- Departamento de Biología, Facultad de Ciencias Naturales, Universidad del Rosario, Bogotá, Colombia
The monodominant forests of Polylepis quadrijuga, endemic to the páramos of the eastern cordillera in the Colombian Andes, are among the most threatened in South America due to fragmentation and anthropogenic degradation. Despite their role in regulating water flow and forming biodiverse, endemic biotic communities, there are few studies on their functional responses to stress caused by anthropogenic disturbance and climate change. In this study, we evaluate how six different populations of P. quadrijuga with distinct levels of anthropogenic disturbance (low and high) change 11 foliar, stem, and root functional traits. Also, the physicochemical properties of the soils were analyzed, and mycorrhizal colonization was quantified to evaluate how each population responds to the stress conditions. The results indicated that populations with lower disturbance levels exhibit a conservative leaf trait configuration, whereas those with higher disturbance levels adopt an acquisitive strategy, potentially making them more vulnerable. Additionally, we found no functional coordination between above- and belowground traits. The results also highlight a lower percentage of arbuscular mycorrhizarl fungi (AMF) colonization in sites with a high level of disturbance. Our findings show P. quadrijuga’s sensitivity to anthropogenic disturbance and its resilience, demonstrated by high plasticity in aboveground traits. Conservation efforts for this endangered species should focus on fragmented populations and those under stress from grazing or agriculture, aiming to create connectivity and promote its establishment
1 Introduction
The mountainous region of the Andes is a center of biodiversity and endemism (Myers et al., 2000). In particular, the high Andean forests and páramos contain a high number of plant species in a small area, displaying unique adaptations to extreme environmental conditions (Valencia et al., 2020; Caballero-Villalobos et al., 2021). However, the plants inhabiting these ecosystems are highly threatened by changes in hydrological cycles, temperature increases, and habitat loss, due to anthropogenic activities such as land use change and expansive agriculture (Espitia Villarraga, 2018; Caballero-Villalobos et al., 2021). These activities have also been magnified worldwide by the effects of climate change (Toivonen et al., 2011; Montalvo et al., 2018; Chacón-Moreno et al., 2021; Singh et al., 2021).
Polylepis quadrijuga Bitter is a native tree of the Eastern Cordillera of Colombia and is cataloged as critically endangered (Boza Espinoza, 2023). These plants grow above the treeline (ca. 3,200–3,600 m.a.s.l), below or interspersed with the páramo ecosystem, and despite their limited geographical distribution, they form monodominant but highly diverse forests. It has been documented that these forests contain a variety of endemic and endangered avifauna, such as Diglossa gloriosissima or the yellow-eared parrot (Leptosittaca branickii) (Peña and Rangel, 2007). The trunks of Polylepis harbor numerous species of bryophytes (mosses) and lichens (Pulido Herrera, 2016). Additionally, species of Polylepis provide ecosystem services, acting as carbon sinks, protecting water sources through runoff regulation, reducing soil erosion, and aiding in the retention of nutrients and sediments (Peña and Rangel, 2007; Cuyckens and Renison, 2018; Montalvo et al., 2018; Boza Espinoza and Kessler, 2022).
Despite their importance, Polylepis forests have been exploited for centuries for dendroenergetic purposes (Cuyckens and Renison, 2018; Boza Espinoza and Kessler, 2022). Additionally, the ongoing expansion of agricultural and livestock frontiers has led to the fragmentation and isolation of species such as P. quadrijuga (Ramos et al., 2013; Caballero-Villalobos et al., 2021). These relicts or man-made patches (Toivonen et al., 2011; Rangel and Arellano Peña, 2010) are highly susceptible to climate change due to the stressful conditions caused by high altitudes, low temperatures, and high humidity (Macek et al., 2009; Kessler et al., 2014; Caballero-Villalobos et al., 2021). One approach to understand and identify how different populations of P. quadrijuga respond to the current climatic conditions and anthropogenic disturbance is through the study of functional traits (Violle et al., 2007; Garnier et al., 2015).
Functional traits are morphological, physiological or phenological characteristics that are inherited and that influence fitness (Garnier et al., 2015). Therefore, functional traits help us understand how populations respond to the biotic and abiotic factors of a specific habitat. For instance, it has been found that altitudinal gradients (Rozman et al., 2013; Pandey et al., 2018), fragmentation (Ramos et al., 2013), mining, agricultural and livestock activities (Ding et al., 2012; Soteras et al., 2016; Ji et al., 2022), and the composition and interactions of soil microbiological communities can alter the functional diversity (Soteras et al., 2016; Gazol et al., 2017) and increase the intraspecific variability of species (Aubin et al., 2016; Gazol et al., 2022). Additionally, anthropogenic disturbances, such as land use changes and fragmentation, significantly impact abiotic conditions such as soil nutrients and moisture, temperature, and light levels (Vitousek et al., 1997). These disturbances can favor species or individuals with specific functional traits and reduce trait diversity in a population or community, which can in turn, affect ecosystem functioning and increase competitive exclusion (e.g., Flynn et al., 2011; Laliberté and Tylianakis, 2012; Mouillot et al., 2013).
Previous studies on functional traits in Polylepis species, such as P. tarapacana (distributed in Peru, Chile, Bolivia, and Argentina), which has a near threatened conservation status (Boza Espinoza, 2023), have found a complex interplay between water stress, elevation (Hertel and Wesche, 2008; Macek et al., 2009; Toivonen et al., 2018), nutrient availability (Toivonen et al., 2014) and seasonality (Kessler et al., 2014). These factors have a greater effect on trees than anthropogenic disturbance (e.g., fire, fragmentation and/or agricultural practices; Soteras et al., 2015; Soteras et al., 2016). Polylepis tarapacana is distributed in countries with stronger temperature seasonality and in drier conditions, therefore, we expect a different functional response compared to P. quadrijuga. Additionally, the severe fragmentation experienced by P. quadrijuga populations, will likely cause a different functional response, compared to P. tarapacana. Plants exposed to higher abiotic stress in response to low temperatures and high solar radiation, rather than recent anthropogenic disturbance (Ramos et al., 2013; Weemstra et al., 2016) will show conservative trait values. This would be reflected in higher specific leaf area (SLA) (Weemstra et al., 2016), wood density (WD) (Garnier and Navas, 2012; Reich, 2014), leaf thickness (LT) (Macek et al., 2009) and leaf dry matter content (LDMC) (Garnier and Navas, 2012), and lower values of leaf area (LA) (Ramos et al., 2013; Macek et al., 2009) and branching frequency (BF) (Semchenko et al., 2018). Conversely, plants associated with anthropogenic disturbance will tend to have acquisitive functional trait values, such as high values of specific root length (SRL) (Wright et al., 2004; Weemstra et al., 2016; Bergmann et al., 2020), root tip abundance (RTA) (Hertel and Wesche, 2008), root branching index (RBI) (Pierick et al., 2021) and low values of root diameter (ARD) (Ma et al., 2018; Bergmann et al., 2020) and root tissue density (RTD) (Bergmann et al., 2020) (Supplementary Table S1).
There are also substantial knowledge gaps regarding the belowground symbiosis in the genus Polylepis, such as the association with mycorrhizae, which has only been assessed in P. australis (Menoyo et al., 2007; Menoyo et al., 2009; Soteras et al., 2013; Soteras et al., 2014; Soteras et al., 2015; Soteras et al., 2016). Colonization by symbionts, such as N-fixing bacteria and mycorrhizae are key to enhance the nutrient uptake capacity of plants and is considered as a belowground functional trait (Pérez-Harguindeguy et al., 2013). In P. australis, the arbuscular mycorrhizal fungi (AMF) communities are present under various types of disturbances, such as grazing or fire, and no significant differences in colonization have been found between these disturbances. However, other studies in tropical mountains have shown that anthropogenic disturbance, such as intensive land use (Barnola and Montilla, 1997) and fertilization (Jefwa et al., 2012), significantly reduces fungal communities and colonization (Ji et al., 2022). This could decrease, in turn, the nutrient uptake by plants and affect their growth and survival (e.g., Begum et al., 2019).
Regardless of whether populations experience human disturbance, we expect individuals of P. quadrijuga to exhibit a coordinated functional response (e.g., Messier et al., 2017; Carvalho et al., 2020). This expectation is supported by the fact that traits are interlinked in a plant’s growth, survival and reproduction, and these share similar environmental pressures. Therefore, both sets of traits should respond in unison (Violle et al., 2007; Reich, 2014; Messier et al., 2017). Previous studies at the local scale (Hu et al., 2019) have shown a coordination between leaf and root traits, aligning with the “do it yourself” strategy (Bergmann et al., 2020). These traits have proven to be reliable indicators of plant metabolic activity in response to environmental factors such as temperature in temperate regions (Ding et al., 2024) and the degree of succession (Hu et al., 2019). Generally, traits tend to be more conservative in colder climates and later successional stages.
In this study, we aim to evaluate the intraspecific functional trait variability between different populations of Polylepis quadrijuga (Rosaceae) with two levels of disturbance (measured as the proximity to anthropogenic activity and fragmentation) and conservation in high-Andean forests of the Colombian eastern mountain range. We expect to find (1) a functional conservation strategy for populations with high abiotic stress and low anthropogenic disturbance, and an acquisitive strategy for high anthropogenic disturbance sites. (2) We also hypothesize that AMF colonization will be impacted by anthropogenic disturbance, similar to what has been shown in species from tropical mountains. (3) We anticipate a functional coordination between above- (leaves and stems) and belowground traits (roots) of P. quadrijuga. This knowledge is critical in the establishment of conservation strategies for an endangered species such as P. quadrijuga, taking into account the anthropogenic pressures experienced by different populations.
