- 1College of Forestry, Guangxi University, Nanning, China
- 2Guangxi Key Laboratory of Forest Ecology and Conservation, Nanning, China
- 3Guangxi Colleges and Universities Key Laboratory for Cultivation and Utilization of Subtropical Forest Plantation, Nanning, China
Introduction: The production of Eucalyptus, a principal economic tree genus in China, is faced with challenges related to soil phosphorus (P) limitations. In this study, we explore variations in phosphorus content, storage, and transformation in Eucalyptus forests. We hypothesize that mixed forests augment soil aggregate stability and P content and that microaggregates are pivotal in determining P differences between mixed and pure forests. Additionally, we posit that mixed forests foster P transformation, enhancing its efficacy in the soil. Current research on the distribution and transformation of soil total P (TP) and P fractions at the soil aggregate level is limited.
Methods: In this study, we selected soil from a Eucalyptus-Mytilaria laosensis Lecomte mixed forest, Eucalyptus-Erythrophleum fordii Oliv mixed forest, and pure Eucalyptus forest in Chongzuo County, Guangxi, China, as the research objects. Using a dry-sieving method, we divided the soil collected in situ from the 0–40 cm layer into aggregates of >2, 1–2, 0.25–1, and <0.25 mm particle sizes, measured the TP and P fractions (resin-extractable inorganic P, bicarbonate-extractable inorganic P, bicarbonate-extractable organic P, sodium hydroxide-extractable inorganic P, sodium hydroxide-extractable organic P, dilute hydrochloric acid-extractable P, concentrated hydrochloric acid extractable inorganic P, concentrated hydrochloric acid-extractable organic P and residue-P) in different aggregates, and used redundancy analysis and PLS SEM to reveal key factors affecting soil P accumulation and transformation.
Results: The results showed that compared to pure Eucalyptus forests, mixed Eucalyptus forests significantly enhanced the stability of soil aggregates and the content and storage of phosphorus, especially the Eucalyptus-Mytilaria laosensis mixed forest. The content of total soil phosphorus and its fractions decreased with increasing aggregate particle size, while the opposite trend was observed for stored P, with aggregates <0.25 mm being the main fraction influencing soil phosphorus accumulation. The transformation process of P fractions was primarily constrained by dissolution rates, mineralization rates, biological activity, including the action of microbes, fungi, and plant–root interactions, and other factors.
Discussion: Mixed forests increased the transformation of phosphorus in soil aggregates, effectivel enhancing the availability of soil phosphorus. In summary, this study provides important evidence for the systematic management of subtropical artificia Eucalyptus forests and the sustainable utilization of soil resources.
1 Introduction
Plantations are considered one of the most widely distributed forest types worldwide, these forests play a crucial role in alleviating land degradation, mitigating the impacts of climate change, reducing air pollution, and curtailing the loss of biodiversity (Yang et al., 2021). Among different plantations, Eucalyptus, which is characterized by rapid growth rates, high productivity, and strong adaptability, is cultivated worldwide to meet the global demand for timber and wood products. Eucalyptus species are grown in more than 90 countries, with China ranking second globally in terms of plantation area, next only to Brazil (Wei et al., 2022; Hutapea et al., 2023). The Guangxi Zhuang Autonomous Region in China has become a principal region for Eucalyptus plantations in China due to its advantageous geographical location and favorable climatic conditions (Zhao et al., 2018). At present, these plantations are mainly monocultures, with intensive management involving short rotations and continuous rotations (Huang et al., 2017). However, continuous cropping has led to severe soil nutrient losses, especially with respect to phosphorus (P) (Liu et al., 1998; Sicardi et al., 2004). Given that P significantly affects timber production efficiency, high amounts of phosphate fertilizers are often used to ensure high yield and sustainable forest production (Laclau et al., 2013; Bazani et al., 2014). However, this increases economic costs and leads to ecological problems such as soil acidification and groundwater pollution, generating negative environmental impacts (Goncalves et al., 1997). To address ongoing challenges associated with declining soil quality and reduced productivity in Eucalyptus plantations, experts advocate for a transition from monocultures to mixed forests (Guo et al., 2022). Specifically, incorporating native tree species, such as Mytilaria laosensis and Erythrophleum fordii, alongside Eucalyptus has been shown to be an effective approach. Recent studies highlight that these mixed plantations not only improve soil chemical properties by enriching the soil with vital nutrients but also enhance soil physical structure, leading to improved water retention and reduced soil erosion (Luo et al., 2015; Dawud et al., 2016; Zhang Q. et al., 2021; Zhang Y. et al., 2021). Furthermore, these mixed ecosystems have demonstrated markedly higher microbial diversity relative to monoculture systems, which results in improved nutrient cycling and overall soil health (Xu et al., 2020; Zhang et al., 2022; Formaglio et al., 2023).
Phosphorus is an essential macronutrient for forest ecosystems, with timber yields largely depending on the fulfilment of forestland P demands (Yang et al., 2019). Weathered soils in subtropical regions typically supply several times the amount of unstable inorganic phosphorus (Pi) needed by vegetation annually (Yang et al., 2011). Phosphorus is considered one of the nutrients that most limits the productivity of tropical and subtropical forests (Sullivan et al., 2014). This is because approximately 95%–99% of soil total P exists in insoluble or unavailable forms that plants cannot directly utilize (Richardson and Simpson, 2011). To better study soil P and improve plant P utilization rates, Hedley et al. (1982) developed a method for continuous P extraction or fractionation to characterize the different organic and inorganic fractions of P in soil based on solubility. This method has become widely accepted and used for characterizing P forms in soil (Condron and Newman, 2011). Later, Tiessen and Moir (2007) improved Hedley’s et al. method, categorizing P into nine fractions. Among these, resin-extractable inorganic P (resin-Pi) is generally considered the most readily available form of P in soil for plant absorption and use, as it exists in the soil solution and can be directly absorbed by plant roots (Costa et al., 2016). Therefore, the content and availability of resin-Pi are important for plant growth. If the soil contains high amounts of resin-Pi, Eucalyptus can usually obtain sufficient P to grow and develop (Yang et al., 2011). After resin-Pi is consumed, it can be replenished from lower solubility P pools through desorption, dissolution, and mineralization (Tiessen and Moir, 2007). Bicarbonate-extractable inorganic P (NaHCO3-Pi) is another form of inorganic P that is easily absorbed by plants. If the soil contains ample NaHCO3-Pi, then plants can obtain sufficient P, which can stimulate growth (Yang et al., 2011). Bicarbonate-extractable organic P (NaHCO3-Po) must be microbially decomposed before it can be utilized by plants. Its impact on Eucalyptus growth depends on the decomposition rate of organic P and the plant’s P demand (Tiessen and Moir, 2007). Sodium hydroxide-extractable inorganic P (NaOH-Pi) and sodium hydroxide-extractable organic P (NaOH-Po) typically exist as iron phosphates and aluminum phosphates (Hunt et al., 2007). They have smaller impacts on Eucalyptus growth than resin-Pi, NaHCO3-Pi and NaHCO3-Po, as these P compounds have low solubility and require a certain period of desorption, dissolution, and mineralization to be available for plant use (Bunemann, 2008). Dilute hydrochloric acid-extractable P (Dil. HCl-P) represents native mineral P pools bound to calcium, which can be utilized by plants after a long period of weathering (Yang et al., 2011). Other P fractions have low solubility and represent the most inaccessible occluded P pools for plants, requiring long-term weathering for plant uptake (Condron and Newman, 2011). Research has underscored that within mixed forests, Mytilaria laosensis and Erythrophleum fordii possess the capacity to access phosphorus from deep soil strata due to their expansive and deep root systems (Luo et al., 2015; Zhang Q. et al., 2021; Zhang Y. et al., 2021). However, the reasons for the enhanced phosphorus acquisition efficiency of these woodlands is still poorly understood. In this context, the enhanced Hedley method can be used to study diverse phosphorus fractions in soil and provide insights into phosphorus efficacy in subtropical forest ecosystems, such as Eucalyptus plantations (Johnson et al., 2003).
Notably, current research on P in mixed Eucalyptus forests mainly focuses on entire soil layers, and studies at the microscopic level of soil aggregates are relatively limited. Soil aggregates are the basic units of the soil structure, affecting its physical, chemical, and biological properties (Six et al., 2004). The quantity and quality of soil aggregates reflect the soil’s nutrient supply and storage capabilities (Mustafa et al., 2020). The stability of soil aggregates is an important index that is closely related to soil P storage and nutrient recovery (Song et al., 2019). According to the formation and stabilization mechanisms of soil aggregates, they are divided into large aggregates (>0.25 mm) and microaggregates (≤0.25 mm) (Alag and Yilmaz, 2009). Aggregates serve as an important compartment for soil P storage and effective utilization. Aggregates of different particle sizes show significant differences in P adsorption, retention, and supply capabilities, which in turn affect the transformation and distribution of different P fractions (Song et al., 2019). Much research has been focused on soil aggregates and organic carbon (Cao et al., 2021; Li et al., 2021), but the related features of P fractions in aggregates are not clear. While a few studies exist on the effects of aggregate size on P, they are not comprehensive (Sun and Xiong, 1998; Rubk et al., 1999). Furthermore, the formation and stabilization processes of aggregates are influenced by both natural conditions and human activities, leading to differences in the number and quality of soil aggregates in different regions (Six et al., 2002; Cooper et al., 2020; Liu et al., 2021). Therefore, evaluating differences in P fraction content and transformation in the soil of subtropical pure and mixed Eucalyptus forests from the perspective of aggregates has important practical value for elucidating the retention and release mechanisms of P in soil aggregates during the management of Eucalyptus plantations.
In this study, variations in phosphorus content, storage, and transformation within soil aggregates were examined across various Eucalyptus forest types. Based on previous research, this study proposes three hypotheses: (1) mixed forests show higher stability of soil aggregates and the content and storage of P in soil aggregates relative to pure forests; (2) microaggregates are the main factors leading to differences in total P and P fraction content between mixed forest and pure forest soils; and (3) mixed forests promote P transformation to enhance the effectiveness of P in the soil. In response to these hypotheses, we set the following research objectives: (1) to determine the impact of mixed forests on the stability, content, and storage of P in soil aggregates; (2) to elucidate the role of microaggregates in driving differences in total P and its fractions between mixed and pure forest soils; and (3) to analyze the degree and mechanisms through which mixed forests affect P transformation. To achieve these objectives, we performed a meticulous and systematic evaluation of data collected from a comprehensive set of field surveys and laboratory experiments.
2 Materials and methods
2.1 Study site
The study area is located in the Qing Mountain Experimental Field of the Experimental Center of Tropical Forestry, Chinese Academy of Forestry in Pingxiang County, Guangxi Zhuang Autonomous Region, China (21°57′-22° 19’N, 106°39′-106° 59′E) (Figure 1). The climate of the region is mainly subtropical monsoon, with an annual average rainfall of 1,500 mm and an average annual temperature of 18.8°C. The topography is dominated by low mountains and hills, with a slope of 20–22° and an altitude of 500–900 m. The soil type of this region is mainly Latosol (IUSS Working Group, 2014), with sedimentary rocks as the native rock. Currently, the Tropical Forestry Center has 6,000 hectares of experimental and demonstration forests with valuable and high-quality broad-leaved tree species, mainly Eucalyptus and Pinus massoniana Lamb., as well as tree species such as Mytilaria laosensis, Erythrophleum fordii, Castanopsis hystrix, Tectona grandis, Betula alnoides, Michelia gioii, and Dalbergia odorifera.
