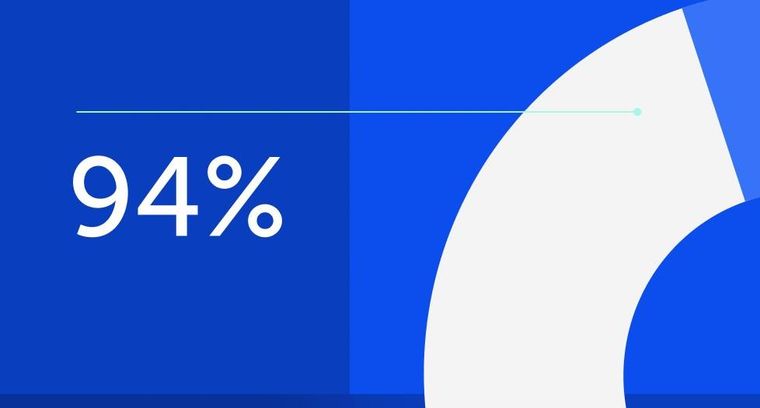
94% of researchers rate our articles as excellent or good
Learn more about the work of our research integrity team to safeguard the quality of each article we publish.
Find out more
ORIGINAL RESEARCH article
Front. For. Glob. Change, 17 July 2024
Sec. Forest Management
Volume 7 - 2024 | https://doi.org/10.3389/ffgc.2024.1359361
This article is part of the Research TopicChanging Driving Mechanisms of Climate Change and Human Management on Forest and Other Vegetation EcosystemsView all 8 articles
Investigating functional traits among mountain species with differing altitude requirements is integral to effective conservation practices. Our study aims to investigate the structural and chemical characteristics of Daphniphyllum macropodum leaves at three altitudes (1100 m, 1300 m, and 1500 m) across southern China to provide insight into changes in leaf functional traits (LFT) as well as plant adaptations in response to changing environmental conditions. Leaf structural characteristics include leaf thickness (LT), leaf area (LA), specific leaf area (SLA), and leaf tissue density (LD), respectively, while chemical properties include carbon-nitrogen-phosphorus (C:N:P) contents and ratios, such as C/N, C/P, and N/P. Our findings demonstrated the significant effect of altitude on both structural (LT, SLA, LD) and chemical aspects (N, C/N, N/P) of LFT. In particular, leaves at 1100 and 1300 m differed greatly, with 1300 m having lower SLA values than 1100 m. Observable trends included an initial increase followed by a decline as the altitude rose. Notable among them were the LT, LD, N, and N/P values at both locations. Traits at 1300 m were significantly higher than at 1100 m; SLA and C/N values displayed an inverse trend, with their lowest values occurring at 1300 m. Furthermore, this research demonstrated various degrees of variation among LFT, with structural traits exhibiting greater fluctuation than chemical traits. Robust correlations were observed among certain traits, such as LT, LD, and SLA. Furthermore, the interdependency relationships between N and C/N, as well as P and C/P, demonstrated interconnectedness. Redundancy analysis indicated that soil factors, specifically P content, exerted the strongest impact on LFT. At 1100 m, D. macropodum employed acquisition strategies; however, at 1300 m, conservation strategies emerged, showing a shift from acquisition strategies at lower altitudes to conservative strategies at higher ones.
Climate change has caused extreme weather events that threaten plant survival and growth (Hai et al., 2023; Huang et al., 2023; Muhammad et al., 2024). Plant Functional Traits (PFTs) are highly sensitive and adaptable to environmental changes (Madsen-Hepp et al., 2023; Wang J. et al., 2023), such as leaf morphology, photosynthetic properties, nutrient content, water relations, mechanical characteristics, reproductive traits, root characteristics, phenological traits, and flowering time (Wang J. et al., 2023). Leaf functional traits (LFT), an integral component of PFT, can adapt to environmental fluctuations (Morffi-Mestre et al., 2023; Islam et al., 2024). LFT characteristics may serve as useful indicators of plant responses to climate change (Niinemets, 2023; Sandoval-Granillo and Meave, 2023; Shi X. M. et al., 2023). LFT characteristics are closely tied to plant survival strategies and effective resource use (Siefert and Laughlin, 2023). As a result of these changes, LFT characteristics often vary significantly, reflecting genetic diversity and plasticity within individuals (Bai et al., 2023; Haber et al., 2023). Leaf attributes exhibit significant variation both within and among populations, particularly with regards to altitudinal changes; additionally, leaf nutrient concentrations differ among different populations (Chen et al., 2023; Ramesh et al., 2023). As in nature, dissimilar species’ leaves exhibit significant variation depending on their population (Wang X. et al., 2023; Xing et al., 2023). This is apparent in the size, specific leaf area, thickness, leaf tissue density, and leaf weight differences between populations (Wang X. et al., 2023). An additional study by Guo et al. (2023) also demonstrated significant variations in leaf morphology and leaf physiology among and within broad-leaved plant populations. Other studies also demonstrated considerable variance in LFT between populations of broad-leaved plants (Griebel et al., 2023). Most research indicates that more detailed and precise investigations will likely reveal significant variances among populations rather than within them (Kermavnar et al., 2023; Koleszár et al., 2023).
Environmental influences play a pivotal role in plant development traits, specifically water, radiation, and temperature—three key variables that have a significant bearing on LFT characteristics (Chen et al., 2022). Modifying these conditions and optimizing resource efficiency are both key to plant survival (Arif et al., 2023; Hidalgo-Triana et al., 2023; Rauf et al., 2023). Morphological features (Cox et al., 2023; Liu L. B. et al., 2023; Rius et al., 2023), such as leaf area and shape, play an integral part in water consumption and photosynthesis efficiency. Kermavnar et al. (2023) and Long et al. (2023) provide insight into a plant’s ability to endure physical stress through various mechanical characteristics like leaf dry matter content and carbon content, among other metrics. Economic indicators such as specific leaf area, leaf nitrogen content, and leaf phosphorus content provide a comprehensive picture of a plant’s ability to adapt and respond to environmental conditions as well as nutritional stability and limitations (Wright et al., 2004; Khan et al., 2023; Liu X. R. et al., 2023). Under conditions such as drought or low temperature exposures, leaves often decrease their specific leaf area and leaf nitrogen content (Felix et al., 2023; Nomura et al., 2023; Wang Y. N. et al., 2023). Such adjustments optimize water use and photosynthesis efficiency. These changes could enhance water use efficiency and photosynthesis efficiency by improving both mechanisms simultaneously.
