- 1Jiangxi Provincial Key Laboratory for Restoration of Degraded Ecosystems and Watershed Ecohydrology, Nanchang Institute of Technology, Nanchang, China
- 2Fujian Forestry Prospect and Design Institute, Fuzhou, China
- 3Pushang State-Owned Forest Farm in Shunchang, Nanping, China
- 4Chinese Fir Engineering Technology Research Center of the State Forestry and Grassland Administration, Fujian Agriculture and Forestry University, Fuzhou, China
Introduction: Thinning has a crucial impact on the carbon dynamics of forest ecosystems. The inconsistencies about the effects of thinning on carbon stocks in previous studies may be attributed to different thinning plans that designed to achieve different management goals.
Methods: This study focuses on Cunninghamia lanceolata as the research object and investigates three treatments: crop tree release (CTR), traditional thinning (TM), and no thinning (CK). The different thinning methods (CTR and TM) had different management objectives and selection of crop trees principle, although with the same thinning density. The short-term effects of CTR and TM treatments on tree growth and carbon storage in Cunninghamia lanceolata plantations were analyzed and compared.
Results: Both CTR and TM were beneficial to the growth of individual trees when compared to CK, with CTR being more beneficial. The average annual increments in DBH, TH, and volume of a single tree in CTR increased by 62.50%, 61.29%, and 74.07% higher than CK, respectively. So CTR was the best for large-diameter timber yield, which was 77.40% higher than CK, whereas CK had a higher proportion of medium- and small-diameter timber yield. However, CTR had an insignificant short-term boosting effect on stand volume, and its timber volume was considerably higher than that in TM treatment, but not as high as in CK. Therefore, the carbon storage in the tree layer, litter, and different soil layers under different treatments showed that CTR and TM were significantly lower than CK. And the total carbon storage in the three treatments showed that CK > CTR > TM, with CTR showing a 13.07% higher than TM. On the contrary, thinning could effectively improve understory vegetation carbon storage. The carbon storage in the herb and shrub layers under CTR increased by 47.77% and 183.44%, respectively, compared to CK.
Discussion: Although thinning could significantly promote the growth of individual trees and understory vegetation, their total carbon storage in the short term was lower than that in CK. CTR showed a higher carbon sequestration and sink capacity than TM. It suggests that CTR, as a special type of thinning method, should be encouraged to selected because it has the chance to achieve a faster carbon sequestration rate than traditional thinning and it also can produce higher-quality timber.
1. Introduction
Forest ecosystems play a vital role in providing forest products, increasing carbon sinks, and improving the ecological environment (Pan et al., 2011). Among them, forest ecosystems are the largest organic carbon reservoir in terrestrial ecosystems (Gräfe and Köhl, 2020), storing about 76–98% of organic carbon and playing a major role in the carbon cycle (Baccini et al., 2017). With the sharp decline in natural forest area and the continual expansion in planted forest area, plantations are becoming an increasingly important element of the global carbon cycle (Wei et al., 2013; Liang et al., 2022). The management of plantations has a crucial impact on the carbon dynamics of forest ecosystems.
Studies have indicated that carbon storage in plantations is influenced by a variety of factors, such as site conditions, thinning intensity, management mode, forest age, and tree species (Marín-Spiotta et al., 2007). Among them, the effect of tending and thinning on forest carbon storage is still controversial. Some studies suggest that periodic tending and thinning affect stand structure, suppress stand productivity, reduce stand biomass, and decrease stand carbon storage (Pearson et al., 2014). However, untreated control forests show no significant change in carbon storage due to the decomposition of dry branches and fallen leaves in the process of natural mortality, which restores nutrients to the soil (Neil et al., 2019). On the contrary, several studies have demonstrated that tending and thinning open up the growth area of forest stands, reducing the competitive pressure among trees and facilitating their growth. At the same time, tending and thinning improve the light conditions in the forest, enhance the utilization of light by understory plants, and increase the biomass of understory vegetation, resulting in increased carbon storage (Ma et al., 2018). Is it possible that different methods of tending and thinning may be one of the reasons for these controversies?
The crop tree release (CTR) approach, developed based on the concept of close-to-nature forest management, focuses on cultivating a portion of high-quality forest trees that can be harvested and utilized after reaching the required diameter. Its basic objective is to select high-quality crop trees, cut down interference trees that compete with the crop trees, and promote the growth of crop trees by reducing competition between tree canopies and freeing stand space (Lu, 2006). In contrast to the traditional tending and thinning management goals in our country, CTR management focuses on crop tree species, primarily to improve the stability and productivity of forests, whereas traditional tending management focuses on the average tree of the stand to maximize the total timber yield per unit area (Shen, 2001). Therefore, in the thinning process, traditional tending and thinning adhere to the principle of “cutting small trees and retaining large ones, cutting dense trees and retaining sparse ones, cutting inferior trees and retaining superior ones, cutting curved trees and retaining straight ones” (Abetz and Kladtke, 2002); the objects of thinning are mostly below-canopy trees with relatively poor growth (He et al., 2014). On the other hand, the main objects of CTR are trees that are adjacent to crop trees and hinder their growth and development (Abetz and Kladtke, 2002), while other general trees in the stand (including small-diameter trees) are left to natural regulation. There are significant differences between the two types of tending and thinning in aspects such as management objectives, stand structure, and selection of crop trees in practical application. Is it these differences that significantly affect carbon storage in the stand?
In light of this, the main research object of this study was Cunninghamia lanceolata (Chinese fir), one of the main afforestation species in southern China. Cunninghamia lanceolata (Chinese fir) was then used to set two different thinning modes in a 17-year-old middle-aged Cunninghamia lanceolata plantation in Pushang State-owned Forestry Farm, Shunchang County, Fujian Province, namely, CTR (10 plants.400 m−2) and traditional tending and thinning. The modes were based on the intensity of crop tree release number (retention density of 600 plants/hm2), with no thinning as the control treatment. Comparative analyses of CTR and traditional thinning management were conducted in terms of individual tree growth, timber yield, stand structure, and stand carbon storage. The aim was to provide a scientific basis for optimizing stand management measures and improving the capacity of carbon sequestration and sink enhancement in Cunninghamia lanceolata plantations.
2. Methods
2.1. Study areas
The study area is located in the Pushang State-owned Forest Farm in Shunchang of Fujian Province, the central production area of Cunninghamia lanceolata in China (geographical coordinates: 117°47′5′′E, 26°56′8′′N) (Figure 1). The area falls within the Wuyi Mountains’ low hills and hilly terrain. The climate is subtropical oceanic monsoon weather, with an average annual temperature of 18.5°C, an average annual precipitation of 1,880 mm, an average annual sunshine duration of 1,699 h, and a frost-free period of more than 260 d. Shunchang is a key forestry county in southern China. The county has a forested area of 1,527 km2, with a total forest volume of 14 million m3 and a forest coverage rate of 82.8%, of which the forest area of Cunninghamia lanceolata is 753 km2 and forest volume is 10.28 million m3. The predominant soil type is red soil, characterized by deep soil layers and fertility. The vegetation includes natural Cunninghamia lanceolata, and Phyllostachys heterocycla, as well as Cunninghamia lanceolata plantations, Pinus massoniana, and mixed coniferous and broad-leaved forests.
The experimental site was located at Hedun, Pushang State-owned Forestry Farm, Fujian Province. The afforestation was carried out in 2001, covering an experimental forest area of 780 hm2. The land preparation for afforestation was performed by digging holes, with a hole size of 50 cm × 50 cm × 40 cm, using seedlings at an initial planting density of 2,500 plants/hm2. The understory vegetation mainly includes Indocalamus tessellatus, Angiopteris fokiensis, Parathelypteris glanduligera, Allantodia metteniana, Woodwardia japonica, Miscanthus sinensis, Dicranopteris pedata, Callicarpa bodinieri, Clerodendrum cyrtophyllum, Dryopteris sparsa, Odontosoria chinensis, etc.
