- 1Department of Biological, Geological, and Environmental Sciences, Cleveland State University, Cleveland, OH, United States
- 2Department of Environmental Sciences, Federal University of São Carlos, São Carlos, Brazil
- 3Department of Botany and Forest Habitats, Faculty of Forestry and Wood Technology, Poznań University of Life Sciences, Poznań, Poland
- 4Mechanical Engineering Department, Cleveland State University, Cleveland, OH, United States
Knowledge of the processes and impacts associated with the canopy’s partitioning of rainfall into stemflow (water that drains to the base of tree stems) and throughfall (water that drips through gaps and from canopy surfaces) has expanded in recent years. However, the effect of canopy interactions on the fundamental physical properties of rainwater as it travels through the canopy to the soil, particularly surface tension, remains understudied. To discuss specific hypotheses within this context and their relevance to ecohydrological theory, the surface tension of rainwater samples was examined directly. Over a period of 9 months, open rainwater, throughfall and stemflow samples were collected during 20 storms from 12 study trees located in Secrest Arboretum (about 2.5 km outside Wooster, Ohio). Study trees were selected to highlight a range of canopy characteristics, with each tree being from a unique deciduous species. Surface tension was measured using pendant drop goniometry, and measurements were analyzed for variation across study trees and correlation with event air temperature and rain intensity. In general, surface tension was reduced in throughfall and stemflow compared to measurements made for event rainwater, with median surface tension changes of −0.446 mN m−1 and −0.595 mN m−1 for throughfall and stemflow, respectively. The extent of this reduction varied among study trees (with changes as great as −6.5 to −5.5 mN m−1), and storm event characteristics were directly and indirectly correlated with surface tension changes in select cases. Hypothetically, a number of mechanisms may account for the observed reduction (and variation in this reduction) in surface tension, including differences in tree surface properties, canopy microenvironments, and microbiomes, and each warrant further research. Testing these hypotheses may advance broader ecohydrological theory as surface tension changes will influence wetting, absorption, and solute exchange processes within the canopy which, in turn, may affect related surface processes.
1 Introduction
Rainfall over forested landscapes must pass through tree canopies before reaching the ground. With a combined leaf and bark surface area estimated to exceed one billion km2 globally (Vorholt, 2012; Van Stan et al., 2020), these surfaces and their deposited materials significantly alter rainwater, thereby influencing related hydrological (Savenije, 2004, 2018), ecological (Aubrey, 2020; Mendieta-Leiva et al., 2020), and biogeochemical processes (Ponette-González et al., 2020; Qualls, 2020; Stubbins et al., 2020). Canopy-rainfall interactions also provide ecosystem services, such as runoff reduction in urban areas due to the evaporation of rainfall, resulting in financial benefits at both city and watershed scales (Nowak et al., 2020). As rain passes through plant canopies, it is partitioned into three pathways. Some is evaporated by the canopy through a process called interception, which influences regional precipitation patterns (van der Ent et al., 2014) and regional-to-global energy balances (Davies-Barnard et al., 2014). The remaining rainfall either falls through gaps and drips from the canopy (as throughfall) or drains down stems (as stemflow), creating heterogenous spatiotemporal patterns of net precipitation (Van Stan et al., 2021). As rainwater drains through the canopy, it will exchange nutrients or become enriched in pollutants (Parker, 1983; Klučiarová et al., 2008) and abiotic particles (Le Mellec et al., 2010), or supply the canopy floor with biota such as bacteria (Bittar et al., 2018), fungi (Magyar et al., 2021), and metazoans (Ptatscheck et al., 2018; Guidone et al., 2021).
Interest in and knowledge of about throughfall and stemflow have grown in recent years, particularly concerning their magnitudes and patterns (Magliano et al., 2019; Sadeghi et al., 2020; Van Stan and Friesen, 2020), their ecosystem manifestations at various scales (Whitworth-Hulse et al., 2021; Yue et al., 2021), and their contents (Ponette-González et al., 2020). Despite these advances, knowledge of the underlying physical properties of these flows remains elusive. Examining these physical properties is important, as current theory suggests that they can govern how throughfall and stemflow interact with tree surfaces and the litter and soils they eventually permeate (Zhang et al., 2022 and references therein). Hypothetically, this may affect associated ecological functions, like nutrient leaching from the canopy to rainwater (Levia and Herwitz, 2000), or soil and litter biogeochemical functions reliant on moisture retention (Bachmann and van der Ploeg, 2002; Kim et al., 2023).
Though some research has cast light on the optical properties of throughfall and stemflow (Stubbins et al., 2020), other physical characteristics are usually discussed as side notes or in an otherwise qualitative manner. Instances include Singh’s et al. (2014) mention of “sticky, resin-like, material” in springtime throughfall, and Levia and Herwitz’s (2000) hypothesis that “kinematic viscosity and surface tension of (winter) intercepted precipitation” affects stemflow chemistry by “increasing the contact time of meltwater with the bark tissue.” In other instances, physical properties of intercepted rainwater were indirectly investigated, such as studies that report influences of temperature on rainwater retention for leaves (Klamerus-Iwan and Błońska, 2018). This study revealed that increases in rainfall temperature decreased droplet contact angle on leaves, increasing their canopy storage capacity by 0.3–0.5 g-water per g-leaf dry weight for every 1°C increase. In each of these examples, the discussed alterations are related to a key physical property of water: surface tension.
Surface tension is the underlying force that binds water droplets together, working at the air-water boundary to limit the liquid’s surface area. Internally, molecules within a droplet experience equal intermolecular forces from all surrounding directions. However, those at the droplet’s periphery must interface with the air and, as a result, only experience intermolecular forces from their neighbors (and those deeper inside the droplet). The “absence” of these forces on the air-exposed side creates a consistent inward tug on surface molecules, manifesting as surface tension (Riba and Esteban, 2014). Ultimately, a droplet’s form is governed by an interplay of forces, inclusive of surface tension, but also considering factors like inertia, viscosity, viscoelasticity, and electrical forces (Zhang et al., 1994). The presence of resin-like materials in springtime throughfall (Singh et al., 2014) could alter the chemical composition and consequently the surface tension of the water. A modified surface tension can lead to the noted “stickiness.” In winter, decreasing temperatures can reduce the kinetic energy of water molecules in stemflow rivulets, leading to stronger hydrogen bonds and increased cohesion (i.e., greater surface tension), which may slow droplet movement, amplifying contact time and influencing stemflow chemistry (per Levia and Herwitz, 2000). Conversely, increasing the temperature of intercepted waters may decrease surface tension, altering the shape of droplets such that contact angle diminishes, increasing a leaf’s ability to retain water (Klamerus-Iwan and Błońska, 2018). In each of these cases, subtle alterations in surface tension, influenced by environmental factors or the droplet’s constituents, have profound implications for how water interacts with forest canopies.
Here, we present a discussion of theory and hypotheses regarding the possible mechanisms that may alter the surface tension of rainfall as it travels through the canopy to become throughfall and stemflow. Although investigations of the physical interactions of purified water droplets with the surfaces of leaves and bark have been made (Konrad et al., 2012; Holder, 2020; Tonello et al., 2021), no observations known to the authors have been reported on the surface tension of throughfall and stemflow water itself. Thus, to inform this discussion, we report observations of rainfall, throughfall, and stemflow surface tension using the pendant drop method for 12 trees of different canopy traits from a site in Northeastern Ohio (United States).
2 Methods and materials
The study was conducted using isolated urban tree canopies at the Secrest Arboretum in Wooster, OH (United States) in their “shade plot” (40°46′41.9″N 81°55′06.2″W, 311 m a.s.l.). The shade plot is ~2.5 km southeast of downtown Wooster and the surrounding area consists of developed landscapes. A four-lane state highway is ~1 km to the north of the shade plot, and a two-lane state highway is ~0.25 km to the east. Apart from the city, most surrounding land is agricultural, with croplands, orchards, and dairy farms accounting for approximately 70% of the land area in the county for which Wooster is the central, county seat (USGS GAP/LANDFIRE). The climate is considered hot-summer humid continental (Köppen Dfa) and the area has a mean annual temperature and precipitation of 10.6°C and 1,750 mm y−1, respectively (The Ohio State University, 2023). Precipitation is relatively evenly distributed throughout the year, with 990 mm y−1 being rainfall and 760 mm y−1 being snowfall (primarily occurring December through April). The highest and lowest mean monthly temperatures are 22.9°C in July and − 3°C in January, respectively. Shade trees of species commonly used in urban forests were planted on a grassy field with moderately well-drained soils classified as Wooster-Riddles silt loams: WuB with 2%–6% slope and WuC with 6%–12% slope (The Natural Resources Conservation Service, 2023). Study trees were selected to represent 12 deciduous species commonly found in urban forests with visually contrasting bark and branching structures (summarized in Table 1).
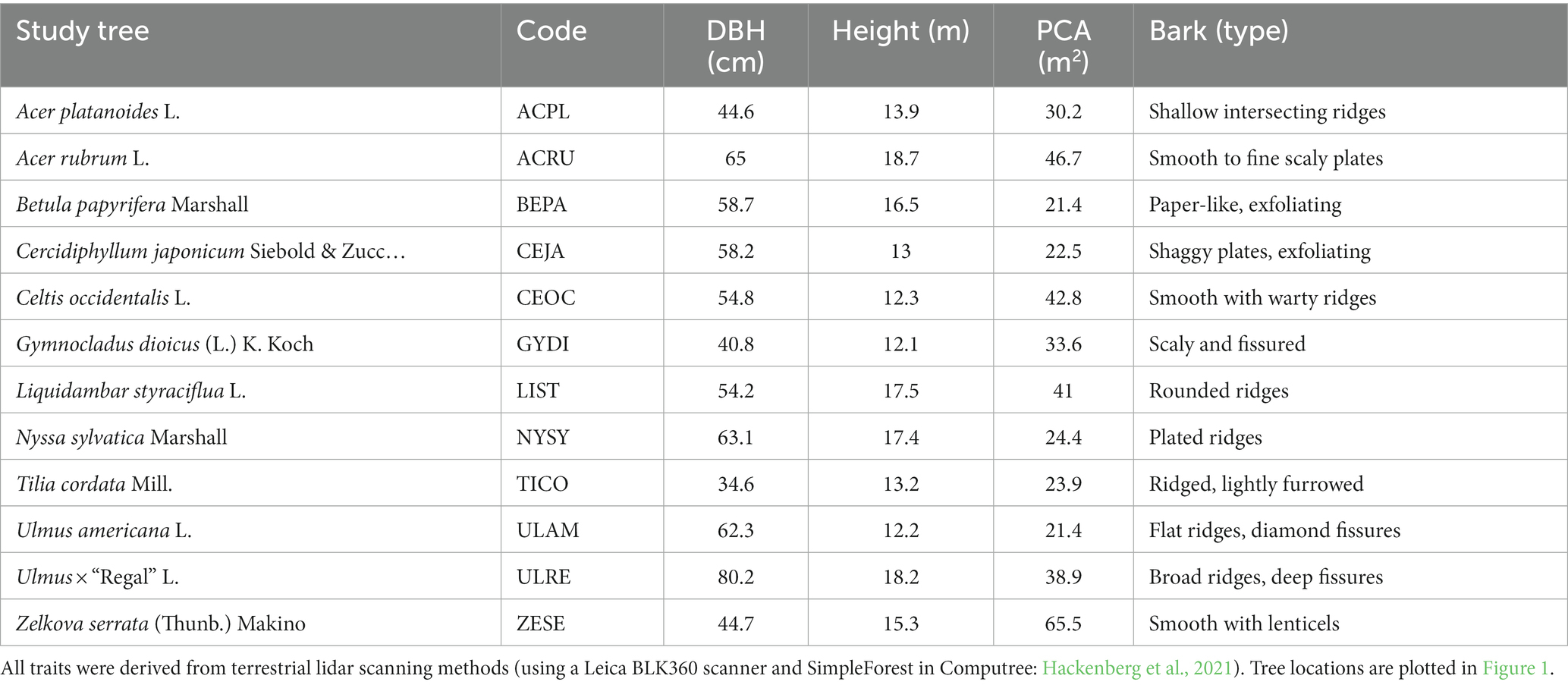
Table 1. Study tree species, the code used in other plots, their stem diameter at breast height (DBH), tree height, projected crown area (PCA), and a brief qualitative description of their stem bark.
