- 1Key Laboratory of Geographical Processes and Ecological Security in Changbai Mountains, Ministry of Education, School of Geographical Sciences, Northeast Normal University, Changchun, China
- 2Swiss Federal Institute for Forest, Snow and Landscape Research WSL, Birmensdorf, Switzerland
- 3School of Life Science, Hebei University, Baoding, China
Introduction: Root hairs increase the surface area of a plant’s root system that is in contact with the soil, thus facilitating plant water and nutrient uptake. However, little is known about the characteristics of the root hairs of herbaceous and woody plants and their specific response patterns to biotic and abiotic variables from the perspective of resource acquisition strategies in the context of global change.
Methods: Here, we analyzed 74 published case studies with 1074 observations of root hair traits to identify patterns of root hair length, density and diameter in relation to soil (e.g., soil pH, nutrient levels), growing environments (e.g., greenhouse, field) and climatic factors (e.g., air temperature), as well as genome size and plant age.
Results: Root hairs were longer, denser and thicker in woody plants compared with herbaceous plants, and the length and diameter of root hairs in herbaceous plants increased with genome size. With increasing plant age, woody plants had significantly longer and thicker root hairs, while root hair density and diameter declined significantly for herbaceous plants. Soil-cultured plants had longer root hairs than solution-cultured plants. The length and density of root hairs were greater in greenhouse-cultured plants than in field-grown plants, and the latter had thicker root hairs than the former. As soil pH increased, root hair length increased but diameter decreased in woody plants, while root hair density increased in herbaceous plants. Increased soil total nitrogen (N) and potassium (K) significantly increased root hair length, density and diameter in herbaceous plants, while soil total N significantly decreased root hair density in woody plants. Root hair length increased significantly, while root hair density decreased significantly, with higher mean annual temperature and greater precipitation seasonality, while the opposite pattern was true for a wider annual temperature range.
Discussion: Our findings emphasize the life-form-specific responses of root hairs to soil and climatic variables. These findings will help deepen our understanding of resource acquisition strategies and their mechanisms in different plant forms under global climate change.
1 Introduction
Root hairs are the outward protrusions of epidermal cells in the mature zone with polar growth characteristics (Bibikova and Gilroy, 2002; Libault et al., 2010; Zhang et al., 2023b). As short-lived organs for absorbing water and nutrients, root hairs can increase exploration of the soil by increasing the root surface area in contact with the soil (Gilroy and Jones, 2000; Foreman and Dolan, 2001; Lippold et al., 2022). Moreover, root hairs improve soil resource uptake efficiency with very low carbon construction and maintenance costs relative to other root morphological features (Fohse et al., 1991; Bates and Lynch, 2001; Waddell et al., 2016). Thus, the growth and development of root hairs are strongly dependent on soil nutrient and water availability, as well as soil structure and soil texture (Krasilnikoff et al., 2003; Duddek et al., 2023b). Root hair traits are often associated with the uptake and accumulation of soil mineral elements by roots, especially for the less mobile nutrients, such as inorganic phosphate (Brown et al., 2012; Duddek et al., 2023a). Therefore, the growth status and vigor of root hairs directly affect the nutritional status and biomass of aboveground parts, playing an important role in plant growth and yield formation.
Root hair traits vary among plant species and are strongly controlled by intrinsic genetic factors and genotype (Burak et al., 2021; Marin et al., 2021; Cai et al., 2022). The length, diameter and density of root hairs are important functional traits, indicating the degree of root hair development (Zygalakis et al., 2011). The length of root hairs can generally range from a few to several millimeters, depending on the plant species, its growth stages, and the reproductive environment (Caradus, 1980; Ruiz et al., 2020). Recent studies have highlighted the species specificity of the water uptake capacity of root hairs (Keyes et al., 2017; Cai et al., 2021; Adu et al., 2023). Moreover, root hair density is a phosphorus-regulated and phosphorus-responsive trait (Nestler et al., 2016; Cui et al., 2018; Bichara et al., 2021), showing greater variability among genotypes than root hair length (Vandamme et al., 2013). A significant negative correlation between root hair density and length has been observed in several plant species, such as Zea mays and Glycine max (Wang et al., 2004; Adu et al., 2017), suggesting that a limited exploration ability of shorter root hairs may be compensated by higher root hair density (Stetter et al., 2015; Adu et al., 2023). Accordingly, the morphological characteristics of root hairs are determined by the interactions between the plant’s genetic potential and its response to environmental factors (Jungk, 2001; Datta et al., 2011; Pang et al., 2017).
Life forms are key to understanding changes in species composition and vegetation types and their functional traits (Matsuo et al., 2023). Different life forms, such as woody and herbaceous plants, vary in woodiness, longevity, size, form and physiology, leading to distinct responses to the environment changes (Grytnes and Felde, 2014; Pérez-Harguindeguy et al., 2016). Generally, woody plants have higher water-carbon exchange rates than herbaceous plants because of the greater resource availability in the deeper soil where their roots are located and their greater tolerance to environment stress (Chandrasekaran et al., 2014; Wang et al., 2020c). By contrast, herbaceous roots possess greater plasticity, enhancing their environmental adaptability (Damian et al., 2020; Pu et al., 2023; Zu et al., 2023). Therefore, herbaceous and woody plants may employ different root foraging strategies (e.g., contrasting root hair densities) to cope with changing water and nutrient patterns (Graves et al., 2006; Wang et al., 2019; Xing et al., 2024). In addition, woody plants tend to be more climate sensitive than herbaceous plants (Golivets et al., 2024). Trees, for instance, are often more sensitive to climate warming than herbaceous plants (Graves et al., 2006; Hassan et al., 2023) and changes in light (Xu et al., 2023), due to long-term environmental selection (Beidler et al., 2015; Ma and Chen, 2018; Hassan et al., 2023).
Collectively, the effects of soil conditions and climate changes on root hairs may depend on differences in root hair development between herbaceous and woody plants (Tawaraya, 2003; Ohri, 2005; Yu et al., 2014; Hwang et al., 2017). Heterogeneity in soil structure leads to spatial variation in soil oxygen, water and nutrient concentrations and in soil biological activity (Michael, 2001; Lippold et al., 2021). Root hair growth has been shown to increase under limited soil moisture conditions (Schnall and Quatrano, 1992), with root hairs of soil-cultivated plants being longer and denser than those of solution-cultivated plants (Datta et al., 2011). However, root hairs tend to be shorter and more globular in response to drought (Zou et al., 2017). Nutrient availability is one of the most important factors affecting root hair development (Barrow, 1977; Cui et al., 2018). Modification of root hair architecture is an effective strategy for plants to obtain nutrients from nutrient-poor soils. For example (Schmidt and Gaudin, 2017) found that environmental nutrient deficiencies triggered plants to build a “compensatory” mechanism for facilitating resource acquisition by increasing root hair length or density (Haling et al., 2010; Vissenberg et al., 2020). Additionally, uptake of sparingly soluble nutrients, such as phosphorus, at the root surface may exceed the diffusion rates of these nutrients in soil, resulting in depletion zones surrounding the roots (Segal et al., 2008; Zhang et al., 2023a). Root hairs extend the root uptake surface beyond the existing concentration loss zone and increase the nutrient mass flow around the roots, thus minimizing the ionic diffusivity limitation of uptake rates (Robinson and Rorison, 1987; Segal et al., 2008; Zhang et al., 2023a).
In general, woody plants have a deeper and wider rooting range than herbaceous plants, and the rooting range is deeper in dry and nutrient-poor environments than in moist and nutrient-rich ones (Tumber-Dávila et al., 2022). Little is known about how root hairs vary with plant life form (e.g., woody versus herbaceous plants) and how they respond to changes in growth conditions. We therefore investigated the patterns of root hair traits in woody and herbaceous plants and their associations with soil water and nutrient availability, to better understand the response characteristics of root hairs of different plant life forms to resource variations. We hypothesized that: (1) the morphological structure of root hairs would differ between herbaceous and woody plants, with root hairs of herbaceous plants being shorter and finer but denser than those of woody plants, and (2) the responses of root hairs to soil and climate factors would vary between herbaceous and woody plants, due to their contrasting resource uptake strategies.
2 Materials and methods
2.1 Data collection
We collected papers published between 1937 and December 2022 from the Web of Science and the China National Knowledge Infrastructure (CNKI). We used the keywords: “root hair,” “life form,” “soil” and “climate change” to find 74 papers that contained complete attributes of plant root hairs. The root hair traits we selected were: root hair length (μm), root hair density (number of root hairs per cm of root length), and root hair diameter (average diameter of root hair cross-section, μm). In all the original studies, morphometric measurements of root hairs at randomly distributed points within the apical zone on root samples were performed with the help of microscopes (Caradus, 1980; Adu et al., 2017). Based on the species information provided by the authors of the original studies, we categorized the dataset into woody communities consisting only of woody species (shrubs and trees) and herbaceous communities consisting only of herbaceous species. We also extracted the plant ages from the original studies. Based on the latitude and longitude information of each field-grown plant species provided in the original literature, we extracted the corresponding soil and climate data using the extract function in the raster package (Hijmans, 2018) in R version 4.2.3 (R Core Team, 2021, http://www.R-project.org). For soil factors, we extracted total soil N and total soil K contents and soil pH from the global soil dataset (http://globalchange.bnu.edu.cn/research/soilw) at a spatial resolution of 1 km. For climate factors, we extracted mean annual temperature, mean annual precipitation, seasonal variation in precipitation, and temperature annual range at a spatial resolution of 30-s from the WorldClim database (https://worldclim.org/data/worldclim21.html). As a biodiversity trait, genome size varies greatly across plant species (Hwang et al., 2017). We defined the 1C-value of DNA content as a measurement of genome size, which we obtained from the latest version of the Kew Plant DNA C-values Database (https://cvalues.science.kew.org/search; Greilhuber, 2005; Shao et al., 2021).
