- 1Department of Geoscience, Soil Science and Geomorphology, Institute of Geography, University of Tübingen, Tübingen, Germany
- 2Nees Institute for Biodiversity of Plants, University of Bonn, Bonn, Germany
Forests cover one-third of the global land and are important components of carbon and nitrogen cycling. Anthropogenic disturbances, such as forest road systems or skid trails for timber harvesting, can dramatically change the nutrient cycling in these ecosystems. Skid trails increase soil erosion and thus the displacement of soil organic carbon (SOC) and total nitrogen (Nt). Additionally, runoff transports high amounts of dissolved organic carbon (DOC), which can have a negative impact on aquatic ecosystems. One of the most important countermeasures against soil erosion is the quick recolonization of vegetation. To date, the extent to which natural vegetation succession influences the relocation of SOC and Nt, and in particular the role of mosses in this context, has not been well investigated. This study investigates the influence of natural vegetation succession and in particular of mosses on the displacement process of SOC and Nt as well as DOC caused by soil erosion. To this end, we combine the results of a field study using in-situ rainfall simulations with small-scale runoff plots in skid trails of the Schönbuch Nature Park in southwestern Germany with the results of ex-situ rainfall simulation experiments with infiltration boxes containing the substrate from the respective skid trails. The eroded sediments of skid trails were on average enriched in SOC by 16% and in Nt by 35% compared to the original soil, which lead to a decrease of the C/N ratio in sediments. As vegetation succession progressed, the displacement of SOC and Nt was reduced, confirmed by a negative correlation between the enrichment ratios of SOC (ERSOC), Nt and total vegetation cover. However, mosses tended to reduce ERSOC more than vascular plants. Additionally, mosses significantly decreased DOC concentration in surface runoff compared to bare soils, while no difference in DOC concentration in percolated water could be observed. Future research should explore the role of mosses in the storage of SOC and Nt in the soil and their impact on soil stability. Thus, utilizing mosses could potentially minimize environmental impacts from soil disturbances in forests.
1 Introduction
Worldwide, approximately 2,157 to 2,293 Pg of carbon are stored in the top 100 cm of the soil, making soil the largest terrestrial carbon sink, of which about 1,462 to 1,548 Pg is organic carbon (Batjes, 1996). Soil organic carbon (SOC) plays an essential role in food security and climate change mitigation (Lal, 2004) and there is a huge potential for carbon sequestration with the help of sustainable soil management strategies (Batjes, 2019; FAO, 2022). Additionally, SOC improves the availability of nutrients in the soil (Tiessen et al., 1994; Gerke, 2022), therefore SOC and nitrogen (N) are positively linked, meaning that an increase in SOC improves the availability of N (Oldfield et al., 2019), and land use is a key factor for the amount of SOC and N stored in an ecosystem (Beillouin et al., 2023; Kim et al., 2023). For example, forests cover 31% of the global land (FAO, 2020) and store a total of 662 Pg of carbon, of which 45% is SOC (FAO, 2020). However, anthropogenic activities, such as logging and land-use changes, can disturb the delicate equilibrium of these forest ecosystems, leading to alterations in soil properties and nutrient dynamics (Pan et al., 2011; Wei et al., 2014; Wilson et al., 2021). Such forest disturbances are particularly susceptible to soil erosion (Zemke, 2016; Gall et al., 2022), as the forest floor and also its protective layer of coniferous and deciduous litter or understorey vegetation is destroyed when heavy machinery is used for timber harvesting (Picchio et al., 2020). When creating skid trails, for example, the average increase in sediment discharge on bare wheel tracks can be 22 times higher than on the undisturbed forest floor, as demonstrated by erosion from 30-min rainfall simulations with an intensity of 45 mm h−1 (Gall et al., 2022).
Water erosion also has a major influence on the distribution of SOC and N (Lal, 2003; Holz and Augustin, 2021). Lal (2003) estimated that 4.0–6.0 Pg of SOC is relocated globally due to water erosion under the assumption of a delivery ratio of 10% (proportion of sediment produced over land that enters a major river system (Walling and Webb, 1983)), a sediment load of 20 billion Mg, and SOC content of 2–3%. Moreover, in a meta-study, Holz and Augustin (2021) showed that eroded sediments contain on average 51.3% more SOC and 50.6% more N compared to the original soil, which clearly changes the C and N dynamics along a slope. In general, the displacement of SOC and N depends on the grain size distribution and the initial contents of SOC and N in the original soil (Alberts et al., 1983; Koiter et al., 2017; Hofbauer et al., 2023). Fine-grained soil particles are preferentially transported by water erosion, whereby this effect of particle size selectivity is greater with low rainfall intensities and higher slope gradients (Sun et al., 2023) and is also influenced by further original soil properties such as soil moisture and vegetation cover (Koiter et al., 2017). As fine-grained soil particles usually bind more SOC and N, displaced sediments are often enriched in SOC and N compared to the original soil (Yang et al., 2014; Koiter et al., 2017), although a high initial content of SOC in the soil tends to have an erosion-reducing effect (Hofbauer et al., 2023). In order to determine the loss of SOC and N due to soil erosion, enrichment ratios (ER) are generally used in literature, which indicate the proportion of nutrients in the sediment to the proportion in the original soil (Massey and Jackson, 1952; Yang et al., 2014; Holz and Augustin, 2021).
Revegetation is an important measure to prevent soil erosion, which also applies to forest skid trails (Zemke, 2016; McEachran et al., 2018). However, the type of vegetation plays a decisive role here, as Gall et al. (2022) found, for example, that bryophytes tend to reduce soil erosion in skid trails to a greater extent than a vascular plant layer. Logically, the erosion-reducing effect of vegetation also reduces the displacement of SOC and N, but few studies have measured this relationship. For example, Liang et al. (2021) showed that the transport of SOC due to erosion along a slope was significantly reduced by vegetation restoration. Furthermore, Gou et al. (2022) presented in a Revised Universal Soil Loss Equation (RUSLE)-based modelling approach that the displacement of SOC follows similar patterns as soil erosion, such that vegetation restoration has reduced the displacement of SOC in the study area on the Loess Plateau. However, it is still unclear to what extent SOC and N are displaced in skid trails due to soil erosion and how this process is influenced or mitigated by natural succession. Of particular interest in this context is the influence of bryophytes, which are known to fix large amounts of SOC in the soil and thus contribute to soil stability (Kasimir et al., 2021; Xiao et al., 2023).
During erosion processes, organic carbon is not only transported with sediments but also with runoff as dissolved organic carbon (DOC). DOC plays a major role in the displacement of SOC by water erosion (He et al., 2023). For example, Manninen et al. (2023) discovered that in a clayey agricultural soil, 35% of organic carbon and 20% of N are discharged with sediment, while the remaining much larger proportion is transported in dissolved forms. As a result, not only are large quantities of SOC lost in terrestrial ecosystems, leading to a reduction in soil quality (Hua et al., 2014; Manninen et al., 2023), but they are also introduced into aquatic ecosystems, where they can have serious consequences for freshwater biota and drinking water quality (Evans et al., 2005).
This study aims to quantify the displacement of SOC and total nitrogen (Nt) by water erosion in skid trails of a temperate forest and how it is affected by natural vegetation succession driven by mosses. In this context, the impact of mosses on the displacement processes were surveyed over the course of 1 year using in-situ rainfall simulations with micro-scale runoff plots. Furthermore, the study investigates how moss covers affect DOC fluxes with generating runoff and percolation in forest soil substrates of the same ecosystem by performing an ex-situ rainfall simulation. Overall, we tested the following hypotheses:
1. The relocation of SOC and Nt by water erosion in forest skid trails is reduced due to vegetation succession and moss-dominated vegetation shows a stronger impact than vascular vegetation (in-situ experiment).
2. The displacement of SOC caused by erosion and percolation through forest soil substrates is reduced due to moss cover (ex-situ experiment).
2 Methodology
2.1 Experimental setup
The present study utilizes rainfall simulations to analyse the relocation of SOC and Nt in eroded sediments of disturbed forest soils and DOC in surface runoff and percolated water. For this purpose, data of two individual experiments were analysed: An in-situ rainfall simulation in skid trails (Gall et al., 2022) and an ex-situ rainfall simulation with soil substrates from the same skid trails (Gall et al., 2023).
This study was conducted in the Schönbuch Nature Park in southwestern Germany, located in Triassic hills with sandstones, marlstones, and claystones, as well as some Lower Jurassic shales and limestones, whereby the Lower Jurassic plateaus are often covered by a loess layer (Einsele and Agster, 1986). Schönbuch Nature Park, with its highest peak at 583 meters (Bromberg), is a low-altitude, hilly (69% with slopes ≤3° and 14% with slopes >15°), and predominantly forested (86%) area in the sub-Atlantic temperate climate zone (Arnold, 1986; Einsele and Agster, 1986). The mean annual temperature is 8.3°C, with an average precipitation of 740 mm (mean annual values from 1979 to 1984 at the Herrenberg climate station; DWD Climate Data Center (2021c)), which is similar to Germany’s long-term average (DWD Climate Data Center, 2021a, 2021b). We selected four skid trails in the Schönbuch Nature Park, which differ in terms of parent material, soil properties and vegetation characteristics and distinguished them according to the geological formation of their parent material: Angulatensandstein (AS), Psilonotenton (PT), Löwenstein (LS) and Trossingen (TS; Einsele and Agster, 1986). The skid trails are described in detail in Gall et al. (2022).
