- 1Instituto de Investigaciones Sobre los Recursos Naturales, Universidad Michoacana de San Nicolás de Hidalgo (UMSNH), Morelia, Mexico
- 2Laboratorio Nacional CONAHCyT de Biología del Cambio Climático, Mexico City, Mexico
- 3Investigadoras e Investigadores por México, Consejo Nacional de Humanidades, Ciencia y Tecnología, Ciudad de México, Mexico City, Mexico
- 4Instituto de Investigaciones Agropecuarias y Forestales, Universidad Michoacana de San Nicolás de Hidalgo (UMSNH), Morelia, Michoacán, Mexico
- 5Instituto de Investigaciones en Ecosistemas y Sustentabilidad, Universidad Nacional Autónoma de México, Morelia, Mexico
- 6Kalamalka Forestry Centre, Forest Improvement and Research Management Branch, British Columbia Ministry of Forests, Vernon, BC, Canada
- 7Facultad de Biología, (UMSNH), Morelia, Mexico
- 8Instituto de Ciencias Agropecuarias y Rurales, Universidad Autónoma del Estado de México, Toluca, Mexico
Climate change projections suggest a precarious future for the Monarch butterfly (Danaus plexippus) as the suitable climatic habitat of its exclusive overwintering host Abies religiosa (oyamel, Sacred fir, a conifer endemic to Mexico) inside the Monarch Butterfly Biosphere Reserve (MBBR) is expected to disappear by 2090. Since the upper elevation limit of A. religiosa is approximately 3,500 m and the summits of mountains within the MBBR are ca. 3,550 m, we tested the feasibility of establishing A. religiosa at four locations outside its current geographic range in the MBBR, on a geographically close volcano, Nevado de Toluca at 4000 (timberline, an extreme site), 3,800, and 3,600 m (to test species range expansion upward in elevation), and at 3400 m (a reference site, slightly lower than the upper elevation limit of A. religiosa). Using existing shrubs as nurse plants to protect the seedlings from extreme temperatures, at each site we planted five to eight populations, originating between 3,100 and 3,500 m within the MBBR. After three growing seasons in the field (6 years after sowing), we found that: (a) survival and height increment declined steeply with test site elevation; (b) even at the highest sites (3,800 and 4,000 m), survival was acceptable, at 68 and 44%, respectively, although the growth was very poor at 4000 m; (c) populations responded similarly to transfer; (d) transfer effects were best accounted for by annual dryness index; (e) to compensate for the expected 2.3°C increase in mean annual temperature or 0.009 √°Cmm−1 increase of annual dryness index from the reference period (1961–1990) to the decade centered in 2060, it would be necessary to shift populations approximately 500 m to higher elevations; and (f) upward transfers to compensate for the 2.3°C increase in mean annual temperature are expected to result in height increment and survival that are approximately 47 and 21% lower, respectively, than values expected at zero transfer distance. We conclude that the establishment of A. religiosa at 3600 and 3,800 m is feasible and that planted stands could eventually serve as overwintering sites for the Monarch butterfly under projected future climates.
1 Introduction
Unusual tree mortality linked to anthropogenic climate change (Allen et al., 2010) likely places temperate forests at or near a tipping point. This is because droughts are now more frequent, intense, longer (Munson et al., 2018; Ritchie et al., 2021), and hotter (Hammond et al., 2022). The ongoing acceleration of climate change, driven by the synergic combination of the 2023–2024 El Niño (warm Pacific Ocean Equatorial current; also known as El Niño-Southern Oscillation cycle, ENSO) and other factors (reduction of suspended particles and upper atmospheric sulfur particles; Hansen et al., 2023), is making it increasingly evident that temperate forests around the world may reach and even surpass a tipping point for forest decline (Sáenz-Romero, 2024).
Some forests face a challenge to recover after recent disturbances linked to climate change, such as the 18 million hectares burned by forest fires in Canada in 2023 (Natural Resources Canada, 2023). Fires also risk converting the iconic temperate and boreal forests of Canada from a carbon source to a carbon sink (Council of Canadian Academies, 2022). Boreal Eurasian Larix decidua forests are also approaching a tipping point, due to increasing maximum temperatures (Rao et al., 2023).
Abies religiosa (Kunth) Schltdl. and Cham. (oyamel, Sacred fir) is a conifer endemic to Mexico, distributed mainly on moist (mean annual precipitation 900 to 1,500 mm) and cold sites (mean annual temperature 9 to 14°C), at high elevations (2,800 to 3,500 m), mostly along the central-Mexico Trans-Mexican Volcanic Belt, an East–West mountain range between 19° and 20° north latitude, with the highest volcanoes in México (De Rzedowski and Rzedowski, 2005; Benavides-Meza et al., 2011; Sáenz-Romero et al., 2012; Gómez-Pineda et al., 2020). It is a shade-tolerant species, found mainly on northern aspects and in deep soils (Sanchez-Velasquez et al., 1991; Rzedowski, 2006). A map of its contemporary suitable habitat and locations of the natural populations identified in the Mexican National Forest Inventory is available in Sáenz-Romero et al. (2012).
High elevation (3,000 to 3,500 m), pure, and dense stands of Abies religiosa within the Monarch Butterfly Biosphere Reserve (MBBR; with an area of 56,259 ha) in central Mexico (see Figure 1) act as an over-wintering host for migratory populations of the Monarch butterfly (Danaus plexippus) from November to March each year. The Monarch butterfly migratory populations overwinter exclusively on the crowns and stems of large, mature, A. religiosa trees, historically inside of the MBBR, because the dense crown of this species protects the butterfly from extreme temperatures and precipitation (Anderson and Brower, 1996). Rain followed by freezing temperatures in thinned stands of A. religiosa sites has been associated with significant Monarch mortality events, suggesting the need for fully occupied stands to protect migratory overwintering colonies.
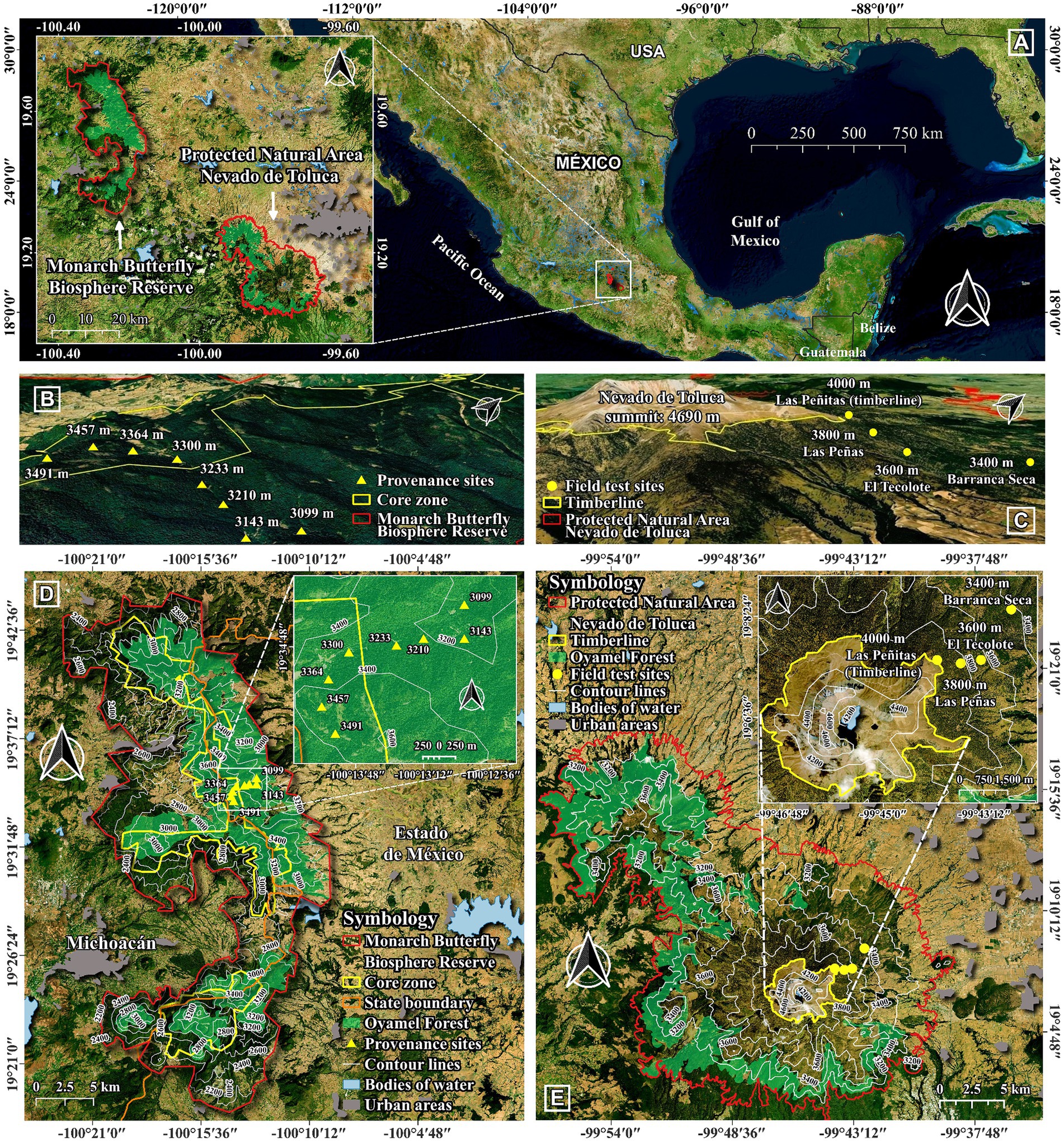
Figure 1. (A) Geographic location of the Monarch Butterfly Biosphere Reserve (MBBR) where A. religiosa is found, and the Protected Natural Area Nevado de Toluca where the provenance trials were established, in central Mexico. In the left inserted panel, Protected Area limits are indicated with a red contour line. (B–E) The core zones of both Protected Natural Areas (coincidental with the timberline at Nevado de Toluca) are indicated with yellow contour lines. (B,D) Provenance locations and elevations along an elevational transect in the MBBR (yellow triangles), where seed was collected. (C,E) Provenance test site locations (yellow circle symbols) and elevations in the Nevado de Toluca Protected Natural Area, including at the timberline (4,000 m elevation). (D,E) Actual distribution of Abies religiosa (layer colored in light green) based on Comisión Nacional Forestal (2018). Note that A. religiosa is absent above approximately 3,500 m (E).
The size of the Monarch butterfly migratory populations has been decreasing, with the winter 2023–2024 population count being the second lowest in history (Comisión Nacional de Áreas Naturales Protegidas, 2024). Migratory populations were recently identified as endangered on the IUCN list (Walker et al., 2022), despite the MBBR being an example of relatively successful (at least in the Mexican socio-ecological context) in situ conservation, having almost completely stopped illegal logging while developing controlled ecotourism (Rendón-Salinas et al., 2023).
Climatic niche modeling under future climate change scenarios suggests that the suitable climatic habitat for A. religiosa inside the MBBR will move upward in elevation, before disappearing entirely by the end of the century (Sáenz-Romero et al., 2012). Loss of climatic niche is exacerbated by increased bark beetle outbreaks in A. religiosa stands (Gómez-Pineda et al., 2023) linked to drought stress (Sáenz-Romero et al., 2023). Unfortunately, such projected decoupling between the sites occupied by native A. religiosa populations and locations where their suitable climate will occur is associated with extreme drought stress, particularly at the xeric limit (low altitude) of the contemporary natural range distribution. Dendrochronological analysis and recent measurements using the Normalized Difference Vegetation Index (NDVI), indicate that A. religiosa stands are highly dependent on winter–spring precipitation (Vivar-Vivar et al., 2021) and very sensitive to drought stress. This appraisal seems to be confirmed by recent field observations of rapid defoliation and decay of natural stands due to the now warmer and drier springs (Flores-Nieves et al., 2011; Sáenz-Romero et al., 2012). Also, induced drought stress experiments on A. religiosa provenances in nursery and common garden tests, suggest that genetic differentiation among populations for drought tolerance is not statistically significant (Cruzado-Vargas, 2017; Zamora-Sánchez, 2019).
