- 1State Key Laboratory of Soil and Sustainable Agriculture, Institute of Soil Science, Chinese Academy of Sciences, Nanjing, China
- 2School of Economic Geography, Hunan University of Finance and Economics, Changsha, China
- 3State Key Laboratory of Subtropical Silviculture, Zhejiang Agriculture & Forestry University, Hangzhou, China
Phyllostachys praecox is a shallow-rooted bamboo that often encounters hypoxia conditions which could be induced by long-term organic material mulching or flooding. It is important to uncover the effect of mulching and flooding on soil nutrient, ammonia-oxidizing microbes, and bacterial diversity. We set up field pot experiments with three treatments (control, mulching, and flooding) under P. praecox. Mulching or flooding altered soil conditions significantly, and both increased ammonium-nitrogen (NH4+-N), total phosphorus (TP), available P (AP), and available potassium (AK) concentrations, and decreased oxygen (O2) concentrations over control. Flooding increased pH and decreased nitrate-nitrogen (NO3−-N), while mulching decreased soil pH and NO3−-N. As O2 content decreased, archaeal 16S rRNA, amoA gene copies of ammonia-oxidizing archaea (AOA) and ammonia oxidizing bacteria (AOB) increased. Mulching and flooding decreased Shannon, ACE and Chao 1 diversity when compared with the control, and as the O2 contents decreased, bacterial diversity decreased. Redundancy Analysis revealed O2, NO3−-N, AK, AP, and pH were the major factors driving bacterial community structure. Correlation Analysis showed AK and O2 contents were highly correlated with bacterial community structure. In addition, structural equation modeling indicated that O2 facilitated efficient soil N use mainly through soil pH, AK content, and bacterial diversity. Mulching or flooding exerted great effects on environment factor and bacterial community structure, which could be exploited to facilitate the regulation of soil O2 conditions for sustainable P. praecox production.
1 Introduction
Plants are frequently exposed to low oxygen (O2) levels in soil attributable to various external factors, which can drastically affect plant growth and development (Kozlowski, 1997; Dat et al., 2006). Phyllostachys praecox is a shallow-rooted bamboo susceptible to soil O2 content (Evans et al., 2012; Gao et al., 2023). The major factors influencing soil O2 content are gaseous exchange between soil and the atmosphere, soil water content, and soil microbial O2 consumption rate (Bray and Brawley, 2002). Previous studies have demonstrated that the bamboo often experiences two types of soil hypoxia, one caused by flooding, as soil voids are occupied by water, and another caused by organic material mulching, which reduces gaseous exchange and increases O2 consumption via organic matter decomposition (Chen et al., 2005; Xu et al., 2017). The two types of hypoxias could have distinct effects on soil properties and microbial community structure.
Soil properties are key factors influencing healthy plant growth (Jiao et al., 1997; Deng et al., 2014). In bamboo production, mulching is used to stimulate shoot dormancy breaking following organic matter decomposition and increase in temperature (Lin et al., 2020). However, over time, soil nutrient balance is affected by mulching, severe rotting occurs, and yield decreases (Yuan, 2009). Previous studies have reported that the primary reasons for bamboo forest deterioration under mulching are organic matter decomposition and O2 depletion, which cause the roots to rise to the soil surface, leading to bamboo mortality. Qian et al. (2022) observed that 3–4 years of mulch induced soil acidification and limited soil nutrient utilization. In addition, Chen et al. (2014) observed that soil nitrogen (N) N: phosphorus (P) and potassium (K): P decreased significantly and the correlations among soil nutrients were weakened significantly following long-term mulching management. In semi-arid orchards, mulching increased soil organic matter, total N (TN), and nitrate-N (NO3−-N) concentrations (Shen, 2019).
Flooding, conversely, alters soil water, gas, and temperature conditions, and reduces soil redox potential. Specifically, flooding impairs soil ventilation, increases temperature, causes N loss, reduces available N (AN) content, and influences soil nutrient morphology and transformation (Li et al., 2006; Shen et al., 2016). For example, as flooding time is extended, soil available P (AP) concentration increases dramatically (Liang, 1996). Moreover, the P release from rice soil decreases after flooding, whereas the exchangeable K level in soil remains high (Dobermann et al., 1996; Zhang et al., 2002). In addition, flooding can reduce the fixation of exogenous K and increase AK content in soil (Guo et al., 2003).
Soil microorganisms participate in various biochemical reaction processes and are the main decomposing agents of organic matter (Wu et al., 2024). Soil microorganisms store effective plant nutrients and regulate the effectiveness of soil C and N nutrients and their cycling characteristics (Spehn et al., 2000). Nitrification is a key process in the global N cycle, with conversion of ammonia to nitrite by ammonia oxidizing bacteria (AOB) as the rate-limiting step (Qin et al., 2012). Ammonia-oxidizing archaea (AOA) and AOB are the major microbes involved in the process (Guo and Peng, 2008). Mulching increased AOA and AOB abundance and promoted soil N cycling (Shen, 2019). In addition, Cao et al. (2019) observed that long-term rice flooding resulted in low O2 stress to ammoxic microbes in paddy soil, and selectively promoted AOA growth. At the same time, it was found that AOA had a wider range of ammonium-N (NH4+-N) substrates than AOB. In addition, Jiang et al. (2009) observed that AOA were more abundant than AOB in oxic lake water, with an opposite trend in anoxic sediments. Furthermore, a study showed that mulch increased soil bacterial community abundance and diversity and altered its structure, with an increase in dominance of Proteobacteria, Acidobacteria, and Actinomyces (Shen, 2019). However, flooding changed the abundance of bacteria and the diversity of the dominant taxa, resulted in new dominant phyla: Firmicutes and Bacteroidetes (Kan et al., 2014). Currently, our understanding of bacterial adaptation to mulching or flooding remains poor, and investigation of the varied responses could provide insights and a theoretical basis for regulation of soil O2 and facilitate sustainable bamboo production.
In the present study, field pot experiments were conducted under P. praecox to investigate the effects of mulching or flooding on the bacterial community structure, AOA and AOB abundance, and soil physicochemical characteristics. We hypothesized that different hypoxia conditions would lead to different changes in soil microbial communities, thus affecting soil nutrient utilization. The results of the present study could elucidate the mechanisms via which soil respond to various hypoxia under P. praecox and provide a reference for effective management of P. praecox plantations.
2 Materials and methods
2.1 Experimental site
The experiments were performed at the Panmugang Modern Forestry Demonstration Base of Zhejiang Agriculture and Forestry University, Zhejiang Province, China (119°58′ E, 30°29′ N). The area has a subtropical monsoon climate with an average annual temperature of 17.8°C, an average annual relative humidity of 70.3%, an annual precipitation of 1,454 mm, a frost-free period of 234 d, and 1,765 h of sunshine per year. The agricultural area has a hilly environment with hills typically less than 150 m high. The soil is classified as a Ferralsol since its source material is mostly quaternary sandstone. Natural precipitation and soil water storage are the primary sources of water used for agricultural production (Xu et al., 2017).
2.2 Experimental design
Field pot experiments were conducted including (1) control, (2) mulching, and (3) flooding, under P. praecox. There were three replicates per treatment and nine seedlings per replicate. We planted 81 seedlings, a diameter at breast of 3.89 cm were planted in pots (bottom diameter 32 cm, height 35 cm), for each treatment then buried them in bamboo groves. Finally, three samples were taken randomly for measurement. Control treatments were normal growth, uncovered and unflooded bamboos. The flooding water was 5 cm above the soil surface and the mulching procedure was as follows. At first, 0.45 kg/m2 of chicken manure was sprinkled on the soil surface. Subsequently, the chicken manure was covered with straw (0.375 kg/m2). Afterward, rice bran (41.25 kg/m2) was sprinkled on top to a height of 15 cm above the soil surface. The experiments were initiated on17th December, 2020, and ran for 20 days. Samples were collected on March 7, 2021. In each pot, rhizosphere soil was sampled from a depth of 20–30 cm and transported to the laboratory for analysis. The rhizosphere soil was collected as follows: (1) Bulk soils of the root were shaken off in the ultra-clean bench, and about 1 mm attached to the roots as rhizosphere soil (Edwards et al., 2015). (2) The root samples were transferred into a 50 mL sterile centrifuge tube containing 20 mL 10 mM PBS solution and placed in a shaker at 120 rpm for 20 min at room temperature (Beckersz et al., 2017). (3) Roots were removed in 50 mL centrifuge tubes using sterile forceps and the remaining suspension was centrifuged at high speed (6000 × g, 4°C, 20 min) to collect the rhizosphere soil (Qin et al., 2018). (4) The collected rhizosphere soil samples were frozen in liquid nitrogen and then stored in an ultra-low temperature refrigerator of −80°C.
2.3 Soil physicochemical properties
The Kjeldahl method was used to determine TN (Bremner, 2009). NH4+-N and NO3−-N contents in soil were measured using indophenol blue colorimetry and dual wavelength colorimetry, respectively (Bao, 2000). TP and AP were determined using a colorimetric method (Lu, 2000); TK and AK were determined using flame photometry (Xiong et al., 2008). According to Gao et al. (2023), soil O2 concentration was measured using a fiber-optic O2 meter.
2.4 DNA extraction and real-time quantitative PCR
Total genomic DNA was extracted from each soil sample according to the manufacturer’s instructions (FastDNA & SPIN Kit, MP, United States). The purity and quantity of the isolated DNA were determined using 1% agarose gel electrophoresis and a spectrophotometer (NanoDrop® 1,000, Thermo Fisher Scientific, United States).
