- 1Anhui Provincial Key Laboratory of Forest Resources and Silviculture, Anhui Agricultural University, Hefei, China
- 2School of Forestry and Landscape Architecture, Anhui Agricultural University, Hefei, China
- 3College of Urban Construction, Zhejiang Shuren University, Hangzhou, China
- 4College of Environmental Engineering, Xuzhou University of Technology, Xuzhou, China
Introduction: Land-use changes significantly impact soil properties in forests, which is an area of concern. Therefore, the effects of changing forest types on soil microbial communities and their functions in northern subtropical forest regions need to be further researched.
Methods: We used 16S rDNA sequencing and Functional Annotation of Prokaryotic Taxa (FAPROTAX) to assess the variation of soil bacterial communities and potential functions related to carbon (C) and nitrogen (N) cycling in two soil layers (0–10 and 10–30 cm) after the conversion of the secondary masson pine (Pinus massoniana, PM) forest to plantations of slash pine (Pinus elliottii, PE) and Chinese fir (Cunninghamia lanceolata, CL) located in Jingde County, Anhui Province, China.
Results: The study found that converting coniferous secondary forests to coniferous plantations resulted in a notable increase in soil pH and a decrease in nitrate nitrogen and organic carbon contents. Additionally, soil microbial diversity increased significantly, and microbial community structure changed, particularly in the topsoil. These changes might affect the C- and N-cycling mediated by soil bacteria. The analysis revealed a significant decrease in the abundance of functional groups associated with C-cycling and a significant increase in the abundance of functional groups associated with N-cycling, particularly those associated with denitrification. Soil organic carbon, pH, and ammonium nitrogen were the most critical variables affecting changes in the soil microbial community.
Discussion: These findings provide valuable information for ecological restoration and future sustainable forest management.
1 Introduction
The global area of naturally regenerating forests is about 3.737 billion hectares, which decreased at a declining rate of loss since 1990 (FAO, 2020). Rapid social and economic development and the expansion of agribusiness activities have resulted in ecosystem degradation and widespread conversion of primary and secondary forests into agricultural fields and tree plantations (Drescher et al., 2016; Tateishi et al., 2021). Generally, primary forests (including secondary forests) are naturally occurring forests that have not been cultivated or less disturbed by humans, and in primary forests, there are companion tree species, young trees and other ground covers in addition to dominant species. Their species richness is usually higher than those in plantations (Hua et al., 2022). The plantation forest has a specific business purpose, commonly with intensive management practices such as tending and intermediate harvesting. Moreover, plantations are generally even-aged with a low species composition and a simple hierarchical structure. The conversion of secondary forests to monoculture plantations has great impacts on ecosystem functions and services, including a reduction in species diversity and changes in microbial communities (Diaz-Vallejo et al., 2021). In southeastern China, large areas of natural secondary forests have been cut down and burned for planting economic conifers, especially Chinese fir (Cunninghamia lanceolata), to renovate and improve the existing inefficient forest ecosystems and to increase their productivity (Zhou et al., 2016). This conversion through a series of management measures (e.g., logging, refining mountains, land preparation) is an essential form of land use change (Keenan et al., 2015), which resulted in intensive disturbance of belowground ecosystems (Paz-Ferreiro and Fu, 2016; Lyu et al., 2017). Thus, forest conversion is a major cause of influencing the soil ecological environment (Bruun et al., 2015). It also affects the cycling of major bioelements in the terrestrial ecosystem (Camponi et al., 2022; Zhu et al., 2022). For example, Mayer et al. (2020) showed that forest management practices, such as site preparation, restoration harvesting, and species replacement, significantly affect the capacity of soil organic carbon (SOC) stock. As SOC decreased, microbial nitrogen (N) turnover also decreased, which in turn reduced N mineralization rates (Burton et al., 2007). In addition, an experiment conducted in a subtropical forest in southern China showed that forest conversion reduced soil water content, available phosphorus (AP), total nitrogen (TN), microbial biomass, and enzyme activity (urease, protease, and acid phosphatase) (Guo et al., 2022). It could result from soil disturbances and/or organic matter input from litterfall production and fine root turnover (Sewerniak, 2020; Ni et al., 2021). Therefore, the study of forest conversion on soil C and N cycling is crucial in subtropical forest ecosystems.
Soil microorganisms play an active role in forest biogeochemical cycles (Bardgett and Van der Putten, 2014). As the most dominant microbial group, bacteria are vital in the cycling of basic elements such as C, N, and P (Dennis et al., 2013). They are particularly sensitive to microenvironment changes and thus can serve as an indicator to reflect changes in soil habitat (Wu et al., 2014). For example, Proteobacteria is one of the most diverse microbiota on Earth (Bradley and Pollard, 2017). It exhibits a huge functional library, including phototrophic, autotrophic, and heterotrophic (Zhou Z. et al., 2020). It is an ideal lineage to study the link between genome diversification and environmental adaptation. In addition, Proteobacteria can regulate soil organic matter mineralation (González et al., 2000). While Actinobacteria can produce a variety of secondary metabolites. The effects of Actinobacteria on other microorganisms varied significantly under different nutrient conditions, with neutral effects dominating the interactions. It accounted for 68% under eutrophic conditions, indicating that high nutrient levels promoted the active metabolism of Actinobacteria. Whereas, the effect under poor nutrient conditions was an indication of overlapping ecological niches (Alvarez et al., 2017; Yan et al., 2021). Different tree species composition can affect soil properties, nutrient cycling, and soil microbiota diversity (Liu J. L. et al., 2018; Nakayama et al., 2019; Kitson and Bell, 2020). Therefore, changes in forest types and species composition strongly influence the quality and quantity of forest litterfall inputs (Yang et al., 1997; Wang J. et al., 2020), thereby altering soil bacterial community properties and functions. Conversion of tropical rainforests to plantations altered soil bacteria composition, decreasing Alphaproteobacteria and increasing Acidobacteria (Kerfahi et al., 2016; Berkelmann et al., 2018). Forest conversion significantly altered soil bacterial beta-diversity, and this variation had significant seasonal differences (Liu S. G. et al., 2018). It is evident that forest conversion is an important driver of changes in soil bacterial composition and diversity (Nakayama et al., 2019).
Soil microorganisms and their functional groups are crucial for maintaining biogeochemical cycle, particularly in the period of forest succession (Paula et al., 2014; Tian et al., 2019). C and N are important elements in the biogeochemical cycle. It has been widely noticed that the C- and N-cycling processes are mediated by soil microorganisms (Rivett and Bell, 2018; Castro et al., 2019; Chen and Sinsabaugh, 2021). The conversion of natural broadleaf forests to plantations has led to a decrease in the taxa abundance of soil carbon fixation, carbon degradation, and methane metabolism, which may be due to the reduction of microbial biomass carbon (MBC), dissolved organic carbon (DOC), and pH by forest conversion (Luo et al., 2022). In the process of N-cycling, microorganisms are mainly involved in nitrate reduction, nitrogen fixation, nitrification, denitrification, and ammonia oxidation (Levy-Booth et al., 2014; Wang S. Y. et al., 2020). Merloti et al. (2019) showed that forest conversion altered microbial composition and function and increased nitrification and denitrification in agricultural lands. However, due to the complexity and spatial heterogeneity of soil microbial communities in natural environments, particularly in mountain forests, there is limited researches on the potential mechanisms of forest conversion on soil ecological functions. In addition, most of the studies on forest conversion were from natural or secondary forests to coniferous plantations, and rarely from coniferous secondary forests to coniferous plantations. Therefore, further studies are necessary to comprehensively understand the effect of coniferous forest conversion on soil bacterial communities and their functional groups.
In this study, the primary objective was to evaluate the effects of forest conversion on soil microbial communities, especially those potential functional groups associated with C- and N-cycling, in the northern subtropical region in east China. We used 16S rDNA sequencing technology and the FAPROTAX platform to examine alterations in both the structure and functional groups of soil bacteria in different soil layers following the conversion of secondary masson pine (Pinus massoniana, PM) forest to plantations of slash pine (Pinus elliottii, PE) and Chinese fir (Cunninghamia lanceolata, CL). We had three main hypotheses: Firstly, changes in soil properties led to significant changes in soil bacterial community composition and diversity. Secondly, reduced nutrient availability in plantations increased the abundance of bacterial functional groups associated with N-cycling. Finally, soil pH and N availability may be the most critical factors influencing soil bacterial communities and potential functional structure after forest conversion.
2 Methods and materials
2.1 Site description
The experimental site is located in the Caijiaqiao Forest Farm (118°29′8″ E, 30°20′53″ N), which is a State-owned forest covering a total area of 3,577 hectares in Jingde County, Anhui, China. This area belongs to the subtropical humid monsoon climate zone. The average annual temperature is 16.7°C and the average annual precipitation is 1476 mm. The primary soil type is yellowish-red soil, corresponding to the typic Ultisols in soil taxonomy (USDA Classification). The zonal vegetation at the study site is subtropical forest dominated by evergreen broad-leaved tree species, such as Castanopsis eyrei (Champ. ex Benth.) Tutcher, C. sclerophylla (Lindl.) Schottky, Quercus glauca Thunb. The secondary forest is dominated by Pinus massoniana Lamb. with deciduous and evergreen broad-leaved species (Liquidambar formosana Hance, Sassafras tzumu (Hemsl.) Hemsl., Q. variabilis Blume, and C. sclerophylla) covering large area in this forest farm. Since the 1980s, a large number of secondary P. massoniana forests has been harvested, and replaced by coniferous plantations, such as Cunninghamia lanceolata (Lamb.) Hook., Pinus elliottii Engelm. for the timber production.
The sampling forests included the secondary masson pine forest (P. massoniana, PM; 39 years old), plantation forests of Chinese fir (C. lanceolata, CL; 10 years old) and slash pine (P. elliottii, PE; 11 years old) converted from the secondary masson pine forest.
2.2 Sampling
On August 23, 2020, we collected soil samples from the above-mentioned forest stands. A randomized block design (9 replicates) was arranged, with each replicate plot of 20 m × 20 m in size spaced at least 100 m apart to avoid mutual interference. Supplementary Appendix Table 1 contains the standing conditions of the sample plots. The management measures were consistent for different forest types.
In each replicate plot, soil samples were collected from 0–10 to 10–30 cm layers after removing surface litterfall. Five cores were randomly mixed to form a composite sample from each layer. All sampling tools were sterilized at high temperatures before sampling. 54 samples were collected (3 treatments, 2 layers, and 9 replicates). They were immediately transferred to the laboratory in a cooler box with ice. In the laboratory, samples were further homogenized and screened (2 mm) and then split into two. One portion was stored at −80°C for subsequent microbial extraction, while the other was air-dried for soil property analysis.
2.3 Soil properties analysis
Soil pH was measured in a soil and water suspension with a pH meter, using a ratio of 1 part soil to 2.5 parts water. The soil organic carbon (SOC) and total nitrogen (TN) concentrations were analyzed by dry combustion on an elemental analyzer (EA 3000, Vector, Italy). Ammonium and nitrate nitrogen concentration were extracted using 1 mol ⋅ L–1 KCl, and total phosphorus (TP) was digested with mixed acids (HNO3: HClO4, 3: 1 by volume) and subsequently determined using a flow injection analyzer (Alliance Futura, France). The previous study provided detailed measurements (Teng et al., 2020).
2.4 Soil samples DNA extraction and bioinformatics analysis
The total genomic DNA of the soil was extracted with a soil DNA kit (D4015, Omega, Inc., USA). Afterward, agarose gel electrophoreses and UV spectrophotometers were used to detect the quality and quantify DNA samples, respectively. The v3-v4 variable regions of the 16S rDNA gene were then amplified by primers 341F (5′-CCTACGGGGNGGCWGCAG-3′) and 805R (5′GACTACHVGGGTATCTAATCC-3′). Amplification products were purified and quantified. The final amplicon library was sequenced on the NovaSeq PE250 platform (Teng et al., 2018).
The raw data were obtained after the up-sequencing was completed. Paired-end reads were spliced using overlap, and quality control and filtering were performed to obtain high-quality clean data. Noise reduction of the sequence was performed by calling DADA2 (Divisive Amplicon Denoising Algorithm), which recovered the true microbial sequence in the sample through two steps: error correction and denoising. Error correction utilized machine learning methods to build an error model and used that model to correct the sequencing data. Denosing utilized Bayesian methods to make denoising decisions for each ASV to obtain the final Feature table and characterized sequences. The SILVA (release 132) reference dataset was used to train and annotate the feature sequences. QIIME2 was utilized to calculate the alpha and beta diversity of the soil bacterial community. At last, the 16S rDNA sequencing results were in turn annotated by the FAPROTAX platform to clarify the potential functions of biogeochemical cycles (Louca et al., 2017).
