- 1Key Laboratory of Agro-Ecological Processes in Subtropical Region, Institute of Subtropical Agriculture, Chinese Academy of Sciences, Changsha, China
- 2University of Chinese Academy of Sciences, Beijing, China
- 3Huanjiang Observation and Research Station for Karst Ecosystem, Chinese Academy of Sciences, Huanjiang, China
Introduction: Plant diversity and soil chemical properties are important factors affecting the plant growth. We sought to compare the explanatory rates of diversity and soil chemical properties in explaining the variation of basal area in karst forests, and also sought to compare the relative importance of the niche complementarity and mass ratio hypotheses.
Methods: On the basis of linear regression and structural equation modelling, we examined the correlation between the basal area of plant communities and species diversity, functional diversity, phylogenetic diversity, the community-weighted mean (CWM) of traits, and soil chemical properties, using data obtained from 35 monitoring plots in southwest China.
Results: Species, functional, and phylogenetic diversities were all significantly correlated with the basal area of the plant community, among the indices of which, Faith’s phylogenetic diversity was found to have the greatest explanatory power for basal area. These plant diversity indices can better explain the variation in basal area than the CWM of traits, suggesting the niche complementarity hypothesis is more applicable than the mass ratio hypothesis. Moreover, soil chemical properties also have an equal important impact. Different chemical properties were found to show significant positive correlations with basal area, and their total effects on basal area were shown to be greater than the CWM of traits.
Discussion: Attention should be paid to diversity and soil chemical properties. This study provides theoretical guidance for understanding biodiversity maintenance mechanisms and protecting karst forests.
1 Introduction
The relationship between biodiversity and ecosystem service functions is an active focal area and controversial topic in ecology (García et al., 2018; Ouyang et al., 2019). Although the level of plant productivity is an important manifestation of ecosystem function, it is typically difficult to study, owing to the complexity of the relationships between biodiversity and ecosystem service functions (Lasky et al., 2014; Tilman et al., 2014; Wang et al., 2022). Most of the early studies in this field tended to consider only the influence of species diversity, as represented by species richness, on ecosystem processes (Hooper et al., 2005; Maestre et al., 2012). However, as research has progressed, it has become established that whereas species diversity is similar within the same community types, it is generally associated with different ecosystem functions (Hooper and Vitousek, 1997). This thus tends to indicate that the effects of biodiversity on ecosystems are not exclusively associated with the quantity and relative abundance of species (Dı’az and Cabido, 2001). Some researchers have also found that changes in certain functional traits of species can better explain the influence of diversity on ecosystems and effectively clarify the role of biodiversity in ecosystem processes (Mason et al., 2013). Among these, the range, value, and distribution of functional traits of plant community species are known as functional diversity (Díaz et al., 2011). However, although the measurement of functional traits is relatively intuitive, the selection of functionally meaningful traits is readily influenced by human subjectivity (Bradley and Katharine, 2013). Related species may have similar functional traits, and the pedigree structure can be used to distinguish the similarity of functional traits among related species, particularly in circumstances in which certain key traits are missing. The index of phylogenetic diversity can be introduced to explain variations in productivity (Pennington et al., 2006; Cadotte et al., 2009). To date, there has been considerable controversy regarding which among plant species, functional, and phylogenetic diversity can best explain variations in biomass (Hao et al., 2018; Huang et al., 2020; Gao et al., 2021).
There are two main mechanisms by which diversity affects ecosystem functions: the mass ratio hypothesis and the niche complementarity hypothesis. The mass ratio hypothesis was proposed by Grime, who believed that ecosystem function was mainly determined by the characteristics and functional traits of the most abundant species. The hypothesis is usually represented by the community-weighted mean (CWM) of individual trait (Grime, 1998). Under conditions in which the aboveground biomass is closely associated with the CWM, this mechanism is considered to play a major role (Ali et al., 2017). The niche complementarity hypothesis posits that, with an increase in diversity, there in an enhancement of species interactions, thereby promoting niche differentiation, and thus increasing the optimal utilization efficiency of resources and space by species, alleviating competition, and enhancing productivity (Tilman, 1997; Petchey and Gaston, 2006). This hypothesis is generally represented by three indices, namely, species, functional, and phylogenetic diversities (Tilman et al., 2014). At present, there is still considerable debate as to the dominant contribution of these two mechanisms in the processes whereby diversity affects ecosystem function. Although some scholars believe that the niche complementarity and mass ratio hypotheses can jointly explain the factors that determine aboveground biomass (Ali et al., 2017; Cadotte, 2017). In a study of the relationship between plant diversity and productivity in broad-leaved Korean pine forests, Wen and Jin found that the explanatory percentage of functional diversity is higher than that of CWM, thereby indicating that the niche complementarity hypothesis can provide a better explanation of the variation in productivity than can the mass ratio hypothesis (Wen and Jin, 2019). In addition, studies have identified soil chemical properties as potential factors limiting forest growth, either directly or indirectly affecting tree mortality and turnover in forest ecosystems (Schoenholtz et al., 2000; Quesada et al., 2012). Consequently, the relative importance of biotic and abiotic factors in ecosystem productivity needs to be further verified (Zhang et al., 2015).
When assessing the relationships between biodiversity and plant productivity, most scholars tend to select grassland ecosystems that are readily manipulated and show relatively rapid responses, the biomass of which can be measured using direct harvesting methods (Chamagne et al., 2017). Comparatively, obtaining biomass measurements in forest ecosystems is time-consuming and laborious. The most commonly adopted approach used for the estimation of forest biomass entails establishing a model based on estimates of trunk diameter, tree height factors, wood specific gravity, and parameters that are known to be highly correlated with forest biomass (Chave et al., 2014; Sullivan et al., 2018). Karst forests are a unique type of non-zonal vegetation that develops in specific environments, such as karst landforms and limestone, and measurement of the associated biomass tends to be more difficult (Liu et al., 2021). For example, given the fragility of the karst ecosystem, conventional analytical wood sampling is highly destructive and difficult to recover (Maia Araújo et al., 1999). Moreover, there are numerous species endemic to karst areas, along with considerable differences with respect to wood density, plant morphology, and configuration, for which suitable biomass models are currently lacking. In forests with high canopy density, it is difficult to accurately measure tree height, and the allometric growth equation cannot be used to accurately estimate the forest biomass in this area (Larjavaara and Muller-Landau, 2013). Farahat et al. have proposed that basal area is a reliable independent variable for predicting the aboveground biomass production of tree species that provides an easy-to-measure index for karst forest biomass estimation (Farahat et al., 2012).
Worldwide, the distribution of karst ecosystems accounts for approximately 15% of the land surface area, whereas in China, approximately one-third of the land area is covered by karst formations. Southwest China, comprising the provinces of Guangxi, Yunnan, and Guizhou, is recognized as the core area of karst ecosystems worldwide (Wang et al., 2004). However, given its ecological fragility and increasing disturbance attributable to human activities, there has been an increase in the extent of rocky desertification, an intensification of soil erosion, a sharp decline of biodiversity, and disruption of the regional ecological balance (Zou et al., 2020). To date, most research on karst forests has tended to focus on the eco-stoichiometry and plant functional traits of these forests, although in addition, there have been a little study on the plant diversity of karst forest communities during the process of restoration (Firn et al., 2019; Zhang et al., 2022a). However, vegetation restoration is a fundamental approach to controlling karst desertification, lack of diversity research is not conducive to understanding the maintenance of biodiversity and plant growth protection mechanisms in karst areas (Wang et al., 2022). This study comprehensively considered the species, functional, and phylogenetic diversity, to study the effects of multi-dimensional diversity on basal area. Moreover, diversity was found to explain biodiversity and ecosystem function(BEF) relationships in better than functional traits, elucidating the driving mechanism of karst forest ecosystem function.
In this study, which was conducted within a karst region of southwestern China, we measured various parameters in 35 sample plots within the karst forest ecosystem. These parameters included tree basal area, soil chemical properties, species diversity, functional diversity, phylogenetic diversity, and community-weighted mean traits. By using linear equations and structural equation models, we attempted to answer the following three scientific questions. (1) Which component of plant diversity, namely species, functional, or phylogenetic diversity, can best explain the changes in basal area? (2) Which of the two mechanisms, the mass ratio and the niche complementarity hypotheses, plays a dominant role in diversity by influencing basal area? (3) Do soil chemical properties have a prominent influence on plant diversity and basal area? The aim of the study was to clarify the relationships between basal area and plant diversity and to elucidate the mechanisms underlying the maintenance of diversity, which may provide a scientific basis for enhancing the productivity and service functions of degraded ecosystems.
2 Materials and methods
2.1 Study site
The study site is located in Huanjiang Maonan Autonomous County, Guangxi Province, China, in an area characterized by a mid-subtropical monsoon climate (Figure 1). The annual average temperature is between 15.0 and 18.7°C, and the average annual rainfall is between 1,530 and 1,820 mm. Rainfall is mainly concentrated in the period between April and September, and the relative humidity is comparatively high. The area of soil coverage is relatively small, and the soil is of a simple calcareous type. Within the overall study area, we established 35 sample plots in two areas: Baidan (25°08′N, 108°04′E) and Bannan (25°09′N, 107°58′E). Sample plots in the 1-hectare Baidan area were numbered 1 to 25, with the average elevation of the sample plots being 578 m and a soil pH value of 7.4. Within this area, we investigated a total of 105 plant species, among which, Bridelia tomentosa and Pittosporum kwangsiense were the dominant species, and the associated tree species were Brassaiopsis glomerulata, Maesa japonica, Cornus macrophylla, and Lindera communis. Sample plots in the Bannan area were numbered 26 to 35. The average elevation of these plots is 478 m and the average soil pH is 7.2. In this area, we surveyed 28 plant species, among which, the dominant species was Pterospermum heterophyllum, with Decaspermum gracilentum and Lindera communis as associated tree species. The forest in the study area is a typical subtropical evergreen deciduous broad-leaved forest characterized by rich and diverse vegetation, which has both important scientific research and conservation value with respect to understanding karst forest ecosystems.
