- 1Independent, El Rocio SA, Santo Pipo, Argentina
- 2Department of Production, Instituto Linea Cuchilla (ILC), Ruiz de Montoya, Argentina
- 3Department of Agricultural Extension, Asesor Productores Yerba Mate SCL, Santo Pipo, Argentina
- 4Asesor Técnico de la Dirección General de Yerba Mate y Té, Ministerio del Agro y la Producción, Posadas, Argentina
- 5Instituto Nacional de Tecnología Agropecuaria (INTA), Estación Experimental Agropecuaria Montecarlo, Montecarlo, Argentina
- 6Instituto Nacional de Tecnología Agropecuaria (INTA), Centro Regional Misiones, Posadas, Misiones, Argentina
In this work, we advocate agroforestry as a sustainable agricultural method that leverages biodiversity and ecosystem services, simultaneously tackling the problems of adaptation and mitigation to climate change, and of land restoration for sustainable agriculture across scales. While the rise of industrial agriculture has been instrumental in addressing the food demands of an expanding global population, enhancing food quality, yield, productivity, and efficiency, we must now reckon with the consequences. This advancement, which prioritizes simplification, specialization, and external inputs, has escalated detrimental externalities including deforestation, biodiversity loss, soil degradation, pollution, and an increase in greenhouse gases, contributing significantly to global warming and to exacerbated environmental crises. These demand urgent attention. In response, various agricultural methodologies such as organic, biodynamic, ecological, and biological farming have emerged, attempting to propose alternatives. However, these methods have yet to significantly alter the trajectory of mainstream agriculture. For over two decades, we have devoted our efforts to developing and refining a multispecies integrated agroforestry system for the sustainable cultivation of Ilex paraguariensis, “yerba mate,” in the subtropical north-east of Argentina. With “integrated” we mean that the trees are planted within the I. paraguariensis distribution, not between alleys as in “alley cropping” or “hedgrow intercropping.” The experimental work we present here was designed and implemented to enable data comparisons across consociations of multiple species of trees, at a relevant experimental scale. We achieve soil preservation and restoration, productivity comparable to or exceeding monocultures, and a significant increase in resiliency, particularly evidenced during the extreme climate events of spring and summer 2021 and 2022. These results underscore agroforestry’s potential for climate change mitigation and adaptation.
1 Introduction
The Northeast of Argentina is a region that extends into the subtropical forests it shares with Paraguay to the North, Brazil to the Northeast, and Uruguay to the Southeast (Figure 1, left inset; Google Maps, 2024). The whole region was first colonized by the Jesuits (Roca, 2017) who, starting in 1609, built settlements throughout a vast territory that today is part of Uruguay, Paraguay, Brazil, and Argentina (Roca, 2017). In Argentina, most of the missions are in the province of Misiones (Roca, 2017). The Jesuits were pioneers in cultivating the tree I. paraguariensis to produce the infusion popularly known as “mate (UNESCO’s World Heritage Centre, 2023; Heck and De Mejia, 2007; Gawron-Gzella et al., 2021; Bracesco et al., 2011).” The plant is popularly known as “yerba mate (UNESCO’s World Heritage Centre, 2023; Heck and De Mejia, 2007; Gawron-Gzella et al., 2021; Bracesco et al., 2011)” and its crop became in main economic activity of the Guarani territories in the 17th century (UNESCO’s World Heritage Centre, 2023), spreading throughout southern South America. Mate gained further diffusion during the independence wars against the Spanish crown and subsequent conflicts involving Paraguay, Uruguay, Brazil, and Argentina (UNESCO’s World Heritage Centre, 2023; Sarreal, 2023).
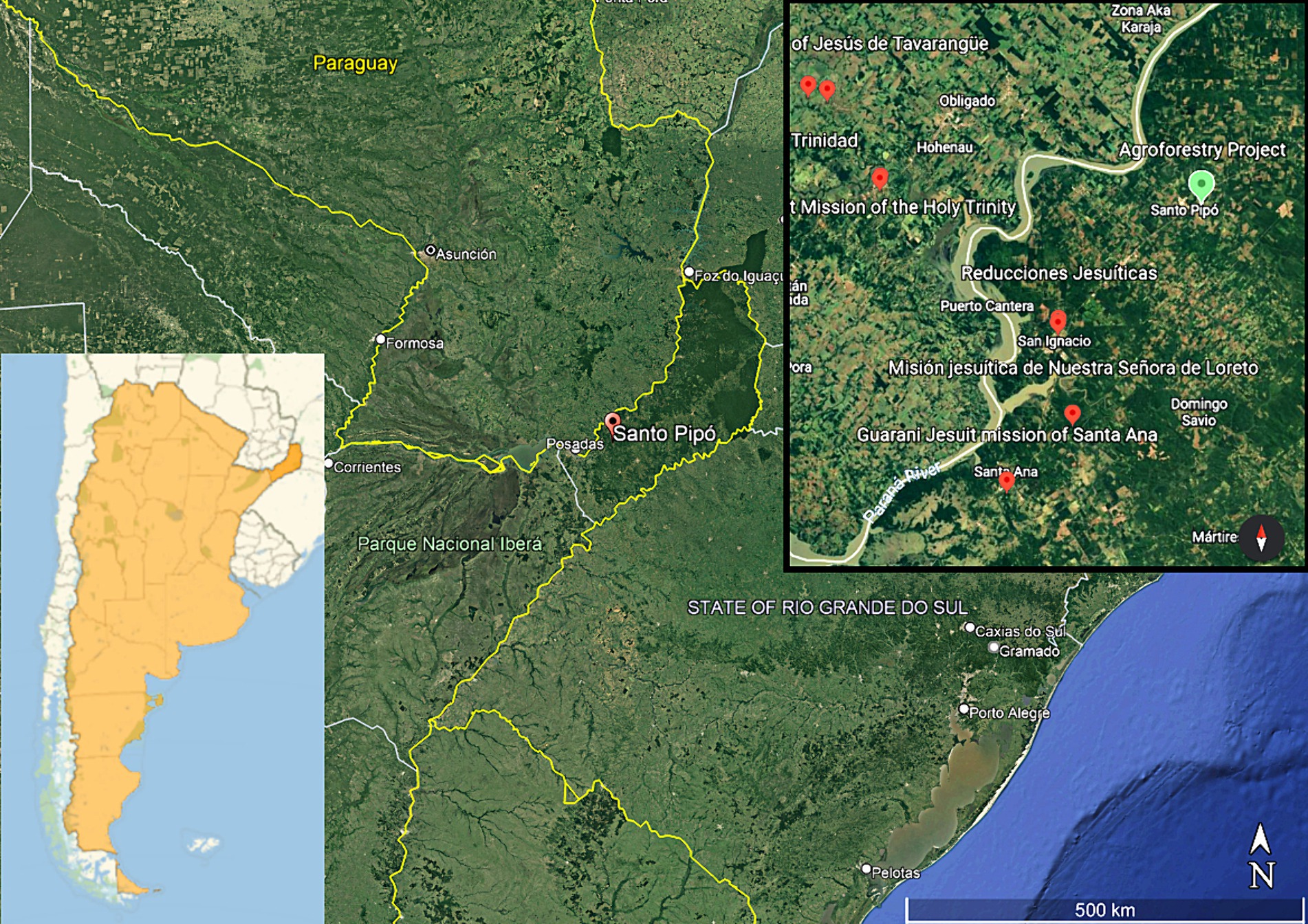
Figure 1. Left inset: map of Argentina emphasizing the province of Misiones. Main, map of the north-east of Argentina. The red pin marks the village of Santo Pipo, Misiones, site of this agroforestry project. Political boundaries between Argentina, Paraguay, Uruguay, and Brazil as yellow lines (Google Maps, 2024). Right inset: A blue pin marks the location of the agroforestry project presented here, and the closest red “pin” marks the location of the Jesuit ruin San Ignacio Mini (Roca, 2017). The other red pins mark other Jesuit ruins.
The region is defined by its humid subtropical climate without dry season and its soils are red oxysols with a very high clay content (Pereyra, 2012). While much of Paraguay and southern Brazil have cleared their jungles for cereals, grains, and cattle ranching, the Argentinian part of this territory has retained a mix of native forests and planted exotic forests, intersperse with agriculture dominated by perennial species. This fact is immediately apparent in low resolution satellite maps of the region, in which Argentina appears with a much darker green colour than Brazil and Paraguay, the contrast of forests versus arable land cultivated with grains (Figure 1; Google Maps, 2024).
Although I. paraguariensis is a native tree species of the subtropical forests of the region, the industrialized production of mate lead to the adoption of the highly mechanized agricultural practices developed for monocultures, using land made available by deforestation. Other agricultural and forestry activities, including, tea plantations, commercial planted forests, annual crops, and animal husbandry, also led to deforestation. There are inherent limits to the expansion of deforestation, primarely because forests are a finite resource. Yet, although the region is well-suited for sustainable practices such as agroforestry and agrosilvopastoral systems (for animal husbandry), these have not been adopted on a transformative scale.
The advent of more frequent and intense climate events, often dramatic in nature, has started to shift perceptions significantly. Throughout most of 2021, the region experienced a shortfall in precipitation, with drought periods in May–June and August–September. By early summer, surface temperatures were consistently above historical averages, culminating in record highs of 42.5°C on January 24, 2022. These extreme conditions, persisting through March 2022, resulted in substantial losses in agricultural output, destruction of young, planted perennials, and damage to both ancient forests and newer planted forests. The persistent drought and unprecedented high temperatures led to severe wildfires, further exacerbating the crisis. The combined effect of these events not only devastated local ecosystems but also disrupted both wildlife and human communities, altering public perceptions about climate change and the resilience of traditional agricultural practices in the face of such climate shocks. This crisis has underscores the urgent need for agricultural practices that integrate and leverage the natural complexity of the environment, enhancing resilience against climate variability and promoting sustainability for the future.