2.2 Statistical analysis
We grouped plant species according to life form (woody versus herbaceous), culture medium (soil versus solution), and culture environment (greenhouse versus field). We performed a non-parametric Kruskal-Wallis test of the stats package (R Core Team, 2021) to determine the effects of life form, culture medium and culture environment on root hair traits. We generated box plots and scatter plots of root hair length, density and diameter using the ‘boxplot’ and ‘scatter’ functions of the ggplot2 package in R, respectively (Wickham, 2011). We used a general linear model to assess the relationships between root hair morphological traits and climate (R Core Team, 2021) and soil-related factors, as well as plant age, using the ‘lmer’ function in the lme4 package in R (Bates et al., 2015). All statistical analyses in this paper were completed in R version 4.2.3. http://www.R-project.org.
3 Results
There were significant differences in the length, density and diameter of root hairs between woody and herbaceous plants. Specifically, woody plants had longer root hair lengths (884 ± 313 μm for woody plants versus 572 ± 103 μm for herbaceous plants, mean ± SE), higher root hair densities (463 ± 55 hairs cm−1 for woody plants versus 269 ± 24 hairs cm−1 for herbaceous plants), and larger root hair diameters compared with herbaceous plants (12.5 ± 0.34 μm for woody plants versus 9.2 ± 0.41 μm for herbaceous plants; Figures 1A,D,G). Plants in soil cultures had longer root hair lengths (655 ± 194 μm) than those in solution cultures (607 ± 109 μm; Figure 1B). No difference was found for root hair density or diameter between culture types (Figures 1E,H). Moreover, root hair length (682 ± 116 μm) and density (335 ± 25 number cm−1) of plants cultured in the greenhouse were greater than the corresponding metrics of field-grown plants (257 ± 18.6 μm for root hair length and 69 ± 21 hairs cm−1 for root hair density; Figures 1C,F). By contrast, field-grown plants had root hair diameters (11.2 ± 0.51 μm) similar to those of plants cultured in greenhouses (10.9 ± 0.32 μm, Figure 1I).
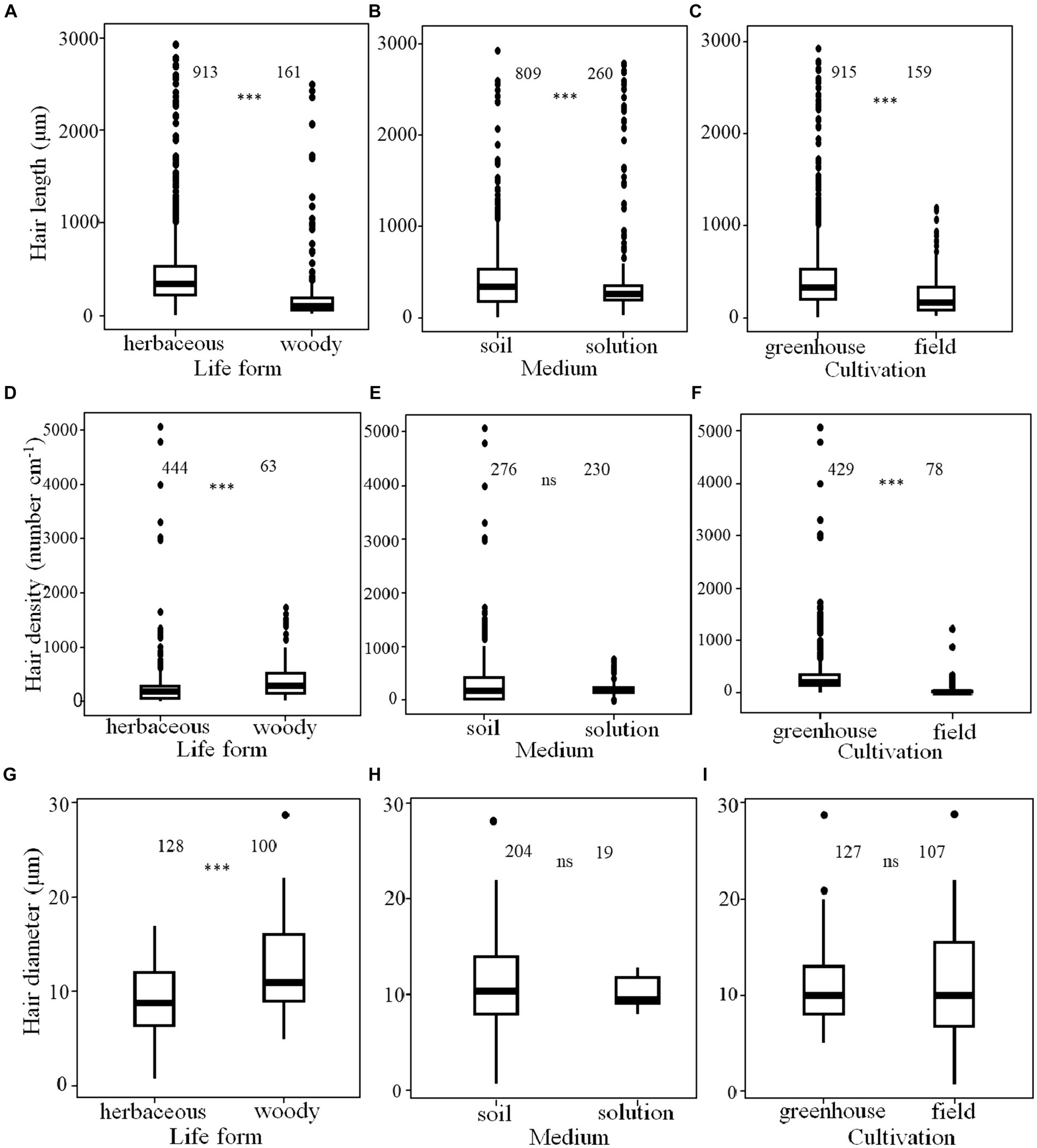
Figure 1. Root hair length, density and diameter among plant life forms (A,D,G), culture media (B,E,H) and cultivation types (C,F,I). The number above each boxplot in each panel is the number of observations. The boxplots summarize the distribution of data points for each group: median (bold horizontal line in the box); interquartile range (box); 10th and 90th percentiles (lower and upper error bars), and outliers (individual points outside the box). Significant differences are indicated with *** (p < 0.001); ns, not significant.
Root hair length and diameter increased significantly with increasing root hair density (Figures 2A,B). By contrast, there was a negative correlation between root hair length and root hair diameter (Figure 2C). The root hair length of woody plants and the root hair density of herbaceous plants were both positively correlated with pH (Figures 3A,D). By contrast, the root hair diameter of woody plants decreased significantly with increasing soil pH (Figure 3G). For herbaceous plants, root hair length (Figures 3B,C), density (Figures 3E,F) and diameter (Figures 3H,I) all increased significantly with increasing soil total N and K contents. For woody plants, root hair length was positively correlated with soil total K content, while root hair density was negatively correlated with soil total N content (Figures 3C,E). Moreover, the root hair length and diameter of woody plants increased significantly but the root hair density and diameter of herbaceous plants decreased significantly with increasing plant age (Supplementary Figure S1).
