- Institute for Ecosystem Research, Kiel University, Kiel, Germany
In past decades, ash dieback has caused a rapid decline of European ash (Fraxinus excelsior) in temperate forests of Europe. Numerous studies focus on mitigating the negative impacts of ash dieback to forest ecosystems or identifying resistant genotypes. The role of natural selection toward genotypes withstanding ash dieback for ash regeneration has been less frequently studied with experimental means to date. This is, however, necessary in times of global change, because the preservation of ash in Europe’s forests will depend, above all, on the adaptability of the future generations of ash trees. To quantify the extent and effects of ash dieback severity for ash regeneration we selected five forest stands moderately damaged and five forest stands highly damaged by ash dieback, in Schleswig-Holstein, Germany. We reciprocally transplanted naturally regenerated ash seedlings sampled in the field between these 10 sites. A shading treatment added to each half of the plots per site was meant to test for effects of altered light conditions in the herb layer due to canopy opening caused by ash dieback. With this approach, we tested seedling survival, performance and fungal infection for an interacting effect of origin and target site in regard to ash dieback severity and environmental factors over 2 years and recorded leaf traits (specific leaf area, leaf dry matter content) in the second year. Reduced light conditions under the shading nets had strong effects, influencing first year performance and infection probability as well as second year survival, growth and leaf trait characteristics. Soil conditions had only a marginal influence on transplanted seedlings. Transplantation direction between moderately and highly damaged sites affected infection marginally during the first year and survival as well as leaf traits significantly during the second year. Most notably, seedlings transplanted from moderately damaged to severely damaged sites exhibited the highest infection probability and lowest SLA, while seedlings transplanted vice versa were least likely to be infected and exhibited the highest SLA. Results hint at a first filtering effect by the ash dieback history of a forest stand and might indicate a transition from ecologically to evolutionary driven differentiation of ash seedling responses.
1 Introduction
Against the background of ongoing global change, forests are subjected to drastic transformation due to interacting imprints of climatic alteration, land use change, pollution and exploitation of resources (Dale et al., 2001; Pautasso et al., 2015). Aside from the most notable effects of increasing frequency and intensity of climate extremes (e.g., drought, heavy storms) (Seidl et al., 2017; Venäläinen et al., 2020) and increasing fragmentation (Estreguil et al., 2012; Fischer et al., 2021), the introduction of alien species still adds to the pressure on ecosystem functioning and challenges faced by forest managers. The accumulation of alien species in forests often involves highly competitive plant species, which can quickly become invasive in their new environment (e.g., van Kleunen et al., 2015). Moreover, fungi (and microbes) are subject to intercontinental translocation as well and can act as pathogens in their new ranges (Desprez-Loustau et al., 2007; Santini et al., 2013; Panzavolta et al., 2021). In the past decades, there have been many examples of invasive forest pathogens, some with detrimental effects on their new host species and entire forest ecosystems (Thakur et al., 2019). For example, in the late 1800s, Cryphonectria parasitica (Murr.) Barr was introduced to North America and after only 50 years, the disease, commonly known as chestnut blight, had decimated the majority of mature American chestnut trees [Castanea dentata (Marsh.) Borkh.] (Anagnostakis, 2001). Ecological implications involved major changes in tree species composition (Woods and Shanks, 1959; Myers et al., 2004) leading to changes in the leaf litter composition, often decreasing leaf decomposition and mineralization, and generally altering nutrient cycling and carbon processing (Smock and MacGregor, 1988). Further down the cascade succeeding this major forest disturbance event impacts were also seen in other trophic groups (Haney et al., 2001) and associated ecosystems (Wallace et al., 2001). Another prominent example is the spread of the Dutch elm disease in the early 1900s. The disease swept across the northern hemisphere in two major outbreaks by Ophiostoma ulmi (Buisman) Nannfeldt and only a few years later by the aggressive Ophiostoma novo-ulmi Brasier, which resulted in the loss of hundreds of millions elm trees (Brasier, 2001). In Europe, major impacts have been seen in riparian forests where the replacement of Ulmus minor Mill. was difficult, as only few other tree species are suitable for sites characterized by frequent flooding as well as summer water deficits (Martín et al., 2010).
European ash (Fraxinus excelsior L.), a tree species overlapping with elm in its ecological niche (Marigo et al., 2000), has also recently been affected by an introduced tree pathogen causing the ash dieback disease. The ascomycete Hymenoscyphus fraxineus (T. Kowalski) Baral, Queloz & Hosoya, was first detected as the causal agent in the early 1990s (Kowalski, 2006) and has since spread across the entire distribution range of F. excelsior. Direct impacts of the fungal pathogen include crown defoliation and stem necrosis (Kirisits et al., 2009; Schumacher et al., 2010; Gross et al., 2014), which not only lead to reduced growth and loss of tree vitality but to high mortality of ash in most temperate European forest stands (Marçais et al., 2017; Coker et al., 2018). Subsequent changes in forest structure can range from small gaps quickly being taken over by other tree species to large gaps forming where drastic changes in light regime and nutrient availability often favor the establishment of highly competitive forest-edge plants in the understory (Turczański et al., 2020). Accordingly, for the next few decades, Hultberg et al. (2020) predict an extinction cascade of ash-associated species which is even accelerated by the concurrent endangerment of ecologically similar elm species, since ash and elm species are considered mutual substitutes (Mitchell et al., 2016; Thomas et al., 2018; Hultberg et al., 2020).
Apart from immediate ecological effects, the introduction of invasive forest pathogens might also involve evolutionary consequences (e.g., Budde et al., 2016; Zenni et al., 2016). Basically, a host species has a long-term coevolution with its antagonist which is driven by complex processes varying on multiple spatiotemporal scales from the genetic to the landscape level (Burdon and Thrall, 2009; Dickie et al., 2017). This might also be evident in trees, seeing that even with the short-lived pathogens advantage over trees in terms of generation time, a rapid onset of adaptive evolutionary processes induced by the strong selection pressure of an invasive pathogen has been observed (Heiniger and Rigling, 1994; Ahrens et al., 2019). Aside from adaptive processes of the host tree species itself, such as natural selection toward most tolerant genotypes or phenotypes, the evolutionary potential of an affected tree species can also be determined by viral or other biological antagonists to the pathogen as seen in Dutch elm disease as well as chestnut blight (Heiniger and Rigling, 1994; Brasier, 2001).
Predictions of evolutionary consequences are still vague for European ash due to the comparably short time that has passed since initial disease outbreak and the long generation time of trees. While it was possible to show a genetic basis of resistance toward H. fraxineus involving medium to high heritability under controlled conditions in planted provenance trials or seed orchards (Pliūra et al., 2011; Kjær et al., 2012; Muñoz et al., 2016), the situation under natural heterogeneous conditions is a substantially different one. For example, there was no correlation found between the damage intensity of mature ash trees and their offspring as revealed in a parentage analysis in a natural ash stand (Wohlmuth et al., 2018). However, the authors admit that the inspected sites represent neither typical nor highly affected ash stands, therefore, the data set was most likely not able to comprehensively encompass the complexity of differences in susceptibility toward H. fraxineus. In contrast, Semizer-Cuming et al. (2021) observed ash regeneration in a near-natural mixed forest stand which had been moderately affected by ash dieback and found that healthy ash trees were overrepresented as parents of young natural regeneration, thus suggesting incipient selection toward more tolerant ash individuals.
To what extent these selection processes are affected by the heterogeneity of natural forest stands has not yet been addressed in empirical research. While ecotypic differentiation in regard to climatic conditions is well known for trees (Kawecki and Ebert, 2004; Davis et al., 2005; Jump et al., 2006; Savolainen et al., 2007; Alberto et al., 2013; Vitasse et al., 2013), it is still debatable to what extent tree performance and fitness also rely on local adaptation to abiotic edaphic or even biotic environmental conditions. Manzanedo et al. (2018) studied Fagus seedling performance of three geographical origins in a reciprocal soil transplant experiment: there was a strong signal of plant × soil origin interactions to affect the performance of transplanted seedlings, but there was no evidence for a home soil advantage for seedlings grown in their own soil. However, the authors only tested for soil biota effects and thus rather suggested maladaptation to local soil biota including specialized antagonists. In contrast, their results showed that seedlings transplanted from populations in the central part of a species’ range can still be strongly differentiated despite presumably high levels of gene flow that hamper ecotypic differentiation.
There is increasing evidence that processes of local adaptation or even maladaptation to antagonists also apply to invasive forest pathogens e.g., (see Ahrens et al., 2019). Besides a genetic basis of resistance (Budde et al., 2016), higher tolerance to an invasive pathogen could also be due to mechanisms of disease avoidance, e.g., by early leaf senescence in ash trees (McKinney et al., 2011; Muñoz et al., 2016), or interaction with the associated endophytic community (Kosawang et al., 2018; Becker et al., 2020; Griffiths et al., 2020). In addition, heritability of tolerance traits can be affected by strong gene × environment interactions and thus vary under different environmental conditions (Velásquez et al., 2018; Ahrens et al., 2019). At the same time, biotic factors, such as soil biota, endophytes or even the pathogen itself, could play a role in a local differentiation in the susceptibility of the host species. For F. excelsior, evidence is provided that tolerance to the ash dieback pathogen can depend on its infection pressure—some ash individuals that are healthy at low pathogen infection pressure may become diseased when the infection pressure is high (Pliūra et al., 2011, 2014). There is high spatial variation in the severity of ash dieback symptoms with some ash stands showing little damage while other stands have completely collapsed (e.g., Enderle et al., 2018). Generally, abiotic site conditions and especially soil moisture have been identified as major contributing factors to these differences (Erfmeier et al., 2019; Haupt et al., 2022; Cracknell et al., 2023). Especially in areas with highly fragmented forest patches, there might also be temporal differences in disease progression. However, the extent as to which these differences in ash dieback severity and environmental conditions affect natural ash regeneration is still unknown, although the question of the natural regenerative capacity of ash-rich stands is such an obvious one. Reciprocal common garden studies have proven to be appropriate tools to test for local adaptation to climatic (Muffler et al., 2021; Lortie and Hierro, 2022), abiotic (Smith et al., 2012), and also biotic factors (Manzanedo et al., 2018).
We make use of the current infestation situation of ash-rich forests in fragmented Northern German woodlands to test for the impact of the disease status of mature stands on offspring development. In a full reciprocal transplant experiment, we examined survival, performance and infection status of ash regeneration in forest stands of differing pre-existing ash dieback severity. Here, we distinguish between seedling performance during first year establishment and the second year early growth phase. Origin and target forest sites are classified as showing either moderate or high damage caused by ash dieback. Considering light as the environmental condition, which is most drastically altered in forest stands affected by ash dieback, artificial shading was added as a treatment while also taking abiotic site factors into account.
Addressing effects of environments and origins, we hypothesize that (1) moderately damaged target stands involve a favorable environment and promote a better survival and performance of ash regeneration. At the same time, (2) we assume that an increased selection pressure caused by a higher ash mortality will contribute to a better performance of ash regeneration originating from highly damaged forest stands. Looking at the transplantation direction as a combination of these target and origin effects to quantify interaction effects, (3) more precisely, we expect origin × target site interactions that show seedlings from highly damaged stands (which are more likely to be tolerant to ash dieback) transplanted into moderately damaged ash stands (which offer more favorable site conditions) will outperform all other transplant combinations.
2 Materials and methods
2.1 Site description
The experimental sites are distributed on the eastern coast of Schleswig-Holstein, Germany (Figure 1). A mean annual temperature of 9.3°C and a mean annual precipitation of 812.8 mm (1991–2020) classify the climate in this northern-most part of Germany as a sub-oceanic one [Deutscher Wetterdienst (DWD), 2023]. As a recent moraine landscape formed by glaciers during the Weichselian glaciation, the region is characterized by a hilly topography, where the prevailing soil types are Luvisols replaced by Stagnosols and Gleysols in areas with higher soil moisture or groundwater influence. Bearing a forest cover of only 11% (173,412 ha) of the total area, Schleswig-Holstein is the federal state with the least amount in Germany. The forests are largely fragmented and thus comprise a mosaic of small ancient forest remnants and reforested stands.
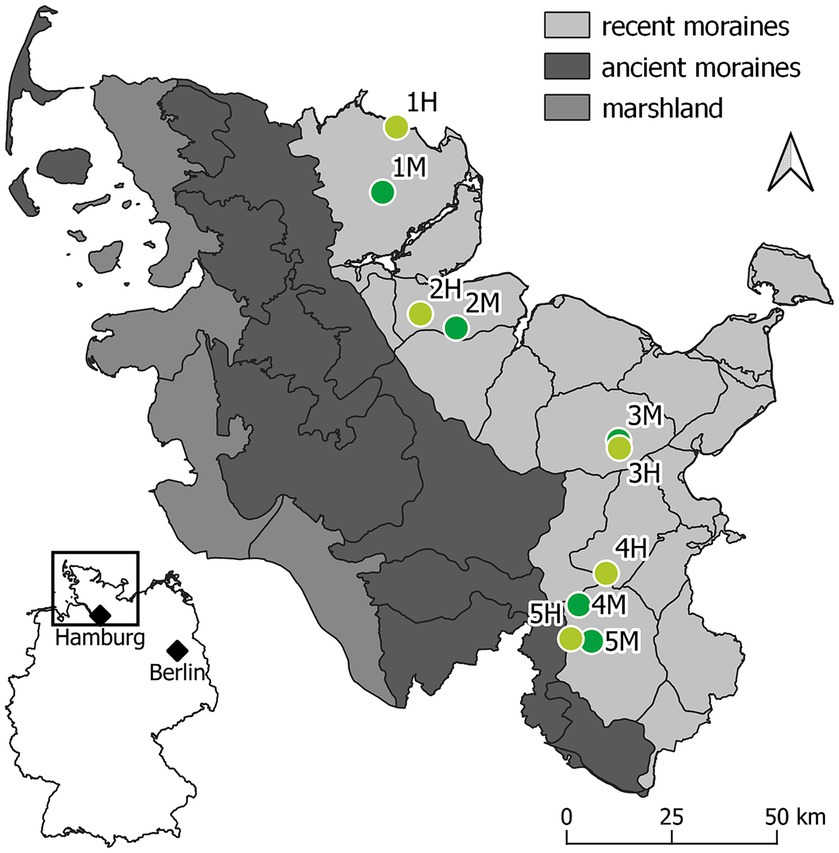
Figure 1. Location of the 10 experimental sites. The map shows the three major landscape types of Schleswig-Holstein, in Germany: recent moraines (light gray), ancient moraines (dark gray), and marshland (anthracite). All experimental sites are arranged in pairs of each one moderately (light green) and one highly (dark green) damaged ash-rich forest stand on the recent moraines of the Schleswig-Holstein east coast.