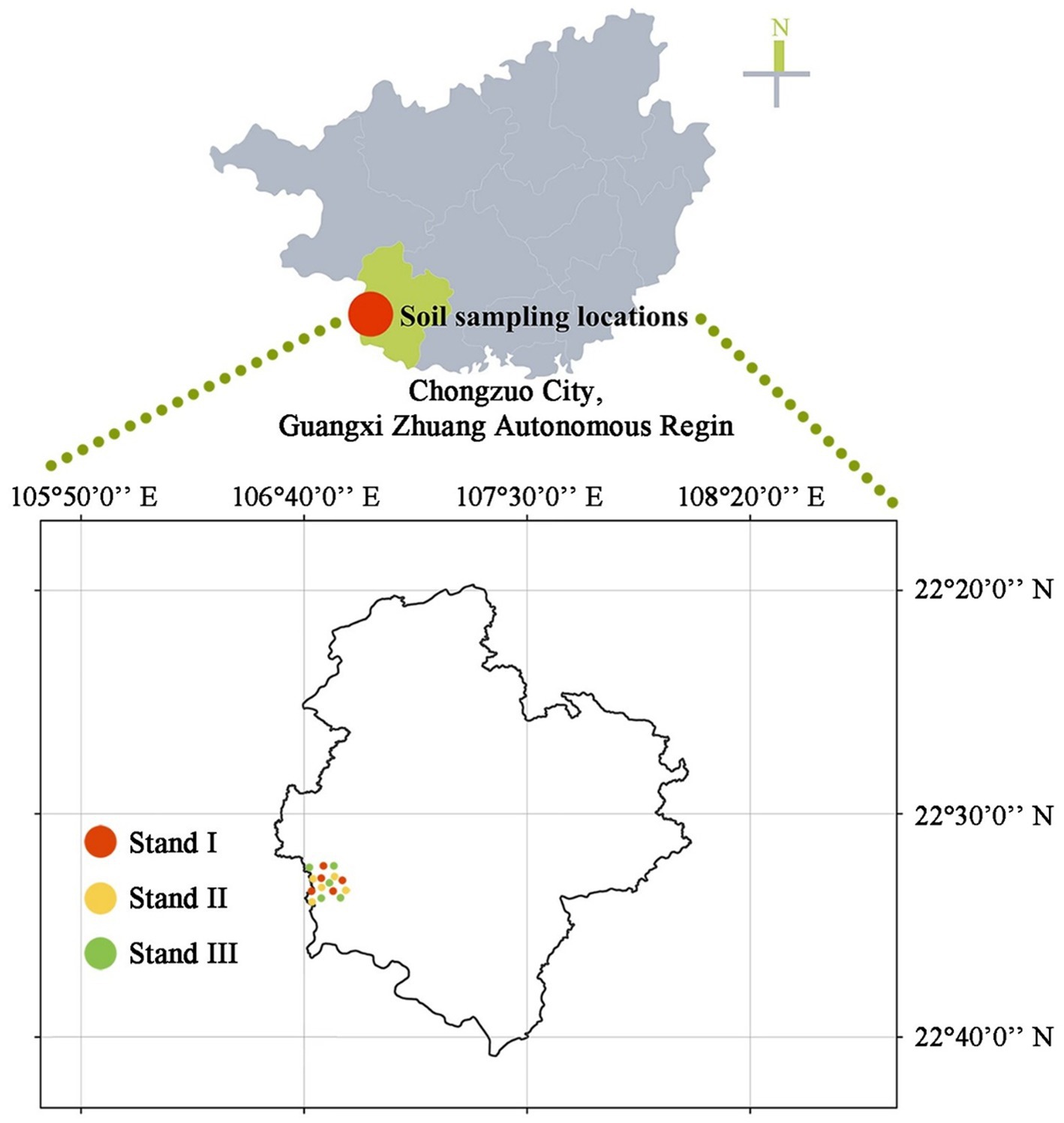
Figure 1. The location of the study area. Stand I: the mixed Eucalyptus and Mytilaria laosensis forest; stand II: the mixed Eucalyptus and Erythrophleum fordii forest; stand III: the pure Eucalyptus stand.
2.2 Experimental design
After field surveys, we selected a Eucalyptus-Mytilaria laosensis mixed forest (stand type I), a Eucalyptus-Erythrophleum fordii mixed forest (stand type II), and a pure Eucalyptus forest (stand type III) (Table 1) with similar site conditions with respect to soil type, parent material, altitude, slope, and aspect. All three types of forests were established on barren forestland in March 2012 by full reclamation and afforestation, with a planting density of approximately 1,666 plants·ha−1. The ratio of Eucalyptus to other mixed species in the mixed forests was 2:1, with a spacing of approximately 2 m × 3 m. For the first 3 years after afforestation, fertilization and weeding were carried out once per year, with a fertilizer application of 840 kg·ha−1 (fertilizer composition: N: 31%, P: 10%, K: 10%, B: <0.5% and Zn: <0.5%). Afterward, the plantation managers employed a near-natural management strategy, emphasizing natural tree regeneration, minimal chemical intervention, and selective thinning to closely simulate natural forest conditions and limit human interference. A completely randomized design was adopted in this study: five 20 m × 20 m standard plots were set up in each of the three stand types, totaling 15 plots (3 stand types × 5 repetitions) (Figure 1). The distance between adjacent plots was greater than 1,000 m to prevent pseudoreplication and to reduce spatial autocorrelation. In each plot, a subplot (S = 20 m × 20 m) was randomly established at a distance >50 m from the forest edge.
2.3 Litter and soil sampling
Sampling was conducted in October 2021 for this study. Before soil sampling, 5 litter samples were collected from the surface of each of 5 random subplots (1 m × 1 m) and placed in plastic bags. Then, the 5 litter samples were combined into a composite litter sample. The 15 composite litter samples were dried at 80°C to a constant weight and were weighed after drying. Soil samples were collected from the same locations as the litter samples; 5 soil samples were collected with a spade and combined into a composite soil sample. The 30 (3 types of forest × 2 soil layers × 5 replicates) composite soil samples were carefully separated into natural aggregates. Then, a 5 mm sieve was used to remove small stones, coarse roots, and animal remains. Finally, the sieved soil aggregates (<5 mm) were further used for the classification of aggregates of various particle sizes. From each plot, 5 soil samples from each soil layer within 5 small subplots were collected with a ring knife (depth = 50 mm, Ø = 50.46 mm, V = 100 cm−3) to measure bulk density, pH, and P fraction content.
2.4 Soil aggregate separation
The Savinov dry-sieving method (Wang et al., 2021) was used for soil aggregate separation. Air-dried soil samples (500 g) were sieved using sieves with apertures of 2 mm, 1 mm, and 0.25 mm that were vertically oscillated at a rate of 5 cm s−1 for 20 min. This resulted in the separation of aggregates of four grain sizes, namely, large soil aggregates (>2 mm), medium aggregates (1–2 mm), fine aggregates (0.25–1 mm), and microaggregates (<0.25 mm) (Table 2). The contents of clay particles and P fractions in the aggregates of each particle size were determined.
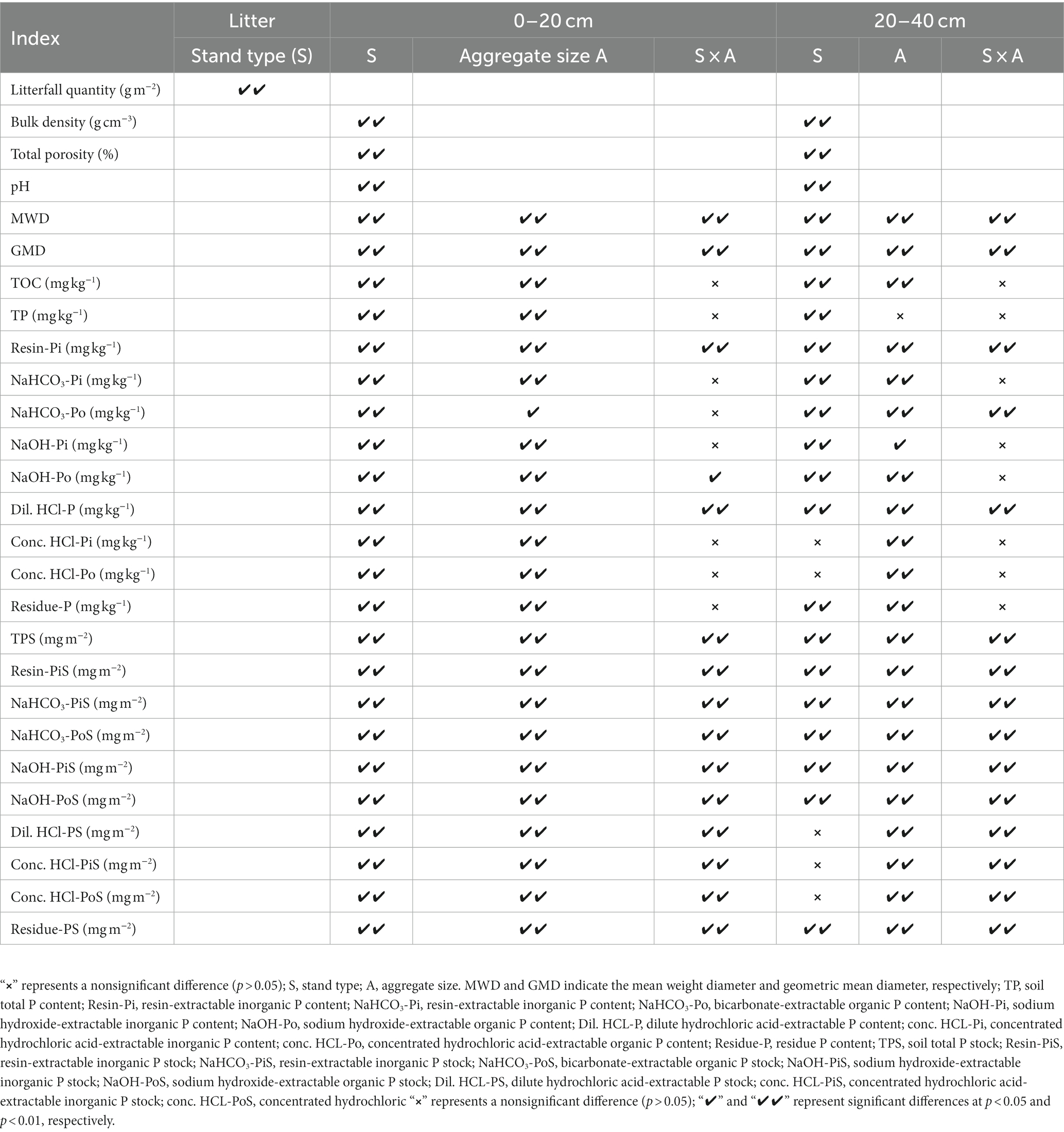
Table 2. Litterfall and soil properties as influenced by aggregate size and stand type in Eucalyptus plantations.
2.5 Soil property analyses
Before analyzing soil properties, we air-dried the soil samples at room temperature and then weighed them. A cutting ring was used to determine the soil bulk density (Lu, 2000). Soil porosity was measured using the ring knife method (Lu, 2000). Soil pH was determined by a potentiometric method (Lu, 2000). A pycnometer (TM 85, Veichi, China) was used to measure soil clay content (Lu, 2000). Determination of soil organic carbon content was performed using the potassium dichromate-external heating method (Lu, 2000).
The sequential fractionation of P fractions was carried out in reference to the meth Sequential fractionation of P fractions was carried out based on the methods of Hedley et al. (1982) and Tiessen and Moir (2007), in which P is separated into various forms through a series of extractions. The extraction process was as follows: 0.5 g of soil was sequentially added to 30 mL H2O and a 2 cm2 ion-exchange resin film, followed by different extractants, i.e., 0.5 mol L−1 NaHCO3, 0.1 mol L−1 NaOH, 1 mol L−1 HCl, and 11.3 mol L−1 HCl. Each extract was shaken at 60 rpm for 16 h and then centrifuged at 4,000 rpm for 20 min. The supernatant was collected as the sample to be tested for these fractions. Then, the next extractant was added to the precipitated soil sample. Thus, the following soil fractions were sequentially extracted: resin-P, NaHCO3-Pi, NaHCO3-Po, NaOH-Pi, NaOH-Po, Dil. HCl-P, concentrated hydrochloric acid-extractable inorganic P (conc. HCl-Pi), concentrated hydrochloric acid-extractable organic P (conc. HCl-Po) and residue P.
2.6 Statistical analysis
The mean weight diameter (MWD, mm) and geometric mean diameter (GMD, mm) are key indicators to evaluate soil aggregate stability, and the calculation formulas for MWD and GMD are as follows (Zhang Q. et al., 2021; Zhang Y. et al., 2021):
where is the average diameter of aggregates in each grade (mm) and is the weight percentage of aggregates in each grade (%).
