- State Key Laboratory of Eco-Hydraulics in Northwest Arid Region of China, Xi’an University of Technology, Xi’an, China
The dissolved organic matter (DOM) in forest ecosystems significantly impacts soil carbon cycling due to its active turnover characteristics. However, whether different plantation forest soil profiles exhibit distinct DOM characteristics remains unclear. Hence, utilizing fluorescence spectroscopy and the parallel factor analysis (PARAFAC) method, a 1-meter soil profile analysis was carried out on three distinct artificial forests (Pinus tabuliformis (PT), Quercus crispula (QC), and a mixed forest of PT and QC (MF)), concurrently assessing the impact of soil chemical properties and enzyme activity on dissolved organic matter (DOM). The findings indicated that the mean concentration of dissolved organic carbon (DOC) was greatest in the MF and lowest in PT, exhibiting considerable variation with soil depth, suggesting that mixed tree species may promote the discharge of organic matter. The fluorescence spectra revealed two distinct peaks: humic-like fluorescence peaks (Peaks A and C) and a protein-like fluorescence peak (Peak T), with the most intense fluorescence observed in MF soil. As the soil depth increased, the fluorescence intensity of Peaks A and C steadily declined, while the intensity of Peak T rose. Four DOM components were identified in three types of plantations forests: surface soil was dominated by humic acid-like fluorescent components (C1 and C2), while the deep soil was primarily characterized by protein-like fluorescence components (C3 and C4). Different soil profile fluorescence parameter indices indicated that the source of DOM in the surface soil (i.e., 0–20 cm) was mainly allochthonous inputs, whereas, in the deep soil (i.e., 60–100 cm), it was mainly autochthonous, such as microbial activity. The findings from the partial least squares path modeling (PLS-PM) revealed that TP, aP, NH4+-N, and the combined impact of soil enzymes were influential in shaping the diversity of DOM attributes. Put differently, alterations in DOM concentration were concomitantly influenced by forest classification, soil characteristics, and depth. It has been demonstrated that, in contrast to monoculture forests, the establishment of mixed forest models has been more advantageous in enhancing the soil dissolved organic matter (DOM). These discoveries offer innovative perspectives on the dynamic characteristics of DOM in soil profiles and its influencing factors under different plantations forest planting patterns.
Highlights
• The dissolved organic carbon (DOC) content in order was MF (Quercus acutissima mixed forest) > QC (Quercus acutissima) > PT (Pinus tabulaeformis).
• Surface soil DOM was mainly humic acid substances, while deep soil DOM was mainly tryptophan substances.
• Mixed forest species were more beneficial for increasing soil organic matter than single species forests.
1 Introduction
Forest-derived dissolved organic matter (DOM) has various chemical compositions and heterogeneous physical properties (Smith et al., 2013; Xu and Guo, 2017). DOM participates a significant in the cyclical changes of carbon of forest ecosystems and facilitates the migration, transformation, cycling of organic matter in the entire ecological environment (Bolan et al., 2011; Batjes, 2014). In forest ecosystems, DOM originates from the decay and degradation of organic matter, substances produced by animal and microbial metabolism, secretions from plant roots, and litter produced during plant growth (Jaffrain et al., 2010; Kaiser and Kalbitz, 2012). Owing to the unique hydrological pathways of forest ecosystems, rainwater percolates through penetrates the surface litter layer, inevitably exerting a strong influence on the further downward migration of DOM (Thieme et al., 2019). Additionally, different forest stand types are important factors influencing the origin of DOM. For example, DOM in coniferous forests primarily originates from the decomposition of plant biomass, such as leaves, bark, and branches, whereas DOM in broadleaf forests is mainly derived from leaf litter decomposition and root exudates (Kiikkilä et al., 2006). Therefore, it is vital to characterize the DOM components of different forest species and the vertical depth of soil to understand the carbon cycling processes in this region.
Decaying debris, root systems, and microbial secretions in forests are potential nutrient sources for soil microbes, promoting microbial proliferation, and thus releasing DOM into the soil (Schmidt et al., 2011). Different soil minerals have varying abilities and selectivity for the adsorption of DOM, which ultimately affects the stability and decomposition rate of organic matter (Saidy et al., 2013; Liu et al., 2023a). However, different vegetation types have significantly different effects on soil enzymes. Research by He et al. (2020) indicated that, compared to coniferous tree species, broadleaf tree species had higher levels of C-cycle-related enzymes (β-glucosidase, BG). Guo et al. (2023) emphasized through meta-analysis that the mixture of tree species had a positive impact on the soil nutrients and enzyme activity of fir trees. The physicochemical properties of the soil, metabolic processes of soil microorganisms, and catalytic effects of soil enzymes are key driving factors in the differentiation of DOM profiles in the soil (Hu et al., 2021; Li H. et al., 2022). The availability of soil microbiota and enzymes is usually influenced by soil depth (Goberna et al., 2005; Eilers et al., 2012; Stone et al., 2014), possibly due to oxygen limitation and soil properties (Ko et al., 2017). A recent study used parallel factor analysis (PARAFAC) and found that soil physicochemical properties are important factors affecting DOM, whereas microbial metabolism and enzyme activity were identified as factors that further influenced DOM at a deeper level (Li W. et al., 2022). Thieme et al. (2019) observed that the composition of DOM varies based on the tree species, while investigating coniferous, deciduous, and unmanaged birch forests. Li W. et al. (2022) identified that the dominant component of DOM in the surface soil of Larix principis-rupprechtii in North China was humic substances, which originated from plant residues. Although these results indicate that the migration of dissolved organic matter (DOM) in soil profiles is influenced by plant inputs, microbial processing, and re-synthesis, limited studies have only focused on the DOM characteristics in soils of single tree species. The effects brought about by mixed forest planting patterns are increasingly evident, such as improving forest structure and increasing species diversity. However, it remains unclear whether this pattern affects DOM. Moreover, data on different DOM characteristics in soil profiles of artificial forests, which are important forest resources, are still limited.
The Loess Plateau, an important ecological security barrier in China, is one of the world’s most ecologically fragile areas. To improve land productivity and safeguard the living environment of the people, the government has implemented large-scale afforestation policies and conducted extensive reforestation efforts (Wang et al., 2013; Meng et al., 2022). Studying the characteristics of DOM in the soils of plantation forests in this region provides insights into the impact of plantation forests on soil properties and carbon cycling. Common plantations tree species in here include Pinus tabuliformis (PT, coniferous) and Quercus acutissima Carruth (QC, broad-leaved), both of which have significantly different effects on soil. Coniferous tree leaves are difficult to decompose, resulting in soil acidification (Jílková et al., 2019). Compared with needle leaves, broad leaves are more easily decomposed, resulting in the enrichment of soil organic matter (SOM) and an increase in soil fertility (Song et al., 2016). However, mixed forests of coniferous and broad-leaved trees typically have high SOM accumulation and low organic matter decomposition rates, leading to carbon sequestration (Li et al., 2022). Consequently, exploring the characteristics of DOM in plantation forests is crucial for a deeper understanding of the properties of plantation forest soils and their impact on carbon cycling, especially in the Loess Plateau region.
The aims of this study are to (1) investigate the vertical distribution characteristics of soil DOM spectra in different forest stands, and (2) explore the response of soil DOM to the interaction between soil physicochemical properties and soil enzymes. This study provides novel insights into the changes and potential driving factors of soil DOM under different planting modes and helps to facilitate future studies on soil fertility and carbon storage in plantation forests.
2 Materials and methods
2.1 Study description
The soil samples were collected the northern part of the Shuanglong State-owned Ecological Experimental Forest Farm, Huangling County, Yan’an City, China. The geographical coordinates are 108°45′32″ E—109°1′21″ E and 35°33′7″ N—35°49′30″ N. This region has a warm-temperate continental monsoon climate. The soil is classified as grey-brown, with less noticeable soil calcification and silicification, which creates favourable conditions for tree growth. The predominant tree species include Pinus tabulaeformis, Chamaecyparis fortunei, Larix gmelinii, Quercus spp., Betula platyphylla, Robinia pseudoacacia, Populus davidiana, and deciduous and evergreen hardwoods. The trees in the plantation are approximately 30 years. The locations of the watersheds under study are depicted in Figure 1, while Table 1 provides essential information about the study plots.
