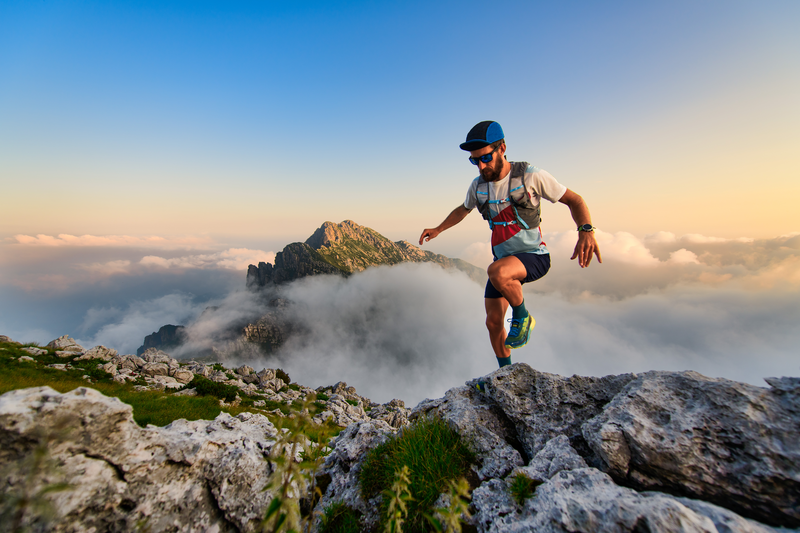
94% of researchers rate our articles as excellent or good
Learn more about the work of our research integrity team to safeguard the quality of each article we publish.
Find out more
PERSPECTIVE article
Front. For. Glob. Change , 05 September 2023
Sec. Forest Disturbance
Volume 6 - 2023 | https://doi.org/10.3389/ffgc.2023.1219685
This article is part of the Research Topic Biotic Pest Disturbance - Risk, Evaluation, and Management in Forest Ecosystems View all 14 articles
The expanding world population demands superior forest protection to fulfil feasible environmental certainty. The persistent pest infestations negatively influence forest health and cause substantial economic losses. In contrast, the traditional use of conventional pesticides results in a loss of soil microbial biodiversity, a drop in the population of pollinators, and adverse effects on other non-target organisms, including humans. Global forestry is looking for solutions to reduce the adverse environmental effects of current chemical pesticides. RNAi-nanotechnology has recently drawn much attention for its use in pest management. The advantages of engineered RNAi-chitosan nano-formulations in terms of simple digestion and dissolution, non-toxicity, high adsorption power, potential biodegradation in nature, and widespread availability and cost-effectiveness, have been well documented for pest management in agroecosystems. However, deploying such control strategies in forest ecosystems is still pending and demands further research. Hence, we highlight the putative uses of RNAi-chitosan biopesticides and their preparation, characterization, and putative application methods for forest pest management. We also discussed potential environmental risks and plausible mitigation strategies.
Given the ongoing increase in global population, many countries have lost forests and facing climate change (Ritchie and Roser, 2021).1 Forestry is a crucial industry in many developing countries, and it can produce food and gross income as a domestic product for both people and animals, as well as contributes to balancing the environmental conditions, respectively. However, a variety of biotic factors like insect pests (i.e., bark beetles, weevils, chewing, sucking, and foliage-feeding insects) and diseases caused by pathogens (i.e., tree leaves diseases, pine needle diseases, hardwood leaf diseases, tree bark diseases, and tree root diseases) limit forest growth and tend to get worse with a growing human population (Kan et al., 2023). Consequently, to address pest-related issues, pesticides (insecticides, fungicides, herbicides, etc.) have been overused and often misused, which has had fatal short and long-term effects on humans and other life forms (Chhipa, 2017). Pesticide resistance is common in pest insects, and their preexisting adaptive capabilities facilitate quick resistance in field conditions (Bras et al., 2022). With the accessibility of new technologies, superior approaches to controlling insect pests and disease-caused pathogens can be considered. RNA interference (RNAi) technology and nanotechnology have recently captured the interest and imagination of scientists and researchers due to recent advancements in the discipline. Delivering RNAi biopesticides with the use of nanotechnology in the forestry sector is a quick, innovative, and promising field (Shang et al., 2019; Joga et al., 2021; Silver et al., 2021; Mogilicherla et al., 2022).
Polymeric nanoparticles are non-toxic, economical, environmentally friendly, and most significant controlled-release formulations, so researchers are interested in the feasibility of their application in different sectors (Prajapati et al., 2022). Nonetheless, employing some polymeric nanoparticles at higher concentrations demonstrates a phytotoxicity effect on plants, and it depends on initial material selection, nanoparticle preparation methods, and the impact varies according to plant species (Jogaiah et al., 2021). Remarkably, no instances of phytotoxicity have been reported concerning RNAi-polymeric nanoparticles. Chitin is the second-most common natural polymer after cellulose and is obtained mainly from shrimps, crabs, lobsters, and crawfish by-products (Figure 1; Faqir et al., 2021). Chitin is a linear, poly-(1,4)-N-acetyl-D glucosamine that appears in nature as organized crystalline microfibrils called α-chitin, β-chitin, and γ-chitin (Vani and Stanley, 2013). Chitosan is a partly deacetylated polymer of N-acetyl glucosamine produced by the alkaline deacetylation of chitin (Figure 1). Chitosan has several unique features due to the amine and hydroxyl groups, making it useful in many contexts and accessible for chemical reactions (Chouhan and Mandal, 2021). Since it may produce safe and non-toxic complexes through electrostatic interaction with its positive cationic group and the negative anionic group of the RNAi molecules (dsRNA/siRNA), it enhances the stability of RNAi molecules (Gurusamy et al., 2020a; Sandal et al., 2023). A natural process of RNAi converts dsRNA into 21-25-nucleotide-long siRNAs, which are then recruited to the RNA-induced silencing complex (RISC), which then finds and degrades the mRNA (Fire et al., 1998; Agrawal et al., 2003; Yu et al., 2013). RNAi has demonstrated considerable potential for formulating new pest control practices because of its species specificity and high efficacy (Zhu and Palli, 2020; Joga et al., 2021). However, it is underexploited in the forestry sector (Joga et al., 2021; Mogilicherla et al., 2022).
Figure 1. Scheme illustrating the RNAi-chitosan biopesticides formulations and their applications for forest insect pest management. (A) Synthesis of chitosan nanoparticles: marine by-products will produce chitin, which has been partially deacetylated and yields chitosan. Chitosan nanoparticles produced by chitosan dissolved in acetic acid under spontaneous mechanical churning at room temperature. (B) Production of RCNPs: RCNPs can be created via the adsorption, encapsulation, and electrostatic interaction approaches. Also, chitosan can be used as a coating material for dsRNA-expressed symbiotic microbes. RCNPs can be characterized in terms of size, zeta potential, and shape. (C) Putative application of RNCPs: RCNPs can be applied to forests to control forest pests and diseases using the foliar application, trunk injection, and soil drenching approaches leading to the species-specific killing of forest insect pests (bark beetles, termites, ants). (D) Environmental impact: deploying RNCPs will reduce the application of commercial pesticides.