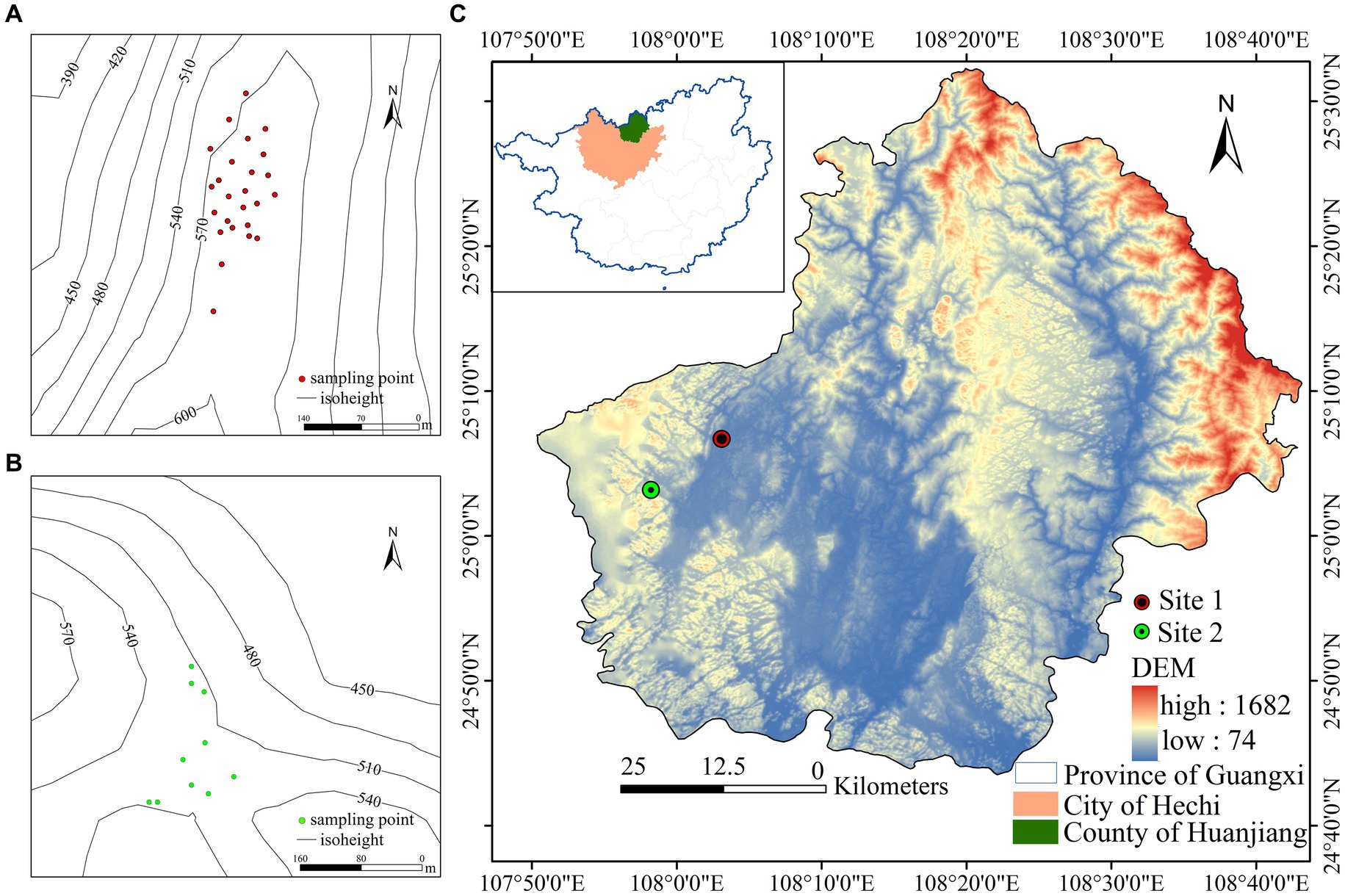
Figure 1. (A) Distribution map of sampling points in Baidan area. (B) Distribution map of sampling points in Bannan area. (C) Study area overview map.
2.2 Sample site survey
For the purpose of a vegetation resource survey, we established thirty-five 20 m × 20 m sampling quadrats in the Baidan and Bannan areas, each of which was divided into 16 sub-samples of 5 m × 5 m in area (Condit, 1995). According to the monitoring standards for fixed plots, we surveyed all woody plants with a diameter at breast height ≥1 cm in each plot. For each of the assessed trees, we established the species, and recorded crown width, plant height, and diameter at breast height. With respect to shrubs and herbs, we recorded species names, percentage coverage, and plant height. In each quadrat, soil samples were collected from each of the 16 sub-plots along an S-shaped route using a 3 cm diameter soil sampler. Samples collected at multiple points within a sub-plot were mixed to give representative composite soil samples, which transferred to the laboratory for subsequent analysis. Topographic factors, such as elevation, slope, slope aspect, and percentage rock exposure, were also measured. The average elevation and slope direction were measured using a Garmin GPS map 60CS× device (Garmin Ltd., Olathe, United States) and an electronic tachometer (NTS-302R; SOUTH Ltd., China), respectively. The slope angle was measured using an inclinometer (BR-CX-3; Borui, China) and the percentage rock exposure was defined as the ratio of exposed rock area to total area. The average percentage rock exposure of the four sub-quadrats on the diagonal was taken be representative of the overall rock exposure of each plot (Du et al., 2015; Zhang et al., 2022a).
2.3 Determination of plant leaf traits and soil chemical properties
In this study, as the main indicators, we selected the following 12 plant leaf functional traits most closely associated with plant diversity and growth: leaf length-width ratio, leaf area, leaf dry weight, leaf thickness, fresh leaf weight, branch tissue density, specific leaf area, leaf dry matter content, leaf density, total nitrogen, total phosphorus, and total carbon (Finegan et al., 2015; Wen and Jin, 2019; Zhang et al., 2022b). Mature leaves of all surveyed species were collected in each quadrat and brought back to the laboratory for the measurement of leaf functional traits. To reflect the photosynthetic capacity and transpiration rate of different species, we measured the ratio of leaf length to width and leaf area using a Yaxin-1241 scanner (Yaxin, China). Fresh leaves were placed in an oven and dried at 60–70°C for 48 h, after which they were weighed on a 1/1000 electronic balance (BSM-220.4; Zhuojing, China). To gain an insight on leaf transpiration and water storage capacity, we measured leaf thickness using Vernier calipers (SF2000; Guilin, China) (Zhang et al., 2022b). The trait branch tissue density is associated with nutrient and water transport and distribution. Leaf dry matter content (the ratio of leaf dry weight to leaf fresh weight) reflects the capacity of leaves to obtain resources (Wen and Jin, 2019). The total nitrogen, phosphorus, and potassium contents of leaves are closely associated with nutrient absorption and ecosystem function (Zhang et al., 2018), among which, the total nitrogen and total carbon contents of leaves were measured using a fully automatic elemental analyser (CN802; Aunion, China), and total phosphorus content was measured spectrophotometrically (Li et al., 2010).
Having initially removed gravel and roots, soil samples were placed in an airy place to dry naturally. The chemical properties of all soil samples were determined using the technical specifications for soil analysis (Table 1). Soil pH was determined using a soil-to-water ratio of 1:5 with a pH meter, and organic matter content was determined using the potassium dichromate capacity-external heating method (Li et al., 2023). Available potassium and hydrolysable nitrogen were measured using (NH4)2CO3 extraction-flame spectrophotometry and the diffusion–absorption method, respectively. Available phosphorus was extracted using NaHCO3 molybdenum antimony spectrophotometry (Du et al., 2015). Total nitrogen content was determined using a semi-trace Kjeldahl method, total phosphorus content was determined using the NaOH melting-molybdenum antimony anti-colorimetric method, and total potassium content was determined using NaOH melting-flame photometry (Zhang et al., 2022a).
2.4 The community-weighted means of traits and plant diversity
Values of the descriptive variables associated with plant diversity and the community-weighted mean (CWM) of traits are presented in Tables 2, 3, respectively. For the purposes of this study, we used plant diversity indices to represent the niche complementarity hypothesis and the CWM of traits to represent the mass ratio hypothesis. The processes proposed by niche complementarity hypothesis are considered to play a major role when the basal area is associated plant diversity. However, when the CWM provides better explanation for the observed change in the basal area, the mass ratio hypothesis is considered more appropriate (Ali et al., 2017; Wen and Jin, 2019). Plant diversity is a composite measure, comprising species, functional, and phylogenetic diversities. Among these, species diversity estimated were obtained using the vegan package (Oksanen, 2015) in R4.0.3 (R core team, 2022), which includes species richness, the Simpson index, the Shannon index, and the evenness index (Li et al., 2018). Using the functional diversity (FD) package (Mammola et al., 2021) in R4.0.3, we calculated the functional diversity of various plots, which included the functional richness index, functional divergence index, functional dispersion index, functional evenness index, and Rao’s quadratic entropy index (Laliberte and Legendre, 2010; Ouyang et al., 2023). With respect to phylogenetic diversity, we calculated Faith’s phylogenetic diversity, the mean pairwise phylogenetic distance, and the mean nearest phylogenetic distance using the picante package (Kembel et al., 2010) in R4.0.3 (Zhang et al., 2019).
