- 1Instituto de Pesquisa Ambiental da Amazônia, Brasília, DF, Brazil
- 2Programa de Pós-graduação em Biodiversidade e Conservação, Universidade Federal do Maranhão, São Luís, Maranhão, Brazil
- 3Department of Geography, School of Environment Education and Development, The University of Manchester, Manchester, United Kingdom
- 4BeZero Carbon, London, United Kingdom
- 5Departamento de Biologia, Facultad de Ciencias, Universidad Nacional de Colombia, Bogotá, DC, Colombia
- 6Programa de Pós-graduação em Geografia, Natureza e Dinâmica do Espaço, Universidade Estadual do Maranhão, São Luís, Maranhão, Brazil
- 7Faculty of Environment, Science and Economy, The University of Exeter, Exeter, United Kingdom
Editorial on the Research Topic
Remote sensing of environmental changes in the Neotropical region
Remote sensing is a crucial tool for monitoring environmental changes in large-scale (Wulder et al., 2022). It allows the acquisition of geospatial data in a comprehensive way. Using sensors on orbital and non-orbital platforms makes it possible to observe and analyze changes on the Earth's surface, such as vegetation cover, land use, surface temperature, and other environmental conditions. Remote sensing plays a fundamental role in understanding environmental changes, allowing a wide range of assessments, from monitoring human activities such as deforestation to quantifying the negative impacts of climate change (Hansen et al., 2013; Vancutsem et al., 2021). Furthermore, it assists in the sustainable management of natural resources and in determining the best strategies for responding to disasters. Therefore, remote sensing is crucial for monitoring regions such as the Neotropics, where the rate of change in land use and land cover is increasing rapidly.
The Neotropical region encompasses an expansive biogeographic area that stretches from Central America to South America, including the Caribbean islands. This region has a significant range of climates, soils, and topographies, leading to a remarkable diversity of ecosystems. The Neotropics are home to the world's largest rainforest, the Amazon, and the most biodiverse savanna, the Brazilian Cerrado. Nonetheless, human activities have led to adverse environmental changes. Deforestation, degradation, and climate change are pushing the region's ecosystems into a cycle of loss of carbon stocks and biodiversity (Barlow et al., 2016; Silva-Junior et al., 2021; Lapola et al., 2023).
Using the Web of Science database, we searched with the terms “Global Change” and “Neotropical Region or Neotropics” to identify articles published (Figure 1). The database search showed that between 1991 and 2022, 1,216 published articles were found. Between 1991 and 2022, the number of articles published grew at ~26 documents per year, or 4% per year. Brazilian institutions were involved in about 46% of all articles published between 1991 and 2022, followed by Mexico (10%) and Argentina (7%), all countries in the Neotropics. Across the same period, countries from the Global North were involved in ~50% of all articles published. The United States (34%) led the rank, followed by England (9%) and Spain (7%).
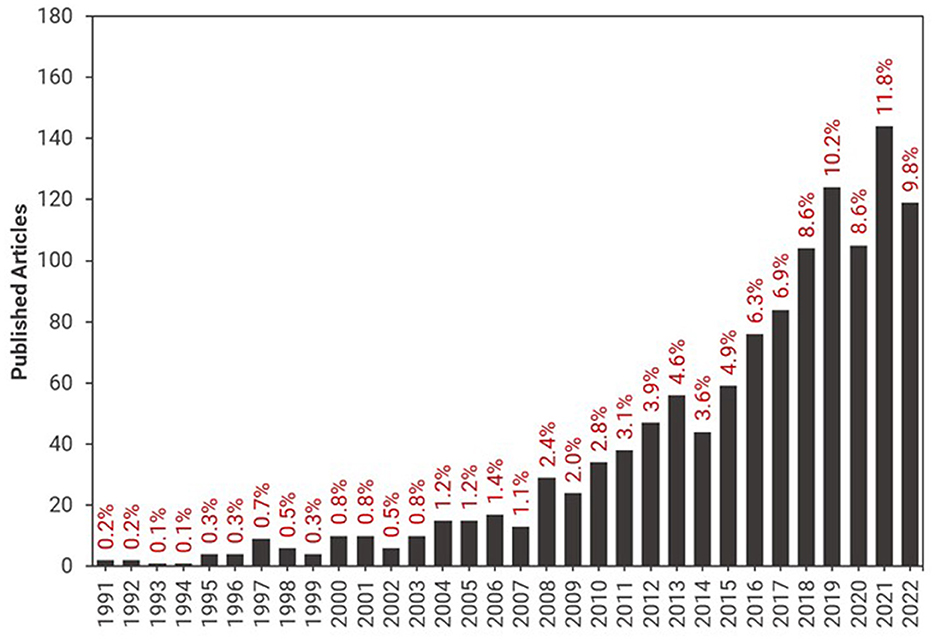
Figure 1. Yearly published articles in the 1991–2022 period of search with the terms “Global Change” and “Neotropical Region or Neotropics” in the Web of Science Core Collection database. We highlight that the search terms used here identified only a tiny proportion of the relevant articles, as many may have used different terms.
