracted to α-pinene and ethanol (Schroeder et al., 2020). However, as it has been demonstrated that a closely related species, T. fuscum, responds to a blend of five monoterpenes that are released from stressed spruce trees (Sweeney et al., 2004), it is possible that also T. gabrieli is attracted to host volatiles. Males of T. gabrieli are thought to release a pheromone (fuscumol) as it was found to attract both males and females (Schroeder et al., 2020). Three other Tetropium species are attracted to fuscumol and the pheromone has been demonstrated to be released by the males of two of these species (Silk et al., 2007; Sweeney et al., 2010).
2.2 Study area and stand selection
The study was conducted in Denmark (in the regions Zealand, Funen, South Denmark, Mid and North Jutland), and Southern Sweden (in the regions Skåne, Småland, Blekinge, Kalmar and Halland), during 2020. Both I. cembrae and T. gabrieli had previously been detected in many of the regions included in the current study (Isacsson, unpublished data; Ravn and Harding, 1995; Ravn, 2012; Lindelöw et al., 2015; Schroeder et al., 2020). With the above mentioned regions, we refer to the administrative entities in Denmark and southern Sweden, except for the island of Funen, which, although administratively belongs to Southern Jutland, we defined as its own entity due to its isolated location. The number of study locations differed between regions spanning from one (Funen) to 14 (Skåne). The first records of T. gabrieli in Denmark dates from 1890, with a few subsequent sightings, but with a limited distribution (Hansen, 1996). The first report of I. cembrae in Denmark dates from 1995 (Ravn and Harding, 1995) and it is currently established over large parts of the country (Ravn, 2012). The first record of T. gabrieli in Sweden occurred in 2007 in the province of Blekinge (Ericson, 2010). Ips cembrae was first reported in Sweden in 2011 in the province of Skåne (Lindelöw et al., 2015).
Forty-two stands of hybrid larch were selected, ranging from 0.79 to 9.74 ha in size. All stands were monocultures except for five stands, which had undergrowth trees, consisting mainly of spruce. A subset of the stands had previously been included in a monitoring program for I. cembrae conducted by Swedish Forest Agency (Lindelöw et al., 2015) while others were selected from larch stand data provided by forest owners. The minimum distance between selected stands was 1.8 km. For each stand the diameter at 1.3 m height of 40 randomly selected trees (10 trees around each trap position, see below) were measured. Only one standing tree, successfully attacked by T. gabrieli in the previous year (2019), was found in two of our focal stands (both in Sweden, one in Blekinge and one in Kalmar region). In the remaining stands, there were no signs of any local hibernating populations of neither I. cembrae nor T. gabrieli. In the 42 stands, the relative population density of I. cembrae and T. gabrieli were estimated with pheromone-baited traps. In addition, in 34 stands colonization success was assessed by felling larch trees in early spring that subsequently were inspected for attacks.
To analyze the relationship between amount of larch in the adjacent landscape and the relative population density of I. cembrae and T. gabrieli, we used two buffer zones with a radius of 500 and 1,000 m respectively, from the center of each focal stand. We chose 1,000 m as the maximum buffer radius not to violate the independence of our observations (i.e., overlapping buffers). In addition to the focal stand, the quantity of larch (in hectares) in the created buffer zones was calculated. We used the quantity of larch as a proxy for presence of suitable breeding material like weakened standing trees, wind-felled trees and logging residues. The mean, standard deviations and range of stand and landscape variables are provided in Table 1. For all stands in Denmark, and a subset of ten stands in Sweden, the forest owners provided detailed maps with all the larch stands around the focal stands. For the remaining stands in Sweden maps were lacking and we estimated the amount of larch based on aerial images Google maps and maps from the Swedish Forest Agency website.1 The estimates from aerial images were accurate as demonstrated by a check against the detailed maps provided by the ten forest owners in Sweden.
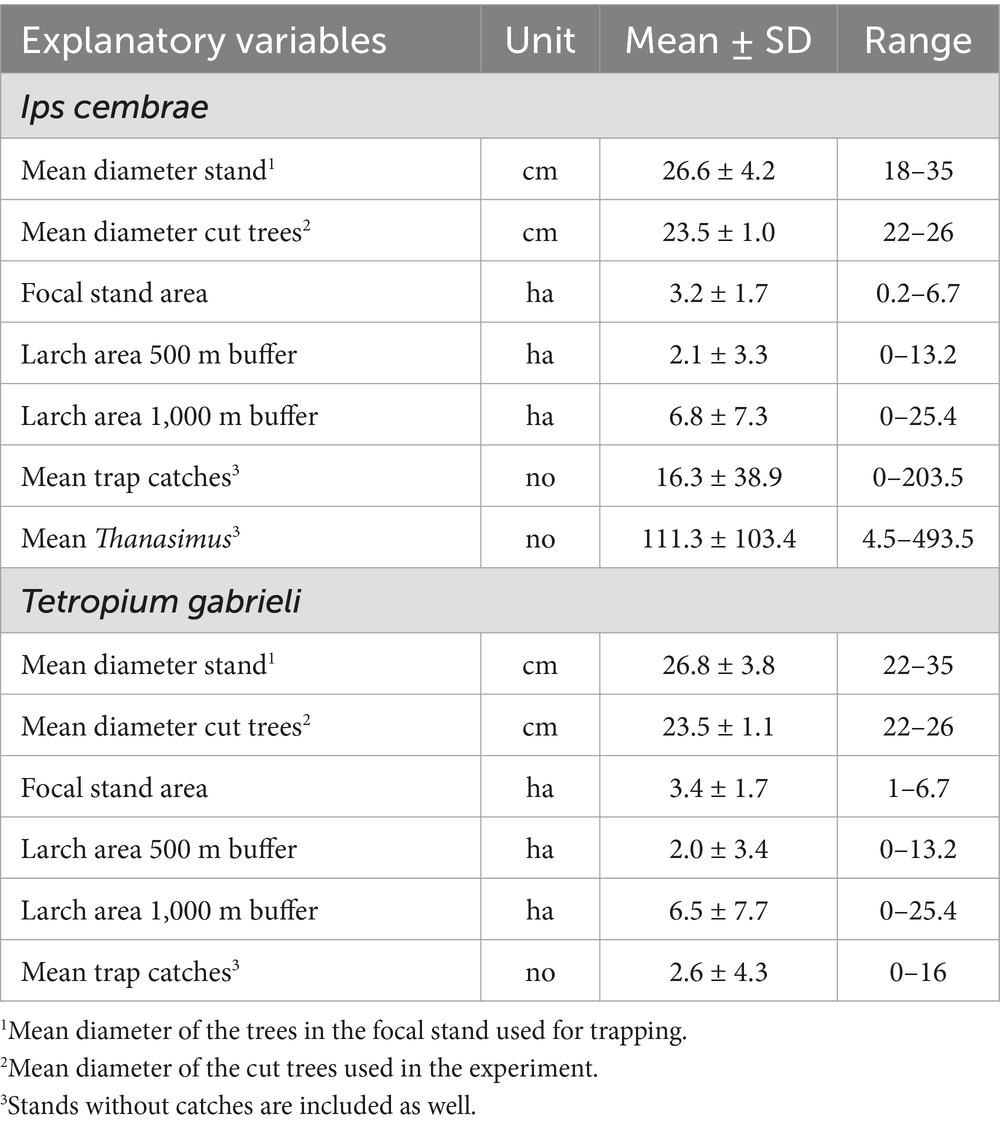
Table 1. Means ± SD, and ranges of the stand variables, landscape variables and trap catches of Ips cembrae and Tetropium gabrieli for the stands included in the statistical analyses.
2.3 Relative population density
To estimate the relative population density of I. cembrae and T. gabrieli, for each of the selected stands, we used two pheromone-baited traps (ECONEX MULTIFUNNEL-12®, Econex SL, Murcia, Spain) for each species. The trapping was standardized by placing the traps inside larch stands, at least 10 m from the stand edge with a minimum distance of 50 m between them. The traps were hung from two 1.8 m wooden poles. A 1:1 mixture of propylene glycol and water was used in the trap jars. Cemsan dispensers (Flügel Gmbh, Germany; active substance 3-Methyl-3-buten-1-ol), were used for attracting I. cembrae and aPhinity BSLB dispensers (racemic E-fuscumol, Andermatt Biocontrol, Switzerland) for attracting T. gabrieli. The lures were not changed during the experiment since the attraction of the lures should last for the duration of an entire trapping season based on the pheromone data sheet provided by the manufacturer. All traps were baited between 13th and 19th of April 2020 and emptied four times between 12th of May and 27th of July 2020, at approximately 3 weeks intervals. This period includes the major part of the flight activity of T. gabrieli as shown in an earlier trapping study conducted in the study area (Schroeder et al., 2020). There is no study on seasonal flight activity of I. cembrae from Scandinavia but the considerably slower development rate, and higher temperature requirements, compared with I. typographus (Schebeck and Schopf, 2017) suggests that most of the flight has occurred during the trapping period (c.f. Fritscher and Schroeder, 2022). As the important predator of I. cembrae, Thanasimus spp., is attracted to bark beetle pheromone, the funnel traps also provided an estimate of their relative population density.
Ips cembrae adults were determined based on morphological characteristics using the identification key from Douglas et al. (2019), and sexed by inspecting the genitalia through dissection. Tetropium gabrieli adults were determined based on Pfeffer (1995), and Ehnström and Holmer (2007), and sexed by inspection of the tip of the abdomen (Yanega, 1996). As in many Cerambycidae, a portion of the sixth abdominal tergite is visible beyond the pygidium of males.