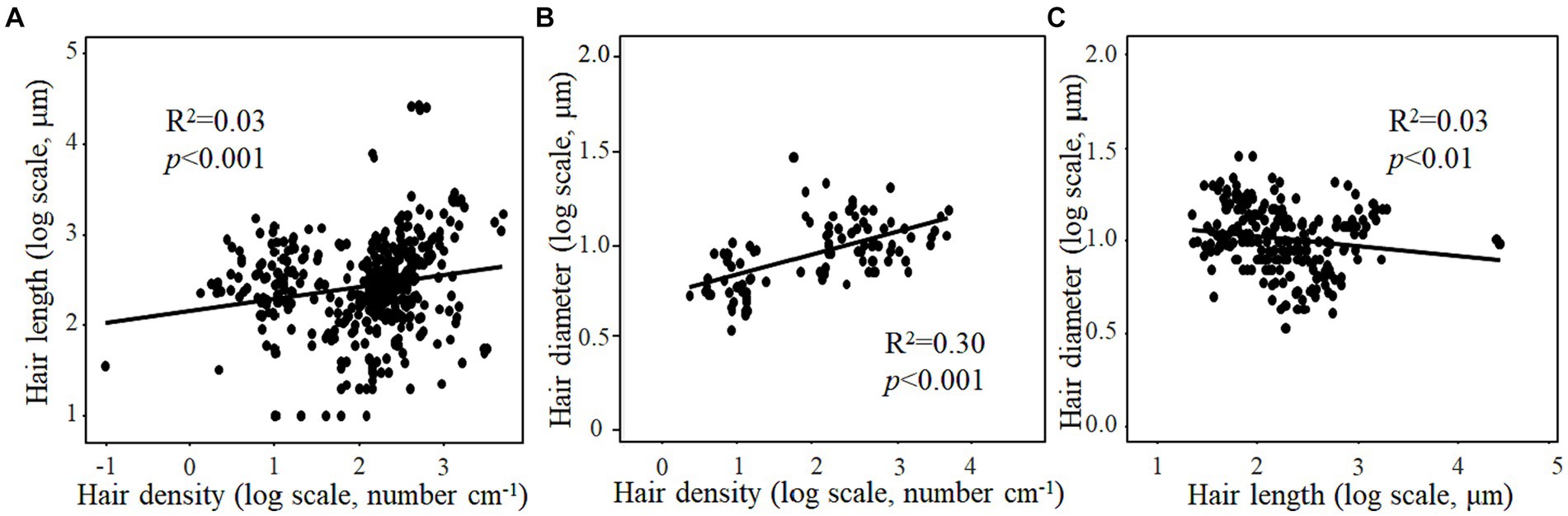
Figure 2. Relationships of root hair density with length (A) and diameter (B), as well as the relationship between root hair length and diameter (C).
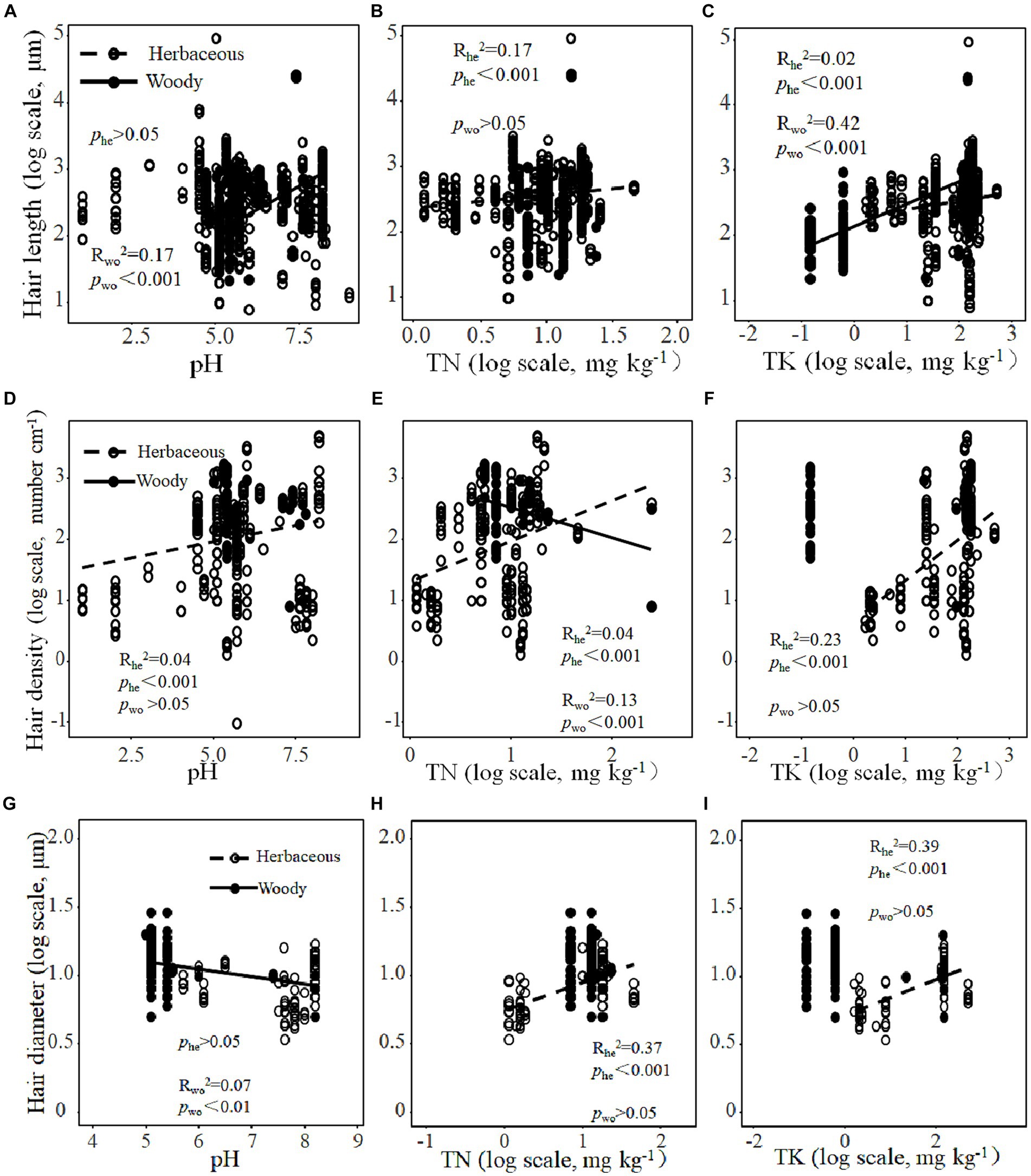
Figure 3. Relationships of root hair length (A), density (D) and diameter (G) with soil pH, relationships of root hair length (B), density (E) and diameter (H) with soil total nitrogen content (TN), and relationships of root hair length (C), density (F) and diameter (I) with soil total potassium content (TK) for herbaceous (he) and woody plants (wo).
The root hair length and diameter of herbaceous plants and the root hair diameter of woody plants increased considerably with increasing genome size (Table 1). Root hair length increased significantly but root hair density decreased significantly with increasing mean annual temperature, while the opposite was true for annual temperature range (except for root hair diameter; Table 2). Root hair density was significantly negatively related to mean annual precipitation and precipitation seasonality, while root hair length and diameter were significantly related to precipitation seasonality, with a positive correlation for root hair length and a negative correlation for root hair diameter (Table 2).

Table 1. Results of a general linear model of the relationships of root hair length, density and diameter with genome size across plant life forms.

Table 2. Results of a general linear model of the relationships of root hair length, density and diameter with climatic factors. ***p < 0.001.
4 Discussion
We found that herbaceous plants had shorter, thinner and sparser root hairs than woody plants, which is partly consistent with our first hypothesis. This discrepancy in the morphological characteristics of root hairs between the two life forms may indicate their contrasting soil resource uptake mechanisms and capacities to adapt to their habitats (Camacho-B et al., 1974; Yang et al., 2017; Golivets et al., 2024). Some studies on roots have shown that herbaceous species generally have thinner root systems compared with those of woody species, which have thicker roots with a highly lignified columnar organization and higher root tissue density (Zangaro et al., 2005; Wang et al., 2020a). In addition, most herbaceous plants have a short life cycle and fast root growth over a short period to complete their life cycle, whereas most woody plants are long-lived and have conservative root phenotypes and rooting strategies (Van der Waal et al., 2009; Wang et al., 2020a; Xing et al., 2024).
In addition, we observed plasticity of root hair patterning in response to plant age for herbaceous and woody plants. Specifically, the root hair density and diameter of herbaceous plants decreased significantly with greater plant age, while the root hair length and diameter of woody plants increased significantly. This divergence in root hair patterning with age for different plant life forms may be associated with their contrasting nutritional requirements in the different stages of plant development. Roots of most herbaceous plants are located near the soil surface, making them vulnerable to damage from unfavorable conditions such as drought and freeze–thaw cycles (Gulden and Vessey, 2000; Stetter et al., 2015). In contrast, the roots of most woody plants are located deeper in the soil profile, allowing plants to obtain water and nutrients from deeper soils (Gilroy and Jones, 2000; Bengough et al., 2016; Wang et al., 2020c; Xu et al., 2021). Root hair growth may be the most directly favorable morphological responses of the root system to nutrient stress, and the morphological structure of the root hair system in different life forms is related to differences in the strategies for obtaining nutrients from the soils.
Moreover, we found that root hair length and/or diameter increased significantly with genome size. In fact, earlier cell-based studies demonstrated that genome size was positively correlated with the size of different plant tissues (Schweiger et al., 1995; Ohri, 2005; Beaulieu et al., 2008; Stetter et al., 2015). Longer or more abundant root hairs can increase exploration of the soils by increasing the root surface area in contact with the soils (Lopez-Bucio et al., 2003; Zhu et al., 2020). Additionally, longer root hairs are more efficient at extracting water and nutrients from mineral soils by expanding the zone of depletion, and exhibit higher soil and water holding capacity than short root hairs (Gahoonia and Nielsen, 2004; Sandral et al., 2019). Plant species with longer root hairs also tend to have a greater specific root length and nutrient foraging potential, maintaining a larger biomass (Fort et al., 2015; Zhang et al., 2023b). Therefore, the structure of root hairs in herbaceous and woody plants is influenced by genetic factors that help the plants adapt to their specific growing conditions and develop their specific absorption mechanisms.
