- 1United States Department of Agriculture (USDA) Forest Service, Office of Sustainability and Climate, Olympia, WA, United States
- 2USDA Forest Service, Northern Institute of Applied Climate Science, Duluth, MN, United States
- 3USDA Forest Service, State, Private, and Tribal Forestry, West Lafayette, IN, United States
- 4USDA Forest Service, Eastern Region, Milwaukee, WI, United States
- 5USDA Forest Service, Northern Research Station, Grand Rapids, MI, United States
- 6USDA Forest Service, Northern Research Station, Irvine, PA, United States
- 7USDA Forest Service, Forest Management, Washington, DC, United States
- 8USDA Forest Service, Forest Management, Lytle Creek, CA, United States
- 9USDA Forest Service, Office of Sustainability and Climate, St. Paul, MN, United States
Recent and projected changes in climate over this century pose an unprecedented threat to the health, diversity, and productivity of forest ecosystems. Forests have migrated and adapted to long-term changes in climate over thousands to millions of years; however, natural migration rates and adaptive responses of tree populations cannot match the rapid pace of current climate change. Consequently, more climate-informed approaches to reforestation are needed as current reforestation strategies using local seed sources may no longer be adequate to meet forest management objectives. Assisted migration is a climate change adaptation technique that can help maintain the ecosystem services and economic value that forests provide. Forestry assisted migration (FAM) focusses on the movement of populations of widespread, commercially, or ecologically important forest tree species within or just beyond their current ranges as a way to maintain forest productivity and health in the face of climate change. Although the forestry community recognizes FAM as a reforestation tool, guidance for planning and implementation of FAM is lacking and a framework that provides this guidance can prove useful to land managers with limited time and resources available who want to undertake FAM. We developed a practical framework (the FAM Framework) to provide a structured approach to ensure the most important considerations and best available science are utilized by land managers wanting to implement FAM on their land base. The FAM Framework incorporates multiple factors for the application of FAM in four sequential phases: assessment and analysis, climate-based plant material selection, seed procurement and deployment, and documentation and monitoring. The FAM Framework was tested by developing an assisted migration plan for the Superior National Forest, Minnesota (MN), and lessons learned from the development of this specific plan were used to revise and improve the FAM Framework for suitability across all lands. While originally designed to meet the needs of National Forest System land managers, it is relevant and applicable across the spectrum of land ownership because it incorporates consideration of critical elements in planning and implementing FAM on any landscape while facilitating adaptive management for active learning and future implementation.
1 Introduction
There is overwhelming evidence that the earth’s climate is changing at a rate unprecedented in the last 2,000 years (USGCRP, 2023). Mean global surface temperatures have increased by ∼1.1°C from 1850–1900 to 2011–2020 and are projected to increase by 1.4 to 4.4°C by 2100 (IPCC, 2023). The rapid rate and magnitude of these climatic shifts may exceed both the adaptive capacity of local tree populations and rates of natural seed and pollen dispersal to more climatically appropriate regions (Aitken et al., 2008). As tree populations become increasingly stranded in unsuitable climates, forest health and the sustained provisioning of ecosystem services are at risk (Allen et al., 2010; van Mantgem et al., 2009). The harmful impacts of climate change on forest ecosystems are already evident in forest health declines and tree mortality episodes (Anderegg et al., 2012; Betzen et al., 2021; Breshears et al., 2005; Fettig et al., 2019; Hartmann et al., 2022; Hennon et al., 2016; Mohan et al., 2009; van Mantgem et al., 2009). Moreover, in some locations long-term forest inventory data and modeling exercises both suggest seedling recruitment at range margins lags behind climatically suitable habitat shifts, further constraining the ability of forests to adapt and shift in response to climatic changes (Boisvert-Marsh et al., 2022; Dobrowski et al., 2015; Woodall et al., 2018; Zhu et al., 2012).
Tree planting is the primary tool for re-aligning populations and species with shifting climatic conditions on the landscape to sustain healthy and productive forest ecosystems. In the face of climate change, conventional regeneration strategies, including a reliance on natural regeneration or locally sourced seedlings grown as nursery stock, may no longer be adequate, (Hancock et al., 2023). Forest geneticists have recognized the need to adapt reforestation and seed movement guidelines in the face of changing climates for over 30 years (Ledig and Kitzmiller, 1992). Because natural selection reduces the prevalence of genotypes that are poorly adapted to their local environment, most plant populations, especially those of long-lived trees, are usually considered to be locally adapted (Linhart and Grant, 1996). Foresters recognized this early on after plantation failures with non-local seed and developed seed movement guidelines and seed zones to ensure the use of local seed sources—operating under a “local is best” paradigm (Buck et al., 1970; Cunningham, 1975; Fowells, 1946; McCall, 1939; Pike et al., 2020; Randall, 1996; Randall and Berrang, 2002; Rudolph, 1956; Schmidtling, 2001; Schubert and Pitcher, 1973.).
Increasing evidence suggests that the rate of climate change relative to the long lifespan of trees imparts an intergenerational adaptation lag where individuals are better adapted to the climate in which they germinated, decades to centuries in the past, than contemporary climatic conditions (Rehfeldt et al., 2012). For example, Gray and Hamann (2013) found that due to climate change that has occurred since the reference period of 1961–1990, climatic niches for 15 tree species of major commercial value in western North America, lagged behind their optimum by an average of 130 km or approximately 1.5°C. They projected that this lag would more than double before the mid-21st century. The assumption that local is best may still be conceptually valid but interpreting “local” in the wide-sense rather than the strict sense (Alía et al., 2022). This means using materials from locations with similar climates even if they are geographically distant, rather than using materials only from at or near the deployment site. Therefore, climate change creates an opportunity to reevaluate and potentially decouple seed collection zones and seed deployment zones through the use of assisted migration.
Assisted migration is a general term for the deliberate movement of genotypes, populations, or species to locations or areas outside of their current ranges to maintain biological diversity or ecosystem function in response to climate change (Richardson et al., 2009; Schwartz et al., 2012). Assisted migration actions can be divided into three broad categories: assisted population migration (APM), assisted range expansion (ARE), and assisted species migration (ASM) (Williams and Dumroese, 2013; Figure 1). Contemporary forestry assisted migration (FAM) (Pedlar et al., 2012), focusses on APM and ARE, whereas ASM moves species well outside of their native ranges (Figure 1). Although ASM may be used in targeted circumstances, [for example in a research context (Nagel et al., 2017; Palik et al., 2022; Royo et al., 2023) or for the conservation of a rare, threatened, or endangered species (USFWS, 2024)], it is generally not under broad consideration by the United States Forest Service (USFS) at the landscape scale because it is the most controversial of the three assisted migration types with the highest possible risks (Figure 1). However, it should be noted that due to the lack of standardized terminology for assisted migration the distinction between range expansion and species migration is not clear-cut due to uncertainty on locations of species range limits and varying opinions on what constitutes a natural migration barrier for a given species, or what distance is great enough to make an action ASM. The focus of the framework presented here is on APM and ARE, but this does not necessarily preclude movements that are bordering on species migration when appropriate.
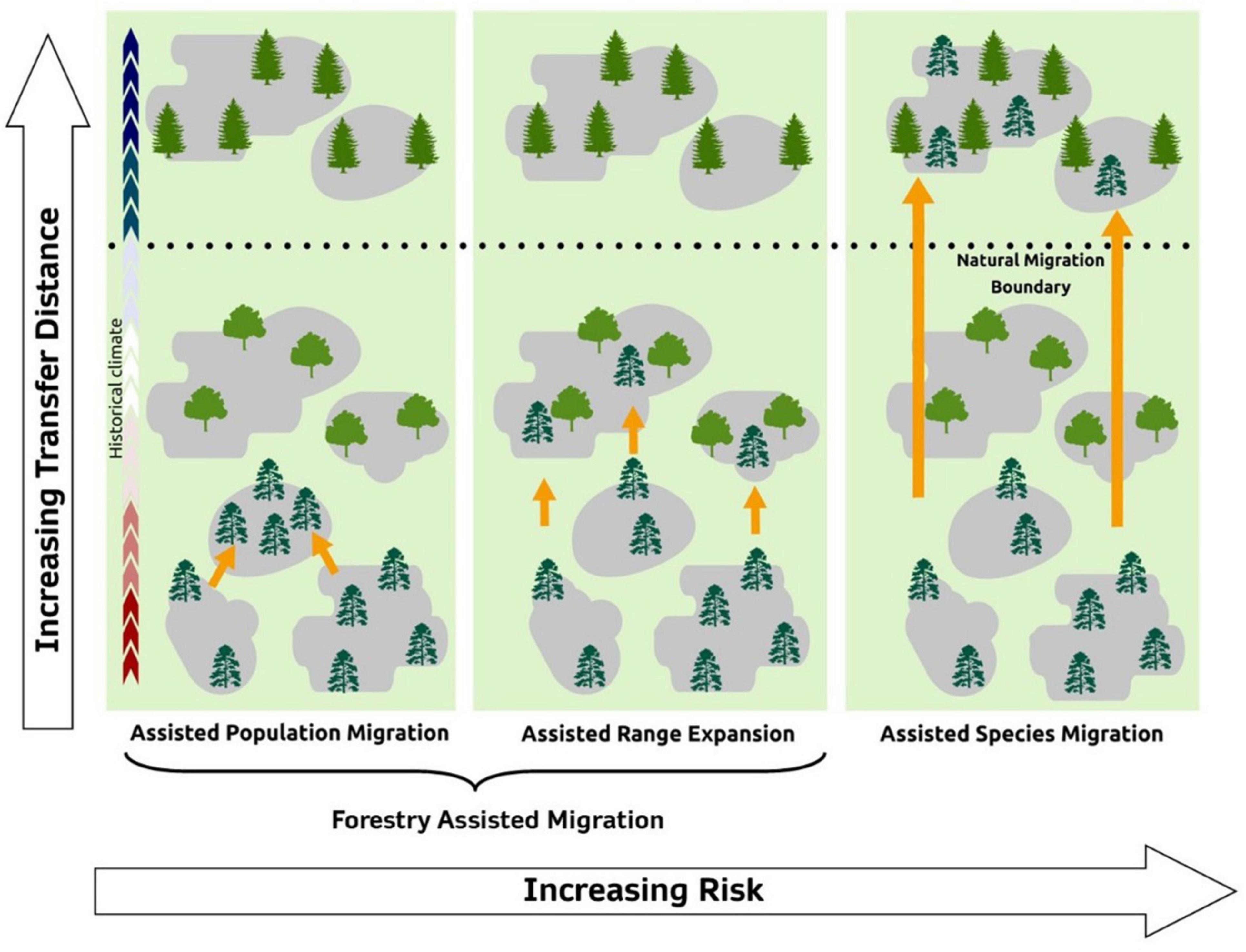
Figure 1. Three types of assisted migration where different tree symbols represent different species ranges with historical climates ranging from warm (red) to cool (blue). Gray areas represent tree populations and colored arrows indicate human assisted movement of plant materials. Risks associated with assisted migration generally increases with transfer distance (from USDA Climate Hubs, 2024a).
