- 1Department of Botany, Rabindra Mahavidyalaya, Champadanga, West Bengal, India
- 2Forest Microbiome Team, EVA 4.0 Unit, Faculty of Forestry and Wood Sciences, Czech University of Life Sciences Prague, Prague, Czechia
- 3Forest Molecular Entomology Laboratory, EXTEMIT-K, EVA 4.0, Faculty of Forestry and Wood Sciences, Czech University of Life Sciences Prague, Prague, Czechia
Forests form rich biodiversity hubs that act as large reservoirs of natural carbon. The spatial and temporal heterogeneity of these complex habitats of forest floors provides ecological services of immense socio-economic importance. However, these socio-economic ecological hotspots are incessantly exposed to multifarious abiotic, biotic, and anthropogenic disturbances, amongst which unpredictable forest pest (i.e., bark beetle) outbreak account for the loss of vegetation and microbiome of measurable quantum. The importance of the microbiome in forming an inseparable functional unit of every host and shaping its interaction with other partners has been well realized. Interestingly, forest pests, including bark beetles, are also reported to rely on their endosymbiotic microbial partners to manipulate tree defense machinery. In contrast, the microbiome forming the holobiont of trees also regulates the overall function and fitness of the host and significantly contributes to tackling these challenging situations. Nevertheless, how the holobiont of trees directly or indirectly influence beetle holobiont is still an enigma. The present review shall elaborate on the role of microbial tools in enhancing tree performance and fitness, which helps counter beetle damage. Besides, it shall also emphasize exploiting the role of microorganisms in acting as biocontrol agents in shielding the trees against beetle destruction. The application of endosymbiont-mediated RNA interference (RNAi) in working with two-tier specificity for controlling beetle devastations shall be discussed as new-age technological advances. All explanations are expected to put forth the potential of the microbial toolbox in offering better and more sustainable beetle management strategies in the future.
1. Introduction
Forests are immensely important due to their socio-economic and ecosystem services (Pan et al., 2013). They serve the ecosystem as a large sink of atmospheric carbon, supporting wildlife habitats and timber industries. Over the years, the resilience and functionality of forests have been under constant threat due to rise in temperature, drought stress, windthrows, wildfire outbreaks, and pest infestation (Hlásny et al., 2019). Amongst them, pest infestation is reported to account for measurable losses and hence has drawn the concern of forest scientists. Climate-driven intensification in the frequency, severity, and cyclicity of coleopteran forest pest outbreaks has already been documented (Haynes et al., 2014; Hlásny et al., 2021). Besides, bark beetles have expanded their geographic range by exploiting native hosts previously unexplored due to low temperatures (Ramsfield et al., 2016; Howe et al., 2021). Such insect range expansion also causes widespread tree mortality, decreasing forest productivity and carbon storage and enhancing discharges from the decayed dead wood. Frequent pest outbreaks cause severe forest depletion triggering trajectories outside the resilience limits of forest ecosystems, causing irreversible ecosystem regime shifts (Dhar et al., 2016). With global climate change, beetles have become a formidable threat to forest health worldwide (Hlásny et al., 2021). The most common aggressive tree-killing coleopteran beetles that have caused widespread damage to millions of trees across Europe and North America include Emerald Ash Borer (EAB) [Agrilus planipennis (Fairmaire)] (Coleoptera: Buprestidae), Asian long-horned beetle (ALB) [Anoplophora glabripennis (Motschulsky)] (Coleoptera: Cerambycidae), and bark beetles (Coleoptera: Curculionidae: Scolytinae); ambrosia beetle, mountain pine beetle (Dendroctonus ponderosae Hopkins), Eurasian spruce bark beetle [Ips typographus (L.)]. Several conventional approaches, such as sanitation felling (Seidl et al., 2016), removal of wind-felled trees (Leverkus et al., 2018), and deployment of pheromone-baited and poisoned log tripod traps (Wermelinger, 2004) have been deployed for the last few decades to manage the pest population levels within endemic limits. However, the success of all these approaches remains questionable in managing the recent outbreaks (Hlásny et al., 2019). Several synthetic pesticides have been used to suppress forest beetles over the past years (Holmes and MacQuarrie, 2016; Liebhold and Kean, 2019). However, many of these compounds caused other problems, such as environmental pollution, detrimental effects on non-target organisms, and caused widespread pesticide resistance (Billings, 2011; Bras et al., 2022; Avanasi et al., 2023; Kenko et al., 2023). Therefore, questions have been raised about the effectiveness of conventional phytosanitary measures. Hence, eco-friendly alternatives for controlling these devastating tree-killing forest beetles have become the utmost priority in the Anthropocene.
Very interestingly, the associations between the forest beetles and their bacterial and fungal endosymbionts are reported to add to the host fitness by aiding them to feed on recalcitrant bark tissues, provide protection against pathogens and increase intra and intercellular communicability that helps to overpower host defense and build up pest population (Cheng et al., 2018; Chakraborty et al., 2020a,b). Besides, forest flora, predominantly comprised of trees, house a huge population of diverse microorganisms both above and belowground. These forests exhibit a wide and dynamic spatial and temporal heterogeneity ranging from short-term seasonal events to long-term standing developments, allowing diverse microorganisms to thrive in these variable habitats and form an ecosystem of rich bio-resources (Baldrian, 2017). Soil microbiome and plant-associated above and below-ground microbiome, also called the “plant holobiont,” are believed to directly influence plant metabolism and defense that ultimately demarcate the expanses and limitations of an ecosystem (Soldan et al., 2022). Soil microbial profiles are often similar to gut microbial profiles of herbivorous insect pests suggesting a direct link between soil and insect pest microbiome, which are often independent of tree host microbiome (Hannula et al., 2019). On the contrary, studies conducted on forests and woodlands have shown spruce beetle infestation to modify soil microbial assemblage by altering edaphic properties (Custer et al., 2020). Such resemblance of microbial composition across several microbiomes indicates bottom-up and top-down connectivity amongst food webs. However, how these microbial assemblies add to the functionality of diverse holobionts is still an enigma. Research on the role of plant and pest holobionts and their microbial exchange is increasing (Pirttilä et al., 2023). Few knowledge bases are being translated from laboratory benchtops to agricultural fields. However, owing to the vastness of forests, comparative analyses and similar studies conducted on forests are notably less (Figure 1). Research on agriculture is prioritized as it meets the growing population’s demand and ensures food security. Hence, forest research is trailing behind (Figure 1), but it has recently gained more interest. The beneficial impact of a less polluted environment is vivid after COVID-19 pandemic. Forests play a major role in our environment, and saving forest ecosystemss from insect pests are crucial. However, limited studies have been conducted on forest pests and their microbiomes (Figure 1). There are a handful of forest pest genomes published so far. Besides, how the edaphic and other environmental cues alter the soil biodiversity and biogeochemical performance in forest ecosystems is also understudied (Chen et al., 2019). The present review shall look into the different aspects of tree-beetle interaction, emphasizing the role of forest beetles and their associated microorganisms that directly or indirectly influence the host-beetle-microbiome tripartite relationships. Using such knowledge for future forest beetle management is also debated (Figure 2).
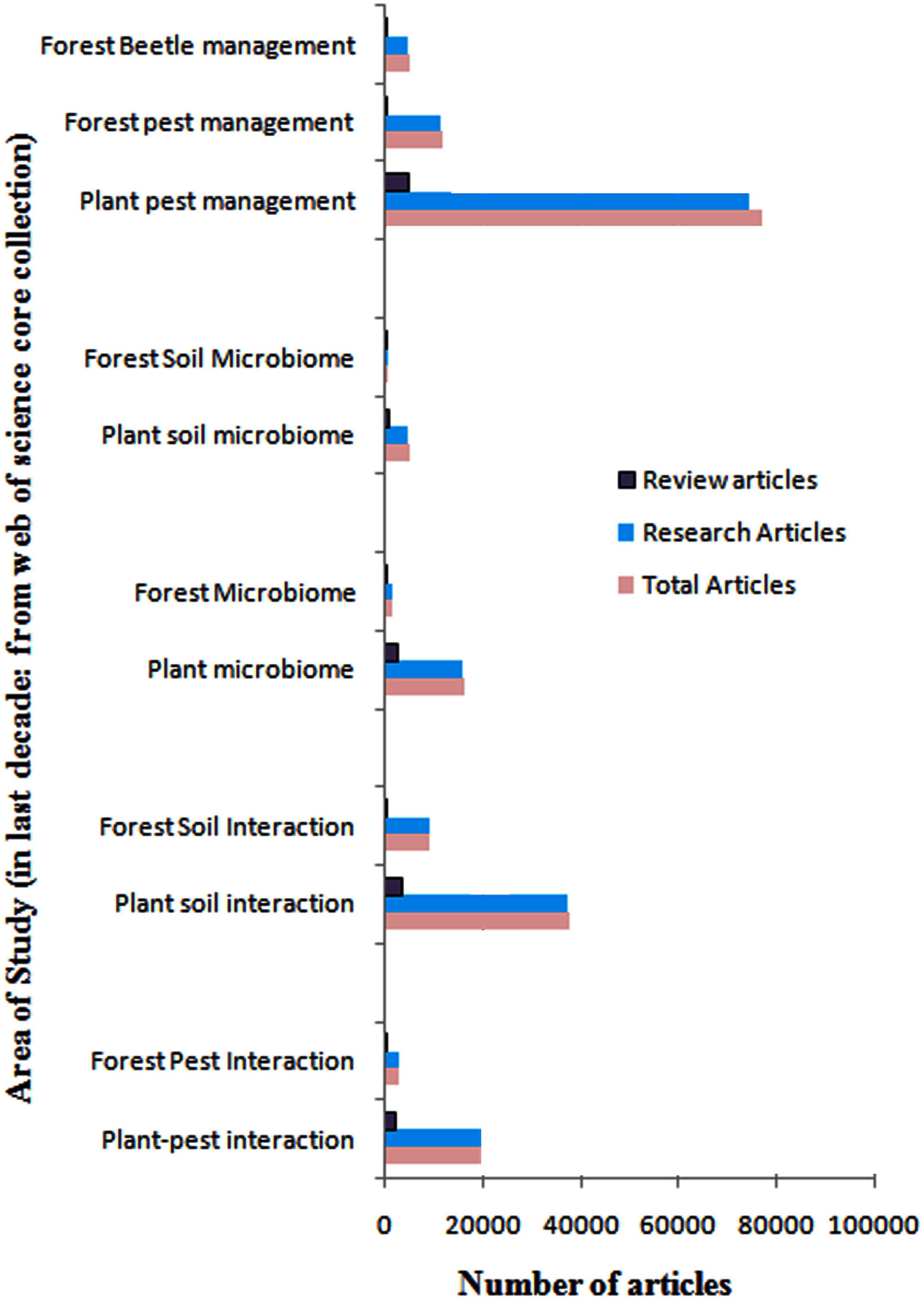
Figure 1. Comparative analyses of numerical data of studies on plant-microbe-pest interaction of typical habitats versus forest areas over the past decade. The source of publications is core collections indexed in the Web of Science (January 2023).
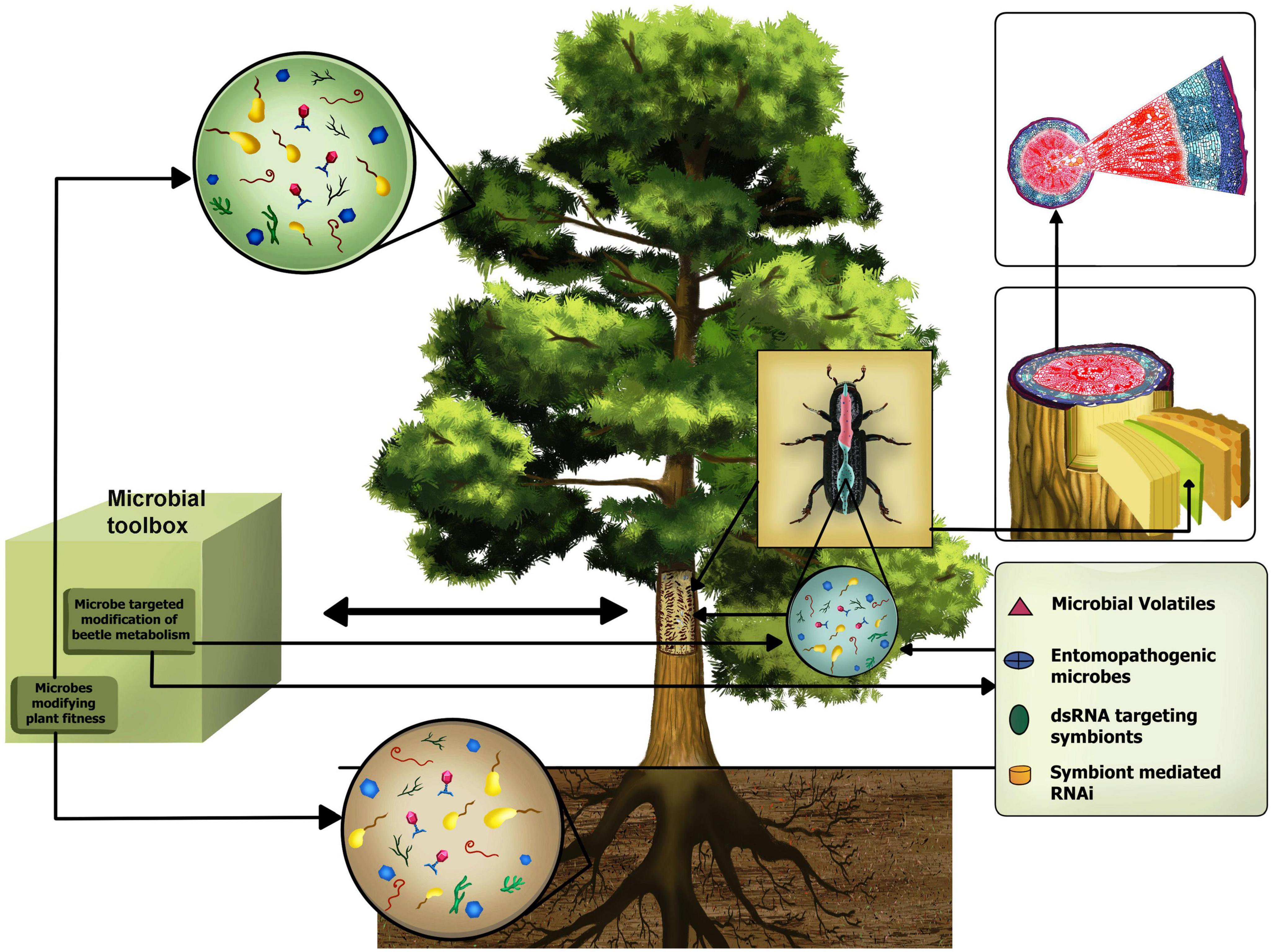
Figure 2. Schematic illustration projecting the probable roles of the microbial toolbox in developing a direct or indirect defense of forest trees against beetle infestation.
2. Coleopteran insect pests: a constant threat to forest flora
Forest beetles, such as Asian longhorn beetles, EAB, bark beetles, etc., have been reported to have taken advantage of current climate change and caused substantial damage to the forests worldwide (Aukema et al., 2011; Meng et al., 2015; Hlásny et al., 2019). EAB is a tree-killing forest insect pest from northeastern Asia that recently entered North America via solid wood packaging material (Poland and McCullough, 2006). EAB has killed millions of North American Ash (Fraxinus sp.) and is recognized as one of the costliest insect pest invaders in American history (Aukema et al., 2011). EAB larvae disrupt the translocation of water and essential nutrients in the attacked plants during phloem and cambium feeding (cambio-xylophages), killing Ash trees within 3–4 years of infestation (Haack et al., 2002). Like EAB, the Asian longhorn beetle (A. glabripennis), native to China and Korea, is a globally known invader with the potential to thrive on more than 100 species of trees (Meng et al., 2015). If uncontrolled, the ALB population is believed to cause tremendous economic losses (in billions of dollars) (Pedlar et al., 2020). Fascinatingly, the trunk injection of imidacloprid (systemic insecticide) was documented to be effective against ALB infestation. However, the cost and environmental impact of using chemical pesticides jeopardize such strategies, which demand better eco-friendly alternatives for ALB management (Avanasi et al., 2023).
The forest beetles, primarily bark beetles (Coleoptera: Curculionidae: Scolytinae), feed as larvae and adults in the phloem of trees and woody shrubs (Coulson, 1979), have caused widespread coniferous tree mortality and severe economic losses around the globe (Sun et al., 2013). Bark beetles are thought to destroy more trees than all other natural factors combined (Franceschi et al., 2005). Furthermore, these insects are enormous profiteers of global climate change, as increasing temperatures have contributed to historically unprecedented beetle outbreaks, killing hundreds of millions of conifer trees (Raffa et al., 2008). One important tree-killing species is I. typographus, which has decimated more than 170 million m3 of spruce in Europe over the last 65 years (Økland et al., 2016). During outbreaks, the beetle population surpasses a critical threshold and overwhelms the defensive capacity of individual trees in pheromone-mediated mass-attacks (Wermelinger, 2004). Males are the pioneering sex that selects trees, tunnels into the bark to make a mating chamber and produces aggregation pheromones while detoxifying fierce spruce defenses. Females are attracted to the pheromones and ingest large quantities of well-defended spruce tissues as they chew out ∼10 cm long tunnels in the inner bark where they deposit their eggs (Wermelinger, 2004; Schebeck et al., 2023). Despite their enormous economic and ecological importance, the exact mechanisms by which these tiny insects can overwhelm the defenses of giant conifer trees are still unknown (Krokene, 2015). Only limited studies provided molecular insights into bark beetle adaptive strategies (Liu et al., 2022; Naseer et al., 2023; Tholl et al., 2023; Zaman et al., 2023).
3. Microbial symbiosis: ecological benefits
The recipe for such adaptive success intended for thriving under the bark is often hinged on the association with microbial mutualists that benefit its host by feeding on recalcitrant plant material, protecting against pathogens, and enhancing the inter-and intraspecific communications (Chakraborty and Roy, 2021; Singh et al., 2021; Picciotti et al., 2023). Interestingly, microorganisms exhibit extremely short generation times and thus possess rapid evolutionary rates compared to multicellular organisms. Hence, they can evolve various metabolic pathways and adapt to diverse ecological niches. Multiple studies suggest mutualistic associations with microbes in coleopteran forest pests; however, rigorous experimental validation remains lacking. Thus, the exact mechanisms or the molecular dialogue during symbiosis enabling the spectacular destructiveness of tree-killing forest beetles is still unknown (García-Fraile, 2018). However, it is already established that microorganisms could give their host a substantial advantage over non-symbiotic competitors in the severe interspecies competition. Insects, including forest beetles, cannot survive without their associated microbes. Hence, such microorganisms can be alternative targets for controlling forest beetles (García-Fraile, 2018; Rupawate et al., 2023). Although, in most cases, these symbiotic associations are species-specific and fine-tuned on the ecological requirements of their host, targeting symbionts for pest management reduces the chances of non-target effects (Douglas, 2007; Chung et al., 2018).
