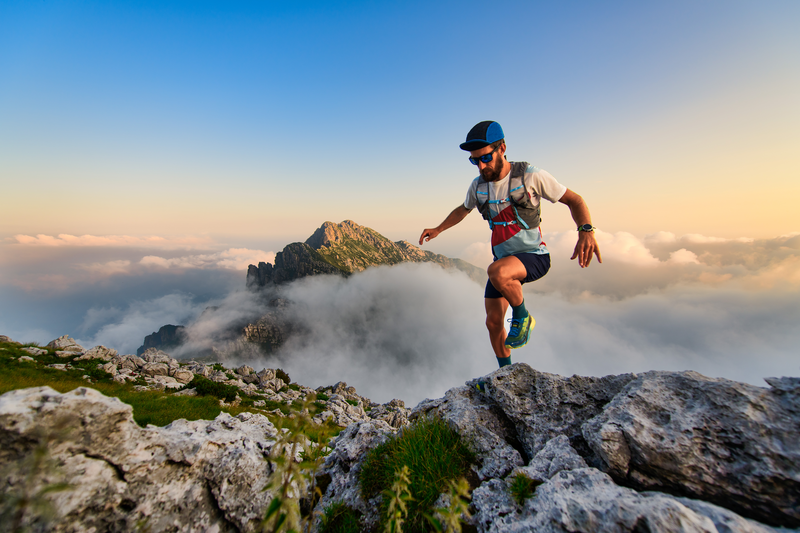
94% of researchers rate our articles as excellent or good
Learn more about the work of our research integrity team to safeguard the quality of each article we publish.
Find out more
ORIGINAL RESEARCH article
Front. For. Glob. Change , 28 January 2025
Sec. Forest Hydrology
Volume 7 - 2024 | https://doi.org/10.3389/ffgc.2024.1457522
This article is part of the Research Topic Community Series in Bark-Water Interactions - Volume II View all 4 articles
The movement of water between xylem and inner bark (phloem and associated tissues), mostly driven by water potential differences, forms a key part of the diel transpiration cycle. It is not known how the use of water stored in bark at the diel transpiration cycle may influence the isotopic composition of xylem water. Understanding these possible effects is a major challenge for the identification of tree water sources and the interpretation of water use patterns using isotopes. Here, we examined the variation in the isotopic composition of water in inner bark and xylem at the diel scale and assessed how this varied in relation to traits and water use strategies on nine tree species in a tropical rainforest at the end of the dry season. We measured δ2H and δ18O in bark and xylem at two shallow depths: ‘outer xylem’ and ‘inner xylem’ (up to ~0.5 cm and ~ 1 cm from inner bark, respectively) collected at predawn, morning and midday. Considering all species together, the average isotopic composition of water in bark and outer xylem was similar at predawn and midday, suggesting water exchange between these tissues was reflected at these times, but differed significantly in the morning during increased transpiration. Results suggest that bark-xylem water exchange throughout the diel transpiration cycle affects the isotopic composition of xylem water in tropical rainforest trees. Furthermore, variations in δ2H and δ18O between xylem and bark were more pronounced in a deep-rooted, more isohydric species with dense wood than in a shallow-rooted, more anisohydric species with low wood density. This may suggest differences related to traits and hydraulic strategies in the reliance of bark-stored water across the diel cycle to buffer changes in xylem water potential. We discuss implications for interpreting tropical tree water sources in relation to water use strategies.
From which soil depths trees draw water, and how water is stored in woody tissues and used over short-or long-term periods, are fundamental questions for understanding ecophysiological and ecohydrological cycles and constraints. We examined these questions with a focus on the natural abundance of water isotopic composition in mature tropical rainforest trees with contrasting physiologies and water use strategies.
Stem water storage and the exchange of water between xylem and inner bark1 are key for tree hydraulic functioning (Pfautsch et al., 2015a; Steppe et al., 2012), contributing to daily transpiration even during abundant water availability conditions (Pfautsch et al., 2015a; Zweifel et al., 2001). A variety of water pools along the stem, roots, branches or leaves serve as water storage (Gaines et al., 2016), acting as capacitors from which water can flow into the transpiration stream to buffer changes in xylem water potential after rapid increases in transpiration (Gaines et al., 2016; Pfautsch et al., 2015b; Steppe et al., 2012) and drought (Pineda-García et al., 2013). In the stem, generally, capillary storage water (e.g., intercellular spaces and dead xylem cells) is released first, while elastic storage water (e.g., intracellular spaces and in living cells of bark and xylem) is released as potentials become more negative (Jupa et al., 2016; Knipfer et al., 2019; Tyree and Yang, 1990). Furthermore, Knipfer et al. (2019) found that inner bark— the largest water storage site after sapwood (Čermák et al., 2007)— required less negative water potentials than xylem to shrink and thus release stored water into the transpiration stream early during dry conditions.
Pfautsch et al. (2015a) described a “pulse” or cycle of diel variations in water potential between xylem and inner bark, driven by transpiration and environmental conditions, which results in the radial (and bidirectional) transfer of water between these tissues. When transpiration increases in the morning, more water is lost than enters the transpiration stream, causing water to be released from inner bark into xylem as the xylem potential decreases (Pfautsch et al., 2015b). Typically, stored water is used for transpiration in the morning and under high radiation in the early afternoon (Phillips et al., 2003), decreasing stem diameter, and is replenished through the afternoon and night, increasing stem diameter (Goldstein et al., 1998; Phillips et al., 2003; Meinzer et al., 2004) and under low vapour pressure deficit (VPD) (Goldstein et al., 1998), as stomata close and transpiration is reduced (Pfautsch et al., 2015b).
These daily exchanges of water between xylem and bark, inherent to the transpiration cycle, may lead to isotopic variations in xylem water potentially affecting its interpretation. Although stable water isotope (δ2H and δ18O) methods have advanced understanding of water use patterns and sources, plant-in addition to soil-structure and processes may bias the interpretation of water sources (Beyer and Penna, 2021; von Freyberg et al., 2020). The isotopic composition of xylem water may not only reflect the plant’s water sources (Martín-Gómez et al., 2017), resulting in spatial and temporal isotopic heterogeneities in xylem water (von Freyberg et al., 2020). One of these processes is stem water storage, which may influence water residence time in trees from root water uptake (Gaines et al., 2016; James et al., 2003; Meinzer et al., 2006). Even at the diel scale, overnight stagnation may be reflected in the isotopic composition of xylem water at predawn (Martín-Gómez et al., 2017). Recent studies have proposed that isotopic differences between xylem and soil water may be related to phloem-xylem exchange (Barbeta et al., 2019; De Deurwaerder et al., 2020), or mixing with storage water under low transpiration in dry conditions (Barbeta et al., 2020). In an experiment with potted Fagus sylvatica saplings, Barbeta et al. (2020) found that observed isotopic differences between xylem and soil water became smaller as soils reached the permanent wilting point, suggesting greater mixing of xylem water and water stored in non-conductive stem tissues under low transpiration.
While the use of stem storage water is a key part of the diel transpiration cycle (Goldstein et al., 1998; Pfautsch et al., 2015a; Scholz et al., 2007), how the use of water stored in stem tissues and the water exchange between bark and xylem may impact the isotopic composition of xylem water (Berry et al., 2017; Beyer and Penna, 2021; De Deurwaerder et al., 2020; Penna et al., 2018), and how these processes may vary between species or trees with differing hydraulic strategies (Nehemy et al., 2021) is currently not understood. Several studies have, however, used isotopic tracers to examine radial water movement in the stem (e.g., James et al., 2003; Meinzer et al., 2006; Treydte et al., 2021), and others have discussed these effects to explain findings with potted plants (e.g., Barbeta et al., 2020) or single trees (e.g., Nehemy et al., 2021). Knighton et al. (2020) modeled the effect of stem-stored water on the isotopic composition of xylem water in trees and found that including stem storage water yielded closer estimates to the observed xylem water isotopic composition. The authors concluded that considering water storage and mixing in the interpretation of xylem water isotopic composition should improve estimations of the depth of root water uptake. More recently, Nehemy et al. (2021) found that phloem water in a Salix viminalis tree was more depleted than xylem water and hypothesized that, especially during times of water stress, water release from phloem into xylem could potentially lead to more depleted xylem water isotopic composition. In another recent study using isotopic tracer injections in two eucalypt species, Treydte et al. (2021) found differences in tracer presence across the stem that were related to the hydraulic architecture of the species.
Despite these advances, there is currently little understanding of how the use of stored water in bark, in association to the diel transpiration cycle, may influence the isotopic composition of xylem water temporally and spatially. Furthermore, it is unknown to what extent this occurs in natural environments: is it widespread or limited to species sharing certain traits or water use strategies? Here, we assessed the potential effects of these dynamics, and how they may relate to traits and water use strategies, on 19 mature trees of 9 species in a tropical rainforest plot and tested the null hypothesis that all species would show the same pattern. We investigated the isotopic composition of water in the inner bark as an important water storage site, in relation to the isotopic variation of xylem water across key times at the diel transpiration scale. To address how variation in xylem water isotopic composition may be related to the exchange of water between bark and xylem throughout the day, we examined the isotopic composition of water in bark and xylem at two radial depths at predawn, morning and midday. We expected similar isotopic composition between xylem and bark to be reflected at predawn, resulting from the radial transfer of water from xylem to bark throughout the afternoon and night, as the stem refills when transpiration decreases and water potentials between these tissues equilibrate (Pfautsch et al., 2015a) (Figure 1). While the greatest use of storage water should occur in the morning when transpiration and water potential differences between xylem and bark increase (Pfautsch et al., 2015b), if the water pools of these tissues are isotopically similar at predawn, then, the movement of water from bark into xylem at the onset of transpiration should not be initially reflected as isotopic variation. The greatest difference in isotopic composition between xylem and bark was instead expected to occur during the morning, following increased transpiration. At midday, as sap flow rates may decrease due to increased water stress (Goldstein et al., 1998; Martín-Gómez et al., 2017; Pfautsch et al., 2015a), water movement from xylem to bark was expected to result in isotopic similarity again. We thus expected isotopic composition between xylem and bark to be similar at predawn and midday, but not in the morning (Figure 1). To assess how the effects of bark-xylem exchange dynamics may relate to traits and water use strategies, because tree hydraulic strategies are coordinated at the whole tree level (Matheny et al., 2015; Matheny et al., 2017; Mursinna et al., 2018), we compared wood density, tree size, leaf water potential and rooting depth. We also assessed tendencies of isohydricity versus anisohydricity, i.e., tighter versus less regulation of water potentials during drought stress (Martínez-Vilalta and Garcia-Forner, 2016) by assessing the relationship of predawn and midday leaf water potentials of the studied trees (Martínez-Vilalta et al., 2014). We further incorporated data on sap flow and stem diameter variations from a subset of trees of the two main subject species, which previous root water uptake studies found to have contrasting water use strategies (Sohel, 2018). We thus expected these dynamics to vary depending on water use strategies, with more isohydric species potentially relying more on bark water to buffer changes in xylem water potential. Note that for the purpose of this study, we used the concept of water use strategy, or hydraulic strategy, as defined by Matheny et al. (2017), where it is determined by the combination of hydraulic traits at the leaf, stem and root levels. Finally, we discuss implications for the interpretation of xylem water isotopic composition when identifying tree water sources through isotope analyses.
