- College of Landscape and Horticulture, Yunnan Agricultural University, Kunming, China
Rhododendron delavayi Franch. predominantly thrives in broad-leaved evergreen forests and bushes at altitudes of 1,200–3,200 meters. It favors a cool, moist climate and thrives in acidic soils. Due to its high ornamental, medicinal, and scientific values, understanding its ecological needs and optimal cultivation ranges is crucial. This study analyzing the key environmental factors and their thresholds affecting its distribution and predicts the future potential habitats of R. delavayi using existing distribution data alongside current and projected climate data. The results indicate that the primary environmental influencers are soil pH (4.9–5.4), precipitation in the driest month (10–20 mm), and altitude, contributing 41.8, 24.1, and 18.3%, respectively. It also shows a declining trend in suitable habitats: from 27.75 × 104 km2 under current conditions, to 3.69 × 104 km2 by the 2050s, and further to 2.65 × 104 km2 by the 2070s.
1 Introduction
The Southwest region of China is a pivotal distribution and origination hub for the Rhododendron genus, housing many superior alpine varieties and emphasizing the scaled and industrial production of these plants as a key focus area (Cai et al., 2015). Rhododendron delavayi Franch., notable for its robust, majestic form and vivid, large red flowers, is highly valued for both its ornamental and medicinal properties (Yang and Xia, 2014). Despite their stunning appearance, high-altitude Rhododendrons face considerable challenges in adapting to lower altitudes, which limits their use in urban landscaping and impacts the diversity of both urban and rural green ecosystems. Consequently, understanding the environmental factors that inhibit the “descent” of R. delavayi is crucial.
Research on the interaction between plants and their environments is enhanced by utilizing species distribution points and ecological niche theory (Lai and Sun, 2004; Wang, 2013). The Maximum Entropy Model (MaxEnt), widely used in biology, geology, and other fields, employs a mathematical approach to deduce unknown probability distributions from limited known data (Phillips et al., 2006; Xing and Hao, 2011). Developed by American researchers Phillips and colleagues, MaxEnt is noted for its rapid modeling capabilities, minimal data requirements, and high accuracy, making it a popular choice for predicting species’ future trends (Phillips et al., 2006). R. delavayi primarily inhabits the mountainous regions of Southwest China, especially in provinces like Yunnan, Guizhou, Sichuan, Guangxi, and Tibet, and is a dominant species within the subtropical alpine evergreen broad-leaved forests of the Baili Rhododendron National Forest Park (Li and Chen, 2005).
The spatial distribution pattern of a species, a significant indicator in plant ecology, results from long-term environmental influences (Li et al., 2020; Xiao et al., 2020; Yu et al., 2021). Understanding habitat suitability based on environmental factors is essential for the conservation and utilization of species like R. delavayi, which thrives in acidic soils at high altitudes and exhibits a clustered distribution (Xu et al., 2022). These clusters, often formed by wind-dispersed seeds accumulating near the parent plant or along natural barriers, provide advantages during early succession stages, enhancing adaptation and resistance. This study uses MaxEnt modeling based on integrated data to simulate potential suitable habitats for R. delavayi, significantly impacting its future distribution and aiding its introduction and domestication.
In this paper, we used the MaxEnt model to simulate the potential distribution areas of R. delavayi under different climatic backgrounds, focusing on the following questions: (1) What are the main factors affecting the distribution of R. delavayi (2) How does the potential distribution pattern of R. delavayi change in different climate change backgrounds? The results of this study will provide a theoretical basis for the conservation, management, and development of R. delavayi resources.
2 Materials and methods
2.1 Species distribution data
The original data for R. delavayi specimen distribution points have sourced from the Chinese Virtual Herbarium.1 A total of 74 distribution points data of Rhododendron maenas selected for this study were screened and used for this study. The distribution points were screened using the ENMTools tool, and duplicate points have eliminated according to the raster size (5 km × 5 km) of the climatic factors, and only one point was retained in the raster, retaining only one point per grid (Figure 1). This process ensures the accuracy and reliability of the data used for ecological niche modeling and subsequent analyses.
