- 1Charles Darwin Research Station, Charles Darwin Foundation, Santa Cruz, Galápagos, Ecuador
- 2Galapagos National Park Directorate, Santa Cruz, Galápagos, Ecuador
Island forests are becoming increasingly fragmented and colonized by invasive species, which can eventually lead to local species extinctions. In the Galapagos Islands, invasive species pose a serious extinction threat to the endemic daisy tree Scalesia pedunculata, formerly the dominant habitat-forming species of the unique Scalesia forest. This forest has been reduced to fragments due to land use changes in the past and is now increasingly invaded by introduced plants. We conducted a field experiment on Santa Cruz Island to assess the impacts of blackberry (Rubus niveus) and two other invasive plant species, Cestrum auriculatum and Tradescantia fluminensis, as well as the effects of the removal of two of these (R. niveus and C. auriculatum) on cover, composition and diversity of the resident plant communities. Particular attention was paid to effects of the invasive species on the S. pedunculata population. Annual vegetation monitoring was carried out in a total of 34 permanent plots (10 m × 10 m) over 10 years (2014–2023), using the line-intercept method. Seventeen of these plots were established in an invaded area and 17 plots in an area with continuous invasive plant removal since 2014. Results indicated that there were significant changes in both the species composition of the plant communities and average percent cover of species over time, comparing removal plots with invaded plots. Species composition in removal plots changed significantly more than in invaded plots, towards a plant community with greater percent cover of endemic species. A significant negative relationship between the three invasive species and cover of S. pedunculata suggested that multiple invader species may have additive negative impacts. Natural recruitment of S. pedunculata by seeds was observed in the removal but not in the invaded plots. These results, as well as the striking decrease of 71% in cover of adult S. pedunculata in the invaded plots indicate that this threatened species will be driven to local extinction on Santa Cruz Island, Galapagos, in less than 20 years if invasive plant species are not removed on a large scale.
Introduction
Biological invasions threaten global ecosystem structure, function and delivery of ecosystem services, and they are increasing geographically and in frequency (Vilà and Hulme, 2017; Pyšek et al., 2020). This is especially the case on islands, which are hotspots of both endemic and alien species richness (Lenzner et al., 2020). Geographic isolation and vacant niches that promoted evolutionary speciation on oceanic islands are the same features that make endemic species on islands highly vulnerable to anthropogenic impacts (Fordham and Brook, 2010; Fernández-Palacios et al., 2021). Land-use change and invasive species are the primary drivers of the decline of oceanic island biota; they can result in extinctions of island endemics (Harter et al., 2015). Invasive species are also a major problem in native forests, which are increasingly perturbed by anthropogenic disturbances (Waddell et al., 2020), including the long-term press disturbance of climate change (Seidl et al., 2017). In response to this situation, ecosystem restoration, defined as the process of assisting the recovery of ecosystems that have been degraded, damaged or destroyed, has become a global priority (SER, 2004; Löf et al., 2019). Long-term monitoring of the plant communities under restoration can provide important insight about the success or failure of management practices (Lindenmayer et al., 2012). A large number of invasive plant removal experiments have been short term, involved only a few species in small plots and have been conducted mainly in grassland and herbaceous communities (Kettenring and Adams, 2011 and citations herein). Long-term data are essential for providing a baseline to evaluate the effects of disturbance and climate change effects on community structure and function because they allow enough time for the effects of species interactions to play out (Hanski and Ovaskainen, 2002; Lindenmayer et al., 2012).
Despite global recognition of the Galapagos Islands as locus of adaptive radiation and speciation, it is not widely known that endemic species are threatened in Galapagos terrestrial ecosystems (Jäger et al., 2009; Rentería et al., 2012; Riegl et al., 2023). This situation occurs especially in the unique humid highland ecosystems, which have undergone massive losses of plant biodiversity and abundance due to their transformation into pastures and agricultural lands (Watson et al., 2009). The remaining forest fragments are exposed to progressive degradation due to the synergistic effects of invasive plant species and climate change (Trueman et al., 2010; Gardener et al., 2013). For example, the extent of the native forest in the humid zone of Santa Cruz formed by Scalesia pedunculata is now only 3% (ca. 300 ha) of its historical distribution, due to past land use change and more recently, to invasions of non-native plant species (Riegl et al., 2023). The worst invasive plant is Rubus niveus (blackberry, Rosaceae), which grows vigorously and prevents recruitment of native species, thus changing the surrounding plant community (Rentería et al., 2012). However, other invasive species such as Cestrum auriculatum and Tradescantia fluminensis are also very abundant in the highlands. Over more than two decades, the Galapagos National Park Directorate (GNPD) has manually and chemically controlled invasive plant species at Los Gemelos, a site in the highlands of Santa Cruz Island. An experimental removal program was established at Los Gemelos in 2014 (Figure 1) to study the effects of R. niveus and other invasive plant species as well as the effects of the intensive management on resident plant community recovery.
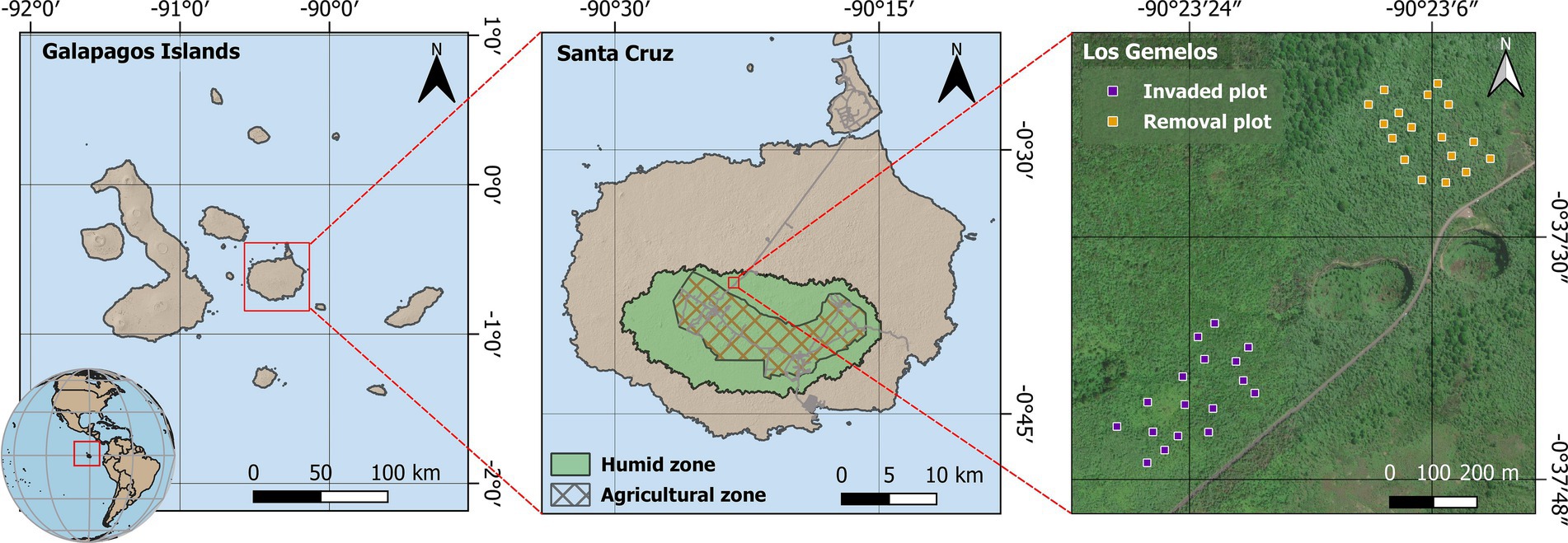
Figure 1. Map of the study site at Los Gemelos on Santa Cruz Island, Galapagos, with 34 (10 m × 10 m) plots: 17 plots in the invaded area (purple squares) and 17 plots where invasive plant species removal is been continuously carried out since 2014 (orange squares).
Our study aimed to investigate the effects of invasive plant species, mainly R. niveus, and the ecological consequences of invasive species management on plant communities, with emphasis on their impact on endemic habitat forming species, specifically Scalesia pedunculata. To achieve this, we conducted a comprehensive assessment that included temporal changes in plant species cover, composition and diversity. We examined how the presence of invasive species, particularly R. niveus, and their subsequent removal, influenced resident plant species over time in both invaded and removal plots. Secondly, the impacts of invasive plant species and impacts of their removal on cover and recruitment of the endemic tree S. pedunculata were studied. Examining both of these aspects will allow us to better understand interactions between invasive, native and endemic plant species within the plant community structure. We predicted that R. niveus (and other invasive plant species) would suppress S. pedunculata and its natural regeneration, and that removal of these plants would increase the cover and diversity of native and endemic plant species. This study assesses the effect of multi-species plant invasions, which while common in nature, are less commonly studied (Brandt et al., 2023).