2 Materials and methods
2.1 Study area
The study was conducted at six páramo sites located in the Eastern Cordillera of the Colombian Andes (Figure 1): Santuario de Fauna y Flora Guanentá alto Río Fonce (SFF), surroundings of the Parque Nacional Natural (PNN) El Cocuy, Laguna de Palchacual and La Rusia Páramo Complex in the Departments of Boyacá and Santander, as well as Sumapaz and Guerrero páramos in the Department of Cundinamarca. At the Cocuy site we collected in two different areas: Parada de Romero and Laguna de San Pablín (less than 10 km away). Collections were carried out during the rainy season between April–May and September–November 2022. Areas with a high density of adult individuals of P. quadrijuga forming closed-canopy forests (control areas) or located along roadsides and pastures (anthropogenic disturbance) were selected for sampling. The sampling was conducted at elevations ranging from 3,400 to 4,000 m.a.s.l. in at least 0.3 ha per site (Table 1).
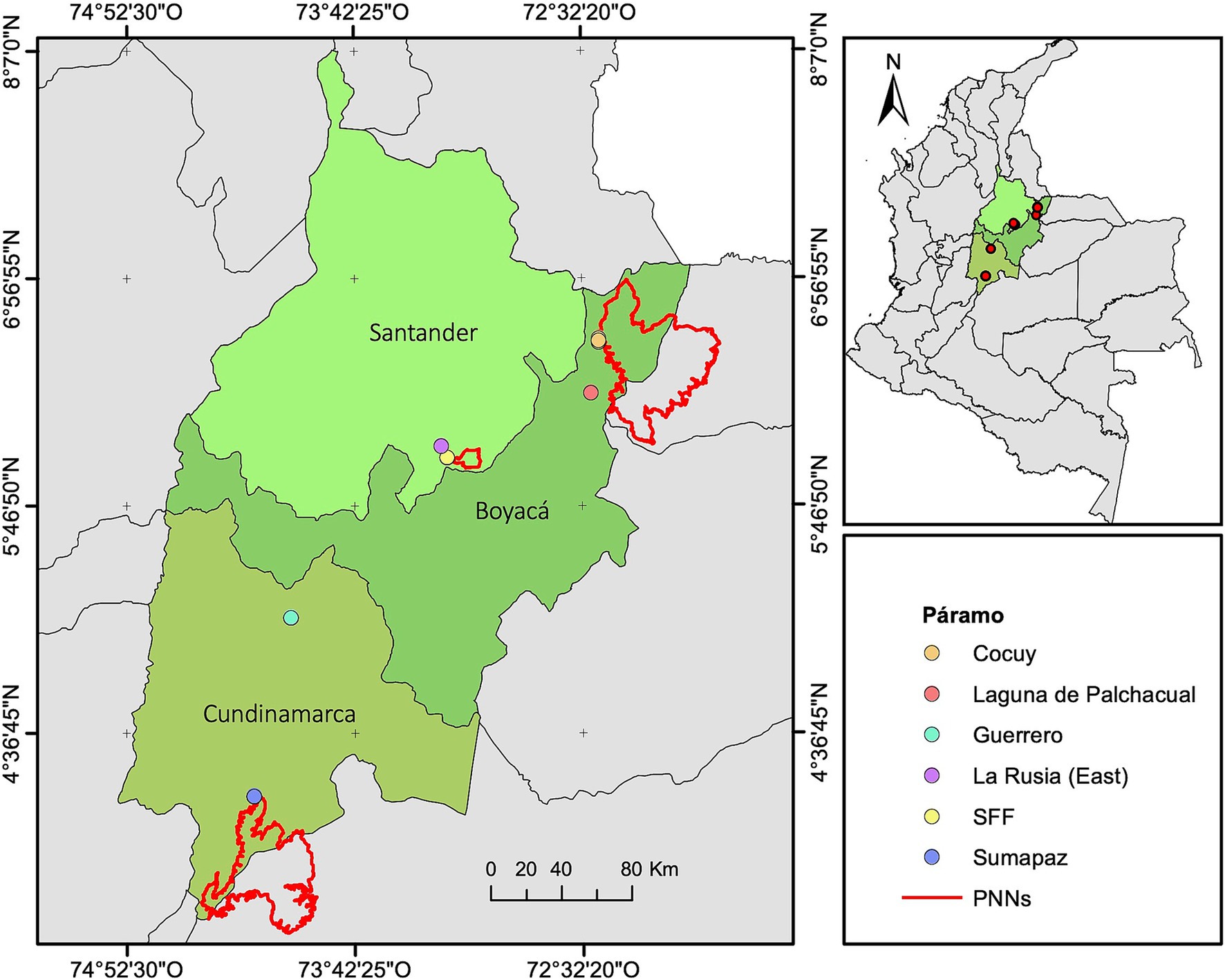
Figure 1. Populations of Polylepis quadrijuga sampled in this study. The symbols and colors represent the location of each páramo site, and the red lines represent the boundary of the protected areas: Parque Nacional Natural (PNN) Sumapaz, PNN Cocuy and Santuario de Fauna y Flora (SFF) Guanentá-Alto Río Fonce. The maps were created based on the Colombian administrative maps obtained from the HUMANITARIAN DATA EXCHANGE (https://data.humdata.org/dataset/cod-ab-col; Creative Commons Attribution 4.0 International license) and from the Parques Nacionales Naturales (PNN) de Colombia website (https://www.parquesnacionales.gov.co/). The PNN shapefiles are licensed under the terms specified at: https://portaldatosabiertos-pnnc.hub.arcgis.com/pages/trminos-y-condiciones.
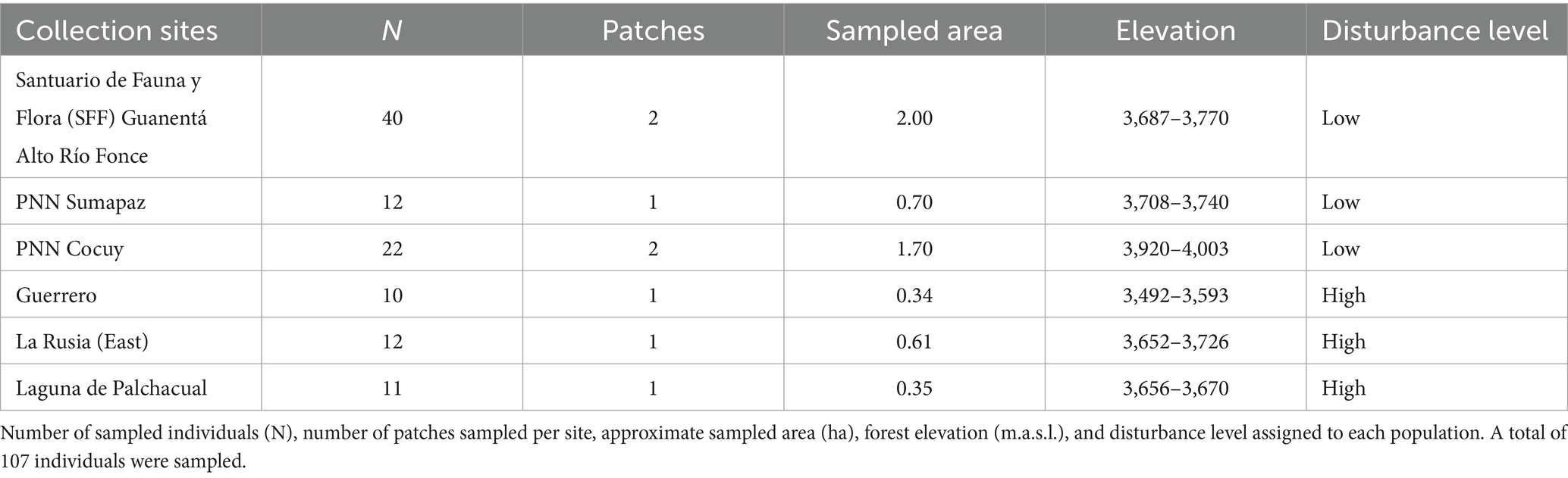
Table 1. Collection sites for the study of intraspecific functional trait variability in Polylepis quadrijuga (Figure 2).
2.2 Description of Polylepis quadrijuga
Polylepis quadrijuga is a tree endemic to the eastern Andean Cordillera of Colombia, found at an elevational range between 2,200 to 4,000 m.a.s.l. It is usually found in fairly humid areas near water bodies (Boza Espinoza and Kessler, 2022). This species can reach ca. 10 m in height, and flowering occurs approximately in August, while fruiting occurs in October (Velez et al., 1998). Polylepis quadrijuga growth rate (like that of other species of the genus) is slow, growing approximately 1 mm per year, and its germination is characterized by a long dormancy and by being thermally dependent (maximum germination at 20°C) (Boza Espinoza and Kessler, 2022).