Soil factors (e.g., soil texture, pH and nutrient contents) have strong influences on root hair traits, such as length and diameter (Schenk, 2006; Karlova et al., 2021). In this study, we found that the root hair length of plants cultured in soil was greater than that of plants cultured in solution. This result supports findings from previous experiments, in which root hairs were observed on roots cultured in sandy soil but not on roots cultured in solution (Gahoonia et al., 1997; Nestler et al., 2016; Zhang et al., 2023b). Given the unidirectional growth of root hairs, the high penetration resistance represented by sand and other hard particles, as well as soil compactness and the resulting mechanical resistance, stimulate the development of root hairs under natural conditions (Genc et al., 2007; Lippold et al., 2022). This could explain the result in our study that soil-grown plants had longer root hairs than solution-grown plants. Furthermore, it has been observed previously that the higher sand content of the soil, the greater length and density of root hairs (Bailey and Scholes, 1997). The occurrence of root hairs is an indirect response not only to different soil textures, but also to different water and nutrient availabilities in the soils (Bates and Lynch, 2001; Kiswara et al., 2009; Pacheco et al., 2023). Soils with different textures have different chemical and physical properties, providing contrasting habitats for plants (Meisner and Karnok, 1991; Duddek et al., 2023b). Longer root hairs can help plant root systems to explore a larger volume of soils, promoting better plant growth compared with shorter root hairs (Lazarević et al., 2018). In this study, we also found that plants cultured in soils had larger root hair diameters than those cultured in solution. Thick root hairs may confer the great advantage to plant root penetration and growth in high-compacted soil zones (Kidd et al., 2016). Thus, our findings indicate that, as an adaptation to soil resistance, long and thick root hairs can provide plants with sufficient tensile strength for anchoring root tips, to aid in root penetration into high-compacted layers.
Our results showed that root hair length in woody plants and root hair density in herbaceous plants were positively correlated with soil pH. Root hairs require precise control of the interaction between surface pH and the extracellular reactive oxygen species balance to achieve normal morphogenesis by increasing cell wall acidification in specific zones, such as the apical and elongation zones (Tatiana et al., 1998; Stéger and Palmgren, 2022; Pacheco et al., 2023). Low soil pH may directly affect plasma membrane proteins, such as pH-sensitive K channels, thereby disrupting the development of root hairs and inhibiting water uptake and transport by root hairs (Konno et al., 2006; Yuan et al., 2009). Several studies have demonstrated that all growing root hairs and their tips break off within 10 min when the pH of the medium is below 4.5 (Schnall and Quatrano, 1992; Monshausen et al., 2007; Stéger and Palmgren, 2022). Bailey and Scholes (1997) applied lime to neutralize the acidity in acidic soils, to eliminate the toxic effects of H+ and Al3+ on plants, and they reported improved development of root hairs and root sheaths (Bailey and Scholes, 1997). Furthermore, the growth of root hairs has also been found to be inhibited when the pH of the medium is alkalized to 8 or higher (Monshausen et al., 2007; Haling et al., 2010; Pacheco et al., 2023), which explains our results that the diameter of root hairs in woody plants decreased significantly with increasing soil pH. Accordingly, our findings indicate that the growth pattern of root hairs is closely related to the physical contact of the medium and the changes in pH of the root hair surface.
It is well established that root hair development is regulated by the availability of soil nutrients such as N, K, and phosphorus (P) (Krasilnikoff et al., 2003; Haling et al., 2013). In our study, the root hair length and diameter of herbaceous plants tended to increase with increasing soil N and K. Our finding of longer root hairs in nutrient-rich soils is supported by the results from several previous studies (Vatter et al., 2015; Canales et al., 2017; Cui et al., 2018). Plant absorption of mobile nutrients is directly proportional to root hair length because soil nutrients more easily reach the roots with greater root hair lengths (Bates and Lynch, 2001; Evans, 2012). For woody plants, by contrast, we only observed that root hair length was positively related to soil K. These results indicate that morphological traits of the root hairs of herbaceous plants were more closely related to soil nutrients than those of woody plants, suggesting that herbaceous plants undergo more active morphological adjustments than woody plants in this respect (Bibikova and Gilroy, 2002; Matsuo et al., 2023). Specifically, herbaceous plants depend primarily on root proliferation for resource foraging, while woody plants depend mainly on mycorrhizal foraging (Michael, 2001; Tawaraya, 2003; Golivets et al., 2024). The strong adaptation and active adjustment of herbaceous plants to soil nutrient availability are directly manifested by root hair traits, i.e., plant uptake of mobile nutrients is proportional to root hair length, density and diameter (Bates and Lynch, 2001; Pu et al., 2023). Accordingly, our results suggest that herbaceous plants in fertile habitats produced root systems with the longer and thicker root hairs to occupy more soil space, providing sufficient nutrients and water for plant growth and development.
In this study, we found that the root hair density of herbaceous plants increased significantly with soil N and K contents, but the root hair density of woody plants decreased with soil N content, supporting our second hypothesis of different responses of root hairs between herbaceous and woody plants to soil factors. It is well known that the root growth of herbaceous plants differs from that of woody plants. Specifically, herbaceous plants have a higher capacity to absorb resources per unit of biomass, due to their finer root hairs (Beidler et al., 2015; Ma et al., 2018; Bergmann et al., 2020). A higher density of root hairs in herbaceous plants may allow them to directly explore large amounts of soil to rapidly occupy more resource patches (Zangaro et al., 2005; Liu et al., 2019; Wang et al., 2023). On the other hand, herbaceous plants have shallower root systems compared with woody plants (Van der Waal et al., 2009; Wang et al., 2023; Golivets et al., 2024). The vertical distribution of the root system is directly related to their efficiency in absorbing soil resources (Matsuo et al., 2023). Consequently, woody plants are subjected to more nutrient stress than herbaceous plants (Wang et al., 2020a). Woody root systems produce a greater number of root hairs to accommodate the decrease in nutrient availability. However, over-dense root hairs may in turn limit nutrient assimilation by roots (Jungk, 2001; Zygalakis et al., 2011). The decreasing root hair density in woody plants with increasing soil nutrient contents may be a “compensatory” mechanism for the excessive overlap of nutrient-poor zones around root hairs (Morris and Myerscough, 1991; Schenk, 2006; Karlova et al., 2021).
We found that root hair length was positively correlated with mean annual temperature and negatively correlated with annual temperature range, which was completely opposite to the trends for root hair density. Increases in the transpiration rate of plants under higher temperature conditions promote the metabolic rate and stimulate the differentiation of a more developed root hair system, causing the root hairs to develop in the direction of sturdiness (Hoffmann and Jungk, 1995; Michael, 2001; Kemp et al., 2003; Zhang et al., 2020a; Karlova et al., 2021). Previous studies have indicated that the acclimation of maize from tropical to temperate regions is accompanied by a characteristic reduction in root hair growth (Saengwilai et al., 2021). Moreover, it has been proposed that root hair density may be more sensitive to the magnitude of annual temperature change (Fan et al., 2022; Ding et al., 2023). In addition, we found that the root hair length and density of greenhouse-cultured plants were higher than the corresponding values for field-grown plants. Greenhouse cultivations are characterized by good thermal insulation and light transmission (Hwang et al., 2011), which create an optimal environment for plant growth and development (Ceasar et al., 2020). Therefore, the root hair trait characteristic of greenhouse-cultured plants tends to be longer and denser. Accordingly, we can speculate that multiple environmental factors (e.g., background climate, light intensity and experimental duration) influence the responses of root hair morphological characteristics to temperature changes (Hoffmann and Jungk, 1995; Karlova et al., 2021; Zhang et al., 2023b).
Moreover, as important structures for water absorption, root hairs are very sensitive to changes in precipitation (Graves, 1991; Ruiz et al., 2020; Xing et al., 2024). In this study, although the length of root hairs was not associated with mean annual precipitation, it was positively correlated with seasonal variations in precipitation. Drought conditions affect the genes responsible for root hair formation (Cheng et al., 2016; Zhang et al., 2023b), which negatively regulate root hair growth in response to osmotic stress (Wang et al., 2020b). Thus, the change in root hair length is a key factor in plant drought tolerance (Zhang et al., 2020b). Moreover, root hair density was negatively correlated with mean annual precipitation and seasonal variations in precipitation in our study, suggesting that higher root hair densities largely compensate for shorter root hairs in drought-exposed soils. These results indicate the ability of plant root systems to buffer a certain range of precipitation (Siddique et al., 2015; Zhou et al., 2019; Xing et al., 2024). This ability ensures that the plant root system has access to resources in the face of variable soil resources, thereby stabilizing the growth and development of plants in the field to withstand changes in precipitation (Wang et al., 2020a; Xing et al., 2024). Therefore, different strategies of plant root hair systems to cope with changing temperature and water patterns are very important for our understanding of the effects of environmental changes on root growth and plant communities.
