- 1Aukema Forest Entomology Laboratory, Department of Entomology, University of Minnesota, St. Paul, MN, United States
- 2Idaho Department of Lands, Forest Health, Coeur d'Alene, ID, United States
- 3United States Forest Service, R1/R4 Forest Health Protection, Missoula, MT, United States
Eastern larch beetle Dendroctonus simplex LeConte has been outbreaking for the past two decades in the Great Lakes region of North America, impacting approximately 400,000 hectares of eastern larch Larix laricina (tamarack) forest in Minnesota, United States, alone. Range expansions of some Dendroctonus species driven by climate change have occurred across North America as rising temperatures increase climatically suitable habitats across latitudes and elevations. The range of eastern larch beetle is currently sympatric to that of its host, eastern larch, but the insect could, in theory, eventually access ranges of other species of Larix native to North America. We compared host suitability of two potential novel hosts, western larch (Larix occidentalis Nutt.) and subalpine larch (Larix lyalli Parl.) against the beetle’s native host, eastern larch, using a reproductive success experiment conducted in cut bolts of all three species. We measured fertility, maternal gallery length, and offspring size and lipid content as metrics of reproductive success. We found beetles could reproduce in both novel Larix species, with subalpine larch having high levels of success compared to the beetle’s native host, eastern larch. These findings suggest that subalpine larch may be at risk should a range expansion of eastern larch beetle occur.
1 Introduction
Bark beetles (Coleoptera: Curculionidae: Scolytinae) live and reproduce in the phloem of trees. Often species specific, the relationships between beetle and host tree species have evolved over millions of years (Labandeira et al., 2001; Raffa, 2014). These relationships are apparent through the complex chemical signals and receptors by which bark beetles find host trees and mates (Byers, 1989; Francke et al., 1995), the presence of symbiotic fungi, and the physical structures by which some species carry fungal spores (Paine et al., 1997; Six and Wingfield, 2011), and the physical and chemical defenses that host trees have evolved to evade death by bark beetles (Raffa and Berryman, 1983, 1987; Krokene, 2015). In recent decades, anthropogenic-driven climate change has facilitated range expansions of bark beetles that have resulted in new relationships with novel and naïve host tree species. Insect life cycles are highly temperature dependent and rising temperatures have facilitated range expansions of several species of bark beetles northwards and/or to higher elevations (Bentz and Jönsson, 2015; Pureswaran et al., 2018). Naive host tree species along these expanding range margins may be at increased risk of mortality if they have not co-evolved defenses against beetle attack or if they have lost defensive capabilities over time (Logan and Powell, 2001; Raffa et al., 2013).
Range expansion of some species of Dendroctonus have occurred and has the potential to occur for three more species as a result of climate change (Pureswaran et al., 2022). The range of mountain pine beetle (Dendroctonus ponderosae Hopkins) has expanded northward and eastward over the continental divide (Safranyik et al., 2010; de la Giroday et al., 2012; Rosenberger et al., 2017a). The range of mountain pine beetle has also expanded higher in elevation, increasing exposure to high-elevation five-needle pines such as whitebark pine Pinus albicaulis Engelm (Logan and Powell, 2001; Raffa et al., 2013; Six et al., 2021; United States Fish and Wildlife Service, 2022). Southern pine beetle (Dendroctonus frontalis Zimmermann) has moved northwards as increasing winter temperatures have permitted survival in new habitats (Ungerer et al., 1999; Lesk et al., 2017). Another example is spruce beetle (Dendroctonus rufipennis Kirby), which will see an increase in climatically suitable habitat containing its host, Engelmann spruce (Picea engelmannii Parry ex Engelm.), as temperatures rise with climate change (DeRose et al., 2013). Moreover, rising temperatures and increasing drought negatively impact host tree defenses, making trees more susceptible to beetle attack (Bentz et al., 2010; Kolb et al., 2019).
Native to North America, the eastern larch beetle Dendroctonus simplex LeConte has a range sympatric to that of its host, the eastern larch (tamarack) Larix laricina (Du Roi) K. Koch. Eastern larch grows across northeastern North America down into the Great Lakes region and west into northwestern Canada with disjunct populations in Alaska (Figure 1) (Johnston, 1990; Seybold et al., 2002). Dendroctonus simplex was first recorded by A. D. Hopkins in 1909 as less damaging than other Dendroctonus species due to its preference for dead and dying larch (Hopkins, 1909). However, landscape-level outbreaks occurring in the 1970s and 1980s in northeastern North America and Alaska prompted reconsideration of D. simplex as a primary forest pest (Werner, 1986; Langor and Raske, 1989). These early outbreaks typically lasted 2–6 years before resolving and were often preceded by other biotic and abiotic host stressors such as defoliation by the larch sawfly Pristiphora erichsonii Hartig (Hymenoptera: Tenthredinidae), larch casebearer Coleophora laricella Hübner (Lepidoptera: Coleophoridae), larch budmoth Zeiraphera sp. (Lepidoptera: Tortricidae), or drought and flooding (Langor and Raske, 1989).
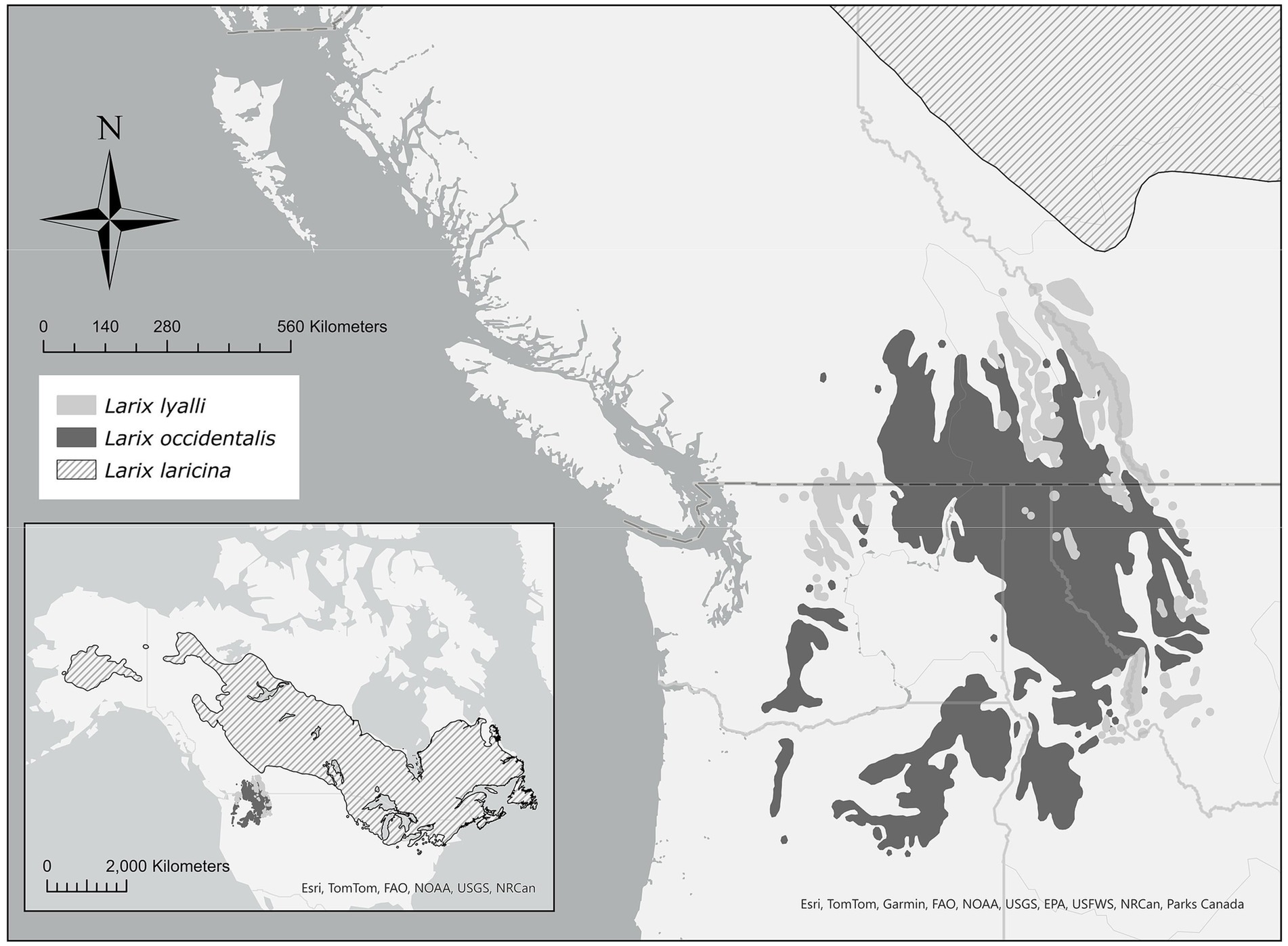
Figure 1. Range map of North American Larix species (Little, 1971); inset displays extent of eastern larch Larix laricina throughout North America. Scale bar displays planar distance.