CWM values for each trait were calculated using the FD package (Mammola et al., 2021) in R4.0.3, including values for leaf length to width ratio (CWMratio), leaf area (CWMLA), leaf dry weight (CWMLDW), leaf thickness (CWMLT), fresh leaf weight (CWMFLW), branch tissue density (CWMBTD), specific leaf area (CWMSLA), leaf dry matter content (CWMLDMC), leaf tissue density (CWMLTD), total nitrogen (CWMTN), total phosphorus (CWMTP), and total carbon (CWMTC). The CWM of a given trait was calculated as the average trait value in the community weighted by species abundance (Garnier et al., 2004).
2.5 Data analysis
As independent variables, we used the functional diversity, species diversity, phylogenetic diversity, and CWM of each trait, and soil chemical properties, whereas basal area was used as the dependent variable. Basal area refers to the cross-sectional area of a tree trunk at 1.3 meters from the ground, and the calculation formula for the basal area is 1/4π multiplied by the square of breast diameter. The vegan package in R4.0.3 was used to calculate the species diversity indices in each plot. The functional diversity indices and CWM for each trait were measured using the FD package. The Picante package (Kembel et al., 2010) was used to calculate phylogenetic diversity. Using SPSS 26.0 (IBM, United States), linear fitting in Origin 8.0 software (OriginLab, United States) was used to establish a linear regression equation to facilitate analysis of the correlations between variables and the basal area.
SPSS 26.0 was used to carry out stepwise multiple regression. Given the multicollinearity between certain factors, which can influence the explanatory ability of an independent variable with respect to the dependent variables, we screened independent variables using stepwise regression, and non-significant variables were removed to reduce the influence of these variables on the regression equation. This ensured that each variable in the regression model was meaningful and thereby enabled us to establish the most relevant explanatory equation.
Amos 20.0.0 software (IBM, United States) and Visio 2016 (Microsoft, United States) were used to analyse the relationships among the CWM of plant traits, plant diversity, soil chemical properties, and basal area based on structural equation model (SEM). In SEM, the fit of the model is poor when assessing large numbers of variables, and consequently, it is necessary to select the most suitable variables via variable screening to establish the optimal model. To fit the SEM, we used the maximum likelihood method, and the critical value of the goodness-of-fit was a chi-square value (CMIN/DF < 3), compare fit index (CFI > 0.9), adjusted goodness-of-fit index (AGFI > 0.9), goodness of fit index (GFI > 0.9), approximate root mean square error (RMSEA < 0.05), and significance probability value (p > 0.05). The standardized coefficients of each path were calculated using the SEM, which were used to express the explanatory rate of the diversity index, the CWM of traits, and soil chemical properties with respect to the changes in basal area. To assess the relative importance of the niche complementarity and mass ratio hypotheses in explaining the changes in basal area within the karst forest community, we used different interpretation rates of the diversity index and CWM. If the diversity indices explain a greater proportion of the variation in basal area, this is taken to be indicative of a dominant niche complementarity hypothesis. In contrast, if the CWM explains a greater proportion, this means that the mass ratio hypothesis has a higher relative importance.
3 Results
3.1 Relationships between basal area and diversity, the community-weighted mean of traits, and soil chemical properties
Values obtained for species richness, Shannon index, Simpson index, functional richness index, Faith’s phylogenetic diversity, and mean nearest taxon phylogenetic distance index were found to be extremely significantly correlated with the basal area (p < 0.001), and evenness, functional divergence, functional dispersion, Rao’s quadratic entropy, and mean pairwise phylogenetic distances indices were significantly correlated with basal area (p < 0.05). Among these indices, we established that Faith’s phylogenetic diversity had the greatest explanatory power for basal area (R2 = 0.751, p < 0.001), followed by species richness (R2 = 0.729, p < 0.001) (Figure 2). The CWM values of leaf length-to-width ratio, leaf thickness, and leaf total carbon content were found to be extremely significantly correlated with basal area (p < 0.001), whereas the CWM values of branch tissue density, specific leaf area, and leaf total nitrogen content were significantly correlated with basal area (p < 0.05), and the CWM values of other traits showed no significant correlation with basal area (p > 0.05) (Figure 3). Among the soil chemical property factors, pH, and the contents of hydrolysable nitrogen, available potassium, organic carbon, total nitrogen, and total phosphorus showed extremely significant correlations with basal area (p < 0.001), and the contents of available phosphorus and total potassium were significantly correlated with basal area (p < 0.05) (Figure 4).
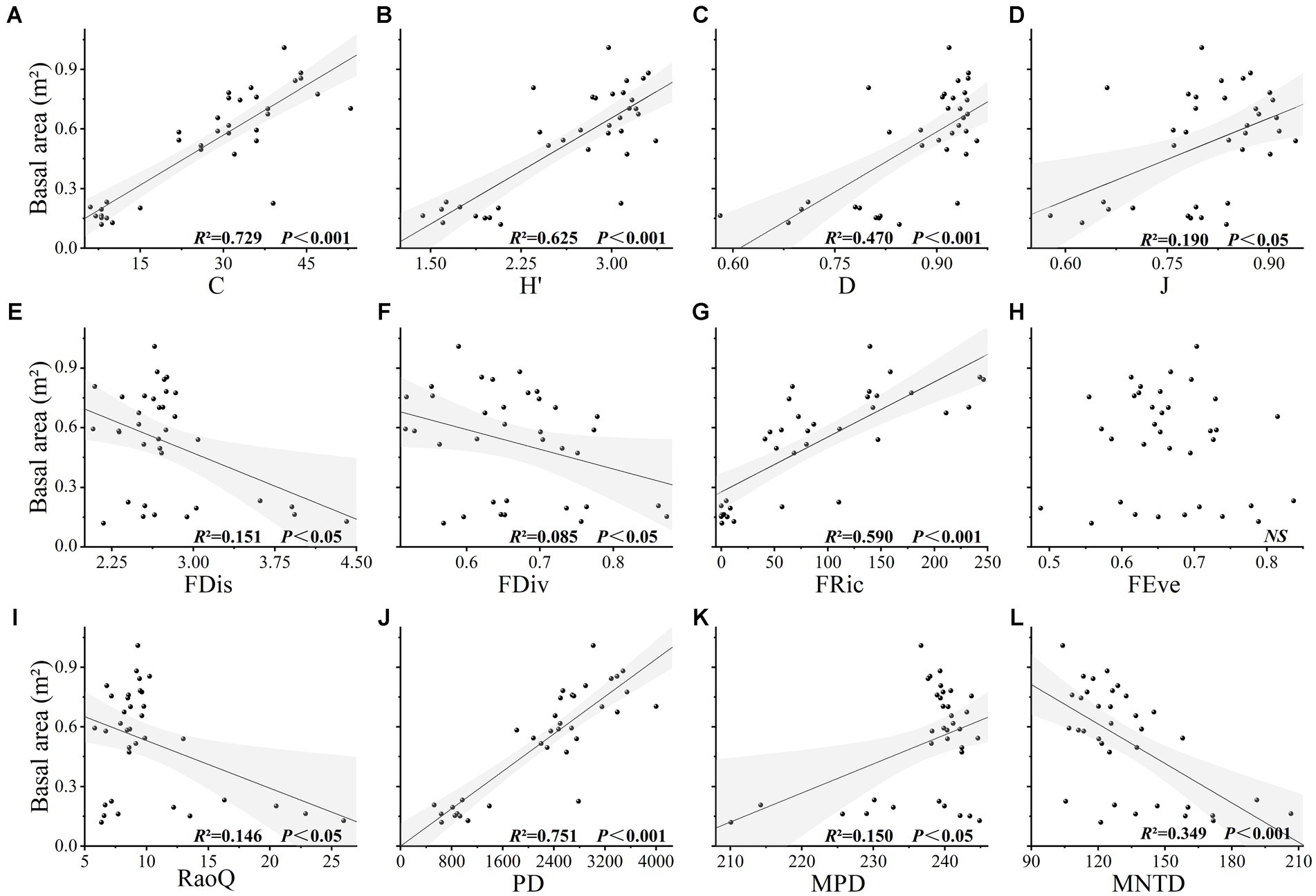
Figure 2. (A-L) The relationship between basal area and plant diversity in karst forest community. C, H, D, and J, respectively, represent species richness, Shannon index, Simpson index and evenness index in species diversity. FDis, FDiv, FRic, FEve, and RaoQ, respectively, represent the functional dispersion index, functional divergence index, functional richness index, functional evenness index, and Rao’s quadratic entropy index. PD, MPD, and MNTD, respectively, represent Faith’s phylogenetic diversity, mean pairwise phylogenetic distance, and mean nearest taxon phylogenetic distance.