Figure 1. Diagram adapted from Pfautsch et al. (2015a). Increasing transpiration (‘onset’) and decreasing xylem water potential cause water to move from bark into xylem. Through the afternoon and night – here represented under ‘midday’ to match our study’s sampling times – storage tissues are refilled as transpiration decreases and xylem water potential increases, causing water movement from xylem into bark. We hypothesize that that water isotopic composition in xylem and bark becomes similar throughout the afternoon and night and is reflected at predawn. Thus, at the onset of transpiration, water movement from bark into xylem is not reflected in the isotopic composition of xylem water. In contrast, greater isotopic differences between xylem and bark should occur in the morning, after transpiration rates have increased, while at midday, increasing stress causes sap flow to decrease, and water isotopic composition to become more similar again.
The study was conducted in ‘Experiment 78’ (Plot 1), a 200×20 m long-term rainforest plot in the Danbulla South Forest Reserve in the Wet Tropics of North Queensland, Australia, established in 1948 by the Queensland Department of Forestry. Trees were sampled from the NE section of the plot (~80×20 m area). The site has a mean annual rainfall of 1,370–1,650 mm (Wills et al., 2018), with a wet season from December to March (BOM), and an elevation of 680–790 masl. Soil texture is mainly sandy clay loam to clay loam (Sohel et al., 2021).
Sampling was conducted at the end of the dry season, where the trees were expected to be most water stressed due to average conditions of highest annual VPD along with low soil water content. Between October 4–9, 2020, stem samples were collected from 19 trees at predawn (4:00 am), morning (9:00 am) and midday (12:00 pm). Two main species (A. peralatum and D. photiniphylla) – the most common in the plot – had five replicate trees, a third species had three replicates, and six additional trees of different species were sampled (Table 1). Of these trees, three replicates of the two main species were equipped with plant water use monitoring instruments (described in Section 2.5). Stem samples were collected ~10 cm from DBH height (diameter at breast height, 1.3 m) using a cordless drill with a 25 mm Forstner bit and sealed in 12 mL double-wadded Exetainer glass vials (Labco Limited, UK). Outer bark was discarded, and inner bark was collected into a vial. Immediately, drilling was continued to collect new ‘outer xylem’ at a shallow depth (up to ~0.5 cm from inner bark) and ‘inner xylem’ (for an additional ~0.5 cm) into separate vials, wiping drill bit between samples. Note that in the sampled trees, these tissues were clearly distinguishable due to marked contrasts in color and structure. This differentiation, as well as the determination of sampling depths, were based on previous observations via drilling or coring of either the same trees or trees of the same species on the site. In cases where additional material was needed, parallel holes were drilled. In total, collection of stem samples took 2–3 min per tree.
To collect rainwater for isotope analysis, a cylinder rain gauge was installed on site on Oct 3, 2020, and fitted with a plastic table tennis ball to prevent evaporation. Using a hand-held auger, soil samples were collected at 0–5, 5–10, 10–20, 20–40, 40–60, 60–80, 80–100 cm from eight random locations in the NE section of the plot. Between October 4–9, samples were collected throughout the day to obtain the average soil water isotopic composition per depth. From each depth, soil samples were also collected in Ziplock bags for gravimetric water content analysis. On October 12, two undisturbed soil cores were collected down to 4.8 m depth on the edge of the forest near the entrance to the plot with a truck-mounted soil auger. From each core, samples were collected at 100–150, 150–200, 250–300, 350–400, 450–480 cm. On Oct 6, a rainwater sample (2 mL) was collected at 5:30 am, the only rainfall that occurred during the sampling campaign. All samples were collected in Exetainer vials and kept at room temperature before sending for laboratory analysis.
Water was extracted from samples through cryogenic vacuum extraction as per Koeniger et al. (2011). Extractions were conducted at 180°C and ~ 8 mbar, with 15 min runs for soils and 24 min for plant samples. Off-Axis Integrated Cavity Output Spectroscopy (Los Gatos Research OA-ICOS CA, USA) (accuracy of ≤ ±0.5‰ for δ2H and ± 0.2‰ for δ18O) was used to analyze soil water and rainwater. Stem water was analyzed with Isotope Ratio Mass Spectrometry (Elementar Isoprime IRMS, accuracy of ±2.0‰ for δ2H and ± 0.2‰ for δ18O), due to possible interference from co-extracted wood organic compounds (Millar et al., 2018). All analyses were carried out in the McDonnell Hillslope Hydrology Lab at the University of Saskatchewan, Canada (see Supplementary materials). Values are reported as parts per thousand (‰) according to Vienna Standard Mean Ocean Water-Standard Light Antarctic Precipitation (VSMOW-SLAP) scales, with standard δ notation: δ18O = [(18O/16Osample - 18O/16Ostandard) /18O/16Ostandard] x 1,000.
To assess how isotopic effects of bark-xylem exchange dynamics may be related to traits and overall water use strategies, data on plant traits, and from plant water use and environmental monitoring (Section 2.5) was collected. DBH, wood density, predawn and midday leaf water potential were measured per tree. Wood density of individual trees was measured from wood cores collected from shallow xylem (~1 cm from inner bark) near DBH level. Cores were immediately wrapped in parafilm and aluminium foil and kept cool until processing three days later. Fresh volume was measured through the water displacement method. Samples were oven dried at 60°C for 72 h. Wood density was calculated as dry weight over fresh volume. Proportions of soil water uptake depths were estimated from isotopic composition of xylem water samples (as detailed in Section 2.6). Previous studies (Sohel, 2018; Sohel et al., 2021) conducted on the two main species were also used to inform classification into deep or shallow water users.
To measure leaf water potential, a branch was collected from each tree on the same day it was sampled for isotope analyses at pre-dawn (4–5:00 am) and at midday (12:00–1:00 pm). Branches were collected from the shaded, lower part of the crown. Immediately, 3–5 twigs were cut from the branch and sealed in plastic bags with a humid paper towel, covered in aluminium foil and stored in a dark bag. The bags were immediately transported ~200 m in the forest for leaf water potential measurements. Each leaf was cut from the twig before inserting on a portable PMS Model 1,000 pressure chamber and immediately before measurement. Leaf water potential was measured on at least 3 leaves per tree. The average of predawn leaf water potential (predawn ψ) and midday leaf water potential (midday ψ) measurements per tree were taken for analyses.
On the site, six trees of the two main subject species, A. peralatum and D. photiniphylla, were instrumented with one sap flow meter and one dendrometer band each (SFM1 Sap Flow Meter and DBL60 Stand-Alone Logging Dendrometer, ICT International Pty Ltd., Armidale, NSW, Australia). Soil moisture content data (20, 40, 60, 80 and 100 cm) is monitored in two locations in the plot (MP406 ICT International). Rainfall, VPD and solar radiation data were obtained from the Robson Creek OzFlux station (Liddell, 2013), ~7 km from site on the same forest. On October 5, 2020, a temperature and relative humidity logger (Onset HOBO) was installed on the plot.
Outliers and data resulting from instrument problems were removed from the time series before analyses. Sap flow rates and sap velocity were calculated in ICT Sap Flow Tool Software (ICT International Pty Ltd., Armidale, NSW, Australia). Dendrometer data were processed using the R package treenetproc (Haeni et al., 2020; Knüsel et al., 2021), and remaining outliers were removed after visual scanning. All further processing and analyses were conducted in R. As it was the most complete dataset for the study period (September–October), averaged data per hour from 2019 on sap flow, dendrometer, soil moisture, rainfall and VPD was used to assess variations among trees. Due to instrument problems, data from the exact sampling dates in October 2020 was not available, however, data prior to sampling dates, and from previous years for the same periods, was used to provide general diagnostics on overall variations. See Supplementary material for additional details regarding sap flow, dendrometer, soil moisture and environmental data processing and analysis.
Relative sap flow and stem diameter variations (sap flow and stem diameter variations as a percentage of the maximum values per tree observed for the study period) were used to assess responses to VPD among trees. Night and day periods were determined based on solar radiation (W/m2 < or > 1), which resulted the day period being defined as 6 am – 7 pm. The refilling of storage tissues overnight was estimated as done by several studies (e.g., Doronila and Forster, 2013; Fisher et al., 2007; Pfautsch and Adams, 2013; Rosado et al., 2012; Yi et al., 2017). Storage recharge was distinguished from night-time transpiration by regressing and comparing the relationship of night-time sap flow with VPD (Fisher et al., 2007). Refilling of stored water was calculated as the night-time proportion of total sap flow in a 24-h period. The relative contribution of stored water to transpiration was assessed by comparing these proportions among trees (Meinzer et al., 2004; Yi et al., 2017).