Fraxinus excelsior occurs on a wide range of site conditions in Schleswig-Holstein (Härdtle, 1995; Haupt et al., 2022), therefore the experiment was established under conditions involving fresh to moist and meso- to eutrophic soils. All experimental sites are situated in ash-rich forest stands currently with differing proportions of F. excelsior and high proportions of Fagus sylvatica L. and/or Quercus robur L. and a low abundancy of other tree species like Acer pseudoplatanus L. (Table 1). Plant communities at all sites can be ascribed to the beech forest community Hordelymo-Fagetum with some transitions to Galio odorati-Fagetum on sandy to loamy soils (Härdtle et al., 2008).
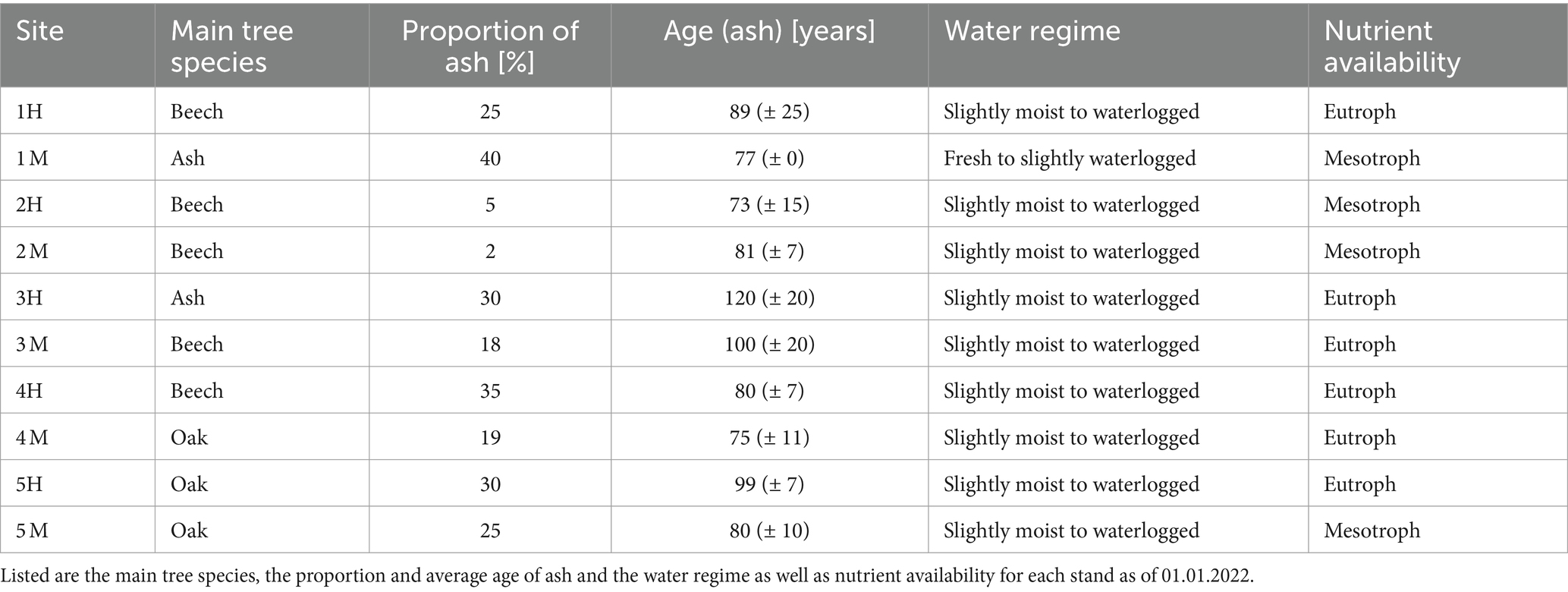
Table 1. Stand properties extracted from the Schleswig-Holstein State Forest databank for the forest stands in which the 10 experimental sites are located.
In Schleswig-Holstein, ash dieback was first recorded in 2002 (Enderle et al., 2017). Sites were selected in separate forest stands according to the ash dieback severity of the remaining mature ash trees and the overall damage status of the stand (Figure 2). Along the moraine landscape, five pairs of forest stands were selected, each with one moderately and one severely damaged stand (Figure 1). The sampling in pairs was intended to ensure that the variation in climatic conditions within the two groups of infestation situation is comparable. An area of approximately 150 m2 of each of these experimental sites was selected for experimental implementation and fenced in order to ensure exclusion of wildlife.
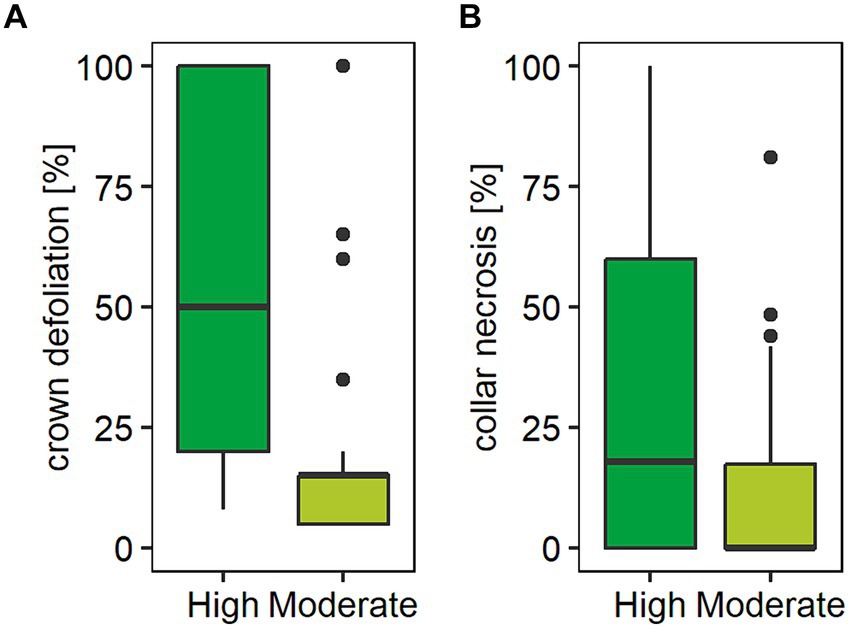
Figure 2. Boxplots showing (A) crown damage and (B) collar necrosis by forest stand damage class (High / Moderate). Crown damage was determined as the proportion of loss of crown foliage and collar necrosis as proportion of necrotic tissue of the total stem diameter measured at 15 cm above ground level. In each forest stand, five standing mature ash trees (BHD > 15 cm) closest to the experimental site were evaluated in summer 2020. Graph (A) shows a median crown defoliation of 15% (mean 19.4%) in forest stands characterized as moderately damaged compared to a median loss of crown foliage of 50% (mean 56.5%) in stands characterized as highly damaged. Graph (B) shows a median collar necrosis of 0% (mean 13.6%) in forest stands characterized as moderately damaged and 17.9% (mean 34.2%) in stands characterized as highly damaged.
2.2 Experimental design
The study was set up as a reciprocal transplant experiment across 10 sites with naturally rejuvenated 1–2-year old ash seedlings obtained directly from each of these sites. In September 2019, naturally rejuvenated ash seedlings were harvested from each of the 10 forest stands (henceforth addressed as sites of “origin”). Seedlings were kept moist and dark during transport. Within the day of harvest, roots were carefully but thoroughly washed with water and seedlings were potted in Quickpot trays (QuickPot QP 96 T) using standard peat culture substrate. For the first few days seedlings were kept in a greenhouse to ensure that they can take root before being moved to a common garden area of Kiel University covered by a shading net (65% shading) to simulate forest-like light conditions. In preparation for planting, seedlings were rearranged within the Quickpot trays. Only individuals lacking any signs of ash dieback or any other damage were transplanted to the forest sites (henceforth addressed as “target” sites) in October 2019.
The reciprocal transplantation included each of six seedling individuals from 10 sites of origin to be (re-)planted into each of the 10 target sites, yielding 600 individuals in total. At each site, six rectangular experimental plots were established as replicates. Each plot included one seedling of each origin planted at a distance of 0.5 m from each other in a 3 × 4 rectangle (long side facing north, with no plant in the north-east and the south-west corner; Figure 3). Assignment of seedling origins to planting positions was randomized for every plot. In May 2020, dead seedlings were replaced with healthy individuals from the same harvest cohort, which had been cultivated over winter in the common garden area.
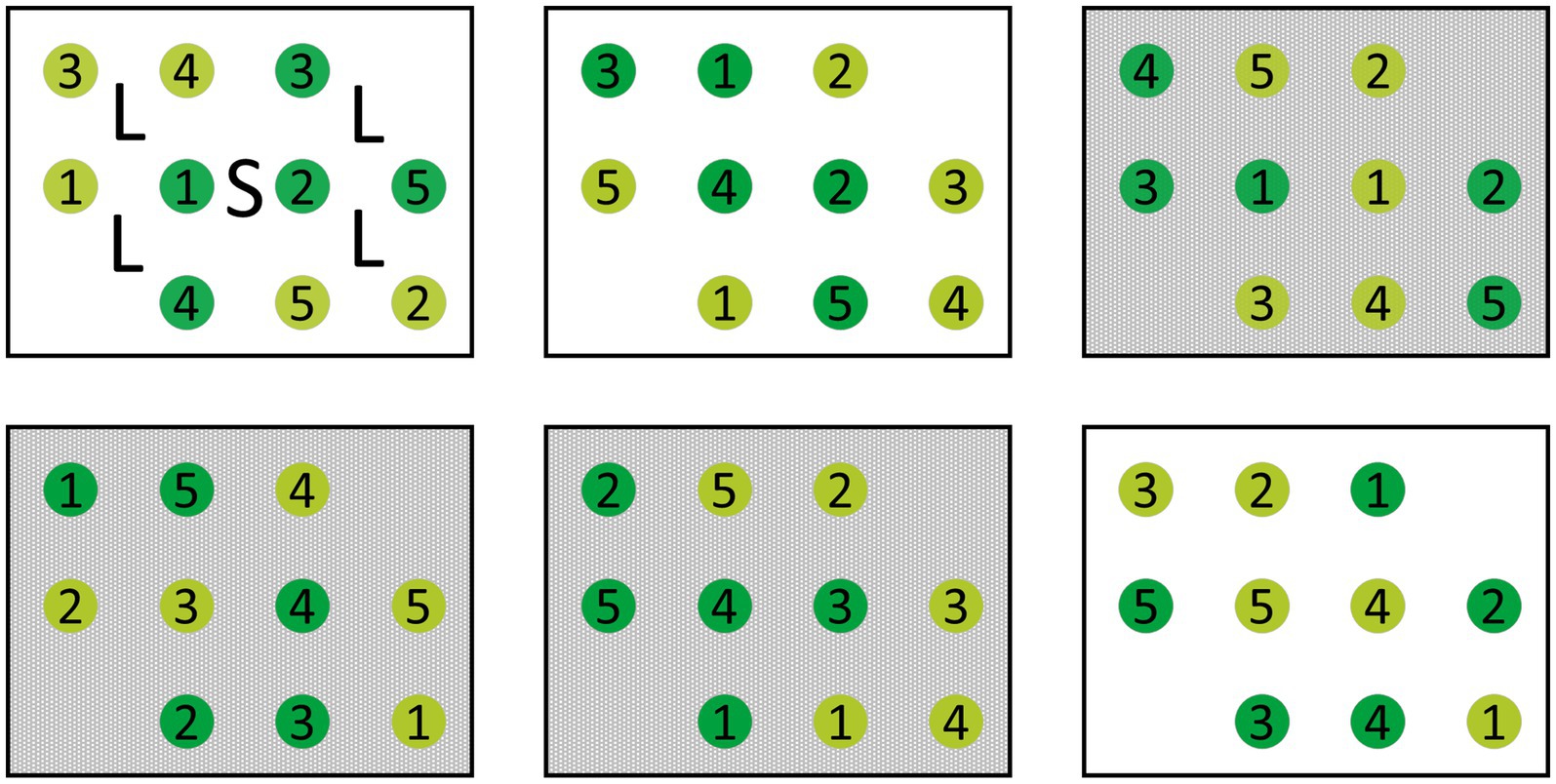
Figure 3. Schematic of an experimental site. Each site contains each three plots with (gray) and without (white) shading treatment. In every plot, one individual of each of the 10 sites of origin was planted. Light green circles depict individuals from moderately damaged, dark green from highly damaged sites. Numbers indicate the site of origin. Markings in the upper left plot depict the locations where light measurements (L) and soil samples (S) were taken in every experimental plot.
2.3 Shading treatment
Three plots per site were randomly selected and assigned a 65% shading treatment resulting in a total of 300 shaded and 300 non-shaded ash seedlings. Shading nets were installed on a wooden frame of 0.5 m height. The nets were set up in April before bud break each year and removed at the end of each vegetation period. Since light conditions generally differed between forest stands due to differences in forest composition and amount of leaf loss caused by ash dieback, light conditions were measured once per summer in 2020 and 2021 each at standardized positions. Four point measurements of photosynthetic active radiation (PAR) (LI-1500, LI-COR) were recorded in each plot at seedling height (approximately 15–20 cm) while an additional sensor logged reference levels in an open field near the forest stand (Figure 3). From this, relative light was calculated and averaged over the four measurements per plot.
2.4 Data acquisition
Shoot length as the total length of the main shoot and basal diameter at ground level were measured directly after planting in October 2019 as baseline information. In the following years, growth (as increase in shoot length and basal diameter) and infection with H. fraxineus was recorded at the end of each vegetation period (December 2020, October 2021). In addition, growth and infection was repeatedly monitored each month from May to August during the first year. Infection by the ash dieback pathogen is visible as necrotic tissue on leaves and shoots, the former appearing typically in mid to late summer and the latter during the following winter or spring. In 2020, we recorded seedlings showing any amount of ash dieback symptoms as infected without distinguishing between leaf and shoot infection [thereby recorded as presence (1)/absence (0)]. Thus, a seedling that was recorded as infected in 2020 might have exhibited leaf infection, shoot infection or both. In 2021, the infection status was assessed only once at the end of the vegetation period and represents only shoot infection. Functional leaf traits were determined once, in June 2021 on all individuals. One fully developed leaf per seedling was harvested, quickly stored in a cooler box for transport, scanned, analyzed (WinFOLIA, Regent Instruments, Québec, Canada), weighed, dried (at least 48 h at 110°C) and weighed again to determine specific leaf area (SLA) and leaf dry matter content (LDMC) at the Environmental Lab, Kiel University.