Variable RNAi efficiency among insects has been linked to several mechanisms, including dsRNA degradation in the hemolymph and midgut lumen, decreased dsRNA uptake by cells, decreased induction of RNAi components upon exposure to dsRNA, missing components in the RNAi pathway, and accumulation of dsRNA in endosomes (Katoch et al., 2013; Shukla et al., 2016; Singh et al., 2017; Yoon et al., 2017; Cooper et al., 2019). The last 10 years have spotted the development and implementation of a chitosan-based dsRNA delivery method that boosts the possibility of RNAi applications in insect pest management (Table 1; Zhang et al., 2010; Das et al., 2015; Gurusamy et al., 2020a; Kolge et al., 2021). In order to prevent insect pests and diseases, chitosan-RNAi is utilized in the field of agriculture (Reglinski et al., 2004; Fitza et al., 2013; Bharani et al., 2014; Sahab et al., 2015; Silva-Castro et al., 2018; Ingle et al., 2022) and can also be used for forest protection (Joga et al., 2021; Mogilicherla et al., 2022). This succinct perspective discusses the synthesis of RNAi-chitosan nanopesticides (RCNPs) and characterization, as well as the evaluation of their effectiveness and biocompatibility against insect pests and microbes from a forest insect pest management and forest health point of view (Figure 1).
Chitosan is a polycationic polysaccharide that occurs naturally and is produced when chitin is partially deacetylated (Figure 1). Chitosan has several physicochemical characteristics, including molecular weight, viscosity, degree of deacetylation, and crystallinity (Kas, 1997; Riseh et al., 2022). A primary amine group with a pKa value of around 6.5 is present in every deacetylated subunit of chitosan; as a result, chitosan is soluble in acidic pH, like acetic acid but insoluble in neutral and alkaline pH. The amount of chitosan© deacetylation, molecular weight, ionic strength of the solution, and pH significantly impact its solubility (Mao et al., 2010). Chitosan dissolved in acetic acid and spontaneous mechanical churning at room temperature leads to caused nanoparticles. In addition, adjusting the chitosan-to-stabilizer ratio altered the particle size and surface charge (Hosseini et al., 2015). Several methods have been described for synthesizing RCNPs, such as electrostatic interaction, encapsulation, and adsorption (Figure 1). When chitosan is dissolved in acidic circumstances, the degree of chitosan deacetylation (DCDA) value influences the positive charge density; more DCDA results in an enhanced positive charge, allowing a better dsRNA/siRNA binding capacity (Liu et al., 2007; Mao et al., 2010). The ionotropic gelation method uses the electrostatic contact between a negatively charged group of nucleotides (e.g., in dsRNA) and the amine group of chitosan and self-assembled to form the polyelectrolyte complex (PEC) as a result of a decrease in hydrophilicity caused by charge neutralization between the cationic polymer and dsRNA. When dsRNA is added to chitosan (in acetic acid) solution and with continuous stirring at room temperature, the RCNPs can be produced spontaneously (Figure 1). Also, chitosan© molecular weight affects the physicochemical characteristics of RCNPs, including their size, zeta potential, shape, and complex stability (Mao et al., 2010). The surface charge of the RCNPs is dependent on the molar ratio of chitosan nitrogen (N) to dsRNA phosphate (P) (N/P ratio), which affects the particle capacity to efficiently condense dsRNA and interact with negatively charged cells, which in turn affects the transfection efficiency (Köping-Höggård et al., 2001; Huang et al., 2005; Jeong et al., 2007; Nafee et al., 2007). The chitosan salt form also impacted the RCNPs, such as chitosan glutamate, which had a larger molecular weight, created smaller complexes with dsRNA/siRNA, and had a higher siRNA loading efficiency than chitosan hydrochloride (Katas and Alpar, 2006). The amount of dsRNA at a certain point within the RCNPs plays a fundamental role in host cell transfection efficiency, whereas more concentration of dsRNA will increase the diameter of the particles and form an aggregation, and will decline the transfection (MacLaughlin et al., 1998; Romøren et al., 2003; Zhao et al., 2006; Mao et al., 2010). Chitosan can be employed as a dsRNA-chitosan complex as well as a coating material for symbiotic microbes that express dsRNA to provide a flexible technology platform for the management of forest insect pests (Figure 1; Mao et al., 2010; Joga et al., 2021; Riseh et al., 2022).
RNAi-chitosan nanopesticides extend to precision use due to their minuscule dimensions, high surface area, enhanced permeability, thermal stability, dispersion, and biodegradability to improve forest yield and to control target action based on insect pests or microbes infection (Figure 1; Adisa et al., 2019; Kumar et al., 2019). For applying RCNPs in forestry, several methods like foliar application, trunk injection, and soil drenching can be considered (Figure 1; Joga et al., 2021; Mogilicherla et al., 2022). Chitosan nanoparticle-mediated RNAi has been developed over the last 10 years as an alternative to traditional pest control methods (Table 1).
The formulations of RCNPs have significant potential to control the attack of several common pests like aphids, moths, and beetles (Sahab et al., 2015; Gurusamy et al., 2020a). Silencing of the CHS1, CHS2, semaphorin-1a, and vestigial (vg) genes by feeding chitosan-dsRNA nanoparticles to mosquitoes (Anopheles gambiae and Aedes aegypti) showed more pesticide-susceptible (Zhang et al., 2010, 2015; Mysore et al., 2013; Kumar et al., 2016). Our group and colleagues successfully knocked down the target genes (CAD, AMN, CHS, JHAMT, AMY, V-ATPase, IAP1, V-ATPase B, Sec23, SNF7, and SRC) using chitosan-dsRNA nanoparticles and observed decent mortality in A. aegypti and Spodoptera frugiperda (Das et al., 2015; Gurusamy et al., 2020a). Also, the complexes of chitosan-sodium tripolyphosphate-dsRNA (CS-TPP-dsRNA) showed improved mortality in A. aegypti (Dhandapani et al., 2019). In another study, the chitosan-dsRNA nanopesticides showed good stability, cellular uptake, and mortality in Chilo suppressalis (Wang et al., 2020). Helicoverpa armigera was significantly controlled when RCNPs were applied topically to chickpea plants (Kolge et al., 2021). Additionally, RCNPs were stable for 5 days on leaf surfaces, effectively protected from nuclease degradation and insect gut pH, and efficiently knocked down the targeted genes (JHAMT and AChE), resulting in 100% insect mortality, whereas the non-targeted insects like Spodoptera litura and Drosophila melanogaster were unaffected and showed no signs of toxicity (Kolge et al., 2021, 2023). A recent study demonstrated that topically applying dsRNA-coated with rosin-modified PEG and chitosan (dsRNA/ROPE@C) to Nilaparvata lugens (Brown plant hopper) causes excellent gene knockdown and mortality (Lyu et al., 2023). Recently, our team created chitosan-dsRNA nanopesticides, fed them to bollworms (Earias vittella), and observed considerable target gene knockdown and mortality (Sandal et al., 2023). Additionally, the price drop from $12500 to $2 for 1 g of dsRNA has increased the likelihood that RNAi technology will be applied in the field (Zotti et al., 2018). Our colleagues successfully applied bacterially expressed dsRNA in a tropical setting and observed a significant reduction in Colorado potato beetle (CPB) infection (Máximo et al., 2020; Petek et al., 2020). Most recently, researchers developed an RNAi-based biopesticide known as “ledprona” against the CPB, which inhibits enzyme expression, facilitates protein breakdown, and ultimately causes mortality (Pallis et al., 2023). These investigations could pave the path for creating and using RCNPs as a safe, effective, and novel way to protect crops and forest trees.
