Corrigendum: High emissions or carbon neutral? Inclusion of “anthropogenic” forest sinks leads to underreporting of forestry emissions
- 1School of Environmental Science, University of Guelph, Guelph, ON, Canada
- 2International Program, Natural Resources Defense Council, New York, NY, United States
- 3Department of Physical and Environmental Sciences, University of Toronto, Toronto, ON, Canada
- 4Faculty of Forestry and Environmental Management, University of New Brunswick Fredericton, Fredericton, NB, Canada
Recent research has shown forest-related emissions reported in national greenhouse gas inventories are much lower than global estimates from models summarized in Intergovernmental Panel on Climate Change reports. A substantial part of this discrepancy could be explained by conceptual differences in what is counted as part of the anthropogenic forest carbon sink and the way countries report on their forest harvesting sectors. With Canada as a case study, we used published National Inventory Report and Common Reporting Format tables to isolate emissions and removals directly associated with forestry from those associated with forests more broadly. Forestry-related factors that affect CO2 emissions and removals include tree harvesting, post-harvest forest regeneration and growth, and carbon storage in long-lived harvested wood products. We found that between 2005 and 2021, forestry in Canada represented a net source of carbon (annual mean = 90.8 Mt. CO2e), and that total area logged was a significant predictor of net forestry emissions. In contrast, Canada’s NIR reported a small net carbon sink during the same time period (annual mean = −4.7 Mt. CO2e). We show this discrepancy can be explained by Canada’s GHG reporting approach that claims GHG emissions from wildfires are natural, but GHG removals from forests at the age of commercial maturity, despite being primarily natural disturbance origin, are anthropogenic. This reporting approach may lead to climate mitigation policies that are ineffectual or detrimental to reducing net carbon in the global atmosphere.
1 Introduction
Climate change is an urgent global issue that requires immediate, effective action. With the increasing frequency and intensity of extreme weather events, rising temperatures, and the loss of critical ecosystems, it is imperative for all countries to reduce their greenhouse gas (GHG) emissions across all sectors (Ripple et al., 2022).
Forests play an important role in climate change mitigation due to their large and dynamic carbon stocks. For Canada, which is a country with one of the largest quantities of forest cover in the world (nearly 9% of the world’s total forest area), forest carbon dynamics play a particularly significant role in its national contribution to global GHG emissions. Canada, along with Brazil, China, the Democratic Republic of the Congo, Russia, and the United States, account for 51% of global gross forest carbon emissions and 56% of global gross removals (Harris et al., 2021). In 2021, leaders from 141 countries signed the Glasgow Leaders’ Declaration on Forests and Land Use. Under the Declaration, signatories committed to work collectively to halt and reverse forest loss and land degradation by 2030 (United Nations Climate Change Conference UK, 2021), recognizing the role the world’s forests play in GHG emissions and removals is critical to achieving international climate targets.
As stated in Article 2 of the United Nations Framework Convention on Climate Change (United Nations Conference on Environment and Development, 1992), all countries should be working toward “stabilization of GHG concentrations in the atmosphere at a level that would prevent dangerous anthropogenic interference with the climate system.” Parties to the United Nations Framework Convention on Climate Change must submit national greenhouse gas inventories (NGHGI) quantifying emissions and removals occurring within their geographic territories. NGHGIs help inform opportunities for policy to mitigate emissions in sectors under human control. The Intergovernmental Panel on Climate Change (IPCC) has developed guidelines for estimating anthropogenic GHG emissions and removals (IPCC, 2006, 2014a, b) to help countries address the challenge of distinguishing between anthropogenic and non-anthropogenic GHG sources and sinks associated with land management (Ogle et al., 2018). In 2006, the IPCC adopted the Managed Land Proxy (MLP) method. Under the MLP, a country identifies land that it considers “managed” and then reports all emissions and removals from that land, including both anthropogenic and natural flows. Annex 1 of the IPCC guidelines defines “managed land” as “land where human interventions and practices have been applied to perform production, ecological, or social functions” (IPCC, 2022, p. 1795). In 2019, the IPCC refined the methodology, allowing countries to disaggregate (i.e., exclude and report elsewhere) emissions and removals associated with natural disturbances, in order to help reduce the interannual variability in GHG flux reporting. The IPCC indicated, however, that it is good practice for countries adopting this disaggregated approach to still report all emissions and removals from managed lands, and to ensure that disaggregation of natural disturbance emissions is carried out in a balanced way (IPCC, 2019).
Recent research has shown large deviations between emissions reported in NGHGIs versus modeled atmospheric impacts. For example, Grassi et al. (2018) found that NGHGI estimates compiled from reporting countries were 4.3 GtCO2e yr.−1 lower than global estimates from models summarized in IPCC reports. This discrepancy is significant-larger than the total annual carbon emissions of India (Grassi et al., 2018). While there are inherent discrepancies and uncertainties associated with different models (e.g., bookkeeping models, dynamic vegetation models, and national greenhouse gas inventories) to estimate global land use, the authors suggest a substantial part of this discrepancy (approximately 3.2 GtCO2e yr.−1) may be explained by conceptual differences in what is counted as part of the anthropogenic forest carbon sink and the way countries report on their forest harvesting sectors (Harris et al., 2021; Grassi et al., 2023).
Transparently and accurately reporting GHG emissions from the forestry sector is necessary to inform effective forestry-related policies and nature-based climate solutions (e.g., Drever et al., 2021; Moomaw and Law, 2023). Our objective here is to quantify conceptual biases in how GHG emissions and removals are attributed to the forestry sector, using Canada as a case study. To address our research question, we calculated emissions directly associated with the forestry sector on Forest Land Remaining Forest Land (FLFL) using data reported in Canada’s NGHGI (known as the National Inventory Report or NIR) and common reporting format (CRF) tables (United Nations Framework Convention on Climate Change (UNFCCC), 2022b). Our analysis considers carbon emissions (sources) driven by tree harvesting, and net carbon removals (sinks) driven by post-harvest forest regeneration, and by the carbon stored in long-lived wood products. This study has international importance, as transparent NGHGIs are critical to informing strategies that governments use to meet emissions reduction targets. Furthermore, these findings concerning emissions impacts of Canada’s forestry sector point to opportunities for reducing land-use sector emissions in many other countries.
2 Materials and methods
2.1 Study area
This study covers Canada’s “managed forest” as delineated by Natural Resources Canada (NRCan; see Ogle et al., 2018; Commissioner of the Environment and Sustainable Development to the Parliament of Canada, 2023). NRCan defines the managed forest as: (i) lands managed for the sustainable harvest of wood fiber (e.g., saw logs and pulpwood) or wood-based bioenergy, (ii) lands under intensive protection from natural disturbances (e.g., fire and insect suppression), and (iii) protected areas, such as national and provincial parks that are managed to conserve ecological values (Figure 1). Managed forests in Canada tend to have a more uniform, structured landscape than unmanaged forests (Kurz et al., 2008). In the managed forest, areas allocated for industrial timber harvesting, usually through forest management plans, can be subjected to a range of post-harvest silvicultural techniques, including planting, seeding, thinning, and aerial herbicide spraying, and can also be left for natural regeneration. In contrast, the unmanaged forest has not been logged at an industrial scale, nor is industrial logging planned in this zone. It tends to be primarily shaped by natural disturbance, including wildfire, insect outbreaks, and storm events (Landry and Ramankutty, 2015). Much of the unmanaged forest is north of the managed boreal zone in Canada and has low productivity and is largely inaccessible.