To assess the proportions of soil water sources contributing to xylem water of the studied trees, Bayesian mixing models were performed, with both δ2H and δ18O together, using the R package ‘MixSIAR’, which estimates probable distributions for the proportion of sources contributing to a mixture (Stock et al., 2018). Isotopic composition of soil water was compared per depths using Kruskal-Wallis followed by the Dunn test (Benjamini-Hochberg p-value adjustment). Based on statistical differences and visual inspection of dual isotope plot and boxplots, soil depths were grouped into 0–20, 20–100 and 100–400 cm source depths. Source correlation plots showed that sources were mostly well separated, i.e., distinguishable by the model, although the 0–20 and 20–100 cm depths were relatively highly correlated (Supplementary Figure 1). The means of source values were used, and the sample size artificially increased (n = 1,000) in the models, which fixes the source mean at the sample mean (Stock et al., 2018). To estimate water uptake depth proportions per individual tree and species, models were run using samples only from the ‘outer xylem’ depth sampled at 9 am, with “tree” and “species” as factors. Error structure was set to ‘process only’ and models were run at “very long” (chain length 1,000,000, discarding the first 500,000), with discrimination factors set to zero.
R version 4.4.1 (R Core Team, 2024), using packages “ggpubr” (Kassambara, 2023a), “rstatix” (Kassambara, 2023b). The overall variation in δ2H and δ18O in stem tissues (‘bark’, ‘outer xylem’ and ‘inner xylem’) across sampling times was assessed in the dual isotope plots and in boxplots. Because of non-normal distributions and non-linear relationships, Spearman rank correlations were used to assess trait–trait correlations, the correlation of δ2H and δ18O between stem tissues per sampling time, and the correlations of the calculated isotopic differences (Δ2H and Δ18O) between stem tissues (i.e., bark - outer xylem, outer xylem - inner xylem) with individual traits, per sampling time, including the proportions of water use depth estimated through Bayesian mixing models.
The data obtained from the measurement of plant traits, plant water use monitoring, environmental data, and estimates of soil water use proportions (Sections 2.2–2.6) were used to help characterise water use strategies of the studied trees. To evaluate isohydric-anisohydric tendencies, we assessed the relationship between predawn and midday leaf water potentials as done by Martínez-Vilalta et al. (2014). To assess the relationship of isotopic differences across stem tissues with isohydric tendencies, δ2H and δ18O in stem tissues, per sampling time, were plotted against the slope of the linear regression between predawn and midday leaf water ψ (for species with replicates) and with the difference between predawn and midday leaf water ψ (Δψ) per tree. Daily and nightly sap flow and stem diameter variations were regressed against VPD and soil moisture content per depths to assess environmental responses.
To assess the effect of traits on the differences in δ2H and δ18O between stem tissues per sampling time, permutational analysis of variance (PERMANOVA) with the ‘adonis2’ function in the vegan package (Oksanen et al., 2024) was used, due to deviations from normal distributions, non-linearity and non-homogeneity of variance in the relationship of δ2H and δ18O with traits. To achieve this, a dissimilarity matrix was first conducted using the ‘vegdist’ function to obtain pairwise distances of the isotopic composition (δ2H and δ18O) between stem tissues (bark, outer xylem and inner xylem). This distance matrix was used as input data in the PERMANOVA models described below. To restrict permutations within each sampling time, ‘sampling time’ was set as blocking variable across models, reducing the need to account for repeated measures.
First, to assess the differences in δ2H and δ18O between stem tissues (i.e., the pairwise distances) per sampling time, a model was run by including ‘sampling time’ as blocking variable and ‘tree’ and ‘species’ as fixed factors. Then, to consider how the differences in δ2H and δ18O between stem tissues per sampling time may be explained by traits, models were run with ‘sampling time’ as blocking variable and ‘wood density’, ‘Δψ’ and ‘DBH’ as fixed factors. All models were fit “by terms,” where the model considers the additional variation in δ2H and δ18O between stem tissues that is explained by a factor after the variation explained by the preceding factor has been accounted for. The Spearman rank correlations between individual traits and the calculated isotopic differences between stem tissues (Section 2.7) were used to inform the selection and order of traits fit in the models. Finally, for the subset of trees with sensors, the same model was run additionally including ‘mean nightly refill’ as factor. For simplicity, only the results for bark - outer xylem are discussed in the text, while full results are provided in the Supplementary materials.
Homogeneity of variance in δ2H and δ18O between stem tissues per sampling time, between species was determined by the ‘betadisper’ function in the vegan package (similar to Levene’s test). The ‘pairwise.adonis2’ function from the pairwiseAdonis package (Martinez Arbizu, 2017), which was used to test significant differences in δ2H between stem tissues per time of sampling.
The variation in proportions of soil water source use estimated through Bayesian mixing models (MixSIAR) was similarly explained by species (median 2.36) and by individual trees (median 2.77). Considering all sampled trees together, the shallow soil (0–20 cm) source depth contributed the most to xylem water isotopic composition [median 57.1% credible interval (11.2, 91.4)], followed by the mid soil (20–100 cm) source depth [31.5% (0.04, 79.1)], while the deep (100–400 cm) soil depth contributed the least [5.3% (0.00, 50.0)]. However, the large credible intervals implied a great level of overlap, making the estimates highly uncertain, which probably resulted from the high correlations between the 0–20 and 20–100 cm depths (Supplementary Figures 1, 2; Supplementary Table 1).
Among the focus species, D. photiniphylla used the greatest amount of shallow soil water [98.5% (56.4, 100.0)], with negligible use from the middle [0.5% (0, 36.4)] and deep soil water sources [0.3% (0, 11.9)] (Supplementary Figure 2). While also showing mostly shallow soil water use [79.8% (12.7, 99.6)], A. peralatum showed more variation in estimated proportions, with 9.2% (0, 82.2) from the mid soil water source and 2.7% from the deep soil water source (0, 53.8). In contrast, S. claviflorum used 24.5% (0, 95.2) from shallow soil, with most water use from the mid soil source depth [70.1% (3.4, 99.9)] and negligible use from the deep soil water source [0.5% (0, 28.6)]. These overall patterns were reflected with some variation among the trees monitored with sensors (Supplementary Figure 2). The contrasting water use depths between the focus species was also evident in the dual isotope space (Supplementary Figure 3).
Greater variation in sap velocity in trees of A. peralatum was explained by VPD (R2 ranging from 0.71–0.78 in the day and 0.43–0.45 at night), as compared to trees of D. photiniphylla (R2 0.23–0.35 day, R2 0.00–0.22 night). All regressions were significant for A. peralatum, but not for D. photiniphylla at night (Supplementary Table 2). D. photiniphylla had generally higher relative sap velocity at night than did A. peralatum, suggesting the refilling of storage tissues at night. In fact, mean nightly refill of storage water was overall greater in D. photiniphylla than in A. peralatum (Supplementary Table 2), corresponding with the previous study, where D. photiniphylla was found to store more water than A. peralatum (Sohel et al., 2021).
Spearman rank correlations showed that while wood density and DBH were moderately correlated (ρ = 0.46, p < 0.001), they had opposite relationships with Δψ, PDψ and MDψ (Supplementary Table 3). DBH was negatively correlated with MDψ (ρ = −0.31, p < 0.001), and Δψ (ρ = −0.2, p < 0.05), while not being correlated with PDψ (ρ = −0.03, p = 0.7). In contrast, wood density was positively correlated with MDψ (ρ = 0.24, p < 0.01) and PDψ (ρ = 0.26, p < 0.01), and positively, but not significantly, correlated with Δψ (ρ = 0.18, p = 0.05). Mean night storage refill was not correlated to either wood density or DBH but was positively correlated with Δψ and PDψ (ρ = 0.42, p < 0.05 for both), and strongly correlated with MDψ (ρ = 0.96, p < 0.001).
Despite shallow soil water being the most used source overall, it was negatively correlated only with wood density (ρ = −0.26, p = 0.001) (Supplementary Table 3). While all other traits were negatively correlated with deep soil water use, mean nightly storage refill was most strongly negatively correlated with the middle (ρ = −0.94, p < 0.001) and deep soil water use (ρ = −0.72, p < 0.001), suggesting that recharge of storage tissues was greater in trees using greater proportions of shallow soil water.
On average, across species, stem tissues (bark, inner xylem and outer xylem) were most similar at predawn and differed the most in the morning (Figure 2). While δ2H in outer xylem was strongly significantly correlated (p < 0.001) with δ2H in bark across all sampling times, it was most strongly correlated at predawn (ρ = 0.91), followed by midday (ρ = 0.81) and morning (ρ = 0.76) (Supplementary Figure 4). δ18O in outer xylem was also most strongly correlated with δ18O in bark at predawn (ρ = 0.63, p = 0.004), followed by morning (ρ = 0.52, p = 0.03), and not significantly at midday (ρ = 0.35, p = 0.14). δ2H and δ18O between inner and outer xylem were strongly, significantly correlated at all sampling times, most strongly at predawn (ρ = 0.95, p < 0.001 and ρ = 0.96, p < 0.001, respectively), followed by midday (ρ = 0.86, p < 0.001 and ρ = 0.71, p = 0.001, respectively) and morning (ρ = 0.81, p < 0.001 and ρ = 0.73, p = 0.001, respectively).
Figure 2. Boxplots showing the variation in δ2H and δ18O in bark, outer xylem and inner xylem in all trees at predawn, in the morning, and at midday. Pairwise comparisons (‘pairwiseAdonis’) showed that bark and outer xylem significantly differed only in the morning. No other significant differences were found.
Pairwise comparison by ‘pairwiseAdonis’ showed that overall, bark and outer xylem significantly differed in isotopic composition in the morning (p = 0.02), while not differing at midday (p = 0.35) or at predawn (p = 0.30). In contrast, inner xylem did not significantly differ from outer xylem at predawn, in the morning or at midday (p = 0.64, p = 0.33 and p = 0.37, respectively). The ‘betadisper’ function showed that stem tissues did not significantly differ in their variance at predawn, morning or midday (p = 0.68, p = 0.99, p = 0.49, respectively), which implies that PERMANOVA results are due to differences between stem tissues, and not to dispersion within each stem tissue.