2.2. Study design
The research object for this study was a 17-year-old Cunninghamia lanceolata plantation, Two thinning operations were carried out in the 8th and 14th years after initial planting, with the retention density of 1950 plants/hm2 and 1,050 plants/hm2 respectively. The information of different stands are showed as Table 1 and the average density was 1,000 plants/hm2. From 2017 to 2020, continuous and site-specific observations were conducted. The completely randomized block design method was adopted to set three different treatments (Table 2), i.e., crop tree release (10 plants.400 m−2, CTR), traditional tending and thinning based on the intensity of crop tree release number (retention density of 600 plants/hm2, TM), and no thinning (control, CK). Each treatment was replicated three times, resulting in a total of nine experimental plots. To ensure experimental accuracy, Cunninghamia lanceolata plantations with essentially the same conditions, such as slope position, slope direction, and soil, were selected for establishing the experimental sample plots. If possible, each plot covered an area of 400 m2 (20 m × 20 m) at an interval of about 5 m between the sample plots.
According to the experimental design, a tally was conducted in April 2017 (before cutting), mainly investigating tree species, diameter at breast height (DBH), canopy width, and tree height. Thinning operations were conducted in May. CTR was conducted mainly based on the growth state of individual trees and competition with neighboring trees to determine the interference tree (Raymond et al., 2018). Crop trees were determined by evenly selecting 10 dominant trees in a dominant position from the sample plot exhibiting strong vitality, vigorous growth, a straight stem, good wood quality, and no damage. Interference trees were selected among trees adjacent to the crop trees that negatively affected their growth (e.g., the canopy squeezed the crop tree by more than 1/3), with relatively poor shape and quality such as curved stems, thick branches, poor canopy shape, or obvious damages on the trunk. To avoid excessive disturbance to the harvested stand, the selective cutting intensity of interference trees was controlled within 20% (Wang et al., 2014), and trees with canopies that did not inhibit the growth of crop trees were retained as auxiliary trees (Ward et al., 2017), Traditional thinning management was performed by low thinning; oppressed trees with poor growth and small DBH in the sample plot were cut down based on the set retention density. The relevant research and investigation were conducted in May 2020, three years after the deployment of various thinning treatments.
2.3. Measurements
2.3.1. Determination of individual tree growth of Cunninghamia lanceolata plantation
Cunninghamia lanceolata trees within the sample plots were labeled using spray-painting and tagging. Measurements were taken for each tree in both 2017 and 2020 to determine their individual growth rates. Ultrasonic altimeter (Haglof Sweden) and DBH ruler were used to measure the tree height and DBH, respectively. The individual volume of Cunninghamia lanceolata stand was determined using the formula for Cunninghamia lanceolata volume in Fujian Province (Liu and Tong, 1996), as shown in Eq. (1):
Where: Di denotes the average DBH in the i-th diameter class, and Hi denotes the fitted tree height in the i-th diameter class.
2.3.2. Timber assortment structure calculation in Cunninghamia lanceolata plantation
The DBH of Cunninghamia lanceolata standing timber in the stand was divided by 2 cm as a diameter class. Each assortment in the stand was differentiated by the diameter class to calculate the standing timber yield in each diameter class. The proportions of each wood species within each diameter class of the standby timber assortment were consolidated based on the proportion shown in Table 3 (Zhang et al., 2013).
After dividing the number of plants of each timber species in each diameter class, the timber yield of each assortment and the total timber yield of the stand were calculated according to the dimensional lumber and non-dimensional lumber (small strip timber), respectively (Liu et al., 1995), as shown in Eq. (2) and Eq. (3):
2.3.3. Investigation of carbon storage in Cunninghamia lanceolata plantation stands with different management
The aboveground carbon storage in this study was calculated according to Eq. (4):
2.3.3.1. Determination of carbon storage in the tree layer
In order to prevent the disruption of long-term observation sample plots, the carbon conversion coefficient (0.5095) in this experiment was derived from the model for the average carbon content rate of middle-aged Cunninghamia lanceolata plantations in Fujian Province (Lin et al., 2016). The biomass of different biomass components (leaves, branches, bark, peeled trunk, stumps and roots) were calculated based on the model for estimation of the biomass of individual trees in Cunninghamia lanceolata plantation (Li et al., 2010) (Li et al., 2010) (Table 4). The carbon storage of different biomass components were determined according to Eq. ( ). The carbon storage of individual tree was obtained by summing up the carbon storage of its biomass components and the carbon storage of the tree layer was obtained by summing up the carbon storage of individual tree.
2.3.3.2. Determination of carbon storage in shrubs, herbs, and litter
The biomass of shrubs, herbs, and litter was measured using the harvest method (collecting all samples above and below ground). Nine 2 m × 2 m small quadrats were established in the upper, middle, and lower parts of the sample plots to determine the biomass of the shrub layer. Within each 2 m × 2 m quadrat, a 1 m × 1 m and a 0.5 m × 0.5 m sub-quadrats were designated to determine the biomass of the herb layer and litter, separately. The collected samples of the shrub layer, herb layer, and litter were weighed for fresh mass, and a certain amount of samples were taken back to the laboratory after thoroughly mixing the samples from the same plot. Fresh plant samples were subjected to enzyme deactivation at 105°C for 30 min and then dried at 85°C to a constant weight to determine the moisture content. The dried samples were crushed and sieved through a 0.149 mm pore sieve, and the carbon content was determined using automatic carbon and nitrogen analyzer (Elementar Vario Max, Germany).
2.3.3.3. Determination of soil organic carbon density (SOCD)
In order to obtain the SOCD data, a soil pit was excavated in the upper, middle, and lower slopes of each sample plot, respectively, to collect samples until the 100 cm depth. Prior to taking the soil samples, the surface leaf litter (including undecomposed and partially decomposed) and moss (if any) were carefully removed from the ground at each sampling point. Along the profile, undisturbed soil samples were collected using a standard container (ring cutting sampler with 100 cm3 in volume) for bulk density measurements at four layers of depth: 0–10 cm, 10–20 cm, 20–40 cm, and 40–60 cm (Tesfaye et al., 2016). All of the 108 soil samples were then transported to the laboratory for the determination of bulk density. Disturbed soil samples were taken with a hand auger (5 cm in diameter) at four layers of depth: 0–10, 10–20, 20–40, and 40–60 cm. In each plot, soil samples were taken at 5 points that were uniformly distributed along the two diagonal lines. For each layer, all samples from the 5 sampling points were effectively pooled into one composite sample and then a subsample around 300 g was taken by coning and quartering for laboratory analysis. All of the soil samples were then transported to the laboratory for soil organic carbon (SOC) concentration analysis.
Each undisturbed soil core was oven-dried at 105°C to determine the bulk density. Visible rock fragments (> 2 mm) were collected to determine the proportion percentage of rock fragments. The rock fragments and roots were removed from each of the disturbed soil samples, following which, the soil samples were air-dried, ground, and passed through a 0.25 mm mesh. The SOC concentrations were determined by elemental analyzer (Elementar Vario EL, Germany). Based on the field sampling and laboratory analysis for soil profile layers in each plot, the site-specific value of SOCD within 60 cm depth was calculated using Eq. (5) (Guan et al., 2019).
Where: S SOCD denotes soil organic carbon density, t/hm2; C i denotes soil organic carbon content, g/kg; D i denotes soil bulk density, g/cm3; H i denotes soil thickness, cm; S i denotes the percentage of gravel (>2 mm) in the soil layer, %.
2.4. Data analysis
Data processing was performed using Microsoft Excel 2019. One-way ANOVA was conducted using SPSS 25.0 software. Figures were plotted using Origin 2018.
3. Results
3.1. Comparison of individual tree growth in Cunninghamia lanceolata plantation under different thinning modes
The comparative analysis of the average annual growth increment of individual trees in Cunninghamia lanceolata plantation over different treatment periods shows that the average annual increments in DBH (Figure 2A), tree height (Figure 2B), and volume of a single tree (Figure 2C) showed a consistent change pattern. The growth increment of individual trees under CTR treatment was significantly higher than that under TM and CK (p < 0.05). Compared to CK, which had the lowest growth increment, the average annual increments in DBH, TH, and volume of a single tree in CTR increased by 62.50, 61.29, and 74.07% higher, respectively. The growth increment of individual trees under TM was lower than in CTR, and its average annual increments in DBH, TH, and volume of a single tree were 17.00, 28.21, and 20.51% lower than that in CTR, respectively.