For our in-situ experiment, we conducted rainfall simulations with micro-scale runoff plots (ROP, 0.4 × 0.4 m; Seitz (2015)) at 4 times (March 2019, July 2019, October 2019, and February 2020) with different degrees of soil cover of vascular plants and mosses resulting from natural succession in the skid trails. A total of 32 ROPs were installed, with 8 ROPs per skid trail, repeating 4 in wheel tracks and 4 in centre tracks; due to the 4 measurement times, 128 rainfall simulations were obtained. In the immediate vicinity of the individual ROPs, soil samples of the topsoil (1–2 cm depth) were taken in July 2019 as a reference for the original soil (Figure 1).
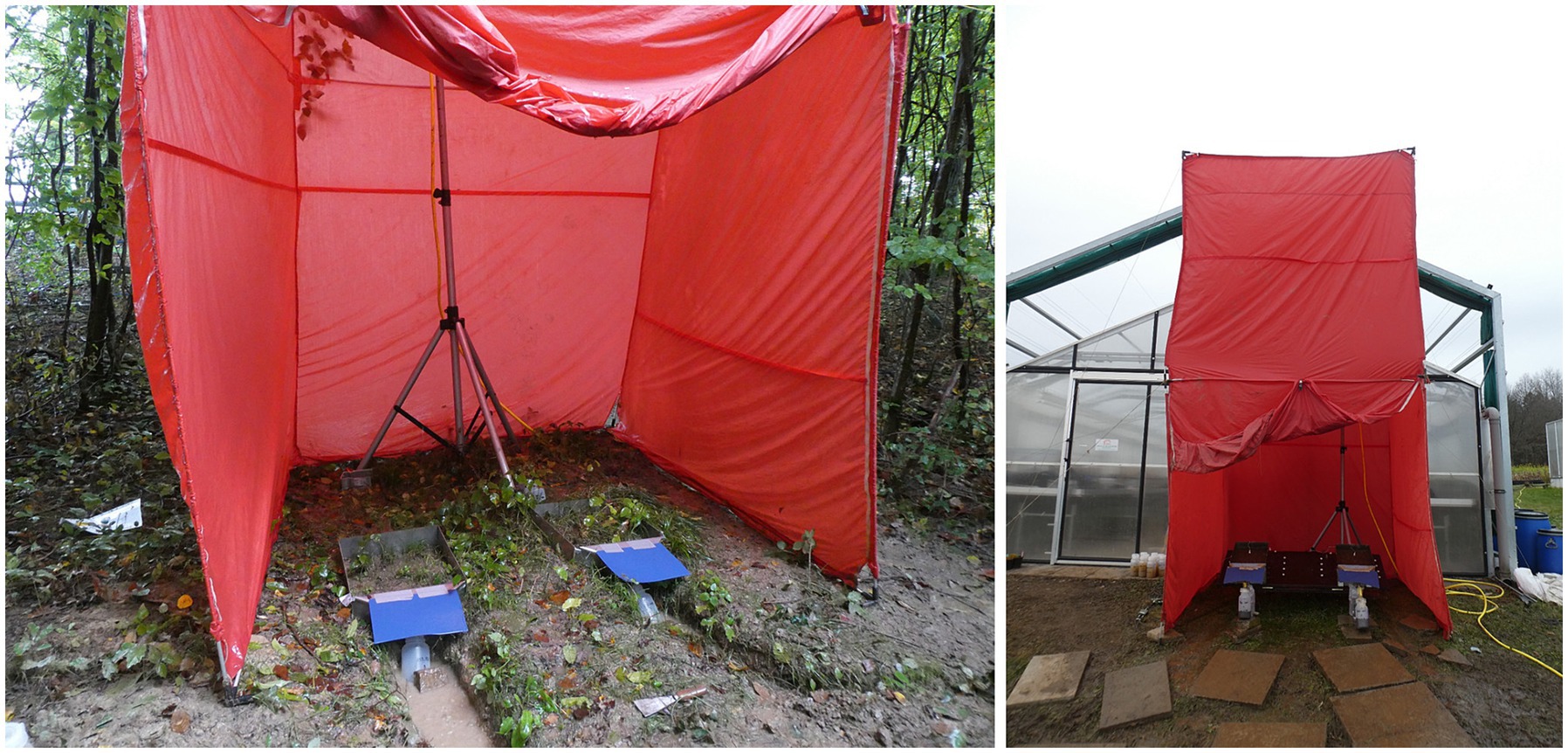
Figure 1. In-situ rainfall simulation in the skid trails (left) and ex-situ rainfall simulation with soil substrates in infiltration boxes (right).
For our ex-situ experiment, soil substrates were sampled from the topsoil of the four skid trails to a depth of 10 cm. Afterwards, they were air dried, sieved by 6.3 mm and filled into infiltration boxes (40 × 30 × 15 cm) up to a height of 6.5 cm by installing a substructure of perforated metal. The substructure served to reduce the time required for percolation, which would have taken more than an hour if the infiltration boxes had been completely filled with the clayey soil substrates. First, rainfall simulations were carried out for bare and air-dried substrates (bare&dry) and again 24 h later for wet substrates (bare&wet). Then moss samples were placed onto the substrates and stored in a shady place outside for 5 months to ensure adaption and growth. Detailed information on the selected moss species can be found in Thielen et al. (2021). Second, rainfall simulations were performed for dry moss treatments (moss&dry) and 24 h later for wet moss treatments (moss&wet). A total of 64 rainfall simulations were completed.
All rainfall simulations were realized with the Tübingen rainfall simulator (Iserloh et al., 2013; Seitz, 2015), which has a drop falling height of 3.5 m and was set to a mean rainfall intensity of 45 mm h−1 with a duration of 30 min. During rainfall simulations surface runoff, sediment (soil transported with the surface runoff) and—in case of the ex-situ experiment—percolated water were collected in 1 L sample bottles. For the ex-situ experiment, the amount of DOC in the liquid samples, previously filtered with a 0.45 μm filter, was determined using a total organic carbon analyzer (vario TOC cube, Elementar Analysensysteme GmbH, Langenselbold, Germany). SOC and Nt were measured in all sediment and soil samples with an elemental analyzer (element analyzer vario EL II, Elementar Analysensysteme GmbH, Langenselbold, Germany).
2.2 Data preparation
In order to compare SOC and Nt of the original soil with the eroded sediment, we used enrichment ratios (ER) that are commonly used in the literature (Massey and Jackson, 1952; Schiettecatte et al., 2008; Yang et al., 2014; Nie et al., 2015). The enrichment ratios of SOC (ERSOC) and Nt (ERN) were calculated as follows:
where SOCsediment is the total organic carbon [%] in the sediment, SOCsoil is the total organic carbon [%] in the original soil, Nsediment is the total nitrogen [%] in the sediment, and Nsoil is the total nitrogen [%] in the original soil.
In addition, we have determined the total SOC concentration (SOCcon) using the following formula:
where SOCtot is the total mass of organic carbon [mg] in the eroded sediment and runoff is the amount of surface runoff [L] by which the sediment was transported. We used this parameter for comparison with the DOC concentrations in surface runoff and percolation.
2.3 Data analysis
Data were analysed with R software version 4.0.4 (R Core Team, 2021). Normality was examined with Shapiro–Wilk test prior to all statistical tests, and homoscedasticity was verified with Levene’s test. As three sediment samples showed extreme SOC and Nt contents, we declared these as outliers and removed them from the dataset, as it was highly probable that organic material was measured in these cases. These outliers were also confirmed by the Rosner’s Outlier Test, even if the conditions for normality were not met. To test for significant differences, Kruskal-Wallis tests were conducted. The post hoc tests used were either Wilcoxon rank-sum tests for independent measurements or Wilcoxon signed-rank tests for related measurements (R package “stats”). For all mean values described, the standard deviation was also given (mean ± standard deviation). The datasets compiled and analysed in this study are available on figshare (Gall et al., 2024).
3 Results
3.1 Displacement of SOC and Nt due to water erosion in temperate forest skid trails
Overall, the original soil contains an average of 5.69 ± 1.93% SOC for all skid trails, while the sediment has an average SOC content of 6.28 ± 1.80%. Similarly, the average Nt content was higher in the sediment (0.39 ± 0.09%) compared with the original soil (0.30 ± 0.09%). In summary, the eroded sediment contained 16.46 ± 30.01% more SOC and 35.36 ± 33.88% more Nt compared with the original soil sample (Figure 2). Furthermore, we found that the C/N ratio was reduced by −13.48 ± 11.18% compared with the original soil. This means that the C/N ratio has decreased from an average of 18.82 ± 2.36 in the original soil to 16.17 ± 1.93 in the eroded sediment.