2.3 Experimental design and data collection
In each páramo site, we randomly selected at least 10 adult individuals (defined by their height and/or the presence of inflorescences) per site (Table 1), and growing more than 2 m away from each other. A level of disturbance (low or high) was also assigned based on the anthropogenic accessibility (Figure 2). Accessibility refers to the distance from the individuals’ growth location to anthropogenic activities such as livestock, agriculture and fertilization, mining, fragmentation by roads, and housing. The surrounding vegetation of the site was also taken into account because monodominant forests did not form on sites with a high level of disturbance. We considered the proximity to the outer limits of National protected areas, such as national parks (PNN), as an additional indicator of disturbance. In this context, sites categorized as having low disturbance levels were located close (< 2 km) to PNN boundaries (PNN Cocuy and Sumapaz) or within a protected area (SFF Guantentá Alto Río Fonce). If a group of individuals was growing at the edge of a road with a matrix of pastures and other trees around them, a “high” level of disturbance was assigned; for example, at the Guerrero site (Table 1 and Figure 2F). Each individual plant sampled was georreferenced.
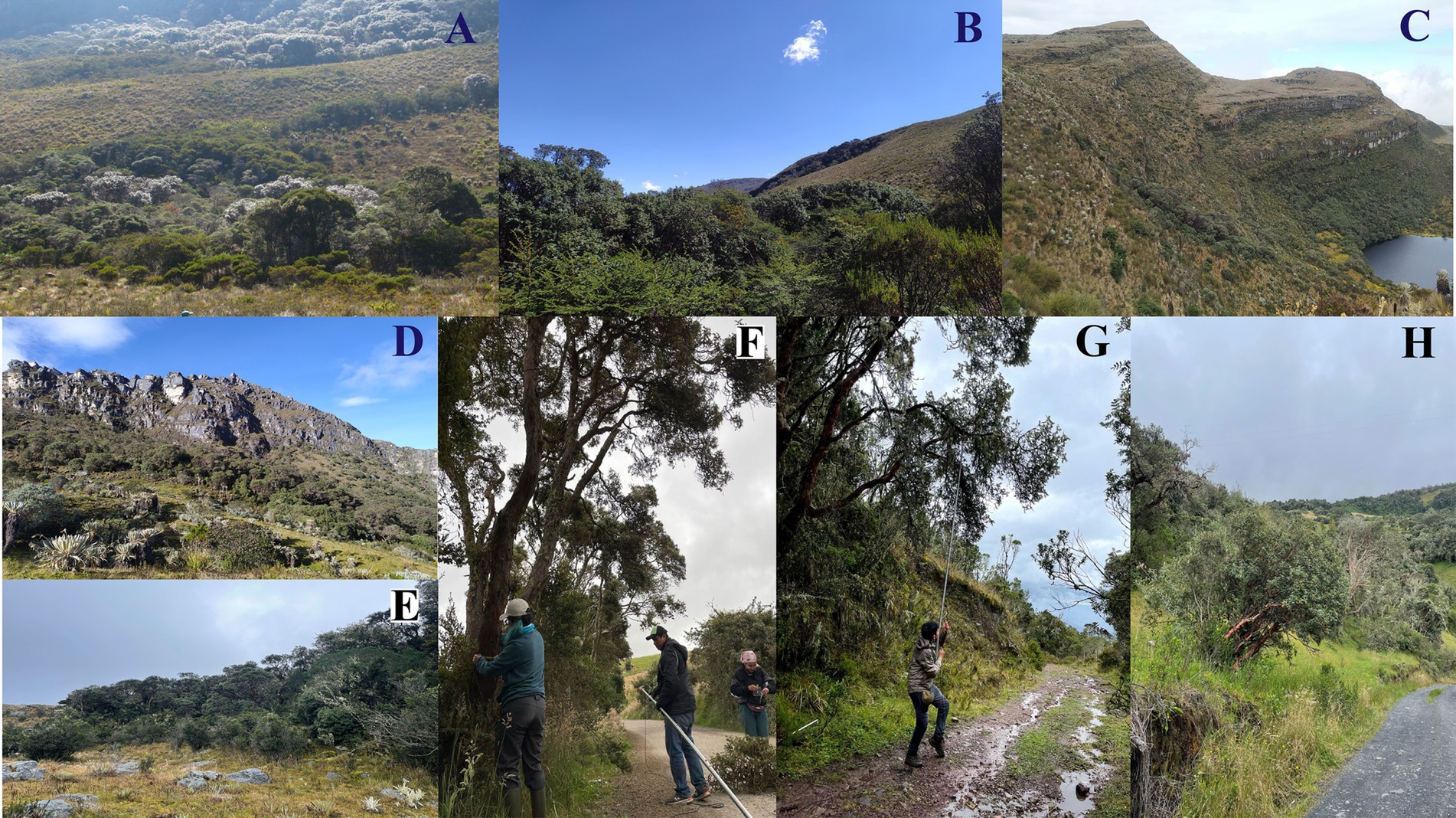
Figure 2. Photos of the different study sites: (A) Forest near Laguna Agua Clara within the Santuario de Fauna y Flora Guanentá Alto Río Fonce; (B) Forest near Laguna de Media Luna within the Santuario de Fauna y Flora Guanentá Alto Río Fonce; (C) Forest located west of Laguna Cajitas in the Sumapaz complex; (D) Forest located near La Parada de Romero in the municipality of Güicán; (E) Patch located near the Laguna San Pablín in the municipality of Güicán; (F) Individuals located along the road in the Guerrero complex; (G) Individuals located along the road on the east side of the La Rusia páramo complex, near the Laguna de Patos; (H) Individuals located along the road near the Laguna Palchacual in the municipality of Cocuy. Sites from (F-H) correspond to high disturbance. Photographs (C, D, F-G) and (H) by Adriana Sanchez; (B, E) by Yessica Hoyos; (A) by Camilo Márquez.
2.4 Sampling and functional traits measurement
Samples of leaves, trunks, and roots were collected, and the diameter at breast height (DBH) was recorded in 107 adult individuals. A total of 11 functional traits related to plant growth and survival (Supplementary Table S1) were measured using standardized protocols (Pérez-Harguindeguy et al., 2013).
Four foliar traits were measured: leaf area (LA, mm2), specific leaf area (SLA, mm2 mg−1), leaf thickness (LT, mm), and leaf dry matter content (LDMC, mg g−1) and the measurements were taken on three healthy, sun-exposed leaves per individual (excluding the petiole). We weighed leaves in the field. To calculate LA, we took photos of the leaves using a tripod and processed them with ImageJ software (Schneider et al., 2012). The SLA value was determined by dividing LA by the dry weight of the leaf. The leaves were dried at 70°C for 48 h and weighed immediately after removing them from the oven. Using a Vernier caliper, we measured LT values in the field. Since P. quadrijuga has compound leaves, three leaflets per leaf were chosen for LT measurements. These leaflets always corresponded to one closest to the petiole, one from the middle, and the tip leaflet. We always measured LT in the middle of each leaflet. For LDMC, the dry weight value was calculated based on the fresh weight measured in the field. In the stem, we measured wood density (WD, mg mm−3) and was calculated based on three stem pieces per individual. These were dried at 70°C for 96 h to weigh and calculate WD as the ratio of their dry weight to volume.
For the six root traits average root diameter (ARD, mm), specific root length (SRL, m g−1), root tip abundance (RTA, n mg−1), root tissue density (RTD, g mm−3), branching frequency (BF, mm) and root branching index (RBI, n cm−1), we sampled fine roots (10–25 cm depth) per individual and stored them in Ziplock bags. Subsequently, we scanned the samples with an Epson Perfection V19 scanner at a resolution of 300 DPI and dried them in an oven at 70°C for 48 h. The images were analyzed using RhizoVision Explorer V2.0.3 (Seethepalli et al., 2020), where each sample was divided into three categories based on its diameter (0–2 mm, 2–5 mm, and > 5 mm). In this study, we only used fine roots (0–2 mm) to estimate functional traits. Following this criterion, we eliminated five individuals with roots >2 mm. Root traits were therefore analyzed in 102 individuals. Measurements of root length (RL), fine root volume (RV), the number of tips (NT), BF, and ARD were taken based on the software. SRL was calculated as the ratio of RL to its dry weight; RTA as the ratio of NT to its dry weight; RTD as the ratio of dry weight to RV, and RBI as the ratio of NT to RL.
2.5 Arbuscular mycorrhizal colonization (AMF)
Colonization by bacteria and/or fungi has also been considered as a functional trait (“Nutrient uptake strategy” in Pérez-Harguindeguy et al., 2013). Given that disturbed areas could impact the colonization by symbionts (Barnola and Montilla, 1997; Jefwa et al., 2012; Ji et al., 2022), and that the symbiosis with P. quadrijuga had not been described, we explored the fungal colonization and included an estimate of fungal colonization. We used two fine-root samples per individual (10 per each páramo site), removed soil and organic particles from the roots collected for colonization, stored them in alcohol (90%) and later cleared and stained for observation (Grace and Stribley, 1991). We cleared the roots with 10% KOH (20 min at 90°C) and rinsed the sample three times with water. We bleached the roots with 3% H2O2 (15 min at 90°C) and rinsed the sample twice with water. Then, we acidified them with 1% HCl (5 min at room temperature) and stained them with 0.05% black ink (5% acetic acid +0.05% black ink). Finally, we rinsed three times with water and stored them with a destain solution (50% glycerol +45% water +5% HCl). We mounted each sample for examination under a LEICA-DM750 4-100x microscope. We employed the grid line intersection method (Giovannetti and Mosse, 1980) to record the presence of arbuscules, vesicles, and hyphae as well as the absence of mycorrhizal structures at a magnification of x40 and a 6 × 5 grid line. For estimating colonization percentage (Col %), we calculated the total number of infected roots divided into the total number of roots intersecting grid lines.
