- 1Institute of Forestry and Engineering, Estonian University of Life Sciences, Tartu, Estonia
- 2Latvian State Forest Research Institute “Silava”, Salaspils, Latvia
- 3Department of Forest Resources and Center for Forest Ecology, University of Minnesota, St Paul, MN, United States
- 4Department of Environmental and Biological Sciences, University of Eastern Finland, Joensuu, Finland
- 5Ministry of Climate, Tallinn, Estonia
- 6Natural Resources Institute Finland (Luke), Helsinki, Finland
Trees that survive disturbances are important biological legacies that facilitate forests’ recovery and enhance their structural and species diversity, substantially contributing to the resilience of these ecosystems. The dynamic pattern of legacy syndromes sets the understudied aspects of survivors of wind disturbance into focus. Several factors at tree, stand, and landscape scales alter the susceptibility of the remnant trees, and affect their potential to recover and survive subsequent disturbances. The characteristics of the survivors interact with direct stress and mortality drivers such as changed environmental conditions and pressure by pests and pathogens. Climate change further enhances the post-storm vulnerability of the remaining stand. This literature review analyzes the impact of disturbance parameters (e.g., severity, seasonal timing) and characteristics of the affected forest (e.g., tree species composition, successional stage of a forest stand) on the conditions of survivors through post-windthrow stand development. We attempted to reveal the main agents and processes driving the fate of remnant trees and linked delayed mortality patterns to the main stand-scale wind disturbance regimes in Eurasian and North American boreal and temperate forests: (1) stand-replacing, (2) partially stand-replacing, and (3) fine-scale gap disturbance. We found that after stand-replacing wind disturbance, the spatial location of the remaining trees largely determines their onward fate, whereas these survivors are generally more susceptible to subsequent mortality compared to trees that survived less severe events. After partially stand-replacing wind disturbance, the structure of the remnant stand as well as characteristics of the individual remnant trees (e.g., species, age, size) largely determine their survival probability. Following a fine-scale gap disturbance, the trees at the gap edge are more likely to die, compared to the trees situated in the stand interior, but the mortality-causing processes usually operate on a longer time scale. Our findings contribute to the current knowledge on post-windthrow stand development and offer insights into temporal stability of these increasingly important biological legacies.
1 Introduction
Wind is the primary naturally occurring agent of forest turnover in humid climates where wildfires are infrequent (Ulanova, 2000; Frelich, 2002). For instance, windstorms were responsible for 53% of the total damage caused by natural disturbances in European forests over the period 1950–2000 (Schelhaas et al., 2003). In a compilation covering 29 European countries, Seidl et al. (2014a) estimated that wind damaged 32.3 million m3·yr.−1 of timber during the first decade of the 21st century. This estimate excludes subsequent damage caused by bark beetles and other interacting disturbance agents. Wind disturbance has remained the most important disturbance agent in Europe in the past 20 years as well, although a slight proportional decrease has occurred due to doubling of bark beetle disturbance (Patacca et al., 2023). The severity of wind-induced effects varies from scattered tree mortality, i.e., complex cohort- or fine-scale mosaics of living and dead trees to very large windthrow areas with near-total overstory destruction (Schaetzl et al., 1989; Everham and Brokaw, 1996; McCarthy, 2001; Angelstam and Kuuluvainen, 2004; Shorohova et al., 2008), resulting in several potential pathways for onward stand development (Seidl et al., 2011a; Meigs et al., 2017). Interaction between wind and trees is complex; understanding the process and response requires integration of multiple disciplines such as soil science, physics, physiology, ecology, meteorology, and climatology (Quine and Gardiner, 2007).
Trees that survive disturbance are an important biological component of ecosystem legacy, affecting the resilience and complexity of forest ecosystems (Franklin et al., 2000; Seidl et al., 2014b; Jõgiste et al., 2017). Different initial states after disturbance (e.g., species, age, coverage and spatial configuration of surviving trees) affect eventual dominance, size differentiation, degree of mingling and aggregation of trees, thus resulting in communities with different structures and compositions (Turner et al., 1998; Seidl et al., 2014b; Bāders et al., 2021).
Several potential concurrent factors can predispose a tree to spiral into decline. The decline-disease spiral model is a well-known concept originally proposed by Manion (1981). It explains how the cumulative impact of predisposing, inciting, and contributing factors can lead to tree mortality. Following a wind event, a dynamic interplay sets up between ecosystem legacies (e.g., surviving trees, deadwood, pit-and-mound complexes) and altered environmental conditions (e.g., light, temperature, soil, water regime) while forests are susceptible to subsequent disturbances (Paine et al., 1998; Buma, 2015). Pathogens, insect pests, and herbivores also respond to these changes and can cause further cascading effects (Gandhi et al., 2007; Buma, 2015; Cannon et al., 2017). Delayed mortality of trees damaged by wind may occur for decades after wind events (Harmon and Pabst, 2019).
Many studies have focused on the consequences of windstorms for forest ecosystems in temperate and boreal biomes (e.g., Skvortsova et al., 1983; Everham and Brokaw, 1996; Ulanova, 2000; Shorohova et al., 2008; Vodde et al., 2011; Mitchell, 2013; Peterson, 2019a). Few studies, however, provide insights into the onward fate of the remnant trees after wind disturbance. The ability to predict onward mortality patterns after wind disturbance of various severities would significantly contribute to our understanding of stand dynamics. Disturbance management is increasingly important as the frequency of windstorms is expected to increase under climate change (IPCC, 2014).
The main aim of this literature review was to summarize current knowledge on the prospects of remnant trees in wind-disturbed stands located in boreal and temperate biomes. Susceptibility of surviving trees to subsequent disturbance agents was estimated for three main stand-scale wind disturbance regimes inherent in boreal and temperate forests: (1) stand-replacing (SR), (2) partially stand-replacing (PR), and (3) fine-scale gap (FS) disturbance. We expected to find that the variety of survival patterns include aspects that make forests particularly vulnerable to subsequent disruptive events. Acknowledging tree survival patterns predominating after wind disturbance in boreal and temperate forests, we aspired to find an answer to the questions: (1) which factors affect survival chances of trees under different patterns, during and after wind disturbance; and (2) what disturbance types are the most likely subsequent risk factors to remnant trees?
Literature was retrieved through searches in the Web of Science and Google Scholar. We used different combinations of search terms in the title, abstracts, and keywords, using Boolean operators, (e.g., remnant trees OR surviving trees OR residual trees) AND (boreal OR hemiboreal OR temperate) AND (wind OR windthrow OR storm). Additional references were identified through cross-references and the authors’ knowledge. The extensive literature cited in this review reflects complex patterns of forests’ response to the highly variable wind disturbance regimes.
2 Surviving trees and their susceptibility to subsequent disturbance agents
2.1 General vulnerability and development of wind-disturbed stands
Disturbances are more likely to occur in previously damaged stands (Hanewinkel et al., 2011; Ruel et al., 2023). Surviving trees have often encountered mechanical damage to roots, xylem, and crown, stressing them and increasing their susceptibility (White and Pickett, 1985; Puhe, 2003; Seidl and Blennow, 2012; Harmon and Pabst, 2019). Stress from injuries and abrupt changes in environmental conditions might cause growth retardation. Seidl and Blennow (2012) found that the increment of Norway spruce (Picea abies L. Karst) dropped significantly in forests affected by the highly destructive winter storm Gudrun, compared to pre-storm levels. Exposed remnant trees experience physiological adaptations including thickening of trunks and increased root growth; response rate depends on growth conditions (Stathers et al., 1994). Trees may need several years to acclimate to an altered wind environment and regain mechanical stability. Mortality from other agents often remains high during these years (Schroeder and Lindelöw, 2002; Köster et al., 2009; Shibuya and Ishibashi, 2019) as trees allocate resources to repair mechanical damages at the expense of defensive mechanisms, thereby increasing their susceptibility to insect pests and pathogens (Schowalter, 1985; Baier et al., 2002; Wermelinger, 2004; Huang et al., 2020; Puglielli et al., 2023).
Delayed mortality can strike trees without any visible damage. Ueda and Shibata (2004) associated tree decline and dieback of apparently healthy-looking remnant trees in a typhoon-damaged forest stand with increased aboveground hydraulic resistance caused by a large reduction in trunks’ water conducting area. Although delayed mortality mostly occurs within a few years following the disturbance, Harmon and Pabst (2019) observed delayed mortality long after the damaging wind event—of 319 surviving wind-damaged trees, 144 (about 45%) died within 35 years. Mortality of the damaged trees was highest within the first 5 years, but approximately 11% of mortality cases occurred after a 10–25-year delay.
Wind-damaged trees can sometimes resprout, rebuild injured crowns or develop secondary shoots from downed stems, even despite severe damage (Peterken, 1996; Cooper-Ellis et al., 1999; Peterson, 2000; Canham et al., 2001). Sprouting ability is substantially affected by several tree traits (e.g., species, age, size) as well as by the level of pre-disturbance competition between trees (Peterson and Carson, 1996; Peterson and Rebertus, 1997; Cooper-Ellis et al., 1999; Peterson, 2000; Dietze and Clark, 2008; Matula et al., 2019). Type of damage (stem breakage versus uprooting) is also important—uprooted trees sprout more often than broken trees (Dyer and Baird, 1997). As most conifers do not sprout, stand regeneration via vegetative recovery is usually unattainable in wind-damaged conifer-dominated stands, while in situations where species prone to sprouting make up a large proportion of the forest, the role of sprouting in re-establishment of the forest structure could be substantial (Peterson, 2000; Dietze and Clark, 2008).
Peterson and Pickett (1991) reported that 25% of broken trees sprouted in the four growing seasons after a tornado in an old-growth hemlock-hardwood forest; the tendency to sprout varied significantly among species. Of those broken trees that sprouted, 68% were alive at the end of the fourth growing season. Cooper-Ellis et al. (1999) reported that a surprisingly large amount (approximately 80%) of damaged trees survived and sprouted in the first growing season in an experimentally pulled down 75-yr-old temperate Quercus rubra–Acer rubrum forest. After 4 years, survival of uprooted and broken trees remained above 40% (Foster et al., 1997). In a follow-up study, Plotkin et al. (2013) concluded that the presence of abundant advance regeneration, root suckers and stump sprouts, understory vegetation, and, most importantly, surviving trees allowed the forest to resist changes in ecosystem processes and functions. However, when a forest stand is mainly recovered via sprouting, an abrupt shift in tree-community structure and composition can occur, due to the differential ability of species to sprout (Peterson and Pickett, 1991; Everham and Brokaw, 1996). Moreover, sprouts (and trees that originate from sprouts), may be more susceptible to wood-rotting organisms and further mechanical damage, affecting the future development of the stand (True and Tryon, 1966; Putz and Sharitz, 1991; Del Tredici, 2001).
Characteristics of a wind event also affect the condition of remnant trees. A simulation of wind damage to old-growth boreal stands of mixed species showed that wind speed was more important than the number of events for relatively low wind speeds (equal to or < 20 m s−1), but at higher wind speeds, the number of events had greater impact on severity (i.e., damage done to the forest in terms of proportion of trees downed, loss in basal area, loss in above-ground biomass; Anyomi et al., 2016). For long duration events, damage propagates until all susceptible trees in a stand are windthrown. Similarly, frequent high wind speed events generally clear an area more rigorously of weaker trees than do low wind speed events, regardless of their frequency. Short duration high wind speed events, however, create patches of damage that are bordered by stand edges that survived only because the wind speed dropped. These edges remain vulnerable to subsequent winds of similar magnitude (Anyomi et al., 2016).
Downbursts are a special case of wind disturbance that can result in distinct patterns of wind damage. A downburst is a rapid, localized, straight-direction surface wind caused by a strong downdraft from the base of convective thundershowers and thunderstorms (Xi and Peet, 2011). Contrary to other types of wind disturbances, in case of a downburst, the trees sheltered by other trees or topographic features may not have advantages over the trees that have more exposed positions, as all trees in a path of a downburst regardless of their level of exposure are similarly vulnerable to the downward facing wind pressure (Foster et al., 1998; Lin et al., 2004). Thus, downburst often create sharp edges between intact forest and completely windthrown areas (Foster et al., 1998).
2.2 Susceptibility to next wind event
Wind conditions within the first years after the initial event are critical in determining the long-term survival of remnant trees as air flow at higher speeds in more exposed stands may cause further damage to crowns, stems, or root systems of remnants (Hunter, 1995; Mitchell, 2013). For instance, Kitenberga et al. (2021) found that birch trees with prior damage had a significantly higher probability (odds ratio 4.32, i.e., four times as likely) of further wind damage. Vulnerability of the remnant stand to further wind damage generally increases with increasing severity of the initial event, whereas the level of damage from following wind event can be much higher than expected for the level of winds experienced (Gardiner, 2021).
The timing of the next wind event is important. If the wind event takes place between growing seasons, conifers are more susceptible to windthrow than deciduous broadleaves due to needle retention (Zeng et al., 2010). Moreover, root anchorage strength is increased by soil freezing and reduced by soil saturation (Gardiner et al., 2010), thus trees are more susceptible to uprooting if wind disturbance takes place when the ground is saturated but not yet frozen. Precipitation during a windstorm also increases susceptibility to wind damage as water adds extra weight to the canopy, increasing the bending forces onto the stem when the stem is displaced by the wind (Gardiner et al., 2008).
Long-term disturbance and land use history affect the susceptibility of the survivors to further wind damage. For instance, even though susceptibility to wind damage generally increases with increasing age and size of trees (Foster, 1988; Whitney, 1989), sometimes the surviving trees may be the oldest and largest ones in the stand (Everham and Brokaw, 1996; Peterson, 2000). Very large trees can be open-grown trees from previous land use or trees that have had time to develop open-grown characteristics when they became canopy emergents due to the gradual death of surrounding trees. Such combinations of legacies that often contribute to increased resilience of the forest are termed ‘legacy syndromes’ (Jõgiste et al., 2017). On a single tree scale, heavy snow or ice loading on the crown, which occurs primarily in forests located at higher latitudes or high altitudes, increases windthrow susceptibility due to increased canopy mass and an increase in the drag coefficient (Valinger et al., 1993; Stathers et al., 1994). Concomitant factors, such as falling of adjacent trees or branches can also cause bark, root, crown, and sapwood damage, in some cases leading to fatality (Webb, 1989; Cooper-Ellis et al., 1999).
2.3 Susceptibility to fire
The increased amounts of CWD associated with wind disturbances increase the probability and severity of a subsequent wildfire; wind–fire interactions vary with climatic conditions, with regional differences, and with the intensity or severity of individual disturbances (Kulakowski and Veblen, 2007; Cannon et al., 2017). Wind disturbance may interact with fire not only through the addition of fuel, but also through more subtle changes in fuel composition, consumption, and arrangement (Cannon et al., 2014). Moreover, wind damage may increase fire intensity or severity indirectly as well, by changing species composition and/or forest structure toward more flammable ones (Cannon et al., 2017; Anoszko et al., 2022).
2.4 Susceptibility to insect outbreaks
Insect pests are the most significant biotic mortality-causing agents following a wind disturbance. Increased amounts of CWD in wind-disturbed forests are prime breeding habitats for potentially greatly damaging subcortical insects (Gandhi et al., 2007). In turn, insect pests can vector fungal infections that aid in the depletion of host defenses (Paine et al., 1997; Krokene and Solheim, 1998; Faccoli and Santini, 2016; Vogt et al., 2020). Bacteria also may play a role in neutralizing tree defenses (Boone et al., 2013).
Tree-killing bark beetles can have a profound ecological effect on wind-disturbed remnant stands, influencing their species composition, age structure, density, woody debris inputs etc. (Raffa et al., 2015). At stand scale, local context regarding insect pests (e.g., presence and abundance of the pest, climatic conditions influencing reproduction rate of pests as well as susceptibility of a host) is clearly important; presence and abundance of antagonists, i.e., predators and parasites of the pest, may also make a considerable difference (Massey and Wygant, 1954; Osetrov, 2002; Bouget and Duelli, 2004; Wermelinger, 2004; Eriksson et al., 2007; Kärvemo et al., 2014a; Jan Weslien et al., 2024). Location of the disturbed forest can influence the severity of infestation as voltinism (number of broods or generations of an organism within a year) of insect pests depends on latitude and elevation, with a greater number of generations occurring in milder climates (Lange et al., 2006; Jönsson A M, et al., 2007). Species composition of the disturbed stand is of high importance, as pests and pathogens are usually host-specific (Bouget and Duelli, 2004; Tedersoo et al., 2019). There is a strong positive correlation between stand-scale host tree volume and risk of bark beetle infestation (Kärvemo et al., 2014b). Among bark beetles that colonize live trees, most have evolved adaptations to exploit conifers in the Pinaceae family, despite remarkable defenses that these trees can mount (Sequeira et al., 2000; Franceschi et al., 2005; Raffa et al., 2015). Species composition can have more complex effects on vulnerability as well, for example, Baier et al. (2002) found that spruces in mixed stands had a higher primary resin flow as a response to bark beetle attack, compared to trees in pure spruce stands. Moreover, it has been found that volatiles released by non-host trees disturb bark beetle response to pheromones (Byers et al., 1998; Jactel et al., 2001; Zhang, 2003), thus possibly improving the resistance of mixed forests.
On a single tree scale, the level of damage the tree was subjected to during wind disturbance determines the fate of a remnant tree to a substantial extent, as bark beetles can detect cues associated with stress physiology of their host plants (Raffa et al., 2015). Attacks by several insect species can occur simultaneously, causing cumulative effect (Göthlin et al., 2000). Higher entry rates have been observed in response to root infection, defoliation, fire- or lightning injury, old age, and other stresses (Christiansen, 1991; Jakuš et al., 2011; Lindgren and Raffa, 2013). However, once the pest population has reached the epidemic phase, host defenses no longer play a major role since the insects are abundant enough to overcome the defense mechanism in all but the most vigorous or genetically resistant trees (Lindgren and Raffa, 2013). The age of a tree is a significant factor, as several age-related parameters (thickness and texture of bark, thickness of phloem, vitality of the host) affect its susceptibility to bark beetles (Långström, 1983; Ferrenberg and Mitton, 2014; Takei et al., 2021), whereas different species have dissimilar host requirements (Göthlin et al., 2000). For instance, the lower limit of the thickness of bark which can be colonized by Ips typographus L. is 2.5 mm, which is usually attained when the DBH of the host tree has reached about 15 cm (Grunwald, 1986; Seidl and Rammer, 2017). Dendroctonus rufipennis Kirby prefers large, mature trees as a host, but may attack trees with DBH as small as 5 cm when larger trees become depleted and the beetle population has become excessively abundant (Massey and Wygant, 1954). Some highly damaging bark beetles prefer a breeding substrate exposed to the sun; Jakuš et al. (2011) found that trees with a higher level of stem shading (longer crown length) had a higher probability of surviving the I. typographus outbreak. Some observations of potentially highly damaging insect pests associated with wind disturbances in temperate and boreal biomes are described in Table 1. Several landscape and stand-scale parameters (e.g., elevation, aspect, initial stand density) additionally affect the predisposition of the remnant stand to insect outbreaks (Tables 2, 3).