The projected disappearance of the suitable climatic habitat of A. religiosa due to climate change (a predicted loss of 96.5% of its suitable climatic habitat nationwide by the end of the century; Sáenz-Romero et al., 2012) is not a problem exclusive to this species. It is a trait shared by many high-elevation forest tree species, for which their proximity to their respective mountain summits represents a risk of population extirpation, as natural migration to higher elevations might not be an option. Such is the case for the Mexican timberline species Pinus hartwegii (Gómez-Pineda et al., 2020), the western United States and Canada timberline species Pinus albicaulis (McLane and Aitken, 2012), the highly endangered Picea mexicana and Picea martinezii (Ledig et al., 2010; Mendoza-Maya et al., 2022), among several other North America forest tree species (Seliger et al., 2021).
High-elevation and tree-line tree species are not alone in being challenged to find suitable habitats at elevations higher than their upper elevation range limit. Rare microendemic alpine plant species found above the timberline—Arenaria bryoides, Chionolaena lavandulifolia, Castilleja tolucensis, Draba nivicola, and Plantago tolucensis—are also expected to lose significant proportions or their entire realized niche due to lack of habitat at higher elevations as climate warms (Ramírez-Amezcua et al., 2016).
Under such grim future scenarios, forest management must adapt. In situ conservation measures are insufficient. Pro-active ex situ conservation is urgently required, including exploring the establishment of stands of Abies religiosa at locations above its upper elevation limit in the Mexican Transvolcanic Belt (MTVB) to recouple the species with its historic climate. Movement of the species beyond its current natural distribution is referred to as “assisted range expansion,” one form of assisted migration of the forest – the intentional establishment of forest plantations with seed sources (provenances) from climates slightly warmer than that of the planting site to help maintain forest productivity, health, and ecosystem services in the face of rapid climate change (Winder et al., 2011).
Recoupling could be achieved by planting seedlings grown from seeds taken from the MBBR in locations where MBBR-like climates are expected to occur in the near future (Sáenz-Romero et al., 2016; Richardson et al., 2024). Assisted migration is used throughout British Columbia, Canada (O'Neill et al., 2017) and is proposed as an effective conservation measure for endangered timberline species such as Pinus albicaulis (McLane and Aitken, 2012; Sáenz-Romero et al., 2021) or the extremely rare Picea mexicana (Ledig et al., 2010; Mendoza-Maya et al., 2022).
The success of assisted migration efforts can be significantly impacted by the magnitude of climatic distance that the populations are moved (i.e., by the migration distance: the difference between the site climate minus the seed source climate): short migration distances may be ineffective, while long migration distances may result in early mortality due to frost damage. Selection of suitable migration distances can be achieved by establishing plantations with populations whose historic climate matches that of the plantation climate expected at some future date (O’Neill and Degner, 2024). However, the lack of empirical evidence of optimum future plantation climate and historic population climate represents a knowledge gap in assisted migration initiatives. Field tests, particularly, those with elevational reciprocal transplants, may provide insight into this issue.
The mountain summits within the MBBR are approximately coincidental with the upper elevation of the natural distribution of A. religiosa. It is therefore necessary to establish the species outside the MBBR. Even if such an enterprise were to succeed, there is no guarantee that the Monarch butterfly migratory population would overwinter in the new host plantation sites. This is due to an astonishing phenomenon: the descendants of a fourth generation of the monarchs that stayed in Mexican overwintering sites can return to approximately the same sites every winter. However, we consider inaction not to be an option and prefer to explore novel adaptive forest management options that could offer an opportunity for the survival of the migratory Monarch butterfly populations under future climates. Although the challenges associated with A. religiosa establishment are well known, recent evidence suggests that planting under the shade of a nurse shrub can significantly increase the probability of survival (Carbajal-Navarro et al., 2019).
The objective of this study was to assess the ability of Abies religiosa to establish and grow at elevations higher than its upper elevation natural range limit outside the MBBR. We hypothesize that acceptable seedling survival and growth rate of A. religiosa populations can be achieved in locations higher (and thus colder) than the species’ current upper elevation distribution limit. We therefore initiated a species trial of A. religiosa using populations from disparate seed source elevations within the MBBR and planted them under nurse shrubs at locations outside the MBBR and at elevations higher than the natural range limit of the species.
2 Materials and methods
2.1 Seed sources
Cones were collected in December 2017 from eight stands of A. religiosa, at 50 m intervals along an elevation transect from 3,100 to 3,500 m within the MBBR (Table 1). In each stand, cones were collected from 10 randomly selected open-pollinated mother trees located at least 30 m apart to reduce the possibility of inbreeding.
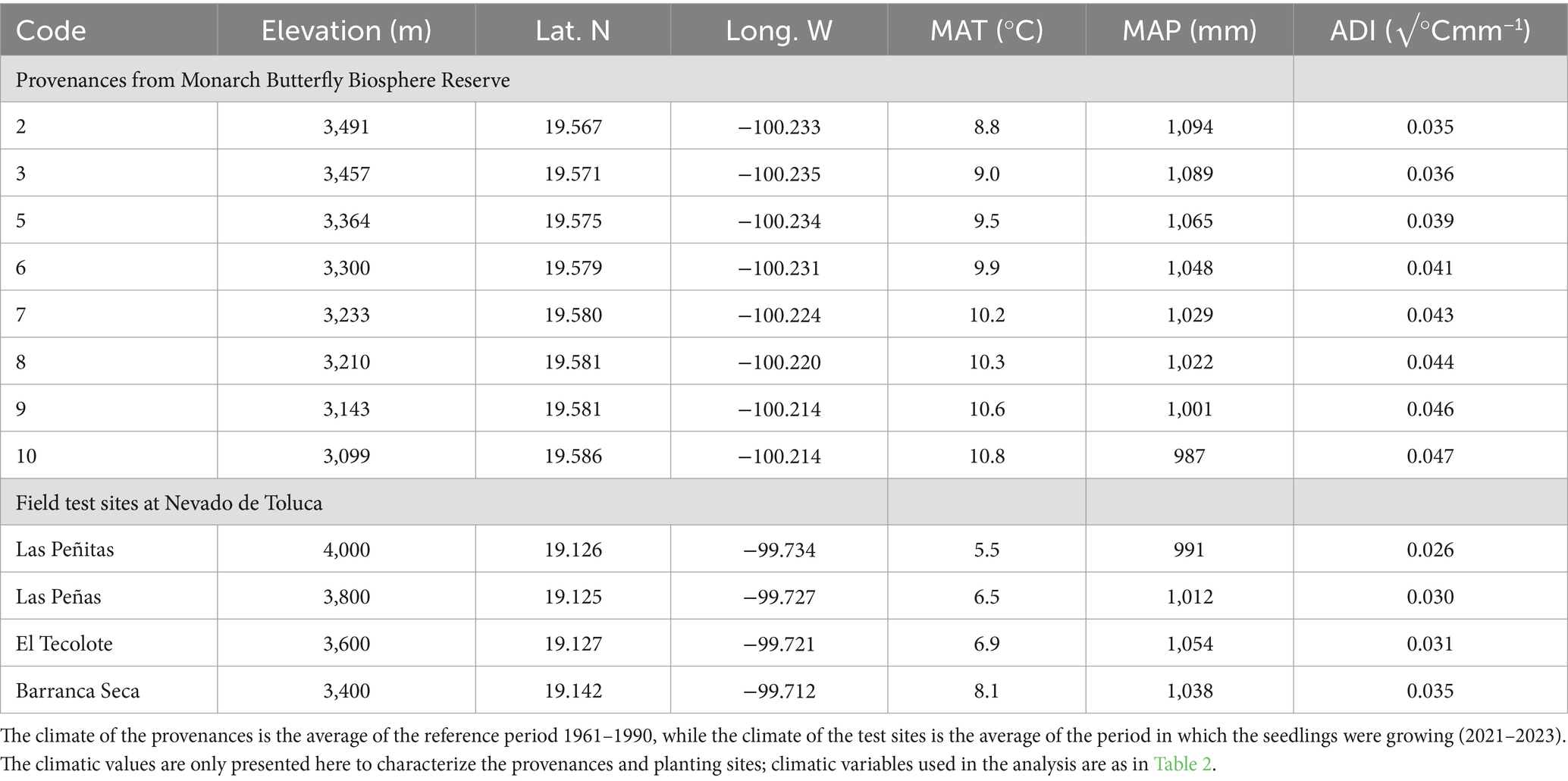
Table 1. Geographic coordinates, elevation, mean annual temperature (MAT), mean annual precipitation (MAP), and annual dryness index (ADI) of Abies religiosa provenances and test sites.
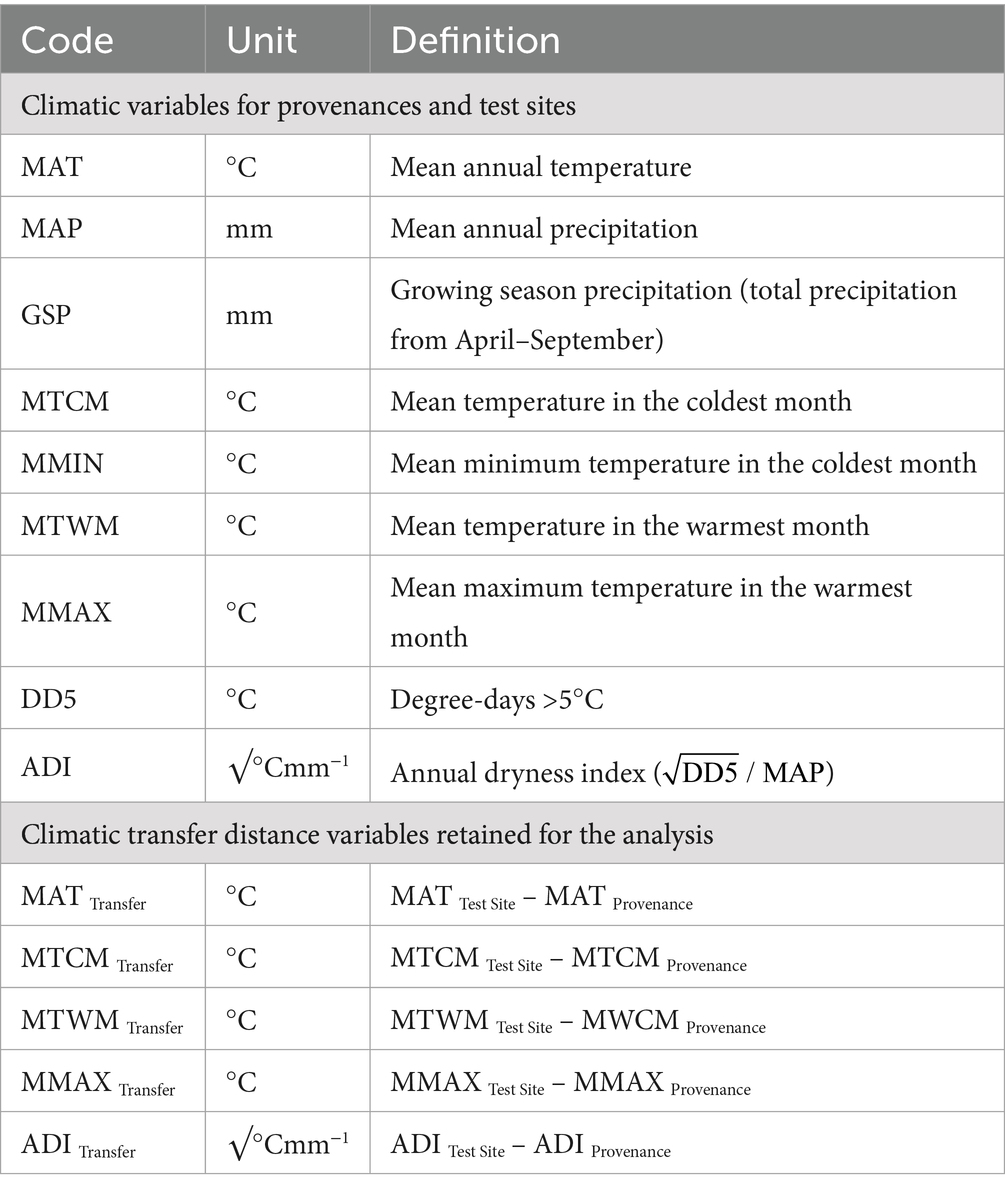
Table 2. Climatic variables estimated for each provenance and for each test site, and climatic transfer distance variables retained as candidate variables for the analysis with the final mixed model.
