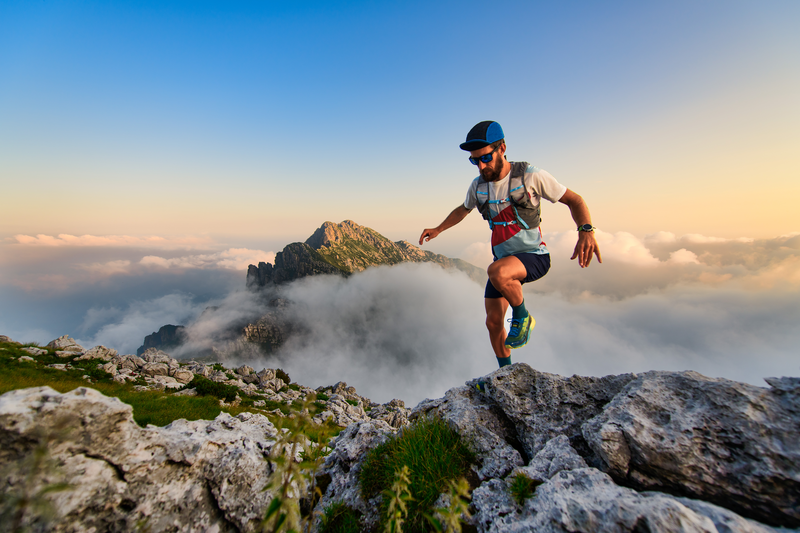
95% of researchers rate our articles as excellent or good
Learn more about the work of our research integrity team to safeguard the quality of each article we publish.
Find out more
ORIGINAL RESEARCH article
Front. For. Glob. Change , 17 July 2023
Sec. Temperate and Boreal Forests
Volume 6 - 2023 | https://doi.org/10.3389/ffgc.2023.1229661
Forest biomass and biodiversity are the most important elements of forest functions and ecosystem services. In this study, we explore the possibilities and ways to enhance ecosystem functions and services related to biomass and biodiversity. Biotic drivers (i.e., species, phylogenetic and functional diversity, stand structural attributes, and community-weighted mean of trait values), abiotic drivers (i.e., topography and climate), and stand age were extracted as independent variables to explain aboveground biomass (AGB). Using South Korea’s 7th National Forest Inventory data, we analyzed 2,070 plots belonging to the natural forests consisting of 394 plots (19.0%) of coniferous stands, 829 plots (40.0%) of broadleaved stands, and 847 plots (40.9%) of mixed stands. Multimodel inference test and model-averaging approaches were conducted to determine the most significant control variables on AGB in each stand type, and piecewise structural equation modeling was conducted to quantify the relationships and directions between the variables. Abiotic drivers, including stand age and climate moisture index, control tree size variation in all stand types, but biotic drivers control AGB through different mechanisms depending on the stand type. Our results show that there were differences in the composition of variables for controlling AGB among stand types. Across all forest types and total stands, we found that increasing the tree size variation is the key driver of increasing AGB as stand age increases. Our study suggests that forest carbon accumulation by stand type can be enhanced if the key drivers of each stand type are properly managed across forest succession, and different forest management plans that consider different regulation factors among stand types are required. Moreover, it is important to adapt resource use patterns for each stand type with considering environmental conditions to maintain healthy and sustainable forests.
In terrestrial ecosystems, forests play an important role as storehouses of biodiversity and constituent parts of the global carbon cycle (Gao et al., 2021). In addition, by preventing degradation and deforestation or improving forest management and conservation, forests support climate change mitigation, which is one of the ecosystem’s services (Njana et al., 2021). Biomass, biodiversity, and abiotic drivers (e.g., climatic and edaphic drivers) within forest systems are the most important components of a forest’s functions and services (Ali et al., 2019a). Specifically, forests account for 44% of the biomass of terrestrial ecosystems and about two-thirds of biodiversity (Kim, 2012). For decades, research has been intense on the relationships between the facets of biodiversity and aboveground biomass (AGB) in forest ecosystems (Van Con et al., 2013; Ali and Yan, 2017), and on how biotic and abiotic drivers simultaneously regulate AGB, which determines the amount of carbon accumulated above ground (Chen et al., 2023). However, there is still a lack of understanding of the mechanisms that control these relationships (Ali and Yan, 2017).
Generally, AGB is controlled by abiotic and biotic drivers in different forest types including tropical, subtropical, and temperate forests (McEwan et al., 2011; Poorter et al., 2015; Ali et al., 2019b; Chun et al., 2020). Abiotic drivers include topography (e.g., slope, aspect, and elevation), climate (e.g., mean annual precipitation and temperature), edaphic conditions (e.g., clay, sand, silt, cation exchange capacity, and pH), and forest succession (e.g., species interaction) and disturbance (e.g., typhoon and wildfire). Biotic drivers such as species diversity, functional trait diversity, functional trait identity, and stand structural complexity simultaneously influence forest ecosystems and AGB through multiple pathways (Becknell and Powers, 2014; Poorter et al., 2017). Topographic heterogeneity affects abiotic and biotic drivers due to variations in light availability, microclimate, and soil chemistry along topographical gradients (Ullah et al., 2021). Furthermore, climate and edaphic conditions are important factors for controlling biotic drivers in forests. For example, an appropriate temperature and precipitation range are important for plant growth through respiration and photosynthesis (Michaletz et al., 2014), and a high climatic water availability through high precipitation could lengthen the growing season of vegetation, thereby increasing AGB in forests (Ali et al., 2019b). Forest stand age, as a proxy for forest successional and development stage, also directly and indirectly affects AGB through changes in the distribution of functional traits and the composition of forest communities (Becknell and Powers, 2014). The contribution of biotic drivers to AGB is explained by two effects: (1) niche complementarity and (2) mass ratio hypotheses (Fotis et al., 2018; Chun et al., 2020). The niche complementarity hypothesis states that resource complementarity between coexisting species is often regarded as a mechanism for supporting the positive relationships between forest tree diversity (e.g., taxonomic and functional diversity) and AGB in forests (Ali et al., 2019a). On the other hand, the mass ratio hypothesis implies that functional traits of the dominant tree species, which are driven by the community-weighted mean (CWM) of trait values, are the critical drivers of AGB and forest productivity (Grime, 1998). Stand structural complexity refers to vertical diversity and horizontal heterogeneity, demographic structure, and the distribution and diversity of diameter at breast height (DBH); tree maximum height and crown width also affect the AGB of forests (Fotis et al., 2018; Yuan et al., 2019). It has also been recognized that AGB and biodiversity are affected by stand types (e.g., coniferous, broadleaved, and mixed forests) in the same forest type as important variations, and several studies have suggested that different stand types with different dominant tree species cause differences in biodiversity and AGB (Lee et al., 2013; Ullah et al., 2021). Differences in the species composition of different forest stands are reflected in the differences in plant traits and ecosystem function, including photosynthetic capacity, leaf structure, tissue composition, and hydraulic network. Therefore, changes in species composition as a result of climate change are considered to have to have a direct or indirect impact on forest growth and the cycling of carbon, water, and soil nutrients (Augusto et al., 2015). As the importance of our response to climate change increases, the need to monitor vegetation distribution and establish forest management plans based on stand types have become important (Lee et al., 2013). Hence, understanding the relationships between abiotic and biotic drivers and AGB among stand types is important to provide empirical bases for sustainable forest management in each stand type. However, few studies have investigated the relationship between AGB and environmental conditions, forest development stage (i.e., stand age), and biotic drivers among different stand types (Ullah et al., 2021; Wang et al., 2023).