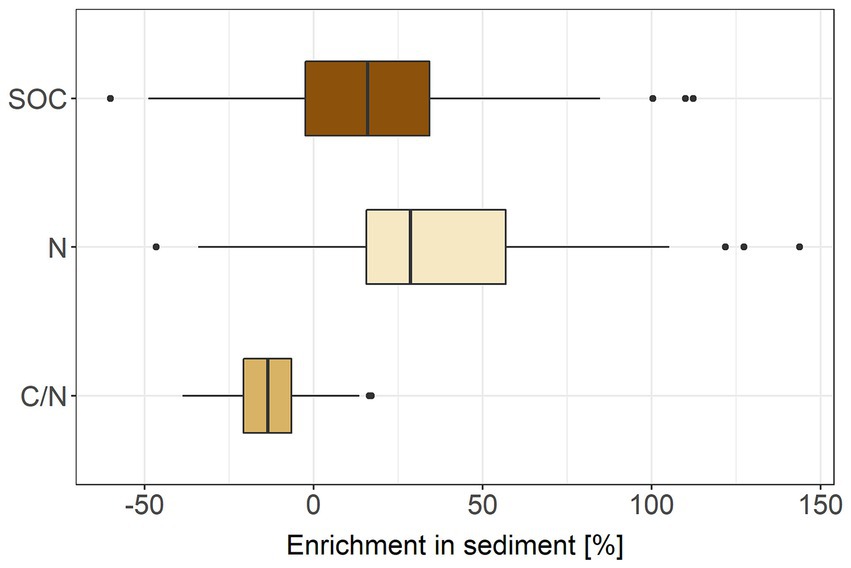
Figure 2. Enrichment of soil organic carbon (SOC), total nitrogen (Nt), and C/N ratio in the eroded sediment compared to the original soil. The lines inside the box show the median, and the bottom and the top of the box represent the third quartiles, while whiskers extend up to 1.5 times the interquartile range (IQR) of the data. Outliers are defined as more than 1.5 times the IQR and are displayed as dots.
3.2 Influence of natural succession on the displacement of SOC and Nt in skid trails of a temperate forests
In all skid trails, the ERSOC was higher than one. The highest average ERSOC was achieved with 1.28 ± 0.27 in AS, and the lowest with 1.01 ± 0.37 in TS, with the difference being significant (p < 0.01), where AS and TS refer to different sites of skid trails. On average for all skid trails, the ERSOC was highest in March 2019 (1.32 ± 0.30), when skid trails were newly established and mostly bare (7.47 ± 13.04%), with a significant difference to July 2019 (1.18 ± 0.36; p < 0.05), October 2019 (1.05 ± 0.27; p < 0.01) and February 2020 (1.12 ± 0.20; p < 0.01), when vegetation cover was significantly higher compared to March 2019 (54.69 ± 42.54%, 67.91 ± 40.23% and 57.41 ± 37.74%; all p < 0.01). The individual examination of the skid trails regarding ERSOC did not reveal any significant differences between the measurement times (Figure 3).
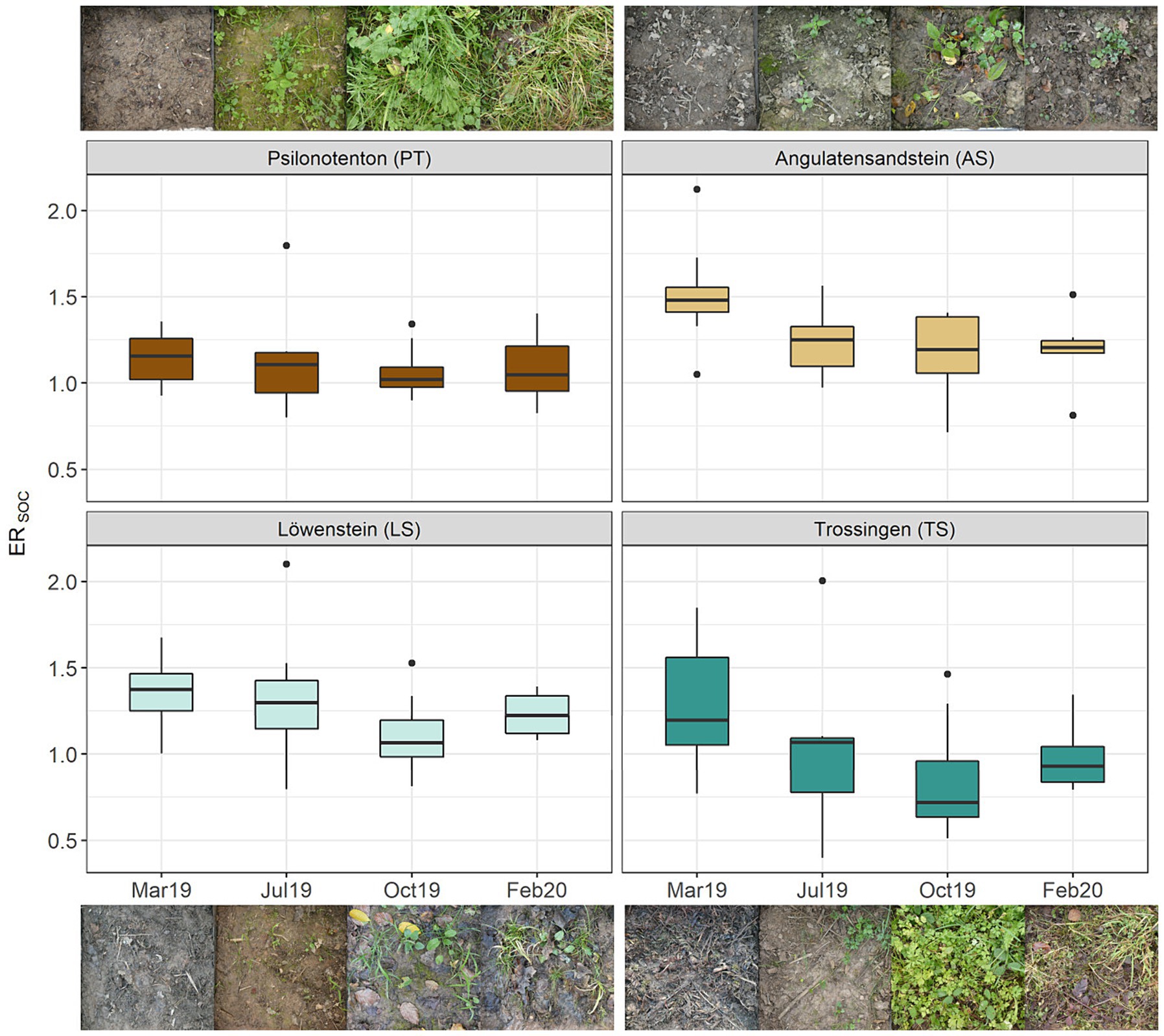
Figure 3. Enrichment ratio for soil organic carbon (ERSOC) in sediments at four times during natural succession in four skid trails (n = 8). The images show exemplary vegetation succession at the soil surface in the runoff plots of the wheel tracks. The lines inside the box show the median, and the bottom and the top of the box represent the third quartiles, while whiskers extend up to 1.5 times the interquartile range (IQR) of the data. Outliers are defined as more than 1.5 times the IQR and are displayed as dots.
Although ERN for the four skid trails are not explicitly presented here, they are congruent with the ERSOC values with significant differences between the measurement times in March 2019 (1.52 ± 0.36), July 2019 (1.29 ± 0.37, p < 0.001), October 2019 (1.30 ± 0.33, and p < 0.01), and February 2020 (1.31 ± 0.25, p < 0.001). In general, there is a strong positive correlation between ERSOC and ERN (Spearman’s correlation ρ = 0.87, p < 0.001). However, the correlation between ERSOC and ERN varies with regard to the four skid trails (Figure 4). In the skid trail AS, ERSOC and ERN exhibit the strongest correlation, while LS shows the weakest correlation.