Materials and methods
Study site
Field work was carried out in one of the only two remaining Scalesia forest patches in the highlands of Santa Cruz Island, Galapagos, Ecuador; at an altitude of about 400–550 m a.s.l., near the twin volcanic sinkholes “Los Gemelos” (00°37′ 20′′ S, 90°23′00′′ W) within the Galapagos National Park (Figure 1). Annual precipitation during the 10-year study period ranged from 1,036 mm in 2014 to 745 mm in 2022. The mean for 2014–2022 was lower (854 mm) than the yearly average from 1987 to 2022 (1,326 mm) (Supplementary Figure S1). Average annual temperature in the study area from 1987 to 2022 was 22.0°C and during the study period (2014–2022), was 20.7°C (Supplementary Figure S1). The remnants of the Scalesia forest on Santa Cruz are composed of the endemic tree species Scalesia pedunculata, which grows up to 15 m in height (Hamann, 2001). It is no longer the dominant species and it competes with aggressive invasive plants, like Rubus niveus (blackberry), Cedrela odorata (Cuban cedar), Cestrum auriculatum (sauco) and Tradescantia fluminensis. To protect S. pedunculata, the Galapagos National Park Directorate (GNPD) has been applying manual and chemical measures to control these invasive plant species for more than 20 years (Jäger et al., 2017; Riegl et al., 2023).
To study the impacts of invasive plant species and the impacts of the removal of these species on the plant communities, we defined two study areas at Los Gemelos. In 2014, we established 34 permanent 10 m × 10 m plots in collaboration with the GNPD. All plots contained R. niveus and other dominant invaders, such as C. auriculatum and T. fluminensis at the onset. Of the 34 plots, 17 were within a 6-ha area where R. niveus and C. auriculatum were continuously removed (removal plots) and 17 were within an 8-ha area that was left untouched (invaded plots). In the removal plots, R. niveus (starting in 2014) and C. auriculatum (starting in 2017) were manually cut down to 5 cm above the ground with a machete and one month later, the regrowth was sprayed with a mixture of the herbicides glyphosate and a combination of metsulfuron-methyl and picloram, a standard control procedure carried out by the GNPD (Jäger et al., 2017). This same treatment was repeated twice more in one-month intervals (three herbicide applications in total). Thereafter, resprouts and germinated blackberry seedlings were manually removed every 3 months from 2015 to 2018 and every 6 months from 2019 on.
Since the GNPD needed to focus removal efforts in a specific area, we were limited in the area where we could set up permanent plots. This led to spatial clustering of removal plots rather than random interspersion of removal and control plots. The two groups of plots were only ~500 m apart and had comparable biotic and abiotic conditions (slopes, tree densities, sun exposure, etc.), thus there was no indication of confounding factors such as differences in microclimate or soil-type (Jäger et al., 2017; Filek et al., 2018; Cimadom et al., 2019). In addition, at the onset of this experiment, the mean combined percent cover of all plant species in the removal and invaded plots was nearly identical and up to 160% of this was comprised of invasive species (Figure 2).
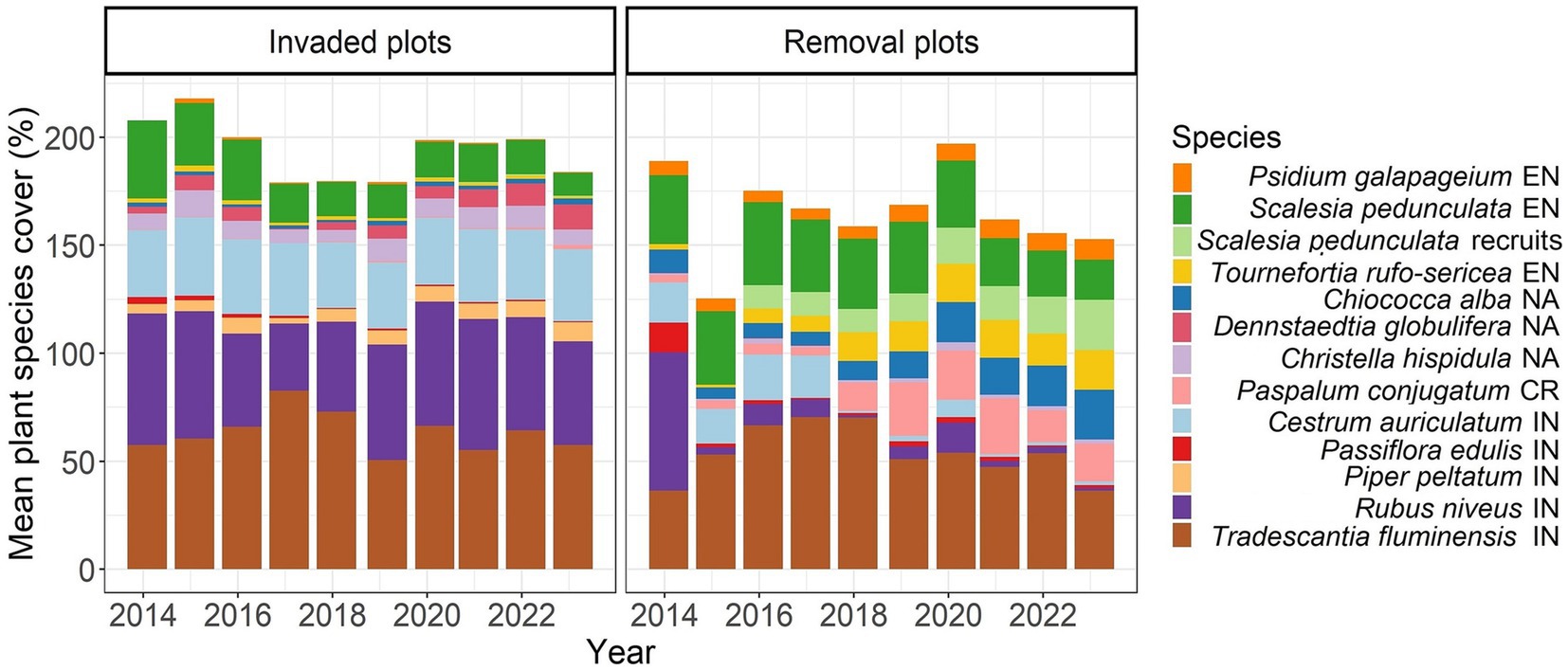
Figure 2. Mean plant species cover (%) of the 13 most common species across years in invaded and removal plots. Origin of species: EN, endemic; NA, native; IN, introduced; CR, cryptogenic. Rubus niveus removal started after vegetation sampling in 2014, and Cestrum auriculatum removal after vegetation monitoring in 2017. Please note that several vegetation strata were measured and therefore, total cover can exceed 100%.
Vegetation monitoring
Vegetation monitoring was carried out once before the removal of invasive plant species commenced (February 2014), and then again annually from 2015 to 2023, always in February/March, at the invaded and removal plots. Monitoring of the vascular plant species was carried out along three parallel 10-m transects, 5 m apart, in each of the 34 permanent plots. The vegetation cover for each species was estimated using the line-intercept method and was always conducted by the same investigator. To account for rare species, the areas between transects were searched and species not recorded along the transects were assigned the minimum cover value (10 cm) (Jäger et al., 2009). The species percentage cover of each plot was calculated by averaging the three transect covers. Since cover was combined across several vegetation strata, cover of grouped species may exceed 100%. Plant species nomenclature and origin follows the database of the Charles Darwin Foundation (CDF, 2023) and the World Flora Online (WFO, 2023).
Statistical analyses
All analyses were performed in R statistical software version 4.2.1 (R Development Core Team, 2022). To show the mean cover of dominant species and to visualize changes by site (invaded vs. removal plots) and year, we first selected the five species with the highest cover per site and year. We obtained a list of 13 species and plotted their mean percent cover stacked per year, separately for invaded and removal plots. To quantify changes in species composition and relative percent cover between treatments across years, we used the codyn package (Hallett et al., 2016) for comparisons of rank abundance curves. We used the multivariate_change() function to quantify dissimilarity between the composition of a sampling over time, which compares and quantifies shifts in the centroids of the community (i.e., compositional change). Finally, we compared the compositional change between sites with a Wilcoxon test.
To assess the community trajectories in invaded and removal plots, we used changes in multivariate dispersion, following Matthews and Spyreas (2010) and Catano et al. (2022). Multivariate dispersion measures the distance from each plot to the centroid (spatial median in principal coordinate space) of all plots in a given year (Anderson et al., 2006). We first calculated Bray–Curtis dissimilarity, using the vegdist() function in vegan package (Oksanen et al., 2022). Species relative cover values were used for pairs of invaded and removal plots, separately for each year. Then, changes in vegetation composition in invaded and removal plots were displayed using non-metric multidimensional scaling (NMDS) of Bray–Curtis dissimilarity matrices through metaMDS() function. We tested whether the centroids for the groups of invaded and removal plots changed over time relative to one another, using Permutational Multivariate Analysis of Variance (PERMANOVA) (McArdle and Anderson, 2001). Also, we used the envfit() function to identify species that may be driving the distribution pattern of the sites.
We assessed the effect of invasive plant species removal on the percent cover of plant species across years, comparing the mean percent cover of endemic, native (non-endemic) and introduced species in invaded and removal plots. Due to the segregated sampling design and to avoid pseudoreplication, we tested differences in endemic plant species cover and number of species in 2014 and in 2023 with generalized linear mixed models (GLMM) in glmmTMB package (Brooks et al., 2023) in invaded and removal plots. For all models, we included plot nested within site as random effects to control for spatial autocorrelation. We determined the effect of treatment and year on the log percentage cover of endemic plant species with a type-II ANOVA, performed on a mixed-effects Gaussian regression. We included random effects for plot/site and species. Due to nesting, the random effects variance was close to zero, so we adjusted the tolerance to 1−10 to get the conditional R2. We used a similar approach for the number of endemic species but fitted a GLMM with a Poisson error distribution with counts of endemic species observed in a plot as the response. We included the site and the year as fixed-effects predictors. Plot number nested in treatment was included as a random effect. To assess the variation explained by each model, we obtained marginal (R2 m ; fixed effects) and conditional (R2 c ; fixed and random effects) R2 values for both models.