2.5 Statistical analysis
Two-way repeated measures analysis of variance (ANOVA) was used to analyze significant differences among soil layers (0–10 cm and 10–30 cm) and three stands (masson pine, slash pine, and Chinese fir), respectively, and the LSD test for pairwise comparisons using SPSS 22.0 software. The Galaxy platform1 was used to perform linear discriminant analysis Effect Size (LDA) to detect differences in bacterial taxa and functional groups among forest types, with significance at p < 0.05 and LDA > 3. Principal coordinate analysis (PCoA) was used to analyze soil bacterial compositions. Spearman correlation and redundancy analysis (RDA) were used to evaluate the relationship between soil properties, soil bacterial compositions, and functional groups. The statistical analyses were performed using SPSS version 22.0, and Origin 2019b was used for plotting. Statistical significance was considered at p < 0.05 in all analyses.
3 Results
3.1 Soil properties
The variation in soil properties was influenced by both forest type and soil layer (Table 1). Compared with the secondary forests, the pH and NH4+-N concentration in the plantations were significantly increased, while NO3–-N and SOC were significantly decreased (p < 0.05). Furthermore, a significant decrease in SOC, TN, and NH4+-N was detected with soil depth in all three stands (p < 0.05). A similar significant decrease in TP and NO3–-N was observed with soil depth in the secondary forest and Chinese fir plantation (p < 0.05).
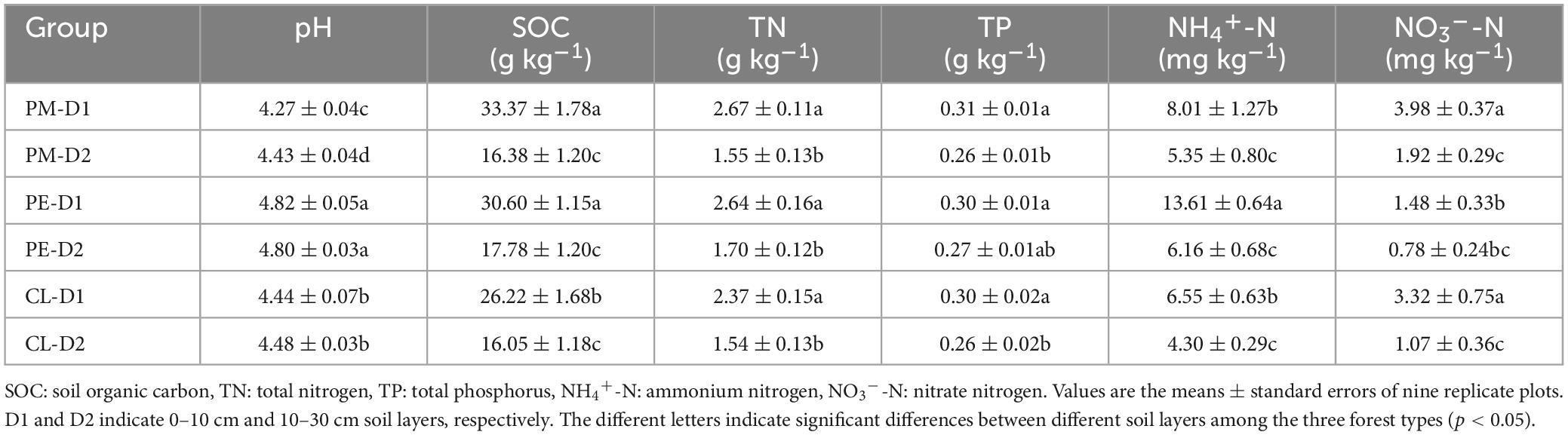
Table 1. Soil chemical properties among the secondary Pinus massoniana forest (PM), the plantation forests of Pinus elliottii (PE), and Cunninghamia lanceolata (CL).
3.2 Composition and diversity of soil bacterial community
There were notable differences in soil bacterial community structure between secondary and plantation forests (Figure 1). The Adonis test confirmed that forest conversion altered the soil bacterial community structure (p < 0.05, Table 2). The alpha-diversity indices (Shannon and Simpson indices) and soil bacterial community richness (Observed species and Chao1) demonstrated a significant increase in the slash pine plantation compared to the secondary forests. No significant differences were found between the two plantations. The alpha-diversity indices and community richness of the secondary forest varied between soil layers (0–10 cm, 10–30 cm), while no statistically significant differences were found in the plantation forests (Figure 2).
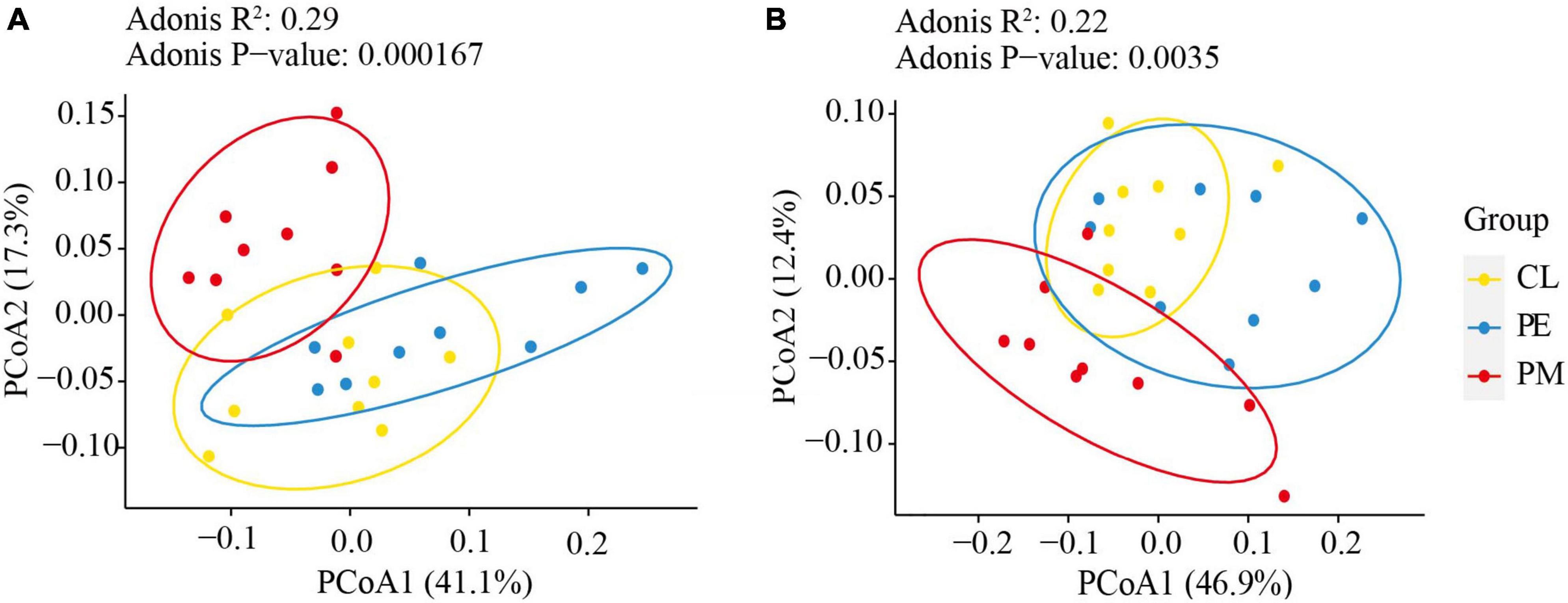
Figure 1. The bacterial communities in 0–10 cm (A) and 10–30 cm (B) among the secondary forest of Pinus massoniana (PM), the plantation forests of Pinus elliottii (PE) and Cunninghamia lanceolata (CL) were analyzed using principal coordinate analysis (PCoA) based on Weighted UniFrac distances.
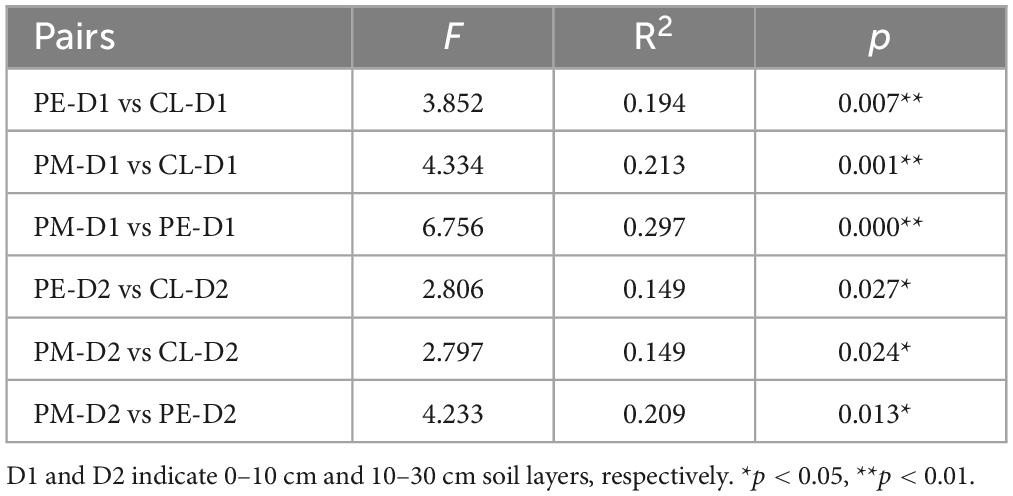
Table 2. Differences in soil bacterial community composition among the secondary forest of Pinus massoniana (PM), the plantation forests of Pinus elliottii (PE) and Cunninghamia lanceolata (CL) based on Adonis analysis.
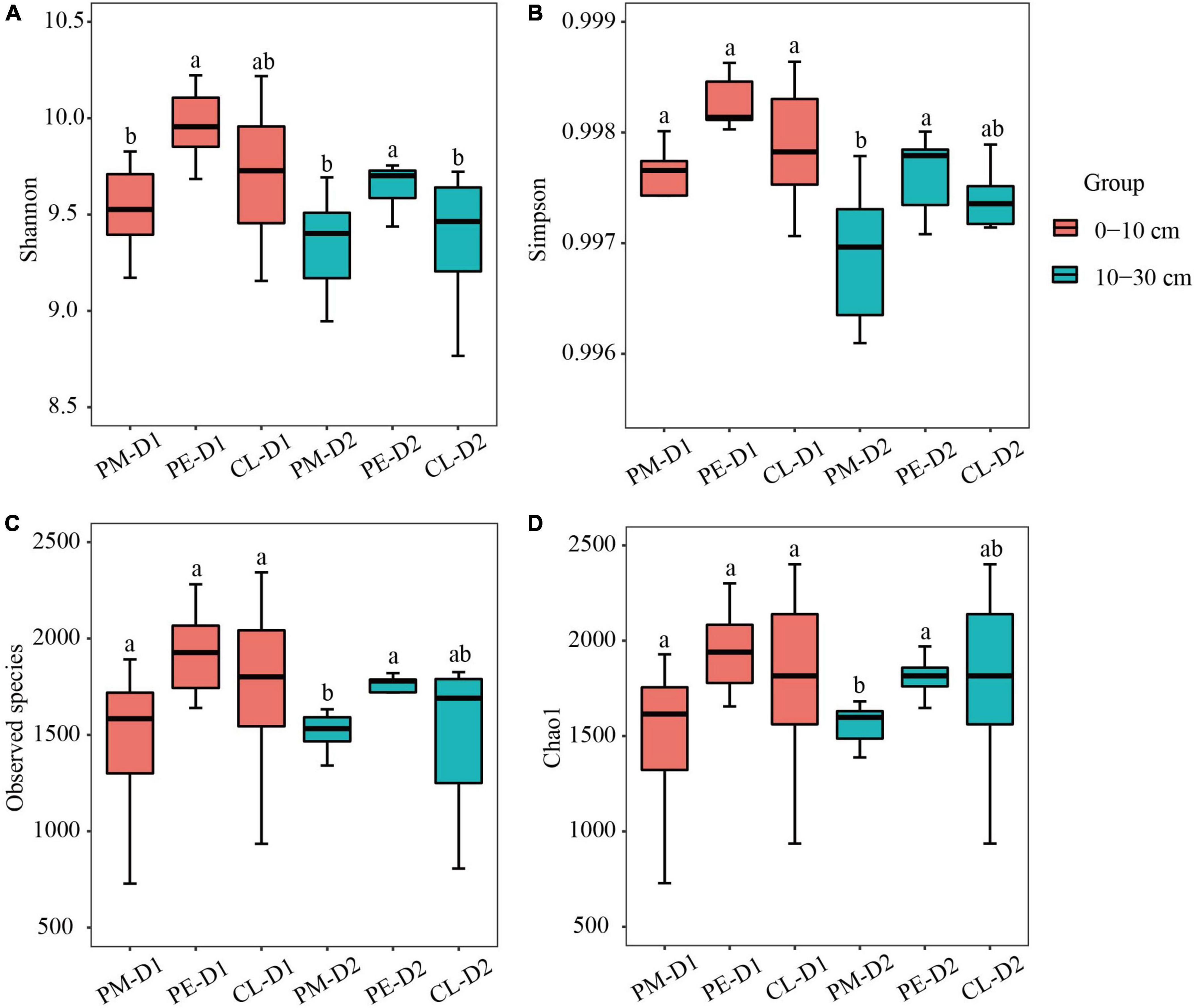
Figure 2. Diversity (A,B) and richness (C,D) measurements of soil bacterial communities among the secondary Pinus massoniana forest (PM), the plantation forests of Pinus elliottii (PE), and Cunninghamia lanceolata (CL). D1 and D2 indicate 0–10 cm and 10–30 cm soil layers, respectively. Error bars represent the standard error of nine independent replicates. The different letters indicate significant differences between different soil layers among the three forest types (p < 0.05).
