- 1College of Agronomy and Agricultural Engineering, Liaocheng University, Liaocheng, China
- 2College of Forestry, Henan Agricultural University, Zhengzhou, China
- 3Country Shandong Territorial Spatial Planning Institute, Jinan, China
Monitoring changes to growth-survival strategies is beneficial during plant growth and development to understand the dynamics of community succession. We measured key leaf traits and calculated competition, stress-tolerance, and ruderals ecological strategy scores for trees and seedlings in different successional stages in tropical lowland rain forests. We analyzed functional trait patterns and CSR strategies with plant growth and development through the different succession stages. We found that trees used strategies that were shifted from S/CS and CS strategies to CS/CSR strategies along the succession. However, seedlings maintained the use of the S/CSR strategy. Seedlings showed lower leaf dry matter content, higher specific leaf area, and their leaf area was relatively conservative. We also observed that the functional traits of seedlings and large trees showed basically consistent changes through each succession stage. Using the standard deviation of C-, S-, and R-scores, we found that the ecological strategy width of trees is smaller, while seedlings have a wider range of ecological strategies. Together, this information can be used to define plant succession changes with functional plant trait changes using different CSR ecological strategies in tropical rainforests that are a threated due to human activities. The research results provide scientific basis for understanding the strategic change of plant growth and predicting the direction of forest function succession, and also provide theoretical support for the management of tropical lowland rainforest in China.
1. Introduction
During the process of plant development, plant functional traits will change during different growth stages due to constant environmental change and self-regulation (Osunkoya, 1996; Lu et al., 2014; Henn and Damschen, 2021). In this process, there is also continuous competition for the use of limited resources between the different functional plant organs (Westley, 1993; Craine and Dybzinski, 2013). In order to adapt to the environment, the distribution of plant resources need to be optimized for the plant to grow, reproduce, and survive. Plant functional traits reflect physiological trade-offs that correspond to different resource acquisition strategies and environmental challenges (Falster et al., 2018; Hanisch et al., 2020). Therefore, quantifying functional traits is a powerful index used to measure the life cycle strategies of plants (Grime, 1974, 1977, 2001; Westoby and Wright, 2003). We can learn more about the functional transformation of the plant growth process and the functional traits of the community in the succession process by locating plant ecological strategy.
Many biological processes change with the growth of plants. Previous studies have generally shown that leaf traits change significantly during ontogeny (Souza et al., 2021; Mediavilla et al., 2022). Compared with late succession species, specific leaf area (SLA) decrease during development is obvious in pioneer species (Chelli et al., 2022). SLA reflects the ability of leaves to obtain resources. High SLA is beneficial to the expansion of plants, but it reduces the defensive ability of leaves. The constant change of plant functional traits reflects the life strategy of plants to obtain resources for conservative survival, expanding plant scale or reproductive evolution. If the environmental factors are arranged as a resource axis, at one end of the resource axis, there are plants that respond quickly to the functional performance of resources, and at the other end, there are plants that respond highly to the functional performance of resources. With the axis of resources, a series of functional features represent different ecological strategies. The different functional traits and combinations of plants reflect the different environmental atmosphere and the distribution and utilization of the obtained resources by plants themselves, and then reflect the results of plant community construction, which is of great significance for maintaining biodiversity. Thus, many functional traits are considered to play an important role in the performance and strategies of plants (Diaz et al., 2004; Díaz et al., 2016). Pierce et al. (2017a) found that the quantitative relationship between three key leaf functional traits in plants, namely specific leaf area, leaf dry matter content and leaf area, are used to judge the ecological strategies of an individual or group of plants (Pierce et al., 2013, 2017a). Since the three leaf traits are applicable to all vascular plant life worldwide, they represent a wide range of plant functions including (1) leaf area, which largely determines the light-intercepting capacity of plants (Díaz et al., 2016), and constitutes an indicator of the plant organ size spectrum, and (2) The higher leaf dry matter content and lower specific leaf area helps to classify the conservative strategies and the opposite, in the other extreme, an acquisitive strategy (Wright et al., 2004; Kattge et al., 2011). The results from different studies show that the intraspecific variability of competition, stress-tolerance, and ruderals strategies in trees is also different during the developmental process, which may show complex changes between ontogeny stages (Giupponi, 2020). The plant trait changes play important roles in understanding how plants adjust their life history strategies to adapt to the environment during ontogenetic development. However, there are few reports that have explored the changes of functional traits and ecological strategy during the growth of plants. Investigating these plant trait changes during ontogeny will play an important role in understanding how plants adjust their life history strategies to adapt to the environment (Rusman et al., 2020).
The CSR strategies of plants are divided into three fundamental types: competitive (C), stress-tolerant (S) and ruderal (R), and there are also some intermediate types (Grime, 1974, 1977, 2001; Hodgson et al., 1999). Among them, the plants with C- competition strategy in the CSR strategy theory will quickly monopolize the capture of resources through the dynamic search of roots and stems in space. These traits only appear in habitats with abundant resources, no interference, and no fierce competition, and they are difficult to show in habitats with poor resources or long-term interference. Plants with S-tolerant strategy usually resist the bite of herbivores by maintaining tissue and alleviating the stress caused by mineral nutrition deficiency in the environment, which is easy to happen in harsh habitats. Plants adopting R- ruderal strategy have a short life history, and usually put the acquired resources into reproduction, which is suitable for long-term survival in the environment with frequent interference (Pierce et al., 2017a). Therefore, this method can link the ecological strategy of plants with the variation space of functional traits, and make use of the scale flexibility of traits to apply CSR strategy analysis to ecological research at different levels, such as species, communities, and ecosystems (Negreiros et al., 2014; Araujo da Costa et al., 2020; Han et al., 2022b; Moeneclaey et al., 2022).
The succession of plant communities is governed by changes in the community assembly process and species traits (Bhaskar et al., 2014). A deeper understanding of the mechanisms that drive succession could help to mitigate losses of biodiversity and ecosystem function. Functional traits have also been used to understand and predict community and ecosystem functions (Diaz et al., 2004), as well as to understand the patterns of functional diversity and the coexistence of species along successional gradients (Hu et al., 2014; Zhang et al., 2018; White et al., 2022). However, there are few studies on the changes of plant functional traits in the process of succession, which is of great significance for understanding forest development and predicting the direction of forest functional changes. Thus, understanding the functional traits of seedlings and trees in different successional stages and ecological strategies can provide insights that support community assembly and help to expand research on ecosystem function.
Although both seedlings and trees of tropical forest woody plants exhibit the same growth-survival trade-off patterns (Capers et al., 2005; Comita et al., 2007; Lu et al., 2014), it is not clear whether these species occupy the same niche at different growth and developmental stages. In the history of plant life, the seedling stage is the period when individual plants are most vulnerable to growth and most sensitive to habitat changes (Hanley et al., 2004). However, previous studies have shown that seedlings show higher SLA and LA and lower LDMC, which indicates that they have a stronger ability to cope with high-level interference (Dayrell et al., 2018; Zhang and Wang, 2021). Most likely, there is little habitat filtration in the early succession stage that shows greater differences in CSR ecological strategies (Raevel et al., 2012). However, trees undergo the filtration of temperature, light, soil moisture, and soil nutrients for a longer time, so their functions are more convergent. And finally form the S strategy with high construction cost and slow benefits (Han et al., 2022a). Therefore, habitat filtering will lead to different ecological strategies of the same species in different habitats and development stages (de Paula et al., 2015). Previous research showed that the intraspecific changes of C, S, and R strategy scores greatly reflect the variability of ecological strategy breadth in the growth and development of seedlings or trees (May et al., 2017). Therefore, we should pay more attention to intraspecific variation in the study of plant ecological strategies based on functional traits.