Three rainfall samplers were deployed in a nearby clearing, immediately adjacent to the Secrest Aboretum shade plot. Each tree was outfitted with three throughfall samplers and a single stemflow sampler. Rainfall and throughfall samplers were constructed similarly, using a trough method. Troughs consisted of two white schedule 40 PVC pipes with outer and inner diameters of 60.3 mm and 52.0 mm, respectively, that were angled toward a central drainage point. For throughfall troughs, the pipe lengths were cut to exactly the canopy radius dimensions of each study tree. For rain troughs, each pipe length was 3.048 m. Pipes were supported above the ground by electrical conduits and metal hardware.
To capture rain or throughfall, slits were made in the pipe using an oscillating tool (M18 FUEL Multi-Tool, Milwaukee, WI, United States) and the troughs were positioned at a 15-degree angle to facilitate drainage. At the low point, where the troughs came together, a PVC fitting was placed over the pipe end which had a hose barb, allowing the connection of a flexible vinyl tube that transferred rainfall or throughfall to an HDPE reservoir for sampling.
For each study tree, the throughfall trough system included three of these troughs deployed in an orientation to collect throughfall from a similar fraction of the total projected canopy area. The troughs and slits were arranged so that every meter along the canopy radii was sampled by three consecutive slits. Slit width varied with distance from the trunk to ensure proportional canopy area sampling. For example, slits between 4 and 5 meters from the trunk captured 432.7 cm2, which is 35.9% of the total collection area (1,202 cm2) for one trough system. This proportion was maintained regardless of tree size or canopy area. Thus, collection areas for each trough system (per direction) were as follows: 432.7 cm2 from 4–5 m, 336.6 cm2 from 3–4 m, 240.4 cm2 from 2–3 m, 144.2 cm2 from 1–2 m, and 48.1 cm2 from 0–1 m away from the stem. For instance, a tree with three 5 m long collection systems had a total throughfall collection area of 3,606 cm2 beneath a canopy area of ~78.5 m2 (i.e., the trough system is sampling from 0.46% of the projected canopy area), while a tree with three 4 m long collection systems had a total throughfall collection area of 2,308 cm2 beneath a canopy area of ~50.3 m2 (which is also equal to 0.46% of that tree’s canopy area). Photograph of trough provided (inset, Figure 1C).
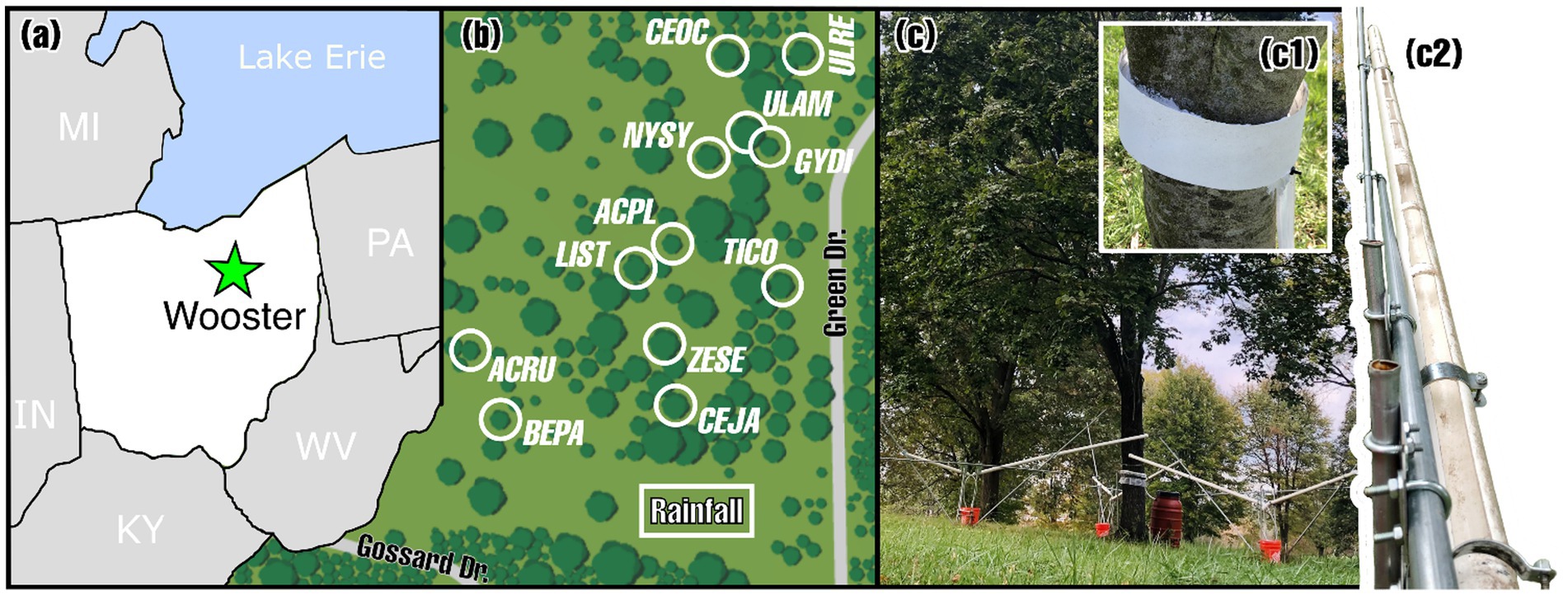
Figure 1. (a) Location of Wooster (OH, United States) where the (b) Secrest Arboretum’s shade tree plot is located. The location of the rainfall sampling plot and the 12 study trees (abbreviations related to Latin species names in Table 1). (c) An example of the throughfall and stemflow water sampling collection devices with insets (c1) of stemflow collar and (c2) of throughfall trough (photos: John T. Van Stan).
Each study tree was outfitted with a stemflow collector per the design of Mabrouk et al. (2022) consisting of three components: (1) a collar to act as a gutter that captures the stemflow water draining down the outside of the stem bark; (2) tubing to direct captured stemflow from the collar to the third component; (3) a collection bin from which stemflow may be sampled. The base of each stemflow collar was constructed from adhesive-backed expandable waterproofed foam insulation tape: 3.8 cm × 3.8 cm Platinum Expandable Foam Weatherseal (M-D Building Products, Inc., Oklahoma City, OK, United States). Before installation, the foam tape was unpackaged and allowed to expand to its full width and height. Sections of the foam tape were cut to lengths slightly smaller than each study tree’s circumference, permitting the collar base to be installed at a moderate slope around the stem and leaving a small opening at the lowest point for a 2.54 cm (internal diameter, 3.18 cm outer diameter) flexible silicone tube. To guide the collar placement, the silicone tubing was zip-tied to the tree stem at a location convenient for placing a collection bin. To prevent stemflow water from flowing off the side edges of the foam tape, it was wrapped with flexible 8 mm thick plastic sheets (Arrow Home Products Co., Elk Grove Village, IL, United States). Silicon sealant was applied to all connection points (bark-to-foam tape and foam tape-to-plastic sheeting) and the top of the foam tape. The entire process was noninvasive. The tubing was connected to a 220 L HDPE storage bin with a screw-on lid (mirainbarrel, Taylor, MI, United States). Collar photo included in Figure 1C reproduced from Mabrouk et al. (2022). The collars, tubing, and collection bins were inspected weekly and repaired if needed.
All rainfall, throughfall, and stemflow water samples were collected 24–48 h after the end of a discrete storm event. A discrete storm event was defined as any rainfall exceeding 2 mm after an antecedent dry period longer than 12 h. In the field, 50 mL of each sample were immediately filtered through a 0.45 μm syringe filter (25 mm PES filters, Biomed Scientific, China) into sterile 50 mL capacity HDPE centrifuge tubes (Thermo Fisher Scientific, Waltham, MA, United States). Filtered samples were immediately put in a cooler and transported to the Wet Plant Lab in nearby Cleveland (OH), and stored in the dark in a refrigerator until analysis for surface tension.
Twenty storms of varying intensity and air temperature were sampled from March until November 2022. The median storm duration was 13.5 h and ranged from 2.1 to 41.0 h. Total rainfall values across all discrete storms had a median of 11.6 m and ranged from 2.0 to 52.4 mm. Storm intensity values ranged from 0.2 to 5.8 mm h−1 and exhibited a median of 1.2 mm h−1. All rainfall event characteristics (duration, total rainfall, and intensity) were positively skewed (skewness = 0.91, 1.12, and 1.13, respectively), indicating storms tended to be shorter and less intense than would be indicated by the average.