2.2 Environmental data and preprocessing
2.2.1 Climate data
Bioclimatic variables include indicators of annual trends (mean annual temperature and precipitation), seasonality (annual range of temperature and precipitation), and extreme or limiting environmental factors. This study utilizes 19 bioclimatic variables from the WorldClim database,2 as outlined in Table 1. The dataset encompasses historical climate data from 1970 to 2000 and projections for the 2050s (2041–2060) and 2070s (2061–2080). The CCSM4 climate model, known for its robust climate simulation capabilities, was used for future climate scenarios, specifically under the RCP 4.5 pathway—a scenario with moderate global CO2 emissions (Xiao et al., 2021). The spatial resolution of this dataset was set at 2.5 arc minutes.
2.2.2 Soil data
The soil variables considered in this study include soil pH (T-PH-H2O), available water capacity (AWC-CLASS), and topsoil texture (T-TEXTURE). These variables were obtained from the Harmonized World Soil Database (HWSD).3 The resolution of this soil data is 30 arc-seconds, providing detailed information essential for understanding the soil-related ecological requirements and constraints for the species.
2.2.3 Terrain data
Terrain data, specifically elevation (Ele), was sourced from the WorldClim database (see text footnote 2). This elevation data, derived from the Shuttle Radar Topography Mission (SRTM), is available at a spatial resolution of 2.5 arc minutes. Such detailed topographic information is crucial for assessing the effects of altitude on the species’ distribution and ecological preferences.
2.2.4 Data preprocessing
To mitigate the risk of over-fitting in the model due to multicollinearity among environmental factors, which can compromise model accuracy (Li et al., 2019; Sheng-Lin et al., 2019), a selective approach was adopted for climate, topographic, and soil factors. The Band Collection Statistics tool in ArcGIS was utilized to perform a correlation analysis on all environmental factors. Factors with a correlation coefficient (r-value) greater than 0.8, indicating high correlation, were excluded. Only those variables with the highest contribution rates among the highly correlated variables were retained. This strategy effectively minimized the risk of overfitting, enhancing the reliability of the simulation results. Consequently, eight environmental factors were selected for modeling the potential suitable habitats for R. delavayi, as detailed in Table 2.
2.3 Method
2.3.1 Modeling
Geographical coordinates of Rhododendron delavayi distribution points and eight selected environmental variables were imported into the MaxEnt model for analysis. Within the model’s parameter settings interface, the Jackknife test was activated to evaluate the significance of each environmental factor. Additionally, response curves were generated to depict the relationships between R. delavayi and the environmental factors. The model output was configured to logistic format. A test set proportion of 25% was selected, with 10 random model runs to ensure robustness (Cong et al., 2018). Settings were adjusted to include options such as ‘Write plot data’ and ‘Random seed,’ with the repetition type set to ‘Subsample.’ This configuration aims to accurately predict the spatial distribution of suitable habitats for R. delavayi under both current and projected future climatic conditions (Liang et al., 2017; Sun et al., 2019).
2.3.2 Model accuracy verification
The accuracy of the model was assessed using the area under the curve (AUC) of the receiver operating characteristic curve (ROC). An AUC value greater than 0.9 is classified as ‘excellent,’ indicating high model accuracy, while a value between 0.8 and 0.9 is considered ‘good’ (Swets, 1988). The study conducted 10 iterations to robustly evaluate the model’s performance, providing a reliable measure of the model’s capability to predict suitable habitats for R. delavayi.
2.3.3 Classification of suitable living areas
The output from the MaxEnt model was exported in ASCII format and subsequently imported into ArcGIS 10.8 for detailed spatial analysis specific to China. This process involved masking and extracting over the defined Chinese region, followed by a reclassification of the layers. The reclassification utilized the natural break method to categorize the suitability areas into four distinct levels: non-suitable areas (p < 0.04), low suitability areas (0.04 ≤ p < 0.20), medium suitability areas (0.20 ≤ p < 0.46), and high suitability areas (p ≥ 0.46) (Xiao et al., 2021). After reclassification, a raster-to-polygon conversion was performed, resulting in numerous polygons. These polygons were then merged based on their suitability levels to create unified areas of similar environmental suitability for R. delavayi.
3 Result and analysis
3.1 Model validity
During the model construction, a testing dataset comprising 25% of the data was used, and the model was run 10 times randomly to produce a Receiver Operating Characteristic (ROC) curve for accuracy evaluation. The area under the curve (AUC) value for the potential distribution of R. delavayi was 0.959. An AUC value above 0.9 indicates that the model has performed well (see Figure 2), demonstrating high accuracy in predicting the future potential suitable habitats for R. delavayi (Zhang, 2015; Liu et al., 2016; Yan et al., 2016).
