- Global Environmental Change Lab, Department of Geography, Portland State University, Portland, OR, United States
Altered fire regimes, combined with a warmer and drier climate, have been eroding the resilience of temperate rainforests and peatlands worldwide and leading to alternative post-fire vegetation communities. Chronic anthropogenic burning of temperate rainforests at the forest-peatland ecotone in western Patagonia appears to have shifted vegetation communities in poorly-drained sites from forests dominated by the threatened conifer, Pilgerodendron uviferum, to peat-accumulating wetlands covered by Sphagnum mosses. We collected and modeled post-reburn field data using ordinations and hierarchical Bayesian regressions to examine mechanisms through which P. uviferum forests may recover following fire or become locked into alternative development pathways by comparing biophysical factors of a reburned ecotone to those of an unburned (control) ecotone. We found that, (1) the significantly higher densities of P. uviferum trees and seedlings in the forested patches at both the reburned and control sites were associated with significantly lower seasonal water tables, lower cover of Sphagnum mosses and higher cover of other mosses (i.e., not in the Sphagnum or Dicranaloma genera); (2) despite abrupt boundaries in vegetation at both sites, successive fires homogenized the environment at the reburned site; and (3) the distinct life forms and individual species that characterized the understory plant communities across the ecotones affected seedling abundance by shaping microtopography and the substrates available for establishment. Together, our results suggest that fire can push edaphically wet P. uviferum-dominated sites towards a non-forested state by reducing the diversity of microsite structure and composition, thereby placing P. uviferum seedlings in direct competition with Sphagnum mosses and potentially limiting the availability of microsites that are protected from both seasonal inundation and seasonal drought. If wildfires continue under increasingly warmer and drier conditions, the forest-peatland ecotone of western Patagonia may be susceptible to large-scale transformation towards a non-forested state.
1 Introduction
Ecosystems worldwide are experiencing heightened frequencies, spatial extents and severities of wildfire due to the interactive effects of climate change and land use practices (McDowell et al., 2020; Duane et al., 2021; Pausas and Keeley, 2021). Such is the case in landscapes dominated by temperate forests in both the Northern and Southern Hemisphere where the increasing intensity and length of drought conditions coupled with changes in the frequency of human-based ignitions of wildland fire have caused seemingly irreversible shifts from forested to non-forested patches (e.g., Odion et al., 2010; Wood and Bowman, 2012; Holz et al., 2015; Paritsis et al., 2015; Whitlock et al., 2015; Donato et al., 2016; Kitzberger et al., 2016; Tepley et al., 2016; Sommerfeld et al., 2018). Given repeated patterns in how ecosystems are shifting over time (Wilson and Agnew, 1992; Suding and Hobbs, 2009; Pausas and Bond, 2020), the potentially wide geographical scales of such transformations (e.g., Coop et al., 2020), and that they can happen rapidly and with little advance warning (Scheffer et al., 2001; Scheffer and Carpenter, 2003), understanding the drivers and mechanisms underlying shifts in ecosystem states, including post-fire vegetation trajectories, is a major theme of theoretical and applied research (Ratajczak et al., 2018; Falk et al., 2022; Seidl and Turner, 2022).
Repeated patterns in ecosystem transition are observed not just over time, but also across space. “Ecotones” (di Castri et al., 1988; Yarrow and Marín, 2007) may be the best places in which to pursue questions regarding ecological resilience or ecological change (Desjardins et al., 2015) given their sensitivity to altered climate (Allen and Breshears, 1998) and disturbance regimes (Myster, 2012). Franklin et al.’s (2021) recent synthesis on forest edges highlights the notion that ecotones can be ‘inherent’ or ‘induced’. That is, transitions between ecological systems across space can occur due to overlying climatic gradients or underlying biophysical gradients (e.g., those observed at latitudinal and altitudinal treelines, respectively; Risser, 1995), but ecotones can also be induced by the external forcing of natural or anthropogenic disturbances (Franklin et al., 2021). Thus, studying how boundaries are generated and maintained between the ecological systems that form long-standing, widely-occurring spatial ecotones may help us understand the causes and likelihood of persistence of newly-induced shifts in ecological system states (Walker et al., 2010; Scheffer et al., 2012).
Shifts in the location and abundance of forest-non-forest ecotones as the result of altered fire regimes have been investigated from patch (e.g., Kitzberger et al., 2016) to landscape (e.g., Lindenmayer et al., 2011) to biome (e.g., Oliveras and Malhi, 2016) scales across different parts of the globe. However, temperate forest-peatland ecotones (especially those of the Southern Hemisphere) have generally received less attention than other forest-non-forest ecotones (Arroyo et al., 2005; Joosten, 2016; León et al., 2021), despite increasing concern about the impacts of persistent temperate forest loss on the one hand and persistent peatland loss on the other. Investigating ecological dynamics across forest-peatland ecotones may be particularly relevant to understanding feedbacks between climate, fire and vegetation given that changes in precipitation and temperature regimes can alter local environmental conditions such that peatlands or forests expand or contract (Crawford, 2003; Ratcliffe et al., 2017; Morris et al., 2018; van der Velde et al., 2021), with concurrent effects on carbon sequestration or release (Hartshorn et al., 2003; Simard et al., 2007; Running, 2008; Turetsky et al., 2014; Goodrich et al., 2017), flammability (Turetsky et al., 2014), and water storage (Waddington et al., 2014; Perez-Quezada et al., 2023).
Our research takes place in the temperate forest-peatland ecotone of western Patagonia in southern South America (ca. 47°S), where the impact of Euro-Chilean settlers and climate change on its fire regimes and vegetation dynamics has been pervasive (Huber and Markgraf, 2003; Szeicz et al., 2003; Holz and Veblen, 2011; Holz et al., 2012a,b, 2016). The current mosaic of mesic temperate rainforest patches and sparsely- to non-forested wetland sites (many of which are peatlands, or at least peat-accumulating ecosystems or “mires”; sensu Rydin and Jeglum, 2006) differs from what it had been historically as evidenced by charred snags and stumps that rise from carpets of orange peat moss (Sphagnum magellanicum). These observations, in combination with an apparent lack of regeneration of the keystone wetland conifer Pilgerodendron uviferum (Don) Florín, Cupressaceae (ciprés de las Guaitecas) since the 1970s (Holz, 2009), sometimes even in the presence of surviving seed sources, suggests that a transition away from tree dominance has occurred in at least some burned areas (Supplementary Figure 1). The question, then, is whether P. uviferum forests will ultimately recover following fire (and under the drought conditions projected for Patagonia; Boisier et al., 2018 and Garreaud, 2018), reassemble to altered states of forest structure and/or composition (Falk et al., 2022; Seidl and Turner, 2022), or be replaced by non-forest systems entirely (e.g., those dominated by Sphagnum mosses).
Research on P. uviferum forest dynamics conducted further north in warmer south-central Chile (e.g., Bannister et al., 2012, 2017; Galindo et al., 2021) and investigations of conifer regeneration in the presence of Sphagnum spp. in wet forests in other parts of the world (e.g., Hörnberg et al., 1997; Roy et al., 2000; Camill et al., 2010), indicate that the life history characteristics of these plants (especially their contrasting growth rates) and their different physiological responses to environmental conditions such as water table depth (Gengarelly and Lee, 2005; Waddington et al., 2014; Holmgren et al., 2015), light levels and climatic conditions (Fenton et al., 2007; Heijmans et al., 2013; Pacé et al., 2018; Bengtsson et al., 2021), may drive post-fire trajectories in the forest-peatland ecotone of western Patagonia. More specifically, dynamics across the ecotone may be controlled by feedbacks that are mediated by climatic conditions and disturbance severity (Figure 1): (1) A water table depth-sun exposure-Sphagnum feedback initiated by allogenic disturbances (e.g., fire), which kill trees thus promoting water-logging (Díaz et al., 2007) and high light levels. Such conditions favor rapid surface colonization by Sphagnum mosses (Díaz and Armesto, 2007), whose growth habit raises the surface relative to the water table so that the survival of young tree seedlings may be compromised during the summer growing season due to desiccation (Bannister et al., 2019). 2) An evapotranspiration-shading-tree recruitment feedback whereby as the density of trees encroaching upon forest-peatland boundaries increases, interception and evapotranspiration reduces saturation and reinforces tree establishment. The lower light levels generated through canopy closure hamper Sphagnum moss development, further promoting tree establishment.
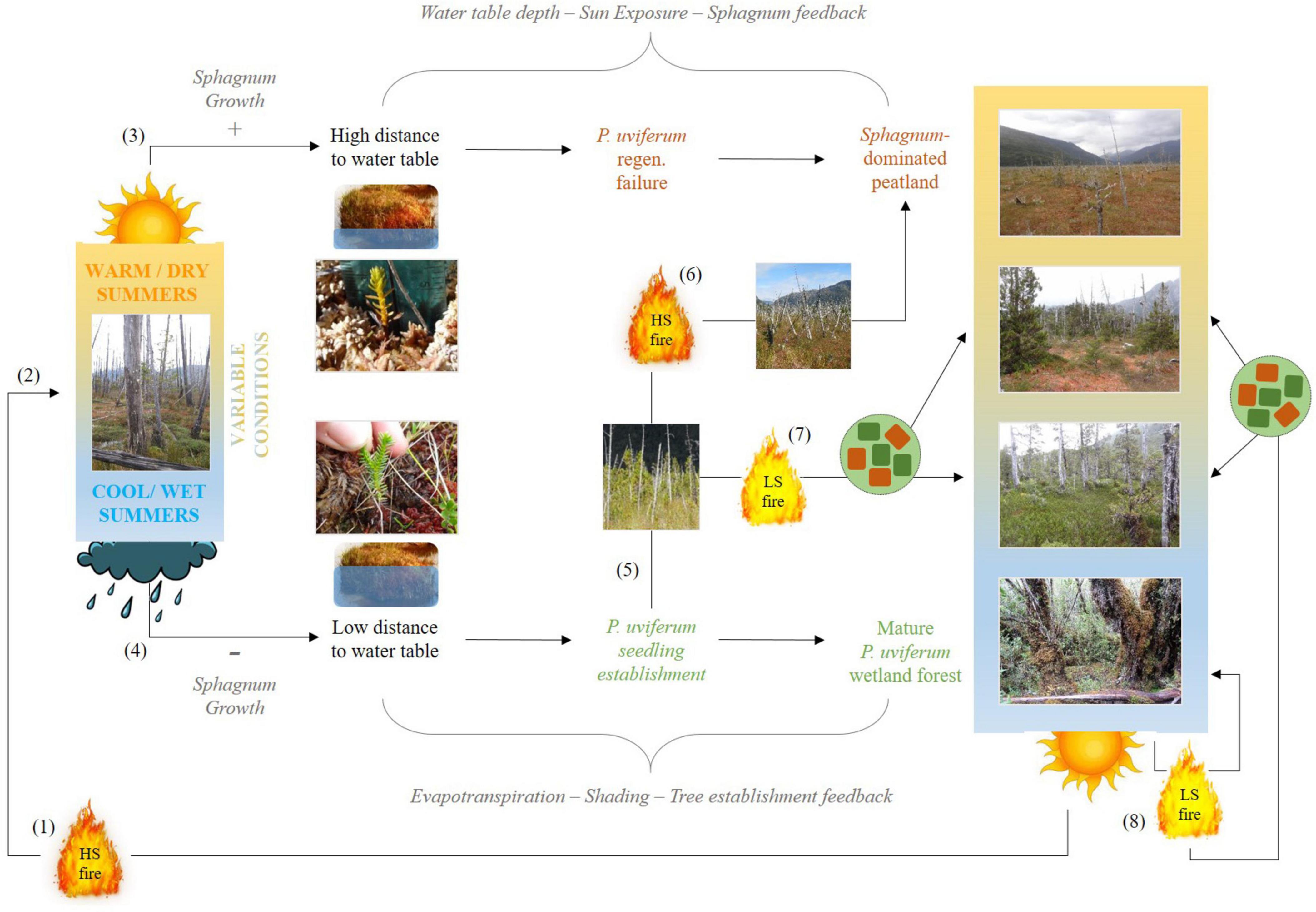
Figure 1. Conceptual model of proposed mechanisms and feedbacks between climate (warm/dry, cool/wet or variable summer conditions), fire severity (high severity, HS, or low severity, LS), abiotic site conditions (distance to water table, sun exposure) and vegetation dominance within the forest-peatland ecotone of SW Patagonia. (1) High severity fire (driven by severe, widespread drought and enhanced by human ignitions; Holz and Veblen, 2009, 2011) removes the forest canopy and the majority of understory plants, which reduces interception (Díaz et al., 2007) and evapotranspiration and increases the duration and/or extent of surface inundation and saturation, and the degree of sun exposure, thus creating conditions for Sphagnum magellanicum release across the burned area (Díaz and Armesto, 2007) (2), especially in the first growing season following fire. Subsequent summers characterized by warm temperatures (3), will encourage S. magellanicum growth, deepening moss carpets or hummocks relative to the position of the water table, and stranding emerging P. uviferum seedlings in desiccation-prone microsites. This water table depth – sun exposure – Sphagnum feedback will stabilize S. magellanicum dominance. If summers following high severity fire are instead cool (4), S. magellanicum growth will be slower and P. uviferum seedling roots will have access to the water table throughout the growing season. As the density and size of tree seedlings increase over time (5), they will begin to lower the water table via evapotranspiration and to create shadier, cooler microsite conditions less favorable to S. magellanicum. Given a relatively long fire-free period, this evapotranspiration – shading – tree establishment feedback will lead to the development and maintenance of a mature, relatively closed canopy P. uviferum forest. However, fire occurrence during the tree establishment phase could either (6) tip a site toward Sphagnum dominance via drought and high severity fire, or (7) cause reorganization towards the development of a more open P. uviferum forest via less extreme fire weather conditions and low severity fire (which were historically more common in P. uviferum wetlands; Holz and Veblen, 2009). Low severity fire (7, 8) will generate heterogeneous vegetation composition across a site, which could lead to more open or closed P. uviferum forests depending on the structure of the forest and composition and distribution of microsites at the time of fire and postfire climatic conditions.
Successful P. uviferum seedling establishment following fire, including multiple instances of burning across the same area (i.e., “reburns”), may thus be the most critical process for determining the structure and composition of the ecosystems that develop afterward, and this may depend on the abundance of microsites that are suitable for germination, growth and survival given the species’ physiological constraints under the prevailing climatic and edaphic conditions. Due to dynamic interactions between water tables and seasonal climatic patterns in wet temperate environments, microsites that promote tree regeneration must be “safe” from both long-term inundation and seasonal drought (Hörnberg et al., 1997; Albornoz et al., 2013). Biotic interactions (e.g., competition or facilitation) with understory plants including and in addition to Sphagnum mosses may also affect the likelihood that P. uviferum seedlings can grow and survive on microsites characterized by more extreme environmental conditions (Kroiss and HilleRisLambers, 2015).
Besides shedding light on forest resilience in climatically and edaphically wet places, understanding the mechanisms driving successful post-fire or post-reburn P. uviferum regeneration in wetland areas is key to determining the conservation and management needs (González et al., 2014) of this long-lived, slow-growing (i.e., < 1 mm/year), globally-threatened (Souto et al., 2013) and culturally significant (Zaret, 2011) conifer species. In particular, land managers need to know whether or which restoration activities are feasible and effective in P. uviferum habitats (e.g., Galindo et al., 2021) or if alternative ecosystem services should be encouraged following fire (e.g., the extraction of peat moss, or the protection of freshwater reservoirs and potential carbon pools encompassed by Sphagnum-dominated sites; León et al., 2016, 2021; Iturraspe and Urciuolo, 2021).
Given the above observations and concerns, the overarching aim of our study was to explore whether similar mechanisms maintain a transition from forested to non-forested conditions across a forest-peatland ecotone induced by reburns versus a long-standing forest-peatland ecotone in western Patagonia. We examined whether and why P. uviferum forests may recover following fire or may become locked into alternative development pathways by comparing abiotic and biotic factors of the reburned ecotone to those of the unburned (i.e., proxy of, and hereafter, “control”) ecotone. Specifically, we asked:
(a) How do the properties of air temperature, vapor pressure deficit, photosynthetically active radiation, water table depth, substrate and understory plant community differ between reburned patches that are characterized by varying densities of P. uviferum trees and seedlings (i.e., forested vs. non-forested)? Do the same properties differ similarly across patches at the control site?
(b) Which of the above variables best predict P. uviferum seedling abundance at the microsite scale, and how do these compare between the reburned and control sites?
Overall, we expected that forested patches (i.e., patches dominated by live P. uviferum individuals greater than 5 cm in diameter at 1.3 m in height) would differ from non-forested patches in consistent ways (e.g., cooler, moister and darker conditions, water tables further below the surface, and substrates and understory plant communities characterized by non-Sphagnum mosses and other temperate rainforest taxa) regardless of fire history. Based on our conceptual model (Figure 1), we expected P. uviferum seedling abundance to be most strongly associated with light level, water table and substrate variables, and we expected to find patch-level effects within sites rather than major differences in predictors between the reburned and control sites. Most importantly, similarities in the above abiotic and biotic properties between the non-forested patches would indicate that the once-forested but now Sphagnum-dominated patch at the reburned site was on a trajectory towards a peatland rather than forest state, with associated implications for ecosystem functioning and potential for resource use/management.
To address our research questions, we collected data using automated sensors and field observations. The sensors yielded hourly readings of water level, air temperature, and relative humidity across multi-week to multi-month periods, thus offering a larger temporal context in which to interpret the snapshot observations of environmental conditions that were made manually. We used non-metric multidimensional scaling to explore differences in understory plant communities, analysis of variance to test for differences in select variables and seedling counts between patches within and across sites, and hierarchical Bayesian regression models to identify the factors most strongly associated with seedling abundance. Ultimately, our findings contribute much-needed information about the ecological dynamics of understudied high-latitude but non-boreal forest-peatland ecotones (Davies et al., 2013; Joosten, 2016) and offer land managers insight into which characteristics of P. uviferum habitats should be targeted for monitoring following fire(s) or to evaluate a site for its restoration potential.
2 Materials and methods
2.1 Field study sites
We investigated P. uviferum patches and seedling abundance across two temperate forest-peatland ecotones in coastal areas of the Aysén Region of Chile in western Patagonia (Figure 2). Given the landscape-scale burning that was used to clear land during colonization of the Aysén District, very few sites encompassing the forest-peatland ecotone remain that are accessible and represent historic (i.e., prior to Euro-Chilean settlement) conditions where Indigenous burning existed but was comparatively minimal (Holz and Veblen, 2011; Holz et al., 2016). Thus, our control (unburned) and reburned (hereafter referred to simply as ‘burned’) sites were approximately 445 km apart on a north-south gradient. Both sites were located to the west of the Andes Mountains where the climate is temperate hyperhumid, strongly influenced by westerly winds off the Pacific Ocean and orographic uplift, and characterized by a seasonal cycle whereby the warmest temperatures and least precipitation occur during the austral summer (December through February) and the coldest temperatures and greatest precipitation occur during the winter (June through August; Garreaud et al., 2013). The control and burned sites were subject to somewhat different temperature and precipitation regimes given their latitudinal positions and locations relative to the ocean and Patagonian icefields (details below), and consequently were characterized by slightly different ecoregions (Veblen et al., 1995) within the Temperate Broadleaf and Mixed Forest biome (Dinerstein et al., 2017). However, both sites experienced strong year-round maritime hyperhumidity, and the total local precipitation observations at our control site (2,617 mm/year) were only around 4% higher than those of the burned site (2,515 mm/year; Dirección General de Aguas [DGA], 2015, 2022). In addition, both sites were found in similar topo-edaphic conditions: they were located in valley bottoms shaped by glacial, fluvioglacial and fluvial processes and characterized by imperfectly to poorly drained soils (Pfeiffer et al., 2010; Casanova et al., 2013).
