- 1Department of Environmental Biology, Sapienza University of Rome, Rome, Italy
- 2Biotechnical Faculty, University of Ljubljana, Ljubljana, Slovenia
- 3Department of Forest Botany, Dendrology and Geobiocoenology, Faculty of Forestry and Wood Technology, Mendel University in Brno, Brno, Czechia
- 4Centre for Academic Heritage and Archives and Ghent University Botanical Garden, Ghent University, Ghent, Belgium
The Socotra Archipelago (Yemen) is an interesting biodiversity hotspot, with a significant proportion of endemic species that have evolved to survive in an arid subtropical environment, inscribed as a World Heritage Site by UNESCO. The terrestrial ecosystems of Socotra face several threats, including climate change, overgrazing and soil degradation. Socotra Island has four endemic species of the genus Commiphora (Burseraceae). Little is known about their local distribution and ecology, yet these trees could be useful indicator species. Our study focuses on the distribution and niche characterisation of the four endemic Commiphora species of Socotra and how climate change may affect them. The aim is to improve insights into their habitats and to provide an essential basis for future local management plans and ecological restoration. We compared the current distribution with the forecasted potential distribution under a CMIP6 (Coupled Model Intercomparison Project) climate scenario, allowing us to define target conservation areas and assess potential local extinction risks. To achieve this, we collected distribution data in the field throughout Socotra Island, covering the current distribution ranges of the four species. To assess the potential distribution of these species, we applied three models (GAM, MaxEnt, RandomForest) using bioclimatic, topographic and soil variables. Forecasts under a climate change scenario were made using bioclimatic variables from the CMCC-CESM2 climate model for two different socioeconomic pathways. The distribution of three endemic Socotran Commiphora is mainly correlated to clay content in the soil and winter precipitation, while C. socotrana is affected by seasonal precipitation and temperature. Under different potential future climate scenarios, the distribution of C. ornifolia is predicted to remain stable or increase, while C. parvifolia distribution could increase, yet C. planifrons and C. socotrana are predicted to undergo a strong reduction of suitable areas and an upward shift in the mountains. Our results highlight that it is essential to conserve the unique terrestrial ecosystems in Socotra and to preserve these endemic trees which have a wide range of ecosystem services. Updates on the predicted extinction risk assessment are fundamental to understand conservation priorities and strategize future actions to ensure the persistence of Socotran myrrh trees and other endangered endemic tree taxa on the island.
1. Introduction
After land use changes, climate change is one of the major threats to the conservation of plant species, along with invasive species and air pollution, affecting the conservation status of many species and stressing an already precarious natural balance (McCarty, 2001; Corlett, 2016). According to the Global Tree Assessment (Newton et al., 2015), which aims to assess the conservation status of all tree species globally (Beech et al., 2017), a third of world’s tree species are threatened with extinction. The most endangered species are the endemic ones with restricted habitats, occurring in confined areas, such as small isolated islands or other insular habitats such as mountains (Lavergne et al., 2004; Wulff et al., 2013; Rivers et al., 2022).
An archipelago with particularly fragile and endangered terrestrial ecosystems with a high proportion of endemics among plants, is the Socotra Archipelago (Yemen) situated in the western Indian Ocean, just east of the Horn of Africa. This biodiversity sanctuary and UNESCO Natural World Heritage Site is often described as the “Galápagos of the Indian Ocean” (Van Damme and Banfield, 2011) and about 37% of the plant species are endemic (Van Damme and Banfield, 2011; Maděra and Van Damme, 2022). The family Burseraceae stands out as having the highest rate of endemism among the plants in the Socotra Archipelago, with at least 15 taxa present in the genera Boswellia (11 taxa, all endemic) and Commiphora (5 species, 4 endemic) (Miller and Morris, 2004; Brown and Mies, 2013; Thulin, 2020). Although there have been several studies on the taxonomy, distribution, conservation and biology of the Socotran Boswellia (Miller and Morris, 2004; Attorre et al., 2011; Lvončík et al., 2013, 2020; Thulin, 2020; Hamdiah et al., 2022), comparably little is known about the archipelago’s endemic Commiphora species.
The genus Commiphora comprises trees and shrubs that are widespread in (sub)tropical zones of the Old World, from India throughout Africa; the genus is represented in the New World by only one species (Gillett, 1980). It is estimated to count ∼200 species with a high level of endemism and with a wide ecological range, from dry arid zones to humid tropical and even equatorial zones with different regimes of rainfall (Mahr, 2012). Despite the taxonomic problems that this genus poses, illustrated by the difficulties of dividing it into subgenera or subtribes (Weeks and Simpson, 2007; Gostel et al., 2016), the centre of differentiation is clearly in the Horn of Africa, from where the species probably migrated to other continents (Soromessa, 2013). Commiphora trees provide a wide range of important ecosystem services, first and foremost as a vital economic resource in many different cultures, a use which has persisted for thousands of years through the incense and myrrh trade (Blom et al., 2006; Hassan et al., 2019). The gum resin collected from the trees by tapping, known as “myrrh,” has been used as incense but also as an important medicine, toothpaste, and industrial uses such as glue and sealer (Shen et al., 2012; Eslamieh, 2016).
Adjacent to the radiation centre of the genus, is the Socotra Archipelago, where five Commiphora species are present (Miller and Morris, 2004): C. kua, C. ornifolia, C. parvifolia, C. planifrons, and C. socotrana. Only the first one, which is a shrub-like species, is not endemic, since it is also present in the Arabian Peninsula (Miller and Morris, 2004). Three out of the four endemic species are considered a clearly separate clade (Spinescens-clade) as described by Gostel et al. (2016), while the phylogenetic position of C. ornifolia has not been investigated yet. All four endemics are large shrubs to proper trees in Socotra (although different growth-forms are present). From an ethnobotanical point of view, these are historically highly valuable trees in Socotra, which remain an important part of the Socotri people’s cultural identity. Commiphora resin has been used, and is still used on the island, as an important medicine to treat wounds and stomach pains; in addition, some of the endemic Commiphora serve as major local sources of incense and during dry periods these trees (branches are cut by shepherds) are used as additional livestock fodder (Miller and Morris, 2004).
The vulnerable terrestrial ecosystems in which the endemic Socotran Commiphora trees occur, are facing a wide range of threats. Several challenges to Socotra’s biodiversity affect entire habitats including the shrublands, woodlands and forests, the latter of which are relatively limited in distribution (Riccardi et al., 2020). The rich terrestrial flora of Socotra is strongly impacted by overgrazing which has started centuries ago and which has partly shaped the plant communities by counter-acting regeneration of palatable species, leading to a general decline of habitat- and soil quality (Van Damme and Banfield, 2011; Attorre and Van Damme, 2020; Maděra and Van Damme, 2022). Affected by overgrazing, environmental factors such as soil and climatic factors play major roles in shaping the current distribution of trees in Socotra (Riccardi et al., 2020). Recently, a rapid deterioration of vegetation can be witnessed in the Socotran highlands and valley slopes (Maděra et al., 2019a; Lvončík et al., 2020; Rezende et al., 2022). A direct decline of some populations of endemic tree species has been observed and this effect can be directly attributed to extreme weather events as a result of climate change (Maděra et al., 2019b; Lvončík et al., 2020; Maděra and Van Damme, 2022). Besides local droughts, recent extreme weather events included two subsequent violent cyclones in 2015 (cyclones Chapala and Megh), which had a directly negative impact on the Socotran woodlands. For example, a recent study clearly illustrates the rapid decline of a local forest of the endemic frankincense tree, Boswellia elongata (in Homhil Nature Sanctuary on the Socotran eastern limestone plateaus). After a steady decline due to overmaturity and overgrazing since the 1950s, 38% of the individual trees of this population was destroyed directly by the 2015 cyclones and another 29% died in the following two years due to weakened health and attacks by bark beetles (Lvončík et al., 2020). In addition to overgrazing and climate impacts, wood collection has increased in Socotra (Maděra and Van Damme, 2022), which could be dangerous for overexploitation in the future (Brown and Mies, 2013). In general, the current threats affecting Socotra’s endemic Commiphora are still to be re-assessed in detail, as much has changed in these islands since the last assessment of 2004 (e.g., Miller, 2004; Miller and Morris, 2004), including new climate impacts. In other Burseraceae such as Boswellia, the incense trade (in combination with the aforementioned overgrazing and climate effects), is a threat that is leading to a rapid decline on a global scale (Bongers et al., 2019).
However, for the Socotran Commiphora these threats have not been evaluated in detail yet. The four endemic species have been classified as Near Threatened in the last IUCN Red List Assessment (Miller and Morris, 2004), and despite their high cultural importance in a biodiversity hotspot, no reassessments have been carried out since 2004. The ecological degradation processes as described above, are still ongoing, therefore a proper evaluation of the poorly known ecology, distribution, and potential threats of Socotran Commiphora are urgently needed, leading to the current study. This information is crucial for developing effective conservation strategies and ecological restoration works (Gann et al., 2019). The first step is to investigate the distribution and the ecological needs of a species; in this way it is possible to identify the limits and vulnerabilities of the taxa studied.
Promising tools for studying the distribution and ecological niche of tree taxa are the Species Distribution Models (SDMs) which are often used for addressing ecological vegetation restoration projects (Zellmer et al., 2019; Frans et al., 2022; Rezende et al., 2022) since they can help to choose the optimal sites for restoration and to explore newly suitable areas for the species. However, one aspect that is often neglected in vegetation restoration/conservation projects is the effect of climate change on the future distributional pattern of the species, which can give information on which areas are most at risk and where the species will most likely disappear.
Living in a changing world, especially climate-wise, has led researchers to conduct several studies on how climate may impact plant ecology (Thuiller et al., 2008; Piao et al., 2019). Fortunately, climate models can help in investigating the effects of present and future climate scenarios. Among them, is the model suite of the 6th Coupled Model Intercomparison Project (CMIP6, Eyring et al., 2016) with improvements and increased resolution in comparison to earlier models (Voldoire et al., 2019; Chen et al., 2020). Since the future climate is a result of several factors (such as politics, economics, land-use change), these factors have been considered in updated models, along with radiative forcing achieved by the year 2100 (similar to the old RCP scenarios) in order to create four Shared Socioeconomic Pathways (SSP) which describe different development paths of society, from the more sustainable to the more CO2-emitting (O’Neill et al., 2016; Riahi et al., 2017). Such tools are useful to determine the effects of climate change on organisms under different future (global) scenarios.
In this study, we investigated the current distribution of the Socotran endemic Commiphora spp. based on thorough field inventories, described the ecological niche, and we modelled the potential distribution (including forecasting under different scenarios) to investigate current threats, future population trends, and suitable areas for possible ecological restoration in the future. By evaluating the impact of different climate change scenarios, we assess the potential extinction risk, and we identify areas that could potentially be unsuitable for these endemic tree species in the future.
2. Materials and methods
2.1. Commiphora data collection
We focussed on the four endemic Commiphora species occurring in Socotra Island (C. ornifolia, C. parvifolia, C. planifrons, C. socotrana; see Figure 1). Location points of individual trees were taken in the field by hand using a GPS through a custom-designed application via mobile phone using ArcGIS Field Maps, which was also used for the field inventory of Dracaena in Oman (Vahalík et al., 2020). The island was investigated during field surveys in 2022 in all known areas of the distribution of the four species to cover the actual distribution, but also to cover new areas with the intention to improve our knowledge of the geographic distribution. During the surveys we encountered many individuals exhibiting morphological features intermediate between different species of Commiphora, including probable hybrids. These individuals were not included in the database to not introduce any bias in the model processing. Location points were then filtered by a grid of 50 m to avoid overprediction of the species distribution models (Mendes et al., 2020).