The most abundant phyla (mean abundance > 1%) in all soil samples were Acidobacteria, Proteobacteria, Chloroflexi, Actinobacteria, Verrucomicrobia, Planctomycetes, Gemmatimonadetes, WPS-2, and Rokubacteria (Figure 3), which accounted for more than 95.85% of the taxon tags. In the 0–10 cm layer, the relative abundance of Verrucomicrobia, Rokubacteria, and Chloroflexi was significantly higher in the plantations than in the secondary forest (p < 0.05, LDA > 3, Supplementary Appendix Figure 1), while the abundance of Proteobacteria and Actinobacteria was significantly lower. In the 10–30 cm layer, the abundance of Planctomycetes and Rokubacteria was significantly higher while Actinobacteria was significantly lower in plantations than in the secondary forest (p < 0.05, LDA > 3, Supplementary Appendix Figure 1). Significant differences were detected among the predominant phyla Acidobacteria, Proteobacteria, and Chloroflexi in the two soil layers of the three stands (Supplementary Appendix Figure 2).
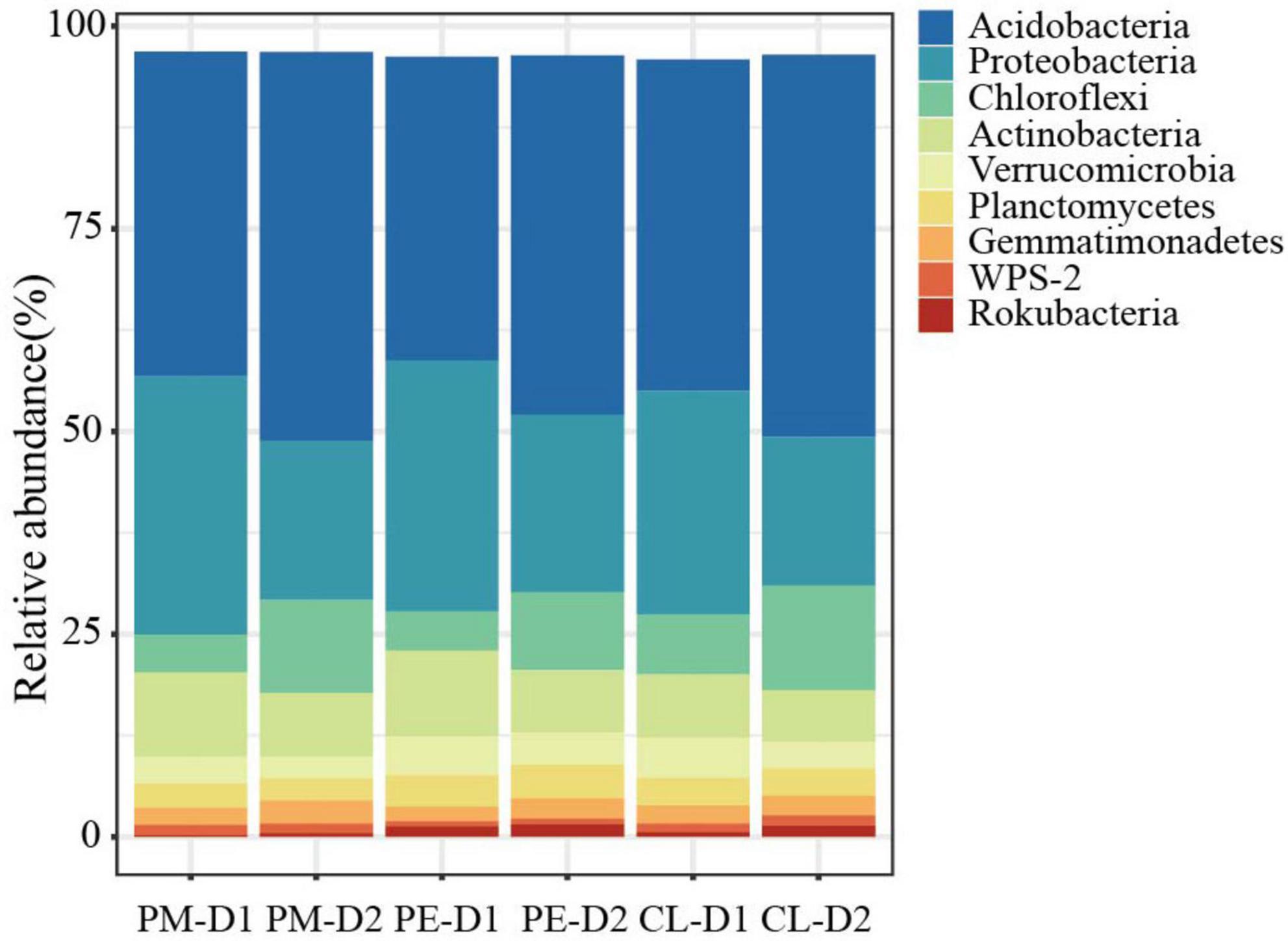
Figure 3. Changes of the relative abundance of the 9 most dominant phyla among the secondary Pinus massoniana forest (PM), the plantation forests of Pinus elliottii (PE) and Cunninghamia lanceolata (CL) (mean abundance > 1%). D1 and D2 indicate 0–10 cm and 10–30 cm soil layers, respectively.
3.3 Functional prediction of microbial community related to C- and N-cycling
With the annotation results of 16S rDNA sequences, 4150 features (10.11% of the total) were categorized into 92 functional groups using FAPROTAX. These functional groups were predominantly associated with biogeochemical cycles. The potential functions of soil bacterial communities in the various forest stands are presented in Supplementary Appendix Table 2.
The study focused on functional groups associated with C- and N-cycling. The relative abundance of functional groups participating in C- and N-cycling varied across stands (Figure 4). In the C-cycling functional groups, the abundance of chemoheterotrophy (23.65%), aerobic chemoheterotrophy (23.28%), and cellulolysis (9.63%) was significantly lower in the plantations than in the secondary forest in the 0–30 cm soil layer. In contrast, the presence of predatory or exoparasitic (1.74%) and animal parasites or symbionts (1.66%) were significantly higher in the plantations than in the secondary forests (p < 0.05, LDA > 3, Supplementary Appendix Figure 3). In the soil profile, the relative abundance of cellulolysis, animal parasites or symbionts in the secondary forest, and chemoheterotrophy, aerobic chemoheterotrophy in plantation forests were significantly elevated in deeper soil (Supplementary Appendix Figure 4). Furthermore, based on the analysis of functional group annotated pathways, the predominant bacterial groups involved in the C-cycling were found to be Verrucomicrobia, Actinobacteria, Proteobacteria, and Acidobacteria (Supplementary Appendix Figure 5). Of these, Bacteroidaceae, Acidobacteriaceae, and Solibacteraceae_(Subgroup_3) were all specialized anaerobic or heterotrophic aerobic types that metabolize carbohydrates.
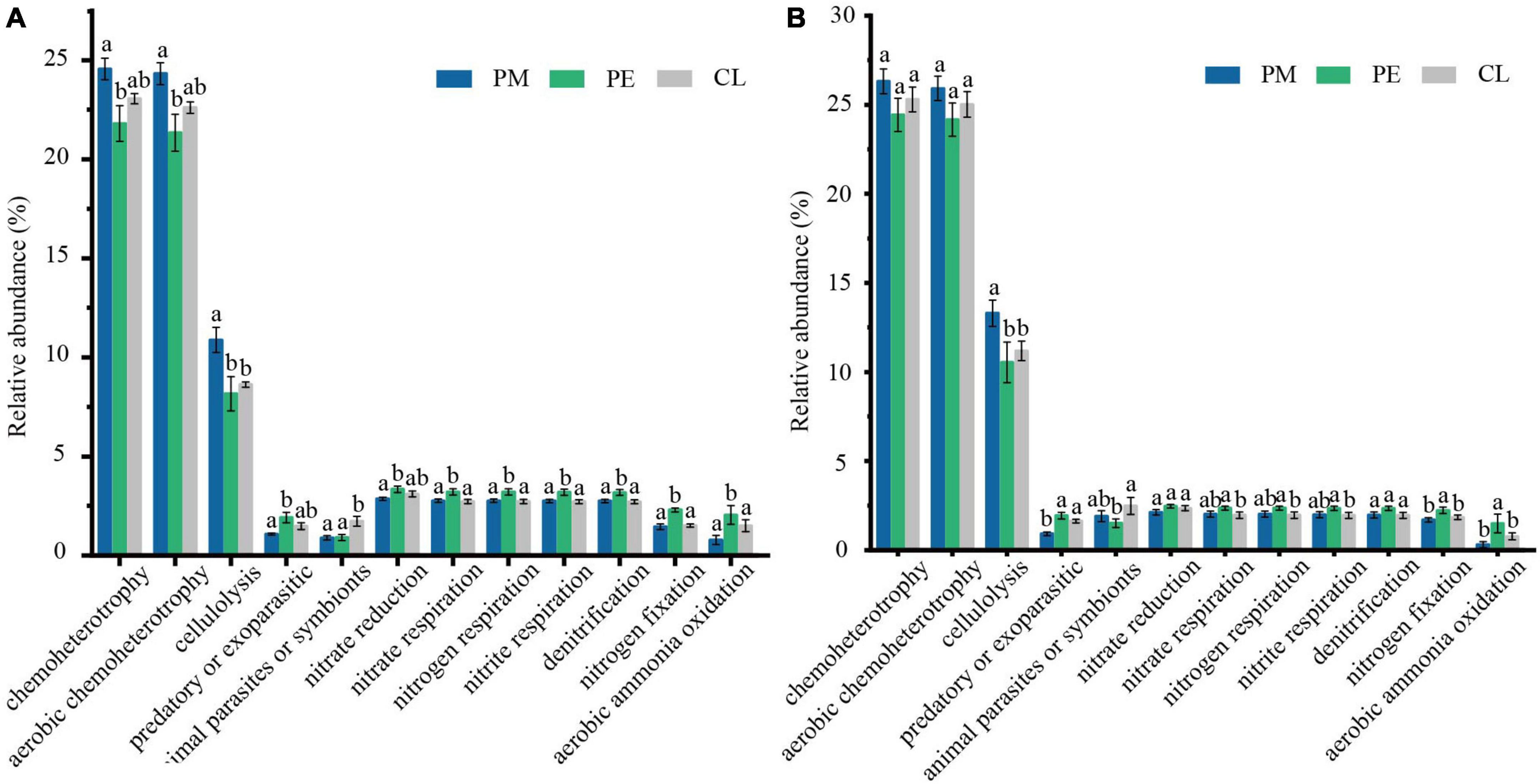
Figure 4. The significantly distinct functional groups in 0–10 cm (A) and 10–30 cm (B) involved in C- and N-cyclings among the secondary Pinus massoniana forest (PM), the plantation forests of Pinus elliottii (PE) and Cunninghamia lanceolata (CL). Chemoheterotrophy, aerobic chemoheterotrophy, cellulolysis, predatory or exoparasitic, and animal parasites or symbionts are functional groups related to C-cycling, while the others related to N-cycling (relative abundance > 5%). Error bars represent the standard error of nine independent replicates.
On the other hand, the abundance of functional groups participating in nitrate reduction (2.81%), nitrate respiration (2.56%), nitrogen respiration (2.56%), nitrite respiration (2.55%), denitrification (2.54%), nitrogen fixation (1.97%), and aerobic ammonia oxidation (1.45%) in the N-cycling was significantly higher in the plantations than in the secondary forest in the 0–30 cm layer (p < 0.05, LDA > 3, Supplementary Appendix Figure 3). In the soil profile, the relative abundance of nitrite respiration, denitrification, nitrate reduction, nitrate respiration, and nitrogen respiration decreased significantly in all three stands (Supplementary Appendix Figure 4). With further analysis, it was found that Proteobacteria is the main contributor to the N-cycling in our study (Supplementary Appendix Figure 5). The most active among them were the Bradyrhizobiaceae, Hyphomicrobiaceae, and Acetobacteraceae, both belonging to the b Proteobacteria family, which can perform nitrogen fixation, nitrate respiration, and aerobic ammonia oxidation, and play important roles in the N-cycling process.
