- Department of Forest and Conservation Sciences, Faculty of Forestry, The University of British Columbia, Vancouver, BC, Canada
Downed woody debris is important for biodiversity, forest regeneration, and carbon, nutrient, and water cycling, and past studies have examined how the coarse fraction is affected by climate or harvesting. In a field study in Douglas-fir dominated forests, we expand existing knowledge by investigating the interacting effects of climate and harvesting on downed woody debris of all sizes. Across a 900-km long latitudinal gradient in British Columbia, we found that coarse woody debris (CWD, >7.5 cm diameter) in humid climates contained 700% greater carbon stocks, had 500% greater volume, and was more diverse than in arid climates. Pre- and post-harvest, small and fine woody debris comprised a higher proportion of total woody debris carbon stocks in arid than moist climates, especially after clearcutting and seed tree treatments. Harvesting generally decreased total CWD volume, but it was not depleted on any site. Harvesting substantially reduced the volume of large, highly decomposed CWD except at the two most arid sites, and losses of large CWD increased with increasing tree removal. These losses were accompanied by a pulse of fresh, small diameter CWD and SWD which are short-term organic nutrient sources but have less habitat value than larger pieces and contribute to fuel loads. Because CWD was less abundant in arid than humid mature forests, care must be taken on arid sites to avoid its depletion during harvesting, especially clearcutting, where future woody debris inputs will not occur for decades.
Introduction
Downed woody debris is a crucial structural and functional forest component that positively contributes to biodiversity, carbon stocks and other values, but also has negative features including its role as a wildfire fuel, an impediment to planting, host material for bark beetles, and a source of CO2 emissions (Harmon et al., 1986; Caza, 1993; Stevens, 1997; Arsenault, 2002). Detailed inventories of woody debris are scarce but are crucial in establishing baselines in natural and managed stands across climatic regions against which different harvesting methods can be evaluated (Clark et al., 1998; Korboulewsky et al., 2021). As climate changes and partial retention harvesting provides a favorable ecological alternative to clearcutting (Lindenmayer et al., 2012), it will be necessary to evaluate their interacting effects on woody debris decay class, size, and species distributions across a broad range of forest ecosystems (Province of British Columbia, 2010).
Climate is recognized as an important driver of downed woody debris dynamics in forests through its combined influence on tree productivity and wood decomposition rate (Gould et al., 2008; Woodall and Liknes, 2008; Zell et al., 2009; Garbarino et al., 2015; Smith et al., 2021), although this has not been demonstrated in all studies (Oettel et al., 2020). Many studies have investigated the effects climate or forest harvesting have on woody debris volume and carbon stocks, but not the interaction between these two variables.
A shortcoming of many investigations of downed woody debris volume, biomass, and carbon stocks is a focus on the coarse fraction (CWD) (Riffell et al., 2011; Korboulewsky et al., 2021), although fine woody debris of all sizes is included in national inventories in the United States (Woodall et al., 2019). The rationale for the focus on CWD includes its greater ecological value than smaller downed wood due to its greater longevity, its ability to hold more moisture, and its role in providing useable structures for more organisms (Arsenault, 2002; Bunnell et al., 2002). However, small pieces also have ecological effects, and in some forests comprise a substantial portion of the total downed wood volume and carbon storage (e.g., Teissier du Cros and Lopez, 2009). Korboulewsky et al. (2021) recommend accurate inventorying of all sizes of downed woody debris down to pieces with a diameter approaching zero.
We designed a large-scale, replicated field experiment in which we measured downed woody debris of all sizes before and after five levels of tree retention were applied in nine climatic regions across British Columbia (B.C.), Canada. The overall objective of our study was to examine the interacting effects of climate and forest harvesting method on the characteristics of downed wood debris. Woody debris amount and type is affected by forest stand variables such as stand origin and disturbance history (Clark et al., 1998; Herrero et al., 2014), age and successional stage (Clark et al., 1998), basal area and density (Castagneri et al., 2010), and canopy composition (Hély et al., 2000; Krankina et al., 2001; Banaś et al., 2014), although this is not supported by all studies (e.g., Böhl and Brӓndli, 2007). We held these variables as constant as practically possible given that tree species, basal area, and stand density naturally vary with climate. We controlled logging method, utilization standard, time between harvesting and measurement, sampling methodology, and the definition of woody debris. We focused on Douglas-fir because of its commercial importance, wide natural latitudinal distribution (19–55 oN) and predicted increase in range with climate change (Wang et al., 2016). Our specific objectives were to examine the effects of climate and harvesting treatment on: (1) the amount of carbon (Mg ha−1) stored in downed coarse, small, and fine woody debris, (2) the volume of CWD (m3 ha−1) and its diversity in terms of species, decay class, and size class, and (3) the number pieces per hectare of CWD. We discuss the ecological implications of our findings.
Methods
Study area
The study took place in B.C., Canada at eight interior and one coastal location situated along a 900-km climate gradient (Figure 1). The interior locations are within the current range of interior Douglas-fir (P. menziesii var. glauca) in B.C. and are situated from south of Cranbrook (49.21oN, 115.37oW) north to the John Prince Research Forest near Fort St James (54.65oN, 124.43oW) (Table 1). Mean annual temperature at the interior locations varies from 2.3 to 7.7o C and mean annual precipitation from 398 to 1,059 mm. The study locations are in the Interior Douglas-fir (IDF), Interior Cedar-Hemlock (ICH), and Sub-boreal Spruce (SBS) biogeoclimatic zones. The coastal location (mean annual temperature 8.0°C; mean annual precipitation 2,701 mm) is situated in the Coastal Western Hemlock (CWH) zone about 60 km east of Vancouver, B.C. (49.32oN, 122.54oW) and is within the range of the coastal variety of Douglas-fir (P. menziesii var. menziesii). Climate data for each location was obtained from ClimateWNA, based on latitude, longitude, and elevation, using the 1981–2010 climate normal dataset (Wang et al., 2016).
Douglas-fir occupied a dominant position in the canopy of the mature forests and there were one to eight tree species per location. (Table 2). The stands were mature (68–128 years-old), fully stocked prior to harvesting, and originated from natural regeneration following wildfire. Tree density by diameter class is illustrated in Figure 2. The sites have mesic soil moisture regimes (relative to their respective biogeoclimatic zone), south or west aspects, gentle slope gradients (<30 percent), and mid-slope positions. Elevations vary from 540 to 1,450 m.
Experimental design and harvesting treatments
The interaction between regional climate and harvesting treatment was tested in a randomized block design. The regional climate factor was represented by nine forest locations. Each location encompassed one to three 20-ha sites (reps), and each rep was divided into five 4-ha treatment blocks, with tree retention levels randomly assigned to the blocks (Figure 3). In this paper we use the terms “location” and “climatic region” interchangeably, meaning a set of one to three reps in a certain climate. Harvesting treatments were applied in 2017 to 2018. They were: (i) clearcut (0% tree retention); (ii) seed tree (10% tree retention; retention of 25 large, well-distributed Douglas-fir stems ha−1); (iii) small patch retention (30% retention; retention of 30% of the stand area in Approx. 30-m wide unconnected patches, with all trees cut in the remaining 70% of the stand); (iv) large patch retention (60% retention; retention of 60% of the stand area with all trees cut in the remaining 40% of the stand); and (v) uncut control (100% retention). The 60% retention blocks were thinned from below by reaching into the uncut patches with a feller-buncher and removing the smaller stems. Harvesting was primarily carried out using feller-bunchers but trees with sizes exceeding machine capabilities were hand-felled. Whole trees were skidded on trails from stumps to landings with rubber-tired skidders. Woody debris was not re-distributed on the blocks following harvesting.
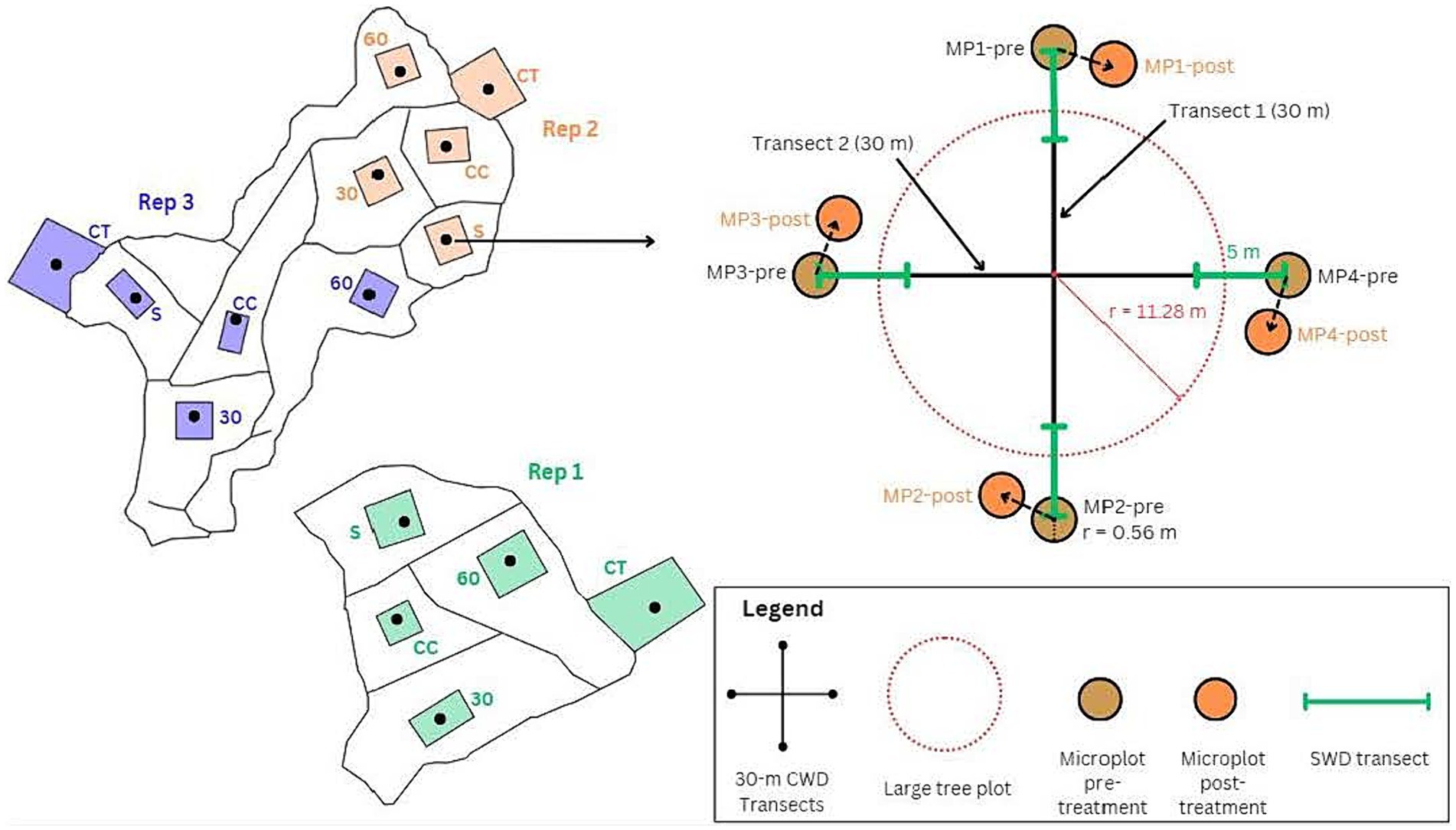
Figure 3. An example of the layout of three replicates at a single location (left side of diagram), and a close-up of the layout of an NFI measurement plots (right side of diagram). The NFI plots are marked on the diagram with black dots located near the center of approx. 1-ha plots squares or rectangles, within approx. 4-ha treatment units. The squares and rectangles were established as locations for field measurements. Treatments were randomly assigned (CC, clearcut; S, seed tree; 30, 30% retention; 60, 60% retention; CT, control). All woody debris data collection took place in the circular NFI plots shown on the right side of the diagram.