Studying of growth-survival strategy changes during plant growth and development is helpful to understand the dynamic processes of these communities. There are secondary forests and undisturbed aged forest communities in different succession stages after slash-and-burn disturbance in tropical lowland rainforest in Bawangling, Hainan. These forests provide a good study site to understand functional traits and ecological strategies from the perspective of seedlings and trees through varied successional stages. Here, our goal was to investigate the developmental shifts in plant ecological strategies by assessing the mean and the variability of CSR scores of species at two developmental stages. We compared the seedling and tree stages of coexisting species in four successional forests to investigate the following questions: (a) What are the CSR ecological strategies of seedlings and trees in different succession stages? and (b) How do the interspecific variation of seedlings and trees change? Our hypothesis was that the functional traits of coexisting tree species will change at different growth stages and different succession stages. As the tree has experienced a longer period of biomass accumulation, we predicted that with the growth, the leaf area of the tree is higher than that of the seedling. Seedlings are more fragile and have poor resistance. We predicted that the specific leaf area of seedlings is larger and the dry matter content of leaves is smaller than trees. With the succession, the specific leaf area first increased and then decreased, and the dry matter content first decreased and then increased. Seedlings are inclined to R-selection, while trees are more inclined to C-S strategies. We predict that compared to trees, seedlings have more diverse functions and wider ecological strategies. Ultimately, these results will provide fundamental plant functional trait characterizations for CSR ecological strategies through different sessional stages.
2. Materials and methods
2.1. Study site
This study was carried out in the Bawangling Forest Region on Hainan Island (18°52′-19°12′ N, 108°53′-109°20′ E), China (Ding and Zang, 2009). The study focused on the tropical lowland rainforest (<800 m asl) where the mean annual temperature is 23.6°C, and the annual precipitation is 1,677 mm. We have established 8 sample plots of 1 hm2 (100 m × 100 m) tropical lowland rain forest community, including four succession stages. Two in the secondary forest abandoned 18 years ago (LSA1, LSA2), two in the secondary forest abandoned 30 years ago (LSB1, LSB2), two in the secondary forest abandoned 60 years ago (LSD1, LSD2), and two in no-disturbance old-growth forest (LOG1, LOG2). Secondary forests were naturally recovered after shifting cultivation. The secondary succession of forest vegetation and its environment have not recovered to the level of old forest. The historical data of land use can be found in the management records of Bawangling Forestry Bureau of Hainan Province.
Each plot is subdivided into 25 subplots (20 m × 20 m) for community investigation and environmental factor determination. The species name, diameter at 1.3 m height (DBH), and height of all the standing woody stems (excluding lianas; ≥1 cm DBH) were recorded in each plot. At the center of each plot, we established a 2 × 2 m seedling plot within that were tagged, measured, and identified for all the woody seedlings (<1 cm DBH).
2.2. Data collection
Through the statistical classification of four succession stages of species, 13 co-existing tree species were found in this study. Thirteen successional co-existing tree species were taken as the research objects for the follow-up study of this paper. See Supplementary Table 1 for co-existing species information. To compare individual changes in leaf traits and CSR strategies for different succession stages, we sampled seedlings and trees in 8 plots of 1 hm2. We defined woody plants as seedlings when the DBH was less than 1 cm, and trees were defined as woody plants whose DBH was greater than 1 cm. According to the individual development stages of woody plants, the leaves of seedlings and trees were sampled extensively (n = 13 species in total, including n = 5 shrubs, n = 6 trees, and n = 2 lianas).
To compare developmental stages, we also targeted large trees of the same species (when possible) and sampled at least n = 8 in areas where young trees were also sampled. In all, we measured the functional characteristics of n = 13 trees and n = 6 seedlings. In the early stages of succession, forests had sufficient light resources and stable environment, often finding many large trees and seedlings of the same species in the same place. However, the patterns presented by species in the middle and late stages of succession were not the same, with very few species having at least 8 individuals in our sampling area, which resulted in a smaller sample size of seedlings after succession. In our sampling work, only 6 species of seedlings were found in the middle and late stages of succession (Supplementary Table 1).
2.3. Functional traits collection
We collected three main leaf functional traits, leaf area (LA, mm2), specific leaf area (SLA, mm2 mg–1) and leaf dry matter content (LDMC,%). We collected 2–5 recently expanded, non-senescent leaves from multiple branches following the methods and criteria from Pérez-Harguindeguy et al. (2013) for each individual. Leaf area was measured for each leaf or leaflet using ImageJ (from the US National Institutes of Health; http://www.nih.gov/, accessed 22 August 2021) after scanning. The leaf fresh weight (LFW, mg) is weighed by a balance with an accuracy of one ten thousandth. Each leaf was then placed in a drying oven for a minimum of 48 h at 80°C and dry them to a constant weight, then the final dry mass was recorded (LDW, mg). From these measurements, we calculated leaf dry matter content (LDMC,%) and specific leaf area (SLA, mm2 mg–1).
2.4. Statistical analyses
“StrateFy,” a tool for analyzing CSR strategies, has been developed to compare these different strategies in plants. The advantage of this method is that only three easily accessible leaf traits (LA, LDMC, SLA) are used to represent the extremes of a trade-off between large size and conservative versus acquisitive economics (Pierce et al., 2017a). This method is applicable to vascular plants all over the world and can be used to compare the functional traits of different ecological scales, such as communities (Cerabolini et al., 2016; Pierce et al., 2017a; Zanzottera et al., 2021; Han et al., 2022b), species (Pierce et al., 2013; Rosado and Mattos, 2017; Wen et al., 2022), individuals (May et al., 2017; Dayrell et al., 2018), and succession stages (Caccianiga et al., 2006; Chai et al., 2016; Chen et al., 2023) to calculate their ecological strategies. Therefore, “StrateFy” is considered to be an important tool to detect the variation of plant functional characters (Pierce et al., 2017b; Zanzottera et al., 2020). We used “StrateFy” to calculate C-, S- and R-selection scores for each individual based on their SLA, LDMC, and LA values and visualized the distribution pattern of ecological strategies for trees and seedlings in differ succession stage by “SigmaPlot” 10.0 (Systat Software, Inc., Chicago, IL, USA). The values of leaf traits and CSR strategy for each stage and species are in Supplementary Table 2. In order to compare the differences of functional traits and ecological strategies between seedlings and trees, non-parametric Kruskal–Wallis and Wilcoxon tests (“ggbarplot” function in “ggpubr” package in R) (Kassambara, 2023) were implemented.
We used linear mixed-effect models to test whether two developmental stages from the 4 successional stages exhibited different leaf trait values (log-transformed SLA, log-transformed LA and LDMC) and C-, S-, and R-values. We have constructed a set of models including the fixed effects for development stages, succession, and their interactions, and the random effects of plant species. The significance was estimated by comparing a minimum model with the null model. The random effect was included in order to control for non-accounted differences between groups. We then generated a full set of models using the “dredge” function in the “MuMIn” package (Bartoń, 2022). These models were ranked using the Akaike information criterion corrected for small sample size (AICc), and the model with the lowest AICc value was selected as the best model.
After, we used the standard deviation (SD) of the C-, S-, and R- values to obtain ecological strategy width for each species at each developmental stage as a measurement of variation in functional strategies within groups of trees or seedlings to assess ontogeny- and succession-related shifts in the width of ecological strategies. According to the method of Dayrell et al. (2018), the lower the SD value of CSR score, the smaller the width of ecological strategy of plants. We used Paired test (“ggwithinstats” function in “ggstatsplot” package in R) (Patil, 2021) to compare the niche differences of conspecific seedlings and trees. All statistical analyses were performed in R 4.0.3 (R Core Team, 2022).