3.4 Factors driving the variation of soil bacterial community structure and composition
According to the Spearman and RDA analysis, our findings indicate that soil bacterial phyla were significantly influenced by SOC, NO3–-N, NH4+-N, and pH (Figures 5, 6). In the 0–10 cm layer, soil pH was positively correlated with Verrucomicrobia, Planctomycetes, and Rokubacteria, while was negatively correlated with Gemmatimonadetes and WPS-2. NO3–-N was positively correlated with Gemmatimonadetes and WPS-2, and negatively correlated with Verrucomicrobia. NH4+-N was positively correlated with Actinobacteria. TN was negatively correlated with Acidobacteria. SOC was positively correlated with Actinobacteria. RDA analysis revealed that NH4+-N (11.8%) was the most significant driver affecting the soil bacterial phyla (Figure 6A and Table 3). In the 10–30 cm layer, soil pH was found to be positively correlated with Planctomycetes and Rokubacteria, whereas it showed a negative correlation with WPS-2. NO3–-N was negatively correlated with Planctomycetes and Rokubacteria. SOC was positively correlated with Actinobacteria, and negatively correlated with Acidobacteria. According to the RDA result, SOC (13.3%) had a more significant contribution than the other environmental factors (Table 3).
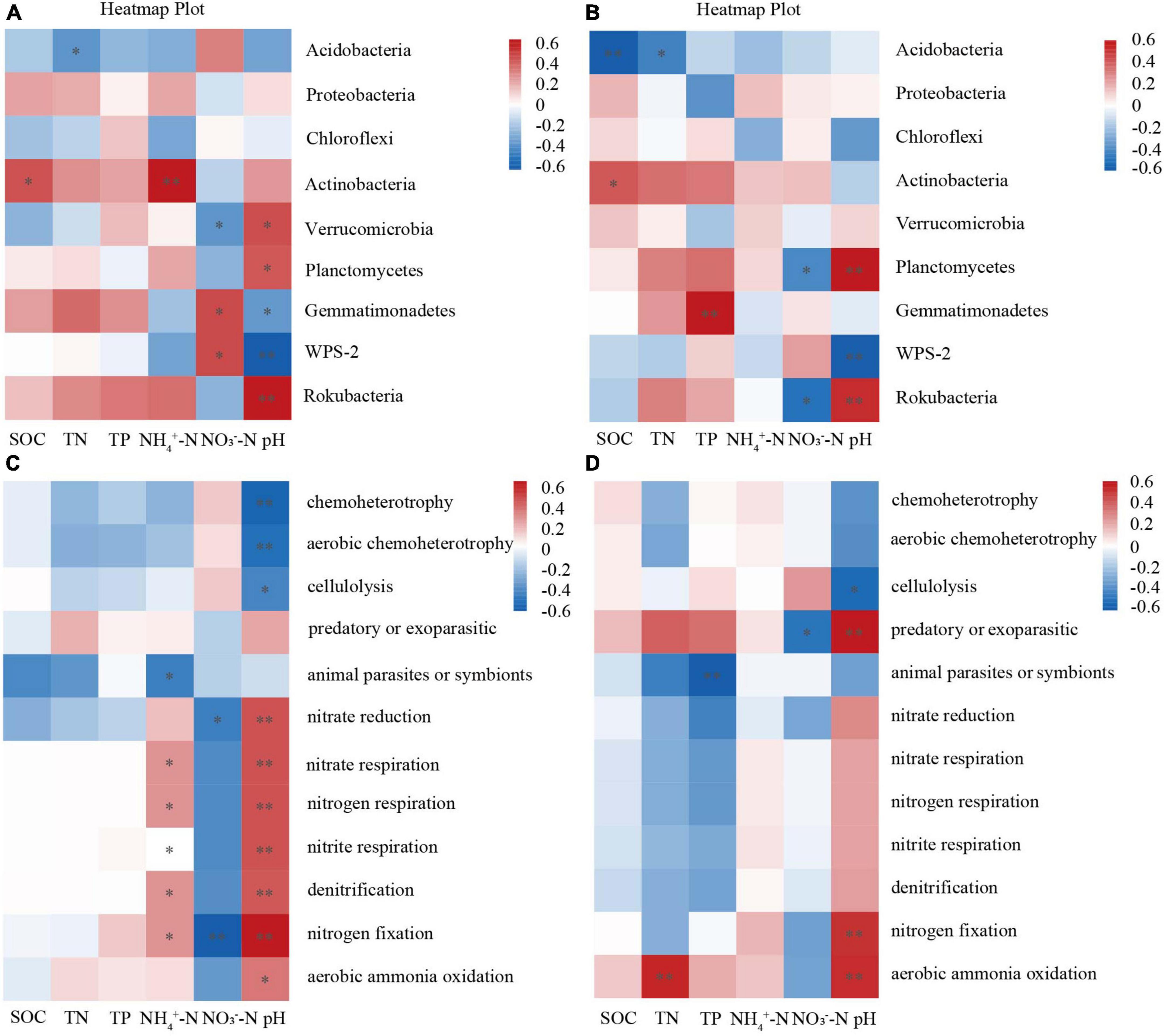
Figure 5. Spearman correlation analysis of bacterial phyla and soil properties (A: 0–10 cm, B: 10–30 cm), functional groups and soil properties (C: 0–10 cm, D: 10–30 cm) among the secondary Pinus massoniana forest (PM), the plantation forests of Pinus elliottii (PE) and Cunninghamia lanceolata (CL).
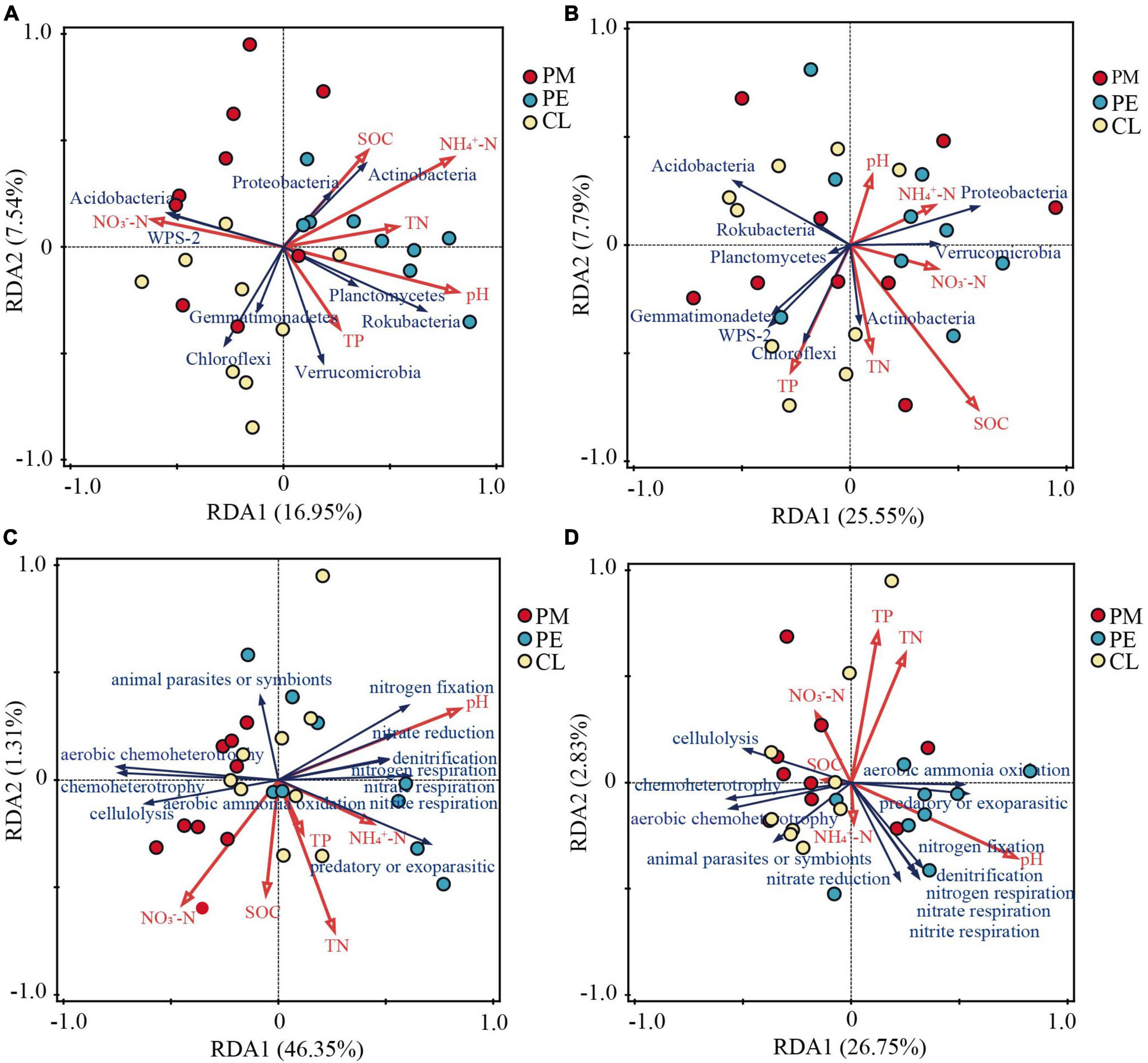
Figure 6. Redundancy analysis (RDA) of bacterial phyla and soil properties (A: 0–10 cm, B: 10–30 cm), functional groups and soil properties (C: 0–10 cm, D: 10–30 cm) among the secondary Pinus massoniana forest (PM), the plantation forests of Pinus elliottii (PE) and Cunninghamia lanceolata (CL).
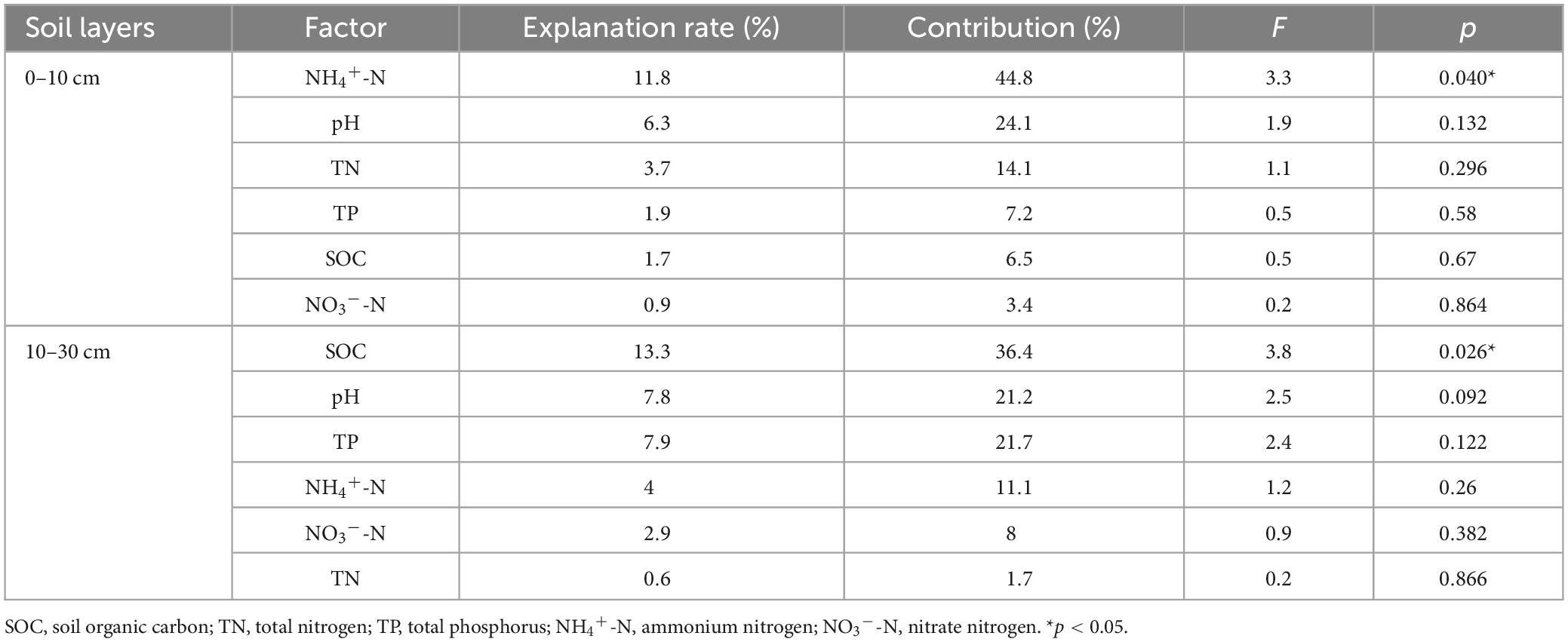
Table 3. Distance-based RDA determines the explanation rate of different environmental factors on soil bacterial phyla.
