- 1Dipartimento di Agraria, Università degli Studi di Napoli Federico II, Portici, Italy
- 2Dipartimento di Scienze e Tecnologie Ambientali Biologiche e Farmaceutiche, Università degli Studi della Campania Luigi Vanvitelli, Caserta, Italy
- 3Laboratoire sur les Écosystèmes Terrestres Boréaux, Département des Sciences Fondamentales, Université du Québec à Chicoutimi, Chicoutimi, QC, Canada
In the Mediterranean region of southern Europe, Castanea sativa Mill. (Castanea) coppice stands are being invaded by non-native pioneer tree species due to recurrent coppicing and wildfire disturbances. There is a need to control the spread of non-native tree species in Castanea coppices due to their pivotal socio-economic role, however, to facilitate this, further research into invasive strategies and their interactions with disturbances is required. The non-native Robinia pseudoacacia L. (Robinia) has widely colonized the overaged and unmanaged Castanea coppice forests in Vesuvius National Park, which were disturbed by the low-severity fires in the summer of 2017. Here, we aimed to assess the functional traits conferring a competitive advantage to the Robinia over Castanea and the changes in spatial stand patterns driven by wildfire disturbance. To achieve this we assessed the spatial stem patterns, regeneration strategies, and conducted field-assessments and remote sensing of the spring leaf phenology of both Castanea and Robinia in five 300–600 m2 plots. After the 2017 wildfire, root suckers constituted 72% (±12) of the Robinia vegetative living sprouts and occurred up to a distance of 10 m from the adult parent trees under the canopy of the Castanea trees. NDVI-based estimates at the start of the growing season (sos) in Castanea occurred over a 7 day period, from DOY 118–124, increasing at a rate (rsp) of 0.10–0.16, in agreement with the field-based assessment of spring leaf phenology. In Robinia, the sos estimated at DOY 109 from the NDVI seasonal trajectory disagreed with the field-based observations, which started later than Castanea, after approximately DOY 133. Here, the high percentage cover (∼90%) of the understory species influenced NDVI-based estimates of Robinia leaf spring phenology. The results suggest that low-severity wildfires increased the invasiveness of Robinia, and that vegetative regeneration strategies, rather than earlier spring phenology, conferred a competitive advantage in the fire-disturbed Castanea coppice stands. The results thus suggest that appropriate management of invasive Robinia trees to conserve the continuous canopy cover of Castanea stands should guide nature-based solutions to control the spread of non-native tree species.
1. Introduction
The anthropogenic migration of species beyond their native ranges has substantially increased in the last century, and there does not appear to be any signs of global saturation (Seebens et al., 2017). In the Mediterranean Basin, the ecological and socioeconomic impacts of exotic species invasions has drastically increased in recent decades (Kourantidou et al., 2021), and this frequently has detrimental effects on ecosystem services and human well-being (Stoett et al., 2019). Biological invasions and climate change are considered the key drivers threatening the conservation of native flora in protected areas around the world, and this is jeopardizing efforts to halt biodiversity loss (Gallardo et al., 2017). The impacts are particularly dramatic in the protected areas of southern Europe around the Mediterranean, where losses of biodiversity occur more frequently as a consequence of the wide and diffuse socioeconomic activities, coupled with the ongoing issues regarding climate and land use changes (Nadal-Sala et al., 2019; Fois et al., 2020).
Conservation and restoration of disturbed native habitats and plant communities requires an understanding of the mechanisms and patterns by which invasive species outperform native species. The spread of invasive plants is mainly linked to their functional traits (e.g., growth rate, early leaf onset, and resprouting ability) (Van Kleunen et al., 2010) and to key processes (biotic and abiotic filters) of ecosystems at local and landscape scales that promote colonization and establishment (e.g., disturbances, resource availability, empty niches) (Theoharides and Dukes, 2007). Among the strategies for tree invasion, vegetative regeneration is one of the major drivers of tree species colonization (Nunez-Mir et al., 2019). A large proportion of invasive trees spread and persist outside their native range via vegetative regeneration strategies such as resprouting (i.e., from root fragments and basal stems), layering, and suckering (Reichard and Hamilton, 1997).
Concurrently, plant traits related to phenology also contribute significantly to the spread of non-native species (Fridley, 2012), emphasizing their invasiveness (Chuine and Beaubien, 2001) and inequality between non-native and native invasive species (Wolkovich and Cleland, 2014). Early leaf growth can enable non-native species to take advantage of light availability and carbon assimilation while native species remain leafless, explaining their persistence in non-native habitats (Harrington et al., 1989; Wolkovich and Cleland, 2011). Despite efforts to identify the common traits of invasive plants, knowledge of the key functional traits for invasive non-native tree species is still lacking (Nunez-Mir et al., 2019). Understanding the traits conferring invasiveness allows for the proper management of established non-native tree species (Mathakutha et al., 2019) and can help to predict whether and how non-native species will potentially become invasive when introduced into a new ecosystem. Therefore, assessing the key functional traits that enable non-native tree species to become invasive is crucial for implementing appropriate management programs in invaded local vegetation communities (Gallagher et al., 2015), especially in forest areas where environmental resources can be deliberately manipulated (Funk et al., 2016).
In European Mediterranean landscapes, Castanea sativa Mill. (hereafter Castanea) stands are the most frequent human-made forest type, replacing native deciduous forests in the lowland mountain belt (Allevato et al., 2012). Currently, in Europe, Castanea forests cover approximately two million hectares, and 40% of this growth is in Italy, from plain to hilly topographies (INFC, 2005). Castanea stands are prone to invasion from Robinia pseudoacacia L. (hereafter Robinia), and this is exacerbated by natural and anthropogenic disturbances, especially coppicing, as it spreads easily in both managed and unmanaged coppice stands (Radtke et al., 2013; Marcolin et al., 2020). Robinia is a North American, nitrogen-fixing, and light-demanding pioneer tree species that frequently occurs in forest and non-forest sites in Europe (Cierjacks et al., 2013). In Mediterranean mountainous forests, its past spread has been encouraged mainly by extensive planting for afforestation, wood provisioning, and to prevent and control erosion along mountain slopes (Vítková et al., 2017, 2020), which highlights its potential role in regulating ecosystem services (Castro-Díez et al., 2019).
Outside its native range, Robinia is considered an invasive tree species that colonizes and persists in new environmental habitats (Richardson and Pyšek, 2006). Its spread has been recognized in many protected areas from riparian to hilly landscapes (Motta et al., 2009), often replacing native forest vegetation and impacting mostly on the plant-soil biotic community traits analyzed (Lazzaro et al., 2018; De Marco et al., 2023). Due to its invasiveness, Robinia is currently listed as a dangerous invasive tree and has been targeted for controlled management in European and Asian countries (Lazzaro et al., 2020). In southern Switzerland and northern Italy, Robinia has replaced entire valleys of European Castanea coppice forests (Sabo, 2000; Sitzia et al., 2012) and further Castanea forests in Italy are expected to be invaded (Benesperi et al., 2012). Its capability to spread beyond places of introduction and to establish forest habitats with conservation objectives requires active management to counteract its invasiveness (Kleinbauer et al., 2010). Understanding how to effectively counteract the invasiveness of Robinia, especially in protected forest areas, requires useful insights into its autecology, ecophysiological traits, and the site features of colonized forest community types.
The evolutionary dynamics of Castanea coppice forests along the Italian peninsula are affected by various human-mediated disturbances. In this context, Castanea coppices represent an opportunity to shed light on the invasive strategies displayed by the non-native Robinia species and on the management options required to cope with its spread in fire-disturbed stands. Past eruption episodes repeatedly destroyed the native vegetation on the slopes of the Somma-Vesuvius stratovolcano, the last of which occurred in 1944 (De Vivo et al., 2019). However, charcoal and archeological analyses attested to the presence of Castanea before the Roman Period (Di Pasquale et al., 2010). In the last century, the bare slopes of the Somma-Vesuvius volcano has been prone to soil erosion and has thus been reforested with a number of species, including Robinia and shrub Genista etnensis (Raf.) DC. (Stinca et al., 2015) to mitigate the hydrogeological hazards of andic soil. Since its plantation in the southeastern and northeastern flanks of Mount Somma, Robinia has spread in broadleaved forests, especially in Castanea coppice stands. Moreover, recurrent wildfires have disturbed the forests of Vesuvius National Park, especially in the summer of 2017, as approximately 88% of the total forested area was burned, causing serious damage to the existing biodiversity and ecosystem services supply (Silvestro et al., 2021; Cervelli et al., 2022). In the summer of 2017, low-severity wildfires experienced by Castanea coppice forests on the northern slopes (Saulino et al., 2020) increased Robinia’s invasiveness, posing relevant issues for post-fire management. Considering the recent increase in the number and severity of wildfires observed in the Mediterranean basin (San-Miguel-Ayanz et al., 2021), we believe that assessing the role of fire disturbances on the spread of invasive tree species (Keeley, 2006) could provide useful insights into the post-fire management of Mediterranean Castanea coppice stands.
In an overaged and unmanaged Mediterranean Castanea coppice stand of the Vesuvius National Park affected by the 2017 summer wildfires, we assessed (i) the functional traits conferring competitive advantages to invasive Robinia over Castanea trees, and (ii) the changes in stem spatial patterns driven by fire disturbances. We hypothesized that the early spring phenology and root sprouting would be identified as functional traits that confer a competitive advantage to Robinia in the colonization of Castanea coppice stands. Mediterranean chestnut coppice forests offer a useful and yet unexplored system for examining the role of species-specific functional traits which allow invasive Robinia to exploit empty niches following wildfire disturbances.
2. Materials and methods
2.1. Study site
Vesuvius National Park is a geo-site in southern Italy, covering 8,250 ha, including the Somma-Vesuvius volcanic complex, which is still active in mainland Europe (De Vivo et al., 2019). The slopes of the volcano are covered by mixed broadleaved, monospecific, and mixed Mediterranean coniferous and shrubland forests. On the northern slope of Mt. Somma, the Castanea forest covers 646 ha, distributed from a minimum elevation of 350 m a.s.l. to a maximum of 1,120 m a.s.l. (Figure 1). According to the European forest type classification EFTs (Barbati et al., 2014), the Mt. Somma Castanea forest belong to the type level “8.7 Chestnut forest” in the category level “8. Thermophilous deciduous forest.”