The surface tension of all water samples was measured via the pendant drop method using a goniometer (Ramé-Hart, Model 100-00, Succasunna, NJ, United States). The goniometer is outfitted with a three-axis leveling stage, fiber optic illuminator backlight, an automated imaging kit using a U4 Series SuperSpeed digital camera, and a central tower with a chamber to contain the drop and syringe, temperature controlled using thermocouples (model 100-50-01) with a 0.1°C resolution. The camera and light are placed on either side of the central tower to create a backlit image of the syringe and droplet. Drops suspended from the 22-gauge flat-tipped needle (attached to a 3 mL syringe) were observed on a computer monitor via Ramé-Hart’s DROPimage Pro software. Observations of surface tension were made using the surface tension tool where the external phase was set to air and the internal phase was set to water. This tool requires crosshairs to be set to the center of the triple interface between the syringe tip, the liquid, and air at the top of the pendant drop for measurement.
The following procedure was followed for the analysis of each storm event. Before any stormwater sample was measured, the syringe and needle were triple rinsed using deionized water, whereafter, a blank surface tension measurement was obtained, and the syringe was then emptied of any remaining deionized water. Prior to making measurements of rain, throughfall, or stemflow, the syringe was triple rinsed with sample water and filled with ~0.5 mL of the sample. Between each sample, the syringe was triple rinsed with deionized water, then the measurement procedure was repeated. While observing the live image of the drop on DROPImage Pro, pressure was applied to the syringe plunger until the drop was near maximum volume, indicated by a change in the interaction of light with the top of the pendant near the tip of the syringe. Five replicate measurements were taken for each sample and deionized water blank. As this procedure was repeated for each storm event, a set deionized blank measurements was generated for each storm. Across all samples, droplet volume was consistently maintained around (average ± standard deviation) 11.3 ± 1.1 μL, and droplet area was consistently maintained around 24.2 ± 1.7 mm2.
The pendant drop surface tension, area, and volume measurements for all replicates were exported for each rain event and organized into comma-delimited (csv) files according to tree species ID and sample type (i.e., open rainwater, throughfall, stemflow). Data within each group (species and sample type) were normally distributed. Because relevant changes in surface tension may be small relative to the overall surface tension of water in the standard units (mN m−1) (this is further contextualized in section 3.1), results are presented as units of change between the rainfall and the canopy-derived waters from each storm event. Thus, for each storm event, surface tension observations for rainwater (γR), throughfall (γT), stemflow (γS), and deionized water (γDI) were reduced by the mean γR of that storm. Changes in the surface tension of rainwater (ΔγR), throughfall (ΔγT), stemflow (ΔγS), and deionized water (ΔγDI) were plotted in box and whisker plots. Note that ΔγR and ΔγDI values are computed by comparing individual surface tension measurements from a single storm event to the mean surface tension of rainwater measurements for the storm event in which they were measured. Thus, a set of deionized measurements were retrieved for each storm, as described in the previous paragraph, however these ΔγDI measures were simply blanks generated for procedural/method-check purposes.
Since species were not replicated, interspecific statistical comparisons were not performed. Statistical examination of variability in response to storm characteristics was done. Each storm event consists of different amounts (mm) and duration (h), thus the relationship between storm intensity (mm h−1) and the change in surface tension with canopy interaction was examined using correlation analysis. Additionally, the relationship between average temperature (°C) and the change in surface tension was examined. Rainfall measurements and temperature readings at 300 cm above ground were retrieved in five-minute increments from the Wooster Station of the Ohio State University’s CFAES weather system (The Ohio State University, 2023). All data analysis was performed using the following Python packages: Pandas, Matplotlib, Scipy, Statsmodel, and Seaborn.
3 Results and discussion: flux comparison
In general, surface tension of throughfall and stemflow samples was reduced in comparison to rainwater samples from the same storm, suggesting that rainwater-canopy interactions lower rainwater surface tension (Figure 2). Median surface tension of rainwater was 70.93 mN m−1, similar to deionized water (median = 71.12 mN m−1). Differences in surface tension (Δγ) between rainfall and both throughfall and stemflow samples were apparent (Figure 2). Median (interquartile range: IQR) ΔγR for all storms was near zero: −0.046 (−0.222 to 0.223) mN m−1 (Figure 2). Throughfall surface tension across all trees and storms clearly decreased compared to rainwater, with a median (IQR) ΔγT of −0.446 (−0.806 to −0.195) mN m−1 (Figure 2). A greater reduction was observed for stemflow, showing a median (IQR) ΔγS of −0.595 (−0.971 to −0.333) mN m−1 (Figure 2).
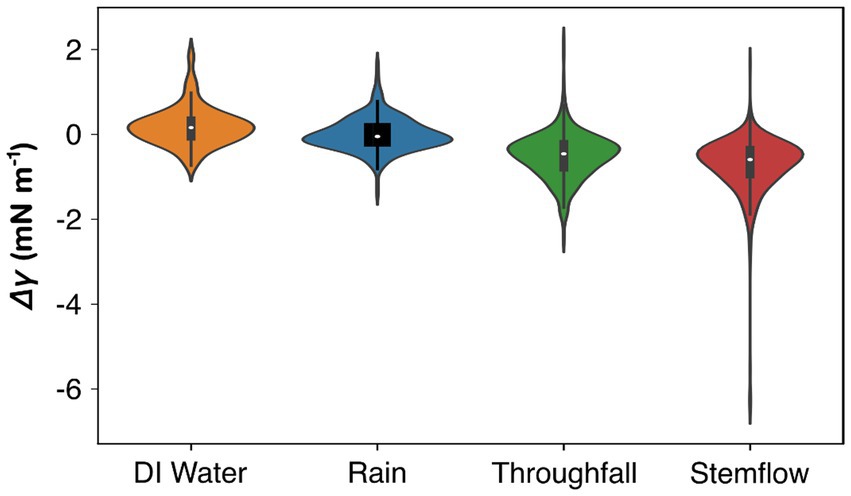
Figure 2. Violin plots illustrating the differences in surface tension (Δγ) between rainfall, throughfall, and stemflow samples across all 20 storms for all 12 trees, next to reference values from deionized (DI) water. Dots are medians, boxes are interquartile ranges, lines are non-outlier ranges, and violins represent the kernel density plot.
Some stemflow and throughfall samples had very low surface tension relative to most other samples. For instance, during a moderately intense storm (5.8 mm h−1) on August 23, 2022, multiple study trees (C. occidentalis, Ulmus × Regal, G. dioicus, T. cordata, and A. rubrum) produced outlier ΔγT observations between −2.5 and −1.5 mN m−1. During a low intensity (0.4 mm h−1) storm on July 7, 2022, ΔγS observations from C. japonicum were extremely low compared to other observations in the dataset, with values ranging from −6.5 to −5.5 mN m−1 (hence the long tail in Figure 2). In the presentation of surface tension data for each study tree (Figure 3), outliers were not plotted (to enhance figure interpretability); however, these outliers are provided in the Supplementary Table S1.
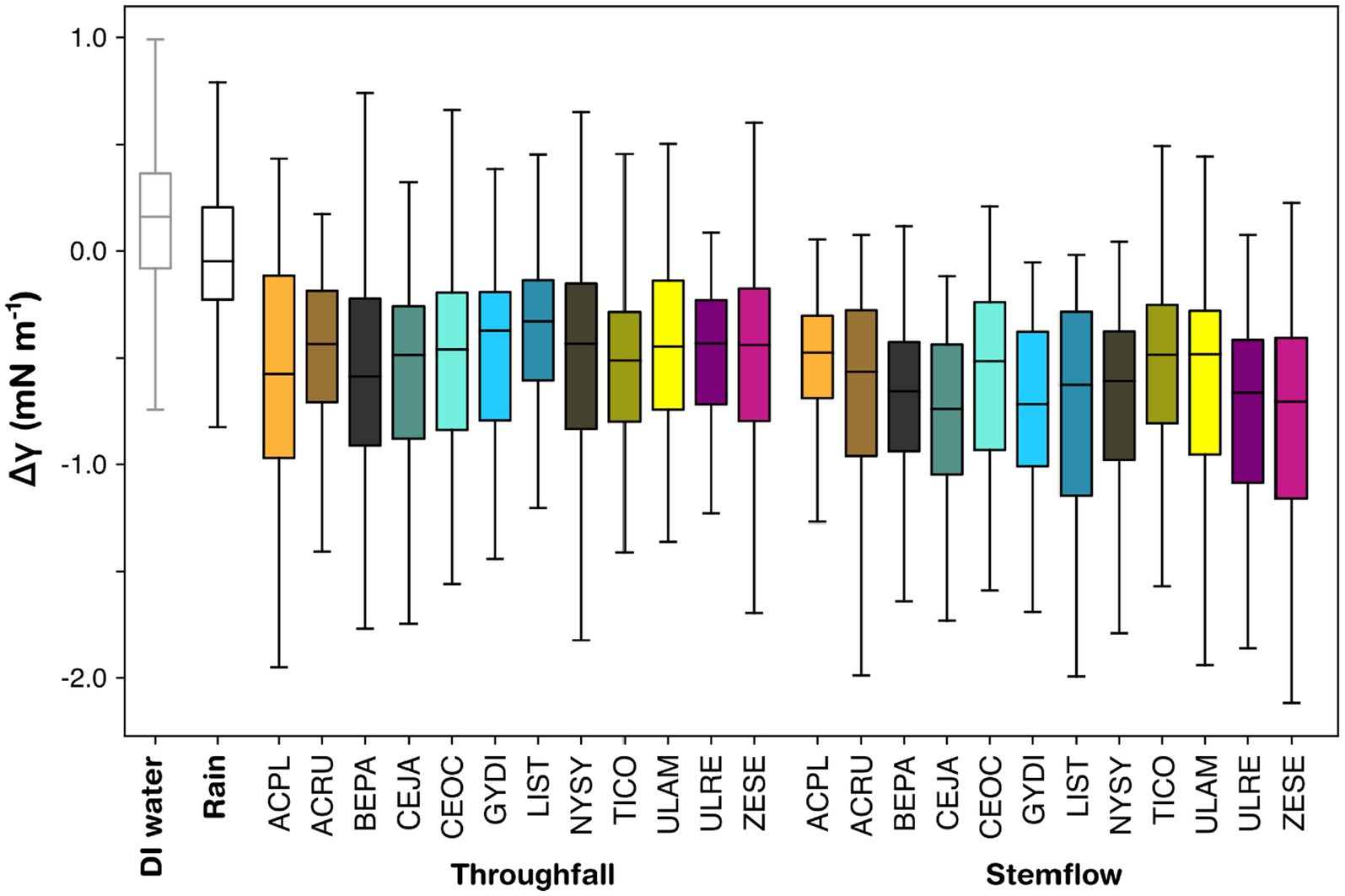
Figure 3. Box plots showcasing surface tension differences (Δγ) for throughfall and stemflow for each species studied, with rainfall and deionized (DI) water for reference. Lines are medians, boxes are interquartile ranges, and lines are non-outlier ranges. Outliers are provided in the Supplementary Table S1. Full tree species names are provided beside the species codes in Table 1.