2.4 Species colonization success
Both I. cembrae and T. gabrieli are known to colonize felled trees (Lindelöw et al., 2015; Resnerová et al., 2020; Schroeder et al., 2020). Five larch trees were felled in early spring (2–8 March in Denmark and 16–22 March 2020, in Sweden) in each of the 34 selected stands. Stands with felled trees had a minimum size of 1.8 ha. The minimum distance between the felled trees within stands was 30 m and with a minimum distance of 50 m from the pheromone-baited traps. The trees to be felled were randomly selected (although the distance to the previous felled tree was kept to a minimum of 30 meters). At each trap emptying, the whole length of the trunk, and branches with a diameter exceeding 3 cm of the felled trees, were inspected for the presence of boring dust and entrance holes, indicating possible attacks of I. cembrae. If boring dust was found, the bark was removed and species present were determined. At the final inspection (15–25 September), on every half meter of the trunk, pieces of bark were peeled off with an axe, to check for possible larval galleries of Tetropium (not possible to determine to species level from galleries) and I. cembrae attacks, as well as other non-target bark- and wood-boring beetles.
From each tree with I. cembrae or Tetropium attacks, one 1.2 m log was cut for rearing of adult beetles and enemies. As few trees were colonized by I. cembrae no data from rearing of this species is presented. All collected logs were placed in insect nets and stored outside the laboratory in Uppsala during winter. On 30 and 31 March 2021, one 60 cm stem section was cut from each log and waxed at both ends to prevent desiccation. Each stem section was hung under a translucent roof outside, in a cotton sack, with a plastic collection funnel attached to a collection jar located at the bottom of the sack. The collection jars were emptied throughout the summer. The data collection was completed by the end of August when all the logs were debarked, and dead adults and larvae were recorded. The only Tetropium species emerging was T. gabrieli. Emerging parasitoids belonged to either Braconidae or Ichneumonidae family based on morphological characteristics (see Schroeder et al., 2020 for more details). Parasitism rates of T. gabrieli larvae was estimated for each stem section by dividing the number of emerging parasitoids by the sum of emerging T. gabrieli adults and parasitoids [we assume that each emerged parasitoid killed one larva, based on the life history of the species found to parasitize T. gabrieli previously (see Schroeder et al., 2020)].
2.5 Statistical analyses
All statistical analyses were performed using R ver. 4.3.3 (R Core Team, 2024). For the analyses, locations without any catches or lacking colonization by either species were excluded. These locations were assumed to be outside the distribution area of the species based on the results from this and previous studies (Ravn and Harding, 1995; Ravn, 2012; Lindelöw et al., 2015). Thus, for the analyses of trap catches, 36 locations remained for I. cembrae and 32 locations for T. gabrieli. For the analyses of tree colonization, 30 locations remained for I. cembrae and 26 for T. gabrieli.
2.5.1 How does habitat availability affect the relative population density?
To test the effect of focal stand characteristics, and the amount of larch in the surrounding landscape, on the relative population density (i.e., trap catches), generalized linear mixed models with negative binomial distribution were fitted. Two separate models were run for each species. For each model, location was the level of replication, with 36 replicates for I. cembrae models and 32 for T. gabrieli. Size of the focal stand and the quantity of larch within a 500 m buffer or a 1000 m buffer were explanatory variables for the response variable trap catches. The negative binomial distribution was used instead of Poisson distribution as overdispersion was detected when inspecting the residuals (dispersion_glmer; blmeco package; Korner-Nievergelt et al., 2015). In all the models, Region was included as a random factor to account for potential difference in the trap catches related to different forestry practices between regions (glmer.nb(); lme4 package, Bates et al., 2015). Because we encountered problems with model convergence we fitted the model with adaptive Gauss-Hermite quadrature instead of Laplace approximation and (nAGQ) was set to zero (Olver et al., 2010). Anova type II Wald chi-square test was used to test for significance (Anova() function; car package; Fox and Weisberg, 2019).
2.5.2 How does colonization probability relate to the relative population density?
To examine the effect of mean tree diameter of the cut trees on the mean number of trapped beetles, as well as the mean number of trapped females and males (in three separate models), generalized linear models with a negative binomial distribution were applied. Location was the level of replication, with 30 replicates for I. cembrae models and 26 for T. gabrieli. Again, the negative binomial distribution was used instead of Poisson distribution as overdispersion was detected when inspecting the residuals, (dispersiontest; AER package; Kleiber and Zeileis, 2008). Anova type II Wald chi-square test was used to test for significance (Anova() function; car package; Fox and Weisberg, 2019). Unpaired t-test was used to test whether there was a difference in the mean population size between the locations with colonized trees compared to the locations without colonization för I. cembrae. For the reared Tetropium, chi-square test was used to test whether there was a difference in the sex ratio of emerged beetles.
2.5.3 What is the relationship between the relative population density of beetles and enemy pressure?
To assess the relationship between the relative population density of I. cembrae and of its enemy Thanasimus spp. (from trap catches) we fitted a generalized linear model with quasipoisson distribution. Quasipoisson distribution was used instead of Poisson as overdispersion was detected when inspecting the residuals (dispersiontest; AER package; Kleiber and Zeileis, 2008). To assess the relationship between the numbers of T. gabrieli larvae in the stem sections and proportion parasitism we fitted a generalized linear model with quasibinomial distribution, where proportion of parasitized individuals was used as the response variable and the number of emerged beetles plus emerged parasitoids as the explanatory variables. A similar model was used to test the relationship between the relative population density of T. gabrieli (trap catches) and proportions parasitized in stem sections. Each log was seen as an individual replicate. For the second model on the relationship between the proportion parasitized and the local population from trap catches, the proportion parasitized beetles from the logs from the same location were pooled against the average trap catch from the respective location. Anova type II test was used to test for significance (Anova() function; car package; Fox and Weisberg, 2019).
3 Results
In total, 1185 I. cembrae were caught in 19 larch stands out of the total 36 used in the analyses for trapping (Figure 1A). Of these 800 beetles were sexed that resulted in 46% trapped males and 54% trapped females. The mean (±SE) number of trapped beetles per trap and location was 16.3 (±6.5). At two different locations I. cembrae individuals (two males and six females) were caught in the traps baited with T. gabrieli pheromone (hence cross attraction was negligible). These individuals were not included in the analyses.
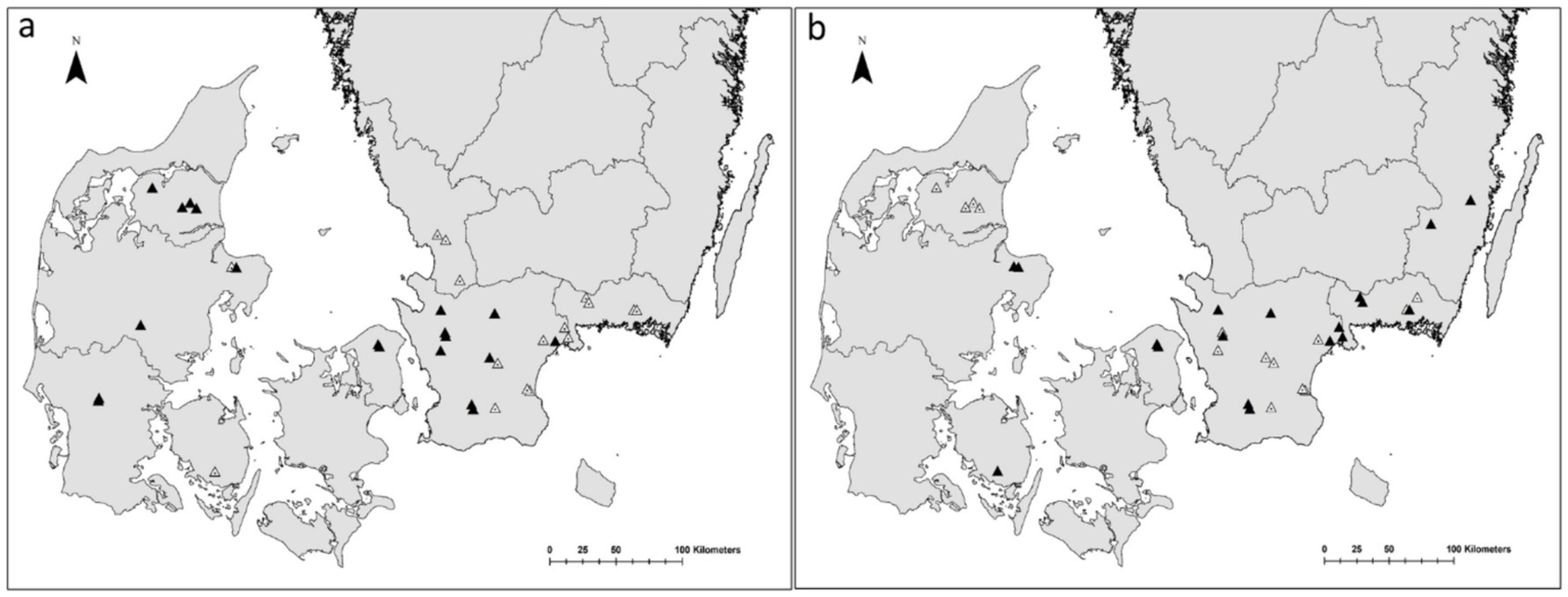
Figure 1. Locations of experimental stands with pheromone traps in Denmark and Sweden for (A) Ips cembrae (36 replicates) and (B) Tetropium gabrieli (32 replicates) which were considered to be within the species distribution areas and thus included in the analyzes. Black triangles represent locations with trapped beetles, empty triangles represent locations with no beetles trapped.