We also assessed the changes in mean cover of Scalesia pedunculata in invaded and removal plots, taking into account the cover of adults and recruits (i.e., new individuals since 2014) separately. To assess the effects of invasive plant species on the percent cover of the endemic S. pedunculata over years, we arcsine-square-root transformed the mean percent covers and then performed linear models separately for each year. First, we assessed the effects of two invasive and widespread plant species (the shrub R. niveus and the tree C. auriculatum) on S. pedunculata adult trees in invaded plots. Then, using data from the removal plots, we evaluated the effect of the invasive herb Tradescantia fluminensis on the percent cover of S. pedunculata recruits.
Results
We recorded a total of 115 plant species in the 34 permanent plots from 2014–2023. The majority were of native origin (56 species), followed by introduced (31 species), endemic (20 species), and eight cryptogenic species. At the site of the removal plots, 20 endemic plant species were recorded, 75% of which were also recorded at the site of the invaded plots (15 species). More information on these species is provided in the Supplementary Table S1.
Temporal changes in plant species cover, composition and diversity
There were pronounced differences in the abundance of plant species between the invaded and removal plots (Figure 2). In 2023, the most abundant species in the invaded plots were Rubus niveus (shrub, introduced), Tradescantia fluminensis (herb, introduced), Cestrum auriculatum (tree, introduced) and Dennstaedtia globulifera (fern, native); while in the removal plots, T. fluminensis, Scalesia pedunculata (tree, endemic), Chioccoca alba (shrub, native) and Tournefortia rufo-sericea (shrub, endemic) were the most abundant. As a consequence of the removal of R. niveus and C. auriculatum, invaded and removal plots showed opposite patterns for the percent cover of these species. For example, both sites had similar R. niveus cover in 2014, but after invasive species control, R. niveus cover was maintained below 14% in removal plots from 2015 on. In the invaded plots, R. niveus maintained a mean cover of around 60% (reaching a maximum percent cover of 98% for one of the plots in 2023). The invasive C. auriculatum had not been a target of removal until 2017 and therefore, mean percent cover in 2017 was 20%, whereas in 2023, it was only 1.8%; but in invaded plots it covered 33% on average in 2023 (up to 57% in one of the plots). Invasive T. fluminensis had never been removed, however, mean cover was significantly higher in invaded plots compared to removal plots (57.4% vs. 36.5% in 2023, W = 212, p = 0.02). Cover of some shrub species, like the native C. alba, the endemic Psychotria rufipes, and the endemic T. rufo-sericea, significantly increased over time in the removal plots, whereas in the invaded plots, cover of some native ferns, such as D. globulifera, significantly increased over time (Figure 2).
Plant species of different origins were differently affected by ongoing invasive plant species control since 2014. As a logical result of the control of invasive R. niveus and C. auriculatum, mean percent cover of all introduced species decreased significantly in the removal plots from 135.8% in 2014 to 46.4% in 2023 (Figure 3A). In the invaded plots, mean percent cover of all introduced species did not change significantly over time and oscillated between 147% in 2019 and 166% in 2020. According to GLMM results, mean endemic plant species cover changed differently over time at invaded and removal plots (R2c = 0.491, R2m = 0.044, p < 0.001). The sites (plot types) had a similar total cover of all endemic species in 2014 (p = 0.38) but in 2023, it was significantly different (p < 0.001). There was a significant interaction between site and year (p < 0.001), indicating that in removal plots, the endemic species cover increased significantly from 53.7% in 2014 to 86.2% in 2023, while it decreased significantly in the invaded plots from 47.5% in 2014 to 17.3% in 2023 (Figure 3B). Mean cover of endemic plant species mainly increased because of an increase in the naturally recruited S. pedunculata from 0% in 2014 to 23.2% in 2023 (see detailed information in the following section). On the other hand, the reduction of endemic plant species cover in invaded plots was mainly caused by the decrease in adult S. pedunculata cover by almost 26%. Mean native species cover did not change much, neither over time nor between sites (Figure 3C).
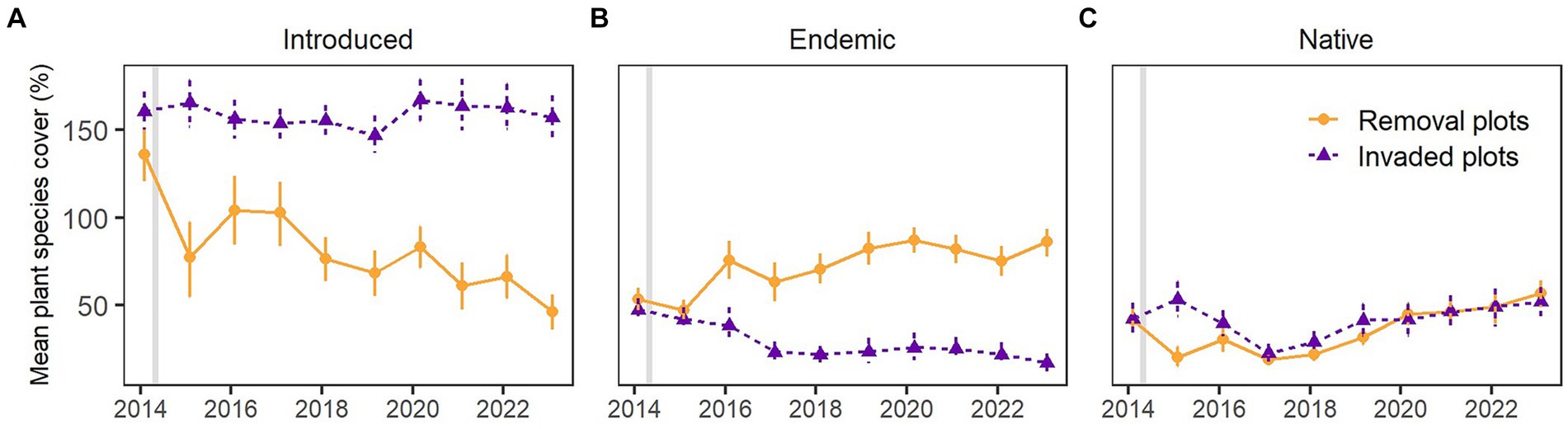
Figure 3. Mean percent cover (±95% CI) of all (A) introduced, (B) endemic, and (C) native plant species in invaded (purple) and removal (orange) plots at Los Gemelos, Santa Cruz, Galapagos. Vertical gray bar indicates when invasive plant species removal started (after first vegetation monitoring in 2014).
With the exception of 2015, when plant species richness was higher in the invaded plots, the number of plant species was similar over time in invaded compared to removal plots (Figure 4). Invaded plots averaged 26.3 species per 100 m2 in 2014 and 26.9 species in 2023, meanwhile removal plots averaged 27.6 species in 2014 and 26.4 species in 2023. There was a decrease in the number of species at both sites in 2017. Although the diversity remained higher in the removal plots, the Shannon Diversity Index (H) exhibited no significant differences between invaded and removal plots over most of the years. However, in 2015, it was significantly higher in invaded plots (W = 47, p < 0.001), probably due to the removal of almost the entire understory by manual and chemical control in removal plots in 2014 (W = 04, p = 0.041, Figure 5). In 2019 and 2020, H was significantly higher in removal plots (W = 219, p = 0.009, Figure 5), which could be an indication of recovery of the plant species community in the removal plots, since in 2021–2023, H was also higher here, but not significantly so.
