- 1Center for Biodiversity Conservation and Management, GB Pant National Institute of Himalayan Environment, Almora, Uttarakhand, India
- 2Department of Zoology and Environmental Science, Gurukula Kangri (Deemed to be University), Haridwar, Uttarakhand, India
- 3Department of Forestry and Natural Resources, Hemvati Nandan Bahuguna Garhwal University, Srinagar, Garhwal, India
Unprecedented seasonal forest fires pose a significant threat to the carbon stocks of diverse ecosystems, particularly in regions like Uttarakhand, west Himalaya. Understanding the impact of varying fire frequencies on different forest types is crucial for effective conservation and management strategies. This study aims to assess the loss of carbon stock in three distinct forest types—Sal, Pine, and Mixed across an elevation gradient in Uttarakhand, facing unprecedented seasonal forest fires. By investigating pre- and post-fire conditions, analyzing biomass dynamics, and mapping fire frequencies, the research aims to provide insights into the complex interplay of fire regimes and forest resilience. The investigation covers vegetation analysis, biomass assessment, and fire frequency mapping. Biomass and carbon stock calculations were carried out using a non-destructive sampling method. Fire frequency maps were generated using Landsat satellite imagery spanning a decade, integrating MODIS hotspot data for classification. The study reveals distinct patterns in biomass changes across Sal, Pine, and Mixed forests in response to varying fire frequencies. Sal forests exhibit resilience to low-intensity fires, while Pine forests show higher sensitivity. Carbon stock contributions of dominant species varied significantly, with Sal and Chir-Pine forests emerging as crucial contributors. High fire frequencies lead to substantial carbon stock reduction in all forest types. The findings emphasize the sensitivity of aboveground biomass to fire frequency, with significant carbon stock loss observed in higher fire frequency classes. The study underscores the importance of nuanced conservation strategies tailored to distinct forest types and species characteristics. This research provides valuable insights for policymakers, forest managers, and conservationists in formulating targeted conservation and management approaches.
Introduction
Forests provide a wide array of essential goods and services that sustain life (Bisht et al., 2018), encompassing fuel, timber, food (Joshi and Negi, 2011), bioproducts (Shmulsky and Jones, 2019), greenhouse gas regulation (Ribeiro-Kumara et al., 2020), air quality (Baró et al., 2014), water supply (Cassiano and Ferraz, 2022), carbon storage (Fahey et al., 2010; Rai et al., 2018), nutrient cycling (Sharma and Sharma, 2004), and genetic and species diversity (Connell and Orias, 1964; de Groot et al., 2002; Singh, 2002). The preservation of forests has become a prominent policy objective for environmental safeguarding (Koskela and Karppinen, 2021), and sustenance of their critical ecological and climatic contributions (Humpenoder et al., 2014; Capellesso et al., 2021; Maren and Sharma, 2021). Uttarakhand Himalaya, known for its rich floral and faunal diversity (Anthwal et al., 2010), geology, and ecology, also considered a repository of biological (Bargali et al., 2022a) and cultural diversity (Negi, 2010), warranting conservation and sustainable management for future generations (Kumar et al., 2015; Mathela et al., 2020).
However, according to the India State of Forest Report (FSI, 2021), the forest cover in Uttarakhand is only 45.44% (Kumar and Hole, 2021; Bargali et al., 2022a), significantly below then the recommended forest cover (66%) for hill states in India (National Forest Policy, 1952). The prevalence of forest fires in the most hill states is one of the contributing factors to forest degradation (Babu et al., 2016; Ranjan, 2018; Babu, 2019; Bargali et al., 2022). Fire, a natural and recurrent phenomenon (Vasconcelos et al., 2017), exerts both positive and negative effects on forests (Certini, 2005). Positive outcomes involve enhancing biodiversity and stimulating plant growth (Bhatt et al., 2019; He et al., 2019), while negative impacts encompass habitat destruction, alterations in soil chemistry, and reduction in forest biomass (Bargali et al., 2017; Bowd et al., 2021). Grasping the consequences of fires on forests is vital for their conservation and management (Naveh, 1994; Bowman and Balch, 2013), especially in regions where fires occur frequently and with intensity (Bargali et al., 2022a). In Uttarakhand Himalaya, fires are traditionally perceived as disruptive and harmful (Rawat et al., 2017), with their role in local forest ecosystems historically marginalized (Gururani, 1996; Niklasson et al., 2010; Bargali et al., 2020).
Studies of paleoecology and fire history indicate that human presence in the landscape has increased the frequency of fire events in the past (Bahuguna and Upadhay, 2002; Whitlock et al., 2010; Negi and Dhyani, 2012; Abrams and Nowacki, 2019). Fire is recognized as one of the Earth’s most powerful agents of ecological change (Allendorf and Hard, 2009; Stenseth and Dunlop, 2009), with far-reaching ecological implications (Harrison et al., 2010). Forest fires entail significant losses (Hansen et al., 2010), encompassing human lives (Pausas and Keeley, 2009) economic consequences (De Mendonça et al., 2004), depletion of forest resources (Sadowska et al., 2021), destruction of biogeocenosis (Zhukov, 1976), and severe impacts on soil (Verma and Jayakumar, 2015) leading to loss and erosion post-fire (Scott et al., 2009; Jhariya et al., 2012). Additionally, forest fires contribute to greenhouse gas emissions, changes in climate patterns (Kurniawan et al., 2022), and the loss of ecosystem values (Lecina-Diaz et al., 2021) and environmental services (Lee et al., 2015). Key impacts include reduced floral productivity (Flower and Gonzalez-Meler, 2015) changes in regeneration rates (Deb and Sundriyal, 2008; Sathya and Jayakumar, 2017; Boucher et al., 2020), and the loss of various endemic and endangered fauna species (Laurance and Useche, 2009; Yule, 2010; Sati and Bandooni, 2018; Ward et al., 2020).
In Uttarakhand Himalaya, forest fire intensities are anticipated to increase due to rising temperatures and global warming (Ahmad and Goparaju, 2018). Most forest fires in this region are of anthropogenic origin (Bhandari et al., 2012), stemming from both accidental and intentional sources (Semwal and Mehta, 1996; Chauhan et al., 2018; Fulé et al., 2021). Fire behavior, which involves the ignition of fuels (Burgan, 1984), flame development (Vahabi et al., 2015), and fire spread (Countryman, 1964), is influenced by the interplay of fuels (Bessie and Johnson, 1995) weather, and topography in forest fires (Agee, 1996). Additionally, the type of forest, calorific value of fuels, dead plant matter, and litter plays a significant role (Hakkila and Parikka, 2002; Cornelissen et al., 2017).
In recent decades, forest fires have become more frequent and widespread in the Himalayan state of Uttarakhand (Singh et al., 2016; Bargali et al., 2017; Ahmad and Goparaju, 2018; Fulé et al., 2021). These forests have historically experienced fires for thousands of years (Gadgil, 1992; Anthwal et al., 2010; Bargali et al., 2024), with fire being a significant influence on landscape patterns and species diversity (Delcourt and Delcourt, 1997; Bhandari et al., 2012; Bargali et al., 2022b). The prevalence of plant populations offers insight into species’ capacity to respond to disturbances, including forest fires (Rodríguez-Trejo, 2014; Miller et al., 2019). In Uttarakhand, the dominance of Chir-pine (Pinus roxburghii) covers approximately 28% of the forest area (Fulé et al., 2021), contributing to the acceleration of forest fires (Kumar et al., 2019; Ray et al., 2019). The altitudinal zone of Chir pine forests, between 1,000 and 1800 meters above sea level, is considered a fire-prone area in the study region (Negi, 2019). Anthropogenic activities, such as the collection of non-timber forest products and agricultural practices, have also played a role in forest fires in the study area (Bargali et al., 2020; Bhandari and Bijlwan, 2020; Saha et al., 2023).