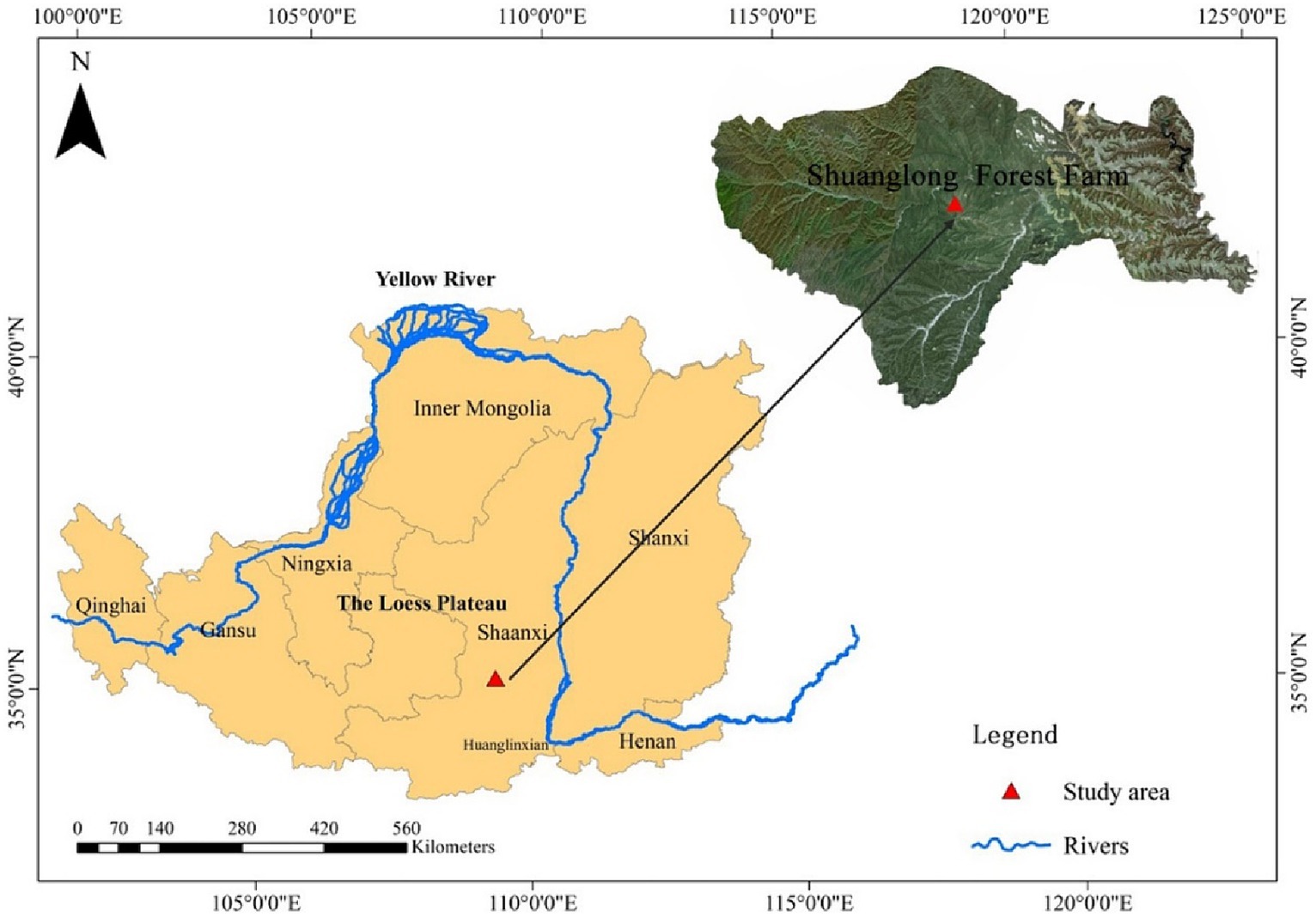
Figure 1. Schematic illustration of soil sampling locations in plantations forests on the Loess Plateau.
2.2 Soil sampling
In March 2023, sampling was conducted in three representative plantation forests within the study area, with a distance of ≥50 m between each site. These forests included Pinus tabuliformis forest (PT), Quercus acutissima Carruth forest (QC), and mixed forests of Pinus tabuliformis and Quercus acutissima Carruth (MF). In each type of plantations forest, profile sampling was conducted at a depth of 1 m, divided into three depth levels: 0–20 cm, 20–60 cm, and 60–100 cm. This process was repeated three times for each treatment group. To prevent cross-contamination, 27 samples were divided into two groups and placed in sterile bags. One group of soil samples was stored at 4°C after handling (air-drying at room temperature and passing through a 2 mm and 0.15 mm sieve). The other group was stored in a − 20°C refrigerator to determine soil enzyme activities.
2.3 Laboratory analyses
The filtrate was analysed using a Vario TOC Cube Select Total Organic Carbon Analyser (Elementar, Germany). Total phosphorus (TP), available phosphorus (aP), and ammonia nitrogen (NH4+-N) were analysed using an automated discontinuous chemical analyser (Clever Chem 200, Germany). Total N (TN) was analysed using a FOSS automatic Kjeldahl nitrogen analyser (Kjeltec 8400, United States). All specific measurement methods for the chemical properties are described in Supplementary Text S1.
According to Sinsabaugh et al. (2010) and Parham and Deng (2000), six soil enzymes involved in C, N, and P cycling were selected (Table 2). Soil enzyme activity was measured using a 96-well microplate assay, as described by Saiya-Cork et al. (2002) and Xiao et al. (2023). 1.0 g of fresh soil was suspended in a buffered solution and shaken for 1 h to create a suspension. An aliquot of the suspension and corresponding fluorescent substrates specific to the soil enzymes were added to the microplate. Fluorescent substrates and buffers were added as blanks, with six replicates for each sample. The microplate was incubated containing soil enzymes at 25°C for a specified period of time (Xiao et al., 2020).
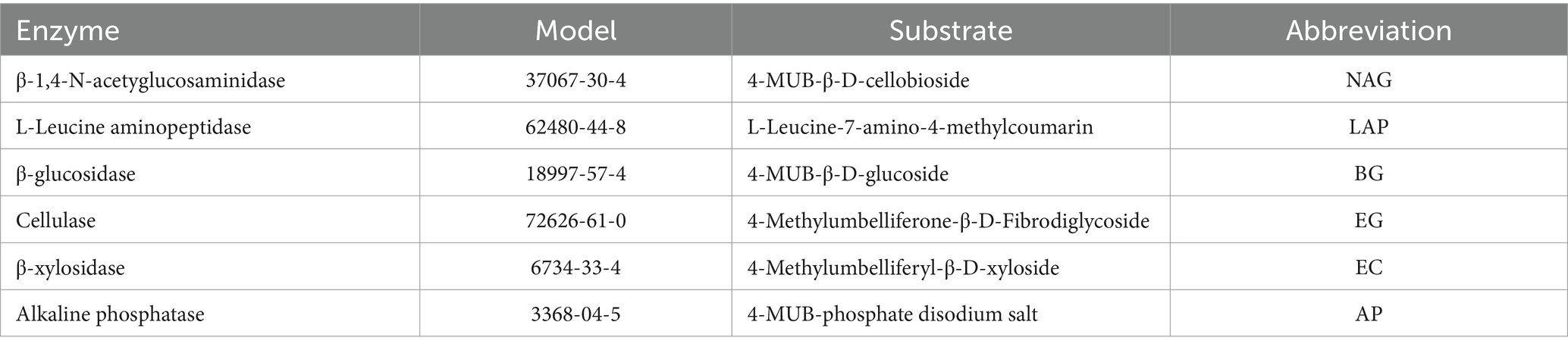
Table 2. Six types of soil enzyme types and their abbreviation (He et al., 2021).
The dissolved organic carbon (DOC) solution was obtained by mixing soil with deionized water at a ratio of 1:5 w/v (Jones and Willett, 2006). After oscillation and centrifugation, it was filtered through a 0.45 μm organic membrane to obtain the DOC filtrate for measurement. The DOC filtrate was diluted with ultrapure water to obtain a DOM solution, to ensure that the concentration of soluble organic matter was within the desired range (using an absorbance of 0.10 at 254 nm as a reference point) and reducing the fluorescence quenching effect. An Aqualog fluorescence spectrophotometre (Horiba, Tokyo, Japan) was used. The excitation and emission wavelength ranges were from 230 to 600 nm, with a 3 nm interval and a 1-s integration time of 1 s. The Aqualog system automatically corrected for filter and Rayleigh scattering to provide corrected data.
2.4 Spectral parameters and PARAFAC modeling
To further investigate the spectral and source characteristics of DOM, we calculated various fluorescence spectral parameters (Table 3 and Supplementary Text S2). To address the issue of overlapping spectra and to quantitatively analyze the composition of DOM, the PARAFAC method was used to analyze the fluorescence spectroscopy data (Supplementary Text S3). The reliability of the results was verified using a nuclear consistency function and half-analysis (Stedmon and Bro, 2008).