For characterization of edaphic conditions, soil samples were taken from the uppermost mineral horizon at the center of each plot in November 2019. Soil samples were sieved and air-dried. Soil pH values were determined in a 1 N KCl solution using a single rod glass electrode [pH-meter Lab 860 Sen Tix HW (Schott Instruments)]. Total carbon (C) and nitrogen (N) contents were analyzed following the Dumas method using the C/N-Analyzer EURO EA 3000 (HEKAtech GmbH, Wegberg, Germany). In October 2021, soil types were determined from a drill stick profile according to the German soil classification (Boden, 2005) in the middle of each of the 60 plots. For each soil profile, field capacity at medium bulk density of each soil type was added up to a depth of 1 m (Boden, 2005).
2.5 Statistical analysis
To characterize abiotic site conditions on all experimental plots, a non-metric multidimensional scaling of pH, C:N ratio, field capacity and relative light in the first year was calculated.
In order to distinguish between effects on plant establishment and effects on early growth, we separated the data set into first year data after planting as the establishment phase and second year data as the early growth phase, and analyzed the establishment and early growth phases separately. For each phase, mixed models were built using survival (1|0), performance [relative growth rates (RGR), SLA, and LDMC] and infection (1|0) as response variables. Models for SLA and LDMC were only available for the second phase. Seedlings which were dead at the end of the first year were removed from second year survival analysis. Performance analysis was conducted only for seedlings alive at the end of each year, resulting in a model N of 524 for year 1 and 450 for year 2. Performance analysis included relative growth rates of basal diameter (BD) and shoot length (SL) as response variables. Prior to analysis a square root-transformation was used to obtain a normal distribution, therefore all RGR equal to or smaller than zero were set to 0.001 to avoid a zero. For infection in the first phase, all monitoring assessments between June and December of the first year were summarized into a binomial variable showing if a seedling was either alive and infected (1) or alive and not infected (0) at any one or multiple points during the year. Second phase infection data reflect a single assessment at the end of 2021.
To test whether damage status and site conditions of origin and target sites had an effect on survival, performance and infection of the transplanted seedlings, we determined four transplantation treatments based on the direction of transplantation between the moderately and highly damaged stands: two treatments with origin in low damage stands, i.e., from moderately into moderately damaged stands (M2M), from moderately into highly damaged stands (M2H), and two treatments with an origin in highly damaged stands, i.e., from highly into moderately damaged stands (H2M) and from highly into highly damaged stands (H2H). In the full model, we considered direction of transplantation and shading treatment (1|0) as fixed factors and included relative light, pH and C:N ratio in the upper soil and field capacity as a proxy for soil moisture as covariates. For first year survival analysis only, basal diameter and shoot length at time of planting (initial plant BD|SL) were included as covariates in two separate models.
To account for potential spatial autocorrelation between plots, sites and the regions, which include one site from each of the two damage classes, a nested random structure of plot nested in site nested in region was included in all models. In a few cases, a singularity in the nested random structure prevented further model inference. In those cases, the causal random factor was removed from the model and these changes were indicated in the model inference tables (Table 2; Supplementary Table S4). Few extreme values for single sites or even plots resulted in correlations between environmental parameters and transplantation direction. Since correlation was low to moderate and we detected no problems in model evaluation, such as multicollinearity, we included both transplantation direction and environmental parameters in our models. All covariates were tested for correlation. To account for correlation between covariates, e.g., light incidence and shading treatment, as well as chemical soil properties, models were selected by their fit. Results presented refer to models including pH and shading treatment (which generally showed the best model fit) but deviations in model inference are indicated where appropriate. For results of alternative models, refer to the Supplementary material.
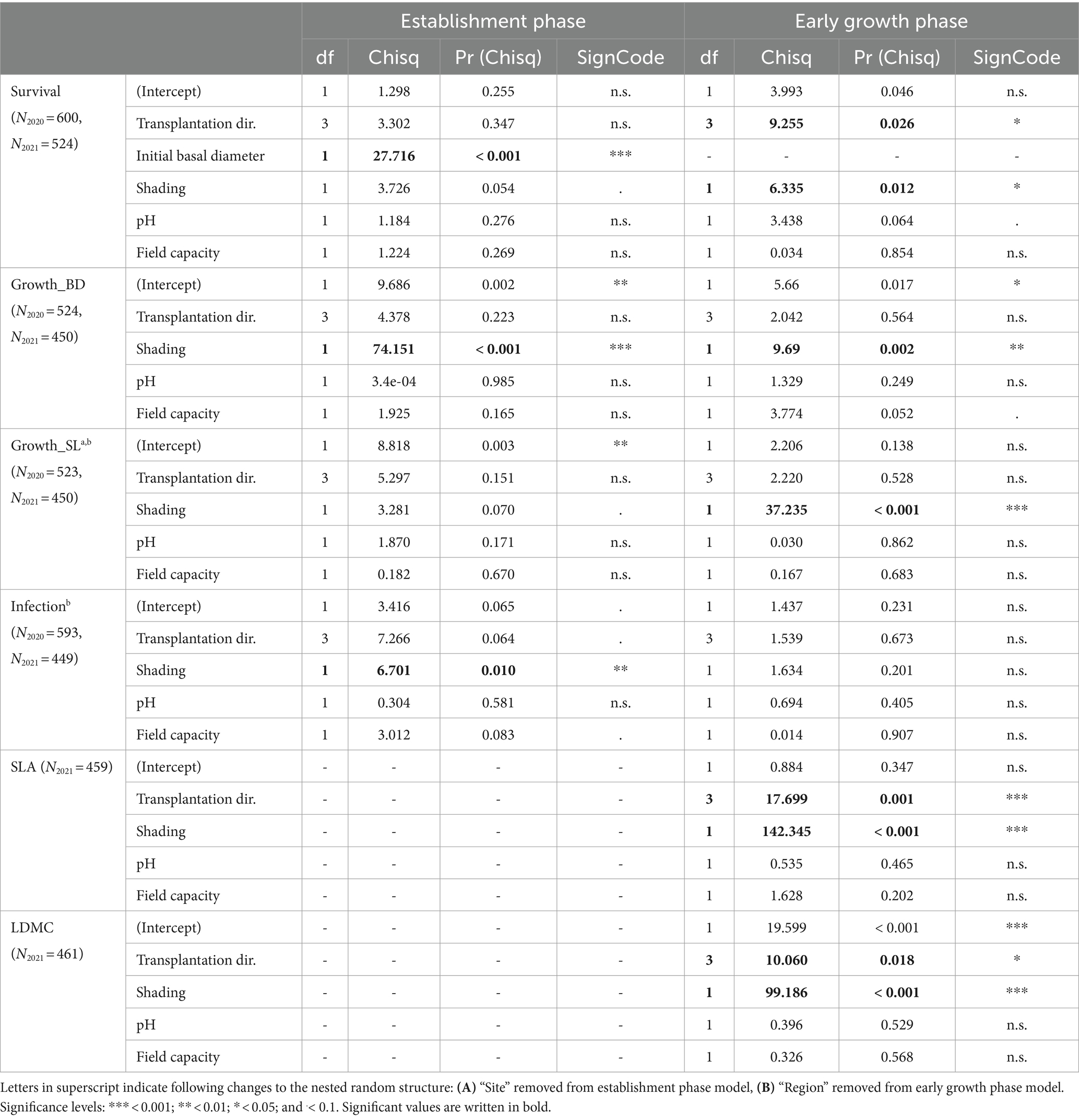
Table 2. Type III ANOVA statistics on the generalized linear mixed effect models predicting survival, RGR of basal diameter and shoot length, and infection of the seedlings in the establishment phase (2020) as well as the early growth phase (2021) and generalized linear mixed effect models predicting the functional plant traits SLA and LDMC in the early growth phase (2021) only.
Survival and infection were analyzed with generalized linear mixed models (GLMM) fitted with a binomial error distribution and cloglog-link function. Performance and functional traits (SLA, LDMC) were analyzed with linear mixed models (LMM) and final models were fit with restricted maximum likelihood. All data analysis was performed in R (v4.3.1) (R Core Team, 2023). NMDS of abiotic site conditions was calculated with vegan (v2.6-4) (Oksanen et al., 2022). GLMMs were built with glmmTMB (v1.1.7) (Brooks et al., 2017). LMMs were calculated with lme4 (v1.1-34) (Bates et al., 2015). We used the R packages DHARMa (v0.4.6) (Hartig, 2022) and performance (v0.10.5) (Lüdecke et al., 2021) to check model diagnostics, emmeans (v1.8.8) (Lenth, 2023) for post-hoc comparisons, Car::Anova (v3.1-2) (Fox and Weisberg, 2019) and insight (v0.19.5) (Lüdecke et al., 2019) for model interpretation and ggeffects (v1.3.1.3) (Lüdecke, 2018) for model predictions. Plots were created using ggplot2 (v3.4.3) (Wikham, 2016).
3 Results
3.1 Abiotic site conditions
Upper soil pH varied between 3.14 and 6.22 across all plots with a median of 3.76 while C:N ratios displayed a range between 11.7 and 19.4 with a median of 13.7. Soil moisture characteristics also differed between sites but generally showed high field capacities from 140 to 177 mm with a median of 156 mm. In 2020, relative light availability ranged from 0.2 to 27.2% at seedling height. In 2021, light conditions were slightly lower but overall similar to the first year with relative light availability between 0.1 and 21%. Most sites were characterized by low light conditions below 15% with a mean relative PAR of 5.5% for non-shaded and 2.1% for shaded plots in the first year and a mean relative PAR of 4.5% for non-shaded and 1.5% for shaded plots in the second year (Supplementary Table S1; Supplementary Figures S1A–E).
The NMDS revealed that the types of forest stands differed in their chemical soil properties with highly damaged sites exhibiting more alkaline conditions and lower C:N ratios in the upper soil (Figure 4). However, there is some overlay where plots from both damage classes show similar site conditions. Sites that have been classified as highly damaged by ash dieback are more differentiated especially in their light conditions compared to moderately damaged sites. Overall, the magnitude of plot differentiation varied strongly between sites.
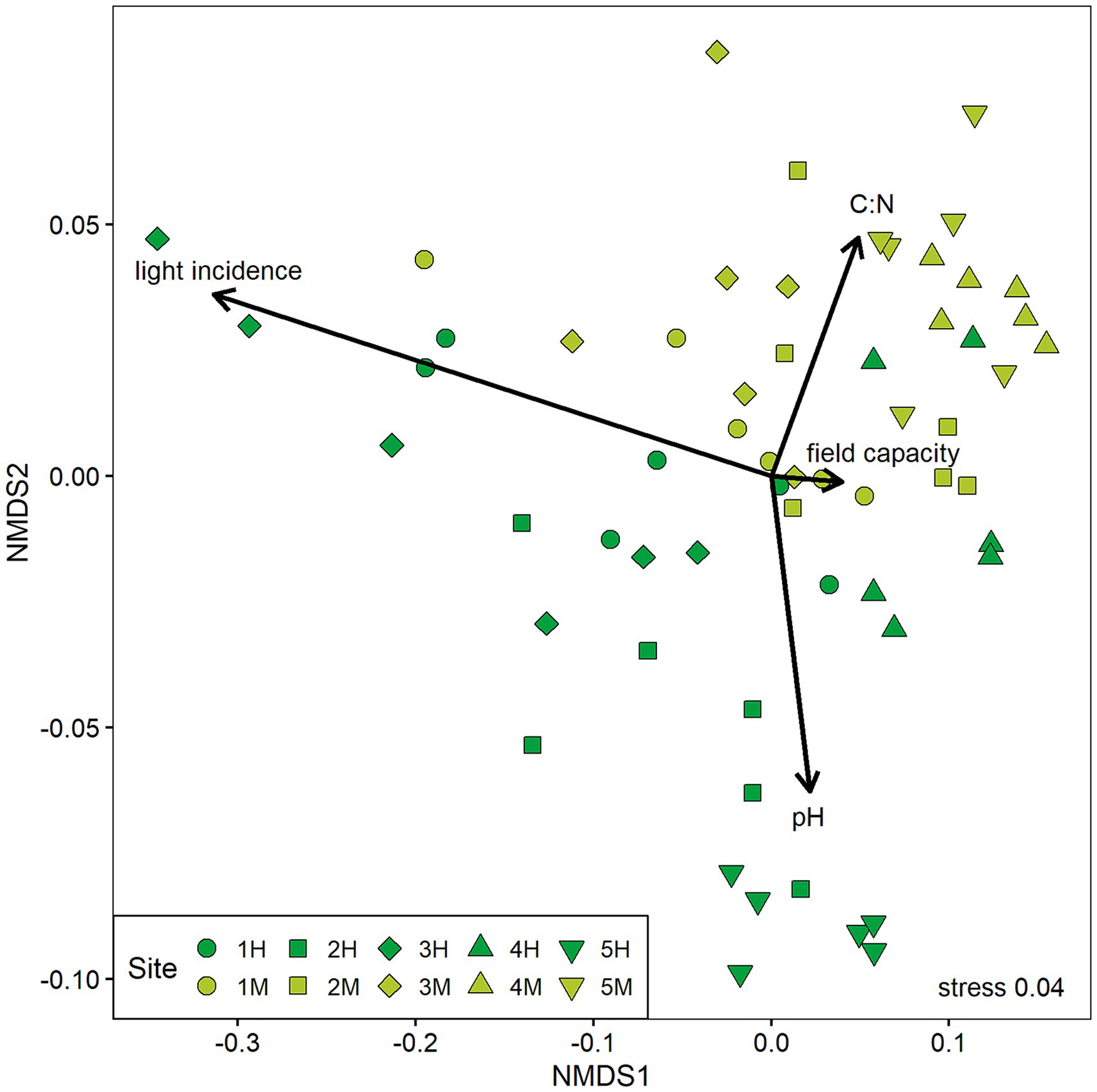
Figure 4. Result of the non-metric multidimensional scaling (NMDS) of abiotic site conditions on all experimental plots (n = 60). Variables included are pH and C:N ratio of the upper soil, field capacity, and light incidence measured in 2020. The two forest stand damage classes are marked in different colors (dark green = highly damaged, light green = moderately damaged) and geographically paired sites are depicted in the same shape.