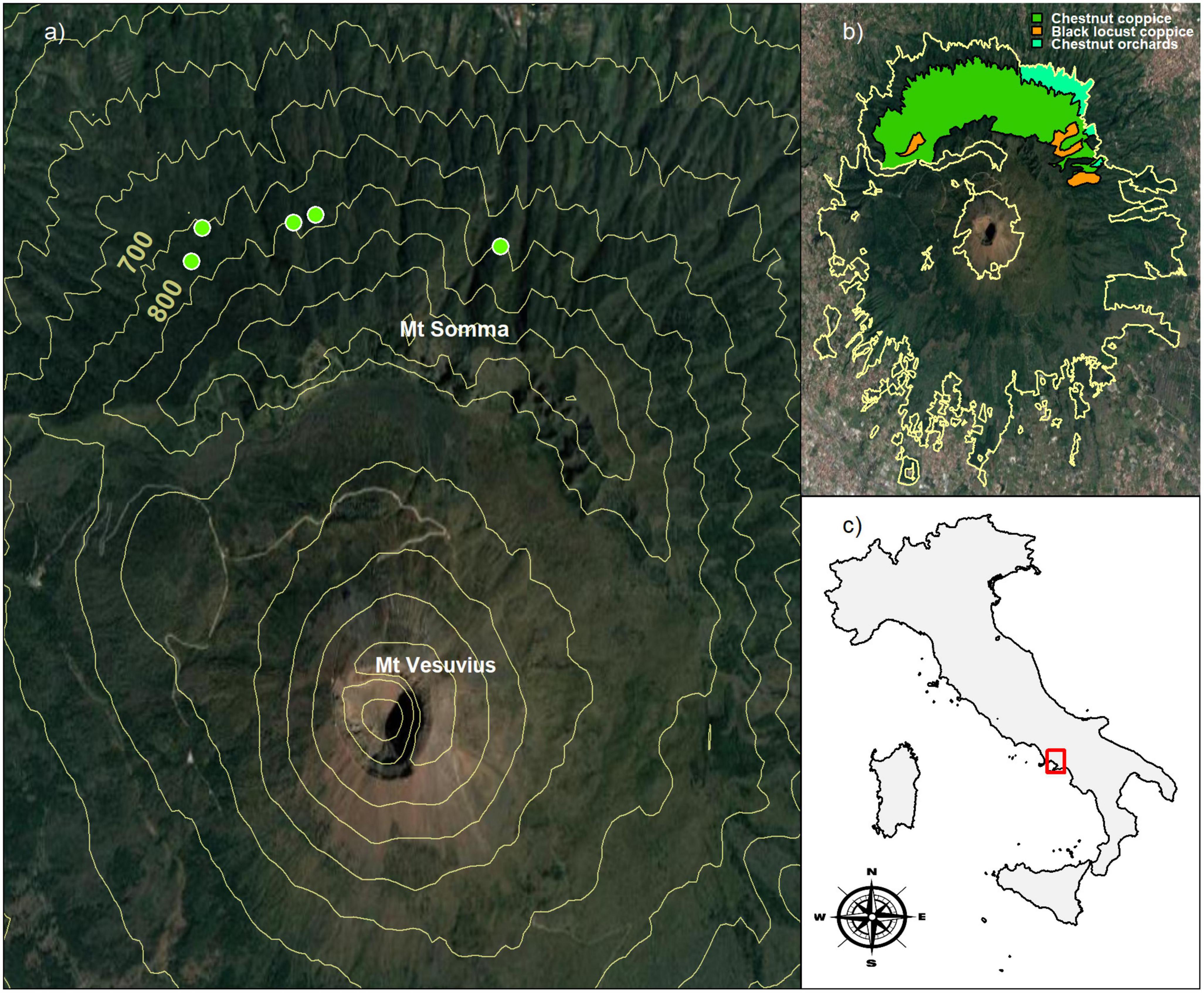
Figure 1. Location of the study sites in Vesuvius National Park, Southern Italy. (a) The green circular markers indicate the geospatial position of permanent plots, while the solid yellow contour lines represent 100 m elevation intervals; (b) the yellow boundary line represents the forest perimeter of Vesuvius National Park, while colored polygons indicate the forest types on Mt. Somma: chestnut coppice (green), chestnut orchards (light-green) and black locust coppice (orange). (c) The red square identifies the geographic position of the Vesuvius National Park. Aerial images were obtained from Google Maps Platform APIs service.
In the last century, most of Castanea stands have been managed as coppices with standards for timber production to obtain small-to medium-sized pole assortments. Since the establishment of the National Park (1995), Castanea coppices have been unmanaged and frequently colonized by Robina tree sprouts. Other native tree species include Acer opalus Mill. subsp. obtusatum (Waldst. & Kit. ex Willd.) Gams, Fraxinus ornus L. subsp. ornus, Quercus ilex L. subsp. ilex occurs sporadically and mainly in the understory layer of Castanea coppice stands. Native shrub species are common in coppice stands, particularly Rubus ulmifolius Schott and Rubus hirtus Waldst. & Kit. groups, Cytisus scoparius (L.) Link subsp. scoparius, Sambucus nigra L., Hedera helix subsp. helix, and Emerus major Mill. subsp. emeroides (Boiss & Spruner), Soldano & F. Conti (Supplementary Material 1). However, on the northeastern slope of Mt. Somma (<600 m a.s.l.), Castanea orchards are managed for fruit production, with a limited surface area of 72 ha.
The area has a Mediterranean climate with a cold winter and a relatively hot and dry summer (Stinca et al., 2015). In the summer, the average monthly temperature is 21.6°C whereas in the winter it is 5.7°C. The average annual rainfall is 1100 mm and this is asymmetrically distributed with 73 % occurring during the autumn-winter season and 27% in the spring-summer season.
2.2. Stem spatial pattern, size attributes, and plant diversity sampling
In the winter-spring 2022, synchronic sampling approach was applied to investigate Castanea stands invaded by the non-native species Robinia across multiple sites burned at same points in time, in the summer 2017. Such sampled plots were retrospectively organized to construct a Robinia invasion gradient. Synchronic approach is commonly used to compare and investigate non-native species invasion strategies in forest communities (Walker and Smith, 1997), especially when the bi-temporal - before-after disturbance approach (or diachronic sampling) - cannot be applied (Pino et al., 2009).
The spatial positions of stems and their size attributes were collected in five permanent plots ranging from 300 to 600 m2 (Figures 2A–E). Here, the variability of plot size is imposed by the topography constraints of volcanic slope. The plots were positioned on the north face of the Somma-Vesuvius mountain, from 680 to 780 m a.s.l., with slope ranging from 25 to 30° (Figure 1A). In all plots, the forest soil developed on falls of ash and lapilli, and belongs to Molli-Vitric Andosols class according to the world reference base classification system (IUSS Working Group WRB, 2022), with a variable depth ranging from 100 to 150 cm.
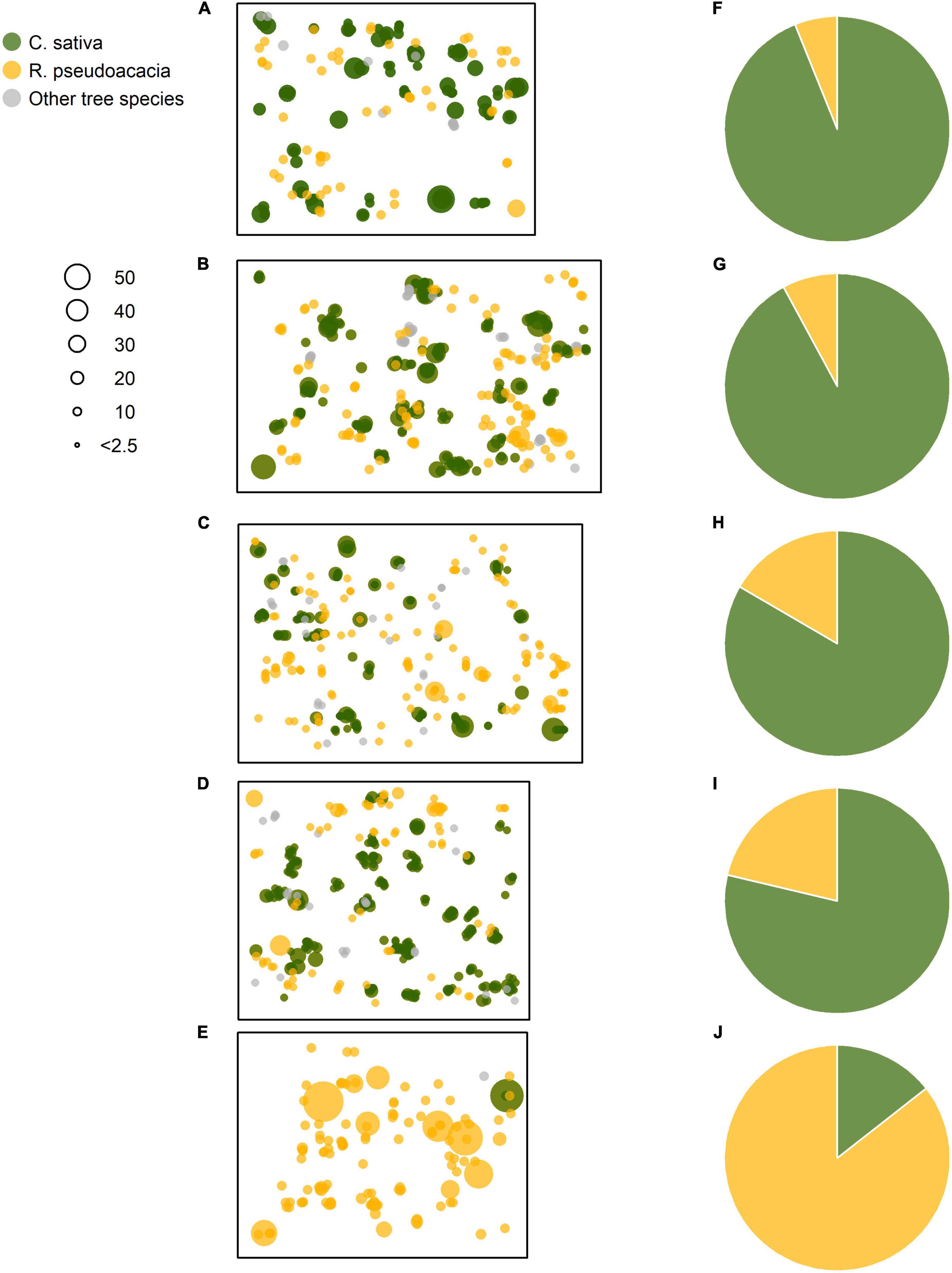
Figure 2. Spatial distributions of the sprouted stems and their sizes for the Castanea, Robinia, and other Mediterranean tree species. (A–E) The plots were ordered by decreasing basal area values of the standing Castanea sprouts belonging to the overstory layer. (F–J) Represent the basal area percentage (%) for the Castanea and Robinia sprouts belonging to the overstory layer. Point sizes are proportional to diameter at breast height (DbH, cm). (A–E) Correspond to the plots C1–R5 of Table 1.
In each plot, the relative spatial coordinates of all standing (live and dead) stem sprouts of Castanea, Robinia, and the other native tree species were recorded. For each stem, the Xi and Yi Cartesian coordinates (m) were measured using a Vertex ultrasound hypsometer (Haglöf® Vertex Laser VL400). The diameter at breast height (DbH, cm) 1.30 m above-ground was measured for each stem sprout using a caliper. Total height (TH, m) and crown base height (CBH, m) were measured in standing trees belonging to the dominant and intermediate positions using a Vertex ultrasound hypsometer.
In the winter-spring 2022, the diversity of the plant communities was assessed in terms of coverage of the different layers and all individual plant species. Vascular species cover was visually assessed according to the Braun-Blanquet scale [i.e., (r) rare; (+) <1%; (1) 1–5%; (2a) 6%–15%; (2b) 16–25%; (3) 26–50%; (4) 51–75%; and (5) 76–100%] and transformed into an ordinal scale according to Van der Maarel (1979). Nomenclature and taxon delimitation followed recent checklists of Italian vascular flora (Bartolucci et al., 2018; Galasso et al., 2018). The taxonomic identification of the species is provided in Supplementary Material 1.