In these contexts, this study focuses on identifying the multiple effects of abiotic (i.e., three climatic and five topographic drivers, and stand age) and biotic (three taxonomic, eight phylogenetic, and five functional trait diversity; seven CWM traits; and five stand structural attributes) drivers that regulate AGBs among coniferous, broadleaved, mixed, and total stands using South Korea’s 7th National Forest Inventory (NFI) data. On the basis of conceptual models (Figure 1), we propose the following: (1) The process of forest succession with increasing forest age will lead to changes in the diversity of species and functional traits, which will alter the primary productivity and AGB of the forest depending on the dominant traits of a few key tree species in the stand. (2) The relative contributions of abiotic and biotic drivers to AGB variation will vary depending on the stand types across forest succession. (3) Forest stand age affects stand structural complexity (i.e., stand structural variation) and is probably an important driver in increasing AGB in each stand type. In sum, we suggest the possibilities and ways for the sustainable management of the forest to increase biodiversity and the AGB based on the stand types.
Figure 1. Conceptual models for testing the hypothesized effects of biotic and abiotic drivers on aboveground biomass (AGB) among stand types in the temperate forests of South Korea.
This study was carried out in the entire forest area of South Korea; by the end of 2020, the forests occupied about 63% of the total area of the country (Korea Forest Service, 2022). About 81% of the forest area is comprised of stands between 31 and 50 years old in forest age. The survey also showed that the forest area was made up of 37% coniferous, 32% broadleaved, and 26% mixed forests (Korea Forest Service, 2022). For the coniferous forests, Pinus densiflora Siebold and Zucc., Pinus rigida Mill., and Pinus thunbergii Parl, which all belong to the Pinaceae family, were the most abundant tree species. Quercus mongolica Fisch. Ex Ledeh., Quercus variabilis Blume, and Quercus serrata Murray, which all belong to the Fagaceae family, were the most abundant tree species for the broadleaved forests, while Q. mongolica Fisch. Ex Ledeh. and P. densiflora Siebold and Zucc. were the most abundant for the mixed forests. Under temperate climate zones, the mean annual temperature (MAT) of South Korea ranges between 7 and 15°C and the mean annual precipitation (MAP) ranges between 1,011 and 1,921 mm (Korean Meteorological Administration, 2021).
In this study, the NFI data collected from 2016 to 2020 were used to analyze the drivers that control AGB in each stand type (i.e., broadleaved, coniferous, and mixed stands) and total stands (i.e., the combination of with coniferous, broadleaved, and mixed stands). In the NFI, plots are located at the intersection of grids dividing South Korea into 4 km × 4 km areas and one plot consists of four 400-m2 circular subplots (Korea Forest Service, 2017). In other words, the area of a circular subplot is 0.04 ha, and the area of a plot is 0.16 ha (Supplementary Figure 1). The 2,070 plots belonging to natural forests were finally selected and comprised 394 plots (19.0%) of coniferous forests, 829 plots (40.0%) of broadleaved forests, and 847 plots (40.9%) of mixed forests (Figure 2). In the vegetation survey, the tree species name and DBH were measured for all individuals over 6 cm DBH within the four subplots. Overall, the mean age of the forests ranged from 4.2 to 154.25 years (Supplementary Table 1). As proposed in previous studies, species-specific allometric equations based on DBH were used to calculate the AGB of each plot by the summation of AGBs of all individuals (Supplementary Table 2).
Figure 2. Location of 2,070 study plots, including 394 plots (19.0%) of coniferous stands, 829 plots (40.0%) of broadleaved stands, and 847 plots (40.9%) of mixed stands in temperate forests in South Korea.
Species, phylogenetic, and functional trait diversity; CWM trait values; and stand structural attributes (Fotis et al., 2018; Ali et al., 2019b; Chun et al., 2020) were used as biotic drivers. For species diversity, species richness (SR), Shannon H index (SD), and Pielou species evenness (SE) were used. Phylogenetic trees for each of the three stand types and for the total stands were formed to quantify phylogenetic diversity using the investigated tree species and a megatree approach in R’s “V.PhyloMaker” package, as suggested in earlier studies (Jin and Qian, 2019; Chun et al., 2020). In all, we quantified phylogenetic species diversity (PD), phylogenetic SR, phylogenetic species clustering, phylogenetic species variability, phylogenetic species evenness (PSE), standard effective size of phylogenetic diversity (SES PD), standardized effect size of mean pairwise phylogenetic distance (SES MPD), and standardized effect size of mean nearest taxon distances (SES MNTD; Helmus et al., 2007; Chun et al., 2020) for each stand type.