PFT covers essential characteristics that relate to plant colonization, survival, growth, and mortality (Shi X. Z. et al., 2023). PFT data provide valuable insight into the responses and adaptation strategies of individual plants to environmental changes as well as longitudinal variations (Siefert and Laughlin, 2023). LFT encompasses structural (specific leaf area, dry matter content, and leaf carbon content) and chemical traits (leaf carbon content and nitrogen content), both closely associated with biomass production, resource acquisition, and utilization (Manohan et al., 2023). As part of their ability to adapt to shifting climatic conditions, plants dynamically alter LFT (Dong et al., 2023). Altitude-induced variations in environmental factors, including light intensity, temperature, and soil types, can influence PFT and LFT ratios (Manohan et al., 2023). PFTs such as leaf area, specific leaf area, specific leaf weight, and nitrogen content serve as indicators of plant resource allocation for growth or storage (McIntire, 2023). Researchers utilize such indicators extensively when investigating the responses of plants to environmental changes and potential growth strategies. As altitude increases, plants typically adapt by creating defensive tissue structures—reducing leaf area, specific leaf area, and specific leaf weight—while simultaneously increasing specific leaf weight, dry matter content, and nitrogen content—in order to survive high radiation environments and low temperatures (He et al., 2023). Unfortunately, research findings on the relationship between altitude and LFT remain inconclusive.
Melis et al. (2023) have found that specific leaf area increased with altitude while leaf area declined, yet no significant correlation was detected between leaf nitrogen content and altitude gradient. Research by other investigators demonstrated an increase in specific leaf weight with increasing altitude (Xu S. W. et al., 2023). While nitrogen levels showed decreasing trends with altitude increase, phosphorus content and nitrogen-to-phosphorus ratio (N/P) did not significantly change due to altitude change (Zhang X. et al., 2023). Researchers found varying responses of evergreen and deciduous trees as altitude gradients increased (He et al., 2023), thus rendering patterns of LFT changes along altitude gradients inconclusive. Studies on the relationships between soil factors and LFT along altitude gradients in southern China are limited; observing their changing patterns helps develop our knowledge of plant responses to environmental change and adaptive mechanisms (Liu et al., 2022).
Mao’er Mountain Nature Reserve (MMNR), located in Guilin, Guangxi Province, China, presents visitors with a subtropical climate. Here, vegetation varies with altitude gradient and vertical arrangement (Chen et al., 2024). Daphniphyllum macropodum Miq. is found in subtropical broad-leaved forests at altitudes between 600 and 1700 m, where its role in maintaining forest ecosystem stability and function plays an essential part (Gao et al., 2023). Field investigations also suggest that concentrations of D. macropodum within the 1100–1500 m altitude range display “bell-shaped” population distribution patterns (Yang et al., 2022). MMNR and subtropical mountains make excellent experimental sites for studying how elevation affects plant traits. Currently, most research on concatenated D. macropodum concentrates on its photosynthetic traits (Eljounaidi et al., 2024) and population traits (Yoichi et al., 2023). Few studies have examined LFT, altitude variation, or environmental factors that influence their correlations. This makes it challenging to understand how this species adapts to its environment from the perspective of plant functional traits. Therefore, our study investigates the structural and chemical characteristics of D. macropodum leaves at different altitudes within MMNR with the aim of (1) understanding their functional traits and chemical characters across altitudes and (2) investigating any relationships between soil characteristics and LFT.
The sampled sites are located within MMNR (Figure 1), in the northern part of Guilin, Guangxi, China (110°20′ ∼ 110°35′ E, 25°48′ ∼ 25°58′ N). Located in South China, Mao’er Mountain has an elevation of 2,141.5 m and is the highest peak in the region. The subtropical mountain climate is prevalent in the region, with average annual temperatures ranging from 16.4 to 18.1 °C and 2600 mm of precipitation. The study area features an expansive elevation drop, creating an altitude gradient with varied vegetation types at every elevation level. Some of these ecosystems include evergreen broad-leaved forest, evergreen and deciduous broad-leaved mixed forest, evergreen coniferous and broad-leaved mixed forest, and hilltop coppice forest (Yang et al., 2022; Gao et al., 2023). This area consists of mountainous red and yellow soils formed by granite.
Figure 1. Location (A–C) of sample sites (D) across different altitudes (E) in southern mountainous regions of China.
In order to determine whether the functional traits of D. macropodum leaves were different at different elevations, we chose 27 plots, 9 of which were at 1100 m, 1300 m, and 1500 m, as shown in Table 1. These plots were used to demonstrate any differences in functional traits between those growing at higher and lower altitudes of this species. During the peak growth season of D. macropodu in July 2022, we performed leaf sampling on 20 m × 20 m quadrats with relatively high concentrations in middle-aged forests at each altitude plot. The sampling was conducted at 9 quadrats per altitude plot, with an average of 5–8 trees per quadrat. The sample collection included 40 healthy and undamaged leaves from various parts of the upper, middle, and lower crowns of adjacent plants to collect samples free of pests and diseases. This was intended to eliminate neighborly effects within a population. Our team selected 40 leaves from the southeast, northwest, and east parts of the canopy of other non-adjacent plants. We mixed the harvested leaves, selected 10 leaves using the quarter method, and then transported them back to the laboratory for analysis. At each sample plot site, 27 locations were randomly chosen for obtaining soil samples using the five-point sampling method of plum blossom. After removing the litter and humus layers, we extracted topsoil (0–10 cm) with a 100 cm3 ring knife. Once collected, soil samples were mixed thoroughly to form repeat samples. These samples were labeled and packaged into self-sealing bags before being transported back to the laboratory at a low temperature for further study. We collected 27 soil samples in total.