The total P storage (TPS, g m−2) calculation formula is (Wang et al., 2020):
where is the mass ratio of aggregates of different sizes to total aggregates; TP i is the total P content of the ith aggregate size (g kg−1); B is the soil bulk density (g cm−3); H is the soil layer thickness (cm); and 10 is the unit conversion factor. Similarly, stored soil resin-Pi, NaHCO3-Pi, NaHCO3-Po, NaOH-Pi, NaOH-Po, Dil-HCl-P, conc. HCl-Pi, conc. HCl-Po, and residue-P was calculated in the same way.
We identified two main variables in this study: stand type and aggregate size. Therefore, statistical analysis was conducted according to soil depth. Statistical analysis was performed using SPSS 22.0 (SPSS Inc., Chicago, IL, United States). The Shapiro–Wilk test was used to check the normality of the data before variance analysis was conducted. The Levene method was used to test variance homogeneity. One-way analysis of variance (ANOVA) was used to test the impact of stand type on litter mass and soil physicochemical properties. Two-way ANOVA was used to test the impact of stand type, aggregate size, and their interaction on soil aggregate physicochemical characteristics. Subsequently, the Tukey HSD post hoc test was used to test the significance of differences. p < 0.05 indicated that the difference was statistically significant. CANOCO 5.0 (CANOCO Inc., Shanghai, China) was used to conduct redundancy analysis to test the impact of soil aggregate parameters on P content and storage in the soil. In addition, a partial least squares structural equation model was constructed using Smart PLS 3.0 (SmartPLS GmbH Inc., Oststeinbek, Germany) to predict the P transformation patterns in different stand types. We used the coefficient of determination (R2) and cross-validated redundancy (Q2) to evaluate the structural model. R2 measures the relationship between the explained variance of latent variables and total variance (Chin, 1998). R2 values of 0.670, 0.333, and 0.190 indicate high, medium, and low explanatory rates, respectively (Diamantopoulos and Siguaw, 2006). Q2 measures the predictive relevance of a specific structural model (An et al., 2010; Hair et al., 2019).
3 Results
3.1 Litter mass and overall soil physicochemical properties
The litter mass and total soil porosity of stand types I and II were significantly higher than those of stand type III (p < 0.05), whereas soil bulk density and pH showed the opposite trend (Table 3). In the 0–20 cm soil layer, the contents of TOC, TP, resin-Pi, and NaHCO3-Pi in stand type I were significantly higher than those in stand types II and III. The contents of NaHCO3-Po, NaOH-Pi, NaOH-Po, Dil. HCl-P, conc. HCl-Pi, conc. HCl-Po and residue-P in stand types I and II were significantly higher than those in stand type III (p < 0.05). In the 20–40 cm soil layer, the TP, resin-Pi, NaHCO3-Pi, and NaHCO3-Po contents in stand type I were significantly higher than those in stand types II and III. The contents of NaOH-Pi, NaOH-Po, and residue-P in stand types I and II were significantly higher than those in stand type III (p < 0.05). However, there were no significant differences in the contents of Dil. HCl-P, conc. HCl-Pi, and conc. HCl-Po among the three types of forests. Additionally, the total soil porosity, TP, and P fraction contents of all three stand types decreased with increasing soil depth, while the soil bulk density and pH increased with increasing soil depth.
3.2 Distribution and stability characteristics of soil aggregates
In both the 0–20 cm and 20–40 cm soil layers, the proportion of aggregates with a diameter of >2 mm in stand types I and II was significantly higher than that in stand type III (p < 0.05), while the proportion of aggregates with a diameter of <0.25 mm exhibited the opposite trend (Table 4). All three stand types were predominantly characterized by aggregates >2 mm in diameter, with proportions significantly different from those of other aggregates (p < 0.05), ranging from 54.10% to 69.58%. Simultaneously, in both soil layers, the mean weight diameter (MWD) and geometric mean diameter (GMD) of stand types I and II were significantly higher than those of stand type III (p < 0.05).
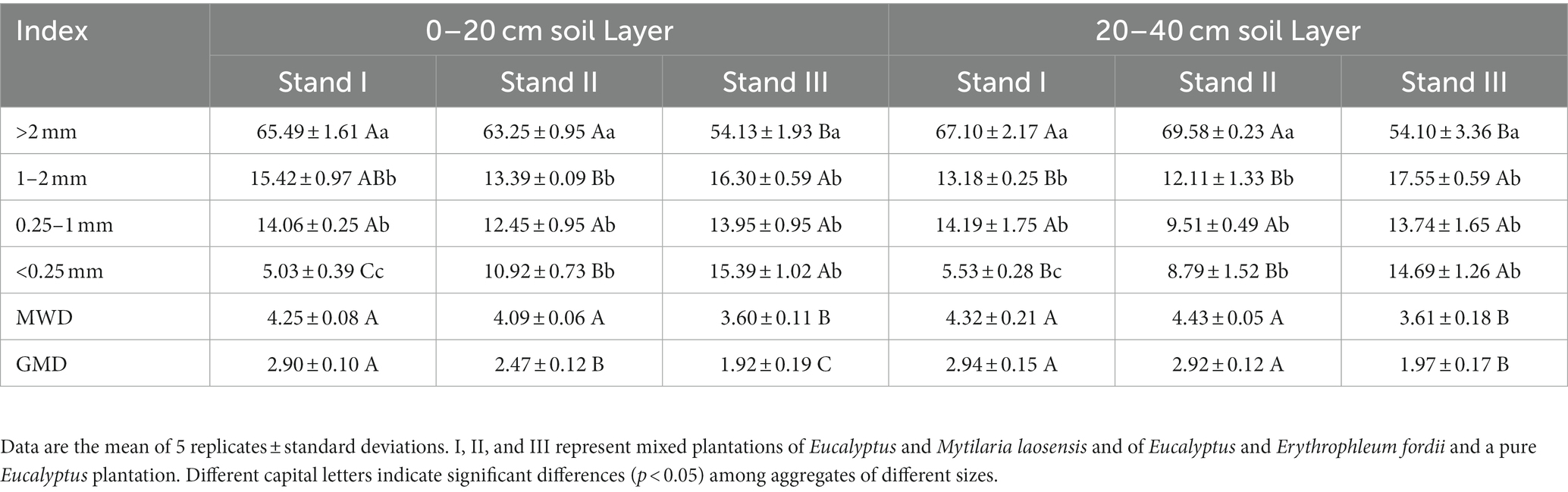
Table 4. Distribution and stability of soil aggregates as influenced by stand type in Eucalyptus plantations.
3.3 Total P and its fractions in soil aggregates: content, storage and contribution
In this study, we undertook an in-depth analysis to elucidate the distribution and storage of multiple phosphorus components across varying forest stand types and soil particle sizes. At the aggregate level, our experimental findings suggest that the content of diverse phosphorus fractions is principally confined to 0.25–1 mm aggregates in each type of forest stand (Figure 2). However, phosphorus storage exhibits a different distribution pattern; reserves are primarily localized in aggregates greater than 2 mm for all stand types under consideration. Regarding the relative contribution of aggregate sizes to phosphorus content, it was observed that aggregates larger than 2 mm in size play a significantly more prominent role than their smaller-sized counterparts, although there are particular phosphorus components that show exceptions to this trend (p < 0.05) (Figure 3). From a stand type perspective, our data consistently demonstrate that stand type I exhibits appreciably higher levels of both phosphorus content and storage compared to stand type III. This marked discrepancy is observable across two distinct soil strata, namely, the 0–20 cm and 20–40 cm layers. One commonality that was observed across all stand types and soil depths was a consistent decline in both phosphorus content and storage with increasing soil depth.
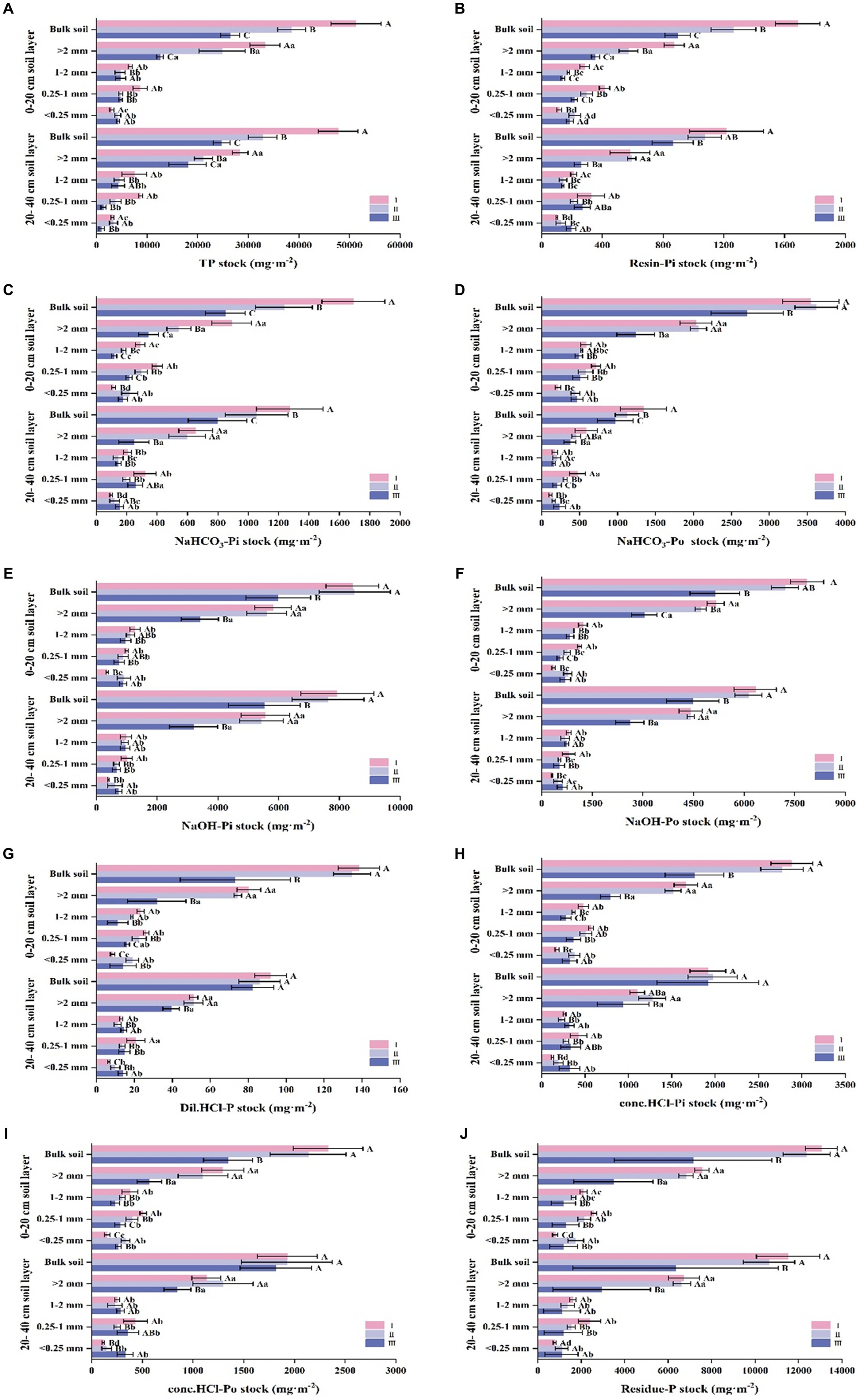
Figure 2. Stock of phosphorus components in soil aggregates of different Eucalyptus forest types. In different types of artificial Eucalyptus forest stands, the stocks of aggregate-related resin-extractable inorganic P (resin-Pi), bicarbonate-extractable inorganic P (NaHCO3-Pi), bicarbonate-extractable organic P (NaHCO3-Po), sodium hydroxide-extractable inorganic P (NaOH-Pi), sodium hydroxide-extractable organic P (NaOH-Po), dilute hydrochloric acid-extractable P (Dil. HCl-P), concentrated hydrochloric acid-extractable inorganic P (conc. HCl-Pi), concentrated hydrochloric acid-extractable organic P (conc. HCl-Po), and residual P (residue-P) were examined. Different uppercase letters indicate significant differences between different stand types (p < 0.05), and different lowercase letters indicate significant differences in aggregate particle size within the same stand type (p < 0.05).
