- 1Department of Ecological Engineering and Forest Hydrology, Faculty of Forestry, University of Agriculture in Krakow, Krakow, Poland
- 2Department of Geomorphology, Institute of Geography and Spatial Management, Faculty of Geography and Geology, Jagiellonian University in Krakow, Krakow, Poland
- 3Chrzanów Forest Inspectorate, Chrzanów, Poland
Woody debris (WD) stabilizes riverbeds, creates habitats, supports biodiversity, and enhances water quality in ecosystems. This study investigates the impact of WD on sediment characteristics and particulate organic matter (POM) accumulation in the Roztoka stream, located within a forested catchment in the Polish Carpathians. The focus is on the influence of different WD types: coarse (CWD, length > 1 m and diameter > 10 cm), fine (FWD, length < 1 m and diameter < 10 cm), and mixed (MWD, a combination of CWD and FWD) on sediment differentiation and POM accumulation. Stand age, ranging from 20 to over 120 years (in 20-year intervals), was also analyzed as a factor affecting WD structure and POM deposition. Results indicate that MWD had the greatest effect on sediment differentiation, with mean grain size (Mz) ranging from −3.91 phi to −0.95 phi. Stand age significantly influenced POM accumulation, with the highest content (19.51%) observed in age classes III and VI. Older stands (> 120 years) had a diminished effect on both WD structure and POM accumulation. This study underscores the critical role of WD in sediment differentiation and provides insights for improving the ecological function of natural streams. Although the findings are region-specific, they have broader implications for similar temperate mountainous forests.
1 Introduction
Woody debris (WD) plays a fundamental role in forest ecosystems, influencing not only the physical structure of aquatic habitats but also contributing to essential biogeochemical and nutrient cycles (Gurnell, 2013). As a key component of forest dynamics, WD enhances stand productivity by slowly decomposing organic matter, thereby releasing vital nutrients into the environment and improving soil fertility. In streams and rivers, WD contributes to carbon sequestration and regulates nutrient cycling, serving as a substrate for microbial communities that facilitate nutrient processing (Beckman and Wohl, 2014). Additionally, it creates habitat complexity, benefiting a range of organisms from microorganisms to higher trophic levels. These ecosystem functions underscore the importance of WD in maintaining the health and productivity of forested landscapes, making its study critical for forest management and conservation efforts.
River and stream channels in forested mountain catchments, as well as in lowland ones commonly contain woody debris (WD). In studies on the subject, WD is typically divided into large (or coarse) woody debris (CWD), and fine woody debris (FWD). CWD consists of whole trees or their fragments (logs, trunks, main branches, stumpwood) whose length exceeds 1 m while their diameter at mid-length exceeds 10 cm (Wyżga et al., 2003; Galia et al., 2018). FWD consists of trees, trunks, roots and branch fragments smaller than CWD, fallen leaves, needles, cones, bark and finer organic fractions (Kałuża and Radecki-Pawlik, 2014). A heterogeneous mixture CWD and FWD in streams forms mixed type woody debris (MWD). The supply of WD to watercourse channels depends on the terrain conditions within the catchment, varying over time and space. Sources of WD are various, including natural tree mortality, competition within forest communities, pest outbreaks, industrial pollution weakening stand health, wind events (windblows, windbreaks), avalanches, landslides, rock debris flows, water erosion on the slope and in the watercourse channel, animal activities (mainly from beavers), and forest management practices in the catchment area (Wyżga et al., 2003). The occurrence of FWD in watercourse channels may also be related to phenological changes in vegetation (Wang et al., 2019). From an ecohydrological perspective, WD in watercourse channels not only provides a foodsource for aquatic fauna and flora, but also enhances habitat diversity and availability within the channel (Gurnell et al., 1995; Gregory et al., 2003), benefiting the development of ichthyofauna (Crisp, 2000).
In streams with a small channel width, WD tends to accumulate near its source due to the hydrodynamic conditions that limit its transport (Curran and Wohl, 2003). In the case of CWD, when the length of logs and branches exceeds the channel width, it may becomes fully or partly suspended above the channel. WD deposited in the river and stream channel can be transported, as a result of which it undergoes mechanical processing and biological decomposition (Braudrick and Grant, 2000; Wyżga et al., 2003; Wohl et al., 2023). Along with the growth of channel width and the strength of the current, the chance of mobility of WD increases (Piégay and Gurnell, 1997). FWD, though smaller in size compared to coarse woody debris (CWD), plays a similarly important role in forest and stream ecosystems. FWD contributes to nutrient cycling, organic matter accumulation, and habitat complexity, particularly for smaller organisms such as invertebrates and microorganisms. As it decomposes more rapidly than CWD, FWD provides a faster turnover of nutrients, supporting microbial activity and enhancing soil fertility (Zhou et al., 2007). Additionally, in streams, FWD can influence water flow, sediment deposition, and POM retention, particularly in smaller headwater streams where its abundance is higher. The presence of FWD is crucial for the overall energy flow and material transport within forest ecosystems, complementing the larger-scale effects of CWD.
Many studies have demonstrated that WD can play an important role in shaping channel morphology, particularly in mountain areas (Montgomery and Piégay, 2003; Gurnell, 2013; Wohl and Scott, 2016). Blockages of WD determine the forking of lines of the current and may contribute to intensification of lateral erosion, which results in the local growth of channel width (Montgomery and Piégay, 2003). Among various other WD roles, studies also show the direct dependence of channel width on the mass of WD present in the channel (Wyżga et al., 2012). As a result, the ratio of the length of WD to channel width is a parameter that is accepted as decisive regarding the possibility of WD transport, as well as the retention capacity of mineral material (Swanson et al., 1998). Woody debris occurring in stream channels enables maximizing the resistance of the flow and effective dispersion of the energy of flood water (Assani and Pêtit, 1995, Gippel, 1995; Merten et al., 2010; Wohl and Scott, 2016). The partitioning of smaller watercourses with tree dams leads to the occurrence of the stepped longitudinal profile of channels, with a system of steps and accompanying step pools (Keller et al., 1995; Nickolotsky and Pavlowsky, 2007). Woody debris significantly increases the roughness of the channel bed (Zimmermann and Church, 2001), reduces the speed of water flow and distracts the kinetic energy of the flowing water. This promotes the formation of erosion and deposition zones. Sediment deposition locally changes the longitudinal gradient of the channel, changing its width and, consequently, reducing its depth (Nakamura and Swanson, 1993). The presence of WD significantly affects the conditions of sediment transport throughout the longitudinal profile of the channel and sediment diversity (Mikos, 1994; Cadol and Wohl, 2011; Osei et al., 2015; Słowik-Opoka et al., 2018).
The influence of channel-deposited WD on channel morphology, sediment deposition, and transport, along with the characteristics of woody debris itself (i.e., its width and height), depend on its location within the watercourse channel (Galia and Hradecký, 2014; Galia et al., 2018). The CWD that reaches the bedrock can force the formation of alluvial sections of the channel (Montgomery and Piégay, 2003). Woody debris blockages oriented obliquely relative to the channel axis favor sediment deposition upstream and downstream of the blockage (Keller and Swanson, 1979; Smith et al., 1993; Montgomery and Buffington, 1997).
In larger channels, the presence of WD stabilizes alluvial channel formations, protects rooted vegetation from flood impacts, and enhances the succession of riparian zones and floodplain biodiversity (Wyżga et al., 2003). The ability of CWD to structurally block and retain dead plant materials within stream channels makes it an important food reservoir for small benthic organisms. However, sudden large influxes of organic matter to water channels can alter habitat proportions and, potentially, transform the trophic relations of aquatic communities on a large scale (Webster et al., 1990; Lemly and Hilderbrand, 2000; Stelzer et al., 2014). Furthermore, extensive WD positioned high in the channel can act as a natural barrier to the migration of certain fish species (Bylak, 2018). Mountain rivers and streams in forested areas accumulate fine organic matter (Hoover et al., 2010; Akcay et al., 2022; Wohl and Scamardo, 2022) in the form of twigs, cones, needles, foliage and bark, which are often trapped by CWD.
Although an extensive research literature exists on WD deposition in watercourses (Verdonschot and Verdonschot, 2024), studies on organic matter accumulation in fluvial sediments taking into account the stand age remain scarce. The main objective of this study is to examine the influence of WD on the characteristics of alluvial sediments and the POM accumulation in the small forested mountain catchment in the Polish Carpathians. Two main hypotheses are tested. H1 posits that the type of WD within the channel affects the variability of the alluvial sediment’s granulometric features. H2 suggests that forest stand age is a major factor determining the accumulation of POM in alluvial sediments. Specifically, the research aims to determine how different types of WD (CWD, FWD, and MWD) affect sediment granulometry and POM accumulation. Additionally, the study investigates how stand age influences the structure of WD and its role in sediment deposition and organic matter accumulation. By addressing these objectives, this study aims to contribute new insights into the ecological functions of WD in the stream channel.
2 Materials and methods
2.1 Study area
This research was performed in the small mountain catchment of the Roztoka Stream, with the surface area of 4.11 km2, located in the southwest of Poland in the Polish Carpathians (Figure 1). The topographic boundary of the catchment area runs from the north-eastern ridge of Kiczera Mt (827 m above sea level, asl) to the summits of Cisowa Grapa (810 m asl) and Wielka Cisowa Grapa (853 m asl.) as well as the northern ridge of Kocierz Mt (879 m asl), to the western slope of Okręgiełek Mt (627 m asl). Catchment relief reaches 450 m; there is a dominant occurrence of slopes with an inclination of 27% (15°) (75% of the catchment surface area), and there also occur areas with slopes exceeding 58% (30°). The springs of the Roztoka Stream flow out below the Przysłop Cisowy Pass at 792 m asl, this is the left-side tributary of the Wielka Puszcza Stream, into which it flows at 435 m asl. The length of the Roztoka Stream is 3.18 km and its gradient equals 11‰. The main stream (MS) has 6 right-side tributaries (RST1−RST6) and two left-side tributaries (LST1, LST2). The length of each tributary (in km) are, respectively: RST1−0.91, RST2−0.96, RST3−0.80, RST4−0.69, RST5−0.98, RST6−0.38, LST1−1.43 and LST2−1.47. The density of the stream network is 2.63 (km ⋅ km–2) and the length of permanent streams totals 10.8 km.