2.6 Soil analyses
We collected soil samples near each individual plant to create a composite soil sample (~1,000 g) for each páramo site (two for SFF and Cocuy, one for the other sampling sites; Table 1). The soils were air-dried for a week and then sieved. The physicochemical analysis was conducted by Agrilab Environmental and Agricultural Services (Bogotá, Colombia) for the following variables: pH and electrical conductivity (EC, dS m−1) were measured using the conductimetry technique, with a saturation paste as the extractant; oxidizable organic carbon (COOX, %) was obtained through a colorimetric analysis using potassium dichromate as the extractant; average humidity saturation (Humidity, %) was measured using a gravimetric analysis with a saturation paste as the extractant; effective cation exchange capacity (ECEC, meq/100 g), organic matter (OM, %), total nitrogen (N, %), and apparent density (DA, g cm−3) were calculated; potassium (K, mg kg−1), calcium (Ca, mg kg−1), magnesium (Mg, mg kg−1), and sodium (Na, mg kg−1) were obtained using inductively coupled plasma optical emission spectrometry (ICP-OES) with ammonium acetate as the extractant; phosphorus (P, mg kg−1) was also obtained through a colorimetric technique using Bray II solution as the extractant; sulfur (S, mg kg−1) was measured using turbidimetry, with monobasic calcium phosphate as the extractant; and finally, exchangeable acidity (EA) was determined using volumetric analysis with 1 N potassium chloride as the extractant.
2.7 Statistical analysis
In order to have a general climatic characterization of the sites and compare between disturbance levels, we used WorldClim1 with a spatial resolution of 30 s (~1 km2). We included seven environmental variables that describe the conditions of each site: precipitation seasonality (Pr_season), temperature seasonality (TM_season), wet season precipitation (Pr_Wet), dry season precipitation (Pr_Dry), maximum temperature in the hottest month (MaxTM_Hot), minimum temperature in the coldest month (MinTM_Cold), and annual mean precipitation (Annual_Pr). A Principal Component Analysis (PCA) was performed using the FactoMineR v1.34 package (Husson et al., 2008) and factoextra v1.0.7 package (Kassambara and Mundt, 2020) in R v4.2.0 (R Core Team, 2020). In each of the páramo sites we sampled multiple individuals, and in some cases more than one patch (Table 1). These individuals were all georreferenced.
Additionally, we performed two additional PCAs to observe trends and patterns for each disturbance level in: (1) Aboveground functional traits and (2) belowground functional traits. We also compared the physicochemical soil properties between highly and less disturbed sites using a pairwise test (Student’s t-test or U Mann–Whitney), depending on normality and homoscedasticity.
For each functional trait, we checked for normality and homoscedasticity. For RTA, we normalized values using the logarithmic function to conduct parametric comparison tests (Student’s t-test) between disturbance levels (high and low). A Wilcoxon test was conducted when the transformation did not meet the assumptions of normality and/or homoscedasticity. We also performed a logistic model to evaluate which functional traits were significantly affected by the level of disturbance: 1 = low level of disturbance and 0 = high level of disturbance (R Core Team, 2020). Then, we used the function “stepAIC” from the MASS v7.3–58.1 package (Venables and Ripley, 2002) to perform a stepwise model selection by Akaike Information Criterion (AIC). The best model with the lowest AIC (56.37) (Equation 1) was:
Where p is the probability that the dependent variable “Level of Disturbance” is equal to 1, βX are the coefficients of the model which are multiplied by each of the independent variables that contributed significantly to the model.
3 Results
3.1 Functional variability between levels of disturbance
The principal component analysis (PCA) showed climatic differences between the páramo sites, but not between disturbance levels (Supplementary Figure S1). We also found that P. quadrijuga exhibited high intraspecific functional trait variability. The clustering pattern of aboveground functional traits, which explained 70.1% of the variability (44.9 and 25.2% for each axis, respectively), showed a different response depending on the disturbance level (Figure 3A). In contrast, we found that the clustering pattern of belowground functional traits was similar between different disturbance levels (Figure 3B). This PCA explained 65.8% of the variation (43.2 and 22.6%, respectively).
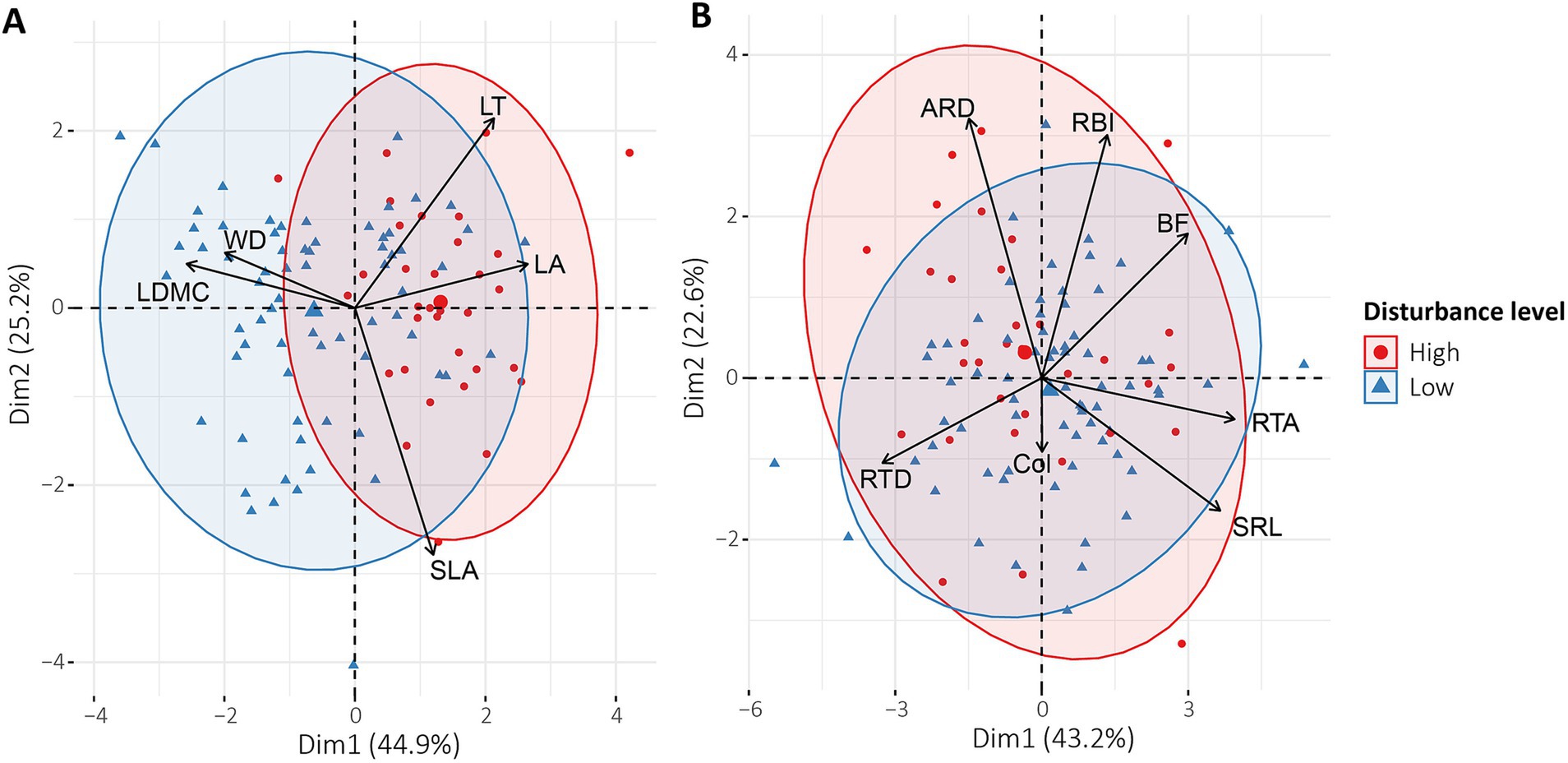
Figure 3. PCAs showing the relationships between functional traits of Polylepis quadrijuga (A) Aboveground functional traits from two disturbance levels: leaf area (LA, mm2), specific leaf area (SLA, mm2 g−1), leaf thickness (LT, mm), leaf dry matter content (LDMC, mg g−1) and wood density (WD, mg mm−3). (B) Belowground functional traits from two levels of disturbance: average root diameter (ARD, mm), specific root length (SRL, m g−1), root tip abundance (RTA, n mg−1), root tissue density (RTD, g mm−3), branching frequency (BF, mm), root branching index (RBI, n cm−1) and colonization percentage (Col %).
Populations with high disturbance levels had significantly higher values of LT, LA, and SLA and populations with low disturbance levels had high WD and LDMC values (Figure 4; Supplementary Table S2; p < 0.05). Although belowground traits showed high overlap in the PCA, there were significant differences in ARD, BF, and Col % between disturbance levels (Figure 4; Supplementary Table S2; p < 0.05). Populations with lower disturbance had lower ARD values but higher BF compared to high disturbance sites (Figure 4). The other functional traits evaluated had no significant differences (SRL, RTA, RTD, RBI; p > 0.05).