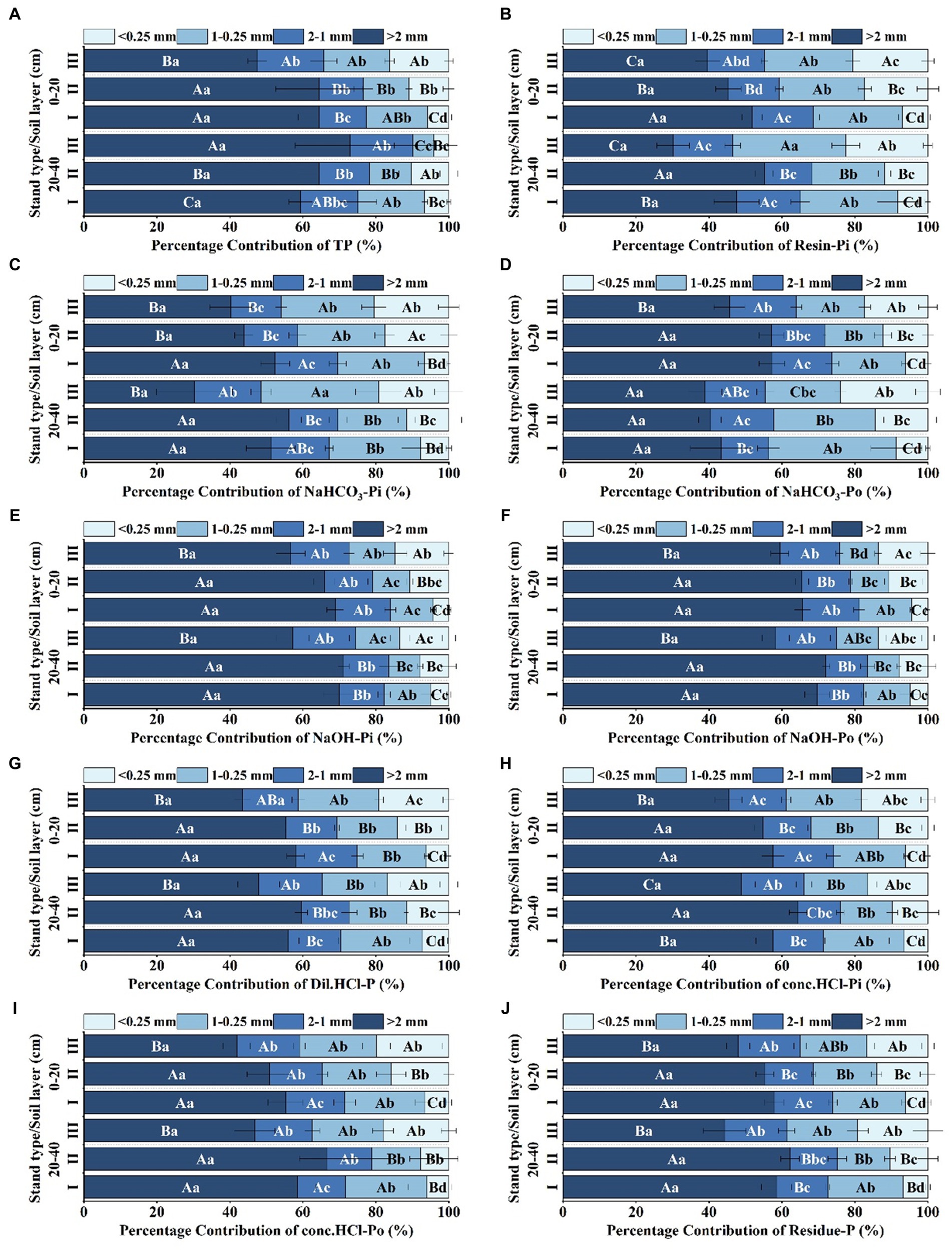
Figure 3. Contribution rate of phosphorus components in soil aggregates of different Eucalyptus forest types The contribution rate of the aggregate reserves of resin-extractable inorganic P (resin-Pi), bicarbonate-extractable inorganic P (NaHCO3-Pi), bicarbonate-extractable organic P (NaHCO3-Po), sodium hydroxide-extractable inorganic P (NaOH-Pi), sodium hydroxide-extractable organic P (NaOH-Po), dilute hydrochloric acid-extractable P (Dil. HCl-P), concentrated hydrochloric acid-extractable inorganic P (conc. HCl-Pi), concentrated hydrochloric acid-extractable organic P (conc. HCl-Po), and residual P (residue-P) in aggregates of different particle sizes across various artificial Eucalyptus forest stand types. Different uppercase letters indicate significant differences between different stand types (p < 0.05), and different lowercase letters indicate significant differences in aggregate particle size within the same stand type (p < 0.05).
3.4 Relationship between soil aggregate characteristics and P content
The RDA results showed (Figure 4) that in the two soil layers studied, soil aggregate characteristics explained 93.83% and 98.41% of the soil P content. Aggregates with a diameter of <0.25 mm were the most critical factor affecting the soil P content in the 0–20 cm layer, explaining 82.70% of the variance, followed by aggregates with diameters of 0.25–1 mm and the MWD. Aggregates with a diameter of <0.25 mm were also the most critical factor affecting the soil P content in the 20–40 cm layer, explaining 30.70% of the variance, followed by aggregates with diameters of 1–2 mm and the MWD. Additionally, in the upper soil stratum of 0–20 cm, there was a positive correlation between each phosphorus component and parameters such as GMD and MWD, as well as with aggregates larger than 2 mm. In the 20–40 cm layer, a similar positive correlation was observed for most phosphorus components with GMD, MWD, and aggregates exceeding 2 mm in size. However, it is noteworthy that only Dil. HCl-P and Conc. HCl-Po exhibited a positive association with aggregates sized between 0.25–1 mm at this depth.
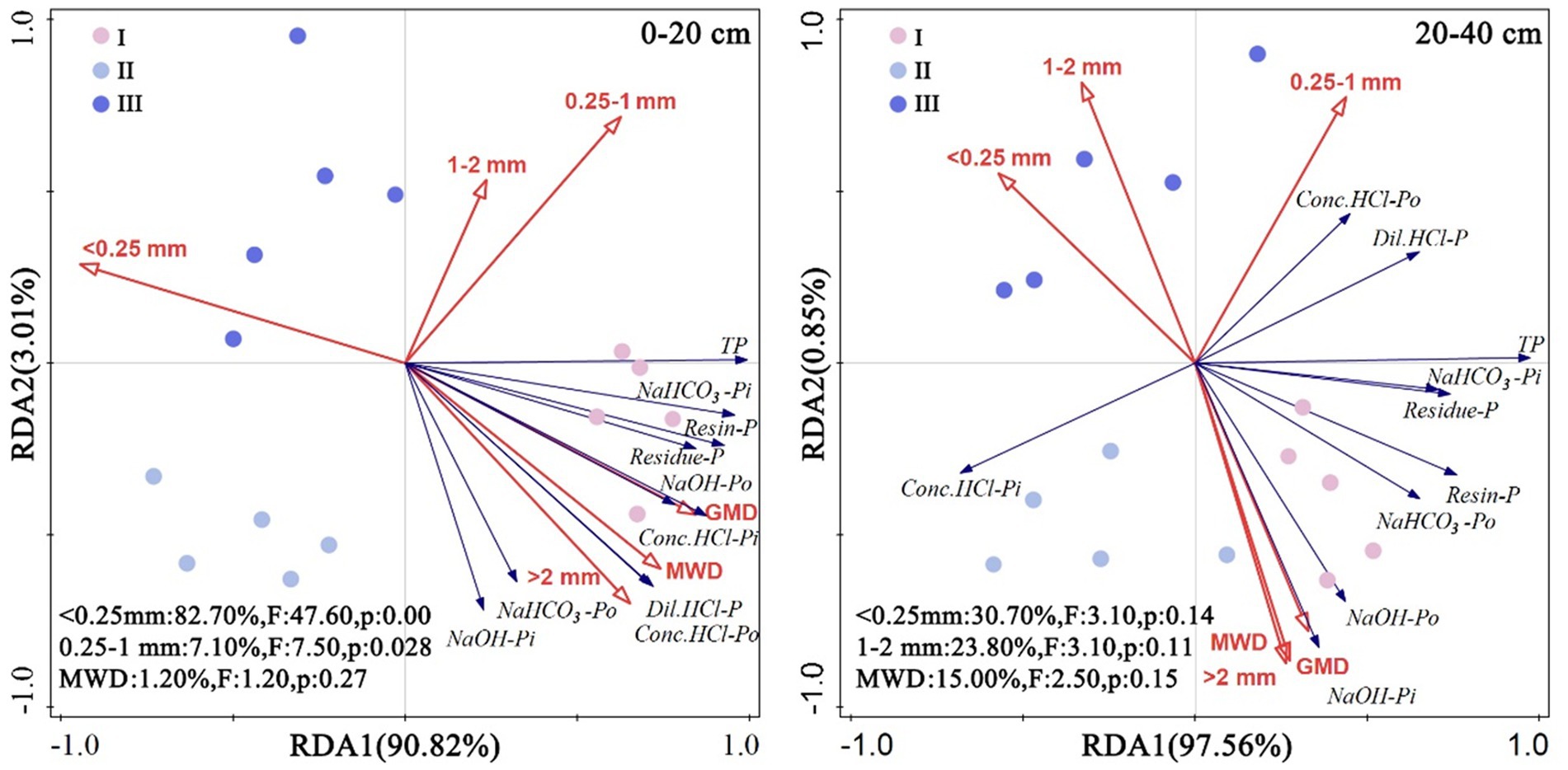
Figure 4. Redundancy analysis of chemical characteristics and soil aggregate distribution characteristics in different artificial Eucalyptus forest stands at soil depths of 0–20 cm and 20–40 cm. TP, resin-Pi, NaHCO3-Pi, NaHCO3-Po, NaOH-Pi, NaOH-Po, Dil. HCl-P, conc. HCl-Pi, conc. HCl-Po and residue-P represent the total P, resin-extractable inorganic P, bicarbonate-extractable inorganic P, bicarbonate-extractable organic P, sodium hydroxide-extractable inorganic P, sodium hydroxide-extractable organic P, dilute hydrochloric acid-extractable P, concentrated hydrochloric acid-extractable inorganic P, concentrated hydrochloric acid-extractable organic P, and residual P contents in the soil, respectively. MWD and GMD represent the mean weight diameter and geometric mean diameter, respectively. The proportions of microaggregates (<0.25 mm), small aggregates (0.25–1 mm), medium aggregates (1–2 mm), and large aggregates (>2 mm) are represented.
3.5 P fraction transformation characteristics in different stand types
Based on the PLS-SEM results, the direct and indirect impacts of various P fractions on resin-Pi in the three stand types were identified, each showing different P fraction transformation characteristics (Figure 5). For instance, in stand type I, resin-Pi was directly and positively influenced by NaHCO3-Pi (total effect = 0.659), NaHCO3-Po (total effect = 0.459), and Dil. HCl-P (total effect = 0.758) and directly and negatively influenced by NaOH-Po (total effect = −0.245). In stand type II, resin-Pi was directly and positively influenced by NaHCO3-Po (total effect = 0.559) and NaOH-Po (total effect = 0.506) and directly and negatively influenced by NaHCO3-Pi (total effect = −0.483). In stand type III, resin-Pi was directly and positively influenced by NaHCO3-Pi (total effect = 0.666), NaHCO3-Po (total effect = 0.203), and occluded-P (total effect = 0.649) and directly and negatively influenced by NaOH-Po (total effect = −0.483). Other P fractions indirectly affected resin-Pi through differential regulation. Furthermore, apart from the impacts of various P fractions on resin-Pi, other fixed P transformation pathways existed in the three stand types. For example, occluded-P transformed into Dil. HCl-P and NaOH-Pi, Dil. HCl-P transformed into NaOH-Po, and NaOH-Po transformed into NaHCO3-Po. In the three stand types, the R2 values of the P fractions all followed the trend of stand type I > stand type II > stand type III and were all above 0.670. The mixed forest model had a higher explanatory rate than the pure forest model, and the Q2 value displayed a similar pattern.