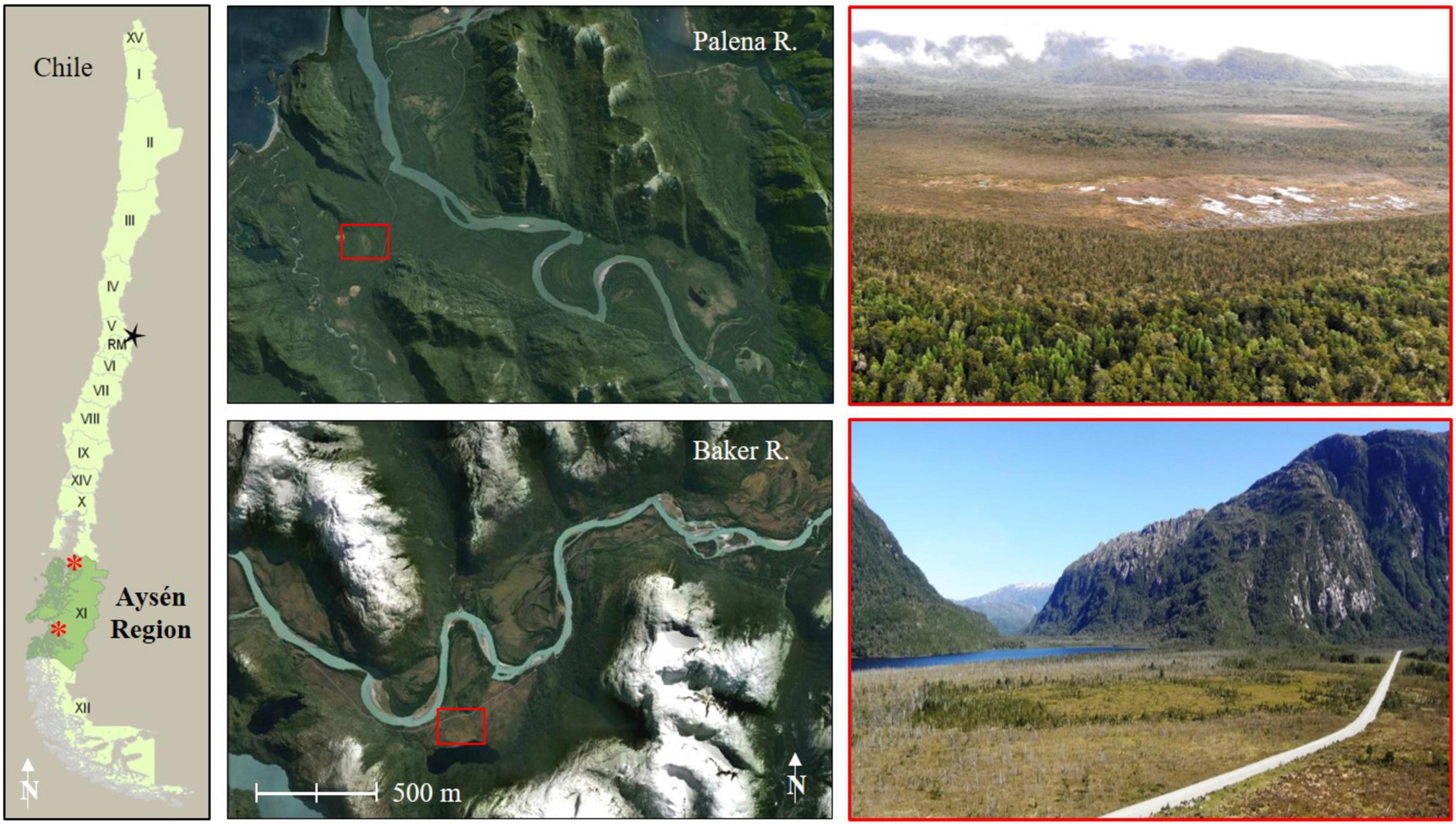
Figure 2. Location of the control site (Palena River; 43°52′34″S, 72°57′36″W, top panels) and burned site (Baker River; 47°49′2″S, 73°25′38″W, bottom panels) in the Aysén District, Chile. The top right photo was taken toward the west in April 2019; photo credit: Rodrigo Poliche. The bottom right photo was taken toward the southwest in July 2013.
The control site (43°52′34″S, 72°57′36″W) was situated near the town of Puerto Raúl Marín Balmaceda (RMB) and about 5 km from the open ocean in a portion of the Palena River watershed that has experienced relatively little land use disturbance since settlement began in the 1910s (Holz and Veblen, 2011). The river valley has been traversable by road only since 2009, and local livelihoods were historically oriented around fishing and fine-scale timber harvest, though they have more recently (since the 1990s) turned to aquaculture and tourism. While long-term instrumental data are lacking, meteorological records from 2001 through 2018 show that average annual precipitation at the RMB station was 2617 mm, with an annual minimum of 1,759 mm and a maximum of 5,582 mm (Dirección General de Aguas [DGA], 2015, 2022). Modeled temperatures for Chaitén, 100 km to the north along the coast, indicate an average annual temperature of 7.4°C from 2001 through 2018, with an average summer temperature of 14.5°C and average winter temperature of 2.8°C (Alvarez-Garreton et al., 2018). The vegetation mosaic of the lower Palena River valley is characterized by North Patagonian rainforests (Veblen et al., 1995), with scattered marshes, mires and peatlands. P. uviferum occupies emergent positions in drier substrate with dominant and codominant Nothofagus nitida, N. betuloides, Drymis winteri, and several myrtles from the Myrtaceae family, and dominant and codominant positions with the tall, multi-stemmed shrub Tepualia stipularis in saturated bog-forests ecotones. Many of these latter sites are virtually inaccessible given the density of the surrounding forest; for example, it can take two hours to travel 600 meters by foot. Only one single fire-scarred tree was observed within the study site, and it is likely that no major fire events have affected the area in the last ∼200–300 years based on ages of P. uviferum trees (Holz et al., in review). The historic fire regime was characterized by mixed severity fires with mostly patchy, fine-scale events (Holz and Veblen, 2011).
The burned study site was adjacent to Lake Cute (pronounced, ‘kú-teh’; 47°49′2″S, 73°25′38″W) near the town of Caleta Tortel in the lower Baker River watershed. Like the control site, the burned site is influenced by maritime conditions; the site is 3 km from an inlet in the fjords and about 90 km from the open ocean. The meteorological station at Tortel recorded average annual precipitation of 2,515 mm from 2006 through 2018 (excluding 2013 due to a dearth of data), with an annual minimum of 1,611 mm and a maximum of 3122 mm (DGA 2022). Modeled temperatures for a location 50 km to the northeast (i.e., more continental conditions; 47°30′15.25″S, 72°58′47.37″W) indicate an annual average of 5.2°C from 2001 through 2018, with an average summer temperature of 9.5°C and average winter temperature of 0.6°C (Alvarez-Garreton et al., 2018). Euro-Chilean settlers began arriving and establishing in the area in the 1920s, and fire was the primary tool by which they cleared forested areas to create homesteads and grazing lands (Holz and Veblen, 2011). Thus, much of the lower Baker River valley bottom has been affected by fire since then. While there are remnant forest stands characteristic of the transition zone between North Patagonian and Magellanic temperate rainforests (Veblen et al., 1995), much of the low-lying, flat terrain is now characterized by extensive lawns of S. magellanicum with charred snags of P. uviferum. Aerial imagery reveals the extent to which the vegetation mosaic of the lower Baker River valley has been affected by fire (e.g., Figure 2, lower right image). Fire scar dates from the burned study site indicate that at least 23 fires (mostly human-initiated burns) occurred between the 1920s and early 2000s, with the most recent fire in 2004 and a mean fire return interval (MFI) of 3.5 years (Holz, 2009). Prior to European settlement this area experienced a mixed-severity fire regime similar to that of the control site (Holz and Veblen, 2011). That the site once had a more extensive forest cover than presently is reflected in historical imagery (Supplementary Figure 2) and soil profiles: Rodriguez (2015) found shallower and more thoroughly decomposed organic soils under remnant patches of tree cover. In addition to multiple burns, the site−which is located on an old meander bend of the Baker River (Rodriguez, 2015)−has been affected by glacial lake outburst floods (GLOFs; Rodriguez, 2015). At least 23 GLOFs have occurred between 2008 and 2020 (Vandekerkhove et al., 2021), but neither the total number of historic events nor those that have affected the hydrology of the burned site it known.
2.2 Data collection
The sampling in the control (unburned) and burned sites were stratified by patch type based on vegetation structure and composition and hydrologic regime using information obtained via aerial and satellite imagery interpretation and analysis (e.g., ESRI World Imagery, Google Earth, and high-resolution multi-spectral imagery) and on-site reconnaissance in 2016 and earlier (see Zaret, 2011). At both sites, three patch types were identified that appeared to encompass the historic (in the case of the burned site) transition from forest to peatland. These included the Forest, Bog Forest and Bog patches at the control site, and the Burned Forest, Transition, and Cushion patches at the burned site (Supplementary Figures 3, 4). Field sampling was conducted in 2017 and 2018.
2.2.1 Seasonal water level and microclimate
To understand how abiotic environmental properties varied across the forest-peatland ecotone, we used sensors to continuously measure water level, air temperature and relative humidity over multi-week to multi-month periods. Sensors were only available for some combinations of patch, site and field season dates. Our goal was to capture high temporal resolution data across key periods of the growing season at each site and to use these data to aid our interpretations of one-time measurements made within microsites, as discussed in more detail in subsequent sections.
Two water level monitoring wells (Supplementary material) were established in the control and burned site in the patches with the greatest and least tree cover. In the burned site, wells were established in the Transition and Burned Forest patches, and in the control site, they were established in the Forest and Bog patches (Supplementary Table 1). Continuous one-hour interval readings were collected using In-Situ Rugged TROLL 100 water level sensors.
We used iButton Hygrochron Temperature/Humidity Loggers by Maxim Integrated to measure air temperature and relative humidity at hour intervals. These were suspended under plastic cones to protect them from precipitation, while allowing for air flow, and the cones were hung from wooden stakes at about 30 cm from the ground to better capture the conditions experienced by understory plant communities and juvenile trees. iButtons were deployed in all patches of the control and burned sites during both the 2017 and 2018 field seasons (Supplementary material). To examine differences in light levels between patches, measurements of photosynthetically active radiation (PAR) were made using a hand-held LI-COR Light Sensor Logger at the same locations where iButtons were deployed; readings per site were obtained on the same day under uniform weather conditions.
2.2.2 Understory vegetation, Pilgerodendron tree and seedling abundance and microsite conditions
Plots of 10 x 10 m were placed along transects ca. 40 m from each other that crossed the relatively abrupt forest-peatland ecotone at each site. At the control site, the first transect was established haphazardly to center fieldwork on the eastern side of the site and along the presumed gradient in hydrology. All remaining transects crossed two vegetation types, as Bog plots were clumped to the north and Bog Forest plots to the south, while Forest plots were established roughly in the middle of that patch type, away from the edge of the narrow band of transitional forest to the west or the edge of the riparian gallery forest to the east (Supplementary Figure 3). At the burned site, the first transect was established haphazardly to center fieldwork along the transition from the open bog (i.e., the ‘Cushion’ patch) to the burned forest on the eastern side of the site, thus following gradients in hydrology and slope (i.e., from W to E) (Supplementary Figure 4). Due to time constraints and low evidence of fire effects, the Cushion patch was not sampled for seedling abundance or vegetation composition, but seasonal air temperature and humidity were investigated.
Within each 100 m2 plot (n = 27 at the control site, n = 18 at the burned site), the height and diameter at breast height (DBH at 1.3 m) of all P. uviferum trees ≥ 5 cm were recorded. Along the centerline of each plot, four 80 cm diameter (2 m2) circular subplots were established (n = 108 subplots at the control site, n = 72 subplots at the burned site; Supplementary Figure 7) to collect the following data pertaining to P. uviferum regeneration and abiotic and biotic factors that may influence seedling establishment and performance: (1) counts and height measurements for P. uviferum seedlings (all individuals < 5 cm DBH were tallied; heights were recorded for all individuals > 5 cm height); (2) photosynthetically active radiation (PAR); (3) distance to the water table (DWT); (4) percent cover of individually identified vascular plant species; and (5) percent cover of select ‘substrate types’ (i.e., pool, bare ground, litter, wood, trunk, cushion-forming plants, Sphagnum spp., Dicranaloma spp., and other moss species (i.e., those not in the aforementioned moss genera). To assess differences in PAR at the short and tall seedling height, PAR was measured at two heights (0.3 m and 1.3 m) at each of three locations per subplot using a LI-COR LI-1500 Light Sensor Logger. DWT was measured at the same locations as PAR and represented the distance from the top of whichever substrate would have been first hit when dropping a pin from breast height down to the water table; distances were recorded as negative values if the substrate top was below the water table (i.e., if there was surface water), otherwise as positive values. If the water table was not evident at or above the surface within the subplot, a bore hole was created adjacent to the subplot using a 2.75 inch diameter Eijkelkamp Edelman soil auger and metal rulers with magnetic levels were used to measure DWT at the select within-subplot locations. Of the substrate types, “wood” referred to relatively flat, thin pieces of wood scattered on the ground surface, whereas “trunk” referred to live or dead, vertical, less-than-vertical or fallen tree trunks.
2.3 Data analysis
2.3.1 Seasonal water level and microclimate
Water level, temperature and relative humidity data were downloaded and corrected (as needed) per the instruments’ standard software procedures (Supplementary material). The “lubridate” (Grolemund and Wickham, 2011), “dplyr” (Wickham et al., 2021), “ggplot2” (Wickham, 2016), “FSA” (Ogle et al., 2022), and “stats” software packages in R 4.1.2 (R Core Team, 2021) were used to aggregate hourly water level depths, temperature, and relative humidity readings to mean daily values, derive and test for differences between summary statistics, and plot results. Instrumental records of daily precipitation were downloaded (Dirección General de Aguas [DGA], 2022) for the Caleta Tortel and Raul Marín Balmaceda meteorological stations and plotted to facilitate interpretation of water level data. We statistically compared mean water table depths across patches and seasons using Welch’s one-way analysis of variance (ANOVA) and Games-Howell post-hoc tests due to unequal variances across those groups (Ruxton and Beauchamp, 2008). Temperature and relative humidity data were used to estimate vapor pressure deficit (VPD) following Jones (1992). To explore microclimatic conditions most representative of seasonal drought, we identified the 10% warmest and driest hours, respectively, per iButton per patch (i.e., the highest temperatures and VPD values) and then tested for statistical differences between their means using Kruskal-Wallis one-way ANOVA due to non-normal distributions but equal variances across groups. We conducted group-wise comparisons using Dunn’s tests with “Benjamini-Hochberg” corrections to increase power and control the false detection rate given small sample sizes per patch (Benjamini and Hochberg, 1995). Since seasonal water level and microclimate data were collected during different time periods per site, we were most interested in comparing the relationships between parameters within sites and patch types.
2.3.2 Understory vegetation
Non-metric multidimensional scaling (NMDS) was conducted to examine differences in vegetation species assemblages among subplots in the patch types per site. For the vegetation ordinations, only species that occurred in two or more subplots per site were included in the analysis, resulting in a total of 42 species for the control (unburned) site and 30 species for the burned site (i.e., leaving 3 and 13 species out of further analyses from the control and burned sites, respectively).
NMDS ordinations were conducted using PC-ORD version 6.22 (McCune and Mefford, 2011) following Peck (2010). For both sites, ordinations were run using Bray-Curtis dissimilarity measures. Based on the results of autopilot stress tests, a final 3-dimensional solution was chosen for the ordination for the control site while a final 2-dimensional solution was chosen for the burned site. Multi-response permutation procedure (MRPP) was used to test the null hypothesis of no significant difference in vegetation species assemblages among patches per site. To identify the understory plant types most characteristic of each patch, indicator species analysis (ISA) was run using the Donoso (1993), Peck (2010). Vector fitting based on Pearson’s r-squared and Kendall’s tau rank correlations between explanatory variables and ordination axes was conducted to identify abiotic and biotic factors associated with differences in species assemblages among subplots.
2.3.3 Seedling abundance and microsite conditions
We investigated the relationships between P. uviferum regeneration and abiotic and biotic components of the microsite environment at two different scales. At the patch scale, we summarized field data across subplots to the plot level (if applicable) and then tested for differences between patches within and across sites using one-way ANOVA (Supplementary material). We were particularly interested in whether select variables differed significantly between forested and non-forested patches, as defined by the presence of live P. uviferum trees of at least 5 cm DBH at the time of sampling (i.e., Forest vs. Bog patches at the control site, or Transition vs. Burned Forest patches at the burned site), indicating that patterns in microsite composition and distribution may help to maintain those patch boundaries (Peters et al., 2006).
To identify the microsite-scale variables that were most strongly associated with seedling counts, Bayesian hierarchical models were used to examine the response of P. uviferum seedling counts to alternative combinations of abiotic and biotic predictors. We chose to use a Bayesian rather than frequentist statistical approach to propagate uncertainty across the levels of our models, thus increasing the accuracy of parameter estimates given our relatively small sample sizes per site (Muth et al., 2018). To investigate whether the relationships between P. uviferum seedling abundance and microsite characteristics differed given local site histories and legacies of natural disturbance and land use (e.g., fire, surface inundation, ditching, road construction), we fit separate series of candidate models to data from the control and burned site, respectively.
Following Gelman et al. (2020), we used a Bayesian workflow to construct, check the fit of, validate, and compare models within each series per site. Our approach to model-building was one of iterative model expansion: from the set of variables hypothesized to be associated with P. uviferum seedling abundance, we used Spearman rank correlations to determine which had stronger associations with seedling counts while minimizing multicollinearity within sites and allowing us to include the same suite of predictors in models for both sites (Supplementary Figures 8, 9). Prior to model fitting, PAR was log-transformed and all quantitative predictors were standardized. Patch was included as a nominal variable with three levels for the control site (Forest, Bog Forest, and Bog) and two levels for the burned site (Transition, Burned Forest).
While differing in their fixed effects, all of the models that we constructed contained a multilevel structure in which plots were included as random effects to account for the lack of independence and any resulting autocorrelation inherent in our study design (i.e., four subplots nested within each plot; Zuur et al., 2009). In addition, all models predicted the shape (i.e., overdispersion) parameter as a function of patch (given the high numbers of zeros in the Bog and Burned Forest patches), and all model parameters were specified with weakly informative prior distributions (Lemoine, 2019; Supplementary material). As discussed in more detail in the Supplementary material (see Models of Seedling Counts), we first fit a series of simple, single-predictor candidate models and then used those results to build more complex models. Ultimately, we selected one or two models from each site for interpretation and comparison to our expectations regarding the relationships between P. uviferum seedling abundance and individual predictors (Supplementary Table 2). All data exploration and analysis were conducted using R 4.1.2 (R Core Team, 2021); models were fit using the ‘brms’ package (Bürkner, 2021). The ‘tidybayes’ package (Kay, 2022) was used to summarize and plot parameter estimates for the final model(s) per site.