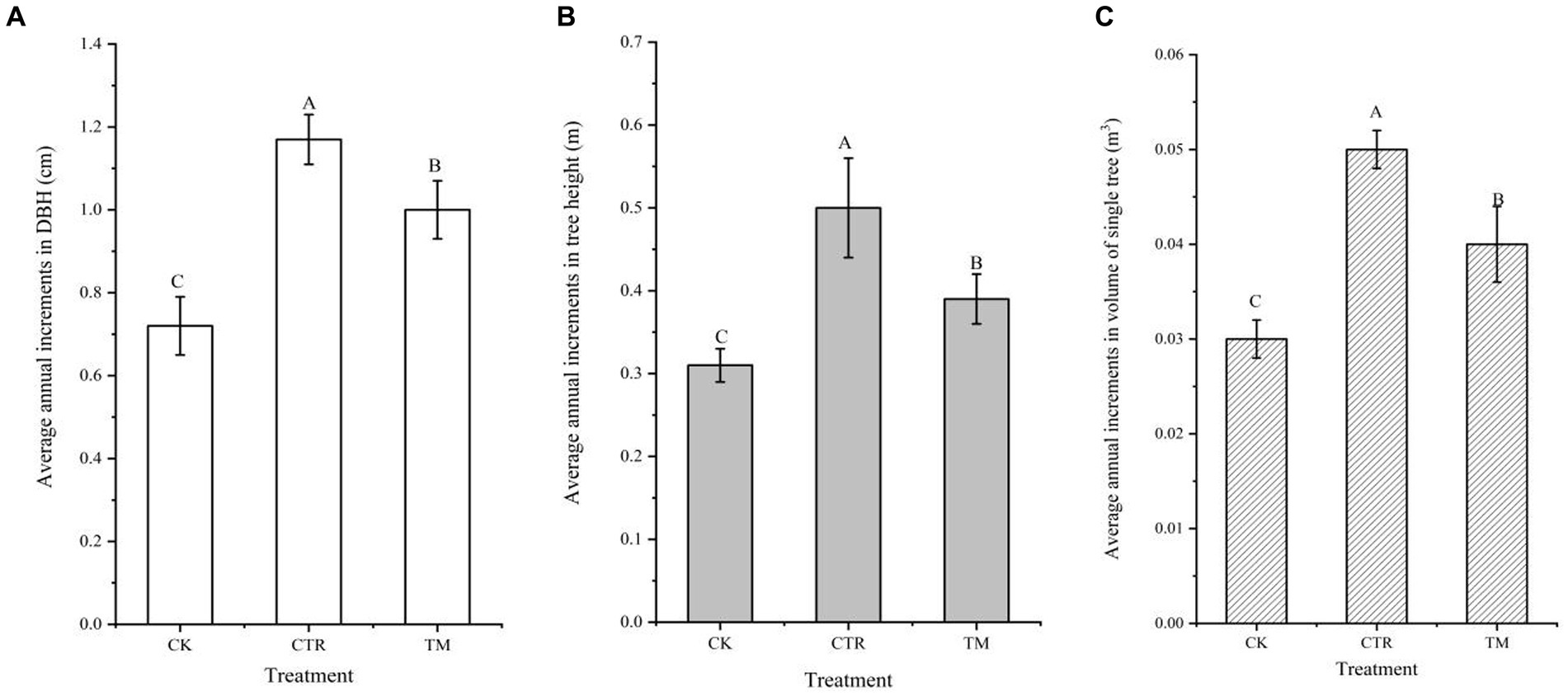
Figure 2. Comparison of average annual growth of Chinese fir under different treatments. (A) DBH, (B) tree height, (C) volume of a single tree. Error bars represent standard error. Different uppercase letters represent different treatments of the same indicator to achieve significant differences at p < 0.05 level.
3.2. Comparison of timber assortment structure in Cunninghamia lanceolata plantation under different thinning modes
The comparison of the timber yield of different assortments (Figure 3) indicates that in CTR and TM, the yield of large-diameter timber was the highest, which was significantly higher than that of other diameter assortments (p < 0.05), followed by medium-diameter timber, and the lowest being the small-diameter timber and small strip timber. Conversely, the CK treatment showed no significant difference in the timber yield between medium-diameter timber and large-diameter timber (p > 0.05), both of which were significantly higher than the yield of small-diameter timber and small strip timber (p < 0.05). The comparison of different treatments indicated that the large-diameter timber yield was CTR > TM > CK. The timber yield for large-diameter timber under CTR treatment was 77.40% higher than that under CK treatment, while the medium-diameter timber was significantly higher in CK treatment than that in CTR and TM treatment (p < 0.05). From the analysis of total timber yield, it can be concluded that the total timber yield in CTR is similar to that in CK and significantly higher than that in TM. Moreover, the timber volume among the three treatments exhibits a consistent pattern (Figure 4).
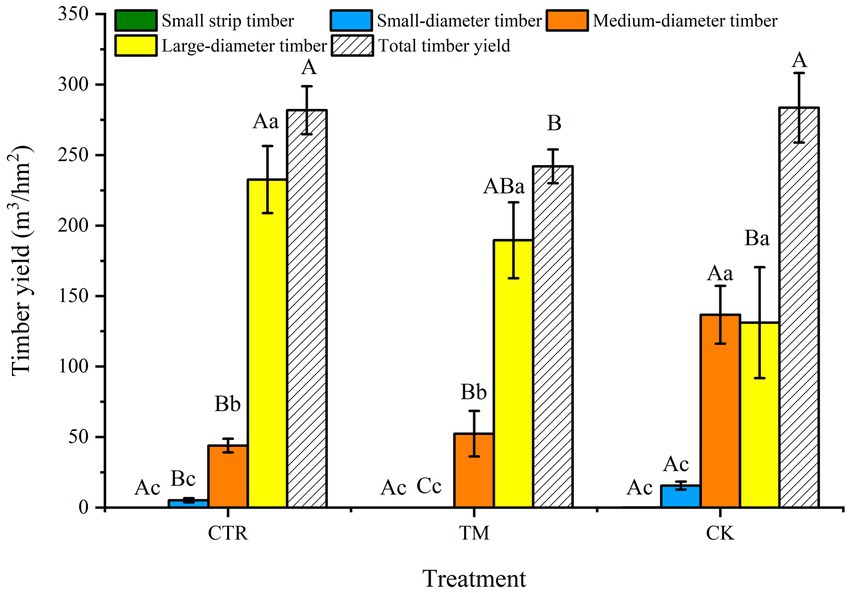
Figure 3. Comparison of timber yields of various assortments in Cunninghamia lanceolata plantation under different treatments. Error bars represent standard error. Different uppercase letters represent timber yield of the same diameter assortment under different treatments achieve significant differences at p < 0.05 level. Different lowercase letters indicate significant differences (p < 0.05) of timber yield of different diameter assortments under the same treatment.
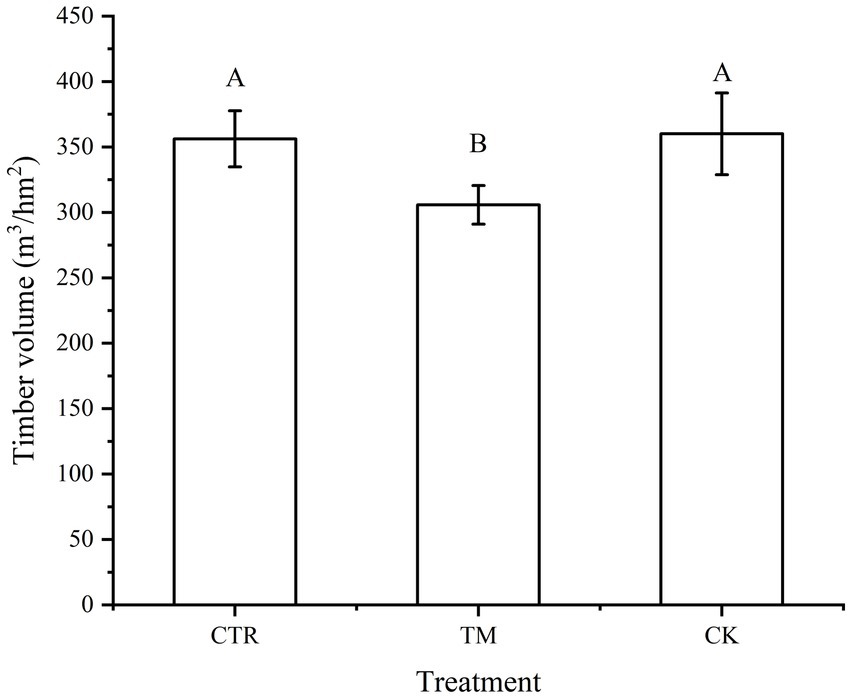
Figure 4. Comparison of timber volume in Cunninghamia lanceolata plantation under different treatments. Error bars represent standard error. Different uppercase letters represent different treatments of the timber volume to achieve significant differences at p < 0.05 level.