In total 165 T. gabrieli were caught in 18 out of 32 larch stands (Figure 1B). The mean number of trapped beetles per trap and location was 2.6 (±0.8). No T. gabrieli was caught in the traps baited with I. cembrae pheromones. Both native Tetropium species, the brown spruce longhorn beetle T. fuscum (F.) and the European longhorn beetle T. castaneum (L.) were caught in the traps as well, however, none of the species colonized any of the cut trees. In total, 157 T. fuscum and 359 T. castaneum were caught.
3.1 How does habitat availability affect the relative population density?
For I. cembrae, no significant correlation was found between any of the explanatory variables and the size of the local population in the model including the 500 m buffer zone. However, a positive significant relationship was found between relative population density and the amount of larch within the 1,000 m buffer (Table 2). Region explained some of the variance in both the 500 and 1,000 m model (between 0.88 and 9.06%, see Table 2). In both models for T. gabrieli, the relative population density showed a significant positive relationship with the size of the focal stand (Table 2), but not with the quantity of larch in the surrounding landscape.
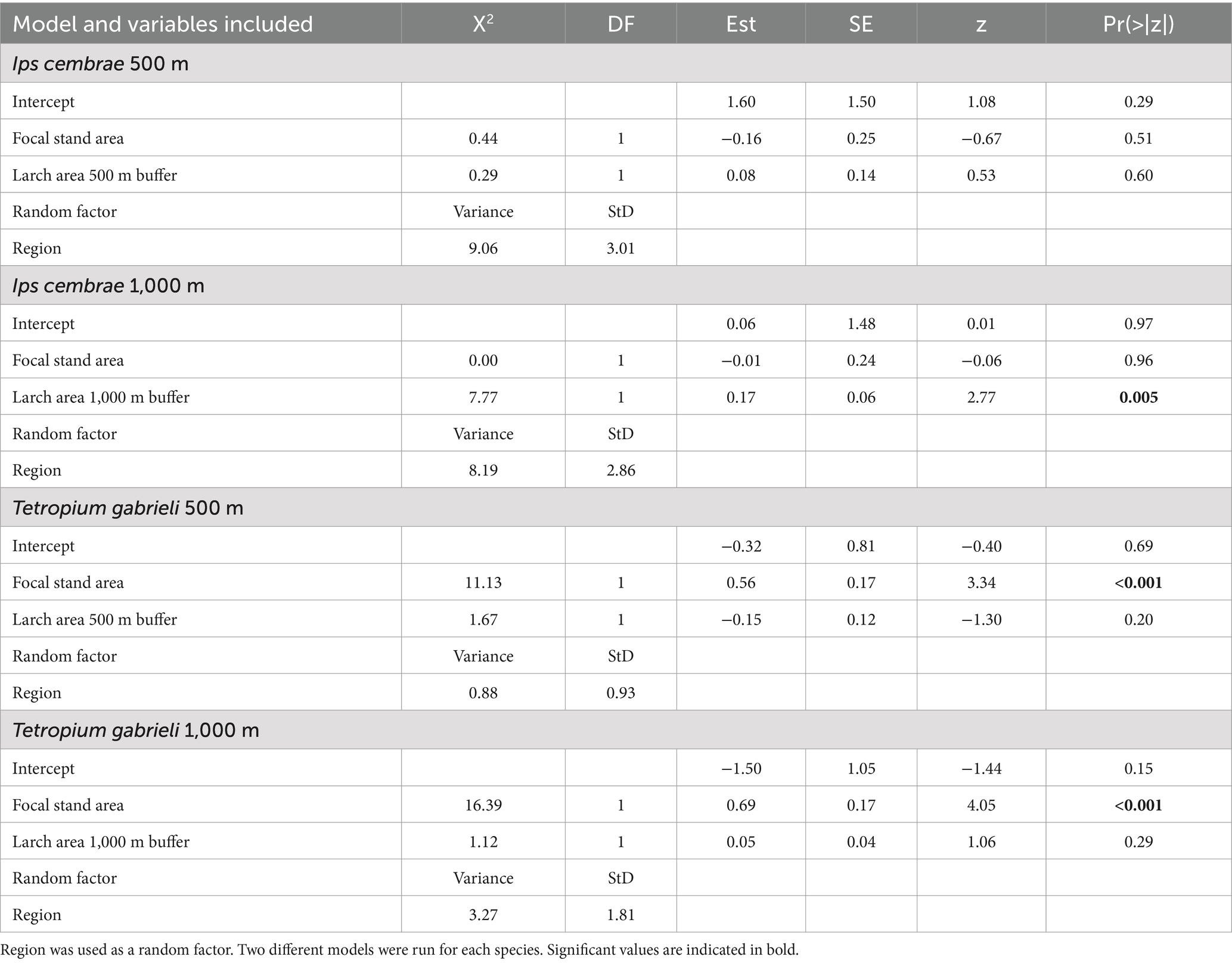
Table 2. Anova (type II test) and summary table for generalized linear mixed models testing the difference in number of beetles (Ips cembrae and Tetropium gabrieli) caught in traps against the size of the focal area and the total amount of larch within 500 and 1,000 m around the focal stand.
3.2 How does colonization probability relate to the relative population density?
Ips cembrae colonized four felled larch trees, distributed among two of the 30 stands included in the analyses (Figure 2A). The two locations with colonized trees were those with the highest trap catches (Figure 2B) and, there was a significant difference in the mean (±SE) number of trapped beetles between the two locations with colonized trees and the 17 locations without colonized trees (287.5 ± 59.7 versus 35.4 ± 5.8, t-test, p < 0.001). No colonization occurred in stands with mean trap catches below 80 beetles. No standing trees were attacked by either of the species in the focal stands in the year of the experiment.
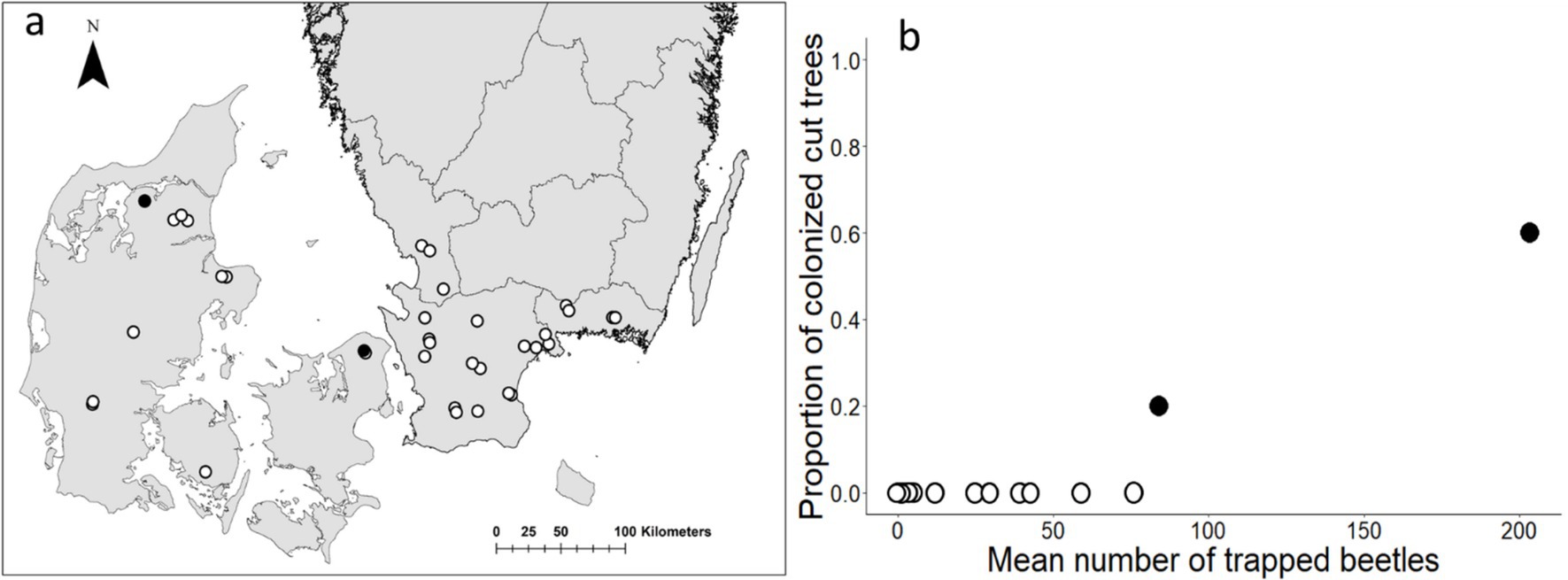
Figure 2. (A) Locations of experimental stands with cut trees in Denmark and Sweden, which were considered to be within the distribution area of Ips cembrae. Black circles represent locations with colonized cut trees; white circles represent locations without colonized cut trees. (B) Relationship between the mean number of trapped I. cembrae and the proportion of colonized cut trees per location (30 replicates).
