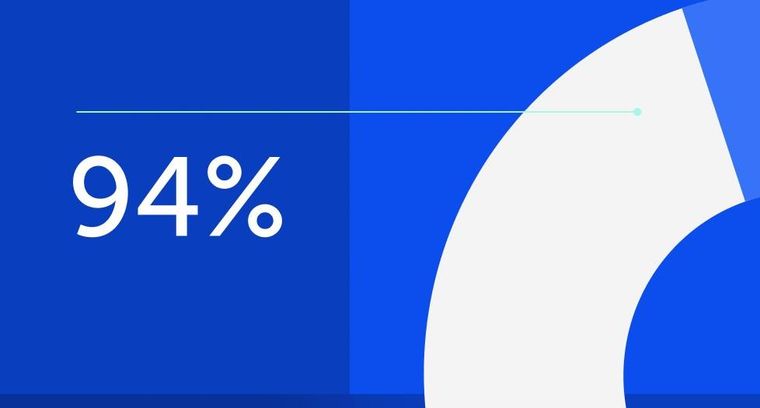
94% of researchers rate our articles as excellent or good
Learn more about the work of our research integrity team to safeguard the quality of each article we publish.
Find out more
ORIGINAL RESEARCH article
Front. For. Glob. Change, 01 July 2024
Sec. Forest Ecophysiology
Volume 7 - 2024 | https://doi.org/10.3389/ffgc.2024.1435659
Abies spectabilis (East Himalayan Fir), a dominant timber-producing species in the Himalayas, plays a dynamic role in ecological balance and sustains various habitats across temperate to subalpine altitudes. Himalayan montane forests express signs of changes in the growth, regeneration, and population density of forest tree species due to ongoing climate change, including its upper distribution limit. The research was conducted at 30°11´02˝N and 79°39′36˝E, ranging from 3,100 to 3,500 m asl elevation in the treeline ecotone of western Himalaya. The study presents a comprehensive perspective on how water availability and its intricate interactions with climatic and ecological variables shape the dynamics of treeline ecosystems. The study’s phytosociological analysis of treeline sites revealed the complex interplay between species composition, adaptability, and regeneration potential. The eco-physiological aspects of water balance were thoroughly explored, underscoring how plants employ various strategies to cope with changing water availability. The soil moisture content ranged from 32.17 to 73.50%. The dynamic nature of water potential (pre-dawn (ΨPD) and mid-day (ΨMD)) across seasons and years reflects and varies between −0.13 and − 1.25 MPa, the species’ ability to adjust and manage water balance according to environmental shifts. Osmotic potential at full turgor varied from −0.72 to −1.77 MPa, these adjustments are crucial for key life cycle events of vegetative and reproductive phases of species. The strong correlation of water potential (Ψ) and phenophases emphasizes the importance of water status in regulating these vital processes. Furthermore, the study delved into the maturation and germination processes, highlighting the significance of Ψ and moisture content in seed development and germination success. The maximum germination of 46.33% was observed when the seed moisture content was 30.90 ± 3.11%. The observed correlations between cone characteristics and seed parameters indicate the existence of trade-offs that impact seed size, weight, and germination capacity, contributing to the overall fitness of the species.
Species distributions, productivity, phenology, and vegetation dynamics, are the features of tree growth and forest ecosystems that have been greatly affected by climate change (IPCC, 2014; Gaire et al., 2020). Climate change’s impact on higher altitude regions has been the subject of extensive research, particularly in treeline areas (Roush, 2009; Zhang et al., 2009). Numerous studies have examined, how climate change would impact distinct biomes’ tree species, mainly in the Himalayas, where temperature-limited circumstances have been observed to increase tree growth (Borgaonkar et al., 2011). Understanding the consequences of climate change on higher altitudes, particularly near treeline areas, has become an area of extensive research. In mountainous regions, these impacts have been discovered to have a more noticeable effect on the ecophysiology aspect of trees and their population dynamics (Körner, 2012). Climate change has certain effects on the treeline, which is the boundary above which trees cannot grow. However, the responses of trees to climate change can differ depending on species and climatic settings (Gaire et al., 2020). While some trees show positive responses to changes in precipitation or drought indices (Yang et al., 2013; Gaire et al., 2020) extreme climate situations such as severe droughts can also negatively affect tree growth, even in areas that are naturally controlled by temperature conditions (Gaire et al., 2019). The eco-physiological responses of trees to climate change can be similar or fluctuate across elevation gradients. The environmental factors including, temperature, moisture, light, nutrients, and gases, influence the parent plant during growth and development, affect morphological, physiological, biochemical, and molecular aspects of seeds (LeFait and Qaderi, 2022) and changes are portrayed by the seed size, shape, mass (Qaderi et al., 2003; Qaderi, 2023) and germinability (Gutterman, 2000; Qaderi et al., 2003). The growth and development of trees close to treeline regions or higher elevations are generally limited by temperature variations, whereas at lower elevations it is influenced by precipitation (Gaire et al., 2020).
Tree species growing in the western Himalayan region exposed positive relationships with precipitation and negative responses to pre-monsoon temperature (Gaire et al., 2020). The Himalayan treeline is characterized by a broad gradient of bioclimatic zones and diverse forests along elevation gradients and is one of the world’s most vulnerable regions to global warming (Rai et al., 2020) and warming-induced drought stress. Studying the germination responses of seeds to water and temperature can provide insights into a species’ adaptability to different habitats (Zhang et al., 2020). Water potential can influence the timing of phenological phases by acting as a cue for the plant to transition between dormancy and active growth. Adequate water availability supports the various growth and reproductive stages of the tree. The water potential (Ψ) of a plant, which refers to the water’s potential energy in the plant cells, is closely correlated with the phenology and germination of seeds of plants. The tree water relations play a substantial role in various phenological events as well as seed germination in plants. Insufficient water due to drought stress can delay or inhibit the overall health and vigor of the tree, which can impact its reproductive processes. Water stress might lead to reduced flower production and poor pollination, affecting reproductive success.