3. Results
For the entire succession cycle, there were significant differences in LA, SLA, and LDMC between seedlings and trees (Figures 1A–C), and seedlings showed higher leaf economic structure (higher SLA and lower LDMC; Figures 1B, C), and their leaves were relatively similar in size (Figure 1A). In contrast, we also observed that the functional traits of seedlings and trees showed basically consistent changes through each succession stage. SLA of trees and seedlings showed an upward trend, while LDMC showed a downward trend. Both seedlings and trees had higher LA in 60 yr forest (Figure 1A), while the LDMC was lower (Figure 1C). The SLA of the trees were higher in the 60 yr forests (Figure 1B), but the seedlings were higher in the OG stage (Figure 1B).
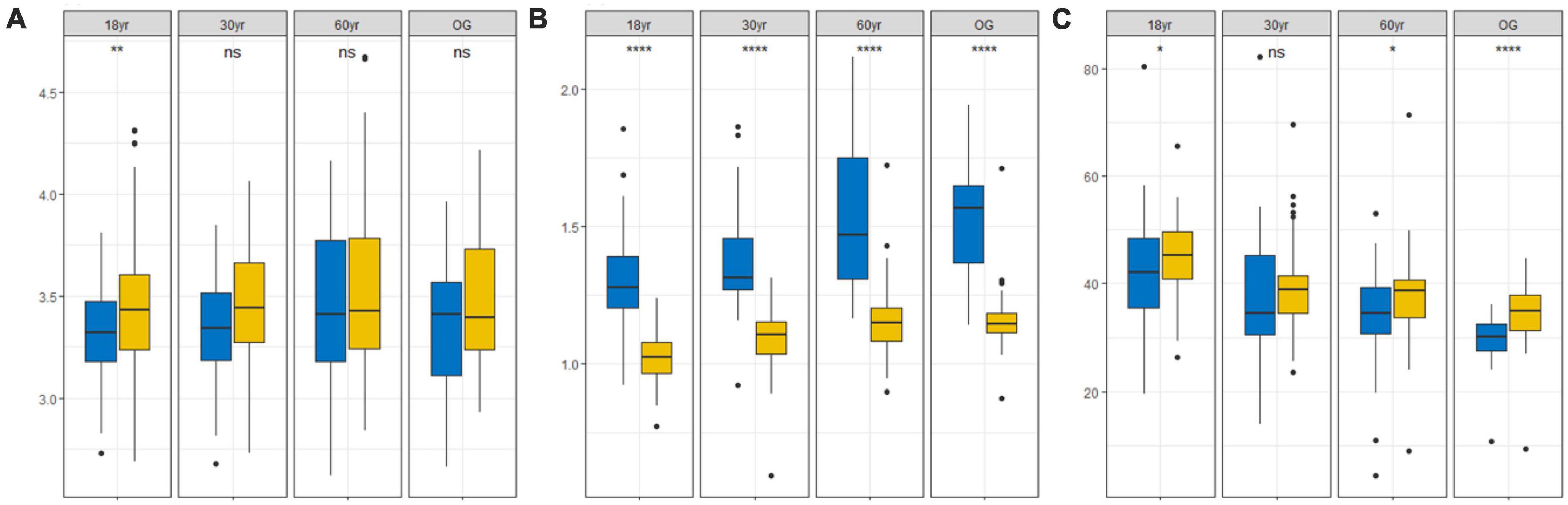
Figure 1. Ontogenetic shifts in leaf traits for seedlings (blue box) and trees (yellow box) from four successional stages in the tropical lowland rainforests. Comparisons of functional trait values between succession and developmental stages of: (A) leaf area (LA; mm2), (B) specific leaf area (SLA; mm2 mg– 1), and (C) leaf dry matter content (LDMC;%) (18 yr, 18-year-old forest; 30 yr, 30-year-old forest; 60 yr, 60-year-old forest; OG, old-growth forest). Within each individual plot, Kruskal–Wallis tests and Wilcoxon tests were conducted where ns (p > 0.05) indicates non-significant differences between the different stages, * (p < 0.05), ** (p < 0.01), and **** (p < 0.0001) indicates a significant difference among stages.
We also found that trees from the 18 yr forest was mainly concentrated around the S/CS region of the triangle (mean C: S: R strategy = 34:63:3%; Figures 2A, 3), while seedlings were mainly S/CSR (28: 53: 19%; Figures 2A, 3). Further, trees from the 30 yr forest were mainly S/CS (31: 63: 5%; Figures 2B, 3), and the seedlings showed S/CSR (30: 55: 15%; Figures 2B, 3) strategies. Interestingly, we found that trees from the 60 yr forest exhibited a mean strategy of CS/CSR (39:49:11%; Figures 2C, 3), whereas seedlings showed S/CSR (30: 54: 15%; Figures 2C, 3). Trees from OG forest exhibited a mean strategy of CS/CSR (39: 49: 12%; Figures 2D, 3), seedlings were mainly concentrated around the S/CSR region of the triangle (mean C:S: R strategy = 25: 57: 18%; Figures 2D, 3). From these results, we found that the strategic positioning of trees were along the C-S axis of the CSR triangle, and the R component contributed little (Figure 2). However, the C- and S- value of seedlings seemed to decrease, and the R value was higher than trees (Figures 2, 3).
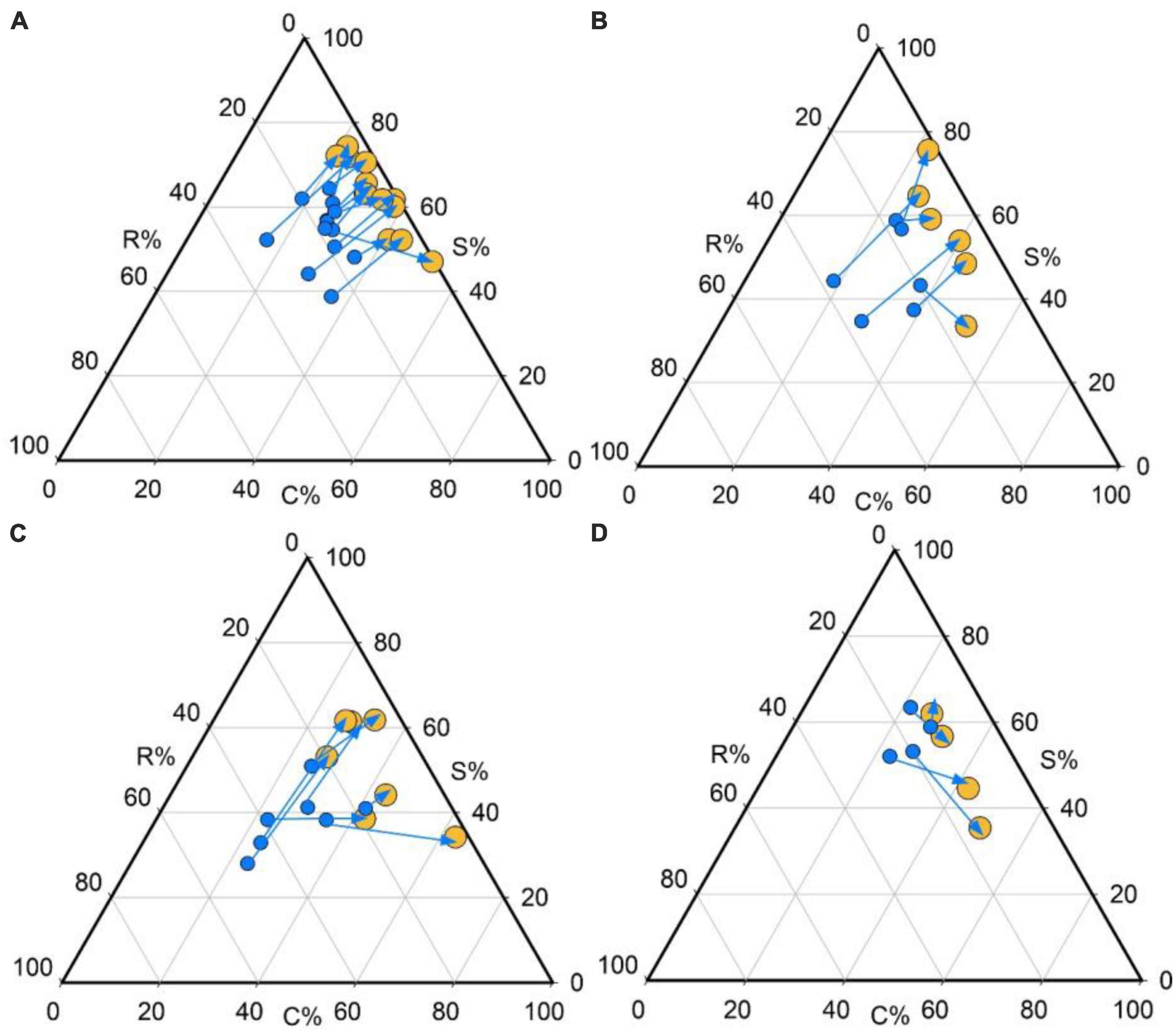
Figure 2. Individual development shifts in CSR strategies for the four successional stages in tropical lowland rainforests. Shifts in the relative proportion of C-, S-, and R-selection are represented by the direction of the arrows [from seedlings (blue dots) to trees (yellow dots)] in the ternary plot. Plant species from: (A) 18 years old forest, (B) 30 years old forest, (C) 60 years old forest, and (D) old growth forest.
