- 1Natural Resources Canada, Canadian Forest Service – Great Lakes Forestry Centre, Sault Ste. Marie, ON, Canada
- 2Forest Gene Conservation Association, Kemptville, ON, Canada
Introduction: Forest assisted migration has been proposed as a means to align tree populations with shifting climate habitats under climate change. Here we report on the growth and survival of oak species and seed sources at five assisted migration trials in southern Ontario – an important transition zone between boreal and temperate ecosystems.
Methods: Each trial featured one or more oak species – including red oak (Quercus rubra), white oak (Q. alba), burr oak (Q. macrocarpa), and swamp white oak (Q. bicolor) – and seed sources from Ontario, Pennsylvania, and/or Tennessee. The trials were measured for survival and height at between 7 and 13 years after planting.
Results: For several trials and species, southern seed sources performed nearly as well as local sources. However, southern seed sources of burr oak performed significantly worse than local sources at 2 trials in eastern Ontario. These outcomes may have been influenced by reduced quality of southern seed source planting stock at these trials.
Discussion: Our findings generally support previous work that suggests northward movements of seed sources of several hundred kilometers may be safe for assisted migration efforts involving broadleaf tree species. Notably, the trial sites were located at the northern range limits of two oak species in this study (Q. alba and Q. bicolor), suggesting the potential for modest range expansions in this boreal-temperate transition zone. These findings help forest managers to better understand potential assisted migration outcomes under climate change.
1 Introduction
Climate change is impacting forests in a variety of ways, including both direct impacts on tree growth (Wang et al., 2023; Girardin et al., 2016) and mortality (Hartmann et al., 2022), and indirect impacts on forest disturbance agents such as insects (Johnson and Haynes, 2023) and fire (Hanes et al., 2019). Given these many drivers of forest change, it is not surprising that global forests are starting to show signs of declining health and resilience (Hammond et al., 2022; Forzieri et al., 2022). Consequently, forest managers are increasingly tasked with adopting policies and practices to help maintain healthy and productive forests – and associated ecosystem functions – as climate change unfolds over the coming decades (Janowiak et al., 2014).
A variety of climate change adaptation techniques have been proposed in the forest management context (Williamson et al., 2019; Peterson et al., 2011). One such approach is assisted migration, wherein planting materials are moved (typically poleward or upslope) to climatically appropriate locations within and/or beyond existing range limits (McLachlan et al., 2007). The goal of such movements is to maintain a reasonable match between the climate at the planting site and the climate to which planting materials are adapted. Several types of assisted migration have been distinguished along a gradient of movement distance (Ste-Marie et al., 2011; Williams and Dumroese, 2013), including: (1) assisted population migration (movement of populations within existing range limits); (2) assisted range expansion (movement of species just beyond existing range limits); and (3) assisted species migration (movement of species far outside existing range limits – typically on the scale of hundreds to thousands of kilometers).
While the concept of assisted migration has existed for some time, empirical examples have been slower to emerge. In the context of commercial forestry, the movement of seed sources within existing range limits has become standard practice in some regions (e.g., O’Neill et al., 2017; van Kerkhof et al., 2022). Modest range expansions have also been proposed for a number of tree species in both forestry (O’Neill et al., 2008) and conservation (McLane and Aitken, 2012) settings. Results from assisted migration field trials have recently started to be reported for a number of species and locations (Pedlar et al., 2023; Wang et al., 2019; Sáenz-Romero et al., 2020; Etterson et al., 2020), but further information is needed to better understand the implications of these movements.
Oaks contribute significant economic and ecological benefits to forest ecosystems in North America (Pedlar et al., 2020). Genetic studies have shown high, range-wide genetic variation and modest population differentiation in oaks (Borkowski et al., 2017). Kriebel (1993) summarized early provenance results and reported potential growth gains associated with seed source movements of several hundred kilometers for a variety of oak species. Furthermore, drought tolerance is widely associated with oaks due to traits such as deep roots and thick leaves (Abrams, 1990; Kaproth and Cavender-Bares, 2016). This combination of features makes various oak species reasonable candidates for assisted migration efforts.
Southern Ontario is an important ecological transition zone between the boreal and temperate forest ecosystems. In this region, characteristic boreal species can be found in remnant populations at their southern range limits, while Carolinian broadleaf tree species reach their northern range limits. As such, this region provides an ideal setting for studying the northward expansion of southern tree species.
Here we report on the early growth and survival of several species of oak at assisted migration trials in southern Ontario, Canada. These trials were established to better understand assisted migration outcomes for these species, several of which are at – or beyond – their northern range limits at the study site locations. Ultimately, this work aims to assist forest managers with climate change adaptation efforts in an era of rapid climate change.
2 Materials and methods
2.1 Seed origins
Our aim was to incorporate provenances that would be reasonable selections for assisted migration efforts. Selection of planting stock origin (seed sources) was guided by a climate similarity analysis using Seedwhere (McKenney et al., 1999; https://cfs.cloud.nrcan.gc.ca/seedwhere/), which identified candidate seed source locations with recent historical climate that is projected to be similar to future climate at each planting site. We employed 1961–1990 as the recent historical climate period because it coincides with peak weather station numbers in Canada and precedes recent, rapid changes in climate. For future climate, we employed a composite of 4 climate models for the 2041–2070 period under the RCP4.5 and 8.5 scenarios [see McKenney et al. (2011) for details on the climate models]. Based on this approach and using a Seedwhere climatic similarity threshold of 0.9, stock at each trial was sourced from two or more locations along a temperature/latitudinal gradient, including: (1) Ontario (ON) seed zones 32, 36, 37, and 38, (2) Indiana County, Pennsylvania (PA), and (3) Warren County, Tennes(see TN) (Figure 1). All planting stock was derived from bulk seed collections in Ontario seed zones or US counties.
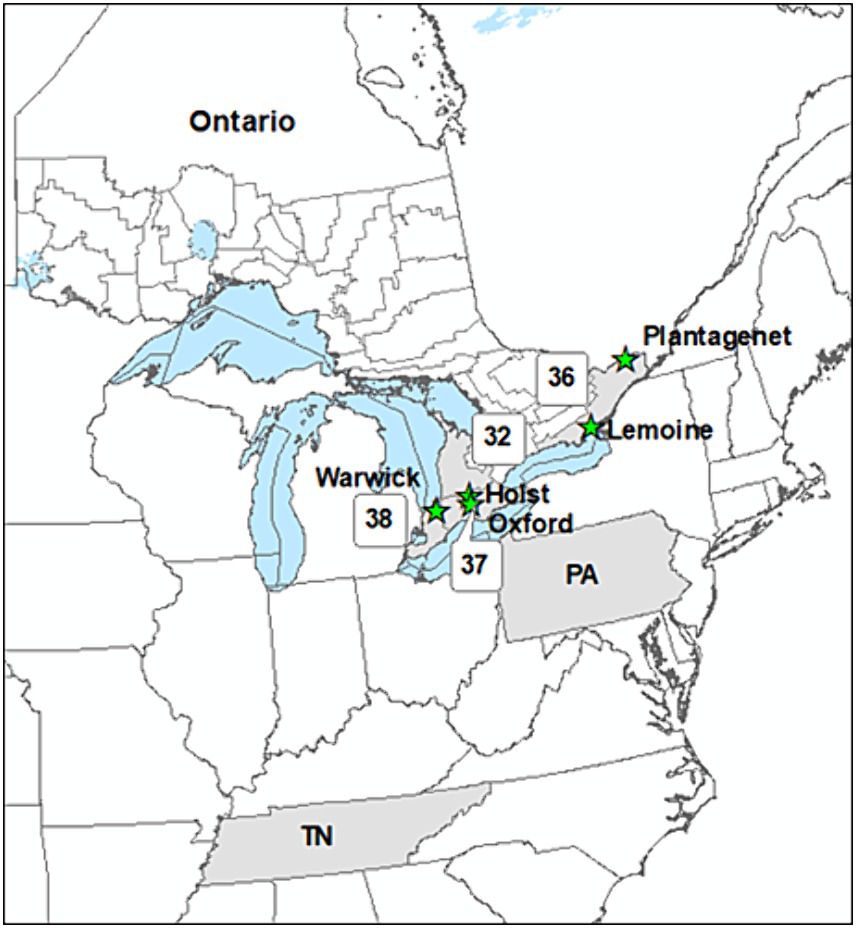
Figure 1. Location of seed sources (shaded Ontario seed zones and U.S. states) planted in various combinations at five assisted migration trials (green stars) in southern Ontario.
2.2 Trial sites
All trials were located in southern Ontario (Figure 1). Temperatures in this region average approximately 7.1°C, with cool winters (−6.0°C on average) and warm summers (19.3°C on average). Annual precipitation averages 886 mm, with similar amounts in summer (222 mm) and winter (210 mm). A significant amount of precipitation in winter falls as snow, with accumulations commonly reaching >20 cm. A summary of key trial site features is provided in Table 1 and maps are provided that show trial locations in relation to each species’ distribution (Supplementary Figures S1–S5).