Forests act as the key carbon pool (C) and store more carbon per unit area compared to other terrestrial ecosystems (Zhao et al., 2014). Forest cover is around 30% of the Earth’s total surface area, and contains 19% of the Earth’s overall biomass and carbon pool (Kindermann et al., 2008; FAO and UNEP, 2020); forests having greater carbon in biomass and soil than in any natural ecosystem and atmosphere (Pan et al., 2011; Zhao et al., 2014; Jiang et al., 2022). Tropical and temperate forests are global centers of biodiversity, which play an important role in the regulation of the global and regional C cycles (Poorter et al., 2015). Among various forests, tropical forests fixes higher biomass, and serve as a major potential sink of carbon due to high species diversity and high net primary production (Malhi et al., 1999; Kothandaraman et al., 2020). Approximately 767 million hectares (25%) area covered by temperate forest globally of the total land surface, and they storage about 14% of total carbon (Pan et al., 2011). Currently, climate change is a global concern, and forests plays vital role in climate change regulation and mitigation through reducing CO2 concentrations in the atmosphere (Streck and Scholz, 2006; Scholz and Huber, 2017; Bracki, 2019; Ali et al., 2020; Burman et al., 2021). Thus, estimation of carbon stocks of different forest ecosystems would help in appropriate decision-making on carbon (C) management. This kind of information also contributes toward atmospheric carbon reduction targets as part of international obligations (UNFCCC, 2014; Sahu et al., 2016; Mayer et al., 2020). The amount of total biomass stored in a forest indicates the quantity of C that can be sequestered to meet the emission targets (Brown et al., 1999; Raha et al., 2020).
Carbon sequestration is among the most important ecosystem services provided by forest ecosystems that play an important role in global climate mitigation. For example, in a recent study Tolangay and Moktan (2020) reported that the Indian Himalayan region (IHR) sequesters about 65 million tonnes of carbon each year. This has leaded to conclude that ‘the Himalayan forests have the potential to mitigate climate change and global warming’. We hypothesize that seasonal forest fires in Uttarakhand Himalaya lead to significant losses in carbon stocks within forest ecosystems. This hypothesis is based on the understanding that forest fires contribute to habitat degradation, alter vegetation dynamics, and release stored carbon into the atmosphere, thereby impacting the overall carbon sequestration capacity of the forests. With the above background and hypothesis in mind, a first-of-its-kind study was conducted in the Himalayan region on forest fires, it was aimed to analyze the loss of carbon stock in the forest of Uttarakhand due to unprecedented seasonal forest fires.
Materials and methods
Study area
The investigation encompassed three primary forest types along the elevation gradient (300–1700 m a.s.l) in Uttarakhand state of Western Himalaya, India. Among the chosen forest stands, the Motichur, Haridwar site (Site-I) was characterized by the dominance of Shorea robusta Gaertn. (Sal), while the Mona, Nainital range (Site-II) was dominated by Pinus roxburghii Roxb. Chir-Pine and the Dhaulchina, Almora site (Site-III) was primarily dominated by Quercus leucotrichophora A. Camus (Banj-oak). Geologically, all the sites (stands) were situated in the lesser Himalayan region (Valdiya, 1980). All the selected forests are managed by the forest department. A comprehensive overview of the geographical and ecological characteristics of the study sites are provided in Table 1.
Vegetation analysis
Vegetation analysis was conducted by deploying random quadrats within identified forest stands both before and after a fire event. A total of 30 (10 × 10 m) quadrats were randomly placed for the investigation. The Circumference at Breast Height (CBH) of each tree species was measured at 1.37 m above the ground. The collected field data underwent analysis for various phytosociological parameters, including density, frequency, basal area, and species richness (Mishra, 1968). Standard phytosociological methods, as outlined by Ellenberg and Muller-Dombois (1974), were employed for obtaining field data. Microsoft Excel 2019 was used to analyze phytosociological data.
Biomass analysis
Biomass and productivity were assessed using a non-harvesting sampling method, with 1.0 ha areas sampled in each site. All trees with a diameter less than 30 cm in the sampled quadrat were recorded and measured for Circumference at Breast Height (CBH) at 1.37 m above the ground. Yellow paint was used to mark all trees for long-term monitoring. Carbon stock was considered as approximately half (0.475) of biomass, and carbon sequestration was estimated as half of productivity, following the approach by Magnussen and Reed (2004). Trees measured in permanent plots during the first year (2017) were re-measured in the subsequent years (2018 and 2019).
Biomass for different components (bole, branch, twig, foliage, stump root, and fine roots) in the first year (Y1), second year (Y2), and third year (Y3) was calculated using the regression equation of the allometric method (Rawat and Singh, 1988; Rana et al., 1989; Basuki et al., 2009; Navar, 2009; Dabi et al., 2021): Y = a + b·lnX. Here, ln denotes the natural log, Y represents the dry weight of the component (kg), X is CBH (cm), a is the y-intercept, and b is the slope of the regression (Table 2). Net productivity was determined by the difference in biomass between 2 years: ΔY=Y2 − Y1. Where ΔY is the net primary productivity, Y1 is the biomass in the first year, and Y2 is the biomass in the second year. Biomass and carbon stock were computed in Megagrams per hectare (Mg ha−1). Carbon stock and sequestration were assumed to be half of the total estimated biomass of each tree species, following the methodology by Magnussen and Reed (2004): stock = Biomass·0.475; sequestration = NPP·0.475 stock = Biomass·0.475; C sequestration = NPP·0.475.
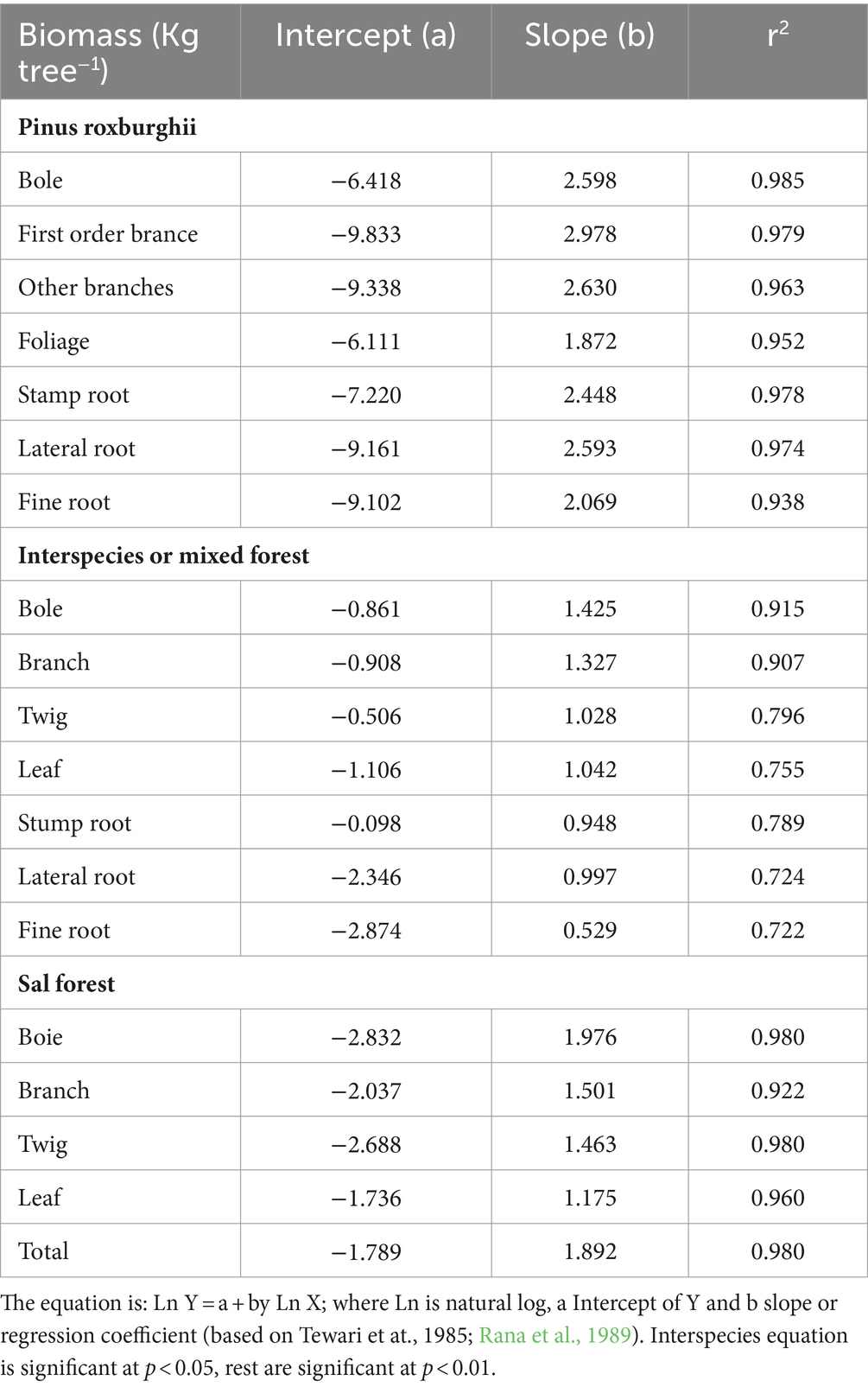
Table 2. Allometric relationships between the biomass of tree components (Y, kg−1 tree) and circumference of tree at breast height (X, cm at 1.37 m height) for different tree used.