Discussions of the various potential benefits and risks of assisted migration are covered in depth elsewhere both generally (Hunter, 2007; McLachlan et al., 2007; Ricciardi and Simberloff, 2009a,b; Stanturf et al., 2024; Vitt et al., 2009; Vitt et al., 2010; Xu and Prescott, 2024) and specific to FAM (Palik et al., 2022; Pedlar et al., 2012). In brief, by utilizing seed sources pre-adapted to projected future climates, FAM seeks to maintain forest health and productivity in the face of climate change. This is accomplished by reducing the likelihood of maladaptation and thereby sustaining the full range of ecosystem services that forests provide such as wildlife habitat, erosion prevention, and carbon uptake and storage (Pedlar et al., 2012; Xu and Prescott, 2024). Healthy forests also take up and store more carbon than unhealthy forests and, thus, are a powerful tool counteracting atmospheric carbon dioxide (CO2) increases that cause climate change (Bastin et al., 2019). Therefore, it is important that tree planting campaigns are conducted in a climate-informed manner. Climate-informed reforestation explicitly incorporates the best available science to plan for, monitor, tend, and adaptively manage naturally seeded or planted tree seedlings so they successfully establish under current climates and persist under a range of projected future climates (USDA Forest Service, 2024a). It considers both challenges and opportunities to foster resilient, healthy, and productive forests that sustain ecosystem services into the future. FAM is one element of climate-informed reforestation that can help to ensure that the right tree (both source population and species) is planted in the right place at the right time. Assisted migration is not without risks, however, with the type and degree of risk varying among the three types of assisted migration (Figure 1). There is also an inherent risk in doing nothing resulting in maladaptation and reduced forest health, diversity, and productivity; so there are risks on all sides of the assisted migration debate.
There is a growing literature on FAM covering its potential benefits, risks, and implementation in large-scale forest management operations and in research (Benomar et al., 2022; Twardek et al., 2023; Xu and Prescott, 2024). Previous frameworks have been developed to address various aspects of assisted migration, including the need for and type of assisted migration (Hällfors et al., 2017 and McLachlan et al., 2007) and the threats and vulnerabilities to species posed by climate change (Potter et al., 2017, Thomas et al., 2010), but often these frameworks are in the context of conservation (Chen et al., 2022, USFWS, 2024). Palik et al. (2022) present a conceptual framework for FAM intended to examine its role in the context of a range of climate adaptation strategies, and they identify a range of institutional barriers to its wide-scale adoption. Royo et al. (2023) present a research framework designed to address some of the uncertainties that likewise limit the implementation of FAM through the use of modeling to identify seed sources, experimentally testing these sources in greenhouses and growth chambers as well as silvicultural field tests, and then using these results in forest dynamics models to forecast the long-term outcomes of FAM. However, what is lacking is an applied framework designed to directly translate the principles of FAM into practice to assist land managers. The focus of this paper is to build upon the recommendations of Palik et al. (2022) and provide a practical framework to facilitate the planning and routine implementation of FAM on a landscape scale.
Our practical approach, the FAM Framework, a process model by which to implement management is comprised of four phases, each incorporating multiple factors: (1) assessment and analysis, (2) climate-based plant material selection, (3) seed procurement and deployment, and (4) documentation and monitoring (Figure 2). The benefit of the FAM framework is that it is science-based and utilizes a structured approach to ensure the most important considerations are utilized by land managers wanting to implement FAM. Use of the FAM Framework should lead to scientifically defensible planning and socially acceptable implementation of FAM as a climate adaptation technique. This is important because as pointed out by Palik et al. (2022) and Royo et al. (2023), FAM is still in its infancy, and many questions, uncertainties, and barriers exist to its application at the landscape scale. To maintain forest health and productivity, changes to the status quo are needed now, and this will require planning prior to implementation and monitoring after implementation in order to enable adaptive management in the future.
The Superior National Forest (SNF) in Minnesota, USA, used the FAM Framework in the development of an assisted migration plan for their forest, the first such plan developed for a national forest. The insights gained from this pilot effort were used to refine the final version of the FAM Framework presented here. We present the FAM Framework through this case study and provide details for how the SNF implemented each phase below.
2 Case study–Superior National Forest
The Superior National Forest is in northeastern Minnesota, along and to the west of Lake Superior and within the 1854 Ceded Territory of the Grand Portage Band of Lake Superior Chippewa, the Fond du Lac Band of Lake Superior Chippewa, and the Bois Forte Band of Chippewa (Figure 3). It has a mix of boreal and temperate forest ecosystems and associated tree species. The SNF encompasses a transition zone between boreal and temperate ecological regions, where several important tree species are near their southern range limits, including white spruce (Picea glauca) and jack pine (Pinus banksiana), along with significant boreal wildlife species that are rare in other Great Lakes states, such as the moose (Alces alces). Other trees are at the northern extent of their current natural distribution on the SNF, such as sugar maple (Acer saccharum), yellow birch (Betula alleghaniensis), and northern red oak (Quercus rubra). The SNF uses tree planting on a large scale (approximately 1 million seedlings across > 1,200 hectares per year) to meet forest management objectives. Climate change is expected to have significant effects on growing conditions on the SNF (Handler et al., 2014) and will likely cause shifts in species composition across its landscape (Iverson et al., 2019). Aware of these projected changes and the unique value of the SNF’s boreal characteristics for diverse communities that use the forest, the forest leadership team requested that the SNF become the first national forest within the United States Department of Agriculture (USDA) Forest Service to pilot the FAM Framework (Frerker et al., 2023; Handler et al., 2022).
To develop the plan, the SNF formed an initial core team. This core team engaged a broad group of over 20 partner organizations and 100 individuals from state and local governments, local Tribal Nations, academic researchers, and private industry. Nine working groups contributed to various components of the plan (Table 1). The final plan was published in November of 2023 (Frerker et al., 2023) and an Implementation Guide was developed for SNF use that will be updated as needed as knowledge is gained through experience with FAM implementation (see Supplementary material).
2.1 Phase 1–Assessment and analysis
The assessment and analysis phase of the FAM Framework is an appropriate starting point for developing a plan for FAM and helps determine where, when, and what type of FAM is appropriate. This phase comprises (1) a science-based assessment of the need for FAM, (2) analysis of risk to determine the most appropriate type of FAM, (3) Tribal engagement to understand Tribal priorities and perspectives, (4) community/partner engagement, (5) and policy and legal considerations.
2.1.1 Science-based assessment
An important first step is to evaluate the past, current, and projected future climate to determine whether the use of local seed sources based on current seed zones or seed movement guidelines is likely to result in a forest that is maladapted to its future climate. Mature trees that produce the seed used for reforestation germinated from seeds in the past, and survived because they were adapted to the climatic conditions at that time. However, the current climate where a particular tree is growing may have measurably changed from the climate at the time when the seed first germinated and began to grow. Online tools such as ClimateNA1 (Wang et al., 2016) can be used to determine how much the current climate at a given location departs from its historical climate. These comparisons between the past and current climate can indicate the extent to which local seed sources are suitable for use in reforestation. For example, on the SNF from the 1961–1990 to the 1991–2020 time period mean annual temperature has increased nearly 0.5°C and mean coldest month (winter) temperature has increased > 1°C. This analysis can be extended to projected future climate of the intended planting site (see “2.2.3 Climate-matching tools and resources” below). In addition to climate, other factors are important when assessing the suitability of FAM, including stand management history; protected areas and land classification; physiography; ecological, social/cultural values, and edaphic factors; biological interactions, including insect and disease threats and wildlife issues; fire history and future fire risk; and economic values.
Decision support tools such as flowcharts, checklists, or decision trees can be useful to assist land managers in asking the right questions and incorporating the necessary information to determine when, where, and what type of FAM to use. For example, the SNF used USDA Forest Service Forest Inventory and Analysis (FIA) plot data to determine tree species abundance for the suite of species they commonly plant to determine which type of FAM would be applicable to which species. Using Hierarchy of Ecological Units Sections and Subsections as the geographic boundary and FIA abundance values as summarized in the Climate Change Tree Atlas (Peters et al., 2020), a species was determined to be abundant, common, rare, or absent in the Section or Subsection where the planting site is located. If a species was abundant or common, APM of that species was considered. If the species is absent, this was considered ARE or ASM depending on how far away the seed source is located. If a species is “rare” on the landscape, the SNF agreed to coordinate with Tribal Nations to ensure planting of the species considers Tribal priorities. The SNF then developed decision trees that considered site conditions (e.g., land classification, soil and slope parameters, tree species vulnerability, past management), insect and disease issues, and wildlife and other species of interest to help guide which type of FAM to implement on a given planting site (see Supplementary material). These decision trees referenced geospatial data in a mapping exercise that factored in forest type, soil characteristics, and slope together as a tool to assess how climate change risk is distributed across the landscape. In addition, there are other post-fire restoration tools that can be used to prioritize areas for tree planting and inform the choices involved in FAM implementation such as the Southern Rockies Regeneration Tool (Rodman et al., 2022), Regen Mapper (Holden et al., 2022), and the Postfire Conifer Reforestation Planning Tool (Stewart et al., 2021).
2.1.2 Analysis of risk
Assessing the risk of various assisted migration strategies and predicting how well each addresses the desired land management objectives will help managers determine what approach may be appropriate in terms of implementing FAM. Risk can be considered as the probability of a less-than-desired outcome and the severity of the associated impact (USFWS, 2024). A risk assessment identifies relevant risk factors for a variety of risk types (see Karasov-Olson et al., 2021), whether those factors are present or not, and the potential consequences or impact of that risk. A risk assessment also considers ways to reduce the risk of undesirable outcomes and describes the uncertainty associated with various alternatives being assessed, including not implementing FAM (USFWS, 2024). The extent and level of detail of the risk assessment will depend on both the context and the level of identified risk considering all management alternatives. A risk assessment can be done either using a formal quantitative approach (Karasov-Olson et al., 2021) or using a more informal qualitative guide or checklist to ensure that all types of risks are addressed appropriately (National Invasive Species Council [NISC], 2024). In addition to assessing the potential risks associated with FAM, the risk posed by not implementing FAM is an important consideration. A key risk of not implementing FAM is “adaptation lag” or maladaptation as local adaptation is decoupled from the selective pressures of the environment to which a population is adapted (Jordan et al., 2024) resulting in continued, and potentially irreversible, decline in forest health and productivity. As the earth’s climate continues to change, the risk of not implementing FAM has the potential to outweigh the risks its use may pose (Palik et al., 2022).