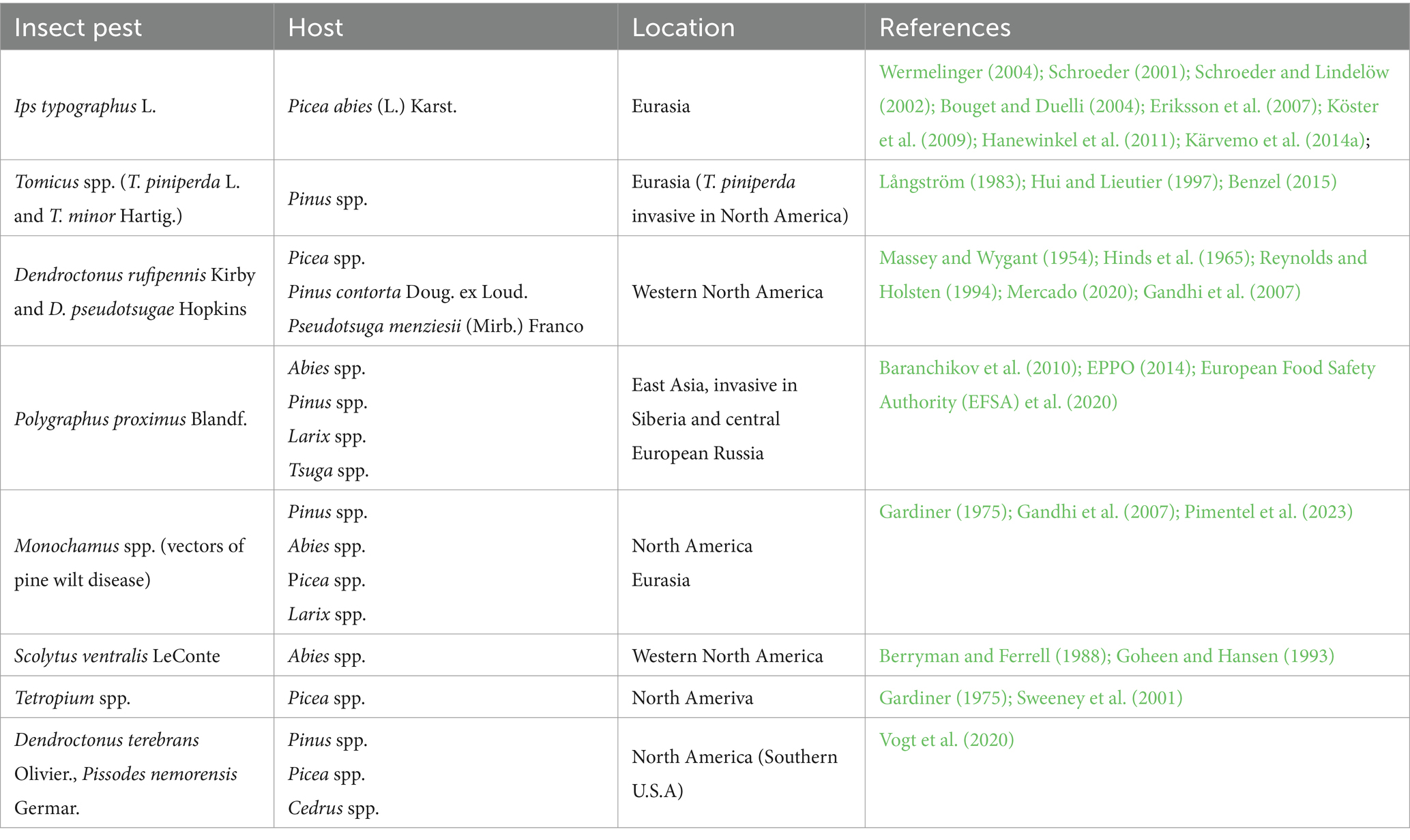
Table 1. Observations of highly damaging insect pests associated with wind disturbances in boreal and temperate forests.
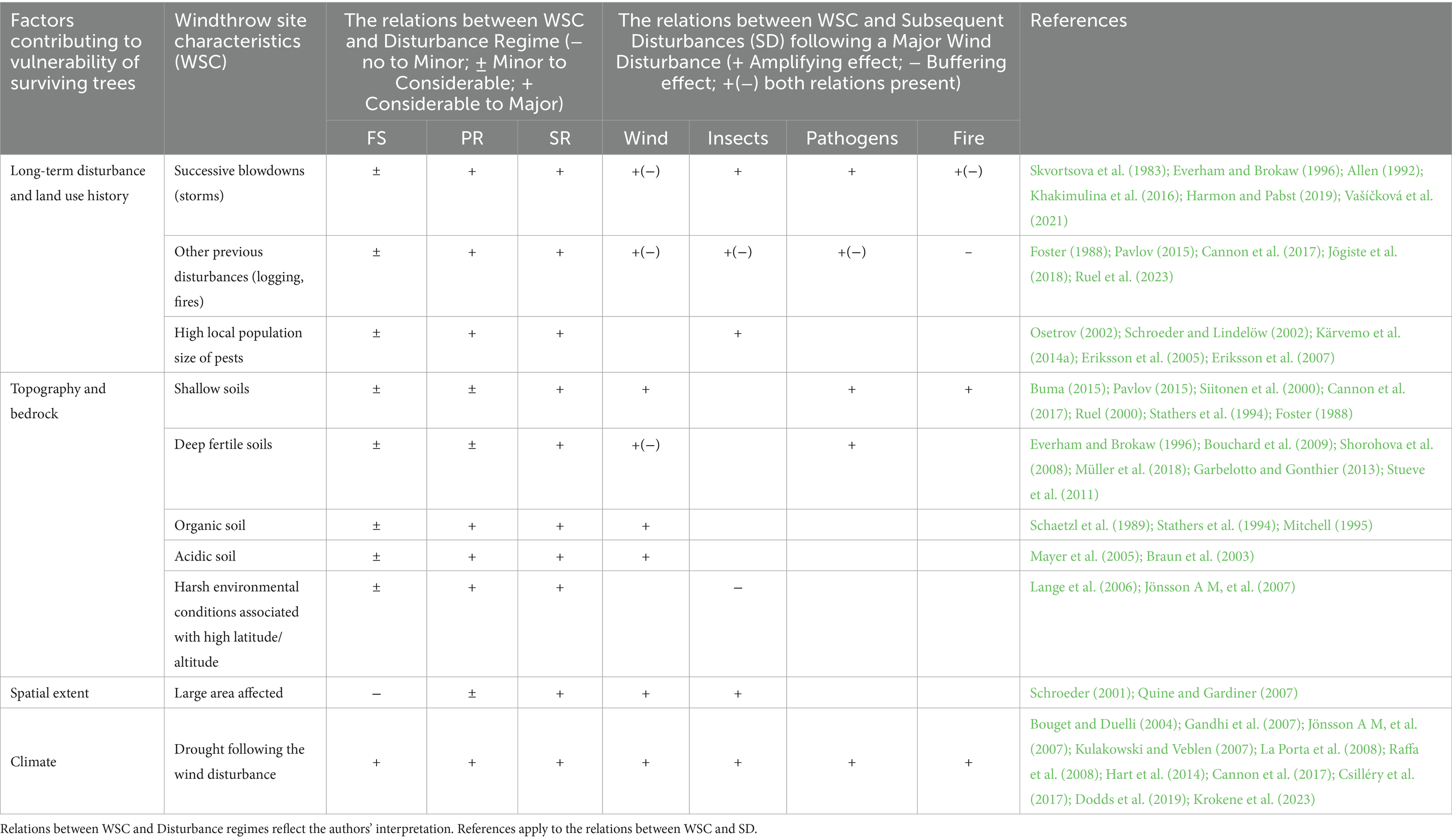
Table 2. Summary table describing landscape-scale factors that affect post-windthrow vulnerability of surviving trees to subsequent disturbances (SD) based on relations revealed between Windthrow Site Characteristics (WSC) and wind disturbance regimes.
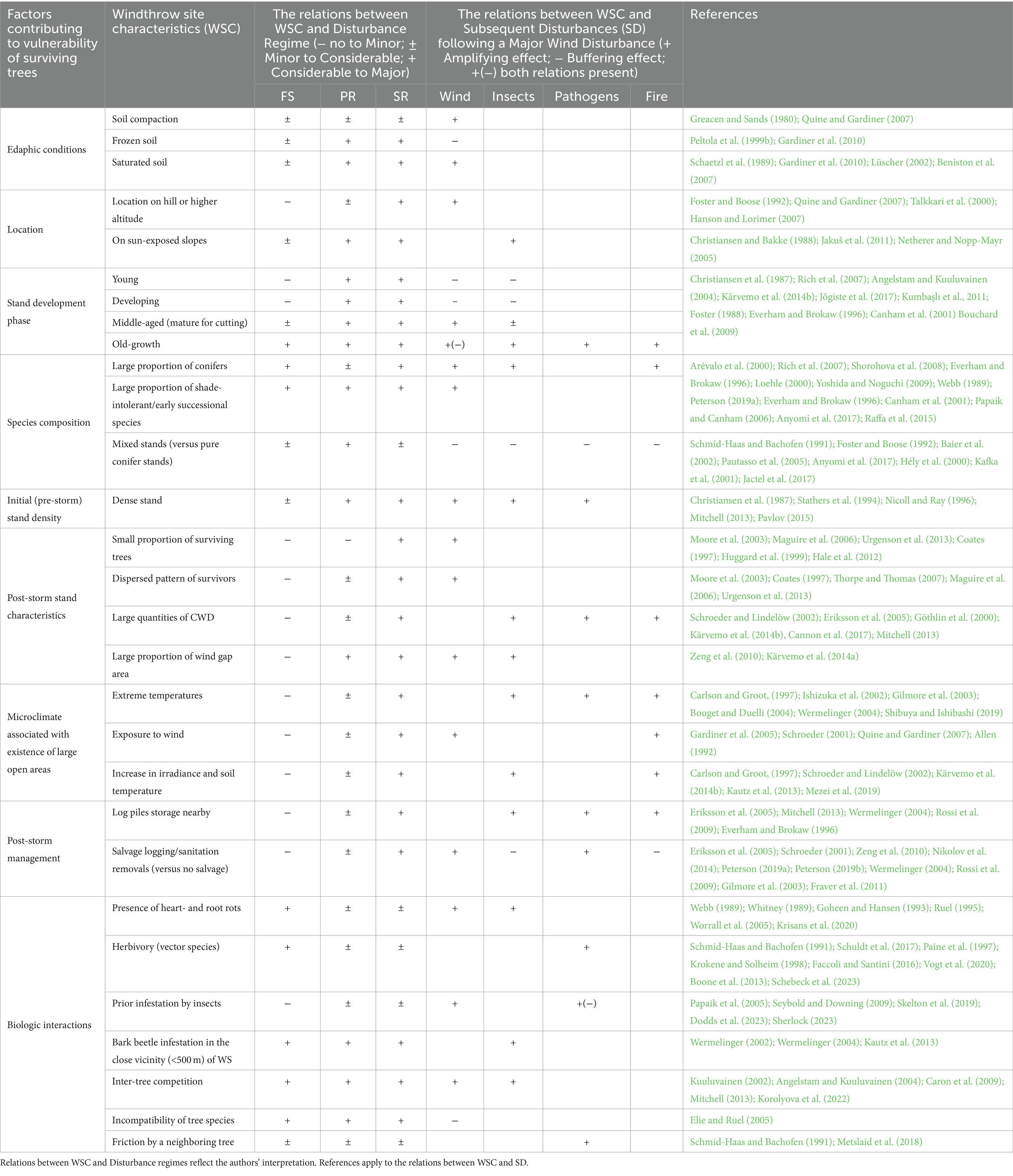
Table 3. Summary table describing stand-scale factors that affect post-windthrow vulnerability of surviving trees to subsequent disturbances (SD) based on relations revealed between Windthrow Site Characteristics (WSC) and wind disturbance regimes.
The decline-disease spiral model concept applies in regard to insect pest outbreaks, as all large-scale wind disturbances within the range of severe pests such as D. rufipennis or I. typographus do not result in subsequent outbreaks, indicating that some other factors need to co-occur for a large-scale infestation to emerge; drought has been suggested to be the most influential contributing factor (Jönsson A M, et al., 2007; Raffa et al., 2008; Hart et al., 2014; Netherer et al., 2015; Dodds et al., 2019). For instance, after World War II, catastrophic insect outbreaks occurred when large cuttings for reparations that generated huge amounts of litter and debris were followed by a hot and dry summer in 1946 (Hanewinkel et al., 2011).
2.5 Susceptibility to pathogenic fungi
The presence of rot-causing fungi such as Heterobasidion spp., Armillaria spp. and Phellinus spp. is known to harm the mechanical stability of infected trees, thus increasing the risk of wind damage (Whitney, 1989; Quine and Gardiner, 2007). In turn, wind disturbance may facilitate the spread of pathogenic fungi by creating infection courts on residual trees from collision and abrasion from falling trees, by fracturing the roots, and by fungal spore dissemination (Schmid-Haas and Bachofen, 1991; Hennon, 1995; Worrall et al., 2005; Krisans et al., 2020). Moreover, wind disturbances can facilitate fungal pathogens via altered microclimatic conditions (e.g., temperature, relative humidity) which can increase abundance and infection success of many pathogenic species (Cobb and Metz, 2017; Idbella et al., 2023). Additionally, wind disturbance can substantially alter the communities of symbiotic fungi (Idbella et al., 2023; Venice et al., 2023), which can affect seedling establishment (Nara, 2006; Liang et al., 2020) and decrease stand’s resistance to abiotic and biotic stressors (Van Der Heijden et al., 2008; Anthony et al., 2022). However, the impact of wind disturbance on fungal communities is not a subject of many research papers, thus the magnitude and causality of these interactions is still largely unclear.
Due to different susceptibility of tree species, a forest stand with diverse tree species composition is less vulnerable to fungal pathogens, compared to monospecific stands (Pautasso et al., 2005). Some studies have shown that root and butt rot (caused by Heterobasidion spp. and other pathogens) frequency in Norway spruce is higher in fertile sites (e.g., Garbelotto and Gonthier, 2013; Müller et al., 2018).
Bark beetles that colonize living conifers are frequently associated with pathogenic fungi that are introduced into the tree during the attack process (Paine et al., 1997; Krokene and Solheim, 1998; Netherer et al., 2021). These symbiotic fungi are important allies to the pest as they produce beetle semiochemicals and degrade host toxins, helping to exhaust tree defenses (Netherer et al., 2021). Among the fungal associates of spruce bark beetles in Eurasia, a blue stain fungus Endoconidiophora polonica (formerly known as Ceratocystis polonica), primarily transmitted by I. typographus, is the most virulent (Solheim, 1988; Christiansen, 1991; Krokene and Solheim, 1998.). Another blue stain fungus Grosmannia penicillata is also a noteworthy subsidiary to the pest (Schebeck et al., 2023; Netherer et al., 2024). In North America, Leptographium abietinum is associated with Dendroctonus spp., causing additive damage to members of the Pinaceae, especially Picea spp. (Jacobs et al., 1998). Tomicus spp. have several fungal associates as well, for instance they are an active transmitter of the tracheomycotic genus Ophiostoma, inducing blue stain in Pinus yunnanensis Franchet in China (Pan et al., 2017) and Pinus sylvestris in Sweden (Solheim and Långström, 1991). In northern Spain, Tomicus piniperda has been found to vector Fusarium circinatum, the causal agent of pitch canker disease, in Pinus radiata D. Don (Bezos et al., 2015).
3 Patterns under different wind disturbance regimes
3.1 Stand-scale wind disturbance regimes in boreal and temperate forests
Disturbance size and severity are inversely related to event frequency (Foster and Reiners, 1986; Turner et al., 1998; Angelstam and Kuuluvainen, 2004; Hanewinkel et al., 2011). This relationship creates a gradient from infrequent, coarse-grained stand-replacing disturbances (SR) to moderately frequent, medium-grained disturbances (partially stand-replacing disturbances, PR), to very frequent fine-grained single (or few) tree gap disturbances (fine-scale gap disturbances, FS), as depicted in Figure 1. These regimes usually create even-aged stands, multi-aged stands, and all-aged stands, respectively (Frelich, 2002), according to the prevailing local disturbance regime. Within the framework of this study, we consider a wind disturbance event to be stand-replacing when >75% of the upper strata (i.e., canopy trees and emergent trees) are downed during the incident, as proposed by Bouchard et al. (2009). Fine-scale gap disturbance occurs when small gaps are formed by one or a few fallen trees, this disturbance regime is inherent in late-successional forests (Runkle, 1985; Kuuluvainen, 1994). Gap size for single treefalls mostly ranges from 50 to 200 m2 (McCarthy, 2001). Discriminating FS from PR is challenging, as the threshold between the severity levels is often unclear (Hart and Kleinman, 2018). Within the framework of this review, we consider a wind disturbance to be partially stand-replacing when at least 15% (but less than 75%) of the trees in the upper and mid canopy layers have been downed during the event. Our delineation is based on a review article (McCarthy, 2001) in which gap characteristics of gap-disturbed forests were examined. We acknowledge that setting such a threshold is arbitrary, thus, if possible, we also took origin of the gaps into consideration: partially stand replacing disturbances are abrupt exogeneous events, while fine-scale gap disturbances are more gradual and mostly driven by endogenous agents such as fungi, insects, and inter-tree competition; wind appears as the final agent that fells the predisposed trees.