We use the term ‘population’ to represent the genotypes represented by these stand samples and ‘provenance’ to refer to the geographic origin of the populations. However, in the tree breeding domain, populations and provenances are sometimes used interchangeably.
2.2 Seedling production
After exposing the cones to sunlight, seeds were extracted manually and stratified at 4°C for 14 days. Equal numbers of seeds from each of the 10 mother trees were bulked to form each seed lot (population). Seedlings were grown for 2 years in a shade-house (35% shade mesh) in 380 cm3 rigid containers at the Instituto de Investigaciones sobre los Recursos Naturales (INIRENA), in Morelia (1900 m), Michoacán, Mexico. (Additional seedling production details in Cruzado-Vargas et al., 2021). Seedlings were then transplanted to larger rigid containers (1,000 mL) and transferred to a communal nursery at 3000 m at the Ejido La Mesa, San José del Rincón, Estado de México, near the MBBR where they remained for 1 year to harden before planting.
2.3 Test sites and experimental design
The populations were planted at four field provenance test sites along an elevational gradient on the northeast slope of Nevado de Toluca, an extinct volcano and Protected Natural Area (officially named Flora and Fauna Protection Area Nevado de Toluca), in July 2021 at the start of the rainy season. The test site location was selected because it is the closest mountain to the MBBR with a summit (4,680 m) substantially higher than the Abies religiosa upper elevation limit in the MBBR (3,550 m) and because Abies religiosa is found most frequently on the same aspect. Furthermore, we chose to situate the provenance test sites within a forest owned by the indigenous community of Calimaya, of the Matlatzincas ethnic group, due to their exceptional forest management. Experience has shown that collaboration with local communities provides site surveillance, capitalizes on traditional ecological knowledge, and engages participants in a way that leads to more effective outcomes, such as the eventual adoption of novel forest management practices.
The four test sites were located at the following elevations: (a) a reference site at 3400 m, an elevation close to that of the upper natural distribution of A. religiosa populations at both Nevado de Toluca and the MBBR; (b) 3,600 m, located just above the upper elevation limit of A. religiosa, which is around 3,550 m; (c) 3,800 m, to test the feasibility of a significant upward shift beyond the natural upper limit of A. religiosa. This shift of about 300 m upward in elevation could compensate for a mean annual temperature increase of 1.5°C, considering the lapse rate of -0.5°C for each 100 m increase in elevation (Sáenz-Romero et al., 2010); and (d) 4,000 m (at the timberline), to test at an extreme cold site to identify the upper elevation limit of the species’ fundamental niche. At all four sites, the surrounding forest is dominated by Pinus hartwegii, although the site at 3400 m also strongly features Alnus jorullensis. More detail of the test site locations is presented in Table 1 and Figure 1.
The experimental design consisted of eight populations planted at each of the four test sites in a randomized complete block design with 30 blocks per site, and a single seedling per population per block. Due to insufficient numbers of seedlings, only 5, 7, and 5 populations were planted at the 3,400, 3,600, and 4,000 m sites, respectively. Thus, we gave priority to the site at 3800 m located 300 m higher than the species’ upper elevation limit (equivalent to a coldward shift of approximately 1.5°C in mean annual temperature) where we planted 30 blocks in each site, except at the 4,000 m site, where we planted only 16 blocks. Where a population was absent in an incomplete block, it was replaced by a surplus seedling of another population to ensure that all blocks contained eight seedlings (except for site at 4000 m, where blocks had five seedlings).
To enable trial seedlings to be shaded, shrubs with crowns large enough to provide shade to all eight seedlings were identified. Seedlings were planted in a circle around the main stem, 50 cm from the stem (Figure 2), based on a previous positive experience of using pre-existing shrubs as nurse plants (see Carbajal-Navarro et al., 2019). The 30 blocks at each test site were positioned in a non-contiguous manner since the nurse plants were distributed in an irregular pattern. At the 3,400, 3,600, and 3,800 m sites, the dominant shrub available, Senecio cinerarioides, was used as the nurse plant. At the 4,000 m site, where S. cinerarioides was nearly absent, the dominant shrub, Lupinus montanus, and in a few cases small P. hartwegii trees, were used as nurse plants.
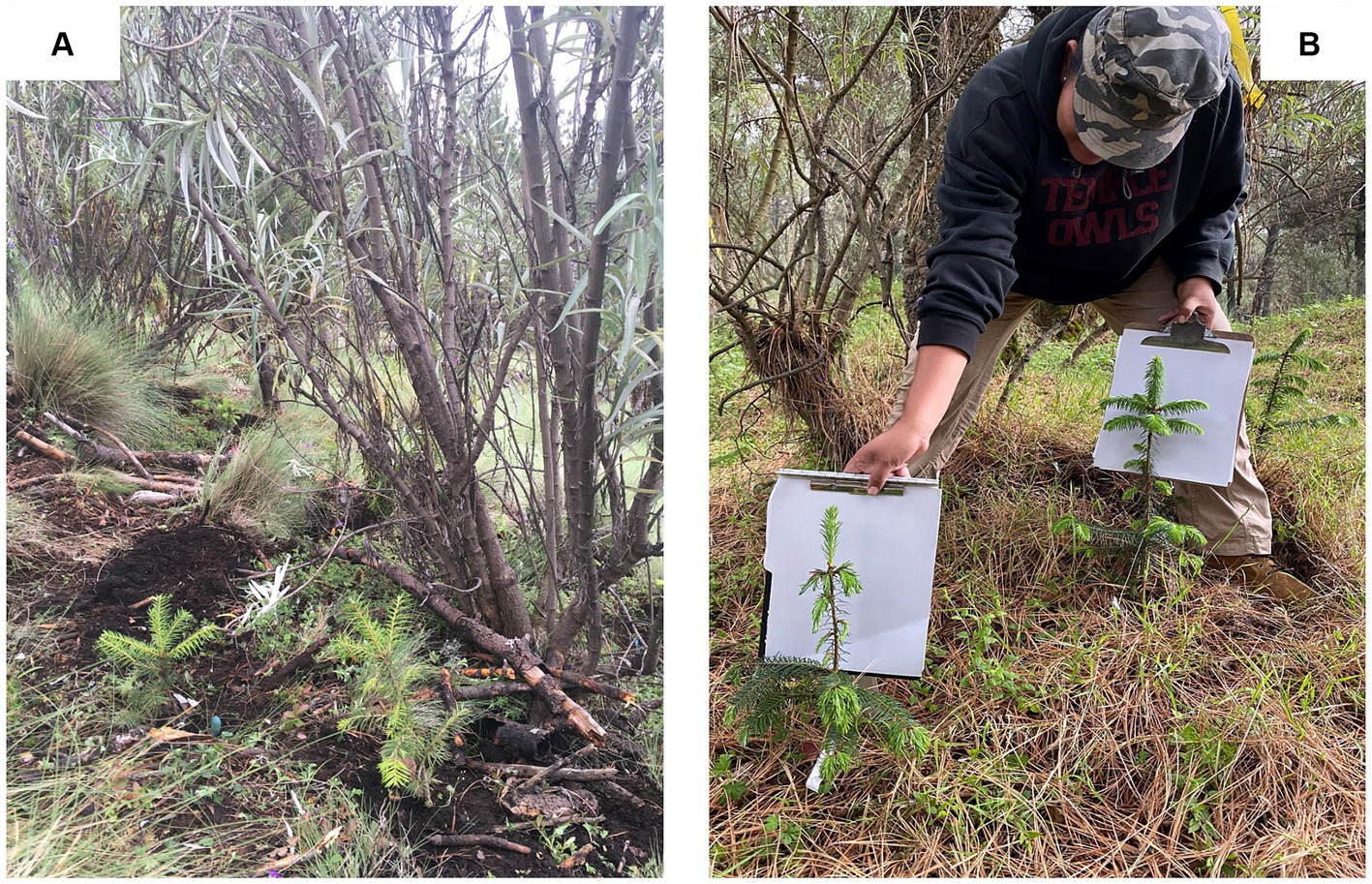
Figure 2. Abies religiosa seedlings planted in a circle consisting of eight seedlings (one seedling per population) under the shade of pre-existing shrubs (Senecio cinerarioides), with the latter serving as a protective nurse plant. The group of eight A. religiosa seedlings constitute a block. (A) A. religiosa seedlings just after planting at the 3,800 m test site. (B) A. religiosa seedlings at the 3,400 m test site 2 years after planting.
2.4 Field measurements
Seedling height (HT) and survival (SURV) were assessed bimonthly (from September 2021) during the growing season and basal diameter was recorded every 6 months. Final measurements were taken in December 2023 at the end of the third field growth season, nearly 6 years after sowing. Plant height was measured to ±1 mm from ground level to the tip of the apical bud. Basal diameter (DIAM; also known as root-collar diameter) was measured at the base of the stem with a digital vernier (KNOVA®, Cupertino, CA, United States) to ±0.1 mm.
Seedling height increment (HTincr) was obtained by subtracting the first measurement from the final measurement. Aerial biomass (BIOMASS) of each tree was estimated from final HT and DIAM using a relationship developed through destructive sampling of seedlings of the populations used in the current project (Equation 1 Cruzado-Vargas et al., 2021):
Where: BIOMASS is the aerial dry biomass (g), HT is the total seedling height (cm), and DIAM is the basal diameter (mm).
As SURV was scored as a binary trait, and blocks contained (in general) a single seedling per population, mean SURV was calculated as proportion of seedlings alive per population per site; consequently, the term ‘block’ was omitted from the model when analyzing SURV.
As the primary goal of this initiative is conservation, not timber production, we considered survival to be the most important response variable. However, seedling height and aerial biomass (the latter often reflects the growth capacity when considering the abundance of foliage) were also recorded since competition of seedlings with grasses and shrubs at high elevation can eventually compromise success.
2.5 Climate data
Climate data of the seed provenances were obtained for the reference period (1961–1990, i.e., the recent historic climate) from climate spline models (Rehfeldt, 2006; Rehfeldt et al., 2006; Sáenz-Romero et al., 2010; Crookston and Rehfeldt, 2024).1 The recent historic climate of the provenances is considered the climate to which the populations have evolved and are adapted (Sáenz-Romero et al., 2015).