Tetropium gabrieli colonized 46 felled larch trees, distributed over 12 of the 26 stands included in the analyses (Figure 3A). The number of colonized trees varied from one to five per location. All five trees were colonized in 30% of the locations. Colonization occurred at mean catches as low as 0.5 beetles per stand, and took place in all stands with mean trap catches of two beetles or more (Figure 3B).
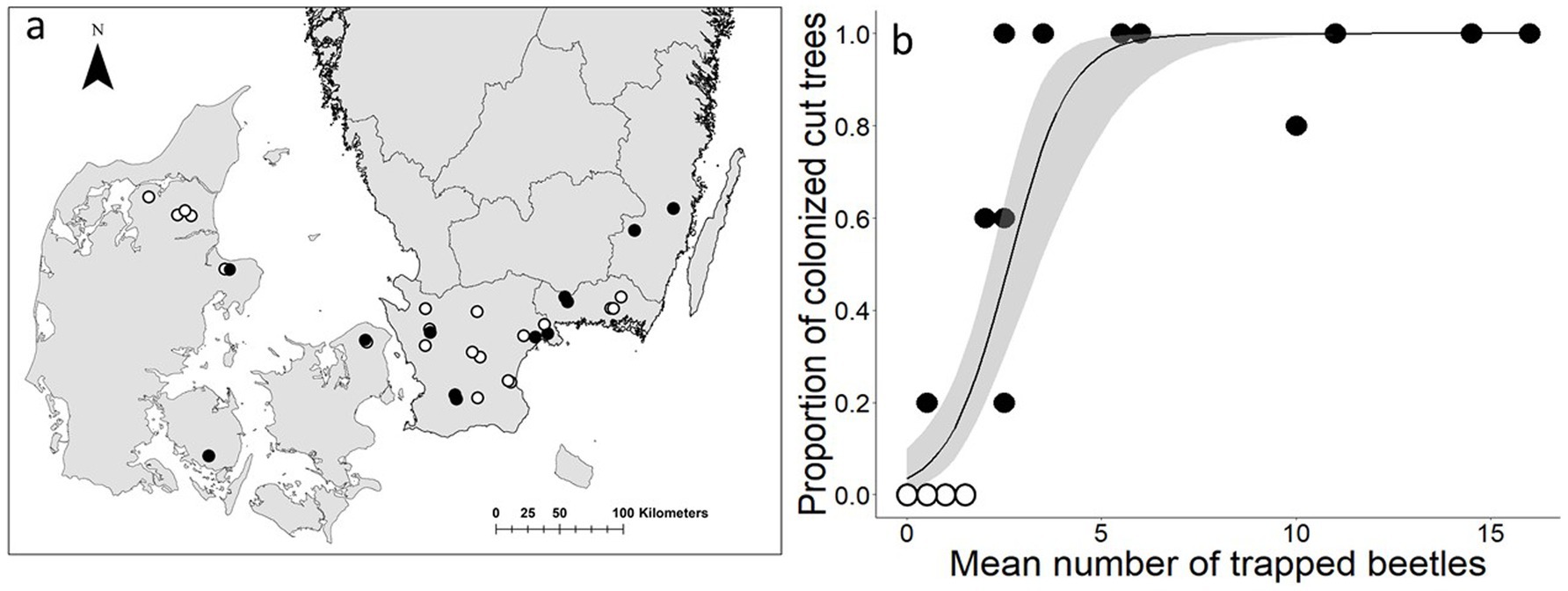
Figure 3. (A) Locations of experimental stands with cut trees in Denmark and Sweden which were considered to be within the distribution area of Tetropium gabrieli. Black circles represent locations with colonized cut trees; white circles represent locations without colonized cut trees. (B) Relationship between the mean number of trapped T. gabrieli and the proportion of colonized cut trees per location (26 replicates). Grey shaded areas represent the 95% confidence intervals.
The number of felled trees colonized by T. gabrieli was significantly and positively related to the mean number of trapped individuals, and the mean number of trapped females and males (Table 3). We found a negative significant relationship between mean diameter of the cut trees and the number of colonized trees in the models including the mean number of trapped individuals and the mean number of trapped males.
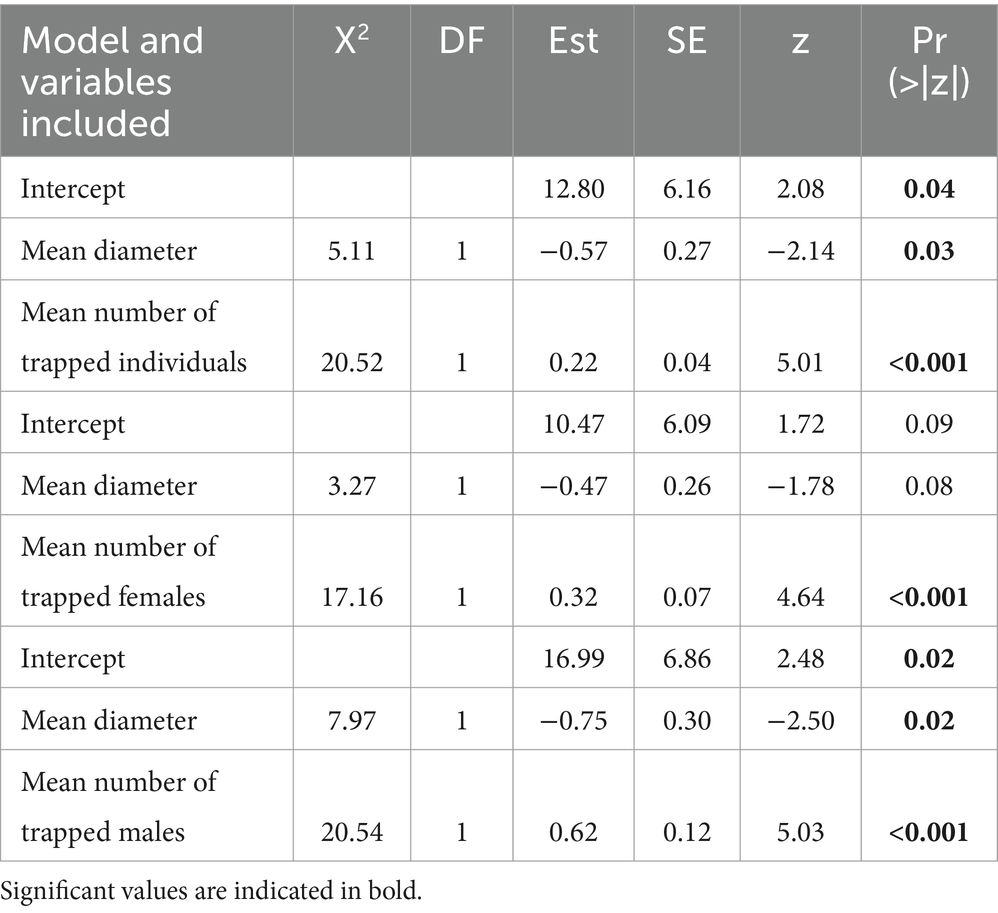
Table 3. Anova (type II test) and summary table for generalized linear models testing the difference in the number of colonized trees by Tetropium gabrieli against the mean number of trapped individuals, mean number of trapped females and males and mean diameter of the cut trees.
A total of 676 T. gabrieli emerged from 42 stem sections out of the total 46 used in the rearing experiment. The mean (±SE) rate of emergence per stem section was 16.1 (±3.2) beetles. There was no significant difference between the number of emerged males and females (p = 0.16, chi-square test).
3.3 What is the relationship between the relative population density of beetles and enemy pressure?
A total of 7684 Thanasimus beetles (7649 T. formicarius and 35 T. femoralis), were caught in the I. cembrae traps at the 36 locations. There was no significant relationship between the mean numbers of trapped I. cembrae and Thanasimus (Figure 4). The ratio between trapped Thanasimus/I. cembrae was 6.6 to 1. One location was removed from the model as it was considered an outlier; however it is included in the figure.