2.5 Data analysis and statistics
We assessed significant differences in parameters across sampling points parameters using two-way ANOVA in SPSS Statistics software (version 21.0). Pearson correlation analysis was performed in R 4.0.3 to evaluate the correlation between the parameters. Origin 2021 (Northampton, Massachusetts, OriginLab Inc.) was used to analyse and plot the changes in soil chemical properties, soil enzyme activity, and spectral data. PLS-PM was used to predict the impact of soil enzymes and chemical properties on DOM.
3 Results
3.1 Soil properties and DOM content
The depth of the soil and the type of vegetation had a significant impact on the soil chemical properties (Figure 2). The chemical properties of the soil (TN, NH4+-N, TP, and aP) varied significantly at different depths. For different soil profiles within the same vegetation type, the soil chemical properties (TN, NH4+-N, and TP) decreased as the soil depth increased across all three types. Among different soil types within the same profile, the TN values were highest in MF (0.71–2.30 mg/kg), followed by QC (0.63–1.71 mg/kg) and PT (0.57–1.38 mg/kg). The sequence of NH4+-N variations was QC < MF < PT, with NH4+-N values ranging from 6.82 to 10.11 mg/kg in QC, 6.57 to 9.37 mg/kg in MF, and 4.95 to 8.36 mg/kg in PT. The average TP values in PT and QC soils were significantly higher than in MF. In the surface layer (0–20 cm), the TP concentration in QC was significantly higher than in the other two forests (PT and MF), at 221.92 mg/kg. The aP values of the three forest types fluctuated across different profiles, with the highest average concentration of aP in the 0–20 cm layer of MF soil (51.81 mg/kg). The concentration of aP decreased in the 20–60 cm layer but increased in the 60–100 cm layer. The trend of aP concentration variations in the PT profile was consistent with that of MF.
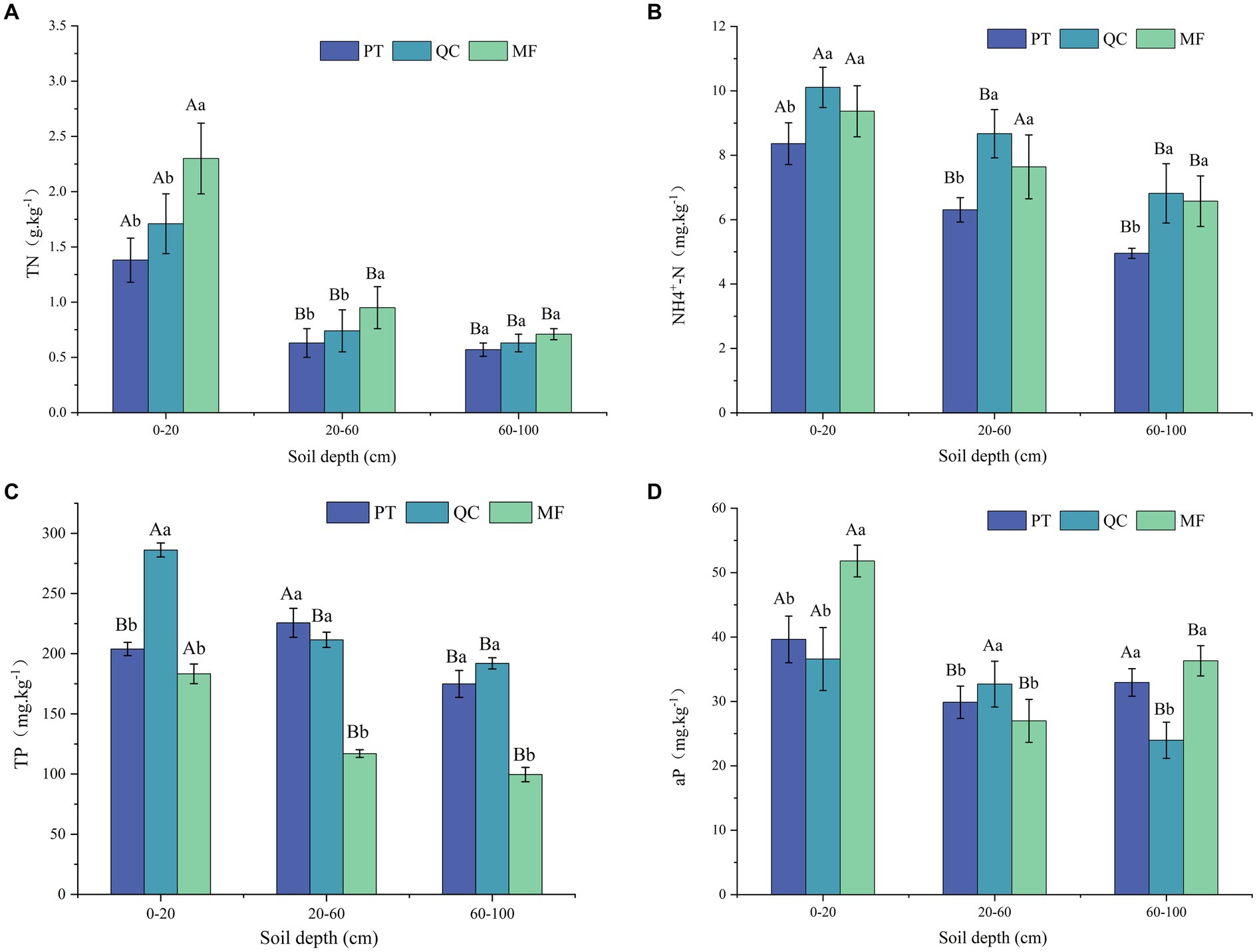
Figure 2. Characteristics of chemical properties of three forest types of soil vertical sections. (A) Total nitrogen(TN). (B) Ammonia nitrogen (NH4+-N). (C) Total phosphorus (TP). (D) Available phosphorus (aP). Capital letters denote marked enzyme activity differences in profiles of the same tree species; lowercase indicates significant variations among species within a profile (p < 0.05).
The DOC content of the three vegetation types decreased with increasing soil depth (Table 4). The average DOC content was highest in MF and QC forests, exceeding that of PT (16.18–24.25 mg/L). Additionally, the variations in the a(355) values along the soil profile were consistent with the changes in DOC concentration. However, for different soil types within the same profile, the decreasing order of DOC content at 0–20 cm and 20–60 cm was: QC < PT < MF. This indicates that the biological characteristics of different forest types result in significant differences in soil DOC content.
3.2 Changes in soil enzyme activity concentration
As shown in Figure 3, the activities of three soil enzymes related to carbon cycling (BG, EC, and EG) and soil microbial secretion of enzymes for nitrogen and phosphorus acquisition (NAG, LAP, and AP) all sharply decreased with depth. Additionally, there were significant differences in soil enzyme activities among the depths of the three vegetation types. In the surface layer (0–20 cm) and (20–60 cm) of soil, except for EC and LAP enzymes, the soil enzyme activities in the MF forest were at least twice as high as those in the QC and PT forests, following a decreasing pattern of PT < QC < MF. In the 60–100 cm soil depth, the enzyme activity in the QC forest was the highest, which may be related to the litter production, decomposition rate, and root biomass of different forest types.