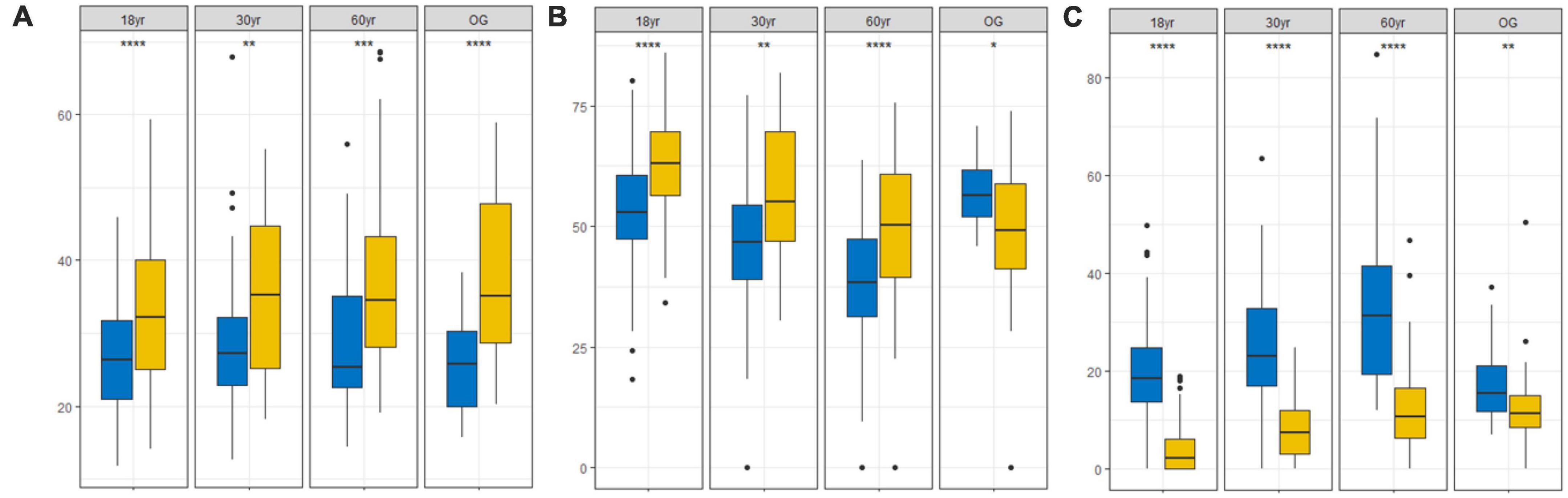
Figure 3. Changes in C-, S-, and R-values of trees (yellow box) and seedlings (blue box) in different succession stages in tropical lowland rainforests in Hainan Island, China. (A) C component (C;%), (B) S component (S;%), and (C) R component (R;%) (18 yr, 18-year-old forest; 30 yr, 30-year-old forest; 60 yr, 60-year-old forest; OG, old-growth forest). * (p < 0.05), ** (p < 0.01), *** (p < 0.001), and **** (p < 0.0001) indicate significant differences according to Wilcoxon tests.
A higher SD in CSR score indicates a wider strategic scope, while a lower SD indicates a smaller strategic width. Our results showed that the width of ecological strategy depends on the succession stage and individual development. Figure 4 showed that there were significant differences between 18 yr and 30 yr forests in CSR standard deviations (Figures 4A–F, P < 0.05). The SD in R-score of seedlings was approximately 4–6 times and 1–2 times higher than trees from the same plant, respectively (Figures 4C, F), but C- and S-components was no significant difference. In 60 yr forests, there is no obvious difference in ecological strategy breadth between seedlings and trees (Figures 4G–I). In OG forest, due to the small sample size, we give a set of mean SD, and the S-SD of seedlings was 2–4 times that of trees, showing significant differences.
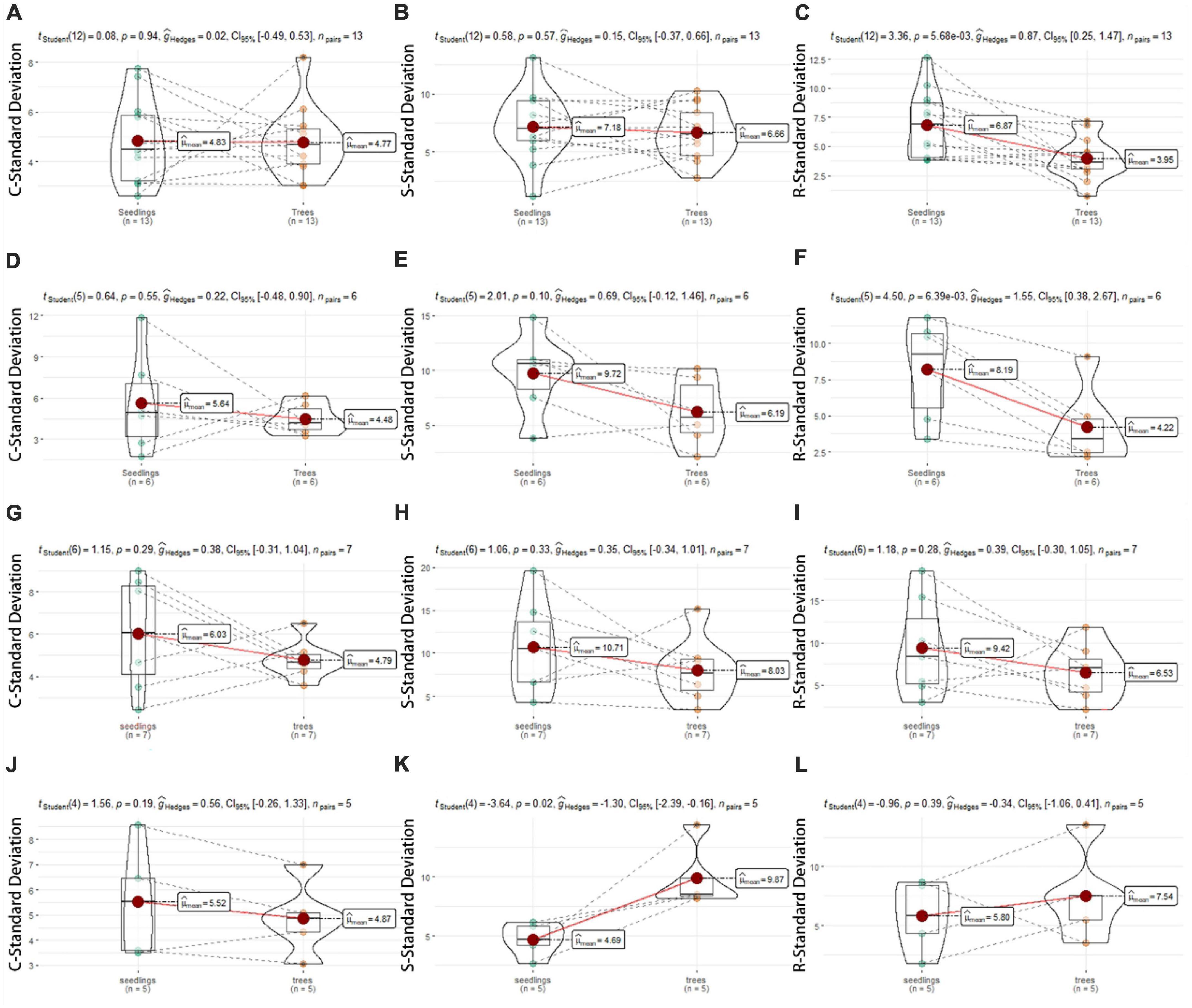
Figure 4. Standard deviation (SD) values in C-, S-, and R- component compared between seedlings (green) and trees (orange) in four successional forests. [18 yr, (A–C); 30 yr, (D–F); 60 yr, (G–I); OG, (J–L)]. The dotted line connects SD values of conspecific seedlings and trees.