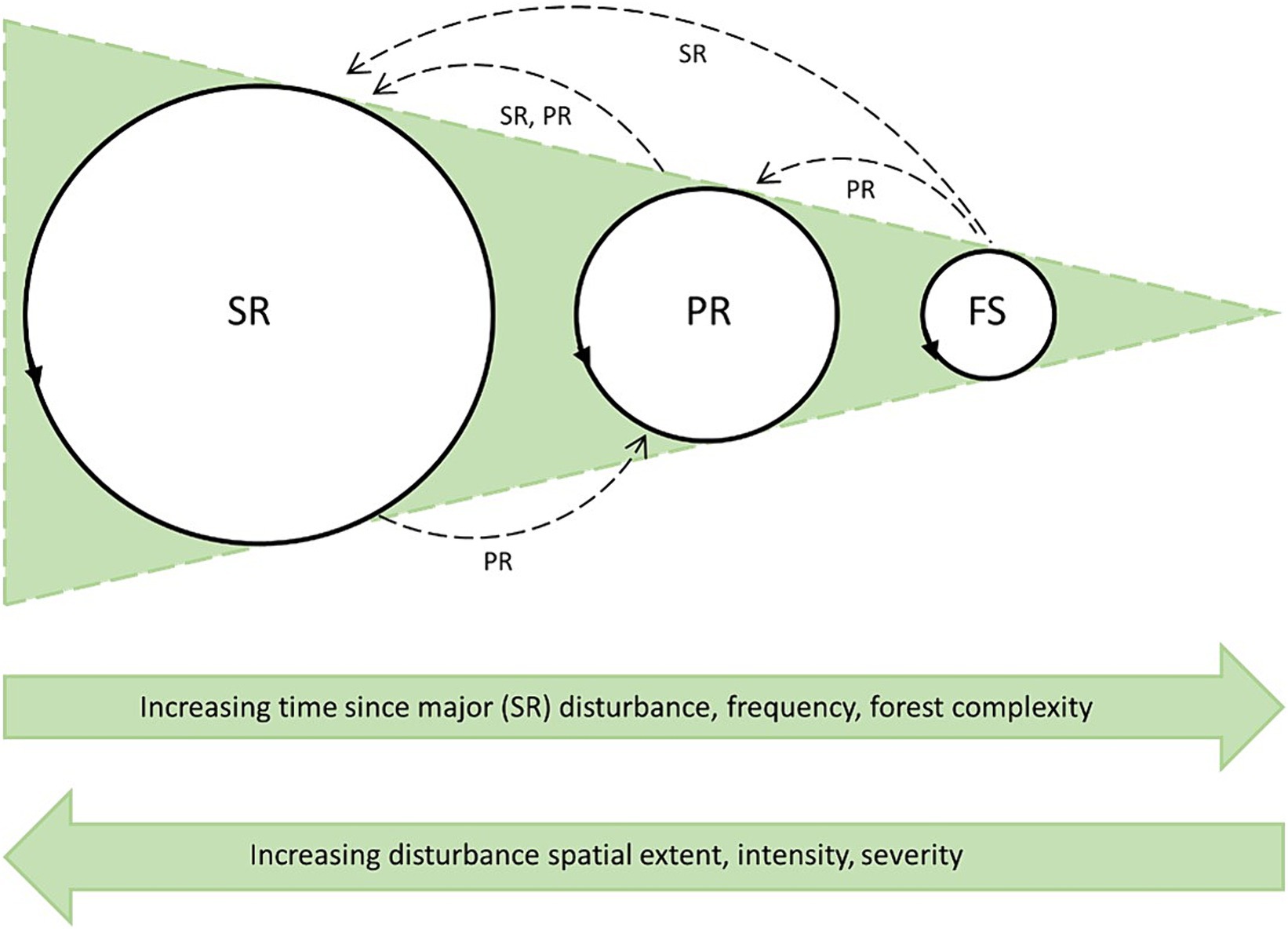
Figure 1. Schematic succession cycles under different disturbance regimes (circles, circle size represents cycle length). FS, Fine-scale dynamics occur most frequently and have the shortest cycle; PR, Partially stand replacing events generally have a lower frequency and hence a longer cycle; SR, Stand replacing disturbance events have the longest cycle. Partially stand replacing and stand replacing disturbance events (arrows with dashed lines), depending on the current regime, atypical disturbance severity and frequency, may accelerate or delay succession in the process, and temporarily shift disturbance regime.
3.2 Vulnerability after stand-replacing disturbances
Long-term estimates regarding the fate of remnant trees are rarely documented, but Shibuya and Ishibashi (2019) reported a cumulative 56% mortality rate of remnant trees 60 years after a wind event that downed 93% of the basal area (85% of trees) in a conifer-dominated study plot located in Hokkaido. In a mixed species study plot nearby where the same wind event had downed 86% of the basal area (82% of trees), the cumulative post-windthrow mortality added up to 60% 37 years after the wind disturbance (mortality was not tracked from there on). In both study plots, mortality was particularly high during the first 5 years following the windthrow event, especially for conifers. It must be kept in mind that after an extended period of time, tree death cannot always be clearly associated with wind disturbance anymore, as with an expanding timescale, other interacting factors (e.g., other unrelated abiotic and biotic disturbance agents, tree senescence) become increasingly influential (Harmon and Bell, 2020).
The mortality probability of a remnant stand depends on the severity of the disturbance; remnants from stand-replacing disturbances are generally more susceptible to subsequent disturbances than remnants from less severe disturbances. Evidence regarding high correlation between disturbance severity and growth retardation of remnant trees (Seidl and Blennow, 2012) indicates that trees endure more stress after high-severity wind disturbance, compared to a less severe event. The authors found that for every 10% of the standing timber volume structurally damaged by storm Gudrun, a 6.7% growth reduction occurred on average in the 3 years following the storm.
Severe large disturbances create more variability in successional pathways than small disturbances, providing opportunities for the initiation of multiple stable states, while abundance and spatial arrangement of remnants is a key factor in determining the outcome (Turner et al., 1998). Wind disturbances are rarely spatially uniform; thus, the heterogeneity of storm impacts can cause the spatial pattern of survivors to be more aggregated compared to a pre-disturbance state, whereas aggregation is likely to peak at high but not extreme levels of severity (Woods, 2004; Peterson, 2020). For instance, Wolf et al. (2004) observed strongly aggregated mortality patterns at scales of 5–50 m as a result of the 1999 extratropical cyclone in a Danish mixed-species deciduous forest. Allen et al. (2012) also reported that a severe storm greatly altered the spatial pattern of a Pinus strobus stand, producing a more clumped distribution of surviving trees. Such development was not clear in an oak-dominated (Quercus borealis) stand nearby that was similar in topographic and soil conditions but had endured less severe wind damage. The tendency for the survivors to form aggregated patterns can be important, because numerous studies on variable retention systems have demonstrated that in general, the proportion of residual trees left and their onward wind-caused mortality are inversely related, while highly dispersed trees have higher mortality than the trees clustered in aggregates (e.g., Moore et al., 2003; Maguire et al., 2006; Thorpe and Thomas, 2007; Lavoie et al., 2012; Urgenson et al., 2013). Similarly, Peterson and Cannon (2021) found that support from neighboring trees reduces the force that a given tree must withstand, thus increasing critical wind speed required to down the tree.
Survivors growing at the formerly open edges of a wind-damaged stand are better acclimated and generally less susceptible to the next wind, while newly exposed trees that previously grew in the stand interior are not accustomed to the exposure and thus more susceptible (Ennos, 1997; Brüchert and Gardiner, 2006; Gardiner et al., 2008). Static wind load on survivors is positively correlated with the size of a canopy opening; the increase of maximum static wind load is up to 14 times higher than the load in an undisturbed forest, thus possibly leading to onward windthrow mortality at the downwind gap edges (Quine and Gardiner, 2007; Panferov and Sogachev, 2008). Moreover, the maximum static wind load is experienced at the downwind gap edge independently of gap size, the maximum turbulent kinetic energy, however, shows the apparent shift toward the lateral and even to the leeward gap edges with the increasing gap diameter above a size of 15 times canopy height (Panferov and Sogachev, 2008), making all remnant trees at newly formed edges of a large gap susceptible to next heavy intense wind event. On the other hand, recurring windstorms may have only a little effect on a forest if the previous storm has already removed most of the susceptible trees (Webb, 1989).
High-severity wind disturbances are accompanied by a greater amount of breeding material with non-existent or weak defenses and more favorable microclimatic conditions for bark beetles, compared to less severe disturbances (Göthlin et al., 2000; Schroeder and Lindelöw, 2002; Wermelinger, 2002; Kärvemo et al., 2014b). Some hazardous forest pests such as I. typographus attack windthrown spruces in gaps more frequently than they attack trees along edges, which in turn are preferred over trees in closed stands (Göthlin et al., 2000; Schroeder and Lindelöw, 2002; Bouget and Duelli, 2004). Mortality caused by I. typographus usually peaks in the second or third summer after severe wind damage—insects migrate from gap interior to the forest edge where they first colonize the most susceptible trees, but later, when the bark beetle population size has reached epidemic level, they may migrate to trees without any visible damage (Schroeder, 2001; Osetrov, 2002; Schroeder and Lindelöw, 2002; Kärvemo et al., 2014a). For instance, Köster et al. (2009) found that only 25% of the initial Norway spruce remnants that survived at the edges of the largest wind gaps were alive 5 years after the wind event, whereas I. typographus caused most of the subsequent tree deaths. South-facing newly formed gap edges have been found to be twice as vulnerable to I. typographus attacks, compared to north-facing gap edges (Kautz et al., 2013).
The magnitude of bark beetle damage can be immense—in western Colorado, after a severe windstorm of 1939, a severe outbreak of D. rufipennis followed, destroying an additional 10.1 million m3 of timber by 1951 (Massey and Wygant, 1954). The outbreaks of D. pseudotsugae can be similarly destructive—after a series of windstorms between 1949 and 1953 in western Oregon and southwestern Washington that resulted in 27 million m3 of windthrown trees, an additional 8.3 million m3 of standing trees were killed by D. pseudotsugae (Gandhi et al., 2007).
Depending on spatial and temporal factors, wind-disturbed forests may be highly vulnerable to subsequent fire disturbance (Tables 2–4); vulnerability increases with increasing severity of wind damage (Cannon et al., 2017). For instance, due to the increase in CWD, modeled fire burn times and the extent of the fire were found to increase dramatically with increasing blowdown severity (Buma and Wessman, 2011). Similarly, Kulakowski and Veblen (2007) found that high blowdown severity was strongly spatially correlated with high fire severity. The risk of fire substantially increases if wind disturbance is followed by periods of dry, hot, windy weather (Kulakowski and Veblen, 2007; Mitchell, 2013).
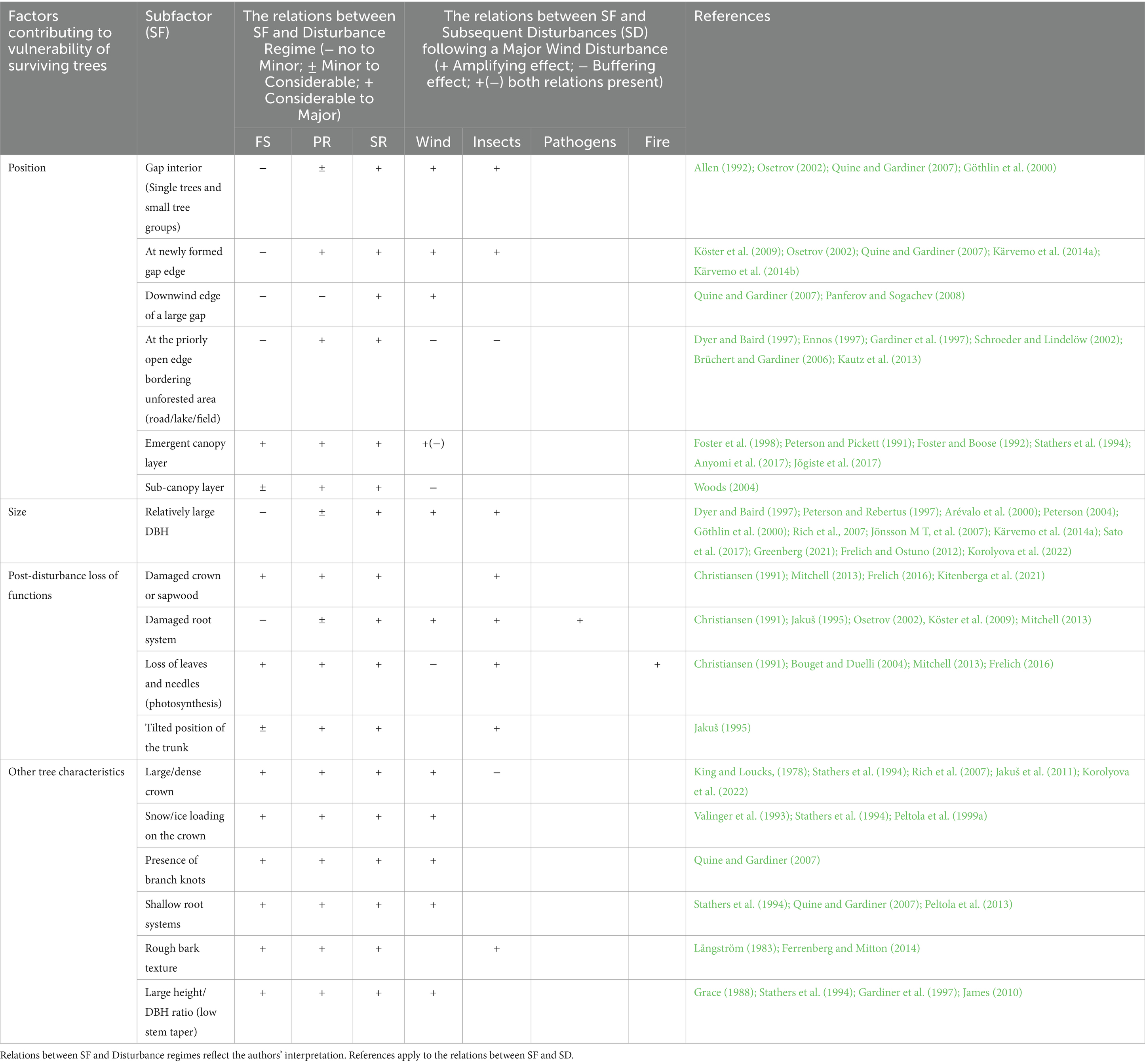
Table 4. Summary table describing tree-scale factors that affect post-windthrow vulnerability of surviving trees to subsequent disturbances (SD) based on relations revealed between subfactors (SF) and wind disturbance regimes.
3.3 Vulnerability after partially stand-replacing disturbances
Large areas of boreal and temperate forests are naturally regulated by moderate-severity disturbances, leading to heterogeneous and dynamic stand and landscape structures (Frelich and Lorimer, 1991; Papaik and Canham, 2006; Kuuluvainen, 2009; Stueve et al., 2011; Koivula et al., 2014; Khakimulina et al., 2016; Hart and Kleinman, 2018; Meigs and Keeton, 2018). Generally, structural complexity peaks when wind disturbance is of intermediate severity (Peterson, 2019b). Greater stand-scale structural and compositional diversity enables spreading risk among survivors that have different susceptibility to various stressors and disturbance agents (Jactel et al., 2017; Mitchell et al., 2023). Thus, if such heterogeneity is evident following a moderate-severity wind disturbance, the survivors are generally more resistant to subsequent disturbance agents such as wind, insects, pathogenic fungi, and fire (Everham and Brokaw, 1996; Hély et al., 2000; Kafka et al., 2001; Pautasso et al., 2005; Griess and Knoke, 2011; Seidl et al., 2011b; Jactel et al., 2017), compared to remnant stands with homogeneous structure and composition.
Partially stand-replacing disturbances have some characteristics similar to SR, although to a lesser degree. With increasing size of the openings, surviving trees become more susceptible to subsequent mortality. Wind loading increases very rapidly with increasing size of the opening, up to a gap diameter of twice the height of the edge trees (Quine and Gardiner, 2007). Windthrow increases the proportion of edge habitats. New edges created by wind are susceptible to further wind damage due to sudden changes in wind loading that the trees are not acclimated to (Stathers et al., 1994; Gardiner et al., 1997; Peltola et al., 1999a). Zeng et al. (2010) found that when the proportion of gap areas increased from 10 to 20%, the length of edges at risk increased by 77–80% for different age classes. The total length of edges at risk reached a maximum when about 50% of the landscape was open gaps. The characteristics of the newly formed edges matter, as abruptness and density of the edges strongly influence wind loading (Stathers et al., 1994; Gardiner et al., 1997). Structural contrast between the forested and non-forested areas generally increases with increasing disturbance severity (Foster et al., 1998; Stueve et al., 2011), thus uneven sparse stand- and gap edges often resulting from moderate windthrows may be very vulnerable to further windthrow (Stathers et al., 1994; Gardiner et al., 1997).
Severity and extent of wind disturbance affect remnant stands’ vulnerability to bark beetle outbreaks: Schroeder and Lindelöw (2002) found that tree mortality caused by I. typographus peaked earlier in smaller stands with fewer wind-felled trees than in the larger stands with more wind-felled trees. They also found that the total number of wind-felled spruces and the area of the wind-disturbed forest were significantly correlated with the number of trees subsequently killed by I. typographus. Similarly, Kärvemo et al. (2014a) found that at the landscape level, the area of storm gaps was a significant factor affecting tree mortality from I. typographus. Sometimes after a moderate-severity disturbance, insect pests may exhibit a bimodal response—first they colonize the windthrown and dying trees and build up populations, then there may be some decline, but the abundance still stays at relatively high levels, and then the pest responds positively again as the remnant trees are further weakened and colonized over time (Vogt et al., 2020). It is also possible that medium-severity wind disturbances can result in higher beetle populations than high-severity events because extensive mortality can lead to abrupt collapse in suitable habitat provision when the dead trees become too dry for bark beetle colonization, whereas intermediate-severity disturbances can maintain suitable habitats longer (Stadelmann et al., 2013; Kärvemo et al., 2014a; Vogt et al., 2020). Moreover, the death of host trees exposes nonhost remnant trees to higher wind loads, making them more vulnerable to wind (Ruel et al., 2023).
Greenberg (2021) observed recovery dynamics following moderate-severity wind disturbance in a temperate upland hardwood forest. Formed gaps were 0.166–1.08 ha in size, an average of 24.0% of trees (41.1% BA) were windthrown, and 1.0% of trees (1.1% BA) died standing during wind disturbance within the gaps. Over the 21 years after the hurricane, 15.4% (16% BA) of trees additionally died in gaps, while mortality was 15.1% (14.1% BA) in the control plots. Although in gaps more trees died additionally due to windthrow (8.2% in gaps versus 3.1% in control), these long-term results indicate that overall delayed tree mortality was not substantially accelerated in gaps following the initial “pulse” of hurricane-related mortality. Similarly, Szwagrzyk et al. (2017) reported very low subsequent mortality 13 years after wind disturbance (plots with different severities pooled) in a mixed forest dominated by Scots pine; many quite heavily damaged (e.g., strongly bent or leaning) trees had survived. However, Sato et al. (2017) observed high delayed mortality following a moderate-severity typhoon disturbance in a natural mixed forest dominated by Sakhalin fir (Abies sachalinensis (F.Schmidt) Mast.). During a 7-year post-disturbance period, the annual mortality was approximately 2–4%, with averages of 3.5 and 2.8% during the periods of 1–2 years later and 3–7 years later, respectively, significantly exceeding the level recorded during the pre-disturbance period (average of 0.9%). The main tree species A. sachalinensis had especially high delayed mortality levels; during the 7-year post-disturbance period, additionally damaged basal area reached approximately 80% of that which occurred during the disturbance. Post-disturbance deaths of A. sachalinensis exhibited a spatial pattern of gradual expansion around A. sachalinensis trees that had died during the disturbance.
Several factors may account for large variations in mortality, including contingency—remnant trees may or may not experience another heavy wind. Similarly, weather conditions prior to, during, and after partial wind disturbance may or may not facilitate insect pests. Moreover, pest infestation and wildfire do not necessarily occur after wind disturbance, even if weather conditions favor such events (Szwagrzyk et al., 2017). Soil conditions (e.g., periodically waterlogged or hydromorphic soils) also add to the variation in mortality (Mitchell, 1995; Vašíčková et al., 2021).
On a single tree scale, the characteristics of a survivor such as species, age, and size largely affect its fate following PR (Table 4). The level of damage caused to its stem, roots and canopy during wind disturbance clearly influences its prospects as well (Webb, 1989; Everham and Brokaw, 1996; Arévalo et al., 2000). Arévalo et al. (2000) studied tree damage and mortality over 14 years following a moderate severity (approximately 50% mortality during the wind event; two forest types pooled) windthrow in permanent plots in an oak forest and a pine forest in central Minnesota. They found that the probability of a tree dying varied with respect to damage type and severity—mortality probability was approximately 0.18 for undamaged trees and 0.38 for lightly damaged trees. At the same time, for trees with broken stems the mortality probability increased to 0.84 and uprooted trees had only marginal chances (mortality probability 0.98) to survive throughout the study period. Interestingly, Vašíčková et al. (2021) noticed that storm Herwart (2017) selectively impacted the conifer population in the Czech Republic. They found that trees that germinated under the canopy and experienced several periods of suppression and release were more likely to survive the storm. Thus, the authors proposed that individuals with a more varied disturbance history with accompanying denser wood are more likely to survive recurring wind disturbances.