2.3. Standing stems classification and post-fire scars assessment
The stems were classified as (i) basal sprouts for both Castanea and Robinia and (ii) root-suckerings only in Robinia, according to the definition provided by Del Tredici (2001). The root-suckerings of Robinia were classified based on their distance from the adult parent tree. However, root suckerings were manually dug in doubtful cases to exclude their seed origin. Leeward fire scars on the basal portions of the stem sprouts were assessed by the scorched height (Gutsell and Johnson, 1996), about 5 years after the 2017 wildfires. Therefore, pre-fire stand structure was built excluding basal stem sprouts and root-suckers having basal stem diameter ≤4 cm, corresponding in turn to an age ≤ 5 years. Age was determinate by visual counting of tree rings stem cross-section on six Castanea and six Robinia sprouts randomly sampled. Standing stem basal or root-suckering sprouts were categorized as live not-scorched, dead not-scorched, live-scorched, and dead-scorched. The category dead-not scorched includes standing Castanea stem basal resprouts died for the spread of Cryphonectria parasitica, and exacerbated by the leaf area reduction of Dryocosmus kuriphilus, while Robinia root-suckers died for self-thinning.
2.4. Spatial patterns and structural analysis
The spatial aggregation pattern of the standing trees and their stem diameter differentiations were assessed using two second-order spatially explicit procedures: (i) the normalized mark differentiation function τ(r) (Stoyan and Stoyan, 1994; Hui and Pommerening, 2014) and (ii) the nearest neighbor distance distribution function G(r) (Cressie, 2015). In the function τ(r), the diameter at breast height (DbH, cm) was used as a quantitative marker. The Epanechnikov kernel method (Pommerening and Grabarnik, 2019) was employed to estimate the mark differentiation function τ(r), applying a bandwidth value of 0.8 m and implementing the translation edge correction method to minimize bias. Bandwidth was estimated according to the formula developed by Illian et al. (2008) for planar Poisson processes. In the estimation of the function G(r), the spatial Kaplan-Meier correction method was implemented to reduce the bias associated with the edge effect (Baddeley and Gill, 1997). Management and analysis of the spatial point patterns was performed in R using the “spatstat” package (Baddeley et al., 2015).
2.5. Remote sensed NDVI data
We used the recently improved Landsat Collection 2 products (USGS, 2021) available from the Google Earth Engine (GEE) open cloud-platform database (Gorelick et al., 2017). We selected Landsat 7 ETM+ (LE07) and 8 OLI/TIRS (LC08) Surface Reflectance Collection 2, Tier 1, Level 2 datasets (C02/T1_L2), spanning from January 4, 2005, to August 31, 2022, for Landsat 7 ETM+, and from April 24, 2013, to August 31, 2022, for Landsat 8 OLI/TIRS. The collection C02/T1_L2 includes data that are radiometrically and geometrically corrected and inter-calibrated across different Landsat instruments.1 Before using the C02/T1_L2 data, the surface reflectance bands were scaled by applying a scale factor of 0.0000275 and an additional offset of -0.2 per pixel.2
The quality assessment mask to exclude pixels with clouds, shadow, water, and snow was produced by applying a multi-pass algorithm called “CFMask” (Foga et al., 2017) and employing the Landsat quality assessment band (QA_PIXEL) (Supplementary Material 2).3
For each plot (see below), the aforementioned Landsat datasets (C02/T1_L2) were used to assess the normalized difference vegetation index (NDVI) (Zhang et al., 2018). NDVI values were calculated per pixel across the stack of valid pixels (e.g., cloud and snow-free pixels). Prior to computing the NDVI, a filter that selected Landsat images with a sun elevation of >10° was applied. Near-infrared and red surface reflectance bands from both Landsat sensors were used in the computation of the NDVI: B4 and B3 reflectance bands from Landsat LE07, B5, and B4 for Landsat LC08.
2.6. Satellite- and field based assessment of phenology
A double logistic function developed by Beck et al. (2006) was applied to model the annual NDVI trends. The function models the NDVI as a function of time (t) using six parameters: the winter NDVI (mn) and the maximum NDVI of the growing season (mx), two inflection points at the start of the growing season (sos) and the end of the growing season (eos), and two slopes, the spring increasing rate (rsp) and the autumn decreasing rate (rau).
Prior to fitting the Beck function, the NDVI data were pre-processed using a two-step filtering procedure, consisting of the removal of (i) the altered observation related to the multiple wildfires that occurred in the summer of 2017 (i.e., from July 2, 2017, to December 31, 2017) (Supplementary Figure 1 in Supplementary Material 3) and (ii) incongruous/anomalous observations with the time of acquisition and expected phenological stage, especially low and high NDVI recordings that occurred later during the growing period or early in the dormant season. In the second step, we applied a stand-specific filtering procedure for each plot, pre-fitting the NDVI data, and computing the absolute residues for each NDVI value and the timing of the observations. All NDVI recordings with absolute residues (i.e., | NDVIpredicted – NDVIrecorded|) higher than 0.2 were discarded (Supplementary Figure 2 in Supplementary Material 3). After pre-processing, for each plot, a double logistic curve was fitted to the filtered NDVI recordings.
In the spring of 2022, the vegetative phenology of Castanea and Robinia stem sprouts growing in the understory and overstory layers was visually assessed using a digital camera. The gradual vegetative process from bud burst to leaf unfolding was assessed using the BBCH phenological scale (Meier, 1997).
The parameters of the model function were estimated using the iterative nonlinear last square method (Bates and Watts, 1988), employing the Levenberg-Marquardt optimization algorithm. Statistical differences among the estimated parameters were tested by applying the Wald test procedure using the Bonferroni multiplicity adjustment method, followed by Tukey’s multiple comparison test (Bretz et al., 2011). Management and analysis of the NDVI data were performed in R using “minpack.lm” (Elzhov et al., 2022) and “aomisc” (Onofri, 2020) packages. All data analysis were performed at significance level p = 0.05.
3. Results
3.1. Coppice stand structures and spatial sprout patterns
In all five plots, Castanea and Robinia stems grew in spatially restricted aggregations (Figures 2A–E). Castanea was the dominant tree species in the stands, with the basal area of these living trees accounting for 78–94% of the overstory layer (Figures 2F–I). In contrast, Robinia contributed much less to the overstory layer, with a basal area ranging from 6 to 22% (Figures 2F–I). In the coppice stand, where Robinia was the dominant tree species accounting for 87% of the basal area, Castanea contributed just 13% of the basal area (Figure 2J).
Where Castanea dominates the overstory layer, its stool density ranges from 450 to 767 stools ha–1 (Table 1). The average number of live basal sprouts ranged from 4 (±3) to 8 (±2) per stool sample. However, there was a minimum value of 33 stools ha–1 for Castanea, containing only two living basal sprouts, which were observed in the coppice stand dominated by Robinia. Stool density and the number of living basal sprouts per stool with the Robinia was lower when compared with the Castanea, ranging from 67 to 340 stools ha–1 and from 2 (±1) to 3 (±2) sprouts per stool, respectively. A comparable value of 600 stools per hectare was observed in a coppice stand dominated by Robinia.
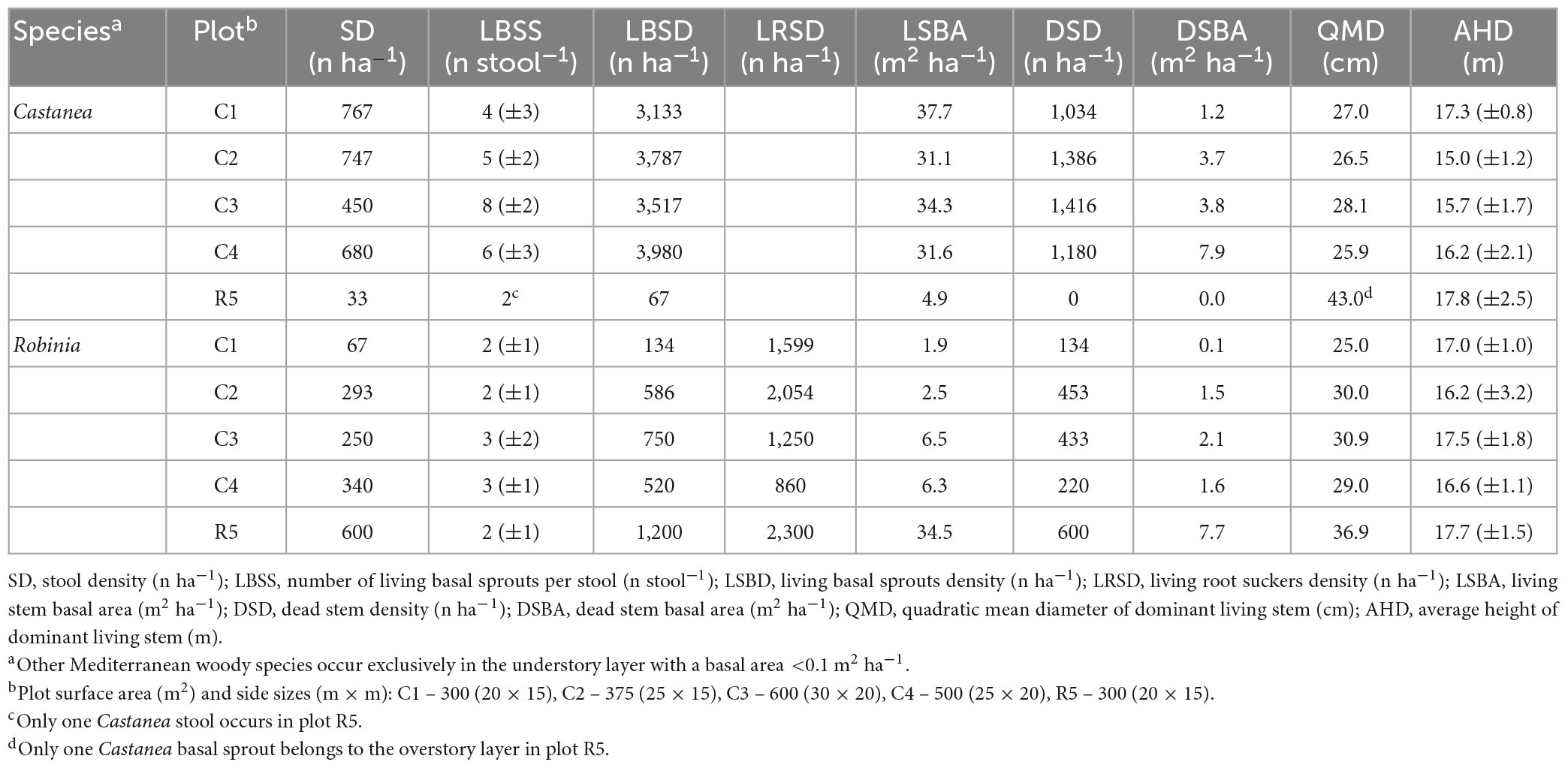
Table 1. Post-fire stand structure attributes split by tree species (Castanea and Robinia) and plots.
After the 2017 wildfire, in stands where Castanea was dominant in the overstory layer, living basal sprouts accounted for 74% (±2.6), ranging from 3,133 to 3,980 n ha–1(Table 1). Among them, the average basal sprouts with no fire scars accounted for 12% (±4.3) of the total sprouts for the species. Such basal sprouts are small, exhibiting an average stem diameter (DbH) of 3.9 (±1.2) cm. The basal area ranges from 31.1 to 37.7 m2 ha–1, accounting for an average of 89% (±7%) of the total Castanea basal area. The dead stem density varied from 1,034 to 1,416 n ha–1, accounting for 11% (±7%) of the total Castanea stand basal area, ranging from 1.2 to 7.9 m2 ha–1. These dead stems exhibited an average DbH of 8.9 (±6.3) cm. The average number of dead sprouts with no basal fire scars accounted for only 4% (±1%) of the total dead basal sprouts.