LFT were determined by measuring leaf thickness (LT/mm) at 27 random locations with a 1% thickness variance and then calculating the average value as an indication of leaf thickness (Hai et al., 2023; Shi X. Z. et al., 2023). A scanner was used to scan the leaves, and MATLAB image analysis software (MathWorks, USA) was used to calculate the leaf area (LA/cm2). We determined the leaf saturated fresh weight (SFW/g) and leaf dry weight (DW/g) using a 1/1000 electronic balance. The potassium dichromate high-temperature external heating method was employed to determine the leaf carbon content (LC/mg⋅g–1) and soil organic carbon (SOC/mg⋅g–1). Leaf nitrogen content (LN/mg⋅g–1), leaf phosphorus content (LP/mg⋅g–1), soil total nitrogen (LN/mg⋅g–1), and soil total phosphorus (STP/mg⋅g–1) were conducted using continuous flow analyzers. The soil pH was determined using a pH meter. The formula for calculating the LFT is as follows:
The effect of altitude on LFT was assessed by using a one-way analysis of variance (ANOVA), with the least significant difference (LSD) applied to examine differences in the same trait across altitudes. In order to determine whether LFT are correlated, Pearson correlation analysis was used. A redundancy analysis (RDA) was also performed to determine the relationship between LFT and soil nutrient factors. We conducted the RDA analysis using Canoco 5.0 (Biometris & Petr Šmilauer) and performed the one-way ANOVA and LSD multiple comparative analysis using SPSS 24.0 (IBM, USA).
In addition to leaf area, altitude exerted a highly significant influence on leaf thickness, specific leaf area, leaf dry matter content, and leaf tissue density (Figure 2, P < 0.01). Across the altitude gradients examined, leaf thickness, leaf dry matter content, and leaf tissue density exhibited an initial increase followed by a subsequent decrease with increasing elevation. Specific leaf areas displayed a trend of an initial decrease followed by an increase with elevation. Notably, at 1300 m, leaf thickness, leaf dry matter content, and leaf tissue density were significantly higher than those at 1100 m (P < 0.05). Conversely, the specific leaf area at 1100 m was significantly higher than that at 1300 m (P < 0.05).
Figure 2. Leaf structural traits (A–E) of Daphniphyllum macropodum at different altitudes within sampled sites. Different letters (a, b, and c) indicate significant differences in leaf structural traits among different altitudes (P < 0.05). The y-axis denotes the mean scores, and the vertical line represents the standard error.
Except for specific leaf area at 1300 m, both leaf area and specific leaf area exhibited a coefficient of variation greater than 10% at different altitudes, indicating a higher degree of variation compared to other structural traits (Figure 3). The overall degree of variation in leaf structural traits was ranked from large to small as follows: leaf area > specific leaf area > leaf tissue density > leaf dry matter content > leaf thickness.
Figure 3. The coefficient of variation of leaf structural traits of Daphniphyllum macropodum at different altitudes within sampled sites. Abbreviations in the figure define leaf thickness (LT), leaf area (LA), specific leaf area (SLA), leaf dry matter content (LDMC), and leaf tissue density (LD).
The interaction among leaf structural traits was robust (Figure 4, P < 0.01** and P < 0.001***). Leaf thickness exhibited a negative correlation with specific leaf area (r = −0.60***). Specific leaf area demonstrated a significant negative correlation with leaf dry matter content (r = −0.62***) and leaf tissue density (r = −0.91***). Meanwhile, leaf dry matter content showed a positive correlation with leaf tissue density (r = 0.78***).
Figure 4. Heat map of Pearson’s correlation between leaf structural traits of Daphniphyllum macropodum. A dark color indicates a strong relationship, whereas a light color represents a weak relationship. Abbreviations in the figure define leaf thickness (LT), leaf area (LA), specific leaf area (SLA), leaf dry matter content (LDMC), and leaf tissue density (LD). *** Correlation is significant at the 0.001 level (two-tailed); ** Correlation is significant at the 0.01 level (two-tailed).
Altitude exerted significant effects on nitrogen content, C/N ratio, and N/P ratio (Figure 5, P < 0.05). As altitude increased, leaf nitrogen and N/P exhibited a trend of initial increases followed by a subsequent decrease. Leaf nitrogen at 1300 m was significantly higher than at 1100 m and 1500 m (P < 0.05), while N/P at 1300 m was considerably higher than at 1500 m (P < 0.05). Conversely, the leaf C/N ratio showed a trend of initial decrease followed by an increase with increasing elevation, with the C/N ratio at 1300 m significantly lower than at 1100 m and 1500 m (P < 0.05).
Figure 5. Leaf chemical traits (A–F) of Daphniphyllum macropodum at different altitudes within sampled sites. Different letters (a, b, and c) indicate significant differences in leaf chemical traits among different altitudes (P < 0.05). The y-axis denotes the mean scores, and the vertical line represents the standard error.
The coefficient of variation for leaf phosphorus and C/P ratio at all altitudes exceeded 10%, indicating a higher degree of variation, while the coefficient of variation for leaf carbon was the smallest, remaining below 5%. The overall degree of variation in leaf chemical traits was ranked as follows: C/P > leaf phosphorus > N/P > leaf nitrogen > C/N > leaf carbon (Figure 6).
Figure 6. The coefficient of variation of leaf chemical traits of Daphniphyllum macropodum at different altitudes within sampled sites. Abbreviations in the figure define leaf carbon content (LC), leaf nitrogen content (LN), leaf phosphorus content (LP), carbon/nitrogen ratio (C/N), carbon/phosphorus ratio (C/P), and nitrogen/phosphorus ratio (N/P).
Correlation analysis revealed a negative correlation (r = −0.98***) between leaf nitrogen and C/N, and leaf phosphorus exhibited a negative correlation (r = −0.99***) with C/P (Figure 7, P < 0.001***). Apart from these two associations, others exhibited correlation strength but lacked statistical significance.
Figure 7. Heat map of Pearson’s correlation between leaf chemical traits of Daphniphyllum macropodum. A dark color indicates a strong relationship, whereas a light color represents a weak relationship. Abbreviations in the figure define leaf carbon content (LC), leaf nitrogen content (LN), leaf phosphorus content (LP), carbon/nitrogen ratio (C/N), carbon/phosphorus ratio (C/P), and nitrogen/phosphorus ratio (N/P). *** Correlation is significant at the 0.001 level (two-tailed).
Based on the RDA analysis, the interpretation of the relationship between functional leaf traits and soil factors in the first two axes accounted for 50.94% (refer to Figure 8). Notably, soil phosphorus emerged as the primary determinant, explaining LFT variation. Specifically, soil phosphorus exhibited positive correlations with leaf thickness, leaf dry matter content, and leaf tissue density of leaf structural traits. In addition, soil total phosphorus exhibited positive correlations with leaf nitrogen, leaf phosphorus, and the N/P of leaf chemical traits. Conversely, it demonstrated negative correlations with leaf area and specific leaf area of leaf structural traits and leaf carbon, C/N, and C/P of leaf chemical traits. This analysis provides valuable insights into the intricate connections between soil factors and the diverse functional traits exhibited by leaves.