Measurement and sampling methods
Woody debris data was collected before and 1 year after logging using permanent 0.04 ha National Forest Inventory (NFI) plots positioned at the center of the 4-ha treatment blocks (total 108 NFI plots measured). Canadian NFI ground sampling methodology was followed (Canadian Forest Inventory Canadian Forest Inventory Committee & Canadian Forest Service, 2008), which includes measuring the stand and ecological properties of each NFI plot and collecting samples for carbon analysis. The NFI methodology defines CWD as aboveground woody pieces >7.5 cm in diameter at the point where it crosses the transect line and includes fallen trees (logs), large downed dead branches and fragments of wood. Small woody debris (SWD) is defined as fallen trees, branches, and wood fragments 1–7.5 cm in diameter. Fine woody debris (FWD) includes twigs and wood fragments <1 cm in diameter and does not include the litter (L) layer of the forest floor. For this paper, we excluded stumps and dead standing trees from the woody debris inventory.
CWD was measured along the same transects before and after harvesting, using the line intersect method (Marshall et al., 2000). A 30-m transect was established at a randomly chosen bearing with the mid-point at the center of the NFI plot. A second 30-m transect, also intersecting the plot center at its mid-point, was established perpendicular to the first oneDiameter, length, species, decay class, and tilt angle of each “round” CWD piece intersecting the transect were recorded. Each “odd-shaped” piece (i.e., non-round, such as slabs) was assessed for horizontal and vertical depth, length, species, and decay class. Species was recorded as “unknown” where missing bark and branches or advanced decay made identification unreliable. Pieces were assigned to one of five wood decay classes, varying from hard and intact (Class 1) to highly decomposed (Class 5) (British Columbia Ministry of Forests and Range & British Columbia Ministry of Environment, 2010) (Supplementary Table S1). The number of SWD pieces that intersected the CWD transects were counted by diameter class (1–3 cm; 3.1–5 cm, and 5.1–7.5 cm) along two 5-m subsections of each 30-m transect, and their average decay class for the transect was recorded. At the pre-logging assessment, all FWD was collected from the surface of a circular 1 m2 microplot at each end of the CWD transects (four microplots per NFI plot). Because FWD sampling was destructive, the microplot position was moved 1.5 m clockwise for the post-logging assessment, maintaining a 15-m distance from the plot center. The FWD samples were transported to the laboratory where they were oven dried at 70oC for 72 h, then weighed and discarded.
Data analysis
Pre- and one-year post-harvest woody debris volume, biomass, and carbon content were calculated according to the National Forest Inventory (2021) and as outlined in Supplementary material S1. Summary statistics for these variables were calculated for each NFI plot, then NFI plots receiving the same treatment were averaged for each location. Woody debris volume was calculated by decay class, diameter class, and species. CWD density (pieces per hectare) was calculated for large (≥20 cm diameter and ≥ 10 m long), and medium pieces (<20 cm diameter and/or < 10 m long). For SWD and FWD, carbon content was calculated as biomass x 0.50 (Harmon et al., 2013).
A pre- and post-harvest “dead wood diversity index” was calculated as the number of combinations of tree species, decay class, 10 cm diameter classes and two length classes (<10 m and ≥10 m) at each NFI plot. This follows Siitonen et al. (2000) and Kunttu et al. (2015) except we added length class to the calculation.
Statistical analyses were conducted with R version 4.1.2 (R Core Team, 2022). Results were considered statistically significant at p < 0.05. We investigated the influence of climatic factors on carbon stocks and composition of downed woody debris in intact Douglas-fir forests, as well as the response of woody debris in these forests to the interaction between climatic factors and harvest intensity. Our response variable ‘forest woody debris’ was quantified in five dimensions: (1) carbon stocks including relative contributions of coarse, small and fine woody debris; (2) volume per hectare of coarse and small woody debris; (3) number and size of pieces; (4) decay class, diameter class, species and distribution; and (5) diversity index. Climate factors tested were mean annual precipitation, summer precipitation, mean annual temperature, summer mean maximum temperature, and aridity [AHM, annual heat moisture index = (mean annual temperature + 10)/(mean annual precipitation/1000)].
For analysis of carbon, volume, pieces per hectare and diversity we fit linear mixed-effects models (LMMs) using restricted maximum likelihood with the ‘lmer’ function from package lme4 (Bates et al., 2015). Before analysis, most response variables were transformed using log10 or square root to meet the assumptions of the models. Climate and harvest intensity were included as interacting effects, while location and replicate were nested random factors. Models were compared using the Akaike information criterion (function ‘AIC’ in package stats) and model fit checked using adjusted likelihood-ratio based pseudo-R2 (Bartoń, 2022). Significance of fixed effects were tested using Wald chi-square tests with function ‘Anova’ in package car (Fox and Weisberg, 2019), and contrasts between levels of fixed effects were tested using the Tukey method and function ‘emmeans’ in package emmeans (Lenth, 2022).
Results
Distribution of carbon amongst coarse, small, and fine woody debris
More carbon was stored in CWD than SWD + FWD combined in mature forests at all locations except Jaffray and Two-bit Creek (Figure 4). CWD comprised 48–85%, SWD 10–35%, and FWD 3–19% of the total pre-harvest woody debris carbon stocks. One-year post-harvest, CWD comprised 21–82%, SWD 14–60%, and FWD 2–27% of the total woody debris carbon stocks. One-year after harvesting, SWD + FWD tended to comprise a higher proportion of the total woody debris carbon in the more arid climates (AHM 24.7–36.5) than in cool or moist climates, as well as in the clearcut and seed tree versus the 30 and 60% retention treatments.
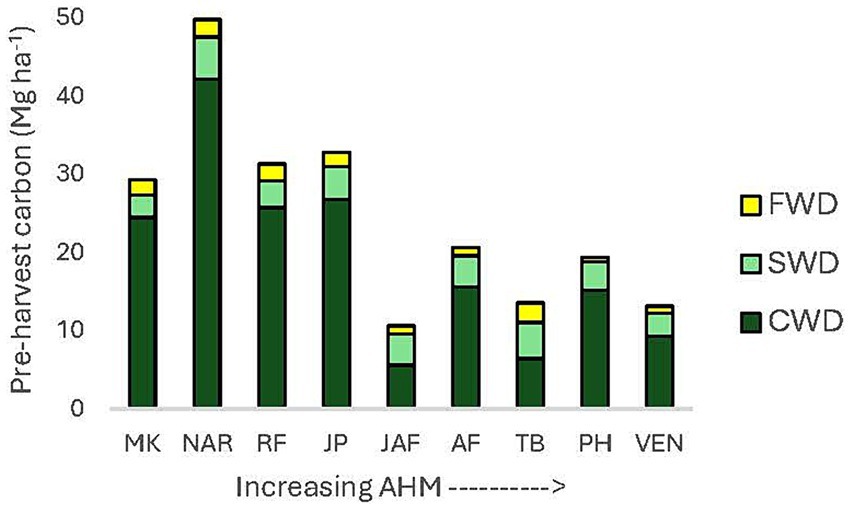
Figure 4. Pre-harvest carbon stocks in coarse, small, and fine woody debris by climatic region. Sites are arranged from lowest to highest aridity (AHM). MK, Malcolm Knapp; NAR, Narrows Creek; RF, Redfish Creek; JP, John Prince; JAF, Jaffray; AF, Alex Fraser; TB, Two-bit Creek; PH, Peterhope Lake; VEN, Venables.
Influence of climate and harvesting treatment on woody debris carbon stocks
In the mature forests, average carbon stocks in CWD ranged from 5.6 ± 3.0 to 42.2 Mg ha−1 (Table 3) and increased with decreasing AHM (p = 0.0096, data log10 transformed). The ratio of post to pre-harvest CWD carbon stocks was correlated with the interaction between mean annual temperature and treatment (p = 0.0265; data log10 transformed). CWD carbon stocks decreased or were essentially unchanged following all harvesting treatments at Alex Fraser, Narrows Creek, Redfish Creek, Venables, and Peterhope Lake as well as clearcutting at John Prince, seed tree at Malcolm Knapp, and all treatments except clearcutting at Jaffray. CWD carbon losses following harvesting were generally <10 Mg ha−1, except in the 30% retention treatment at the two interior wet belt locations (Narrows Creek and Redfish Creek) where 25–50 Mg ha−1 (40–75% of the pre-harvest CWD carbon) was lost. At the arid locations, percentage losses of CWD carbon were considerable (up to 40%), but the absolute amount relatively small (1–13 Mg ha−1).