Seven functional traits were selected to calculate functional trait diversity and CWM trait values, including leaf dry matter content (LDMC, g), specific leaf area (SLA, mm2 g–1), wood density (WD, g cm–3), leaf nitrogen content (N, mg g–1), leaf phosphorus content (P, mg g–1), seed mass (mg), and maximum tree height (H, m), which are recognized as being important traits for the survival and growth of the plant and directly correlated with the functioning of the ecosystem such as AGB and stand dynamics as demonstrated in previous studies (Poorter et al., 2017; Yuan et al., 2018; Ali et al., 2019a; Chun et al., 2020). We obtained the functional traits from the published literature and open data sources or obtained the data by directly analyzing the leaf and wood samples (i.e., leaves for LDMC, SLA, N, P, and wood pieces for WD) of tree species at Dr. Chang-Bae Lee’s Laboratory following standardized protocols (Perez-Harguindeguy et al., 2013). We adapted the mean value at the same genus level for the species when species level data were absent for a species.
Functional dispersion, functional richness (FRic), functional divergence (FDiv), and functional evenness as a proxy for functional trait diversity were quantified using the R “FD” package (Laliberté et al., 2014) in R version 4.2.3 (R Development Core Team, 2023) for seven functional traits. To quantify CWM values, each trait value was calculated by weighing plot mean trait values by the relative basal area for each species (Chun et al., 2020). To describe the structural characteristics of the stand, we used the mean DBH (DBH mean), standard deviation of DBHs (DBH STD), coefficient of variation of DBHs (DBH CV), Shannon H index of DBH classes with 2-cm-diameter interval (DBH SD), skewness of DBH (DBH Skew), and stem density (Stand density) in each plot.
For each stand type and total stands, three climatic and five topographic drivers and stand age were selected to quantify the effect of abiotic drivers on AGB. The climatic drivers including MAT, MAP, and climate moisture index (CMI) were quantified in each plot from WorldClim,1 and Global Aridity Index and Potential Evapotranspiration Climate Database.2 In our study plots, MAT ranges from 5.6 to 16°C, MAP ranges from 618 to 3,870 mm, and CMI ranges from 0.1 to 0.68. Topographic drivers such as elevation, slope, topographic position index, topographic wetness index, and topographic ruggedness index were constructed using a digital elevation model with ArcGIS Pro version 3.1.0 spatial analyst tools. Annual rings were collected using cores from the five dominant trees in each subplot and then averaged across each plot as the stand age for that plot. The comparisons of the biotic and abiotic drivers and AGB along the three stand types and total stands are shown in Supplementary Figures 2–6.
To improve normality and linearity, the abiotic and biotic variables and AGB were log or square root transformed before implementing statistical procedures. The variables were standardized to unify the different units (Chun et al., 2020). For all variables, Pearson correlation analysis was conducted to remove highly correlated (| r| ≥ 0.7) explanatory variables (Supplementary Figures 7–10). In addition, to check for multicollinearity, we calculated the variance inflation factor (VIF) of each variable in the multiple regression model (Graham, 2003). Typically, the explanatory power of the model is reduced by a VIF value above 10, but in this study, multicollinearity had no effect on the results of the model as the VIF values were <3 in all models.
To compare the spatial and non-spatial model fitness, we calculated generalized least squares models (Legendre and Legendre, 2012). Spatial models include the latitudinal and longitudinal coordinates, whereas non-spatial models do not contain the coordinates for each plot. By comparing the suitability of the spatial and non-spatial models within the Akaike Information Criterion (AIC), the effects of spatial autocorrelation were found in several variables (Supplementary Table 3). Hence, we applied latitudinal and longitudinal coordinates for further multimodel inference tests and piecewise structural equation modeling (pSEM; Lefcheck, 2016) to explain spatial autocorrelation.
To select the most significant variable for AGB in each stand type and total stands, we employed multimodel inference tests as proposed in previous studies (Chun et al., 2020). As a result, the variables within the highest standardized regression coefficients (β) for AGB in each category of abiotic and biotic drivers were selected (Supplementary Figure 11).
Based on the basic conceptual model (Figure 1), we conducted pSEM (Lefcheck, 2016) to assess the multiple pathways between abiotic and biotic drivers of AGB. In this study, we implemented four pSEMs including three major stands and one total stand: (1) coniferous stand model, (2) broadleaved stand model, (3) mixed stand model, and (4) total stand model. For each model, we first constructed a model that included all pathways and then we removed the insignificant ones (Yi et al., 2021). Fisher’s C statistic, P-value, and AIC were calculated for all models to evaluate fitness.
In the coniferous stand model (Figure 3A), AGB increased significantly along with the direct effects of elevation (β = 0.231, P < 0.001), stand age (β = 0.140, P < 0.001), DBH STD (β = 0.592, P < 0.001), and CWM LDMC (β = 0.109, P = 0.002). Stand age also had positive indirect effects on AGB through its influence on DBH STD and CWM LDMC. CMI had an indirect positive effect on AGB through DBH STD. Additionally, elevation had an indirect effect on AGB through its negative influence on CWM LDMC.
Figure 3. Piecewise structural equation models for testing the multiple pathways of biotic and abiotic drivers on AGB for (A) coniferous, (B) broadleaved, (C) mixed, and (D) total stands in the temperate forests of South Korea. Blue and red arrows indicate positive and negative paths, respectively. Gray arrows represent the estimated covariance. Solid arrows represent significance (P < 0.05). For each arrow and covariance, standardized coefficients are presented. CMI, climate moisture index; MAT, mean annual temperature; SR, species richness; PSE, phylogenetic species evenness; SES MPD, standardized effect size of mean pairwise phylogenetic distance; DBH STD, standard deviation of diameter at breast height (DBH); FDiv, functional divergence; FRic, functional richness; CWM LDMC, community-weighted mean of leaf dry matter content; CWM P, community-weighted mean of leaf phosphorus content. Significance levels are *P < 0.05, **P < 0.01, and ***P < 0.001.
The broadleaved stand model (Figure 3B) demonstrated that MAT (β = 0.107, P < 0.001), stand age (β = 0.188, P < 0.001), and DBH STD (β = 0.725, P < 0.001) had positive direct effects on AGB, but SES MPD (β = −0.049, P = 0.045), FRic (β = −0.090, P < 0.001), and CWM P (β = −0.102, P < 0.001) had negative direct effects on AGB. Moreover, MAT had an indirect effect on AGB through SES MPD and DBH STD. Stand age showed a positive direct effect on DBH STD (β = 0.492, P < 0.001) and had a positive indirect effect on AGB through its influence on DBH STD.