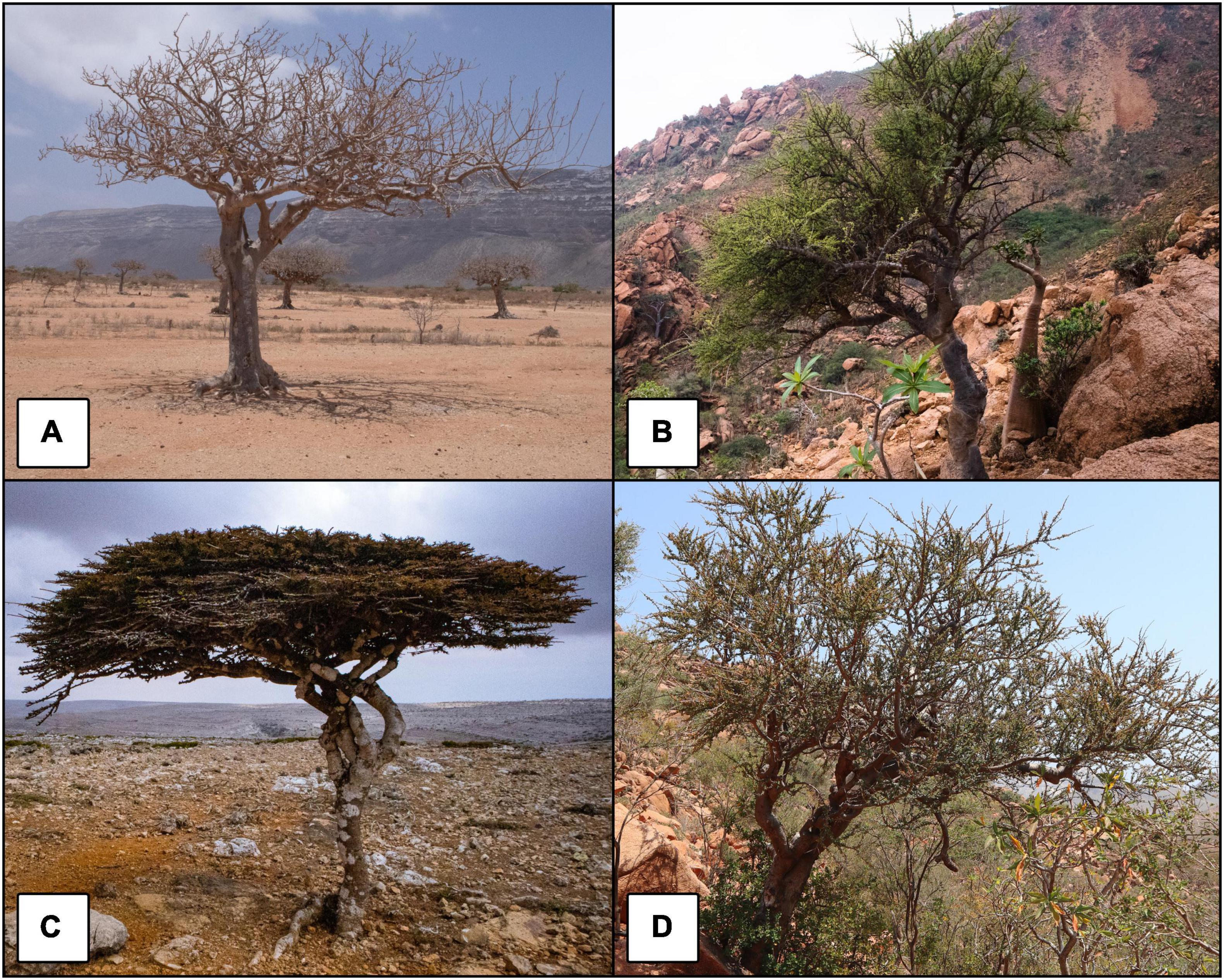
Figure 1. The four Commiphora species (myrrh trees) endemic to Socotra Island (Yemen): (A) Commiphora ornifolia; (B) Commiphora parvifolia; (C) Commiphora planifrons; (D) Commiphora socotrana.
Since it is impossible to take absence points on the island (i.e., for example, we do not know if the recent extinction events such as extreme climate and overgrazing in Socotra have destroyed trees that were recently present), a number of pseudo-absence points equal to the number of available presence points were randomly generated in RStudio platform (R Core Team, 2022), to have balanced datasets (Barbet-Massin et al., 2012).
2.2. Environmental layers processing
Different environmental layers were used for the modelling of the species distribution in RStudio platform (R Core Team, 2022). Topographic, bioclimatic, and edaphic variables were used as predictors of the potential distribution (Table 1). Altitude, slope and aspect have been assessed using a DTM at 30 m resolution from the SRTM (Farr et al., 2007) and for the climate, we used WorldClim V.2 bioclimatic variables at a resolution of 1 km (Fick and Hijmans, 2017). Finally, SoilGrid variables (Hengl et al., 2017) were used for the edaphic conditions. While we know that these are global data layers and local differences are possible (e.g., local microclimates), these are currently the best variables available (e.g., there is no detailed soil map for Socotra). Several of these layers were used in recent studies to update land classes, understand tree cover, potential distribution, and productivity of (tree) vegetation (Rezende et al., 2020, 2022; Riccardi et al., 2020).
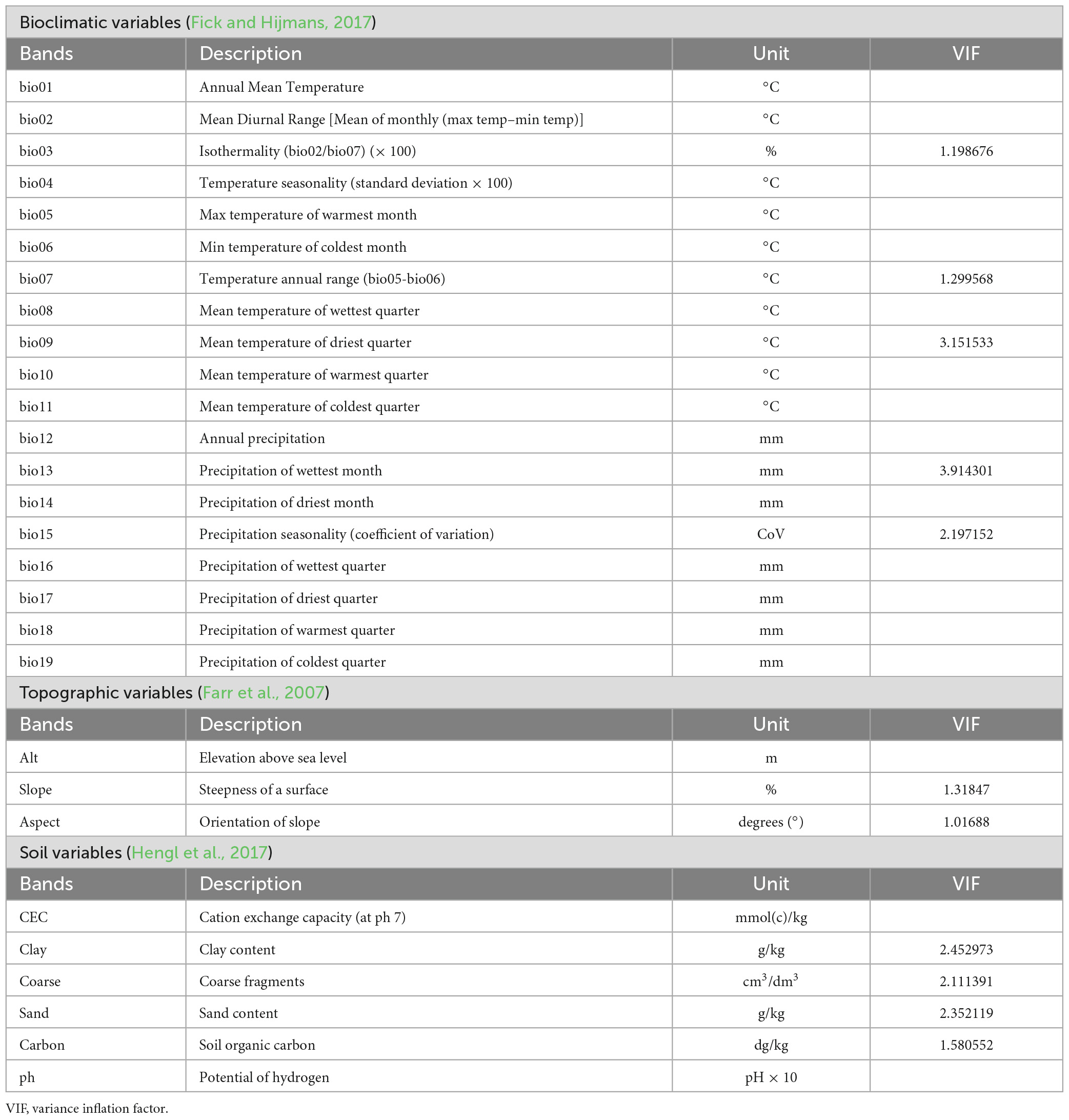
Table 1. Data layers (bioclimatic, topographic, and soil variables) used in this study with references of the sources and multicollinearity test results conducted with the variance inflation factor, showing the variables selected with no autocorrelation and with values <5.
We harmonised the different resolutions of the environmental layers with a bicubic interpolation neighbours using the software Google Earth Engine (Gorelick et al., 2017). It creates high-resolution layers and allows the selection of the final resolution. We chose a 50 m resolution corresponding to the cell size used to filter GPS points of Commiphora species.
Multicollinearity is a problem for the species distribution models since the environmental variables should be independent in order not to weaken the model’s statistical power. For this reason, to detect any correlation between the variables, a Variance Inflation Factor (VIF) test was conducted for each species on RStudio (R Core Team, 2022) using the “usdm” package (Naimi et al., 2014). This step excludes the highly correlated variables through stepwise processing. All the environmental variables with a threshold ≤5 were selected for the elaboration of the model (Table 1).
2.3. Climate change scenarios and climate models
The CMCC-CESM2 from CMIP6 (Coupled Model Intercomparison Project) was downloaded from WorldClim (Fick and Hijmans, 2017) and used as a global climate model since it works more accurately than others in this geographic area, considering the effects of the winter and summer monsoons (Mohan and Bhaskaran, 2019). The same bioclimatic, topographic and edaphic variables (Table 1) were employed for each species to train the model, jointly with the layers harmonisation conducted in Google Earth Engine software mentioned before.
We compare two different standard scenarios, the SSP126 (Sustainable and “green” pathway with 2.6 W/m2 radiative force by the year 2100) and SSP585 (Fossil-fuelled Development with 8.5 W/m2 radiative force by the year 2100). The selected time range is 2081–2100 (mean 2090).
2.4. Data analysis—Species distribution modelling
All the following analyses were performed on RStudio (R Core Team, 2022) using the package “sdm” (Naimi and Araújo, 2016). The datasets were split into 70% for training and 30% for testing the species distribution model. For each dataset of species occurrences, a distribution model was produced. Three modelling approaches–GAM (Hastie and Tibshirani, 1990), Random Forest (Breiman, 2001), and MaxEnt (Phillips et al., 2006)–were used, and the results were averaged in an ensemble model (Araujo and New, 2007). Sometimes using single models based on different assumptions, makes it difficult to choose which algorithm performs best. Therefore, ensemble models have been used to combine and average predictions in different ways (Thuiller et al., 2009) but also to provide information about the overall trend (Guisan et al., 2017; Thuiller et al., 2019). The models were run with 25 replicates for each method (for a total of 75 replicates), using the “subsampling” partitioning method. Before doing it, a seed (9505) was set to ensure the analysis’s replicability. The variable importance and response curves were elaborated with the same R package.