3.2 Establishment phase (year 1)
3.2.1 Survival
One year after planting 524 out of the 600 ash seedlings (87%) were still alive. Generalized linear mixed effects models showed that only initial plant size (BD or SL at time of planting) significantly affected survival during the initial establishment phase (Table 2; Supplementary Tables S4, S5) with a higher survival probability for larger seedlings (Figure 5A). However, in the establishment phase, none of these models was able to explain more than 17% of the variance in survival (Supplementary Table S6).
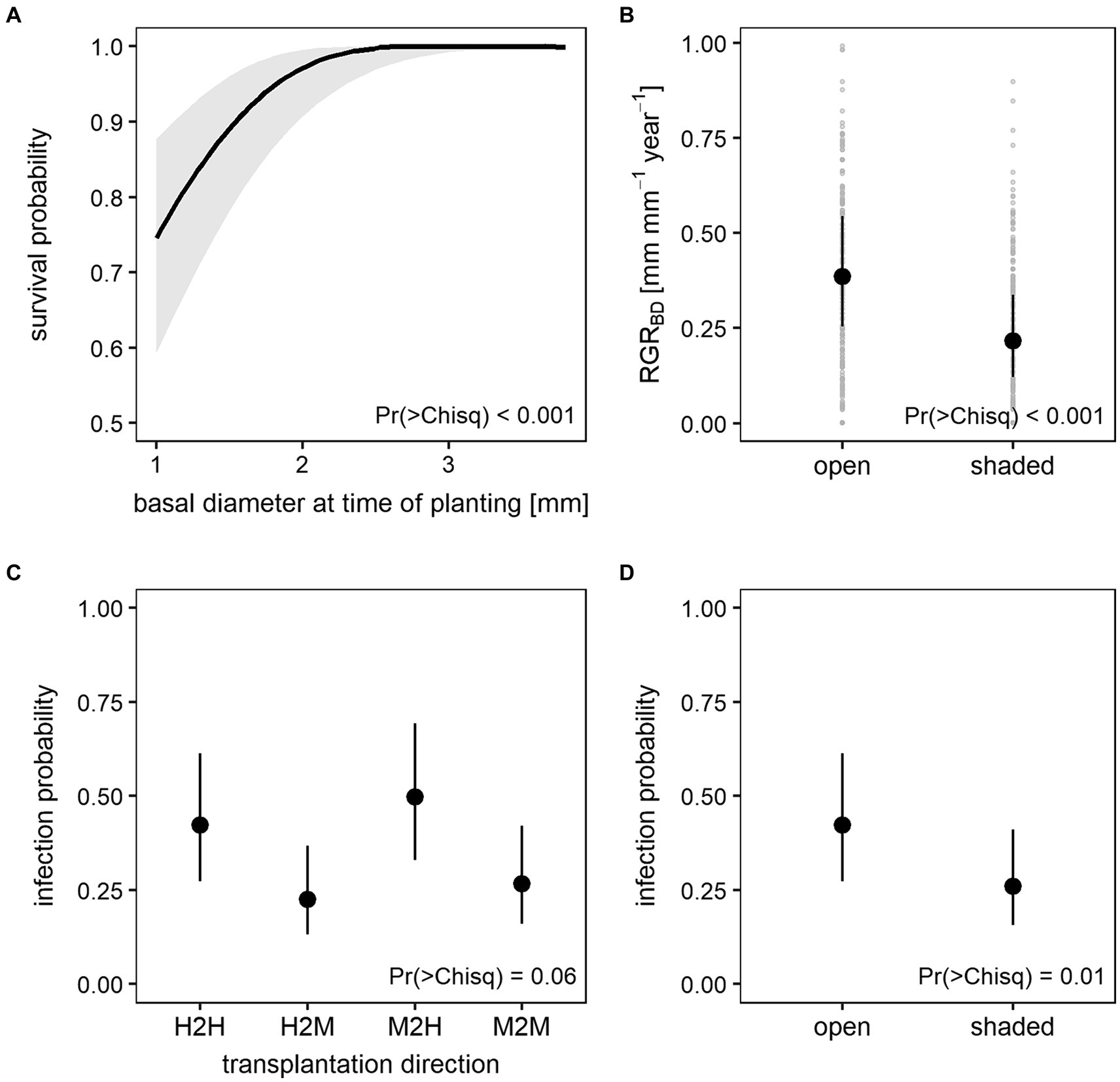
Figure 5. Predicted mean values and 95% confidence intervals of the establishment phase generalized linear mixed models for (A) survival probability in response to basal diameter at time of planting, (B) relative growth rate of the basal diameter (RGRBD) in response to shading treatment, and infection probability in response to (C) transplantation direction and (D) shading treatment. Plot (B) additionally shows variation of RGRBD data in light gray.
3.2.2 Growth
At time of planting, basal diameter of the seedlings varied from 1.0 to 3.8 mm (mean 1.8 mm) and shoot length from 37 to 139 mm (mean 83 mm). After 1 year, seedlings had grown to a mean basal diameter of 2.5 mm with a maximum of 4.6 mm while the lowest basal diameter of all living seedlings remained 1.0 mm. Mean shoot length increased to 111 mm with the smallest and the tallest seedling measuring 57 and 183 mm, respectively (Supplementary Table S2). When disregarding negative values as no growth (i.e., setting negative values to zero), mean RGR was calculated at 0.29 mm mm−1 year−1 for both basal diameter and shoot length. However, basal diameter exhibited a higher maximum RGR with 0.99 mm mm−1 year−1 compared to 0.82 mm mm−1 year−1 for shoot length. Basal diameter growth during the establishment phase was affected by light conditions with significantly lower RGR on shaded plots compared to open plots (Figure 5B). The model has a conditional R2 (considering both fixed and random effects) of 0.42 and a marginal R2 (providing variances explained by fixed effects only) of 0.19 (Supplementary Table S6). No such effect was found for shoot growth (Table 2).
3.2.3 Infection
Throughout the first year, ash dieback symptoms were recorded on 187 living seedlings on at least one occasion. This may have been leaf infection as well as shoot infection. During the establishment phase, infection was significantly affected by shading treatment (Table 2). Shading decreased infection probability significantly (Figure 5D). The effect of transplantation direction on infection was only marginally significant. Seedlings transplanted from moderately to highly damaged stands showed the highest and seedlings transplanted from highly to moderately damaged stands the lowest infection probability (Figure 5C). The GLMM for infection has a conditional R2 of 0.32 and a marginal R2 of 0.12 (Supplementary Table S6).
Although infection probability differed significantly between treatment levels with lower probabilities under shading nets, this effect is not confirmed in models including light incidence (Supplementary Table S4). Here, no such effect of light conditions on infection probability was found.
3.3 Early growth phase (year 2)
3.3.1 Survival
After the second year, overall survival rate dropped to 75% when referred to the beginning of the experiment (450 out of 600 seedlings). When referred to those individuals, which survived the first year alone, survival in the early growth phase was 86% (450 out of the 524 individuals). Results of the mixed models show significantly lower survival under shading nets during the early growth phase (Figure 6B). While transplantation direction showed a significant effect on survival, differences between the levels of transplantation direction were only close to significance (Supplementary Table S7). At the 10% significance margin, seedlings transplanted from moderately into highly damaged sites were more likely to survive compared to seedlings transplanted from highly damaged sites into either moderately or highly damaged sites (Figure 6A). Models could overall only explain 17% of total variation in the survival data (Supplementary Table S6).
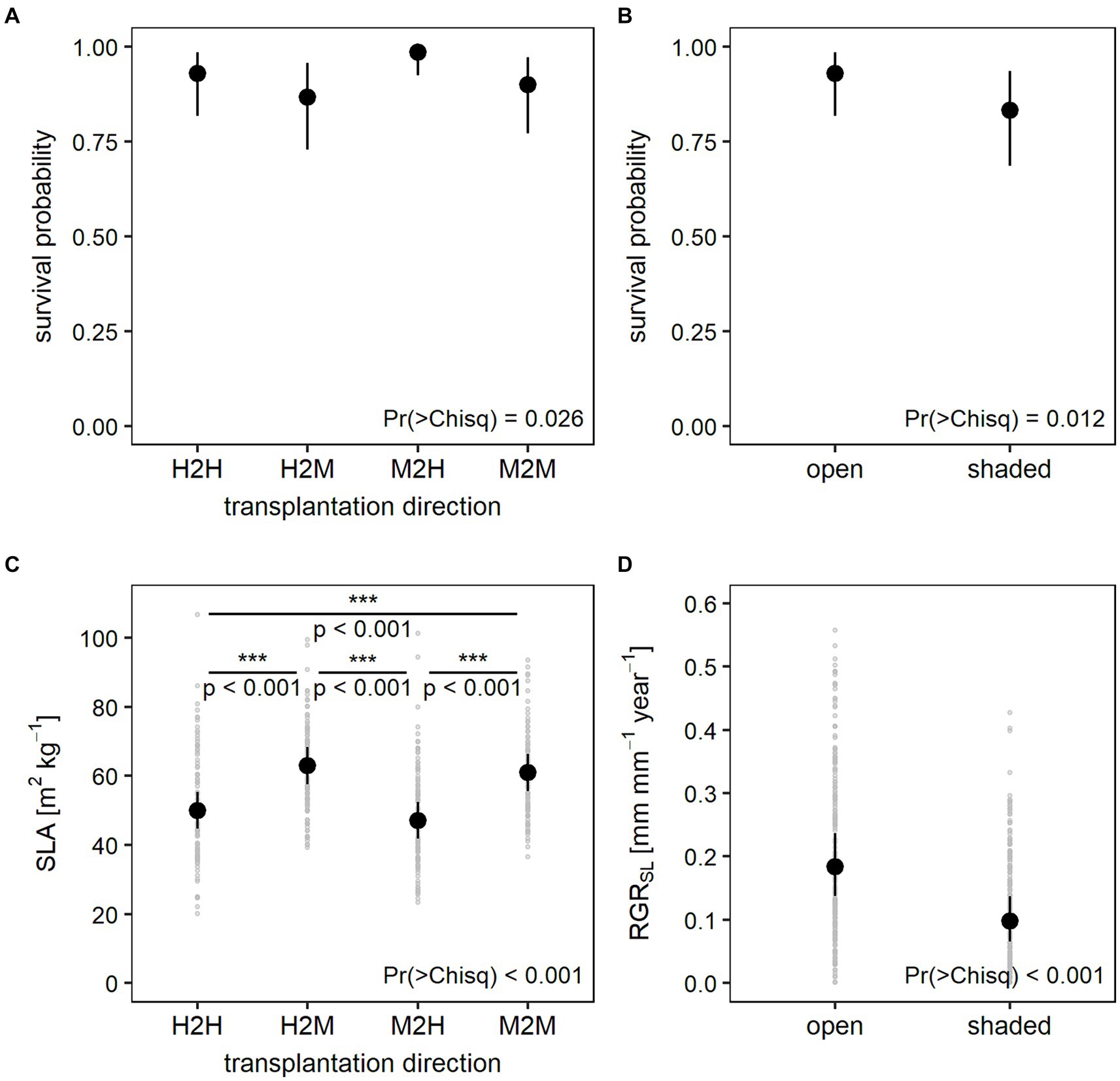
Figure 6. Predicted values and 95% confidence intervals of the early growth phase generalized linear mixed models for (A) survival probability in response to transplantation direction, (B) survival probability in response to shading treatment, (C) specific leaf area (SLA) in response to transplantation direction including light incidence instead of shading treatment, and (D) relative growth rate of the shoot length (RGRSL) in response to shading treatment. p values depicted in plot (C) were derived from post-hoc pairwise comparisons between all transplantation levels. Plots (C,D) additionally show variation in RGRSL and SLA in light gray.
3.3.2 Growth
In the early growth phase, growth was much lower than in the establishment phase with seedlings reaching a mean RGR of 0.12 and 0.16 for basal diameter and shoot length, respectively. At the end of the second year, basal diameters measured between 1.0 and 6.1 mm with a mean of 2.8 mm while shoot length measured between 11 and 261 mm with a mean of 132 mm (Supplementary Table S2). Overall, mixed effects models for growth showed the same effect as during establishment, i.e., RGR both for basal diameter and shoot length was significantly lower in shaded plots (Figure 6D). However, early growth models for basal diameter and shoot length could explain less variance with a conditional R2 of 0.37 and 0.34 and a marginal R2 of 0.09 and 0.14, respectively (Supplementary Table S6).
3.3.3 Infection
At the end of the second year, 30 out of the living 450 seedlings showed symptoms of ash dieback. During the early growth phase, infection was not affected by any parameters included in the mixed models (Table 2). Even though conditional R2 of 0.61 was higher compared to first year infection models, marginal R2 and thus the variance explained by fixed effects only was much lower with 0.07 (Supplementary Table S6).
3.3.4 Functional traits (SLA and LDMC)
Specific leaf area (SLA) of the ash seedlings varied between 20.1 and 158.8 m2 kg−1 with a mean of 55.5 m2 kg−1 while leaf dry matter content (LDMC) ranged from 59.9 to 325.9 mg g−1 with a mean of 186.6 mg g−1. Both functional leaf traits were significantly affected by the shading treatment and transplantation direction (Table 2). Seedlings growing under lower light conditions showed higher SLA and lower LDMC. Even though transplantation direction was identified as an influencing factor for both leaf traits in the mixed models, differences between the single levels of transplantation direction were only significant for the SLA model including light incidence (Figure 6C; Supplementary Table S7). At the 10% significance margin, seedlings that had been transplanted from moderately to highly damaged stands (M2H) also differed in their SLA from seedlings transplanted from highly to moderately (H2M) damaged stands when including shading treatment as a measure of light conditions in the model (Supplementary Table S7). Leaf trait models have a conditional R2 of 0.63 and 0.67 and a marginal R2 of 0.36 and 0.24 for SLA and LMDC, respectively (Supplementary Table S6).
