- 1Institute for Alpine Environment, Eurac Research, Bolzano, Italy
- 2Department of Geography, University of Innsbruck, Innsbruck, Austria
- 3Faculty of Agricultural, Environmental and Food Sciences, Free University of Bolzano-Bozen, Bolzano, Italy
- 4Centre for Climate Change and Transformation, Eurac Research, Bolzano, Italy
- 5Forest Ecology, Institute of Terrestrial Ecosystems, Department of Environmental Systems Science, ETH Zürich, Zürich, Switzerland
- 6Department of Ecological Modelling, Helmholtz Centre for Environmental Research–UFZ, Leipzig, Germany
Protection forests play a key role in protecting settlements, people, and infrastructures from gravitational hazards such as rockfalls and avalanches in mountain areas. Rapid climate change is challenging the role of protection forests by altering their dynamics, structure, and composition. Information on local- and regional-scale impacts of climate change on protection forests is critical for planning adaptations in forest management. We used a model of forest dynamics (ForClim) to assess the succession of mountain forests in the Eastern Alps and their protective effects under future climate change scenarios. We investigated eleven representative forest sites along an elevational gradient across multiple locations within an administrative region, covering wide differences in tree species structure, composition, altitude, and exposition. We evaluated protective performance against rockfall and avalanches using numerical indices (i.e., linker functions) quantifying the degree of protection from metrics of simulated forest structure and composition. Our findings reveal that climate warming has a contrasting impact on protective effects in mountain forests of the Eastern Alps. Climate change is likely to not affect negatively all protection forest stands but its impact depends on site and stand conditions. Impacts were highly contingent to the magnitude of climate warming, with increasing criticality under the most severe climate projections. Forests in lower-montane elevations and those located in dry continental valleys showed drastic changes in forest structure and composition due to drought-induced mortality while subalpine forests mostly profited from rising temperatures and a longer vegetation period. Overall, avalanche protection will likely be negatively affected by climate change, while the ability of forests to maintain rockfall protection depends on the severity of expected climate change and their vulnerability due to elevation and topography, with most subalpine forests less prone to loosing protective effects. Proactive measures in management should be taken in the near future to avoid losses of protective effects in the case of severe climate change in the Alps. Given the heterogeneous impact of climate warming, such adaptations can be aided by model-based projections and high local resolution studies to identify forest stand types that might require management priority for maintaining protective effects in the future.
1. Introduction
In the Alpine region, the protective role of forests against gravitational natural hazards such as rockfall and avalanches is an essential ecosystem service (Motta and Haudemand, 2000; Schirpke et al., 2019; Stritih et al., 2021). There is a growing need to protect an increasing number of human infrastructures and settlements (Sebald et al., 2019) and the presence of forest can avoid the need for artificial defensive structures or reduce their maintenance costs. In the case of rockfall, the effectiveness of a protection forest, however, depends on multiple environmental factors, namely related to the topography (e.g., slope, initial fall height, terrain shape), the characteristics of the forest (e.g., structure, composition, presence of lying trees), and the rock properties (size, shape, and density) (Dorren et al., 2004). Differently, in the case of avalanches, protection forests mostly prevent snow masses from releasing (Bebi et al., 2001; Schuler et al., 2017), reducing the risk of small to medium avalanches (with a released volume < 1,000 m3 and between 1,000 and 10,000 m3 volume, respectively) (Brang et al., 2006; Teich et al., 2012; Feistl et al., 2014), by stabilizing the snow layer through different mechanisms such as evaporation, sublimation, and snow interception (Teich et al., 2012; Bebi et al., 2022).
Climate change is expected to rapidly alter the key processes driving tree and forest dynamics in the Alps (Maroschek et al., 2009). Mountain areas such as the Alps are particularly vulnerable to climate change, where temperatures have been increasing faster than the global average (Leonelli et al., 2011; Pepin et al., 2015) and with a further acceleration observed over the recent decades (Kotlarski et al., 2023). With a rapidly warming climate, these ecosystems will likely experience an acceleration of their dynamics (Albrich et al., 2022; Thom and Seidl, 2022), such as changes in their regeneration, growth, and mortality processes (Allen et al., 2010; Morán-Ordóñez et al., 2020). Numerous studies have shown negative effects of increasing temperatures and drought-stress on the provision of multiple ecosystem services of mountain forests (Rasche et al., 2012, 201; Elkin et al., 2013; Seidl et al., 2019). For example, studies in the Austrian Alps showed an expected decrease in number of large trees with increasing higher temperatures, as well as significant changes in forest composition, which may affect protective effects in Alpine forests (Albrich et al., 2020; Caduff et al., 2022). Comparable results were also registered in the Swiss Alps, where Norway spruce (Picea abies [L.] Karst) was foreseen to experience a strong decline under a warming future climate (Huber et al., 2020). However, the impact of climate change on multiple ecosystem services provided by mountain forests has been shown to be highly heterogeneous due to the large variability in site and climatic conditions (Mina et al., 2017a).
Since forests are long-living ecosystems characterized by slow dynamics (Lindner et al., 2010; Thom and Seidl, 2016; Albrich et al., 2018), evaluations of future potential impacts of a changing climate on their development and protective effects can only be addressed with simulation models. Models of forest dynamics (Bugmann and Seidl, 2022) are tools that can give useful indications of the long-term effects of climate change on forest ecosystems, particularly if they include the most important ecological processes influencing forest dynamics under changing environmental conditions (Bosela et al., 2022). Among them, forest succession (gap) models have been proven to be flexible tools capable to accurately simulate stand dynamics at the local scale, considering both changes in the climate and in the environmental condition, but also being useful for improving decision-making strategies and decision supporting systems (Rasche et al., 2012; Searle et al., 2021). Several studies applied forest succession models to evaluate forest responses to climate change and ecosystem services provision (Shugart et al., 2018), often including protection against gravitational hazards assessed with indicators expressing the protective effects of forests, based on stand characteristics such as structure and composition (Elkin et al., 2013; Mina et al., 2017b; Thrippleton et al., 2020). Only a few studies, however, have explicitly investigated the protective effects of forests under changing climate using models of forest dynamics (Cordonnier et al., 2008; Moos et al., 2021; Moos and Lischke, 2022). So far, only forests in the Northern Alps (i.e., Switzerland) were investigated on this key aspect (Rasche et al., 2012; Huber et al., 2020; Thrippleton et al., 2020), while a few examples of studies on protection forests in the Italian Alps exist but excluding climate change (Scheidl et al., 2020; Costa et al., 2021). Most importantly, dynamic forest models like the one applied in this study (see below) have never been tested nor applied in the Italian Alps (Vacchiano et al., 2012). Investigating whether and how the protective effects of mountain forests characterized by different environmental condition is likely to be affected by a changing climate is of crucial importance to support adaptations in management strategies and to increase long-term forest resilience.
In the present study, we applied the model of forest dynamics ForClim (Bugmann, 1996) to examine the impact of climate change on future forest structure and composition, and how these affect protective effects in mountain forests of the Eastern Alps. We selected representative sites distributed across a study area to cover different climatic and environmental conditions, as well as species composition that are typical for montane and subalpine protection forests and addressed the following questions: (1) how will different climate change scenarios affect the dynamics of mountain forests in the Eastern Italian Alps until the end of the 21st century? (2) On the basis of assumed climate change scenarios, what long-term effects will the structural and compositional changes of forest stands have on their protective effects?
2. Materials and methods
2.1. Study area
The study area covers the Autonomous Province of Bozen/Bolzano, South Tyrol (hereafter South Tyrol), in northern Italy (Figure 1). The climate is heterogeneous because it is influenced by air masses coming from both continental and Mediterranean areas (Zentralanstalt fr Meteorologie und Geodynamik (ZAMG), Abteilung Brand- und Zivilschutz – Autonome Provinz Bozen, Agenzia Regionale per la Prevenzione e Protezione Ambientale del Veneto (ARPAV), 2015). Mean annual temperature varies largely with elevation (from 12.5°C at 246 m a.s.l. in Bozen/Bolzano to 3.3°C at 1,903 m a.s.l. in Sulden/Solda in the inner Alps) while precipitation pattern is highly influenced by topography (from 505 mm/year in dry inner alpine valleys such as the Vinschgau/Venosta to 1,299 mm/year in the Dolomites) (Crespi et al., 2021). More than half of the study area‘s surface (375,351 ha, 51%) is covered by forests, mainly dominated by conifers such as Norway spruce (Picea abies), European larch (Larix decidua), Scots pine (Pinus sylvestris), Swiss stone pine (Pinus cembra), and silver fir (Abies alba) (Gasparini et al., 2022). Broadleaved trees cover a marginal portion of the overall forested area (2 % of standing volume) but species such as European beech (Fagus sylvatica) and oaks (Quercus pubescens, Q. petraea) play an important role in the southern part of the province. The montane elevational belt, ranging from ca. 1,000 to 1,600 m a.s.l., is widely dominated by spruce, while from the subalpine elevation belt (1,600–2,300 m a.s.l.), spruce is gradually replaced by larch and Swiss stone pine (Autonomous Province of Bolzano/Bozen, 2010). More than 90% of the forest area is subject to forestry-hydrogeological constraint (i.e., national legislation that limits the intensity of silvicultural interventions to avoid soil denudation that could trigger erosion and loss of stability of mountain slopes; cf. (Floris and Di Cosmo, 2022) and about 58% of forests are considered protection forests, of which 24% have a direct protective effects, i.e., they are directly placed above infrastructures (roads, rail network) and settlements protecting them from natural hazards (Kössler Aichner et al., 2021, 81).
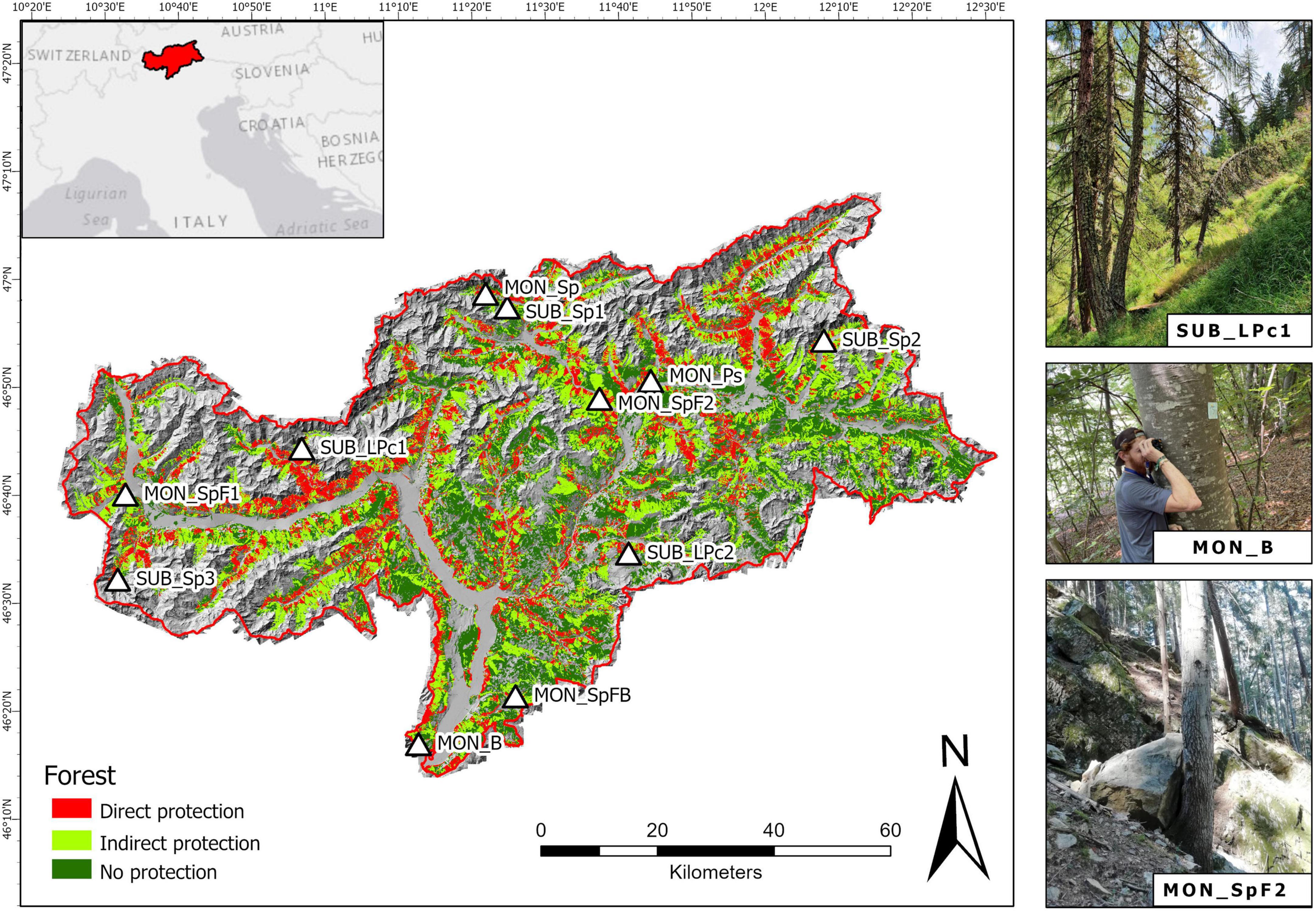
Figure 1. Overview of the study area and the study sites (white triangles, see Table 1 for explanations of codes). Colored layers indicate area covered by forests with different levels of protection: direct protection (i.e., forest area actively protecting settlements or infrastructures from rockfall and/or avalanches), indirect protection (protection of soil from erosion, landslide) (Autonomous Province of Bolzano/Bozen, 2007, 2013). Photos show three sites as an example: a subalpine larch-Swiss stone pine stand (SUB_LPc1), a mature beech forest (MON_B) and a boulder stopped by a tree in a montane spruce-fir stand (MON_SpF2; credits: L. Hillebrand, M. Mina).