Disturbance of moderate severity might lower the incidence of diseases affecting relatively old trees for a given species. For example, balsam firs (Abies balsamea) in the southern boreal forest of North America become more susceptible to spruce budworm (Choristoneura fumiferana Clem.) attacks as they age due to declining concentrations of defensive secondary foliar compounds (Kumbașlı et al., 2011). Although other factors besides host age also influence population dynamics of C. fumiferana, windstorms that blow down older cohorts every few decades may reduce the incidence of this insect at stand- and landscape-scales.
3.4 Vulnerability of remnant stands under gap dynamics
Following FS, the affected stands are generally less vulnerable to subsequent wind disturbances as a result of the local high frequency-low intensity wind disturbance regime (McCarthy, 2001; Angelstam and Kuuluvainen, 2004). The relatively frequent winds enhance the adaptation of trees in the entire stand, including the trees at gap edges. For instance, Shorohova et al. (2009) concluded that 0.2–10.4% basal area removal by windthrow does not significantly increase subsequent mortality in boreal forests.
Runkle (1998) found that nearly half of the gaps (46%) expanded during a 14-year study period in a southern Appalachian old-growth hardwood-dominated forest. Mortality rates were higher for larger stems; American beech (Fagus grandifolia) exhibited greatest mortality rate (1.18% y−1). The average mortality rate of canopy trees bordering gaps (0.60% y−1) was, however, not significantly higher than canopy-tree mortality in general, suggesting that the presence of gaps did not influence tree mortality rates. Nevertheless, storms with higher wind speeds can cause significant expansion of gaps due to increased exposure to wind of trees at gap edges. Foster and Reiners (1986) found that stepwise gap expansion caused by windthrow and coalescence of gaps into larger gaps were common processes in the virgin subalpine forests located at Crawford Notch, New Hampshire. Bottero et al. (2011) also recognized gap expansion to be an important process in a temperate mixed old-growth forest in Bosnia and Herzegovina, whereas wind and snow were suggested to be the main agents of onward mortality. In another study from Bosnia and Herzegovina, Nagel and Svoboda (2008) found that 70% of gaps showed evidence of subsequent expansion after their initial formation in a mountainous forest reserve dominated by European beech (Fagus sylvatica L.) and silver fir (Abies alba Miller).
Pathogenic fungi are highly influential mortality agents in forests driven by gap dynamics (Hennon, 1995; Angelstam and Kuuluvainen, 2004). As infected trees fall, they often injure nearby trees, thereby creating entry points for new infections and contributing to the persistence of decay fungi in the stand (Hennon, 1995). Worrall et al. (2005) stated that gap expansion is a prominent feature of the disturbance regime of Picea rubens-Abies balsamea forests in the northern Appalachians mountains, as gap expansion was found to occur more frequently than gap initiation in these forests. The genus Abies was strongly associated with gap expansion; stem breakage under wind-loading was the most commonly identified onward mortality agent, whereas fungi causing root and butt rots were the most frequent biotic mortality agents, followed closely by spruce beetles (D. rufipennis).
The spatial and temporal dynamics of bark beetles and their natural enemies are linked to the disturbance regime. Forests that are primarily shaped by gap dynamics exhibit relatively constant and diverse supply of dead and dying trees (Bouget and Duelli, 2004; Vogt et al., 2020), ensuring the continuous presence of natural enemies and competitors of bark beetles (Bouget and Duelli, 2004). Nevertheless, the potential still exists for insect outbreak if there is a source of infestation nearby (Osetrov, 2002; Wermelinger, 2004; Kärvemo et al., 2014a), given that the stand contains suitable host trees. For instance, in Switzerland, a major bark beetle (mainly I. typographus) outbreak that started in 1993 in a 120-year-old Norway spruce stand presumably was a consequence of some scattered trees felled by storm Vivian. This outbreak culminated in almost total death of the stand by 1995 (Wermelinger, 2002). Described disturbance regimes and most likely subsequent disturbance agents associated with them are depicted in Figure 2. Summarized effects of windthrow on subsequent disturbances classified by landscape, stand and tree spatial scales are described in Tables 2–4.
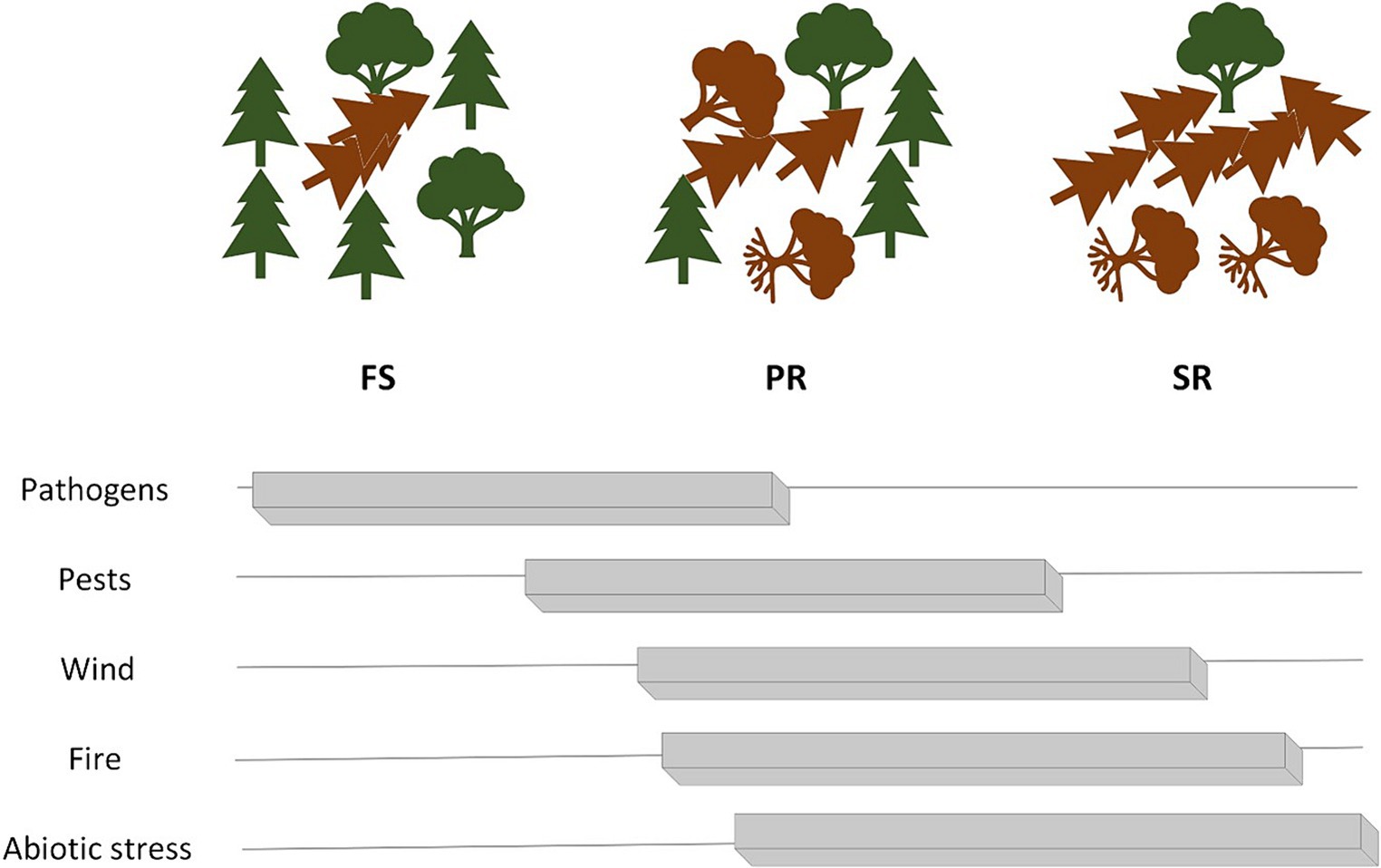
Figure 2. A conceptual diagram visualizing wind disturbance regimes and the generally most likely subsequent disturbance agents affecting the survivors. Relationships between disturbance regimes and subsequent disturbance agents reflect the authors’ interpretation. Environmental and meteorological factors have a strong influence on these processes, thus extreme values of these factors can cause deviations from general patterns.
4 Cumulative effects of climate change on post-storm vulnerability
Climate change alters natural disturbance regimes and the relevance of disturbance agents; multiple compounding disturbance events, such as wind followed by fire, or multiple windstorms within a short period of time, are predicted to become more common (Seidl and Rammer, 2017; Seidl et al., 2020; Anoszko et al., 2022). Compounded disturbances within the normative recovery time of the community may have serious ecological consequences—resilience of the ecosystem might decrease, possibly leading to altered states and disruptions in ecosystem functioning (Paine et al., 1998; Buma and Wessman, 2011; Seidl et al., 2014b; Johnstone et al., 2016).
Wind disturbance events are expected to increase in frequency and intensity in the future (IPCC, 2014). Vulnerability of forests to wind disturbance is also expected to increase due to decreased periods of frozen soils that provide strong root anchorage (Peltola et al., 1999b). Moreover, storms may be accompanied by heavier rainfall, leading to more saturated soils, thus further increasing vulnerability to uprooting (Beniston et al., 2007). Droughts, which are expected to set in quicker and become more intense due to climate change (Mukherjee et al., 2018), also weaken trees and make them more prone to stem breakage under wind-loading (Csilléry et al., 2017; Krokene et al., 2023) and to bark beetle attacks (Jönsson A M, et al., 2007; Hart et al., 2014; Dodds et al., 2019).
Disturbances from fire, insect pests, and pathogens are likely to become more frequent and more intense under climate change (Dale et al., 2001; Jönsson A M, et al., 2007; Seidl and Rammer, 2017). Wildfire frequency and seasonality are projected to increase concomitantly with higher temperatures and more frequent drought occurrences (Flannigan et al., 2000; Liu et al., 2010; Whitman et al., 2019). Such trend is already evident, as fire impact has increased significantly in the last decades across Europe (Patacca et al., 2023). The vulnerability of forests to fungal pathogens such as Heterobasidion spp. and Armillaria spp. is expected to increase due to the increased sporulation and mycelial growth of pathogens in a warmer climate and due to decreased resistance of trees under drought and heat stress (e.g., Gonthier et al., 2005; La Porta et al., 2008; Klopfenstein, 2009; Müller et al., 2018). Drought can also affect the complex interactions between host trees, bark beetles and associated microorganisms—Netherer et al. (2024) demonstrated decreased resistance of P. abies to the infection of bark beetle fungal mutualists in response to simulated drought conditions. Outbreaks of insect pests are expected to become more frequent and more severe, for direct (i.e., greater swarming activity and development rate, reduced overwintering mortality) as well as indirect (i.e., drought, more susceptible host trees due to increased wind disturbances) reasons (Lange et al., 2006; Jönsson A M, et al., 2007; Seidl et al., 2008; Hart et al., 2014). Since 2000, the magnitude of bark beetle disturbance has increased drastically, doubling its share of the total disturbance damage, evidencing the amplifying effect of climate change (Patacca et al., 2023). Several pests and pathogens may expand their range and colonize new areas due to climate warming (Dale et al., 2001; Lange et al., 2006). For example, the mountain pine beetle (Dendroctonus ponderosae) has already spread from its historic range and now affects boreal Pinus forests in North America (Safranyik et al., 2010). Moreover, introduced pests or pathogens can shift hosts, threatening local species, whereas genetic resistance of the new host to such diseases might be limited or absent due to the lack of coevolutionary history (Budde et al., 2016). As a result, past outbreak management methods will not be sufficient to counteract climate-mediated increases in bark beetle disturbance (Dobor et al., 2020).
Single disturbance events, unless of extreme intensity, severity, or both, tend to leave plentiful ecosystem legacies that ensure continuity of composition (Turner et al., 1998; Frelich et al., 2020). Compounding disturbances, however, not only lessen the effect of initial survivors on ecosystem dynamics, but the agents are themselves affected by the amount and distribution of legacies from previous disturbances (Seidl et al., 2014b).
Although there is much evidence for interactive effects when the first disturbance increases the probability or severity of a subsequent disturbance (e.g., Paine et al., 1998), disturbance legacies may also increase ecosystem resistance or resilience to a subsequent disturbance (Simard et al., 2011; Buma, 2015; Cannon et al., 2017). For instance, the presence of wind-damaged trees may increase the risk of wildfire due to greater potential fuel load, but at the same time, the storm legacy might reduce the risk of crown fires (Gardiner, 2021). The extent to which the changes in disturbance regimes and concomitant interactions will affect forest ecosystems is uncertain. Disturbance legacies, including surviving remnant trees, determine the recovery dynamics and successional pathway of the stand (Seidl et al., 2014b). However, due to changed disturbance regimes under climate change and intensive disturbance management practices, resilience-enhancing legacies can be lost or diminished (Johnstone et al., 2016; Frelich et al., 2020).
5 Managing remnant stands after wind disturbance
Forest management practices such as planting conifer monocultures, increasing growing stock, thinning operations etc. can have considerable influence on the susceptibility of forests to strong winds (Gardiner et al., 1997, 2010; Jactel et al., 2017). Part of the recent intensification of disturbance regimes in Europe can be related to management-driven changes in forest structure and composition (Seidl et al., 2011b). For instance, trees in a dense stand experience a smaller turning moment (i.e., are less vulnerable) for a given wind speed than more exposed trees (Hale et al., 2012). In dense stands, however, trees generally allocate few resources to radial growth that provides mechanical stability while they allocate more resources in maximizing upwards growth to access light (Dekker et al., 2009; Bigler, 2016). For this reason, extensive damage can occur in formerly dense stands that are heavily thinned, especially if dominants are removed and the residual trees are tall and slender (Stathers et al., 1994). While naturally developing stands usually also go through a self-thinning (i.e., stem-exclusion) phase (Oliver et al., 1996; Chen and Popadiouk, 2002), the process proceeds slowly, allowing the surviving trees time to adapt to new conditions. Similarly, weakened spruce trees in overstocked, monospecific plantations established outside of P. abies’ natural range provide perfect conditions for bark beetle outbreaks (Hlásny et al., 2021). Thus, promoting concepts that emulate development and functioning of natural systems is of great importance.
Following wind disturbance, disturbance legacies are important as they provide possibilities for ecosystem reorganization and recovery (Franklin et al., 2000; Vodde et al., 2011; Jõgiste et al., 2017) and facilitate sustaining biodiversity within the ecosystem (Lindenmayer et al., 2004; Swanson et al., 2011; Bāders et al., 2021; Mitchell et al., 2023). However, disturbance legacies can also increase vulnerability to subsequent disturbances. Large quantities of deadwood that accompany wind disturbances greatly enhance the risk of intense bark beetle attack and fire hazard, which might lead the ecosystem beyond the point of no return, i.e., to lose its resilience. For instance, following a strong windstorm, uncleared wind-damaged sites triggered a serious bark beetle outbreak in the Tatra National Park; additional damage caused by the beetles eventually exceeded that of the storm (Nikolov et al., 2014).
Salvage logging of disturbed forests is thought to be indispensable to control population levels of bark beetles (Gandhi et al., 2007). However, salvage logging does not necessarily ensure total prevention of bark beetle damage (Ravn, 1985; Schroeder and Lindelöw, 2002; Kautz et al., 2013). Salvage cutting is effective for pest control only when conducted in time. To decrease the risk of a spruce bark beetle outbreak, wind-felled spruces should be removed from the area before the following midsummer, and potential breeding substrate at the edges of larger storm gaps should be given the highest priority (Göthlin et al., 2000). Dobor et al. (2020) found that salvage logging in the vicinity of roads created a “fire break effect” on bark beetle spread and was moderately efficient in reducing landscape-scale bark beetle disturbance. They further suggested that in Central Europe, removal of >80% of all wind-felled trees is required to substantially reduce bark beetle disturbances.
Management may be needed at different times after a medium-severity disturbance due to several pulses of tree dieback (Vogt et al., 2020). When the disturbance is too catastrophic for harvesting all the windthrown trees during the first year, sanitation operations should first be applied in small gaps and scattered treefalls, because in large, sunlit gaps, downed trees dry more quickly than in small gaps, thus becoming unattractive for beetles (Bouget and Duelli, 2004). Wind-felled trees are suitable as breeding material for bark beetles for a limited time period only (Wermelinger, 2004), whereas uprooted trees (as opposed to broken trees) may remain habitable for a longer time (Göthlin et al., 2000). Dead trees remain attractive to bark beetles for 1–3 years, depending on the weather, altitude and latitude (Wermelinger, 2002; Modlinger and Novotný, 2015). Thus, when the bark of the dead trees has become too dry for beetle colonization, foresters should focus on salvage cutting of newly attacked living trees and leave the older stems for the benefit of saproxylic organisms (Wermelinger, 2002).
However, outbreaks of severe forest pests like I. typographus or D. rufipennis do not always occur following a wind disturbance, even if the area is categorically at high risk of beetle outbreak, as other factors (e.g., occurrence of drought at the time of wind disturbance, timing of the disturbance event in relation to the beetle flight period) determine infestation initiation as well (Kulakowski and Veblen, 2003; Wermelinger, 2004; Hart et al., 2014; Dodds et al., 2019). Eriksson et al. (2007) suggest that in Finland, at endemic I. typographus population levels, it is safe to leave fewer than 20 wind-felled spruces in a managed forest. The risk of bark beetle infestation can be reduced by using some of the wind-felled host trees as trap trees (Schroeder and Lindelöw, 2002). Kulakowski and Veblen (2007) also state that mitigation efforts such as salvage logging following wind disturbance do not always reduce the likelihood of extensive and severe fires. The threat of further wind damage should be considered as well. Salvaging that results in exposing previously protected stand edges to strong winds may lead to windfall events, which in turn may intensify or prolong a bark beetle outbreak (Modlinger and Novotný, 2015).
Sanitary clear-cutting after moderate- or high-severity natural events such as windstorms can have a negative impact on a forest ecosystem, due to the cumulative severity of the two events and because of the loss of disturbance legacies (Peterson and Leach, 2008). For instance, the regulating effect of antagonistic species of insect pests may be inhibited in salvaged stands (Bouget and Duelli, 2004). Retaining the survivors after wind disturbance enables formation or maintenance of structurally complex stands (Meigs and Keeton, 2018; Peterson, 2019b) that are more resilient to disturbances and climate change (Messier et al., 2013). Windthrow legacies may also impede ungulate access, thereby protecting survivors and saplings from browsing damage (Rammig et al., 2007; Bosley-Smith et al., 2024). Moreover, in mountain forests, not removing logs after a storm can temporarily protect against natural hazards (e.g., rockfall and avalanches; Wohlgemuth et al., 2017) while root systems of surviving trees are important for stabilizing soils developed on unstable parent material or steep slopes (Reubens et al., 2007). Root systems of the survivors also sustain and support the re-establishment of belowground life and function; retaining the survivors may mitigate soil carbon losses as well (Seidl et al., 2014a; Prescott and Grayston, 2023).