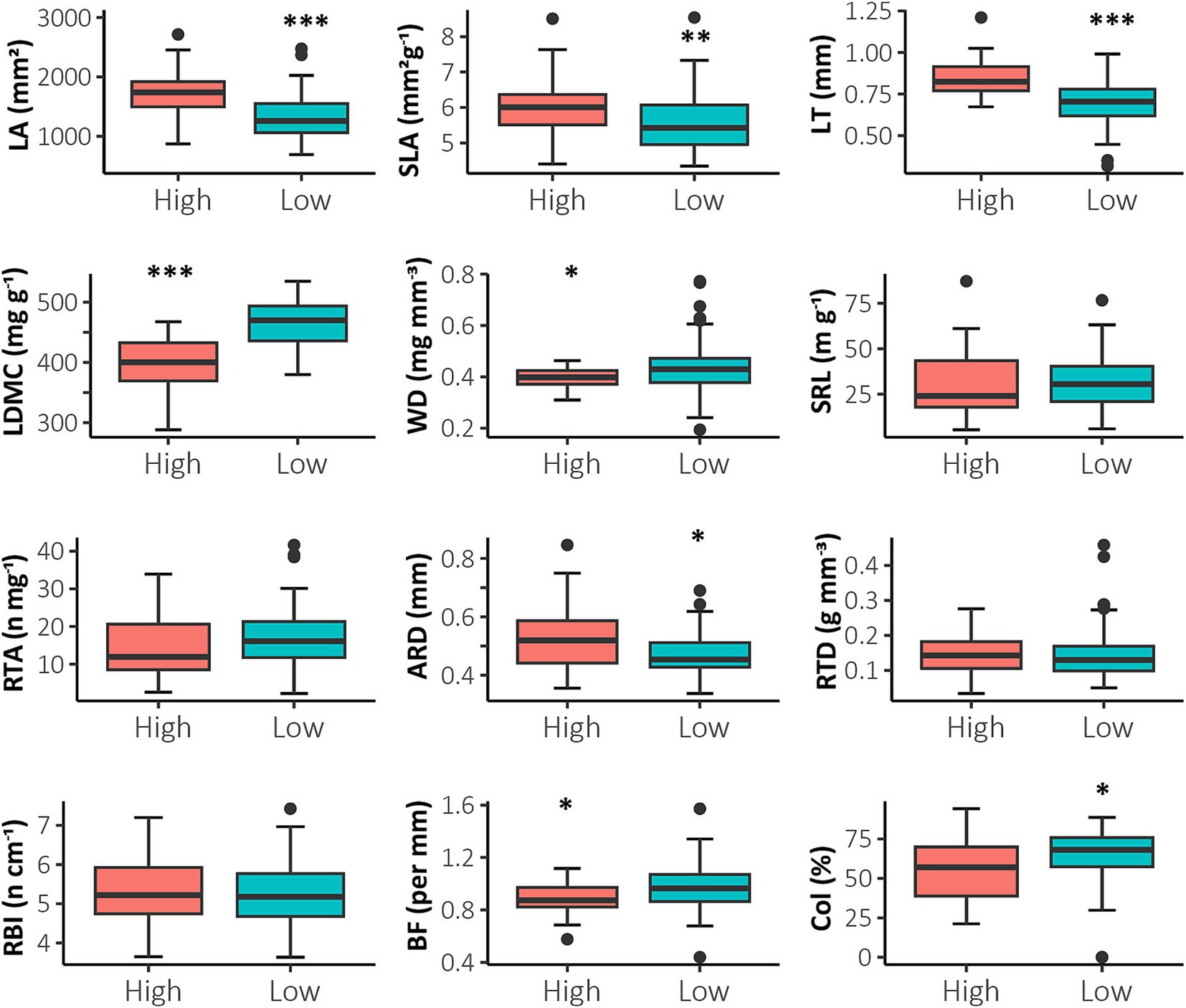
Figure 4. Comparison of Polylepis quadrijuga functional traits between the different disturbance levels (High, Low) using boxplots. The median value of each trait and its corresponding patch is shown (box: standard error; whisker: standard deviation): leaf area (LA, mm2), specific leaf area (SLA, mm2 g−1), leaf thickness (LT, mm), leaf dry matter content (LDMC, mg g−1), wood density (WD, mg mm−3), average root diameter (ARD, mm), specific root length (SRL, m g−1), root tip abundance (RTA, n mg−1), root tissue density (RTD, g mm−3), branching frequency (BF, mm), root branching index (RBI, n cm−1) and colonization percentage (Col, %). Significant differences between the two different disturbance levels are indicated as follows: p < 0.001: ***; p < 0.01: **; p < 0.05: *.
AMF colonization (Col %) was significantly higher in lower disturbance (mean = 65.27, p = 0.026; Figure 4) and lower in higher disturbance sites (mean = 56.10; Figure 4). Also, we found a vesicle percentage of 13.11%, arbuscles of 0.14%, and hyphae of 42.50% for high-disturbance sites. For low disturbance sites we found a vesicle percentage of 15.64%, arbuscles of 0.38%, and hyphae of 46.36%.
We also found that the functional traits that significantly influence the differentiation between high and low disturbance sites in the logarithmic model were: LA, LDMC, SRL, RTD, ARD, BF, Col and LT. Although LT did not have a significant influence, the best model included it as a variable (Supplementary Table S3). In summary, we found that functional traits such as lower LA, SLA, LT, and ARD were associated with sites experiencing low disturbance levels and reflect a conservative strategy. In contrast, sites with high disturbance level showed functional traits such as lower LDCM, WD, BF, and colonization percentage (Col), which indicates an acquisitive strategy.
3.2 Physicochemical properties of soils with high and low levels of disturbance
We found a significant difference in three of the fifteen physicochemical properties analyzed between sites with high and low levels of disturbance (Supplementary Table S4). Soils from high disturbance sites were characterized by being less acidic (mean = 4.52, t = 2.302, p = 0.06) than low disturbance (mean = 4.08). In contrast, sites with lower disturbance levels had higher EC (t = −3.078, p = 0.021), and higher humidity (77.1 vs. 48.5 in high disturbance) (t = −3.167, p = 0.019).
4 Discussion
Our analyses show that Polylepis quadrijuga responds to different environmental changes caused by anthropogenic disturbance. According to our first hypothesis, we expected that individuals under high disturbance (road fragmentation, near crops and livestock) would have trait values reflecting an acquisitive strategy. Based on our results, populations exposed to a high disturbance level mainly differed in aboveground functional traits and had higher values of leaf area (LA) and specific leaf area (SLA), and lower values of leaf dry matter content (LDMC) and wood density (WD), compared to populations with a low disturbance level. These traits are characteristic of an acquisitive functional strategy (Wright et al., 2004; Reich, 2014), which is in line with our hypothesis. In the second hypothesis, we expected less AMF colonization in highly disturbed sites in response to land use change, which our results corroborated (Figure 4). For the last hypothesis, we had proposed that there would be coordination between above- and belowground traits. We found that these traits are not coordinated, and root traits are more similar between disturbance levels (Figure 3).
Climate was similar for páramo sites that were located nearby (Supplementary Figure S1). Therefore, locations such as SFF-La Rusia (East) and Sumapaz-Guerrero only differed in their levels of disturbance (low and high, respectively), yet displayed varying responses in both above- and belowground functional traits. This response aligns with findings from previous studies in Polylepis (Toivonen et al., 2014), indicating that functional traits not only respond to shifts in climate but also to the specific requirements of each species (Martínez-Vilalta et al., 2010). In this case, to stress conditions that may be caused by anthropogenic activities. Likewise, increased disturbance levels in tropical mountain ecosystems (e.g., shifting cultivation and logging) intensify the impact of abiotic filters, leading to the emergence of species with traits tailored to such disturbances (Ding et al., 2012; Feng et al., 2014; Singh et al., 2021). In this regard, P. quadrijuga populations exhibit a functional response to the anthropogenic alterations they have undergone in recent decades, including deforestation (Caballero-Villalobos et al., 2021) and fragmentation for agricultural purposes (Ramos et al., 2013; Boza Espinoza and Kessler, 2022). As a result of these anthropogenic activities, most Polylepis forests are currently restricted to areas that are difficult to access (Kessler et al., 2014; Toivonen et al., 2018).
On the one hand, individuals in sites with high disturbance levels may exhibit significantly different functional traits, potentially facilitating rapid growth and reproduction (an acquisitive strategy). This could be attributed to intense trait filtering, which enables them to mitigate the diverse environmental changes induced by the disturbance (Schellenberger Costa et al., 2017). High trait values of LA, SLA and LT had been reported in fragmented populations of P. quadrijuga as a strategy for light harvesting (Velez et al., 1998; Ramos et al., 2013). Individuals exposed to the edge also changed their leaf composition by increasing the lignin content in the parenchyma, antioxidant and flavonoids substance to reduce photo-oxidation damage (Velez et al., 1998; Ramos et al., 2013). Nevertheless, the trend of increasing leaf size with leaf thickness represents a unique functional pattern observed in P. quadrijuga. In other Polylepis species, an increase in leaf size is typically accompanied by a decrease in leaf thickness (Macek et al., 2009; Toivonen et al., 2014).
On the other hand, sites with low disturbance have conservative functional traits: plants invest more carbon in leaf and stem tissues, so their growth is slow as a response to the abiotic filters of their environment (Reich, 2014; Firn et al., 2019). Populations of P. quadrijuga in these sites also tend to have thinner leaves (Figure 4: LA). Interestingly, this pattern contrasts with previous findings where low SLA and high LDMC were associated with low water and nutrient availability in P. rugulosa, P. tarapacana and P. tomentella (Macek et al., 2009). However, recent studies have shown that SLA is not a good indicator of the nutrient amount in the soil because it also changes with light intensity (Hodgson et al., 2011; Singh and Negi, 2018; Firn et al., 2019). The observed high LDMC values in this study may represent a response to environmental conditions that could potentially cause damage, such as wind, sun, or hail (Pérez-Harguindeguy et al., 2013). Structural responses to environmental harshness such as low temperatures, rather than by anthropogenic disturbance, have also been observed in P. subsericans (Toivonen et al., 2018), P. racemosa, P. pauta, P. sericea, P. pepei (Kessler et al., 2014), P. tomentella, P. rugulosa and P. tarapacana (Macek et al., 2009).
