- Department of Ecology and Evolutionary Biology, University of California, Santa Cruz, Santa Cruz, CA, United States
Diel activity is one main feature of animal‘s behavior and is often an intrinsic trait characterizing distinct taxonomic groups. Abiotic conditions such as temperature may influence the diel activity patterns of arthropod communities associated with a particular ecosystem or habitat. Similarly, biotic factors, such as resource availability, affect arthropod activity. In addition, diel activity is thought to be an important factor in niche partitioning of arthropod communities. As part of a larger beetle survey in a lowland tropical rainforest in southern Venezuela, I analyzed the diel activity of an arboreal beetle community collected from 23 canopy-tree species over a cumulative year. Diel activity was observed in 535 beetle species, comprising 5,948 individuals, using a canopy crane installed in the study area. Of the 535 beetle species, 198 (37%) showed diurnal activity, and 281 (52.5%) showed nocturnal activity. In contrast, the proportions of nocturnal (n = 2,024, 34%) and diurnal (n = 1,983, 33.3%) individuals were balanced. Most of the observed beetles occurred only during the activity phase in their host trees. This particularly applies to extrafloral nectary- and flower-visiting beetle species. Flowering trees attracted different proportions of diurnal and nocturnal species according to flowering syndrome, whereas extrafloral nectaries were mainly visited at night. Thus, the beetle communities associated with single tree species showed distinct compositions of nocturnal and diurnal species.
1 Introduction
Tropical rainforest canopies are in harsh environments. They receive high solar insolation during the day (Parker, 1995; Hallé, 1998), but exhibit high levels of water condensation at night (Blanc, 1990). As a result, the relative humidity and air temperature fluctuate significantly in the upper canopy (Pinker, 1980; Parker, 1995; Walsh, 1996; Szarzynski and Anhuf, 2001; Turton and Siegenthaler, 2004; Ulyshen, 2011). Nevertheless, adult insects are often more abundant and specious in the canopy than in the understory (Basset et al., 2001, 2015). Beetles associated with tree canopies are often highly diverse and abundant (Erwin, 1982; Stork, 1991; Basset and Arthington, 1992; Allison et al., 1993; Floren and Linsenmair, 1999). Like many other arthropods, most beetle species have a small body size; therefore, they are strongly influenced by microclimatic conditions at the plant surface (Pincebourde and Woods, 2020; Pincebourde et al., 2021).
Beetles inhabiting this life zone are expected to develop specific strategies to overcome hygrothermal stress associated with rainforest canopies. One possibility is the use of different strata during the day and night; consequently, beetles may be present in the upper canopy only during their activity. Distinct patterns in the activities of arthropod communities are observed in different rainforest canopies. For instance, the canopy of a subtropical rainforest in Australia exhibits significantly higher activity of associated herbivores during the day (Basset and Springate, 1992). In contrast, Basset (1991) found high activity in an Australian subtropical rainforest tree species during the first part of the night. In Sulawesi, malaise trap samples revealed an extremely low level of night flight activity in major insect groups (Hammond, 1990). Basset et al. (2003) summarized more arthropod activity in the canopy during the day than at night. Generally, diel activity is correlated with habitat characteristics, such as temperature (Giménez-Gómez et al., 2018). The flight activity of dung beetles, for instance, is principally diurnal in the northernmost European regions (Koskela, 1979), whereas there are more crepuscular-nocturnal species in Mediterranean climates (Mena et al., 1989). In tropical and subtropical environments, the numbers of nocturnal and diurnal species are relatively similar (Cambefort, 1991; Gill, 1991). However, Feer and Pincebourde (2005) found that diurnal species were approximately twice as numerous and abundant as either nocturnal or crepuscular species in French Guiana, whereas other dung beetle communities showed dominance of nocturnal species in rainforests (Halffter and Matthews, 1966; Howden et al., 1991).
Organisms have evolved circadian clocks based on predictable 24-h environmental cycles (Paranjpe and Sharma, 2005; Rosbash, 2009; Brady et al., 2021). In insects, circadian clocks control the rhythmicity of several behaviors and physiological features, including locomotion and feeding (Saunders, 2002; Tomioka and Matsumoto, 2010; Franco et al., 2018). Thus, diel activity in insects is separated into hours of day and night (Fullard et al., 2000; Feer and Pincebourde, 2005). The diel activity of insects is often an intrinsic trait that causes uniform patterns in a single taxon (Kronfeld-Schor and Dayan, 2003; Boulter et al., 2005). Otherwise, diel activity may be adaptive and vary in accordance with the biotic conditions of the habitat. Diel activity can be affected by the quantity and quality of trophic resources (Martín-Piera et al., 1994). Brady et al. (2021) observed synchronization between the circadian clocks of Lepidoptera and their host plants. The variable caterpillar growth rate depends on diurnal feeding behavior in relation to the production of jasmonic acid in plants (Reymond et al., 2000; Jander et al., 2001; Kessler and Baldwin, 2002; Howe and Jander, 2008). Jasmonic acid triggers plant herbivore defense and shows peak accumulation in the middle of the day (Goodspeed et al., 2012).
Likewise, the diel activity patterns of communities may be the result of resource partitioning and facilitate coexistence (Hanski and Cambefort, 1991; Caveney et al., 1995; Palmer, 1995; Sowig, 1997; Krell-Westerwalbesloh et al., 2004). Niche partitioning has been discussed as one of the major reasons for high species diversity (Gause, 1934; Mayr, 1979). Consequently, temporal changes in community assemblages may be a common feature in many ecosystems, particularly in species-rich tropical rainforests. For instance, the turnover between diurnal and nocturnal ant assemblages supports the coexistence of competitors (Albrecht and Gotelli, 2001; Hossaert-McKey et al., 2001; Luna et al., 2018).
In this study, I analyzed the diel activity of a canopy-inhabiting beetle community to clarify: (1) if the diel activity of canopy beetles is related to specific taxonomic groups indicating intrinsic traits; (2) if diel activity is linked to resource availability; and (3) if the diel activity of canopy beetles is linked to strata use.
2 Material and methods
2.1 Study area
The study site is located in the upper Orinoco region (Venezuela, state of Amazonas) and is part of the Man and Biosphere Reserve Alto Orinoco-Casiquiare. The region is a transitional zone between the Guyana-Highlands and the lowlands of the Río Negro-Casiquiare Basin (Winkler and Listabarth, 2003). The landscape is dominated by gently undulating peneplains interrupted by table mountains to the north. The Orinoco depression primarily contains Pliocene to recent alluvial deposits as well as denudation products of the Guyana-Highland (Gonzales et al., 1980; Gibbs and Barron, 1993). The soil structure and texture in the study plot are heterogeneous (Winkler and Listabarth, 2003) with ferralic arenosols and plinthichumic ferralsols (FAO-UNESCO, 1988), which prevail in the northern part.
The average annual precipitation in the study area is approximately 3,100 mm with year-to-year fluctuations of roughly 500 mm (Anhuf et al., 1999). A strong peak in annual precipitation occured from May to July, and a lower peak occurred in September and October. The canopy intercepted circa 18% of the annual precipitation in the study plot (Anhuf and Rollenbeck, 2001; Szarzynski and Anhuf, 2001) with large variations between the rainy and dry seasons, attaining values of 5% and 56%, respectively. The average annual temperature in the study area was approximately 26°C. Maximum temperatures during the day may reach 30.5°C and drop to only 20–21°C during the night. While the canopy receives the most solar radiation during the daytime, heat is lost through radiation and sensible and latent heat via condensation during the night. Accordingly, the temperature fluctuates by nearly 10°C in the upper canopy and only slightly near the ground (Anhuf and Rollenbeck, 2001; Szarzynski and Anhuf, 2001).
To access the tree tops, a large tower crane was installed in the study area close to the black water river Surumoni (3°10′N, 65°40′W; 105 m asl), a tributary of the Orinoco River. The canopy-crane system, 42 m in height, ran on a 120 m rail track. It covered an area of about 1.4 ha with its 40 m long swing. Using remote control, scientists could reach trees up to a maximum height of 35 m in a gondola. The crane was operated between 1995 and 2000.
2.2 Vegetation
The Surumoni area belongs to the Japura/Negro moist forest ecoregion (Dinerstein et al., 1995) or Imerí province (Morrone, 2014) which extends from Brazil to southern Venezuela, Colombia, and Peru. The study region is covered by a mosaic of lowland rainforests, swamp forests, and savannas (Winkler and Listabarth, 2003). The vegetation of the crane plot is that of a moist lowland tropical rainforest classified as terra firme (Prance, 1979). The upper canopy usually ranges from 25 to 27 m in height, including a few emergent trees up to 35 m.
Altogether, 322 species of higher plants were identified in the 1.4 ha crane plot belonging to 208 genera from 78 families (Wesenberg, 2004). The species richest Spermatophyta were Fabaceae (23 species), Orchidaceae (19 species), Araceae (16 species), Rubiaceae (15 species), Lauraceae (13 species), and 10 species of Annonaceae and Chrysobalanaceae. The stem density of the area was 546 living trees per hectare, with an estimated 92 species per hectare. In 1999, there were 781 living trees ≥ 10 cm in diameter at breast height (DBH) belonging to 141 tree species within the crane plot. Frequent species in the tree fraction with a DBH ≥ 10 cm were Goupia glabra Aubl. (Goupiaceae), Oenocarpus bacaba Mart. (Arecaceae), Dialium guianense (Aubl.) Sandwith (Fabaceae), Ocotea aff. amazonica (Meisn.) Mez (Lauraceae), or Ruizterania trichanthera (Spruce ex Warm.) Marc.-Berti (Vochysiaceae) (Wesenberg, 2004). The most abundant species in the upper layer were G. glabra, R. trichanthera, and D. guianense. The abundant species in the middle layer were, for instance, Couma utilis (Mart.) Müll. Arg. (Apocynaceae), O. bacaba, and Podocalyx loranthoides Klotzsch (Picrodendraceae) (Wesenberg, 2004). Epiphytes and hemiepiphytes comprised 778 individuals from 53 species in 1996 (Engwald et al., 2000).
2.3 Beetle collection and observation
The sampling and observation of beetles comprised the following periods: September–November 1997, May–August and December 1998, and January–April 1999, thus enabling cumulative data collection for the aspect of a year. In addition, trap collection in October 1999 targeted a single tree species. License and authorization were issued under the number 15-1277 by Servicio Autonoma de Fauna, Ministerio del Ambiente y de los Recursos Naturales Renovables, Caracas, Venezuela.