2.2.1 Oxford County
This trial is located on Municipal property southeast of Ingersoll, Ontario at 43.001°N and − 80.8016°W. The site was previously used for agricultural cropping and is classified as moist, with silty texture. Microtopography within the site resulted in variable moisture conditions, which appeared to impact seedling performance throughout. The site was hand planted in April 2011 with 2 + 0 bareroot seedlings. Several hardwood species were planted at the site, but results reported here are limited to three seed sources (Ontario Seedzone 37, Pennsylvania, and Tennessee) of Q. macrocarpa – a test of assisted population migration (Supplementary Figure S1). The trial employed a randomized complete block design, consisting of: 12 blocks, each of which contained 4 plots (one for each of the Q. macrocarpa seed sources, plus one for the remaining hardwood species not presented here); which in turn contained 36 seedlings planted in a 6 × 6 layout at a 1.8 m x 3.6 m spacing (for a total of 1,296 Q. macrocarpa trees). Competing vegetation was controlled by a combination of plastic mulch and mowing between rows. Height and survival measurements for the current study were carried out in winter 2023, 12 growing seasons after planting. No thinning had been carried out at time of measurement.
2.2.2 Holst site
This trial is located on private property northwest of Woodstock Ontario at 43.1935°N and − 80.8121°W. The site is classified as prime agricultural land, with well-drained soils predominantly comprised of glacial deposits (till). Soil textures are predominantly Clay-Loam. The site was disced in fall 2009 and hand planted in April 2010 with 2 + 0 bareroot seedlings. The trial included 2 species (Q. alba and Q. rubra) and 3 seed sources (Ontario Seedzone 37, Pennsylvania, and Tennessee). Note that the test site is at the northern range limit for Q. alba (i.e., assisted range expansion; Supplementary Figure S2), but is well south of the northern limits for Q. rubra (Supplementary Figure S3). The two species were planted on adjoining plots of land following identical randomized complete block designs, consisting of: 6 blocks, each of which contained 3 plots (one for each seed source), which in turn contained 36 seedlings planted in a 6 × 6 layout at a 1.7 m x 3.7 m spacing (for a total of 648 trees per species). Competing vegetation was controlled by a combination of plastic mulch and mowing between rows. Tree densities in each plot were thinned by approximately 50% in summer 2019. Height and survival measurements for the current study were carried out in winter 2019 for Q. rubra and winter 2023 for Q. alba, 9 and 13 growing seasons after planting, respectively. Note that, at time of measurement, it was determined that approximately 33% of the Q. alba stock from TN was actually blackjack oak (Quercus marilandica) and/or hybrids of this species. Measurements of these trees were not included as part of the formal analysis for this trial but are summarized in the results to indicate how well this species performed at the site, which is located approximately 500 km north of the contiguous northern range limit for Q. marilandica (Supplementary Figure S4).
2.2.3 Warwick conservation area
This trial is located on Conservation Area land approximately 40 km east of Sarnia, Ontario at 42.9948°N and − 81.9445°W. The site was previously used for agricultural cropping and is classified as moist, with coarse loamy textured soils. Three seed sources (Ontario Seedzone 37, Pennsylvania, and Tennessee) of two oak species, Q. rubra (assisted population migration; Supplementary Figure S3) and Q. bicolor (assisted range expansion; Supplementary Figure S5), were hand planted at the site in April 2016. For both species, seedlings from Tennessee were 1 + 0 bareroot seedlings, while those from ON and PA were 2 + 0 bareroot seedlings. The two species were planted on adjoining plots of land following identical randomized complete block designs, consisting of: 6 blocks, each of which contained 3 plots (one for each seed source), which in turn contained 36 seedlings planted in a 6 × 6 layout at a 1.8 m x 3.6 m spacing (for a total of 648 trees per species). Competing vegetation was controlled by mowing between rows. Height and survival measurements for the current study were carried out in winter 2023, 7 growing seasons after planting. At time of measurement, it was determined that all Q. bicolor seedlings from Pennsylvania were actually black oak (Q. velutina); thus, results reported here for Q. bicolor are limited to the Ontario and Tennessee seed sources. No thinning had been carried out at time of measurement.
2.2.4 Lemoine point conservation area
This trial is located on Conservation Area land near Kingston, Ontario at 44.220041°N and − 76.607709°W. The site was previously used for hay production but had been fallow for several years prior to planting. It is classified as a wet site, with heavy clay soils. The site was originally planted in 2012, but due to high levels of mortality, was replanted in April 2016, at which time four Q. macrocarpa (assisted population migration; Supplementary Figure S1) seed sources (Ontario Seedzones 36 and 38, Pennsylvania, and Tennessee) were hand planted using 2 + 0 bareroot seedlings. The trial employed a randomized block design, consisting of: 7 blocks, each containing 4 plots (one for each seed source), a further 7 blocks containing 3 plots (one for each seed source except Pennsylvania), and a final block containing 2 plots (one for each of the Ontario seed sources). All plots contained 36 seedlings planted in a 6 × 6 layout at a 1.8 m x 3.6 m spacing (for a total of 1908 trees). Competing vegetation was controlled by mowing between rows, and a fence was installed to reduce deer predation. Height and survival measurements for the current study were carried out in winter 2023, 7 growing seasons after replanting.
2.2.5 Plantagenet
This trial is located on Municipal property in the town of Plantagenet, approximately 50 km east of Ottawa, Ontario at 45.539372°N and − 74.986774°W. The site was previously used for agricultural cropping but had been fallow for several years prior to planting. It is classified as a moist site, with clay textured soils. Five Q. macrocarpa (assisted population migration; Supplementary Figure S1) seed sources (Ontario Seedzones 32, 36, and 37, Pennsylvania, and Tennessee) were hand planted at the site in May 2014. Seedlings from Ontario were 1 + 0 bareroot seedlings, while those from Pennsylvania and Tennessee were 2 + 0 bareroot seedlings. The trial employed a randomized block design, consisting of: 3 blocks, each containing 5 plots (one for each seed source), and 3 further blocks, each containing 4 plots (one for each seed source except Ontario Seedzone 36). All plots contained 36 seedlings planted in a 6 × 6 layout at a 1.8 m x 3.6 m spacing (for a total of 972 trees). Competing vegetation was controlled by a combination of mulch mats (1-m diameter) and mowing between rows. Height and survival measurements for the current study were carried out in winter 2022, 8 growing seasons after planting. There was significant vole damage to seedlings during the first few years after establishment, which impacted survival and growth at the trial.
2.3 Climate data
Climate estimates were obtained by interrogating spatial climate models covering Canada and the United States (McKenney et al., 2011). These thin-plate spline-based models are spatially continuous, allowing them to be queried at any location for which geographic coordinates and elevations are known. Specifically, climate values were generated at seed source origins for each year over the 1961–1990 period – a period prior to rapid climate change that may approximate conditions to which the seed sources are adapted – and at each planting site for each year between establishment and measurement (see Table 1). Climate variables selected for comparison included growing season average temperature and precipitation, and annual climate moisture index, which measures the balance between precipitation and potential evapotranspiration (Hogg, 1994). Variables associated with extreme climate events, which can greatly impact young plantation success, are also provided, including annual minimum and maximum temperatures and growing season start date. Due to space limitations, summaries of these variables are provided as supplementary material.
2.4 Statistical approach
Two sample t-tests (R Core Team, 2021) were used to test for differences between the historical climate at a given seed source and the climate experienced at each trial site where it was planted. A bonferroni correction was used to adjust the initial significance cutoff (i.e., p < 0.05) to account for multiple comparisons at each test site.
Given that, at certain sites, the age and size of planting stock was not entirely consistent across seed sources, we recognize that calculating relative growth rates (sensu Hunt, 1982) would provide a more accurate measure of growth performance. However, immediate post-planting height measurements were not available for these trials, so such an analysis was not possible. Thus, we analyzed tree heights at each site using a mixed effects model:
where, μ is the overall mean, αi is the (fixed) effect of seed origin, γj is the (random) effect of block, δk(j) is the (random) effect of plot nested within block, and ϵijk is the model error. The analysis was performed in SAS 9.4 (SAS Institute Inc., 2013) using PROC GLIMMIX, with a residual pseudo-likelihood estimation method and Kenward-Roger correction to the denominator degrees of freedom. The model specified in equation 1 was also used to analyze tree survival, but in this case the logit link and binary distribution were specified in the call to PROC GLIMMIX. Note that, at sites with multiple species (i.e., the Holst and Warwick sites), separate analyses were carried out for each species as the experiments were not designed to incorporate species as an analysis factor.
The R2 metric, which is a well-known estimate of the proportion of variance explained by normal linear models, is not typically provided for the generalized linear mixed models used here. Thus, we employed a published SAS Macro routine (Jaeger et al., 2017) to obtain R2 values for the fixed effects in each of our models.