Furthermore, researchers used the chitosan domain to encapsulate metal-based nanoparticles (Ag, Au, Fe, Co, Cu, TiO2, ZnO, SiO2, and CaCO3) to increase plant resilience to salt, drought, and heavy metal environments (Souri et al., 2017; Behboudi et al., 2019; Sen et al., 2020; Ali et al., 2021; Sheikhalipour et al., 2021) and improve their health for protecting themselves from other biotic stresses (Naidu et al., 2023). The previous studies successfully used double-layered hydroxide (LDH), carbon quantum dots (CQD), branched amphiphilic peptide capsules (BAPCs), and lipid nanoparticle-based dsRNA formulations to address biotic stress caused by insects (Mitter et al., 2017; Christiaens et al., 2020; Gurusamy et al., 2020b; Kaur et al., 2020). Such findings encourage researchers to adopt similar approaches to improve forest health. However, the above-mentioned nanomaterials have some limitations, i.e., manufacturing synthetic nanomaterials is expensive, and excessive nanoparticle concentrations may negatively impact forest soil health and microfauna. Dedicated studies can evaluate the feasibility of these nanoparticles in forest protection.
Chitosan is frequently utilized as a carrier for encasing microbial agents because of its ability to take the form of particles, films, capsules, gels, fibres, and porous forms and its unquestionable success in field applications (Lakkis, 2016; Saberi Riseh et al., 2021). Three potential methods (diffusion, osmotic burst, and erosion or breakdown) will work separately or together and release the microbial substances from chitosan encapsulations. Encapsulating chitosan-microbes (chitosan-ATCC393 and chitosan-139S1) can protect against several environmental challenges (Li et al., 2011; Vejan et al., 2019). Moreover, the Harpinpss-chitosan, BMI-chitosan, B. thuringiensis-chitosan, B. cereus-chitosan, E. fergusonii-chitosan, B. thuringiensis-chitosan, and Pseudomonas-chitosan encapsulations tested on tomato, soybean, cotton, tobacco, bean, corn, and Hyaloptera peroni plants showed a reduction in egg-laying in female insects, thereby reducing the population and insect damage (Badawy and El-Aswad, 2012; Zeng et al., 2012; Chandrashekharaiah et al., 2015; Sahab et al., 2015; Badawy and Rabea, 2016; Kitherian, 2017; Ureña Saborío et al., 2017; Nadendla et al., 2018; De Oliveira et al., 2021). Based on the aforementioned findings, RNAi molecules expressed in microbes that can be encapsulated with chitosan are a viable technology and can be used as RNAi-biopesticides in forest pest management (Figure 1). However, such potential demands further dedicated studies and pilot field experiments.
SMR is a potent tool, and researchers have developed endogenous symbionts to express target dsRNAs for insect control (Chen et al., 2015; Hu and Wu, 2016; Whitten et al., 2016; Hu and Xia, 2019). Recent research identified the bacterial symbionts, used them to express dsRNA effectively, and controlled the two evolutionarily divergent insect species (R. prolixus and F. occidentalis) (Whitten et al., 2016). Additionally, entomopathogenic fungi were identified and used to induce fungal-induced gene silencing (FIGS) in the insects B. tabaci and L. migratoria (Chen et al., 2015; Hu and Xia, 2019). Our colleagues from the United States are deploying fungal-induced gene silencing (FIGS) technology to manage bark beetles, i.e., genetically modifying the bark beetle-associated yeast Ogataea pini, to generate specific dsRNA molecules that target Ips calligraphus (information based on personal communication). Our team has also successfully identified and isolated insect-symbiotic bacteria and fungi (Chakraborty et al., 2020a,b, 2023b) and may use them as a CSMR for tropical application to control the bark beetles and termites (Gupta et al., 2023). Recently, our group identified 69 core bacterial genera and 19 fungal genera among six bark beetles (Ips typographus, Ips duplicatus, Ips cembrae; Ips sexdentatus, Ips acuminatus, and Polygraphus poligraphus). Notably, the most abundant bacterial genera were Erwinia, Sodalis, Serratia, Tyzzerella, Raoultella, Rahnella, Wolbachia, Spiroplasma, Vibrio, and Pseudoxanthomonas whereas the most abundant fungal genera belong to the phylum Ascomycota (Chakraborty et al., 2020a,b, 2023a). Further, our group focused on exploring how varying ages of Norway spruce wood and different terpene concentrations affect the microbial compositions associated with two termite species, Reticulitermes flavipes and Microcerotermes biroi (Chakraborty et al., 2023b). In termite-infested wood samples, the relative abundance of bacterial genera like Pseudomonas, Massilia, and Rhizobium was high, and Spirochaeta and Treponema revealed notable changes in relative abundance between these two species. Moreover, within termite-infested wood, fungal communities affiliated with the Eurotiales, Sordariales, Hypocreales, Trichospornales, and Ophiostomatales orders were identified, notably, the fungal genera Apiotrichum, Fusarium, Hawksworthiomyces, Lasiodiplodia, Sporothrix, Trichosporon, and Trichoderma displayed substantial prevalence in the termite-infested wood. As described thoroughly in our recent review, some identified microbial associates of bark beetle or termites can be good candidates for Symbiont-mediated RNAi or SMR (Gupta et al., 2023). Nevertheless, SMR technology can be considered for its potential in forest conservation; additional refinements are necessary before applications.
In order to increase forest production and health, RCNPs will be utilized more frequently in forestry and dispersed into the environment. Due to their biodegradable nature, these substances may not harm non-target organisms; they may not bioaccumulate and not interact with other environmental contaminants and dissolved organic matter, which means they will not harm the environment as well as humans and animals (Chandy and Sharma, 1990; Aspden et al., 1997; Rao and Sharma, 1997). RNAi-biopesticides made of chitosan are easily dissolved in nature and unable to accumulate in the food chain, stunt plant growth, or potentially harm people and animals. Although studies have shown that nanoparticles pose a risk to the environment, they have also sparked much interest in environmental cleanup (Roy et al., 2021). Therefore, more research is necessary to comprehend RCNPs and their relationship to the environment thoroughly. Understanding the characteristics of various RNAi-chitosan bioformulation is crucial, as is making comparisons between pure active ingredients and both nanoformulations and traditional formulations to see how the behaviour of the active components changes (Kah et al., 2018).