In Minnesota, an eastern larch beetle outbreak that began in 2000 continues to present. The outbreak has impacted just over four hundred thousand hectares of eastern larch forest type to date (MN DNR Forest Health Team, 2024). There are no apparent predisposing biotic external factors that can account for the spatial and temporal scale of the outbreak, with defoliation by larch casebearer only occurring in approximately 5% of impacted area between 2000 and 2017 (Ward and Aukema, 2019a). Eastern larch beetle was previously thought to be univoltine with an obligate, overwintering adult reproductive diapause (Hopkins, 1909; Langor and Raske, 1987a). Work conducted in the early 2010s, however, demonstrated that some adults in Minnesota were capable of reproducing without overwintering in laboratory conditions (McKee and Aukema, 2015b), and evidence for a second generation was observed in the field (McKee, 2015). Therefore, the ongoing outbreak is attributed to increased beetle populations as a result of an ameliorating climate (Venette and Walter, 2008; McKee, 2015).
Eastern larch beetle, to date, has never been recorded infesting allopatric species of North American Larix such as western larch, Larix occidentalis Nutt., or subalpine larch, Larix lyalli Parl. Both of these species are free of potential phloem-inhabiting specialist competitors, save for the larch engraver Scolytus laricis Blackman (Coleoptera: Curculionidae) that is typically restricted to pole-sized, distressed trees and slash (Furniss and Carolin, 1977). Western larch is found within western Montana, eastern and central Washington, northeastern Oregon, and southeastern British Columbia (Figure 1). It is a major timber species in western North America and provides ecological benefits (Schmidt and Shearer, 1990). A shade intolerant pioneer species, western larch rapidly regenerates in the bare soils of burned and logged areas. Root diseases are extremely prevalent tree mortality agents in the inland northwest, and western larch is considered to be one of the most tolerant native tree species to root diseases such as Armillaria ostoyae, Heterobasidion annosum, and laminated root rot Phellinus weirii (Shaw and Kile, 1991; Thies and Sturrock, 1995; Hagle et al., 2003). Due to its high commercial value, key role in forest restoration of root diseased areas, and limited insect and disease problems, land management agencies have prioritized planting of western larch (Tyler Nelson, Idaho Department of Lands Forest Management Program, personal communication). However, this emphasis on western larch has resulted in tens of thousands of hectares of monocultures, raising concerns about forest resilience in the face of a changing climate that could bring about novel insect and disease issues.
Subalpine larch (Larix lyalli) is found within the Northern Rockies and Cascades at high elevations, typically 2,300 m or greater, but it can be found as low as 1,520 m in the Cascades (Arno and Habeck, 1972; Arno, 1990). Although separated by an elevation gradient of 150–300 m, the ranges of western and subalpine larch overlap geographically and the two species sometimes hybridize in western Montana (Carlson and Blake, 1969; Arno and Habeck, 1972; Figure 1). Subalpine larch has occasionally been found growing in lower elevations with western larch on sites that have experienced disturbance (Arno and Habeck, 1972; Arno, 1990). As a high-elevation species, subalpine larch will likely experience increasing stress due to rising temperatures as a result of climate change.
The goal of this study was to compare the suitability of eastern, western and subalpine larches for reproduction of eastern larch beetle, motivated by the ongoing outbreak in the Great Lakes region. Spread of Larix pests from eastern to western hosts has occurred in the past, as seen with the invasive, needle-mining larch casebearer Coleophora laricella (Ryan et al., 1987; Ward and Aukema, 2019b). Work in the 1970s found that a small number of eastern larch beetle can successfully reproduce in cut bolts of western larch (Furniss, 1976), but reproduction of eastern larch beetle has never been tested in subalpine larch. The present study was conducted using cut bolts and thus we can only assess host suitability and not host susceptibility, as introducing beetles to live trees outside of the insect’s native range is unwise (Cale et al., 2015; Rosenberger et al., 2017b). We compared the reproductive success of standardized densities of D. simplex in western and subalpine larch to eastern larch, the beetle’s native host. Reproductive success was evaluated in terms of fertility and reproductive gallery length of introduced females, as well as lipid content and size of progeny. By evaluating host suitability of western and subalpine larch, we hope to determine if the eastern larch beetle may pose a threat to these species as global change continues to facilitate range expansions and invasions of forest insects.
2 Methods
2.1 Materials
Twelve trees (n = four/species) were harvested between 30 June and 10 July, 2023. Western larch were harvested 30 June within Coeur d’Alene National Forest, Idaho, United States (47°41′20.4”N, 116°36′54.0”W). Subalpine larch were harvested 4 July from the Beaverhead-Deerlodge National Forest, Montana, United States (46°04′30.0”N, 113°16′19.2”W). Eastern larch were harvested 10 July from the Hill River State Forest, Minnesota, USA (46°58′22.8”N, 93°26′49.2”W). All trees were between 15.2–18.5 cm (6–7.3 in) DBH (diameter at breast height; 1.4 m) with phloem a minimum of 2 mm thick to allow reproduction of eastern larch beetle (Langor and Raske, 1987b). Once felled, trees were sectioned to bolts 30.5 cm (12 in) in length. Each bolt’s position within each tree was recorded. Phloem thickness was measured using calipers (three measurements, evenly spaced, around top and bottom surfaces) and cut surfaces were sealed with paraffin wax to reduce desiccation. Bolts were stored in plastic body bags under ambient conditions (Waterproof Cadaver Bag, Ejoyous, Amazon), separated by species, while being driven from field to laboratory to prevent colonization by any insects. Bolts were stored at room temperature prior to use.
Live eastern larch beetle adults were collected using Lindgren funnel traps baited with seudenol lures (release rate = 7.5 mg/day at 30°C; Synergy Semiochemical, British Columbia, Canada) from five field locations distributed across two sites in Northern Minnesota (Sax-Zim Bog: 47°04′31.2”N 92°39′42.7”W, 47°02′03.8”N 92°40′16.8”W, 47°01′40.3”N 92°39′42.7”W; and Beltrami Island State Forest: 48°46′13.3”N 95°10′16.8”W, 48°35′51.6”N 95°20′44.0”W) between 10 and 20 July 2023. Beetles were collected from the Sax-Zim traps four times over ten days (July 10, 12, 13 and 20) while Beltrami Island beetles were collected on July 15th. Adults were triple-sexed by listening for stridulation (Rosenberger et al., 2016), stored at room temperature, and used within 5 days of collection. Only vigorous, walking, intact beetles were used in the experiment.
2.2 Infesting and monitoring bolts
Bolts were selected at random for infestation, with the total number of bolts evenly distributed across the three species (n = 49; 17 eastern larch, 16 subalpine larch, 16 western larch). Prior to infestation, the weight and moisture content of each bolt was recorded. Five equidistant (3–3.5 cm) entrance holes were drilled to the phloem around each bolt approximately 5 cm from the top and labeled. Females were placed dorsal-side up in 1.5 mL microcentrifuge tubes. The tubes were then gently pressed into each entrance hole to allow females confined access to the phloem and bolt. Tunneling was gauged by the presence of frass within the centrifuge tube. Inactive females were replaced after 24 h. Any introduction points without evidence of tunneling activity within 72 h of initial introduction were discontinued without replacement. One male was added to each microcentrifuge tube 24–72 h after presumed successful female introduction. As with female introductions, inactive males were replaced after 24–72 h. For one introduction point for one bolt of eastern larch, however, a lack of male beetles resulted in a delay of 4 days. If after two attempts to replace a male no evidence of tunneling activity was observed, the introduction point was discontinued without replacement.
While we took care to harvest and use only trees that were visually free of insect/pathogen damage, one of the four subalpine larch harvested was found to have white pocket rot in the lower bole after felling. Samples were cultured, isolated, and later identified via sequencing as Onnia (Innonotus) tomentosa (Dr. Robert A. Blanchette, University of Minnesota Department of Plant Pathology, personal communication). Tomentosus root rot typically causes decay within the lower bole of spruce, Doug-fir, and other tree species (Hansen and Lewis, 1997; Trummer, 1999). Pathologists currently studying the Onnia root disease species believe this is the first record of Onnia (Innonotus) tomentosa in subalpine larch (Dr. Patrick Bennett, United States Forest Service Rocky Mountain Research Station, personal communication). We used three bolts from the upper bole of this tree in the study as rot was not visible in these bolts and the bolts appeared to be in otherwise good condition.
Once beetles had accepted all entrance points on a bolt or the entrance points had been discontinued, bolts were placed in cardboard emergence tubes (61 cm long, 30.5 cm diameter) with a glass collection jar on the end and held at ambient temperature (24.7°C). Based on the known developmental rate from egg to adults at this temperature (McKee and Aukema, 2015a), we decided to peel the bark from one bolt from each species to assess experimental progression and insect development, if any, approximately 30 days after males were introduced. Due to this step, our sample size for emerging brood adults decreased to 46 bolts (16 eastern larch, 15 subalpine larch, 15 western larch). The collection jars on the emergence tubes were examined daily and all insects found in jars collected. Brood adults were counted and frozen at −20°C. Once emergence dropped below an average of 0.5 beetles/species/day for a two-week period (day 53), the experiment concluded.
All bolts were then debarked, with a post-infestation weight and moisture reading taken prior to bark removal. Any remaining beetles found under the bark were extracted and counted. Immature life forms (larvae and pupae) were stored in ethanol while adults were frozen at −20°C. The lengths of maternal galleries were measured using a digital plan measure tool (Calculated Industries, Scalemaster Classic). Only maternal galleries that had larval tunnels radiating from them were included in analyses; unsuccessful maternal galleries were rare and evenly distributed between the three species.