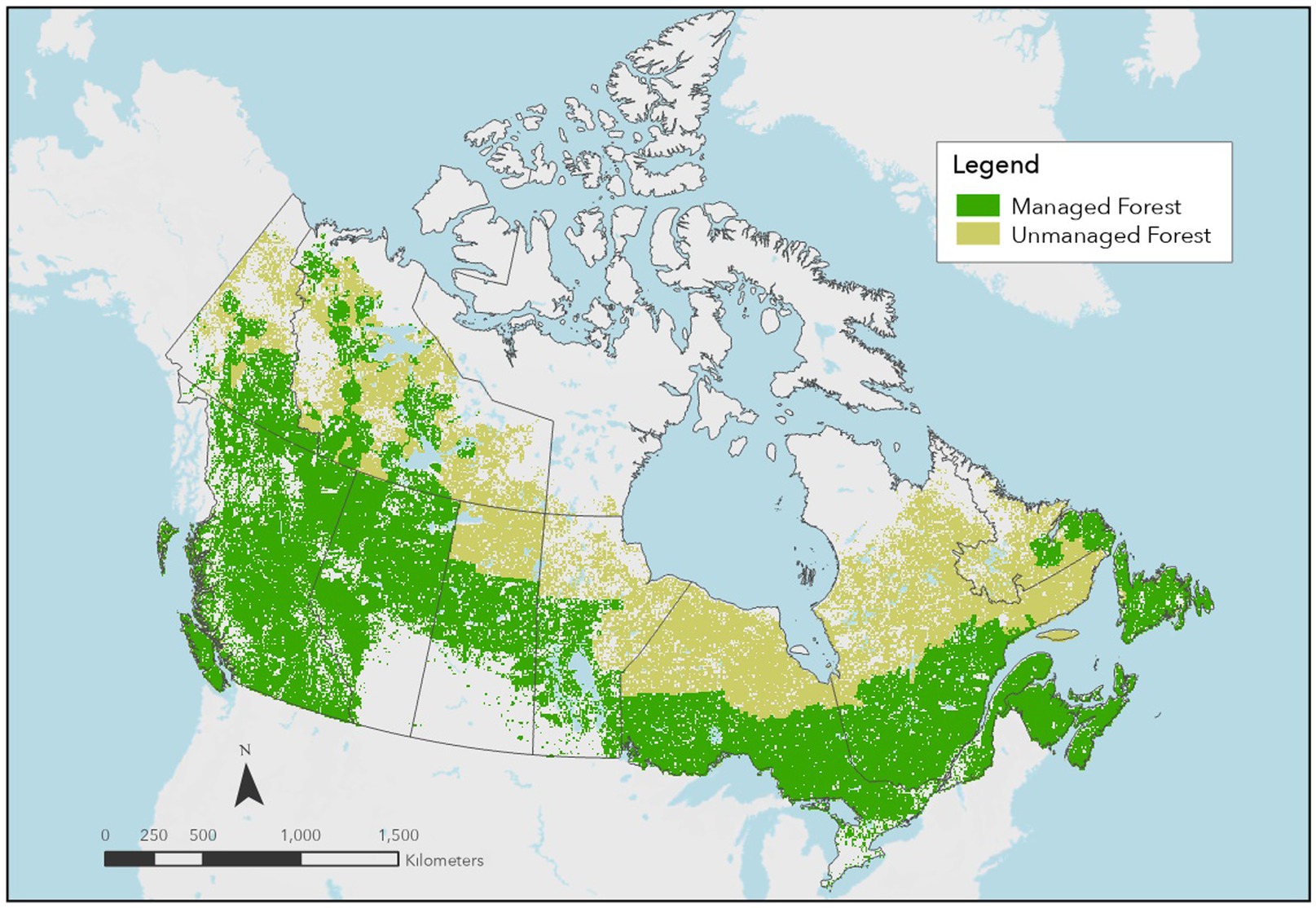
Figure 1. Approximate distribution of managed forest (dark green) and unmanaged forest (light green) in Canada. Managed forest and unmanaged forest in Canada cover approximately 232 million hectares and 115 million hectares, respectively (Natural Resources Canada, 2023).
The managed forest varies by province and territory across Canada. In British Columbia, Alberta, New Brunswick, Nova Scotia, and Prince Edward Island, all forest land is included in the managed forest, whereas in Saskatchewan, Manitoba, Ontario and Quebec, forested land north of the boundary of fire suppression is excluded (Ogle et al., 2018). All forests on the island of Newfoundland are included, but forested lands in Labrador that are not managed for timber harvesting are excluded. In the Yukon and the Northwest Territories, only areas designated for forest harvesting operations and fire suppression zones around communities are included. Nunavut does not have any land considered managed forest (Ogle et al., 2018). The managed forest in Canada overall - approximately 226 million hectares (in the year 2015) – contains an estimated 50.6 Petagrams of carbon (Pg C; Metsaranta et al., 2017) and an estimated carbon density of 224 Megagrams of carbon per hectare (Mg C/ha; Stinson et al., 2011). The total area of unmanaged forest in Canada is approximately 118 million hectares located primarily in northern regions of the country where there is limited or no road access.
2.2 Data collection
We collected and compiled data from the 2023 NIR and associated CRF tables [Environment and Climate Change Canada (ECCC), 2023] associated with the managed forest in Canada. Specific data used in this study are provided as Supplementary Tables S1–S5. The CRF tables, used by the United Nations Framework Convention on Climate Change, provide a consistent format for countries to report their GHG emissions and removals. When data were reported as mass of carbon, we converted it to mass of CO2 by multiplying it by the molecular weight of CO2 (44) and dividing by the atomic weight of carbon (12; IPCC, 2006, 2019). We used GHG emission and removal information for 2005–2021 to calculate annual net forestry emissions from Canada’s managed forest.
2.3 Determining net forestry emissions
Our definition of annual net emissions associated with forestry comprises the sum of the flows that can reasonably be directly attributed to forestry activities on FLFL (see Bramley and Saul, 2022), either immediate or delayed, including: carbon harvested from the forest in a given year, the net carbon stored in long-lived wood products, and the net carbon sequestered by forest regeneration (‘net’ refers to the balance between emissions and removals associated with regeneration and growth after harvesting disturbance; see Table 1).
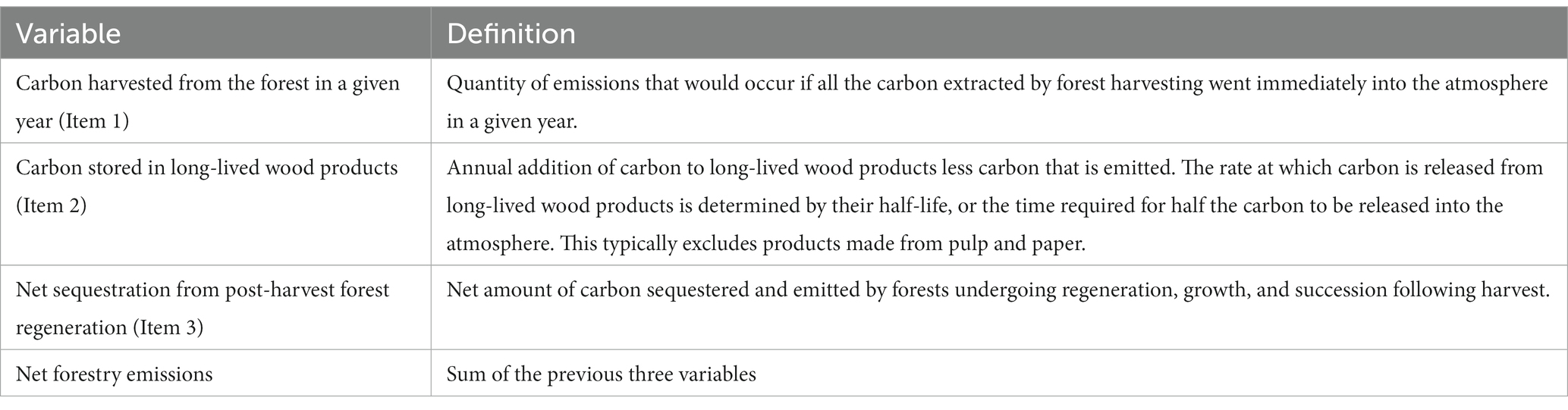
Table 1. Definitions of variables used in calculations of net forestry emissions (see text for details).
The net carbon in long-lived wood products derives from the difference between the amount of carbon that is added to the product pool in a given year minus the carbon released. The carbon that is eventually released from long-lived wood products is based on the half-life of the wood product (i.e., the amount of time it takes for one-half of the carbon to be released into the atmosphere) and generally excludes pulp and paper products [see Table A3.5–7 of the NIR; Environment and Climate Change Canada (ECCC), 2023].
2.4 Data analyses
2.4.1 Estimating CO2e in material harvested from the forest in a given year (item 1)
We calculated the total amount of carbon harvested from FLFL by forestry operations in a given year; namely, the “potential” emissions that would occur if all carbon in the material harvested from the forest was instantly oxidized in a given year (Table 2). This value is positive as it represents an input of CO2e to the atmosphere. The carbon in all FLFL harvested wood in a given year was determined using data in Supplementary Table S1. Specifically, this value is the sum of the total harvested wood product (HWP) both produced and used domestically and exported internationally. As our analysis was restricted to FLFL, we excluded carbon in wood that resulted from land use change and from firewood removed from non-forested land.