Extreme climate events have become more common worldwide, heightening public awareness of global warming (AghaKouchak et al., 2020; Fischer et al., 2021; Zhou et al., 2023; Walker and Van Loon, 2023; Yuan et al., 2023). As global temperatures continue to rise, we can expect more frequent and severe climate hazards (AghaKouchak et al., 2020). The measures we adopt to mitigate global warming will influence the likelihood and severity of these unprecedented extremes (Fischer et al., 2021), as well as their geographical extent (Zhou et al., 2023). With the global population increasing and urban areas expanding, more people are at risk from these climate events. Additionally, the likelihood of simultaneous climate extremes, which significantly affect human welfare and ecosystem sustainability, is expected to increase (Zhou et al., 2023). For instance, concurrent large-scale wildfires, driven by hot and dry conditions, can exceed firefighting capacities, leading to extensive damage and significant ecological consequences (Zhou et al., 2023). Flash droughts, which arise from a sudden deficit in precipitation coupled with increased evapotranspiration, rapidly deplete soil moisture (Walker and Van Loon, 2023; Yuan et al., 2023), making them exceptionally destructive to ecosystems, which cannot adapt swiftly. Projections indicate that under higher emission scenarios, flash droughts could become more frequent and begin more abruptly, posing substantial challenges for climate adaptation strategies (Walker and Van Loon, 2023; Yuan et al., 2023).
How human societies use, manage, and interact with land plays a pivotal role in addressing sustainability challenges and mitigating climate change impacts (Meyfroidt et al., 2022; UN Environment Program, 2023). Addressing the complexities of global climate change requires efforts that extend well beyond reducing atmospheric CO2 levels (UN Environment Program, 2023). Agroforestry emerges as a viable strategy in this context, notably because it can be initiated and maintained from the bottom up, enabling stakeholders who cannot afford to wait for top-down global solutions to take immediate action. Extreme climate events have starkly highlighted the biophysical effects of deforestation, reforestation, and afforestation, underscoring the local benefits these practices offer, aligned with the self-interest of society.
When we plant a tree, we hope that it will grow tall and straight; that it will have a full, healthy crown with strong, well-spaced branches; that it will cast a broad expanse of sheltering shade; that it will resist damage from wind and ice; and that it will be easy to maintain. Without proper pruning, however, a tree can become unhealthy and expensive to maintain. An unmaintained or poorly maintained tree is more likely to become hazardous, with branches that break during storms, and weak, unsightly shoots. In a multispecies agroforestry system, all the above challenges are present, with the added complexity of managing and calibrating the integration of the trees with the agricultural activity. Our objective is to present the design and implementation of a scalable multispecies integrated agroforestry system capable of sustaining and restoring soil fertility, providing long-term self-sustainable economic results, and contributing to resilience against climate change, insect attacks, and diseases.
2 Materials and methods
2.1 Experimental site
The study was conducted in a 10 has Trial Lot spanning “Lots 1 and 3” (Lote 1 and Lote 3) within “Lote XII” (⁓98 has), cadastre of the municipality of Santo Pipo, province of Misiones, Argentina, (Google Earth, https://earth.google.com/; 27° 8′30.47′′S, 55°23′32.72′′W and 27° 8′34.77′′S, 55°23′37.18′′W respectively). The location is at approximately 175 m above mean sea level. The soils of this region are red oxysols with a very high clay content (Ultisol soil; Pereyra, 2012). The region is defined by its humid subtropical climate without dry season. From year 1967 to year 2020 the average annual rainfall was 1,998 mm, with a minimum of 1,120 (2004) mm and a maximum of 3,034 (2014) mm. Towards the end of 2020 and during 2021 and 2022 the weather phenomenon called “El Niño” brought extremes of heat and drought, while during 2023 “La Niña” brought extremes of rainfall. The total rainfall for 2020, 2021, 2022, and 2023 was 1,310, 1,536, 2,136, and 1,900 mm, respectively. Typically, temperatures range from a minimum just above zero °C to approximately 36°C. The minimum historical temperature was recorded on July 18, 2017, with −8°C at 5 cm above the soil, and the maximum historical temperature on January 24, 2022, was of 42.5°C (Roset, 2022).
2.2 Equipment
During 1993 a Taeda pine or loblolly pine forest was installed for fallow. These trees were fell approximately a year ahead of the plantation of the system in 2010, to allow for the preparation of the land. The remnants of tree crowns were cut down manually with chainsaws, and the field was plowed mechanically. Burning was entirely avoided. Lastly, the fields were marked as described below and a subsoiler was passed tracking the future lines of the plantation. Seedlings were implanted using a shovel, on the track made by the subsoiler, following the distances as planned in the design described below. Field measurements of widths and heights were made using a measuring tape and a telescopic rod, during the month of February of each year (summer). Photosynthetically active radiation (PAR) measurements were performed with a one-meter-long rod ceptometer during the month of September (spring). Soil samples were obtained using a borer or ring sampler of 0.25 m in diameter at 0.2 m of depth, and 1 kg plastic bags for sample transfers. Samples were collected along the rows of each tree species and within the rows of mate (displaced from each tree), for each block of the “trial lot.” During 2013 the samples were collected in April and May, with 3 samples at each sampling point (1st, 2nd, and 3rd row of mate away from a tree), that were kept individually separated resulting in 107 soil tests. During 2021 the samples were collected during September, also for each block, but binned at each sampling point, resulting in 36 soil tests. All soil tests análisis were carried out at the Instituto Nacional de Tecnología Agropecuaria (INTA), Estación Experimental Agropecuaria Cerro Azul (EEA Cerro Azul), Ruta Nacional 14. Km. 836, Cerro Azul, Misiones C. P. (3313). The method used for estimations of soil readily oxidizable carbon (ROC %) is based by the chromic acid titration method by Walkley and Black*, standardized in Argentina by the Instituto Argentino de Normalización y Certificación (IRAM) with norm “IRAM-SAGyP 29571-3:2016 Parte 3: Determinación de Carbono orgánico oxidable por mezcla oxidante fuerte- microescala.” Oxydazable Organic Matter (OOM) and Total Organic Matter (TOM) are obtained from ROC by multiplicative factors that depend on the context (Walkley and Black, 1934). The values reported were computed from 36 and 107 individual samples for 2013 and 2021, respectively. Soil tests for each individual species have been averaged in aggregate. On-site materials and equipment are described below within Methods as appropriate.
2.3 Methodology
The objective is to establish an experimental multispecies agroforestry system within a 10-ha trial lot. The design of this system will facilitate precise measurements and generate self-consistent data sets. This will allow for meaningful comparisons between different species, ranging from individual plant growth metrics to their contributions to soil health. All I. paraguariensis seedlings derived from the seeds created at Estación Experimental Agropecuaria Cerro Azul INTA (EEA Cerro Azul), Ruta Nacional 14. Km. 836, Cerro Azul, Misiones C.P. (3313), Argentina. For the exotic species, seedlings were obtained from local seed orchards, where they undergo selective breeding to enhance specific traits, ensuring a degree of genetic advancement. The seedlings for native species as listed in Table 1 were made in-house from seeds that were meticulously collected from trees within the remnants of native forests in the local region, selected based on their phenotypic characteristics. The nine species were Lapacho negro (Handroanthus heptaphyllus), Petiribi o Loro negro (Cordia trichotoma), Araucaria (Araucaria angustifolia), Cañafístola (Peltophorum dubium), Anchico Colorado (Parapiptadenia rigida), Guatambú (Balfourodendron riedelianum), Toona or Australian cedar (Toona ciliata), Grevillea (Grevillea robusta), and Kiri (Paulownia sp). Figure 2 shows images of the in-house nursery located in “Lote 6A, municipality of Santo Pipo, Misiones, Argentina (https://earth.google.com/; 27° 7′45′′S, 55°25′07′′W). The seedlings are routinely taken to the field in pots 6 to 8 months after a preselection.