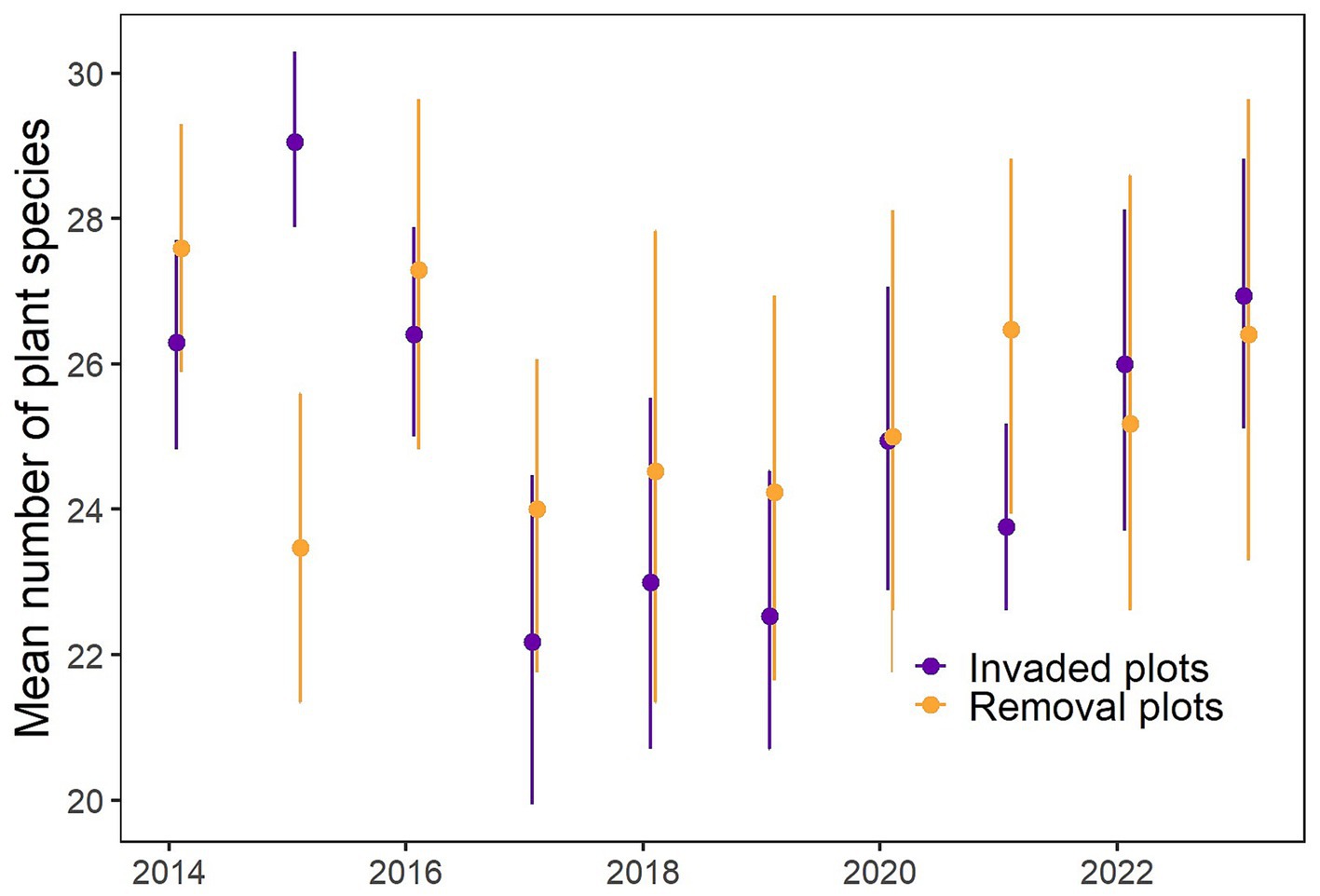
Figure 4. Mean number of all plant species in invaded (purple) and removal (orange) plots from 2014 to 2023. Bars indicate 95% confidence intervals.
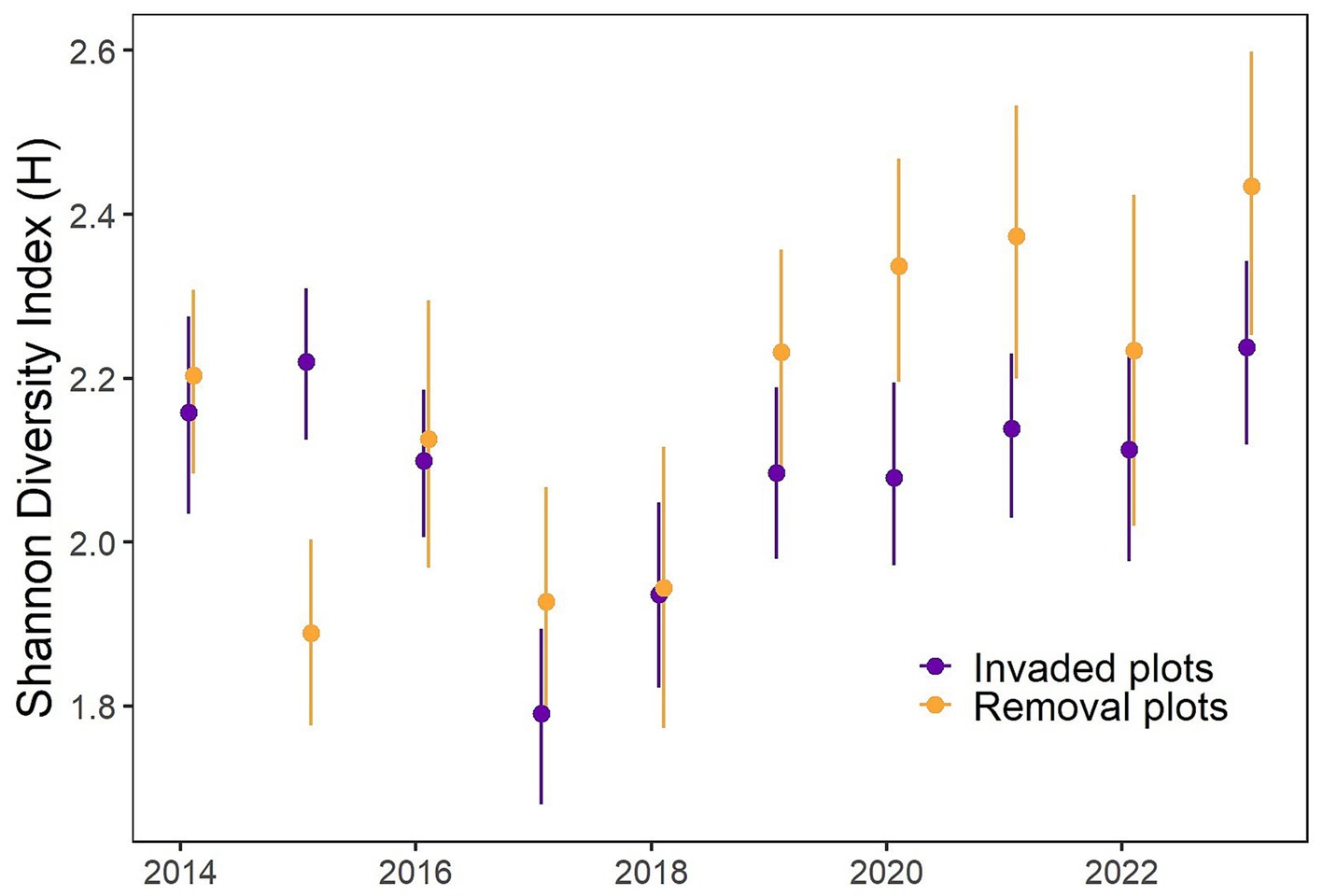
Figure 5. Mean Shannon Diversity Index (H) for all plant species (including dominant invasive species and Scalesia pedunculata) in invaded (purple) and removal (orange) plots. Bars indicate 95% confidence intervals.
Comparison of the multivariate changes in plant species composition for invaded and removal plots from 2014 to 2023, using a Wilcoxon test, showed that species composition over time changed significantly more in removal plots compared to invaded plots (W = 11, p = 0.008, Figure 6). When assessing the plant species community trajectories, invaded and removal plots were well-separated along the first NMDS axis over time (Figure 7). The PERMANOVA showed that the central tendency (centroid; i.e. spatial median in multivariate space) of species composition differed between invaded and removal sites (R2 = 0.22, p = 0.001) and the composition of both groups shifted over time (R2 = 0.11, p = 0.001); with an interaction between plots and year (R2 = 0.10, p = 0.001; Supplementary Table S2). The species that seemed to be driving the site distribution pattern in invaded plots were the invasive species R. niveus, C. auriculatum, T. fluminensis and native ferns, like D. globulifera. In the removal plots, it seemed that endemic species, like S. pedunculata, P. rufipes, T. rufo-sericea, T. pubescens (shrub), and native species like C. alba and Commelina diffusa were mainly driving the site distribution pattern (R2 > 0.25 and p < 0.05, Supplementary Table S3).
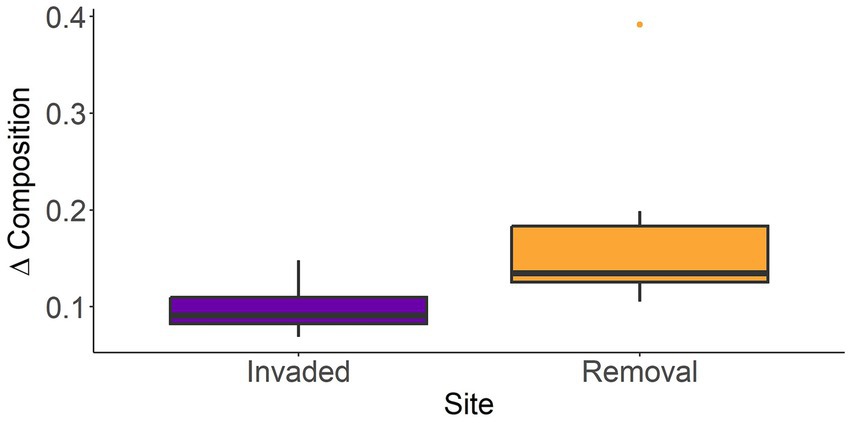
Figure 6. Wilcoxon test for the comparison of the multivariate changes in plant species composition for invaded (purple) and removal (orange) plots at Los Gemelos, Santa Cruz, Galapagos from 2014–2023. Species composition over time changed significantly more in removal plots compared to invaded plots (W = 11, p = 0.008). Median (horizontal line) and 1st and 3rd quartiles (box) shown.