Similarly, the correlation between soil properties and bacterial functional groups was analyzed (Figures 5C, D). The result revealed that RDA explained 47.66% and 29.58% of the total variation in the different soil layers, respectively (Figures 6C, D). Soil pH was the primary driver of the total variation of the bacterial functional groups (Table 4). In the 0–10 cm layer, soil pH explained 33.2% of the variation, while in the 10–30 cm layer, it explained 16.1% (Table 4).
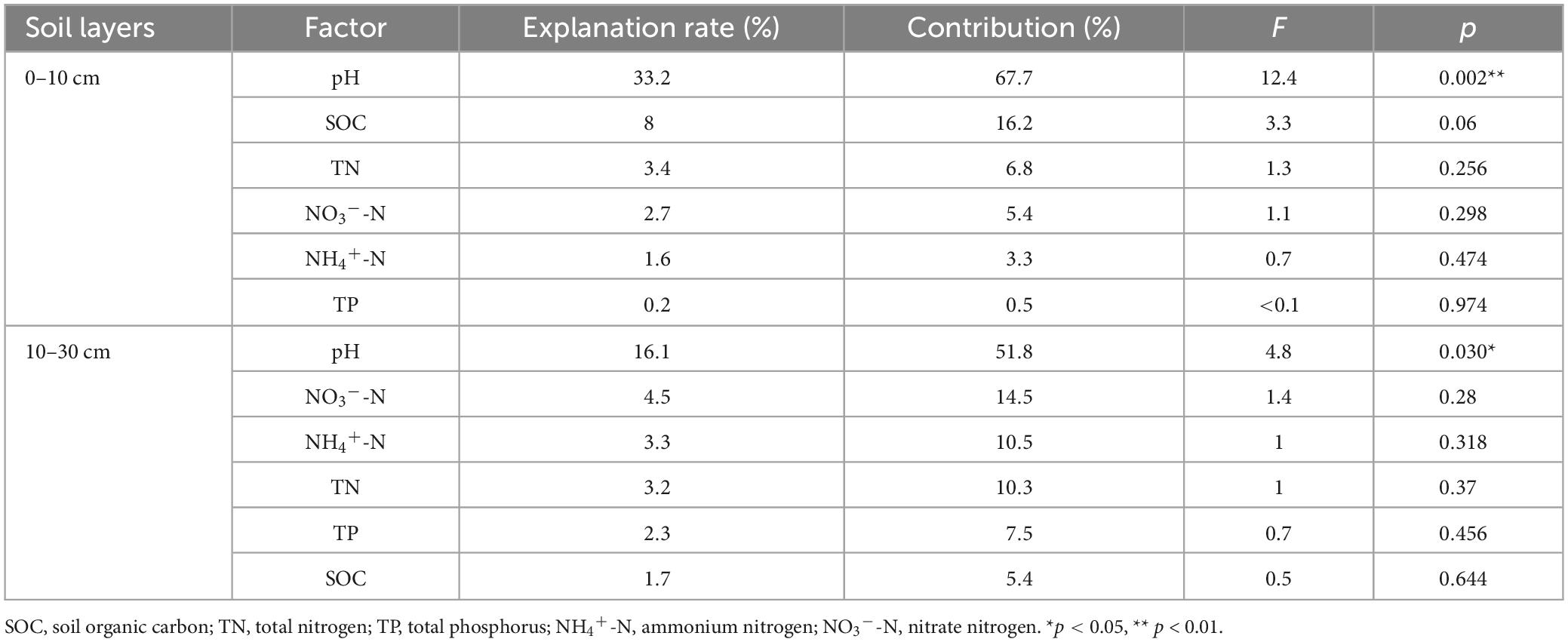
Table 4. Distance-based RDA determines the explanation rate of different environmental factors on soil bacterial functional groups.
4 Discussion
4.1 Forest conversion on soil properties
The conversion of natural or secondary forests to plantations strongly influenced soil properties, particularly soil C, N, and pH (Wang et al., 2017; Peng et al., 2021). In this study, compared with secondary forests, the contents of SOC in the Chinese fir plantation and NO3–-N in the slash pine plantation were significantly reduced. The soil pH was significantly higher in the surface soil layers in plantation stands than in the secondary forest (Table 1). These changes could be attributable to site preparations, such as slash burning and land reclamation (Berthrong et al., 2009; Huang et al., 2013). These management practices could result in the loss of soil nutrient particularly during the early stage of the plantation establishment (Jandl et al., 2007). According to Yang et al. (2019), the first year after forest conversion resulted in a significant loss of organic carbon, 10% of which was through erosion, 11% through fire-related volatilization, and 7% due to increased soil microbial respiration after burning. On the other hand, in comparison to the secondary forest, the vegetation coverage of the planted forests decreased, and the litterfall input to the soil decreased after forest conversion (Ni et al., 2021). These changes altered soil temperature and humidity, which accelerated the decomposition of litterfall and organic matter, leading to a decrease in SOC and NO3–-N content in the plantations (Xu et al., 2014). This is consistent with the results of other studies (Beillouin et al., 2023; Wang et al., 2023). According to Zhu et al. (2022), the average annual litterfall yield was significantly higher in natural forests than in monoculture rubber plantations, and temperature and light were the main driving factors for the change in litterfall yield. The increase in soil pH after conversion was probably related to the slash burning and the decreased litterfall input from coniferous tree species (Yang et al., 1997). As a result, changes in soil C and N content and pH after forest conversion can be due to a variety of factors, such as litterfall amount, fine root biomass, etc. (Ni et al., 2021; Peng et al., 2021).
Owing to the continuously high atmospheric deposition, soil N deficiency is less severe in most of the natural ecosystems. Unlike soil N, the significance of soil P as a limiting nutrient element has markedly escalated in recent decades (Zhang et al., 2016; Tahovska et al., 2018). Generally, the conversion of natural forests to plantations leads to a reduced availability of soil nutrient (Yang et al., 2019). However, our results showed no statistically significant difference in soil P content between the secondary forest and plantations without external phosphorus fertilizer input. P is known to be an essential nutrient element and less available to plants under most soil conditions. Previous studies suggested that a reduction in litterfall following forest-type conversion might lead to a decrease in P input to soils and thus affect the P pool (Yang et al., 2021). Zhang et al. (2016) showed that soil P had not significantly changed in a secondary succession of tropical forest with significant changes in P fractions in different stages. The significant correlation between the potency of soil phosphorus and microbial activity, as well as its chemical properties, is undeniable (Zaidi et al., 2009; Zhang et al., 2014).
4.2 Forest conversion on soil bacterial community diversity
Soil microbial community diversity largely depended on the above-ground vegetation in the ecosystem (Meng et al., 2019). Studies have shown that soil bacterial diversity is positively correlated with plant diversity (Lange et al., 2015; Steinauer et al., 2015). With the increase of plant diversity, the biomass and activity of soil microorganisms increased significantly (Sewerniak, 2020). In this study, bacterial diversity increased significantly after forest conversion, with different effects between slash pine and Chinese fir plantations (Figure 2). On the one hand, this may be related to differences in plant species (Xu et al., 2015). Plant species is a key factor influencing soil bacterial diversity (Meng et al., 2019). Ding et al. (2021) found an increase in bacterial diversity in bamboo forests despite a decrease in above-ground plant diversity in southeastern China. This result also supports this point of view. On the other hand, according to Zhou Z. et al. (2020), soil pH can also be used to explain changes in microbial diversity due to forest types conversion. Studies have shown that the conversion of natural or secondary forests into plantations increased microbial richness and diversity (Liu et al., 2020), and these may have something to do with the rising soil pH. Soil pH is a key factor in regulating soil microbial diversity (Bárcenas-Moreno et al., 2016). It may be due to the reason that soil pH plays an important role in the stabilization of cell membrane proton pumps and proteins, thus directly exerting physiological stresses on microorganisms, and when soil pH is outside a certain range (ecological niche), the net growth of unviable individual taxa decreases, which may alter the outcome of the competition (Zhou Z. C. et al., 2020; Hu et al., 2024). That is appropriate soil pH promotes microbial activity and bacterial growth. Conversely, soil acidification would inhibit microbial activity and bacterial growth. Soil disturbance also raised bacterial diversity in plantations (Wang H. et al., 2020). Moreover, the surface and subsurface soil microbial communities showed a clear stratification because of the strong ecological function of filtration in the soil vertical space (Eilers et al., 2012). It is due to the huge accumulation of litterfall on the soil surface in forest ecosystems. Therefore, owing to differences in nutrient availability and the involvement of rhizosphere soil microorganisms, substrates in surface and subsurface soils differ significantly leading to relatively high microbial diversity in surface soil (Xu et al., 2014).
The land-use change affects the soil bacterial community structure, increasing the relative abundance of oligotrophic taxa (e.g., Acidobacteria and Chloroflexi) and decreasing the relative abundance of eutrophic taxa (e.g., Proteobacteria and Actinobacteria) (Lee-Cruz et al., 2013; Wang J. et al., 2020). Our results showed that the dominant groups were Acidobacteria, Proteobacteria, Chloroflexi, and Actinobacteria, which was consistent with previous results on the soil bacterial community composition after forest conversion (Merloti et al., 2019). The predominant bacterial phyla are ubiquitous and abundant in various terrestrial ecosystems (Ranjan et al., 2015). Acidobacteria, Proteobacteria, and Actinobacteria have been reported to dominate in coniferous forest ecosystems (Baldrian et al., 2012). This result could be potentially explained by the copiotroph/oligotroph functional classification model (Li et al., 2022). Soil C, N, and pH were considered as the main factors influencing the variation of soil bacterial community composition (Meng et al., 2019; Wang et al., 2021). The findings demonstrated that the relative abundances of Verrucomicrobia and Rokubacteria were notably elevated in slash pine and Chinese fir plantations compared to the secondary masson pine forest. Some of the genes of Verrucomicrobia have biological nitrogen-fixing capability (Khadem et al., 2010). Additionally, The distribution of the Verrucomicrobia community was found to be associated with alteration in soil C dynamics (Ranjan et al., 2015). Verrucomicrobia were relatively slow-growing taxa and thrived under nutrient-limited conditions (Fierer et al., 2013). On the other hand, Verrucomicrobia can be capable of degrading polysaccharide by sulfate under eutrophic conditions (Martinez-Garcia et al., 2012), and increase soil mineral nutrients by facilitating mineral solubilization through plant-microbe interactions as well (Nixon et al., 2019). This result also supports our findings. Clearly, Verrucomicrobia plays a key role in terrestrial ecosystems. Rokubacteria was also prevalent in terrestrial ecosystems (Becraft et al., 2017). Prior studies indicated that the relative abundance of Verrucomicrobia and Rokubacteria was positively correlated with soil pH (Shen et al., 2017; Ivanova et al., 2022). It is consistent with our findings (Figures 5A, B). The abundance of eutrophic groups decreased as the content of SOC decreased (Figure 5). Actinobacteria belonging to eutrophic groups observed a significant decrease in their relative abundance after forest conversion. In conclusion, forest conversion leads to a transition from the eutrophic to the oligotrophic system due to changes in pH and nutrient availability.
4.3 Forest conversion on the microbial function
Soil C cycling is one of the most important and complex metabolic processes in the biogeochemical cycles (Lynn et al., 2017; Wang J. et al., 2020), regulating global climate change (Bardgett et al., 2008). In forest ecosystems, the abundance of the functional groups involved in soil microbial C-cycling is closely correlated with plant species and soil properties (Wang et al., 2019). Their variation affects the carbon metabolism function of microbial communities (Wang et al., 2021). Our study showed that the most metabolized functional groups of microorganisms in soils were chemoheterotrophic, aerobic chemoheterotrophic, and cellulolysis associated with C-cycling (Figure 4). The conversion of the secondary masson pine forests to slash pine and Chinese fir plantations significantly reduced the relative abundance of these functional groups. These functional microorganisms are mainly heterotrophic microorganisms with low carbon sequestration efficiency. This is because heterotrophic microorganisms can only take in readily available organic matter from the outside world as a source of energy to sustain their growth and metabolic activities. Among the microbial taxa involved in soil C-cycling were mainly Verrucomicrobia, Actinobacteria, Proteobacteria, and Acidobacteria (Supplementary Appendix Figure 5). They are also the most abundant of all the bacteria phyla and the main C-fixing group. With the conversion of secondary forests to plantations, the relative abundance of Proteobacteria and Actinobacteria decreased significantly. The abundance of C-cycling functional groups tends to be positively correlated with SOC content, which is mainly derived from microbial carbon sequestration and its residues (Tang et al., 2015). The study confirmed that abundant carbon sources can satisfy microbial growth, and the abundance of C-cycling functional groups can characterize the carbon sequestration capacity of soil microorganisms (Chen et al., 2021). The finding of Luo et al. (2022) revealed a significant reduction in the abundance of genes associated with C-fixation, methane metabolism, and C-degradation, following the conversion of natural forests to plantations. Therefore, it is seen that the conversion of forest types reduces the C input, stability, and turnover of litterfall production and fine root turnover, which in turn affects the soil bacterial functional groups (Wei et al., 2014). It was found that soil NH4+-N, NO3–-N, and pH might be the main factors regulating the functional groups (Deng et al., 2022). These findings suggest that changes in bacteria associated with C-cycling may be more influenced by environmental factors and soil properties (Wang et al., 2023). pH is a key factor influencing soil bacterial diversity, and high concentrations of H+ inhibit bacterial growth. The pH of plantations was significantly higher than that of secondary forests (Table 1). pH indirectly affects organic matter decomposition and nutrient release, leading to changes in the abundance of microbial populations and their functional groups, which are more sensitive to habitat changes. As abovementioned, this forest conversion led to a reduction in vegetation litterfall and C input and thus caused significant changes in soil organic carbon and pH. These changes accounted for the notable reduction in the relative abundance of C-cycling functional groups. Besides, forest conversion significantly increased the relative abundance of predatory or exoparasitic related to the transformation of soil organic matter (Sokol et al., 2022). This is not without the role of Actinobacteria.