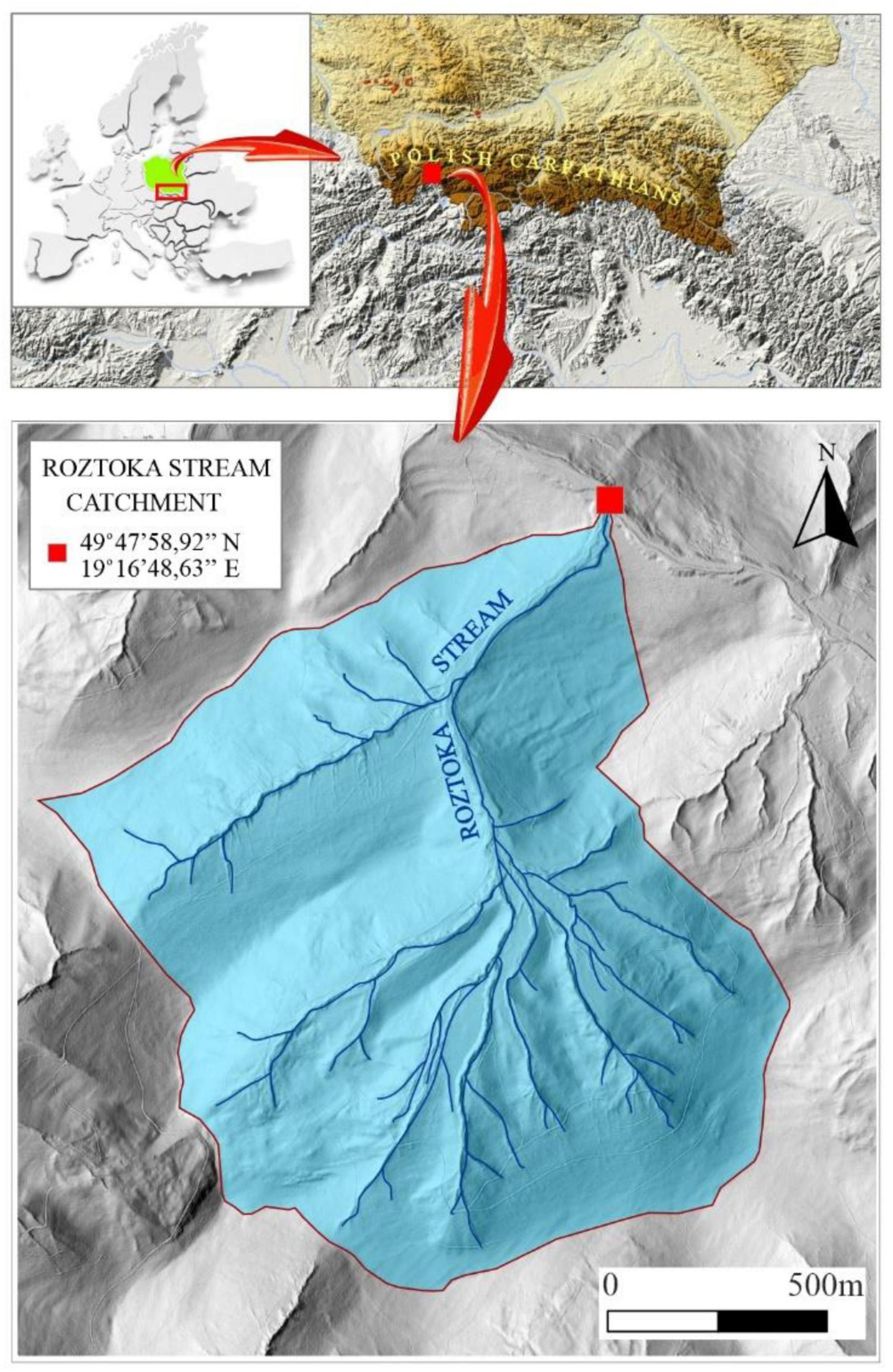
Figure 1. Location of the Roztoka Stream catchment where the study was performed. The inset map (below) provides a view of the catchment area, highlighting the stream network and topography.
In terms of the climatic conditions, the catchment area of the Roztoka Stream belongs to the Mesoregion of the Silesian and Little Beskids (VIII Carpathian Nature and Forest Land) (Zielony and Kliczkowska, 2012). The climate in the study area corresponds to the Dfb category of the Köppen-Geiger climate classification (Błaś and Ojrzyńska, 2024), indicating a humid continental climate with warm summers and no dry season, with an average annual temperature ranging from 4°C to 6°C. Annual rainfall totals change with the altitude and slope, on average, approx. 1100 mm. In 2023, there were 173 days with precipitation (47.4% of the days of the year) and the highest monthly rainfall totals occur in June and July. The period of presence of the snow cover in the analyzed area ranges from 110 to 140 days. Snow cover lingered for 73 days of the year (20% of the days of the year). The average length of the vegetation period is 200 days (PUL, 2015).
The Roztoka Stream catchment is characterized by the flysh structure, typical of the Outer Carpathians. The research area consists of deposits of the Silesian series, i.e., godula sandstone (thinly- and thick-bedded) as well as medium- and lower-level slates, locally separated by layers of clay shales (PUL, 2015). At the valley bottom, there are alluvial deposits with varied sizes: boulders, gravel, sand as well clays and loams. The foothills display the presence of colluvium and alluvium, varied in terms of fractions.
The forest cover in the catchment area amounts to 97% (PUL, 2015). The dominant forest species is European beech (Fagus sylvatica L.), along with a share of silver fir (Abies alba Mill.) and Norway spruce (Picea abies (L.) H. Karst), with an admixture of European larch (Larix decidua), sycamore maple (Acer pseudoplatanus L.), wild cherry (Prunus avium L.) or species growing in the stream valley: European ash (Fraxinus excelsior L.), black alder (Alnus glutinosa (L.) Gaertn.) and grey alder [Alnus incana (L.) Moench]. The majority of plant communities are constituted by stands aged over 60 years and older. The stand age classes (in years): I−up to 20, II−from 21 to 40, III−from 41 to 60, IV−from 61 to 80, V−from 81 to 100, VI−from 101 to 120, VII−120 and more years, according to the classification in force in Poland (PUL, 2015), were considered. Forest habitat types occurring in the research area include fresh mountain broadleaved forest (fertile beech, 97% of the forest surface area) and fresh mixed mountain broadleaved forest (poor beech connected with district cambisol, about 3% of the forest surface area). The stands in the catchment area are dominated by the plant communities of fertile Carpathian beech forests (Dentario glandulosae-Fagetum), the remaining area is occupied by acidic mountain beech forest (Luzulo luzuloidis-Fagetum); small acreage is occupied by a group of riverside mountain alders (Alnetum incanae).
2.2 Field work
2.2.1 Inventory-taking of woody debris in the channel
Field measurements were conducted in August 2023 as part of a 10-day measurement campaign. In the course of field research, no intensive rainfall, windbreaks or floods were noted. We inventoried all WD sites occurring in the Roztoka Stream channel, in the main watercourse (MS) and in the channels of its right-side tributaries (RST) and left-side ones (LST). The position of each site of WD was located with the use of gps, with the numbers ascribed in the direction from the springs to the mouth. The factors determined in situ included: the type of woody debris (CWD, FWD, MWD), the location of the debris relative to the stream bed axis and, whenever possible, the dominant tree species. As the presence of WD in watercourses causes changes in the nature of water flow and channel morphology (Curran and Wohl, 2003), the width (W) and depth (H) of the watercourse channel were also measured. Simultaneously, the stand covering the slopes in the zone close to the streambed (i.e., in the direct neighborhood of woody debris sites) was characterized and photographed in order to confirm the lack of differences in the riparian composition.
2.2.2 Alluvial sediment sampling
While inventorying WD sites in the channels, alluvial sediment samples were collected upstream (U) and downstream (D) of each WD site. Sediment samples were obtained using the conventional surface hand-picking method (Książek et al., 2008). Surface particles in the form of gravels and stones were hand-picked from an area of 0.5 × 0.5 m, delineated with a frame. The finer deposits of the sandy fractions were collected using the riverbed sediment catcher of the Van Veen type (Eijkelkamp). The deposits were obtained from the depositional zone at a distance of approximately 0.3 m upstream and downstream of each WD site. Sediment samples were transported to the laboratory for the purpose of further research.
2.3 Laboratory tasks
2.3.1 Alluvial sediment analysis
The presence of WD in streams plays an important role in the process of stream sediment transport. During the movement of sediments in the watercourse channel, what changes is not only their size (diameter) but also shape (Malarz, 2004). The size and shape of grains determines, among others, the coefficient of flow resistance or shear stresses. When these values are exceeded, grains of a specific type of size and shape are selectively or massively included in the transport (Heitmuller and Hudson, 2009).
Alluvial sediment samples collected in the field underwent the sieve analysis in order to find out the diversity of their granulometric composition. We determined the degree of sediment sorting and calculated the values of diameter d50 and basic sedimentological indicators: mean grain diameter Mz (in phi-scale), standard deviation (σ1), skewness (Sk), kurtosis (Kg), according to Folk and Ward (1957). For Mz, the unit phi is converted from metric units according to the formula: phi = log2d, where d is the diameter of the grain expressed in millimetres. The result is a logarithmic scale of grain size extremely useful in graphical interpretation of sediments with a large range of grains from the boulder fraction to the clay fraction. The shape of gravels and stones was determined using the method proposed by Zingg (1935) and Bala (2016). The length of each of the three main axes of gravels and stones was measured with calipers with an accuracy of 0.01 mm. Next, the following relationships were determined: (1) the ratio of the length of the medium axis (b) to the longest axis (a) (the so-called elongation measure) and and (2) the ratio of the length of the shortest axis (c) to length (b) (the so-called flattening measure) in order to develop Zingg diagrams and to assign the particles to one of 4 shape categories: sphere-shaped (S), disc-shaped (D), blade-shaped (B) and rod-shaped (R). The stones and gravels transported in the channel undergo defragmentation and attrition/abrasion, as a result of which there appear mineral river sediments of the sandy fraction, often containing significant quantities of POM (Ward and Aumen, 1986). Hence, due to observed differences in sandstone grain consistency, the stones and gravels were additionally grouped into particles of fine-grained sandstone (FG) and coarse-grained sandstone (CG).
The proportion of fine organic matter in the alluvial sediments of the sandy fraction was determined using the roasting method (Myślińska, 2016) and was calculated according to the following formula (1):
where : Iż−losses after roasting (in %),mst−the weight of the crucible with the sample dried to solid mass (g), mu −the weight of the crucible with the sample roasted to solid mass (g), mt −the weight of the crucible after roasting (g).
2.4 Statistical data elaboration
Statistical analyses were performed using R software (R Core Team, 2020 v. 4.3.3) with the use of car packages (Fox and Weisberg, 2019) and emmeans (Lenth et al., 2019). We performed the analysis of skewness of the distributions studied, along with the Shapiro-Wilk test, Spearman’s rho correlation analysis and analysis of variance for independent tests (ANOVA). For important effects in ANOVA, we performed the post-hoc analysis with Holm’s correction for multiple comparisons. The significance level adopted was α = 0.05.
3 Results
3.1 Characteristics of woody debris structure in the main channel and in tributaries
The slopes of the Roztoka catchment are characterized by a significant gradient (Figure 2A), which favors water erosion and the delivery of WD to valley floors and channel beds. In the channel of the main stream (MS) and in the channels of the tributaries (RST, LST), a total of 542 sites of woody debris (WD) were inventoried (Figure 2B), including 196 CWD sites (36%), 159 FWD sites (29%), and 187 MWD sites (35%). The majority of WD sites were found in the main stream channel (77), while the fewest (36) were found in the shortest right-side tributary (RST6). Nearly half of the inventoried WD sites consist of accumulations arranged parallel to the channel axis, while the remainder are arranged obliquely or perpendicularly to the channel axis.
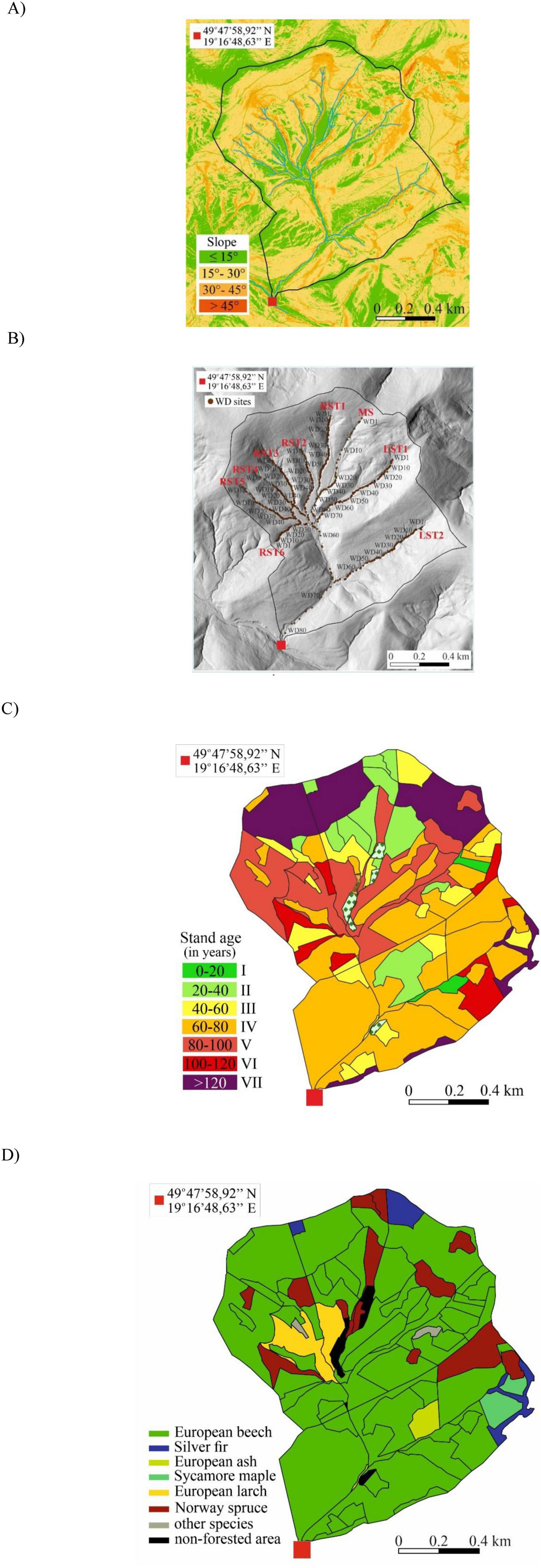
Figure 2. The Roztoka Stream catchment: (A) map of terrain slopes, (B) inventoried woody debris sites (WD) in the main channel (MS) and in right-side (RST) and left-side (LST) tributaries, (C) stand age classes (in years): I–up to 20, II–from 21 to 40, III–from 41 to 60, IV–from 61 to 80, V–from 81 to 100, VI–from 101 to 120, VII–120 and more years, (D) tree species.