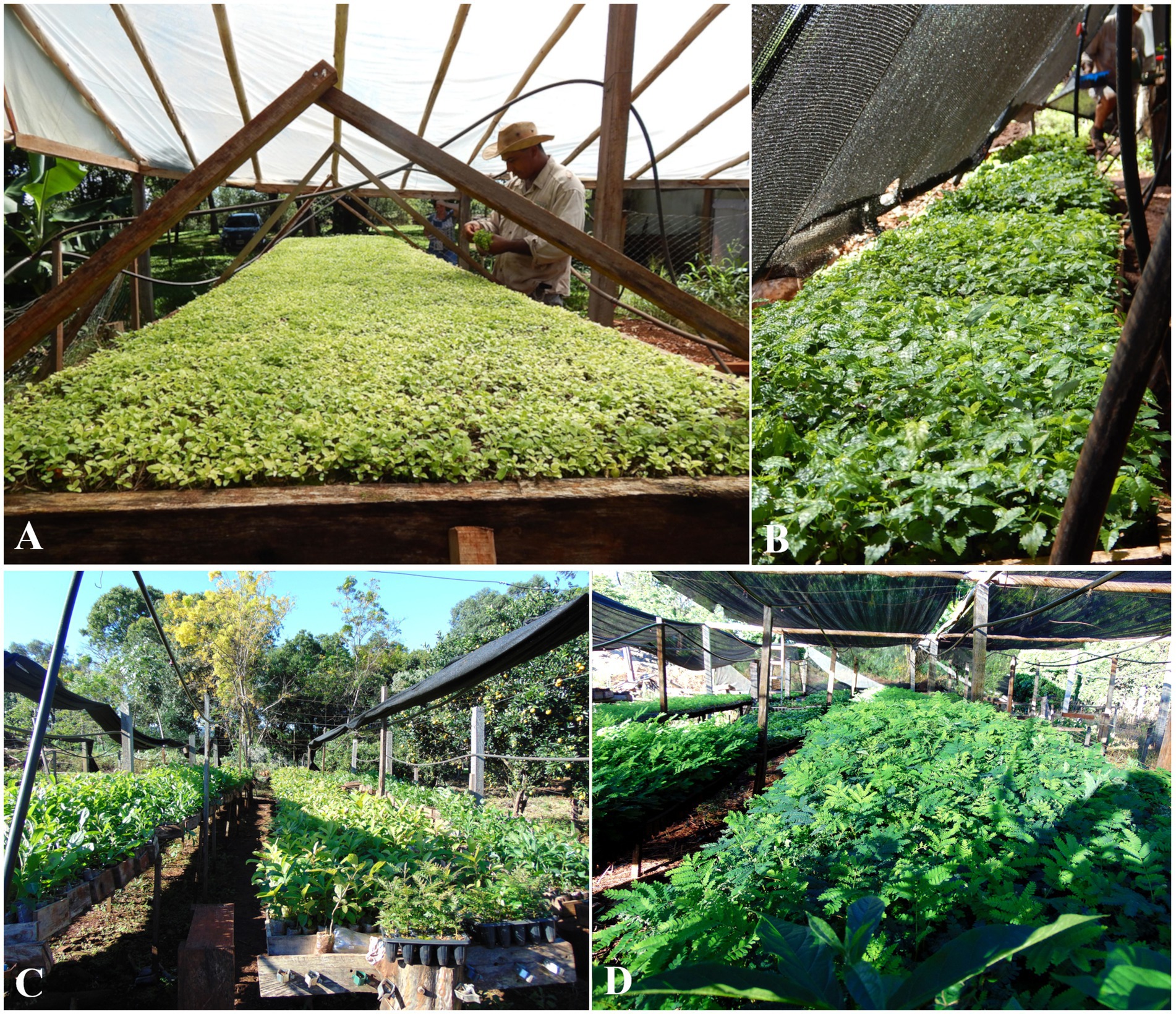
Figure 2. View of the seedlings of native spaces and I. paraguariensis. In-house production of seedlings to ensure good quality can be achieved with low cost, ordinary means. Benches in (A) are seedlings of yerba mate (I. paraguariensis) being transferred to pots; (B) Lapacho negro (Handroanthus heptaphyllus); (C) I. paraguariensis in pots approximately 7 months later than (A) and ready for planting in the field; (D) are seedlings of Cañafístola (Peltophorum dubium).
2.4 Experimental design (a scalable model for self-sustainable, integrated agroforestry)
The development of our agroforestry project unfolded gradually, starting in the 1990s. The first step was the soil restoration of a 40-ha swath of highly degraded yerba mate plantations, established in 1934 and managed with conventional methods, by transitioning them into monoculture forest lots of pine (Pinus elliottis and Taeda). This transformation achieved a relevant level of soil restoration through a process of fallowing, in anticipation of a future redevelopment into diversified yerba mate plantations that would integrate various tree species (Comolli et al., 2023). At the heart of this initiative was the creation of a 10-ha agroforestry pilot, or ‘trial lot,’ carved out from the initially restored 40 has in 2010, as detailed in Chart 1. The pilot project was meticulously designed to enable a comparative analysis of different tree species and to contrast these with control lots that remained treeless. The implementation of the experimental trial lot is the subject of this manuscript. On the basis of this experience and motivated by these results, our agroforestry effort was expanded to 200+ has increasing the number of species as possible, but not keeping this rigorous design for data acquisition and quantitative comparisons. The overall characterization of the 200 + has agroforestry project is explained in a manuscript presented in the open source site agriRxiv (Comolli et al., 2023).
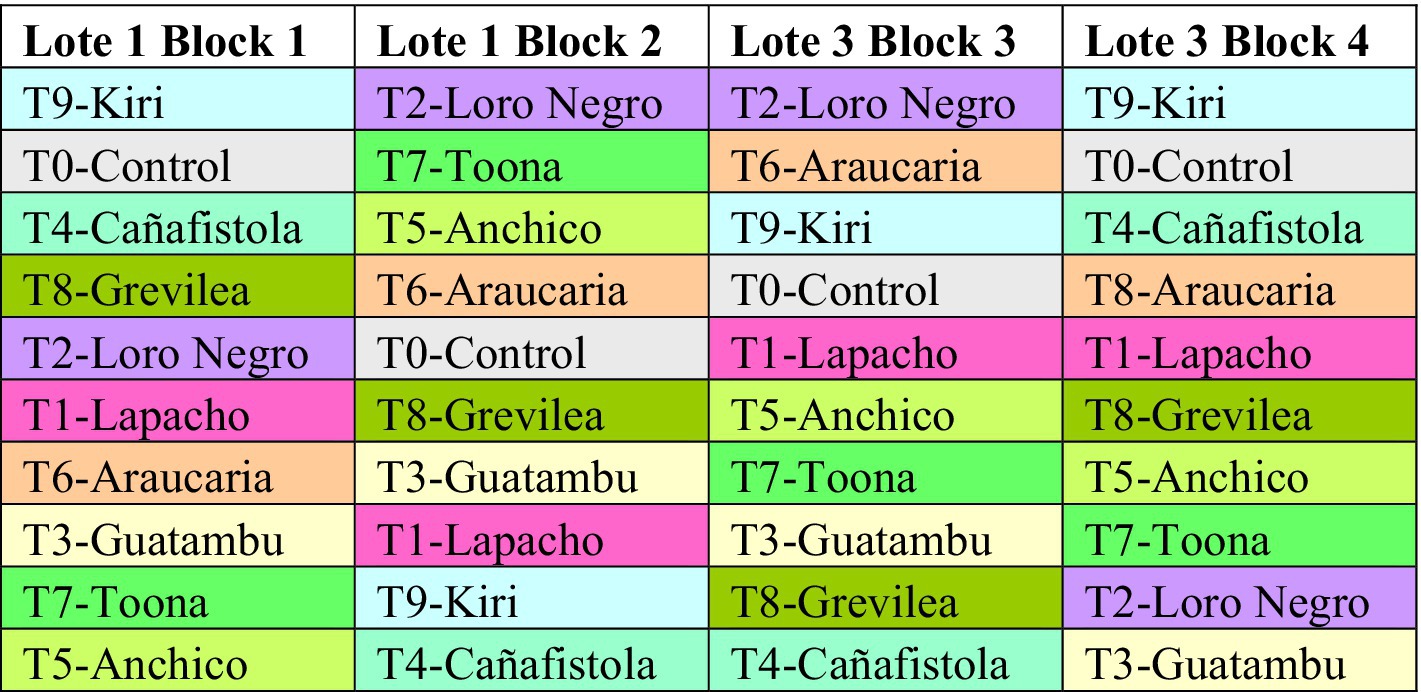
CHART 1. Randomized installation of nine tree species for the creation of the multispecies agroforestry system trial lot spanning 10 has in four repeated blocks. Distribution of tree species planted in four repeated blocks, B1, B2, B3, and B4, allotted to “Lote 1 and Lote 3,” spanning a total of 10 has. T1, T2, …, T9 refers to “treatment.” A similar chart with this information is also included in an article about general and complementary, but less technical, aspects of the system for divulgation (Comolli et al., 2023).
2.5 Planted density of yerba mate and of trees
The planting strategy in our agroforestry lots was meticulously designed for optimal interaction between yerba mate (I. paraguariensis) and various tree species. The design of the trial lot consists of the randomized installation of nine tree species, providing nine “treatments,” spanning 10 has in four repeated blocks. The initial planting density and subsequent adjustments were based on empirical observations and aimed at achieving the best possible synergy between the components of the system. The agroforestry trial lot was created with a high density of yerba mate (I. paraguariensis) and trees following the diagram illustrated in Chart 2. The spacing was 1.5 m between plants of yerba mate in 5 adjacent lines or rows separated by 1.5 m, making for paired 5-fold lines. These where then spaced by 3 m “farm roads” for machinery. We call these rows “paired” or “composite” rows. This architecture in groups of 5 adjacent rows separated by a space of 3 m results in 3,700 plants per ha. The trees were planted in one row at the centre of each composite row, with 1.5 m of separation between trees (of a given species for a given group of neighbouring rows). The rows of trees were thus separated by 9 m, resulting in 741 trees per ha (Table 1). Visual examples are shown in Supplementary materials. The whole trial lot, therefore, comprehends approximately 37,000 plants of yerba mate (I. paraguariensis) and 7,410 trees of 9 species in equal numbers.
The development of the system was during the first few years, since the seedlings were implanted until the trees had acquired crowns and stems of adult trees, was monitored with great attention to the vitality and adaptability of various species within the integrated setup, and to the lessons we could learn for future work. The most commonly used measures of tree growth (Sterck and Bongers, 1998; Sumida et al., 2013) are stem diameter at breast height (DBH) and tree height (H), which were measured during the fourth and fifth year of the integrated agricultural agroforestry creation, verifying a very satisfactory evolution of the system since its inception, see Charts 3, 4 below. The most significant disparities were observed between Kiri and Araucaria respect to the development of the other species.