The advent of high throughput omics technology such as genomics, metagenomics, meta-transcriptome, meta-proteomics, and metabolomics has provided exciting insights into the function of the microbiome in forest beetle ecology and evolution. It has been possible to characterize the full spectrum of biological diversity of previously hidden habitats, including inside plants and insects. This has opened up fascinating new research fields, such as the study of phytobiomes, i.e., all microbial diversity in and around plants (Ledford, 2015), and endomicrobiomes, i.e., primarily gut symbionts of different animals (Adams et al., 2013). Many studies suggest that microbes in and around an insect’s body are more critical to its success than was once believed (Picciotti et al., 2023). Symbiotic bacteria and fungi have been found to play essential roles in many processes, including the breakdown of food (Scully et al., 2014; Lee et al., 2015), protection against plant defenses (Ceja-Navarro et al., 2015; Howe and Herde, 2015) and fungal pathogens (Cardoza et al., 2006), and even affecting insect reproduction and development (Vavre and Kremer, 2014). For instance, in some North American bark beetle species, the endomicrobiome is critical for the digestion of plant tissues, breakdown of plant defenses (especially terpenes), and parts of pheromone production (Adams et al., 2013; Boone et al., 2014). Adams et al. (2013) conducted a shotgun metagenomic sequencing analysis of microbiota in the mountain pine beetle, a severe pest of pine, and detected enrichment in bacterial genes involved in diterpene degradation. Also, most of these genes were derived from Gamma-proteobacteria and Beta-proteobacteria (genus Burkholderia) (Adams et al., 2013). Coupled with this culture-independent analysis, they isolated terpenoid-degrading bacteria from mountain pine beetle gut belonging to the bacterial genera Serratia, Rahnella, and Brevundimonas.
Moreover, symbiont-mediated terpenoid degradation in the large pine weevil, Hylobius abietis (L.) (Coleoptera: Curculionidae), in vitro and in vivo analyses is already reported (Berasategui et al., 2017). In conjunction with physiological confirmations, the metagenome and metatranscriptome investigations have identified microbial genes involved in the detoxifying symbiosis in beetle systems (Itoh et al., 2018). This opens up the opportunities to search for essential microbial genes within tree-killing beetles, give vital survival services, detoxify terpenes, and aggregate pheromone production in EAB, ALB, and other targets beetles that pose a constant threat to forests globally under the influence of climatic changes. Such genes of microbial origin can be targeted to formulate eco-friendly strategies to control the beetles via symbiont management (Rupawate et al., 2023). For instance, targeting horizontally transferred genes to manage whitefly, Bemisia tabaci (Gennadius) (Hemiptera: Aleyrodidae) (Wang and Luan, 2023). The work on genetic engineering of honeybee gut microbiota shows the feasibility of such an approach (Leonard et al., 2018; Chhun et al., 2023). Although there is increasing scientific literature on the importance of symbiotic microorganisms in forest beetles that evolved from our perspective of viewing them as a holobiont, the idea of decoding such knowledge into developing forest pest management tools is still limited.
4. Tree defense against coleopteran forest beetles
Statistical records suggest that tree mortality is caused by the sporadic outbreaks of the beetle population, which under natural environmental conditions, remain confined within endemic limits (Raffa et al., 2008). Such restrain in the beetle population is attributed to the intrinsic surveillance and defense system of the host trees (Krokene et al., 2013; Whitehill and Bohlmann, 2019). Minor fractions of aggressive beetles that usually thrive on dead and decayed wood tissues are known to succeed in overwhelming host defense and colonizing tree trunks under conducive conditions, while semi-aggressive and facultative parasitic members complete their life cycle primarily on weak and/or dead trees (Krokene et al., 2013). Beetles are reported to entrap the host immune system by employing pheromone-mediated mass attack. Symbiotic microbes residing in the beetle gut collaborate with the host beetle in devastating tree defense (Six and Wingfield, 2011). Beetle mass outbreaks are reported predominantly in coniferous forests where colonization is predominantly found on the bark tissues. Successful establishment of the beetles on its prospective hosts is achieved in a phase-dependent manner where the first stage is to inhibit the pest by the preformed defenses. The second stage is to compartmentalize the attackers by inducible defenses. The third stage means sealing damaged areas, while the last stage imparts systemic acquired resistance to tackle consecutive outbreaks (Eyles et al., 2010). Heavy impregnation of lignin and suberin polymers in the periderm of bark regions are reported to provide preformed constitutive defense against invasion and establishment of beetle pests, while the inducible defense is offered by chemicals such as tree metabolites, proteins and enzymes (Franceschi et al., 2005; Keeling and Bohlmann, 2006). The conifer stem anatomy offers a highly structured natural barrier for the invading beetles (Figure 3). The bark is the primary site of conifer defense. The bark tissue is known to impart mechanical support and also supply photosynthates. Besides, the specialized cells of the inner secondary phloem named polyphenolic parenchyma cells (PP), lignified sclerenchyma cells, cells with calcium oxalate crystals, resin ducts and the connecting radial cells, contribute to providing resistance against beetle aggression (Franceschi et al., 2005). The sap wood also contains a few resin ducts that help act as sources of inducible chemical defenses (Krokene et al., 2013; Whitehill and Bohlmann, 2019). The defenseless vascular cambium plays a pivotal role in tissue differentiation and supplying needful cells to outer bark region and inner sapwood region. Hence, damage of the said region due to beetle infestation leads to irreversible loss and ultimately death of the host conifer (Franceschi et al., 2005).
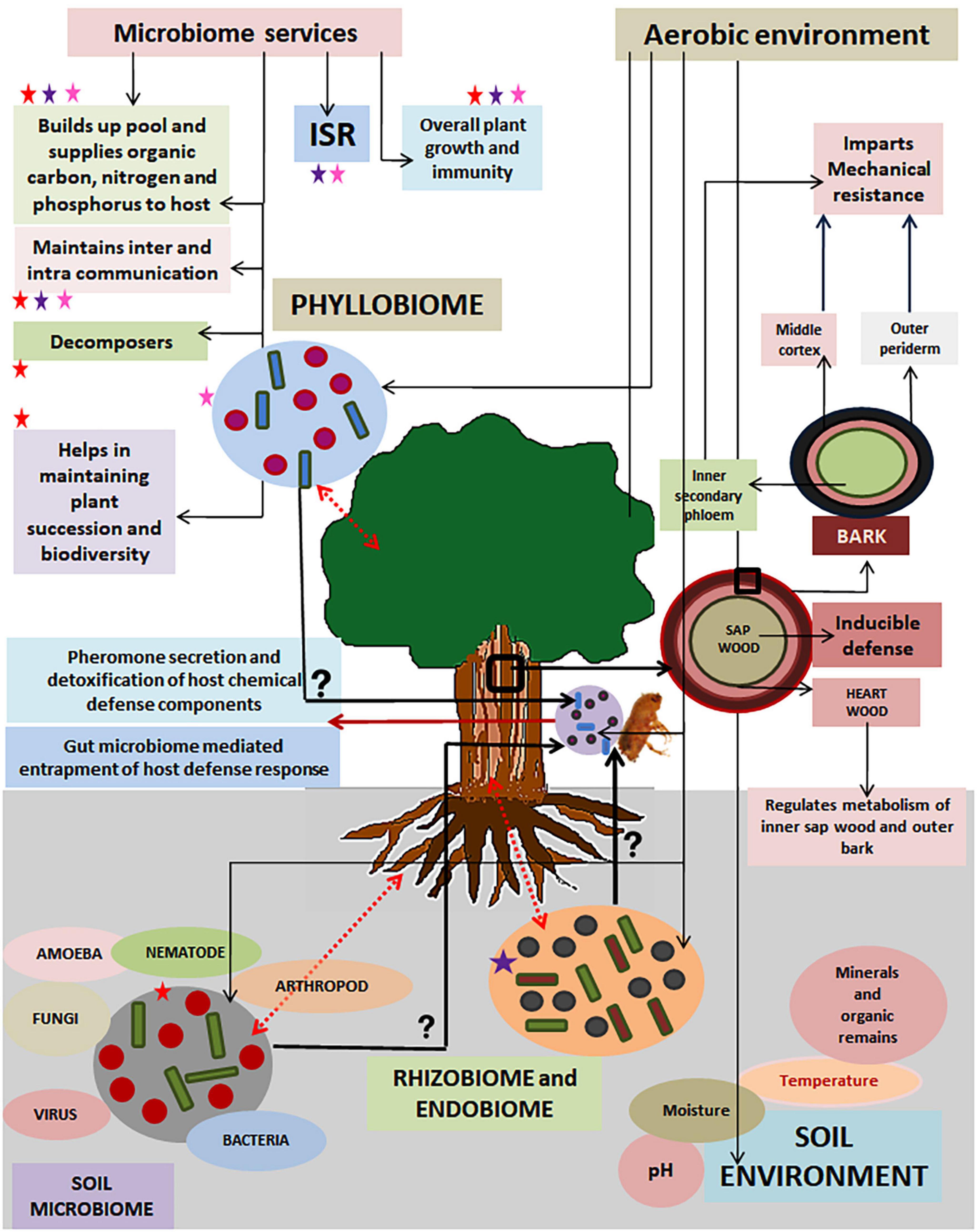
Figure 3. A schematic graphic illustration shows tree defense against forest beetle destruction and the role of soil microbiome and tree holobiont in providing indirect host resistance in a forest ecosystem. The unidirectional dashed arrows show the unidirectional regulation of the aerobic environment with its different biotic and abiotic components of soil, the tree host, tree holobiont, and beetle holobiont. The bidirectional arrows show bidirectional regulation between the soil microbiome tree holobiont and the host tree. The interrogation sign shows unknown roles and regulations of the tree holobiont and soil microbiome with beetle endosymbiont. Red, purple, and pink stars indicate soil, rhizosphere/endosphere, and phyllosphere microbiome, respectively. These star indicators have been used to mark the microbiome services they provide in a forest ecosystem. The small square-marked boxes of tree trunks have been enlarged to highlight the different layers of tree trunk sections. The roles of different layers of bark in providing defense against attacking beetles are elaborated in brief.
Chemical signaling has been known to impact almost all facets of species-species interaction. Amongst several chemicals, terpenoids, and phenolics stored in resin ducts, PP cells and secondary phloem are considered to modulate beetle-tree interaction (Keeling and Bohlmann, 2006). Terpenoids are reported to have mutually exclusive bidirectional roles of acting as flavoring and venomous agents for beetles from time to time. On one hand, they assist the beetles in locating compromised hosts and exhaust their immune system, while on the other hand, they form a formidable barrier against their mass colonization and attract their predators and competitors (Gitau et al., 2013; Zhang et al., 2013). Besides terpenoids, tree phenolics are also known to induce immune responses upon beetle infestation (Krokene et al., 2013). Different secondary phenolic compounds, such as soluble stilbenes and flavonoids, condensed tannins, polyphenols, and structural lignin polymers, modulate host defense. Majority of these secondary metabolites are produced via the shikimic acid pathway, where carbohydrate metabolism is connected to producing the primary precursor aromatic amino acid of all secondary phenolics, the phenyl alanine (Ralph et al., 2006).
Over the past two decades, research has defined different types of acquired resistance mechanisms in forest trees, especially conifers, that have made them resistant to consecutive attacks (Whitehill and Bohlmann, 2019; Mageroy et al., 2020). Two such defense mechanisms are reported in conifer hosts, one induced upon fungal attack while the other upregulated following treatment with the phytohormone methyl jasmonate (MeJA) (Wilkinson et al., 2019). Although defense priming has provided experimental evidence in sufficiently equipping the immunogenic memory of a host plant, similar studies showing comparable results in real-world ecological contexts of forest regions are still not adequate. Besides, the long generation time of forest trees makes it hard to quantify the fitness versus allocation costs (Neilson et al., 2013). Moreover, the priming-induced decline in a symbiotic relationship that otherwise imposes additional fitness costs on trees by increasing their vulnerability toward pathogenic attack also questions the sustainability of priming as a defense measure in forest backdrops (Martinez-Medina et al., 2016). Despite of highly structured defense system of forest trees, the devastating bark beetles manage to bring on mass killings mainly by exploiting their own bacterial and fungal symbionts (Krokene, 2015; Zaman et al., 2023). In contrast, tree hosts are also shelter-providers to a large number of microorganisms referred to as the “tree holobiont”(Soldan et al., 2022). These microorganisms are also supposed to significantly contribute to shaping “tree health” and “immunity.”
5. Role of microbiome in boosting tree fitness: indirect protection against beetle damage
The role of microbiome in shaping the entire biological world and its communication with all other biotic entities have been well established (Berg et al., 2020; Singh et al., 2021). Plants depend on beneficial microorganisms to enhance their stress tolerance and pathogen resistance. The discovery and refinement of technological tools as well as continuing upgradation of understanding has provided re-definitions of “microbiome” and “microbiota” where any and every eukaryote is considered to have an inseparable unit referred to as “second genome” or “holobiont” or “meta-organism,” and likewise, death of any and every organism is considered as “dysbiosis” and loss of biodiversity comprising of its entire meta-organism (Lakshmanan et al., 2014; Stegen et al., 2018; Berg et al., 2020). Hence, the addition and/or alteration of microbial entities could result in significant alterations in the immune signaling cascade and lead to a paradigm shift in the health and productivity of the primary host (Bello et al., 2018). The following sections shall elaborate on the potential roles of the microbiome in developing tree resistance against beetle destruction.
5.1. The role of the below-ground microbiome and its potential for forest insect pests management
Plants being stationary, are predominantly dependent on their roots for their development from the onset of the seed emergence stage. These roots are exposed to a plethora of microorganisms both externally (by soil and rhizospheric organisms) as well as internally (by endophytic organisms) (Leach et al., 2017). Among the external members, soil microbes influence almost all spheres of below and aboveground ecosystems directly by altering the plant performance and/or indirectly by modulating the mutualistic or pathogenic interactions which ultimately impact host fitness (Pieterse et al., 2016).
The belowground microbiome of a forest comprises biotic entities present in various habitats ranging from soil, in close proximity to tree roots and within their rhizosphere, in litter, in deadwood, on the rock surfaces, inside rock clefts, etc. (Baldrian, 2017). Amongst all habitats, forest soils are known to regulate microbial behavior to a large extent. The spatial diversity of microorganisms of forest top soils is controlled by primarily two driving factors (1) soil and litter chemistry that regulate the primary metabolism of soil bacteria and fungi; and (2) the attributes of above-ground vegetation that alter the performance and efficiency of soil microorganisms (Tedersoo et al., 2016). Spatial variation and the relative effects of the above-mentioned two driving factors cause wide diversity in microbial abundance and activity, often restricting them to form “activity hotspots” comprising of the rhizosphere, the detritusphere and biopores which altogether regulate the soil ecosystem of vast expanses (Baldrian, 2014; Kuzyakov and Blagodatskaya, 2015). Soil microbiota comprises predominantly fungal and bacterial members. Fungal members belong to the ectomycorrhizal class (EMF), which forms almost one-third of the soil microbial biomass and adds to 50% of dissolved organic carbon (Ekblad et al., 2013). These EMFs not only supply nitrogen and phosphorus from the dead organic remains to their symbiotic hosts but also form communication networks with different tiers of vegetation. Besides, they also form mats and establish interaction with other saprophytic fungi, endobacteria belonging to the Alphaproteobacteria group, amoeba, nematodes, microarthropods, enchytraeids, etc., that influence the mycelial growth to a large extent (Bertaux et al., 2005; Anderson et al., 2014). Archaebacteria of class Thaumarchaeota, known to recycle nitrogen, are dominantly found in acidic soils with low ammonia (Levy-Booth et al., 2014). However, soil microorganisms exhibit variation across soil profiles, natural seasonal variation, as well as variation due to abrupt climatic eruptions referred to as “hot moments” of climatology and also variation due to anthropogenic disturbances (Kuzyakov and Blagodatskaya, 2015; Žifčáková et al., 2016). The saprophytic basidiomycetes solely or in combination with ascomycetes microfungi and bacterial members belonging to Proteobacteria and Bacteroidetes act as decomposers of the recalcitrant plant remains (Eichlerová et al., 2015). Deadwood, rock surfaces and rock clefts harbor cord-forming saprophytic basidiomycete decomposers, low pH tolerant bacteria, macrophages, commensal bacterial population, lignicolous lichens, and EMF members. These microorganisms aid in the decomposition and/or weathering process of organic matter or rock surfaces and deliver essential mineral nutrients to the host trees (Johnston et al., 2016). Metagenomic data of the forest soil samples from the Lipova region of the Czech Republic showed a predominance of Ectomycorrhiza (ECM), fungal basidiomycetes population, and acidophilic bacterial members are known to control nitrogen-supply mediated metabolical processes of aboveground vegetation (Chakraborty et al., 2023). However, the assemblage and activity of microorganisms of bulk soil and/or soil adjoining specialized habitats are exposed to constant modification during soil degradation, changing climatic conditions and throughout anthropogenic disturbances to provide resilience against any form of opposing selection pressure (Liu et al., 2019). On the contrary, the climatic alteration cause changes in above ground vegetation which in turn modify their root exudations of antimicrobials and/or semiochemicals to attract suitable microbes of the soil toward their rhizospheric zones and/or beyond to the endospermic regions to help the host trees tackle the stressful situation by triggering induced systemic responses (ISR) and recruiting antagonistic/parasitic approaches against pathogens and pests (Rolfe et al., 2019).