Using the tower crane, crowns of tree species in the upper (approximately 25–30 m height) and middle canopy (approximately 18–25 m height) were searched regularly for Coleoptera during the day and night. A spotlight was used to enable nighttime observations. The plant parts sampled included leaves, small twigs, flowers, and fruits. The inventory of this canopy-beetle assemblage included 23 canopy-tree species (45 trees, 23 genera, 13 plant families). The observed beetles were captured by net, hand, branch, and foliage beating. However, these methods do not yield quantitative data. Additionally, aerial traps were used to collect flying beetles (Basset et al., 1997). These window traps consisted of two clear acrylic panels fixed over a cross, each with a length of 30 cm and height of 25 cm. A plastic tube, ending in a container, was placed beneath the panels. The container was filled with water mixed with surface-tension-diminishing detergent. The beetles were removed every two days. These traps provided semi-quantitative sampling results. A detailed description of the methodology and the trees selected for the survey was published in Kirmse (in prep.).
The collected beetles were stored in 70% ethanol. The beetles were assigned to morphospecies (hereafter species) and later, in part, identified by specialists. A complete list of all experts has been published in Kirmse (in prep.). Named genera in this study were determined by Alistair S. Ramsdale (Lycidae), Ubirajara R. Martins (Cerambycidae), Wills Flowers (eumolpine Chrysomelidae), Sergio Antonio Vanin (Curculionidae), and Brett C. Ratcliffe (Scarabaeidae). Carabidae, Dermestidae, and Meloidae were identified using Reichardt (1977), Pinto and Bologna (1999), and Háva (2004). Family group names follow Bouchard et al. (2011). Voucher specimens of the collected beetles were deposited at the Museo del Instituto de Zoología Agrícola “Francisco Fernandez Yepez,” Maracay, Venezuela.
2.4 Diel activity of beetles
The beetle species were ecologically characterized according to canopy data and observations. Adult beetles and species were considered, regardless of the number of individuals observed and collected. The diel activity of the species was distinguished as either nocturnal or diurnal if all observed beetles showed the same activity. Species comprising individuals that were active during the day and night were categorized as “indifferent.” Species with unknown activity (e.g., caught only in traps) were classified as “unknown.” Activity includes all behaviors except passive (quiescent) resting, for example, locomotion including walking and flying, feeding, mating, or cleaning.
To characterize the entire community, I generalized the diel activity in a second step for single taxa as diurnal or nocturnal if all members (n ≥ 2 spp.) were found to be active during either the day or night. This applies to two families (Buprestidae, Elateridae) and 11 genera (Carabidae: Agra Fabricius; Cerambycidae: Acanthoderes Audinet-Serville, Agaone Pascoe, Chrysoprasis Audinet-Serville; Chrysomelidae: Entomochirus Lefévre, Nycterodina Bechyné; Curculionidae: Heilipus Germar; Dermestidae: Cryptorhopalum Guérin-Meneville; Lycidae: Idiopteron Bourgeois, Plateros Bourgeois; Scarabaeidae: Isonychus Mannerheim).
Furthermore, the diel activity of all observed species was related to the diet used in the canopy. I distinguished general flower visitors, visitors to small white flowers with a generalized morphology, visitors to extrafloral nectaries, species utilizing extrafloral nectar and flowers, leaf feeders as well as other or mixed diets as diverse diets. The assignment of these “feeding guilds” was consistent with the plant parts sampled. A detailed description was published in Kirmse (in prep.).
The flowers were distinguished according to their commonly recognized flower syndromes (Faegri and Van der Pijl, 1979) to clarify possible specific associations between beetles and floral traits. Typical beetle flowers are open during the day and night and often award their visitors enormous quantities of pollen (Howe and Westley, 1986, 1988). Although the cantharophilous syndrome encompasses a broad range of floral structures (Irvine and Armstrong, 1990; Bernhardt, 2000), typical beetle flowers are commonly described as either flat or cup-shaped and of radial symmetry, allowing open access to their rewards (Howe and Westley, 1986; Frame, 2003). Their colors are often dull, white, or cream (Faegri and Van der Pijl, 1979; Howe and Westley, 1986; Willmer, 2011). I found seven tree species bearing this type of flower with a generalized morphology, whereas 13 tree species had dissimilar flowers.
I further analyzed the diel activity patterns of beetle communities associated with distinct phenological seasons of several tree species (flowering and leaf-flushing with active extrafloral nectaries) and beetle communities collected from distinct tree species for a cumulative year. I selected all of the most species-rich assemblages comprising most of the observed species. The inclusion criterion for the samples was at least 30 observed species comprising more than 40 individuals. To avoid bias caused by manual collection, I provided only data for trap samples for the most speciose communities. Other host-tree-related samples combined data from hand and trap collections.
Beetle communities visiting extrafloral nectaries were analyzed from two tree species of Chrysobalanaceae: Licania hebantha Mart. ex Hook. f. (one tree, two seasons) and Moquilea subarachnophylla (Cuatrec.) Sothers and Prance (five trees, one season) (Kirmse and Chaboo, 2019). These data combine trap and hand collection. The samples over a cumulative year included the following three tree species: Goupia glabra (two trees: trap sample), Ruizterania trichanthera (two trees: combined trap and hand collection), and Senna cf. silvestris (Vell.) H. S. Irwin and Barneby (Fabaceae) (one tree: combined trap and hand collection).
I separated flower-visiting beetle communities into two different groups according to flowering syndrome. The first group comprised four tree species with generalist small white flowers: Hymenopus heteromorphus (Benth.) Sothers and Prance var. heteromorphus (Chrysobalanaceae) (one tree, one season: trap sample) (Kirmse and Chaboo, 2020), Matayba guianensis Aubl. (Sapindaceae) (two trees, one season: trap sample), and Tachigali guianensis (Benth.) Zarucchi and Herend. (Fabaceae) (one tree, one season: trap sample) (Kirmse et al., 2003). Hymenopus heteromorphus, M. guianensis, and T. guianensis were mass-flowering, producing a very high number of flowers over a short period (Heinrich and Raven, 1972; Frankie, 1975). The fourth species, Ocotea aff. amazonica (six trees: trap sample), did not exhibit mass-flowering. The second group comprises tree species with flowers of other syndromes, such as Balizia pedicellaris (DC.) Barneby and J.W. Grimes (Fabaceae) (one tree, two seasons: trap sample), Podocalyx loranthoides (one tree, three seasons: combined trap and hand collection), and Qualea paraensis Ducke (Vochysiaceae) (one tree, one season: combined trap and hand collection).
3 Results
3.1 Diel activity of the beetle community
In total, 6,738 adult beetles were collected from 23 canopy-tree species. These beetles were assigned to 862 (morpho-)species belonging to 45 families. During the entire survey, I observed the activity of 535 beetle species (62.1%) representing 5,948 individuals (88.3%) in the canopy, while the activity of 327 species remained unknown (Figure 1). Of the 535 species with assigned diel activities, 198 (37%) were diurnal. In contrast, 281 beetle species (52.5%) were found to be active at night. In 56 beetle species (10.5%), I found both individuals active during the day and night. The 198 diurnal species comprise 1,983 individuals (33.3%), whereas the 281 nocturnal species include 2,024 individuals (34%). The proportion of individuals representing indifferent species in the canopy sample was 32.6% (1,941 individuals).
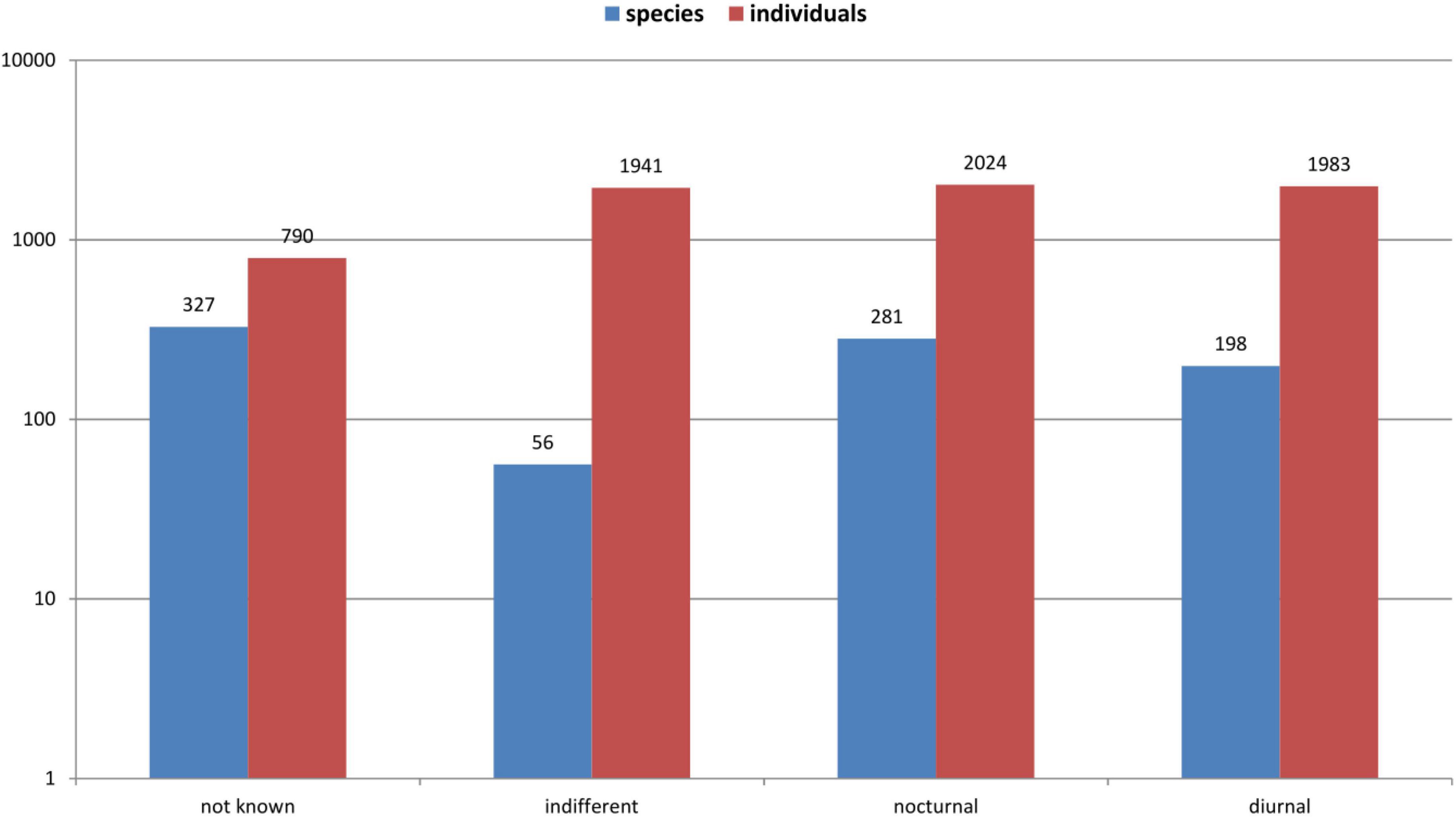
Figure 1. Distribution of diel activity in 862 beetle species comprising 6,738 individuals observed and collected on 23 tree species in a lowland rainforest canopy, Venezuela, for a cumulative year between 1997 and 1999.