The largest number of woody debris sites in the channels was inventoried in the part of the catchment area covered by stands of age class V. Channel sections near forests of age class I are dominated by MWD. In areas with age class IV stands, there is a noticeable increase in the proportion of CWD and MWD, while in areas near age class VII stands, the oldest, CWD sites are predominant. In stream sections surrounded by young trees and saplings (of age class I) as well as stands of age class II (Figure 2C) the channel is dominated by MWD in the form of mixtures of small fragments of wood, thicker branches and shorter fragments of logs. In contrast, channel sections surrounded by pole-timber forest or a thin stand (age class III) are dominated by FWD sites (48%), then by CWD sites (approx. 30%) and−to still a lesser extent−by MWD sites (22%). In channel sections within which there occur stands of age class IV (and higher), there is a noticeable increase of CWD and MWD sites as well as a reduction of the number of FWD sites (from 22 to 30%). Presumably, the dominant share of CWD results from: the smaller crown closure of trees in older stands (a higher age class), an increased susceptibility to damage triggered by the force of wind, and from biotic factors. In sections of the watercourse surrounded by the oldest stands (age class VII), what prevails is the share of CWD sites (55%).
Considering the species of trees, both in the main stream channel (MS) of the Roztoka Stream and in its tributaries (RST, LST) there was a dominant share of fragments of dead beech wood (Fagus sylvatica L.) (22% of all sites) (Figures 2D, 3A). In 10% of the inventoried woody debris sites, the tree species could not be identified due to a high degree of wood decomposition. The structure of woody debris was dominated by branches and logs (Figure 3B).
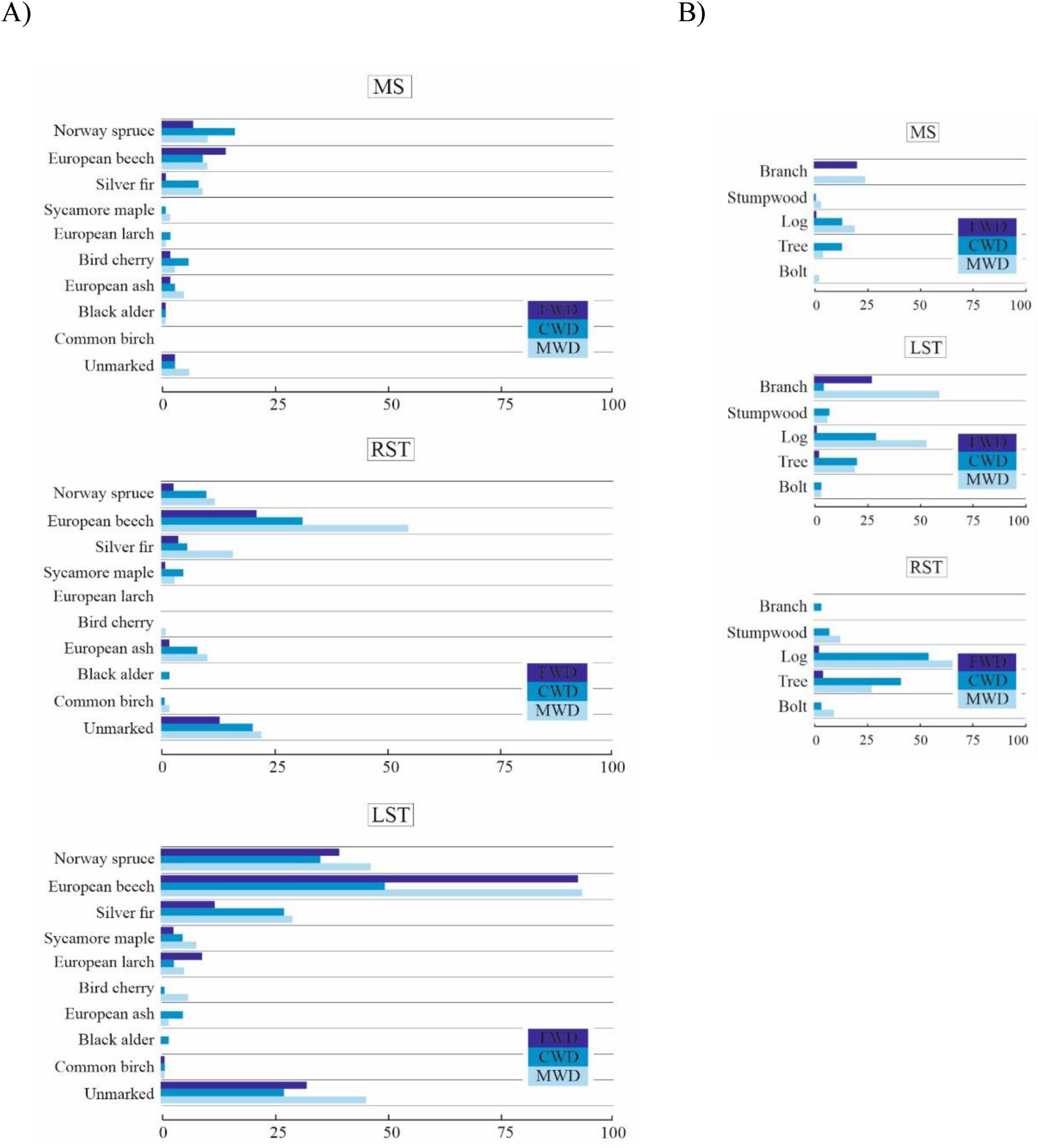
Figure 3. Characteristics of woody debris (FWD, CWD and MWD) in the main channel (MS), right-side tributary (RST), and left-side tributary (LST) (in frequency numbers): The fraction of (A) identified tree species constituting woody debris, and (B) elements of woody debris.
3.2 Differentiation of the granulometric features of alluvial sediments
At all steps along the longitudinal profile of the Roztoka Stream channel, the sediments are characterized by a similar differentiation of the granulometric composition (Table 1). A similar range of mean diameters for grains was present for both the sediments deposited upstream (Mz from −4.41 to −1.72 phi) and downstream of the WD (Mz from −4.44 to −0.92 phi), regardless of its type (CWD, FWD, MWD). This regularity is also confirmed by a similar differentiation of the diameter of grains d50 in sediments deposited upstream (d50 from 3.99 mm to 25.68 mm) and downstream of woody debris (d50 from 2.19 mm to 28.11 mm). Sediments deposited upstream of woody debris, regardless of the type of debris, contain from 61.3 to 98.66% of grains > 2 mm in size, while downstream of woody debris these values are slightly lower, i.e., from 52.25 to 97.16%.
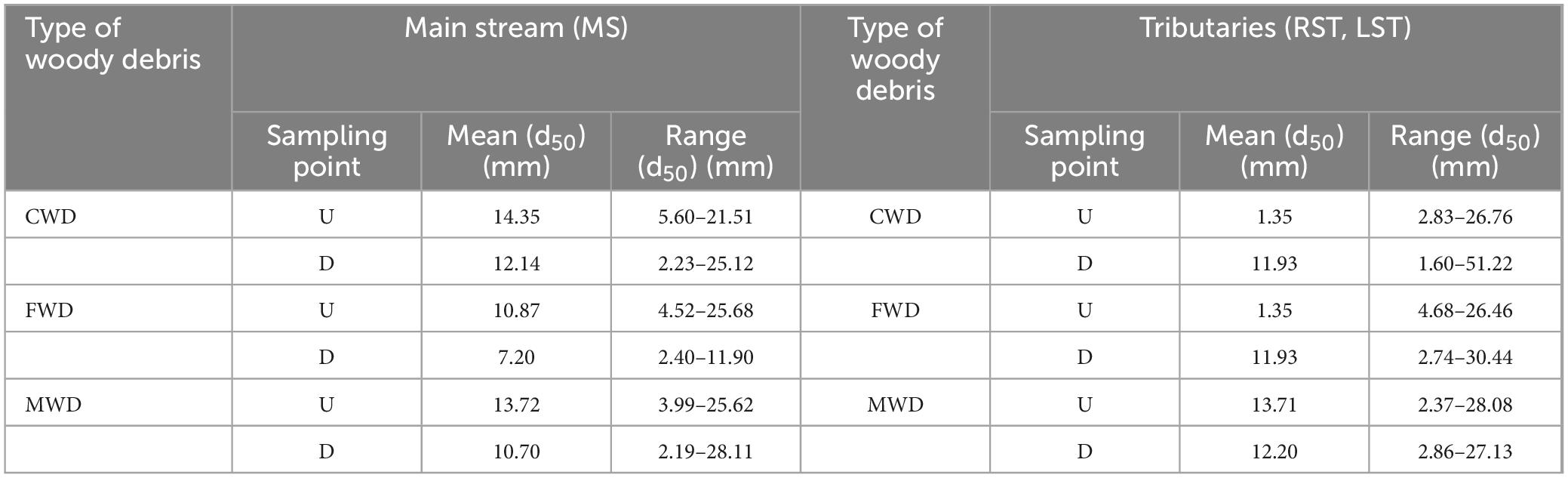
Table 1. Characteristics of sediment diameter (d50%) of alluvium taken from main stream (MS), right-side tributaries (RST) and left-side tributaries (LST), upstream (U) and downstream (D) of coarse woody debris (CWD), fine woody debris (FWD) and mixed-type woody debris (MWD).
3.2.1 Alluvial sediments accumulated within CWD
In the case of sediments sampled along the longitudinal profile of the main channel (MS), upstream and downstream of coarse woody debris sites (CWD), the share of grains with a diameter > 2 mm is, on average, 82.89%. The grain diameter d50% shows values ranging from 2.23 mm to 25.12 mm (average 13.25 mm) (Table 1). Skewness assumes positive values, which is characteristic of the conditions of deposition or periodic transit. Sediment sorting is very weak, weak, less often moderate, which indicates sediment transport in short sections of the channel. Mz values range from −4.44 to −0.92 phi (sediments transported in traction), which suggests that these are the sediments of the channel’s streambed armoring (upper flow regime). This is also confirmed by an analysis of the diagram of the relation between Mz and the degree of sorting (Figure 4), where one can notice better sorting of sediments along with the increase of the mean diameter (Mz).