In addition, forest conversion resulted in a decrease in the abundance of the C-cycling functional groups, while the abundance of the N-cycling functional groups increased. The active participation of soil bacteria in N-cycling processes, such as nitrogen fixation, ammonification, nitrification, and denitrification (Canfield et al., 2010), is critical. Specific functional groups of microorganisms determine their ability to facilitate the transformation of different forms of N. In this study, we found that the relative abundance of nitrate reduction, nitrate respiration, nitrite respiration, denitrification, nitrogen fixation, and aerobic ammonia oxidation related to N-cycling exhibited significant elevation in plantation forests compared to secondary forests. This suggests that forest conversion might have enhanced microbial nitrification and denitrification, as well as nitrogen fixation. One explanation for this might be the higher availability of microbial nutrients in plantation forests. This is supported by the higher soil available nitrogen and SOC content in plantation forests (Table 1). Interestingly, all these functional groups were significantly and positively correlated with soil pH (Figure 5). It has been reported that soil environmental conditions, particularly NH4+-N, NO3–-N, and pH, have a significant impact on the distribution of N-cycling functional groups (Liang et al., 2020). This may be attributed to the increasing pH after forest conversion that promotes N mineralization, affecting N availability in the soil (Zhang et al., 2019). Meanwhile, the shift from eutrophic to oligotrophic taxa also promoted N mineralization to meet the N demand (Wang Y. et al., 2020). According to the nitrogen metabolism pathway, the functional groups involved in the N-cycling are mainly from Proteobacteria (Supplementary Appendix Figure 5). Proteobacteria are very sensitive to N availability, and play the most dominant role in organic matter decomposition. Among them, Bradyrhizobiaceae, Hyphomicrobiaceae, and Acetobacteraceae were more involved, all of which were engaged in nitrogen fixation, nitrate nitrogen respiration, and aerobic ammonia oxidation. It is known that ammonia-oxidizing bacteria and nitrite-oxidizing bacteria belong to Proteobacteria (Daims et al., 2016; Gao et al., 2016). Xu et al. (2021) concluded that Proteobacteria, Chloroflexi, Actinobacteria, and Verrucomicrobia were the dominant functional microorganisms involved in nitrate reduction, nitrogen fixation, and ammonia oxidation processes in the Napa Sea Plateau wetland ecosystem. Our findings corroborate those of Lammel et al. (2015) and Merloti et al. (2019), who also observed that land use change augments the abundance of microbial functional groups associated with N-cycling, particularly denitrifying bacteria. Denitrifying bacteria, a group of bacteria that reduce nitrate into N2. In an agroforestry system, the denitrification process is mainly accomplished by microbial respiration, and the relevant microorganisms are usually found in anoxic environments (Zumft, 1997). Therefore, the abundance of denitrifying bacteria can be used to detect soil fertility status. An increase in the abundance of denitrifying bacteria following forest conversion may pose a potential issue. This is primarily because a high abundance of denitrifying bacteria could elevate N2O emission (Li et al., 2017). Alterations in soil properties and the abundance of denitrifying bacteria might indicate a potential source of N2O following forest conversion. Further research is warranted in this regard.
5 Conclusion
In summary, this study examines how forest conversion affects soil properties, bacterial communities, and functional groups in both surface and subsurface layers. The results indicated that converting coniferous secondary forests to coniferous plantations led to a significant increase in soil pH and a concurrent significant decrease in soil organic carbon and nitrate. After forest conversion, soil microbial diversity increased significantly, and the community composition changed significantly, especially in the topsoil. The abundance of functional groups related to C-cycling decreased significantly, while significantly increased in the abundance of functional groups related to N-cycling, particularly those involved in denitrification. Soil microbial community variation was primarily driven by soil pH, SOC, and NH4+-N. The study highlights the impact of converting secondary coniferous forests to plantations on C and N cycling in forest ecosystems and provides useful information for sustainable management of plantation forestry.
Data availability statement
The datasets presented in this study can be found in online repositories. The names of the repository/repositories and accession number(s) can be found below: https://dataview.ncbi.nlm.nih.gov/object/PRJNA1037004?
Author contributions
XC: Writing – original draft, Visualization, Methodology, Investigation, Formal analysis. KX: Writing – original draft, Visualization, Methodology, Investigation, Formal analysis, Conceptualization. HZ: Writing – review and editing. PD: Writing – review and editing, Investigation. ZT: Writing – review and editing, Methodology. XX: Writing – review and editing, Supervision, Investigation, Funding acquisition, Conceptualization.
Funding
The author(s) declare that financial support was received for the research, authorship, and/or publication of this article. This work was supported by the National Natural Science Foundation of China (Grant No. 31770672) and the National Key Research and Development Program of China (Grant No. 2016YFD0600304-03).
Conflict of interest
The authors declare that the research was conducted in the absence of any commercial or financial relationships that could be construed as a potential conflict of interest.
Publisher’s note
All claims expressed in this article are solely those of the authors and do not necessarily represent those of their affiliated organizations, or those of the publisher, the editors and the reviewers. Any product that may be evaluated in this article, or claim that may be made by its manufacturer, is not guaranteed or endorsed by the publisher.
Supplementary material
The Supplementary Material for this article can be found online at: https://www.frontiersin.org/articles/10.3389/ffgc.2024.1331672/full#supplementary-material
Supplementary Appendix Figure 1 | The LEfSe analysis of soil bacterial groups in different forest types at the phyla level. PM, secondary Pinus massoniana forest; PE, Pinus elliottii plantation; CL, Cunninghamia lanceolata plantation. D1 and D2 indicate 0–10 cm and 10–30 cm soil layers, respectively. The threshold value of the log LDA score for discriminating features was 3.0. LDA scores represent the degree of influence of significantly different species between different groups.
Supplementary Appendix Figure 2 | The LEfSe analysis of bacterial groups in different soil layers at the phyla level. PM, secondary Pinus massoniana forest; PE, Pinus elliottii plantation; CL, Cunninghamia lanceolata plantation. D1 and D2 indicate 0–10 cm and 10–30 cm soil layers, respectively. The threshold value of the log LDA score for discriminating features was 3.0. LDA scores represent the degree of influence of significantly different species between different groups.
Supplementary Appendix Figure 3 | The LEfSe analysis of soil bacterial groups in different forest types. PM, secondary Pinus massoniana forest; PE, Pinus elliottii plantation; CL, Cunninghamia lanceolata plantation. D1 and D2 indicate 0–10 cm and 10–30 cm soil layers, respectively. The threshold value of the log LDA score for discriminating features was 3.0. LDA scores represent the degree of influence of significantly different species between different groups.
Supplementary Appendix Figure 4 | The LEfSe analysis of bacterial functional groups in different soil layers. PM, secondary Pinus massoniana forest; PE, Pinus elliottii plantation; CL, Cunninghamia lanceolata plantation. D1 and D2 indicate 0–10 cm and 10–30 cm soil layers, respectively. The threshold value of the log LDA score for discriminating features was 3.0. LDA scores represent the degree of influence of significantly different species between different groups.
Supplementary Appendix Figure 5 | The number of OTUs assigned to functional groups related to dominant phyla.
Supplementary Appendix Table 1 | Basic situation of the sampling stands. PM: secondary forest of Pinus massoniana; PE: Pinus elliottii plantation; CL: Cunninghamia lanceolata plantation.
Supplementary Appendix Table 2 | The relative abundance of functional groups in the different forest types (PM, PE, and CL). The functional groups with average relative abundance > 0.5% are displayed. PM: secondary forest of Pinus massoniana; PE: Pinus elliottii plantation; CL: Cunninghamia lanceolata plantation.
Footnotes
References
Alvarez, A., Saez, J. M., Costa, J. S. D., Colin, V. L., Fuentes, M. S., Cuozzo, S. A., et al. (2017). Actinobacteria: Current research and perspectives for bioremediation of pesticides and heavy metals. Chemosphere 166, 41–62. doi: 10.1016/j.chemosphere.2016.09.070
Baldrian, P., Kolařík, M., Štursová, M., Kopecký, J., Valášková, V., Větrovský, T., et al. (2012). Active and total microbial communities in forest soil are largely different and highly stratified during decomposition. ISME J. 6, 248–258.
Bárcenas-Moreno, G., Baath, E., and Rousk, J. (2016). Functional implications of the pH-trait distribution of the microbial community in a re-inoculation experiment across a pH gradient. Soil Biol. Biochem. 93, 69–78. doi: 10.1016/j.soilbio.2015.10.024
Bardgett, R. D., Freeman, C., and Ostle, N. J. (2008). Microbial contributions to climate change through carbon cycle feedbacks. ISME J. 2, 805–814. doi: 10.1038/ismej.2008.58
Bardgett, R. D., and Van der Putten, W. H. (2014). Belowground biodiversity and ecosystem functioning. Nature 515, 505–511. doi: 10.1038/nature13855
Becraft, E. D., Woyke, T., Jarett, J., Ivanova, N., Poulton, N., Brown, J. M., et al. (2017). Rokubacteria: Genomic giants among the uncultured bacterial phyla. Front. Microbiol. 8:2264. doi: 10.3389/fmicb.2017.02264
Beillouin, D., Corbeels, M., Demenois, J., Berre, D., Boyer, A., Fallot, A., et al. (2023). A global meta-analysis of soil organic carbon in the Anthropocene. Nat. Commum. 14:3700. doi: 10.1038/s41467-023-39338-z
Berkelmann, D., Schneider, D., Engelhaupt, M., Heinemann, M., Christel, S., Wijayanti, M., et al. (2018). How rainforest conversion to agricultural systems in Sumatra (Indonesia) affects active soil bacterial communities. Front. Microbiol. 9:02381. doi: 10.3389/fmicb.2018.02381
Berthrong, S. T., Jobbagy, E. G., and Jackson, R. B. (2009). A global meta-analysis of soil exchangeable cations, pH, carbon, and nitrogen with afforestation. Ecol. Appl. 19, 2228–2241. doi: 10.1890/08-1730.1
Bradley, P. H., and Pollard, K. S. (2017). Proteobacteria explain significant functional variability in the human gut microbiome. Microbiome 5:36. doi: 10.1186/s40168-017-0244-z
Bruun, T. B., Elberling, B., and Magid, J. (2015). Organic carbon dynamics in different soil types after conversion of forest to agriculture. Land Degrad. Dev. 26, 272–283. doi: 10.1002/ldr.2205
Burton, J., Chen, C. R., Xu, Z. H., and Ghadiri, H. (2007). Gross nitrogen transformations in adjacent native and plantation forest’s of subtropical Australia. Soil Biol. Biochem. 39, 426–433. doi: 10.1016/j.soilbio.2006.08.011
Camponi, L., Cardelli, V., Cocco, S., Serrani, D., Salvucci, A., Cutini, A., et al. (2022). Effect of coppice conversion into high forest on soil organic C and nutrients stock in a Turkey oak (Quercus cerris L.) forest in Italy. J. Environ. Manage. 312:114935. doi: 10.1016/j.jenvman.2022.114935
Canfield, D. E., Glazer, A. N., and Falkowski, P. G. (2010). The evolution and future of Earth’s nitrogen cycle. Science 330, 192–196. doi: 10.1126/science.1186120
Castro, S. P., Cleland, E. E., Wagner, R., Al Sawad, R., and Lipson, D. A. (2019). Soil microbial responses to drought and exotic plants shift carbon metabolism. ISME J. 13, 1776–1787. doi: 10.1038/s41396-019-0389-9
Chen, H., Wang, F., Kong, W. D., Jia, H. Z., Zhou, T. Q., Xu, R., et al. (2021). Soil microbial CO2 fixation plays a significant role in terrestrial carbon sink in a dryland ecosystem: A four-year small-scale field-plot observation on the Tibetan Plateau. Sci. Total Environ. 761:143282. doi: 10.1016/j.scitotenv.2020.143282
Chen, J., and Sinsabaugh, R. L. (2021). Linking microbial functional gene abundance and soil extracellular enzyme activity: Implications for soil carbon dynamics. Glob. Chang Biol. 27, 1322–1325. doi: 10.1111/gcb.15506
Daims, H., Luecker, S., and Wagner, M. (2016). A new perspective on microbes formerly known as nitrite-oxidizing bacteria. Trends Microbiol. 24, 699–712. doi: 10.1016/j.tim.2016.05.004
Deng, P. F., Fan, W., Wang, H. L., Ti, J. H., and Xu, X. N. (2022). Chinese Torreya agroforestry alters the Rhizosphere soil bacterial communities and potential functions. Appl. Soil Ecol. 177:104504. doi: 10.1016/j.apsoil.2022.104504
Dennis, P. G., Newsham, K. K., Rushton, S. P., Ord, V. J., O’Donnell, A. G., and Hopkins, D. W. (2013). Warming constrains bacterial community responses to nutrient inputs in a southern, but not northern, maritime Antarctic soil. Soil Biol. Biochem. 57, 248–255. doi: 10.1016/j.soilbio.2012.07.009
Diaz-Vallejo, E. J., Seeley, M., Smith, A. P., and Marin-Spiotta, E. (2021). A meta-analysis of tropical land-use change effects on the soil microbiome: Emerging patterns and knowledge gaps. Biotropica 53, 738–752.