Fire frequency mapping
Forest fire frequency maps were created for the state of Uttarakhand by using Landsat 5, 7, and 8 satellite imagery spanning a decade (2011–2020). The MODIS hotspot data (MOD14) from NASA’s Fire Information for Resource Management System (FIRMS) official website were downloaded and integrated with shape-file data representing fire points (Bargali et al., 2023). The satellite images for specific sites were extracted, geo-corrected, and classified into four frequency classes—namely, low fire, moderate fire, and high fire frequency classes (Figure 1). Supervised classification was applied to categorize the extracted and geo-corrected satellite images of chosen study sites, including Sal Forest, Chir-Pine Forest, and Mixed Forest. Using the data management tool in ArcMap software, a fishnet was generated, and fire points and raster data were spatially joined to produce the fire frequency map. A total of 16,206 fire points were recorded and classified into four fire frequency classes for the entire state. To exclude fire points in settlements, the settlement layer was overlaid onto the fire point layer, ensuring that only fire points within forest regions were considered for analysis. The fire frequency was further divided into four classes—low fire, moderate fire, and high fire frequency classes, as illustrated in Figure 1. To maintain objectivity, a 1 hectare area for each forest type was selected within each fire frequency class.
Data analysis
The statistical analysis involved paired t-tests independently for both aboveground and belowground carbon stock components. Using Statistical Package for the Social Sciences (IBM, SPSS) Statistics 26 package.
Results
The paired t-test analysis was conducted on the pre-fire and post-fire carbon stock of three forest types: Sal Forest, Chir-Pine Forest, and Mixed Forest. For the aboveground carbon stock, the mean differences and their respective standard errors (SE or ±) were calculated. In Sal Forest, the mean difference was 12 Mg ha−1 with an SE (±) of 8 Mg ha−1, resulting in a t-statistic of 1.29 [t(2) = 1.29, p = 0.29], indicating a lack of statistical significance. However, in Chir-Pine Forest, the mean difference was 20 Mg ha−1 with an SE (±) of 10 Mg ha−1, yielding a t-statistic of 2.00 [t(2) = 2.00, p = 0.12], which was statistically significant. Conversely, Mixed Forest showed a mean difference of 8 Mg ha−1 with an SE (±) of 4 Mg ha−1, also not reaching statistical significance [t(2) = 2.00, p = 0.12]. Moving to Belowground carbon stock, Sal Forest had a mean difference of 4 Mg ha−1 (SE = 2) and a t-statistic of 2.00 [t(2) = 2.00, p = 0.12], indicating no significant difference. Chir-Pine Forest exhibited a mean difference of 6 Mg ha−1 (SE = ± 4) with a t-statistic of 1.50 [t(2) = 1.50, p = 0.23], also not statistically significant. However, Mixed Forest showed a notable mean difference of 7 Mg ha−1 (SE ± 2) with a significant t-statistic of 3.50 [t(2) = 3.50, p = 0.04], indicating a significant change in Belowground carbon stock post-fire.
Impact of pre-and-post fire frequencies on different forest types
Sal forest
Pre-fire, tree biomass in the Sal forest ranged from 270 Mg ha−1 (Low fire) to 219 Mg ha−1 (High fire) (Figure 2). Among all fire classes, aboveground biomass was highest in the low fire class (270 Mg ha−1), followed by moderate fire (250 Mg ha−1), and high fire (230 Mg ha−1). Post-fire, the tree biomass in the Sal forest exhibited changes, ranging from 265 Mg ha−1 (Low fire) to 219 Mg ha−1 (High fire) (Figure 2). Aboveground biomass remained highest in the low fire class (265 Mg ha−1), followed by moderate fire (243 Mg ha−1), and high fire (219 Mg ha−1).
Chir-Pine forest
Pre-fire, tree biomass in the Chir-Pine forest ranged from 200 Mg ha−1 (Low fire) to 122 Mg ha−1 (High fire) (Figure 2). Among all fire classes, aboveground biomass was highest in the low fire class (200 Mg ha−1), followed by moderate fire (160 Mg ha−1), and high fire (130 Mg ha−1). Post-fire, the tree biomass in the Chir-Pine Forest displayed changes, ranging from 198 Mg ha−1 (Low fire) to 123 Mg ha−1 (High fire) (Figure 2). Aboveground biomass remained highest in the low fire class (198 Mg ha−1), followed by moderate fire (155 Mg ha−1), and high fire (123 Mg ha−1).
Mixed forest
Pre-fire, tree biomass in the Mixed forest ranged from 170 Mg ha−1 (Low fire) to 123 Mg ha−1 (High fire) (Figure 2). Among all fire classes, aboveground biomass was highest in the low fire class (170 Mg ha−1), followed by moderate fire (150 Mg ha−1), and high fire (135 Mg ha−1). Post-fire, the tree biomass in the Mixed Forest showed changes, ranging from 164 Mg ha−1 (Low fire) to 123 Mg ha−1 (High fire) (Figure 2). Aboveground biomass remained highest in the low fire class (164 Mg ha−1), followed by moderate fire (142 Mg ha−1), and high fire (123 Mg ha−1).
Sal forest
The analysis of carbon stock changes in the Sal forest, categorized by different fire frequency classes, revealed distinct patterns in the aftermath of fires. In the low fire frequency class, a relatively minor reduction of 5 MgC in carbon stock was observed. This suggests that low-intensity fires have a limited impact on carbon storage within the Sal forest. Moving to the moderate fire frequency class, a more substantial decrease of 7 MgC in carbon stock was noted. This indicates that as the fire intensity increases, the magnitude of the impact on carbon stocks also increases. Notably, in the high fire frequency class, the most significant reduction was observed, with a noteworthy decline of 11 MgC in carbon stock. This underscores the heightened vulnerability of the Sal forest to carbon loss in the presence of frequent and intense fires (Figure 3).
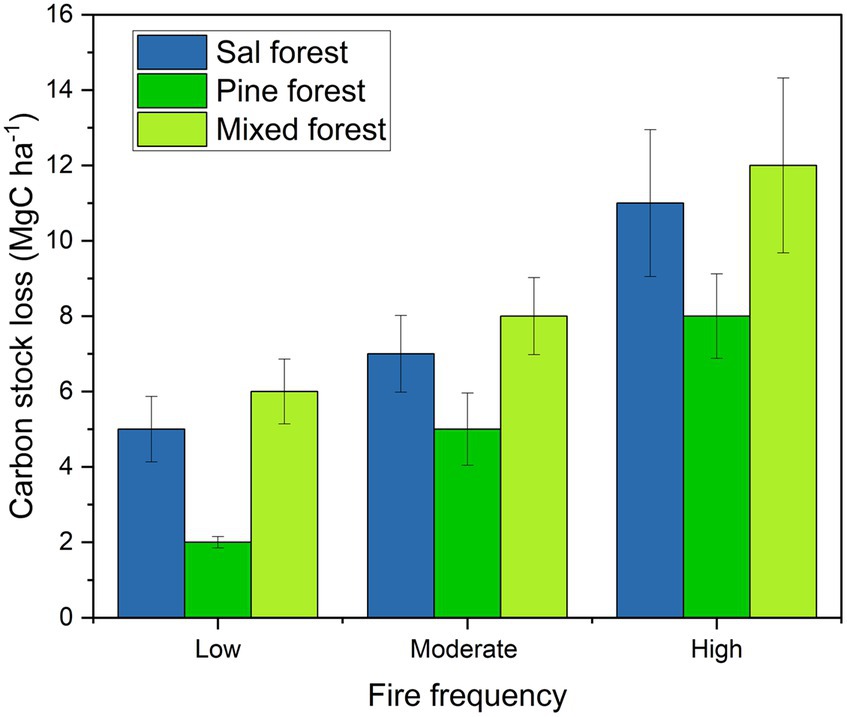
Figure 3. Carbon losses due to seasonal forest fire in the selected forest types in Uttarakhand, western Himalaya Carbon loss due to forest fire in selected forest types and fire frequencies.