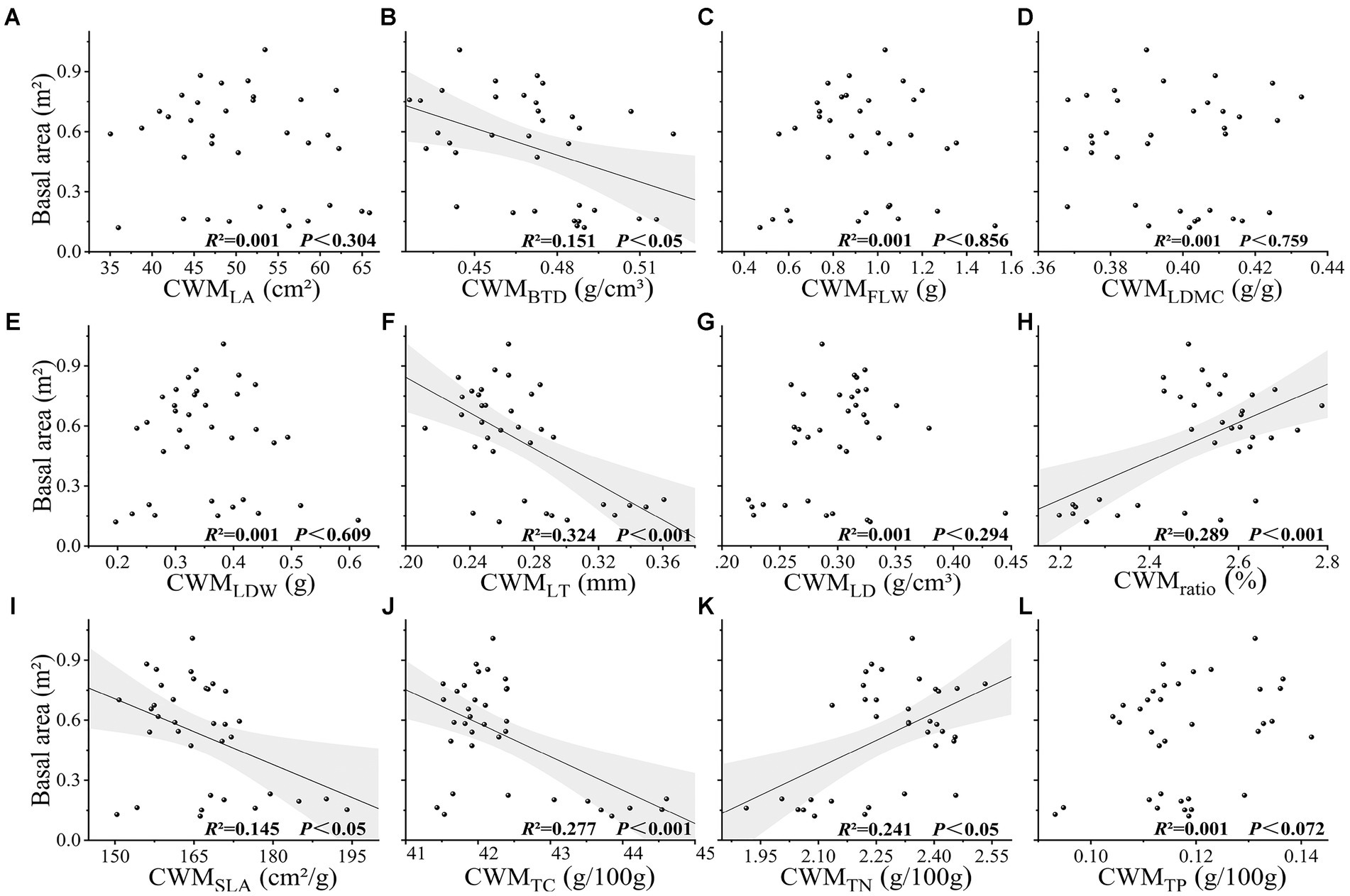
Figure 3. (A-L) Relationship between basal area and CWM of leaf traits in karst forest community. CWM represents the community-weighted mean of traits. LA leaf area, BTD branch tissue density, FLW fresh leaf weight, LDMC leaf dry matter content, LDW leaf dry weight, LT leaf thickness, LD leaf density, ratio represents leaf length to width ratio, SLA specific leaf area, TC leaf total carbon content, TN leaf total nitrogen content, TP leaf total phosphorus content.
3.2 Main variables explaining the variation in basal area
Multivariate stepwise analysis indicated that species richness was extremely significantly positively correlated with basal area (p < 0.001), explaining 72.9% of the change in basal area (Table 4). Functional richness was highly significantly positively correlated with basal area (p < 0.001), whereas the functional dispersion index was highly significantly negatively correlated with it (p < 0.001). Faith’s phylogenetic diversity was positively and extremely significantly correlated with basal area (p < 0.001), explaining 75.1% of the variation. The CWM values of both leaf thickness and branch tissue density were shown to be highly significantly negatively correlated with basal area (p < 0.001). Similarly, the soil contents of total phosphorus and total potassium showed an extremely significant positive correlation (p < 0.001) and an extremely significant negative correlation (p < 0.001) with basal area, respectively (Table 4). In the full model, which includes plant diversity, the CWM of traits, soil chemical properties, and basal area, Faith’s phylogenetic diversity was introduced as the first factor and found to show a significant positive correlation with basal area, thereby indicating that phylogenetic diversity was the most important factor influencing the change in basal area. Stepwise regression revealed that the determination coefficients of diversity could be ordered as follows: phylogenetic diversity (R2 = 0.751) > species diversity (R2 = 0.729) > functional diversity (R2 = 0.637). Furthermore, we found that the determination coefficients of the stepwise regression equation between the three plant diversity indices and basal area (R2 = 0.729; 0.637; and 0.751) were higher than those of the CWM of traits and basal area (R2 = 0.509).
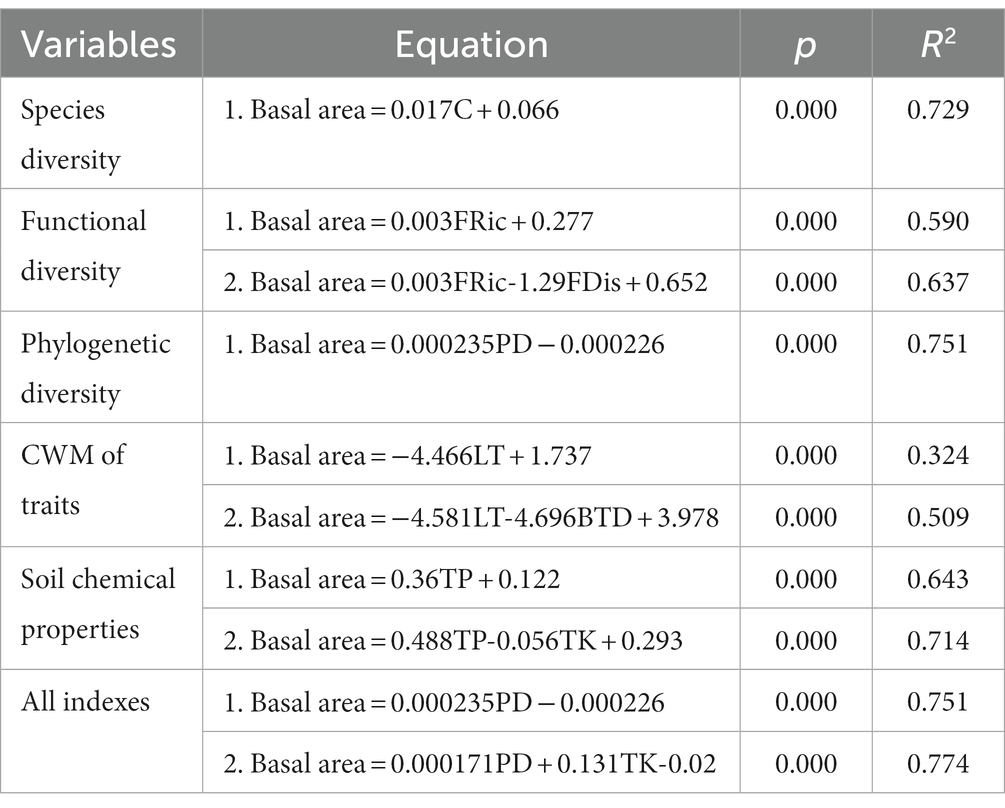
Table 4. Stepwise multiple regression (SMR) equations of community variables and basal area in karst forests.
3.3 Direct and indirect effects of different factors on basal area
The species richness, Shannon, Faith’ phylogenetic diversity, and functional richness indices, which were established to be the most closely associated with basal area growth, were selected to represent plant diversity. Three CWM traits, namely, CWMratio, CWMSLA, and CWMTC, were found to be the most closely associated with basal area. Soil organic carbon, available phosphorus, and total carbon contents were selected as the main variables of soil chemical properties and included in the Structural equation modelling (SEM). The statistical values of the optimal model were as follows: CMIN/DF = 0.647, GFI = 0.906, CFI = 1.000, RMSEA < 0.001, and p = 0.941. SEM revealed that plant diversity indices had a highly significantly direct effect on the basal area (p < 0.001), with a path coefficient of 0.80. However, the CWM of traits had no significant direct effects on basal area (p > 0.05), for which the path coefficient was only −0.01 (Figure 5). The CWM of traits mainly had indirect effects on the basal area, mediated via plant diversity, with an indirect effect of 0.208. Soil chemical properties also influenced the change in basal area, which had an indirect influence on plant diversity and the CWM of traits, with a path coefficient of 0.57. The direct effect of soil chemical properties on basal area was also non-significant (p > 0.05), with a path coefficient of 0.12. The total effect (sum of the direct and indirect effects) of plant diversity on basal area was the largest, with total effect of 0.824. In addition, the total effects of soil chemical properties and the CWM of traits on basal area were 0.689 and 0.198, respectively (Figures 5, 6).