Ding, X. X., Liu, G. L., Fu, S. L., and Chen, H. Y. H. (2021). Tree species composition and nutrient availability affect soil microbial diversity and composition across forest types in subtropical China. Catena 201:105224.
Drescher, J., Rembold, K., Allen, K., Beckschafer, P., Buchori, D., Clough, Y., et al. (2016). Ecological and socio-economic functions across tropical land use systems after rainforest conversion. Philos. T. R. Soc. B. 372:20150275. doi: 10.1098/rstb.2015.0275
Eilers, K. G., Debenport, S., Anderson, S., and Fierer, N. (2012). Digging deeper to find unique microbial communities: The strong effect of depth on the structure of bacterial and archaeal communities in soil. Soil Biol. Biochem. 50, 58–65. doi: 10.1016/j.soilbio.2012.03.011
FAO (2020). Global Forest Resources Assessment 2020 – Key Findings. Rome: Food and Agriculture Organization of the UN, doi: 10.4060/ca8753en
Fierer, N., Ladau, J., Clemente, J. C., Leff, J. W., Owens, S. M., Pollard, K. S., et al. (2013). Reconstructing the microbial diversity and function of pre-agricultural tallgrass prairie soils in the United States. Science 342, 621–624. doi: 10.1126/science.124376
Gao, J. F., Fan, X. Y., Pan, K. L., Li, H. Y., and Sun, L. X. (2016). Diversity, abundance and activity of ammonia-oxidizing microorganisms in fine particulate matter. Sci. Rep. 6:38785. doi: 10.1038/srep38785
González, J. M., Simó, R., Massana, R., Covert, J. S., Casamayor, E. O., Pedrós-Alió, C., et al. (2000). Bacterial community structure associated with a dimethylsulfoniopropionate-producing North Atlantic algal bloom. Appl. Environ. Microbial 66, 4237–4246.
Guo, J. H., Feng, H. L., Roberge, G., Feng, L., Pan, C., McNie, P., et al. (2022). The negative effect of Chinese fir (Cunninghamia lanceolata) monoculture plantations on soil physicochemical properties, microbial biomass, fungal communities, and enzymatic activities. For. Ecol. Manag. 519:120297. doi: 10.1016/j.foreco.2022.120297
Hu, Z. K., Delgado-Baquerizo, M., Fanin, N., Chen, X. Y., Zhou, Y., Du, G. Z., et al. (2024). Nutrient-induced acidification modulates soil biodiversity-function relationship. Nat. Commun. 15:2858. doi: 10.1038/s41467-024-47323-3
Hua, F. Y., Bruijnzeel, L. A., Meli, P., Martin, P. A., Zhang, J., Nakagawa, S., et al. (2022). The biodiversity and ecosystem service contributions and trade-offs of forest restoration approaches. Science 376:839. doi: 10.1126/science.abl4649
Huang, Z. Q., He, Z. M., Wan, X. H., Hu, Z. H., Fan, S. H., and Yang, Y. S. (2013). Harvest residue management effects on tree growth and ecosystem carbon in a Chinese fir plantation in subtropical China. Plant Soil. 364, 303–314. doi: 10.1007/s11104-012-1341-1
Ivanova, A. A., Oshkin, I. Y., Danilova, O. V., Philippov, D. A., Ravin, N. V., and Dedysh, S. N. (2022). Rokubacteria in northern peatlands: Habitat preferences and diversity patterns. Microrganisms 10:11. doi: 10.3390/microorganisms10010011
Jandl, R., Lindner, M., Vesterdal, L., Bauwens, B., Baritz, R., Hagedorn, F., et al. (2007). How strongly can forest management influence soil carbon sequestration? Geoderma 137, 253–268. doi: 10.1016/j.geoderma.2006.09.003
Keenan, R. J., Reams, G. A., Achard, F., de Freitas, J. V., Grainger, A., and Lindquist, E. (2015). Dynamics of global forest area: Results from the FAO Global Forest Resources Assessment 2015. For. Ecol. Manage. 352, 9–20. doi: 10.1016/j.foreco.2015.06.014
Kerfahi, D., Tripathi, B. M., Dong, K., Go, R., and Adams, J. M. (2016). Rainforest conversion to rubber plantation may not result in lower soil diversity of bacteria, fungi, and nematodes. Microb. Ecol. 72, 359–371. doi: 10.1007/s00248-016-0790-0
Khadem, A. F., Pol, A., Jetten, M. S. M., and Op den Camp, H. J. M. (2010). Nitrogen fixation by the Verrucomicrobial methanotroph ’Methylacidiphilum fumariolicum’ SolV. Microbiology 156, 1052–1059. doi: 10.1099/mic.0.036061-0
Kitson, E., and Bell, N. G. A. (2020). The response of microbial communities to peatland drainage and rewetting. a review. Front. Microbiol. 11:582812. doi: 10.3389/fmicb.2020.582812
Lammel, D. R., Nüsslein, K., Tsai, S. M., and Cerri, C. C. (2015). Land use, soil and litter chemistry drive bacterial community structures in samples of the rainforest and Cerrado (Brazilian Savannah) biomes in Southern Amazonia. Eur. J. Soil Biol. 66, 32–39. doi: 10.1016/j.ejsobi.2014.11.001
Lange, M., Eisenhauer, N., Sierra, C. A., Bessler, H., Engels, C., and Griffiths, R. I, et al. (2015). Plant diversity increases soil microbial activity and soil carbon storage. Nat. Commun. 6:6707.
Lee-Cruz, L., Edwards, D. P., Tripathi, B. M., and Adams, J. M. (2013). Impact of logging and forest conversion to oil palm plantations on soil bacterial communities in Borneo. Appl. Environ. Microbiol. 79, 7290–7297.
Levy-Booth, D. J., Prescott, C. E., and Grayston, S. J. (2014). Microbial functional genes involved in nitrogen fixation, nitrification and denitrification in forest ecosystems. Soil Biol. Biochem. 75, 11–25.
Li, S., Song, L., Gao, X., Jin, Y., Liu, S., Shen, Q., et al. (2017). Microbial abundances predict methane and nitrous oxide fluxes from a windrow composting system. Front. Microbiol. 8:245780. doi: 10.3389/fmicb.2017.00409
Li, W., Wu, Z., Zong, Y., Wang, G. G., Chen, F., Liu, Y., et al. (2022). Tree species mixing enhances rhizosphere soil organic carbon mineralization of conifers in subtropical plantations. For. Ecol. Manage. 516:120238. doi: 10.1016/j.foreco.2022.120238
Liang, S. C., Deng, J. J., Jiang, Y., Wu, S. J., Zhou, Y. B., and Zhu, W. X. (2020). Functional distribution of bacterial community under different land use patterns based on FaproTax function prediction. Pol. J. Environ. Stud. 29, 1245–1261. doi: 10.15244/pjoes/108510
Liu, J. L., Dang, P., Gao, Y., Zhu, H. L., Zhu, H. N., Zhao, F., et al. (2018). Effects of tree species and soil properties on the composition and diversity of the soil bacterial community following afforestation. For. Ecol. Manage. 427, 342–349. doi: 10.1016/j.foreco.2018.06.017
Liu, S. G., Wang, H., Deng, Y., Tian, P., and Wang, Q. K. (2018). Forest conversion induces seasonal variation in microbial β-diversity. Environ. Microbiol. 20, 111–123. doi: 10.1111/1462-2920
Liu, T., Wu, X. H., Li, H. W., Alharbi, H., Wang, J., Dang, P., et al. (2020). Soil organic matter, nitrogen and pH driven change in bacterial community following forest conversion. For. Ecol. Manage. 477:118473. doi: 10.1016/j.foreco.2020.118473
Louca, S., Jacques, S. M. S., Pires, A. P. F., Leal, J. S., Gonzalez, A. L., Doebeli, M., et al. (2017). Functional structure of the bromeliad tank microbiome is strongly shaped by local geochemical conditions. Environ. Microbiol. 19, 3132–3151. doi: 10.1111/1462-2920.13788
Luo, X. Z., Wen, D. Z., Hou, E. Q., Zhang, L. L., Li, Y., and He, X. J. (2022). Changes in the composition of soil microbial communities and their carbon-cycle genes following the conversion of primary broadleaf forests to plantations and secondary forests. Land Degrad. Dev. 33, 974–985.