3 Results
3.1 Seasonal water level and microclimate
3.1.1 Seasonal water level
In general, changes in water table depth (WTD) at each of the four monitoring wells followed peaks and troughs in daily precipitation and trends in monthly precipitation (Figures 3A–D). For example, at the burned site, WTD values were lowest in both patches in June 2016, which followed 2.5 months of consistently low to zero precipitation; and in the control (unburned) site, the abrupt peak in precipitation in November 2018 was followed by low daily values and WTDs in both patches responded in kind.
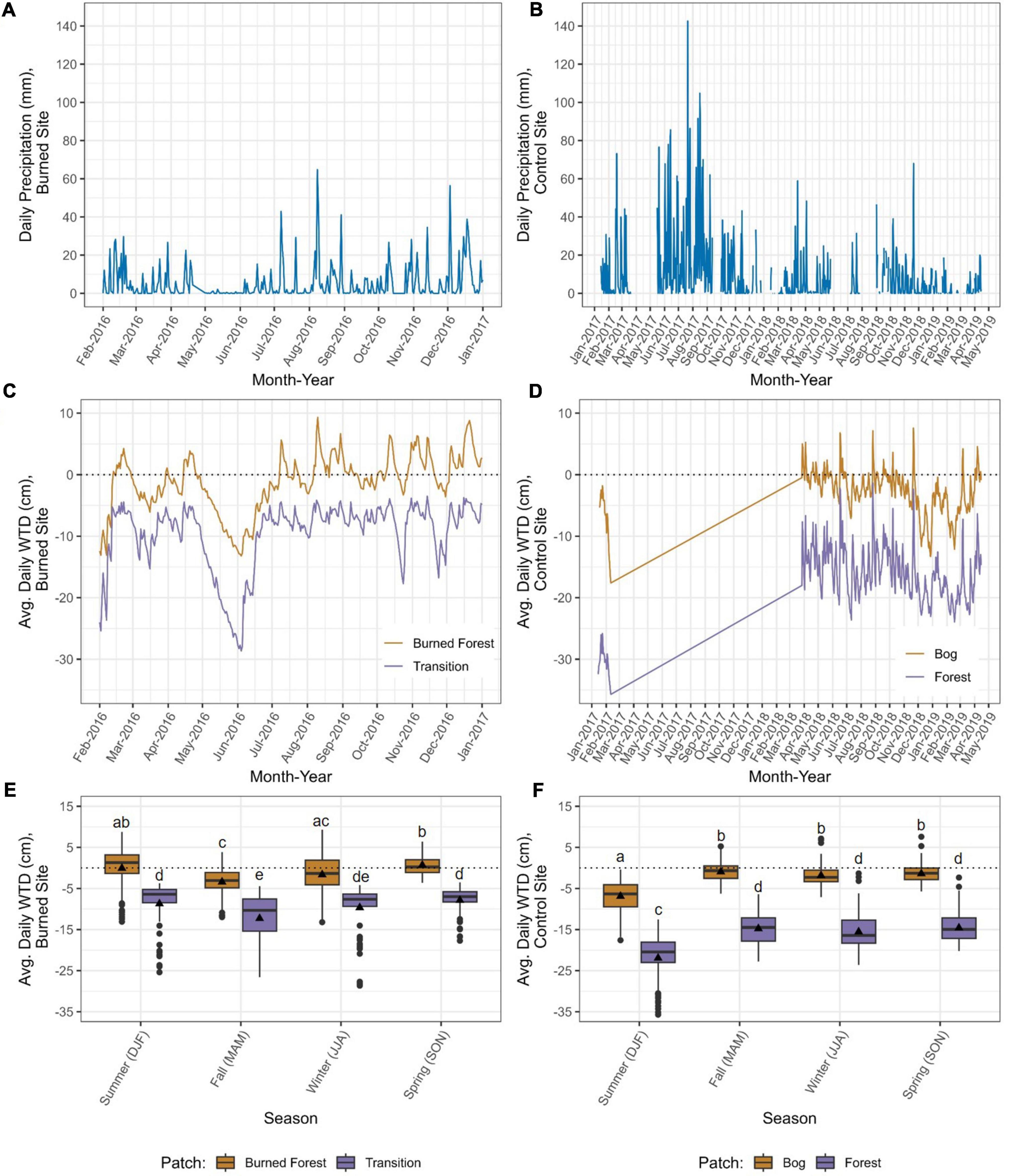
Figure 3. Daily precipitation (Dirección General de Aguas [DGA], 2022, Caleta Tortel and Raul Marin Balmaceda meteorological stations) (A,B), daily average water table depths (WTDs) (C,D), and daily average WTDs summarized across seasons (Summer = DJF, Fall = MAM, Winter = JJA, Spring = SON) (E,F) for the burned site (left) and control (unburned) site (right). Water table depths were recorded at hour intervals by data loggers suspended in PVC wells in the vegetation patches in each site with the highest (Transition, Forest) and lowest (Burned Forest, Bog) densities of live tree cover. Different letters indicate significant differences between means using Welch’s one-way ANOVA and post-hoc Games-Howell tests (P < 0.05).
Despite their similar responsiveness to the precipitation regime, WTDs differed between the two sites as follows: Water tables tended to be closer to the surface at the burned site, as reflected in daily (Figures 3C, D) and seasonal (Figures 3E, F) averages. There also tended to be a smaller difference in WTDs between the patches in the burned site than between those in the control site (average differences plus or minus the standard deviation, of 8.5 ± 1.7 and 14.64 ± 3.35 cm, respectively).
Water table dynamics at both sites were patch-dependent. Mean WTDs differed significantly between the two patches within each site across all seasons. In addition, patches within sites differed in their hydropatterns (i.e., depth and duration of surface inundation). Within the burned site, the water table was above the surface in the Burned Forest patch during 43% of the days sampled and in all months except May and June 2016; the average depth of surface water (i.e., WTDs > 0 cm) was 2.34 ± 1.1 cm (Supplementary Table 12). In contrast, the water table was never above the surface in the Transition patch; rather, the WTD in that patch seemed to frequently return to about 5 cm below the ground surface, never rising far above that level and only dropping substantially lower during periods of low to no precipitation (Supplementary Table 13). At the control site, the average surface water depth in the Bog patch was 1.69 ± 1.03 cm (Supplementary Table 14). The water table was only above the surface in the Bog patch during 19% of the days sampled; it never rose above the surface during January and February 2017 or December 2018 through February 2019, and it reached the lowest levels (i.e., greatest depths below the ground surface) during those months. The Forest patch was never inundated and the subsurface water table was variable, with monthly averages of −12.7 to −29.3 cm (Supplementary Table 15).
3.1.2 Seasonal microclimate
Microsite climatic conditions during the 2017 austral summer tended to differ substantially across patches at the control site while they were relatively uniform at the burned site. Within the Forest patch at the control site, we found no statistical differences in temperature or VPD, respectively, between microsites with abundant P. uviferum regeneration and those with no regeneration; thus we lumped and plotted those data together. At the control site, temperatures were warmest in the Bog patch, intermediate in the Bog Forest patch and lowest in the Forest patch, and this trend held across the temporal resolutions at which we examined the data. Of the 10% hottest hours recorded during the summer sampling period, the average maximum temperature ( ± SD) was significantly higher in the Bog patch (30.6 ± 1.6°C) than in the Forest patch (23.6 ± 4.0°C; P < 0.05), while the average maximum temperature in the Bog Forest (27.2 ± 2.3°C) was not significantly different from the others (Figure 4A). The above trends were also observed over time for mean daily temperatures (Figure 4C). At the burned site, there were no significant differences between the average 10% hottest hours in the Burned Forest, Transition and Cushion patches, which was also reflected in daily temperatures (Figures 4B, D).
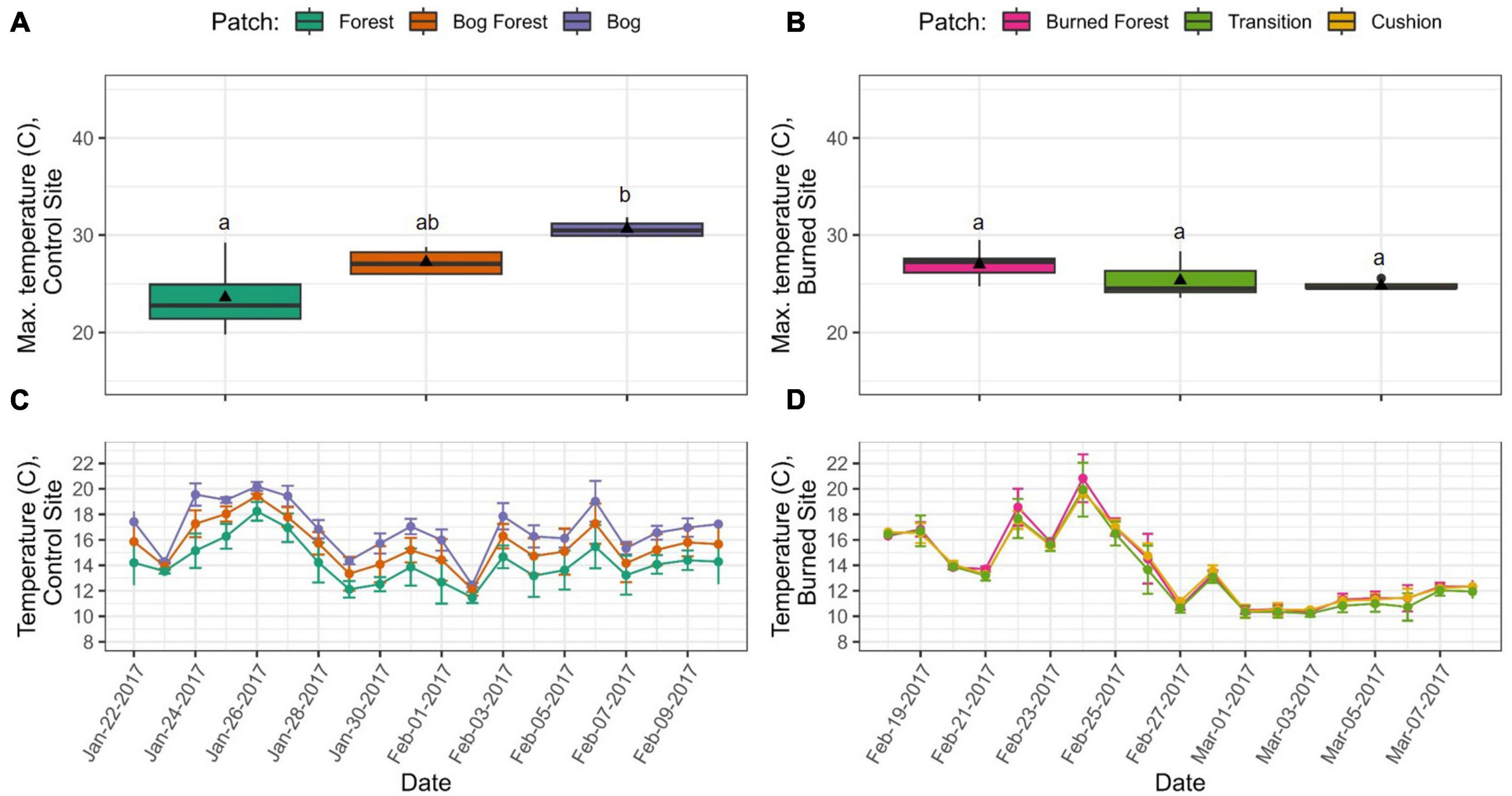
Figure 4. Comparison of summer temperatures within and across sites (control left, burned right). Boxplots in (A,B) represent the 10% hottest hours recorded per iButton per patch across the sampling period (n = 48 hours control site, n = 46 hours burned site). Different letters indicate significant differences between means within sites using Kruskal-Wallis one-way ANOVA and post-hoc Dunn’s tests (P < 0.05). Time series (C,D) are of average daily temperature ± 2 standard deviations for each treatment or patch. Temperature data were recorded at hour intervals by iButtons: n = 12, Forest; n = 4, Bog Forest and Bog; n = 7, Burned Forest and Transition; n = 6, Cushion.
Within site trends in vapor pressure deficit (VPD) were similar to those observed for temperature during the austral summer at the control site (Supplementary Figure 15). However, in the burned site, rather than uniform values across the three patches, the maximum average VPD in the Burned Forest patch (2.09 ± 0.94 kPa) was significantly higher than that of the Cushion patch (1.54 ± 0.49 kPa; P < 0.05), while neither were significantly different from the Transition patch (1.74 ± 0.92 kPa). This pattern only applied to the warmest and driest hours of the day; there was substantial overlap in mean daily VPD values across the three patches.
During the 2018 austral autumn sampling period, trends in microsite temperatures and VPD were somewhat different from those observed in the austral summer. In the control site, there was substantial overlap between the temperatures recorded in the Bog and Bog Forest patches, whose average 10% hottest hours were significantly higher than those in the Forest patch (Supplementary Figure 16). In the burned site, there was substantial overlap in mean daily temperatures across the three patches, but of the 10% hottest hours, the maximum average temperatures in the Burned Forest and Transition patches were 17.1 ± 2.1°C and 16.8 ± 3.1°C, respectively, which were significantly higher than that of the Cushion patch (14.8 ± 1.8°C; P < 0.05). Regarding VPD in the control site, trends between patches mirrored those observed for temperature: of the 10% warmest/driest hours, the average VPD for the Forest patch was close to zero (0.06 ± 0.06 kPa) and significantly lower than the average VPDs for the Bog and Bog Forest patches (0.93 ± 0.25 kPa and 0.75 ± 0.33 kPa, respectively, P < 0.05; Supplementary Figure 17). In the burned site, there were significant differences between the average 10% maximum VPD values for the Burned Forest and Cushion patches (0.95 ± 0.30 kPa, 0.74 ± 0.24, respectively; P < 0.05), but these trends did not hold across all days sampled.
3.2 Understory vegetation
For both the control and burned sites, vegetation species assemblages at the subplot level were clustered in ordination space by patch type (Figure 5). In the control site, subplots from the three patch types were most clearly grouped along the first of three orthogonal axes (see Supplementary Figures 18, 19 for additional biplots). Understory plant communities in subplots from the Forest patch were most tightly clustered and were located at the end of Axis 1 that was strongly correlated with higher abundances of live P. uviferum trees (r = 0.74), and the percent cover of moss species not in the Sphagnum or Dicranaloma genera (r = 0.80), ferns (r = 0.67) and taller shrubs (i.e., those that did not form dense, low-lying mats; r = 0.63; Supplementary Table 16). The understory plant communities in the Bog subplots were located at the other end Axis 1, which was correlated with PAR at 0.3 m above the ground (r = −0.69) and the percent cover of low-lying, mat-forming shrubs (r = −0.62). Vegetation community assemblages from the Bog Forest patch were generally situated between those of the other patches. They tended to be either, (1) clustered near the Forest patch assemblages along Axis 1 or at the end of Axis 2 that was correlated with the percent cover of understory trees (excluding P. uviferum; r = −0.60), or (2) closer to the Bog vegetation assemblages along Axis 1 and clustered toward the end of Axis 3 that was most strongly correlated with Sphagnum spp. (r = 0.63). Subplots within patches were more similar to one another than expected by chance alone, as indicated by the statistic of multi-response permutation procedure (MRPP) within-group agreement, which was greater than zero at the P < 0.001 significance level for each pairwise comparison between patches for the control site vegetation ordination (Forest vs. Bog Forest, A = 0.164; Forest vs. Bog, A = 0.215; Bog Forest vs. Bog, A = 0.100). The top two indicator species (identified to at least genus) for each of the three patches, respectively, were as follows: Philesia magellanica (a climbing evergreen shrub) and Gaultheria antarctica (a short-statured evergreen shrub in the heath family) for the Forest patch; Schoenoplectus californicus (a widely-distributed bulrush in the sedge family) and Dicranaloma spp. (mat-forming mosses associated with forest floors and tree trunks) for the Bog Forest patch; and Myrteola nummularia (a short-statured, mat-forming evergreen shrub in the myrtle family) and Tetroncium magellanicum (a member of the arrowgrass family) for the Bog patch; (Supplementary Table 17).
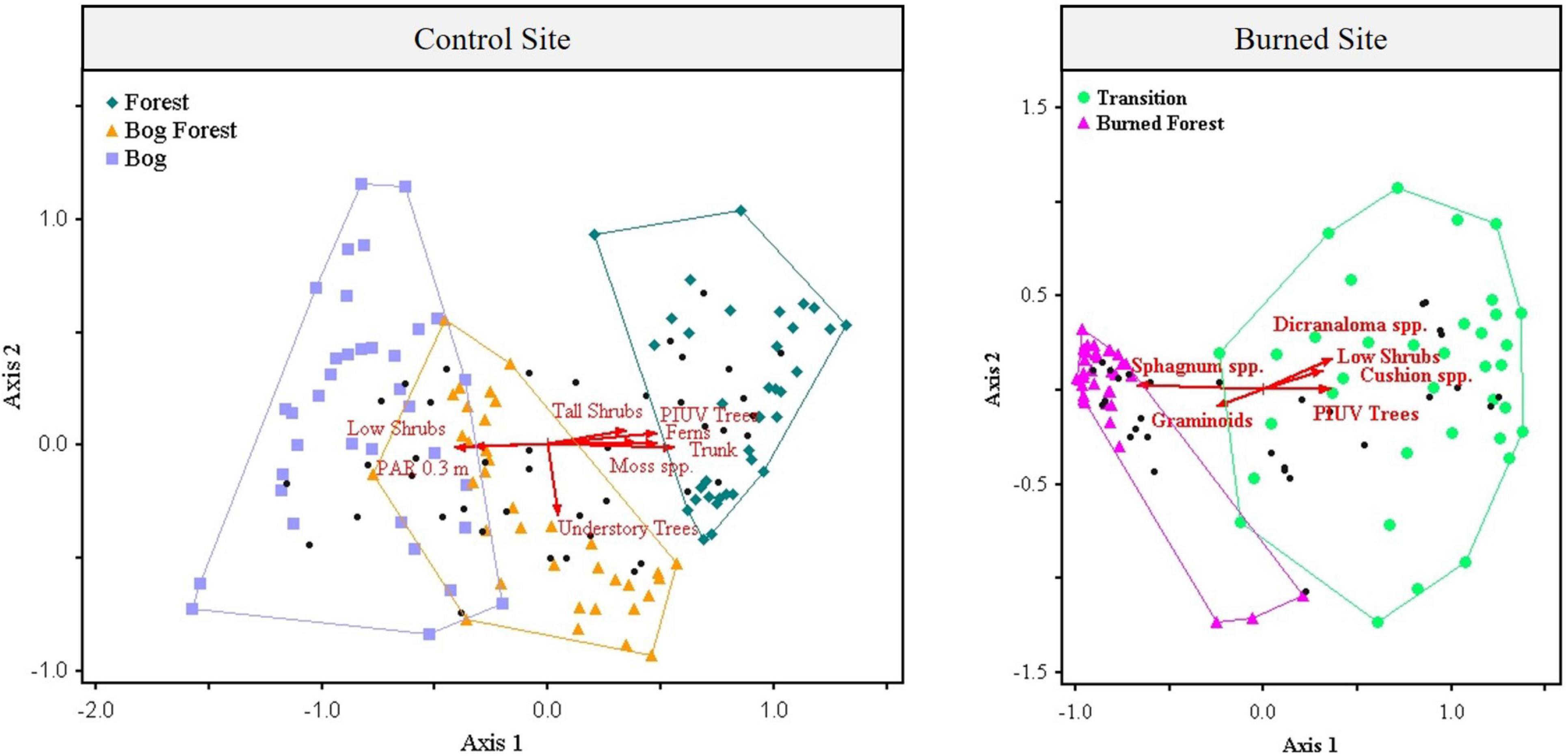
Figure 5. Biplots of first two (of three) orthogonal ordination axes produced through non-metric multidimensional scaling (NMDS) of the percent cover of 42 vegetation species in 108 2 m2 subplots (36 subplots per Forest, Bog Forest or Bog patches) at the control site, and two orthogonal ordination axes produced through NMDS of the percent cover of 30 vegetation species in 72 2 m2 subplots (36 subplots per Burned Forest or Transition habitat patches) at the burned site. Each ordination was conducted using a Bray-Curtis distance matrix; the mean stress of 250 runs with real data for the control site NMDS was 12.912, that for the burned site was 14.375. The vegetation analysis included the following 19 explanatory variables: DWT (distance to the water table from the top of substrates), PAR 0.3 (log-transformed photosynthetically active radiation at 0.3 m above the ground), PAR 1.3, PIUV Trees (count of live P. uviferum trees > 5 cm DBH), and the percent cover of Sphagnum spp., Dicranaloma spp., other moss species (i.e., those not in the Sphagnum or Dicranaloma genera), cushion plants, graminoids, forbs, ferns, low/mat-forming shrubs, other/taller shrubs, understory trees, pool, bare ground, litter, wood, and trunk (i.e., of standing live or dead trees). Vectors in red (scaled to 50%) represent those explanatory variables with Pearson coefficient of determination of at least 0.35.
