- 1College of Forestry, Hainan University, Haikou, China
- 2College of Life and Pharmaceutical Sciences, Hainan University, Haikou, China
Identifying soil characteristics associated with the plant’s resource use and acquisition strategy at different scales could be a crucial step to understanding community assembly and plant strategy. There is an increasing trend that plant functional properties can be an important driver of ecosystem functioning. However, major knowledge gaps exist about how soil abiotic properties, shape species diversity, above-ground biomass (AGB) and plant functional diversity in the Bawangling tropical forest (TCF) of Hainan island. Hence we hypothesized that plant functional traits and above-ground biomass would be strongly associated with soil abiotic factors given their direct relationship to soil resource acquisition and use. Here, we used 12 plant functional traits (FTs), above-ground biomass (AGB), and five soil nutrients in the Bawangling tropical cloud forest of Hainan Island by using a polynomial regression model and multivariate correlations to show relationship and identify how plants allocate their limited resources to adapt to their surroundings. Various phytosociological attributes were assessed and an Importance Value Index (IVI) value was calculated for each species to determine the dominant species. More than half of the total variations could be attributed to interspecific variations in H, DBH, LA, LMA, and LDW. From a taxonomic perspective; we found that species-level variance was more significant for plant functional traits and soil nutrients like TN, AP, TP, and OM. On the other hand, variation in specific stem density (SSD), leaf thickness (LT), leaf phosphorus (LP) and leaf soluble sugar (LS) was an exception for these tendencies. Among soil nutrients, soil nitrogen and phosphorus significantly impact the species and functional traits. Furthermore, the soil AN and TP we also found to have a comparatively strong positive relationship with above-ground biomass (AGB) as compared with other soil nutrients. The morpho-physiological functional traits showed a trade-off between conservative and acquisitive resource usage. These variations suggested that the relationships of functional traits, AGB, and species with soil nutrients mainly AN and TP in tropical cloud forests can directly affect the growth, reproduction, and survival of the species and are beneficial for the species co-existence and maintenance of biodiversity.
Introduction
Forests are the primary component of terrestrial ecosystems, offering diverse ecological services and ensuring people’s safety (Borma et al., 2022). There is a high level of biodiversity in the tropical forests, and strongly weathered soil is needed for the growth of these forests. There is a high diversity in the distribution of plant species and soils within a tropical region (Fujii et al., 2018). The tropical cloud forest has a distinct community structure, remarkable species, and distinctive habitats. Functional traits and phylogeny have been studied by several researchers (Luna-Vega and Magallón, 2010; Long et al., 2011, 2015a; Kang et al., 2017). In the humid tropics of Africa, America, and Asia, all the higher-elevation forests are covered mainly by mist or clouds (Bubb et al., 2004). Tropical cloud forests have more deformed trees covered by epiphytes than low-altitudinal tropical forests. When forests are compared at lower altitudes, their stand environment is defined by stronger winds, higher amounts of UV radiation, more frequent fogs and lower air temperatures. The preservation and stability of species variety in the community is the foundation of forest ecosystems’ stability and versatility of line of sight (Maestre et al., 2012). The species preservation strategy from a geographical pattern, functional features, and pedigree structure is revealed at equal angles (Kraft et al., 2008; Paine et al., 2011; Pearse et al., 2013). In Chinese vegetation, secondary forests are mostly found in Yunnan and Hainan Island (Bubb et al., 2004). In the previous findings, species richness and composition of the TCF were recognized in Yunnan (Shi and Zhu, 2009). Hainan Island’s tropical cloud forest community structure was also studied in the past on TCF’s community structure and species richness. Still, there was no comprehensive research on the Association and ordination between soil nutrients and functional diversity in a tropical cloud forest of Hainan Island. Hence, further research is needed to fill this gap.
Ecologists have studied the community-building mechanism using functional and phylogenetic diversity over the last ten years (Webb et al., 2002; Swenson et al., 2012; Swenson, 2013). The functional diversity of plants is known by their response under environmental stress, renewal reproduction, and resource acquisition stratifies. Plant functional characteristics (PFT) also indicate a plant’s evolutionary response to climate, competition, carbon gain, defense, life cycle and water relations (Wright et al., 2004). Ecologists increasingly recognize variation in PFT as a factor in community formation (Weiher and Keddy, 1999; McGill et al., 2006). PFTs significantly influence ecosystem processes, can anticipate ecosystem responses to environmental shifts and are crucial in defining how plant species interact with their surroundings (Cornelissen et al., 2003). The prevalent trait variations origin can be understood using strategies focusing on the significance of functional variations between species (Weiher and Keddy, 1998) and individuals (Paine et al., 2011). Systematic collection of characteristic features to determine the usefulness of certain traits in co-existing species can be assessed using different typical techniques such as field data and null models (Weiher et al., 1998; Kraft et al., 2008).
The functional traits are divided into different categories within individual plant organs, i.e., leaves (Wright et al., 2004; Li et al., 2015) and stem (Chave et al., 2009; Zanne et al., 2010) for supporting a limited set of possible trait combination; we have collected empirical evidence. According to the other studies, the plant organs are mostly inconsistent and are geographically or taxonomically very rare. However, it is unclear how the whole plant’s function and form are restricted. The SSD considers the trade-off between morality risk and the growth potential caused by the hydraulic failure. The Leaf area (LA) directly affects water and leaf energy balance LN and the LMA are the conversation strategies and the many features of leaf resource achievement. Leaf Mass Area reflects the trade-off between carbon gaining and longevity. Similarly, Nmass indicates the trade-off between the benefits of the photosynthetic capability and the expense of collecting nitrogen and suffering herbivory (Díaz et al., 2016; Yaseen et al., 2022).
In contrast to low-altitude tropical forests, tropical cloud forests (TCF) experience an unusual interaction of abiotic variables (Long et al., 2011). Individuals, species, and communities are affected by abiotic influences, species distribution, and the basic composition of communities (Gao et al., 2023). For instance, research has demonstrated that, despite severe abiotic constraints such as insufficient availability of soil nutrients and strong winds, facilitative interactions in tropical cloud forests facilitate the assembly and coexistence of tree species (Long et al., 2013). Similar abiotic factors will probably influence systems for allocating resources to individual species, interspecies relationships, and communities. Phosphate and nitrogen are key factors affecting species richness, Evenness, and community composition in soil. According to Augusto et al. (2017), the amount of soil N is positively correlated with the plant Simpson index, and according to Medvigy et al. (2019), soil P is crucial for plant diversity production and maintenance. Insufficient soil nutrients may generally be a limiting factor for species diversity reduction (Liu et al., 2020). For example, soil qualities are mechanistically linked to plant biomass (Fayolle et al., 2012). In contrast, Plant growth has been limited in Neotropical forests due to differences in soil P (John et al., 2007).
Above-ground productivity of a forest community is positively connected to soil formation, air purification, and the entire ecosystem where tree species predominated (Baró et al., 2014; Grace et al., 2016; Pesola et al., 2017). Tropical forests are well-known for their higher biodiversity and above-ground biomass and the fact that they grow on strongly weathered soils. A diverse range of plant species and soil types exist even in a tropical region (Fujii et al., 2018). Tree biomass is influenced by abiotic factors, such as water availability, although other factors also play a role in tropical regions (Poorter et al., 2016). Abiotic factors affecting tree biomass include soil nutrients and management history (Lohbeck et al., 2015; Poorter et al., 2016; Ali and Yan, 2017; Van Der Sande et al., 2017). Studying leaf morphological and physiological plasticity in response to altering soil properties such as soil nitrogen (N) and phosphorus (P) availability is significant and necessary for predicting carbon (C) dynamics in tropical forests worldwide (Mo et al., 2020). However, there are several studies on above-ground biomass worldwide, but very little research has been focused on the factors or drivers of biomass in tropical cloud forests, Hainan Island.
Most early community-building research focused on changes in species diversity, where all species are viewed as ecological equivalents of autonomous evolution. However, they cannot describe species’ evolutionary history and functional characteristic information and adequately expose the roots of community development (Webb et al., 2002; Swenson et al., 2012). Besides, there is limited knowledge about the construction mechanism of tropical cloud forest communities and different variations and correlations in FTs of plants in tropical cloud forests. We studied 12 functional traits and five soil nutrients of 73 tree species in Bawangling Nature Reserve (BNR), in the Hainan Island tropical cloud forest situation, and conducted multivariate analyses to examine their relationships and variations. In particular, the elevation is founded on the leaf and the stem functional traits associated with resource gain plus protection (Wright et al., 2004; Agrawal and Fishbein, 2006). Preserving the species (community building) is often regarded as the most challenging ecological concern to address.
The multivariate relationships among functional traits reflect how plants adjust to environments through limited resource allocation. These relationships are well studied across species, but whether the nature of these trait relationships changes across soil abiotic factors is rarely explored in the tropical cloud forest of Bawangling, Hainan Island. We hypothesized that plant functional traits and above-ground biomass would be strongly associated with soil abiotic factors given their direct relationship to soil resource acquisition and use. We also aim (a) how soil abiotic factors influence species diversity that allocate resources based on functional traits and soil nutrients in the tropical cloud forests of Bawangling and (b) whether morphological traits were linked with leaf nutrient concentration.
Materials and methods
Site conditions
Bawangling Nature Reserve (18°50′ –19°05’N, 109°05′ –109°25′ E) is one of the tropical cloud forests of Hainan Island. The terrain is high in the middle and low in the surroundings, with higher rainfall in the east. The west is less, and the south is warmer than the north; the southwest and west are affected by the southwestern season Wind influences; the dry season, which runs from November until April the following year, is accentuated by the wind; the central mountainous region has more clouds and fog and lower temperatures. While the average daily relative humidity ranges from 87.88 to 100% during the rainy season (May to October), the average daily air temperature ranges from 17.6 to 24.8°C. Notably, during the rainy season (May–October), the average daily temperature varies between 17.6 and 24.8°C, while relative humidity averages between 87.88 and 100%. The soils are montane meadow soils made from sandstone parent material, and the terrain may be steep with slopes ranging from 3° to 65° (Hu and Li, 1992; Long et al., 2011). In the cloud forests at BNR, the average tree height was 4.79 ± 2.80 m, with 9,633 stems per hectare of trees. Despite significant variations in tree density, all sites shared a similar species composition, with Exbucklandia tonkinensis, Osmanthus hainanensis, Polyspora axillaris, Machilus velutina, Illicium ternstroemioides, Rhododendron moulmainense, Syzygium odoratum, Osmanthus didymopetalus, Podocarpus neriifolius, and Michelia mediocris.
Data collection
In the Bawangling Nature Reserve, we collected data from randomly located 20 m x 20 m plots in tropical cloud forests (BNR: 21 plots). Each 400 m2 field was divided into four subplots of 10 × 10 m and 16 quadrats of 5 × 5 m using the neighbor grid method. The spacing across plots was kept higher than 50 meters. Flora Reipublicae Popularis Sinicae was used for taxonomic identification (Editorial Committee of Flora China, 2004). The forest where the sample plot is located consists of primitive old forest (undisturbed), mainly distributed at an altitude of 1,250 m above the summit or ridge. The DBH information was collected using the measuring tape for all the sampled trees, and the tree height was measured using a laser rangefinder (Long et al., 2022). Overall, we investigated 118 species having 9,728 individuals in the Bawangling tropical cloud forest of Hainan Island. The height of all trees with a dbh ≥ 1 was recorded using a clinometer.