The measurement of seed water content during seed development is valuable insight in understanding the stages of maturity and determining the optimal time for seed collection, storage, and planting. The seed water content provides essential information about seed quality, viability, and potential dormancy, which is used to determination of the physiological maturity (Castro et al., 2021). Adequate water potential is crucial for the development of cones and the maturation of seeds. Water stress during cone development can result in reduced seed set and lower seed quality. Seeds with low base water potentials (Ψb) germinate better at low Ψ than seeds with high Ψb (Zhang et al., 2020). Successful seed germination and succeeding seedling formation depend on optimum Ψ conditions. Species adapted to humid environments may exhibit greater sensitivity to water stress compared to those adapted to arid environments. The germination and emergence stages are critical in a plant’s life as they regulate the efficient utilization of available nutrients and water resources (Gan, 1996). A limited study has explored the differential responses of seeds from different populations of the same species to water stress during the germination stage.
Abies spectabilis (D. Don.), is a conifer species endemic to the Himalayan region, that plays a substantial role in high-elevation forest ecosystems (Gaire et al., 2020), this species also holds economic value as a timber species. The local communities rely on it for their livelihoods, making it an essential resource (Gaire et al., 2020) and also an ecologically, economically, and culturally important tree species. Unfortunately, A. spectabilis forests have suffered severe reduction through logging and deforestation, resulting in a population decline of approximately 25% over the past three generations (Kunwar et al., 2021). The species is adapted to a temperate to sub-alpine climate and is capable of surviving in areas with varying water availability and is generally considered moderately drought-tolerant once established, but it prefers well-drained soils and consistent moisture. Moreover, insufficient regeneration has emerged as a significant challenge in A. spectabilis within mountain forests (Krauchii et al., 2000). However, research on eco-physiological and seed-related aspects of A. spectabilis under current warming climatic conditions in the treeline region of the Himalayas is limited. The purpose of the present research was: (i) to observe water relations of the A. spectabilis tree in the Himalaya treeline region; (ii) to determine the impact of temperature and precipitation on cone developmental phases of A. spectabilis, and (iii) to investigate the cone and seed maturation time and germination status of A. spectabilis.
The study was conducted at Tungnath treeline areas, situated in the western Indian Himalayas. The investigated site is located between 3,145 to 3,355 m above sea level at 30029’45˝N latitude and 79013′24˝E longitude and falls within the sub-alpine and alpine zones. The soil in these regions has a characteristic brown hue and possesses a sandy loam texture, characterized by a high concentration of sand and silt particles, and exhibits acidity, as indicated by pH values ranging from 4.0 to 5.0 (Singh et al., 2019a). The climate of the research region is impacted by the monsoon, which is characterized by extended periods of harsh winters and brief periods of mild summers. The mean monthly minimum temperature varied between −6.02 ± 0.23 and 10.36 ± 0.43°C, the mean monthly maximum temperature varied between 3.08 ± 0.76 and 13.71 ± 1.03°C and the total monthly precipitation was 13.0 ± 1.16 and 541.0 ± 4.37 mm during 2017–2020 at Tungnath treeline site (IHTP, 2021) (Figure 1). These treeline areas mostly experience anthropogenic disturbance from migratory grazing and tourist activities. Other causes include the removal of litter by locals in the area and the felling of trees for fuel, timber, and fodder (Singh et al., 2019a).
Figure 1. The mean monthly data of temperature and precipitation at Tungnath study site during 2017–2020 (Source: 20).
In the study area 30 (10 × 10 m) quadrats have been placed to conduct the phytosociological assessment of treeline tree species. The samples’ size and number were determined (Curtis and McIntosh, 1950). Quadrats were distributed on a random basis (Mueller-Dombois and Ellenberg, 1974). The density, total basal area, and the important value index (IVI) were calculated (Curtis and McIntosh, 1950; Mueller-Dombois and Ellenberg, 1974) (Figure 2).
At these marked areas, 20 representative mature trees that had clear boles, well-developed crowns, free of disease, enough cones, and no buttresses, varying circumferences ranging from 130 to 160 cm, were randomly selected and marked for tree twig water potential (Ψtwig), tree twig water potential components (Ψtwig components), phenological observation and seed maturation study. The Ψtwig, Ψtwig components measurements were taken on a seasonal interval basis: pre-monsoon (March–May), monsoon (June – September), post-monsoon (October – November), and winter (December – February).
Figure 2. Layout of the experimental setup for phytosociological assessment, water potential, phenological studies, and seed-related studies in the treeline sites.
To estimate soil moisture, a thorough soil sampling approach was employed, focusing on three distinct depths: the upper layer (0-15 cm), the middle layer (15-30 cm), and the lower layer (30-45 cm) at seasonally. This was done at five representative locations within each site, utilizing a stainless-steel soil corer manufactured by Vienna Scientific Instruments in Vienna, Austria. In the field, a quantity of soil weighing, fifty grams was measured using a battery-operated digital weighing balance. The soil samples were subjected to a drying process at a temperature of 100°C until a consistent weight was achieved (Jackson, 1958).
The Ψ of A. spectabilis tree twigs was assessed using a Pressure chamber (PMS Instrument Co. model 1,000, range 70 bars) at two specific time points: pre-dawn (ΨPD) from 5:30–6:30 h and mid-day (ΨMD) from 13:30–14:30 h. These measurements were conducted on previously marked individual trees at regular intervals throughout the seasons (Zobel et al., 2001; Tewari et al., 2018; Singh et al., 2023). The theoretical and practical aspects of the above-mentioned technique have been provided (Ritchie and Hinckley, 1975; Garkoti et al., 2003). The estimation of Ψtwig was conducted by collecting samples from a vertical distance of 2-3 m. These samples were roughly 15 cm in length and had a diameter of 1-2 mm. The severed portion of the twigs was introduced through a perforated rubber stopper, with about 1.0 cm of the twigs extending beyond the stopper. Subsequently, the chamber valve was unsealed to facilitate the ingress of gas into the chamber, while concurrently modifying the pace of pressure escalation. Subsequently, the lid was affixed onto the chamber and securely fastened by twisting it. The chamber valve was activated, and further adjustments were made to the rate control and control needle valve to achieve a controlled increase in pressure inside the chamber, ranging from 0.05 to 0.25 bars per second. The N2 gas was externally pressurized until a pronounced bubbling of water was detected at the severed end of the twig. The pressure expressed in Bars/MPa is often referred to as Balance Pressure (BP), where a negative BP is interpreted as the water potential of a tree (Ψ = -BP) (Zobel et al., 2001; Garkoti et al., 2003; Tewari et al., 2018; Singh et al., 2023, 2024).