For the mixed stand model (Figure 3C), elevation (β = 0.185, P < 0.001), stand age (β = 0.184, P < 0.001), and DBH STD (β = 0.632, P < 0.001) had positive direct effects on AGB, whereas CMI (β = −0.168, P = 0.006), PSE (β = −0.053, P = 0.027), and CWM P (β = −0.077, P = 0.002) had negative direct effects. Elevation had a positive indirect effect on AGB through PSE, but a negative indirect effect on AGB through CWM P. CMI had a positive indirect effect on AGB through DBH STD and CWM P. Stand age had a positive indirect effect on AGB through DBH STD.
In the total stand model (Figure 3D), slope (β = 0.084, P < 0.001), stand age (β = 0.157, P < 0.001) and DBH STD (β = 0.692, P < 0.001) had positive direct effects on AGB, whereas FRic (β = −0.058, P < 0.001) and CWM P (β = −0.070, P < 0.001) had negative direct effects. Slope had an indirect effect on AGB through FRic and CWM P, while CMI had a positive indirect effect on AGB through DBH STD but a negative indirect effect on AGB through CWM P. Moreover, stand age had a positive indirect effect on AGB through DBH STD and CWM P. The bivariate relationships supporting the pSEMs are shown in Figures 4, 5.
Figure 4. Bivariate relationships between AGB and abiotic drivers in (A) coniferous, (B) broadleaved, (C) mixed, and (D) total stands. Fitted regressions were significant (P < 0.05). Abbreviations to variables are presented in Figure 3.
Figure 5. Bivariate relationships between AGB and biotic drivers in (A) coniferous, (B) broadleaved, (C) mixed, and (D) total stands. Fitted regressions were significant (P < 0.05). Abbreviations to variables are presented in Figure 3.
The models tested in this study provide new and additional insights into the relationship between biodiversity and ecosystem function across forest stand types. The main findings of this study were as follows: (1) In terms of total stands, AGB was mainly determined by stand age as a proxy for forest successional and developmental stage, and tree size variation induced by stand age. (2) Multiple abiotic and biotic drivers simultaneously control AGBs through a variety of biological processes, with different mechanisms depending on stand types.
In three major and total stands, we found that a higher variation in tree size with increasing stand age led to a higher AGB. Based on niche complementarity effects, it appears that tree size variation acts as a primary regulatory mechanism for promoting positive effects on the AGB (Zhang and Chen, 2015; Ullah et al., 2021). For instance, variations in tree size occurring within and between different species facilitate higher packing densities of diverse canopy heights, promoting the capture of above ground light, and resulting in improved light use efficiency (Zhang and Chen, 2015). In forest ecosystems, tree stands pass through successional stages over time, with the early stages characterized by a higher density of smaller, faster growing trees, and the later stages consisting of larger, slower growing trees (Lienard et al., 2015). As the stand progresses through these stages, the variation in tree size increases, leading to a wider range of tree sizes within niche complementarity and consequently an increase in AGB (Zhang and Chen, 2015; Matsuo et al., 2021). In temperate forests, most successional series require a minimum of 100 years to progress to a climax stage (Choung et al., 2020). However, most forest stands in South Korea are less than 50 years old (Korea Forest Service, 2022) due to historical forest degradation events such as the Japanese colonization of the Korean Peninsula and the Korean War, and large areas have been reforested in a short time with the three main forest types: coniferous, broadleaved, and mixed stands (Korea Forest Research Institute, 2016). In our study, across all forest types, the increase in tree size variation in the early stages of forest succession is the main factor that drives the increase in AGB, but over time, coniferous stands will decline and be replaced by the next stand type (i.e., Quercus broadleaved forests; Choung et al., 2020), so it is crucial to consider not only stand structural diversity but also biotic and abiotic drivers for increasing AGB in forest types at each forest succession stage (Qianwen et al., 2022).
In coniferous stands, we found that as stand age increased, SR decreased and CWM LDMC increased. High leaf LDMC means few spaces between the cells and resistance of the mesophyll to the diffusing gases (Bussotti and Pollastrini, 2015), which conserves resources by increasing leaf dry matter at higher construction cost to reduce water loss in coniferous stands (Qin and Shangguan, 2019). This suggests that the dominant species within specific functional trait values play an influential role in species-poor coniferous stands, in accordance with the selection effect (Poorter et al., 2015; Ullah et al., 2021). CMI, as a climate driver, had a positive indirect effect on AGB through DBH STD. Since variability in potential evapotranspiration and precipitation indicates the prominent role of soil moisture as a driver of tree growth and site productivity, the availability of moisture from higher levels of precipitation could promote greater tree growth and subsequently result in greater tree size heterogeneity in coniferous stands (Pompa-García et al., 2021). We also found that elevation increases AGB in coniferous stands. Forest carbon uptake is believed to increase along an elevation–climate environmental gradient, where greater resource availability (water and soil nutrients) occurs in temperate forests (Chun et al., 2020; Yuan et al., 2021). This is consistent with our findings of a high correlation between CMI and elevation in coniferous stands (Supplementary Figure 7). When considering the negative relationship between elevation and CWM LDMC, earlier studies have revealed that with increasing drought stress, increasing values of LDMC were observed in different tree species along an ecological gradient (Bussotti and Pollastrini, 2015). Thus, elevation, which is highly correlated with precipitation, would have had a negative direct effect on CWM LDMC in coniferous stands. For these reasons, biotic drivers and AGB are regulated by abiotic conditions, among which tree size variation and certain functional traits increase AGB through complex mechanisms in temperate coniferous stands.
Our results showed that SES MPD had a negative direct effect on AGB in temperate broadleaved stands. Generally, low SES MPD indicates that the communities are composed of species that are more phylogenetically clustered and have relatively recent common ancestors (Edwards et al., 2017). Due to the evolutionary conservatism of many species traits within a lineage, the presence of a phylogenetically clustered community structure is considered to be the most important evidence for the verification of the phylogenetic effect of environmental filtering (Asefa et al., 2017). Our results showed that MAT had a negative effect on SES MPD. Based on previous studies that have shown a strong correlation between high temperatures and growth rates and leaf nitrogen productivity in temperate broadleaved forests (Tang et al., 2018; Chen et al., 2023), these climatic conditions have created an environment conducive to specific phylogenetic groups (Ali et al., 2020), resulting in increased AGB in broadleaved stands where phylogenetic clustering occurred.