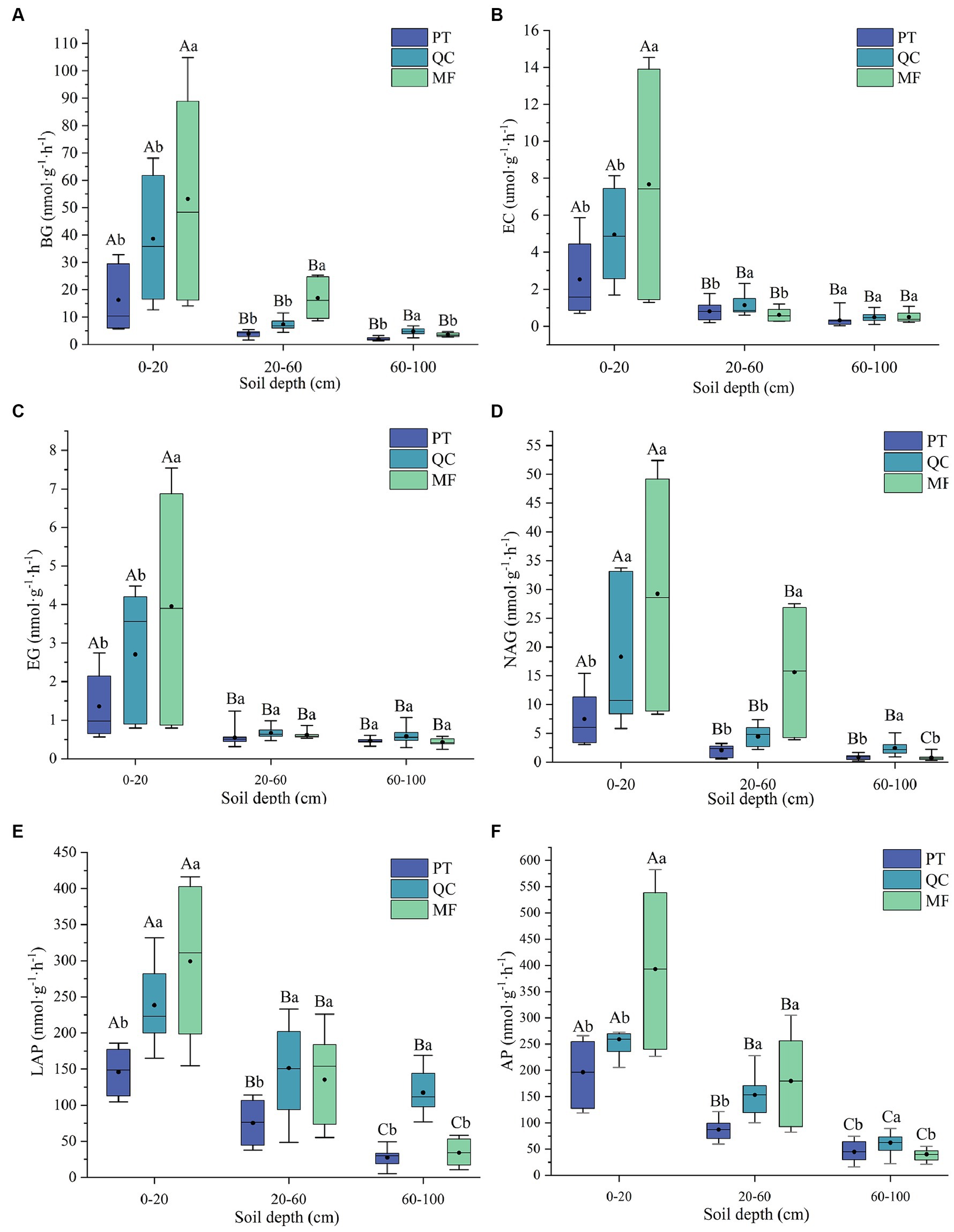
Figure 3. Vertical variation characteristics of soil enzyme activity in three forest types. (A) Activities of β-glucosidase (BG). (B) Activities of β-xylosidase (EC). (C) Activities of Cellulase (EG). (D) Activities of β-1,4-N-acetyglucosaminidase (NAG). (E) Activities of L-Leucine aminopeptidase (LAP). (F) Activities of Alkaline phosphatase (AP). Capital letters denote marked differences in enzyme activity between profiles of the same tree species, while lowercase indicates significant differences among tree species within a profile (p < 0.05).
3.3 Spectroscopic properties of DOM
The fluorescence spectral characteristics of DOM at different soil depths in the three forest types were identified. The samples had two fluorescence peaks, namely a humus type fluorescence peak (peak A and peak C), and a protein-like fluorescence peak (peak T), as shown in Supplementary Figure S1. In the soils of the three forest types, the fluorescence intensity revealed a consistent pattern with all fluorescence peaks following the same pattern: MF > PT > QC (Figure 4). Simultaneously, with the increase in soil depth, the fluorescence intensity of A peak and C peak gradually decreased, while the intensity of T peak increased. This is related to the properties of the litter, compared with broad-leaved litter, coniferous litter contains significant amounts of cellulose and lignin, which are difficult to decompose, cellulose and lignin, these substances decompose slowly, accumulating recalcitrant materials that can polymerize into humus in the soil.
The PARAFAC model resolved four components of samples (Figure 5). C1 is a humic substance, represented by humic acids (Yamashita et al., 2010). C2 is a high molecular weight aromatic amino acid and is a fulvic-like acid components (Stedmon et al., 2003). C3 is a typical protein-like fluorescent component associated with microbial activity. C4 is a component of tryptophan-like substances (Zhang et al., 2022). C1 and C2 are fluorescent components that could reflect the quality of SOM, whereas C3 and C4 was a type of fluorescent components related to protein in soil, and is generally associated with microbial protein metabolites (Stedmon et al., 2003; He et al., 2014). In the surface soil (0–20 cm), only C1 and C2 were present, while in the deeper soil, C3 and C4 components were also present. It is worth noting that the proportion of C3 and C4 increased with increasing soil depth.
3.4 Changes in DOM fluorescence parameters
To further distinguish and compare the sources and properties of the DOM components, we calculated fluorescence parameter indicators for different soil profiles separately, as shown in Figure 6. The FI values of the three forest types showed that below a depth of 60 cm, were below 1.4, indicating that the input from soil leaching was the main factor. In the soil profile of the 60–100 cm layer, the FI value ranged from 1.4 to 1.9, indicating that the DOM in the soil was influenced by both allochthonous and autochthonous inputs. The HIX and BIX indicators exhibited opposite trends along the soil profile. The HIX continued to decrease as the soil depth increased, whereas the BIX showed the opposite trend. This indicates that at a depth of 0–20 cm, the DOM had strong humification and weak endogenous characteristics. However, as the soil depth increased, DOM showed strong autochthonous characteristics. SUVA254 values indicated a significant decrease in DOM aromaticity in deep soil layers, with a consistent pattern observed across the three soil types. In addition, the SUVA254 value of PT was greater, suggesting a greater degree of humification in the PT. Overall, in the three soil types, the source of DOM in the source of DOM in the surface soil (0–20 cm) was mainly allochthonous, whereas in deep soil (60–100 cm) originating mainly from endogenous sources, such as microbial activity.
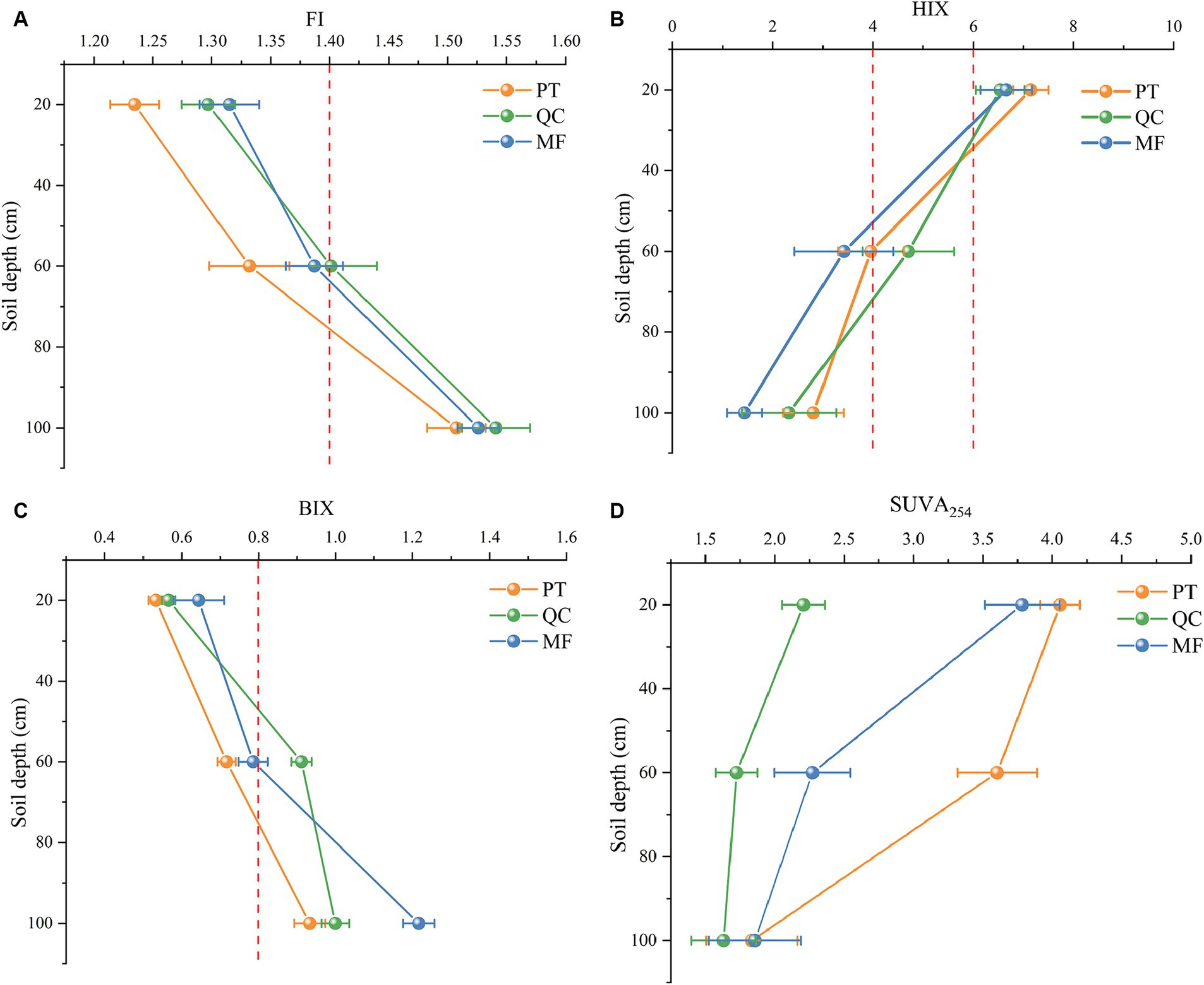
Figure 6. Optical indices of soil DOM of the three plantations forest types (PL, QC, and MF) at depths of 20, 60, and 100 cm. (A) Fluorescence Index (FI). (B) Humification index (HIX). (C) Autogenic index (BIX). (D) Parameters indicating the level of aromaticity (SUVA254).