3 Results
3.1 Climate comparisons
For the US seed sources, historical growing season temperatures in Tennessee were significantly warmer than recent temperatures at all trial sites, while those in Pennsylvania did not differ signficantly from temperatures experienced at the trial sites (Figure 2). For the Ontario seed sources, historical growing season temperatures were significantly lower than recent temperatures experienced at the trial sites where they were planted (Figure 2). Historical growing season precipitation was higher at the two US seed sources than at any of the trial sites, but did not differ significantly between Ontario seed sources and trial sites (Figure 2). Annual climate moisture index (CMI) did not vary greatly between seed sources and trial sites, though historical CMI at seed zones 36 and 37 was significantly lower than CMI experienced at the Plantagenet site, while seed zone 38 was historically drier than the Lemoine Point site (Figure 2).
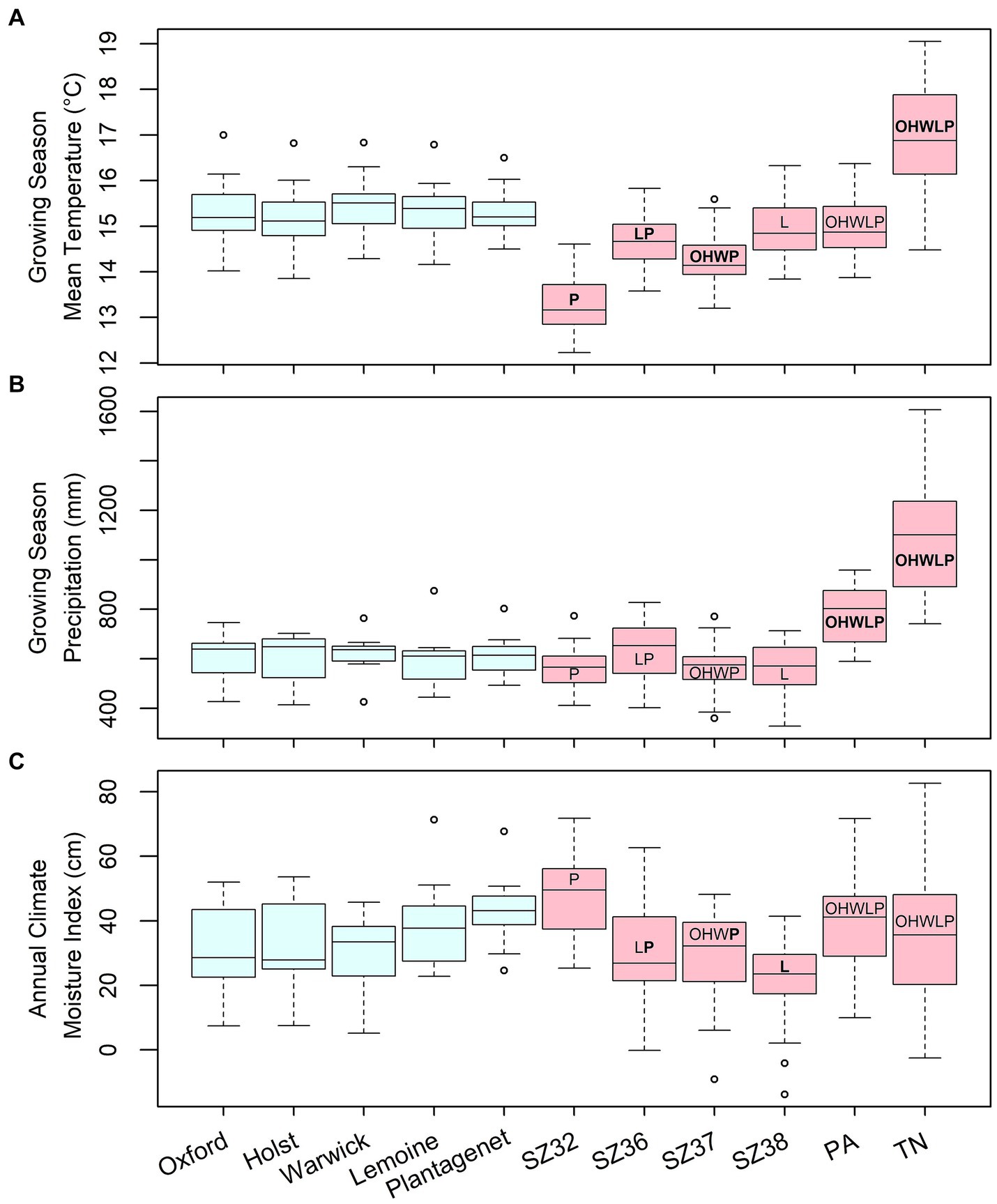
Figure 2. Summaries of (A) growing season mean temperature, (B) growing season precipitation, and (C) annual climate moisture index at assisted migration test sites (blue boxes) and seed origins (red boxes) used in the current study. Seed source climate values are for an historical period (1961–1990) that precedes recent rapid climate change; test site values are for the period between planting and measurement (see Table 1 for details). Letters on the seed source boxes indicate the first initial of the trial sites at which they were planted; bold letters indicate significant differences between seed source and trial site climates.
Extreme minimum and maximum temperatures were significantly warmer, and growing seasons started significantly earlier, at the Tennessee and Pennsylvania seed sources relative to the trial sites (Supplementary Figure S6). These metrics were not significantly different between many of the Ontario seed sources and trial sites, though seed zone 32 had lower temperature extremes and later growing season start dates than those experienced at the Plantagenet site, while seed zone 38 historically had warmer temperatures and a longer growing season than the Lemoine Point site where it was planted (Supplementary Figure S6).
3.2 Plantation performance
Due to inconsistencies across trials (e.g., different species, provenances, establishment and measurement dates, and site conditions), we present results separately for each assisted migration trial below, but attempt to identify emergent patterns across trials to support key findings from the work.
After 12 growing seasons at the Oxford County site, Q. macrocarpa heights averaged 3.68 ± 0.99 m and survival averaged 95.5 ± 5.1%. Neither metric varied significantly across the three seed sources (TN, PA, and ON seed zone 37) planted at the site (Table 2; Figure 3).
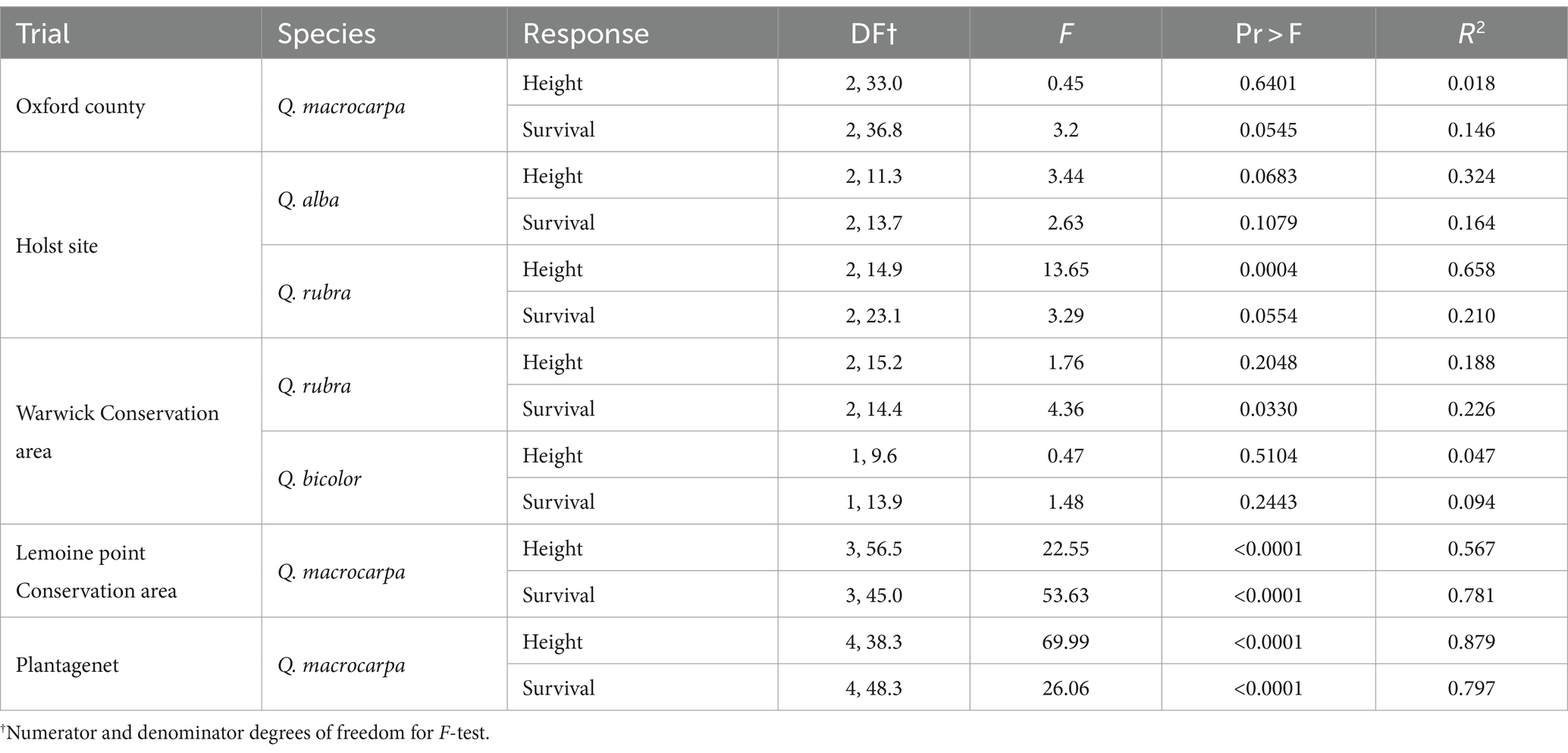
Table 2. Significance tests for mixed models relating height and survival of various oak species to seed origin at five assisted migration trials in southern Ontario.
