- 1Faculty of Forestry and Wood Sciences, Czech University of Life Sciences Prague, Prague, Czechia
- 2Max Planck Institute for Chemical Ecology, Jena, Germany
Introduction: In recent years, Norway spruce (Picea abies L.) forests in Central Europe have faced escalating threats from bark beetles, primarily Ips typographus (L.), and other species, such as I. duplicatus (Sahlberg). Outbreaks are partially attributed to weakened tree defense resulting from drought periods induced by climate change. This study examines Norway spruce’s physiological and metabolic reactions to acute drought stress during the growing season and evaluates its susceptibility to I. typographus and I. duplicatus.
Methodology: In order to induce drought stress, mature Norway spruces had their roots covered with a roof in April 2021, depriving them of water. Control trees were left with free access to natural rainwater. Over 5 months of the growing season, soil water potential, bark temperature, tree trunk circumference, and sap flow were monitored. Roofed trees and controls were sampled in July, August, and September and analyzed for non-structural carbohydrates and the two classes of defensive compounds, phenolics and terpenes. Furthermore, two different bioassays in tubes and boxes were performed using adult I. typographus and I. duplicatus beetles to assess host choice and acceptance.
Results: Roofed trees exhibited signs of stress as early as July, resulting in decreased tree trunk and a consequent increase in non-structural carbohydrate content. Defensive metabolites remained largely unaffected except for an increase in diterpenes in September. In bioassays, I. typographus preferred boring into the bark of roofed trees in August in tubes and in September in boxes. This increased tree acceptance correlated with increased levels of soluble carbohydrates in the phloem. I. typographus and I. duplicatus beetles showed higher mobility in boxes in August and September on roofed trees but not in July, even though bark surface temperatures were elevated in roof-covered trees during all three bioassay periods.
Conclusion: The study revealed rapid physiological responses of trees to acute drought stress, although not many changes were observed in defense traits. Despite the absence of natural bark beetle attacks, drought trees were more accepted by I. typographus than naturally watered trees. This response may indicate the beetles’ preference for trees with phloem of higher nutritional quality induced by the acute drought stress conditions.
1 Introduction
Over the last few years, the increasing frequency and severity of bark beetle outbreaks in conifer forests worldwide has been ascribed primarily to climate change. Rising temperatures, with an anticipated global increase of 2–4°C in the next 50 years, are projected to lead to more frequent and severe droughts (Allen et al., 2010; Liu et al., 2018). Droughts affect insect population dynamics and also impact tree growth and defense mechanisms in ways that can enhance beetle outbreaks (Hart et al., 2014; Raffa et al., 2015). While conifer species vary in their ability to withstand drought stress, Norway spruce (Picea abies L.), the most economically significant conifer in Europe, lacks efficient mechanisms to cope with varying water regimes, rendering it more susceptible to bark beetle infestations (Christiansen et al., 1987). Over the past decade, Central Europe has experienced severe outbreaks of spruce bark beetles (Curculionidae, Scolytinae), primarily Ips typographus (L.), and, to a lesser extent, other species such as I. duplicatus. These outbreaks exploit weakened tree defenses triggered by drought stress, which has become increasingly significant in recent years (Holusa et al., 2010; Fernandez-Carrillo et al., 2020; Hlásny et al., 2021). Since 2015, bark beetle colonization, coupled with fungal infestation, has resulted in extensive Norway spruce mortality and reduced wood quality across thousands of hectares in Central Europe, leading to substantial economic losses (Hlásny et al., 2019, 2021; Fernandez-Carrillo et al., 2020). In Czechia, outbreaks peaked in 2019 and 2020, with salvage logging reaching approximately 15 million m3, gradually decreasing to 5.5 million m3 in subsequent years (Lubojácký et al., 2023).
The less hazardous Northern spruce bark beetle, I. duplicatus, originating from boreal forests, has become invasive across Europe (Wermelinger et al., 2020). It coexists with I. typographus on Norway spruces but favors the upper stem below the canopy, preferring less thick bark due to its smaller size. I. duplicatus competes with I. typographus for space and resources on the same tree (Grodzki, 2012; Kavčič et al., 2023). However, even though it has a postponed generation peak, it is often suppressed by the high population density of the more aggressive I. typographus.
Trees exhibit different responses to sudden, severe water deficits, termed acute drought stress, and long-term water deficits, referred to as chronic drought stress. Both significantly affect tree physiology, altering carbon balances and the spectrum of primary and secondary tree metabolites produced (Erbilgin et al., 2021; Netherer et al., 2021). The original hydrologically based theories suggest a link between tree carbon balance and secondary metabolism, influencing resistance against biotic invaders (Loomis, 1932; Lorio, 1986; Herms and Mattson, 1992). During moderate acute drought stress, Luxmoore et al. (1995) noted that conifer trees increase carbohydrate storage during minimal growth activity. Potentially, more recent studies have confirmed that trees prioritize the storage of carbon reserves during drought stress at the expense of growth to sustain respiration and defense (McDowell and Sevanto, 2010; Berini et al., 2018). If respiratory costs exceed carbon assimilation rates, trees may experience a negative carbon balance, necessitating the use of stored non-structural carbohydrates (NSCs) such as glucose and starch to support metabolism (Lombardero et al., 2000; McDowell et al., 2008).
Conifers defend against bark beetle attacks through a complex network of biochemical mechanisms, producing secondary defense metabolites, including terpenes, the main component of conifer resin, and soluble phenolics. These metabolites are stored constitutively, or their biosynthesis is induced after attacks by herbivores and pathogens (Franceschi et al., 2005). The production of these defense metabolites depends on regulatory mechanisms that balance carbon resources, primarily NSC substrates, among the processes involved in growth, respiration, storage, and defense (Huang et al., 2020a,b; Netherer et al., 2021). In drought conditions, conifers redirect NSCs towards the stress repair and biosynthesis of defense metabolites (Hartmann et al., 2018; Huang et al., 2019). However, when trees weakened by drought face biotic intruders, such as bark beetles or their symbiotic fungi, the availability and allocation of NSCs for defense metabolites may be compromised (Netherer et al., 2021).
The resin in Norway spruce contains monoterpene hydrocarbons, such as α-pinene, β-pinene, β-phellandrene, myrcene, limonene, Δ3-carene, and camphene, accompanied by trace amounts of their oxygenated derivatives, with high biological activity (Netherer et al., 2021). Sesquiterpenes, less volatile than monoterpenes, occur in smaller amounts. Additionally, the resin contains abundant diterpenes and oxygenated diterpenes, which have a high viscosity (Zeneli et al., 2006; Zhao et al., 2011; Schiebe, 2012). Terpenes in Norway spruce are synthesized in epithelial cells of constitutive or traumatic resin ducts and then loaded under pressure into the ducts (Celedon and Bohlmann, 2019).
The defense function of resin is believed to arise from the toxicity of terpenes to adult bark beetles, their larvae, and their symbiotic fungi. In addition, the diterpenes may act as a viscous mechanical barrier, preventing beetles from entering the bark (Franceschi et al., 2005). The levels of trace oxygenated monoterpenes vary with the physiological condition and age of the tree. The content of these compounds increases after biotic and abiotic stresses, such as infestation by beetles (Stříbrská et al., 2023), fungi (Kandasamy et al., 2023), or in felled trees (Schiebe et al., 2019).
Terpenes in the bark beetle-Norway spruce interaction are also proposed to function as signals for beetles, potentially aiding in selecting suitable habitats (Netherer et al., 2021) or identifying weakened trees as potential targets during their dispersal flight (Lehmanski et al., 2023). The major monoterpene hydrocarbons, such as the pinenes, can enhance the attraction of bark beetle aggregation pheromones in a pheromone trap when used in high doses, but they will not attract beetles if used alone in the trap (Erbilgin et al., 2007). The minor oxygenated monoterpenes, long neglected by researchers, have recently been found to be selectively detected by beetle antennae (Schiebe et al., 2019). Some, such as eucalyptol (1,8-cineole) and trans-4-thujanol, inhibit the attraction of beetles to I. typographus aggregation pheromones (Jirošová et al., 2022), while others, such as isopinocamphone, exhibit the opposite effect, as shown by field trapping experiments (Moliterno et al., 2023). Volatile terpenes are hypothesized to facilitate beetle navigation to spruce first based on the perception of the whole complex mixture to recognize suitable habitats with a high abundance of conifers and later to recognize weakened over healthy trees. The impact of drought on terpene concentrations and emissions varies across Pinaceae species (Lewinsohn et al., 1993; Holopainen et al., 2018). Severe drought stress might impair the resin defenses of conifer trees (Gaylord et al., 2013) and affect photosynthesis and the allocation of carbon resources into the production of terpenoids (McDowell, 2011).
In addition to terpenes, Norway spruce produces soluble but nonvolatile phenolics, such as stilbenes and flavonoid defense compounds. These substances are believed to be synthesized in phloem parenchyma cells (Franceschi et al., 2005) and can be activated by various biotic stressors (Mageroy et al., 2020). Certain flavonoids act directly as antifeedants against beetles (Faccoli and Schlyter, 2007; Hammerbacher et al., 2019) and serve as fungicides combating fungi associated with bark beetles (Brignolas et al., 1998; Hammerbacher et al., 2011). In an experiment involving the roofing of mature Norway spruce conducted by Netherer et al. (2024), an increase in total phenolic content was observed during June of the second year, with the stilbene glucoside isorhapontin exhibiting an elevated concentration during this period. Additionally, the volatile phenylpropanoid estragole (4-allylanisole) has been found to inhibit the attraction of I. typographus species to its aggregation pheromone (Moliterno et al., 2023).
Previous research has revealed much about how water stress affects the interaction of Norway spruce with I. typographus, including primary attraction, host acceptance, and beetle development (Netherer et al., 2015, 2024). However, the precise relationships between the severity and duration of drought stress, alterations in tree physiology and metabolism, and susceptibility to bark beetle attacks remain incompletely understood (Erbilgin et al., 2021; Netherer et al., 2021). Based on the hydrological balance hypothesis, we hypothesize that mature Norway spruce trees exposed to acute drought stress should decrease their growth and increase their NSC content. With the increased NSC content available for the biosynthesis of defense metabolites, the defensive capabilities of spruce against bark beetles are anticipated to strengthen accordingly. We also hypothesize that two spruce bark beetle species, naturally sharing the same host tree but living on separate parts of the stem, will exhibit different preferences for their host at different stages of acute drought stress.
To investigate these hypotheses, we studied mature Norway spruce trees subjected to acute drought stress by blocking all soil water recharge from April to September 2021. Roofs to cover tree roots were employed as an efficient method for inducing drought stress in forest stands, as established in previous work (Netherer et al., 2015, 2024). The roots of 10 trees were covered just above soil level before the growing season in April 2021. As a control, naturally watered trees were monitored and sampled from two plots in the same locality. We measured the effect of these two treatments on growth, sap flow, non-structural carbohydrate content, and levels of defense compounds in the phloem. Defense compounds included representatives of all classes of terpenes and phenolics, namely stilbenes and flavonoids and their glucosides. To assess the response of these characterized trees to two different spruce bark beetle susceptibility, we also monitored natural attacks and conducted two types of bioassays with living beetles of two species, I. typographus and I. duplicatus.
2 Materials and methods
2.1 Experimental site and set-up
An experimental site was carefully selected within a 100-year-old Norway spruce forest growing under suboptimal physiological conditions in the Prague-East district of Kostelec nad Černými Lesy, Czechia, on the property of the Company Forest CZU (Figure 1). The site was divided into three plots. One of these plots, labeled “Roof” (coordinates: 49.94783294, 14.91734061), underwent a specialized treatment involving the construction of a wooden and plastic roofing structure in April 2021, covering an area of 30 × 15 m. The primary purpose of this roof was to provide a protective cover for tree roots, shielding them from rainwater. The remaining two plots, Control 1 (CTRL1; coordinates: 49.94846209, 14.9178258) and Control 2 (CTRL2; coordinates: 49.94824598, 14.91786334), were left to be naturally watered by rainfall. Each plot contained 10 trees, six of which were monitored by sensors (Supplementary Table S1).