The research area’s trees are relatively mature in stature, which allowed us to precisely measure the H, DBH, LA, LSA, LC, LT, LDW, SSD, LMA, LP, LN, and LSS for each of the tree species occurring plots. Response characteristics and impact traits were selected as functional features to illustrate how plants develop, endure, adjust to interference, and use environmental resources (Chave et al., 2009; Manek, 2023) (Table 1). Taking the one-sided area of a fresh leaf as an example, LMA is the dry mass divided by the one-sided area. It is believed that variations in LMA reflect the number of resources each species allocates to leaf development (Poorter et al., 2009). A plant’s capacity to receive nutrients and alter its pace of development is also influenced by LMA (Castro-Díez, 2012). As a measure of a plant’s capacity to strive for space and light (Long et al., 2015a), H measures the shortest distance from the plant stem base to the tallest sucker or shoot in the canopy. As a result, H is associated with acquiring carbon (Falster and Westoby, 2003).
Five newly sprouted leaves from each species were collected from each plot 400 m2 plot. To measure leaf thickness, a Vernier caliper with digital displays was used. Similarly, the leaf area (LA) was also measured using a leaf area meter. For each tree, a digital balance was used to weigh the leaves. The leaf is weighed on an Ohaus Adventurer AR2140 Analytical Balance, Hayward, CA, U.S.A. after it has been dried for at least 72 h at 70 degrees Celsius to a constant weight to determine the leaf Mass Area (mg mm2) and SLA (Specific Leaf Area) (mm2 mg−1).
Plant central stem portion oven-dry mass is measured over by the portion of the volume of the same plant when it is still fresh to determine SSD it is shown as (mg mm−3). The same type of plants should be indicated for both leaf quality and height, i.e., mature, healthy plants with leaves exposed to full sunshine. A small sample size of five individuals per species was taken with thinner main stems (diameter < 6 cm) and a 10 cm long section at least cut out at the height or length of one-third of the stems (Reyes et al., 1992; Cornelissen et al., 2003).
Kjeldahl technique was used to measure nitrogen concentration. After samples were broken down using the HClO4-H2SO4 digestion procedure, the phosphorus level was assessed using the molybdenum blue colorimetric method (Yang et al., 2021) and the anthrone colorimetric technique was used to determine the amount of soluble sugar (Cheng et al., 2019).
Soil analysis
Each 5m × 5 m takes off all of the soil’s surface litter in the tiny sample square, 20 cm from top to bottom. We collected soil samples from different study sites, mixed them thoroughly, and marked them for further analysis such as to determine the soil’s organic matter (OM), total nitrogen (TN), total phosphorus (TP), available nitrogen (AN), and adequate phosphorus (AP) contents after it dries naturally. The ability of potassium dichromate to measure the amount of organic matter in soil The material is first broken down using the HClO4-H2SO4 digestion process before being measured using the molybdenum antimony colorimetric method, which is a quantitative procedure. Use the hydrochloric acid-ammonium technique to calculate the effective phosphorus content. Alkaline solution diffusion was used to calculate the available nitrogen concentration (China Professional Council of Agricultural Chemistry, 1983).
Statistical analysis
The mean values of functional traits (H, DBH, SLA, LA, LDM, LC, LT, LMA, SSD, LP, LN, and LSS) were determined in Bawangling Nature Reserve (BNR) present in 21 plots of 20 × 20 m (400 m2) (Long et al., 2020). A multivariate correlation analysis was performed by using “psych” package and “pairs.panels” function. Furthermore, the p-value, t-value, standard error (SE) and correlation (R-values) were quantified by using corr.test function in RStudio 4.3.1. A multivariate principal component analysis (PCA) was performed to evaluate correlations between soil nutrients and dominant species using OriginPro 2021 software. After that, we also quantified the polynomial bivariate regression to determine the non-linear relationship between above-ground biomass and soil nutrients. We chose polynomial regression because the distribution of the data was not significantly linear. To calculate the above-ground biomass (AGB), we used the equation of Kenzo et al. (2009) developed for the tropical cloud forest with tree DBH >5cm.
Whereas dbh represents the diameter at breast height and H represents the plant height (Equation 1).
To calculate the importance value (IVI) of each tree species we first calculated the relative frequency, relative density and relative dominance of each species in the study area (Equations 2–5).
Moreover, the common diversity indices such as Pielou’s evenness index (E) (Pielou, 1966) Shannon–Wiener index (H), (Shannon and Weaver, 1963), Simpson’s diversity (D1), Simpson’s dominance (D2), (Simpson, 1949) were used to describe the general properties of the species diversity in the tropical cloud forest of Bawangling.
Results
Relationship between morphological and leaf nutrient concentration
The multivariate analysis was performed to show the correlation between the morphological and leaf physiological functional traits (Figure 1). The leaf chlorophyll contents (chl) were significantly positively correlated with the morphological functional traits such as plant height (r = 0.77), dbh (r = 0.60), LMA (0.74), SLA (r = 0.44), LA (r = 0.30) and LDW (r = 0.33). This association confirms that leaf structure modulates leaf chlorophyll and leaf morphological traits association. Secondly, the leaf total nitrogen (LTN) was significantly positively correlated with SLA (r = 0.39) and significantly negatively with LMA (r = −0.47), and LTh (r = −0.33). However, no significant association was found with the rest of the morphological traits. The correlation of leaf total phosphorus (LTP) and leaf soluble sugar (LS) with morphological functional traits was found non-significant, indicating that both LTP and LS were less defined by the morphological functional traits. Moreover, morphological functional traits were highly correlated with one another. Together, this set of leaf traits has been called the ‘worldwide leaf economics spectrum’ (LES) because this correlated suite of traits reflects the trade-off between the rapid acquisition of resources and the conservation of captured resources. The results of the correlation test such as standard error, correlation value and probability value for each trait were provided in (Supplementary Tables S2–S4).
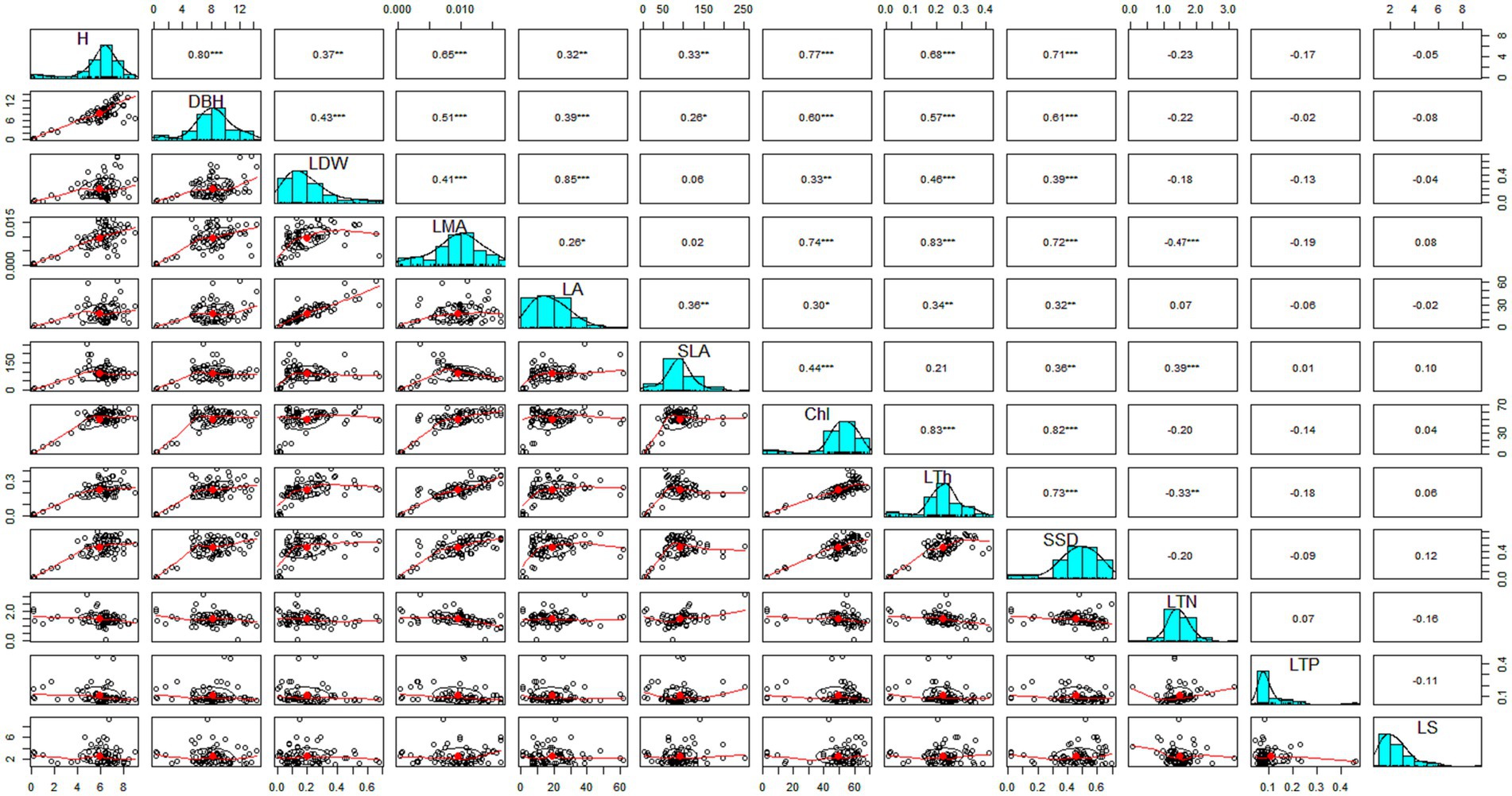
Figure 1. Showing the Pearson correlations between the morphological traits and leaf nutrient concentration.
Functional traits determined by soil nutrients
The multivariate analysis was performed to identify the correlation between the functional traits and soil nutrients (Figure 2). We found that OM was significantly positively correlated with SLA (r = 0.26, p = 0.05), but negatively associated with LTP (r = −0.02), SSD (r = −0.01), LA (r = −0.02), LDW (r = −0.13) and H (r = −0.3). The soil total phosphorus (TP) was significantly correlated with SLA (r = 0.25, p = 0.05), but negatively associated with LDW (r = −0.01). However, it was positively but weakly associated with the rest of the functional traits. The soil available nitrogen (AN) was significantly positively correlated with LTN (r = 0.25, p = 0.5), and SLA (r = 0.38, p = 0.005), while significantly negatively associated with LDW (r = −0.23, p = 0.5). Similarly, the soil available (AP) was significantly correlated with SLA (r = 0.23, p = 0.5) but negatively with LTP (r = −0.12), LA (r = −0.05), LDW (r = −0.15), dbh (r = −0.02), and H (r = −0.02). Moreover, we found that the soil total nitrogen (TN) was not significantly related to any of the functional traits indicating that plant functional traits were affected by soil TN. The results of the multiple correlation tests such as standard error, correlation value and probability value for each functional trait and soil nutrient were provided in (Supplementary Tables S5–S7).