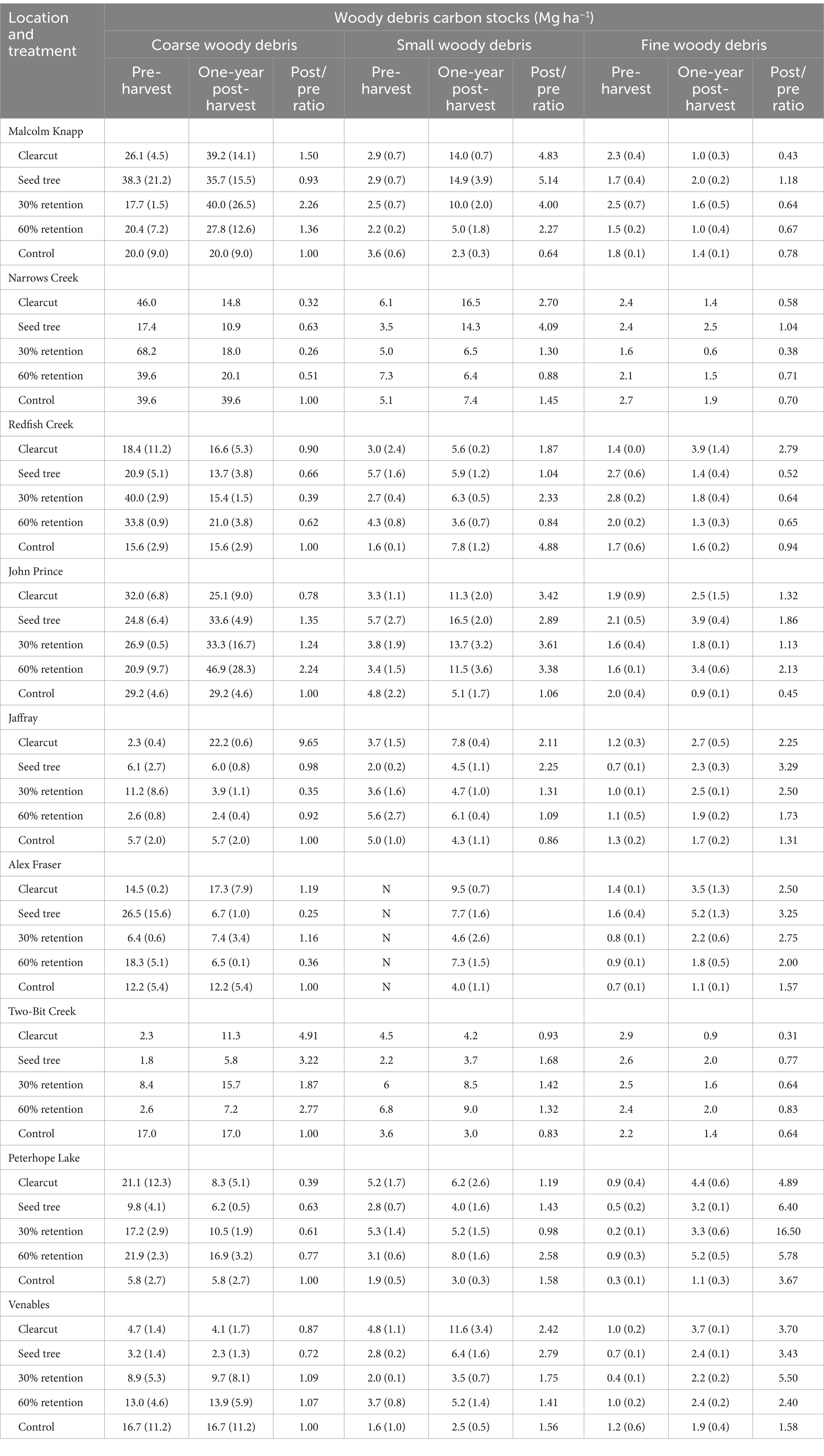
Table 3. Carbon stocks (averages with standard error in parentheses) in coarse, small, and fine woody debris before and 1 year after harvesting, and the ratio of post to pre-harvest carbon.
Increases in CWD carbon stocks occurred after all harvesting treatments at Two-bit Creek, where stocks increased by 4.0–9.0 Mg ha−1 (two to five times). A 700% increase in CWD carbon stocks occurred following clearcutting at Jaffray only because some large trees were felled and left on site after skidding was completed. Apart from Jaffray, the largest absolute gain of CWD carbon was about 25 Mg ha−1, which occurred in the 30% retention treatment at Malcolm Knapp and the 60% retention treatment at John Prince.
Average pre-harvest SWD carbon stocks ranged from 1.6 ± 0.1 to 7.1 ± 0.0 Mg ha−1 and were not correlated with climatic variables (p ≥ 0.05). Average post-harvest SWD carbon stocks ranged from 2.5 ± 0.5 to 16.5 ± 2.0 Mg ha−1. Treatment interacted with AHM to affect the ratio of post to pre-harvest SWD carbon stocks (p = 0.0005; data log10 transformed). Malcolm Knapp and John Prince had the highest ratios of post to pre-logging SWD carbon stocks, increasing 4–5 times in all treatments at Malcolm Knapp except 60% retention, and about 3–3.5 times at John Prince. At these two locations, SWD increases averaged about 10 Mg ha−1 which was not enough to offset decreases in CWD carbon stocks. Arid locations gained 1–7 Mg ha−1 of SWD carbon after harvesting.
The pre-harvest FWD carbon pool ranged from 0.2 ± 0.1 Mg ha−1 to 2.9 ± 0.0 Mg ha−1 and increased with decreasing AHM when the data were square root transformed (p < 0.05). Post-harvest FWD carbon stocks ranged from 0.6 ± 0.0 to 5.2 ± 0.5 Mg ha−1. The ratio of post to pre-harvest FWD stocks increased with AHM (p = 0.0128). FWD carbon stocks increased by up to 3.6 Mg ha−1 after harvesting where AHM was 20.8–36.5 but decreased or stayed about the same at moister to humid locations (AHM 6.6–19.4), except in the clearcut at Redfish Creek.
Influence of climate and harvesting treatment on CWD volume
Average pre-harvest total CWD volume ranged from 15 to 663 m3 ha−1 across the climate gradient and increased with decreasing AHM, when the data was log10 transformed (p = 0.0063) (Figure 5). Average post-harvest CWD volume was 12–243 m3 ha−1 and was lower in the clearcut and seed tree than the 30 and 60% retention treatments (p < 0.0001). Clearcut and seed tree harvesting decreased CWD volume to about half of the pre-harvest volume in most locations. The ratio of post to pre-harvest CWD volume for each location and treatment is shown in Figure 6. Ratios were highest at Two-bit Creek, the most arid location, where there was up to a four-fold (50 m3 ha−1) increase in total CWD volume after harvesting. Total CWD volume decreased or was unchanged following all harvesting treatments everywhere else except in the 60% retention treatment at John Prince where it increased by 1.5 times.
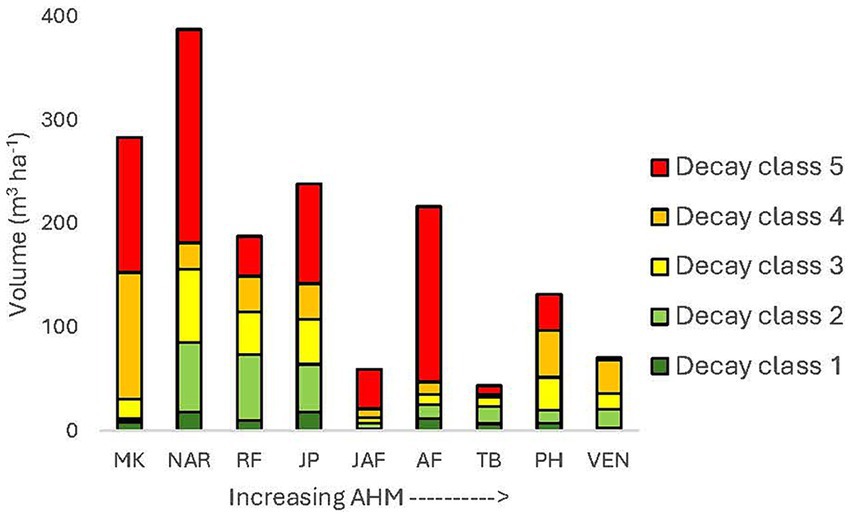
Figure 5. Pre-harvest coarse woody debris volume per hectare by decay class and location. Sites are arranged from lowest to highest aridity (AHM). Class 1 is the least decomposed and class 5 is highly decomposed. MK, Malcolm Knapp; NAR, Narrows Creek; RF, Redfish Creek; JP, John Prince; JAF, Jaffray; AF, Alex Fraser; TB, Two-bit Creek; PH, Peterhope Lake; VEN, Venables.
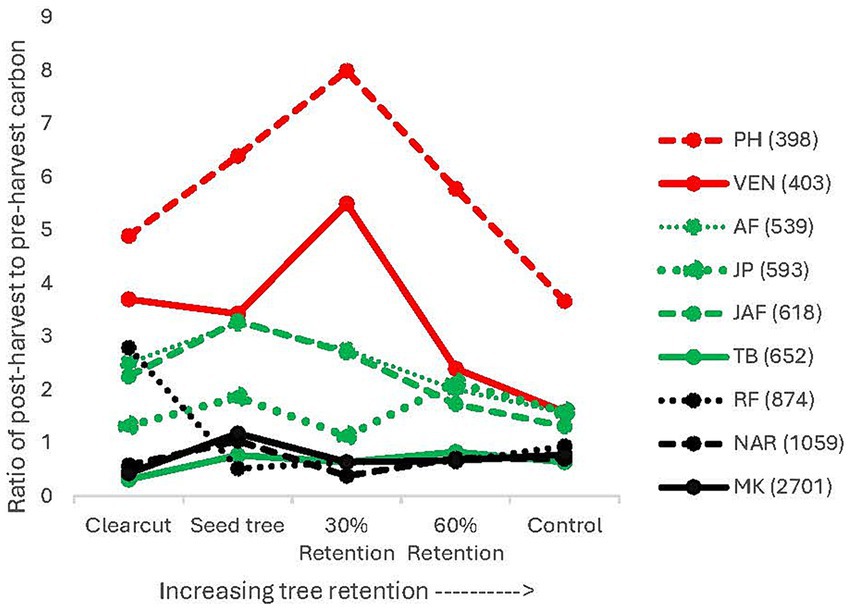
Figure 6. Ratio of post to pre-logging CWD volume. Numbers in parenthesis in the legend are summer heat: moisture index, with a higher number representing a more arid climate.
Influence of climate and harvesting treatment on CWD diversity index
The CWD diversity index in the mature forests differed amongst climatic regions (p < 0.0001) (Supplementary Table S2). Sites in the more arid climates (Peterhope Lake, Venables, Two-bit-Creek and Jaffray) had lower CWD diversity (average 6.0) than moist interior locations (Narrows Creek, 23.4; and Redfish Creek, 14.2) and the cold northern location (John Prince, 20.7). Malcolm Knapp had a lower index (10.6) than John Prince and Narrows Creek but did not significantly differ from the other locations. The ratio of post- to pre-harvest CWD diversity index increased with mean annual temperature (p = 0.0405) and was correlated with the interaction between treatment and summer mean maximum temperature (p = 0.0041). Following the harvesting treatments, ratios ranged from 0.36 to 3.50, decreasing or remaining constant at six locations, but increasing following all treatments at Two-bit Creek and Malcolm Knapp, and the clearcut and seed tree treatments at Jaffray.