Living root suckers of Robinia accounted for 72% (±12%), compared to 28% (±11%) of the basal sprouts in the total living vegetative sprouts (Table 1). Among them, the basal fire-scorched living root suckers accounted for 10% (±3%), exhibiting a DbH of 6.2 (±1.2) cm. In contrast, living root suckers with no fire scars accounted for 90% (±3%). Such root suckers had a small diameter at breast height (DbH < 4 cm) up to a distance of 10 m from the Robina adult parent ramets (Figure 3 in the Supplementary Material 3). The basal area of the living vegetative sprouts ranged from 1.9 to 6.3 m2 ha–1 in Castanea dominated coppice stands, while in the Robina-dominated coppice stands they accounted for 34.5 m2 ha–1. The remaining vegetative sprouts of the Robinia regenerated from the basal stems, and accounted for an average of 28% (±12%) of the total living vegetative sprouts. In Robinia, fire-scorched stems accounted for 100% of the dead vegetative sprouts, showing a basal area ranging from 0.1 to 7.7 m2 ha–1 (Table 1).
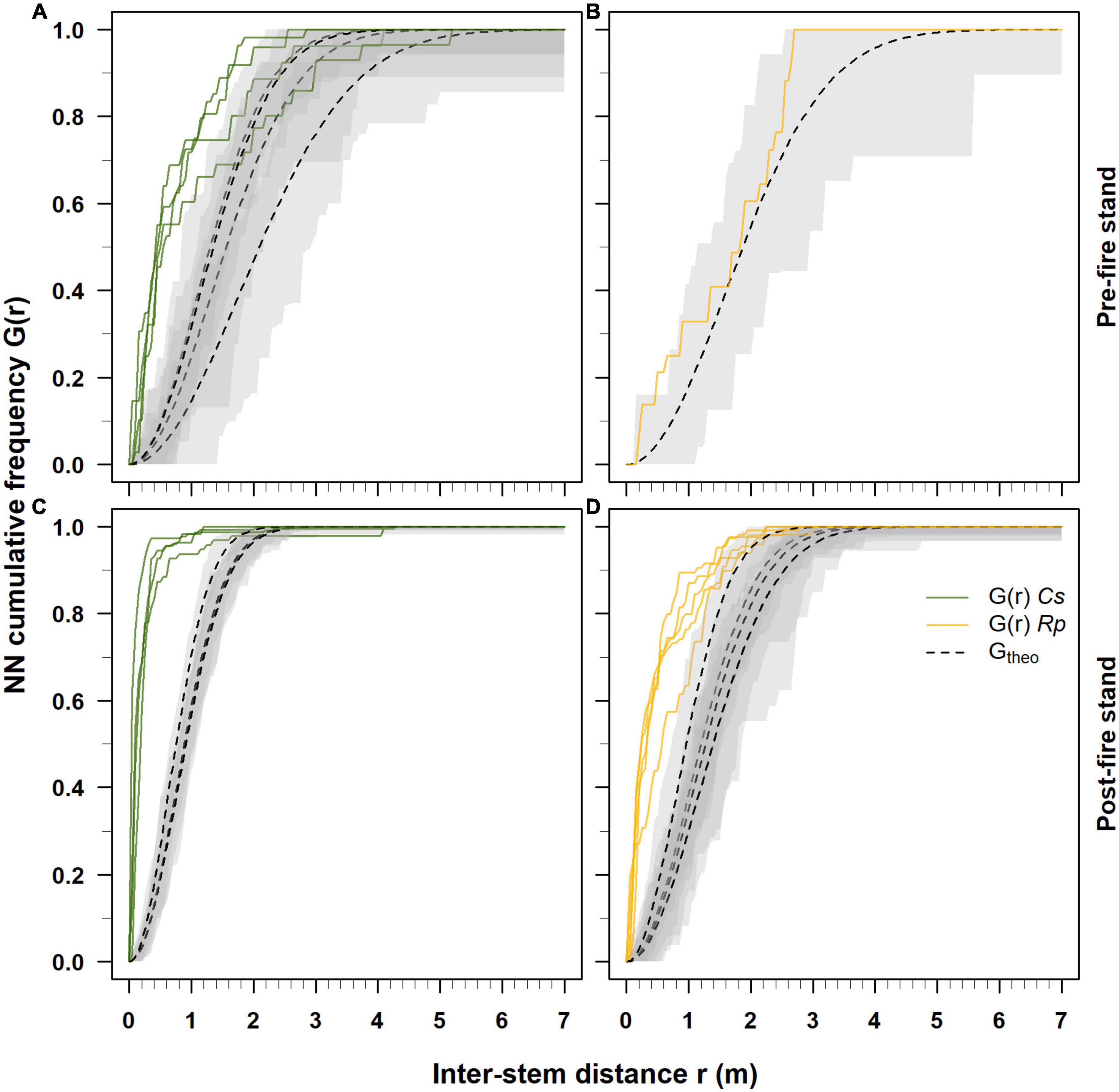
Figure 3. Cumulative frequency distribution of the nearest-neighbor (NN) distances estimated respectively for Castanea (Cs) and Robinia (Rp) in pre-2017 (A,B) and post-2017 fire event (C,D) coppice stands. Solid green and yellow lines indicate the estimated NN cumulative distribution functions G(r) for Castanea and Robinia, respectively. Dotted black lines represent the cumulative NN distribution function Gtheo with the CSR hypothesis, and gray bands are 95% envelope bands.
The average total height of the dominant Castanea sprouts range from 15.0 (±1.2) to 17.8 (±2.5) m, while in Robinia they ranged from 16.2 (±3.2) to 17.7 m (±1.5) (Table 1). In both tree species, the crown base of the overstory layer was 10.5 (±1.7) m above the ground.
In each plot where Castanea was dominant, the pre- and post-fire 2017 event nearest-neighbor NN cumulative distribution functions G(r) indicated a clustered spatial pattern of the coppice stands (Figures 3A, C). However, the estimation of the NN cumulative distribution functions G(r) was hampered in the plots where the occurrence of Castanea (plot R5) and Robinia (C1-C4) stems was low (Table 1). In the 2017 pre-fire stands, 70–90% of the Castanea stems exhibited a spatial aggregation pattern with inter-stem distance close to 2 m (Figure 3A). Five years after the wildfire event, 95% of the Castanea neighboring stems exhibited a clustered spatial assemblage with an inter-stem distance of up to 0.5 m (Figure 3C).
In contrast, in the 2017 pre-fire stand where Robinia was dominant, its stems showed random spatial patterns with inter-distances not different from those expected when using the hypothesis of complete spatial randomness (Figure 3B). Five years after the wildfire event, Robinia largely exhibited a clustered spatial pattern, with 65% of the stems having an inter-distance of <0.5 m and the remaining 35% with an inter-distance >0.5 m (Figure 3D). Above an inter-distance of 1 m, the stems showed random patterns that were not different from those expected under the hypothesis of complete spatial randomness (Figure 3D).
In post-fire stands, the estimated spatial distribution of the normalized stem diameter differentiation functions τ(r) indicates that clusters of different and similar-sized Castanea and Robinia stems were spatially proximal and alternate in the coppice stands (Figures 4A–E).
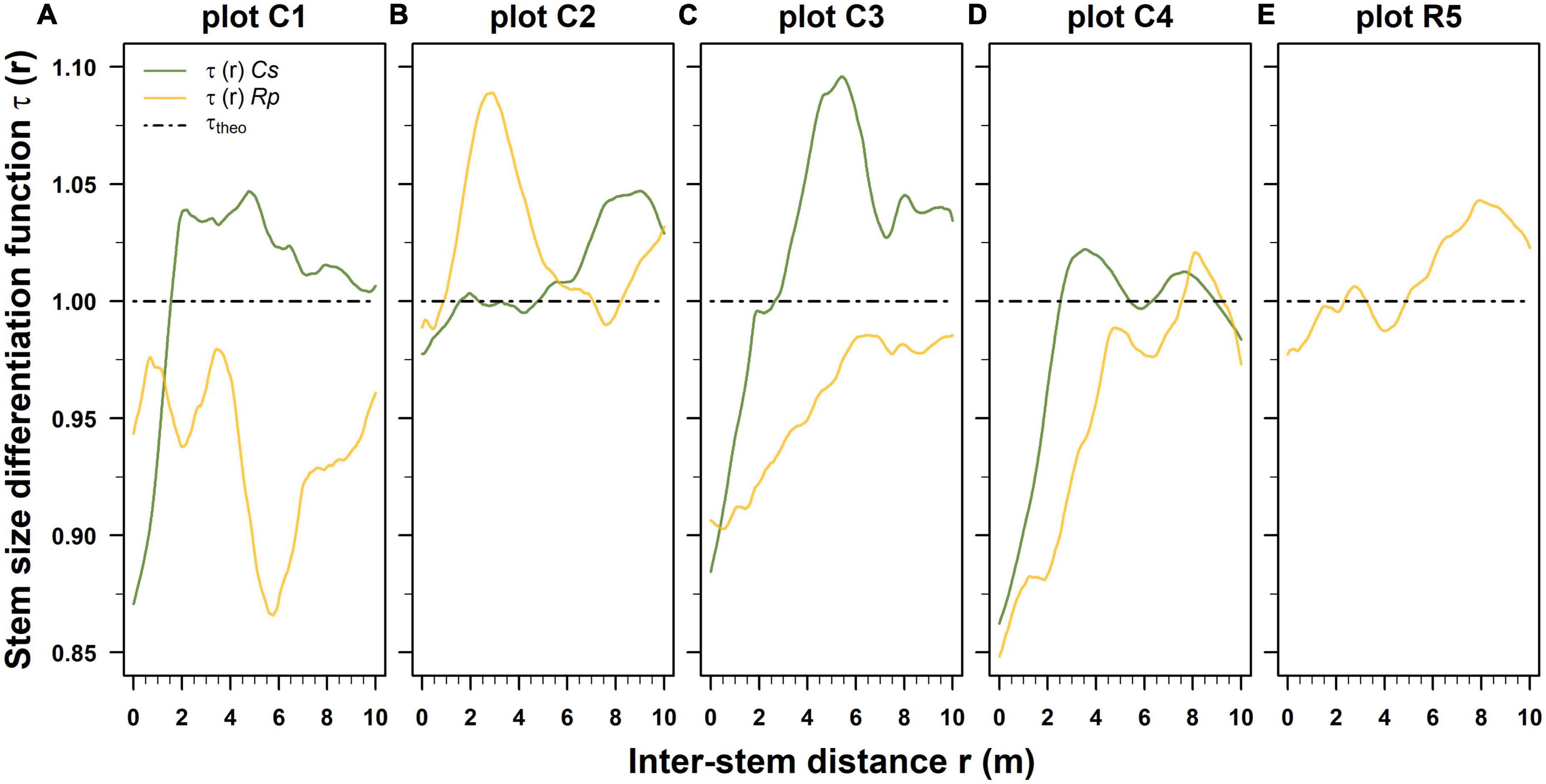
Figure 4. Post-fire normalized stem diameter differentiation function τ(r) estimated separately for Castanea (Cs) and Robinia (Rp) for each permanent plot, using the Epanechnikov kernel method and a bandwidth of 0.8 m. The dash-dotted black horizontal lines denote the condition of spatial uncorrelation among the stem diameters (τtheo). Panels (A–E) were ordered by decreasing basal area values of standing Castanea tree sprouts belonging to the overstory layer. (A–E) Correspond to the plots C1–R5 of Table 1.