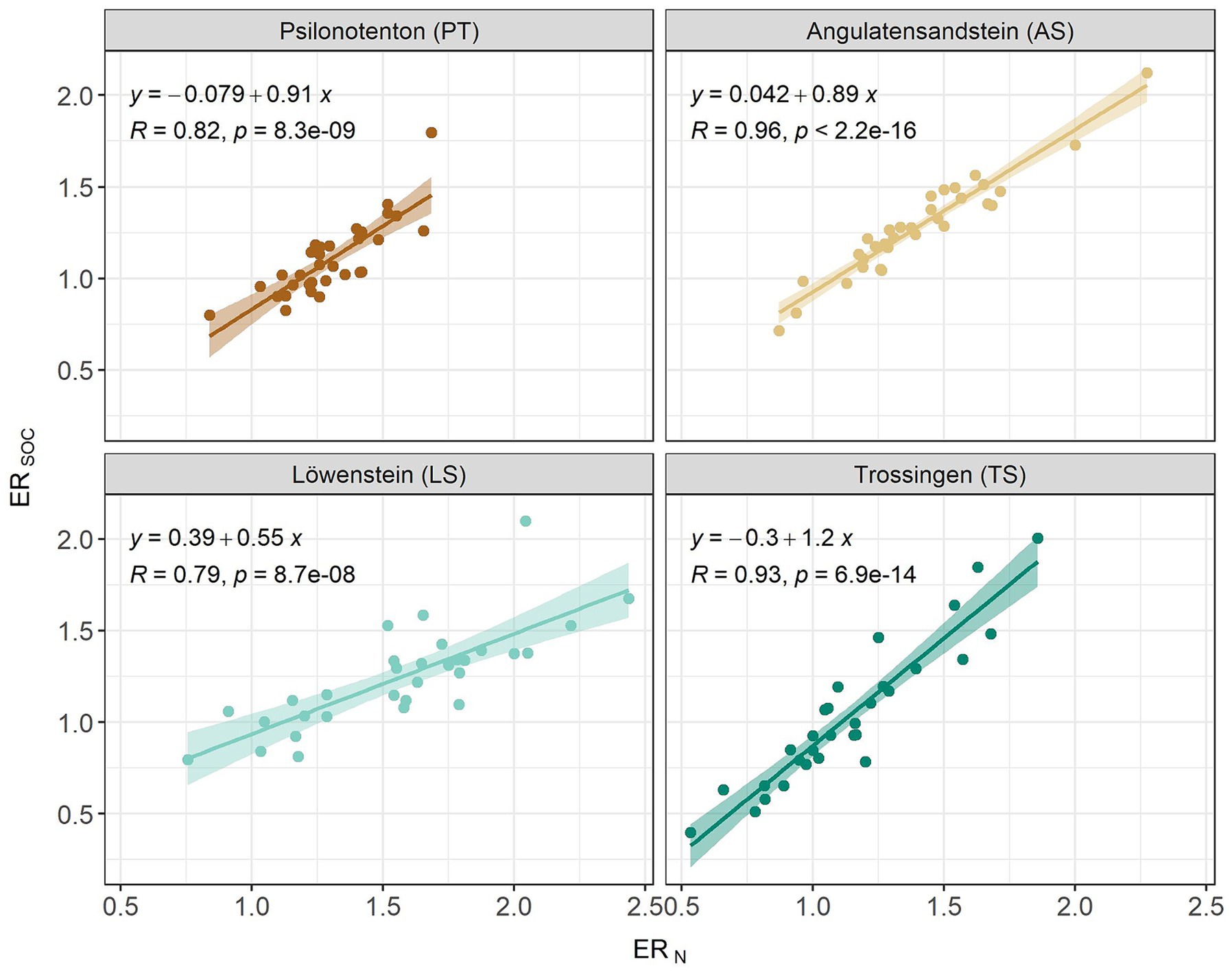
Figure 4. Scatterplots of enrichment ratio for soil organic carbon (ERSOC) and total nitrogen (ERN) with regression lines for four skid trails. The formulas of the regression lines, the Spearman correlation coefficient (R) and the corresponding p-values are shown.
The relationships between the vegetation characteristics and the displacement of SOC and Nt (Figure 5) demonstrate that both ERSOC and ERN have a significant negative correlation with the respective initial content of SOC and Nt in the original soil. Surprisingly, there is no significant correlation between ERSOC and ERN and sediment discharge, while SOC, Nt in the original soil and all vegetation parameters have a negative correlation with sediment discharge. Additionally, there is a slight negative correlation between ERSOC and total vegetation cover, with a slightly stronger negative correlation between ERSOC and moss cover compared to vascular plant cover. Moreover, we discovered a strong positive correlation between total SOC concentration and total vegetation cover.
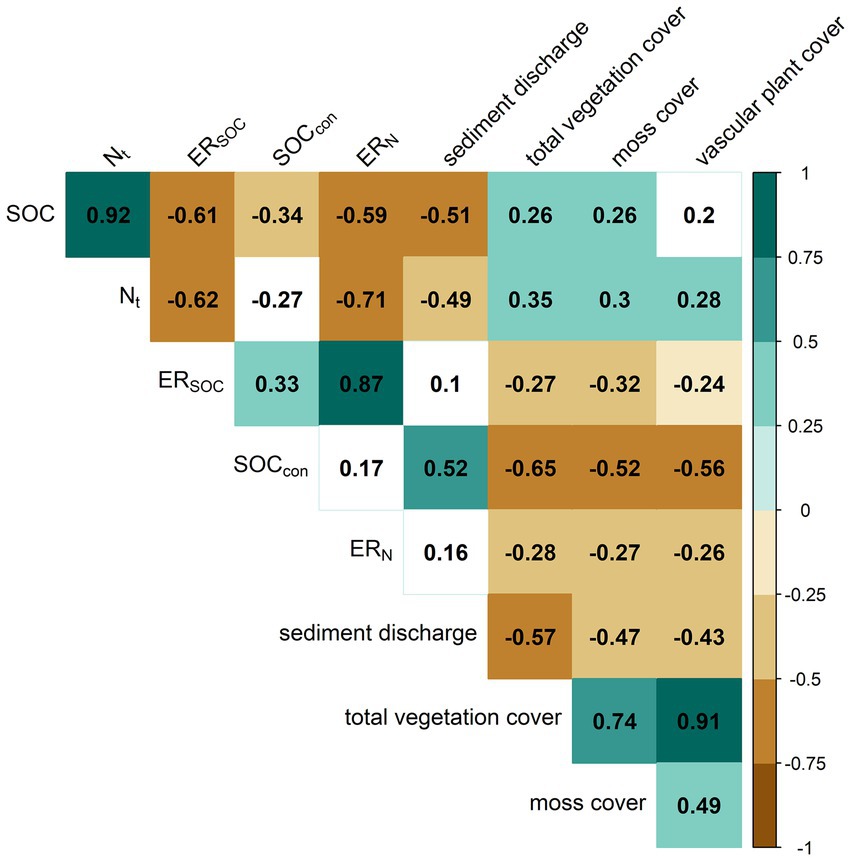
Figure 5. Correlation matrix for carbon and nitrogen parameters, sediment discharge, and vegetation parameters. Turquois colors indicate positive, brown colors negative correlations, and blank squares mean non-significant correlations (p < 0.05). SOC, total organic carbon in the original soil; Nt, total nitrogen in the original soil; ERSOC, enrichment ratio of soil organic carbon; SOCcon, total SOC concentration; ERN, enrichment ratio of total nitrogen; sediment discharge, sediment discharge; total vegetation cover, photogrammetrically derived total vegetation cover; moss cover, field estimated moss cover; vascular plant cover, photogrammetrically derived total vegetation cover—field estimated moss cover.
3.3 Influence of mosses on the displacement of SOC due to erosion and percolation through forest soil substrates
Considering all displacement pathways (runoff, sediment, and percolation) in the ex-situ experiment, the two bare treatments (bare&dry + bare&wet) had a factor of 5.5 higher displacement of SOC compared to the two moss treatments (moss&dry + moss&wet; p < 0.01). This difference is particularly large, since almost no sediment was produced in the moss treatments (Figure 6). Nevertheless, on average for all skid trails the DOC concentration in surface runoff was also significantly lower in moss treatments (14.43 ± 17.96 mg L−1) compared to bare treatments (173.26 ± 137.81 mg L−1, p < 0.01). While there was no difference between bare&dry and bare&wet treatments regarding DOC concentration in surface runoff, we found a significantly higher DOC concentration in surface runoff of moss&dry (23.10 ± 22.17 mg L−1) compared to moss&wet treatments (5.77 ± 3.84 mg L−1, p < 0.001). Regarding the average DOC concentration in percolated water there was no difference between bare (bare&dry + bare&wet) and moss (moss&dry + moss&wet) treatments. However, DOC concentration in percolated water was significantly higher in bare&wet (220.37 ± 117.37 mg L−1) compared to bare&dry (52.30 ± 26.91 mg L−1, p < 0.001), moss&dry (22.29 ± 11.84 mg L−1, p < 0.001), and moss&wet (13.89 ± 5.91 mg L−1, p < 0.001) treatments. Moreover, the difference between moss&dry and moss&wet was also significant (p < 0.05), whereby the direction of this effect was dependent on the substrate.