Association between soil nutrients and dominant species
The principal component analysis was performed to show the visual relationship and dimensionality between the soil abiotic factors and dominant species. The two axes of PCA accounted for 87.3% of the variance in the soil nutrients and dominant species. The first PC showed 77.95% variation and is dominated by Distylium racemosum (IVI = 14.32), Michelia mediocris (IVI = 5.4), Hypserpa nitida (IVI = 5.92), Rhododendron moulmainense (IVI = 6.03), Tabernaemontana bovina (IVI = 7.55), Psychotria asiatica (IVI = 11.39), Symplocos poilanei (IVI = 7.54) and Symplocos lancifolia (IVI = 7.2). In comparison, the second PC showed 9.35% variation and is dominated by Cyclobalanopsis disciformis (IVI = 5.73), Illicium ternstroemioides (IVI = 4.43), Elaeocarpus howii (IVI = 4.68), Polyspora axillaris (IVI = 5.03), Machilus velutina (IVI = 3.63) (Figure 3).
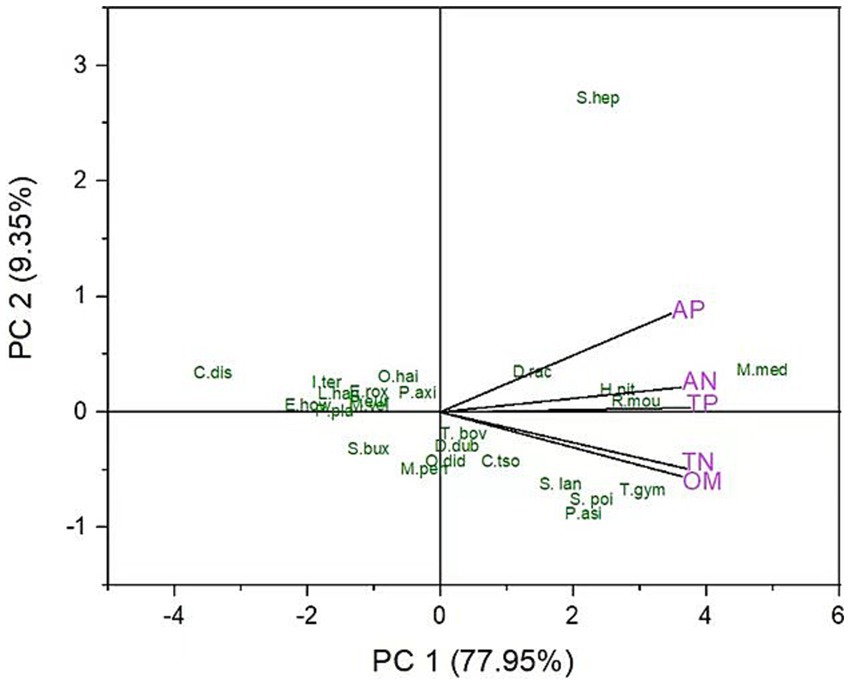
Figure 3. The principal component analysis shows the relationship between dominant species and soil nutrients.
Relationship between above-ground biomass and soil abiotic factors
The polynomial regression fit was used to identify a nonlinear relationship between AGB and soil nutrients. A non-significant (p > 0.05) relationship existed between soil abiotic factors and above-ground biomass. In tropical cloud forests of Bawangling, we found a relatively high and positive correlation of AGB with soil AN (r2 = 0.031) followed by TP (r2 = 0.0.014) and TN (r2 = 0.013), but a positively weak relationship of AGB with OM (r2 = 0.008), and AP (r2 = 0.006) (Figure 4).
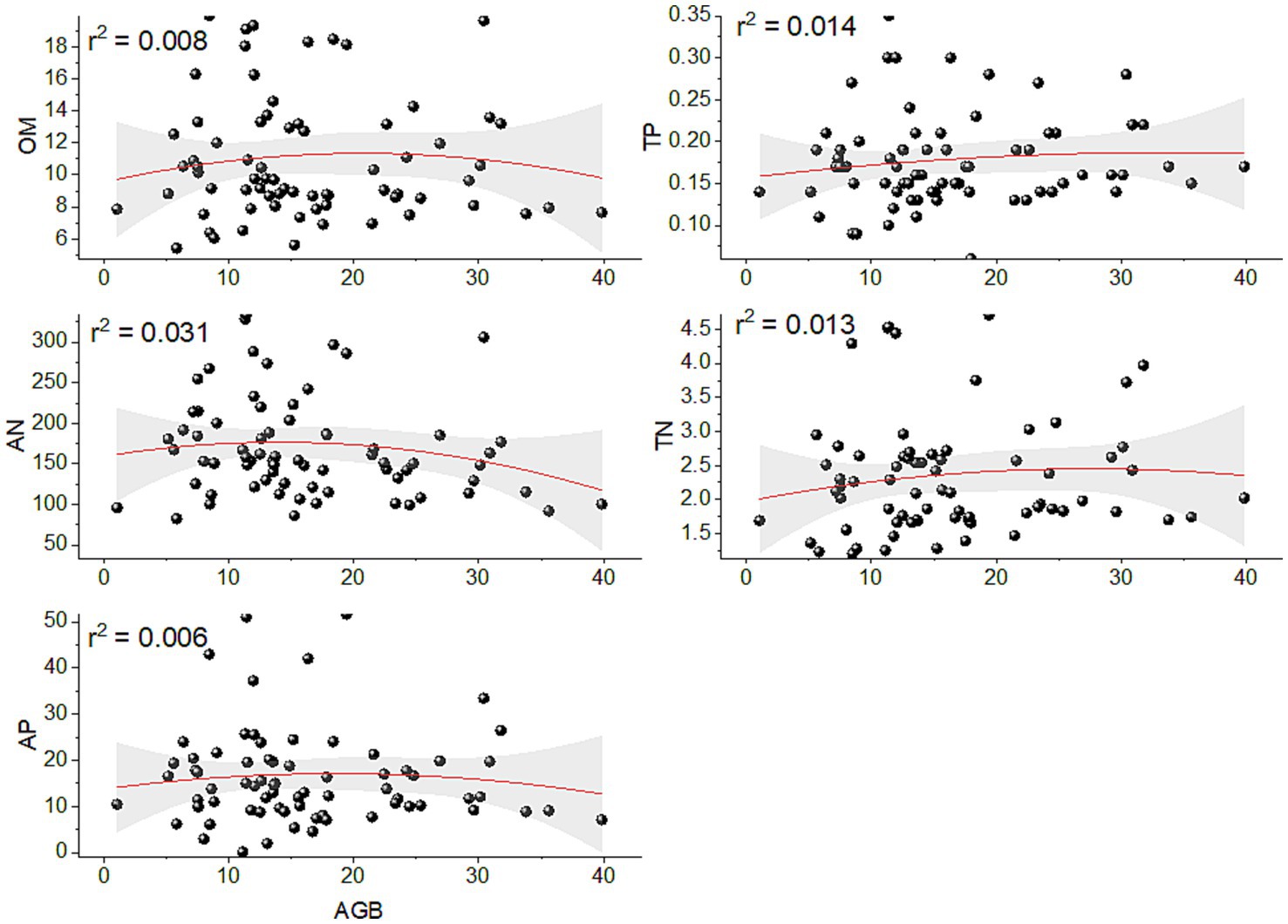
Figure 4. Showing the polynomial regression model to identify the non-linear correlation between above-ground biomass and soil nutrients. OM with AGB, soil TP with AGB, Soil AN with AGB, Soil TN with AGB and soil AP with AGB.
Phytosociological attributes and diversity indices
In BWL, tropical cloud forest structure and composition were quantified using various phytosociological indices such as frequency, density, and basal area analysis. The relative frequency, density, and basal area values were also determined. The species with a high importance value index (IVI) were called the dominant species. The maximum IVI was reported for Cyclobalanopsis disciformis (7.53), Cyclobalanopsis poilanei (8.83), Dacrydium pectinatum (4.94), Exbucklandia tonkinensis (4.84), Ilex hainanensis (3.58), Illicium ternstroemioides (4.43), Lithocarpus hancei (3.98), Melastoma penicillatum (6.37), Syzygium buxifolium (10.08), Tabernaemontana bovina (7.74) and Psydrax dicoccos (2.75). The phytosociological investigation would help us understand the vegetation dynamics of BWL forest vegetation and indicate the status of various species in the respective study sites. Diversity indices are the indicators of the species richness and health of vegetation in a particular area at a particular time. The diversity indices of the analyzed species showed higher values of Simpson diversity index (0.9581), Shannon_H, Shannon diversity index (H’e) (3.649), Evenness (0.3257) and the Fisher alpha diversity (18.89) was recorded in the tropical cloud forest of Bawangling (Figure 5).
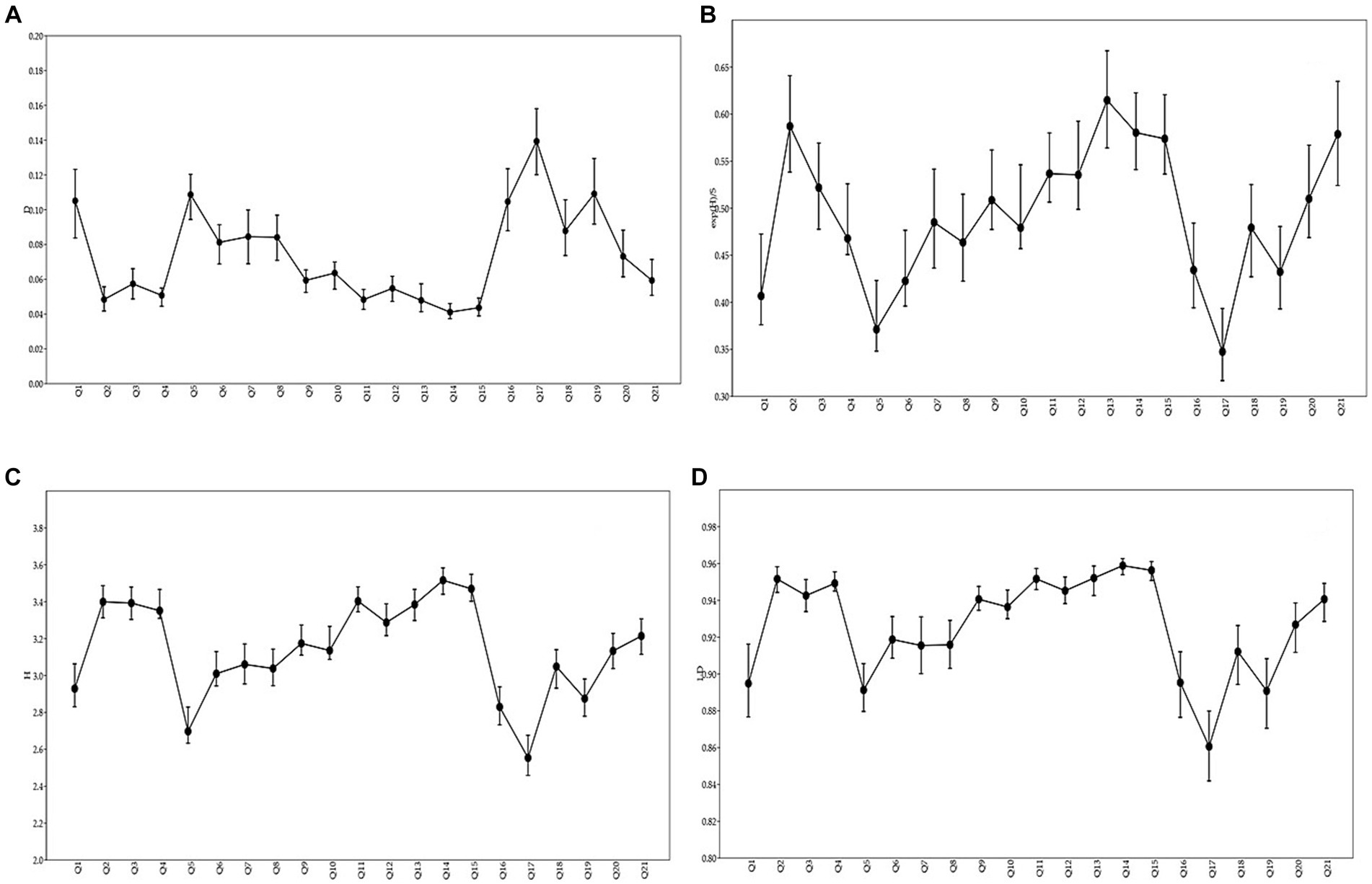
Figure 5. Showing Simpson’s dominance (A), Evenness (B), Shannon diversity index (C), and Simpson diversity (D).