Influence of climate and harvesting treatment on CWD decay class
Prior to logging, decay classes 4 and 5 combined (highly decomposed logs) comprised 31–88% of the total CWD volume and decay class 1 (fresh logs) comprised <15% (Supplementary Table S3). One year after logging, decay classes 1 and 2 dominated total CWD volume. Volume in decay classes 4 and 5 was greatly reduced at the wet, moist, and northern locations except at Redfish Creek (Figure 7). At the more arid locations (e.g., Interior Douglas-fir zone) losses of decomposed logs were less (Figure 8) but pre-harvest volumes were also generally lower. The losses of decomposed CWD volume were greater than gains in fresh pieces.
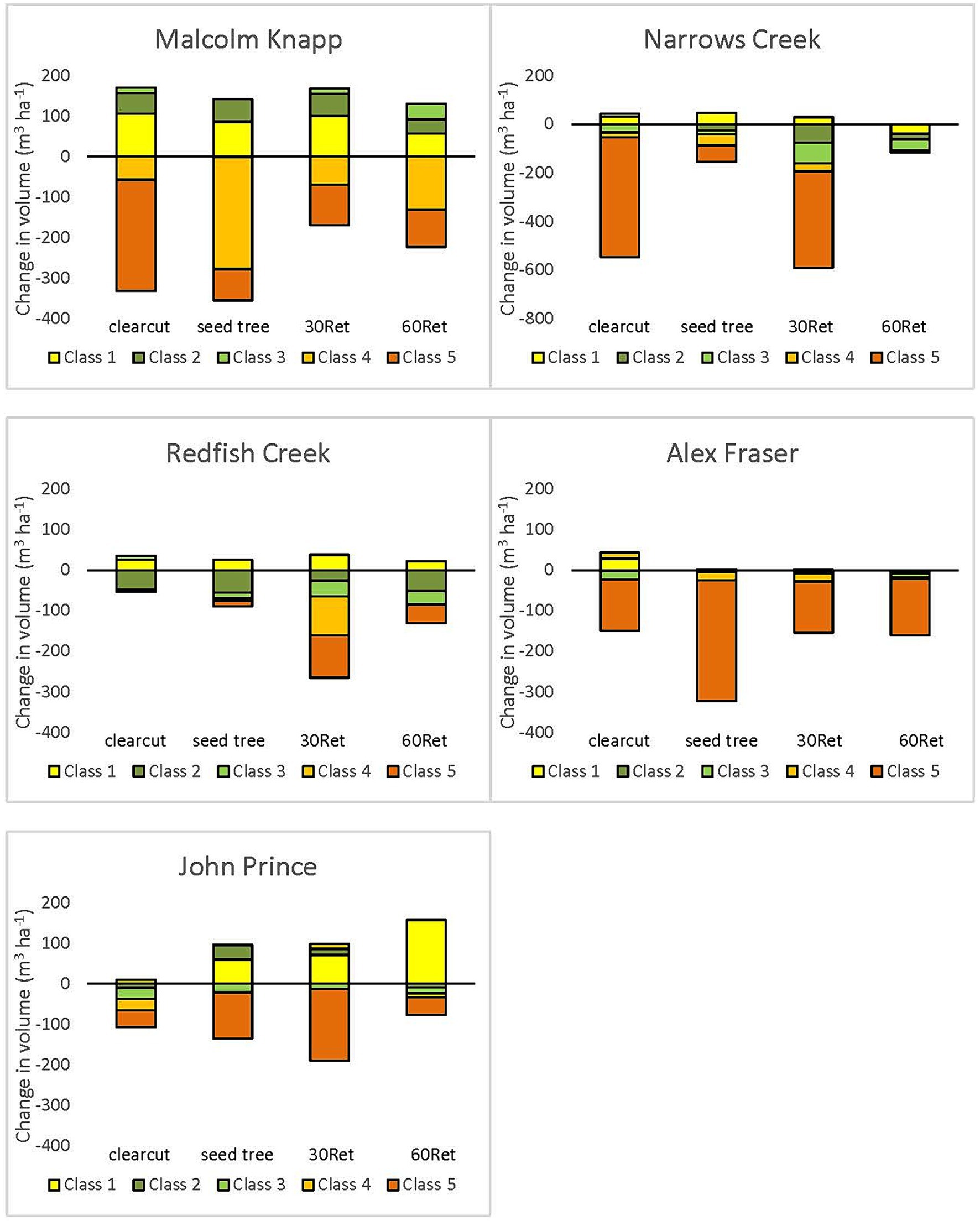
Figure 7. Change in coarse woody debris volume per hectare by decay class, from pre- to post-harvest at wet (Malcolm Knapp), moist (Redfish Creek; Narrows Creek), and northern (John Prince; Alex Fraser) locations. Boxes above the zero line indicate an increase in volume following harvesting for that decay class, and boxes below the zero line indicate a decrease in volume for that decay class. Decay classes that are not shown had no change in volume from pre- to post-harvest.
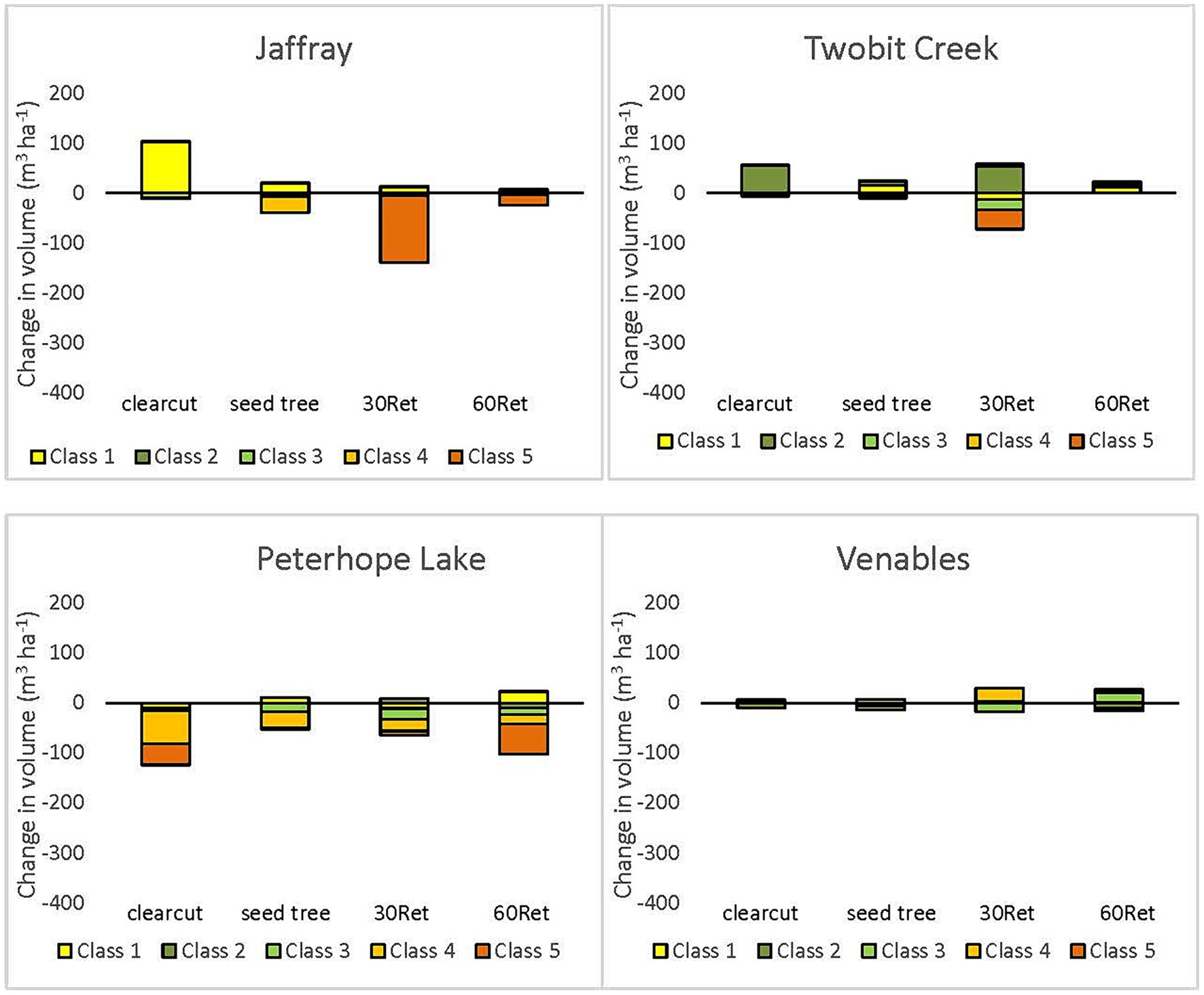
Figure 8. Change in coarse woody debris volume per hectare by decay class, from pre- to post-harvest at warm and dry locations (Jaffray; Two-bit Creek; Peterhope Lake; Venables). Boxes above the zero line indicate an increase in volume following harvesting for that decay class, and boxes below the zero line indicate a decrease in volume for that decay class. Decay classes that are not shown had no change in volume from pre- to post-harvest.
Influence of climate and harvesting treatment on CWD size
In all mature forests, the most CWD volume was in large (≥20 cm diameter) pieces (Supplementary Table S4). Volume distribution by diameter class tended to become more even following harvesting, due to increases in the volume of small intact pieces and decreases in the volume of large, decomposed pieces. Mature forests with the largest trees (humid coastal, moist interior, and cool northern locations) tended to have a greater abundance of large CWD pieces after harvesting than smaller stands on arid sites.
Influence of climate and harvesting treatment on pieces per hectare of CWD
Neither climate nor treatment was significantly correlated with the number of large (≥20 cm diameter and ≥ 10 m long) or medium (<20 cm diameter and/or < 10 m long) pieces per hectare before or after harvesting (p ≥ 0.05). There was a non-significant trend of fewer large pieces in the more arid climates (Interior Douglas-fir zone) versus the other climatic regions both before and after harvesting. The ratio of post- to pre-harvest pieces per hectare of large CWD increased with tree retention (p = 0.0031). Across the climate and harvesting gradients, there were substantially more medium than large CWD pieces both before and after harvesting. In mature forests, large CWD density was 3–117 pieces/ha, and 1 year after harvesting 0–60 pieces/ha (Table S5). The density of medium CWD was 93–3,700 pieces/ha in the pre-harvest mature forests and 312–5,163 pieces/ha after harvesting.