Applications for RCNP in the forest have numerous obstacles, including developing different delivery strategies for various microbes and insect pests, facilitating plant uptake and in planta systemic movement of RCNPs, looking for synergistic effects, such as dsRNAs targeting multiple genes and combining RNAi with other pest control methods, and establishing a congruent confluence, building a regulatory framework that is widely accepted for the commercialization, therefore lowering the price for their widespread use. Recently, RCNPs were used in the field, and the result demonstrated their compact size, cationic charge, effective loading, resistance to degradation, effective cellular uptake, stability, and adhesion to leaf surfaces (Petek et al., 2020; Kolge et al., 2021, 2023). Few RNAi-based insecticides have so far been licensed and will soon be available on the market (Li et al., 2023; Pallis et al., 2023).
The advancement of RNAi-nanotechnology has been beneficial to forestry. However, strict controls are in place for forests to ensure the security of feed and food sources, possible risks to human and animal health, non-target organisms and beneficial microbes, and the long-term environmental effects of the deliberate release of RNAi-nanomaterials (Kumar et al., 2019; Gilbertson et al., 2020; Hofmann et al., 2020; Mogilicherla et al., 2022). The European Union is developing regulatory rules for engineered RNAi-nanomaterials in forestry food safety (Lowry et al., 2019). Preparing regulatory guidelines for RNAi-nanomaterials is more difficult due to several factors, including the difficulty in defining nanomaterials, tracing their sources and transport pathways, quantifying them in environmental samples, assessing their bioavailability, and interpreting their toxicity (Lai et al., 2018; Hofmann et al., 2020; Gottardo et al., 2021). Under such circumstances, creating cutting-edge analytical methods for regulatory purposes is necessary.
The capacity of researchers and scientists to develop forest pest-specific dsRNAs will increase as more omics data for forest insects, helpful microorganisms, and non-target organisms become accessible and help to reduce possible risks. Fortunately, our group and CZU colleagues (CZU, Prague) have recently involved forest insects (bark beetles and termites) genome and transcriptome sequencing and their symbiotic microbes transcriptome sequencing, which along with other excellent efforts from colleagues worldwide, will significantly enhance sequence information on forest insect pests and facilitate future species-specific RNAi-based biopesticides development.
RCNPs may replace currently used pesticides since they are biodegradable, biocompatible, and low toxicity (Figure 1). Chitosan nanoparticles can encapsulate different RNAi molecules (dsRNA/siRNA) and RNAi-symbiotic microbes and form RCNPs. They are more effective and have better bioavailability, a longer half-life, and a higher surface-to-volume ratio and act as a bio-stimulant used to combat microbial illnesses and insect pests in forest management. RCNPs can be applied in forests using various techniques, including foliar application, trunk injection, and soil drenching. Based on current findings, using RCNPs can also increase forest productivity, protect forests from insect pests, and extend their commercialization. However, research on product development and technique optimization is required before commercial manufacture and environmental application. Nevertheless, this perspective will provide new direction to the research community working on forest protection and enhance their interest in using alternative approaches, such as deploying molecular toolboxes against forest insect pests.
The original contributions presented in this study are included in the article, further inquiries can be directed to the corresponding author.
AR contributed to the conceptualization. KM wrote the first draft. Both authors contributed to the figure preparation, reviewing, and finalizing the draft.
AR and KM are supported by grant no. CZ.02.1.01/0.0/0.0/15_003/0000433; Grant No. CZ.02.1.01/0.0/0.0/16_019/0 000803 financed by OP RDE and “Excellent team” grant 2023-24 from FLD, CZU.
We appreciate the helpful feedback provided by two reviewers and the handling editor.
The authors declare that the research was conducted in the absence of any commercial or financial relationships that could be construed as a potential conflict of interest.
All claims expressed in this article are solely those of the authors and do not necessarily represent those of their affiliated organizations, or those of the publisher, the editors and the reviewers. Any product that may be evaluated in this article, or claim that may be made by its manufacturer, is not guaranteed or endorsed by the publisher.
A. aegypti, Aedes aegypti; AchE, acetylcholine esterase; AMN, Aminopeptidase; AMY, Alpha-amylase; A. gambiae, Anopheles gambiae; A. solani, Alternaria solani; BMI, bacterial metabolic infiltrates; CAD, Cadheri; CHS, Chitin synthase; CHS1, chitin synthase 1; CHS2, chitin synthase 2; CPB, Colorado potato beetle; D. melanogaster, Drosophila melanogaster; DCDA, degree of chitosan deacetylation; dsRNA, double-stranded RNA; E. vittella, Earias vittella; H. armigera, Helicoverpa armigera; IAP1, Inhibitor of apoptosis 1; JHAMT, juvenile hormone methyltransferase; mRNA, complementary messenger RNA; N. lugens, Nilaparvata lugens; P. grisea, Pyricularia grisea; PEC, polyelectrolyte complex; PRR, pattern recognization receptor; PSTV, Potato spindle tuber virus; RCNPs, RNAi-chitosan nanopesticides; RISC, RNA-induced silencing complex; Sec23, Sec23 homolog A; SNF7, ESCRT-III subunit protein SNF7; SRC, SRC proto-oncogene; S. frugiperda, Spodoptera frugiperda; S.litura, Spodoptera litura; S. lycopersicum, Solanum lycopersicum; siRNA, small interfering RNA; TBSV, bean/tomato bushy stunt virus; TNV, tobacco necrosis virus; Vg, vestigial; V-ATPase, V-type proton ATPase; V-ATPase B, Vacuolar-type ATPase B; SMR, symbiont mediated RNAi.
Adisa, I. O., Pullagurala, V. L. R., Peralta-Videa, J. R., Dimkpa, C. O., Elmer, W. H., Gardea-Torresdey, J. L., et al. (2019). Recent advances in nano-enabled fertilizers and pesticides: a critical review of mechanisms of action. Environ. Sci. 6, 2002–2030. doi: 10.1039/C9EN00265K
Agrawal, N., Dasaradhi, P., Mohmmed, A., Malhotra, P., Bhatnagar, R. K., and Mukherjee, S. K. (2003). RNA interference: biology, mechanism, and applications. Microbiol. Mol. Biol. Rev. 67, 657–685. doi: 10.1128/MMBR.67.4.657-685.2003
Ali, E., El-Shehawi, A., Ibrahim, O., Abdul-Hafeez, E., Moussa, M., and Hassan, F. (2021). A vital role of chitosan nanoparticles in improvisation the drought stress tolerance in Catharanthus roseus (L.) through biochemical and gene expression modulation. Plant Physiol. Biochem. 161, 166–175. doi: 10.1016/j.plaphy.2021.02.008
Aspden, T. J., Mason, J. D., Jones, N. S., Lowe, J., Skaugrud, Ø, and Illum, L. (1997). Chitosan as a nasal delivery system: the effect of chitosan solutions on in vitro and in vivo mucociliary transport rates in human turbinates and volunteers. J. Pharm. Sci. 86, 509–513. doi: 10.1021/js960182o
Badawy, M. E., and El-Aswad, A. F. (2012). Insecticidal activity of chitosans of different molecular weights and chitosan-metal complexes against cotton leafworm Spodoptera littoralis and oleander aphid Aphis nerii. Plant Protect. Sci. 48, 131–141. doi: 10.17221/67/2010-PPS
Badawy, M. E., and Rabea, E. I. (2016). “Chitosan and its derivatives as active ingredients against plant pests and diseases,” in Chitosan in the preservation of agricultural commodities, eds S. Bautista-Baòos, G. Romanazzi, and A. Jiménez-Aparicio (Amsterdam: Elsevier). doi: 10.1016/B978-0-12-802735-6.00007-0
Behboudi, F., Tahmasebi-Sarvestani, Z., Kassaee, M. Z., Modarres-Sanavy, S. A. M., Sorooshzadeh, A., and Mokhtassi-Bidgoli, A. (2019). Evaluation of chitosan nanoparticles effects with two application methods on wheat under drought stress. J. Plant Nutr. 42, 1439–1451. doi: 10.1080/01904167.2019.1617308
Bharani, R. A., Namasivayam, S. K. R., and Shankar, S. S. (2014). Biocompatible chitosan nanoparticles incorporated pesticidal protein beauvericin (CSNp-BV) preparation for the improved pesticidal activity against major groundnut defoliator Spodoptera litura (Fab.)(Lepidoptera. Noctuidae). Int. J. ChemTech. Res. 6, 5007–5012.