The Research Topic “Remote sensing of environmental changes in the Neotropical region” showed the importance of integrating Earth observation with field-collected datasets. We also highlight that the Research Topic encouraged scientists from the Global South to publish in a high-impact international journal. All seven articles published in the Research Topic collection had the participation of institutions from the Global South; six of them were led by authors affiliated with institutions from this region.
In Jalisco (Mexico), Wheeler et al. address human-induced forest degradation, an important yet overlooked issue in tropical forests. This degradation is defined as a harmful change in forest conditions, affecting factors such as carbon storage and biodiversity, either temporarily or over the long term. Considering the challenges of detecting degradation caused by activities like removing small trees for firewood or clearing the understory for agricultural purposes, which are not easily discernible through Earth observation dataset analyses, the authors have devised a novel forest plot protocol specifically designed for monitoring forest degradation. This innovative method enables establishing and measuring numerous plots across a landscape. In a demonstration network of plots based and measured using this proposed method in Jalisco, the authors successfully identified degradation, specifically the loss of above-ground biomass exceeding 10%. The researchers concluded that this protocol holds significant potential for supporting networks of forest plots dedicated to degradation monitoring, whether used in conjunction with Earth observation analyses or independently.
In Madre de Dios (Peru), Montoya-Zumaeta et al. tackled an emerging topic: the effectiveness of REDD+ projects (Reducing Emissions from Deforestation and Forest Degradation). The authors analyzed a REDD+ project implemented in Brazil nut concessions in the southeastern Peruvian Amazon. They employed a before-after control-intervention study design to achieve this goal, combining remote sensing data with household surveys. Their study aimed to assess two key aspects: (1) the impacts of the project between 2012 and 2018 on deforestation, forest degradation, and participant wellbeing and (2) the potential complementary effects of on-the-ground law enforcement inspections on the project's outcomes. The authors discovered that the REDD+ initiative had no discernible effect on deforestation and forest degradation. However, they did confirm the restraining impact of field inspection measures on forest loss. They argue that the lack of significant REDD+ effects may be attributed to delays in the disbursement of cash incentives to concessionaires involved in the project, insufficient provision of benefits to participants, and limited enforcement of project conditionalities. Most participants reported a diminished subjective sense of wellbeing, possibly reflecting dashed expectations associated with project implementation.
Continuing the discussion on REDD+, Andoh et al. assessed the primary drivers of deforestation and forest degradation in 29 countries participating in REDD+ and the Forest Carbon Partnership Facility (15 countries). They aimed to inform policymakers and donors about the importance of investing in REDD+ and the necessity for participating countries to allocate funds to crucial activities for the effective and efficient implementation of REDD+. The authors discovered that agricultural expansion was the leading cause of deforestation, while logging was the primary driver of forest degradation in most analyzed countries. They noted that out of the 15 countries surveyed, more than US$702 million was allocated for REDD+ preparedness activities, whereas the remaining countries received < US$10 million for similar activities. Furthermore, they emphasized a significant disparity in funding between sub-Saharan African countries and their counterparts in Asia and Latin America, notably Indonesia and Peru, respectively. These countries allocated relatively modest amounts to capacity building and training. In conclusion, they argue that fund donors should prioritize strengthening institutional capacities and establishing effective forest governance structures in participating REDD+ countries. Additionally, they should facilitate collaborative platforms between donors and participating countries to address the primary drivers of deforestation and forest degradation.