2.3 Lipid analysis of offspring
Lipid analysis was performed on a subset of emerged and manually-extracted adults (N = 252), with sampling proportional to the total number of emerged and manually-extracted adults from each bolt (n emerged beetles = 131 (40 eastern larch, 47 subalpine larch, 44 western larch); n manually-extracted beetles = 121 (35 eastern larch, 45 subalpine larch, 41 western larch)). Beetles were sexed by examining the 7th tergite for medial tines (Lyon, 1958) and their pronotal widths measured to the nearest 0.001 mm using a digital micrometer (Leica LED3000). Beetles were dried at 55°C overnight, then weighed to the nearest 0.01 mg (Metler Toledo AX105 DeltaRange). Lipids were extracted via petroleum ether using a Soxhlet apparatus for 8 h (Hansen and Bentz, 2003; McKee and Aukema, 2015a). Beetles were then dried for a minimum of 12 h at 55°C to remove any remaining petroleum ether and then weighed a second time. In total, five runs were performed to process all beetles. Total lipid content was calculated as the difference in pre- and post-extraction weight. Percent lipid content was calculated by dividing the final lipid weight by pre-extraction weight.
2.4 Statistical analysis
All analyses were performed in R v4.3.3 (R Core Team, 2024). Average reproduction per mating pair was calculated by dividing the total number of emerged beetles from each bolt by the number of successful reproductive galleries and then standardized by dividing by the total surface area of the bolt (dm2), as there were small variations in surface area between species (mean +/− SE: eastern larch 14.8 +/− 0.2 dm2, subalpine larch 16.4 +/− 0.7 dm2, western larch 13.9 +/− 0.4 dm2; F2, 43 = 6.88, p = 0.0026). Fertility (number of viable eggs laid) was calculated by dividing the total number of all offspring (i.e., emerged beetles, manually-extracted beetles, pupae and larvae) by the number of successful reproductive galleries and standardized by surface area for each bolt.
The effect of tree species on mean beetle reproductive gallery length, fertility, and lipid content was likewise determined using linear mixed effects ANOVA models, with a term for species as a fixed effect and a term for tree as a random effect. A log (y + 1) transformation of the fertility response variable was used to meet assumptions of normality and homogeneity of residuals. Where ANOVAs noted differences between each species, Tukey’s post-hoc pairwise comparisons were used to evaluate differences between groups. Effect of species on lipid content (reported as dry weight to the nearest 0.01 mg and percent dry beetle mass) was modeled with a linear mixed effects model with terms for extraction run, tree, and bolt within tree as nested random effects. An angular transformation [arcsin sqrt(y)] was performed on lipid percent dry beetle mass to satisfy model assumptions as above. Likelihood ratio tests were used to simplify models by testing whether the lowest nested levels of hierarchical random effects explained significant variation relative to higher models, with the simplest, most parsimonious model reported. Additionally, we tested if the following had effects on lipid content: adult offspring size (pronotal width), sex, and if the beetle had emerged on its own or was manually extracted when bolts were debarked.
We evaluated whether phloem thickness, phloem moisture, and weight of bolts varied among species using ANOVA as a quality control check to assess similar sizes and conditions of host material. No differences were observed in phloem moisture content nor weight of bolts between species, so we do not present further results. Likewise, we do not further examine phloem thickness measurements in beetle reproductive success analyses. All trees harvested met the minimum phloem thickness requirement of 2 mm and we visually observed the phloem was of similar thickness on all species, but felt that some measurements >2 mm on the first two species harvested (western and subalpine larch) might be high due to measurement techniques on unfamiliar species.
We analyzed whether reproduction of eastern larch beetle in the subalpine larch with the O. tomentosa fungus was significantly different than the other subalpine larch trees in terms of starting moisture, and the gallery lengths, fertility, and lipid contents of eastern larch beetles using ANOVA. We recognize this analysis is by definition pseudoreplication, as there was only one tree so affected, but offer it anyways with a reader caution to avoid extending inference to other trees potentially affected by O. tomentosa in the future. Metrics were similar in all respects so we included these data within our full analyses described above.
Additionally, we evaluated the sex ratio of the beetles that emerged from bolts to see if a difference occurred between species, and to determine if a given species might serve as a beetle source or sink. We used a generalized linear mixed effects model with bolt as a random effect to compare the mean percentages of females that emerged from each species overall. Then, we multiplied the percent of females by the average number of beetles that emerged from each bolt within a species to determine the average number of females emerging from a given bolt of each species overall. If that number was greater than or equal to the average number of maternal galleries per bolt for a species (representative of successfully tunneling female colonizers), the species was considered a source and thus a viable host.
3 Results
Beetles were able to reproduce in cut bolts of all three species of North American Larix, although western larch did not appear to be a highly suitable host (Figure 2). Over a 53-day period, 2019 beetles emerged from bolts of eastern larch, 1,147 beetles from bolts of subalpine larch, and only 153 beetles from bolts of western larch. The differences in numbers of emerged beetles between species were not simply due to differences in the numbers of females that successfully entered the bolts, mated, and laid eggs, as these numbers were similar across species (overall mean ± SE = 4.3 ± 0.1, F2,43 = 0.12, p = 0.885).
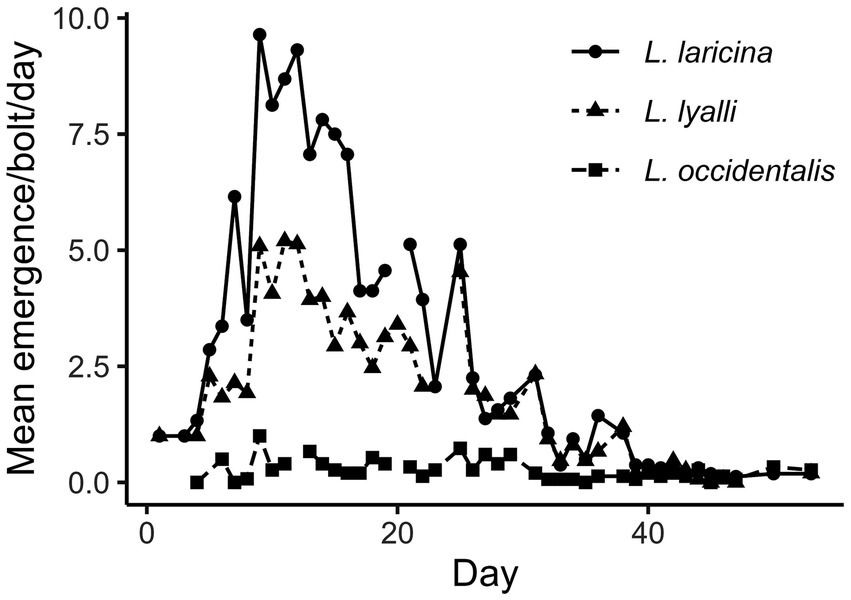
Figure 2. Mean number of eastern larch beetles emerging each day by species, where day 1 represents the first observed emergence from any bolt. The first bolts were infested 34 days prior (n = 15–16 bolts/species; summer 2023).
Indeed, the number of offspring that emerged per female, once standardized for bolt area, varied between species (F2,43 = 18.52, p < 0.0001; Figure 3A). Almost twice as many beetles emerged from bolts of eastern larch (1.9 ± 0.3 beetles/female/dm2) than bolts of subalpine larch (1.0 ± 0.2 beetles/female/dm2); however, this difference was insignificant. Emergence from bolts of western larch (0.2 ± 0.05 beetles/female/dm2) was almost 10 times lower than emergence from bolts of eastern larch. However, there was no significant difference in sex ratio of emerged beetles by species (x2 = 1.094, df = 2, p = 0.579). The average percentage of females emerging from eastern larch was 40.0 ± 0.33%, 51.1 ± 0.44% from subalpine larch, and 47.7 ± 0.45% from western larch. On average, 119 ± 20.2 beetles emerged from eastern larch bolts, 72 ± 11.8 beetles from subalpine larch bolts, and 9 ± 2.4 beetles emerged from western larch bolts.