To demonstrate a correlation between the constituents of Ψ and relative water content (RWC%), Pressure-Volume (PV) curves were generated by the use of a Pressure chamber. The PV curves were generated using the bench drying process. To generate PV curves, a total of 20 twigs were procured from 10 distinct trees at the designated study location. These twigs were thereafter stored inside an insulated container for preservation purposes. The specimens were conveyed to the laboratory and afterward re-sectioned at the severed extremity (4-5 cm) while submerged in water. They were then left undisturbed overnight to regain their saturation. The techniques used in this study to calculate osmotic potential at full turgor (OP full), osmotic potential at zero turgor (OP zero), and RWC% at turgor loss point (RWCz) were determined from the PV curves (Pallardy et al., 1991). The investigation included the computation of osmotic adjustment, namely the decrease in Ψπ, for each of the various seasons.
A phenological study was conducted to observe five phenophases in A. spectabilis: leafing, flowering, fruiting, seed fall, and leaf fall. From all the quadrats, twenty-five individual trees were randomly selected and marked at the studied treeline sites for phenological observations. During the low activity period, observations were made fortnightly to monthly, while during the peak activity period, observations were conducted weekly. Each selected tree was monitored, and the presence or absence of specific phenological phases was observed and recorded (Singh et al., 2015). To consider an event as active, it had to be present and observed in at least 20% of observed tree crowns; otherwise, the events were recorded as absent (Singh and Mittal, 2019). The assessment of the crown was conducted through direct observation from a terrestrial vantage point. A phenophase was just launched if it was active and seen in 5–10% of the individuals (Singh and Negi, 2018). Furthermore, the species was considered to stay in that phenophase as long as it was represented by at least 5–10% of the individuals. In addition, ΨPD of twigs measurements were taken during each phenological event and correlated with the water status parameters of the trees (Singh et al., 2019b). This allowed for an assessment of the relationship between the ΨPD and the overall water status of the trees during different phenological stages.
A. spectabilis cone collection began from the initiations of cones to the availability of cones in trees. Direct cone harvesting from the tree was followed by seed germination in the laboratory. Three replicates of 10 cones and 100 seeds each were used to determine the cone parameters. The parameters that were measured were the weight of 10 cones and 100 seeds as well as the number of cones and seeds per 100 g and per 10 g. “Digital vernier calipers, model numbers CD-6” accuracy +0.02 mm Mitutoyo Co. and PGB 301 accuracy +0.001 mg Wensar,” were the tools used for the size and weight parameters, respectively. For seed mass, the collected seed was dried in the air until its weight remained consistent (Harel et al., 2011). Cone and seed moisture content were calculated (International Seed Testing Association, 1981).
At each collection date, the seed had been removed manually from the cone, and germination was observed in a seed germinator. Four replicates of 100 seeds each were applied for germination (Tamta and Singh, 2018). In a seed germinator, seeds were sown and allowed to germinate at a temperature of 25°C on top of the germination paper, and germination was counted daily when the radical protruded visibly (1 mm). As needed during the experiment, water was added.
The obtained data were analyzed using the statistical software SPSS version 2016 for the analysis of variance (ANOVA) test with a 95 and 99% significant level. Variables of ANOVA were sites, seasons, and years of their interaction.
The total tree density and total basal area at the investigated treeline sites were 590 ind. ha−1 and 59.50 m2 ha−1, respectively. A. spectabilis had a tree density of 170 ind. ha−1, while the seedling and sapling layers had no remnants of the species. The species had a total basal area of 21.11 m2 ha−1, and its IVI was 71.25.
The soil moisture (Sm) data collected from different depths showed significant variability (p < 0.05). In the first year (Yr1), Sm ranged from 32.17 ± 1.02 to 73.50 ± 1.29%, and in the second year (Yr2), it ranged from 31.56 ± 1.98 to 66.06 ± 1.19% across all seasons. These results indicate that the Sm fluctuated over the study period and exhibited variations across the different seasons. The maximum Sm values were observed at a depth of 0–15 cm in the monsoons and post-monsoon season. On the other hand, the minimum Sm values were found at a depth of 30–45 cm in the winter season (Figure 3).
Figure 3. Mean soil moisture content (%) of the studied sites, seasons, and years. Error bars indicate ± SE while the bars in different colors indicate the soil depths and year.
In A. spectabilis, pre-dawn twig water potential (ΨPD) ranged from −0.13 ± 0.09 to −0.80 ± 0.07 MPa, and mid-day twig water potential (ΨMD) varied from −0.23 ± 0.10 to −1.19 ± 0.12 MPa across all seasons in Yr1. In Yr2, ΨPD was from −0.13 ± 0.09 to −0.89 ± 0.13 MPa and ΨMD was between −0.22 ± 0.09 and − 1.25 ± 0.08 MPa across all seasons. The least negative values were measured in monsoon seasons, while the most negative values were in pre-monsoon and winter seasons (Figure 4). ANOVA revealed significant variations in ΨPD across sites and seasons, but not between study years. ΨMD showed significant variations across sites, years, and seasons. Interactions were also significant for both parameters (p < 0.05).
Figure 4. Mean ΨPD and ΨMD of A. spectabilis trees twig across all the seasons. Error bars indicate ± SE.
In A. spectabilis, seasonal change or variation (ΔΨ = ΨMD - ΨPD) in tree twig water potential varied between 0.10 ± 0.02 to 0.65 ± 0.03 MPa in Yr1 and 0.09 ± 0.01 to 0.69 ± 0.03 MPa in Yr2. Maximum seasonal variation was in the winter season and post-monsoon season, while the minimum occurred during the monsoon season. Seasonal change was generally high from pre-monsoon to post-monsoon, except during the monsoon season (Figure 5). The ANOVA results indicated that ΔΨ varied significantly across the seasons, sites, and years (p < 0.05). This suggests that the seasonal fluctuations in tree twig Ψ were influenced by environmental conditions and varied across different locations and times of the year.
In A. spectabilis, OP full varied from −0.72 ± 0.05 to −1.76 ± 0.02 MPa and − 0.75 ± 0.03 to −1.77 ± 0.02 MPa between the years across all seasons. Similarly, OP zero ranged from −1.15 ± 0.05 to −2.10 ± 0.03 MPa and − 1.05 ± 0.01 to −2.12 ± 0.01 MPa. Both OP full and OP zero were highly negative in winter season for both years and least negative in pre-monsoon and monsoon seasons (Figure 6). Pressure potential at full turgor (PP full) was 0.89 ± 0.02 to 1.49 ± 0.07 MPa in Yr1 and 0.87 ± 0.01 to 1.56 ± 0.03 MPa in Yr2 across all seasons, with maximum in winter and minimum in pre-monsoon season (Figure 6). RWC% ranged from 52.18 ± 0.74 and 91.08 ± 0.43% in Yr1 and 53.13 ± 0.47 and 90.77 ± 0.51% in Yr2 across all seasons, with minimum in pre-monsoon and maximum in winter (Figure 6). ANOVA revealed significant variations in OP full, OP zero, PP full and RWC% across seasons, but not between study years. Significant interaction between species and seasons was observed (p < 0.05).