For the burned site, subplot vegetation species assemblages were grouped by patch along the first of two orthogonal ordination axes (Figure 5). In the Transition patch, they were generally associated with the end of Axis 1 that was most strongly correlated with counts of live P. uviferum trees (r = 0.69), and the percent cover of Dicranaloma spp. (r = 0.70), cushion plants (r = 0.70) and low, mat-forming shrubs (r = 0.66; Supplementary Table 19). The majority of the vegetation assemblages in the Burned Forest subplots were tightly clustered at the opposite end of Axis 1, which was strongly correlated with the percent cover of Sphagnum spp. (r = −0.95). The MRPP within-group agreement statistic was greater than zero for vegetation species assemblages in the Transition vs. Burned Forest patches (A = 0.325, P < 0.001), and the top two indicator species for each of the two patches, respectively, were Dicranaloma spp. and Lepidothamnus fonckii (a dwarf conifer in the Podocarpaceae family) for the Transition patch; and Marsippospermum grandiflorum (a species of rush) and S. magellanicum for the Burned Forest patch (Supplementary Table 20).
3.3 Seedling abundance and microsite conditions
3.3.1 Patch scale
P. uviferum seedling abundances differed across forested and non-forested patches and tended to be highly variable within patches (Figure 6 and Table 1; see Supplementary Table 22 for count estimates per hectare). At the control site, median ( ± MAD) seedling counts in 2 m2 microsites were highest in the Forest patch (18 ± 14 seedlings), followed by the Bog Forest (10 ± 8 seedlings) and Bog (0 ± 0 seedlings) patches. At the burned site, counts were higher in the Transition patch (11 ± 9 seedlings) than the Burned Forest patch (1 ± 1 seedling). Analysis of variance and post-hoc multiple comparison tests failed to reject the null hypotheses of no significant differences between median seedling counts amongst the Forest, Bog Forest and Transition patches, while seedling counts in those patches were significantly higher than those in the Bog and Burned Forest patches.
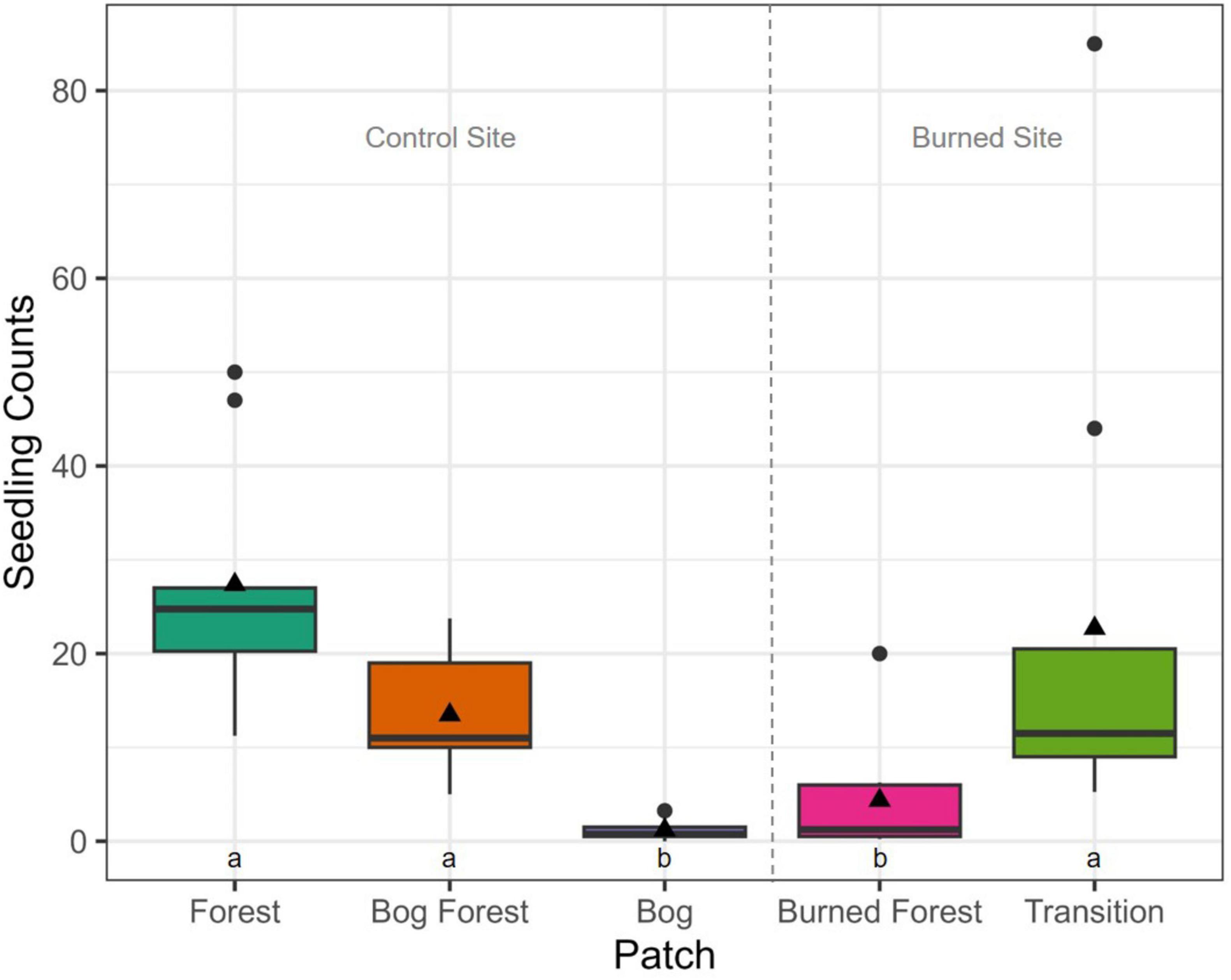
Figure 6. Boxplots of P. uviferum seedling (DBH < 5 cm; all heights) counts per patch: Forest, Bog Forest and Bog patches in the control site, Burned Forest and Transition patches in the burned site. Seedlings were tallied in four 2 m2 subplots per plot, nine plots per patch. Boxes represent the interquartile range of the data summarized to plot and patch; thick horizontal lines and black triangles represent median and mean seedling counts, respectively; and vertical lines indicate variability in seedling counts outside of the interquartile range with black circles are outliers. Different letters indicate significant differences between median counts across sites using Kruskal-Wallis one-way ANOVA and post-hoc Dunn’s tests (P < 0.05).
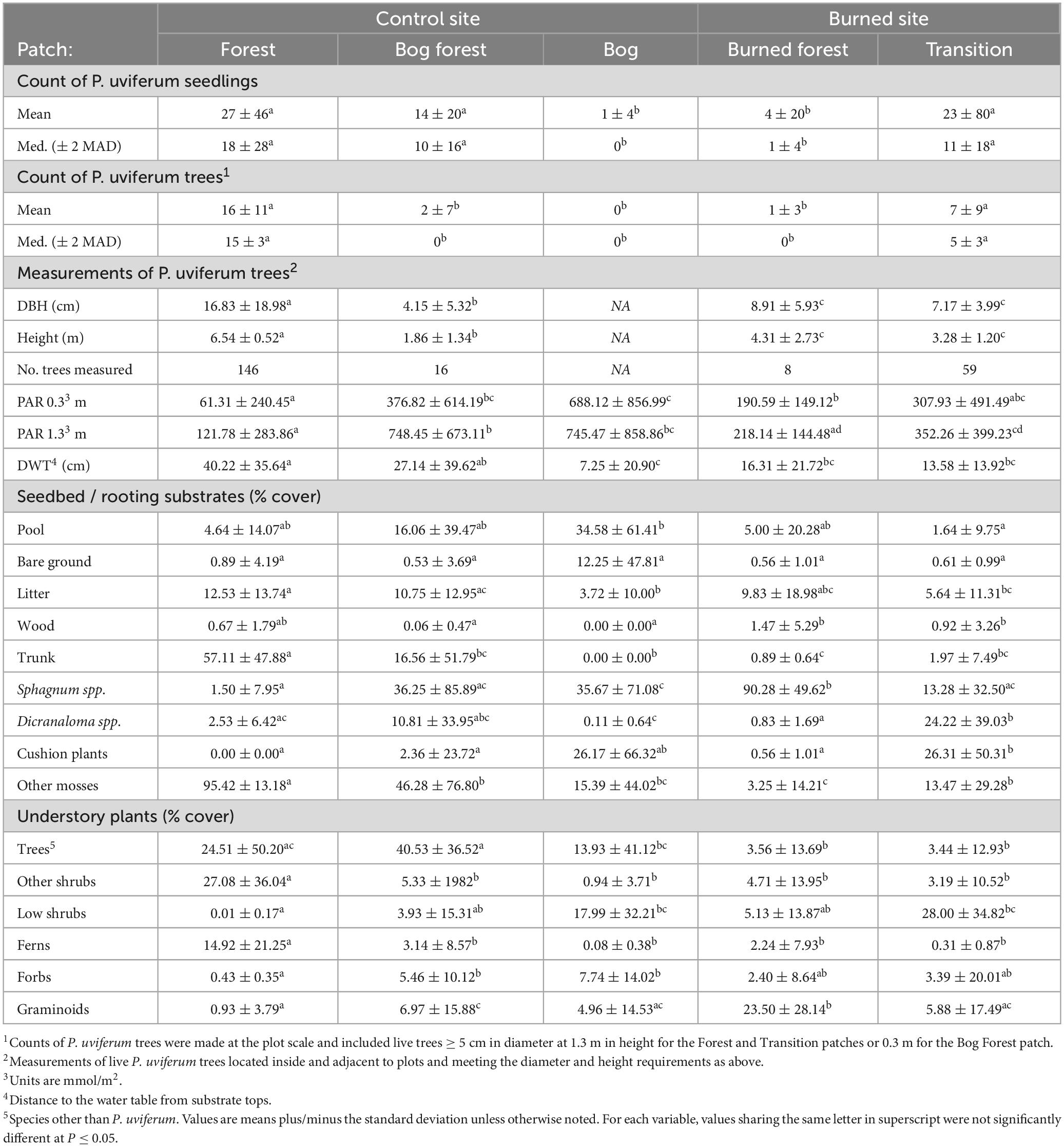
Table 1. Summary statistics by patch for P. uviferum seedling counts and quantitative variables observed in subplot microsites.
Counts of P. uviferum trees and the percent cover of Sphagnum spp. also differed significantly between patches at both the control and burned site (Figures 7, 8). There were greater numbers of trees and lower coverages by Sphagnum spp. within the forested Forest and Transition patches as compared to the non-forested Bog and Burned Forest patches; however, the percent cover of Sphagnum spp. ranged from low to high values across microsites in the Bog Forest patch. In contrast to the above variables, PAR and DWT measurements were significantly different between forested and non-forested patches at the control site, but relatively uniform across patches at the burned site (Figures 9, 10; see also Supplementary Figures 20, 21).
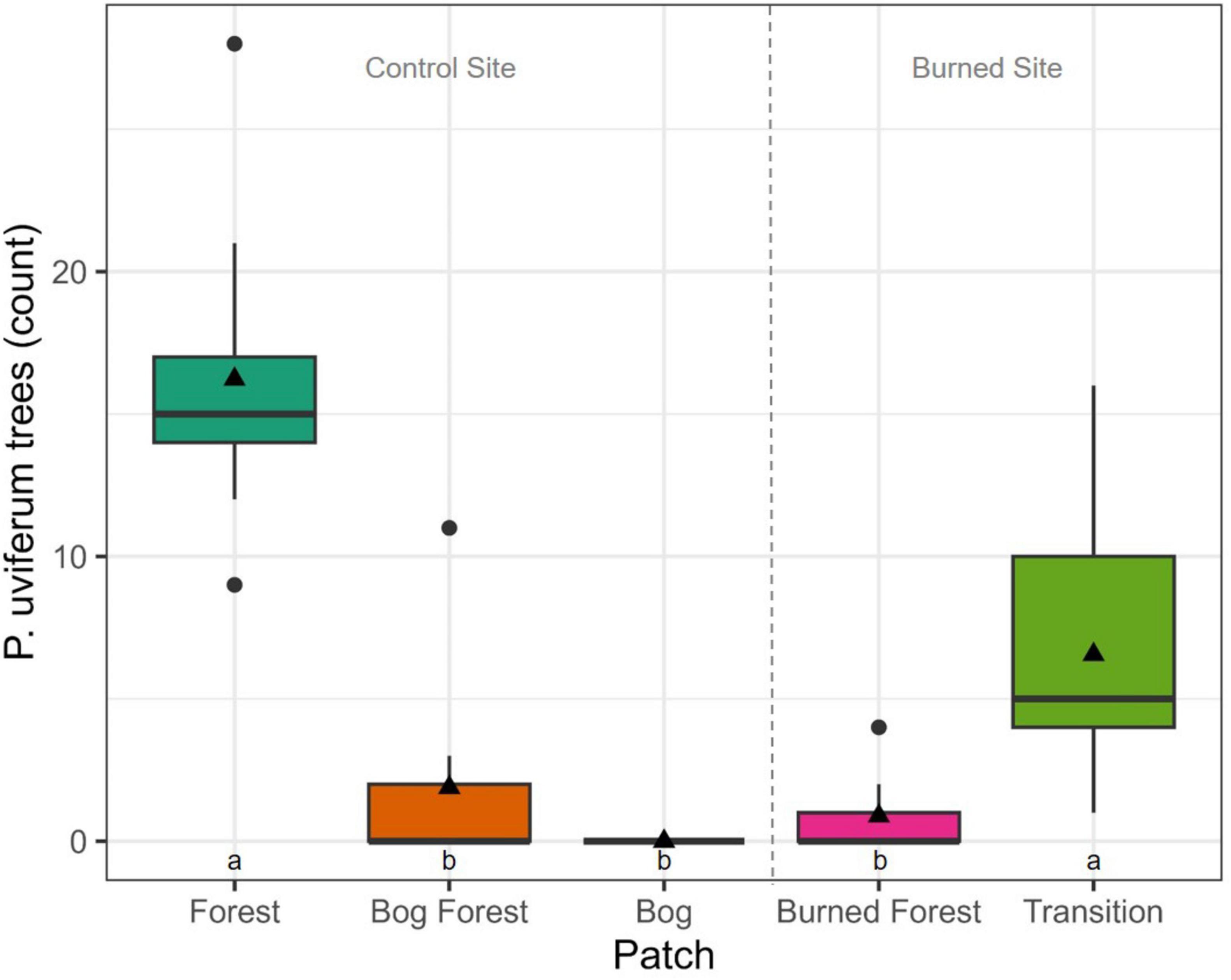
Figure 7. Boxplots of live P. uviferum tree (DBH ≥ 5 cm) counts per patch: Forest, Bog Forest and Bog patches in the control site, Burned Forest and Transition patches in the burned site. Trees were tallied in nine 100 m2 plots per patch. Boxes represent the interquartile range of the data; thick horizontal lines and black triangles represent median and mean counts, respectively; and vertical lines indicate variability in counts outside of the interquartile range with black circles are outliers. Different letters indicate significant differences between median counts across sites using Kruskal-Wallis one-way ANOVA and post-hoc Dunn’s tests (P < 0.05).
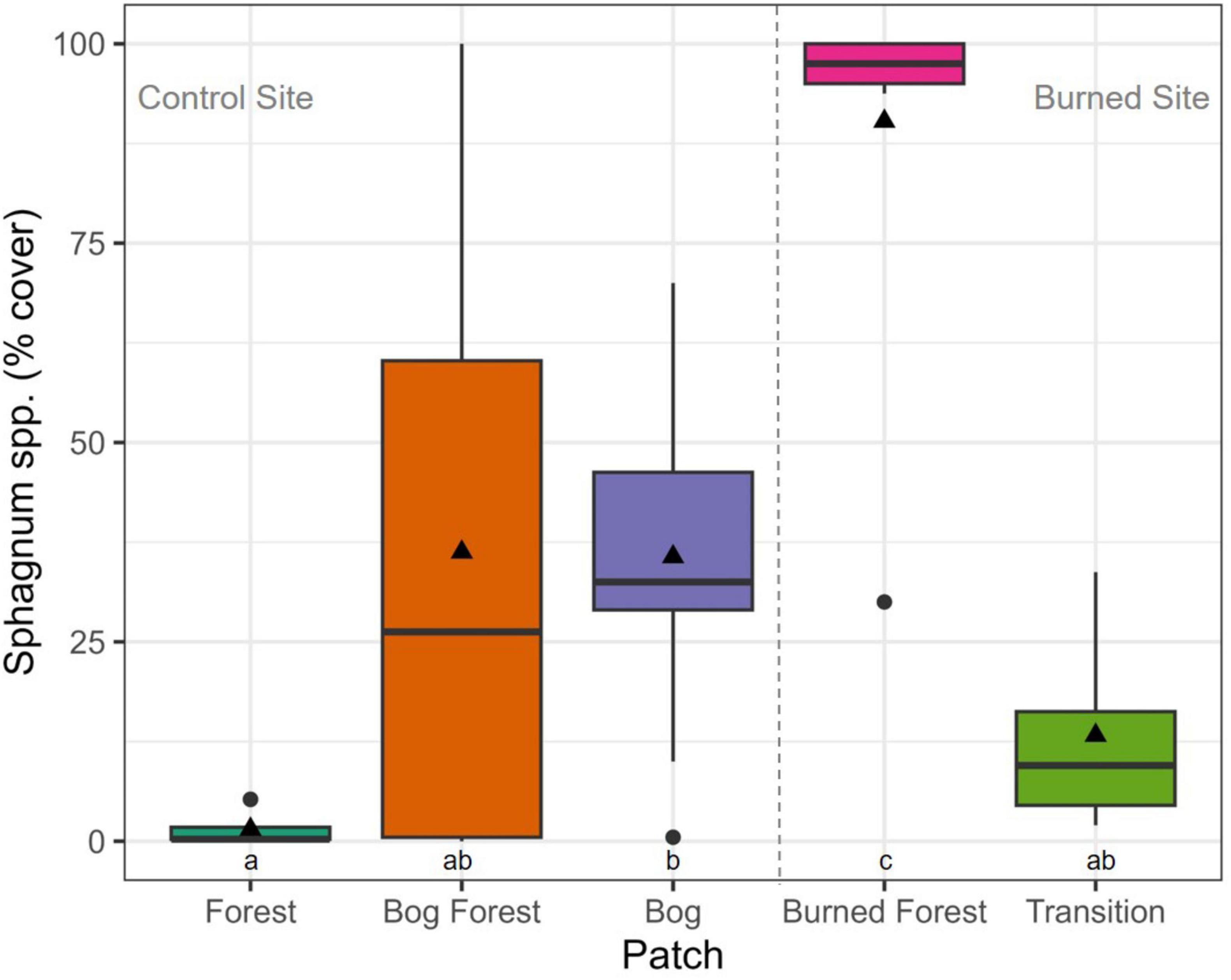
Figure 8. Boxplots of the percent cover of Sphagnum spp. per patch: Forest, Bog Forest and Bog patches in the control site, Burned Forest and Transition patches in the burned site. Percent cover was estimated across four 2 m2 subplots per plot, nine plots per patch. Boxes represent the interquartile range of the data summarized to plot and patch; thick horizontal lines and black triangles represent median and mean percent cover, respectively; and vertical lines indicate variability in percent cover outside of the interquartile range with black circles are outliers. Different letters indicate significant differences between mean percent cover across sites using Welch’s one-way ANOVA and post-hoc Games-Howell tests (P < 0.05).