The correlation range extended beyond 10 m for both the Castanea and Robinia stems. Up to an inter-distance of 2.1 m, and the stem size differentiation in Castanea was lower than expected for the uncorrelated stem diameters (Figures 4A–E). However, with >2 m, the inter-distance differentiation was substantially higher, with an irregular sequence of local minimum and maximum stem diameter differentiations among the neighboring stems (Figures 4A–E). In contrast, Robinia exhibited a more variable and complex spatial stem size differentiation than Castanea (Figures 4A–E). Robinia predominantly exhibited lower size differentiation (Figures 4A, C, D), although in a few cases it exhibited higher size differentiation τ(r) at variable inter-stem distance intervals (1.8 m < r < 6.0 m; 8.0 m < r < 10.0 m) (Figures 4B, D) than expected under the hypothesis of uncorrelated stem diameters. In the stand where Robinia was dominant, up to an inter-distance of 3.0 m, stem size differentiation among sprouts was lower than expected, while it was higher with an inter-distance >3.0 m (Figure 4E).
3.2. Curve-fitting and NDVI-based phenological parameters
The Beck double logistic function accurately modeled Landsat NDVI trajectories showing high pseudo R2 values ranging from 0.88 to 0.91 (Figures 5A–E). All estimated NDVI-based phenological parameters were statistically significant at p < 0.001 (Supplementary Table 1 in Supplementary Material 3). Where Castanea was the dominant tree species in the overstory layer, the estimated spring phenological parameters (sos and rsp) differed significantly (p < 0.05) from the parameters estimated for stands where Robinia dominated the overstory layer (Figures 6A, B). In the Castanea stand, the NDVI-based start of the growing season (sos) occurred in a time window of 7 days, from a minimum DOY of 118 (±1) (28 April) to a maximum of 124 (±1) (4 May) (Figure 6A). In contrast, in the stand where Robinia was dominant and the degree of cover by the understory species was approximately 90%, the estimated NDVI-based sos occurred on DOY 109 (±3), 9–15 days early than the Castanea stand (Figures 5J, 6A). Additionally, significant differences were observed for the sos estimates of the Castanea in stands where the understory shrub species cover was higher than 20% (Figures 5F–I, 6A). The NDVI-based spring increasing rate parameter (rsp) estimated for Castanea was significantly lower than that estimated for Robinia (Figure 6B). A lower value of rsp account for 0.04 (±0.001) in the Robinia coppice stand, in which the cover of the understory species was approximately 90% (Figures 5J, 6B). No significant differences were observed in the NDVI-based estimates for the rsp in stands dominated by the Castanea.
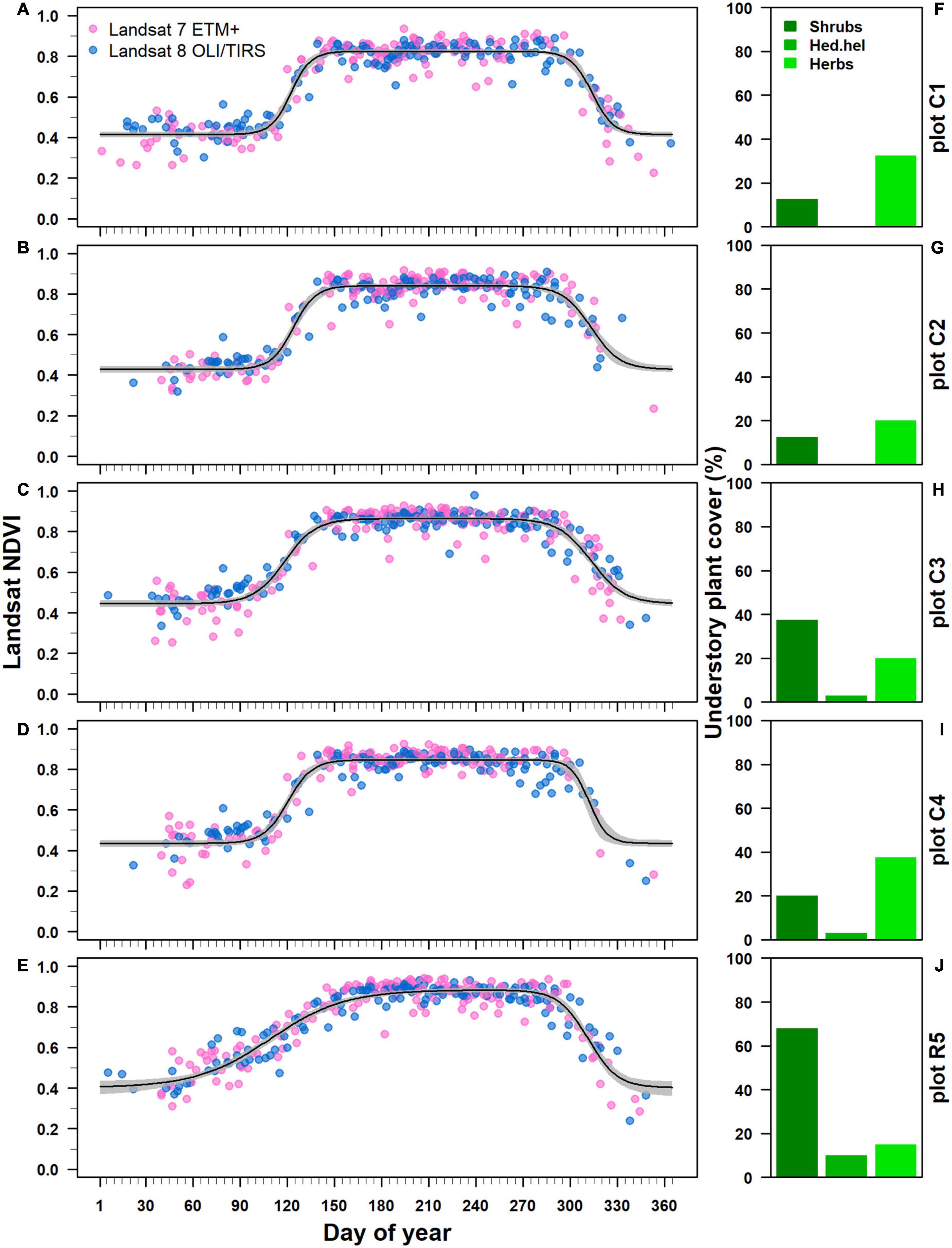
Figure 5. Pixel-based Landsat NDVI trajectory for Castanea and Robinia coppice forest stands. (A–E) The NDVI trajectories were ordered by decreasing basal area values for the standing Castanea tree sprouts belonging to the overstory layer. Each black line represents the Back double logistic function fitted to NDVI values derived from both Landsat 7 ETM+ (blue points) and Landsat 8 OLI/TIRS (pink points) satellites. Gray bands represents 95% confidence intervals. (F–J) Show the percentage cover of understory species: shrub species (shrubs), Hedera helix subsp. helix (Hed.hel) and herbaceous species (Herbs).
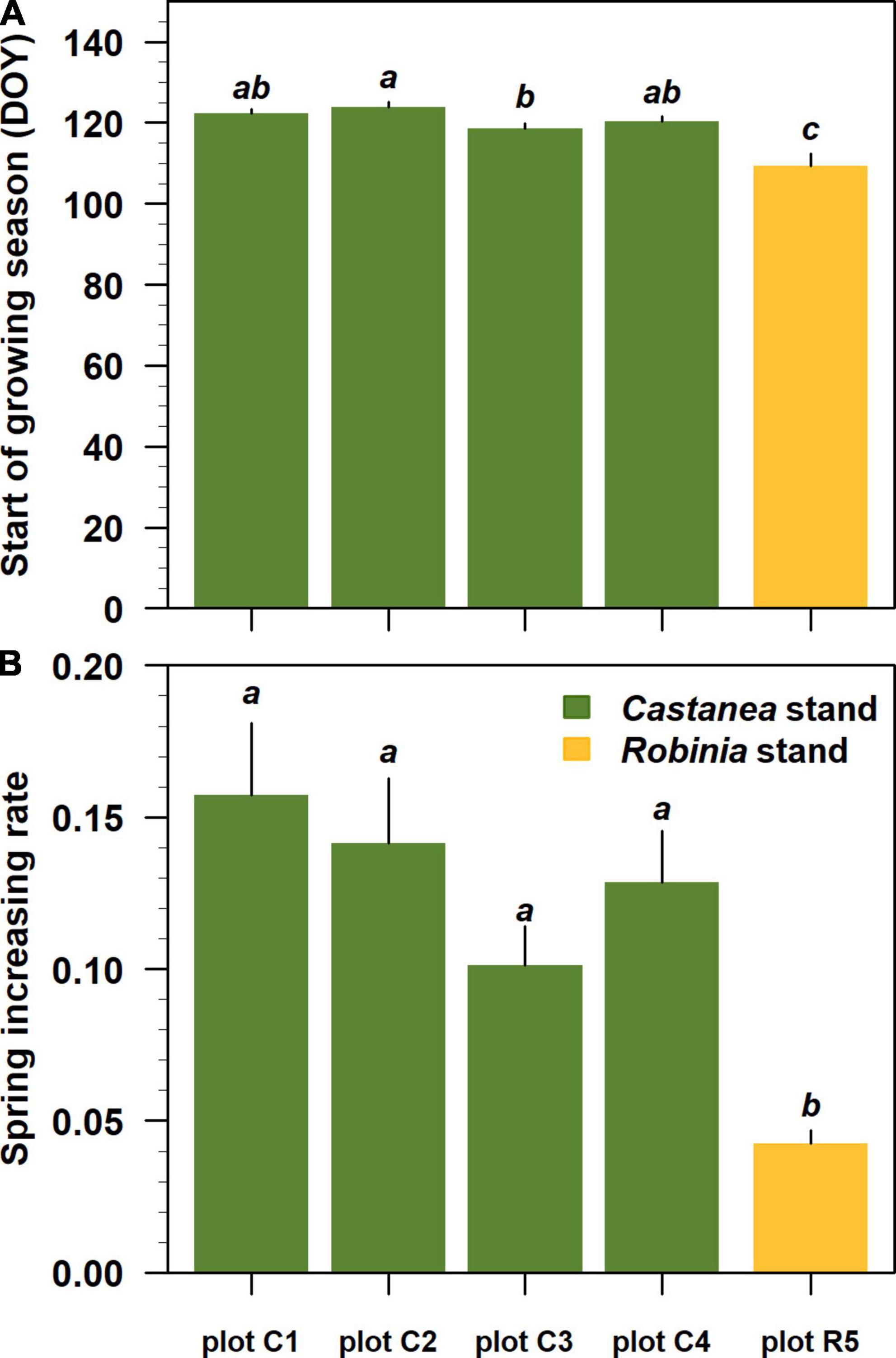
Figure 6. Spring phenological parameters estimated for each stand dominated by Castanea and Robinia trees sprouts. From plot C1 to plot R5, phenological parameters were ordered by decreasing basal area values for the standing Castanea tree sprouts belonging to the overstory layer. In each panels (A,B), lower case letters indicate significant differences at p-level < 0.05 following Tukey’s multiple comparison test. Black vertical bars represent the standard error of the estimates.
Field-based observations of the start of the growing season (sos) differed from the NDVI-based estimates in Robinia, while the results were accurate for the Castanea stands. On DOY 133 (May 13, 2022), on the northern slope of Mt. Somma, Robinia vegetative sprouts belonging to both the understory and overstory layers, exhibited a delay in leaf phenology when compared to Castanea (Supplementary Figure 4 in the Supplementary Material 3). Here, Robinia was mostly in the early stages of bud burst with buds slightly swollen and brown colored, while Castanea exhibited green leaves on >50% of their maximum laminar expansions (Supplementary Figure 4 in Supplementary Material 3).