The Area Under the Receiver Operating Characteristic Curve (AUC) was used as a model performance indicator. When the AUC values were <0.6, 0.6–0.7, 0.7–0.8, 0.8–0.9, or 0.9–1.0, the predictions were considered as invalid, poor, fair, good, or excellent, respectively (Swets, 1988).
Instead of using the arithmetic mean or median, the models were ensembled with a weighted average, in particular applying the predictive performance of the True Skill Statistic (TSS), a measure of omission and commission errors and among the other accuracy measures is the more realistic and practical (Shabani et al., 2018). Finally, we selected an occurrence threshold through the sensitivity-specificity sum maximisation on the ensemble models (Manel et al., 2001) to create the Habitat Suitability maps. To have an idea of the potential distribution of the species in the future and under different climate change scenarios, the ensemble model was run with the global climate model chosen using the same parameters for the ensemble model as before.
2.5. IUCN criterion B assessment
The results achieved through this work, together with additional threats assessments during the surveys (unpublished data), will allow us to reassess the IUCN conservation status of the four endemic Socotran Commiphora species according to the Guidelines for Using the IUCN Red List Categories and Criteria (IUCN Standards and Petitions Committee, 2022). Since we only discuss distributional data in this study, the species are assessed for now by criterion B (yet only partly); this is only a first step and preliminary because we do not include the exact population estimates and fragmentation of the species in this study (which may alter the final IUCN Red List reassessment). The calculation of Extent of Occupancy (EOO) and Area of Occurrence (AOO) was made with the “redlistR” package in RStudio (Lee et al., 2019).
3. Results
3.1. Actual distribution
The data collected during the fieldwork, allowed us to explore the actual (current) distribution of the four endemic species of Commiphora on the island. We collected 345 occurrences of C. ornifolia, and, as shown in Figure 2, it is widely distributed with a preference for the central and eastern parts. Similarly, C. parvifolia seems to prefer the central and north-eastern parts with few isolated populations on the west and for a total of 279 individuals recorded. C. planifrons records (208 occurrences) are more frequent in mountainous areas of the island, especially in the Haggeher Mountains, however, there are many records of the species on the high plains in the western part (Ma’alah and Qatariyah). The species has no geographical preference, but it is present at higher altitudes, with some exceptions in the northwest part where it is present between 200 and 300 m a.s.l. Finally, C. socotrana is concentrated in the northern part of the island, with a relatively lower number of occurrences (179 records), and one southern population, in the Zahr Basin.
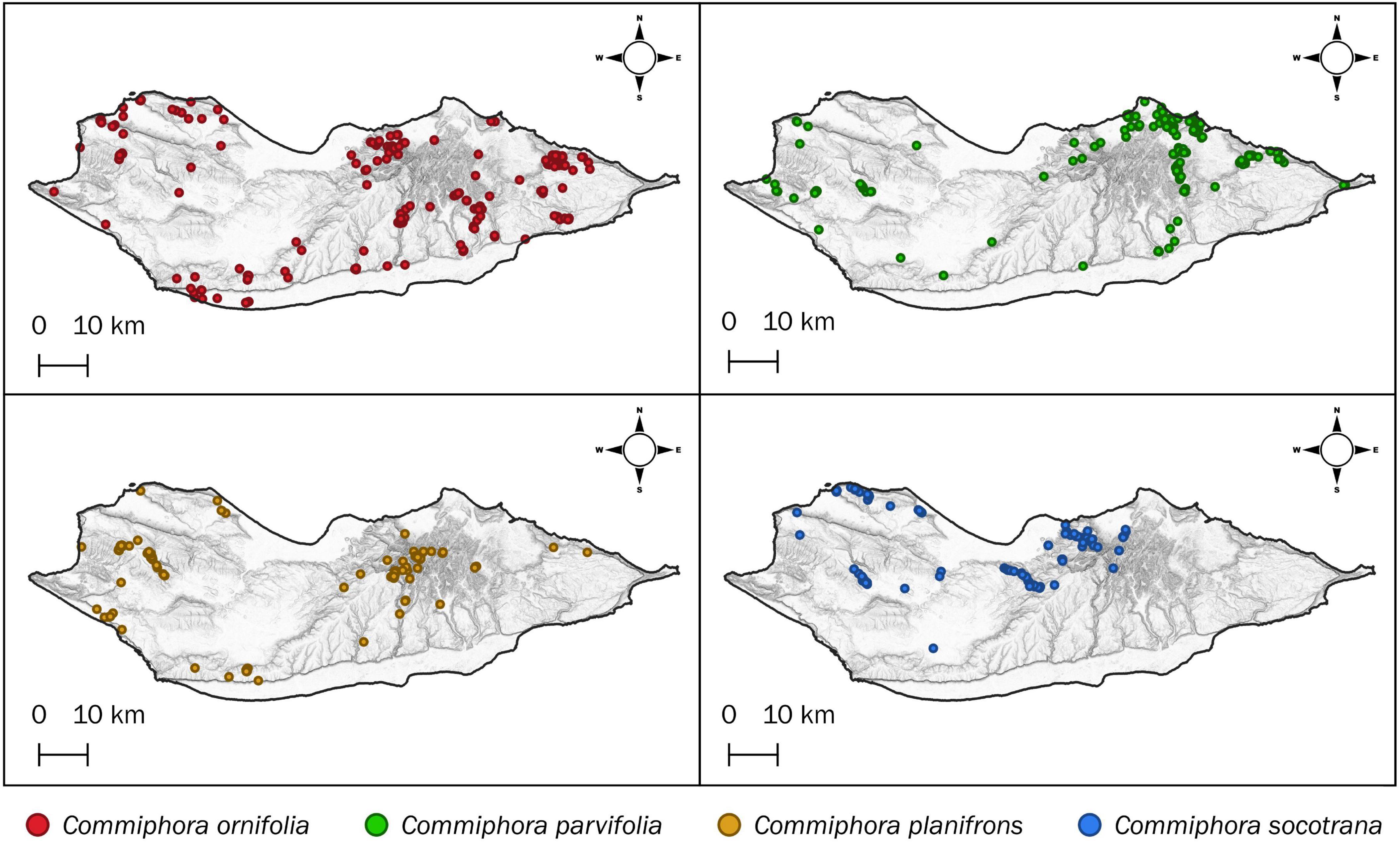
Figure 2. Actual distribution of the four endemic Commiphora species of Socotra Island (as observed by field surveys).
3.2. Importance of the environmental variables
Averaging all the results from each model (Figure 3), it turned out that the most important variable explaining the distribution potentiality is bio03 (isothermality; Table 1) which is shared between three species (C. ornifolia, C. parvifolia, and C. socotrana) with a relative importance of 19.7, 42.9, and 45.1%, respectively. The second shared variable is bio13 (precipitation of wettest month) which turned out to be important for C. ornifolia (23.1%), C. parvifolia (11.6%), and C. planifrons (13%).
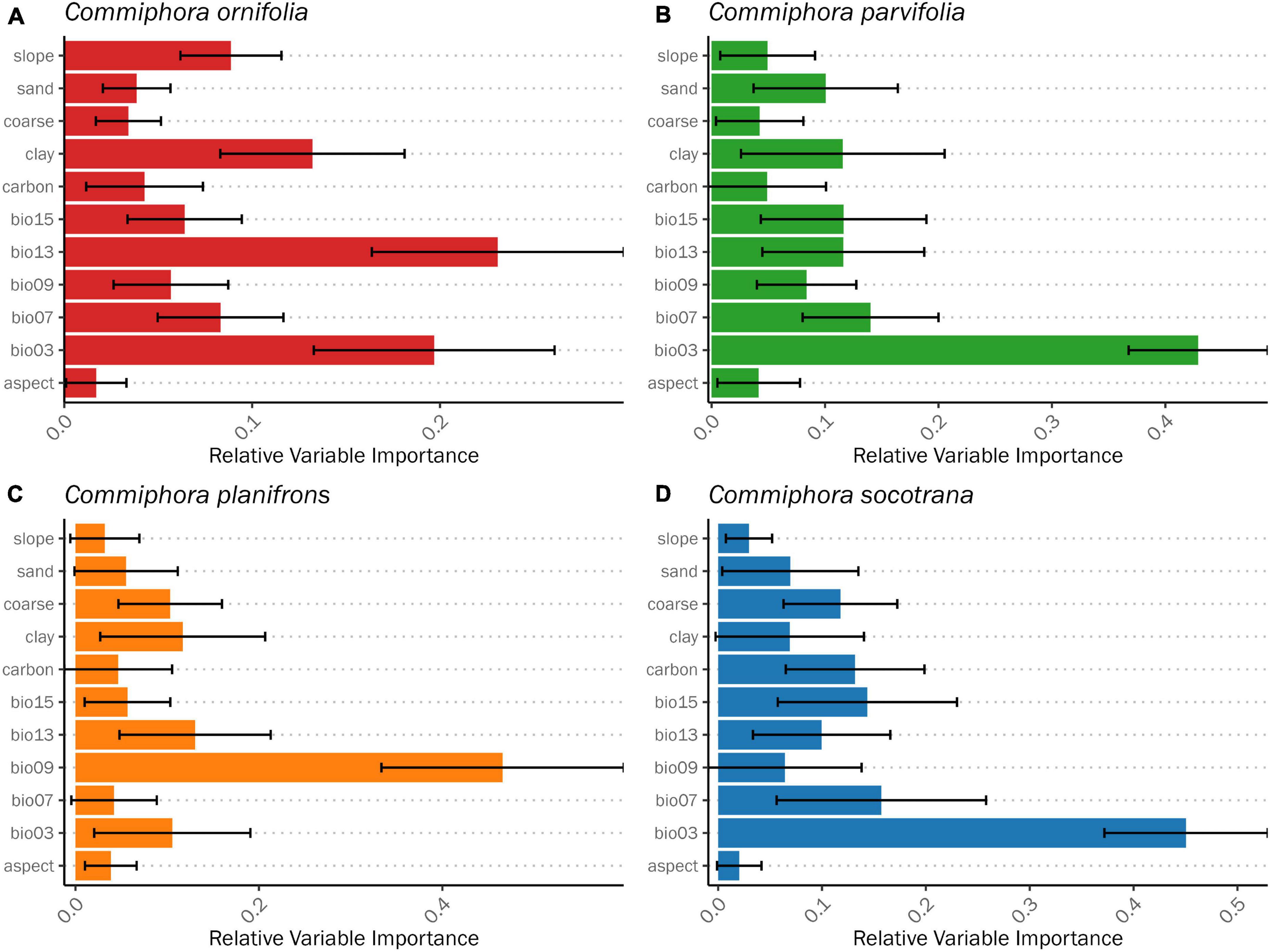
Figure 3. Average relative variable importance (permutation importance) of environmental variables to the final species distribution models for Commiphora species: (A) Commiphora ornifolia, (B) Commiphora parvifolia, (C) Commiphora planifrons, and (D) Commiphora socotrana. The error bar indicates the standard deviation of all the models taken into account.