The lack of coordination between the leaf economic spectrum (LES) and root economic spectrum (RES) observed in our study could be attributed to root traits responding multidimensionally to biotic and abiotic factors (Weemstra et al., 2016). Interestingly, sites with low disturbance levels have more compact soils (high apparent density) and display roots with lower root diameter (ARD) and higher branching frequency (BF) values (Ma et al., 2018; Semchenko et al., 2018). Such traits are typically suited for soils that are easy to explore, which is not ideal for compact soils (Weemstra et al., 2016; Bergmann et al., 2020). Consequently, the roots of these populations may rely on the colonization of arbuscular mycorrhizae (AMF) to acquire the necessary nutrients (Barnola and Montilla, 1997). Therefore, root responses may not align as clearly with the LES gradient. Additionally, of the seven belowground functional traits evaluated, only three showed significant differences between high and low disturbance sites. This finding is consistent with other studies on Polylepis, which have noted that belowground traits do not tend to vary as much as the aboveground and do not clearly align with a RES strategy (Hertel and Wesche, 2008; Toivonen et al., 2014; Valverde-Barrantes, et al., 2016).
Previous findings have shown that AMF colonization does not necessarily correlate with an increase in ARD (Maherali, 2014) and, in more acidic soils, AMF plays an important role in nitrogen (N) uptake (Camenzind et al., 2016). Although, in our study there was no evidence of differences in soil N content between sites, there is a greater limitation of N at higher elevations in tropical mountains (Soethe et al., 2008; Fisher et al., 2013). Additionally, we found a lower percentage of AMF colonization in sites with a high level of disturbance. It is possible that the colonization of AMF in P. quadrijuga is less resilient to anthropogenic disturbance compared to P. australis (Soteras et al., 2015; Soteras et al., 2016). One possible reason for this difference could be the specific environmental conditions of tropical mountains where P. quadrijuga is found. Previous studies have shown that AMF colonization in tropical mountains subjected to agrochemicals, tillage, or human management significantly decreases their colonization rate (Barnola and Montilla, 1997; Jefwa et al., 2012).
This is the first study (we know of) exploring the intraspecific functional trait variability of P. quadrijuga under different disturbance levels. Our findings underscore the sensitivity of this species to anthropogenic disturbance, but also its resilience. Polylepis quadrijuga exhibits high plasticity in its aboveground functional traits, enabling it to adjust its ecological performance in response to both abiotic and anthropogenic disturbances, such as land use change and fragmentation. This plasticity may be crucial for resisting stress and invasive species (Ferrero et al., 2022; Kaushik et al., 2022). We recommend conducting more detailed studies on the effects of anthropogenic activities such as cattle ranching, fire, or mining on the functional traits and interactions between foliar and root traits of each Polylepis species. In addition, it is important to study the AMF associated with P. quadrijuga and to evaluate how the identity of the fungi changes with disturbance. This information could be key in restoration efforts of P. quadrijuga’s monodominant forests. Further research is also needed to enhance our understanding of the microclimate in these populations. Studies on P. quadrijuga have used platforms like WorldClim (Fajardo-Gutiérrez et al., 2018; Caballero-Villalobos et al., 2021; Bedoya-Canas et al., 2024), which may not yield precise results for an accurate climatic characterization of these populations. Therefore, it is important to use on-site climatic sensors to understand the microclimatic conditions of these populations. As observed in this and other studies in Polylepis (e.g., Hertel and Wesche, 2008; Macek et al., 2009; Toivonen et al., 2011; Toivonen et al., 2014; Ramos et al., 2013), the functional traits of P. quadrijuga adjust to various biotic and abiotic conditions. It is crucial to determine and implement conservation and restoration strategies for these forests, which provide numerous ecosystem services. Conservation efforts for P. quadrijuga should prioritize fragmented populations or those facing stressful conditions such as livestock grazing or agricultural activities. These strategies should aim to create microhabitats that promote the establishment of this endangered species.
Data availability statement
The datasets presented in this study can be found in online repositories. The names of the repository/repositories and accession number(s) can be found below: https://doi.org/10.5281/zenodo.13377113.
Author contributions
YH: Conceptualization, Data curation, Formal analysis, Investigation, Methodology, Writing – original draft, Writing – review & editing. NV: Methodology, Writing – original draft, Writing – review & editing. AS: Conceptualization, Data curation, Formal analysis, Investigation, Writing – original draft, Writing – review & editing.
Funding
The author(s) declare that financial support was received for the research, authorship, and/or publication of this article. This research was funded by the Universidad del Rosario, through funds awarded by the Research and Innovation Center (Adriana Sanchez) and the Faculty of Natural Sciences.
Acknowledgments
We would like to acknowledge S. Acero, J. Diaz, C. Marquez, A. Moreno, C. Peralta, J.J. Pinzon, J.D. Sanchez, M. Salamanca, V. Vargas, for helping with field data collection and data processing. We are also grateful to the Santuario de Fauna y Flora (SFF) Guanentá Alto Río Fonce (in particular to Fabio Muñoz) for their support during the field work, and to Roberto Ariano and Omar Elicio López for recommending the best places to sample Polylepis around PNN Cocuy. All field trips and collections were under ANLA permits 0530–2014 and 0061–2016, and the research permit # 20222000003193 (SFF). We would also like to thank the reviewers that revised our manuscript. We used ChatGTP (GPT- 4o Mini, version 2024.11, source OpenAI) for grammar checks and for improving readability.
Conflict of interest
The authors declare that the research was conducted in the absence of any commercial or financial relationships that could be construed as a potential conflict of interest.
Publisher’s note
All claims expressed in this article are solely those of the authors and do not necessarily represent those of their affiliated organizations, or those of the publisher, the editors and the reviewers. Any product that may be evaluated in this article, or claim that may be made by its manufacturer, is not guaranteed or endorsed by the publisher.
Supplementary material
The Supplementary material for this article can be found online at: https://www.frontiersin.org/articles/10.3389/ffgc.2024.1488389/full#supplementary-material
Footnotes
1. ^http://www.worldclim.org, accessed in February 2023
References
Aubin, I., Munson, A. D., Cardou, F., Burton, P. J., Isabel, N., Pedlar, J. H., et al. (2016). Traits to stay, traits to move: a review of functional traits to assess sensitivity and adaptive capacity of temperate and boreal trees to climate change. Environ. Rev. 24, 164–186. doi: 10.1139/er-2015-0072
Barnola, L. G., and Montilla, M. G. (1997). Vertical distribution of Mycorrhizal colonization, root hairs, and belowground biomass in three contrasting sites from the tropical High Mountains, Mérida, Venezuela. Arct. Alp. Res. 29, 206–212. doi: 10.2307/1552047
Bedoya-Canas, L. E., López-Hernández, F., and Cortés, A. J. (2024). Climate change responses of high-elevation Polylepis forests. Forests 15:811. doi: 10.3390/f15050811
Begum, N., Qin, C., Ahanger, M. A., Raza, S., Khan, M. I., Ashraf, M., et al. (2019). Role of Arbuscular Mycorrhizal Fungi in plant growth regulation: implications in abiotic stress tolerance. Front. Plant Sci. 10:1068. doi: 10.3389/fpls.2019.01068
Bergmann, J., Weigelt, A., Van Der Plas, F., Laughlin, D. C., Kuyper, T. W., Guerrero-Ramirez, N., et al. (2020). The fungal collaboration gradient dominates the root economics space in plants. Sci. Adv. 6:eaba3756. doi: 10.1126/sciadv.aba3756
Boza Espinoza, T. E. (2023). IUCN red list of threatened species: Polylepis tarapacana. IUCN Red List of Threatened Species. Available at:. (https://www.iucnredlist.org/en).
Boza Espinoza, T. E., and Kessler, M. (2022). A monograph of the genus Polylepis (Rosaceae). Phytokeys 203, 1–274. doi: 10.3897/phytokeys.203.83529
Caballero-Villalobos, L., Fajardo-Gutiérrez, F., Calbi, M., and Silva-Arias, G. A. (2021). Climate change can drive a significant loss of suitable habitat for Polylepis quadrijuga, a Treeline species in the Sky Islands of the northern Andes. Front. Ecol. Evol. 9:1550. doi: 10.3389/fevo.2021.661550
Camenzind, T., Homeier, J., Dietrich, K., Hempel, S., Hertel, D., Krohn, A., et al. (2016). Opposing effects of nitrogen versus phosphorus additions on mycorrhizal fungal abundance along an elevational gradient in tropical montane forests. Soil Biol. Biochem. 94, 37–47. doi: 10.1016/j.soilbio.2015.11.011
Carvalho, B., Bastias, C. C., Escudero, A., Valladares, F., Benavides, R., et al. (2020). Intraspecific perspective of phenotypic coordination of functional traits in scots pine. PLoS One 15:e0228539. doi: 10.1371/journal.pone.0228539
Chacón-Moreno, E., Rodríguez-Morales, M., Paredes, D., Suárez del Moral, P., and Albarrán, A. (2021). Impacts of global change on the spatial dynamics of Treeline in Venezuelan Andes. Front. Ecol. Evol. 9:223. doi: 10.3389/fevo.2021.615223
Cuyckens, G. A. E., and Renison, D. (2018). Ecología y conservación de los bosques montanos de Polylepis: Una introducción al número especial. Ecol. Austral 28, 157–162. doi: 10.25260/EA.18.28.1.1.766
Ding, J., Wang, Q., Ge, W., Liu, Q., Kong, D., and Yin, H. (2024). Coordination of leaf and root economic space in alpine coniferous forests on the Tibetan plateau. Plant Soil 496, 555–568. doi: 10.1007/s11104-023-06381-0
Ding, Y., Zang, R., Letcher, S. G., Liu, S., and He, F. (2012). Disturbance regime changes the trait distribution, phylogenetic structure and community assembly of tropical rain forests. Oikos 121, 1263–1270. doi: 10.1111/j.1600-0706.2011.19992.x
Espitia Villarraga, E. A. (2018). Bosques de Polylepis (Rosaceae): biodiversidad, distribución y vulnerabilidad en la cordillera oriental de Colombia. Bogotá, DC: Universidad de los Andes.