Chir-Pine forest
The examination of carbon stock dynamics within the Chir-Pine forest, classified by varying fire frequency classes, revealed distinctive trends in the aftermath of fires. In the low fire frequency class, a minimal reduction of 2 MgC in carbon stock implies that low-intensity fires have a limited impact on carbon storage within the Chir-Pine forest. Transitioning to the moderate fire frequency class, a more pronounced decrease of 5 MgC in carbon stock suggests an escalating impact as fire intensity increases. Notably, in the high fire frequency class, the most substantial reduction was observed, with an 8 MgC decline in carbon stock (Figure 3).
Mixed forest
Examining the carbon stock dynamics in the mixed forest across different fire frequency classes reveals distinctive patterns prior to and after fire incidents. In the low fire frequency class, there was a marginal reduction of 6 MgC in carbon stock, indicating a relatively modest impact of low-intensity fires on carbon storage within the mixed forest. Moving to the moderate fire frequency class, a more significant decrease of 8 MgC in carbon stock was observed, suggesting an escalating impact as fire intensity increases. Notably, in the high fire frequency class, the most substantial reduction was evident, with a noteworthy decline of 12 MgC in carbon stock (Figure 3).
Species wise contribution of carbon stock
Sal forest
The carbon stock contributions of different tree species in the Sal forest are presented in the Figure 4. In the Low fire, Shorea robusta exhibited the highest carbon stock contribution among the species with a value of 36 MgC ha−1, followed by Mallotus philippensis with 16 MgC ha−1. Syzygium cumini, Butea monosperma, and Aegle marmelos contributed 18, 12, and 14 MgC ha−1, respectively. Under Moderate fire conditions, S. robusta maintained its leading position, contributing 33 MgC ha−1, followed by M. philippensis with 13 MgC ha−1. S. cumini, B. monosperma, and A. marmelos contributed 14, 9, and 7 MgC ha−1, respectively. In the High fire class, the contribution of S. robusta declined to 12 MgC ha−1, while M. philippensis became the highest contributor with 14 MgC ha−1. S. cumini, B. monosperma, and A. marmelos contributed 16, 16, and 6 MgC ha−1, respectively.
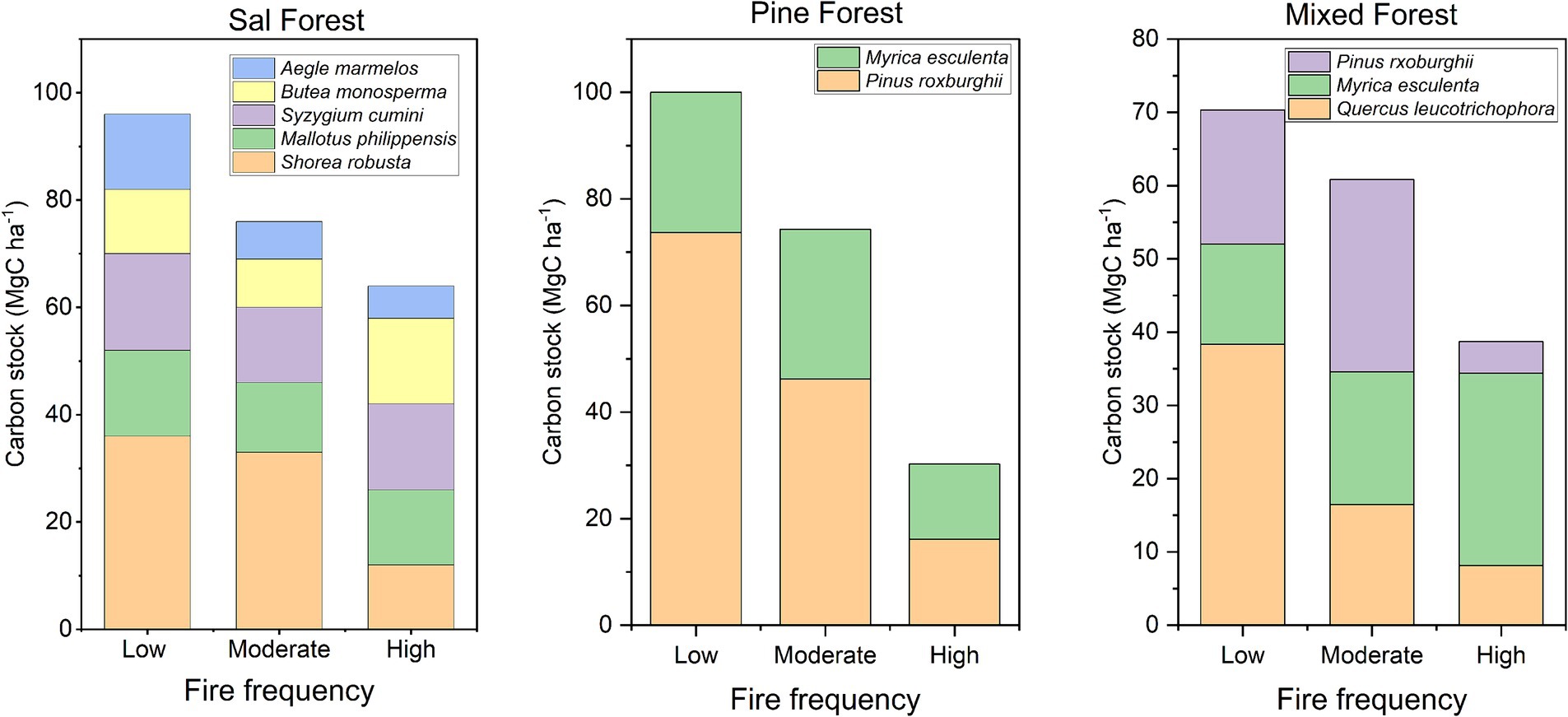
Figure 4. Species-wise contribution (dominant species) of above ground biomass (MgC ha−1) in different fire classes.
Chir-Pine Forest
In the Chir-Pine forest, the carbon stock contributions of two dominant tree species, Pinus roxburghii and Myrica esculenta, are outlined in the Figure 4. Under Low fire conditions, P. roxburghii dominated the carbon stock contribution with a value of 73.68 MgC ha−1, while M. esculenta contributed 26.31 MgC ha−1. In the Moderate fire scenario, P. roxburghii maintained a substantial carbon stock contribution of 46.18 MgC ha−1, surpassing M. esculenta, which contributed 28.12 MgC ha−1. In the High fire class, both species experienced a reduction in carbon stock contributions. P. roxburghii contributed 16.12 MgC ha−1, while M. esculenta contributed 14.13 MgC ha−1.
Mixed forest
In the mixed forest, the carbon stock contributions of three dominant tree species—Quercus leucotrichophora, Myrica esculenta, and Pinus roxburghii—are detailed in the Figure 4. Under Low fire conditions, Q. leucotrichophora emerged as the primary contributor to carbon stock with 38.35 MgC ha−1. M. esculenta and P. roxburghii contributed 13.69 and 18.26 MgC ha−1, respectively. In the Moderate fire class, the dynamics shifted, and P. roxburghii took the lead with a carbon stock contribution of 26.26 MgC ha−1. M. esculenta followed closely with 18.1 MgC ha−1, while Q. leucotrichophora’s contribution decreased to 16.46 MgC ha−1. In the High fire scenario, M. esculenta became the top contributor and yielded a carbon stock of 26.26 MgC ha−1. Q. leucotrichophora and P. roxburghii contributed 8.16 and 4.26 MgC ha−1, respectively.