4 Discussion
4.1 Habitat quality and ash seedling performance
Habitat quality, most notably determined by soil humidity, soil acidity, nutrient availability and light availability, is a main driver for seedling survival and establishment (Messaoud and Houle, 2006; Major et al., 2013; Muffler et al., 2021). Small-scale spatial heterogeneity of the abiotic environment can determine survival and successful establishment of tree seedlings (Mejía-Domínguez et al., 2012; Magee et al., 2021). However, effects of soil properties recorded in our study were not found to be significant either during the establishment phase or during the early growth phase. At the same time, there is huge variation in the data, which is mirrored by high explanatory values of the random structure of our models and which is largely due to the variation of sites and plots therein. In Northern Germany, ash-rich forest sites are typically situated in very small-scale transitional areas between fresh beech dominated habitats and wet alder forests (Haupt et al., 2022). For the present study, we explicitly constrained the range of the factor soil moisture, which is considered most influential to differences in ash dieback severity (Marçais et al., 2016; Timmermann et al., 2017; Chumanová et al., 2019; Erfmeier et al., 2019; Klesse et al., 2021), by selecting sites of similar soil moisture. While field capacity, as a proxy for soil moisture, was indeed not correlated with ash dieback severity in the experimental stands, moderately and highly affected stands could clearly be separated by differences in their top soil chemistry. In fact, there is evidence that abiotic site conditions, such as soil pH, significantly affect ash dieback severity and ash mortality (Havrdová et al., 2017; Turczański et al., 2020, 2021). However, although we included soil properties with careful consideration in all final models, no significant effects on ash seedling establishment and growth were found. Since we restricted site selection to only a limited range of habitat diversity, correlation between transplantation direction and upper soil pH could mainly be attributed to extreme values of single sites or even plots rather than strong overall differences between the two damage classes (Supplementary Figure S1A).
While we did not identify edaphic variables to contribute to explaining ash seedling performance, in contrast, light conditions significantly affected almost all plant properties in both establishment and early growth phase. This is very much in accordance with observational studies that attribute a strong impact of light availability on ash regeneration displaying high correlation of ash juvenile density and performance with light availability as approximated by tree layer cover (Tabari et al., 1998; Petriţan et al., 2009; Turczański et al., 2022). Compared to soil conditions, light is altered more severely and rapidly by ash dieback. Continuous dieback of ash crowns as well as loss of mature ash trees quickly opens up the forest canopy. While damage classes did not differ in their overall light conditions, most highly damaged sites showed more heterogeneous light conditions compared to less damaged sites, which reflects increasing canopy opening due to ash dieback in our study sites. In Schleswig-Holstein, F. excelsior is often admixed as a co-dominant species in low to medium proportions. In consequence, even in ash-rich forest stands, crown dieback or loss of dominant ash trees mostly results in multiple small rather than large canopy gaps, which contribute to an overall increase in heterogeneity of the light conditions. Expanding crowns of neighboring trees, which in many cases intercept more light compared to ash, will then often close these small gaps and create darker conditions, to which juvenile ash have to respond.
Accordingly, the shading treatment as well as relative light incidence significantly reduced basal stem growth during the establishment phase, whereas shoot growth did not show such an effect. In the early growth phase, however, the positive effect of light on seedling performance was then even more pronounced for shoot growth compared to basal growth. This is in line with current knowledge that ash will typically invest heavily in its root system, thus increasing its basal stem diameter, during establishment to support an increased shoot growth in the following years (Kerr and Cahalan, 2004; Eisen, 2007). In seedlings, basal growth generally correlates with growth of the root system, which is one of the main factors influencing early seedling establishment since root expansion allows the seedling early provisioning of resources such as nutrients and water and reduces susceptibility toward drought and other stresses (Burdett, 1990; Grossnickle, 2012). Accordingly, survival in the establishment phase was affected by seedling size at the time of planting. Larger seedlings would likely have a more pronounced root system, which in turn affects physiological attributes, such as drought stress resistance and nutritional status of the plant, giving them an advantage during establishment. In the same way, higher basal growth in unshaded plots translate directly to a better establishment, which is then conveyed into higher survival rates on these plots in the following year. However, low light conditions in many plots could explain the overall reduced growth performance of the ash seedlings during the early growth phase as well (see Annighöfer et al., 2017; Xu et al., 2023). This is a typical growth strategy of many light demanding tree species, initially exhibiting minimal growth under a closed canopy, which can be maintained for long periods of reduced light conditions, but that will rapidly turn into increased growth once light incidence rises due to gap formation in the canopy (Tabari et al., 1998; Petriţan et al., 2007, 2009). However, light conditions might also be too dark for ash seedling survival since especially under the shading nets some light measurements did not reach the light compensation point of ash seedlings (5 ± 3 μmol m−2 s−1) (Thomas, 2016) in one or both years. Darkening of ash-rich forest stands due to replacement of F. excelsior with more shade-producing species such as F. sylvatica could thus further endanger survival and establishment of ash natural regeneration.
A significant shading effect is also visible for infection. While decreasing light incidence under the forest canopy usually goes along with more sheltered and humid conditions (De Frenne et al., 2019; Zellweger et al., 2019; Leuschner et al., 2023), we would accordingly assume a higher infection on shaded than on open plots as the pathogen favors humid conditions (Chumanová et al., 2019; Marçais et al., 2023). It is well known that ash dieback is more severe in moist to waterlogged stands (Marçais et al., 2016; Pušpure et al., 2017; Erfmeier et al., 2019; Klesse et al., 2021) where higher humidity facilitates ideal conditions for fruiting and sporulation of H. fraxineus and subsequent infection of ash (Timmermann et al., 2011; Dvořák et al., 2016; Burns et al., 2022). Conversely, trees under more open conditions seem to be less affected by ash dieback (Havrdová et al., 2017; Grosdidier et al., 2020), leading to the assumption that seedlings on open, non-shaded plots should exhibit less ash dieback symptoms. This might also be in line with a ventilation and thinning effect: Since a lower infection rate at lower light conditions was not evident when including light incidence instead of shading treatment in our models, we also need to consider a caging effect of the shading nets. While ascospore distribution is generally wind-dependent, the active ascospore discharge mechanism of H. fraxineus (see Hietala et al., 2013) suggests that wind turbulence is not needed for near-ground transmission under the shading nets so it is more likely that differences in the amount of infectious material here indicate a caging effect. Even though we ensured presence of infectious material on all plots by removing the shading nets during winter, the majority of ash foliage would fall before net removal. Thus, it is likely that the shading nets acted as a mechanical barrier in two ways: by preventing the entry of ash leaves (i.e., the rachis that serves as the substrate for pathogen ascomata formation after overwintering) before net removal, and by preventing the deposition of pathogen ascospores formed by ascomata located outside the shaded areas. This would substantially decrease infection pressure on shaded plots and result in the observed lower infection probability.
4.2 Ash dieback damage as a driver of selection
In both, the establishment and the early growth phase, seedlings showed differences between transplantation treatments. During establishment, seedlings which originated from highly damaged stands and were transplanted into moderately damaged stands displayed a significantly lower infection probability compared to seedlings which were transplanted from moderately damaged stands into highly damaged ones. A similar effect was detected in SLA during the early growth phase with lower SLA in individuals transplanted from moderately into highly damaged stands compared to seedlings originating from highly damaged stands. While one might argue that these effects reflect overall differences in ash vitality due to contrasting habitat quality between moderately and highly damaged forest stands, all models accounted for effects due to environmental variation and assigned no significant effect to edaphic conditions tested. Transplantation treatment showed an opposing significant effect on survival during the early growth phase. However, post-hoc pairwise testing revealed only marginally significant differences between transplantation directions, which are most likely caused by unrecorded biotic or abiotic factors that were not addressed by our experimental setup, such as rodent damage (e.g., clipping the main shoot at the root collar or rooting out the seedling).
Differences in ash seedling performance and susceptibility between transplantation directions also display a signature of the transplants’ origin. This involves different intensities of pathogen-induced damage, which might correlate with disease history, i.e., how long the pathogen H. fraxineus has been present in the respective forest stands. In fact, there is increasing evidence of ongoing natural selection processes in ash-rich forest stands, thereby conferring wild grown offspring some advantage in withstanding subjection to pathogen pressure or an infection. Multiple studies showed that natural ash regeneration is in general not as severely affected by ash dieback compared to planted trials (Lygis et al., 2014; Enderle et al., 2015; Dietrich, 2016; Turczański et al., 2021; Osewold and Nagel, 2023). In a Danish forest, Semizer-Cuming et al. (2021) were able to show that seedlings originating from symptom free parent trees were overrepresented in the natural regeneration compared to seedlings from highly susceptible parents. Varying degrees of selection pressure in highly and moderately damaged stands could indeed partially explain the significant differences in infection probabilities between transplantation directions we found during the establishment phase. On the one hand, seedlings from moderately damaged stands, which have not been subjected to a strong selection pressure, are more often infected when subjected to the strong selection pressure on highly damaged sites. On the other hand, seedlings originating from highly damaged stands might already have undergone a filtering process and are more likely a less susceptible genotype. While the existing literature most often ascribes patterns of adaptive divergence to abiotic drivers (Leimu and Fischer, 2008; Solarik et al., 2018), local adaptation in tree populations might also be due to biotic factors (Manzanedo et al., 2018)—such as pathogens.
An additive resistance in some ash trees, which is strongly under genetic control, was confirmed in planted trials with a well-known genetic background (Sollars et al., 2017; Stocks et al., 2019) and several studies revealed moderate to high heritability of susceptibility traits in ash (Pliūra et al., 2011; Kjær et al., 2012; Lobo et al., 2015; Muñoz et al., 2016; Stener, 2018). Equally, there is evidence that mechanisms behind the variation in susceptibility in ash trees are more complex. Even in clonal trials, susceptibility to ash dieback varied between genetically identical individuals (McKinney et al., 2012; de Vries and Kopinga, 2017) and a study focusing on natural ash regeneration was not able to find a correlation between ash dieback severity in parent trees and their offspring (Wohlmuth et al., 2018). Under natural, more heterogonous conditions, phenotypic plasticity in phenological responses might play a more important role in ash susceptibility. In fact, we found significant variation in leaf traits, which reflects a portion of the phenotypic variation in a tree: in the early growth phase, SLA differed between transplantation directions similar to the transplantation effect in infection found during the establishment phase. On the one hand, seedlings originating from moderately damaged stands developed low SLA leaves when transplanted into highly damaged stands, which is a typical reaction to high stress conditions (Poorter et al., 2009). On the other hand, seedlings from highly damaged stands transplanted into moderately damaged stands formed low-cost and short-lived leaves, presented as a higher SLA and conforms to the leaf economic spectrum balancing leaf construction costs against growth potential (Díaz et al., 2016). The formation of tender and short-lived leaves is typical for well supplied sites (Poorter et al., 2009), but could also reflect a defense mechanism to ash dieback. There is evidence that differences in leaf traits can affect pathogen resistance in trees (González-Teuber et al., 2021; Wang et al., 2022). In regard to ash dieback, multiple studies have explored possible mechanisms of plant-pathogen interaction in ash leaves (Agan et al., 2020; Griffiths et al., 2020; Ulrich et al., 2020; Nielsen et al., 2022). Apart from differing SLA between transplantation directions, we noticed much lower infection rates in winter, when only the shoot was available for assessment, compared to summer, when the assessment was based on the whole plant, including leaves. During establishment, continuous monitoring showed that infection peaked at almost 27% in August and dropped to under 3% at the end of the year (Supplementary Table S3). This discrepancy cannot solely be attributed to seedling mortality but rather shows that infection must have mostly been located on leaves and not the shoot during summer. It is common that symptom appearance in the shoot is delayed from infection and arises during late winter or spring (Gross et al., 2014). Thus, shoot symptoms can also be interpreted as signs of infection in the previous year. However, during the only assessment at the end of the following year, only 7% of the living seedlings showed necrotic tissue. While our data does not allow us to distinguish between leaf and shoot necrosis, these discrepancies heavily imply that leaf infection did not necessarily lead to shoot colonization by the ash pathogen in a lot of infected individuals. This is in accordance with other observations that leaf infection does not always translate into shoot necrosis (de Vries and Kopinga, 2017) and underlines the fact that leaf traits and the phenological response of a tree might play a vital role in ash dieback susceptibility. Multiple studies observed a lower susceptibility of ash trees that exhibit early leaf shedding (McKinney et al., 2011, 2012) or early spring flushing (Cleary et al., 2017; Nielsen et al., 2017; Pliūra et al., 2017; Rozsypálek et al., 2017). Fussi and Konnert (2014) even found a higher proportion of heterozygotic ash trees, which generally have a higher plasticity, in the group with lower susceptibility to ash dieback. This might also translate onto the evolutionary selection processes through maternal effects, which are strongly expressed in the offspring’s phenotype and fitness. Maternal effects can be triggered when the mother tree is subjected to biotic stress and can result in an increased resistance or tolerance in offspring subjected to the same biotic stress (Vivas et al., 2020). Thus, in addition to the estimated 1% ash trees considered resistant (see, e.g., Enderle et al., 2019), maternal effects and plastic responses might facilitate survival of ash natural regeneration in the early stages of establishment and growth.