Influence of climate and harvesting treatment on CWD species
The species composition of CWD before and 1 year after harvesting reflected the species composition of standing trees in the mature forests. A total of 17 species of CWD were identified across the experiment (Supplementary Table S6). Douglas-fir was the dominant CWD species in all climatic regions except Malcolm Knapp and John Prince, where western redcedar and spruce predominated, respectively. Coniferous CWD was much more common than broadleaf CWD in all climatic regions before and after harvesting.
Discussion
Influence of climate and harvesting treatment on woody debris carbon
The CWD carbon stocks in our mature forests fell within the pool size reported by Pregitzer and Euskirchen (2004) for the temperate biome. We found that the greatest amount of downed woody debris occurred in wet (coastal), moist (interior wet belt), and cool (northern) climates and the least amount in arid climates, including the Interior Douglas-fir zone. This agrees with analyses of forests across the USA conducted by Smith et al. (2021) and Woodall and Liknes (2008), who note that moist, cool climates favor high productivity and deadwood accumulation, and slow wood decomposition. Decomposition rates of woody debris increase with temperature (Woodall and Liknes, 2008; Berbeco et al., 2012; Shorohova and Kapitsa, 2014; Finér et al., 2016; Rinne-Garmston et al., 2019; Harmon et al., 2020) but the interaction of temperature and moisture also affects decay rate (Gould et al., 2008; Forrester et al., 2012) such that decomposition may be inhibited on very wet sites (Harmon et al., 1986). High CWD carbon stocks in the most humid climatic region of our study may also reflect that Douglas-fir was mixed with western redcedar, the latter of which is more resistant to decay than other species (Harmon et al., 2008; Woodall and Liknes, 2008). At our cool northern site, high amounts of CWD may be related to both slow decomposition and past episodic storms, rather than the presence of decay-resistant species. Most CWD there was Douglas-fir and interior spruce whose decay rates are similar (Harmon et al., 2008).
The variable impact of harvesting on total CWD carbon stocks across the climatic gradient in our study demonstrates that broad generalizations about harvesting effects on CWD cannot be made. Other studies have reported increases (Payne et al., 2019) or decreases (Fredeen et al., 2005; Krueger et al., 2017) in the CWD carbon pool after harvesting, with outcomes dependent upon the forest type (Brassard and Chen, 2008; Oettel et al., 2020), silviculture system (Matsuzaki et al., 2013), logging method (Simmons et al., 2014; Thiffault et al., 2014), and utilization standard (Simmons et al., 2014; Berg et al., 2016). Estimates of harvesting effects are further influenced by woody debris definition, which is not internationally standardized (En-Rong et al., 2006), lag time between disturbance and measurement (Martin et al., 2005), and sampling methodology. All of these factors were held constant in our study except forest type, which naturally varied with climate from open, nearly pure Douglas-fir to closed mixed stands of Douglas-fir mixed with other species.
Our finding that SWD carbon stocks in mature forests were not influenced by climate agrees with Woodall and Liknes (2008), who found only a weak correlation between climate and carbon stocks in woody debris <7.62 cm in diameter. That the ratio of post to pre-harvest SWD carbon stocks was higher in our humid forests than in semi-arid, open forests probably reflects the higher pre-harvest tree volume and density in humid forests, which is expected to leave more small debris behind. Similarly, the greatest increases in SWD were in treatments where most or all trees were cut, reflecting the greater number of felled trees contributing slash. FWD carbon stocks increased after all harvesting treatments in arid and cool climates and because of the high flammability of FWD, fire hazard is expected to have increased.
Contributions of coarse, small, and fine woody debris to carbon stocks
Small and fine woody debris comprised a higher proportion of the total downed woody debris carbon in arid than moister climates, whether before or after harvesting. This agrees with Woodall and Liknes (2008), who found more carbon held in <7.62 cm diameter pieces than in larger pieces at latitudes less than 32.66o in the U.S., while the reverse was true in less arid forests occurring in latitudes >32.66o. We found the highest proportions of small and fine pieces in clearcut and seed tree treatments, yet on an arid site, even the 60% retention harvesting resulted in 80% of the downed woody debris carbon in small and fine pieces. This contrasts with many older studies in the USA (Harmon, 1980; Triska and Cromack, 1980; Brown and See, 1981), where larger woody debris usually comprises >80% of the total woody debris biomass.
Influence of climate and harvesting treatment on CWD volume
Volume of CWD is highly correlated with the richness of deadwood-dependent organisms, and thus has been considered a more useful indicator than CWD carbon for biodiversity studies (Stokland et al., 2012). Our results agree with Gould et al. (2008) who found that CWD volume was greater in moist than dry climates in temperate forests. This can be explained by higher tree productivity and denser stands where precipitation is high, leading to more trees available for recruitment to CWD, as well as more self-thinning in dense stands. We found relatively high variability in volume within climatic regions in agreement with many other studies (e.g., Bond-Lamberty et al., 2002).
In our study, harvesting had variable effects on CWD volume as it did for carbon. Decreases in CWD volume following clearcutting have been reported by many others, particularly in Nordic countries where wood recovery rates are high (Sippola et al., 1998; Fridman and Walheim, 2000; Gibb et al., 2005; Ekbom et al., 2006), but also in lodgepole pine forests in the United States (Tinker and Knight, 2000) and boreal forests in Canada (Pedlar et al., 2002; Brassard and Chen, 2008). Clearcutting has increased CWD volume in other locations and forest types, including hardwood stands in the USA (Idol et al., 2001), southern taiga stands in Russia (Krankina et al., 2001), and hemiboreal stands in Estonia (Rosenvald et al., 2018). In contrast, Stevenson et al. (2006) found that clearcutting had no significant effect on volume in temperate forests in the ICH zone in B.C. Partial cutting has also resulted in declines in CWD volume (e.g., in boreal forests in Sweden, Gibb et al., 2005; hardwood forests in the eastern United States and Canada, Vanderwel et al., 2008; Bolton and D’Amato, 2011; and in Estonia, Rosenvald et al., 2018). Volume remained the same following partial cutting near the timberline in Finnish Lapland (Sippola et al., 1998), in boreal forests in Finland and Russia (Rouvinen et al., 2011), and in the ICH zone in B.C. (Stevenson et al., 2006). Our result is not easily compared with these other studies because of differences in harvesting practices, forest type, and other factors.
Influence of climate and harvesting treatment on CWD diversity index
The low CWD diversity index before and after harvesting at our arid locations is explained by low tree diversity and low total CWD volume. Kunttu et al. (2015) found that diversity index is positively correlated with total volume. The relatively low pre-harvest index in our mature coastal forests reflects the scarcity of decay class 1 and 2 pieces, probably due to few recent natural disturbances. Harvesting increased diversity index only at the coastal and warmest interior locations, due to up to four-fold increases in total volume at the interior location, and the addition of fresh pieces of several species at the coast. The primary reason that the diversity index decreased after harvesting at our other locations is that highly decayed and large diameter pieces were lost due to breakage, crushing, and scattering by equipment.
Influence of climate and harvesting treatment on decay class
The large proportion of highly decomposed wood in mature forests across our climatic gradient is similar to in the Scots pine-dominated stands studied by Linder et al. (1997) in northern Sweden. In studies from various other places, however, intermediate decay classes comprise the largest fraction of CWD biomass, while the most and least decayed classes comprise the smallest fraction (Harmon et al., 1986; Spetich et al., 1999; Lombardi et al., 2008; Herrero et al., 2014). Our results agree with other reports that decay class distributions of CWD are substantially altered by harvesting (Idol et al., 2001; Krankina et al., 2001; Fraver et al., 2002; Pedlar et al., 2002; Gibb et al., 2005; Ekbom et al., 2006; Stevenson et al., 2006), with a pulse of undecomposed CWD inputs after logging accompanied by a decrease in highly decayed CWD volume due to disturbance by equipment. We did not observe this response in our arid climates probably because the open stands had fewer trees to harvest, resulting in less disturbance by skidders.
Influence of climate and harvesting treatment on size of CWD
Diameter distribution of CWD changed after harvesting across our entire climatic gradient, agreeing with Pedlar et al. (2002) and Gibb et al. (2005). Average diameter decreased due to the input of fresh pieces too small to utilize with current manufacturing facilities, combined with the destruction of large, highly decayed pieces. That losses of large CWD increased with harvesting intensity is likely associated with greater equipment traffic and crushing of large, decomposed pieces where more pieces were felled and skidded. Because our pre-harvest stands were mature and healthy, most large, felled trees were merchantable and skidded off the blocks, limiting the introduction of large new pieces. The loss of large pieces is of particular concern on clearcuts where there are no residual trees (except at the forest edge) to provide future CWD inputs.
Ecological implications of our findings
The mature, naturally regenerated forests in our study provide a baseline for assessing ecological impacts of changes in downed woody debris following harvesting. Downed woody debris in all ages of forests is important for soils and forest productivity, is a regeneration substrate, and is a critical habitat feature for cryptogams, fungi, and invertebrates, as well as mammals, amphibians, and reptiles which go in or under it to den, hide, forage, nest, or hibernate (Freedman et al., 1996; Bunnell et al., 2002). CWD is also important for geomorphology of streams and slopes and long-term carbon storage (Stevens, 1997).
All types of downed woody debris have ecological value, and healthy forest ecosystems require a variety of decay classes, sizes, and species, so that organisms with varying habitat requirements are supported and multiple ecological processes are favored (Province of British Columbia, 2010; Bouget et al., 2013; Kunttu et al., 2015). At our locations, the downed wood diversity index decreased after most harvesting treatments at seven of the nine climatic regions, suggesting an overall negative ecological impact of harvesting on diversity of organisms and ecological processes.
Except at the semi-arid locations, the decay class distribution of CWD changed after harvesting with a large decrease in rotten wood and an increase in fresh, intact pieces. This shift will likely reduce the amount of nitrogen, phosphorus, and sulfur available for use by regenerating plants for several decades (Idol et al., 2001), reduce habitat for mycorrhizal and saprophytic fungi, which are ecologically important in nutrient cycling and plant nutrition, reduce substrate for bryophytes, reduce the abundance of rotting “nurse” logs upon which seedlings of several tree and plant species establish, reduce availability of soft logs which small mammals burrow into, which subsequently provide amphibians and reptiles access to the inner log, and reduce availability of habitat for many invertebrates that salamanders and other vertebrates feed upon (see reviews by Harmon et al., 1986; Rose et al., 2001; Bunnell et al., 2002). The increase in hard, undecomposed logs may increase availability of perches and runways (Maser et al., 1979). Rose et al. (2001) note that all decay classes of downed woody debris are used by wildlife, and habitat functions may encompass several decay classes, thus generalizations regarding which decay classes are most important for habitat must be made cautiously.