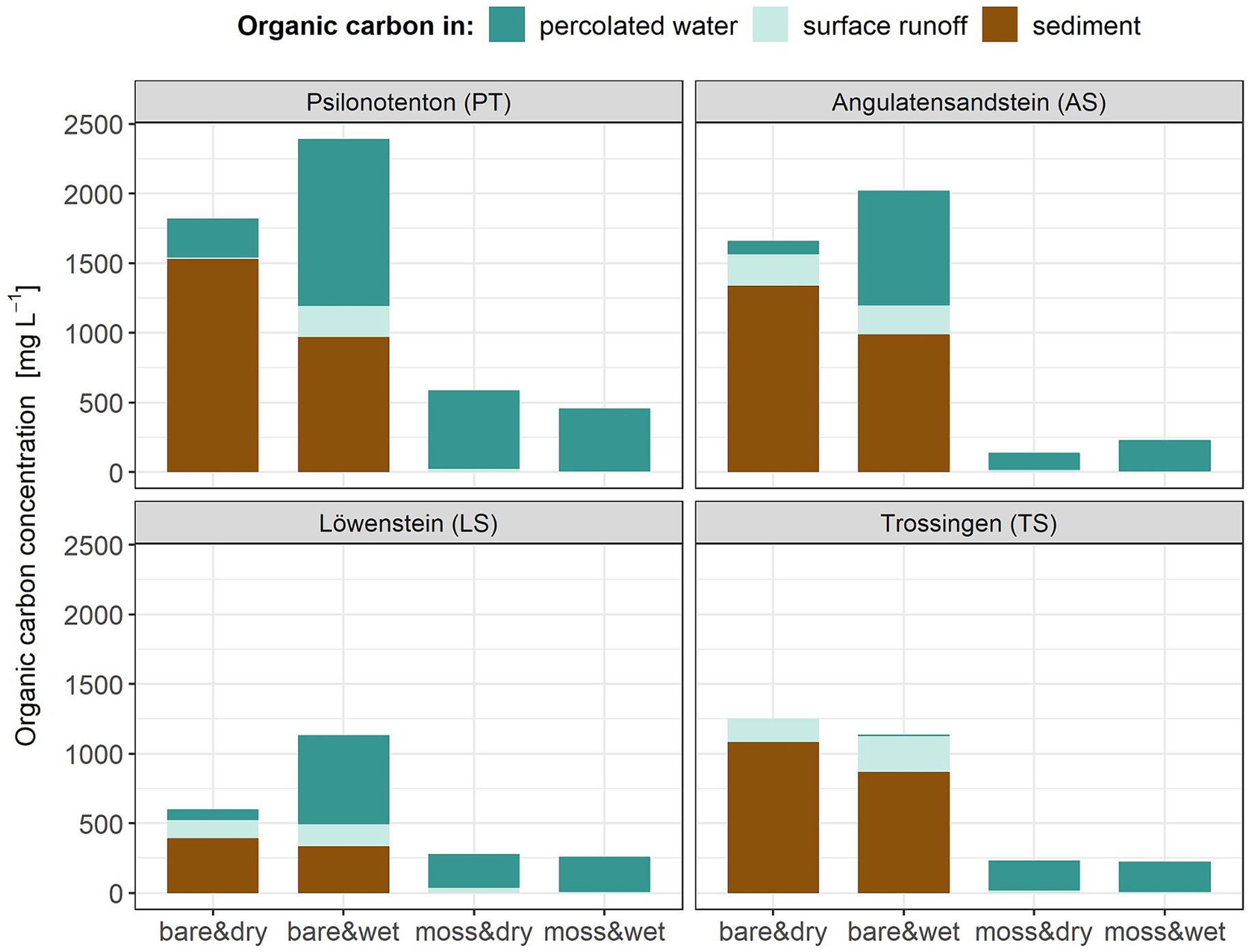
Figure 6. Total organic carbon concentrations in percolated water, surface runoff and sediment for four substrates. Displayed are stacked mean values.
4 Discussion
4.1 Displacement of SOC and Nt due to water erosion in temperate forest skid trails
In skid trails of this temperate forest ecosystem (Schönbuch Nature Park), SOC and Nt were preferentially displaced by soil erosion and enriched in the sediment compared to the original soil. This phenomenon of SOC and Nt enrichment in eroded sediment confirms several other studies (Girmay et al., 2009; Chartier et al., 2013). An interesting observation was, however, that the enrichment of Nt in the sediment was more pronounced than that of SOC, which contradicts the results of Holz and Augustin (2021), who found a slightly higher enrichment of SOC compared to Nt in the eroded sediment in a global meta-study. These differences may be due to the fact that the meta-study of Holz and Augustin (2021) included a large number of different research sites worldwide, while our study focused on a single research site with less investigated bryophyte-dominated vegetation communities.
As a consequence of the differential enrichment of SOC and Nt, there is a reduction in the C/N ratio in the eroded sediment compared to the original soil. The results suggest that erosion in skid trails not only leads to an increased transport of SOC and Nt in the eroded sediment but also alters the relative proportions of these elements. A decrease in the C/N ratio could have implications for microbial community structure and its activity (Wan et al., 2015), thus influencing nutrient cycling and soil quality. In their study, Holz and Augustin (2021) also concluded that the process of soil erosion leads to a change in the spatial variability of C and N along slopes and that this is crucial to capture the erosion-related changes in soil quality. The C/N ratio of the organic and mineral topsoil in turn influences N leaching processes in forest ecosystems (Dise et al., 1998; Gundersen et al., 2009), with a tendency for lower C/N ratios to favor N leaching (Dise et al., 1998). Presumably, therefore, the lower C/N ratio in the eroded sediment increases the probability that the forest ecosystem will become depleted in N. However, since N leaching processes depend on a variety of other factors, such as tree species and soil type, the C/N ratio cannot be used as a general indicator of N mobilization in forest ecosystems (Gundersen et al., 2009; Cools et al., 2014).
4.2 Influence of natural succession on the displacement of SOC and Nt in skid trails of temperate forests
The ERSOC in the skid trails of the Schönbuch Nature Park is consistently higher than one, indicating that there is a proportionally greater amount of SOC in the eroded sediment compared to the original soil. The highest ERSOC was reached, when skid trails were newly established and mostly bare. According to Gall et al. (2022), this was also the measurement time when sediment discharge in the skid trails was highest. In all other measurement times in which the skid trails had significantly higher vegetation cover, the ERSOC was lower, indicating that with progressive vegetation succession, the displacement of SOC is reduced. This impact is also illustrated by the negative correlation between ERSOC and total vegetation cover. In contrast, Koiter et al. (2017) found that although vegetation cover was an important factor in reducing soil loss in agricultural landscapes, it did not significantly affect ERSOC. This contradiction can be explained by considering that the mobilization processes of SOC in forest ecosystems are different from those in agricultural landscapes. For example, forest soils generally contain more SOC compared to agricultural soils, which has a positive effect on soil aggregate stability and thus soil erodibility (Lan, 2021). This can be seen from the clear negative correlation between SOC in the original soil and sediment discharge, but is also demonstrated in a couple of other studies (Le Bissonnais and Arrouays, 1997; Xue et al., 2019; Dou et al., 2020).
Moss covers tend to reduce ERSOC more than vascular plant covers, although the difference is small. This slight difference can probably be attributed to the fact that mosses have a positive effect on the SOC content (García-Carmona et al., 2020) and can reduce general erosion rates slightly more than vascular plants (Gall et al., 2022). Biological soil crust (biocrust) research shows that moss-dominated biocrusts in particular reduce soil erodibility and ascribe the effect to an increase in soil organic matter (SOM; Gao et al., 2017). In addition, Yang et al. (2022) showed that moss-dominated biocrusts increased the mean weight diameter (MWD), an index of soil aggregate stability, at 0–2 cm, 2–5 cm, and 5–10 cm soil depth of an abandoned farmland. This effect was accompanied by a strong positive effect of SOM at the depths 0–2 cm and 2–5 cm, but was absent at the depth level 5–10 cm, although the moss biocrusts still had a clear positive effect on MWD in this level (Yang et al., 2022). Hence, it has not yet been conclusively investigated whether the effects of moss cover on soil stability are determined by factors other than the increase in SOC. Nevertheless, vascular plant covers, such as grass covers, are also extremely effective in reducing soil erosion and the associated displacement of SOC, which can also be explained by aggregate stability (Zheng et al., 2021). Future research should focus on highlighting the differences between moss cover and vascular plant cover in terms of SOC and N displacement, to use the most effective measures for vegetation restoration in disturbed forest ecosystems.
There was a variation in ERSOC between the different skid trails, with the highest average of ERSOC observed in AS and the lowest in TS. Among other things, this can be explained by the negative correlation between SOC in the original soil and ERSOC, as AS has the lowest SOC content and TS the highest. A negative correlation between SOC in the original soil and ERSOC was also demonstrated in the studies by Koiter et al. (2017) and Holz and Augustin (2021). An even stronger negative correlation was found between ERN and Nt in the original soil. This means that in soils with a low SOC and Nt content, a larger proportion of SOC and Nt is transported with the sediment due to soil erosion, which has previously been observed by Holz and Augustin (2021). Consequently, soils with poor fertility are particularly at risk of SOC and N translocation, and it is often precisely these soils that are not the focus of soil conservation, but this should be reconsidered based on these results, especially as the input of SOC and soil nutrients can cause damage to aquatic ecosystems (Evans et al., 2005).