For our simulation experiment, we selected forest sites distributed across the entire study area (Figure 1 and Table 1). To do so, we overlapped the mapped distribution of the forest types of the region (Autonomous Province of Bolzano/Bozen, 2010) with the map of the forest’s protective effects and the map of known occurrences of rockfalls or avalanches (Autonomous Province of Bolzano/Bozen, 2013). Since the ForClim model required to be initialized with forest data at the single-tree resolution, we looked for recent and available forest stand data from two datasets, such as the Biodiversity Monitoring South Tyrol (Hilpold et al., 2023) and the local forest management plans of the Provincial Forest Services (Autonomous Province of Bolzano/Bozen, 2010)–see section “2.2.4. Forest stand data and forest management” and section “S1.2. Forest stand initialization.” We eventually selected eleven sites with available forest stand data that fulfilled the above mentioned criteria (Figure 1). The sites ranged from 950 to 2,000 m a.s.l., with mean annual temperature spanning from 2.9 to 9.2°C and annual precipitation ranging from 589 mm in the inner alpine valleys to 1,144 mm at the northernmost sites bordering Austria (Table 1 and Supplementary Figure 5). Exposition varied from north to south-south-west and all sites had a slope of above 30°. The sites included all the dominant tree species found across the study area, with a prevalence of Norway spruce commonly mixed with European beech and silver fir, but also European larch and Swiss stone pine dominating the subalpine belts, and Scots pine often forming pure stands in south-exposed slopes.
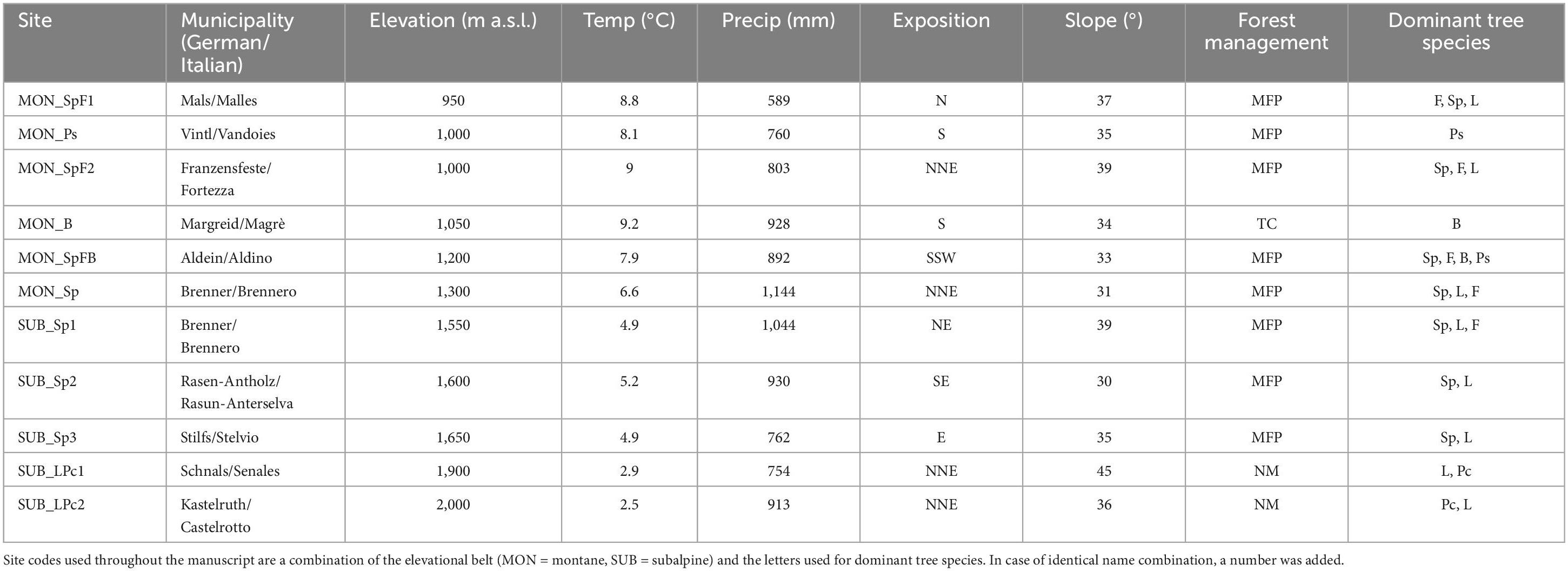
Table 1. List of the forest sites with: name of the municipality, elevation (rounded to 50 m), mean annual temperature and precipitation sum, exposition, slope, type of forest management regime (MFP, mountain forest plentering; TC, target cutting; NM, unmanaged), dominant tree species in order of basal area abundance (legend: B = European beech, Sp = Norway spruce, F = Silver fir, Ps = Scots pine, L = European larch, Pc = Swiss stone pine).
2.2. Simulation modeling
2.2.1. Forest model ForClim
ForClim is a climate-sensitive forest succession model developed to simulate stand-scale forest dynamics over a wide range of environmental conditions (Bugmann, 1996; Shugart and Smith, 1996). Following the assumptions of gap models and the ecological concept of patch dynamics (Botkin et al., 1972), ForClim simulates forest succession on multiple independent patches with a size that represents a small area of land (Bugmann, 2001). Within each patch, processes such as establishment and growth of cohorts (i.e., trees with same age and species) are modeled based on environmental inputs and internal state variables such as available light, soil moisture, browsing pressure, soil nutrients, growing season temperatures and winter temperature. Tree diameter and height are calculated based on the principle of growth-limiting factors (Moore, 1989) while mortality derives from combined age- and stress-related components (Bircher et al., 2015; Huber et al., 2020).
ForClim requires a variety of input data and parameters to simulate forest dynamics, from data on site biophysical conditions (e.g., topographic and soil information) to climate variables and species-specific parameters denoting the ecological characteristics of tree species (Gutiérrez et al., 2016; Huber et al., 2018). The model also requires climatic inputs such as monthly means of mean air temperature and precipitation sum, and it uses an internal weather generator that creates climatic series for the desired simulation timespan by sampling stochastically from a normal distribution for temperature and a log-normal distribution for precipitation (Bircher et al., 2015). A management submodel (Rasche et al., 2011) allows the application of a wide range of silvicultural interventions, from clear cuts to shelterwood felling, thinning to more complex management regimes such as removals by relative and absolute diameter classes (Mina et al., 2017b) and mountain forest plentering (Thrippleton et al., 2020). The model is parameterized for > 30 European tree species (see Supplementary Table 4 for parameters used in the current study) and it has been applied to multiple temperate forests worldwide (Gutiérrez et al., 2016; Mina et al., 2017b; Martin-Benito et al., 2022), undergoing several evaluations and validation routines under different site conditions (Rasche et al., 2012; Huber et al., 2018). In this study we used ForClim v 4.0.1 (Huber et al., 2020). As comparing different model variants to account for uncertainties in processes formulation (i.e., background mortality and maximum establishment rate) was beyond the scope of our study, we used only one variant for our simulations [variant 1 from Huber et al. (2021)].
2.2.2. Current climate and climate change scenarios
For current climatic conditions (hereafter baseline), monthly time series of average temperature (°C) and precipitation sum (mm) from 1980 to 2010 were derived from a gridded dataset of daily temperature and precipitation covering Trentino/South Tyrol at 250 m resolution for the period 1980–2018 (Crespi et al., 2021). It was obtained by interpolating a quality-checked and homogenized archive of weather station records covering the region and surrounding countries. For South Tyrol, about 95 (for temperature) and 80 (precipitation) stations were included in the observation database. The accuracy of interpolated daily fields was assessed through a leave-one-out validation procedure against station observations, which reported no systematic biases and an average absolute error of 1.5°C for temperature and 1.1 mm for precipitation (Crespi et al., 2021). Monthly averages, standard deviations and cross-correlations of temperature and precipitation, needed as an input for the ForClim stochastic weather generator, were calculated at each site, with annual mean temperature ranging from 2.5 to 9.2°C and total annual precipitation from 589 to 1,144 mm (Supplementary Figure 5).
Future climate change scenarios were derived from CHELSA V2.1 (Karger et al., 2017, 2020). This dataset provides a preselected, bias corrected combination of downscaled global climate models (GCMs) and emissions scenarios driven by different socioeconomic assumptions, so-called shared socio-economic pathways (SSPs), from the Coupled Model Intercomparison Project Phase 6 (Karger et al., 2021). We selected two out of the five featured GCMs in CHELSA, GFDL-ESM4 and UKESM1-0-LL, as they were given the highest priorities following the ISIMIP3b protocol. Priority was assessed according to the models’ performance in the historical period (1850–2014), as well as availability of required data (Lange, 2021). We then selected two scenarios as representative for moderate and severe climate change, namely SSP1-RCP2.6 and SSP3-RCP7 (O’Neill et al., 2017). We excluded SSP5-RCP8.5 as it is often deemed as highly unlikely worst case scenario that has recently caused controversy and debates among scholars on its application in simulation models (Hausfather and Peters, 2020; Hausfather et al., 2022). CHELSA provides 30-year averages of monthly temperature and precipitation for different time periods (1981–2010, 2011–2040, 2041–2070, and 2071–2100) at 1 km spatial resolution. For each scenario and model we calculated the differences in seasonal mean temperature and precipitation between subsequent 30-year periods, using the last period as reference for the next one and including the historical period (1981–2010) which was used as the first reference period. Lastly, season-specific delta values for each future climate scenario were used as inputs for the simulation. When the long-term changes for 2071–2100 were computed with respect to current conditions (1981–2010), all future scenarios and models showed temperature increases in all seasons, while shifts in precipitation varied depending on the scenario and season (Figure 2). Climate change scenarios were ranked and renamed as “CC1” for SSP1 and “CC2” for SSP3, with letters indicating the climate model (a = GFDL_ESM4; b = UKESM1-0-LL).
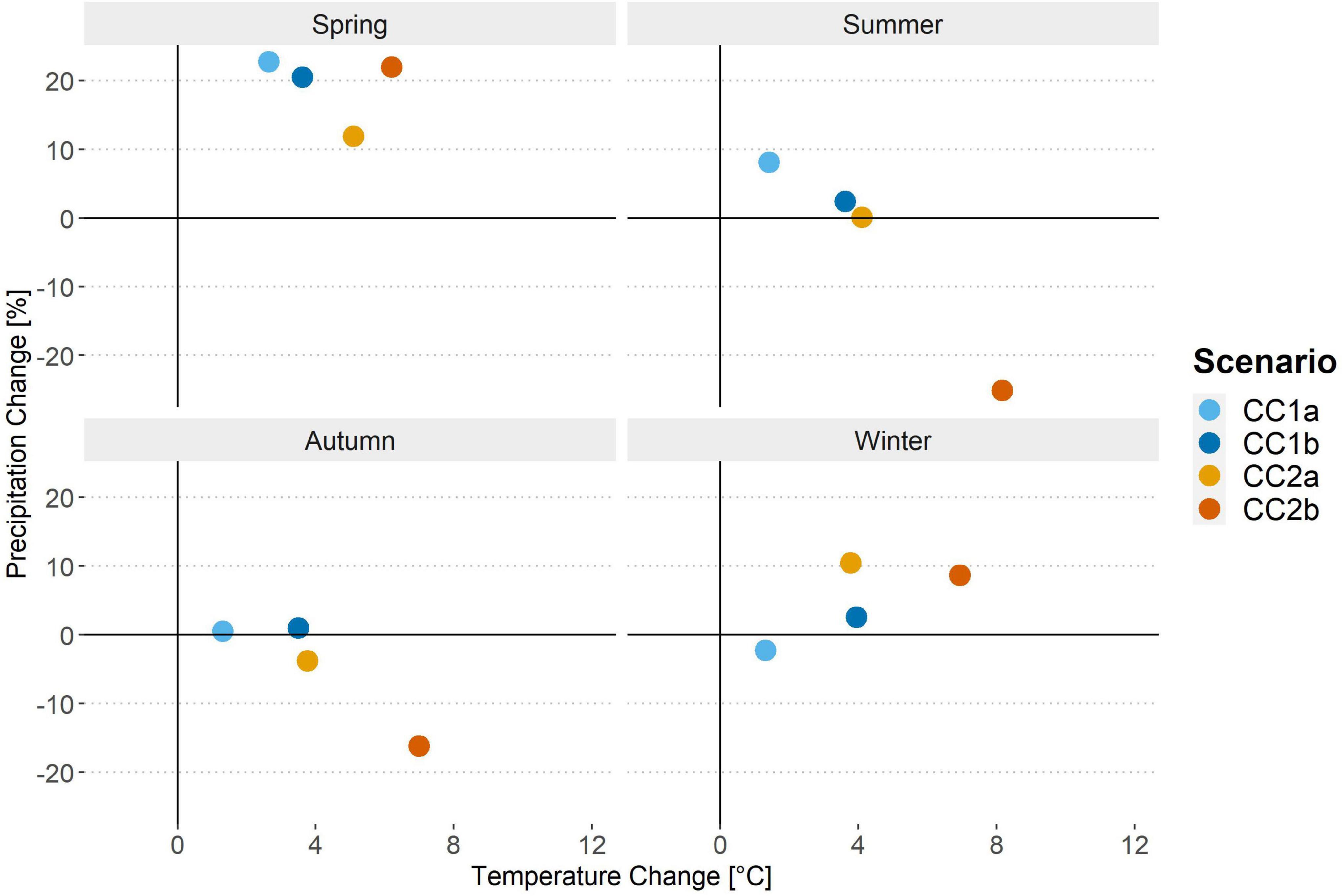
Figure 2. Climate change delta values for temperature and precipitation averaged over all study sites per season (spring = March to May; summer = June to August; autumn = September to November; winter = December to February). Values are differences between the period 2071–2100 and the CHELSA historical period (1981–2010). Scenario combinations: CC1a = SSP126/GFDL_ESM4, CC1b = SSP126/UKESM1_0_LL, CC2a = SSP370/GFDL_ESM, CC2b = SSP370/UKESM1_0_LL.