Fajardo-Gutiérrez, F., Infante-Betancour, J., and Cabrera-Amaya, D. M. (2018). Modelización de la distribución potencial del género Polylepis en Colombia y consideraciones para su conservación. Ecol. Austral 28, 202–215. doi: 10.25260/EA.18.28.1.1.585
Feng, G., Svenning, J.-C., Mi, X., Jia, Q., Rao, M., Ren, H., et al. (2014). Anthropogenic disturbance shapes phylogenetic and functional tree community structure in a subtropical forest. For. Ecol. Manag. 313, 188–198. doi: 10.1016/j.foreco.2013.10.047
Ferrero, M. C., Tecco, P. A., and Gurvich, D. E. (2022). Is intraspecific variability an advantage in mountain invasions? Comparing functional trait variation in an invasive and a native woody species along multiple environmental gradients. Biol. Invasions 24, 1393–1412. doi: 10.1007/s10530-021-02722-1
Firn, J., McGree, J. M., Harvey, E., Flores-Moreno, H., Schütz, M., Buckley, Y. M., et al. (2019). Leaf nutrients, not specific leaf area, are consistent indicators of elevated nutrient inputs. Nat. Ecol. Evol. 3, 400–406. doi: 10.1038/s41559-018-0790-1
Fisher, J. B., Malhi, Y., Torres, I. C., Metcalfe, D. B., van de Weg, M. J., Meir, P., et al. (2013). Nutrient limitation in rainforests and cloud forests along a 3,000-m elevation gradient in the Peruvian Andes. Oecologia 172, 889–902. doi: 10.1007/s00442-012-2522-6
Flynn, D. F. B., Mirotchnick, N., Jain, M., Palmer, M. I., and Naeem, S. (2011). Functional and phylogenetic diversity as predictors of biodiversity–ecosystem-function relationships. Ecology 92, 1573–1581. doi: 10.1890/10-1245.1
Garnier, E., and Navas, M.-L. (2012). A trait-based approach to comparative functional plant ecology: concepts, methods and applications for agroecology. A review. Agron. Sustain. Dev. 32, 365–399. doi: 10.1007/s13593-011-0036-y
Garnier, E., Navas, M.-L., and Grigulis, K. (2015). Plant functional diversity: organism traits, community structure, and ecosystem properties. Oxford: Oxford Academic.
Gazol, A., Camarero, J. J., Igual, J. M., González de Andrés, E., Colangelo, M., and Valeriano, C. (2022). Intraspecific trait variation, growth, and altered soil conditions at tree species distribution limits: from the alpine treeline to the rear edge. Agric. For. Meteorol. 315:108811. doi: 10.1016/j.agrformet.2022.108811
Gazol, A., Moiseev, P., and Camarero, J. J. (2017). Changes in plant taxonomic and functional diversity patterns following treeline advances in the south Urals. Plant Ecol. Divers. 10, 283–292. doi: 10.1080/17550874.2017.1400126
Giovannetti, M., and Mosse, B. (1980). An evaluation of techniques for measuring vesicular arbuscular mycorrhizal infection in roots. New Phytol. 84, 489–500. doi: 10.1111/j.1469-8137.1980.tb04556.x
Grace, C., and Stribley, D. P. (1991). A safer procedure for routine staining of vesicular-arbuscular mycorrhizal fungi. Mycol. Res. 95, 1160–1162. doi: 10.1016/S0953-7562(09)80005-1
Hertel, D., and Wesche, K. (2008). Tropical moist Polylepis stands at the treeline in East Bolivia: the effect of elevation on stand microclimate, above- and below-ground structure, and regeneration. Trees 22, 303–315. doi: 10.1007/s00468-007-0185-4
Hodgson, J. G., Montserrat-Martí, G., Charles, M., Jones, G., Wilson, P., Shipley, B., et al. (2011). Is leaf dry matter content a better predictor of soil fertility than specific leaf area? Ann. Bot. 108, 1337–1345. doi: 10.1093/aob/mcr225
Hu, Y., Pan, X., Yang, X., Liu, G., Liu, X., Song, Y., et al. (2019). Is there coordination of leaf and fine root traits at local scales? A test in temperate forest swamps. Ecol. Evol. 9, 8714–8723. doi: 10.1002/ece3.5421
Husson, F., Josse, J., and Lê, S. (2008). FactoMineR: an R package for multivariate analysis. J. Stat. Softw. 25, 1–18. doi: 10.18637/jss.v025.i01
Jefwa, J. M., Okoth, S., Wachira, P., Karanja, N., Kahindi, J., Njuguini, S., et al. (2012). Impact of land use types and farming practices on occurrence of arbuscular mycorrhizal fungi (AMF) Taita-Taveta district in Kenya. Ecosyst. Environ. 157, 32–39. doi: 10.1016/j.agee.2012.04.009
Ji, L., Yang, X., Zhu, C., Ma, L., Chen, Y., Ling, N., et al. (2022). Land-use changes alter the arbuscular mycorrhizal fungal community composition and assembly in the ancient tea forest reserve. Agric. Ecosyst. Environ. 339:108142. doi: 10.1016/j.agee.2022.108142
Kassambara, A., and Mundt, F. (2020). Factoextra: extract and visualize the results of multivariate data analyses.. (https://cran.r-project.org/web/packages/factoextra/index.html).
Kaushik, P., Pati, P. K., Khan, M. L., and Khare, P. K. (2022). Plant functional traits best explain invasive species’ performance within a dynamic ecosystem - a review’. Trees For. People 8:100260. doi: 10.1016/j.tfp.2022.100260
Kessler, M., Toivonen, J. M., Sylvester, S. P., Kluge, J., and Hertel, D. (2014). Elevational patterns of Polylepis tree height (Rosaceae) in the high Andes of Peru: role of human impact and climatic conditions. Front. Plant Sci. 5:194. doi: 10.3389/fpls.2014.00194
Laliberté, E., and Tylianakis, J. M. (2012). Cascading effects of long-term land-use changes on plant traits and ecosystem functioning. Ecology 93, 145–155. doi: 10.1890/11-0338.1
Ma, Z., Guo, D., Xu, X., Lu, M., Bardgett, R. D., Eissenstat, D. M., et al. (2018). Evolutionary history resolves global organization of root functional traits. Nature 555, 94–97. doi: 10.1038/nature25783
Macek, P., Macková, J., and de Bello, F. (2009). Morphological and ecophysiological traits shaping altitudinal distribution of three Polylepis treeline species in the dry tropical Andes. Acta Oecol. 35, 778–785. doi: 10.1016/j.actao.2009.08.013
Maherali, H. (2014). Is there an association between root architecture and mycorrhizal growth response? New Phytol. 204, 192–200. doi: 10.1111/nph.12927
Martínez-Vilalta, J., Mencuccini, M., Vayreda, J., Retana, J., et al. (2010). Interspecific variation in functional traits, not climatic differences among species ranges, determines demographic rates across 44 temperate and Mediterranean tree species. J. Ecol. 98, 1462–1475. doi: 10.1111/j.1365-2745.2010.01718.x
Menoyo, E., Becerra, A. G., and Renison, D. (2007). Mycorrhizal associations in Polylepis woodlands of Central Argentina. Can. J. Bot. 85, 526–531. doi: 10.1139/B07-042
Menoyo, E., Renison, D., and Becerra, A. G. (2009). Arbuscular mycorrhizas and performance of Polylepis australis trees in relation to livestock density. For. Ecol. Manag. 258, 2676–2682. doi: 10.1016/j.foreco.2009.09.031
Messier, J., Lechowicz, M. J., McGill, B. J., Violle, C., and Enquist, B. J. (2017). Interspecific integration of trait dimensions at local scales: the plant phenotype as an integrated network. J. Ecol. 105, 1775–1790. doi: 10.1111/1365-2745.12755
Montalvo, J., Minga, D., Verdugo, A., López, J., Guazhambo, D., Pacheco, D., et al. (2018). Características morfológico-funcionales, diversidad arbórea, tasa de crecimiento y de secuestro de carbono en especies y ecosistemas de Polylepis del sur de Ecuador. Ecol. Austral 28, 249–261. doi: 10.25260/EA.18.28.1.1.557
Mouillot, D., Graham, N. A., Villéger, S., Mason, N. W., and Bellwood, D. R. (2013). A functional approach reveals community responses to disturbances. Trends Ecol. Evol. 28, 167–177. doi: 10.1016/j.tree.2012.10.004
Myers, N., Mittermeier, R. A., Mittermeier, C. G., da Fonseca, G. A. B., and Kent, J. (2000). Biodiversity hotspots for conservation priorities. Nature 403, 853–858. doi: 10.1038/35002501
Pandey, A., Badola, H. K., Rai, S., and Singh, S. P. (2018). Timberline structure and woody taxa regeneration towards treeline along latitudinal gradients in Khangchendzonga National Park, Eastern Himalaya. PLoS One 13:e0207762. doi: 10.1371/journal.pone.0207762
Peña, H. A., and Rangel, J. O. (2007). Los bosques de Polylepis en Colombia: riqueza y amenazas para su conservación. Available at: https://www.researchgate.net/publication/313671233 (Accessed May 5, 2024).