These results from the mixed model show that the succession stage, developmental stage, and their interaction explain C-, S-, R-selection and LDMC values. This trend indicates that many factors need to be considered in their coexistence mechanism, and any difference in light, temperature, moisture, soil, and other factors may have a direct impact on community construction. However, it is worth noting that the final model for LA and SLA included only the developmental stage and succession stage (Table 1).
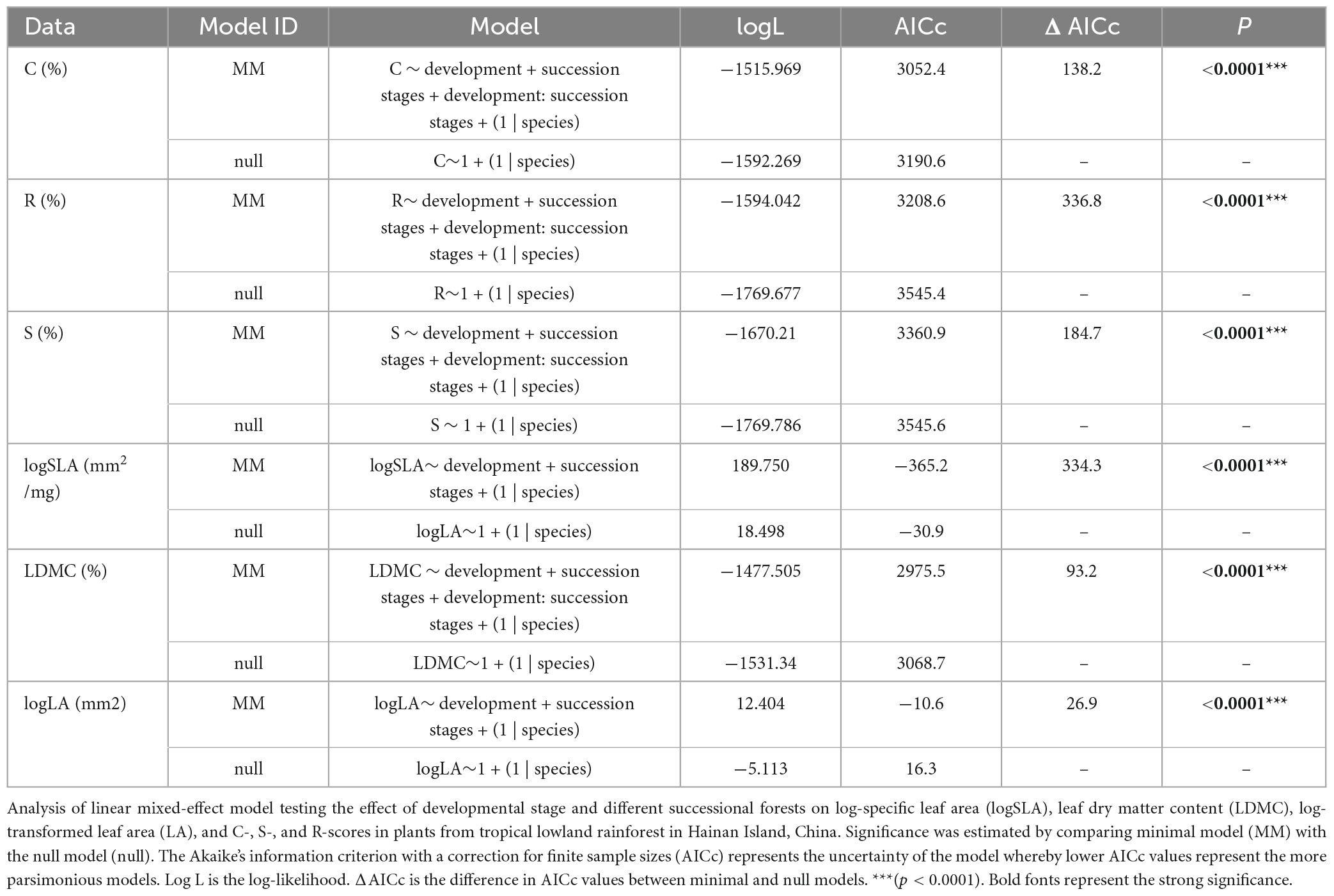
Table 1. Linear mixed-effects models for plant ecological strategy values and functional traits for each individual’s development.
4. Discussion
Trait-based ecology is a powerful method to understand the strategies that support the life history of species and the mechanism of community and ecosystem dynamics. Our research showed that ecological strategy’s quantification integrates many traits, allows the transferability and predictive ability of the results, and has important ramifications for forest regeneration and the maintenance of species diversity. Plants arranged according to the basic trade-offs described by CSR theory will change for different individuals with each ecological strategy in the succession stages. The ecological strategies of the plants are related to the variation space of the functional traits, which allows us to study their changes during growth and development under CSR theory (Grime, 1977; Zhang and Wang, 2021). The flexibility of functional traits makes CSR strategy analysis applied to different levels of ecological research, such as species, communities, and ecosystems, which brings us a new perspective on functional traits research. This trait-based method can provide an assessment of ecological strategy breadth for many potential species so that it is possible to understand and predict species ecological strategy width changes under environmental changes. In this work, we evaluated the functional traits of coexisting species and the transformation mode of ecological strategies in the process of forest succession and growth. We found that succession has a significant effect on the functional traits of trees and seedlings. There are significant differences in functional traits of plants in the two growth stages, and the combination of functional traits, that is, the way to balance and utilize resources, has also changed. Based on the coexisting species, we also found that the ecological strategies have differentiated within conspecific species. We also found that the functional traits and ecological strategies of seedlings are more differentiated than those of trees.
The findings demonstrated that functional traits between seedlings and trees at various phases of succession varied significantly. Many ecologists study community association rules from the perspective of plant functional traits (McGill et al., 2006; Reich, 2014). However, there are few reports that have explored the changes of functional traits during the growth of plants. Our studies have shown that seedlings exhibit smaller LDMC and higher SLA than trees, which has been confirmed by other studies (Mason et al., 2013; Lu et al., 2015; Ye et al., 2023). Previous research has shown that as plants develop from seedlings (higher SLA) to trees (higher LDMC), they tend to put more effort into fortifying their defenses and fending off physical harm (Lu et al., 2015; Dayrell et al., 2018; Falster et al., 2018; Ochoa-López et al., 2020). With the change in the succession stage, our studies found the LA of seedlings steadily increased, although it was still much lower than that of tree-layer plants at the same time. The SLA of seedlings gradually increased with the increase of the succession stage, which was significantly higher than tree layer vegetation. LDMC gradually decreased with succession, which was considerably lower than the tree layer. When plants transition from seedlings (high SLA is beneficial to their rapid growth) to trees (high LDMC), plants often invest more in building defenses and resisting physical injuries in their later years. This can be explained by the transition of plants into tissues with more conservative traits. This demonstrated that different developmental stages of plant communities have varying levels of resource adaptability and can select the optimum ecological strategy to adapt to shifting environmental conditions (Grime, 1974, 1977). Moreover, the ecological strategy width difference between seedlings and trees can only describe species differentiation in multiple ecological dimensions by the trade-off of traits (Wang et al., 2022).