Nonetheless, there is a lot of evidence that ash dieback severity is affected by a multitude of variable and interacting factors (e.g., Marçais et al., 2016; Havrdová et al., 2017; Pušpure et al., 2017; Turczański et al., 2020) such as stand characteristics (Marçais et al., 2017; Grosdidier et al., 2020; Madsen et al., 2021) or time of disease presence (Díaz-Yáñez et al., 2020; George et al., 2022), which might contribute to differences in ash dieback damage severity between experimental sites and thus affect the transplanted seedlings. However, most studies connecting site and stand characteristics to ash dieback severity were conducted on mature ash trees and ignored effects on seedlings and saplings and thereby on the regeneration. Dietrich (2016) has already reported that ash regeneration smaller than 1.3 m in height was generally less affected by ash dieback. Disease severity in this height class was not related to canopy cover while canopy cover significantly affected seedlings larger than 1.3 m. The author theorized that even though spore concentration of H. fraxineus is significantly higher at near-ground levels (Chandelier et al., 2014; Dvořák et al., 2023), small seedlings might simply escape infection more easily. Lower infection probabilities in stands only moderately affected by ash dieback would thus point toward a lower infection pressure and consequentially a higher proportion of seedlings that escape infection in these stands. However, ash dieback severity and infection pressure are usually not directly positively correlated since disease progression and not infection itself is hampered in less susceptible individuals. Marçais et al. (2023) recently confirmed that ash trees exhibiting no or only slight ash dieback symptoms still function as carriers of H. fraxineus. Hence, a less susceptible tree with only a little crown defoliation can still produce large amounts of infectious material compared to a highly affected ash tree with only a little foliage left (Grosdidier et al., 2020; Marçais et al., 2023) leading to a possibly higher infection pressure in less damaged stands. This relation between inoculum level and disease severity can thus be complex and measuring the amount of infected rachis in the litter could help interpreting data in future studies. In addition, one has to take into consideration that any selection pressure due to pathogen load might differ across season and sites (Hietala et al., 2013; Grosdidier et al., 2018; Burns et al., 2022). While disease escape can explain the overall low proportion of infected seedlings in our study, it cannot solely explain differences in infection probability between the transplantation directions. A longer experimental period would allow seedlings to grow taller, which might go along with an increase in the prevalence of ash dieback symptoms and allow for a clearer detection of factors influencing ash dieback severity.
5 Conclusion
Fully reciprocal transplant experiments are often considered as the gold standard in testing for local adaption to climatic, abiotic as well as biotic site conditions (Kawecki and Ebert, 2004; Brady et al., 2019; Johnson et al., 2022). Capturing processes under multivariate environmental conditions and complex interactions of an ecosystem is one major advantage of this approach, compared to, e.g., common garden transplant setups. At the same time, the limited possibility to control for environmental factors is a considerable difficulty. Both are especially evident in the case of ash dieback in the experimental region. While highly damaged sites were more differentiated in their abiotic site conditions compared to only moderately damaged ash stands, the influence of soil conditions on the survival, growth and infection of ash natural regeneration was only marginal. In contrast, ash dieback related transformations of forest sites as expressed through changes in light conditions due to rapid crown defoliation or loss of ash trees, affected the performance of the transplanted ash natural regeneration. Together with the hint of a filtering effect by the ash dieback history of a forest stand already in the first year after planting, this might indicate evolution in response to ash dieback. Further quantifying selection effects at the ecosystem level would require to control for the amount of effective infection pressure on the stands, involving annual recording of leaf and shoot symptoms and consideration of the habitat conditions over a longer experimental period. Ecological and evolutionary processes are likely to change during dynamic phases of regeneration in the presence of new agents (Dietz and Edwards, 2006). At this point in time, however, studying ash regeneration is just in the middle of a transition from ecologically driven to evolutionary shaped differentiation in seedling responses.
Data availability statement
The raw data supporting the conclusions of this article will be made available by the authors upon reasonable request.
Author contributions
KH: Data curation, Formal analysis, Investigation, Writing – original draft, Visualization. KM: Investigation, Methodology, Writing – review & editing. JL: Investigation, Writing – review & editing. PM: Investigation, Writing – review & editing. MS: Investigation, Writing – review & editing. JS: Funding acquisition, Writing – review & editing. AE: Conceptualization, Funding acquisition, Methodology, Project administration, Supervision, Writing – review & editing.
Funding
The author(s) declare that financial support was received for the research, authorship, and/or publication of this article. This study was conducted within the FraDiv project. The FraDiv project (German title “Bedeutung des Eschentriebsterbens für die Biodiversität von Wäldern und Strategien zu ihrer Erhaltung”) is funded within the Federal Program for Biodiversity with means from the Federal Ministry for the Environment, Nature Conservation, Nuclear Safety and Consumer Protection (Funding No. 3518685010) and by the Ministry for Energy Transition, Climate Protection, Environment and Nature of Schleswig-Holstein. We acknowledge financial support by Land Schleswig-Holstein within the funding program Open Access Publication Fund.
Acknowledgments
We are grateful to the Schleswig-Holstein State Forest who provided access to their forests and supported the establishment of all sites. The authors specially thank V. Zajonc and all student helpers for their help during fieldwork. We also thank the Environmental Lab at Kiel University, in particular I. Meyer and M. Beckers, for their support in the soil analysis.
Conflict of interest
The authors declare that the research was conducted in the absence of any commercial or financial relationships that could be construed as a potential conflict of interest.
Publisher’s note
All claims expressed in this article are solely those of the authors and do not necessarily represent those of their affiliated organizations, or those of the publisher, the editors and the reviewers. Any product that may be evaluated in this article, or claim that may be made by its manufacturer, is not guaranteed or endorsed by the publisher.
Supplementary material
The Supplementary material for this article can be found online at: https://www.frontiersin.org/articles/10.3389/ffgc.2024.1355098/full#supplementary-material
References
Agan, A., Drenkhan, R., Adamson, K., Tedersoo, L., Solheim, H., Børja, I., et al. (2020). The relationship between fungal diversity and invasibility of a foliar niche: the case of ash dieback. J. Fungi 6:150. doi: 10.3390/jof6030150
Ahrens, C. W., Mazanec, R. A., Paap, T., Ruthrof, K. X., Challis, A., Hardy, G., et al. (2019). Adaptive variation for growth and resistance to a novel pathogen along climatic gradients in a foundation tree. Evol. Appl. 12, 1178–1190. doi: 10.1111/eva.12796
Alberto, F. J., Aitken, S. N., Alía, R., González-Martínez, S. C., Hänninen, H., Kremer, A., et al. (2013). Potential for evolutionary responses to climate change: evidence from tree populations. Glob. Chang. Biol. 19, 1645–1661. doi: 10.1111/gcb.12181
Anagnostakis, S. L. (2001). American chestnut sprout survival with biological control of the chestnut-blight fungus population. For. Ecol. Manag. 152, 225–233. doi: 10.1016/S0378-1127(00)00605-8
Annighöfer, P., Petritan, A. M., Petritan, I. C., and Ammer, C. (2017). Disentangling juvenile growth strategies of three shade-tolerant temperate forest tree species responding to a light gradient. For. Ecol. Manag. 391, 115–126. doi: 10.1016/j.foreco.2017.01.010
Bates, D., Mächler, M., Bolker, B. M., and Walker, S. (2015). Fitting linear mixed-effects models using lme4. J. Stat. Softw. 67, 1–48. doi: 10.18637/jss.v067.i01
Becker, R., Ulrich, K., Behrendt, U., Kube, M., and Ulrich, A. (2020). Analyzing ash leaf-colonizing fungal communities for their biological control of Hymenoscyphus fraxineus. Front. Microbiol. 11:590944. doi: 10.3389/fmicb.2020.590944
Boden, AG. (2005). Bodenkundliche Kartieranleitung. 5th Edn. Hannover: Bundesanstalt für Geowissenschaften und Rohstoffe.
Brady, S. P., Bolnick, D. I., Barrett, R. D. H., Chapman, L., Crispo, E., Derry, A. M., et al. (2019). Understanding maladaptation by uniting ecological and evolutionary perspectives. Am. Nat. 194, 495–515. doi: 10.1086/705020
Brasier, C. M. (2001). Rapid evolution of introduced plant pathogens via interspecific hybridization. Bioscience 51:123. doi: 10.1641/0006-3568(2001)051[0123:REOIPP]2.0.CO;2
Brooks, M. E., Kristensen, K., van Benthem, K. J., Magnusson, A., Berg, C. W., Nielsen, A., et al. (2017). glmmTMB balances speed and flexibility among packages for zero-inflated generalized linear mixed modeling. R J. 9, 378–400. doi: 10.32614/RJ-2017-066
Budde, K. B., Nielsen, L. R., Ravn, H. P., and Kjær, E. D. (2016). The natural evolutionary potential of tree populations to cope with newly introduced pests and pathogens: lessons learned from forest health catastrophes in recent decades. Curr. Forestry. Rep. 2, 18–29. doi: 10.1007/s40725-016-0029-9
Burdett, A. N. (1990). Physiological processes in plantation establishment and the development of specifications for forest planting stock. Can. J. For. Res. 20, 415–427. doi: 10.1139/x90-059
Burdon, J. J., and Thrall, P. H. (2009). Coevolution of plants and their pathogens in natural habitats. Science 324, 755–756. doi: 10.1126/science.1171663
Burns, P., Timmermann, V., and Yearsley, J. M. (2022). Meteorological factors associated with the timing and abundance of Hymenoscyphus fraxineus spore release. Int. J. Biometeorol. 66, 493–506. doi: 10.1007/s00484-021-02211-z
Chandelier, A., Helson, M., Dvorak, M., and Gischer, F. (2014). Detection and quantification of airborne inoculum of Hymenoscyphus pseudoalbidus using real-time PCR assays. Plant Pathol. 63, 1296–1305. doi: 10.1111/ppa.12218
Chumanová, E., Romportl, D., Havrdová, L., Zahradník, D., Pešková, V., and Černý, K. (2019). Predicting ash dieback severity and environmental suitability for the disease in forest stands. Scand. J. For. Res. 34, 254–266. doi: 10.1080/02827581.2019.1584638
Cleary, M., Nguyen, D., Stener, L. G., Stenlid, J., and Skovsgaard, J. P. (2017). “Ash and ash dieback in Sweden: a review of disease history, current status, pathogen and host dynamics, host tolerance and management options in forests and landscapes” in Dieback of European ash (Fraxinus spp.). (eds.) R. Vasaitis and R Enderle, 195–208.
Coker, T. L. R., Rozsypálek, J., Edwards, A., Harwood, T. P., Butfoy, L., and Buggs, R. J. A. (2018). Estimating mortality rates of European ash (Fraxinus excelsior) under the ash dieback (Hymenoscyphus fraxineus) epidemic. Plants People Planet 1, 48–58. doi: 10.1002/ppp3.11
Cracknell, D. J., Peterken, G. F., Pommerening, A., Lawrence, P. J., and Healey, J. R. (2023). Neighbours matter and the weak succumb: ash dieback infection is more severe in ash trees with fewer conspecific neighbours and lower prior growth rate. J. Ecol. 111, 2118–2133. doi: 10.1111/1365-2745.14191
Dale, V. H., Joyce, L. A., McNulty, S., Neilson, R. P., Ayres, M. P., Flannigan, M. D., et al. (2001). Climate change and forest disturbances. Bioscience 51:723. doi: 10.1641/0006-3568(2001)051[0723:CCAFD]2.0.CO;2
Davis, M. B., Shaw, R. G., and Etterson, J. R. (2005). Evolutionary responses to changing climate. Ecology 86, 1704–1714. doi: 10.1890/03-0788
De Frenne, P., Zellweger, F., Rodríguez-Sánchez, F., Scheffers, B. R., Hylander, K., Luoto, M., et al. (2019). Global buffering of temperatures under forest canopies. Nat. Ecol. Evol. 3, 744–749. doi: 10.1038/s41559-019-0842-1
de Vries, S. M.G., and Kopinga, J. (2017). Differences in susceptibility to Hymenoscyphus fraxineus (dieback of ash) of selections of common ash (Fraxinus excelsior) in the Netherlands: report of the observations and results of 2012 and 2015 in dieback of European ash (Fraxinus spp.). eds. Vasaitis, R. and Enderle, R., 238–248.
Desprez-Loustau, M.-L., Robin, C., Buée, M., Courtecuisse, R., Garbaye, J., Suffert, F., et al. (2007). The fungal dimension of biological invasions. Trends Ecol. Evol. 22, 472–480. doi: 10.1016/j.tree.2007.04.005
Deutscher Wetterdienst (DWD) (2023). Time series and trends. Available at: https://www.dwd.de/EN/ourservices/zeitreihen/zeitreihen.html (Accessed September 11, 2023).
Díaz, S., Kattge, J., Cornelissen, J. H. C., Wright, I. J., Lavorel, S., Dray, S., et al. (2016). The global spectrum of plant form and function. Nature 529, 167–171. doi: 10.1038/nature16489
Díaz-Yáñez, O., Mola-Yudego, B., Timmermann, V., Tollefsrud, M. M., Hietala, A. M., and Oliva, J. (2020). The invasive forest pathogen Hymenoscyphus fraxineus boosts mortality and triggers niche replacement of European ash (Fraxinus excelsior). Sci. Rep. 10:5310. doi: 10.1038/s41598-020-61990-4
Dickie, I. A., Bufford, J. L., Cobb, R. C., Desprez-Loustau, M.-L., Grelet, G., Hulme, P. E., et al. (2017). The emerging science of linked plant-fungal invasions. New Phytol. 215, 1314–1332. doi: 10.1111/nph.14657
Dietrich, M. (2016). The impact of ash dieback on ash regeneration in the forest reserve Dalby Söderskog. Master's thesis. Swedish University of Agricultural Sciences, Alnarp.
Dietz, H., and Edwards, P. J. (2006). Recognition that causal processes change during plant invasion helps explain conflicts in evidence. Ecology 87, 1359–1367. doi: 10.1890/0012-9658(2006)87[1359:RTCPCD]2.0.CO;2
Dvořák, M., Rotková, G., and Botella, L. (2016). Detection of airborne inoculum of Hymenoscyphus fraxineus and H. albidus during seasonal fluctuations associated with absence of apothecia. Forests 7:1. doi: 10.3390/f7010001
Dvořák, M., Štoidl, P., and Rost, M. (2023). Vertical spread of Hymenoscyphus fraxineus propagules. NeoBiota 84, 231–246. doi: 10.3897/neobiota.84.90981
Eisen, A.-K. (2007). Growth and photosynthesis of ash Fraxinus excelsior and beech Fagus sylvatica seedlings in response to a light gradient following natural gap formation. Ecol. Bull. 52, 147–165.
Enderle, R., Fussi, B., Lenz, H. D., Langer, G., Nagel, R., and Metzler, B. (2017). “Ash dieback in Germany: research on disease development, resistance and management options” in Dieback of European Ash (Fraxinus spp.). eds. R. Vasaitis and R. Enderle, 89–105.
Enderle, R., Kändler, G., and Metzler, B. (2015). Eschentriebsterben in Waldzustandsbericht 2015 für Baden-Württemberg, ed. Forstliche Versuchs-und Forschungsanstalt Baden-Württemberg (FVA). (Freiburg), 46–54.