Figure 6. Osmotic potential at full turgor, osmotic potential at zero turgor, pressure potential at full turgor, and relative water content of A. spectabilis trees twig across all seasons for 2 years of study period. Error bars indicate ± SE.
In A. spectabilis, the new leafing arose from the first week of May and was completed by the last week of July, while flowering started in the second week of May and finished in the last week of June. New cones were observed on only 8% of individuals from the third week of May to the second week of August, while 35% of trees had previous year cones. Seed/cone dispersal took place from the third week of September to the third week of December. Leaf fall began in the first week of June and ended in the fourth week of October (Figure 7).
Figure 7. Phenological events and their relationship with ΨPD of A. spectabilis. (I-IV) weeks of each month. L = Leafing; FL = Flowering; FR = Fruiting; SF = Seed fall; LF = Leaf fall. Error bars indicate ± SE.
The species did not experience severe water stress, as the ΨPD of tree twig remained above −1.04 ± 0.14 MPa throughout the phenological activities. The ΨPD of twig during leafing and flowering was generally high and was −0.88 ± 0.02 to −0.97 ± 0.14 MPa, and as the leaves matured, the twig water potential marginally declined in May and June, then increased continuously in the monsoon season. Pre-dawn water potential of twigs was most negative during the starting of fruiting and leaf fall time between May and June, with ΨPD declining from −1.04 ± 0.14 to −0.14 ± 0.05 MPa between May and July, and continuously declining till December. Seed dispersal (September to December) saw a gradual decline in twig ΨPD ranging between −0.32 ± 0.05 and − 0.67 ± 0.11 MPa (Figure 7).
The cone colour of A. spectabilis during the first collection was dark green and in the final collection, it changed to brownish green. The mean cone size varied by 5312.25 mm2 throughout the collection period, with measurements ranging from 1955.50 ± 18.24 to 7267.74 ± 12.50 mm2. Additionally, the average weight of 10 cones exhibited a variation of 1086.58 g, with values ranging from 414.03 ± 15.33 to 1500.60 ± 5.56 g. The average mass of 10 cones also showed a variation of 1076.09 g, ranging from 410.05 ± 5.13 to 1486.14 ± 2.14 g during the collection period. Cones per kg were collected at an average of 25.67 ± 1.20 during the first collection, then dropped to 6.00 ± 0.00 at the end. Similar to this, the starting moisture content of the cone was 69.39 ± 0.91%, and by the time it was collected, it had decreased to 50.85 ± 0.17% (Figure 8). ANOVA indicated that there were differences in the cone size, weight of 10 cones, number of cones/kg, and moisture content of the cones between the collection dates (p < 0.05). At a 95% confidence level, a correlation between the number of cones/kg and the moisture content (r = 0.736) and between cone size and the number of cones per kg (r = 0.882) as well as between cone size and moisture content (r = 0.699) was observed.
Figure 8. Variations in physical attributes of A. spectabilis cones over the collection period. Error bars indicate ± SE.
The seed colour of A. spectabilis during the first collection was greenish brown and in the final collection it changed to brown. The difference in seed size throughout the course of the collection period was 7.07 mm2, ranging from 47.44 ± 6.11 to 54.50 ± 2.10 mm2 between the initial and final collections. 100 seeds had a weight difference of 4.06 g between the first and last collection dates, or 2.22 ± 0.55 and 6.28 ± 0.04 g, respectively. The variation in average mass of 100 seeds was 4.66 g, ranging from 2.18 ± 0.54 and 6.84 ± 0.05 g between the collections. The number of seeds per 100 g was 3966.67 ± 15.59 during the initial collection, but it gradually decreased until it was 2721.67 ± 12.14 at the end (Figure 9). ANOVA revealed that whereas seed size and moisture content did not vary among collection dates at a 0.05% significant level (p < 0.05), the weight, number, mass, and germination of seeds did. At a 0.05% significant level, it was found that there was a correlation between the weight and the mass of seeds (r = 0.890), the weight of seeds and germination (r = 0.867), between the weight of seeds and the number of seeds (r = 0.909), and the number of seeds and germination (r = 0.822).
Figure 9. Variations in physical attributes of A. spectabilis seeds over the collection period. Error bars indicate ± SE.
During the first collection the mean germination was 34.50 ± 1.04% which gradually increased with each collection date. The maximum germination was 46.33 ± 2.67% during the third collection after that the germination started to decline and reached 42.00 ± 2.32 at the final collection. Over the collection, the moisture content percent varied from 28.28 ± 2.41 to 35.05 ± 2.10%. The peak germination was observed when the seed moisture content was 30.90 ± 3.11%. It was observed that the seeds of A. spectabilis attained maturity in the third week of October when it showed the highest germination percentage (Figure 10).
Figure 10. Correlation between moisture content and germination percentage of A. spectabilis seed over the collection dates.
The structure and functioning of forests are significantly influenced by variations in precipitation rates, which impact soil humidity and the water status of plants (Miranda et al., 2011). The seasonal variations in tree water status may serve as a causal relation between climatic seasonality and phenology (Borchert, 1998; Miranda et al., 2011). Temperature and precipitation patterns are considered the principal factors controlling phenological patterns and germination of species (Reich and Borchert, 1984; Miranda et al., 2011). Water stress is believed to be the principal cause, though not the only cause of leaf fall (Borchert and Rivera, 2001). However, from an eco-physiological perspective, understanding only external factors is insufficient to draw definitive conclusions about the influence of water scarcity on individual plants (Miranda et al., 2011). Only the plant itself can reliably indicate when and where water scarcity becomes a stress factor (Larcher, 2000).