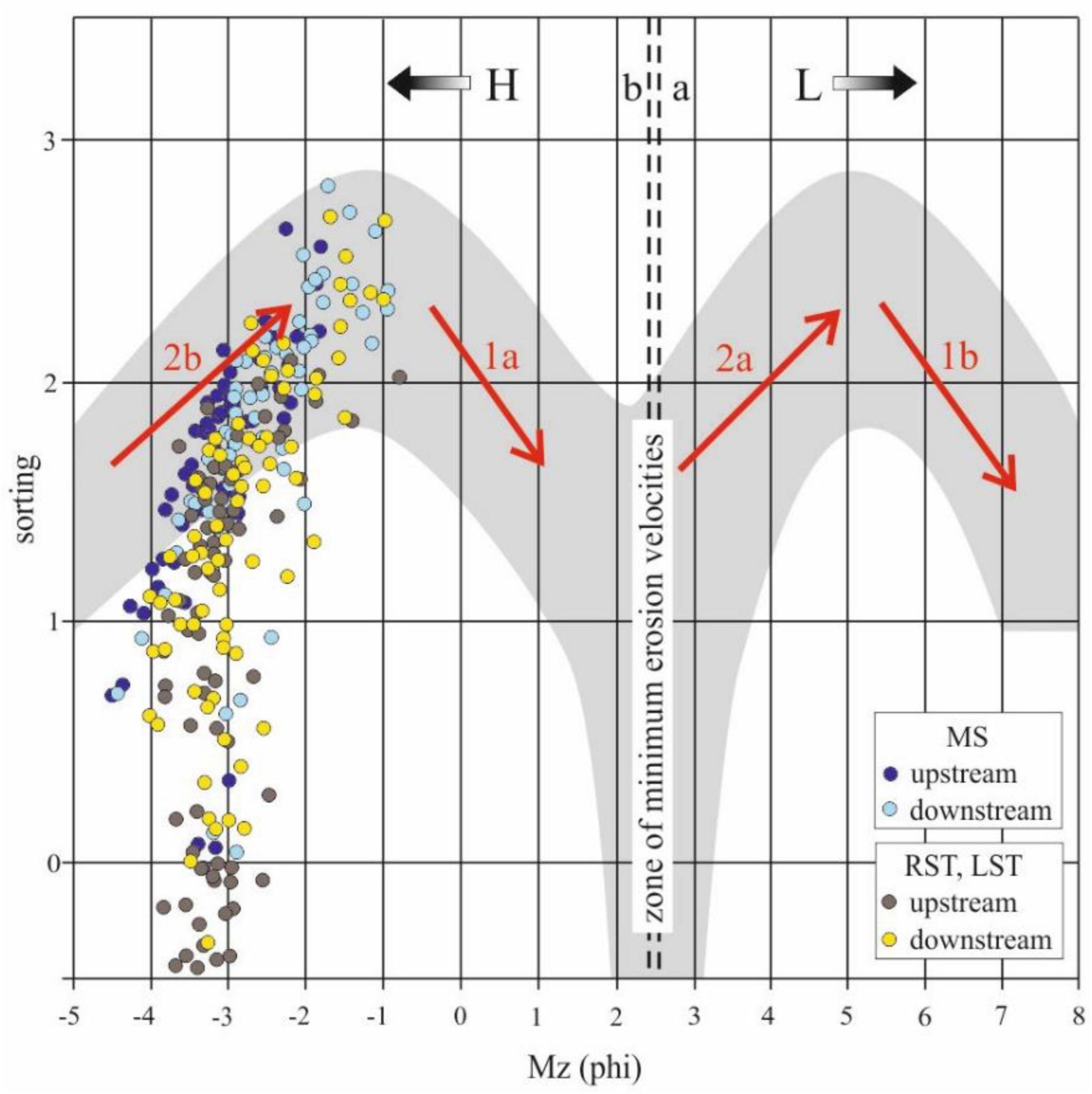
Figure 4. Relationship between mean grain size and sorting, on the basis of flow regime, tendency lines relative to sediment genesis after Mycielska-Dowgiałło (2007): H–high energy regime, L–low energy regime, a–maximum kurtosis value, b–skewness divided, 1a, b–tendency 1–line of sorting decreasing along with increasing mean grain size in 1a river channel, fluvioglacial and eolian deposits, and also 1b suspended filling of palaeochannel deposits (after Ludwikowska-Kędzia, 2000); 2a, b–tendency 2–line of sorting increasing along with decreasing mean grain size in overbank deposits, eolian-desert and deluvial deposits, 2b–channel lag deposits.
In the channel tributaries (RST, LST), alluvial sediments deposited upstream of coarse woody debris (CWD) are characterized by Mz values ranging from −3.88 phi to −1.46 phi; whereas those found downstream of those sites range from −3.86 phi to −1.01 phi. In both cases, the values of Mz indicate that these are the sediments of streambed armoring, transported by traction. Slightly less varied are sediments accumulated upstream of coarse woody debris, and containing, on average, 85.39% of grains with a diameter > 2 mm (from 63.43 to 98.13%). Downstream of CWD, the share of this fraction is slightly smaller (on average 82.84%) (the range from 44.93 to 97.33%). The diameter d50 for all sediments, i.e., upstream and downstream of CWD, reveals variations ranging from 1.60 mm to 51.22 mm (on average 12.64 mm). Upstream of WD, d50 adopts values from 2.83 mm to 26.76 mm (Table 1) and downstream of steps from 1.60 mm to 51.22 mm (on average 11.93 mm). The diameter d50 adopts slightly higher values in sediments deposited upstream of CWD, but maximum values of d50 (51.22 mm) apply to sediment samples deposited below CWD. Skewness (Sk) adopts mostly positive values, the exception being only 9 samples where Sk has negative values, which indicates sediment redeposition or washing. In the case of 10 out of 23 CWD sites, the degree of sediment sorting collected upstream and downstream of CWD is similar. However, in the case of sediments collected from 8 CWD sites, sediment sorting became reduced downstream of places of CWD accumulations. On the remaining sites, the degree of sorting does not change.
3.2.2 Alluvial deposits accumulated within FWD
The mean diameter Mz in sediments sampled from the main stream channel (MS) adopts values from −4.41 phi to −1.15 phi (sediments transported in traction) (Figure 4). Sediments in all samples collected upstream and downstream of FWD throughout the longitudinal profile of the MS channel contain from 29.61 to 95.11% of grains > 2 mm (average 50.69%). The diameter of grains (d50) adopts values ranging from 2.40 mm to 25.68 mm (on average 9 mm). Skewness adopts positive values, which proves that these are sediments originating in transit or deposition. The sorting of sediments is very weak and weak, which means that they were transported along short channel sections or there occurred their dynamic deposition. An exception is constituted by two samples of sediments with moderate sorting (R66U, R67U), deposited in the lower section of MS. In the case of the main diameter of grains (d50), a slightly larger differentiation of values was recorded in sediments sampled upstream of FWD sites. Skewness, as is the case of CWD sediments, adopts positive values. Sediment sorting accumulated downstream of FWD is weak, only in a few cases the sorting was better than upstream of FWD, i.e., a change was observed in the degree of sorting from very weak to weak or from weak to moderate. In the remaining cases, the sorting of sediments captured upstream and downstream of given sites of fine woody debris (FWD) is the same upstream; in several (seven) samples there were found a change from very weak to weak sorting, and in one case a change from very good to moderate. To sum up, sediments accumulated downstream of FWD are characterized by lower sorting compared to sediments accumulated upstream of FWD.
In the tributaries, the mean diameter (Mz) of sediments deposited upstream and downstream of FWD adopts values ranging from −4.0 phi to −1.09 phi, and is very close to the range of Mz values for sediments sampled from CWD. Less varied are sediments accumulated upstream of FWD. As shown in the diagram (Figure 4), these are the sediments of streambed armoring, transported by traction. Skewness (Sk) assumes mostly positive vsalues; only in the case of a few FWD sites does Sk adopt negative values. In DP01 and DP05 tributaries, none of the analyzed sediments display any negative Sk values, where, for comparison, in the case of CWD in the channel of each of the tributaries, there singly occurred negative Sk values. In the case of 16 out of 23 FWD sites, the sorting of sediments deposited in the channel downstream of FWD underwent decreasing. What is interesting is a significant change in the degree of sediment sorting, e.g. from very good (sediments upstream of FWD) to very weak (sediments below FWD). The degree of sorting underwent particularly significant worsening in sediment samples downstream of FWD, where, for comparison, in the case of CWD no deterioration was noticed in the degree of sorting of the sediments deposited downstream of this type of debris; the sorting of those sediments was more often slightly better.
3.2.3 Alluvial sediments accumulated within MWD
The mean diameter of the grains (Mz) of alluvial sediments deposited in the channel of the Roztoka main stream, within mixed woody debris (MWD), adopts values in the range from −3.91 phi to −0.95 phi (sediments transported in traction). This feature is also confirmed by an analysis of the diagram of relation between Mz and the degree of sorting (Figure 4), where the degree of sorting increases along with the increase of the mean grain diameter. Similarly, as in the case of sediments accumulated at CWD and FWD, these are the deposits of streambed armoring, i.e. of the upper flow regime. Grain size, expressed as the conclusive diameter (d50) (Table 1), is more evidently similar to CWD deposits, especially the sediments detained upstream of this type of debris. Upstream of MWD, the sediments contained from 45.17 to 87.48% of grains with a diameter > 2 mm, on average 62.84%. Skewness, except two sediment samples, adopts positive values. Only in three cases, the sediments deposited downstream of MWD are sorted better than the upstream ones (a change in the degree of sorting from very weak to weak, or from weak to moderate). In the remaining cases, the sorting of sediments is the same upstream and downstream of MWD, or slightly worse downstream (8 cases).
Mz for sediments in the channels of the tributaries deposited in the vicinity of MWD adopts values ranging from −4.01 phi to −0.85 phi. Similarly as in the case of sediments from CWD and FWD, these are the sediments of streambed armoring. When comparing the range of Mz values upstream of MWD, it is slightly higher than the range of Mz for sediments of the remaining types of woody debris; sediments accumulated upstream of MWD are slightly more varied granulometrically than sediments upstream of CWD and FWD. Alluvial sediments contain on average 86.79% of grains with a diameter > 2 mm. The conclusive diameter (d50) of all sediments from MWD (upstream and downstream) adopts varied values ranging from 2.37 mm to 28.02 mm (on average, d50 = 12.96 mm) and its mean (d50) is lower than the diameter of the sediments accumulated within CWD. In most cases, skewness adopts positive values; only 4 cases reveal negative values. No negative Sk values were found only in the case of sediments sampled from the channel in tributaries: DP02, DP03, DP04 and DL01, where Sk values are positive. The degree of sorting shows large variation compared to features of sediments accumulated downstream and upstream of MWD in the main channel.
3.2.4 Grain shape analysis
Analyzing the developed diagrams which help determine the shape of grains, one can notice that there were more gravels and stones in the sediments accumulated upstream of CWD, and that the share of disc-shaped (D) and blade-shaped (B) particles was dominant. In sediments accumulated downstream of FWD sites, similarly to gravels and stones sampled within large woody debris, there was also a smaller share of gravels and stones. However, one can note that, compared to CWD, the share of sphere-shaped (S), disc-shaped (D), blade-shaped (B) and rod-shaped (R) particles is comparable (Figure 5). The share of clasts of the gravel or stone fraction built of fine-grained sandstone (FG) in sediments sampled upstream and downstream of woody debris sites was 74% and 61%, respectively, while for those made of coarse-grained sandstone (CG), it was 39% and 25%, respectively. Blade-shaped particles made of fine grain sandstone (FG) constituted half (50%) of all clasts made of this type of sandstone in the sediments sampled upstream of woody debris sites, and 48% in sediments collected downstream of those sites. Observed was a numerous occurrence of disc-shaped particles (D)−upstream of woody debris sites, the gravels and sandstone rocks (FG) in this shape accounted for 31%, whereas downstream−33% of all particles. The share of sphere-shaped clasts (S) and rod-shaped clasts (R) made of sandstone (FG) was smaller and, upstream and downstream of the sites, amounted to 4% and 15%, respectively.