The strong positive correlation between ERSOC and ERN due to soil erosion in skid trails indicates that the transport of SOC and Nt in eroded sediment is closely linked, suggesting a consistent pattern of co-transport of these elements during erosion events. A close positive relationship between the enrichment ratios for C and N was also demonstrated by Chartier et al. (2013) in grazed semi-arid rangelands in north-eastern Patagonia. However, when considering individual skid trails, variations in the correlation between ERSOC and ERN were observed, indicating that the degree of coupling between the transport of SOC and Nt is site-dependent.
Originally, we hypothesized that the enrichment on SOC and Nt in eroded sediments is mainly driven by the amount of sediment discharge, albeit we could not proof this hypothesis with our measurements. There was no significant correlation between sediment discharge and ERSOC or ERN. Similar findings were reported by Girmay et al. (2009) who found a significantly higher sediment discharge in cultivated land, but this did not result in a higher SOC or Nt enrichment ratio. This was justified by the fact that, in addition to the total sediment discharge, other parameters determine the displacement of SOC and Nt, such as the initial SOC and Nt contents in the original soil (Girmay et al., 2009), which could also play a major role in our study, as we have demonstrated a strong correlation between ERSOC and SOC, or ERN and Nt in the original soil.
4.3 Influence of mosses on the displacement of SOC due to erosion and percolation through forest soil substrates
Overall, the bare treatments (bare&dry + bare&wet) showed a 5.5 times higher displacement of SOC compared to moss treatments (moss&dry + moss&wet), demonstrating a substantial impact of moss covers on the relocation of SOC. This can mainly be attributed to the fact that significantly less sediment was discharged in the moss treatments compared to the bare treatments (Gall et al., 2023). The erosion-reducing effect of the mosses therefore also reduces the risk of SOC displacement. In this context, Xiao et al. (2023) showed that removing moss covers in a subtropical karst area lead to a reduction of SOC in the first two centimetres of the topsoil after 1 year, which could probably also be induced by soil erosion. Additionally, Wang et al. (2022) demonstrated that moss-dominated biocrusts incorporated into an alfalfa maize intercropping system massively reduced SOC loss by 94.7% compared to bare land, which is why they are recommended as a nature-based solution for degraded agricultural soils to increase carbon sequestration.
Moss treatments exhibited significantly lower DOC concentration in surface runoff compared to bare treatments. It is already known from biocrust research that with intact biocrust covers less SOC is lost in surface runoff through DOC compared to disturbed or removed biocrusts, however, mainly cyanobacteria-and lichen-dominated biocrusts were considered here (Barger et al., 2006; Chamizo et al., 2017). Nevertheless, a difference between biocrust types could be demonstrated in both Barger et al. (2006) and Chamizo et al. (2017), according to which the late-successional lichen-dominated biocrusts resulted in less DOC in the surface runoff compared to the cyanobacteria-dominated biocrusts. In line with this trend, more developed moss-dominated biocrusts or mature moss covers could lead to even further reductions in DOC losses with surface runoff, as indicated by our results. The reason for the low DOC leaching in moss covers could be due, among other things, to their high water storage capacity (Thielen et al., 2021) and their ability to increase surface roughness (Wang et al., 2017), which was previously hypothesized by Xiao et al. (2023).
Surprisingly, there was no significant difference in the average DOC concentration in percolated water between bare (bare&dry + bare&wet) and moss (moss&dry + moss&wet) treatments. Due to the significantly higher amount of percolated water in the moss treatments compared to bare treatments (Gall et al., 2023), we would have expected a difference in DOC leaching, but this was not the case. However, in our approach, extractable DOC was not determined in the substrate directly after the experiment. In this context, Young et al. (2022) demonstrated that moss-dominated biocrusts (2 cm depth) increased the DOC content of the leachate compared to the references light and dark biocrusts dominated by cyanobacteria and thus more extractable DOC was found in the subsoil (2–10 cm depth) than in the two reference samples. The occurrence of desiccation cracks in our moss treatments as described in Gall et al. (2023) has certainly influenced our measurements of DOC leaching with percolated water.
In summary, our results suggest that moss covers have a substantial impact on reducing SOC displacement and DOC concentration in surface runoff, highlighting the potential role of moss in mitigating environmental impacts associated with soil disturbance.
5 Conclusion
In this study, we have shown that eroded sediments of skid trails in a temperate forest are significantly enriched in SOC and Nt. Our study area is characterized by a stronger enrichment of Nt compared to SOC, so that the C/N ratio in the sediment decreases compared to the original soil. Accordingly, soil erosion in skid trails modifies the relative proportions of SOC and Nt, which can have a negative impact on soil fertility. With regard to our hypotheses, we made the following conclusions:
1. As vegetation succession progresses in the skid trails, the displacement of SOC and Nt is reduced. This was confirmed by the negative correlation between ERSOC as well as ERN and total vegetation cover. Moss covers tend to reduce ERSOC more than vascular plant covers, which can be explained by the positive influence of mosses on SOC in the original soil and thus aggregate stability.
2. Mosses have a significant effect on the reduction of SOC displacement associated with sediment and DOC concentration in surface runoff compared to bare soils, while there was no difference in DOC concentration of percolated water.
Based on our results, future research should focus on understanding the role of mosses in storing SOC and Nt in the soil and their impact on soil stability. With this in mind, it would be conceivable to use mosses in the future, to minimize the environmental impacts of soil disturbances in forests, as already demonstrated by Wang et al. (2022) for degraded soils in agriculture.
Data availability statement
The datasets presented in this study can be found in online repositories. The names of the repository/repositories and accession number(s) can be found below: the dataset compiled and analysed in this study is available on figshare at https://doi.org/10.6084/m9.figshare.25102787.v1.
Author contributions
CG: Conceptualization, Formal analysis, Investigation, Visualization, Writing – original draft. MN: Conceptualization, Investigation, Supervision, Writing – review & editing. TS: Resources, Supervision, Writing – review & editing. SS: Conceptualization, Funding acquisition, Investigation, Project administration, Supervision, Writing – review & editing.
Funding
The author(s) declare that financial support was received for the research, authorship, and/or publication of this article. This research has been funded by the Deutsche Forschungsgemeinschaft (DFG SE 2767/2-1, “MesiCrust”) and the Federal Ministry of Food and Agriculture/Federal Ministry for the Environment, Nature Conservation, Nuclear Safety and Consumer Protection via Fachagentur Nachwachsende Rohstoffe e.V. (FNR 2220WK67A4, “AnKliMoos”). We acknowledge support by Open Access Publishing Fund of University of Tübingen.
Acknowledgments
First, we would like to thank Michael Sauer for his support and expertise in collecting and identifying moss species, which was essential for this study. Secondly, we would like to thank all those who helped with field and laboratory work, which made this work possible: Antonia Tertelmann, Laura Bindereif, Lena Grabherr, Helena Obermeier, Philipp Gries, Delia Maas, Stefanie Gotterbarm, Giulia König, Alexander Maurer, Gesche Weiß, Nicolás Riveras-Muñoz, Sascha Scherer, Matthew A. Bowker, Daniel Schwindt, and all students of GEO51 in winter semester 2019/20. Many thanks to Sabine Flaiz, Rita Mögenburg and Peter Kühn, who were always available to help with questions about laboratory work. We extend our gratitude to Daniel Buchner for facilitating DOC measurements at the University of Tübingen’s Center of Applied Geosciences laboratory and the plant ecology working group at the University of Tübingen for providing us with space in their greenhouse. Furthermore, we would like to thank the State Forests of Baden-Württemberg (ForstBW) for their continuous support. During the preparation of this work, ChatGPT-4 by OpenAI was used for refining language and grammar of the manuscript in individual cases.
Conflict of interest
The authors declare that the research was conducted in the absence of any commercial or financial relationships that could be construed as a potential conflict of interest.
Publisher’s note
All claims expressed in this article are solely those of the authors and do not necessarily represent those of their affiliated organizations, or those of the publisher, the editors and the reviewers. Any product that may be evaluated in this article, or claim that may be made by its manufacturer, is not guaranteed or endorsed by the publisher.
References
Alberts, E. E., Wendt, R. C., and Piest, R. F. (1983). Physical and chemical properties of eroded soil aggregates. Trans. ASAE 26, 0465–0471. doi: 10.13031/2013.33959
Arnold, W. (1986). “Der Wald im Naturpark Schönbuch” in Das landschaftsökologische Forschungsprojekt Naturpark Schönbuch: Wasser-und Stoffhaushalt, Bio-, Geo-und Forstwirtschaftliche Studien in Südwestdeutschland. ed. G. Einsele, vol. 1 (Weinheim: VCH Verlagsgesellschaft).