3.1 Different factors for different fluxes?
The reduction in median surface tension among these fluxes may seem subtle, but a decrease of 0.2 to 1.0 mN m−1 from rainfall to throughfall or stemflow is worth contextualizing. For example, each 1°C increase in the temperature of pure water can decrease its surface tension by ~0.15 mN m−1 (Pallas and Harrison, 1990), indicating that the observed reduction between these fluxes is equivalent to a temperature increase of approximately 1.3°C to 6.6°C in pure water. Changes like those observed for C. japonicum stemflow, as high as −6.5 mN m−1, are analogous to a >40°C change in the temperature of pure water. Importantly, these analogies rely on a general value in pure water, and these relationships can vary based on the specific conditions and measurements used.
Stemflow’s lower surface tension compared to throughfall may hypothetically be due to their interactions with distinct tree surfaces. While throughfall originates from a mixture of gap rainfall and rainwater influenced by a blend of leaf and branch-bark surfaces, stemflow is predominantly derived from flow over the bark of branches and the trunk. These surfaces, being components of different organs with different functions, differ in physicochemical composition. Bark, with its rough texture and sorptive properties, can better retain water and selectively exchange solutes (Ponette-González, 2021). In contrast, the leave’s cuticle, composed of long chain aliphatic compounds, resists wettability (Fernández et al., 2014). These contrasting surface attributes likely influence the chemical enrichment—and consequently the surface tension—of canopy rainwater (Parker, 1983). It’s worth noting that Rai et al. (2023) found that stembark-derived saponins reduced surface tension in water more compared to those from leaves, though the extent to which this would affect rainwater is not yet known. (Chemical relationships to surface tension in throughfall and stemflow will be discussed in the proceeding section.) Notably, stemflow has often been reported to form bubbles from a diversity of tree types and settings (Figure 4)—likely a result of lowered surface tension.
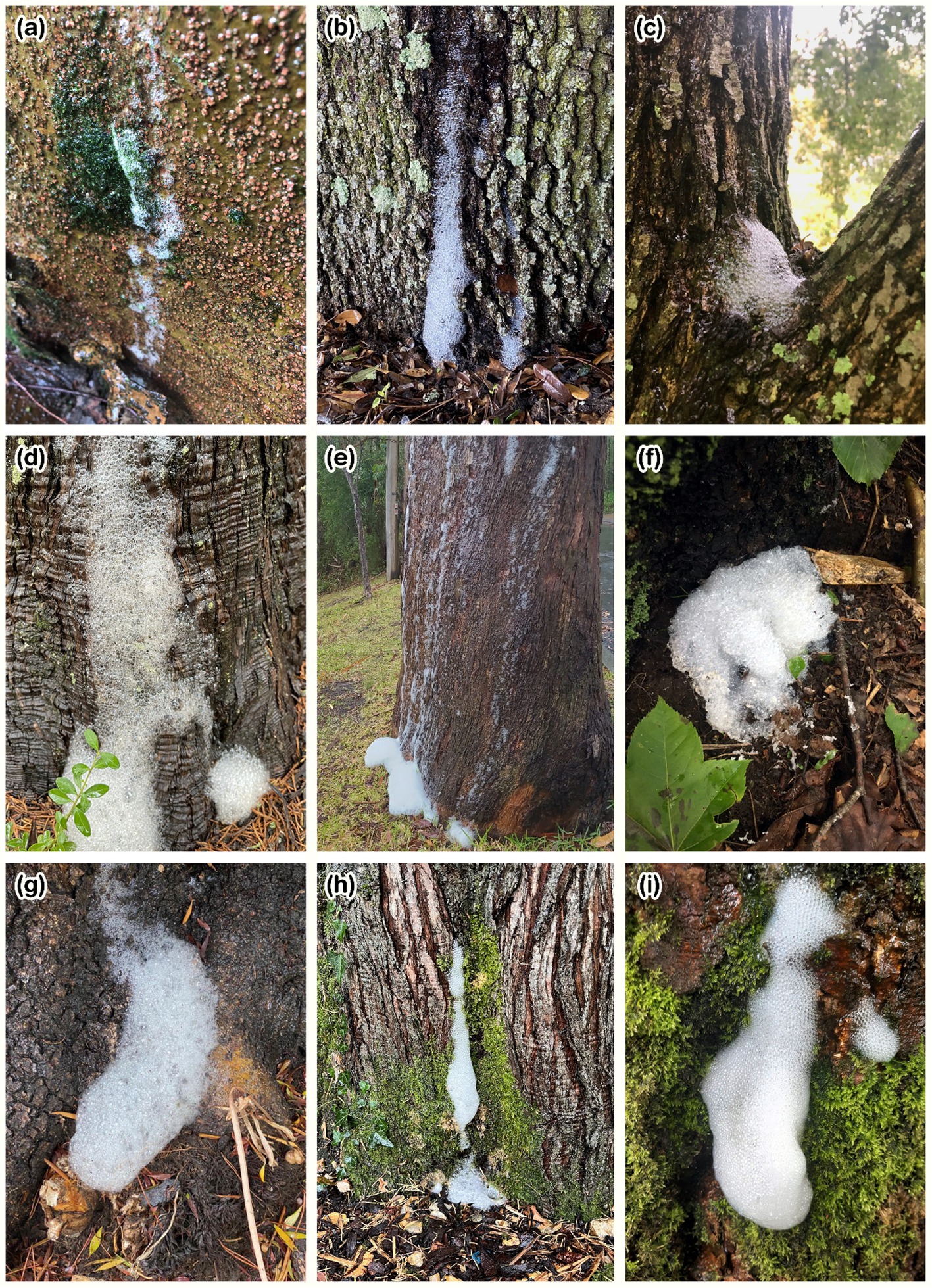
Figure 4. Bark “bubble baths” in stemflow rivulets, occurring on multiple bark types and in various geographic locations: (a) Celtis occidentalis (OH, United States, J. T. Van Stan); (b) Quercus virginiana (SC, United States, C. L. Cooper); (c) Quercus nigra (GA, United States, J. T. Van Stan); (d) Juniperus ashei (TX, United States, R. Schumann); (e) Eucalyptus piperita (Sydney, Australia, V. S. A. Mella); (f) Fagus crenata (Fukushima, Japan, Yuzuru Yoshida); (g) Unknown sp. (Gibraltar, Ornithological and Natural History Society); (h) Quercus macrocarpa (England, I. Garland); (i) Acer pseudoplatanus (Irvine, Scotland, Ranger Jo).
Leaf and bark surfaces also foster unique microhabitats, leading to distinct aerosol depositional dynamics and microbiomes. Few studies have compared the capture of aerosol particulates on bark and leaves; yet, Xu et al. (2019) found stembark, branchbark, and leaves accumulated different amounts and types of particulate matter. Larger, less water-soluble particles accumulated in stembark compared to other surfaces, while greater finer particle fractions accumulated on leaves and branch bark. The surface structure and relative temporal stability (i.e., it does not shed seasonally) of bark, allows substantial organic matter accumulation compared to leaves (Catinon et al., 2009). In addition, the microbial and epiphytic communities on leaves can differ substantially from those on bark (Lambais et al., 2014; Mendieta-Leiva et al., 2020), especially regarding fungi (Magyar, 2008). Variances in microbiomes could hypothetically lead to differences in microbial contributions, potentially impacting the chemical profile and surface tension of draining precipitation.
These different surface structures, microenvironments, and microbiomes can also influence rainwater drainage times on trees. This may alter surface tension due to prolonged contact and heightened exchange time with these surfaces. Two studies in the Pacific Northwest found mean throughfall residence times ranged from 4 to 52 min under relatively low rain intensities (Keim and Skaugset, 2004; Keim and Link, 2018). Given the higher rainfall intensities at our study site, it is conceivable that throughfall residence times might be shorter. While exact stemflow residence times remain elusive in current literature, it is theorized that drainage durations for stemflow exceed throughfall under equivalent rainfall scenarios due to the extended pathlength and reported average stemflow velocities being ~0.1–0.6 m s−1 (Zhang et al., 2022). Given that the nature of contact with a canopy surface likely influences the chemical enrichment of rainwater, differences in residence times between throughfall and stemflow could play a role in the observed disparity in surface tension measurements.
3.2 Influence of throughfall and stemflow chemistry
The observed surface tension reductions could be explained by variability in chemical composition caused by canopy-rainfall interactions. For instance, throughfall and stemflow typically have higher electrical conductivity, ionic concentrations, and acidity than rainwater (Parker, 1983; Ponette-González et al., 2020). These properties could affect surface tension through the interplay of ions with water molecules at the air-water interface. According to the Gibbs adsorption isotherm equation, which describes how a component’s concentration affects surface tension, when the surface tension of an electrolyte solution is lower than pure water, ions should remain hydrated and distant from the air-water interface. Yet, this rule considers the entire ion profile perpendicular to the interface, meaning ions may still locally concentrate near the interface despite overall depletion. This behavior could paradoxically increase surface tension, consistent with thermodynamic principles [see discussion in Kunz (2006)]. Notably, ion polarizability largely affects ion-water interactions and the orientation of water molecules at the interface, rather than ion-air or ion-ion interactions. Consequently, further work may employ a primitive model approach, incorporating parameters from simulations that focus on the water molecule arrangement and water profile near the interface. Note that, to the knowledge of the authors, properties specific to the water profile of stemflow rivulets—and for the branch flows that generate throughfall drip points—has not yet been reported.