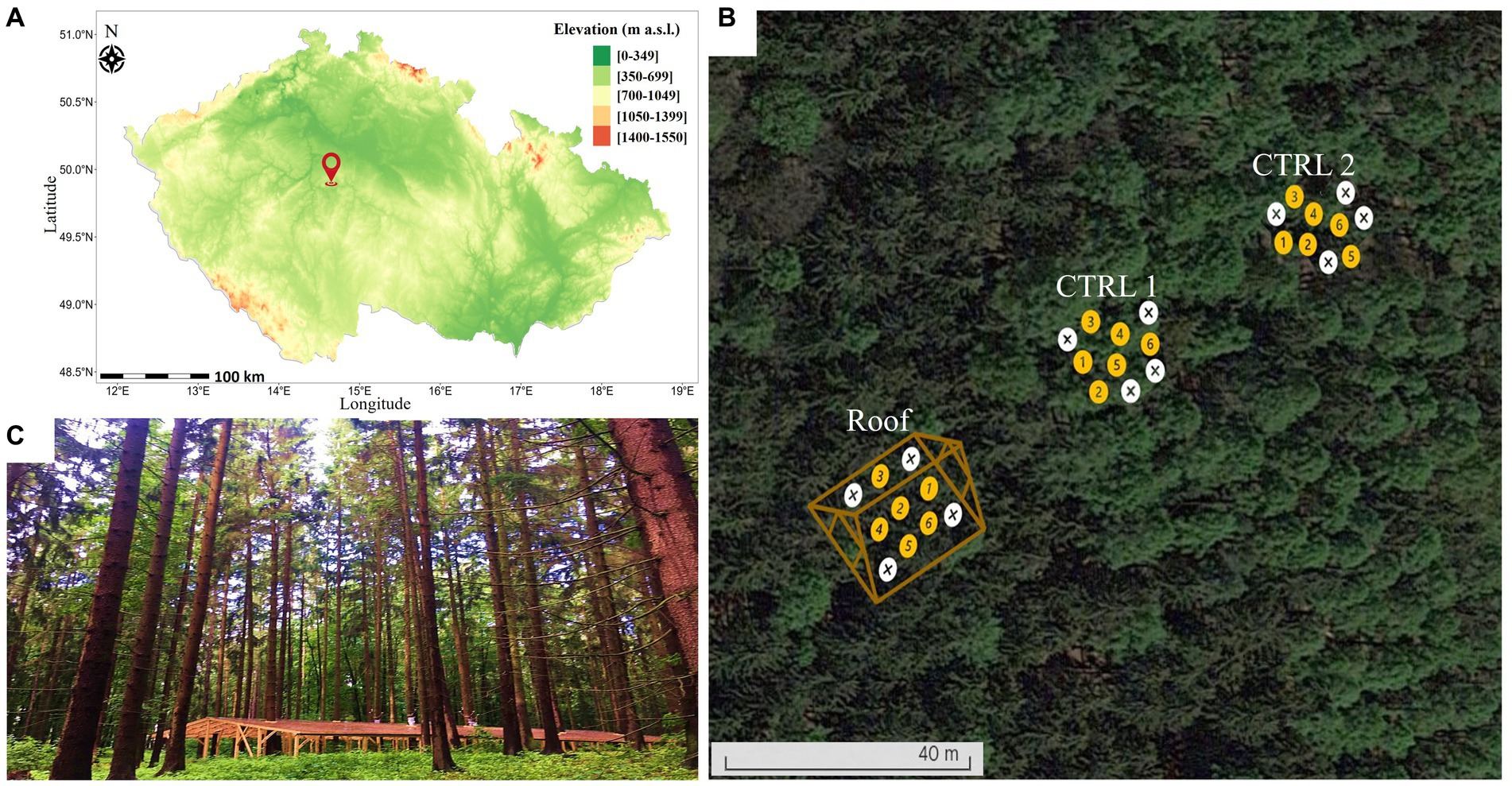
Figure 1. (A) Location of the study area in Kostelec nad Černými Lesy, Czechia. (B) Experimental plots set-up within the study area. Roof plot: trees’ roots were covered by a wooden and plastic roof in April 2021; CTRL plots: trees were naturally watered by rainfall and used as control groups. Yellow circles indicate trees with sensors for measuring physiological parameters, while white circles indicate trees without sensors. (C) Photo of the roof covering roots.
2.2 Measurement of hydrological and meteorological conditions
To quantify soil water availability for trees, we monitored soil water potential in three subplots from May to September 2021. Data was recorded hourly using five sensors (Teros 21, Meter Group, München, Germany) strategically placed 20 cm below the ground surface. Subplot data were averaged from the five sensors per hour and 24-h period.
The meteorological conditions during the vegetation season 2021 (Supplementary Table S2) were recorded by a meteorological station placed in the area. Air temperature and humidity were measured by (Minikin QTHi, EMS Brno, Czech Republic). Minikin ERi recorded precipitation with a Pronamic Pro Rain gauge (EMS Brno), and the photosynthetically active radiation (PAR) was measured by a Quantum Sensor EMS 12 (EMS Brno). Bark surface temperature was measured on the south side of the stem, where bioassays with beetles were conducted. Infrared thermometers (Apogee Instruments, Logan, UT) were installed at a height of 3 m. Data were collected hourly, and daily averages were calculated for the collection period (Stříbrská et al., 2022).
2.3 Measurement of physiological parameters of experimental trees
On all monitored trees, we measured sap flow and tree trunk circumference increment following the methods presented by Stříbrská et al. (2023). Data were stored in a singular data logger (GreyBox N2N3P; EMS Brno, Czech Republic) and linked to a cloud system through GSM connectivity. To facilitate statistical analysis, we averaged continuously recorded data per collection day or specific time period, enabling integration with discretely measured characteristics to illustrate concurrent changes.
Sap flow was assessed using the trunk heat balance method (EMS 81; EMS Brno, Czechia) at 10 min intervals. Post-processing involved baseline correction, and sap flow rates were recalculated as kg/h for each sampling date.
Trunk circumference was monitored with a DR26E Band dendrometer (DR26E, EMS Brno, Czechia) 3.5 m above ground, recording circumference changes every 5 min. After error correction, data were converted to hourly averages. The beginning of the growing season was determined by the initiation of an increase in trunk circumference and sap flow, with circumference increases measured from the start of the 2021 growing season, using a 1-week average preceding each sampling date for data acquisition.
2.4 Sample collection for analysis of metabolites
On July 15th, August 11th, and September 8th, 2021, five bark sections (ø 8 mm) were collected from each monitored tree, spaced at least 50 cm from locations of bioassays with beetles and 15 cm apart at the height of 2 m on the south side of the trunk. Samples were immediately frozen in liquid nitrogen and stored at −80°C in the laboratory until extraction. The phloem, including the cambium, was dissected from the bark sections, pooled, ground into a fine powder in liquid nitrogen, and divided into three 100 mg parts for analysis of non-structural carbohydrates (NSCs), soluble phenolics, and terpenes.
2.5 Non-structural carbohydrate analysis
A 2 mL test tube was filled with 20 mg of freeze-dried homogenized phloem and 1.5 mL of a methanol: water solution (80:20 v/v) was added. The tube was vortexed for a couple of seconds and then placed in a thermoshaker for 30 min at 60°C and 1,000 rpm. Then, samples were centrifuged at 20°C and 13,500 rpm for 5 min. The supernatant was collected and filtered through a PVDF syringe filter (0.22 μm) before LC-qTOF-MS/MS analyses.
NSC concentrations were determined using LC-qTOF-MS/MS (Agilent, United States) analysis, according to Šulc et al. (2021). Analysis was performed on Agilent 1,290 Infinity II LC coupled with an Agilent 6,546 LC/MS QTOF system. Chromatographic separation was performed on a Supelco apHera NH2 Polymer column (150 × 2 mm, 5 μm) operated at 30°C. The mobile phase was composed of acetonitrile (A) and water (B). Gradient elution was performed with the initial conditions of mobile phase 80:20 (A:B), followed by 0.5–13 min 55:45 (A:B), and 14–15 min 80:20 (A:B) with a flow rate of 0.2 mL min−1 (Madsen et al., 2015). The volume for injection was set as 1 μL. The system was operated in negative ionization mode. The QTOF parameters were optimized using standards (glucose, sucrose, fructose, raffinose, mannitol, and trehalose) and were as follows: scan range 100–1,000 m/z; drying gas temperature, 280°C; sheath gas flow rate, 12.0 L/min; sheath gas temperature, 400°C; capillary voltage, 2.0 kV; fragmentor, 120 V; collision energy at 10, 20 and 40 eV. MS/MS data were acquired at a 50–800 m/z scan range. The analysis continuously measured two reference masses, 112.9855 m/z and 922.0098 m/z, for mass correction. The data collection was carried out using Agilent Mass Hunter Acquisition software. The data analysis was performed using Qualitative Analysis 10.0 and Q-TOF Quantitative analysis, Agilent Mass Hunter. Compounds were identified and quantified by calibration curves for individual carbohydrates.
2.6 Analysis of soluble phenolic compounds
Thirty mg of freeze-dried and homogenized samples were accurately weighed, and 500 μL of methanol: water (70:30 v/v) was added to each sample. Then, samples were well-mixed using a vortex. Afterward, the samples were placed into an ultrasonic bath with ice for 10 min. Subsequently, the tubes were centrifuged at 13000 rpm for 10 min at 4°C. The supernatant was filtered using a 0.22 μm PTFE syringe filter before LC-qTOF-MS/MS analysis.
LC-qTOF-MS/MS analysis was carried out using an Agilent 1,290 Infinity II LC coupled with an Agilent 6,546 LC/MS QTOF system (Agilent, United States) and a Zorbax Eclipse Plus C18 column (2.1 × 50 mm, 1.8 μm), (Agilent, United States). Mobile phase A contained 0.05% formic acid, and mobile phase B consisted of acetonitrile. The gradient elution was: 0–1 min, 100% A; 1–7 min, 35% A; 7–10 min, 100% A. The flow rate of the mobile phase was set at 1.1 mL min−1 (Huang et al., 2020b). The column temperature was set at 35°C. The injection volume was 1 μL. The system was operated in negative ionization mode. The qTOF parameters were previously optimized using the standards (catechin, procyanidin, epicatechin, taxifolin, quercetin, resveratrol, piceatannol, isorhapontin, astringin). The qTOF parameters were as follows: scan range 100–1,000 m/z; drying gas temperature, 350°C; sheath gas flow rate, 12.0 L/min; sheath gas temperature, 400°C; capillary voltage, 5.0 kV; nozzle voltage, 0.9 kV; fragmentor, 140 V; collision energy at 10, 20 and 40 eV. MS/MS data were acquired at a scan range of 50–800 m/z. The analysis continuously measured two reference masses, 112.9855 m/z, and 966.0007 m/z, for mass correction. Data collection was carried out using Agilent Mass Hunter Acquisition software. The data analysis was performed using Qualitative Analysis 10.0 and Q-TOF Quantitative analysis. Compounds were identified by standards and quantified by calibration curves measured for standards.
2.7 Analysis of terpenes
A 100 mg quantity of phloem was extracted using 1 mL of n-hexane, filtered, and then injected into a two-dimensional gas chromatograph coupled with a time of flight mass spectrometer (GC × GC-TOF–MS) (Pegasus 4D, Leco, United States). The injector (275°C) was operated in split mode (10:1 split ratio). Separation of compounds takes place on two chromatographic columns connected in a consumable-free modulator for the first dimension, HP-5 MS UI column (0.25 mm i.d., 0.25 μm film thickness); for second-dimensional separation VF-17 MS (1.5 m, 0.1 mm i.d., 0.1 μm film thickness), both from Agilent (United States). The temperature program for separation started at 40° C with a hold time of 2 min, followed by a gradient of 10°C/min to 100°C and then at 5°C/min to 320°C with a hold time of 3 min. The secondary oven and modulator had temperature offsets of 5°C and 15°C, respectively. A 5-s modulation period was used. Separated compounds were ionized in the ion source of MSD at 70 eV, and full spectral (35–500 Da) information was recorded 100 times a second. After measurement, data were processed. Deconvolution and peak alignment options were used. Only signals with intensity higher than 25 (S/N) appearing in the chromatogram in the same (+ − 5 s) retention time were aligned. The area of unique quantification mass for each signal was provided in the aligned table. Data were exported into MS Excel for statistical analysis. Compounds were identified using retention indexes and mass spectra similarity and evaluated automatically with the NIST 2018 library. Terpene abundance was calculated as the proportion of the peak area of each identified terpene relative to the sum of peak areas of all identified terpenes evaluated in this study.