4. Discussion
4.1. Stand spatial structures and vegetative regeneration strategies
Vegetative regeneration, rather than earlier spring phenology, confers a competitive advantage to Robinia over Castanea basal sprouts after a fire disturbance. The production of basal sprouts in both Castanea and Robinia, as well as root-suckers in Robinia, was found to be stimulated by fire disturbance, due to the presence of scorched stems that were <2 m in the study area and the documented occurrence of low-severity wildfires at the same site by Saulino et al. (2020). Hence, a double vegetative regeneration strategy enables Robinia to take advantage of wildfire disturbances by exploiting empty niches and thus persisting in Castanea coppice stands. Vegetative regeneration enables most tree species to cope with disturbances, such as wildfires or coppicing (Del Tredici, 2001). Furthermore, many root-suckering species have shallow horizontal roots that produce extensive clonal growth from endogenous uninjured buds and exogenous reparative buds after injury or senescence of the roots (Del Tredici, 2001). In this investigation the allocation of resources to the shallow horizontal roots allowed Robinia to spatially advance in the soil up to 10 m away from the parent plant in adjacent coppice stands, appearing as single root-suckers in the understory layer of the Castanea stands 5 years after the fire.
Under suppressed conditions, the aboveground root sucker portions of the plant exhibited a low stem size with only a few thin branches (personal observation). This latent stage is probably metabolically dependent on the adult parent trees, allowing Robinia to persist until the next disturbance in the overstory, which modifies the near-ground light regime. Indeed, Robinia occurred as a cluster of stems spatially alternating with clusters of Castanea stems or as belts at the margins of the Castanea coppice stand, in canopy gaps generated by single trees dying, wildfires, wind throw, or uprooting, which consequently reduced the competition for light resources (Cierjacks et al., 2013). However, the spatial patterns of the young Robina suckers that sprout from sub-superficial roots could be related to physiological integration processes (Zhang et al., 2006). Jung et al. (2009) found that Robinia suckers are interconnected through shallow horizontal roots to parent trees, highlighting their role in the allocation of photosynthates to the horizontal roots until they are capable of sprouting new suckers. The Robinia root suckers take advantage of the shared nutritive resources among the connected adult parent ramets, and this influences their survival and ecophysiological responses to changing environmental growth conditions (Zhang et al., 2006). Repeated human disturbances (e.g., wildfires and clear-cutting) favor spatially fixed basal sprouts and the ubiquitous and far-reaching root suckers of Robinia, amplify their invasiveness over Castanea basal sprouts, as was recently observed in similar Castanea coppice forests in northeastern Italy (Radtke et al., 2013; Marcolin et al., 2020).
Robinia seedlings were not observed under the fire-disturbed Castanea canopy cover. It is well known that light-demanding Robinia does not occur as seedling (Vítková et al., 2017), because its ripe seeds exhibit physical dormancy (Baskin and Baskin, 2014) which varies at the individual tree level and with the persistence of seedpods in the crown during the winter (Masaka and Yamada, 2009). This seed trait favors a persistent soil seed bank that lowers the germination rate in the absence of thermal or mechanical scarification (Giuliani et al., 2015). However, the lack of Robinia seedlings suggests that the heat flux in the soil during the low-intensity wildfire, as documented by the height of the scorched basal stems (<2 m), stimulated the basal stem and root bud banks, rather than the soil seed bank.
Additionally, Robinia takes advantage from a lower susceptibility to diseases and pests (Cierjacks et al., 2013) than the Castanea trees. In the unmanaged coppice forest, we observed mortality of Castanea stem basal sprouts related to Cryphonectria parasitica and exacerbated by leaf area reduction caused by Dryocosmus kuriphilus attacks in the last decades (Gehring et al., 2018). Therefore, the lower susceptibility to diseases and pests exhibited by Robinia could represent an advantageous trait, allowing it to invade and persist in Castanea coppice stands.
Disturbances such as fire and coppicing promote fluctuating and spatial heterogeneous light environmental conditions which stimulate both basal and root resprout of the light-demanding Robinia. In our protected context, like in other Mediterranean areas which shared similar disturbances, coppicing has been discontinuous for decades and has favored senescence of Robinia (Motta et al., 2009), while mixed-severity fires (Saulino et al., 2020) represent the main physical stimulus for the spatial advancement of Robinia by root-suckering. Consequently, all measures of fire suppression in chestnut stands with and without Robinia in the canopy layer or colonized only by its root-suckering in the suppressed layer, are effective against the invasion of Robinia in canopy gaps after the dominant trees of both species are killed by fire. Nature-based solutions (Gann et al., 2019) such as salvage logging restricted to areas with charred Robinia trees, burned wood chips and mulching in the influence area of roots could reduce light availability for root-suckering and may aid the recovery of Castanea resprouts. In undisturbed dense and close stands, incomplete girdling (see Nicolescu et al., 2020) and pollarding of dominant trees in the dormant season could be mechanical control techniques to be verified for their effectiveness. In our study site, stem and crown of dominant Robinia trees growing on the slope, carried the root-climbing liana Hedera helix more frequently compared to Castanea. The overload induces a reduction of leaf surface in the crown and mechanical instability with uprooting, suggesting a biological control technique which could favor colonization by bird disperser (mainly Columba palumbus, Snow and Snow, 1988), rather than eliminating this liana species. Finally, the evergreen native Rubus spp. shrub cover prevents the formation of root-suckering by reducing light on the ground (Warne, 2016) which, in the long run can be obtained also by planting under the canopy native already present shade-tolerant evergreen broadleaved tree and shrub species.
Although we investigated spring phenology and regeneration strategies of both Robinia and Castanea in small plots in a small Mediterranean chestnut forest, our results well clarify their different role played in spatial niche exploitation and in competitive interaction between non-native and native tree species, where vegetative regeneration from the roots rather than advanced spring phenology allows Robinia to take advantage of recurrent wildfire disturbances and spreads in to Castanea coppice forest.
4.2. Divergence in the spring leaf phenology
Relevant phenological attributes related to leaf phenology have been observed in invasive plants (Fridley, 2012). However, the advanced spring phenology is recognized as a trait that confers a competitive advantage during the colonization of exotic species (Polgar et al., 2014). In our study, the start of the growing season for the exotic Robinia was found to differ from that of Castanea in both young and adult sprouts. In the field, we observed that the growing season occurred later in Robinia than in Castanea, suggesting that success in the colonization of Castanea stands by Robinia is unrelated to the competitive advantage of an advanced spring phenology. Spring phenology and its close interaction with climate and genotype are well-recognized in the literature (Murray et al., 1989; Silvestro et al., 2019). However, the combination of a winter chilling, photoperiod, and spring warming (forcing) is strongly involved in the triggering of bud-break and leaf-out in tree species (Polgar and Primack, 2011; Rossi and Isabel, 2017). Nevertheless, winter chilling has been identified as a necessary requirement for leaf spring phenology in most invasive species (Laube et al., 2014; Polgar et al., 2014). The late spring phenology observed in Robinia suggests that it probably requires a higher level of winter chilling to achieve leaf out in the spring season (Laube et al., 2014; Fu et al., 2015) when compared with Castanea. However, a high chilling requirement in winter can signi?cantly reduce the probability that invasive species such as Robinia will gain an additional competitive advantage under ongoing climate warming. Nevertheless, in Robinia, later leaf-out could be interpreted as an adaptive trait useful for maximizing the length of the growing season and avoiding the occurrence of leaf damage due to late spring frost events (Allevato et al., 2019).
This species-specific phenological pattern provides interesting insights into the interpretation of satellite-derived spring phenological parameters. Indeed, the observed divergences between field and remote sensing assessments of the Robinia spring phenology were related to the degree of understory cover from the shrub [mainly Rubus spp. and Cytisus scoparius (L.) Link subsp. scoparius] and herbaceous [Sesleria autumnalis (Scop.) F.W.Schultz, Luzula sylvatica (Huds.) Gaudin s.l., Drymochloa drymeja (Mert. & W.D.J.Koch) Holub subsp. exaltata (C.Presl) Foggi & Signorini)] species. In the low-elevation mountains in the southern Mediterranean, such understory species are characterized by an early and progressive bud break that starts approximately in the middle of February. Moreover, the winter green habitus of herbaceous understory species and of root-climbing liana (Hedera helix subsp. helix) contributed to maintaining the high winter NDVI (0.42 approximately), as was recently observed in other stratified Mediterranean forests (Helman et al., 2015; Helman, 2018). Therefore, in the spring, from the beginning of March to the middle of April, when Robinia exhibits dormant buds with no signs of vegetative activity, NDVI-based satellite assessment of its phenology is influenced by the cover and phenology of common understory vegetation assemblages under a continuous canopy dominated by Robinia. Once established, Robinia trees increase the soil nitrogen stock (Boring and Swank, 1984; De Marco et al., 2023), enhancing the eutrophication of understory community species by establishing nitrophytic, ruderal, and non-native plant species (Von Holle et al., 2013). Nevertheless, Castanea spring phenology was appropriately assessed from the NDVI-based satellite, without divergence between the field and remote assessments at the start of the growing season and the increasing rate in the spring. Indeed, in the coppice stand where Castanea is dominant in the overstory layer, understory vegetation cover consistently differs from that in the coppice stand where Robinia dominates in the overstory layer. Differences in species composition suggest that ground recognition is an essential requirement in the validation of satellite-retrieved spring phenology, especially in forest stands where shrubs and herbaceous cover are both abundant. Indeed, in both evergreen and deciduous stratified forests, changes in understory species cover and composition within a pixel results in changes to the time series for the remote-sensed spectral signals because of the distinct phenology of the plant species involved (Ryu et al., 2014; Helman et al., 2015). These represent cases in which the changes in the NDVI signal could be misinterpreted as a spring phenological change, while it is actually related to changes in species composition and phenology within the pixel (Helman, 2018).
5. Conclusion
Robinia is progressively colonizing the ancient Castanea coppice forest in Vesuvius National Park using two vegetative regeneration strategies: basal stem sprouting and root suckering from endogenous uninjured buds and exogenous reparative buds after injury or senescence of the shallow horizontal roots. We found that vegetative regeneration rather than early spring phenology, allows Robinia to spatially advance, colonize, and persist in patches of coppice stands that have been disturbed by fire. The results of this study suggest that even with low severity wildfires, the invasiveness of Robinia will increase, as the fires will still stimulate the resprouting of suckers from roots. Although our study falls in a small area within the distribution of Mediterranean Castanea coppices, our results agree with the observed vegetative regeneration strategies adopted by Robinia in the invasion of chestnut forests. Furthermore, advanced spring phenology of exotic species conferring them a competitive advantage over native species, in Robinia plays, instead, a marginal role, suggesting that vegetative regeneration is more effective for the invasion of the Mediterranean Castanea forests. More broadly, although the strategies and patterns of spatial invasion of the alien species Robinia are site-specific, the methodology approach proposed in the present study is replicable in other sites where the disturbances (mainly fire and coppicing) threaten the existence of a culturally valuable Mediterranean landscape such as the Castanea coppice. From a management perspective, additional studies on root-sucker clonality in Robinia could provide further ecological and evolutionary insights into the ecophysiological relationship between parent trees and root suckers. An improved understanding of the functional traits that allow exotic trees to be invasive would help to provide reliable guidelines for the management of Castanea coppice stands under changing climatic and land use conditions. Our findings highlight that appropriate control methods are required for invasive Robinia tree sprouts in order to conserve the continuous canopy cover in Castanea stands. However, increasing insights into vegetative regeneration strategies based on species-specific phenological traits could support forest managers in developing appropriate nature-based solutions to control the spread of non-native tree species, especially in protected areas where chemical control methods are forbidden.