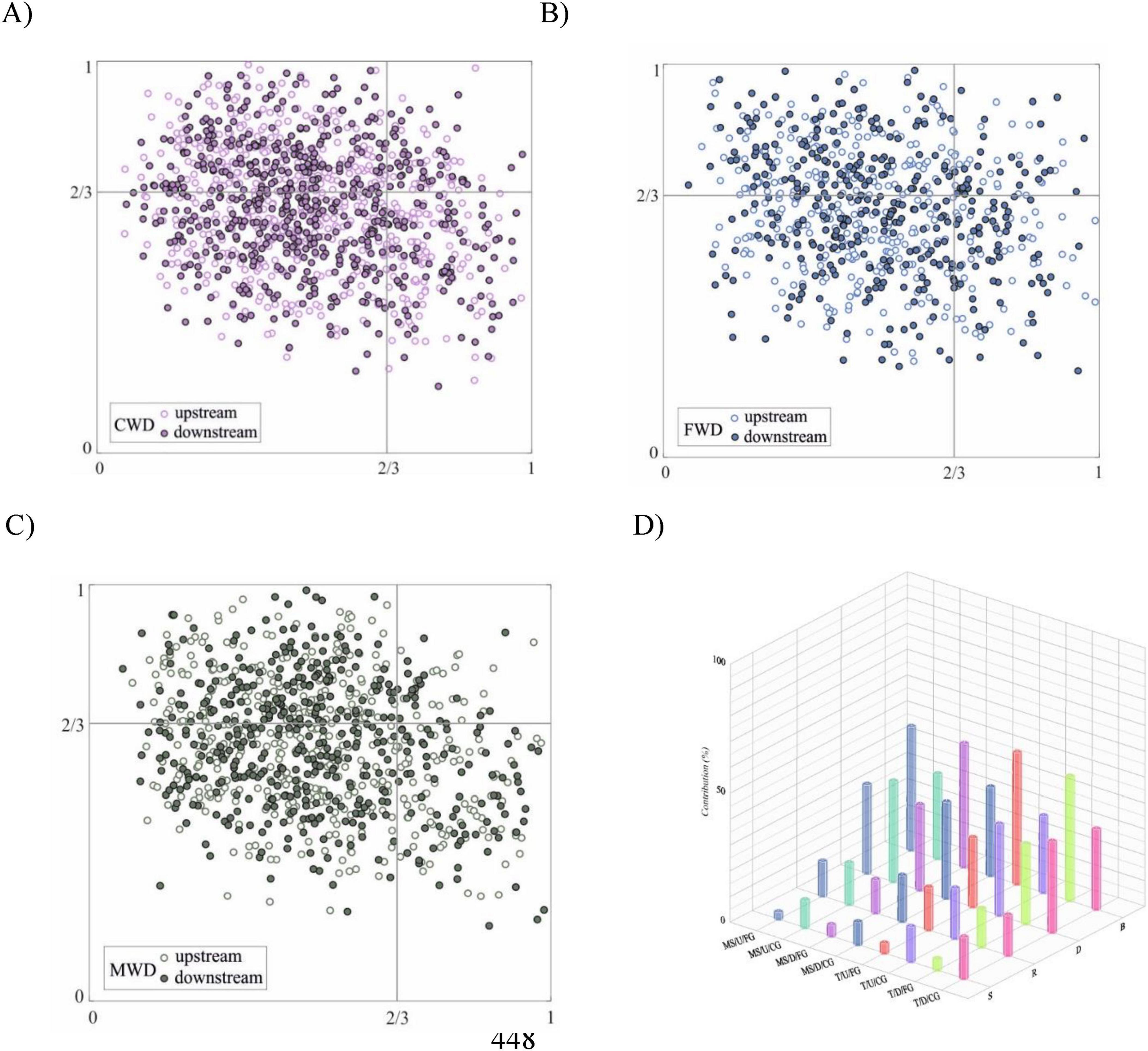
Figure 5. Shape of gravels in sediments taken from: CWD (A), FWD (B) and MWD (C) upstream (U) and downstream (D) of woody debris; (D) Share (in %) of particles of finen-grained sandstone (FG) and coarse- grained sandstone (CG) with a given shape (S–sphere-shaped, R–rod-shaped, D–disc-shaped, B–blade-shaped), sampled upstream (U) and downstream (D) of sites: CWD, FWD and MWD in the main channel of the stream (MS) and in the tributaries (RST, LST).
Among gravels and stones of coarse-grained sandstone (CG), there was a significant share of disc-shaped particles (D)−37% (upstream and downstream of WD) and of blade-shaped particles (B)−32% (upstream and downstream of WD). The prevalence of blade-shaped particles and disc-shaped particles (D) may be related to water erosion observed in the Roztoka Stream channel, resulting in the supply of fresh rock material from lateral erosion undercuts (no rounding in the short transport). The share (in alluvial sediments) of gravels and stones which are sphere-shaped (S) and rod-shaped (R) was greater than in the case of particles built of fine grain sandstone (FG). Rod-shaped gravels and rocks of coarse-grained sandstone (CG) upstream of the sites accounted for 18% and approximately 16% on sites downstream of those sites. In contrast, the share of sphere-shaped particles (S) was twice higher, and amounted to 13% both upstream and downstream of debris sites.
3.3 Accumulation of particular organic matter (POM) in alluvial sediments
Examination of the influence of independent variables, i.e. the type of woody debris (CWD, FWD, MWD), the place of sediment deposition (upstream, downstream), and verification of the hypothesis (H2) that stand age is a factor significantly influencing the accumulation of POM in alluvial sediments was performed with the use of a one-way analysis of variance (ANOVA). The model did not test interaction effects and only included the main effects of the above-mentioned variables (Table 2). The analysis showed a significant effect of stand age on the accumulation of POM in sediments (Figure 6). Post-hoc analysis revealed that in the channel sections surrounded by stands of age class VI, a higher share of POM was found in alluvial sediments than in the case of sediments deposited in the stream channel adjacent to the stands of age class II [t (259) = 4.88, p < 0.001] and age class V [t (259) = 5.32, p < 0.001] (Table 3). The analysis was performed on POM values transformed with a logarithmic function.

Table 2. Descriptive statistics of particular organic matter POM (%) grouped according to the stand age classes from I do VII (in years): I−up to 20, II−from 21 to 40, III−from 41 to 60, IV−from 61 to 80, V−from 81 to 100, VI−from 101 to 120, VII−120 and more years; n−sample size.
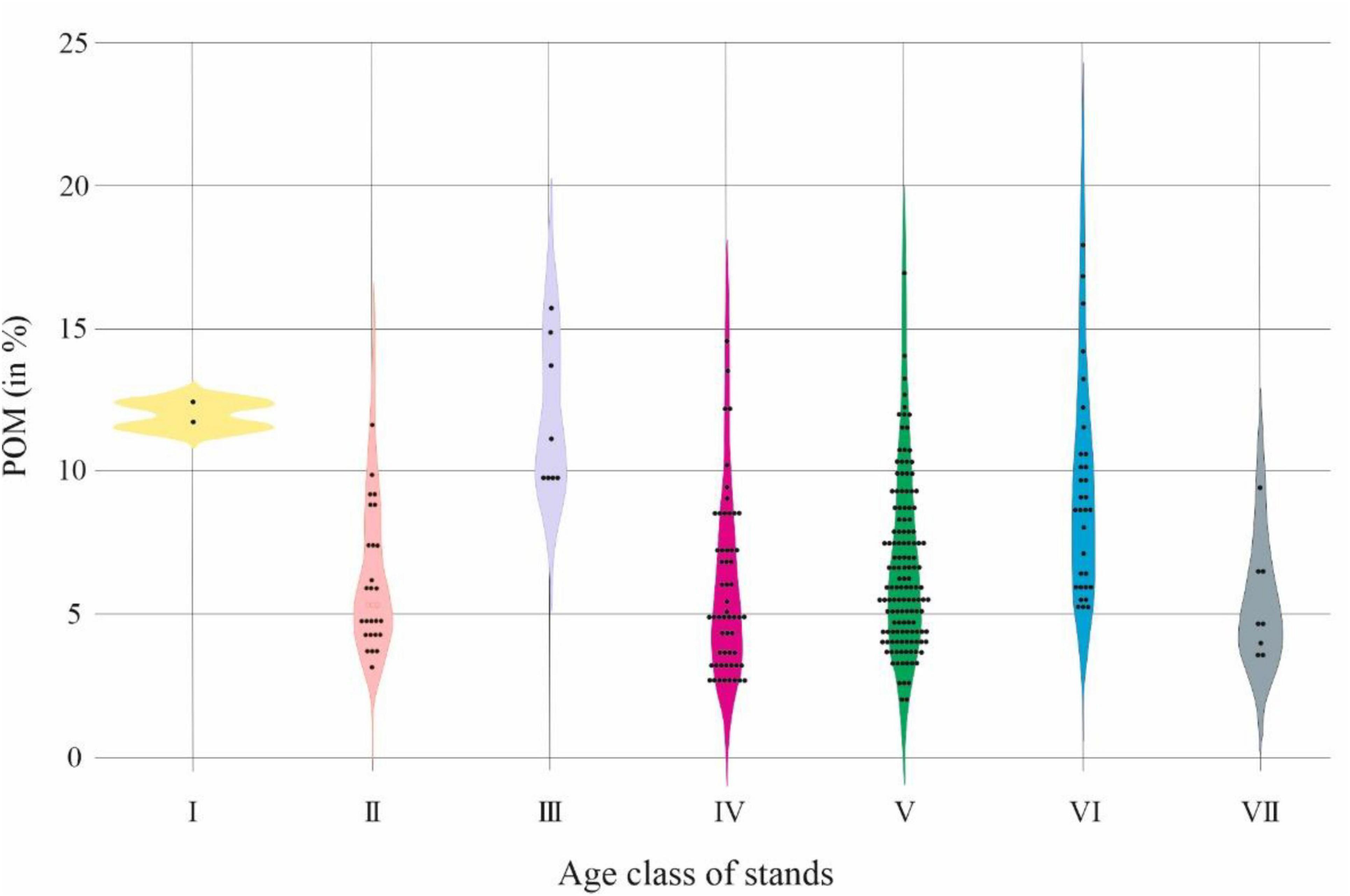
Figure 6. Share of POM (%) in sediments, taking into account the age class of stands (in years): I–up to 20, II–from 21 to 40, III–from 41 to 60, IV–from 61 to 80, V–from 81 to 100, VI–from 101 to 120, VII–120 and more years.
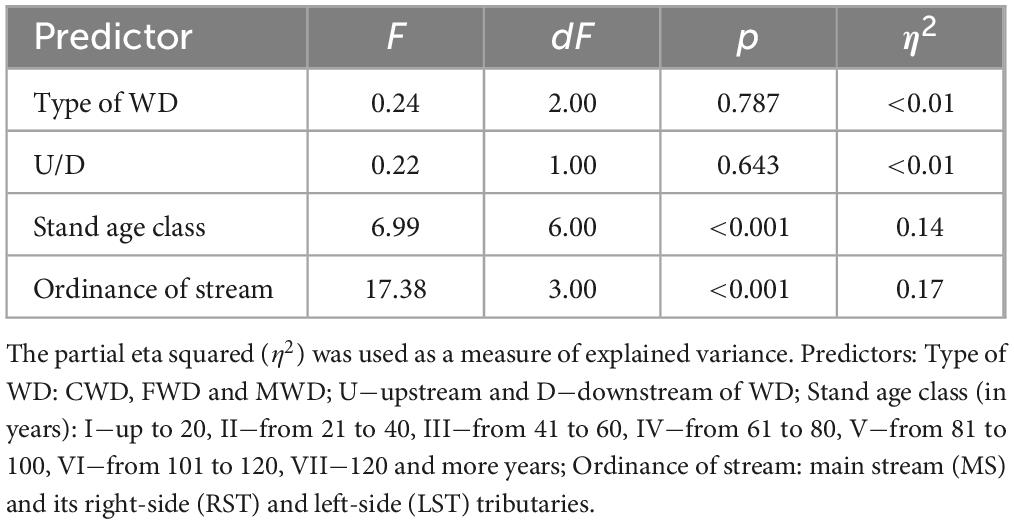
Table 3. The results of the analysis of variance for a particular organic matter (POM) in %, F−F statistic, dF−degrees of freedom, p−relevance.
The Kruskal-Wallis test (at the significance level α = 0.05) confirmed important differences between the functions of distribution of the compared groups. Multiple rank comparison for all attempts indicated important differences in the share of POM accumulated in alluvial sediments sampled upstream (U) and downstream (D) of the woody debris accumulated in the vicinity of stands of age class IV and VI (Figure 6). In the case of sediments sampled in the vicinity of stands of other age classes, differences in the share of POM in sediments were not considered significant statistically.
A significant share of particulate organic matter in alluvial sediments was found in the sediments sampled within the woody debris sites situated in the channel section surrounded by stands of age class III (median: U−9.29%; D−7.98%) and age class IV (median: U−8.77%; D−8.52). In samples of sediments collected on the sites of woody debris deposited in places where slopes are covered by the stand of the oldest age class VII, a reduction was noted in the share of particulate organic matter in sediments (median: U−4.50%; D−5.86%), which may be due to abiotic disorders occurring in the stands. Older forest communities, with a smaller density of trees, and a moderate or intermittent crown closure, are more susceptible to damage, mainly due to the activity of wind.