Barger, N. N., Herrick, J. E., Van Zee, J., and Belnap, J. (2006). Impacts of biological soil crust disturbance and composition on C and N loss from water erosion. Biogeochemistry 77, 247–263. doi: 10.1007/s10533-005-1424-7
Batjes, N. H. (1996). Total carbon and nitrogen in the soils of the world. Eur. J. Soil Sci. 47, 151–163. doi: 10.1111/j.1365-2389.1996.tb01386.x
Batjes, N. H. (2019). Technologically achievable soil organic carbon sequestration in world croplands and grasslands. Land Degrad. Dev. 30, 25–32. doi: 10.1002/ldr.3209
Beillouin, D., Corbeels, M., Demenois, J., Berre, D., Boyer, A., Fallot, A., et al. (2023). A global meta-analysis of soil organic carbon in the Anthropocene. Nat. Commun. 14:3700. doi: 10.1038/s41467-023-39338-z
Chamizo, S., Rodríguez-Caballero, E., Román, J. R., and Cantón, Y. (2017). Effects of biocrust on soil erosion and organic carbon losses under natural rainfall. Catena 148, 117–125. doi: 10.1016/j.catena.2016.06.017
Chartier, M. P., Rostagno, C. M., and Videla, L. S. (2013). Selective erosion of clay, organic carbon and total nitrogen in grazed semiarid rangelands of northeastern Patagonia, Argentina. J. Arid Environ. 88, 43–49. doi: 10.1016/j.jaridenv.2012.08.011
Cools, N., Vesterdal, L., De Vos, B., Vanguelova, E., and Hansen, K. (2014). Tree species is the major factor explaining C:N ratios in European forest soils. For. Ecol. Manag. 311, 3–16. doi: 10.1016/j.foreco.2013.06.047
Dise, N. B., Matzner, E., and Forsius, M. (1998). Evaluation of organic horizon C:N ratio as an indicator of nitrate leaching in conifer forests across Europe. Environ. Pollut. 102, 453–456. doi: 10.1016/S0269-7491(98)80068-7
Dou, Y., Yang, Y., An, S., and Zhu, Z. (2020). Effects of different vegetation restoration measures on soil aggregate stability and erodibility on the Loess Plateau, China. Catena 185:104294. doi: 10.1016/j.catena.2019.104294
DWD Climate Data Center (2021a). Annual regional averages of air temperature (annual mean) in °C (2 m above ground). Available at: https://opendata.dwd.de/climate_environment/CDC/regional_averages_DE/annual/air_temperature_mean/regional_averages_tm_year.txt (Accessed July 31, 2024).
DWD Climate Data Center (2021b). Annual regional averages of precipitation height (annual sum) in mm. Available at: https://opendata.dwd.de/climate_environment/CDC/regional_averages_DE/annual/precipitation/regional_averages_rr_year.txt (Accessed July 31, 2024).
DWD Climate Data Center (2021c). Jahresmittel der Stationsmessungen der Lufttemperatur in 2 m Höhe in °C und Jahressumme der Niederschlagshöhe für Deutschland. Available at: https://opendata.dwd.de/climate_environment/CDC/observations_germany/climate/annual/kl/historical/ (Accessed July 31, 2024).
Einsele, G., and Agster, G. (1986). “Überblick zur Geologie und Morphologie des Schönbuchs” in Das landschaftsökologische Forschungsprojekt Naturpark Schönbuch: Wasser-und Stoffhaushalt, Bio-, Geo-und Forstwirtschaftliche Studien in Südwestdeutschland. ed. G. Einsele, vol. 1 (Weinheim: VCH Verlagsgesellschaft).
Evans, C. D., Monteith, D. T., and Cooper, D. M. (2005). Long-term increases in surface water dissolved organic carbon: observations, possible causes and environmental impacts. Environ. Pollut. 137, 55–71. doi: 10.1016/j.envpol.2004.12.031
FAO (2022). Global soil organic carbon sequestration potential map – GSOCseq v.1.1 technical report. Rome, Italy: FAO.
Gall, C., Nebel, M., Quandt, D., Scholten, T., and Seitz, S. (2022). Pioneer biocrust communities prevent soil erosion in temperate forests after disturbances. Biogeosciences 19, 3225–3245. doi: 10.5194/bg-19-3225-2022
Gall, C., Nebel, M., Scholten, T., and Seitz, S. (2024). Relocation of SOC and N due to soil erosion in a temperate forest.
Gall, C., Nebel, M., Scholten, T., Thielen, S. M., and Seitz, S. (2023). Water’s path from moss to soil Vol. 2: how soil-moss combinations affect the soil water balance and soil erosion. Biologia. doi: 10.21203/rs.3.rs-3268666/v1
Gao, L., Bowker, M. A., Xu, M., Sun, H., Tuo, D., and Zhao, Y. (2017). Biological soil crusts decrease erodibility by modifying inherent soil properties on the Loess Plateau, China. Soil Biol. Biochem. 105, 49–58. doi: 10.1016/j.soilbio.2016.11.009
García-Carmona, M., Arcenegui, V., García-Orenes, F., and Mataix-Solera, J. (2020). The role of mosses in soil stability, fertility and microbiology six years after a post-fire salvage logging management. J. Environ. Manag. 262:110287. doi: 10.1016/j.jenvman.2020.110287
Gerke, J. (2022). The central role of soil organic matter in soil fertility and carbon storage. Soil Syst. 6:33. doi: 10.3390/soilsystems6020033
Girmay, G., Singh, B. R., Nyssen, J., and Borrosen, T. (2009). Runoff and sediment-associated nutrient losses under different land uses in Tigray, northern Ethiopia. J. Hydrol. 376, 70–80. doi: 10.1016/j.jhydrol.2009.07.066
Gou, F., Liang, W., Yan, J., Sun, S., Chen, Z., Zhang, W., et al. (2022). Decrease in Erosion-induced soil organic carbon as a result of vegetation restoration in the Loess Plateau, China. J. Geophys. Res. Biogeosci. 127:e2022JG006917. doi: 10.1029/2022JG006917
Gundersen, P., Sevel, L., Christiansen, J. R., Vesterdal, L., Hansen, K., and Bastrup-Birk, A. (2009). Do indicators of nitrogen retention and leaching differ between coniferous and broadleaved forests in Denmark? For. Ecol. Manag. 258, 1137–1146. doi: 10.1016/j.foreco.2009.06.007
He, Z., Xu, H., He, S., Liang, X., Zheng, Z., Luo, Z., et al. (2023). Characteristics of soil organic carbon (SOC) loss with water Erosion in sloping farmland of southwestern China during maize (Zea mays L.) growth stages. Agronomy 13:738. doi: 10.3390/agronomy13030738
Hofbauer, M., Kincl, D., Vopravil, J., Kabelka, D., and Vráblík, P. (2023). Preferential Erosion of soil organic carbon and fine-grained soil particles— an analysis of 82 rainfall simulations. Agronomy 13:217. doi: 10.3390/agronomy13010217
Holz, M., and Augustin, J. (2021). Erosion effects on soil carbon and nitrogen dynamics on cultivated slopes: a meta-analysis. Geoderma 397:115045. doi: 10.1016/j.geoderma.2021.115045
Hua, K., Zhu, B., and Wang, X. (2014). Dissolved organic carbon loss fluxes through runoff and sediment on sloping upland of purple soil in the Sichuan Basin. Nutr. Cycl. Agroecosyst. 98, 125–135. doi: 10.1007/s10705-014-9601-5
Iserloh, T., Ries, J., Arnáez, J., Boix-Fayos, C., Butzen, V., Cerdà, A., et al. (2013). European small portable rainfall simulators: a comparison of rainfall characteristics. Catena 110, 100–112. doi: 10.1016/j.catena.2013.05.013
Kasimir, Å., He, H., Jansson, P.-E., Lohila, A., and Minkkinen, K. (2021). Mosses are important for soil carbon sequestration in forested peatlands. Front. Environ. Sci. 9:680430. doi: 10.3389/fenvs.2021.680430
Kim, D.-G., Kirschbaum, M. U. F., Eichler-Löbermann, B., Gifford, R. M., and Liáng, L. L. (2023). The effect of land-use change on soil C, N, P, and their stoichiometries: a global synthesis. Agric. Ecosyst. Environ. 348:108402. doi: 10.1016/j.agee.2023.108402
Koiter, A. J., Owens, P. N., Petticrew, E. L., and Lobb, D. A. (2017). The role of soil surface properties on the particle size and carbon selectivity of interrill erosion in agricultural landscapes. Catena 153, 194–206. doi: 10.1016/j.catena.2017.01.024
Lal, R. (2003). Soil erosion and the global carbon budget. Environ. Int. 29, 437–450. doi: 10.1016/S0160-4120(02)00192-7
Lal, R. (2004). Soil carbon sequestration impacts on global climate change and food security. Science 304, 1623–1627. doi: 10.1126/science.1097396