This study found significant differences in ecological strategies in two developmental stages along four succession stages. We also found that the ecological strategy of trees seems to be dominated by C/S selection, and only the component R contributed little, which has also been found in previous studies (Han et al., 2021; Wen et al., 2022; Chen et al., 2023). Yet, compared to trees, seedlings’ ecological strategy focuses more on S/CSR and has a higher R component. It may be because, in 18 and 30-year-old forests, seedlings are easily disturbed and destroyed, the forest is in the early stages of succession, and it is resource-rich but also subject to significant environmental change. These characteristics make R-strategy species with robust reproductive capacities more likely to survive. Our research has also demonstrated that the R component of trees increases with succession, which indicates that trees invest more in growth rate in the early stage of development to cope with higher levels of disturbance (Zhang and Wang, 2021). Therefore, higher LDMC (Wright et al., 2004; Donovan et al., 2011) for trees is maintained to balance the input of leaf defense structure to prevent water loss and retain nutrients by acquiring more carbon, nitrogen, and phosphorus resources to cope with unfavorable conditions (Grime, 2001). Seedlings are the most competitive in 60 yr forests, which may be because they use strategies to accumulate biomass faster in juveniles to improve plant fitness so that they are less susceptible to disturbances. Previous research has shown that the S-strategy prioritizes plant survival and aims to maintain primary metabolism. We can see that the S strategy of seedlings reaches the maximum in OG forest. Therefore, seedlings will show typical stress resistance in long-term poor habitats (Negreiros et al., 2014).
Exploring the changes in plant traits during individual development stages plays a vital role in understanding how plants adjust their life history strategies to adapt to the environment. Previous studies have shown that plants choose different ecological strategies from different available resources. For example, studies on Arabidopsis (Arabidopsis thaliana) (May et al., 2017), the Alps mountain wind bells (Giupponi, 2020), and the mountain dianthus species (Dianthus pseudocrinitus and Dianthus polylepis) (Behroozian et al., 2020) all show intraspecific variation. These intraspecific variations further help evaluate species’ ecological strategy breadth and the diverse ecological strategy categories (Pierce et al., 2013). Together, they contribute to better understanding of how plants adapt to environmental heterogeneity (Jung et al., 2010). Individual development is considered the main source of intraspecific variation (Ochoa-López et al., 2020), but it is rarely evaluated whether trait variation increases or decreases during development. We found that when CSR-SD difference was used to represent ecological strategy breadth, the function of trees converged, and the variability of ecological strategies decreased after long-term habitat filtration. However, seedlings have not been filtered by substantial habitat, they have high functional diversity, and their niche is broader. Our study also showed that the R-SD of seedlings is higher than conspecific trees. It may be that trees were long-term filtered by the environment in the succession process and survived the environmental changes. Our results show that the choice of functional traits that depend on succession is the main driving force of individual transformation. The diversity of ecological strategy within species can enhance the adaptability of species to the environment, while the diversity of ecological strategy between species is conducive to maintaining biodiversity and ecosystem functions, which is the basis of species coexistence.
5. Conclusion
This study assessed, for the first time, variation in ecological strategies of co-existing tree species during the succession of tropical lowland rainforests and niche breadth in different development stages. We found that trees and seedlings adopted different strategies to adapt to growth and succession. Trees are mainly along the C-S axis, while seedlings are mostly located in the R axis of the CSR triangle, and the niche breadth of seedlings is broader than that of trees, indicating that long-term environmental filtration makes the functions of plants converge. This work is beneficial to understand the transformation of plant adaptation strategies caused by succession and different development stages, and the transformation of ecological strategies of tree communities in succession. Our results provide a scientific basis for the conservation and management of forest ecosystems.
Data availability statement
The original contributions presented in this study are included in the article/Supplementary material, further inquiries can be directed to the corresponding author.
Author contributions
YW: conceptualization, methodology, formal analysis, and writing—original draft. CC: investigation, methodology, visualization, and writing—review and editing. TS: methodology and writing—review and editing. YY: investigation and methodology. XH and BH: investigation and visualization. XL: conceptualization, methodology, data curation, project administration, writing—review and editing, supervision, and funding acquisition. All authors contributed to the article and approved the submitted version.
Funding
This study was funded by the Key Research and Development Program of Liaocheng City (2021GY01), the Open Project of Liaocheng University Landscape Architecture (319462212), the National Natural Science Foundation of China (31901210), the Doctoral Research Foundation of Liaocheng University (318051822), and the Innovation Training Program for College Students in Liaocheng University (CXCY2021238).
Acknowledgments
We are grateful to the many people who have contributed to this study, especially Xiusen Yang and Yuecai Tang at the Bawangling Nature Reserve for their assistance in specimen identification and work in the field investigation. We would like to thank Dr. Kelly Dunham for her assistance with the English language.
Conflict of interest
The authors declare that the research was conducted in the absence of any commercial or financial relationships that could be construed as a potential conflict of interest.
Publisher’s note
All claims expressed in this article are solely those of the authors and do not necessarily represent those of their affiliated organizations, or those of the publisher, the editors and the reviewers. Any product that may be evaluated in this article, or claim that may be made by its manufacturer, is not guaranteed or endorsed by the publisher.