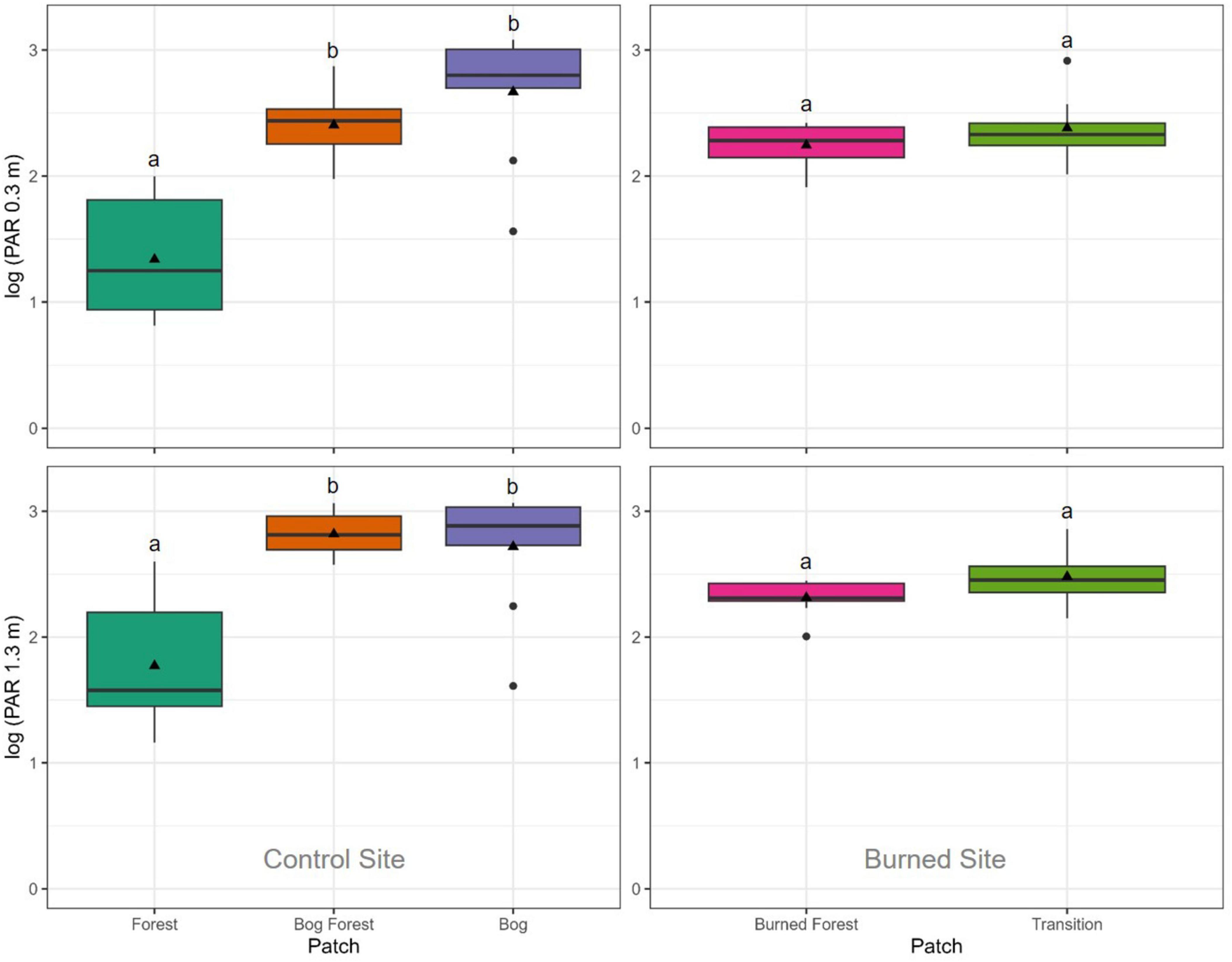
Figure 9. Boxplots of log-transformed photosynthetically active radiation (PAR; millimoles/m2) measured at 0.3 and 1.3 m above the ground surface in the Forest, Bog Forest and Bog patches of the control site and the Burned Forest, Transition and Cushion patches of the burned site. Photosynthetically active radiation was measured using a hand-held light sensor at three systematic locations per 2 m2 subplot (four subplots per plot, nine plots per patch). Boxes represent the interquartile range of the data summarized to plot and patch; thick horizontal lines and black triangles represent median and mean PAR readings, respectively; and vertical lines indicate variability in PAR outside of the interquartile range with black circles are outliers. Different letters indicate significant differences between mean PAR readings within sites using Kruskal-Wallis one-way ANOVA and post-hoc Dunn’s tests (P < 0.05).
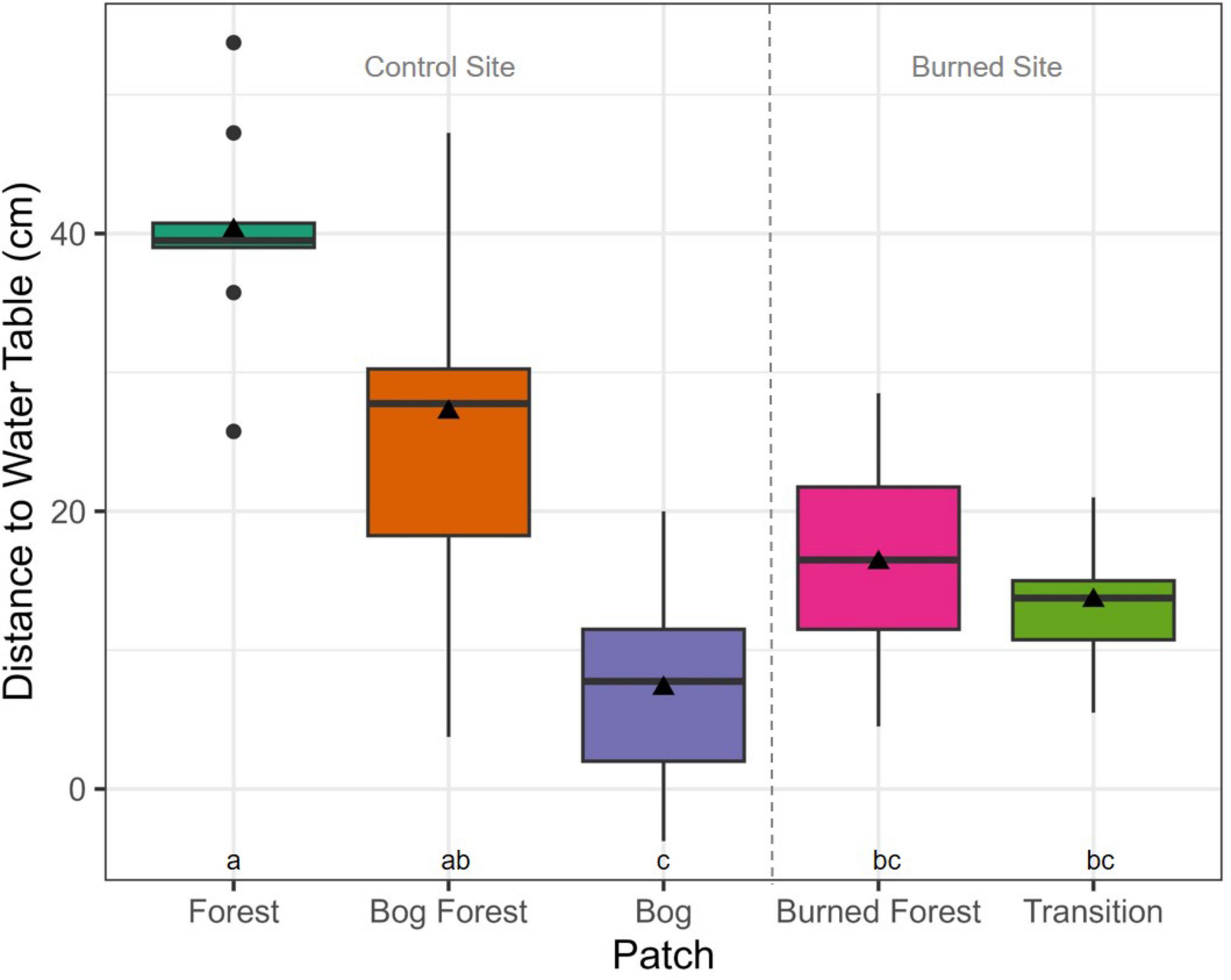
Figure 10. Boxplots of distance to the water table from substrate tops per patch: Forest, Bog Forest and Bog patches in the control site, Burned Forest and Transition patches in the burned site. Values are the average of measurements made in three systematic locations per 2 m2 subplots (four subplots per plot, nine plots per patch). Boxes represent the interquartile range of the data summarized to plot and patch; thick horizontal lines and black triangles represent median and mean values, respectively; and vertical lines indicate variability in DWT outside of the interquartile range with black circles are outliers. Different letters indicate significant differences between mean values of DWT across sites using Kruskal-Wallis one-way ANOVA and post-hoc Dunn’s tests (P < 0.05).
3.3.2 Microsite Scale
Within microsites, different sets of covariates better predicted P. uviferum seedling counts at the control versus burned site (Figure 11). For the control site, our final model included the following predictors: patch, interactions between patch and DWT and log (PAR 1.3), the number of nearby P. uviferum trees, the percent cover of Sphagnum spp. and cushion plants, and the interaction between patch and the percent cover of ‘other’ shrubs (i.e., those that were not mat-forming and generally taller in stature). Of these, the ‘Bog’ level of patch had the greatest effect on seedling counts relative to the reference condition of ‘Forest’ (median point estimates of −4.43 and 4.51, 90% and 95% credible intervals, CI, respectively). Subplots in the Bog Forest patch were also predicted to have fewer seedlings than those in the Forest patch, though the marginal effect size was smaller than for the Bog patch (−1.79, 95% CI). Within the Bog and Bog Forest patches, seedling counts were predicted to be further reduced by increasing levels of PAR at 1.3 m, with the greatest effect in the Bog patch (−1.51 and −0.74, respectively; 95% CI). In contrast, there was a positive effect of PAR at 1.3 m on seedling counts in the Forest patch (0.43, 95% CI). In the Forest patch, seedling counts were also associated, albeit negatively, with the percent cover of other shrubs (−0.33, 95% CI). The percent cover of cushion plants had a positive effect (0.40, 95% CI) on seedling counts across the pooled data. Parameter estimates for the count of P. uviferum trees and percent cover of Sphagnum spp. did not exclude zero with at least 80% probability and were thus considered “not significant” (Supplementary Table 23). Regarding the overdispersion and plot-level components of the model: seedling counts in the Bog patch (−1.68, 95% CI) were overdispersed compared to those in the Forest patch (0.97, 95% CI; the shape parameter is inversely related to the variance, so the larger the negative value, the greater the variance and overdispersion), and there was a slight positive random effect of plot on seedling counts across patches (0.20, 95% CI).
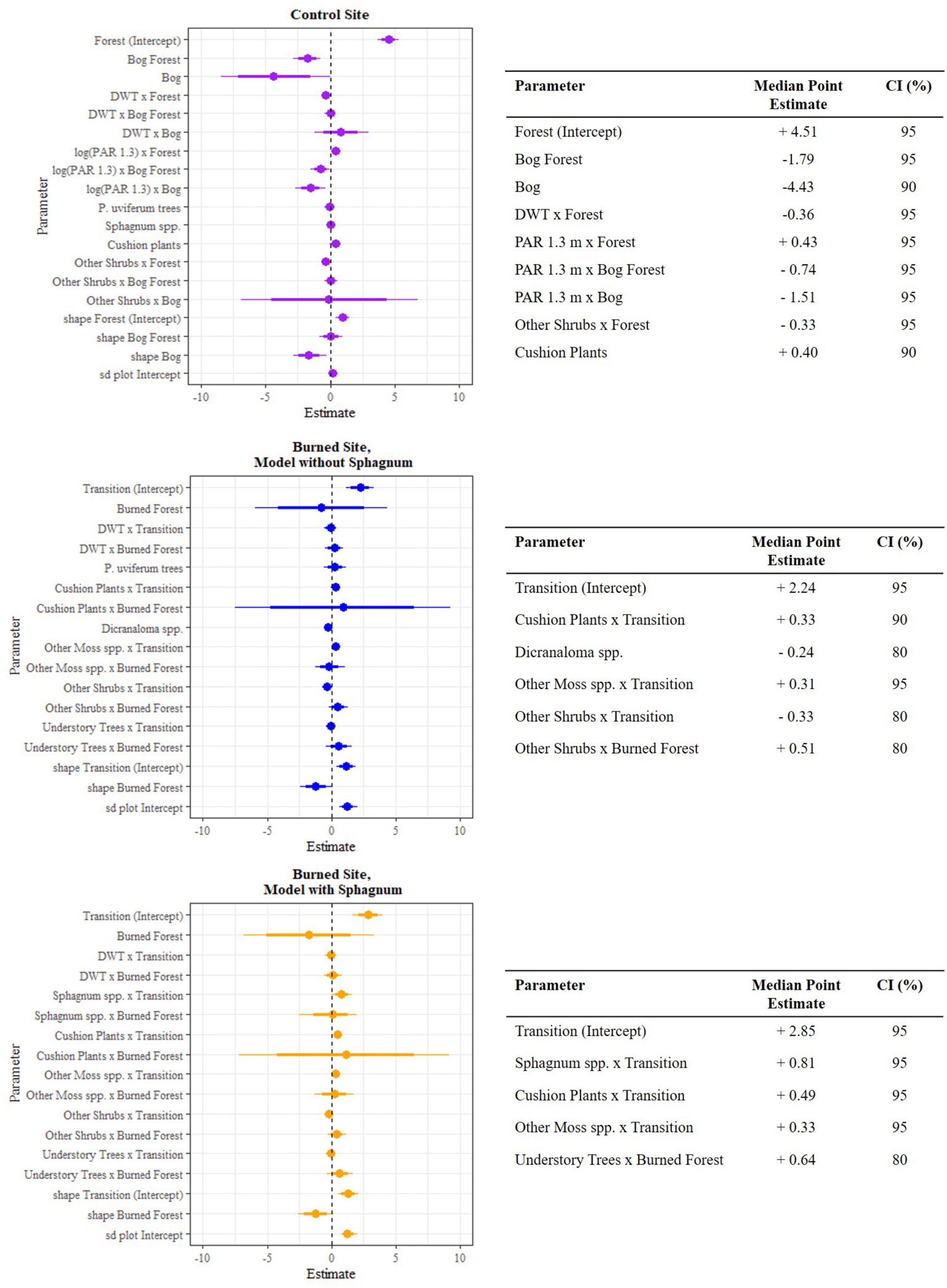
Figure 11. Posterior estimates of parameters from final Bayesian hierarchical models of P. uviferum seedling counts for the control site (top) and burned site (middle and bottom) with 80% and 95% credible intervals. Parameters with estimates that excluded zero with at least 80% probability (except the shape and plot parameters) are shown in the tables with their widest non-zero credible interval. Estimates are on the log scale.
For the burned site, we chose to examine two final models P. uviferum seedling counts. These differed in whether the percent cover of Sphagnum spp. was included as a predictor. The first model was fit using the following covariates: patch, the interaction between patch and DWT, count of P. uviferum trees, percent cover of Dicranaloma spp., and interactions between patch and the percent cover of cushion plants, other moss species, other shrubs and understory trees. We decided to fit and interpret a second model that included Sphagnum spp. given (1) our strong expectation of an association between the percent cover of the mosses and P. uviferum seedling counts, (2) inclusion of the predictor in the final control site model, (3) a trend toward significant marginal effects of Sphagnum spp. by patch in one of the simple candidate models for the burned site (Supplementary Table 11), and (4) the idea that microsites not dominated by Sphagnum may be characterized by a different suite of factors that affect P. uviferum seedling germination, growth and survival than those dominated by the peat moss. To avoid the potential for autocorrelation among covariates, Sphagnum spp. could only be included if the count of P. uviferum trees and percent cover of Dicranaloma spp. were removed (Supplementary material).
According to the model without Sphagnum spp., P. uviferum seedling abundance was most strongly affected by the percent cover of other/taller shrubs. This marginal effect was strongest and positive in the Burned Forest patch (median point estimate of 0.51, 80% CI), while it was negative in the Transition patch (−0.33, 80% CI). Seedling counts in the Burned Forest patch also tended to be positively associated with the percent cover of understory trees, but the parameter estimates did not exclude zero with 80% probability (median point estimate of 0.57). Seedling counts in the Transition patch were positively affected by the percent cover of cushion plants (0.33, 90% CI) and other mosses (0.31, 95%), but neither had a significant effect in the Burned Forest patch. The percent cover of Dicranaloma spp. was negatively associated with seedlings across patches (−0.24, 80% CI). There was no evidence for significant effects of the interaction between patch and DWT or the number of P. uviferum trees on seedling abundance (Supplementary Table 24).
The signs of the parameter estimates for the model with Sphagnum spp. were similar to the first model with regard to the predictors that they had in common, though the strengths and the confidence intervals of the parameter estimates differed. For example, the effect of cushion plants on seedling abundance in the Transition patch was stronger in the model with Sphagnum spp. (0.49, 95% CI), while the effect sizes for the interactions between other shrubs and either patch decreased and their variability increased such that their confidence intervals no longer excluded zero with at least 80% probability. In this model, the percent cover of Sphagnum spp. had a stronger effect than any other predictor in either of the models: there was a positive effect on P. uviferum seedling counts in the Transition patch (0.81, 95%), though no significant effect was observed in the Burned Forest patch (Supplementary Table 25). There was also a relatively strong effect of the percent cover of understory trees on seedling counts in the Burned Forest patch (0.64, 80% CI). In both of the models, seedling counts in the Burned Forest patch were overdispersed; however there was no overall “significant effect” of “Burned Forest” on seedling counts.