The number of amoA copies in the bacterial (AOB) and archaeal (AOA) kingdoms were used to calculate the concentrations of ammonia mono-oxygenase. Functional marker genes and 16S rRNA were measured using fluorescent dye Ultra SYBR Mixture (Takara, RR820A, Japan) and gene-specific primers. The QuantStudio 6 Real-Time PCR instrument (Thermo Scientific, MA, United States) was used for the qPCR analysis. The thermal programs and reaction mixtures were in accordance with the manufacturers’ recommendations. The gene-specific qPCR primers are listed in Supplementary Table S1. The PCR reaction solution is listed in Supplementary Table S2. The procedures of soil microbe analyze in real-time PCR are listed in Supplementary Table S3. The MIQE criteria were used to assess and examine the qPCR findings (Bustin et al., 2009). R2 > 0.99 indicated that the target gene was amplified with a rate ranging from 96 to 105%.
2.5 High-throughput gene sequencing and bioinformatics analysis
Three duplicate samples of each soil type were used to sequence the V3–V4 regions of the 16S rRNA. Amplicon samples were examined on an Illumina MiSeq instrument using the industry-standard procedures. An Illumina® MiSeq Sequencer (Illumina, Inc., United States) was used to examine soil microbial community structure. The 16S rRNA genes were sequenced using MiSeq and the ubiquitous primers 338F (5′- ACTCCTACGGGAGGCAGCAG-3′) and 806R (5’-GGACTACHVGGGTWTCTAAT-3′) (Peiffer et al., 2013; Li et al., 2020). The PCR reaction system and amplification procedures were as described previously (Zhang et al., 2019). Prior to measurement with a QuantiFluor®-ST Fluorometer, the PCR products were separated on 2% agarose gel and purified using an AxyPrepTM DNA Gel Extraction Kit (Axygen Scientific Inc., USA) (Promega Corp., United States). Afterward, Majorbio Bio-Pharm Technology Co. Ltd. (Shanghai, China) carried out cluster formation, 250-bp pair-end sequencing, and Illumina pair-end library preparation.
Short and poor-quality reads, singletons, triplicates, and chimeras were removed, obtaining 553,381 clean reads. Based on a 97% similarity, a total of 3,799 Operational Taxonomic Units (OTUs) were found. The raw readings were deposited in the NCBI Sequence database (Accession Number: SUB12955876).
QIIME v1.701 was used to depolymerize, quality filter, and treat the unprocessed Illumina FASTQ sequence data. To obtain accurate tag data, any discovered chimera sequences were matched to those within the reference Genomes Online Database. UPARSE v7.12 was used to classify non-repeating gene sequences as OTUs using a fixed 97% threshold. To gather species annotation information, the RDP Classifier method was used to compare OTU representative sequences against the SILVA (SSU123) 16S rRNA database.
2.6 Statistical analysis
Principal Co-ordinates Analysis (PCoA), based on Bray-Curtis distance measurements, was used to examine changes in bacteria community composition, which were illustrated using the “Vegan” and “ggplot2” packages in R v4.0.2 (R Foundation for Statistical Computing, Vienna, Austria) (Buza et al., 2019). To quantify differences between the control and other treatments, the Bray–Curtis dissimilarity index was determined using the “vegdist” function in the “vegan” package. The index was generated by calculating the average pairwise comparison between plots subjected to mulching or flooding treatment and the control treatment (Canarini et al., 2021). Pearson correlation analysis of dominant phyla and genera and soil parameters was performed in R v4.0.2 (R Foundation for Statistical Computing) and visualized using the “pheatmap” package. Redundancy analysis (RDA) was carried out using CANOCO 5.0 (Microcomputer Power, Ithaca, NY, USA) to investigate the connections between bacterial community structure and soil characteristics. The main function of MonteCarlo tests is to test the significance of the constrained ranking method (Julian and Peter, 1989). The impacts of mulching and aeration on soil characteristics and bacterial community structure were examined using one-way Analysis of Variance using IBM SPSS Statistics 20 (IBM Corp., Armonk, NY, USA). Tukey’s multiple range test was used for post-hoc analysis, with a significance threshold of p < 0.05. Partial least squares path modeling (PLS-PM) was conducted using the “plspm” package in R (Sanchez, 2013) to reveal the effects of O2 content, pH, AK, N available (NH4+-N, NO3−-N), bacterial diversities. Bacterial diversity was indicated by the richness and Shannon index.
3 Results
3.1 Soil properties influenced by mulching and flooding
As shown in Figure 1, compared with control, the mulching treatment increased TN (13.9%), NH4+-N (48.4%), NO3−-N (36.7%), AP (11.7%), and AK (99.1%) significantly, and decreased pH (4.9%), TK (14.3%), and O2 content (30%) significantly. In addition, the flooding treatment increased pH (4.9%) and NH4+-N (16.6%), TP (25%), AP (27.6%), and AK (106.5%) contents significantly, and decreased NO3−-N (16.2%) and O2 levels (1248%) significantly. Moreover, the flooding treatment increased pH, AP, TK, and AK significantly, and reduced TN, NH4+-N, NO3—N, and O2 signifiacntly, when compared with the mulching treatment. The greatest differences between the mulching and flooding treatments were observed in pH and NO3−-N and O2 contents.
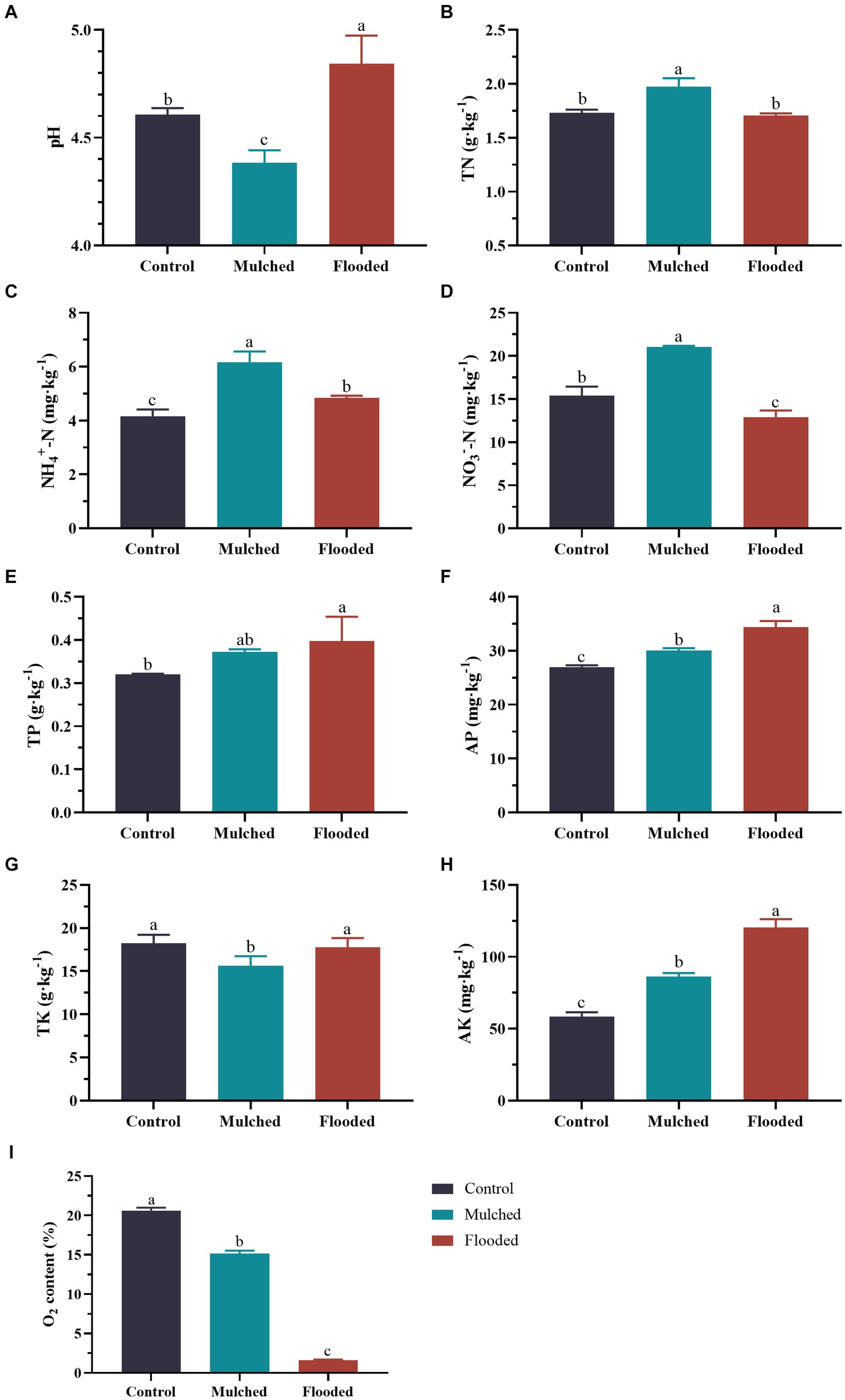
Figure 1. Effects of mulching and flooding treatments on soil properties under Phyllostachys praecox. pH (A); total nitrogen (TN) (B); ammonium nitrogen (NH4+-N) (C); nitrate nitrogen (NO3−-N) (D); total phosphorus (TP) (E); available phosphorus (AP) (F); total potassium (TK) (G); available potassium (AK) (H); oxygen (O2) (I) contents.
3.2 Abundances of AOA, AOB, archaeal and bacterial 16S rRNA
The mulching or flooding treatments increased archaea 16S rRNA, AOA and AOB amoA gene copies significantly and decreased bacteria 16S rRNA significantly, when compared with the control treatment (Figure 2). Moreover, the flooding treatment decreased bacteria 16S rRNA significantly and increased archaea 16S rRNA, AOA, and AOB amoA gene copies significantly, when compared with the mulching treatment. Overall, bacterial 16S rRNA, AOA, and AOB amoA gene copies increased with a decrease in O2 concentration, and bacterial 16S rRNA increased with a decrease in O2 concentration.