Other important predictors of the actual species distribution are: bio09 (mean temperature driest quarter) for C. planifrons (46%), bio07 (temperature annual range) for C. socotrana (15.7%) and C. parvifolia (14%). Regarding soil variables, clay plays a role for three species, C. ornifolia (13.2%), C. parvifolia (11.6%) and C. planifrons (11.7%).
3.3. Potential optimum conditions
The potential optimal ecological conditions for each species are shown in Figure 4. The occurrence of C. ornifolia is related to clay, bio03 (isothermality) and bio13 (precipitation of wettest month). The occurrence probability increases when the amount of clay in the soil is higher than 200 g/kg, the isothermality is lower than 60% and the precipitation of the wettest month is between 25 and 30 mm (Figure 4A). Regarding C. parvifolia (Figure 4B), we have the same response to the clay variable, with a higher occurrence when the clay is higher than 200 g/kg. The occurrence probability sharply increases where bio13 (precipitation of wettest month) is 22 mm and when bio07 (temperature annual range) reaches values between 10.5°C and 12.5°C. In C. planifrons (Figure 4C) the optimum conditions occur when bio09 (mean temperature of driest quarter) is 21°C and bio13 (precipitation of wettest month) is higher than 50 mm, as well as the amount of clay in the soil which increases the occurrence when it is 300 g/kg. Finally, C. socotrana (Figure 4D) shows an increase in occurrence when bio03 (isothermality) and bio07 (temperature annual range) are 55% and 13°C, respectively. On the other hand, the optimum for bio15 (precipitation seasonality) is between 60 and 70%.
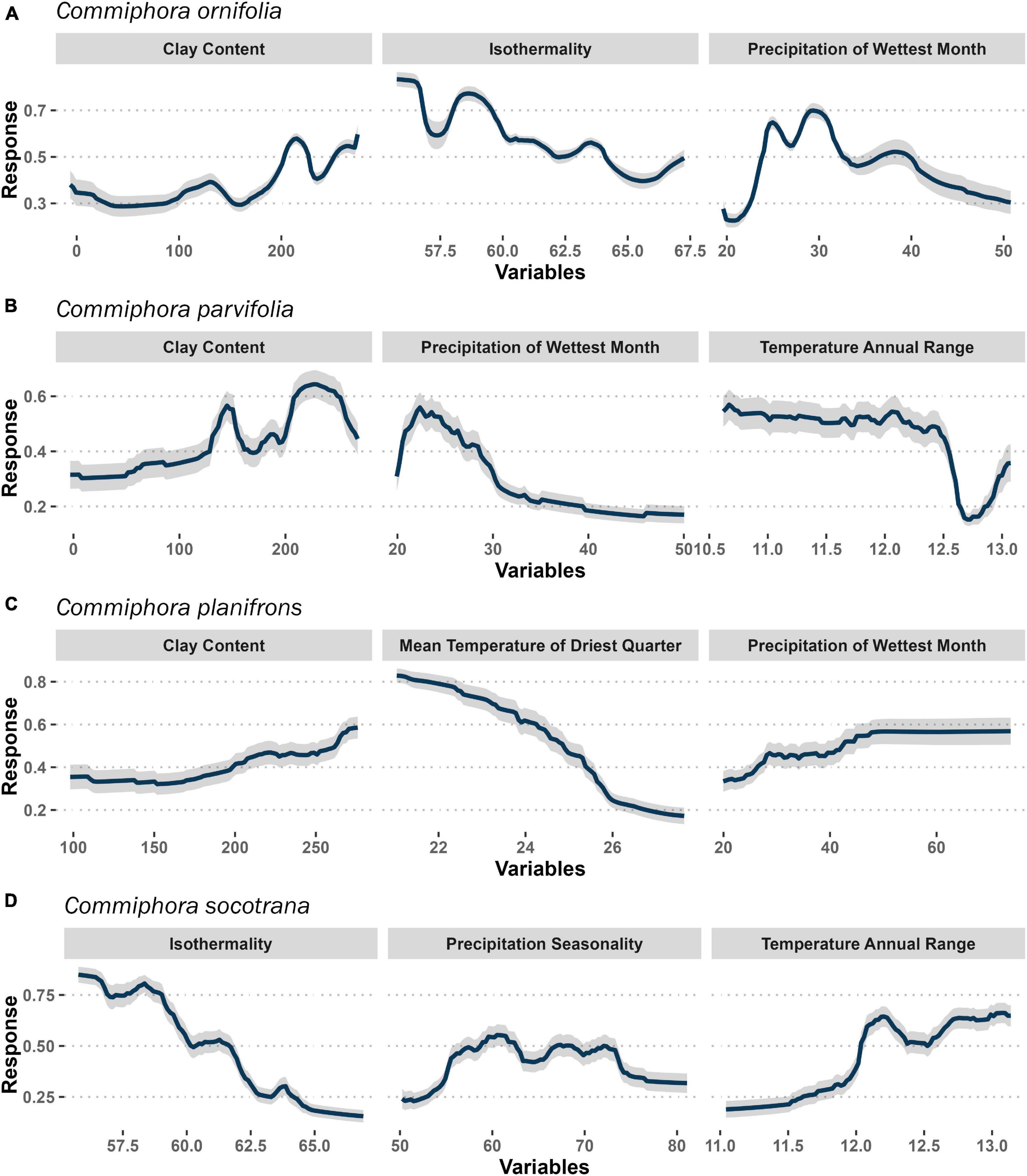
Figure 4. Partial dependence curves showing the response of Commiphora species [(A) C. ornifolia; (B) C. parvifolia; (C) C. planifrons; (D) C. socotrana] to three variables mostly responsible for their spatial distribution. The higher the response values (vertical axis), the more suitable conditions for particular Commiphora species. The grey area is the standard deviation.
3.4. Potential distribution according to the ecological niche
The potential distribution (niche modelling according to the current environmental variables), i.e., all suitable areas for the actual, current occurrence of the four species, is shown in Figure 5. From the actual records and potential distribution of C. ornifolia, we found that this species occupies mostly wadi/canyon areas (Figure 5). However, is not unusual to find the latter species in elevated zones, on high slopes. There is only an exception in the southern part of the island where it is recorded on the coastal plains of Noged (Figure 2). In total the potential calculated distribution of the species is 751.2 km2 and it currently occupies 23% of the suitable areas.
Commiphora planifrons can be found in different areas (Figure 2). The model extends the potential distribution in the eastern and western areas (Figure 5), following the topographic conditions needed by the species, reaching a potential coverage on the island of 611.8 km2 of which 28% is currently occupied.
The last two species share a similar distribution, mostly concentrated on the northern side of the island. Nevertheless, there are differences since records of C. parvifolia can be found in the south and C. socotrana in the central plain of the island (Figure 2). Concerning the extent of potential distribution, they cover 494.1 and 271.1 km2 respectively (Table 3), while the actual distribution cover only 17% for C. parvifolia and 33% for C. socotrana which has the highest percentage.
Relating to the performance of the species distribution models, they have been classified as very good for C. planifrons and C. socotrana and excellent for C. ornifolia and C. parvifolia with a mean testing AUCs of 0.859, 0.870, 0.912, and 0.959, respectively (Table 2 and Supplementary Table).
3.5. Future potential distribution
Among the four species, only two show a clear reduction of their distribution in the future in both climate scenarios (SSP126, SSP585; Table 3). Commiphora socotrana is the only species that could potentially disappear in 2090 in a SSP585 scenario, and in the same case, C. planifrons will encounter a remarkably high reduction of its potential distribution (−97.9%).
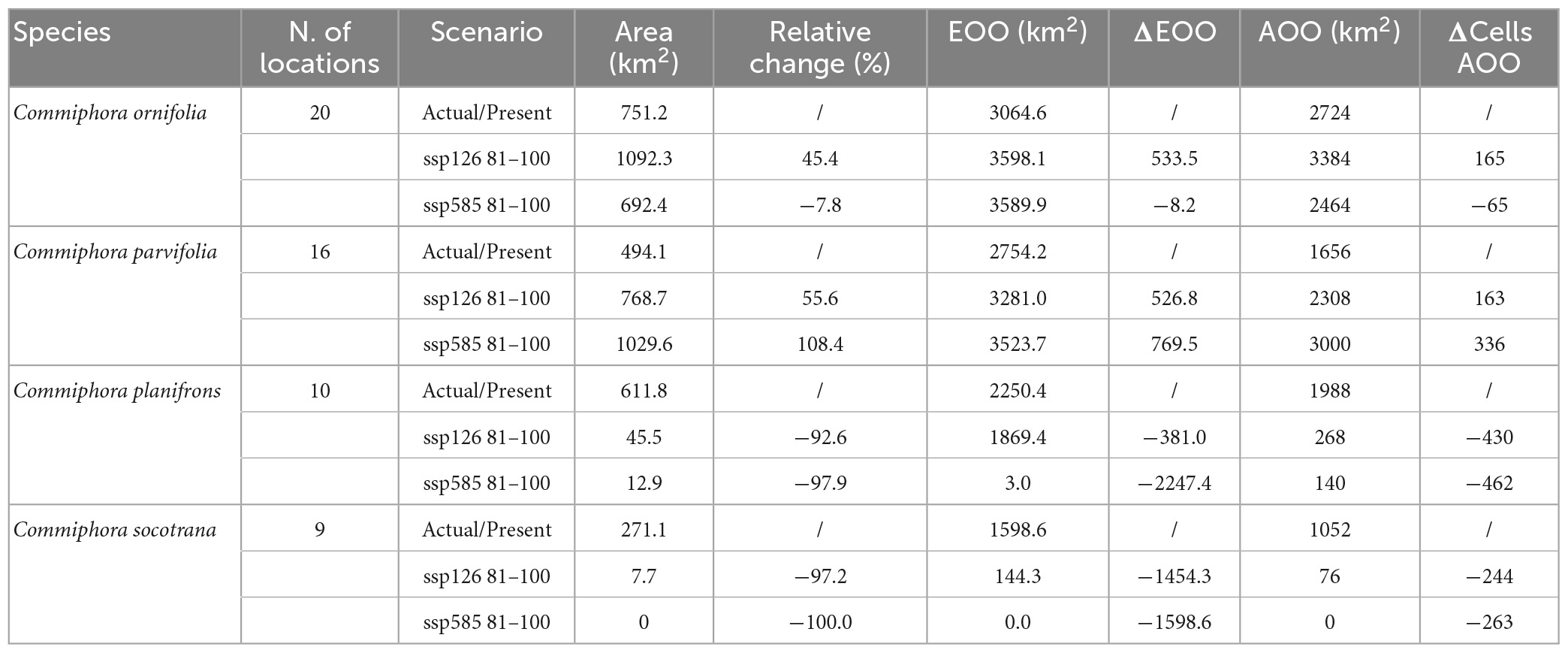
Table 3. Changes in four Commiphora sp. potential coverage until 2100 according to two contrasting climate change scenarios, together with fundamental information for IUCN reassessment.
The situation for these two species does not change in the “better” scenario (SSP126) since habitat suitability is forecasted to decrease extremely, by −97.2% for C. socotrana and −92.6% for C. planifrons (Table 3).
The species least affected by the climate change scenarios seems to be C. parvifolia. In both scenarios, the species shows an actual increase in its potential distribution, more in the worst-case scenario where it could potentially reach an increase of 108.4% by 2090 (Table 3).
Commiphora ornifolia shows a different behaviour in the two scenarios (Table 3). In the “better” one (SSP126), the potential distribution will increase by 45.4% by 2090 whilst in the worst case (SSP585) it will slightly decrease by −7.8%.