The SNF did not do a formal risk analysis but did analyze risk through the development of the flow charts and mapping climate change risk (see Supplementary material) combined with a series of Tribal roundtables described in section “2.1.3 Tribal engagement”. Ultimately the SNF determined there was a need to be proactive in their approach to implementing assisted migration because of the vulnerability of the forest’s boreal ecosystems to climate change (Handler et al., 2014). The map, flowcharts, and roundtable information led them to develop an overall approach of pursuing APM where possible and implementing ARE and ASM with caution, and only after careful consideration of risk categories and formal consultation with Tribal Nations.
2.1.3 Tribal engagement
An important step in the assessment and analysis phase of FAM projects is to understand Tribal perspectives and priorities. Land management decisions on public lands have the potential to affect Tribal sovereignty, treaty rights, and cultural values of local or removed/relocated Indigenous communities including the relationships between plants, animals, water, and people. Tribal engagement can occur through formal government to government consultation and/or more informal collaboration. The SNF developed a model for engaging local Tribal Nations to hear their perspectives on FAM before drafting their plan. To do this, a series of roundtable discussions were convened with a network of Tribal staff and community members. Each roundtable focused on a set of discussion questions/topics (Box 1) and information from these sessions ultimately informed the type and depth of Tribal consultation that would be required for FAM actions (see section “3.2.1 - Consider tribal perspectives early”). An additional resource that provides a framework to integrate Tribal perspectives in FAM planning is Dibaginjigaadeg Anishinaabe Ezhitwaad–A Tribal Climate Adaptation Menu (Tribal Adaptation Menu Team, 2019). This resource provides some guiding principles for Tribal engagement and contains an organized collection of general climate change adaptation actions that reflect Indigenous perspectives which may be applicable for FAM.
BOX 1 Sample questions and discussion topics for Tribal engagement. |
Some suggested questions and topics include: |
• What climate impacts are you most concerned with and why? |
• What are our best opportunities for collaboration? |
• What are the climate change effects to culturally important species? |
• What are the concerns about AM regarding culturally important species? |
• What are some potential effects of AM on these species? (positive or negative) |
• How should we be mindful of relationships between these species and other beings or other values? |
• Are you already doing, or planning, other or other complementary climate adaptation action(s) for these species? |
2.1.4 Community/partner engagement
Regardless of land ownership, there are potentially multiple relevant community groups and partners that may have an interest in FAM. Forest management projects of any size on public lands require engagement with the public to elicit ideas, gain support, and ultimately be implemented. This may be especially true for FAM, which is an emerging issue. Key interest groups could include adjacent public and/or private/industry landowners and local communities that use forest lands that could potentially be impacted by FAM. The successful implementation of FAM may benefit from collaboration or partnership with other landowners and all activities on public lands require appropriate environmental analyses and public scoping. Community engagement and partnerships from the earliest stages of planning can help to build support and approval proactively rather than responding to objections at later stages of planning. The SNF actively engaged many partners from the onset of the development of their AM Plan by inviting these stakeholders to provide input and participate in the core team and be active participants in the development of the plan (Table 1).
2.1.5 Policy and legal considerations
Policy regarding use of FAM likely differs among land ownership and land management organizations, and potential restrictions on using non-local seed need to be considered during planning. There may be policies restricting the use of non-local seed sources that organizations may need to revise before FAM can be implemented operationally. Moreover, it is important to consider how any implementation of FAM aligns with land management goals even if it may not be explicitly addressed in existing planning documents. For example, the SNF Land Management Plan (LMP) states that desired conditions include native vegetation communities that are diverse, productive, healthy, and resilient, and that ecological conditions are maintained or restored at multiple landscape scales (USDA Forest Service, 2004). The SNF determined that that there was no language in their LMP that would prohibit them from implementing FAM and its use is consistent both with current and proposed Agency policy and is in alignment with achieving their LMP’s desired future conditions.
2.2 Phase 2–Climate-based plant material selection
The success of reforestation is determined by a variety of biotic and abiotic factors at the planting site and hinges on the ability for seedlings to synchronize their growth to the local climate. This phase of the FAM Framework comprises the selection of plant materials that will be needed to ensure seedlings will be adapted to their planting site under a changing climate, including: (1) considering transfer limits and seed transfer guidelines, (2) assessing species choice, and (3) utilizing tools and resources to identify climate-matched seed source locations.
2.2.1 Seed transfer guidelines
Many tree species of commercial value have been extensively studied in common gardens replicated across different environments to develop seed transfer guidelines (Bower and Aitken, 2008; Rehfeldt, 1994; Rehfeldt and Jaquish, 2010; Savva et al., 2007; Schmidtling, 2001; Sorensen, 1992, 1994; St. Clair et al., 2005). These transfer limits and guidelines indicate whether a species is a climatic specialist or generalist and how adaptive genetic diversity is distributed throughout a species’ range. For species where species-specific seed zones based on empirical research are not available, provisional seed zones can be used (Bower et al., 2014; Pike et al., 2020). Provisional seed zones are delineated based on areas with climatic conditions that fall within set thresholds. The assumption is that because individuals are adapted to the climate within their local seed zone they can be transferred to planting sites within that zone with limited risk of maladaptation (Bower et al., 2014). However, when implementing FAM, seed will likely need to be moved outside its local seed zone because of mismatches with the projected future climate. For example, seed zones for the SNF were originally developed in 1970 (USDA Forest Service, 2006), and seed for reforestation was collected solely from local stands. Those seed collection zones were updated in 2019 to account for climate change (Berrang, 2019), and climate-matched seed collection areas for future reforestation are in areas to the south of the forest with almost no overlap with SNF forest boundaries (Frerker et al., 2023).
2.2.2 Species choice
Although implementation of FAM involves lower risk movement of populations within or just beyond their current species ranges, species choice will still be a consideration if FAM is employed. Tree species vary in their vulnerability to the impacts of climate change (Potter et al., 2017) and nearly all forest tree species are projected to experience changes in their distribution (Iverson et al., 2019; Peters et al., 2020). If a planting site is near a contracting (i.e., warmer, in most cases lower latitude or elevation) range margin, it may mean that this species, even if currently abundant, may not be well suited to projected future climates at that location (Aitken et al., 2008). In these cases, it may be desirable to focus reforestation efforts on other species present at the site that are not at the potentially contracting edge of their distribution. Conversely, populations of tree species that are near the edge of a distribution projected to expand under climate change may be good candidates for ARE as new suitable habitat becomes available. Populations of trees that exist within the core area of their species range are likely the best candidates for APM (Aitken et al., 2008).
In addition to climate change impacts, some tree species also have important disease or insect considerations that may need to be taken into account when selecting seed sources [e.g., white pines (Pinus spp. subgenus Strobus) (Keane et al., 2022), Port-Orford-cedar (Chamaecyparis lawsoniana) (Sniezko et al., 2020), and eastern hemlock (Tsuga canadensis) (Evans et al., 2011)]. For some species where disease or insect resistant seed sources are available these may be the best-adapted even if they are at a higher risk of climate maladaptation. For other species, the presence of insects or diseases in some parts of the range may preclude or complicate seed and seedling movement. Additional resources are available that may be helpful to assess potential future risks from insect and disease incidence increases due to climate change, for example the National Insect & Disease Risk Map (USDA Forest Service, 2018).
The SNF is in a unique ecological position at the transition between the boreal forest to the north and temperate hardwood forest to the south. Depending on the species, any of the three types of assisted migration could potentially be appropriate in this kind of situation. The SNF used species abundance values calculated from FIA data, predicted species distribution maps from the Climate Change Tree Atlas (Peters et al., 2020), as well as feedback from Tribal engagement sessions to choose species for FAM planning. In addition, they also incorporated other considerations such as insect and disease issues {e.g., emerald ash borer (Agrilus planipennis) and spruce budworm (Choristoneura fumiferana) as well as culturally significant species [e.g., moose, northern white cedar (Thuja occidentalis), sugar maple, and paper birch (Betula papyrifera)]} in their species choice decisions.
2.2.3 Climate-matching tools and resources
One of the primary justifications of implementing FAM is to prevent a decrease in forest health and productivity due to maladaptation. Global circulation models can project the values of a large suite of climate variables for future time periods and online tools such as ClimateNA (Wang et al., 2016), CHELSA (Karger et al., 2021), and Climate Toolbox (Hegewisch and Abatzoglou, 2024) are available to obtain past, current, and future climate data for the site to be planted and the seed source locations. Simple calculations of the difference between the climate of the seed source location and the planting site provide the climatic transfer distance (St. Clair et al., 2022) and can be used to evaluate the risk of climatic maladaptation. Under the assumption associated with clinal genetic variation that fitness gradually decreases as climatic transfer distance increases, climatic transfer distances that exceed a pre-selected threshold would indicate that this seed would likely be maladapted for a future projected climate. Beyond calculating climatic transfer distance, several climate-matching tools have been developed to assist in matching seed sources with planting sites (e.g., the Seedlot Selection Tool and Climate Adapted Seed Tool) (St. Clair et al., 2022; Stewart et al., 2023). These tools are powerful and can be extremely useful, but they also require varying levels of user input.
When using modeled future climate data, it is important to select the appropriate future time period in order to assess the magnitude of climatic differences and potential maladaptation. Time periods in the near future (e.g., through 2040) may lead to selection of seed sources that will still become maladapted as the climate continues to change beyond that timeframe. Time periods too far into the future (e.g., 2071–2100) may lead to selection of seed sources that are maladapted to current conditions (Aitken and Bemmels, 2016). For example, if seed sources are chosen for much warmer future climates projected at the end of the century, they could suffer frost damage during the critical establishment phase because they are poorly adapted to current temperatures. Mid-century (2041–2070) time period balances the risks of under- or over-estimating future projected climate change (Aitken and Bemmels, 2016). For example, British Columbia uses a climatic transfer that matches the seed source climate prior to significant anthropogenic climate change (1931–1960) with the climate projected for the planting site at a quarter of the rotation age or approximately 15 years after planting (O’Neill and Degner, 2024). This accounts for climate change that has already occurred with a conservative buffer for expected future projected climate change.