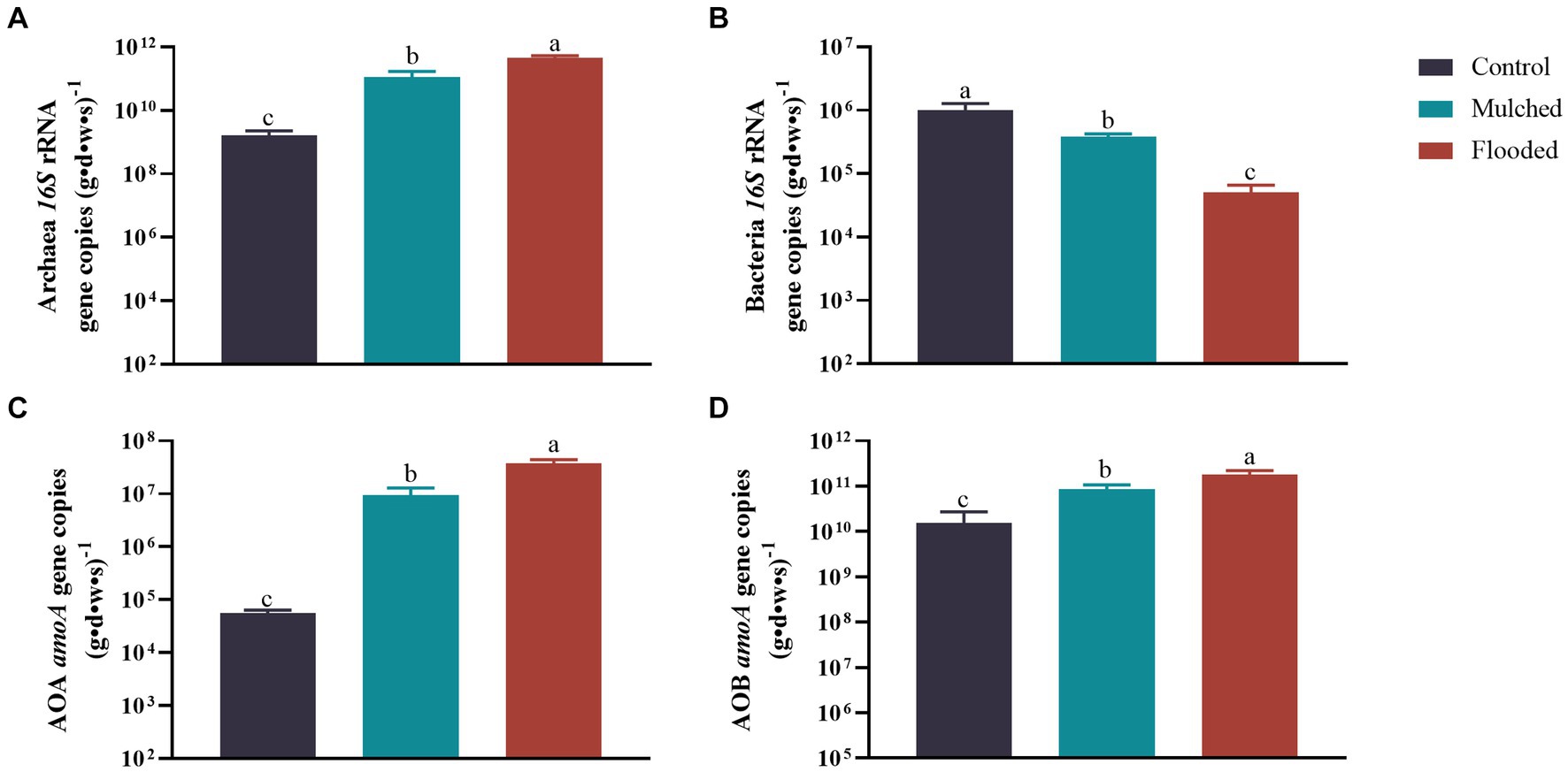
Figure 2. Effects of mulching and flooding treatments on Archaeal (A) and Bacterial 16S rRNA (B), ammonia-oxidizing archaea (AOA) (C) and ammonia-oxidizing bacteria (AOB) (D) abundance, under Phyllostachys praecox.
3.3 Soil bacterial diversity
As illustrated in Figure 3, the Shannon, ACE, and Chao 1 indices were 5.19–6.51, 1,568–2,646, and 1,579–2,646, respectively, under the three hypoxia treatments. The mulching and flooding treatments decreased the bacterial Shannon, ACE and Chao 1 diversity indices when compared with those in the control. Conversely, the flooding treatment increased bacterial Shannon, ACE, and Chao 1 diversity indices significantly when compared with the mulching treatment. Overall, bacterial diversity index decreased as O2 concentration decreased.
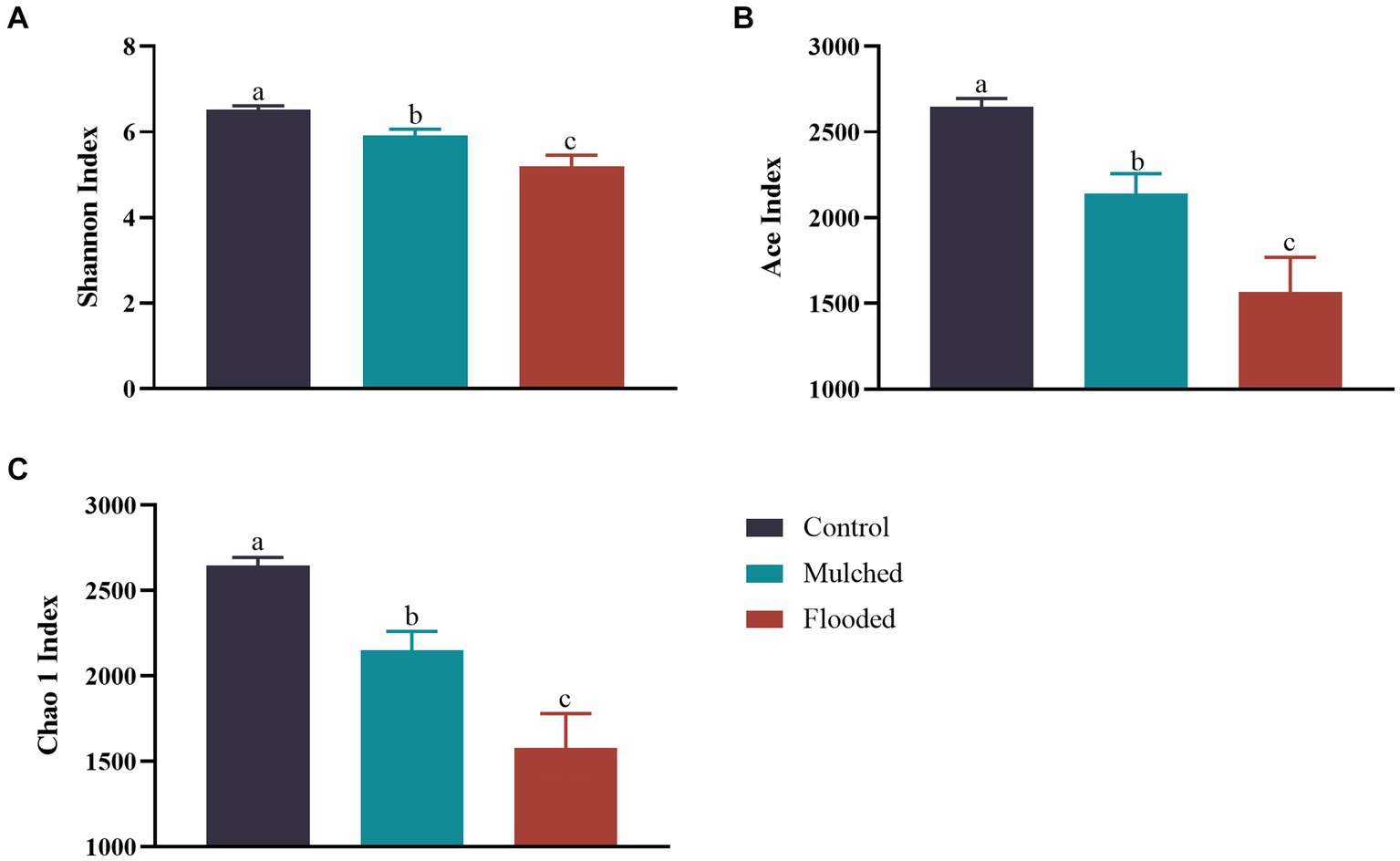
Figure 3. Effects of mulching and flooding on Shannon (A), Ace (B) and Chao 1 (C) indexes under Phyllostachys praecox.
3.4 Soil bacterial community composition
The dominant phyla under the three hypoxia treatments were Proteobacteria, Actinobacteriota, Acidobacteriota, Chloroflexi, and Firmicutes (Figure 4A). Compared with the control, the mulching treatment increased Chloroflexi and Firmicutes abundance, and decreased Acidobacteriota abundance. Conversely, the flooding treatment increased Proteobacteria and Firmicutes abundance, and decreased Acidobacteriota and Chloroflexi abundance, when compared with the control and mulching treatments.