5 Conclusion and limitations
Plant root hairs are key structures for acquiring soil resources. Findings from the present study confirm that herbaceous and woody plants have different root hair morphologies in terms of length, diameter and density, and that the responses of root hairs to changes in soil and climatic factors depend on plant life form (herbaceous versus woody plants). The age-dependent patterns of root hair traits may permit a better understanding of the changing dynamics of root hairs as they age in herbaceous and woody plants. Our findings further indicate differences in soil resource acquisition strategies between woody and herbaceous plants, which implies that plant life forms coexisting in a community/ecosystem will differ in their ability to compete for soil resources in the face of future environmental change.
We acknowledge that several potential factors potentially limit the generality of our conclusions on the patterns of root hairs. For instance, most of the data used in this synthesis originated from experiments of herbaceous plants in greenhouses (Figure 1), with rather contrasting growth conditions compared with natural ones in the field. Additionally, our results may be biased by the inconsistency in experiment methodology used for root hair investigations across large space and time scales, given that root hairs are strongly influenced by small-scale soil heterogeneity (e.g., rhizosphere environmental conditions) and root growth seasonality. Thus, increasing the number of field studies within a unified research framework, especially to include more data from woody plants in forests, would greatly enhance our comprehensive understanding of the underlying variations in root hair traits and their potential responses to changing environments.
Data availability statement
Existing datasets are available in a publicly accessible repository: Publicly available datasets were analyzed in this study. This data can be found here: Novel data collected at in this study and compiled from FRED are available in the Supplementary materials and through FRED (Iversen et al., 2017, 2021; https://doi.org/10.25581/ornlsfa.014/1459186). Further inquiries can be directed to the corresponding author/s.
Author contributions
YZ: Conceptualization, Data curation, Investigation, Methodology, Software, Supervision, Writing – original draft. CW: Conceptualization, Data curation, Formal analysis, Funding acquisition, Methodology, Project administration, Resources, Software, Supervision, Writing – original draft, Writing – review & editing. QT: Software, Validation, Writing – original draft. JW: Methodology, Validation, Writing – original draft. M-HL: Conceptualization, Formal analysis, Methodology, Project administration, Supervision, Writing – review & editing.
Funding
The author(s) declare that financial support was received for the research, authorship, and/or publication of this article. This work was financially supported by the Natural Science Foundation of China (41971052 and 42171051).
Acknowledgments
The Authors thank Melissa Dawes for help editing the manuscript and improving the language.
Conflict of interest
The authors declare that the research was conducted in the absence of any commercial or financial relationships that could be construed as a potential conflict of interest.
Publisher’s note
All claims expressed in this article are solely those of the authors and do not necessarily represent those of their affiliated organizations, or those of the publisher, the editors and the reviewers. Any product that may be evaluated in this article, or claim that may be made by its manufacturer, is not guaranteed or endorsed by the publisher.
Supplementary material
The Supplementary material for this article can be found online at: https://www.frontiersin.org/articles/10.3389/ffgc.2024.1324405/full#supplementary-material
References
Adu, M. O., Asare, P. A., Yawson, D. O., Ackah, F. K., Amoah, K. K., Nyarko, M. A., et al. (2017). Quantifying variations in rhizosheath and root system phenotypes of landraces and improved varieties of juvenile maize. Rhizosphere 3, 29–39. doi: 10.1016/j.rhisph.2016.12.004
Adu, M. O., Zigah, N., Yawson, D. O., Amoah, K. K., Afutu, E., Atiah, K., et al. (2023). Plasticity of root hair and rhizosheath traits and their relationship to phosphorus uptake in sorghum. Plant Direct 7:e521. doi: 10.1002/pld3.521
Bailey, C., and Scholes, M. (1997). Rhizosheath occurrence in south African grasses. S. Afr. J. Bot. 63, 484–490. doi: 10.1016/S0254-6299(15)30803-6
Barrow, N. J. (1977). Phosphorus uptake and utilization by tree seedlings. Aust. J. Bot. 25, 571–584. doi: 10.1071/BT9770571
Bates, D., Mächler, M., Bolker, B. M., and Walker, S. C. (2015). Fitting linear mixed-effects models using lme4. J. Stat. Softw. 67, 1–48. doi: 10.18637/jss.v067.i01
Bates, T. R., and Lynch, J. P. (2001). Root hairs confer a competitive advantage under low phosphorus availability. Plant Soil 236, 243–250. doi: 10.1023/A:1012791706800
Beaulieu, J. M., Leitch, I. J., Patel, S., Pendharkar, A., and Knight, C. A. (2008). Genome size is a strong predictor of cell size and stomatal density in angiosperms. New Phytol. 179, 975–986. doi: 10.1111/j.1469-8137.2008.02528.x
Beidler, K. V., Taylor, B. N., Strand, A. E., Cooper, E. R., Schonholz, M., and Pritchard, S. G. (2015). Changes in root architecture under elevated concentrations of CO2 and nitrogen reflect alternate soil exploration strategies. New Phytol. 205, 1153–1163. doi: 10.1111/nph.13123
Bengough, A. G., Loades, K., and McKenzie, B. M. (2016). Root hairs aid soil penetration by anchoring the root surface to pore walls. J. Exp. Bot. 67, 1071–1078. doi: 10.1093/jxb/erv560
Bergmann, J., Weigelt, A., van der Plas, F., Laughlin, D. C., Kuyper, T. W., Guerrero-Ramirez, N., et al. (2020). The fungal collaboration gradient dominates the root economics space in plants. Sci. Adv. 6:eaba3765. doi: 10.1126/sciadv.aba3756
Bibikova, T., and Gilroy, S. (2002). Root Hair Development. J. Plant Growth Regul. 21, 383–415. doi: 10.1007/s00344-003-0007-x
Bichara, S., Mazzafera, P., and de Andrade, S. A. L. (2021). Root morphological changes in response to low phosphorus concentration in eucalypt species. Trees 35, 1933–1943. doi: 10.1007/s00468-021-02161-4
Brown, L. K., George, T. S., Thompson, J. A., Wright, G., Lyon, J., Dupuy, L., et al. (2012). What are the implications of variation in root hair length on tolerance to phosphorus deficiency in combination with water stress in barley (Hordeum vulgare)? Ann. Bot. 110, 319–328. doi: 10.1093/aob/mcs085
Burak, E., Quinton, J. N., and Dodd, I. C. (2021). Root hairs are the most important root trait for rhizosheath formation of barley (Hordeum vulgare), maize (Zea mays) and Lotus japonicus (Gifu). Ann. Bot. 128, 45–57. doi: 10.1093/aob/mcab029
Cai, G., Ahmed, M. A., and Gifford, M. (2022). The role of root hairs in water uptake: recent advances and future perspectives. J. Exp. Bot. 73, 3330–3338. doi: 10.1093/jxb/erac114
Cai, G., Carminati, A., Abdalla, M., and Ahmed, M. A. (2021). Soil textures rather than root hairs dominate water uptake and soil–plant hydraulics under drought. Plant Physiol. 187, 858–872. doi: 10.1093/plphys/kiab271
Camacho-B, S. E., Hall, A. E., and Kaufmann, M. R. (1974). Efliciency and regulation of water transport in some Woody and Herbaceous species. Plant Physiol. 54, 169–172. doi: 10.1104/pp.54.2.169
Canales, J., Contreras-Lopez, O., Alvarez, J. M., and Gutierrez, R. A. (2017). Nitrate induction of root hair density is mediated by TGA1/TGA4 and CPC transcription factors in Arabidopsis thaliana. Plant J. 92, 305–316. doi: 10.1111/tpj.13656
Caradus, J. R. (1980). Distinguishing between grass and legume species for efficiency of phosphorus use. N. Z. J. Agric. Res. 23, 75–81. doi: 10.1080/00288233.1980.10417847
Ceasar, S. A., Ramakrishnan, M., Vinod, K. K., Roch, G. V., Upadhyaya, H. D., Baker, A., et al. (2020). Phenotypic responses of foxtail millet (Setaria italica) genotypes to phosphate supply under greenhouse and natural field conditions. Pub. Lib. Sci. One 15:e0233896. doi: 10.1371/journal.pone.0233896
Chandrasekaran, M., Boughattas, S., Hu, S., Oh, S.-H., and Sa, T. (2014). A meta-analysis of arbuscular mycorrhizal effects on plants grown under salt stress. Mycorrhiza 24, 611–625. doi: 10.1007/s00572-014-0582-7
Cheng, S., Zhou, D. X., and Zhao, Y. (2016). WUSCHEL-related homeobox gene WOX11 increases rice drought resistance by controlling root hair formation and root system development. Plant Signal. Behav. 11:e1130198. doi: 10.1080/15592324.2015.1130198
Cui, S., Suzaki, T., Tominaga-Wada, R., and Yoshida, S. (2018). Regulation and functional diversification of root hairs. Semin. Cell Dev. Biol. 83, 115–122. doi: 10.1016/j.semcdb.2017.10.003
Damian, X., Ochoa-Lopez, S., Gaxiola, A., Fornoni, J., Dominguez, C. A., and Boege, K. (2020). Natural selection acting on integrated phenotypes: covariance among functional leaf traits increases plant fitness. New Phytol. 225, 546–557. doi: 10.1111/nph.16116
Datta, S., Kim, C. M., Pernas, M., Pires, N. D., Proust, H., Tam, T., et al. (2011). Root hairs: development, growth and evolution at the plant-soil interface. Plant Soil 346, 1–14. doi: 10.1007/s11104-011-0845-4
Ding, J., Yin, H., Kong, D., Liu, Q., Zhang, Z., Wang, Q., et al. (2023). Precipitation, rather than temperature drives coordination of multidimensional root traits with ectomycorrhizal fungi in alpine coniferous forests. J. Ecol. 111, 1935–1949. doi: 10.1111/1365-2745.14151
Duddek, P., Ahmed, M. A., Javaux, M., Vanderborght, J., Lovric, G., King, A., et al. (2023a). Do roots need a good haircut for water uptake? New Phytol. 240, 2484–2497.