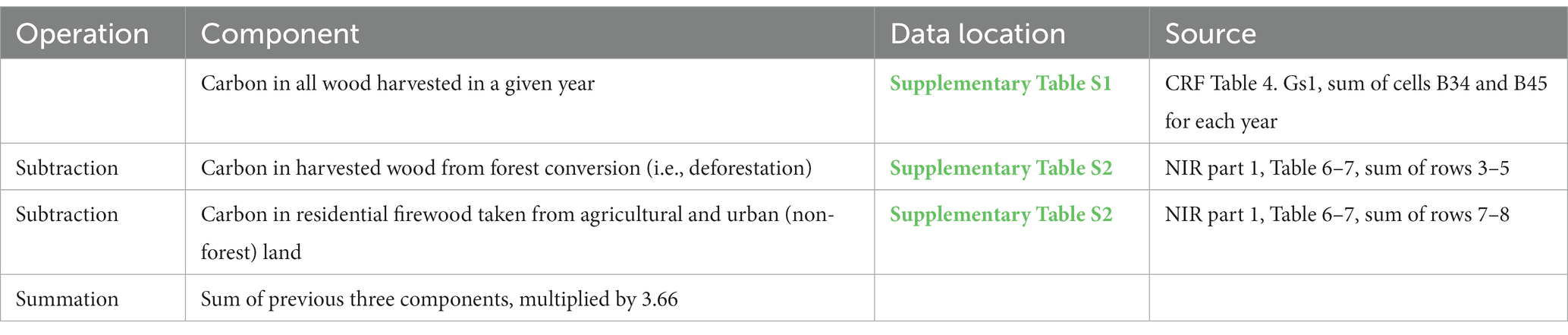
Table 2. The components of Item 1: estimating CO2e in material harvested from the forest in a given year (see text for details).
Supplementary Table S2 provides emissions data for residential firewood obtained from forest land, cropland, and settlements, respectively. To effectively capture the emissions on FLFL, we removed emissions data from firewood that was taken from cropland or settlements. The total carbon stock of HWP from land use change to croplands, wetlands, or settlements (i.e., LUC) can be found in Supplementary Table S2 as the sum of the rows listing forest conversion amounts. These values represent the carbon stock in the HWP pool derived from forest conversion to these different land use forms and hence are excluded from FLFL (Table 2).
2.4.2 Estimating CO2e storage in long-lived wood products (item 2)
Item 2 represents the net CO2e transferred to and stored in the harvested wood carbon pool from FLFL that is being kept out of the atmosphere in the form of long-lived wood products. This value is typically negative, as it represents a removal of CO2e. This pool of carbon is dynamic: on an annual basis, carbon is stored in harvested wood, while a certain amount of this carbon is emitted back to the atmosphere as the wood decays, based on the reported half-life of HWPs. To reflect this dynamic relationship, Item 2 was calculated as the difference between the actual calculated end-of-life emissions (described below) and the ‘potential’ emissions (equivalent to Item 1 and indicative of hypothetical carbon oxidation from the forest in a given year).
To calculate the actual end-of-life emissions, we took the total emissions from end-of-life HWP (Supplementary Table S3) and subtracted emissions from HWP originating from forest conversion (Supplementary Table S4), and carbon in residential firewood taken from agricultural and urban (non-forest) land (Supplementary Table S2). Carbon stock inputs for residential firewood are obtained from forest land, cropland, and settlements, respectively in the NIR. Since the study is restricted to emissions on FLFL, we removed emissions data from firewood that was taken from cropland or settlements. A description of the variables utilized to calculate ‘end-of-life’ and ‘potential’ emissions can be found in Table 3. Notably, this does not include the fossil fuel emissions attributed to the cutting and hauling activities associated with harvest to produce HWPs.
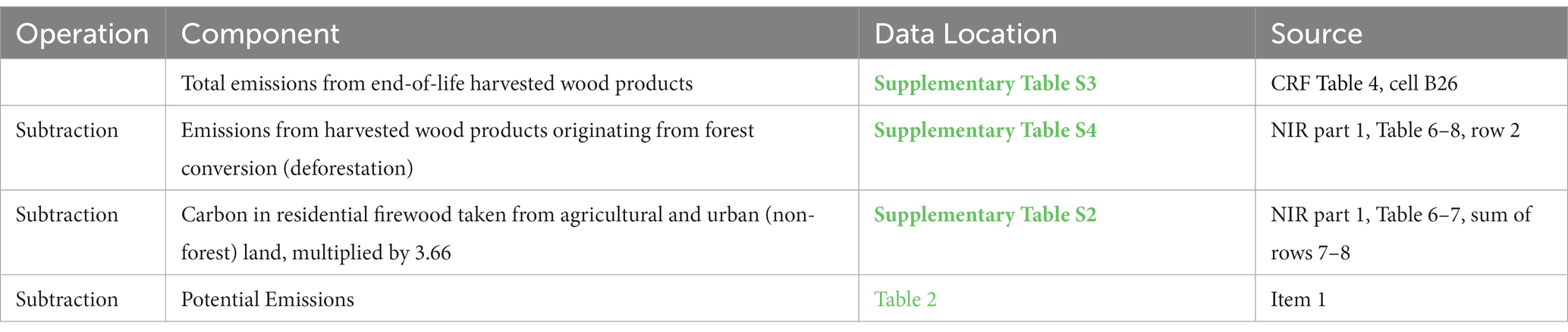
Table 3. The components of Item 2: estimating CO2e storage in long-lived wood products (see text for details).
2.4.3 Estimating CO2e sequestration from forest regeneration on FLFL after forest harvesting (item 3)
The final variable constituting net forestry emissions is the CO2e flux from FLFL after forest harvesting operations occur (Table 4). This value is negative as it represents a removal of CO2e. This value comprises the difference between CO2e removals from the atmosphere by tree regrowth in the years following forest harvest and the post-harvest emissions from the decay of dead wood, litter, and soil organic matter, as well as smaller amounts of emissions from certain activities directly related to forest regeneration, namely controlled burning and soil drainage (controlled burning emits methane and nitrous oxide, which means that the total is a CO2e rather than a pure CO2 figure), as calculated by Natural Resources Canada’s Carbon Budget Model (Kull et al., 2016). The value for forest regeneration was obtained by utilizing the “Anthropogenic component, past forest management activities” reported in Supplementary Table S5.

Table 4. The component for Item 3: estimating CO2e sequestered by forest regeneration on FLFL after forest harvesting (see text for details).
2.4.4 Estimating net CO2e forestry emissions
Overall net emissions from forestry are calculated as the sum of the three items detailed above; that is:
Here “Net Forestry Emissions” represents our estimate of the emissions that can be attributed to forestry sector activities, where: “Item 1” represents the carbon in materials harvested from forests in a given year; “Item 2” represents the net carbon added to the long-lived product pool, and ‘Item 3’ represents the net flux attributed to post-harvest forest regeneration. Where Item 1 is a positive value, Items 2 and 3 are typically negative values.
Further, to understand trends in net forestry emissions over time, we used data from NRCan on total area harvested per year. We used simple linear regression to evaluate the relationship between net forestry emissions and harvested area using the statistical software R (v. 3.4.2; R Core Development Team, 2017). We also compared and contrasted our net emissions from forestry activities to the reported NIR values.
3 Results
3.1 Net CO2e emissions and removals attributed to forest harvesting
Annual emissions from forest harvesting on FLFL lands (Figure 2A) varied between 120 and 200 Mt. CO2e over the 16-year sample period (mean = 154.3 Mt. CO2e). The highest amount was in the first year of the record (2005), with the lowest amount during the economic downturn of 2009. The pool of carbon in HWP (Figure 2B) was greatest in 2005–2007 and was only a net source in 2009 (5.9 Mt. CO2e; mean = −21.3 Mt. CO2e). Net carbon removals from post-harvest regrowth (Figure 2C) were lowest during 2005–2007, with more carbon progressively being removed via regrowth through 2021 (p < 0.001, R2 = 0.80, n = 16). This net regrowth represented a substantial net carbon sink throughout the study period (mean = −42.2 Mt. CO2e). Overall, net forestry emissions (Figure 2D) were a net source of carbon to the atmosphere in every year (mean = 90.8 Mt. CO2e). Net forestry harvest emissions were highest during 2005–2007 but experienced a significant declining trend over time (p < 0.001, R2 = 0.67, n = 16).