3.5 Correlation index analysis and PLS-PM
DOC exhibited significant positive correlations (p < 0.01) with TN, aP and a(355), as well as with the four enzymes LAP, NAG, BG, and AP (Figure 7A). However, TN was negatively correlated with TP, and the soil enzyme indicators showed no significant correlation with TP. Building upon the results of the correlation analysis, a PLS-SEM model was adopted to investigate the effects of soil depth, chemical properties, and enzymes on DOM. The arrows in Figure 7B indicate the direction of influence of one variable on another, and the adjacent numbers indicate the standardised path coefficients. DOM received a positive contribution from soil enzymes in all models; however, while chemical properties had a positive impact on DOM, this impact did not reach a significant level. Soil depth had a negative direct path coefficient for both the chemical properties and soil enzymes. The effect of soil chemical properties on DOM was mainly achieved indirectly through various soil enzymes related to the soil C, N, and P cycles.
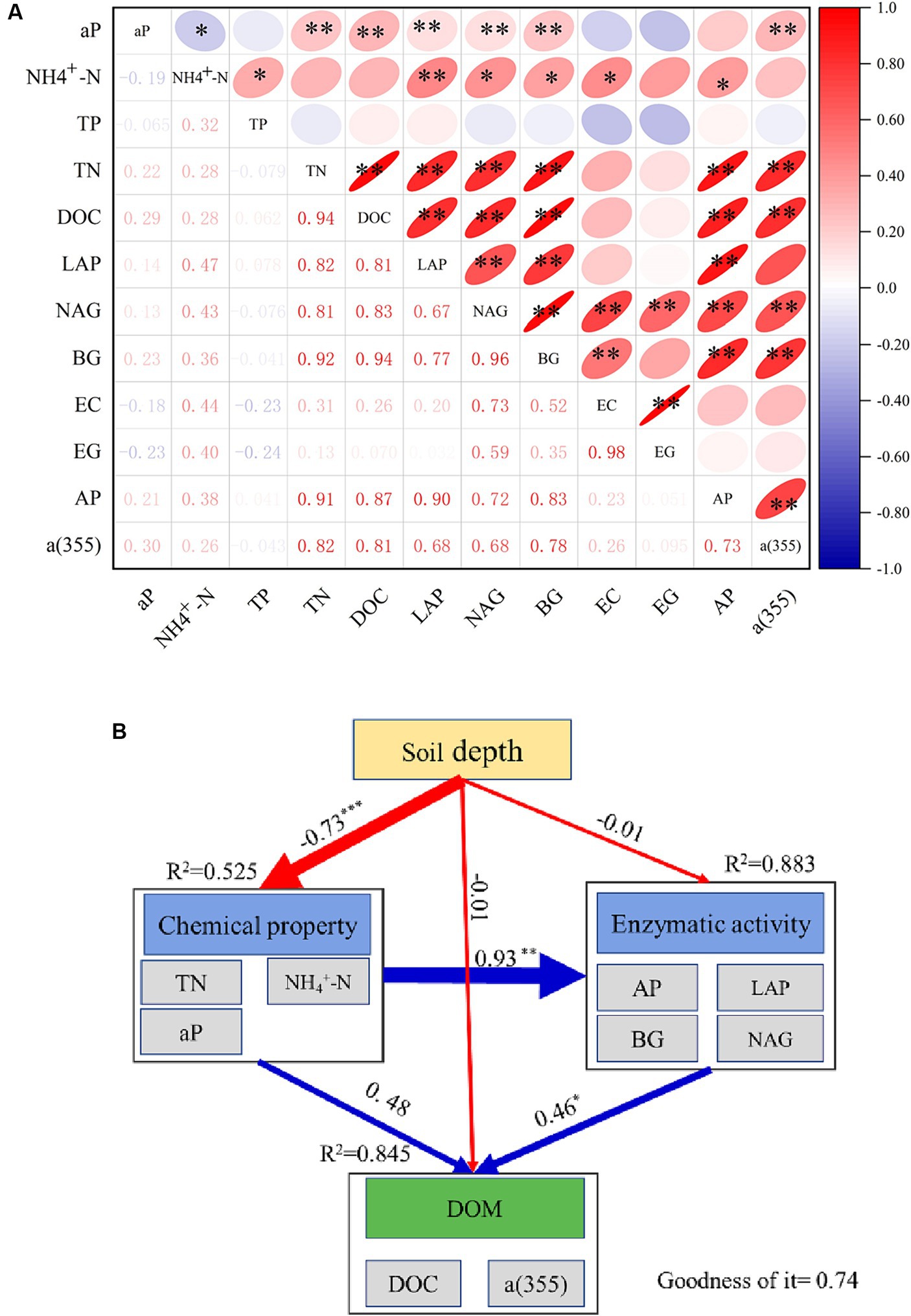
Figure 7. Correlation analysis and PLS-PM models. (A) Correlations among the reflecting indicators; **p < 0.01, *p < 0.05. (B) Results of the PLS-PM models for DOM. The reflecting indicators of the chemical property were a:NH4+-N, TN, TP and aP. The reflecting indicators of the enzymatic activity were AP, LAP, BG, NAG; *p < 0.05, **p < 0.01, ***p < 0.005.
4 Discussion
4.1 Differences in DOM storage among three forest types
DOC directly or indirectly influences the biogeochemical cycling in forest soil. Its component dynamics and turnover are mainly controlled by changes in the forest vegetation type, litter input type, soil temperature, and humidity (Gruba and Socha, 2019). DOC, an important component of DOM, is typically used to characterize the concentration of DOM (Zhang et al., 2017). The DOC values of the soil at depths of 0–20 cm (22.07–46.32 mg/L) and 20–60 cm (9.47–11.15 mg/L) in the area were comparable to the values of subtropical forest tree species (0–20 cm:37.10–47.40 mg/L; 20–60 cm:4.80–9.50 mg/L) (Tu et al., 2011). DOC distribution and changes in soil profiles have certain regularities (McDowell and Likens, 1988; Gabor et al., 2014; McDowell, 2022), and the content of DOC in the surface soil layer is usually greater than that in the bottom soil layer. The elevated levels of DOC in the upper layers of forest soil mainly arise from the leachates of the canopy and litter; however, the decrease in DOC levels may be related to losses caused by adsorption and decomposition during downward migration in soil minerals, leading to a decrease in DOC levels with increasing depth (Tu et al., 2011). Therefore, the three types of forest soils exhibited consistent patterns in vertical depth. This aligns with the research findings regarding dissolved organic carbon (DOC) in the coniferous, broad-leaved, and mixed conifer-broadleaf forests of Shennongjia Forest and Dinghu Mountain Forest (Gu et al., 2023). Furthermore, the surface-level concentrations of DOC were notably elevated in MF and QC compared to PT, due in part to the greater levels of litter products, root exudates, and microbial biomass in the MF and QC forests (Weintraub et al., 2007), leading to the generation of more DOC.