Discussion
Aboveground biomass (AGB) stands as a crucial quantitative parameter for assessing the health and sustainability of forest ecosystems (Joshi et al., 2022). AGB reflects the cumulative biomass of trees and vegetation above the soil surface and serves as a key indicator of the ecosystem’s carbon storage capacity. Our investigation into AGB changes in three diverse forest types—Sal, Chir-Pine, and Mixed—in response to varying fire frequencies provides valuable insights into the complex interplay of factors influencing forest dynamics. The observed alterations in tree biomass were influenced by a multitude of factors inherent to forest ecosystems. These include stand age, species composition, forest type, size class of trees, site conditions, rainfall patterns, edaphic factors, and elevation (Peichl and Arain, 2006; Gairola et al., 2011). Each of these factors contributed uniquely to the overall dynamics of AGB, shaping the structure and function of the forest. Our results affirmed that fire, whether occurring as a low frequency event or high frequency, exerts a discernible influence on AGB. The observed changes in AGB following fires in the Sal, Chir-Pine, and Mixed forests underscore the sensitivity of this parameter to variations in fire frequency. The impact of fire on AGB was particularly pronounced in higher fire frequency classes, as demonstrated by the substantial reductions in carbon stock. The Sal forest, known for its resilience to lower intensity fires, exhibited a gradient of AGB loss corresponding to increasing fire frequency. The Chir-Pine forest, characterized by higher sensitivity to fire, displayed more substantial biomass reductions across all fire frequency classes. In the Mixed forest, the diversity of tree species contributed to varied responses to fire and further emphasized the complexity of factors influencing AGB dynamics. The paired t-test analysis also revealed significant changes in the carbon stock dynamics post-fire, with Chir-Pine Forest showing a notable shift in Aboveground carbon stock and Mixed Forest exhibiting a significant change in Belowground carbon stock. The comparison of carbon stock contributions across the three distinct forest types—Sal, Pine, and Mixed—revealed interesting patterns and species-specific dynamics in response to varying fire intensities. Examining the data collectively provides valuable insights into the overall carbon sequestration capacity and highlights the contributions of individual tree species in each forest type. In the Sal forest, S. robusta consistently emerged as a significant contributor to carbon stock, leading the pack across all fire frequencies. Its remarkable resilience in maintaining high carbon stock levels showcased its importance in carbon sequestration, underscoring the need for its preservation in forest management strategies. Contrastingly, the Chir-Pine forest displayed a different pattern, with P. roxburghii playing a pivotal role in carbon stock contributions. This species demonstrated a robust ability to sequester carbon, particularly evident in low and moderate fire scenarios. While contributing substantially, M. esculenta does not match the carbon stock levels of P. roxburghii. The mixed forest presented a unique scenario, where Q. leucotrichophora, M. esculenta, and P. roxburghii showcased varying contributions across different fire intensities. Q. leucotrichophora dominated in low fire frequency, whereas P. roxburghii took the lead in moderate fires, and M. esculenta became the primary contributor in high fire frequency. It was evident that the species response to fire intensity played a crucial role in determining their carbon sequestration capacity. Across all forests, P. roxburghii consistently exhibited significant carbon stock contributions, showcasing its resilience in the face of different fire regimes. This emphasized the importance of understanding the unique characteristics of each species for effective forest management and conservation.
Conclusion
Our findings emphasize the need for a comprehensive understanding of the interactions between fire, forest type, and above ground biomass (AGB) dynamics. The identification of specific fire frequency classes associated with minimum and maximum biomass losses provides critical information for targeted conservation strategies. Acknowledging the multifaceted nature of AGB changes which contributes to the development of nuanced and effective forest management approaches, ensuring the resilience and sustainability of these diverse ecosystems in the face of evolving fire regimes. The findings suggest that preserving and promoting certain key/local/native species, such as Shorea robusta in Sal forests and Pinus roxburghii in Chir-Pine forests, can contribute significantly to maintaining or enhancing carbon rather than new species. These insights will be instrumental for policymakers, forest managers, and conservationists in devising strategies to ensure the sustainability and resilience of diverse forest ecosystems in the context of changing fire dynamics and global climate patterns.
Data availability statement
The raw data supporting the conclusions of this article will be made available by the authors, without undue reservation.
Author contributions
HB: Conceptualization, Data curation, Investigation, Methodology, Software, Writing – original draft, Writing – review & editing. AP: Supervision, Writing – original draft, Writing – review & editing. DB: Supervision, Writing – review & editing. RS: Writing – review & editing.
Funding
The author(s) declare that no financial support was received for the research, authorship, and/or publication of this article.
Acknowledgments
The authors extend their appreciation to the Director of G. B. Pant National Institute of Himalayan Environment (NIHE) for institutional support. We also recognize the Department of Zoology and Environment Science at Gurukula Kangri (Deemed to be University), Haridwar, for their logistical assistance. Special thanks are given to Rohit Kumar, IIT, Roorkee for his valuable suggestions in the fire frequency map.
Conflict of interest
The authors declare that the research was conducted in the absence of any commercial or financial relationships that could be construed as a potential conflict of interest.
Publisher’s note
All claims expressed in this article are solely those of the authors and do not necessarily represent those of their affiliated organizations, or those of the publisher, the editors and the reviewers. Any product that may be evaluated in this article, or claim that may be made by its manufacturer, is not guaranteed or endorsed by the publisher.
References
Abrams, M. D., and Nowacki, G. J. (2019). Global change impacts on forest and fire dynamics using paleoecology and tree census data for eastern North America. Ann. For. Sci. 76, 1–23,
Agee, J. K. (1996). The influence of forest structure on fire behavior. In Proceedings of the 17th annual forest vegetation management conference, 52–68
Ahmad, F., and Goparaju, L. (2018). Climate change and its impact on forest fire in the state of Himachal Pradesh and Uttarakhand states of India: remote sensing and GIS analysis. Contemp. Trends. Geosci 7, 229–246. doi: 10.2478/ctg-2018-0016
Ali, A., Ashraf, M. I., Gulzar, S., and Akmal, M. (2020). Estimation of forest carbon stocks in temperate and subtropical mountain systems of Pakistan: implications for REDD+ and climate change mitigation. Monit. Assess. 192, 1–13,
Allendorf, F. W., and Hard, J. J. (2009). Human-induced evolution caused by unnatural selection through harvest of wild animals. Proc. Natl. Acad. Sci. USA 106, 9987–9994. doi: 10.1073/pnas.0901069106
Anthwal, A., Gupta, N., Sharma, A., Anthwal, S., and Kim, K. H. (2010). Conserving biodiversity through traditional beliefs in sacred groves in Uttarakhand Himalaya, India. Resour. Conserv. Recycl. 54, 962–971. doi: 10.1016/j.resconrec.2010.02.003
Babu, K. V. S (2019). Developing forest fire danger index using geo-spatial techniques. PhD thesis, 1–131
Babu, K. V. S., Roy, A., and Prasad, P. R. (2016). Forest fire risk modeling in Uttarakhand Himalaya using Terra satellite datasets. Eur. J. Remote Sen. 49, 381–395. doi: 10.5721/EuJRS20164921
Bahuguna, V. K., and Upadhay, A. (2002). Forest fires in India: policy initiatives for community participation. I. Forest. Review. 4, 122–127. doi: 10.1505/IFOR.4.2.122.17446
Bargali, H., Bhatt, D., Sundriyal, R. C., Uniyal, V. P., Pandey, A., and Ranjan, R. (2023). Effect of forest fire on tree diversity and regeneration in the forests of Uttarakhand, Western Himalaya, India. Front. For. Glob. Change. 6:1198143. doi: 10.3389/ffgc.2023.1198143
Bargali, H., Calderon, L. P. P., Sundriyal, R. C., and Bhatt, D. (2022b). Impact of forest fire frequency on floristic diversity in the forests of Uttarakhand, western Himalaya. Trees, For. People 9:100300. doi: 10.1016/j.tfp.2022.100300
Bargali, H., Gupta, S., Malik, D. S., and Matta, G. (2017). Estimation of fire frequency in Nainital district of Uttarakhand state by using satellite images. J. Remote Sens. GIS. 6, 1–5. doi: 10.4172/2469-4134.1000214
Bargali, H., Kumar, A., and Singh, P. (2022a). Plant studies in Uttarakhand, Western Himalaya: a comprehensive review. Trees For. People :100203. doi: 10.1016/j.tfp.2022.100203
Bargali, H., Pandey, A., Bhatt, D., Sundriyal, R. C., and Uniyal, V. P. (2024). Forest fire management, funding dynamics, and research in the burning frontier: A comprehensive review. Trees For. People.. 16:100526. doi: 10.1016/j.tfp.2024.100526
Bargali, H., Singh, P., and Bhatt, D. (2020). Role of Chir pine (Pinus roxburghii Sarg.) in the forest fire of Uttarakhand Himalaya. ENVIS Bull. Himalayan Ecol 28, 82–85,
Baró, F., Chaparro, L., Gómez-Baggethun, E., Langemeyer, J., Nowak, D. J., and Terradas, J. (2014). Contribution of ecosystem services to air quality and climate change mitigation policies: the case of urban forests in Barcelona, Spain. Ambio 43, 466–479,
Basuki, T. M., Van Laake, P. E., Skidmore, A. K., and Hussin, Y. A. (2009). Allometric equations for estimating the above-ground biomass in tropical lowland dipterocarp forests. For. Ecol. Manag. 257, 1684–1694. doi: 10.1016/j.foreco.2009.01.027
Bessie, W. C., and Johnson, E. A. (1995). The relative importance of fuels and weather on fire behavior in subalpine forests. Ecology 76, 747–762. doi: 10.2307/1939341
Bhandari, B. S., and Bijlwan, K. (2020). “Vulnerability of Forest vegetation due to anthropogenic disturbances in Western Himalayan region of India” in Research anthology on ecosystem conservation and preserving biodiversity (IGI Global), 1297–1312.