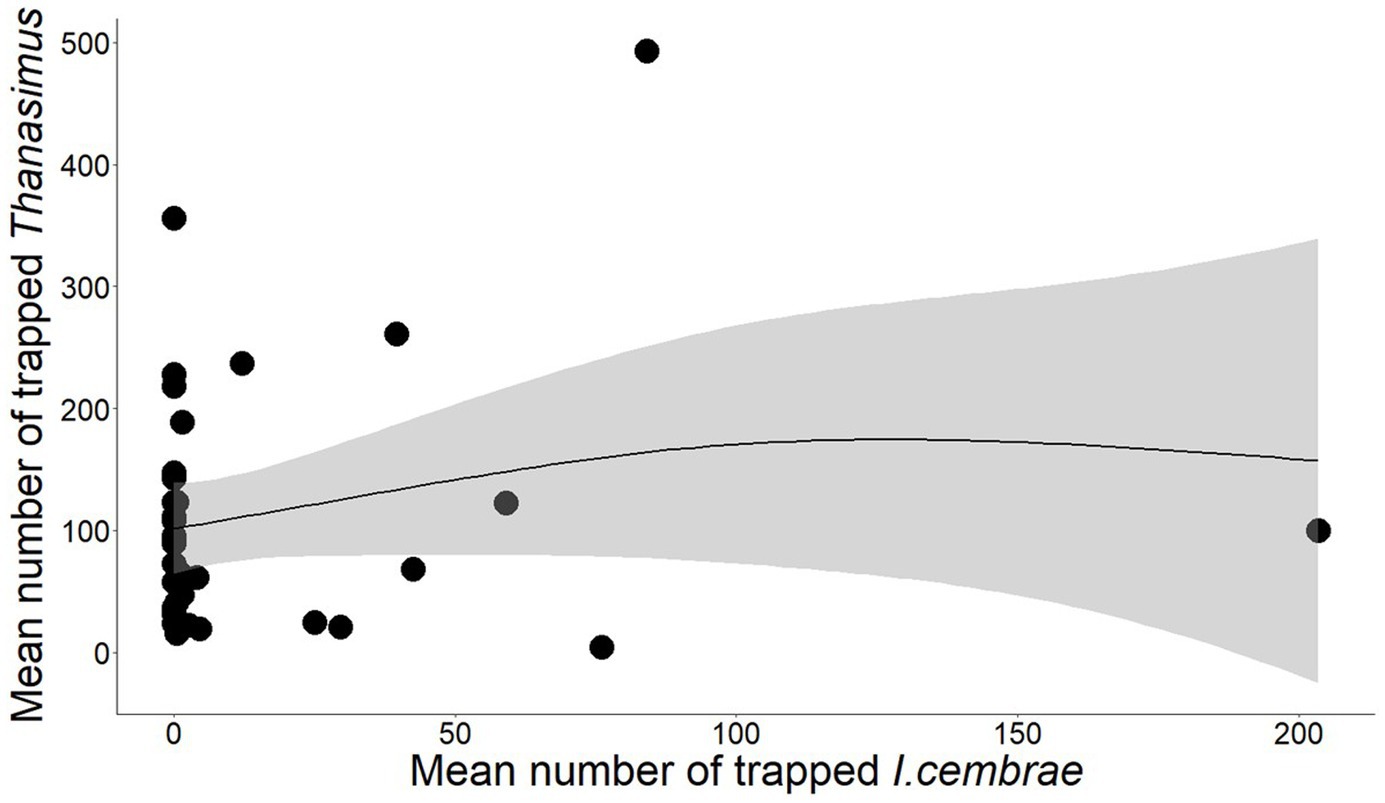
Figure 4. Relationship between the mean number of trapped Thanasimus spp. and the mean number of trapped Ips cembrae. Only locations with both I. cembrae and Thanasimus spp. were included in the graph. Each dot represents a different location. The location on the right hand side of the graph was considered an outlier, thus removed from the analysis. Grey shaded areas represent the 95% confidence intervals.
A total of 184 parasitoids emerged from the stem sections colonized by T. gabrieli. The average rate of parasitism was 30.1% (SE = 5.0%). There was no significant relationship between the proportion of parasitized T. gabrieli larvae and the density of larvae (Figure 5A) or the proportion of parasitized larvae and the mean number of trapped T. gabrieli (Figure 5B).
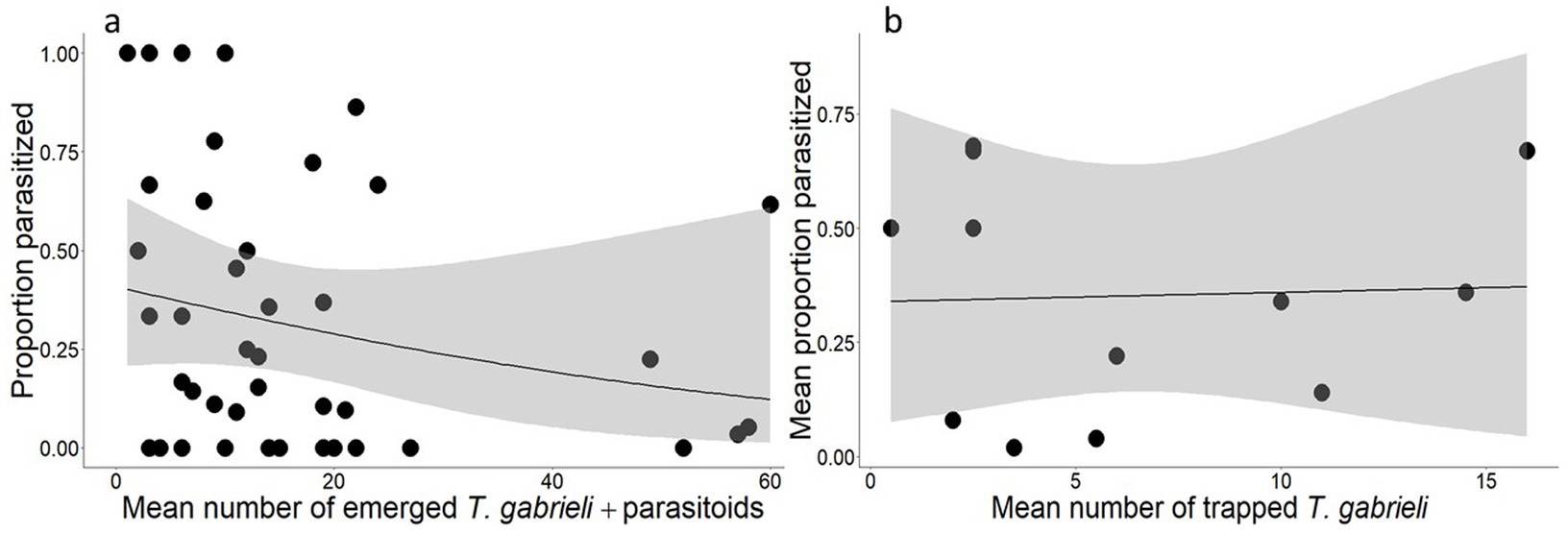
Figure 5. Relationship between (A) the number of emerging T. gabrieli plus emerging parasitoids and the proportion of parasitized Tetropium gabrieli larvae from the rearing experiment (each dot represent one stem section), and (B) mean number of trapped T. gabrieli per stand and the mean proportion of parasitized T. gabrieli larvae from the rearing experiment (each dot represent the mean values for stands with colonization).
Three other bark- and wood-boring species emerged from the T. gabrieli colonized stem sections, Serropalpus barbatus (Schaller), (Coleoptera, Melandryidae), Urocerus gigas (L), (Hymenoptera, Siricidae) and Cryphalus sp. (Coleoptera, Scolytinae). A total of 121 S. barbatus adults emerged from 13 of the 47 stem sections, 28 U. gigas emerged from seven of the sections and two adults of Cryphalus sp. were found after debarking one stem section at the end of the rearing experiment.
4 Discussion
The aim of the study was to compare the colonization success of I. cembrae and T. gabrieli, two non-native beetle species with markedly different reproductive strategies. Our results provide important insights into the relationship between the relative population density and habitat availability, and how colonization probability is related to the relative population density. The relative population density of both I. cembrae and T. gabrieli was, as hypothesized, positively influenced by the amount of habitat but at different spatial scales. For both species colonization probability increased with relative population density. For I. cembrae a considerably higher population size was required for colonization than for T. gabrieli, which was according to our predictions, and may be explained by differences in reproductive strategy. As expected, there was no relationship between population sizes of the beetles and their enemies, likely because both studied species are mainly affected by generalist predators.
4.1 How does habitat availability affect relative population density?
Our results show that relative population density of both species was positively related to habitat availability (see also Schroeder, 2013; Kautz et al., 2016; Gohli et al., 2024). However, the species differed in the spatial scale of their response to habitat quantity: Tetropium gabrieli showed a positive response to the size of the focal stand, in both the 500 m and 1,000 m buffer models. Whereas I. cembrae showed a positive response to the quantity of larch at the 1,000 m buffer. The result for I. cembrae might be explained by the presence of local populations in larch stands within the 1,000 m radius resulting from the previous year. The positive relationship of T. gabrieli with the size of the focal stand was not only due to the local hibernating populations in two of the stands. Tetropium gabrieli still showed a positive response to the size of the focal stand even after removing the only two localities with hibernating populations. Research has shown that responses to habitat availability at different spatial scales is often species specific due to different life history traits (Holland et al., 2004).
4.2 How does colonization probability relate to the relative population density?
Both species showed a positive relationship between the relative population density and the probability of colonization of the cut trees. At too low population sizes no colonization occurred. This indicates the presence of the component Allee effects for host finding (i.e., finding of suitable breeding material) and/or mate finding for both species. For the bark beetle I. cembrae, a much higher relative population density was required for colonization than for the longhorn beetle T. gabrieli. At the same time, the mean population size of I. cembrae in the colonized localities was significantly higher than in localities without colonization. The large difference in minimum relative population density (i.e., trap catch) required for colonization events strongly indicates that lower population density is required for T. gabrieli than for I. cembrae. Reproductive strategy could be an explanation for this difference. Tetropium gabrieli females can, after mating, oviposit in many substrates independent of males. Whereas, for I. cembrae, a male must first find the object and then successfully attract a female (i.e., the female cannot by herself colonize an object). In addition, each female colonizes potentially two objects. However, when comparing the trap catches of the two species, we need to be aware that it is hard to know if both species are equally attracted to the pheromone bait used. Regardless of the magnitude of the trap catch colonization of the felled logs is, however, a good indication of reproductive activity for both species.