Figure 8. Redundancy analysis (RDA) with a two-dimensional ordination diagram of the relationship between leaf functional traits and the soil factors for Daphniphyllum macropodum. RDA axes 1 and 2 factor loadings explained 50.94% of the total variance. Abbreviations in the figure are defined as leaf thickness (LT), leaf area (LA), specific leaf area (SLA), leaf dry matter content (LDMC), leaf tissue density (LD), leaf carbon content (LC), leaf nitrogen content (LN), leaf phosphorus content (LP), carbon/nitrogen ratio (C/N), carbon/phosphorus ratio (C/P), and nitrogen/phosphorus ratio (N/P).
Specific leaf area is a key leaf structural trait that reflects the ability of a plant to intercept light and is related to environmental acclimation strategies and resource allocation (Xu R. J. et al., 2023). Temperature has clear positive effects on leaf area changes and variation of specific leaf area with increasing temperature. Specific leaf areas tend to increase slightly under high temperature conditions (Peguero et al., 2023; Xu R. J. et al., 2023; Wang et al., 2024). Based on our study, we observed that the specific leaf area of D. macropodum had a higher value at the lower altitude (at 1100 m) compared to the middle altitude (at 1300 m) and high altitude (at 1500 m). The smallest specific leaf area was at middle altitude (Figure 2). This finding is consistent with other research on various mountain ecosystems (e.g., Yang et al., 2022; Gao et al., 2023), where specific leaf areas tended to first decrease and then increase with altitude. In another study for another species, the specific leaf area initially increases and subsequently decreases with elevation (Yu et al., 2023); however, this stands in contrast to our conclusion in this study. Leaf area response to temperature and altitude is usually very complicated and differs among plant species and ecotypes under varied growth conditions. Therefore, its effect can be variable in different places due to environmental conditions.
As the altitude increases and the temperature drops, UV rays reach higher levels. Plants also tend to increase the density of leaf mesophyll cells to form leaves with a higher thickness and a smaller surface area to cope with low temperatures and strong UV radiation (Zheng et al., 2023). The findings reinforced this hypothesis, showing an increase in plant quality (e.g., tissue composition) at middle (1300 m) and high (1500 m) altitudes compared to low (1100 m) altitudes in terms of leaf thickness, tissue density, and leaf area. Given its direct correlation with plant resource acquisition, the specific leaf area could serve as a crucial functional trait. This could offer insights into plant acquisition mechanisms. Existing and conservative strategies divide resource acquisition modes, with a specific leaf zone serving as the concealment area. Plants will have many leaves, which capture a lot of light energy used in photosynthesis. They have high acquisition speed, rapid growth, and a high acquisition-type resource utilization strategy (Cao et al., 2023). According to Melis et al. (2023) and Poggiato et al. (2023), plants with the lowest specific leaf area tend to prioritize structural protection, such as building new cell walls, over life-growth, adopting a “conservative” resource use strategy that balances growth and resource conservation in the harsh conditions of an arid climate. This study speculated that an “accommodative” strategy may have been adopted in response to relatively wet and cold environmental conditions at middle and higher altitudes by increasing leaf thickness and tissue density while simultaneously decreasing leaf area and specific leaf area in order to adapt.
Plants adapt to their environment through PFTs and combinations thereof, such as specific leaf area, specific leaf weight, and dry matter content (Yao et al., 2023). Together, these measures demonstrate their strategies for resource acquisition and conservation (Yao et al., 2023). Our study revealed a strong negative correlation between specific leaf area, and dry matter content. Therefore, as specific leaf area increased, dry matter content and weight also rose. This result was in line with other studies (Rahman et al., 2023). Leaf dry matter content can serve as an indicator of environmental resource use and plant water content in their natural state. In addition, there has been an inverse relationship between leaf dry matter content and specific leaf areas. Altitude increases leaf dry matter and allows plants to adjust to habitat changes by decreasing leaf area per plant and reinforcing structural organization. The LFT coefficient demonstrates a plant’s adaptation potential in relation to environmental conditions (Zhao et al., 2023). Studies showed varying degrees of variation among leaf structure traits at various altitudes, suggesting that functional traits adapt differently to altitude changes. Overall, the variation coefficients and degrees for leaf area at various altitudes were the highest (Figure 3), reflecting strong plasticity. Meanwhile, leaf thickness and leaf dry matter content varied under 10%, indicating strong internal stability.
Leaf carbon, nitrogen, and phosphorus and their stoichiometric ratios play a crucial role in plant growth and metabolism, providing valuable insights into plant adaptability to the environment and the impact of environmental changes (Pulido et al., 2023). Except for leaf nitrogen content, C/N and N/P ratios, and N/P ratios, we observed no significant variations between altitudes for any other chemical traits (Figure 5). Leaf nitrogen and C/N, as well as leaf phosphorus and C/P, exhibited a highly significant negative correlation, while no significant correlation was observed for other leaf chemical traits (Figure 7). Although leaf carbon and leaf phosphorus content did not show significant differences, leaf nitrogen and its stoichiometric ratios, L:C and L:P, were more sensitive to altitude changes. Leaf nitrogen initially increased and then decreased with elevation, consistent with prior research findings (Raymundo et al., 2023; Sánchez-Bermejo et al., 2023). Our results support the temperature-plant physiology hypothesis, which holds that temperature governs plant growth and metabolic rates while necessitating higher nitrogen and phosphorus content to offset decreased physiological efficiency under low temperature conditions.