Lynn, T. M., Ge, T. D., Yuan, H. Z., Wei, X. M., Wu, X. H., Xiao, K. Q., et al. (2017). Soil carbon-fixation rates and associated bacterial diversity and abundance in three natural ecosystems. Microb. Ecol. 73, 645–657. doi: 10.1007/s00248-016-0890-x
Lyu, M. K., Xie, J. S., Ukonmaanaho, L., Jiang, M. H., Li, Y. Q., Chen, Y. M., et al. (2017). Land use change exerts a strong impact on deep soil C stabilization in subtropical forests. J. Soil Sediments 17, 2305–2317. doi: 10.1007/s11368-016-1428-z
Martinez-Garcia, M., Brazel, D. M., Swan, B. K., Arnosti, C., Chain, P. S. G., Reitenga, K. G., et al. (2012). Capturing single cell genomes of active polysaccharide degraders: An unexpected contribution of Verrucomicrobia. PLoS One 7:e35314. doi: 10.1371/journal.pone.0035314
Mayer, M., Prescott, C. E., Abaker, W. E., Augusto, L., Cécillon, L., Ferreira, G. W., et al. (2020). Tamm Review: Influence of forest management activities on soil organic carbon stocks: A knowledge synthesis. For. Ecol. Manage. 466:118127. doi: 10.1016/j.foreco.2020.118127
Meng, M. J., Lin, J., Guo, X. P., Liu, X., Wu, J. S., Zhao, Y. P., et al. (2019). Impacts of forest conversion on soil bacterial community composition and diversity in subtropical forests. Catena 175, 167–173. doi: 10.1016/j.catena.2018.12.017
Merloti, L. F., Mendes, L. W., Pedrinho, A., de Souza, L. F., Ferrari, B. M., and Tsai, S. M. (2019). Forest-to-agriculture conversion in Amazon drives soil microbial communities and N-cycle. Soil Biol. Biochem. 137:107567. doi: 10.1016/j.soilbio.2019.107567
Nakayama, M., Imamura, S., Taniguchi, T., and Tateno, R. (2019). Does conversion from natural forest to plantation affect fungal and bacterial biodiversity, community structure, and co-occurrence networks in the organic horizon and mineral soil? For. Ecol. Manage. 446, 238–250. doi: 10.1016/j.foreco.2019.05.042
Ni, X., Lin, C., Chen, G., Xie, J., Yang, Z., Liu, X., et al. (2021). Decline in nutrient inputs from litterfall following forest plantation in subtropical China. For. Ecol. Manage. 496:119445. doi: 10.1016/j.foreco.2021.119445
Nixon, S. L., Daly, R. A., Borton, M. A., Solden, L. M., Welch, S. A., Cole, D. R., et al. (2019). Genome-resolved metagenomics extends the environmental distribution of the Verrucomicrobia phylum to the deep terrestrial subsurface. mSpsere 4:e00613-19. doi: 10.1128/mSphere.00613-19
Paula, F. S., Rodrigues, J. L. M., Zhou, J. Z., Wu, L. Y., Mueller, R. C., Mirza, B. S., et al. (2014). Land use change alters functional gene diversity, composition and abundance in Amazon forest soil microbial communities. Mol. Ecol. 23, 2988–2999. doi: 10.1111/mec.12786
Paz-Ferreiro, J., and Fu, S. L. (2016). Biological indices for soil quality evaluation: Perspectives and limitations. Land Degrad. Dev. 27, 14–25. doi: 10.1002/ldr.2262
Peng, S. M., Liu, W., Xu, G., Pei, X. J., Millerick, K., and Duan, B. L. (2021). A meta-analysis of soil microbial and physicochemical properties following native forest conversion. Catena 204:105447. doi: 10.1016/j.catena.2021.105447
Ranjan, K., Paula, F. S., Mueller, R. C., Jesus, E. D., Cenciani, K., Bohannan, B. J., et al. (2015). Forest-to-pasture conversion increases the diversity of the phylum Verrucomicrobia in Amazon rainforest soils. Front. Microbiol. 6:155163. doi: 10.3389/fmicb.2015.00779
Rivett, D. W., and Bell, T. (2018). Abundance determines the functional role of bacterial phylotypes in complex communities. Nat. Microbiol. 3, 767–772. doi: 10.1038/s41564-018-0180-0
Sewerniak, P. (2020). Plant species richness or soil fertility: Which affects more the productivity of Scots pine in Central Europe? Ann. For. Res. 63, 57–73. doi: 10.15287/afr.2020.2003
Shen, C. C., Ge, Y., Yang, T., and Chu, H. Y. (2017). Verrucomicrobial elevational distribution was strongly influenced by soil pH and carbon/nitrogen ratio. J. Soils Sediments 17, 2449–2456. doi: 10.1007/s11368-017-1680-x
Sokol, N. W., Slessarev, E., Marschmann, G. L., Nicolas, A., Blazewicz, S. J., Brodie, E. L., et al. (2022). Life and death in the soil microbiome: How ecological processes influence biogeochemistry. Nat. Rev. Microbiol. 20, 415–430. doi: 10.1038/s41579-022-00695-z
Steinauer, K., Tilman, D., Wragg, P. D., Cesarz, S., Cowles, J. M., Pritsch, K., et al. (2015). Plant diversity effects on soil microbial functions and enzymes are stronger than warming in a grassland experiment. Ecology 96, 99–112. doi: 10.1890/14-0088.1
Tahovska, K., Capek, P., Santruckova, H., and Kopacek, J. (2018). In situ phosphorus dynamics in soil: Long-term ion-exchange resin study. Biogeochemistry 139, 307–320. doi: 10.1007/s10533-018-0470-x
Tang, Z. X., Fan, F. L., Wan, Y. F., Wei, W., and Lai, L. M. (2015). Abundance and diversity of RuBisCO genes responsible for CO2 fixation in arid soils of Northwest China. Pedosphere 25, 150–159. doi: 10.1016/S1002-0160(14)60085-0
Tateishi, H. R., Bragagnolo, C., and de Almeida, A. N. (2021). Forest, agriculture and land conversion: Environmental efficiency in Brazilian Amazon rainforest. For. Policy Econ. 133:102615. doi: 10.1016/j.forpol.2021.102615
Teng, Z., Cui, J., Wang, J. J., Fu, X. L., and Xu, X. N. (2018). Effect of exogenous nitrogen and phosphorus inputs on the microbe-soil interaction in the secondary Castanopsis sclerophylla forest in east China. iForest 11, 794–801. doi: 10.3832/ifor2673-011
Teng, Z., Fan, W., Wang, H. L., Cao, X. Q., and Xu, X. N. (2020). Monitoring soil microorganisms with community-level physiological profiles using Biolog EcoPlates in Chaohu lakeside wetland, east China. Eurasian Soil Sci. 53, 1142–1153. doi: 10.1134/S1064229320080141
Tian, P., Liu, S. G., Wang, Q. K., Sun, T., and Blagodatskaya, E. (2019). Organic N deposition favours soil C sequestration bydecreasing priming effect. Plant Soil 445, 439–451. doi: 10.1007/s11104-019-04331-3
Wang, H., Liu, S., Kuzyakov, Y., Zhan, P., Wang, Q., Hettenhausen, C., et al. (2020). Differentiating microbial taxonomic and functional responses to physical disturbance in bulk and rhizosphere soils. Land Degrad. Dev. 31, 2858–2871. doi: 10.1002/ldr.3679
Wang, J., Zou, Y., Di Gioia, D., Singh, B. K., and Li, Q. (2020). Conversion to agroforestry and monoculture plantation is detrimental to the soil carbon and nitrogen cycles and microbial communities of a rainforest. Soil Biol. Biochem. 147:107849. doi: 10.1016/j.soilbio.2020.107849
Wang, J. C., Ren, C. Q., Cheng, H. T., Zou, Y. K., Bughio, M. A., and Li, Q. F. (2017). Conversion of rainforest into agroforestry and monoculture plantation in China: Consequences for soil phosphorus forms and microbial community. Sci. Total Environ. 595, 769–778. doi: 10.1016/j.scitotenv.2017.04.012
Wang, S. S., Chen, D. M., Liu, Q. F., Zang, L. P., Zhang, G. Q., Sui, M. Z., et al. (2023). Dominant influence of plants on soil microbial carbon cycling functions during natural restoration of degraded karst vegetation. J. Environ. Manage. 345:118889. doi: 10.1016/j.jenvman.2023.118889
Wang, S. Y., Zhu, G. B., Zhuang, L. J., Li, Y. X., Liu, L., Lavik, G., et al. (2020). Anaerobic ammonium oxidation is a major N-sink in aquifer systems around the world. Isme J. 14, 151–163. doi: 10.1038/s41396-019-0513-x
Wang, Y., Chen, L., Xiang, W. H., Ouyang, S., Zhang, T. D., Zhang, X. L., et al. (2021). Forest conversion to plantations: A meta-analysis of consequences for soil and microbial properties and functions. Glob. Change Biol. 27, 5643–5656. doi: 10.1111/gcb.15835
Wang, Y., Zhang, C., Zhang, G. N., Wang, L. Z., Gao, Y., Wang, X. L., et al. (2019). Carbon input manipulations affecting microbial carbon metabolism in temperate forest soils - A comparative study between broadleaf and coniferous plantations. Geoderma 355:113914. doi: 10.1016/j.geoderma.2019.113914
Wang, Y., Zheng, H., Chen, F. L., Zeng, J., Zhou, J. Z., and Ouyang, Z. Y. (2020). Stabilities of soil organic carbon and carbon cycling genes are higher in natural secondary forests than in artificial plantations in southern China. Land Degrad. Dev. 31, 2986–2995. doi: 10.1002/ldr.3649
Wei, X. R., Shao, M. G., Gale, W., and Li, L. H. (2014). Global pattern of soil carbon losses due to the conversion of forests to agricultural land. Sci. Rep. 4:4062. doi: 10.1038/srep04062
Wu, L. H., Liu, J. L., and Zhao, J. (2014). Pyrosequencing-based assessment of bacterial community structure in Wuliangsuhai Wetland. Adv. Mater. Res. 955-959, 459–462.
Xu, Q. F., Jiang, P. K., Wu, J. S., Zhou, G. M., Shen, R. F., and Fuhrmann, J. J. (2015). Bamboo invasion of native broadleaf forest modified soil microbial communities and diversity. Biol. Invasions 17, 433–444. doi: 10.1007/s10530-014-0741-y
Xu, W., Li, W., Jiang, P., Wang, H., and Bai, E. (2014). Distinct temperature sensitivity of soil carbon decomposition in forest organic layer and mineral soil. Sci. Rep. 4, 1–6. doi: 10.1038/srep06512
Xu, Z. W., Chen, X. M., Wei, Y. L., Qi, Z., and Ji, X. L. (2021). Macrogenomic-based analysis of microorganisms and their carbon and nitrogen metabolism diversity in the Napa Sea plateau wetlands. Chin. J. Biotrch. 37, 3276–3292. doi: 10.13345/j.cjb.200658
Yan, B. F., Liu, N., Liu, M. H., Du, X. Y., Shang, F., and Huang, Y. (2021). Soil actinobacteria tend to have neutral interactions with other co-occurring microorganisms, especially under oligotrophic conditions. Environ. Microbial. 23, 4126–4140. doi: 10.1111/1462-2920.15483
Yang, L., Yang, Z., Zhong, X., Xu, C., Lin, Y., Fan, Y., et al. (2021). Decreases in soil P availability are associated with soil organic P declines following forest conversion in subtropical China. Catena 205:105459. doi: 10.1016/j.catena.2021.105459
Yang, Y. S., He, Z. M., Ma, X. Q., and Yu, X. T. (1997). View on the advantages and disadvantages of the effects of controlled burning on the ecological system of Chinese fir plantation and the countermeasures. J. Resour. Ecol. 12, 153–159.
Yang, Z. J., Chen, S. D., Liu, X. F., Xiong, D. C., Xu, C., Arthur, M. A., et al. (2019). Loss of soil organic carbon following natural forest conversion to Chinese fir plantation. For. Ecol. Manage. 449:117476. doi: 10.1016/j.foreco.2019.117476
Zaidi, A., Khan, M. S., Ahemad, M., Oves, M., and Wani, P. A. (2009). “Recent advances in plant growth promotion by phosphate-solubilizing microbes,” in Microbial Strategies for Crop Improvement, eds M. S. Khan, A. Zaidi, and J. Musarrat (Berlin: Springer), 23–50. doi: 10.1007/978-3-642-01979-1_2
Zhang, H. Z., Shi, L. L., Wen, D. Z., and Yu, K. L. (2016). Soil potential labile but not occluded phosphorus forms increase with forest succession. Biol. Fertil. Soils 52, 41–51. doi: 10.1007/s00374-015-1053-9
Zhang, L., Ding, X., Chen, S., He, X., Zhang, F., and Feng, G. (2014). Reducing carbon: Phosphorus ratio can enhance microbial phytin mineralization and lessen competition with maize for phosphorus. J. Plant Interact. 9, 850–856.
Zhang, Y., Tigabu, M., Yi, Z., Li, H., Zhuang, Z., Yang, Z., et al. (2019). Soil parent material and stand development stage effects on labile soil C and N pools in Chinese fir plantations. Geoderma 338, 247–258.
Zhou, L. L., Shalom, A. D. D., Wu, P. F., He, Z. M., Liu, C. H., and Ma, X. Q. (2016). Biomass production, nutrient cycling and distribution in age-sequence Chinese fir (Cunninghamia lanceolate) plantations in subtropical China. J. For. Res. 27, 357–368. doi: 10.1007/s11676-015-0167-0
Zhou, Z., Wang, C., and Luo, Y. (2020). Meta-analysis of the impacts of global change factors on soil microbial diversity and functionality. Nat. Commun. 11, 1–10. doi: 10.1038/s41467-020-16881-7
Zhou, Z. C., Tran, P. Q., Kieft, K., and Anantharaman, K. (2020). Genome diversification in globally distributed novel marine Proteobacteria is linked to environmental adaptation. ISME J. 14, 2060–2077. doi: 10.1038/s41396-020-0669-4
Zhu, X., Jiang, X., Singh, A. K., Zeng, H., Chen, C., Lu, E., et al. (2022). Reduced litterfall and decomposition alters nutrient cycling following conversion of tropical natural forests to rubber plantations. Ecol. Indic. 138:108819. doi: 10.1016/j.ecolind.2022.108819
Keywords: forest conversion, coniferous plantation, soil layers, bacterial community, potential function
Citation: Cao X, Xia K, Zhao H, Deng P, Teng Z and Xu X (2024) Soil organic carbon, pH, and ammonium nitrogen controlled changes in bacterial community structure and functional groups after forest conversion. Front. For. Glob. Change 7:1331672. doi: 10.3389/ffgc.2024.1331672
Received: 01 November 2023; Accepted: 22 April 2024;
Published: 15 May 2024.
Edited by:
Henry Joseph Oduor Ogola, Jaramogi Oginga Odinga University of Science and Technology, KenyaReviewed by:
Ramganesh Selvarajan, Chinese Academy of Sciences (CAS), ChinaGrace Nkechinyere Ijoma, University of South Africa, South Africa
Copyright © 2024 Cao, Xia, Zhao, Deng, Teng and Xu. This is an open-access article distributed under the terms of the Creative Commons Attribution License (CC BY). The use, distribution or reproduction in other forums is permitted, provided the original author(s) and the copyright owner(s) are credited and that the original publication in this journal is cited, in accordance with accepted academic practice. No use, distribution or reproduction is permitted which does not comply with these terms.
*Correspondence: Hongfei Zhao, aGZ6aGFvQHpqc3J1LmVkdS5jbg==; Xiaoniu Xu, eG54dTIwMDdAYWhhdS5lZHUuY24=
†These authors have contributed equally to this work