Apart from soil microbes, rhizospheric and endophytic microbes significantly promote plant growth and immunity to above-ground vegetation. Besides, they are known for their crucial role in manipulating belowground microbial assembly, building up “activity hotspots” and controlling ecological parameters of vast expanses (Baldrian, 2014; Kuzyakov and Blagodatskaya, 2015). Comparative studies carried out with temperate, boreal and Mediterranean forest trees revealed that apart from fungal members belonging to EM class, Ascomycetes and saprophytic Basidiomycetes, bacterial members belonging to Actinobacteria, Acidobacteria, Firmicutes, Bacteroidetes, Proteobacteria, etc. were found in the rhizosphere, endosphere, and root adjoining soil samples. All of this microbiota, in a concerted manner, played essential roles in nutrient acquisition, proper allocation, and recycling within host trees, thus helping maintain their vigor and vitality (Pettifor and McDonald, 2021). Detailed analyses conducted on the belowground microbiota and its effect on tree health using both conventional (community-based physiological fingerprinting methods) and advanced molecular approaches (epifluorescence-based assays and meta-omics techniques), explained multidimensional beneficial roles of belowground microorganisms (Mercado-Blanco et al., 2018). The mycorrhizal fungi [EMF and arbuscular mycorrhiza fungi (AMF)], the endophytic bacterial and fungal members and diazotrophs secrete different volatile organic compounds (VOCs), which altogether alter soil attributes for promoting soil-water-tree growth relations, increase soluble phosphate and availability of iron by synthesizing siderophores, increase root absorbing surface area by hundred to thousand manifold, control the invasion of pathogens and alien species, increase hormonally mediated tree tolerance toward both abiotic and biotic stressors, manipulate internal ROS levels to decrease self-decay, influencing primary and secondary metabolic signaling to balance optimum growth of host trees, etc. Besides, these microorganisms also account for properly restoring destroyed forest lands, upholding plant succession and maintaining above and belowground biodiversity (Rasmann et al., 2017; Mercado-Blanco et al., 2018). In addition, the belowground microbiome is also known to contribute to induced priming and inheritance of above-ground host plants (Tiwari et al., 2022). However, similar generalized interpretations in the case of forest trees are complex since plant-soil-microbe inter-chemistry is still not very clearly understood in different forest ecosystems. Moreover, studies on Arabidopsis spp. and other uncultivated species showed that belowground microbiomes controlled above-ground herbivores and cell-feeding pests through complex plant-soil-feedback (PSF) (Pineda et al., 2020). However, such PSF studies in forest backgrounds are still challenging due to the unavailability of growth and survival models of complex and bio-diverse microbial populations that are perpetually exposed to dynamic environmental factors. Considering the established role of the belowground microbiome, including soil, rhizosphere, and endospermic members in well-studied agricultural backdrops, it is time to think about how this information can aid in boosting tree resistance against biotic stresses, including beetle attacks. Preparation of beneficial soil microbial formulations for boosting tree health and stress resistance and testing them in the experimental forest can be a good starting point, and inspirations can be taken from agricultural set-ups (Naik et al., 2020; Tyagi et al., 2023). However, the primary task will be ensuring the survival and establishment of soil microbial inoculants in the forest (Kaminsky et al., 2019).
5.2. The role of above-ground microbiome and its potential for forest insect pests management
In the forest ecosystem, above ground microbiome comprises of foliar parts, stem and bark regions, and reproductive parts (Sieber, 2019). The foliar microbiome consists of beneficial and pathogenic fungi (epiphytic and endophytic), bacteria (pathogenic, commensal, and symbiotic) and invertebrates. All these organisms are known to help in hormone-mediated plant growth, nitrogen uptake, plant protection through antibiosis and control secretion of organic volatiles, thus regulating inter and intra-communication signaling and overall host metabolism. Studies on forest regions of temperate zones suggest that epiphytic fungi belonging to Ascomycetes and Basidiomycetes classes mainly colonize on foliar surfaces. These epiphytic fungi depend on host exudations, spores, pollens, organic aerosols, and honeydew released from sucking pests for their nutrition (Menkis et al., 2019). Besides, endophytic and pathogenic fungi also comprise a large portion of the foliar microbiome. However, the segregation between the endophyte and pathogen often becomes inconspicuous as many pathogenic fungi (for example, rust, mildew, and snow mold-forming fungi) remain as harmless endophytes for long periods, while many defined pathogens restrict themselves as local endophytes under unfavorable conditions (Schlegel et al., 2018; Sieber, 2019). The climatic conditions, location, precipitation amounts, host specificity, site specificity, host, and tissue age, interaction with other symbiotic and/or pathogenic entities and anthropogenic hindrances define the etiology of the endophyte/pathogen (Sieber, 2021).
In forests, the phyllosphere is inhabited by many bacterial members who reside in phylloplane and inside the phyllotelma region (Doan and Leveau, 2015). They belong to class Proteobacteria, Firmicutes, Acidobacteria, Actinobacteria, Firmicutes, Saccharibacteria, etc. (Doan and Leveau, 2015). The phyllosphere forms an extreme habitat for the bacterial population because of its variable phylloplane topology, trichome physiology, uneven nutrient distribution within its internal segments, and direct exposure to extreme environmental factors. The phyllosphere bacteria depend on minimal amounts of carbon, nitrogen, volatiles, etc., for nutrition (Vorholt, 2012). Some bacteria may be transitory, while others may be permanent residents (Suslow, 2002). However, the biodiversity of the bacterial population supporting selection or drift is driven by co-evolutionary parameters of both the phyllobiome and the host (Vacher et al., 2016). The phyllobacteria are known to influence host metabolism and fitness either by producing or by altering the production of host phytohormones IAA (indole acetic acid), cytokinins, ABA (abscisic acid), etc., which control host growth, transportation of nitrogen, and regulate the opening and closure of stomata thus controlling the entry of pest and pathogens (Holland, 2011; Munemasa et al., 2015; Romero et al., 2016). On the other hand, Pseudomonas syringae and many other epiphytic bacteria are known to emit bio-surfactants that form biofilm and help in their uniform distribution on the host phylloplane. Besides, they alter cuticle permeability and increase water and sugar availability, supporting their growth (van der Wal and Leveau, 2011). Besides, the phyllobacteria are also known to enhance the abiotic stress tolerance of the host, prime the host against biotic stressors and promote ISR-mediated resistance response (Schlechter et al., 2019). However, research regarding how these phyllofungi and phyllobacteria and their community dynamics shape host health is still being extensively performed (Gong and Xin, 2021).
In addition to the above, the microorganisms (fungi and bacteria) associated with reproductive structures are also known to contribute remarkably to transgenerational microbiome transfer (Ren et al., 2021). The microbiome (mainly endophytes) associated with reproductive structure may result from vertical transfer from the maternal parent or environment through horizontal transfer (Perlmutter and Bordenstein, 2020). Maternal effects of the reproductive microbiome are reported to impact offspring physiology and its relation with other abiotic and biotic factors, especially in choosing pollinators (Vannette and Fukami, 2016). It also helps in nullifying the ill effects of climatic change (Burgess and Marshall, 2014). Interestingly, the reproductive microbiome inherited through vertical transfer is also known to bring about epigenetic modifications such as histone methylation and modulate the expression of many stress-induced host tree genes (Vivas et al., 2015). In the case of horizontal microbiome transfer, the role of soil in transferring microbes to seeds, the role of sap-sucking and non-sap sucking insects and animal pollinators in introducing and/or relocating microbes to visiting floral parts as well as agents (wind, water, human interference, etc.) influencing the pollination appears to be very important in building up of tree holobiont (Ren et al., 2021).
Experimental studies involving multitrophic interaction comprising microbial pathogens, symbionts, higher-order pests, and predators showed that complex, stressful situations induced localized and systemic defensive responses in tree hosts and enhanced their metabolic performance compared to trees exposed to symbionts only (Saleem et al., 2017). This view was further supported by dysbiosis of Pseudomonas population during insect herbivory, which enhanced immune responses in Cardamine hirsuta (Humphrey and Whiteman, 2020). Such information at the level of above-ground microbial interactions with trees can be used to formulate strategies to boost tree resistance against pests and pathogens using microbes (Ajayi and Olufolaji, 2023). For instance, priming inducible defenses can protect Norway spruce (fungus-inoculated trees) against tree-killing bark beetles (Mageroy et al., 2020). However, more research endeavors toward delineating the role of microbes at tree holobiont in various forest ecosystems define the feasibility of such a management approach. A better understanding of the defense priming of forest trees and its impact on establishing beneficial microbial communities and vice versa will be an essential starting point for assessing the ecological implication of forest tree defense priming. Such knowledge is vital to employ defense priming as an effective pest management strategy in forestry.
6. Biological control of coleopteran forest pests: exploring the microbial toolbox
6.1. Entomopathogenic control of coleopteran beetles
Coleopteran forest insect pests cause economic damage and impact the environment globally. Chemical pesticides have been a primary measure for pest control for several decades. Extensive use of these pesticides and the constant evolutionary dynamics of insects have introduced pesticide resistance in target species and have killed several non-target beneficial insects (Usta, 2013; Bras et al., 2022). Therefore, integrated pest management (IPM) strategies have emerged to address these issues and promote environmentally friendly, economically viable, socially acceptable, and sustainable alternatives to managing insect pests (Dara, 2019). Classical biological control involves the introduction of living organisms such as parasitoids, predators, and entomopathogenic microbes to reduce pest populations (Eilenberg et al., 2001; Heimpel and Mills, 2017). Entomopathogenic microbes are the natural regulators that significantly control insect populations (Roy and Cottrell, 2008). Entomopathogens as pest regulators controlling insect pest populations efficiently and are environmentally safe for non-target organisms (Hajek and Bauer, 2009). Several invasive forest pests belonging to the order Coleoptera, Hemiptera, Hymenoptera, and Lepidoptera have been a target for management practices, including using microbes as a control agent.
Over the years, several entomopathogenic microbes, including viruses, bacteria, fungi, and nematodes, have been commercially produced and used augmentatively as microbial pesticides (Lacey et al., 2015). Baculoviridae has been commercially developed significantly among the different viral pesticides (Moscardi et al., 2011). For instance, the naturally occurring host-specific virus Lymantria dispar multiple nucleopolyhedrovirus (LdMNPV) successfully controls the outbreak gypsy moth (L. dispar L.) population in the United States, Canada, and Germany (Boukouvala et al., 2022). Similarly, other nucleopolyhedroviruses are used to control forest pests, including balsam fir sawfly (Neodiprion abietis Harris), pine sawfly (Neodiprion sertifer Geoffroy), and Douglas-fir tussock moth (Orgyia pseudotsugata McDunnough) (Moscardi, 1999). Compared to viral pesticides, entomopathogenic bacteria are commercially less available (Jurat-Fuentes and Jackson, 2012). For example, Bacillus thuringiensis var. kurstaki (Btk) is commercially used worldwide to suppress defoliate forest insects and crop pest outbreaks (Hajek and Van Frankenhuyzen, 2017). However, suppressing the pest population is temporary and requires repeated application of Btk during the outbreak event (Hajek and Van Frankenhuyzen, 2017). Btk is extensively used to control forest pests, including spruce budworm (Choristoneura fumiferana Clemens); gypsy moth (L. dispar), and other lepidopteran forest defoliators (Polanczyk et al., 2017). The phytophagous beetle Chrysomela tremulae Paykull is also susceptible to Btk, but large-scale field trials have not yet been conducted against these forest pests (Génissel et al., 2003). Other commercially used entomopathogenic bacteria include Serratia entomophila, Paenibacillus spp., and Chromobacterium subtsugae (Lacey et al., 2015). Besides entomopathogenic viruses and bacteria, fungal entomopathogens or mycoinsecticidal products from entomopathogenic fungi are widely used as microbial control agents for managing insect populations, including many bark beetle species belonging to genera Dendroctonus, Ips, and Polygraphus (Mann and Davis, 2021). Several fungal species, including Beauveria bassiana, Metarhizium anisopliae, Hirsutella guignardii, Isaria farinosa, and Lecanicillium lecanii are applied to manage bark beetle populations (Mann and Davis, 2021). These fungi species are considered generalist pathogens that are virulent against arthropods that occupy trees, vegetation, and forest soil. For instance, Metarhizium brunneum is deployed against Asian longhorn beetles in field trials in China and North America (Hajek and Bauer, 2009). Similarly, the isolate of B. bassiana is virulent against the EAB (Hajek and Bauer, 2009). These entomopathogenic fungi can be isolated from different sources and cultured to produce a large number of spores for potential commercial applications. In contrast to other entomopathogenic organisms that kill the insects after their entry through wounds or after ingestion, the entomopathogenic fungi infect the insect host by penetrating directly into their exoskeleton (Lacey et al., 2015). Furthermore, host-associated microbes from genera Wolbachia spp., Arsenophonus spp., Cardinium spp., Rickettsia spp., and Spiroplasma spp. often influence host reproduction by inducing cytoplasmic incompatibility parthenogenesis, feminizing genetic males, or male-killing (Lv et al., 2021). Using such reproductive manipulators may be an alternative strategy for controlling insect pests (Chakraborty and Roy, 2021).
Despite extensive research on entomopathogenic microbes as biological control agents, their large-scale commercial field application is limiting due to several abiotic and biotic factors, including susceptibility to ultraviolet light, low moisture, temperature, plant secondary metabolites, and competition with other microorganisms that impact their pathogenicity (Mann and Davis, 2021). The colonization of entomopathogens is achieved mainly by foliar spraying, injections, seed soaking, and soil drenching (Peña-Peña et al., 2015; Qayyum et al., 2015; Jaber and Araj, 2018; Rondot and Reineke, 2018). Direct spraying with entomopathogens or their products to the affected area is the most common and straightforward application practice that requires less equipment. Nevertheless, such an approach is not consistently successful in the field application against wood-boring insects due to their susceptibility to environmental conditions. Hence, adding protectants such as polymeric matrix, plant oils, clays, and humic acid increases the viability of the entomopathogenic microbes under laboratory and field conditions without compromising their pathogenic efficiency (Barta et al., 2018; Kaiser et al., 2019). However, spraying an entire forest to control pest infestation is not feasible. Alternatively, using an auto dissemination device to control pests is a promising strategy. The semiochemical baited traps connected to an auto-inoculative device attract insect pests and then contaminate them with the entomopathogen before returning to the environment to infect other pests horizontally or vertically (Dimbi et al., 2003; Ekesi et al., 2007). Such a dissemination approach can protect entomopathogens from UV exposure and rainfall (Srei et al., 2017). For instance, B. bassiana is introduced into a spruce bark beetle, I. typographus population, via an auto-inoculative device (Kreutz et al., 2004). Although this approach is reported to be successful against bark beetles, the studies are done on relatively smaller areas and for a shorter period. Hence, large-scale field applications need to be done to assess the feasibility of such a strategy further. Additionally, the formulations used should effectively kill all the developmental stages of the bark beetle. The formulation targeting only adults will not effectively control the beetle population unless they die before reproduction (Mann and Davis, 2021). Another approach is establishing entomopathogenic fungi as plant endophytes through colonization through seed and root coatings (Brownbridge et al., 2012). For example, studies reported that soil fungi, B. bassiana, colonize the pine trees via root and are pathogenic against invasive bark beetle species (Hylastes ater Paykull and Hylurgus ligniperda Fabricius) in New Zealand (Brownbridge et al., 2012).
Until recently, the identification of potential pathogens was limited to microscopy-based methods. With the advancement in genome sequencing and other molecular tools like RNA interference (RNAi), the construction of recombinant entomopathogenic microbial strains with enhanced virulence can be achieved (Chen et al., 2015; Wang et al., 2020). The advent of “omics” technologies can provide new insights into interactions among entomopathogens, host insects, and their host trees (Akinola and Babalola, 2020). Identifying new diverse host-specific entomopathogens and improvements in mass production, formulation, and their application will increase the commercial application of entomopathogens for efficient and sustainable use in pest management. Furthermore, deploying entomopathogens in combination with inorganic insecticides will help mitigate the risk of resistance and reduce environmental pollution caused due to excessive use of chemical pesticides (Al-Ani et al., 2021). An alternative strategy is integrating entomopathogens with semiochemicals and other natural enemies of insect pests that successfully manage insects (Sharma et al., 2019). A similar concept was recently used to protect agricultural and forest crops from the Arvicolinae rodents in Sweden (Villalobos et al., 2022). Although the application of entomopathogens or their products serves as a promising alternative to chemical pesticides, more research is still needed in this field to delve into their services thoroughly. Moreover, the policy and regulations for applying entomopathogens must be addressed apart from the laboratory and field trials.
6.2. Microbial volatile organic compounds as biocontrol agents
Like plants and animals, microbes also emit a plethora of VOCs in their metabolic processes (Bitas et al., 2013; Binyameen et al., 2021). These microbial volatile organic compounds (MVOCs) belong to different chemical classes, including alkenes, alcohols, ketones, benzenoids, pyrazines, sulfides, and terpenes (Kanchiswamy et al., 2015; Schmidt et al., 2015; Lemfack et al., 2018). The volatile compounds are perceived as chemical signals to communicate with each other, partaking in innumerable interactions and contributing significantly to multitrophic interaction (Schulz-Bohm et al., 2017). Insect chemoreception of microbial emissions plays a major role in insect-microbe interactions. Apart from the gustatory, tactile, and visual cues, insects have evolved complex chemosensory systems influencing their behavior. Insects are primarily sensitive to odors that endorse nutrient resources, predators, competitors, potential mates, and suitable habitats (Price et al., 2011; Davis et al., 2013). Although the ability of odor to influence animal behavior is long studied, relatively little is known about MVOCs as behavioral cues. MVOCs have been shown to influence insect behavior, such as stimulating insect oviposition, signaling food sources, and attracting or repelling insects (Davis et al., 2011). Furthermore, insects possess pheromones that are emitted by or associated with microbial symbionts (Tillman et al., 1999; Wertheim et al., 2005). Studies reported that gut symbionts could produce compounds used as pheromones by their hosts (Hunt and Borden, 1990; Zhao et al., 2015). For instance, yeasts, Hansenula capsulata Wickerham, and Pichia pini (Holst) Phaff are associated with the mountain pine beetle, D. ponderosae. They can convert cis and trans-verbenol to the anti-aggregation pheromone verbenone in female beetles (Frühbrodt et al., 2023). The anti-aggregation pheromone signals subcortical population density and terminates aggregation and mass attack on host trees (Hunt and Borden, 1990). Mycangial fungi and yeast Candida nitrophila play a similar role in the southern pine beetle and spruce beetle, respectively (Brand et al., 1976; Leufvén et al., 1984). Nevertheless, the mutualistic fungus, Grosmannia clavigera, associated with D. ponderosae produces sterols that act as a precursor for the biosynthesis of aggregation pheromones by the females (Bentz and Six, 2006). Interestingly, fungal volatiles also increased the attractiveness of host-mimic volatiles to the invasive ambrosia beetle, serving as insect lures. Despite the importance of microbes in insect life, combining chemical, and microbial ecology in entomological research is rarely explored. Integrating these approaches in entomological research, harnessing the MVOCs, and targeting these candidates is a promising avenue for controlling insect pests (Baig et al., 2023). Currently, less than 10% of MVOCs have been functionally described (Lemfack et al., 2018), suggesting the immense potential of MVOCs in sustainable pest management.