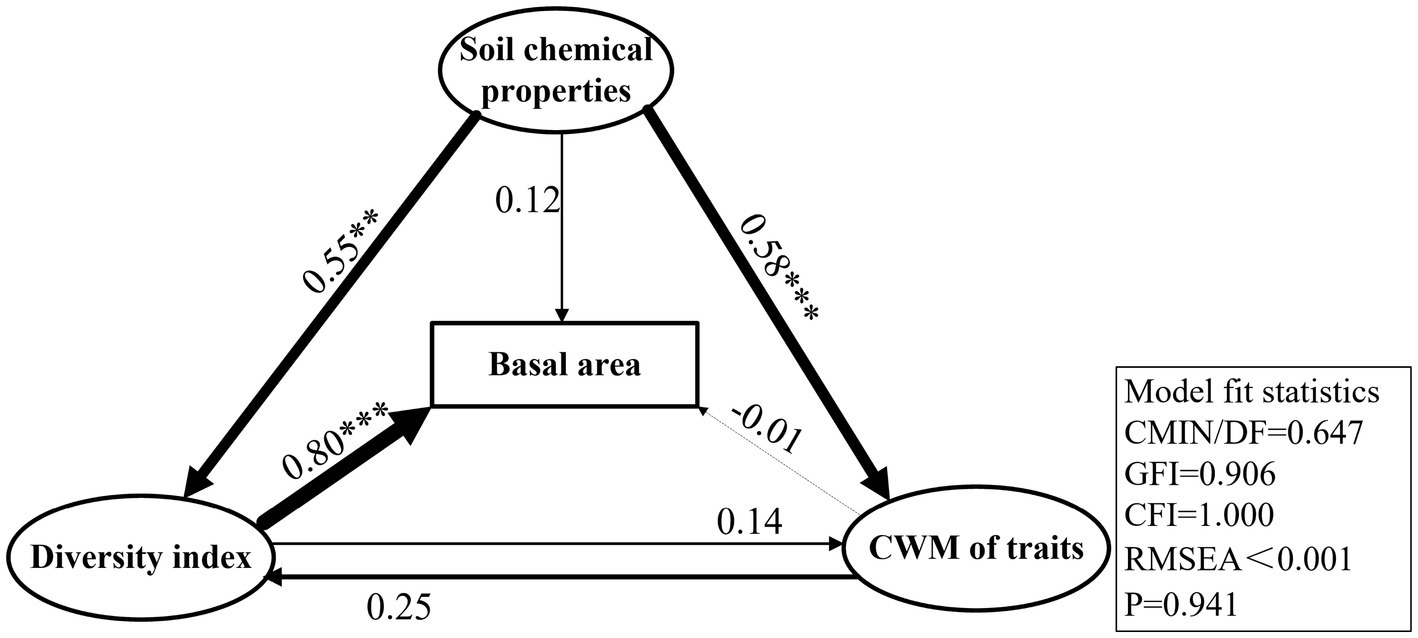
Figure 5. The best-fit structural equation model (SEM) was used to analyze the effects of the community-weighted mean (CWM) of traits, plant diversity and soil chemical properties on the basal area of karst forest. The thickness of the line segment in the figure indicated the size of the correlation, the solid line indicated the positive correlation, and the dotted line indicated the negative correlation, values on each line represent normalized path coefficients with associated statistical significance, ***p < 0.001, **p < 0.01, and *p < 0.05, the data in the box was the model fit value.
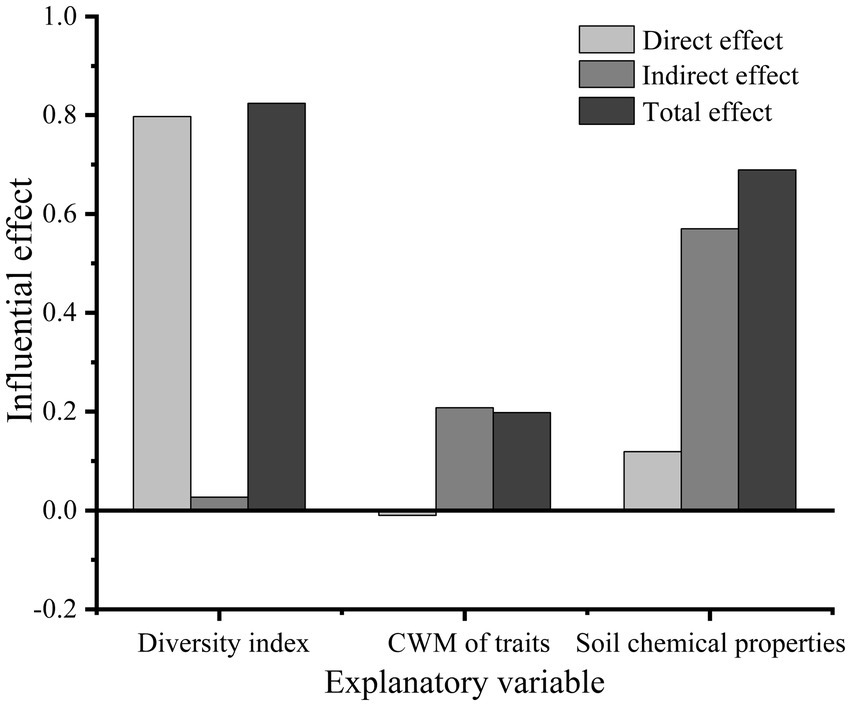
Figure 6. The direct effect, indirect effect and total effect of relevant explanatory variables of plant diversity index, community-weighted mean of traits and soil chemical properties on basal area.
4 Discussion
4.1 Phylogenetic diversity can better explain the changes in basal area than either species or functional diversity
Plant communities develop as a consequence of the interactions between ecological processes and evolutionary history, and taking into account the functional traits and phylogenetic relationships of species at the community level provides a more accurate reflection of the changes in community structure and the underlying mechanisms (Swenson et al., 2011; Wang et al., 2013). Numerous studies have begun to focus on the relationships between phylogenetic diversity and ecosystem function, and have found that phylogenetic diversity can be used as a highly effective predictor of the changes in community productivity of biomass, with superior performance compared with that of either species or functional diversity. Moreover, it has also been found that an increase in phylogenetic diversity contributes to an increase in community ecosystem functions, including biomass (Maherali and Klironomos, 2007; Cadotte et al., 2008; Flynn et al., 2011; Tan et al., 2012; Pu et al., 2014), which is consistent with our findings in the present study. In this study of karst areas, we combined species, functional, and phylogenetic diversity indices to examine the relationship between these indices and the basal area of the forest communities in these areas. Among these indices, we identified Faith’s phylogenetic diversity index (showing a positive correlation) as being the most important factor influencing the change in tree basal area, and we accordingly established that compared with other diversity indices, phylogenetic diversity can better explain the changes in basal area (Figure 2 and Table 4).
We speculate that these differences in predictive performance can be ascribed to the fact that a consideration of species diversity tends to overlook differences in functional traits, treating the contribution of all species as equal, and functional and phylogenetic diversity can better capture the characteristics of species within a community (Hao et al., 2018). However, functional diversity cannot measure all the efficient traits related to the ecological strategies and physiological processes of a species, and consequently, certain important functional traits can be readily overlooked (Yang et al., 2014). Moreover, functional traits do not effectively express the historical evolutionary information harboured by the phylogenetic interspecies of the community, and as such, there are certain limitations in explaining community changes and ecosystem functions (Cadotte et al., 2008). Given the importance of studying species composition and community development from an evolutionary perspective, assessing phylogenetic diversity is of particular value, in that it can reflect the genetic relationships among species. In addition, phylogenetic diversity is unaffected by changes in the taxonomic status of a species and can better reflect community-related species traits, niche diversity, and ecosystem function (Cadotte et al., 2008; Rosauer et al., 2009; Flynn et al., 2011). Indeed, it has been established that the higher the phylogenetic diversity of a community, the more stable is the community and the higher its productivity (Dinnage et al., 2012). With respect to karst areas, the soil layer is thin and the environmental conditions and availability of resources tend to be relatively poor, moreover, species with close kinship inhabiting these areas often have similar ecological niches, thereby intensifying competition and limiting coexistence (Swenson, 2013). However, communities with higher phylogenetic diversity exploit a broader range of ecological niches, thereby maximizing the allocation and utilization of resources, enhancing complementarity, and increasing productivity by maximizing total resource uptake, which in turn contributes to higher levels of ecosystem functioning (Cavender-Bares et al., 2009). Phylogenetic diversity also has a positive influence on the biomass production of microbial communities and the decomposition of organic matter, thereby promoting the coexistence of a more diverse range of fungal species, and thus increasing the biomass of plant communities (Maherali and Klironomos, 2007; Pu et al., 2014).