2.2.3. Local soil conditions
Parameters expressing soil conditions such as soil water holding capacity and available nitrogen were derived from the descriptions for each forest type where our forest sites were located (Supplementary Figure 1 and Supplementary Table 1). Browsing pressure affecting seedling survival probability after browsing (Didion et al., 2011) was derived from assessments made in the field during data collection (i.e., severity of damage from ungulate to regeneration). Other needed site-specific inputs such as exposition, slope and latitude were retrieved from the available information from the original datasets and verified with a digital elevation model. Additional details on the derivation of the site-specific parameters are provided in section “S1.1. Site-specific inputs.”
2.2.4. Forest stand data and forest management
The forest stands were initialized using forest data from two province-wide datasets: Biodiversity Monitoring South Tyrol (Hilpold et al., 2023) and the local forest management plans (Cullotta and Maetzke, 2009) by the South Tyrol’s Provincial Forest Services (Autonomous Province of Bolzano/Bozen, 2010). The first is a long-term monitoring program of biodiversity, which includes survey in South Tyrol’s forests following the field protocol by fixed-area sample plot of the Italian National Forest Inventory (Supplementary Figure 2; Gasparini et al., 2022). The second include stand-level information and angle-count sampling data (Supplementary Figure 3 and Supplementary Table 2) that are being collected in the framework of the renewal of forest management plans on forests under public ownership or large private properties across the province. The year of survey of forest data ranged from 2016 to 2022 (Supplementary Table 1). The two datasets used for initialization differed in terms of callipering thresholds and sampling area; all details about the forest stand initialization, as well as model testing, are given in the sections “S1.2. Forest stand initialization” and “S1.3. Model testing and parameterization” (Supplementary Figure 4).
Information on current forest management strategies were obtained from the forest management plans and the forest types of South Tyrol (Autonomous Province of Bolzano/Bozen, 2010, 2021). Following the principles of sustainable forest management outlined in the Provincial Forest Strategy (Autonome Provinz Bozen - Südtirol, 2011), periodic harvested volume should not exceed stand increment to allow long-term sustainability. For protection forests, usually located in steep terrain and at high elevation, forests management options become increasingly constrained, and interventions are executed mainly to assure sufficient regeneration (Frehner et al., 2005). A typical management regime is mountain forest plentering (MFP), which represents small-scale felling of groups of trees with the aim of promoting regeneration by enhancing light and temperature at the forest floor (Allgaier Leuch et al., 2017). Thus, on most of our sites we applied the MFP harvesting algorithm in ForClim, which mimic the harvesting of tree groups above a defined target diameter in small patches that is typical of protection forests in the Alps (Thrippleton et al., 2020) while on a montane site dominated by beech we applied the target cutting harvesting function (Rasche et al., 2012). On the two subalpine sites at the highest elevation, no management interventions such as harvesting or planting were scheduled according to information gained from the Forest Services, thus we did not simulate any silvicultural treatment (Table 1). Further details about the ForClim management module and implementation of forest management scenarios are found in “section S1.4. “Forest management scenario (Supplementary Table 3).”
2.3. Experimental design and analysis
We ran our simulations of forest dynamics for baseline climate (i.e., no climate change) and four climate scenarios representing moderate (CC1a and CC1b) and severe climate change (CC2a and CC2b). All simulations included current forest management regime that was defined for each a site as above and were run with a selected number of tree species according to the site (see section “S1.2. Forest stand initialization”). Simulations were designed to start the year following the survey of the forest stand data and were run on 200 forest patches with a size of 531 m2 until the year 2100. To evaluate expected changes in forest structure and composition, we analyzed species-specific and stand-level model outputs such as stem number, basal area and biomass. We also calculated an index of tree size inequality (Gini index), which expresses size diversity in forest stands and ranges from 0–perfect equality of individual tree’s basal area–and 1 for maximum theoretical inequality (Cordonnier and Kunstler, 2015; Bourdier et al., 2016).
To assess protective effects against gravitational hazards we calculated dimensionless protection indexes: the rockfall protection index (RPI) and the avalanche protection index (API). The rockfall protection index (Cordonnier et al., 2014; Mina et al., 2017a) was calculated by dividing the current energy dissipation of the stand by the dissipating maximal energy, expressed as the probable residual hazard (PRH). Dissipating maximal energy is thereby a function of stand (percentage of evergreen and deciduous species, average DBH), rock (volume and mass of rock) and terrain features (slope angle and fall height at start). Depending on a threshold of basal area (10 m2/ha), Eq. 1 or Eq. 2 is used. PRH (Eq. 3a) is then used to calculate the RPI (Eq. 3b), which ranges from 0 to 1, denoting the percentage of rocks stopped by the forest, and having a minimum value of 0.01 (i.e., 1%).
where Φrock = rock diameter [m], N = stems/ha, slope = slope angle [°], EvG = percentage of evergreen species (on basal area level), DcD = percentage of deciduous species (on basal area level), = average DBH [cm], ρ = rock density [kg/mł], Fih = initial fall height [m] (Cordonnier et al., 2014). Rock types for each site (Supplementary Table 1) were derived from geological maps in the scale of 1:1,000 from the Italian Institute for Environmental Protection and Research (ISPRA) of the Italian Government. The rock densities were subsequently derived from a table published by the University of Berlin (Carmichael, 1984; Kobranova, 1989; Schön, 2015). In order to evaluate rockfall protection from different rock size, we calculated three different RPIs expressing protection from boulders with different volume and equivalent diameter: RPI1 for small rocks with volume 1 m3 and equivalent diameter 1.24 m, RPI2.5 for medium rocks with volume 2.5 m3 and diameter 1.68 m, and RPI5 for large rocks with volume 5 m3 and diameter 2.12 m. Additional description on the formulation of the RPI can be found in Cordonnier et al. (2014).
The avalanche protection index (Elkin et al., 2013; Thrippleton et al., 2020) was calculated based on the ratio between current stand and ideal protection parameters according to knowledge developed in the project “Sustainability and monitoring of performance in protection forests” by Frehner et al. (2005) in Switzerland. The API (Eq. 4) uses a combination of both interception and stability, which are key processes when it comes to the effective protection of forests against avalanches (Berger, 1997). Interception depends on stand leaf area index (LAI) and the fraction of evergreen trees (Eq. 5), while stability is contingent on the density of the stand, which varies depending on the steepness of the slope (for slopes ≥ 40> Eq. 6 is used and Eq. 7 for slopes > 40°).
All analysis and visualization were performed with the R language and environment for statistical computing, version 4.0.1 (R Core Team, 2022).
3. Results
3.1. Changes in forest structure and composition
Both montane and subalpine study sites showed changes in forest structure and composition under the different climate scenarios (Figure 3). Overall, a general decreasing trend in stem number, basal area and biomass under climate change was recognizable in montane forests with exceptions in both elevational belts.
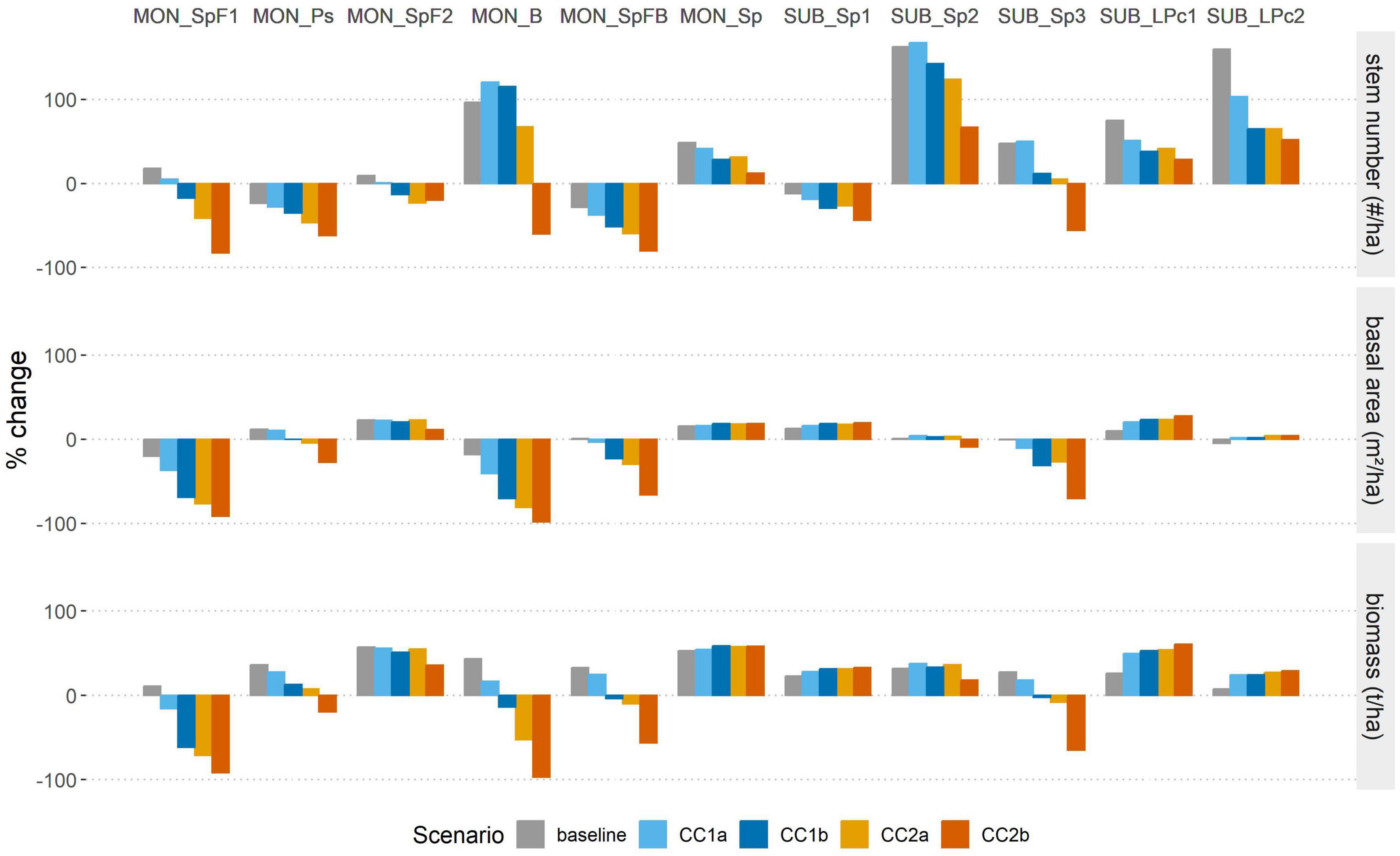
Figure 3. Percent change in stem number, basal area and biomass under baseline and climate change scenarios relative to current conditions.