2.8 Origin of Ips typographus and I. duplicatus beetles used in bioassays
Norway spruce logs (±30 cm diameter x ± 40 cm length), aged between 80 to 100 years, naturally infested by I. typographus and I. duplicatus, were collected 4 weeks before the bioassay at the Forest CZU in Kostelec nad Černými Lesy. The infested logs were stored in insect-rearing cages (60 × 60 × 90 cm; 25 ± 2°C) to complete the development of beetles, which naturally emerged.
Adults of both species (F0 generation) were collected by manually peeling the infested logs and sexed under a stereomicroscope, as Schlyter and Cederholm (1981) described for I. typographus and Nunberg (1954) for I. duplicatus. Only males without body damage were selected and acclimated individually into a 2 mL Eppendorf tube filled with a strip of filter paper for 1–2 h before the bioassays. Each Eppendorf tube was drilled with several holes to provide ventilation.
2.9 No-choice bioassay with Ips typographus and I. duplicatus males in Eppendorf tubes
A no-choice feeding assay was conducted to assess the differences in acceptance of trees under roofs versus controls. The bioassay was carried out in August and September 2021, concurrently with a collection of samples for chemical analyses, following the methods outlined by Stříbrská et al. (2022) and Turcani and Nakládal (2007). Ten Eppendorf tubes, each containing a selected single male specimen of either I. typographus or I. duplicatus, were affixed with tape at the height of 1.60 m above the ground on both drought-stressed and control trees.
After 24 h, beetle activity was assessed by observing whether they were actively feeding on the tree or were inactive, following the method described by Stříbrská et al. (2022).
2.10 Attack box bioassay with Ips typographus and I. duplicatus
The attack box method, initially outlined by (Netherer et al., 2015) and adapted by Stříbrská et al. (2022), was used to periodically assess the host choice and acceptance of both species of spruce bark beetles. Two boxes (50 × 25 × 20 cm) with front plexiglass windows were affixed per tree, one above the other at a height of 4 meters on the south-facing side of the trunk. Each box contained 20 unsexed I. typographus or I. duplicatus beetles. Bioassays were conducted on July 15th, August 11th, and September 8th, starting between 10 am and 12 pm, concurrent with sample collection, and lasting for 24 h. Initially, theunsexed individuals of either species were placed in start bottles mounted to the side of the attack boxes. Over the next 24 h, beetles could choose between leaving the start bottle, entering the attack box, and potentially boring into the tree bark. The beetle movement was monitored by observing how many remained in the starting bottle, and boring into the bark was recorded by counting the number of boring holes.
2.11 Data analysis
All the statistical analyses were performed using Statistica 14.0 software for Windows and GraphPad Prism 9 software for MacOS.
The Mann–Whitney U Test was employed to assess the significance of differences between two independent groups, accompanied by a continuity correction to evaluate all chemical analyses and for the physiological parameters. This non-parametric test is suitable for ordinal data or when assumptions of normality are not met.
The activity of beetles was evaluated using the Chi quadrat test. Furthermore, we conducted Spearman correlation analysis to explore the potential linear relationship between NSC and phenolic content with the acceptance of beetles in individual trees.
3 Results
3.1 Roofed trees had higher bark surface temperatures
Since April, trees whose roots had been covered by roofs had bark surface temperatures significantly higher than those of control trees during the July, August, and September analyses (Figure 2). The mean bark surface temperatures on the south side of the tree were 19.2 ± 0.6°C on roofed trees and 18.2 ± 0.3°C on control trees in July, 23.5 ± 0.2°C on roofed trees and 22.8 ± 0.7°C on control trees in August, and 22.5 ± 0.2°C on roofed trees and 21.8 ± 0.6°C on control trees in September. The mean air temperatures during the three measurement periods were 18.3°C in June, 22.8°C in August, and 21.8°C in September.
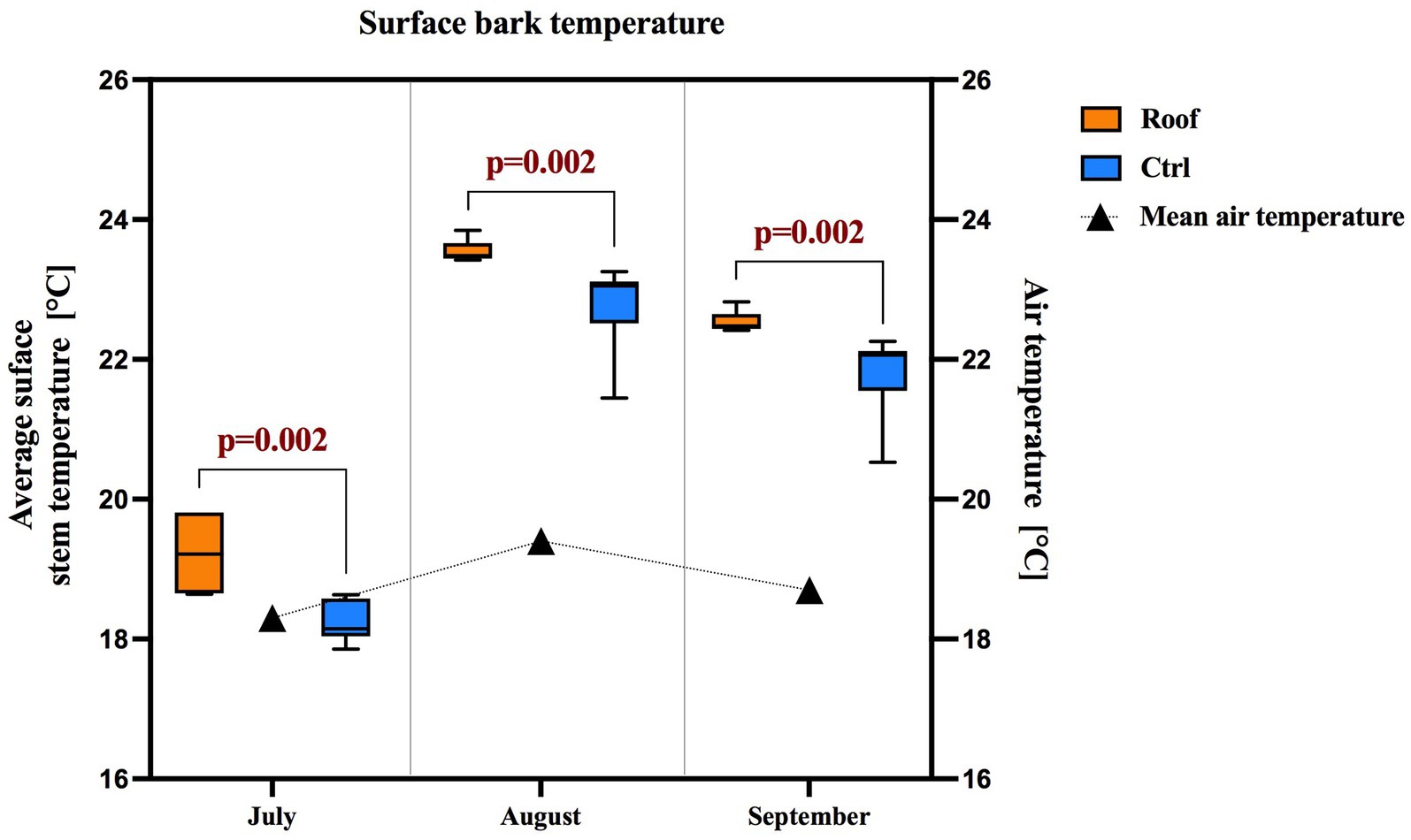
Figure 2. The surface stem temperature of roofed and control trees and air temperature in the experimental site were measured during data collection in 2021. The box plots show the median and quartile ranges.
3.2 Roofs decreased soil water potential
In the study plot with roofs covering the roots, soil water potential (SWP) began to decline below-500 kPa as early as June and continued to decline throughout measurements ending in September. By contrast, in the control plots, a decline below-500 kPa was observed first in August (Figure 3A). In the roofed plot, SWP had decreased below the wilting point (−1,500 kPa) by July, indicating that the roofed trees were experiencing drought stress. Conversely, the SWP in the control plots never reached values indicating drought stress for trees.
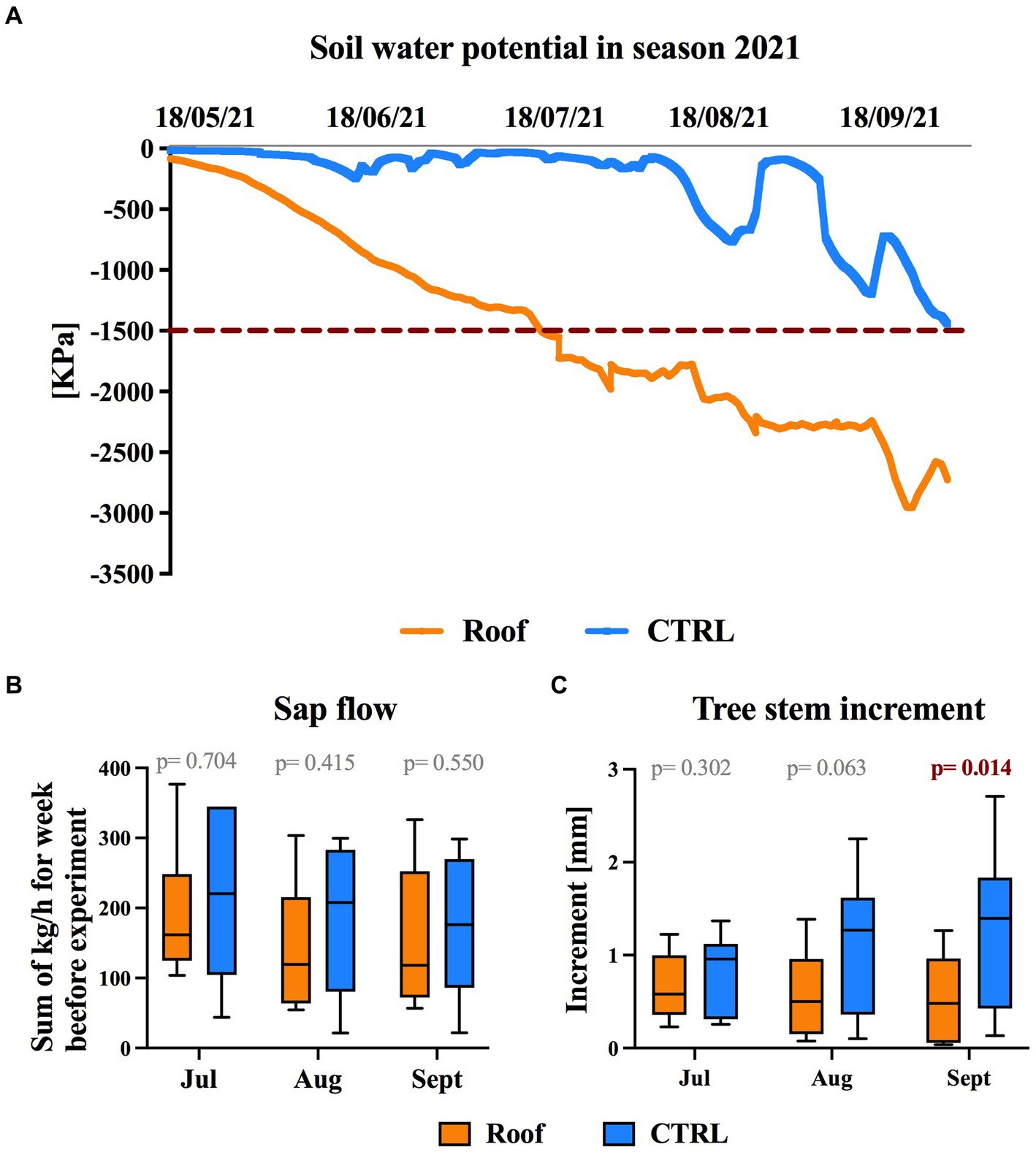
Figure 3. Effect of drought on soil water potential, sap flow, and tree stem growth increment. (A) Seasonal measurement of soil water potential in roofed (orange line) and control (blue line) plots. (B) The sap flow of roofed (orange) and control (blue) trees, represented as the sums of recorded sap flow in kg/h per week before experimental days. (C) Tree stem increment of roofed (orange) and control (blue) trees was calculated as the weekly average of daily increments. The box plots show the median and quartile ranges.