Furthermore, bark beetle-associated ophiostomatoid fungi release many volatile compounds such as terpenoids, aliphatic alcohols, fusel alcohols, and aromatic compounds, which positively or negatively influence the behavior of bark beetle, I. typographus (Kanchiswamy et al., 2015; Kandasamy et al., 2016; Tanin et al., 2021). Hence, they can potentially be used in bark beetle control (Table 1). For instance, studies revealed that the symbiotic fungi (ophiostomatoid fungi such as Grosmannia europhioides, Endoconidiophora polonica, Grosmannia penicillata, Ophiostoma bicolor, and Ophiostoma piceae) of I. typographus modify the spruce bark volatile profiles by converting the major spruce monoterpenes into a blend of oxygenated derivatives that are more attractive to bark beetles (Kandasamy et al., 2023). Other interesting fungal associates of bark beetles from the genera Geosmithia spp. (e.g., Geosmithia morbida), and Fusarium spp. (e.g., Fusarium circinatum), might serve as a nutrient source for the beetles and exhibit antimicrobial activity, but their role in ecological implications is unknown (Teetor-Barsch and Roberts, 1983; Bezos et al., 2018; Zhang et al., 2022). Interestingly, bark beetle species vector primarily either Geosmithia spp. or ophiostomatoid fungi, but not both. The less water content of the tree substrate facilitates Geosmithia association (Williams and Ginzel, 2021). Several independent studies have proven Geosmithia species as stable and dominant symbionts of many bark beetles worldwide, as described thoroughly in a recent comprehensive review article (Kolaøík and Hulcr, 2023). Interestingly, Geosmithia is an ecological complement to the ophiostomatoid fungi and co-evolved with bark beetle vectors (Kirschner, 2001). However, the molecular basis underlying Geosmithia–bark beetle symbiosis and the potential of volatile compounds (MVOCs, Table 1) identified from Geosmithia for bark beetle management demands further investigation (Blood et al., 2018; Kolaøík and Hulcr, 2023).
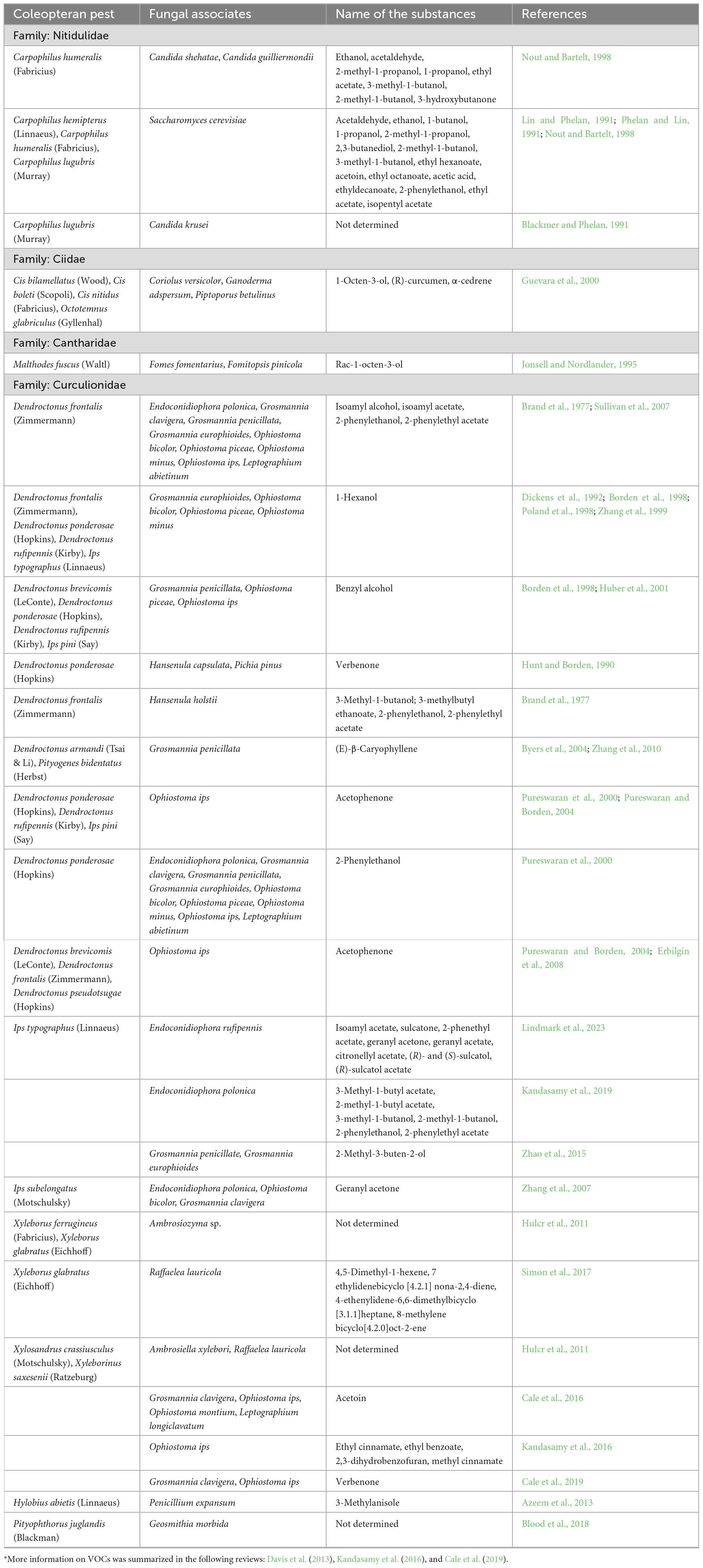
Table 1. A list of fungal volatile organic compounds (VOCs)* from different coleopteran beetles that can be applied for management practices.
The MVOCs, recognized by beetles to aid in host finding, are currently being tested along with aggregation pheromones in the forests in Czechia. In this study, the fungal volatile and aggregation pheromone used in the dispensers worked synergistically to attract I. typographus (Figure 4; Jirošová et al., 2022). However, such preliminary observations must be further experimentally validated by performing similar field experiments across different forest locations under different forest management regimes. It is worth mentioning here that beetle symbiosis is very complex, and the behavior of the bark beetles varies depending on the bacterial or fungal species, host tree, and stage of the beetle life cycle (Popa et al., 2012; Briones-Roblero et al., 2017; Netherer et al., 2021). Further research on the bark beetle symbiosis by taking advantage of affordable omics and electrophysiological methodologies will facilitate the deployment of MVOCs as management tools. Identifying and characterizing next-generation semiochemicals from microbial origin, their emission pattern, and the influence of target forest pests, including bark beetles, will ensure the sustainability of the pest management products based on MVOCs. It is interesting to point out that the influence of such MVOCs on the natural enemies of the coleopteran insect pests needs to be evaluated for their greater exploitation for safeguarding conifer and other broadleaved forests. Nevertheless, local laws and the government must approve the ecological consequences and environmental risks of introducing fungal or bacterial cultures and other potential pathogenic microbes into the forest ecosystem via traps and dispensers. Deploying fungal MVOCs, in general, may avoid these issues as they can be used in pheromone dispensers similar to the field trials in Czechia. Such deployments of MVOCs as attractants or deterrents (i.e., push and pull strategy) alone or in combination with other types of existing semiochemicals could substantially improve our chances against forest pests.
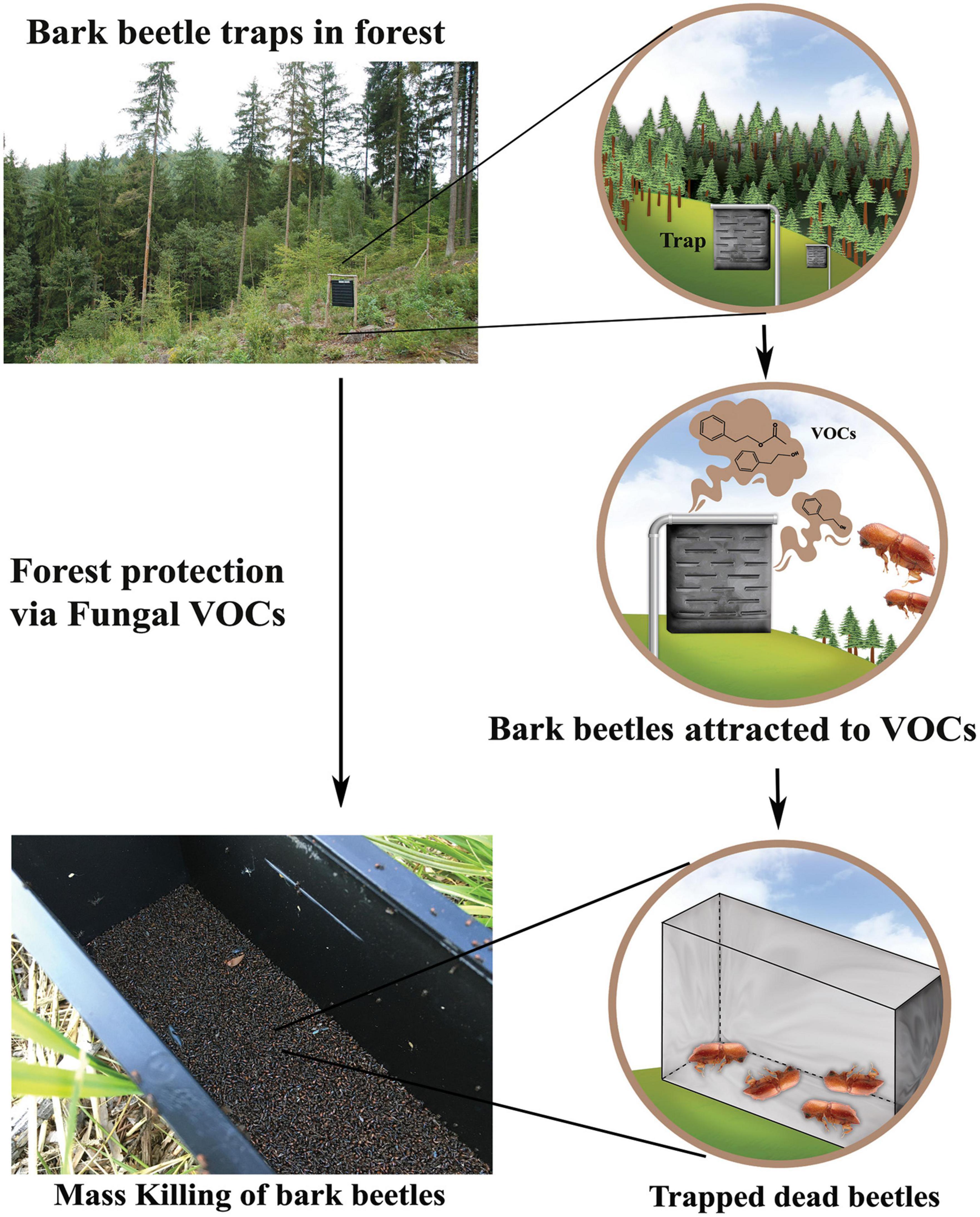
Figure 4. Using MVOCs in bark beetle (Coleoptera: Curculionidae: Scolytinae) management. A typical way of trapping bark beetles in the forests by using pheromone traps comprising fungal VOCs with or without beetle pheromones. Using MVOCs could enhance bark beetle capture substantially.
7. Molecular control of coleopteran insect pests: a new age technological development
7.1. Targeting coleopteran forest insect pest symbionts using RNAi
A surge of recent studies highlighted the contribution of the symbiotic microbiome underlying insect adaptations, ecological niche expansion, and invasion. However, symbionts can constrain the evolutionary potential by fostering niche specialization; for instance, symbiont-aided detoxification of caffeine by the gut bacteria (Pseudomonas fulva) enables coffee berry borer beetles (Hypothenemus hampei Ferrari) (Coleoptera: Curculionidae: Scolytinae) to thrive on unique caffeine-rich coffee beans (Ceja-Navarro et al., 2015). There are copious examples where symbionts aided their insect hosts to detoxify entomotoxic compounds from plant origin from different insect orders (Hosokawa et al., 2007; Xia et al., 2017; Cheng et al., 2018). It is also documented that detoxifying symbionts confer pesticide resistance in pest insects (Kikuchi et al., 2012; Cheng et al., 2017; Bras et al., 2022). In the case of forest insect pests (Coleoptera), symbiont-mediated detoxification of monoterpenoids such as limonene or diterpene acids is also reported. Mountain pine beetle (Coleoptera: Curculionidae), causing catastrophic damage to pine forests (Pinus contorta and hybrid P. contorta × Pinus banksiana), showed high expression of genes from the microbial origin involved in diterpene degradation after shotgun DNA sequencing (Adams et al., 2013). Most of these genes for diterpene degradation originated from bacterial genera Serratia, Erwinia, Rahnella, and Pseudomonas under Gammaproteobacteria and genus Burkholderia under Betaproteobacteria (Adams et al., 2013). Symbionts-mediated terpenoid degradation was also documented in the H. abietis (L) (Coleoptera: Curculionidae) based on in vitro and in vivo investigations (Berasategui et al., 2016, 2017). Serratia, Erwinia, and Rahnella were found within the core bacterial community in H. abietis (L). Interestingly, a gut bacteriome study on conifer-feeding bark beetles from the genus Ips (Coleoptera; Curculionidae; Scolytinae) also documented the same bacteria (Serratia, Erwinia, and Rahnella) within the core microbial community (Chakraborty et al., 2020a). Hence, it can be predicted that these symbiotic microbes perform a conserved function, i.e., detoxification of host allelochemicals, in conifer-feeding insect pests.
There are also reported symbionts offering defensive functions for their insect hosts by protecting against pathogens. For instance, filamentous actinobacteria are documented as defensive symbionts in many insects due to their capacity to produce secondary metabolites with antibiotic properties (Kaltenpoth, 2009; Seipke et al., 2012; Qin et al., 2017; Rupawate et al., 2023). Symbiotic bacteria (Streptomyces sp.) in southern pine beetle (Dendroctonus frontalis Zimmermann) (Coleoptera, Curculionidae) produce antifungal compounds against antagonistic fungi, Ophiostoma minus (Scott et al., 2008). Furthermore, microbial symbionts can protect their insects even against natural enemies (Flórez et al., 2017; Wang and Rozen, 2018; Muhammad et al., 2019).
With the increase in the information about detoxifying and defensive symbionts in pest insects, including forest insect pests (Coleoptera), it is necessary to use that information to formulate novel species-specific pest management opportunities. With increasing genomes available for major forest pests (Powell et al., 2021; Gagalova et al., 2022; Keeling et al., 2022) and microbial genome sequencing advancements, the feasibilities for conducting in-depth symbiosis studies, i.e., metatranscriptomic and metaproteomic level, increased manifold. Such advanced studies can delineate the metabolic exchange between beetles and their symbionts (Liu et al., 2022; Salem and Kaltenpoth, 2022) and offer exciting targets for future forest insect pest management via molecular tools such as RNAi, a post-transcriptional gene silencing mechanism suppressing protein formation by introducing environmental RNA (Montgomery and Fire, 1998). Technically, dsRNA can knock down key functional genes from symbionts to intrude symbiosis, making the target pest more susceptible to plant toxins or natural enemies (Chung et al., 2018). RNAi targeting symbiont genes is an intriguing idea worth dedicated research efforts (Wang and Luan, 2023). However, there is a significant concern about the environmental stability and biosafety of RNAi-based wood-boring pest control products (Joga et al., 2021; Mogilicherla et al., 2023; Singewar and Fladung, 2023). Although the contribution of symbionts in the evolution of bark beetles is unquestionable, targeting the symbionts inside the beetles, completing most of their life underneath the bark, is challenging and needs some intelligent strategy. Developing a sophisticated, eco-friendly, species-specific delivery system for dsRNA to reach its target will be crucial. Protection of dsRNA can be obtained by coating it inside nanostructures such as Chitosan, Carbon Quantum Dot, Silica nanoparticles, BioClay, etc. (Das et al., 2015; Pugsley et al., 2021; Jain et al., 2022; Sandal et al., 2023). Recent studies also documented the development of catechin, poly-L-lysine, for optimizing dsRNA delivery against target pests (Dhandapani et al., 2021; Yan et al., 2021). Such biodegradable nano formulations open a possibility of safe and efficient delivery of dsRNA to targets and often give protection against dsRNases (i.e., cationic polymers) inside the pest. However, spraying the nanoparticle-coated dsRNA is undoubtedly not the most favorable option in forestry applications at the landscape level.
Interestingly, using microbes (i.e., bacterial minicells) as dsRNA carriers and for mass production seems a promising alternative to pesticides (Guan et al., 2021; Whitten et al., 2023; Xue et al., 2023). Unfortunately, investigation on these vesicles for dsRNA delivery is limited; however, specific companies, e.g., AgroSpheres,1 delve into such technology for dsRNA or siRNA delivery for agricultural field applications. Another attractive option might be the co-expression of viral-like particles (VLPs) (Xue et al., 2023) and dsRNA inside the bacterial cell to use in the forest or isolate dsRNA-containing particles using the capacity of VLPs to self-assemble in vitro. Replicating engineered viruses permit superior cellular uptake and protection of the dsRNA in the extracellular environments in the forest (Kroemer et al., 2015; Sun, 2015; Xue et al., 2023), but after the COVID-19 pandemic, the approval of such alternatives might encounter hurdles to receive societal acceptance.
7.2. Symbiont-mediated RNAi: two-tier specificity for enhanced coleopteran forest insect pests control
A novel way to exploit the symbiotic associations for managing forest pests (Coleoptera) is symbiont-mediated RNAi (SMR) (Whitten et al., 2016). Strategies like feeding and injections are labor-intensive, posing a significant limitation for practical application. Alternatively, expressing dsRNA targeting specific pest insects inside recombinant bacteria or yeast lacking RNase III activity is a rewarding alternative when applied to the food source (Tian et al., 2009; Zhang et al., 2019; Ma et al., 2023). SMR is an advancement of this approach using symbiotic microbes as a carrier for the species-specific dsRNA delivery against target pests (Whitten et al., 2016; Xue et al., 2023). SMR has selective advantages over other strategies. Recombinant bacteria can fail to establish themselves after entering the target pest as they are alien inside the insect body (i.e., gut), and the possibility of inducing an immune response is also high. This limits the sustainable application as a minimum bacterial cell number is required to stimulate a decent RNAi response inside target pests. SMR technology will not have such issues. After establishment, it helps continuous dsRNA synthesis inside the pest, eliminating the necessity for repeated application for longer living pest insects because the amplification of interfering RNA is not possible due to the lack of RNA-dependent RNA polymerases (RdRP) in an insect leading to transient RNAi (Whitten and Dyson, 2017). It also facilitates the escape from dsRNA degrading enzymes expressed in saliva and gut of many pest insects based on the localization of SMR bacteria, or the dsRNA produced continuously by symbionts can overwhelm the activities of dsRNA degrading enzymes. There are already documented successes for SMR against short and long-lived insects (Whitten et al., 2016; Whitten and Dyson, 2017). Furthermore, SMR can be transmitted horizontally to other members, i.e., engineered Rhodococcus rhodnii expressing dsRNA transmitted horizontally to other kissing bugs via ingestion of feces (Whitten and Dyson, 2017).