Pérez-Harguindeguy, N., Diaz, S., Garnier, E., Lavorel, S., Poorter, H., Jaureguiberry, P., et al. (2013). New handbook for standardized measurement of plant functional traits worldwide. Aust. J. Bot. 61, 167–234. doi: 10.1071/BT12225
Pierick, K., Leuschner, C., and Homeier, J. (2021). Topography as a factor driving small-scale variation in tree fine root traits and root functional diversity in a species-rich tropical montane forest. New Phytol. 230, 129–138. doi: 10.1111/nph.17136
Pulido Herrera, K. L. (2016). Efecto de borde en la distribución de líquenes y el contenido de clorofilas en fragmentos de Polylepis quadrijuga (Rosaceae) en el páramo de La Rusia (Boyacá-Colombia). Rev. Biol. Trop. 64. doi: 10.15517/rbt.v64i4.22735
R Core Team (2020). R: A language and environment for statistical computing. Vienna, Austria: R foundation for statistical computing.
Ramos, C., Buitrago, S. P., Pulido, K. L., and Vanegas, L. J. (2013). Variabilidad ambiental y respuestas fisiológicas de Polylepis quadrijuga (Rosaceae) en un ambiente fragmentado en el Páramo de la Rusia (Colombia). Rev. Biol. Trop. 61, 351–361. doi: 10.15517/rbt.v61i1.11134
Rangel, J., and Arellano Peña, H. (2010). Bosques de Polylepis: un tipo de vegetación condenado a la extinción. Available at: https://www.researchgate.net/publication/313693515 (Accessed December 10, 2023).
Reich, P. B. (2014). The world-wide “fast–slow” plant economics spectrum: a traits manifesto. J. Ecol. 102, 275–301. doi: 10.1111/1365-2745.12211
Rozman, A., Diaci, J., and Batič, F. (2013). Functional analysis of vegetation on alpine treeline ecotone in the Julian and Kamnik-Savinja Alps in Slovenia. Eur. J. For. Res. 132, 579–591. doi: 10.1007/s10342-013-0691-4
Schellenberger Costa, D., Gerschlauer, F., Pabst, H., Kühnel, A., Huwe, B., Kiese, R., et al. (2017). Community-weighted means and functional dispersion of plant functional traits along environmental gradients on Mount Kilimanjaro. J. Veg. Sci. 28, 684–695. doi: 10.1111/jvs.12542
Schneider, C. A., Rasband, W. S., and Eliceiri, K. W. (2012). NIH image to ImageJ: 25 years of image analysis. Nat. Methods 9, 671–675. doi: 10.1038/nmeth.2089
Seethepalli, A., Guo, H., Liu, X., Griffiths, M., Almtarfi, H., Li, Z., et al. (2020). RhizoVision crown: an integrated hardware and software platform for root crown phenotyping. Plant Phenomics 2020, 1–15. doi: 10.34133/2020/3074916
Semchenko, M., Lepik, A., Abakumova, M., and Zobel, K. (2018). Different sets of belowground traits predict the ability of plant species to suppress and tolerate their competitors. Plant Soil 424, 157–169. doi: 10.1007/s11104-017-3282-1
Singh, S. P., Bhattacharyya, A., Mittal, A., Pandey, A., Tewari, A., Latwal, A., et al. (2021). Indian Himalayan timberline ecotone in response to climate change – initial findings. Curr. Sci. 120:859. doi: 10.18520/cs/v120/i5/859-871
Singh, P., and Negi, G. C. S. (2018). Treeline species phenology: shoot growth, leaf characteristics and nutrient dynamics. Trop. Ecol. 59, 297–311.
Soethe, N., Lehmann, J., and Engels, C. (2008). Nutrient availability at different altitudes in a tropical montane forest in Ecuador. J. Trop. Ecol. 24, 397–406. doi: 10.1017/S026646740800504X
Soteras, F., Coutinho Moreira, B., Grilli, G., Pastor, N., Carneiro Mendes, F., Ruela Mendes, D., et al. (2016). Arbuscular mycorrhizal fungal diversity in rhizosphere spores versus roots of an endangered endemic tree from Argentina: is fungal diversity similar among forest disturbance types? Appl. Soil Ecol. 98, 272–277. doi: 10.1016/j.apsoil.2015.09.003
Soteras, F., Grilli, G., Cofré, M. N., Marro, N., and Becerra, A. (2015). Arbuscular mycorrhizal fungal composition in high montane forests with different disturbance histories in Central Argentina. Appl. Soil Ecol. 85, 30–37. doi: 10.1016/j.apsoil.2014.09.004
Soteras, F., Renison, D., and Becerra, A. G. (2013). Growth response, phosphorus content and root colonization of Polylepis australis bitt. Seedlings inoculated with different soil types. New For. 44, 577–589. doi: 10.1007/s11056-013-9364-x
Soteras, F., Renison, D., and Becerra, A. G. (2014). Restoration of high altitude forests in an area affected by a wildfire: Polylepis australis bitt. Seedlings performance after soil inoculation. Trees 28, 173–182. doi: 10.1007/s00468-013-0940-7
Toivonen, J. M., Gonzales-Inca, C. A., Bader, M. Y., Ruokolainen, K., and Kessler, M. (2018). Elevational shifts in the topographic position of Polylepis Forest stands in the Andes of southern Peru. Forests 9:7. doi: 10.3390/f9010007
Toivonen, J. M., Horna, V., Kessler, M., Ruokolainen, K., and Hertel, D. (2014). Interspecific variation in functional traits in relation to species climatic niche optima in Andean Polylepis (Rosaceae) tree species: evidence for climatic adaptations. Funct. Plant Biol. 41, 301–312. doi: 10.1071/FP13210
Toivonen, J. M., Kessler, M., Ruokolainen, K., and Hertel, D. (2011). Accessibility predicts structural variation of Andean Polylepis forests. Biodivers. Conserv. 20, 1789–1802. doi: 10.1007/s10531-011-0061-9
Valencia, J. B., Mesa, J., León, J., Madriñán, S., and Cortés, A. (2020). Climate vulnerability assessment of the Espeletia Complex on Páramo Sky Islands in the northern Andes. Front. Ecol. Evol. 8:565708. doi: 10.3389/fevo.2020.565708
Valverde-Barrantes, O. J., Horning, A. L., Smemo, K. A., and Blackwood, C. B. (2016). Phylogenetically structured traits in root systems influence arbuscular mycorrhizal colonization in woody angiosperms. Plant Soil 404, 1–12. doi: 10.1007/s11104-016-2820-6
Velez, V., Cavelier, J., and Devia, B. (1998). Ecological traits of the tropical treeline species Polylepis quadrijuga (Rosaceae) in the Andes of Colombia. J. Trop. Ecol. 14, 771–787. doi: 10.1017/S026646749800056X
Violle, C., Navas, M., Vile, D., Kazakou, E., Fortunel, C., Hummel, I., et al. (2007). Let the concept of trait be functional! Oikos 116, 882–892. doi: 10.1111/j.0030-1299.2007.15559.x
Vitousek, P. M., Mooney, H. A., Lubchenco, J., and Melillo, J. M. (1997). Human domination of Earth’s ecosystems. Science 277, 494–499. doi: 10.1126/science.277.5325.494
Weemstra, M., Mommer, L., Visser, E. J. W., van Ruijven, J., Kuyper, T. W., Mohren, G. M. J., et al. (2016). Towards a multidimensional root trait framework: a tree root review. New Phytol. 211, 1159–1169. doi: 10.1111/nph.14003
Keywords: abiotic factors, disturbance, fragmentation, functional coordination, nutrients, treeline
Citation: Hoyos YD, Vargas N and Sanchez A (2024) Different functional responses in populations of Polylepis quadrijuga (Rosaceae) as a consequence of anthropogenic disturbance. Front. For. Glob. Change. 7:1488389. doi: 10.3389/ffgc.2024.1488389
Edited by:
Manfred J. Lexer, University of Natural Resources and Life Sciences Vienna, AustriaReviewed by:
Aseesh Pandey, Govind Ballabh Pant National Institute of Himalayan Environment and Sustainable Development, IndiaMariasole Calbi, University of Florence, Italy
Copyright © 2024 Hoyos, Vargas and Sanchez. This is an open-access article distributed under the terms of the Creative Commons Attribution License (CC BY). The use, distribution or reproduction in other forums is permitted, provided the original author(s) and the copyright owner(s) are credited and that the original publication in this journal is cited, in accordance with accepted academic practice. No use, distribution or reproduction is permitted which does not comply with these terms.
*Correspondence: Yessica D. Hoyos, eWVzc2ljYS5ob3lvc0B1cm9zYXJpby5lZHUuY28=; Adriana Sanchez, YWRyaWFuYS5zYW5jaGV6QHVyb3NhcmlvLmVkdS5jbw==