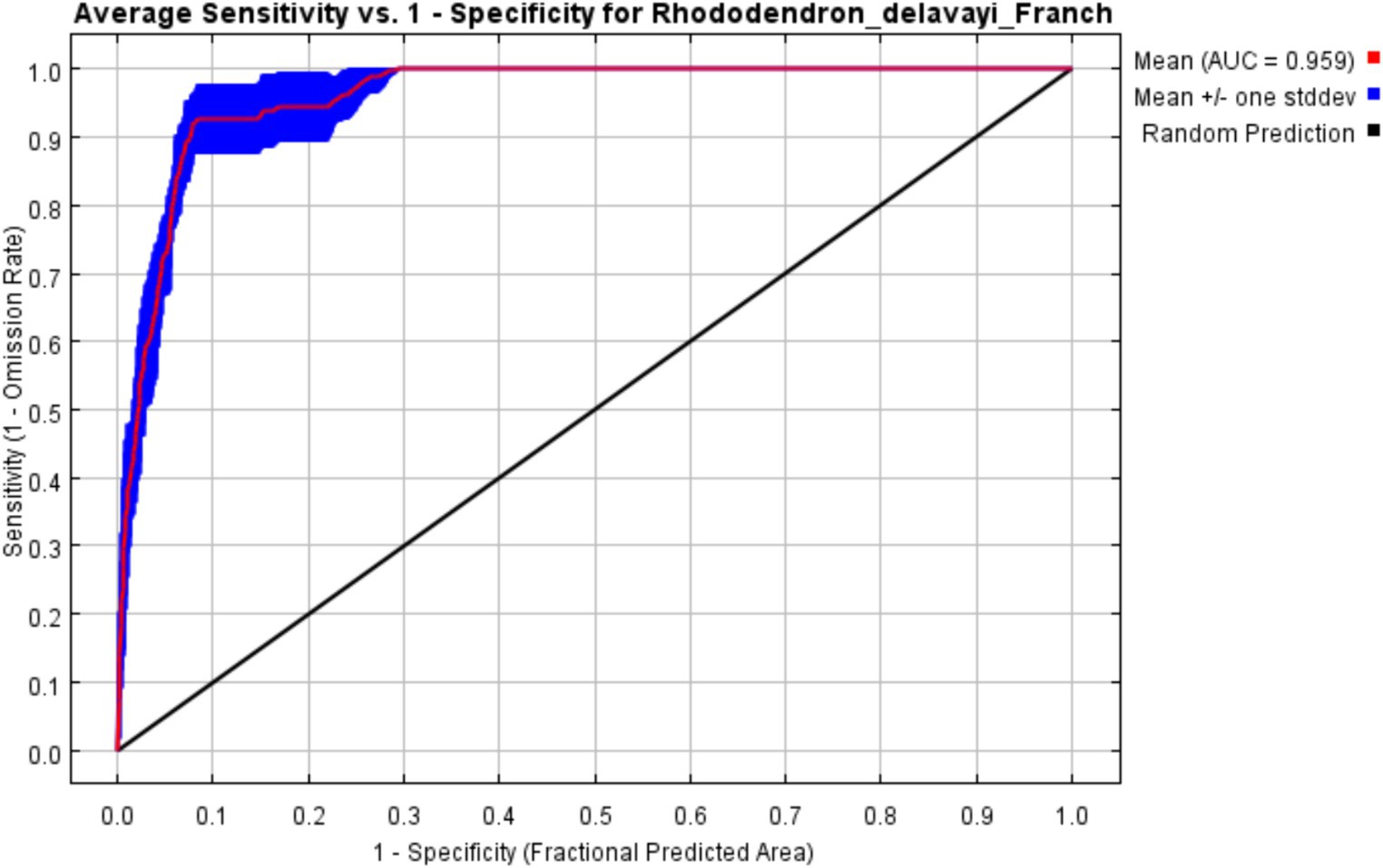
Figure 2. Predicted ROC curves for the westward distribution of the current climatic conditions of R. delavayi.
3.2 Suitable range of climate factors
Utilizing the Jackknife method to assess the importance of each environmental factor (Xiang et al., 2020), the analysis revealed that the most influential variable when used alone was soil pH (Efron and Stein, 1981). The primary environmental factors affecting the distribution of R. delavayi are primarily soil pH, contributing 41.8%, precipitation in the driest month (bio14) at 24.1%, elevation at 18.3%, the minimum temperature of the coldest month (bio6) at 8.4%, isothermality (bio3) at 4.5%, and precipitation of the wettest quarter (bio16) at 2.5%, cumulatively accounting for 99.6% of the model’s predictive power. Precipitation and temperature were identified as key factors influencing the potential distribution patterns of R. delavayi (Figure 3).