For individual traits, Spearman rank correlations showed that, considering all sampled trees, but not sampling time, the calculated isotopic differences (Δ2H and Δ18O) between bark and outer xylem were significantly correlated only with Δψ (ρ = 0.49, p < 0.001 for Δ2H and ρ = 0.53, p < 0.001 for Δ18O), while not being correlated between inner and outer xylem (ρ = −0.05, p = 0.74 for Δ2H and ρ = −0.2, p = 0.17 for Δ18O). No other significant correlations were found when not considering sampling time. However, when separating per sampling time, Δ2H and Δ18O between bark and outer xylem were moderately correlated with Δψ in the morning (ρ = 0.61, p = 0.02 and ρ = 0.60, p = 0.03, respectively) and at midday (ρ = 0.56, p = 0.03 and ρ = 0.58, p = 0.02, respectively), but not at predawn (ρ = 0.26, p = 0.33 and ρ = 0.29, p = 0.27, respectively) (Supplementary Table 4; Figure 3). Δ18O between bark and outer xylem was strongly correlated with MDψ (ρ = 0.74, p < 0.01), while no other significant correlations were found.
Figure 3. Dual isotope plots showing the average δ2H and δ18O per stem tissue across sampling times for A. peralatum, D. photiniphylla and S. claviflorum.
While Δ2H and Δ18O between stem tissues overall were not correlated with wood density, when considering only the trees instrumented with sensors, Δ18O between inner and outer xylem was strongly, negatively correlated with wood density at midday (ρ = −0.89, p = 0.03) and moderately correlated with DBH (ρ = 0.55, p = 0.02) in the morning. Δ2H and Δ18O between bark and outer xylem in the trees instrumented with sensors showed strong but not significant correlations with mean nightly refill at predawn (ρ = 0.90, p = 0.08 for both), morning (ρ = 0.80, p = 0.33 for both) and midday (ρ = 0.70, p = 0.23 for Δ2H and ρ = 0.50, p = 0.45 for Δ18O). Among the monitored trees, Δ2H and Δ18O between sample types tended to increase with Δψ, with A. peralatum, which appeared to be more isohydric, showing smaller Δ2H and Δ18O (Figure 4). In contrast, D. photiniphylla, which generally appeared to be more anisohydric, showed greater Δ2H and Δ18O between stem tissues.
Figure 4. Spearman rank correlation of Δ2H and Δ18O between bark and outer xylem (dark grey) and between inner and outer xylem (light grey) with the Δψ between predawn and midday for all the study trees.
In contrast with the individual trait correlations, the PERMANOVA models to assess the effect of traits on δ2H and δ18O showed that mean nightly storage refill-in the subset of trees instrumented with sensors-explained 43.04% (F = 30.18, p < 0.05) and 31.71% (F = 6.68, p < 0.05) of the variation in δ2H and δ18O, respectively, between bark and outer xylem per sampling time (Supplementary Table 5). This was followed by Δψ (29.67%, F = 20.62, p < 0.05 for δ2H and 27.82%, F = 5.86, p < 0.05 for δ18O), and wood density (16.87%, F = 11.73, p < 0.05 for δ2H and 7.24%, F = 1.52, p > 0.05 for δ18O). However, considering all trees, wood density overall had the greatest effect size and explained most variation in δ2H and δ18O between stem tissues per sampling time. Between bark and outer xylem, most of the variation in δ2H was explained by wood density (22.6%, effect size F = 22.50, p = 0.001) followed by DBH (10.7%, F = 10.64, p < 0.01 for δ2H), and Δψ (5.6%, F = 5.61, p < 0.05), while the interaction of wood density and Δψ explained 21.2% (F = 21.08, p = 0.001) (Supplementary Table 5). For δ18O, most of the variation between bark and outer xylem was explained by wood density (10.90%, F = 6.02, p < 0.05) and the interaction of wood density and Δψ (10.97%, F = 6.06, p < 0.05), while Δψ explained 6.61% (F = 3.66, p < 0.05).
As shown by PERMANOVA, tree explained 83.67% (F = 10.25, p = 0.001) of the difference in δ2H between bark and outer xylem, while the effect of species was negligible (Supplementary Table 5). After removing ‘tree’ from the model, ‘species’, explained 72.06% (F = 14.83, p = 0.001) of the difference in δ2H between bark and outer xylem. Among the focus species, pairwise comparisons showed that Δ2H and Δ18O between stem tissues at each sampling time were significantly different (p = 0.002) between A. peralatum and D. photiniphylla, and between S. claviflorum and D. photiniphylla (p = 0.001), but not between S. claviflorum and A. peralatum (p = 0.65).
Visual assessments in the dual isotope plots showed that species appeared to have more similar isotopic composition between bark and outer xylem in the morning or at midday (Figure 5; Supplementary Figures 5, 6). The dual isotope plots of species-level means for bark, outer xylem and inner xylem per time of sampling (Figure 5), and per tree (Figure 6), suggested different patterns between A. peralatum and D. photiniphylla. In A. peralatum, bark and outer xylem were most similar at midday and at predawn than in the morning, while in contrast, inner xylem did not vary across sampling times. While there was no apparent pattern in D. photiniphylla, bark isotopic composition always remained separate from inner and outer xylem, which may indicate negligible water exchange between xylem and bark in this species. In S. claviflorum, all stem tissues appeared to follow a similar pattern, while outer xylem became more enriched in the morning, probably reflecting sap flow. At midday, all stem tissues in this species appeared to become more similar again.
Figure 5. δ2H and δ18O per stem tissue across sampling times for A. peralatum (top) and D. photiniphylla (bottom). Each panel represents a tree.
Figure 6. Top: Plot of predawn (PDψ) and midday (MDψ) leaf water potential showing a tendency of isohydric versus anisohydric behaviour, although more measurements would be needed to conclude iso- or anisohydricity in the studied trees and species. Dots show the average value of all the sampled leaves per tree, and size indicates each tree’s DBH. Trees above and to the right are consistent with more anisohydric behaviour, while trees below and to the left are consistent with more isohydric behaviour. Bottom: Δ2H and Δ18O tend to increase with the difference between predawn and midday leaf water potential (Δψ), with A. peralatum, more isohydric, showing smaller offsets than D. photiniphylla, more anisohydric, showing greater offsets.
In this study, we examined the variation in the isotopic composition of water in inner bark and xylem across the diel transpiration cycle in trees of nine tropical rainforest species. On average across species, the isotopic composition of water in bark and outer xylem was similar at predawn and at midday, suggesting that water exchange between these tissues occurring overnight and at times of reduced transpiration was reflected as more similar isotopic composition at predawn and midday. In contrast, inner and outer xylem remained similar across all sampling times. However, this general pattern varied in relation to traits and water use strategies. A. peralatum, a high-wood density, deep-rooted, more isohydric species, showed more marked effects of potential bark-xylem water exchange than D. photiniphylla, a low wood density, shallow-rooted, more anisohydric species.
Overall, our findings suggested that in the tropical rainforest species studied, water exchange between bark and outer xylem occurring throughout the afternoon and night may have been reflected in xylem water isotopic composition. Considering all sampled trees together, isotopic composition of water in bark and outer xylem was significantly different only in the morning, under high transpiration, and was most similar at predawn and midday, when water exchange between these tissues was expected to be reflected. Trees showed greater similarities between bark and outer xylem at predawn, midday, or both (Figure 6; Supplementary Figures 5, 6). In contrast, inner and outer xylem remained similar across all sampling times, which would be expected if the isotopic composition in these tissues reflects sap flow. δ2H and δ18O between bark and outer xylem were most strongly correlated at predawn, suggesting that water exchange occurred between these tissues, leading to more similar isotopic compositions being reflected at predawn.
The greater similarities in δ2H and δ18O found between bark and outer xylem at predawn suggest possible water exchange between these tissues, which may have occurred overnight or throughout the previous afternoon. This may have been due to the movement of water from xylem into bark to refill storage tissues, as transpiration decreased throughout the preceding afternoon, or to overnight xylem water stagnation leading to isotopic mixing between xylem and bark storage water (Martín-Gómez et al., 2017). Isotopic composition appeared to return to predawn levels at midday (Figure 6; Supplementary Figures 5, 6), and δ2H and δ18O were similar between bark and outer xylem at predawn and midday but not in the morning, which may suggest water moving from xylem into bark during the afternoon. If this movement or mixing is reflected as similar isotopic compositions at predawn, water transfer from bark into xylem at the onset of transpiration would not be initially reflected as a change in isotopic composition between tissues. Instead, increasing sap flow rates around the morning led to the only significant difference between bark and outer xylem. These results support the occurrence of isotopic exchange between these tissues following changes in xylem water potential linked to the diel transpiration cycle (Pfautsch et al., 2015a), with outer xylem and bark differing only in the morning when transpiration rates were increasing, contrasting with the isotopic similarity between these tissues observed at predawn and midday.
Overall, Δψ appeared to be a key trait in correlation with the calculated isotopic differences (Δ2H and Δ18O) between bark and outer xylem. Greater calculated isotopic differences (Δ2H and Δ18O) between bark and outer xylem were shown by more anisohydric trees, corresponding to higher values of Δψ (Figure 4). Furthermore, Δ2H and Δ18O between bark and outer xylem were significantly correlated in the morning and midday with Δψ and with MDψ, while not being significantly correlated with any other trait (Supplementary Table 4). In contrast, Δ2H and Δ18O between inner and outer xylem were never correlated with Δψ (Figure 3). In the morning, under higher sap flow, Δ18O between inner and outer xylem was instead moderately correlated with tree size (DBH). This suggests that the difference in isotopic composition between inner and outer xylem may have been associated, as may be expected, to sap flow variations, while between bark and outer xylem, the difference may have reflected xylem-bark water exchange due to Δψ.