We also found that CWM P had a direct negative effect on AGB. Generally, leaf phosphorus is essential for tree metabolism and influences the distribution of photosynthate by participating in the transport of plant carbohydrates, which controls biomass accumulation (Tang et al., 2018; Yu et al., 2022). In this study, a lower CWM P was associated with a higher AGB in broadleaved forest stands. This is probably because broadleaved stands with low leaf phosphorus content strategically allocate available phosphorus resources to maximize AGB production, resulting in lower leaf phosphorus concentrations but higher total biomass, thereby maximizing their competitive advantage (Yu et al., 2022).
Our study concludes that warm climate conditions in broadleaved stands promote the formation of phylogenetically clustered communities through environmental filtering effects and that these communities employ resource allocation strategies that contribute to their high AGB in temperate forests (Liu et al., 2023).
We found that elevation had positive direct effects on AGB and CWM P, whereas it had a negative effect on PSE in the plots of mixed stands. Previous studies have shown that broadleaved tree species are known to have higher phosphorus content in their leaves than coniferous tree species (Yuan and Chen, 2009; Tang et al., 2018). Coniferous tree species mostly have a smaller SLA compared to broadleaved tree species, which limits their ability to absorb nutrients such as phosphorus (Khan et al., 2022). In contrast, broadleaved tree species tend to absorb and accumulate more phosphorus in their tissues due to their faster growth rate and higher leaf turnover, which can lead to higher AGB (Rahman and Tsukamoto, 2013). However, our results showed that forest stands dominated by broadleaved tree species with higher phosphorous content at high elevations could reduce AGB in temperate mixed stands. In natural forests, the growth of trees can be constrained by various site-specific factors including temperature, water availability, and the accessibility of available nutrients (Elser et al., 2007). In nutrient-poor environments, coniferous tree species exhibit higher growth rates compared to broadleaved tree species despite their lower biomass growth and photosynthetic rates (Sawada et al., 2016). Moreover, under less favorable conditions, such as at higher elevations, coniferous tree species can outcompete broadleaved tree species due to their higher survival rate, higher growth rate, and more efficient use of nutrients (Augusto et al., 2015). Thus, forest stands dominated by deciduous tree species with high leaf phosphorous content at higher elevations would have a low AGB in mixed stands. Moreover, we found that PSE decreased as elevation increased in mixed stands. As mentioned earlier, a higher elevation could create a more favorable environment for certain tree species to grow, environmental filtering could occur under these conditions, and phylogenetically associated species with unequal abundance could contribute to high AGB in mixed stands (Kunwar et al., 2021; Qin et al., 2022).
We also found that CMI and stand age had positive direct and indirect effects on AGB through CWM P and DBH STD in temperate mixed stands. Since elevation and CMI are positively correlated but also negatively correlated with MAT, mixed stands with enough CMI and at high elevation would have been dominated by coniferous tree species with lower leaf phosphorus content (Zhou et al., 2018). Moreover, coniferous tree species that adapt to cold and harsh environments would have better resource utilization, resulting in greater tree size variation (Ali et al., 2020). However, our pSEM results showed that CMI had a negative direct effect on AGB, which is in contrast to the results of the bivariate analysis (Figure 4C). This is expected to be due to the negative indirect effect of CMI on AGB masking the direct positive effect.
Under these conditions, as the stand age increases, mixed stands dominated by taxonomically related coniferous species that exhibit environmental filtering effects were expected to have a higher AGB in temperate mixed stands.
In total stands, we found that slope had a positive direct effect on AGB (Figure 3D). In general, low slopes tend to increase AGB in forests because gentle slopes tend to reduce soil erosion and nutrient leaching. However, about 51% of the total area of forests in South Korea are steep with slopes of 20° or more (National Geography Information Institute, 2020), and the average slope across our study plots was 28.7° (Supplementary Table 1). The forest stands distributed on the higher slopes could avoid the effects of human activities and could be better preserved, contributing to a higher plant growth rate that promotes biomass accumulation (Xu et al., 2013). We also found that slope and CMI had positive direct effects on CWM P. Generally, steep slopes are often washed by rainfall, making it difficult for vegetation to grow due to nutrient limitations and faster nutrient leaching; however, if suitable moisture is present, this can be beneficial for tree growth (Wang et al., 2020). Steep slopes induce plants to adapt by conserving and efficiently utilizing available nutrients such as phosphorus and allocating a greater proportion of these nutrients to leaves for growth and metabolic processes (Yan et al., 2016). This adaptive strategy leads to higher leaf phosphorus content as plants optimize nutrient utilization to overcome nutrient constraints on steep slopes.
In total stands, stand age had positive direct and indirect effects on AGB through DBH STD and CWM P. This is identical to the results of the coniferous forest pSEM. In line with the selection effect, this suggests that forest stands dominated by productive species with low leaf phosphorus content play an influential role in temperate forests (Poorter et al., 2015; Ullah et al., 2021). A consistent mechanism across the stand types was suggested by the observation of an increase in tree size variation with increasing stand age and suitable environmental conditions for plant growth in the three major stands in temperate forests (Koch et al., 2022).
In this study, we showed how abiotic and biotic drivers simultaneously control AGBs through multiple pathways in three major temperate forest stands in South Korea. In particular, we found that tree size variation with increasing stand age increases AGBs across all stand types under conditions of adequate precipitation. Under these environmental conditions, we also found that biotic drivers control AGB through different mechanisms depending on the stand types. Each forest type has its own ecological characteristics and provides the basis for the formation of specific species and ecosystem structures. The biodiversity of these forest types is important for maintaining the stability and function of the ecosystem. Therefore, it is necessary to conserve and maintain the diversity of ecosystems by adopting forest management practices appropriate for each forest type: (1) For the coniferous stands, given sufficient precipitation levels or moisture conditions may result in increased tree growth and greater variation in tree size, so it is important to control water stress in management practices under environmental conditions. (2) In broadleaved stands, appropriate cutting and vegetation management should be done to increase AGB and induce the formation of phylogenetically clustered communities through natural regeneration. (3) Different management practices that are appropriate to the dominant species in the natural mixed stands should be encouraged to maintain biodiversity and their role as carbon sinks through successional stages over time. In addition, to maintain healthy and sustainable forests, it is necessary to form different resource utilization patterns for each forest type according to the local resource characteristics and environmental conditions. Accordingly, resources can be optimally utilized by introducing appropriate forest management practices, such as focusing on timber production in certain forest types while emphasizing ecosystem conservation in others. This study may provide a good reference for sustainable forest management measures according to forest types.