It is also important to choose climatic variables carefully, considering local and/or specialized knowledge of the planting site and species of interest. Plant distributions are generally driven by the annual supply of energy (temperature) and water (precipitation) (Stephenson, 1990) and for many temperate forest trees, past research has shown that temperature (both mean annual temperature and winter temperature) is a driving force in local adaptation of populations (Aitken and Bemmels, 2016; Bower and Aitken, 2008; St. Clair et al., 2005). Both mean annual temperature and winter temperature are projected to increase to varying degrees in most locations (IPCC, 2023; Marvel et al., 2023). Projections of precipitation have greater uncertainty both annually and seasonally. However, when precipitation is combined with increased temperatures, it is projected that moisture deficit (either annual or summer) is likely to increase in many locations. Additionally, more extreme events are projected, and many areas may experience both an increase in heavy rain events and dry periods (IPCC, 2023; Marvel et al., 2023). Therefore, it is important to include a measure of precipitation or aridity when determining climate analogs.
The SNF used seed collection zones mapped to climate analogs based on the Seedlot Selection Tool (SST) to identify climate-matched seed collection locations before 2040 and from 2041 to 2070. They combined this information with species-specific transfer distance recommendations to determine the best location for future seed collection. For example, the SNF assisted migration plan includes shifting seed sourcing of some species (e.g., white spruce) to existing orchards that roughly match the Forest’s climate analog zone as identified by the SST, while identifying new collection areas within climate-adapted seed zones for species that need to be collected every year [e.g., bur oak (Quercus macrocarpa)].
2.3 Phase 3–Seed procurement, management, and deployment
Tree seed is a critical resource of reforestation efforts. Insufficient seed availability is creating constraints for reforestation as well as for implementing FAM. This phase comprises the suite of considerations relevant to the reforestation pipeline including: (1) considerations for seed use planning and seed procurement, (2) seed inventory management, and (3) considering various strategies for mixed provenancing of seed sources during deployment.
2.3.1 Seed procurement considerations
Implementation of FAM will require seed sourced from geographic areas that match the future climate analog, which may mean procuring non-local seed or seedlings from seed zones matched to the climate of the target project area. These non-local sources may, in some cases, include adjacent lands under different jurisdictions or ownership. Land managers that plan to implement FAM will benefit from developing a multi-year seed planning effort to identify locations of appropriate non-local sources to procure sufficient seed ahead of time. Surplus seeds collected for most conifers and hardwood tree species with seeds that can be dried and frozen can be stored for years or decades. Tree species with large seeds that cannot survive drying and freezing, however, would require seed collection areas or seed orchards to ensure dependable annual seed inventories. Several geospatial products are currently available to estimate future seed needs based on forestland risk to disturbances and future climates including the LANDFIRE Fire Return Interval (La Puma, 2023), the USDA Forest Service Climate Risk Viewer (USDA Forest Service, 2024b) and Climate Change Vulnerability Assessments (USDA Forest Service, 2024c), among others.
In the case of the SNF, a portion of the plan is dedicated to identifying seed sourcing cooperators in the event that climate-matched seed is not available from within the boundaries of the forest. The list identifies other national forests, Tribal Nations, state, and private cooperators and information such as species available, the cooperator’s ability to collect seed and point-of-contact for seed collection are listed.
2.3.2 Seed inventory management
Climate-informed reforestation requires precise knowledge of seed origin to match the projected climate with a planting site. Where collections are identified only at a coarse scale level (e.g., seed zone, breeding zone, county), an average value (e.g., the climatic center, median value) for climatic variables is used but at the expense of climatic accuracy compared to more precise location identification (e.g., GPS acquired latitude and longitude). This may be especially problematic in areas with steep elevational gradients. Information on seed source location is most useful when conserved at the most descriptive level available so that seed can be deployed in the most precise manner possible. Seed sources that reside in storage but are designated using imprecise source location information from older or improper documentation methods create a challenge for geneticists to align current seed inventory with climate models, making it difficult to provide fine-scale guidance for AM deployment by managers. If source location information is not tracked for a seedlot, this could preclude the use of that seed for FAM because the source climate cannot be determined.
New accessions would benefit from being labeled with the most refined location information available (latitude, longitude, and elevation). This information is also necessary to understand the potential factors affecting planting outcomes (e.g., seedling survival) over time for both FAM projects and reforestation more broadly. In the western US, because of the complex topography, seed collection for FAM will only be useful if collections are separated into accessions that represent relatively small geographic areas, recording the latitude and longitude of the stand of origin. In the eastern US, such as the SNF, tracking seed to the county or counties of origin may provide enough information in many areas for assisted migration where the seed is bulked by state and seed collection zone. Currently, standards for defining seed accessions vary depending on the collector and requirements set by the nursery or other buyer. Improved communication of seed-source identification standards, including seed origin labeling, between seed collectors and nurseries or buyers will help improve the availability of source-identified seed for FAM use on all lands.
2.3.3 Strategies for mixed provenancing
There are benefits and risks to mixing seed sources (e.g., increasing genetic diversity vs. potential outbreeding depression) (Bucharova et al., 2019). The proportion of seedlings that will be from local vs. non-local sources is a consideration when planning FAM. This mixing of seed sources employs portfolio theory (Crowe and Parker, 2008) to minimize risk by increasing genetic diversity to buffer against the impacts of climate change. There are different strategies that have been developed for determining how to mix various seed sources based on planning and land management objectives (Figure 4). A variety of different provenancing strategies have been described elsewhere (Figure 4A), including composite provenancing, which aims to mimic natural patterns of gene flow by mixing seed from multiple source locations, but with a larger proportion of local seed, and progressively smaller amounts of seed as the distance of the collection site from the planting site increases (Broadhurst et al., 2008); admixture provenancing which also mixes seed source locations, but aims to maintain a wide genetic base, without regard to the location of the source population relative to the planting site (Breed et al., 2013); predictive provenancing where seed from areas where the current climate matches a future predicted climate of the planting site (Sgrò et al., 2011); and climate-adjusted provenancing where the seed sources are biased in the direction of projected future climates (Byrne et al., 2013). Both predictive provenancing and climate-adjusted provenancing account for just a single projected future climate at a time. Prober et al. (2015) state that although future climates cannot be predicted without uncertainty the general trend is robustly predicted and the climate-adjusted provenancing approach incorporates a mix of seed sources from a climatic gradient biased toward the direction of predicted climate change. However, it is important to consider a diverse range of potential future climates based on a suite of models and climate scenarios to account for uncertainty. Managing for specific future climates, even if based on ensemble projections may be easier operationally, but this can provide a false sense of certainty and security. Portfolio climate-adjusted provenancing is a variation that simultaneously considers a diverse range of plausible future climates (Figure 4B). This approach can be used in a practical manner to bracket the range of plausible futures considering different representative concentration pathways (RCPs), climate models, time-periods, and climate variables. It establishes sideboards on the range for which seed sources can be collected and/or used, but also ensures the entire range is considered. Managing for the range of potential future climates is more complicated but intentionally and purposefully provides for designing seed mixes to be robust to the range of future climates. This will require land managers to use professional judgement to select the sources to include in seed mixes.
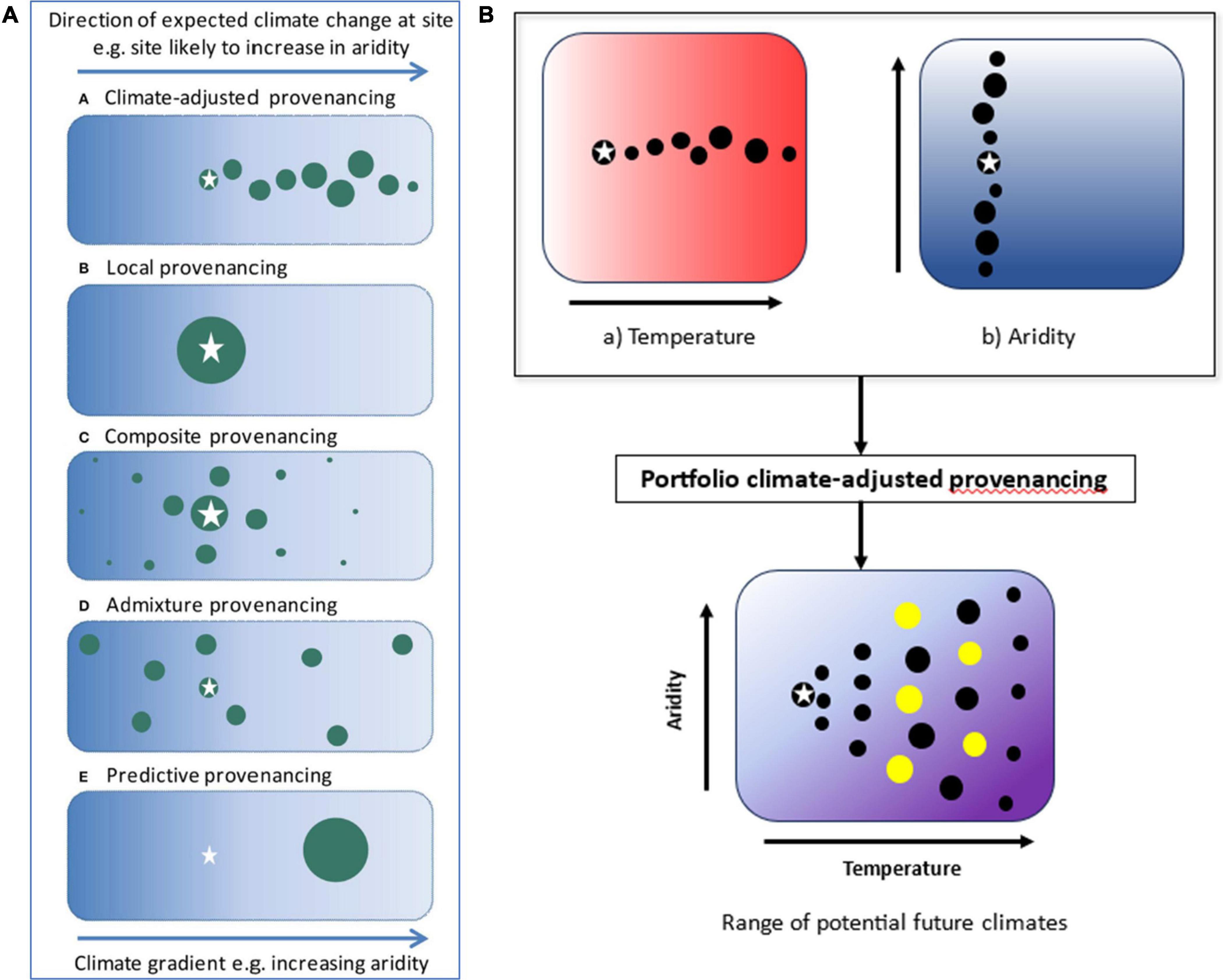
Figure 4. (A) From Prober et al. (2015) (used with permission). The star indicates the planting site and the green circles represent seed sources used for reforestation. The size of the circles indicates the relative quantities of each seed source used at the planting site. (B) Portfolio climate-adjusted provenancing. In this example climatic variables are (a) temperature, which increases from left to right, and (b) aridity which increases from bottom to top. When using portfolio climate-adjusted provenancing, multiple future climates (RCPs and time-periods) are considered to identify a diverse range of potentially adapted seedlots. Yellow colored circles indicate a potential set of seed sources for a seed mix designed to be robust to a range of possible future climates.