Simulations under baseline climate resulted with the highest increase in stem number in all montane forests except for MON_B, where moderate climate change (i.e., CC1a, CC1b) induced higher increases in tree density than baseline (CC1a with + 120%). The largest decrease in stem number for montane sites was observed under the most severe climate change scenario (CC2b; maximum of −83% in MON_SpF1) in all but one site, MON_SpF2, where relative changes were less pronounced and the largest decrease was found under CC2a. Four montane sites (i.e., MON_Sp, MON_B, MON_SpF1, and MON_SpF2) showed a (partial) increase in stem number under climate change scenarios, with decreasing trends mainly under CC2b. In the subalpine forests, instead, we observed a general increase in stem number, which was consistently the highest under baseline (except at sites SUB_Sp2 and SUB_Sp3 where the highest increases were observed under CC1a) and the lowest under severe climate change. Only in SUB_Sp1 stem number declined under all scenarios, while in SUB_Sp3 a sudden reduction was observed only under severe climate change (−56% under CC2b). Overall, if we only compare climate change scenarios, stem number was always the highest under the mildest climate change projection (CC1a) and the lowest under the most severe one (CC2b), suggesting a general decline in tree number with more pronounced future temperature changes. Stand basal area increased only on two (MON_SpF2, MON_Sp) out of six montane forests. In three sites (MON_SpF1, MON_B, MON_SpFB) we observed a decline in basal area, which was larger the more severe the climate change scenario (e.g., up to −98% in site MON_B under CC2b). Overall, the reductions in basal area were more intense than the increases, which were rather modest (e.g., max +23% in MON_SpF2 under CC2a). On the contrary, in the majority of the subalpine forests (four out of five), basal area generally increased under climate change, although not in a large amount (max + 27% in SUB_LPc1 under CC2b). The sole exceptions were SUB_Sp3, where basal area declined regardless the climate change scenario (up to −70% under CC2b), and SUB_Sp2, where a reduction in basal area occurred only under CC2b.
In terms of forest biomass, our results showed a heterogeneous trend depending on the climate scenarios. Biomass generally increased in every site under baseline and under the mildest climate change, likely due to establishment of new cohorts and accelerated forest dynamics (see Discussion) but often showed a drastic reduction under the most severe climate change scenario (e.g., down to −97% in MON_B under CC2b), although in a few montane forests biomass increased even under CC2b (e.g., MON_SpF2, MON_Sp). Consistently with the trend for basal area, biomass in subalpine forests generally increased under climate change. This variable sometimes reached its maximum under CC2b (e.g., as in SUB_Sp1 and SUB_LPc1) with the exception of SUB_Sp3, where biomass increased under the mildest climate scenario (CC1a) but drastically declining under severe climate change (−65% under CC2b). Size diversity was also affected differently according to the forest site and climate scenarios (Supplementary Figure 7). In most of the montane sites (e.g., MON_SpF1, MON_B), tree size diversity was projected to increase under baseline and mild climate change compared to current conditions but it declined when climate change was simulated to be more severe. In some cases, however, montane forests were projected to become generally more heterogeneous in terms of diameter distribution (MON_Sp, MON_SpFB). Size diversity in subalpine forests, instead, was less affected by climate change, showing future increases in size heterogeneity compared to current conditions (SUB_Sp2, SUB_Sp3) or no major changes (SUB_LPc1, SUB_LPc2) but overall no substantial differences among the climate scenarios.
Temporal pattern of species-specific changes in basal area differed substantially among sites (Figure 4 and Supplementary Figure 6), especially due to differences in initial species composition. Although we did not observe drastic changes in projected species composition across our forest stands, some species were more affected by climate change than other. This, however, differed depending on the site and climate scenario. For example, European larch in MON_SpF1 and MON_SpFB persisted until 2100 under baseline climate, but it gradually declined until being completely absent from those stands under climate change, with steepest reductions observed under severe climate change.
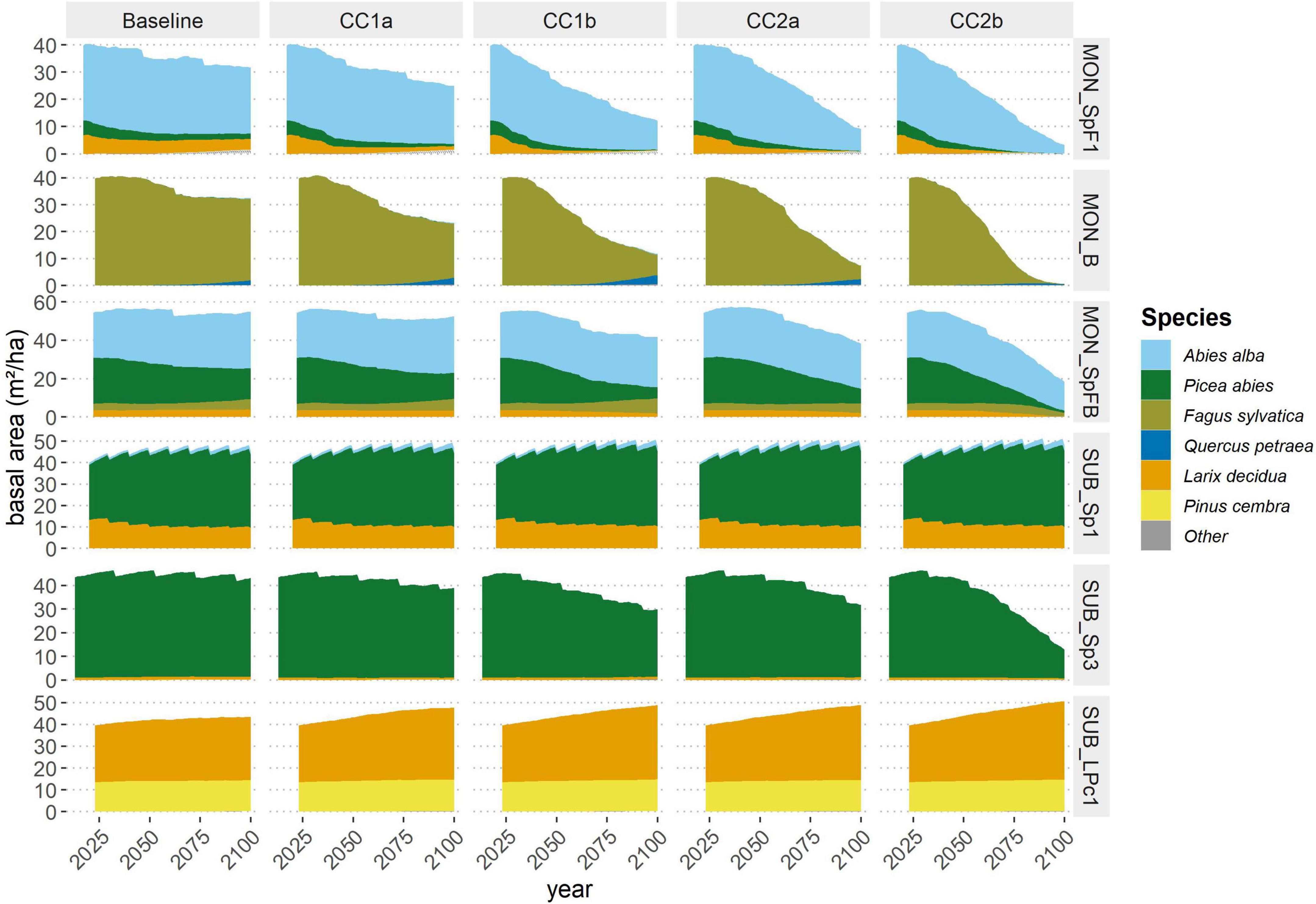
Figure 4. Simulated changes in species-specific basal area under baseline and climate change scenarios for selected forest sites. Results for the other sites are given in Supplementary Figure 6.
3.2. Protective effects
All montane sites experienced a decline in avalanche protection under climate change (Figure 5 and Supplementary Figure 8). API was generally higher under current conditions and was projected to decrease in the future under baseline climate and climate change scenarios. Severe climate change (CC2b) often induced drastic reductions of avalanche protection, as in sites MON_SpF1, MON_SpFB and MON_B (API as low as 0.03 in 2100 under CC2b) but not in site MON_Sp where API reductions were projected to be minimal. In the subalpine sites too, avalanche protection was generally higher under current conditions and was projected to reduce under climate change. While in some sites projected changes of the API were minimal (e.g., SUB_Sp1, SUB_Sp2) in others avalanche protection declined with intensifying climate change (SUB_Sp3). In one site (SUB_LPc1), API was very low also under current conditions (0.29), showing that the structure of this forest is at present time not suitable to protect against avalanches.
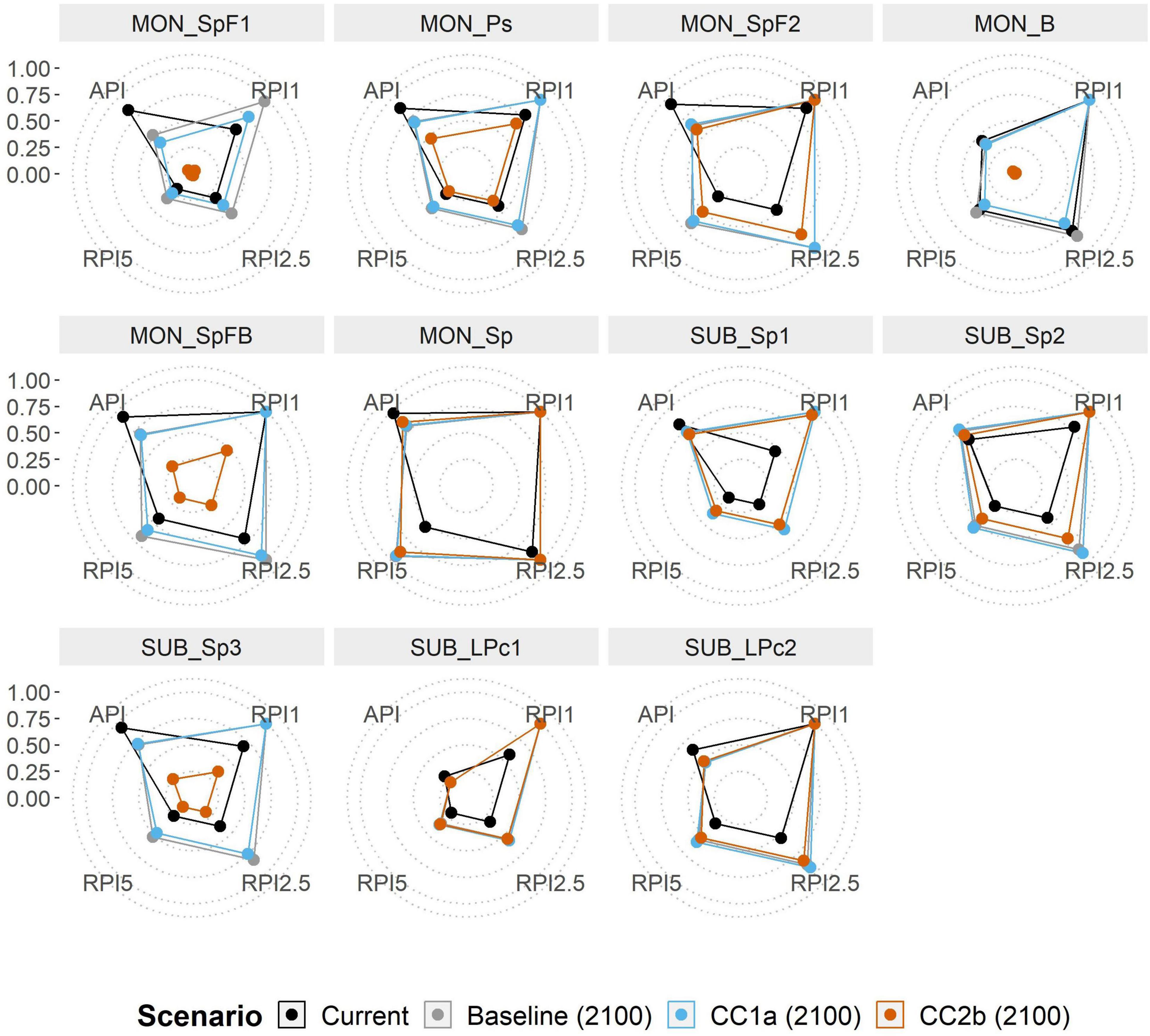
Figure 5. Projected changes in protection indexes for the forest sites under baseline climate and selected climate change scenarios (mildest CC1a, most severe CC2) compared to simulation start (Current). API = Avalanche Protection Index, RPI = Rockfall Protection Index (see section “2.3. Experimental design and analysis” for explanations of differences between RPI1, RPI2.5 and RPI5).