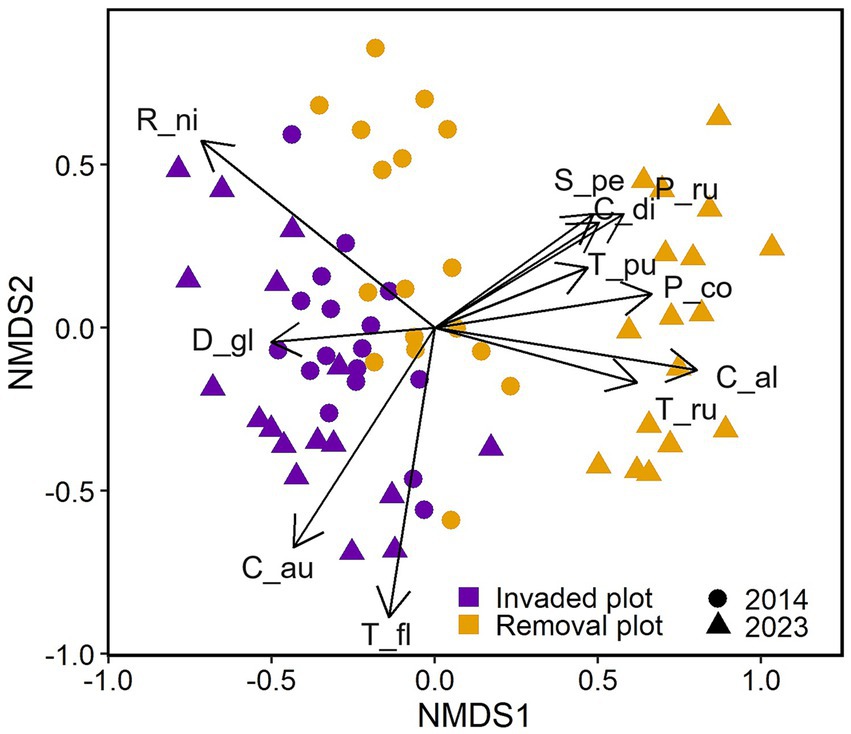
Figure 7. Non-metric multidimensional scaling (NMDS) of plant species composition for each of the 34 permanent plots (17 invaded plots in purple, 17 removal plots in orange) on Santa Cruz, Galapagos (stress = 0.14). Circles represent the communities before removal of invasive species started (2014) and triangles represent the same communities 10 years later (2023). Species composition significantly changed over time only in the removal plots. Species shown are those mainly driving the composition patterns (R2 > 0.25 and p < 0.05). R_ni, Rubus niveus; D_gl, Dennstaedtia globulifera; C_au, Cestrum auriculatum; T_fl, Tradescantia fluminensis; T_ru, Tournefortia rufo-sericea; C_al, Chioccoca alba; P_co, Paspalum conjugatum; T_pu, Tournefortia pubescens; C_di, Commelina diffusa; P_ru, Psychotria rufipes; S_pe, Scalesia pedunculata.
Impacts of invasive plant species and impacts of their control on cover and recruitment of the endemic tree Scalesia pedunculata
The mean cover of adult S. pedunculata trees, which had been present since the onset of the study, decreased significantly from 36.2% in 2014 to 10.5% in 2023 in the invaded plots (R2c = 0.624, R2m = 0.184, p < 0.001) and from 31.9 to 18.5% in the removal plots (R2c = 0.702, R2m = 0.121, p < 0.001, Figures 2, 8). The decrease in adult S. pedunculata cover was lower in the removal plots, compared to the invaded plots. Cover of the naturally recruited S. pedunculata in the removal plots greatly increased over time after the initial invasive plant species removal actions in 2014, from 0% in 2015 to 23.2% in 2023 (Figures 2, 8). In the invaded plots, S. pedunculata seeds were only able to germinate on the trails that had been cut into the R. niveus thicket to access the plots, and consequently, only few S. pedunculata recruits emerged there, barely reaching a cover of 0.5% (Figure 8).
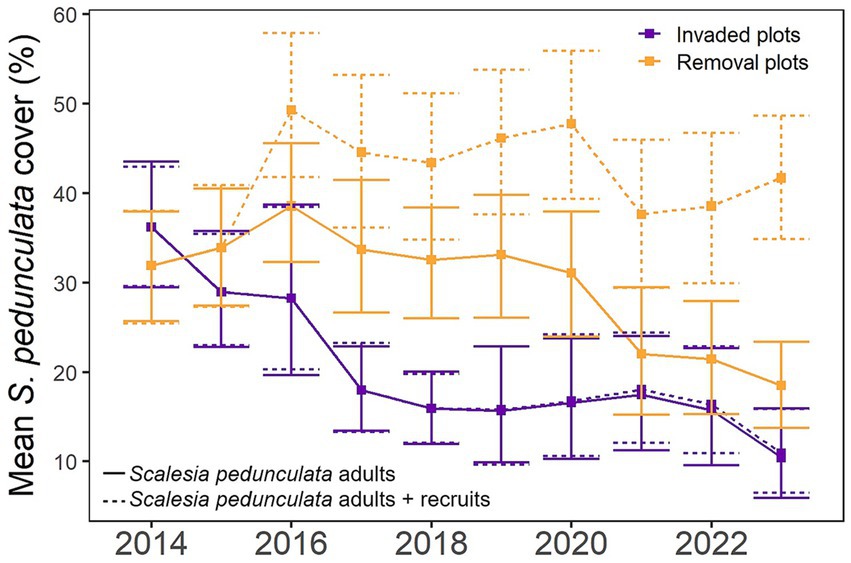
Figure 8. Mean cover of Scalesia pedunculata from 2014 to 2023 in invaded (purple) and removal (orange) plots. Continuous lines indicate cover of adult trees and dotted lines indicate the sum of cover of adult trees and recruits (bars indicate 95% confidence intervals).
In the invaded plots, linear model analyses by year indicated that the combined cover of two dominant invasive plant species (C. auriculatum and R. niveus) had significant negative effects (R2 > 0.27, p < 0.03) on the cover of the endemic S. pedunculata during half of the study years: in 2016, 2017, 2018, 2022, and 2023 (Figure 9; Supplementary Table S4). In the remaining years, there were also negative effects but they were not significantly different from zero. The cover of the invasive ground-covering herb T. fluminensis had a significantly negative effect on the cover of S. pedunculata recruits from 2016 to 2018 (R2 > 0.38, p < 0.008), but not in the other years of the investigation (Figure 9; Supplementary Table S5).
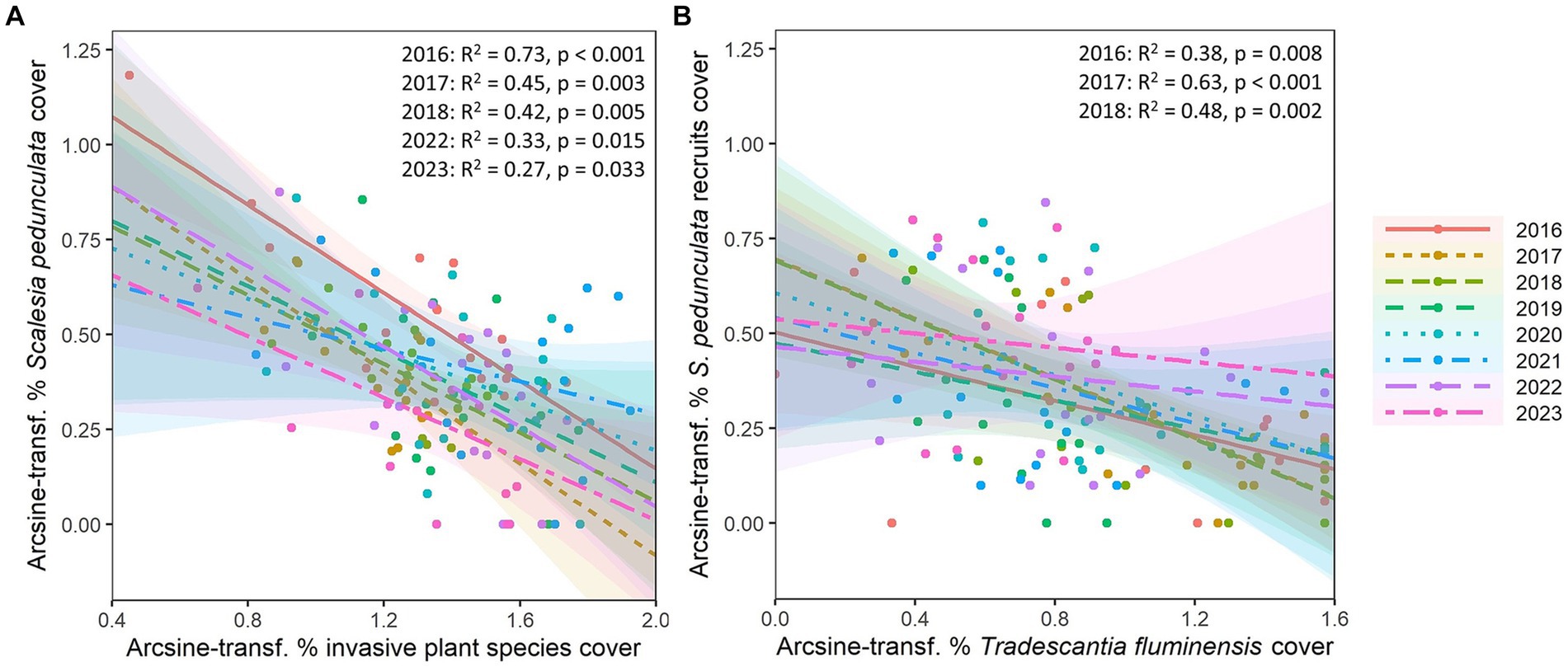
Figure 9. Linear models of the annual effect of (A) cover of two woody dominant invasive plant species combined (Cestrum auriculatum, Rubus niveus) on cover of the endemic Scalesia pedunculata in 17 invaded plots, and (B) effect of the cover of the invasive herb Tradescantia fluminensis on cover of Scalesia pedunculata recruits in removal plots. Axes show the arcsine-sqrt transformed percentage cover. Details of significant relationships are indicated as p-values (p < 0.05) in the top right corner along with R2, variance explained by the model.