While wood density is typically negatively correlated with stem water storage and the ability to release it, (Meinzer et al., 2009; Oliva Carrasco et al., 2015; Scholz et al., 2007), we did not find it was correlated with mean nightly storage refill. Despite this, PERMANOVA models showed that wood density and mean nightly storage refill had the largest effect sizes among traits and explained the greatest amount of variation in δ2H and δ18O between stem tissues. Although available only for a subset of trees, mean nightly storage refill explained >40 and > 30% of the variation in δ2H and δ18O between bark and outer xylem per sampling time in the instrumented trees, followed by Δψ (~30% for both δ2H and δ18O) and wood density (>15% for δ2H and > 5% for δ18O). However, considering the whole data set, it was wood density, and the interaction of wood density and Δψ, that best explained the variation in δ2H and δ18O between bark and outer xylem (~20% each). Thus, while wood density individually did not correlate with nightly storage refill or with the isotopic differences between bark and outer xylem, the more holistic PERMANOVA approach showed that along with other traits, wood density contributed substantially to the differences in δ2H and δ18O between bark and outer xylem. Together, these results suggest that it may not be a single trait alone, but a combination of traits, shaping water use strategies, which may have driven water exchanges leading to isotopic differences between bark and outer xylem.
We expected that water transfer from xylem to bark through the afternoon and night would result in similar isotopic composition between these tissues at predawn and that this would be observed in all nine species. On average, this appeared to be a general pattern across all species. However, our results showed variations among species which may ultimately depend on traits and water use strategies. Between the focus species, A. peralatum and D. photiniphylla, the Δ2H and Δ18O between stem tissues per sampling time differed significantly. While D. photiniphylla showed great isotopic differences between bark and outer xylem across all sampling times, indicating little mixing between these tissues, A. peralatum showed larger isotopic differences between bark and xylem in the morning, under high transpiration, and less at predawn and midday, suggesting greater use of bark stored water or greater water movement between these tissues (Figures 5, 6). These differences were probably related to the water use patterns of these species (Sohel, 2018), which appear to be at opposing ends of a water use strategy gradient. Sohel (2018) showed that A. peralatum, a mature succession, slow-growing species, had high wood density, low stem water storage, high sap flow rates and mostly deep soil water use. In contrast, D. photiniphylla, a fast-growing pioneer species, had low wood density, high stem water storage, low sap flow rates and used mostly shallow soil water (Sohel, 2018). Our results also showed that A. peralatum used greater proportions of deep soil water than D. photiniphylla (Supplementary Figures 1, 2; Supplementary Table 1), and that nightly storage refill was greater in D. photiniphylla than in A. peralatum. While in A. peralatum there was a stronger response of sap velocity to VPD, D. photiniphylla had higher sap velocity at night, despite no relationship with nightly VPD, which suggests greater storage water recharge at night. Furthermore, the opposite trends in Δψ (Figure 4) between these species suggested contrasting anisohydric-isohydric behaviors. The contrasting isotopic variations between stem tissues observed in these species may have therefore resulted from opposing water use patterns including differences in storage water use.
Our findings suggest, at least for the species studied, that the isotopic effect of bark-xylem water exchange, as part of the diel transpiration cycle, may be more evident on more isohydric species with high wood density and deep roots, such as A. peralatum. The small Δψ (Figure 4) and greater isotopic similarities shown at predawn (Figure 6) by A. peralatum support findings by Knipfer et al. (2019), who showed that only small water potential differences were required for water to be released from bark. This also suggests that high wood density and more isohydric species (e.g., A. peralatum) may rely more on bark-stored water to buffer changes in water potential, compared to low wood density species (e.g., D. photiniphylla) which may use stored water from sapwood. A reliance on sapwood water storage in D. photiniphylla may be suggested by the generally greater isotopic similarities between inner and outer xylem observed at predawn and the greater differences at midday (with bark always different), contrasting with the similarities between bark and outer xylem observed both at predawn and at midday in A. peralatum and S. claviflorum.
As A. peralatum appeared to be more isohydric (Figure 4), it may tend to close stomata to maintain water potentials under increasing VPD, reducing sap flow. Note, however, that this is an oversimplification of the relationship of stomatal and water potential regulation, which has been demonstrated to be complicated (Martínez-Vilalta et al. 2014). In contrast, it is possible that the isotopic effect of storage refill may be observed later in the day for anisohydric species such as D. photiniphylla than for isohydric species such A. peralatum. As we did not sample later than midday, we were not able to observe this. However, some trees of D. photiniphylla showed more similar isotopic compositions between inner and outer xylem at predawn than in the morning or midday, suggesting that mixing with storage water in sapwood occurred later in the afternoon or throughout the night. It is therefore possible that, at predawn and midday, if greater storage water use from bark is reflected in similar isotopic compositions between bark and outer xylem, reliance on stored water in sapwood may be reflected in greater isotopic similarity between inner and outer xylem (Figure 1).
Although it has been shown that hydraulic traits are correlated from root to leaf level, with sensitivity to drought being related to rooting depth, wood density and isohydricity (Mursinna et al., 2018), how the relationships between these traits may define water use strategies is not well understood (Martínez-Vilalta and Garcia-Forner, 2016). In this regard, our findings add to the current understanding of water use trade-offs between rooting depths, wood density and water storage in the buffering of changes in water potential. It is thought that low wood density species buffer these changes by using stored water (Meinzer et al., 2009; Scholz et al., 2007), while in high wood density species, deep soil water access helps maintain water potentials (Martínez-Vilalta and Garcia-Forner, 2016; Matheny et al., 2017). However, our results suggested that storage water use to buffer changes in water potential at the diel cycle is important regardless of wood density, but the tissue where stored water is drawn from may differ for species depending on wood density, rooting depth and isohydric tendency.
There is little understanding of how much mixing there is between water stored in the stem and more mobile, recently sourced water (Berry et al., 2017; De Deurwaerder et al., 2020; Knighton et al., 2020; Penna et al., 2018). It is not known how stored water at longer, seasonal scales (e.g., Allen et al., 2019), or even at diel scales of quicker turn-over (e.g., De Deurwaerder et al., 2020; Martín-Gómez et al., 2017; Pfautsch et al., 2015a), can proportionally contribute to the isotopic composition of xylem water. The isotopic composition of water stored in the stem may reflect that of soil water before the time of sampling (De Deurwaerder et al., 2020). However, it is possible that isotopic composition does not remain intact as water moves along the stem from the moment of uptake (original isotopic signal from soil) to the storage site, from which it is released back into transpiration. It is further possible that water in the stem has undergone several cycles of storage and release (Meinzer et al., 2006), or of exchange between bark and xylem, re-mixing several times throughout its path along the stem. While the greatest amounts of stored water are used at the onset of transpiration (Goldstein et al., 1998; Pfautsch et al., 2015a), during times of decreases in sap flow rates during the day, additional exchange between bark and xylem may occur. Sap flow, tracking VPD, radiation, and variations in soil water content and potential, may ‘pause’ and ‘resume’ throughout the day, or show sudden variations (Meinzer et al., 2006). It is therefore possible that this causes frequent bark-xylem exchange along the stem, occurring several times in a diel cycle. Furthermore, Treydte et al. (2021) showed that water can move circumferentially around the stem, finding evidence of injected deuterium enriched water around the stem opposite to injection sites, while Fabiani et al. (2022) found that isotopic composition variabilities with sapwood depth and between sapwood and heartwood were related to low sap flow periods. This re-mixing of water with transport along stem may result in isotopic variations, although these may be small and not necessarily be reflected in xylem water. However, especially when sampling twigs, this effect may become more important, leading to larger isotopic differences, due to the greater proportional use of stored water in the upper stem (Čermák et al., 2007) and because greater residence times in tall trees may allow more xylem-phloem mixing to occur (Cernusak et al., 2005). In this regard, although the variations found in our study were relatively small, consequent cycles of storage and release, either in a day or throughout longer periods, will cause deviations from the original isotopic composition from the time of root water uptake.
We found that overall, bark was more depleted than xylem, except in δ18O at predawn (Figure 2), where it was slightly more enriched than both outer and inner xylem. This is contrary to findings by, e.g., Ellsworth and Williams (2007) and Cernusak et al. (2005), where bark was more enriched than xylem water, while coinciding with findings by Nehemy et al. (2021) and Treydte et al. (2021). Our finding supports proposed explanations by Barbeta et al. (2020), who suggested that isotopic fractionation between plant and soil water observed by recent studies (e.g., Barbeta et al., 2019; Brooks et al., 2010; Evaristo et al., 2017; Vargas et al., 2017) may be partly caused by stem storage water being more depleted than xylem vessel water and soil water. The authors suggested that possible of effects of stem water storage on soil-xylem offsets should be therefore be investigated by differentiating between isotopic composition of water in vessels and other stem tissues (Barbeta et al., 2020). While we did not separate water in vessels from bulk stem water in this study, we found water in inner bark, an important storage site from which water is drawn into the transpiration stream throughout the day, to be more depleted than water in xylem. Following this, to improve the understanding of isotopic variations between these tissues, it would be useful to investigate how does inner bark water isotopic composition vary with tree physiology and functional traits across species.
Martín-Gómez et al. (2017) recommended that stem should be sampled at times of highest transpiration in order to avoid stagnation effects from reduced sap flow conditions that occur at predawn and in the afternoon. This is partly supported by our results, which suggested that the exchange between bark and xylem water was reflected in isotopic composition at predawn and midday. However, variation in the dual isotope space appeared generally smaller at predawn than in the morning or midday (Supplementary Figure 7), which may result in smaller isotopic offsets between soil and xylem at predawn. De Deurwaerder et al. (2020) used a model to show that variations in xylem water isotopic composition resulted from root water uptake variations throughout the day, which would additionally pick up heterogeneities in soil isotopic composition. Coinciding with their study, our results suggest larger variation in xylem water isotopic composition in the day compared to predawn. It is therefore possible that variations in xylem water isotopic composition reflected throughout the day may be homogenized throughout the afternoon and night through the radial exchange of water between xylem and bark.