Regardless of the numerous benefits of maintaining remnant trees, several other aspects need to be considered. Depending on site conditions and severity of the disturbance, surviving canopy trees may facilitate germination, but they may also limit light levels (along with shading by upwards-turned root plates and fallen stems) and reduce the availability of soil resources due to root competition, making establishment conditions suitable only for shade-tolerant and frugal species (Cooper-Ellis et al., 1999; Palik et al., 2003). In recreational areas, retaining damaged trees can be a safety hazard (Royo et al., 2016). Thus, the decision whether, when and to what extent to salvage the windthrow should be carefully considered, with aspects like severity, delayed mortality, possibility of sprouting and recovering of damaged trees, possibility of secondary disturbances and their potential influence on nearby stands, public safety, ecologic and economic expediency in mind. If the wind-disturbed forest is affected by a pathogen or pest with a restricted or defined host range, it may be possible to increase the percentage of trees more resistant to the agent.
6 Conclusion
The risk of tree mortality due to wind disturbance, as well as its susceptibility to a subsequent disturbance, is influenced by multiple interacting factors. Besides severity of the initial event, contingency along with numerous contributing factors interrelating at tree, stand and landscape scales (e.g., species composition of the survivors, local pest and pathogen context, weather conditions during and after the wind event) that influence the onward turn of events make it difficult to suggest valid outcome patterns or quantify risk dynamics for variable forest types occurring in boreal and temperate biomes. More conclusive findings could be detected for a set of narrower predefined factors, but currently the data regarding the fate of remnant trees is very limited.
We found that in areas with severe wind damage (SR), the location of the remaining trees largely determines their onward fate, whereas the survivors are generally more susceptible to subsequent mortality compared to trees that survived moderate to low severity damage (PR and FS). Highly dispersed trees have higher mortality than the trees clustered in aggregates and the survivors situated in the wind gap interior are more susceptible to subsequent disturbance agents than the trees along the gap edges.
In a forest stand that has experienced a moderate-severity wind disturbance (PR), the characteristics of the remnant stand largely determine the onward fate of the survivors. If the remnant stand displays high variability in terms of age, size and species, the survivors are more resistant to subsequent disturbance agents. Coniferous remnant trees have a higher risk of mortality, as in boreal and temperate biomes, most of the destructive insect pests strongly associated with wind disturbance have coniferous hosts.
Following a fine-scale gap disturbance (FS), the trees situated along gap edges can be more likely to die, compared to the trees situated in stand interior, but the mortality-causing processes operate on a longer time scale, often allowing the initial gap to close before the delayed mortality occurs.
Differences in the duration or magnitude of windstorms in future climates could affect the severity and propagation of wind damage, with concurrent impacts on biological legacies, thus possibly changing post-disturbance successional trajectories. The impact of more frequent and compounded disturbances on post-windthrow stand development requires further study to find the best management methods, as past management methods may no longer be appropriate. There is little opportunity for humans to alter wind disturbance regimes directly, but the impact of wind may be changed by altering stand and landscape characteristics. More heterogeneous patterns in terms of tree age and species will make the ecosystem more resistant to disturbances or at least increase recovery opportunities. Survivors play important roles in stand recovery and buildup of future resilience. The fate of remnant trees and salvage retention (similar aspects to retention forestry) need to be further investigated to find a balance between the threat that CWD presents, timber management plans, and goals for ecosystem services set by society at large, such as biodiversity, wildlife habitat and carbon sequestration.
Author contributions
KP-H: Conceptualization, Investigation, Methodology, Visualization, Writing – original draft, Writing – review & editing. FV: Conceptualization, Visualization, Writing – original draft, Writing – review & editing. JS: Conceptualization, Writing – review & editing. KJ: Supervision, Funding acquisition, Writing – review & editing. KK: Conceptualization, Writing – review & editing. ES: Conceptualization, Writing – review & editing. LF: Conceptualization, Writing – review & editing. MM: Conceptualization, Funding acquisition, Writing – review & editing. OP: Writing – review & editing. MS: Writing – review & editing. EB: Writing – review & editing.
Funding
The author(s) declare that financial support was received for the research, authorship, and/or publication of this article. This work was supported by the Estonian Research Council grant (PRG1586) and European Regional Development Fund (EE-LV00001).
Acknowledgments
The authors are grateful to the editor and two reviewers for their time and energy in providing helpful comments that have improved the manuscript.
Conflict of interest
The authors declare that the research was conducted in the absence of any commercial or financial relationships that could be construed as a potential conflict of interest.
The author(s) declared that they were an editorial board member of Frontiers, at the time of submission. This had no impact on the peer review process and the final decision.
Publisher’s note
All claims expressed in this article are solely those of the authors and do not necessarily represent those of their affiliated organizations, or those of the publisher, the editors and the reviewers. Any product that may be evaluated in this article, or claim that may be made by its manufacturer, is not guaranteed or endorsed by the publisher.
Abbreviations
SR, stand-replacing wind disturbance; PR, partially stand-replacing wind disturbance; FS, fine-scale gap disturbance; CWD, coarse woody debris; DBH, diameter at breast height; BA, basal area; ha, hectare; y, year
References
Allen, J. R. L. (1992). Trees and their response to wind: mid Flandrian strong winds, Severn estuary and inner Bristol Channel, Southwest Britain. Philos. Trans. R. Soc. Lond. Ser. B Biol. Sci. 338, 335–364.
Allen, M. S., Thapa, V., Arévalo, J. R., and Palmer, M. W. (2012). Windstorm damage and forest recovery: accelerated succession, stand structure, and spatial pattern over 25 years in two Minnesota forests. Plant Ecol. 213, 1833–1842. doi: 10.1007/s11258-012-0139-9
Angelstam, P., and Kuuluvainen, T. (2004). Boreal forest disturbance regimes, successional dynamics and landscape structures: a European perspective. Ecol. Bull. 51, 117–136. doi: 10.2307/20113303
Anoszko, E., Frelich, L. E., Rich, R. L., and Reich, P. B. (2022). Wind and fire: rapid shifts in tree community composition following multiple disturbances in the southern boreal forest. Ecosphere 13:e3952. doi: 10.1002/ecs2.3952
Anthony, M. A., Crowther, T. W., van der Linde, S., Suz, L. M., Bidartondo, M. I., and Cox, F. (2022). Forest tree growth is linked to mycorrhizal fungal composition and function across Europe. ISME J. 16, 1327–1336. doi: 10.1038/s41396-021-01159-7
Anyomi, K. A., Mitchell, S. J., Perera, A. H., and Ruel, J. C. (2017). Windthrow dynamics in boreal Ontario: a simulation of the vulnerability of several stand types across a range of wind speeds. Forests 8:233. doi: 10.3390/f8070233
Anyomi, K. A., Mitchell, S. J., and Ruel, J. C. (2016). Windthrow modelling in old-growth and multi-layered boreal forests. Ecol. Model. 327, 105–114. doi: 10.1016/j.ecolmodel.2016.02.003
Arévalo, J. R., DeCoster, J. K., McAlister, S. D., and Palmer, M. W. (2000). Changes in two Minnesota forests during 14 years following catastrophic windthrow. J. Veg. Sci. 11, 833–840. doi: 10.2307/3236553
Bāders, E., Jõgiste, K., Elferts, D., Vodde, F., Kiviste, A., Luguza, S., et al. (2021). Storm legacies shaping post-windthrow forest regeneration: learnings from spatial indices in unmanaged Norway spruce stands. Eur. J. For. Res. 140, 819–833. doi: 10.1007/s10342-021-01368-x
Baier, P., Führer, E., Kirisits, T., and Rosner, S. (2002). Defence reactions of Norway spruce against bark beetles and the associated fungus Ceratocystis polonica in secondary pure and mixed species stands. For. Ecol. Manag. 159, 73–86. doi: 10.1016/S0378-1127(01)00711-3
Baranchikov, Y., Akulov, E., and Astapenko, S. (2010). Bark beetle Polygraphus proximus: a new aggressive far eastern invader on Abies species in Siberia and European Russia. In Proceedings, 21st US Department of Agriculture interagency research forum on invasive species (pp. 12–15).
Beniston, M., Stephenson, D. B., Christensen, O. B., Ferro, C. A., Frei, C., and Goyette, S. (2007). Future extreme events in European climate: an exploration of regional climate model projections. Clim. Chang. 81, 71–95. doi: 10.1007/s10584-006-9226-z
Benzel, J. S. (2015). Screening aid: Pine shoot beetles, Tomicus spp. US: Identification Technology Program (ITP), USDAAPHIS-PPQ-S&T, Fort Collins, CO, 6.
Berryman, A. A., and Ferrell, G. T. (1988). “The fir engraver beetle in Western States,” in Dynamics of forest insect populations. Population ecology. ed. A. A. Berryman (Boston, MA: Springer), 555–577.
Bezos, D., Martínez-Álvarez, P., Diez, J. J., and Fernández, M. M. (2015). The pine shoot beetle Tomicus piniperda as a plausible vector of Fusarium circinatum in northern Spain. Ann. For. Sci. 72, 1079–1088. doi: 10.1007/s13595-015-0515-4
Bigler, C. (2016). Trade-offs between growth rate, tree size and lifespan of mountain pine (Pinus montana) in the Swiss National Park. PLoS One 11:e0150402. doi: 10.1371/journal.pone.0150402
Boone, C. K., Keefover-Ring, K., Mapes, A. C., Adams, A. S., Bohlmann, J., and Raffa, K. F. (2013). Bacteria associated with a tree-killing insect reduce concentrations of plant defense compounds. J. Chem. Ecol. 39, 1003–1006. doi: 10.1007/s10886-013-0313-0
Bosley-Smith, C., Fraver, S., D'Amato, A. W., Rogers, N. S., Tabak, N., and Wason, J. (2024). The natural ‘exclosure effect’ and tree regeneration following post-windstorm salvage logging. J. Appl. Ecol. 61, 260–270. doi: 10.1111/1365-2664.14560
Bottero, A., Garbarino, M., Dukic, V., Govedar, Z., Lingua, E., Nagel, T. A., et al. (2011). Gap-phase dynamics in the old-growth forest of Lom (Bosnia-Herzegovina). Silva fennica 45, 875–887. doi: 10.14214/sf.76
Bouchard, M., Pothier, D., and Ruel, J. C. (2009). Stand-replacing windthrow in the boreal forests of eastern Quebec. Can. J. For. Res. 39, 481–487. doi: 10.1139/X08-174
Bouget, C., and Duelli, P. (2004). The effects of windthrow on forest insect communities: a literature review. Biol. Conserv. 118, 281–299. doi: 10.1016/j.biocon.2003.09.009
Braun, S., Schindler, C., Volz, R., and Flückiger, W. (2003). Forest damages by the storm ‘Lothar’ in permanent observation plots in Switzerland: the significance of soil acidification and nitrogen deposition. Water Air Soil Pollut. 142, 327–340. doi: 10.1023/A:1022088806060
Brüchert, F., and Gardiner, B. (2006). The effect of wind exposure on the tree aerial architecture and biomechanics of Sitka spruce (Picea sitchensis, Pinaceae). Am. J. Bot. 93, 1512–1521. doi: 10.3732/ajb.93.10.1512
Budde, K. B., Nielsen, L. R., Ravn, H. P., and Kjær, E. D. (2016). The natural evolutionary potential of tree populations to cope with newly introduced pests and pathogens—lessons learned from forest health catastrophes in recent decades. Curr. For. Rep. 2, 18–29. doi: 10.1007/s40725-016-0029-9
Buma, B. (2015). Disturbance interactions: characterization, prediction, and the potential for cascading effects. Ecosphere 6, 1–15. doi: 10.1890/ES15-00058.1
Buma, B., and Wessman, C. A. (2011). Disturbance interactions can impact resilience mechanisms of forests. Ecosphere 2, art64–art13. doi: 10.1890/ES11-00038.1
Byers, J. A., Zhang, Q. H., Schlyter, F., and Birgersson, G. (1998). Volatiles from nonhost birch trees inhibit pheromone response in spruce bark beetles. Science of Nature 85, 557–561. doi: 10.1007/s001140050551
Canham, C. D., Papaik, M. J., and Latty, E. F. (2001). Interspecific variation in susceptibility to windthrow as a function of tree size and storm severity for northern temperate tree species. Can. J. For. Res. 31, 1–10. doi: 10.1139/x00-124
Cannon, J. B., O’Brien, J. J., Loudermilk, E. L., Dickinson, M. B., and Peterson, C. J. (2014). The influence of experimental wind disturbance on forest fuels and fire characteristics. For. Ecol. Manag. 330, 294–303. doi: 10.1016/j.foreco.2014.07.021
Cannon, J. B., Peterson, C. J., O'Brien, J. J., and Brewer, J. S. (2017). A review and classification of interactions between forest disturbance from wind and fire. For. Ecol. Manag. 406, 381–390. doi: 10.1016/j.foreco.2017.07.035
Carlson, D. W., and Groot, A. (1997). Microclimate of clear-cut, forest interior, and small openings in trembling aspen forest. Agric. For. Meteorol. 87, 313–329. doi: 10.1016/S0168-1923(95)02305-4
Caron, M. N., Kneeshaw, D. D., De Grandpré, L., Kauhanen, H., and Kuuluvainen, T. (2009). “Canopy gap characteristics and disturbance dynamics in old-growth Picea abies stands in northern Fennoscandia: is the forest in quasi-equilibrium?” in Annales Botanici Fennici, vol. 46 (Helsinki: Finnish Zoological and Botanical Publishing Board), 251–262.
Chen, H. Y., and Popadiouk, R. V. (2002). Dynamics of north American boreal mixedwoods. Environ. Rev. 10, 137–166. doi: 10.1139/a02-007
Christiansen, E. (1991). “Ips typographus and Ophiostoma polonicum versus Norway spruce: joint attack and host defense,” in Forest Insect Guilds: Patterns of Interaction with Host Trees; 1989 August 13-17; Abakan, Siberia, U.S.S.R. Gen. Tech. Rep. NE-153. ed. Y. N. Baranchikov (Radnor, PA: U.S. Department of Agriculture, Forest Service, Northeastern Forest Experiment Station), 321-334.
Christiansen, E., and Bakke, A. (1988). “The spruce bark beetle of Eurasia” in Dynamics of forest insect populations: Patterns, causes, implications. ed. A. A. Berryman (Boston, MA: Springer US), 479–503.
Christiansen, E., Waring, R. H., and Berryman, A. (1987). Resistance of conifers to bark beetle attack: searching for general relationships. For. Ecol. Manag. 22, 89–106. doi: 10.1016/0378-1127(87)90098-3
Coates, K. D. (1997). Windthrow damage 2 years after partial cutting at the date creek silvicultural systems study in the interior cedar hemlock forests of northwestern British Columbia. Can. J. For. Res. 27, 1695–1701. doi: 10.1139/x97-132
Cobb, R. C., and Metz, M. R. (2017). Tree diseases as a cause and consequence of interacting forest disturbances. Forests 8:147. doi: 10.3390/f8050147
Cooper-Ellis, S., Foster, D. R., Carlton, G., and Lezberg, A. (1999). Forest response to catastrophic wind: results from an experimental hurricane. Ecology 80, 2683–2696. doi: 10.1890/0012-9658(1999)080[2683:FRTCWR]2.0.CO;2
Csilléry, K., Kunstler, G., Courbaud, B., Allard, D., Lassègues, P., Haslinger, K., et al. (2017). Coupled effects of wind-storms and drought on tree mortality across 115 forest stands from the Western Alps and the Jura mountains. Glob. Chang. Biol. 23, 5092–5107. doi: 10.1111/gcb.13773
Dale, V. H., Joyce, L. A., Mcnulty, S., Neilson, R. P., Ayres, M. P., and Flannigan, M. D. (2001). Climate change and forest disturbances: climate change can affect forests by altering the frequency, intensity, duration, and timing of fire, drought, introduced species, insect and pathogen outbreaks, hurricanes, windstorms, ice storms, or landslides. Bioscience 51, 723–734. doi: 10.1641/0006-3568(2001)051[0723:CCAFD]2.0.CO;2
Dekker, M., Sass-Klaassen, U., Den Ouden, J., and Goedhart, P. W. (2009). The effect of canopy position on growth and mortality in mixed sapling communities during self-thinning. Eur. J. For. Res. 128, 455–466. doi: 10.1007/s10342-009-0293-3
European Food Safety Authority (EFSA)de la Peña, E., Kinkar, M., and Vos, S. (2020). Pest survey card on Polygraphus proximus. EFSA Supporting Pub. 17:1780E. doi: 10.2903/sp.efsa.2020.EN-1780
Del Tredici, P. (2001). Sprouting in temperate trees: a morphological and ecological review. Bot. Rev. 67, 121–140. doi: 10.1007/BF02858075
Dietze, M. C., and Clark, J. S. (2008). Changing the gap dynamics paradigm: vegetative regeneration control on forest response to disturbance. Ecol. Monogr. 78, 331–347. doi: 10.1890/07-0271.1
Dobor, L., Hlásny, T., Rammer, W., Zimová, S., Barka, I., and Seidl, R. (2020). Spatial configuration matters when removing windfelled trees to manage bark beetle disturbances in central European forest landscapes. J. Environ. Manag. 254:109792. doi: 10.1016/j.jenvman.2019.109792
Dodds, K. J., DiGirolomo, M. F., and Fraver, S. (2019). Response of bark beetles and woodborers to tornado damage and subsequent salvage logging in northern coniferous forests of Maine, USA. For. Ecol. Manag. 450:117489. doi: 10.1016/j.foreco.2019.117489
Dodds, K. J., Sweeney, J., and Allison, J. D. (2023). “Woodborers in Forest stands” in Forest entomology and pathology: Volume 1: Entomology. eds. D. J. Allison, T. D. Paine, B. Slippers, and M. J. Wingfield (Cham: Springer International Publishing), 361–415.
Dyer, J. M., and Baird, P. R. (1997). Wind disturbance in remnant forest stands along the prairie-forest ecotone, Minnesota, USA. Plant Ecol. 129, 121–134. doi: 10.1023/A:1009761013081
Elie, J. G., and Ruel, J. C. (2005). Windthrow hazard modelling in boreal forests of black spruce and jack pine. Can. J. For. Res. 35, 2655–2663. doi: 10.1139/x05-189
Ennos, A. R. (1997). Wind as an ecological factor. Trends Ecol. Evol. 12, 108–111. doi: 10.1016/S0169-5347(96)10066-5
Eriksson, M., Neuvonen, S., and Roininen, H. (2007). Retention of wind-felled trees and the risk of consequential tree mortality by the European spruce bark beetle Ips typographus in Finland. Scand. J. For. Res. 22, 516–523. doi: 10.1080/02827580701800466
Eriksson, M., Pouttu, A., and Roininen, H. (2005). The influence of windthrow area and timber characteristics on colonization of wind-felled spruces by Ips typographus (L.). For. Ecol. Manag. 216, 105–116. doi: 10.1016/j.foreco.2005.05.044
Everham, E. M., and Brokaw, N. V. (1996). Forest damage and recovery from catastrophic wind. Bot. Rev. 62, 113–185. doi: 10.1007/BF02857920
Faccoli, M., and Santini, A. (2016). “Dutch elm disease and elm bark beetles: Pathogeninsect interaction,” in Vector-mediated transmission of plant pathogens: American Phytopathology Society. ed. J. K. Brown (St. Paul, MN: APS Publications), 73–86.