3.3. Comparison of carbon storage in Cunninghamia lanceolata plantations under different thinning modes
3.3.1. Comparison of carbon storage in the tree layer of Cunninghamia lanceolata plantations under different thinning modes
The effects of various treatments on carbon storage in the tree layer are shown in Figure 5. The total carbon storage in the tree layer under different treatments indicates CK was significantly higher than the two thinning treatments (p<0.05) (Figure 5A), in which the total carbon storage in the tree layer was 7.09% higher under CTR than under TM. Further analysis of carbon storage in different biomass components (Figure 5B) indicates that, similar to the total carbon storage, the carbon storage in each biomass component under CK was significantly higher than that under CTR and TM (p<0.05), while the difference between CTR and TM did not reach the level of significance (p > 0.05). A comparison of carbon storage in different biomass components indicates that the overall performance was as follows: peeled trunk > bark > stump > root > leaf > branch. Peeled trunk accounted for the largest proportion of total carbon storage, with 56.2, 55.8, and 55.8% in CK, CTR, and TM, respectively, followed by bark, accounting for 13.8% of total carbon storage in the three treatments. The differences in carbon storage among roots, branches, and leaves were not significant (p > 0.05), accounting for the lowest proportion of total carbon storage.
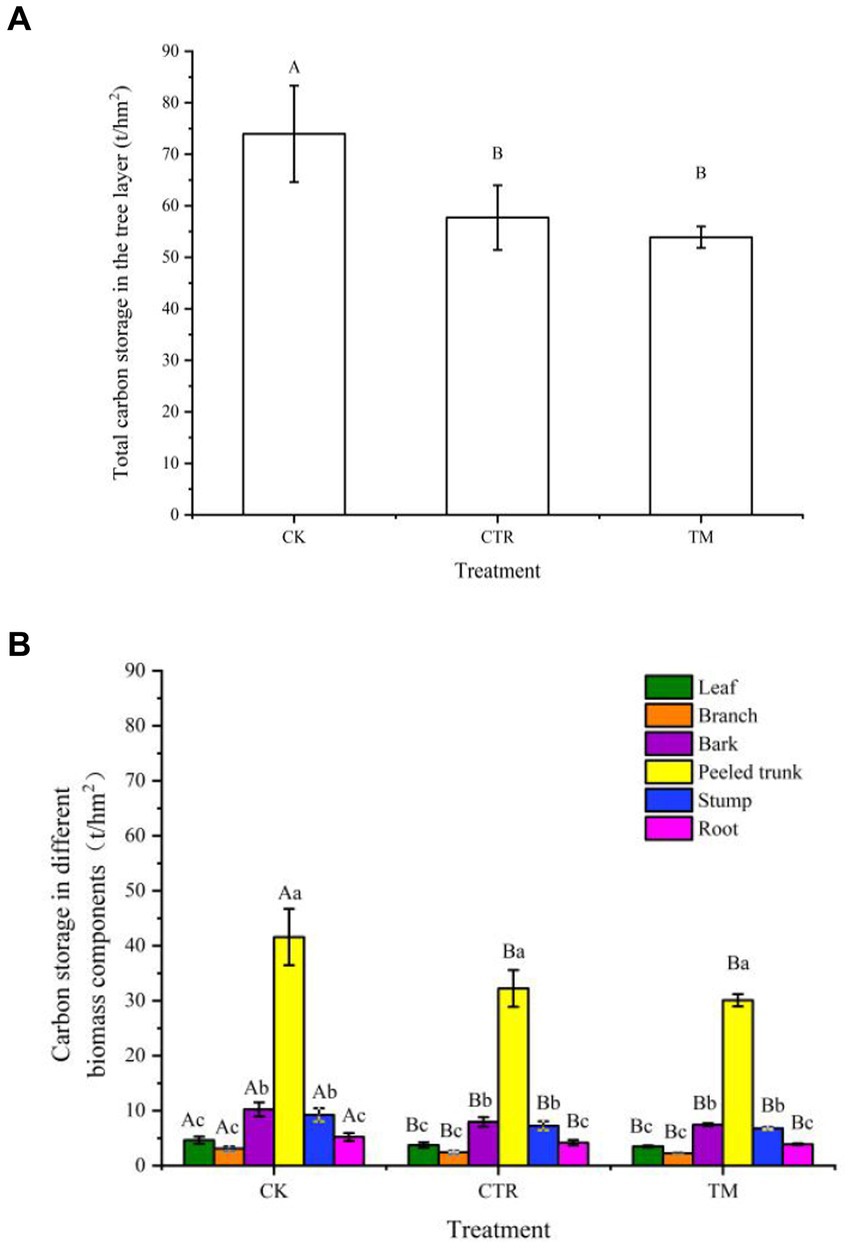
Figure 5. Comparison of carbon storage in the tree layer by different managements (t/hm2). (A) Total carbon storage in the tree layer, (B) carbon storage in different biomass components of the tree layer. Error bars represent standard error. Different uppercase letters represent total carbon storage and carbon storage in different biomass components of the tree layer under different treatments achieve significant differences at p < 0.05 level. Different lowercase letters in (B) indicate significant differences (p < 0.05) of carbon storage in different biomass components under the same treatment.
3.3.2. Comparison of carbon storage in understory vegetation and litter of Cunninghamia lanceolata plantation under different thinning modes
Comparative analysis of carbon storage in understory vegetation under different treatments indicates that CTR treatment significantly affects the carbon storage in the herb and shrub layers of understory vegetation (Figure 6). The carbon storage in both herb and shrub layers shows that CTR was significantly higher than TM and CK (p<0.05), with carbon storage in the herb layer being increased by 47.77% compared with the lowest value in CK. The carbon storage in the shrub layer also showed CTR had the highest value, reaching 2.83 times the lowest value in CK. In contrast to the understory vegetation, the carbon storage in the litter layer indicates that the value of CK was significantly higher than that of CTR and TM, but the difference between CTR and TM was not significant (p > 0.05).
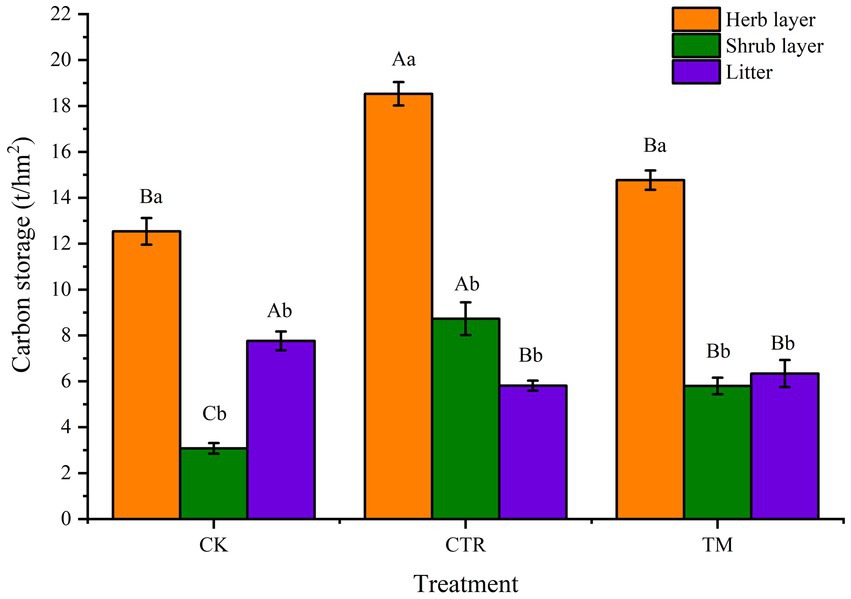
Figure 6. Comparison of carbon storage of understory vegetation and litter of different managements (t/hm2). Error bars represent standard error. Different uppercase letters represent carbon storage of the understory vegetation and litter under different treatments achieve significant differences at p < 0.05 level. Different lowercase letters represent carbon storage of the different layers under the same treatment achieve significant differences at p < 0.05 level.