Data availability statement
The original contributions presented in this study are included in the article/Supplementary material, further inquiries can be directed to the corresponding author.
Author contributions
LS and AnS: conceptualization, methodology, and writing—original draft. LS, AdS, and GL: data curation. LS: formal analysis, software, and visualization. LS, AnS, GL, AdS, and AR: investigation. All authors contributed to writing—review and editing and approved the submitted version.
Funding
This work was supported by the Technical-Scientific Agreement between the National Park Cilento Vallo di Diano e Alburni and the Department of Agricultural Sciences of the University of Naples Federico II (CUP: E77G22000440005), granted to AnS.
Acknowledgments
We are grateful to E. S. Ziccardi and S. Somma for their valuable support in the field sampling. We thank the staff at Raggruppamento Carabinieri Biodiversità di Caserta for providing authorization to access the Riserva Forestale di Protezione Tirone Alto Vesuvio. This study was carried out within the Agritech National Research Center and received funding from the European Union Next-Generation EU (Piano Nazionale di Ripresa e Resilienza (PNRR) – MISSIONE 4 COMPONENTE 2, INVESTIMENTO 1.4 – D.D. 1032 17/06/2022, CN00000022).
Conflict of interest
The authors declare that the research was conducted in the absence of any commercial or financial relationships that could be construed as a potential conflict of interest.
Publisher’s note
All claims expressed in this article are solely those of the authors and do not necessarily represent those of their affiliated organizations, or those of the publisher, the editors and the reviewers. Any product that may be evaluated in this article, or claim that may be made by its manufacturer, is not guaranteed or endorsed by the publisher.
Author disclaimer
This manuscript reflects only the authors’ views and opinions, neither the European Union nor the European Commission can be considered responsible for them.
Supplementary material
The Supplementary Material for this article can be found online at: https://www.frontiersin.org/articles/10.3389/ffgc.2023.1177551/full#supplementary-material
Footnotes
- ^ https://www.usgs.gov/landsat-missions/landsat-collection-2-level-2-science-products
- ^ https://www.usgs.gov/faqs/how-do-i-use-scale-factor-landsat-level-2-science-products
- ^ https://code.earthengine.google.com/67d924911e9e2526e56d981ee064dadf
References
Allevato, E., Buonincontri, M., Vairo, M., Pecci, A., Cau, M. A., Yoneda, M., et al. (2012). Persistence of the cultural landscape in Campania (Southern Italy) before the AD 472 Vesuvius eruption: Archaeoenvironmental data. J. Archaeol. Sci. 39, 399–406. doi: 10.1016/j.jas.2011.09.026
Allevato, E., Saulino, L., Cesarano, G., Chirico, G. B., D’Urso, G., Falanga Bolognesi, S., et al. (2019). Canopy damage by spring frost in European beech along the Apennines: effect of latitude, altitude and aspect. Remote Sens. Environ. 225, 431–440. doi: 10.1016/j.rse.2019.03.023
Baddeley, A., and Gill, R. D. (1997). Kaplan-Meier estimators of interpoint distance distributions for spatial point processes. Ann. Stat. 25, 263–292.
Baddeley, A., Rubak, E., and Turner, R. (2015). Spatial Point Patterns: Methodology and Applications with R. London: Chapman and Hall, doi: 10.1201/b19708
Barbati, A., Marchetti, M., Chirici, G., and Corona, P. (2014). European Forest Types and Forest Europe SFM indicators: Tools for monitoring progress on forest biodiversity conservation. For. Ecol. Manage. 321, 145–157. doi: 10.1016/j.foreco.2013.07.004
Bartolucci, F., Peruzzi, L., Galasso, G., Albano, A., Alessandrini, A., Ardenghi, N. M. G., et al. (2018). An updated checklist of the vascular flora native to Italy. Plant Biosyst. 152, 179–303. doi: 10.1080/11263504.2017.1419996
Baskin, C. C., and Baskin, J. M. (2014). Seeds: Ecology, biogeography, and, evolution of dormancy and germination, 2nd Edn. Cambridge, MA: Academic Press, doi: 10.1016/C2013-0-00597-X
Bates, D. M., and Watts, D. G. (1988). Nonlinear Regression Analysis and Its Applications. Hoboken, NJ: John Wiley & Sons, Inc, doi: 10.1002/9780470316757
Beck, P. S. A., Atzberger, C., Høgda, K. A., Johansen, B., and Skidmore, A. K. (2006). Improved monitoring of vegetation dynamics at very high latitudes: A new method using MODIS NDVI. Remote Sens. Environ. 100, 321–334. doi: 10.1016/j.rse.2005.10.021
Benesperi, R., Giuliani, C., Zanetti, S., Gennai, M., Mariotti Lippi, M., Guidi, T., et al. (2012). Forest plant diversity is threatened by Robinia pseudoacacia (black-locust) invasion. Biodivers. Conserv. 21, 3555–3568. doi: 10.1007/s10531-012-0380-5
Boring, L. R., and Swank, W. T. (1984). The role of black locust (Robinia pseudoacacia) in forest succession. J. Ecol. 72, 749. doi: 10.2307/2259529
Bretz, F., Hothorn, T., and Westfall, P. (2011). Multiple comparisons using R. Boca Raton, FL: CRC Press Taylor & Francis Group, doi: 10.1201/9781420010909
Castro-Díez, P., Vaz, A. S., Silva, J. S., van Loo, M., Alonso, Á, Aponte, C., et al. (2019). Global effects of non-native tree species on multiple ecosystem services. Biol. Rev. 94, 1477–1501. doi: 10.1111/brv.12511
Cervelli, E., Pindozzi, S., Allevato, E., Saulino, L., Silvestro, R., and Scotto, et al. (2022). Landscape Planning Integrated Approaches to Support Post-Wildfire Restoration in Natural Protected Areas: The Vesuvius National Park Case Study. Land 11:1024. doi: 10.3390/land11071024
Chuine, I., and Beaubien, E. G. (2001). Phenology is a major determinant of tree species range. Ecol. Lett. 4, 500–510. doi: 10.1046/j.1461-0248.2001.00261.x
Cierjacks, A., Kowarik, I., Joshi, J., Hempel, S., Ristow, M., von der Lippe, M., et al. (2013). Biological flora of the british isles: Robinia pseudoacacia. J. Ecol. 101, 1623–1640. doi: 10.1111/1365-2745.12162
Cressie, N. A. C. (2015). Statistics for spatial data revised edition. Hoboken, NJ: Wiley, doi: 10.1002/9781119115151
De Marco, A., Napoletano, P., Panico, S. C., Memoli, V., Santorufo, L., Ruggiero, A. G., et al. (2023). Combined effect of black locust invasion and fire on soils of Mediterranean shrublands and pine forests. CATENA 220, 106656. doi: 10.1016/J.CATENA.2022.106656
De Vivo, B., Belkin, H. E., and Rolandi, G. (2019). “Introduction to Vesuvius, Campi Flegrei, and Campanian Volcanism,” in Vesuvius, Campi Flegrei, and Campanian Volcanism, eds B. De Vivo, H. E. Belkin, and G. Rolandi (Amsterdam: Elsevier), doi: 10.1016/B978-0-12-816454-9.00001-8
Del Tredici, P. (2001). Sprouting in Temperate Trees: A Morphological and Ecological Review. Bot. Rev. 67, 121–140.
Di Pasquale, G., Allevato, E., Ermolli, E. R., Coubray, S., Lubritto, C., Marzaioli, F., et al. (2010). Reworking the idea of chestnut (Castanea sativa Mill.) cultivation in Roman times: New data from ancient Campania. Plant Biosyst. 144, 865–873. doi: 10.1080/11263504.2010.491974
Elzhov, T. V., Mullen, K. M., Spiess, A.-N., and Bolker, B. (2022). minpack.lm: R Interface to the Levenberg-Marquardt Nonlinear Least-Squares Algorithm Found in MINPACK, Plus Support for Bounds. R Packag. version 1.2-2. Vienna: R Foundation for Statistical Computing.
Foga, S., Scaramuzza, P. L., Guo, S., Zhu, Z., Dilley, R. D., Beckmann, T., et al. (2017). Cloud detection algorithm comparison and validation for operational Landsat data products. Remote Sens. Environ. 194, 379–390. doi: 10.1016/j.rse.2017.03.026
Fois, M., Podda, L., Médail, F., and Bacchetta, G. (2020). Endemic and alien vascular plant diversity in the small Mediterranean islands of Sardinia: Drivers and implications for their conservation. Biol. Conserv. 244, 108519. doi: 10.1016/j.biocon.2020.108519
Fridley, J. D. (2012). Extended leaf phenology and the autumn niche in deciduous forest invasions. Nature 485, 359–362. doi: 10.1038/nature11056
Fu, Y. H., Piao, S., Vitasse, Y., Zhao, H., De Boeck, H. J., Liu, Q., et al. (2015). Increased heat requirement for leaf flushing in temperate woody species over 1980-2012: Effects of chilling, precipitation and insolation. Glob. Chang. Biol. 21, 2687–2697. doi: 10.1111/gcb.12863
Funk, J. L., Standish, R. J., Stock, W. D., and Valladares, F. (2016). Plant functional traits of dominant native and invasive species in mediterranean-climate ecosystems. Ecology 97, 75–83. doi: 10.1890/15-0974.1
Galasso, G., Conti, F., Peruzzi, L., Ardenghi, N. M. G., Banfi, E., Celesti-Grapow, L., et al. (2018). An updated checklist of the vascular flora alien to Italy. Plant Biosyst. 152, 556–592. doi: 10.1080/11263504.2018.1441197
Gallagher, R. V., Randall, R. P., and Leishman, M. R. (2015). Trait differences between naturalized and invasive plant species independent of residence time and phylogeny. Conserv. Biol. 29, 360–369. doi: 10.1111/cobi.12399
Gallardo, B., Aldridge, D. C., González-Moreno, P., Pergl, J., Pizarro, M., Pyšek, P., et al. (2017). Protected areas offer refuge from invasive species spreading under climate change. Glob. Chang. Biol. 23, 5331–5343. doi: 10.1111/gcb.13798
Gann, G. D., McDonald, T., Walder, B., Aronson, J., Nelson, C. R., Jonson, J., et al. (2019). International principles and standards for the practice of ecological restoration Second edition. Restor. Ecol. 27, S1–S46. doi: 10.1111/rec.13035
Gehring, E., Bellosi, B., Quacchia, A., and Conedera, M. (2018). Assessing the impact of Dryocosmus kuriphilus on the chestnut tree: Branch architecture matters. J. Pest Sci. 91, 189–202. doi: 10.1007/s10340-017-0857-9
Giuliani, C., Lazzaro, L., Mariotti Lippi, M., Calamassi, R., and Foggi, B. (2015). Temperature-related effects on the germination capacity of black locust (Robinia pseudoacacia L. Fabaceae) seeds. Folia Geobot. 50, 275–282. doi: 10.1007/s12224-015-9224-x
Gorelick, N., Hancher, M., Dixon, M., Ilyushchenko, S., Thau, D., and Moore, R. (2017). Google Earth Engine: Planetary-scale geospatial analysis for everyone. Remote Sens. Environ. 202, 18–27. doi: 10.1016/j.rse.2017.06.031
Gutsell, S. L., and Johnson, E. A. (1996). How fire scars are formed: Coupling a disturbance process to its ecological effect. Can. J. For. Res. 26, 166–174. doi: 10.1139/x26-020
Harrington, R. A., Brown, B. J., and Reich, P. B. (1989). Ecophysiology of exotic and native shrubs in Southern Wisconsin - I. Relationship of leaf characteristics, resource availability, and phenology to seasonal patterns of carbon gain. Oecologia 80, 356–367. doi: 10.1007/BF00379037
Helman, D. (2018). Land surface phenology: What do we really ‘see’ from space? Sci. Total Environ. 618, 665–673. doi: 10.1016/J.SCITOTENV.2017.07.237
Helman, D., Lensky, I. M., Tessler, N., and Osem, Y. (2015). A phenology-based method for monitoring woody and herbaceous vegetation in mediterranean forests from NDVI time series. Remote Sens. 7, 12314–12335. doi: 10.3390/rs70912314
Hui, G., and Pommerening, A. (2014). Analysing tree species and size diversity patterns in multi-species uneven-aged forests of Northern China. For. Ecol. Manage. 316, 125–138. doi: 10.1016/j.foreco.2013.07.029
Illian, J., Penttinen, A., Stoyan, H., and Stoyan, D. (2008). Statistical Analysis and Modelling of Spatial Point Patterns. Hoboken, NJ: John Wiley & Sons, Ltd, doi: 10.1002/9780470725160
INFC (2005). “L’Inventario Nazionale delle Foreste e dei serbatoi forestali di Carbonio INFC 2005. Secondo inventario forestale nazionale italiano. Metodi e Risultati,” in Edagricole-Il Sole 24 ore, ed. T. G. Bologna (Augsburg: INFC).