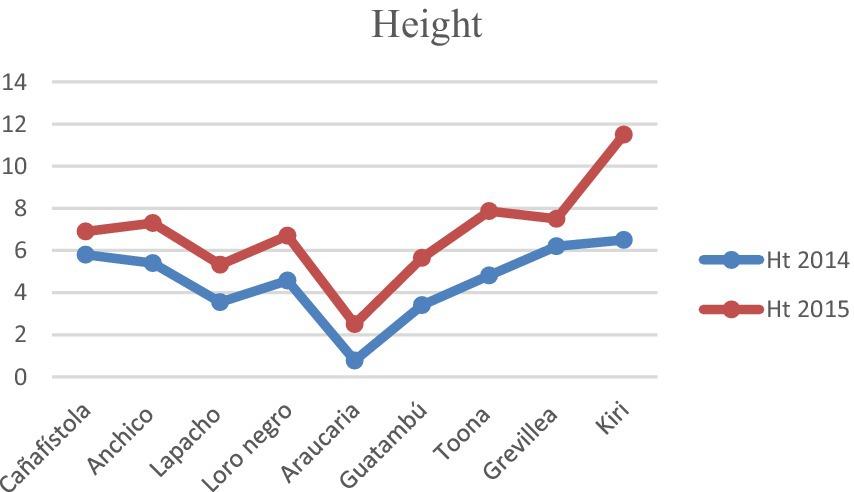
CHART 4. Heights for 2014 and 2015. Diameter at breast height, or DBH (m), and heights, Ht (m), for the tree species in the integrated agroforestry system at the age of 4 and 5 years. These measurements were of key relevance in the creation of criteria for the management of the system. The differences in measured values are statistically significant using a p-value of 0.05, except for the DBH of Araucaria. See also Supplementary Table 1.
2.6 Treatment details
The tree distribution was subsequently thinned at an age of 5 years to a space of 4.5 m between trees within each row as their growth and uniformity exceeded expectations; see Supplementary Figures 1, 2. The remaining trees had the lower branches trimmed to reduce the direct physical competition for space with I. paraguariensis, and to provide more space for the cultural manual work during the harvest. This thinning brought the density of trees to approximately 246 trees per ha and provided valuable material to the soil. It also improved growth conditions for I. paraguariensis (Table 1). A second intervention of thinning, taking every other tree out was done at an age of 8 years, in parallel with the trimming of the remaining tree crowns. This intervention left a space of 9 m between adult trees, with a density of trees of approximately 123 trees per ha and, and practically no branches at a height lower than 4.5 m (Table 1; Figure 3). The regrowth of the trees is being managed, and any dead tree replanted, so that when the new trees reach a good size, the older trees are cut down, alternating in this fashion to always have young trees and decomposing logs and branches on the ground as shown in Figure 4. In Figures 3A,B, we clearly see the size differences between adult trees and the regrowth from the shoots of the trees that were fell in the interventions.
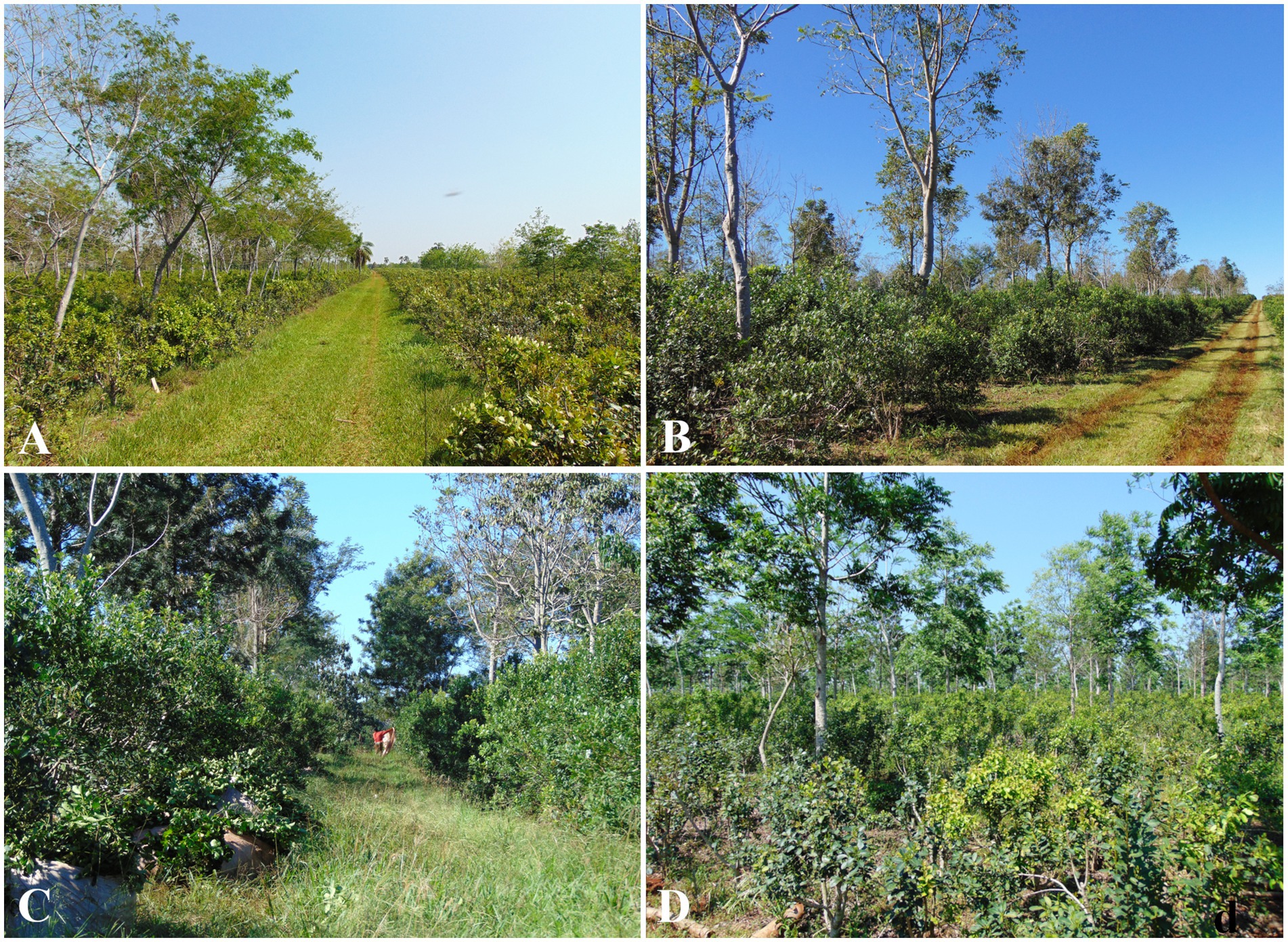
Figure 3. View of the experimental lot in February 2018 (A) and May 2019 (B–D). Panel (A) Anchico; (B) Toona the first two lines or rows, and Loro negro rows further back; (C) Toona left of road, and behind there are Lapacho negro and Anchico, (D) Toona front plane, Lapacho negro, and Guatambu backwards.
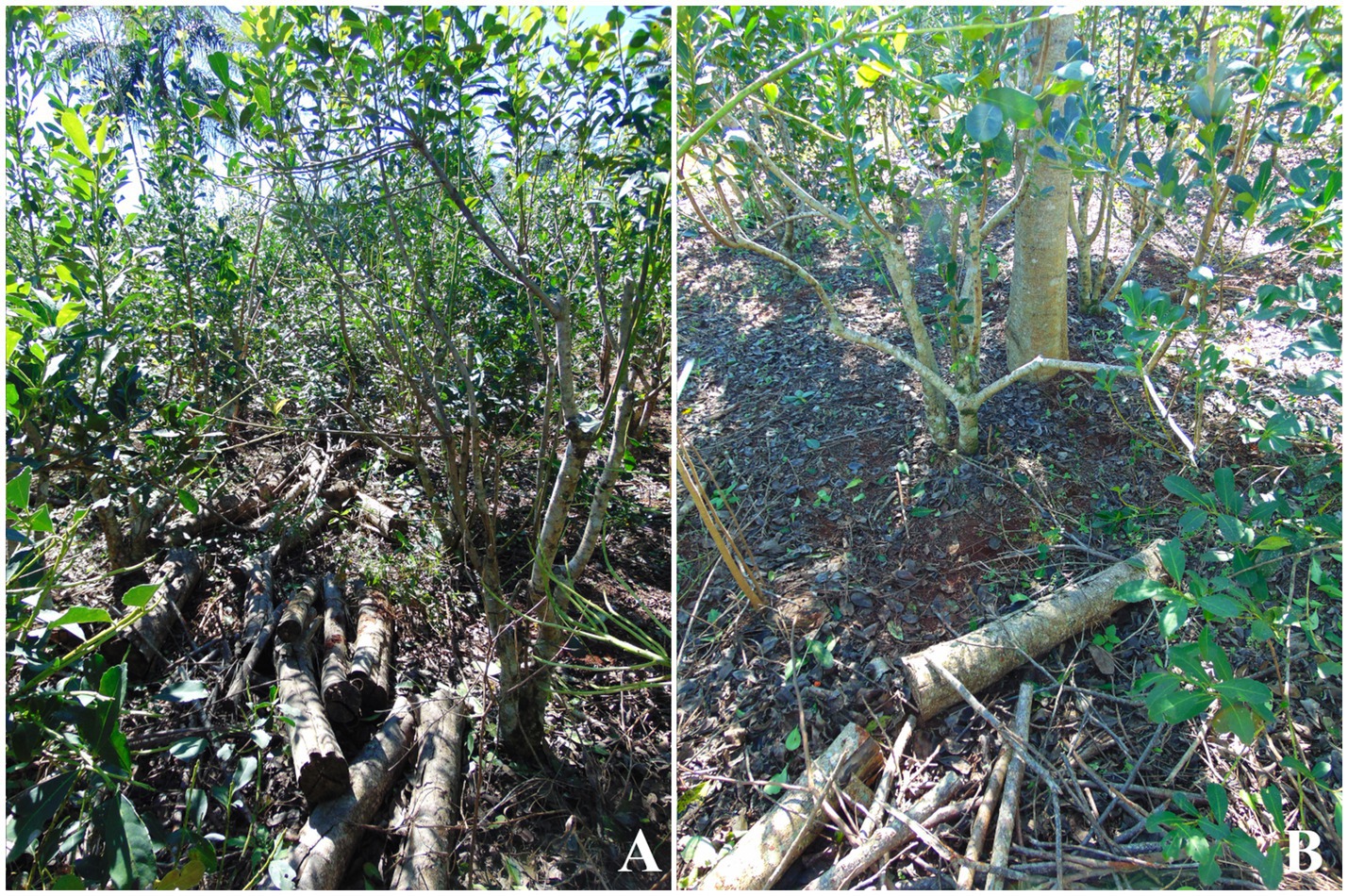
Figure 4. Integration of part of the biomass created to the soil. These logs were produced with the thinning that increased the distance between trees from 4.5 to 9 m during spring of 2018, and these images were acquired in May 2019.