3.3.3. Comparison of carbon storage in the soil layer of Cunninghamia lanceolata plantations under different thinning modes
Different treatments had a certain effect on soil carbon density in different soil layers of Cunninghamia lanceolata plantation (Figure 7). Compared to CK, the soil carbon density in different soil layers was significantly lower (p < 0.05) in both CTR and TM treatments. Among them, TM had the lowest soil carbon density in different soil layers, where the soil carbon densities in 0–10 cm, 10–20 cm, 20–40 cm, and 40–60 cm were 51.46, 54.23, 60.72, and 32.70% lower than those in CK, respectively. Compared with TM, CTR showed overall higher soil carbon densities, which were 33.74, 16.34, 5.18, and 2.79% higher in the four layers of 0–10 cm, 10–20 cm, 20–40 cm, and 40–60 cm than TM treatment, respectively. It can be observed that the differences between the two treatments decrease significantly with increasing soil layer depth.
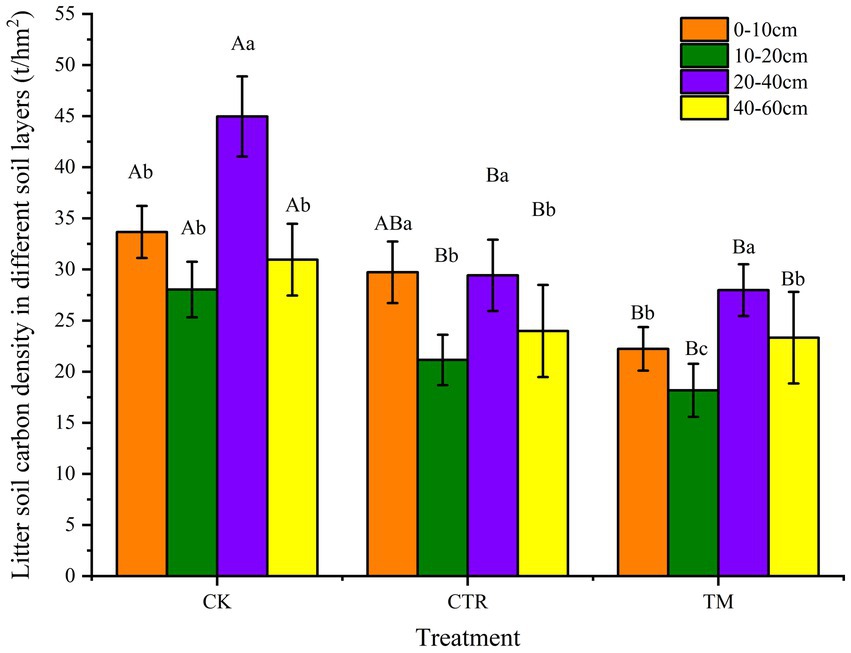
Figure 7. Comparison of carbon density in soil of different managements (t/hm2). Error bars represent standard error. Different uppercase letters represent carbon density in the same soil layer under different treatments achieve significant differences at p < 0.05 level. Different lowercase letters represent carbon density in the different soil layers under the same treatment achieve significant differences at p < 0.05 level.
3.3.4. Comparison of total carbon storage in Cunninghamia lanceolata plantations under different thinning modes
The comparison of total carbon storage under different treatments indicates that the total carbon storage in CK was significantly higher than in CTR and TM (p<0.05) (Figure 8A). The total carbon storage in the three treatments showed CK > CTR > TM, with CTR showing a 13.07% higher value than TM. However, the difference between the two treatments was not statistically significant (p > 0.05). The proportion of carbon storage in different layers to the total carbon storage in the stand was analyzed (Figure 8B). An analysis of the proportion of carbon storage in the stand under different treatments showed that the soil layer was the highest, followed by the tree layer, whereas the proportion of carbon storage in the understory vegetation and litter varied among treatments, with herb layer > litter > shrub layer in CK and TM, and herb layer > shrub layer > litter in CTR. A comparison of different treatments indicates that the carbon storage in the soil layer, tree layer, and litter were similar. However, the carbon storage in the herb layer and shrub layer under CTR and TM was significantly higher than that under CK. The proportion of carbon storage in the herb layer under the two treatments was about 2 times higher than that under CK, while the carbon storage in the shrub layer was 3–4 times higher than that under CK.
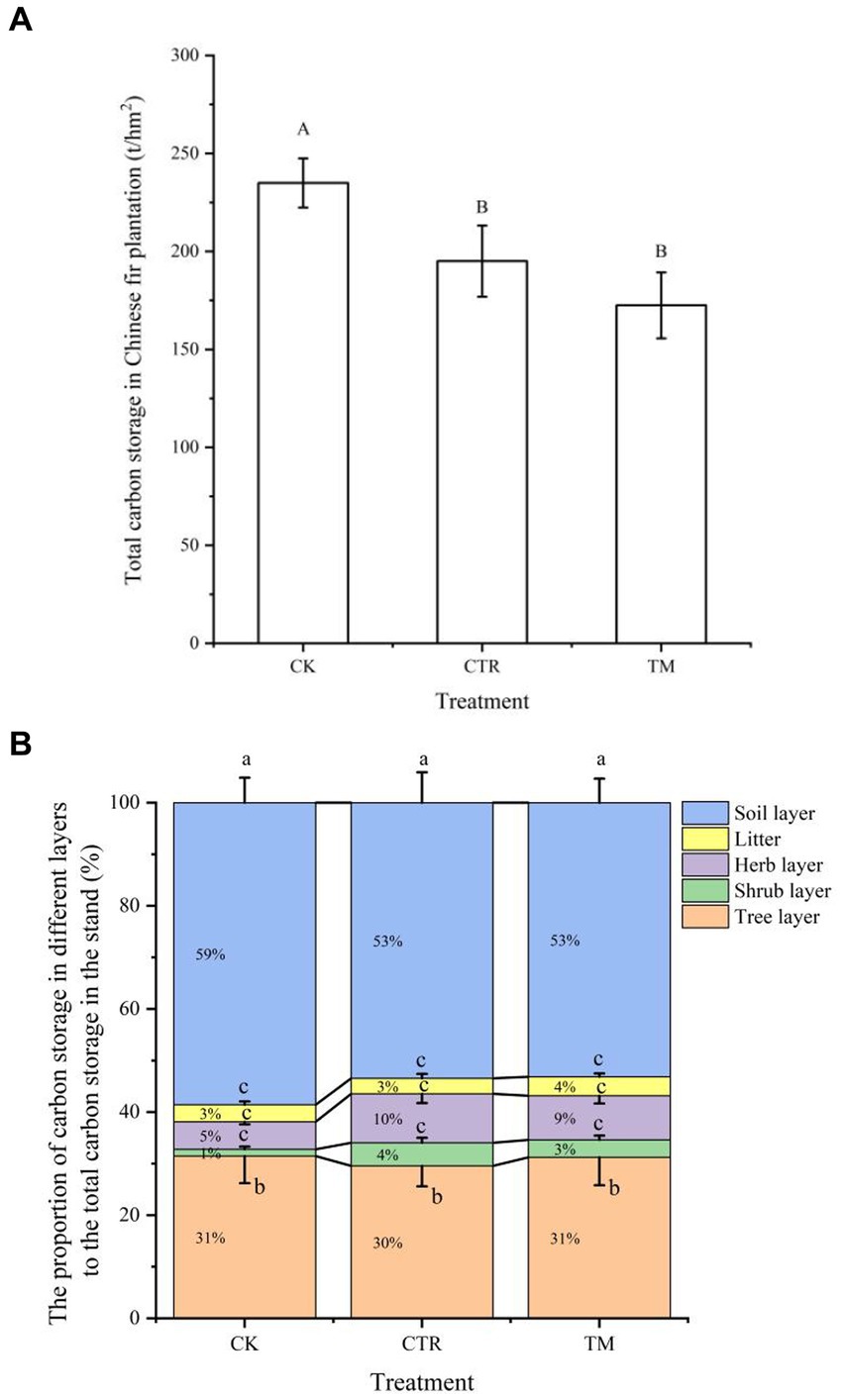
Figure 8. Comparison of total carbon storage in Cunninghamia lanceolata plantation under different managements.(A) Total carbon storage in the stand (B) The proportion of carbon storage in different layers to the total carbon storage in the stand. Different uppercase letters in (A) represent total carbon storage of the Chinese fir plantations under different treatments achieve significant differences at p < 0.05 level. Different lowercase letters in (B) represent the proportion of carbon storage in different layers to the total carbon storage in the stand under the same treatment achieve significant differences at p < 0.05 level.