Lan, J. (2021). Changes of soil aggregate stability and erodibility after cropland conversion in degraded karst region. J. Soil Sci. Plant Nutr. 21, 3333–3345. doi: 10.1007/s42729-021-00609-7
Le Bissonnais, Y., and Arrouays, D. (1997). Aggregate stability and assessment of soil crustability and erodibility: II. Application to humic loamy soils with various organic carbon contents. Eur. J. Soil Sci. 48, 39–48. doi: 10.1111/j.1365-2389.1997.tb00183.x
Liang, Y., Li, X., Zha, T., and Zhang, X. (2021). Vegetation restoration alleviated the soil surface organic carbon redistribution in the hillslope scale on the Loess Plateau, China. Front. Environ. Sci. 8:614761. doi: 10.3389/fenvs.2020.614761
Ma, Y., Woolf, D., Fan, M., Qiao, L., Li, R., and Lehmann, J. (2023). Global crop production increase by soil organic carbon. Nat. Geosci. 16, 1159–1165. doi: 10.1038/s41561-023-01302-3
Manninen, N., Kanerva, S., Lemola, R., Turtola, E., and Soinne, H. (2023). Contribution of water erosion to organic carbon and total nitrogen loads in agricultural discharge from boreal mineral soils. Sci. Total Environ. 905:167300. doi: 10.1016/j.scitotenv.2023.167300
Massey, H. F., and Jackson, M. L. (1952). Selective Erosion of soil fertility constituents. Soil Sci. Soc. Am. J. 16, 353–356. doi: 10.2136/sssaj1952.03615995001600040008x
McEachran, Z. P., Slesak, R. A., and Karwan, D. L. (2018). From skid trails to landscapes: vegetation is the dominant factor influencing erosion after forest harvest in a low relief glaciated landscape. For. Ecol. Manag. 430, 299–311. doi: 10.1016/j.foreco.2018.08.021
Nie, X., Li, Z., He, J., Huang, J., Zhang, Y., Huang, B., et al. (2015). Enrichment of organic carbon in sediment under field simulated rainfall experiments. Environ. Earth Sci. 74, 5417–5425. doi: 10.1007/s12665-015-4555-8
Oldfield, E. E., Bradford, M. A., and Wood, S. A. (2019). Global meta-analysis of the relationship between soil organic matter and crop yields. Soil 5, 15–32. doi: 10.5194/soil-5-15-2019
Pan, Y., Birdsey, R. A., Fang, J., Houghton, R., Kauppi, P. E., Kurz, W. A., et al. (2011). A large and persistent carbon sink in the World’s forests. Science 333, 988–993. doi: 10.1126/science.1201609
Picchio, R., Mederski, P. S., and Tavankar, F. (2020). How and how much, do harvesting activities affect forest soil, regeneration and stands? Curr. For. Rep. 6, 115–128. doi: 10.1007/s40725-020-00113-8
R Core Team (2021). AR: A language and environment for statistical computing. Vienna, Austria: R Foundation for Statistical Computing. Available at: https://www.R-project.org/ (Accessed August 08, 2024).
Schiettecatte, W., Gabriels, D., Cornelis, W. M., and Hofman, G. (2008). Enrichment of organic carbon in sediment transport by Interrill and rill erosion processes. Soil Sci. Soc. Am. J. 72, 50–55. doi: 10.2136/sssaj2007.0201
Seitz, S. (2015). Mechanisms of soil erosion in subtropical chinese forests - effects of species diversity, species identity, functional traits and soil fauna on sediment discharge. Dissertation, Universitätsbibliothek Tübingen. Available at: http://dx.doi.org/10.15496/publikation-8010.
Sun, L., Dai, Q., and Feng, Z. (2023). Effects of multiple factors on particle size selectivity under artificial extreme rainfall events on simulated Gobi surface. Sci. Rep. 13:23049. doi: 10.1038/s41598-023-50136-x
Thielen, S. M., Gall, C., Ebner, M., Nebel, M., Scholten, T., and Seitz, S. (2021). Water’s path from moss to soil: a multi-methodological study on water absorption and evaporation of soil-moss combinations. J. Hydrol. Hydromech. 69, 421–435. doi: 10.2478/johh-2021-0021
Tiessen, H., Cuevas, E., and Chacon, P. (1994). The role of soil organic matter in sustaining soil fertility. Nature 371, 783–785. doi: 10.1038/371783a0
Walling, D. E., and Webb, B. W. (1983). “Patterns of sediment yield” in Background to palaeohydrology. ed. K. J. Gregory. 1st ed (New York: John Wiley & Sons).
Wan, X., Huang, Z., He, Z., Yu, Z., Wang, M., Davis, M. R., et al. (2015). Soil C:N ratio is the major determinant of soil microbial community structure in subtropical coniferous and broadleaf forest plantations. Plant Soil 387, 103–116. doi: 10.1007/s11104-014-2277-4
Wang, L., Zhang, G., Zhu, L., and Wang, H. (2017). Biocrust wetting induced change in soil surface roughness as influenced by biocrust type, coverage and wetting patterns. Geoderma 306, 1–9. doi: 10.1016/j.geoderma.2017.06.032
Wang, W., Zhou, R., Wang, B.-Z., Zhao, L., Zhao, Z.-Y., Sheteiwy, M. S., et al. (2022). Biocrust as a nature-based strategy (NbS) to restore the functionality of degraded soils in semiarid rainfed alfalfa (Medicago sativa L.) field. J. Clean. Prod. 336:130378. doi: 10.1016/j.jclepro.2022.130378
Wei, X., Huang, L., Xiang, Y., Shao, M., Zhang, X., and Gale, W. (2014). The dynamics of soil OC and N after conversion of forest to cropland. Agric. For. Meteorol. 194, 188–196. doi: 10.1016/j.agrformet.2014.04.008
Wilson, N., Bradstock, R., and Bedward, M. (2021). Comparing forest carbon stock losses between logging and wildfire in forests with contrasting responses to fire. For. Ecol. Manag. 481:118701. doi: 10.1016/j.foreco.2020.118701
Xiao, L., Zhang, W., Hu, P., Vesterdal, L., Zhao, J., Tang, L., et al. (2023). Mosses stimulate soil carbon and nitrogen accumulation during vegetation restoration in a humid subtropical area. Soil Biol. Biochem. 184:109127. doi: 10.1016/j.soilbio.2023.109127
Xue, B., Huang, L., Huang, Y., Zhou, F., Li, F., Kubar, K. A., et al. (2019). Roles of soil organic carbon and iron oxides on aggregate formation and stability in two paddy soils. Soil Tillage Res. 187, 161–171. doi: 10.1016/j.still.2018.12.010
Yang, Y., Ye, Z., Liu, B., Zeng, X., Fu, S., and Lu, B. (2014). Nitrogen enrichment in runoff sediments as affected by soil texture in Beijing mountain area. Environ. Monit. Assess. 186, 971–978. doi: 10.1007/s10661-013-3431-9
Yang, K., Zhao, Y., and Gao, L. (2022). Biocrust succession improves soil aggregate stability of subsurface after “grain for green” project in the hilly Loess Plateau, China. Soil Tillage Res. 217:105290. doi: 10.1016/j.still.2021.105290
Young, K. E., Ferrenberg, S., Reibold, R., Reed, S. C., Swenson, T., Northen, T., et al. (2022). Vertical movement of soluble carbon and nutrients from biocrusts to subsurface mineral soils. Geoderma 405:115495. doi: 10.1016/j.geoderma.2021.115495
Zemke, J. J. (2016). Runoff and soil erosion assessment on forest roads using a small scale rainfall simulator. Hydrology 3:25. doi: 10.3390/hydrology3030025
Keywords: soil organic carbon, dissolved organic carbon, soil erosion, rainfall simulation, bryophytes
Citation: Gall C, Nebel M, Scholten T and Seitz S (2024) The effect of mosses on the relocation of SOC and total N due to soil erosion and percolation in a disturbed temperate forest. Front. For. Glob. Change. 7:1379513. doi: 10.3389/ffgc.2024.1379513
Edited by:
Hongxi Liu, Beijing Normal University, ChinaReviewed by:
Jörg Luster, Swiss Federal Institute for Forest, Snow and Landscape Research (WSL), SwitzerlandChao Guo, Changjiang River Scientific Research Institute (CRSRI), China
Copyright © 2024 Gall, Nebel, Scholten and Seitz. This is an open-access article distributed under the terms of the Creative Commons Attribution License (CC BY). The use, distribution or reproduction in other forums is permitted, provided the original author(s) and the copyright owner(s) are credited and that the original publication in this journal is cited, in accordance with accepted academic practice. No use, distribution or reproduction is permitted which does not comply with these terms.
*Correspondence: Corinna Gall, Y29yaW5uYS5nYWxsQHVuaS10dWViaW5nZW4uZGU=