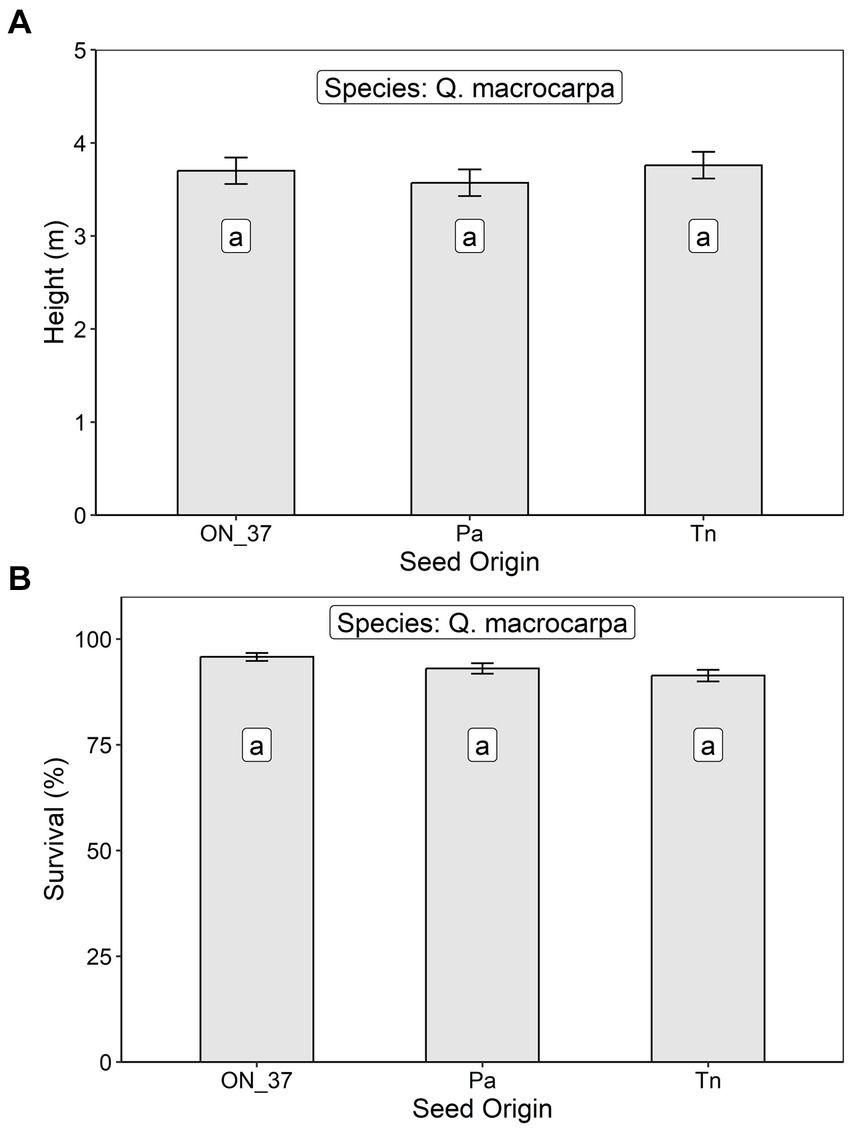
Figure 3. Least squares mean (A) height and (B) survival at age 12 (± S.E.) of Q. macropcarpa trees at an assisted migration trial in Oxford County, Ontario in relation to seed origin (ON_37, Ontario Seed Zone 37, Pa, Pennsylvania, and Tn, Tennessee). Differences between seed origins not sharing the same letter are statistically significant (i.e., p < 0.05).
After 9 growing seasons at the Holst site, height of Q. rubra trees averaged 6.01 ± 1.43 m, with the Ontario seed source growing significantly better than both U.S. seed sources (Table 2; Figure 4A). Survival of Q. rubra trees averaged 95.2 ± 4.9%, with no significant differences between seed sources (Table 2; Figure 4B). For Q. alba, at 13 growing seasons after planting, height averaged 5.95 ± 1.34 m and survival averaged 75.9 ± 15.2%; there were no significant differences between seed sources for either of these response variables (Table 2; Figure 4).
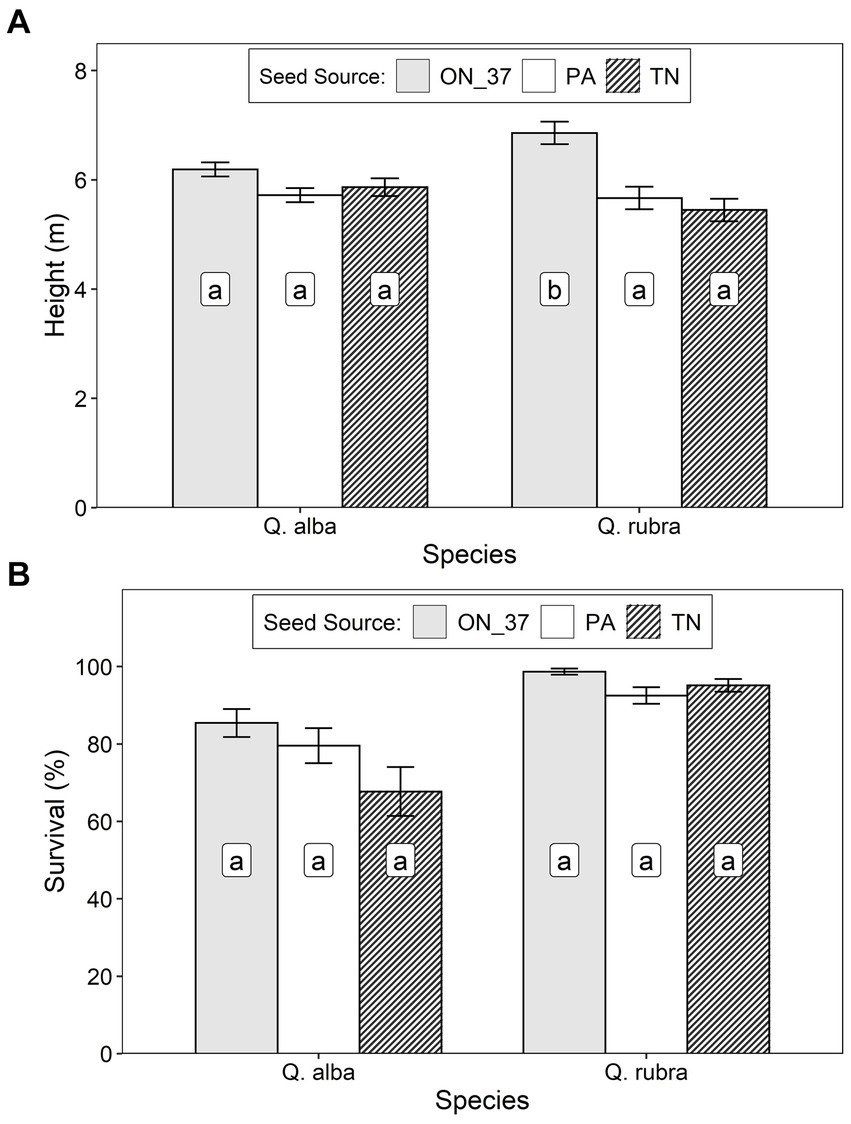
Figure 4. Least squares mean (± S.E.) (A) height and (B) survival of Q. rubra and Q. alba trees – at age 9 and 13 years, respectively – for 3 seed sources (ON_37, Ontario Seed Zone 37, Pa, Pennsylvania, and Tn, Tennessee) at an assisted migration trial near Woodstock, Ontario. Differences between seed origins not sharing the same letter are statistically significant (i.e., p < 0.05).