Discussion
Relationship between morphological and leaf physiological traits
Results of the multivariate analysis of morphological and physiological functional traits indicated a significant interaction. The observed functional traits were first categorized into two groups, morphological (H, DBH, LT, LA, SLA) and leaf physiological functional traits (LTN, LS, LC, LTP). These functional traits were chosen because they are recognized as reflecting important plant growth and survival strategies (Chave et al., 2009). The trees in the tropical cloud forest of Bawangling showed a disproportionate scaling relationship between morphological functional and leaf physiological traits. In our data set, LT showed a clear pattern of weak positive association with SLA indicating that low-SLA plants, with differential allocation to storage and defense, and more typical of resource-poor habitats tended to have thicker leaves than high-SLA plants with preferential allocation to photosynthesis and growth, more typical of resource-rich habitats. This is in agreement with Roderick et al. (2000). However, in the case of the Argentine dataset, LT could be as useful as SLA as an indicator of plant resource-use strategy. However, Wilson et al. (1999) have suggested a non-monotonic relationship between LT and plant resource-use strategy in the Northern European flora. The LT can vary for reasons related more to light availability than the use of soil resources (Roderick et al., 2000). This suggests that the usefulness of LT as an indicator of resource use can vary from region to region, and for different ecological reasons. Some other studies also showed that LT reflects the mechanical strength of leaves and is related to nutrient storage and resistance to adverse environmental conditions (Witkowski and Lamont, 1991; Onoda et al., 2011). Plants living in conditions of intense light and drought or in infertile soil conditions tend to have leaves with a greater thickness (Grady et al., 2013). Moreover, SLA is one of the fundamental functional characteristics that reflect trade-offs between resource acquisition and conservation (Conti et al., 2018). The SLA appears as a trait with more direct ecological interpretation in comparative studies. Therefore, among the leaf traits analyzed in this study, SLA appears to be the best candidate for inclusion in large screening programs oriented to regional to global-scale comparisons. The plant height (H) and diameter at breast height (dbh) are important traits describing the tropical forest stand. In our data set, we found significantly positive relations between height (H) and diameter at breast height (DBH). This is an agreement with Mugasha et al. (2013). The trees with large DBH were taller and had more expansive canopies. Moreover, tree dbh and height had a great significance in forest inventory, management, as well as forest carbon-stock and biomass estimation (Liu et al., 2018).
We also found a positive correlation of leaf chlorophyll contents (Chl) with leaf thickness (LT) and SLA. It is well known, that water stress causes greater thickness of outer walls of the leaf epidermis and cuticle, and leaf thickness has been shown to affect leaf chlorophyll contents (Wood et al., 1993). As a way of resource allocation within leaves, SLA profoundly influences the efficiency of assimilating photosynthetic active radiation and ultimately plant photosynthetic capacity (Green et al., 2003). Moreover, exposed leaves in the canopy are thicker, more vertically inclined, and have lower chlorophyll content per unit weight and a higher chlorophyll a/b ratio compared to understory leaves (Poorter et al., 1995). Some other studies documented the relationship between LMA-LTh and LMA-Chl relationships, indicating that these relationships clarify plant strategies that balance responses to stress with maximizing growth in tropical cloud forests. In addition, a positive relation between LMA-WD and LDW-LMA was observed in the present study that corroborated with the previous findings of Long et al. (2020). The positive relationship between LMA and WD indicates that plants augment the accumulation of carbon-rich chemicals in leaves and stems, increasing the lignin and carbon density of the cell wall, and contributing to the thickening and hardening of those walls. According to Hanley et al. (2007), some functional traits, such as WD, are universally regarded as essential functional features responsible for plant growth rates. A high WD reflects a highly competitive tolerance and a strong competitive impact associated with a slow prospective development rate. The LMA is the dry mass divided by the one-sided area of a fresh leaf and can reflect the investment strategy of carbon and other nutrients in leaves (Wright et al., 2004; Poorter et al., 2009). The LMA is also related to a plant’s ability to obtain nutrients and change growth rate (Castro-Díez, 2012).
Some previous studies reported a positive correlation between morphological (SLA) and leaf physiological traits (LN and LP) for a wide range of species richness and biomes (Wright and Westoby, 2001; Lamont and Wiens, 2003; Yang et al., 2021; Yaseen et al., 2022) which supports our findings. Several factors affect the distribution and variation of nutrients between organs of the plant. For example, it depends upon plant functional groups, environmental control, and evolutionary history (Han et al., 2011; Song et al., 2016; Yang et al., 2018). Soil nutrients and climate are the main factors controlling the change of LPC and LNC and their proportion. In addition, SLA is closely associated with other important traits including leaf nitrogen concentration, lifespan and plant relative growth rate (Wright et al., 2004), representing one of the main ecological strategies of plants (Westoby, 1998; Wright et al., 2004) that are in line with our study. Some other studies showed a significantly positive correlation between TN and LPC (Zhang et al., 2020). This is because the phosphorus cycle is mainly influenced by climate and soil (Elser et al., 2010). Moreover, phosphorus is produced by rock weathering. It is, therefore, more dependent on climate change. The primary component of nucleic acid, ATP, sugar phosphate, and phospholipids in plants is phosphorus since these processes are directly tied to photosynthesis (Rao and Terry, 1995). Phosphorus-rich plants generally have increased stomatal conductance Thomas et al. (2006), while stomata function as a control interface for regulating plant water loss. Approximately 40% of the precipitation that falls on land surfaces is returned to the atmosphere via transpiration. The increase in soil temperature benefits the available nitrogen (AN) because it positively impacts the decomposition of litter and nutrient circulation. Under high AN conditions, plants may take more nitrogen, increasing the LN contents, as there is no inhibition from the soil components. With increased LN contents, photosynthesis and respiratory enzymes are activated, resulting in a greater CO2 exchange in leaves (Noguchi and Terashima, 2006).
In our data set, we found a positive correlation between morphological traits (SLA, LA, LT) and nonstructural carbohydrates (NSCs). The nonstructural carbohydrates (NSCs) are stored primarily as soluble sugars and starch in tree species and play important roles in the metabolic processes (photosynthesis, respiration, production) of plants and represent important physiological functional traits in plant’s adaptation to external environment (Fischer and Höll, 1991; Quentin et al., 2015). According to Dietze et al. (2014), soluble sugar can be used as a direct carbon source for transportation and plant use. Thus, soluble sugar indicates the plant’s ability to buffer environmental fluctuations (Zhang et al., 2021) by reflecting the balance between carbon intake and consumption (Würth et al., 2005). In the same way, soluble sugars influence the growth and development of plant tissue, both generative and vegetative. The leaf sugar content increased as the plants were flowering (Yang et al., 2022). Some other studies reported that SLA, NCS and LN concentrations were important predictors of leaf respiration across species and populations (Lee et al., 2005). The content of NSCs and their composition reflects the balance between carbon sources and sinks in plants (Li et al., 2016), while their allocation ratios in leaves, roots, and stems are considered as an eco-physiological attribute to evaluate the carbon budgets and adaptive strategies of plant species. Hence, these morpho-physiological traits suggest that these type trait relationships in tropical cloud forests could directly affect the growth, reproduction, and survival of the species in the tropical cloud forest.
Association between functional traits and soil nutrients
In this attempt to quantify relationships between functional traits and soil nutrients for the tropical cloud forest of Bawangling, we found several consistent, significant relationships. For example, OM was significantly positively correlated with SLA, but negatively associated with LTP, SSD, LA, LDW and H. Contrary to this, the relationship between SLA and soil organic carbon (TOC) displays an opposite pattern. This is probably because more nitrogen was locked up in relatively recalcitrant soil OM and therefore less available for uptake by plants, and also because of stoichiometric constraints (Ordoñez et al., 2009). The soil total phosphorus (TP) was significantly correlated with SLA and LTP. These findings were in agreement with the previous study of Ordoñez et al. (2009). Similarly, the soil available (AP) was also significantly correlated with SLA and is considered to be a limiting nutrient for plant growth and development all over the world (Vance et al., 2003). However, some other studies found an opposite trend indicating that the SLA and soil AP were not related (He et al., 2018). Although soil total P is not directly associated with actual P availability (Hinsinger, 2001), it still seemed to be a robust indicator of phosphorus fertility and was related to both leaf N and leaf P. Similarly, the soil available nitrogen (AN) was significantly positively correlated with LTN. The leaf nitrogen was the only leaf trait related to indicators of both N and P. This effect of soil P on leaf N seems determined by a tight coupling of leaf N and leaf P (Güsewell, 2004). Moreover, we found that the soil total nitrogen (TN) was positively related to SLA. Some previous studies revealed that nitrogen fertilization can increase SLA (Al Haj Khaled et al., 2005), while high nitrogen availability favors the dominance of high-SLA species (Craine et al., 2001). Likewise, other reports also indicated that SLA was positively related and responsive to soil nitrogen concentration (Niklas et al., 2005; He et al., 2018). Moreover, Ordoñez et al., (2009) revealed that the relations of soil total N with SLA, LP were not significant and those with LNC were in the opposite direction which supports our finding. Overall, the soil nitrogen and phosphorus were significantly correlated with leaf traits, while leaf traits such as SLA, LN, and LP are positively related to plant relative growth rates, leaf carbon assimilation rates, and energy supply.