3 Results
The first and unexpected result was the vigor and uniformity of growth of all the species of planted trees during the first year, with less than 5% of failures, height variations within less than 10% within species, and the high rate of development of the trees throughout the years (Supplementary Figures 1–3). The species that presented a greater challenge in terms of cultural agricultural maintenance were Kiri and Grevillea, due to their susceptibility to the ‘water mold’ Phytophthora and to ants, respectively. The seedlings are routinely taken to the field in pots after a preselection (see Methods). Besides, the system is constantly managed with interventions that modify the width of tree crowns and number of trees per planted line, see below, so we cannot derive conclusions about “selective pressures” or “fitness” across the species used in this context. Araucaria trees have a significantly slower growth rate compared to other native species and species commonly used in commercial forestry, whereas Kiri trees grow very quickly. Yerba mate, on the other hand, grows even more slowly than Araucaria. Consequently, all these species develop a canopy that provides shade, beneficial for the Yerba mate plants which are still young and undeveloped. Typically, Yerba mate plants are trimmed for the first time in their third year to shape them. This trimming process is repeated in the fourth year, a time when Yerba mate’s yield remains low.
The canopy developed by Kiri, and the size and weight of the trunks, rapidly exceeds all other species (Charts 3, 4), and the balance needed for positive synergies with yerba mate. While Kiri has a positive impact on the growth of yerba mate during the first 4 years, beyond 5 years the competition created by the shade is detrimental (Supplementary Figure 2). The trimming and thinning the Kiri trees causes more widespread damage to mate plants than the other species, affecting more plants, as it must be done very early when the trees are at high uniform density. In contrast, all the other species reach a comparable, large size (exceeding the optimal size range for this system) after many more years. By this time the tree density has been reduced by a factor of 6 or more leading to more limited damage from the thinning these trees, and several seasons of positive synergies have been accomplished. Due to these factors, Kiri was quickly discarded as a candidate species for our system, and it largely vanished except for small patches that retained the original density (see Supplementary Figure 2C) for a few more years. All Kiri trees died by 2020. However, it has been subsequently used by other producers of yerba mate attending to its short-term high commercial value which provides a countercyclical activity to their operations.
The evolution of the system into adult trees and mate plants was very favourable, with a steady and consistent development (see Figure 3), and vigorous growth of the tree species. The canopy was managed heuristically by direct human observation through pruning, trimming, and thinning, towards a photosynthetically active radiation (PAR) permeability of at least approximately 50% or above. During the first 6 years interventions were limited to the trimming of lower tree branches, with the dual purpose of enhancing the trees apical growth and diminishing their physical competition for space. Supplementary Figure 2 shows the overall aspect of several tree species at the age of 6 years. After 7 and 9 years the number of trees was reduced as described in the section “Treatment Details.” The branches and trunks were systematically cut down in size and distributed along the lines of the plantation to enrich the soil with organic carbon, as shown in Figure 4. In this agroforestry agricultural system, pruning and trimming yerba mate plants accentuates the growth disparity between the canopy of the trees and the mate itself, establishing a lower (mate) and a higher (tree crowns) stratum, and guiding the heuristic management of the trees, as clearly seen in Figure 3.
Table 2 presents a list of stem diameter at breast height (DBH) and tree height (H), which are the most commonly used measures of tree growth (Sterck and Bongers, 1998; Sumida et al., 2013), at the ages of 7 and 12 years. The highest values for DBH and height at year 7 were obtained for Kiri, 37 cm and 11 m respectively, which was phased out of this system. For the rest of the species, the highest values of DBH were measured in Toona, Grevilea, Loro negro, and Cañafístola, with 20, 16, 16, and 15 cm, respectively. The tallest trees were Grevilea, Toona, Loro negro, and Guatambu, with 11, 8.5, 8, and 7.5 m of height, respectively. The smallest values were measured in Lapacho negro, Guatambu, and Anchico, with 11, 10, and 9 cm, respectively. The smallest heights were Guatambu, Lapacho negro, and Anchico with 3.8, 3.5, and 3.5 m, respectively. The allometric relationship, which assesses how these two growth metrics, DBH and H, correlate across different species, appears consistent. This implies that species with higher DBH measurements tend to also be taller, indicating a proportional growth pattern among these species. The smallest DBH measurements at year 7 were observed in Lapacho negro, Guatambu, and Anchico, with correspondingly low heights, marking them as slower-growing species in the early years. By the year 2022, notable increases in both DBH and height are observed for all species, indicating continued growth. Toona showed exceptional growth, reaching a DBH of 37.4 cm and a height of 15 m, marking it as the fastest-growing species in this period. Araucaria shows moderate growth by 2022, confirming the slow growth rate of this species.
Measurements of photosynthetic active radiation (PAR) reaching the lower stratus after 7 years gave similar results in the range between 60 and 71% for Cañafístola, Toona, Grevilea, Guatambu, Lapacho negro, and Loro negro, see Table 2. For Anchico the PAR measurement was 53%, which, for practical purposes, aligns closely with the other species while transmitting slightly less sun light to yerba mate. For the remaining trees of Kiri (e.g., Supplementary Figure 2C) PAR measurements were 31%, validating the elimination of the species from the candidate list for this type of agroforestry system. The differences in recorded PAR values is due to tree canopy structure. On the other hand, due to their slow growth rate, Araucaria trees remained comparatively small and very thin. Even after 12 years, their height was still less than that of Cañafístola and Grevilea at 7 years, as detailed in Table 2. They require several more years to create a canopy stratum at a higher level. Consequently, PAR measurements for Araucaria were considered not relevant at this stage, as they are statistically undistinguishable from open sky measurements.
As illustrated in Table 3 and Chart 5, there is a relevant increase in total organic matter (TOM), from an average value of 3.06% in 2013 to 3.33% in 2021. Nonetheless, to fully account for the contributions to soil organic matter (SOM) by the trees, it is essential to consider the complete decomposition of the thick trunks of the thinned trees. This process is still ongoing at the time of writing this report and contributes incrementally to the soil’s organic content. This fact stands in stark contrast to the degradation of the soil typical of traditional, monoculture, yerba mate agriculture (Capellari et al., 2017). However, contrasting contributions are observed among the species studied. Loro negro and Araucaria have negatively impacted SOM, decreasing it by 0.16 and 0.46%, respectively. This reduction correlates with their narrow crowns and, in the case of Araucaria, its coniferous nature with less leafy, triangular-shaped needles. These features likely reduce the litter fall compared to species with broader crowns. All other species contributed to an increase in organic matter.
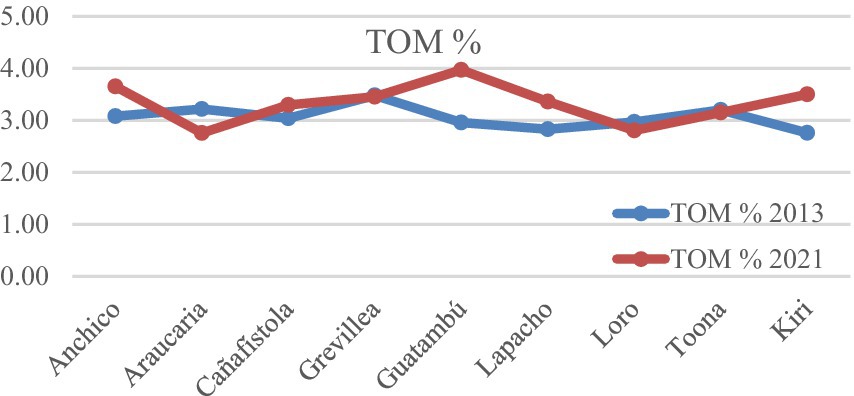
CHART 5. Comparative evolution of the total organic matter per tree species. The values of TOM for Toona and Grevilea are very similar in 2013 and 2021, and their p-values are 0.758 and 0.776. All the other differences in values for TOM (%) between 2021 and 2013 are different with a statistical significance better than a p-value of 0.05 for these samples (including the loss of SOM for Loro negro and Araucaria).
These results are consistent, as all these species feature well-developed, wide-spreading crowns that contribute extensive canopy coverage. Guatambu, an evergreen tree which features a dense roundish crown, continuously sheds, and renews leaves, contributed the highest increase in SOM over the observed period. We sampled the Kiri sublots where the trees that that were let die in place had fell and decomposed, and the result is an increase in total organic matter as expected. The positive effects on soil from various species consociated with yerba mate are well-documented, leading to the enrichment of micronutrients and key elements such as phosphorous, calcium, nitrogen, and magnesium (Fernandez et al., 1997).