CWD plays important roles in maintaining healthy soils and forest productivity (Stevens, 1997). Woody material improves soil structure moisture-carrying capacity. It provides a substantial nutrient storage pool and is the most important organic matter source over a stand rotation. It provides habitat for decomposer organisms and sites for nitrogen-fixing bacteria which can aid in improving ecosystem nutrient deficiencies. By holding moisture in dry periods, CWD provides a refuge for ectomycorrhizal roots and their associated soil organisms. In some forests, large logs are the most important sites for conifer germination, particularly where thick understory vegetation restricts light, or where the ground is very wet (Stevens, 1997). At our sites, regeneration occurred on both logs and other substrates, suggesting that losses of large CWD due to harvesting would negatively impact regeneration, but not as severely as in some other forest types. The abundance of CWD influences the type and extent of animal use (Maser et al., 1979), and a decline in downed dead wood volume after harvesting has been associated with a decrease in species population size or fitness. We therefore expect a negative impact of our clearcutting and seed tree treatments, which generally reduced CWD volume to about half of the pre-harvest value. However, many terrestrial vertebrates including most birds do not require downed wood but rather use it opportunistically (Bunnell et al., 2002).
Large pieces (diameter and length) of woody debris last longer and generally have more potential uses as wildlife habitat than small pieces (Lee and Sturgess, 2001). The loss of large pieces following our harvesting treatments everywhere except the most arid climate negatively affects hiding, denning, and foraging structures for mammals, substrate quality for bryophytes and lichens and refuge sites for plants that are prone to herbivory when growing on the forest floor (see reviews by Harmon et al., 1986; Freedman et al., 1996). Very large diameter logs (≥100 cm) contribute disproportionately to ecosystem function (Lutz et al., 2012, 2013) but were uncommon in our mature and harvested forests.
A range of CWD species is advantageous in forests of all ages because different tree species decay at different rates (Kahl et al., 2017), resulting in a range of decay classes over time, and thus a greater range of functional roles. Across all climates and treatments, CWD species distributions on our sites changed little after harvesting so in terms of CWD species the effect of harvesting was neutral.
SWD loads increased considerably following clearcutting and seed tree treatments at humid locations and increased to a lesser degree after all treatments in the interior. All treatments in the interior increased ground FWD fuel loads compared to pre-harvest. That the density of small and fine downed wood increased after logging has positive ecological effects including provision of habitat for small mammals (Manning and Edge, 2008), fungi (Nordén et al., 2004), and arthropods (Castro and Wise, 2009), increased cryptogam diversity (Kruys and Jonsson, 1999), and creation of a short-term nutrient supply (Rittenhouse et al., 2012), However, it is uncertain if these benefits outweigh the fact that smaller woody pieces are more flammable and a greater contributor to intense forest fire behavior than CWD (Brown et al., 2003).
Legislation and guidance for post-logging woody debris in British Columbia
The Forest Act and subsequent agreements require licensees to carry out waste and residue assessments, and fines are issued if an excessive volume of debris remains after logging (British Columbia Ministry of Forests, 2019a,b). At the same time, the Forest Act specifies that “an agreement holder who carries out timber harvesting must retain at least the following logs on a cutblock: (a) if the area is on the coast, a minimum of 4 logs per hectare, each being a minimum of 5 m in length and 30 cm in diameter at one end; (b) if the area is in the interior, a minimum of 4 logs per hectare, each being a minimum of 2 m in length and 7.5 cm in diameter at one end. These minimum conditions for CWD retention were met at our sites. The Chief Forester of British Columbia recommended a median value of at least 4, 5, 9, and 23 large CWD pieces per hectare on harvested sites in the IDF, ICH, and SBS zones and wet subzones of the CWH zone, respectively (Province of British Columbia, 2010). In our study, all harvesting treatments met this guideline in the CWH and SBS zones. In the ICH zone, up to three of the four harvesting treatments met the guideline, with the 30% retention treatment most successful. In the IDF zone, only the 60% retention treatment was consistently successful.
Management interpretations
We found that total downed woody debris carbon stocks, volume, and diversity were much lower in arid mature forests (e.g., dry Interior Douglas-fir subzones) than humid coastal or interior wet belt forests of B.C. This suggests that particular care must be taken on arid sites to avoid woody debris depletion during harvesting, especially clearcutting where no legacy trees are available to provide future recruitment of downed wood for many decades. A key goal in woody debris management is protection of non-merchantable decayed and large CWD during harvesting, or if this is not feasible, retention of some large legacy trees and snags to provide future inputs of woody debris over time. If forests are managed for relatively short rotation repeated harvesting, large logs, which are particularly important to ecosystem functioning, may be eradicated over time if large legacy trees are not retained. Climate change predictions for B.C. of warmer temperatures and summer droughts are already occurring, which in the long term is expected to result in drier, more open forests, with faster decomposition rates which are associated with less woody debris. On the other hand, drought-induced mortality may increase woody debris stocks temporarily when the dead trees fall. Management for diverse CWD species must begin when stands are young by applying reforestation and stand tending treatments that promote a diversity of tree species. Woody debris is naturally variable and dynamic over time and space in part due to local episodic disturbances, thus caution is needed in extrapolating our inventory results to other sites. We recommend that future work include studies in other forest types that include assessment of woody debris dynamics across a harvesting and climatic gradient.
Data availability statement
The raw data supporting the conclusions of this article will be made available by the authors, without undue reservation.
Author contributions
WR: Conceptualization, Methodology, Project administration, Writing – original draft, Writing – review & editing. SS: Conceptualization, Funding acquisition, Investigation, Methodology, Project administration, Writing – review & editing. ES: Formal analysis, Writing – review & editing.
Funding
The author(s) declare that financial support was received for the research, authorship, and/or publication of this article. Site selection was funded by a Natural Sciences and Engineering Research Council of Canada Strategic Project Grant to SS. Field data collection and lab work was funded by the Forest Enhancement Society of B.C. (grant to WR) and the Forest Carbon Initiative (B.C. Ministry of Forests) (grant to SS). Funding for the preparation of this article was provided by the Forest Carbon Initiative.
Acknowledgments
We thank Brian Pickles, the B.C. Ministry of Forests, the University of British Columbia, and each of the local partners (Alex Fraser Research Forest, B.C. Timber Sales, Canfor, Cooks Ferry Indian Band, Harrop-Procter Community Forest, John Prince Research Forest, Kalesnikoff Lumber Co., and Malcolm Knapp Research Forest) for their contributions to locating the study sites, block layout and harvesting. We also thank the many members of the Simard Lab who assisted in collecting the field data.
Publisher’s note
All claims expressed in this article are solely those of the authors and do not necessarily represent those of their affiliated organizations, or those of the publisher, the editors and the reviewers. Any product that may be evaluated in this article, or claim that may be made by its manufacturer, is not guaranteed or endorsed by the publisher.
Supplementary material
The Supplementary material for this article can be found online at: https://www.frontiersin.org/articles/10.3389/ffgc.2024.1397142/full#supplementary-material
References
Arsenault, A. (2002). Managing coarse woody debris in British Columbia’s forests: A cultural shift for professional foresters? Berkeley, CA: Pacific Southwest Forest and Range Experiment Station, 10.
Banaś, J., Bujoczek, L., Zieba, S., and Drozd, M. (2014). The effects of different types of management, functions, and characteristics of stands in polish forests on the amount of coarse woody debris. Eur. J. For. Res. 133, 1095–1107. doi: 10.1007/s10342-014-0825-3
Bartoń, K. (2022). MuMIn: Multi-Model Inference. R package version 46.0. Available at: https://CRAN.R-project.org/package=MuMIn
Bates, D., Maechler, M., Bolker, M., and Walker, S. (2015). Fitting linear mixed-effects models using lme4. J. Stat. Softw. 67, 1–48. doi: 10.18637/jss.v067.i01
Berbeco, M. R., Melillo, J. M., and Orians, C. M. (2012). Soil warming accelerates decomposition of fine woody debris. Plant Soil 356, 405–417. doi: 10.1007/s11104-012-1130-x
Berg, E. C., Morgan, T. A., Simmons, E. A., Zarnoch, S. J., and Scudder, M. G. (2016). Predicting logging residue volumes in the Pacific northwest. For. Sci. 62, 564–573. doi: 10.5849/forsci.15-176
Böhl, J., and Brӓndli, U. B. (2007). Deadwood volume assessment in the third Swiss National Forest Inventory: methods and first results. Eur. J. For. Res. 126, 449–457. doi: 10.1007/s10342-007-0169-3
Bolton, N. W., and D’Amato, A. W. (2011). Regeneration responses to gap size and coarse woody debris within natural disturbance-based silvicultural systems in northeastern Minnesota. USA. For. Ecol. Manag. 262, 1215–1222. doi: 10.1016/j.foreco.2011.06.019
Bond-Lamberty, B., Chuankuan, W. C., and Gower, S. T. (2002). Annual carbon flux from woody debris for a boreal black spruce fire chronosequence. J. Geophys. Res. 107, 1–10. doi: 10.1029/2001JD000839
Bouget, C., Larrieu, L., Nusillard, B., and Parmain, G. (2013). In search of the best local habitat drivers for saproxylic beetle diversity in temperate deciduous forests. Biodivers. Conserv. 22, 2111–2130. doi: 10.1007/s10531-013-0531-3
Brassard, B. W., and Chen, H. Y. N. (2008). Effects of forest type and disturbance on diversity of coarse woody debris in boreal forest. Ecosystems 11, 1078–1090. doi: 10.1007/s10021-008-9180-x
Braumandl, T. F., and Curran, M. P. (1992). A field guide for site identification and interpretation for the Nelson Forest region. Victoria, B.C: B.C. Ministry of Forests.
British Columbia Ministry of Forests (2019a). Provincial logging residue and waste measurement procedures manual – Interior version. Victoria, B.C.: Timber Pricing Branch.
British Columbia Ministry of Forests. (2019b), Provincial logging residue and waste measurement procedures manual – Coast version. Timber Pricing Branch. Victoria, B.C.R Core Team.2022). R: A language and environment for statistical computing. R Foundation for Statistical Computing, Vienna, Austria. Available at: https://www.R-project.org/
British Columbia Ministry of Forests and Range & British Columbia Ministry of Environment (2010). Field manual for describing terrestrial ecosystems. 2nd Edn. Victoria, BC: B.C. Ministry of Forests and Range and B.C. Ministry of Environment Resource Inventory Branch.