The significant presence of A. spectabilis tree density and IVI suggests its importance in the tree community, possibly due to its adaptability to the treeline conditions, but the seedlings and saplings of this species were absent in this study sites, this could be due to the absent of good seed crop every year, extraction of immature cone, anthropogenic reasons or climatic factors. R. campanulatum and R. arboreum showed good regeneration status while all other treeline species including A. spectabilis exhibited poor regeneration observed in similar treeline sites (Singh et al., 2019b). Local environmental conditions including microclimate, edaphic, and topographic factors as well as disturbance in the form of grazing responsible for the low regeneration and mortality of seedlings in these treeline areas coupled with stressful winter conditions (Tewari et al., 2020). Species distributions are influenced by the availability of water, with complex interactions between community composition and physiological responses of individual species to a given environmental change (Aguirre-Gutiérrez et al., 2019). In habitats with pronounced seasons, plants have evolved specialized mechanisms to acquire, conserve, and utilize water. This adaptation ensures a positive water balance even during periods of limited water availability (Oliveira et al., 2014). Among various parameters, ΨPD is considered the most reliable indicator of a plant’s water status. Unlike variables like evapotranspiration and stomatal closure, which can fluctuate throughout the day, ΨPD remains stable (Singh et al., 2023).
Throughout the study, the variations in ΨPD and ΨMD across seasons and years were − 0.13 and − 1.25 MPa, indicating the species’ ability to adjust its water balance in response to environmental changes. The most negative ΨPD and ΨMD were observed during the dry period. In the Himalayas, and across much of the rest of the Indian subcontinent, the pre- and post-monsoon period is particularly dry, and may adversely affect tree growth (Singh et al., 2006). The pronounced fluctuations in water potential between seasons and across years underline the species’ adaptation to varying water availability. An increase in the proportion of rainfall to snowfall suggests that trees are likely to experience more drought stress throughout their growth period (Tewari et al., 2023). In our study, we observed distinct differences in seasonal change patterns from 0.09 to 0.69 MPa. The high ΔΨ during spring to post-monsoon, excluding the monsoon season, demonstrates the dynamic nature of water relations, likely influenced by temperature and moisture conditions. Higher ΔΨ values were normally related to elevated transpiration rates, negative leaf/twig water potential, and increased photosynthetic activity. Treeline species exhibited lower ΔΨ because of precise climatic circumstances, such as precipitation, temperature, soil dampness, plant composition, and moisture (Singh et al., 2023).
The OP full, OP zero, PP full, and RWC% reflect the species’ strategies for water conservation and stress tolerance. The OP full ranged from −0.72 to −1.77 MPa and OP zero was −1.05 to −2.12 MPa across all seasons and years. The most negative values of OP during winter align with potential dehydration avoidance strategies, while the least negative values in pre-monsoon and monsoon seasons may indicate active osmotic adjustment (OA) for water balance. A. spectabilis exhibited a relatively smaller adjustment from post-monsoon to winter, with a more significant decrease of −1.22 MPa at zero osmotic potential (OP) from winter and spring (Tewari et al., 2023). These variations emphasize the species’ capacity to regulate its Ψ under changing circumstances. All the species at the treeline reduced their OP during the spring, coinciding with the onset of phenological processes (Tewari et al., 2023). The RWCz was notably high, ranging between 52.18 and 91.08%. The ability to maintain a high RWC% even under conditions of water deficit was also identified (Zlatev, 2005; Gorai et al., 2010; Tewari et al., 2018). The high water content is crucial for the tree’s metabolic and physiological processes, such as nutrient transportation and photosynthesis.
The water status assumes a central role in regulating the phenological phases of trees (Singh et al., 2019b). The recorded phenophases such as leafing, flowering, cone development, and seed dispersal provide valuable insights into the species’ reproductive and growth patterns. In the present observation, the leafing, flowering, and cone development occurred during the pre-monsoon period (April–May) when the temperature had become higher after winters, and cone/seed dispersal and leaf fall occurred during the dry season when the Ψ was more negative. Phenological adjustments might represent one of the most crucial mechanisms through which trees can respond to and manage rapid environmental changes (Linares et al., 2012), tree water status plays an important role in this process (Singh et al., 2023). The rise in ΨPD coupled with temperature are the important factors triggering the leafing and flowering of Himalayan species (Tewari et al., 2016). Within temperate forests, the annual sequence of phenological stages in woody plants is intricately connected to temperature and photoperiod, indicating a fine adjustment of tree physiology as a response to the seasonal changes in climate (Linares et al., 2012). The correlation between phenophases and Ψ reveals how A. spectabilis manages its water status during key life cycle events. The observed timing of these events aligns with the species’ adaptation to local climatic cues, enabling optimal resource allocation and reproductive success. The observed high ΨPD of twigs during leafing and flowering indicates the species’ capability to maintain water availability during energetically demanding phases. The decline in Ψ of twigs during fruiting and leaf fall suggests water investment toward reproductive processes. Abies pinsapo populations during drought conditions reveal a significant phenological variability in twig growth and litterfall (Linares et al., 2012).
The phenomenon of colour change has long been associated with seed maturity (Tewari et al., 2017¸ 2020). In our study, the transition of A. spectabilis cones from dark green to brownish green, and seed colour from greenish brown to brown, proved to be an effective maturity indicator. Seed mass is a critical trait that profoundly influences a species’ reproductive biology and ecology. In general, larger seeds with higher nutritional reserves tend to exhibit better survival during the seedling phase, especially in nutrient, light, or water-restricted environments (Coelho et al., 2016). Our study revealed A. spectabilis seed mass ranging from 2.18 to 6.84 g, similar to Pinus sylvestris, which reported seed mass between 7.23 and 14.2 g (Castro et al., 2008). Furthermore, fruit and seed size and weight have been associated with the maturation time of various species (Tewari et al., 2020; Singh et al., 2021). In our study, during the maximum seed germination period, the size of A. spectabilis cones was between 5368.21 and 7267.74 mm2, with a weight of 10 cones ranging from 1213.94 to 1500.0 g. Furthermore, the size of seeds was approximately 51.50 mm2, with a weight of 100 seeds being 6.33 g. Similar studies on seed moisture content between 36.4–38.4% for maximum seed germination 59.6–56.75% is a maturity indicator in Quercus leucotrichophora (Tewari et al., 2017). A study on moisture content of 31.0–32.0% can be associated with the optimum germination of 23.33–25.67% for Betula utilis (Tewari et al., 2020). The maximum germination in R. campanulatum was observed to be 39.57% when the moisture content was 29.0% (Singh et al., 2021). The observed changes in cone color, size, weight, moisture content, and seeds per kg highlight the progression of cone development and maturation. The correlations among cone characteristics and seed parameters indicate potential trade-offs between seed size, weight, and germination capacity.