3.6. Preliminary IUCN reassessment
The actual and expected Areas Of Occupancy and Extent Of Occurrence (AOO and EOO) are shown in Table 3, while the maps of EOO are in the Supplementary Figures 1–11. Notes on the assessment of the species (only using criterion B) suggests a change in the IUCN Red List status for at least two species (Table 4). Commiphora planifrons and C. socotrana could be listed as “Vulnerable” while C. ornifolia and C. parvifolia would remain in the same category (Near Threatened). However, a future reassessment is still likely to change because all these species have, besides limited occurrence, strongly fragmented subpopulations with severe risks of decline due to lack of regeneration (because of overgrazing). A more detailed threats assessment and detailed population size results are part of ongoing studies.
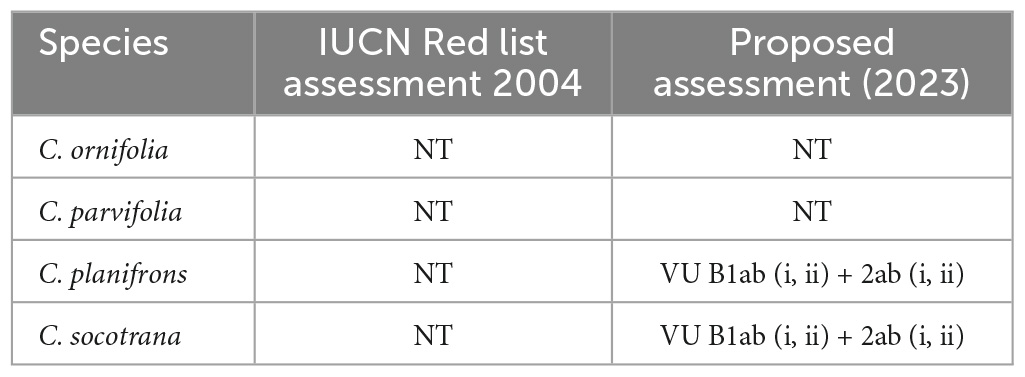
Table 4. Past IUCN assessment (Miller, 2004) and preliminary notes on conservation according to criterion B.
4. Discussion
4.1. Ecological needs and potential distribution of the species
In our work, we explored the current and potential distribution of four endemic Commiphora species of Socotra Island using an ensemble of three modelling approaches. This work confirms the predictive power of the method in investigating the potential distribution of species. Ensemble models are shown to have a better predictive performance compared to single models, reducing the uncertainty and the inevitable problems of over-fitting and under-fitting (Marmion et al., 2009; Battini et al., 2019). The potential distribution of this genus has been analysed only by Kulloli and Kumar (2014) for C. wightii in Rajasthan (India), however, no one has covered the potential future impact of climate change for other species of the genus. Despite this, the impact has been studied and highlighted in other similar works with focus on the trees of Socotra. Many of these studies are limited to the application of a single SDM method, however, yielding useful results (Attorre et al., 2007; Rezende et al., 2022).
Highlighting the ecological factors which shape the potential distribution, the species in our study have some similarities with the Indian C. wightii only in terms of seasonal factors, such as the precipitation seasonality, which it has in common with C. socotrana. The Socotran and Indian species likely have a different phytogeographical history, even though the section to which they belong originated at the beginning of the Miocene (Gostel et al., 2016). The island of Socotra was in contact with the Arabian Peninsula before rifting started in Oligo-Miocene times, and it is possible that Commiphora species colonised before this (Brown and Mies, 2013). Commiphora wightii probably arrived in India through the Arabian Peninsula being a potential divergence area (Lakhanpal, 1970). However, the ecological characteristics of the endemic Commiphora of Socotra can hardly be compared with species in other continents, because of the specific local conditions and potential local radiation in Socotra, which is often the case for island endemics (unusual morphologies and/or ecological characteristics), and because of the wide range of niches in which Commiphora may occur worldwide.
Comparing the current and potential distribution of the Socotran Commiphora according to their ecology, we found that these four species occur in many of the areas predicted as currently suitable, shown by the high values of the sensitivity index. Another interesting aspect is how the prediction for C. socotrana conserves a prevalent northern distribution, whilst C. ornifolia does not seem to prefer lowland areas, although in the south there is a significant population of this species, as shown by the current distribution. This population could be the remnant of an ancient lowland forest, yet there is also a possibility that some trees may have been planted by local communities in previous times because of its ethnobotanical value. Population genetic analyses could help to understand if the distribution is entirely natural. Thinking about the differences between the actual and potential distribution, the concept of realised and fundamental niche comes to mind (Peterson, 2006). The actual distribution is how the species interacted with biotic interactions and/or geographical/historical constraints. Consequently, the extent is inferior to the potential distribution, which corresponds to the fundamental niche.
Predictions of actual vs. potential niche occupancy have been made for other endemic trees of Socotra, for example the Dragon’s Blood Tree Dracaena cinnabari (Attorre et al., 2007; Rezende et al., 2022). However, there is a clear difference between the wider actual occupancy of Commiphora vs. Dracaena, the latter covering only 5.5% of its potential distribution in Socotra Island (Attorre et al., 2007), despite the fact that both genera are facing similar local pressures of overgrazing and climate impacts, as well as being used for resin extraction (Maděra et al., 2019a). This is likely primarily a result of a difference in biology and age-structure of the populations of these endemic trees. Dracaena cinnabari is extremely slow-growing (Maděra et al., 2019a) and the populations of Commiphora could be relatively younger. In general, suitable areas were studied for trees as well as reptiles in Socotra using similar ecological niche modelling (Attorre et al., 2013; Fasola et al., 2020).
Our study reveals key details about the Socotran Commiphora species, as we identified their optimal ecological conditions for the first time. Whereas the climatic variables provide us with information about the optimal temperature and precipitation ranges for a given species, the edaphic ones tell us about species ecology. We found that relatively higher levels of clay in the soil correspond to a higher probability of occurrence of C. ornifolia, C. parvifolia, and C. planifrons. Clay is an important soil component because it helps the hydric retention and nutrients release through the cation exchange for the plants (Rakshit et al., 2021). Unfortunately, the ongoing soil degradation in Socotra due to overgrazing does not allow the formation of stable vegetation communities (Pietsch and Morris, 2010; Van Damme and Banfield, 2011) and leads to more erosion and a loss of potentially suitable areas for these species in the future, threatening them further with extinction. Suitable soil substrates are not only crucial for Commiphora, but also for other endemics living in Socotra’s terrestrial ecosystems, such as the Boswellia elongata woodlands, which are undergoing a degradation phase as shown in some areas (Lvončík et al., 2020), and the reptiles, where types of substrates and vegetation play a crucial role in their ecology (Fasola et al., 2020). Such detailed ecological information and identifying suitable areas has been used in other species to identify proper management, for example in reptiles where a large part of the endemic endangered species and vulnerable populations were not covered by the areas with highest protection in Socotra (nature sanctuaries) (Vasconcelos et al., 2018). With the current information, this can now be also evaluated for the Socotran Burseraceae in the future (reserve design).
4.2. Future distribution: are the Commiphora species at risk of extinction?
Insular ecosystems are more vulnerable to the effects of climate change (Duvat et al., 2017; Cartwright, 2019) even more if other pressures such as invasive species and land use change are added (Reaser et al., 2007; Shao et al., 2017). This is why more studies are urgently needed to understand the potential effects of climate change on the endangered tree species endemic to Socotra. The two different (SSP) scenarios which we examined give us some idea about the reaction of the species in the next 60 years of climate effects, taking into account the current ecological needs of these taxa to observe how potential distribution changes in the future. A similar study was conducted in the New Caledonia Archipelago, a biodiversity hotspot rich in endemics, where changes in potential distribution of tree species were assessed (Pouteau and Birnbaum, 2016).
Commiphora ornifolia seems to be relatively less vulnerable to predicted climate change impacts since the future potential habitat area will actually increase by over 45.4% until 2090 according to SSP126, whilst according to SSP585 the species’ habitat will decrease by just 8%. In any case, the actual distribution of the species on the island is relatively large since the local communities still use it as a food source (e.g., due to fruit properties to lessen thirst) and several local communities have replanted it in their villages (Miller and Morris, 2004). On the contrary, C. parvifolia seems to be favoured by in a general climate change scenario with an increase in the future potential habitat suitability by 2090 of 55 and 108% for SSP126 and SSP585, respectively. This enlargement of the distribution may be due to the species’ tolerance for low precipitation regimes (optimum precipitation during the wettest month is 22 mm), driving it to resist and thrive under changing climate effects. The last two species, C. planifrons and C. socotrana share a similar fate, with a decrease in the potential distribution in both SSP scenarios, making them the relatively more vulnerable Commiphora species on the island. The first one clearly shows a future likely upward shift to the higher parts of the island, in search of higher precipitation and colder temperature during the dry season, disappearing in many areas of the island with a decrease of its distribution of 98% in the worst scenario (Figure 6).
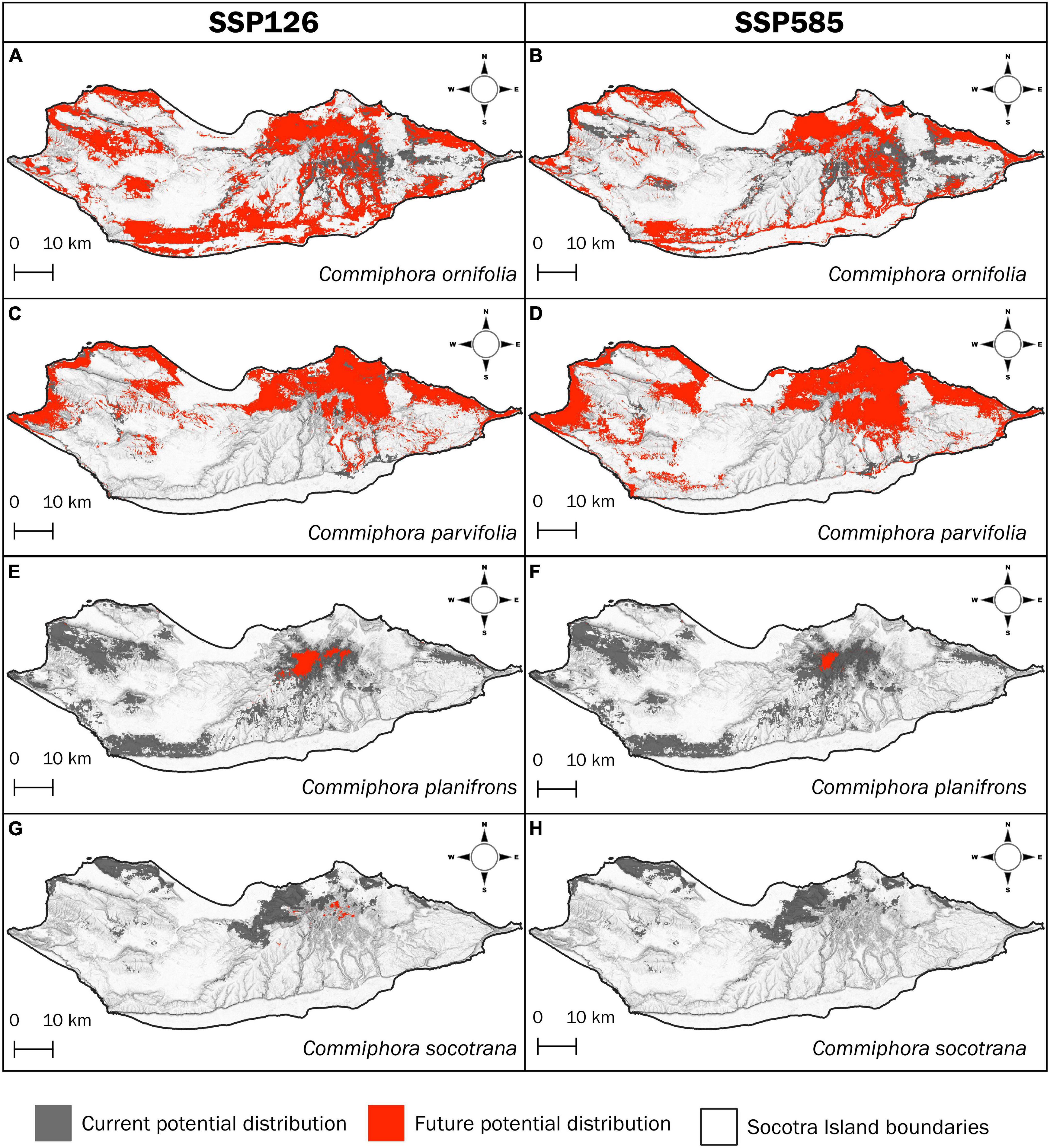
Figure 6. Current potential distributions (in grey; according to the ecological niche modelling) and future (forecasted) potential distributions under climate change models SSP126 and SSP585 (in red), respectively, for C. ornifolia (A,B), C. parvifolia (C,D), C. planifrons (E,F), and C. socotrana (G,H).