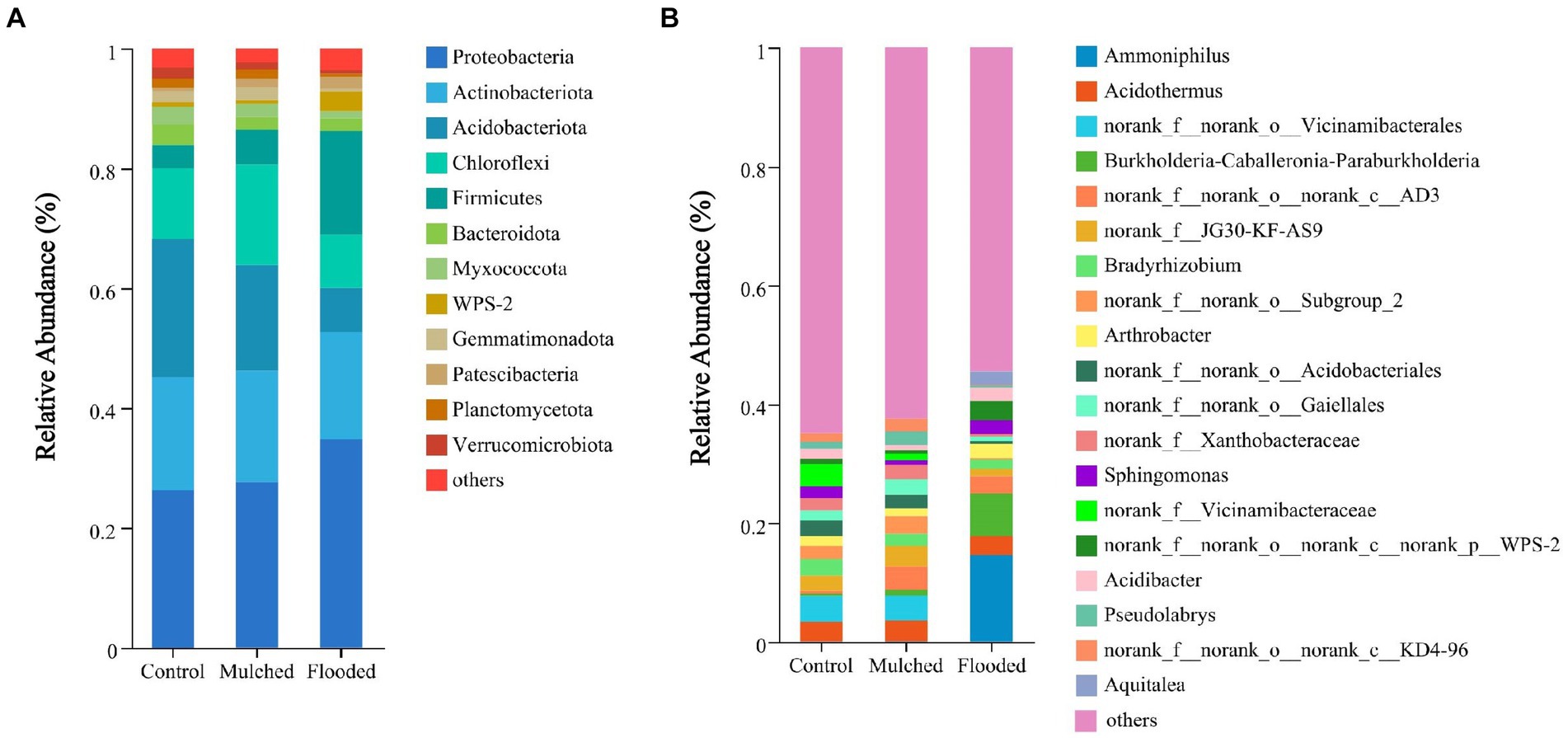
Figure 4. Relative abundances of bacteria at the phylum (A) and genus (B) levels under mulching and flooding treatments.
At the genus level, the dominant genera in the three treatments were Ammoniphilus, Acidothermus, Vicinamibacterales, Burkholderia-Caballeronia-Paraburkholderia, AD3, and JG30-KF-AS9. Ammoniphilus was the endemic genus in the flooding treatment and vicinamibacterales was the endemic order in the control and mulching treatments (Figure 4B).
3.5 Relationships among soil bacterial community structure and soil properties
In the PCoA ordination plot, the three treatment groups formed different circles, with the control and the mulching treatments closer together and the flooded group further away, at 47.39 and 14.4% for Axis 1 and 2, respectively (Figure 5A). We conducted RDA to investigate if there was any commonality between treatments based on bacterial diversity and soil nutrient contents (O2, NO3−-N, NH4−-N, TN, TP, AP, AK, and pH) (Figure 5B), and we observed interactions between bacterial diversity and soil nutrient contents. O2, AP, and pH explained the variation in bacterial diversity the most. In addition, most nutrient contents excluding NH4−-N content, influenced bacterial composition significantly, with O2, NO3−-N, AK, AP, and pH (p < 0.01) having the most significant effects. RDA1 and RDA2 exhibited differences across all treatments, accounting for 74.32 and 8.70% of the variation, respectively (Figure 5B).
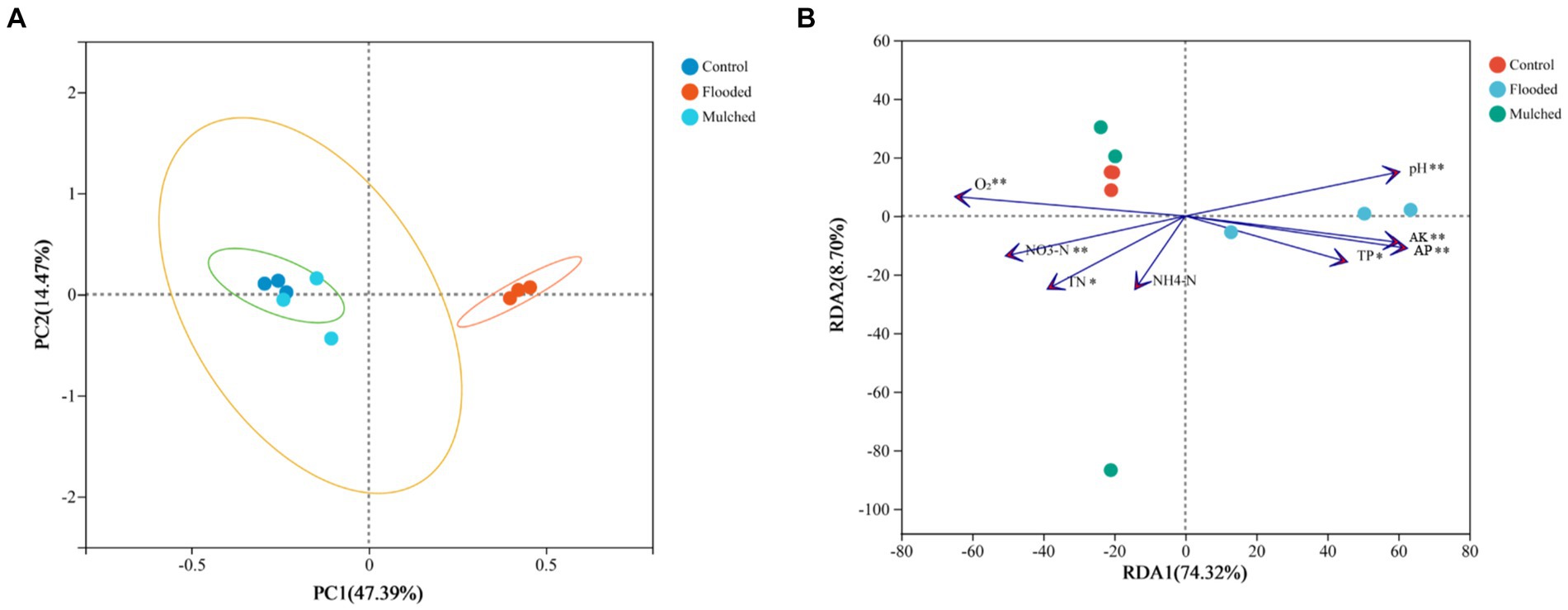
Figure 5. Principal Co-ordinates Analysis (PCoA) ordination plot for the 16S rRNA gene under different hypoxia treatments (A); Redundancy Analysis (RDA) of the correlation between soil properties and bacterial community structure (B). TN, total nitrogen; TP, Total Phosphorus; NH4+-N, Ammonium Nitrogen; NO3−-N, Nitrate Nitrogen; AP, Available Phosphorus; AK, Available Potassium. *, p < 0.05, ** p < 0.01, *** p < 0.001.
In terms of phylum, pH was negatively correlated with Gemmatimonadota and positively correlated with WPS-2 (Figure 6A). In contrast, NO3−-N was positively correlated with Gemmatimonadota and negatively correlated with WPS-2. TN was negatively correlated with WPS-2 and positively correlated with Chloroflexi. In addition, O2 was negatively correlated with Patescibacteria, Proteobacteria, and Firmicutes, and positively correlated with Myxococcota and Acidobacteriota. AP was positively correlated with Firmicutes and negatively correlated with Myxococcota and Acidobacteriota. Firmicutes and positively correlated with Myxococcota and Acidobacteriota.