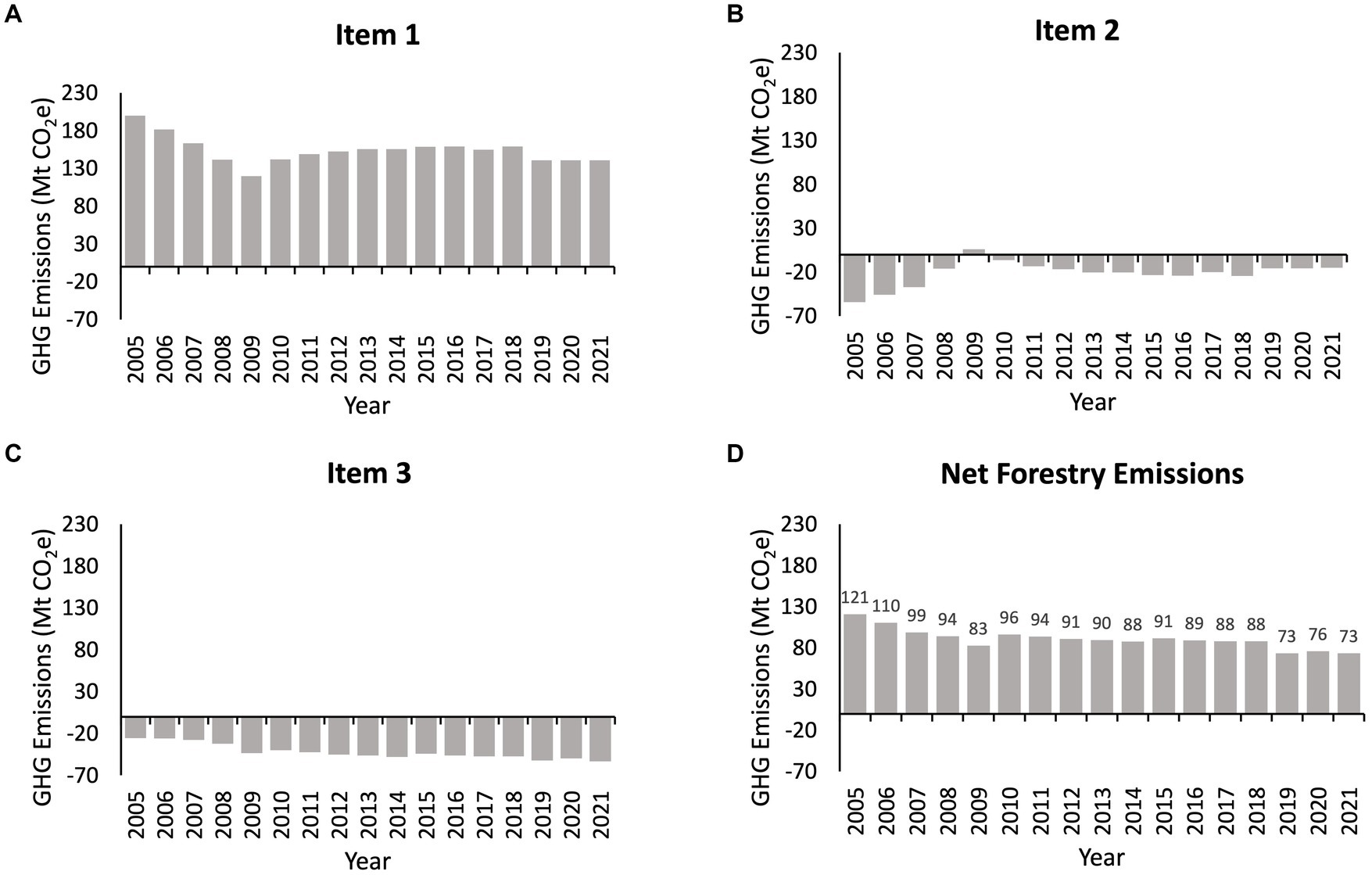
Figure 2. Emissions and sinks due to forestry activities in Canada. (A) The amount of CO₂e extracted from “forest land remaining forest land” (FLFL) via forest harvesting, (B) the CO₂e of the pool of carbon in wood harvested from FLFL that is being kept out of the atmosphere in the form of long lived wood products, (C) net amount of CO₂e removed from the atmosphere by tree regrowth on FLFL, and (D) the total net forest harvest emissions by year (2005–2021) as a sum of the values from figures (A–C). Negative values indicate a carbon sink while positive values indicate a carbon source.
3.2 Comparing net forestry sector emissions to reported NIR values
When comparing net forestry sector emissions estimated in this study to the combined net emissions from FLFL and HWP reported in the NIR there were large discrepancies. The mean value for net forestry sector emissions in this study was 90.8 Mt. CO2e, representing a large net source of carbon to the atmosphere, whereas mean values reported in the NIR indicated a slight sink of −4.7 Mt. CO2e. The largest value reported by the NIR (one of only 3 years in this study where the NIR reported a positive CO2e value) came in 2005, where the NIR reported a value of 9.1 Mt. CO2e. This year also represented the largest discrepancy between the reported NIR value, and the value calculated in this study (119.8 Mt. CO2e), with a difference of 110.7 Mt. CO2e. The largest sink reported by the NIR came in 2009 with a value of −20.3 Mt. CO2e. In contrast, the value we report for this year was 78.7 Mt. CO2e (more than 99 Mt. larger than the NIR estimate). Year-to-year variation in the values reported by the NIR (with a range of 29.5 Mt. CO2e) were less than those reported in this study (with a range of 47.4 Mt. CO2e; Figure 3).
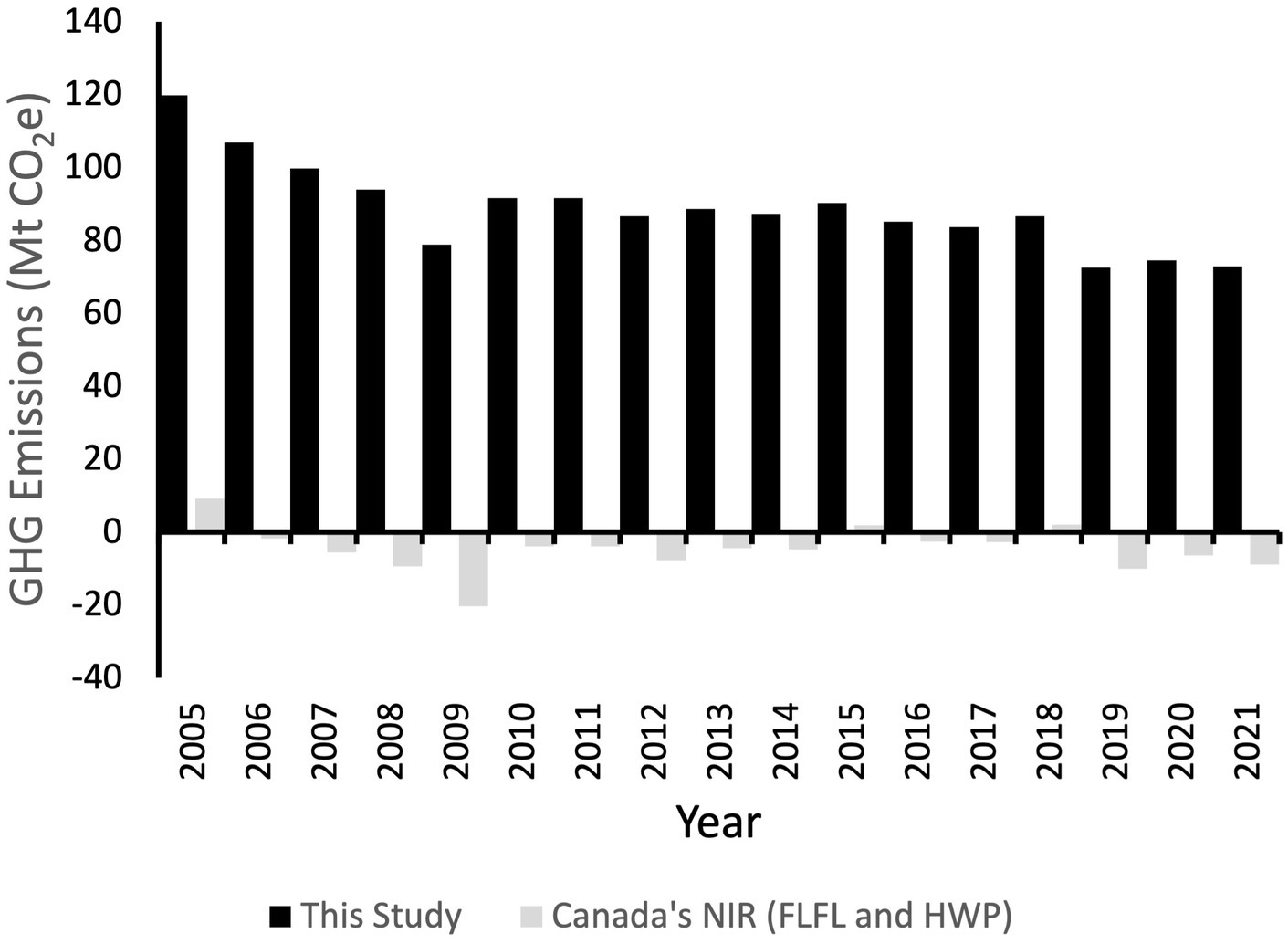
Figure 3. Comparison between net forest harvest emissions calculated in this study (black) and the combined values for the emissions attributed to forest land remaining forest land (FLFL) and harvested wood products (HWP) as reported in Canada’s National Inventory Report (gray).