The extreme climate event spanning the spring of 2021 and summer of 2022 brought the ecosystem services provided by agroforestry system into stark contrast with the fragility of yerba mate monocultures. A comparison between yerba mate plants closely consociated with various tree species and those planted further away from trees as shown in Figure 5 reveals that plants consociated to trees experience significantly less stress. Trees provided shade, moderated the temperature, and help conserve moisture in the soil. In the months following the end of the extreme weather event, plants within the agroforestry system returned to produce sprouts and to grow, while plants in the monoculture control required a much longer period and human intervention to prune dry branches. While the harvest of yerba mate in the agroforestry system decrease approximately 20% for the year, the monoculture decreased by more than 30%. Before the extreme climate events of droughts and heat the differences between mate production with or without trees were smaller and subtler, as these are perennial species, and the effects accrue throughout years.
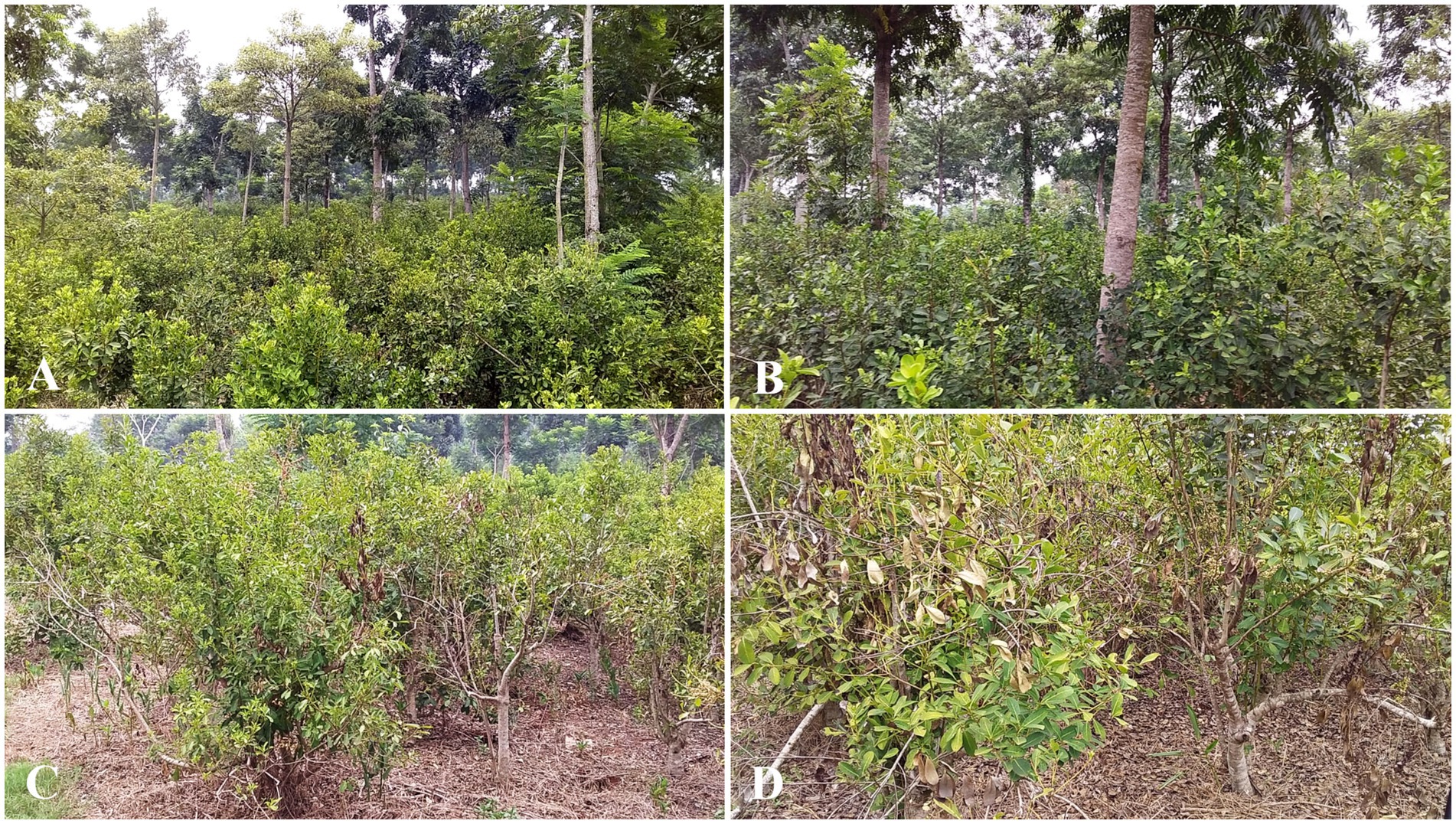
Figure 5. View of the experimental lot in 2022. A comparison between yerba mate plants consociated with trees, and plants without intercalated trees within the same lot. These are views within the agroforestry experimental pilot lot of 10 has planted in 2010, and an adjacent lot planted in 2011, on the same soil with the same history. (A) The tree-planted cultivation of yerba mate; (B) a close-up view of yerba mate plants in close association with trees. Species in the field of view include Toona, Grevilea, Loro negro, and Cañafístola; (C) the control lot without trees, an area immediately adjacent to that shown in (B); (D) close-up view of the state of yerba mate plants in (C), within the control area free of trees of the trial lot. There is a mix of species including Toona, Lapacho, and Loro negro more visible from the background. Photos taken in January 2022.
4 Discussion
4.1 What our results show
In this study, we have successfully designed and implemented a multispecies agroforestry system specifically tailored for the perennial cultivation of yerba mate (I. paraguariensis) in a subtropical region. To assess the viability of agroforestry in the production of yerba mate, we tested the suitability of several species of trees in a systematic way. All species were tested in small single species lots, randomized, and repeated four times by design. Trees were integrated with the cultivated species, not along boundary alleys, leveraging inter-species interactions. With a size of 10 has, the experimental lot has the adequate size to isolate causes and effects from the wider context. This integrated multispecies system is proposed as a viable and superior self-sustainable alternative to traditional monoculture practices, in full agreement with previous work (Capellari et al., 2017; Fernandez et al., 1997; Day et al., 2011; Montagnini et al., 2011; Montagnini et al., 2006). This system not only supports the growth of yerba mate but also promotes biodiversity (Comolli et al., 2023), enhances soil quality, and improves ecological resilience.
An important result is that well-chosen agroforestry systems can sustain higher yields of yerba mate harvests while also increasing SOM and improving the working environment. Specifically, yerba mate harvest yields were higher beneath the tree canopy compared to the control plot for species such as Loro negro, Lapacho negro, Toona, and Cañafístola. However, yields were slightly lower for Anchico, Grevilea, and Guatambu. Interestingly, despite its slower growth, Araucaria-associated yerba mate yields also surpassed those of the control after 12 years. This variance in yield over time can be attributed to the differing levels of competition posed by each species, highlighting the dynamic nature of their influence on yerba mate productivity. The biodiversity created by the multispecies integrated system also contributed to a significantly lower incidence of detrimental insects relative to yerba mate traditional monocultures. These results are further discussed in another paper (Comolli et al., 2023) that compiles complementary information for a more general readership and divulgation of the value of agroforestry.
The system prevents soil degradation and irreversible loss of fertility and helps to restore already degraded lands. While the management of the trees through trimming and thinning provides organic matter, their roots help keep the permeability of the soil. Rainfall water runoff is considerably decreased, increasing soil water retention at higher depths. For optimal results, it is recommended that several of the tested species—excluding Kiri—be combined in alternating sequences. This approach would allow for more uniform permeability to photosynthetically active radiation (PAR), create a more natural habitat for birds, and support a broader range of biodiversity. Two species, Araucaria and Loro negro, did not prevent the loss of soil organic carbon typical of yerba mate monocultures (Capellari et al., 2017), a result consistent with their small and narrow crowns The intercalation of multiple species will solve this problem by spreading the contributions to the soil across them. Another important result was the performance of the system as climate change mitigation and adaptation strategy. During the extreme weather events of the spring and summer of 2021 and 2022, the system proved to be significantly more resilient. Plants within the system suffered only a delay in their evolution but no significant damages, while monocultures were devastated. The system harbours birds, and detrimental insect counts reveal average populations to be half, or lower, than outside the system in monoculture lots (Comolli et al., 2023). During the climate shock it was more common to find small animals within the slightly more benign environment provided by the agroforestry system (Comolli et al., 2023), which served a bit as a sanctuary.
4.2 Previous work and context
The effects of trees on the soil of yerba mate cultivation are well documented (Fernandez et al., 1997; Day et al., 2011; Montagnini et al., 2011; Montagnini et al., 2006). The consociation of trees across several species has a significant impact increasing micronutrients in the soil. In addition to organic matter, different species have measurable impact on the content of, e.g., phosphorus, nitrogen, calcium, magnesium (Fernandez et al., 1997; Day et al., 2011; Montagnini et al., 2011). In our case, we fertilize the system with standard, commercial triple nitrogen (N), phosphorus (P) and potassium (K; NPK) at the rate of 50 kg per 1,000 kg of harvested yerba mate distributed uniformly across the whole lot. As we cannot accurately deconvolute the contributions from both sources, we do not report them as absolute values here, but we observe variations across species analogous as the reported variations in organic matter. For example, Toona, Anchico, and Araucaria sublots have higher nitrogen content than the rest, Lapacho, Loro negro, and Toona higher phosphorous content, followed closely by Cañafístola and Anchico while Grevilea has a lesser improvement in values and Guatambu a decrease in values (see Supplementary Chart 1).