The original contributions presented in this study are included in the article/Supplementary material, further inquiries can be directed to the corresponding author.
Y-JL: methodology, formal analysis, data curation, visualization, and writing—original draft preparation. C-BL: conceptualization, funding acquisition, methodology, supervision, and writing—review and editing. M-KL: visualization and formal analysis. All authors had read and agreed to the published version of the manuscript.
This study was carried out with the support of R&D Program for Forest Science Technology (Project No. 2019150C10-2323-0301) and also was supported by the National Research Foundation of Korea (NRF) grant funded by the Korea Government (MIST) (No. 2020R1A2C2011226).
The authors declare that the research was conducted in the absence of any commercial or financial relationships that could be construed as a potential conflict of interest.
All claims expressed in this article are solely those of the authors and do not necessarily represent those of their affiliated organizations, or those of the publisher, the editors and the reviewers. Any product that may be evaluated in this article, or claim that may be made by its manufacturer, is not guaranteed or endorsed by the publisher.
The Supplementary Material for this article can be found online at: https://www.frontiersin.org/articles/10.3389/ffgc.2023.1229661/full#supplementary-material
Ali, A., and Yan, E. R. (2017). Relationships between biodiversity and carbon stocks in forest ecosystems: a systematic literature review. Tropic. Ecol. 58, 1–14.
Ali, A., Chen, H. Y., You, W. H., and Yan, E. R. (2019a). Multiple abiotic and biotic drivers of aboveground biomass shift with forest stratum. For. Ecol. Manag. 436, 1–10. doi: 10.1016/j.foreco.2019.01.007
Ali, A., Lin, S. L., He, J. K., Kong, F. M., Yu, J. H., and Jiang, H. S. (2019b). Climate and soils determine aboveground biomass indirectly via species diversity and stand structural complexity in tropical forests. For. Ecol. Manag. 432, 823–831. doi: 10.1016/j.foreco.2018.10.024
Ali, A., Sanaei, A., Nalivan, O. A., Ahmadaali, K., Pour, M. J., Valipour, A., et al. (2020). Environmental filtering, predominance of strong competitor trees and exclusion of moderate-weak competitor trees shape species richness and biomass. Sci. Total Environ. 723:138105. doi: 10.1016/j.scitotenv.2020.138105
Asefa, M., Cao, M., Zhang, G., Ci, X., Li, J., and Yang, J. (2017). Environmental filtering structures tree functional traits combination and lineages across space in tropical tree assemblages. Sci. Rep. 7:132. doi: 10.1038/s41598-017-00166-z
Augusto, L., De Schrijver, A., Vesterdal, L., Smolander, A., Prescott, C., and Ranger, J. (2015). Influences of evergreen gymnosperm and deciduous angiosperm tree species on the functioning of temperate and boreal forests. Biol. Rev. 90, 444–466. doi: 10.1111/brv.12119
Becknell, J. M., and Powers, J. S. (2014). Stand age and soils as drivers of plant functional traits and aboveground biomass in secondary tropical dry forest. Can. J. For. Res. 44, 604–613. doi: 10.1139/cjfr-2013-0331
Bussotti, F., and Pollastrini, M. (2015). Evaluation of leaf features in forest trees: methods, techniques, obtainable information and limits. Ecol. Indic. 52, 219–230. doi: 10.1016/j.ecolind.2014.12.010
Chen, G., Cai, Q., Ma, S., Feng, Y., Fang, W., Ji, C., et al. (2023). Climate and forest attributes influence above-ground biomass of deciduous broadleaf forests in China. J. Ecol. 111, 495–508. doi: 10.1111/1365-2745.14042
Choung, Y., Lee, J., Cho, S., and Noh, J. (2020). Review on the succession process of Pinus densiflora forests in South Korea: progressive and disturbance-driven succession. J. Ecol. Environ. 44, 1–17. doi: 10.1186/s41610-020-00157-8
Chun, J. H., Ali, A., and Lee, C. B. (2020). Topography and forest diversity facets regulate overstory and understory aboveground biomass in a temperate forest of South Korea. Sci. Total Environ. 744:140783. doi: 10.1016/j.scitotenv.2020.140783
Edwards, D. P., Massam, M. R., Haugaasen, T., and Gilroy, J. J. (2017). Tropical secondary forest regeneration conserves high levels of avian phylogenetic diversity. Biol. Conserv. 209, 432–439. doi: 10.1016/j.biocon.2017.03.006
Elser, J. J., Bracken, M. E., Cleland, E. E., Gruner, D. S., Harpole, W. S., Hillebrand, H., et al. (2007). Global analysis of nitrogen and phosphorus limitation of primary producers in freshwater, marine and terrestrial ecosystems. Ecol. Lett. 10, 1135–1142. doi: 10.1111/j.1461-0248.2007.01113.x
Fotis, A. T., Murphy, S. J., Ricart, R. D., Krishnadas, M., Whitacre, J., Wenzel, J. W., et al. (2018). Above-ground biomass is driven by mass-ratio effects and stand structural attributes in a temperate deciduous forest. J. Ecol. 106, 561–570. doi: 10.1111/1365-2745.12847
Gao, W. Q., Lei, X. D., Gao, D. L., and Li, Y. T. (2021). Mass-ratio and complementarity effects simultaneously drive aboveground biomass in temperate Quercus forests through stand structure. Ecol. and Evol. 11, 16806–16816. doi: 10.1002/ece3.8312
Graham, M. H. (2003). Confronting multicollinearity in ecological multiple regression. Ecology 84, 2809–2815. doi: 10.1890/02-3114
Grime, J. P. (1998). Benefits of plant diversity to ecosystems: immediate, filter and founder effects. J. Ecol. 86, 902–910. doi: 10.1046/j.1365-2745.1998.00306.x
Helmus, M. R., Bland, T. J., Williams, C. K., and Ives, A. R. (2007). Phylogenetic measures of biodiversity. Am. Natural. 169, E68–E83. doi: 10.1086/511334
Jin, Y., and Qian, H. (2019). V. PhyloMaker: an R package that can generate very large phylogenies for vascular plants. Ecography 42, 1353–1359. doi: 10.1111/ecog.04434
Khan, A., Yan, L., Hasan, M. M., Wang, W., Xu, K., Zou, G., et al. (2022). Leaf traits and leaf nitrogen shift photosynthesis adaptive strategies among functional groups and diverse biomes. Ecol. Indic. 141:109098. doi: 10.1016/j.ecolind.2022.109098
Kim, E. (2012). A study on the role of the forest sector in response to climate change. Sustain. Stud. 3, 1–20.