Brown, J. K., Reinhardt, E. D., and Kramer, K. A. (2003). Coarse woody debris: Managing benefits and fire hazard in the recovering forest. Ogden, UT: Rocky Mountain Research Station, 16.
Brown, J. K., and See, T. E. (1981). Downed dead woody fuel and biomass in the northern Rocky Mountains. Ogden, UT: Intermountain Forest and Range Experiment Station, 48.
Bunnell, F. L., Houde, I., Johnston, B., and Wind, E. (2002). How dead trees sustain live organisms in western forests. Berkeley, CA: Pacific Southwest Forest and Range Experiment Station, 27.
Canadian Forest Inventory Committee & Canadian Forest Service. (2008). Canada’s National Forest Inventory: Ground sampling guidelines, Ver. 5.0. Available at: www.worldcat.org/oclc/606580128 (Accessed November 10, 2023)
Castagneri, D., Garbarino, M., Berretti, R., and Motta, R. (2010). Site and stand effects on coarse woody debris in montane mixed forests of eastern Italian Alps. For. Ecol. Manag. 260, 1592–1598. doi: 10.1016/j.foreco.2010.08.008
Castro, A., and Wise, D. H. (2009). Influence of fine woody debris on spider diversity and community structure in forest leaf litter. Biodivers. Conserv. 18, 3705–3731. doi: 10.1007/s10531-009-9674-7
Caza, C. (1993). Woody debris in the forests of British Columbia: a review of the literature and current research. Victoria, BC: B.C. Ministry of Forests.
Clark, D. F., Kneeshaw, D. D., Burton, P. J., and Antos, J. A. (1998). Coarse woody debris in sub-boreal spruce forests of west-Central British Columbia. Can. J. For. Res. 28, 284–290. doi: 10.1139/x97-208
Delong, C., Tanner, D., and Jull, M. J. (1993). A field guide for site identification and interpretation for the southwest portion of the Prince George Forest region. Victoria, BC: B.C. Ministry of Forests.
Ekbom, B., Schroeder, L. M., and Larsson, S. (2006). Stand specific occurrence of coarse woody debris in a managed boreal forest landscape in Central Sweden. For. Ecol. Manag. 221, 2–12. doi: 10.1016/j.foreco.2005.10.038
En-Rong, Y., Xihua, W., and Jianjun, H. (2006). Concept and classification of coarse woody debris in forest ecosystems. Front. Biol. China 1, 76–84. doi: 10.1007/s11515-005-0019-y
Finér, L., Jurgensen, M., Palviainen, M., Piirainen, S., and Page-Dumroese, D. (2016). Does clear-cut harvesting accelerate initial wood decomposition? A five-year study with standard wood material. For. Ecol. Manag. 372, 10–18. doi: 10.1016/j.foreco.2016.03.060
Forrester, J. A., Mladenoff, D. J., Gower, S. T., and Stoffel, J. L. (2012). Interactions of temperature and moisture with respiration from coarse woody debris in experimental forest canopy gaps. For. Ecol. Manag. 265, 124–132. doi: 10.1016/j.foreco.2011.10.038
Fox, J., and Weisberg, S. (2019). An {R} companion to applied regression. Third Edn. Thousand Oaks CA: Sage.
Fraver, S., Wagner, R. G., and Day, M. (2002). Dynamics of coarse woody debris following gap harvesting in the Acadian forests of Central Maine, U.S.A. Can. J. For. Res. 32, 2094–2105. doi: 10.1139/X02-131
Fredeen, A. L., Bois, C. H., Janzen, D. T., and Sanborn, P. T. (2005). Comparison of coniferous forest carbon stocks between old-growth and young second-growth forests on two soil types in Central British Columbia, Canada. Can. J. For. Res. 35, 1411–1421. doi: 10.1139/X05-074
Freedman, B., Zelazny, V., Beaudette, D., Fleming, T., Johnson, G., Flemming, S., et al. (1996). Biodiversity implications of changes in the quantity of dead organic matter in managed forests. Environ. Rev. 4, 238–265. doi: 10.1139/a96-013
Fridman, J., and Walheim, M. (2000). Amount, structure, and dynamics of dead wood on managed forest land in Sweden. For. Ecol. Manag. 131, 23–36. doi: 10.1016/S0378-1127(99)00208-X
Garbarino, M., Marzano, R., Shaw, J. D., and Long, J. N. (2015). Environmental drivers of deadwood dynamics in woodlands and forests. Ecosphere 6, 1–24. doi: 10.1890/ES14-00342.1
Gibb, H., Ball, J. P., Johansson, T., Atlegrim, O., Hjältén, J., and Danell, K. (2005). Effects of management on coarse woody debris volume and composition in boreal forests in northern Sweden. Scand. J. For. Res. 20, 213–222. doi: 10.1080/02827580510008392
Gould, W. A., Gonzales, G., Hudak, A. T., Hollingsworth, T. N., and Hollingsworth, J. (2008). Forest structure and downed woody debris in boreal, temperate, and tropical forest fragments. Ambio 37, 577–587. doi: 10.1579/0044-7447-37.7.577
Green, R. N., and Klinka, K. (1994). A field guide to site identification and interpretation for the Vancouver Forest Region. Victoria, BC: B.C. Ministry of Forests.
Harmon, M. E. (1980). The distribution and dynamics of forest fuels in the low elevation forests of Great Smoky Mountains National Park. Res. Resour. Manage.
Harmon, M. E., Fasth, B., Woodall, C. W., and Sexton, J. (2013). Carbon concentration of standing and downed woody detritus: effects of tree taxa, decay class, position, and tissue type. For. Ecol. Manag. 291, 259–267. doi: 10.1016/j.foreco.2012.11.046
Harmon, M. E., Fasth, B. G., Yatskov, M., Kastendick, D., Rock, J., and Woodall, C. W. (2020). Release of coarse woody detritus-related carbon: a synthesis across forest biomes. Carbon Balance Manag. 15:1. doi: 10.1186/s13021-019-0136-6
Harmon, M. E., Franklin, J. F., Swanson, F. J., Sollins, P., Gregory, S. V., Lattin, J. D., et al. (1986). “Ecology of coarse woody debris in temperate ecosystems” in Advances in ecological research. eds. A. MacFadyens and E. D. Ford (Orlando, FL: Academic Press Inc.), 133–302.
Harmon, M. E., Woodall, C. W., Fasth, B., and Sexton, J. (2008). Woody detritus density and density reduction factors for tree species in the United States: A synthesis. USDA Forest Service gen. Newtown Square, PA: Northern Research Stations, 84.
Hély, C., Bergeron, Y., and Flannigan, M. D. (2000). Coarse woody debris in the southeastern Canadian boreal forest: composition and load variations in relation to stand replacement. Can. J. For. Res. 30, 674–687. doi: 10.1139/cjfr-30-5-674
Herrero, C., Krankina, O., Monleon, V. J., and Bravo, F. (2014). Amount and distribution of coarse woody debris in pine ecosystems of North-Western Spain, Russia and the United States. iForest 7, 53–60. doi: 10.3832/ifor0644-006
Idol, T. W., Figler, R. A., Pope, P. E., and Ponder, F. (2001). Characterization of coarse woody debris across a 100 year chronosequence of oak-hickory forests. For. Ecol. Manag. 149, 153–161. doi: 10.1016/S0378-1127(00)00536-3
Kahl, T., Arnstadt, T., Baber, K., Bässler, C., Bauhus, J., Borken, W., et al. (2017). Wood decay rates of 13 temperate tree species in relation to wood properties, enzyme activities and organismic diversities. For. Ecol. Manag. 391, 86–95. doi: 10.1016/j.foreco.2017.02.012
Korboulewsky, N., Bilger, I., and Bessaad, A. (2021). How to evaluate downed fine woody debris including logging residues? Forests 12:881. doi: 10.3390/f12070881
Krankina, O. N., Treyfeld, R. E., Harmon, M. E., Spycher, G., and Povarov, E. D. (2001). Coarse woody debris in the forests of the St. Petersburg region, Russia. Ecol. Bull. 49, 93–104. doi: 10.2307/20113267
Krueger, I., Shulz, C., and Borken, W. (2017). Stocks and dynamics of soil organic carbon and coarse woody debris in three managed and unmanaged temperate forests. Eur. J. For. Res. 136, 123–137. doi: 10.1007/s10342-016-1013-4
Kruys, N., and Jonsson, B. G. (1999). Fine woody debris is important for species richness on logs in managed boreal spruce forests of northern Sweden. Can. J. For. Res. 29, 1295–1299. doi: 10.1139/x99-106
Kunttu, P., Junninen, K., and Kouki, J. (2015). Dead wood as an indicator of forest naturalness: a comparison of methods. For. Ecol. Manag. 353, 30–40. doi: 10.1016/j.foreco.2015.05.017
Lee, P. C., and Sturgess, K. (2001). The effects of logs, stumps, and root throws on understory communities within 28-year-old aspen-dominated boreal forests. Can. J. Bot. 79, 905–916. doi: 10.1139/b01-072
Lenth, R. V. (2022). Emmeans: estimated marginal means, aka least-squares means. R package version 1.8.2. Available at: https://CRAN.R-project.org/package=emmeans
Lindenmayer, D. B., Franklin, J. F., Lõhmus, A., Baker, S. C., Bauhus, J., Beese, W., et al. (2012). A major shift to the retention approach for forestry can help resolve some global forest sustainability issues. Conserv. Lett. 5, 421–431. doi: 10.1111/j.1755-263X.2012.00257.x
Linder, P., Elfving, B., and Zackrisson, O. (1997). Stand structure and successional trends in virgin boreal forest reserves in Sweden. For. Ecol. Manag. 98, 17–33. doi: 10.1016/S0378-1127(97)00076-5
Lloyd, D., Angove, K., Hope, G., and Thompson, C. (1990). A guide to site identification and interpretation for the Kamloops Forest region B.C : Ministry of Forests, Victoria, BC.
Lombardi, F., Lasserre, B., Tognetti, R., and Marchetti, M. (2008). Deadwood in relation to stand management and forest type in central Apennines (Molise, Italy). Ecosystems 11, 882–894. doi: 10.1007/s10021-008-9167-7
Lutz, J. A., Larson, A. J., Freund, J. A., Swanson, M. E., and Bible, K. J. (2013). The importance of large-diameter trees to forest structural heterogeneity. PLoS One 8:e82784. doi: 10.1371/journal.pone.0082784
Lutz, J. A., Larson, A. J., Swanson, M. E., and Freund, J. A. (2012). Ecological importance of large-diameter trees in a temperate mixed-conifer forest. PLoS One 7:e36131. doi: 10.1371/journal.pone.0036131
Manning, J. A., and Edge, W. D. (2008). Small mammal responses to fine woody debris and forest fuel reduction in Southwest Oregon. J. Wildl. Manag. 72, 625–632. doi: 10.2193/2005-508
Marshall, P. L., Davis, G., and LeMay, V. M. (2000). Using line intersect sampling for coarse woody debris. Vancouver, BC: Vancouver Forest Region.