Although this project is not about planting tree forests, it is aligned and consistent with work showing much greater response ratios for planted forests made of a variety of different species than mono species (Gurevitch, 2022; Hua et al., 2022; Feng et al., 2022). Forest restoration projects based on multispecies achieve considerably higher results than monospecies using, as metrics, biomass production, above ground biomass, water retention, erosion control, species specific abundance, and biodiversity (Gurevitch, 2022; Hua et al., 2022; Feng et al., 2022). Indeed, multispecies restoration projects can approach values typical of native forests for these metrics (Gurevitch, 2022; Hua et al., 2022; Feng et al., 2022).
4.3 Climate change, adaptation, and mitigation
This conceptual framework also addresses the linked crisis of biodiversity loss, and climate change adaptation and mitigation. As the world continues to warm, climate hazards are expected to increase in frequency and intensity. The impacts of extreme events will also be more severe due to the increased exposure (growing population and development) and vulnerability (aging infrastructure) of human settlements (AghaKouchak et al., 2020). We all depend on nature for our food, air, water, energy, and raw materials. Nature and biodiversity make life possible, provide health and social benefits and drive our economy. Healthy ecosystems can also help us cope with the impacts of climate change. However, natural ecosystems and their vital services are under pressure from urban sprawl, intensive agriculture, pollution, invasive species, and climate change (Feng et al., 2022; Our World in Data, 2021; World Resources Institute, 2019; Food and Agriculture Organization of the United Nations (FAO), 2024; Intergovernmental Science-Policy Platform on Biodiversity and Ecosystem Services (IPBES), 2016). Integrated sustainable agroforestry systems provide a high intrinsic value independently of hypothetical scenarios of biodiversity and ecosystem services, and leverage Nature’s Contributions to People (NCP; Food and Agriculture Organization of the United Nations (FAO), 2024; Intergovernmental Science-Policy Platform on Biodiversity and Ecosystem Services (IPBES), 2016; Wilson and Lovell, 2016; van Noordwijk et al., 2021; van Noordwijk, 2021). They provide a set of strategies with the flexibility to incorporate a diversity of values, forms of knowledge or “ways of knowing,” which top-down solutions cannot provide (Meyfroidt et al., 2022; UN Environment Program, 2023; Wilson and Lovell, 2016; van Noordwijk et al., 2021; van Noordwijk, 2021).
Ecological and Conservation goals are not simply about “combating climate change,” adaptation and mitigation. Nor about aesthetic notions of nature and conservation, although there is wisdom as well in such views. Henry D. Thoreau (Cunningham, 2019) had already formulated a modern environmental philosophy suited for the sustainable development goals (SDGs) in the context of climate change. Observing the environmental degradation wrought by technological development and economic growth, in the second half of the 1800s he called for a revaluation of the natural world as having intrinsic worth (Cunningham, 2019). Indeed, the genetic codes of living organisms constitute the digital library of evolutionary adaptations of all life on Earth, a vast knowledge. A good deal of conservation should perhaps be done also for scientific reasons. We simply do not know enough about specific biological entities and about the web of connections that underpins our biosphere. What is clear however, is that we do not “extract” resources from an infinity pool, nor do we dump “externalities” into infinite sinks. We set agencies in motion as our actions cause reactions or responses.
Agroforestry projects based on an extensive search for maximum possible complexity do halt and reverse soil degradation, help reverse biodiversity loss, and provide some of the benefits, to a degree, provided by forests such as local climate modulation (Meyfroidt et al., 2022). Healthier ecosystems with richer biodiversity also yield greater long-term benefits such as more fertile soils, bigger yields of timber, and larger stores of greenhouse gases (UN Environment Program, 2023; Food and Agriculture Organization of the United Nations (FAO), 2024; Intergovernmental Science-Policy Platform on Biodiversity and Ecosystem Services (IPBES), 2016; Wilson and Lovell, 2016; van Noordwijk et al., 2021; van Noordwijk, 2021). We will always need farmland and infrastructure on land that was once forest. The open question is how ecosystems, like societies, will adapt to a changing climate (Our World in Data, 2021; World Resources Institute, 2019). If significant extensions of farmland and extensive free land area subject to human-induced degradation were converted to ambitious agroforestry schemes across scales, based on a sufficiently high number of consociated species instead of monocultures as we show in this work, a high impact in carbon sequestration, local climate modulation, land, and biodiversity restoration, could be achieved (Wilson and Lovell, 2016; van Noordwijk et al., 2021; van Noordwijk, 2021; Cunningham, 2019; Reith et al., 2020; Rodrigues et al., 2023). Actions that help reduce losses of biodiversity are found to also benefit the climate (Shin et al., 2022), and we have today unprecedented technological innovations including a digital infrastructure that could be leveraged towards SDGs (TWI2050, 2019; TWI2050, 2020).
The long-term sustainability and resilience of the system hinge on how the soil evolves and how the system responds to the increasingly frequent climate shocks and extreme climate events occurring worldwide. Our findings demonstrate the system’s effectiveness in conserving soil fertility and highlight its substantial benefits in mitigating the impacts of extreme climate events. In our view, these are two of the most critical results of our research.
5 Conclusion
Our findings illustrate the robustness of this agroforestry model in integrating diverse plant species with yerba mate cultivation. We demonstrate that incorporating trees into the production of yerba mate leverages the natural synergies between different species and provides significant benefits. The system has the capacity to sustain and restore soil fertility and create a viable, sustainable long-term cultivation strategy. It also helps to restore part of the biodiversity historically typical of the region and enhances resilience to climate change.
The scale of the experiment adequately enables the acquisition of relevant data and the formulation of conclusions of general validity. Our experimental system is scalable, capable of being expanded or reduced in size, and can be managed with or without mechanization, depending on the scale. Additionally, the experiment resulted in the creation of sufficient experience to further expand this initiative. This type of knowledge can help improve land management and reverse land abandonment in the region and elsewhere (Rodrigues et al., 2023). Implementing such agroforestry systems can significantly impact carbon sequestration, local climate modulation, and land and biodiversity restoration. As climate hazards increase in frequency and intensity due to global warming, integrated sustainable agroforestry systems provide a high intrinsic value, supporting Nature’s Contributions to People (NCP) and offering flexible strategies that incorporate diverse values and knowledge forms.
Overall, our study shows that well-designed agroforestry systems can sustain higher yields of yerba mate harvests while increasing SOM and improving the working environment. They prevent soil degradation, help restore degraded lands, and support greater biodiversity. These systems represent a superior, sustainable alternative to traditional monoculture practices, offering significant ecological, economic, and social benefits.
Data availability statement
The original contributions presented in the study are included in the article/Supplementary material, further inquiries can be directed to the corresponding author.
Author contributions
LC: Conceptualization, Data curation, Formal analysis, Funding acquisition, Investigation, Methodology, Project administration, Resources, Software, Supervision, Validation, Visualization, Writing – original draft, Writing – review & editing. ES: Formal analysis, Methodology, Project administration, Supervision, Writing – review & editing. CI: Conceptualization, Data curation, Investigation, Methodology, Supervision, Writing – review & editing. NM: Conceptualization, Data curation, Investigation, Methodology, Writing – review & editing. HF: Conceptualization, Formal analysis, Investigation, Methodology, Visualization, Writing – review & editing. AW: Conceptualization, Formal analysis, Investigation, Methodology, Writing – review & editing. NB: Writing – review & editing. PG: Data curation, Formal analysis, Investigation, Methodology, Writing – review & editing. SB: Investigation, Writing – review & editing, Data curation, Formal analysis, Methodology. MG: Conceptualization, Investigation, Resources, Writing – review & editing. FW: Conceptualization, Investigation, Methodology, Resources, Supervision, Writing – review & editing.
Funding
The author(s) declare that financial support was received for the research, authorship, and/or publication of this article. The whole project is funded by El Rocio S.A., a primary agricultural producer, solely dedicated to the activity of raw yerba mate production in previously degraded lands. No branding, marketing, or proprietary commercial activities are involved. All the yerba mate production is sold as an undifferentiated raw, green harvest, to the local cooperative Productores de Yerba Mate de Santo Pipó SCL.
Acknowledgments
LC, ES, and CI acknowledge the generous time given to this project by all members of the Instituto Nacional de Tecnología Agropecuaria (INTA) whose encouragement, enthusiasm, knowledge and moral support made this project possible.
Conflict of interest
The authors declare that the research was conducted in the absence of any commercial or financial relationships that could be construed as a potential conflict of interest.
The author(s) declared that they were an editorial board member of Frontiers, at the time of submission. This had no impact on the peer review process and the final decision.
Publisher’s note
All claims expressed in this article are solely those of the authors and do not necessarily represent those of their affiliated organizations, or those of the publisher, the editors and the reviewers. Any product that may be evaluated in this article, or claim that may be made by its manufacturer, is not guaranteed or endorsed by the publisher.
Supplementary material
The Supplementary material for this article can be found online at: https://www.frontiersin.org/articles/10.3389/ffgc.2024.1424174/full#supplementary-material
References
AghaKouchak, A., Chiang, F., Huning, L. S., Love, C. A., Mallakpour, I., Mazdiyasni, O., et al. (2020). Climate extremes and compound hazards in a warming world. Annu. Rev. Earth Planet. Sci. 48, 519–548. doi: 10.1146/annurev-earth-071719-055228
Bracesco, N., Sanchez, A. G., Contreras, V., Menini, T., and Gugliucci, A. (2011). Recent advances on Ilex paraguariensis research: minireview. J. Ethnopharmacol. 136, 378–384. doi: 10.1016/j.jep.2010.06.032
Capellari, P. L., Valentín, L., and Ricardo DanielIcon, M. (2017). Yerba mate, reseña histórica y estadística, producción e industrialización en el siglo XXI. 1st Edn. Consejo Federal de Inversiones: Ciudad Autónoma de Buenos Aires, 310.