Bhandari, B. S., Mehta, J. P., and Semwal, R. L. (2012). “Forest fire in Uttarakhand Himalaya: an overview” in Glimpses of forestry research in the Indian Himalayan region (Almora: GB Pant Institute of Himalayan Environment & Development), 167–175.
Bhatt, R., Negi, S. S., and Bhatt, J. P. (2019). Fire affects regeneration potential of moist temperate forests in the western Himalaya. Sci. Rep. 9:12130. doi: 10.1038/s41598-019-48592-6
Bisht, S., Adhikari, B. S., and Srivastava, A. K., (2018). Assessment of forest communities and dependence of local people on NTFPs in Askot landscape, Uttarakhand, Phase 1–Report, Wildlife Institute of India.
Boucher, D., Gauthier, S., Thiffault, N., Marchand, W., Girardin, M., and Urli, M. (2020). How climate change might affect tree regeneration following fire at northern latitudes: a review. New For. 51, 543–571. doi: 10.1007/s11056-019-09745-6
Bowd, E. J., Banks, S. C., Bissett, A., May, T. W., and Lindenmayer, D. B. (2021). Direct and indirect disturbance impacts in forests. Ecol. Lett. 24, 1225–1236. doi: 10.1111/ele.13741
Bowman, D. M. J. S., and Balch, J. K. (2013). Fire in the earth system. Nature 501, 1–10. doi: 10.1038/nature12540
Bracki, D (2019). Forests and climate change. In: Proceedings of background study prepared for the fourteenth session of the United Nations forum on forests
Brown, S. L., Schroeder, P., and Kern, J. S. (1999). Spatial distribution of biomass in forests of the eastern USA. For. Ecol. Manag. 123, 81–90. doi: 10.1016/S0378-1127(99)00017-1
Burgan, R. E. (1984). Behave: fire behavior prediction and fuel modeling system, fuel subsystem. Ogden, UT: USDA Forest Service General Technical Report INT–167.
Burman, P. K. D., Launiainen, S., Mukherjee, S., Chakraborty, S., Gogoi, N., Murkute, C., et al. (2021). Ecosystem-atmosphere carbon and water exchanges of subtropical evergreen and deciduous forests in India. For. Ecol. Manag. 495:119371,
Capellesso, E. S., Cequinal, A., Marques, R., Sausen, T. L., Bayer, C., and Marques, M. C. M. (2021). Co-benefits in biodiversity conservation and carbon stock during forest regeneration in a preserved tropical landscape. For. Ecol. Manag. 492:119222. doi: 10.1016/j.foreco.2021.119222
Cassiano, C. C., and Ferraz, S. F. D. B. (2022). Fast-growing forest management to regulate the balance between wood production and water supply. Sci. Agric. 80:e20210418.
Certini, G. (2005). Effects of fire on properties of forest soils: a review. Oecologia 143, 1–10. doi: 10.1007/s00442-004-1788-8
Chauhan, J. S., Gautam, A. S., and Negi, R. S. (2018). Natural and anthropogenic impacts on forest structure: a case study of Uttarakhand state. Open Environment. Research J. 11, 38–46. doi: 10.2174/1874213001811010038
Connell, J. H., and Orias, E. (1964). The ecological regulation of species diversity. Am. Nat. 98, 399–414. doi: 10.1086/282335
Cornelissen, J. H., Grootemaat, S., Verheijen, L. M., Cornwell, W. K., van Bodegom, P. M., van der Wal, R., et al. (2017). Are litter decomposition and fire linked through plant species traits? New Phytol. 216, 653–669. doi: 10.1111/nph.14766
Countryman, C. M. (1964). Mass fires and fire behavior, vol. 19: Pacific Southwest Forest and Range Experiment Station, Forest Service, US Department of Agriculture, 53.
Dabi, H., Bordoloi, R., Das, B., Paul, A., Tripathi, O. P., and Mishra, B. P. (2021). Biomass, carbon stock and soil physicochemical properties in plantation of east Siang district. Arunachal Pradesh, India. Environmental. Challenges 4:100191,
Deb, P., and Sundriyal, R. C. (2008). Tree regeneration and seedling survival patterns in old-growth lowland tropical rainforest in Namdapha National Park, north-East India. For. Ecol. Manag. 255, 3995–4006. doi: 10.1016/j.foreco.2008.03.046
De Groot, R. S., Wilson, M. A., and Boumans, R. M. J. (2002). A typology for the classification, description and valuation of ecosystem functions, goods and services. Ecol. Econ. 41, 393–408. doi: 10.1016/S0921-8009(02)00089-7
Delcourt, H. R., and Delcourt, P. A. (1997). Pre Columbian native American use of fire on southern Appalachian landscapes. Cons. Biol. 11, 1010–1014. doi: 10.1046/j.1523-1739.1997.96338.x
De Mendonça, M. J. C., Diaz, M. D. C. V., Nepstad, D., da Motta, R. S., Alencar, A., Gomes, J. C., et al. (2004). The economic cost of the use of fire in the Amazon. Ecol. Econ. 49, 89–105. doi: 10.1016/j.ecolecon.2003.11.011
Ellenberg, D., and Mueller-Dombois, D. (1974). Aims and methods of vegetation ecology. New York: Wiley.
Fahey, T. J., Woodbury, P. B., Battles, J. J., Goodale, C. L., Hamburg, S. P., Ollinger, S. V., et al. (2010). Forest carbon storage: ecology, management, and policy. Front. Ecol. Environ. 8, 245–252. doi: 10.1890/080169
Flower, C. E., and Gonzalez-Meler, M. A. (2015). Responses of temperate forest productivity to insect and pathogen disturbances. Annu. Rev. Plant Biol. 66, 547–569. doi: 10.1146/annurev-arplant-043014-115540
FSI (2021). India State of Forest Report ; Ministry of Environment and Forests. Available at: https://fsi.nic.in/forest-report-2021details
Fulé, P. Z., Garkoti, S. C., and Semwal, R. L. (2021). Frequent burning in chir pine forests, Uttarakhand, India. Fire Ecol. 17:20,
Gadgil, M. (1992). Conserving biodiversity as if people matter: a case study from India. Ambio 21, 266–270,
Gairola, S., Sharma, C. M., Ghildiyal, S. K., and Suyal, S. (2011). Live tree biomass and carbon variation along an altitudinal gradient in moist temperate valley slopes of the Garhwal Himalaya (India). Curr. Sci. 100, 1862–1870.
Gururani, S. (1996). Fuel, fodder, and forests: Politics of forest use and abuse in Uttarakhand Himalaya, India. India: Syracuse University.
Hakkila, P., and Parikka, M. (2002). “Fuel resources from the forest” in Bioenergy from sustainable forestry: Guiding principles and practice. (eds.) Richardson, J., Bjorheden, R., Hakkila, P., Lowe, A. T., and Smith, C. T. (Kluwer Academic Publishers, London: Springer Netherlands), 19–48.
Hansen, M. C., Stehman, S. V., and Potapov, P. V. (2010). Quantification of global gross forest cover loss. Proc. Natl. Acad. Sci. 107, 8650–8655. doi: 10.1073/pnas.0912668107
Harrison, S. P., Marlon, J. R., and Bartlein, P. J. (2010). Fire in the earth system. Netherlands: Springer, 21–48.