Current rockfall protection across all sites was the highest for small rocks (RPI1 higher than 0.59 for 9 out of 11 sites) than for medium (RPI2.5) or large rocks (RPI5). Rockfall protection was projected to generally increase–or to remain constant–in the future under baseline climate. However, climate change impact on this indicator differed according to the site and to the intensity of climate change. For montane sites, mild climate change induced no or little differences of RPIs compared to current conditions (e.g., see MON_B, MON_SpFB, MON_SpF1) but severe climate change (CC2b) caused drastic declines of rockfall protection (Figure 5), which in some cases did not occur under the second strongest climate scenario (CC2a for MON_SpFB in Supplementary Figure 8). In some sites (MON_SpF2, MON_Sp), all three rockfall protection indices increased under climate change scenarios, in line with the projected increases of tree size diversity (Supplementary Figure 7). However, in MON_SpFB rockfall protection declined strongly under CC2b despite an increase in tree size diversity likely because average stand DBH decreased drastically. For subalpine sites, rockfall protection was generally projected to increase under baseline and climate change scenarios. All three RPIs increased in every site under all climate scenarios compared to current conditions, except in SUB_Sp3 where rockfall protection decreased only under the most severe climate change scenario–which is in line with the simulated decline in stem number (Figure 3) despite an increase in tree size diversity (Supplementary Figure 7).
4. Discussion
This study reveals a heterogeneous impact of climate change on protective effects in mountain forests of the Eastern Italian Alps. Overall, changing climate did not show having negative effects on all kind of protection forests but its impact depends on site and stand conditions (i.e., elevation, species composition, geographical location) as well as on the intensity of projected climate change. In the long term, however, expected changes of forest structure (i.e., reductions in basal area and/or biomass) and composition (i.e., reduction of Norway spruce) might jeopardize the role of mountain forest to protect against avalanches and rockfall.
4.1. Future development of montane and subalpine forests under climate change
We found that elevation and topography–altering local climate in a mountain environment–as well as current forest composition–often altered by past management–play an important role in determining the projected severity of climate change impacts. Our simulations indicate that stands at lower elevations (montane belt) are the ones that are likely to be more strongly affected by climate change. Future drier and hotter conditions like those projected under our most severe scenario (−25% annual precipitation by 2100) caused drastic stress-induced tree mortality in our simulations, resulting in decreases in forest biomass and basal area. This is particularly the case of spruce and spruce-fir forests located within dry inner Alpine valleys or in the Prealps, which might become maladapted to future climate conditions due to increased drought-induced mortality (Jolly et al., 2005; Honkaniemi et al., 2020). This coincides with a study conducted by Falk and Hempelmann (2013) who transferred the relation between species occurrence and climate to projected future climatic conditions, showing a regression of Norway spruce in large parts of the Alps. Other studies carried out in the Swiss Alps have also shown that low-elevation stands will likely be impacted by drought-induced mortality in the future (Thrippleton et al., 2020; Huber et al., 2021).
Contrarily, climate change had a minor or no impact on simulated forest dynamics and structural characteristics of most forest stands located at high elevations (i.e., subalpine forests). However, even within subalpine forests the trend in our results were not consistent across all sites, indicating that elevation alone is not the only determinant of local climate in a topographically complex region such as South Tyrol. For example, Norway spruce-dominated subalpine forests located in dry, inner Alpine valleys seem to be more sensitive to climate change than similar stands located in the transitional Alps or Prealps. This likely because of notable differences in precipitation regimes and increasing evapotranspiration trends under dryer climate (Jolly et al., 2005; Van Der Maaten-Theunissen et al., 2013; Ponocná et al., 2016). This further confirms that local-specific conditions (inner Alps vs. Prealps) and species composition (i.e., stands in which Norway spruce has been promoted in the past) are pivotal factors when it comes to the sensitivity to climate change, with spruce’s forests being more vulnerable at the trailing dry edge of their distribution (Lévesque et al., 2013). These results are in line with previous studies showing a high climate sensitivity of this species, particularly at its xerothermic range limit (Boden et al., 2014; Honkaniemi et al., 2020).
Subalpine larch- and Swiss stone pine-dominated forest stands located in the proximity of dry inner Alpine valleys (e.g., stand SUB_LPc1) experience a precipitation regime that is similar to dry inner Alpine valley spruce stands. However, being located at a higher elevation they also face a shortened vegetation period. The reason that these stands did not experience similar mortality in our simulations as the subalpine Norway spruce stand are twofold: (1) European larch and Swiss pine are more drought-tolerant than Norway spruce at higher elevations (Obojes et al., 2020); (2) vegetation period is shorted and here the main factor limiting growth is temperature rather than drought. Studies in the same geographical area demonstrated different behaviors of these species toward climate warming, for instance the ability of European larch to profit from a warmer temperature (Obojes et al., 2022), at the expense of Swiss pine growth close to the tree line (Motta et al., 2006).
Climate change also seems to have a stronger impact on those forests located in the Prealps, such as those sites at the southern edge of our study region (e.g., montane beech MON_B and mixed spruce-fir-beech MON_SpFB). Our projections here showed reductions in basal area in both stands, with drastic declines the more severe the climate change scenario, reaching as low as 1 m2/ha in the beech dominated stand. In this site, our results under mild climate change scenarios showed an increase in simulated stem number, indicating that large-diameter beech trees might experience a high mortality rate–and compensated by new regeneration cohorts–even under the most optimistic changes in climatic regime. In the south-located montane site with a mixture of spruce-fir-beech, our simulation instead showed a decrease in both stem number and basal area under all climate change scenarios despite being at a slightly higher elevation than other sites that instead showed gains in basal area (e.g., MON_SpF2 in the transitional inner Alps). This further indicates that geographical position within the Alpine mountain range and exposure to different precipitation regimes due to topography has an important influence on the development of mountain forests (Mina et al., 2017b). According to past studies, at some location in the Prealps current cumulative precipitation is barely sufficient for the growth of the main occurring tree species such as silver fir, European beech and Norway spruce (Lyr, 1992; de Rigo et al., 2016).
Current stand conditions and species composition also appeared to be a crucial factor influencing the future impact of climate change on forest dynamics. For instance, our only Scots pine-dominated stand (MON_Ps) experienced only a very minor decrease in basal area and biomass, which became apparent only toward the end of the simulated period under the most severe climate change scenario. This is assumingly due to the high drought-tolerance of Scots pine compared to other late-successional species such as spruce and beech (de Rigo et al., 2016). A dendroclimatological study in the Austrian Alps also found Scots pine to be better adapted to drought than Norway spruce (Schuster and Oberhuber, 2013), suggesting that at some sites this species could be promoted via silvicultural interventions as an alternative (or mixed) to spruce. In drier, low-elevation sites, past studies have shown that even Scots pine might be affected by drought-induced mortality, and that it will be likely replaced by pubescent oaks (Q. pubescens) (Rigling et al., 2013). However, in our experiment we did not consider forests at low elevation (i.e., below 1,000 m a.s.l.) or close to the valley bottom, which certainly deserve further investigation. Compared to spruce and beech, Silver fir also appears to be better adapted to cope with a warmer future climate (Vitasse et al., 2019). While other species in our simulations experienced a clear reduction under climate change–confirming that they will likely be maladapted to future climate–silver fir generally exhibited a minor decline, partially even gaining in relative share in basal area at intermediate elevations (but not in the montane site within dry inner Alpine valleys). Van Der Maaten-Theunissen et al. (2013) came to a similar conclusion, stating that the growth of silver fir is limited by water availability only at very low elevation. This is consistent with previous studies, showing that this species might be more drought-tolerance under a warmer climate (up to 5–7°C) if annual precipitation do not fall below a certain threshold (Tinner et al., 2013; Hartl-Meier et al., 2014).
Another factor worth mentioning and playing a role in defining vulnerability of a forest stand to climate change is exposition due to topography. Among our stands, NNE and NE exposed sites showed lower sensitivity in terms of simulated changes in forest structural characteristics due to climatic changes, the only exception being the N-exposed, low-elevation spruce-fir forest (MON_SpF1), because located in a dry, inner Alpine valley and already prone to drought-stress. Finally, current forest management–as we designed and implemented in the simulations–only had a negligible influence in future forest dynamics, but the aim of our investigation was on climate change impacts rather than designing and evaluating alternative management scenarios.
4.2. Impacts on protective effects
As a consequence of projected changes in forest structure, the protective effect of forests showed a heterogeneous trend under different site conditions and climate scenarios. Yet, we could identify some general patterns from our findings for our study area and considering our methodology: (1) avalanche protection will likely be negatively affected by climate change; (2) the ability of montane forests to maintain rockfall protection depends on the severity of climate change; (3) most subalpine forests will still be able to maintain rockfall protection under climate change.
Most of the stands in our study showed reductions of avalanche protection compared to current state, decreases that were also observed under baseline climate. This is likely due to simulated reductions in stems with a diameter greater than 8 cm due to competition for light and perhaps partly to management, which is a major factor in the calculation of stand stability within the avalanche protection index. Even if in some cases tree size heterogeneity was projected to increase–denoting a higher diversity in diameter distribution–future avalanche protection decreased. Although our methodology does not take into account the upward shift of the forest (see also further below), this indicates that the ability of a forest to stabilize a mountain slope against avalanche release depends on multiple aspects than merely an uneven distribution in stem diameter classes (Bebi et al., 2022). Also, although our simulations showed increases in stem number due to regeneration, not all newly established trees might reach the 8 cm threshold, creating gaps in DBH distributions of the simulated stands. Similar findings were reported by Maroschek et al. (2015) for a mountainous forest district in Austria, where avalanche protection was also found to be negatively impacted by climate change [but see Bebi et al. (2012) who found a potential positive impact of continuing recent temperature trend on subalpine avalanche protection forests]. Temperli et al. (2020) also projected a decreased avalanche protection across Swiss forests, although their assessment was more related to future disturbance effects rather than the direct effect of climate change on forest dynamics.
Our findings indicate that rockfall protection can be generally maintained–or even increased–under the most optimistic climate scenarios. This is due to the simulated increase in average DBH following the growth development of forest stands, and to which rockfall protection is positively related (see section “2.3. Experimental design and analysis”). As long as climate does not change drastically, Alpine forests are likely to experience higher tree growth and therefore higher basal area and biomass leading to a generic increase in protective effects against rockfall (Lingua et al., 2020). As forests in the Alps might experience a climate-induced acceleration in their dynamics even under the mildest climate scenarios (Thom and Seidl, 2022), maintaining a stable stand structure for protection via management might be problematic in the future (Brang, 2001). However, the simulated climate change effects under the most severe scenario (CC2b) can have pronounced consequences for the ability of montane forest stands (and even of some subalpine) to protect against rockfall. Concerning this aspect, our findings are consistent with results from previous modeling studies (Elkin et al., 2013; Mina et al., 2017a; Thrippleton et al., 2020) and additional expertise projections (Essl and Rabitsch, 2013). Moos et al. (2021) also found that the protective effects of forests against rockfall may substantially decline at sites particularly prone to drought stress. It is also possible that other tree species more adapted to a warmer climate, e.g., tree of heaven (Ailanthus altissima) might migrate and replace current dominant ones in the future. Although the presence of neophytes might not compromise the potential rockfall protection (Moos et al., 2019), we acknowledge that this aspect was not considered here.
On the other hand, we also found that most subalpine forests will likely experience an increase in rockfall protective effects. This is due to negligible simulated changes in species composition and increased average stand DBH thanks to warming that will induced a longer vegetation period at high elevation, where temperature is currently the main limiting factor to tree growth (Tranquillini, 1979; Holtmeier, 1993; Lenz et al., 2013; Körner, 2021). However, our outcomes also demonstrate that not all subalpine forests will be able to maintain rockfall protection. For example, spruce-dominated subalpine stands in dry inner alpine valleys (as site SUB_Sp3) might also be affected by drought-induced mortality if climate change will shift beyond a specific threshold of severity (e.g., CC2b). In this case, high local resolution studies like the one here presented can help identifying forest stand types that might require management priority (e.g., harvesting to improve structure, assisted tree species migration along elevational gradients) for maintaining protective effects in the future. Additionally, warming climate might also promote forest expansion at the upper elevations, where, combined with crops and pastures abandonment, forest can theoretically provide increased protection against rockfall and avalanche hazards (Berger et al., 2013). Although of high interest, the topic of timberline expansion should be better investigated using spatially-explicit forest landscape models (Shifley et al., 2017).
4.3. Methodological aspects
The model applied in the present study has been widely tested at multiple locations across the Alps (Rasche et al., 2011; Elkin et al., 2013; Bugmann et al., 2017; Mina et al., 2017a). ForClim has also been compared to other models of forest dynamics, showing rather good performances in reproducing forest development when assessed against inventory records (Irauschek et al., 2021). However, there are factors and aspects that are not included in the model or were not considered in our experiment. ForClim, for example, does not explicitly simulate herbaceous vegetation, thus competition between tree regeneration and herbaceous layer–that can delay establishment and therefore forest dynamics as shown by Thrippleton et al. (2018)–was not considered. It is therefore possible that our simulations might have overestimated tree establishment at some sites, like in the subalpine larch–Swiss pine dominated site (Angulo et al., 2019; Vacek et al., 2021). Another aspect to consider is the sudden decline of basal area and biomass for the two most southern study sites under climate change. Such high mortality might be partly overestimated due to fact that the temporal resolution of the climate input data (monthly) and the transformation of precipitation values into the log naturalis in ForClim were possibly not able to accurately capture the variation of precipitation that typically occur within a month, thus overestimating the impact of drought, which can cause the growth reduction function within the model to reach zero, in some cases. This aspect has been circumvented in past studies by weighting the annual index considering the different influence of seasons based on tree ring data (Mina et al., 2016) or by constraining growth reduction based on species-specific tree ring width observation collected in the field (Zamora-Pereira et al., 2021). Additionally, more robust approaches to initialize forest models with representative stand data such as the one presented by Mey et al. (2021) would have been helpful to overcome the limitation of using truncated data from small forest samples. We herewith acknowledge such limitations and recommend further investigations on this aspects for future studies on protective effects on mountain forests.