4.2 The niche complementarity hypothesis may be more suitable than the mass ratio hypothesis in explaining the variation in basal area
Although the verity of both the niche complementarity hypothesis (Tilman, 1997) and the mass ratio hypothesis (Grime, 1998) have been validated in ecosystem studies, the two hypotheses tend to differ in their relative importance according to environment (Diaz et al., 1998; Mouillot et al., 2011; Potvin et al., 2011). For example, in temperate deciduous forests in the eastern United States, Fotis found that aboveground biomass was positively correlated with traits such as maximum tree height and minimum root depth, thereby providing support for the mass ratio hypothesis (Fotis et al., 2018). Conversely, Mensah found that functional diversity can influence aboveground carbon storage to a greater extent than functional dominance in South African forests, they tending to indicate that the niche complementarity hypothesis provides a better explanation of the variation in aboveground biomass and productivity compared with the mass ratio hypothesis (Mensah et al., 2016). Our finding in the present study tends to be similar to those obtained by Mensah et al., with species richness, Shannon, functional richness, and faith’s phylogenetic diversity indices all being found to show an extremely significantly positively correlation with basal area, which were significantly superior to the CWMs of traits (Figures 2, 3). The structural equation model (SEM) we developed indicated that the total effect of diversity indices on the basal area (0.824) was considerably greater than that of the CWM (0.198) (Figure 6). These findings accordingly indicate that the basal area of the forest community in karst areas of southwest China is primarily determined by plant diversity indices, and that the performance of the niche complementarity hypothesis is superior to that of the mass ratio hypothesis in explaining the variation in basal area.
These two explanatory mechanisms are characterized by different responses in different environments. The mass ratio hypothesis more effectively explains the vegetation-productivity relationship under general habitat conditions (Diaz et al., 1998), whereas the niche complementarity hypothesis is more suitable for explaining the effects of harsh conditions under intense environmental stress (Laio et al., 2001). Karst landforms, which are widely distributed in the study area, are characterized by complex topographical features, such as a thin soil layer and poor water retention, with clear spatial and temporal heterogeneity. The deficiencies in soil and water, along with alkaline habitat conditions, contribute to the harsh conditions of the karst landscape, thereby markedly limiting plant growth, this study area belongs to the latter relatively severe environment (Guo et al., 2011). With an increase in plant diversity, differences in the functional characteristics of tree species within the community also increase. Highly diverse communities tend to be characterized by less intense interspecific competition and optimization of the utilization of limited resources, thereby facilitating higher growth and productivity (Hao et al., 2018). Notably, beneficial complementary interactions between species may be more important for ecosystem processes in nutrient-poor and climatically unfavourable forest environments (Paquette and Messier, 2011). The niche complementarity effect can indirectly reduce the adverse effects of host-specific pathogens and herbivores, reduce biological mortality, and promote growth (Schnitzer et al., 2011). Although in the present study, we established the niche complementarity effect to be more important, we should not overlook the potential influence of either dominant species or dominant traits, given that community-dominant species and their characteristics can also contribute to shaping ecosystem functions (Grime, 1998).
4.3 Plant diversity and community basal area are influenced by soil chemical properties
It has been established that plant diversity and biomass are positively influenced by the availability of resources, as represented by soil chemical properties, together with biotic factors to determine the composition and diversity of plant communities (Tilman, 1997; Zhang et al., 2015). In the present study, we detected significantly positive correlations between different soil chemical properties and basal area in the karst forest community, among which, the pH and total phosphorus content of soil had the highest explanatory power for basal area (Figure 4). Structural equation modelling revealed that soil chemical properties have a direct influence on diversity indices and CWM values, with effects of 0.55 and 0.58, respectively. Whereas the direct effects of soil chemical properties on basal area were relatively low at 0.12, the indirect effects, mediated via diversity and CWM, were as high as 0.57. These findings thus indicated that soil chemical properties are important factors influencing plant diversity and basal area, the contributions of which are more pronounced that those of the CWM of each trait (Figures 5, 6).
Biodiversity is also influenced by abiotic factors, including soil chemical properties, which indicates that these are not independent variables, and that their associations with productivity are dependent on environmental interactions (Zhang et al., 2015). Environmental conditions can modify the complementarity among species and contribute to the development of biodiversity-productivity relationships via complex plant-soil feedback loops. Consequently, soil chemical properties may be served as important factor influencing biodiversity-productivity relationship (Hao et al., 2018). Soil fertility has been identified as a major driver of biomass increases in humid tropical forests, and changes in soil chemical properties have been established to promote changes in species diversity and community productivity, which in turn results in richer soils, thereby contributing to more vigorous and higher turnover rates, and thus a higher community productivity (Slik et al., 2010). In karst ecosystems characterized by nutrient-poor soils, the lack of resources, particularly phosphorus, will result in a direct decline in productivity, which will limit maximal productivity, thereby detrimentally influencing the forest ecosystem, and even material cycling (Paoli and Curran, 2007). A stable nutrient pool can effectively ensure the maintenance of nutrient and water cycles in the ecosystem, enhance plant growth, and have a positive effect on tree productivity (Prado-Junior et al., 2016). Consequently, soil chemical properties play important roles in plant growth, directly influencing community composition and determining ecosystem function and productivity levels.
5 Conclusion
Our findings show that diversity, community-weighted mean of traits, and soil chemical properties all contribute to the basal area in karst forest. Among diversity indices, phylogenetic diversity can better explain the changes in basal area. And diversity had large effects on basal area than community-weighted mean of traits, thus the niche complementarity hypothesis is more suitable. Most important, soil chemical properties also have an equally important effect on basal area. This study helps us better understand the driving mechanism of basal area in karst forest and how diversity affects this function. Ecosystems can provide multiple functions simultaneously, and considering only one function can easily overlook the complementarity and balance between other functions. In addition, we only considered the three diversity dimensions of aboveground plants, while ignoring underground biodiversity. In the future, we can simultaneously consider how aboveground and underground biodiversity affects multifunctional ecosystems.
Data availability statement
The raw data supporting the conclusions of this article will be made available by the authors, without undue reservation.
Author contributions
LL: Formal analysis, Methodology, Writing – original draft. FZ: Methodology, Resources, Supervision, Writing – review & editing. ZZ: Methodology, Supervision, Validation, Writing – review & editing. HD: Methodology, Resources, Visualization, Writing – review & editing. CZ: Investigation, Methodology, Resources, Writing – review & editing. HZ: Funding acquisition, Methodology, Project administration, Resources, Writing – review & editing.
Funding
The author(s) declare financial support was received for the research, authorship, and/or publication of this article. This research was supported by the National Natural Science Foundation of China (31870712, 32071846 and 42071073), Key Research and Development Program of Guangxi Province (GuikeAB23026129), State Key Laboratory of Environmental Geochemistry (SKLEG2021207), and Hechi City Program of Distinguished Experts in China.
Acknowledgments
We are grateful for the support from Huanjiang Observation and Research Station for Karst Ecosystem and help from Xiaoxiao Zou, Qingzhi Long, and Ziwen Lian for their useful suggestion and kind assistance for data analysis.
Conflict of interest
The authors declare that the research was conducted in the absence of any commercial or financial relationships that could be construed as a potential conflict of interest.
Publisher’s note
All claims expressed in this article are solely those of the authors and do not necessarily represent those of their affiliated organizations, or those of the publisher, the editors and the reviewers. Any product that may be evaluated in this article, or claim that may be made by its manufacturer, is not guaranteed or endorsed by the publisher.
References
Ali, A., Yan, E., Chang, S. X., Cheng, J., and Liu, X. (2017). Community-weighted mean of leaf traits and divergence of wood traits predict aboveground biomass in secondary subtropical forests. Sci. Total Environ. 574, 654–662. doi: 10.1016/j.scitotenv.2016.09.022
Bradley, J. B., and Katharine, N. S. (2013). Single-trait functional indices outperform multi-trait indices in linking environmental gradients and ecosystem services in a complex landscape. J. Ecol. 101:12013. doi: 10.1111/1365-2745.12013
Cadotte, M. W. (2017). Functional traits explain ecosystem function through opposing mechanisms. Ecol. Lett. 20, 989–996. doi: 10.1111/ele.12796
Cadotte, M. W., Cardinale, B. J., and Oakley, T. H. (2008). Evolutionary history and the effect of biodiversity on plant productivity. Proc. Natl Acad. Sci. 105, 17012–17017. doi: 10.1073/pnas.0805962105
Cadotte, M. W., Cavender-Bares, J., Tilman, D., and Oakley, T. H. (2009). Using phylogenetic, functional and trait diversity to understand patterns of plant community productivity. PLoS One 4:e5695. doi: 10.1371/journal.pone.0005695
Cavender-Bares, J., Kozak, K. H., Fine, P. V. A., and Kembel, S. W. (2009). Merging of community ecology and phylogenetic biology. Ecol. Lett. 12, 693–715. doi: 10.1111/j.1461-0248.2009.01314.x
Chamagne, J., Tanadini, M., Frank, D., Matula, R., Paine, C. E. T., Philipson, C. D., et al. (2017). Forest diversity promotes individual tree growth in central European forest stands. J. Appl. Ecol. 54, 71–79. doi: 10.1111/1365-2664.12783
Chave, J., Réjou-Méchain, M., Búrquez, A., Chidumayo, E., Colgan, M. S., Delitti, W. B. C., et al. (2014). Improved allometric models to estimate the aboveground biomass of tropical trees. Glob. Change Biol. 20, 3177–3190. doi: 10.1111/gcb.12629
Condit, R. (1995). Research in large, long-term tropical forest plots. Trends Ecol. Evol. 10, 18–22. doi: 10.1016/S0169-5347(00)88955-7
Dı’az, S, and Cabido, M, (2001). Vive la différence: plant functional diversity matters to ecosystem processes. Elsevier Ltd, Oxford, 646.