Ferrenberg, S., and Mitton, J. B. (2014). Smooth bark surfaces can defend trees against insect attack: resurrecting a ‘slippery’hypothesis. Funct. Ecol. 28, 837–845. doi: 10.1111/1365-2435.12228
Flannigan, M. D., Stocks, B. J., and Wotton, B. M. (2000). Climate change and forest fires. Sci. Total Environ. 262, 221–229. doi: 10.1016/S0048-9697(00)00524-6
Foster, D. R. (1988). Species and stand response to catastrophic wind in Central New England, USA. J. Ecol. 76, 135–151. doi: 10.2307/2260458
Foster, D. R., Aber, J. D., Melillo, J. M., Bowden, R. D., and Bazzaz, F. A. (1997). Forest response to disturbance and anthropogenic stress. Bioscience 47, 437–445. doi: 10.2307/1313059
Foster, D. R., and Boose, E. R. (1992). Patterns of forest damage resulting from catastrophic wind in Central New England, USA. J. Ecol. 80, 79–98. doi: 10.2307/2261065
Foster, D. R., Knight, D. H., and Franklin, J. F. (1998). Landscape patterns and legacies resulting from large, infrequent forest disturbances. Ecosystems 1, 497–510. doi: 10.1007/s100219900046
Foster, J. R., and Reiners, W. A. (1986). Size distribution and expansion of canopy gaps in a northern Appalachian spruce-fir forest. Vegetatio 68, 109–114. doi: 10.1007/BF00045062
Franceschi, V. R., Krokene, P., Christiansen, E., and Krekling, T. (2005). Anatomical and chemical defenses of conifer bark against bark beetles and other pests. New Phytol. 167, 353–376. doi: 10.1111/j.1469-8137.2005.01436.x
Franklin, J. F., Lindenmayer, D., MacMahon, J. A., McKee, A., Magnuson, J., Perry, D. A., et al. (2000). Threads of continuity. Conserv. Biol. Pract. 1, 8–17. doi: 10.1111/j.1526-4629.2000.tb00155.x
Fraver, S., Jain, T., Bradford, J. B., D'Amato, A. W., Kastendick, D., Palik, B., et al. (2011). The efficacy of salvage logging in reducing subsequent fire severity in conifer-dominated forests of Minnesota, USA. Ecol. Appl. 21, 1895–1901. doi: 10.1890/11-0380.1
Frelich, L. E. (2002). Forest dynamics and disturbance regimes: Studies from temperate evergreen-deciduous forests. Cambridge, United Kingdom: Cambridge University Press.
Frelich, L. E., Jõgiste, K., Stanturf, J., Jansons, A., and Vodde, F. (2020). Are secondary forests ready for climate change? It depends on magnitude of climate change. Landscape Diversity and Ecosyst. Legacies. Forests 11:965. doi: 10.3390/f11090965
Frelich, L. E., and Lorimer, C. G. (1991). Natural disturbance regimes in hemlock-hardwood forests of the upper Great Lakes region. Ecol. Monogr. 61, 145–164. doi: 10.2307/1943005
Frelich, L. E., and Ostuno, E. J. (2012). Estimating wind speeds of convective storms from tree damage. E-Journal of Severe Storms Meteorology 7, 1–19. doi: 10.55599/ejssm.v7i9.45
Gandhi, K. J., Gilmore, D. W., Katovich, S. A., Mattson, W. J., Spence, J. R., and Seybold, S. J. (2007). Physical effects of weather events on the abundance and diversity of insects in north American forests. Environ. Rev. 15, 113–152. doi: 10.1139/A07-003
Garbelotto, M., and Gonthier, P. (2013). Biology, epidemiology, and control of Heterobasidion species worldwide. Annu. Rev. Phytopathol. 51, 39–59. doi: 10.1146/annurev-phyto-082712-102225
Gardiner, B. (2021). Wind damage to forests and trees: a review with an emphasis on planted and managed forests. J. For. Res. 26, 248–266. doi: 10.1080/13416979.2021.1940665
Gardiner, B. A., Stacey, G. R., Belcher, R. E., and Wood, C. J. (1997). Field and wind tunnel assessments of the implications of respacing and thinning for tree stability. Forestry: Int. J. Forest Res. 70, 233–252. doi: 10.1093/forestry/70.3.233
Gardiner, B., Blennow, K., Carnus, J. M., Fleischner, P., Ingemarson, F., Landmann, G., et al. (2010). Destructive storms in European forests: Past and forthcoming impacts. Finland: Final report to European Commission-DG Environment, European Forest Institute.
Gardiner, B., Byrne, K., Hale, S., Kamimura, K., Mitchell, S. J., Peltola, H., et al. (2008). A review of mechanistic modelling of wind damage risk to forests. Forestry 81, 447–463. doi: 10.1093/forestry/cpn022
Gardiner, B., Marshall, B., Achim, A., Belcher, R., and Wood, C. (2005). The stability of different silvicultural systems: a wind-tunnel investigation. Forestry 78, 471–484. doi: 10.1093/forestry/cpi053
Gardiner, L. M. (1975). Insect attack and value loss in wind-damaged spruce and jack pine stands in northern Ontario. Can. J. For. Res. 5, 387–398. doi: 10.1139/x75-053
Gilmore, D.W., Kastendick, D.N., Zasada, J.C., and Anderson, P.J. (2003). Alternative fuel reduction treatments in the gunflint corridor of the superior National Forest: second-year results and sampling recommendations. Res. Note NC-381. U.S. Department of Agriculture, Forest Service, north central Research Station: St. Paul, MN, USA, 8p.
Goheen, D. J., and Hansen, E. M. (1993). “Effects of pathogens and bark beetles on forests” in Beetle-pathogen interactions in conifer forests. eds. T. D. Schowalter and G. M. Filip (San Diego: Academic Press), 175–196.
Gonthier, P., Garbelotto, M. M., and Nicolotti, G. (2005). Seasonal patterns of spore deposition of Heterobasidion species in four forests of the western Alps. Phytopathology 95, 759–767. doi: 10.1094/PHYTO-95-0759
Göthlin, E., Schroeder, L. M., and Lindelöw, A. (2000). Attacks by Ips typographus and Pityogenes chalcographus on windthrown spruces (Picea abies) during the two years following a storm felling. Scand. J. For. Res. 15, 542–549. doi: 10.1080/028275800750173492
Grace, J. (1988). 3. Plant response to wind. Agric. Ecosyst. Environ. 22-23, 71–88. doi: 10.1016/0167-8809(88)90008-4
Greacen, E. L., and Sands, R. (1980). Compaction of forest soils. A review. Soil Res. 18, 163–189. doi: 10.1071/SR9800163
Greenberg, C. H. (2021). Long-term recovery dynamics following hurricane-related wind disturbance in a southern Appalachian forest. For. Ecol. Manag. 502:119704. doi: 10.1016/j.foreco.2021.119704
Griess, V. C., and Knoke, T. (2011). Growth performance, windthrow, and insects: meta-analyses of parameters influencing performance of mixed-species stands in boreal and northern temperate biomes. Can. J. For. Res. 41, 1141–1159. doi: 10.1139/x11-042
Grunwald, M. (1986). Ecological segregation of bark beetles (Coleoptera, Scolytidae) of spruce 1, 2. J. Appl. Entomol. 101, 176–187. doi: 10.1111/j.1439-0418.1986.tb00846.x
Hale, S. E., Gardiner, B. A., Wellpott, A., Nicoll, B. C., and Achim, A. (2012). Wind loading of trees: influence of tree size and competition. Eur. J. For. Res. 131, 203–217. doi: 10.1007/s10342-010-0448-2
Hanewinkel, M., Hummel, S., and Albrecht, A. (2011). Assessing natural hazards in forestry for risk management: a review. Eur. J. For. Res. 130, 329–351. doi: 10.1007/s10342-010-0392-1
Hanson, J. J., and Lorimer, C. G. (2007). Forest structure and light regimes following moderate wind storms: implications for multi-cohort management. Ecol. Appl. 17, 1325–1340. doi: 10.1890/06-1067.1
Harmon, M. E., and Bell, D. M. (2020). Mortality in forested ecosystems: suggested conceptual advances. Forests 11:572. doi: 10.3390/f11050572
Harmon, M. E., and Pabst, R. J. (2019). The long-term effects of wind disturbance on a Sitka spruce-western hemlock forest. Forests 10:119. doi: 10.3390/f10020119
Hart, J. L., and Kleinman, J. S. (2018). What are intermediate-severity forest disturbances and why are they important? Forests 9:579. doi: 10.3390/f9090579
Hart, S. J., Veblen, T. T., Eisenhart, K. S., Jarvis, D., and Kulakowski, D. (2014). Drought induces spruce beetle (Dendroctonus rufipennis) outbreaks across northwestern Colorado. Ecology 95, 930–939. doi: 10.1890/13-0230.1
Hély, C., Bergeron, Y., and Flannigan, M. D. (2000). Effects of stand composition on fire hazard in mixed-wood Canadian boreal forest. J. Veg. Sci. 11, 813–824. doi: 10.2307/3236551
Hennon, P. E. (1995). Are heart rot fungi major factors of disturbance in gap-dynamic forests? Northwest Sci. 69, 284–293.
Hinds, T. E., Hawksworth, F. G., and Davidson, R. W. (1965). Beetle-killed Engelmann spruce its deterioration in Colorado. J. For. 63, 536–542.
Hlásny, T., König, L., Krokene, P., Lindner, M., Montagné-Huck, C., Müller, J., et al. (2021). Bark beetle outbreaks in Europe: state of knowledge and ways forward for management. Curr. For. Rep. 7, 138–165. doi: 10.1007/s40725-021-00142-x
Huang, J., Kautz, M., Trowbridge, A. M., Hammerbacher, A., Raffa, K. F., Adams, H. D., et al. (2020). Tree defence and bark beetles in a drying world: carbon partitioning, functioning and modelling. New Phytol. 225, 26–36. doi: 10.1111/nph.16173
Huggard, D. J., Klenner, W., and Vyse, A. (1999). Windthrow following four harvest treatments in an Engelmann spruce-subalpine fir forest in southern interior British Columbia, Canada. Can. J. For. Res. 29, 1547–1556. doi: 10.1139/x99-135
Hui, Y., and Lieutier, F. (1997). Shoot aggregation by Tomicus piniperda L (col: Scolytidae) in Yunnan, southwestern China. Ann. Sci. For. 54, 635–641. doi: 10.1051/forest:19970704
Hunter, M. G. (1995). Residual trees as biological legacies. Cascade Center for Ecosystem Manag. Commun. 2:13.
Idbella, M., Stinca, A., Abd El-Gawad, A. M., Motti, R., Mazzoleni, S., and Bonanomi, G. (2023). Windstorm disturbance sets off plant species invasion, microbiota shift, and soilborne pathogens spread in an urban Mediterranean forest. For. Ecol. Manag. 540:121058. doi: 10.1016/j.foreco.2023.121058
IPCC (2014). Climate change 2014: Synthesis report summary chapter for policymakers. Geneva, Switzerland: IPCC.
Ishizuka, M., Ochiai, Y., and Utsugi, H. (2002). “Microenvironments and growth in gaps” in Diversity and interaction in a temperate Forest Community: Ogawa Forest Reserve of Japan. eds. T. Nakashizuka and Y. Matsumoto (Tokio, Japan: Springer-Verlag), 229–244.
Jacobs, K., Wingfield, M. J., Crous, P. W., and Harrington, T. C. (1998). Leptographium engelmannii, a synonym of Leptographium abietinum, and description of Leptographium hughesii sp. nov. Can. J. Bot. 76, 1660–1667.
Jactel, H., Bauhus, J., Boberg, J., Bonal, D., Castagneyrol, B., Gardiner, B., et al. (2017). Tree diversity drives forest stand resistance to natural disturbances. Curr. For. Rep. 3, 223–243. doi: 10.1007/s40725-017-0064-1
Jactel, H., Van Halder, I., Menassieu, P., Zhang, Q. H., and Schlyter, F. (2001). Non-host volatiles disrupt the response of the stenographer bark beetle, Ips sexdentatus (Coleoptera: Scolytidae), to pheromone-baited traps and maritime pine logs. Integr. Pest Manag. Rev. 6, 197–207. doi: 10.1023/A:1025775419193
Jakuš, R. (1995). Bark beetle (Col., Scolytidae) communities and host and site factors on tree level in Norway spruce primeval natural forest. J. Appl. Entomol. 119, 643–651. doi: 10.1111/j.1439-0418.1995.tb01352.x
Jakuš, R., Edwards-Jonášová, M., Cudlín, P., Blaženec, M., Ježík, M., Havlíček, F., et al. (2011). Characteristics of Norway spruce trees (Picea abies) surviving a spruce bark beetle (Ips typographus L.) outbreak. Trees 25, 965–973. doi: 10.1007/s00468-011-0571-9
James, K. R. (2010). A dynamic structural analysis of trees subject to wind loading. Melbourne: University of Melbourne, Melbourne School of Land and Environments.
Jan Weslien,, Öhrn, P., Rosenberg, O., and Schroeder, M. (2024). Effects of sanitation logging in winter on the Eurasian spruce bark beetle and predatory long-legged flies. For. Ecol. Manag. 554:121665. doi: 10.1016/j.foreco.2023.121665
Jõgiste, K., Frelich, L. E., Laarmann, D., Vodde, F., Baders, E., Donis, J., et al. (2018). Imprints of management history on hemiboreal forest ecosystems in the Baltic States. Ecosphere 9:e02503. doi: 10.1002/ecs2.2503
Jõgiste, K., Korjus, H., Stanturf, J. A., Frelich, L. E., Baders, E., Donis, J., et al. (2017). Hemiboreal forest: natural disturbances and the importance of ecosystem legacies to management. Ecosphere 8:e01706. doi: 10.1002/ecs2.1706
Johnstone, J. F., Allen, C. D., Franklin, J. F., Frelich, L. E., Harvey, B. J., Higuera, P. E., et al. (2016). Changing disturbance regimes, ecological memory, and forest resilience. Front. Ecol. Environ. 14, 369–378. doi: 10.1002/fee.1311
Jönsson, A. M., Harding, S., Bärring, L., and Ravn, H. P. (2007). Impact of climate change on the population dynamics of Ips typographus in southern Sweden. Agric. For. Meteorol. 146, 70–81. doi: 10.1016/j.agrformet.2007.05.006
Jönsson, M. T., Fraver, S., Jonsson, B. G., Dynesius, M., Rydgård, M., and Esseen, P. A. (2007). Eighteen years of tree mortality and structural change in an experimentally fragmented Norway spruce forest. For. Ecol. Manag. 242, 306–313. doi: 10.1016/j.foreco.2007.01.048
Kafka, V., Gauthier, S., and Bergeron, Y. (2001). Fire impacts and crowning in the boreal forest: study of a large wildfire in western Quebec. Int. J. Wildland Fire 10, 119–127. doi: 10.1071/WF01012
Kärvemo, S., Rogell, B., and Schroeder, M. (2014a). Dynamics of spruce bark beetle infestation spots: importance of local population size and landscape characteristics after a storm disturbance. For. Ecol. Manag. 334, 232–240. doi: 10.1016/j.foreco.2014.09.011
Kärvemo, S., Van Boeckel, T. P., Gilbert, M., Grégoire, J. C., and Schroeder, M. (2014b). Large-scale risk mapping of an eruptive bark beetle–importance of forest susceptibility and beetle pressure. For. Ecol. Manag. 318, 158–166. doi: 10.1016/j.foreco.2014.01.025
Kautz, M., Schopf, R., and Ohser, J. (2013). The “sun-effect”: microclimatic alterations predispose forest edges to bark beetle infestations. Eur. J. For. Res. 132, 453–465. doi: 10.1007/s10342-013-0685-2
Khakimulina, T., Fraver, S., and Drobyshev, I. (2016). Mixed-severity natural disturbance regime dominates in an old-growth Norway spruce forest of Northwest Russia. J. Veg. Sci. 27, 400–413. doi: 10.1111/jvs.12351
King, D., and Loucks, O. L. (1978). The theory of tree bole and branch form. Radiat. Environ. Biophys. 15, 141–165.
Kitenberga, M., Šņepsts, G., Vuguls, J., Elferts, D., Jaunslaviete, I., and Jansons, Ā. (2021). Tree-and stand-scale factors shape the probability of wind damage to birch in hemiboreal forests. Silva Fennica 55, 1–15. doi: 10.14214/sf.10483
Klopfenstein, N. B. (2009). Approaches to predicting potential impacts of climate change on forest disease: An example with Armillaria root disease. Forest Service, Rocky Mountain Research Station: US Department of Agriculture.
Koivula, M., Kuuluvainen, T., Hallman, E., Kouki, J., Siitonen, J., and Valkonen, S. (2014). Forest management inspired by natural disturbance dynamics (DISTDYN)–a long-term research and development project in Finland. Scand. J. For. Res. 29, 579–592. doi: 10.1080/02827581.2014.938110
Korolyova, N., Buechling, A., Ďuračiová, R., Zabihi, K., Turčáni, M., Svoboda, M., et al. (2022). The last trees standing: climate modulates tree survival factors during a prolonged bark beetle outbreak in Europe. Agric. For. Meteorol. 322:109025. doi: 10.1016/j.agrformet.2022.109025
Köster, K., Voolma, K., Jõgiste, K., Metslaid, M., and Laarmann, D. (2009). “Assessment of tree mortality after windthrow using photo-derived data” in Annales Botanici Fennici, vol. 46 (Helsinki, Finland: Finnish Zoological and Botanical Publishing Board), 291–298.
Krisans, O., Matisons, R., Rust, S., Burnevica, N., Bruna, L., Elferts, D., et al. (2020). Presence of root rot reduces stability of Norway spruce (Picea abies): results of static pulling tests in Latvia. Forests 11:416. doi: 10.3390/f11040416
Krokene, P., Børja, I., Carneros, E., Eldhuset, T. D., Nagy, N. E., Volařík, D., et al. (2023). Effects of combined drought and pathogen stress on growth, resistance, and gene expression in young Norway spruce trees. Tree Physiol. 43, 1603–1618. doi: 10.1093/treephys/tpad062
Krokene, P., and Solheim, H. (1998). Pathogenicity of four blue-stain fungi associated with aggressive and nonaggressive bark beetles. Phytopathology 88, 39–44. doi: 10.1094/PHYTO.1998.88.1.39
Kulakowski, D., and Veblen, T. T. (2003). Subalpine forest development following a blowdown in the Mount Zirkel Wilderness, Colorado. J. Veg. Sci. 14, 653–660. doi: 10.1111/j.1654-1103.2003.tb02197.x
Kulakowski, D., and Veblen, T. T. (2007). Effect of prior disturbances on the extent and severity of wildfire in Colorado subalpine forests. Ecology 88, 759–769. doi: 10.1890/06-0124
Kumbașlı, M., Bauce, É., Rochefort, S., and Crépin, M. (2011). Effects of tree age and stand thinning related variations in balsam fir secondary compounds on spruce budworm Choristoneura fumiferana development, growth and food utilization. Agric. For. Entomol. 13, 131–141. doi: 10.1111/j.1461-9563.2010.00505.x
Kuuluvainen, T. (1994). Gap disturbance, ground microtopography, and the regeneration dynamics of boreal coniferous forests in Finland: a review. Ann. Zool. Fenn. 9, 35–51.