Given the significant role of fire in causing forest degradation and contributing to greenhouse gas emissions in the Amazon, Ferreira et al. investigated the primary factors influencing fire occurrence and its changing suitability in the MAP region (a transnational area shared by Brazil, Peru, and Bolivia). The researchers employed the MaxEnt (Maximum Entropy) model in conjunction with satellite products, including active fires, climate variables, and land use and cover mapping for 2005, 2010, 2016, and 2020. Their findings revealed that, on average, more than 38% of the study region exhibited conditions suitable for fire occurrence during the study period. Notably, most fire-prone areas, comprising 74%, were located within Brazil, specifically in the State of Acre. The authors also emphasized that highly fragmented landscapes combined with extreme climatic conditions (such as severe droughts) led to an increasing vulnerability to fire occurrence in the southwestern Amazon. In conclusion, the authors suggest that their proposed approach, which combines the MaxEnt model with Earth observation data, can be a valuable tool for predicting locations suitable for fire occurrence and, consequently, help mitigate negative regional impacts.
To address climate change effectively, it is crucial to comprehend the various methods used to estimate atmospheric carbon emissions. In the Brazilian Amazon, Tejada et al. conducted a comparison of two approaches for estimating the Brazilian Amazon carbon budget: the top-down approach (which combines atmospheric carbon measurements with atmospheric transport information) and the bottom-up approach (based on land use and land cover change data—LULC—derived from remote sensing methods). The authors integrated LULC datasets with emission and removal factors to calculate the bottom-up carbon balance. Then, they compared these results with top-down carbon balance estimates based on atmospheric measurements from 2010 to 2018. Their analysis revealed that, in the bottom-up approach, deforestation and forest degradation were the primary contributors to CO2 emissions, accounting for 58% (4.3 Pg) and 37% (2.7 Pg), respectively. Mature forests exhibited absorption of −5.9 Pg CO2 (representing 79% of the total absorbed), while secondary forests absorbed −1.2 Pg CO2 (constituting 17% of the total absorbed). However, the authors demonstrated that emissions exceeded removals during 2010–2018 when employing the top-down approach. Both approaches estimated the highest CO2 emissions in 2016—an extreme drought year in the region. In conclusion, the authors emphasize that a comprehensive understanding of these different approaches is essential for their improvement and for enhancing our comprehension of the carbon cycle in the Brazilian Amazon.
Also in the Brazilian Amazon, Souza et al. propose a new approach to characterize and measure regional land cover dynamics. The researchers used a combination of compositional abundance data from Landsat subpixel information—including Green Vegetation (GV), Non-Photosynthetic Vegetation, Soil, and Shade—and the application of the machine learning algorithm random forest. Ten land use land cover classes were mapped: Forest, Flooded Forest, Shrubland, Natural Grassland, Pastureland, Cropland, Outcrop, Bare and Impervious, Wetland, and Water. In 2022, the authors found that Forest covered 78.6% (331.9 Mha) of the Amazon biome, with 1.4% of secondary regrowth over 5 years old. Total herbaceous covered 15.6% of the area, with the majority of Pastureland (13.5%) and the remainder Natural Grassland. Water was the third largest Land Cover class with 2.4%, followed by Cropland (1.2%) and Shrubland (0.4%). The maps showed 89% overall accuracy. Most forest change has been driven by the conversion of pasture and agricultural land, and there are signs that climate change is the primary driver of the loss of aquatic ecosystems.
Transitioning from the Amazon to the Yucatan region in Mexico, Cinco-Castro et al. assessed carbon stocks in mangroves with different ecological types. To accomplish this, the authors followed the guidelines outlined in the IPCC (Intergovernmental Panel on Climate Change) Good Practices Supplement for wetlands and utilized standardized methods commonly employed in mangrove studies worldwide. Using these methods, they estimated the total carbon stock and examined whether carbon storage varied among the different ecological types of mangroves within the northern Ria Celestun Biosphere Reserve. The study revealed that mangroves associated with freshwater springs, referred to as “petenes” or hammock mangroves, exhibited a higher total ecosystem carbon stock (683.7 Mg C ha-1) in comparison to fringe, basin, and dwarf mangrove ecological types (429, 385, and 214 Mg C ha-1, respectively). Additionally, there were variations in both above-ground and below-ground carbon stocks among the different mangrove ecological types—the observed differences in carbon stock were linked to environmental hydrological factors, including flooding levels, interstitial water salinity, and redox potential. In conclusion, the authors argue that these findings can serve as a valuable tool for developing specific management strategies at regional or local scales to promote the restoration and conservation of mangrove ecosystems.