Bras, A., Roy, A., Heckel, D. G., Anderson, P., and Karlsson Green, K. (2022). Pesticide resistance in arthropods: Ecology matters too. Ecol. Lett. 25, 1746–1759. doi: 10.1111/ele.14030
Chakraborty, A., Ashraf, M. Z., Modlinger, R., Synek, J., Schlyter, F., and Roy, A. (2020a). Unravelling the gut bacteriome of Ips (Coleoptera: Curculionidae: Scolytinae): identifying core bacterial assemblage and their ecological relevance. Sci. Rep. 10, 18572. doi: 10.1038/s41598-020-75203-5
Chakraborty, A., Modlinger, R., Ashraf, M. Z., Synek, J., Schlyter, F., and Roy, A. (2020b). Core mycobiome and their ecological relevance in the gut of five Ips bark beetles (Coleoptera: Curculionidae: Scolytinae). Front. Microbiol. 11, 568853. doi: 10.3389/fmicb.2020.568853
Chakraborty, A., Purohit, A., Khara, A., Modlinger, R., and Roy, A. (2023a). Life-stage and geographic location determine the microbial assemblage in Eurasian spruce bark beetle, Ips typographus L.(Coleoptera: Curculionidae). Front. For. Global Change 6, 1176160. doi: 10.3389/ffgc.2023.1176160
Chakraborty, A., Šobotník, J., Votýpková, K., Hradecký, J., Stiblik, P., Synek, J., et al. (2023b). Impact of wood age on termite microbial assemblages. Appl. Environ. Microbiol. 89, e361–e323. doi: 10.1128/aem.00361-23
Chandrashekharaiah, M., Kandakoor, S. B., Basana Gowda, G., Kammar, V., and Chakravarthy, A. (2015). Nanomaterials: a review of their action and application in pest management and evaluation of DNA-tagged particles. New Delhi: Springer. doi: 10.1007/978-81-322-2089-3_12
Chandy, T., and Sharma, C. P. (1990). Chitosan-as a biomaterial. Biomater. Artif. Cells Artif. Organs 18, 1–24. doi: 10.3109/10731199009117286
Chen, X., Li, L., Hu, Q., Zhang, B., Wu, W., Jin, F., et al. (2015). Expression of dsRNA in recombinant Isaria fumosorosea strain targets the TLR7 gene in Bemisia tabaci. BMC Biotechnol. 15, 753790. doi: 10.1186/s12896-015-0170-8
Chhipa, H. (2017). Nanopesticide: current status and future possibilities. Agric. Res. Technol. 5, 1–4. doi: 10.19080/ARTOAJ.2017.05.555651
Chouhan, D., and Mandal, P. (2021). Applications of chitosan and chitosan based metallic nanoparticles in agrosciences-A review. Int. J. Biol. Macromol. 166, 1554–1569. doi: 10.1016/j.ijbiomac.2020.11.035
Christiaens, O., Petek, M., Smagghe, G., and Taning, C. N. T. (2020). “The use of nanocarriers to improve the efficiency of RNAi-based pesticides in agriculture,” in Nanopesticides: From research and development to mechanisms of action and sustainable use in agriculture, eds L. F. Fraceto, V. L. de Castro, R. Grillo, D. Ávila, H. Caixeta Oliveira, and R. Lima (Cham: Springer International Publishing). doi: 10.1111/1744-7917.12822
Cooper, A. M., Silver, K., Zhang, J., Park, Y., and Zhu, K. Y. (2019). Molecular mechanisms influencing efficiency of RNA interference in insects. Pest Manage. Sci. 75, 18–28. doi: 10.1002/ps.5126
Cooper, A. M., Song, H., Shi, X., Yu, Z., Lorenzen, M., Silver, K., et al. (2020). Molecular characterizations of double-stranded RNA degrading nuclease genes from Ostrinia nubilalis. Insects 11:652. doi: 10.3390/insects11100652
Das, S., Debnath, N., Cui, Y., Unrine, J., and Palli, S. R. (2015). Chitosan, carbon quantum dot, and silica nanoparticle mediated dsRNA delivery for gene silencing in Aedes aegypti: a comparative analysis. ACS Appl. Mater. Interfaces 7, 19530–19535. doi: 10.1021/acsami.5b05232
De Oliveira, J. L., Fraceto, L. F., Bravo, A., and Polanczyk, R. A. (2021). Encapsulation strategies for bacillus thuringiensis: From now to the future. J. Agric. Food Chem. 69, 4564–4577. doi: 10.1021/acs.jafc.0c07118
Dhandapani, R. K., Gurusamy, D., Howell, J. L., and Palli, S. R. (2019). Development of CS-TPP-dsRNA nanoparticles to enhance RNAi efficiency in the yellow fever mosquito, Aedes aegypti. Sci. Rep. 9, 8775. doi: 10.1038/s41598-019-45019-z
Faqir, Y., Ma, J., and Chai, Y. (2021). Chitosan in modern agriculture production. Plant Soil Environ. 67, 679–699. doi: 10.17221/332/2021-PSE
Fire, A., Xu, S., Montgomery, M. K., Kostas, S. A., Driver, S. E., and Mello, C. C. (1998). Potent and specific genetic interference by double-stranded RNA in Caenorhabditis elegans. Nature 391, 806–811. doi: 10.1038/35888
Fitza, K. N., Payn, K., Steenkamp, E. T., Myburg, A. A., and Naidoo, S. (2013). Chitosan application improves resistance to Fusarium circinatum in Pinus patula. South Afr. J. Bot. 85, 70–78. doi: 10.1016/j.sajb.2012.12.006
Gilbertson, L. M., Pourzahedi, L., Laughton, S., Gao, X., Zimmerman, J. B., Theis, T. L., et al. (2020). Guiding the design space for nanotechnology to advance sustainable crop production. Nat. Nanotechnol. 15, 801–810. doi: 10.1038/s41565-020-0706-5
Gottardo, S., Mech, A., Drbohlavová, J., Małyska, A., Bøwadt, S., Sintes, J. R., et al. (2021). Towards safe and sustainable innovation in nanotechnology: State-of-play for smart nanomaterials. NanoImpact 21, 100297. doi: 10.1016/j.impact.2021.100297
Gupta, S., Chakraborty, A., and Roy, A. (2023). Prospects for deploying microbes against tree-killing beetles (Coleoptera) in Anthropocene. Front. For. Global Change 6, 1182834. doi: 10.3389/ffgc.2023.1182834
Gurusamy, D., Mogilicherla, K., and Palli, S. R. (2020a). Chitosan nanoparticles help double-stranded RNA escape from endosomes and improve RNA interference in the fall armyworm, Spodoptera frugiperda. Arch. Insect Biochem. Physiol. 104, e21677. doi: 10.1002/arch.21677