The movement of species to higher altitudes and latitudes as refuge areas is recorded worldwide. It is widespread in mountainous areas (Parmesan, 2006; Engler et al., 2011; Zu et al., 2021) and in insular ecosystems (Pouteau and Birnbaum, 2016), where it could lead the species to extinction since they cannot find other suitable mountainous areas due to the topographic constraints. Even though fluctuations are normal, our climate is now changing faster than before (Jansen et al., 2007). Species moving in newly suitable areas sometimes may create “climate refuges” (Hegazy and Lovett-Doust, 2016), places where isolated populations of a migrating species remain stuck in small areas with suitable conditions but surrounded by unsuitable ones. In fact, the mountains of Socotra are already “wet refugia” for many plants, which are thought to have had a wider distribution in the past (Miller and Morris, 2004). This leads us to think that the populations of C. planifrons in Socotra’s central granite mountains (the Haggeher mountains) could further become a relict population (and even more endangered than now).
On the other hand, C. socotrana could probably face a different path. On Socotra Island, the species is not so widespread at present, but it forms populations with a high density of individuals. Despite this, the species potentially will decrease by 2090 of 100% of its potential distribution (Figure 6H), indicating that the species is at a high level of extinction risk. It seems to be affected by seasonal factors, while a major role is played by the winter and summer monsoons that hit the island, causing different rainfall and temperature regimes between the northern and the southern part (Scholte and De Geest, 2010; Brown and Mies, 2013). The possible extinction of C. socotrana on the island by 2090 could be related to the strengthening of the winter monsoon due to the effects of the Arabian Sea warming on the atmospheric circulation, which will increase extreme events, e.g., cloudbursts, high-speed winds, etc. (Mishra et al., 2022).
However, all the modelled potential distributions under different climate scenarios are based only on climatic, edaphic and topographic parameters, which are absolutely fundamental for the ecological niche of a species (Franklin, 2010; Wan et al., 2021), but they do not take into account the availability of suitable habitats and the current and future anthropogenic pressure on these species. On Socotra, these two aspects are strictly interdependent. In fact, thousands of years of humans living there, with introduced livestock, have definitely changed the pristine habitats (Scholte and De Geest, 2010; Van Damme and Banfield, 2011). What we are seeing now is just the result of a prolonged nature disturbance activity (Van Damme and Banfield, 2011). At present, climate, soil, temperature, and altitude are stronger predictions of forest landscapes in Socotra, relatively more than anthropogenic effects (Riccardi et al., 2020), however, from suitable niche modelling it is clear that several tree species do not cover their full potential and are relictual populations already, e.g., Dracaena (Rezende et al., 2022). In addition, our applied climate prediction models do not consider the rapid degradation of woodlands and forests in Socotra due to cyclones (Lvončík et al., 2020), the effects of overgrazing, increase of landslides and erosion in the highlands and slopes (Rezende et al., 2020), and economic-driven threats such as wood collection (Maděra and Van Damme, 2022). Therefore, our predictions with the current models could be under-estimations: considering the likelihood of more cyclones, and ongoing trends of soil degradation, overgrazing and woodland degradation, as well as the lack of regeneration, the rate of potential decline of some very vulnerable species (e.g., Commiphora socotrana) is likely much faster. Such species should be not seen separately from their plant communities, but as local indicator species in Socotra to understand the threats for entire habitats.
Many areas are still in good conservation condition but are threatened by the grazing activity of goats (Van Damme and Banfield, 2011; Brown and Mies, 2013). Overgrazing poses under pressure the plant communities in several ways. One is the change in plant composition since goats eat only the palatable plants, with no chance of survival, highly stressed and with development problems (Trlica and Rittenhouse, 1993; Herrero-Juregui and Oesterheld, 2018), whilst the others are totally untouched. Another issue is the lack of natural regeneration from seeds, due to the grazing pressure which leads the goats to eat whatever plants they encounter in particular small seedlings, except for those situated in inaccessible areas or in a protected location (e.g., rocky slopes, surrounded by stones), as seen for Boswellia spp. (Lvončík et al., 2020; Hamdiah et al., 2022) or Dracaena cinnabari (Habrová and Pavlis, 2017; Maděra et al., 2019a). Ultimately, an indirect effect of overgrazing is land degradation and soil erosion. The lack of plant regeneration due to the high number of goats on the island, together with drought periods, exacerbate the soil erosion through strong wind and surface runoff, leading to a loss of suitable habitats for Commiphora spp. and other taxa (Brown and Mies, 2013).
In general, the new insights of current and potential distribution of the endemic Commiphora species of Socotra Island provided in our study, as well as a prediction of changes under climate change scenarios, can be used to design specific species management plans for the future and strategize direct conservation actions, prioritising the most vulnerable species. The ethnobotanical uses of these species locally (Miller and Morris, 2004) is a starting point to work with indigenous communities for their conservation. With the current information on specific ecology and sensitivity of Socotra’s endemic Commiphora, and suitable areas for the future, well-informed conservation actions can be taken to protect these culturally relevant insular species.
5. Conclusion and potential next steps
The ecology of species is important to have a deep understanding of an organism in all possible aspects. Our work has revealed which are the main factors that determine the distribution of endemic Commiphora species in Socotra Island, and how their distributions would likely change in response to climate change. The ensemble model performed well on the predictions with high performance, confirming it as a useful tool for the island. With the present results and information now is possible to lay the foundations for choosing the best suitable areas for the survival of the species and focussing on the ones exposed to higher extinction risk (e.g., C. socotrana), providing a starting point for the IUCN reassessment.
Furthermore, this paper suggested the need for an in-depth study of the ecological features of other Commiphora species widespread in the tropical and subtropical parts of the world, to find similarities and connect them with genetic studies to understand how species with apparent different phytogeographical histories can instead have in common ecological and genetic features.
In any case, fragmentation of the populations can determine the survival or extinction of a species, and this limiting factor has not been taken into consideration in this work. Possible future research could explore this aspect through the genetic diversity of Commiphora spp. populations on the island, and other biological factors should be investigated such as seed viability (e.g., to estimate regeneration potential and potential cross-pollination of the populations, since these species are dioecious). All this would allow us to understand whether future conservation actions should aim to connect different populations or not to intervene to favour natural processes of speciation (given the potential presence of individuals with phenotypic variations like interspecific hybrids, as in Boswellia). This will be explored with the final reassessment of the conservation status of these species, which will allow the prioritisation of conservation actions, helping to avoid the danger of extinction of the myrrh trees in Socotra.
Data availability statement
The raw data supporting the conclusions of this article will be made available by the authors, without undue reservation.
Author contributions
DLM, MDS, FA, and KVD conceived the research. DLM, MDS, SH, PM, PV, and KVD collected the data. DLM, LM, and PV analysed the data. KVD, PM, and FA supervised the work. KVD, PM, and FA obtained the funding and administrated the project. All authors contributed to the writing of the manuscript with a final review by FA, PM, and KVD. All authors contributed to the article and approved the submitted version.
Funding
This research was funded by the Franklinia Foundation (2020–2023) “Conservation of the endangered endemic Boswellia trees on Socotra Island (Yemen)” Phase I (Grant Number: 2020-03) and Phase II (Grant Number: 2023-03).
Acknowledgments
We thank all the Socotran people who helped us with their incomparable experience during the field work, in particular to the local team (Amar M.; Keybani S.; Shanayeghen M.; and Keybani M.). We are grateful also to the local authorities of the Socotra Archipelago (the Governor and the Environmental Protection Authority) for facilitating and showing interest in the scientific work. Finally we also thank G. Grippo and M. Stefanelli for their support and help. DLM acknowledges the Ministry of Academia and Research (Programma Operative Nazionale) for making this research possible as part of a Ph.D. project.
Conflict of interest
The authors declare that the research was conducted in the absence of any commercial or financial relationships that could be construed as a potential conflict of interest.
Publisher’s note
All claims expressed in this article are solely those of the authors and do not necessarily represent those of their affiliated organizations, or those of the publisher, the editors and the reviewers. Any product that may be evaluated in this article, or claim that may be made by its manufacturer, is not guaranteed or endorsed by the publisher.