The climate experienced by the seedlings while in the field (2021–2023) was used to represent the test site climate. Two HOBO data loggers located at each site (Onset Computer Corporation®, Bourne, MA, United States) recorded ambient temperature every 30 min. Monthly precipitation was measured by capturing rainwater in two rainwater traps per site (20 L buried containers with an exterior funnel). The two temperature and precipitation observations at each site were averaged and monthly mean (maximum, average, and minimum) temperatures as well as precipitation were calculated and used to derive nine climatic variables of relevance to plant growth (Rehfeldt et al., 2006; Table 2).
To estimate the impact of a climatic transfer distance on seedling growth and survival, future climatic values were obtained using an ensemble of global climate models with an intermediate level of climate forcing (6.0 Watts/m3) in https://charcoal2.cnre.vt.edu/climate/ (Crookston and Rehfeldt, 2024).
2.6 Statistical analysis
For each population at each test site, transfer distances (site minus provenance) were calculated for each of the nine climatic variables (the ones from Table 2). Data from the four test sites were pooled and transfer functions relating transfer distances to each derived response trait (HTincr, BIOMASS and SURV) were then developed with a quadratic function to assess the effect of the climatic or elevational transfer.
A mixed model was used in Procedure Mixed of SAS Institute (2014) to account for both the random design and fixed climatic factors (Cruzado-Vargas et al., 2021). The fixed effects considered three components of the effect of climate as a selective force that produces genetic differentiation among populations, or phenotypic plasticity (also considered genotype × environment interaction; Sáenz-Romero et al., 2017): the climate of the provenance (C); the climatic transfer distance (T), and the interaction between the climate of the provenance and climatic transfer distance (C * T). Random effects include factors associated with the experimental design: site, population, block, and the interaction between the site and the population. Details regarding the rationale behind this model are described in Leites et al. (2012a), Leites et al. (2012b), and Sáenz-Romero et al. (2017).
The following mixed model (Equation 2) was initially fitted with the data from all four sites:
where is the value of the response variable corresponding to the lth tree for the jth provenance in the kth block in the ith test site. is the intercept. is the climatic transfer distance of the jth provenance at the ith test site. is the value of the climatic variable of the jth provenance. is the interaction between the climatic transfer distance for the jth provenance at the ith test site and the climatic variable of the jth provenance. is the effect of the ith test site; is the effect of the jth provenance; is the effect of the kth block nested within the ith test site; and is the error term.
Before developing the full model above, nine reduced models (one for each climatic transfer distance variable) were developed for each response trait (HTincr, BIOMASS, and SURV) to identify the most influential climatic transfer distance variables for use in selecting the strongest full model (Leites et al., 2012a; Sáenz-Romero et al., 2017). The reduced model omitted the terms for Cj and from the full model. For each response variable, the five climatic transfer distance variables with the lowest Akaike Information Criterion (AIC) value were identified and included in the subsequent full models. Models that produced a positive quadratic term coefficient (β2) were omitted to ensure the biological validity of the models (Rehfeldt et al., 2001; Leites et al., 2012a).
Subsequently, for each response variable, 9 × 5 = 45 full “competing” models were run, which included all possible combinations of the nine provenance climatic variables (those listed in Table 2) and the five climatic transfer distance variables selected in the previous step [after selecting the best climatic transfer distance variables with the reduced model (the model without climate of the provenance explained above); also enlisted in Table 2]. For each response variable, the best model was selected based on AIC value (the smallest the AIC the better) and the significance of the climatic variables (with a F tests, using the option “Solution” of Procedure Mixed; SAS Institute, 2014).
After selecting the best full model for each response variable, we dropped non-significant terms. We then ran all the competing models again to confirm that the final model with non-significant terms removed remained superior to the competing models. Thus, the final model was simplified, retaining only the climate of the provenance and climatic transfer distance as fixed terms, as well as their interaction (Equation 3):
Fitted curves were estimated for each response variable from regression parameter coefficients to visualize the effect of climatic transfer distance and seed source climate on the responses. Finally, the impact of using assisted migration (i.e., the migration distance or climatic transfer distance) on each response variable was estimated by substituting the migration distance and seed source climate into the final fitted equation. To balance the need for adaptation at the time of plantation establishment and at plantation maturity, the migration distance used in these calculations considered the decade centered on the year 2060 as the date to which we expect the plantation climate to match the seed source historic climate (1961–1990). Furthermore, by 2060, the trees would be around 40 years old (from germination), likely providing a minimum acceptable crown size for the overwintering Monarch butterfly. Impacts were expressed relative to the height increment or aerial biomass expected at the zero transfer distance, or at a site with 100% expected survival (projected by the fitted model).
3 Results
Annual Dryness Index (ADI) emerged as the transfer distance variable that produced the strongest final full model for HTincr (p = 0.0064) and BIOMASS (p = 0.0595; Table 3). Although ADI was not significant (at the p > 0.05 level) as a climatic transfer distance term for SURV (p = 0.4327), it was still the variable that yielded the best fit in the full model (Table 3).
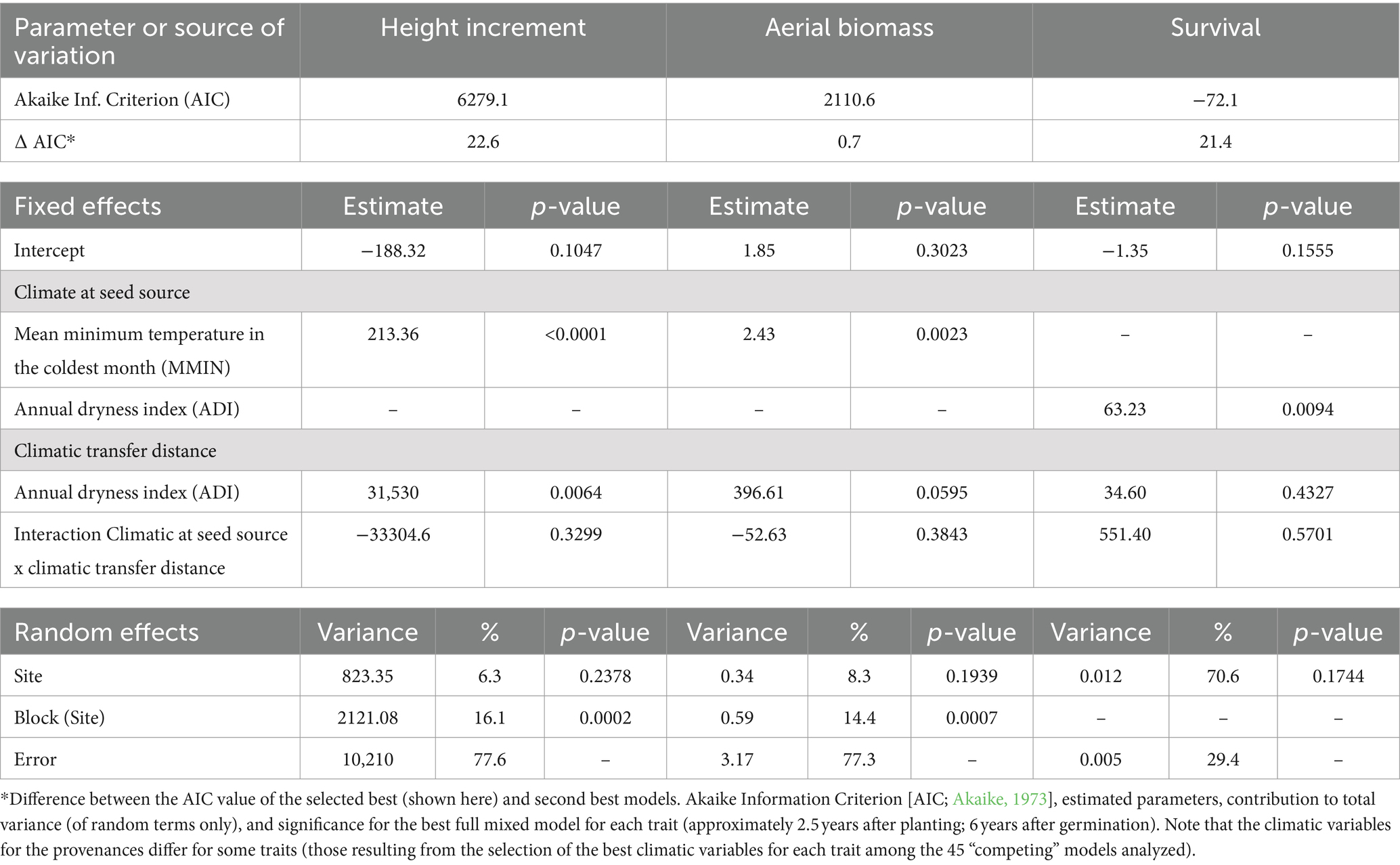
Table 3. Mixed model analysis [using the terms of the final model (Equation 3)] for increment in seedling height, aerial biomass, and survival.
The fitted climatic transfer curves indicated that seedling performance decreases as transfer distance increases. Specifically, HTincr, SURV, and BIOMASS decreased as the populations were transferred to colder (higher elevation) environments (Figure 3). Although the quadratic response curve had a slightly stronger fit than a linear regression model, we dropped the quadratic term because it was not significant in analyses involving any the climatic variables fitted to the three response variables examined (p > 0.05). Thus, the final model was simplified, retaining only the climate of the provenance and climatic transfer distance as fixed terms, as well as their interaction (Equation 3).
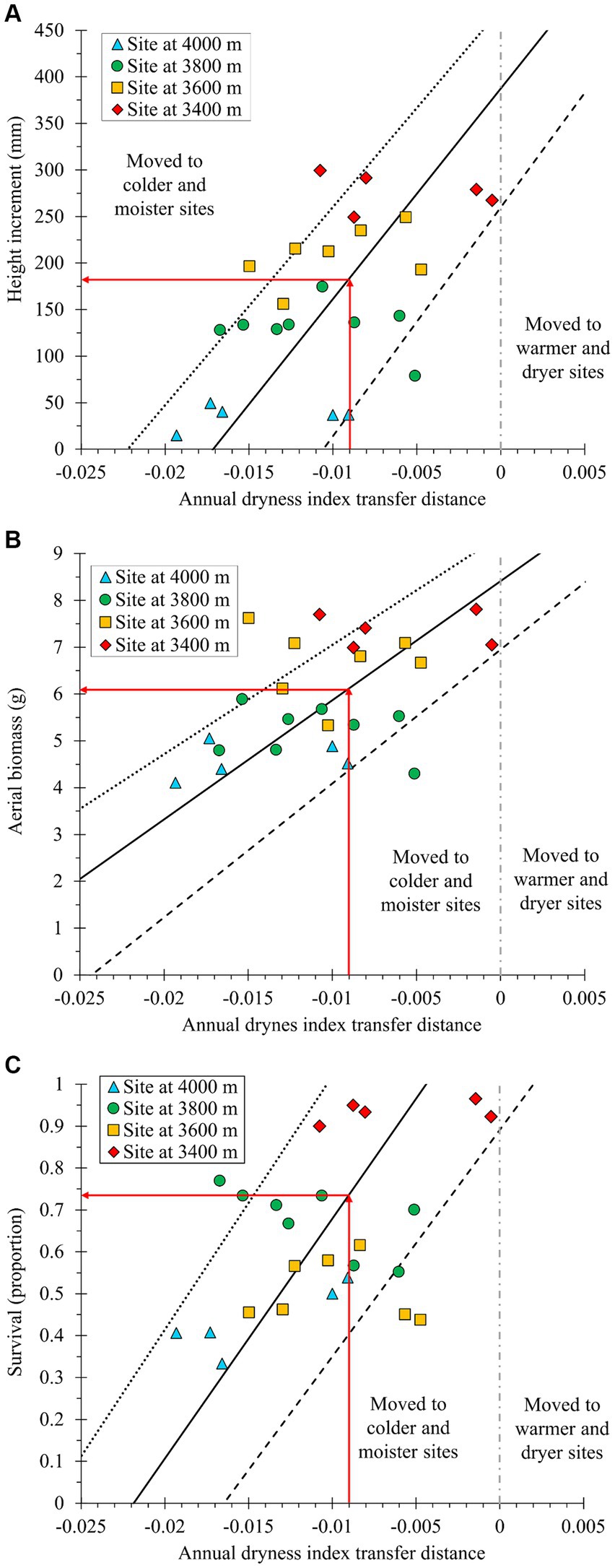
Figure 3. Observed response of increment in seedling height (A), aerial biomass (B), and survival proportion (C) to the transfer distance for Annual Dryness Index (ADI). On the X-axis, more negative values signify moving the seed sources toward colder and moister sites, while a value of zero (vertical gray dashed line) indicates transfer to a test site with a climate similar to that of the seed source (the provenance). Symbols are averages per population per site. Predicted response curves were constructed using the parameters of the fixed terms after fitting the best mixed model (Table 3). The solid curve was estimated using the average climate at the seed sources; the dashed line used the climate of the coldest provenance and the dotted line used that of the warmest provenance. Red arrows indicate the impact on the response variable when the transfer distance is 0.009 √°Cmm−1 ADI transfer difference, the value required to compensate for the amount of climate change expected by year 2060 at the seed source locations.