We also explored trends in the forestry sector in the context of total area harvested in the managed forest. Across the time period of 2005–2021 (Figure 4), we found that the total area harvested in the managed forest was a significant predictor of net forestry emissions (p < 0.001; R2 = 0.62; F = 25.05). Both net forestry sector and area harvested showed a strong decrease up to c. 2009, but then remained approximately consistent thereafter.
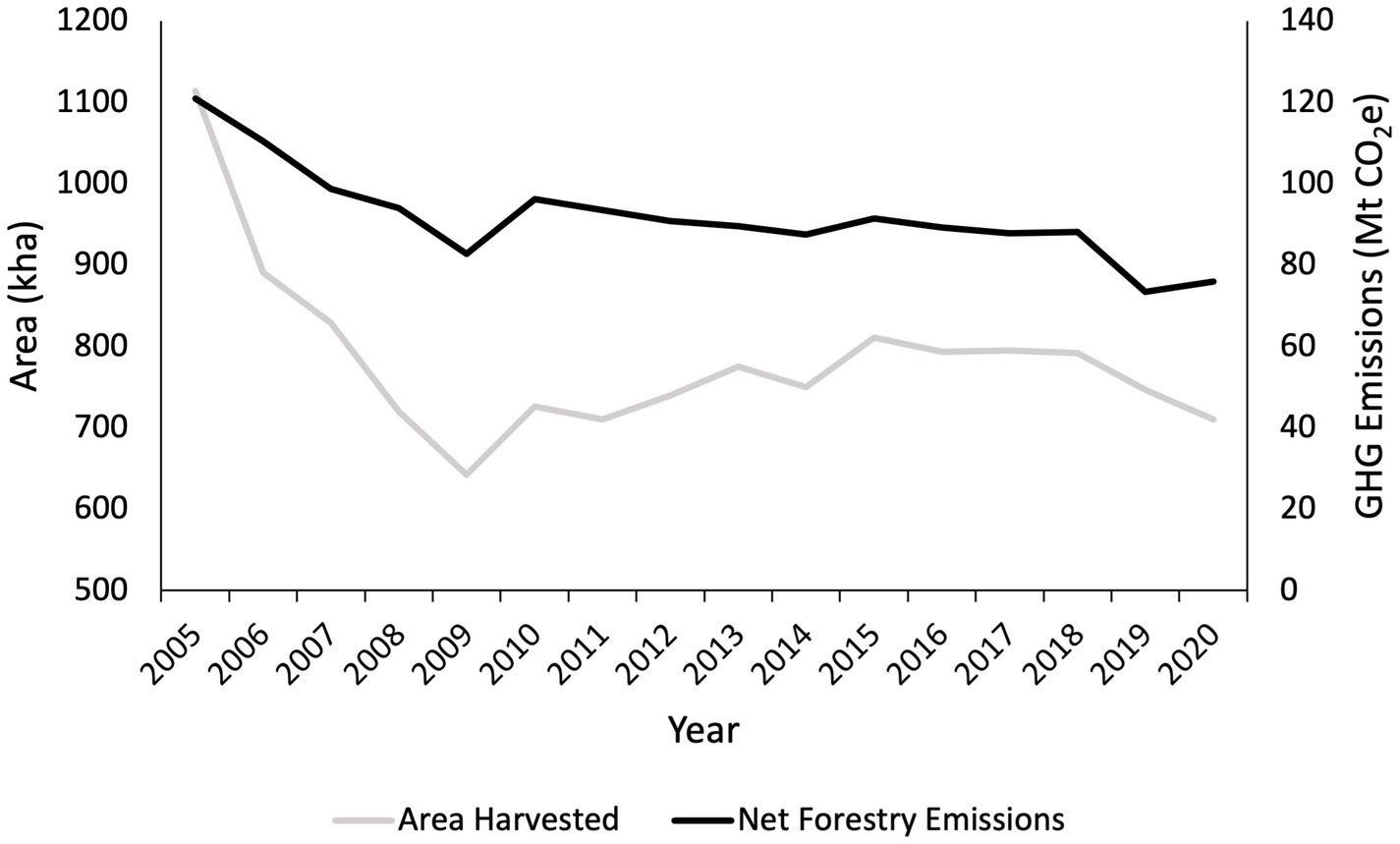
Figure 4. Trends between net forestry emissions (this study) and area harvested in Canada’s managed forest from 2005 to 2020. Statistical data provided by the Canadian forest service on forests and forestry is only currently available until 2020. The downward trend in net forest harvest emissions (circa 2009) is correlated with reduction in harvest levels.
4 Discussion
We calculated the net emissions attributed to the forestry sector in the Canadian managed forest from 2005 to 2021 and found that the sector was a sizable net source of emissions to the atmosphere, with annual mean GHG emissions of 90.8 Mt. CO2e. These emissions are broadly comparable to other high-emitting national sectors such as electricity, agriculture, and show considerably higher net emissions than those reported in the NIR for combined net flux from forest land and harvested wood products, which averaged −4.7 Mt. CO2e per year during the same time period.
We propose that this discrepancy primarily results from Canada’s approach to reporting wildfire GHG sources as “natural” emissions and GHG sinks associated with forests after the age of commercial maturity as “anthropogenic” removals. According to Canada’s reporting methods, if a stand in the managed forest has attained commercial maturity (and therefore is actively considered for logging), that forest’s carbon removals can be reported as anthropogenic [Environment and Climate Change Canada (ECCC), 2023]. Since 2018, the NIR has used regionally specific rotation age based on factors such as forest management practices, species distributions, and stand dynamics. The resulting estimated minimum return period to commercial maturity ranges from 45 to 99 years, with a national average of 76 years. The designation of these forest sinks after commercial maturity as ‘anthropogenic’ obfuscates emissions and removals directly related to the forestry sector.
Canada’s NIR excludes natural disturbances to reduce large year-to-year fluctuations in emissions caused by wildfires and insect outbreaks (IPCC, 2019). In the 2019 NIR, nearly 25% of the managed forest area was excluded based on this criterion, with stand-replacing wildfires being the most significant contributor to excluded emissions. Canada’s 2022 inventory report indicates that between 2005 and 2021, mean annual emissions from major wildfires excluded from the inventory were 114 Mt. CO2e, while mean annual removals from post-fire regrowth up to commercial maturity (also excluded from the inventory) were − 37 Mt. CO2e. Thus, between 2005 and 2021, a net annual average of 77 Mt. CO2e were excluded from the NIR reporting (Figure 5).