The ratio of carbon, nitrogen, and phosphorus elements strongly correlates with plant growth rate. Plant C/N and C/P ratios reflect plant nitrogen and phosphorus utilization efficiency (Chen et al., 2022). In this study, C/N and C/P at medium and high altitudes were higher than those at low altitudes. This indicates lower photosynthetic and growth rates at middle and high altitudes. This result is in line with specific leaf area results, which indicate that more resources are devoted to building defensive tissue structures at middle and high altitudes to adapt to low-temperature environments (Rauf et al., 2023). The N/P ratio is also used to infer the nutrient availability (especially N and P) of plants in the environment. When the N/P ratio is less than 14, nitrogen plays a role in controlling plant growth; when the N/P ratio is greater than 16, phosphorus dominates in limiting plant growth; and when the N/P ratio is between 14 and 16, nitrogen and phosphorus are both limiting factors for plant growth (Chen et al., 2022). However, the N/P ratios of leaves at all three elevations are between 11 and 14 (all are < 14, Figure 5). This indicated that N was probably limiting tree growth in the study area. The coefficient of variation for leaf chemical characteristics at different altitudes demonstrated changes in leaf chemical traits with elevation, in contrast to the coefficient of variation for plant resources at various elevations, which varied based on the chemical properties of different chemicals (Figure 6). The ecological stoichiometric ratio coefficient of variation for leaf phosphorus was much higher than those for leaf nitrogen content, carbon content, and C/N. This showed that it changed more quickly in response to changes in elevation and was more adaptable to changes in elevation than structural traits (Zhang X. et al., 2023). Contrary to chemical traits, structural traits exhibited less variance, suggesting that they are more susceptible to environmental changes.
Peguero et al. (2023) and Chen et al. (2024) have shown the importance of soil environments as sources of nutrients for plants. Changes in nutrient availability can also impact the PFT and, consequently, its performance (Zhang H. et al., 2023). The RDA demonstrated that soil phosphorus content played a critical role in explaining LFT score variations. The variance partitioning analysis revealed that soil phosphorus explained 50.94% of LFT variation, making it the key soil factor that affected LFT (Figure 7). A study by Zhang X. et al. (2023), however, demonstrated a positive correlation between soil phosphorus levels and leaf phosphorus concentration. Most of these traits are rate traits that change in magnitude over a plant’s life cycle. As we know, plants absorb nutrients from the soil through their roots. Soil phosphorus fluctuations can affect plant leaf phosphorus. It is an essential constituent of plant organs and tissues (Yang et al., 2023). Results showed that soil phosphorus enrichment at middle and high altitudes is an important factor in determining leaf phosphorus content. As a result, leaf thickness increased, dry matter in leaves increased, and leaf tissue density and specific weight increased. However, D. macropodum gained eco-safety by increasing soil phosphorus concentrations (higher leaf thickness and higher dry matter content). This adaptation makes it possible for the plant to grow under environmental conditions at middle and high altitudes.
At various altitudes, D. macropodum leaves displayed distinct structural and chemical characteristics. Significant variations were identified in key structural traits (specific leaf area, leaf area, thickness, dry matter content, and tissue density) and chemical attributes of leaves such as nitrogen content, C/N ratio, and N/P ratio. D. macropodum in this region demonstrated remarkable adaptation to changing environmental conditions linked to elevation gain by adjusting leaf structure and chemical composition. Results demonstrated that soil factors explained 50.94% of LFT variation. Particularly notable was that changes in soil phosphorus content variations emerged as potential drivers of LFT variations, providing adaptation strategies against elevation-induced environmental changes. Leaves at mid (1300 m) and high (1500 m) elevations appeared to prefer resource conservation strategies, while those at 1100 m elevations appeared to adopt resource acquisition ones. Our study focused only on LFT changing with altitude; further investigation should explore their effects on other organs (branches, roots, or combinations) at various altitudes and how these organs interplay physiologically with one another. Understanding this relationship will shed light on plants’ mechanisms for adapting to altitude-induced changes.
The original contributions presented in the study are included in the article/supplementary material, further inquiries can be directed to the corresponding author.
ZC: Conceptualization, Data curation, Formal analysis, Funding acquisition, Investigation, Methodology, Project administration, Resources, Software, Supervision, Validation, Visualization, Writing−original draft, Writing−review and editing. QL: Data curation, Formal analysis, Investigation, Methodology, Software, Validation, Visualization, Writing−review and editing. ZJ: Data curation, Formal analysis, Investigation, Methodology, Software, Validation, Visualization, Writing−review and editing. PY: Data curation, Formal analysis, Investigation, Methodology, Software, Validation, Visualization, Writing−review and editing. MA: Conceptualization, Data curation, Formal analysis, Funding acquisition, Investigation, Methodology, Project administration, Resources, Software, Supervision, Validation, Visualization, Writing−original draft, Writing−review and editing.
The author(s) declare financial support was received for the research, authorship, and/or publication of the article. This work was supported by the Research Project of the Scientific Research Team of the School of Tourism Ecology and Environment at Guilin Tourism University in China (No. STKY-2024001); the 2024 Annual Young and Middle-aged Teachers’ Basic Research Capability Improvement Project in Guangxi Universities (2024KY0847); the 2024 Guangxi Higher Education Undergraduate Teaching Reform Engineering Project (2024JGB406); and the 2022 School-Enterprise Cooperation Demonstration Course Construction Project of Guilin Tourism University (2022XQHZ010).
During the preparation of this work, the author(s) used Wordtune in order to fix grammatical errors and enhance understanding of context and meaning. After using this tool/service, the author(s) reviewed and edited the content as needed and take(s) full responsibility for the content of the publication.
The authors declare that the research was conducted in the absence of any commercial or financial relationships that could be construed as a potential conflict of interest.
All claims expressed in this article are solely those of the authors and do not necessarily represent those of their affiliated organizations, or those of the publisher, the editors and the reviewers. Any product that may be evaluated in this article, or claim that may be made by its manufacturer, is not guaranteed or endorsed by the publisher.