Gurusamy, D., Mogilicherla, K., Shukla, J. N., and Palli, S. R. (2020b). Lipids help double-stranded RNA in endosomal escape and improve RNA interference in the fall armyworm, Spodoptera frugiperda. Arch. Insect Biochem. Physiol. 104, e21678. doi: 10.1002/arch.21678
Hofmann, T., Lowry, G. V., Ghoshal, S., Tufenkji, N., Brambilla, D., Dutcher, J. R., et al. (2020). Technology readiness and overcoming barriers to sustainably implement nanotechnology-enabled plant agriculture. Nat. Food 1, 416–425. doi: 10.1038/s43016-020-0110-1
Hosseini, S. F., Rezaei, M., Zandi, M., and Farahmandghavi, F. (2015). Fabrication of bio-nanocomposite films based on fish gelatin reinforced with chitosan nanoparticles. Food Hydrocolloids 44, 172–182. doi: 10.1016/j.foodhyd.2014.09.004
Hu, J., and Xia, Y. (2019). Increased virulence in the locust-specific fungal pathogen Metarhizium acridum expressing dsRNAs targeting the host F1F0-ATPase subunit genes. Pest Manage. Sci. 75, 180–186. doi: 10.1002/ps.5085
Hu, Q., and Wu, W. (2016). Recombinant fungal entomopathogen RNAi target insect gene. Bioengineered 7, 504–507. doi: 10.1080/21655979.2016.1146833
Huang, M., Fong, C.-W., Khor, E., and Lim, L.-Y. (2005). Transfection efficiency of chitosan vectors: effect of polymer molecular weight and degree of deacetylation. J. Control. Release 106, 391–406. doi: 10.1016/j.jconrel.2005.05.004
Ingle, P. U., Shende, S. S., Shingote, P. R., Mishra, S. S., Sarda, V., Wasule, D. L., et al. (2022). Chitosan nanoparticles (ChNPs): A versatile growth promoter in modern agricultural production. Heliyon 8, e11893. doi: 10.1016/j.heliyon.2022.e11893
Jeong, J. H., Kim, S. W., and Park, T. G. (2007). Molecular design of functional polymers for gene therapy. Prog. Polym. Sci. 32, 1239–1274. doi: 10.1016/j.progpolymsci.2007.05.019
Joga, M. R., Mogilicherla, K., Smagghe, G., and Roy, A. (2021). RNA interference-based forest protection products (FPPs) against wood-boring coleopterans: Hope or hype? Front. Plant Sci. 12, 733608. doi: 10.3389/fpls.2021.733608
Jogaiah, S., Paidi, M. K., Venugopal, K., Geetha, N., Mujtaba, M., Udikeri, S. S., et al. (2021). Phytotoxicological effects of engineered nanoparticles: An emerging nanotoxicology. Sci. Total Environ. 801, 149809. doi: 10.1016/j.scitotenv.2021.149809
Kah, M., Kookana, R. S., Gogos, A., and Bucheli, T. D. (2018). A critical evaluation of nanopesticides and nanofertilizers against their conventional analogues. Nat. Nanotechnol. 13, 677–684. doi: 10.1038/s41565-018-0131-1
Kan, S., Chen, B., Persson, U. M., Chen, G., Wang, Y., Li, J., et al. (2023). Risk of intact forest landscape loss goes beyond global agricultural supply chains. One Earth 6, 55–65. doi: 10.1016/j.oneear.2022.12.006
Kas, H. S. (1997). Chitosan: properties, preparations and application to microparticulate systems. J. Microencapsul. 14, 689–711. doi: 10.3109/02652049709006820
Katas, H., and Alpar, H. O. (2006). Development and characterisation of chitosan nanoparticles for siRNA delivery. J. Control. Release 115, 216–225. doi: 10.1016/j.jconrel.2006.07.021
Katoch, R., Sethi, A., Thakur, N., and Murdock, L. L. (2013). RNAi for insect control: current perspective and future challenges. Appl. Biochem. Biotechnol. 171, 847–873. doi: 10.1007/s12010-013-0399-4
Kaur, R., Gupta, M., Singh, S., Joshi, N., and Sharma, A. (2020). Enhancing RNAi efficiency to decipher the functional response of potential genes in Bemisia tabaci AsiaII-1 (Gennadius) through dsRNA feeding assays. Front. Physiol. 11, 123. doi: 10.3389/fphys.2020.00123
Kitherian, S. (2017). Nano and bio-nanoparticles for insect control. Research J. Nanosci. Nanotechnol. 7, 1–9. doi: 10.3923/rjnn.2017.1.9
Kolge, H., Kadam, K., Galande, S., Lanjekar, V., and Ghormade, V. (2021). New frontiers in pest control: Chitosan nanoparticles-shielded dsRNA as an effective topical RNAi spray for Gram Podborer biocontrol. ACS Appl. Bio Mater. 4, 5145–5157. doi: 10.1021/acsabm.1c00349
Kolge, H., Kadam, K., and Ghormade, V. (2023). Chitosan nanocarriers mediated dsRNA delivery in gene silencing for Helicoverpa armigera biocontrol. Pest. Biochem. Physiol. 189, 105292. doi: 10.1016/j.pestbp.2022.105292
Köping-Höggård, M., Tubulekas, I., Guan, H., Edwards, K., Nilsson, M., Vårum, K. M., et al. (2001). Chitosan as a nonviral gene delivery system. Structure–property relationships and characteristics compared with polyethylenimine in vitro and after lung administration in vivo. Gene Therapy 8, 1108–1121. doi: 10.1038/sj.gt.3301492
Kumar, D. R., Kumar, P. S., Gandhi, M. R., Al-Dhabi, N. A., Paulraj, M. G., and Ignacimuthu, S. (2016). Delivery of chitosan/dsRNA nanoparticles for silencing of wing development vestigial (vg) gene in Aedes aegypti mosquitoes. Int. J. Biol. Macromol. 86, 89–95. doi: 10.1016/j.ijbiomac.2016.01.030
Kumar, S., Nehra, M., Dilbaghi, N., Marrazza, G., Hassan, A. A., and Kim, K.-H. (2019). Nano-based smart pesticide formulations: Emerging opportunities for agriculture. J. Control. Release 294, 131–153.
Lai, R. W., Yeung, K. W., Yung, M. M., Djurišić, A. B., Giesy, J. P., and Leung, K. M. (2018). Regulation of engineered nanomaterials: current challenges, insights and future directions. Environ. Sci. Pollut. Res. 25, 3060–3077.