Comolli, L. R., Schegg, E., Infuleski, C., Fassola, H., von, A., Bulfe, N., et al. (2023). A sustainable integrated agroforestry system. agriRxiv 2023. doi: 10.31220/agriRxiv.2023.00180
Cunningham, J. (2019). Thoreau's surprisingly modern environmental philosophy. Medium. Available at: https://medium.com/@jackcunningham_21334/thoreaus-surprisingly-modern-environmental-philosophy-a2c7cac7cd54 (Accessed April 18, 2024).
Day, S., Montagnini, F., and Eibl, B. (2011). Effects of native trees in agroforestry systems on the soils and yerba mate in Misiones, Argentina, in ration. Eds. Montagnini, F., Francesconi, W. and Rossi, E. Nova New York: Science Publishers. 99–112.
Feng, Y., Schmid, B., Loreau, M., Forrester, D., Fei, S., Zhu, J., et al. (2022). Multispecies forest plantations outyield monocultures across a broad range of conditions. Science 376, 865–868. doi: 10.1126/science.abm6363
Fernandez, R., Montagnini, F., and Hamilton, H. (1997). The influence of five native tree species on soil chemistry in a subtropical humid forest region of Argentina. J Trop Forest Sci. 10, 188–196.
Fischer, E. M., Sippel, S., and Knutti, R. (2021). Increasing probability of record-shattering climate extremes. Nat. Clim. Chang. 11, 689–695. doi: 10.1038/s41558-021-01092-9
Food and Agriculture Organization of the United Nations (FAO) (2024). Agroforestry. Available at: https://www.fao.org/forestry/agroforestry/en/ (Accessed April 18, 2024).
Gawron-Gzella, A., Chanaj-Kaczmarek, J., and Cielecka-Piontek, J. (2021). Yerba mate—a long but current history. Nutrients 13:3706. doi: 10.3390/nu13113706
Google Maps (2024). Available at: https://www.google.com.ar/maps/@-27.142163,-55.393091,2268m/data=!3m1!1e3?hl=en; https://maps.app.goo.gl/9zSb4Qc6bdwq2iHZ8 (Accessed April 18, 2024).
Gurevitch, J. (2022). Managing forests for competing goals. Science 376, 792–793. doi: 10.1126/science.abp8463
Heck, C. I., and De Mejia, E. G. (2007). Yerba mate tea (Ilex paraguariensis): a comprehensive review on chemistry, health implications, and technological considerations. J. Food Sci. 72, R138–R151. doi: 10.1111/j.1750-3841.2007.00535.x
Hua, F., Bruijnzeel, L., Meli, P., Martin, P., Zhang, J., Nakagawa, S., et al. (2022). The biodiversity and ecosystem service contributions and trade-offs of forest restoration approaches. Science 376, 839–844. doi: 10.1126/science.abl4649
Intergovernmental Science-Policy Platform on Biodiversity and Ecosystem Services (IPBES) (2016). Scenarios and models. Available at: https://ipbes.net/scenarios-models (Accessed April 18, 2024).
Meyfroidt, P., de Bremond, A., Ryan, C. M., Archer, E., Aspinall, R., Chhabra, A., et al. (2022). Ten facts about land systems for sustainability. PNAS 119:e2109217118. doi: 10.1073/pnas.2109217118
Montagnini, F., Eibl, B., and Fernández, R. A. (2006). Rehabilitation of degraded lands in Misiones, Argentina. Bois et Forets des Trop. 288, 51–65. doi: 10.19182/bft2006.288.a20317
Montagnini, F., Francesconi, W., and Rossi, E. (2011). Agroforestry as a tool for landscape restoration. New York: Nova Biomedical, 201.
Our World in Data: Hannah Ritchie, Fiona Spooner and Max Roser (2021). “Forests and Deforestation” Published online at OurWorldInData.org. Retrieved from: https://ourworldindata.org/forests-and-deforestation [Online Resource] (Accessed April 18, 2024).
Pereyra, F. X. (2012). Suelos de la Argentina. Buenos Aires, Argentina: Ed. INTA-SEGEMAR-AACS-GAEA, ANALES N° 50 178 pp. Available at: https://core.ac.uk/download/pdf/287302379.pdf (Accessed April 18, 2024).
Reith, E., Gosling, E., Knoke, T., and Paul, C. (2020). How much agroforestry is needed to achieve multifunctional landscapes at the forest frontier?—coupling expert opinion with robust goal programming. Sustain. For. 12:6077. doi: 10.3390/su12156077
Roca, M. V. (2017). “Archaeology, heritage, and development in two south American colonial sites: the Guarani-Jesuit Missions (1610–1767)” in Collision or collaboration. eds. P. Gould and K. Pyburn (Cham: Springer), 117–135.
Rodrigues, C. I. D., Brito, L. M., and Nunes, L. J. R. (2023). Soil carbon sequestration in the context of climate change mitigation: a review. Soil Syst. 7:64. doi: 10.3390/soilsystems7030064
Roset, P. (2022). Sequía e incendios se agravan en el norte del país. SLT – Sobre La Tierra, Facultad de Agronomía, Universidad de Buenos Aires. Available at: http://sobrelatierra.agro.uba.ar/sequia-e-incendios-se-agravan-en-el-norte-del-pais/ (Accessed April 18, 2024).
Sarreal, J. (2023). Yerba mate: the drink that shaped a nation. Berkeley: University of California Press, 394.
Shin, Y. J., Midgley, G. F., Archer, E. R. M., Arneth, A., Barnes, D. K. A., Chan, L., et al. (2022). Actions to halt biodiversity loss generally benefit the climate. Glob. Chang. Biol. 28, 2846–2874. doi: 10.1111/gcb.16109
Sterck, F. J., and Bongers, F. (1998). Ontogenetic changes in size, allometry, and mechanical design of tropical rain forest trees. Am. J. Bot. 85, 266–272. doi: 10.2307/2446315
Sumida, A., Miyaura, T., and Torii, H. (2013). Relationships of tree height and diameter at breast height revisited: analyses of stem growth using 20-year data of an even-aged Chamaecyparis obtusa stand. Tree Physiol. 33, 106–118. doi: 10.1093/treephys/tps127
TWI2050 (2019). The digital revolution and sustainable development: opportunities and challenges. International Institute for Applied Systems Analysis (IIASA), Laxenburg, Austria; Available at: http://pure.iiasa.ac.at/id/eprint/15913/; http://pure.iiasa.ac.at/id/eprint/15913/1/TWI2050-for-web.pd (Accessed April 18, 2024).
TWI2050 (2020). Innovations for sustainability. Pathways to an efficient and post-pandemic future. International Institute for Applied Systems Analysis (IIASA), Laxenburg, Austria; Available at: http://pure.iiasa.ac.at/id/eprint/16533/; http://pure.iiasa.ac.at/id/eprint/16533/1/TWI2050web%20%281%29.pdf (Accessed April 18, 2024).
UN Environment Program (2023). Decade on restoration. Available at: https://www.decadeonrestoration.org/ (Accessed April 18, 2024).
UNESCO’s World Heritage Centre (2023). Yerba Mate Cultural Landscape (PCYM). Available at: https://whc.unesco.org/en/tentativelists/6612/ (Accessed April 18, 2024).
van Noordwijk, M. (2021). Agroforestry-based ecosystem services: reconciling values of humans and nature in sustainable development. Land 10:699. doi: 10.3390/land10070699
van Noordwijk, M., Coe, R., Sinclair, F. L., Luedeling, E., Bayala, J., Muthuri, C. W., et al. (2021). Climate change adaptation in and through agroforestry: four decades of research initiated by Peter Huxley. Mitig. Adapt. Strateg. Glob. Chang. 26:18. doi: 10.1007/s11027-021-09954-5
Walker, D. W., and Van Loon, A. F. (2023). Droughts are coming on faster. Science 380, 130–132. doi: 10.1126/science.adh3097
Walkley, A. J., and Black, I. A. (1934). Estimation of soil organic carbon by the chromic acid titration method. Soil Sci. 37, 29–38.
Wilson, M. H., and Lovell, S. T. (2016). Agroforestry—the next step in sustainable and resilient agriculture. Sustain. For. 8:574. doi: 10.3390/su8060574
World Resources Institute (2019). Road to restoration. Available at: https://www.wri.org/research/road-restoration (Accessed April 18, 2024).
Yuan, X., Wang, Y., Ji, P., Wu, P., Sheffield, J., and Otkin, J. A. (2023). A global transition to flash droughts under climate change. Science 380, 187–191. doi: 10.1126/science.abn6301
Keywords: agroforestry, multispecies, sustainability, restoration, biodiversity, adaptation, mitigation
Citation: Comolli LR, Schegg E, Infuleski C, Munareto N, Fassola H, von Wallis A, Bulfe NM, González P, Barth SR, Gauchat ME and Wyss F (2024) Implementing a sustainable integrated agroforestry system for the cultivation of Ilex paraguariensis. Front. For. Glob. Change. 7:1424174. doi: 10.3389/ffgc.2024.1424174
Edited by:
Yashmita Ulman, Narendra Dev University of Agriculture and Technology, IndiaReviewed by:
Francis Dube, University of Concepción, ChileTim Payn, New Zealand Forest Research Institute Limited (Scion), New Zealand
Copyright © 2024 Comolli, Schegg, Infuleski, Munareto, Fassola, von Wallis, Bulfe, González, Barth, Gauchat and Wyss. This is an open-access article distributed under the terms of the Creative Commons Attribution License (CC BY). The use, distribution or reproduction in other forums is permitted, provided the original author(s) and the copyright owner(s) are credited and that the original publication in this journal is cited, in accordance with accepted academic practice. No use, distribution or reproduction is permitted which does not comply with these terms.
*Correspondence: Luis R. Comolli, bHJjb21vbGxpQGdtYWlsLmNvbQ==