Duddek, P., Ahmed, M. A., Javaux, M., Vanderborght, J., Lovric, G., King, A., et al. (2023b). The effect of root hairs on root water uptake is determined by root–soil contact and root hair shrinkage. New Phytol. 240, 2484–2497. doi: 10.1111/nph.19144
Evans, P. S. (2012). Comparative root morphology of some pasture grasses and clovers. N. Z. J. Agric. Res. 20, 331–335. doi: 10.1080/00288233.1977.10427343
Fan, C., Hou, M., Si, P., Sun, H., Zhang, K., Bai, Z., et al. (2022). Response of root and root hair phenotypes of cotton seedlings under high temperature revealed with RhizoPot. Front. Plant Sci. 13:1007145. doi: 10.3389/fpls.2022.1007145
Fohse, D., Claassen, N., and Jungk, A. (1991). Phosphorus efficiency of plants II. Significance of root radius, root hairs and cation-anion balance for phosphorus influx in seven plant species. Plant Soil 132, 261–272. doi: 10.1007/BF00010407
Foreman, J., and Dolan, L. (2001). Root hairs as a model system for studying plant cell growth. Ann. Bot. 88, 1–7. doi: 10.1006/anbo.2001.1430
Fort, F., Cruz, P., Catrice, O., Delbrut, A., Luzarreta, M., Stroia, C., et al. (2015). Root functional trait syndromes and plasticity drive the ability of grassland Fabaceae to tolerate water and phosphorus shortage. Environ. Exp. Bot. 110, 62–72. doi: 10.1016/j.envexpbot.2014.09.007
Gahoonia, T. S., Care, D., and Nielsen, N. E. (1997). Root hairs and phosphorus acquisition of wheat and barley cultivars. Plant Soil 191, 181–188. doi: 10.1023/A:1004270201418
Gahoonia, T. S., and Nielsen, N. E. (2004). Root traits as tools for creating phosphorus efficient crop varieties. Plant Soil 260, 47–57. doi: 10.1023/b:plso.0000030168.53340.bc
Genc, Y., Huang, C. Y., and Langridge, P. (2007). A study of the role of root morphological traits in growth of barley in zinc-deficient soil. J. Exp. Bot. 58, 2775–2784. doi: 10.1093/jxb/erm142
Gilroy, S., and Jones, D. L. (2000). Through form to function: root hair development and nutrient uptake. Trends Plant Sci. 5, 56–60. doi: 10.1016/S1360-1385(99)01551-4
Golivets, M., Knapp, S., Essl, F., Lenzner, B., Latombe, G., Leung, B., et al. (2024). Future changes in key plant traits across Central Europe vary with biogeographical status, woodiness, and habitat type. Sci. Total Environ. 907:167954. doi: 10.1016/j.scitotenv.2023.167954
Graves, J. H., Peet, R. K., and White, P. S. (2006). The influence of carbon—nutrient balance on herb and woody plant abundance in temperate forest understories. J. Veg. Sci. 17, 217–226. doi: 10.1111/j.1654-1103.2006.tb02440.x
Graves, W. R. (1991). Influence of hydroponic culture method on morphology and hydraulic conductivity of roots of honey locust. Tree Physiol. 11, 205–211. doi: 10.1093/treephys/11.2.205
Greilhuber, J. (2005). Intraspecific variation in genome size in angiosperms: identifying its existence. Ann. Bot. 95, 91–98. doi: 10.1093/aob/mci004
Grytnes, J. A., and Felde, V. A. (2014). Diversity patterns in a diversity hotspot. Appl. Veg. Sci. 17, 381–383. doi: 10.1111/avsc.12109
Gulden, R. H., and Vessey, J. K. (2000). Penicillium bilaii inoculation increases root-hair production in field pea. Can. J. Plant Sci. 80, 801–804. doi: 10.4141/P99-171
Haling, R. E., Brown, L. K., Bengough, A. G., Young, I. M., Hallett, P. D., White, P. J., et al. (2013). Root hairs improve root penetration, root-soil contact, and phosphorus acquisition in soils of different strength. J. Exp. Bot. 64, 3711–3721. doi: 10.1093/jxb/ert200
Haling, R. E., Richardson, A. E., Culvenor, R. A., Lambers, H., and Simpson, R. J. (2010). Root morphology, root-hair development and rhizosheath formation on perennial grass seedlings is influenced by soil acidity. Plant Soil 335, 457–468. doi: 10.1007/s11104-010-0433-z
Hassan, T., Gulzar, R., Hamid, M., Ahmad, R., Waza, S. A., and Khuroo, A. A. (2023). Plant phenology shifts under climate warming: a systematic review of recent scientific literature. Environ. Monit. Assess. 196:36. doi: 10.1007/s10661-023-12190-w
Hoffmann, C., and Jungk, A. (1995). Influence of soil temperature and soil compaction on growth and P uptake of sugar beet. J. Plant Nutr. Soil Sci. 159, 263–270. doi: 10.1002/jpln.1996.3581590307
Hwang, S. F., Ahmed, H. U., Zhou, Q., Strelkov, S. E., Gossen, B. D., Peng, G., et al. (2011). Influence of cultivar resistance and inoculum density on root hair infection of canola (Brassica napus) by Plasmodiophora brassicae. Plant Pathol. 60, 820–829. doi: 10.1111/j.1365-3059.2011.02457.x
Hwang, Y., Choi, H. S., Cho, H. M., and Cho, H. T. (2017). Tracheophytes contain conserved orthologs of a basic helix-loop-helix transcription factor that modulate root hair specific genes. Plant Cell 29, 39–53. doi: 10.1105/tpc.16.00732
Iversen, C. M., and McCormack, M. L. (2021). Filling gaps in our understanding of belowground plant traits across the world: an introduction to a Virtual Issue. New Phytol 231, 2097–2103. doi: 10.1111/nph.17326
Iversen, C. M., McCormack, M. L., Powell, A. S., Blackwood, C. B., Freschet, G. T., Kattge, J., et al. (2017). A global Fine‐Root Ecology Database to address below‐ground challenges in plant ecology. New Phytol 215, 15–26. doi: 10.1111/nph.14486
Jungk, A. (2001). Root hairs and the acquisition of plant nutrients from soil. J. Plant Nutr. Soil Sci. 164, 121–129. doi: 10.1002/1522-2624(200104)164:2<121::AID-JPLN121>3.0.CO;2-6
Karlova, R., Boer, D., Hayes, S., and Testerink, C. (2021). Root plasticity under abiotic stress. Plant Physiol. 187, 1057–1070. doi: 10.1093/plphys/kiab392
Kemp, E., Adam, P., and Ashford, A. E. (2003). Seasonal changes in hair roots and mycorrhizal colonization in Woollsia pungens (Cav.) F. Muell. (Epacridaceae). Plant Soil 250, 241–248. doi: 10.1023/A:1022896014205
Keyes, S. D., Zygalakis, K. C., and Roose, T. (2017). An explicit structural model of root hair and soil interactions parameterised by synchrotron X-ray computed tomography. Bull. Math. Biol. 79, 2785–2813. doi: 10.1007/s11538-017-0350-x
Kidd, D. R., Ryan, M. H., Haling, R. E., Lambers, H., Sandral, G. A., Yang, Z., et al. (2016). Rhizosphere carboxylates and morphological root traits in pasture legumes and grasses. Plant Soil 402, 77–89. doi: 10.1007/s11104-015-2770-4
Kiswara, W., Behnke, N., van Avesaath, P., Huiskes, A. H. L., Erftemeijer, P. L. A., and Bouma, T. J. (2009). Root architecture of six tropical seagrass species, growing in three contrasting habitats in Indonesian waters. Aquat. Bot. 90, 235–245. doi: 10.1016/j.aquabot.2008.10.005
Konno, M., Ooishi, M., and Inoue, Y. (2006). Temporal and positional relationships between Mn uptake and low-pH-induced root hair formation in Lactuca sativa cv. Grand Rapids seedlings. J. Plant Res. 119, 439–447. doi: 10.1007/s10265-006-0006-7
Krasilnikoff, G., Gahoonia, T., and Nielsen, N. E. (2003). Variation in phosphorus uptake efficiency by genotypes of cowpea (Vigna unguiculata) due to differences in root and root hair length and induced rhizosphere processes. Plant Soil 251, 83–91. doi: 10.1023/A:1022934213879
Lazarević, B., LoŠÁk, T., and Manschadi, A. M. (2018). Arbuscular mycorrhizae modify winter wheat root morphology and alleviate phosphorus deficit stress. Plant Soil Environ. 64, 47–52. doi: 10.17221/678/2017-PSE
Libault, M., Brechenmacher, L., Cheng, J., Xu, D., and Stacey, G. (2010). Root hair systems biology. Trends Plant Sci. 15, 641–650. doi: 10.1016/j.tplants.2010.08.010