4 Discussion
This study shows that the significantly higher densities of P. uviferum trees and seedlings in the forested patches at both, burned and control, sites (as compared to the non-forested patches) were associated with significantly lower seasonal water tables, lower cover of Sphagnum spp. moss and higher cover of mosses that were not in the Sphagnum or Dicranaloma genera. S. magellanicum was in fact an indicator species of non-forested patches in the burned and control sites. We also found distinct understory plant communities across patches and sites in response to different factors: differences in vegetation structure and composition were associated with gradients in abiotic conditions at the control site, whereas the environment had become homogenized across the repeatedly-burned site even though patches were separated by abrupt boundaries in vegetation. A scale-dependent influence of microsite structures and environments (within patches) at both sites on tree seedling establishment was identified and was characterized by intricate and multifarious effects, sometimes via abiotic conditions and/or biotic elements that differed from those that were most prevalent at the larger patch scale (Figure 12). We discuss our findings in more detail below in the context of post-reburn P. uviferum forest reorganization under drought conditions and their implications for the maintenance of forest-peatland ecotone boundaries (i.e., reproduction, establishment, modification of abiotic conditions, and biotic interactions).
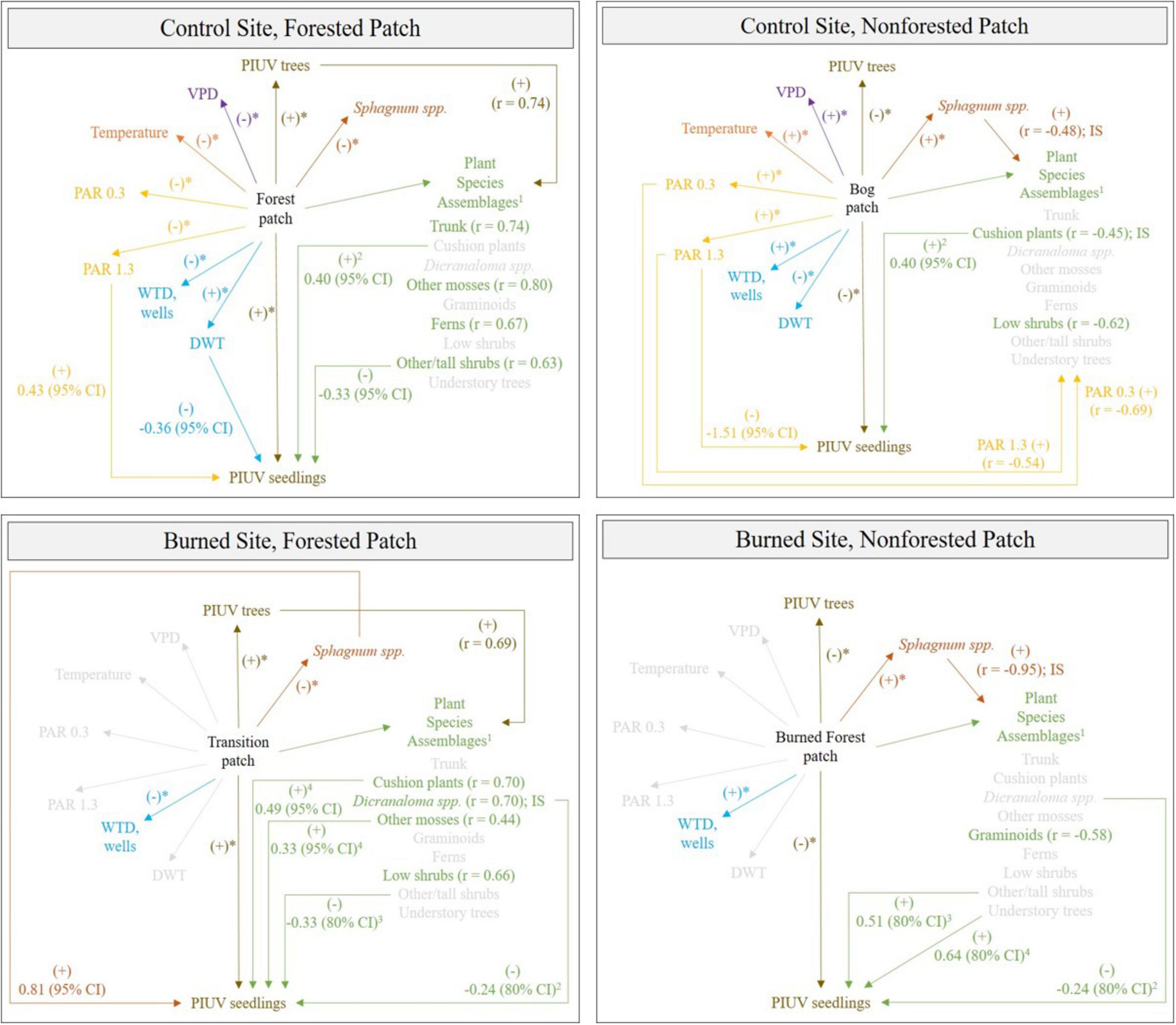
Figure 12. Abiotic and biotic factors associated with patch, plant species assemblages and P. uviferum (PIUV) seedling abundance at the control and burned site. Significantly different median (tree and seedling counts) or mean (vapor pressure deficit [VPD], temperature, photosynthetically active radiation [PAR], water table depth [WTD], distance from substrate tops to the water table [DWT], and Sphagnum spp.) values between the forested and non-forested patches at each site separately were determined via one-way analysis of variance and indicated with an asterisk (*P ≤ 0.05). Plant species assemblages, their predictors and indicator species (IS) were identified via non-metric multidimensional scaling; only the predictors that were also associated with seedling counts via regression analysis were included (1); Pearson’s correlations are shown if the predictor was associated with the same region of ordination space as the plant species assemblages for that patch (i.e., same end of axis 1). Parameter estimates and credible intervals (CI) were generated from Bayesian hierarchical linear regression models of seedling abundance; some parameter effects were pooled across patches (2); at the burned site, final models excluded (3) or included (4) Sphagnum spp. as a predictor, but only the strongest effect is shown here.
4.1 Post-reburn reorganization
4.1.1 Reproduction
At the repeatedly-burned site, the patch with the greatest abundance of P. uviferum seedlings also had the greatest abundance of seed trees, as was found at the unburned control site. Reproductively-mature trees that remain within or near disturbance perimeters can be important biological legacies (Bannister et al., 2012) that initiate forest regeneration via seed dispersal for tree species that lack re-sprouting capabilities, like P. uviferum. In a comparative study of P. uviferum regeneration on burned bog or upland sites on Chiloé Island (ca. 42° S), Bannister et al. (2012) only observed regeneration where seed trees survived fire or established shortly thereafter (and were sexually mature at the time of their study). Within the forested patch of the burned site (i.e., the Transition patch), mature P. uviferum individuals may have survived repeated burning given the wet conditions of the adjacent cushion bog to the west coupled with westerly winds, which could have reduced fire severity and contributed to patchy fire effects (Holz and Veblen, 2009, 2011). Low-severity fires typically have spatially heterogeneous effects, which might also have allowed seedlings to establish and mature between successive fires (Holz, 2009; Holz et al., in review). This in turn seems likely given the relatively young ages of the trees in the forested patch in the burned site: the oldest minimum age observed was 118 years with 51 years as the average minimum age; in contrast, the oldest minimum age in the forested patch at the control site (i.e., the Forest patch) was 517 years, with an average minimum age of 235 years (Zaret, 2011). While the density of potential seed trees and seedlings tended to be lower within the forested patch at the burned site than the forested patch at control site, seed availability does not appear to be limiting the potential for at least a portion of the burned site to recover to a P. uviferum forest similar in structure to that of the control site. The time needed for that to happen likely depends on multiple factors, including masting cycles, dispersal capabilities, substrate, and hydroclimatic patterns. For instance, successful P. uviferum establishment was found to be strongly associated with wetter conditions (Holz et al., in review), but its growth has been observed to be substantially slower on saturated as compared to well-drained soils on burned sites (e.g., requiring on average 70 vs. 40 years to reach a root collar diameter of 5 cm; Bannister et al., 2012). Under forecasted warmer and drier conditions (and in the absence of future burning), tree growth rates could increase and canopy closure may occur faster than historically (Holz et al., 2018).
P. uviferum’s ability to colonize new sites or to re-colonize disturbed sites via sexual reproduction may be hampered by limited dispersal distances even in instances where seed trees are nearby (and even numerous): though seeds have wings, seedling recruitment is most abundant within 20 m of seed trees, and the majority of seedlings and saplings are found clustered within 5 m of such trees (Bannister et al., 2014; Galindo et al., 2021). Seedlings were less abundant in the non-forested patch of the control site (i.e., the Bog patch) than in that of the burned site (i.e., the Burned Forest patch), perhaps due to differences in the direction of the predominant westerly winds coupled with distances to seed trees. At the burned site the non-forested patch was downwind of the seed trees located in the adjacent forested patch; in contrast, the non-forested patch at the control site was upwind of potential seed trees. Despite the favorable wind direction, seed rain into the non-forested patch of the burned site is likely irregular (following masting cycles of 7–9 years; Donoso, 1993; Galindo et al., 2021) and may also be limited by the relatively short stature of the slow-growing mature, potentially cone-bearing trees in the adjacent forested patch (average of 3.28 ± 1.20 m, n = 59; Table 1). Thus, forest regeneration may proceed within the non-forested patch of the repeatedly-burned site, but at a slow rate as sets of successive seed trees establish, mature and produce seed along a shifting edge into the patch. Ultimately, a ‘restructured’ forest may develop (i.e., one still dominated by P. uviferum, but with lower density). Such a forest could differ in understory plant composition, abiotic conditions and biotic interactions as compared to the P. uviferum forest at the control site (see below).
4.1.2 Establishment
Short fire-return intervals relative to the slow growth of P. uviferum would seem to be a major limitation to seedling establishment (in the event that seed production is successful). However, direct seedling mortality from fire does not appear to be the sole factor limiting tree recruitment due to the following observations: (1) evidence of historic post-fire cohorts (Holz et al., in review; Zaret, 2011), which indicate that P. uviferum forests have successfully recovered from fire in the past; and (2) the absence of large-scale fire activity for more than a decade at the time this study was undertaken, but still the lack of widespread seedling establishment. As discussed below, our findings indicate that feedbacks between fire, abiotic conditions and the biotic community together limit P. uviferum forest regeneration.
4.1.3 Modification of abiotic conditions
As noted above, repeated burning, through its effects of restructuring the abundance and size classes of P. uviferum trees and other co-dominant tree species (likely Nothofagus spp., Drimys winteri, Podocarpus nubigenus, Weinmannia trichospera, etc.; Veblen et al., 1995), appears to have also disrupted some of the gradients in abiotic conditions that were observed across the forest-peatland ecotone at the control site, instead creating uniform conditions across the burned site. Though P. uviferum trees (≥ 5 cm DBH) were present in both the regenerating forest patch at the burned site (i.e., the Transition patch) and the old-growth forest at the control site (i.e., the Forested patch), there were substantially fewer trees in the forested patch at the burned site and they were significantly smaller in DBH and height (Figure 7 and Table 1). Because of their very slow growth rate and the relatively recent history of reburns in the site (Holz and Veblen, 2011; Holz et al., 2018), canopies of slow-growing P. uviferum individuals in the forested patch were still sparse and short, and their understory microclimatic and light conditions were similar to those in the non-forested patch (Figures 4, 9, and Supplementary Figures 15–17). As a result, no effect of PAR on seedling abundances was found for either patch within the burned site (Supplementary Table 7). In contrast, light levels decreased with increasing tree density across the forest-peatland ecotone at the control site (which was especially evident when PAR was measured across patches on the same day under consistent weather conditions; Supplementary Figure 21). For the control site, our best-fit model of seedling abundance included a positive parameter estimate for PAR in the forested patch, but negative parameter estimates in the other patches. This finding corresponds with research conducted by Bannister et al. (2013), who documented negative effects of light levels on shoot elongation and foliar phosphorous and nitrogen concentrations in P. uviferum seedlings planted in open conditions; it also suggests that relationships between light levels and P. uviferum establishment, growth and/or survival may be non-linear (Supplementary material). Differences in forest structure across the forest-peatland ecotone at the control site were also associated with different distances to the water table (DWT), which increased with increasing P. uviferum tree abundance across the three patches. At the burned site, however, DWT did not differ significantly between the forested and non-forested patches (Figure 10), indicating that repeated burning can reduce spatial variability in microtopography, effectively homogenizing that dimension of microsite structure across an entire burned area.
Though evidence from the control site indicates that tree regeneration is lacking in the non-forested patch due to sunnier, warmer, and drier conditions and/or excessive soil moisture (Figure 12), these factors by themselves did not explain why P. uviferum seedling abundance was prolific within the Transition patch but not in the adjacent Burned Forest patch at the burned site. Ultimately, seasonal water table depths (WTD) were the only environmental condition that differed significantly between the forested (lower/further from the surface) and non-forested (higher/closer to the surface) patches at both sites, though there tended to be smaller differences in WTD between patches at the burned site. That greater tree densities were associated with lower water tables supports the idea of an evapotranspiration-tree establishment feedback (Figure 1). However, we did not find evidence that microsites characterized by lower distances to the water table were negatively associated with seedling abundance in more exposed patches (Figure 11, Supplementary Table 2); water tables in the forested patches at the burned and control site were usually within 5 cm and 25 cm of the substrate surface, respectively, (well within the rooting zone); and soils were continuously saturated (regardless of water table depth) in both forested patches. Our findings, like those of other researchers, were thus ambiguous regarding the effects of high water tables on P. uviferum regeneration and subsequent forest development pathways.
Past research in climatically more moderate (i.e., seasonally warmer and drier) northwestern Patagonia has suggested that waterlogging limits tree regeneration on secondary shrublands (Díaz et al., 2007) or anthropogenic peatlands (Díaz et al., 2008) that develop following logging and/or fire. However, a negative relationship between high water tables and P. uviferum has not been demonstrated experimentally or through field observation (e.g., Díaz and Armesto, 2007; Bannister et al., 2013). In fact, of the 12 tree species observed by Díaz and Armesto (2007), only P. uviferum seedlings were observed in the middle and most saturated portion of the site under investigation. Rather, P. uviferum is often referred to in association with “poorly-drained conditions” (e.g., Rovere et al., 2002), and the species is believed to be found in greatest abundance in lowland sites given its capacity to withstand wet and nutrient-poor conditions better than potential angiosperm competitors (Cruz and Lara, 1981), potentially due to a lack of vessels and associated resource conservation (Bond, 1989; Brodribb et al., 2012; Piper et al., 2019). Bannister et al. (2012) found evidence for continuous P. uviferum regeneration in undisturbed upland and wetland forests on Chiloé Island, and Esse et al. (2021) identified two main types of P. uviferum forests in the fjords of SW Patagonia, which differed from other forest communities in terms of high water tables. Thus, the species appears to be capable of regeneration under a wide range of naturally-occurring soil moisture conditions (i.e., regeneration niches; Grubb, 1977). In terms of post-disturbance establishment, Soto and Figueroa (2008) found evidence of post-disturbance cohorts of P. uviferum following high disturbance levels (e.g., repeated fire, logging and/or grazing), and they concluded, for its northernmost and warmest/driest populations, that the species needs open sites with wet soils in order to regenerate and persist. On Chiloé Island, Bannister et al. (2012) actually documented greater post-fire seedling density in bog forests compared to upland forests, and Mansilla (2008) observed P. uviferum seedlings on burned bogs on the colder and drier Brunswick Peninsula in far southern Patagonia. Rather than high water tables, per se, there may be interactions between water tables and substrate types, especially those that are biotic (e.g., living mosses rather than bare soil or trunks) that most strongly determine the likelihood of P. uviferum establishment and ongoing growth and survival, which we explore in more detail below.
4.1.4 Biotic interactions
Differing abundances of P. uviferum trees and seedlings across the forest-peatland ecotones at the burned and control site were also associated with distinct understory plant communities (Figures 5, 12). The cool, moist, dark understory of the forested patch at the control site was characterized by species commonly found in temperate rainforests of the area (e.g., Luebert and Pliscoff, 2017), but the forested patch at the burned site was characterized by species that have been documented in naturally-occurring and disturbance-induced peatlands elsewhere in Chile (Díaz et al., 2008; Supplementary Tables 16–20). The understory communities in the non-forested patches at both sites were also typical of open peatlands, though Sphagnum spp. was a more important member of the community at the burned site, and both communities were readily distinguishable in ordination space, as were plant assemblages for each patch across both sites (Supplementary Figure 23). Thus, despite evidence that forest structure may be recovering across a portion of the burned site, understory composition differs notably from that of the control site.
The distinct life forms and individual plant species that characterized the understories of each patch type (and contributed to microsite composition and structure) appeared to affect seedling abundance via facilitative or competitive interactions, thereby having the potential to influence forest development over the long-term. For example, understory trees and taller shrubs were predicted to increase seedling abundance in the non-forested patch of the burned site (Figure 11), perhaps ameliorating any negative impacts of higher light levels, as discussed earlier, and supporting findings of shrub-nursery effects on P. uviferum seedlings in disturbed, open areas further north (Bannister et al., 2019). In contrast, taller shrubs were negatively associated with seedling abundance in the patches in which woody plants were more abundant or under an already-established tree canopy, as in the forested patches at both sites. That the direction of these interactions are mediated by light levels (PAR had a relatively strong correlation with understory plant assemblages; Figure 5), highlights the importance of biotic-abiotic feedbacks in determining dynamics across the forest-peatland ecotones under investigation.
When we took a closer look at interactions between P. uviferum seedling abundance and microsite composition to determine whether they were mediated by DWT, we found that the majority of microsites in the non-forested patch of the burned site (where few seedlings were observed) were dominated by S. magellanicum, while those in the forested patch were composed of a diversity of plants (often including cushion species, Dicranaloma spp., S. magellanicum and other mosses, with no one type covering more than 50% of the area; Supplementary Figure 24). These results align with previous work on P. uviferum regeneration with regard to substrate type and Sphagnum mosses in particular. Bannister et al. (2013) observed significantly higher survival rates of seedlings planted on artificially created mounds of moss as compared to those planted on naturally-occurring Sphagnum spp. lawns or on swaths of mineral soil created by removing Sphagnum mosses. Galindo et al. (2021) found significantly greater numbers of P. uviferum seedlings on substrates dominated by cushion plants (i.e., Donatia spp. and Astelia spp.) and accompanied by a small percent cover of mosses and the least number of seedlings on microsites dominated by Sphagnum spp. Likewise, our regression models for the burned site predicted positive effects of other mosses, cushion species and Sphagnum. spp. on seedling abundances in the forested patch (Figure 11, Transition patch results). Thus, it may be that the presence of Sphagnum spp. can facilitate P. uviferum establishment, growth and/or survival under conditions that limit the moss species’ growth rate or lateral spread (e.g., drier or darker microsites or via competition with other ground-covering plants), but when peat moss cover predominates, i.e., under wetter and/or more open conditions, it will likely have detrimental effects on tree seedlings via overtopping or competition for nutrients.