4. Discussion
4.1. Effects of different thinning modes on the growth of individual trees in Cunninghamia lanceolata plantation
As one of the essential management modes of forest cultivation, thinning involves making certain adjustments to the density and structure of the stand and cutting down some short or dead trees to provide more growth space for the remaining trees in the stand aiming to achieve optimal cultivation outcomes (Pretzsch and Schütze., 2015; Pommerening and Grabarnik, 2019). Traditional thinning aims to adjust the stand structure, enhance tree quality, and increase the stand’s stability (Wang et al., 2014). As a special type of thinning, CTR thinning involves selecting a few crop trees for cultivation and management and removing the interference trees that affect the crop trees, it intends to reduce competition around selected trees so that they can improve in vigor, remain competitive in the stand, and provide desired future benefits (Pommerening and Grabarnik, 2019).
In this study, compared with CK, both CTR and TM were conducive for the growth of individual Cunninghamia lanceolata. Among them, the individual tree growth in CTR was better. Compared to CK, which had the lowest growth increment, the average annual increments in DBH, TH, and volume of a single tree in CTR increased by 62.50, 61.29, and 74.07% higher, respectively. And the growth increment of individual trees under TM was also lower than in CTR, its average annual increments in DBH, TH, and volume of a single tree were 17.00, 28.21, and 20.51% lower than that in CTR, respectively. This is consistent with the results of previous studies. Chen et al. (2017) indicated in their study of Spruce-fir mixed broadleaf-conifer forest that the growth volume and growth rate of individual tree DBH and volume in the stands treated by CTR showed substantial increase, with a particularly significant boosting effect on the growth of crop trees. Periodic growth in DBH and volume in Betula lenta under CTR over an 8-year period was about twice that in CK (Schuler, 2006); the periodic growth rates of the stand section area and volume in Quercus mongolica secondary forest over a 3-year period were about 2.5 and 3.6 times that in the control plot (Zhang et al., 2020). Cutting down interference trees can enhance the density of newly planted crop trees in the sample plots, which is conducive to the growth of crop trees (Ward, 2009; Steinauer et al., 2016) by reducing the competition with general trees for light, water, and nutrient resources (Caellas et al., 2004).
The analysis of timber yield under different treatments also indicated that CTR and TM were more conducive to the yield of large-diameter timber, while the proportion of small-diameter timber yield was relatively high in CK. The timber yield for large-diameter timber under CTR treatment was 77.40% higher than that under CK treatment, and the large-diameter timber yield of different treatments presented as CTR > TM > CK. Therefore, we believe that the proportion of dominant trees in the stand increased after CTR, and the differentiation of forest trees in superiority and inferiority became more evident. This is consistent with the research conclusions of other scholar (Ward, 2017; Vogel et al., 2022). It can be explained that the average competition index within the forest was reduced more significantly after CTR, in which interfering trees were selectively cut, significantly reducing the competitive pressure on environmental resources of the retained trees and enhancing the growth potential of trees (Wang et al., 2014; Forrester, 2019). In addition, this result also reflects the different management objectives of CTR and traditional thinning. The focus of traditional tending management is on the average tree of the stand, aiming to maximize the total yield of timber per unit area, whereas CTR aims at crop tree species, primarily to improve the stability and productivity of forests (Lu et al., 2009; Zhu et al., 2022).
4.2. Effects of different thinning modes on carbon storage in Cunninghamia lanceolata plantation
Our results indicate that both CTR and TM were beneficial to the growth of individual trees when compared to CK, with CTR being more beneficial. However, for the stand, CTR did not have a significant effect on promoting stand volume in the short term. The total timber yield and volume in CTR were significantly higher than TM but not as high as CK. So it also found that the total carbon storage in the tree layer and the carbon storage in each biomass component were significantly lower in CTR and TM than in CK. Although thinning can significantly promote the stand growth, the biomass and carbon storage of the stand decrease in the short term. This is due to the fact that some of the trees are removed from the stand after thinning, leading to a decrease in productivity and carbon storage within the stand (Schaedel et al., 2017). Thornley and Cannell (2000) believed that tending and thinning reduced stand density, decreased the competition of trees within the stand, and increased their growth. However, the short-term effect on the increase in stand volume was not significant. In this study, CTR has demonstrated a certain promoting effect on stand volume. The analysis of the total timber yield and volume in different treatments indicates that the total timber yield and volume in CTR were lower than but close to that in CK. There was no significant difference between the two, and they were significantly higher than that in TM. Related studies suggest that the carbon storage of the tree layer under CTR can restored by cutting the interference trees within a certain period. Wu Jianqiang’s research also found that the carbon storage of individual trees can compensate for a certain proportion of stand carbon storage in the short term after thinning (Wu et al., 2015).
The carbon storage of understory vegetation and litter, as one of the important indicators of aboveground carbon storage in a forest stand, can reflect the ability of aboveground carbon sequestration in a forest stand (Tue et al., 2014). In this study, thinning effectively increased the carbon storage of understory vegetation within the stand, unlike the tree layer, where CTR showed a more significant enhancement effect. Compared to the lowest value in CK, CTR increased the carbon storage of the herb and shrub layers by 47.77 and 183.44%, respectively. The primary reason for this improvement is that tending and thinning open up the growth space of the forest stand, improve the light conditions within the forest, and increase the utilization of light by understory plants, thus increasing the carbon storage of understory vegetation (Ma et al., 2018). CTR is a special type of tending and thinning that can effectively improve the light transmission rate of the forest stand, thus promoting the increase of carbon storage in the herb and shrub layers (Aponte et al., 2020). In contrast to understory vegetation, the carbon storage in the litter was significantly lower in CTR and TM than in CK. This could be due to the reduction of biomass in the tree layer caused by tending thinning, leading to decreased litter input. It might also be due to increased light availability in the forest after thinning and the effective improvement of environmental factors such as air temperature, surface temperature, and soil water content. It not only promotes the growth of understory vegetation and improves the composition of dry branches and fallen leaves, but also provides favorable conditions for litter decomposition, improving soil microbial activity and accelerating litter decomposition rates (Taki et al., 2010).
Soil carbon storage, as an important indicator of belowground carbon storage in forest stands, is negatively correlated with artificial interference (Li et al., 2012). Studies have suggested that tending and thinning affect soil surface carbon storage (Magnússon et al., 2016; Agevi et al., 2017). After CTR, soil carbon storage gradually decreases with increasing soil depth, and is lower than the control in each soil layer (Dang et al., 2017). Hu et al. (2014) found that the carbon storage in the soil layer of the forest stand decreased by 10.09% compared with that of the control 5 years after tending and thinning in a Cunninghamia lanceolata plantation. The results of this study are consistent with this finding that the soil carbon density was significantly lower (p < 0.05) in different soil layers under CTR and TM compared to that under CK. Existing studies generally agree that after tending and thinning, the increased biological activity and soil respiration due to increased light in the forest accelerate the decomposition and release of soil organic matter, which is a key reason for the decrease in forest soil carbon storage (Nilsen and Strand, 2008). However, compared with TM, the soil carbon density in different soil layers under CTR was higher than that under TM. This indicates that while thinning treatments accelerate soil carbon output, the input of carbon from vegetation within the stand also directly influences soil carbon density (Agevi et al., 2017).
Currently, most research reports on CTR focus on crop tree growth (Wang et al., 2014), understory species diversity (Li et al., 2022), regeneration (Barsoum et al., 2016), and other aspects after the implementation of CTR. In this study, CTR was combined with traditional thinning based on different cutting tree selection principles and thinning objectives. Comparative analyses of different thinning modes on the productivity and carbon storage of Cunninghamia lanceolata plantation were conducted. The aim of this study was to explore whether the adoption of thinning treatments would significantly affect the productivity and carbon storage of Cunninghamia lanceolata plantations. Although the thinning treatment significantly promoted the growth of individual trees and understory vegetation, the short-term carbon storage in Cunninghamia lanceolata plantations under the thinning treatment was lower than that under CK, with the total carbon storage of the forest stands being CK > CTR > TM. The comparison of the two thinning modes showed that the carbon sequestration capacity of CTR was higher than that of TM, but the effect was not significant at the initial treatment stage. Therefore, future research can further validate these findings by incorporating additional thinning intensities and conducting in-depth studies over longer time scales.