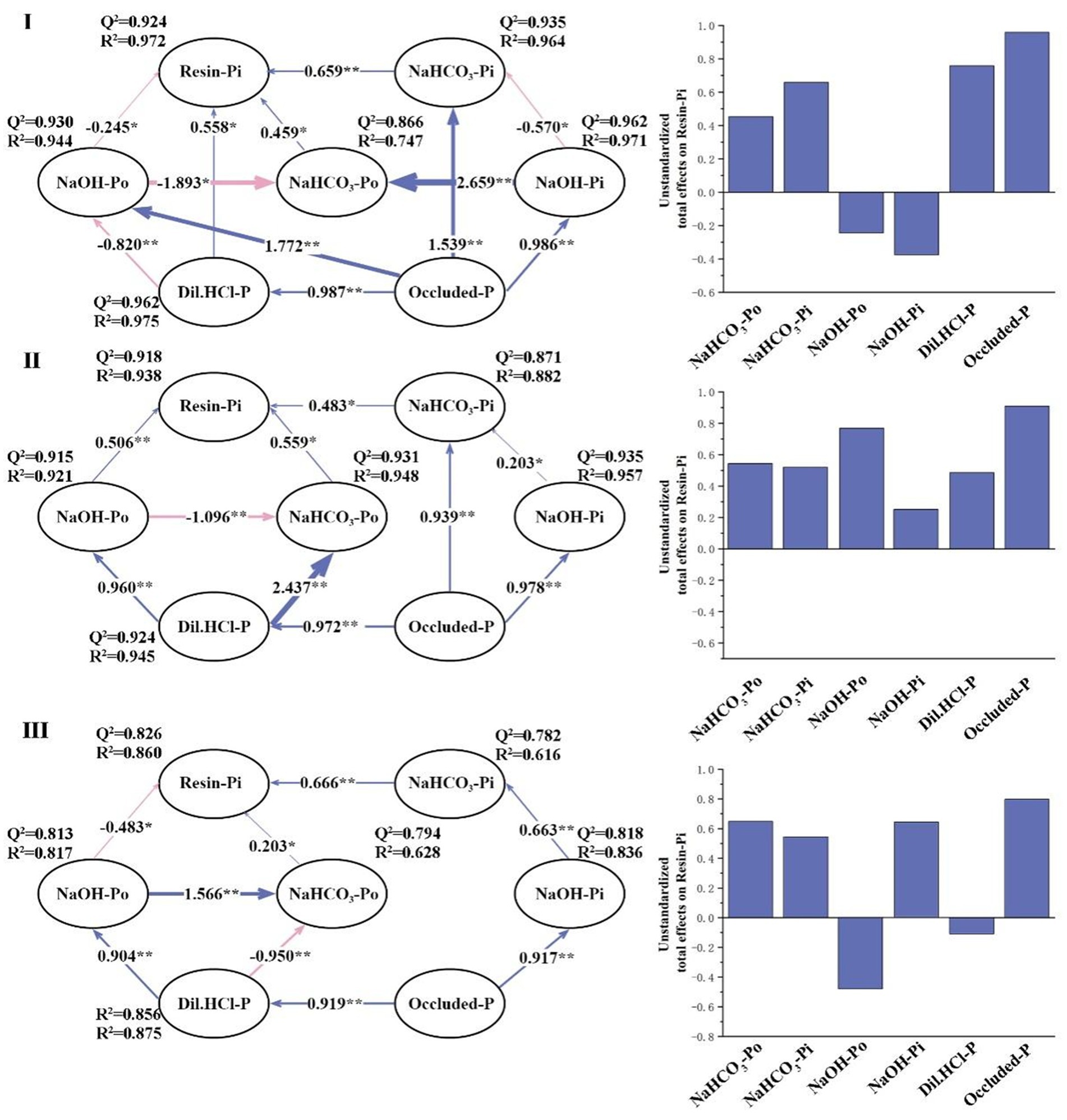
Figure 5. PLS-SEM analysis of P dynamics in diverse Eucalyptus plantation systems. TP, resin-Pi, NaHCO3-Pi, NaHCO3-Po, NaOH-Pi, NaOH-Po, Dil. HCl-P, conc. HCl-Pi, conc. HCl-Po and residue-P represent resin-extractable inorganic P, bicarbonate-extractable inorganic P, bicarbonate-extractable organic P, sodium hydroxide-extractable inorganic P, sodium hydroxide-extractable organic P, dilute hydrochloric acid-extractable P, concentrated hydrochloric acid-extractable inorganic P, concentrated hydrochloric acid-extractable organic P, and the residual P content in soil, respectively. A purple line signifies a positive association, whereas a pink line indicates a negative association. The thickness of the line is proportional to the size of the path coefficient. ** and * represent significant correlations at p < 0.01 and p < 0.05, respectively.
4 Discussion
4.1 Effects of mixed forests on soil aggregate stability and P distribution
Soil aggregates are the basic units of soil structure, and their distribution determines the size distribution of soil pores, which in turn influences the cycling of material and energy at the aggregate scale. Well-aggregated soil fosters good water cycling, absorption and plant growth and enables the sustainability of forest plantations (Dal Ferro et al., 2012; Gao et al., 2022). Aggregate composition and stability are critical for assessing soil structure, which in turn affects nutrient supply, storage, and erosion resistance (Fattet et al., 2011). Larger aggregates improve soil stability and pore structure, while an increase in microaggregates can elevate the risk of soil erosion (Zhang et al., 2008; Wang et al., 2022).
In our study, we found that mixed Eucalyptus-Mytilaria laosensis and Eucalyptus-Erythrophleum fordii forests have better soil aggregate stability than pure Eucalyptus forests. This is due to higher TOC and litter levels, which enhance large aggregate formation and reduce erosion. Consequently, these mixed forests showed improved soil quality metrics, such as higher MWD and GMD of aggregates (Al-Kaisi et al., 2014; Giumbelli et al., 2020) (Table 4). The physical and chemical attributes of soil structure and biological factors play a pivotal role in determining soil stability (Wang et al., 2022). In mixed Eucalyptus forests, increased plant diversity often supports a broader range of soil fauna and flora. This biodiversity can lead to enhanced soil aggregation through the combined action of various organisms (He et al., 2023). For instance, the burrowing activities of larger soil animals, such as earthworms, can create channels in soils, facilitating root growth and water movement (Mao et al., 2022). Furthermore, in mixed forests, the presence of diverse plants can lead to richer fungal networks or mycorrhizae, which help to bind soil particles into stable aggregates (Tang and Wang, 2022). Mixed forests also play a pivotal role in addressing the triple planetary crises. The introduction of diverse mixed plantations can contribute to reducing biodiversity losses by providing varied habitats and promoting ecosystem balance. Additionally, increased carbon sequestration in both the biomass and well-aggregated soil of mixed plantations directly contributes to the mitigation of climate change effects. While in our research, we did not directly investigate the effects of mixed forests on air pollution, it is worth noting that healthy forests can act as filters, capturing pollutants and improving air quality.
P is an essential nutrients for plant growth, and soil P levels determine the growth status of plants (Yang et al., 2019). At the aggregate level, this study revealed that TP, resin-Pi, NaHCO3-Pi, NaHCO3-Po, Dil. HCl-P, conc. HCl-Pi, conc. HCl-Po, and residue-P contents were mainly distributed in aggregates 0.25–1 mm and <0.25 mm in size (Figure 6). This may indicate that smaller aggregates have a stronger adsorption capacity for P than NaOH-Pi and NaOH-Po, as smaller aggregates have larger specific surface areas and provide more binding sites for P (Egan et al., 2018). In addition, the fine-grained minerals in soil, mainly contained in small aggregates, provide protection for most organic matter through physical retention, thereby enhancing the adsorption capacity of small aggregates for organic P (Adesodun et al., 2007). NaOH-Pi and NaOH-Po are primarily distributed in >2 mm and 1–2 mm aggregates (Figure 6) because they are easily precipitated or adsorbed by amorphous Al and Fe in the soil (Tiessen et al., 1984; Barrow, 2015). Studies report that Al3+ and Fe2+ in the soil are mainly distributed within these aggregates (Wang et al., 2022). Many studies have shown that mixed forests significantly improve soil nutrient conditions, including soil P content (Huang et al., 2013; He et al., 2023). Our study also showed that the total P and its fractions in the soil of the Eucalyptus-Mytilaria laosensis and Eucalyptus-Erythrophleum fordii mixed forests were generally significantly higher than those in the pure Eucalyptus forest (Figure 6). This is because decomposition of plant litter is a major source of soil P, which not only directly replenishes P in the soil but also promotes P mineralization and weathering in the soil by promoting soil microbial proliferation and enzyme activity (Stewart and Tiessen, 1987; Frossard et al., 2000). Moreover, mixed forests have more diverse litter, which often provides a greater array of enzymes and microbial communities than litter from pure forests, further enhancing nutrient cycling (Vitousek et al., 2010; Kerdraon et al., 2020; Sayer et al., 2020). Beyond chemical interactions, dynamic interactions among biological processes—including root activity, intricate microbial associations, and soil fauna behavior—come together in mixed forests. This diversity enriches the soil environment, amplifying P availability (Turner and Engelbrecht, 2011; Gao et al., 2022). Moreover, the heterogeneous root architectures and penetration depths inherent to mixed plantations enable plant access to varied P stores throughout the soil strata, facilitating holistic nutrient cycling (Liu and Liu, 2012; He et al., 2023; Yan et al., 2023). Furthermore, the intertwined relationships that exist between the physical, chemical, and biological factors involved in soil health are magnified in mixed-species plantations. The combined effects of varied root systems, diverse microbial communities, and soil fauna activities result in a robust and resilient soil environment that is optimal for supporting forest plantations (Zhao and Hu, 2022). For instance, a balanced C:P ratio, influenced by decomposition rates and microbial activities, indicates effective nutrient cycling, and studies have pointed to the crucial role it plays in determining the mineralization capacity of soil P (Zeng et al., 2015; Zhang et al., 2023). Our study results indicate that the C:P ratio in the mixed forests was lower than that in the pure forest, which increases the soil’s P mineralization capacity (Table 3). According to a study by Six et al. (2004), changes in soil bulk density and porosity affect aeration and the permeability of soil. Excessively high soil bulk density or soil porosity that is very low can indirectly lead to a decrease in soil P content. In this study, creating mixed forests reduced soil bulk density and increased soil porosity (Table 3), which also resulted in a higher P content in mixed forests than in pure forests. In heterogeneous forest ecosystems, such as mixed forests, the activities of soil arthropods, such as centipedes and woodlice, have a significant impact on soil structural integrity. Centipedes, while hunting for prey, navigate through soil particles, inadvertently aerating the soil and creating minute passageways. Moreover, woodlice, by feeding on decaying plant material, contribute to the formation of stable soil aggregates by excreting fine particulate organic matter. This combination of activities from diverse fauna results in a well-structured soil with enhanced porosity, which in turn facilitates better water storage, gas exchange, and root exploration, fortifying ecosystem resilience and health (Kuperman, 1996; Yang et al., 2023).