Discussion
Temporal changes in plant species cover, composition and diversity
At the end of the 10-year monitoring period, the percent cover of all introduced plant species in the invaded plots had not changed (157% in 2014 vs. 148% in 2023) and the plant community was dominated by the same introduced and invasive species, mainly Rubus niveus, Cestrum auriculatum and Tradescantia fluminensis. Over the same period in the removal plots, percent cover of all introduced species decreased significantly from 136 to 46% due to continuous control actions, with cover of R. niveus decreasing from 60 to 14% and that of Cestrum auriculatum from 20 to 1.8%. The vegetation there was dominated by the native and endemic species Scalesia pedunculata, Chioccoca alba, Tournefortia rufo-sericea and Psychotria rufipes, but also by T. fluminensis (Figures 2, 3). These results contrasted with those of a comprehensive meta-study on the success and impacts of controlling invasive species on the resident plant community, which indicated that gains in native species were mainly small (Kettenring and Adams, 2011). Few of these studies evaluated results over more than 2 years, which could have contributed to limited knowledge about resident species recovery (Kettenring and Adams, 2011). However, in a decade long study in a Hawaiian lowland wet forest, researchers also found little recovery of the native biodiversity despite a constant control of invasive plant species (Cordell et al., 2016). Potential explanations for the success of control actions in the Scalesia forest remnant could be related to the abundant seed production and fast growth of endemic and native species, especially S. pedunculata (Walentowitz et al., 2021), allowing fast regeneration.
Our results indicated that invasive plants and their removal affected plant species differently depending on their origin, with endemic species being the most affected. The cover of all endemic species increased significantly from 54 to 86% in the removal plots, while it decreased significantly from 48 to 17% in invaded plots over the 10-year study. In comparison, cover of native plant species did not change, suggesting that endemic species had been most suppressed by the invaders. The reduction of endemic plant species cover in invaded plots was mainly driven by the decrease in adult S. pedunculata cover by almost 26%. There was a decrease in cover of native and endemic species right after the initial removal event in 2015, which could have been caused by the use of herbicides and manual control methods. However, cover increased again and negative effects seemed ephemeral, which coincides with a restoration study from the Seychelles that documented short-term detrimental effects of herbicides on native plant species (Kaiser-Bunbury et al., 2015).
Contrary to our expectations, the number of plant species as well as the Shannon Diversity index (H) were similar over time in invaded compared to removal plots (Figures 4, 5). Both the number of species and H were significantly lower in the removal plots compared to the invaded plots in 2015, likely as a result of the removal of almost the entire understory (Cimadom et al., 2019). In 2017, number of species and H were lower compared to the previous year in both the invaded and removal plots, which coincided with a dry year in 2016 (average 794 mm, Supplementary Figure S1). The Shannon Diversity was always higher in removal plots compared to invaded plots, except in 2015, but only significantly so in 2019 and 2020. While there were more endemic species present in the removal plots compared to the invaded plots in 2023 (20 vs. 15), the invaded plots accounted for a larger number of native fern species that seemed to manage to grow and even thrive under the thick R. niveus bush. In addition, we recorded annual, ephemeral species that thrive in disturbed areas, like Conyza bonariensis, Adenostemma platyphyllum and other introduced species, which probably contributed to keeping H in the invaded plots at a level similar compared to the removal plots. This underscores the importance of taking species identification into account, which otherwise can mask changes in the communities (Hillebrand et al., 2018). The increased number of fern species under the invasive cover thicket could be an indication that R. niveus facilitates the establishment and growth of fern species by providing a constantly moist environment, a similar effect observed under the invasive quinine tree Cinchona pubescens in Galapagos (Jäger et al., 2009).
Non-metric multidimensional scaling analysis showed that species composition changed significantly over the study period only in the removal plots (Figure 6). Successional trajectories in the removal plots were altered and might be shifting towards a plant community more typical of the original Scalesia forest structure. Here, S. pedunculata, T. rufo-sericea and C. alba, which were the most abundant species, were also the main species driving the plant composition patterns in these plots (Figure 7). In the invaded plots, the most abundant species were also the species driving the plant composition change patterns, namely R. niveus, T. fluminensis and C. auriculatum, as well as the native fern Dennstaedtia globulifera (Figure 7). A study in a different S. pedunculata forest remnant invaded by the introduced Cedrela odorata (Cuban cedar) revealed similar results in the way that this invasive tree had significantly altered the plant species assemblage and forest structural characteristics in this ecosystem (Rivas-Torres et al., 2018). In our study, apart from the dramatic decline of S. pedunculata, the remaining plant community in the invaded plots did not change much over the study period (Figure 7), which could be an indication that the species composition had already been altered by invasive plant species at the onset of this study. The description of the plant species in the Scalesia forest prior to 2001 by Hamann (2001), indicates a plant community more similar to what we now encounter in the removal plots. Importantly, this suggests that changes caused by invasive species are reversible if removal of these harmful species is carried out, as shown by our results.
Since both R. niveus and C. auriculatum were removed in the removal plots, response of plant community cannot be attributed to one or the other species but rather both taken together. Since R. niveus had always been more abundant in the invaded plots, it is likely that it is mainly responsible for the shift in the plant species composition. Despite the constant removal of R. niveus over 10 years and C. auriculatum over 6 years, these species were still present at low percent covers in the removal plots (14 and 1.8% respectively). This may be explained by several factors including the presence of viable seeds in the seedbank, vegetative resprouting by both species after cutting, or re-invasion by seed dispersal from the surrounding invaded areas, as both species are dispersed by animals, particularly birds (Buddenhagen and Jewell, 2006). In Galapagos, R. niveus produces fruits almost all year long that contain a large number of seeds and form large seedbanks of up to 7,000 seeds m2 (Landázuri, 2002). In addition to its large seedbank, a life history strategy involving more rapid and abundant germination than native species makes R. niveus a successful competitor for resources and space (Renteria et al., 2021). In terms of management, this suggests that long-term follow-up control of these invaders is needed to preserve the restored plant community.
Impacts of invasive plant species and impacts of their control on cover and recruitment of the endemic tree Scalesia pedunculata
We also examined the effects of invasive plant species presence and removal on the endemic habitat-forming Scalesia pedunculata. In the invaded plots, linear models indicated that the combined cover of two dominant invasive plant species (C. auriculatum and R. niveus) had significant negative effects on the cover of adult S. pedunculata trees in half of the years: in 2016, 2017, 2018, 2022, and 2023 (Figure 9A; Supplementary Table S4). For the remaining years, there were also negative effects but they were not significantly different from zero. These results are in line with a meta-analysis that showed that an increasing abundance of invasive species has marked negative impacts on populations and communities of native species (Bradley et al., 2019). In fact, another study in the Scalesia forest remnant of Santa Cruz postulated that a R. niveus cover of 60% might be a threshold for adverse effects on the native plant community (Rentería et al., 2012), which is exactly what we found in our study: a mean cover of 60% in the invaded plots made it impossible for the S. pedunculata seeds to germinate (Figure 2). Cover of the invasive ground-covering herb Tradescantia fluminensis significantly decreased the cover of Scalesia pedunculata recruits from 2016 to 2018 but not in the remaining years (Figure 9B; Supplementary Table S5). The likely reason for this is that the S. pedunculata recruits are most vulnerable to shading by T. fluminensis while they are still small. Once surpassing T. fluminensis height, S. pedunculata is able to outgrow the invasive herb. Tradescantia fluminensis is known to be a severe invader elsewhere and has been shown to alter nutrient availability in temperate forests and to hinder native forest regeneration (Standish et al., 2004). We found a multi-species invader effect that could be an additive effect (Brandt et al., 2023) and suggests that more work needs to be done on the interactive effects of co-occurring invasive species (Kuebbing et al., 2013).
In the removal plots, cover of naturally recruited S. pedunculata greatly increased over time after the initial invasive plant species removal actions in 2014, from 0% in 2015 to 23.2% in 2023 (Figures 2, 8). These results are in line with the previously mentioned study that tracked the number of adult S. pedunculata trees and naturally recruited seedlings over a shorter period (8 years, Jäger et al., 2023; Riegl et al., 2023). As in these studies, natural recruitment of S. pedunculata only took place on the access trails that were cut into the R. niveus thicket to be able to access the permanent plots and thus technically grew outside the plots (but their crowns reached into the plots and therefore, cover was measured), barely reaching a cover of 0.5% (Figure 8). The applied aspect of this finding is that even cutting narrow trails into dense R. niveus can spark the germination of the light-dependent small S. pedunculata seeds (Riegl et al., 2023). This suggests that R. niveus creates shade atypical for S. pedunculata, which leads to further dominance of the invader and strong inhibition of this endangered species (Reinhart et al., 2006). To assess impacts of invasive plants and impacts of their removal on soil parameters, we took soil samples in both plot types that are currently being analyzed.