The species producing a good seed year in a decade resulted in the absence of regeneration is a significant concern, which could potentially worsen due to global warming, thereby increasing the risk of regional extinction for various tree species (Dirnböck et al., 2003). Harsh climatic conditions in the alpine zone might restrict the regeneration and survival of treeline tree species. Temperature and precipitation play essential roles in seed germination and seedling growth across plant species (Sanehkoori et al., 2021). Seed tolerance to water stress emerges from a combination of multiple environmental factors, but not just a single factor (Zhang et al., 2022). The critical role of moisture in seed development cannot be emphasized enough; water uptake initiates the germination process and subsequent growth, helping as a primary factor. Ψ strongly influences seed germination, as it regulates the amount of water capable of acting work within a solution. In the current study, the twig Ψ of A. spectabilis started to decline from −0.32 to −0.67 MPa during seed maturation and germination process from a physiological perspective, the decrease in Ψ leads to reduced germination capacity as well as germination rate (Bradford, 1995). The seed moisture content of 30.90% and tree twig ΨPD of −0.50 MPa coincided with maximum germination in the present study, indicating its crucial role in seed maturation and germination success. The gradual increase and subsequent decline in germination percentages align with the species’ adaptation to seasonal cues (Singh et al., 2020).
This comprehensive study sheds light on the intricate relationship between temperature, precipitation, water availability, and the ecological dynamics of Abies forests, particularly at treeline sites. The observed variations are shown to significantly impact forest structure and functioning, primarily through their effects on soil moisture and tree water status. The dynamic nature of water potential across seasons and years reflects the species’ ability to adjust and manage water balance according to environmental shifts. The absence of seedlings and saplings points toward challenges in regeneration. These findings highlight the critical role of water stress in influencing various aspects of tree behavior, from phenology to seedling regeneration of A. spectabilis. The findings underscore the sensitivity of these ecosystems to climatic changes and emphasize the importance of understanding such complexities for effective conservation and management strategies. As global warming continues to influence these fragile environments, the insights provided by this study could contribute to our ability to predict, mitigate, and adapt to the challenges ahead, ensuring the persistence of these unique forest communities in the face of changing climates. In a broader perspective, conducting further long-term research across diverse regions with varying climatic and ecological conditions across various treeline regions in the Himalayas and other mountain ranges would be required to contribute to a more accurate understanding of the effects of climate change on this species and its ecosystems and it would also provide a detailed view for understanding the role of water potential for future investigation.
The raw data supporting the conclusions of this article will be made available by the authors, without undue reservation.
NS: Conceptualization, Data curation, Formal analysis, Investigation, Methodology, Supervision, Writing – original draft, Writing – review & editing. AM: Data curation, Methodology, Writing – review & editing. AT: Conceptualization, Investigation, Methodology, Supervision, Validation, Writing – review & editing. ShS: Conceptualization, Data curation, Methodology, Writing – review & editing. ZW: Writing – review & editing. SaS: Funding acquisition, Writing – review & editing.
The author(s) declare that financial support was received for the research, authorship, and/or publication of this article. The authors extend their appreciation to the Deanship of Research and Graduate Studies at King Khalid University for funding this work through Large Research Project under grant number (RGP2/49/45).
The authors are thankful to the Head, Department of Forestry, Kumaun University, Nainital for providing laboratory facilities.
The authors declare that the research was conducted in the absence of any commercial or financial relationships that could be construed as a potential conflict of interest.
All claims expressed in this article are solely those of the authors and do not necessarily represent those of their affiliated organizations, or those of the publisher, the editors and the reviewers. Any product that may be evaluated in this article, or claim that may be made by its manufacturer, is not guaranteed or endorsed by the publisher.
The Supplementary material for this article can be found online at: https://www.frontiersin.org/articles/10.3389/ffgc.2024.1435659/full#supplementary-material
Aguirre-Gutiérrez, J., Oliveras, I., Rifai, S., Fauset, S., Adu-Bredu, S., Affum-Baffoe, K., et al. (2019). Drier tropical forests are susceptible to functional changes in response to a long-term drought. Ecol. Lett. 22, 855–865. doi: 10.1111/ele.13243
Borchert, R. (1998). Responses of tropical trees to rainfall seasonality and its long-term changes. Clim. Chang. 39, 381–393. doi: 10.1023/A:1005383020063
Borchert, R., and Rivera, G. (2001). Photoperiodic control of seasonal development and dormancy in tropical stem-succulent trees. Tree Physiol. 21, 213–221. doi: 10.1093/treephys/21.4.213
Borgaonkar, H. P., Sikder, A. B., and Ram, S. (2011). High altitude forest sensitivity to the recent warming: a tree-ring analysis of conifers from Western Himalaya, India. Quat. Int. 236, 158–166. doi: 10.1016/j.quaint.2010.01.016
Bradford, K. J. (1995). “Water relations in seed germination” in Seed development and germination (Marcel Dekker, Inc), 351–396.
Castro, J., Aguirre, L., and Distel, R. (2021). Seed quality as related to harvest time in three key perennial grasses native to Puna tussock rangelands of Peru. Int. J. Ecol. 2021:4346186.