Association between soil nutrients and dominant species
In tropical coastal forests in southern China, species richness is strongly correlated with soil nutrients, TP, TN, and OM By increasing the concentration of OM and TN in soil, species diversity and dominant species richness can be maintained (Whittaker et al., 1989; Long et al., 2018). Our findings also showed that soil nutrients significantly influenced dominant species, corroborated by previous studies (Neri et al., 2012). For example, Distylium racemosum (14.32), Hypserpa nitida (5.92), Rhododendron moulmainense (6.03), Tabernaemontana bovina (7.55), Psychotria asiatica (11.39), Symplocos poilanei (7.54) and Symplocos lancifolia (7.2) is positively and closely associated with AN, TP, TN, and OM Furthermore, in the deciduous forests of western India, the data showed a clear correlation between soil nitrogen and phosphorus concentrations as well as soil carbon concentrations and tree species richness (Kumar et al., 2010). Thus, dominant species and their impacts on their environments are key to predicting ecosystem diversity patterns.
Association between above-ground biomass and soil abiotic factors
The relationship between above-ground biomass and soil nutrients is fundamental to ecosystem functioning. Nutrients such as nitrogen (N), phosphorus (P), and organic matter (OM) are essential for plant growth and development, and their availability in the soil directly influences the productivity and composition of above-ground biomass. Additionally, organic matter in the soil plays a crucial role in nutrient cycling and availability. Above-ground biomass and productivity in forest ecosystems are influenced by changes in functional attributes as well as tree sizes, and by environmental factors (such as soils and topography) (Paquette et al., 2015; Zhang and Chen, 2015; Prado-Junior et al., 2016; Yuan et al., 2016). Our findings showed that TP was weakly but positively correlated with AGB, while soil phosphorus is an important growth-limiting resource and a vital driver of AGB in tropical forests (Cleveland et al., 2011). Some other studies indicated that soil phosphorus cannot be the significant determinant of AGB in tropical cloud forests, these findings were approximately support our findings (Lavorel and Gamier, 2002). It is very likely that many other soil nutrients are also essential and may be co-limiting tree growth. Contrary to our findings, a significantly positive association was found between soil TP and plant biomass (Fayiah et al., 2019). Soil P is the primary nutrient balancing plant productivity and better development in the forest ecosystem (Chen et al., 2000). We also found that TN was positively correlated with AGB, which corroborates with the study of Fayiah et al. (2019). A strong positive correlation between available nitrogen and above-ground biomass, indicating that higher availability of N promotes increased biomass production Lambers et al. (2008). Soil properties are essential in determining plant relationships because soil nitrogen availability can predict plant biomass across different ecosystems (Dingaan et al., 2016). According to Tiessen et al. (1994), organic matter content in the soil was positively correlated with above-ground biomass. A strong positive relationship between AGB and OM was found in the study of Zhao et al. (2023). In line with this, we also found the same results for AGB and OM. In terms of organic matter, it serves as a source of nutrients and plays a vital role in nutrient cycling within ecosystems. In conclusion, we found a positive but weak relationship between above-ground biomass and soil nutrients, including total N, total P, available N, available P, and organic matter. The availability of nutrients in the soil directly influences above-ground biomass production and composition. These findings underscore the importance of nutrient management and preserving organic matter to ensure sustainable ecosystem functioning and productivity.
Phytosociological attributes and species diversity
Various phytosociological attributes were evaluated and quantified for Bawangling vegetation stands, and it was found that most of the species were distributed contagiously (>0.025). In contrast, few species were distributed randomly, such as Casearia membranacea, Elaeocarpus sylvestris, Exbucklandia tonkinensis and Ficus variolosa. In Riparian vegetation, the structure and composition of the forest were also investigated through density frequency and abundance analysis (Curtis and McIntosh, 1950; Kujur et al., 2022) and abundance to frequency ratio (A/F ratio). This ratio represents both random (0.025–0.05) and contagious (>0.05) distributions (Curtis and Cottam, 1962; Kujur et al., 2022). Furthermore, species distribution patterns can be inferred from the A/F ratio. In optimal conditions, there may not be enough opportunities for seed dispersal or survival of saplings and such distributions are caused by phytogeographical restrictions (Long et al., 2015b; Kumar et al., 2017). In some cases, there was a clumping pattern of the species. Alternatively, this clumping may result from insufficient species seed dispersal (Richards, 1996) or the loss of trees allowing for the growth of several saplings simultaneously. Nevertheless, in Bawangling, the high tree density is responsible for the clumping of species distribution. Bawangling trees were dispersed in different directions and had different densities, indicating significant effects of disturbance regimes (Jhariya et al., 2019; Khan et al., 2020). Lyon and Gross (2005) suggest that disturbance regimes can regulate species composition and diversity patterns. Heterogeneity is likely the result of site conditions and geomorphological processes, which significantly determine species diversity, distribution, and abundance. The species (Distylium racemosum, Pinus fenzeliana, Psychotria asiatica) having IVI value >10 reflect their dominance in the form of frequency and density value of the species in Bawangling TCF Moreover, in the tropical cloud forests of Yunnan Province, the Lauraceae and Fagaceae (Shi and Zhu, 2009) and in Costa Rica, the Lauraceae (Nadkarni et al., 1995) are dominant families. Moreover, in Mexico’s tropical cloud forests, Symphytum species are the dominant species; the other dominant genera are variable (Alcántara and LunaI, 2002). In addition, over time, species diversity has increased; it may be possible to identify what tree species will be most suitable for restoring coastal windbreaks in the area (Yaseen et al., 2022). Moreover, the appearance and structure of communities are significantly different due to different environments and climates. As for the species with the lowest IVI values, they are fragile and may threaten biodiversity. In the study site, fragmentation is responsible for degenerating indigenous species, which alters their structure, composition and diversity (Ramakrishnan et al., 2000). The diversity indices are indicators of species richness and vegetation health in particular areas at certain times. The diversity indices of the analyzed species indicated higher values of Simpson diversity index (0.9581), Shannon diversity index (H’e) (3.649), Evenness (0.3257) and the Fisher alpha diversity (18.89) were recorded in the TCF of Bawangling. There are several ways of expressing plant diversity, but the most intuitive way is through species diversity. It represents the number and distribution of species within a community, a reflection of the resource-based coexistence (Jung, 2014; Wang et al., 2016).
Many ecological complexities within a plant community evolve in a specific location over time from different plant species. According to Wang et al. (2001), it is formed by the long-term interactions and relationships between plants and other species before moving on to the environment. Community species’ structure and composition are the basis for ecosystem activities and functions. The composition and structure of the community can be analyzed to learn more about coexistence guidelines and mechanisms that lead to species formation (Loreau et al., 2001). Our study confirms the significant role of soil abiotic factors in helping plant species assemble into communities in tropical cloud forests (TCF). The leaf economic spectrum (LES) of the Bawangling tropical cloud forest produces resource acquisition methods with distinct trade-offs between morphological and nutritional traits.
Conclusion
The soil nutrients that determine species diversity and above-ground biomass in the Bawangling tropical cloud forest of Hainan Island have been investigated. The functional traits were categorized into two groups such as morphological (H, DBH, LT, LA, SLA) and leaf physiological functional traits (LTN, LS, LC, LTP) showing a trade-off between conservative and acquisitive resource usage. These morpho-physiological traits suggest the inter-trait relationships in tropical cloud forests that directly affect the growth, reproduction, and survival of the species. This study highlights the critical role of local variation in soil nutrients primarily soil nitrogen and phosphorus for regulating trait selection and species distributions that support our hypothesis. However, the above-ground biomass (AGB) was not significantly influenced by soil abiotic factors that partially support our hypothesis. Our research also revealed that soil nutrients significantly affect several dominant species such as Distylium racemosum, Hypserpa nitida individuals, Rhododendron moulmainense, Tabernaemontana bovina, Psychotria asiatica, Symplocos poilanei and Symplocos lancifolia in which soil phosphorus and avaliable nitrogen shared the highest. Based on these findings, plants in this forest may use various developmental strategies connected to functional qualities and soil characteristics to adapt to local environmental conditions equally. Using several phytosociological attributes such as frequency density and basal area, the tropical forest showed more heterogeneity regarding species composition and community structure. Hence, these morpho-physiological traits and ABG associated with soil nutrients suggest that these trait relationships in tropical cloud forests can directly affect growth performance, reproduction, and survival of the species in the tropical cloud forest and contribute to a better understanding of plant ecological services, species co-existence, maintenance of the biodiversity and functioning of the tropical cloud forests.
Data availability statement
The original contributions presented in the study are included in the article/Supplementary material, further inquiries can be directed to the corresponding author.
Author contributions
FB: Formal analysis, Methodology, Writing – original draft. SB: Formal analysis, Methodology, Software, Writing – review & editing. WL: Conceptualization, Data curation, Funding acquisition, Investigation, Project administration, Resources, Supervision, Validation, Visualization, Writing – review & editing.
Funding
The author(s) declare financial support was received for the research, authorship, and/or publication of this article. This research was funded by the NSFC (32171772).
Acknowledgments
We thank our College of Forestry lab mates for their assistance in fieldwork. We also thank the National Positioning Observation and Research Station of Forest Ecosystem and Bawangling Branch of Hainan Tropical Rainforest National Park Administration.
Conflict of interest
The authors declare that the research was conducted in the absence of any commercial or financial relationships that could be construed as a potential conflict of interest.
Publisher’s note
All claims expressed in this article are solely those of the authors and do not necessarily represent those of their affiliated organizations, or those of the publisher, the editors and the reviewers. Any product that may be evaluated in this article, or claim that may be made by its manufacturer, is not guaranteed or endorsed by the publisher.