Arif, M., Jie, Z., Behzad, H. M., and Changxiao, L. (2023). Assessing the impacts of ecotourism activities on riparian health indicators along the three Gorges reservoir in China. Ecol. Indic. 155:111065. doi: 10.1016/j.ecolind.2023.111065
Bai, R., Hu, H. W., Zhou, M., Sheng, J., Xiong, C., Guo, Y. M., et al. (2023). Effects of long-term mowing on leaf- and root-associated bacterial community structures are linked to functional traits in 11 plant species from a temperate steppe. Funct. Ecol. 37, 1787–1801. doi: 10.1111/1365-2435.14343
Cao, W., Muhammad, A., Cui, Z., and Li, C. (2023). Effects of different trace elements on ecophysiological characteristics of Ligustrum obtusifolium saplings. Forests 14:972. doi: 10.3390/f14050972
Chen, K. Q., Pan, Y. F., Li, Y. Q., Cheng, J. Y., Lin, H. L., Zhuo, W. H., et al. (2023). Slope position- mediated soil environmental filtering drives plant community assembly processes in hilly shrublands of Guilin, China. Front. Plant Sci. 13:1074191. doi: 10.3389/fpls.2022.1074191
Chen, Z., Jiang, Z., Li, Q., Tan, Y., Yan, P., and Arif, M. (2024). Examining the stoichiometry of C:N:P:K in the dynamics of foliar-litter-soil within dominant tree species across different altitudes in southern China. Glob. Ecol. Conserv. 51:e2885. doi: 10.1016/j.gecco.2024.e02885
Chen, Z., Song, H., Muhammad, A., and Li, C. (2022). Effects of hydrological regime on Taxodium ascendens plant decomposition and nutrient dynamics in the three Gorges reservoir riparian zone. Front. Environ. Sci. 10:990485. doi: 10.3389/fenvs.2022.990485
Cox, A. J. F., González-Caro, S., Meir, P., Hartley, I. P., Restrepo, Z., Villegas, J. C., et al. (2023). Variable thermal plasticity of leaf functional traits in Andean tropical montane forests. Plant Cell Environ. 25, 1–20. doi: 10.1111/pce.14778
Dong, N., Dechant, B., Wang, H., Wright, I. J., and Prentice, I. C. (2023). Global leaf-trait mapping based on optimality theory. Glob. Ecol. Biogeogr. 32, 1152–1162. doi: 10.1111/geb.13680
Eljounaidi, K., Radzikowska, B. A., Whitehead, C. B., Taylor, D. J., Conde, S., Davis, W., et al. (2024). Variation of terpene alkaloids in Daphniphyllum macropodum across plants and tissues. New Phytol. 243, 299–313. doi: 10.1111/nph.19814
Felix, J. A., Stevenson, P. C., and Koricheva, J. (2023). Plant neighbourhood diversity effects on leaf traits: A meta-analysis. Funct. Ecol. 37, 3150–3163. doi: 10.1111/1365-2435.14441
Gao, W. N., Lotfollahi, P., and Xue, X. F. (2023). Four new Diptilomiopus mite species (Acari: Diptilomiopidae) from China. Syst. Appl. Acarol. 28, 1080–1094. doi: 10.11158/saa.28.6.6
Griebel, A., Boer, M. M., Blackman, C., Choat, B., Ellsworth, D. S., Madden, P., et al. (2023). Specific leaf area and vapour pressure deficit control live fuel moisture content. Funct. Ecol. 37, 719–731. doi: 10.1111/1365-2435.14271
Guo, H. F., Duan, D. T., Lei, H. Y., Chen, Y., Li, J. T., Albasher, G., et al. (2023). Environmental drivers of landscape fragmentation influence intraspecific leaf traits in forest ecosystem. Forests 14:1875. doi: 10.3390/f14091875
Haber, L. T., Atkins, J. W., Bond-Lamberty, B. P., and Gough, C. M. (2023). Dynamic subcanopy leaf traits drive resistance of net primary production across a disturbance severity gradient. Front. For. Glob. Change 6:1040839. doi: 10.3389/ffgc.2023.1150209
Hai, X. Y., Shangguan, Z. P., Peng, C. H., and Deng, L. (2023). Leaf trait responses to global change factors in terrestrial ecosystems. Sci. Total Environ. 898:5572. doi: 10.1016/j.scitotenv.2023.165572
He, X., Arif, M., Zheng, J., Ni, X., Yuan, Z., Zhu, Q., et al. (2023). Plant diversity patterns along an elevation gradient: The relative impact of environmental and spatial variation on plant diversity and assembly in arid and semi-arid regions. Front. Environ. Sci. 11:1157. doi: 10.3389/fenvs.2023.1021157
Hidalgo-Triana, N., Pérez-Latorre, A. V., Adomou, A. C., Rudner, M., and Thorne, J. H. (2023). Adaptations to the stressful combination of serpentine soils and Mediterranean climate drive plant functional groups and trait richness. Front. Plant Sci. 14:1040839. doi: 10.3389/fpls.2023.1040839
Huang, C. S., Xu, Y., and Zang, R. G. (2023). Environmental correlates of tree functional diversity with different leaf habits across subtropical evergreen broadleaved forests. J. Veg. Sci. 34:183. doi: 10.1111/jvs.13183
Islam, T., Hamid, M., Nawchoo, I. A., and Khuroo, A. A. (2024). Leaf functional traits vary among growth forms and vegetation zones in the Himalaya. Sci. Total Environ. 906:274. doi: 10.1016/j.scitotenv.2023.167274
Kermavnar, J., Kutnar, L., Marinsek, A., and Babij, V. (2023). Are ecological niche optimum and width of forest plant species related to their functional traits? Flora 301:2247. doi: 10.1016/j.flora.2023.152247
Khan, A., Karim, M. R., Kibria, M. G., Sinha, K., Sultana, F., Mukul, S. A., et al. (2023). Anthropogenic disturbance modifies tree functional traits in the only remnant swamp forest of Bangladesh. Front. Ecol. Evol. 11:1062764. doi: 10.3389/fevo.2023.1062764
Koleszár, G., Szabó, S., Kékedi, L., Löki, V., Botta-Dukát, Z., and Lukács, B. A. (2023). Intraspecific trait variability is relevant in assessing differences in functional composition between native and alien aquatic plant communities. Hydrobiologia 1–14. doi: 10.1007/s10750-023-05313-4
Liu, L. B., Xia, H. J., Quan, X. H., and Wang, Y. Q. (2023). Plant trait-based life strategies of overlapping species vary in different succession stages of subtropical forests, Eastern China. Front. Ecol. Evol. 10:1103937. doi: 10.3389/fevo.2022.1103937
Liu, X. R., Wang, X., Chen, H. X., Chen, K. X., Mo, W. Y., Yuan, Y. Q., et al. (2023). Is the multi-species variation in leaf anatomical traits along the environmental gradient modulated by herbaceous functional groups? Ecol. Indic. 154:880. doi: 10.1016/j.ecolind.2023.110880