Based on the simulation results from the MaxEnt model, response curves were plotted to analyze the impact of various environmental factors on the distribution of R. delavayi (Figure 4). This analysis also identified environmental thresholds conducive to the growth of R. delavayi, focusing on conditions where the distribution probability exceeded 0.5 R. delavayi predominantly grows in acidic soils. The response curve indicated an optimal soil pH range of 4.9–5.4 for the species (Figure 4A), with a contribution rate of 41.8%. The precipitation range during the driest month conducive to its growth is 10–20 mm (Figure 4B), contributing 24.1% to the model. The suitable elevation range for R. delavayi was between 1,300 m and 2,800 m (Figure 4C), accounting for 18.3% of the model’s accuracy. The minimum temperature of the coldest month ideally between 0 and 5°C (Figure 4D) was also significant for its growth, contributing 8.4%. Isothermality within the range of 44–52 has a contribution rate of 4.5%. Precipitation during the wettest quarter should be between 490 mm and 750 mm, contributing 2.5%, and precipitation seasonality (coefficient of variation) between 61 and 90 contributes 0.5% to the model’s predictive power.
3.3 The potential suitable habitats for Rhododendron delavayi under current climatic conditions
Using MaxEnt modeling, the potential suitable habitats for R. delavayi across China under current climatic conditions (1970–2000) were identified (Figure 5). The main distribution of these habitats is in the southwestern region of China, particularly in Yunnan and Guizhou provinces. The area of highly suitable habitats for R. delavayi is estimated to be 27.75 × 104 km2. The moderately suitable habitats cover an area of 23.63 × 104 km2, while the low suitability areas span 77.08 × 104 km2.
The areas with a high suitability for R. delavayi mainly encompass 16 prefecture-level cities in Yunnan, including Zhaotong, Qujing, Honghe, Wenshan, Kunming, Yuxi, Chuxiong, Dali, Lijiang, Shangri-La, Nujiang, Baoshan, and others. However, the southwestern parts of Xishuangbanna, Pu’er, Dehong, and the southwest of Lincang in the southwestern region of Yunnan are less suitable due to their lower latitudes and higher temperatures, which are not conducive to the growth of R. delavayi. Beyond Yunnan Province, this species is also distributed in western Sichuan, central and western Guizhou, and the northwestern part of Guangxi.
3.4 The potential suitable habitats for Rhododendron delavayi under future climatic conditions
Predictions for the suitable habitats of R. delavayi under future climate scenarios for the 2050s and 2070s indicate significant changes. Under the 2050s climate scenario, the area of highly suitable habitat is projected to be 3.69 × 104 km2, with moderately suitable habitats covering 9.92 × 104 km2, and low suitability areas extending over 38.95 × 104 km2 (Figure 6). In the 2050s, the highly suitable habitats in Yunnan Province are expected to decrease substantially, with distribution primarily in the Zhaotong and eastern Qujing areas, and scattered distribution in the Hengduan Mountains region of western Yunnan. In Guizhou Province, the distribution is anticipated to be limited to a small area in the western part.
Under the 2070s climate scenario, the area of highly suitable habitat for R. delavayi is forecasted to be 2.65 × 104 km2, with the moderately suitable habitat covering 9.23 × 104 km2, and the low suitability area encompassing 37.13 × 104 km2 (Figure 7). In Yunnan Province, the area of highly suitable habitat is expected to further diminish, with distribution mainly in the eastern part of Zhaotong and the western regions of Guizhou that border Zhaotong. There will also be scattered distribution in the northwestern Hengduan Mountains area of Yunnan Province.
Compared to current climatic conditions, there is a trend of decreasing area in the highly suitable habitats for R. delavayi nationwide, shrinking from 27.75 × 104 km2 to 3.69 × 104 km2 in the 2050s and further to 2.65 × 104 km2. Meanwhile, the areas of moderate and low suitability are gradually expanding.
According to the predictions of the MaxEnt model, global warming and the associated rise in global temperatures are the primary factors causing a significant reduction in the most suitable habitats for this species. As the planet warms, R. delavayi’s habitats are expected to retreat to areas of higher latitude and elevation, where conditions remain conducive to its growth and survival.