Tree-derived dissolved organic matter (tree-DOM) can reach high concentrations in canopy-exposed rainwater, >100 mg C L−1 (Van Stan and Stubbins, 2018). The few studies that delve into the composition of tree-DOM indicate it mainly consists of carbohydrates, aromatics, and aliphatic compounds (Stubbins et al., 2017, 2020). Some of these tree-derived compounds may be easily soluble, affecting surface tension in a Gibbs-type manner; however, other compounds may be larger and less soluble while having surfactant qualities, like bark-derived saponins (Böttcher and Drusch, 2017; Penfold et al., 2018; Rai et al., 2021). Surfactants, due to their molecular structure, have a propensity to align at liquid-air interfaces which can change the surface tension of the liquid. The Langmuir adsorption isotherm may be applied to begin characterizing this behavior for insoluble compounds or surfactants that do not dissolve completely in throughfall and stemflow but tend to adsorb onto interfaces. The Szyszkowski equation, an evolution of the Langmuir isotherm (Chang and Franses, 1995; Eastoe and Dalton, 2000), may offer a refined lens to study tree-DOM’s impact on the surface tension of throughfall and stemflow. It provides a quantitative linkage between solute concentration and changes in surface tension, capturing the nuances of saturation behavior at the air-water interface. Tailored for electrolyte solutions, the equation can represent tree-DOM behavior alongside its varied ionic and polar compounds. This framework also facilitates the estimation of parameters, such as adsorption constants, to decode the behavior of tree-DOM components. Moreover, its foundation can pave the way for advanced models that accommodate the multifaceted nature of tree-DOM. Lastly, adopting the Szyszkowski equation aligns the study of tree-DOM with other systems—which includes precipitation (Mazurek et al., 2006) and, due to recent interests in per-and-polyfluoroalkyl substances (PFAS), groundwater (Brusseau and Guo, 2021) and soil water (Sima and Jaffé, 2021). This should enable meaningful comparisons and a richer understanding within a broader context.
Given this, surfactant-like compounds present in tree-DOM may have a substantial influence on the reduced surface tension observed in throughfall and stemflow. Beyond affecting surface tension, these surfactants might also tweak the wettability and adhesion of water on leaf surfaces, thereby steering the path and volume of water that funnels into stemflow [as hypothesized by Levia and Herwitz (2000)]. It’s also worth mentioning that the specific composition of tree-DOM could be influenced by myriad factors, including the tree’s functional traits, age, and health as well as the surrounding environmental conditions (Stubbins et al., 2020). This variability might manifest as a spectrum of surface tension values across different tree species or even within a single species under distinct conditions. Hence, the next section will examine tree-to-tree variability in our dataset.
4 Results and discussion: tree-to-tree variability
Variability in ΔγT and ΔγS was evident across the study trees (Figure 3). The median ΔγT across all storms was lowest for A. platanoides (−0.615 mN m−1) and highest for L. styraciflua (−0.320 mN m−1), although G. dioicus was similarly high (−0.328 mN m−1). Most trees’ median ΔγT values fell between −0.4 and − 0.5 mN m−1 (in order of increasing magnitude): Ulmus × Regal, N. sylvatica, A. rubrum, Z. serrata, C. occidentalis, C. japonicum (Figure 3). For B. papyrifera and T. cordata, median ΔγT was between −0.5 and −0.6 mN m−1 (Figure 3). Throughfall variability (IQR) was consistent across study trees, generally spanning 0.5 to 0.7 mN m−1. The narrowest ΔγT IQR was observed for L. stryraciflua (−0.599 to −0.134 mN m−1)—though T. cordata and Ulmus × Regal also achieved ΔγT IQR <0.5—and largest for A. platanoides (−1.008 to −0.117 mN m−1).
For the same study tree, median ΔγT was typically less negative than median ΔγS; with most ΔγS medians being 0.1 to 0.3 mN m−1 lower (Figure 3). However, a few study trees had marginal differences between median ΔγT and ΔγS values (i.e., <0.1 mN m−1): T. cordata, U. americana, C. occidentalis, B. papyrifera (Figure 3). Lowest median ΔγS was for C. japonicum (−0.752 mN m−1), with G. dioicus producing a comparable reduction in surface tension (−0.716 mN m−1). T. cordata exhibited the highest median ΔγS (−0.469 mN m−1), though this was similar to U. americana (−0.482 mN m−1). Three of the study trees (A. rubrum, C. occidentalis, and A. platanoides) displayed median ΔγS values between −0.5 and −0.6 mN m−1. Median ΔγS values for the five remaining study trees were between −0.6 and −0.7 mN m−1. Generally, ΔγS IQRs were similar to those reported for ΔγT (Figure 3). Trees like T. cordata produced a ΔγS IQR of −0.760 to −0.244 mN m−1, similar to its ΔγT IQR (−0.798 to −0.308 mN m−1). However, trees like A. platanoides, which had the greatest variability in ΔγT measurements, exhibited the smallest ΔγS IQR (−0.696 to −0.309 mN m−1). Similarly, despite producing the narrowest ΔγT IQR, L. styraciflua had the greatest ΔγS IQR (−0.376 to −1.049 mN m−1). Most of the remaining sample trees produced ΔγS IQRs between 0.5 and 0.7 mN m−1.
4.1 Why may different trees release more tension in rainwater?
This study selected tree species with visibly distinct canopy architectures, highlighting potential differences in key traits like bark structure and roughness, branch geometry, and crown density and shape. Past studies have established that such morphological characteristics can play a role in throughfall and stemflow volumes (Ford and Deans, 1978; Schooling and Carlyle-Moses, 2015; Sadeghi et al., 2020) and the solute concentrations of these fluxes (Parker, 1983; Staelens et al., 2006; Pypker et al., 2011; Oka et al., 2021a,b). As previously discussed, the chemistry of throughfall and stemflow can influence surface tension. The impact of these traits on throughfall and stemflow chemistry has been previously understood through indirect, direct, and community interactions. Some canopy traits may passively and indirectly influence solute concentrations, through the deposition of materials during dry periods (Sæbø et al., 2012; Xu et al., 2019, 2020; Oka et al., 2021a) and their subsequent wash-off and dilution during storms (Kazda, 1990; Van Stan et al., 2017; Xu et al., 2017). Other traits may directly modify the chemistry of throughfall and stemflow through physiological processes that uptake, leach, or transform soluble materials transported over plant organs during rainfall (Long et al., 1956; Tukey, 1966; Oka et al., 2021b). A less discussed but significant mechanism involves the exchange of solutes with resident animal and plant communities on the leaf and bark surfaces (Mabrouk et al., 2022). These community traits also offer an additional layer for trait-based investigations of canopy biogeochemistry (sensu Powell et al., 2013; Malik et al., 2020) and its related variability in surface tension of throughfall and stemflow.
Traits that increase aerosol capture—like canopy architectures with large roughness (and micro-roughness) parameters (Petroff et al., 2008)—can also increase the water storage capacity (Klamerus-Iwan et al., 2020), thereby, reducing the volumes of water available to drain as throughfall and stemflow (Sadeghi et al., 2020), ultimately limiting dilution effects and increasing solute concentrations. Alternatively, traits which tend to reduce water storage capacity (smoother bark, steeper-inclined branches and hydrophobic leaves) tend to both reduce aerosol capture efficiency and capacity per unit surface area and increase the potential for dilution (Sadeghi et al., 2020). These variations in water storage and dilution may influence surface tension, depending on the type of solutes present. Solutes can be categorized as kosmotropes, which reinforce the hydrogen-bonding network of water, or chaotropes, which disrupt this structure (dos Santos et al., 2010). For instance, when considering sea salt aerosol, NaCl, dissolved in throughfall or stemflow: Na+ typically acts as a kosmotrope while Cl− acts as a chaotrope. Canopies, like soils, may retain Na+ while leaching Cl−, causing throughfall and stemflow to have higher Cl− concentrations compared to Na+ (Eaton et al., 1973; Heartsill-Scalley et al., 2007; Thimonier et al., 2008). Consequently, waters enriched with chaotropic Cl− ions would likely exhibit reduced surface tension (if they accumulated in the water-air interface), if not balanced by an equivalent rise in kosmotropic ions. In this way, tree traits (and settings) that capture greater sea salt aerosols, retain Na+, and leach Cl− may contribute to reduced surface tension.
If one considers commonly reported physiological influences on ion concentrations of throughfall and stemflow, they point to an increase in chaotropic ions, like K+ (in fact, this is the generally the most concentrated ion reported in throughfall and stemflow studies per literature reviews) (Levia and Frost, 2006; Levia and Germer, 2015). Moreover, weakly kosmotropic ions (like Mg2+) are reported at high concentrations (Levia and Frost, 2006; Levia and Germer, 2015) and strongly kosmotropic ions, like NH4+ and Ca2+, have been reported to decrease due to uptake by plants (Macinnis-Ng et al., 2012; Van Stan et al., 2012). The exact physiological traits that may explain these uptake and leaching observations are currently unknown. A recent study examining various bark anatomical features and their relationship with lab-derived leachate chemical composition shed some insight, suggesting that K+ concentration may be linked to the abundance of cellular structures associated with nutrient storage, like parenchyma (Oka et al., 2021b). The selective uptake of kosmotropic ions and leaching of chaotropic ions by canopies might affect the overall “structuring” of water and thereby its surface tension, which could in turn influence processes like water flow, absorption, and even evaporation rates.
5 Results and discussion: variability with storm conditions
Throughfall and stemflow data across all study trees were analyzed for correlations between changes in surface tension (Δγ) and storm parameters, specifically rain intensity and mean air temperature. Notable relationships were observed. Both C. occidentalis and U. americana exhibited correlations between Δγ in stemflow (ΔγS) and event rainfall intensity (Figures 5A,B). This correlation was notably stronger for U. americana. Additionally, five correlations were identified between canopy-derived waters and mean air temperature. ΔγS was indirectly correlated with air temperature for three species—N. sylvatica, U. americana, and T. cordata—with the strength of correlation increasing in that order (Figures 5C–E). For changes in surface tension in throughfall (ΔγT), two indirect correlations with air temperature were observed, namely for L. styriciflua and U. americana (Figures 5D,F).