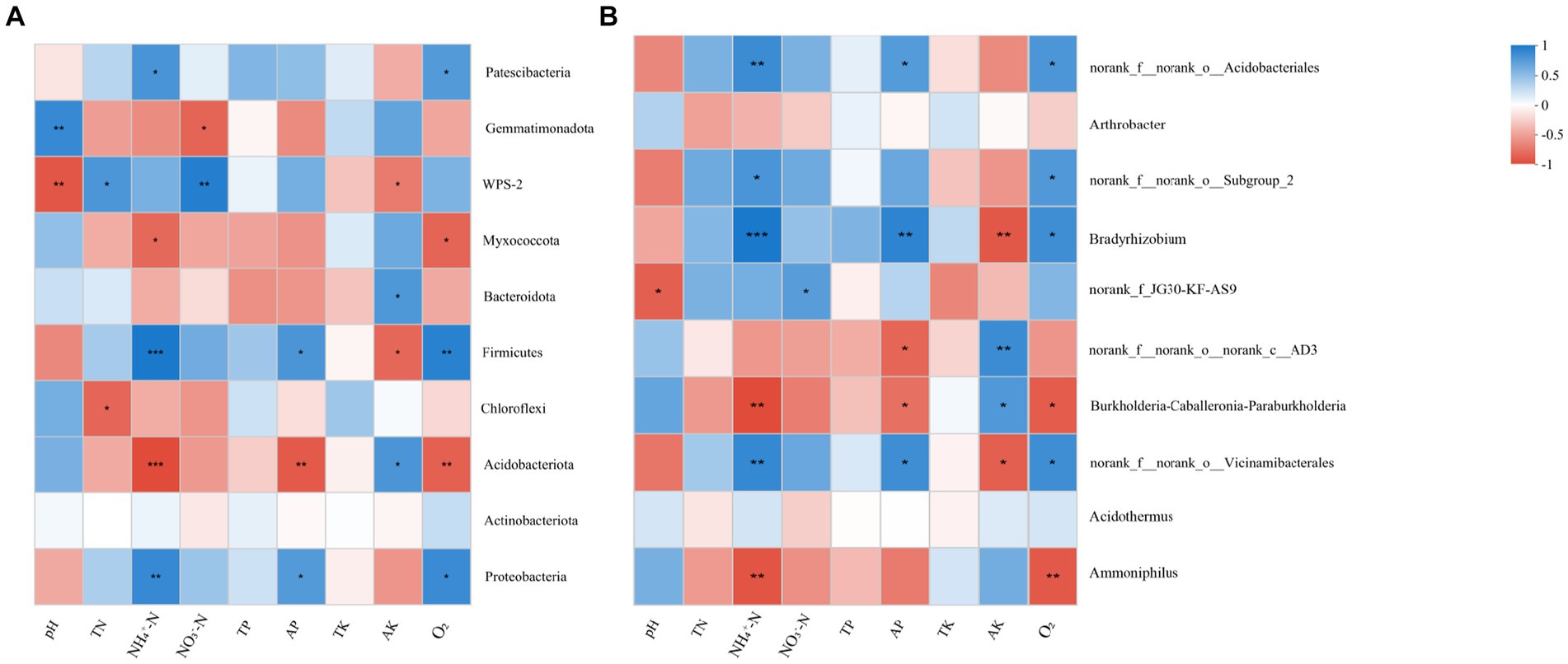
Figure 6. Spearman correlation coefficients among soil physicochemical properties and keystone phyla (A) and genera (B). Red represents a positive correlation, blue represents a negative correlation, and the larger the circle and the darker the color, the stronger the correlation. The rough arrows represent environmental factors, and the dotted lines represent biological factors. The angle between them represents their correlation. TN, Total Nitrogen; AN, Available Nitrogen; NO3—N, Nitrate Nitrogen; NH4+-N, Ammonium Nitrogen; AP, Available Phosphorus; AK, Available Potassium. *, p < 0.05.
At the genus level, pH was positively correlated with Ammoniphilus and negatively correlated with Subgroup_2 (Figure 6B). O2 was positively correlated with Acidobacteriota, Subgroup_2, Bradyrhizobium, and Vicinamibacterales, and negatively correlated with Ammoniphilus. TP was negatively correlated with Bradyrhizobium and Vicinamibacterales. AP was negatively correlated with Acidobacteriates and Bradyrhizobium, and positively correlated with Ammoniphilus.
3.6 Bacterial function prediction
The soil bacterial communities were classified into 60 functional groups using Functional Annotation of Prokaryotic Taxa (Figure 7). The dominant functional groups were chemoheterotrophy (29.2%), aerobic_chemoheterotrophy (27.8%), cellulolysis (5.5%), and nitrogen_fixation (4.1%). Compared with the control treatment, the flooding treatment increased N cycling-related functions, such as N respiration, nitrate reduction, and N fixation, significantly, and decreased photosynthetic autotrophy-related functions, such as respiration_of_sulfur_compounds, anoxygenic_photoautotrophy_S_oxidizing, and aerobic anoxygenic_photoautotrophy, significantly. However, compared with the flooded treatment, the mulching treatment significantly increased dark_sulfide_oxidation and reduced functions associated with N cycling, such as N respiration, nitrate reduction, and N fixation.
3.7 Structural equation modeling of the impacts of mulching and flooding
To better understand the effects of mulching or flooding on bacterial community structure and nutrient status, Partial Least Squares-Path Modelling (PLS-PM) was performed (Figure 8). O2 concentration (path coefficient = 1.005) and pH (path coefficient = 0.089) had positive effects on bacterial diversity and negative effects on AK. Moreover, O2 concentration had a significant and negative effect on pH (path coefficient = −0.663). Bacterial diversity (path coefficient = −0.820), pH (path coefficient = −1.164), and AK (path coefficient = −0.192) had negative effects on N availability, with the largest path coefficient for pH. In addition, N availability had positive effects on both NH4+-N (path coefficient = 0.927) and NO3−-N (path coefficient = 0.933).

Figure 8. Partial Least Squares-Path Models (PLS-PM) of the drivers of bacterial diversity and soil condition. The red and blue lines represent positive and negative effects, respectively. The full and dashed lines indicated the significant correlations (p < 0.05) and no correlations (p > 0.05), respectively. Significance levels are indicated: *, p < 0.05; ***, p < 0.001. NO3—N, Nitrate Nitrogen; NH4+-N, Ammonium Nitrogen; AK, Available Potassium.
4 Discussion
4.1 Impacts of mulching or flooding on soil nutrient availability
P. praecox is a shallow-rooted bamboo species susceptible to soil anoxia stress, and the effects of anoxia on soil nutrients vary under mulching and flooding conditions (Qian et al., 2022). According to our results, compared with control treatments, mulching increased soil nutrient content significantly, which is consistent with the results of Zhang et al. (2013). In addition, mulching and flooding treatments increased AK contents, with the effect of flooding being significantly higher than that of mulching, which could be attributed to soil water content directly affecting K fixation and release, flooding promoting AK release, and exchange K remaining at a high level, promoting the conversion of slow K into AK and increasing AK content (Zhang X. et al., 2018; Zhang Y. et al., 2018). Both hypoxic stress treatments lowered O2 content when compared with that in the control, which is in line with previous research (Guenet et al., 2012; Qian et al., 2021). Furthermore, both mulching and flooding treatments affected soil O2 diffusion and transport, and the effects of flooding were more severe as the O2 concentration decreased. The mulching and flooding treatments had considerable differences in pH and NO3−-N. Under anaerobic conditions, three major N transformations occur: (i) ammonification (conversion of organic N into NH4+-N), (ii) nitrate reduction (conversion of NO3−-N into NH4+-N), or (iii) denitrification (conversion of NO3−-N into N2) (Reddy and Patrick, 1975; Reddy et al., 1984). In a previous study, NH4+-N production (ammonification and nitrate reduction) and NO3−-N loss (nitrate reduction and denitrification) occurred under the flooding conditions, with the highest values observed at 5 weeks of flooding (Kozlowski, 1997; Unger et al., 2009). The results are consistent with our findings. In the present study, mulching increased NO3−-N content, which could be attributed to organic mulch introducing high amounts of nutrients to plants, especially NO3−-N and AP input, which influence bamboo shoot yield considerably (Zhang et al., 2021) (Figure 5). We also observed that pH increased in the flooding treatment and decreased in the mulching treatment. (Tang and Huang, 2005) demonstrated that pH in flooded soil was significantly higher than that in the un-flooded control. High pH is linked to a series of reducing processes that occur when the soil lacks O2 (Reynolds et al., 1999). Nitrate is a strong acid ion found in NO3−-N. If the soil contains a high concentration of NO3−-N, the pH would be low; conversely, if NO3−-N decreases considerably or is eliminated, pH tends to rise (Reddy and Patrick, 1975). Denitrifying bacteria convert nitrates in soil to N molecules in the absence of O2 (Narteh and Sahrawat, 1999). However, long-term mulching reduces soil pH, and soil acidification increasingly emerges over time (Zhai et al., 2017). The results of the present study also demonstrated a clear influence of pH on N uptake. Structural equation modelling illustrates that pH has a significant negative impact on N utilization (Figure 8).
4.2 Impacts of hypoxia and soil nutrient conditions on AOA and AOB, and archaeal and bacterial 16S rRNA genes
The amoA gene is frequently used as a genetic marker in studies of ammonia-oxidizing microbe abundance, community distribution, and phylogeny in environmental samples (Sinigalliano et al., 1995). The amoA copy numbers of AOA and AOB can reveal the N conversion pathways (Munroe et al., 2016). In the present study, we observed significant increases in the abundance of 16S RNA, AOA, and AOB in the mulching and flooding treatments when compared with the control (Figures 2A,B). Chen et al. (2021) and Cerecetto et al. (2021) showed that archaea 16S, AOA, and AOB abundances could be increased by mulching. In addition, according to Liu (2021), reduction in bacterial 16S gene abundance under mulching conditions was due to soil degradation and reduced microbial diversity caused by mulching for many years, whereas archaea were more likely to survive under anoxic conditions caused by mulching (Lehours et al., 2007). Conversely, there were more bacteria in the mulching treatment than in the flooding treatment, which may be attributed to the higher soil O2 level in the mulching treatment than in the flooding treatment. Consequently, there were more types of bacteria and fewer types of archaea and ammonia-oxidizing microbes in the mulching treatment than in the flooding treatment. Yu et al. (2020) observed that archaea abundance decreased with an increase in O2, which may be due to the inhibitory effect of O2 on some specialized anaerobic microorganisms.
4.3 Impacts of mulching or flooding on soil bacterial diversity
Bacterial diversity is influenced considerably by soil O2 content (Waid, 1999). In the present study, the mulching or flooding treatments reduced bacterial Shannon, ACE and Chao 1 diversity indexes when compared with the control (Figure 3). PLS-PM results also revealed that soil O2 content had a direct and significant effect on bacterial diversity, potentially because most bacteria prefer O2-rich environments (Maisch et al., 2007; Baez and Shiloach, 2014). Conversely, mulching increased bacterial Shannon, ACE and Chao 1 indexes significantly when compared with in the flooding treatment. Although the mulching treatment reduced soil O2 content, it was still greater than that of the flooding treatment, and it altered the soil microenvironment. Nevertheless, the mulching could provide rich substrates for soil microbial communities, weakening the inhibition effect of soil hypoxia (Ghanbari and Khajoei-Nejad, 2021).