The SNF decided on a staggered process of FAM implementation that combines provenancing strategies outlined above. The SNF will gradually use up local seed caches while new protocols are developed for collecting seeds in climate analog zones. Full conversion of the reforestation program to APM stock is anticipated by 2050. ARE will be implemented on a case-by-case basis only after formal Tribal Consultation.
2.4 Phase 4–Documentation and monitoring
In the US, tens of millions of hectares of both public and private land are in need of reforestation (Fargione et al., 2021) and the increasing size and severity of wildfires is increasing the number of hectares in need of reforestation through planting (Fargione et al., 2021; USDA Forest Service, 2022). As public land managers strive to scale up and optimize climate-informed reforestation to meet land management goals (e.g., Executive Order 14072), it will be critical to establish clear protocols and guidelines to ensure that the necessary data are collected to determine the outcomes of management work. Only monitoring can ascertain when FAM approaches are working and when they are not, to ensure forests continue to provide the ecological benefits on which Tribes, communities, and stakeholders rely. The final phase of the FAM Framework comprises (1) documentation of activities at the time of planting and (2) monitoring of plantings to guide adaptive management.
2.4.1 Documentation at the time of planting
Planting is a critical time to ensure robust documentation and data archiving because of that information’s importance to tracking the success of FAM over time. It is helpful to plan and track information about the seedlings and how seedlings are planted as well as identify information needs about the planting site to inform future monitoring. During FAM plantings, the design of the physical layout of the planting may require tradeoffs between achieving silvicultural objectives and the ease of future monitoring. It is important to track the seedling sources to compare growth and survival among different seed sources, especially when multiple seed sources are planted on a single site. Comparisons of growth and survival rates can help managers identify which seed sources are performing well and can highlight under which circumstances the local (i.e., status quo) seed source is showing maladaptation or performing poorly relative to a FAM source. It typically is easiest to track seed sources if blocks are planted from a single seed source. The risk from this layout is that if a seed source proves to be unsuitable for a location, the whole block is more likely to fail. To buffer against having large areas with failed establishment or poor survival, seedlings from different seed sources could be intermixed in a planting. However, with multiple sources, unless seedlings are labeled by source at the time of planting, it will be impossible to track seed source over time. With a modest amount of pre-planning, it is possible to incorporate a small monitoring plot within operational plantings where seedlings are labeled by source location. Ideally, the plot is representative of the broader planting site so that it can be utilized for monitoring throughout the lifespan of the stand and reliably inform future management decisions.
When implementing FAM, it will be important to not only plan for the layout of the planting, but also to determine the information to be collected and recorded at the time of planting and where to store that information for future use. Data standards and stewardship are critical for future use of the data to inform adaptive management of the stand and future seed selection and procurement across the ecological region. Data can be maintained in both spatial and tabular databases, and in operational use, it is important to develop minimum data requirement guidelines and distinguish any optional elements. It is critical that the protocol captures data and information necessary to determine the success of FAM while simultaneously minimizing the impact on the field-going staff. A minimum standard set of core data for FAM will require components related to seed sources, planting, and initial survival. When possible, FAM efforts can leverage existing systems and practices to streamline adoption and determine what augmentations to those systems are needed to ensure FAM can be identified and tracked. For example, the SNF identified places in an existing agency database, the Forest Service Activity Tracking System, that could record when and where FAM took place at the time of planting, and developed protocols for properly monumenting FAM seedlings in the field.
2.4.2 Monitoring
Monitoring provides feedback and forms the critical link to close the loop in our FAM Framework to inform adaptive management so that based on lessons learned as FAM is implemented, future implementation can be adjusted. The data gathered through monitoring provides the information to initiate the Assessment and Analysis phase for future FAM projects and ensure (if not improve) their success.
A monitoring protocol will help ensure that relevant information is collected at appropriate intervals to inform future analyses. Important monitoring information for FAM plantings is data related to survival and health for each seed source, and land managers may also wish to collect climate data (e.g., temperature, precipitation, occurrence of extreme events) for additional analysis. Depending on the planting site’s location, local weather stations may be sufficient, or this information could be supplemented with local meteorological data collection in remote locations or when additional precision is needed. The seedling stage is when trees are most vulnerable to abiotic stressors such as cold injury or drought stress (Aitken and Bemmels, 2016), and collecting data on survival at frequent intervals can be particularly informative during early years of stand development. One-year and three-year post planting assessments are suggested to detect potential impacts of planting technique and to respond in the event of failure. After initial establishment, seedlings should be free-to-grow, and monitoring of both growth and survival could shift to longer intervals (e.g., every five years). The number of trees monitored is an important consideration in the monitoring protocol. If FAM is implemented over hundreds or thousands of hectares, it will be impractical or even impossible to monitor all trees or even all stands. This is where monitoring plots established at the time of planting may be most efficient, effective, and valuable. An additional consideration is who will be responsible for carrying out monitoring over time. When local staff and technicians are unable to accommodate the increased workload, it is worth considering collaboration with external partners to provide staff and/or contracting capacity for monitoring. In some instances, researchers may want to partner with managers where FAM provides opportunities to address outstanding research questions [e.g., The Experimental Network for Assisted Migration and Establishment Silviculture (USDA Forest Service, 2023)]. No matter who is assigned the role and responsibility for monitoring, it will also be important to ensure any data collected during monitoring be incorporated into appropriate databases so that it can be linked back to the planting information and be queried for future planning analyses and to inform future management decisions. For example, the Superior NF devised a monitoring protocol that built on established protocols, modifying the existing survey techniques to be conducted at a higher intensity and with additional information collected on tree condition and competition from surrounding vegetation. In addition to monitoring survival and condition of seedlings, climate conditions from the nearest weather station will be monitored to help determine potential causes of seedling mortality.
3 Discussion
Applying an early draft of the FAM Framework in the development of the first Assisted Migration Plan for a national forest, the SNF gained considerable insights into each of the four phases of the FAM Framework. These insights helped to refine the final FAM Framework presented here. During each phase of the FAM Framework, it became apparent that a one-size-fits-all approach for planning and implementing FAM is not possible, but rather, the broad FAM Framework can be adapted and tailored to suit local needs. A key outcome of implementing the FAM Framework was interpersonal networking between SNF staff, the Minnesota Department of Natural Resources, county governments, and others who will have important roles in procuring climate-adapted tree seed for the SNF. The roundtables that were conducted also fostered relationship building and provided key information to the Core Team on what Tribal community members and natural resources staff did and did not want to see from the SNF’s FAM efforts. Following are some key insights gained from the development of the SNF Assisted Migration Plan.
3.1 Key insights - assessment and analysis
3.1.1 Consider Tribal perspectives early
Climate change impacts and decisions related to FAM implementation will undoubtedly affect the ability of Tribal citizens to fully exercise their formal treaty rights within the 1854 Ceded Territory within which the SNF is located. Utilizing the FAM Framework, the SNF engaged with local Tribal Bands at the onset of the Assessment and Analysis phase to ensure FAM would be considered in a respectful manner. It immediately became apparent that there was a difference in opinion on how proactive the SNF should be with FAM on the landscape. The SNF felt a great urgency to begin shifting species compositions on the landscape in order to adapt to future climates and was initially pushing for an aggressive approach that considered both APM and ARE strategies. However, Tribal engagement via the roundtables revealed that there was discomfort from the Tribal Nations around these aggressive types of FAM because of the cultural value that current species and species assemblages on the landscape hold. As a result of these early dialogs, the final approach to FAM outlined in the SNF plan is more conservative, focusing on APM of the entire planting stock (∼1 million seedlings annually) by 2050. The SNF plan outlines requirements to receive consent for ARE and ASM actions through formal consultation meetings. APM actions, by contrast, may proceed with informal Tribal collaboration.
3.1.2 Define a process for distinguishing between the three FAM types—scale matters
When considering FAM for different tree species, it is important to acknowledge the different risk levels associated with each type of AM (Figure 1). However, distinguishing between APM, ARE, and ASM in practice and on the ground is less clear-cut than the definitions on paper. Through Tribal roundtable discussions, it became apparent that there was comfort with implementation of APM on the SNF but discomfort with ARE and ASM on the landscape. Further conversation revealed that it can be hard to distinguish where a particular species range begins or ends on the edge of species distribution limits like on the SNF. For example, a SNF staff member may consider moving a species from a neighboring state onto the forest a form of ARE, a Tribal Band member may consider ARE to be moving a species from a neighboring county. To resolve this difference in perception of scale and develop a way to clearly articulate when each type of FAM was being implemented, the SNF developed a process for distinguishing between the three types, based on species abundance at the planting site location.
3.2 Key insights - climate-based plant material selection
3.2.1 Consider species genetics
In order to select the appropriate species and populations within species for use in FAM, it was important to review information regarding genetic diversity, population structure, and any existing seed zones and/or seed transfer guidelines developed for species commonly used in reforestation. This information is critical to ensuring the appropriate choice of seed source. It is helpful to consult information available on these topics for the species of interest when planning FAM. The SNF provided a team of geneticists with a list of 16 tree species of interest (most commonly planted or ones they were interested in introducing in the future) and asked them to provide recommendations on transfer distances (Pike and Haase, 2024). This information can be found for many commercially important species in the published literature and will be included in the forthcoming update to the Silvics of North America (USDA Climate Hubs, 2024b).