Species specificity of RNAi is one of the key advantages of RNAi over other pest control strategies. SMR technology further complements it as it enhances the specificity of RNAi. SMR introduces two-tier specificity for RNAi; the first tier is from the species-specific target dsRNA design, and the second is by selecting the symbiont specific to the target pest. The inefficiency of true symbionts outside the environment also minimizes the concern for biosafety issues and their effect on non-target organisms. Although SMR is intriguing, some key issues must be alleviated before taking it to forest application (Elston et al., 2023; Whitten et al., 2023). Understanding the true nature of insect-symbiont relationships is fundamental for the success of SMR, as the optimal selection of the symbiont for delivery of dsRNA is the key to success. The information about the symbiont’s colonization potential (Engel and Moran, 2013), cell number per insect and association with different insect life stages is cardinal for the success of SMR technology. It is worth mentioning that holometabolous insects (e.g., coleopterans, dipterans, and lepidopterans) undergo a metamorphosis, implying the loss and re-acquisition of symbionts that can limit the success of SMR. However, with coleopteran forest pests, this might not be an issue. The issue here might be the lack of studies on forest pest symbiosis. For instance, only a few studies revealed the gut microbial assemblage of bark beetles (Chakraborty et al., 2020a,b; Cheng et al., 2023; Moussa et al., 2023). More in-depth studies are necessary at the functional level, such as metatranscriptomics or metaproteomics, to understand the molecular basis of symbiosis within forest pests (Salem and Kaltenpoth, 2022). Identifying symbionts with the possibility of in vitro culturing (Liu et al., 2022) and manipulation is fundamental. The advent of genome sequence data for a wide range of pest insects and their symbionts undoubtedly facilitates in-depth studies on symbiosis. However, more genome sequencing efforts for forest pests (Powell et al., 2021; Gagalova et al., 2022; Keeling et al., 2022) and their symbionts (Liu et al., 2022; Cheng et al., 2023) are required to develop SMR as a forest pest management tool in future. Another major limitation of SMR is that often true symbionts are due to genome reduction as a consequence of symbiotic tie-ups with their insect host (McCutcheon and Moran, 2012; Boscaro et al., 2017; Noh, 2021); hence they are not culturable and cannot be engineered to express dsRNA. The technological development in this area is impending and crucial for SMR against forest pests.
8. Understanding of holistic tree-beetle-microbe dialogue for futuristic IPM
The rapid advancement of sequencing methodologies has enormously increased microbiology research in the past few decades. However, a vast number of the studies (approximately 50%) lack adequate details of experimental design (information source: ESA 2020 conference presentation, titled: A synthesis of insect-microbiome research). Thus, the requirement for standard norms or protocols of metagenomic, metatranscriptomics, and metaproteomic studies that can ensure the reliability and reusability of the sequencing data is crucial. Besides, depositing the obtained data in the appropriate public data repository is also essential. Above all in-depth understanding of the plant holobiont, beetle holobiont, and/or “plant-beetle-microbiome-environment” interaction pyramid for protecting and improving plant performance and managing beetle destruction is undoubtedly critical. In addition, analytical evaluations of factors that are likely to cause variation in data generation, such as nucleic acid extraction method, sequencing platform used, primer pairs used (i.e., 16S, ITS, and 18S studies) in microbial omics study, insect handling procedure and material (whole body/gut, surface-sterilized or not) and ecological factors such as phylogeny, sex, life stage, diet, treatment, origin, etc., that are known to influence the microbial community of insects, need to be sufficiently described. Hence, mentioning the above information in the published report is a prerequisite for accurately understanding the symbiosis mechanism and using the information for management purposes. The lack of good-quality bacterial and fungal databases for microbial studies at a functional level is also considered a void in forest-beetle-microbiome research.
Technological innovations such as SMR for the environmental application of RNAi using symbiotic microbes as carriers (Zhang et al., 2019) appear promising. Hence, bio-engineered microbes could be applied in the forest for pest control and tree health development in the near future. While we are at the onset of such intriguing possibilities, the biosafety aspect for the short- and long-term environmental impact of deploying such genetically engineered microbes must be formulated under a defined regulatory framework and evaluated in forest habitation. Although risk assessment protocols exist for RNAi-based GM crops or RNAi-based biocontrol products (De Schutter et al., 2022), no published risk assessment protocol for GM microorganisms obtained through targeted delivery of dsRNA has been reported. Thus, certified regulatory agencies must formulate appropriate risk assessment protocols for forest microbiome research. Such protocols should also incorporate the short and long-term impact of GM microbes on the forest ecosystem.
9. Conclusion
Even though safer GM microbes hold an excellent perspective of the application instead of utilizing synthetic pesticides in agricultural ecosystems, translation of the knowledge base from agricultural fields to the forest is challenging because of landscape enormity. Besides, knowledge of plant-soil feedbacks that largely shape the soil and host microbiome in the forest is lacking. Steering soil microorganisms in forest floors to assemble all good things may be practically attainable only after extensive in-depth experimentation. Thus, exploring and exploiting the role of holobionts of forest tree hosts and their associated beetle pests and implementing targeted bioengineering of beetle-associated symbiotic microorganisms within the regulated legal framework could help devise better management stratagem for tackling the problem of Coleopteran insect pests-mediated forest destruction in future.
Author contributions
AR and AC: conceptualization. SG, AC, and AR: manuscript writing and figure preparation. All authors approved the final version of the manuscript.
Funding
AR and AC were supported by “EVA 4.0,” No. CZ.02.1.01/0.0/0.0/16 019/0000803 and “EXTEMIT – K,” No. CZ.02.1.01/0.0/0.0/15_003/0000433 financed by OP RDE. AR and AC were also supported by “Excellent Team Grants” (2023–2024) from the Faculty of Forestry and Wood Sciences, Czech University of Life Sciences, Prague, Czechia.
Acknowledgments
We appreciate the helpful feedback provided by the reviewers and especially the handling editor.
Conflict of interest
The authors declare that the research was conducted in the absence of any commercial or financial relationships that could be construed as a potential conflict of interest.
Publisher’s note
All claims expressed in this article are solely those of the authors and do not necessarily represent those of their affiliated organizations, or those of the publisher, the editors and the reviewers. Any product that may be evaluated in this article, or claim that may be made by its manufacturer, is not guaranteed or endorsed by the publisher.
Footnotes
References
Adams, A. S., Aylward, F. O., Adams, S. M., Erbilgin, N., Aukema, B. H., Currie, C. R., et al. (2013). Mountain pine beetles colonizing historical and naive host trees are associated with a bacterial community highly enriched in genes contributing to terpene metabolism. Appl. Environ. Microbiol. 79, 3468–3475. doi: 10.1128/AEM.00068-13
Ajayi, A. M., and Olufolaji, D. B. (2023). “The use of plant growth promoting microorganisms in the management of soil-borne plant pathogenic organisms,” in Plant Growth Promoting Microorganisms of Arid Region, eds R. Mawar, R. Z. Sayyed, S. K. Sharma, and K. S. Sattiraju (Singapore: Springer), 195–212.
Akinola, S. A., and Babalola, O. O. (2020). The importance of adverse soil microbiomes in the light of omics: implications for food safety. Plant Soil Environ. 66, 421–430.
Al-Ani, L. K. T., Aguilar-Marcelino, L., Salazar-Vidal, V. E., Becerra, A. G., and Raza, W. (2021). “Role of useful fungi in agriculture sustainability,” in Recent trends in mycological research: volume 1: agricultural and medical perspective, ed. A. N. Yadav (Cham: Springer), 1–44.
Anderson, I. C., Genney, D. R., and Alexander, I. J. (2014). Fine-scale diversity and distribution of ectomycorrhizal fungal mycelium in a S cots pine forest. N. Phytol. 201, 1423–1430. doi: 10.1111/nph.12637
Aukema, J. E., Leung, B., Kovacs, K., Chivers, C., Britton, K. O., Englin, J., et al. (2011). Economic impacts of non-native forest insects in the continental United States. PLoS One 6:e24587. doi: 10.1371/journal.pone.0024587
Avanasi, R., Glover, A., Lord, C., Macari, J., Munday, M., McKillican, C., et al. (2023). How protective is the pesticide risk assessment and registration process to humans in the United States? J. Regul. Sci. 11, 1–29.
Azeem, M., Rajarao, G. K., Nordenhem, H., Nordlander, G., and Borg-Karlson, A. K. (2013). Penicillium expansum volatiles reduce pine weevil attraction to host plants. J. Chem. Ecol. 39, 120–128. doi: 10.1007/s10886-012-0232-5
Baig, F., Farnier, K., Ishtiaq, M., and Cunningham, J. P. (2023). Volatiles produced by symbiotic yeasts improve trap catches of Carpophilus davidsoni (Coleoptera: Nitidulidae): an important pest of stone fruits in Australia. J. Econ. Entomol. 116:toad027. doi: 10.1093/jee/toad027
Baldrian, P. (2014). Distribution of extracellular enzymes in soils: spatial heterogeneity and determining factors at various scales. Soil Sci. Soc. Am. J. 78, 11–18.
Baldrian, P. (2017). Forest microbiome: diversity, complexity and dynamics. FEMS Microbiol. Rev. 41, 109–130.
Barta, M., Kautmanová, I., Čičková, H., Ferenčík, J., Florián, Š, Novotnı, J., et al. (2018). The potential of Beauveria bassiana inoculum formulated into a polymeric matrix for a microbial control of spruce bark beetle. Biocontrol Sci. Technol. 28, 718–735.
Bello, M. G. D., Knight, R., Gilbert, J. A., and Blaser, M. J. (2018). Preserving microbial diversity. Science 362, 33–34.
Bentz, B. J., and Six, D. L. (2006). Ergosterol content of fungi associated with Dendroctonus ponderosae and Dendroctonus rufipennis (Coleoptera: Curculionidae, Scolytinae). Ann. Entomol. Soc. Am. 99, 189–194.
Berasategui, A., Axelsson, K., Nordlander, G., Schmidt, A., Borg-Karlson, A. K., Gershenzon, J., et al. (2016). The gut microbiota of the pine weevil is similar across Europe and resembles that of other conifer-feeding beetles. Mol. Ecol. 25, 4014–4031. doi: 10.1111/mec.13702
Berasategui, A., Salem, H., Paetz, C., Santoro, M., Gershenzon, J., Kaltenpoth, M., et al. (2017). Gut microbiota of the pine weevil degrades conifer diterpenes and increases insect fitness. Mol. Ecol. 26, 4099–4110. doi: 10.1111/mec.14186
Berg, G., Rybakova, D., Fischer, D., Cernava, T., Vergés, M.-C. C., Charles, T., et al. (2020). Microbiome definition re-visited: old concepts and new challenges. Microbiome 8:103.
Bertaux, J., Schmid, M., Hutzler, P., Hartmann, A., Garbaye, J., and Frey-Klett, P. (2005). Occurrence and distribution of endobacteria in the plant-associated mycelium of the ectomycorrhizal fungus Laccaria bicolor S238N. Environ. Microbiol. 7, 1786–1795. doi: 10.1111/j.1462-2920.2005.00867.x
Bezos, D., Martínez-Álvarez, P., Sanz-Ros, A. V., Martín-García, J., Fernandez, M. M., and Diez, J. J. (2018). Fungal communities associated with bark beetles in Pinus radiata plantations in Northern Spain affected by Pine Pitch Canker, with special focus on Fusarium species. Forests 9:698.
Billings, R. F. (2011). “Use of chemicals for prevention and control of southern pine beetle infestations,” in Southern pine beetle II. General technical reports SRS-140”, Vol. 285, eds R. Coulson and K. Klepzig (Asheville, NC: Department of Agricultural Forest Service, Southern Research Station), 367–379.
Binyameen, M., Ali, Q., Roy, A., and Schlyter, F. (2021). “Plant volatiles and their role in insect olfaction,” in Plant-pest interactions: from molecular mechanisms to chemical ecology: chemical ecology, eds I. K. Singh, and A. Singh (Singapore: Springer) 127–156.
Bitas, V., Kim, H.-S., Bennett, J. W., and Kang, S. (2013). Sniffing on microbes: diverse roles of microbial volatile organic compounds in plant health. Mol. Plant Microbe Interact. 26, 835–843. doi: 10.1094/MPMI-10-12-0249-CR
Blackmer, J., and Phelan, P. (1991). Effect of physiological state and fungal inoculation on chemically modulated host-plant finding by Carpophilus hemipterus and Carpophilus lugubris. Entomol. Exp. Applic. 61, 33–43.
Blood, B., Klingeman, W., Paschen, M., Hadžiabdić, Ð, Couture, J., and Ginzel, M. (2018). Behavioral responses of Pityophthorus juglandis (Coleoptera: Curculionidae: Scolytinae) to volatiles of black walnut and Geosmithia morbida (Ascomycota: Hypocreales: Bionectriaceae), the causal agent of thousand cankers disease. Environ. Entomol. 47, 412–421. doi: 10.1093/ee/nvx194
Boone, C. K., Six, D. L., Zheng, Y., and Raffa, K. F. (2014). Parasitoids and dipteran predators exploit volatiles from microbial symbionts to locate bark beetles. Environ. Entomol. 37, 150–161. doi: 10.1603/0046-225x(2008)37[150:padpev]2.0.co;2
Borden, J. H., Wilson, I. M., Gries, R., Chong, L. J., Pierce, J., Harold, D., et al. (1998). Volatiles from the bark of trembling aspen, Populus tremuloides Michx.(Salicaceae) disrupt secondary attraction by the mountain pine beetle, Dendroctonus ponderosae Hopkins (Coleoptera: Scolytidae). Chemoecology 8, 69–75.
Boscaro, V., Kolisko, M., Felletti, M., Vannini, C., Lynn, D. H., and Keeling, P. J. (2017). Parallel genome reduction in symbionts descended from closely related free-living bacteria. Nat. Ecol. Evol. 1, 1160–1167.
Boukouvala, M. C., Kavallieratos, N. G., Skourti, A., Pons, X., Alonso, C. L., Eizaguirre, M., et al. (2022). Lymantria dispar (L.)(Lepidoptera: Erebidae): current status of biology, ecology, and management in Europe with notes from North America. Insects 13:854. doi: 10.3390/insects13090854
Brand, J., Bracke, J., Britton, L., Markovetz, A., and Barras, S. (1976). Bark beetle pheromones: production of verbenone by a mycangial fungus of Dendroctonus frontalis. J. Chem. Ecol. 2, 195–199.
Brand, J., Schultz, J., Barras, S., Edson, L., Payne, T., and Hedden, R. (1977). Bark-beetle pheromones: enhancement of Dendroctonus frontalis (Coleoptera: Scolytidae) aggregation pheromone by yeast metabolites in laboratory bioassays. J. Chem. Ecol. 3, 657–666.
Bras, A., Roy, A., Heckel, D. G., Anderson, P., and Karlsson Green, K. (2022). Pesticide resistance in arthropods: ecology matters too. Ecol. Lett. 25, 1746–1759. doi: 10.1111/ele.14030
Briones-Roblero, C. I., Hernández-García, J. A., Gonzalez-Escobedo, R., Soto-Robles, L. V., Rivera-Orduña, F. N., and Zúñiga, G. (2017). Structure and dynamics of the gut bacterial microbiota of the bark beetle, Dendroctonus rhizophagus (Curculionidae: Scolytinae) across their life stages. PLoS One 12:e0175470. doi: 10.1371/journal.pone.0175470
Brownbridge, M., Reay, S. D., Nelson, T. L., and Glare, T. R. (2012). Persistence of Beauveria bassiana (Ascomycota: Hypocreales) as an endophyte following inoculation of radiata pine seed and seedlings. Biol. Control 61, 194–200.
Burgess, S. C., and Marshall, D. J. (2014). Adaptive parental effects: the importance of estimating environmental predictability and offspring fitness appropriately. Oikos 123, 769–776.
Byers, J. A., Zhang, Q.-H., and Birgersson, G. (2004). Avoidance of nonhost plants by a bark beetle, Pityogenes bidentatus, in a forest of odors. Naturwissenschaften 91, 215–219. doi: 10.1007/s00114-004-0520-1
Cale, J. A., Collignon, R. M., Klutsch, J. G., Kanekar, S. S., Hussain, A., and Erbilgin, N. (2016). Fungal volatiles can act as carbon sources and semiochemicals to mediate interspecific interactions among bark beetle-associated fungal symbionts. PLoS One 11:e0162197. doi: 10.1371/journal.pone.0162197
Cale, J. A., Ding, R., Wang, F., Rajabzadeh, R., and Erbilgin, N. (2019). Ophiostomatoid fungi can emit the bark beetle pheromone verbenone and other semiochemicals in media amended with various pine chemicals and beetle-released compounds. Fungal Ecol. 39, 285–295.
Cardoza, Y. J., Klepzig, K. D., and Raffa, K. F. (2006). Bacteria in oral secretions of an endophytic insect inhibit antagonistic fungi. Ecol. Entomol. 31, 636–645.
Ceja-Navarro, J. A., Vega, F. E., Karaoz, U., Hao, Z., Jenkins, S., Lim, H. C., et al. (2015). Gut microbiota mediate caffeine detoxification in the primary insect pest of coffee. Nat. Commun. 6:7618. doi: 10.1038/ncomms8618
Chakraborty, A., and Roy, A. (2021). “Microbial influence on plant–insect interaction,” in Plant-pest interactions: From molecular mechanisms to chemical ecology: Chemical ecology, eds I. K. Singh, and A. Singh (Singapore: Springer), 337–363.