4.4 Impacts of mulching or flooding on bacterial community structure and function
In the present study, the dominant clades were Proteobacteria, Acidobacteriota, Chloroflexi, and Firmicutes. Similar findings have been reported by Zhai et al. (2017). Flooding increased Proteobacteria and Firmicutes abundance, and decreased Acidobacteriota and Chloroflexi abundance, when compared with in the control. This may be due to the fact that Proteobacteria is mostly found in shallow lakes, substrate sediments and inter-rooted plant soils, where it has an excellent nitrogen and phosphorus removal effect (Tu et al., 2019). Firmicutes accounted for the largest proportion of bacteria under flooding, mainly including Bacillus and Clostridium, which may be due to their ability to produce spores, strong adaptability and reproduction capacity, and ability to withstand environmental pressures caused by waterlogging (Sar et al., 2013). In the present study, Ammoniphilus (Firmicutes) was determined to be endemic to the flooding treatment. Acidobacteriota and Chloroflexi are parthenogenic anaerobic microbes that can grow under both aerobic and anaerobic environments, but grow better in the presence of O2 although they can survive in the absence of O2 (Wang et al., 2016). Therefore, their growth and reproduction are inhibited when O2 levels are reduced in the later stages (Kan, 2015; Liu et al., 2022).
In the present study, the mulching treatment increased Chloroflexi and Firmicutes abundance and decreased Acidobacteriota abundance. First, mulching decreases O2 concentrations but not to very low levels. Secondly, Thermobacillus, Lactobacillus, Bacillus, and Ammoniphilus in the phylum Firmicutes are bacterial genera associated with lignocellulose degradation in aerobic composting (Pan et al., 2022). Thirdly, Chloroflexi particpates in the cycling of elements such as carbon (C), N and sulfur, via processes such as CO2 fixation and cellulose macromolecule degradation. Additionally, Chloroflexi is generally tolerant of high temperature conditions, so that mulching increases soil warming and Chloroflexi abundance (Xian et al., 2020; Pan et al., 2022). The decreases in soil pH and acidification with increasing mulching years should theoretically help acidophilic bacteria to multiply. However, the decline in Acidobacteria due to mulching in the present study may be related to changes in other soil environmental factors, which have greater inhibitory effects on Acidobacterial growth than the positive effect of soil acidification. Acidobacter populations prefer soil environments with low soluble organic C contents (Kielak et al., 2009); therefore, the decline in Acidobacteria following prolonged mulching may indicate an increase in soil fugitive C content and a decrease in soil C sequestration capacity (Zhai et al., 2017). Furthermore, we observed Vicinamibacteria to be a class endemic to the mulching environment, and similar observations have been made by (Lin et al., 2020).
4.5 Impacts of mulching or flooding on relationships among soil bacterial communities and soil properties
According to the RDA results, pH and O2 explained the highest and most significant variation in bacterial community structure. Some studies have similar findings to the present study. There was a significant positive correlation between pH and WPS-2, and that WPS-2 is adapted to low soil pH environments (Ward et al., 2019; Qu et al., 2020). Myxococcota and Acidobacteriota are aerobic oligotrophic microbes that are influenced by O2 conditions significantly (Murphy et al., 2021; Lin and Lin, 2022).
The functional prediction results showed that flooding reduced photosynthesis and autotrophism-related functions when compared with the control, which could be due to insufficient light under inundation. In the present study, the flooding treatment increased relative N cycling-related functions compared to the control, which may be due to the inhibition of nitrification by flooding, which is consistent with the study (Zhang X. et al., 2018; Zhang Y. et al., 2018). Nitrifying microorganisms are aerobic microorganisms. Soil flooding inhibits O2 diffusion and transport into the soil, which is detrimental to the growth and reproduction of nitrifying bacteria (Song et al., 2021). In addition, NH4+-N fixation and nitrification are two processes that compete for common substrates (Drury et al., 1991), flooding enhances soil NH4+-N fixation, thus reducing nitrification by limiting substrate availability. Conversely, mulching increased dark_sulfide_oxidation and suppresses N cycling related activities compared to flooding. With less light penetration and higher O2, C, and N levels, mulched soils modified microbial structures to adapt to increased N than flooding treatments.
5 Conclusion
O2 levels significantly influenced soil characteristics. NH4+-N, TP, AP and AK increased in both mulching and flooding treatments while O2 decreased. Mulching resulted in a reduction in NO3−-N and pH, whereas flooding led to an increase in pH and a decrease in NO3−-N. Copies of archaeal and bacterial 16S rRNA genes, AOA and AOB amoA genes increased with decreasing O2 content. Both mulching and flooding decreased soil bacterial diversity, as indicated by lower Shannon, ACE, and Chao 1 indices. Soil bacterial community structures were notably altered due to changes in O2, NO3−-N, AK, AP, and pH levels. Soil nutrient accessibility, particularly AK and O2, was closely related to bacterial community structure. PLS-PM suggested that O2 was mainly influenced by soil pH, and AK content along with bacterial diversity influenced the efficient utilization of soil nitrogen. In conclusion, hypoxia significantly affected soil properties and bacterial communities, with more intense hypoxia exerting a greater impact on these factors.
Data availability statement
The datasets presented in this study can be found in online repositories. The names of the repository/repositories and accession number(s) can be found below: https://www.ncbi.nlm.nih.gov/, PRJNA945320.
Author contributions
JG: Conceptualization, Data curation, Formal analysis, Investigation, Methodology, Project administration, Writing – original draft. CY: Conceptualization, Data curation, Investigation, Methodology, Project administration, Resources, Writing – review & editing. SZ: Conceptualization, Funding acquisition, Resources, Supervision, Visualization, Writing – review & editing. RG: Conceptualization, Methodology, Project administration, Supervision, Validation, Visualization, Writing – review & editing.
Funding
The author(s) declare that financial support was received for the research, authorship, and/or publication of this article. This work was supported by the National Natural Science Foundation of China grant number (41671296) and the Central Guidance for Local Science and Technology Development Projects (20231ZDD02003-2).
Acknowledgments
The authors would like to express their gratitude to Renyi Gui for supplying us with bamboo planting bases throughout the study effort.
Conflict of interest
The authors declare that the research was conducted in the absence of any commercial or financial relationships that could be construed as a potential conflict of interest.
Publisher’s note
All claims expressed in this article are solely those of the authors and do not necessarily represent those of their affiliated organizations, or those of the publisher, the editors and the reviewers. Any product that may be evaluated in this article, or claim that may be made by its manufacturer, is not guaranteed or endorsed by the publisher.
Supplementary material
The Supplementary material for this article can be found online at: https://www.frontiersin.org/articles/10.3389/ffgc.2024.1411297/full#supplementary-material
Footnotes
References
Baez, A., and Shiloach, J. (2014). Effect of elevated oxygen concentration on bacteria, yeasts, and cells propagated for production of biological compounds. Microb. Cell Factories 13:181. doi: 10.1186/s12934-014-0181-5
Beckersz, B., De Beeck, M. O., Weyens, N., Boerian, W., and Vangronsveld, J. (2017). Structuralvariability and niche diferentiation in the rhizosphere and endosphere bacterial microbiome of field-grown poplar trees. Microbiome 5:25. doi: 10.1186/s40168-017-0241-2
Bray, S. R., and Brawley, L. R. (2002). Role efficacy, role clarity, and role performance effectiveness. Small Group Res. 33, 233–253. doi: 10.1177/104649640203300204
Bremner, J. M. (2009). Determination of nitrogen in soil by the Kjeldahl method. J. Agric. Sci. 55, 11–33. doi: 10.1017/S0021859600021572
Bustin, S. A., Benes, V., Garson, J. A., Hellemans, J., Huggett, J., Kubista, M., et al. (2009). The MIQE guidelines: minimum information for publication of quantitative real-time PCR experiments. Clin. Chem. 55, 611–622. doi: 10.1373/clinchem.2008.112797
Buza, T. M., Tonui, T., Stomeo, F., Tiambo, C., Katani, R., Schilling, M., et al. (2019). iMAP: an integrated bioinformatics and visualization pipeline for microbiome data analysis. BMC Bioinformatics 20:374. doi: 10.1186/s12859-019-2965-4
Canarini, A., Schmidt, H., Fuchslueger, L., Martin, V., Herbold, C. W., Zezula, D., et al. (2021). Ecological memory of recurrent drought modifies soil processes via changes in soil microbial community. Nat. Commun. 12:5308. doi: 10.1038/s41467-021-25675-4
Cao, Y. Q., Qiang, Z. H., Mo, Y. L., Wang, M., and Jiang, X. J. (2019). Effects of fertilization and water management on abundance of ammonia-oxidizing microoganisms in paddy soils. Acta Pedol. Sin. 56, 1004–1011. doi: 10.11766/trxb201805250121
Cerecetto, V., Smalla, K., Nesme, J., Garaycochea, S., Fresia, P., Sørensen, S. J., et al. (2021). Reduced tillage, cover crops and organic amendments affect soil microbiota and improve soil health in Uruguayan vegetable farming systems. FEMS Microbiol. Ecol. 97:fiab023. doi: 10.1093/femsec/fiab023
Chen, S., Chen, S. L., Guo, Z. W., and Fan, Y. R. (2014). Correlations between soil nutrient contents and 459 nutrient characteristics of Phyllostachys violascens leaves under mulching management. Guihaia 34, 793–798. doi: 10.3969/j.issn.1000-3142.2014.06.011
Chen, S., Gao, R., Xiang, X., Yang, H., Ma, H., Zheng, T., et al. (2021). Straw mulching and nitrogen application altered ammonia oxidizers communities and improved soil quality in the alkaline purple soil of Southwest China. AMB Express 11:52. doi: 10.1186/s13568-021-01211-x
Chen, H., Qualls, R. G., and Blank, R. R. (2005). Effect of soil flooding on photosynthesis, carbohydrate partitioning and nutrient uptake in the invasive exotic Lepidium latifolium. Aquat. Bot. 82, 250–268. doi: 10.1016/j.aquabot.2005.02.013
Dat, J. F., Folzer, H., Parent, C., Badot, P. M., and Capelli, N. (2006). “Hypoxia stress: current understanding and perspectives” in Floriculture ornamental & plant biotechnology advances & topical issues. Global Science Books.