Several different pathways of radial water transfer between xylem and bark have been found, including bulk water movement, as opposed to diffusion, and mainly through a symplastic route through ray parenchyma cells (Pfautsch et al., 2015b), or through an apoplastic route as well as through aquaporins (Steppe et al., 2012). It is possible that transfer through the different pathways may result in variations in isotopic composition, leading to fractionation of xylem water from that of root water uptake. In their study of fractionation during root water uptake in halophytic and xerophytic species, Ellsworth and Williams (2007) found that uptake through a symplastic route led to fractionation of xylem water, while Poca et al. (2019) proposed that aquaporins were responsible for observed fractionation during root water uptake in saplings of Acacia caven, which was enhanced by presence of mycorrhizal fungi. Although this has been discussed in isotope-based plant water use studies in the context of root water uptake, it is possible that similar effects may occur in relation to bark-xylem exchange along the stem. In fact, recently, in their study of the relationship of tree water deficit on water source partitioning, Nehemy et al. (2021) hypothesized that findings of more depleted phloem water with respect to xylem water could have resulted from radial movement of preferentially lighter isotopes through aquaporins from xylem into phloem during storage recharge at night. Meanwhile, Treydte et al. (2021) found spatial variations in the presence of injected isotopic tracer in tree stems which showed differences in radial versus vertical water movement between species that were related to sapwood and hydraulic architecture. Furthermore, both intra and extracellular water from different parts of the stem can be released into the transpiration stream (e.g., De Schepper et al., 2012; Jupa et al., 2016; Knipfer et al., 2019; Tyree and Yang, 1990), while as drought progresses, water may be moved from capillary storage in dead tissues into elastic storage in living cells (Knipfer et al., 2019). All of these dynamics may have different effects on xylem water isotopic composition and implications regarding the ‘mobile’ versus ‘immobile’ or intra-versus extracellular water in xylem sampled by different methods.
In this study, stem tissue samples, as well as sap flow and stem diameter measurements were collected at a single point in the tree stem, which may not provide a full representation of variations along the tree. Although the lower part of the tree stem has the largest stored water content, the use of stored water is proportionally greater in the upper stem (Čermák et al., 2007; Pfautsch et al., 2015a). It is therefore possible that the isotopic differences found among tissues, sampled near the base of the stem at DBH level, may have been greater if sampling further along the tree or in the upper part of the stem. Future studies should consider comparing the stem tissues sampled here across different heights along the tree, e.g., at the base of the stem and near crown level, or across locations spaced vertically over several centimetres, at each sampling time. In this way, variations in the isotopic composition of radial samples could be compared to variations due to water movement up the stem. This could further be improved by using continuous in situ isotopic measurements (e.g., Volkmann et al., 2016; Marshall et al., 2020; Kühnhammer et al., 2022; Kübert et al., 2023). In addition, it would be useful for these more detailed follow-up studies to compare how including samples obtained from bark in the model estimations of soil water uptake depths may impact on the estimates.
In this study we used cryogenic vacuum extraction, which extracts all water in a sample and not just mobile water from the transpiration stream (Millar et al., 2018), and which has been found to produce methods-related effects on the sampled isotopic compositions (e.g., Chen et al., 2020; Haberstroh et al., 2024). Although we cannot distinguish methods-related effects in our study, using both cryogenic extraction and continuous in situ vapour methods, or offset corrections (e.g., Duvert et al., 2024; Sobota et al., 2024; Younger et al., 2024), would help clarify how spatiotemporal isotopic variations across stem tissues, such as the effects of water exchange between bark and xylem, may be differently reflected through the two methods. In addition, since water for transpiration can be drawn from capillary, elastic, intercellular and intracellular storages at different times during the day (e.g., De Schepper et al., 2012; Jupa et al., 2016; Knipfer et al., 2019), it would be useful to further compare the two methods, while targeting specific storage compartments-perhaps assessed via microscopic imaging-paired with stem diameter variations measured via point dendrometers, instead of the band dendrometers used here. This would allow to track isotopic variations in phloem and xylem linked to the exact timings of bark or xylem-related variations in stem diameter throughout the day. If further paired with a model decoupling stem diameter variations between tension-related versus osmotic-related elastic changes in bark water content (such as in Mencuccini et al., 2017), this could provide very useful insight into the extent of impacts on xylem water isotopic composition.
Finally, while current understanding is that the depletion and refilling of bark tissues is the main driver of changes in stem diameter (e.g., Dietrich et al., 2018; Pfautsch et al., 2015b; Sevanto et al., 2011; Steppe et al., 2015; Zweifel et al., 2001), the use of band dendrometers in our study did not allow to distinguish between bark and xylem-related diameter changes. The patterns observed could reflect expansion or contraction of either bark or xylem (Treydte et al., 2021). Regardless, these variations are principally a result of the radial movement of water between the tissues (Sevanto et al., 2011; Zweifel et al., 2014).
Our findings suggest that exchange of stored water between inner bark and xylem, occurring throughout the afternoon and night and in association to the diel transpiration cycle may affect the isotopic composition of xylem water in tropical rainforest species, with the greatest similarity between these tissues observed at predawn. Although this varied among trees, species, and traits, we found that on average, the isotopic composition of water in inner bark and shallow outer xylem was similar at predawn and midday and significantly differed only in the morning, under high transpiration. In contrast, inner and outer xylem remained similar across all sampling times. These findings build on previous studies which have confirmed the role of inner bark as an important storage site from which water can be drawn into xylem and back and show that the exchange between these tissues can be reflected in the isotopic composition of xylem water.
We further found that greater isotopic differences between bark and outer xylem were associated with larger differences between predawn and midday leaf water potential. A mature successional phase species and a pioneer species of opposing water use strategies-wood density, storage water recharge, leaf water potential regulation and soil water uptake depth-showed different trends in isotopic offsets between bark and outer xylem, with stronger isotopic effects in the high wood density species. This adds to current understanding of trade-offs between rooting depths, wood density and storage water use, suggesting that storage water use through the diel transpiration cycle is an important component of hydraulic strategy regardless of wood density, while the tissue where stored water is drawn from may differ depending on wood density, rooting depth and hydraulic regulation.
Taken together, we found evidence that the exchange between bark and xylem throughout the diel transpiration cycle is impacted by water use strategies and may affect the isotopic composition of xylem water, and possibly its interpretation. Thus, understanding of water use strategies, the covariation between traits, and how they are related to the use and exchange of stored water between bark and xylem throughout the day and night is important to appropriately interpret xylem water isotopic composition and to understand tree water use responses to environmental changes.
The raw data supporting the conclusions of this article will be made available by the authors, without undue reservation.
AV: Conceptualization, Data curation, Formal analysis, Funding acquisition, Investigation, Methodology, Software, Visualization, Writing – original draft, Writing – review & editing. JH: Conceptualization, Funding acquisition, Project administration, Resources, Supervision, Validation, Writing – review & editing. SS: Conceptualization, Resources, Supervision, Validation, Writing – review & editing. JM: Conceptualization, Resources, Supervision, Validation, Writing – review & editing.
The author(s) declare that financial support was received for the research, authorship, and/or publication of this article. This work was supported by an Australian Government Research Training Program Scholarship Stipend, an Australian Government Research Training Program Tuition Fee Offset Scholarship, the Wet Tropics Management Authority, the Skyrail Rainforest Foundation and the Tropical Forests and People Research Centre.
We thank the Queensland Parks and Wildlife rangers (Australian Government’s Department of Environment and Science) for providing access to the site and for supporting our work there. We are especially grateful to Grahame Applegate for his invaluable help, support and guidance in the preparation and conduction of field work, and to Micah Scudder and Nestor Gregorio for their invaluable contribution to field work during challenging conditions. We deeply thank Kim Janzen (Global Institute for Water Security, University of Saskatchewan) for her incredible support in sample analysis and advice. This article includes material that first appeared as part of the doctoral thesis “Water use of tropical trees: plant traits and water isotope relationships” by Adriana Vega Grau.
The authors declare that the research was conducted in the absence of any commercial or financial relationships that could be construed as a potential conflict of interest.
All claims expressed in this article are solely those of the authors and do not necessarily represent those of their affiliated organizations, or those of the publisher, the editors and the reviewers. Any product that may be evaluated in this article, or claim that may be made by its manufacturer, is not guaranteed or endorsed by the publisher.
The Supplementary material for this article can be found online at: https://www.frontiersin.org/articles/10.3389/ffgc.2024.1457522/full#supplementary-material
1. ^Although treating the tissues in inner bark, or phloem, as a “single unit” ignores physiological and ecological characteristics (Romero, 2014), for simplicity, the term “inner bark” (bark) is used here to include phloem, parenchyma, cambium and related tissues [e.g., De Schepper et al. (2012), Knipfer et al. (2019), and Pfautsch et al. (2015a)]. Although we did not distinguish between these tissues in our sampling, we recognize that the dynamics of water storage and release may involve different compartments in bark and xylem.