We also recognize that our simulations only considered the direct impact of climate change on forest stands, as we did not include natural disturbances. Biotic and abiotic disturbances such as insect outbreaks, fires, windstorms are likely to increase with a warming climate (Bebi et al., 2017; Jakoby et al., 2019; Hlásny et al., 2021) causing an increased mortality due to a reduced resistance of trees (Netherer et al., 2015). These may have even stronger impacts on protective effects against natural hazards (Caduff et al., 2022). However, these effects, as well as the direct interactions between gravitational disturbances and forest conditions should be better explored with spatially-explicit forest landscape models coupled with three-dimensional rockfall and avalanche module (Zurbriggen et al., 2014; Moos and Lischke, 2022). Lastly, the climate change scenarios used for this study feature temperature and precipitation values up until the year 2100 (Karger et al., 2017, 2020). As no climate projections were available beyond this year, instead of assuming a stabilization of climate at the end of the century as often done with simulation models (Seidl et al., 2019; Mina et al., 2022) we limited our simulation experiment until 2100 although climate change might continue to affect forest dynamics on a much longer term. Hence, changes in forest structure, as well as its effect on protective effects should be investigated for longer timeframes, when possible, and on a larger array of climate scenarios to better account for the uncertainty in future climatic projections.
5. Conclusion
This study presents an assessment at high local resolution on the potential impact of climate change on protection forests in the Eastern Italian Alps. Such investigations can be highly relevant to identify forest stand types that are–or will be–more vulnerable of losing the provision of specific ecosystem services such as protection against gravitational hazards. Knowing in advance what type of protection forests are more prone to mortality because likely maladapted to future climate change can aid decisions in forest management, so that targeted interventions can be planned in the long-term to enhance forest adaptability and resilience to climate change.
For our study region, key conclusions were that (1) the protective effects of lower elevation, montane forests–which often provide imminent direct protection to human settlements and infrastructures–are likely to be jeopardized by climate change, but their vulnerability depends on how severely climate will change as well as local site- and forest conditions. These protection forests should receive special priority in forest planning, and proactive measurements in management should be taken in the near future to avoid losses of protective effects in the case of severe climate change. (2) Most subalpine forests will still be able to maintain protection against natural hazards, but some of them might also be exposed to adverse climate change impacts, particularly those located in dry inner Alpine valleys and spruce-dominated. Given the potential risk of natural disturbances (herewith not considered), we recommend further model-based investigations protection forests of the Italian Alps. Finally, this study remarks the need of rapidly abating climate-altering emissions to avoid worst-case climate scenarios that could induce severe damages on forest ecosystems and the key services they provide, including protection.
Data availability statement
The datasets presented in this study can be found in online repositories. The repository can be accessed at the following link: https://doi.org/10.5281/zenodo.8131674.
Author contributions
MM and LH conceptualized the research and the methodology. LH prepared the data, setup scenarios, ran simulations, analyzed outputs, and wrote the original draft. AC provided the historical and climate change data. UH provided the training and software support. MM provided the supervision and funding. MM and SM wrote the final draft. All authors contributed to reviewing and editing and approved the submitted version.
Funding
MM acknowledges funding from the European Union’s Horizon 2020 research and innovation program under the Marie Skłodowska-Curie framework (grant no. 891671, REINFORCE project), which also funded LH internship at the Institute for Alpine Environment at Eurac Research. We also acknowledge funding from the Biodiversity Monitoring South Tyrol project that covered fieldwork expenses and the Department of Innovation, Research and University of the Autonomous Province of Bozen/Bolzano for covering the Open Access publication costs.
Acknowledgments
We are grateful to the Forest Service of the South Tyrol Province, in particular to Marco Pietrogiovanna and Hannes Markart, for providing forest management data and other useful information. We thank Harald Bugmann and Gina Marano for sharing expertise on model testing and application, and to Clemens Geitner for the academic supervision of LH’s MSc thesis. We are also grateful to colleagues of the Institute for Alpine Environment, in particular to Nikolaus Obojes and to Francesca Rigo, for helping the data collection in the field.
Conflict of interest
The authors declare that the research was conducted in the absence of any commercial or financial relationships that could be construed as a potential conflict of interest.
Publisher’s note
All claims expressed in this article are solely those of the authors and do not necessarily represent those of their affiliated organizations, or those of the publisher, the editors and the reviewers. Any product that may be evaluated in this article, or claim that may be made by its manufacturer, is not guaranteed or endorsed by the publisher.
Supplementary material
The Supplementary Material for this article can be found online at: https://www.frontiersin.org/articles/10.3389/ffgc.2023.1240235/full#supplementary-material
References
Albrich, K., Rammer, W., and Seidl, R. (2020). Climate change causes critical transitions and irreversible alterations of mountain forests. Glob. Change Biol. 26, 4013–4027. doi: 10.1111/gcb.15118
Albrich, K., Rammer, W., Thom, D., and Seidl, R. (2018). Trade-offs between temporal stability and level of forest ecosystem services provisioning under climate change. Ecol. Appl. 28, 1884–1896. doi: 10.1002/eap.1785
Albrich, K., Seidl, R., Rammer, W., and Thom, D. (2022). From sink to source: Changing climate and disturbance regimes could tip the 21st century carbon balance of an unmanaged mountain forest landscape. For. Int. J. For. Res. 96:cac022. doi: 10.1093/forestry/cpac022
Allen, C. D., Macalady, A. K., Chenchouni, H., Bachelet, D., McDowell, N., Vennetier, M., et al. (2010). A global overview of drought and heat-induced tree mortality reveals emerging climate change risks for forests. For. Ecol. Manag. 259, 660–684. doi: 10.1016/j.foreco.2009.09.001
Allgaier Leuch, B., Streit, K., and Brang, P. (2017). Naturnaher waldbau im klimawandel. Available online at: https://www.dora.lib4ri.ch/wsl/islandora/object/wsl%3A13997/ (accessed April 21, 2023).
Angulo, M. A., Ninot, J. M., Peñuelas, J., Cornelissen, J. H. C., and Grau, O. (2019). Tree sapling responses to 10 years of experimental manipulation of temperature, nutrient availability, and shrub cover at the pyrenean treeline. Front. Plant Sci. 9:1871. doi: 10.3389/fpls.2018.01871
Autonomous Province of Bolzano/Bozen (2007). Regioni d’Europa [Map]. Ufficio Pianificazione territoriale e cartografia. Available online at: https://geoservices1.civis.bz.it/geoserver/p_bz-AdministrativeUnits/wms? (accessed November 30, 2022).
Autonomous Province of Bolzano/Bozen (2010). Tipologie forestali dell’ Alto Adige/Waldtypisierung Südtirol - Volume 1 and Volume 2. Available online at: https://www.provincia.bz.it/agricoltura-foreste/bosco-legno-malghe/studi-progetti/tipologie-forestali-in-alto-adige.asp (accessed November 30, 2022).
Autonomous Province of Bolzano/Bozen (2013). Map of protective function of the forest/Carta indicativa della funzione protettiva del bosco. Forest Planning Office Bolzano/Bozen. Available online at: https://data.civis.bz.it/dataset/forestecarta-indicativa-della-funzione-protettiva-del-bosco1 (accessed November 30, 2022).
Autonomous Province of Bolzano/Bozen (2021). Landesforstplan. Available online at: https://www.provinz.bz.it/land-forstwirtschaft/wald-holz-almen/downloads/Landesforstplan.pdf (accessed November 30, 2022).
Bebi, P., Bast, A., Helzel, K., Schmucki, G., Brozova, N., and Bühler, Y. (2022). “Avalanche protection forest: From process knowledge to interactive maps,” in Protective forests as ecosystem-based solution for disaster risk reduction (Eco-DRR), eds M. Teich, C. Accastello, F. Perzl, and K. Kleemayr (London: IntechOpen), doi: 10.5772/intechopen.99514
Bebi, P., Kienast, F., and Schönenberger, W. (2001). Assessing structures in mountain forests as a basis for investigating the forests’ dynamics and protective function. For. Ecol. Manag. 145, 3–14. doi: 10.1016/S0378-1127(00)00570-3
Bebi, P., Seidl, R., Motta, R., Fuhr, M., Firm, D., Krumm, F., et al. (2017). Changes of forest cover and disturbance regimes in the mountain forests of the Alps. For. Ecol. Manag. 388, 43–56. doi: 10.1016/j.foreco.2016.10.028
Bebi, P., Teich, M., Schwaab, J., Krumm, F., Walz, A., and Grêt-Regamey, A. (2012). Entwicklung und Leistungen von Schutzwäldern unter dem Einfluss des Klimawandels. Schlussbericht im Rahmen des Forschungsprogramms “Wald und Klimawandel”. Eidg. Forschungsanstalt für Wald, Schnee und Landschaft WSL, Bern, Bundesamt für Umwelt.
Berger, F. (1997). Interaction forêt de montagne-risques naturels. Détermination de Zones d’Interventions Forestières Prioritaires–L’exemple du département de la Savoie, Ph.D. thesis. Paris: University ENGREF.
Berger, F., Dorren, L., Kleemayr, K., Maier, B., Planinsek, S., Bigot, C., et al. (2013). “Eco-engineering and protection forests against rockfalls and snow avalanches,” in Management strategies to adapt alpine space forests to climate change risks, ed. G. Cerbu (London: InTech), doi: 10.5772/56275
Bircher, N., Cailleret, M., and Bugmann, H. K. M. (2015). The agony of choice: Different empirical mortality models lead to sharply different future forest dynamics. Ecol. Appl. 25, 1303–1318. doi: 10.1890/14-1462.1
Boden, S., Kahle, H.-P., Wilpert, K. V., and Spiecker, H. (2014). Resilience of Norway spruce (Picea abies (L.) Karst) growth to changing climatic conditions in Southwest Germany. For. Ecol. Manag. 315, 12–21. doi: 10.1016/j.foreco.2013.12.015
Bosela, M., Merganičová, K., Torresan, C., Cherubini, P., Fabrika, M., Heinze, B., et al. (2022). “Modelling future growth of mountain forests under changing environments,” in Climate-smart forestry in mountain regions managing forest ecosystems, eds R. Tognetti, M. Smith, and P. Panzacchi (Cham: Springer International Publishing), 223–262. doi: 10.1007/978-3-030-80767-2_7
Botkin, D. B., Janak, J. F., and Wallis, J. R. (1972). Some ecological consequences of a computer model of forest growth. J. Ecol. 60, 849–872. doi: 10.2307/2258570
Bourdier, T., Cordonnier, T., Kunstler, G., Piedallu, C., Lagarrigues, G., and Courbaud, B. (2016). Tree size inequality reduces forest productivity: An analysis combining inventory data for ten European species and a light competition model. PLoS One 11:e0151852. doi: 10.1371/journal.pone.0151852
Brang, P. (2001). Resistance and elasticity: Promising concepts for the management of protection forests in the European Alps. For. Ecol. Manag. 145, 107–119. doi: 10.1016/S0378-1127(00)00578-8
Brang, P., Schönenberger, W., Frehner, M., Schwitter, R., and Wasser, B. (2006). Management of protection forests in the European Alps: An overview. Snow Landsc. Res. 80, 23–44.
Bugmann, H. (2001). A review of forest gap models the description, understanding and prediction of the long-term dynamics of forest ecosystems has fascinated ecologists for a long time (cf. Clements, 1916; Watt, 1925; Gleason, 1926; Tansley, 1936; Whittaker, 1953. Clim. Change 59, 259–305.
Bugmann, H., Cordonnier, T., Truhetz, H., and Lexer, M. J. (2017). Impacts of business-as-usual management on ecosystem services in European mountain ranges under climate change. Reg. Environ. Change 17, 3–16. doi: 10.1007/s10113-016-1074-4
Bugmann, H. K. M. (1996). A simplified forest model to study species composition along climate gradients. Ecology 77, 2055–2074. doi: 10.2307/2265700
Bugmann, H., and Seidl, R. (2022). The evolution, complexity and diversity of models of long-term forest dynamics. J. Ecol. 110, 2288–2307. doi: 10.1111/1365-2745.13989
Caduff, M. E., Brožová, N., Kupferschmid, A. D., Krumm, F., and Bebi, P. (2022). How large-scale bark beetle infestations influence the protective effects of forest stands against avalanches: A case study in the Swiss Alps. For. Ecol. Manag. 514:120201. doi: 10.1016/j.foreco.2022.120201
Cordonnier, T., Berger, F., Elkin, C., Lämas, T., and Martiney, M. (2014). ARANGE deliverable D2. 2: Models and linker functions (indicators) for ecosystem services. Available online at: http://www.arange-project.eu/wp-content/uploads/ARANGE-D2.2_Models-and-linker-functions.pdf (accessed November 30, 2022).