Approximately 70 suspected Q. marilandica seedlings were unintentionally planted at the Holst site along with the Q. alba stock from Tennessee. Though not included in the statistical analysis reported above, these trees were measured in 2023 and averaged 3.36 ± 1.12 m in height and survived at a rate of 63.9 ± 20.3%. It is noteworthy that, following cold events in fall 2020/spring 2021, many of these trees exhibited at least modest dieback (e.g., death of branch tips and buds), with about 14% exhibiting severe dieback (e.g., death of 2–5 cm diameter branches). Many of these trees, including those that experienced severe dieback, managed to survive and grow well in future years. None of the other species/seed sources at this site exhibited comparable levels of dieback in relation to these climatic events.
After 7 growing seasons at the Warwick site, height of Q. rubra trees averaged 1.97 ± 1.09 m, with no significant differences between seed sources (Table 2; Figure 5A), despite noticeably lower growth associated with the Tennessee seed source. The lack of statistical significance in this case likely reflects the relatively high within-group variation in growth at the site and modest power of the test. Survival of Q. rubra trees averaged 68.5 ± 17.6% and the Tennessee seed source exhibited significantly lower survival than the Pennsylvania source, with the Ontario source intermediate between the two (Figure 5B). For Q. bicolor, height averaged 2.47 ± 0.79 m and survival averaged 94.0 ± 2.7%; there were no significant differences between seed sources for either of these response variables (Table 2; Figure 5).
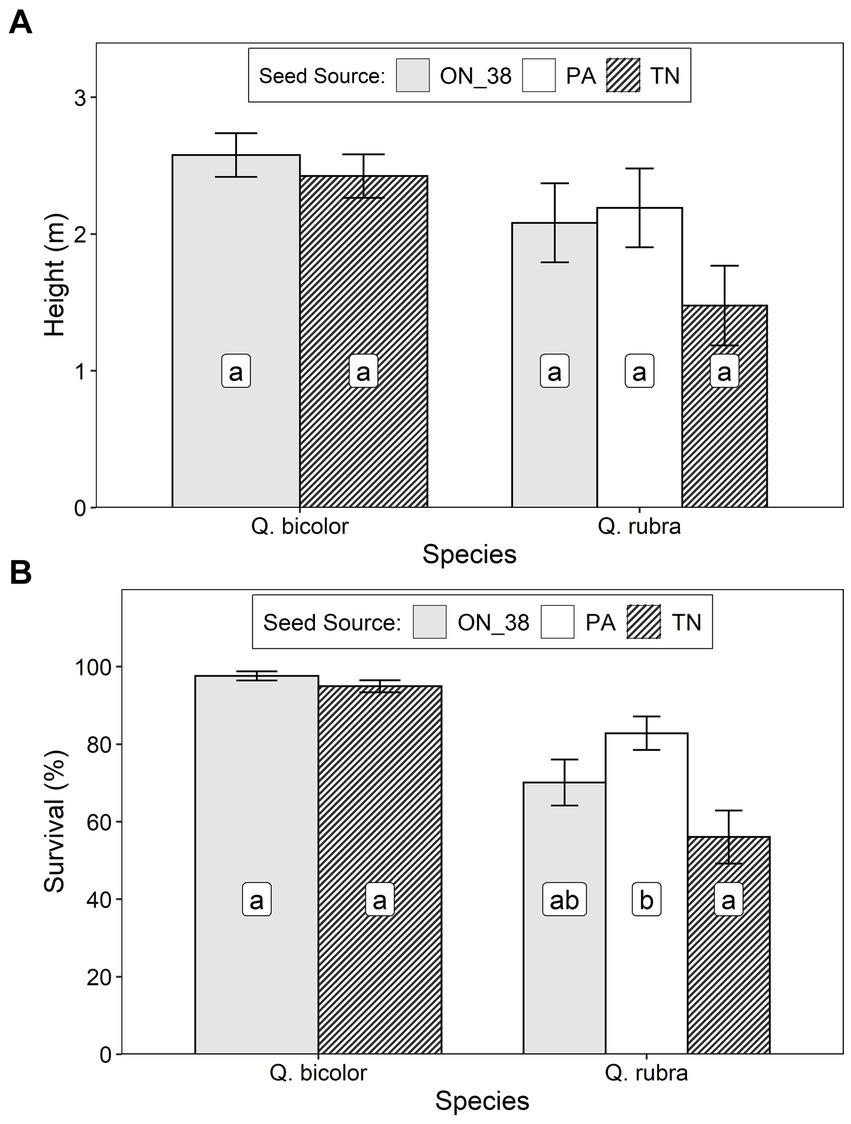
Figure 5. Least squares mean (± S.E.) (A) height and (B) survival of Q. rubra and Q. bicolor trees at age 7 for 3 seed sources (ON_38 = Ontario Seed Zone 38, Pa, Pennsylvania, and Tn, Tennessee) at an assisted migration trial at the Warwick Conservation Area near Sarnia, Ontario. Differences between seed origins not sharing the same letter are statistically significant (i.e., p < 0.05).
At the Lemoine Point site, after seven growing seasons, height of Q. macrocarpa trees averaged 1.78 ± 0 0.69 m, with the two Ontario seed sources growing significantly better than the two U.S. sources (Table 2; Figure 6A). Survival averaged 68.1 ± 30.5%, with the Pennsylvania seed source exhibiting the lowest survival, the Tennessee source intermediate, and the two Ontario sources highest (Table 2; Figure 6B).
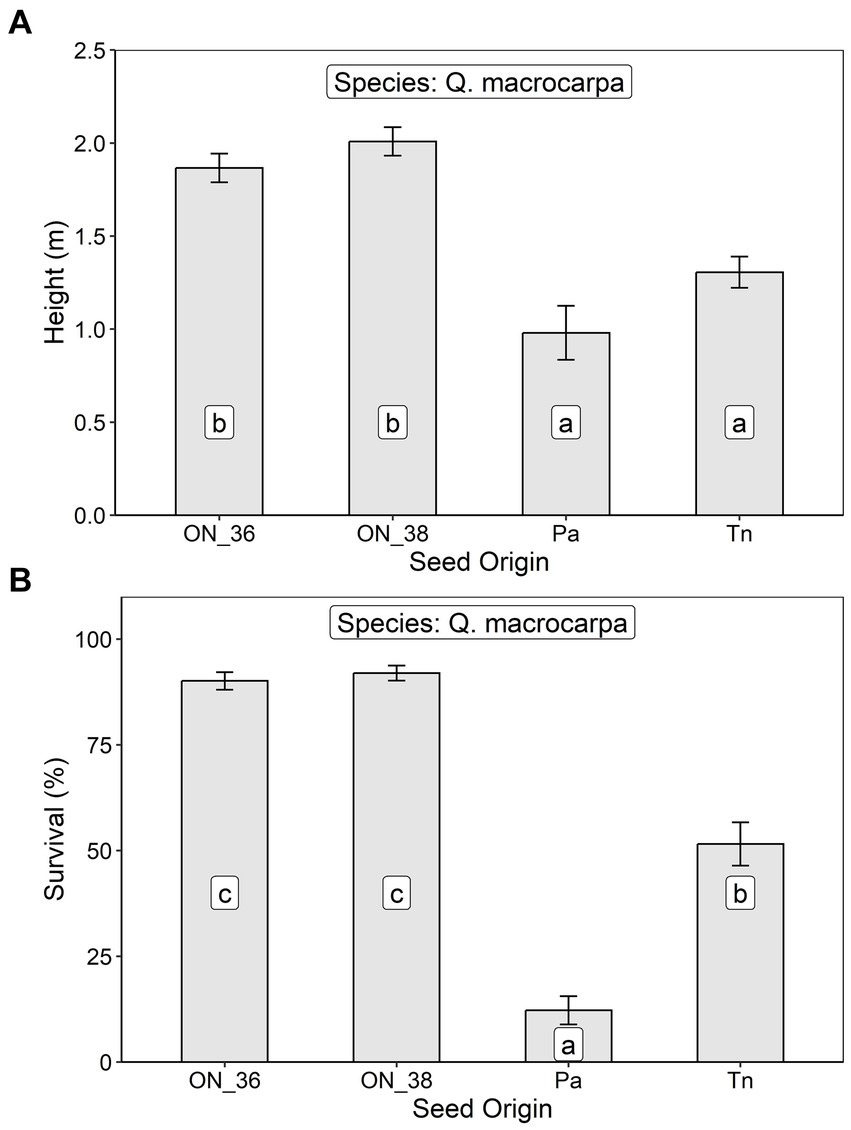
Figure 6. Least squares mean (A) height and (B) survival at age 7 (± S.E.) of Q. macrocarpa trees in relation to seed origin (ON_36, Ontario Seed Zone 36, ON_38, Ontario Seed Zone 38, Pa, Pennsylvania, and Tn, Tennessee) at an assisted migration trial in the Lemoine Point Conservation Area near Kingston, Ontario. Differences between seed origins not sharing the same letter are statistically significant (i.e., p < 0.05).
After 8 growing seasons at the Plantagenet site, height of Q. macrocarpa trees averaged 2.43 ± 0.89 m, with the Seed Zone 37 source growing best and the two U.S. sources growing worst (Table 2; Figure 7A). Survival averaged 72.8 ± 26.5%, with the US seed sources exhibiting significantly lower survival than the three Ontario sources (Table 2; Figure 7B).