3.3 Roofing decreased stem circumference increment but did not significantly affect sap flow
Trunk circumference increments measured on the main trunk during the week preceding the sampling days did show a noticeable difference between the roofed and control trees in August, and by September, the trunk circumference increments of roofed trees were significantly lower than those of well-watered trees (p = 0.014) (Figure 3C). However, in July, there was no significant reduction in trunk circumference increment for the roofed trees compared to the control trees. The trunk diameters of trees in September were even lower than the July measurements for the same trees, indicating trunk shrinkage.
The sap flow dynamics of trees of roofed and control trees were measured by summing the sap flow measurements from the week preceding each sampling collection. While there was a trend towards lowered sap flow in roofed trees, no significant differences existed between the trees covered by the roof and the control plots (Figure 3B).
3.4 Roofing increased the levels of some soluble non-structural carbohydrates (NSCs) in phloem
Acute water stress induced by 5 months without soil water recharge after roofing had a noticeable effect on phloem carbohydrate concentrations in the trunk. Carbohydrates were analyzed only in August and September. The sum of all measured carbohydrates (excluding the alcohol mannitol) was significantly higher in both months (Supplementary Figure S1). The main contribution was made by glucose and fructose, which were significantly higher in the roofed trees in August compared to the control group (G: p = 0.031; F: p = 0.010), with a similar pattern observed in September (G: p = 0.007; F: p = 0.049). For the trisaccharide raffinose, there was a significant elevation in roofed trees compared to the control (p = 0.004 in September). However, the roofing treatment did not affect the concentration of the disaccharide sucrose. The concentration of the monosaccharide alcohol mannitol was consistently low, approximately 1,000 times lower than soluble saccharides, and exhibited high variability between trees (Figure 4). When correlating the content of monosaccharides with tree stem increment using Spearman’s rank-order correlation in individual trees, a nonsignificant negative correlation was observed between glucose and trunk circumference increment (−0.41), as well as between fructose and trunk circumference increment (−0.5) in September.
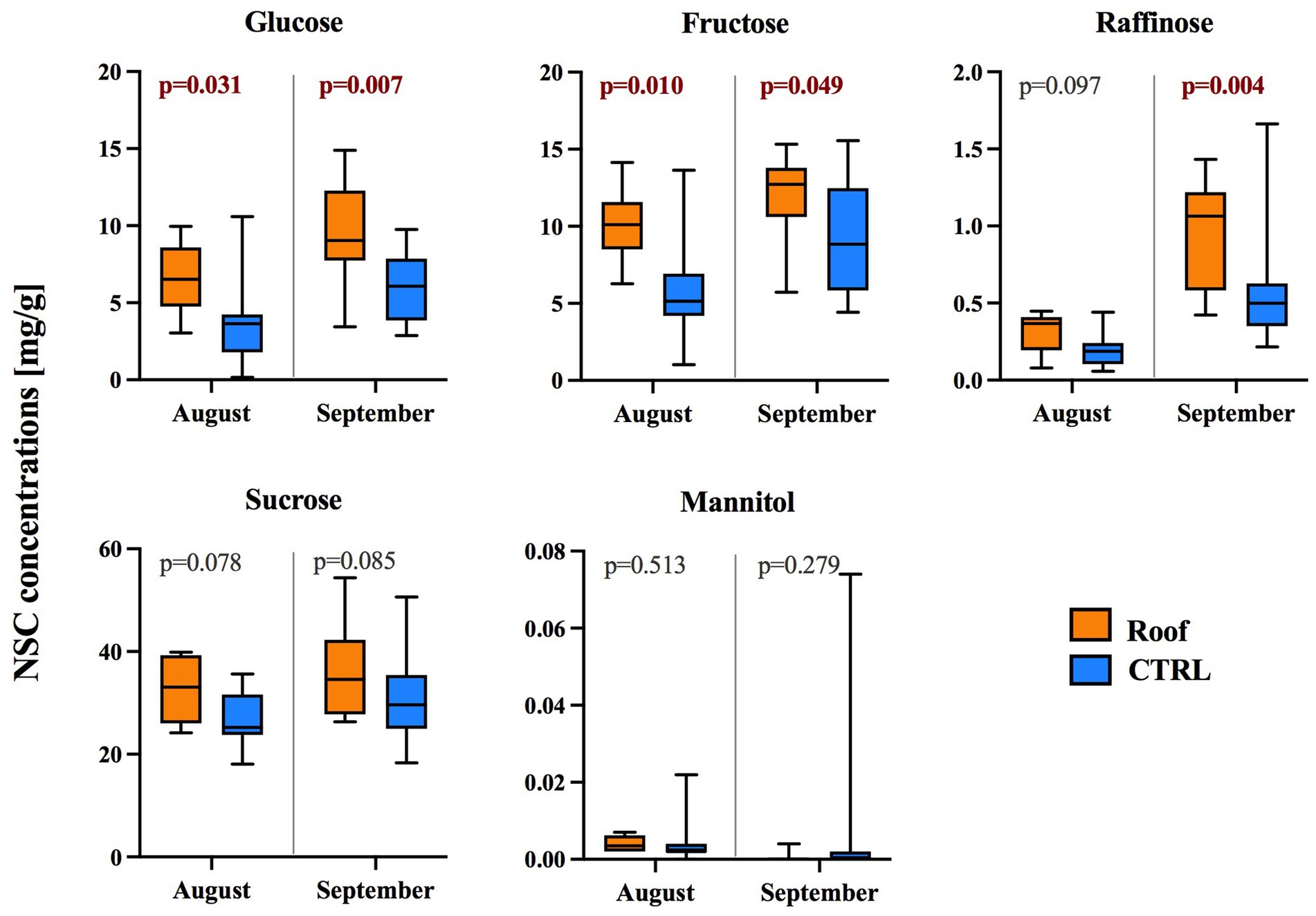
Figure 4. Concentration (mg/g dry weight) of selected soluble non-structural carbohydrates and the carbohydrate alcohol mannitol in phloem samples collected from the stems of roofed (orange) and control (blue) trees at a height of 1.3 m on the stem. The box plots illustrate the median and quartile ranges for each group.
3.5 Roofing altered levels of some phloem soluble phenolic compounds
The effect of drought treatment on the concentrations of nine soluble phenolics was measured in August and September (Figure 5). These phenolics were categorized into four structural classes: three flavan-3-ols (catechin, procyanidin, and epicatechin), two (dihydro) flavonols (taxifolin and quercetin), two stilbenes (resveratrol and piceatannol), and two stilbene glucosides (isorhapontin and astringin). The sum of these measured phenolics was higher in roofed trees in September (Supplementary Figure S2), primarily due to the very high content of stilbene glucosides, which sum was significantly higher in September (Supplementary Table S3).
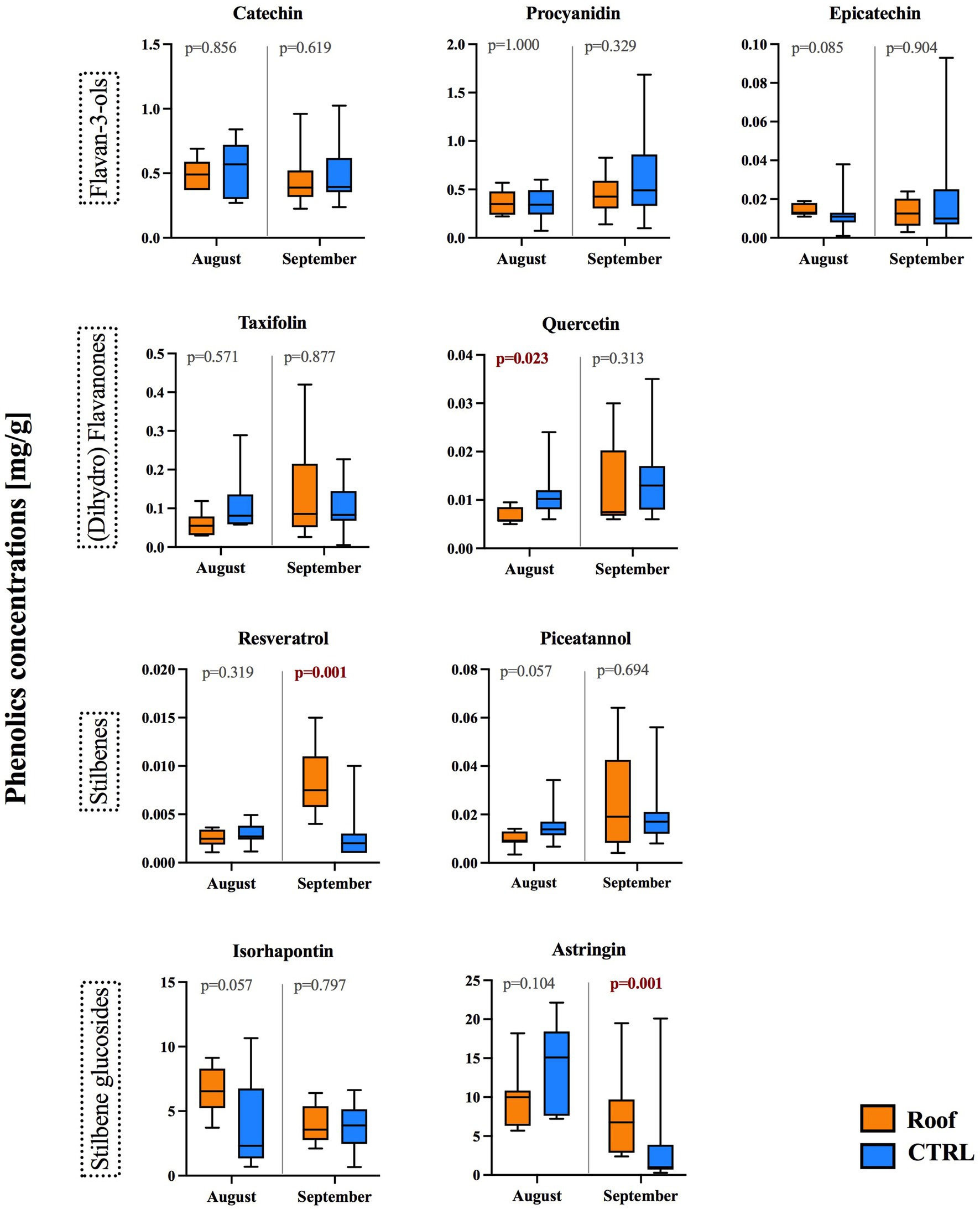
Figure 5. Concentration (mg/g dry weight) of selected soluble phenolics, stilbenes, and stilbene glucosides in phloem samples collected from the stems of roofed (orange) and control (blue) trees at a height of 1.3 m on the stem. The box plots show the median and quartile ranges for each category.
The flavan-3-ols did not exhibit any changes in roofed trees compared to unroofed controls in either August or September.
Among the (dihydro) flavonols, quercetin exhibited a significant decrease in roofed trees, specifically in August (p = 0.023), while taxifolin showed a declining trend in August, but it was not significant.
Concerning stilbenes, there was a notable increase in the aglycone resveratrol in roofed trees in September (p = 0.001), while piceatannol exhibited a decreasing trend in August (p = 0.057). However, the stilbene glucosides, more abundant than the aglycones, generally showed an increase in roofed trees for isorhapontin in August (p = 0.057) and astringin in September (p = 0.001).
3.6 Roofing mildly decreases phloem terpene contents but has little impact on the composition
The sum of chromatographic responses of selected terpenes (recorded on specific masses for each compound) extracted from non-dried tissue was lower in roofed trees compared to naturally watered trees (control). In July, it was 1.5 times lower; in August, it was 1.2 times lower; and in September, it was 1.4 times lower, but the differences were not statistically significant (Supplementary Figure S3).
The water content in extracted phloem tissues from roofed trees was 54 ± 10% in August and September, while in control trees, it was 60 ± 10% in August and 59 ± 10% in September. The water content was lower in tissue from roofed trees than in control trees, about 5% in August and 4% in September.
The relative fraction of abundance of various terpene classes, including monoterpenes, oxygenated monoterpenes, oxygenated sesquiterpenes, sesquiterpenes, oxygenated diterpenes, and diterpenes, did not exhibit many statistically significant differences between the roofed and control trees during any of the sample collection months, as depicted in Figure 6. Abundances were calculated for peak areas of selected compounds relative to the sum of peak areas of all selected terpenes for any given tree. An exception was observed in September, where roofed trees displayed a significantly higher fraction of abundance of diterpenes (p = 0.022). The abundance of monoterpenes, oxygenated monoterpenes, sesquiterpenes, and oxygenated sesquiterpenes did not differ between roofed trees and control throughout the measurement. This was also true for specific oxygenated monoterpenes with proven biological activity for I. typographus, such as eucalyptol, trans-4-thujanol, bornyl acetate, and the phenylpropanoid estragole.