Allen, S. T., Kirchner, J. W., Braun, S., Siegwolf, R. T. W., and Goldsmith, G. R. (2019). Seasonal origins of soil water used by trees. Hydrol. Earth Syst. Sci. 23, 1199–1210. doi: 10.5194/hess-23-1199-2019
Barbeta, A., Gimeno, T. E., Clavé, L., Fréjaville, B., Jones, S. P., Delvigne, C., et al. (2020). An explanation for the isotopic offset between soil and stem water in a temperate tree species. New Phytol. 227, 766–779. doi: 10.1111/nph.16564
Barbeta, A., Jones, S. P., Clavé, L., Wingate, L., Gimeno, T. E., Fréjaville, B., et al. (2019). Unexplained hydrogen isotope offsets complicate the identification and quantification of tree water sources in a riparian forest. Hydrol. Earth Syst. Sci. 23, 2129–2146. doi: 10.5194/hess-23-2129-2019
Berry, Z. C., Evaristo, J., Moore, G., Poca, M., Steppe, K., Verrot, L., et al. (2017). The two water worlds hypothesis: addressing multiple working hypotheses and proposing a way forward. Ecohydrology. doi: 10.1002/eco.1843
Beyer, M., and Penna, D. (2021). On the Spatio-temporal under-representation of isotopic data in Ecohydrological studies. Front. Water 3:16. doi: 10.3389/frwa.2021.643013
Brooks, J. R., Barnard, H. R., Coulombe, R., and McDonnell, J. J. (2010). Ecohydrologic separation of water between trees and streams in a Mediterranean climate. Nat. Geosci. 3, 100–104. doi: 10.1038/ngeo722
Čermák, J., Kučera, J., Bauerle, W. L., Phillips, N., and Hinckley, T. M. (2007). Tree water storage and its diurnal dynamics related to sap flow and changes in stem volume in old-growth Douglas-fir trees. Tree Physiol. 27, 181–198. doi: 10.1093/treephys/27.2.181
Cernusak, L., Farquhar, G., and Pate, J. S. (2005). Environmental and physiological controls over oxygen and carbon isotope composition of Tasmanian blue gum, Eucalyptus globulus. Tree Physiol. 25, 129–146. doi: 10.1093/treephys/25.2.129
Chen, Y., Helliker, B. R., Tang, X., Li, F., Zhou, Y., and Song, X. (2020). Stem water cryogenic extraction biases estimation in deuterium isotope composition of plant source water. Proc. Natl. Acad. Sci. 117, 33345–33350. doi: 10.1073/pnas.2014422117
De Deurwaerder, H., Visser, M. D., Detto, M., Boeckx, P., Meunier, F., Zhao, L., et al. (2020). Causes and consequences of pronounced variation in the isotope composition of plant xylem water. Biogeosciences 17, 4853–4870. doi: 10.5194/bg-17-4853-2020
De Schepper, V., van Dusschoten, D., Copini, P., Jahnke, S., and Steppe, K. (2012). MRI links stem water content to stem diameter variations in transpiring trees. J. Exp. Bot. 63, 2645–2653. doi: 10.1093/jxb/err445
Dietrich, L., Zweifel, R., and Kahmen, A. (2018). Daily stem diameter variations can predict the canopy water status of mature temperate trees. Tree Physiol. 38, 941–952. doi: 10.1093/treephys/tpy023
Doronila, A. I., and Forster, M. A. (2013). Performance measurement via sap flow monitoring of three Eucalyptus species for mine site and dryland salinity phytoremediation. Int. J. Phytoremediation 17, 101–108. doi: 10.1080/15226514.2013.850466
Duvert, C., Barbeta, A., Hutley, L. B., Rodriguez, L., Irvine, D. J., and Taylor, A. R. (2024). Cavitron extraction of xylem water suggests cryogenic extraction biases vary across species but are independent of tree water stress. Hydrol. Process. 38:e15099. doi: 10.1002/hyp.15099
Ellsworth, P. Z., and Williams, D. (2007). Hydrogen isotope fractionation during water uptake by woody xerophytes. Int. J. Plant Soil Relation 291, 93–107. doi: 10.1007/s11104-006-9177-1
Evaristo, J., McDonnell, J. J., and Clemens, J. (2017). Plant source water apportionment using stable isotopes: a comparison of simple linear, two-compartment mixing model approaches. Hydrol. Process. 31, 3750–3758. doi: 10.1002/hyp.11233
Fabiani, G., Penna, D., Barbeta, A., and Klaus, J. (2022). Sapwood and heartwood are not isolated compartments: consequences for isotope ecohydrology. Ecohydrology 15:e2478. doi: 10.1002/eco.2478
Fisher, J. B., Baldocchi, D. D., Misson, L., Dawson, T. E., and Goldstein, A. H. (2007). What the towers don’t see at night: nocturnal sap flow in trees and shrubs at two Ameri flux sites in California. Tree Physiol. 27, 597–610. doi: 10.1093/treephys/27.4.597
Gaines, K. P., Meinzer, F. C., Duffy, C. J., Thomas, E. M., and Eissenstat, D. M. (2016). Rapid tree water transport and residence times in a Pennsylvania catchment. Ecohydrology 9, 1554–1565. doi: 10.1002/eco.1747
Goldstein, G., Andrade, J. L., Meinzer, F. C., Holbrook, N. M., Cavelier, J., Jackson, P., et al. (1998). Stem water storage and diurnal patterns of water use in tropical forest canopy trees. Plant Cell Environ. 21, 397–406. doi: 10.1046/j.1365-3040.1998.00273.x
Haberstroh, S., Kübert, A., and Werner, C. (2024). Two common pitfalls in the analysis of water-stable isotopologues with cryogenic vacuum extraction and cavity ring-down spectroscopy. Analytical Sci. Adv. 2024:2300053. doi: 10.1002/ansa.202300053
Haeni, M., Knüsel, S., Wilhelm, M., Peters, R. L., and Zweifel, R. (2020). Treenetproc-clean : Process and Visualise Dendrometer Data. R package version 0.1.4. Github repository: https://github.com/treenet/treenetproc
James, S. A., Meinzer, F. C., Goldstein, G., Woodruff, D., Jones, T., Restom, T., et al. (2003). Axial and radial water transport and internal water storage in tropical forest canopy trees. Oecologia 134, 37–45. doi: 10.1007/s00442-002-1080-8
Jupa, R., Plavcová, L., Gloser, V., and Jansen, S. (2016). Linking xylem water storage with anatomical parameters in five temperate tree species. Tree Physiol. 36, 756–769. doi: 10.1093/treephys/tpw020
Kassambara, A . (2023a). ggpubr: ‘ggplot2’ Based Publication Ready Plots.. R package version 0.6.0, Available at: https://CRAN.R-project.org/package=ggpubr
Kassambara, A . (2023b). rstatix: Pipe-Friendly Framework for Basic Statistical Tests. R package version 0.7.2. Available at: https://CRAN.R-project.org/package=rstatix
Knighton, J., Kuppel, S., Smith, A., Soulsby, C., Sprenger, M., and Tetzlaff, D. (2020). Using isotopes to incorporate tree water storage and mixing dynamics into a distributed ecohydrologic modelling framework. Ecohydrology 13:e2201. doi: 10.1002/eco.2201
Knipfer, T., Reyes, C., Earles, J. M., Berry, Z. C., Johnson, D. M., Brodersen, C. R., et al. (2019). Spatiotemporal coupling of vessel cavitation and discharge of stored xylem water in a tree Sapling1[OPEN]. Plant Physiol. 179, 1658–1668. doi: 10.1104/pp.18.01303
Knüsel, S., Peters, R. L., Haeni, M., Wilhelm, M., and Zweifel, R. (2021). Processing and extraction of seasonal tree physiological parameters from stem radius time series. Forests 12:765. doi: 10.3390/f12060765
Koeniger, P., Marshall, J. D., Link, T., and Mulch, A. (2011). An inexpensive, fast, and reliable method for vacuum extraction of soil and plant water for stable isotope analyses by mass spectrometry. Rapid Commun. Mass Spectrom. 25, 3041–3048. doi: 10.1002/rcm.5198
Kübert, A., Dubbert, M., Bamberger, I., Kühnhammer, K., Beyer, M., van Haren, J., et al. (2023). Tracing plant source water dynamics during drought by continuous transpiration measurements: an in-situ stable isotope approach. Plant Cell Environ. 46, 133–149. doi: 10.1111/pce.14475
Kühnhammer, K., Dahlmann, A., Iraheta, A., Gerchow, M., Birkel, C., Marshall, J. D., et al. (2022). Continuous in situ measurements of water stable isotopes in soils, tree trunk and root xylem: field approval. Rapid Commun. Mass Spectrom. 36:e9232. doi: 10.1002/rcm.9232
Liddell, M. (2013). Robson Creek OzFlux tower site OzFlux: Australian and New Zealand Flux Research and Monitoring.