4 Discussion
4.1 Simulation accuracy
This study employed the MaxEnt model and ArcGIS software to predict the potential suitable habitats for Rhododendron delavayi across China. The model’s accuracy, confirmed by a Receiver Operating Characteristic (ROC) curve with an Area Under the Curve (AUC) value greater than 0.9, indicates reliable and effective simulation results. However, some discrepancies exist between the predicted results and actual species distributions; the ROC curve reflects the model’s accuracy to a certain extent only (Pan et al., 2021). The precision of the model was improved by using the Band Collection Statistics tool in ArcGIS, which helped eliminate highly correlated factors among the selected climatic, soil, and elevation variables. Consequently, the model’s predictions should be viewed as a reference rather than an exact projection of the future habitats for R. delavayi.
According to the “Flora of China” and the Chinese Virtual Herbarium, R. delavayi is found in northwestern Guangxi, southwestern Sichuan, western Guizhou, throughout Yunnan, and southern Tibet, typically thriving in evergreen broad-leaved forests or shrubberies at altitudes of 1,200–3,200 meters. This natural distribution corresponds with the areas predicted to be highly suitable in the study. With its demonstrated high accuracy, the model is valuable for supplementing the distribution data of R. delavayi in the Yunnan region.
In reality, the distribution of species is influenced by a combination of biotic and abiotic factors, which drive phenotypic variation and resource utilization efficiency under different environmental conditions (Yao et al., 2023). This research modeled the potential distribution of R. delavayi based solely on climatic, soil, and elevation factors. However, additional influences such as human disturbance, interspecies interactions, extreme climate events, soil fauna, slope, and aspect also significantly impact its distribution (Fu et al., 2022). Soil fauna are essential in transforming soil structure, accumulating organic carbon, and maintaining plant health, while the slope and aspect influence the spatial distribution of soil nutrients, with steeper slopes experiencing more significant organic carbon and nitrogen loss due to surface runoff, leading to richer soil conditions in valleys and gentler slopes (Xue et al., 2023).
4.2 Key factors affecting potential distribution
Heat and moisture are key determinants of plant distribution on a large geographic scale (Lu et al., 2020). The results of this study indicate that soil pH, precipitation in the driest month (bio14), elevation, and the minimum temperature of the coldest month are the primary environmental factors influencing the growth of R. delavayi. This species typically thrives in cool, moist conditions, and in acidic soils rich in humus, loose, and moist. It is drought-tolerant but vulnerable to excessive sun exposure and dry environments. In this model, an annual precipitation range of 490–750 mm meets the moisture requirements of R. delavayi during its growth phase. A soil pH range of 4.9–5.4 is optimal for R. delavayi, fulfilling its need for acidic soil conditions.
4.3 Distribution of highly suitable growth areas
Under historical, 2050s, and 2070s climate scenarios, the most suitable habitats for R. delavayi are predominantly concentrated in the northeastern part of the Yunnan-Guizhou Plateau. This region, located in Southwest China, features a typical subtropical monsoon climate. During the summer, it experiences abundant rainfall due to the influence of warm and moist air currents from the Bay of Bengal. In winter, it is affected by the dry and cold northeast monsoon, but the intensity of these winds is weakened due to the region’s distance from the winter monsoon source and the barrier effect of the terrain. Despite its relatively low latitude at approximately 25°N, the Yunnan-Guizhou Plateau’s high altitude results in small temperature variations between summer and winter, with moderate summer temperatures.
The loss of biodiversity and global warming are significant global challenges, with biodiversity loss exacerbating the process of climate change (Feng, 2023). The trend of shrinking highly suitable habitats for R. delavayi is primarily attributed to global warming, as this species prefers shady, moist, and cooler environments. Liupanshui in Guizhou Province, known as “China’s Cool City” as awarded by the China Meteorological Society, represents such an environment. In the context of global warming, the highly suitable habitats predicted by the model could become the most optimal regions for the growth of this species.