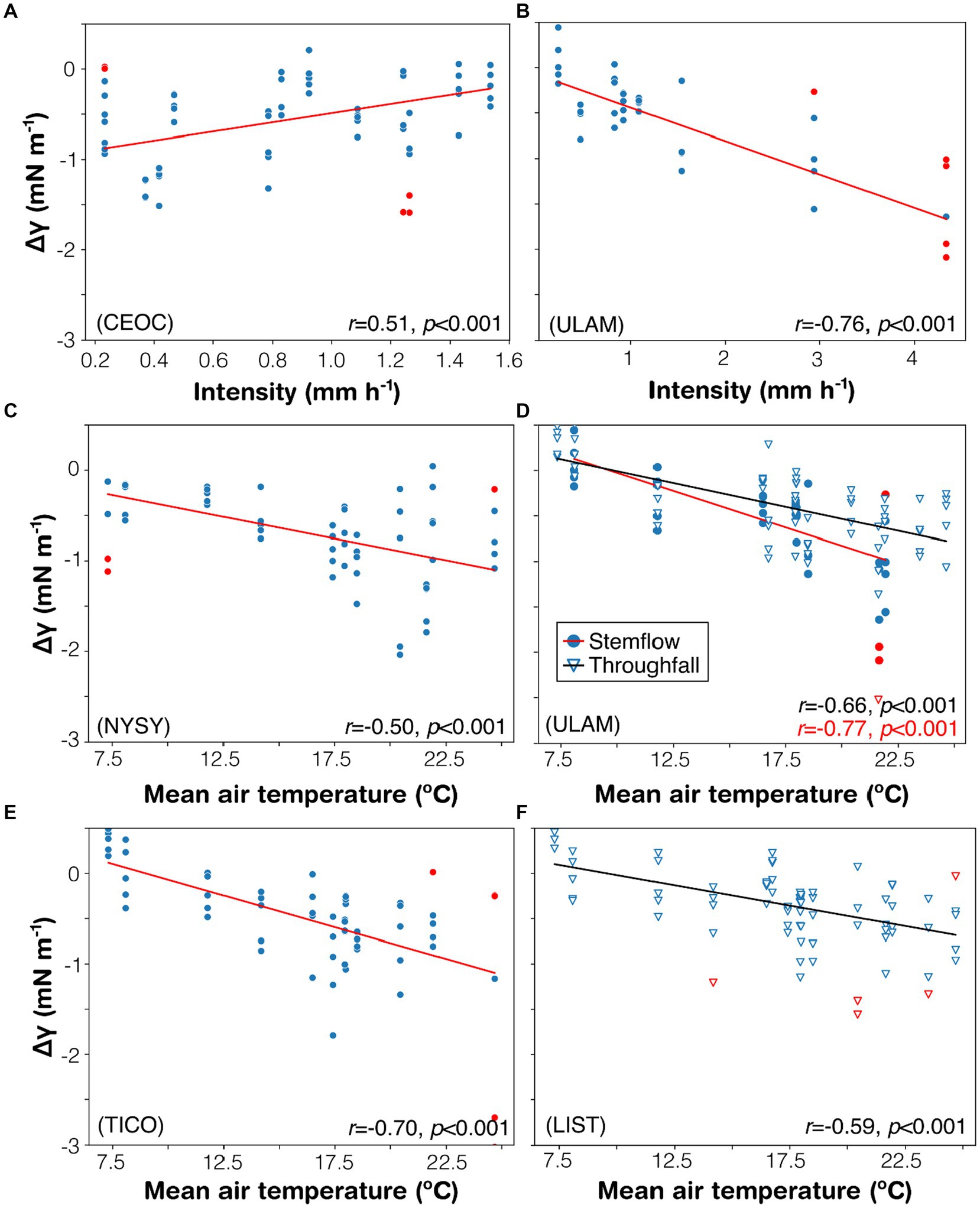
Figure 5. Scatterplots and regression results for any significant result relating (A,B) rainfall intensity and (C–F) mean event air temperature to changes in surface tension (Δγ) for throughfall (triangles) and stemflow (dots). Each panel is labelled with its species identifier (linked to Table 1). Leverage points are in red and not included in the regression statistics.
5.1 How might storm conditions affect throughfall and stemflow surface tension?
Few significant statistical correlations were observed between storm conditions and changes in surface tension, particularly for throughfall. This suggests that various fluxes may have unique influencing factors that alter surface tension. We examined storm variables that are theoretically connected to hydraulic residence time (rain intensity) and surface-area interactions (mean event air temperature). Intuitively, more intense rainfall could decrease residence time in the canopy (Keim and Skaugset, 2004; Keim and Link, 2018), thereby reducing the opportunity for canopies to passively enrich throughfall and stemflow with chaotropes while absorbing kosmotropes. If this were the case, one would expect a direct relationship between Δγ and rain intensity—meaning that Δγ would approach the mean rainwater surface tension (or zero) with increasing intensity. However, this was only weakly observed for the stemflow from one tree species (Figure 5A). Surprisingly, the most significant correlations between surface tension changes and rain intensity were negative (Figure 5B), for which we currently have no hypothesized mechanism to explain why increased rain intensity would reduce surface tension. Regarding the influence of mean event air temperature on Δγ, the indirect relationships observed for all statistically significant fluxes across all tree species could be linked to greater surface area interaction with rainfall. Generally, as dilute water (such as rainwater) warms, its surface tension decreases, making the water more prone to spreading. This could impact various processes, including wetting, absorption, and solute exchange (Adriaenssens et al., 2011; Wang et al., 2015; Berry and Goldsmith, 2020). (It is important to note that the mean air temperature during a storm event could not have directly influenced our surface tension measurements, as all samples were analyzed at a constant, controlled temperature in the lab.)
6 Conclusions and relevance to forest ecohydrology
Changes in surface tension between open rainfall and the net rainfall fluxes beneath urban tree canopies were of large enough magnitude to potentially influence related ecohydrological processes. This is because surface tension affects wetting, absorption, and solute exchange processes for leaf, bark and epiphyte surfaces. Assuming that rainwater entrained on these surfaces maintains the generally higher surface tension of open rainfall could lead to biases in estimating related states, like water storage capacity, and fluxes, such as leaching and washoff. This might also affect laboratory methodologies, which often use purified water to study leaf or bark hydrophobicity and wettability. These concerns could similarly impact surface processes, with the typically lower surface tension of throughfall and stemflow affecting interactions with litter layers and subsequent soil infiltration (Fletcher, 1949; Nimmo and Shillito, 2023). Despite all study trees showcasing lower surface tension values in throughfall and, more markedly, in stemflow compared to open rainfall, the extent of these reductions varied by species and, at times, weather conditions. Thus, our results highlight a diversity in surface tension modifications across tree species, shaped by their unique canopy traits and interactions with weather conditions. Hypothetically, variations in tree surface properties, canopy microenvironments, and microbiomes contribute to these differences, and merit future research attentions. Correlations were found between surface tension and storm conditions (temperature and rain intensity) in a small selection of cases. Although this supports the hypothesis that distinct, likely canopy-related, factors are more consequential in regulating the mechanisms that alter rainfall surface tension, it also hints at the possible varied impacts of climate change-induced modifications to rainfall for different tree species. The potential for stemflow to exhibit lower surface tension than throughfall due to differences in surface characteristics opens new avenues for research into the chemical enrichment and hydrological consequences of these fluxes. In conclusion, our findings emphasize the imperative for an in-depth understanding of the myriad physical and ecological factors governing canopy-rainwater interactions. The diverse alterations in surface tension amongst different tree species, especially in the face of changing weather conditions, necessitate focused attention and research for comprehensive insight into ecological and hydrological processes.
Data availability statement
The original contributions presented in the study are included in the article/Supplementary material, further inquiries can be directed to the corresponding author.
Author contributions
BN: Conceptualization, Data curation, Formal analysis, Investigation, Methodology, Visualization, Writing – original draft, Writing – review & editing. NL: Data curation, Formal analysis, Resources, Writing – review & editing. KT: Conceptualization, Supervision, Writing – review & editing. AI: Conceptualization, Supervision, Writing – review & editing. JV: Conceptualization, Formal analysis, Funding acquisition, Investigation, Methodology, Project administration, Resources, Supervision, Visualization, Writing – original draft, Writing – review & editing.
Funding
The author(s) declare financial support was received for the research, authorship, and/or publication of this article. BN and JV were supported by US-NSF DEB-2213623. KT was supported by FAPESP 2021/11697-9.
Acknowledgments
The authors gratefully acknowledge the staff at Secrest Arboretum, including Jason Veil, Paul Snyder, and Roger Hamilton; Professor Kevin Mueller and Sarah Blair for establishing the study site at Secrest; Professor Nolan Holland for his assistance in setting up the goniometer and for permissions to work in his lab space; and Jillian Bastock for an occasional helping hand in the field.
Conflict of interest
The authors declare that the research was conducted in the absence of any commercial or financial relationships that could be construed as a potential conflict of interest.
The author(s) declared that they were an editorial board member of Frontiers, at the time of submission. This had no impact on the peer review process and the final decision.
Publisher’s note
All claims expressed in this article are solely those of the authors and do not necessarily represent those of their affiliated organizations, or those of the publisher, the editors and the reviewers. Any product that may be evaluated in this article, or claim that may be made by its manufacturer, is not guaranteed or endorsed by the publisher.