Kuuluvainen, T. (2002). Natural variability of forests as a reference for restoring and managing biological diversity in boreal Fennoscandia. Silva fennica 36, 97–125. doi: 10.14214/sf.552
Kuuluvainen, T. (2009). Forest management and biodiversity conservation based on natural ecosystem dynamics in northern Europe: the complexity challenge. AMBIO: a journal of the human. Environment 38, 309–315. doi: 10.1579/08-A-490.1
Lange, H., Økland, B., and Krokene, P. (2006). Thresholds in the life cycle of the spruce bark beetle under climate change. Interj. Complex Syst. 1648, 1–10.
Långström, B. (1983). Within-tree development of Tomicus minor (Hart.)(Col., Scolytidae) in wind-thrown scots pine. Acta Entomologica Fennica 42, 42–46.
la Porta, N., Capretti, P. I. M. T., Thomsen, I. M., Kasanen, R., Hietala, A. M., and von Weissenberg, K. (2008). Forest pathogens with higher damage potential due to climate change in Europe. Can. J. Plant Pathol. 30, 177–195. doi: 10.1080/07060661.2008.10540534
Lavoie, S., Ruel, J. C., Bergeron, Y., and Harvey, B. D. (2012). Windthrow after group and dispersed tree retention in eastern Canada. For. Ecol. Manag. 269, 158–167. doi: 10.1016/j.foreco.2011.12.018
Liang, M., Johnson, D., Burslem, D. F., Yu, S., Fang, M., Taylor, J. D., et al. (2020). Soil fungal networks maintain local dominance of ectomycorrhizal trees. Nat. Commun. 11:2636. doi: 10.1038/s41467-020-16507-y
Lin, Y., Hulting, M. L., and Augspurger, C. K. (2004). Causes of spatial patterns of dead trees in forest fragments in Illinois. Plant Ecol. 170, 15–27.
Lindenmayer, D. B., Foster, D. R., Franklin, J. F., Hunter, M. L., Noss, R. F., Schmiegelow, F. A., et al. (2004). Salvage harvesting policies after natural disturbance. Science 303:1303. doi: 10.1126/science.1093438
Lindgren, B. S., and Raffa, K. F. (2013). Evolution of tree killing in bark beetles (Coleoptera: Curculionidae): trade-offs between the maddening crowds and a sticky situation. Canadian Entomologist 145, 471–495. doi: 10.4039/tce.2013.27
Liu, Y., Stanturf, J., and Goodrick, S. (2010). Trends in global wildfire potential in a changing climate. For. Ecol. Manag. 259, 685–697. doi: 10.1016/j.foreco.2009.09.002
Loehle, C. (2000). Strategy space and the disturbance spectrum: a life-history model for tree species coexistence. Am. Nat. 156, 14–33. doi: 10.1086/303369
Lüscher, P. (2002). Humus dynamics and changes in rooting patterns in windthrow areas. For Snow Landsc Res 77, 49–59.
Maguire, D. A., Mainwaring, D. B., and Halpern, C. B. (2006). Stand dynamics after variable-retention harvesting in mature Douglas-fir forests of Western North America. Allg. Forst-u. J.-Ztg. 177, 120–131.
Massey, C. L., and Wygant, N. D. (1954). Biology and control of the Engelmann spruce beetle in Colorado, vol. No. 944. Washington, D.C.: US Department of Agriculture.
Matula, R., Šrámek, M., Kvasnica, J., Uherková, B., Slepička, J., Matoušková, M., et al. (2019). Pre-disturbance tree size, sprouting vigour and competition drive the survival and growth of resprouting trees. For. Ecol. Manag. 446, 71–79. doi: 10.1016/j.foreco.2019.05.012
Mayer, P., Brang, P., Dobbertin, M., Hallenbarter, D., Renaud, J. P., Walthert, L., et al. (2005). Forest storm damage is more frequent on acidic soils. Ann. For. Sci. 62, 303–311. doi: 10.1051/forest:2005025
McCarthy, J. (2001). Gap dynamics of forest trees: a review with particular attention to boreal forests. Environ. Rev. 9, 1–59. doi: 10.1139/a00-012
Meigs, G. W., and Keeton, W. S. (2018). Intermediate-severity wind disturbance in mature temperate forests: legacy structure, carbon storage, and stand dynamics. Ecol. Appl. 28, 798–815. doi: 10.1002/eap.1691
Meigs, G. W., Morrissey, R. C., Bače, R., Chaskovskyy, O., Čada, V., Després, T., et al. (2017). More ways than one: mixed-severity disturbance regimes foster structural complexity via multiple developmental pathways. For. Ecol. Manag. 406, 410–426. doi: 10.1016/j.foreco.2017.07.051
Mercado, J. E. (2020). Improved identification and new records of Dendroctonus bark beetles attacking Pinus contorta in the subalpine forest of the southern Rocky Mountains. Forests 11:656. doi: 10.3390/f11060656
Messier, C., Puettmann, K. J., and Coates, K. D. (2013). Managing forests as complex adaptive systems: Building resilience to the challenge of global change. Milton Park, Abingdon, Oxfordshire: Routledge.
Metslaid, M., Granhus, A., Scholten, J., Fjeld, D., and Solheim, H. (2018). Long-term effects of single-tree selection on the frequency and population structure of root and butt rot in uneven-sized Norway spruce stands. For. Ecol. Manag. 409, 509–517. doi: 10.1016/j.foreco.2017.11.050
Mezei, P., Potterf, M., Škvarenina, J., Rasmussen, J. G., and Jakuš, R. (2019). Potential solar radiation as a driver for bark beetle infestation on a landscape scale. Forests 10:604. doi: 10.3390/f10070604
Mitchell, J. C., Kashian, D. M., Chen, X., Cousins, S., Flaspohler, D., Gruner, D. S., et al. (2023). Forest ecosystem properties emerge from interactions of structure and disturbance. Front. Ecol. Environ. 21, 14–23. doi: 10.1002/fee.2589
Mitchell, S. J. (1995). The windthrow triangle: a relative windthrow hazard assessment procedure for forest managers. For. Chron. 71, 446–450. doi: 10.5558/tfc71446-4
Mitchell, S. J. (2013). Wind as a natural disturbance agent in forests: a synthesis. Forestry: Int. J. Forest Res. 86, 147–157. doi: 10.1093/forestry/cps058
Modlinger, R., and Novotný, P. (2015). Quantification of time delay between damages caused by windstorms and by Ips typographus. Clinical dental roentgenology; technic and interpretation including Roentgen studies of the child and young adult 61, 221–231. doi: 10.1515/forj-2015-0030
Moore, J. R., Mitchell, S. J., Maguire, D. A., and Quine, C. P. (2003). Wind damage in alternative silvicultural systems: review and synthesis of previous studies. In Proceedings of an international conference on wind effects on trees (pp. 16–18).
Mukherjee, S., Mishra, A., and Trenberth, K. E. (2018). Climate change and drought: a perspective on drought indices. Curr. Clim. Change Rep. 4, 145–163. doi: 10.1007/s40641-018-0098-x
Müller, M. M., Kaitera, J., and Henttonen, H. M. (2018). Butt rot incidence in the northernmost distribution area of Heterobasidion in Finland. For. Ecol. Manag. 425, 154–163. doi: 10.1016/j.foreco.2018.05.036
Nagel, T. A., and Svoboda, M. (2008). Gap disturbance regime in an old-growthFagus–Abiesforest in the Dinaric Mountains, Bosnia-Herzegovina. Can. J. For. Res. 38, 2728–2737. doi: 10.1139/X08-110
Nara, K. (2006). Ectomycorrhizal networks and seedling establishment during early primary succession. New Phytol. 169, 169–178. doi: 10.1111/j.1469-8137.2005.01545.x
Netherer, S., Kandasamy, D., Jirosová, A., Kalinová, B., Schebeck, M., and Schlyter, F. (2021). Interactions among Norway spruce, the bark beetle Ips typographus and its fungal symbionts in times of drought. J. Pest. Sci. 94, 591–614. doi: 10.1007/s10340-021-01341-y
Netherer, S., Lehmanski, L., Bachlehner, A., Rosner, S., Savi, T., Schmidt, A., et al. (2024). Drought increases Norway spruce susceptibility to the Eurasian spruce bark beetle and its associated fungi. New Phytol. 242, 1000–1017. doi: 10.1111/nph.19635
Netherer, S., Matthews, B., Katzensteiner, K., Blackwell, E., Henschke, P., Hietz, P., et al. (2015). Do water-limiting conditions predispose Norway spruce to bark beetle attack? New Phytol. 205, 1128–1141. doi: 10.1111/nph.13166
Netherer, S., and Nopp-Mayr, U. (2005). Predisposition assessment systems (PAS) as supportive tools in forest management—rating of site and stand-related hazards of bark beetle infestation in the high Tatra Mountains as an example for system application and verification. For. Ecol. Manag. 207, 99–107. doi: 10.1016/j.foreco.2004.10.020
Nicoll, B. C., and Ray, D. (1996). Adaptive growth of tree root systems in response to wind action and site conditions. Tree Physiol. 16, 891–898. doi: 10.1093/treephys/16.11-12.891
Nikolov, C., Konôpka, B., Kajba, M., Galko, J., Kunca, A., and Janský, L. (2014). Post-disaster Forest Management and Bark Beetle Outbreak in Tatra National Park, Slovakia. Mt. Res. Dev. 34, 326–335. doi: 10.1659/MRD-JOURNAL-D-13-00017.1
Oliver, C. D., Larson, B. C., and Oliver, C. D. (1996). Forest stand dynamics. New York: John Wiley.
Osetrov, A.V. (2002). Transformation of bark-beetle complexes (Coleoptera, Scolytidae) of coniferous stands weakened by intensive windthrows. PhD thesis. Saint-Petersburg, Russia. 22 p.
Paine, R. T., Tegner, M. J., and Johnson, E. A. (1998). Compounded perturbations yield ecological surprises. Ecosystems 1, 535–545. doi: 10.1007/s100219900049
Paine, T. D., Raffa, K. F., and Harrington, T. C. (1997). Interactions among scolytid bark beetles, their associated fungi, and live host conifers. Annu. Rev. Entomol. 42, 179–206. doi: 10.1146/annurev.ento.42.1.179
Palik, B., Mitchell, R. J., Pecot, S., Battaglia, M., and Pu, M. (2003). Spatial distribution of overstory retention influences resources and growth of longleaf pine seedlings. Ecol. Appl. 13, 674–686. doi: 10.1890/1051-0761(2003)013[0674:SDOORI]2.0.CO;2
Panferov, O., and Sogachev, A. (2008). Influence of gap size on wind damage variables in a forest. Agric. For. Meteorol. 148, 1869–1881. doi: 10.1016/j.agrformet.2008.06.012
Pan, Y., Chen, P., Lu, J., Zhou, X. D., and Ye, H. (2017). First report of blue-stain in Pinus yunnanensis caused by Ophiostoma tingens associated with Tomicus minor in China. J. Plant Pathol. 99:805.
Papaik, M. J., and Canham, C. D. (2006). Species resistance and community response to wind disturbance regimes in northern temperate forests. J. Ecol. 94, 1011–1026. doi: 10.1111/j.1365-2745.2006.01153.x
Papaik, M. J., Canham, C. D., Latty, E. F., and Woods, K. D. (2005). Effects of an introduced pathogen on resistance to natural disturbance: beech bark disease and windthrow. Can. J. For. Res. 35, 1832–1843. doi: 10.1139/x05-116
Patacca, M., Lindner, M., Lucas-Borja, M. E., Cordonnier, T., Fidej, G., Gardiner, B., et al. (2023). Significant increase in natural disturbance impacts on European forests since 1950. Glob. Chang. Biol. 29, 1359–1376. doi: 10.1111/gcb.16531
Pautasso, M., Holdenrieder, O., and Stenlid, J. (2005). Susceptibility to Fungal Pathogens of Forests Differing in Tree Diversity. Ecolog. Stud. 176:263. doi: 10.1007/3-540-26599-6_13
Pavlov, I. N. (2015). Biotic and abiotic factors as causes of coniferous forests dieback in Siberia and Far East. Contemp. Probl. Ecol. 8, 440–456. doi: 10.1134/S1995425515040125
Peltola, H., Gardiner, B., and Nicoll, B. (2013). Mechanics of wind damage. Agric. For. Meteorol. 151, 328–344.
Peltola, H., Kellomäki, S., and Väisänen, H. (1999b). Model computations of the impact of climatic change on the windthrow risk of trees. Clim. Chang. 41, 17–36. doi: 10.1023/A:1005399822319
Peltola, H., Kellomäki, S., Väisänen, H., and Ikonen, V. P. (1999a). A mechanistic model for assessing the risk of wind and snow damage to single trees and stands of scots pine, Norway spruce, and birch. Can. J. For. Res. 29, 647–661. doi: 10.1139/x99-029
Peterken, G. F. (1996). Natural woodland: Ecology and conservation in northern temperate regions. Cambridge, United Kingdom: Cambridge University Press.
Peterson, C. J. (2004). Within-stand variation in windthrow in southern boreal forests of Minnesota: Is it predictable? Can. J. For. Res. 34, 365–375. doi: 10.1139/x03-257
Peterson, C. J. (2000). Damage and recovery of tree species after two different tornadoes in the same old growth forest: a comparison of infrequent wind disturbances. For. Ecol. Manag. 135, 237–252. doi: 10.1016/S0378-1127(00)00283-8
Peterson, C. J. (2019a). Twenty-five years of aboveground biomass and carbon accumulation following extreme wind damage in an old-growth forest. Forests 10:289. doi: 10.3390/f10030289
Peterson, C. J. (2019b). Damage diversity as a metric of structural complexity after forest wind disturbance. Forests 10:85. doi: 10.3390/f10020085
Peterson, C. J. (2020). Change in tree spatial pattern after severe wind disturbance in four north American northern hardwood and sub-boreal forests. Front. Forests and Global Change 3:57. doi: 10.3389/ffgc.2020.00057
Peterson, C. J., and Cannon, J. B. (2021). Modelling Wind Damage to Southeastern U.S. Trees: Effects of Wind Profile, Gaps, Neighborhood Interactions, and Wind Direction. Front. Forests and Global Change 4:719813. doi: 10.3389/ffgc.2021.719813
Peterson, C. J., and Carson, W. P. (1996). Generalizing forest regeneration models: the dependence of propagule availability on disturbance history and stand size. Can. J. For. Res. 26, 45–52. doi: 10.1139/x26-005
Peterson, C. J., and Leach, A. D. (2008). Limited salvage logging effects on forest regeneration after moderate-severity windthrow. Ecol. Appl. 18, 407–420. doi: 10.1890/07-0603.1
Peterson, C. J., and Pickett, S. T. A. (1991). Treefall and resprouting following catastrophic windthrow in an old-growth hemlock-hardwoods forest. For. Ecol. Manag. 42, 205–217. doi: 10.1016/0378-1127(91)90025-Q
Peterson, C. J., and Rebertus, A. J. (1997). Tornado damage and initial recovery in three adjacent, lowland temperate forests in Missouri. J. Veg. Sci. 8, 559–564. doi: 10.2307/3237207
Pimentel, C., Rahman Khan, M., Zheng, Y., and Quintanilla, M. (2023). “Nematode problems in forests and their sustainable management,” in Nematode diseases of crops and their sustainable management. eds. M. R. Khan, and M. Quintanilla (Cambridge, MA: Academic Press), 457–493.
Plotkin, A. B., Foster, D., Carlson, J., and Magill, A. (2013). Survivors, not invaders, control forest development following simulated hurricane. Ecology 94, 414–423. doi: 10.1890/12-0487.1
Prescott, C. E., and Grayston, S. J. (2023). TAMM review: continuous root forestry—living roots sustain the belowground ecosystem and soil carbon in managed forests. For. Ecol. Manag. 532:120848. doi: 10.1016/j.foreco.2023.120848
Puglielli, G., Laanisto, L., Gori, A., and Cardoso, A. A. (2023). Woody plant adaptations to multiple abiotic stressors: where are we? Flora 299:152221. doi: 10.1016/j.flora.2023.152221
Puhe, J. (2003). Growth and development of the root system of Norway spruce (Picea abies) in forest stands—a review. For. Ecol. Manag. 175, 253–273. doi: 10.1016/S0378-1127(02)00134-2
Putz, F. E., and Sharitz, R. R. (1991). Hurricane damage to old-growth forest in Congaree Swamp National Monument, South Carolina, U.S.A. Can. J. For. Res. 21, 1765–1770. doi: 10.1139/x91-244
Raffa, K. F., Aukema, B. H., Bentz, B. J., Carroll, A. L., Hicke, J. A., Turner, M. G., et al. (2008). Cross-scale drivers of natural disturbances prone to anthropogenic amplification: the dynamics of bark beetle eruptions. Bioscience 58, 501–517. doi: 10.1641/B580607
Raffa, K. F., Grégoire, J. C., and Staffan Lindgren, B. (2015). “Natural history and ecology of bark beetles” in Bark beetles. eds. F. E. Vega and R. W. Hofstetter (Cambridge, MA: Academic Press), 1–40.
Rammig, A., Fahse, L., Bebi, P., and Bugmann, H. (2007). Wind disturbance in mountain forests: simulating the impact of management strategies, seed supply, and ungulate browsing on forest succession. For. Ecol. Manag. 242, 142–154. doi: 10.1016/j.foreco.2007.01.036
Ravn, H. P. (1985). Expansion of the populations ofIps typographus(L.) (Coleoptera, Scolytidae) and their local dispersal following gale disaster in Denmark1. Z. Angew. Entomol. 99, 26–33. doi: 10.1111/j.1439-0418.1985.tb01955.x
Reubens, B., Poesen, J., Danjon, F., Geudens, G., and Muys, B. (2007). The role of fine and coarse roots in shallow slope stability and soil erosion control with a focus on root system architecture: a review. Trees 21, 385–402. doi: 10.1007/s00468-007-0132-4
Reynolds, K. M., and Holsten, E. H. (1994). Relative importance of risk factors for spruce beetle outbreaks. Can. J. For. Res. 24, 2089–2095. doi: 10.1139/x94-268
Rich, R. L., Frelich, L. E., and Reich, P. B. (2007). Wind-throw mortality in the southern boreal forest: effects of species, diameter and stand age. J. Ecol. 95, 1261–1273. doi: 10.1111/j.1365-2745.2007.01301.x
Rossi, J. P., Samalens, J. C., Guyon, D., van Halder, I., Jactel, H., Menassieu, P., et al. (2009). Multiscale spatial variation of the bark beetle Ips sexdentatus damage in a pine plantation forest (Landes de Gascogne, Southwestern France). For. Ecol. Manag. 257, 1551–1557. doi: 10.1016/j.foreco.2008.12.012
Royo, A. A., Peterson, C. J., Stanovick, J. S., and Carson, W. P. (2016). Evaluating the ecological impacts of salvage logging: can natural and anthropogenic disturbances promote coexistence? Ecology 97, 1566–1582. doi: 10.1890/15-1093.1
Ruel, J. C. (1995). Understanding windthrow: silvicultural implications. For. Chron. 71, 434–445. doi: 10.5558/tfc71434-4
Ruel, J. C. (2000). Factors influencing windthrow in balsam fir forests: from landscape studies to individual tree studies. For. Ecol. Manag. 135, 169–178. doi: 10.1016/S0378-1127(00)00308-X
Ruel, J. C., Wermelinger, B., Gauthier, S., Burton, P. J., Waldron, K., and Shorohova, E. (2023). “Selected examples of interactions between natural disturbances” in Boreal forests in the face of climate change: Sustainable management. ed. M. M. Girona (Cham: Springer International Publishing), 123–141.