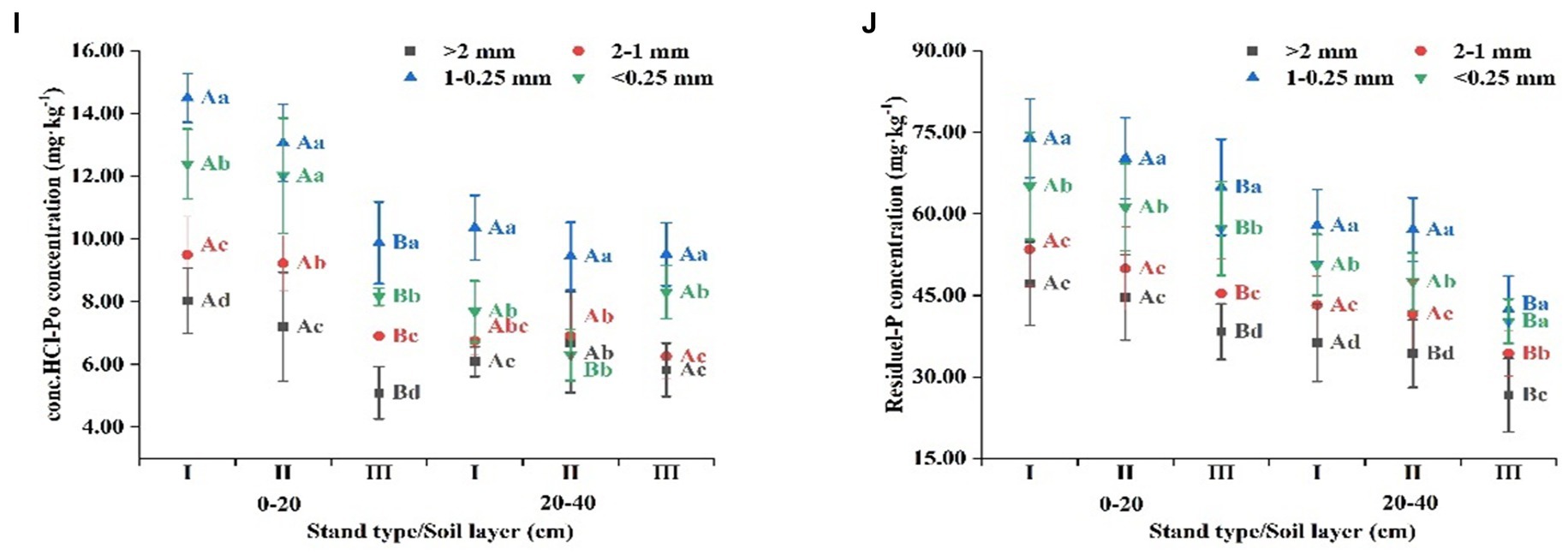
Figure 6. In different types of artificial Eucalyptus forest stands, the concentrations of aggregate-related resin-extractable inorganic P (resin-Pi), bicarbonate-extractable inorganic P (NaHCO3-Pi), bicarbonate-extractable organic P (NaHCO3-Po), sodium hydroxide-extractable inorganic P (NaOH-Pi), sodium hydroxide-extractable organic P (NaOH-Po), dilute hydrochloric acid-extractable P (Dil. HCl-P), concentrated hydrochloric acid-extractable inorganic P (conc. HCl-Pi), concentrated hydrochloric acid-extractable organic P (conc. HCl-Po), and residual P (residue-P) were examined. Different uppercase letters indicate significant differences between different stand types (p < 0.05), and different lowercase letters indicate significant differences in aggregate particle size within the same stand type (p < 0.05).
The distribution of P reserves are closely related to the P content of soil aggregates of different particle sizes, the composition ratio of soil aggregates, and soil bulk density (Tamura et al., 2017). Therefore, in this study, total P (TP) and its reserves in all three types of forests exhibited a pattern in which they were significantly higher in the mixed forests than in the pure forest, and the reserves were mainly concentrated in aggregates with particle sizes >2 mm. Except for the 20–40 cm soil layer in stand type III, where the contribution rates of resin-Pi and NaHCO3-Pi in the >2 mm aggregates were not significantly different from those in the 0.25–1 mm aggregates, the P fractions all showed that the contribution rate of the >2 mm aggregates was significantly higher than that of other particle sizes (p < 0.05). This indicates that in all types of forests, >2 mm aggregates were the main carriers of stored P, which is consistent with the research results of He et al. (2023). The total P and P reserves in >2 mm aggregates in stand types I and II were generally significantly higher than those in stand type III. The above results indicate that mixed planting improved the stability of soil aggregates and increased the content and reserves of total P and its fractions in soil aggregates. The above results support hypothesis (1) in this study.
4.2 The influence of mixed forests on P accumulation
Soil P content is closely related to the formation and stability of soil aggregates. In this study, mixed forests had a positive impact on soil P accumulation by affecting the formation and stability of soil aggregates (Wang et al., 2021). Some research has revealed that microaggregates contain more active organic P and easily mineralizable organic P (Rubk et al., 1999). In red soil, organic P mainly exists in particles <0.005 mm, and the content of different organic P fractions increases with decreasing particle size. Expanding on this concept, it is plausible that the biological complexity of mixed forests, characterized by varied root systems and rich microbial diversity, is particularly favorable for facilitating the formation of microaggregates. Root exudates, coupled with varied microbial interactions, can dynamically modify the soil physicochemical landscape. Such alterations can, in turn, promote the formation of microaggregates, thereby amplifying a soil’s potential for P sequestration. Therefore, it is believed that increasing the proportion of soil microaggregates can have a positive impact on soil P accumulation (Sun and Xiong, 1998).
However, the results of this study present the opposite trends. The RDA (Figure 4) results showed that in both soil layers, the proportion of aggregates with particle sizes <0.25 mm had a negative impact on soil P accumulation and was the most significant influential factor. This is because large aggregates endow the soil with larger pore structures and are rich in organic matter; therefore, they are capable of forming a microenvironment suitable for P retention and accumulation, while the role of aggregates with particle sizes of <0.25 mm is the opposite (Zhang et al., 2008).
Furthermore, aggregates with particle sizes <0.25 mm increase the risk of soil erosion. Under rainfall or surface runoff, P in a soil is more likely to be lost (Giumbelli et al., 2020). However, the RDA (Figure 4) results showed that mean weight diameter (MWD) was the factor with the greatest positive impact on soil P accumulation. This is because the MWD is related to soil consolidation, erosion resistance, aeration, permeability, etc., and soils with larger particle sizes generally have lower degrees of consolidation and good permeability, which is beneficial for the activity of soil microorganisms and root growth. On the one hand, a good soil structure is conducive to the storage and accumulation of P, and on the other hand, microbial activity and root growth promote the decomposition of organic matter in the soil, thereby increasing P content (Song et al., 2019). Therefore, mixed forests had a positive effect on soil P accumulation by reducing the proportion of aggregates with particle sizes <0.25 mm and by improving the soil MWD value. The above results support hypothesis (2).
4.3 The influence of mixed forests on soil P transformation
Currently, there is limited research on how mixed forests influence soil P transformation. Thus, we attempted to explore this through partial least squares structural equation modeling (PLS-SEM). In research by Tiessen and Moir (2007), the P in soil was divided into nine fractions according to solubility. Resin-Pi and NaHCO3-Pi represent the two fractions that have the highest solubility and can be directly absorbed and utilized by plants. Their contents are directly related to plant growth (Yang et al., 2011). The remaining fractions, including NaHCO3-Po, NaOH-Pi, NaOH-Po, Dil. HCl-P, and occluded-P (conc. HCl-Pi, conc. HCl-Po, residue-P), have decreased solubility, and not all of these fractions can be directly absorbed and utilized by plants—they need to be desorbed, dissolved, and mineralized into fractions with higher solubility to be utilized by plants (Condron and Newman, 2011).
We conducted PLS-SEM based on this, and according to evaluations of the model, all three models showed excellent interpretability and predictability. The R2 values were generally higher than 0.67, indicating that the model had strong explanatory power for the data. The Q2 values were generally higher than 0.8, indicating that the model had good predictive ability (Figure 5). Overall, these results demonstrate that our model is effective and reliable.
Our findings revealed that in comparison to NaHCO3-Pi, NaHCO3-Po has a lower degree of total influence on resin-Pi. This differential effect arises because NaHCO3-Po must be microbially decomposed prior to its conversion into resin-Pi (Reed et al., 2011, 2015). Notably, soil microbiota, particularly fungi and bacteria, play a pivotal role in these transformation processes. Certain soil fungi, including mycorrhizal associations, have been shown to enhance the mobilization and uptake of phosphorus fractions, including those bound organically (Olander and Vitousek, 2004; Tiessen and Moir, 2007; Spohn and Kuzyakov, 2013).
Among the three models analyzed, the influence of NaHCO3-P in mixed forests surpassed that in pure forests. This suggests that mixed forestry practices amplify microbial decomposition rates, thereby accelerating the conversion of NaHCO3-Po into resin-Pi. Notably, microbial communities enriched by the diversity of mixed forests could expedite this transformation. For example, bacterial communities, particularly phosphobacteria, are known to release specific enzymes, such as phosphatases, which facilitate the hydrolysis of organic P, making it available for plant uptake (Wan et al., 2020).
NaOH-Pi and NaOH-Po had minimal direct impacts on resin-Pi and instead indirectly influenced resin-Pi through NaHCO3-Pi or NaHCO3-Po. This is primarily due to two reasons: one is the low solubility of NaOH-P, which limits its transformation rate (Bunemann, 2008), and the second is that most of the P released by mineralization is absorbed by Al/Fe minerals in the soil (Tiessen et al., 1984; Barrow, 2015).
Mixed forests evidently mitigate the effect of Al/Fe minerals on the transformation of NaOH-P. The enhanced soil aeration and permeability within these mixed forests favor the weathering of highly insoluble P fractions, such as Dil. HCl-P and occluded-P (Song et al., 2019). The diverse root exudates and microbial interactions within these forests, including those with Miscanthus and Cassia, can result in the production of organic acids and enzymes, modulating soil properties and in turn augmenting the solubility of P fractions (Liu and Liu, 2012; Gao et al., 2022; Yan et al., 2023).
Fractions with extremely low solubility, Dil. HCl-P and occluded-P, in forests I and II directly impacted resin-Pi or NaHCO3-Pi, whereas in forest III, the influence was only indirect via NaOH-P. This is because compared to pure forests, aeration and permeability are better in mixed forest soil, which facilitates the weathering of P fractions with extremely low solubility (Song et al., 2019). Additionally, the diverse microbial community that exists within mixed forests could be pivotal in mineralizing organic P forms. Bacterial phosphatases and fungal exoenzymes play a synergistic role in the mineralization of organic P, making it accessible to plants (Zhou et al., 2020).
In summary, mixed forests promote the transformation of soil P fractions into resin-Pi, increasing the effectiveness of soil P. These results support our third hypothesis.
5 Conclusion
Our research results clearly demonstrate that mixed forest cultivation significantly improved the stability of soil aggregates and the content and reserves of P, especially in the case of mixed Eucalyptus-Mytilaria laosensis forests. Additionally, soil aggregates with a particle size of less than 0.25 mm played a key role in influencing soil P accumulation. The mixed forests promoted the transformation of P in soil aggregates, thereby effectively enhancing the availability of soil P.
Notably, the positive influence of mixed forest cultivation is promising for the restoration of degraded lands, addressing the pervasive issue of soil degradation that affects vast areas globally. By stabilizing soil structures and enhancing nutrient content, mixed plantations can play a significant role in rehabilitating such lands, offering a solution to this pressing environmental challenge.
Overall, these results not only contribute to improving soil fertility management but also promote progress in soil P research, particularly on the distribution and transformation of P and its fractions at the soil aggregate level. In future investigations, diverse mixed forest combinations should be considered to provide a more holistic understanding of the influence of species mixing on soil P dynamics, and biological factors influencing these mixed plantations should also be explored in greater detail. By integrating insights from the complex interplay of soil microbes, fungi, and their interactions with plant roots, we can further refine and optimize management strategies for Eucalyptus plantations. It is imperative to consider these biological elements to truly create a sustainable forest management framework that promotes both soil health and overall environmental well-being.
Data availability statement
The original contributions presented in the study are included in the article/supplementary material, further inquiries can be directed to the corresponding author.
Author contributions
YC: Writing – review & editing, Conceptualization, Data curation, Formal analysis, Investigation, Methodology, Project administration, Software, Supervision, Validation, Visualization, Writing – original draft. YY: Conceptualization, Data curation, Investigation, Methodology, Supervision, Validation, Visualization, Writing – review & editing. SW: Methodology, Supervision, Validation, Writing – review & editing, Formal analysis. HZ: Formal analysis, Methodology, Supervision, Validation, Writing – review & editing, Investigation, Software. YH: Formal analysis, Investigation, Methodology, Supervision, Validation, Writing – review & editing. CJ: Investigation, Writing – review & editing. RF: Investigation, Writing – review & editing. SY: Writing – review & editing, Funding acquisition, Resources.
Funding
The author(s) declare financial support was received for the research, authorship, and/or publication of this article. This research was supported by the National Natural Science Foundation of China (Grant number: 32260382).
Acknowledgments
Insightful comments from American Journal Experts (www.aje.cn) helped us improve the grammar and phrasing in this manuscript.