Supplementary material
The Supplementary material for this article can be found online at: https://www.frontiersin.org/articles/10.3389/ffgc.2024.1342135/full#supplementary-material
References
Agrawal, A. A., and Fishbein, M. (2006). Plant defense syndromes. Ecology 87, S132–S149. doi: 10.1890/0012-9658(2006)87[132,PDS]2.0.CO;2
Al Haj Khaled, R., Duru, M., Theau, J. P., Plantureux, S., and Cruz, P. (2005). Variation in leaf traits through seasons and N-availability levels and its consequences for ranking grassland species. Plant Sci. 16, 391–398. doi: 10.1111/j.1654-1103.2005.tb02378.x
Alcántara, O., and LunaI, V. A. (2002). Altitudinal distribution patterns of Mexican cloud forests based upon preferential characteristic genera. Plant Ecol. 161, 167–174. doi: 10.1023/A:1020343410735
Ali, A., and Yan, E. R. (2017). The forest strata-dependent relationship between biodiversity and above-ground biomass within a subtropical forest. For. For. Ecol. Manag. 401, 125–134. doi: 10.1016/j.foreco.2017.06.056
Augusto, L., Achat, D. L., Jonard, M., Vidal, D., and Ringeval, B. (2017). Soil parent material—a major driver of plant nutrient limitations in terrestrial ecosystems. Glob. Chang. Biol. 23, 3808–3824. doi: 10.1111/gcb.13691
Baró, F., Chaparro, L., Gómez-Baggethun, E., Langemeyer, J., Nowak, D. J., and Terradas, J. (2014). Contribution of ecosystem services to air quality and climate change mitigation policies: the case of urban forests in Barcelona, Spain. Ambio 43, 466–479. doi: 10.1007/s13280-014-0507-x
Borma, L. S., Costa, M. H., da Rocha, H. R., Arieira, J., Nascimento, N. C. C., Jaramillo-Giraldo, C., et al. (2022). Beyond carbon: the contributions of south American tropical humid and subhumid forests to ecosystem services. Rev. Geophys. 60:e2021RG000766. doi: 10.1029/2021RG000766
Castro-Díez, P. (2012). Functional traits analyses: scaling-up from species to community level. Plant Soil 357, 9–12. doi: 10.1007/s11104-012-1185-8
Chave, J., Coomes, D., Jansen, S., Lewis, S. L., Swenson, N. G., and Zanne, A. E. (2009). Towards a worldwide wood economics spectrum. Ecol. Lett. 12, 351–366. doi: 10.1111/j.1461-0248.2009.01285.x
Chen, C. R., Condron, L. M., Davis, M. R., and Sherlock, R. R. (2000). Effects of afforestation on phosphorus dynamics and biological properties in a New Zealand grassland soil. Plant Soil 220, 151–163. doi: 10.1023/A:1004712401721
Cheng, Y., Zhang, H., Wang, X., Long, W., Li, C., Fang, Y., et al. (2019). Effects of functional diversity and phylogenetic diversity on the tropical cloud forest community assembly. Chin. J. Plant Ecol. 43, 217–226. doi: 10.17521/cjpe.2019.0003
China Professional Council of Agricultural Chemistry, (1983). Agricultural chemical routine analysis methods of soil. Beijing, China: Science Press.
Cleveland, C. C., Townsend, A. R., Taylor, P., Alvarez-Clare, S., Bustamante, M. M., Chuyong, G., et al. (2011). Relationships among net primary productivity, nutrients and climate in tropical rain forest: a pan-tropical analysis. Ecol. Lett 14, 939–947. doi: 10.1111/j.1461-0248.2011.01658.x
Conti, L., Block, S., Parepa, M., Münkemüller, T., Thuiller, W., Acosta, A. T., et al. (2018). Functional trait differences and trait plasticity mediate biotic resistance to potential plant invaders. J. Ecol 106, 1607–1620. doi: 10.1111/1365-2745.12928
Cornelissen, J. H. C., Lavorel, S., Garnier, E., Díaz, S., Buchmann, N., Gurvich, D. E., et al. (2003). A handbook of protocols for standardized and easy measurement of plant functional traits worldwide. Aust. J. Bot. 51, 335–380. doi: 10.1071/BT02124
Coste, S., Baraloto, C., Leroy, C., Marcon, É., Renaud, A., Richardson, A. D., et al. (2010). Assessing foliar chlorophyll contents with the SPAD-502 chlorophyll meter: a calibration test with thirteen tree species of tropical rainforest in French Guiana. Ann. For. Sci. 67:607. doi: 10.1051/forest/2010020
Craine, J. M., Froehle, J., Tilman, D. G., Wedin, D. A., and Chapin, F. S. III. (2001). The relationships among root and leaf traits of 76 grassland species and relative abundance along fertility and disturbance gradients. Oikos 93, 274–285. doi: 10.1034/j.1600-0706.2001.930210.x
Curtis, J. T., and Cottam, G. (1962). Plant ecology workbook: laboratory field and reference manual. 3rd Edn. Pennsylvania State University: Burgess Publishing Company, 193.
Curtis, J. T., and McIntosh, R. P. (1950). The interrelations of certain analytic and synthetic phytosociological characters. Ecology 31, 434–455. doi: 10.2307/1931497
Díaz, S., Kattge, J., Cornelissen, J. H., Wright, I. J., Lavorel, S., Dray, S., et al. (2016). The global spectrum of plant form and function. Nature 529, 167–171. doi: 10.1038/nature16489
Dietze, M. C., Sala, A., Carbone, M. S., Czimczik, C. I., Mantooth, J. A., Richardson, A. D., et al. (2014). Nonstructural carbon in woody plants. Annu. Rev. Plant Biol. 65, 667–687. doi: 10.1146/annurev-arplant-050213-040054
Dingaan, M. N. V., Walker, S., Tsubo, M., and Newby, T. (2016). Influence of grazing on plant diversity-productivity relationship in semiarid grassland of South Africa. Appl. Ecol. Environ. Sci. 14, 1–13. doi: 10.15666/aeer/1404_001013
Elser, J. J., Fagan, W. F., Kerkhoff, A. J., Swenson, N. G., and Enquist, B. J. (2010). Biological stoichiometry of plant production: metabolism, scaling and ecological response to global change. New Phytol. 186, 593–608. doi: 10.1111/j.1469-8137.2010.03214.x
Falster, D. S., and Westoby, M. (2003). Plant height and evolutionary games. Trends Ecol. Evol. 18, 337–343. doi: 10.1016/S0169-5347(03)00061-2
Fayiah, M., Dong, S., Li, Y., Xu, Y., Gao, X., Li, S., et al. (2019). The relationships between plant diversity, plant cover, plant biomass and soil fertility vary with grassland type on Qinghai-Tibetan plateau. Agric. Ecosyst. Environ. 286:106659. doi: 10.1016/j.agee.2019.106659
Fayolle, A., Engelbrecht, B., Freycon, V., Mortier, F., Swaine, M., Réjou-Méchain, M., et al. (2012). Geological substrates shape tree species and trait distributions in African moist forests. PLoS One 7:e42381. doi: 10.1371/journal.pone.0042381
Fischer, C., and Höll, W. (1991). Food reserves of scots pine (Pinus sylvestris L.). I. Seasonal changes in the carbohydrate and fat reserves of pine needles. Trees 5, 187–195. doi: 10.1007/BF00227524
Fujii, K., Shibata, M., Kitajima, K., Ichie, T., Kitayama, K., and Turner, B. L. (2018). Plant–soil interactions maintain biodiversity and functions of tropical forest ecosystems. Ecol. Res. 33, 149–160. doi: 10.1007/s11284-017-1511-y
Gao, C., Bezemer, T. M., van Bodegom, P. M., Cornelissen, H. C., van Logtestijn, R., Liu, X., et al. (2023). Plant community responses to alterations in soil abiotic and biotic conditions are decoupled for above-and belowground traits. J. Ecol. 111, 903–914. doi: 10.1111/1365-2745.14070
Grace, J. B., Anderson, T. M., Seabloom, E. W., Borer, E. T., Adler, P. B., Harpole, W. S., et al. (2016). Integrative modelling reveals mechanisms linking productivity and plant species richness. Nature 529, 390–393. doi: 10.1038/nature16524
Grady, K. C., Laughlin, D. C., Ferrier, S. M., Kolb, T. E., Hart, S. C., Allan, G. J., et al. (2013). Conservative leaf economic traits correlate with fast growth of genotypes of a foundation riparian species near the thermal maximum extent of its geographic range. Funct. Ecol. 27, 428–438. doi: 10.1111/1365-2435.12060
Green, D. S., Erickson, J. E., and Kruger, E. L. (2003). Foliar morphology and canopy nitrogen as predictors of light-use efficiency in terrestrial vegetation. Agric. For. Meteorol. 115, 163–171. doi: 10.1016/S0168-1923(02)00210-1
Güsewell, S. (2004). N:P ratios in terrestrial plants: variation and functional significance. New Phytol. 164, 243–266. doi: 10.1111/j.1469-8137.2004.01192.x
Han, W. X., Fang, J. Y., Reich, P. B., Ian Woodward, F., and Wang, Z. (2011). Biogeography and variability of eleven mineral elements in plant leaves across gradients of climate, soil and plant functional type in China. Ecol. Lett. 14, 788–796. doi: 10.1111/j.1461-0248.2011.01641.x
Hanley, M. E., Lamont, B. B., Fairbanks, M. M., and Rafferty, C. M. (2007). Plant structural traits and their role in anti-herbivore defence. Perspect. Plant Ecol. Evol. Syst. 8, 157–178. doi: 10.1016/j.ppees.2007.01.001
He, D., Chen, Y., Zhao, K., Cornelissen, J. H. C., and Chu, C. (2018). Intra-and interspecific trait variations reveal functional relationships between specific leaf area and soil niche within a subtropical forest. Ann. Bot. 121, 1173–1182. doi: 10.1093/aob/mcx222
Hinsinger, P. (2001). Bioavailability of soil inorganic P in the rhizosphere as affected by root-induced chemical changes: a review. Plant Soil 237, 173–195. doi: 10.1023/A:1013351617532
Hu, YJ, and Li, YX (1992). TropicalrainforestinHainanIsland.GuangdongHigherEducationPress,Guangzhou. 1–332. (in Chinese with English abstract).
Jhariya, M. K., Banerjee, A., Meena, R. S., and Yadav, D. K. (2019). Sustainable agriculture, Forest and Environmental Management. Singapore: Springer. 1:606.
John, R., Dalling, J. W., Harms, K. E., Yavitt, J. B., Stallard, R. F., Mirabello, M., et al. (2007). Soil nutrients influence spatial distributions of tropical tree species. PNAS 104, 864–869. doi: 10.1073/pnas.0604666104
Jung, S. H. (2014). Stratified Fisher’s exact test and its sample size calculation. Biom. J. 56, 129–140. doi: 10.1002/bimj.201300048
Kang, Y., Deng, Z., Zang, R., and Long, W. (2017). D.N.A. barcoding analysis and phylogenetic relationships of tree species in tropical cloud forests. Sci. Rep. 7:12564. doi: 10.1038/s41598-017-13057-0
Kenzo, T., Furutani, R., Hattori, D., Kendawang, J. J., Tanaka, S., Sakurai, K., et al. (2009). Allometric equations for accurate estimation of above-ground biomass in logged-over tropical rainforests in Sarawak. Malaysia. J. Res. 14, 365–372. doi: 10.1007/s10310-009-0149-1
Khan, N., Jhariya, M. K., Yadav, D. K., and Banerjee, A. (2020). Herbaceous dynamics and CO2 mitigation in an urban setup- a case study from Chhattisgarh. India Environ. Sci. Poll. Res. 27, 2881–2897. doi: 10.1007/s11356-019-07182-8
Kraft, N. J. B., Valencia, R., and Ackerly, D. D. (2008). Functional traits and niche-based tree community assembly in an Amazonia Forest. Science 322, 580–582. doi: 10.1126/science.1160662
Kujur, E., Jhariya, M. K., Yadav, D. K., and Banerjee, A. (2022). Phytosociological attributes and regeneration potential of riparian vegetation in northern Chhattisgarh. India Environ. Dev. Sustain. 24, 2861–2886. doi: 10.1007/s10668-021-01557-z
Kumar, A., Jhariya, M. K., Yadav, D. K., and Banerjee, A. (2017). Vegetation dynamics in Bishrampur collieries of northern Chhattisgarh, India: eco-restoration and management perspectives. Environ. Monit. Assess. 189:371. doi: 10.1007/s10661-017-6086-0
Kumar, J. N., Kumar, R. N., Bhoi, R. K., and Sajish, P. R. (2010). Tree species diversity and soil nutrient status in three sites of tropical dry deciduous forest of western India. Trop. Ecol. 51, 273–279. doi: 10.6165/tai.2019.64.28
Lambers, H., Chapin, F. S., and Pons, T. L. (2008). Plant physiological ecology (Vol. 2, pp. 11–99). New York: Springer.