Broken branches, both large and small, or fragments of whole trees deposited in watercourse channels form a specific kind of dams, or blockages, which reduce the speed of water flowing in the channel, and this facilitates the deposition of transported alluvial sediments and particulate organic matter. Forests of age class VII present in the catchment area of the Roztoka Stream are represented by stands in the class of forest regeneration. These are old trees, growing in large dispersion, with often intensively developing undergrowth, therefore the possibility of delivering tree fragments of significant sizes to the watercourse channel is not highly difficult in this ecosystem.
It was found that the distribution of individual types of woody debris accumulations, including the criterion of the hierarchy of watercourses (the main stream and its tributaries) was diversified. The largest number of sites of fine woody debris (FWD) and mixed woody debris (MWD) are located in watercourse channels in the area of springs (of the first order); characterized by a smaller width and a larger longitudinal inclination (Figure 7). In contrast, in the channel of the main watercourse, i.e., the one with a significantly larger channel width and a smaller terrain inclination, we identified mainly the sites of CWD in the form of logs or their fragments, whose weight and length prevent their transport during floods.
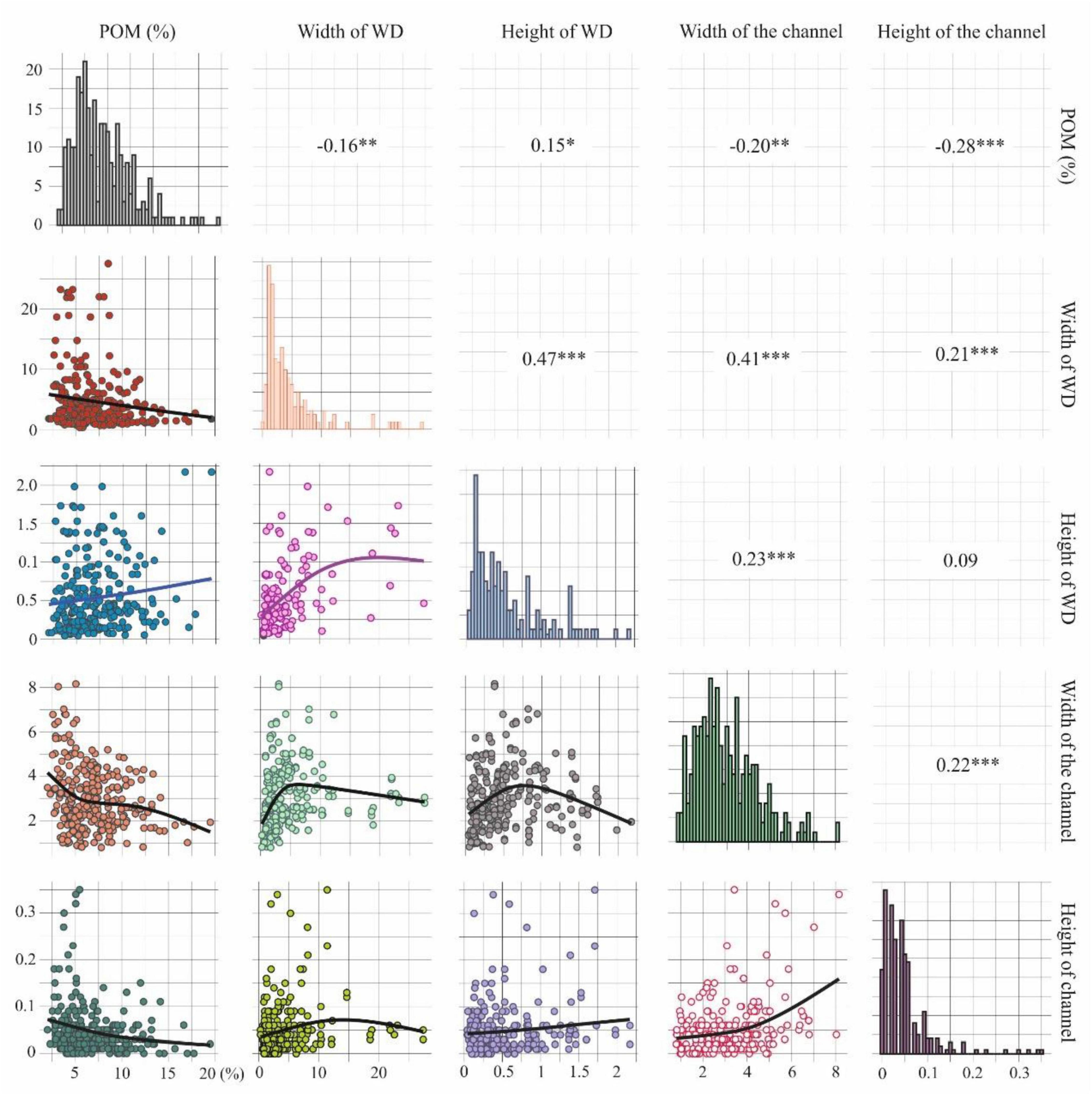
Figure 7. Correlation matrix of the study variables: the lower part of the matrix presents correlation plots of the raw data, the middle part plots the distribution of the variables, while the upper part presents Spearman’s rho correlation coefficients together with the significance of the test *p ≤ 0,05, **p < 0,01, ***p < 0,001.
4 Discussion
Mountain catchments with natural, forested stream channels facilitate WD deposition in the channels and the accumulation of particulate organic matter in alluvial sediments (Roberts et al., 2022).
This study provides strong evidence supporting the hypothesis that woody debris (WD), particularly mixed woody debris (MWD), plays a significant role in influencing the differentiation of alluvial sediments and particulate organic matter (POM) accumulation in the Roztoka Stream. Our findings align with prior research on the ecological and hydrological functions of WD, demonstrating its critical influence on sediment structure and nutrient retention (Gurnell, 2013).
The data indicate that stand age significantly affects the type of WD in the stream channel, with older stands contributing more CWD, which in turn has a greater impact on sediment differentiation (size and shape) and POM accumulation. This research within the Roztoka stream catchment identified over 500 sites of woody debris in stream channels. A larger number of these sites is located in the main channel due to its length and a more diversified morphodynamic structure, which is related, among others, to the occurrence of lateral erosion undercuts and a larger intensity of alluvial processes, especially during events of greater energy (Montgomery and Piégay, 2003).
Our results show that the number and structure of woody debris in the Roztoka’s main and tributary channels are influenced by the age of the stand on the slopes. Older stands, especially those over 100 years old, are characterized by lower crown density and increased deadwood volume. Data on stand density and volume by age class indicate that these older forests are more susceptible to natural disturbances, such as windthrow, which increases the likelihood of deadwood delivery to stream channels (Enrong et al., 2006). Conducting studies that include analysis of tree crown structure and its correlation with WD accumulation in stream channels across other temperate forests would significantly expand our understanding of these dynamics.
The largest quantities of fine organic matter were found in alluvial sediments sampled from channels with stands of age classes III and VI, while the smallest quantity was found in sediments sampled near stands of age class VII, which predominantly contained coarse woody debris (CWD). The increase in the share of fine organic matter in alluvial sediments which is typically due to the falling of leaves is a phenomenon characteristic of forested catchments (King et al., 1987; Delong and Brusven, 1993). Phenophases of tree species in temperate forests, such as beech and fir, affect the seasonal availability of woody debris (Roberts et al., 2022). In Poland, the growing season ranges from 180 days in the northern and central regions to 230 days in the southern part of the country, lasting from mid-October to early December. The end of the growing season is marked by the leaf fall of chestnuts and birches (Bartold, 2018), while beeches and oaks end their growing season somewhat later. Commonly, blockages made of WD, occurring in channels, are regarded as the most effective factor which retains organic matter in mountain streams (Speaker et al., 1984; Webster et al., 1990; Quinn et al., 2007). The catchment of the Roztoka Stream is located in commercial stands (timberlands) where planned silvicultural treatments (e.g., clearing, thinning) involving the removal of trees are carried out. As a result, the number of trees and the associated supply of organic matter in the form of foliage decrease, while the growth of the remaining trees increases due to improved light conditions and greater availability of water and essential nutrients (Bujoczek, 2012). In beech stands from age classes II to V, foliage fall occurs at a similar level. In age classes VI and VII, the renewal process begins, which in the case of beech forests is usually carried out using a shelterwood system. This involves removing trees every 5 years or so over a period of 20 years (Rozwałka, 2003), which reduces the supply of foliage and increases the supply of coarse wood elements (branches, boughs, logs).
The presence of WD in stream channels not only regulates water flow, enhances channel stability, and reduces the energy of floodwaters but also influences sediment retention. This finding is consistent with research conducted in similar mountainous regions, where WD is crucial for creating channel complexity and promoting ecological resilience (Gurnell, 2013). Our results further suggest that WD is essential for sediment differentiation and organic matter accumulation in small forested catchments. In the catchment area of the Roztoka Stream, all of the analyzed sites revealed the presence of coarse-grained deposits, transported in conditions of the so-called upper flow regime, but sediments sampled downstream of woody debris sites were characterized by a smaller diversity of granulometric composition than sediments sampled upstream of these sites. Therefore, these results confirm the research done by other authors (Haschenburger and Rice, 2004; Petts et al., 2000), where it was found that woody debris occurring in channels may influence the differentiation of the characteristics of sediments deposited upstream and downstream of channel blockages. This conclusion is particularly interesting as the present research was done in a small, forested mountain catchment which constitutes the initial section of a larger alluvial system. This supports our first hypothesis that woody debris occurring in the studied channels differentiates the alluvial sediments there (downstream and upstream of woody debris sites), although no large diversity was noted in the granulometric composition of the sediments relative to the type of WD – all sites consisted of coarse-grained deposits, transported by traction.
Factors that influence the location of woody debris deposition, the dominant forms of WD accumulation and the stability of these forms change with the increasing size of the channel (Piégay and Gurnell, 1997). The growing size of the channel also modifies the influence of woody debris on channel morphology (Nakamura and Swanson, 1993). The present research reveals an interesting regularity regarding the diversity of size of the conclusive diameter (d50). For alluvial sediments on the analyzed sites, the values of this diameter decrease along with the growth of the watercourse ordering, mainly due to larger inclinations of the channel in the area of the springs and as a result of mechanical processing of rocks along the longitudinal profile. The only exception is constituted by one watercourse (of the 4th order), where the locally occurring undercuts of lateral erosion supply to the channel some fresh clastic material in the form of larger rock fragments.
In the channels of mountain rivers and streams in which woody debris is deposited in various forms, the activation and transport of the bottom rock debris receives less energy of flood waters than in streams devoid of WD. Getting the rock debris into motion requires more energy, and therefore larger, and at the same time less frequent values of the flow (Assani and Pêtit, 1995). Such rivers and streams are characterized by a smaller intensity of the transport of the bottom rubble, and there is a tendency towards the appearance of various forms of accumulation of bottom material (Smith et al., 1993). The accumulation of coarse-grained sediments affects the dispersion of the flow energy, which translates to processes of transport and erosion in channels (Reid and Hickin, 2008). Wallerstein and Thorne (2004) report that woody debris intercepting the transported sediments significantly contributes to the restoration of riverbed stability, especially in channels with the dominance of deep erosion. The occurrence of woody debris in the channels influences sediment differentiation of the longitudinal channel profile and the location of erosion and deposition zones, principally by aiding to “armor” the channel (Mosley, 1981; Marston, 1982; Curran and Wohl, 2003; Kail, 2003). In stream channels, the presence of woody debris on which the riverbed material is retained allows such channels to easily absorb a large quantity of violently-delivered rubble (e.g., as a result of landslides, rubble flows) and to gradually release this material to a lower section in the alluvial system (Keller et al., 1995). In the Roztoka’s catchment, the characteristics of sediment sorting and sedimentological indicators suggest that these are the sediments of channel armor, transported during floods over short distances and then deposited abruptly, which is evident in their typicaly weak-to-very weak sorting. Despite the small length of the analyzed channels, the role of woody debris significantly affects sediment transport along their longitudinal profile. Coarse-grained sediments are retained within the catchment area and are rarely subject to transfer to the part of the alluvial system situated downstream.