Lippold, E., Lucas, M., Fahrenkampf, T., Schlüter, S., and Vetterlein, D. (2022). Macroaggregates of loam in sandy soil show little influence on maize growth, due to local adaptations of root architecture to soil heterogeneity. Plant Soil 478, 163–175. doi: 10.1007/s11104-022-05413-5
Lippold, E., Phalempin, M., Schlüter, S., and Vetterlein, D. (2021). Does the lack of root hairs alter root system architecture of Zea mays? Plant Soil 467, 267–286. doi: 10.1007/s11104-021-05084-8
Liu, R., Li, Y., Wang, Y., Ma, J., and Cieraad, E. (2019). Variation of water use efficiency across seasons and years: different role of herbaceous plants in desert ecosystem. Sci. Total Environ. 647, 827–835. doi: 10.1016/j.scitotenv.2018.08.035
Lopez-Bucio, J., Cruz-Ramirez, A., and Herrera-Estrella, L. (2003). The role of nutrient availability in regulating root architecture. Curr. Opin. Plant Biol. 6, 280–287. doi: 10.1016/s1369-5266(03)00035-9
Marin, M., Feeney, D. S., Brown, L. K., Naveed, M., Ruiz, S., Koebernick, N., et al. (2021). Significance of root hairs for plant performance under contrasting field conditions and water deficit. Ann. Bot. 128, 1–16. doi: 10.1093/aob/mcaa181
Matsuo, T., van der Sande, M. T., Amissah, L., Dabo, J., Mohammed Abdul, S., and Poorter, L. (2023). Herbaceous species and dry forest species have more acquisitive leaf traits than woody species and wet forest species. Funct. Ecol. 38, 194–205. doi: 10.1111/1365-2435.14477
Ma, X., Zarebanadkouki, M., Kuzyakov, Y., Blagodatskaya, E., Pausch, J., and Razavi, B. S. (2018). Spatial patterns of enzyme activities in the rhizosphere: effects of root hairs and root radius. Soil Biol. Biochem. 118, 69–78. doi: 10.1016/j.soilbio.2017.12.009
Ma, Z., and Chen, H. Y. H. (2018). Positive species mixture effects on fine root turnover and mortality in natural boreal forests. Soil Biol. Biochem. 121, 130–137. doi: 10.1016/j.soilbio.2018.03.015
Meisner, C. A., and Karnok, K. J. (1991). Root hair occurrence and variation with environment. Agron. J. 83, 814–818. doi: 10.2134/agronj1991.00021962008300050008x
Michael, G. (2001). The control of root hair formation: suggested mechanisms. J. Plant Nutr. Soil Sci. 164, 111–119. doi: 10.1002/1522-2624(200104)164:2<111::Aid-jpln111>3.0.Co;2-a
Monshausen, G. B., Bibikova, T. N., Messerli, M. A., Shi, C., and Gilroy, S. (2007). Oscillations in extracellular pH and reactive oxygen species modulate tip growth of Arabidopsis root hairs. Proc. Natl. Acad. Sci. USA 104, 20996–21001. doi: 10.1073/pnas.0708586104
Morris, E. C., and Myerscough, P. J. (1991). Self-thinning and competition intensity over a gradient of nutrient availability. J. Ecol. 79, 903–923. doi: 10.2307/2261088
Nestler, J., Keyes, S. D., and Wissuwa, M. (2016). Root hair formation in rice (Oryza sativa L.) differs between root types and is altered in artificial growth conditions. J. Exp. Bot. 67, 3699–3708. doi: 10.1093/jxb/erw115
Ohri, D. (2005). Climate and growth form: the consequences for genome size in plants. Plant Biol. 7, 449–458. doi: 10.1055/s-2005-865878
Pacheco, J. M., Gabarain, V. B., Lopez, L. E., Lehuede, T. U., Ocaranza, D., and Estevez, J. M. (2023). Understanding signaling pathways governing the polar development of root hairs in low-temperature, nutrient-deficient environments. Curr. Opin. Plant Biol. 75:102386. doi: 10.1016/j.pbi.2023.102386
Pang, J., Ryan, M. H., Siddique, K. H. M., and Simpson, R. J. (2017). Unwrapping the rhizosheath. Plant Soil 418, 129–139. doi: 10.1007/s11104-017-3358-y
Pérez-Harguindeguy, N., Díaz, S., Garnier, E., Lavorel, S., Poorter, H., Jaureguiberry, P., et al. (2016). Corrigendum to: new handbook for standardised measurement of plant functional traits worldwide. Aust. J. Bot. 64:715. doi: 10.1071/bt12225_co
Pu, Z., Zhang, R., Wang, H., Li, Q., Zhang, J., and Wang, X. X. (2023). Root morphological and physiological traits and arbuscular mycorrhizal fungi shape phosphorus-acquisition strategies of 12 vegetable species. Front. Plant Sci. 14:1150832. doi: 10.3389/fpls.2023.1150832
R Core Team (2021). R: A language and environment for statistical computing Vienna, Austria: R Foundation for Statistical Computing.
Robinson, D., and Rorison, I. H. (1987). Root hairs and plant growth atlow nitrogen availabilities. New Phytol. 107, 681–693. doi: 10.1111/j.1469-8137.1987.tb00906.x
Ruiz, S., Koebernick, N., Duncan, S., Fletcher, D. M., Scotson, C., Boghi, A., et al. (2020). Significance of root hairs at the field scale - modelling root water and phosphorus uptake under different field conditions. Plant Soil 447, 281–304. doi: 10.1007/s11104-019-04308-2
Saengwilai, P., Strock, C., Rangarajan, H., Chimungu, J., Salungyu, J., and Lynch, J. P. (2021). Root hair phenotypes influence nitrogen acquisition in maize. Ann. Bot. 128, 849–858. doi: 10.1093/aob/mcab104
Sandral, G. A., Price, A., Hildebrand, S. M., Fuller, C. G., Haling, R. E., Stefanski, A., et al. (2019). Field benchmarking of the critical external phosphorus requirements of pasture legumes for southern Australia. Crop Pasture Sci. 70:1080. doi: 10.1071/cp19014
Schenk, M. K. (2006). Nutrient efficiency of vegetable crops. Acta Hortic. 700, 21–34. doi: 10.17660/ActaHortic.2006.700.1
Schmidt, J. E., and Gaudin, A. C. M. (2017). Toward an integrated root ideotype for irrigated systems. Trends Plant Sci. 22, 433–443. doi: 10.1016/j.tplants.2017.02.001
Schnall, J. A., and Quatrano, R. S. (1992). Abscisic acid elicits the water-stress response in root hairs of Arabidopsis thaliana. Plant Physiol. 100, 216–218. doi: 10.1104/pp.100.1.216
Schweiger, P. F., Robson, A. D., and Barrow, N. J. (1995). Root hair length determines beneficial effect of a Glomus species on shoot growth of some pasture species. New Phytol. 131, 247–254. doi: 10.1111/j.1469-8137.1995.tb05726.x
Segal, E., Kushnir, T., Mualem, Y., and Shani, U. (2008). Water uptake and hydraulics of the root hair rhizosphere. Vadose Zone J. 7, 1027–1034. doi: 10.2136/vzj2007.0122
Shao, C., Li, Y., Luo, A., Wang, Z., Xi, Z., Liu, J., et al. (2021). Relationship between functional traits and genome size variation of angiosperms with different life forms. Biodivers. Sci. 29, 575–585. doi: 10.17520/biods.2020450
Siddique, K. H. M., Chen, Y. L., and Rengel, Z. (2015). Efficient root system for abiotic stress tolerance in crops. Procedia Environ. Sci. 29:295. doi: 10.1016/j.proenv.2015.07.269
Stéger, A., and Palmgren, M. (2022). Root hair growth from the pH point of view. Front. Plant Sci. 13, 1–12. doi: 10.3389/fpls.2022.949672
Stetter, M. G., Schmid, K., and Ludewig, U. (2015). Uncovering genes and ploidy involved in the high diversity in root hair density, length and response to local scarce phosphate in Arabidopsis thaliana. PLoS One 10:e0120604. doi: 10.1371/journal.pone.0120604
Tatiana, N. B., Tobias, J., Ingo, D., and Simon, G. (1998). Localized changes in apoplastic and cytoplasmic pH are associated with root hair development in Arabidopsis thaliana. Development 125, 2925–2934. doi: 10.1242/dev.125.15.2925
Tawaraya, K. (2003). Arbuscular mycorrhizal dependency of different plant species and cultivars. Soil Sci. Plant Nutr. 49, 655–668. doi: 10.1080/00380768.2003.10410323
Tumber-Dávila, S. J., Schenk, H. J., du, E., and Jackson, R. B. (2022). Plant sizes and shapes above and belowground and their interactions with climate. New Phytol. 235, 1032–1056. doi: 10.1111/nph.18031