Supplementary material
The Supplementary material for this article can be found online at: https://www.frontiersin.org/articles/10.3389/ffgc.2023.1315936/full#supplementary-material
References
Adriaenssens, S., Staelens, J., Wuyts, K., de Schrijver, A., Van Wittenberghe, S., Wuytack, T., et al. (2011). Foliar nitrogen uptake from wet deposition and the relation with leaf wettability and water storage capacity. Water Air Soil Pollut. 219, 43–57. doi: 10.1007/s11270-010-0682-8
Aubrey, D. P. (2020) J. T. StanVan, E. Gutmann, and J. Friesen. Relevance of precipitation partitioning to the tree water and nutrient balance. Precipitation partitioning by vegetation Springer Cham, 147–162
Bachmann, J., and van der Ploeg, R. R. (2002). A review on recent developments in soil water retention theory: interfacial tension and temperature effects. J. Plant Nutr. Soil Sci. 165:468. doi: 10.1002/1522-2624(200208)165:4<468::AID-JPLN468>3.0.CO;2-G
Berry, Z. C., and Goldsmith, G. R. (2020). Diffuse light and wetting differentially affect tropical tree leaf photosynthesis. New Phytol. 225, 143–153. doi: 10.1111/nph.16121
Bittar, T. B., Pound, P., Whitetree, A., Moore, L. D., and Van Stan, J. T. (2018). Estimation of Throughfall and Stemflow bacterial flux in a subtropical oak-cedar Forest. Geophys. Res. Lett. 45, 1410–1418. doi: 10.1002/2017gl075827
Böttcher, S., and Drusch, S. (2017). Saponins—self-assembly and behavior at aqueous interfaces. Adv. Colloid Interf. Sci. 243, 105–113. doi: 10.1016/j.cis.2017.02.008
Brusseau, M. L., and Guo, B. (2021). Air-water interfacial areas relevant for transport of per and poly-fluoroalkyl substances. Water Res. 207:117785. doi: 10.1016/j.watres.2021.117785
Catinon, M., Ayrault, S., Boudouma, O., Asta, J., Tissut, M., and Ravanel, P. (2009). The inclusion of atmospheric particles into the bark suber of ash trees. Chemosphere 77, 1313–1320. doi: 10.1016/j.chemosphere.2009.09.039
Chang, C.-H., and Franses, E. I. (1995). Adsorption dynamics of surfactants at the air/water interface: a critical review of mathematical models, data, and mechanisms. Colloids Surf. A 100, 1–45. doi: 10.1016/0927-7757(94)03061-4
Davies-Barnard, T., Valdes, P. J., Jones, C. D., and Singarayer, J. S. (2014). Sensitivity of a coupled climate model to canopy interception capacity. Clim. Dyn. 42, 1715–1732. doi: 10.1007/s00382-014-2100-1
dos Santos, A. P., Diehl, A., and Levin, Y. (2010). Surface tensions, surface potentials, and the Hofmeister series of electrolyte solutions. Langmuir 26, 10778–10783. doi: 10.1021/la100604k
Eastoe, J., and Dalton, J. S. (2000). Dynamic surface tension and adsorption mechanisms of surfactants at the air–water interface. Adv. Colloid Interf. Sci. 85, 103–144. doi: 10.1016/S0001-8686(99)00017-2
Eaton, J. S., Likens, G. E., and Bormann, F. H. (1973). Throughfall and stemflow chemistry in a northern hardwood forest. J. Ecol. 61, 495–508. doi: 10.2307/2259041
Fernández, V., Sancho-Knapik, D., Guzmán, P., Peguero-Pina, J. J., Gil, L., Karabourniotis, G., et al. (2014). Wettability, polarity and water absorption of Quercus ilex leaves: effect of leaf side and age. Plant Physiol. 166:242040, 168–180. doi: 10.1104/pp.114.242040
Fletcher, J. E. (1949). Some properties of water solutions that influence infiltration. Trans. Am. Geophys. Union 30, 548–554. doi: 10.1029/TR030i004p00548
Ford, E. D., and Deans, J. D. (1978). The effects of canopy structure on stemflow, throughfall and interception loss in a young Sitka spruce plantation. J. Appl. Ecol. 15, 905–917. doi: 10.2307/2402786
Guidone, M., Gordon, D. A., and Van Stan, J. T. (2021). Living particulate fluxes in throughfall and stemflow during a pollen event. Biogeochemistry 153, 323–330. doi: 10.1007/s10533-021-00787-7
Hackenberg, J., Calders, K., Demol, M., Raumonen, P., Piboule, A., and Disney, M. (2021). SimpleForest—a comprehensive tool for 3d reconstruction of trees from forest plot point clouds. bioRxiv. Available at: https://doi.org/10.1101/2021.07.29.454344
Heartsill-Scalley, T., Scatena, F. N., Estrada, C., McDowell, W. H., and Lugo, A. E. (2007). Disturbance and long-term patterns of rainfall and throughfall nutrient fluxes in a subtropical wet forest in Puerto Rico. J. Hydrol. 333, 472–485. doi: 10.1016/j.jhydrol.2006.09.019
Holder, C. D. (2020). “Advances and future research directions in the study of leaf water repellency” in Forest-water interactions. eds. D. F. Levia, D. E. Carlyle-Moses, S. Iida, B. Michalzik, K. Nanko, and A. Tischer (Cham: Springer), 261–278.
Kazda, M. (1990). “Sequential stemflow sampling for estimation of dry deposition and crown leaching in beech stands” in Nutrient cycling in terrestrial ecosystems: field methods, application and interpretation. eds. A. F. Harrison, P. Ineson, and O. W. Heal (London: Elsevier), 46–55.
Keim, R. F., and Link, T. E. (2018). Linked spatial variability of throughfall amount and intensity during rainfall in a coniferous forest. Agric. For. Meteorol. 248, 15–21. doi: 10.1016/j.agrformet.2017.09.006
Keim, R. F., and Skaugset, A. E. (2004). A linear system model of dynamic throughfall rates beneath forest canopies. Water Resour. Res. 40:W05208. doi: 10.1029/2003WR002875
Kim, T., Kim, J., Lee, J., Kim, H. S., Park, J., and Im, S. (2023). Water retention capacity of leaf litter according to field lysimetry. Forests 14:478. doi: 10.3390/f14030478
Klamerus-Iwan, A., and Błońska, E. (2018). Canopy storage capacity and wettability of leaves and needles: the effect of water temperature changes. J. Hydrol. 559, 534–540. doi: 10.1016/j.jhydrol.2018.02.032
Klamerus-Iwan, A., Link, T. E., Keim, R. F., and Van Stan, J. T. (2020). “Storage and routing of precipitation through canopies” in Precipitation partitioning by vegetation: a global synthesis, Cham, Switzerland: Springer Nature. 17–34.
Klučiarová, D., Márton, P., Pichler, V., Márton, E., and Túnyi, I. (2008). Pollution detection by magnetic susceptibility measurements aided by the Stemflow effect. Water Air Soil Pollut. 189, 213–223. doi: 10.1007/s11270-007-9569-8
Konrad, W., Ebner, M., Traiser, C., and Roth-Nebelsick, A. (2012). Leaf surface wettability and implications for drop shedding and evaporation from forest canopies. Pure Appl. Geophys. 169, 835–845. doi: 10.1007/s00024-011-0330-2
Kunz, W. (2006). Specific ion effects in liquids, in biological systems, and at interfaces. Pure Appl. Chem. 78, 1611–1617. doi: 10.1351/pac200678081611
Lambais, M. R., Lucheta, A. R., and Crowley, D. E. (2014). Bacterial community assemblages associated with the phyllosphere, dermosphere, and rhizosphere of tree species of the Atlantic forest are host taxon dependent. Microb. Ecol. 68, 567–574. doi: 10.1007/s00248-014-0433-2
Le Mellec, A., Meesenburg, H., and Michalzik, B. (2010). The importance of canopy-derived dissolved and particulate organic matter (DOM and POM)—comparing throughfall solution from broadleaved and coniferous forests. Ann. Sci. For. 67:411. doi: 10.1051/forest/2009130
Levia, D. F., and Frost, E. E. (2006). Variability of throughfall volume and solute inputs in wooded ecosystems. Prog. Phys. Geogr. 30, 605–632. doi: 10.1177/0309133306071145
Levia, D. F., and Germer, S. (2015). A review of stemflow generation dynamics and stemflow-environment interactions in forests and shrublands. Rev. Geophys. 53, 673–714. doi: 10.1002/2015RG000479
Levia, D. F. Jr., and Herwitz, S. R. (2000). Physical properties of water in relation to stemflow leachate dynamics: implications for nutrient cycling. Can. J. For. Res. 30, 662–666. doi: 10.1139/x99-244
Long, W. G., Sweet, D. V., and Tukey, H. B. (1956). Loss of nutrients from plant foliage by leaching as indicated by radioisotopes. Science 123, 1039–1040. doi: 10.1126/science.123.3206.1039-a
Mabrouk, A. I., Gordon, D. A., Gotsch, S. G., and Van Stan, J. T. (2022). Conceptual analysis: what signals might plant canopies send via stemflow? Front. Water 4:1075732. doi: 10.3389/frwa.2022.1075732
Macinnis-Ng, C. M. O., Flores, E. E., Müller, H., and Schwendenmann, L. (2012). Rainfall partitioning into throughfall and stemflow and associated nutrient fluxes: land use impacts in a lower montane tropical region of Panama. Biogeochemistry 111, 661–676. doi: 10.1007/s10533-012-9709-0
Magliano, P. N., Whitworth-Hulse, J. I., and Baldi, G. (2019). Interception, throughfall and stemflow partition in drylands: global synthesis and meta-analysis. J. Hydrol. 568, 638–645. doi: 10.1016/j.jhydrol.2018.10.042
Magyar, D., Van Stan, J. T., and Sridhar, K. R. (2021). Hypothesis and theory: fungal spores in stemflow and potential bark sources. Front. For. Glob. Change 4:19. doi: 10.3389/ffgc.2021.623758
Malik, A. A., Martiny, J. B. H., Brodie, E. L., Martiny, A. C., Treseder, K. K., and Allison, S. D. (2020). Defining trait-based microbial strategies with consequences for soil carbon cycling under climate change. ISME J. 14, 1–9. doi: 10.1038/s41396-019-0510-0
Mazurek, A. Z., Pogorzelski, S. J., and Kogut, A. D. (2006). A novel approach for structure quantification of fatty acids films on rain water. Atmos. Environ. 40, 4076–4087. doi: 10.1016/j.atmosenv.2006.03.021
Mendieta-Leiva, G., Porada, P., and Bader, M. Y. (2020). “Interactions of epiphytes with precipitation partitioning” in Precipitation partitioning of vegetation: a global synthesis (Cham: Springer), 133–146.
Nimmo, J., and Shillito, R. (2023). “Infiltration of water into soil” in Oxford research encyclopedia of environmental science (Oxford: Oxford University Press)
Nowak, D. J., Coville, R., Endreny, T. A., Abdi, R., and Van Stan, J. T. (2020). “Valuing urban tree impacts on precipitation partitioning” in Precipitation partitioning by vegetation: a global synthesis (Cham: Springer), 253–268.
Oka, A., Takahashi, J., Endoh, Y., and Seino, T. (2021a). Bark effects on Stemflow chemistry in a Japanese temperate Forest I. The role of bark surface morphology. Front. For. Glob. Change 4:654375. doi: 10.3389/ffgc.2021.654375
Oka, A., Takahashi, J., Endoh, Y., and Seino, T. (2021b). Bark effects on Stemflow chemistry in a Japanese temperate Forest II. The role of bark anatomical features. Front. For. Glob. Change 4:657850. doi: 10.3389/ffgc.2021.657850
Pallas, N. R., and Harrison, Y. (1990). An automated drop shape apparatus and the surface tension of pure water. Colloids Surf. 43, 169–194. doi: 10.1016/0166-6622(90)80287-E
Parker, G. G. (1983). Throughfall and stemflow in the forest nutrient cycle. Adv. Ecol. Res. 13, 57–133. doi: 10.1016/S0065-2504(08)60108-7
Penfold, J., Thomas, R. K., Tucker, I., Petkov, J. T., Stoyanov, S. D., Denkov, N., et al. (2018). Saponin adsorption at the air–water interface—neutron reflectivity and surface tension study. Langmuir 34, 9540–9547. doi: 10.1021/acs.langmuir.8b02158
Petroff, A., Mailliat, A., Amielh, M., and Anselmet, F. (2008). Aerosol dry deposition on vegetative canopies. Part I: review of present knowledge. Atmos. Environ. 42, 3625–3653. doi: 10.1016/j.atmosenv.2007.09.043
Ponette-González, A. G. (2021). Accumulator, transporter, substrate, and reactor: multidimensional perspectives and approaches to the study of bark. Front. For. Glob. Change 4:716557. doi: 10.3389/ffgc.2021.716557
Ponette-González, A. G., Van Stan, J. T., and Magyar, D. (2020). “Things seen and unseen in throughfall and stemflow” in Precipitation partitioning by vegetation: a global synthesis (Cham: Springer), 71–88.