We conclude this Editorial by highlighting the critical role of scientists and institutions from the Global South in advancing Science in this region. Moreover, we acknowledge that ground-based collection of environmental and social data is irreplaceable in enhancing our comprehension of information acquired from space through remote sensing approaches. As we move forward, let us champion global cooperation and equitable access to data, fostering a collective effort toward a more sustainable and informed world.
Author contributions
CS-J: Writing – original draft. CS: Writing – review & editing. SA: Writing – review & editing. TR: Writing – review & editing. PB: Writing – review & editing.
Funding
The author(s) declare that no financial support was received for the research, authorship, and/or publication of this article.
Acknowledgments
The editors thank all contributors and referees for this Research Topic.
Conflict of interest
CS was employed by BeZero Carbon.
The remaining authors declare that the research was conducted in the absence of any commercial or financial relationships that could be construed as a potential conflict of interest.
Publisher's note
All claims expressed in this article are solely those of the authors and do not necessarily represent those of their affiliated organizations, or those of the publisher, the editors and the reviewers. Any product that may be evaluated in this article, or claim that may be made by its manufacturer, is not guaranteed or endorsed by the publisher.
References
Barlow, J., Lennox, G. D., Ferreira, J., Berenguer, E., Lees, A. C., Nally, R., et al. (2016). Anthropogenic disturbance in tropical forests can double biodiversity loss from deforestation. Nature 535, 144–147. doi: 10.1038/nature18326
Hansen, M. C., Potapov, P. V., Moore, R., Hancher, M., Turubanova, S. A., Tyukavina, A., et al. (2013). High-resolution global maps of 21st-century forest cover change. Science 342, 850–853. doi: 10.1126/science.1244693
Lapola, D. M., Pinho, P., Barlow, J., Aragão, L. E. O. C., Berenguer, E., Carmenta, R., et al. (2023). The drivers and impacts of Amazon forest degradation. Science 379, eabp8622. doi: 10.1126/science.abp8622
Silva-Junior, C. H. L., Carvalho, N. S., Pessôa, A. C. M., Reis, J. B. C., Pontes-Lopes, A., Doblas, J., et al. (2021). Amazonian forest degradation must be incorporated into the COP26 agenda. Nat. Geosci. 14, 634–635. doi: 10.1038/s41561-021-00823-z
Vancutsem, C., Achard, F., Pekel, J.-F., Vieilledent, G., Carboni, S., Simonetti, D., et al. (2021). Long-term (1990–2019) monitoring of forest cover changes in the humid tropics. Sci. Adv. 7, eabe1603. doi: 10.1126/sciadv.abe1603
Keywords: remote sensing, socio-environmental changes, REDD+, forest degradation and deforestation, ecosystem restoration, climate change
Citation: Silva-Junior CHL, Silva C, Alvarado ST, Rosan TM and Bispo PC (2023) Editorial: Remote sensing of environmental changes in the Neotropical region. Front. For. Glob. Change 6:1330489. doi: 10.3389/ffgc.2023.1330489
Received: 30 October 2023; Accepted: 27 November 2023;
Published: 07 December 2023.
Edited and reviewed by: John Robert Healey, Bangor University, United Kingdom
Copyright © 2023 Silva-Junior, Silva, Alvarado, Rosan and Bispo. This is an open-access article distributed under the terms of the Creative Commons Attribution License (CC BY). The use, distribution or reproduction in other forums is permitted, provided the original author(s) and the copyright owner(s) are credited and that the original publication in this journal is cited, in accordance with accepted academic practice. No use, distribution or reproduction is permitted which does not comply with these terms.
*Correspondence: Celso H. L. Silva-Junior, celsohlsj@gmail.com
†These authors have contributed equally to this work