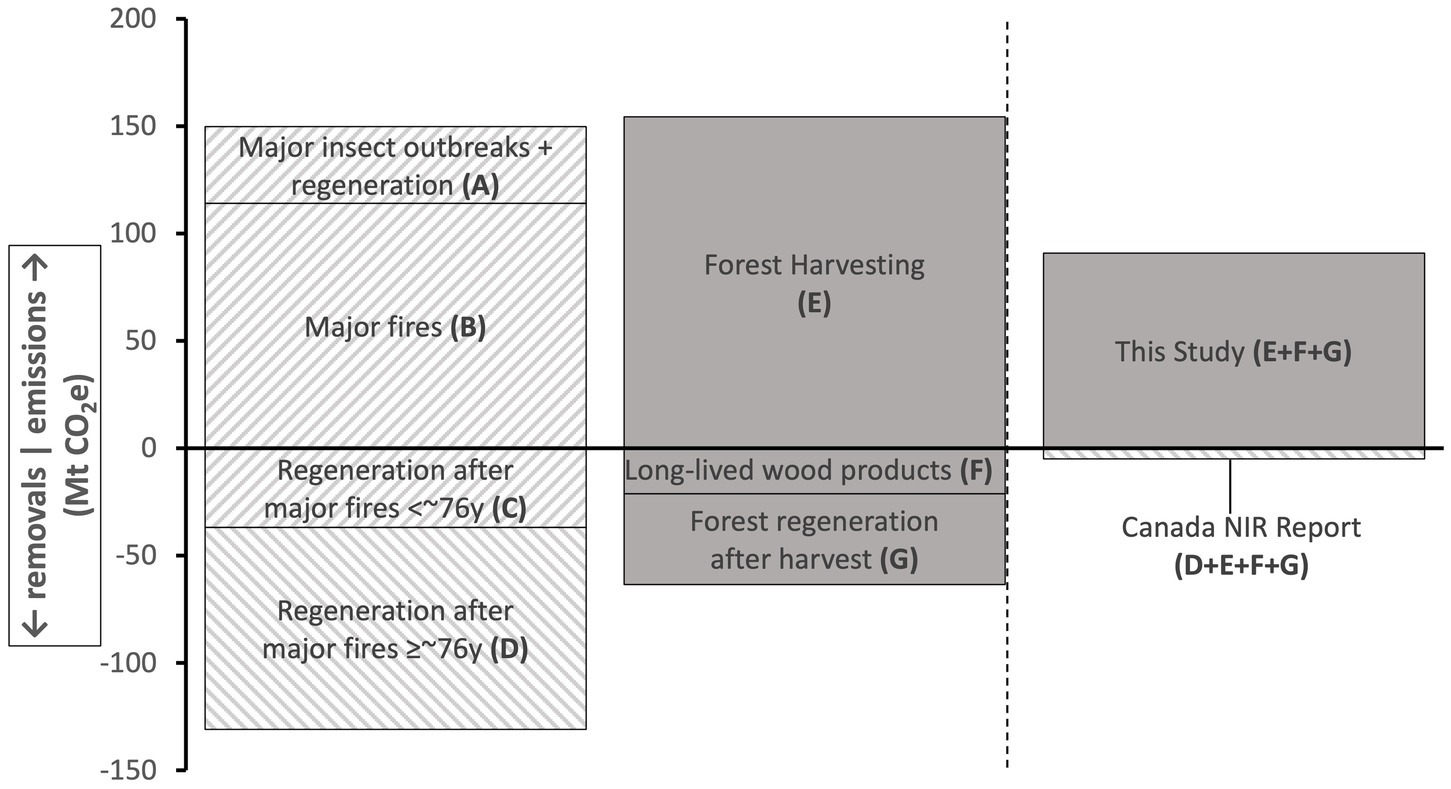
Figure 5. The components of forest carbon flows in Canada’s NIR over the 16-year study period and their average values. The left-hand column shows major wildfires, insect outbreaks, and the subsequent removals from regrowth. The middle column shows emissions and removals directly attributable to forestry. The right column shows net calculations in this study and in Canada’s NIR reporting. NIR emissions portray the managed forest as almost carbon neutral due to the NIR’s disaggregation of significant natural disturbance emissions, while still including natural removals from forest regeneration and growth.
The IPCC guidelines describe two methodological approaches to estimate forest carbon stock changes in NGHGI: the stock-change and gain-loss methods (IPCC, 2006). Countries following IPCC’s stock-change reporting approach (e.g., US, Sweden, Japan, Spain, Norway, and Germany) implicitly account for CO2e emissions due to wildfires and other natural disturbances because the net change in carbon stocks is estimated from successive measurements and estimates. Wildfires (and other natural disturbances) decrease carbon stocks in the forest, and the inventory data already reflects the carbon loss from disturbances because only carbon remaining in the forest is estimated. Countries following the gain-loss approach estimate emissions by multiplying the area of different land use change classes (characterized as activity data) by the responses of carbon stocks for those classes (described as emission factors; McRoberts et al., 2018). Canada and Australia follow the gain-loss reporting approach. They are also the only countries to exclude emissions from natural disturbances such as wildfires, insect damage and windstorms, in their NGHGIs (Grassi et al., 2023). According to Grassi et al. (2023), the average net emissions that were disaggregated for 2000–2020 were approximately 104 Mt. CO2e yr.−1 in Canada and 39 Mt. CO2e yr.−1 in Australia.
Disaggregation of natural emissions is consistent with the 2019 refinements of the 2006 IPCC guidelines, provided that a balanced approach is employed (IPCC, 2019). This means that if lands with wildfire emissions are excluded from the inventory’s calculation of anthropogenic emissions/removals, those lands should only be re-inserted into the total when removals from subsequent tree regrowth have fully balanced the wildfire emissions (IPCC, 2019 refinement, 2.72). Based on our analysis, this balance is not achieved in Canada’s NIR, with a net average of 77 Mt. CO2e emissions. While Canada excludes stand-replacing wildfire emissions from its NGHGI, it includes removals from post-fire tree regrowth in its annual inventory for stands that have reached commercial maturity. This approach adds a large carbon sink to the reporting, and as a result, the forestry sector as a whole is assumed to be a small carbon sink. Canada’s application of the natural disturbance provision in the 2019 refinement of IPCC guidelines thus places it as an outlier regarding GHG reporting practices of other Annex I countries.
Our analysis focuses on the importance of how anthropogenic sinks are defined and reported in the NIR (not whether or not some or all wildfire emissions need necessarily be reported as anthropogenic), however, we suggest this decision also requires more investigation. A UNFCCC expert review team found that Canada’s 2021 NIR did not provide a satisfactory justification for the assumption that all stand-replacing wildfire emissions in managed forests are natural [United Nations Framework Convention on Climate Change (UNFCCC), 2022a,b]. While these findings are variable across the country (Taylor et al., 2020), it is estimated that almost half of the wildfires in Canada are started by human activity, whether through negligence, accident or on purpose (Tymstra et al., 2020). There is also uncertainty about the extent to which forest management activities contribute to wildfire, for example, by creating forest access roads for hunters, anglers, and recreationists (Taylor et al., 2020). Fire suppression strategies also play an essential role in wildfire-related emissions (Tymstra et al., 2020). Hanes et al. (2019) argued that only approximately 10% of the area burned is attributable to human activity in Canada, despite the importance of human-caused ignition of wildfires, because there is usually little or no suppression of wildfires that ignite in isolated areas where there aren’t many people. In 2023, Canada’s GHG emissions associated with wildfire were unprecedented, releasing an estimated 2 billion tones of CO2 (with a preliminary estimate of 850 million tones from the managed forest). The increasing frequency and intensity of wildfires anticipated to occur due to climate change further underscores the need for an accurate understanding of forests’ role in GHG emissions and removals and which of these emissions we have the greatest control to manage (Jones et al., 2020; Dahl et al., 2023).
The reporting of emissions associated with the forestry sector are further complicated by how combustion of fossil fuels from logging, hauling, processing, and shipping are reported in the NIR. Specifically, the CO2e we calculated do not include emissions attributed to the combustion of fossil fuels from forestry operations. While these operational and production emissions appear to be accounted for in the NIR, they cannot be explicitly linked to the forestry sector as they are reported in other categories. For example, the fossil fuel emissions attributed to pulp, paper, and print were 6,860 kt CO2e in 2021, but these were reported in the energy sector of the NIR (Section 3.2.5.1).
Additionally, there is an ongoing debate on the real or potential substitution role of HWP, yet many studies assessing forest management or products for their climate change mitigation potential depend on a suite of assumptions that the literature either does not support or only partially supports (Howard et al., 2021). Recent analyses suggest the actual mitigation benefits of HWP substitution may be overestimated by 2–100 times due to these assumptions (Harmon, 2019; Howard et al., 2021). For example, assumptions related to substitution effects include that (a) changes in harvest or production rates will lead to a corresponding change in wood product consumption, as well as an opposite response in concrete, steel, or fossil fuel use, (b) wood building products are substitutable for concrete and steel, (c) the same mix of products could be produced from increased harvest rates of a given area, and (d) there are no market responses to increased wood use (e.g., that a subsequent fall in the price of steel and concrete does not increase the use of such materials elsewhere; Howard et al., 2021). Since perceived HWP substitution benefits are often incorporated into forestry sector GHG accounting and life cycle analyses, overestimated substitution effects could lead to policies misaligned with GHG mitigation goals. An improved understanding of HWP substitution dynamics is needed to ensure mitigation policies based on forestry sector GHG accounting reflect the most appropriate, scientifically supported strategies.