Deng, L., Zhang, Z., and Shangguan, Z. (2014). Long-term fencing effects on plant diversity and soil properties in China. Soil Till Res. 137, 7–15. doi: 10.1016/j.still.2013.11.002
Dobermann, A., Cassman, K. G., Sta.Cruz, P. C., Adviento, M. A. A., and Pampolino, M. F. (1996). Fertilizer inputs, nutrient balance and soil nutrient supplying power in intensive, irrigated rice system. III. Phosphorus. Fertilizer Res. 46, 111–125. doi: 10.1007/BF00704311
Drury, C. F., Voroney, R. P., and Beauchamp, E. G. (1991). Availability of NH4+-N to microorganisms and the soil internal N cycle. Soil Biol. Biochem. 23, 165–169. doi: 10.1016/0038-0717(91)90130-C
Edwards, J., Johnson, C., Santos-Medellin, C., Podishetty, N. K., Bhatnagar, S., Eisen, J. A., et al. (2015). Structure, variation, and assembly of the root-associated microbiomes of rice. Proc. Natl. Acad. Sci. USA 112, E911–E920. doi: 10.1073/pnas.1414592112
Evans, C. R. W., Krzic, M., Broersma, K., and Thompson, D. J. (2012). Long-term grazing effects on grassland soil properties in southern British Columbia. Can. J. Soil Sci. 92, 685–693. doi: 10.4141/CJSS2011-070
Gao, J., Zhuang, S., and Gui, R. (2023). Subsurface aeration mitigates organic material mulching-induced anaerobic stress via regulating hormone signaling in Phyllostachys praecox roots. Front. Plant Sci. 14:1121604. doi: 10.3389/fpls.2023.1121604
Ghanbari, J., and Khajoei-Nejad, G. (2021). Integrated nutrient management to improve some soil characteristics and biomass production of saffron. Ind. Crop. Prod. 166:113447. doi: 10.1016/j.indcrop.2021.113447
Guenet, B., Lenhart, K., Leloup, J., Giusti-Miller, S., Pouteau, V., Mora, P., et al. (2012). The impact of long-term CO2 enrichment and moisture levels on soil microbial community structure and enzyme activities. Geoderma 170, 331–336. doi: 10.1016/j.geoderma.2011.12.002
Guo, J. H., Han, B. W., and Xing, Z. (2003). The potassium fixed and effect on cotton of depletion soil. North China J. Agric. 18, 94–96. doi: 10.3321/.issn:1000-7091.2003.01.026
Guo, J. H., and Peng, Y. Z. (2008). Heterotrophic nitrification, anaerobic ammonia oxidation and archaeal ammonia oxidation in a new nitrogen cycle. Acta Sci. Circumst. 28, 1489–1498. doi: 10.13671/j.hjkxxb.2008.08.006
Jiang, H., Dong, H., Yu, B., Lv, G., Deng, S., Berzins, N., et al. (2009). Diversity and Abundance of Ammonia-Oxidizing Archaea and Bacteria in Qinghai Lake, Northwestern China. Geomicrobiol. J. 26, 199– 211. doi: 10.1080/01490450902744004
Jiao, R. Z., Yang, C. D., Tu, X. N., and Sheng, W. T. (1997). The change of undergrowth, soil microorganism, enzyme activity and nutrient in different developing stage of the Chinese fir plantation. For. Res. 10, 373–379. doi: 10.1007/BF02951625 (In Chinese)
Julian, B., and Peter, C. (1989). Generalized Monte Carlo significance tests. Biometrika 76, 633–642. doi: 10.1093/biomet/76.4.633
Kan, J. B. (2015). Response of abundance and community structure of iron-reducing bacteria to flooding time in paddy soil. Master Thesis. Yangling, Shaanxi, China: Northwest Agriculture and Forestry University.
Kan, J. B., Li, L. N., Qu, D., and Wamg, B. L. (2014). Changes in bacterial abundance and community structure associated with flooding in paddy soil. Biodivers. Sci. 22, 508–515. doi: 10.3724/SP.J.1003.2014.14053
Kielak, A., Pijl, A. S., van Veen, J. A., and Kowalchuk, G. A. (2009). Phylogenetic diversity of Acidobacteria in a former agricultural soil. ISME J. 3, 378–382. doi: 10.1038/ismej.2008.113
Kozlowski, T. T. (1997). Responses of woody plants to flooding and salinity. Tree Physiol. 17:490. doi: 10.1093/treephys/17.7.490
Lehours, A.-C., Evans, P., Bardot, C., Joblin, K., and Gerard, F. (2007). Phylogenetic diversity of archaea and bacteria in the anoxic zone of a meromictic lake (lake Pavin, France). Appl. Environ. Microbiol. 73, 2016–2019. doi: 10.1128/AEM.01490-06
Li, Y., Niu, W., Zhang, M., Wang, J., and Zhang, Z. (2020). Artificial soil aeration increases soil bacterial diversity and tomato root performance under greenhouse conditions. Land Degrad. Dev. 31, 1443–1461. doi: 10.1002/ldr.3560
Li, Y. L., Zhang, Y. L., Hu, J., and Shen, Q. R. (2006). Spatiotemporal variations of nitrification in rhizosphere soil for two different rice cultivars at the seedling stage growing under waterlogged conditions. Acta Ecol. Sin. 26, 1461–1467. doi: 10.3321/j.issn:1000-0933.2006.05.022
Liang, J. L. (1996). Studies on the composed form and releasing amount of phosphorus in podzoluvisol under submerge condition. Heilongjiang Agric. Sci. 3, 9–11.
Lin, G., and Lin, X. (2022). Bait input altered microbial community structure and increased greenhouse gases production in coastal wetland sediment. Water Res. 218:118520. doi: 10.1016/j.watres.2022.118520
Lin, G. Y., Xiao, J., Wu, Y. X., Huang, X. D., Yang, S. D., and Qu, D. C. (2020). Effects of straw mulching on soil biological properties and bacterial community structure in mulberry plantation. J. Southern Agric. 51, 2339–2347. doi: 10.3969/j.issn.2095-1191.2020.10.004
Liu, X. Z. (2021). Effects of highly intensive management on soil physicochemical properties and bacterial community diversity of Moso bamboo forest. Master Thesis. Hangzhou, Zhejiang, China: Zhejiang Agriculture and Forestry University.
Liu, Y. H., Fang, B. Z., Gao, L., Li, L., Wang, S., Jiang, H. C., et al. (2022). Community structure and ecological functions of soil microorganisms in the degraded area of Barkol Lake. Acta Microbiol Sin. 62, 2053–2073. doi: 10.13343/j.cnki.wsxb.20220269
Lu, R. K. (2000). “Methods of soil agrochemical analysis” in Methods of agrochemical analysis of soils. China Agricultural Science and Technology Press.
Maisch, T., Baier, J., Franz, B., Maier, M., Landthaler, M., Szeimies, R. M., et al. (2007). The role of singlet oxygen and oxygen concentration in photodynamic inactivation of bacteria. Proc. Natl. Acad. Sci. USA 104, 7223–7228. doi: 10.1073/pnas.0611328104
Munroe, J. W., McCormick, I., Deen, W., and Dunfield, K. E. (2016). Effects of 30 years of crop rotation and tillage on bacterial and archaeal ammonia oxidizers. J. Environ. Qual. 45, 940–948. doi: 10.2134/jeq2015.06.0331
Murphy, C. L., Yang, R., Decker, T., Cavalliere, C., Andreev, V., Bircher, N., et al. (2021). Genomes of novel Myxococcota reveal severely curtailed machineries for predation and cellular sifferentiation. Appl. Environ. Microbiol. 87:e0170621. doi: 10.1128/AEM.01706-21
Narteh, L. T., and Sahrawat, K. L. (1999). Influence of flooding on electrochemical and chemical properties of west African soils. Geoderma 87, 179–207. doi: 10.1016/S0016-7061(98)00053-6
Pan, Z. Z., Chen, Z., Liu, B., Liu, X., Xiao, R. F., Ruan, C. Q., et al. (2022). Characteristics of microbial diversity membrane-covered high temperature aerobic composting. Fujian Agric. Technol. 53, 8–18. doi: 10.13651/j.cnki.fjnykj.2022.06.002
Peiffer, J. A., Spor, A., Koren, O., Jin, Z., Tringe, S. G., Dangl, J. L., et al. (2013). Diversity and heritability of the maize rhizosphere microbiome under field conditions. PNAS 110, 6548–6553. doi: 10.1073/pnas.1302837110
Qian, Z., Zhuang, S., Gao, J., Tang, L., Harindintwali, J. D., and Wang, F. (2022). Aeration increases soil bacterial diversity and nutrient transformation under mulching-induced hypoxic conditions. Sci. Total Environ. 817:153017. doi: 10.1016/j.scitotenv.2022.153017
Qian, Z., Zhuang, S., Gui, R., and Tang, L. (2021). Effect of soil aeration treatment on the physiological and biochemical characteristics of Phyllostachys praecox under the organic material mulching. Plant Soil 459, 357–369. doi: 10.1007/s11104-020-04770-3
Qin, H., Liu, B. R., Xu, Q. F., Bai, J. F., Li, Y. F., and Li, Y. C. (2012). Effects of long-term intensive management on soil ammonia oxidizing archaea community under Phyllostachys praecoxstands. Acta Ecol. Sin. 32, 6076–6084. doi: 10.5846/stxb201108251240
Qin, Y., Pan, X. Y., Jin, W., Chen, L. Q., and Yuan, Z. L. (2018). Comparison of four extraction methods of soil microbiome in poplar plantation. Sci. Silvae Sin. 54, 169–176. doi: 10.11707/j.1001-7488.20180919
Qu, Z. L., Liu, B., Ma, Y., Xu, J., and Sun, H. (2020). The response of the soil bacterial community and function to forest succession caused by forest disease. Funct. Ecol. 34, 2548–2559. doi: 10.1111/1365-2435.13665
Reddy, K. R., and Patrick, W. H. (1975). Effect of alternate aerobic and anaerobic conditions on redox potential, organic matter decomposition and nitrogen loss in a flooded soil. Soil Biol. Biochem. 7, 87–94. doi: 10.1016/0038-0717(75)90004-8
Reddy, K. R., Patrick, W. H., and Broadbent, F. E. (1984). Nitrogen transformations and loss in flooded soils and sediments. Crit. Rev. Environ. Control 13, 273–309. doi: 10.1080/10643388409381709
Reynolds, J. G., Naylor, D. V., and Fendorf, S. E. (1999). Arsenic aorption in phosphate-amended soils during flooding and subsequent aeration. Soil Sci. Soc. Am. J. 63, 1149–1156. doi: 10.2136/sssaj1999.6351149x
Sar, P., Kazy, S. K., Paul, D., and Sarkar, A. (2013). Metal bioremediation by thermophilic microorganisms. Thermophilic Microbes Environ. Ind. Biotechnol., 6, 171–201. doi: 10.1007/978-94-007-5899-5_6
Shen, P. F. (2019). Effects of different mulching measures on soil physical and chemical properties, microbial community characteristics in Weibei apple orchard. Master Thesis, Yangling, Shaanxi, China: Northwest Agriculture and Forestry University.