Unlike at the burned site, there did appear to be interactions between seedling abundance, microsite diversity and DWT at the control site (Supplementary Figure 25). In the non-forested patch, the microsites with the greatest DWTs were composed almost exclusively by Sphagnum spp., while microsites with more intermediary DWTs were associated with relatively diverse assemblages of biotic substrates (most including both cushion species and Sphagnum spp.) and also the greatest numbers of P. uviferum seedlings. This relationship was more notable in the intermediary (i.e., Bog Forest) patch of the control site: microsites were generally composed by either a variety of substrates (e.g., other non-Sphagnum mosses, trunks and/or pools) or S. magellanicum. The former tended to be closer to the water table and to have greater numbers of seedlings, while the latter had the opposite relationships (i.e., drier with fewer seedlings), which supports our proposed water table depth−sun exposure−Sphagnum feedback (Figure [conceptual model]). This result also supports the idea that the Bog Forest represents an intermediary successional stage in terms of forest structure (Figures 6, 7), understory composition (Figure 5) and abiotic conditions (Figures 4, 10 and Supplementary Figure 21).
In summary, the forest-peatland ecotone at the control site seems to represent a gradient in forest structure, where environmental conditions and biotic interactions are controlled, at least in part, by tree density, and where regeneration-safe microsites tend to be heterogeneous in their composition and structure. In contrast, the forest-peatland ecotone at the burned site seems to represent a gradient in disturbance history, where the current structure and composition of patches have been shaped by fire, which has opened the forest canopy and disrupted gradients in most abiotic conditions. While legacy effects associated with less frequent or less severe burning (e.g., spatially patchy consumption of trees, understory vegetation and/or microsite features like downed logs or moss hummocks) appear to have supported the regeneration of a new tree cohort in part of the site, almost no tree regeneration is occurring where all older/larger trees were removed by past fires and where microsites are now characterized by a nearly-homogenous cover of Sphagnum moss. The regenerating tree cohort will likely recover to a forest similar in structure to that of the control site unless forest development is disrupted by future fires and/or other disturbances. Intensifying drought conditions across western Patagonia may increase that possibility. On the other hand, in these hyperhumid forests warmer, drier conditions in the absence of fire may quicken canopy closure (by increasing P. uviferum growth rates), and shift the composition of the understory plant community towards species more representative of temperate rainforests and less like those of non-forested peatlands. Whether trees can recolonize the previously-forested portion of the burned site (that now more closely resembles the bog of the control site) may depend on how climatic patterns simultaneously affect water table dynamics, Sphagnum mosses, and their interactive effects on P. uviferum establishment, growth and survival. Ultimately, intentional interventions could be needed to restore P uviferum trees to severely- or repeatedly burned patches.
4.2 Forest restoration
To date, active post-fire restoration efforts have demonstrated that P. uviferum seedlings planted in bog environments have relatively high survival rates after 10 years depending upon the substrates used as growth media and/or the presence of shade plants (Bannister et al., 2019; Galindo et al., 2021). This is supported by preliminary results from an experimental plantation established at our burned site under the present study: planted seedlings may overcome some limitations of post-reburn abiotic conditions and/or biotic composition, but taller seedlings should be selected to re-forest carpets of Sphagnum spp. in order to avoid differences in growth rates that can result in overtopping by the peat moss (Supplementary Figures 26, 27). The prospects for P. uviferum restoration to burned sites in western Patagonia are encouraged by relatively widespread recognition of the tree species as an important part of natural and cultural heritage, which could be leveraged to foster support for community-based restoration efforts on private and state-owned lands.
4.3 Limitations and opportunities
As with other investigations based on space-for-time substitutions (e.g., Lovell et al., 2023), our study was limited by its timing relative to the onset of disturbance and its short-term duration.
Stochastic factors like legacy effects (e.g., the preservation or generation of a diverse spatial array of microsites), priority effects (e.g., immediate post-fire colonization by Sphagnum mosses), and rare events (e.g., abnormally wet or dry climatic conditions coupled with a mast year) may have important roles in determining the likelihood of successful P. uviferum regeneration. This suggests that the best approach for investigating the forest-peatland ecotone of western Patagonia should be longitudinal and include field data collection that begins directly following disturbance and continues through early succession and into late succession. Given the extended time frame (especially with a focal species as slow-growing and long-lived as P. uviferum), the logistics for supporting this type of investigation could be modeled on Long-term Ecological Research efforts (e.g., Carmona et al., 2010).
Long-term study would contribute toward understanding how dynamic interactions between top-down factors like climate and bottom-up factors like local environmental conditions affect tree regeneration at ecotonal boundaries. Climate differentially affects the growth of P. uviferum trees across the species’ extensive range (39°35′–54°20′ S, ca. 1600 km; Holz et al., 2018), and recent work suggests similar effects on the conifer’s establishment: high precipitation was associated with higher establishment of P. uviferum on more temperate Chiloé Island and in the Baker River watershed, but the opposite trend was suggested in the colder and wetter Pascua River watershed (Holz et al., in review). Climate may have direct effects on reproduction (e.g., through influencing mast cycles and seed rain). It may also interact with soil moisture and substrate types to promote asexual over sexual reproduction, such as through the formation of adventitious roots and layering, which is more likely under harsh and wet conditions (Cooper, 1911; Del Tredici, 2001) and has been observed previously in P. uviferum (Bannister et al., 2012) and in the present study. Future research on P. uviferum should include careful quantification of sexually- vs. vegetatively-produced stems to determine how the importance of legacy trees to the process of post-disturbance reproduction changes under varying climatic and local conditions.
Finally, long-term, fine-scale quantitative measurements of P. uviferum habitat hydroperiods (e.g., ‘flood duration’ or ‘flood frequency’; Mitsch and Gosselink, 2015) are needed to fully understand the species’ regeneration dynamics on edaphically wet sites. That is, such habitats should be investigated using methods appropriate for forested wetlands rather than simply saturated forests. Determining ongoing interactions between climate and hydroperiod on all aspects of P. uviferum establishment (e.g., germination, growth, and survival) is key to identifying the conservation and restoration needs of this threatened species, the world’s southernmost conifer, and anticipating future shifts in vegetation dominance across the forest-peatland ecotone of western Patagonia.
Data availability statement
The raw data supporting the conclusions of this article will be made available by the authors, without undue reservation.
Author contributions
KZ: Conceptualization, Formal analysis, Funding acquisition, Investigation, Methodology, Visualization, Writing−original draft, Writing−review and editing. AH: Conceptualization, Funding acquisition, Methodology, Supervision, Writing−review and editing.
Funding
The author(s) declare financial support was received for the research, authorship, and/or publication of the article. Funding for this research was provided by the National Science Foundation (NSF awards 1806037 and 1832483). Publication of this article in an open access journal was funded by the Portland State University Library’s Open Access Fund.
Acknowledgments
We thank L. Mielke, A. Nicholson, G. Vandas, C. Westfall, N. Smith and D. Arriagada for their assistance in collecting field data and performing data entry. E. Buhle and T. Veblen provided feedback that improved the quality of this work. Support and field site access in Chile were graciously provided by P. Hartmann, G. Agnetti, and numerous individuals from the communities of Caleta Tortel and Cochrane.
Conflict of interest
The authors declare that the research was conducted in the absence of any commercial or financial relationships that could be construed as a potential conflict of interest.
Publisher’s note
All claims expressed in this article are solely those of the authors and do not necessarily represent those of their affiliated organizations, or those of the publisher, the editors and the reviewers. Any product that may be evaluated in this article, or claim that may be made by its manufacturer, is not guaranteed or endorsed by the publisher.
Supplementary material
The Supplementary Material for this article can be found online at: https://www.frontiersin.org/articles/10.3389/ffgc.2024.1385506/full#supplementary-material
References
Albornoz, F. E., Gaxiola, A., Seaman, B. J., Pugnaire, F. I., and Armesto, J. J. (2013). Nucleation-driven regeneration promotes post-fire recovery in a Chilean temperate forest. Plant Ecol. 214, 765–776. doi: 10.1007/s11258-013-0206-x
Allen, C. D., and Breshears, D. D. (1998). Drought-induced shift of a forest-woodland ecotone: Rapid landscape response to climate variation. Proc. Natl. Acad. Sci. U.S.A. 95, 14839–14842. doi: 10.1073/pnas.95.25.14839
Alvarez-Garreton, C., Mendoza, P. A., Boisier, J. P., Addor, N., Galleguillos, M., Zambrano-Bigiarini, M., et al. (2018). The CAMELS-CL dataset: Catchment attributes and meteorology for large sample studies – Chile dataset. Hydrol. Earth Syst. Sci. 22, 5817–5846. doi: 10.5194/hess-22-5817-2018
Arroyo, M. T. K., Pliscoff, P., Mihoc, M., and Arroyo-Kalin, M. (2005). “The Magellanic moorland,” in The world’s largest wetlands: Ecology and conservation, eds L. H. Fraser and P. A. Keddy (Cambridge: Cambridge University Press), 424–445.
Bannister, J. R., Donoso, P. J., and Bauhus, J. (2012). Persistence of the slow growing conifer Pilgerodendron uviferum in old-growth and fire-disturbed southern bog forests. Ecosystems 15, 1158–1172. doi: 10.1007/s10021-012-9574-7
Bannister, J. R., Kremer, K., Carrasco-Farías, N., and Galindo, N. (2017). Importance of structure for species richness and tree species regeneration niches in old-growth Patagonian swamp forests. Forest Ecol. Manag. 401, 33–44. doi: 10.1016/j.foreco.2017.06.052
Bannister, J. R., Travieso, G., Galindo, N., Acevedo, M., Puettmann, K., and Salas-Eljatib, C. (2019). Shrub influences on seedling performance when restoring the slow-growing conifer Pilgerodendron uviferum in southern bog forests. Restor. Ecol. 28, 396–407. doi: 10.1111/rec.13090
Bannister, J. R., Wagner, S., Donoso, P. J., and Bauhus, J. (2014). The importance of seed trees in the dioecious conifer Pilgerodendron uviferum for passive restoration of fire disturbed southern bog forests: Passive restoration in P. uviferum bog forests. Austral Ecol. 39, 204–213. doi: 10.1111/aec.12060
Bannister, J., Coopman, R., Donoso, P., and Bauhus, J. (2013). The Importance of microtopography and nurse canopy for successful restoration planting of the slow-growing conifer Pilgerodendron uviferum. Forests 4, 85–103. doi: 10.3390/f4010085
Bengtsson, F., Rydin, H., Baltzer, J. L., Bragazza, L., Bu, Z., Caporn, S. J. M., et al. (2021). Environmental drivers of Sphagnum growth in peatlands across the Holarctic region. J. Ecol. 109, 417–431. doi: 10.1111/1365-2745.13499
Benjamini, Y., and Hochberg, Y. (1995). Controlling the false discovery rate: A practical and powerful approach to multiple testing. J. R. Stat. Soc. 57, 289–300.
Boisier, J. P., Alvarez-Garretón, C., Cordero, R. R., Damiani, A., Gallardo, L., Garreaud, R. D., et al. (2018). Anthropogenic drying in central-southern Chile evidenced by long-term observations and climate model simulations. Elem. Sci. Anth. 6:74. doi: 10.1525/elementa.328
Bond, W. J. (1989). The tortoise and the hare: Ecology of angiosperm dominance and gymnosperm persistence. Biol. J. Linnean Soc. 36, 227–249.
Brodribb, T. J., Pittermann, J., and Coomes, D. A. (2012). Elegance versus speed: Examining the competition between conifer and angiosperm trees. Int. J. Plant Sci. 173, 673–694. doi: 10.1086/666005
Bürkner, P.-C. (2021). Bayesian item response modeling in R with brms and Stan. J. Stat. Soft. 100, 1–54. doi: 10.18637/jss.v100.i05
Camill, P., Chihara, L., Adams, B., Andreassi, C., Barry, A., Kalim, S., et al. (2010). Early life history transitions and recruitment of Picea mariana in thawed boreal permafrost peatlands. Ecology 91, 448–459. doi: 10.1890/08-1839.1
Carmona, M. R., Aravena, J. C., Bustamante-Sánchez, M. A., Celis-Diez, J. L., Charrier, A., Díaz, I. A., et al. (2010). Estación biológica senda darwin: Investigación ecológica de largo plazo en la interfase ciencia-sociedad. Rev. Chil. Hist. Nat. 83:7. doi: 10.4067/S0716-078X2010000100007
Casanova, M., Salazar, O., Seguel, O., and Luzio, W. (2013). Soils of Chile. New York, NY: Springer.
Coop, J. D., Parks, S. A., Stevens-Rumann, C. S., Crausbay, S. D., Higuera, P. E., Hurteau, M. D., et al. (2020). Wildfire-driven forest conversion in western north American landscapes. BioScience 70, 659–673. doi: 10.1093/biosci/biaa061
Crawford, R. M. M. (2003). Paludification and forest retreat in Northern oceanic environments. Ann. Bot. 91, 213–226. doi: 10.1093/aob/mcf185
Cruz, G., and Lara, A. (1981). Tipificación, cambio de estructura y normas de manejo para Ciprés de las Guaitecas (Pilgerodendron uviferum D. Don Florin) en la isla Grande de Chiloé. Santiago: Universidad de Chile.
Davies, G. M., Gray, A., Rein, G., and Legg, C. J. (2013). Peat consumption and carbon loss due to smouldering wildfire in a temperate peatland. Forest Ecol. Manag. 308, 169–177. doi: 10.1016/j.foreco.2013.07.051
Del Tredici, P. (2001). Sprouting in temperate trees: A morphological and ecological review. Botan. Rev. 67, 121–140. doi: 10.1007/BF02858075
Desjardins, E., Barker, G., Lindo, Z., Dieleman, C., and Dussault, A. C. (2015). Promoting resilience. Q. Rev. Biol. 90, 147–165. doi: 10.1086/681439
di Castri, F., Hansen, A. J., and Holland, M. M. (1988). A new look at ecotones, emerging international projects on landscape boundaries. Biol. Intl. 17, 1–163.
Díaz, M. F., and Armesto, J. J. (2007). Limitantes físicos y bióticos de la regeneración arbórea en matorrales sucesionales de la Isla Grande de Chiloé. Rev. Chilena Hist. Nat. 80, 13–26.
Díaz, M. F., Bigelow, S., and Armesto, J. J. (2007). Alteration of the hydrologic cycle due to forest clearing and its consequences for rainforest succession. Forest Ecol. Manag. 244, 32–40. doi: 10.1016/j.foreco.2007.03.030
Díaz, M. F., Larraín, J., Zegers, G., and Tapia, C. (2008). Caracterización florística e hidrológica de turberas de la Isla Grande de Chiloé, Chile. Rev. Chilena Hist. Nat. 81, 2. doi: 10.4067/S0716-078X2008000400002
Dinerstein, E., Olson, D., Joshi, A., Vynne, C., Burgess, N. D., Wikramanayake, E., et al. (2017). An ecoregion-based approach to protecting half the terrestrial realm. BioScience 67, 534–545. doi: 10.1093/biosci/bix014
Dirección General de Aguas [DGA] (2015). Análisis y modelación hidrológica cuenca del Río Palena: Gobierno de Chile. Santiago: Informe Final.
Dirección General de Aguas [DGA] (2022). Información Oficial Hidrometeorológica y de Calidad de Aguas en Línea: Gobierno de Chile. Available online at: https://snia.mop.gob.cl/BNAConsultas/reportes (accessed April 22, 2022).
Donato, D. C., Harvey, B. J., and Turner, M. G. (2016). Regeneration of montane forests 24 years after the 1988 Yellowstone fires: A fire-catalyzed shift in lower treelines? Ecosphere 7, 1–16.
Donoso, C. (1993). Bosques templados de Chile y Argentina. Variación, estructure y dinámica. Santiago: Editorial Universitaria S.A.
Duane, A., Castellnou, M., and Brotons, L. (2021). Towards a comprehensive look at global drivers of novel extreme wildfire events. Clim. Change 165, 1–21. doi: 10.1007/s10584-021-03066-4
Dufrene, M., and Legendre, P. (1997). Species assemblages and indicator species: The need for a flexible asymmetrical approach. Ecol. Monogr. 67, 345–366.
Esse, C., Correa-Araneda, F., Acuña, C., Santander-Massa, R., De Los Ríos-Escalante, P., Saavedra, P., et al. (2021). Structure, diversity, and environmental determinants of high-latitude threatened conifer forests. Forests 12:775. doi: 10.3390/f12060775
Falk, D. A., van Mantgem, P. J., Keeley, J. E., Gregg, R. M., Guiterman, C. H., Tepley, A. J., et al. (2022). Mechanisms of forest resilience. Forest Ecol. Manag. 512:120129. doi: 10.1016/j.foreco.2022.120129
Fenton, N. J., Béland, C., De Blois, S., and Bergeron, Y. (2007). Sphagnum establishment and expansion in black spruce (Picea mariana) boreal forests. Can. J. Bot. 85, 43–50. doi: 10.1139/b06-148
Franklin, C. M. A., Harper, K. A., and Clarke, M. J. (2021). Trends in studies of edge influence on vegetation at human-created and natural forest edges across time and space. Can. J. For. Res. 51, 274–282. doi: 10.1139/cjfr-2020-0308
Galindo, N., Bannister, J. R., and Laage, K. (2021). Monitoreo a corto y largo plazo en ensayos de restauración de la conífera longeva y de lento crecimiento Pilgerodendron uviferum. Bosque (Valdivia) 42, 217–229. doi: 10.4067/S0717-92002021000200217
Garreaud, R. D. (2018). Record-breaking climate anomalies lead to severe drought and environmental disruption in western Patagonia in 2016. Clim. Res. 74, 217–229.
Garreaud, R., Lopez, P., Minvielle, M., and Rojas, M. (2013). Large-scale control on the patagonian climate. J. Clim. 26, 215–230. doi: 10.1175/JCLI-D-12-00001.1
Gelman, A., Vehtari, A., Simpson, D., Margossian, C. C., Carpenter, B., Yao, Y., et al. (2020). Bayesian workflow. arXiv [Preprint]. arXiv: 2011.01808.
Gengarelly, L. M., and Lee, T. D. (2005). The role of microtopography and substrate in survival and growth of Atlantic white-cedar seedlings. Forest Ecol. Manag. 212, 135–144. doi: 10.1016/j.foreco.2005.03.009
González, M. E., Amoroso, M., Lara, A., Veblen, T. T., Donoso, C., Kitzberger, T., et al. (2014). “Ecologia de disturbios y su influencia en los bosques templados de Chile y Argentina,” in Ecología forestal: Bases para el manejo sustentable y conservación de los bosques nativos de Chile, eds C. Donoso, M. González, and A. Lara (Valdivia: Ediciones Universidad Austral de Chile), 411–502.
Goodrich, J. P., Campbell, D. I., and Schipper, L. A. (2017). Southern Hemisphere bog persists as a strong carbon sink during droughts. Biogeosciences 14, 4563–4576. doi: 10.5194/bg-14-4563-2017
Grolemund, G., and Wickham, H. (2011). Dates and times made easy with lubridate. J. Stat. Softw. 40, 1–25.
Grubb, P. J. (1977). The maintenance of species-richness in plant communities: The importance of the regeneration niche. Biol. Rev. 52, 107–145.