Lastly, global land use CO2e fluxes are calculated according to various models and quantification methods including bookkeeping models and dynamic vegetation models. As the methodologies behind each model differ, so does the respective uncertainty with the CO2e flux estimates. The bookkeeping models provide a relatively simple representation of anthropogenic land use change impacts, treating only direct human-induced effects as contributing to net CO2e fluxes. Key sources of uncertainty in bookkeeping models arise from uncertain model parameters and inputs, spatial and temporal variability, and linkage across complex systems like climate and land use (Harris et al., 2021). In contrast, dynamic vegetation models estimate natural ecosystem processes like photosynthesis, respiration, and vegetation dynamics in more detail but do not fully represent key land management activities in their simulations of future scenarios. For example, dynamic vegetation models may not fully capture the effects of agriculture, deforestation, afforestation, or forest management practices on CO2 fluxes. The incomplete representation of anthropogenic land use change and management limits the ability of dynamic vegetation models to estimate human-induced CO2 emissions and uptake from managed lands accurately (Harris et al., 2021).
5 Conclusion
Our analysis demonstrates that the current approach used for accounting forest carbon sinks in Canada may, in part, explain why forest-related emissions reported in national greenhouse gas inventories are much lower than global estimates from models summarized in IPCC reports. More specifically, we demonstrate that Canada’s use of the IPCC’s natural disturbance provision creates a dubious anthropogenic forest carbon sink that leads to a bias toward underestimating the GHG emissions directly attributable to the forestry sector. This bias has important implications for meeting Canada’s enhanced Paris Agreement climate targets (to reduce its economy-wide greenhouse gas emissions by 40–45% below 2005 levels by 2030 and net-zero by 2050) and can be used as support for misaligned policy incentives. For example, arguments that forests better serve emissions reduction targets when logged and put into harvested wood products or burned as biomass depend on several assumptions, including that the forestry sector has nearly zero or negative emissions (Malcolm et al., 2020; Peng et al., 2023). In contrast, our analyses show that, in Canada, forestry activities are a relatively large source of GHG emissions (roughly equivalent to emissions from the energy sector).
How GHG emissions are reported in national inventory reports may play a pivotal role in determining whether countries adopt the necessary policies to mitigate climate change effectively. In its 2023 Synthesis Report, the IPCC urged mitigation measures “across all sectors” to meet global climate targets. It is thus imperative for governments to develop forest carbon accounting practices that clearly articulate the GHG emissions directly attributable to the forestry sector, and to use these reports to incentivize the development and implementation of emissions reductions strategies (Mackey et al., 2022; Moomaw and Law, 2023). If the forestry sector is recognized as a GHG emitter, policies and management approaches could more readily shift to include, for example, strategies such as longer harvest rotations, silvicultural methods that maintain more on-site biomass after harvests, reductions in harvest levels, and a decrease in the production of short-lived forest products. Further, regulating these forestry sector emissions could assist in driving economic innovation, such as the development of long-term and secure financial pathways for nature-based climate solutions (Seddon et al., 2021). Forests and other natural ecosystems provide the largest share of the “Land Use, Land-Use Change and Forestry” mitigation potential between 2020 and 2050. As a result, examining countries’ accounting practices in this sector is critical to capitalize on opportunities that encourage the ambition and transformational action needed to achieve global climate goals.
Data availability statement
The original contributions presented in the study are included in the article/Supplementary material, further inquiries can be directed to the corresponding author.
Author contributions
DB: Formal analysis, Investigation, Writing – original draft, Writing - review & editing. JB: Project administration, Supervision, Visualization, Writing – original draft, Writing – review & editing. JM: Validation, Visualization, Writing – review & editing. AT: Validation, Visualization, Writing – review & editing.
Funding
The author(s) declare financial support was received for the research, authorship, and/or publication of this article. This work was supported, in part, by the Frank E. and Seba B. Payne Foundation.
Acknowledgments
We thank our colleague, Matthew Bramley, who gave so generously his time and talents to this effort over 2 years, despite overwhelming health challenges. He played a pivotal role in developing the analysis presented in this paper. We dedicate this paper to Dr. Bramley, his legacy, and his family, with deep gratitude for his lifelong commitment to advancing effective climate action, promoting scientific integrity, and ensuring a livable future for all. Bramley passed away on September 24, 2022.
Conflict of interest
The authors declare that the research was conducted in the absence of any commercial or financial relationships that could be construed as a potential conflict of interest.
Publisher’s note
All claims expressed in this article are solely those of the authors and do not necessarily represent those of their affiliated organizations, or those of the publisher, the editors and the reviewers. Any product that may be evaluated in this article, or claim that may be made by its manufacturer, is not guaranteed or endorsed by the publisher.
Supplementary material
The Supplementary material for this article can be found online at: https://www.frontiersin.org/articles/10.3389/ffgc.2023.1297301/full#supplementary-material
References
Bramley, M. J., and Saul, G. (2022). Net Greenhouse Gas Emissions from Logging in Canada? Available online at: http://naturecanada.ca/report/what-are-net-ghgemissions-from-logging-in-canada (Accessed May 1, 2023).
Commissioner of the Environment and Sustainable Development to the Parliament of Canada. (2023). Forests and climate change. Available at: https://www.oag-bvg.gc.ca/internet/docs/parl_cesd_202304_01_e.pdf
Dahl, K. A., Abatzoglou, J. T., Phillips, C. A., Ortiz-Partida, J. P., Licker, R., Merner, L. D., et al. (2023). Quantifying the contribution of major carbon producers to increases in vapor pressure deficit and burned area in western US and southwestern Canadian forests. Environ. Res. Lett. 18:064011. doi: 10.1088/1748-9326/acbce8
Drever, C. R., Cook-Patton, S. C., Akhter, F., Badiou, P. H., Chmura, G. L., Davidson, S. J., et al. (2021). Natural climate solutions for Canada. Sci. Adv. 7:eabd6034. doi: 10.1126/sciadv.abd6034
Environment and Climate Change Canada (ECCC). (2023). National Inventory Report 1990–2021: Greenhouse gas sources and sinks in Canada. Retrieved from https://www.canada.ca/en/environment-climate-change/services/climate-change/greenhouse-gas-emissions/inventory.html
Grassi, G., Pilli, R., House, J., Federici, S., and Kurz, W. A. (2018). Science-based approach for credible accounting of mitigation in managed forests. Carbon Balance Manag. 13, 1–16. doi: 10.1186/s13021-018-0096-2
Grassi, G., Schwingshackl, C., Gasser, T., Houghton, R. A., Sitch, S., Canadell, J. G., et al. (2023). Harmonising the land-use flux estimates of global models and national inventories for 2000–2020. Earth System Sci. Data 15, 1093–1114. doi: 10.5194/essd-15-1093-2023
Hanes, C. C., Wang, X., Jain, P., Parisien, M. A., Little, J. M., and Flannigan, M. D. (2019). Fire-regime changes in Canada over the last half century. Can. J. For. Res. 49, 256–269. doi: 10.1139/cjfr-2018-0293
Harmon, M. E. (2019). Have product substitution carbon benefits been overestimated? A sensitivity analysis of key assumptions. Environ. Res. Lett. 14, 065008. doi: 10.1088/1748-9326/ab1e95
Harris, N. L., Gibbs, D. A., Baccini, A., Birdsey, R. A., De Bruin, S., Farina, M., et al. (2021). Global maps of twenty-first century forest carbon fluxes. Nat. Clim. Chang. 11, 234–240. doi: 10.1038/s41558-020-00976-6
Howard, C., Dymond, C. C., Griess, V. C., Tolkien-Spurr, D., and van Kooten, G. C. (2021). Wood product carbon substitution benefits: a critical review of assumptions. Carbon Balance Manag. 16, 1–11. doi: 10.1186/s13021-021-00171-w
IPCC (2006). “2006 IPCC guidelines for national greenhouse gas inventories” in Agriculture, Forestry and Other Land Use, Vol. 4. eds. H. S. Eggleston, L. Buendia, K. Miwa, T. Ngara, and K. Tanabe (Japan: GES, Prepared by the National Greenhouse Gas Inventories Programme).