3.3 Key insights - seed procurement and deployment
3.3.1 Partnerships are critical
Based on climate-matching analyses and established seed transfer guidelines, a series of maps of analog zones were produced for potential future seed collection locations for the SNF. It was immediately apparent that none of these locations were located within the SNF boundary. Some collection zones before 2040 overlapped other national forest boundaries in the area, but by mid-century the analog zones have little to no overlap with USDA Forest Service National Forest Systems lands (Berrang, 2019). This means that partnerships will be critical to the SNF for obtaining FAM seed, emphasizing the importance of collaboration with state, county, Tribal, private, and non-profit organizations to gather and obtain seed. Obtaining seed for FAM on national forests in the Eastern US poses additional challenges because of the varied land ownership. Administrative boundaries likely will be a consideration when identifying suitable seed sources regardless of whether neighboring lands are other national forests or under a different jurisdiction. Therefore, establishing partnerships to ensure the species of interest are available and accessible in off-site locations and establishing a mechanism for obtaining the seed (SNF seed collections crews or purchasing of seed from the landowner) are logistics that are important to address in the near-term.
3.4 Key insights - documentation and monitoring
3.4.1 Timing and communication of FAM seed availability are critical
FAM implementation is only beginning on the SNF, however, it is apparent that detailed documentation and continuous communication are necessary for success. There are many unknowns related to when a stand will be harvested and ready for planting and when the desired seed stock will be ready for deployment in the field. Because of this unpredictability related to timing, continual feedback on the process is critical to make sure the proper FAM stock is available for planting when the SNF needs it and that it is tracked and recorded once it is placed in the ground to allow for future monitoring.
4 Conclusion–toward national-scale implementation
Land managers on the SNF and elsewhere are eager to implement FAM on the ground, but thoughtful planning is important to ensure this adaptation technique is implemented in a climate-informed and a culturally and ecologically respectful manner. Before implementing FAM at the landscape scale, it is important to consider each of the elements in the FAM Framework so that managers can work in a consistent and coordinated approach that fully accounts for cultural (Tribal, wildlife, and other social values), ecological (species ranges, genetic diversity and structure, and climate change) and operational factors specific to their local landscape. Further, the burden of figuring out the logistics for selecting, procuring, deploying, and monitoring FAM seedlings cannot be placed on one individual land manager, and local policy and management guidelines for the landowner/manager will also be important to consider. Also, FAM is one just tool in the climate adaptation toolbox and can be employed alongside other climate adaptation treatments and practices that can be selected and combined to fit local challenges, concerns, and situations (Halofsky et al., 2018; Janowiak et al., 2014; Nagel et al., 2017; Ontl et al., 2018).
In a time of rapid climate change, there is no clear guidebook on how to implement FAM and professional judgement will be critical. The FAM framework is a structured approach to ensure the most important considerations and best available science are utilized in the planning and implementation of FAM. The practical FAM Framework developed here follows a logical cycle from planning, to implementation, to follow-up monitoring that is intended to facilitate the application of FAM in a scientifically, culturally, and ecologically defensible way. The piloting of the FAM Framework by the SNF provided valuable insights and helped to refine the final version presented here, and in addition, the SNF developed some useful tools and resources to accompany the FAM Framework. Using lessons learned from this pilot, it is hoped that this framework can be applied to other national forests and public lands more generally.
As Aitken and Bemmels (2016) state: “it’s time to get moving.” Over three decades ago Ledig and Kitzmiller (1992) predicted the need for deploying non-local seed and promoted the idea of broader deployment of species, seed sources, and families. Today, FAM is still not widely implemented outside the context of research. Given the current rapid pace of change, land managers do not have the luxury of waiting another 30 years to implement these practices. Land managers can use the FAM Framework to apply FAM and learn as we go. Documentation and monitoring will be crucial to allow adaptive management as we learn from our collective experience. Every instance of reforestation using FAM will have its own nuances, but our FAM Framework seeks to overcome some of the institutional barriers to wide-scale adoption of FAM identified by Palik et al. (2022). Ultimately, our FAM Framework is relevant and applicable across the spectrum of land ownership because it incorporates consideration of critical elements in planning and implementing FAM on any landscape while facilitating adaptive management for active learning and future implementation.
Author contributions
AB: Conceptualization, Writing – original draft, Writing – review & editing. KF: Writing – original draft, Writing – review & editing. CP: Writing – original draft, Writing – review & editing. NL: Writing – original draft, Writing – review & editing. BP: Writing – original draft, Writing – review & editing. AR: Writing – original draft, Writing – review & editing. SA: Writing – original draft, Writing – review & editing. AF: Writing – review & editing. LB: Writing – review & editing.
Funding
The authors declare that no financial support was received for the research, authorship, and/or publication of this article.
Acknowledgments
The authors would like to thank Jeanne Barker for creating the FAM Framework figure, and Chris Swanston, David Peterson, Stephen Handler, Holly Prendeville, Nicole Balloffet, and Stephanie Miller for reviews of earlier versions of this manuscript. We would like to thank the Grand Portage Band of Lake Superior Chippewa, the Fond du Lac Band of Lake Superior Chippewa, and the Bois Forte Band of Chippewa for sharing their knowledge and teaching us how to implement assisted migration respectfully—chi-miigwech. Thanks also to the staff of the Superior National Forest for their efforts applying the AM Framework and providing valuable feedback helping us to improve the FAM Framework.
Conflict of interest
The authors declare that the research was conducted in the absence of any commercial or financial relationships that could be construed as a potential conflict of interest.
The authors declared that they were an editorial board member of Frontiers, at the time of submission. This had no impact on the peer review process and the final decision.
Publisher’s note
All claims expressed in this article are solely those of the authors and do not necessarily represent those of their affiliated organizations, or those of the publisher, the editors and the reviewers. Any product that may be evaluated in this article, or claim that may be made by its manufacturer, is not guaranteed or endorsed by the publisher.
Supplementary material
The Supplementary Material for this article can be found online at: https://www.frontiersin.org/articles/10.3389/ffgc.2024.1454329/full#supplementary-material
Footnotes
References
Aitken, S., and Bemmels, J. (2016). Time to get moving: Assisted gene flow of forest trees. Evol. Appl. 9, 271–290. doi: 10.1111/eva.12293
Aitken, S., Yeaman, S., Holliday, J., Wang, T., and Curtis-McLane, S. (2008). Adaptation, migration or extirpation: Climate change outcomes for tree populations. Evol. Appl. 1, 95–111.
Allen, C., Macalady, A., Chenchouni, H., Bachelet, D., McDowell, N., Vennetier, M., et al. (2010). A global overview of drought and heat-induced tree mortality reveals emerging climate change risks for forests. For. Ecol. Manag. 259, 660–684.
Alía, R., Notivol, E., Climent, J., Pérez, F., Barba, D., Majada, J., et al. (2022). Local seed sourcing for sustainable forestry. PLoS One 17:e0278866. doi: 10.1371/journal.pone.0278866
Anderegg, W., Berry, J., Smith, D., Sperry, J., Anderegg, L., and Field, C. (2012). The roles of hydraulic and carbon stress in a widespread climate-induced forest die-off. Proc. Natl. Acad. Sci. U S A. 109, 233–237. doi: 10.1073/pnas.1107891109
Bastin, J., Finegold, Y., Garcia, C., Mollicone, D., Rezende, M., Routh, D., et al. (2019). The global tree restoration potential. Science 365, 76–79.
Benomar, L., Elferjani, R., Hamilton, J., O’Neill, G., Echchakoui, S., Bergeron, Y., et al. (2022). Bibliometric analysis of the structure and evolution of research on assisted migration. Curr. For. Rep. 8, 199–213.
Berrang, P. (2019). Eastern region guidebook: Tree seed program. USDA Forest Service Eastern Region. Unpublished Document, Milwaukee, WI, 58.
Betzen, J., Ramsey, A., Omdal, D., Ettl, G., and Tobin, P. (2021). Bigleaf maple, acer macrophyllum pursh, decline in western Washington. USA. For. Ecol. Manag. 501:11.
Boisvert-Marsh, L., Pedlar, J., de Blois, S., Le Squin, A., Lawrence, K., McKenney, D., et al. (2022). Migration-based simulations for Canadian trees show limited tracking of suitable climate under climate change. Diversity Distrib. 28, 2330–2348.
Bower, A., and Aitken, S. (2008). Ecological genetics and seed transfer guidelines for pinus albicaulis (pinaceae). Am. J. Bot. 95, 66–76. doi: 10.3732/ajb.95.1.66
Bower, A. D., St. Clair, B., and Erickson, V. (2014). Generalized provisional seed zones for native plants. Ecol. Appl. 24, 913–919. doi: 10.1890/13-0285.1
Breed, M., Stead, M., Ottewell, K., Gardner, M., and Lowe, A. (2013). Which provenance and where? Seed sourcing strategies for revegetation in a changing environment. Conserv. Genet. 14, 1–10.
Breshears, D., Cobb, N., Rich, P., Price, K., Allen, C., Balice, R., et al. (2005). Regional vegetation die-off in response to global-change-type drought. Proc. Natl. Acad. Sci. U S A. 102, 15144–15148.
Broadhurst, L., Lowe, A., Coates, D., Cunningham, S., McDonald, M., Vesk, P., et al. (2008). Seed supply for broadscale restoration: Maximizing evolutionary potential. Evol. Appl. 1, 587–597. doi: 10.1111/j.1752-4571.2008.00045.x
Bucharova, A., Bossdorf, O., Hölzel, N., Kollmann, J., Prasse, R., and Durka, W. (2019). Mix and match: Regional admixture provenancing strikes a balance among different seed-sourcing strategies for ecological restoration. Conserv. Genet. 20, 7–17.
Buck, J., Adams, R., Cone, J., Conkle, M., Libby, W., Eden, C., et al. (1970). California Tree Seed Zones. San Francisco, CA: Department of Agriculture, Forest Service.
Byrne, M., Prober, S., McLean, L., Steane, D., Stock, W., Potts, B., et al. (2013). Adaptation to Climate in Widespread Eucalypt Species. Gold Coast: National Climate Change Adaptation Research Facility, 86.