Visitors to flowers and extrafloral nectaries were regularly found only during their phases of activity on their host trees. Commonly, these beetle guilds do not rest on their host trees. An exception were beetle species associated with dense inflorescences or infructescences of Oenocarpus bacaba. These beetles often remained inside the resources during both the day and night. In contrast to most flower and extrafloral nectary visitors, foliage feeders usually occur both day- and nighttime on their host trees. Among the leaf feeders, I found both strictly diurnal species (all Buprestidae) and species active during the day and night, such as a species of Naupactus Dejean (Entiminae).
3.2 Diel activity of taxa
Of all identified genera comprising at least two species with observed activity (n = 72), species of 47 genera (65.3%) showed uniform diel activity in the canopy, whereas in 25 genera (34.7%) species showed both diurnal and nocturnal activity. Among the 11 well-observed beetle families comprising at least 10 species (Brentidae, Buprestidae, Cantharidae, Carabidae, Cerambycidae, Chrysomelidae, Curculionidae, Elateridae, Mordellidae, Scarabaeidae, and Tenebrionidae), Buprestidae showed clear diurnal activity, and Elateridae were found in general active during the night in the canopy. Carabidae (54 spp. nocturnal, 2 spp. diurnal, 1 sp. indifferent) and Tenebrionidae (17 spp. nocturnal, 1 sp. diurnal) were mainly nocturnal, whereas Mordellidae (14 spp. diurnal, 1 sp. nocturnal) were commonly diurnal. In contrast, Brentidae, Cantharidae, Cerambycidae, Chrysomelidae, Curculionidae, and Scarabaeidae did not exhibit uniform diel activity patterns among their respective species and genera. For instance, Chrysomelidae comprised 25 diurnal and 44 nocturnal species, Curculionidae comprised 53 diurnal and 59 nocturnal species, and Scarabaeidae nine diurnal and 20 nocturnal species.
Generalizing the diel activity in two beetle families and 11 genera, the beetle community collected on the 23 canopy-tree species comprised 568 species including 6,082 individuals. Of these 568 species, 215 (37.9%) were diurnal, whereas 298 (52.5%) showed nocturnal activity. Diurnal species comprised 2,055 individuals (33.8%), and nocturnal species 2,087 individuals (34.3%). Fifty-five species (9.7%) with 1,940 individuals (31.9%) remained indifferent.
3.3 Diel activity and diet
Of the 535 beetle species observed, diel activity was analyzed in relation to the plant diet commonly utilized in the canopy. A diet was assigned to 482 beetle species, comprising 242 nocturnal species (1,980 individuals), 185 diurnal species (1,969 individuals), and 55 indifferent species (1,937 individuals) (Figure 2). Among the diurnal species, visitors to small white flowers prevailed (128 spp., 69.2%; 1,327 individuals, 67.4%). They were followed by visitors to diverse flowers (36 spp., 19.5%; 440 individuals, 22.3%). Although indifferent species contained an almost equal proportion of individuals visiting small white flowers with a generalized morphology (1,335 individuals, 68.9%), they comprised only 16 species (29.1%). This group was followed by species that utilized diverse diets (15 spp., 27.3%; 226 individuals, 11.7%) and visitors to diverse flowers (10 spp., 18.2%; 181 individuals, 9.3%). In contrast, nocturnal beetle species were observed most often, utilizing more parts of the trees or other than flowers and extrafloral nectaries (66 spp., 27.3%; 705 individuals, 35.6%). These species with diverse diets were followed in similar proportions by species utilizing flowers and extrafloral nectaries (45 spp., 18.6%; 407 individuals, 20.6%), visitors to diverse flowers (44 spp., 18.2%; 478 individuals, 24.1%), visitors to small white flowers (40 spp., 16.5%; 227 individuals, 11.5%), and extrafloral nectary visitors (43 spp., 17.8%; 135 individuals, 6.8%).
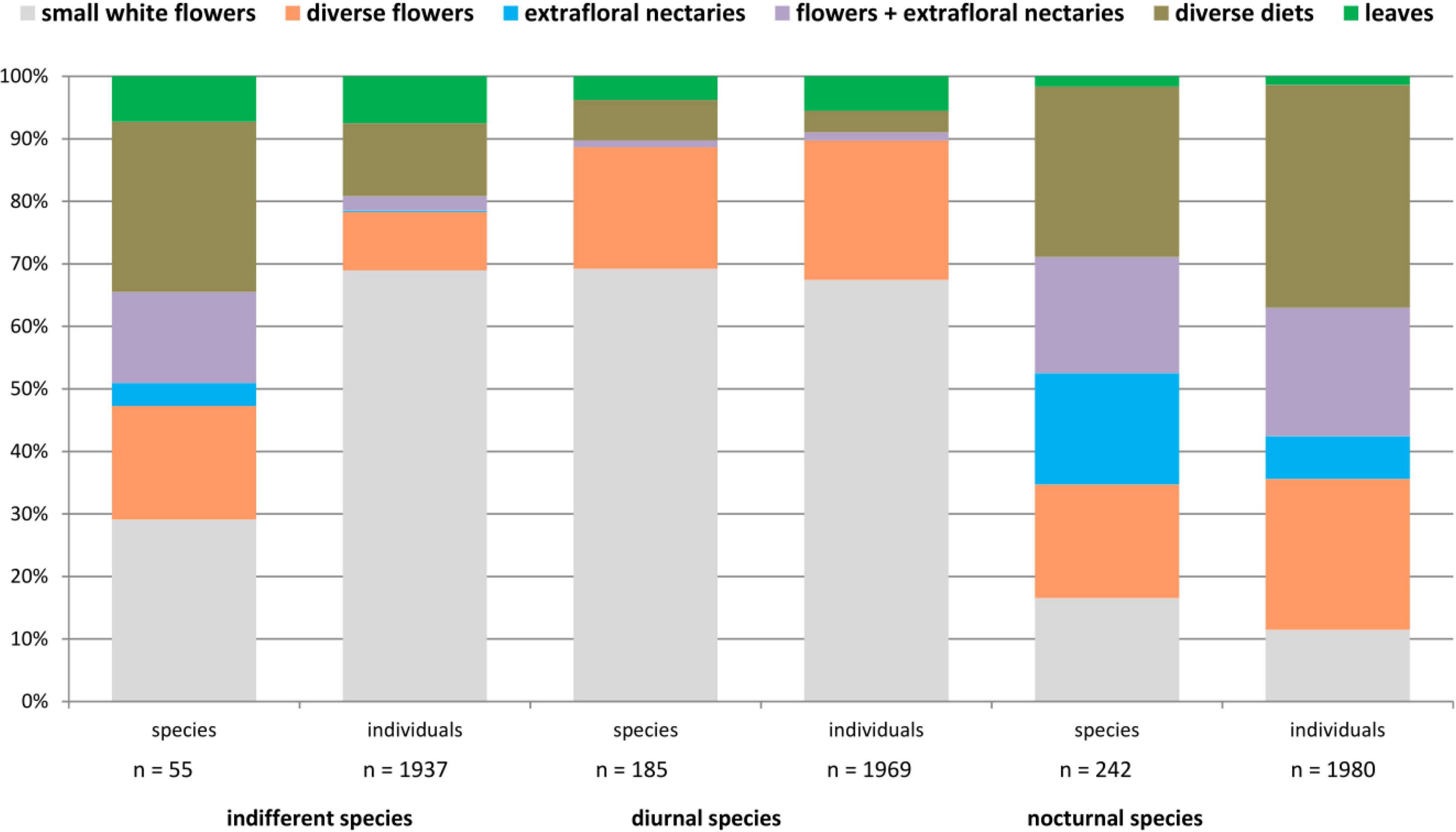
Figure 2. Plant parts utilized in relation to the diel activity in 482 beetle species observed and collected on 23 tree species in a lowland rainforest canopy, Venezuela, for a cumulative year between 1997 and 1999.
3.4 Diel activity and food resources
The diel activity of the beetle communities on the tree species varied, but showed distinct patterns associated with the phenological seasons and available food resources. Diurnal species were particularly represented in flowering trees (Figures 3–6). The highest proportion of diurnal flower visitors was trapped on the three mass-flowering tree species H. heteromorphus (47.4% of the species), M. guianensis (71.8% of the species), and T. guianensis (52.9% of the species). These three tree species also had the highest proportions of diurnal individuals. The other flowering tree species, O. aff. amazonica, B. pedicellaris, P. loranthoides, and Q. paraensis, attracted more nocturnal than diurnal species and individuals. The three year-aspect samples of G. glabra, R. trichanthera, and S. cf. silvestris contained approximately five times more nocturnal than diurnal species. The dominance of nocturnal individuals in these tree species was expressed as at least three times higher numbers. The beetle communities collected on L. hebantha and M. subarachnophylla were observed exclusively during night feeding on extrafloral nectar secreted from emerging foliage for about three weeks.