He, T., Lamont, B. B., and Pausas, J. G. (2019). Fire as a key driver of Earth's biodiversity. Biol. Rev. 94, 1983–2010. doi: 10.1111/brv.12544
Humpenoder, F., Popp, A., Dietrich, J. P., Klein, D., Lotze-Campen, H., Bonsch, M., et al. (2014). Investigating afforestation and bioenergy CCS as climate change mitigation strategies. Environ. Res. Let. 9:064029. doi: 10.1088/1748-9326/9/6/064029
Jhariya, M. K., Bargali, S. S., Swamy, S. L., and Kittur, B. (2012). Vegetational structure, diversity and fuel load in fire affected areas of tropical dry deciduous forests in Chhattisgarh. Int. J. Plant Res. 25, 210–224,
Jiang, B., Yin, Z., Yam, M. C., Zhang, J., and Wang, L. (2022). Influence of rotational restraints on response of H-section steel columns under fire. J. Constr. Steel Res. 190:107104. doi: 10.1016/j.jcsr.2021.107104
Joshi, G., and Negi, G. C. (2011). Quantification and valuation of forest ecosystem services in the western Himalayan region of India. Int. J. Biodivers. Sci. Ecosys. Service Manage. 7, 2–11. doi: 10.1080/21513732.2011.598134
Joshi, V. C., Bisht, D., Sundriyal, R. C., and Pant, H. (2022). Species richness, diversity, structure, and distribution patterns across dominating forest communities of low and mid-hills in the central Himalaya. Geol. Ecol. Landscape., 7, 329–339. doi: 10.1080/24749508.2021.2022424
Joshi, V. C., Sundriyal, R. C., and Arya, D. (2021). Forest floor diversity, distribution and biomass pattern of oak and Chir-pine forest in the Indian Western Himalaya. Ind. J. Ecol. 48, 232–237,
Kindermann, G., Obersteiner, M., Sohngen, B., Sathaye, J., Andrasko, K., Rametsteiner, E., et al. (2008). Global cost estimates of reducing carbon emissions through avoided deforestation. Proc. Natl. Acad. Sci. 105, 10302–10307. doi: 10.1073/pnas.0710616105
Kodandapani, N., Cochran, M. A., and Sukumar, R. (2008). A comparative analysis of spatial, temporal, and ecological characteristics of forest fires in seasonally dry tropical ecosystems in the Western Ghats, India. For. Ecol. Manage. 256, 607–617. doi: 10.1016/j.foreco.2008.05.006
Koskela, T., and Karppinen, H. (2021). Forest owners’ willingness to implement measures to safeguard biodiversity: values, attitudes, ecological worldview and forest ownership objectives. Small-Scale Forestry 20, 11–37. doi: 10.1007/s11842-020-09454-5
Kothandaraman, S., Dar, J. A., Sundarapandian, S., Dayanandan, S., and Khan, M. L. (2020). Ecosystem-level carbon storage and its links to diversity, structural and environmental drivers in tropical forests of Western Ghats. India. Sci. Rep. 10, 1–15,
Kumar, A., Mitra, M., Adhikari, B. S., and Rawat, G. S. (2015). Depleting indigenous knowledge of medicinal plants in cold-arid region of Nanda Devi biosphere reserve, Western Himalaya. Medic. Aromat. Plants. 4:195. doi: 10.4172/2167-0412.1000195
Kumar, M., Kalra, N., Khaiter, P., Ravindranath, N. H., Singh, V., Singh, H., et al. (2019). PhenoPine: a simulation model to trace the phenological changes in Pinus roxhburghii in response to ambient temperature rise. Ecol. Model. 404, 12–20. doi: 10.1016/j.ecolmodel.2019.05.003
Kumar, S., and Hole, R. M. (2021). Geospatial modelling of soil erosion and risk assessment in Indian Himalayan region—a study of Uttarakhand state. Environment. Adv. 4:100039,
Kurniawan, K., Supriatna, J., Sapoheluwakan, J., Soesilo, T. E. B., Mariati, S., and Gunarso, G. (2022). The analysis of forest and land fire and carbon and greenhouse gas emissions on the climate change in Indonesia. AgBioforum 24, 1–11,
Laurance, W. F., and Useche, D. C. (2009). Environmental synergisms and extinctions of tropical species. Conserv. Biol. 23, 1427–1437. doi: 10.1111/j.1523-1739.2009.01336.x
Lecina-Diaz, J., Martínez-Vilalta, J., Alvarez, A., Vayreda, J., and Retana, J. (2021). Assessing the risk of losing forest ecosystem services due to wildfires. Ecosystems, 24, 1–15.
Lee, C., Schlemme, C., Murray, J., and Unsworth, R. (2015). The cost of climate change: ecosystem services and wildland fires. Ecol. Econ. 116, 261–269. doi: 10.1016/j.ecolecon.2015.04.020
Malhi, Y., Baldocchi, D. D., and Jarvis, P. G. (1999). The carbon balance of tropical, temperate and boreal forests. Plant Cell Environ. 22, 715–740. doi: 10.1046/j.1365-3040.1999.00453.x
Maren, I. E., and Sharma, L. N. (2021). Seeing the wood for the trees: carbon storage and conservation in temperate forests of the Himalayas. For. Ecol. Manag. 487:119010. doi: 10.1016/j.foreco.2021.119010
Mathela, M., Bargali, H., Sharma, M., Sharma, R., and Kumar, A. (2020). Brainstorming on the future of the highly threatened medicinal plants of the Western Himalaya, India. Curr. Sci. 118, 1485–1486,
Mayer, M., Prescott, C. E., Abaker, W. E., Augusto, L., Cecillon, L., and Ferreira, G. W. (2020). Tamm review: influence of forest management activities on soil organic carbon stocks: a knowledge synthesis. For. Ecol. Manag. 466, 118–127,
Miller, R. G., Tangney, R., Enright, N. J., Fontaine, J. B., Merritt, D. J., Ooi, M. K., et al. (2019). Mechanisms of fire seasonality effects on plant populations. Trends Ecol. Evol. 34, 1104–1117. doi: 10.1016/j.tree.2019.07.009
Navar, J., (2009). Allometric equations for tree species and carbon stocks for forests of north-western Mexico. For. Ecol. Manage. 257, 427–434.
Naveh, Z. (1994). “The role of fire and its management in the conservation of Mediterranean ecosystems and landscapes” in The role of fire in Mediterranean-type ecosystems. (eds.) Moreno, J. M., and Oechel, W. C. (New York, NY: Springer New York), 163–185.
Negi, C. S. (2010). Traditional culture and biodiversity conservation: examples from Uttarakhand, Central Himalaya. Mountain Res. Develop. 30, 259–265. doi: 10.1659/MRD-JOURNAL-D-09-00040.1
Negi, G. C. S. (2019). Forest fire in Uttarakhand: causes, consequences and remedial measures. I. J. Ecol. Environ. Sci. 45, 31–37,
Negi, G. C. S., and Dhyani, P. P. (2012). Glimpses of forestry research in the Indian Himalayan region. Almora: G.B. Pant Institute of Himalayan Environment & Development, 184.
Niklasson, M., Zin, E., Zielonka, T., and Feijen, M. (2010). A 350-year tree-ring fire record from Bialowieza primeval Forest, Poland: implications for central European lowland fire history. J. Ecol. 98, 1319–1329. doi: 10.1111/j.1365-2745.2010.01710.x
Pan, Y., Birdsey, R., Fang, J., Houghton, R., Kauppi, P., Kurz, W. A., et al. (2011). Carbon Sink in the World’s Forests. Wildl. Res. 8.
Pausas, J. G., and Keeley, J. E. (2009). A burning story: the role of fire in the history of life. Bioscience 59, 593–601. doi: 10.1525/bio.2009.59.7.10
Peichl, M., and Arain, M. A. (2006). Above-and belowground ecosystem biomass and carbon pools in an age-sequence of temperate pine plantation forests. Agr. For. Meteor. 140, 51–63,
Poorter, L., van der Sande, M. T., Thompson, J., Arets, E. J. M. M., Alarcón, A., Javier Álvarez-Sánchez, N., et al. (2015). Diversity enhances carbon storage in tropical forests. Glob. Ecol. Biogeogr. 24, 1314–1328. doi: 10.1111/geb.12364
Raha, D., Dar, J. A., Pandey, P. K., Lone, P. A., Verma, S., Khare, P. K., et al. (2020). Variation in tree biomass and carbon stocks in three tropical dry deciduous forest types of Madhya Pradesh, India. Carbon Manag. 11, 109–120. doi: 10.1080/17583004.2020.1712181
Rai, S., Pandey, A., and Badola, H. K. (2018). Biomass and carbon stock estimation across the timberline of Khangchendzonga National Park, Eastern Himalaya, India. Taiwania 63.