Koch, N. M., Stanton, D., Müller, S. C., Duarte, L., Spielmann, A. A., and Lücking, R. (2022). Nuanced qualitative trait approaches reveal environmental filtering and phylogenetic constraints on lichen communities. Ecosphere 13:e4042. doi: 10.1002/ecs2.4042
Korea Forest Research Institute, (2016). The 6th national forest inventory report. Seoul: Korea Forest Research Institute.
Korea Forest Service (2017). Guide to the seventh national forest resources Inventory and field survey of forest ecosystem health and vitality. Incheon: Korea Forest Service.
Korea Forest Service. (2022). Korea Forest Service: Statistical yearbook of forest. Available from https://www.forest.go.kr. (in Korean).
Korean Meteorological Administration (2021). Weather information. Available online at: https://data.kma.go.kr (accessed November 1, 2022).
Kunwar, S., Wang, L. Q., Chaudhary, R., Joshi, P. R., and Ali, A. (2021). Evolutionary diversity and species richness predict aboveground biomass better than tree size variation in local-scale tropical forest types of Nepal. For. Ecol. Manag. 490:119146. doi: 10.1016/j.foreco.2021.119146
Laliberté, E., Legendre, P., and Shipley, B. (2014). FD: Measuring functional diversity from multiple traits, and other tools for functional ecology. R Package Version 1.0-12.
Lee, C., Chun, J., and Cho, H. (2013). Elevational patterns and determinants of plant diversity in the Baekdudaegan Mountains, South Korea: species vs. functional diversity. Chinese Sci. Bull. 58, 3747–3759. doi: 10.1007/s11434-013-5957-1
Lefcheck, J. S. (2016). piecewiseSEM: piecewise structural equation modelling in r for ecology, evolution, and systematics. Methods Ecol. Evol. 7, 573–579. doi: 10.1111/2041-210X.12512
Lienard, J., Florescu, I., and Strigul, N. (2015). An appraisal of the classic forest succession paradigm with the shade tolerance index. PLoS One 10:e0117138. doi: 10.1371/journal.pone.0117138
Liu, L., Xia, H., Quan, X., and Wang, Y. (2023). Plant trait-based life strategies of overlapping species vary in different succession stages of subtropical forests, Eastern China. Front. Ecol. Evol. 10:1103937. doi: 10.3389/fevo.2022.1103937
Matsuo, T., Martínez-Ramos, M., Bongers, F., van der Sande, M. T., and Poorter, L. (2021). Forest structure drives changes in light heterogeneity during tropical secondary forest succession. J. Ecol. 109, 2871–2884. doi: 10.1111/1365-2745.13680
McEwan, R. W., Lin, Y. C., Sun, I. F., Hsieh, C. F., Su, S. H., Chang, L. W., et al. (2011). Topographic and biotic regulation of aboveground carbon storage in subtropical broad-leaved forests of Taiwan. For. Ecol. Manag. 262, 1817–1825. doi: 10.1016/j.foreco.2011.07.028
Michaletz, S. T., Cheng, D., Kerkhoff, A. J., and Enquist, B. J. (2014). Convergence of terrestrial plant production across global climate gradients. Nature 512, 39–43. doi: 10.1038/nature13470
National Geography Information Institute (2020). The national atlas of Korea. Suwon-si: National Geography Information Institute.
Njana, M. A., Mbilinyi, B., and Eliakimu, Z. (2021). The role of forests in the mitigation of global climate change: emprical evidence from Tanzania. Environ. Challenges 4:100170. doi: 10.1016/j.envc.2021.100170
Perez-Harguindeguy, N., Diaz, S., Garnier, E., Lavorel, S., Poorter, H., Jaureguiberry, P., et al. (2013). New handbook for standardised measurement of plant functional traits worldwide. Aust. Bot. 61, 167–234. doi: 10.1071/BT12225
Pompa-García, M., Camarero, J. J., Colangelo, M., and González-Cásares, M. (2021). Inter and intra-annual links between climate, tree growth and NDVI: improving the resolution of drought proxies in conifer forests. Int. J. Biometeorol. 65, 2111–2121. doi: 10.1007/s00484-021-02170-5
Poorter, L., van der Sande, M. T., Arets, E. J., Ascarrunz, N., Enquist, B. J., Finegan, B., et al. (2017). Biodiversity and climate determine the functioning of Neotropical forests. Glob. Ecol. Biogeogr. 26, 1423–1434. doi: 10.1111/geb.12668
Poorter, L., van der Sande, M. T., Thompson, J., Arets, E. J., Alarcón, A., Álvarez-Sánchez, J., et al. (2015). Diversity enhances carbon storage in tropical forests. Glob. Ecol. Biogeogr. 24, 1314–1328. doi: 10.1111/geb.12364
Qianwen, G., Arif, M., Zhongxun, Y., Jie, Z., Xinrui, H., Dongdong, D., et al. (2022). Plant species composition and diversity along successional gradients in arid and semi-arid regions of China. For. Ecol. Manag. 524:120542. doi: 10.1016/j.foreco.2022.120542
Qin, J., and Shangguan, Z. (2019). Effects of forest types on leaf functional traits and their interrelationships of Pinus massoniana coniferous and broad-leaved mixed forests in the subtropical mountain, Southeastern China. Ecol. Evol. 9, 6922–6932. doi: 10.1002/ece3.5259
Qin, Y., He, X., Lei, X., Feng, L., Zhou, Z., and Lu, J. (2022). Tree size inequality and competition effects on nonlinear mixed effects crown width model for natural spruce-fir-broadleaf mixed forest in northeast China. For. Ecol. Manag. 518:120291. doi: 10.1016/j.foreco.2022.120291
Rahman, M. M., and Tsukamoto, J. (2013). Leaf traits, litter decomposability and forest floor dynamics in an evergreen-and a deciduous-broadleaved forest in warm temperate Japan. Forestry 86, 441–451. doi: 10.1093/forestry/cpt015