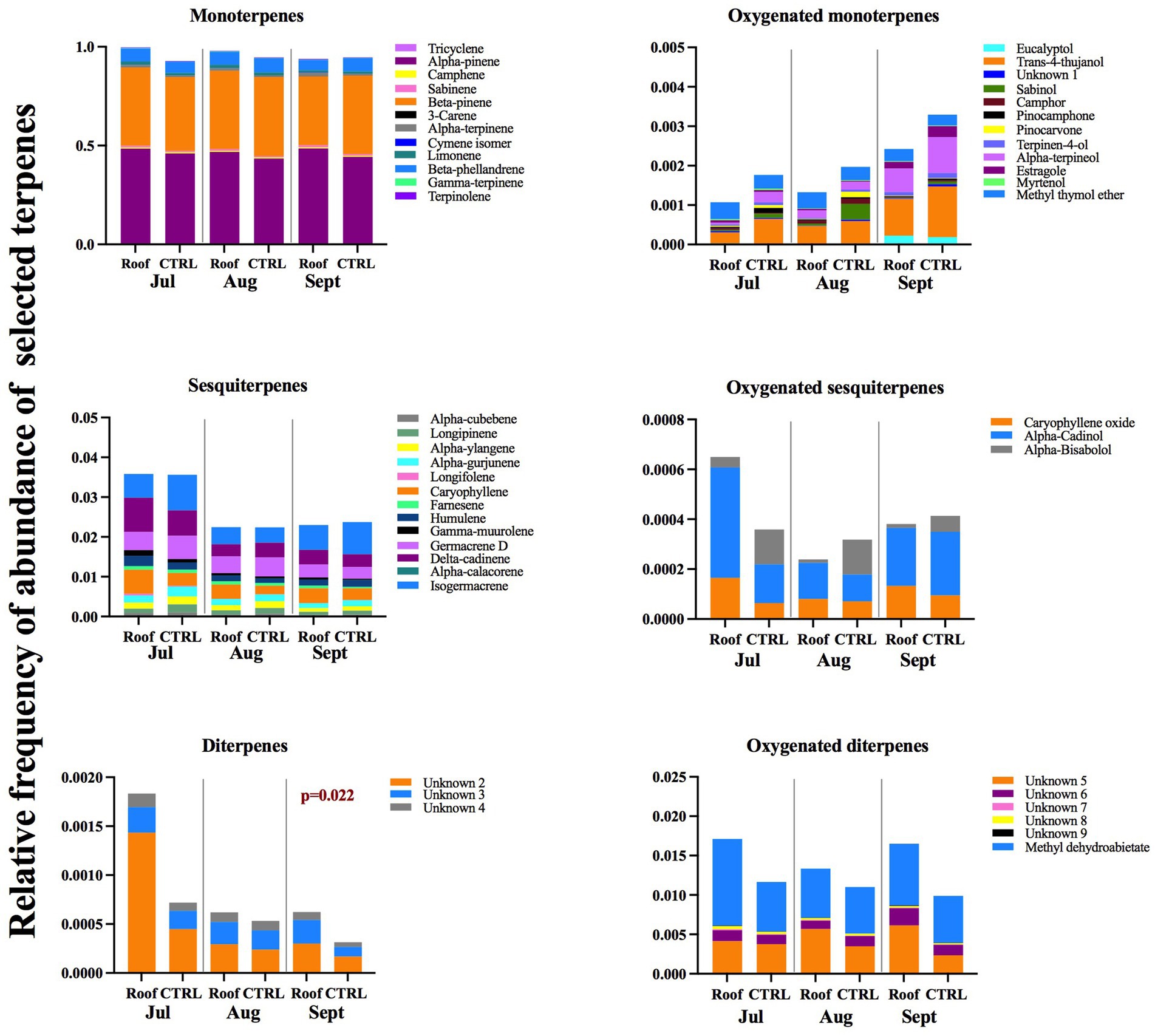
Figure 6. The mean of the relative frequency of selected terpenes abundance identified in phloem samples collected from the stems of roofed and control trees at the height of 1.3 m. Fraction abundances were calculated for peak areas relative to the sum of peak areas of all selected terpenes. Identified terpenes were categorized into classes: monoterpenes, oxygenated monoterpenes, oxygenated sesquiterpenes, sesquiterpenes, oxygenated diterpenes, and diterpenes.
3.7 Roofs increase host tree acceptance by I. typographus but not by I. duplicatus in individual bioassay in Eppendorf tubes
When placed in Eppendorf tubes at the height of 1.3 m on the trunk for 1 day, I. typographus males significantly preferred roofed trees over control trees in August (p = 0.001) but not in September. However, in the case of I. duplicatus, no significant difference in host tree acceptance was observed between roofed and control trees (Figure 7A). The number of I. typographus males actively boring or attempting to bore into the tree bark was two to ten times higher for both roofed and control trees compared to the number of boring I. duplicatus males.
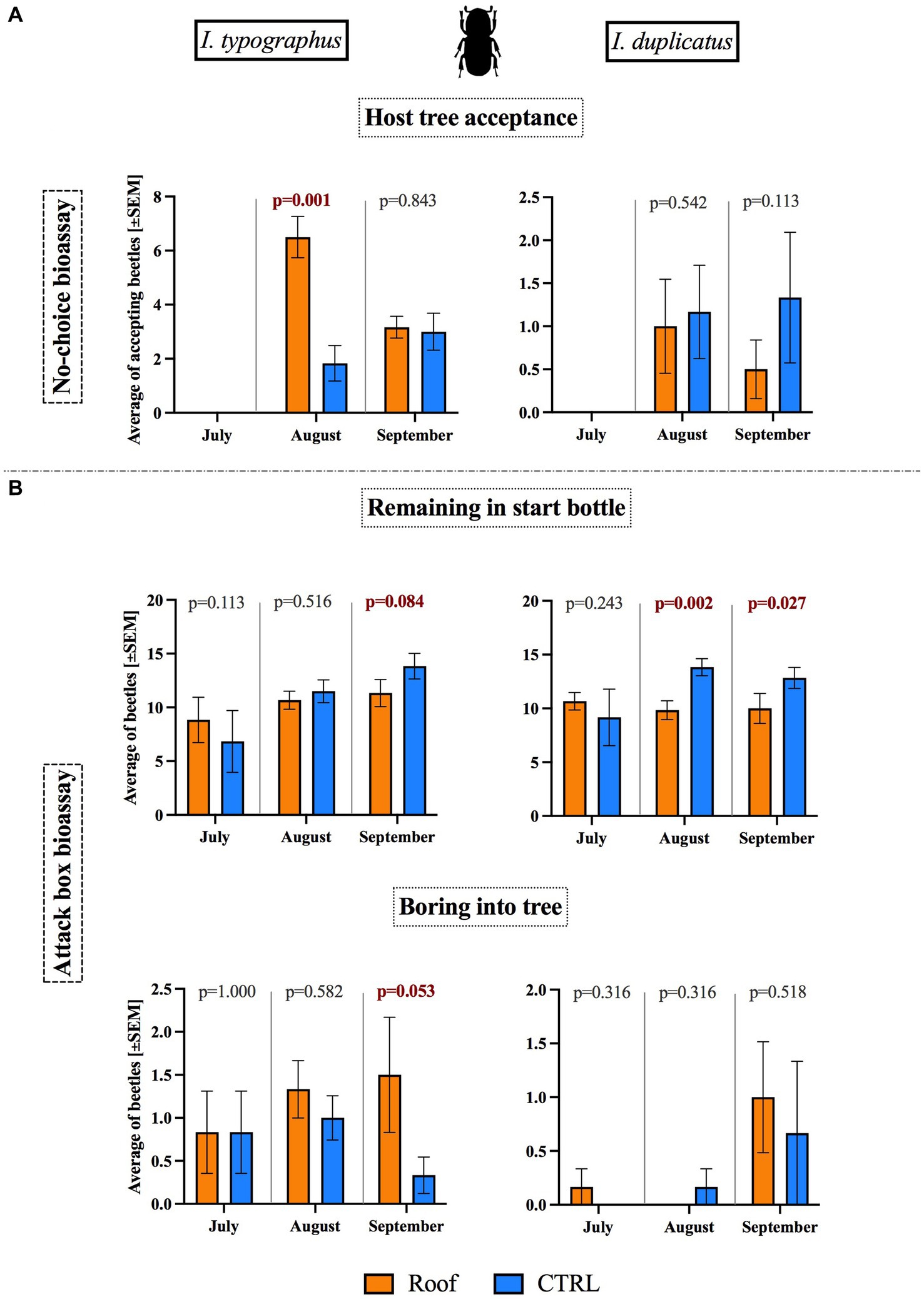
Figure 7. Bioassays with beetles. (A). Host tree acceptance in a no-choice bioassay conducted in Eppendorf tubes. (B). The number of beetles remaining in the start bottle and the number that bored into the tree in a choice bioassay conducted in attack boxes.
3.8 Roofs increased bark beetle movement in the attack box assay and increased boring attempts into the tree
During the attack box bioassays, the beetles could remain in their start bottles or move into the attack box and bore into the tree. The percentage of beetles remaining in the start bottles within the 24 h of the experiment comprised 50 to 60% on average of all individuals placed there. Most of those who left the starting bottles remained in the attack box. Only 5 to 7.5% of I. typographus beetles and 0 to 5% (only in September) of I. duplicatus beetles in the attack box attempted to bore into the bark.
Over the season, the presence of the roofs showed an increasing effect on beetle movement out of the start bottle for both species. In July, beetle movement out of the bottle did not differ between roofed and control trees. However, in August, more beetles emerged from the start bottle on roofed trees (significantly in I. duplicatus, p = 0.002), and in September, more beetles emerged from start bottles on roofed trees for both species (I. typographus, p = 0.084; I. duplicatus, p = 0.027).
Beetle mobility from the start bottles did not correlate with either air or bark surface temperature in any of the three experiments, even though the bark surface temperatures of roofed trees were significantly higher than those of control trees during each monthly bioassay.
The number of beetles of both species that started to bore into the bark of either roofed or control trees was the highest from three seasonal repetitions in September. For I. typographus, there was a noticeable trend of more beetles boring into trees in boxes installed on roofed trees than on controls, starting in August and becoming significant in September (p = 0.053). However, in I. duplicatus, there was no significant difference between roofed trees and controls (Figure 7B).
3.9 Bark beetle boring was correlated positively with NSCs content and negatively with monoterpene content
The number of individual I. typographus males boring into the bark in the non-choice bioassay was positively correlated with the content of measured soluble carbohydrates. This correlation was significant in August for glucose and fructose (R2 = 0.7 for both) and in September for raffinose (R2 = 0.6). No correlation was observed for I. duplicatus.
In the attack box bioassay, significantly more I. typographus individuals started to bore into the bark of trees with higher sucrose content in September (R2 = 0.6). A weak correlation was also observed for other soluble carbohydrates. Similarly, a weak correlation was observed between I. duplicatus and the content of these compounds in September. However, no visible trend was observed for either species in August.
In the attack box bioassays, a weak negative correlation existed between the number of I. typographus that bored into the bark and the monoterpene content (R2 = 0.4 in July and R2 = 0.3 in September).
4 Discussion
Droughts are often implicated in bark beetle outbreaks, yet the underlying mechanisms remain poorly understood. In this study, we found that acute drought increased spruce bark beetle (I. typographus) acceptance of their Norway spruce (P. abies) host trees, probably due to an increase in the soluble sugars in the phloem rather than any significant decline in terpenoid or phenolic chemical defenses. Our results are consistent with theories (Luxmoore et al., 1995; Berini et al., 2018) predicting that cessation of growth under drought increases storage of non-structural carbohydrates (NSCs) that may be used for respiration, defense, or later regrowth. We observed high variability in the measured characteristics of the mature trees covered by the roof. This variability could be due to some trees being able to reach a water source despite the roof coverage. Additionally, the unknown genetic origin and stress history of the investigated trees may have contributed to this variability. However, notable trends in trunk circumference growth and carbohydrate content were observed. Blocking soil water recharge caused acute drought in Norway spruce.
The roofs built above soil level in our experimental plots prevented rainwater from reaching the soil and decreased soil water potential so drastically that it dropped below the wilting point (−1,500 kPa) only 3 months after the roofs were installed. In contrast, the soil water potential of control plots remained above the wilting point throughout the entire growing season.