Supplementary material
The Supplementary Material for this article can be found online at: https://www.frontiersin.org/articles/10.3389/ffgc.2023.1183858/full#supplementary-material
References
Araujo, M. B., and New, M. (2007). Ensemble forecasting of species distributions. Trends Ecol. Evol. 22, 42–47. doi: 10.1016/j.tree.2006.09.010
Attorre, F., and Van Damme, K. (2020). Twenty years of biodiversity research and nature conservation in the Socotra Archipelago (Yemen). Rend. Lincei. Sci. Fis. Nat. 31, 563–569. doi: 10.1007/s12210-020-00941-7
Attorre, F., De Sanctis, M., Farcomeni, A., Guillet, A., Scepi, E., Vitale, M., et al. (2013). The use of spatial ecological modelling as a tool for improving the assessment of geographic range size of threatened species. J. Nat. Conserv. 21, 48–55. doi: 10.1016/j.jnc.2012.10.001
Attorre, F., Francesconi, F., Taleb, N., Scholte, P., Saed, A., Alfo, M., et al. (2007). Will dragonblood survive the next period of climate change? Current and future potential distribution of Dracaena cinnabari (Socotra, Yemen). Biol. Conserv. 138, 430–439. doi: 10.1016/j.biocon.2007.05.009
Attorre, F., Taleb, N., De Sanctis, M., Farcomeni, A., Guillet, A., and Vitale, M. (2011). Developing conservation strategies for endemic tree species when faced with time and data constraints: Boswellia spp. on Socotra (Yemen). Biodivers. Conserv. 20, 1483–1499. doi: 10.1007/s10531-011-0039-7
Barbet-Massin, M., Jiguet, F., Albert, C. H., and Thuiller, W. (2012). Selecting pseudo-absences for species distribution models: How, where and how many? Methods Ecol. Evol. 3, 327–338. doi: 10.1111/j.2041-210X.2011.00172.x
Battini, N., Farías, N. E., Giachetti, C., Schwindt, E., and Bortolus, A. (2019). Staying ahead of invaders: Using species distribution modeling to predict alien species’ potential niche shifts. Mar. Ecol. Prog. Ser. 612, 127–140. doi: 10.3354/meps12878
Beech, E., Rivers, M., Oldfield, S., and Smith, P. P. (2017). GlobalTreeSearch: The first complete global database of tree species and country distributions. J. Sustain. For. 36, 454–489. doi: 10.1080/10549811.2017.1310049
Blom, R. G., Crippen, R., Elachi, C., Clapp, N., Hedges, G. R., and Zarins, J. (2006). “Southern arabian desert trade routes, frankincense, myrrh, and the ubar legend,” in Remote Sensing in Archaeology, eds J. Wiseman, and F. El-Baz (New York, NY: Springer New York), 71–87. doi: 10.1007/0-387-44455-6_3
Bongers, F., Groenendijk, P., Bekele, T., Birhane, E., Damtew, A., Decuyper, M., et al. (2019). Frankincense in peril. Nat. Sustain. 2, 602–610. doi: 10.1038/s41893-019-0322-2
Brown, G., and Mies, B. A. (2013). Vegetation ecology of socotra. Dordrecht/Heidelberg/New York/London: Springer. doi: 10.1007/978-94-007-4141-6
Cartwright, J. (2019). Ecological islands: Conserving biodiversity hotspots in a changing climate. Front. Ecol. Environ. 17:331–340. doi: 10.1002/fee.2058
Chen, H., Sun, J., Lin, W., and Xu, H. (2020). Comparison of CMIP6 and CMIP5 models in simulating climate extremes. Sci. Bull. 65, 1415–1418. doi: 10.1016/j.scib.2020.05.015
Corlett, R. T. (2016). Plant diversity in a changing world: Status, trends, and conservation needs. Plant Divers. 38, 10–16. doi: 10.1016/j.pld.2016.01.001
Duvat, V. K. E., Magnan, A. K., Wise, R. M., Hay, J. E., Fazey, I., Hinkel, J., et al. (2017). Trajectories of exposure and vulnerability of small islands to climate change. WIREs Clim. Change 8:e478. doi: 10.1002/wcc.478
Engler, R., Randin, C. F., Thuiller, W., Dullinger, S., Zimmermann, N. E., Araújo, M. B., et al. (2011). 21st century climate change threatens mountain flora unequally across Europe. Glob. Chang. Biol. 17, 2330–2341. doi: 10.1111/J.1365-2486.2010.02393.X
Eslamieh, J. (2016). The genus commiphora - preservation through horticulture. British Columbia, BC: A Book’s Mind.
Eyring, V., Bony, S., Meehl, G. A., Senior, C. A., Stevens, B., Stouffer, R. J., et al. (2016). Overview of the coupled model intercomparison project phase 6 (CMIP6) experimental design and organization. Geosci. Model Dev. 9, 1937–1958. doi: 10.5194/gmd-9-1937-2016
Farr, T. G., Rosen, P. A., Caro, E., Crippen, R., Duren, R., Hensley, S., et al. (2007). The shuttle radar topography mission. Rev. Geophys. 45:RG2004. doi: 10.1029/2005RG000183
Fasola, M., Razzetti, E., Sindaco, R., Ziliani, U., Delle Monache, D., Pellitteri-Rosa, D., et al. (2020). Ecological preferences of the endemic reptile community of Socotra. Rend. Lincei. Sci. Fis. Nat. 31, 687–701. doi: 10.1007/s12210-020-00922-w
Fick, S. E., and Hijmans, R. J. (2017). WorldClim 2: New 1-km spatial resolution climate surfaces for global land areas. Int. J. Climatol. 37, 4302–4315. doi: 10.1002/joc.5086
Franklin, J. (2010). Mapping species distributions. Cambridge: Cambridge University Press. doi: 10.1017/CBO9780511810602
Frans, V. F., Augé, A. A., Fyfe, J., Zhang, Y., McNally, N., Edelhoff, H., et al. (2022). Integrated SDM database: Enhancing the relevance and utility of species distribution models in conservation management. Methods Ecol. Evol. 13, 243–261. doi: 10.1111/2041-210X.13736
Gann, G. D., Mcdonald, T., Walder, B., Aronson, J., Nelson, C. R., Hallett, J. G., et al. (2019). International standards and principles for the practice of Ecological Restoration. 2nd edition: November 2019. Restor. Ecol 27, 1–46.
Gillett, A. J. B. (1980). Commiphora (Burseraceae) in South America and its relationship to Bursera. Bibliography 34, 569–587.
Gorelick, N., Hancher, M., Dixon, M., Ilyushchenko, S., Thau, D., and Moore, R. (2017). Google earth engine: Planetary-scale geospatial analysis for everyone. Remote Sens. Environ. 202, 18–27. doi: 10.1016/j.rse.2017.06.031
Gostel, M. R., Phillipson, P. B., and Weeks, A. (2016). Phylogenetic reconstruction of the myrrh genus, Commiphora (Burseraceae), reveals multiple radiations in Madagascar and clarifies infrageneric relationships. Syst. Bot. 41, 67–81. doi: 10.1600/036364416X690598
Guisan, A., Thuiller, W., and Zimmermann, N. E. (2017). Habitat suitability and distribution models. Cambridge: Cambridge University Press. doi: 10.1017/9781139028271
Habrová, H., and Pavlis, J. (2017). Dynamic response of woody vegetation on fencing protection in semi-arid areas. Case study: Pilot exclosure on the Firmihin Plateau, Socotra Island. Saudi J. Biol. Sci. 24, 338–346. doi: 10.1016/J.SJBS.2015.09.030
Hamdiah, S., Karas, L., Houšková, K., Van Damme, K., Attorre, F., Vahalík, P., et al. (2022). Seed viability and potential germination rate of nine endemic Boswellia Taxa (Burseraceae) from Socotra Island (Yemen). Plants 11:1418. doi: 10.3390/plants11111418
Hassan, B. A., Glover, E. K., Luukkanen, O., Kanninen, M., and Jamnadass, R. (2019). Boswellia and Commiphora species as a resource base for rural livelihood security in the horn of Africa: A systematic review. Forests 10:551. doi: 10.3390/f10070551
Hegazy, A., and Lovett-Doust, J. (2016). Plant ecology in the middle east. Oxford: Oxford University Press.
Hengl, T., De Jesus, J. M., Heuvelink, G. B. M., Gonzalez, M. R., Kilibarda, M., Blagotić, A., et al. (2017). SoilGrids250m: Global gridded soil information based on machine learning. PLoS One 12:e0169748. doi: 10.1371/journal.pone.0169748
Herrero-Juregui, C., and Oesterheld, M. (2018). Effects of grazing intensity on plant richness and diversity: A meta-analysis. Oikos 127, 757–766. doi: 10.1111/OIK.04893
IUCN Standards and Petitions Committee (2022). Guidelines for Using the IUCN Red List Categories and Criteria. Version 15.1. Available online at: https://www.iucnredlist.org/documents/RedListGuidelines.pdf
Jansen, E., Overpeck, J., Briffa, K. R., Duplessy, J.-C., Joos, F., Masson-Delmotte, V., et al. (2007). “Palaeoclimate,” in Climate change 2007: The physical science basis. Contribution of working group i to the fourth assessment report of the intergovernmental panel on climate change, eds S. Solomon, D. Qin, M. Manning, Z. Chen, M. Marquis, K. B. Averyt, et al. (Cambridge: Cambridge University Press).
Kulloli, R. N., and Kumar, S. (2014). Comparison of bioclimatic, NDVI and elevation variables in assessing extent of Commiphora wightii (Arnt.) Bhand. Int. Arch. Photogramm. Remote Sens. Spat. Inf. Sci. 8, 589–595. doi: 10.5194/isprsarchives-XL-8-589-2014
Lakhanpal, R. N. (1970). Tertiary floras of India and their bearing on the historical geology of the region. Taxon 19, 675–694. doi: 10.2307/1219280
Lavergne, S., Thompson, J. D., Garnier, E., and Debussche, M. (2004). The biology and ecology of narrow endemic and widespread plants: A comparative study of trait variation in 20 congeneric pairs. Oikos 107, 505–518. doi: 10.1111/j.0030-1299.2004.13423.x
Lee, C. K. F., Keith, D. A., Nicholson, E., and Murray, N. J. (2019). Redlistr: Tools for the IUCN red lists of ecosystems and threatened species in R. Ecography 42, 1050–1055. doi: 10.1111/ecog.04143
Lvončík, S., Maděra, P. M., Volařík, D., Vrškový, B., and Habrová, H. (2013). First proposal of seed regions for Frankincense Trees (Boswellia Spp.) on Socotra Island. J. Landsc. Ecol. 6, 35–45. doi: 10.2478/jlecol-2014-0002
Lvončík, S., Vahalík, P., Bongers, F., Peijnenburg, J., Hušková, K., van Rensburg, J. J., et al. (2020). Development of a population of Boswellia elongata Balf. F. in Homhil nature sanctuary, Socotra island (Yemen). Rend. Lincei. Sci. Fis. Nat. 31, 747–759. doi: 10.1007/s12210-020-00936-4
Maděra, P., and Van Damme, K. (2022). “Socotra archipelago (Yemen)” in Imperiled: The encyclopedia of conservation, eds D.A. DellaSala and M.I. Goldstein (Elsevier), 267–281. doi: 10.1016/b978-0-12-821139-7.00009-x
Maděra, P., Habrová, H., Šenfeldr, M., Kholová, I., and Lvončík, S. (2019a). Growth dynamics of endemic Dracaena cinnabari Balf. f. of Socotra Island suggest essential elements for a conservation strategy. Biologia 74, 339–349. doi: 10.2478/s11756-018-0152-0
Maděra, P., Volařík, D., Patočka, Z., Kalivodová, H., Divín, J., Rejžek, M., et al. (2019b). Sustainable land use management needed to conserve the dragon’s blood tree of Socotra Island, a vulnerable endemic umbrella species. Sustainability 11:3557. doi: 10.3390/su11133557
Mahr, D. (2012). Commiphora: An introduction to the genus. Cactus Succul. J. 84, 140–154. doi: 10.2985/0007-9367-84.3.140
Manel, S., Williams, H. C., and Ormerod, S. J. (2001). Evaluating presence-absence models in ecology: The need to account for prevalence. J. Appl. Ecol. 38, 921–931. doi: 10.1046/j.1365-2664.2001.00647.x
Marmion, M., Parviainen, M., Luoto, M., Heikkinen, R. K., and Thuiller, W. (2009). Evaluation of consensus methods in predictive species distribution modelling. Divers. Distrib. 15, 59–69. doi: 10.1111/j.1472-4642.2008.00491.x
McCarty, J. P. (2001). Ecological consequences of recent climate change. Conserv. Biol. 15, 320–331. doi: 10.1046/j.1523-1739.2001.015002320.x
Mendes, P., Velazco, S. J. E., de Andrade, A. F. A., and De Marco, P. (2020). Dealing with overprediction in species distribution models: How adding distance constraints can improve model accuracy. Ecol. Modell. 431:109180. doi: 10.1016/j.ecolmodel.2020.109180
Miller, A. G. (2004). Commiphora planifrons. The IUCN red list of threatened species. Available online at: https://dx.doi.org/10.2305/IUCN.UK.2004.RLTS.T30417A9547043.en (accessed January 10, 2023).