Due to the large number of woody debris, occurring in the channel of the Roztoka Stream and in the channels of its tributaries, sediments are varied in terms of the degree of roundness, despite their similar size. The largest share in the stream rubble belongs to blade-shaped particles (B), the next are disc-shaped particles (D) and rod-shaped ones (R). Spherical particles (S) have the smallest share. Sediments are differentiated in shape also because of their location relative to woody debris and its type. Downstream of the accumulations of coarse woody debris (CWD) in the watercourse channel, the share of blade-shaped particles (B) increases, while downstream of the accumulations of fine woody debris (FWD) there is an increase in the number of rod-shaped particles (R). The type of woody debris accumulations is more differentiated by the shape of particles than by their size. Taking into account the criterion of grain size variation in sandstone, in the case of river rubble particles built of fine-grained sandstone (FG), the dominant particles are blade-shaped (B), whereas in the case of coarse-grained sandstone (CG), disc-shaped particles (D) have a dominant share. The results obtained in this study regarding the grain size of sediments deposited upstream and downstream of WD sites are consistent with the findings of other authors (Keller et al., 1995; Montgomery et al., 2003) and demonstrate that WD accumulation in the stream channel promotes differentiation in fluvial sediment characteristics. The grain sizes of alluvial sediments collected upstream of WD sites were generally larger than those of sediments collected downstream of WD. Roundness is extremely sensitive to abrasion, and angular particles change rapidly in their roundness during bedload transport (Krumbein, 1941). Due to the abundance of WD in the channel and the selective transport of sediment over short distances, it is highly difficult for flat and sharp-edged particles to achieve a spherical shape. The proportion of flat-shaped grains downstream of WD sites was similar, which may be because these particles, unlike spherical ones, are far more difficult to incorporate into sediment transport (Giriat et al., 2010). Our results demonstrate that the presence of WD significantly alters sediment transport dynamics by creating zones of deposition and reducing flow velocity. This confirms the findings of Wohl and Scott (2016), who showed that WD enhances channel roughness and retains coarse sediments. In the Roztoka stream catchment, these effects are particularly pronounced in areas with mixed WD, which supports more diverse sediment granulometry.
The presented study did not track the precise origin of WD or how it was delivered to the Roztoka stream channel, nor did it examine temporal changes in WD input. Future research could involve long-term monitoring of WD dynamics to better understand the spatial and temporal distribution of WD in forested catchments. Additionally, expanding the study to include more detailed measurements of stand structure, such as canopy density, would provide valuable insights for forest management.
The variation in POM accumulation with stand age has direct implications for nutrient cycling and stream habitat quality. Older stands, which contribute more coarse woody debris (CWD), also facilitate greater organic matter retention, enhancing microbial activity and supporting benthic communities (Hoover et al., 2010). This organic matter provides a continuous source of nutrients, contributing to higher biodiversity in streams. Further research could explore how these dynamics influence carbon sequestration in temperate forest streams.
The presence of WD in streams increases bed roughness, modifies water flow velocity, and promotes water retention, which is particularly important in small forested catchments that are part of larger river systems. WD in streams plays a significant role in the functioning of the hyporheic zone -the interface between groundwater and surface water, which is crucial for the functioning of certain types of aquatic ecosystems. WD influences fluvial processes and promotes the accumulation of POM in sediments, which can significantly contribute to carbon deposition in the environment. Additionally, WD in streams enhances habitat diversity within the stream itself as well as in adjacent areas.
Given the hydrological and ecological importance of WD in streams, proper management is recommended, including avoiding the removal of WD from stream channels and allowing trees in riparian zones to age and die naturally to ensure a continuous supply of WD to the stream.
5 Conclusion
The present research results confirm that woody debris deposited in the watercourse channel affects the differentiation of alluvial sediment features. The granulometric composition of alluvial sediments has a dominant share of gravels and stones and a smaller share of the sandy fraction. Sediments sampled downstream of woody debris sites are characterized by a smaller diversity of granulometric composition than sediments collected upstream of these sites. Most sites of fine and mixed woody debris are located in the stream section located in the area of springs, characterized by a smaller channel width and a larger longitudinal inclination. In contrast, in the main watercourse channel, whose width is much larger and whose longitudinal inclination is smaller, the dominant sites are those consisting of coarse woody debris in the form of tree trunks whose weight and length prevent transport during floods. It was found that the age of the stand growing on the valley slopes does affect the structure of woody debris supplied to the watercourse channel, which constitutes important information from the perspective of watercourse hydromorphology. In channel sections located in the surroundings of forest of age class I, there prevail mixed-type woody debris sites (MWD); in the surroundings of forest of age class IV, there is an increased share of coarse and mixed-type woody debris; while in the case of stands of age class VII, there prevail coarse woody debris sites (CWD). Stand age also affects the accumulation of particulate organic matter in sediments. The largest quantity of particulate organic matter (POM) was found in alluvial sediments sampled from the stream channel within the forest of age classes III and VI; the smallest quantity of POM was noted in age class VII. Most of the particulate organic matter was found in sediments collected upstream of woody debris sites located in tributaries, and the smallest amount in sediments sampled in the main stream channel. These research results extend the knowledge of the significance of woody debris deposition in stream channels for the differentiation of alluvial sediment characteristics as well as the knowledge of the role of woody debris in forming the alluvial sediment circulation in small forested mountain catchments.
Data availability statement
The raw data supporting the conclusions of this article will be made available by the authors, without undue reservation.
Author contributions
ES-O: Conceptualization, Formal analysis, Investigation, Methodology, Resources, Validation, Visualization, Writing – original draft, Writing – review and editing. AM: Formal analysis, Methodology, Validation, Visualization, Writing – original draft, Writing – review and editing. AJ: Investigation, Visualization, Writing – original draft.
Funding
The author(s) declare financial support was received for the research, authorship, and/or publication of this article. This research was funded by the President of the University of Agriculture in Krakow.
Acknowledgments
We express their gratitude to the editorial board and reviewers for the efforts for suggestion and reviewing this paper. We also appreciate the editor for his cooperation during the review process.
Conflict of interest
The authors declare that the research was conducted in the absence of any commercial or financial relationships that could be construed as a potential conflict of interest.
Publisher’s note
All claims expressed in this article are solely those of the authors and do not necessarily represent those of their affiliated organizations, or those of the publisher, the editors and the reviewers. Any product that may be evaluated in this article, or claim that may be made by its manufacturer, is not guaranteed or endorsed by the publisher.
References
Akcay, I., Tugrul, S., and Ozhan, K. (2022). Effects of river inputs on particulate organic matter composition and distributions in surface waters and sediments of the Mersin Bay, Northeastern Mediterranean Sea. Reg. Stud. Marine Sci. 52:102316. doi: 10.1016/j.rsma.2022.102316
Assani, A., and Pêtit, F. (1995). Log-jam effects on bedload for experiment conducted in the small gravel-bed forest ditch. Catena 25, 117–126. doi: 10.1016/0341-8162(95)00004-C
Bala, E. (2016). Textural features of the beach sediments of Wast Water Lake, Northwest England. Miscellanea Geogr. 20, 46–53. doi: 10.1515/mgrsd-2016-0013
Bartold, M. (2018). Determining the onset and the end of the growing season of the broad-leaved forests over Poland using long-term SPOT Vegetation and Proba-V satellite observations. Wydawnictwa Uniwers. Warszawskiego 63, 7–31.
Beckman, N. D., and Wohl, E. (2014). Carbon storage in mountainous headwater streams: The role of old-growth forest and logjams. Water Resour. Res. 50, 2376–2393. doi: 10.1002/2013WR014167
Błaś, M., and Ojrzyńska, H. (2024). “The climate of Poland,” in Landscapes and landforms of Poland. World geomorphological landscapes, eds P. Migoń and K. Jancewicz (Cham: Springer), doi: 10.1007/978-3-031-45762-3_3
Braudrick, C. A., and Grant, G. E. (2000). When do logs move in rivers? Water Resour. Res. 36, 571–583. doi: 10.1029/1999WR900290
Bujoczek, L. (2012). Decomposition of coarse woody debris in forest ecosystems with particular reference to spruce, beech and fir. Sylwan 156, 208–217.
Bylak, A. (2018). Wpływ niskich barier na ichtiofaunę – przykłady z potoków Karpackich. Rocz. Nauk. PZW 31, 5–19. doi: 10.12823/sapaa.0860-648X.18001
Cadol, D., and Wohl, E. (2011). Coarse sediment movement in the vicinity of a logjam in a neotropical gravel-bed stream. Geomorphology 71, 48–60. doi: 10.1016/j.geomorph.2011.01.007
Crisp, D. T. (2000). Trout and Salmon: Ecology, conservation and rehabilitation. Oxford: Blackwell, 224.
Curran, J., and Wohl, E. (2003). Large woody debris and flow resistance in step-pool channels, Cascade Range, Washington. Geomorphology 51, 141–157. doi: 10.1016/S0169-555X(02)00333-1
Delong, M. D., and Brusven, M. A. (1993). Storage and decomposition of particulate organic matter along the longitudinal gradient of an agriculturally-impacted stream. Hydrobiologia 262, 77–88. doi: 10.1007/BF00007508
Enrong, Y., Xihua, W., and Jianjun, H. (2006). Concept and classification of coarse woody debris in forest ecosystems. Front. Biol. China 1:76–84. doi: 10.1007/s11515-005-0019-y
Folk, R. L., and Ward, W. C. (1957). Brazos River bar: A study in the significance of grain size parameters. J. Sediment. Pet. 27, 3–26.
Fox, J., and Weisberg, S. (2019). An R companion to applied regression, 3rd Edn. Thousand Oaks CA: Sage Publications Ltd.
Galia, T., and Hradecký, J. (2014). Morphological patterns of headwater streams based in flysch bedrock: Examples from the outer western carpathians. Catena 119, 174–183. doi: 10.1016/j.catena.2014.02.013
Galia, T., Ruiz-Villanueva, V., Tichavský, R., Šilhán, K., Horáček, M., and Stoffel, M. (2018). Characteristics and abundance of large and small instream wood in a Carpathian mixed-forest headwater basin. Forest Ecol. Manag. 424, 468–482. doi: 10.1016/j.foreco.2018.05.031
Gippel, C. (1995). Environmental hydraulics of large woody debris in streams and rivers. J. Environ. Eng. 121, 388–395. doi: 10.1061/(ASCE)0733-9372(1995)121:5(388)
Giriat, D., Kosieradzka, K., and Roszczęda, U. (2010). Ułożenie i kształt otoczaków a mikroformy korytowe typu pebblecluster. Acta Sci. Polonor. Form. Circumiectus 9, 3–22.
Gregory, S. V., Boyer, K. L., and Gurnell, A. M. (2003). Ecology and management of wood in world rivers, American fisheries society symposium 37. Bethesda, MD: American Fisheries Society, 431.
Gurnell, A. M., Gregory, K. J., and Petts, G. E. (1995). The role of coarse woody debris in forest aquatic habitats: Implications for management. Aquat. Conserv. Mar. Freshwat. Ecosyst. 5, 143–166. doi: 10.1002/aqc.3270050206
Haschenburger, J. K., and Rice, S. P. (2004). Changes in woody debris and bed material texture in a gravel-bed channel. Geomorphology 60, 241–267. doi: 10.1016/j.geomorph.2003.08.003
Heitmuller, F. T., and Hudson, P. F. (2009). Downstream trends in sediment size and composition of channel-bed, bar, and bank deposits related to hydrologic and lithologic controls in the Llano River watershed, central Texas, USA. Geomorpology 112, 246–260. doi: 10.1016/j.geomorph.2009.06.010
Hoover, T. M., Marczak, L. B., Richardson, J. S., and Yonemitsu, N. (2010). Transport and settlement of organic matter in small streams. Freshwater Biol. 55, 436–449. doi: 10.1111/j.1365-2427.2009.02292.x
Kail, J. (2003). Influence of large woody debris on the morphology of six central European streams. Geomorphology 51, 207–223. doi: 10.1016/S0169-555X(02)00337-9
Kałuża, T., and Radecki-Pawlik, A. (2014). Influence of coarse and fine plant debris on river channel hydrodynamics. Acta Sci. Pol. Formatio Circumiectus 13, 67–80.