Castro, J., Reich, P. B., Sánchez-Miranda, A., and Guerrero, J. D. (2008). Evidence that the negative relationship between seed mass and relative growth rate is not physiological but linked to species identity: a within-family analysis of scots pine. Tree Physiol. 28, 1077–1082. doi: 10.1093/treephys/28.7.1077
Coelho, G. C., Benvenuti-Ferreira, G., Schirmer, J., and Lucchese, O. A. (2016). Survival, growth and seed mass in a mixed tree species planting for Atlantic Forest restoration. AIMS Environ. Sci. 3, 382–394. doi: 10.3934/environsci.2016.3.382
Curtis, J. T., and McIntosh, R. P. (1950). The interrelationship of certain analytic and synthetic characters. Ecology 31, 434–455. doi: 10.2307/1931497
Dirnböck, T., Dullinger, S., and Grabherr, G. (2003). A regional impact assessment of climate and land-use change on alpine vegetation. J. Biogeogr. 30, 401–417. doi: 10.1046/j.1365-2699.2003.00839.x
Gaire, N. P., Dhakal, Y. R., Shah, S. K., Fan, Z. X., Bräuning, A., Thapa, U. K., et al. (2019). Drought reconstruction of trans-Himalayan region of central Himalaya using Pinus wallichiana tree-rings. Palaeogeogr. Palaeoclimatol. Palaeoecol. 514, 251–264. doi: 10.1016/j.palaeo.2018.10.026
Gaire, N. P., Fan, Z. X., Bräuning, A., Panthi, S., Rana, P., Shrestha, A., et al. (2020). Abies spectabilis shows stable growth relations to temperature, but changing response to moisture conditions along an elevation gradient in the central Himalaya. Dendrochronologia 60:125675. doi: 10.1016/j.dendro.2020.125675
Gan, Y. (1996). Evaluation of select nonlinear regression models in quantifying seedling emergence rate of spring wheat. Crop Sci. 36, 165–168. doi: 10.2135/cropsci1996.0011183X003600010029x
Garkoti, S. C., Zobel, D. B., and Singh, S. P. (2003). Variation in drought response of Sal (Shorea robusta) seedlings. Tree Physiol. 23, 1021–1030. doi: 10.1093/treephys/23.15.1021
Gorai, M., Hachef, A., and Neffati, M. (2010). Differential response in growth and water relation of Medicago sativa (L.) and Astragalus gombiformis (pom.) under water-limited conditions. Emir. J. Food Agric. 22, 1–12. doi: 10.9755/ejfa.v22i1.4902
Gutterman, Y. (2000). Environmental factors and survival strategies of annual plant species in the Negev Desert, Israel. Plant Species Biol. 15, 113–125. doi: 10.1046/j.1442-1984.2000.00032.x
Harel, D., Holzapfel, C., and Sternberg, M. (2011). Seed mass and dormancy of annual plant populations and communities decrease with aridity and rainfall predictability. Basic Appl. Ecol. 12, 674–684. doi: 10.1016/j.baae.2011.09.003
IHTP (2021). Meteorological data were collected from Tungnath by Joshi R., under the project “Timberline and altitudinal gradient ecology of Himalayas, and human use sustenance in a warming climate”, MoEF&CC, Government of India (2016-2021).
International Seed Testing Association (1981). Moisture content and equipment Wkg group. In report of the Forest tree seed committee. Seed Sci. Technol. 9, 101–108.
IPCC (2014). “Climate change: synthesis report” in Core writing team. eds. R. K. Pachauri and L. A. Meyer (Geneva, Switzerland: Contribution of Working Groups I, II and III to the Fifth Assessment Report of the Intergovernmental Panel on Climate Change).
Körner, C. (2012). Treelines will be understood once the functional difference between a tree and a shrub is. Ambio 41, 197–206. doi: 10.1007/s13280-012-0313-2
Krauchii, N., Brang, P., and Schonenberger, W. (2000). Forests of mountainous regions: gaps in knowledge and research needs. For. Ecol. Manag. 132, 73–82. doi: 10.1016/S0378-1127(00)00382-0
Kunwar, R. M., Sher, H., and Bussmann, R. W. (2021). Ethnobotany of the Himalayas : Springer Nature.
Larcher, W. (2000). Temperature stress and survival ability of Mediterranean sclerophyllous plants. Plant Biosyst. 134, 279–295. doi: 10.1080/11263500012331350455
LeFait, B. M., and Qaderi, M. M. (2022). Maternal environmental effects of temperature and exogenous gibberellic acid on seed and seedling traits of four populations of evening primrose (Oenothera biennis). Seeds 1, 110–125. doi: 10.3390/seeds1020010
Linares, J. C., Covelo, F., Carreira, J. A., and Merino, J. A. (2012). Phenological and water-use patterns underlying maximum growing season length at the highest elevations: implications under climate change. Tree Physiol. 32, 161–170. doi: 10.1093/treephys/tps003
Miranda, J. D., Armas, C., Padilla, F. M., and Pugnaire, F. I. (2011). Climatic change and rainfall patterns: effects on semi-arid plant communities of the Iberian southeast. J. Arid Environ. 75, 1302–1309. doi: 10.1016/j.jaridenv.2011.04.022
Mueller-Dombois, D., and Ellenberg, H. (1974). Aims and methods of vegetation science. New York: J. Wiley and Sons, 547.
Oliveira, P. T. S., Nearing, M. A., Moran, M. S., Goodrich, D. C., Wendland, E., and Gupta, H. V. (2014). Trends in water balance components across the Brazilian Cerrado. Water Resour. Res. 50, 7100–7114. doi: 10.1002/2013WR015202
Pallardy, S. D., Pereira, J. S., and Parker, W. C. (1991). “Measuring the state of water in tree systems” in Techniques and approaches in Forest tree ecophysiology (27–76). eds. J. Lassoie and T. M. Hinckley (Boca Raton, FL: CRC Press).
Qaderi, M. M. (2023). Environmental regulation of weed seed dormancy and germination. Seeds 2, 259–277. doi: 10.3390/seeds2030020
Qaderi, M. M., Cavers, P. B., and Bernards, M. A. (2003). Pre- and post-dispersal factors regulate germination patterns and structural characteristics of scotch thistle (Onopordum acanthium) cypselas. New Phytol. 159, 263–278. doi: 10.1046/j.1469-8137.2003.00777.x
Rai, S., Dawadi, B., and Wang, Y. (2020). Growth response of Abies spectabilis to climate along an elevation gradient of the Manang valley in the Central Himalayas. J. For. Res. 31, 2245–2254. doi: 10.1007/s11676-019-01011-x
Reich, P. B., and Borchert, R. (1984). Water stress and tree phenology in a tropical dry forest in the lowland of Costa Rica. J. Ecol. 72, 61–74. doi: 10.2307/2260006
Ritchie, G. A., and Hinckley, T. M. (1975). “The pressure chamber as an instrument for ecological research” in Advances in ecological research. ed. A. Macfadyen (Academic Press, London: England), 165–254.
Roush, W. M. (2009). A substantial upward shift of the alpine Treeline ecotone in the southern Canadian Rocky Mountains. Master's thesis: University of Victoria.