Chen, Z., Grossfurthner, L., Loxterman, J., Masingale, J., Richardson, B., Seaborn, T., et al. (2022). Applying genomics in assisted migration under climate change: Framework, empirical applications, and case studies. Ecol. Appl. 15, 3–21. doi: 10.1111/eva.13335
Crowe, K., and Parker, W. (2008). Using portfolio theory to guide reforestation and restoration under climate change scenarios. Clim. Change. 89, 355–370.
Cunningham, R. (1975). Provisional Tree and Shrub Seed Zones for the Great Plains. Res. Pap. RM-150. Fort Collins, CO: USDA Forest Service, 15.
Dobrowski, S., Swanson, A., Abatzoglou, J., Holden, Z., Safford, H., Schwartz, M., et al. (2015). Forest structure and species traits mediate projected recruitment declines in western US tree species. Glob. Ecol. Biogeogr. 24, 917–927.
Evans, D., Aust, W., Dolloff, C., Templeton, B., and Peterson, J. (2011). Eastern hemlock decline in riparian areas from Maine to Alabama. Northern J. Appl. For. 28, 97–104.
Fargione, J., Haase, D., Burney, O., Kildisheva, O., Edge, G., Cook-Patton, S., et al. (2021). Challenges to the reforestation pipeline in the United States. Front. For. Glob. Change. 4:18. doi: 10.3389/ffgc.2021.629198
Fettig, C., Mortenson, L., Bulaon, B., and Foulk, P. (2019). Tree mortality following drought in the central and southern Sierra Nevada, California, US. For. Ecol. Manag. 432, 164–178. doi: 10.1002/eap.2002
Fowells, H. (1946). Forest Tree Seed Collection Zones in California. San Francisco, CA: U.S. Department of Agriculture.
Frerker, K., Bower, A., Brandt, L., Gregory, C., Handler, S., LaBonte, N., et al. (2023). Superior National Forest Assisted Migration Plan. Duluth, MN: USDA Forest Service, 1–96.
Gray, L., and Hamann, A. (2013). Tracking suitable habitat for tree populations under climate change in western North America. Clim. Change 117, 289–303.
Hällfors, M., Aikio, S., and Schulman, L. (2017). Quantifying the need and potential of assisted migration. Biol. Conserv. 205, 34–41.
Halofsky, J., Andrews-Key, S., Edwards, J., Johnston, M., Nelson, H., Peterson, D., et al. (2018). Adapting forest management to climate change: The state of science and applications in Canada and the United States. For. Ecol. Manag. 421, 84–97.
Hancock, N., Encinas-Viso, F., and Broadhurst, L. (2023). A documented paradigm shift in seed sourcing: Attitudinal changes to using local native seed for ecological restoration. Restoration Ecol. 31:e13845.
Handler, S., Duveneck, M., Iverson, L., Peters, E., Scheller, R., Wythers, K., et al. (2014). Minnesota Forest Ecosystem Vulnerability Assessment and Synthesis: A Report From the Northwoods Climate Change Response Framework Project. Washington, DC: U.S. Department of Agriculture.
Handler, S., Brandt, L., Frerker, K., Gilkeson, J., Gregory, G., Labonte, N., et al. (2022). “Developing an assisted migration plan for the superior national forest,” in Foundational Concepts in Silviculture with Emphasis on Reforestation and Early Stand Improvement—2022 National Silviculture Workshop. Proc. RMRS-P-80, eds T. B. Jain and T. M. Schuler (Fort Collins, CO: U.S. Department of Agriculture).
Hartmann, H., Bastos, A., Das, A., Esquivel-Muelbert, A., Hammond, W., and Martínez-Vilalta, J. (2022). Climate change risks to global forest health: Emergence of unexpected events of elevated tree mortality worldwide. Annu. Rev. Plant Biol. 73, 673–702. doi: 10.1146/annurev-arplant-102820-012804
Hegewisch, K., and Abatzoglou, J. (2024). The Climate Toolbox. Available online at: https://climatetoolbox.org/ (accessed May 1, 2024).
Hennon, P., McKenzie, C., D’Amore, D., Wittwer, D., Mulvey, R., Lamb, M., et al. (2016). A Climate Adaptation Strategy for Conservation and Management of Yellow-Cedar in Alaska. Gen. Tech. Rep. PNW-GTR-917. Portland, OR: U.S. Department of Agriculture.
Holden, Z., Warren, D., Davis, K., Jungck, E., Maneta, M., Dobrowski, S., et al. (2022). “Regen mapper: A web-based tool for predicting postfire conifer regeneration and prioritizing reforestation efforts in the western united states,” in Foundational Concepts in Silviculture with Emphasis on Reforestation and Early Stand Improvement - 2022 National Silviculture Workshop. Proc. RMRS-P-80, eds T. B. Jain and T. M. Schuler (Fort Collins, CO: U.S. Department of Agriculture).
Hunter, M. (2007). Climate change and moving species: Furthering the debate on assisted colonization. Conserv. Biol. 21, 1356–1358. doi: 10.1111/j.1523-1739.2007.00780.x
IPCC (2023). Climate Change 2023: Synthesis Report. Contribution of Working Groups i, ii and iii to the Sixth Assessment Report of the Intergovernmental Panel on Climate Change. Geneva: IPCC.
Iverson, L., Prasad, A., Peters, M., and Matthews, S. (2019). Facilitating adaptive forest management under climate change: A spatially specific synthesis of 125 species for habitat changes and assisted migration over the eastern United States. Forests 10:989.
Janowiak, M., Swanston, C., Nagel, L., Brandt, L., Butler, P., Handler, S., et al. (2014). A practical approach for translating climate change adaptation principles into forest management actions. J. For. 112, 424–433.
Jordan, R., Harrison, P., and Breed, M. (2024). The eco-evolutionary risks of not changing seed provenancing practices in changing environments. Ecol. Lett. 27:e14348.
Karasov-Olson, A., Schwartz, M., Olden, J., Skikne, S., Hellmann, J., and Allen, S. (2021). Ecological Risk Assessment of Managed Relocation as a Climate Change Adaptation Strategy. Natural Resource Report NPS/NRSS/CCRP/NRR—2021/2241. Fort Collins, CO: National Park Service.
Karger, D., Lange, S., Hari, C., Reyer, C., and Zimmermann, N. (2021). Chelsa-w5e5 v1.0: W5e5 v1.0 downscaled with chelsa v2.0.
Keane, R., Schoettle, A., and Tomback, D. (2022). Effective actions for managing resilient high elevation five-needle white pine forests in western North America at multiple scales under changing climates. For. Ecol. Manag. 505, 119–136.
La Puma, I. P. (2023). LANDFIRE Technical Documentation: U.S. Geological Survey Open-File Report 2023–1045. Virginia: USGS.
Ledig, F., and Kitzmiller, J. (1992). Genetic strategies for reforestation in the face of global climate change. For. Ecol. Manag. 50, 153–169.
Linhart, Y., and Grant, M. (1996). Evolutionary significance of local genetic differentiation in plants. Annu. Rev. Ecol. Syst. 27, 237–277.
Marvel K, Su W, Delgado R, Aarons S, Chatterjee A, Garcia M et al. (2023). “Climate trends,” in Fifth National Climate Assessment, D.R, Easterling, K.E and Kunkel, B.C, eds. U.S. Global Change Research Program, Washington, DC
McCall, M. (1939). Forest tree seed policy of the U.S. department of agriculture. J. For. 37, 820–821.
McLachlan, J., Hellmann, J., and Schwartz, M. (2007). A framework for debate of assisted migration in an era of climate change. Conserv. Biol. 21, 297–302. doi: 10.1111/j.1523-1739.2007.00676.x
Mohan, J., Cox, R., and Iverson, L. (2009). Composition and carbon dynamics of forests in northeastern North America in a future, warmer world. Can. J. For. Res. 39, 213–230.
Nagel, L., Palik, B., Battaglia, M., D’Amato, A., Guldin, J., Swanston, C., et al. (2017). Adaptive Silviculture for Climate Change: A national experiment in manager-scientist partnerships to apply an adaptation framework. J. For. 115, 167–178.
National Invasive Species Council [NISC] (2024). Considering the Risks of Managed Relocation. Washington, DC: National Invasive Species Council.
O’Neill, G., and Degner, J. (2024). Seed trek 2.0: The next generation of seed transfer systems. Tree Planters Notes 67, 56–63.
Ontl, T., Swanston, C., Brandt, L., Butler, P., D’Amato, A., Handler, S., et al. (2018). Adaptation pathways: Ecoregion and land ownership influences on climate adaptation decision-making in forest management. Clim. Change 146, 75–88.
Palik, B., Clark, P., D’Amato, A., Swanston, C., and Nagel, L. (2022). Operationalizing forest-assisted migration in the context of climate change adaptation: Examples from the eastern USA. Ecosphere 13:19.
Pedlar, J., McKenney, D., Aubin, I., Beardmore, T., Beaulieu, J., Iverson, L., et al. (2012). Placing forestry in the assisted migration debate. BioScience 62, 835–842.
Peters, M., Prasad, A., Matthews, S., and Iverson, L. (2020). Climate Change Tree Atlas, version 4. Delaware, OH: U.S. Forest Service. Available at https://www.nrs.fs.fed.us/atlas (accessed May 13, 2024).
Pike, C. C., and Haase, D. L. (eds). (2024). “Seed-transfer guidelines for important tree species in the Eastern United States,” Agriculture handbook 801 (Washington, DC: US Department of Agriculture, Forest Service), 135.
Pike, C., Potter, K., Berrang, P., Crane, B., Baggs, J., Leites, L., et al. (2020). New seed-collection zones for the eastern United States: The eastern seed zone forum. J. For. 118, 444–451.
Potter, K., Crane, B., and Hargrove, W. (2017). A United States national prioritization framework for tree species vulnerability to climate change. New For. 48, 275–300.
Prober, S., Byrne, M., McLean, E., Steane, D., Potts, B., Vaillancourt, R., et al. (2015). Climate-adjusted provenancing: A strategy for climate-resilient ecological restoration. Front. Ecol. Evol. 3:65. doi: 10.3389/fevo.2015.00065
Randall, W. (1996). Forest Tree Seed Zones for Western Oregon. Salem, OR: Oregon Department of Forestry.
Randall, W., and Berrang, P. (2002). Washington Tree Seed Transfer Zones. Olympia WA: Washington Department of Natural Resources.
Rehfeldt, G. (1994). Genetic-structure of western red-cedar populations in the interior west. Can. J. For. Res. 24, 670–680.