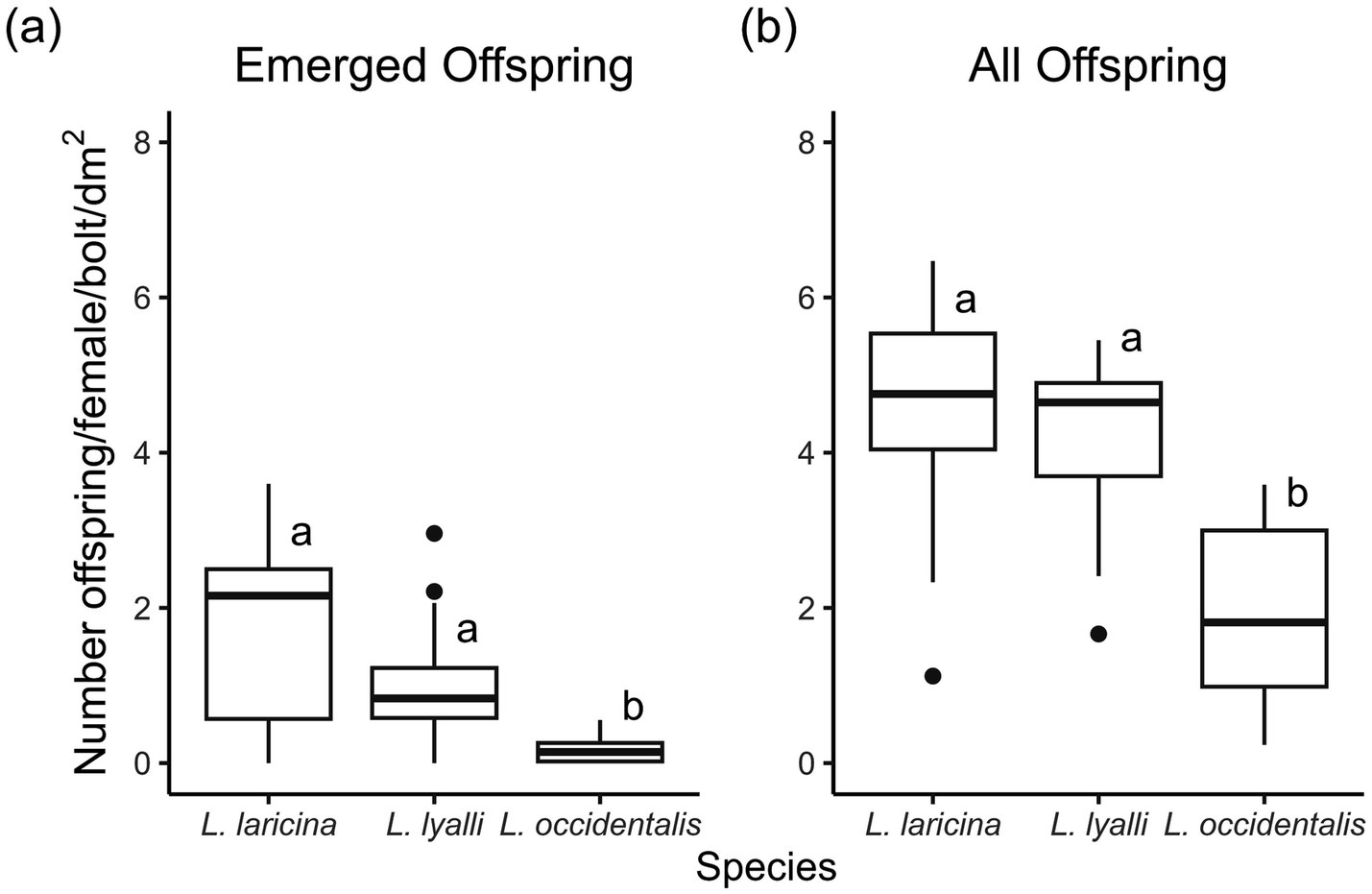
Figure 3. Effect of species on (a) number of emerged eastern larch beetles per female, standardized by surface area of bolt (n = 15–16 bolts per species) (b) emerged eastern larch beetles plus all progeny found after removing the bark, per female, standardized by surface area of bolt (n = 16–17 bolts per species; experiment conducted summer 2023). On this and subsequent plots: the upper/lower whiskers indicate the maximum/minimum values as the third quartile added to/subtracted from 1.5 times the interquartile range. The middle line of each box and whisker plot represents the median of the data set. Dots represent data points beyond plus or minus 1.5 times the interquartile range.
Mean maternal gallery length varied by species (Figure 4; F2, 43 = 23.68, p < 0.0001). On average, females tunneled the farthest in subalpine larch (60.4 ± 4.2 cm). This distance was approximately double the length of galleries noted in both eastern larch (36.6 ± 2.2 cm) and western larch (32.7 ± 2.6 cm). In eastern larch, the average number of galleries per bolt was 4.2 ± 0.2, comparable to subalpine larch (4.4 ± 0.2 galleries) and western larch (4.2 ± 0.2 galleries). Multiplying the overall species sex ratios by the mean number of emerged beetles from each bolt within a species, we calculated that, on average, 47.5 females emerged from each eastern larch bolt, 36.6 females came from each bolt of subalpine larch, and 4.6 beetles from each western larch bolt. In all three species, the calculated average of emerged females was greater than the average number of females that successfully colonized each bolt.
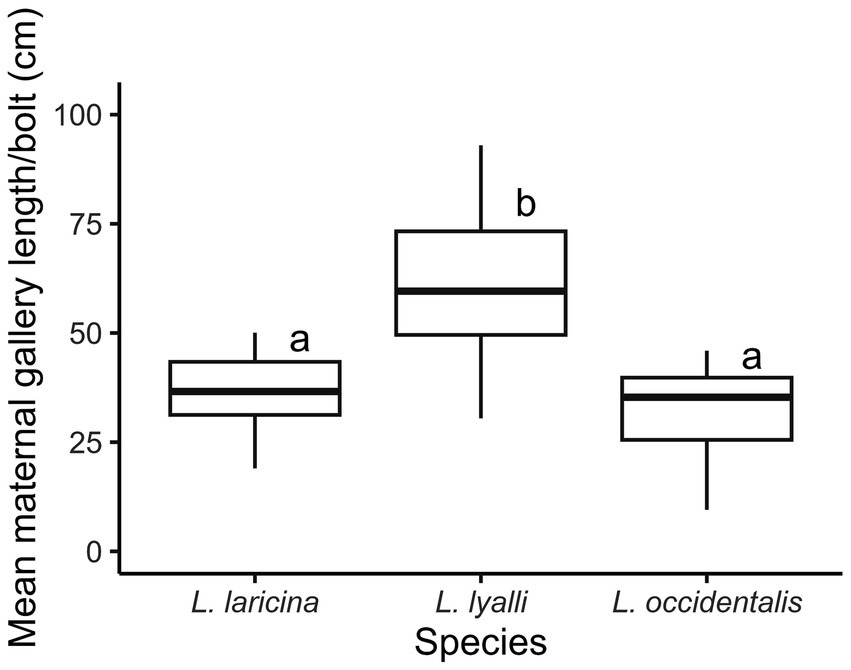
Figure 4. Effect of species on eastern larch beetle maternal gallery length (n = 16–17 bolts per species; summer 2023).
When evaluating fertility in terms of total number of offspring (i.e., including all individuals found at debarking), results were similar to above (F2, 43 = 21.11, p < 0.0001; Figure 3B). Mating pairs in eastern larch produced 4,798 progeny, those in subalpine larch produced 4,684 progeny, and those in western larch produced 1,638 progeny. A higher proportion of emerged adults versus manually-extracted adult beetles occurred in eastern larch (2019:2604 beetles) than subalpine larch (1,147:3235 beetles), which in turn was higher than the proportion that emerged from western larch (153:1357 beetles). Standardized by bolt size, we found females in bolts of eastern larch produced an average of 4.5 ± 0.3 progeny per dm2. These numbers were comparable to females in subalpine larch, which on average produced approximately 4.2 ± 0.3 progeny per dm2. In western larch, however, reproductive output dropped by just over half, as each female only produced an average of 1.9 ± 0.3 offspring per dm2.
The lipid content in the beetles that were manually extracted from underneath the bark after emergence concluded was higher than the lipid content of beetles that emerged naturally to collection jars (F1,194 = 176.79, p < 0.0001), so we display these results separately (Figure 5). The mean lipid weight in emerged beetles was 0.495 ± 0.026 mg, while lipid weight for manually extracted beetles averaged 1.377 ± 0.053 mg. Despite these differences in lipid content among emergence status, the effect of species on lipid weight was consistent (F2,124 = 34.31, p < 0.0001 for emerged beetles, Figure 5A; F2, 115 = 16.99, p < 0.0001 for manually extracted beetles, Figure 5B). Beetles that emerged from bolts of eastern and subalpine larch had approximately double the lipid content of insects developing in western larch. This species effect was consistent whether lipid content was measured in dry weight or as a percentage of total body weight (F2, 124 = 37.11, p < 0.0001 for emerged beetles, Figure 5C; F2, 115=15.60, p < 0.0001 for extracted beetles, Figure 5D). Mean percent lipid content varied between 9.23 and 20.70% by species for naturally emerging beetles, but was lowest in western larch (Figure 5C). Likewise, the mean percent lipid content varied between 26.71 and 37.47% by species for beetles harvested upon debarking, but was lowest for beetles developing in western larch (Figure 5D).