Conflict of interest
The authors declare that the research was conducted in the absence of any commercial or financial relationships that could be construed as a potential conflict of interest.
Publisher’s note
All claims expressed in this article are solely those of the authors and do not necessarily represent those of their affiliated organizations, or those of the publisher, the editors and the reviewers. Any product that may be evaluated in this article, or claim that may be made by its manufacturer, is not guaranteed or endorsed by the publisher.
References
Adesodun, J. K., Adeyemi, E. F., and Oyegoke, C. O. (2007). Distribution of nutrient elements within water-stable aggregates of two tropical agro-ecological soils under different land uses. Soil Tillage Res. 92, 190–197. doi: 10.1016/j.still.2006.03.003
Alag, Z. Z., and Yilmaz, E. (2009). Effects of different sources of organic matter on soil aggregate formation and stability: a laboratory study on a lithic Rhodoxeralf from Turkey. Soil Tillage Res. 103, 419–424. doi: 10.1016/j.still.2008.12.006
Al-Kaisi, M. M., Douelle, A., and Kwaw-Mensah, D. (2014). Soil micro-aggregate and macroaggregate decay over time and soil carbon change as influenced by different tillage systems. J. Soil Water Conserv. 69, 574–580. doi: 10.2489/jswc.69.6.574
An, S., Mentler, A., Mayer, H., and Blum, W. E. H. (2010). Soil aggregation, aggregate stability, organic carbon and nitrogen in different soil aggregate fractions under forest and shrub vegetation on the Loess Plateau, China. Catena 81, 226–233. doi: 10.1016/j.catena.2010.04.002
Barrow, N. (2015). A mechanistic model for describing the sorption and desorption of phosphate by soil. Eur. J. Soil Sci. 66, 9–18. doi: 10.1111/ejss.12198_2
Bazani, J. H., Gonçalves, J. L. M., and Rocha, J. H. T. (2014). Nutrição fosfatada em plantações de eucalipto. Info. Agron. 148, 1–11. doi: 10.11606/d.11.2014.tde-11112014-160156
Bunemann, E. K. (2008). Enzyme additions as a tool to assess the potential bioavailability of organically bound nutrients. Soil Biol. Biochem. 40, 2116–2129. doi: 10.1016/j.soilbio.2008.03.001
Cao, S., Zhou, Y. Z., Zhou, Y. Y., Zhou, X., and Zhou, W. (2021). Soil organic carbon and soil aggregate stability associated with aggregate fractions in a chronosequence of citrus orchards plantations. J. Environ. Manag. 293:112847. doi: 10.1016/j.jenvman.2021.112847
Chin, W. W. (1998). “The partial least squares approach to structural equation modeling” in Modern methods for business research. ed. G. A. Marcoulides (Mahwah, NJ: Lawrence Erlbaum Associates), 1295–1336.
Condron, L. M., and Newman, S. (2011). Revisiting the fundamentals of phosphorus fractionation of sediments and soils. J. Soils Sediments 11, 830–840. doi: 10.1007/s11368-011-0363-2
Cooper, J., Greenberg, I., Ludwig, B., Hippich, L., Fischer, D., Glaser, B., et al. (2020). Effect of biochar and compost on soil properties and organic matter in aggregate size fractions under field conditions. Agric. Ecosyst. Environ. 295:106882:106882. doi: 10.1016/j.agee.2020.106882
Costa, M. G., Gama-Rodrigues, A. C., Gonçalves, J. L. M., Gama-Rodrigues, E., Sales, M., and Aleixo, S. (2016). Labile and non-labile fractions of phosphorus and its transformations in soil under Eucalyptus plantations, Brazil. Forests 7:15. doi: 10.3390/f7010015
Dal Ferro, N., Berti, A., Francioso, O., Ferrari, E., Matthews, G. P., and Morari, F. (2012). Investigating the effects of wettability and pore size distribution on aggregate stability: the role of soil organic matter and the humic fraction. Eur. J. Soil Sci. 63, 152–164. doi: 10.1111/j.1365-2389.2012.01427.x
Dawud, S. M., Raulund-Rasmussen, K., Domisch, T., Finér, L., Jaroszewicz, B., and Vesterdal, L. (2016). Is tree species diversity or species identity the more important driver of soil carbon stocks, C/N ratio, and pH? Ecosystems 19, 645–660. doi: 10.1007/s10021-016-9958-1
Diamantopoulos, A., and Siguaw, J. A. (2006). Formative versus reflective indicators in organizational measure development: a comparison and empirical illustration. Br. J. Manag. 17, 263–282. doi: 10.1111/j.1467-8551.2006.00500.x
Egan, G., Crawley, M. J., and Fornara, D. A. (2018). Effects of long-term grassland management on the carbon and nitrogen pools of different soil aggregate fractions. Sci. Total Environ. 614, 810–819. doi: 10.1016/j.scitotenv.2017.09.165
Fattet, M., Fu, Y., Ghestem, M., Ma, W., Foulonneau, M., Nespoulous, J., et al. (2011). Effects of vegetation type on soil resistance to erosion: relationship between aggregate stability and shear strength. Catena 87, 60–69. doi: 10.1016/j.catena.2011.05.006
Formaglio, G., Krusche, A. V., Mareschal, L., Bouillet, J. P., Gonçalves, J. L. M., Nouvellon, Y., et al. (2023). Planting nitrogen-fixing trees in tropical Eucalyptus plantations does not increase nutrient losses through drainage. For. Ecol. Manag. 537:120940. doi: 10.1016/j.foreco.2023.120940
Frossard, E., Condron, L. M., Oberson, A., Sinaj, S., and Fardeau, J. C. (2000). Processes governing phosphorus availability in temperate soils. J. Environ. Qual. 29, 15–23. doi: 10.2134/jeq2000.00472425002900010003x
Gao, G., Huang, X., Xu, H., Wang, Y., Shen, W., Zhang, W., et al. (2022). Conversion of pure Chinese fir plantation to multi-layered mixed plantation enhances the soil aggregate stability by regulating microbial communities in subtropical China. For. Ecosyst. 9:100078. doi: 10.1016/j.fecs.2022.100078
Giumbelli, L. D., Loss, A., Ventura, B. S., dos Santos, E., Almeida, J., de Cássia Piccolo, M., et al. (2020). Aggregation index, carbon, nitrogen, and natural abundance of 13C and 15N in soil aggregates and bulk soil cultivated with onion under crop successions and rotations. Soil Res. 58:622. doi: 10.1071/SR19346
Goncalves, J. L. M., Barros, N. F., and Nambiar, E. K. S. “Soil and stand management for short-rotation plantations” in Management of Soil, nutrients and water in tropical plantation forests. eds. E. K. S. Nambiar and A. G. Brown (Canberra: Australian Centre for International Agricultural Research), 379–418.
Guo, J. H., Feng, H. L., McNie, P., Wang, W., Peng, C., Feng, L., et al. (2022). The effect of the conversion from natural broadleaved forests into Chinese fir (Cunninghamia lanceolata (Lamb.) Hook.) plantations on soil microbial communities and nitrogen functional genes. Forests 13:158. doi: 10.3390/f13020158
Hair, J. F., Risher, J. J., Sarstedt, M., and Ringle, C. M. (2019). When to use and how to report the results of PLS-SEM. Eur. Bus. Rev. 31, 2–24. doi: 10.1108/EBR-11-2018-0203
He, Y., Zhang, Q., Jiang, C., Lan, Y., Zhang, H., and Ye, S. (2023). Mixed planting improves soil aggregate stability and aggregate-associated C-N-P accumulation in subtropical China. Front. For. Glob. Change 6:1141953. doi: 10.3389/ffgc.2023.1141953
Hedley, M. J., Stewart, J. W. B., and Chauhan, B. S. (1982). Changes in inorganic and organic soil-phosphorus fractions induced by cultivation practices and by laboratory incubations. Soil Sci. Soc. Am. J. 46, 970–976. doi: 10.2136/sssaj1982.03615995004600050017x
Huang, W. J., Liu, J. X., Wang, Y. P., Zhou, G., Han, T., and Li, Y. (2013). Increasing phosphorus limitation along three successional forests in southern China. Plant Soil 364, 181–191. doi: 10.1007/s11104-012-1355-8
Huang, X., Liu, S., You, Y., Wang, H., and Wang, J. (2017). Microbial community and associated enzymes activity influence soil carbon chemical composition in Eucalyptus urophylla plantation with mixing N2-fixing species in subtropical China. Plant Soil 414, 199–212. doi: 10.1007/s11104-016-3117-5
Hunt, J. F., Ohno, T., He, Z., Honeycutt, C. W., and Dail, D. B. (2007). Inhibition of phosphorus sorption to goethite, gibbsite, and kaolin by fresh and decomposed organic matter. Biol. Fertil. Soils 44, 277–288. doi: 10.1007/s00374-007-0202-1
Hutapea, F. J., Weston, C. J., Mendham, D., and Volkova, L. (2023). Sustainable management of Eucalyptus pellita plantations: a review. For. Ecol. Manag. 537:120941. doi: 10.1016/j.foreco.2023.120941
IUSS Working Group (2014). “World reference base for soil resources 2014” in International soil classification system for naming soils and creating legends for soil maps. World soil resources reports no. 106 (Rome: FAO)
Johnson, A. H., Frizano, J., and Vann, D. R. (2003). Biogeochemical implications of labile phosphorus in forest soils determined by the Hedley fractionation procedure. Oecologia 135, 487–499. doi: 10.1007/s00442-002-1164-5
Kerdraon, D., Drewer, J., Chung, A. Y. C., Majalap, N., Slade, E. M., Bréchet, L., et al. (2020). Litter inputs, but not litter diversity, maintain soil processes in degraded tropical forests-across-continental comparison. Front. For. Glob. Change. 2:90. doi: 10.3389/ffgc.2019.00090
Kuperman, R. G. , (1996) Relationships between soil properties and community structure of soil macroinvertebrates in oak-history forests along an acidic deposition gradient. United States: UNT Digital Library.
Laclau, J. P., Goncalves, J. L. D., and Stape, J. L. (2013). Perspectives for the management of eucalypt plantations under biotic and abiotic stresses. For. Ecol. Manag. 301, 1–5. doi: 10.1016/j.foreco.2013.03.007
Li, Y. P., Wang, J., and Shao, M. A. (2021). Application of earthworm cast improves soil aggregation and aggregate-associated carbon stability in typical soils from Loess Plateau. J. Environ. Manag. 278:111504. doi: 10.1016/j.jenvman.2020.111504
Liu, D. D., Ju, W. L., Jin, X. L., Li, M., Shen, G., Duan, C., et al. (2021). Associated soil aggregate nutrients and controlling factors on aggregate stability in semiarid grassland under different grazing prohibition timeframes. Sci. Total Environ. 777:146104. doi: 10.1016/j.scitotenv.2021.146104
Liu, S. R., Li, X. M., and Niu, L. M. (1998). The degradation of soil fertility in pure larch plantation in the northeastern part of China. Ecol. Eng. 10, 75–86. doi: 10.1016/S0925-8574(97)10024-6
Liu, E., and Liu, S. R. (2012). The research of carbon storage and distribution feature of the Mytilaria laosensis plantation in south sub-tropical area. Acta Ecol. Sin. 32, 5103–5109. doi: 10.5846/stxb201108141190
Lu, R. , (2000). Analysis of soil agrochemistry. Chinese Agricultural Science and Technology Press, Beijing.