Of the competing models, mean minimum temperature in the coldest month (MMIN) was the seed source climatic variable that accounted for the most variation in both HTincr (p < 0.0001) and BIOMASS (p = 0.0023) in the final model, while ADI was the seed source climatic variable that accounted for the most variation in the final model for SURV (p = 0.0094; Table 3). The random term ‘population’ (resulting from non-climatic factors) was not significant (p > 0.05) for any response variable and was therefore dropped from the final model. In contrast, block was significant for all response variables except SURV, for which the term was absent.
Population responses to climatic transfer were relatively weak within each site (Figure 3). In contrast, site climate had a strong effect on the responses, and was the main factor shaping the overall trend of the results, as illustrated in a scatterplot of test site mean annual temperature (MAT) versus population HTincr means at each site (R2 adjusted = 0.88; p < 0.0001; Figure 4). Notably, at the 4,000 m elevation site, the average HTincr approached zero and numerous seedlings displayed frost damage. In contrast, at the 3,400 m site, HTincr was large and no frost damage was observed (Figures 3A, 4).
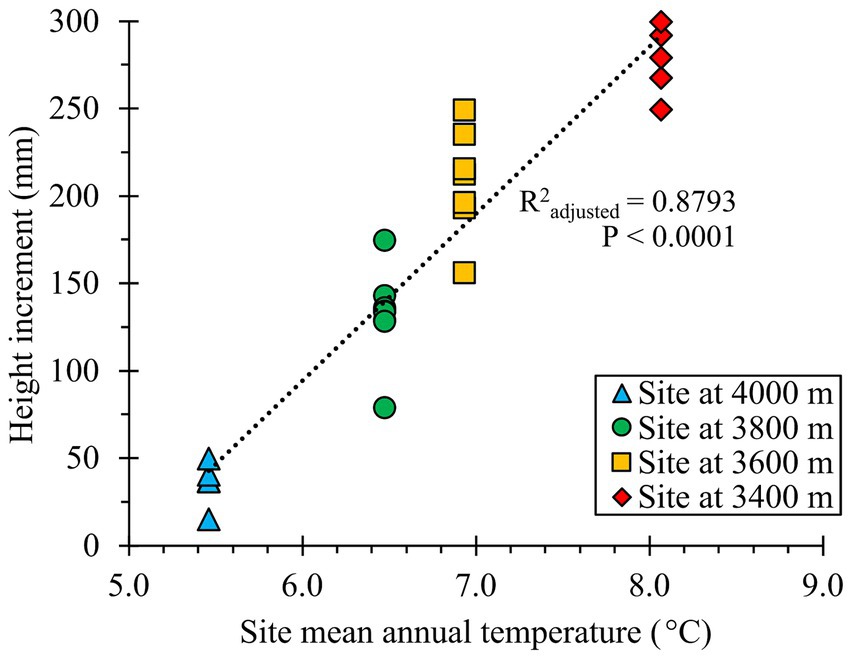
Figure 4. Regression of population mean height increment at each test site as a function of test site mean annual temperature (MAT, °C; average 2021–2023). The number of populations tested at the 3,400, 3,600, 3,800, and 4,000 m test sites were 5, 7, 8, and 5, respectively.
The ADI of the seed sources is expected to increase by 0.009 √°Cmm−1 (a warmer and dryer climate) by 2060, relative to the historic (1961–1990) mean ADI. Therefore, to ensure that planted A. religiosa populations will experience their historic ADI in 2060, it is necessary to establish plantations on sites that currently have an ADI that is 0.009 units smaller (colder and moister) than the historic climates of the seed sources. In other words, planted seedlings should originate from provenances that are slightly warmer and dryer than the plantation sites. Such a climatic transfer distance would result in seedlings with approximately 54% slower growth rate (Figure 3A), 27% less aerial biomass (Figure 3B), or 27% lower survival compared to that expected in a population at zero climatic transfer distance (Figure 3C).
To translate these results to a more intuitive climatic variable, the response to the assisted migration is more easily appreciated when plotting the response variables against MAT transfer distance (Figure 5). When establishing plantations with seed sources from climates 2.3°C in MAT warmer than the plantation site to compensate for warming between the reference period (1961–1990) and the climate matching the target date (2060), the projected loss is 47% in HTincr (Figure 5A) and 21% in SURV (Figure 5B).
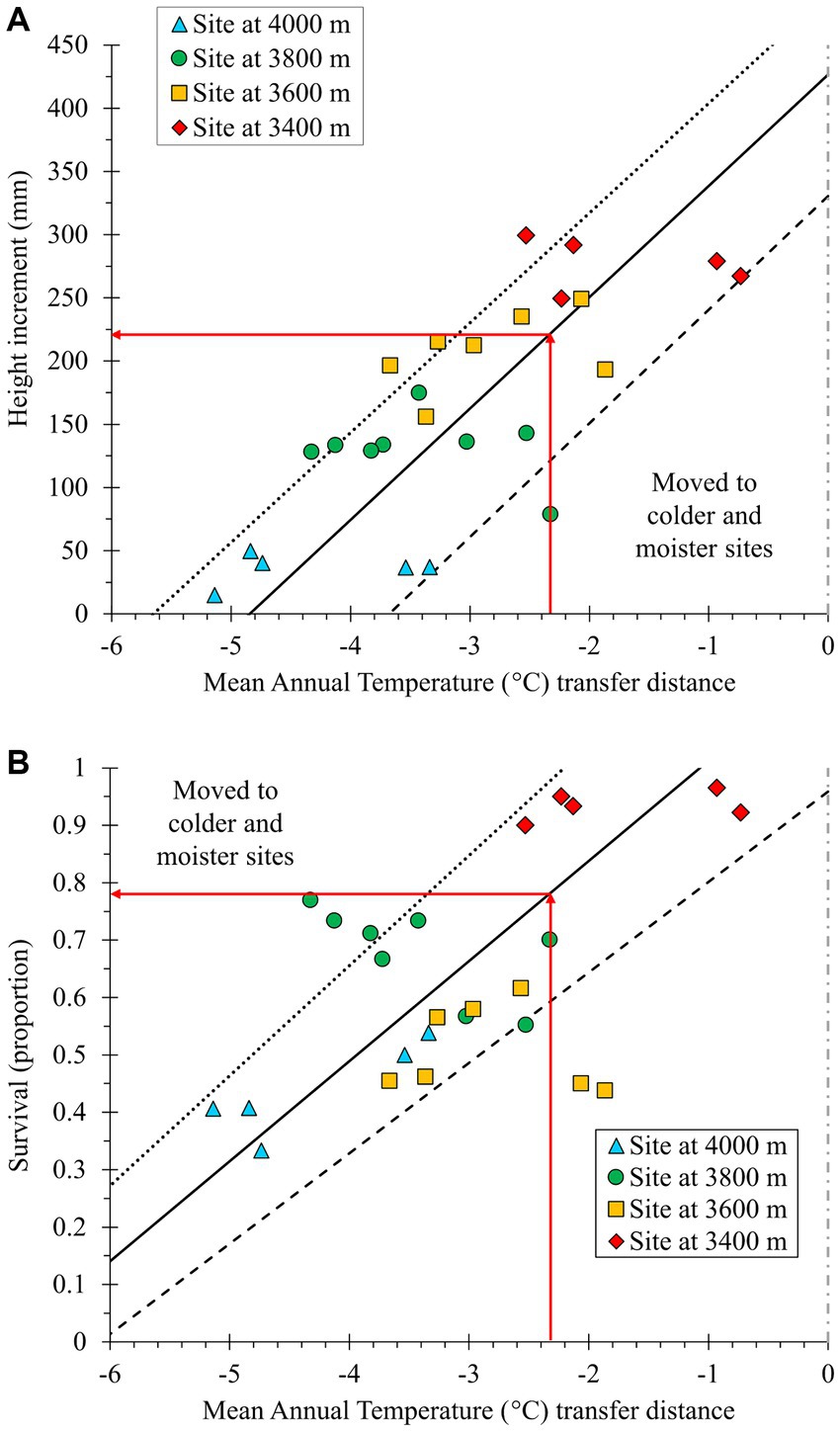
Figure 5. Transfer function relating height increment (A) and survival (B) of Abies religiosa populations to mean annual temperature (MAT, °C) climatic transfer distance. Negative transfer values indicate seed source movement toward colder sites (higher altitudes). A transfer distance of zero indicates transfer to a test site with a climate similar to that of the seed source (dashed gray vertical line). Predicted relationships were constructed using the parameters of the fixed terms of the final full model (Equation 3). Solid, dotted, and dashed curves were estimated using the mean, minimum, and maximum MAT of the seed source, respectively. Red arrows indicate the impact on the response variable when populations are transferred by 2.3°C MAT, the amount required to account for expected climatic change by year 2060 at the seed source locations.
Our fitted elevation transfer functions suggested that a 500 m upward elevation transfer would yield seedlings having a HTincr that is half that of a local seed source (R2 adjusted = 0.65; p < 0.0001; Figure 6).