Liu, X., Arif, M., Wan, Z., and Zhu, Z. (2022). Dynamic evaluation of coupling and coordinating development of environments and economic development in key state-owned forests in Heilongjiang Province, China. Forests 13:2069. doi: 10.3390/f13122069
Long, Q. Z., Du, H., Su, L., Zeng, F. P., Lian, Z. W., Peng, W. X., et al. (2023). Patterns in leaf traits of woody species and their environmental determinants in a humid karstic forest in southwest China. Front. Ecol. Evol. 11:1230819. doi: 10.3389/fevo.2023.1230819
Madsen-Hepp, T. R., Franklin, J., McFaul, S., Schauer, L., and Spasojevic, M. J. (2023). Plant functional traits predict heterogeneous distributional shifts in response to climate change. Funct. Ecol. 37, 1449–1462. doi: 10.1111/1365-2435.14308
Manohan, B., Shannon, D. P., Tiansawat, P., Chairuangsri, S., Jainuan, J., and Elliott, S. (2023). Use of functional traits to distinguish successional guilds of tree species for restoring forest ecosystems. Forests 14:1075. doi: 10.3390/f14061075
McIntire, C. D. (2023). Physiological impacts of beech leaf disease across a gradient of symptom severity among understory American beech. Front. For. Glob. Change 6:6742. doi: 10.3389/ffgc.2023.1146742
Melis, R., Morillas, L., Roales, J., Costa-Saura, J. M., Lo Cascio, M., Spano, D., et al. (2023). Functional traits related to competition for light influence tree diameter increments in a biodiversity manipulation experiment. Eur. J. For. Res. 142, 709–722. doi: 10.1007/s10342-023-01552-1
Morffi-Mestre, H., Angeles-Pérez, G., Powers, J. S., Andrade, J. L., Feldman, R. E., May-Pat, F., et al. (2023). Leaf litter decomposition rates: Influence of successional age, topography and microenvironment on six dominant tree species in a tropical dry forest. Front. For. Glob. Change 6:2233. doi: 10.3389/ffgc.2023.1082233
Muhammad, A., Petrosillo, I., and Changxiao, L. (2024). Effects of changing riparian topography on the decline of ecological indicators along the drawdown zones of long rivers in China. Front. For. Glob. Change 7:1293330. doi: 10.3389/ffgc.2024.1293330
Niinemets, U. (2023). Variation in leaf photosynthetic capacity within plant canopies: Optimization, structural, and physiological constraints and inefficiencies. Photosynth. Res. 158, 131–149. doi: 10.1007/s11120-023-01043-9
Nomura, Y., Matsuo, T., Ichie, T., Kitayama, K., and Onoda, Y. (2023). Quantifying functional trait assembly along a temperate successional gradient with consideration of intraspecific variations and functional groups. Plant Ecol. 224, 669–682. doi: 10.1007/s11258-023-01329-x
Peguero, G., Coello, F., Sardans, J., Asensio, D., Grau, O., Llusià, J., et al. (2023). Nutrient-based species selection is a prevalent driver of community assembly and functional trait space in tropical forests. J. Ecol. 111, 1218–1230. doi: 10.1111/1365-2745.14089
Poggiato, G., Gaüzere, P., Martinez-Almoyna, C., Deschamps, G., Renaud, J., Violle, C., et al. (2023). Predicting combinations of community mean traits using joint modelling. Glob. Ecol. Biogeogr 32, 1409–1422. doi: 10.1111/geb.13706
Pulido, C. F., Rasmussen, J., Eriksen, J., and Abalos, D. (2023). Cover crops for nitrogen loss reductions: Functional groups, species identity and traits. Plant Soil 1–14. doi: 10.1007/s11104-023-05895-x
Rahman, N. E. B., Smith, S. W., Lam, W. N., Chong, K. Y., Chua, M. S. E., Teo, P. Y., et al. (2023). Leaf decomposition and flammability are largely decoupled across species in a tropical swamp forest despite sharing some predictive leaf functional traits. New Phytol. 238, 598–611. doi: 10.1111/nph.18742
Ramesh, A. S., Cheesman, A. W., Flores-Moreno, H., Preece, N. D., Crayn, D. M., and Cernusak, L. A. (2023). Temperature, nutrient availability, and species traits interact to shape elevation responses of Australian tropical trees. Front. For. Glob. Change 6:1089167. doi: 10.3389/ffgc.2023.1089167
Rauf, Z., Zarif, N., Khan, A., Siddiqui, S., Fatima, S., Iqbal, W., et al. (2023). The Western Himalayan fir tree ring record of soil moisture in Pakistan since 1855. Int. J. Biometeorol. 67, 1477–1492. doi: 10.1007/s00484-023-02517-0
Raymundo, D., Lehmann, C. E. R., de Oliveira-Neto, N. E., Martini, V. C., Altomare, M., Prado, J., et al. (2023). Temporal changes in the dominance of tree functional traits, but no changes in species diversity during woody plant encroachment in a Brazilian savanna. J. Veg. Sci. 34:178. doi: 10.1111/jvs.13178
Rius, B. F., Darela, J. P., Fleischer, K., Hofhansl, F., Blanco, C. C., Rammig, A., et al. (2023). Higher functional diversity improves modeling of Amazon forest carbon storage. Ecol. Model. 481:323. doi: 10.1016/j.ecolmodel.2023.110323
Sánchez-Bermejo, P. C., Davrinche, A., Matesanz, S., Harpole, W. S., and Haider, S. (2023). Within-individual leaf trait variation increases with phenotypic integration in a subtropical tree diversity experiment. New Phytol. 240, 1390–1404. doi: 10.1111/nph.19250
Sandoval-Granillo, V., and Meave, J. A. (2023). Leaf functional diversity and environmental filtering in a tropical dry forest: Comparison between two geological substrates. Ecol. Evol. 13:10491. doi: 10.1002/ece3.10491
Shi, X. M., Qi, J. H., Liu, A. X., Zakari, S., and Song, L. (2023). Leaf phenotypic plasticity coupled with integration facilitates the adaptation of plants to enhanced N deposition. Environ. Pollut. 327:1570. doi: 10.1016/j.envpol.2023.121570
Shi, X. Z., Wang, L. Y., Sun, J. W., Lucas-Borja, M. E., and Wang, J. Q. (2023). Nitrogen cycling-related functional genes exhibit higher sensibility in soil than leaf phyllosphere of different tree species in the subtropical forests. Plant Soil 493, 173–185. doi: 10.1007/s11104-023-06223-z