Chakraborty, A., Ashraf, M. Z., Modlinger, R., Synek, J., Schlyter, F., and Roy, A. (2020a). Unravelling the gut bacteriome of Ips (Coleoptera: Curculionidae: Scolytinae): identifying core bacterial assemblage and their ecological relevance. Sci. Rep. 10:18572. doi: 10.1038/s41598-020-75203-5
Chakraborty, A., Modlinger, R., Ashraf, M. Z., Synek, J., Schlyter, F., and Roy, A. (2020b). Core mycobiome and their ecological relevance in the gut of five Ips bark beetles (Coleoptera: Curculionidae: Scolytinae). Front. Microbiol. 11, 568853. doi: 10.3389/fmicb.2020.568853
Chakraborty, A., Zádrapová, D., Dvořák, J., Faltinová, Z., Zacek, P., Cajthaml, T., et al. (2023). Impact of 30-year precipitation regime differences on forest soil physiology and microbial assemblages. Front. For. Glob. Change 6:1142979. doi: 10.3389/ffgc.2023.1142979
Chen, X., Dunfield, K., Fraser, T., Wakelin, S., Richardson, A., and Condron, L. (2019). Soil biodiversity and biogeochemical function in managed ecosystems. Soil Res. 58, 1–20.
Chen, X., Li, L., Hu, Q., Zhang, B., Wu, W., Jin, F., et al. (2015). Expression of dsRNA in recombinant Isaria fumosorosea strain targets the TLR7 gene in Bemisia tabaci. BMC Biotechnol. 15:64. doi: 10.1186/s12896-015-0170-8
Cheng, C., Wickham, J. D., Chen, L., Xu, D., Lu, M., and Sun, J. (2018). Bacterial microbiota protect an invasive bark beetle from a pine defensive compound. Microbiome 6:132. doi: 10.1186/s40168-018-0518-0
Cheng, D., Guo, Z., Riegler, M., Xi, Z., Liang, G., and Xu, Y. (2017). Gut symbiont enhances insecticide resistance in a significant pest, the oriental fruit fly Bactrocera dorsalis (Hendel). Microbiome 5:13. doi: 10.1186/s40168-017-0236-z
Cheng, T., Veselská, T., Křížková, B., Švec, K., Havlíček, V., Stadler, M., et al. (2023). Insight into the genomes of dominant yeast symbionts of European spruce bark beetle, Ips typographus. Front. Microbiol. 14:1108975. doi: 10.3389/fmicb.2023.1108975
Chhun, A., Zoppi, F., Moriano-Gutierrez, S., Cabirol, A., Engel, P., and Schaerli, Y. (2023). Engineering a symbiont as a biosensor for the honey bee gut environment. bioRxiv [Preprint]. doi: 10.1101/2023.02.02.526826
Chung, S. H., Jing, X., Luo, Y., and Douglas, A. E. (2018). Targeting symbiosis-related insect genes by RNAi in the pea aphid-Buchnera symbiosis. Insect Biochem. Mol. Biol. 95, 55–63. doi: 10.1016/j.ibmb.2018.02.004
Coulson, R. N. (1979). Population dynamics of bark beetles. Annu. Rev. Entomol. 24, 417–447. doi: 10.1146/annurev.en.24.010179.002221
Custer, G. F., van Diepen, L. T., and Stump, W. L. (2020). Structural and functional dynamics of soil microbes following spruce beetle infestation. Appl. Environ. Microbiol. 86, e1984–e1919. doi: 10.1128/AEM.01984-19
Dara, S. K. (2019). The new integrated pest management paradigm for the modern age. J. Integr. Pest Manag. 10:12.
Das, S., Debnath, N., Cui, Y., Unrine, J., and Palli, S. R. (2015). Chitosan, carbon quantum dot, and silica nanoparticle mediated dsRNA delivery for gene silencing in Aedes aegypti: a comparative analysis. ACS Appl. Mater. Interfaces 7, 19530–19535. doi: 10.1021/acsami.5b05232
Davis, T. S., Crippen, T. L., Hofstetter, R. W., and Tomberlin, J. K. (2013). Microbial volatile emissions as insect semiochemicals. J. Chem. Ecol. 39, 840–859.
Davis, T. S., Hofstetter, R. W., Foster, J. T., Foote, N. E., and Keim, P. (2011). Interactions between the yeast Ogataea pini and filamentous fungi associated with the western pine beetle. Microb. Ecol. 61, 626–634. doi: 10.1007/s00248-010-9773-8
De Schutter, K., Taning, C. N. T., Van Daele, L., Van Damme, E. J., Dubruel, P., and Smagghe, G. (2022). RNAi-based biocontrol products: market status, regulatory aspects, and risk assessment. Front. Insect Sci. 1:818037. doi: 10.3389/finsc.2021.818037
Dhandapani, R. K., Gurusamy, D., and Palli, S. R. (2021). Development of catechin, poly-l-lysine, and double-stranded RNA nanoparticles. ACS Appl. Bio Mater. 4, 4310–4318. doi: 10.1021/acsabm.1c00109
Dhar, A., Parrott, L., and Hawkins, C. D. (2016). Aftermath of mountain pine beetle outbreak in British Columbia: stand dynamics, management response and ecosystem resilience. Forests 7:171.
Dickens, J., Billings, R., and Payne, T. (1992). Green leaf volatiles interrupt aggregation pheromone response in bark beetles infesting southern pines. Experientia 48, 523–524.
Dimbi, S., Maniania, N. K., Lux, S. A., Ekesi, S., and Mueke, J. K. (2003). Pathogenicity of Metarhizium anisopliae (Metsch.) Sorokin and Beauveria bassiana (Balsamo) Vuillemin, to three adult fruit fly species: Ceratitis capitata (Weidemann), C. rosa var. fasciventris Karsch and C. cosyra (Walker)(Diptera: Tephritidae). Mycopathologia 156, 375–382. doi: 10.1023/b:myco.0000003579.48647.16
Doan, H. K., and Leveau, J. H. (2015). Artificial surfaces in phyllosphere microbiology. Phytopathology 105, 1036–1042.
Douglas, A. E. (2007). Symbiotic microorganisms: untapped resources for insect pest control. Trends Biotechnol. 25, 338–342. doi: 10.1016/j.tibtech.2007.06.003
Eichlerová, I., Homolka, L., Žifčáková, L., Lisá, L., Dobiášová, P., and Baldrian, P. (2015). Enzymatic systems involved in decomposition reflects the ecology and taxonomy of saprotrophic fungi. Fungal Ecol. 13, 10–22.
Eilenberg, J., Hajek, A., and Lomer, C. (2001). Suggestions for unifying the terminology in biological control. Biocontrol 46, 387–400.
Ekblad, A., Wallander, H., Godbold, D. L., Cruz, C., Johnson, D., Baldrian, P., et al. (2013). The production and turnover of extramatrical mycelium of ectomycorrhizal fungi in forest soils: role in carbon cycling. Plant Soil 366, 1–27.
Ekesi, S., Dimbi, S., and Maniania, N. (2007). “The role of entomopathogenic fungi in the integrated management of fruit flies (Diptera: Tephritidae) with emphasis on species occurring in Africa,” in Use entomopathogenic fungi in biological pest management, eds S. Ekesi, and N. K. Maniania (Kerala: Research Signpost), 239–274.
Elston, K. M., Maeda, G. P., Perreau, J., and Barrick, J. E. (2023). Addressing the challenges of symbiont-mediated RNAi in aphids. PeerJ 11, e14961. doi: 10.7717/peerj.14961
Engel, P., and Moran, N. A. (2013). The gut microbiota of insects–diversity in structure and function. FEMS Microbiol. Rev. 37, 699–735. doi: 10.1111/1574-6976.12025
Erbilgin, N., Gillette, N. E., Owen, D. R., Mori, S. R., Nelson, A. S., Uzoh, F., et al. (2008). Acetophenone superior to verbenone for reducing attraction of western pine beetle Dendroctonus brevicomis to its aggregation pheromone. Agric. For. Entomol. 10, 433–441.
Eyles, A., Bonello, P., Ganley, R., and Mohammed, C. (2010). Induced resistance to pests and pathogens in trees. N. Phytol. 185, 893–908.
Flórez, L. V., Scherlach, K., Gaube, P., Ross, C., Sitte, E., Hermes, C., et al. (2017). Antibiotic-producing symbionts dynamically transition between plant pathogenicity and insect-defensive mutualism. Nat. Commun. 8:15172. doi: 10.1038/ncomms15172
Franceschi, V. R., Krokene, P., Christiansen, E., and Krekling, T. (2005). Anatomical and chemical defenses of conifer bark against bark beetles and other pests. N. Phytol. 167, 353–376.
Frühbrodt, T., Schebeck, M., Andersson, M. N., Holighaus, G., Kreuzwieser, J., Burzlaff, T., et al. (2023). Verbenone—the universal bark beetle repellent? Its origin, effects, and ecological roles. J. Pest Sci. doi: 10.1007/s10340-023-01635-3
Gagalova, K. K., Whitehill, J. G., Culibrk, L., Lin, D., Lévesque-Tremblay, V., Keeling, C. I., et al. (2022). The genome of the forest insect pest Pissodes strobi reveals genome expansion and evidence of a Wolbachia endosymbiont. G3 12:jkac038. doi: 10.1093/g3journal/jkac038
García-Fraile, P. (2018). Roles of bacteria in the bark beetle holobiont–how do they shape this forest pest? Ann. Appl. Biol. 172, 111–125.
Génissel, A., Leplé, J.-C., Millet, N., Augustin, S., Jouanin, L., and Pilate, G. (2003). High tolerance against Chrysomela tremulae of transgenic poplar plants expressing a synthetic cry3Aa gene from Bacillus thuringiensis ssp tenebrionis. Mol. Breed. 11, 103–110.
Gitau, C., Bashford, R., Carnegie, A., and Gurr, G. (2013). A review of semiochemicals associated with bark beetle (Coleoptera: Curculionidae: Scolytinae) pests of coniferous trees: a focus on beetle interactions with other pests and their associates. For. Ecol. Manag. 297, 1–14.
Gong, T., and Xin, X. F. (2021). Phyllosphere microbiota: community dynamics and its interaction with plant hosts. J. Integr. Plant Biol. 63, 297–304.
Guan, R., Chu, D., Han, X., Miao, X., and Li, H. (2021). Advances in the development of microbial double-stranded RNA production systems for application of RNA interference in agricultural pest control. Front. Bioeng. Biotechnol. 9:753790. doi: 10.3389/fbioe.2021.753790
Guevara, R., Hutcheson, K. A., Mee, A. C., Rayner, A., and Reynolds, S. (2000). Resource partitioning of the host fungus Coriolus versicolor by two ciid beetles: the role of odour compounds and host ageing. Oikos 91, 184–194.
Haack, R. A., Jendak, E., Houping, L., Marchant, K. R., Petrice, T. R., Poland, T. M., et al. (2002). The emerald ash borer: a new exotic pest in North America. Newsletter Michigan Entomol. Soc. 47, 1–5.
Hajek, A. E., and Bauer, L. S. (2009). “Use of entomopathogens against invasive wood boring beetles in North America,” in Use of microbes for control and eradication of invasive arthropods (A. E. Hajek, T.R. Glare, and M. O’Callaghan (Dordrecht: Springer), 159–179. doi: 10.1016/j.jip.2012.05.008
Hajek, A., and Van Frankenhuyzen, K. (2017). “Use of entomopathogens against forest pests,” in Microbial Control of Insect and Mite Pests, ed. L A. Lacey (Amsterdam: Elsevier), 313–330.
Hannula, S. E., Zhu, F., Heinen, R., and Bezemer, T. M. (2019). Foliar-feeding insects acquire microbiomes from the soil rather than the host plant. Nat. Commun. 10:1254. doi: 10.1038/s41467-019-09284-w
Haynes, K. J., Allstadt, A. J., and Klimetzek, D. (2014). Forest defoliator outbreaks under climate change: effects on the frequency and severity of outbreaks of five pine insect pests. Glob. Change Biol. 20, 2004–2018. doi: 10.1111/gcb.12506
Hlásny, T., König, L., Krokene, P., Lindner, M., Montagné-Huck, C., Müller, J., et al. (2021). Bark beetle outbreaks in Europe: state of knowledge and ways forward for management. Current Forestry Reports 7, 138–165.
Hlásny, T., Krokene, P., Liebhold, A., Montagné-Huck, C., Müller, J., Qin, H., et al. (2019). Living with bark beetles: impacts, outlook and management options. Joensuu: European Forest Institute.
Holland, M. A. (2011). “Nitrogen: give and take from phylloplane microbes,” in Ecological aspects of nitrogen metabolism in plants, eds J. C. Polacco and C. D. Todd (London: Wiley-Blackwell), 217–230.
Holmes, S. B., and MacQuarrie, C. J. (2016). Chemical control in forest pest management. Can. Entomol. 148, S270–S295.
Hosokawa, T., Kikuchi, Y., Shimada, M., and Fukatsu, T. (2007). Obligate symbiont involved in pest status of host insect. Proc. R. Soc. B Biol. Sci. 274, 1979–1984.
Howe, G. A., and Herde, M. (2015). Interaction of plant defense compounds with the insect gut: new insights from genomic and molecular analyses. Curr. Opin. Insect Sci. 9, 62–68. doi: 10.1016/j.cois.2015.03.004
Howe, M., Carroll, A., Gratton, C., and Raffa, K. F. (2021). Climate-induced outbreaks in high-elevation pines are driven primarily by immigration of bark beetles from historical hosts. Glob. Change Biol. 27, 5786–5805. doi: 10.1111/gcb.15861
Huber, D. P., Borden, J. H., and Stastny, M. (2001). Response of the pine engraver, Ips pini (Say)(Coleoptera: Scolytidae), to conophthorin and other angiosperm bark volatiles in the avoidance of non-hosts. Agric. For. Entomol. 3, 225–232.
Hulcr, J., Mann, R., and Stelinski, L. L. (2011). The scent of a partner: ambrosia beetles are attracted to volatiles from their fungal symbionts. J. Chem. Ecol. 37, 1374–1377. doi: 10.1007/s10886-011-0046-x
Humphrey, P. T., and Whiteman, N. K. (2020). Insect herbivory reshapes a native leaf microbiome. Nat. Ecol. Evol. 4, 221–229. doi: 10.1038/s41559-019-1085-x
Hunt, D., and Borden, J. (1990). Conversion of verbenols to verbenone by yeasts isolated from Dendroctonus ponderosae (Coleoptera: Scolytidae). J. Chem. Ecol. 16, 1385–1397. doi: 10.1007/BF01021034
Itoh, H., Tago, K., Hayatsu, M., and Kikuchi, Y. (2018). Detoxifying symbiosis: microbe-mediated detoxification of phytotoxins and pesticides in insects. Natural Product Rep. 35, 434–454. doi: 10.1039/c7np00051k
Jaber, L. R., and Araj, S.-E. (2018). Interactions among endophytic fungal entomopathogens (Ascomycota: Hypocreales), the green peach aphid Myzus persicae Sulzer (Homoptera: Aphididae), and the aphid endoparasitoid Aphidius colemani Viereck (Hymenoptera: Braconidae). Biol. Control 116, 53–61.
Jain, R., Fletcher, S., and Mitter, N. (2022). Effective RNAi-mediated control of the crop pest whitefly. Nat. Plant 8, 461–462.
Jirošová, A., Modlinger, R., Hradecký, J., Ramakrishnan, R., Beránková, K., and Kandasamy, D. (2022). Ophiostomatoid fungi synergize attraction of the Eurasian spruce bark beetle, Ips typographus to its aggregation pheromone in field traps. Front. Microbiol. 13: 980251. doi: 10.3389/fmicb.2022.980251
Joga, M. R., Mogilicherla, K., Smagghe, G., and Roy, A. (2021). RNA interference-based forest protection products (FPPs) against wood-boring coleopterans: Hope or hype? Front. Plant Sci. 12:733608. doi: 10.3389/fpls.2021.733608
Johnston, S. R., Boddy, L., and Weightman, A. J. (2016). Bacteria in decomposing wood and their interactions with wood-decay fungi. FEMS Microbiol. Ecol. 92:fiw179.
Jonsell, M., and Nordlander, G. (1995). Field attraction of Coleoptera to odours of the wood-decaying polypores Fomitopsis pinicola and Fomes fomentarius. Ann. Zool. Fenn. 32, 391–402.
Jurat-Fuentes, J. L., and Jackson, T. A. (2012). Bacterial entomopathogens,” in Insect Pathology, eds H. Kaya and F. Vera (Amsterdam: Elsevier), 265–349.
Kaiser, D., Bacher, S., Méne-Saffrané, L., and Grabenweger, G. (2019). Efficiency of natural substances to protect Beauveria bassiana conidia from UV radiation. Pest Manag. Sci. 75, 556–563. doi: 10.1002/ps.5209
Kaltenpoth, M. (2009). Actinobacteria as mutualists: general healthcare for insects? Trends Microbiol. 17, 529–535. doi: 10.1016/j.tim.2009.09.006
Kaminsky, L. M., Trexler, R. V., Malik, R. J., Hockett, K. L., and Bell, T. H. (2019). The inherent conflicts in developing soil microbial inoculants. Trends Biotechnol. 37, 140–151. doi: 10.1016/j.tibtech.2018.11.011
Kanchiswamy, C. N., Malnoy, M., and Maffei, M. E. (2015). Chemical diversity of microbial volatiles and their potential for plant growth and productivity. Front. Plant Sci. 6:151. doi: 10.3389/fpls.2015.00151
Kandasamy, D., Gershenzon, J., and Hammerbacher, A. (2016). Volatile organic compounds emitted by fungal associates of conifer bark beetles and their potential in bark beetle control. J. Chem. Ecol. 42, 952–969. doi: 10.1007/s10886-016-0768-x
Kandasamy, D., Gershenzon, J., Andersson, M. N., and Hammerbacher, A. (2019). Volatile organic compounds influence the interaction of the Eurasian spruce bark beetle (Ips typographus) with its fungal symbionts. ISME J. 13, 1788–1800. doi: 10.1038/s41396-019-0390-3
Kandasamy, D., Zaman, R., Nakamura, Y., Zhao, T., Hartmann, H., Andersson, M. N., et al. (2023). Conifer-killing bark beetles locate fungal symbionts by detecting volatile fungal metabolites of host tree resin monoterpenes. PLoS Biol. 21:e3001887. doi: 10.1371/journal.pbio.3001887
Keeling, C. I., and Bohlmann, J. (2006). Genes, enzymes and chemicals of terpenoid diversity in the constitutive and induced defence of conifers against insects and pathogens. N. Phytol. 170, 657–675.