4.2 Fluorescent component characteristics of DOM
Proteins in soil organic matter can provide nutrition and energy to microorganisms, which can promote the maintenance and improvement of soil fertility (McFarland et al., 2010). In fact, biochemical processes driven by microorganisms can easily break down the proteins used by microorganisms into monomeric molecules, which can then be polymerised to form humus through aggregation processes (Yan et al., 2019). We found that the fluorescence peak value of the humus type gradually decreased with increasing soil depth, whereas the protein fluorescence peak exhibited the opposite trend. This result indicates that the decayed branches and leaves in the forest were preserved on the soil surface, hence, the humus content was higher. The aromatic substances produced by trees can chemically react with soil and are stably preserved in soil (Wei et al., 2020). The fluorescence peaks A and C, formed by the input of exogenous humic-acid and fulvic acid, have stable structures (Ussiri and Johnson, 2003). Furthermore, the PARAFAC model showed that in surface soil (0–20 cm), only humic components C1 and C2 existed. This is mainly because of the long-term accumulation of forest litter, which provides a material basis for microbial growth, effectively promotes the accumulation of C1 and C2 substances in the soil. C3 and C4 accumulate in deep soil, indicating a gradual increase in microbial products. A possible explanation for this phenomenon could be that the surface soil DOM was easily influenced by the vegetation type (Kaiser and Kalbitz, 2012). As the soil depth increased, DOM was retained through adsorption and precipitation. The microbial community could consume and decompose key components of DOM while involving the generation of new molecules, leading to changes in DOM composition. Therefore, the dominant components of deep soil were C3 and C4 plants (Roth et al., 2019). This is similar to previous findings on the characteristics of DOM of different tree species in plantations forests (Ye et al., 2020). In addition, the three indicators FI, HIX, and BIX showed that with increasing soil depth (Figure 5), the self-generated sources caused by microbial activity became more significant. The SUVA254 values displayed that the aromaticity of DOM decreased with soil depth, and the molecular weight of the DOM was accompanied by low-quality aromatics. In temperate grassland soils, the same phenomenon has also been recorded (Roth et al., 2019), indicating that fresh plant-derived organic matter is rapidly degraded and low-quality polyphenols are rapidly consumed. Therefore, in the vertical migration of soil layers, DOM was a process of continuous decomposition, adsorption, and transformation, closely related to soil properties and microbial activity.
4.3 The interaction between physicochemical properties and soil enzymes is essential for understanding soil DOM
Different vegetation types produce different qualities of litter and root exudates, resulting in differences in soil chemical properties (Weiss et al., 2016; Liu et al., 2023b), and these physical and chemical properties can indirectly affect soil enzyme activity by influencing the composition and species of soil microorganisms. Since each enzyme has a specific substrate and the ability to catalyze biochemical reactions, it inevitably affects Soil Organic Carbon (SOC) pools (Chandra et al., 2016). During our research, DOM in forest soils was closely correlated with soil enzyme activity. The enzymes associated with C, N, and P cycling were found to have a highly significant correlation with DOC (Figure 7). PLS-PM analysis showed that soil chemical properties mainly influenced DOM through the activity of various enzymes. Owing to differences in litter input, enzyme activity, and soil microbes among the different forest types, the concentration of DOM varies. Soil enzyme activity was limited by vegetation type, which exerts a considerable influence on enzyme activity in the soil. Consistent with findings from other studies on forested areas, mixed forests were able to promote an increase in soil enzyme activity (Zhang et al., 2008; He et al., 2020). This suggests that mixed tree species stimulate soil enzyme activity, which may be attributed to three potential mechanisms. First, mixed forests alleviate soil acidification by decomposing alkaline cations in fallen leaves and branches (Hong et al., 2018); second, mixed forests have a lower bulk density, thereby improving soil aeration (Qian et al., 2022); third, higher physicochemical properties increase soil enzyme activity, which in turn promotes the release of organic matter (Singh et al., 2012). Therefore, mixed-species plantations forests are more effective at accumulating soil organic matter. In addition, a single forest type causes biodiversity loss, seriously affecting the carbon sequestration function of the soil (Lu et al., 2018; Islam et al., 2022). In contrast, mixed-species forests have a positive effect on ecosystem carbon storage (Hulvey et al., 2013; Gong et al., 2022). In conclusion, mixed-species forests are more beneficial for increasing soil organic matter compared to monoculture forests. In afforestation practices, increasing the number of mixed species can most effectively improve soil environmental quality and enhance soil ecological functions, without considering economic limitations.
5 Conclusion
The study revealed that the DOC content followed the sequence of MF > QC > PT, with a significant decrease observed in the deeper soil layers. This may be due to varying litter production and root exudate levels among the forest types. The vertical distribution pattern of soil enzyme activity was consistent with the DOC pattern, indicating that mixed species could stimulate soil enzyme activity, leading to an increase in soil enzyme activity and subsequently promoting the release of organic matter. Additionally, the dominant components in the surface soil were C1 and C2, while in the deep soil, C3 and C4 were predominant. This suggests that the vertical migration of DOM in the soil profile is a continuous process of decomposition and adsorption of new substances. Furthermore, the three indices (FI, HIX, and BIX) indicated that the influence of microbially induced local sources on the lower layer DOM became more prominent. PLS-PM analysis revealed that the impact of soil chemical properties on DOM was mainly mediated through various soil enzymes. In conclusion, mixed tree species are more conducive to improving soil organic matter compared to single tree species. Future research should continue to emphasize the interactions among soil chemical properties, soil enzymes, and DOM under different artificial forest planting patterns.
Data availability statement
The raw data supporting the conclusions of this article will be made available by the authors, without undue reservation.
Author contributions
YM: Writing – review & editing, Writing – original draft, Software, Investigation, Formal analysis, Conceptualization. PL: Writing – review & editing, Methodology. LX: Writing – review & editing. BH: Writing – original draft, Software. CZ: Writing – review & editing, Data curation. SY: Writing – original draft, Software. JL: Writing – original draft, Software. BZ: Writing – review & editing, Data curation.
Funding
The author(s) declare financial support was received for the research, authorship, and/or publication of this article. This work was supported by the Key Research and Development Plan of Shaanxi Province (No. 2023-ZDLSF-65), National Natural Science Foundation of China (Nos. U2243201, U 42277359 and 42007070).
Conflict of interest
The authors declare that the research was conducted in the absence of any commercial or financial relationships that could be construed as a potential conflict of interest.
Publisher’s note
All claims expressed in this article are solely those of the authors and do not necessarily represent those of their affiliated organizations, or those of the publisher, the editors and the reviewers. Any product that may be evaluated in this article, or claim that may be made by its manufacturer, is not guaranteed or endorsed by the publisher.
Supplementary material
The Supplementary material for this article can be found online at: https://www.frontiersin.org/articles/10.3389/ffgc.2024.1344784/full#supplementary-material
Abbreviations
DOM, Dissolved organic matter; DOC, Dissolved organic carbon; TN, Total nitrogen; TP, Total phosphorus; NH4+-N, Ammonia nitrogen; aP, available phosphorus; NAG, β-1,4-N-acetyglucosaminidase; LAP, L-Leucine aminopeptidase; BG, β-glucosidase; EG, Cellulase; EC, β-xylosidase; AP, Alkaline phosphatase.