Lamont, B. B., and Wiens, D. (2003). Are seed set and speciation ratesalways low among species that resprout after fire, and why? Evol. Ecol. 17, 277–292. doi: 10.1023/A:1025535223021
Lavorel, S., and Gamier, E. (2002). Predicting changes in community composition and ecosystem functioning from plant traits: revisiting the holy grail. Funct. Ecol. 16, 545–556. doi: 10.1046/j.1365-2435.2002.00664.x
Lee, T. D., Reich, P. B., and Bolstad, P. V. (2005). Acclimation of leaf respiration to temperature is rapid and related to specific leaf area, soluble sugars and leaf nitrogen across three temperate deciduous tree species. Funct. Ecol. 19, 640–647. doi: 10.1111/j.1365-2435.2005.01023
Li, N., He, N., Yu, G., Wang, Q., and Sun, J. (2016). Leaf non-structural carbohydrates regulated by plant functional groups and climate: evidences from a tropical to cold-temperate forest transect. Ecol. Indic. 62, 22–31. doi: 10.1016/j.ecolind.2015.11.017
Li, L. E., McCormack, M. L., Ma, C., Kong, D., Zhang, Q., Chen, X., et al. (2015). Leaf economics and hydraulic traits are decoupled in five species-rich tropical-subtropical forests. Ecol. Lett. 18, 899–906. doi: 10.1111/ele.12466
Liu, H., Chen, Q., Liu, X., Xu, Z., Dai, Y., Liu, Y., et al. (2020). Variation patterns of plant composition/diversity in Dacrydium pectinatum communities and their driving factors in a biodiversity hotspot on Hainan Island, China. Glob. Ecol. Conserv. 22:e01034. doi: 10.1016/j.gecco.2020.e01034
Liu, G., Wang, J., Dong, P., Chen, Y., and Liu, Z. (2018). Estimating individual tree height and diameter at breast height (DBH) from terrestrial laser scanning (TLS) data at plot level. Forests 9:398. doi: 10.3390/f9070398
Lohbeck, M., Poorter, L., Martínez-Ramos, M., and Bongers, F. (2015). Biomass is the main driver of changes in ecosystem process rates during tropical forest succession. Ecology 96, 1242–1252. doi: 10.1890/14-0472.1
Long, W. X., Schamp, B. S., Zang, R. G., Ding, Y., Huang, Y. F., and Xiang, Y. Z. (2015a). Community assembly in a tropical cloud forest related to specific leaf area and maximum species height. J. Veg. Sci. 26, 513–523. doi: 10.1111/jvs.12256
Long, W. X., Xiong, M. H., Zang, R. G., Schamp, B. S., Yang, X. B., Ding, Y., et al. (2015b). Changes in patterns of species co-occurrence across two tropical cloud forests differing in soil nutrients and air temperature. Biotropica 47, 416–423. doi: 10.1111/btp.12235
Long, C., Yang, X., Long, W., Li, D., Zhou, W., and Zhang, H. (2018). Soil nutrients influence plant community assembly in two tropical coastal secondary forests. Trop. Conserv. Sci. 11:194008291881795. doi: 10.1177/1940082918817956
Long, W., Zang, R. G., Ding, Y., and Huang, Y. F. (2013). Effects of competition and facilitation on species assembly in two types of tropical cloud forest. PLoS One 8:e60252. doi: 10.1371/journal.pone.0060252
Long, W., Zang, R., Schamp, B. S., and Ding, Y. (2011). Within-and among-species variation in specific leaf area drive community assembly in a tropical cloud forest. Oecologia 167, 1103–1113. doi: 10.2307/41500021
Long, W., Zang, R., Wang, X., and Bahadur, S. (2022). “Environmental characteristics in tropical cloud forests” in Tropical cloud Forest ecology in Hainan Island (Singapore: Springer Nature Singapore), 3–12.
Long, W., Zhou, Y., Schamp, B. S., Zang, R., Yang, X., Poorter, L., et al. (2020). Scaling relationships among functional traits are similar across individuals, species, and communities. J. Veg. Sci. 31, 571–580. doi: 10.1111/jvs.12893
Loreau, M., Naeem, S., Inchausti, P., Bengtsson, J., Grime, J. P., Hector, A., et al. (2001). Biodiversity and ecosystem functioning: current knowledge and future challenges. Science 294, 804–808. doi: 10.1126/science.1064088
Luna-Vega, I., and Magallón, S. (2010). Phylogenetic composition of angiosperm diversity in the cloud forests of Mexico. Biotropica 42, 444–454. doi: 10.1111/j.1744-7429.2009.00606.x
Lyon, J., and Gross, N. M. (2005). Patterns of plant diversity and plant–environmental relationships across three riparian corridors. For. Ecol. Manag. 204, 267–278. doi: 10.1016/j.foreco.2004.09.019
Maestre, F. T., Quero, J. L., Gotelli, N. J., Escudero, A., Ochoa, V., Delgado-Baquerizo, M., et al. (2012). Plant species richness and ecosystem multifunctionality in global drylands. Science 335, 214–218. doi: 10.1126/science.1215442
Manek, H. (2023). “Plant functional traits: mountainous soil function and ecosystem services” in Understanding soils of mountainous landscapes (Understanding Soils of Mountainous Landscapes, Elsevier), 347–373.
McGill, B. J., Enquist, B. J., Weiher, E., and Westoby, M. (2006). Rebuilding community ecology from functional traits. Trends Ecol. Evol. 21, 178–185. doi: 10.1016/j.tree.2006.02.002
Medvigy, D., Wang, G., Zhu, Q., Riley, W. J., Trierweiler, A. M., Waring, B. G., et al. (2019). Observed variation in soil properties can drive large variation in modelled forest functioning and composition during tropical forest secondary succession. New Phytol. 223, 1820–1833. doi: 10.1111/nph.15848
Mo, Q., Wang, W., Chen, Y., Peng, Z., and Zhou, Q. (2020). Response of foliar functional traits to experimental N and P addition among overstory and understory species in a tropical secondary forest. Glob. Ecol. Conserv. 23:e01109. doi: 10.1016/j.gecco.2020.e01109
Morgenroth, J., Nowak, D. J., and Koeser, A. K. (2020). DBH distributions in America’s urban forests—an overview of structural diversity. Forests 11:135. doi: 10.3390/f11020135
Mugasha, W. A., Bollandsås, O. M., and Eid, T. (2013). Relationships between diameter and height of trees in natural tropical forest in Tanzania. South. For. 75, 221–237. doi: 10.2989/20702620.2013.824672
Nadkarni, N. M., Matelson, T. J., and Haber, W. A. (1995). Structural characteristics and floristic composition of a neotropical cloud forest, Monteverde, Costa Rica. J. Trop. Ecol 11, 481–495. doi: 10.1017/S0266467400009020
Neri, A. V., Schaefer, C. E. G. R., Silva, A. F., Souza, A. L., Ferreira-Junior, W. G., and Meira-Neto, J. A. A. (2012). The influence of soils on the floristic composition and community structure of an area of Brazilian Cerrado vegetation. Edinb. J. Bot. 69, 1–27. doi: 10.1017/S0960428611000382
Niklas, K. J., Owens, T., Reich, P. B., and Cobb, E. D. (2005). Nitrogen/phosphorus leaf stoichiometry and the scaling of plant growth. Ecol. Lett. 8, 636–642. doi: 10.1111/j.1461-0248.2005.00759.x
Noguchi, K. O., and Terashima, I. (2006). Responses of spinach leaf mitochondria to low N availability. Plant Cell Environ. 29, 710–719. doi: 10.1111/j.1365-3040.2005.01457.x
Onoda, Y., Westoby, M., Adler, P. B., Choong, A. M., Clissold, F. J., Cornelissen, J. H., et al. (2011). Global patterns of leaf mechanical properties. Ecol. Lett. 14, 301–312. doi: 10.1111/j.1461-0248.2010.01582.x
Ordoñez, J. C., Van Bodegom, P. M., Witte, J. P. M., Wright, I. J., Reich, P. B., and Aerts, R. (2009). A global study of relationships between leaf traits, climate and soil measures of nutrient fertility. Glob. Ecol. Biogeogr. 18, 137–149. doi: 10.1111/j.1466-8238.2008.00441.x
Paine, C. T., Baraloto, C., Chave, J., and Hérault, B. (2011). Functional traits of individual trees reveal ecological constraints on community assembly in tropical rain forests. Oikos 120, 720–727. doi: 10.1111/j.1600-0706.2010.19110.x
Paquette, A., Joly, S., and Messier, C. (2015). Explaining forest productivity using tree functional traits and phylogenetic information: two sides of the same coin over evolutionary scale? Ecol. Evol. 5, 1774–1783. doi: 10.1002/ece3.1456
Pearse, W. D., Jones, A., and Purvis, A. (2013). Barro Colorado Island’s phylogenetic assemblage structure across fine spatial scales and among clades of different ages. Ecology 94, 2861–2872. doi: 10.1890/12-1676.1
Pesola, L., Cheng, X., Sanesi, G., Colangelo, G., Elia, M., and Lafortezza, R. (2017). Linking above-ground biomass and biodiversity to stand development in urban forest areas: a case study in northern Italy. Landsc. Urban Plan. 157, 90–97. doi: 10.1016/j.landurbplan.2016.06.004
Phalla, T., Ota, T., Mizoue, N., Kajisa, T., Yoshida, S., Vuthy, M., et al. (2017). The importance of tree height in estimating individual tree biomass while considering errors in measurements and allometric models. AGRIVITA J Agricul. Sci. 40, 131–140. doi: 10.17503/agrivita.v40i1.1730
Pielou, E. C. (1966). The measurement of diversity in different types of biological collections. J. Theor. Biol. 13, 131–144. doi: 10.1016/0022-5193(66)90013-0
Poorter, L., Bongers, F., Aide, T. M., Almeyda Zambrano, A. M., Balvanera, P., Becknell, J. M., et al. (2016). Biomass resilience of Neotropical secondary forests. Nature 530, 211–214. doi: 10.1038/nature16512
Poorter, H., Niinemets, Ü., Poorter, L., Wright, I. J., and Villar, R. (2009). Causes and consequences of variation in leaf mass per area (LMA): a meta-analysis. New Phytol. 182, 565–588. doi: 10.1111/j.1469-8137.2009.02830.x
Poorter, L., Oberbauer, S. F., and Clark, D. B. (1995). Leaf optical properties along a vertical gradient in a tropical rain forest canopy in Costa Rica. Am. J. Bot. 82, 1257–1263. doi: 10.1002/j.1537-2197.1995.tb12659.x
Prado-Junior, J. A., Schiavini, I., Vale, V. S., Arantes, C. S., van der Sande, M. T., Lohbeck, M., et al. (2016). Conservative species drive biomass productivity in tropical dry forests. J. Ecol. 104, 817–827. doi: 10.1111/1365-2745.12543
Quentin, A. G., Pinkard, E. A., Ryan, M. G., Tissue, D. T., Baggett, L. S., Adams, H. D., et al. (2015). Non-structural carbohydrates in woody plants compared among laboratories. Tree Physiol. 35, tpv073–tpv1165. doi: 10.1093/treephys/tpv073
Ramakrishnan, P. S., Chandrashekara, U. M., Elouard, C., Chaumette, M., and De Pommery, H. (2000). Mountain biodiversity, Landuse dynamics, and traditional ecological knowledge. Oxford and I.B.H. Publishing Co. Pvt. Ltd., New Delhi.