The distribution and growth of Rhododendron plants are influenced by both geographical and soil factors. Variations in altitude within the same location lead to different water and thermal conditions, while the physical and chemical properties of the soil, along with temperature, humidity, and light levels, are critical environmental factors affecting Rhododendron growth. Soil thickness and pH significantly impact the moisture and nutrient content of the soil (Fu, 2023). Historical changes in the distribution of these species indicate that global warming is causing the optimal habitat range to shrink. As the middle and low latitudes become warmer and drier, the environmental conditions suitable for these plants are altering, reducing the range where they can thrive. For instance, while theoretically, Shangri-La in northwestern Yunnan could support this species, the high altitude may prevent the establishment of a large population due to suboptimal water and heat conditions. Consequently, only northeastern Yunnan (including eastern Zhaotong and northeastern Qujing), with its moderate latitude and altitude, offers a conducive environment for Rhododendron delavayi.
For the conservation and effective utilization of R. delavayi, outdoor cultivation and breeding are recommended. A comparative study of healthy, unpruned seven-year-old R. delavayi plants in and outside the greenhouses at the Royal Botanic Garden Edinburgh showed that outdoor plants are superior in terms of stem thickness, crown width, fullness of form, and leaf count (Chen, 2000).
4.4 Model application
The Maximum Entropy (MaxEnt) model, grounded in the principle of maximum entropy, is a species geographic distribution model widely used in invasion biology, conservation biology, global climate change impact studies, and evolutionary biology. As global warming progresses, understanding how species adapt to climate change remains a pivotal concern in biodiversity research. The MaxEnt model effectively captures species’ response patterns to varying climatic conditions over time, providing a robust scientific framework for the study of Rhododendron delavayi.
Rhododendron delavayi, a distinctive alpine Rhododendron species native to Southwest China, is celebrated for its ornamental attributes. Yet, it faces significant challenges in adapting to lower altitudes, a process often referred to as “descending the mountains.” This research highlights soil pH, precipitation in the driest month (bio14), and elevation as critical factors that restrict its distribution. By predicting suitable habitats, the MaxEnt model aids in optimizing environmental conditions for the introduction and transplantation of R. delavayi, thereby facilitating assessments of its growth and confirming the model’s practical utility.
The predictions from the MaxEnt model suggest that thermohydric factors, soil pH, and elevation are decisive in shaping the geographic distribution of R. delavayi. The model’s simulated distribution not only guides field surveys but also emphasizes the importance of safeguarding its native habitats. Conservation strategies aimed at enhancing the species’ survival should avoid causing anthropogenic damage. Furthermore, the simulation data underscore the need to bolster monitoring and conservation efforts for wild populations of R. delavayi to preserve its genetic resources, which are essential for its sustainable utilization and transplantation.
5 Conclusion
The main environmental factors affecting the distribution of R. delavayi are soil pH, precipitation in the driest month (bio14) and elevation. Altitude, as the main factor affecting the distribution of R. delavayi, is mainly due to the difference in water and heat conditions caused by the difference in altitudinal gradient, with higher altitude resulting in lower temperatures and relatively higher humidity. With the intensification of global warming, the potential habitat of the R. delavayi has been gradually reduced, and it has migrated from low latitude and low altitude areas to high latitude and high altitude areas. In the migration process of R. delavayi is not only subjected to changes occurring in the hydrothermal conditions, soil conditions are also an important factor that cannot be ignored. Soils rich in organic matter, high soil maturity and acidic soils are more suitable for the growth of R. delavayi.
Data availability statement
The original contributions presented in the study are included in the article/supplementary material, further inquiries can be directed to the corresponding authors.
Author contributions
JD: Data curation, Investigation, Methodology, Validation, Visualization, Writing – original draft, Writing – review & editing. TB: Writing – review & editing. Z-sG: Writing – review & editing. S-wY: Writing – review & editing. J-lZ: Conceptualization, Funding acquisition, Project administration, Supervision, Writing – review & editing. Y-wW: Writing – review & editing.
Funding
The author(s) declare financial support was received for the research, authorship, and/or publication of this article. This study was supported by the Key Project of the Agricultural Joint Special Program of the Yunnan Provincial Science and Technology Department (No. 202301BD070001-139) and Yunnan Yiliang County Seedling Industry Science and Technology Delegation (202404BI090014).
Conflict of interest
The authors declare that the research was conducted in the absence of any commercial or financial relationships that could be construed as a potential conflict of interest.
Publisher’s note
All claims expressed in this article are solely those of the authors and do not necessarily represent those of their affiliated organizations, or those of the publisher, the editors and the reviewers. Any product that may be evaluated in this article, or claim that may be made by its manufacturer, is not guaranteed or endorsed by the publisher.