Lakkis, J. M. (2016). Encapsulation and controlled release technologies in food systems. Hoboken, NJ: John Wiley & Sons.
Li, M., Sun, X., Yin, M., Shen, J., and Yan, S. (2023). Recent advances in nanoparticle-mediated co-delivery system: A promising strategy in medical and agricultural field. Int. J. Mol. Sci. 24, 5121. doi: 10.3390/ijms24065121
Li, X. Y., Chen, X. G., Sun, Z. W., Park, H. J., and Cha, D.-S. (2011). Preparation of alginate/chitosan/carboxymethyl chitosan complex microcapsules and application in Lactobacillus casei ATCC 393. Carbohyd. Polym. 83, 1479–1485.
Liu, X., Howard, K. A., Dong, M., Andersen, M. Ø, Rahbek, U. L., Johnsen, M. G., et al. (2007). The influence of polymeric properties on chitosan/siRNA nanoparticle formulation and gene silencing. Biomaterials 28, 1280–1288.
Lowry, G. V., Avellan, A., and Gilbertson, L. M. (2019). Opportunities and challenges for nanotechnology in the agri-tech revolution. Nat. Nanotechnol. 14, 517–522.
Lyu, Z., Xiong, M., Mao, J., Li, W., Jiang, G., and Zhang, W. (2023). A dsRNA delivery system based on the rosin-modified polyethylene glycol and chitosan induces gene silencing and mortality in Nilaparvata lugens. Pest Manage. Sci. 79, 1518–1527. doi: 10.1002/ps.7322
MacLaughlin, F. C., Mumper, R. J., Wang, J., Tagliaferri, J. M., Gill, I., Hinchcliffe, M., et al. (1998). Chitosan and depolymerized chitosan oligomers as condensing carriers for in vivo plasmid delivery. J. Control. Release 56, 259–272.
Mao, S., Sun, W., and Kissel, T. (2010). Chitosan-based formulations for delivery of DNA and siRNA. Adv. Drug Deliv. Rev. 62, 12–27.
Máximo, W. P., Howell, J. L., Mogilicherla, K., Basij, M., Chereddy, S. C., and Palli, S. R. (2020). Inhibitor of apoptosis is an effective target gene for RNAi-mediated control of Colorado potato beetle, Leptinotarsa decemlineata. Arch. Insect Biochem. Physiol. 104, e21685. doi: 10.1002/arch.21685
Mitter, N., Worrall, E. A., Robinson, K. E., Xu, Z. P., and Carroll, B. J. (2017). Induction of virus resistance by exogenous application of double-stranded RNA. Curr. Opin. Virol. 26, 49–55.
Mogilicherla, K., Chakraborty, A., Taning, C. N. T., Smagghe, G., and Roy, A. (2022). RNAi in termites (Isoptera): current status and prospects for pest management. Entomol. Gener. 43, 1638.
Mysore, K., Flannery, E. M., Tomchaney, M., Severson, D. W., and Duman-Scheel, M. (2013). Disruption of Aedes aegypti olfactory system development through chitosan/siRNA nanoparticle targeting of semaphorin-1a. PLoS Neglect. Trop. Dis. 7, e2215. doi: 10.1371/journal.pntd.0002215
Nadendla, S. R., Rani, T. S., Vaikuntapu, P. R., Maddu, R. R., and Podile, A. R. (2018). HarpinPss encapsulation in chitosan nanoparticles for improved bioavailability and disease resistance in tomato. Carbohyd. Polym. 199, 11–19. doi: 10.1016/j.carbpol.2018.06.094
Nafee, N., Taetz, S., Schneider, M., Schaefer, U. F., and Lehr, C.-M. (2007). Chitosan-coated PLGA nanoparticles for DNA/RNA delivery: effect of the formulation parameters on complexation and transfection of antisense oligonucleotides. Nanomedicine 3, 173–183. doi: 10.1016/j.nano.2007.03.006
Naidu, S., Pandey, J., Mishra, L. C., Chakraborty, A., Roy, A., Singh, I. K., et al. (2023). Silicon nanoparticles: Synthesis, uptake and their role in mitigation of biotic stress. Ecotoxicol. Environ. Safety 255, 114783.
Pallis, S., Alyokhin, A., Manley, B., Rodrigues, T., Barnes, E., and Narva, K. (2023). Effects of low doses of a novel dsRNA-based Biopesticide (Calantha) on the Colorado potato beetle. J. Econ. Entomol. 116, toad034. doi: 10.1093/jee/toad034
Petek, M., Coll, A., Ferenc, R., Razinger, J., and Gruden, K. (2020). Validating the potential of double-stranded RNA targeting Colorado potato beetle mesh gene in laboratory and field trials. Front. Plant Sci. 11, 1250. doi: 10.3389/fpls.2020.01250
Prajapati, D., Pal, A., Dimkpa, C., Singh, U., Devi, K. A., Choudhary, J. L., et al. (2022). Chitosan nanomaterials: A prelim of next-generation fertilizers; existing and future prospects. Carbohyd. Polym. 288, 119356. doi: 10.1016/j.carbpol.2022.119356
Rao, S. B., and Sharma, C. P. (1997). Use of chitosan as a biomaterial: studies on its safety and hemostatic potential. J. Biomed. Mater. Res. 34, 21–28.
Reglinski, T., Taylor, J., and Dick, M. (2004). Chitosan induces resistance to pitch canker in Pinus radiata. N. Zeal. J. For. Sci. 34, 49–58.
Riseh, R. S., Tamanadar, E., Hajabdollahi, N., Vatankhah, M., Thakur, V. K., and Skorik, Y. A. (2022). Chitosan microencapsulation of rhizobacteria for biological control of plant pests and diseases: Recent advances and applications. Rhizosphere 23, 100565.
Romøren, K., Pedersen, S., Smistad, G., Evensen, Ø, and Thu, B. J. (2003). The influence of formulation variables on in vitro transfection efficiency and physicochemical properties of chitosan-based polyplexes. Int. J. Pharm. 261, 115–127. doi: 10.1016/s0378-5173(03)00301-6
Roy, A., Sharma, A., Yadav, S., Jule, L. T., and Krishnaraj, R. (2021). Nanomaterials for remediation of environmental pollutants. Bioinorgan. Chem. Applic. 2021, 1764647.
Saberi Riseh, R., Ebrahimi-Zarandi, M., Gholizadeh Vazvani, M., and Skorik, Y. A. (2021). Reducing drought stress in plants by encapsulating plant growth-promoting bacteria with polysaccharides. Int. J. Mol. Sci. 22, 12979. doi: 10.3390/ijms222312979
Sahab, A., Waly, A., Sabbour, M., and Nawar, L. S. (2015). Synthesis, antifungal and insecticidal potential of Chitosan (CS)-g-poly (acrylic acid)(PAA) nanoparticles against some seed borne fungi and insects of soybean. Int. J. Chem. Tech. Res. 8, 589–598.