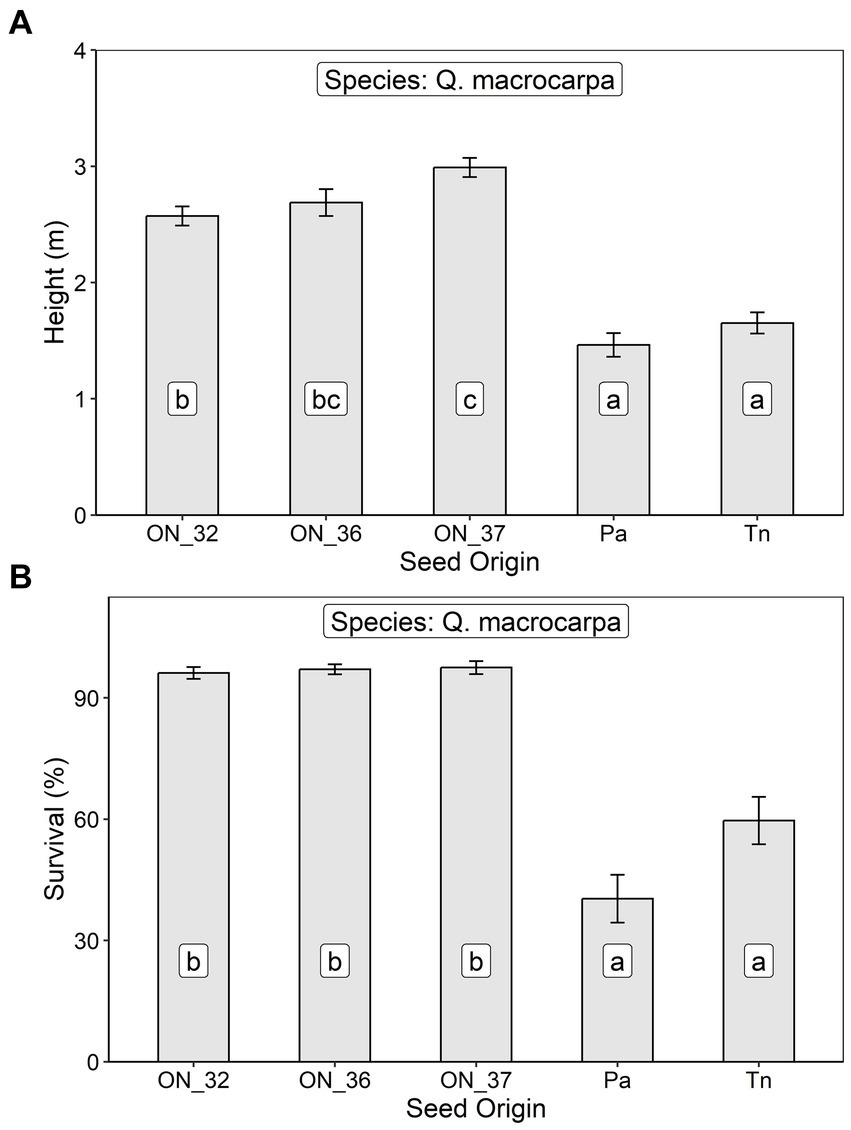
Figure 7. Least squares mean (A) height and (B) survival at age 8 (± S.E.) of Q. macrocarpa trees in relation to seed origin (ON_32, Ontario Seed Zone 32, ON_36, Ontario Seed Zone 36, ON_37 = Ontario Seed Zone 37, Pa, Pennsylvania, and Tn, Tennessee) at an assisted migration trial near Plantagenet, Ontario. Differences between seed origins not sharing the same letter are statistically significant (i.e., p < 0.05).
4 Discussion
This study examines growth and survival of various oak species and seed sources at five trial sites across southern Ontario. Seed sources were selected to cover a range of (primarily northward) movement distances to simulate assisted migration activities under climate change – including movements of some species to locations at, or beyond, northern range limits. Growing season temperatures experienced by trees at the trial sites were warmer than historical conditions associated with many of the Ontario seed sources, but were similar to historical temperatures in Pennsylvania and cooler than those in Tennessee. Extreme temperature conditions followed similar patterns, but in this case, extremes experienced at the planting sites were significantly cooler than historical values in both Pennsylvania and Tennessee. Growing seasons typically started 2–3 weeks earlier in Tennessee and Pennsylvania relative to the planting sites, which indicates potential for frost damage at these northern planting sites. Growing season precipitation at the trial sites was similar to historical precipitation at the various Ontario seed sources, but was lower than historical precipitation in Pennsylvania and Tennessee. Finally, moisture balance was relatively consistent across trial sites and historical seed source conditions. Overall, these findings confirm that a significant range of climate conditions, including climate extremes, was sampled by the seed sources and planting sites included in the study.
Despite evidence that mismatches may already be developing between temperatures at the trial sites and those to which the local seed sources are adapted, the Ontario sources exhibited high growth and survival at all trial sites. There are several potential reasons for this result. First, the magnitude of temperature change, which was approximately 1°C for mean annual temperature in the southern Ontario region over the 1948–2016 period (Bush and Lemmen, 2019), may not yet be large enough to negatively impact growth and survival as trees have been shown to have high levels of phenotypic plasticity (Benito-Garzón et al., 2019). For example, response curves, which plot seed source performance (e.g., height or survival) as a function of climate at a range of planting sites, typically show good performance across a range of conditions (Wang et al., 2006, 2010). Furthermore, previous work has shown that populations in the northern portion of a species’ range (which includes all the Ontario seed sources in the current study) typically benefit from a modest amount of climate warming (Pedlar and McKenney, 2017). Finally, the trials are still relatively young, so extreme weather events that impact survival and growth (e.g., late frosts, droughts) may have not yet materialized at the sites.
Seed sources from the U.S. presented mixed results when moved northward to southern Ontario planting sites. At the Oxford, Holst, and Warwick trials, differences between U.S. and local seed sources were relatively minor, though there was some evidence for reduced performance of Tennessee seed sources at the Holst and Warwick sites. Previous efforts have reported similarly modest impacts associated with seed movements of several hundred km (or approximately 5°C of mean annual temperature) for both hardwood (Pedlar et al., 2023) and conifer (Pedlar et al., 2021) species. Conversely, the two U.S. sources exhibited significantly lower growth and survival rates at the Lemoine Point and Plantagenet trials. Given the significant differences in precipitation and extreme temperatures between these seed sources and planting sites, it is possible that these results were climate driven; however, we note a number of caveats associated with these findings. First, there were challenges with obtaining planting stock from the U.S. that was entirely comparable to that of the local Ontario seed sources; concerns regarding the quality of U.S. stock were noted at time of planting for both trials in question. Second, there was significant mortality at the Plantagenet site due to vole damage, which may have, by chance, disproportionately affected the U.S. seed sources. There was also high mortality at the Lemoine Point trial due to wet soil conditions, which may have exacerbated any climate-related impacts at the site.
Two species – Q. bicolor and Q. alba – achieved high growth and survival rates at trials located near their northern range limits. These results suggest that there is potential for species to survive and grow well when moved to locations at, or beyond, their current northern range limits (i.e., assisted range expansion) – supporting previous studies that have reported successful northward range expansions in forestry (Etterson et al., 2020), restoration (Truax et al., 2018), and horticultural (van der Veken et al., 2008) settings. While there has been considerable debate regarding the appropriate use of assisted migration as a climate change adaptation tool (McLachlan et al., 2007; Hewitt et al., 2011), modest movements of tree species beyond northern range limits, such as those undertaken here, represent a relatively low risk approach that may facilitate northward shifts to track climate change – particularly in landscapes that have been extensively fragmented by human development. The inadvertent planting of Q. marilandica oak at the Holst site provided some insight into longer-distance range expansions. While this species has persisted at the site, it exhibited lower rates of survival and growth than the other (native) oak species planted there and was adversely affected by extreme cold events in fall 2020/spring 2021 – and potentially during winter as evidenced by the significantly lower annual extreme minimum temperatures at the Holst site relative to those typically experienced at the Tennessee seed source (Supplementary Figure S1). This evidence indicates that, for this species at least, movements upwards of 500 km beyond northern range limits involve significant risk to the survival and growth of the resulting plantation. Comparable within-range movement distances for the other species in the study did not produce similar levels of damage, suggesting modest levels of local climate adaptation across populations, with relatively strong climatic controls at the species level – a pattern that has been reported for numerous species (see review in Copenhaver-Parry et al., 2017).