Luo, D., Shi, Z. M., Wang, W. X., Shirong, L., Lihua, L., Angang, M., et al. (2015). Carbon and nitrogen storage in monoculture and mixed young plantation stands of Erythrophleum fordii and Pinus massoniana in subtropical China. Acta Ecol. Sin. 35:9. doi: 10.5846/stxb201401030018
Mao, L., Ye, S., and Wang, S. (2022). Soil nutrient contents and stoichiometry within aggregate size classes varied with tea plantation age and soil depth in southern Guangxi in China. Soil 8, 487–505. doi: 10.5194/soil-8-487-2022
Mustafa, A., Minggang, X., Ali Shah, S. A., Abrar, M. M., Nan, S., Baoren, W., et al. (2020). Soil aggregation and soil aggregate stability regulate organic carbon and nitrogen storage in a red soil of southern China. J. Environ. Manag. 270:110894. doi: 10.1016/j.jenvman.2020.110894
Olander, L. P., and Vitousek, P. M. (2004). Biological and geochemical sinks for phosphorus in soil from a wet tropical forest. Ecosystems 7, 404–419. doi: 10.1007/s10021-004-0264-y
Reed, S. C., Townsend, A. R., Taylor, P. G., and Cleveland, C. C. (2011). “Phosphorus cycling in tropical forests growing on highly weathered soils” in Phosphorus in action: biological processes in soil phosphorus cycling, soil biology 26. eds. E. K. Bünemann, E. Frossard, and A. Oberson (Berlin, German: Springer), 339–369.
Reed, S. C., Yang, X., and Thornton, P. E. (2015). Incorporating phosphorus cycling into global modeling efforts: a worthwhile, tractable endeavor. New Phytol. 208, 324–329. doi: 10.1111/nph.13521
Richardson, A. E., and Simpson, R. J. (2011). Soil microorganisms mediating phosphorus availability. Plant Physiol. 156, 989–996. doi: 10.1104/pp.111.175448
Rubk, G. H., Guggenberger, G., Zech, W., and Christensen, B. T. (1999). Organic phosphorus in soil size separates characterized by phosphorus-31 nuclear magnetic resonance and resin extraction. Soil Sci. Soc. Am. J. 63, 1123–1132. doi: 10.2136/sssaj1999.6351123x
Sayer, E. J., Rodtassana, C., Sheldrake, M., Bréchet, L. M., Ashford, O. S., Lopez-Sangil, L., et al. (2020). Chapter five-revisiting nutrient cycling by litterfall-insights from 15 years of litter manipulation in old-growth lowland tropical forest. Adv. Ecol. Res. 62, 173–223. doi: 10.1016/bs.aecr.2020.01.002
Sicardi, M., García-Pérchac, F., and Frioni, L. (2004). Soil microbial indicators sensitive to land use conversion from pastures to commercial Eucalyptus grandis (Hill ex Maiden) plantations in Uruguay. Appl. Soil Ecol. 27, 125–133. doi: 10.1016/j.apsoil.2004.05.004
Six, J., Bossuyt, H., Degryze, S., and Denef, K. (2004). A history of research on the link between (micro)aggregates, soil biota, and soil organic matter dynamics. Soil Tillage Res. 79, 7–31. doi: 10.1016/j.still.2004.03.008
Six, J., Conant, R. T., Paul, E. A., and Paustian, K. (2002). Stabilization mechanisms of soil organic matter: implications for C-saturation of soils. Plant Soil 241, 155–176. doi: 10.1023/A:1016125726789
Song, L. Q., Wang, Y. D., Li, D. C., Zhang, H. M., Lou, Y. L., Dou, B. K., et al. (2019). Long-term effects of natural restoration on C∶N∶P ecological stoichiometry in aggregates of red soil. Chin. J. Ecol. 38, 1707–1715. doi: 10.13292/j.1000-4890.201906.011
Spohn, M., and Kuzyakov, Y. (2013). Phosphorus mineralization can be driven by microbial need for carbon. Soil Biol. Biochem. 61, 69–75. doi: 10.1016/j.soilbio.2013.02.013
Stewart, J. W. B., and Tiessen, H. (1987). Dynamics of soil organic phosphorus. Biogeochemistry 4, 41–60. doi: 10.1007/BF02187361
Sullivan, B. W., Alvarez-Clare, S., Castle, S. C., Porder, S., Reed, S. C., Schreeg, L., et al. (2014). Assessing nutrient limitation in complex forested ecosystems: alternatives to large-scale fertilization experiments. Ecology 95, 668–681. doi: 10.1890/13-0825.1
Sun, H., and Xiong, D. X. (1998). Study on organic phosphorus in Shajiang black soil and its organic inorganic complex in southern Shandong. Chin. J. Soil Sci. 2, 14–17. doi: 10.19336/j.cnki.trtb.1998.02.005
Tamura, M., Suseela, V., Simpson, M., Powell, B., and Tharayil, N. (2017). Plant litter chemistry alters the content and composition of organic carbon associated with soil mineral and aggregate fractions in invaded ecosystems. Glob. Change Biol. Bioenergy. 23, 4002–4018. doi: 10.1111/gcb.13751
Tang, L., and Wang, S. (2022). Dynamics of soil aggregate-related C-N-P stoichiometric characteristics with stand age and soil depth in Chinese fir plantations. Land Degrad. Dev. 8:33. doi: 10.1002/ldr.4217
Tiessen, H., and Moir, J. O. (2007). “Characterization of available P by sequential extraction” in Soil sampling and methods of analysis. eds. M. R. Carter and E. G. Gregorich. 2nd ed (Boca Raton, FL: CRC Press), 293–306.
Tiessen, H., Stewart, J., and Cole, C. (1984). Pathways of phosphorus transformations in soils of differing pedogenesis. Soil Sci. Soc. Am. J. 48, 853–858. doi: 10.2136/sssaj1984.03615995004800040031x
Turner, B. L., and Engelbrecht, B. M. J. (2011). Soil organic phosphorus in lowland tropical rain forests. Biogeochemistry 103, 297–315. doi: 10.1007/s10533-010-9466-x
Vitousek, P. M., Porder, S., Houlton, B. Z., and Chadwick, O. A. (2010). Terrestrial phosphorus limitation: mechanisms, implications, and nitrogen-phosphorus interactions. Ecol. Appl. 20, 5–15. doi: 10.1890/08-0127.1
Wan, W. J., Xiang, H., Shun, W., Wang, L., Luo, X., Chen, W., et al. (2020). Soil aggregate fractionation and phosphorus fraction driven by long-term fertilization regimes affect the abundance and composition of P-cycling-related bacteria. Soil Tillage Res. 196:104475. doi: 10.1016/j.still.2019.104475
Wang, S., Huang, Y., and Ye, S. (2021). Distribution of organic carbon and nutrients in soil aggregates under different stand types of Cunninghamia lanceolata in southern Guangxi of China. Soil Sci. Plant Nutr. 67, 427–438. doi: 10.1080/00380768.2021.1932585
Wang, S. Q., Zhang, Z., and Ye, S. M. (2020). Response of soil fertility characteristics in water-stable aggregates to tea cultivation aggregates to tea cultivation age in hilly region of southern Guangxi, China. Catena 191:104578. doi: 10.1016/j.catena.2020.104578
Wang, S., Zhou, P., Luo, B., and Ye, S. (2022). Stoichiometric characteristics of medium-and micro-elements (Ca, Mg, Fe, and Mn) in soil aggregates as affected by stand age in Chinese fir plantations. Land Degrad. Dev. 18, 3991–4003. doi: 10.1002/ldr.4439
Wei, J. X., Wang, C., and He, B.. Research progress on soil microorganisms in eucalypt forests. J. Zhejiang A F Univ. 39, 1144–1154. doi: 10.11833/j.issn.2095-0756.20210701
Xu, H. D., Yuan, H. J., Yu, M. K., and Cheng, X. (2020). Large macroaggregate properties are sensitive to the conversion of pure plantation to uneven-aged mixed plantations. Catena 194:104724. doi: 10.1016/j.catena.2020.104724
Yan, Y., Cui, Y. H., and Fan, R. Y., et al. (2023). Soil aggregate stability and distribution characteristics of organic nitrogen components in Eucalyptus pure and mixed forests. J. Cent. South Univ. For. Technol. 7, 149–158. doi: 10.10.14067/j.cnki.1673-923x.2023.07.015
Yang, X., Chen, X., and Yang, X. (2019). Effect of organic matter on phosphorus adsorption in a black soil from Northeast China. Soil Tillage Res. 187, 85–91. doi: 10.1016/j.still.2018.11.016
Yang, X., Post, W. M., et al. (2011). Phosphorus transformation as a function of pedogenesis: a synthesis of soil phosphorus data using Hedley fractionation method. Biogeosciences 8, 2907–2916. doi: 10.5194/bg-8-2907-2011
Yang, H. B., Vina, A., Winkler, J. A., Chung, M. G., Huang, Q., Dou, Y., et al. (2021). A global assessment of the impact of individual protected areas on preventing forest loss. Sci. Total Environ. 777:145995. doi: 10.1016/j.scitotenv.2021.145995
Yang, Y., Zhang, Y., Yu, X., and Jia, G. (2023). Soil microorganism regulated aggregate stability and rill erosion resistance under different land uses. Catena 228:107176. doi: 10.1016/j.catena.2023.107176
Zeng, Q., Li, X., Dong, Y. H., Li, Y. Y., Cheng, M., An, S. S., et al. (2015). Ecological stoichiometry characteristics and physical-chemical properties of soils at different latitudes on the loess plateau. J. Nat. Resour. 30, 870–879. doi: 10.11849/zrzyxb.2015.05.014
Zhang, D. J., Li, J. J., Huang, Y. M., Huang, Y., Gao, S., and Zhang, J. (2022). Root-soil facilitation in mixed Eucalyptus grandis plantations including nitrogen-fixing species. For. Ecol. Manag. 516:120215. doi: 10.1016/j.foreco.2022.120215
Zhang, Y., Shengzhe, E., Wang, Y., Su, S., Bai, L., Wu, C., et al. (2021). Long-term manure application enhances the stability of aggregates and aggregate-associated carbon by regulating soil physicochemical characteristics. Catena 203:105342. doi: 10.1016/j.catena.2021.105342
Zhang, Q., Wang, S., Huang, Y., Yao, X., He, X., and Ye, S. (2021). Ecological stoichiometry of soil carbon, nitrogen, and phosphorus within soil aggregates of four plantations in different Cunninghamia lanceolata stand types. Acta Bot. Boreali-Occident. Sin. 41:8. doi: 10.7606/j.issn.1000-4025.2021.06.1028
Zhang, Z., Wei, C. F., Xie, D. T., Gao, M., and Zeng, X. (2008). Effects of land use patterns on soil aggregate stability in Sichuan Basin, China. Particuology 6, 157–166. doi: 10.1016/j.partic.2008.03.001
Zhang, W., You, Y., Su, X., Yan, J., Gao, G., Ming, A., et al. (2023). Introducing N2-fixing tree species into Eucalyptus plantations promotes soil organic carbon sequestration in aggregates by increasing microbial carbon use efficiency. Catena 231:107321. doi: 10.1016/j.catena.2023.107321
Zhao, Y. D., and Hu, X. (2022). How do freeze-thaw cycles affect the soil pore structure in alpine meadows considering soil aggregate and soil column scales? J. Soil Sci. Plant Nutr. 22, 4207–4216. doi: 10.1007/s42729-022-01019-z
Zhao, B. C., Xu, J. D., Chen, B. Y., Cao, X. F., Yuan, T. Q., Wang, S. F., et al. (2018). Selective precipitation and characterization of lignin-carbohydrate complexes (LCCs) from Eucalyptus. Planta 247, 1077–1087. doi: 10.1007/s00425-018-2842-9
Keywords: Eucalyptus , mixed forest, soil aggregates, phosphorus fractions, phosphorus transformation
Citation: Cui Y, Yan Y, Wang S, Zhang H, He Y, Jiang C, Fan R and Ye S (2023) Mixed Eucalyptus plantations in subtropical China enhance phosphorus accumulation and transformation in soil aggregates. Front. For. Glob. Change. 6:1269487. doi: 10.3389/ffgc.2023.1269487
Edited by:
Ling Zhang, Jiangxi Agricultural University, ChinaReviewed by:
Lydie-Stella Koutika, CRDPI, Republic of CongoMaurício Zagatto, DungTech Biofertilizers, Brazil
Copyright © 2023 Cui, Yan, Wang, Zhang, He, Jiang, Fan and Ye. This is an open-access article distributed under the terms of the Creative Commons Attribution License (CC BY). The use, distribution or reproduction in other forums is permitted, provided the original author(s) and the copyright owner(s) are credited and that the original publication in this journal is cited, in accordance with accepted academic practice. No use, distribution or reproduction is permitted which does not comply with these terms.
*Correspondence: Shaoming Ye, eXNoYW9taW5nQDE2My5jb20=