Vandamme, E., Renkens, M., Pypers, P., Smolders, E., Vanlauwe, B., and Merckx, R. (2013). Root hairs explain P uptake efficiency of soybean genotypes grown in a P-deficient ferralsol. Plant Soil 369, 269–282. doi: 10.1007/s11104-012-1571-2
van der Waal, C., de Kroon, H., de Boer, W. F., Heitkönig, I. M. A., Skidmore, A. K., de Knegt, H. J., et al. (2009). Water and nutrients alter herbaceous competitive effects on tree seedlings in a semi-arid savanna. J. Ecol. 97, 430–439. doi: 10.1111/j.1365-2745.2009.01498.x
Vatter, T., Neuhauser, B., Stetter, M., and Ludewig, U. (2015). Regulation of length and density of Arabidopsis root hairs by ammonium and nitrate. J. Plant Res. 128, 839–848. doi: 10.1007/s10265-015-0733-8
Vissenberg, K., Claeijs, N., Balcerowicz, D., and Schoenaers, S. (2020). Hormonal regulation of root hair growth and responses to the environment in Arabidopsis. J. Exp. Bot. 71, 2412–2427. doi: 10.1093/jxb/eraa048
Waddell, H. A., Simpson, R. J., Ryan, M. H., Lambers, H., Garden, D. L., and Richardson, A. E. (2016). Root morphology and its contribution to a large root system for phosphorus uptake by Rytidosperma species (wallaby grass). Plant Soil 412, 7–19. doi: 10.1007/s11104-016-2933-y
Wang, H., Wang, Z., and Don, X. (2019). Anatomical structures of fine roots of 91 vascular plant species from four groups in a temperate forest in Northeast China. Public Lib Sci. One 14:e0215126. doi: 10.1371/journal.pone.0215126
Wang, L., Liao, H., Yan, X., Zhuang, B., and Dong, Y. (2004). Genetic variability for root hair traits as related to phosphorus status in soybean. Plant Soil 261, 77–84. doi: 10.1023/B:PLSO.0000035552.94249.6a
Wang, P., Huang, K., and Hu, S. (2020a). Distinct fine-root responses to precipitation changes in herbaceous and woody plants: a meta-analysis. New Phytol. 225, 1491–1499. doi: 10.1111/nph.16266
Wang, T., Dong, L., and Liu, Z. (2023). Climate strengthens the positive effects of stand structure on understory plant diversity in Chinese temperate forests. Forests 14:2138. doi: 10.3390/f14112138
Wang, X., Bi, S., Wang, L., Li, H., Gao, B. A., Huang, S., et al. (2020b). GLABRA2 regulates actin bundling protein VILLIN1 in root hair growth in response to osmotic stress. Plant Physiol. 184, 176–193. doi: 10.1104/pp.20.00480
Wang, Y., Lyu, T., Luo, A., Li, Y., Liu, Y., Freckleton, R. P., et al. (2020c). Spatial patterns and drivers of angiosperm sexual systems in China differ between woody and herbaceous species. Front. Plant Sci. 11, 1–10. doi: 10.3389/fpls.2020.01222
Wickham, H. (2011). ggplot2: elegant graphics for data analysis. J. R. Stat. Soc. Ser. A Stat. Soc. 174, 245–246. doi: 10.1111/j.1467-985X.2010.00676_9.x
Xing, Y., Chen, M., Dao, J., Lin, L., Chen, C., Chen, Y., et al. (2024). Fine-root morphology of woody and herbaceous plants responds differently to altered precipitation: a meta-analysis. For. Ecol. Manag. 552:121570. doi: 10.1016/j.foreco.2023.121570
Xu, C., De Frenne, P., Blondeel, H., De Pauw, K., Landuyt, D., Lorer, E., et al. (2023). Light more than warming impacts understory tree seedling growth in a temperate deciduous forest. For. Ecol. Manag. 549:121496. doi: 10.1016/j.foreco.2023.121496
Xu, L., Wang, S., Liu, Y., Ji, L., Shen, F., and Yang, L. (2021). Responses of mycorrhizal colonization to nitrogen and phosphorus addition in fourteen woody and herbaceous species: the roles of hypodermal passage cells and penetration points. Plant Soil 469, 273–285. doi: 10.1007/s11104-021-05107-4
Yang, Z., Culvenor, R. A., Haling, R. E., Stefanski, A., Ryan, M. H., Sandral, G. A., et al. (2017). Variation in root traits associated with nutrient foraging among temperate pasture legumes and grasses. Grass Forage Sci. 72, 93–103. doi: 10.1111/gfs.12199
Yuan, Y., Liu, Y. J., Huang, L. Q., Cui, G. H., and Fu, G. F. (2009). Soil acidity elevates some phytohormone and β -eudesmol contents in roots of Atractylodes lancea. Russ. J. Plant Physiol. 56, 133–137. doi: 10.1134/S1021443709010191
Yu, P., White, P., Hochholdinger, F., and Li, C. J. (2014). Phenotypic plasticity of the maize root system in response to heterogeneous nitrogen availability. Planta 240, 667–678. doi: 10.1007/s00425-014-2150-y
Zangaro, W., Nishidate, F. R., Camargo, F. R. S., Romagnoli, G. G., and Vandressen, J. (2005). Relationships among arbuscular mycorrhizas, root morphology and seedling growth of tropical native woody species in southern Brazil. J. Trop. Ecol. 21, 529–540. doi: 10.1017/s0266467405002555
Zhang, X., Bilyera, N., Fan, L., Duddek, P., Ahmed, M. A., Carminati, A., et al. (2023a). The spatial distribution of rhizosphere microbial activities under drought: water availability is more important than root-hair-controlled exudation. New Phytol. 237, 780–792. doi: 10.1111/nph.18409
Zhang, X., Kuzyakov, Y., Zang, H., Dippold, M. A., Shi, L., Spielvogel, S., et al. (2020a). Rhizosphere hotspots: root hairs and warming control microbial efficiency, carbon utilization and energy production. Soil Biol. Biochem. 148:107872. doi: 10.1016/j.soilbio.2020.107872
Zhang, X., Mi, Y., Mao, H., Liu, S., Chen, L., and Qin, F. (2020b). Genetic variation in ZmTIP1 contributes to root hair elongation and drought tolerance in maize. Plant Biotechnol. J. 18, 1271–1283. doi: 10.1111/pbi.13290
Zhang, Y., Yang, Z., Zhang, Z., Li, Y., Guo, J., Liu, L., et al. (2023b). Root hair development and adaptation to abiotic sress. J. Agric. Food Chem. 71, 9573–9598. doi: 10.1021/acs.jafc.2c07741
Zhou, M., Wang, J., Bai, W., Zhang, Y., Zhang, W. H., and Weiser, M. (2019). The response of root traits to precipitation change of herbaceous species in temperate steppes. Funct. Ecol. 33, 2030–2041. doi: 10.1111/1365-2435.13420
Zhu, L., Liu, L., Sun, H., Zhang, Y., Liu, X., Wang, N., et al. (2020). The responses of lateral roots and root hairs to nitrogen stress in cotton based on daily root measurements. J. Agron. Crop Sci. 208, 89–105. doi: 10.1111/jac.12525
Zou, Y. N., Wang, P., Liu, C. Y., Ni, Q. D., Zhang, D. J., and Wu, Q. S. (2017). Mycorrhizal trifoliate orange has greater root adaptation of morphology and phytohormones in response to drought stress. Sci. Rep. 7:41134. doi: 10.1038/srep41134
Zu, K., Lenoir, J., Fang, J., Tang, Z., Shen, Z., Ji, C., et al. (2023). Elevational shift in seed plant distributions in China's mountains over the last 70 years. Glob. Ecol. Biogeogr. 32, 1098–1112. doi: 10.1111/geb.13692
Keywords: climate change, life form, nitrogen, root hair morphology, soil nutrient, water
Citation: Zhou Y, Wang C, Tang Q, Wang J and Li M-H (2024) Morphological responses of root hairs to changes in soil and climate depend on plant life form. Front. For. Glob. Change. 7:1324405. doi: 10.3389/ffgc.2024.1324405
Edited by:
Liang Kou, Institute of Geographic Sciences and Natural Resources Research, Chinese Academy of Sciences (CAS), ChinaReviewed by:
Qing-Wei Wang, Institute of Applied Ecology, Chinese Academy of Sciences (CAS), ChinaLingyan Zhou, East China Normal University, China
Copyright © 2024 Zhou, Wang, Tang, Wang and Li. This is an open-access article distributed under the terms of the Creative Commons Attribution License (CC BY). The use, distribution or reproduction in other forums is permitted, provided the original author(s) and the copyright owner(s) are credited and that the original publication in this journal is cited, in accordance with accepted academic practice. No use, distribution or reproduction is permitted which does not comply with these terms.
*Correspondence: Cunguo Wang, wangcg505@nenu.edu.cn