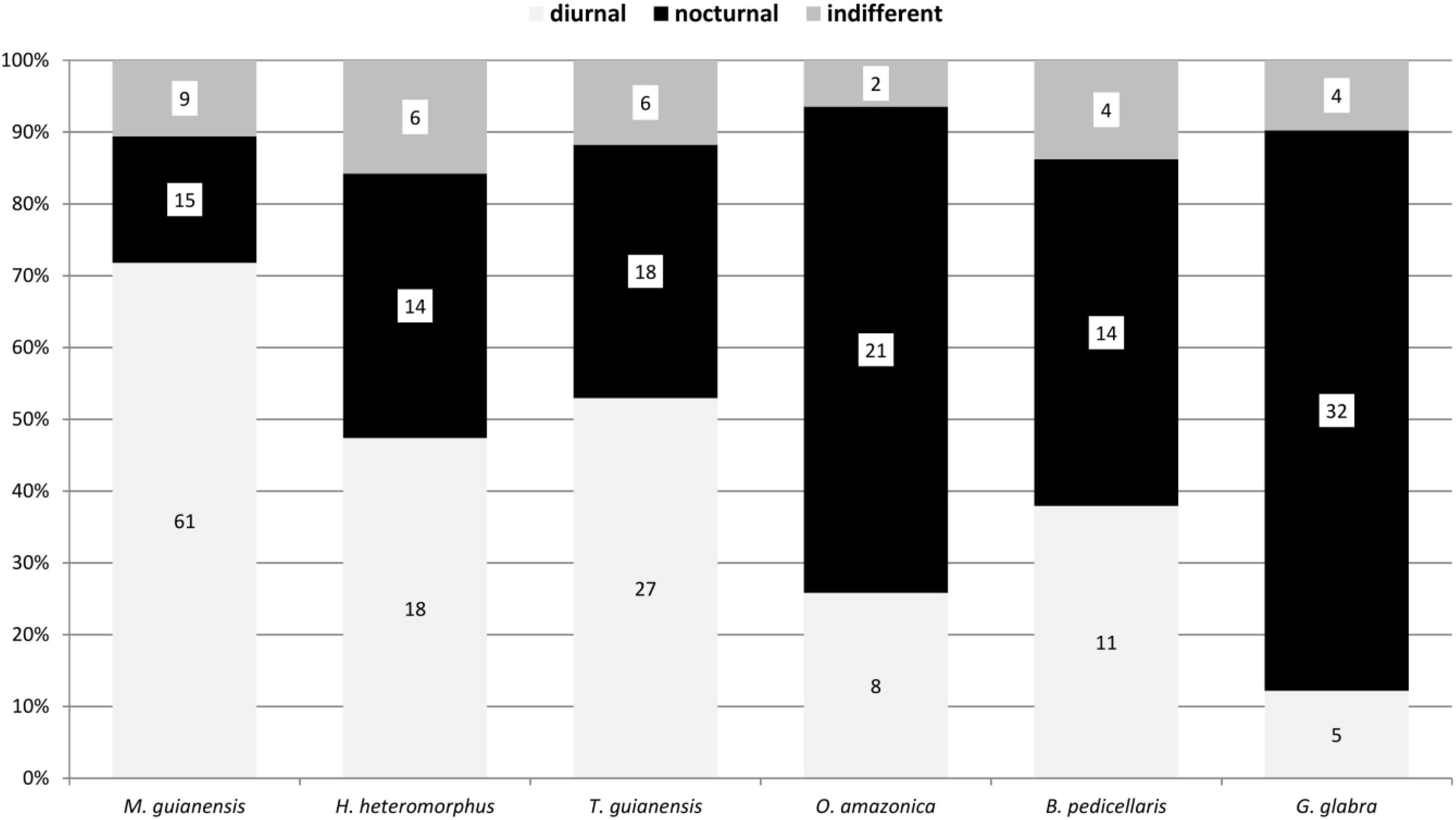
Figure 3. Diel activity of beetle species collected with the window traps in the canopy on six tree species in a lowland rainforest canopy, Venezuela, between 1997 and 1999.
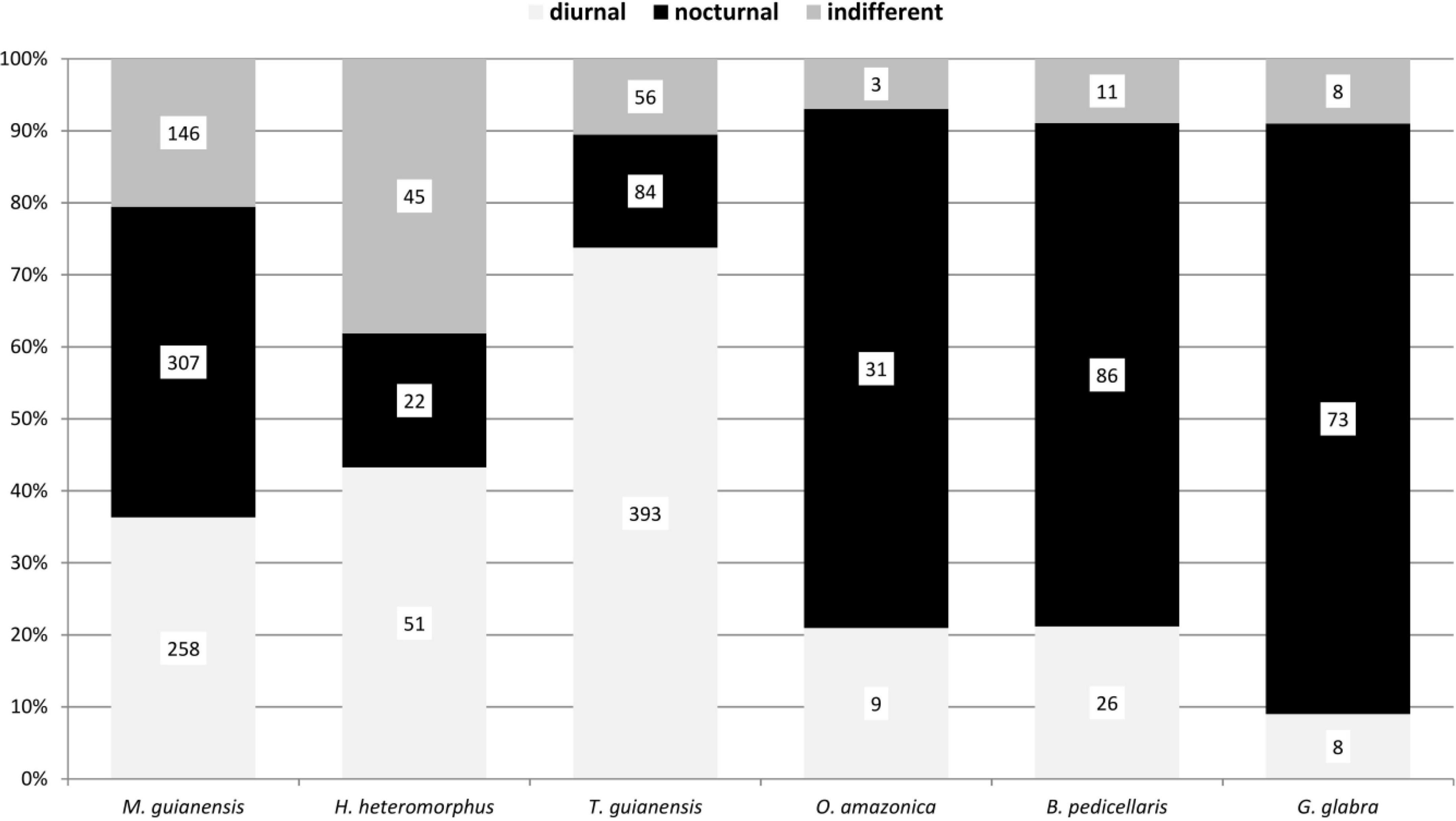
Figure 4. Diel activity of adult beetle individuals collected with the window traps in the canopy on six tree species in a lowland rainforest canopy, Venezuela, between 1997 and 1999.
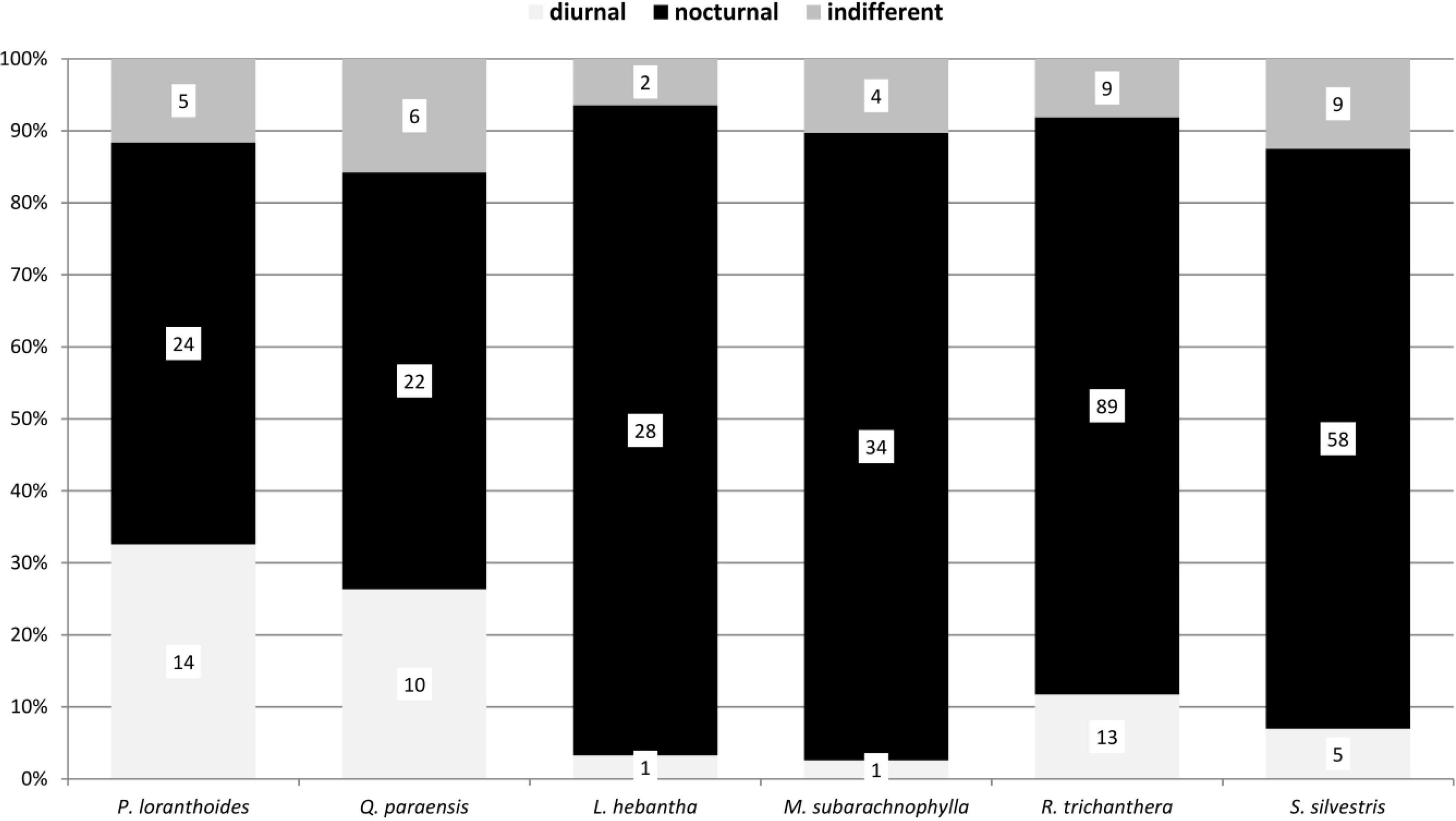
Figure 5. Diel activity of beetle species collected with hand and in the window traps in the canopy on six tree species in a lowland rainforest canopy, Venezuela, between 1997 and 1999.