Significant loss of S. pedunculata cover within plot type over the 10-year study was more pronounced in the invaded than the removal plots (71% vs. 42% respectively, Figures 2, 8). These results are in line with a study that showed a higher mortality rate of adult S. pedunculata trees in the invaded plots at the same sites (Riegl et al., 2023). Scalesia pedunculata is a pioneer species and as such, produced numerous seeds that have a high germination rate, fast growth, a high mortality and a short life-span of 15–20 years (Hamann, 2001; Walentowitz et al., 2021; Riegl et al., 2023). Before the invasion by R. niveus, dying trees would be replaced by natural regeneration of S. pedunculata, but with shade-casting R. niveus thickets, germination of S. pedunculata seeds now has become impossible (Rentería et al., 2012; Riegl et al., 2023). In fact, not a single S. pedunculata seedling was encountered in the invaded plots over the decade of our study, which is very concerning, especially in the face of climate change. Strong El Niños events are predicted to increase in Galapagos, likely increasing precipitation, which would further exacerbate problems with invasive plant species (Paltán et al., 2021). In a meta-analysis of 273 areas of exceptional biodiversity and uniqueness of species, endemic species were shown to be 2.7 to 10 times more impacted by climate change (Manes et al., 2021).
Conclusion
The observed exclusive natural regeneration of S. pedunculata in removal plots highlights the devastating impact that invasive understory cover has on the recruitment of Scalesia pedunculata. Our results clearly indicate that R. niveus could drive a local extinction of S. pedunculata and other associated endemic plant species, especially under climate change scenarios predicted for Galapagos. This study underscores the importance of ongoing efforts to manage invasive plant species, particularly Rubus niveus, in order to preserve vulnerable native and endemic plant communities in island ecosystems. These efforts are vital not only for protecting endangered endemic species like Scalesia pedunculata but also for maintaining the overall biodiversity and ecological integrity of this unique ecosystem. The removal of R. niveus had significantly positive effects on S. pedunculata, by promoting its natural regeneration and by slowing down the death of adult trees. Without invasive plant species control we will lose the Scalesia forest in less than 20 years if no large-scale control of the invaders is carried out (Riegl et al., 2023). Insights from this study will help guide management decisions on invasive plants in Galapagos and in other island ecosystems worldwide.
Data availability statement
The raw data supporting the conclusions of this article will be made available by the authors, without undue reservation.
Author contributions
HJ: Conceptualization, Data curation, Funding acquisition, Investigation, Methodology, Project administration, Supervision, Writing – original draft. MS-J: Data curation, Formal analysis, Methodology, Visualization, Writing – review & editing. CP: Data curation, Formal analysis, Writing – review & editing. RC: Project administration, Resources, Writing – review & editing. CS: Project administration, Resources, Writing – review & editing.
Funding
The author(s) declare that financial support was received for the research, authorship, and/or publication of this article. Funding to carry out the research was provided by the Lindblad Expeditions-National Geographic Fund (LX15-17, LX-01C-18, LX-92247C-21), Keidanren Nature Conservation Fund (15–20–01–1-1, 2016–0043, 2019–0048, 2020–0202), Re:wild (SMA-G00-00250), Galapagos PRO, Azimuth Foundation and Galapagos Conservancy (001–2015).
Acknowledgments
We would like to thank the Galapagos National Park Directorate (GNPD) and the Charles Darwin Foundation (CDF) for their support of this project, as well as M. Loyola and R. Ballesteros for their help in the field, and P. León for providing the map. J. Witman and L. Werden provided statistical advice and J. Witman revised the final version of the manuscript. A special thank you to the reviewers for their valuable comments during the review process. Weather data provided by Rolf Sievers is greatly appreciated. Thanks to the GNPD for issuing research permits PC-67-14, PC-19-15, PC-50-16, PC-42-17, PC-50-18, PC-55-19, PC-26-20, PC-18-21, PC-28-22 and PC-15-23. This publication is contribution number 2581 of the Charles Darwin Foundation for the Galapagos Islands.
Conflict of interest
The authors declare that the research was conducted in the absence of any commercial or financial relationships that could be construed as a potential conflict of interest.
Publisher’s note
All claims expressed in this article are solely those of the authors and do not necessarily represent those of their affiliated organizations, or those of the publisher, the editors and the reviewers. Any product that may be evaluated in this article, or claim that may be made by its manufacturer, is not guaranteed or endorsed by the publisher.
Supplementary material
The Supplementary material for this article can be found online at: https://www.frontiersin.org/articles/10.3389/ffgc.2024.1350498/full#supplementary-material
References
Anderson, M. J., Ellingsen, K. E., and McArdle, B. H. (2006). Multivariate dispersion as a measure of beta diversity. Ecol. Lett. 9, 683–693. doi: 10.1111/j.1461-0248.2006.00926.x
Bradley, B. A., Laginhas, B. B., Whitlock, R., Allen, J. M., Bates, A. E., Bernatchez, G., et al. (2019). Disentangling the abundance–impact relationship for invasive species. Proc. Natl. Acad. Sci. 116, 9919–9924. doi: 10.1073/pnas.1818081116
Brandt, A. J., Png, G. K., Jo, I., McGrannachan, C., Allen, K., Peltzer, D. A., et al. (2023). Managing multi-species plant invasions when interactions influence their impact. Front. Ecol. Environ. 21, 370–379. doi: 10.1002/fee.2658
Brooks, M., Bolker, B., Kristensen, K., Maechler, M., Magnusson, A., McGillycuddy, M., et al. (2023). glmmTMB: Generalized Linear Mixed Models using Template Model Builder. Available at: https://cran.r-project.org/web/packages/glmmTMB/index.html (Accessed June 4, 2023)
Buddenhagen, C., and Jewell, K. J. (2006). Invasive plant seed viability after processing by some endemic Galapagos birds. Ornitol. Neotropical 17, 73–80. Available online: https://digitalcommons.usf.edu/ornitologia_neotropical/vol17/iss1/7
Catano, C. P., Groves, A. M., and Brudvig, L. A. (2022). Community assembly history alters relationships between biodiversity and ecosystem functions during restoration. Ecology 104:e3910. doi: 10.1002/ecy.3910
CDF (2023). Galapagos Species Checklist. Charles Darwin Found. DataZone. Available at: https://www.darwinfoundation.org/en/datazone/checklist (Accessed October 8, 2023)
Cimadom, A., Jäger, H., Schulze, C. H., Hood-Nowotny, R., Wappl, C., and Tebbich, S. (2019). Weed management increases the detrimental effect of an invasive parasite on arboreal Darwin’s finches. Biol. Conserv. 233, 93–101. doi: 10.1016/j.biocon.2019.02.025
Cordell, S., Ostertag, R., Michaud, J., and Warman, L. (2016). Quandaries of a decade-long restoration experiment trying to reduce invasive species: beat them, join them, give up, or start over? Restor. Ecol. 24, 139–144. doi: 10.1111/rec.12321
Fernández-Palacios, J. M., Kreft, H., Irl, S. D. H., Norder, S., Ah-Peng, C., Borges, P. A. V., et al. (2021). Scientists’ warning – the outstanding biodiversity of islands is in peril. Glob. Ecol. Conserv. 31:e01847. doi: 10.1016/j.gecco.2021.e01847
Filek, N., Cimadom, A., Schulze, C. H., Jäger, H., and Tebbich, S. (2018). The impact of invasive plant management on the foraging ecology of the warbler finch (Certhidea olivacea) and the small tree finch (Camarhynchus parvulus) on Galápagos. J. Ornithol. 159, 129–140. doi: 10.1007/s10336-017-1481-4
Fordham, D. A., and Brook, B. W. (2010). Why tropical island endemics are acutely susceptible to global change. Biodivers. Conserv. 19, 329–342. doi: 10.1007/s10531-008-9529-7
Gardener, M. R., Trueman, M., Buddenhagen, C., Heleno, R., Jäger, H., Atkinson, R., et al. (2013). “A pragmatic approach to the Management of Plant Invasions in Galapagos” in Plant invasions in protected areas: Patterns, problems and challenges. eds. L. C. Foxcroft, P. Pyšek, D. M. Richardson, and P. Genovesi (Dordrecht: Springer Netherlands), 349–374.
Hallett, L. M., Jones, S. K., MacDonald, A. A. M., Jones, M. B., Flynn, D. F. B., Ripplinger, J., et al. (2016). Codyn: an r package of community dynamics metrics. Methods Ecol. Evol. 7, 1146–1151. doi: 10.1111/2041-210X.12569
Hamann, O. (2001). Demographic studies of three indigenous stand-forming plant taxa (Scalesia, Opuntia, and Bursera) in the Galápagos Islands, Ecuador. Biodivers. Conserv. 10, 223–250. doi: 10.1023/A:1008952319105
Hanski, I., and Ovaskainen, O. (2002). Extinction debt at extinction threshold. Conserv. Biol. 16, 666–673. doi: 10.1046/j.1523-1739.2002.00342.x
Harter, D. E. V., Irl, S. D. H., Seo, B., Steinbauer, M. J., Gillespie, R., Triantis, K. A., et al. (2015). Impacts of global climate change on the floras of oceanic islands – projections, implications and current knowledge. Perspect. Plant Ecol. Evol. Syst. 17, 160–183. doi: 10.1016/j.ppees.2015.01.003
Hillebrand, H., Blasius, B., Borer, E. T., Chase, J. M., Downing, J. A., Eriksson, B. K., et al. (2018). Biodiversity change is uncoupled from species richness trends: consequences for conservation and monitoring. J. Appl. Ecol. 55, 169–184. doi: 10.1111/1365-2664.12959
Jäger, H., Buchholz, S., Cimadom, A., Tebbich, S., Rodríguez, J., Barrera, D., et al. (2017). Restoration of the blackberry-invaded Scalesia forest: impacts on the vegetation, invertebrates, and birds in Galapagos Report 2015–2016 GNPD, GCREG, CDF, and GC, 142–148.