Supplementary material
The Supplementary Material for this article can be found online at: https://www.frontiersin.org/articles/10.3389/ffgc.2023.1236933/full#supplementary-material
References
Araujo da Costa, H. J., Gurgel, E. S. C., do Amaral, D. D., Vasconcelos, L. V., Rebelo, L. G. B., and Teodoro, G. S. (2020). CSR ecological strategies, functional traits and trade-offs of woody species in Amazon sandplain forest. Flora 273:151710. doi: 10.1016/j.flora.2020.151710
Behroozian, M., Ejtehadi, H., Memariani, F., Pierce, S., and Mesdaghi, M. (2020). Are endemic species necessarily ecological specialists? Functional variability and niche differentiation of two threatened Dianthus species in the montane steppes of northeastern Iran. Sci. Rep. 10:11774. doi: 10.1038/s41598-020-68618-7
Bhaskar, R., Dawson, T. E., and Balvanera, P. (2014). Community assembly and functional diversity along succession post-management. Funct. Ecol. 28, 1256–1265. doi: 10.1111/1365-2435.12257
Caccianiga, M., Luzzaro, A., Pierce, S., Ceriani, R. M., and Cerabolini, B. (2006). The functional basis of a primary succession resolved by CSR classification. Oikos 112, 10–20. doi: 10.1111/j.0030-1299.2006.14107.x
Capers, R. S., Chazdon, R. L., Brenes, A. R., and Alvarado, B. V. (2005). Successional dynamics of woody seedling communities in wet tropical secondary forests. J. Ecol. 93, 1071–1084. doi: 10.1111/j.1365-2745.2005.01050.x
Cerabolini, B. E. L., Pierce, S., Verginella, A., Brusa, G., Ceriani, R. M., and Armiraglio, S. (2016). Why are many anthropogenic agroecosystems particularly species-rich? Plant Biosyst. 150, 550–557. doi: 10.1080/11263504.2014.987848
Chai, Y., Yue, M., Wang, M., Xu, J., Liu, X., Zhang, R., et al. (2016). Plant functional traits suggest a change in novel ecological strategies for dominant species in the stages of forest succession. Oecologia 180, 771–783. doi: 10.1007/s00442-015-3483-3
Chelli, S., Ottaviani, G., Tsakalos, J. L., Campetella, G., Simonetti, E., Wellstein, C., et al. (2022). Intra- and inter-specific leaf trait responses of understorey species to changes in forest maturity. For. Ecol. Manag. 506:119977. doi: 10.1016/j.foreco.2021.119977
Chen, C., Wen, Y., He, B., Yang, Y., Han, X., Sun, T., et al. (2023). Environmental factors driving the succession and differentiation of ecological strategy spectrum in tropical lowland rain forest. Ecol. Indic. 147:110002. doi: 10.1016/j.ecolind.2023.110002
Comita, L., Aguilar, S., Pérez, R., Lao, S., and Hubbell, S. (2007). Patterns of woody plant species abundance and diversity in the seedling layer of a tropical forest. J. Veg. Sci. 18, 163–174. doi: 10.1658/1100-9233(2007)18[163:POWPSA]2.0.CO;2
Craine, J. M., and Dybzinski, R. (2013). Mechanisms of plant competition for nutrients, water and light. Funct. Ecol. 27, 833–840. doi: 10.1111/1365-2435.12081
Dayrell, R. L. C., Arruda, A. J., Pierce, S., Negreiros, D., Meyer, P. B., Lambers, H., et al. (2018). Ontogenetic shifts in plant ecological strategies. Funct. Ecol. 32, 2730–2741. doi: 10.1111/1365-2435.13221
de Paula, L. F. A., Negreiros, D., Azevedo, L. O., Fernandes, R. L., Stehmann, J. R., and Silveira, F. A. O. (2015). Functional ecology as a missing link for conservation of a resource-limited flora in the Atlantic forest. Biodivers. Conserv. 24, 2239–2253. doi: 10.1007/s10531-015-0904-x
Diaz, S., Hodgson, J. G., Thompson, K., Cabido, M., Cornelissen, J. H. C., Jalili, A., et al. (2004). The plant traits that drive ecosystems: Evidence from three continents. J. Veg. Sci. 15, 295–304. doi: 10.1111/j.1654-1103.2004.tb02266.x
Díaz, S., Kattge, J., Cornelissen, J. H. C., Wright, I. J., Lavorel, S., Dray, S., et al. (2016). The global spectrum of plant form and function. Nature 529, 167–171. doi: 10.1038/nature16489
Ding, Y., and Zang, R. (2009). Effects of logging on the diversity of lianas in a lowland tropical rain forest in Hainan Island, South China. Biotropica 41, 618–624. doi: 10.1111/j.1744-7429.2009.00515.x
Donovan, L. A., Maherali, H., Caruso, C. M., Huber, H., and de Kroon, H. (2011). The evolution of the worldwide leaf economics spectrum. Trends Ecol. Evol. 26, 88–95. doi: 10.1016/j.tree.2010.11.011
Falster, D. S., Duursma, R. A., and FitzJohn, R. G. (2018). How functional traits influence plant growth and shade tolerance across the life cycle. Proc. Natl. Acad. Sci. U.S.A. 115, E6789–E6798. doi: 10.1073/pnas.1714044115
Giupponi, L. (2020). Intraspecific variation in functional strategy and leaf shape of Campanula elatinoides reveals adaptation to climate. Flora 268:151605. doi: 10.1016/j.flora.2020.151605
Grime, J. (2001). Plant strategies, vegetation processes, and ecosystem properties. Biol. Conserv. 107:57. doi: 10.1016/S0006-3207(02)00055-1
Grime, J. P. (1974). Vegetation classification by reference to strategies. Nature 250, 26–31. doi: 10.1038/250026a0
Grime, J. P. (1977). Evidence for the existence of three primary strategies in plants and its relevance to ecological and evolutionary theory. Am. Nat. 111, 1169–1194. doi: 10.1086/283244
Han, X., Huang, J., and Zang, R. (2021). Shifts in ecological strategy spectra of typical forest vegetation types across four climatic zones. Sci. Rep. 11:14127. doi: 10.1038/s41598-021-93722-7
Han, X., Xu, Y., Huang, J., and Zang, R. (2022b). Species diversity regulates ecological strategy spectra of forest vegetation across different climatic zones. Front. Plant Sci. 13:807369. doi: 10.3389/fpls.2022.807369
Han, X., Huang, J., and Zang, R. (2022a). Soil nutrients and climate seasonality drive differentiation of ecological strategies of species in forests across four climatic zones. Plant Soil 473, 517–531. doi: 10.1007/s11104-022-05303-w
Hanisch, M., Schweiger, O., Cord, A. F., Volk, M., and Knapp, S. (2020). Plant functional traits shape multiple ecosystem services, their trade-offs and synergies in grasslands. J. Appl. Ecol. 57, 1535–1550. doi: 10.1111/1365-2664.13644
Hanley, M. E., Fenner, M., Whibley, H., and Darvill, B. (2004). Early plant growth: Identifying the end point of the seedling phase. New Phytol. 163, 61–66. doi: 10.1111/j.1469-8137.2004.01094.x
Henn, J. J., and Damschen, E. I. (2021). Plant age affects intraspecific variation in functional traits. Plant Ecol. 222, 669–680. doi: 10.1007/s11258-021-01136-2
Hodgson, J. G., Wilson, P. J., Hunt, R., Grime, J. P., and Thompson, K. (1999). Allocating C-S-R plant functional types: A soft approach to a hard problem. Oikos 85:282. doi: 10.2307/3546494
Hu, G., Jin, Y., Liu, J., and Yu, M. (2014). Functional diversity versus species diversity: Relationships with habitat heterogeneity at multiple scales in a subtropical evergreen broad-leaved forest. Ecol. Res. 29, 897–903. doi: 10.1007/s11284-014-1178-6
Jung, V., Violle, C., Mondy, C., Hoffmann, L., and Muller, S. (2010). Intraspecific variability and trait-based community assembly. J. Ecol. 98, 1134–1140. doi: 10.1111/j.1365-2745.2010.01687.x
Kattge, J., Díaz, S., Lavorel, S. I, Prentice, C., Leadley, P., Bönisch, G., et al. (2011). TRY – a global database of plant traits. Glob. Change Biol. 17, 2905–2935. doi: 10.1111/j.1365-2486.2011.02451.x
Lu, X., Zang, R., and Huang, J. (2015). Relationships between community level functional traits of trees and seedlings during secondary succession in a tropical lowland rainforest. PLoS One 10:e0132849. doi: 10.1371/journal.pone.0132849
Lu, X., Zang, R., Ding, Y., Letcher, S. G., Long, W., and Huang, Y. (2014). Variations and trade-offs in functional traits of tree seedlings during secondary succession in a tropical lowland rain forest. Biotropica 46, 404–414. doi: 10.1111/btp.12125