5. Conclusion
The management of plantations has a crucial impact on the carbon dynamics of forest ecosystems. This study investigates the effects of different thinning modes on productivity and carbon storage in Cunninghamia lanceolata plantations. The results showed that the average annual increments in DBH, tree height, and volume of individual tree under different treatments showed CTR > TM > CK (p < 0.05). Compared with CK, both CTR and TM favored the growth of individual Cunninghamia lanceolate. So we can found that CTR was the most conducive for large-diameter timber yield, which was 77.40% higher than CK, while CK had a higher proportion of small- and medium-diameter timber yield. However, in the short term, CTR did not have a significant effect on promoting stand volume, which was significantly higher than TM but not as high as CK. Therefore, the carbon storage in the tree layer, litter, and different soil layers under different treatments indicated that both CTR and TM treatments had significantly lower carbon storage than the CK treatment. On the contrary, thinning effectively increased the carbon storage of understory vegetation within the stand, where CTR had a more significant enhancement effect. The carbon storage in the herb and shrub layers under CTR increased by 47.77 and 183.44%, respectively, compared to CK. At last, analysis of the total stand carbon storage at the different levels indicates that CK > CTR > TM, indicating that in the short term, the carbon storage in Cunninghamia lanceolata plantations under TM and CTR were lower than that under CK, while the carbon sequestration and sink enhancement capacity of CTR was higher than that of TM. This suggests that even the tree number is decreased, the rapid regeneration of understory vegetation and the fast growth of post-thinning survivors could lead to greater carbon sequestration rates. CTR thinning has the chance to achieve a faster carbon sequestration rate than traditional thinning. It further indicates that CTR is more effective in reducing the competitive pressure among the retained trees than traditional thinning management, leading to the production of higher-quality timber, for which the market demand is increasing.
Data availability statement
The original contributions presented in the study are included in the article/supplementary material, further inquiries can be directed to the corresponding author.
Author contributions
XZ: Data curation, Formal analysis, Funding acquisition, Investigation, Methodology, Writing – original draft, Writing – review & editing. ZZ: Data curation, Formal analysis, Software, Writing – original draft. CY: Data curation, Formal analysis, Investigation, Methodology, Writing – original draft. MY: Data curation, Formal analysis, Software, Writing – original draft. ZG: Data curation, Formal analysis, Writing – original draft. YW: Data curation, Writing – original draft. ZH: Investigation, Supervision, Writing – review & editing. LZ: Supervision, Writing – review & editing. LX: Investigation, Resources, Writing – review & editing. KL: Funding acquisition, Methodology, Project administration, Resources, Supervision, Writing – review & editing.
Funding
The author(s) declare financial support was received for the research, authorship, and/or publication of this article. This work was supported by the National Natural Science Foundation of China (Grant No. 32160361), National Key Research and Development Plan (Grant No. 2016YFD0600301) and National College Students Innovation and Entrepreneurship Training Program (Grant No. 202211319003).
Acknowledgments
We thank those students who assisted with the fieldwork and data collection and the instructor for their constructive comments that helped to improve this study. We would like to thank Daniel Petticord at the University of Cornell for her assistance with English language and grammatical editing.
Conflict of interest
The authors declare that the research was conducted in the absence of any commercial or financial relationships that could be construed as a potential conflict of interest.
Publisher’s note
All claims expressed in this article are solely those of the authors and do not necessarily represent those of their affiliated organizations, or those of the publisher, the editors and the reviewers. Any product that may be evaluated in this article, or claim that may be made by its manufacturer, is not guaranteed or endorsed by the publisher.
References
Abetz, P., and Kladtke, J. (2002). The target tree management system. Forstwiss. Centralbl. 121, 73–82. doi: 10.1046/j.1439-0337.2002.00073.x
Agevi, H., Onwonga, R., Kuyah, S., and Tsingalia, M. (2017). Carbon stocks and stock changes in agroforestry practices: a review. Trop. Subtrop. Agroecosys. 20, 101–109. doi: 10.56369/tsaes.2291
Aponte, C., Kasel, S., Nitschke, C. R., Tanase, M. A., Vickers, H., Parker, L., et al. (2020). Structural diversity underpins carbon storage in Australian temperate forests. Glob. Ecol. Biogeogr. 29, 789–802. doi: 10.1111/geb.13038
Baccini, A., Walker, W., Carvalho, L., Farina, M., Sulla-Menashe, D., and Houghton, R. A. (2017). Tropical forests are a net carbon source based on aboveground measurements of gain and loss. Science 358, 230–234. doi: 10.1126/science.aam5962
Barsoum, N., Coote, L., Eycott, A. E., Fuller, L., Kiewitt, A., and Davies, R. G. (2016). Diversity, functional structure and functional redundancy of woodland plant communities: how do mixed tree species plantations compare with monocultures? For. Ecol. Manag. 382, 244–256. doi: 10.1016/j.foreco.2016.10.005
Caellas, I., del Ro, M., Roig, S., and Montero, G. (2004). Growth response to thinning in Quercus pyrenaica Willd. Coppice stands in Spanish central mountain. Ann. For. Sci. 61, 243–250. doi: 10.1051/forest:2004017
Chen, K., Zhang, H., Lei, X., Lou, M., Wang, Q., and Mao, J. (2017). Effect of thinning on spatial structure of spruce-fir mixed broadleaf-conifer forest base on crop tree management. For. Res. 30, 718–726. doi: 10.13275/j.cnki.lykxyj.2017.05.003
Dang, X., Liu, G., Zhao, L., and Zhao, G. (2017). The response of carbon storage to the age of three forest plantations in the loess hilly regions of China. Catena 159, 106–114. doi: 10.1016/j.catena.2017.08.013
Forrester, D. I. (2019). Linking forest growth with stand structure: tree size inequality, tree growth or resource partitioning and the asymmetry of competition. For. Ecol. Manag. 447, 139–157. doi: 10.1016/j.foreco.2019.05.053
Gräfe, S., and Köhl, M. (2020). Impacts of future crop tree release treatments on forest carbon as REDD+ mitigation benefits. Land. 9, 1–17. doi: 10.3390/land9100394
Guan, J., Deng, L., Zhang, J., He, Q., and Du, S. (2019). Soil organic carbon density and its driving factors in forest ecosystems across a northwestern province in China. Geoderma 352, 1–12. doi: 10.1016/j.geoderma.2019.05.035
He, X., Zhong, S., Duan, Y., and Luo, D. (2014). A brief analysis of divergences between near-natural forest management and traditional forest tending. Chin. For. Econ. 4, 48–50. doi: 10.3969/j.issn.1673-5919.2014.04.013
Hu, Z., He, Z., Huang, Z., Fan, S., Yu, Z., Wang, M., et al. (2014). Effects of harvest residue management on soil carbon and nitrogen processes in a Chinese fir plantation. For. Ecol. Manag. 326, 163–170. doi: 10.1016/j.foreco.2014.04.023
Li, D., Niu, S., and Luo, Y. (2012). Global patterns of the dynamics of soil carbon and nitrogen stocks following afforestation: a meta-analysis. New Phytol. 195, 172–181. doi: 10.1111/j.1469-8137.2012.04150.x
Li, Y., Zhang, J., Duan, A., and Xiang, C. (2010). Selection of biomass estimation models for Chinese fir plantation. Chin. J. Appl. Ecol. 21, 3036–3046. doi: 10.13287/j.1001-9332.2010.0430
Li, Y., Zhao, P., Huang, Y., Zhang, N., Chen, Z., Jia, X., et al. (2022). Growth and species diversity of Larix principis-rupprechtii by target tree management. J. North-east For. Univ. 50, 20–25. doi: 10.13759/j.cnki.dlxb.2022.05.016