Hartshorn, A. S., Southard, R. J., and Bledsoe, C. S. (2003). Structure and function of peatland-forest ecotones in southeastern alaska. Soil Sci. Soc. Am. J. 67:1572. doi: 10.2136/sssaj2003.1572
Heijmans, M. M. P. D., van der Knaap, Y. A. M., Holmgren, M., and Limpens, J. (2013). Persistent versus transient tree encroachment of temperate peat bogs: Effects of climate warming and drought events. Glob Change Biol. 19, 2240–2250. doi: 10.1111/gcb.12202
Holmgren, M., Lin, C.-Y., Murillo, J. E., Nieuwenhuis, A., Penninkhof, J., Sanders, N., et al. (2015). Positive shrub-tree interactions facilitate woody encroachment in boreal peatlands. J. Ecol. 103, 58–66. doi: 10.1111/1365-2745.12331
Holz, A. C. (2009). Climatic and human influences on fire regimes and forest dynamics in temperate rainforests in southern Chile. Boulder, CO: University of Colorado.
Holz, A., and Veblen, T. T. (2009). Pilgerodendron uviferum: The Southernmost tree-ring fire recorder species. Ecoscience 16, 322–329. doi: 10.2980/16-3-3262
Holz, A., and Veblen, T. T. (2011). The amplifying effects of humans on fire regimes in temperate rainforests in western Patagonia. Palaeogeogr. Palaeoclimatol. Palaeoecol. 311, 82–92. doi: 10.1016/j.palaeo.2011.08.008
Holz, A., Haberle, S., Veblen, T. T., De Pol-Holz, R., and Southon, J. (2012a). Fire history in western Patagonia from paired tree-ring fire-scar and charcoal records. Clim. Past 8, 451–466. doi: 10.5194/cp-8-451-2012
Holz, A., Hart, S. J., Williamson, G. J., Veblen, T. T., and Aravena, J. C. (2018). Radial growth response to climate change along the latitudinal range of the world’s southernmost conifer in southern South America. J. Biogeogr. 45, 1140–1152. doi: 10.1111/jbi.13199
Holz, A., Kitzberger, T., Paritsis, J., and Veblen, T. T. (2012b). Ecological and climatic controls of modern wildfire activity patterns across southwestern South America. Ecosphere 3:art103. doi: 10.1890/ES12-00234.1
Holz, A., Méndez, C., Borrero, L., Prieto, A., Torrejón, F., and Maldonado, A. (2016). Fires: The main human impact on past environments in Patagonia? Past Glob. Change Magazine 24, 72–73. doi: 10.22498/pages.24.2.72
Holz, A., Veblen, T. T., Taylor-Rodriguez, D., Zaret, K., and Paritsis, J. (in review). Short-interval anthropogenic burning and drying erodes the resilience of Gondwanan edemic conifer in temperate rainforests in western Patagonia.
Holz, A., Wood, S. W., Veblen, T. T., and Bowman, D. M. J. S. (2015). Effects of high-severity fire drove the population collapse of the subalpine Tasmanian endemic conifer Athrotaxis cupressoides. Glob. Change Biol. 21, 445–458. doi: 10.1111/gcb.12674
Hörnberg, G., Ohlson, M., and Zackrisson, O. (1997). Influence of bryophytes and microrelief conditions on Picea abies seed regeneration patterns in boreal old-growth swamp forests. Can. J. For. Res. 27, 1015–1023. doi: 10.1139/x97-045
Huber, U. M., and Markgraf, V. (2003). European impact on fire regimes and vegetation dynamics at the steppe-forest ecotone of southern Patagonia. Holocene 13, 567–579. doi: 10.1191/0959683603hl647rp
Iturraspe, R. J., and Urciuolo, A. B. (2021). “The ecosystem services provided by peatlands in patagonia,” in Ecosystem services in patagonia, natural and social sciences of patagonia, eds P. L. Peri, G. Martínez Pastur, and L. Nahuelhual (Cham: Springer International Publishing), 155–186.
Jones, H. G. (1992). Plants and microclimate: A quantitative approach to environmental plant physiology, 2nd Edn. Cambridge: Cambridge University Press.
Joosten, H. (2016). “Peatlands Across the Globe,” in Peatland restoration and ecosystem services, ed. A. Bonn, T. Allott, M. Evans, H. Joosten and R. Stoneman (Cambridge: Cambridge University Press), 19–43.
Kitzberger, T., Perry, G., Paritsis, J., Gowda, J., Tepley, A., Holz, A., et al. (2016). Fire–vegetation feedbacks and alternative states: Common mechanisms of temperate forest vulnerability to fire in southern South America and New Zealand. New Zealand J. Bot. 54, 247–272. doi: 10.1080/0028825X.2016.1151903
Kroiss, S. J., and HilleRisLambers, J. (2015). Recruitment limitation of long-lived conifers: Implications for climate change responses. Ecology 96, 1286–1297. doi: 10.1890/14-0595.1
Lemoine, N. P. (2019). Moving beyond noninformative priors: Why and how to choose weakly informative priors in Bayesian analyses. Oikos 128, 912–928. doi: 10.1111/oik.05985
León, C. A., Gabriel, M., Rodríguez, C., Iturraspe, R., Savoretti, A., Pancotto, V., et al. (2021). Peatlands of Southern South America: A review. Mires Peat 27, 1–29. doi: 10.19189/MaP.2020.SNPG.StA.2021
León, C. A., Oliván-Martínez, G., Oliván-Martínez, G., Larraín, J., Larraín, J., Vargas, R., et al. (2016). Patterns of bryophyte and lichen diversity in bogs and Tepualia stipularis forests of Northern Patagonia (Chile): Evidence of a novel ecosystem in southern South America. Bot. Sci. 94:441. doi: 10.17129/botsci.555
Lindenmayer, D. B., Hobbs, R. J., Likens, G. E., Krebs, C. J., and Banks, S. C. (2011). Newly discovered landscape traps produce regime shifts in wet forests. Proc. Natl. Acad. Sci. U.S.A. 108, 15887–15891. doi: 10.1073/pnas.1110245108
Lovell, R. S. L., Collins, S., Martin, S. H., Pigot, A. L., and Phillimore, A. B. (2023). Space-for-time substitutions in climate change ecology and evolution. Biol. Rev. 98, 2243–2270. doi: 10.1111/brv.13004
Luebert, F., and Pliscoff, P. (2017). Sinopsis bioclimática y vegetacional de Chile. Segunda edición. Santiago: Editorial Universitaria.
Mansilla, C. A. (2008). Reclutamiento post-disturbios de Ciprés de las Gauitecas (Pilgerodendron uviferum (D. Don) Florin) en comunidades de turbera, península de Brunswick, Región de Magallanes, Chile. Punta Arenas: Universidad de Magallanes.
McCune, B., and Mefford, M. J. (2011). PC-ORD, multivariate analysis of ecological data. (Version 6.22.) [Computer program]. Gleneden Beach: MjM Software.
McDowell, N. G., Allen, C. D., Anderson-Teixeira, K., Aukema, B. H., Bond-Lamberty, B., Chini, L., et al. (2020). Pervasive shifts in forest dynamics in a changing world. Science 368:eaaz9463. doi: 10.1126/science.aaz9463
Morris, P. J., Swindles, G. T., Valdes, P. J., Ivanovic, R. F., Gregoire, L. J., Smith, M. W., et al. (2018). Global peatland initiation driven by regionally asynchronous warming. Proc. Natl. Acad. Sci. U.S.A. 115, 4851–4856. doi: 10.1073/pnas.1717838115
Muth, C., Oravecz, Z., and Gabry, J. (2018). User-friendly Bayesian regression modeling: A tutorial with rstanarm and shinystan. TQMP 14, 99–119. doi: 10.20982/j.foreco.2018.02.020
Odion, D. C., Moritz, M. A., and DellaSala, D. A. (2010). Alternative community states maintained by fire in the Klamath Mountains. USA Fire alternative community states. J. Ecol. 98, 96–105. doi: 10.1111/j.1365-2745.2009.01597.x
Ogle, D. H., Doll, J. C., Wheeler, P., and Dinno, A. (2022). FSA: Fisheries stock analysis. R package version 0.9.3.
Oliveras, I., and Malhi, Y. (2016). Many shades of green: The dynamic tropical forest–savannah transition zones. Philos. Trans. R. Soc. B Biol. Sci. 371:20150308. doi: 10.1098/rstb.2015.0308
Pacé, M., Fenton, N. J., Paré, D., and Bergeron, Y. (2018). Differential effects of feather and Sphagnum spp. mosses on black spruce germination and growth. Forest Ecol. Manag. 41, 10–18. doi: 10.1016/j.foreco.2018.02.020
Paritsis, J., Veblen, T. T., and Holz, A. (2015). Positive fire feedbacks contribute to shifts from Nothofagus pumilio forests to fire-prone shrublands in Patagonia. J. Veg. Sci. 26, 89–101. doi: 10.1111/jvs.12225
Pausas, J. G., and Bond, W. J. (2020). Alternative biome states in terrestrial ecosystems. Trends Plant Sci. 25, 250–263. doi: 10.1016/j.tplants.2019.11.003
Pausas, J. G., and Keeley, J. E. (2021). Wildfires and global change. Front. Ecol. Environ. 19:387–395. doi: 10.1002/fee.2359
Peck, J. (2010). Multivariate analysis for community ecologists: Step-by-step using PC-ORD. Gleneden Beach: MjM Software Design.
Perez-Quezada, J. F., Trejo, D., Lopatin, J., Aguilera, D., Osborne, B., Galleguillos, M., et al. (2023). Drivers of ecosystem water use efficiency in a temperate rainforest and a peatland in southern South America. Biodiversity and ecosystem function: Terrestrial. EGUsphere [Preprint]. doi: 10.5194/egusphere-2023-1932
Peters, D. P. C., Gosz, J. R., Pockman, W. T., Small, E. E., Parmenter, R. R., Collins, S. L., et al. (2006). Integrating patch and boundary dynamics to understand and predict biotic transitions at multiple scales. Landsc. Ecol. 21, 19–33. doi: 10.1007/s10980-005-1063-3
Pfeiffer, M., Mascayano, C., and Aburto, F. (2010). Soils of Chilean patagonia in glacial and periglacial environments. Eurasian Soil Sci. 43, 1430–1438. doi: 10.1134/S106422931013003X
Piper, F. I., Hoch, G., and Fajardo, A. (2019). Revisiting the relative growth rate hypothesis for gymnosperm and angiosperm species co-occurrence. Am. J. Bot. 106, 101–112. doi: 10.1002/ajb2.1221
R Core Team (2021). R: A language and environment for statistical computing. Vienna: R Foundation for Statistical Computing.
Ratajczak, Z., Carpenter, S. R., Ives, A. R., Kucharik, C. J., Ramiadantsoa, T., Stegner, M. A., et al. (2018). Abrupt change in ecological systems: Inference and diagnosis. Trends Ecol. Evol. 33, 513–526. doi: 10.1016/j.tree.2018.04.013
Ratcliffe, J. L., Creevy, A., Andersen, R., Zarov, E., Gaffney, P. P. J., Taggart, M. A., et al. (2017). Ecological and environmental transition across the forested-to-open bog ecotone in a west Siberian peatland. Sci. Total Environ. 607–608, 816–828. doi: 10.1016/j.scitotenv.2017.06.276
Risser, P. G. (1995). The status of the science examining ecotones. BioScience 45, 318–325. doi: 10.2307/1312492
Rodriguez, A. C. (2015). Hydrogeomorphic classification of mire ecosystems within the Baker and Pascua Basins in the Region Aysén, Chilean Patagonia: A tool for their assessment and monitoring. Berlin: Humboldt University.
Rovere, A. E., Premoli, A. C., and Newton, A. C. (2002). Estado de conservación del ciprés de las Guaitecas (Pilgerodendron uviferum (Don) Florín) en Argentina. Bosque 23, 11–19. doi: 10.4206/bosque.2002.v23n1-02
Roy, V., Ruel, J.-C., and Plamondon, A. P. (2000). Establishment, growth and survival of natural regeneration after clearcutting and drainage on forested wetlands. Forest Ecol. Manag. 129, 253–267. doi: 10.1016/S0378-1127(99)00170-X
Ruxton, G. D., and Beauchamp, G. (2008). Time for some a priori thinking about post hoc testing. Behav. Ecol. 19, 690–693. doi: 10.1093/beheco/arn020
Scheffer, M., and Carpenter, S. R. (2003). Catastrophic regime shifts in ecosystems: Linking theory to observation. Trends Ecol. Evol. 18, 648–656. doi: 10.1016/j.tree.2003.09.002
Scheffer, M., Carpenter, S., Foley, J. A., Folke, C., and Walker, B. (2001). Catastrophic shifts in ecosystems. Nature 413, 591–596. doi: 10.1038/35098000
Scheffer, M., Hirota, M., Holmgren, M., Van Nes, E. H., and Chapin, F. S. (2012). Thresholds for boreal biome transitions. Proc. Natl. Acad. Sci. U.S.A. 109, 21384–21389. doi: 10.1073/pnas.1219844110
Seidl, R., and Turner, M. G. (2022). Post-disturbance reorganization of forest ecosystems in a changing world. Proc. Natl. Acad. Sci. U.S.A. 119, e2202190119. doi: 10.1073/pnas.2202190119
Simard, M., Lecomte, N., Bergeron, Y., and Bernier, P. Y. (2007). Forest productivity decline caused by successional Paludification of boreal soils. Ecol. Appl. 17, 1619–1637. doi: 10.1890/06-1795.1
Sommerfeld, A., Senf, C., Buma, B., D’Amato, A. W., Després, T., Díaz-Hormazábal, I., et al. (2018). Patterns and drivers of recent disturbances across the temperate forest biome. Nat. Commun. 9:4355. doi: 10.1038/s41467-018-06788-9
Soto, D. P., and Figueroa, H. (2008). Efectos de las alteraciones antrópicas sobre la estructura y composición de rodales de Pilgerodendron uviferum en la Cordillera de la Costa de Chile. Ecol. Austral 14:568.
Souto, C., Premoli, A., and Gardner, M. (2013). Pilgerodendron uviferum. The IUCN red list of threatened species 2013: e.T32052A2809552. doi: 10.2305/IUCN.UK.2013-1.RLTS.T32052A2809552.en
Suding, K. N., and Hobbs, R. J. (2009). Threshold models in restoration and conservation: A developing framework. Trends Ecol. Evol. 24, 271–279. doi: 10.1016/j.tree.2008.11.012
Szeicz, J. M., Haberle, S. G., and Bennett, K. D. (2003). Dynamics of North Patagonian rainforests from fine-resolution pollen, charcoal and tree-ring analysis, Chonos Archipelago, Southern Chile. Austral Ecol. 28, 413–422.
Tepley, A. J., Veblen, T. T., Perry, G. L. W., Stewart, G. H., and Naficy, C. E. (2016). Positive feedbacks to fire-driven deforestation following human colonization of the south island of New Zealand. Ecosystems 19, 1325–1344. doi: 10.1007/s10021-016-0008-9
Turetsky, M. R., Benscoter, B., Page, S., Rein, G., van der Werf, G. R., and Watts, A. (2014). Global vulnerability of peatlands to fire and carbon loss. Nat. Geosci. 8, 11–14. doi: 10.1038/ngeo2325
van der Velde, Y., Temme, A. J. A. M., Nijp, J. J., Braakhekke, M. C., van Voorn, G. A. K., Dekker, S. C., et al. (2021). Emerging forest–peatland bistability and resilience of European peatland carbon stores. Proc. Natl. Acad. Sci. U.S.A. 118:e2101742118. doi: 10.1073/pnas.2101742118
Vandekerkhove, E., Bertrand, S., Torrejón, F., Kylander, M. E., Reid, B., and Saunders, K. M. (2021). Signature of modern glacial lake outburst floods in fjord sediments (Baker River, southern Chile). Sedimentology 68, 2798–2819. doi: 10.1111/sed.12874
Veblen, T. T., Burns, B. R., Kitzberger, T., Lara, A., and Villalba, R. (1995). “The ecology of the conifers of Southern South America,” in Ecology of the Southern conifers, eds N. J. Enright and R. S. Hill (Melbourne, VI: Melbourne University Press), 120–155.
Waddington, J. M., Morris, P. J., Kettridge, N., Granath, G., Thompson, D. K., and Moore, P. A. (2014). Hydrological feedbacks in northern peatlands. Ecohydrology 8, 113–127. doi: 10.1002/eco.1493
Walker, L. R., Wardle, D. A., Bardgett, R. D., and Clarkson, B. D. (2010). The use of chronosequences in studies of ecological succession and soil development: Chronosequences, succession and soil development. J. Ecol. 98, 725–736. doi: 10.1111/j.1365-2745.2010.01664.x
Whitlock, C., McWethy, D. B., Tepley, A. J., Veblen, T. T., Holz, A., McGlone, M. S., et al. (2015). Past and present vulnerability of closed-canopy temperate forests to altered fire regimes: A comparison of the pacific northwest, New Zealand, and Patagonia. BioScience 65, 151–163. doi: 10.1093/biosci/biu194
Wickham, H., François, R., Henry, L., and Müller, K. (2021). dplyr: A grammar of data manipulation. R package version 1.0.7.
Wilson, J. B., and Agnew, A. D. Q. (1992). Positive-feedback switches in plant communities. Adv. Ecol. Res. 23, 263–336. doi: 10.1016/S0065-2504(08)60149-X
Wood, S. W., and Bowman, D. M. J. S. (2012). Alternative stable states and the role of fire–vegetation–soil feedbacks in the temperate wilderness of southwest Tasmania. Landsc. Ecol. 27, 13–28. doi: 10.1007/s10980-011-9677-0
Yarrow, M. M., and Marín, V. H. (2007). Toward conceptual cohesiveness: A historical analysis of the theory and utility of ecological boundaries and transition zones. Ecosystems 10, 462–476. doi: 10.1007/s10021-007-9036-9
Zaret, K. (2011). Distribution, use and cultural meanings of ciprés de las Guaitecas in the vicinity of Caleta Tortel, Chile. Missoula, MT: University of Montana.
Keywords: Pilgerodendron uviferum, ciprés de las Guaitecas, post-reburn establishment, Sphagnum, Western Patagonia, temperate rainforests, anthropogenic burning
Citation: Zaret K and Holz A (2024) Exploration of large-scale vegetation transition in wet ecosystems: a comparison of conifer seedling abundance across burned vs. unburned forest-peatland ecotones in Western Patagonia. Front. For. Glob. Change 7:1385506. doi: 10.3389/ffgc.2024.1385506
Received: 12 February 2024; Accepted: 22 July 2024;
Published: 07 August 2024.
Edited by:
Margarita Arianoutsou, National and Kapodistrian University of Athens, GreeceReviewed by:
Panayiotis G. Dimitrakopoulos, University of the Aegean, GreeceFrancisco Castro Rego, University of Lisbon, Portugal
Copyright © 2024 Zaret and Holz. This is an open-access article distributed under the terms of the Creative Commons Attribution License (CC BY). The use, distribution or reproduction in other forums is permitted, provided the original author(s) and the copyright owner(s) are credited and that the original publication in this journal is cited, in accordance with accepted academic practice. No use, distribution or reproduction is permitted which does not comply with these terms.
*Correspondence: Kyla Zaret, kzaret@pdx.edu