4.3 What is the relationship between local population densities of beetles and enemy pressure?
Based on the trap catches, there was no relationship between the relative population density of I. cembrae and the predator Thanasimus spp. Thanasimus formicarius, the dominant species preys on over 20 species, and it is considered one of the most important predator for many bark beetle species, including I. cembrae (Schroeder, 2001; Kenis et al., 2004; Warzée and Grégoire, 2006). Being generalists, Thanasimus spp. densities most likely depend on the presence of other potential prey in the area. At low population densities of the prey generalist predators are thought to be important predators to maintain the population at low densities. In the present study, the average catch of Thanasimus was almost five times higher than that of I. cembrae. When present Thanasimus spp. adults feed on adult bark beetles and their larvae feed on bark beetle larvae under the bark. Thus, one Thanasimus beetle could kill more than one bark beetle and therefore, predation pressure at low density of I. cembrae could be high. In our study, this could mean that the predator may have prevented some colonization in localities with small populations of I. cembrae by being attracted to the felled trees by host volatiles and preying on I. cembrae males prior to boring into the bark. Following the start of pheromone production the predator may also prey on the I. cembrae females before they have managed to enter the mating chamber. Our findings indicate that the potential presence of a component Allee effect of predation pressure could influence successful colonization and establishment of I. cembrae negatively. Using trap catches enables us only to look at the presented relationship, other natural enemies, like parasitoids and woodpeckers, could be important predators as well. Unfortunately, we could not measure this because of the minimum colonization of our felled logs.
For T. gabrieli there was no significant relationship between neither the relative population density and the proportion of larvae parasitized, nor the number of larvae in the stem sections and the proportion of them that were parasitized. Hence, there does not seem to be a component Allee effect of enemy pressure on reproductive success after colonization. Although the rate of parasitism in our study was higher compared to Schroeder et al. (2020), it was still lower than the rate of parasitism reported from its native range (Kenis and Hilszczanski, 2004). In Schroeder et al. (2020) only three parasitoids species, classified as native, were found to parasitize the T. gabrieli larvae. All these species are also associated with the native T. fuscum and T. castaneum. The parasitoids of the present study were not determined to the species level but belonged to the same families as the ones in the study by Schroeder et al. (2020). The limited presence of other species under the bark suggests that T. gabrieli larvae faced no competition for food (phloem) from other species, as S. barbatus and U. gigas larvae feed in the wood instead.
To show a direct effect on population size (the demographic Allee effect) one often needs to investigate those factors that affect population size (component Allee effects) (Lewis and Kareiva, 1993; Berec et al., 2007). In this study, we investigated host location, mate finding and predation pressure as components of the demographic Allee effect. Our study species exhibit distinct ecological differences, and we find that enemy pressure, as well as the mechanisms for host and mate finding, are specific to each species. Based on the natural enemies included in our study, we suggest that component Allee effect involving enemy pressure is more important for I. cembrae than for T. gabrieli in the way it affects their success in population establishment and thus invasion of a new previously uncolonized area with fragmented habitat.
Species establishment and spread are processes that happen over time. Time series collected by the Swedish Forest Agency (Issacson unpublished data) show that trap catches of I. cembrae vary per year and it is general knowledge that based on weather conditions, insect populations can show large variation in densities between years. In that respect, our study is a snapshot in time. To circumvent replication over time, we designed the experiment at a large spatial scale including areas where the species were fully established (Denmark) and areas where evidence of establishment was more tentative (southern Sweden). Based on the geographical scale and the extensive spatial replication, we believe that our results represent those ecological processes influencing population establishment we are aiming to measure.
5 Conclusion
In conclusion, as it has also been shown by other studies (Blackburn et al., 2009; Sekar, 2012; Ducatez and Shine, 2019), species ecology can be a strong indicator for establishment likelihood after introduction. For bark- and wood-boring species with mating strategies where many individuals are required, propagule pressure will be of higher importance than for species where females may disperse when they have already been mated. This species-specific knowledge needs to be integrated in risk assessments for species invasions and can help to prioritize monitoring efforts and subsequent actions to prevent species invasions. However, more studies are needed to fully understand such establishments and spread of non-native species.
Data availability statement
The datasets generated during and/or analyzed during the current study are available from the corresponding author on reasonable request.
Ethics statement
Ethical approval was not required for the study involving animals in accordance with the local legislation and institutional requirements because not required for our studied organisms (beetles).
Author contributions
DC: Conceptualization, Data curation, Formal analysis, Investigation, Methodology, Writing – original draft. MK: Conceptualization, Formal analysis, Methodology, Writing – review & editing. CB: Funding acquisition, Writing – review & editing. HR: Writing – review & editing. MS: Conceptualization, Funding acquisition, Methodology, Writing – review & editing.
Funding
The author(s) declare that financial support was received for the research, authorship, and/or publication of this article. Financial support was provided by the HOMED project from the European Union’s Horizon 2020 (grant agreement No. 771271), and by the Swedish Research Council for Environment, Agricultural Sciences and Spatial Planning (Formas; grant no. 2016–20011 and 2016–01049).
Acknowledgments
We thank Unit for long term field research at SLU Faculty of Forest Science and the forest estates and companies for providing the experimental sites, in both Denmark and Sweden. We thank Helena Hallqvist for the help in the lab, Brage Frick, Ginho Pakeke Haurua, Sammy Jendoubi and Danja Fritscher for the help with the fieldwork. We thank Andrew (Sandy) Liebhold for constructive discussion prior to the execution of the experiment. Eckehard (Ecki) Brockerhoff and four reviewers improved earlier drafts of the manuscript by providing useful comments.
Conflict of interest
The authors declare that the research was conducted in the absence of any commercial or financial relationships that could be construed as a potential conflict of interest.
Generative AI statement
The author(s) declare that no Generative AI was used in the creation of this manuscript.
Publisher’s note
All claims expressed in this article are solely those of the authors and do not necessarily represent those of their affiliated organizations, or those of the publisher, the editors and the reviewers. Any product that may be evaluated in this article, or claim that may be made by its manufacturer, is not guaranteed or endorsed by the publisher.
Footnotes
References
Alexander, K. N. A. (2007). Ips cembrae (Heer) (Scolytinae) in the Forest of dean, Gloucestershire. Coleopterist 16:117.
Allen, W. L., Street, S. E., and Capellini, I. (2017). Fast life history traits promote invasion success in amphibians and reptiles. Ecol. Lett. 20, 222–230. doi: 10.1111/ele.12728
Bates, D., Maechler, M., Bolker, M., and Walker, S. (2015). Fitting linear mixed-effects models using lme4. J. Stat. Softw. 67, 1–48. doi: 10.18637/jss.v067.i01
Berec, L., Angulo, E., and Courchamp, F. (2007). Multiple Allee effects and population management. Trends Ecol. Evol. 22, 185–191. doi: 10.1016/j.tree.2006.12.002
Berec, L., and Mrkvička, T. (2013). Neglecting uncertainty behind Allee effect estimation may generate false predictions of population extinction risk. Oikos 122, 845–856. doi: 10.1111/j.1600-0706.2012.19987.x
Blackburn, T. M., Cassey, P., and Lockwood, J. L. (2009). The role of species traits in the establishment success of exotic birds. Glob. Chang. Biol. 15, 2852–2860. doi: 10.1111/j.1365-2486.2008.01841.x
Brockerhoff, E. G., Bain, J., Kimberley, M., and Knizek, M. (2006). Interception frequency of exotic bark and ambrosia beetles (Coleoptera: Scolytinae) and relationship with establishment in New Zealand and worldwide. Can. J. For. Res. 36, 289–298. https://doi:10.1139/x05-250. doi: 10.1139/x05-250
Brockerhoff, E. G., Kimberley, M., Liebhold, A. M., Haack, R. A., and Cavey, J. F. (2014). Predicting how altering propagule pressure changes establishment rates of biological invaders across species pools. Ecology 95, 594–601. doi: 10.1890/13-0465.1
Brockerhoff, E. G., and Liebhold, A. M. (2017). Ecology of forest insect invasions. Biol. Invasions 19, 3141–3159. doi: 10.1007/s10530-017-1514-1
Bürgi, L. P., Roltsch, W. J., and Mills, N. J. (2015). Allee effects and population regulation: a test for biotic resistance against an invasive leafroller by resident parasitoids. Popul. Ecol. 57, 215–225. doi: 10.1007/s10144-014-0451-4
Caswell, H., Lensink, R., and Neubert, M. G. (2003). Demography and dispersal: life table response experiments for invasion speed. Ecology 84, 1968–1978. doi: 10.1890/02-0100
Chase, K. D., Kelly, D., Liebhold, A. M., and Brockerhoff, E. G. (2023). The role of propagule pressure in experimental bark beetle invasions. J. Appl. Ecol. 60, 342–352. doi: 10.1111/1365-2664.14326
Courchamp, F., and Macdonald, D. W. (2001). Crucial importance of pack size in the African wild dog Lycaon pictus. Anim. Conserv. 4, 169–174. doi: 10.1017/S1367943001001196
Crawshay, G. A. (1907). The life history of Tetropium gabrieli, Ws. – T. fuscum, sharp – T. Crawshayi, sharp, etc. Trans. Entomol. Soc. Lond. 2, 183–212.