Footnotes
References
Cai, Y., Peng, L., Song, J., Zhang, L., Xie, W., and Qu, S. (2015). Photosynthetic response of an alpine plant, Rhododendron delavayi Franch, to water stress and recovery: the role of mesophyll conductance. Front. Plant Sci. 6:1089. doi: 10.3389/fpls.2015.01089
Chen, X. (2000). A comparative study of indoor and outdoor growth of Rhododendron delavayi. Guizhou Sci. Z1, 156–158.
Cong, Y., Yuan, Y., Yongsheng, G., Xianyou, Q., and Yibo, L. (2018). Climate suitability analysis of Dipsacus asper based on MaxEnt model. J. Southwest Univ. 40, 60–67. doi: 10.13718/j.cnki.xdzk.2018.03.009 (In Chinese).
Efron, B., and Stein, C. (1981). The jackknife estimate of variance. Anrals Statistics 9, 586–596. doi: 10.1214/aos/1176345462
Feng, L. (2023). On synergistic governance of biodiversity and climate change in the perspective of international law. Biodivers. Sci. 31:23110. doi: 10.17520/biods.2023110
Fu, T. (2023). Spatial distribution characteristics of Rhododendron delavayi at different age levels and its main influencing factors : Guizhou University.
Fu, S., Liu, M., Zhang, W., and Shao, Y. (2022). A review of recent advances in the study of geographical distribution and ecological functions of soil fauna diversity. Biodivers. Sci. 30:22435. doi: 10.17520/biods.2022435
Lai, E., and Sun, W. (2004). Woody flowering plants. China Architect. Build. Press :145. (in Chinese)
Li, W., and Chen, X. (2005). Preliminary study on the population structure and regeneration of Rhododendron delavayi in Baili Rhododendron Forest Park. Guizhou Sci. 3, 46–49. doi: 10.3969/j.issn.1003-6563.2005.03.011 (In Chinese)
Li, J., Huang, Z., Lu, Y., Qin, X., Huang, Y., Li, H., et al. (2020). Diversity, geographical distribution and species boundary of the Hemiboea subcapitata complex. Guihaia 40, 1477–1490.
Li, N.-N., Zhang, A.-P., Zhang, L., Wang, K.-Q., Luo, H.-Y., and Pan, K.-W. (2019). Predicting potential distribution of two species of spruce in Qinghai-Tibet plateau under climate change. Bull. Botanical Res. 39, 395–406.
Liang, C., Luo, Q., Lu, Z., Xie, Z., Qin, Q., and Huang, X. (2017). Prediction of the geographical distribution of Cymbidium goeringii in China and analysis of key bioclimatic factors based on Maxent and GIS. Northern Horticulture 9, 199–204. doi: 10.11937/bfyy.201709042 (In Chinese)
Liu, X., Yang, Y. F., Song, H. P., Zhang, X. B., Huang, L. Q., and Wu, H. Z. (2016). Study on growth suitability for Coptis chinensis based on ecological factors analysis by MaxEnt and ArcGIS model. China J. Chinese Materia Medica 41, 3186–3193. doi: 10.4268/cjcmm20161712
Lu, S., Chen, Y., Zhou, S., and Yin, X. (2020). Responses of Pinus species to climate change in southwestern China. J. For. Environ. 40, 466–477. doi: 10.13324/j.cnki.jfcf.2020.05.003
Pan, Y., Zhang, H., Zhang, P., Pei, S., and Fan, J. (2021). Prediction of suitable distribution areas for Pseudostellaria heterophylla based on MaxEnt model and ArcGIS. J. Traditi. Chinese Med. Inform. 28, 1–4. doi: 10.19879/j.cnki.1005-5304.202011051 (In Chinese)
Phillips, S. J., Anderson, R. P., and Schapire, R. E. (2006). Maximum en tropy modeling of species geographic distributions. Ecol. Model. 190, 231–259. doi: 10.1016/j.ecolmodel.2005.03.026
Sheng-Lin, W., Yin-Long, L., Li-Juan, Z., Shan, L., and Jing, X. (2019). Thinking on schistosomiasis control under the strategy of China's Yangtze River Economic Belt. Chinese J. Schistosomiasis Control 31:459. doi: 10.16250/j.32.1374.2019225
Sun, J. J., Jiang, B., Qiu, H. J., Guo, J. H., Yuan, W. G., Wu, D. T., et al. (2019). A prediction on the potential suitable areas of Zelkova Schneideriana in Zhejiang Province using maximum entropy model. For. Resour. Manag. 4, 37–45. doi: 10.13466/j.cnki.lyzygl.2019.04.006
Swets, J. A. (1988). Measuring the accuracy of diagnostic system. Science 240, 1285–1293. doi: 10.1126/science.3287615
Wang, G. (2013). Modelling the geographic distribution and study on the ISSR genetic diversity of Rhododendron maculiferum. Master dissertation. Hefei: Anhui Agricultural University.