Sandal, S., Singh, S., Bansal, G., Kaur, R., Mogilicherla, K., Pandher, S., et al. (2023). Nanoparticle-Shielded dsRNA delivery for enhancing RNAi efficiency in cotton spotted bollworm Earias vittella (Lepidoptera: Nolidae). Int. J. Mol. Sci. 24, 9161. doi: 10.3390/ijms24119161
Sen, S. K., Chouhan, D., Das, D., Ghosh, R., and Mandal, P. (2020). Improvisation of salinity stress response in mung bean through solid matrix priming with normal and nano-sized chitosan. Int. J. Biol. Macromol. 145, 108–123. doi: 10.1016/j.ijbiomac.2019.12.170
Shang, Y., Hasan, M. K., Ahammed, G. J., Li, M., Yin, H., and Zhou, J. (2019). Applications of nanotechnology in plant growth and crop protection: a review. Molecules 24, 2558.
Sheikhalipour, M., Esmaielpour, B., Behnamian, M., Gohari, G., Giglou, M. T., Vachova, P., et al. (2021). Chitosan–selenium nanoparticle (Cs–Se NP) foliar spray alleviates salt stress in bitter melon. Nanomaterials 11, 684. doi: 10.3390/nano11030684
Shukla, J. N., Kalsi, M., Sethi, A., Narva, K. E., Fishilevich, E., Singh, S., et al. (2016). Reduced stability and intracellular transport of dsRNA contribute to poor RNAi response in lepidopteran insects. RNA Biol. 13, 656–669. doi: 10.1080/15476286.2016.1191728
Silva-Castro, I., Martín-García, J., Diez, J. J., Flores-Pacheco, J. A., Martín-Gil, J., and Martín-Ramos, P. (2018). Potential control of forest diseases by solutions of chitosan oligomers, propolis and nanosilver. Eur. J. Plant Pathol. 150, 401–411.
Silver, K., Cooper, A. M., and Zhu, K. Y. (2021). Strategies for enhancing the efficiency of RNA interference in insects. Pest Manage. Sci. 77, 2645–2658.
Singh, I. K., Singh, S., Mogilicherla, K., Shukla, J. N., and Palli, S. R. (2017). Comparative analysis of double-stranded RNA degradation and processing in insects. Sci. Rep. 7, 1–12.
Souri, Z., Karimi, N., Sarmadi, M., and Rostami, E. (2017). Salicylic acid nanoparticles (SANPs) improve growth and phytoremediation efficiency of Isatis cappadocica Desv., under As stress. IET Nanobiotechnol. 11, 650–655.
Ureña Saborío, H., Madrigal Carballo, S., Sandoval, J., Vega Baudrit, J., and Rodríguez Morales, A. (2017). Encapsulation of bacterial metabolic infiltrates isolated from different Bacillus strains in chitosan nanoparticles as potential green chemistry-based biocontrol agents against Radopholus similis. J. Renew. Mater. 5, 634119.
Vani, R., and Stanley, S. A. (2013). Studies on the extraction of chitin and chitosan from different aquatic organisms. Adv. Biotech 12, 12–15. doi: 10.3390/molecules13092069
Vejan, P., Abdullah, R., Khadiran, T., and Ismail, S. (2019). Encapsulation of Bacillus salmalaya 139SI using double coating biopolymer technique. Lett. Appl. Microbiol. 68, 56–63. doi: 10.1111/lam.13088
Wang, K., Peng, Y., Chen, J., Peng, Y., Wang, X., Shen, Z., et al. (2020). Comparison of efficacy of RNAi mediated by various nanoparticles in the rice striped stem borer (Chilo suppressalis). Pest. Biochem. Physiol. 165, 104467. doi: 10.1016/j.pestbp.2019.10.005
Whitten, M. M., Facey, P. D., Del Sol, R., Fernández-Martínez, L. T., Evans, M. C., Mitchell, J. J., et al. (2016). Symbiont-mediated RNA interference in insects. Proc. R. Soc. B Biol. Sci. 283, 20160042.
Yoon, J.-S., Gurusamy, D., and Palli, S. R. (2017). Accumulation of dsRNA in endosomes contributes to inefficient RNA interference in the fall armyworm, Spodoptera frugiperda. Insect Biochem. Mol. Biol. 90, 53–60. doi: 10.1016/j.ibmb.2017.09.011
Yu, N., Christiaens, O., Liu, J., Niu, J., Cappelle, K., Caccia, S., et al. (2013). Delivery of dsRNA for RNAi in insects: an overview and future directions. Insect Sci. 20, 4–14. doi: 10.1111/j.1744-7917.2012.01534.x
Zeng, D., Luo, X., and Tu, R. (2012). Application of bioactive coatings based on chitosan for soybean seed protection. Int. J. Carbohyd. Chem. 2012, 104565.
Zhang, X., Mysore, K., Flannery, E., Michel, K., Severson, D. W., Zhu, K. Y., et al. (2015). Chitosan/interfering RNA nanoparticle mediated gene silencing in disease vector mosquito larvae. JoVE 97:e52523. doi: 10.3791/52523
Zhang, X., Zhang, J., and Zhu, K. (2010). Chitosan/double-stranded RNA nanoparticle-mediated RNA interference to silence chitin synthase genes through larval feeding in the African malaria mosquito (Anopheles gambiae). Insect Mol. Biol. 19, 683–693. doi: 10.1111/j.1365-2583.2010.01029.x
Zhao, X., Yu, S.-B., Wu, F.-L., Mao, Z.-B., and Yu, C.-L. (2006). Transfection of primary chondrocytes using chitosan-pEGFP nanoparticles. J. Control. Release 112, 223–228. doi: 10.1016/j.jconrel.2006.01.016
Zhu, K. Y., and Palli, S. R. (2020). Mechanisms, applications, and challenges of insect RNA interference. Annu. Rev. Entomol. 65, 293–311.
Keywords: forestry, RNA interference, nanotechnology, chitosan-RNAi biopesticides, forest insect pest management, forest protection
Citation: Mogilicherla K and Roy A (2023) RNAi-chitosan biopesticides for managing forest insect pests: an outlook. Front. For. Glob. Change 6:1219685. doi: 10.3389/ffgc.2023.1219685
Received: 09 May 2023; Accepted: 21 August 2023;
Published: 05 September 2023.
Edited by:
Milica Zlatkovic, University of Novi Sad, SerbiaReviewed by:
Erika V. S. Albuquerque, Brazilian Agricultural Research Corporation (EMBRAPA), BrazilCopyright © 2023 Mogilicherla and Roy. This is an open-access article distributed under the terms of the Creative Commons Attribution License (CC BY). The use, distribution or reproduction in other forums is permitted, provided the original author(s) and the copyright owner(s) are credited and that the original publication in this journal is cited, in accordance with accepted academic practice. No use, distribution or reproduction is permitted which does not comply with these terms.
*Correspondence: Amit Roy, cm95QGZsZC5jenUuY3o=
Disclaimer: All claims expressed in this article are solely those of the authors and do not necessarily represent those of their affiliated organizations, or those of the publisher, the editors and the reviewers. Any product that may be evaluated in this article or claim that may be made by its manufacturer is not guaranteed or endorsed by the publisher.
Research integrity at Frontiers
Learn more about the work of our research integrity team to safeguard the quality of each article we publish.