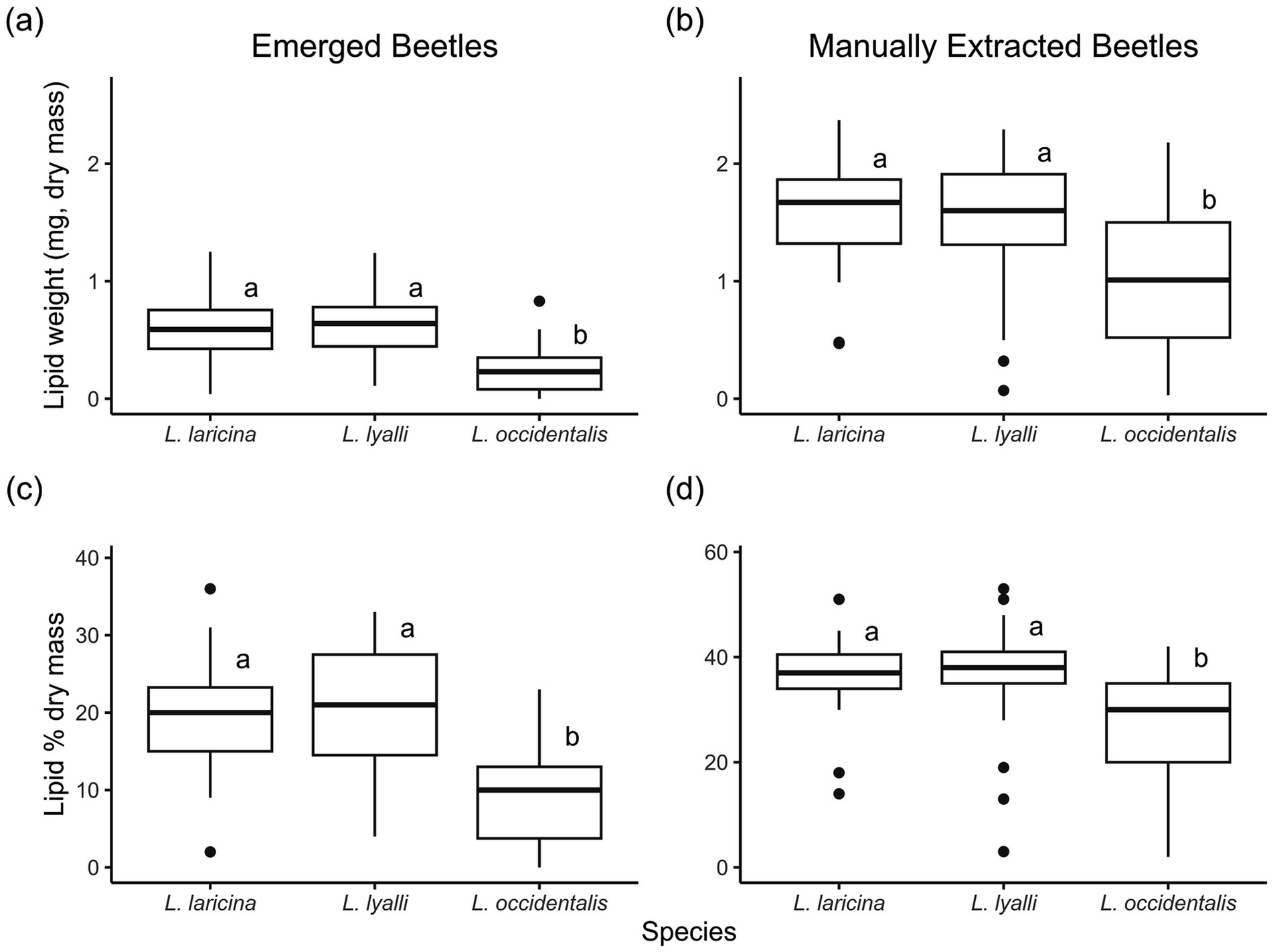
Figure 5. Lipid weight (a, b) and lipid percent dry mass (c, d) by species for n = 131 emerged eastern larch beetles and n = 121 extracted beetles across 46 bolts, fall 2023.
Emerged beetles were approximately 2% larger than manually extracted beetles (Figure 6; F1, 247 = 14.82, p < 0.0001). Among manually extracted beetles, those reared in eastern larch were slightly larger than those reared in subalpine larch, with no difference between subalpine larch- and western larch-reared beetles (F2, 115 = 3.39, p = 0.037; Figure 6B). Sex was not associated with differences in lipid weight (F1, 194 = 0.72, p = 0.39) nor lipid percentage (F1, 194 = 0.34, p = 0.56) when beetle emergence types were pooled. When beetle types were analyzed separately, however, males averaged 0.15 mg (± 0.04 SE) less lipid than females for beetles that had emerged naturally (F1, 91 = 11.46, p = 0.0010).
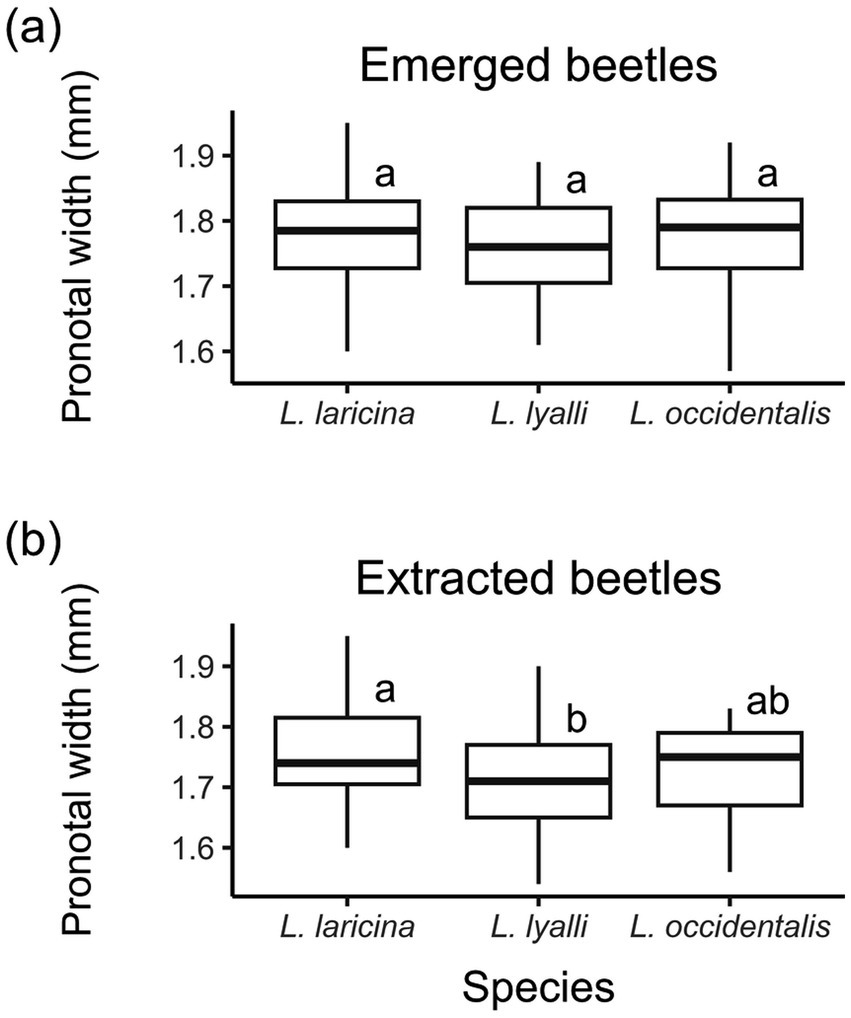
Figure 6. Difference in pronotal width between (a) emerged eastern larch beetles (n = 131) and (b) extracted eastern larch beetles (n = 121), fall 2023.
4 Discussion
Our findings demonstrate that the eastern larch beetle, Dendroctonus simplex LeConte, can successfully reproduce in cut bolts of all three species of North American Larix. These results indicate that if the range of eastern larch beetle were to expand, western and subalpine larch may be at risk from a novel Dendroctonus pest. In all three species, the average number of females emerging from each bolt were greater than the number of females that colonized each bolt, indicating all three species could prove suitable hosts. Two key unknowns are how attractive western and subalpine larch would be to eastern larch beetles in the field, and if attractive, what levels of defenses (constitutive and inducible) these novel hosts might possess. Due to the limitations of using cut bolts vs. live trees, only constitutive effects are manifested in the current study (Rosenberger et al., 2017a). Lower host defenses have been noted in naïve hosts of mountain pine beetle D. ponderosae (Cudmore et al., 2010; Raffa et al., 2013; Rosenberger et al., 2017b).
Host range expansions benefit from accessible geography, insect sources (e.g., outbreaking populations), and active and passive dispersal. Geographically, the range of D. simplex is sympatric to that of its host, eastern larch, and thus relatively close to but not overlapping the ranges of western and subalpine larch. Range maps produced by Little (Figure 1) indicate the ranges of eastern and subalpine larch are closest in southwestern Alberta, just outside of Banff National Park in Canada (Little, 1971). The shortest distance between the two species at their closest point is only 53 km, not accounting for elevation. From these maps, the distance between eastern and western larch at their closest point is 147 km, also in southwestern Alberta. However, recent models based on available soil water holding capacity predict northward range expansion of western larch that would result in eastern and western larch range overlap near Calgary, Alberta (Mathys et al., 2014). From an outbreak standpoint, the present, ongoing outbreak in Minnesota has been attributed, in part, to ameliorating winters (Venette and Walter, 2008) and longer, warmer summers (McKee, 2015). With the predicted increases in global temperatures from 1.5–4.4°C by 2,100 (IPCC, 2023), the potential for outbreak activity in hosts of suitable species, age, and size classes shows no signs of abating in the future.
The dispersal ability of the eastern larch beetle is currently unknown; however, other Dendroctonus species can disperse on mesoscale atmospheric currents up to 30–100 km/day (Jackson et al., 2008), and have been found at elevations up to 3,048 m above sea level (Furniss and Furniss, 1972). Thus, it is possible that eastern larch beetle could disperse to western or subalpine larch stands, particularly if an outbreak were to occur in the southern edge of the eastern larch range and spread to subalpine larch stands (Howe et al., 2021). The ability of western and subalpine larch to hybridize along the elevation gradient dividing the two species could further facilitate spread across elevations (Carlson and Blake, 1969; Arno, 1990). Given the short growing season and harsh winter conditions experienced at high elevations, further research into effects of temperature on eastern larch beetle development is needed to evaluate if the beetle can successfully complete its life cycle in subalpine larch stands. However, since eastern larch beetle adults and larvae are extremely cold-hardy (down to −42°C and − 49°C, respectively; Venette and Walter, 2008), it is likely the beetle would be able to survive winter temperatures in subalpine larch stands, especially with additional climate warming.