A growing number of studies are reporting outcomes from assisted migration trials. Similar to the current study, Pedlar et al. (2023) reported that seed sources of several hardwood species (including several oaks) could be moved hundreds of kilometers northward with little impact on resulting growth and survival. Etterson et al. (2020) examined the growth of southern and northern seed sources for two oak species in northern Minnesota and found that the southern seed sources generally outperformed the local northern sources. Similarly, Toledo-Aceves et al. (2023) reported that, in Mexico’s Veracruz state, populations of the endangered oak, Quercus insignis, could be moved to locations up to 3°C cooler with no negative impacts. Sáenz-Romero et al. (2020) reviewed three different assisted migration trials in Canada and Mexico and reported the following general insights: (1) populations of Picea glauca × P. engelmannii may be safely planted at locations that are 3°C cooler than local (in terms of mean coldest month temperature); (2) Pinus albicaulis can be established outside of its current natural distribution at sites that have climates that are within the species’ modeled historic climatic niche; and (3) Abies religiosa performs well when moved 400 m upward in elevation. Finally, Gómez-Ruiz et al. (2020) translocated two tropical broadleaf species in Mexico and recommended that movements be limited to within 400 m of elevational range limits. These findings generally support those presented here regarding the suitability of seed sources for northward/upslope movements and the potential for range expansion under climate change.
Pike and O’Connor (2024) recommended northward transfer distances for Q. rubra of approximately 300–400 km based on a review of provenance trials and genetic studies. Though not incorporating an extensive range of seed sources, our findings generally support this recommendation for the sample of oak species considered here. Interestingly, Kriebel (1993) reviewed early provenance studies, and concluded that optimal growth of Q. rubra at mid-latitudes in the US could be achieved by moving northern seed sources southward by 250–500 km. Though not tested explicitly here, we did not find strong support for the notion of superior performance by northern provenances of Q. rubra [see also Leites et al. (2019)].
We recognize several caveats related to the current work. The strength of our findings could be improved by the establishment of control plantations at seed source locations as this was not evaluated in the current study. As noted, it was challenging to obtain planting material that was entirely comparable across seed sources. In particular, seedlings from PA and TN were of lower quality than the local seed sources at the Lemoine Point and Plantagenet trials, which likely contributed to the poor performance of these seed sources at these sites. Furthermore, at several of the sites, seedlings for non-target species were mistakenly included in the seedling order. It was several years before trees were large enough to identify these errors, at which point it was too late to replant; thus, replicates were incomplete for certain seed sources at certain sites. Given the relatively young age of the plantations examined here, our findings should be considered preliminary in nature. Provenance studies have reported changes in the ranking of seed source performance over time, which can impact key study outcomes (Rweyongeza, 2016; Kriebel, 1993). Nonetheless, the early years of a plantation are recognized as being critical for tree survival (Lutz and Halpern, 2006), suggesting that important performance metrics may be measured over this period. Finally, we recognize that survival and growth are but two of a suite of metrics that could be measured to assess performance at these trial sites. Phenological measures are particularly important for assisted migration outcomes and may be assessed at these sites in the future.
5 Conclusion
Our findings suggest that oak trees can be moved significant distances (e.g., hundreds of kilometres poleward/hundreds of meters upslope) for assisted migration efforts, which generally supports previous work on this topic. Poor performance by U.S. seed sources at two trial sites was likely due to lower quality planting stock as opposed to climate-related impacts. However, given the potential impacts of extreme weather events such as droughts and late frosts on young plantations, more restrained transfer distances are recommended. This work adds to a growing literature base concerning assisted migration outcomes for oaks. Future efforts at these sites will examine longer-term differences in growth and survival between seed sources and the response of migrated seed sources to extreme climate events.
Data availability statement
The raw data supporting the conclusions of this article will be made available by the authors, without undue reservation.
Author contributions
JP: Conceptualization, Formal analysis, Visualization, Writing – original draft, Writing – review & editing. DM: Conceptualization, Funding acquisition, Project administration, Supervision, Writing – original draft, Writing – review & editing. KS: Conceptualization, Data curation, Investigation, Writing – original draft, Writing – review & editing. HZ: Funding acquisition, Project administration, Supervision, Writing – original draft, Writing – review & editing. KM: Conceptualization, Funding acquisition, Project administration, Supervision, Writing – original draft, Writing – review & editing.
Funding
The author(s) declare that financial support was received for the research, authorship, and/or publication of this article. This research was supported by funding from the Forest Climate Change Program (Natural Resources Canada, Canadian Forest Service).
Acknowledgments
We thank Barb Boysen (retired, FGCA) and Gary Nielsen (retired, OMNRF) for their vision and efforts in establishing these plantations. We also thank the private and municipal property owners who have provided land and technical support for this work.
Conflict of interest
The authors declare that the research was conducted in the absence of any commercial or financial relationships that could be construed as a potential conflict of interest.
Publisher’s note
All claims expressed in this article are solely those of the authors and do not necessarily represent those of their affiliated organizations, or those of the publisher, the editors and the reviewers. Any product that may be evaluated in this article, or claim that may be made by its manufacturer, is not guaranteed or endorsed by the publisher.
Supplementary material
The Supplementary material for this article can be found online at: https://www.frontiersin.org/articles/10.3389/ffgc.2024.1445029/full#supplementary-material
References
Abrams, M. D. (1990). Adaptations and responses to drought in Quercus species of North America. Tree Physiol. 7, 227–238. doi: 10.1093/treephys/7.1-2-3-4.227
Benito-Garzón, M., Robson, T. M., and Hampe, A. (2019). ΔTraitSDMs: species distribution models that account for local adaptation and phenotypic plasticity. New Phytol. 222, 1757–1765. doi: 10.1111/nph.15716
Borkowski, D. S., Hoban, S. M., Chatwin, W., and Romero-Severson, J. (2017). Rangewide population differentiation and population substructure in Quercus rubra L. Tree Genet. Genomes 13, 1–11. doi: 10.1007/s11295-017-1148-6
Bush, E., and Lemmen, D. S. (Eds.) (2019). Canada’s changing climate report. Ottawa, ON: Government of Canada, 444.
Copenhaver-Parry, P. E., Shuman, B. N., and Tinker, D. B. (2017). Toward an improved conceptual understanding of north American tree species distributions. Ecosphere 8:e01853. doi: 10.1002/ecs2.1853
Etterson, J. R., Cornett, M. W., White, M. A., and Kavajecz, L. C. (2020). Assisted migration across fixed seed zones detects adaptation lags in two major north American tree species. Ecol. Appl. 30:e02092. doi: 10.1002/eap.2092
Forzieri, G., Dakos, V., McDowell, N. G., Ramdane, A., and Cescatti, A. (2022). Emerging signals of declining forest resilience under climate change. Nature 608, 534–539. doi: 10.1038/s41586-022-04959-9
Girardin, M. P., Bouriaud, O., Hogg, E. H., Kurz, W., Zimmerman, N. E., Metsaranta, J. M., et al. (2016). No growth stimulation of Canada’s boreal forest under half-century of combined warming and CO2 fertilization. Proc. Natl. Acad. Sci. 113, E8406–E8414. doi: 10.1073/pnas.1610156113
Gómez-Ruiz, P. A., Sáenz-Romero, C., and Lindig-Cisneros, R. (2020). Early performance of two tropical dry forest species after assisted migration to pine–oak forests at different altitudes: strategic response to climate change. J. For. Res. 31, 1215–1223. doi: 10.1007/s11676-019-00973-2
Hammond, W. M., Williams, A. P., Abatzoglou, J. T., Adams, H. D., Klein, T., López, R., et al. (2022). Global field observations of tree die-off reveal hotter-drought fingerprint for Earth’s forests. Nat. Commun. 13:1761. doi: 10.1038/s41467-022-29289-2
Hanes, C. C., Wang, X., Jain, P., Parisien, M. A., Little, J. M., and Flannigan, M. D. (2019). Fire-regime changes in Canada over the last half century. Can. J. For. Res. 49, 256–269. doi: 10.1139/cjfr-2018-0293
Hartmann, H., Bastos, A., Das, A. J., Esquivel-Muelbert, A., Hammond, W. M., Martínez-Vilalta, J., et al. (2022). Climate change risks to global forest health: emergence of unexpected events of elevated tree mortality worldwide. Annu. Rev. Plant Biol. 73, 673–702. doi: 10.1146/annurev-arplant-102820-012804
Hewitt, N., Klenk, N., Smith, A. L., Bazely, D. R., Yan, N., Wood, S., et al. (2011). Taking stock of the assisted migration debate. Biol. Conserv. 144, 2560–2572. doi: 10.1016/j.biocon.2011.04.031
Hogg, E. H. (1994). Climate and the southern limit of the western Canadian boreal forest. Can. J. For. Res. 24, 1835–1845. doi: 10.1139/x94-237
Hunt, R. (1982). Plant growth curves. The functional approach to plant growth analysis. London, UK: Edward Arnold Ltd.