Keller, E. A., and Swanson, F. J. (1979). Effects of large organic material on channel form and fluvial processes. Earth Surf. Process. Landf. 4, 361–380.
Keller, E. A., MacDonald, A., Tally, T., and Merrit, N. J. (1995). Effects of large organic debris on channel morphology and sediment storage in selected tributaries of Redwood Creek, northwestern California. US Geol. Surv. Prof. Pap. 1454, 1–29.
King, J. M., Day, J. A., Davies, B. R., and Henshald-Howard, M. P. (1987). Particulate organic matter in a mountain stream in the south-western Cape South Africa. Hydrobiologia 154, 165–187.
Krumbein, W. C. (1941). The effects of abrasion on the size, shape and roundness of rock fragments. J. Sediment. Res. 11, 64–72. doi: 10.1306/D42690F3-2B26-11D7-8648000102C1865D
Książek, L., Michalik, A., and Śladowski, T. (2008). Skład uziarnienia rumowiska jako wskaźnik lokalnej degradacji koryta rzecznego. Acta Sci. Pol. Form. Circumiectus 7, 3–12.
Lemly, A. D., and Hilderbrand, R. H. (2000). Influence of large woody debris on stream insect communities and benthic detritus. Hydrobiologia 421, 179–185.
Lenth, R., Singmann, H., Love, J., Buerkner, P., and Herve, M. (2019). Estimated marginal means, aka least-squares means. R package, ver. 1.2. Available online at: https://CRAN.R-project.org/package=emmeans
Ludwikowska-Kędzia, M. (2000). Evolution of the middle segment of the Belnianka river valley in the late glacial and Holocene. Warsaw: Dialog Press, 180.
Malarz, R. (2004). The rate of gravel abrasion in the Carpathian rivers. Geogr. Časopis. 56, 99–109.
Marston, R. A. (1982). The geomorphic significance of log steps in forest streams1. Ann. Assoc. Am. Geogr. 72, 99–108. doi: 10.1111/j.1467-8306.1982.tb01386.x
Merten, E. C., Finlay, J., Johnson, L., Newman, R., Stefan, H., and Vondracek, B. (2010). Factors influencing wood mobilization in streams. Water Resour. Res. 46:W10514. doi: 10.1029/2009WR008772
Mikos, M. (1994). “The downstream fining of gravel-bed sediments in the Alpine Rhine River. Earth Science,” in Dynamics and geomorphology of mountain rivers, Vol. 52, eds P. Ergenzinger and K. H. Schmidt (Berlin: Springer Verlag).
Montgomery, D. R., and Buffington, J. M. (1997). Channel reach morphology in mountain drainage basins. Geol. Soc. Am. Bull. 109, 596–611. doi: 10.1130/0016-7606(1997)109<0596:CRMIMD>2.3.CO;2
Montgomery, D. R., and Piégay, H. (2003). Wood in rivers: Interactions with channel morphology and processes. Geomorphology 51, 1–5.
Montgomery, D. R., Massong, T. M., and Hawley, S. C. S. (2003). Influence of debris flows and log jams on the location of pools and alluvial channel reaches. Oregon Coast Range 115, 78–88. doi: 10.1130/0016-7606(2003)115<0078:IODFAL>2.0.CO;2
Mosley, M. P. (1981). The influence of organic debris on channel morphology and bedload transport in a New Zealand forest stream. Earth Surf. Process. Landf. 6, 571–579. doi: 10.1002/esp.3290060606
Mycielska-Dowgiałło, E. (2007). “Research methods fortextural features of clastic deposits and the significance of interpre ational results (in Polish with English summary),” in Badania cech teksturalnych osadów czwartorzędowych i wybrane metody oznaczania ich wieku, eds E. Mycielska-Dowgiałło and J. Rutkowski (Warszawa: WSWPR), 95–189.
Myślińska, E. (2016). Laboratoryjne badania gruntów i gleb. Warszawa: Wydawnictwo Uniwersytetu Warszawskiego.
Nakamura, F., and Swanson, F. J. (1993). Effects of coarse woody debris on morphology and sediment storage of a mountain stream system in western Oregon. Earth Surf. Process. Landforms 18, 43–61. doi: 10.1002/esp.3290180104
Nickolotsky, A., and Pavlowsky, R. T. (2007). Morphology of step-pools in a wilderness headwater stream: The importance of standardizing geomorphic measurements. Geomorphology 83, 294–306. doi: 10.1016/j.geomorph.2006.02.025
Osei, N. A., Gurnell, A. M., and Harvey, G. L. (2015). The role of large wood in retaining fine sediment, organic matter and plant propagules in a small, single-thread forest river. Geomorphology 235, 77–87. doi: 10.1016/j.geomorph.2015.01.031
Petts, G. E., Gurnell, A. M., Gerrard, A. J., Hannah, D. M., Hansford, B., Morrisey, I., et al. (2000). Longitudinal variations in exposed riverine sediments: A context for the ecology of the Fiume Tagliamento, Italy. Aquat. Conserv. Mar. Freshwat. Ecosyst. 10, 249–266. doi: 10.1002/1099-0755(200007/08)10:4<249::AID-AQC410<3.0.CO;2-R
Piégay, H., and Gurnell, A. M. (1997). Large woody debris and river geomorphological pattern: Examples from SE France and S. England. Geomorphology 19, 99–116. doi: 10.1016/S0169-555X(96)00045-1
PUL (2015). Plan urządzenia lasu dla Nadleśnictwa Andrychów: Program ochrony przyrody. Available online at: https://www.gov.pl/attachment/a033b81f-47ad-4c48-8830-2222fe9b16d8
Quinn, J. M., Phillips, N. R., and Parkyn, S. M. (2007). Factors influencing retention of coarse particulate organic matter in streams. Earth Surface Process. Landforms 32, 1186–1203. doi: 10.1002/esp.1547
R Core Team (2020). R: A language and environment for statistical computing. Vienna: R Foundation for Statistical Computing.
Reid, D. E., and Hickin, E. J. (2008). Flow resistance in steep mountain streams. Earth Surf. Process. Landforms 33, 2211–2240. doi: 10.1002/esp.1682
Roberts, B. J., Griffiths, N. A., Houser, J. N., and Mulholland, P. J. (2022). Response of stream metabolism to coarse woody debris additions along a catchment disturbance gradient. Ecosystems 25, 828–842. doi: 10.1007/s10021-021-00687-9
Rozwałka, Z. (2003). Zasady hodowli lasu: PGL Lasy Państwowe. Warszawa: Ośrodek Rozwojowo-Wdrożeniowy Lasów Państwowych w Bedoniu. Available online at: https://cygan.ucoz.ru/LG/ZHL.pdf
Słowik-Opoka, E., Wrońska-Wałach, D., and Michno, A. (2018). Analysis of sediment from steps in a small catchment in the Polish Carpathians in relation to the transition zone between the hillslope and fluvial system. Catena 165, 237–250. doi: 10.1016/j.catena.2018.01.036
Smith, R. D., Sidle, R. C., and Porter, P. E. (1993). Effects on bedload transport of experimental removal of woody debris from a forest gravel-bed stream. Earth Surface Process. Landforms 35, 435–446. doi: 10.1002/esp.3290180507
Speaker, R., Moore, K., and Gregory, S. (1984). Analysis of the processes of retention of organic matter in stream ecosystems. Verhandlungen Int. Verein Limnol. 22, 1835–1841. doi: 10.1080/03680770.1983.11897582
Stelzer, R. S., Scott, J. T., Bartsch, L. A., and Parr, T. B. (2014). Particulate organic matter quality influences nitrate retention and denitrification in stream sediments: Evidence from a carbon burial experiment. Biogeochemistry 119, 387–402. doi: 10.1007/s10533-014-9975-0
Swanson, F. J., Johnson, S. L., Gregory, S. V., and Acker, S. A. (1998). Flood disturbance in a forested mountain landscape. Bioscience 48, 681–689. doi: 10.2307/1313331
Verdonschot, P. F. M., and Verdonschot, R. C. M. (2024). Ecological functions and management of large wood in fluvial systems. Curr. For. Rep. 10, 39–55. doi: 10.1007/s40725-023-00209-x
Wallerstein, N. P., and Thorne, C. R. (2004). Influence of large woody debris on morphological evolution of incised, sand-bed channels. Geomorphology 57, 53–73. doi: 10.1016/S0169-555X(03)00083-7
Wang, H., Tetzlaff, D., Buttle, J., Carey, S. K., Laudon, H., McNamara, J. P., et al. (2019). Climate-phenology-hydrology interactions in northern high latitudes: Assessing the value of remote sensing data in catchment ecohydrological studies. Sci. Total Environ. 656, 19–28. doi: 10.1016/j.scitotenv.2018.11.361
Ward, G. M., and Aumen, N. G. (1986). Woody debris as a source of fine particulate organic matter in coniferous forest stream ecosystems. Can. J. Fish. Aquat. Sci. 43, 1635–1642. doi: 10.1139/f86-202
Webster, J. R., Golladay, S. W., Benfield, E. F., D’Angelo, D. J., and Peters, G. T. (1990). Effects of forest disturbance on particulate organic matter budgets of small streams. J. North Am. Benthol. Soc. 9, 120–140. doi: 10.2307/1467446
Wohl, E., and Scamardo, J. (2022). Patterns of organic matter accumulation in dryland river corridors of the southwestern United States. Sci. Total Environ. 833:155136. doi: 10.1016/j.scitotenv.2022.155136
Wohl, E., and Scott, D. N. (2016). Wood and sediment storage and dynamics of river corridors. Earth Surf. Process. Landf. 42, 5–23. doi: 10.1002/esp.3909
Wohl, E., Uno, H., Dunn, S. B., Kemper, J. T., Marshall, A., Means-Brous, M., et al. (2023). Why wood should move in rivers. River Res Appl. 56, 1–12. doi: 10.1002/rra.4114
Wyżga, B., Kaczka, R. J., and Zawiejska, J. (2003). Gruby rumosz drzewny w ciekach górskich: Formy występowania, warunki depozycji i znaczenie środowiskowe. Folia Geogr. Ser. Geogr. Phys. 3, 117–138.
Wyżga, B., Kaczka, R., and Zawiejska, J. (2012). Zróżnicowanie depozycji grubego rumoszu drzewnego w ciekach górskich o średniej i dużej szerokości. Prace Stud. Geogr. 50, 159–169.
Zhou, L., Dai, L., Gu, H., and Zhong, L. (2007). Review on the decomposition and influence factors of coarse woody debris in forest ecosystem. J. For. Res. 18, 48–54. doi: 10.1007/s11676-007-0009-9
Zielony, R., and Kliczkowska, A. (2012). Regionalizacja przyrodniczo-leśna polski 2010. CILP. Available online at: https://www.lasy.gov.pl/pl/pro/publikacje/copy_of_gospodarka-lesna/hodowla/regionalizacja-przyrodniczo-lesna-polski-2010-1/view
Zimmermann, A., and Church, M. (2001). Channel morphology, gradient profiles and bed stresses during flood in a step - pool channel. Geomorphology 40, 311–327. doi: 10.1016/S0169-555X(01)00057-5
Keywords: temperate forest, woody debris, stand age, alluvial sediments, organic matter
Citation: Słowik-Opoka E, Michno A and Jarosz A (2024) Effects of woody debris on alluvial sediment differentiation and particulate organic matter accumulation in a mountainous forest stream in the Polish Carpathians. Front. For. Glob. Change 7:1456283. doi: 10.3389/ffgc.2024.1456283
Received: 28 June 2024; Accepted: 17 September 2024;
Published: 30 September 2024.
Edited by:
John T. Van Stan, Cleveland State University, United StatesReviewed by:
Christopher Surfleet, California Polytechnic State University, United StatesSeyed Mohammad Moein Sadeghi, University of Florida, United States
Copyright © 2024 Słowik-Opoka, Michno and Jarosz. This is an open-access article distributed under the terms of the Creative Commons Attribution License (CC BY). The use, distribution or reproduction in other forums is permitted, provided the original author(s) and the copyright owner(s) are credited and that the original publication in this journal is cited, in accordance with accepted academic practice. No use, distribution or reproduction is permitted which does not comply with these terms.
*Correspondence: Ewa Słowik-Opoka, ewa.opoka@urk.edu.pl