Lower reproductive success of D. simplex in western larch versus subalpine larch and eastern larch (Figures 2, 3) could be caused by higher constitutive host defenses within western larch bolts. Further evidence suggesting high constitutive defenses in western larch is the inability for Douglas-fir beetle Dendroctonus pseudotsugae to successfully reproduce in live trees, despite being able to do so in cut bolts of western larch (Furniss, 1976). Constitutive defenses can be physical as well as chemical, with variation between chemical compounds occurring within different tissues (Krokene, 2015). Differences between monoterpene composition and concentration vary between eastern, western and subalpine larch as well as between tissues. For example, myrcene, a compound toxic to D. simplex, is present in higher concentrations in the twig and needle oil of western larch than eastern and subalpine larch while the concentrations of limonene, another toxic compound, is present in resin of the latter two species in comparable concentrations (Von Rudloff, 1987; Werner, 1995). An earlier study also found that resin of western larch had demonstrably higher levels of α-pinene compared to resin of eastern larch, with the latter having higher levels of 𝛽-pinene and both species having comparable Δ-3-carene levels (Stairs, 1968). Recent work has found high levels of both α-pinene and Δ-3-carene and relatively low levels of 𝛽-pinene within the phloem of eastern larch (Althoff et al., 2023). Alternatively, differences in reproductive success could be due to differences in moisture content or phloem thickness, although we observed these metrics to be qualitatively similar across all species.
Variation in constitutive chemical defenses across species may also explain why maternal galleries constructed in subalpine larch were approximately 50% longer than maternal galleries constructed in the native host, eastern larch (Figure 4). Factors influencing gallery length in eastern larch beetle include female vigor, availability of unused phloem, and host resin production (Werner, 1986; Langor and Raske, 1987a, 1987b). We used beetles of similar vigor across all species and female densities were similarly standardized. Although there were minor differences in bolt size across species, each bolt had unused areas of phloem, so we do not view crowding as an impediment to gallery construction. The relationship between host conifer defenses and elevation is complex (Moreira et al., 2018), but the elevational gradient in plant defense hypothesis (EGPD) proposes that higher-elevation plants are less-defended than those at lower elevations (Ferrenberg et al., 2017). That the longest galleries were constructed in subalpine larch seem to suggest the trees used in this study had lower constitutive defenses against D. simplex than the other species. Lack of host defenses in high-elevation tree species against Dendroctonus bark beetles has been observed in outbreaks of mountain pine beetle in whitebark pine as well (Raffa et al., 2013). Alternatively, the extended gallery lengths in subalpine larch could be due to lower quality phloem or fewer fungal symbionts (Bentz and Six, 2006), requiring adult beetles to tunnel longer to obtain sufficient nutrition to achieve fertility rates similar to those in eastern larch.
Fertility was similar between beetles reared in eastern versus subalpine larch, but lower than western larch (Figure 3). Similar to maternal gallery length, the pattern seen in fertility may suggest higher constitutive defenses in western larch relative to subalpine and eastern larch, although more study is needed. Genetic relatedness between eastern larch, western and subalpine larch and host range expansion and contraction over time may also explain why beetles performed better in subalpine versus western larch. It is worth noting that eastern and western larch are more genetically similar to one another than to subalpine larch (Gros-Louis et al., 2005), and this relatedness may relate to constitutive host tree defenses. Gene flow within subalpine larch populations is more restricted (Khasa et al., 2006), indicating that bottlenecks occurred during or after establishment of subalpine larch populations. This pattern supports the theory that the discontinuous distribution of subalpine larch is attributed to a shift from warmer to cooler climates, resulting in non-contiguous populations of subalpine larch at high elevations as observed in the current range of the species. Similar to how white pine blister rust resistance factors into whitebark pine restoration efforts, the limited genetic diversity of subalpine larch could pose a conservation challenge, particularly if the species in situ is vulnerable to novel insect threats such as eastern larch beetle.
Size and lipid content are often used to evaluate fitness of offspring (Hansen and Bentz, 2003; McKee and Aukema, 2015a; Esch et al., 2016). Eastern larch-reared beetles were slightly larger than those raised in subalpine larch for beetles extracted upon debarking (Figure 6), but the difference is not likely ecologically meaningful. Beetle size may be positively correlated with phloem thickness (Amman et al., 1986), and smaller beetles may be better suited to reproducing in species with thinner phloem (Safranyik et al., 2010). Size differences between emerging versus extracted beetles overall (Figure 6B) could reflect beetle populations in two different physiological states, but would not indicate that a second generation of beetles was laid during the experiment. Some brood adults emerge and colonize new hosts when the growing season permits while other adults remain within the phloem to overwinter in a putative facultative overwintering diapause (Langor and Raske, 1987a, 1987b; McKee and Aukema, 2015b). Given that it takes approximately 35 days to develop from egg to adult at our rearing temperature of 24.7°C (McKee and Aukema, 2015a), and given that this experiment concluded on day 53, there would not have been sufficient time for a second generation of insects that were smaller in size due to deteriorating phloem conditions.
We note that our results of reproduction across species may be conservative and potentially underestimate reproduction in these different hosts. While emergence was occurring, we found several dozen beetles in the laboratory room outside of the rearing tubes and emergence jars and could not trace their origin. No signs of tunneling through the cardboard sides or the plastic lids of emergence tubes were observed during inspections following the experiment.
The ability of eastern larch beetle to successfully reproduce in cut bolts of all three native North American species of larch indicates that western and subalpine larch could function as suitable hosts, albeit a poor host in the case of western larch. Host suitability is different from susceptibility, and it remains unknown if these insects would be attracted to live western and subalpine larch and how they might fare in live hosts with induced defenses as opposed to cut bolts. Nonetheless, subalpine larch as a high-elevation species appears to be especially at risk, given that beetles exhibit similar fertility and lipid content to those reproducing within the beetle’s native eastern larch host, and construct longer ovipositional galleries. Additional work evaluating the dispersal potential of the beetle, its development at different temperatures (McKee and Aukema, 2015b), its performance in live trees, and susceptibility ratings of stands of subalpine and western larch is necessary to determine ultimate host susceptibility to eastern larch beetle in the future.
Data availability statement
Data will be publically available through DRUM (Data Respository for U of M) https://doi.org/10.13020/9p01-rr31.
Ethics statement
The manuscript presents research on animals that do not require ethical approval for their study.
Author contributions
RP: Data curation, Formal analysis, Investigation, Methodology, Project administration, Visualization, Writing – original draft, Writing – review & editing. EE: Conceptualization, Project administration, Resources, Writing – review & editing. BS: Conceptualization, Project administration, Resources, Writing – review & editing. BA: Conceptualization, Data curation, Formal analysis, Funding acquisition, Methodology, Project administration, Resources, Software, Supervision, Writing – review & editing.
Funding
The author(s) declare that financial support was received for the research, authorship, and/or publication of this article. This work was funded by the AVR Foundation, Minnesota Agricultural Experimental Station Project MIN-17-117, and by the Minnesota Environment and Natural Resources Trust Fund as recommended by the Legislative-Citizen Commission on Minnesota Resources (LCCMR) under appropriation M.L. 2021, First Special Session, Chp. 6, Art. 5, Sec. 2, Subd. 08f for project 2020-047a.
Acknowledgments
We thank Idaho Department of Lands personnel Tom Eckberg, Isabella Valdez and Mikayla Cotton for their assistance procuring and harvesting western larch in Coeur d’Alene. James Steed (US Forest Service) helped source and hand-felled subalpine larch in Montana along with volunteers Joshua Smith (Heritage Forestry), Jennifer McNew (BLM), and Drew Krank (partner of first author). Eric Otto (MN DNR) helped procure the eastern larch. Special thanks to Dr. Robert Blanchette at the University of Minnesota Forest Pathology and Wood Microbiology Research Laboratory for isolating and sequencing the fungal pathogen in our subalpine larch. UMN labmates Abigail Brett, Ian Grossenbacher-McGlamery, Hunter Ness, Emily Althoff, Grace Graham, Sayesha Khanna, and Jessica Rootes provided support in the lab and field. The authors appreciate the insightful comments of Drs. Marcella Windmuller-Campione (UMN) and Robert Venette (US Forest Service) on previous drafts of this manuscript.
Conflict of interest
The authors declare that the research was conducted in the absence of any commercial or financial relationships that could be construed as a potential conflict of interest.
Publisher’s note
All claims expressed in this article are solely those of the authors and do not necessarily represent those of their affiliated organizations, or those of the publisher, the editors and the reviewers. Any product that may be evaluated in this article, or claim that may be made by its manufacturer, is not guaranteed or endorsed by the publisher.
References
Althoff, E. R., O’Loughlin, T. J., Wakarchuk, D. A., Aukema, K. G., and Aukema, B. H. (2023). Monoterpene composition of phloem of eastern larch (Larix laricina (Du Roi) K. Koch) in the Great Lakes region: with what must the eastern larch beetle (Dendroctonus simplex LeConte) contend? Forests 14:566. doi: 10.3390/f14030566
Amman, G. D. (1986). Mountain pine beetle in ponderosa pine: Effects of phloem thickness and egg gallery density, vol. 367. Intermountain Research Station: US Department of Agriculture, Forest Service.