Miller, A. G., and Morris, M. (2004). Ethnoflora of the soqotra archipelago. Edinburgh: Royal botanic garden.
Mishra, A. K., Dubey, A. K., and Das, S. (2022). Identifying the changes in winter monsoon characteristics over the Indian subcontinent due to Arabian Sea warming. Atmos. Res. 273:106162. doi: 10.1016/J.ATMOSRES.2022.106162
Mohan, S., and Bhaskaran, P. K. (2019). Evaluation of CMIP5 climate model projections for surface wind speed over the Indian Ocean region. Clim. Dyn. 53, 5415–5435. doi: 10.1007/s00382-019-04874-2
Naimi, B., and Araújo, M. B. (2016). Sdm: A reproducible and extensible R platform for species distribution modelling. Ecography 39, 368–375. doi: 10.1111/ecog.01881
Naimi, B., Hamm, N. A. S., Groen, T. A., Skidmore, A. K., and Toxopeus, A. G. (2014). Where is positional uncertainty a problem for species distribution modelling? Ecography 37, 191–203. doi: 10.1111/j.1600-0587.2013.00205.x
Newton, A., Oldfield, S., Rivers, M., Mark, J., Schatz, G., Garavito, N. T., et al. (2015). Towards a global tree assessment. Oryx 49, 410–415. doi: 10.1017/S0030605315000137
O’Neill, B. C., Tebaldi, C., Van Vuuren, D. P., Eyring, V., Friedlingstein, P., Hurtt, G., et al. (2016). The scenario model intercomparison project (ScenarioMIP) for CMIP6. Geosci. Model Dev. 9, 3461–3482. doi: 10.5194/gmd-9-3461-2016
Parmesan, C. (2006). Ecological and evolutionary responses to recent climate change. Annu. Rev. Ecol. Evol. Syst. 37, 637–669. doi: 10.1146/annurev.ecolsys.37.091305.110100
Peterson, A. T. (2006). Uses and requirements of ecological niche models and related distributional models. Biodivers. Inform. 3, 59–72. doi: 10.17161/bi.v3i0.29
Phillips, S. J., Anderson, R. P., and Schapire, R. E. (2006). Maximum entropy modeling of species geographic distributions. Ecol. Modell. 190, 231–259. doi: 10.1016/j.ecolmodel.2005.03.026
Piao, S., Liu, Q., Chen, A., Janssens, I. A., Fu, Y., Dai, J., et al. (2019). Plant phenology and global climate change: Current progresses and challenges. Glob. Chang. Biol. 25, 1922–1940. doi: 10.1111/gcb.14619
Pietsch, D., and Morris, M. (2010). “Modern and ancient knowledge of conserving soils in Socotra Island, Yemen,” in Land degradation and desertification: Assessment, mitigation and remediation, ed. P. Zdruli (Dordrecht: Springer Netherlands), 375–386. doi: 10.1007/978-90-481-8657-0_29
Pouteau, R., and Birnbaum, P. (2016). Island biodiversity hotspots are getting hotter: Vulnerability of tree species to climate change in New Caledonia. Biol. Conserv. 201, 111–119. doi: 10.1016/j.biocon.2016.06.031
R Core Team (2022). R: A Language and environment for statistical computing. Available online at: https://www.r-project.org/
Rakshit, A., Singh, S., Abhilash, P. C., and Biswas, A. (eds) (2021). Soil science: Fundamentals to recent advances. Singapore: Springer Singapore. doi: 10.1007/978-981-16-0917-6
Reaser, J. K., Meyerson, L. A., Cronk, Q., De Poorter, M., Eldrege, L. G., Green, E., et al. (2007). Ecological and socioeconomic impacts of invasive alien species in island ecosystems. Environ. Conserv. 34, 98–111. doi: 10.1017/S0376892907003815
Rezende, M., Maděra, P., Vahalík, P., Van Damme, K., Habrová, H., Riccardi, T., et al. (2022). Identifying suitable restoration and conservation areas for Dracaena cinnabari Balf.f. in Socotra. Yemen. Forests 13, 1–12. doi: 10.3390/f13081276
Rezende, M., Riccardi, T., Malatesta, L., Attorre, F., and Van Damme, K. (2020). Land productivity dynamics in Socotra Island (Yemen). Rend. Lincei. Sci. Fis. Nat. 31, 737–746. doi: 10.1007/s12210-020-00928-4
Riahi, K., van Vuuren, D. P., Kriegler, E., Edmonds, J., O’Neill, B. C., Fujimori, S., et al. (2017). The Shared Socioeconomic Pathways and their energy, land use, and greenhouse gas emissions implications: An overview. Glob. Environ. Chang. 42, 153–168. doi: 10.1016/j.gloenvcha.2016.05.009
Riccardi, T., Malatesta, L., Van Damme, K., Suleiman, A. S., Farcomeni, A., Rezende, M., et al. (2020). Environmental factors and human activity as drivers of tree cover and density on the Island of Socotra. Yemen. Rend. Lincei. Sci. Fis. Nat. 31, 703–718. doi: 10.1007/s12210-020-00923-9
Rivers, M., Newton, A. C., and Oldfield, S. (2022). Scientists’ warning to humanity on tree extinctions. Plants People Planet 3:10314. doi: 10.1002/ppp3.10314
Scholte, P., and De Geest, P. (2010). The climate of Socotra Island (Yemen): A first-time assessment of the timing of the monsoon wind reversal and its influence on precipitation and vegetation patterns. J. Arid Environ. 74, 1507–1515. doi: 10.1016/J.JARIDENV.2010.05.017
Shabani, F., Kumar, L., and Ahmadi, M. (2018). Assessing accuracy methods of species distribution models: AUC, specificity, sensitivity and the true skill statistic. Glob. J. Hum. Soc. Sci 18, 6–18.
Shao, X., Jing, C., Qi, J., Jiang, J., Liu, Q., and Cai, X. (2017). Impacts of land use and planning on island ecosystem service values: A case study of Dinghai District on Zhoushan Archipelago, China. Ecol. Process 6:27. doi: 10.1186/s13717-017-0095-3
Shen, T., Li, G. H., Wang, X. N., and Lou, H. X. (2012). The genus Commiphora: A review of its traditional uses, phytochemistry and pharmacology. J. Ethnopharmacol. 142, 319–330. doi: 10.1016/j.jep.2012.05.025
Soromessa, T. (2013). Ecological phytogeography: A case study of Commiphora species. Sci. Technol. Arts Res. J. 2:93. doi: 10.4314/star.v2i3.98910
Swets, J. A. (1988). Measuring the accuracy of diagnostic systems. Sci. Sci. 240, 1285–1293. doi: 10.1126/science.3287615
Thuiller, W., Albert, C., Araújo, M. B., Berry, P. M., Cabeza, M., Guisan, A., et al. (2008). Predicting global change impacts on plant species’ distributions: Future challenges. Perspect. Plant Ecol. Evol. Syst. 9, 137–152. doi: 10.1016/j.ppees.2007.09.004
Thuiller, W., Guéguen, M., Renaud, J., Karger, D. N., and Zimmermann, N. E. (2019). Uncertainty in ensembles of global biodiversity scenarios. Nat. Commun. 10:1446. doi: 10.1038/s41467-019-09519-w
Thuiller, W., Lafourcade, B., Engler, R., and Araújo, M. B. (2009). BIOMOD - a platform for ensemble forecasting of species distributions. Ecography 32, 369–373. doi: 10.1111/j.1600-0587.2008.05742.x
Thulin, M. (2020). “The Genus Boswellia (Burseraceae): The frankincense trees,” in Acta universitatis upsaliensis, ed. M. Thulin Uppsala. Available online at: http://urn.kb.se/resolve?urn=urn:nbn:se:uu:diva-405908
Trlica, M. J., and Rittenhouse, L. R. (1993). Grazing and plant performance. Ecol. Appl. 3, 21–23. doi: 10.2307/1941783
Vahalík, P., Patočka, Z., Drápela, K., Habrová, H., Ehrenbergerová, L., Lengálová, K., et al. (2020). The conservation status and population mapping of the endangered Dracaena serrulata in the Dhofar Mountains. Oman For. 11:322. doi: 10.3390/f11030322
Van Damme, K., and Banfield, L. (2011). Past and present human impacts on the biodiversity of Socotra island (Yemen): Implications for future conservation. Zool. Middle East 54, 31–88. doi: 10.1080/09397140.2011.10648899
Vasconcelos, R., Razgour, O., Tarroso, P., Fasola, M., Carranza, S., and Alves, P. C. (2018). Combining molecular and landscape tools for targeting evolutionary processes in reserve design: An approach for islands. PLoS One 13:e0200830. doi: 10.1371/journal.pone.0200830
Voldoire, A., Saint-Martin, D., Sénési, S., Decharme, B., Alias, A., Chevallier, M., et al. (2019). Evaluation of CMIP6 DECK Experiments With CNRM-CM6-1. J. Adv. Model. Earth Syst. 11, 2177–2213. doi: 10.1029/2019MS001683
Wan, J. N., Mbari, N. J., Wang, S. W., Liu, B., Mwangi, B. N., Rasoarahona, J. R. E., et al. (2021). Modeling impacts of climate change on the potential distribution of six endemic baobab species in Madagascar. Plant Divers. 43, 117–124. doi: 10.1016/J.PLD.2020.07.001
Weeks, A., and Simpson, B. B. (2007). Molecular phylogenetic analysis of Commiphora (Burseraceae) yields insight on the evolution and historical biogeography of an “impossible” genus. Mol. Phylogenet. Evol. 42, 62–79. doi: 10.1016/j.ympev.2006.06.015
Wulff, A. S., Hollingsworth, P. M., Ahrends, A., Jaffré, T., Veillon, J. M., L’Huillier, L., et al. (2013). Conservation priorities in a biodiversity hotspot: Analysis of narrow endemic plant species in New Caledonia. PLoS One 8:e73371. doi: 10.1371/journal.pone.0073371
Zellmer, A. J., Claisse, J. T., Williams, C. M., Schwab, S., and Pondella, D. J. (2019). Predicting optimal sites for ecosystem restoration using stacked-species distribution modeling. Front. Mar. Sci. 6, 1–12. doi: 10.3389/fmars.2019.00003
Keywords: Commiphora, Burseraceae, conservation, ecological niche modelling, Socotra, climate change
Citation: La Montagna D, Attorre F, Hamdiah S, Maděra P, Malatesta L, Vahalík P, Van Damme K and De Sanctis M (2023) Climate change effects on the potential distribution of the endemic Commiphora species (Burseraceae) on the island of Socotra. Front. For. Glob. Change 6:1183858. doi: 10.3389/ffgc.2023.1183858
Received: 10 March 2023; Accepted: 28 April 2023;
Published: 02 June 2023.
Edited by:
Chunyan Lu, Fujian Agriculture and Forestry University, ChinaReviewed by:
Rajendra Mohan Panda, Mississippi State University, United StatesAnastasia Christopoulou, National and Kapodistrian University of Athens, Greece
Copyright © 2023 La Montagna, Attorre, Hamdiah, Maděra, Malatesta, Vahalík, Van Damme and De Sanctis. This is an open-access article distributed under the terms of the Creative Commons Attribution License (CC BY). The use, distribution or reproduction in other forums is permitted, provided the original author(s) and the copyright owner(s) are credited and that the original publication in this journal is cited, in accordance with accepted academic practice. No use, distribution or reproduction is permitted which does not comply with these terms.
*Correspondence: Luca Malatesta, luca.malatesta@uniroma1.it