Sanehkoori, F. H., Pirdashti, H., and Bakhshandeh, E. (2021). Quantifying water stress and temperature effects on camelina (Camelina sativa L.) seed germination. Environ. Exp. Bot. 186:104450. doi: 10.1016/j.envexpbot.2021.104450
Singh, N., and Mittal, A. (2019). Response of phenological events of Aesculus indica Colebr. To climate change along an altitudinal gradient in Kumaun Himalaya. Int. J. Environ. 8, 1–16. doi: 10.3126/ije.v8i1.22069
Singh, P., and Negi, G. C. S. (2018). Treeline species phenology: shoot growth, leaf characteristics and nutrient dynamics. Trop. Ecol. 59, 297–311.
Singh, N., Ram, J., Tewari, A., and Yadav, R. P. (2015). Phenological events along the elevation gradient and effect of climate change of Rhododendron arboreum Sm. In Kumaun Himalaya. Curr. Sci. 108, 106–110.
Singh, N., Tewari, A., Shah, S., and Mittal, A. (2019a). Tree regeneration pattern and size class distribution in Anthropogenically disturbed sub-alpine Treeline areas of Indian Western Himalaya. Int. J. Sci. Technol. Res. 8, 537–546.
Singh, N., Tewari, A., Shah, S., and Mittal, A. (2019b). Water relations and phenological events of two treeline Rhododendron species in Indian western Himalaya. Sylwan 163, 64–76.
Singh, N., Tewari, A., Shah, S., and Mittal, A. (2020). Treeline species: regeneration status and seedling dynamics in Western Himalayan region. Environ. Ecol. 38, 725–732.
Singh, N., Tewari, A., Shah, S., and Mittal, A. (2021). Capsule ripening and seed germination in Rhododendron campanulatum (D. Don) in Aali Treeline area of Western Himalaya, India. Indian J. Ecol. 48, 398–403.
Singh, N., Tewari, A., Shah, S., and Mittal, A. (2023). Seasonal water relations and stress tolerance of Quercus semecarpifolia (smith) in treeline areas of Western Himalaya, India. Vegetos. doi: 10.1007/s42535-023-00665-7
Singh, N., Tewari, A., Shah, S., and Mittal, A. (2024). Phenology and water relations of treeline species of Western Himalaya, India. Braz. J. Bot 47, 343–354. doi: 10.1007/s40415-024-00989-x
Singh, S. P., Zobel, D. B., Garkoti, S. C., Tewari, A., and Negi, C. M. S. (2006). Pattern in water relations of central Himalayan trees. Trop. Ecol. 47, 159–182.
Tamta, K., and Singh, N. (2018). Seed ripening indication in Quercus leucotrichophora A. Camus, in Kumaun, central Himalayan region. Int. J. Adv. Res. Dev. 3, 49–52.
Tewari, A., Bhatt, J., and Mittal, A. (2016). Influence of tree water potential in inducing flowering in Rhododendron arboreum in the central Himalayan region. iForest-Biogeosci. For. 9, 842–846. doi: 10.3832/ifor1525-008
Tewari, A., Mittal, A., and Singh, N. (2017). Seed maturation timing in Quercus leucotrichophora A. camus along an altitudinal gradient in Uttarakhand Himalaya. Environ. Conserv. J. 18, 53–59. doi: 10.36953/ECJ.2017.18307
Tewari, S. N., Shah, A., and Mittal, A. S. (2020). Catkin maturation timing and seed germination in Betula utilis (D. Don) in the western Himalayan treeline area of Uttarakhand. J. Adv. Sci. Res. 11, 145–151.
Tewari, A., Shah, S., Singh, N., and Mittal, A. (2018). Treeline species in western Himalaya are not water stressed: a comparison with low elevation species. Trop. Ecol. 59, 313–325.
Tewari, A., Shah, S., Singh, N., Mittal, A., and Tamta, K. K. (2023). Water relations of the Indian Himalayan Treeline species. Ecol. Himalayan Treeline Ecotone, 395–410. doi: 10.1007/978-981-19-4476-5_16
Yang, W. B., Quan, G. M., and Zhang, J. E. (2013). Effects of environmental factors on seed germination of Bidens pilosa and Bidens bipinnata. Ecol. Environ. 22, 1129–1135.
Zhang, R., Chen, D., and Liu, H. (2022). Effect of temperature and water potential on the germination of seeds from three different populations of Bidens pilosa as a potential cd hyperaccumulator. BMC Plant Biol. 22:487. doi: 10.1186/s12870-022-03876-3
Zhang, R., Luo, K., and Chen, D. L. (2020). Comparison of thermal and hydrotime requirements for seed germination of seven Stipa species from cool and warm habitats. Front. Plant Sci. 11:560714. doi: 10.3389/fpls.2020.560714
Zhang, Y., Xu, M., Adams, J., and Wang, X. (2009). Can Landsat imagery detect treeline dynamics? Int. J. Remote Sens. 30, 1327–1340. doi: 10.1080/01431160802509009
Zlatev, Z. (2005). Effect of water stress on leaf water relations of young bean plants. J. Cent. Eur. Agric. 6, 5–14.
Keywords: Abiesspectabilis, water stress, phenophases, germination, seed maturity, treeline
Citation: Singh N, Mittal A, Tewari A, Shah S, Wani ZA and Siddiqui S (2024) The pivotal role of water potential in phenology and seed germination of Abies spectabilis in the western Himalayan treeline region. Front. For. Glob. Change. 7:1435659. doi: 10.3389/ffgc.2024.1435659
Received: 20 May 2024; Accepted: 19 June 2024;
Published: 01 July 2024.
Edited by:
Jelena Milovanovic, Singidunum University, SerbiaReviewed by:
Jovana Devetaković, University of Belgrade, SerbiaCopyright © 2024 Singh, Mittal, Tewari, Shah, Wani and Siddiqui. This is an open-access article distributed under the terms of the Creative Commons Attribution License (CC BY). The use, distribution or reproduction in other forums is permitted, provided the original author(s) and the copyright owner(s) are credited and that the original publication in this journal is cited, in accordance with accepted academic practice. No use, distribution or reproduction is permitted which does not comply with these terms.
*Correspondence: Nandan Singh, bmFuZGFuZm9yZXN0cnlAZ21haWwuY29t
†Senior authorship
‡These authors share last authorship
Disclaimer: All claims expressed in this article are solely those of the authors and do not necessarily represent those of their affiliated organizations, or those of the publisher, the editors and the reviewers. Any product that may be evaluated in this article or claim that may be made by its manufacturer is not guaranteed or endorsed by the publisher.
Research integrity at Frontiers
Learn more about the work of our research integrity team to safeguard the quality of each article we publish.