Cordonnier, T., Courbaud, B., Berger, F., and Franc, A. (2008). Permanence of resilience and protection efficiency in mountain Norway spruce forest stands: A simulation study. For. Ecol. Manag. 256, 347–354. doi: 10.1016/j.foreco.2008.04.028
Cordonnier, T., and Kunstler, G. (2015). The Gini index brings asymmetric competition to light. Perspect. Plant Ecol. Evol. Syst. 17, 107–115. doi: 10.1016/j.ppees.2015.01.001
Costa, M., Marchi, N., Bettella, F., Bolzon, P., Berger, F., and Lingua, E. (2021). Biological legacies and rockfall: The protective effect of a windthrown forest. Forests 12:1141. doi: 10.3390/f12091141
Crespi, A., Matiu, M., Bertoldi, G., Petitta, M., and Zebisch, M. (2021). A high-resolution gridded dataset of daily temperature and precipitation records (1980–2018) for Trentino-South Tyrol (north-eastern Italian Alps). Earth Syst. Sci. Data 13, 2801–2818. doi: 10.5194/essd-13-2801-2021
Cullotta, S., and Maetzke, F. (2009). Forest management planning at different geographic levels in Italy: Hierarchy, current tools and ongoing development. Int. Forest. Rev. 11, 475–48.
Didion, M., Kupferschmid, A. D., Wolf, A., and Bugmann, H. (2011). Ungulate herbivory modifies the effects of climate change on mountain forests. Clim. Change 109, 647–669. doi: 10.1007/s10584-011-0054-4
Dorren, L. K. A., Berger, F., Imeson, A. C., Maier, B., and Rey, F. (2004). Integrity, stability and management of protection forests in the European Alps. For. Ecol. Manag. 195, 165–176. doi: 10.1016/j.foreco.2004.02.057
Elkin, C., Gutiérrez, A. G., Leuzinger, S., Manusch, C., Temperli, C., Rasche, L., et al. (2013). A 2°C warmer world is not safe for ecosystem services in the European Alps. Glob. Change Biol. 19, 1827–1840. doi: 10.1111/gcb.12156
Essl, F., and Rabitsch, W. eds (2013). Biodiversität und Klimawandel: Auswirkungen und Handlungsoptionen für den Naturschutz in Mitteleuropa. Berlin: Springer, doi: 10.1007/978-3-642-29692-5
Falk, W., and Hempelmann, N. (2013). Species favourability shift in Europe due to climate change: A case study for Fagus sylvatica L. and Picea abies (L.) Karst. Based on an ensemble of climate models. J. Climatol. 2013, 1–18. doi: 10.1155/2013/787250
Feistl, T., Bebi, P., Teich, M., Bühler, Y., Christen, M., Thuro, K., et al. (2014). Observations and modeling of the braking effect of forests on small and medium avalanches. J. Glaciol. 60, 124–138. doi: 10.3189/2014JoG13J055
Floris, A., and Di Cosmo, L. (2022). “Protective function and primary designated management objective,” in Italian National Forest Inventory—Methods and Results of the Third Survey: Inventario Nazionale delle Foreste e dei Serbatoi Forestali di Carbonio—Metodi e Risultati della Terza Indagine, eds P. Gasparini, L. Di Cosmo, A. Floris, and D. De Laurentis (Cham: Springer International Publishing), 469–502. doi: 10.1007/978-3-030-98678-0_11
Frehner, M., Wasser, B., and Schwitter, R. (2005). Nachhaltigkeit und erfolgskontrolle im schutzwald–wegleitung für pflegemassnahmen in wäldern mit schutzfunktion. Bern: Swiss Agency for the Environment, Forests and Landscape (SAEFL) CH-3003 Bern.
Gasparini, P., Di Cosmo, L., Floris, A., and De Laurentis, D. eds (2022). Italian national forest inventory—methods and results of the third survey: Inventario nazionale delle foreste e dei serbatoi forestali di carbonio—metodi e risultati della terza indagine. Cham: Springer International Publishing, doi: 10.1007/978-3-030-98678-0
Gutiérrez, A. G., Snell, R. S., and Bugmann, H. (2016). Using a dynamic forest model to predict tree species distributions. Glob. Ecol. Biogeogr. 25, 347–358. doi: 10.1111/geb.12421
Hartl-Meier, C., Dittmar, C., Zang, C., and Rothe, A. (2014). Mountain forest growth response to climate change in the Northern Limestone Alps. Trees 28, 819–829. doi: 10.1007/s00468-014-0994-1
Hausfather, Z., Marvel, K., Schmidt, G. A., Nielsen-Gammon, J. W., and Zelinka, M. (2022). Climate simulations: Recognize the ‘hot model’ problem. Nature 605, 26–29. doi: 10.1038/d41586-022-01192-2
Hausfather, Z., and Peters, G. P. (2020). Emissions – the ‘business as usual’ story is misleading. Nature 577, 618–620. doi: 10.1038/d41586-020-00177-3
Hilpold, A., Anderle, M., Guariento, E., Marsoner, T., Mina, M., Paniccia, C., et al. (2023). Handbook biodiversity monitoring south Tyrol, Vol. 118 (Bolzano: Eurac Research). doi: 10.57749/2QM9-FQ40
Hlásny, T., König, L., Krokene, P., Lindner, M., Montagné-Huck, C., Müller, J., et al. (2021). Bark beetle outbreaks in Europe: State of knowledge and ways forward for management. Curr. For. Rep. 7, 138–165. doi: 10.1007/s40725-021-00142-x
Holtmeier, F.-K. (1993). “The upper timberline: Ecological and geographical aspects,” in Ecologia delle foreste di alta quota. Pubblicazioni del corso di cultura in ecologia, Vol. 30, eds T. Anfodillo and C. Urbinati (Berlin: Springer), 1–26.
Honkaniemi, J., Rammer, W., and Seidl, R. (2020). Norway spruce at the trailing edge: The effect of landscape configuration and composition on climate resilience. Landsc. Ecol. 35, 591–606. doi: 10.1007/s10980-019-00964-y
Huber, N., Bugmann, H., Cailleret, M., Bircher, N., and Lafond, V. (2021). Stand-scale climate change impacts on forests over large areas: Transient responses and projection uncertainties. Ecol. Appl. 31:e02313. doi: 10.1002/eap.2313
Huber, N., Bugmann, H., and Lafond, V. (2018). Global sensitivity analysis of a dynamic vegetation model: Model sensitivity depends on successional time, climate and competitive interactions. Ecol. Model. 368, 377–390. doi: 10.1016/j.ecolmodel.2017.12.013
Huber, N., Bugmann, H., and Lafond, V. (2020). Capturing ecological processes in dynamic forest models: Why there is no silver bullet to cope with complexity. Ecosphere 11:e03109. doi: 10.1002/ecs2.3109
Irauschek, F., Barka, I., Bugmann, H., Courbaud, B., Elkin, C., Hlásny, T., et al. (2021). Evaluating five forest models using multi-decadal inventory data from mountain forests. Ecol. Model. 445:109493. doi: 10.1016/j.ecolmodel.2021.109493
Jakoby, O., Lischke, H., and Wermelinger, B. (2019). Climate change alters elevational phenology patterns of the European spruce bark beetle (Ips typographus). Glob. Change Biol. 25, 4048–4063. doi: 10.1111/gcb.14766
Jolly, W. M., Dobbertin, M., Zimmermann, N. E., and Reichstein, M. (2005). Divergent vegetation growth responses to the 2003 heat wave in the Swiss Alps. Geophys. Res. Lett. 32:L18409. doi: 10.1029/2005GL023252
Karger, D. N., Conrad, O., Böhner, J., Kawohl, T., Kreft, H., Soria-Auza, R. W., et al. (2017). Climatologies at high resolution for the earth’s land surface areas. Sci. Data 4:170122. doi: 10.1038/sdata.2017.122
Karger, D. N., Schmatz, D. R., Dettling, G., and Zimmermann, N. E. (2020). High-resolution monthly precipitation and temperature time series from 2006 to 2100. Sci. Data 7:248. doi: 10.1038/s41597-020-00587-y
Karger, O. C., Böhner, J., Kawohl, T., Kreft, H., Soria-Auza, R., et al. (2021). Climatologies at high resolution for the earth’s land surface areas. Sci. Data 4:170122. doi: 10.16904/envidat.228
Körner, C. (2021). The cold range limit of trees. Trends Ecol. Evol. 36, 979–989. doi: 10.1016/j.tree.2021.06.011
Kössler Aichner, A., Höller, J., Blasbichler, A., Eisenkeil, N., Raffl, U., Gauly, M., et al. (2021). Agrar- & Forstbericht 2021. Bozen: Autonome Provinz Bozen - Südtirol.
Kotlarski, S., Gobiet, A., Morin, S., Olefs, M., Rajczak, J., and Samacoïts, R. (2023). 21st Century alpine climate change. Clim. Dyn. 60, 65–86. doi: 10.1007/s00382-022-06303-3
Lange, S. (2021). ISIMIP3b bias adjustment fact sheet. Potsdam: Inter-Sectoral Impact Model Intercomparison Project.
Lenz, A., Hoch, G., and Körner, C. (2013). Early season temperature controls cambial activity and total tree ring width at the alpine treeline. Plant Ecol. Divers. 6, 365–375. doi: 10.1080/17550874.2012.711864
Leonelli, G., Pelfini, M., Morra di Cella, U., and Garavaglia, V. (2011). Climate warming and the recent treeline shift in the European Alps: The role of geomorphological factors in high-altitude sites. AMBIO 40, 264–273. doi: 10.1007/s13280-010-0096-2
Lévesque, M., Saurer, M., Siegwolf, R., Eilmann, B., Brang, P., Bugmann, H., et al. (2013). Drought response of five conifer species under contrasting water availability suggests high vulnerability of Norway spruce and European larch. Glob. Change Biol. 19, 3184–3199. doi: 10.1111/gcb.12268
Lindner, M., Maroschek, M., Netherer, S., Kremer, A., Barbati, A., Garcia-Gonzalo, J., et al. (2010). Climate change impacts, adaptive capacity, and vulnerability of European forest ecosystems. For. Ecol. Manag. 259, 698–709. doi: 10.1016/j.foreco.2009.09.023
Lingua, E., Bettella, F., Pividori, M., Marzano, R., Garbarino, M., Piras, M., et al. (2020). “The protective role of forests to reduce rockfall risks and impacts in the alps under a climate change perspective,” in Climate change, hazards and adaptation options climate change management, eds W. Leal Filho, G. J. Nagy, M. Borga, P. D. Chávez Muñoz, and A. Magnuszewski (Cham: Springer International Publishing), 333–347. doi: 10.1007/978-3-030-37425-9_18
Lyr, H. (ed.) (1992). Physiologie und Ökologie der Gehölze: mit 85 Tabellen. Jena Stuttgart: Fischer.
Maroschek, M., Rammer, W., and Lexer, M. J. (2015). Using a novel assessment framework to evaluate protective functions and timber production in Austrian mountain forests under climate change. Reg. Environ. Change 15, 1543–1555. doi: 10.1007/s10113-014-0691-z
Maroschek, M., Seidl, R., Netherer, S., and Lexer, M. J. (2009). Climate change impacts on goods and services of European mountain forests. Unasylva 60, 76–80.