Shen, Y. F., Wang, N., Liu, Z. B., Wang, X. R., Xiao, W. F., and Cheng, R. M. (2016). Effects of waterflooding in water-level-fluctuating zone of three gorges reservoir on transformation of soil nitrogen form and activities of related enzymes. J. Huazhong Agric. Univ. 30, 190–195. doi: 10.13870/j.cnki.stbcxb.2016.03.033
Sinigalliano, C. D., Kuhn, D. N., and Jones, R. D. (1995). Amplification of the amoA gene from diverse species of ammonium-oxidizing bacteria and from an indigenous bacterial population from seawater. Appl. Environ. Microbiol. 61, 2702–2706. doi: 10.1128/aem.61.7.2702-2706.1995
Song, T., Zhang, X., Li, J., Wu, X., Feng, H., and Dong, W. (2021). A review of research progress of heterotrophic nitrification and aerobic denitrification microorganisms (HNADMs). Sci. Total Environ. 801:149319. doi: 10.1016/j.scitotenv.2021.149319
Spehn, E. M., Joshi, J., Schmid, B., Alphei, J., and Körnor, C. (2000). Plant diversity effects on soil heterotrophic activity in experimental grassland ecosystems. Plant Soil 224, 217–230. doi: 10.1023/A:1004891807664
Tang, L. Z., and Huang, B. L. (2005). Dynamics of ferrous iron, redox potential and pH of forested wetland soils. Acta Ecol. Sin. 25, 103–107.
Tu, Y., Li, H. X., Jiang, L., Dong, Q., and Wang, D. Q. (2019). Bacterial communities structure and diversity in rhizosphere of different plants from Huixian wetland, Guangxi. Ecol. Environ. Sci. 28, 252–261. doi: 10.1088/1742-6596/2468/1/012158
Unger, I. M., Motavalli, P. P., and Muzika, R.-M. (2009). Changes in soil chemical properties with flooding: a field laboratory approach. Agric. Ecosyst. Environ. 131, 105–110. doi: 10.1016/j.agee.2008.09.013
Waid, J. S. (1999). Does soil biodiversity depend upon metabiotic activity and influences? Appl. Soil Ecol. 13, 151–158. doi: 10.1016/S0929-1393(99)00030-X
Wang, C., Dong, D., Wang, H., Müller, K., Qin, Y., Wang, H., et al. (2016). Metagenomic analysis of microbial consortia enriched from compost: new insights into the role of Actinobacteria in lignocellulose decomposition. Biotechnol. Biofuels 9, 22–17. doi: 10.1186/s13068-016-0440-2
Ward, L. M., Cardona, T., and Holland-Moritz, H. (2019). Evolutionary implications of anoxygenic phototrophy in the bacterial phylum candidatus eremiobacterota (WPS-2). Front. Microbiol. 10:1658. doi: 10.3389/fmicb.2019.01658
Wu, H., Cui, H., Fu, C., Li, R., Qi, F., Liu, Z., et al. (2024). Unveiling the crucial role of soil microorganisms in carbon cycling: A review. Sci Total Environ. 20, 168627. doi: 10.1016/j.scitotenv
Xian, W. D., Zhang, X. T., and Li, W. J. (2020). Research status and prospect on bacterial phylum Chloroflexi. Acta Microbiol Sin. 60, 1801–1820. doi: 10.13343/j.cnki.wsxb.20200463
Xiong, Y., Xia, H., Li, Z., Cai, X., and Fu, S. (2008). Impacts of litter and understory removal on soil properties in a subtropical Acacia mangium plantation in China. Plant Soil 304, 179–188. doi: 10.1007/s11104-007-9536-6
Xu, M., Zhuang, S., and Gui, R. (2017). Soil hypoxia induced by an organic-material mulching technique stimulates the bamboo rhizome up-floating of Phyllostachys praecox. Sci. Rep. 7, 14353–14356. doi: 10.1038/s41598-017-14798-8
Yu, N., Guo, B., Zhang, Y., Zhang, L., Zhou, Y., and Liu, Y. (2020). Different micro-aeration rates facilitate production of different end-products from source-diverted Blackwater. Water Res. 177:115783. doi: 10.1016/j.watres.2020.115783
Yuan, N. (2009). Soil biological property and changes in covering Phyllostachys violacens stand. Master Thesis. Beijing, China: China Academy of Forestry Science.
Zhai, W. L., Zhong, Z. K., Gao, G. B., and Yang, H. M. (2017). Influence of mulching management on soil bacterial structure and diversity in Phyllostachys praecox stands. Sci. Silvae Sin. 53, 133–142. doi: 10.11707/j.1001-7488.20170916
Zhang, Y., Ding, H., Zheng, X., Cai, Z., Misselbrook, T., Carswell, A., et al. (2018). Soil N transformation mechanisms can effectively conserve N in soil under saturated conditions compared to unsaturated conditions in subtropical China. Biol. Fertil. Soils 54, 495–507. doi: 10.1007/s00374-018-1276-7
Zhang, Y. Y., Fan, L. L., Huang, X., Xiong, T. T., Rong, J. D., Li, S. K., et al. (2021). Effects of eclamation and fertilization on growth and soil nutrient content after strip clear cutting in Phyllostachys Edulis forests. Chin. J. Trop. Crops 42, 1047–1054. doi: 10.3969/j.issn.1000-2561.2021.04.020
Zhang, T. L., Gao, C., and Wu, W. D. (2002). Phosphorus sorption and release of paddy soils: effect of alternation of oxidized and reduced conditions. Acta Pedol. Sin. 39, 542–549. doi: 10.3321/j.issn:0564-3929.2002.04.014
Zhang, T., Li, Y., Chang, S. X., Jiang, P., Zhou, G., Liu, J., et al. (2013). Converting paddy fields to lei bamboo (Phyllostachys praecox) stands affected soil nutrient concentrations, labile organic carbon pools, and organic carbon chemical compositions. Plant Soil 367, 249–261. doi: 10.1007/s11104-012-1551-6
Zhang, D., Yan, D., Fang, W., Huang, B., Wang, X., Wang, X., et al. (2019). Chloropicrin alternated with biofumigation increases crop yield and modifies soil bacterial and fungal communities in strawberry production. Sci. Total Environ. 675, 615–622. doi: 10.1016/j.scitotenv.2019.04.222
Zhang, X., Zhao, X. Y., Guan, Y. X., Wang, X. L., Zhang, X. L., Li, C. H., et al. (2018). Effects of waterlogging stress on available nutrients and maize nutrient absorption and distribution in different soil types at seedling stage. J Maize Sci. 26, 74–82. doi: 10.13597/j.cnki.maize.science.20180112
Keywords: hypoxia, flooding, bamboo, bacterial community, nitrate nitrogen, organic mulch
Citation: Gao J, Yang C, Zhuang S and Gui R (2024) Effects of mulching and flooding on soil nutrients and bacterial community structure under Phyllostachys praecox. Front. For. Glob. Change. 7:1411297. doi: 10.3389/ffgc.2024.1411297
Edited by:
Xiankai Lu, Chinese Academy of Sciences (CAS), ChinaReviewed by:
Jie Chen, Chinese Academy of Forestry, ChinaXiangping Tan, Chinese Academy of Sciences (CAS), China
Copyright © 2024 Gao, Yang, Zhuang and Gui. This is an open-access article distributed under the terms of the Creative Commons Attribution License (CC BY). The use, distribution or reproduction in other forums is permitted, provided the original author(s) and the copyright owner(s) are credited and that the original publication in this journal is cited, in accordance with accepted academic practice. No use, distribution or reproduction is permitted which does not comply with these terms.
*Correspondence: Shunyao Zhuang, syzhuang@issas.ac.cn
†These authors have contributed equally to this work