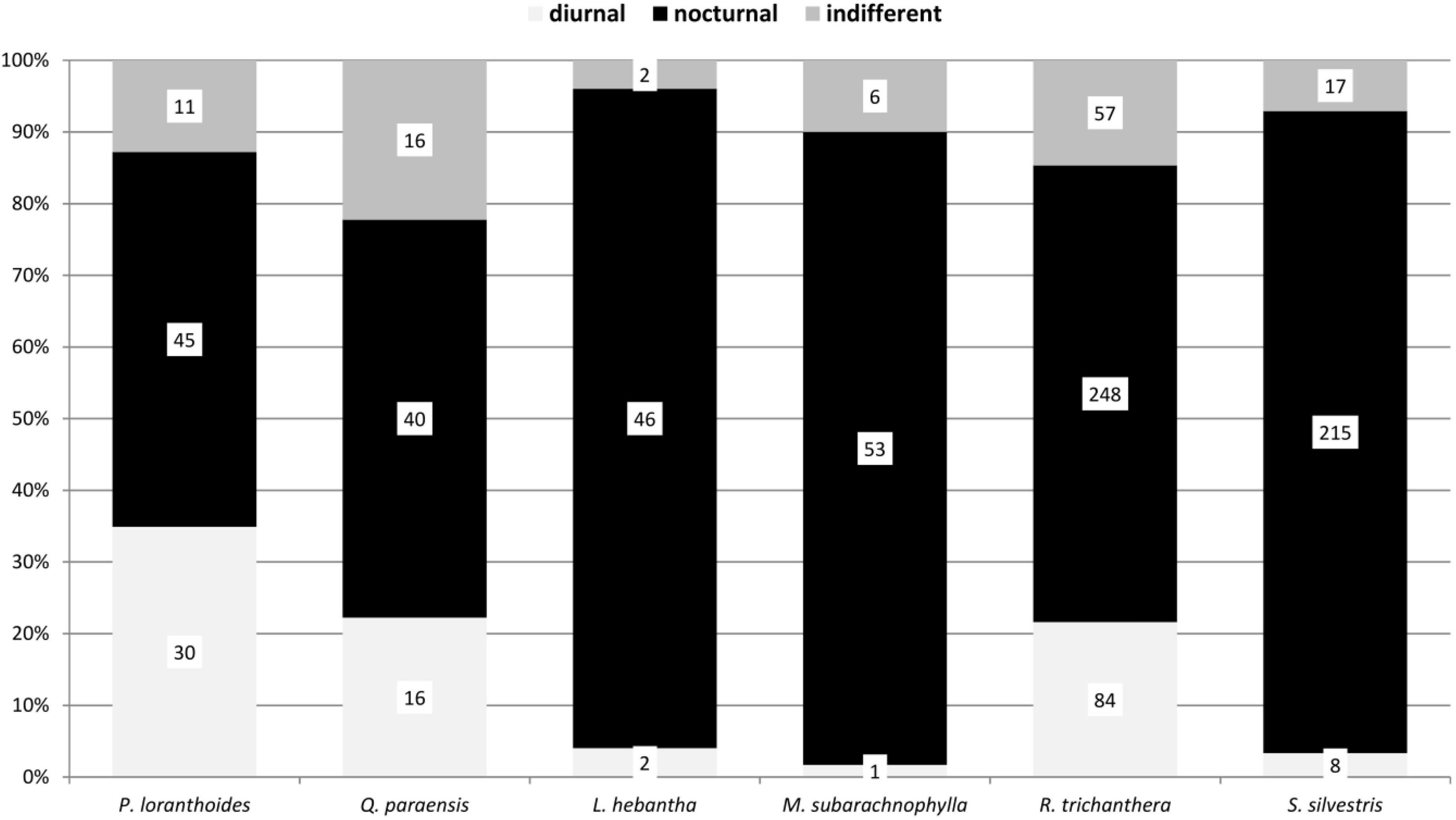
Figure 6. Diel activity of adult beetle individuals collected with hand and in the window traps in the canopy on six tree species in a lowland rainforest canopy, Venezuela, for a cumulative year between 1997 and 1999.
4 Discussion
4.1 Assignment of diel activity
The assignment of diel activity to adult beetles in this canopy survey should be largely correct, as visitors to flowers and extrafloral nectaries were regularly found only during their phases of activity on their host trees. There are only a few exceptions for diurnal beetle species resting in the canopy of neighboring trees at night [e.g., Macraspis festiva Burmeister (Kirmse and Ratcliffe, 2019); Tetraonyx Latreille sp. 1 (Meloidae)]. In a few cases, the spotlight used to enable observations at night might have disturbed diurnal beetles resting in the canopy, possibly leading to a false assignment of diel activity. Resting on the host was observed by Rausher and Fowler (1979) in Chauliognathus distinguendus Waterhouse (Cantharidae), which spent the night in a quiescent state congregating on the leaves and inflorescences of a Polygonaceae in Costa Rica. Similarly, some nocturnal beetle species were quiescent inside dense inflorescences or inside closed flowers during the day, as observed by Listabarth (1992) in bactridine Arecaceae in Peru. The large proportion of indifferent beetles in this survey was mainly due to species associated with dense inflorescences or infructescences of O. bacaba. Among these beetle groups, clear differentiation between diurnal and nocturnal activity was not possible.
4.2 Habitat filtering
Gaston (2019) advised that ecologists are biased toward studying daytime phenomena. Owens et al. (2020) added that this is regrettable, because half of all insect species are nocturnal. This bias was avoided by collecting and observing adult beetles during the day and night on their host trees. I found that the proportions of nocturnal and diurnal individuals were balanced among the 23 canopy-tree species. In contrast to the Venezuelan study, Hernández-Camargo et al. (2017) found that most of the 648 beetle specimens collected from 26 families were active at night (59.87%) in a tropical deciduous forest in Mexico. Unlike the number of individuals, the number of species encountering the Venezuelan canopy at night was with 52.5% higher than the 37% of beetle species that occur during the day. This suggests that more beetle species can cope with the canopy conditions at night. Nonetheless, Hernández-Camargo et al. (2017) collected a similar proportion of nocturnal (41.7%) and diurnal (41.2%) species among 202 beetle species in Mexico. Dealing with both hot and dry conditions in tree canopies during the day requires some kind of stress tolerance. In general, the considerable exchange between nocturnal and diurnal beetle species occurring in the canopy may be largely attributed to the high fluctuations in relative humidity and air temperature by almost 10°C (Walsh, 1996; Szarzynski and Anhuf, 2001). In concordance with the Venezuelan study, Schowalter and Ganio (2003) observed a diurnal change in the presence and relative abundance of arthropods in one species of Cecropiaceae in Puerto Rico, with several taxa being more abundant at night.
Apart from dealing with the harsh microclimatic conditions at the interface between tree tops and the atmosphere, there are possibly many more factors that force the daily exchange of canopy beetles. For instance, predators can be attracted to aggregations of insects that feed on flowers (Wardhaugh et al., 2013). Ants represent the most abundant animal group in the canopy of tropical forests, and thus significantly influence the diversity, structure and dynamics of arboreal arthropod communities (Erwin, 1983; Floren and Linsenmair, 1994; Harada and Adis, 1998; Basset, 2001; Philpott and Armbrecht, 2006; Floren et al., 2014). Novotny et al. (1999) showed that ants were responsible for 77% of the predatory attacks and were three times stronger during the daytime. This may have forced the dominance of nocturnal beetle species in the Venezuelan canopy plot. Wagner (1999) found a significant correlation between chrysomelid beetle percentage and ant abundance in Central Africa. Other predatory arthropods like spiders or hymenopterans have naturally higher abundances and richness in lowland than in highland habitats (Lalisan et al., 2015).
4.3 Diel activity in relation to the taxon
Beetle species in most genera and some families show uniform diel activity in the canopy, suggesting that intrinsic preferences may cause diel activity patterns. In concordance with my survey, Gill et al. (2012) found that Mordellidae were diurnal, and Elateridae were nocturnal. Similarly, Carabidae showed predominantly nocturnal activity in a Mexican study (Hernández-Camargo et al., 2017). This Mexican study revealed almost only nocturnal Scarabaeidae and predominantly diurnal Chrysomelidae, in contrast to my findings. In a subtropical rainforest in Australia, canopy-associated species of Chrysomelidae and Scarabaeidae showed high activity during the night (Basset and Springate, 1992). The activity of Curculionidae was particularly high during the day, whereas my dataset contained approximately as many diurnal and nocturnal Curculionidae.
However, the division of a dung-beetle community in Brazil into nocturnal and diurnal species is related to the coloring of species rather than to taxonomic classification (Hernandez, 2002). Furthermore, there is some evidence that some taxa show differences between geographic ranges. In the United Kingdom, 60% of carabid species are nocturnal and 20% diurnal (Luff, 1978), whereas in a tropical dry forest in Colombia, 80% of ground-dwelling Carabidae are nocturnal and 13.3% show both diurnal and nocturnal activity (Ariza et al., 2021). This may explain, in part, the differences between the results of several studies.
Often, the activity of beetles is not only diurnal or nocturnal but is also defined as distinct hours of the day. For instance, in Borneo, canopy dung beetle activity occurs at either midday or dawn-dusk (Davis et al., 1997). García-López et al. (2011) collected most scarab beetles in tropical forests during the first hours of the night. Most of the bioluminescent species investigated in Brazil are active during twilight and show short nocturnal activity after sunset (Viviani and Santos, 2012). In Brazil, Cyclocephala putrida Burmeister specimens started flying from 18:00 onward and were mostly collected from 20:00 to 22:00 h (Saldanha et al., 2020). However, I did not exactly discriminate the hours of activity due to the rather low abundances and collection of the 23 tree species at different times. Theoretically, this could have caused under-representation of beetles with short activity phases in the sample.
4.4 Diet and diel activity
Diel activity is associated with diet and food resources. Diurnal beetles predominantly visited small white flowers (Figure 2). They dominated the communities of mass-flowering trees with small white flowers of a generalized morphology (Figures 3, 4). In concordance, Ødegaard and Frame (2007) found beetle activity to be highest in the morning on two similar generalist blossoms of canopy trees in a Panamanian lowland forest. Similarly, Küchmeister et al. (1997) observed beetle visitors to Euterpe Mart. (Arecaceae), including Chrysomelidae, Curculionidae, and Staphylinidae only during the day. In a Malaysian Sapindaceae, visits ceased completely after 6 pm (Appanah, 1982). Although many beetle-pollinated plants flower during the day and night (Momose et al., 1998), other flower syndromes attracted more nocturnal than diurnal beetle species and individuals in the Venezuelan canopy plot. Accordingly, Sakai et al. (1999) found three times more beetles at night than during the day in one canopy tree in Malaysia. Species of Annona L. (Annonaceae) are usually visited by nocturnal beetles that escape during the day (Gottsberger, 1989). Inga marginata Willd. (Fabaceae) in Colombia, with a flower syndrome and phenology similar to that of B. pedicellaris was visited exclusively at night (Marin-Gomez et al., 2016).