Enderle, R., Metzler, B., Riemer, U., and Kändler, G. (2018). Ash dieback on sample points of the National Forest Inventory in South-Western Germany. Forests 9:25. doi: 10.3390/f9010025
Enderle, R., Stenlid, J., and Vasaitis, R. (2019). An overview of ash (Fraxinus spp.) and the ash dieback disease in Europe. CAB Rev., 1–12. doi: 10.1079/PAVSNNR201914025
Erfmeier, A., Haldan, K. L., Beckmann, L.-M., Behrens, M., Rotert, J., and Schrautzer, J. (2019). Ash dieback and its impact in near-natural forest remnants: a plant community-based inventory. Front. Plant Sci. 10:658. doi: 10.3389/fpls.2019.00658
Estreguil, C., Caudullo, G., de Rigo, D., and Miguel, J.S. (2012). Forest landscape in Europe: pattern, fragmentation and connectivity. Luxembourg.
Fischer, R., Taubert, F., Müller, M. S., Groeneveld, J., Lehmann, S., Wiegand, T., et al. (2021). Accelerated forest fragmentation leads to critical increase in tropical forest edge area. Sci. Adv. 7:eabg7012. doi: 10.1126/sciadv.abg7012
Fussi, B., and Konnert, M. (2014). Genetic analysis of European common ash (Fraxinus excelsior L.) populations affected by ash dieback. Silvae Genet. 63:158. doi: 10.1515/sg-2014-0026
George, J.-P., Sanders, T. G. M., Timmermann, V., Potočić, N., and Lang, M. (2022). European-wide forest monitoring substantiate the neccessity for a joint conservation strategy to rescue European ash species (Fraxinus spp.). Sci. Rep. 12:4764. doi: 10.1038/s41598-022-08825-6
González-Teuber, M., Palma-Onetto, V., Aguilera-Sammaritano, J., and Mithöfer, A. (2021). Roles of leaf functional traits in fungal endophyte colonization: potential implications for host–pathogen interactions. J. Ecol. 109, 3972–3987. doi: 10.1111/1365-2745.13678
Griffiths, S. M., Galambao, M., Rowntree, J., Goodhead, I., Hall, J., O’Brien, D., et al. (2020). Complex associations between cross-kingdom microbial endophytes and host genotype in ash dieback disease dynamics. J. Ecol. 108, 291–309. doi: 10.1111/1365-2745.13302
Grosdidier, M., Ioos, R., Husson, C., Cael, O., Scordia, T., and Marçais, B. (2018). Tracking the invasion: dispersal of Hymenoscyphus fraxineus airborne inoculum at different scales. FEMS Microbiol. Ecol. 94:fiy049. doi: 10.1093/femsec/fiy049
Grosdidier, M., Scordia, T., Ioos, R., Marçais, B., and Thrall, P. (2020). Landscape epidemiology of ash dieback. J. Ecol. 108, 1789–1799. doi: 10.1111/1365-2745.13383
Gross, A., Holdenrieder, O., Pautasso, M., Queloz, V., and Sieber, T. N. (2014). Hymenoscyphus pseudoalbidus, the causal agent of European ash dieback. Mol. Plant Pathol. 15, 5–21. doi: 10.1111/mpp.12073
Grossnickle, S. C. (2012). Why seedlings survive: influence of plant attributes. New For. 43, 711–738. doi: 10.1007/s11056-012-9336-6
Haney, J. C., Lee, D. S., and Wilbert, M. (2001). A half-century comparison of breeding birds in the southern Appalachians. Condor 103, 268–277. doi: 10.1093/condor/103.2.268
Härdtle, W. (1995). Vegetation und Standort der Laubwaldgesellschaften (Querco-Fagetea) im nördlichen Schleswig-Holstein. Kiel: Arbeitsgemeinschaft Geobotanik in Schleswig-Holstein und Hamburg.
Härdtle, W., Ewald, J., and Hölzel, N. (eds.). (2008). Wälder des Tieflandes und der Mittelgebirge—41 Tabellen. Ökosysteme Mitteleuropas aus geobotanischer Sicht. Stuttgart: Ulmer
Hartig, F. (2022). DHARMa—residual diagnostics for hierarchical (multi-level/mixed) regression. R package version 0.4.6. Available at: https://CRAN.R-project.org/package=DHARMa
Haupt, K. S., Mausolf, K., Richter, J., Schrautzer, J., and Erfmeier, A. (2022). The FraDiv experiment: biodiversity-ecosystem functioning research meets reforestation practice. Ecol. Indic. 144:109497. doi: 10.1016/j.ecolind.2022.109497
Havrdová, L., Zahradník, D., Romportl, D., Pešková, V., and Černý, K. (2017). Environmental and silvicultural characteristics influencing the extent of ash dieback in forest stands. Balt. For. 23, 168–182.
Heiniger, U., and Rigling, D. (1994). Biological control of chestnut blight in Europe. Annu. Rev. Phytopathol. 32, 581–599. doi: 10.1146/annurev.py.32.090194.003053
Hietala, A. M., Timmermann, V., Børja, I., and Solheim, H. (2013). The invasive ash dieback pathogen Hymenoscyphus pseudoalbidus exerts maximal infection pressure prior to the onset of host leaf senescence. Fungal Ecol. 6, 302–308. doi: 10.1016/j.funeco.2013.03.008
Hultberg, T., Sandström, J., Felton, A., Öhman, K., Rönnberg, J., Witzell, J., et al. (2020). Ash dieback risks an extinction cascade. Biol. Conserv. 244:108516. doi: 10.1016/j.biocon.2020.108516
Johnson, L. C., Galliart, M. B., Alsdurf, J. D., Maricle, B. R., Baer, S. G., Bello, N. M., et al. (2022). Reciprocal transplant gardens as gold standard to detect local adaptation in grassland species: new opportunities moving into the 21st century. J. Ecol. 110, 1054–1071. doi: 10.1111/1365-2745.13695
Jump, A. S., Hunt, J. M., Martínez-Izquierdo, J. A., and Peñuelas, J. (2006). Natural selection and climate change: temperature-linked spatial and temporal trends in gene frequency in Fagus sylvatica. Mol. Ecol. 15, 3469–3480. doi: 10.1111/j.1365-294X.2006.03027.x
Kawecki, T. J., and Ebert, D. (2004). Conceptual issues in local adaptation. Ecol. Lett. 7, 1225–1241. doi: 10.1111/j.1461-0248.2004.00684.x
Kerr, G., and Cahalan, C. (2004). A review of site factors affecting the early growth of ash (Fraxinus excelsior L.). For. Ecol. Manag. 188, 225–234. doi: 10.1016/j.foreco.2003.07.016
Kirisits, T., Matlakova, M., Mottinger-Kroupa, S., Cech, T. L., and Halmschlager, E. (2009). “The current situation of ash dieback caused by Chalara fraxinea in Austria” in Proceedings of the conference of IUFRO Working Party. Accessed February 7, 2002
Kjær, E. D., McKinney, L. V., Nielsen, L. R., Hansen, L. N., and Hansen, J. K. (2012). Adaptive potential of ash (Fraxinus excelsior) populations against the novel emerging pathogen Hymenoscyphus pseudoalbidus. Evol. Appl. 5, 219–228. doi: 10.1111/j.1752-4571.2011.00222.x
Klesse, S., Abegg, M., Hopf, S. E., Gossner, M. M., Rigling, A., and Queloz, V. (2021). Spread and severity of ash dieback in Switzerland: tree characteristics and landscape features explain varying mortality probability. Front. For. Glob. Change 4:120. doi: 10.3389/ffgc.2021.645920
Kosawang, C., Amby, D. B., Bussaban, B., McKinney, L. V., Xu, J., Kjær, E. D., et al. (2018). Fungal communities associated with species of Fraxinus tolerant to ash dieback, and their potential for biological control. Fungal Biol. 122, 110–120. doi: 10.1016/j.funbio.2017.11.002
Kowalski, T. (2006). Chalara fraxinea sp. nov. associated with dieback of ash (Fraxinus excelsior) in Poland. For. Path. 36, 264–270. doi: 10.1111/j.1439-0329.2006.00453.x
Leimu, R., and Fischer, M. (2008). A meta-analysis of local adaptation in plants. PLoS One 3:e4010. doi: 10.1371/journal.pone.0004010
Lenth, R. V. (2023). emmeans: estimated marginal means, aka least-squares means. R package version 1.8.8. Available at: https://CRAN.R-project.org/package=emmeans
Leuschner, C., Hohnwald, S., Petriţan, A. M., and Walentowski, H. (2023). Vertical temperature and air humidity gradients in beech and oak forests, and the forest interior climate created by beech. Flora 305:152317. doi: 10.1016/j.flora.2023.152317
Lobo, A., McKinney, L. V., Hansen, J. K., Kjær, E. D., and Nielsen, L. R. (2015). Genetic variation in dieback resistance in Fraxinus excelsior confirmed by progeny inoculation assay. For. Path. 45, 379–387. doi: 10.1111/efp.12179
Lortie, C. J., and Hierro, J. L. (2022). A synthesis of local adaptation to climate through reciprocal common gardens. J. Ecol. 110, 1015–1021. doi: 10.1111/1365-2745.13664
Lüdecke, D. (2018). Ggeffects: tidy data frames of marginal effects from regression models. J. Open Source Softw. 3:772. doi: 10.21105/joss.00772
Lüdecke, D., Ben-Shachar, M., Patil, I., Waggoner, P., and Makowski, D. (2021). Performance: an R package for assessment, comparison and testing of statistical models. J. Open Source Softw. 6:3139. doi: 10.21105/joss.03139
Lüdecke, D., Waggoner, P., and Makowski, D. (2019). Insight: a unified interface to access information from model objects in R. J. Open Source Softw. 4:1412. doi: 10.21105/joss.01412
Lygis, V., Bakys, R., Gustiene, A., Burokiene, D., Matelis, A., and Vasaitis, R. (2014). Forest self-regeneration following clear-felling of dieback-affected Fraxinus excelsior: focus on ash. Eur. J. Forest Res. 133, 501–510. doi: 10.1007/s10342-014-0780-z
Madsen, C. L., Kosawang, C., Thomsen, I. M., Hansen, L. N., Nielsen, L. R., and Kjær, E. D. (2021). Combined progress in symptoms caused by Hymenoscyphus fraxineus and Armillaria species, and corresponding mortality in young and old ash trees. For. Ecol. Manag. 491:119177. doi: 10.1016/j.foreco.2021.119177
Magee, L., Wolf, A., Howe, R., Schubbe, J., Hagenow, K., and Turner, B. (2021). Density dependence and habitat heterogeneity regulate seedling survival in a north American temperate forest. For. Ecol. Manag. 480:118722. doi: 10.1016/j.foreco.2020.118722
Major, K. C., Nosko, P., Kuehne, C., Campbell, D., and Bauhus, J. (2013). Regeneration dynamics of non-native northern red oak (Quercus rubra L.) populations as influenced by environmental factors: a case study in managed hardwood forests of southwestern Germany. For. Ecol. Manag. 291, 144–153. doi: 10.1016/j.foreco.2012.12.006
Manzanedo, R. D., Schanz, F. R., Fischer, M., and Allan, E. (2018). Fagus sylvatica seedlings show provenance differentiation rather than adaptation to soil in a transplant experiment. BMC Ecol. 18:42. doi: 10.1186/s12898-018-0197-5
Marçais, B., Giraudel, A., and Husson, C. (2023). Ability of the ash dieback pathogen to reproduce and to induce damage on its host are controlled by different environmental parameters. PLoS Pathog. 19:e1010558. doi: 10.1371/journal.ppat.1010558
Marçais, B., Husson, C., Caël, O., Dowkiw, A., Saintonge, F.-X., Delahaye, L., et al. (2017). Estimation of ash mortality induced by Hymenoscyphus fraxineus in France and Belgium. Balt. For. 23, 159–167.
Marçais, B., Husson, C., Godart, L., and Caël, O. (2016). Influence of site and stand factors on Hymenoscyphus fraxineus-induced basal lesions. Plant Pathol. 65, 1452–1461. doi: 10.1111/ppa.12542
Marigo, G., Peltier, J. P., Girel, J., and Pautou, G. (2000). Success in the demographic expansion of Fraxinus excelsior L. Trees 15, 1–13. doi: 10.1007/s004680000061
Martín, J. A., Fuentes-Utrilla, P., Gil, L., and Witzell, J. (2010). Ecological factors in Dutch elm disease complex in Europe: a review. Ecol. Bull. 53, 209–224.
McKinney, L. V., Nielsen, L. R., Hansen, J. K., and Kjær, E. D. (2011). Presence of natural genetic resistance in Fraxinus excelsior (Oleraceae) to Chalara fraxinea (Ascomycota): an emerging infectious disease. Heredity 106, 788–797. doi: 10.1038/hdy.2010.119
McKinney, L. V., Thomsen, I. M., Kjær, E. D., and Nielsen, L. R. (2012). Genetic resistance to Hymenoscyphus pseudoalbidus limits fungal growth and symptom occurrence in Fraxinus excelsior. For. Path. 42, 69–74. doi: 10.1111/j.1439-0329.2011.00725.x
Mejía-Domínguez, N. R., Meave, J. A., and Díaz-Ávalos, C. (2012). Spatial structure of the abiotic environment and its association with sapling community structure and dynamics in a cloud forest. Int. J. Biometeorol. 56, 305–318. doi: 10.1007/s00484-011-0434-5
Messaoud, Y., and Houle, G. (2006). Spatial patterns of tree seedling establishment and their relationship to environmental variables in a cold-temperate deciduous forest of eastern North America. Plant Ecol. 185, 319–331. doi: 10.1007/s11258-006-9106-7
Mitchell, R. J., Broome, A., Beaton, J. K., Bellamy, P. E., Ellis, C. J., Hester, A. J., et al. (2016). Challenges in assessing the ecological impacts of tree diseases and mitigation measures: the case of Hymenoscyphus fraxineus and Fraxinus excelsior. Balt. For. 23, 116–140.