Sawada, Y., Aiba, S. I., Seino, T., and Kitayama, K. (2016). Size structure, growth and regeneration of tropical conifers along a soil gradient related to altitude and geological substrates on Mount Kinabalu, Borneo. Plant Soil 403, 103–114. doi: 10.1007/s11104-015-2722-z
Tang, Z., Xu, W., Zhou, G., Bai, Y., Li, J., Tang, X., et al. (2018). Patterns of plant carbon, nitrogen, and phosphorus concentration in relation to productivity in China’s terrestrial ecosystems. Proc. Natl. Acad. Sci. U.S.A. 115, 4033–4038. doi: 10.1073/pnas.1700295114
Ullah, F., Gilani, H., Sanaei, A., Hussain, K., and Ali, A. (2021). Stand structure determines aboveground biomass across temperate forest types and species mixture along a local-scale elevational gradient. For. Ecol. Manag. 486:118984. doi: 10.1016/j.foreco.2021.118984
Van Con, T., Thang, N. T., Khiem, C. C., Quy, T. H., Lam, V. T., Van Do, T., et al. (2013). Relationship between aboveground biomass and measures of structure and species diversity in tropical forests of Vietnam. For. Ecol. Manag. 310, 213–218. doi: 10.1016/j.foreco.2013.08.034
Wang, G., Xie, B., Lv, Y., Yin, J., Zhou, Y., Xu, L., et al. (2023). Interaction effect of stand age and diversity on aboveground wood carbon accumulation in subtropical mixed forests of the Zhejiang Province (China). Forests 14:262. doi: 10.3390/f14020262
Wang, J., Liu, G. Q., Ma, D. L., and Yang, D. F. (2020). Water and soil conservation technology of steep slope based on artificial vegetation restoration. IOP Conf. Ser. Earth Environ. Sci. 446:032044. doi: 10.1088/1755-1315/446/3/032044
Xu, J., Wang, J., Zhang, F., and Ji, B. (2013). Correlation between forest carbon distribution and terrain elements of altitude and slope. J. Zhejiang A F Univ. 30, 330–335.
Yan, Z., Li, P., Chen, Y., Han, W., and Fang, J. (2016). Nutrient allocation strategies of woody plants: an approach from the scaling of nitrogen and phosphorus between twig stems and leaves. Sci. Rep. 6:20099. doi: 10.1038/srep20099
Yi, S., Wu, P., Peng, X., Tang, Z., Bai, F., Sun, X., et al. (2021). Biodiversity, environmental context and structural attributes as drivers of aboveground biomass in shrublands at the middle and lower reaches of the Yellow River basin. Sci. Total Environ. 774:145198. doi: 10.1016/j.scitotenv.2021.145198
Yu, C., Xu, L., Li, M., and He, N. (2022). Phosphorus storage and allocation in vegetation on the Tibetan Plateau. Ecol. Indic. 145:109636. doi: 10.1016/j.ecolind.2022.109636
Yuan, Z., Ali, A., Jucker, T., Ruiz-Benito, P., Wang, S., Jiang, L., et al. (2019). Multiple abiotic and biotic pathways shape biomass demographic processes in temperate forests. Ecology 100:e02650. doi: 10.1002/ecy.2650
Yuan, Z., Ali, A., Sanaei, A., Ruiz-Benito, P., Jucker, T., Fang, L., et al. (2021). Few large trees, rather than plant diversity and composition, drive the above-ground biomass stock and dynamics of temperate forests in northeast China. For. Ecol. Manag. 481:118698. doi: 10.1016/j.foreco.2020.118698
Yuan, Z., and Chen, H. Y. (2009). Global trends in senesced-leaf nitrogen and phosphorus. Glob. Ecol. Biogeogr. 18, 532–542. doi: 10.1111/j.1466-8238.2009.00474.x
Yuan, Z., Wang, S., Ali, A., Gazol, A., Ruiz-Benito, P., Wang, X., et al. (2018). Aboveground carbon storage is driven by functional trait composition and stand structural attributes rather than biodiversity in temperate mixed forests recovering from disturbances. Ann. For. Sci. 75:67. doi: 10.1007/s13595-018-0745-3
Zhang, Y., and Chen, H. Y. (2015). Individual size inequality links forest diversity and above-ground biomass. J. Ecol. 103, 1245–1252. doi: 10.1111/1365-2745.12425
Keywords: aboveground biomass, forest stand type, National Forest Inventory, stand age, tree size variation, piecewiseSEM
Citation: Lee Y-J, Lee C-B and Lee M-K (2023) Tree size variation induced by stand age mainly regulates aboveground biomass across three major stands of temperate forests in South Korea. Front. For. Glob. Change 6:1229661. doi: 10.3389/ffgc.2023.1229661
Received: 26 May 2023; Accepted: 03 July 2023;
Published: 17 July 2023.
Edited by:
Yashwant Singh Rawat, Federal Technical and Vocational Education and Training Institute (FTVETI), EthiopiaReviewed by:
Bhupendra Singh, VCSG Uttarakhand University of Horticulture and Forestry, IndiaCopyright © 2023 Lee, Lee and Lee. This is an open-access article distributed under the terms of the Creative Commons Attribution License (CC BY). The use, distribution or reproduction in other forums is permitted, provided the original author(s) and the copyright owner(s) are credited and that the original publication in this journal is cited, in accordance with accepted academic practice. No use, distribution or reproduction is permitted which does not comply with these terms.
*Correspondence: Chang-Bae Lee, a2Vjb2xlZUBrb29rbWluLmFjLmty
Disclaimer: All claims expressed in this article are solely those of the authors and do not necessarily represent those of their affiliated organizations, or those of the publisher, the editors and the reviewers. Any product that may be evaluated in this article or claim that may be made by its manufacturer is not guaranteed or endorsed by the publisher.
Research integrity at Frontiers
Learn more about the work of our research integrity team to safeguard the quality of each article we publish.