Keeling, C. I., Campbell, E. O., Batista, P. D., Shegelski, V. A., Trevoy, S. A., Huber, D. P., et al. (2022). Chromosome-level genome assembly reveals genomic architecture of northern range expansion in the mountain pine beetle, Dendroctonus ponderosae Hopkins (Coleoptera: Curculionidae). Mol. Ecol. Resourc. 22, 1149–1167. doi: 10.1111/1755-0998.13528
Kenko, D. B. N., Ngameni, N. T., Awo, M. E., Njikam, N. A., and Dzemo, W. D. (2023). Does pesticide use in agriculture present a risk to the terrestrial biota? Sci. Total Environ. 861:160715. doi: 10.1016/j.scitotenv.2022.160715
Kikuchi, Y., Hayatsu, M., Hosokawa, T., Nagayama, A., Tago, K., and Fukatsu, T. (2012). Symbiont-mediated insecticide resistance. Proc. Natl. Acad. Sci. U.S.A. 109, 8618–8622.
Kirschner, R. (2001). “Diversity of filamentous fungi in bark beetle galleries in central Europe,” in Trichomycetes and other fungal groups, eds J. K. Misra, B. W. Horn (Boca Raton, FL: CRC Press), 175–196. doi: 10.1007/s10482-020-01510-6
Kolařík, M., and Hulcr, J. (2023). Geosmithia—widespread and abundant but long ignored bark beetle symbionts. Mycol. Progr. 22, 32.
Kreutz, J., Zimmermann, G., and Vaupel, O. (2004). Horizontal transmission of the entomopathogenic fungus Beauveria bassiana among the spruce bark beetle, Ips typographus (Col., Scolytidae) in the laboratory and under field conditions. Biocontrol Sci. Technol. 14, 837–848.
Kroemer, J., Bonning, B., and Harrison, R. (2015). Expression, delivery and function of insecticidal proteins expressed by recombinant Baculoviruses. Viruses 7, 422–455.
Krokene, P. (2015). “Conifer defense and resistance to bark beetles,” in Bark beetles. Biology and ecology of native and invasive species, eds F. E. Vega and R. W. Hofstetter (San Diego, CA: Elsevier Academic Press), 177–207.
Krokene, P., Fossdal, C. G., Nagy, N. E., and Solheim, H. (2013). “Conifer defense against insects and fungi,” in The Ophiostomatoid fungi: expanding frontier CBS Biodiversity Series 12, eds K. A. Seifert, Z. W. DeBeer, and M. J. Wingfield (Utrecht: CBS Press).
Kuzyakov, Y., and Blagodatskaya, E. (2015). Microbial hotspots and hot moments in soil: concept & review. Soil Biol. Biochem. 83, 184–199.
Lacey, L., Grzywacz, D., Shapiro-Ilan, D., Frutos, R., Brownbridge, M., and Goettel, M. (2015). Insect pathogens as biological control agents: back to the future. J. Invertebr. Pathol. 132, 1–41. doi: 10.1016/j.jip.2015.07.009
Lakshmanan, V., Selvaraj, G., and Bais, H. P. (2014). Functional soil microbiome: belowground solutions to an aboveground problem. Plant Physiol. 166, 689–700. doi: 10.1104/pp.114.245811
Leach, J. E., Triplett, L. R., Argueso, C. T., and Trivedi, P. (2017). Communication in the phytobiome. Cell 169, 587–596.
Lee, F. J., Rusch, D. B., Stewart, F. J., Mattila, H. R., and Newton, I. L. (2015). Saccharide breakdown and fermentation by the honey bee gut microbiome. Environ. Microbiol. 17, 796–815.
Lemfack, M. C., Gohlke, B.-O., Toguem, S. M. T., Preissner, S., Piechulla, B., and Preissner, R. (2018). mVOC 2.0: a database of microbial volatiles. Nucleic Acids Res. 46, D1261–D1265.
Leonard, S. P., Perutka, J., Powell, J. E., Geng, P., Richhart, D. D., Byrom, M., et al. (2018). Genetic engineering of bee gut microbiome bacteria with a toolkit for modular assembly of broad-host-range plasmids. ACS Synth. Biol. 7, 1279–1290. doi: 10.1021/acssynbio.7b00399
Leufvén, A., Bergström, G., and Falsen, E. (1984). Interconversion of verbenols and verbenone by identified yeasts isolated from the spruce bark beetle Ips typographus. J. Chem. Ecol. 10, 1349–1361. doi: 10.1007/BF00988116
Leverkus, A. B., Rey Benayas, J. M., Castro, J., Boucher, D., Brewer, S., Collins, B. M., et al. (2018). Salvage logging effects on regulating and supporting ecosystem services—A systematic map. Can. J. For. Res. 48, 983–1000.
Levy-Booth, D. J., Prescott, C. E., and Grayston, S. J. (2014). Microbial functional genes involved in nitrogen fixation, nitrification and denitrification in forest ecosystems. Soil Biol. Biochem. 75, 11–25.
Liebhold, A. M., and Kean, J. M. (2019). Eradication and containment of non-native forest insects: successes and failures. J. Pest Sci. 92, 83–91.
Lin, H., and Phelan, P. L. (1991). Identification of food volatiles attractive to dusky sap beetle, Carpophilus lugubris (Coleoptera: Nitidulidae). J. Chem. Ecol. 17, 1273–1286. doi: 10.1007/BF01402949
Lindmark, M., Ganji, S., Wallin, E. A., Schlyter, F., and Unelius, C. R. (2023). Semiochemicals produced by fungal bark beetle symbiont Endoconidiophora rufipennis and the discovery of an anti-attractant for Ips typographus. PLoS One 18:e0283906. doi: 10.1371/journal.pone.0283906
Liu, F., Ye, F., Cheng, C., Kang, Z., Kou, H., and Sun, J. (2022). Symbiotic microbes aid host adaptation by metabolizing a deterrent host pine carbohydrate d-pinitol in a beetle-fungus invasive complex. Sci. Adv. 8:eadd5051. doi: 10.1126/sciadv.add5051
Liu, Y., Wang, S., Wang, Z., Zhang, Z., Qin, H., Wei, Z., et al. (2019). Soil microbiome mediated nutrients decline during forest degradation process. Soil Ecol. Lett. 1, 59–71.
Lv, N., Peng, J., Chen, X. Y., Guo, C. F., Sang, W., Wang, X. M., et al. (2021). Antagonistic interaction between male-killing and cytoplasmic incompatibility induced by Cardinium and Wolbachia in the whitefly, Bemisia tabaci. Insect Sci. 28, 330–346. doi: 10.1111/1744-7917.12793
Ma, Z., Zhang, Y., Li, M., Chao, Z., Du, X., Yan, S., et al. (2023). A first greenhouse application of bacteria-expressed and nanocarrier-delivered RNA pesticide for Myzus persicae control. J. Pest Sci. 96, 181–193.
Mageroy, M. H., Christiansen, E., Långström, B., Borg-Karlson, A. K., Solheim, H., Björklund, N., et al. (2020). Priming of inducible defenses protects Norway spruce against tree-killing bark beetles. Plant Cell Environ. 43, 420–430. doi: 10.1111/pce.13661
Mann, A. J., and Davis, T. S. (2021). Entomopathogenic fungi to control bark beetles: a review of ecological recommendations. Pest Manag. Sci. 77, 3841–3846. doi: 10.1002/ps.6364
Martinez-Medina, A., Flors, V., Heil, M., Mauch-Mani, B., Pieterse, C. M., Pozo, M. J., et al. (2016). Recognizing plant defense priming. Trends Plant Sci. 21, 818–822.
McCutcheon, J. P., and Moran, N. A. (2012). Extreme genome reduction in symbiotic bacteria. Nat. Rev. Microbiol. 10, 13–26.
Meng, P., Hoover, K., and Keena, M. (2015). Asian longhorned beetle (Coleoptera: Cerambycidae), an introduced pest of maple and other hardwood trees in North America and Europe. J. Integr. Pest Manag. 6, 1–13.
Menkis, A., Povilaitienė, A., Marčiulynas, A., Lynikienė, J., Gedminas, A., and Marčiulynienė, D. (2019). Occurrence of common phyllosphere fungi of horse-chestnut (Aesculus hippocastanum) is unrelated to degree of damage by leafminer (Cameraria ohridella). Scand. J. For. Res. 34, 26–32.
Mercado-Blanco, J., Abrantes, I., Barra Caracciolo, A., Bevivino, A., Ciancio, A., Grenni, P., et al. (2018). Belowground microbiota and the health of tree crops. Front. Microbiol. 9:1006.
Mogilicherla, K., Chakraborty, A., Taning, C. N. T., Smagghe, G., and Roy, A. (2023). RNAi in termites (Isoptera): current status and prospects for pest management. Entomol. Gen. 43, 55–68.
Montgomery, M. K., and Fire, A. (1998). Double-stranded RNA as a mediator in sequence-specific genetic silencing and co-suppression. Trends Genet. 14, 255–258.
Moscardi, F. (1999). Assessment of the application of baculoviruses for control of Lepidoptera. Annu. Rev. Entomol. 44, 257–289.
Moscardi, F., De Souza, M. L., De Castro, M. E. B., Lara Moscardi, M., and Szewczyk, B. (2011). “Baculovirus pesticides: present state and future perspectives,” in Microbes and microbial technology: agricultural and environmental applications, eds I. Ahmad, F. Ahmad, and J. Pichtel (New York, NY: Springer), 415–445.
Moussa, A., Nones, S., Vannucchi, P. E., Shahzad, G.-R., Dittmer, J., Corretto, E., et al. (2023). The bacterial community of the European spruce bark beetle in space and time. bioRxiv [Preprint]. doi: 10.1101/2023.04.28.538755
Muhammad, A., Habineza, P., Ji, T., Hou, Y., and Shi, Z. (2019). Intestinal microbiota confer protection by priming the immune system of red palm weevil Rhynchophorus ferrugineus Olivier (Coleoptera: Dryophthoridae). Front. Physiol. 10:1303. doi: 10.3389/fphys.2019.01303
Munemasa, S., Hauser, F., Park, J., Waadt, R., Brandt, B., and Schroeder, J. I. (2015). Mechanisms of abscisic acid-mediated control of stomatal aperture. Curr. Opin. Plant Biol. 28, 154–162. doi: 10.1016/j.pbi.2015.10.010
Naik, K., Mishra, S., Srichandan, H., Singh, P. K., and Choudhary, A. (2020). Microbial formulation and growth of cereals, pulses, oilseeds and vegetable crops. Sustain. Environ. Res. 30, 1–18.
Naseer, A., Mogilicherla, K., Sellamuthu, G., and Roy, A. (2023). Age matters: life-stage, tissue, and sex-specific gene expression dynamics in Ips typographus (Coleoptera: Curculionidae: Scolytinae). Front. For. Glob. Change Sec. Pests Pathog. Invas. 6:1124754. doi: 10.3389/ffgc.2023.1124754
Neilson, E. H., Goodger, J. Q., Woodrow, I. E., and Møller, B. L. (2013). Plant chemical defense: at what cost? Trends Plant Sci. 18, 250–258.
Netherer, S., Kandasamy, D., Jirosová, A., Kalinová, B., Schebeck, M., and Schlyter, F. (2021). Interactions among Norway spruce, the bark beetle Ips typographus and its fungal symbionts in times of drought. J. Pest Sci. 94, 591–614. doi: 10.1007/s10340-021-01341-y
Noh, S. (2021). Linear paths to genome reduction in a defensive symbiont. Proc. Nal. Acad. Sci. U.S.A. 118:e2106280118. doi: 10.1073/pnas.2106280118
Nout, M., and Bartelt, R. (1998). Attraction of a flying nitidulid (Carpophilus humeralis) to volatiles produced by yeasts grown on sweet corn and a corn-based medium. J. Chem. Ecol. 24, 1217–1239.
Økland, B., Nikolov, C., Krokene, P., and Vakula, J. (2016). Transition from windfall-to patch-driven outbreak dynamics of the spruce bark beetle Ips typographus. For. Ecol. Manag. 363, 63–73.
Pan, Y., Birdsey, R. A., Phillips, O. L., and Jackson, R. B. (2013). The structure, distribution, and biomass of the world’s forests. Annu. Rev. Ecol. Evo. Syst. 44, 593–622.
Pedlar, J. H., McKenney, D. W., Yemshanov, D., and Hope, E. S. (2020). Potential economic impacts of the Asian longhorned beetle (Coleoptera: Cerambycidae) in Eastern Canada. J. Econ. Entomol. 113, 839–850. doi: 10.1093/jee/toz317
Peña-Peña, A., Santillán-Galicia, M., Hernández-López, J., and Guzmán-Franco, A. (2015). Metarhizium pingshaense applied as a seed treatment induces fungal infection in larvae of the white grub Anomala cincta. J. Invertebr. Pathol. 130, 9–12. doi: 10.1016/j.jip.2015.06.010
Perlmutter, J. I., and Bordenstein, S. R. (2020). Microorganisms in the reproductive tissues of arthropods. Nat. Rev. Microbiol. 18, 97–111.
Pettifor, B. J., and McDonald, J. E. (2021). “Bacterial biota of forest trees,” in Forest microbiology, eds F. O. Asiegbu and A. Kovalchuk (Elsevier: Academic Press), 161–173.
Phelan, P. L., and Lin, H. (1991). Chemical characterization of fruit and fungal volatiles attractive to dried-fruit beetle, Carpophilus hemipterus (L.)(Coleoptera: Nitidulidae). J. Chem. Ecol. 17, 1253–1272. doi: 10.1007/BF01402948
Picciotti, U., Araujo Dalbon, V., Ciancio, A., Colagiero, M., Cozzi, G., De Bellis, L., et al. (2023). “Ectomosphere”: insects and microorganism interactions. Microorganisms 11:440. doi: 10.3390/microorganisms11020440
Pieterse, C. M., de Jonge, R., and Berendsen, R. L. (2016). The soil-borne supremacy. Trends Plant Sci. 21, 171–173. doi: 10.1016/j.tplants.2016.01.018
Pineda, A., Kaplan, I., Hannula, S. E., Ghanem, W., and Bezemer, T. M. (2020). Conditioning the soil microbiome through plant–soil feedbacks suppresses an aboveground insect pest. N. Phytol. 226, 595–608. doi: 10.1111/nph.16385
Pirttilä, A., Brusila, V., Koskimäki, J., Wäli, P., Ruotsalainen, A., Mutanen, M., et al. (2023). Exchange of microbiomes in plant-insect herbivore interactions. Mbio 14:e0321022. doi: 10.1128/mbio.03210-22
Polanczyk, R. A., van Frankenhuyzen, K., and Pauli, G. (2017). The American bacillus thuringiensis based biopesticides market. Bacillus thuringiensis and Lysinibacillus sphaericus: Characterization and use in the field of biocontrol. Berlin: Springer, 173–184.
Poland, T. M., and McCullough, D. G. (2006). Emerald ash borer: invasion of the urban forest and the threat to North America’s ash resource. J. For. 104, 118–124.
Poland, T. M., Borden, J., Stock, A., and Chong, L. (1998). Green leaf volatiles disrupt responses by the spruce beetle, Dendroctonus rufipennis, and the western pine beetle, Dendroctonus brevicomis (Coleoptera: Scolytidae) to attractant-baited traps. J. Entomol. Soc. Br. Columbia 95, 17–24. doi: 10.1603/0022-0493-98.6.2041
Popa, V., Deziel, E., Lavallee, R., Bauce, E., and Guertin, C. (2012). The complex symbiotic relationships of bark beetles with microorganisms: a potential practical approach for biological control in forestry. Pest Manag. Sci. 68, 963–975. doi: 10.1002/ps.3307
Powell, D., Groβe-Wilde, E., Krokene, P., Roy, A., Chakraborty, A., Löfstedt, C., et al. (2021). A highly-contiguous genome assembly of the Eurasian spruce bark beetle, Ips typographus, provides insight into a major forest pest. Commun. Biol. 4:1059. doi: 10.1038/s42003-021-02602-3
Price, P. W., Denno, R. F., Eubanks, M. D., Finke, D. L., and Kaplan, I. (2011). Insect ecology: behavior, populations and communities. Cambridge: Cambridge University Press.
Pugsley, C. E., Isaac, R., Warren, N. J., and Cayre, O. J. (2021). Recent advances in engineered nanoparticles for RNAi-mediated crop protection against insect pests. Front. Agron. 3:652981. doi: 10.3389/fagro.2021.652981
Pureswaran, D. S., and Borden, J. H. (2004). New repellent semiochemicals for three species of Dendroctonus (Coleoptera: Scolytidae). Chemoecology 14, 67–75.
Pureswaran, D. S., Gries, R., Borden, J. H., Pierce, J., and Harold, D. (2000). Dynamics of pheromone production and communication in the mountain pine beetle, Dendroctonus ponderosae Hopkins, and the pine engraver, Ips pini (Say)(Coleoptera: Scolytidae). Chemoecology 10, 153–168.
Qayyum, M. A., Wakil, W., Arif, M. J., Sahi, S. T., and Dunlap, C. A. (2015). Infection of Helicoverpa armigera by endophytic Beauveria bassiana colonizing tomato plants. Biol. Control 90, 200–207.
Qin, Z., Munnoch, J. T., Devine, R., Holmes, N. A., Seipke, R. F., Wilkinson, K. A., et al. (2017). Formicamycins, antibacterial polyketides produced by Streptomyces formicae isolated from African Tetraponera plant-ants. Chem. Sci. 8, 3218–3227. doi: 10.1039/c6sc04265a
Raffa, K. F., Aukema, B. H., Bentz, B. J., Carroll, A. L., Hicke, J. A., Turner, M. G., et al. (2008). Cross-scale drivers of natural disturbances prone to anthropogenic amplification: the dynamics of bark beetle eruptions. Bioscience 58, 501–517.
Ralph, S. G., Yueh, H., Friedmann, M., Aeschliman, D., Zeznik, J. A., Nelson, C. C., et al. (2006). Conifer defence against insects: microarray gene expression profiling of Sitka spruce (Picea sitchensis) induced by mechanical wounding or feeding by spruce budworms (Choristoneura occidentalis) or white pine weevils (Pissodes strobi) reveals large-scale changes of the host transcriptome. Plant Cell Environ. 29, 1545–1570. doi: 10.1111/j.1365-3040.2006.01532.x
Ramsfield, T., Bentz, B., Faccoli, M., Jactel, H., and Brockerhoff, E. (2016). Forest health in a changing world: effects of globalization and climate change on forest insect and pathogen impacts. Forestry 89, 245–252.
Rasmann, S., Bennett, A., Biere, A., Karley, A., and Guerrieri, E. (2017). Root symbionts: powerful drivers of plant above-and belowground indirect defenses. Insect Sci. 24, 947–960. doi: 10.1111/1744-7917.12464
Ren, F., Yan, D. H., and Dong, W. (2021). “Microbiome of reproductive organs of trees,” in Tree Microbiome: Phyllosphere, Endosphere and Rhizosphere. Forest Microbiology, Vol. 1, eds F. O. Asiegbu and A. Kovalchuk (Cambridge, MA: Academic Press), 145–158.