Powell, J. R., Anderson, I. C., and Rillig, M. C. (2013). A new tool of the trade: plant-trait based approaches in microbial ecology. Plant Soil 365, 35–40. doi: 10.1007/s11104-012-1581-0
Ptatscheck, C., Milne, P. C., and Traunspurger, W. (2018). Is stemflow a vector for the transport of small metazoans from tree surfaces down to soil? BMC Ecol. 18:43. doi: 10.1186/s12898-018-0198-4
Pypker, T. G., Levia, D. F., Staelens, J., and Van Stan, J. T. (2011). “Canopy structure in relation to hydrological and biogeochemical fluxes” in Forest hydrology and biogeochemistry (Dordrecht: Springer), 371–388.
Qualls, R. G. (2020). “Role of precipitation partitioning in litter biogeochemistry” in Precipitation Partitioning by vegetation (Cham: Springer), 163–182.
Rai, S., Acharya-Siwakoti, E., Kafle, A., Devkota, H. P., and Bhattarai, A. (2021). Plant-derived Saponins: a review of their surfactant properties and applications. Sci 3:44. doi: 10.3390/sci3040044
Rai, S., Kafle, A., Devkota, H. P., and Bhattarai, A. (2023). Characterization of saponins from the leaves and stem bark of Jatropha curcas L. for surface-active properties. Heliyon 9:e15807. doi: 10.1016/j.heliyon.2023.e15807
Riba, J.-R., and Esteban, B. (2014). A simple laboratory experiment to measure the surface tension of a liquid in contact with air. Eur. J. Phys. 35:055003. doi: 10.1088/0143-0807/35/5/055003
Sadeghi, S. M. M., Gordon, A. G., and Van Stan, J. T. (2020). “A global synthesis of throughfall and stemflow hydrometeorology” in Precipitation partitioning by vegetation: a global synthesis (Cham: Springer), 49–70.
Sæbø, A., Popek, R., Nawrot, B., Hanslin, H. M., Gawronska, H., and Gawronski, S. W. (2012). Plant species differences in particulate matter accumulation on leaf surfaces. Sci. Total Environ. 427–428, 347–354. doi: 10.1016/j.scitotenv.2012.03.084
Savenije, H. H. G. (2004). The importance of interception and why we should delete the term evapotranspiration from our vocabulary. Hydrol. Process. 18, 1507–1511. doi: 10.1002/hyp.5563
Savenije, H. H. G. (2018). Intercepted by lichens. Nat. Geosci. 11, 548–549. doi: 10.1038/s41561-018-0202-9
Schooling, J. T., and Carlyle-Moses, D. E. (2015). The influence of rainfall depth class and deciduous tree traits on stemflow production in an urban park. Urban Ecosyst. 18, 1261–1284. doi: 10.1007/s11252-015-0441-0
Sima, M. W., and Jaffé, P. R. (2021). A critical review of modeling poly- and perfluoroalkyl substances (PFAS) in the soil-water environment. Sci. Total Environ. 757:143793. doi: 10.1016/j.scitotenv.2020.143793
Singh, S., Inamdar, S., Mitchell, M., and McHale, P. (2014). Seasonal pattern of dissolved organic matter (DOM) in watershed sources: influence of hydrologic flow paths and autumn leaf fall. Biogeochemistry 118, 321–337. doi: 10.1007/s10533-013-9934-1
Staelens, J., Schrijver, A.De, Verheyen, K., and Verhoest, N. E. (2006). Spatial variability and temporal stability of throughfall deposition under beech (Fagus sylvatica L.) in relationship to canopy structure. Environ. Pollut. 142, 254–263. doi: 10.1016/j.envpol.2005.10.002
Stubbins, A., Guillemette, F., and Van Stan, J. T. (2020). “Throughfall and stemflow: the crowning headwaters of the aquatic carbon cycle” in Precipitation partitioning by vegetation: a global synthesis, Cham, Switzerland: Springer Nature. 121–132.
Stubbins, A., Silva, L. M., Dittmar, T., and Van Stan, J. T. (2017). Molecular and optical properties of tree-derived dissolved organic matter in throughfall and stemflow from live oaks and eastern red cedar. Front. Earth Sci. 5:22. doi: 10.3389/feart.2017.00022
The Natural Resources Conservation Service (2023). Web soil survey United States Department of Agriculture. https://websoilsurvey.nrcs.usda.gov/app/
The Ohio State University (2023). The College of Food Agricultural and Environmental Sciences Weather System. Wooster, OH. Available at: https://weather.cfaes.osu.edu/weatherarchive/
Thimonier, A., Schmitt, M., Waldner, P., and Schleppi, P. (2008). Seasonality of the Na/Cl ratio in precipitation and implications of canopy leaching in validating chemical analyses of throughfall samples. Atmos. Environ. 42, 9106–9117. doi: 10.1016/j.atmosenv.2008.09.007
Tonello, K. C., Campos, S. D., de Menezes, A. J., Bramorski, J., Mathias, S. L., and Lima, M. T. (2021). How is bark absorbability and wettability related to stemflow yield? Observations from isolated trees in the Brazilian Cerrado. Front. For. Glob. Change 4:650665. doi: 10.3389/ffgc.2021.650665
Tukey, H. B. Jr. (1966). Leaching of metabolites from above-ground plant parts and its implications. Bull. Torrey. Bot. Club, 385–401.
van der Ent, R. J., Wang-Erlandsson, L., Keys, P. W., and Savenije, H. H. G. (2014). Contrasting roles of interception and transpiration in the hydrological cycle–part 2: moisture recycling. Earth Syst. Dynam. 5, 471–489. doi: 10.5194/esd-5-471-2014
Van Stan, J. T., and Friesen, J. (2020). “Precipitation partitioning, or to the surface and back again: historical overview of the first process in the terrestrial hydrologic pathway” in Precipitation partitioning by vegetation: a global synthesis (Cham: Springer), 1–16.
Van Stan, J. T. Jr., Delphis, F. L., Inamdar, S. P., Lepori-Bui, M., and Mitchell, M. J. (2012). The effects of phenoseason and storm characteristics on throughfall solute washoff and leaching dynamics from a temperate deciduous forest canopy. Sci. Total Environ. 430, 48–58. doi: 10.1016/j.scitotenv.2012.04.060
Van Stan, J. T., Morris, C. E., Aung, K., Kuzyakov, Y., Magyar, D., Rebollar, E. A., et al. (2020). “Precipitation partitioning—hydrologic highways between microbial communities of the plant microbiome?” in Precipitation partitioning by vegetation: a global synthesis (Cham: Springer)
Van Stan, J. T., Ponette-González, A. G., Swanson, T., and Weathers, K. C. (2021). Throughfall and stemflow are major hydrologic highways for particulate traffic through tree canopies. Front. Ecol. Environ. 19, 404–410. doi: 10.1002/fee.2360
Van Stan, J. T., and Stubbins, A. (2018). Tree-DOM: dissolved organic matter in throughfall and stemflow. Limnol. Oceanogr. Lett. 3, 199–214. doi: 10.1002/lol2.10059
Van Stan, J. T., Wagner, S., Guillemette, F., Whitetree, A., Lewis, J., Silva, L., et al. (2017). Temporal dynamics in the concentration, flux, and optical properties of tree-derived dissolved organic matter in an epiphyte-laden oak-cedar forest. J. Geophys. Res. Biogeosci. 122, 2982–2997. doi: 10.1002/2017jg004111
Vorholt, J. A. (2012). Microbial life in the phyllosphere. Nat. Rev. Microbiol. 10, 828–840. doi: 10.1038/nrmicro2910
Wang, H., Shi, H., and Wang, Y. (2015). The wetting of leaf surfaces and its ecological significances. Wetting and wettability. London, England: IntechOpen.
Whitworth-Hulse, J. I., Magliano, P. N., Zeballos, S. R., Aguiar, S., and Baldi, G. (2021). Global patterns of rainfall partitioning by invasive woody plants. Glob. Ecol. Biogeogr. 30, 235–246. doi: 10.1111/geb.13218
Xu, X., Xia, J., Gao, Y., and Zheng, W. (2020). Additional focus on particulate matter wash-off events from leaves is required: a review of studies of urban plants used to reduce airborne particulate matter pollution. Urban For. Urban Green. 48:126559. doi: 10.1016/j.ufug.2019.126559
Xu, X., Yu, X., Mo, L., Xu, Y., Bao, L., and Lun, X. (2019). Atmospheric particulate matter accumulation on trees: a comparison of boles, branches and leaves. J. Clean. Prod. 226, 349–356. doi: 10.1016/j.jclepro.2019.04.072
Xu, X., Zhang, Z., Bao, L., Mo, L., Yu, X., Fan, D., et al. (2017). Influence of rainfall duration and intensity on particulate matter removal from plant leaves. Sci. Total Environ. 609, 11–16. doi: 10.1016/j.scitotenv.2017.07.141
Yue, K., Frenne, P.De, Fornara, D. A., Van Meerbeek, K., Li, W., Peng, X., et al. (2021). Global patterns and drivers of rainfall partitioning by trees and shrubs. Glob. Chang. Biol., 27, 3350–3357, doi: 10.1111/gcb.15644
Zhang, X., Harris, M. T., and Basaran, O. A. (1994). Measurement of dynamic surface tension by a growing drop technique. J. Colloid Interface Sci. 168, 47–60. doi: 10.1006/jcis.1994.1392
Keywords: surface tension, ecohydrology, forest hydrology, throughfall, stemflow, biogeochemical processes, urban tree
Citation: Noren BJ, Lewis NR, Tonello KC, Ilek A and Van Stan JT (2023) Hypothesis and theory: Do trees “release the tension” in rainwater? Surface tension reduction in throughfall and stemflow from urban trees. Front. For. Glob. Change. 6:1315936. doi: 10.3389/ffgc.2023.1315936
Edited by:
Daniel Limehouse McLaughlin, Virginia Tech, United StatesReviewed by:
Eleinis Ávila-Lovera, The University of Utah, United StatesSeyed Mohammad Moein Sadeghi, University of Florida, United States
Copyright © 2023 Noren, Lewis, Tonello, Ilek and Van Stan. This is an open-access article distributed under the terms of the Creative Commons Attribution License (CC BY). The use, distribution or reproduction in other forums is permitted, provided the original author(s) and the copyright owner(s) are credited and that the original publication in this journal is cited, in accordance with accepted academic practice. No use, distribution or reproduction is permitted which does not comply with these terms.
*Correspondence: John T. Van Stan II, cHJvZmVzc29yLnZhbnN0YW5AZ21haWwuY29t