Rana, B. S., Singh, S. P., and Singh, R. P. (1989). Biomass and net primary productivity in central Himalayan forests along an altitudinal gradient. For. Ecol. Manag. 27, 199–218,
Ranjan, R. (2018). What drives forest degradation in the Central Himalayas? Understanding the feedback dynamics between participatory forest management institutions and the species composition of forests. Forest Policy Econ. 95, 85–101. doi: 10.1016/j.forpol.2018.07.010
Rawat, N., Purohit, S., Negi, G. S., and Pant, D. (2017). Perception of local people towards blazing mountain due to forest fire in changing climatic scenario of Uttarakhand himalaya: a need for scientific intervention for sensitization and conservation and livelihood sustainability of region. Indian Forester 143, 28–32,
Rawat, Y. S., and Singh, J. S. (1988). Structure and function of oak forests in central Himalaya. I. Dry Matter Dynamics. Ann. Bot. 62, 397–411,
Ray, T., Malasiya, D., Dar, J. A., Khare, P. K., Khan, M. L., Verma, S., et al. (2019). Estimation of greenhouse gas emissions from vegetation fires in Central India. Clim. Chan. Environ. Sustain. 7, 32–38. doi: 10.5958/2320-642X.2019.00005.X
Ribeiro-Kumara, C., Köster, E., Aaltonen, H., and Köster, K. (2020). How do forest fires affect soil greenhouse gas emissions in upland boreal forests? A review. Environ. Res. 184:109328,
Rodríguez-Trejo, D. A. (2014). Incendios de Vegetacion Su Ecología, Manejo e Historia, vol. 1. Mexico: CP, UACh, Semarnat, Conafor, CONANP, PNIP.
Sadowska, B., Grzegorz, Z., and Stępnicka, N. I. N. A. (2021). Forest fires and losses caused by fires–an economic approach. WSEAS Trans. Environ. Dev. 17, 181–191. doi: 10.37394/232015.2021.17.18
Saha, S., Bera, B., Shit, P. K., Bhattacharjee, S., Sengupta, D., Sengupta, N., et al. (2023). Recurrent forest fires, emission of atmospheric pollutants (GHGs) and degradation of tropical dry deciduous forest ecosystem services. Total Environ. Res. Themes 7, 1–12. doi: 10.1016/j.totert.2023.100057
Sahu, S. C., Kumar, M., and Ravindranath, N. H. (2016). Carbon stocks in natural and planted mangrove forests of Mahanadi mangrove wetland. East Coast of India. Nat. Sci. Biol., 8, 125–133.
Sathya, M., and Jayakumar, S. (2017). Post-fire regeneration status of tree species in a tropical dry deciduous forest of southern India. J. Trop. For. Sci. 29, 305–317,
Sati, V. P., and Bandooni, S. K. (2018). Forests of Uttarakhand: diversity, distribution, use pattern and conservation. ENVIS Bull. Himal. Ecol. 26, 21–27,
Scholz, I. G., and Huber, O. (2017). Wildfires and tree mortality in Mediterranean-climate regions. Front. Plant Sci. 8:131. doi: 10.3389/fpls.2017.00131
Scott, D. F., Curran, M. P., Robichaud, P. R., and Wagenbrenner, J. W. (2009). “Soil erosion after forest fire” in Fire effects on soils and restoration strategies (CRC Press), 193–212.
Semwal, R. L., and Mehta, J. P. (1996). Ecology of forest fires in Chir pine (Pinus roxburghii Sarg.) forest of Garhwal Himalaya. Curr. Sci. 70, 426–427,
Sharma, J. C., and Sharma, Y. (2004). Nutrient cycling in forest ecosystems–a review. Agric. Rev. 25, 157–172,
Shmulsky, R., and Jones, P. D. (2019). Forest products and wood science: An introduction. Wiley-Blackwell.
Singh, R. D., Gumber, S., Tewari, P., and Singh, S. P. (2016). Nature of forest fires in Uttarakhand: frequency, size and seasonal patterns in relation to pre-monsoonal environment. Curr. Sci. 111, 398–403. doi: 10.18520/cs/v111/i2/398-403
Singh, S. P. (2002). Balancing the approaches of environmental conservation by considering ecosystem services as well as biodiversity. Curr. Sci. 82, 1331–1335,
Stenseth, N. C., and Dunlop, E. S. (2009). Evolution: unnatural selection. Nature 457, 803–804. doi: 10.1038/457803a
Streck, C., and Scholz, S. M. (2006). The role of forests in global climate change: whence we come and where we go. Inter Affar 82, 861–879,
Tolangay, D., and Moktan, S. (2020). Trend of studies on carbon sequestration dynamics in the Himalaya hotspot region: a review. J. Appl. Nat. Sci. 12, 647–660. doi: 10.31018/jans.v12i4.2426
UNFCCC (2014). UNFCCC detailed annual report. Available at: http://unfccc.int/resource/docs/2014
Vahabi, H., Sonnier, R., and Ferry, L. (2015). Effects of ageing on the fire behaviour of flame-retarded polymers: a review. Polym. Int. 64, 313–328. doi: 10.1002/pi.4841
Valdiya, K. S. (1980). Geology of Kumaun lesser Himalaya. Dehradun, India: Wadia Institute of Himalayan Geology.
Vasconcelos, H. L., Maravalhas, J. B., and Cornelissen, T. (2017). Effects of fire disturbance on ant abundance and diversity: a global meta-analysis. Biodivers. Conserv. 26, 177–188. doi: 10.1007/s10531-016-1234-3
Verma, S., and Jayakumar, S. (2015). Post-fire regeneration dynamics of tree species in a tropical dry deciduous forest, Western Ghats. India. For. Ecol. Manage. 341, 75–82. doi: 10.1016/j.foreco.2015.01.005
Ward, M., Tulloch, A. I., Radford, J. Q., Williams, B. A., Reside, A. E., Macdonald, S. L., et al. (2020). Impact of 2019–2020 mega-fires on Australian fauna habitat. Nat. Ecol. Evol. 4, 1321–1326. doi: 10.1038/s41559-020-1251-1
Whitlock, C., Higuera, P. E., McWethy, D. B., and Briles, C. E. (2010). Paleoecological perspectives on fire ecology: revisiting the fire-regime concept. Open Ecol. J. 3, 6–23.
Yule, C. M. (2010). Loss of biodiversity and ecosystem functioning in Indo-Malayan peat swamp forests. Biodivers. Conserv. 19, 393–409. doi: 10.1007/s10531-008-9510-5
Zhao, J., Kang, F., Wang, L., Yu, X., Zhao, W., Song, X., et al. (2014). Patterns of biomass and carbon distribution across a chrono sequence of Chinese pine (Pinus tabulaeformis) forests. PLoS ONE 9:e94966.
Keywords: forest fire, carbon loss, carbon stock, Himalayan forest, Uttarakhand, Indian Himalayan region
Citation: Bargali H, Pandey A, Bhatt D and Sundriyal RC (2024) Loss of carbon stock in the forests of Uttarakhand due to unprecedented seasonal forest fires. Front. For. Glob. Change. 7:1352265. doi: 10.3389/ffgc.2024.1352265
Edited by:
Gopal Shukla, Uttar Banga Krishi Viswavidyalaya, IndiaReviewed by:
Prashant Sharma, Dr. Yashwant Singh Parmar University of Horticulture and Forestry, IndiaAmit Kumar, Forest Research Institute (ICFRE), India
Copyright © 2024 Bargali, Pandey, Bhatt and Sundriyal. This is an open-access article distributed under the terms of the Creative Commons Attribution License (CC BY). The use, distribution or reproduction in other forums is permitted, provided the original author(s) and the copyright owner(s) are credited and that the original publication in this journal is cited, in accordance with accepted academic practice. No use, distribution or reproduction is permitted which does not comply with these terms.
*Correspondence: Himanshu Bargali, aGltYW5zaHViYXJnYWxpNDRAZ21haWwuY29t; Aseesh Pandey, ZHJhc2Vlc2hwYW5kZXlAZ21haWwuY29t
†ORCID: Himanshu Bargali, orcid.org/0000-0003-1364-2769
Aseesh Pandey, orcid.org/0000-0001-8063-2646