Muffler, L., Schmeddes, J., Weigel, R., Barbeta, A., Beil, I., Bolte, A., et al. (2021). High plasticity in germination and establishment success in the dominant forest tree Fagus sylvatica across Europe. Glob. Ecol. Biogeogr. 30, 1583–1596. doi: 10.1111/geb.13320
Muñoz, F., Marçais, B., Dufour, J., and Dowkiw, A. (2016). Rising out of the ashes: additive genetic variation for crown and collar resistance to Hymenoscyphus fraxineus in Fraxinus excelsior. Phytopathology 106, 1535–1543. doi: 10.1094/PHYTO-11-15-0284-R
Myers, B. R., Walck, J. L., and Blum, K. E. (2004). Vegetation change in a former chestnut stand on the Cumberland plateau of Tennessee during an 80-year period (1921–2000). Castanea 69, 81–91. doi: 10.2179/0008-7475(2004)069<0081:VCIAFC>2.0.CO;2
Nielsen, L. R., McKinney, L. V., and Kjær, E. D. (2017). Host phenological stage potentially affects dieback severity after Hymenoscyphus fraxineus infection in Fraxinus excelsior seedlings. Balt. For. 23, 229–232.
Nielsen, L. R., Nagy, N. E., Piqueras, S., Kosawang, C., Thygesen, L. G., and Hietala, A. M. (2022). Host-pathogen interactions in leaf petioles of common ash and Manchurian ash infected with Hymenoscyphus fraxineus. Microorganisms 10:375. doi: 10.3390/microorganisms10020375
Oksanen, J., Simpson, G. L., Blanchet, F. G., Kindt, R., Legendre, P., Minchin, P. R., et al. (2022). vegan—community ecology package. R package version 2.6-4, Available at: https://CRAN.R-project.org/package=vegan
Osewold, J., and Nagel, R.-V. (2023). Ausprägung des Eschentriebsterbens in der Naturverjüngung in FraxForFuture- Erhalt der Gemeinen Esche (Fraxinus excelsior) als Wirtschaftsbaumart. Berichte Freiburger Forstliche Forschung 106. Forstliche Versuchs- und Forschungsanstalt Baden-Württemberg. Freiburg. 83–87.
Panzavolta, T., Bracalini, M., Benigno, A., and Moricca, S. (2021). Alien invasive pathogens and pests harming trees, forests, and plantations: pathways, global consequences and management. Forests 12:1364. doi: 10.3390/f12101364
Pautasso, M., Schlegel, M., and Holdenrieder, O. (2015). Forest health in a changing world. Microb. Ecol. 69, 826–842. doi: 10.1007/s00248-014-0545-8
Petriţan, A. M., von, L. B., and Petriţan, I. C. (2007). Effects of shade on growth and mortality of maple (Acer pseudoplatanus), ash (Fraxinus excelsior) and beech (Fagus sylvatica) saplings. Forestry 80, 397–412. doi: 10.1093/forestry/cpm030
Petriţan, A. M., von, L. B., and Petriţan, I. C. (2009). Influence of light availability on growth, leaf morphology and plant architecture of beech (Fagus sylvatica L.), maple (Acer pseudoplatanus L.) and ash (Fraxinus excelsior L.) saplings. Eur. J. Forest Res. 128, 61–74. doi: 10.1007/s10342-008-0239-1
Pliūra, A., Bakys, R., Suchockas, V., Marčiulynienė, D., Gustienė, A., Verbyla, V., et al. (2017). “Ash dieback in Lithuania: disease history, research on impact and genetic variation in disease resistance, tree breeding and options for forest management” in Dieback of European Ash (Fraxinus spp.). eds. R. Vasaitis and R. Enderle, 150–165.
Pliūra, A., Lygis, V., Suchockas, V., and Bartkevičius, E. (2011). Performance of twenty-four European Fraxinus excelsior populations in three Lithuanian progeny trials with a apecial emphasis on resistance to Chalara fraxinea. Balt. For. 17, 17–34.
Pliūra, A., Marčiulynienė, D., Bakys, R., and Suchockas, V. (2014). Dynamics of genetic resistance to Hymenoscyphus pseudoalbidus in juvenile Fraxinus excelsior clones. Balt. For. 20, 10–27.
Poorter, H., Niinemets, Ü., Poorter, L., Wright, I. J., and Villar, R. (2009). Causes and consequences of variation in leaf mass per area (LMA): a meta-analysis. New Phytol. 182, 565–588. doi: 10.1111/j.1469-8137.2009.02830.x
Pušpure, I., Matisons, R., Laiviņš, M., Gaitnieks, T., and Jansons, J. (2017). Natural regeneration of common ash in young stands in Latvia. Balt. For. 23, 209–217.
R Core Team (2023). R: A language and environment for statistical computing. R Foundation for Statistical Computing, Vienna, Austria. Available at: https://www.R-project.org/
Rozsypálek, J., Dvořák, M., Longauer, R., Botella, L., Prouza, M., Palovčíková, D., et al. (2017). “Ash and ash dieback in the Czech Republic” in Dieback of European Ash (Fraxinus spp.). eds. R. Vasaitis and R. Enderle, 79–88.
Santini, A., Ghelardini, L., de Pace, C., Desprez-Loustau, M. L., Capretti, P., Chandelier, A., et al. (2013). Biogeographical patterns and determinants of invasion by forest pathogens in Europe. New Phytol. 197, 238–250. doi: 10.1111/j.1469-8137.2012.04364.x
Savolainen, O., Pyhäjärvi, T., and Knürr, T. (2007). Gene flow and local adaptation in trees. Annu. Rev. Ecol. Evol. Syst. 38, 595–619. doi: 10.1146/annurev.ecolsys.38.091206.095646
Schumacher, J., Kehr, R., and Leonhard, S. (2010). Mycological and histological investigations of Fraxinus excelsior nursery saplings naturally infected by Chalara fraxinea. For. Path. 40, 419–429. doi: 10.1111/j.1439-0329.2009.00615.x
Seidl, R., Thom, D., Kautz, M., Martin-Benito, D., Peltoniemi, M., Vacchiano, G., et al. (2017). Forest disturbances under climate change. Nat. Clim. Chang. 7, 395–402. doi: 10.1038/NCLIMATE3303
Semizer-Cuming, D., Chybicki, I. J., Finkeldey, R., and Kjær, E. D. (2021). Gene flow and reproductive success in ash (Fraxinus excelsior L.) in the face of ash dieback: restoration and conservation. Ann. For. Sci. 78:157. doi: 10.1007/s13595-020-01025-0
Smith, D. S., Schweitzer, J. A., Turk, P., Bailey, J. K., Hart, S. C., Shuster, S. M., et al. (2012). Soil-mediated local adaptation alters seedling survival and performance. Plant Soil 352, 243–251. doi: 10.1007/s11104-011-0992-7
Smock, L. A., and MacGregor, C. M. (1988). Impact of the American chestnut blight on aquatic shredding macroinvertebrates. J. North Am. Benthol. Soc. 7, 212–221.
Solarik, K. A., Messier, C., Ouimet, R., Bergeron, Y., and Gravel, D. (2018). Local adaptation of trees at the range margins impacts range shifts in the face of climate change. Glob. Ecol. Biogeogr. 27, 1507–1519. doi: 10.1111/geb.12829
Sollars, E. S. A., Harper, A. L., Kelly, L. J., Sambles, C. M., Ramirez-Gonzalez, R. H., Swarbreck, D., et al. (2017). Genome sequence and genetic diversity of European ash trees. Nature 541, 212–216. doi: 10.1038/nature20786
Stener, L.-G. (2018). Genetic evaluation of damage caused by ash dieback with emphasis on selection stability over time. For. Ecol. Manag. 409, 584–592. doi: 10.1016/j.foreco.2017.11.049
Stocks, J. J., Metheringham, C. L., Plumb, W. J., Lee, S. J., Kelly, L. J., Nichols, R. A., et al. (2019). Genomic basis of European ash tree resistance to ash dieback fungus. Nat. Ecol. Evol. 3, 1686–1696. doi: 10.1038/s41559-019-1036-6
Tabari, M., Lust, M., and Neirynck, J. (1998). Effect of light and humus on survival and height growth of ash (Fraxinus excelsior L.) seedlings. Silva Gandav. 63, 36–49. doi: 10.21825/sg.v63i0.834
Thakur, M. P., van der Putten, W. H., Cobben, M. M. P., van Kleunen, M., and Geisen, S. (2019). Microbial invasions in terrestrial ecosystems. Nat. Rev. Microbiol. 17, 621–631. doi: 10.1038/s41579-019-0236-z
Thomas, P. A. (2016). Biological Flora of the British Isles: Fraxinus excelsior. J. Ecol. 104, 1158–1209. doi: 10.1111/1365-2745.12566
Thomas, P. A., Stone, D., and La Porta, N. (2018). Biological Flora of the British Isles: Ulmus glabra. J. Ecol. 106, 1724–1766. doi: 10.1111/1365-2745.12994
Timmermann, V., Børja, I., Hietala, A. M., Kirisits, T., and Solheim, H. (2011). Ash dieback: pathogen spread and diurnal patterns of ascospore dispersal, with special emphasis on Norway. EPPO Bull. 41, 14–20. doi: 10.1111/j.1365-2338.2010.02429.x
Timmermann, V., Nagy, N. E., Hietala, A. M., Børja, I., and Solheim, H. (2017). Progression of ash dieback in Norway related to tree age, disease history and regional aspects. Balt. For. 23, 150–158.
Turczański, K., Dyderski, M. K., and Andrzejewska, A. (2022). Drivers of ash (Fraxinus excelsior L.) natural regeneration spread into suboptimal sites: refugee or dead end? For. Ecol. Manag. 505:119870. doi: 10.1016/j.foreco.2021.119870
Turczański, K., Dyderski, M. K., and Rutkowski, P. (2021). Ash dieback, soil and deer browsing influence natural regeneration of European ash (Fraxinus excelsior L.). Sci. Total Environ. 752:141787. doi: 10.1016/j.scitotenv.2020.141787
Turczański, K., Rutkowski, P., Dyderski, M. K., Wrońska-Pilarek, D., and Nowiński, M. (2020). Soil pH and organic matter content affects European ash (Fraxinus excelsior L.) crown defoliation and its impact on understory vegetation. Forests 11:22. doi: 10.3390/f11010022
Ulrich, K., Becker, R., Behrendt, U., Kube, M., and Ulrich, A. (2020). A comparative analysis of ash leaf-colonizing bacterial communities identifies putative antagonists of Hymenoscyphus fraxineus. Front. Microbiol. 11:966. doi: 10.3389/fmicb.2020.00966
van Kleunen, M., Dawson, W., Essl, F., Pergl, J., Winter, M., Weber, E., et al. (2015). Global exchange and accumulation of non-native plants. Nature 525, 100–103. doi: 10.1038/nature14910
Velásquez, A. C., Castroverde, C. D. M., and He, S. Y. (2018). Plant-pathogen warfare under changing climate conditions. Curr. Biol. 28, R619–R634. doi: 10.1016/j.cub.2018.03.054
Venäläinen, A., Lehtonen, I., Laapas, M., Ruosteenoja, K., Tikkanen, O.-P., Viiri, H., et al. (2020). Climate change induces multiple risks to boreal forests and forestry in Finland: a literature review. Glob. Chang. Biol. 26, 4178–4196. doi: 10.1111/gcb.15183
Vitasse, Y., Hoch, G., Randin, C. F., Lenz, A., Kollas, C., Scheepens, J. F., et al. (2013). Elevational adaptation and plasticity in seedling phenology of temperate deciduous tree species. Oecologia 171, 663–678. doi: 10.1007/s00442-012-2580-9
Vivas, M., Wingfield, M. J., and Slippers, B. (2020). Maternal effects should be considered in the establishment of forestry plantations. For. Ecol. Manag. 460:117909. doi: 10.1016/j.foreco.2020.117909
Wallace, J. B., Webster, J. R., Eggert, S. L., Meyer, J. L., and Siler, E. R. (2001). Large woody debris in a headwater stream: long-term legacies of forest disturbance. Int. Rev. Hydrobiol. 86, 501–513. doi: 10.1002/1522-2632(200107)86:4/5<501::AID-IROH501>3.0.CO;2-8
Wang, B., Tian, C., and Liang, Y. (2022). Landscape and stand-scale factors drive the infestation of an endemic fungal pathogen: the role of leaf traits. For. Ecol. Manag. 514:120213. doi: 10.1016/j.foreco.2022.120213
Wohlmuth, A., Essl, F., and Heinze, B. (2018). Genetic analysis of inherited reduced susceptibility of Fraxinus excelsior L. seedlings in Austria to ash dieback. Forestry 91, 514–525. doi: 10.1093/forestry/cpy012
Woods, F. W., and Shanks, R. E. (1959). Natural replacement of chestnut by other species in the Great Smoky Mountains National Park. Ecology 40, 349–361. doi: 10.2307/1929751
Xu, C., de Frenne, P, Blondeel, H., Pauw, Kde, Landuyt, D., Lorer, E., et al. (2023). Light more than warming impacts understory tree seedling growth in a temperate deciduous forest. For. Ecol. Manag. 549::121496. doi: 10.1016/j.foreco.2023.121496
Zellweger, F., Coomes, D., Lenoir, J., Depauw, L., Maes, S. L., Wulf, M., et al. (2019). Seasonal drivers of understorey temperature buffering in temperate deciduous forests across Europe. Glob. Ecol. Biogeogr. 28, 1774–1786. doi: 10.1111/geb.12991
Keywords: ash dieback, Hymenoscyphus fraxineus, duration of pathogen exposure, silviculture, temperate forest ecosystems
Citation: Haupt KS, Mausolf K, Lassen J, Music P, Schippmann M, Schrautzer J and Erfmeier A (2024) Ash seedlings in a reciprocal transplant experiment—the extent of damage of mature forest stands affects ash offspring performance. Front. For. Glob. Change. 7:1355098. doi: 10.3389/ffgc.2024.1355098
Edited by:
Barry Alan Gardiner, Institut Européen De La Forêt Cultivée (IEFC), FranceReviewed by:
Ari Mikko Hietala, Norwegian Institute of Bioeconomy Research (NIBIO), NorwayBenoit Marçais, INRA Center Nancy-Lorraine, France
Copyright © 2024 Haupt, Mausolf, Lassen, Music, Schippmann, Schrautzer and Erfmeier. This is an open-access article distributed under the terms of the Creative Commons Attribution License (CC BY). The use, distribution or reproduction in other forums is permitted, provided the original author(s) and the copyright owner(s) are credited and that the original publication in this journal is cited, in accordance with accepted academic practice. No use, distribution or reproduction is permitted which does not comply with these terms.
*Correspondence: Katharina S. Haupt, a2hhdXB0QGVjb2xvZ3kudW5pLWtpZWwuZGU=