Davies, G. D., and Robert Britton, J. (2021). Consistency in the life history traits of four invasive Pseudorasbora parva populations in southern England. J. Appl. Ichthyol. 37, 295–302. doi: 10.1111/jai.14175
Demidko, D. A., Demidko, N. N., Mikhaylov, P. V., and Sultson, S. M. (2021). Biological strategies of invasive bark beetles and borers species. Insects 12:367. doi: 10.3390/insects12040367
Dewhirst, S., and Lutscher, F. (2009). Dispersal in heterogeneous habitats: thresholds, spatial scales, and approximate rates of spread. Ecology 90, 1338–1345. doi: 10.1890/08-0115.1
Douglas, H. B., Cognato, A. I., Grebennikov, V., and Savard, K. (2019). Dichotomous and matrix-based keys to the Ips bark beetles of the world (Coleoptera: Curculionidae: Scolytinae). Canad. J. Arthropod Identif. 38:234. doi: 10.3752/cjai.2019.38
Drake, J. M., and Lodge, D. M. (2006). Allee effects, propagule pressure and the probability of establishment: risk analysis for biological invasions. Biol. Invasions 8, 365–375. doi: 10.1007/s10530-004-8122-6
Ducatez, S., and Shine, R. (2019). Life-history traits and the fate of translocated populations. Conserv. Biol. 33, 853–860. doi: 10.1111/cobi.13281
Ehnström, B., and Holmer, M. (2007). Nationalnyckeln till Sveriges flora och fauna: Coleoptera: Cerambycidae: Skalbaggar: långhorningar. Uppsala: SLU Artdatabanken.
Ericson, B. (2010). Two species of beetles new to Sweden feeding on larch. Entomol. Tidskrift 13, 131–136.
Evans, H. F., Moraal, L. G., and Pajares, J. A. (2004) in Biology, ecology and economic importance of Buprestidae and Cerambycidae. Bark and wood boring insects in living trees in Europe, a synthesis. eds. F. Lieutier, K. R. Day, A. Battisti, J.-C. Grégoire, and H. F. Evans (Dordrecht: Kluwer Academic Publishers).
Everett, R. A. (2000). Patterns and pathways of biological invasions. Trends Ecol. Evol. 15, 177–178. doi: 10.1016/S0169-5347(00)01835-8
Fauvergue, X. (2013). A review of mate-finding Allee effects in insects: from individual behavior to population management. Entomol. Exp. Appl. 146, 79–92. doi: 10.1111/eea.12021
Fox, J., and Weisberg, S. (2019). An {R} companion to applied regression. 3rd Edn. Thousand Oaks, CA: Sage.
Fritscher, D., and Schroeder, M. (2022). Thermal sum requirements for development and flight initiation of new-generation spruce bark beetles based on seasonal change in cuticular colour of trapped beetles. Agric. For. Entomol. 24, 405–421. doi: 10.1111/afe.12503
Gascoigne, J., Berec, L., Gregory, S., and Courchamp, F. (2009). Dangerously few liaisons: a review of mate-finding Allee effects. Popul. Ecol. 51, 355–372. doi: 10.1007/s10144-009-0146-4
Gohli, J., Krokene, P., Flo Heggem, E. S., and Økland, B. (2024). Climatic and management-related drivers of endemic European spruce bark beetle populations in boreal forests. J. Appl. Ecol. 61, 809–820. doi: 10.1111/1365-2664.14606
Grégoire, J.-C., Bonte, J., Bourke, A., Cocos, D., Fielding, N., Gohli, J., et al. (2024). Territorial expansion of the European Ips species in the 20th century – a review. Entomol. Gene. doi: 10.1127/entomologia/2024/2769
Grégoire, J.-C., and Evans, H. F. (2004) in Damage and control of BAWBILT organisms – An overview. Bark and wood boring insects in living trees in Europe, a synthesis. eds. F. Lieutier, K. R. Day, A. Battisti, J.-C. Grégoire, and H. F. Evans (Dordrecht: Kluwer Academic Publishers).
Grodzki, W. (2008). Ips cembrae Heer (Col.: Curculionidae, Scolytinae) in young larch stands - a new problem in Poland. Forstschutz Aktuell 44, 8–9.
Grucmanová, Š., Holuša, J., Trombik, J., and Lukášová, K. (2014). Large larch bark beetle Ips cembrae (Coleoptera: Curculionidae, Scolytinae) in the Czech Republic: analysis of population development and catches in pheromone traps. Lesnicky Casopis 60, 143–149. doi: 10.2478/forj-2014-0015
Grünbaum, D., and Veit, R. R. (2003). Black-browed albatrosses foraging on Antarctic krill: density-dependence through local enhancement? Ecology 84, 3265–3275. doi: 10.1890/01-4098
Haack, R. A. (2006). Exotic bark and wood-boring Coleoptera in the United States: recent establishments and interceptions. Can. J. For. Res. 36, 269–288. doi: 10.1139/x05-249
Hanks, L. M. (1999). Influence of the larval host plant of reproductive strategies of cerambycid beetles. Annu. Rev. Entomol. 44, 483–505. doi: 10.1146/annurev.ento.44.1.483
Hansen, M. (1996). Katalog over Danmarks biller (catalogue of the Coleoptera of Denmark). Entomol. Meddelelser 64, 1–231.
Holland, J. D., Bert, D. G., and Fahrig, L. (2004). Determining the spatial scale of species’ response to habitat. Bioscience 54, 229–235. doi: 10.1641/00063568(2004)054[0227:DTSSOS]2.0.CO;2
Holland, J. D., Fahrig, L., and Cappuccino, N. (2005). Body size affects the spatial scale of habitat–beetle interactions. Oikos 110, 101–108. doi: 10.1111/j.0030-1299.2005.13638.x
Holuša, J., Kula, E., Wewiora, F., and Lukášová, K. (2014). Flight activity, within the trap tree abundance and overwintering of the larch bark beetle (Ips cembrae) in Czhech Republic. Šumarski List 2, 19–27.
Johansson, T. (2012). Höjdutveckling hos hybridlärk – ett snabbväxande barrträd med kort omloppstid. Fakta Skog 11:4.
Johnson, A. R., Wiens, J. A., Milne, B. T., and Crist, T. O. (1992). Animal movements and population dynamics in heterogeneous landscapes. Landsc. Ecol. 7, 63–75. doi: 10.1007/BF02573958
Kahanpää, J. (2017). Tetropium gabrieli Weise, 1905 (Coleoptera: Cerambycidae) found in a larch forest in Finland –. Sahlbergia 23, 7–8.
Kautz, M., Imron, M. A., Dworschak, K., and Schopf, R. (2016). Dispersal variability and associated population-level consequences in tree-killing bark beetles. Mov. Ecol. 4:9. doi: 10.1186/s40462-016-0074-9
Kenis, M., and Hilszczanski, J. (2004) in Natural enemies of Cerambycidae and Buprestidae infesting living trees. Bark and wood boring insects in living trees in Europe, a synthesis. eds. F. Lieutier, K. R. Day, A. Battisti, J.-C. Grégoire, and H. F. Evans (Dordrecht: Kluwer Academic Publishers).
Kenis, M., Wermelinger, B., and Grégoire, J.-C. (2004) in Research on parasitoids and predators of scolytidae – A review. Bark and wood boring insects in living trees in Europe, a synthesis. eds. F. Lieutier, K. R. Day, A. Battisti, J.-C. Grégoire, and H. F. Evans (Dordrecht: Kluwer Academic Publishers).
Kolar, C. S., and Lodge, D. M. (2001). Progress in invasion biology: predicting invaders. Trends Ecol. Evol. 16, 199–204. doi: 10.1016/s0169-5347(01)02101-2
Korner-Nievergelt, F., Roth, T., von Felten, S., Guelat, J., Almasi, B., and Korner-Nievergelt, P. (2015). Bayesian data analysis in ecology using linear models with R BUGS and Stan : Elsevier.
Kullman, L. (1998). Palaeoecological, biogeographical and palaeoclimatological implications of early Holocene immigration of Larix sibirica Ledeb. Into the Scandes Mountains, Sweden. Glob. Ecol. Biogeogr. Lett. 7, 181–188. doi: 10.2307/2997373
Larsson-Stern, M. (2003). Larch in commercial forestry: A literature review to help clarify the potential of hybrid larch (Larix × eurolepis Henry) in southern Sweden. Sweden: Southern Swedish Forest Research Centre Alnarp.
Lewis, M. A., and Kareiva, P. (1993). Allee dynamics and the spread of invading organisms. Theor. Popul. Biol. 43, 141–158. doi: 10.1006/tpbi.1993.1007
Liebhold, A. M., and Tobin, P. C. (2008). Population ecology of insect invasions and their management. Annu. Rev. Entomol. 53, 387–408. doi: 10.1146/annurev.ento.52.110405.091401
Lindelöw, Å., Isacsson, G., Ravn, H. P., and Schroeder, M. (2015). Tetropium gabrieli and Ips cembrae (Coleoptera; Cerambycidae and Curculionidae) – invasion of two potential pest species on larch in Sweden. Entomol. Tidskrift 136, 103–112.
Lockwood, J. L., Cassey, P., and Blackburn, T. (2005). The role of propagule pressure in explaining species invasions. Trends Ecol. Evol. 20, 223–228. doi: 10.1016/j.tree.2005.02.004
Luitjes, J. (1974). Ips cembrae, a new noxious forest insect in the Netherlands. Nederlands Bosbouw Tijdschrift 46, 244–246.