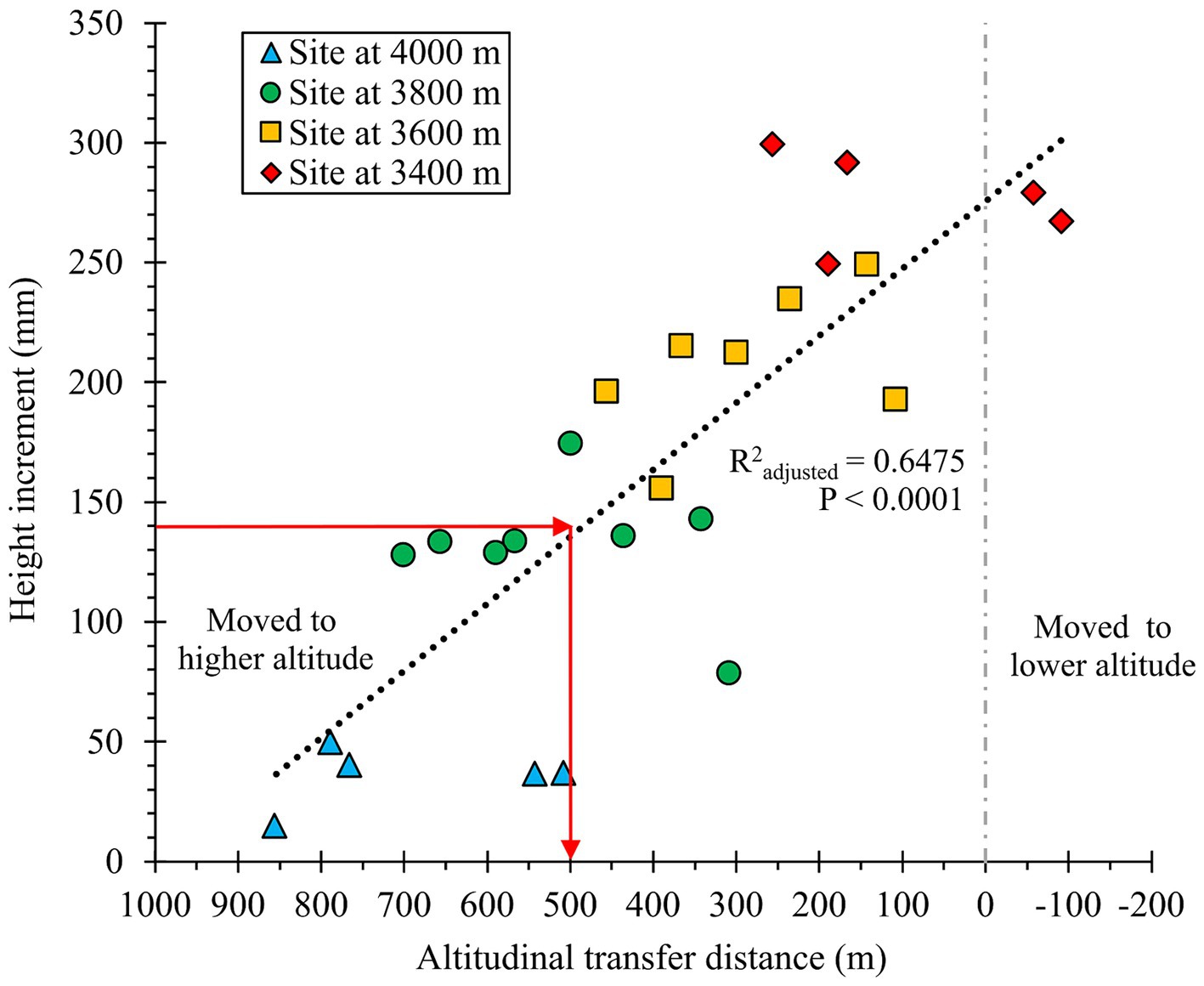
Figure 6. Transfer function relating height increment to elevation transfer distance for populations of Abies religiosa planted at four test sites. Red arrows indicate the maximum safe seed transfer distance – the elevational transfer associated with height increment that is 50% of the expected height increment of a local population. The number of populations tested at the sites at 3400, 3600, 3,800, and 4,000 m in elevation, were 5, 7, 8, and 5, respectively. The adjusted R2 was estimated with a linear regression.
Only two populations (those from 3,457 m and 3,491 m; Table 1) were moved downward in elevation at the 3,400 m test site (Figure 6). Despite being moved downward by only 60 m, these populations showed a -1.0 MAT transfer distance (Figure 5), suggesting that Nevado de Toluca is slightly colder than the MBBR at the same elevation (see Table 1).
4 Discussion
4.1 Relevance of climatic transfer distance variables
The annual aridity index (ADI), reflecting both annual degree day heat sums and annual precipitation, was the climatic variable that most strongly governs the impact of seed transfer or climate change on the growth (seedling height and aerial biomass) and survival of Abies religiosa populations. It was also the climatic variable selected to delineate and match contemporary and future climate seed zones for Mexico (Castellanos-Acuña et al., 2018) and proved to be a critical climatic variable governing the response of Quercus petraea provenances in large multi-site provenance tests (Sáenz-Romero et al., 2017).
The mean minimum temperature of the coldest month (MMIN) was identified as the seed source climatic variable that best accounted for variation in response traits, thus corroborating studies in more controlled provenance test environments (Ortiz-Bibian et al., 2017). Nevertheless, the impact of the colder temperatures at the test sites at Nevado de Toluca appeared to override the expression of genetic differentiation among populations.
The lack of significance of population and site as random variables in the full model could be the result of most of the response trait variation being accounted for by the fixed terms related to seed source climate and climatic transfer distance (Table 3). Thus, the selective force of climate to shape genetic differentiation among populations, and the response of these populations at different sites, appears to be more important than gene flow, demographic history, colonization events, or non-climatic factors of the site, including soil edaphics or topography. However, with only four test sites, and only a single test site at each elevation, site climatic and non-climatic (such as soil fertility, drainage, or aspect) factors are confounded. Thus, it would be advisable to establish future assisted migration experiments with more than one site at each elevation.
4.2 Implications for forest management
Our findings suggest that the establishment of A. religiosa under pre-existing nurse shrubs is feasible above its upper elevation distribution limit (at sites as high as 3,800 m) and outside its geographic range. Coldward transfer to planting sites with an MAT that is 2.3°C colder than the seed source climate, is required to match seed source historic climates with plantation climates projected for 2060 in central Mexico, and is within the range of 2 to 3°C MAT transfer distance suggested by other assisted migration field experiments (Castellanos-Acuña et al., 2015; St Clair et al., 2020; Cruzado-Vargas et al., 2021; Sáenz-Romero et al., 2021). These findings provide a new opportunity to mitigate climate change impacts on A. religiosa and its dependent organism – the Monarch butterfly. As the climate continues to warm, the migration distance required to match the historic climate of seed sources with plantation future climate will increase. Consequently, seed transfer systems developed to implement assisted migration will need to be modified periodically.
In practice, the climatic transfer value selected will depend on how stakeholders and conservation agencies perceive the risk of plantation failure (from over-transfer) versus that of local extirpation (from under-transfer); where local extirpation of the species is anticipated, as is the case with Abies religiosa, up to 47% loss of growth and 21% of loss in survival (impacts expected with 500 m upward elevation transfer) may be perceived as acceptable by stakeholders and conservation agencies.
In our view, the most critical response variable is seedling survival, and a survival rate of 78.6% when transferring to sites 2.3°C colder can be considered very acceptable. Growth rate can also be important when there exists significant vegetative competition at the recipient site. However, as climate change progresses, the planting sites will become more favorable for the translocated populations and growth rates will improve in the future; in contrast, local native populations might be increasingly maladapted to the future warmer climate. Thus, what is absolutely critical for the translocated populations is to survive the cold climate in the early stages after plantation.
It appears feasible to establish A. religiosa at 3800 m on the Nevado de Toluca volcano. Above that elevation, mortality and especially loss of growth become pronounced. Such an appraisal needs to be considered in the context of the fact that Nevado de Toluca has a colder climate than the MBBR at the same elevations, as shown by the lack of populations presenting positive climatic transfer distances (Figure 5), even among the populations that were transferred to lower elevations (those that originating from above 3,400 m; Table 1).
Since Senecio cinerarioides has a very shallow crown, it would be desirable to test the feasibility of upward elevation transfer of other shrub species that have wide crowns. The pre-existing shrub Baccharis conferta has been used successfully as a nurse plant at lower elevations at the MBBR because it has a very dense crown that provides effective protection against an excess of insolation and extreme cold (Carbajal-Navarro et al., 2019). However, B. conferta is nearly absent at 3800 m and completely absent at 4000 m on Nevado de Toluca.
Even if the operational establishment of A. religiosa stands at elevations higher than the upper distribution limit of the species (3,550 m) were successful, there is no guarantee that Monarch butterfly migratory populations would shift their overwintering sites by around 75 km to the southeast. However, the fact that Nevado de Toluca, a historically marginal overwintering site (Pérez-Miranda et al., 2020), became the site with the largest Monarch butterfly colony during the 2023–2024 winter season (Comisión Nacional de Áreas Naturales Protegidas, 2024) is reassuring, particularly given that the new site was at a relatively high elevation (3,400 m) and the Nevado de Toluca sites are colder than sites at the same elevations in the MBBR. The migratory Monarch butterfly populations may now be seeking new overwintering sites with climates similar to the historic climate of their usual overwintering sites, which are becoming warmer.
Seedlings planted today would be approximately 40 years of age (from seed germination) by the year 2060 and should have a crown size acceptable for overwintering Monarch colonies, based on the observation that trees 50 years old are common on the overwintering stands (Sáenz-Ceja and Pérez-Salicrup, 2020; Sáenz-Ceja et al., 2022; Carlón-Allende et al., 2018). Obviously, this trial simply demonstrates the feasibility of establishing Abies religiosa at higher elevations and outside its current natural distribution. A much greater planting effort, including local participation by forest managers and securing of support resources, would be required to provide dense future stands that could serve as suitable overwintering sites. Thus, in this era of the Anthropocene, it may be possible to establish high-elevation A. religiosa stands that can successfully provide overwintering sites for migratory Monarch butterflies in the context of a warmer world.
It is extremely important to note that attempts to provide new colonization areas for the Monarch butterfly and efforts to conserve its current habitat are not mutually exclusive. The eventual establishment of future overwintering sites at higher elevations does not imply that efforts to maintain contemporary stands of A. religiosa would cease or diminish. Reforestation within the MBBR with seedlings originating from seeds collected at lower elevations and planted under the protective shade of nurse plants should continue for ecological restoration of sites perturbed by phytosanitary clear-cuts, forest fires, and illegal logging (Carbajal-Navarro et al., 2019) until climate change precludes such efforts. In other words, promoting healthy A. religiosa stands to be used by Monarch butterfly migratory populations, both inside and outside of the MBBR, should be parallel tasks of maximum priority.
5 Conclusion
We successfully established A. religiosa outside, and at higher elevations, than its current natural distribution limits using pre-existing shrubs as nurse plants. Survival of 68% across all populations at 3800 m, well beyond the natural A. religiosa upper limit distribution of 3,550 m, is an encouraging result. To compensate for an expected warming of 2.3°C of Mean Annual Temperature by the decade centered in 2060 would require an upward shift in elevation of 500 m for each A. religiosa provenance, resulting in a survival of 71% and approximately half the growth rate compared to a zero climatic transfer distance. Thus, it may be possible and highly warranted to establish high-elevation A. religiosa stands to provide overwintering sites to host migratory Monarch butterflies in a future climate change scenario by the year 2060.
Data availability statement
The raw data supporting the conclusions of this article will be made available by the authors, without undue reservation.
Author contributions
CS-R: Conceptualization, Data curation, Formal analysis, Funding acquisition, Investigation, Methodology, Project administration, Supervision, Visualization, Writing – original draft, Writing – review & editing. VO-V: Data curation, Investigation, Writing – review & editing. PH-C: Conceptualization, Data curation, Investigation, Writing – review & editing. LP-C: Data curation, Investigation, Writing – review & editing. MJ-J: Data curation, Writing – review & editing, Investigation. AC-V: Data curation, Formal analysis, Investigation, Visualization, Writing – review & editing. GO’N: Formal analysis, Funding acquisition, Visualization, Writing – review & editing. AZ-C: Investigation, Writing – review & editing. GM-Q: Visualization, Investigation, Writing – review & editing. RL-C: Conceptualization, Funding acquisition, Writing – review & editing. AB-G: Conceptualization, Funding acquisition, Methodology, Writing – review & editing. ÁE-A: Conceptualization, Writing – review & editing. LL-T: Writing – review & editing.