The attraction of either diurnal or nocturnal beetles to different flowers may be due to several reasons. The production of floral nectar may be confined to the day or night, depending on diurnally or nocturnally active pollinator groups associated with a particular plant species (Cruden et al., 1983). The temporal patterns of nectar production dynamics (Pacini and Nepi, 2007) are usually linked to the foraging behavior of visiting animals (Nicolson, 2007). Additionally, plants may release different volatile blends during the day and night, which can attract or repel different visitor groups (De Moraes et al., 2001). In contrast to diurnal species, nocturnal beetles showed a broader spectrum of plant parts utilized in the Venezuelan assemblage (Figure 2). Moreover, many nocturnal beetles consumed extrafloral nectar, in contrast to diurnal beetles. For example, the extrafloral nectaries of R. trichanthera and S. cf. silvestris were visited by many nocturnal beetles (Figures 5, 6; Kirmse, unpub. data). Heil et al. (2004) also found herbivorous beetles on extrafloral nectaries mostly at night. Extrafloral nectaries often exhibit strong variations in nectar flow during the day (O’Dowd, 1979). Nectar production may peak at a distinct time of day or night (Wickers, 1997; Heil et al., 2000; Raine et al., 2002; Heil and McKey, 2003). Elias et al. (1975) indicated that extrafloral nectaries in some plants have nectar production cycles that are correlated with the times of herbivores expected to be most active. Thus, the temporally restricted availability of resources may correspond to the visitor taxon.
5 Conclusion
The majority of beetle species (89.6%) showed either nocturnal or diurnal activity in the canopy of the studied Amazonian rainforest. According to their diel activity, most canopy-beetle species occupied the canopy temporally. This daily interchange may result in the findings of a few canopy specialists as well as the rapid recolonization of trees.
The fact that more than half of the beetle species observed were nocturnal implies that more beetle species can cope with the microclimatic conditions in the canopy during the night than during the day. Still, activity in the canopy is linked to the taxon, with several families active either during the day or night, indicating an intrinsic preference. In contrast, niche partitioning did not seem to play an important role in the canopy, as the beetle assemblages on a single tree species were dominated by diurnal or nocturnal beetles.
Although this beetle survey covered only a small proportion of the diversity of Amazonian tree species, the data suggest that studies on the diel activity of arboreal beetles should incorporate the identity of trees and their phenological seasons.
Data availability statement
The original contributions presented in this study are included in this article, further inquiries can be directed to the corresponding author.
Author contributions
SK: Conceptualization, Funding acquisition, Investigation, Writing – original draft, Writing – review & editing.
Funding
The author declares that financial support was received for the research, authorship, and/or publication of this article. The fieldwork was supported, in part, by grants from the ESF Tropical Canopy Programme and the Stiftung der Deutschen Wirtschaft, Germany. Funding for this study is provided by The Royal Society, London, UK.
Acknowledgments
For the determination of beetles, I thank all the beetle experts indicated in the methods section. I cordially thank Jens Wesenberg for superior assistance in all botanical matters and field work. The Austrian Academy of Sciences and colleagues are gratefully acknowledged for their support and permission to join the Surumoni Project in Venezuela. Finally, but most importantly, the Leopoldina, Germany, generously supported this study through scientific and financial means.
Conflict of interest
The author declares that the research was conducted in the absence of any commercial or financial relationships that could be construed as a potential conflict of interest.
Publisher’s note
All claims expressed in this article are solely those of the authors and do not necessarily represent those of their affiliated organizations, or those of the publisher, the editors and the reviewers. Any product that may be evaluated in this article, or claim that may be made by its manufacturer, is not guaranteed or endorsed by the publisher.
References
Albrecht, M., and Gotelli, N. J. (2001). Spatial and temporal niche partitioning in grassland ants. Oecologia 126, 134–141. doi: 10.1007/s004420000494
Allison, A., Samuelson, G. A., and Miller, S. E. (1993). Patterns of beetle species diversity in New Guinea rain forest as revealed by canopy fogging: Preliminary findings. Selbyana 14, 16–20.
Anhuf, D., and Rollenbeck, R. (2001). Canopy structure of the Surumoni rain forest (Venezuela) and its influence on microclimate. Ecotropica 7, 21–32.
Anhuf, D., Motzer, T., Rollenbeck, R., Schröder, B., and Scarzynski, J. (1999). Water budget of the Surumoni crane site (Venezuela). Selbyana 20, 179–185.
Appanah, S. (1982). Pollination of androdioecious Xerospermum intermedium Radlk. (Sapindaceae) in a rain forest. Biol. J. Linn. Soc. 18, 11–34.
Ariza, G. M., Jácome, J., and Kotze, D. J. (2021). Carabid beetles of tropical dry forests display traits that cope with a harsh environment. Int. J. Trop. Insect Sci. 41, 3011–3021. doi: 10.1007/s42690-021-00493-9
Basset, Y. (1991). Influence of leaf traits on the spatial distribution of insect herbivores associated with an overstory rainforest tree. Oecologia 87, 388–393. doi: 10.1007/BF00634596
Basset, Y. (2001). Invertebrates in the canopy of tropical rain forests: How much do we really know? Plant Ecol. 153, 87–107.
Basset, Y., Aberlenc, H. P., Barrios, H., and Curletti, G. (2003). “Diel activity of arthropods,” in Arthropods of tropical forests. Spatio-temporal dynamics and resource use in the canopy, eds Y. Basset, V. Novotny, S. E. Miller, and R. L. Kitching (Cambridge: Cambridge University Press), 304–314.
Basset, Y., Aberlenc, H.-P., Barrios, H., Curletti, G., Béranger, J. M., Vesco, J. P., et al. (2001). Stratification and diel activity of arthropods in a lowland rain forest in Gabon. Biol. J. Linn. Soc. 72, 585–607.
Basset, Y., and Arthington, A. H. (1992). The arthropod community of an Australian rainforest tree: Abundance of component taxa, species richness and guild structure. Aust. J. Ecol. 17, 89–98.
Basset, Y., and Springate, N. D. (1992). Diel activity of arboreal arthropods associated with a rainforest tree. J. Nat. Hist. 26, 947–952.
Basset, Y., Cizek, L., Cuénoud, P., Didham, R. K., Novotny, V., Ødegaard, F., et al. (2015). Arthropod distribution in a tropical rainforest: Tackling a four dimensional puzzle. PLoS One 10:e0144110. doi: 10.1371/journal.pone.0144110
Basset, Y., Springate, N. D., Aberlenc, H.-P., and Delvare, G. (1997). “A review of methods for sampling arthropods in tree canopies,” in Canopy arthropods, eds N. E. Stork, J. A. Adis, and R. K. Didham (London: Chapman & Hall), 27–52. doi: 10.1098/rspb.2004.3023
Bernhardt, P. (2000). Convergent evolution and adaptive radiation of beetle-pollinated angiosperms. Plant Syst. Evol. 222, 293–320. doi: 10.1007/BF00984108
Blanc, P. (1990). “Bioclimatologie comparée de la canopée et du sous-bois,” in Biologie d’une canopée de forêt équatoriale. Rapport de mission radeau des cimes Octobre-Novembre 1989, Petit Saut – Guyane Française, eds F. Hallé and P. Blanc (Paris: Montpellier II et CNRS-Paris VI), 42–43.
Bouchard, P., Bousquet, Y., Davies, A. E., Alonso-Zarazaga, M. A., Lawrence, J. F., Lyal, C. H. C., et al. (2011). Family-group names in Coleoptera (Insecta). ZooKeys 88, 1–972.
Boulter, S. L., Kitching, R. L., Howlett, B. G., and Goodall, K. (2005). Any which was will do – the pollination biology of a northern Australian rainforest canopy tree (Syzygium sayeri, Myrtaceae). Bot. J. Linn. Soc. 149, 69–84.
Brady, D., Saviane, A., Cappellozza, S., and Sandrelli, F. (2021). The circadian clock in Lepidoptera. Front. Physiol. 12:776826. doi: 10.3389/fphys.2021.776826
Cambefort, Y. (1991). “Dung beetles in tropical savannas,” in Dung beetle ecology, eds I. Hanski and Y. Cambefort (Princeton, NJ: Princeton University Press), 156–178.
Caveney, S., Scholtz, C. H., and McIntyre, P. D. (1995). Patterns of daily flight activity in onitine dung beetles. Oecologia 103, 444–452. doi: 10.1007/BF00328682
Cruden, R. W., Hermann, S. M., and Peterson, S. (1983). “Patterns of nectar production and plant-pollinator coevolution,” in The biology of nectaries, eds B. Bentley and T. S. Elias (New York, NY: Columbia University Press), 80–125.
Davis, A. J., Huijbregts, J., Kirk-Spriggs, A. H., Krikken, J., and Sutton, S. L. (1997). “The ecology and behaviour of arboreal dung beetles in Borneo,” in Canopy arthropods, eds N. E. Stork, J. Adis, and R. K. Didham (London: Chapman and Hall), 417–432.
De Moraes, C. M., Mescher, M. C., and Tumlinson, J. H. (2001). Caterpillar-induced nocturnal plant volatiles repel conspecific females. Nature 410, 577–580. doi: 10.1038/35069058
Dinerstein, E., Olson, D. M., Graham, D. J., Webster, A. L., Primm, S. A., Bookbinder, M. P., et al. (1995). A conservation assessment of the terrestrial ecoregions of Latin America and the Caribbean. Washington, DC: World Bank.
Elias, T. S., Rozich, W. R., and Newcombe, L. (1975). The foliar and floral nectaries of Turnera ulmifolia L. Am. J. Bot. 62, 570–576.
Engwald, S., Schmit-Neuerburg, V., and Barthlott, W. (2000). “Epiphytes in rain forests of Venezuela – diversity and dynamics of a biocenosis,” in Proceedings of the 1st Symposium by the A.F.W Schimper-Foundation – from E. Walter – Hoheneim, Oktober 1998, eds S. W. Breckle, B. Schweizer, and U. Arndt (Stuttgart: Verlag Günter Heimbach), 425–434.
Erwin, T. L. (1982). Tropical forests: Their richness in Coleoptera and other arthropod species. Coleopter. Bull. 39, 74–75.