Marshall, J. D., Cuntz, M., Beyer, M., Dubbert, M., and Kuehnhammer, K. (2020). Borehole equilibration: testing a new method to monitor the isotopic composition of tree xylem water in situ. Frontiers. Plant Sci. 11, 358. doi: 10.3389/fpls.2020.00358
Martinez Arbizu, P. (2017). PairwiseAdonis: Pairwise multilevel comparison using Adonis : R Package. R Package version 0.4. Github repository: https://github.com/pmartinezarbizu/pairwiseAdonis
Martínez-Vilalta, J., and Garcia-Forner, N. (2016). Water potential regulation, stomatal behaviour and hydraulic transport under drought: deconstructing the iso/anisohydric concept. Plant Cell Environ. 40, 962–976. doi: 10.1111/pce.12846
Martínez-Vilalta, J., Poyatos, R., Aguadé, D., Retana, J., and Mencuccini, M. (2014). A new look at water transport regulation in plants. New Phytol. 204, 105–115. doi: 10.1111/nph.12912
Martín-Gómez, P., Serrano, L., and Ferrio, J. P. (2017). Short-term dynamics of evaporative enrichment of xylem water in woody stems: implications for ecohydrology. Tree Physiol. 37, 511–522. doi: 10.1093/treephys/tpw115
Matheny, A. M., Bohrer, G., Garrity, S. R., Morin, T. H., Howard, C. J., and Vogel, C. S. (2015). Observations of stem water storage in trees of opposing hydraulic strategies. Ecosphere 6:art165. doi: 10.1890/ES15-00170.1
Matheny, A. M., Mirfenderesgi, G., and Bohrer, G. (2017). Trait-based representation of hydrological functional properties of plants in weather and ecosystem models. Plant Diversity 39, 1–12. doi: 10.1016/j.pld.2016.10.001
Meinzer, F. C., Brooks, J. R., Domec, J.-C., Gartner, B. L., Warren, J. M., Woodruff, D. R., et al. (2006). Dynamics of water transport and storage in conifers studied with deuterium and heat tracing techniques. Plant Cell Environ. 29, 105–114. doi: 10.1111/j.1365-3040.2005.01404.x
Meinzer, F. C., James, S., and Goldstein, G. (2004). Dynamics of transpiration, sap flow and use of stored water in tropical forest canopy trees. Tree Physiol. 24, 901–909. doi: 10.1093/treephys/24.8.901
Meinzer, F. C., Johnson, D. M., Lachenbruch, B., McCulloh, K. A., and Woodruff, D. R. (2009). Xylem hydraulic safety margins in woody plants: coordination of stomatal control of xylem tension with hydraulic capacitance. Funct. Ecol. 23, 922–930. doi: 10.1111/j.1365-2435.2009.01577.x
Mencuccini, M., Salmon, Y., Mitchell, P., Hölttä, T., Choat, B., Meir, P., et al. (2017). An empirical method that separates irreversible stem radial growth from bark water content changes in trees: theory and case studies. Plant Cell Environ. 40, 290–303. doi: 10.1111/pce.12863
Millar, C., Pratt, D., Schneider, D. J., and McDonnell, J. J. (2018). A comparison of extraction systems for plant water stable isotope analysis. Rapid Commun. Mass Spectrom. 32, 1031–1044. doi: 10.1002/rcm.8136
Mursinna, A., McCormick, E., Van Horn, K., Sartin, L., and Matheny, A. M. (2018). Plant hydraulic trait covariation: a global Meta-analysis to reduce degrees of freedom in trait-based hydrologic models. Forests 9:446. doi: 10.3390/f9080446
Nehemy, M. F., Benettin, P., Asadollahi, M., Pratt, D., Rinaldo, A., and McDonnell, J. J. (2021). Tree water deficit and dynamic source water partitioning. Hydrol. Process. 35:e14004. doi: 10.1002/hyp.14004
Oliva Carrasco, L., Bucci, S. J., Di Francescantonio, D., Lezcano, O. A., Campanello, P. I., Scholz, F. G., et al. (2015). Water storage dynamics in the main stem of subtropical tree species differing in wood density, growth rate and life history traits. Tree Physiol. 35, 354–365. doi: 10.1093/treephys/tpu087
Oksanen, J, Simpson, G, Blanchet, F, Kindt, R, Legendre, P, Minchin, P, et al. (2024). vegan: Community Ecology Package. R package version 2.6–8. Available at: https://CRAN.R-project.org/package=vegan
Penna, D., Hopp, L., Scandellari, F., Allen, S. T., Benettin, P., Beyer, M., et al. (2018). Ideas and perspectives: tracing terrestrial ecosystem water fluxes using hydrogen and oxygen stable isotopes-challenges and opportunities from an interdisciplinary perspective. Biogeosciences 15, 6399–6415. doi: 10.5194/bg-15-6399-2018
Pfautsch, S., and Adams, M. A. (2013). Water flux of Eucalyptus regnans: defying summer drought and a record heatwave in 2009. Oecologia 172, 317–326. doi: 10.1007/s00442-012-2494-6
Pfautsch, S., Hölttä, T., and Mencuccini, M. (2015a). Hydraulic functioning of tree stems—fusing ray anatomy, radial transfer and capacitance. Tree Physiol. 35, 706–722. doi: 10.1093/treephys/tpv058
Pfautsch, S., Renard, J., Tjoelker, M., and Salih, A. (2015b). Phloem as capacitor-radial transfer of water into xylem of tree stems occurs via Symplastic transport in ray parenchyma. Plant Physiol. 167, 963–971. doi: 10.1104/pp.114.254581
Phillips, N. G., Ryan, M. G., Bond, B. J., McDowell, N. G., Hinckley, T. M., and Čermák, J. (2003). Reliance on stored water increases with tree size in three species in the Pacific northwest. Tree Physiol. 23, 237–245. doi: 10.1093/treephys/23.4.237
Pineda-García, F., Paz, H., and Meinzer, F. C. (2013). Drought resistance in early and late secondary successional species from a tropical dry forest: the interplay between xylem resistance to embolism, sapwood water storage and leaf shedding. Plant Cell Environ. 36, 405–418. doi: 10.1111/j.1365-3040.2012.02582.x
Poca, M., Coomans, O., Urcelay, C., Zeballos, S. R., Bodé, S., and Boeckx, P. (2019). Isotope fractionation during root water uptake by Acacia caven is enhanced by arbuscular mycorrhizas. Plant Soil 441, 485–497. doi: 10.1007/s11104-019-04139-1
R Core Team (2024). R: A Language and Environment for Statistical Computing. R Foundation for Statistical Computing, Vienna, Austria. Available at: https://www.R-project.org/
Rosado, B. H. P., Oliveira, R. S., Joly, C. A., Aidar, M. P. M., and Burgess, S. S. O. (2012). Diversity in nighttime transpiration behavior of woody species of the Atlantic rain Forest, Brazil. Agric. For. Meteorol. 158–159, 13–20. doi: 10.1016/j.agrformet.2012.02.002
Scholz, F. G., Bucci, S. J., Goldstein, G., Meinzer, F. C., Franco, A. C., and Miralles-Wilhelm, F. (2007). Biophysical properties and functional significance of stem water storage tissues in Neotropical savanna trees. Plant Cell Environ. 30, 236–248. doi: 10.1111/j.1365-3040.2006.01623.x
Sevanto, S., Hölttä, T., and Holbrook, N. M. (2011). Effects of the hydraulic coupling between xylem and phloem on diurnal phloem diameter variation. Plant Cell Environ. 34, 690–703. doi: 10.1111/j.1365-3040.2011.02275.x
Sobota, M., Li, K., Hren, M., and Knighton, J. (2024). Evidence for variations in cryogenic extraction deuterium biases of plant xylem water across foundational northeastern US trees. Hydrol. Process. 38:e15079. doi: 10.1002/hyp.15079
Sohel, M. S. I. (2018). Spatial and temporal variation of sources of water across multiple tropical rainforest trees. School of Agriculture and Food Sciences: The University of Queensland.
Sohel, M. S. I., Vega Grau, A., McDonnell, J. J., and Herbohn, J. (2021). Tropical forest water source patterns revealed by stable isotopes: a preliminary analysis of 46 neighboring species. For. Ecol. Manag. 494:119355. doi: 10.1016/j.foreco.2021.119355
Steppe, K., Cochard, H., Lacointe, A., and Améglio, T. (2012). Could rapid diameter changes be facilitated by a variable hydraulic conductance? Plant Cell Environ. 35, 150–157. doi: 10.1111/j.1365-3040.2011.02424.x
Steppe, K., Vandegehuchte, M. W., Tognetti, R., and Mencuccini, M. (2015). Sap flow as a key trait in the understanding of plant hydraulic functioning. Tree Physiol. 35, 341–345. doi: 10.1093/treephys/tpv033
Stock, B. C., Jackson, A. L., Ward, E. J., Parnell, A. C., Phillips, D. L., and Semmens, B. X. (2018). Analyzing mixing systems using a new generation of Bayesian tracer mixing models. PeerJ 6:5096. doi: 10.7717/peerj.5096
Treydte, K., Lehmann, M. M., Wyczesany, T., and Pfautsch, S. (2021). Radial and axial water movement in adult trees recorded by stable isotope tracing. Tree Physiol. 41, 2248–2261. doi: 10.1093/treephys/tpab080
Tyree, M. T., and Yang, S. (1990). Water-storage capacity of Thuja, Tsuga and Acer stems measured by dehydration isotherms: the contribution of capillary water and cavitation. Planta 182, 420–426. doi: 10.1007/BF02411394
Vargas, A. I., Schaffer, B., Yuhong, L., and Sternberg, L. D. S. L. (2017). Testing plant use of mobile vs immobile soil water sources using stable isotope experiments. New Phytol. 215, 582–594. doi: 10.1111/nph.14616
Volkmann, T. H. M., Kühnhammer, K., Herbstritt, B., Gessler, A., and Weiler, M. (2016). A method for in situ monitoring of the isotope composition of tree xylem water using laser spectroscopy. Plant Cell Environ. 39, 2055–2063. doi: 10.1111/pce.12725
von Freyberg, J., Allen, S. T., Grossiord, C., and Dawson, T. E. (2020). Plant and root-zone water isotopes are difficult to measure, explain, and predict: some practical recommendations for determining plant water sources. Methods Ecol. Evol. 11, 1352–1367. doi: 10.1111/2041-210X.13461
Wills, J., Herbohn, J., Hu, J., Sohel, S.I., Baynes, J., and Firn, J. (2018). Tree leaf trade-offs are stronger for sub-canopy trees: Leaf traits reveal little about growth rates in canopy trees. Ecol Appli. 28, 1116–1125. doi: 10.1002/eap.1715
Yi, K., Dragoni, D., Phillips, R. P., Roman, D. T., and Novick, K. A. (2017). Dynamics of stem water uptake among isohydric and anisohydric species experiencing a severe drought. Tree Physiol. 37, 1379–1392. doi: 10.1093/treephys/tpw126
Younger, S. E., Blake, J., Jackson, C. R., and Aubrey, D. P. (2024). δ2H isotopic offsets in xylem water measurements under cryogenic vacuum distillation: quantifying and correcting wood-water hydrogen exchange influences. Ecohydrology 17:e 2640. doi: 10.1002/eco.2640
Zweifel, R., Drew, D. M., Schweingruber, F., and Downes, G. M. (2014). Xylem as the main origin of stem radius changes in Eucalyptus. Funct. Plant Biol. 41, 520–534. doi: 10.1071/FP13240
Keywords: bark, plant water use, stable isotopes, ecohydrology, xylem-phloem exchange, transpiration, stem water storage, tropical rainforest
Citation: Vega Grau A, Herbohn J, Schmidt S and McDonnell J (2025) Bark water affects the isotopic composition of xylem water in tropical rainforest trees. Front. For. Glob. Change. 7:1457522. doi: 10.3389/ffgc.2024.1457522
Received: 30 June 2024; Accepted: 24 December 2024;
Published: 28 January 2025.
Edited by:
John T. Van Stan, Cleveland State University, United StatesReviewed by:
Sanna Sevanto, Los Alamos National Laboratory (DOE), United StatesCopyright © 2025 Vega Grau, Herbohn, Schmidt and McDonnell. This is an open-access article distributed under the terms of the Creative Commons Attribution License (CC BY). The use, distribution or reproduction in other forums is permitted, provided the original author(s) and the copyright owner(s) are credited and that the original publication in this journal is cited, in accordance with accepted academic practice. No use, distribution or reproduction is permitted which does not comply with these terms.
*Correspondence: Adriana Vega Grau, YXZlZ2FAdXNjLmVkdS5hdQ==
Disclaimer: All claims expressed in this article are solely those of the authors and do not necessarily represent those of their affiliated organizations, or those of the publisher, the editors and the reviewers. Any product that may be evaluated in this article or claim that may be made by its manufacturer is not guaranteed or endorsed by the publisher.
Research integrity at Frontiers
Learn more about the work of our research integrity team to safeguard the quality of each article we publish.