Siefert, A., and Laughlin, D. C. (2023). Estimating the net effect of functional traits on fitness across species and environments. Methods Ecol. Evol. 14, 1035–1048. doi: 10.1111/2041-210x.14079
Wang, J., Zhao, W. W., Xu, Z. X., Ding, J. Y., Yan, Y., and Ferreira, C. S. S. (2023). Plant functional traits explain long-term differences in ecosystem services between artificial forests and natural grasslands. J. Environ. Manag. 345:8853. doi: 10.1016/j.jenvman.2023.118853
Wang, X., Yuan, Z. Q., Ali, A., Yang, T., Lin, F., Mao, Z. K., et al. (2023). Leaf traits and temperature shape the elevational patterns of phyllosphere microbiome. J. Biogeogr. 50, 2135–2147. doi: 10.1111/jbi.14719
Wang, Y. N., Wang, Y., Yu, F., and Yi, X. F. (2023). Phylogeny more than plant height and leaf area explains variance in seed mass. Front. Plant Sci. 14:1266798. doi: 10.3389/fpls.2023.1266798
Wang, Y., Zhang, C. C., Xiao, X., Wu, H. W., and Zhang, J. H. (2024). Water-use strategies and functional traits explain divergent linkages in physiological responses to simulated precipitation change. Sci. Total Environ. 908:8238. doi: 10.1016/j.scitotenv.2023.168238
Wright, I. J., Reich, P. B., Westoby, M., Ackerly, D. D., Baruch, Z., Bongers, F., et al. (2004). The worldwide leaf economics spectrum. Nature 428, 821–827. doi: 10.1038/nature02403
Xing, H. S., Shi, Z. M., Liu, S., Chen, M., Xu, G. X., Cao, X. W., et al. (2023). Leaf traits divergence and correlations of woody plants among the three plant functional types on the eastern Qinghai-Tibetan Plateau, China. Front. Plant Sci. 14:1128227. doi: 10.3389/fpls.2023.1128227
Xu, S. W., Su, H. X., Ren, S. Y., Hou, J. H., and Zhu, Y. (2023). Functional traits and habitat heterogeneity explain tree growth in a warm temperate forest. Oecologia 203, 371–381. doi: 10.1007/s00442-023-05471-1
Xu, R. J., Qiu, Q., Nong, J. Q., Fan, S. H., and Liu, G. L. (2023). Seasonal patterns and species variability in the leaf traits of dominant plants in the tropical rainforests of Hainan Island, China. Forests 14:522. doi: 10.3390/f14030522
Yang, J. F., Zhang, X. N., Song, D. H., Wang, Y. C., and Tian, J. Y. (2023). Interspecific integration of chemical traits in desert plant leaves with variations in soil water and salinity habitats. Forests 14:1963. doi: 10.3390/f14101963
Yang, S. Q., Yi, L. T., Ye, N. N., Wu, M. Y., and Liu, M. H. (2022). Spatial pattern dynamics of Cyclobalanopsis myrsinifolia in mixed broad-leaved forests on Tianmu Mountain, eastern China, 1996-2012. Silva Fenn. 56:10520. doi: 10.14214/sf.10520
Yao, L. J., Xu, Y., Wu, C. P., Deng, F. Y., Yao, L., Ai, X. R., et al. (2023). Variation in the functional traits of forest vegetation along compound habitat gradients in different climatic zones in China. Forests 14:1232. doi: 10.3390/f14061232
Yoichi, W., Matsuzawa, S., Tamaki, I., Nagano, A. J., and Oh, S. H. (2023). Genetic differentiation and evolution of broad-leaved evergreen shrub and tree varieties of Daphniphyllum macropodum (Daphniphyllaceae). Heredity 131, 211–220. doi: 10.1038/s41437-023-00637-2
Yu, J. B., Li, Q. Y., Wu, X. Y., Zhu, C. L., Huang, S. Q., Yang, F., et al. (2023). Adaptational responses of leaf functional traits of Dicranopteris dichotoma to environmental factors in different vegetational restoration stages. Glob. Ecol. Conserv. 44:e02484. doi: 10.1016/j.gecco.2023.e02484
Zhang, X., Li, B. Y., Penuelas, J., Sardans, J., Cheng, D. L., Yu, H., et al. (2023). Resource-acquisitive species have greater plasticity in leaf functional traits than resource-conservative species in response to nitrogen addition in subtropical China. Sci. Total Environ. 903:6177. doi: 10.1016/j.scitotenv.2023.166177
Zhang, H., Cao, Y. G., Wang, Z. J., Ye, M. X., and Wu, R. L. (2023). functional mapping of genes modulating plant shade avoidance using leaf traits. Plants (Basel) 12:608. doi: 10.3390/plants12030608
Zhao, R., Zhu, Y., Mao, Z. J., Gu, W. P., Zhang, H. G., Long, F. Q., et al. (2023). Predicting fine root decomposition from functional traits in 10 temperate tree species. Forests 14:372. doi: 10.3390/f14020372
Keywords: plant ecology, adaptation traits, morpho-ecological plasticity, plant succession, leaf functional properties, nature reserve
Citation: Chen Z, Li Q, Jiang Z, Yan P and Arif M (2024) Leaf functional traits of Daphniphyllum macropodum across different altitudes in Mao’er Mountain in Southern China. Front. For. Glob. Change 7:1359361. doi: 10.3389/ffgc.2024.1359361
Received: 21 December 2023; Accepted: 02 July 2024;
Published: 17 July 2024.
Edited by:
Jian Tao, Shandong Technology and Business University, ChinaReviewed by:
Lin Zhang, Chinese Academy of Sciences (CAS), ChinaCopyright © 2024 Chen, Li, Jiang, Yan and Arif. This is an open-access article distributed under the terms of the Creative Commons Attribution License (CC BY). The use, distribution or reproduction in other forums is permitted, provided the original author(s) and the copyright owner(s) are credited and that the original publication in this journal is cited, in accordance with accepted academic practice. No use, distribution or reproduction is permitted which does not comply with these terms.
*Correspondence: Muhammad Arif, bXVoYW1tYWRhcmlmQGdsdHUuZWR1LmNu; YXJpZmdyZWVuZXhwZXJ0QG91dGxvb2suY29t
†These authors have contributed equally to this work
Disclaimer: All claims expressed in this article are solely those of the authors and do not necessarily represent those of their affiliated organizations, or those of the publisher, the editors and the reviewers. Any product that may be evaluated in this article or claim that may be made by its manufacturer is not guaranteed or endorsed by the publisher.
Research integrity at Frontiers
Learn more about the work of our research integrity team to safeguard the quality of each article we publish.