Erwin, T. L. (1983). “Beetles and other arthropods of the tropical forest canopies at Manaus, Brasil, sampled with insecticidal fogging techniques,” in Tropical Rain Forests: Ecology, eds Management, S. L. Sutton, T. C. Whitmore, and A. C. Chadwick (Oxford: Blackwell Scientific Publications), 59–75.
Faegri, K., and Van der Pijl, L. (1979). The principles of pollination ecology. Oxford, NY: Pergamon Press.
Feer, F., and Pincebourde, S. (2005). Diel flight activity and ecological segregation within an assemblage of tropical forest dung and carrion beetles. J. Trop. Ecol. 21, 21–30.
Floren, A., and Linsenmair, K. E. (1994). Diversity and recolonization of arboreal Formicidae and Coleoptera in a lowland rain forest in Sabah, Malaysia. Second Int. ESF Workshop Trop. Res. 19, 41–44.
Floren, A., and Linsenmair, K. E. (1999). Changes in arboreal arthropod communities along a disturbance gradient. Selbyana 20, 232–241.
Floren, A., Wetzel, W., and Staab, M. (2014). The contribution of canopy species to overall ant diversity (Hymenoptera: Formicidae) in temperate and tropical ecosystems. Myrmecol. News 19, 65–74.
Frame, D. (2003). Generalist flowers, biodiversity and florivory: Implications for angiosperm origins. Taxon 52, 681–685. doi: 10.2307/4135541
Franco, D. L., Frenkel, L., and Ceriani, M. F. (2018). The underlying genetics of Drosophila circadian behaviors. Physiology 33, 50–62. doi: 10.1152/physiol.00020.2017
Frankie, E. G. (1975). “Tropical forest phenology and pollinator plant coevolution,” in Coevolution of animals and plants, eds L. E. Gilbert and P. H. Raven (London: University of Texas Press), 192–209.
Fullard, J. H., Ortero, L. D., Orellana, A., and Surlykke, A. (2000). Auditory sensitivity and diel flight activity in Neotropical Lepidoptera. Ann. Entomol. Soc. Am. 93, 956–965.
García-López, A., Micó, E., Zumbado, M. A., and Galante, E. (2011). Sampling scarab beetles in tropical forests: The effect of light source and night sampling periods. J. Insect Sci. 11:95. doi: 10.1673/031.011.9501
Gaston, K. J. (2019). Nighttime ecology: The “nocturnal problem” revisited. Am. Nat. 193, 481–502. doi: 10.1086/702250
Gill, B. D. (1991). “Dung beetles in tropical American forest,” in Dung beetle ecology, eds I. Hanski and Y. Cambefort (Princeton, NJ: Princeton University Press), 211–229. doi: 10.1371/journal.pone.0075819
Gill, H. K., Goyal, G., and McSorley, R. (2012). Diel activity of fauna in different habitats sampled at the autumnal equinox. Florida Entomol. 95, 319–325.
Giménez-Gómez, V. C., Lomáscolo, S. B., Zurita, G. A., and Ocampo, F. (2018). Daily activity patterns and thermal tolerance of three sympatric dung beetle species (Scarabaeidae: Scarabaeinae: Eucraniini) from the Monte Desert, Argentina. Neotrop. Entomol. 47, 821–827. doi: 10.1007/s13744-017-0567-2
Gonzales, C. J., Iturraldo, J. M. A., and Picard, C. X. (1980). Geologia de Venezuela y de sus cuencas petrolfferas. Tomo I/II. Caracas: Ediciones Foninves.
Goodspeed, D., Chehab, E., Min-Venditti, A., Braam, J., and Covington, M. F. (2012). Arabidopsis synchronizes jasmonate-mediated defense with insect circadian behavior. Proc. Natl. Acad. Sci. U.S.A. 109, 4674–4677. doi: 10.1073/pnas.1116368109
Gottsberger, G. (1989). Beetle pollination and flowering rhythm of Annona spp. (Annonaceae) in Brazil. Plant Syst. Evol. 167, 165–187.
Halffter, G., and Matthews, E. G. (1966). The natural history of dung beetles of the subfamily Scarabaeinae (Coleoptera: Scarabaeidae). Fol. Ent. Mex. 12-14, 1–312.
Hallé, F. (1998). “Distribution verticale des métabolites secondaires en forêt équatoriale - une hypothèse,” in Biologie d’une canopée de forêt equatoriale – III. Rapport de la Mission d’ exploration scientifique de la canopée de Guyane, Octobre - Décembre 1996, ed. F. Hallé (Paris: Pro-Natura International & Opération Canopée), 129–138.
Hammond, P. M. (1990). “Insect abundance and diversity in the Dumoga-Bone National Park, North Sulawesi, with special reference to the beetle fauna of lowland rain forest in the Toraut region,” in Insects and the rainforests of South East Asia (Wallacea), eds W. J. Knight and J. D. Holloway (London: The Royal Entomological Society of London), 197–254.
Hanski, I., and Cambefort, Y. (1991). “Resource partitioning,” in Dung beetle ecology, eds I. Hanski and Y. Cambefort (Princeton, NJ: Princeton University Press), 330–349.
Harada, A. Y., and Adis, J. A. (1998). Ants obtained from trees of “Jacareúba” (Calophyllum brasiliense) forest plantation in central Amazonia by canopy fogging: First results. Acta Amazonica. 28, 309–318.
Háva, J. (2004). World keys to the genera and subgenera of Dermestidae (Coleoptera), with descriptions, nomenclature and distributional records. Acta Mus. Nat. Pragae Ser. B Hist. Nat. 60, 149–164.
Heil, M., and McKey, D. (2003). Protective ant-plant interactions as model systems in ecological and evolutionary research. Annu. Rev. Ecol. Syst. 34, 425–453. doi: 10.1080/15548627.2015.1100356
Heil, M., Fiala, B., Baumann, B., and Linsenmair, K. E. (2000). Temporal, spatial and biotic variations in extrafloral nectar secretion by Macaranga tanarius. Funct. Ecol. 14, 749–757.
Heil, M., Hilpert, A., Krüger, R., and Linsenmair, K. E. (2004). Competition among visitors to extrafloral nectaries as a source of ecological costs of an indirect defence. J. Trop. Ecol. 20, 201–208.
Heinrich, B., and Raven, P. (1972). Energetics and pollination ecology. Science 176, 597–602. doi: 10.1126/science.176.4035.597
Hernandez, M. I. M. (2002). The night and day of dung beetles (Coleoptera, Scarabaeidae) in the Serra do Japi, Brazil: Elytra colour related to daily activity. Rev. Bras. Entomol. 46, 597–600.
Hernández-Camargo, J. E., Jiménez-Sánchez, E., and Padilla-Ramírez, J. (2017). Diurnal and nocturnal activity of beetles (Insecta: Coleoptera) captured with flight intercept trap in Tonatico, Estado de Mexico, Mexico. Entomol. Mex. 4, 467–472.
Hossaert-McKey, M., Orivel, J., Labeyrie, E., Pascal, L., Delabie, J., and Dejean, A. (2001). Differential associations with ants of three co-occurring extrafloral nectary-bearing plants. Ecoscience 8, 325–335. doi: 10.1080/11956860.2001.11682660
Howden, H. F., Howden, A. T., and Storey, R. (1991). Nocturnal perching of Scarabaeine dung beetles (Coleoptera, Scarabaeidae) in an Australian tropical rain forest. Biotropica 23, 51–57.
Howe, G. A., and Jander, G. (2008). Plant immunity to insect herbivores. Annu. Rev. Plant Biol. 59, 41–66. doi: 10.1146/annurev.arplant.59.032607.092825
Howe, H. F., and Westley, L. C. (1986). “Ecology of pollination and seed dispersal,” in Plant ecology, ed. M. Crawley (Oxford: Blackwells), 185–215.
Howe, H. F., and Westley, L. C. (1988). Ecological relationships of plants and animals. New York, NY: Oxford University Press.
Irvine, A. K., and Armstrong, J. E. (1990). “Beetle pollination in tropical forests of Australia,” in Reproductive ecology of tropical forest plants. Man and the biosphere series 7, eds K. S. Bawa and M. Hadley (Carnforth: UNESCO, Paris and Parthenon Publ), 135–149.
Jander, G., Cui, J., Nhan, B., Pierce, N. E., and Ausubel, F. M. (2001). The TASTY locus on chromosome 1 of Arabidopsis affects feeding of the insect herbivore Trichoplusia ni. Plant Physiol. 126, 890–898. doi: 10.1104/pp.126.2.890
Kessler, A., and Baldwin, I. T. (2002). Plant responses to insect herbivory: The emerging molecular analysis. Annu. Rev. Plant Biol. 53, 299–328. doi: 10.1146/annurev.arplant.53.100301.135207
Kirmse, S., Adis, J., and Morawetz, W. (2003). “Flowering events and beetle diversity in Venezuela,” in Arthropods of tropical forests. Spatiotemporal dynamics and resource use in the canopy, eds Y. Basset, V. Novotny, S. E. Miller, and R. L. Kitching (Cambridge: Cambridge University Press), 256–265.
Kirmse, S., and Chaboo, C. S. (2019). Extrafloral nectaries mediate the arboreal beetle community (Coleoptera) in a Neotropical rainforest. J. Nat. Hist. 53, 1313–1349. doi: 10.1080/00222933.2019.1650211
Kirmse, S., and Chaboo, C. S. (2020). Flowers are essential to maintain high beetle diversity (Coleoptera) in a Neotropical rainforest canopy. J. Nat. Hist. 54, 1661–1696. doi: 10.1080/00222933.2020.1811414
Kirmse, S., and Ratcliffe, B. C. (2019). Composition and host-use patterns of a scarab community (Coleoptera: Scarabaeidae) inhabiting the canopy of a lowland tropical rainforest in southern Venezuela. Coleop. Bull. 73, 149–167. doi: 10.1649/0010-065X-73.1.149
Koskela, H. (1979). Patterns of diel flight activity in dung inhabiting beetles: An ecological analysis. Oikos 33, 419–439. doi: 10.2307/3544330
Krell-Westerwalbesloh, S., Krell, F.-T., and Linsenmair, K. E. (2004). Diel separation of Afrotropical dung beetle guilds –avoiding competition and neglecting resources (Coleoptera: Scarabaeoidea). J. Nat. Hist. 38, 2225–2249. doi: 10.1080/00222930310001618921
Kronfeld-Schor, N., and Dayan, T. (2003). Partitioning time as an ecological resource. Ann. Rev. Ecol. Evol. Syst. 34, 153–181.
Küchmeister, H., Silberbauer-Gottsberger, I., and Gottsberger, G. (1997). Flowering, pollination, nectar standing crop, and nectaries of Euterpe precatoria (Arecaceae), an Amazonian rain forest palm. Plant Syst. Evol. 206, 71–97.