Mason, C. M., McGaughey, S. E., and Donovan, L. A. (2013). Ontogeny strongly and differentially alters leaf economic and other key traits in three diverse Helianthus species. J. Exp. Bot. 64, 4089–4099. doi: 10.1093/jxb/ert249
May, R.-L., Warner, S., and Wingler, A. (2017). Classification of intra-specific variation in plant functional strategies reveals adaptation to climate. Ann. Bot. 119, 1343–1352. doi: 10.1093/aob/mcx031
McGill, B. J., Enquist, B. J., Weiher, E., and Westoby, M. (2006). Rebuilding community ecology from functional traits. Trends Ecol. Evol. 21, 178–185. doi: 10.1016/j.tree.2006.02.002
Mediavilla, S., Martínez-Ortega, M., Andrés, S., Bobo, J., and Escudero, A. (2022). Premature losses of leaf area in response to drought and insect herbivory through a leaf lifespan gradient. J. For. Res. 33, 39–50. doi: 10.1007/s11676-021-01351-7
Moeneclaey, I., Schelfhout, S., Vanhellemont, M., DeCock, E., Van Coillie, F., Verheyen, K., et al. (2022). Species ecological strategy and soil phosphorus supply interactively affect plant biomass and phosphorus concentration. Basic Appl. Ecol. 62, 1–11. doi: 10.1016/j.baae.2022.03.013
Negreiros, D., Le Stradic, S., Fernandes, G. W., and Rennó, H. C. (2014). CSR analysis of plant functional types in highly diverse tropical grasslands of harsh environments. Plant Ecol. 215, 379–388. doi: 10.1007/s11258-014-0302-6
Ochoa-López, S., Damián, X., Rebollo, R., Fornoni, J., Domínguez, C. A., and Boege, K. (2020). Ontogenetic changes in the targets of natural selection in three plant defenses. New Phytol. 226, 1480–1491. doi: 10.1111/nph.16422
Osunkoya, O. O. (1996). Light requirements for regeneration in tropical forest plants: Taxon-level and ecological attribute effects. Aust. J. Ecol. 21, 429–441. doi: 10.1111/j.1442-9993.1996.tb00629.x
Patil, I. (2021). Visualizations with statistical details: The “ggstatsplot” approach. J. Open Source Softw. 6:3167. doi: 10.21105/joss.03167
Pérez-Harguindeguy, Díaz, S., Garnier, E., Lavorel, S., Poorter, H., Jaureguiberry, P., et al. (2013). New handbook for standardised measurement of plant functional traits worldwide. Aust. J. Bot. 61, 167–234. doi: 10.1071/BT12225
Pierce, S., Brusa, G., Vagge, I., and Cerabolini, B. E. L. (2013). Allocating CSR plant functional types: The use of leaf economics and size traits to classify woody and herbaceous vascular plants. Funct. Ecol. 27, 1002–1010. doi: 10.1111/1365-2435.12095
Pierce, S., Negreiros, D., Cerabolini, B. E. L., Kattge, J., Díaz, S., Kleyer, M., et al. (2017a). A global method for calculating plant CSR ecological strategies applied across biomes world-wide. Funct. Ecol. 31, 444–457. doi: 10.1111/1365-2435.12722
Pierce, S., Negreiros, D., Cerabolini, B., Kattge, J., Diaz, S., Kleyer, M., et al. (2017b). StrateFy–The global vascular plant CSR strategy calculator. Funct. Ecol. 31, 444–457. doi: 10.1111/ele.13104
R Core Team (2022). R: A language and environment for statistical computing. Vienna: R Foundation for Statistical Computing.
Raevel, V., Violle, C., and Munoz, F. (2012). Mechanisms of ecological succession: Insights from plant functional strategies. Oikos 121, 1761–1770.
Reich, P. B. (2014). The world-wide ‘fast–slow’ plant economics spectrum: A traits manifesto. J. Ecol. 102, 275–301. doi: 10.1111/1365-2745.12211
Rosado, B. H. P., and Mattos, E. A. (2017). On the relative importance of CSR ecological strategies and integrative traits to explain species dominance at local scales. Funct. Ecol. 31, 1969–1974. doi: 10.1111/1365-2435.12894
Rusman, Q., Lucas-Barbosa, D., Hassan, K., and Poelman, E. H. (2020). Plant ontogeny determines strength and associated plant fitness consequences of plant-mediated interactions between herbivores and flower visitors. J. Ecol. 108, 1046–1060. doi: 10.1111/1365-2745.13370
Souza, M. L., Garcia, L. E., Lovato, M. B., and Lemos-Filho, J. P. (2021). Leaf trait variation during ontogeny in the endangered Brazilian rosewood tree. Plant Biol. 23, 1109–1117. doi: 10.1111/plb.13318
Wang, X.-Z., Sun, S.-W., Sedio, B. E., Glomglieng, S., Cao, M., Cao, K.-F., et al. (2022). Niche differentiation along multiple functional-trait dimensions contributes to high local diversity of Euphorbiaceae in a tropical tree assemblage. J. Ecol. 110, 2731–2744. doi: 10.1111/1365-2745.13984
Wen, Y., Chen, C., He, B., and Lu, X. (2022). CSR ecological strategies and functional traits of the co-existing species along the succession in the tropical lowland rain forest. Forests 13:1272. doi: 10.3390/f13081272
Westley, L. C. (1993). The effect of inflorescence bud removal on tuber production in Helianthus tuberosus L. (Asteraceae). Ecology 74, 2136–2144. doi: 10.2307/1940858
Westoby, M., and Wright, I. J. (2003). The leaf size-twig size spectrum and its relationship to other important spectra of variation among species. Oecologia 135, 621–628. doi: 10.1007/s00442-003-1231-6
White, H. J., Pakeman, R. J., and Buckley, Y. M. (2022). Common species contribute little to spatial patterns of functional diversity across scales in coastal grasslands. J. Ecol. 110, 1149–1160. doi: 10.1111/1365-2745.13858
Wright, I. J., Reich, P. B., Westoby, M., Ackerly, D. D., Baruch, Z., Bongers, F., et al. (2004). The worldwide leaf economics spectrum. Nature 428, 821–827. doi: 10.1038/nature02403
Ye, X., Bu, W., Hu, X., Liu, B., Liang, K., and Chen, F. (2023). Species divergence in seedling leaf traits and tree growth response to nitrogen and phosphorus additions in an evergreen broadleaved forest of subtropical China. J. For. Res. 34, 137–150. doi: 10.1007/s11676-021-01437-2
Zanzottera, M., Dalle Fratte, M., Caccianiga, M., Pierce, S., and Cerabolini, B. (2021). Towards a functional phytosociology: The functional ecology of woody diagnostic species and their vegetation classes in Northern Italy. IForest–Biogeosci. For. 14, 522–530. doi: 10.3832/ifor3730-014
Zanzottera, M., Dalle Fratte, M., Caccianiga, M., Pierce, S., and Cerabolini, B. E. L. (2020). Community-level variation in plant functional traits and ecological strategies shapes habitat structure along succession gradients in alpine environment. Community Ecol. 21, 55–65. doi: 10.1007/s42974-020-00012-9
Zhang, H., Chen, H. Y. H., Lian, J., John, R., Ronghua, L., Liu, H., et al. (2018). Using functional trait diversity patterns to disentangle the scale-dependent ecological processes in a subtropical forest. Funct. Ecol. 32, 1379–1389. doi: 10.1111/1365-2435.13079
Keywords: CSR strategy, plant functional traits, forest succession dynamics, seedlings, leaf traits
Citation: Wen Y, Chen C, Sun T, Yang Y, Han X, He B and Lu X (2023) The change pattern of CSR ecological strategy of trees and seedlings during different succession stages in tropical lowland rainforests. Front. For. Glob. Change 6:1236933. doi: 10.3389/ffgc.2023.1236933
Received: 08 June 2023; Accepted: 24 July 2023;
Published: 09 August 2023.
Edited by:
Wen Xing Long, Hainan University, ChinaReviewed by:
Wande Liu, Chinese Academy of Forestry, ChinaGrazielle Sales Teodoro, Federal University of Pará, Brazil
Copyright © 2023 Wen, Chen, Sun, Yang, Han, He and Lu. This is an open-access article distributed under the terms of the Creative Commons Attribution License (CC BY). The use, distribution or reproduction in other forums is permitted, provided the original author(s) and the copyright owner(s) are credited and that the original publication in this journal is cited, in accordance with accepted academic practice. No use, distribution or reproduction is permitted which does not comply with these terms.
*Correspondence: Xinghui Lu, luxinghui_0@163.com
†These authors have contributed equally to this work and share first authorship