Xiang, L., Chengfu, Z., Shuai, H., Yuqing, W., and Lin, M. (2020). Analysis of research progress on the comprehensive application of MaxEnt model. Green Sci. Technol. 14, 14–17. (In Chinese)
Xiao, J. H., Ding, X., Cai, C. N., Zhang, C. Y., Zhang, X. Y., Li, L., et al. (2021). Simulation of the potential distribution of Phoebe bournei with climate changes using the maximum-entropy (MaxEnt) model. Acta Ecol. Sin. 41, 5703–5712. doi: 10.13207/j.cnki.jnwafu.2020.02.003
Xiao, Z., Wang, W., and Qin, D. (2020). Study on the origin and distribution patterns of Fulgoroidea (Hemiptera: Fulgoromorpha) insects. J. Northwest A&F Univ. 48, 15–25. doi: 10.5846/stxb202008132110 (In Chinese)
Xing, D., and Hao, Z. (2011). The principle of maximum entropy and its applications in ecology. Biodivers. Sci. 19, 295–302. doi: 10.3724/SP.J.1003.2011.08318
Xu, F., Yang, X., Pan, W., Liao, H., Zhang, W., Yang, H., et al. (2022). Analysis of suitable habitats and dominant environmental factors for the precious tree species Fokienia hodginsii based on MaxEnt and ArcGIS. For. Environ. Sci. 38, 29–37. (In Chinese)
Xue, Y., Cheng, A., Li, S., Liu, X., and Li, J. (2023). The effects of environment and species diversity on shrub survival in subtropical forests. Biodivers. Sci. 31:22443. doi: 10.17520/biods.2022443
Yan, H., Zhang, X. B., Zhu, S. D., Qian, D. W., Guo, L. P., Huang, L. Q., et al. (2016). Production regionalization study of Chinese angelica based on MaxEnt model. Zhongguo Zhong Yao Za Zhi 41, 3139–3147. Chinese. doi: 10.4268/cjcmm20161705
Yang, W., and Xia, T. (2014). New compilation of traditional Chinese herbal medicine atlas and classic formulas : Guizhou Science and Technology Publishing House, 303 (in Chinese).
Yao, R., Chen, Y., Lü, X., Wang, J., Yang, F., and Wang, X. (2023). Altitude-related environmental factors shape the phenotypic characteristics and chemical profile of Rhododendron. Biodivers. Sci. 31:22259. doi: 10.17520/biods.2022259
Yu, S., Li, M., Yuan, T., Ren, L., and Bai, X. (2021). Species diversity and geographical distribution of wild Impatiens plants in Guizhou Province. Acta Botan. Boreali Occiden. Sin. 41, 863–871. (In Chinese)
Keywords: Rhododendron delavayi Franch, MaxEnt model, potential distribution, climate change, potential habitat
Citation: Dong J, Bai T, Gao Z-s, Yang S-w, Zhang J-l and Wu Y-w (2024) Prediction of potential habitat areas of Rhododendron delavayi in China based on maximum entropy model MaxEnt. Front. For. Glob. Change. 7:1360823. doi: 10.3389/ffgc.2024.1360823
Edited by:
Aziz Ebrahimi, Purdue University, United StatesReviewed by:
Hafiz Ullah, University of Chitral, PakistanXiaocui Liang, Central South University of Forestry and Technology, China
Copyright © 2024 Dong, Bai, Gao, Yang, Zhang and Wu. This is an open-access article distributed under the terms of the Creative Commons Attribution License (CC BY). The use, distribution or reproduction in other forums is permitted, provided the original author(s) and the copyright owner(s) are credited and that the original publication in this journal is cited, in accordance with accepted academic practice. No use, distribution or reproduction is permitted which does not comply with these terms.
*Correspondence: Jing-li Zhang, jl200812@yeah.net; Ya-wen Wu, yawenwu@ynau.edu.cn
†These authors have contributed equally to this work