Funding
The author(s) declare that financial support was received for the research, authorship, and/or publication of this article. The research project was sponsored with awards to CS-R by the Non-Governmental Organization (NGO) Monarch Butterfly Fund (Madison, WI, United States), the NGO American Forests (Washington DC), jointly with the Fondo de Conservación del Eje Neovolcánico, AC. (F-02-20-002), the North American Forest Commission with funding from the USDA-Forest Service Office of International Programs (Washington, DC; Projects IPC0.017.0635.001 and IPC0.017.1045.001) and the Natural Resources Canada-Canadian Forest Service (Project 3000770810), and the Coordinación de la Investigación Científica of the UMSNH (Funding 8033018); to RL-C by the Universidad Nacional Autónoma de México (PAPIT-IG200221); and to AB-G by Mexican Consejo Nacional de Humanidades, Ciencia y Tecnología (CONAHCyT; project Ciencia Básica-2014-242985). CONAHCyT provided postdoctoral fellowships to GM-Q (CVU 469568) and AZ-C (CVU 550990), and graduate studies fellowships to PH-C (1106671), LP-C (1186935), and AC-V (733822). The Mexican Sistema Nacional de Investigadores provided a research assistant fellowship to MJ-J (20389). AC-V whishes to thank DGAPA-UNAM for a posdoctoral scholarship.
Acknowledgments
This paper is an undertaking of the Forest Genetic Resources Working Group (FGRWG)/North American Forest Commission/Food and Agricultural Organization of the United Nations. We gratefully acknowledge the assistance in seedling production and field test maintenance and evaluation, among others, of Esmeralda Navarro-Miranda, Cecilia Zamora-Sánchez, Erika Gómez-Pineda, José Luis Gallardo-Salazar (UMSNH graduate students), J. Jonathan Aguirre-Zúñiga (Universidad Autónoma del Estado de México graduate student), Jessica S. Ambriz-Guerrero, and Saira Y. Valentín-Estrada (“Jóvenes Construyendo El Futuro” federal program fellows), among others. Special thanks go to Francisco Ramírez-Cruz (deceased), Doña Petra Cruz-Cruz and her family, and Simón Polo Urbina (Comisario Ejidal) from Ejido La Mesa, Municipality of San José del Rincón, Estado de México, for help with seedling production at their forest nursery. José Luis Malvais-Ríos and Bonifacio M. González-Gomorra (current and former President, respectively, of “Consejo de Bienes Comunales”) of the Community of Calimaya, Estado de México, and the community forestry staff, specially Josué E. Carmona-López, Pablo Rosas-Quiñones, Víctor Mendoza-Zarza, and several other forestry staff, for their help with establishment, surveillance, maintenance, and evaluation of the field tests. We thank Amado Fernández-Islas (Director of the MBBR), Mario Fermin Castañeda-Rojas and Dulce María Avendaño-Hernández (former and current Director of Natural Protected Area Nevado de Toluca), and the Comisión Nacional de Areas Naturales Protegidas (CONANP, for its acronym in Spanish), for granting permits to work at the MMBR and Nevado de Toluca, respectively. We thank Fernando Piedra-Gil, José Angel López-López, and others from the Mexican National Forestry Commission (CONAFOR, Guadalajara, Jalisco) for logistic support for transporting the seedlings, and Marco Sánchez, Martel Blanco, and Sergio Valencia, OKRAM (a media company; Morelia, Michoacán) for video documenting the establishment of the experiment. Thanks to film Director Sophie Musgrove and journalist staff of NHNZ Worldwide, New Zealand, for documenting the field experiment on the Serie “Dynamic Planet” of PBS and on “Une Planète Vivante” of ARTE. Finally, we thank Eligio García-Serrano, Director of Fondo de Conservación del Eje Neovolcánico, A.C. (ONG based at Zitácuaro, Michoacán), and Gerald E. Rehfeldt, USDA-Forest Service, Moscow, Idaho, United States, for their help and advice throughout this study. Keith MacMillan provided assistance with the English writing.
Conflict of interest
The authors declare that the research was conducted in the absence of any commercial or financial relationships that could be construed as a potential conflict of interest.
Publisher’s note
All claims expressed in this article are solely those of the authors and do not necessarily represent those of their affiliated organizations, or those of the publisher, the editors and the reviewers. Any product that may be evaluated in this article, or claim that may be made by its manufacturer, is not guaranteed or endorsed by the publisher.
Footnotes
1. ^Available at https://charcoal2.cnre.vt.edu/climate/
References
Akaike, H. (1973). Information theory and an extension of the maximum likelihood principle. Proceedings of the Second International Symposium on Information Theory, pp. 267–281.
Allen, C. D., Macalady, A. K., Chenchouni, H., Bachelet, D., McDowell, N., Vennetier, M., et al. (2010). A global overview of drought and heat-induced tree mortality reveals emerging climate change risks for forests. For. Ecol. Manag. 259, 660–684. doi: 10.1016/j.foreco.2009.09.001
Anderson, J. B., and Brower, L. P. (1996). Freeze-protection of overwintering monarch butterflies in Mexico: critical role of the forest as a blanket and an umbrella. Ecol. Entomol. 21, 107–116. doi: 10.1111/j.1365-2311.1996.tb01177.x
Benavides-Meza, H. M., Gazca Guzmán, M. O., López López, S. F., Camacho Morfín, F., Fernández Grandizo, D. Y., De La Garza López de Lara, M. D. P., et al. (2011). Growth variability in seedlings of eight provenances of Abies religiosa (HBK) Schlecht. et Cham., in nursery conditions. Madera Bosques 17, 83–102. doi: 10.21829/myb.2011.1731144
Carbajal-Navarro, A., Navarro-Miranda, E., Blanco-García, A., Cruzado-Vargas, A. L., Gómez-Pineda, E., Zamora-Sánchez, C., et al. (2019). Ecological restoration of Abies religiosa forests using nurse plants and assisted migration in the Monarch Butterfly Biosphere Reserve, Mexico. Front. Ecol. Evol. 7:421. doi: 10.3389/fevo.2019.00421
Carlón-Allende, T., Villanueva-Díaz, J., Mendoza, M. E., and Pérez-Salicrup, D. R. (2018). Climatic signal in earlywood and latewood in conifer forests in the Monarch Butterfly Biosphere Reserve, Mexico. Tree Ring Res. 74, 63–75. doi: 10.3959/1536-1098-74.1.63
Castellanos-Acuña, D., Lindig-Cisneros, R., and Sáenz-Romero, C. (2015). Altitudinal assisted migration of Mexican pines as an adaptation to climate change. Ecosphere 6, 1–16. doi: 10.1890/ES14-00375.1
Castellanos-Acuña, D., Vance-Borland, K. W., St Clair, J. B., Hamann, A., López-Upton, J., Gómez-Pineda, E., et al. (2018). Climate-based seed zones for Mexico: guiding reforestation under observed and projected climate change. New For. 49, 297–309. doi: 10.1007/s11056-017-9620-6
Comisión Nacional de Áreas Naturales Protegidas (2024). Disminuye la presencia de mariposas Monarca en los bosques mexicanos de hibernación. Ciudad de México. Available at: https://www.gob.mx/conanp/prensa/disminuye-la-presencia-de-mariposas-monarca-en-los-bosques-mexicanos-de-hibernacion (Accessed February 2024).
Comisión Nacional Forestal. (2018). Map of “Formaciones Forestales de México, acorde a los usos y vegetación del suelo de la serie VI de INEGI del año 2017. Escala 1:250,000”. Ciudad de México. Available at: https://idefor.cnf.gob.mx/mviewer/forma_forestal (Accessed July 2024).
Council of Canadian Academies. (2022). Forests Nature-Based Climate Solutions. Ottawa, ON, Canada: The Expert Panel on Canada’s Carbon Sink Potential, CCA.), pp. 40-77. Available at: https://cca-reports.ca/wp-content/uploads/2021/03/Carbon-Sinks_EN_CH-3_Forests.pdf.
Crookston, N. L., and Rehfeldt, G. E. (2024). Research on Forest Climate Change: Potential Effects of Global Warming on Forests and Plant Climate Relationships in Western North America and Mexico. Available at: https://charcoal2.cnre.vt.edu/climate/ (Accessed February 1, 2024).
Cruzado-Vargas, A. L. (2017). Variación de caracteres cuantitativos entre procedencias de Abies religiosa de un gradiente altitudinal en la Reserva de la Biósfera de las Mariposa Monarca: etapa de vivero. Mexico: Master in Forestry Sciences Thesis Dissertation, Universidad Autónoma Chapingo. Chapingo, State of México.
Cruzado-Vargas, A. L., Blanco-García, A., Lindig-Cisneros, R., Gómez-Romero, M., Lopez-Toledo, L., de la Barrera, E., et al. (2021). Reciprocal common garden altitudinal transplants reveal potential negative impacts of climate change on Abies religiosa populations in the Monarch Butterfly Biosphere Reserve overwintering sites. Forests 12:69. doi: 10.3390/f12010069
De Rzedowski, C. G., and Rzedowski, J. (2005). Flora fanerogámica del Valle de México. 2nd Edn. Pátzcuaro, Michoacán: Instituto de Ecología, A.C. y Comisión Nacional para el Conocimiento y Uso de la Biodiversidad (CONABIO), 1406.
Flores-Nieves, P., López-López, M. Á., Ángeles-Pérez, G., De La Isla-Serrano, M. D. L., and Calva-Vásquez, G. (2011). Biomass estimation and distribution models of Abies religiosa (Kunth) Schltdl. et Cham. in decline. Rev. Mex. Cienc. For. 2, 9–20. doi: 10.29298/rmcf.v2i8.543
Gómez-Pineda, E., Sáenz-Romero, C., Ortega-Rodríguez, J. M., Blanco-García, A., Madrigal-Sánchez, X., Lindig-Cisneros, R., et al. (2020). Suitable climatic habitat changes for Mexican conifers along altitudinal gradients under climatic change scenarios. Ecol. Appl. 30:e02041. doi: 10.1002/eap.2041
Gómez-Pineda, E., Sáenz-Romero, C., Salinas-Melgoza, M. Á., and Ramírez, M. I. (2023). Drought occurrence and forest management: Interrelated drivers of sanitary logging in central México temperate forests. Trees Forests People 13:100413. doi: 10.1016/j.tfp.2023.100413
Hammond, W. M., Williams, A. P., Abatzoglou, J. T., Adams, H. D., Klein, T., López, R., et al. (2022). Global field observations of tree die-off reveal hotter-drought fingerprint for Earth’s forests. Nat. Commun. 13:289. doi: 10.1038/s41467-022-29289-2
Hansen, J. E., Sato, M., Simons, L., Nazarenko, L. S., Sangha, I., Kharecha, P., et al. (2023). Global warming in the pipeline. Oxford Open Clim. Change 3:8. doi: 10.1093/oxfclm/kgad008
Ledig, F. T., Rehfeldt, G. E., Sáenz-Romero, C., and Flores-López, C. (2010). Projections of suitable habitat for rare species under global warming scenarios. Am. J. Bot. 97, 970–987. doi: 10.3732/ajb.0900329
Leites, L. P., Rehfeldt, G. E., Robinson, A. P., Crookston, N. L., and Jaquish, B. (2012a). Possibilities and limitations of using historic provenance tests to infer forest species growth responses to climate change. Nat. Resour. Model. 25, 409–433. doi: 10.1111/j.1939-7445.2012.00129.x
Leites, L. P., Robinson, A. P., Rehfeldt, G. E., Marshall, J. D., and Crookston, N. L. (2012b). Height-growth response to climatic changes differs among populations of Douglas-fir: a novel analysis of historic data. Ecol. Appl. 22, 154–165. doi: 10.1890/11-0150.1
McLane, S. C., and Aitken, S. N. (2012). Whitebark pine (Pinus albicaulis) assisted migration potential: testing establishment north of the species range. Ecol. Appl. 22, 142–153. doi: 10.1890/11-0329.1
Mendoza-Maya, E., Gómez-Pineda, E., Sáenz-Romero, C., Hernández-Díaz, J. C., López-Sánchez, C. A., Vargas-Hernández, J. J., et al. (2022). Assisted migration and the rare endemic plant species: the case of two endangered Mexican spruces. PeerJ 10:e13812. doi: 10.7717/peerj.13812
Munson, S. M., Reed, S. C., Peñuelas, J., McDowell, N. G., and Sala, O. E. (2018). Ecosystem thresholds, tipping points, and critical transitions. New Phytol. 218, 1315–1317. doi: 10.1111/nph.15145
Natural Resources Canada. (2023). National Wildland Fire Situation Report. Available at: https://cwfis.cfs.nrcan.gc.ca/report (Accessed December 13, 2023).