Arno, S. F. (1990). Alpine larch. Conifers, Agricultural handbook (United States Department of Agriculture), vol. 1. Ogden, Utah: U.S. Dept. of Agriculture, Forest Service, Intermountain Forest and Range Experiment Station. 281–297.
Arno, S. F., and Habeck, J. R. (1972). Ecology of alpine larch (Larix lyallii Parl.) in the Pacific northwest. Ecol. Monogr. 42, 417–450. doi: 10.2307/1942166
Bentz, B. J., and Jönsson, A. M. (2015). Chapter 13: Modeling bark beetle responses to climate change. In Bark beetles: Biology and ecology of native and invasive species. 533–553. doi: 10.1016/B978-0-12-417156-5.00013-7
Bentz, B. J., Régnière, J., Fettig, C. J., Hansen, E. M., Hayes, J. L., Hicke, J. A., et al. (2010). Climate change and bark beetles of the western United States and Canada: direct and indirect effects. Bioscience 60, 602–613. doi: 10.1525/bio.2010.60.8.6
Bentz, B. J., and Six, D. L. (2006). Ergosterol content of Fungi associated with Dendroctonus ponderosae and Dendroctonus rufipennis (Coleoptera: Curculionidae, Scolytinae). Ann. Entomol. Soc. Am. 99, 189–194. doi: 10.1603/0013-8746(2006)099[0189:ECOFAW]2.0.CO;2
Byers, J. A. (1989). Chemical ecology of bark beetles. Experientia 45, 271–283. doi: 10.1007/BF01951813
Cale, J. A., Taft, S., Najar, A., Klutsch, J. G., Hughes, C. C., Sweeney, J. D., et al. (2015). Mountain pine beetle (Dendroctonus ponderosae) can produce its aggregation pheromone and complete brood development in naïve red pine (Pinus resinosa) under laboratory conditions. Can. J. For. Res. 45, 1873–1877. doi: 10.1139/cjfr-2015-0277
Carlson, C.E., and Blake, G.M., (1969). Hybridization of western and subalpine larch. Montana Forest Experiment Station Bulletin 37. Missoula: University of Montana. 12.
Cudmore, T. J., Björklund, N., Carroll, A. L., and Staffan Lindgren, B. (2010). Climate change and range expansion of an aggressive bark beetle: evidence of higher beetle reproduction in naïve host tree populations. J. Appl. Ecol. 47, 1036–1043. doi: 10.1111/j.1365-2664.2010.01848.x
de la Giroday, H. M. C., Carroll, A. L., and Aukema, B. H. (2012). Breach of the northern Rocky Mountain geoclimatic barrier: initiation of range expansion by the mountain pine beetle. J. Biogeogr. 39, 1112–1123. doi: 10.1111/j.1365-2699.2011.02673.x
DeRose, R. J., Bentz, B. J., Long, J. N., and Shaw, J. D. (2013). Effect of increasing temperatures on the distribution of spruce beetle in Engelmann spruce forests of the interior west, USA. For. Ecol. Manag. 308, 198–206. doi: 10.1016/j.foreco.2013.07.061
Esch, E. D., Langor, D. W., and Spence, J. R. (2016). Gallery success, brood production, and condition of mountain pine beetles (Coleoptera: Curculionidae) reared in whitebark and lodgepole pine from Alberta, Canada. Can. J. For. Res. 46, 557–563. doi: 10.1139/cjfr-2015-0351
Ferrenberg, S., Langenhan, J. M., Loskot, S. A., Rozal, L. M., and Mitton, J. B. (2017). Resin monoterpene defenses decline within three widespread species of pine (Pinus) along a 1530-m elevational gradient. Ecosphere 8:e01975. doi: 10.1002/ecs2.1975
Francke, W., Bartels, J., Meyer, H., Schröder, F., Kohnle, U., Baader, E., et al. (1995). Semiochemicals from bark beetles- new results, remarks and reflections. J. Chem. Ecol. 21, 1043–1063. doi: 10.1007/BF02033807
Furniss, M. M. (1976). Controlled breeding, comparative anatomy and bionomics of Dendroctonus simplex Leconte and Dendroctonus pseudotsugae Hopkins (Coleoptera: Scolytidae). Anniv. Publ. Univ. Ida. Dep. Entomol. 15, 109–120.
Furniss, R.L., and Carolin, V.M. (1977). Western forest insects, Miscellaneous publication - Dept. of Agriculture; no. 1339. Dept. of Agriculture, Forest Service: for sale by the Supt. of Docs., U.S. Govt. Print. Off., Washington, DC.
Furniss, M. M., and Furniss, R. L. (1972). Scolytids (Coleoptera) on snowfields above timberline in Oregon and Washington. Can. Entomol. 104, 1471–1478. doi: 10.4039/Ent1041471-9
Gros-Louis, M.-C., Bousquet, J., Pâques, L. E., and Isabel, N. (2005). Species-diagnostic markers in Larix spp. based on RAPDs and nuclear, cpDNA, and mtDNA gene sequences, and their phylogenetic implications. Tree Genet. Genomes 1, 50–63. doi: 10.1007/s11295-005-0007-z
Hagle, S. K., Gibson, K. E., and Tunnock, S. (2003). Field guide to diseases and insect pests of northern and central Rocky Mountain conifers. Forest Service, State and Private Forestry, Northern Region, Missoula, Mont: U.S. Dept. of Agriculture.
Hansen, E. M., and Bentz, B. J. (2003). Comparison of reproductive capacity among univoltine, semivoltine, and re-emerged parent spruce beetles (Coleoptera: Scolytidae). Can. Entomol. 135, 697–712. doi: 10.4039/n02-109
Hansen, E. M., and Lewis, K. J. (1997). Compendium of conifer diseases, the disease compendium series of the American Phytopathological society. St. Paul, Minn: APS Press, the American Phytopathological Society.
Hopkins, A. D. (1909). The eastern larch beetle. The Douglas fir beetle. Bulletin 83, Part 1, United States Department of Agriculture – Bureau of Entomology, Washington, District of Columbia, United States of America.
Howe, M., Carroll, A., Gratton, C., and Raffa, K. F. (2021). Climate-induced outbreaks in high-elevation pines are driven primarily by immigration of bark beetles from historical hosts. Glob. Chang. Biol. 27, 5786–5805. doi: 10.1111/gcb.15861
IPCC. (2023). Summary for Policymakers. Climate change 2023: Synthesis report. Contribution of working groups I, II and III to the Sixth Assessment Report of the Intergovernmental Panel on Climate Change. eds. H. Lee and J. Romero. IPCC, Geneva, Switzerland, 1–34.
Jackson, P. L., Straussfogel, D., Lindgren, B. S., Mitchell, S., and Murphy, B. (2008). Radar observation and aerial capture of mountain pine beetle,Dendroctonus ponderosaehopk. (Coleoptera: Scolytidae) in flight above the forest canopy. Can. J. For. Res. 38, 2313–2327. doi: 10.1139/X08-066
Johnston, W. F. (1990). Tamarack. Silv. Man. 1. Conifers, Agricultural handbook (United States Department of Agriculture) 1, 260–280.
Khasa, D. P., Jaramillo-Correa, J. P., Jaquish, B., and Bousquet, J. (2006). Contrasting microsatellite variation between subalpine and western larch, two closely related species with different distribution patterns. Mol. Ecol. 15, 3907–3918. doi: 10.1111/j.1365-294X.2006.03066.x
Kolb, T., Keefover-Ring, K., Burr, S. J., Hofstetter, R., Gaylord, M., and Raffa, K. F. (2019). Drought-mediated changes in tree physiological processes weaken tree defenses to bark beetle attack. J. Chem. Ecol. 45, 888–900. doi: 10.1007/s10886-019-01105-0
Krokene, P. (2015). Conifer defense and resistance to bark beetles. Bark Beetles, 177–207. doi: 10.1016/B978-0-12-417156-5.00005-8
Labandeira, C. C., LePage, B. A., and Johnson, A. H. (2001). A Dendroctonusbark engraving (Coleoptera: Scolytidae) from a middle EoceneLarix(Coniferales: Pinaceae): early or delayed colonization? Am. J. Bot. 88, 2026–2039. doi: 10.2307/3558429
Langor, D. W., and Raske, A. G. (1987a). Emergence, host attack, and overwintering behavior of the eastern larch beetle, dendroctonus simplexleconte (coleoptera: scolytidae), in newfoundland. Can. Entomol. 119, 975–983. doi: 10.4039/Ent119975-11
Langor, D. W., and Raske, A. G. (1987b). Reproduction and development of the eastern larch BEETLE, dendroctonus simplexleconte (coleoptera: scolytidae), in newfoundland. Can. Entomol. 119, 985–992. doi: 10.4039/Ent119985-11
Langor, D. W., and Raske, A. G. (1989). The eastern larch beetle, another threat to our forests (Coleoptera: Scolytidae). For. Chron. 65, 276–279. doi: 10.5558/TFC65276-4
Lesk, C., Coffel, E., D’Amato, A. W., Dodds, K., and Horton, R. (2017). Threats to north American forests from southern pine beetle with warming winters. Nat. Clim. Chang. 7, 713–717. doi: 10.1038/nclimate3375
Little, E. L. (1971). Atlas of United States trees, miscellaneous publication / United States Department of Agriculture, vol. no. 1410. Washington, D.C.: U.S. Dept. of Agriculture, Forest Service.