Under drought, trees ceased growth 5 months after roof installation, as measured by trunk circumference, and even started shrinking because of tissue water loss. Similar observations were made on Norway spruce during the drought period in Czechia in 2018 (Krejza et al., 2021). Growth rate declines in conifers have long been closely associated with decreases in water potential (Myers, 1988; Piper et al., 2017).
Despite a rapid decrease in available water for roofed trees, their sap flow did not significantly decline, suggesting the ability of Norway spruce to maintain water transport even under acute drought conditions. However, during longer drought episodes in central Europe, sap flow rates in Norway spruce have been reported to decrease, indicating transpiration deficits (Gartner et al., 2009; Střelcová et al., 2013; Gebhardt et al., 2023).
The bark surface temperature of roofed trees was higher in all experimental periods. This could be a physiological consequence of stress, similar to the temperature increase after bark beetle infestation (Majdák et al., 2021; Stříbrská et al., 2023) or sudden exposure (Baier and Bader, 1997; Stříbrská et al., 2022). However, bark surface temperature is usually heavily influenced by direct radiation and air temperature (Hietz et al., 2005; Stříbrská et al., 2022). It is known that the temperature of the cambium and phloem in a healthy tree with sufficient water content is well-regulated by evaporative cooling due to the water current within the sap flow (Leuzinger and Körner, 2007), and this balance can be disrupted by a lack of water. However, the correlation between this intra-thermal environment of trees and their bark surface temperature has been demonstrated only partially (Powell, 1967; Majdák et al., 2021). Therefore, the increase in surface temperature of our roofed trees could still be due to the sun’s reflectance off the plastic cover on the roofs, especially early in the experimental period when these were not yet covered by litter.
4.1 Drought increased NSCs in phloem but had little impact on the quantity of chemical defense
At the metabolite level, drought caused a significant rise in simple monosaccharides in the phloem tissue of our Norway spruce trees. Such increases have often been noted under drought for conifers and other woody plants, although this varies among organs and with the intensity and duration of drought (Piper et al., 2017; Tomasella et al., 2019; Lehmanski et al., 2023; Li et al., 2023). Elevated NSC concentrations can be seen as a logical consequence of the reduction in growth occurring in drought, which frees up sugars from current photosynthesis or stored reserves for other purposes (Luxmoore et al., 1995). A supply of sugars and other NSCs may be allocated to respiration and defense or stored for later use (McDowell and Sevanto, 2010; Berini et al., 2018; Huang et al., 2020a). Monosaccharides also have an osmotic function to maintain turgor in plant cells under water deficits (Argiolas et al., 2016).
We did not observe a significant increase in plant defense metabolites in the trunk throughout the first growing season after the imposition of drought. Nearly all of the classes of phenolics and terpenoids analyzed did not show any net increase under the roof treatment throughout the experiment. The results are generally consistent with previous studies on drought effects in conifers (Suárez-Vidal et al., 2019; Perreca et al., 2020, 2022;), although exceptions exist (Rissanen et al., 2021). We found an increase in only the diterpenes in September, which corresponds with the findings of Netherer et al. (2024). Severe drought stress on Scots pine and Norway spruce saplings significantly increased the concentrations of monoterpenes, such as α-pinene, limonene, tricyclene, and camphene, along with sesquiterpenes, while diterpene resin acids showed a declining trend (Sancho-Knapik et al., 2017). In other studies, the emission of terpenes (not measured here) was observed to rise with increasing water stress of seedlings but eventually decreased under severe drought conditions (Lieutier, 2004; Holopainen et al., 2018).
In our work, the content of both the stilbene glucosides isorhapontin and astringin and total diterpenes increased under drought treatment during one of our months of measurement, which aligns with the pattern noted for isorhapontin in the recent work of Netherer et al. (2024). Netherer’s study also observed an increase in diterpene resin acids under drought, but only after fungal infection. Furthermore, our results indicated that the compositions of the complex mixtures of monoterpenes remained stable after drought treatment. Even the relative abundance of oxygenated monoterpenes, compounds with proven biological activity against bark beetles (Jirošová et al., 2022), did not show significant changes in roofed trees (Schiebe et al., 2019; Stříbrská et al., 2022).
Our data align with the meta-analysis conducted by Koricheva et al. (1998), which demonstrated that the levels of phenolic and terpene metabolites in woody plants often remain unaffected by drought. However, it is important to note that our study specifically evaluated changes in metabolites within the trunk phloem, the target tissue for bark beetles. The response of tree organisms to drought is complex, involving the relocation of carbohydrates and secondary metabolites between various tree tissues. These broader changes are not within the scope of this article (Herrera-Ramirez et al., 2020; Huang et al., 2020a).
The lack of response of chemical defenses in our experimental trees may be attributed to their drought history. The most recent severe drought in the area occurred relatively recently, in 2018 (Krejza et al., 2021). This previous drought event may have influenced changes in the trees’ physiological, biochemical, and structural parameters, shaping their response when exposed to sudden acute drought 3 years later (Hossain et al., 2018). Stress memory in trees, similar to acquired defense against pathogens, relies on post-transcriptional and epigenetic mechanisms. These mechanisms can allow water-stressed trees to maintain a smaller stomatal aperture (Virlouvet and Fromm, 2015; Peters et al., 2019) or relocate non-structural carbohydrates from branches and reduce xylogenesis (Oberleitner et al., 2022), and the memory of responses to encountered stresses could be passed to the progeny (Matallana-Ramirez et al., 2021; Baldi and La Porta, 2022).
4.2 Ips typographus bark beetles did not naturally infest host trees under acute drought stress but showed increased acceptance in forced feeding bioassays
To determine how bark beetles responded to our drought treatment, we repeatedly conducted a search for incidences of natural bark beetle attacks. However, we found no signs of invasion or invasion attempts on either the acutely drought-stressed trees or the control trees, despite the population of I. typographus being at a transition from epidemic to endemic levels during the 2021 growing season in this area (Lubojácký et al., 2023).
Therefore, we conducted two controlled bioassays: a no-choice bioassay in Eppendorf tubes and a choice bioassay with attack boxes. In both bioassays, I. typographus chose trees under acute drought stress more often than non-drought controls. This corresponds with findings from previous studies where longer-term drought also led to increased acceptance of Norway spruce host trees by I. typographus (Netherer et al., 2015, 2024).
Since there was virtually no significant difference between the roofed trees and controls in the quantity of phenolic and terpene chemical defenses, we surmise that the increased non-structural carbohydrates (NSCs) under drought may have made the drought-stressed trees more acceptable hosts for I. typographus. For bark beetles, as other insects, NSCs have been found to be important nutrients. For example, I. typographus breeding success and the length of maternal galleries were higher when the host tree contained more NSCs (Baier et al., 1998). In addition, the larvae of the bark beetle Dendroctonus valens grew better when their diet was supplied with additional glucose or fructose (Wang et al., 2013). As insects from other orders, beetles readily perceive simple sugars via gustatory receptors (Kent and Robertson, 2009) and might have been able to perceive sugar concentrations in the phloem upon initial entry. However, to prove the hypothesis that the carbohydrate content in phloem is an additional host selection criterion, further research is needed.
However, our results in controlled bioassays with I. duplicatus were quite different. This congener is less of a pest threat than I. typographus and coexists with it while favoring the upper canopy. In our bioassays, I. duplicatus beetles accepted trees in much lower quantities than I. typographus, and in some cases, not at all. This could be explained by the fact that the bioassays were conducted at a height of 1.3 m above the ground. Since this slightly smaller species specializes in the upper crown part of the spruce stem, where the bark is thinnest (Šotola et al., 2021), it may have been unable to penetrate the thicker bark at breast height.
On the other hand, both tested bark beetle species, I. typographus and I. duplicatus, showed increased moving activity in box assays during August and September. This heightened activity could be attributed to the elevated temperatures experienced by the beetles on the bark surface of the roofed trees, consistent with the behavior of ectothermic organisms (Stříbrská et al., 2022). However, this correlation was not observed in July despite similarly elevated roof-tree temperatures. This suggests that factors other than temperature may trigger increased beetle activity. The perception of host volatiles by beetles could be another activating factor for beetles (Netherer et al., 2021). However, in our study, we only analyzed the profile of extractable terpenes, which may influence beetles when they are in direct contact with trees, rather than when terpenes are detected olfactometrically over longer distances.
5 Conclusion
In this study, we demonstrated that imposing an acute five-month drought on Norway spruce trees during the growing season increased the susceptibility of trees to I. typographus bark beetles at close range. The cessation of tree growth was accompanied by an increase in simple carbohydrates in the phloem; however, no substantial changes in terpenoid and phenolic defenses were observed. These rapid physiological and chemical changes demonstrate how even short-term, acute drought can trigger responses in large organisms such as mature Norway spruces. The changes in the profile of nutritional and defense compounds in the trunk may support the bark beetle acceptance of these trees.
Further research, including drought treatments of longer duration and varying severity, the changes in physiology features and in metabolite content and relocation as well as observations of subsequent natural bark beetle infestations, is needed to gain a complete understanding of how drought sequentially affects the metabolism and defense mechanisms of spruces, making them susceptible to bark beetles. Combining this knowledge can help predict spruce vulnerability to bark beetle outbreaks and potentially aid in managing already-infested forests.
Data availability statement
The original contributions presented in the study are included in the article/Supplementary material, further inquiries can be directed to the corresponding author.
Ethics statement
The animal study was approved by we have performed all beetle experiments that comply with the ARRIVE guidelines and were carried out in accordance with (Scientific Procedures) Act, 1986 and associated guidelines, EU Directive 2010/63/EU for animal experiments. The study was conducted in accordance with the local legislation and institutional requirements.
Author contributions
SB: Data curation, Formal analysis, Investigation, Visualization, Writing – original draft, Writing – review & editing. BS: Data curation, Formal analysis, Investigation, Writing – review & editing. AK: Data curation, Investigation, Methodology, Writing – review & editing. JH: Data curation, Investigation, Writing – review & editing. JS: Data curation, Investigation, Writing – review & editing. JG: Methodology, Writing – review & editing. AJ: Conceptualization, Data curation, Formal analysis, Investigation, Methodology, Supervision, Writing – original draft, Writing – review & editing.
Funding
The author(s) declare that financial support was received for the research, authorship, and/or publication of this article. The project was funded by “EXTEMIT-K,” No. CZ.02.1.01/0.0/0.0/15_003/0000433, and Internal Grant Commission [IGA A_14_22, SARA BASILE] at the Faculty of Forestry and Wood Sciences, Czech University of Life Sciences, Prague, Czechia and GAČR No. 23-07916S.
Acknowledgments
We acknowledge the Forest CZU in Kostelec nad Černými Lesy, Czechia, for assistance and support and all students and colleagues for help with fieldwork and sample preparation.
Conflict of interest
The authors declare that the research was conducted in the absence of any commercial or financial relationships that could be construed as a potential conflict of interest.
Publisher’s note
All claims expressed in this article are solely those of the authors and do not necessarily represent those of their affiliated organizations, or those of the publisher, the editors and the reviewers. Any product that may be evaluated in this article, or claim that may be made by its manufacturer, is not guaranteed or endorsed by the publisher.
Supplementary material
The Supplementary material for this article can be found online at: https://www.frontiersin.org/articles/10.3389/ffgc.2024.1436110/full#supplementary-material
References
Allen, C. D., Macalady, A. K., Chenchouni, H., Bachelet, D., Mcdowell, N., Vennetier, M., et al. (2010). Forest ecology and management a global overview of drought and heat-induced tree mortality reveals emerging climate change risks for forests. For. Ecol. Manag. 259, 660–684. doi: 10.1016/j.foreco.2009.09.001
Argiolas, A., Puleo, G. L., Sinibaldi, E., and Mazzolai, B. (2016). Osmolyte cooperation affects turgor dynamics in plants. Sci. Rep. 6:30139. doi: 10.1038/srep30139
Baier, P., and Bader, R. (1997). Gehalt und Emission von Monoterpenen der Fichtenrinde und deren Be-deutung für die Primärattraktion von Borkenkäfern (Coleoptera, Scolyditae). Mitteilungen der Dtsch. Gesellschaft für Allg. und Angew. Entomol. 11, 639–643.
Baier, P., Schafellner, C., Mattanovich, J., and Protection, F. (1998). Norway spruce (picea abies [L.] karst.) on food quality for Ips typographvs L. (scolytidae). Berlin: Springer.