Lynikiene, J., Tamutis, V., Gedminas, A., and Marciulynas, A and, Menkis, A. (2021). First report of the larch longhorn (Tetropium gabrieli Weise, Coleoptera: Cerambycidae: Spondylidinae) on Larix spp. in Lithuania. Insects, 12,:911. doi: 10.3390/insects12100911
Maciel, G. A., and Lutscher, F. (2015). Allee effects and population spread in patchy landscapes. J. Biol. Dyn. 9, 109–123. doi: 10.1080/17513758.2015.1027309
Martin, A. E., and Fahrig, L. (2012). Measuring and selecting scales of effect for landscape predictors in species–habitat models. Ecol. Appl. 22, 2277–2292. doi: 10.1890/11-2224.1
Meurisse, N., Rassati, D., Hurley, B. P., Brockerhoff, E. G., and Haack, R. A. (2019). Common pathways by which non-native forest insects move internationally and domestically. J. Pest. Sci. 92, 13–27. doi: 10.1007/s10340-018-0990-0
Musgrave, J., Girard, A., and Lutscher, F. (2015). Population spread in patchy landscapes under a strong Allee effect. Theor. Ecol. 8, 313–326. doi: 10.1007/s12080-015-0252-1
Nord-Larsen, T., Johannsen, V. K., Riis-Nielsen, T., Thomsen, I. M., and Jørgensen, B. B. (2020). Skovstatistik 2019: Forest statistics (2019). Frederiksberg: Institut for Geovidenskab og Naturforvaltning, Københavns Universitet.
Olver, F. W. J., Lozier, D. M., Boisvert, R. F., and Clark, C. W. (Eds.) (2010). Quadrature: Gauss–Hermite formula, NIST handbook of mathematical functions. Cambridge: Cambridge University Press.
Pfeffer, A. (1995). Zentral- und westpaläarktische Borken und Kernkäfer Scolytidae, Platypodidae Coleoptera Pro Entomologia. Basel: Naturhistorisches Museum.
Ravn, H. P. (2012). Stor laerkebarkbille I Danmark – opdatering af udbredelsen (in Danish) – Skoven, 4, 194–196.
R Core Team (2024). R: A language and environment for stastistical computing. Vienna: R Foundation for Statistical Computing. Available at: https://www.r-project.org/
Resnerová, K., Holuša, J., Surový, P., Trombik, J., and Kula, E. (2020). Comparison of Ips cembrae (Coleoptera: Curculionidae) capture methods: small trap trees caught the Most beetles. Forests 11:1275. doi: 10.3390/f11121275
Rhainds, M. (2010). Female mating failures in insects. Entomol. Exp. Appl. 136, 211–226. doi: 10.1111/j.1570-7458.2010.01032.x
Roy, H. E., Lawson Handley, L. J., Schönrogge, K., Poland, R. L., and Purse, B. V. (2011). Can the enemy release hypothesis explain the success of invasive alien predators and parasitoids? BioControl 56, 451–468. doi: 10.1007/s10526-011-9349-7
Schebeck, M., and Schopf, A. (2017). Temperature-dependent development of the European larch bark beetle, Ips cembrae. J. Appl. Entomol. 141, 322–328. doi: 10.1111/jen.12351
Schroeder, L. M. (2001). Prolonged development time of the bark beetle predator Thanasimus formicarius (Col.: Cleridae) in relation to its prey species Tomicus piniperda (L.) and Ips typographus (L.) (Col.: Scolytidae). Agric. For. Entomol. 1, 127–135. doi: 10.1046/j.1461-9563.1999.00018.x
Schroeder, L. M. (2013). Monitoring of Ips typographus and Pityogenes chalcographus: influence of trapping site and surrounding landscape on catches. Agric. For. Entomol. 15, 113–119. doi: 10.1111/afe.12002
Schroeder, M., Cocoş, D., Johansson, H., and Sweeney, J. (2020). Attraction of the cerambycid beetles Tetropium gabrieli, T. Castaneum and T. fuscum to pheromones and host tree volatiles. Agric. For. Entomol. 23, 203–211. doi: 10.1111/afe.12422
Sekar, S. (2012). A meta-analysis of the traits affecting dispersal ability in butterflies: can wingspan be used as a proxy? J. Anim. Ecol. 81, 174–184. doi: 10.1111/j.1365-2656.2011.01909.x
Silk, P. J., Sweeney, J., Wu, J., Price, J., Gutowski, J. M., and Kettela, E. G. (2007). Evidence for a male-produced pheromone in Tetropium fuscum (F.) and Tetropium cinnamopterum (Kirby) (Coleoptera: Cerambycidae). Naturwissenschaften 94, 697–701. doi: 10.1007/s00114-007-0244-0
Simberloff, D. (2009). The role of propagule pressure in biological invasions. Annu. Rev. Ecol. Evol. Syst. 40, 81–102. doi: 10.1146/annurev.ecolsys.110308.120304
Simberloff, D., Martin, J. L., Genovesi, P., Maris, V., Wardle, D. A., Aronson, J., et al. (2013). Impacts of biological invasions: what's what and the way forward. Trends Ecol. Evol. 28, 58–66. doi: 10.1016/j.tree.2012.07.013
South, A. B., and Kenward, R. E. (2001). Mate finding, dispersal distances and population growth in invading species: a spatially explicit model. Oikos 95, 53–58. doi: 10.1034/j.1600-0706.2001.950106.x
Stephens, P. A., Sutherland, W. J., and Freckleton, R. P. (1999). What is the Allee effect? Oikos 87, 185–190. doi: 10.2307/3547011
Stoakley, J. T., Bakke, A., Renwick, J. A. A., and Vite, J. P. (1978). The aggregation pheromone system of the larch bark beetle, Ips cembrae Heer. Zeitschrift Fur Angewandte Entomol. 86, 174–177. doi: 10.1111/j.1439-0418.1978.tb01925.x
Swedish University of Agricultural Sciences (2022). Forest statistics 2022 - official statistics of Sweden. Umea: Swedish University of Agricultural Sciences.
Sweeney, J., Groot, P., Macdonald, L., Smith, S., Cocquempot, C., Kenis, M., et al. (2004). Host volatile attractants and traps for detection of Tetropium fuscum (F.), Tetropium castaneum (L.), and other longhorned beetles (Coleoptera: Cerambycidae). Environ. Entomol. 33, 844–854. doi: 10.1603/0046-225X-33.4.844
Sweeney, J., Silk, P. J., Gutowski, J. M., Wu, J., Lemay, M. A., Mayo, P. D., et al. (2010). Effect of chirality, release rate, and host volatiles on response of Tetropium fuscum (F.), Tetropium cinnamopterum (Kirby), and Tetropium castaneum (L.) to the aggregation pheromone, Fuscumol. J. Chem. Ecol. 36, 1309–1321. doi: 10.1007/s10886-010-9876-1
Taylor, C. M., and Hastings, A. (2005). Allee effects in biological invasions. Ecol. Lett. 8, 895–908. doi: 10.1111/j.1461-0248.2005.00787.x
Tobin, P. C., Berec, L., and Liebhold, A. M. (2011). Exploiting Allee effects for managing biological invasions. Ecol. Lett. 14, 615–624. doi: 10.1111/j.1461-0248.2011.01614.x
Vilà, M., Basnou, C., Pyšek, P., Josefsson, M., Genovesi, P., Gollasch, S., et al. (2010). How well do we understand the impacts of alien species on ecosystem services? A pan-European, cross-taxa assessment. Front. Ecol. Environ. 8, 135–144. doi: 10.1890/080083
Vilardo, G., Faccoli, M., Corley, J. C., and Lantschner, M. V. (2022). Factors driving historic intercontinental invasions of European pine bark beetles. Biol. Invasions 24, 2973–2991. doi: 10.1007/s10530-022-02818-2
Walter, J. A., Firebaugh, A. L., Tobin, P. C., and Haynes, K. J. (2016). Invasion in patchy landscapes is affected by dispersal mortality and mate-finding failure. Ecology 97, 3389–3401. doi: 10.1002/ecy.1583
Warzée, N., and Grégoire, J.C. (2006). Thanasimus formicarius (Coleoptera: Cleridae): why a large range of prey for a specialized predator? In Proceeding: IUFRO Kanazama 2003 “Forest insect dynamics and host influence” 16–18.
Keywords: Allee effects, habitat fragmentation, larch bark beetles, larch longhorn beetles, life history traits, Ips cembrae , Tetropium gabrieli
Citation: Cocoș D, Klapwijk MJ, Björkman C, Ravn HP and Schroeder M (2024) Factors affecting population density and colonization success of two non-native beetle species with different breeding/life-history strategies. Front. For. Glob. Change. 7:1506158. doi: 10.3389/ffgc.2024.1506158
Edited by:
Bernard Slippers, University of Pretoria, South AfricaReviewed by:
Manuela Branco, Higher Institute of Agronomy, University of Lisbon, PortugalNicolas Meurisse, New Zealand Forest Research Institute Limited (Scion), New Zealand
Albert Mayfield, Southern Research Station, Forest Service (USDA), United States
Copyright © 2024 Cocoș, Klapwijk, Björkman, Ravn and Schroeder. This is an open-access article distributed under the terms of the Creative Commons Attribution License (CC BY). The use, distribution or reproduction in other forums is permitted, provided the original author(s) and the copyright owner(s) are credited and that the original publication in this journal is cited, in accordance with accepted academic practice. No use, distribution or reproduction is permitted which does not comply with these terms.
*Correspondence: Dragoș Cocoș, ZHJhZ29zLmNvY29zQHNsdS5zZQ==
†ORCID: Dragos Cocos, http://orcid.org/0000-0001-9679-2142
Maartje J. Klapwijk, http://orcid.org/0000-0001-7690-045X
Christer Björkman, https://orcid.org/0000-0002-6361-5139
Hans Peter Ravn, http://orcid.org/0000-0001-5090-3273
Martin Schroeder, http://orcid.org/0000-0002-6005-0240