Martin, J. L., Gower, S. T., Plaut, J., and Holmes, B. (2005). Carbon pools in a boreal mixedwood logging chronosequence. Glob. Change Biol. 11, 1883–1894. doi: 10.1111/j.1365-2486.2005.01019.x
Maser, C., Anderson, R. G., Cromack, K., Williams, J. T., and Martin, R. E. (1979). “Dead and down woody material” in Wildlife habitats in managed forests, the Blue Mountains of Oregon and Washington. ed. J. W. Thomas (Washington, DC: USDA For Serv. Agric), 8–96.
Matsuzaki, E., Sanborn, P., Fredeen, A. L., Shaw, C. H., and Hawkins, C. (2013). Carbon stocks in managed and unmanaged old-growth western redcedar and western hemlock stands of Canada’s inland temperate rainforests. For. Ecol. Manag. 297, 108–119. doi: 10.1016/j.foreco.2012.11.042
National Forest Inventory. (2021). Canada’s National Forest Inventory – national standards for ground plots compilation procedures, Ver. 2.4. Available at: http://nfi.nfis.org (Accessed November 10, 2023)
Nordén, B., Ryberg, M., Götmark, F., and Olausson, B. (2004). Relative importance of coarse and fine woody debris for the diversity of wood-inhabiting fungi in temperate broadleaf forests. Biol. Conserv. 117, 1–10. doi: 10.1016/S0006-3207(03)00235-0
Oettel, J., Lapin, K., Kindermann, G., Steiner, H., Schweinzer, K. M., Frank, G., et al. (2020). Patterns and drivers of deadwood volume and composition in different forest types of the Austrian natural forest reserves. For. Ecol. Manag. 463:118016. doi: 10.1016/j.foreco.2020.118016
Payne, N. J., Cameron, D. A., Leblanc, J.-D. D., and Morrison, I. K. (2019). Carbon storage and net primary productivity in Canadian boreal mixedwood stands. J. For. Res. 30, 1667–1678. doi: 10.1007/s11676-019-00886-0
Pedlar, J. H., Pearce, J. L., Venier, L. A., and McKenney, D. W. (2002). Coarse woody debris in relation to disturbance and forest type in boreal Canada. For. Ecol. Manag. 158, 189–194. doi: 10.1016/S0378-1127(00)00711-8
Pregitzer, K. S., and Euskirchen, E. S. (2004). Carbon cycling and storage in worlds forests: biome patterns related to forest age. Glob. Chang. Biol. 10, 2052–2077. doi: 10.1111/j.1365-2486.2004.00866.x
Province of British Columbia (2010). Chief Forester’s guidance on coarse woody debris management. Victoria, B.C.
R Core Team. (2022). R: A language and environment for statistical computing. Ver. 4.1.2. Vienna, Austria: R Foundation for Statistical Computing. Available at: https://www.R-project.org/
Riffell, S., Verschuyl, J., Miller, D., and Wigley, T. B. (2011). Biofuel harvests, coarse woody debris, and biodiversity – a meta-analysis. For. Ecol. Manag. 261, 878–887. doi: 10.1016/j.foreco.2010.12.021
Rinne-Garmston, K. T., Peltoniemi, K., Cheng, J., Peltoniemi, M., Fritze, H., and Makipaa, R. (2019). Carbon flux from decomposing wood and its dependency on temperature, wood N2 fixation rate, moisture and fungal composition in a Norway spruce forest. Glob. Chang. Biol. 25, 1852–1867. doi: 10.1111/gcb.14594
Rittenhouse, T. A. G., MacFarland, D. M., Martin, K. J., and Van Deelen, T. R. (2012). Downed wood associated with roundwood harvest, whole-tree harvest, and unharvested stands of aspen in Wisconsin. For. Ecol. Manag. 266, 239–245. doi: 10.1016/j.foreco.2011.11.029
Rose, C. L., Marcot, B. G., Mellen, T. K., Ohmann, J., Waddell, K. L., Lindley, D. L., et al. (2001). “Decaying wood in Pacific northwest forests: concepts and tools for habitat management” in Wildlife-habitat relationships in Oregon and Washington. eds. D. H. Johnson and T. A. O’Neil (Corvallis: Oregon State University Press), 580–623.
Rosenvald, R., Tullus, H., and Lohmus, A. (2018). Is shelterwood harvesting preferable over clear-cutting for sustaining deadwood pools? The case of Estonian conifer forests. For. Ecol. Manag. 429, 375–383. doi: 10.1016/j.foreco.2018.07.026
Rouvinen, S., Kuulavainen, T., and Karjalainen, L. (2011). Coarse woody debris in old Pinus sylvestris forests along a geographic and human impact gradient in boreal Fennoscandia. Can. J. For. Res. 32, 2184–2200. doi: 10.1139/x02-144
Shorohova, E., and Kapitsa, E. (2014). Influence of the substrate and ecosystem attributes on the decomposition rates of coarse woody debris in European boreal forests. For. Ecol. Manag. 315, 173–184. doi: 10.1016/j.foreco.2013.12.025
Siitonen, J., Martikainen, P., Puntilla, P., and Rauh, J. (2000). Coarse woody debris and stand characteristics in mature managed and old-growth boreal Mesic forests in southern Finland. For. Ecol. Manag. 128, 211–225. doi: 10.1016/S0378-1127(99)00148-6
Simmons, E. A., Morgan, T. A., Berg, E. C., Zarnoch, S. J., Hayes, S. W., and Thompson, M. T. (2014). Logging utilization in Idaho: current and past trends. Rocky Mountain Research Station: Fort Collins, CO
Sippola, A. L., Siitonen, J., and Kallio, R. (1998). Amount and quality of coarse woody debris in natural and managed coniferous forests near the timberline in Finnish Lapland. Scand. J. For. Res. 13, 204–214. doi: 10.1080/02827589809382978
Smith, J. E., Domke, G. M., and Woodall, C. W. (2021). Predicting downed woody material carbon stocks in forests of the conterminous United States. Sci. Total Environ. 803:150061. doi: 10.1016/j.scitotenv.2021.150061
Spetich, M. A., Shifley, S. R., and Parker, G. R. (1999). Regional distribution and dynamics of coarse woody debris in midwestern old-growth forests. For. Sci. 45, 302–313. doi: 10.1093/forestscience/45.2.302
Steen, O., and Coupé, R. A. (1997). A field guide to forest site identification and interpretation for the Cariboo Forest region. B.C. Min. For. Lands. Manag. Handb.
Stevens, V. (1997). The ecological role of coarse woody debris: an overview of the ecological importance of CWD in B.C. forests. B.C. Min. For. Res. Br. Work.
Stevenson, S. K., Jull, M. J., and Rogers, B. J. (2006). Abundance and attributes of wildlife trees and coarse woody debris at three silvicultural systems study areas in the interior cedar-hemlock zone, British Columbia. For. Ecol. Manag. 233, 176–191. doi: 10.1016/j.foreco.2006.06.023
Stokland, J. N., Siitonen, J., and Jonsson, B. G. (2012). Biodiversity in dead wood. New York: Cambridge University Press.
Teissier du Cros, R., and Lopez, S. (2009). Preliminary study on the assessment of deadwood volume by the French national forest inventory. Ann. For. Sci. 66:302. doi: 10.1051/forest/2009007
Thiffault, E., Barrette, J., Paré, D., Titus, B. D., Keys, K., Morris, D. M., et al. (2014). Developing and validating indicators of site suitability for forest harvesting residue removal. Ecol. Indic. 43, 1–18. doi: 10.1016/j.ecolind.2014.02.005
Tinker, D. B., and Knight, D. H. (2000). Coarse woody debris following fire and logging in Wyoming lodgepole pine forests. Ecosystems 3, 472–483. doi: 10.1007/s100210000041
Triska, F. J., and Cromack, K. (1980). “The role of wood debris in forests and streams” in Forests: Fresh perspectives from ecosystem analysis: Proceedings of the 40th annual biology colloquium. ed. R. H. Waring (Corvallis, OR: Oregon State University Press), 171–190.
Vanderwel, M. C., Thorpe, H. C., Shuter, J. L., Caspersen, J. P., and Thomas, S. C. (2008). Contrasting downed woody debris dynamics in managed and unmanaged northern hardwood stands. Can. J. For. Res. 38, 2850–2861. doi: 10.1139/X08-130
Wang, T., Hamann, A., Spittlehouse, D., and Carroll, C. (2016). Locally downscaled and spatially customizable climate data for historical and future periods for North America. PLoS One 11:e0156720. doi: 10.1371/journal.pone.0156720
Woodall, C. W., and Liknes, G. C. (2008). Relationships between forest fine and coarse woody debris carbon stocks across latitudinal gradients in the United States as an indicator of climate change effects. Ecol. Indic. 8, 686–690. doi: 10.1016/j.ecolind.2007.11.002
Woodall, C., Monleon, V., Fraver, S., Russell, M. B., Hatfield, M. H., Campbell, J. L., et al. (2019). The downed and dead wood inventory of forests in the United States. Sci. Data 6:180303. doi: 10.1038/sdata.2018.303
Keywords: aridity, carbon, climate, coarse woody debris, Douglas-fir, fine woody debris, forest harvesting, partial cutting
Citation: Roach WJ, Simard SW and Snyder EN (2024) Downed woody debris varies with climate and harvesting treatment in Douglas-fir forests of British Columbia, Canada. Front. For. Glob. Change. 7:1397142. doi: 10.3389/ffgc.2024.1397142
Edited by:
Rudong Zhao, Chinese Academy of Sciences (CAS), Wuhan, ChinaReviewed by:
Andis Lazdiņš, Latvian State Forest Research Institute Silava (LSFRI), LatviaMaciej Pach, University of Agriculture in Krakow, Poland
Copyright © 2024 Roach, Simard and Snyder. This is an open-access article distributed under the terms of the Creative Commons Attribution License (CC BY). The use, distribution or reproduction in other forums is permitted, provided the original author(s) and the copyright owner(s) are credited and that the original publication in this journal is cited, in accordance with accepted academic practice. No use, distribution or reproduction is permitted which does not comply with these terms.
*Correspondence: Winnifred Jean Roach, jroach12@telus.net