Baldi, P., and La Porta, N. (2022). Toward the genetic improvement of drought tolerance in conifers: an integrated approach. Forests 13:2016. doi: 10.3390/f13122016
Berini, J. L., Brockman, S. A., Hegeman, A. D., Reich, P. B., Muthukrishnan, R., Montgomery, R. A., et al. (2018). Combinations of abiotic factors differentially alter production of plant secondary metabolites in five woody plant species in the boreal-temperate transition zone. Front. Plant Sci. 9:1257. doi: 10.3389/fpls.2018.01257
Brignolas, F., Lieutier, F., Sauvard, D., Christiansen, E., and Berryman, A. A. (1998). Phenolic predictors for Norway spruce resistance to the bark beetle Ips typographus (Coleoptera: Scolytidae) and an associated fungus, Ceratocystis polonica. Can. J. For. Res. 728, 720–728. doi: 10.1139/x98-037
Celedon, J. M., and Bohlmann, J. (2019). Oleoresin defenses in conifers: chemical diversity, terpene synthases and limitations of oleoresin defense under climate change. New Phytol. 224, 1444–1463. doi: 10.1111/nph.15984
Christiansen, E., Waring, R. H., and Berryman, A. A. (1987). Resistance of conifers to bark beetle attack: searching for general relationships. For. Ecol. Manag. 22, 89–106. doi: 10.1016/0378-1127(87)90098-3
Erbilgin, N., Krokene, P., Kvamme, T., and Christiansen, E. (2007). A host monoterpene influences Ips typographus (Coleoptera: Curculionidae, Scolytinae) responses to its aggregation pheromone. Agric. For. Entomol. 9, 135–140. doi: 10.1111/j.1461-9563.2007.00329.x
Erbilgin, N., Zanganeh, L., Klutsch, J. G., Chen, S., Zhao, S., Ishangulyyeva, G., et al. (2021). Combined drought and bark beetle attacks deplete non-structural carbohydrates and promote death of mature pine trees. Plant Cell Environ. 44, 3636–3651. doi: 10.1111/pce.14197
Faccoli, M., and Schlyter, F. (2007). Conifer phenolic resistance markers are bark beetle antifeedant semiochemicals. Agric. For. Entomol. 9, 237–245. doi: 10.1111/j.1461-9563.2007.00339.x
Fernandez-Carrillo, A., Patočka, Z., Dobrovolný, L., Franco-Nieto, A., and Revilla-Romero, B. (2020). Monitoring bark beetle forest damage in Central Europe. A remote sensing approach validated with field data. Remote Sens. 12:3634. doi: 10.3390/rs12213634
Franceschi, V. R., Krokene, P., Christiansen, E., and Krekling, T. (2005). Anatomical and chemical defenses of conifer bark against bark beetles and other pests. New Phytol. 167, 353–376. doi: 10.1111/j.1469-8137.2005.01436.x
Gaylord, M. L., Kolb, T. E., Pockman, W. T., Plaut, J. A., Yepez, E. A., Macalady, A. K., et al. (2013). Drought predisposes piñon-juniper woodlands to insect attacks and mortality. New Phytol. 198, 567–578. doi: 10.1111/nph.12174
Gebhardt, T., Hesse, B. D., Hikino, K., Kolovrat, K., Hafner, B. D., Grams, T. E. E., et al. (2023). Agricultural and Forest meteorology repeated summer drought changes the radial xylem sap flow profile in mature Norway spruce but not in European beech. Agric. For. Meteorol. 329:109285:109285. doi: 10.1016/j.agrformet.2022.109285
Gartner, K., Nadezhdina, N., Englisch, M., Čermak, J., and Leitgeb, E. J. F. E. (2009). Sap flow of birch and Norway spruce during the European heat and drought in summer 2003. For Ecol. Manag. 258, 590–599. doi: 10.1016/j.foreco.2009.04.028
Grodzki, W. (2012). Two types of Norway spruce Picea abies (L.) H. Karst. Infestation by the double spined bark beetle Ips duplicatus CR Sahlb.(Coleoptera: Scolytinae) in southern and North-Eastern Poland. Folia For. Pol. Ser. A. For. 54:109285. doi: 10.5281/zenodo.30734
Hammerbacher, A., Kandasamy, D., Ullah, C., and Schmidt, A. (2019). Flavanone-3-hydroxylase plays an important role in the biosynthesis of spruce phenolic Defenses against bark beetles and their fungal associates. Front. Plant Sci. 10:208. doi: 10.3389/fpls.2019.00208
Hammerbacher, A., Ralph, S. G., Bohlmann, J., Fenning, T. M., Gershenzon, J., and Schmidt, A. (2011). Biosynthesis of the major tetrahydroxystilbenes in spruce, astringin and isorhapontin, proceeds via resveratrol and is enhanced by fungal infection. Plant Physiol. 157, 876–890. doi: 10.1104/pp.111.181420
Hart, S. J., Veblen, T. T., Eisenhart, K. S., Jarvis, D., and Kulakowski, D. (2014). Drought induces spruce beetle (Dendroctonus rufipennis) outbreaks across northwestern Colorado. Ecology 95, 930–939. doi: 10.1890/13-0230.1
Hartmann, H., Moura, C. F., Anderegg, W. R., Ruehr, N. K., Salmon, Y., Allen, C. D., et al. (2018). Research frontiers for improving our understanding of drought-induced tree and forest mortality. New Phytol. 218, 15–28. doi: 10.1111/nph.15048
Herms, D. A., and Mattson, W. J. (1992). The dilemma of plants: to grow or defend. Q. Rev. Biol. 67, 283–335. doi: 10.1086/417659
Herrera-Ramirez, D., Muhr, J., Hartmann, H., Römermann, C., Trumbore, S., and Sierra, C. A. (2020). Probability distributions of non structural carbon ages and transit times provide insights into carbon allocation dynamics of mature trees. New Phytol. 226, 1299–1311. doi: 10.1111/nph.16461
Hietz, P., Baier, P., Offenthaler, I., Führer, E., Rosner, S., and Richter, H. (2005). Tree temperatures, volatile organic emissions, and primary attraction of bark beetles. Phyt. - Ann. Rei Bot. 45, 341–354.
Hlásny, T., König, L., Krokene, P., Lindner, M., Montagné-Huck, C., Müller, J., et al. (2021). Bark beetle outbreaks in Europe: state of knowledge and ways forward for management. Curr. For. Rep. 7, 138–165. doi: 10.1007/s40725-021-00142-x
Hlásny, T., Krokene, P., Liebhold, A., Montagné-Huck, C., Müller, J., Qin, H., et al. (2019). Living with bark beetles: Impacts, outlook and management options. Joensuu: European Forest Institute.
Holopainen, J. K., Virjamo, V., Ghimire, R. P., Blande, J. D., Julkunen-Tiitto, R., and Kivimäenpää, M. (2018). Climate change effects on secondary compounds of forest trees in the northern hemisphere. Front. Plant Sci. 9:1445. doi: 10.3389/fpls.2018.01445
Holusa, J., Lubojacky, J., and Knizek, M. (2010). Distribution of the double-spined spruce bark beetle Ips duplicatus in the Czech Republic: spreading in 1997–2009. Phytoparasitica 38, 435–443. doi: 10.1007/s12600-010-0121-9
Hossain, M., Veneklaas, E. J., Hardy, GE St J., and Poot, P. (2018).Tree host–pathogen interactions as influenced by drought timing: linking physiological performance, biochemical defence and disease severity. Tree Physiol. 39, 6–18. doi: 10.1093/treephys/tpy113
Huang, J., Hammerbacher, A., Weinhold, A., Reichelt, M., Gleixner, G., Behrendt, T., et al. (2019). Eyes on the future – evidence for trade-offs between growth, storage and defense in Norway spruce. New Phytol. 222, 144–158. doi: 10.1111/nph.15522
Huang, J., Kautz, M., Trowbridge, A. M., Hammerbacher, A., Raffa, K. F., Adams, H. D., et al. (2020a). Tree defence and bark beetles in a drying world: carbon partitioning, functioning and modelling. New Phytol. 225, 26–36. doi: 10.1111/nph.16173
Huang, J., Rücker, A., Schmidt, A., Gleixner, G., Gershenzon, J., Trumbore, S., et al. (2020b). Production of constitutive and induced secondary metabolites is coordinated with growth and storage in Norway spruce saplings. Tree Physiol. 40, 928–942. doi: 10.1093/treephys/tpaa040
Jirošová, A., Kalinová, B., Modlinger, R., Jakuš, R., Unelius, C. R., Blaženec, M., et al. (2022). Anti-attractant activity of (+)-trans-4-thujanol for Eurasian spruce bark beetle Ips typographus: novel potency for females. Pest Manag. Sci. 78, 1992–1999. doi: 10.1002/ps.6819
Kandasamy, D., Zaman, R., Nakamura, Y., Zhao, T., Hartmann, H., Andersson, M. N., et al. (2023). Conifer-killing bark beetles locate fungal symbionts by detecting volatile fungal metabolites of host tree resin monoterpenes. PLoS Biol. 21:e3001887. doi: 10.1371/journal.pbio.3001887
Kavčič, A., Devetak, Z., Piškur, B., Groznik, E., and de Groot, M. (2023). First record of the northern spruce bark beetle, Ips duplicatus (Sahlberg, 1836). Slov. Bio Invasions Rec. 12, 699–710. doi: 10.3391/bir.2023.12.3.07
Kent, L. B., and Robertson, H. M. (2009). Evolution of the sugar receptors in insects. BMC Evol. Biol. 9:41. doi: 10.1186/1471-2148-9-41
Koricheva, J, Larsson, S, Haukioja, E, and Keinänen, M. (1998). Regulation of woody plant secondary metabolism by resource availability: hypothesis testing by means of meta-analysis. Oikos 83, 212–226. doi: 10.2307/3546833
Krejza, J., Cienciala, E., Světlík, J., Bellan, M., Noyer, E., Horáček, P., et al. (2021). Evidence of climate-induced stress of Norway spruce along elevation gradient preceding the current dieback in Central Europe. Trees-Struct. Funct. 35, 103–119. doi: 10.1007/s00468-020-02022-6
Lehmanski, L. M., Kandasamy, D., Andersson, M. N., Netherer, S., Alves, E. G., Huang, J., et al. (2023). Addressing a century-old hypothesis–do pioneer beetles of Ips typographus use volatile cues to find suitable host trees? New Phytol. 238, 1762–1770. doi: 10.1111/nph.18865
Leuzinger, S., and Körner, C. (2007). Tree species diversity affects canopy leaf temperatures in a mature temperate forest. Agric. For. Meteorol. 146, 29–37. doi: 10.1016/j.agrformet.2007.05.007
Lewinsohn, E., Savage, T. J., Gijzen, M., and Croteau, R. (1993). Simultaneous analysis of monoterpenes and diterpenoids of conifer oleoresin. Phytochem. Anal. 4, 220–225. doi: 10.1002/pca.2800040506
Li, Q., Liu, X., Sun, X., Zhao, M., Liu, L., Wang, N., et al. (2023). Effects of drought hardening on the carbohydrate dynamics of Quercus acutissima seedlings under successional drought. Front. Plant Sci. 14:1184584. doi: 10.3389/fpls.2023.1184584
Lieutier, F. (2004). “Host Resistance to bark beetles and its variations” in Bark and wood boring insects in living trees in Europe - a synthesis (Netherlands: Springer).
Liu, W., Sun, F., Lim, W. H., Zhang, J., Wang, H., Shiogama, H., et al. (2018). Global drought and severe drought-affected populations in 1. 5 and 2 ° C warmer worlds. Earth Syst. Dynam. 9, 267–283. doi: 10.5194/esd-9-267-2018
Lombardero, M. J., Ayres, M. P., Lorio, P. L., and Ruel, J. J. (2000). Environmental effects on constitutive and inducible resin defences of Pinus taeda. Ecol. Lett. 3, 329–339. doi: 10.1046/j.1461-0248.2000.00163.x
Loomis, W. E. (1932). Growth-differentiation balance vs. carbohydrate-nitrogen ratio. Proc. am. Soc. Hortic. Sci. 29, 240–245.
Lorio, P. L. (1986). Growth-differentiation balance: a basis for understanding southern pine beetle-tree interactions. For. Ecol. Manag. 14, 259–273. doi: 10.1016/0378-1127(86)90172-6
Lubojácký, J., Lorenc, F., Véle, A., and Knížek, M. (2023). Výskyt lesních škodlivých faktorů v Česku v roce 2022. Zprav. Ochr. lesa. Lesn. práce 6, 49–53.