Rehfeldt, G., Crookston, N., Saenz-Romero, C., and Campbell, E. (2012). North American vegetation model for land-use planning in a changing climate: A solution to large classification problems. Ecol. Appl. 22, 119–141. doi: 10.1890/11-0495.1
Rehfeldt, G., and Jaquish, B. (2010). Ecological impacts and management strategies for western larch in the face of climate-change. Mitigation Adaptation Strat. Glob. Change 15, 283–306.
Ricciardi, A., and Simberloff, D. (2009a). Assisted colonization is not a viable conservation strategy. Trends Ecol. Evol. 24, 248–253.
Ricciardi, A., and Simberloff, D. (2009b). Assisted colonization: Good intentions and dubious risk assessment. Trends Ecol. Evol. 24, 476–477.
Richardson, D., Hellmann, J., McLachlan, J., Sax, D., Schwartz, M., Gonzalez, P., et al. (2009). Multidimensional evaluation of managed relocation. Proc. Natl. Acad. Sci. U S A. 106, 9721–9724.
Rodman, K., Fornwalt, P., Chapman, T., Coop, J., Edwards, G., Stevens, J., et al. (2022). SRRT: A Decision support tool to inform postfire reforestation of ponderosa pine and Douglas-fir in the Southern Rocky Mountains. Res. Note RMRS-RN-95. Fort Collins, CO: U.S. Department of Agriculture, Forest Service, Rocky Mountain Research Station, 12. doi: 10.2737/RMRS-RN-95
Royo, A., Raymond, P., Kern, C., Adams, B., Bronson, D., Champagne, E., et al. (2023). Desired regeneration through assisted migration (DREAM): Implementing a research framework for climate-adaptive silviculture. For. Ecol. Manag. 546:13.
Rudolph, P. (1956). “A basis for forest tree seed collection in the lake states,” in Proceedings of the Minnesota Acad. of Sciences, vol 24, (St. Paul, MN).
Savva, Y., Denneler, B., Koubaa, A., Tremblay, F., Bergeron, Y., and Tjoelker, M. (2007). Seed transfer and climate change effects on radial growth of jack pine populations in a common garden in Petawawa, Ontario, Canada. For. Ecol. Manag. 242, 636–647.
Schmidtling, R. (2001). Southern Pine Seed Sources. Gen. Tech. Rep. SRS-44. Asheville, NC: U.S. Department of Agriculture.
Schubert, G., and Pitcher, J. (1973). A Provisional Tree Seed-Zone and Cone-Crop Rating System for Arizona and New Mexico. USDA For. Serv. Res. Pap. RM-105, 8p. Fort Collins, CO: Rocky Mountain Forest and Range Experiment Station.
Schwartz, M., Hellmann, J., McLachlan, J., Sax, D., Borevitz, J., Brennan, J., et al. (2012). Managed relocation: Integrating the scientific, regulatory, and ethical challenges. Bioscience 62, 732–743.
Sgrò, C., Lowe, A., and Hoffmann, A. (2011). Building evolutionary resilience for conserving biodiversity under climate change. Evol. Appl. 4, 326–337.
Sniezko, R., Johnson, J., Reeser, P., Kegley, A., Hansen, E., Sutton, W., et al. (2020). Y genetic resistance to phytophthora lateralis in port-orford-cedar (chamaecyparis lawsoniana) - basic building blocks for a resistance program. Plants People Planet 2, 69–83.
Sorensen, F. (1992). Genetic Variation and Seed Transfer Guidelines for Lodgepole Pine in Central Oregon. Washington, DC: U.S. Department of Agriculture.
Sorensen, F. (1994). Genetic Variation and Seed Transfer Guidelines for Ponderosa Pine in Central Oregon. Washington, DC: U.S. Department of Agriculture.
St. Clair, J. B., Mandel, N. L., and Vance-Boland, K. W. (2005). Genecology of Douglas-fir in western Oregon and Washington. Ann. Bot. 96, 1199–1214. doi: 10.1093/aob/mci278
St. Clair, J. B., Richardson, B. A., Stevenson-Molnar, N., Howe, G. T., Bower, A. D., Erickson, V. J., et al. (2022). Seedlot selection tool and climate-smart restoration tool: Web-based tools for sourcing seed adapted to future climates. Ecosphere 13:e4089.
Stanturf, J., Ivetic, V., and Dumroese, R. (2024). Framing recent advances in assisted migration of trees: A special issue. For. Ecol. Manag. 551:121552.
Stephenson, N. (1990). Climatic control of vegetation distribution - the role of the water-balance. Am. Nat. 135, 649–670.
Stewart, J., Mantgem, P., Young, D., Shive, K., Preisler, H., Das, A., et al. (2021). Effects of postfire climate and seed availability on postfire conifer regeneration. Ecol. Appl. 31:e02280.
Stewart, J., Wright, J., Godfrey, J., O’Neill, G., Ostoja, S., Thorne, J., et al. (2023). Climate Adapted Seed Tool (CAST): An Evidence-Based Tool to Improve Reforestation Outcomes. Davis, CA: La moran reforestation center open house.
Tribal Adaptation Menu Team (2019). Dibaginjigaadeg Anishinaabe Ezhitwaad: A Tribal Climate Adaptation Menu. Odanah, WI: Great lakes Indian Fish and Wildlife Commission.
Thomas, C., Hill, J., Anderson, B., Bailey, S., Beale, C., and Bradbury, R. (2010). A framework for assessing threats and benefits to species responding to climate change. Methods Ecol. Evol. 2, 125–142.
Twardek, W., Taylor, J., Rytwinski, T., Aitken, S., MacDonald, A., Van Bogaert, R., et al. (2023). The application of assisted migration as a climate change adaptation tactic: An evidence map and synthesis. Biol. Conserv. 280:109932.
USDA Climate Hubs (2024a). Northwest Reforestation, Planting to Suit Current and Future Climates. Available online at: https://www.climatehubs.usda.gov/hubs/northwest/topic/northwest-reforestation-planting-suit-current-and-future-climates (accessed April 11, 2024).
USDA Climate Hubs (2024b). Updated Silvics of North America Project. 2024b. Available online at: https://www.climatehubs.usda.gov/hubs/southeast/topic/updated-silvics-north-america-project-usnap (accessed May 13, 2024).
USDA Forest Service (2004). Land and Resource Management Plan - Superior National Forest. Eastern Region. Milwaukee, WI: USDA Forest Service.
USDA Forest Service (2006). Forest Service Handbook, Eastern Region. FSH 24009-17: Silviculture Practices Handbook, Chapter 4 - tree seed. R9 R.O 2409.17-2006-3. Milwaukee, WI: USDA Forest Service.
USDA Forest Service (2018). National Insect & Disease Risk and Hazard Mapping. Milwaukee, WI: USDA Forest Service.
USDA Forest Service (2022). Growing and Nurturing Resilient Forests: A Reforestation Strategy for the National Forest System. Washington, DC: U.S. Department of Agriculture.
USDA Forest Service (2023). Reforestation: Trees for the Future: The Experimental Network for Assisted Migration and Establishment Silviculture (ENAMES). Pacific Northwest Research Station factsheet. Milwaukee, WI: USDA Forest Service.
USDA Forest Service (2024a). FSM-2470 Silvicultural Practices. Washington, D.C: USDA Forest Service.
USDA Forest Service (2024b). Forest Service Climate Risk Viewer (1.0.1). Milwaukee, WI: USDA Forest Service.
USDA Forest Service (2024c). Climate Change Vulnerability Assessments Across the Nation. USDA Forest Service, Office of Sustainability and Climate. Milwaukee, WI: USDA Forest Service.
USFWS (2024). Decision Support Framework for Conservation Introductions: U.S. Fish and Wildlife Service, Pacific Region. Pacific Region Conservation Introductions Working Group. Washington, DC: U.S. Fish and Wildlife Service.
USGCRP (2023). “Fifth national climate assessment,” in U.S. Global Change Research Program, eds C. W. Avery, D. R. Easterling, and K. E. Kunkel (Washington, DC: USGCRP).
van Mantgem, P., Stephenson, N., Byrne, J., Daniels, L., Franklin, J., and Fule, P. (2009). Widespread increase of tree mortality rates in the western United States. Science 323, 521–524.
Vitt, P., Havens, K., and Hoegh-Guldberg, O. (2009). Assisted migration: Part of an integrated conservation strategy. Trends Ecol. Evol. 24, 473–474.
Vitt, P., Havens, K., Kramer, A., Sollenberger, D., and Yates, E. (2010). Assisted migration of plants: Changes in latitudes, changes in attitudes. Biol. Conserv. 143, 18–27.
Wang, T., Hamann, A., Spittlehouse, D., and Carroll, C. (2016). Locally downscaled and spatially customizable climate data for historical and future periods for North America. PLoS One 11:e0156720. doi: 10.1371/journal.pone.0156720
Williams, M., and Dumroese, R. (2013). Preparing for climate change: Forestry and assisted migration. J. For. 111, 287–297.
Woodall, C., Westfall, J., D’Amato, A., Foster, J., and Walters, B. (2018). Decadal changes in tree range stability across forests of the eastern US. For. Ecol. Manag. 429, 503–510.
Xu, W., and Prescott, C. (2024). Can assisted migration mitigate climate-change impacts on forests? For. Ecol. Manag. 556:121738.
Keywords: assisted migration, forest management, reforestation, climate change, climate adaptation, managed relocation
Citation: Bower AD, Frerker KL, Pike CC, Labonte NR, Palik BJ, Royo AA, Anderson SM, Ferreira AR and Brandt LA (2024) A practical framework for applied forestry assisted migration. Front. For. Glob. Change 7:1454329. doi: 10.3389/ffgc.2024.1454329
Received: 25 June 2024; Accepted: 11 October 2024;
Published: 30 October 2024.
Edited by:
Yashwant Singh Rawat, Federal Technical and Vocational Education and Training Institute (FTVETI), EthiopiaReviewed by:
David Ellison, University of Bern, SwitzerlandKamlesh Verma, Central Soil Salinity Research Institute (ICAR), India
Copyright © 2024 Bower, Frerker, Pike, Labonte, Palik, Royo, Anderson, Ferreira and Brandt. This is an open-access article distributed under the terms of the Creative Commons Attribution License (CC BY). The use, distribution or reproduction in other forums is permitted, provided the original author(s) and the copyright owner(s) are credited and that the original publication in this journal is cited, in accordance with accepted academic practice. No use, distribution or reproduction is permitted which does not comply with these terms.
*Correspondence: Andrew D. Bower, YW5kcmV3LmJvd2VyQHVzZGEuZ292