Runkle, J. R. (1985). “Disturbance regimes in temperate forests,” in The ecology of natural disturbance and patch dynamics. eds. S. T. A Pickett and P. S. White (Cambridge, MA: Academic Press), 17–33.
Runkle, J. R. (1998). Changes in southern Appalachian canopy tree gaps sampled thrice. Ecology 79, 1768–1780. doi: 10.1890/0012-9658(1998)079[1768:CISACT]2.0.CO;2
Safranyik, L., Carroll, A. L., Régnière, J., Langor, D. W., Riel, W. G., Shore, T. L., et al. (2010). Potential for range expansion of mountain pine beetle into the boreal forest of North America. Canadian Entomologist 142, 415–442. doi: 10.4039/n08-CPA01
Sato, T., Yamazaki, H., and Yoshida, T. (2017). Extending effect of a wind disturbance: mortality ofAbies sachalinensisfollowing a strong typhoon in a natural mixed forest. J. For. Res. 22, 336–342. doi: 10.1080/13416979.2017.1381492
Schaetzl, R. J., Johnson, D. L., Burns, S. F., and Small, T. W. (1989). Tree uprooting: review of terminology, process, and environmental implications. Can. J. For. Res. 19, 1–11. doi: 10.1139/x89-001
Schebeck, M., Schopf, A., Ragland, G. J., Stauffer, C., and Biedermann, P. H. (2023). Evolutionary ecology of the bark beetles Ips typographus and Pityogenes chalcographus. Bull. Entomol. Res. 113, 1–10. doi: 10.1017/S0007485321000353
Schelhaas, M. J., Nabuurs, G. J., and Schuck, A. (2003). Natural disturbances in the European forests in the 19th and 20th centuries. Glob. Chang. Biol. 9, 1620–1633. doi: 10.1046/j.1365-2486.2003.00684.x
Schmid-Haas, P., and Bachofen, H. (1991). Die Sturmgefährdung von Einzelbäumen und Beständen. [The storm hazard of individual trees and stands.]. Schweiz. Z. Forstwes. 142, 477–504.
Schowalter, T. (1985). “Adaptations of insects to disturbance” in The ecology of natural disturbance and patch dynamics. eds. S. Pickett and P. White (San Diego: Academic Press), 235–252.
Schroeder, L. M. (2001). Tree mortality by the bark beetle Ips typographus (L.) in storm-disturbed stands. Integr. Pest Manag. Rev. 6, 169–175. doi: 10.1023/A:1025771318285
Schroeder, L. M., and Lindelöw, Å. (2002). Attacks on living spruce trees by the bark beetleIps typographus(Col. Scolytidae) following a storm‐felling: a comparison between stands with and without removal of wind‐felled trees. Agric. For. Entomol. 4, 47–56. doi: 10.1046/j.1461-9563.2002.00122.x
Schuldt, A., Hönig, L., Li, Y., Fichtner, A., Härdtle, W., von Oheimb, G., et al. (2017). Herbivore and pathogen effects on tree growth are additive, but mediated by tree diversity and plant traits. Ecol. Evol. 7, 7462–7474. doi: 10.1002/ece3.3292
Seidl, R., and Blennow, K. (2012). Pervasive growth reduction in Norway spruce forests following wind disturbance. PLoS One 7:e33301. doi: 10.1371/journal.pone.0033301
Seidl, R., Fernandes, P. M., Fonseca, T. F., Gillet, F., Jönsson, A. M., Merganičová, K., et al. (2011a). Modelling natural disturbances in forest ecosystems: a review. Ecol. Model. 222, 903–924. doi: 10.1016/j.ecolmodel.2010.09.040
Seidl, R., Honkaniemi, J., Aakala, T., Aleinikov, A., Angelstam, P., Bouchard, M., et al. (2020). Globally consistent climate sensitivity of natural disturbances across boreal and temperate forest ecosystems. Ecography 43, 967–978. doi: 10.1111/ecog.04995
Seidl, R., and Rammer, W. (2017). Climate change amplifies the interactions between wind and bark beetle disturbances in forest landscapes. Landsc. Ecol. 32, 1485–1498. doi: 10.1007/s10980-016-0396-4
Seidl, R., Rammer, W., Jäger, D., and Lexer, M. J. (2008). Impact of bark beetle (Ips typographus L.) disturbance on timber production and carbon sequestration in different management strategies under climate change. For. Ecol. Manag. 256, 209–220. doi: 10.1016/j.foreco.2008.04.002
Seidl, R., Schelhaas, M. J., and Lexer, M. J. (2011b). Unraveling the drivers of intensifying forest disturbance regimes in Europe. Glob. Chang. Biol. 17, 2842–2852. doi: 10.1111/j.1365-2486.2011.02452.x
Seidl, R., Schelhaas, M. J., Rammer, W., and Verkerk, P. J. (2014). Increasing forest disturbances in Europe and their impact on carbon storage. Nat. Clim. Chang. 4, 806–810. doi: 10.1038/nclimate2318
Sequeira, A. S., Normark, B. B., and Farrell, B. D. (2000). Evolutionary assembly of the conifer fauna: distinguishing ancient from recent associations in bark beetles. Proceedings of the Royal Society of London. Series B: Biolog. Sci. 267, 2359–2366.
Seybold, S. J., and Downing, M. (2009). “What risks do invasive bark beetles and woodborers pose to forests of the western United States? A case study of the Mediterranean pine engraver, Orthotomicus erosus,” in The western bark beetle research group: A unique collaboration with forest health protection. Proceedings of a symposium at the 2007 society of American foresters conference. Gen. Tech. Rep. PNW-GTR-784. eds. J. L. Hayes and J. E. Lundquist (Compilers) (Portland, OR: US Department of Agriculture, Forest Service, PNW Research Station), 111–134.
Sherlock, M. A. (2023). Investigation of fungal pathogens and Woodboring beetles of Sugarbush stands in the central Appalachian region. Graduate Theses, Dissertations, and Problem Reports. 11939. Morgantown, U.S.A: West Virginia University.
Shibuya, M., and Ishibashi, S. (2019). Stand-level windthrow patterns and long-term dynamics of surviving trees in natural secondary stands after a stand-replacing windthrow event. Forestry: Int. J. Forest Res. 92, 473–480. doi: 10.1093/forestry/cpz015
Shorohova, E., Fedorchuk, V., Kuznetsova, M., and Shvedova, O. (2008). Wind-induced successional changes in pristine boreal Picea abies forest stands: evidence from long-term permanent plot records. Forestry 81, 335–359. doi: 10.1093/forestry/cpn030
Shorohova, E., Kuuluvainen, T., Kangur, A., and Jõgiste, K. (2009). Natural stand structures, disturbance regimes and successional dynamics in the Eurasian boreal forests: a review with special reference to Russian studies. Ann. For. Sci. 66, 201–220. doi: 10.1051/forest/2008083
Siitonen, J., Martikainen, P., Punttila, P., and Rauh, J. (2000). Coarse woody debris and stand characteristics in mature managed and old-growth boreal Mesic forests in southern Finland. For. Ecol. Manag. 128, 211–225. doi: 10.1016/S0378-1127(99)00148-6
Simard, M., Romme, W. H., Griffin, J. M., and Turner, M. G. (2011). Do mountain pine beetle outbreaks change the probability of active crown fire in lodgepole pine forests? Ecol. Monogr. 81, 3–24. doi: 10.1890/10-1176.1
Skelton, J., Jusino, M. A., Carlson, P. S., Smith, K., Banik, M. T., Lindner, D. L., et al. (2019). Relationships among wood-boring beetles, fungi, and the decomposition of forest biomass. Mol. Ecol. 28, 4971–4986. doi: 10.1111/mec.15263
Skvortsova, E. B., Ulanova, N. G., and Basewich, V. F. (1983). Ekologiccheskaya rol vetrovalov [Ecological role of windthrows]. Moscow: Lesnaya promyshlennost. (In russian)
Solheim, H. (1988). Pathogenicity of some Ips typographus-associated blue-stain fungi to Norway spruce. Norsk institutt for skogforskning 40:11.
Solheim, H., and Långström, B. (1991). Blue-stain fungi associated with Tomicus piniperda in Sweden and preliminary observations on their pathogenicity. Ann. For. Sci. 48, 149–156. doi: 10.1051/forest:19910203
Stadelmann, G., Bugmann, H., Wermelinger, B., Meier, F., and Bigler, C. (2013). A predictive framework to assess spatio-temporal variability of infestations by the European spruce bark beetle. Ecography 36, 1208–1217. doi: 10.1111/j.1600-0587.2013.00177.x
Stathers, R. J., Rollerson, T. P., and Mitchell, S. J. (1994). Windthrow handbook for British Columbia forests. BC Min. For., Victoria 2, 35–51.
Stueve, K. M., Perry, C. H., Nelson, M. D., Healey, S. P., Hill, A. D., Moisen, G. G., et al. (2011). Ecological importance of intermediate windstorms rivals large, infrequent disturbances in the northern Great Lakes. Ecosphere 2, art2–art21. doi: 10.1890/ES10-00062.1
Swanson, M. E., Franklin, J. F., Beschta, R. L., Crisafulli, C. M., DellaSala, D. A., Hutto, R. L., et al. (2011). The forgotten stage of forest succession: early-successional ecosystems on forest sites. Front. Ecol. Environ. 9, 117–125. doi: 10.1890/090157
Sweeney, J., Smith, G., Hurley, J. E., Harrison, K., de Groot, P., and Humble, L., (2001). The brown spruce longhorn beetle in Halifax: Pest status and preliminary results of research. In proceedings of the US Department of Agriculture Interagency Research Forum on gypsy moth and other invasive species. General Tech Report NE-285. Newtown Square, PA, USA. US Department of Agriculture, Forest Service (pp. 6–10).
Szwagrzyk, J., Gazda, A., Dobrowolska, D., Chećko, E., Zaremba, J., and Tomski, A. (2017). Tree mortality after wind disturbance differs among tree species more than among habitat types in a lowland forest in northeastern Poland. For. Ecol. Manag. 398, 174–184. doi: 10.1016/j.foreco.2017.04.041
Takei, S. Y., Köbayashi, K., and Takagi, E. (2021). Distribution pattern of entry holes of the tree-killing bark beetle Polygraphus proximus. PLoS One 16:e0246812. doi: 10.1371/journal.pone.0246812
Talkkari, A., Peltola, H., Kellomäki, S., and Strandman, H. (2000). Integration of component models from the tree, stand and regional levels to assess the risk of wind damage at forest margins. For. Ecol. Manag. 135, 303–313. doi: 10.1016/S0378-1127(00)00288-7
Tedersoo, L., Drenkhan, R., Anslan, S., Morales-Rodriguez, C., and Cleary, M. (2019). High-throughput identification and diagnostics of pathogens and pests: overview and practical recommendations. Mol. Ecol. Resour. 19, 47–76. doi: 10.1111/1755-0998.12959
Thorpe, H. C., and Thomas, S. C. (2007). Partial harvesting in the Canadian boreal: success will depend on stand dynamic responses. For. Chron. 83, 319–325. doi: 10.5558/tfc83319-3
True, R. P., and Tryon, E. H. (1966). “Butt decay in yellow-poplar sprouts in West Virginia,” in West Virginia agricultural and forestry experiment station bulletins. Morgantown, U.S.A: West Virginia University, 541, 1–67.
Turner, M. G., Baker, W. L., Peterson, C. J., and Peet, R. K. (1998). Factors influencing succession: lessons from large, infrequent natural disturbances. Ecosystems 1, 511–523. doi: 10.1007/s100219900047
Ueda, M., and Shibata, E. I. (2004). Why do trees decline or dieback after a strong wind? Water status of Hinoki cypress standing after a typhoon. Tree Physiol. 24, 701–706. doi: 10.1093/treephys/24.6.701
Ulanova, N. G. (2000). The effects of windthrow on forests at different spatial scales: a review. For. Ecol. Manag. 135, 155–167. doi: 10.1016/S0378-1127(00)00307-8
Urgenson, L. S., Halpern, C. B., and Anderson, P. D. (2013). Level and pattern of overstory retention influence rates and forms of tree mortality in mature, coniferous forests of the Pacific northwest, USA. For. Ecol. Manag. 308, 116–127. doi: 10.1016/j.foreco.2013.07.021
Valinger, E., Lundqvist, L., and Bondesson, L. (1993). Assessing the risk of snow and wind damage from tree physical characteristics. Forestry 66, 249–260. doi: 10.1093/forestry/66.3.249
van der Heijden, M. G. A., Bardgett, R. D., and van Straalen, N. M. (2008). The unseen majority: soil microbes as drivers of plant diversity and productivity in terrestrial ecosystems. Ecol. Lett. 11, 296–310. doi: 10.1111/j.1461-0248.2007.01139.x
Vašíčková, I., Šamonil, P., Kašpar, J., Román-Sánchez, A., Chuman, T., and Adam, D. (2021). Dead or alive: drivers of wind mortality initiate multiple disturbance regime in a temperate primeval mountain forest. Forests 12:1599. doi: 10.3390/f12111599
Venice, F., Vizzini, A., Danti, R., Della Rocca, G., and Mello, A. (2023). Responses of a soil fungal community to severe windstorm damages in an old silver fir stand. Front. Microbiol. 14:1246874. doi: 10.3389/fmicb.2023.1246874
Vodde, F., Jõgiste, K., Kubota, Y., Kuuluvainen, T., Köster, K., Lukjanova, A., et al. (2011). The influence of storm-induced microsites to tree regeneration patterns in boreal and hemiboreal forest. J. For. Res. 16, 155–167. doi: 10.1007/s10310-011-0273-6
Vogt, J. T., Gandhi, K. J., Bragg, D. C., Olatinwo, R., and Klepzig, K. D. (2020). Interactions between weather-related disturbance and forest insects and diseases in the southern United States. Forest Service, Southern Research Station: United States Department of Agriculture.
Webb, S. L. (1989). Contrasting Windstorm Consequences in Two Forests, Itasca State Park, Minnesota. Plant world 70, 1167–1180. doi: 10.2307/1941384
Wermelinger, B. (2002). Development and distribution of predators and parasitoids during two consecutive years of anIps typographus(Col., Scolytidae) infestation. J. Appl. Entomol. 126, 521–527. doi: 10.1046/j.1439-0418.2002.00707.x
Wermelinger, B. (2004). Ecology and management of the spruce bark beetle Ips typographus—a review of recent research. For. Ecol. Manag. 202, 67–82. doi: 10.1016/j.foreco.2004.07.018
White, P. S., and Pickett, S. T. A. (1985) in Natural disturbance and patch dynamics: An introduction. The ecology of natural disturbance and patch dynamics. eds. S. T. A. Pickett and P. S. White (San Diego, Calif: Academic Press), 3–13.
Whitman, E., Parisien, M. A., Thompson, D. K., and Flannigan, M. D. (2019). Short-interval wildfire and drought overwhelm boreal forest resilience. Sci. Rep. 9:18796. doi: 10.1038/s41598-019-55036-7
Whitney, R. D. (1989). Root rot damage in naturally regenerated stands of spruce and balsam fir in Ontario. Can. J. For. Res. 19, 295–308. doi: 10.1139/x89-045
Wohlgemuth, T., Schwitter, R., Bebi, P., Sutter, F., and Brang, P. (2017). Post-windthrow management in protection forests of the Swiss Alps. Eur. J. For. Res. 136, 1029–1040. doi: 10.1007/s10342-017-1031-x
Wolf, A., Møller, P. F., Bradshaw, R. H., and Bigler, J. (2004). Storm damage and long-term mortality in a semi-natural, temperate deciduous forest. For. Ecol. Manag. 188, 197–210. doi: 10.1016/j.foreco.2003.07.009
Woods, K. D. (2004). Intermediate disturbance in a late-successional hemlock-northern hardwood forest. J. Ecol. 92, 464–476. doi: 10.1111/j.0022-0477.2004.00881.x
Worrall, J. J., Lee, T. D., and Harrington, T. C. (2005). Forest dynamics and agents that initiate and expand canopy gaps inPicea–Abiesforests of Crawford Notch, New Hampshire, USA. J. Ecol. 93, 178–190. doi: 10.1111/j.1365-2745.2004.00937.x
Xi, W., and Peet, R. K. (2011). “The complexity of catastrophic wind impacts on temperate forests,” in Recent Hurricane research-climate, dynamics, and societal impacts. ed. A. Lupopp (IntechOpen), 503–535.
Yoshida, T., and Noguchi, M. (2009). Vulnerability to strong winds for major tree species in a northern Japanese mixed forest: analyses of historical data. Ecol. Res. 24, 909–919. doi: 10.1007/s11284-008-0566-1
Zeng, H., Garcia-Gonzalo, J., Peltola, H., and Kellomäki, S. (2010). The effects of forest structure on the risk of wind damage at a landscape level in a boreal forest ecosystem. Ann. For. Sci. 67:111. doi: 10.1051/forest/2009090
Keywords: ecosystem legacy, residual trees, windthrow, post-windthrow succession, surviving trees, delayed mortality
Citation: Palm-Hellenurm K, Bāders E, Frelich LE, Köster K, Metslaid M, Polyachenko O, Seedre M, Shorohova E, Stanturf JA, Vodde F and Jõgiste K (2024) The fate of remnant trees after wind disturbances in boreal and temperate forests. Front. For. Glob. Change. 7:1405430. doi: 10.3389/ffgc.2024.1405430
Edited by:
Sigrid Netherer, University of Natural Resources and Life Sciences Vienna, AustriaReviewed by:
Gal Fidej, University of Ljubljana, SloveniaPäivi Lyytikäinen-Saarenmaa, University of Eastern Finland, Finland
Copyright © 2024 Palm-Hellenurm, Bāders, Frelich, Köster, Metslaid, Polyachenko, Seedre, Shorohova, Stanturf, Vodde and Jõgiste. This is an open-access article distributed under the terms of the Creative Commons Attribution License (CC BY). The use, distribution or reproduction in other forums is permitted, provided the original author(s) and the copyright owner(s) are credited and that the original publication in this journal is cited, in accordance with accepted academic practice. No use, distribution or reproduction is permitted which does not comply with these terms.
*Correspondence: Kristiina Palm-Hellenurm, kristiina.palm-hellenurm@emu.ee