Rolfe, S. A., Griffiths, J., and Ton, J. (2019). Crying out for help with root exudates: adaptive mechanisms by which stressed plants assemble health-promoting soil microbiomes. Curr. Opin. Microbiol. 49, 73–82. doi: 10.1016/j.mib.2019.10.003
Romero, F. M., Marina, M., and Pieckenstain, F. L. (2016). Novel components of leaf bacterial communities of field-grown tomato plants and their potential for plant growth promotion and biocontrol of tomato diseases. Res. Microbiol. 167, 222–233. doi: 10.1016/j.resmic.2015.11.001
Rondot, Y., and Reineke, A. (2018). Endophytic Beauveria bassiana in grapevine Vitis vinifera (L.) reduces infestation with piercing-sucking insects. Biol. Control 116, 82–89.
Roy, H. E., and Cottrell, T. E. (2008). Forgotten natural enemies: interactions between coccinellids and insect-parasitic fungi. Eur. J. Entomol. 105, 391–398.
Rupawate, P. S., Roylawar, P., Khandagale, K., Gawande, S., Jaiswal, D. K., and Borgave, S. (2023). Role of gut symbionts of insect pests: a novel target for insect-pest control. Front. Microbiol. 14:1146390. doi: 10.3389/fmicb.2023.1146390
Saleem, M., Meckes, N., Pervaiz, Z. H., and Traw, M. B. (2017). Microbial interactions in the phyllosphere increase plant performance under herbivore biotic stress. Front. Microbiol. 8:41. doi: 10.3389/fmicb.2017.00041
Salem, H., and Kaltenpoth, M. (2022). Beetle–bacterial symbioses: endless forms most functional. Annu. Rev. Entomol. 67, 201–219. doi: 10.1146/annurev-ento-061421-063433
Sandal, S., Singh, S., Bansal, G., Kaur, R., Mogilicherla, K., Pandher, S., et al. (2023). Nanoparticle-shielded dsRNA delivery for enhancing RNAi efficiency in cotton spotted Bollworm Earias vittella (Lepidoptera: Nolidae). Int. J. Mol. Sci. 24:9161. doi: 10.3390/ijms24119161
Schebeck, M., Schopf, A., Ragland, G. J., Stauffer, C., and Biedermann, P. H. (2023). Evolutionary ecology of the bark beetles Ips typographus and Pityogenes chalcographus. Bull. Entomol. Res. 113, 1–10. doi: 10.1017/S0007485321000353
Schlechter, R. O., Miebach, M., and Remus-Emsermann, M. N. (2019). Driving factors of epiphytic bacterial communities: a review. J. Adv. Res. 19, 57–65.
Schlegel, M., Queloz, V., and Sieber, T. N. (2018). The endophytic mycobiome of European ash and sycamore maple leaves–geographic patterns, host specificity and influence of ash dieback. Front. Microbiol. 9:2345. doi: 10.3389/fmicb.2018.02345
Schmidt, R., Cordovez, V., De Boer, W., Raaijmakers, J., and Garbeva, P. (2015). Volatile affairs in microbial interactions. ISME J. 9, 2329–2335.
Schulz-Bohm, K., Martín-Sánchez, L., and Garbeva, P. (2017). Microbial volatiles: small molecules with an important role in intra-and inter-kingdom interactions. Front. Microbiol. 8:2484. doi: 10.3389/fmicb.2017.02484
Scott, J. J., Oh, D.-C., Yuceer, M. C., Klepzig, K. D., Clardy, J., and Currie, C. R. (2008). Bacterial protection of beetle-fungus mutualism. Science 322, 63–63.
Scully, E. D., Geib, S. M., Carlson, J. E., Tien, M., McKenna, D., and Hoover, K. (2014). Functional genomics and microbiome profiling of the Asian longhorned beetle (Anoplophora glabripennis) reveal insights into the digestive physiology and nutritional ecology of wood feeding beetles. BMC Genomics 15:1096. doi: 10.1186/1471-2164-15-1096
Seidl, R., Spies, T. A., Peterson, D. L., Stephens, S. L., and Hicke, J. A. (2016). Searching for resilience: addressing the impacts of changing disturbance regimes on forest ecosystem services. J. Appl. Ecol. 53, 120–129. doi: 10.1111/1365-2664.12511
Seipke, R. F., Kaltenpoth, M., and Hutchings, M. I. (2012). Streptomyces as symbionts: an emerging and widespread theme? FEMS Microbiol. Rev. 36, 862–876. doi: 10.1111/j.1574-6976.2011.00313.x
Sharma, A., Sandhi, R. K., and Reddy, G. V. (2019). A review of interactions between insect biological control agents and semiochemicals. Insects 10:439.
Sieber, T. N. (2019). The endophyte Allantophomopsis cytisporea is associated with snow blight on Calluna vulgaris in the Alps—An effect of climate change? Arctic Antarctic Alpine Res. 51, 460–470.
Simon, A. G., Mills, D. K., and Furton, K. G. (2017). Chemotyping the temporal volatile organic compounds of an invasive fungus to the United States, Raffaelea lauricola. J. Chromatogr. A 1487, 72–76. doi: 10.1016/j.chroma.2017.01.065
Singewar, K., and Fladung, M. (2023). Double-stranded RNA (dsRNA) technology to control forest insect pests and fungal pathogens: challenges and opportunities. Funct. Integr. Genomics 23, 1–22. doi: 10.1007/s10142-023-01107-y
Singh, S., Singh, A., Baweja, V., Roy, A., Chakraborty, A., and Singh, I. K. (2021). Molecular rationale of insect-microbes symbiosis—from insect behaviour to mechanism. Microorganisms 9:2422. doi: 10.3390/microorganisms9122422
Six, D. L., and Wingfield, M. J. (2011). The role of phytopathogenicity in bark beetle–fungus symbioses: a challenge to the classic paradigm. Annu. Rev. Entomol. 56, 255–272. doi: 10.1146/annurev-ento-120709-144839
Soldan, R., Fusi, M., and Preston, G. M. (2022). Approaching the domesticated plant holobiont from a community evolution perspective. Microbiology 168:001188. doi: 10.1099/mic.0.001188
Srei, N., Lavallée, R., and Guertin, C. (2017). Susceptibility of Dendroctonus simplex to Hypocreales fungi: towards the development of a biological control strategy. J. Appl. Entomol. 141, 487–495.
Stegen, J. C., Bottos, E. M., and Jansson, J. K. (2018). A unified conceptual framework for prediction and control of microbiomes. Curr. Opin. Microbiol. 44, 20–27. doi: 10.1016/j.mib.2018.06.002
Sullivan, B. T., Dalusky, M. J., Wakarchuk, D., and Berisford, C. W. (2007). Field evaluations of potential aggregation inhibitors for the southern pine beetle, Dendroctonus frontalis (Coleoptera: Curculionidae). J. Entomol. Sci. 42, 139–149.
Sun, J., Lu, M., Gillette, N. E., and Wingfield, M. J. (2013). Red turpentine beetle: innocuous native becomes invasive tree killer in China. Annu. Rev. Entomol. 58, 293–311. doi: 10.1146/annurev-ento-120811-153624
Sun, X. (2015). History and current status of development and use of viral insecticides in China. Viruses 7, 306–319. doi: 10.3390/v7010306
Suslow, T. (2002). “Production practices affecting the potential for persistent contamination of plants by microbial foodborne pathogens,” in Phyllosphere microbiology, eds S. E. Lindow, E. I. Hecht-Poinar, and V. J. Elliott (St. Paul, MN: APS Press), 241–256.
Tanin, S. M., Kandasamy, D., and Krokene, P. (2021). Fungal interactions and host tree preferences in the spruce bark beetle Ips typographus. Front. Microbiol. 12:695167. doi: 10.3389/fmicb.2021.695167
Tedersoo, L., Bahram, M., Cajthaml, T., Põlme, S., Hiiesalu, I., Anslan, S., et al. (2016). Tree diversity and species identity effects on soil fungi, protists and animals are context dependent. ISME J. 10, 346–362. doi: 10.1038/ismej.2015.116
Teetor-Barsch, G. H., and Roberts, D. W. (1983). Entomogenous Fusarium species. Mycopathologia 84, 3–16.
Tholl, D., Rebholz, Z., Morozov, A. V., and O’Maille, P. E. (2023). Terpene synthases and pathways in animals: enzymology and structural evolution in the biosynthesis of volatile infochemicals. Natural Product Rep. 40, 766–793. doi: 10.1039/d2np00076h
Tian, H., Peng, H., Yao, Q., Chen, H., Xie, Q., Tang, B., et al. (2009). Developmental control of a lepidopteran pest Spodoptera exigua by ingestion of bacteria expressing dsRNA of a non-midgut gene. PLoS One 4:e6225. doi: 10.1371/journal.pone.0006225
Tillman, J. A., Seybold, S. J., Jurenka, R. A., and Blomquist, G. J. (1999). Insect pheromones—an overview of biosynthesis and endocrine regulation. Insect Biochem. Mol. Biol. 29, 481–514. doi: 10.1016/s0965-1748(99)00016-8
Tiwari, M., Pati, D., Mohapatra, R., Sahu, B. B., and Singh, P. (2022). The impact of microbes in plant immunity and priming induced inheritance: a sustainable approach for crop protection. Plant Stress 4:100072.
Tyagi, J., Mishra, A., Kumari, S., Singh, S., Agarwal, H., Pudake, R. N., et al. (2023). Deploying a microbial consortium of Serendipita indica, Rhizophagus intraradices, and Azotobacter chroococcum to boost drought tolerance in maize. Environ. Exp. Bot. 206:105142.
Usta, C. (2013). Microorganisms in biological pest control—a review (bacterial toxin application and effect of environmental factors). Curr. Progr. Biol. Res. 13, 287–317.
Vacher, C., Hampe, A., Porté, A. J., Sauer, U., Compant, S., and Morris, C. E. (2016). The phyllosphere: microbial jungle at the plant–climate interface. Annu. Rev. Ecol. Evol. Syst. 47, 1–24.
van der Wal, A., and Leveau, J. H. (2011). Modelling sugar diffusion across plant leaf cuticles: the effect of free water on substrate availability to phyllosphere bacteria. Environ. Microbiol. 13, 792–797. doi: 10.1111/j.1462-2920.2010.02382.x
Vannette, R. L., and Fukami, T. (2016). Nectar microbes can reduce secondary metabolites in nectar and alter effects on nectar consumption by pollinators. Ecology 97, 1410–1419. doi: 10.1890/15-0858.1
Vavre, F., and Kremer, N. (2014). Microbial impacts on insect evolutionary diversification: from patterns to mechanisms. Curr. Opin. Insect Sci. 4, 29–34. doi: 10.1016/j.cois.2014.08.003
Villalobos, A., Schlyter, F., Birgersson, G., Koteja, P., and Löf, M. (2022). Fear effects on bank voles (Rodentia: Arvicolinae): testing for repellent candidates from predator volatiles. Pest Manag. Sci. 78, 1677–1685. doi: 10.1002/ps.6787
Vivas, M., Kemler, M., and Slippers, B. (2015). Maternal effects on tree phenotypes: considering the microbiome. Trends Plant Sci. 20, 541–544.
Wang, F., Nong, X., Hao, K., Cai, N., Wang, G., Liu, S., et al. (2020). Identification of the key genes involved in the regulation of symbiotic pathways induced by Metarhizium anisopliae in peanut (Arachis hypogaea) roots. 3 Biotech 10, 1–24. doi: 10.1007/s13205-020-2105-x
Wang, T.-Y., and Luan, J.-B. (2023). Silencing horizontally transferred genes for the control of the whitefly Bemisia tabaci. J. Pest Sci. 96, 195–208.
Wang, Y., and Rozen, D. E. (2018). Gut microbiota in the burying beetle, Nicrophorus vespilloides, provide colonization resistance against larval bacterial pathogens. Ecol. Evol. 8, 1646–1654. doi: 10.1002/ece3.3589
Wermelinger, B. (2004). Ecology and management of the spruce bark beetle Ips typographus—a review of recent research. For. Ecol. Manag. 202, 67–82.
Wertheim, B., van Baalen, E.-J. A., Dicke, M., and Vet, L. E. (2005). Pheromone-mediated aggregation in nonsocial arthropods: an evolutionary ecological perspective. Annu. Rev. Entomol. 50, 321–346. doi: 10.1146/annurev.ento.49.061802.123329
Whitehill, J. G., and Bohlmann, J. (2019). A molecular and genomic reference system for conifer defence against insects. Plant Cell Environ. 42, 2844–2859.
Whitten, M. M., Facey, P. D., Del Sol, R., Fernández-Martínez, L. T., Evans, M. C., Mitchell, J. J., et al. (2016). Symbiont-mediated RNA interference in insects. Proc. R. Soc. B Biol. Sci. 283:20160042.
Whitten, M., and Dyson, P. (2017). Gene silencing in non-model insects: overcoming hurdles using symbiotic bacteria for trauma-free sustainable delivery of RNA interference: sustained RNA interference in insects mediated by symbiotic bacteria: applications as a genetic tool and as a biocide. Bioessays 39:1600247. doi: 10.1002/bies.201600247
Whitten, M., Xue, Q., Taning, C. N. T., James, R., Smagghe, G., Del Sol, R., et al. (2023). A narrow host-range and lack of persistence in two non-target insect species of a bacterial symbiont exploited to deliver insecticidal RNAi in Western Flower Thrips. Front. Insect Sci. 3:1093970. doi: 10.3389/finsc.2023.1093970
Wilkinson, S. W., Magerøy, M. H., López Sánchez, A., Smith, L. M., Furci, L., Cotton, T. A., et al. (2019). Surviving in a hostile world: plant strategies to resist pests and diseases. Annu. Rev. phytopathol. 57, 505–529. doi: 10.1146/annurev-phyto-082718-095959
Williams, G. M., and Ginzel, M. D. (2021). Competitive advantage of Geosmithia morbida in low-moisture wood may explain historical outbreaks of thousand cankers disease and predict the future fate of Juglans nigra within its native range. Front. For. Glob. Change 4:725066. doi: 10.3389/ffgc.2021.725066
Xia, X., Gurr, G. M., Vasseur, L., Zheng, D., Zhong, H., Qin, B., et al. (2017). Metagenomic sequencing of diamondback moth gut microbiome unveils key holobiont adaptations for herbivory. Front. Microbiol. 8:663. doi: 10.3389/fmicb.2017.00663
Xue, Q., Avila dos Santos, É, Smagghe, G., Zotti, M. J., and Taning, N. T. C. (2023). Engineering strategies for insect viruses and microbes for dsRNA production and delivery to insects for targeted gene silencing. Entomol. Generalis 43, 31–53. doi: 10.1002/ps.6277
Yan, S., Ren, B. Y., and Shen, J. (2021). Nanoparticle-mediated double-stranded RNA delivery system: a promising approach for sustainable pest management. Insect Sci. 28, 21–34. doi: 10.1111/1744-7917.12822
Zaman, R., May, C., Ullah, A., and Erbilgin, N. (2023). Bark beetles utilize ophiostomatoid fungi to circumvent host tree defenses. Metabolites 13:239. doi: 10.3390/metabo13020239
Zhang, L., Chen, H., Ma, C., and Tian, Z. (2010). Electrophysiological responses of Dendroctonus armandi (Coleoptera: Curculionidae: Scolytinae) to volatiles of Chinese white pine as well as to pure enantiomers and racemates of some monoterpenes. Chemoecology 20, 265–275.
Zhang, P. J., Broekgaarden, C., Zheng, S. J., Snoeren, T. A., van Loon, J. J., Gols, R., et al. (2013). Jasmonate and ethylene signaling mediate whitefly-induced interference with indirect plant defense in Arabidopsis thaliana. N. Phytol. 197, 1291–1299. doi: 10.1111/nph.12106
Zhang, Q.-H., Schlyter, F., and Anderson, P. (1999). Green leaf volatiles interrupt pheromone response of spruce bark beetle, Ips typographus. J. Chem. Ecol. 25, 2847–2861.
Zhang, Q.-H., Schlyter, F., Chen, G., and Wang, Y. (2007). Electrophysiological and behavioral responses of Ips subelongatus to semiochemicals from its hosts, non-hosts, and conspecifics in China. J. Chem. Ecol. 33, 391–404. doi: 10.1007/s10886-006-9231-8
Zhang, X., Li, Y., Si, H., Zhao, G., Kolařík, M., Hulcr, J., et al. (2022). Geosmithia species associated with bark beetles from China, with the description of nine new species. Front. Microbiol. 13:820402. doi: 10.3389/fmicb.2022.820402
Zhang, Y., Xu, L., Li, S., and Zhang, J. (2019). Bacteria-mediated RNA interference for management of Plagiodera versicolora (Coleoptera: Chrysomelidae). Insects 10, 415. doi: 10.3390/insects10120415
Zhao, T., Axelsson, K., Krokene, P., and Borg-Karlson, A.-K. (2015). Fungal symbionts of the spruce bark beetle synthesize the beetle aggregation pheromone 2-methyl-3-buten-2-ol. J. Chem. Ecol. 41, 848–852. doi: 10.1007/s10886-015-0617-3
Keywords: forest microbiome, bark beetles, biocontrol agents, microbial volatile organic compounds (MVOCs), coleopteran forest pest management, RNA interference (RNAi), symbiont-mediated RNAi (SMR)
Citation: Gupta S, Chakraborty A and Roy A (2023) Prospects for deploying microbes against tree-killing beetles (Coleoptera) in Anthropocene. Front. For. Glob. Change 6:1182834. doi: 10.3389/ffgc.2023.1182834
Received: 09 March 2023; Accepted: 10 July 2023;
Published: 26 July 2023.
Edited by:
Milica Zlatkovic, University of Novi Sad, SerbiaReviewed by:
Dhandapani Gurusamy, Kongunadu Arts and Science College, IndiaMilivoj Franjevic, University of Zagreb, Croatia
Magdalena Kacprzyk, University of Agriculture in Krakow, Poland
Copyright © 2023 Gupta, Chakraborty and Roy. This is an open-access article distributed under the terms of the Creative Commons Attribution License (CC BY). The use, distribution or reproduction in other forums is permitted, provided the original author(s) and the copyright owner(s) are credited and that the original publication in this journal is cited, in accordance with accepted academic practice. No use, distribution or reproduction is permitted which does not comply with these terms.
*Correspondence: Amit Roy, roy@fld.czu.cz
†These authors have contributed equally to this work