Diaz, S., Cabido, M., and Casanoves, F. (1998). Plant functional traits and environmental filters at a regional scale. J. Veg. Sci. 9, 113–122. doi: 10.2307/3237229
Díaz, S., Quétier, F., Cáceres, D. M., Trainor, S. F., Pérez-Harguindeguy, N., Bret-Harte, M. S., et al. (2011). Linking functional diversity and social actor strategies in a framework for interdisciplinary analysis of nature’s benefits to society. Proc. Natl. Acad. Sci. 108, 895–902. doi: 10.1073/pnas.1017993108
Dinnage, R., Cadotte, M. W., Haddad, N. M., Crutsinger, G. M., and Tilman, D. (2012). Diversity of plant evolutionary lineages promotes arthropod diversity. Ecol. Lett. 15, 1308–1317. doi: 10.1111/j.1461-0248.2012.01854.x
Du, H., Peng, W. X., Song, T. Q., Zeng, F. P., Wang, K. L., Song, M., et al. (2015). Spatial pattern of woody plants and their environmental interpretation in the karst forest of Southwest China. Plant Biosyst. 149, 121–130. doi: 10.1080/11263504.2013.796019
Farahat, E., Shaltout, K., El-Kady, H., and Shalapy, A. (2012). Allometric equations to predict the total aboveground biomass of tree species in a planted forest in Egypt. Feddes repertorium 123, 27–36. doi: 10.1002/fedr.201100027
Finegan, B., Peña Claros, M., Oliveira, A., Ascarrunz, N., Bret Harte, M. S., Carreño Rocabado, G., et al. (2015). Does functional trait diversity predict above-ground biomass and productivity of tropical forests? Testing three alternative hypotheses. The Journal of ecology 103, 191–201. doi: 10.1111/1365-2745.12346
Firn, J., McGree, J. M., Harvey, E., Flores-Moreno, H., Schütz, M., Buckley, Y. M., et al. (2019). Leaf nutrients, not specific leaf area, are consistent indicators of elevated nutrient inputs. Nat. Ecol. Evol. 3, 400–406. doi: 10.1038/s41559-018-0790-1
Flynn, D. F., Mirotchnick, N., Jain, M., Palmer, M. I., and Naeem, S. (2011). Functional and phylogenetic diversity as predictors of biodiversity – ecosystem-function relationships. Ecology 92, 1573–1581. doi: 10.1890/10-1245.1
Fotis, A. T., Murphy, S. J., Ricart, R. D., Krishnadas, M., Whitacre, J., Wenzel, J. W., et al. (2018). Above-ground biomass is driven by mass-ratio effects and stand structural attributes in a temperate deciduous forest. J. Ecol. 106, 561–570. doi: 10.1111/1365-2745.12847
Gao, W. Q., Lei, X. D., Gao, D. L., and Li, Y. T. (2021). Mass-ratio and complementarity effects simultaneously drive aboveground biomass in temperateQuercus forests through stand structure. Ecol. Evol. 11, 16806–16816. doi: 10.1002/ece3.8312
García, F. C., Bestion, E., Warfield, R., and Yvon-Durocher, G. (2018). Changes in temperature alter the relationship between biodiversity and ecosystem functioning. Proc. Natl. Acad. Sci. 115, 10989–10994. doi: 10.1073/pnas.1805518115
Garnier, E., Cortez, J., Billès, G., Navas, M., Roumet, C., Debussche, M., et al. (2004). Plant functional markers capture ecosystem properties during secondary succession. Ecology 85, 2630–2637. doi: 10.1890/03-0799
Grime, J. P. (1998). Benefits of plant diversity to ecosystems: immediate, filter and founder effects. J. Ecol. 86, 902–910. doi: 10.1046/j.1365-2745.1998.00306.x
Guo, K., Liu, Z. C., and Dong, M. (2011). Ecological adaptation of plants and control of rocky-desertification on karst region of Southwest China. Chin. J. Plant Ecol. 35, 991–999. doi: 10.3724/SP.J.1258.2011.00991
Hao, M., Zhang, C., Zhao, X., and Gadow, K. V. (2018). Functional and phylogenetic diversity determine woody productivity in a temperate forest. Ecol. Evol. 8, 2395–2406. doi: 10.1002/ece3.3857
Hooper, D. U., Chapin, F. S. I., Ewel, J. J., Hector, A., Inchausti, P., Lavorel, S., et al. (2005). Effects of biodiversity on ecosystem functioning: a consensus of current knowledge. Ecol. Monogr. 75, 3–35. doi: 10.1890/04-0922
Hooper, D. U., and Vitousek, P. M. (1997). The effects of plant composition and diversity on ecosystem processes. Science 277, 1302–1305. doi: 10.1126/science.277.5330.1302
Huang, M., Liu, X., Cadotte, M. W., and Zhou, S. (2020). Functional and phylogenetic diversity explain different components of diversity effects on biomass production. Oikos 129, 1185–1195. doi: 10.1111/oik.07032
Kembel, S. W., Cowan, P. D., Helmus, M. R., Cornwell, W. K., Morlon, H., Ackerly, D. D., et al. (2010). Picante: R tools for integrating phylogenies and ecology. Bioinformatics 26, 1463–1464. doi: 10.1093/bioinformatics/btq166
Laio, F., Porporato, A., Fernandez-Illescas, C. P., and Rodriguez-Iturbe, I. (2001). Plants in water-controlled ecosystems: active role in hydrologic processes and response to water stress: IV. Discussion of real cases. Adv. Water Resour. 24, 745–762. doi: 10.1016/S0309-1708(01)00007-0
Laliberte, E., and Legendre, P. (2010). A distance-based framework for measuring functional diversity from multiple traits. Ecology 91, 299–305. doi: 10.1890/08-2244.1
Larjavaara, M., and Muller-Landau, H. C. (2013). Measuring tree height: a quantitative comparison of two common field methods in a moist tropical forest. Methods Ecol. Evol. 4, 793–801. doi: 10.1111/2041-210X.12071
Lasky, J. R., Uriarte, M., Boukili, V. K., Erickson, D. L., John, K. W., and Chazdon, R. L. (2014). The relationship between tree biodiversity and biomass dynamics changes with tropical forest succession. Ecol. Lett. 17, 1158–1167. doi: 10.1111/ele.12322
Li, A., Guo, D. L., Wang, Z. Q., and Liu, H. Y. (2010). Nitrogen and phosphorus allocation in leaves, twigs, and fine roots across 49 temperate, subtropical and tropical tree species: a hierarchical pattern. Funct. Ecol. 24, 224–232. doi: 10.1111/j.1365-2435.2009.01603.x
Li, S. J., Su, P. X., Zhang, H. N., Zhou, Z. J., Xie, T. T., Shi, R., et al. (2018). Distribution patterns of desert plant diversity and relationship to soil properties in the Heihe River basin, China. Ecosphere 9:e2355. doi: 10.1002/ecs2.2355
Li, P., Yan, Y., Li, C., Tang, W. G., Xiong, Z. Q., Tian, Y. H., et al. (2023). Response of rice growth to soil microorganisms and soil properties in different soil types. Agron. J. 115, 197–207. doi: 10.1002/agj2.21239
Liu, Y., Qi, W. C., He, D. N., Xiang, Y. R., Liu, J. C., Huang, H. M., et al. (2021). Soil resource availability is much more important than soil resource heterogeneity in determining the species diversity and abundance of karst plant communities. Ecol. Evol. 11, 16680–16692. doi: 10.1002/ece3.8285
Maestre, F. T., Quero, J. L., Gotelli, N. J., Escudero, A., Ochoa, V., Delgado-Baquerizo, M., et al. (2012). Plant species richness and ecosystem multifunctionality in global drylands. Science 335, 214–218. doi: 10.1126/science.1215442
Maherali, H., and Klironomos, J. N. (2007). Influence of phylogeny on fungal community assembly and ecosystem functioning. Science 316, 1746–1748. doi: 10.1126/science.1143082
Maia Araújo, T., Higuchi, N., De Carvalho, A., and Júnior, J. (1999). Comparison of formulae for biomass content determination in a tropical rain forest site in the state of Pará. Brazil. For. Ecol. Manage. 117, 43–52. doi: 10.1016/S0378-1127(98)00470-8
Mammola, S., Carmona, C. P., Guillerme, T., and Cardoso, P. (2021). Concepts and applications in functional diversity. Funct. Ecol. 35, 1869–1885. doi: 10.1111/1365-2435.13882
Mason, N. W. H., de Bello, F., Wilson, B., and Wilson, B. (2013). Functional diversity: a tool for answering challenging ecological questions. J. Veg. Sci. 24, 777–780. doi: 10.1111/jvs.12097
Mensah, S., Veldtman, R., Assogbadjo, A. E., Glèlè Kakaï, R., and Seifert, T. (2016). Tree species diversity promotes aboveground carbon storage through functional diversity and functional dominance. Ecol. Evol. 6, 7546–7557. doi: 10.1002/ece3.2525
Mouillot, D., Villeger, S., Scherer-Lorenzen, M., and Mason, N. W. (2011). Functional structure of biological communities predicts ecosystem multifunctionality. PLoS One 6:e17476. doi: 10.1371/journal.pone.0017476