Jaeger, B. C., Edwards, L. J., Das, K., and Sen, P. K. (2017). An R2 statistic for fixed effects in the generalized linear mixed model. J. Appl. Stat. 44, 1086–1105. doi: 10.1080/02664763.2016.1193725
Janowiak, M. K., Swanston, C. W., Nagel, L. M., Brandt, L. A., Butler, P. R., Handler, S. D., et al. (2014). A practical approach for translating climate change adaptation principles into forest management actions. J. For. 112, 424–433. doi: 10.5849/jof.13-094
Johnson, D. M., and Haynes, K. J. (2023). Spatiotemporal dynamics of forest insect populations under climate change. Curr. Opin. Insect Sci. 56:101020. doi: 10.1016/j.cois.2023.101020
Kaproth, M., and Cavender-Bares, J. (2016). Drought tolerance and climatic distributions of the American oaks. Int Oaks 27, 49–60.
Kriebel, H. B. (1993). Intraspecific variation of growth and adaptive traits in north American oak species Ann. For. Sci, 50 (Supplement) 153s–165s. doi: 10.1051/forest:19930715
Leites, L. P., Rehfeldt, G. E., and Steiner, K. C. (2019). Adaptation to climate in five eastern North America broadleaf deciduous species: growth clines and evidence of the growth-cold tolerance trade-off. Perspect.Plant Ecol. Evol. Syst. 37, 64–72. doi: 10.1016/j.ppees.2019.02.002
Lutz, J. A., and Halpern, C. B. (2006). Tree mortality during early forest development: a long-term study of rates, causes, and consequences. Ecol. Monogr. 76, 257–275. doi: 10.1890/0012-9615(2006)076[0257:TMDEFD]2.0.CO;2
McKenney, D. W., Hutchinson, M. F., Papadopol, P., Lawrence, K., Pedlar, J., Campbell, K., et al. (2011). Customized spatial climate models for North America. Bull. Am. Meteorol. Soc. 92, 1611–1622. doi: 10.1175/2011BAMS3132.1
McKenney, D. W., Mackey, B. G., and Joyce, D. (1999). Seedwhere: a computer tool to support seed transfer and ecological restoration decisions. Environ. Model Softw. 14, 589–595. doi: 10.1016/S1364-8152(98)00095-4
McLachlan, J. S., Hellmann, J. J., and Schwartz, M. W. (2007). A framework for debate of assisted migration in an era of climate change. Conserv. Biol. 21, 297–302. doi: 10.1111/j.1523-1739.2007.00676.x
McLane, S. C., and Aitken, S. N. (2012). Whitebark pine (Pinus albicaulis) assisted migration potential: testing establishment north of the species range. Ecol. Appl. 22, 142–153. doi: 10.1890/11-0329.1
O’Neill, G. A., Ukrainetz, N. K., Carlson, M. R., Cartwright, C. V., Jaquish, B. C., King, J. N., et al. (2008). Assisted migration to address climate change in British Columbia: recommendations for interim seed transfer standards. BC min. For. Range, res. Br., Victoria. Forest Sci. Branch Tech. Rep. 48.
O’Neill, G., Wang, T., Ukrainetz, N., Charleson, L., McAuley, L., Yanchuk, A., et al. (2017). A proposed climate-based seed transfer system for British Columbia. Technical report-ministry of forests. Tech. Rep. 099. Prov. B.C., Victoria, B.C..
Pedlar, J. H., and McKenney, D. W. (2017). Assessing the anticipated growth response of northern conifer populations to a warming climate. Sci. Rep. 7:43881. doi: 10.1038/srep43881
Pedlar, J. H., McKenney, D. W., and Allen, D. J. (2023). Effect of tree species and seed origin on climate change trial outcomes in southern Ontario. New For. 55, 63–79. doi: 10.1007/s11056-023-09965-x
Pedlar, J. H., McKenney, D. W., Hope, E., Reed, S., and Sweeney, J. (2020). Assessing the climate suitability and potential economic impacts of oak wilt in Canada. Sci. Rep. 10:19391. doi: 10.1038/s41598-020-75549-w
Pedlar, J. H., McKenney, D. W., and Lu, P. (2021). Critical seed transfer distances for selected tree species in eastern North America. J. Ecol. 109, 2271–2283. doi: 10.1111/1365-2745.13605
Peterson, D. L., Millar, C. I., Joyce, L. A., Furniss, M. J., Halofsky, J. E., Neilson, R. P., et al. (2011). Responding to climate change in national forests: a guidebook for developing adaptation options Gen. Tech. Rep. PNW-GTR-855. US Department of Agriculture, Forest Service, Pacific northwest Research Station, Portland.
Pike, C. C., and O'Connor, P. A. (2024). “Quercus rubra (NortherRed oak)” in Seed-transfer guidelines for important tree species in the eastern United States. eds. C. C. Pike and D. L. Haase (USDA Forest Service, Agricultural Handbook 801), 117–123.
R Core Team (2021). R: A language and environment for statistical computing. Vienna, Austria: R Foundation for Statistical Computing.
Rweyongeza, D. M. (2016). A new approach to prediction of the age-age correlation for use in tree breeding. Ann. For. Sci. 73, 1099–1111. doi: 10.1007/s13595-016-0570-5
Sáenz-Romero, C., O’Neill, G., Aitken, S. N., and Lindig-Cisneros, R. (2020). Assisted migration field tests in Canada and Mexico: lessons, limitations, and challenges. Forests 12:9. doi: 10.3390/f12010009
Ste-Marie, C. A., Nelson, E., Dabros, A., and Bonneau, M. E. (2011). Assisted migration: introduction to a multifaceted concept. For. Chron. 87, 724–730. doi: 10.5558/tfc2011-089
Toledo-Aceves, T., Sáenz-Romero, C., Cruzado-Vargas, A. L., and Vásquez-Reyes, V. (2023). Quercus insignis seedling response to climatic transfer distance in the face of climate change. For. Ecol. Manag. 533:120855. doi: 10.1016/j.foreco.2023.120855
Truax, B., Gagnon, D., Fortier, J., Lambert, F., and Pétrin, M. A. (2018). Ecological factors affecting white pine, red oak, bitternut hickory and black walnut underplanting success in a northern temperate post-agricultural forest. Forests 9:499. doi: 10.3390/f9080499
van der Veken, S., Hermy, M., Vellend, M., Knapen, A., and Verheyen, K. (2008). Garden plants get a head start on climate change. Front. Ecol. Environ. 6, 212–216. doi: 10.1890/070063
van Kerkhof, B., Elliott, K. A., Lu, P., McKenney, D. W., Parker, W. C., Pedlar, J. H., et al. (2022). Enhancing forest resilience: advances in Ontario’s wild tree seed transfer policy. For. Chron. 98, 44–53. doi: 10.5558/tfc2022-002
Wang, T., Hamann, A., Yanchuk, A., O'Neill, G. A., and Aitken, S. N. (2006). Use of response functions in selecting lodgepole pine populations for future climates. Glob. Change Biol. 12, 2404–2416. doi: 10.1111/j.1365-2486.2006.01271.x
Wang, T., O'Neill, G. A., and Aitken, S. N. (2010). Integrating environmental and genetic effects to predict responses of tree populations to climate. Ecol. Appl. 20, 153–163. doi: 10.1890/08-2257.1
Wang, Y., Pedersen, J. L., Macdonald, S. E., Nielsen, S. E., and Zhang, J. (2019). Experimental test of assisted migration for conservation of locally range-restricted plants in Alberta, Canada. Glob. Ecol. Conserv. 17:e00572. doi: 10.1016/j.gecco.2019.e00572
Wang, J., Taylor, A. R., and D’Orangeville, L. (2023). Warming-induced tree growth may help offset increasing disturbance across the Canadian boreal forest. Proc. Natl. Acad. Sci. 120:e2212780120. doi: 10.1073/pnas.2212780120
Williams, M. I., and Dumroese, R. K. (2013). Preparing for climate change: forestry and assisted migration. J. For. 111, 287–297. doi: 10.5849/jof.13-016
Keywords: climate change, tree, oak, growth, survival, assisted migration
Citation: Pedlar JH, McKenney DW, Sandvall K, Zurbrigg H and McLaven K (2024) Assisted migration outcomes for oak species and seed sources in southern Ontario, Canada. Front. For. Glob. Change. 7:1445029. doi: 10.3389/ffgc.2024.1445029
Edited by:
Brian J. Palik, Forest Service (USDA), United StatesReviewed by:
Peter Clark, University of Vermont, United StatesTarin Toledo-Aceves, Instituto de Ecología (INECOL), Mexico
Copyright © 2024 Pedlar, McKenney, Sandvall, Zurbrigg and McLaven. This is an open-access article distributed under the terms of the Creative Commons Attribution License (CC BY). The use, distribution or reproduction in other forums is permitted, provided the original author(s) and the copyright owner(s) are credited and that the original publication in this journal is cited, in accordance with accepted academic practice. No use, distribution or reproduction is permitted which does not comply with these terms.
*Correspondence: John H. Pedlar, am9obi5wZWRsYXJAbnJjYW4tcm5jYW4uZ2MuY2E=
†ORCID: John H. Pedlar, orcid.org/0000-0001-5831-1731