References
Batjes, N. H. (2014). Total carbon and nitrogen in the soils of the world. Eur. J. Soil Sci. 47, 151–163. doi: 10.1111/j.1365-2389.1996.tb01386.x
Bolan, N. S., Adriano, D. C., Kunhikrishnan, A., James, T. K., and Senesi, N. (2011). Dissolved organic matter: biogeochemistry, dynamics, and environmental significance in soils. Adv. Agron. 110, 1–75. doi: 10.1016/B978-0-12-385531-2.00001-3
Chandra, L. R., Gupta, S., Pande, V., and Singh, N. (2016). Impact of forest vegetation on soil characteristics: a correlation between soil biological and physico-chemical properties. Biotech 6:188. doi: 10.1007/s13205-016-0510-y
Dilling, J., and Kaiser, K. (2002). Estimation of the hydrophobic fraction of dissolved organic matter in water samples using UV photometry. Water Res. 36, 5037–5044. doi: 10.1016/s0043-1354(02)00365-2
Eilers, K. G., Debenport, S., Anderson, S., and Fierer, N. (2012). Digging deeper to find unique microbial communities: the strong effect of depth on the structure of bacterial and archaeal communities in soil. Soil Biol. Biochem. 50, 58–65. doi: 10.1016/j.soilbio.2012.03.011
Gao, J. K., Liang, C. L., Shen, G. Z., Lv, J., and Wu, H. M. (2017). Spectral characteristics of dissolved organic matter in various agricultural soils throughout China. Chemosphere. 176, 108–116. doi: 10.1016/j.chemosphere.2017.02.104
Gabor, R. S., Eilers, K., McKnight, D. M., Fierer, N., and Anderson, S. P. (2014). From the litter layer to the saprolite: chemical changes in water-soluble soil organic matter and their correlation to microbial community composition. Soil Biol. Biochem. 68, 166–176. doi: 10.1016/j.soilbio.2013.09.029
Goberna, M., Insam, H., Klammer, S., Pascual, J. A., and Sánchez, J. (2005). Microbial community structure at different depths in disturbed and undisturbed semiarid Mediterranean forest soils. Microb. Ecol. 50, 315–326. doi: 10.1007/s00248-005-0177-0
Gong, C., Tan, Q., Liu, G., and Xu, M. (2022). Advantage of mixed trees in the tradeoff between soil water storage and tree biomass: a meta-analysis from artificially planted forests in Chinese loess plateau. Catena 214:106232. doi: 10.1016/j.catena.2022.106232
Gruba, P., and Socha, J. (2019). Exploring the effects of dominant forest tree species, soil texture, altitude, and pH H2O on soil carbon stocks using generalized additive models. For. Ecol. Manag. 447, 105–114. doi: 10.1016/j.foreco.2019.05.061
Gu, J., Bol, R., Wang, Y., and Zhang, H. (2023). Controls on soil dissolved organic carbon along the 4000 km north-south forest transect in eastern China. Catena 220:106691. doi: 10.1016/j.catena.2022.106691
Guo, J., Feng, H., McNie, P., Liu, Q., Xu, X., Pan, C., et al. (2023). Species mixing improves soil properties and enzymatic activities in Chinese fir plantations: a meta-analysis. Catena 220:106723. doi: 10.1016/j.catena.2022.106723
Jones, D. L., and Willett, V. B. (2006). Experimental evaluation of methods to quantify dissolved organic nitrogen (DON) and dissolved organic carbon (DOC) in soil. Soil Biol. Biochem. 38, 991–999. doi: 10.1016/j.soilbio.2005.08.012
He, Q., Wu, Y., Bing, H., Zhou, J., and Wang, J. (2020). Vegetation type rather than climate modulates the variation in soil enzyme activities and stoichiometry in subalpine forests in the eastern Tibetan plateau. Geoderma 374:114424. doi: 10.1016/j.geoderma.2020.114424
He, L. Y., Lu, S. L., Wang, C. G., Mu, J., Zhang, Y. L., and Wang, X. D. (2021). Changes in soil organic carbon fractions and enzyme activities in response to tillage practices in the loess plateau of china. Soil Till. Res. 209:1. doi: 10.1016/j.still.2021.104940
He, X., Xi, B., Cui, D., Liu, Y., Tan, W., Pan, H., et al. (2014). Influence of chemical and structural evolution of dissolved organic matter on electron transfer capacity during composting. J. Hazard. Mater. 268, 256–263. doi: 10.1016/j.jhazmat.2014.01.030
Hong, S., Piao, S., Chen, A., Liu, Y., Liu, L., Peng, S., et al. (2018). Afforestation neutralizes soil pH. Nat. Commun. 9:520. doi: 10.1038/s41467-018-02970-1
Hu, H., Umbreen, S., Zhang, Y., Bao, M., Huang, C., and Zhou, C. (2021). Significant association between soil dissolved organic matter and soil microbial communities following vegetation restoration in the loess plateau. Ecol. Eng. 169:106305. doi: 10.1016/j.ecoleng.2021.106305
Hulvey, K. B., Hobbs, R. J., Standish, R. J., Lindenmayer, D. B., Lach, L., and Perring, M. P. (2013). Benefits of tree mixes in carbon plantings. Nat. Clim. Chang. 3, 869–874. doi: 10.1038/NCLIMATE1862
Islam, W., Saqib, H. S. A., Tayyab, M., Wang, Z., Ding, X., Su, X., et al. (2022). Natural forest chronosequence maintains better soil fertility indicators and assemblage of total belowground soil biota than Chinese fir monoculture in subtropical ecosystem. J. Clean. Prod. 334:130228. doi: 10.1016/j.jclepro.2021.130228
Jaffrain, J., Gérard, F., Meyer, M., and Ranger, J. (2010). Assessing the quality of dissolved organic matter in forest soils using ultraviolet absorption spectrophotometry. Soil Sci. Soc. Am. J. 71, 1851–1858. doi: 10.2136/sssaj2006.0202
Jílková, V., Jandová, K., Sim, A., Thornton, B., and Paterson, E. (2019). Soil organic matter decomposition and carbon sequestration in temperate coniferous forest soils affected by soluble and insoluble spruce needle fractions. Soil Biol. Biochem. 138:107595. doi: 10.1016/j.soilbio.2019.107595
Kaiser, K., and Kalbitz, K. (2012). Cycling downwards – dissolved organic matter in soils. Soil Biol. Biochem. 52, 29–32. doi: 10.1016/j.soilbio.2012.04.002
Kiikkilä, O., Kitunen, V., and Smolander, A. (2006). Dissolved soil organic matter from surface organic horizons under birch and conifers: degradation in relation to chemical characteristics. Soil Biol. Biochem. 38, 737–746. doi: 10.1016/j.soilbio.2005.06.024
Ko, D., Yoo, G., Yun, S., Jun, S., and Chung, H. (2017). Bacterial and fungal community composition across the soil depth profiles in a fallow field. J. Ecol. Environ. 41:34. doi: 10.1186/s41610-017-0053-0
Li, H., Liu, G., Luo, H., and Zhang, R. (2022). Labile carbon input and temperature effects on soil organic matter turnover in subtropical forests. Ecol. Indic. 145:109726. doi: 10.1016/j.ecolind.2022.109726
Li, W., Siddique, M. S., Liu, M., Graham, N., and Yu, W. (2022). The migration and microbiological degradation of dissolved organic matter in riparian soils. Water Res. 224:119080. doi: 10.1016/j.watres.2022.119080
Liu, X. J., Zhang, Y., Xiao, T. Q., and Zhang, L. (2023b). Runoff velocity controls soil nitrogen leaching in subtropical restored forest in southern China. For. Ecol. Manag. 548:121412. doi: 10.1016/j.foreco.2023.121412
Liu, X. J., Zhang, Y., Zhang, L., Fang, X. M., Deng, W. P., and Liu, Y. Q. (2023a). Aggregate-associated soil organic carbon fractions in sub-tropical soil undergoing vegetative restoration. Land Degrad. Dev. 34, 4296–4306. doi: 10.1002/ldr.4777
Lu, D., Wang, G. G., Zhang, J., Fang, Y., Zhu, C., and Zhu, J. (2018). Converting larch plantations to mixed stands: effects of canopy treatment on the survival and growth of planted seedlings with contrasting shade tolerance. For. Ecol. Manag. 409, 19–28. doi: 10.1016/j.foreco.2017.10.058
McDowell, W. H. (2022). DOM in the long arc of environmental science: looking back and thinking ahead. Biogeochemistry 164, 15–27. doi: 10.1007/s10533-022-00924-w
McDowell, W. H., and Likens, G. E. (1988). Origin, composition, and flux of dissolved organic carbon in the Hubbard Brook Valley. Ecol. Monogr. 58, 177–195. doi: 10.2307/2937024
McFarland, J. W., Ruess, R. W., Kielland, K., Pregitzer, K., and Hendrick, R. (2010). Glycine mineralization in situ closely correlates with soil carbon availability across six North American forest ecosystems. Biogeochemistry 99, 175–191. doi: 10.1007/s10533-009-9400-2
Meng, Y., Li, P., Xiao, L., Wang, R., Yang, S., Han, J., et al. (2022). Heavy metal content and pollution assessment in typical check dam sediment in a watershed of loess plateau, China. Sustain. For. 14:8597. doi: 10.3390/su14148597
Ohno, T. (2002). Fluorescence inner-filtering correction for determining the humification index of dissolved organic matter. Environ. Sci. Technol. 36, 742–746. doi: 10.1021/es0155276