IPCC. (2022). Annex II: Glossary [Möller, V., R. van Diemen, J.B.R. Matthews, C. Méndez, S. Semenov, J.S. Fuglestvedt, A. Reisinger (eds.)]. In climate change 2022: Impacts, Adaptation and Vulnerability. Contribution of Working Group II to the Sixth Assessment Report of the Intergovernmental Panel on Climate Change [H.-O. Pörtner, D.C. Roberts, M. Tignor, E.S. Poloczanska, K. Mintenbeck, A. Alegría, M. Craig, S. Langsdorf, S. Löschke, V. Möller, A. Okem, B. Rama (eds.)]. Cambridge University Press, Cambridge, UK and New York, NY, USA, (2897–2930).
IPCC (2014a). “2013 Supplement to the 2006 IPCC Guidelines for National Greenhouse Gas Inventories: Wetlands.” in eds. T. Hiraishi, T. Krug, K. Tanabe, N. Srivastava, J. Baasansuren, and M. Fukuda, et al. Switzerland. https://www.ipcc.ch/site/assets/uploads/2018/03/Wetlands_Supplement_Entire_Report.pdf
IPCC (2014b). “2013 Revised Supplementary Methods and Good Practice Guidance Arising from the Kyoto Protocol.” in eds. T. Hiraishi, T. Krug, K. Tanabe, N. Srivastava, J. Baasansuren, and M. Fukuda, et al. Switzerland. https://www.ipcc.ch/site/assets/uploads/2018/03/Wetlands_Supplement_Entire_Report.pdf
IPCC (2019) “Chapter 12: Harvested wood products”. in 2006 IPCC Guidelines for National Greenhouse Gas Inventories, Volume 4, Agriculture, Forestry and Other Land Use. H.S., Eggleston, L. Buendia, K. Miwa, T. Ngara and K. Tanabe Switzerland. https://www.ipcc.ch/site/assets/uploads/2018/03/Wetlands_Supplement_Entire_Report.pdf
Jones, M. W., Smith, A., Betts, R., Canadell, J. G., Prentice, I. C., and Le Quéré, C. (2020). Climate change increases the risk of wildfires. Sci. Brief Rev. 116:117.
Kull, S.J., Rampley, G.J., Morken, S., Metsaranta, J.M., Neilson, E.T., and Kurz, W.A. (2016). Operational-scale carbon budget model of the Canadian Forest sector (CBM-CFS3) version 1.2: User's guide. 2016.
Kurz, W. A., Stinson, G., Rampley, G. J., Dymond, C. C., and Neilson, E. T. (2008). Risk of natural disturbances makes future contribution of Canada’s forests to the global carbon cycle highly uncertain. Proc. Natl. Acad. Sci. 105, 1551–1555. doi: 10.1073/pnas.0708133105
Landry, J. S., and Ramankutty, N. (2015). Carbon cycling, climate regulation, and disturbances in Canadian forests: Scientific principles for management. Land 4, 83–118. doi: 10.3390/land4010083
Mackey, B., Moomaw, W., Lindenmayer, D., and Keith, H. (2022). Net carbon accounting and reporting are a barrier to understanding the mitigation value of forest protection in developed countries. Environmental Research Letters, 17, 054028. doi: 10.1088/1748-9326/ac661b
Malcolm, J. R., Holtsmark, B., and Piascik, P. W. (2020). Forest harvesting and the carbon debt in boreal east-Central Canada. Clim. Chang. 161, 433–449. doi: 10.1007/s10584-020-02711-8
McRoberts, R. E., Næsset, E., and Gobakken, T. (2018). Comparing the stock-change and gain–loss approaches for estimating forest carbon emissions for the aboveground biomass pool. Can. J. For. Res. 48, 1535–1542. doi: 10.1139/cjfr-2018-0295
Metsaranta, J. M., Shaw, C. H., Kurz, W. A., Boisvenue, C., and Morken, S. (2017). Uncertainty of inventory-based estimates of the carbon dynamics of Canada’s managed forest (1990–2014). Can. J. For. Res. 47, 1082–1094. doi: 10.1139/cjfr-2017-0088
Moomaw, W. R., and Law, B. E. (2023). A call to reduce the carboncosts of forest harvest. Nature 620, 44–45. doi: 10.1038/d41586-023-02238-9
Natural Resources Canada. (2023). Canada’s forest carbon reporting system. Available at: https://natural-resources.canada.ca/climate-change-adapting-impacts-and-reducing-emissions/climate-change-impacts-forests/carbon-accounting/inventory-and-land-use-change/13111
Ogle, S. M., Domke, G., Kurz, W. A., Rocha, M. T., Huffman, T., Swan, A., et al. (2018). Delineating managed land for reporting national greenhouse gas emissions and removals to the United Nations framework convention on climate change. Carbon Balance Manag. 13, 1–13. doi: 10.1186/s13021-018-0095-3
Peng, L., Searchinger, T. D., Zionts, J., and Waite, R. (2023). The carbon costs of global wood harvests. Nature 620, 110–115. doi: 10.1038/s41586-023-06187-1
Ripple, W.J., Wolf, C., Gregg, J.W., Levin, K., Rockström, J., Newsome, T.M., et al. (2022). World scientists’ warning of a climate emergency 2022. Bioscience. 71, 1149–1155. doi: 10.1093/biosci/biac083
Seddon, N., Smith, A., Smith, P., Key, I., Chausson, A., Girardin, C, et al. (2021). Getting the message right on nature‐based solutions to climate change. Glob. Change Biol. 27, 1518–1546. doi: 10.1111/gcb.15513
Stinson, G., Kurz, W. A., Smyth, C. E., Neilson, E. T., Dymond, C. C., Metsaranta, J. M., et al. (2011). An inventory-based analysis of Canada’s managed forest carbon dynamics, 1990 to 2008. Glob. Chang. Biol. 17, 2227–2244. doi: 10.1111/j.1365-2486.2010.02369.x
Taylor, A. R., Mac Lean, D. A., Neily, P. D., Stewart, B., Quigley, E., Basquill, S. P., et al. (2020). A review of natural disturbances to inform implementation of ecological forestry in Nova Scotia. Environmental Rev. 28, 387–414. doi: 10.1139/er-2020-0015
Tymstra, C., Stocks, B. J., Cai, X., and Flannigan, M. D. (2020). Wildfire management in Canada: review, challenges, and opportunities. Progress in Disaster Sci. 5:100045. doi: 10.1016/j.pdisas.2019.100045
United Nations Conference on Environment and Development. (1992). Rio de Janeiro, Brazil. Available at: https://www.un.org/en/conferences/environment/rio1992
United Nations Climate Change Conference UK. (2021). Glasgow leaders declaration on land use and forests. Available at: https://webarchive.nationalarchives.gov.uk/ukgwa/20230418175226/https://ukcop26.org/glasgow-leaders-declaration-on-forests-and-land-use/
United Nations Framework Convention on Climate Change (UNFCCC). (2022a). Report on the individual review of the inventory submission of Canada submitted in 2021. Expert Review Team. Available at: https://unfccc.int/sites/default/files/resource/arr2021_can.pdf
United Nations Framework Convention on Climate Change (UNFCCC). (2022b). Canada. 2022 National Inventory Report (NIR). Available at: https://unfccc.int/documents/461919
Keywords: forest carbon, greenhouse gas emissions, national inventory reports, anthropogenic sinks, wildfire
Citation: Bysouth D, Boan JJ, Malcolm JR and Taylor AR (2024) High emissions or carbon neutral? Inclusion of “anthropogenic” forest sinks leads to underreporting of forestry emissions. Front. For. Glob. Change. 6:1297301. doi: 10.3389/ffgc.2023.1297301
Edited by:
Mohammad Ibrahim Khalil, University College Dublin, IrelandReviewed by:
Victor Rolo, University of Extremadura, SpainBrian Tobin, University College Dublin, Ireland
Copyright © 2024 Bysouth, Boan, Malcolm and Taylor. This is an open-access article distributed under the terms of the Creative Commons Attribution License (CC BY). The use, distribution or reproduction in other forums is permitted, provided the original author(s) and the copyright owner(s) are credited and that the original publication in this journal is cited, in accordance with accepted academic practice. No use, distribution or reproduction is permitted which does not comply with these terms.
*Correspondence: Julee J. Boan, ampib2FuQGxha2VoZWFkdS5jYQ==