O’Neill, G., and Degner, J. C. (2024). Seed Trek 2.0: The Next Generation of Seed-Transfer Systems. Tree Planters Notes 67, 56–63. Available at: https://rngr.net/publications/tpn/67-1/seed-trek-2.0-the-next-generation-of-seed-transfer-systems
O'Neill, G., Wang, T., Ukrainetz, N., Charleson, L., McAuley, L., Yanchuk, A., et al. (2017). A proposed climate-based seed transfer system for British Columbia. Victoria, B.C. Technical Report 099. Available at: www.for.gov.bc.ca/hfd/pubs/Docs/Tr/Tr099.htm.
Ortiz-Bibian, M. A., Blanco-García, A., Lindig-Cisneros, R. A., Gómez-Romero, M., Castellanos-Acuña, D., Herrerías-Diego, Y., et al. (2017). Genetic variation in Abies religiosa for quantitative traits and delineation of elevational and climatic zoning for maintaining Monarch butterfly overwintering sites in Mexico, considering climatic change. Silvae Genet. 66, 14–23. doi: 10.1515/sg-2017-0003
Pérez-Miranda, R., Arriola-Padilla, V. J., and Romero-Sanchez, M. E. (2020). Characterizing new wintering sites for Monarch Butterfly colonies in Sierra Nevada. Mexico. Insects 11:384. doi: 10.3390/insects11060384
Ramírez-Amezcua, Y., Steinmann, V. W., Ruiz-Sanchez, E., and Rojas-Soto, O. R. (2016). Mexican alpine plants in the face of global warming: potential extinction within a specialized assemblage of narrow endemics. Biodivers. Conserv. 25, 865–885. doi: 10.1007/s10531-016-1094-x
Rao, M. P., Davi, N. K., Magney, T. S., Andreu-Hayles, L., Nachin, B., Suran, B., et al. (2023). Approaching a thermal tipping point in the Eurasian boreal forest at its southern margin. Commun. Earth Environ. 4:247. doi: 10.1038/s43247-023-00910-6
Rehfeldt, G. E. (2006). A spline model of climate for the western United States. General Technical Report RMRS-GTR-165. Fort Collins, CO: US Department of Agriculture, Forest Service, Rocky Mountain Research Station.
Rehfeldt, G. E., Crookston, N. L., Warwell, M. V., and Evans, J. S. (2006). Empirical analyses of plant-climate relationships for the western United States. Int. J. Plant Sci. 167, 1123–1150. doi: 10.1086/507711
Rehfeldt, G. E., Wykoff, W. R., and Ying, C. C. (2001). Physiologic plasticity, evolution, and impacts of a changing climate on Pinus contorta. Clim. Chang. 50, 355–376. doi: 10.1023/A:1010614216256
Rendón-Salinas, E., Alonso, A., García-Serrano, E., Valera-Bermejo, A., and Quesada, M. (2023). The monarch butterfly in Mexico: a conservation model. Curr. Opin. Insect Sci. 60:101112. doi: 10.1016/j.cois.2023.101112
Richardson, B. A., Rehfeldt, G. E., Sáenz-Romero, C., and Milano, E. R. (2024). A climate analog approach to evaluate seed transfer and vegetation transitions. Front. Forests Glob. Change 7:264. doi: 10.3389/ffgc.2024.1325264
Ritchie, P. D. L., Clarke, J. J., Cox, P. M., and Huntingford, C. (2021). Overshooting tipping point thresholds in a changing climate. Nature 592, 517–523. doi: 10.1038/s41586-021-03263-2
Rzedowski, J. (2006). Vegetación de México. 1ra. Edición digital. México: Comisión Nacional para el Conocimiento y Uso de la Biodiversidad (CONABIO), p. 504.
Sáenz-Ceja, J. E., Martínez-Ramos, M., Mendoza, M. E., and Pérez-Salicrup, D. R. (2022). Fire scar characteristics in two tropical montane conifer species from central Mexico. Int. J. Wildland Fire 31, 684–692. doi: 10.1071/WF21110
Sáenz-Ceja, J. E., and Pérez-Salicrup, D. R. (2020). Modification of fire regimes inferred from the age structure of two conifer species in a Tropical Montane Forest, Mexico. Forests 11:1193. doi: 10.3390/f11111193
Sáenz-Romero, C. (2024). Arriving at a tipping point for worldwide forest decline due to accelerating climatic change. For. Chron. 100, 1–3. doi: 10.5558/tfc2024-00
Sáenz-Romero, C., Cambrón-Sandoval, V. H., Hammond, W., Méndez-González, J., Luna-Soria, H., Macías-Sámano, J. E., et al. (2023). Abundance of Dendroctonus frontalis and D. mexicanus (Coleoptera: Scolytinae) along altitudinal transects in Mexico: Implications of climatic change for forest conservation. PLoS One 18:e0288067. doi: 10.1371/journal.pone.0288067
Sáenz-Romero, C., Lamy, J. B., Ducousso, A., Musch, B., Ehrenmann, F., Delzon, S., et al. (2017). Adaptive and plastic responses of Quercus petraea populations to climate across Europe. Glob. Chang. Biol. 23, 2831–2847. doi: 10.1111/gcb.13576
Sáenz-Romero, C., Lindig-Cisneros, R. A., Joyce, D. G., Beaulieu, J., St Clair, J. B., and Jaquish, B. C. (2016). Assisted migration of forest populations for adapting trees to climate change. Rev. Chapingo Serie Cienc. Forest. Ambiente 22, 303–323. doi: 10.5154/r.rchscfa.2014.10.052
Sáenz-Romero, C., O'Neill, G., Aitken, S. N., and Lindig-Cisneros, R. (2021). Assisted migration field tests in Canada and Mexico: Lessons, limitations, and challenges. Forests 12:9. doi: 10.3390/f12010009
Sáenz-Romero, C., Rehfeldt, G. E., Crookston, N. L., Duval, P., St-Amant, R., Beaulieu, J., et al. (2010). Spline models of contemporary, 2030, 2060 and 2090 climates for Mexico and their use in understanding climate-change impacts on the vegetation. Clim. Chang. 102, 595–623. doi: 10.1007/s10584-009-9753-5
Sáenz-Romero, C., Rehfeldt, G. E., Duval, P., and Lindig-Cisneros, R. A. (2012). Abies religiosa habitat prediction in climatic change scenarios and implications for monarch butterfly conservation in Mexico. For. Ecol. Manag. 275, 98–106. doi: 10.1016/j.foreco.2012.03.004
Sáenz-Romero, C., Rehfeldt, G. E., Ortega-Rodríguez, J. M., Marín-Togo, M. C., and Madrigal-Sánchez, X. (2015). Pinus leiophylla suitable habitat for 1961-1990 and future climate. Bot. Sci. 93, 709–718. doi: 10.17129/botsci.86
Sanchez-Velasquez, L. R., Pineda-Lopez, M. D. R., and Hernandez-Martinez, A. (1991). Distribución y estructura de la población de Abies religiosa (HBK) Schl. et Cham., en el Cofre de Perote, Estado de Veracruz, México. Acta Bot. Mex. 16, 45–55. doi: 10.21829/abm16.1991.625
SAS Institute (2014). SAS/STAT (Version 9.4) Computer Software. Cary, North Carolina, USA: SAS Institute Inc.
Seliger, B. J., McGill, B. J., Svenning, J.-C., and Gill, J. L. (2021). Widespread underfilling of the potential ranges of North American trees. J. Biogeogr. 48, 359–371. doi: 10.1111/jbi.14001
St Clair, J. B., Howe, G. T., and Kling, J. G. (2020). The 1912 Douglas-Fir heredity study: Long-term effects of climatic transfer distance on growth and survival. J. For. 118, 1–13. doi: 10.1093/jofore/fvz064
Vivar-Vivar, E., Pompa-García, M., Rodríguez-Trejo, D.-A., Leyva-Ovalle, A., Wehenkel, C., Cariilo-Parra, A., et al. (2021). Drought responsiveness in two Mexican conifer species forming young stands at high elevations. Forest Syst. 30:e012. doi: 10.5424/fs/2021303-18371
Walker, A., Oberhauser, K. S., Pelton, E. M., and Peasants, J. M. (2022). Danaus plexippus (errata version published in 2022). The IUCN Red List of Threatened Species 2022: e.T159971A219149911.
Winder, R., Nelson, E., and Beardmore, T. (2011). Ecological implications for assisted migration in Canadian forests. For. Chron. 87, 731–744. doi: 10.5558/tfc2011-090
Keywords: assisted migration, species range expansion, climate change, Abies religiosa, Monarch Butterfly Biosphere Reserve, Nevado de Toluca, mixed model, response curve
Citation: Sáenz-Romero C, Osuna-Vallejo V, Herrejón-Calderón P, Pérez-Cruz LA, Joaquín-Juan MG, Cruzado-Vargas AL, O’Neill GA, Zacarías-Correa AG, Manzanilla-Quijada GE, Lindig-Cisneros R, Blanco-García A, Endara-Agramont ÁR and Lopez-Toledo L (2024) Establishing monarch butterfly overwintering sites for future climates: Abies religiosa upper altitudinal limit expansion by assisted migration. Front. For. Glob. Change. 7:1440517. doi: 10.3389/ffgc.2024.1440517
Edited by:
Paula E. Marquardt, Northern Research Station, Forest Service (USDA), United StatesReviewed by:
Dusan Gomory, Technical University of Zvolen, SlovakiaChristel Kern, Northern Research Station, Forest Service (USDA), United States
Copyright © 2024 Sáenz-Romero, Osuna-Vallejo, Herrejón-Calderón, Pérez-Cruz, Joaquín-Juan, Cruzado-Vargas, O’Neill, Zacarías-Correa, Manzanilla-Quijada, Lindig-Cisneros, Blanco-García, Endara-Agramont and Lopez-Toledo. This is an open-access article distributed under the terms of the Creative Commons Attribution License (CC BY). The use, distribution or reproduction in other forums is permitted, provided the original author(s) and the copyright owner(s) are credited and that the original publication in this journal is cited, in accordance with accepted academic practice. No use, distribution or reproduction is permitted which does not comply with these terms.
*Correspondence: Cuauhtémoc Sáenz-Romero, Y3VhdWh0ZW1vYy5zYWVuekB1bWljaC5teA==