Lalisan, J. A., Dupo, A. L. B., and Nuneza, O. (2015). Diversity of spiders along an elevational gradient in Mt. Pinukis, Zamboanga del Sur, Philippines. JBES 7, 190–201. doi: 10.31396/Biodiv.Jour.2020.11.2.593.610
Listabarth, C. (1992). A survey of pollination strategies in the Bactridinae (Palmae): Bull. Inst. Frétudes Andines 21, 699–714.
Luna, P., Peñaloza-Arellanesa, Y., Castillo-Mezaa, A. L., García-Cháveza, J. H., and Dáttilo, W. (2018). Beta diversity of ant-plant interactions over day-night periods and plant physiognomies in a semiarid environment. J. Arid Environ. 156, 69–76. doi: 10.1016/j.jaridenv.2018.04.003
Marin-Gomez, O. H., Lopez-Garcia, M. M., and Vandehuck, M. G. (2016). Floral visitors of Inga marginata Willd. (Mimosaceae) in a coffee agroecosystem of Quindío. Colombia. Trop. Ecol. 57, 649–654.
Martín-Piera, F., Sanmartín, I., and Lobo, J. M. (1994). Observaciones sobre el ritmo de actividad diaria en escarabeidos telecópridos (Coleoptera, Scarabaeidae). Bull. Soc. Entomol. Fr. 99, 463–470.
Mena, J., Galante, E., and Lumbreras, C. J. (1989). Daily flight activity of Scarabaeidae and Geotrupidae (Col.) and analysis of the factors determining this activity. Ecol. Mediterr. 15, 69–80. doi: 10.3406/ecmed.1989.1635
Momose, K., Yumoto, T., Nagamitsu, T., Kato, M., Nagamitsu, M., Sakai, S., et al. (1998). Pollination biology in a lowland dipterocarp forest in Sarawak, Malaysia. I. Characteristics of the plant – pollinator community in a lowland dipterocarp forest. Am. J. Bot. 85, 1477–1501.
Morrone, J. J. (2014). Monograph biogeographical regionalisation of the Neotropical region. Zootaxa 3782, 1–110. doi: 10.11646/zootaxa.3782.1.1
Nicolson, S. W. (2007). “Nectar consumers,” in Nectaries and nectar, eds S. W. Nicolson, M. Nepi, and E. Pacini (Dordrecht: Springer), 289–342.
Novotny, V., Basset, Y., Auga, J., Boen, W., Dal, C., Drozd, P., et al. (1999). Predation risk for herbivorous insects on tropical vegetation: A search for enemy-free space and time. Aust. J. Ecol. 24, 477–483.
O’Dowd, D. J. (1979). Foliar nectar production and ant activity on a Neotropical tree. Ochroma pyramidale. Oecologia 43, 233–248. doi: 10.1007/BF00344773
Ødegaard, F., and Frame, D. (2007). Generalist flowers and phytophagous beetles in two tropical canopy trees: Resources for multitudes. Taxon 56, 696–706.
Owens, A. C. S., Cochard, P., Durrant, J., Farnworth, B., Perkin, E. K., and Seymoure, B. (2020). Light pollution is a driver of insect declines. Biol, Conserv. 241:108259. doi: 10.1016/j.biocon.2019.108259
Pacini, E., and Nepi, M. (2007). “Chapter 4. Nectar production and presentation,” in Nectaries and nectar, eds S. W. Nicolson, M. Nepi, and E. Pacini (Dordrecht: Springer), 167–214.
Palmer, M. (1995). Testing for a seasonal displacement in a dung beetle guild. Ecography 18, 173–177. doi: 10.1111/j.1600-0587.1995.tb00338.x
Paranjpe, D. A., and Sharma, V. K. (2005). Evolution of temporal order in living organisms. J. Circadian Rhythms 3:7. doi: 10.1186/1740-3391-3-7
Parker, G. G. (1995). “Structure and microclimate of forest canopies,” in Forest canopies, eds M. D. Lowman and N. M. Nadkarni (San Diego, CA: Academic Press), 73–98.
Philpott, S. M., and Armbrecht, I. (2006). Biodiversity in tropical agroforests and the ecological role of ants and ant diversity in predatory function. Ecol. Entomol. 31, 369–377.
Pincebourde, S., and Woods, H. A. (2020). There is plenty of room at the bottom: Microclimates drive insect vulnerability to climate change. Curr. Opin. Insect Sci. 41, 63–70. doi: 10.1016/j.cois.2020.07.001
Pincebourde, S., Dillon, M. E., and Woods, H. A. (2021). Body size determines the thermal coupling between insects and plant surfaces. Funct. Ecol. 35, 1424–1436. doi: 10.1111/1365-2435.13801
Pinto, J. D., and Bologna, M. A. (1999). The new world genera of meloidae (Coleoptera): A key and synopsis. J. Nat. Hist. 33, 569–620. doi: 10.1080/002229399300254
Prance, G. T. (1979). Notes on the vegetation of Amazonia III. The terminology of Amazonian forest types subject to inundation. Brittonia 31, 26–38.
Raine, N. E., Willmer, P., and Stone, G. N. (2002). Spatial structuring and floral avoidance behavior prevent ant-pollinator conflict in a Mexican ant-acacia. Ecology 83, 3086–3096.
Rausher, M. D., and Fowler, N. L. (1979). Intersexual aggression and nectar defense in Chauliognathus distinguendus (Coleoptera: Cantharidae). Biotropica 11, 88–95.
Reichardt, H. (1977). A synopsis of the genera of neotropical Carabidae (Insecta: Coleoptera). Quaest. Entomol. 13, 346–493.
Reymond, P., Weber, H., Damond, M., and Farmer, E. E. (2000). Differential gene expression in response to mechanical wounding and insect feeding in Arabidopsis. Plant Cell. 12, 707–719. doi: 10.1105/tpc.12.5.707
Rosbash, M. (2009). The implications of multiple circadian clock origins. PLoS Biol. 7:e62. doi: 10.1371/journal.pbio.1000062
Sakai, S., Momose, K., Yumoto, T., Kato, M., and Inoue, T. (1999). Beetle pollination of Shorea parvifolia (Section Mutica, Dipterocarpaceae) in a general flowering period in Sarawak, Malaysia. Am. J. Bot. 86, 62–69.
Saldanha, F. G., Rodrigues, S. R., Amaro, R. A., and Fuhrmann, J. (2020). Description of mating behavior, life cycle, and antennal sensilla of Cyclocephala putrida Burmeister, 1847 (Coleoptera, Scarabaeidae, Dynastinae). Biota Neotrop. 20:e20200973. doi: 10.1590/1676-0611-BN-2020-0973
Schowalter, T. D., and Ganio, L. M. (2003). “Diel, seasonal and disturbance-induced variation in invertebrate assemblages,” in Arthropods of tropical forests. Spatiotemporal dynamics and resource use in the canopy, eds Y. Basset, V. Novotny, S. E. Miller, and R. L. Kitching (Cambridge: Cambridge University Press), 315–329.
Sowig, P. (1997). Niche separation in coprophagous beetles: A comparison of two multivariate approaches. Bull. Entomol. Res. 87, 625–631. doi: 10.1017/S0007485300038724
Stork, N. E. (1991). The composition of the arthropod fauna of Bornean lowland rainforest trees. J. Trop. Ecol. 7, 161–180.
Szarzynski, J., and Anhuf, D. (2001). Micrometerological conditions and canopy energy exchanges of a neotropical rain forest (Surumoni–crane project, Venezuela). Plant Ecol. 153, 231–239.
Tomioka, K., and Matsumoto, A. (2010). A comparative view of insect circadian clock systems. Cell Mol. Life Sci. 67, 1397–1406. doi: 10.1007/s00018-009-0232-y
Turton, S. M., and Siegenthaler, D. T. (2004). Immediate impacts of a severe tropical cyclone on the microclimate of a rainforest canopy in north-east Australia. J. Trop. Ecol. 20, 583–586.
Ulyshen, M. D. (2011). Arthropod vertical stratification in temperate deciduous forests: Implications for conservation-oriented management. For. Ecol. Manag. 261, 1479–1489. doi: 10.1016/j.foreco.2011.01.033
Viviani, V. R., and Santos, R. M. (2012). Bioluminescent Coleoptera of biological station of Boracéia (Salesópolis, SP, Brazil): Diversity, bioluminescence and habitat distribution. Biota Neotrop. 12:2125.
Wagner, T. (1999). “Arboreal chrysomelid community structure and faunal overlap between different types of forests in Central Africa,” in Advances in chrysomelid biology 1, ed. M. L. Cox (Leiden: Backhurys Publishers), 247–270.
Walsh, R. P. D. (1996). “Microclimate and hydrology,” in The tropical rain forest an ecological study, ed. P. W. Richards (Cambridge: Cambridge University Press), 206–236.
Wardhaugh, C. W., Edwards, W., and Stork, N. E. (2013). Body size variation among invertebrates inhabiting different canopy microhabitat: Flower visitors are smaller. Ecol. Entomol. 38, 101–111.
Wesenberg, J. (2004). Blühphänologie im kronenraum eines tropischen tieflandregenwaldes am oberen orinoco, Amazonas, Venezuela. Fakultät für biowissenschaften, pharmazie und psychologie. Leipzig: Universität Leipzig.
Wickers, S. (1997). Study of nectariferous secretion in a pioneer plant, Inga thibaudiana, in relation with ants. Acta Bot. Gall. 144, 315–326.
Keywords: diversity, Amazonas, canopy crane, community structure, Venezuela, plant-animal interactions
Citation: Kirmse S (2024) Diel activity patterns of a canopy-inhibiting beetle community (Coleoptera) in a Neotropical rainforest. Front. For. Glob. Change 7:1370044. doi: 10.3389/ffgc.2024.1370044
Received: 13 January 2024; Accepted: 12 April 2024;
Published: 07 May 2024.
Edited by:
Akihiro Nakamura, Chinese Academy of Sciences (CAS), ChinaReviewed by:
Wataru Kojima, Yamaguchi University, JapanJana Růžičková, ELKH-ELTE-MTM Integrative Ecology Research Group, Hungary
Copyright © 2024 Kirmse. This is an open-access article distributed under the terms of the Creative Commons Attribution License (CC BY). The use, distribution or reproduction in other forums is permitted, provided the original author(s) and the copyright owner(s) are credited and that the original publication in this journal is cited, in accordance with accepted academic practice. No use, distribution or reproduction is permitted which does not comply with these terms.
*Correspondence: Susan Kirmse, c3VzYW5raXJtc2VAZ214Lm5ldA==