Liang, B., Wang, J., Zhang, Z., Zhang, J., Zhang, J., Cressey, E. L., et al. (2022). Planted forest is catching up with natural forest in China in terms of carbon density and carbon storage. Funda. Res. 2, 688–696. doi: 10.1016/J.FMRE.2022.04.008
Lin, Z., Wu, C., Hong, W., and Hong, T. (2016). Economic benefits analysis of carbon sequestration and timber and determination of optimal rotation period for a Cunninghamia lanceolata plantation-based on time series model. Scientia Silvae Sinicae. 52, 134–145. doi: 10.11707/j.1001-7488.20161017
Liu, J., Tong, S., Chen, X., Chen, C., Wu, K., and Zhang, L. (1995). Establishment of economic volume table (rate) of Chinese fir pole-timber. For. Res. 8, 402–407. doi: 10.13275/j.cnki.lykxyj.1995.04.008
Liu, J., and Tong, S. (1996). Establishment of tending thinning table for the national Chinese fir plantations. For. Res. 9,152–157. doi:10.13275/j.cnki.lykxyj.1996.02.009
Lu, Y., Zhang, S., Lei, X., Ning, J., and Wang, Y. (2009). Theoretical basis and implementation technigues on close-to-nature transformation of plantations. World For. Res. 22, 20–27. doi: 10.13348/j.cnki.sjlyyj.2009.01.002
Ma, J., Kang, F., Chen, X., and Han, H. (2018). Moderate thinning increases soil nitrogen in a Larix principis-rupprechtii (Pinaceae) plantations. Geoderma 329, 118–128. doi: 10.1016/j.geoderma.2018.05.021
Magnússon, R. Í., Tietema, A., Cornelissen, J. H. C., Hefting, M. M., and Kalbitz, K. (2016). Tamm review: sequestration of carbon from coarse woody debris in forest soils. For. Ecol. Manag. 377, 1–15. doi: 10.1016/j.foreco.2016.06.033
Marín-Spiotta, E., Silver, W. L., and Ostertag, R. (2007). Long-term patterns in tropical reforestation: plant community composition and aboveground biomass accumulation. Ecol. Appl. 17, 828–839. doi: 10.1890/06-1268
Neil, G., Williams, M., and Powers, D. (2019). Carbon storage implications of active management in mature Pseudotsuga menziesii forests of western Oregon. For. Ecol. Manag. 432, 761–775. doi: 10.1016/j.foreco.2018.10.002
Nilsen, P. (2008). Strand, LT. (2008). Thinning intensity effects on carbon and nitrogen stores and fluxes in a Norway spruce (Picea abies (L.) karst.) stand after 33 years. For. Ecol. Manage 256, 201–208. doi: 10.1016/j.foreco.2008.04.001
Pan, Y., Birdsey, R. A., Fang, J., Houghton, R., Kauppi, P. E., Kurz, W. A., et al. (2011). A large and persistent carbon sink in the world's forests. Science 333, 988–993. doi: 10.1126/science.1201609
Pearson, T. R. H., Brown, S., and Casarim, F. M. (2014). Carbon emissions from tropical forest degradation caused by logging. Environ. Res. Lett. 9, 206–222. doi: 10.1088/1748-9326/9/3/034017
Pommerening, A., and Grabarnik, P. (2019). Individual-based methods in forest ecology and management. Switzerland: Springer Cham.
Pretzsch, H., and Schütze, G. (2015). Effect of tree species mixing on the size structure, density, and yield of forest stands. Eur. J. For. Res. 135, 1–22. doi: 10.1007/s10342-015-0913-z
Raymond, P., Royo Alejandro, A., Prévost, M., and Dumais, D. (2018). Assessing the single-tree and small group selection cutting system as intermediate disturbance to promote regeneration and diversity in temperate mixedwood stands. For. Ecol. Manag. 430, 21–32. doi: 10.1016/j.foreco.2018.07.054
Schaedel, M. S., Larson, A. J., Affleck, D. L. R., Belote, R. T., Goodburn, J. M., and Page-Dumroese, D. S. (2017). Early forest thinning changes aboveground carbon distribution among pools, but not total amount. For. Ecol. Manag. 389, 187–198. doi: 10.1016/j.foreco.2016.12.018
Schuler, T. M. (2006). Crop tree release improves competitiveness of northern red oak growing in association with black cherry. North. J. App. For. 23, 77–82. doi: 10.1007/s10086-006-0803-6
Steinauer, K., Jensen, B., Strecker, T., De Luca, E., Scheu, S., and Eisenhauer, N. (2016). Convergence of soil microbial properties after plant colonization of an experimental plant diversity gradient. BMC Ecol. 16:19. doi: 10.1186/s12898-016-0073-0
Taki, H., Inoue, T., Tanaka, H., Makihara, H., Sueyoshi, M., Isono, M., et al. (2010). Responses of community structure, diversity, and abundance of understory plants and insect assemblages to thinning in plantations. For. Ecol. Manag. 259, 607–613. doi: 10.1016/j.foreco.2009.11.019
Tesfaye, M. A., Bravo, F., Ruiz-Peinado, R., Pando, V., and Bravo-Oviedo, A. (2016). Impact of changes in land use, species and elevation on soil organic carbon and total nitrogen in Ethiopian central highlands. Geoderma 261, 70–79. doi: 10.1016/j.geoderma.2015.06.022
Thornley, J. H. M., and Cannell, M. G. R. (2000). Managing forests for wood yield and carbon storage: a theoretical study. Tree Physiol. 20, 477–484. doi: 10.1093/treephys/20.7.477
Tue, N. T., Dung, L. V., Nhuan, M. T., and Omori, K. (2014). Carbon storage of a tropical mangrove forest in Mui ca Mau National Park. Vietnam. Catena. 121, 119–126. doi: 10.1016/j.catena.2014.05.008
Vogel, P. J., Lhotka, J. M., and Stringer, J. W. (2022). Long-term effects of crop tree release on growth and quality in white-oak (Quercus alba L.)-dominated stands. For. Sci. 68, 343–352. doi: 10.1093/forsci/fxac015
Wang, Y., Zhang, S., Lu, Y., Meng, J., and Zeng, J. (2014). Initial effects of crop trees growth after crop tree release on Pinus massoniana plantation. Scientia Silvae Sinicae. 50, 67–73. doi: 10.11707/j.1001-7488.20141009
Ward, J. S. (2009). Intensity of precommercial crop tree release increases diameter growth and survival of upland oaks. Can. J. For. Res. 39, 118–130. doi: 10.1139/X08-165
Ward, J. S. (2017). Twenty-five year response of non-crop trees to partial release during precommercial crop tree management. For. Ecol. Manag. 387, 12–18. doi: 10.1016/j.foreco.2016.05.036
Wei, X., Li, Q., Liu, Y., Liu, S., Guo, X., Zhan, L., et al. (2013). Restoring ecosystem carbon sequestration through afforestation: a sub-tropic restoration case study. For. Ecol. Manag. 300, 60–67. doi: 10.1016/j.foreco.2012.06.018
Wu, J., Wang, Y., Yang, Y., Zhu, T., and Zhu, X. (2015). Effects of crop tree release on stand growth and stand structure of Cunninghamia lanceolata plantation. Chin. J. Appl. Ecol. 26, 340–348. doi: 10.13287/j.1001-9332.20141223.011
Zhang, N., Zhang, H., Lin, H., and Jiang, X. (2013). Visual simulation of growth process in Cunninghamia lanceolata based on competition index. For. Res. 26, 692–697. doi: 10.13275/j.cnki.lykxyj.2013.06.004
Zhang, X., Zhang, H., Lu, J., and Lei, X. (2020). Early effects of crop tree release tending on growth of natural secondary Quercus mongolica forest. Scientia Silvae Sinicae. 56, 83–92. doi: 10.11707/j.1001-7488.20201009
Keywords: tending and thinning, crop tree release, traditional thinning, individual tree growth, carbon storage
Citation: Zou X, Zheng Z, Yang C, Yang M, Guo Z, Wang Y, Huang Z, Zhu L, Xu L and Lin K (2023) Initial effects of crop tree release and traditional thinning on productivity and carbon storage of Cunninghamia lanceolata plantation. Front. For. Glob. Change. 6:1288613. doi: 10.3389/ffgc.2023.1288613
Edited by:
Lingbo Dong, Northeast Forestry University, ChinaReviewed by:
Qiang Liu, Hebei Agricultural University, ChinaPan Wan, Northwest A and F University, China
Copyright © 2023 Zou, Zheng, Yang, Yang, Guo, Wang, Huang, Zhu, Xu and Lin. This is an open-access article distributed under the terms of the Creative Commons Attribution License (CC BY). The use, distribution or reproduction in other forums is permitted, provided the original author(s) and the copyright owner(s) are credited and that the original publication in this journal is cited, in accordance with accepted academic practice. No use, distribution or reproduction is permitted which does not comply with these terms.
*Correspondence: Xianhua Zou, zhouxianhua111@163.com