Martin-Benito, D., Molina-Valero, J. A., Pérez-Cruzado, C., Bigler, C., and Bugmann, H. (2022). Development and long-term dynamics of old-growth beech-fir forests in the Pyrenees: Evidence from dendroecology and dynamic vegetation modelling. For. Ecol. Manag. 524:120541. doi: 10.1016/j.foreco.2022.120541
Mey, R., Stadelmann, G., Thürig, E., Bugmann, H., and Zell, J. (2021). From small forest samples to generalised uni- and bimodal stand descriptions. Methods Ecol. Evol. 12, 634–645. doi: 10.1111/2041-210X.13566
Mina, M., Bugmann, H., Cordonnier, T., Irauschek, F., Klopcic, M., Pardos, M., et al. (2017a). Future ecosystem services from European mountain forests under climate change. J. Appl. Ecol. 54, 389–401. doi: 10.1111/1365-2664.12772
Mina, M., Bugmann, H., Klopcic, M., and Cailleret, M. (2017b). Accurate modeling of harvesting is key for projecting future forest dynamics: A case study in the Slovenian mountains. Reg. Environ. Change 17, 49–64. doi: 10.1007/s10113-015-0902-2
Mina, M., Martin-Benito, D., Bugmann, H., and Cailleret, M. (2016). Forward modeling of tree-ring width improves simulation of forest growth responses to drought. Agric. For. Meteorol. 221, 13–33. doi: 10.1016/j.agrformet.2016.02.005
Mina, M., Messier, C., Duveneck, M. J., Fortin, M., and Aquilué, N. (2022). Managing for the unexpected: Building resilient forest landscapes to cope with global change. Glob. Change Biol. 28, 4323–4341. doi: 10.1111/gcb.16197
Moore, A. D. (1989). On the maximum growth equation used in forest gap simulation models. Ecol. Model. 45, 63–67. doi: 10.1016/0304-3800(89)90100-2
Moos, C., Guisan, A., Randin, C. F., and Lischke, H. (2021). Climate change impacts the protective effect of forests: A case study in Switzerland. Front. For. Glob. Change 4:682923. doi: 10.3389/ffgc.2021.682923
Moos, C., and Lischke, H. (2022). Modeling the effect of rockfall on forest development in a dynamic forest landscape model. Ecosphere 13:e3909. doi: 10.1002/ecs2.3909
Moos, C., Toe, D., Bourrier, F., Knüsel, S., Stoffel, M., and Dorren, L. (2019). Assessing the effect of invasive tree species on rockfall risk–the case of Ailanthus altissima. Ecol. Eng. 131, 63–72. doi: 10.1016/j.ecoleng.2019.03.001
Morán-Ordóñez, A., Ameztegui, A., De Cáceres, M., de-Miguel, S., Lefèvre, F., Brotons, L., et al. (2020). Future trade-offs and synergies among ecosystem services in Mediterranean forests under global change scenarios. Ecosyst. Serv. 45:101174. doi: 10.1016/j.ecoser.2020.101174
Motta, R., and Haudemand, J. (2000). Protective forests and silvicultural stability - An example of planning in the Aosta Valley. Mt. Res. Dev. 20, 180–187.
Motta, R., Morales, M., and Nola, P. (2006). Human land-use, forest dynamics and tree growth at the treeline in the Western Italian Alps. Ann. For. Sci. 63, 739–747. doi: 10.1051/forest:2006055
Netherer, S., Matthews, B., Katzensteiner, K., Blackwell, E., Henschke, P., Hietz, P., et al. (2015). Do water-limiting conditions predispose Norway spruce to bark beetle attack? New Phytol. 205, 1128–1141. doi: 10.1111/nph.13166
Obojes, N., Meurer, A. K., Newesely, C., Tasser, E., Oberhuber, W., Mayr, S., et al. (2022). Swiss stone pine growth benefits less from recent warming than European larch at a dry-inner alpine forest line as it reacts more sensitive to humidity. Agric. For. Meteorol. 315:108788. doi: 10.1016/j.agrformet.2021.108788
Obojes, N., Tasser, E., Newesely, C., Mayr, S., and Tappeiner, U. (2020). Comparing sap flow of European larch with evergreen conifers at different elevations in an inner-alpine dry valley. Acta Hortic. 1300, 113–120. doi: 10.17660/ActaHortic.2020.1300.15
O’Neill, B. C., Kriegler, E., Ebi, K. L., Kemp-Benedict, E., Riahi, K., Rothman, D. S., et al. (2017). The roads ahead: Narratives for shared socioeconomic pathways describing world futures in the 21st century. Glob. Environ. Change 42, 169–180. doi: 10.1016/j.gloenvcha.2015.01.004
Pepin, N., Bradley, R. S., Diaz, H. F., Baraer, M., Caceres, E. B., Forsythe, N., et al. (2015). Elevation-dependent warming in mountain regions of the world. Nat. Clim. Change 5, 424–430. doi: 10.1038/nclimate2563
Ponocná, T., Spyt, B., Kaczka, R., Büntgen, U., and Treml, V. (2016). Growth trends and climate responses of Norway spruce along elevational gradients in East-Central Europe. Trees 30, 1633–1646. doi: 10.1007/s00468-016-1396-3
R Core Team (2022). R: A language and environment for statistical computing. Vienna: R Foundation for Statistical Computing. Available online at: https://www.R-project.org/
Rasche, L., Fahse, L., Zingg, A., and Bugmann, H. (2011). Getting a virtual forester fit for the challenge of climatic change. J. Appl. Ecol. 48, 1174–1186. doi: 10.1111/j.1365-2664.2011.02014.x
Rasche, L., Fahse, L., Zingg, A., and Bugmann, H. (2012). Enhancing gap model accuracy by modeling dynamic height growth and dynamic maximum tree height. Ecol. Model. 232, 133–143. doi: 10.1016/j.ecolmodel.2012.03.004
Rigling, A., Bigler, C., Eilmann, B., Feldmeyer-Christe, E., Gimmi, U., Ginzler, C., et al. (2013). Driving factors of a vegetation shift from Scots pine to pubescent oak in dry Alpine forests. Glob. Change Biol. 19, 229–240. doi: 10.1111/gcb.12038
de Rigo, D., San-Miguel-Ayanz, J., Caudullo, G., Durrant, T. H., and Mauri, A. (eds). (2016). European atlas of forest tree species. Luxembourg: Publications Office of the European Union.
Scheidl, C., Heiser, M., Vospernik, S., Lauss, E., Perzl, F., Kofler, A., et al. (2020). Assessing the protective role of alpine forests against rockfall at regional scale. Eur. J. For. Res. 139, 969–980. doi: 10.1007/s10342-020-01299-z
Schirpke, U., Tappeiner, U., and Tasser, E. (2019). A transnational perspective of global and regional ecosystem service flows from and to mountain regions. Sci. Rep. 9:6678. doi: 10.1038/s41598-019-43229-z
Schön, J. H. (2015). Physical properties of rocks: Fundamentals and principles of petrophysics, 2nd Edn. Amsterdam: Elsevier.
Schuler, L. J., Bugmann, H., and Snell, R. S. (2017). From monocultures to mixed-species forests: Is tree diversity key for providing ecosystem services at the landscape scale? Landsc. Ecol. 32, 1499–1516. doi: 10.1007/s10980-016-0422-6
Schuster, R., and Oberhuber, W. (2013). Drought sensitivity of three co-occurring conifers within a dry inner Alpine environment. Trees 27, 61–69. doi: 10.1007/s00468-012-0768-6
Searle, E. B., Bell, F. W., Larocque, G. R., Fortin, M., Dacosta, J., Sousa-Silva, R., et al. (2021). Simulating the effects of intensifying silviculture on desired species yields across a broad environmental gradient. Forests 12:755. doi: 10.3390/f12060755
Sebald, J., Senf, C., Heiser, M., Scheidl, C., Pflugmacher, D., and Seidl, R. (2019). The effects of forest cover and disturbance on torrential hazards: Large-scale evidence from the Eastern Alps. Environ. Res. Lett. 14:114032. doi: 10.1088/1748-9326/ab4937
Seidl, R., Albrich, K., Erb, K., Formayer, H., Leidinger, D., Leitinger, G., et al. (2019). What drives the future supply of regulating ecosystem services in a mountain forest landscape? For. Ecol. Manag. 445, 37–47. doi: 10.1016/j.foreco.2019.03.047
Shifley, S. R., He, H. S., Lischke, H., Wang, W. J., Jin, W., Gustafson, E. J., et al. (2017). The past and future of modeling forest dynamics: From growth and yield curves to forest landscape models. Landsc. Ecol. 32, 1307–1325. doi: 10.1007/s10980-017-0540-9
Shugart, H. H., and Smith, T. M. (1996). A review of forest patch models and their application to global change research. Clim. Change 34, 131–153.
Shugart, H. H., Wang, B., Fischer, R., Ma, J., Fang, J., Yan, X., et al. (2018). Gap models and their individual-based relatives in the assessment of the consequences of global change. Environ. Res. Lett. 13:033001. doi: 10.1088/1748-9326/aaaacc
Stritih, A., Bebi, P., Rossi, C., and Grêt-Regamey, A. (2021). Addressing disturbance risk to mountain forest ecosystem services. J. Environ. Manage. 296:113188. doi: 10.1016/j.jenvman.2021.113188
Teich, M., Bartelt, P., Grêt-Regamey, A., and Bebi, P. (2012). Snow avalanches in forested terrain: Influence of forest parameters, topography, and avalanche characteristics on runout distance. Arct. Antarct. Alp. Res. 44, 509–519.
Temperli, C., Blattert, C., Stadelmann, G., Brändli, U.-B., and Thürig, E. (2020). Trade-offs between ecosystem service provision and the predisposition to disturbances: A NFI-based scenario analysis. For. Ecosyst. 7:27. doi: 10.1186/s40663-020-00236-1
Thom, D., and Seidl, R. (2016). Natural disturbance impacts on ecosystem services and biodiversity in temperate and boreal forests. Biol. Rev. 91, 760–781. doi: 10.1111/brv.12193
Thom, D., and Seidl, R. (2022). Accelerating mountain forest dynamics in the Alps. Ecosystems 25, 603–617. doi: 10.1007/s10021-021-00674-0
Thrippleton, T., Bugmann, H., and Snell, R. S. (2018). Herbaceous competition and browsing may induce arrested succession in central European forests. J. Ecol. 106, 1120–1132. doi: 10.1111/1365-2745.12889
Thrippleton, T., Lüscher, F., and Bugmann, H. (2020). Climate change impacts across a large forest enterprise in the Northern Pre-Alps: Dynamic forest modelling as a tool for decision support. Eur. J. For. Res. 139, 483–498. doi: 10.1007/s10342-020-01263-x
Tinner, W., Colombaroli, D., Heiri, O., Henne, P. D., Steinacher, M., Untenecker, J., et al. (2013). The past ecology of Abies alba provides new perspectives on future responses of silver fir forests to global warming. Ecol. Monogr. 83, 419–439. doi: 10.1890/12-2231.1
Tranquillini, W. (1979). Physiological ecology of the alpine timberline: Tree existence at high altitudes with special reference to the European Alps. Berlin: Springer.
Vacchiano, G., Magnani, F., and Collalti, A. (2012). Modeling Italian forests: State of the art and future challenges. IForest Biogeosci. For. 5, 113–120. doi: 10.3832/ifor0614-005
Vacek, Z., Prokůpková, A., Vacek, S., Bulušek, D., Šimùnek, V., Hájek, V., et al. (2021). Mixed vs. monospecific mountain forests in response to climate change: Structural and growth perspectives of Norway spruce and European beech. For. Ecol. Manag. 488:119019. doi: 10.1016/j.foreco.2021.119019
Van Der Maaten-Theunissen, M., Kahle, H.-P., and Van Der Maaten, E. (2013). Drought sensitivity of Norway spruce is higher than that of silver fir along an altitudinal gradient in Southwestern Germany. Ann. For. Sci. 70, 185–193. doi: 10.1007/s13595-012-0241-0
Vitasse, Y., Bottero, A., Rebetez, M., Conedera, M., Augustin, S., Brang, P., et al. (2019). What is the potential of silver fir to thrive under warmer and drier climate? Eur. J. For. Res. 138, 547–560. doi: 10.1007/s10342-019-01192-4
Zamora-Pereira, J. C., Yousefpour, R., Cailleret, M., Bugmann, H., and Hanewinkel, M. (2021). Magnitude and timing of density reduction are key for the resilience to severe drought in conifer-broadleaf mixed forests in Central Europe. Ann. For. Sci. 78:68. doi: 10.1007/s13595-021-01085-w
Zentralanstalt fr Meteorologie und Geodynamik (ZAMG), Abteilung Brand- und Zivilschutz – Autonome Provinz Bozen, Agenzia Regionale per la Prevenzione e Protezione Ambientale del Veneto (ARPAV) (2015). Das Klima von Tirol - Sdtirol - Belluno. Vergangenheit – Gegenwart – Zukunft. Available online at: http://www.alpenklima.eu/download (accessed January 11, 2018).
Keywords: climate change, forest modeling, protection forests, ForClim, avalanches, rockfalls, European Alps
Citation: Hillebrand L, Marzini S, Crespi A, Hiltner U and Mina M (2023) Contrasting impacts of climate change on protection forests of the Italian Alps. Front. For. Glob. Change 6:1240235. doi: 10.3389/ffgc.2023.1240235
Received: 14 June 2023; Accepted: 11 August 2023;
Published: 21 September 2023.
Edited by:
Michaela Teich, Austrian Research Centre for Forests (BFW), AustriaReviewed by:
Heike Lischke, Snow and Landscape Research (WSL), SwitzerlandGolo Stadelmann, Snow and Landscape Research (WSL), Switzerland
Copyright © 2023 Hillebrand, Marzini, Crespi, Hiltner and Mina. This is an open-access article distributed under the terms of the Creative Commons Attribution License (CC BY). The use, distribution or reproduction in other forums is permitted, provided the original author(s) and the copyright owner(s) are credited and that the original publication in this journal is cited, in accordance with accepted academic practice. No use, distribution or reproduction is permitted which does not comply with these terms.
*Correspondence: Marco Mina, marco.mina@eurac.edu
†These authors have contributed equally to this work