Ouyang, S., Gou, M., Lei, P., Liu, Y., Chen, L., Deng, X., et al. (2023). Plant functional trait diversity and structural diversity co-underpin ecosystem multifunctionality in subtropical forests. For. Ecosyst. 10:100093. doi: 10.1016/j.fecs.2023.100093
Ouyang, S., Xiang, W., Wang, X., Xiao, W., Chen, L., Li, S., et al. (2019). Effects of stand age, richness and density on productivity in subtropical forests in China. J. Ecol. 107, 2266–2277. doi: 10.1111/1365-2745.13194
Paoli, G. D., and Curran, L. M. (2007). Soil nutrients limit Fine litter production and tree growth in mature lowland Forest of Southwestern Borneo. Ecosystems 10, 503–518. doi: 10.1007/s10021-007-9042-y
Paquette, A., and Messier, C. (2011). The effect of biodiversity on tree productivity: from temperate to boreal forests. Glob. Ecol. Biogeogr. 20, 170–180. doi: 10.1111/j.1466-8238.2010.00592.x
Pennington, R. T., Richardson, J. E., and Lavin, M. (2006). Insights into the historical construction of species-rich biomes from dated plant phylogenies, neutral ecological theory and phylogenetic community structure. New Phytol. 172, 605–616. doi: 10.1111/j.1469-8137.2006.01902.x
Petchey, O. L., and Gaston, K. J. (2006). Functional diversity: back to basics and looking forward. Ecol. Lett. 9, 741–758. doi: 10.1111/j.1461-0248.2006.00924.x
Potvin, C., Mancilla, L., Buchmann, N., Monteza, J., Moore, T., Murphy, M., et al. (2011). An ecosystem approach to biodiversity effects: carbon pools in a tropical tree plantation. For. Ecol. Manag. 261, 1614–1624. doi: 10.1016/j.foreco.2010.11.015
Prado-Junior, J. A., Schiavini, I., Vale, V. S., van der Sande, M. T., Lohbeck, M., Poorter, L., et al. (2016). Conservative species drive biomass productivity in tropical dry forests. J. Ecol. 104, 817–827. doi: 10.1111/1365-2745.12543
Pu, Z., Daya, P., Tan, J., and Jiang, L. (2014). Phylogenetic diversity stabilizes community biomass. J. Plant Ecol. 7, 176–187. doi: 10.1093/jpe/rtt071
Quesada, C. A., Phillips, O. L., Schwarz, M., Czimczik, C. I., Baker, T. R., Patiño, S., et al. (2012). Basin-wide variations in Amazon forest structure and function are mediated by both soils and climate. Biogeosciences 9, 2203–2246. doi: 10.5194/bg-9-2203-2012
R Core Team . (2022) R: A language and environment for statistical computing. Vienna, Austria: R Foundation for Statistical Computing.
Rosauer, D., Laffan, S. W., Crisp, M. D., Donnellan, S. C., and Cook, L. G. (2009). Phylogenetic endemism: a new approach for identifying geographical concentrations of evolutionary history. Mol. Ecol. 18, 4061–4072. doi: 10.1111/j.1365-294X.2009.04311.x
Schnitzer, S. A., Klironomos, J. N., Hillerislambers, J., Kinkel, L. L., Reich, P. B., Xiao, K., et al. (2011). Soil microbes drive the classic plant diversity-productivity pattern. Ecology 92, 296–303. doi: 10.1890/10-0773.1
Schoenholtz, S. H., Miegroet, H. V., and Burger, J. A. (2000). A review of chemical and physical properties as indicators of forest soil quality: challenges and opportunities. For. Ecol. Manag. 138, 335–356. doi: 10.1016/S0378-1127(00)00423-0
Slik, J. W. F., Aiba, S., Brearley, F. Q., Cannon, C. H., Forshed, O., Kitayama, K., et al. (2010). Environmental correlates of tree biomass, basal area, wood specific gravity and stem density gradients in Borneo’s tropical forests. Glob. Ecol. Biogeogr. 19, 50–60. doi: 10.1111/j.1466-8238.2009.00489.x
Sullivan, M. J. P., Lewis, S. L., Hubau, W., Qie, L., Baker, T. R., Banin, L. F., et al. (2018). Field methods for sampling tree height for tropical forest biomass estimation. Methods Ecol. Evol. 9, 1179–1189. doi: 10.1111/2041-210X.12962
Swenson, N. G. (2013). The assembly of tropical tree communities - the advances and shortcomings of phylogenetic and functional trait analyses. Ecography 36, 264–276. doi: 10.1111/j.1600-0587.2012.00121.x
Swenson, N. G., Anglada-Cordero, P., and Barone, J. A. (2011). Deterministic tropical tree community turnover: evidence from patterns of functional beta diversity along an elevational gradient. Proc. R. Soc. B 278, 877–884. doi: 10.1098/rspb.2010.1369
Tan, J., Pu, Z., Ryberg, W. A., and Jiang, L. (2012). Species phylogenetic relatedness, priority effects, and ecosystem functioning. Ecology 93, 1164–1172. doi: 10.1890/11-1557.1
Tilman, D. (1997). Distinguishing between the effects of species diversity and species composition. Oikos 80:185. doi: 10.2307/3546532
Tilman, D., Isbell, F., and Cowles, J. M. (2014). Biodiversity and ecosystem functioning. Annu. Rev. Ecol. Evol. Syst. 45, 471–493. doi: 10.1146/annurev-ecolsys-120213-091917
Wang, Y., Chen, J., Zhang, L., Feng, L., Yan, L., Li, F., et al. (2022). Relationship between diversity and stability of a karst plant community. Ecol. Evol. 12:9254. doi: 10.1002/ece3.9254
Wang, S. J., Liu, Q. M., and Zhang, D. F. (2004). Karst rocky desertification in southwestern China: geomorphology, landuse, impact and rehabilitation. Land Degrad. Dev. 15, 115–121. doi: 10.1002/ldr.592
Wang, X. G., Swenson, N. G., Wiegand, T., Wolf, A., Howe, R., Lin, F., et al. (2013). Phylogenetic and functional diversity area relationships in two temperate forests. Ecography 36, 883–893. doi: 10.1111/j.1600-0587.2012.00011.x
Wen, C., and Jin, G. Z. (2019). Effects of functional diversity on productivity in a typical mixed broadleaved-Korean pine forest. Chin. J. Plant Ecol. 43, 94–106. doi: 10.17521/cjpe.2018.0312
Yang, J., Zhang, G., Ci, X., Swenson, N. G., Cao, M., Sha, L., et al. (2014). Functional and phylogenetic assembly in a Chinese tropical tree community across size classes, spatial scales and habitats. Funct. Ecol. 28, 520–529. doi: 10.1111/1365-2435.12176
Zhang, Y., Chen, H. Y. H., and Coomes, D. (2015). Individual size inequality links forest diversity and above-ground biomass. J. Ecol. 103, 1245–1252. doi: 10.1111/1365-2745.12425
Zhang, X., Huang, Y. T., Liu, S. R., Fu, S. L., Ming, A. G., Li, X. Z., et al. (2019). Mixture of tree species enhances stability of the soil bacterial community through phylogenetic diversity. Eur. J. Soil Sci. 70, 644–654. doi: 10.1111/ejss.12780
Zhang, C., Zeng, F. P., Zeng, Z. X., Du, H., Su, L., Zhang, L. J., et al. (2022b). Impact of selected environmental factors on variation in leaf and branch traits on endangered karst Woody plants of Southwest China. Forests 13:1080. doi: 10.3390/f13071080
Zhang, C., Zeng, F. P., Zeng, Z. X., Du, H., Zhang, L. J., Su, L., et al. (2022a). Carbon, nitrogen and phosphorus stoichiometry and its influencing factors in karst primary Forest. Forests 13:1990. doi: 10.3390/f13121990
Zhang, X. L., Zhou, J. H., Guan, T. Y., Cai, W. T., Jiang, L. H., Lai, L. M., et al. (2018). Spatial variation in leaf nutrient traits of dominant desert riparian plant species in an arid inland river basin of China. Ecol. Evol. 9, 1523–1531. doi: 10.1002/ece3.4877
Keywords: functional diversity, phylogenetic diversity, community-weighted mean, basal area, karst
Citation: Lu L, Zeng F, Zeng Z, Du H, Zhang C and Zhang H (2023) Diversity and soil chemical properties jointly explained the basal area in karst forest. Front. For. Glob. Change. 6:1268406. doi: 10.3389/ffgc.2023.1268406
Edited by:
Akihiro Nakamura, Chinese Academy of Sciences (CAS), ChinaReviewed by:
Shuai Ouyang, Central South University of Forestry and Technology, ChinaYongkuan Chi, Guizhou Normal University, China
Copyright © 2023 Lu, Zeng, Zeng, Du, Zhang and Zhang. This is an open-access article distributed under the terms of the Creative Commons Attribution License (CC BY). The use, distribution or reproduction in other forums is permitted, provided the original author(s) and the copyright owner(s) are credited and that the original publication in this journal is cited, in accordance with accepted academic practice. No use, distribution or reproduction is permitted which does not comply with these terms.
*Correspondence: Hao Zhang, emhhbmdoYW9AaXNhLmFjLmNu