Parham, J. A., and Deng, S. P. (2000). Detection, quantification and characterization of β-glucosaminidase activity in soil. Soil Biol. Biochem. 32, 1183–1190. doi: 10.1016/s0038-0717(00)00034-1
Qian, Z., Zhuang, S., Gao, J., Tang, L., Harindintwali, J. D., and Wang, F. (2022). Aeration increases soil bacterial diversity and nutrient transformation under mulching-induced hypoxic conditions. Sci. Total Environ. 817:153017. doi: 10.1016/j.scitotenv.2022.153017
Roth, V., Lange, M., Simon, C., Hertkorn, N., Bucher, S., Goodall, T., et al. (2019). Persistence of dissolved organic matter explained by molecular changes during its passage through soil. Nat. Geosci. 12, 755–761. doi: 10.1038/s41561-019-0417-4
Saidy, A. R., Smernik, R. J., Baldock, J. A., Kaiser, K., and Sanderman, J. (2013). The sorption of organic carbon onto differing clay minerals in the presence and absence of hydrous iron oxide. Geoderma 209-210, 15–21. doi: 10.1016/j.geoderma.2013.05.026
Saiya-Cork, K. R., Sinsabaugh, R. L., and Zak, D. R. (2002). The effects of long term nitrogen deposition on extracellular enzyme activity in an Acer saccharum forest soil. Soil Biol. Biochem. 34, 1309–1315. doi: 10.1016/s0038-0717(02)00074-3
Schmidt, M. W., Torn, M. S., Abiven, S., Dittmar, T., Guggenberger, G., Janssens, I. A., et al. (2011). Persistence of soil organic matter as an ecosystem property. Nature 478, 49–56. doi: 10.1038/nature10386
Singh, K., Singh, B., and Singh, R. R. (2012). Changes in physico-chemical, microbial and enzymatic activities during restoration of degraded sodic land: ecological suitability of mixed forest over monoculture plantation. Catena 96, 57–67. doi: 10.1016/j.catena.2012.04.007
Sinsabaugh, R. L., Hill, B. H., and Follstad Shah, J. J. (2010). Erratum: Ecoenzymatic stoichiometry of microbial organic nutrient acquisition in soil and sediment. Nature 468:122. doi: 10.1038/nature08632
Smith, C. R., Sleighter, R. L., Hatcher, P. G., and Lee, J. W. (2013). Molecular characterization of inhibiting biochar water-extractable substances using electrospray ionization Fourier transform ion cyclotron resonance mass spectrometry. Environ. Sci. Technol. 47, 13294–13302. doi: 10.1021/es4034777
Song, B., Yan, M., Hou, H., Guan, J., Shi, W., Li, G., et al. (2016). Distribution of soil carbon and nitrogen in two typical forests in the semiarid region of the loess plateau, China. Catena 143, 159–166. doi: 10.1016/j.catena.2016.04.004
Stedmon, C. A., and Bro, R. (2008). Characterizing dissolved organic matter fluorescence with parallel factor analysis: a tutorial. Limnol. Oceanogr. Methods 6, 572–579. doi: 10.4319/lom.2008.6.572
Stedmon, C. A., Markager, S., and Bro, R. (2003). Tracing dissolved organic matter in aquatic environments using a new approach to fluorescence spectroscopy. Mar. Chem. 82, 239–254. doi: 10.1016/s0304-4203(03)00072-0
Stone, M. M., DeForest, J. L., and Plante, A. F. (2014). Changes in extracellular enzyme activity and microbial community structure with soil depth at the Luquillo critical zone observatory. Soil Biol. Biochem. 75, 237–247. doi: 10.1016/j.soilbio.2014.04.017
Thieme, L., Graeber, D., Hofmann, D., Bischoff, S., Schwarz, M. T., Steffen, B., et al. (2019). Dissolved organic matter characteristics of deciduous and coniferous forests with variable management: different at the source, aligned in the soil. Biogeosciences 16, 1411–1432. doi: 10.5194/bg-16-1411-2019
Tu, C., Liu, C., Lu, X., Yuan, J., and Lang, Y. (2011). Sources of dissolved organic carbon in forest soils: evidences from the differences of organic carbon concentration and isotope composition studies. Environ. Earth Sci. 63, 723–730. doi: 10.1007/s12665-010-0741-x
Ussiri, D. A. N., and Johnson, C. E. (2003). Characterization of organic matter in a northern hardwood forest soil by 13C NMR spectroscopy and chemical methods. Geoderma 111, 123–149. doi: 10.1016/S0016-7061(02)00257-4
Wang, Y., Shao, M., and Liu, Z. (2013). Vertical distribution and influencing factors of soil water content within 21-m profile on the Chinese loess plateau. Geoderma 193-194, 300–310. doi: 10.1016/j.geoderma.2012.10.011
Wei, X., Yang, Y., Shen, Y., Chen, Z., Dong, Y., Wu, F., et al. (2020). Effects of litterfall on the accumulation of extracted soil humic substances in subalpine forests. Front. Plant Sci. 11:254. doi: 10.3389/fpls.2020.00254
Weintraub, M. N., Scott-Denton, L. E., Schmidt, S. K., and Monson, R. K. (2007). The effects of tree rhizodeposition on soil exoenzyme activity, dissolved organic carbon, and nutrient availability in a subalpine forest ecosystem. Oecologia 154, 327–338. doi: 10.1007/s00442-007-0804-1
Weiss, C., Weiss, J., Boy, J., Iskandar, I., Mikutta, R., and Guggenberger, G. (2016). Soil organic carbon stocks in estuarine and marine mangrove ecosystems are driven by nutrient colimitation of P and N. Ecol. Evol. 6, 5043–5056. doi: 10.1002/ece3.2258
Xiao, L., Liu, G., Li, P., Li, Q., and Xue, S. (2020). Ecoenzymatic stoichiometry and microbial nutrient limitation during secondary succession of natural grassland on the loess plateau, China. Soil Tillage Res. 200:104605. doi: 10.1016/j.still.2020.104605
Xiao, L., Min, X., Liu, G., Li, P., and Xue, S. (2023). Effect of plant–plant interactions and drought stress on the response of soil nutrient contents, enzyme activities and microbial metabolic limitations. Appl. Soil Ecol. 181:104666. doi: 10.1016/j.apsoil.2022.104666
Xu, H., and Guo, L. (2017). Molecular size-dependent abundance and composition of dissolved organic matter in river, lake and sea waters. Water Res. 117, 115–126. doi: 10.1016/j.watres.2017.04.006
Yamashita, Y., Cory, R. M., Nishioka, J., Kuma, K., Tanoue, E., and Jaffé, R. (2010). Fluorescence characteristics of dissolved organic matter in the deep waters of the Okhotsk Sea and the northwestern North Pacific Ocean. Deep Sea Res. 2 Top. Stud. Oceanogr. 57, 1478–1485. doi: 10.1016/j.dsr2.2010.02.016
Yan, L., Liu, Q., Liu, C., Liu, Y., Zhang, M., Zhang, Y., et al. (2019). Effect of swine biogas slurry application on soil dissolved organic matter (DOM) content and fluorescence characteristics. Ecotoxicol. Environ. Saf. 184:109616. doi: 10.1016/j.ecoenv.2019.109616
Ye, Q., Wang, Y., Zhang, Z., Huang, W., Li, L., Li, J., et al. (2020). Dissolved organic matter characteristics in soils of tropical legume and non-legume tree plantations. Soil Biol. Biochem. 148:107880. doi: 10.1016/j.soilbio.2020.107880
Zhang, P., Tian, X., He, X., Song, F., Ren, L., and Jiang, P. (2008). Effect of litter quality on its decomposition in broadleaf and coniferous forest. Eur. J. Soil Biol. 44, 392–399. doi: 10.1016/j.ejsobi.2008.04.005
Zhang, X., Yu, H., Gao, H., Lu, K., and Liu, D. (2022). Explore variations of DOM components in different land cover areas of riparian zone by EEM-PARAFAC and partial least squares structural equation model. Spectrochim. Acta A Mol. Biomol. Spectrosc. 291:122300. doi: 10.1016/j.saa.2022.122300
Zhang, A., Zhou, X., Li, M., and Wu, H. (2017). Impacts of biochar addition on soil dissolved organic matter characteristics in a wheat-maize rotation system in loess plateau of China. Chemosphere 186, 986–993. doi: 10.1016/j.chemosphere.2017.08.074
Keywords: loess plateau, coniferous and broadleaved mixed forest, dissolved organic matter, soil profile, parallel factor analysis
Citation: Meng Y, Li P, Xiao L, Hu B, Zhang C, Yang S, Liu J and Zhao B (2024) Differences in dissolved organic matter and analysis of influencing factors between plantations pure and mixed forest soils in the loess plateau. Front. For. Glob. Change. 7:1344784. doi: 10.3389/ffgc.2024.1344784
Edited by:
Guangqiang Long, Yunnan Agricultural University, ChinaReviewed by:
William H. McDowell, Florida International University, United StatesHaohao Wu, Nanjing Institute of Geography and Limnology (CAS), China
Copyright © 2024 Meng, Li, Xiao, Hu, Zhang, Yang, Liu and Zhao. This is an open-access article distributed under the terms of the Creative Commons Attribution License (CC BY). The use, distribution or reproduction in other forums is permitted, provided the original author(s) and the copyright owner(s) are credited and that the original publication in this journal is cited, in accordance with accepted academic practice. No use, distribution or reproduction is permitted which does not comply with these terms.
*Correspondence: Peng Li, lipeng74@163.com