Logan, J. A., and Powell, J. A. (2001). Ghost forests, global warming, and the mountain pine beetle (Coleoptera: Scolytidae). Am. Entomol. 47, 160–173. doi: 10.1093/ae/47.3.160
Lyon, R. L. (1958). A useful secondary sex character in Dendroctonus bark beetles. The Canadian entomologist. 90, 582–584. doi: 10.4039/Ent90582-10
Mathys, A., Coops, N. C., and Waring, R. H. (2014). Soil water availability effects on the distribution of 20 tree species in western North America. For. Ecol. Manag. 313, 144–152. doi: 10.1016/j.foreco.2013.11.005
McKee, F. R. (2015). Biology and population dynamics of the eastern larch beetle, Dendroctonus simplex LeConte, and its interactions with eastern larch (tamarack), Larix laricina. [Doctoral dissertation, University of Minnesota].
McKee, F. R., and Aukema, B. H. (2015a). Influence of temperature on the reproductive success, brood development and brood fitness of the eastern larch Beetledendroctonus simplexleconte. Agric. For. Entomol. 17, 102–112. doi: 10.1111/afe.12087
McKee, F. R., and Aukema, B. H. (2015b). Successful reproduction by the eastern larch beetle (Coleoptera: Curculionidae) in the absence of an overwintering period. Can. Entomol. 147, 602–610. doi: 10.4039/tce.2014.81
Moreira, X., Petry, W. K., Mooney, K. A., Rasmann, S., and Abdala-Roberts, L. (2018). Elevational gradients in plant defences and insect herbivory: recent advances in the field and prospects for future research. Ecography 41, 1485–1496. doi: 10.1111/ecog.03184
Paine, T. D., Raffa, K. F., and Harrington, T. C. (1997). Interactions among Scolytid bark beetles, their associated fungi, and live host conifers. Annu. Rev. Entomol. 42, 179–206. doi: 10.1146/annurev.ento.42.1.179
Pureswaran, D. S., Meurisse, N., Rassati, D., Liebhold, A. M., and Faccoli, M. (2022). Climate change and invasions by nonnative bark and ambrosia beetles, in: bark beetle management, ecology, and climate change. Elsevier, pp. 3–30. doi: 10.1016/B978-0-12-822145-7.00002-7
Pureswaran, D. S., Roques, A., and Battisti, A. (2018). Forest insects and climate change. Curr. For. Rep. 4, 35–50. doi: 10.1007/s40725-018-0075-6
R Core Team (2024). R: A language and environment for statistical computing. Vienna, Austria: R Foundation for Statistical Computing.
Raffa, K. F. (2014). Terpenes tell different Tales at different scales: glimpses into the chemical ecology of conifer - bark beetle - microbial interactions. J. Chem. Ecol. 40, 1–20. doi: 10.1007/s10886-013-0368-y
Raffa, K. F., and Berryman, A. A. (1983). The role of host plant resistance in the colonization behavior and ecology of bark beetles (Coleoptera: Scolytidae). Ecol. Monogr. 53, 27–49. doi: 10.2307/1942586
Raffa, K. F., and Berryman, A. A. (1987). Interacting selective pressures in conifer-bark beetle systems: a basis for reciprocal adaptations? Am. Nat. 129, 234–262. doi: 10.1086/284633
Raffa, K. F., Powell, E. N., and Townsend, P. A. (2013). Temperature-driven range expansion of an irruptive insect heightened by weakly coevolved plant defenses. Proc. Natl. Acad. Sci. 110, 2193–2198. doi: 10.1073/pnas.1216666110
Rosenberger, D. W., Aukema, B. H., and Venette, R. C. (2017a). Cold tolerance of mountain pine beetle among novel eastern pines: a potential for trade-offs in an invaded range? For. Ecol. Manag. 400, 28–37. doi: 10.1016/j.foreco.2017.05.031
Rosenberger, D. W., Venette, R. C., and Aukema, B. H. (2016). Sexing live mountain pine Beetles dendroctonus ponderosae: refinement of a behavioral method forDendroctonusspp. Entomol. Exp. Appl. 160, 195–199. doi: 10.1111/eea.12463
Rosenberger, D. W., Venette, R. C., Maddox, M. P., and Aukema, B. H. (2017b). Colonization behaviors of mountain pine beetle on novel hosts: implications for range expansion into northeastern North America. PLoS One 12:e0176269. doi: 10.1371/journal.pone.0176269
Ryan, R. B., Tunnock, S., and Ebel, F. W. (1987). The larch casebearer in North America. J. For. 85, 33–39. doi: 10.1093/jof/85.7.33
Safranyik, L., Carroll, A. L., Régnière, J., Langor, D. W., Riel, W. G., Shore, T. L., et al. (2010). Potential for range expansion of mountain pine beetle into the boreal forest of North America. Can. Entomol. 142, 415–442. doi: 10.4039/n08-CPA01
Schmidt, W. C., and Shearer, R. C. (1990). Western Larch. Silv. Man. Conifers, Agricultural handbook (United States Department of Agriculture) 1, 298–321.
Seybold, S.J., Albers, M.A., and Katovich, S.A., (2002). Eastern larch beetle. Forest insect & disease leaflet no. 175. US Department of Agriculture – Forest Service, Northern Research Station, St Paul, Minnesota.
Shaw, C. G., and Kile, G. A. (1991). Armillaria root disease, agriculture handbook, vol. 691. Washington, DC: Forest Service, U.S. Dept. of Agriculture.
Six, D. L., Trowbridge, A. M., Howe, M., Perkins, D. L., Berglund, E., Brown, P. M., et al. (2021). Growth, chemistry, and genetic profiles of whitebark pine forests affected by climate-driven mountain pine beetle outbreaks. Front. Forest. Glob. Change 4. doi: 10.3389/ffgc.2021.671510
Six, D. L., and Wingfield, M. J. (2011). The role of phytopathogenicity in bark beetle–fungus symbioses: a challenge to the classic paradigm. Annu. Rev. Entomol. 56, 255–272. doi: 10.1146/annurev-ento-120709-144839
Thies, W. G., and Sturrock, R. N. (1995). Laminated root rot in western North America. Forest Service general technical report. Forest Service, Portland: OR (United States). Pacific Northwest Research Station, United States.
Trummer, L. (1999). Tomentosus root rot. United States Forest Service leaflet R10-TP; 80. Alaska Region.
Ungerer, M. J., Ayres, M. P., and Lombardero, M. J. (1999). Climate and the northern distribution limits of dendroctonus frontalis zimmermann (Coleoptera: Scolytidae). J. Biogeogr. 26, 1133–1145. doi: 10.1046/j.1365-2699.1999.00363.x
United States Fish and Wildlife Service (2022). Endangered and threatened wildlife and plants; threatened species status with section 4(d) rule for Whitebark pine. 50 CFR Part 17.
Venette, R. C., and Walter, A. J. (2008). “Connecting overwintering success of eastern larch beetle to health of tamarack” in Forest health monitoring: 2008 National Technical Report, SRS. eds. K. M. Potter and B. L. Conkling (Ashville, NC: USDA Forest Service Southern Research Station), 167–169.
Von Rudloff, E. (1987). The volatile twig and leaf oil terpene compositions of three western north American larches, Larix laricina, Larix occidentalis, and Larix lyallii. J. Nat. Prod. 50, 317–321. doi: 10.1021/np50050a051
Ward, S. F., and Aukema, B. H. (2019a). Anomalous outbreaks of an invasive defoliator and native bark beetle facilitated by warm temperatures, changes in precipitation and interspecific interactions. Ecography 42, 1068–1078. doi: 10.1111/ecog.04239
Ward, S. F., and Aukema, B. H. (2019b). Climatic synchrony and increased outbreaks in allopatric populations of an invasive defoliator. Biol. Invasions 21, 685–691. doi: 10.1007/s10530-018-1879-9
Werner, R.A., (1986). The eastern larch beetle in Alaska. Research paper PNW-357. United States Department of Agriculture – Forest Service, Pacific Northwest Research Station, Fairbanks, Alaska.
Keywords: bark beetle, novel host, host screening, range expansion, Larix laricina
Citation: Picklo RM, Eidson E, Steed B and Aukema BH (2024) Eastern larch beetle (Dendroctonus simplex LeConte) as a potential threat to western (Larix occidentalis Nutt.) and subalpine larches (Larix lyalli Parl.). Front. For. Glob. Change. 7:1490888. doi: 10.3389/ffgc.2024.1490888
Edited by:
Bernard Slippers, University of Pretoria, South AfricaReviewed by:
Kevin J. Dodds, Forest Service (USDA), United StatesDiana L. Six, University of Montana, United States
Copyright © 2024 Picklo, Eidson, Steed and Aukema. This is an open-access article distributed under the terms of the Creative Commons Attribution License (CC BY). The use, distribution or reproduction in other forums is permitted, provided the original author(s) and the copyright owner(s) are credited and that the original publication in this journal is cited, in accordance with accepted academic practice. No use, distribution or reproduction is permitted which does not comply with these terms.
*Correspondence: Rose M. Picklo, cGlja2wwMTFAdW1uLmVkdQ==