Rao, I. M., and Terry, N. (1995). Leaf phosphate status, photosynthesis, and carbon partitioning in sugar beet (IV. Changes with time following increased supply of phosphate to low-phosphate plants). Plant Physiol. 107, 1313–1321. doi: 10.1104/pp.107.4.1313
Reyes, G., Brown, S., Chapman, J., and Lugo, A. E. (1992). Wood densities of tropical tree species—General technical report S0-88. Forest Service southern Forest Station, United States Department of Agriculture, New Orleans, Louisiana.
Richards, P. W. (1996). The tropical Rain Forest: an ecological study (2nd ed.). London: Cambridge University Press.
Roderick, M. L., Berry, S. L., and Noble, I. R. (2000). A framework for understanding the relationship between environment and vegetation based on the surface areas to volume ratio of leaves. Funct. Ecol. 14, 423–437. doi: 10.1046/j.1365-2435.2000.00438.x
Shannon, C. E., and Weaver, W. (1963). The mathematical theory of communication. Univ. Ill. Press 1:102. doi: 10.2307/2489847
Shi, J. P., and Zhu, H. (2009). Tree species composition and diversity of tropical mountain cloud forest in the Yunnan, southwestern China. Ecol. Res. 24, 83–92. doi: 10.1007/s11284-008-0484-2
Song, X., Chen, N., He, M., Li, X., Tian, F., Zhang, K., et al. (2016). Divergent variations in concentrations of chemical elements among shrub organs in a temperate desert. Sci. Rep. 6:20124. doi: 10.1038/srep20124
Swenson, N. G. (2013). The assembly of tropical tree communities–the advances and shortcomings of phylogenetic and functional trait analyses. Ecography 36, 264–276. doi: 10.1111/j.1600-0587.2012.00121.x
Swenson, N. G., Erickson, D. L., Mi, X., Bourg, N. A., Forero-Montaña, J., Ge, X., et al. (2012). Phylogenetic and functional alpha and beta diversity in temperate and tropical tree communities. Ecology 93, S112–S125. doi: 10.1890/11-0402.1
Thomas, D. S., Montagu, K. D., and Conroy, J. P. (2006). Leaf inorganic phosphorus as a potential indicator of phosphorus status, photosynthesis and growth of Eucalyptus grandis seedlings. For. Ecol. Manag. 223, 267–274. doi: 10.1016/j.foreco.2005.11.006
Tiessen, H., Feller, C., and Anderson, J. M. (1994). Carbon sequestration in soils: science, monitoring and beyond. Can. J. Soil Sci. 74, 383–392. doi: 10.1016/j.geoderma.2018.07.026
Van der Sande, M. T., Peña-Claros, M., Ascarrunz, N., Arets, E. J., Licona, J. C., Toledo, M., et al. (2017). Abiotic and biotic drivers of biomass change in a Neotropical forest. J. Ecol. 105, 1223–1234. doi: 10.1111/1365-2745.12756
Vance, C. P., Uhde-Stone, C., and Allan, D. L. (2003). Phosphorus acquisition and use: critical adaptations by plants for securing a nonrenewable resource. New Phytol. 157, 423–447. doi: 10.1046/j.1469-8137.2003.00695.x
Wang, D., Ji, S., and Chen, F. (2001). A review on the species diversity of plant community. Chin. J. Plant Ecol. 4:55.
Wang, X. X., Long, W. X., Yang, X. B., Xiong, M. H., Kang, Y., Huang, J., et al. (2016). Patterns of plant diversity within and among three tropical cloud forest communities in Hainan Island. Chin. J. Plant Ecol. 40, 469–479. doi: 10.17521/cjpe.2016.0021
Webb, C. O., Ackerly, D. D., McPeek, M. A., and Donoghue, M. J. (2002). Phylogenies and community ecology. Annu. Rev. Ecol. Evol. Syst. 33, 475–505. doi: 10.1146/annurev.ecolsys.33.010802.1504
Weiher, E., and Keddy, C. P. A. (1998). Community assembly rules, morphological dispersion, and the coexistence of plant species. Oikos 81, 309–322. doi: 10.2307/3547051
Weiher, E., and Keddy, P. (1999). Assembly rules as general constraints on community composition. Ecol. Assem. Rules 1, 251–271. doi: 10.1017/CBO9780511542237
Westoby, M. (1998). A leaf-height-seed (LHS) plant ecology strategy scheme. Plant Soil 199, 213–227. doi: 10.1023/A:1004327224729
Whittaker, R. J., Bush, M. B., and Richards, K. J. E. M. (1989). Plant recolonization and vegetation succession on the Krakatau Islands, Indonesia. Ecol. Monogr. 59, 59–123. doi: 10.2307/2937282
Wilson, J., Thompson, K., and Hodgson, J. G. (1999). Specific leaf area and leaf dry matter content as alternative predictors of plant strategies. New Phytol. 143, 155–162. doi: 10.1046/j.1469-8137.1999.00427.x
Witkowski, E. T. F., and Lamont, B. B. (1991). Leaf specific mass confounds leaf density and thickness. Oecologia 88, 486–493. doi: 10.1007/BF00317710
Wood, C. W., Reeves, D. W., and Himelrick, D. G. (1993). Relationships between chlorophyll meter readings and leaf chlorophyll concentration, N status, and crop yield: a review. Proceedings of the agronomy society of New Zealand. 23, 1–9.
Wright, I. J., Reich, P. B., Westoby, M., Ackerly, D. D., Baruch, Z., Bongers, F., et al. (2004). The worldwide leaf economics spectrum. Nature 428, 821–827. doi: 10.1038/nature02403
Wright, I. J., and Westoby, M. (2001). Understanding seedling growth relationships through specific leaf area and leaf nitrogen concentration: generalizations across growth forms and growth irradiance. Oecologia 127, 21–29. doi: 10.1007/s004420000554
Würth, M. K., Pelaez-Riedl, S., Wright, S. J., and Körner, C. (2005). Nonstructural carbohydrate pools in a tropical forest. Oecologia 143, 11–24. doi: 10.1007/s00442-004-1773-2
Yang, X. D., Anwar, E., Zhou, J., He, D., Gao, Y. C., Lv, G. H., et al. (2022). Higher association and integration among functional traits in small tree than shrub in resisting drought stress in an arid desert. Environ. Exp. Bot. 201:104993. doi: 10.1016/j.envexpbot.2022.104993
Yang, Y., Liu, B. R., and An, S. S. (2018). Ecological stoichiometry in leaves, roots, litters and soil among different plant communities in a desertified region of northern China. Catena 166, 328–338. doi: 10.1016/j.catena.2018.04.018
Yang, Y., Xiao, C., Wu, X., Long, W., Feng, G., and Liu, G. (2021). Differing trade-off patterns of tree vegetative organs in a tropical cloud Forest. Front. Plant Sci. 12:680379. doi: 10.3389/fpls.2021.680379
Yang, L., Zhao, H., Zuo, Z., Li, X., Yu, D., and Wang, Z. (2021). Generality and shifts in leaf trait relationships between alpine aquatic and terrestrial herbaceous plants on the tibetan plateau. Front. Ecol. Evol. 9:706237. doi: 10.3389/fevo.2021.706237
Yaseen, M., Fan, G., Zhou, X., Long, W., and Feng, G. (2022). Plant diversity and soil nutrients in a tropical coastal secondary forest: association ordination and sampling year differences. Forests 13:376. doi: 10.3390/f13030376
Yaseen, M., Long, W., Khalid, F., Bahadur, S., and Noushahi, H. A. (2022). Shifts in community vegetative organs and their dissimilar trade-off patterns in a tropical coastal secondary Forest, Hainan Island, Southern China. Diversity 14:823. doi: 10.3390/d14100823
Yuan, Z., Wang, S., Gazol, A., Mellard, J., Lin, F., Ye, J., et al. (2016). Multiple metrics of diversity have different effects on temperate forest functioning over succession. Oecologia 182, 1175–1185. doi: 10.1007/s00442-016-3737-8
Zanne, A. E., Westoby, M., Falster, D. S., Ackerly, D. D., Loarie, S. R., Arnold, S. E., et al. (2010). Angiosperm wood structure: global patterns in vessel anatomy and their relation to wood density and potential conductivity. Am. J. Bot. 97, 207–215. doi: 10.3732/ajb.0900178
Zhang, Y., and Chen, H. Y. (2015). Individual size inequality links forest diversity and above-ground biomass. J. Ecol. 103, 1245–1252. doi: 10.1111/1365-2745.12425
Zhang, Z., Fu, X., Sheng, Q., Yin, D., Zhou, Y., and Huang, J. (2021). Effect of rainfall pattern and crack on the stability of a red bed slope: a case study in Yunnan province. Adv. Civ. Eng. 2021, 1–21. doi: 10.1155/2021/6658211
Zhang, S., Zhang, Y., Xiong, K., Yu, Y., and Min, X. (2020). Changes of leaf functional traits in karst rocky desertification ecological environment and the driving factors. Glob. Ecol. Conserv. 24:e01381. doi: 10.1016/j.gecco.2020.e01381
Keywords: Bawangling, functional diversity, soil nutrients, above-ground biomass, principal component analysis
Citation: Batool F, Bahadur S and Long W (2024) Soil nutrients determine leaf traits and above-ground biomass in the tropical cloud forest of Hainan Island. Front. For. Glob. Change. 7:1342135. doi: 10.3389/ffgc.2024.1342135
Edited by:
Ilona Mészáros, University of Debrecen, HungaryReviewed by:
Miguel Ángel Munguía-Rosas, National Polytechnic Institute of Mexico (CINVESTAV), MexicoYonglei Jiang, Yunnan Academy of Tobacco Agricultural Sciences, China
Copyright © 2024 Batool, Bahadur and Long. This is an open-access article distributed under the terms of the Creative Commons Attribution License (CC BY). The use, distribution or reproduction in other forums is permitted, provided the original author(s) and the copyright owner(s) are credited and that the original publication in this journal is cited, in accordance with accepted academic practice. No use, distribution or reproduction is permitted which does not comply with these terms.
*Correspondence: Wenxing Long, oklong@hainanu.edu.cn