Luxmoore, R. J., Oren, R., Sheriff, D. W., and Thomas, R. B. (1995). “Source–sink–storage relationships of conifers” in Resource physiology of conifers (Amsterdam: Elsevier), 179–216.
Madsen, S. R., Kunert, G., Reichelt, M., Gershenzon, J., and Halkier, B. A. (2015). Feeding on leaves of the glucosinolate transporter mutant gtr1gtr2 reduces fitness of Myzus persicae. J. Chem. Ecol. 41, 975–984. doi: 10.1007/s10886-015-0641-3
Mageroy, M. H., Christiansen, E., Långström, B., Borg-Karlson, A. K., Solheim, H., Björklund, N., et al. (2020). Priming of inducible defenses protects Norway spruce against tree - killing bark beetles. Plant Cell Environ. 43, 420–430. doi: 10.1111/pce.13661
Majdák, A., Jakuš, R., and Blaženec, M. (2021). Determination of differences in temperature regimes on healthy and bark-beetle colonised spruce trees using a handheld thermal camera. iForest-Biogeosciences For. 14:203. doi: 10.3832/ifor3531-014
Matallana-Ramirez, L. P., Whetten, R. W., Sanchez, G. M., and Payn, K. G. (2021). Breeding for climate change resilience: a case study of loblolly pine (Pinus taeda L.) in North America. Front. Plant Sci. 12:606908. doi: 10.3389/fpls.2021.606908
McDowell, N. G. (2011). Mechanisms linking drought, hydraulics, carbon metabolism, and vegetation mortality. Plant Physiol. 155, 1051–1059. doi: 10.1104/pp.110.170704
McDowell, N. G., and Sevanto, S. (2010). The mechanisms of carbon starvation: how, when, or does it even occur at all? New Phytol. 186, 264–266. doi: 10.1111/j.1469-8137.2010.03232.x
McDowell, N., Pockman, W. T., Allen, C. D., Breshears, D. D., Cobb, N., Kolb, T., et al. (2008). Mechanisms of plant survival and mortality during drought: why do some plants survive while others succumb to drought? New Phytol. 178, 719–739. doi: 10.1111/j.1469-8137.2008.02436.x
Moliterno, A. A. C., Jakuš, R., Modlinger, R., Unelius, C. R., Schlyter, F., and Jirošová, A. (2023). Field effects of oxygenated monoterpenes and estragole combined with pheromone on attraction of Ips typographus and its natural enemies. Front. For. Glob. Chang. 6:1292581. doi: 10.3389/ffgc.2023.1292581
Myers, B. J. (1988). Water stress integral—a link between short-term stress and long-term growth. Tree Physiol. 4, 315–323. doi: 10.1093/treephys/4.4.315
Netherer, S., Kandasamy, D., Jirosová, A., Kalinová, B., Schebeck, M., and Schlyter, F. (2021). Interactions among Norway spruce, the bark beetle Ips typographus and its fungal symbionts in times of drought. J. Pest. Sci. (2004) 94, 591–614. doi: 10.1007/s10340-021-01341-y
Netherer, S., Lehmanski, L., Bachlehner, A., Rosner, S., Savi, T., Schmidt, A., et al. (2024). Drought increases Norway spruce susceptibility to the Eurasian spruce bark beetle and its associated fungi. New Phytol. 242, 1000–1017. doi: 10.1111/nph.19635
Netherer, S., Matthews, B., Katzensteiner, K., Blackwell, E., Henschke, P., Hietz, P., et al. (2015). Do water-limiting conditions predispose Norway spruce to bark beetle attack? New Phytol. 205, 1128–1141. doi: 10.1111/nph.13166
Nunberg, M. (1954). 516.Korniki-Scolytidae, Wyrynniki-Platypodida. in Klucze Do Oznaczania Hovadów Polski. Warshava: Państwowe Wydawnictwo Naukowe.
Oberleitner, F., Hartmann, H., Hasibeder, R., Huang, J., Losso, A., Mayr, S., et al. (2022). Amplifying effects of recurrent drought on the dynamics of tree growth and water use in a subalpine forest. Plant Cell Environ. 45, 2617–2635. doi: 10.1111/pce.14369
Perreca, E., Eberl, F., Santoro, M. V., Wright, L. P., Schmidt, A., and Gershenzon, J. (2022). Effect of drought and methyl jasmonate treatment on primary and secondary isoprenoid metabolites derived from the MEP pathway in the white spruce Picea glauca. Int. J. Mol. Sci. 23:3838. doi: 10.3390/ijms23073838
Perreca, E., Rohwer, J., González-Cabanelas, D., Loreto, F., Schmidt, A., Gershenzon, J., et al. (2020). Effect of drought on the methylerythritol 4-phosphate (MEP) pathway in the isoprene emitting conifer Picea glauca. Front. Plant Sci. 11:546295. doi: 10.3389/fpls.2020.546295
Peters, R. L., Speich, M., Pappas, C., Kahmen, A., von Arx, G., Graf Pannatier, E., et al. (2019). Contrasting stomatal sensitivity to temperature and soil drought in mature alpine conifers. Plant Cell Environ. 42, 1674–1689. doi: 10.1111/pce.13500
Piper, F. I., Fajardo, A., and Hoch, G. (2017). Single-provenance mature conifers show higher non-structural carbohydrate storage and reduced growth in a drier location. Tree Physiol. 37, 1001–1010. doi: 10.1093/treephys/tpx061
Powell, J. M. (1967). A study of habitat temperatures of the bark beetle Dendroctonus ponderosae Hopkins in lodgepole pine. Agric. Meteorol. 4, 189–201. doi: 10.1016/0002-1571(67)90003-9
Raffa, K. F., Grégoire, J. C., and Lindgren, B. S. (2015). “Natural history and ecology of bark beetles” in Bark Beetles: Biology and Ecology of Native and Invasive Species. eds. F. E. Vega and R. W. Hofstetter (Cambridge, MA: Elsevier Academic Press).
Rissanen, K., Hölttä, T., Bäck, J., Rigling, A., Wermelinger, B., and Gessler, A. (2021). Drought effects on carbon allocation to resin defences and on resin dynamics in old-grown scots pine. Environ. Exp. Bot. 185:104410. doi: 10.1016/j.envexpbot.2021.104410
Sancho-Knapik, D., Sanz, M. Á., Peguero-Pina, J. J., Niinemets, Ü., and Gil-Pelegrín, E. (2017). Changes of secondary metabolites in Pinus sylvestris L. needles under increasing soil water deficit. Ann. For. Sci. 74, 1–10. doi: 10.1007/s13595-017-0620-7
Schiebe, C. (2012). Attraction and resistance in the Picea abies – Ips typographus system. St. Louis, MO: SLU Publication.
Schiebe, C., Unelius, C. R., Ganji, S., Binyameen, M., Birgersson, G., and Schlyter, F. (2019). Styrene, (+)-trans-(1R, 4S, 5S)-4-thujanol and oxygenated monoterpenes related to host stress elicit strong electrophysiological responses in the bark beetle Ips typographus. J. Chem. Ecol. 45, 474–489. doi: 10.1007/s10886-019-01070-8
Schlyter, F., and Cederholm, I. (1981). Separation of the sexes of living spruce bark beetles, Ips typographus (L.), (Coleoptera: Scolytidae) 1. Zeitschrift für Angew. Entomol. 92, 42–47. doi: 10.1111/j.1439-0418.1981.tb01650.x
Šotola, V., Holuša, J., Kuželka, K., and Kula, E. (2021). Felled and lure trap trees with uncut branches are only weakly attractive to the double-Spined bark beetle, Ips duplicatus. Forests 12:941. doi: 10.3390/f12070941
Střelcová, K., Kurjak, D., Leštianska, A., Kovalčíková, D., Ditmarová, Ľ., Škvarenina, J., et al. (2013). Differences in transpiration of Norway spruce drought stressed trees and trees well supplied with water. Biologia (Bratisl) 68, 1118–1122. doi: 10.2478/s11756-013-0257-4
Stříbrská, B., Hradecký, J., Čepl, J., Modlinger, R., Tomášková, I., and Jirošová, A. (2023). Physiological and biochemical indicators in Norway spruces freshly infested by Ips typographus: potential for early detection methods. Front. For. Glob. Chang. 6:1197229. doi: 10.3389/ffgc.2023.1197229
Stříbrská, B., Hradecký, J., Čepl, J., Tomášková, I., Jakuš, R., Modlinger, R., et al. (2022). Forest margins provide favourable microclimatic niches to swarming bark beetles, but Norway spruce trees were not attacked by Ips typographus shortly after edge creation in a field experiment. For. Ecol. Manag. 506:119950. doi: 10.1016/j.foreco.2021.119950
Suárez-Vidal, E., Sampedro, L., Voltas, J., Serrano, L., Notivol, E., and Zas, R. (2019). Drought stress modifies early effective resistance and induced chemical defences of Aleppo pine against a chewing insect herbivore. Environ. Exp. Bot. 162, 550–559. doi: 10.1016/j.envexpbot.2019.04.002
Šulc, M., Tomášková, I., Krejzková, A., Samek, M., Diuzheva, A., Hradecký, J., et al. (2021). Trehalose determination in Norway spruce (Picea abies) roots. Analytics matters. MethodsX 8:101280. doi: 10.1016/j.mex.2021.101280
Tomasella, M., Petrussa, E., Petruzzellis, F., Nardini, A., and Casolo, V. (2019). The possible role of non-structural carbohydrates in the regulation of tree hydraulics. Int. J. Mol. Sci. 21:144. doi: 10.3390/ijms21010144
Turcani, M., and Nakládal, O. (2007). The results of manipulated experiments with inoculation of Ips typographus (L., 1758) to spruce trees under various levels of water stress. J. For. Sci. 53, 25–30. doi: 10.17221/2159-JFS
Virlouvet, L., and Fromm, M. (2015). Physiological and transcriptional memory in guard cells during repetitive dehydration stress. New Phytol. 205, 596–607. doi: 10.1111/nph.13080
Wermelinger, B., Mathis, D. S., Knížek, M., and Forster, B., (2020). Tracking the spread of the northern bark beetle (Ips duplicatus [Sahlb.]) in Europe and first records from Switzerland and Liechtenstein. Alpine Entomol., 4, 179–184. doi: 10.3897/alpento.4.53808
Wang, B., Lu, M., Cheng, C., Salcedo, C., and Sun, J. (2013). Saccharide-mediated antagonistic effects of bark beetle fungal associates on larvae. Biol. Lett. 9:20120787. doi: 10.1098/rsbl.2012.0787
Zeneli, G., Krokene, P., Christiansen, E., Krekling, T., and Gershenzon, J. (2006). Methyl jasmonate treatment of mature Norway spruce (Picea abies) trees increases the accumulation of terpenoid resin components and protects against infection by Ceratocystis polonica, a bark beetle-associated fungus. Tree Physiol. 26, 977–988. doi: 10.1093/treephys/26.8.977
Keywords: drought resistance, Norway spruce, bark beetles, carbohydrates, phenolics, terpenes, tree physiology, host selection
Citation: Basile S, Stříbrská B, Kalyniukova A, Hradecký J, Synek J, Gershenzon J and Jirošová A (2024) Physiological and biochemical changes of Picea abies (L.) during acute drought stress and their correlation with susceptibility to Ips typographus (L.) and I. duplicatus (Sahlberg). Front. For. Glob. Change. 7:1436110. doi: 10.3389/ffgc.2024.1436110
Edited by:
Ari Mikko Hietala, Norwegian Institute of Bioeconomy Research (NIBIO), NorwayReviewed by:
Vytautas Čėsna, Lithuanian Research Centre for Agriculture and Forestry, LithuaniaTeemu Paljakka, University of Helsinki, Finland
Copyright © 2024 Basile, Stříbrská, Kalyniukova, Hradecký, Synek, Gershenzon and Jirošová. This is an open-access article distributed under the terms of the Creative Commons Attribution License (CC BY). The use, distribution or reproduction in other forums is permitted, provided the original author(s) and the copyright owner(s) are credited and that the original publication in this journal is cited, in accordance with accepted academic practice. No use, distribution or reproduction is permitted which does not comply with these terms.
*Correspondence: Anna Jirošová, amlyb3NvdmFhQGZsZC5jenUuY3o=