Jäger, H., Kowarik, I., and Tye, A. (2009). Destruction without extinction: long-term impacts of an invasive tree species on Galápagos highland vegetation. J. Ecol. 97, 1252–1263. doi: 10.1111/j.1365-2745.2009.01578.x
Jäger, H., Sevilla, C., and Riegl, B. (2023). Endemic Galapagos tree daisy Scalesia pedunculata threatened by competition with nonnative blackberry Rubus niveus. Bull. Ecol. Soc. Am. 104:e2074. doi: 10.1002/bes2.2074
Kaiser-Bunbury, C. N., Mougal, J., Valentin, T., Gabriel, R., and Blüthgen, N. (2015). Herbicide application as a habitat restoration tool: impact on native island plant communities. Appl. Veg. Sci. 18, 650–660. doi: 10.1111/avsc.12183
Kettenring, K. M., and Adams, C. R. (2011). Lessons learned from invasive plant control experiments: a systematic review and meta-analysis. J. Appl. Ecol. 48, 970–979. doi: 10.1111/j.1365-2664.2011.01979.x
Kuebbing, S. E., Nuñez, M. A., and Simberloff, D. (2013). Current mismatch between research and conservation efforts: the need to study co-occurring invasive plant species. Biol. Conserv. 160, 121–129. doi: 10.1016/j.biocon.2013.01.009
Landázuri, O. (2002). Distribución, fenología reproductiva y dinámica del banco de semillas de mora (Rubus niveus Thunb) en la parte alta de la isla Santa Cruz, Galápagos. Honors dissertation. Quito, Ecuador: Universidad Central del Ecuador.
Lenzner, B., Latombe, G., Capinha, C., Bellard, C., Courchamp, F., Diagne, C., et al. (2020). What will the future bring for biological invasions on islands? An expert-based assessment. Front. Ecol. Evol. 8:280. doi: 10.3389/fevo.2020.00280
Lindenmayer, D., Likens, G., Andersen, A., Bowman, D., Bull, C., Burns, E., et al. (2012). Value of long-term ecological studies. Austral Ecol. 37, 745–757. doi: 10.1111/j.1442-9993.2011.02351.x
Löf, M., Madsen, P., Metslaid, M., Witzell, J., and Jacobs, D. F. (2019). Restoring forests: regeneration and ecosystem function for the future. New For. 50, 139–151. doi: 10.1007/s11056-019-09713-0
Manes, S., Costello, M. J., Beckett, H., Debnath, A., Devenish-Nelson, E., Grey, K.-A., et al. (2021). Endemism increases species' climate change risk in areas of global biodiversity importance. Biol. Conserv. 257:109070. doi: 10.1016/j.biocon.2021.109070
Matthews, J. W., and Spyreas, G. (2010). Convergence and divergence in plant community trajectories as a framework for monitoring wetland restoration progress. J. Appl. Ecol. 47, 1128–1136. doi: 10.1111/j.1365-2664.2010.01862.x
McArdle, B. H., and Anderson, M. J. (2001). Fitting multivariate models to community data: a comment on distance-based redundancy analysis. Ecology 82, 290–297. doi: 10.1890/0012-9658(2001)082(0290:FMMTCD)2.0.CO;2
Oksanen, J., Simpson, G. L., Blanchet, F. G., Kindt, R., Legendre, P., Minchin, P. R., et al. (2022). vegan: Community Ecology Package. Available at: https://cran.r-project.org/web/packages/vegan/index.html (Accessed March 4, 2023)
Paltán, H. A., Benite, F. L., Rosero, P., Escobar-Camacho, D., Cuesta, F., and Mena, C. F. (2021). Climate and sea surface trends in the Galapagos Islands. Sci. Rep. 11:14465. doi: 10.1038/s41598-021-93870-w
Pyšek, P., Hulme, P. E., Simberloff, D., Bacher, S., Blackburn, T. M., Carlton, J. T., et al. (2020). Scientists’ warning on invasive alien species. Biol. Rev. 95, 1511–1534. doi: 10.1111/brv.12627
R Development Core Team (2022). R: a language and environment for statistical computing. Available at: http://www.R-project.org/ (Accessed February 1, 2023)
Reinhart, K. O., Gurnee, J., Tirado, R., and Callaway, R. M. (2006). Invasion through quantitative effects: intense shade drives native decline and invasive success. Ecol. Appl. 16, 1821–1831. doi: 10.1890/1051-0761(2006)016(1821:ITQEIS)2.0.CO;2
Renteria, J. L., Atkinson, R., Crespo, C., Gardener, M. R., and Grosholz, E. D. (2021). Challenges for the management of the invasive blackberry (Rubus niveus) in the restoration of the Scalesia forest in the Galapagos Islands. Invasive Plant Sci. Manag. 14, 20–28. doi: 10.1017/inp.2021.5
Rentería, J. L., Gardener, M. R., Panetta, F. D., Atkinson, R., and Crawley, M. J. (2012). Possible impacts of the invasive plant Rubus niveus on the native vegetation of the Scalesia forest in the Galapagos Islands. PLoS One 7:e48106. doi: 10.1371/journal.pone.0048106
Riegl, B., Walentowitz, A., Sevilla, C., Chango, R., and Jäger, H. (2023). Invasive blackberry outcompetes the endemic Galapagos tree daisy Scalesia pedunculata. Ecol. Appl. 33:e2846. doi: 10.1002/eap.2846
Rivas-Torres, G., Luke Flory, S., and Loiselle, B. (2018). Plant community composition and structural characteristics of an invaded forest in the Galápagos. Biodivers. Conserv. 27, 329–344. doi: 10.1007/s10531-017-1437-2
Seidl, R., Thom, D., Kautz, M., Martin-Benito, D., Peltoniemi, M., Vacchiano, G., et al. (2017). Forest disturbances under climate change. Nat. Clim. Chang. 7, 395–402. doi: 10.1038/nclimate3303
SER (2004). The SER international primer on ecological restoration. Tucson: Society for Ecological Restoration International
Standish, R. J., Williams, P. A., Robertson, A. W., Scott, N. A., and Hedderley, D. I. (2004). Invasion by a perennial herb increases decomposition rate and alters nutrient availability in warm temperate lowland forest remnants. Biol. Invasions 6, 71–81. doi: 10.1023/B:BINV.0000010127.06695.f4
Trueman, M., Atkinson, R., Guézou, A., and Wurm, P. (2010). Residence time and human-mediated propagule pressure at work in the alien flora of Galapagos. Biol. Invasions 12, 3949–3960. doi: 10.1007/s10530-010-9822-8
Vilà, M., and Hulme, P. E.. (2017). Impact of biological invasions on ecosystem services. Cham: Springer International Publishing
Waddell, E. H., Chapman, D. S., Hill, J. K., Hughes, M., Bin Sailim, A., Tangah, J., et al. (2020). Trait filtering during exotic plant invasion of tropical rainforest remnants along a disturbance gradient. Funct. Ecol. 34, 2584–2597. doi: 10.1111/1365-2435.13679
Walentowitz, A., Manthey, M., Bentet Preciado, M. B., Chango, R., Sevilla, C., and Jäger, H. (2021). Limited natural regeneration of unique Scalesia forest following invasive plant removal in Galapagos. PLoS One 16:e0258467. doi: 10.1371/journal.pone.0258467
Watson, J., Trueman, M., Tufet, M., Henderson, S., and Atkinson, R. (2009). Mapping terrestrial anthropogenic degradation on the inhabited islands of the Galapagos archipelago. Oryx 44, 79–82. doi: 10.1017/S0030605309990226
WFO (2023). World Flora Online. Plant List. Available at: https://wfoplantlist.org/plant-list (Accessed October 18, 2023).
Keywords: cloud forest, unique endemic species, invasive plant species, restoration, ecosystem management, extinction, giant daisy tree, National Park
Citation: Jäger H, San-José M, Peabody C, Chango R and Sevilla C (2024) Restoring the threatened Scalesia forest: insights from a decade of invasive plant management in Galapagos. Front. For. Glob. Change. 7:1350498. doi: 10.3389/ffgc.2024.1350498
Edited by:
Yashwant Singh Rawat, Federal Technical and Vocational Education and Training Institute (FTVETI), EthiopiaReviewed by:
Sudhanshu Kumar, Banaras Hindu University, IndiaPanayiotis G. Dimitrakopoulos, University of the Aegean, Greece
Copyright © 2024 Jäger, San-José, Peabody, Chango and Sevilla. This is an open-access article distributed under the terms of the Creative Commons Attribution License (CC BY). The use, distribution or reproduction in other forums is permitted, provided the original author(s) and the copyright owner(s) are credited and that the original publication in this journal is cited, in accordance with accepted academic practice. No use, distribution or reproduction is permitted which does not comply with these terms.
*Correspondence: Heinke Jäger, aGVpbmtlLmphZWdlckBmY2Rhcndpbi5vcmcuZWM=