IUSS Working Group WRB (2022). World Reference Base for Soil Resources. International soil classification system for naming soils and creating legends for soil maps, 4th Edn. Vienna: International Union of Soil Sciences.
Jung, S. C., Matsushita, N., Wu, B. Y., Kondo, N., Shiraishi, A., and Hogetsu, T. (2009). Reproduction of a Robinia pseudoacacia population in a coastal Pinus thunbergii windbreak along the Kujukurihama Coast, Japan. J. For. Res. 14, 101–110. doi: 10.1007/s10310-008-0109-1
Keeley, J. E. (2006). Fire management impacts on invasive plants in the western United States. Conserv. Biol. 20, 375–384. doi: 10.1111/j.1523-1739.2006.00339.x
Kleinbauer, I., Dullinger, S., Peterseil, J., and Essl, F. (2010). Climate change might drive the invasive tree Robinia pseudacacia into nature reserves and endangered habitats. Biol. Conserv. 143, 382–390. doi: 10.1016/j.biocon.2009.10.024
Kourantidou, M., Cuthbert, R. N., Haubrock, P. J., Novoa, A., Taylor, N. G., Leroy, B., et al. (2021). Economic costs of invasive alien species in the Mediterranean basin. NeoBiota 67, 427–458. doi: 10.3897/neobiota.67.58926
Laube, J., Sparks, T. H., Estrella, N., Höfler, J., Ankerst, D. P., and Menzel, A. (2014). Chilling outweighs photoperiod in preventing precocious spring development. Glob. Chang. Biol. 20, 170–182. doi: 10.1111/gcb.12360
Lazzaro, L., Bolpagni, R., Buffa, G., Gentili, R., Lonati, M., Stinca, A., et al. (2020). Impact of invasive alien plants on native plant communities and Natura 2000 habitats: State of the art, gap analysis and perspectives in Italy. J. Environ. Manage. 274, 111140. doi: 10.1016/j.jenvman.2020.111140
Lazzaro, L., Mazza, G., D’Errico, G., Fabiani, A., Giuliani, C., Inghilesi, A. F., et al. (2018). How ecosystems change following invasion by Robinia pseudoacacia: Insights from soil chemical properties and soil microbial, nematode, microarthropod and plant communities. Sci. Total Environ. 62, 1509–1518. doi: 10.1016/j.scitotenv.2017.10.017
Marcolin, E., Manetti, M. C., Pelleri, F., Conedera, M., Pezzatti, G. B., Lingua, E., et al. (2020). Seed regeneration of sweet chestnut (Castanea sativa Miller) under different coppicing approaches. For. Ecol. Manage. 472, 118273. doi: 10.1016/j.foreco.2020.118273
Masaka, K., and Yamada, K. (2009). Variation in germination character of Robinia pseudoacacia L. (Leguminosae) seeds at individual tree level. J. For. Res. 14, 167–177. doi: 10.1007/s10310-009-0117-9
Mathakutha, R., Steyn, C., le Roux, P. C., Blom, I. J., Chown, S. L., Daru, B. H., et al. (2019). Invasive species differ in key functional traits from native and non-invasive alien plant species. J. Veg. Sci. 30, 994–1006. doi: 10.1111/jvs.12772
Meier, U. (1997). Growth stages of mono- and dicotyledonous plants?: BBCH-monograph. Berlin: Blackwell, doi: 10.5073/20180906-074619
Motta, R., Nola, P., and Berretti, R. (2009). The rise and fall of the black locust (Robinia pseudoacacia L.) in the “Siro Negri” Forest Reserve (Lombardy, Italy): Lessons learned and future uncertainties. Ann. For. Sci. 66, 410–410. doi: 10.1051/forest/2009012
Murray, M. B., Cannell, M. G. R., and Smith, R. I. (1989). Date of budburst of fifteen tree species in Britain following climatic warming. J. Appl. Ecol. 26, 693. doi: 10.2307/2404093
Nadal-Sala, D., Hartig, F., Gracia, C. A., and Sabaté, S. (2019). Global warming likely to enhance black locust (Robinia pseudoacacia L.) growth in a Mediterranean riparian forest. For. Ecol. Manage. 449, 117448. doi: 10.1016/j.foreco.2019.117448
Nicolescu, V. N., Rédei, K., Mason, W. L., Vor, T., Pöetzelsberger, E., Bastien, J. C., et al. (2020). Ecology, growth and management of black locust (Robinia pseudoacacia L.), a non-native species integrated into European forests. J. For. Res. 31, 1081–1101. doi: 10.1007/s11676-020-01116-8
Nunez-Mir, G. C., Guo, Q., Rejmánek, M., Iannone, B. V., and Fei, S. (2019). Predicting invasiveness of exotic woody species using a traits-based framework. Ecology 100, e02797. doi: 10.1002/ecy.2797
Onofri, A. (2020). The broken bridge between biologists and statisticians: a blog and R package. Available online at: http://www.statforbiology.com
Pino, J., Font, X., de Cáceres, M., and Molowny-Horas, R. (2009). Floristic homogenization by native ruderal and alien plants in north-east Spain: the effect of environmental differences on a regional scale. Glob. Ecol. Biogeogr. 18, 563–574. doi: 10.1111/j.1466-8238.2009.00458.x
Polgar, C. A., and Primack, R. B. (2011). Leaf-out phenology of temperate woody plants: From trees to ecosystems. New Phytol. 191, 926–941. doi: 10.1111/j.1469-8137.2011.03803.x
Polgar, C., Gallinat, A., and Primack, R. B. (2014). Drivers of leaf-out phenology and their implications for species invasions: Insights from Thoreau’s Concord. New Phytol. 202, 106–115. doi: 10.1111/nph.12647
Pommerening, A., and Grabarnik, P. (2019). Individual-based methods in forest ecology and management, 1st Edn. Berlin: Springer International Publishing, doi: 10.1007/978-3-030-24528-3
Radtke, A., Ambraß, S., Zerbe, S., Tonon, G., Fontana, V., and Ammer, C. (2013). Traditional coppice forest management drives the invasion of Ailanthus altissima and Robinia pseudoacacia into deciduous forests. For. Ecol. Manage. 291, 308–317. doi: 10.1016/j.foreco.2012.11.022
Reichard, S. H., and Hamilton, C. W. (1997). Predicting invasions of woody plants introduced into North America. Conserv. Biol. 11, 193–203. doi: 10.1046/j.1523-1739.1997.95473.x
Richardson, D. M., and Pyšek, P. (2006). Plant invasions: Merging the concepts of species invasiveness and community invasibility. Prog. Phys. Geogr. 30, 409–431. doi: 10.1191/0309133306pp490pr
Rossi, S., and Isabel, N. (2017). Bud break responds more strongly to daytime than night-time temperature under asymmetric experimental warming. Glob. Chang. Biol. 23, 446–454. doi: 10.1111/gcb.13360
Ryu, Y., Lee, G., Jeon, S., Song, Y., and Kimm, H. (2014). Monitoring multi-layer canopy spring phenology of temperate deciduous and evergreen forests using low-cost spectral sensors. Remote Sens. Environ. 149, 227–238. doi: 10.1016/j.rse.2014.04.015
Sabo, A. E. (2000). Robinia pseudoacacia Invasions and Control in North America and Europe. Restor. Reclam. Rev. 6, 1–9.
San-Miguel-Ayanz, J., Durrant, T., Boca, R., Maianti, P., Libertá, G., Artés-Vivancos, T., et al. (2021). Forest fires in Europe, Middle East and North Africa 2020 EUR 30862 EN. Luxembourg: European Union.
Saulino, L., Rita, A., Migliozzi, A., Maffei, C., Allevato, E., Garonna, A., et al. (2020). Detecting Burn Severity across Mediterranean Forest Types by Coupling Medium-Spatial Resolution Satellite Imagery and Field Data. Remote Sens. 12, 741. doi: 10.3390/rs12040741
Seebens, H., Blackburn, T. M., Dyer, E. E., Genovesi, P., Hulme, P. E., Jeschke, J. M., et al. (2017). No saturation in the accumulation of alien species worldwide. Nat. Commun. 8:14435. doi: 10.1038/ncomms14435
Silvestro, R., Rossi, S., Zhang, S., Froment, I., Huang, J. G., and Saracino, A. (2019). From phenology to forest management: Ecotypes selection can avoid early or late frosts, but not both. For. Ecol. Manage. 436, 21–26. doi: 10.1016/j.foreco.2019.01.005
Silvestro, R., Saulino, L., Cavallo, C., Allevato, E., Pindozzi, S., Cervelli, E., et al. (2021). The footprint of wildfires on mediterranean forest ecosystem services in vesuvius national park. Fire 4:95. doi: 10.3390/fire4040095
Sitzia, T., Campagnaro, T., Dainese, M., and Cierjacks, A. (2012). Plant species diversity in alien black locust stands: A paired comparison with native stands across a north-Mediterranean range expansion. For. Ecol. Manage. 285, 85–91. doi: 10.1016/j.foreco.2012.08.016
Snow, B. K., and Snow, D. (1988). Birds and Berries: A study of an ecological interaction. London: Poyser, doi: 10.5040/9781472597076
Stinca, A., Chirico, G. B., Incerti, G., and Bonanomi, G. (2015). Regime shift by an exotic nitrogen-fixing shrub mediates plant facilitation in primary succession. PLoS One 10:e0123128. doi: 10.1371/journal.pone.0123128
Stoett, P., Roy, H. E., and Pauchard, A. (2019). Invasive alien species and planetary and global health policy. Lancet Planet. Heal. 3, e400–e401. doi: 10.1016/S2542-5196(19)30194-9
Stoyan, D., and Stoyan, H. (1994). Fractals, random shapes, and point fields?: methods of geometrical statistics. Hoboken, NJ: Wiley.
Theoharides, K. A., and Dukes, J. S. (2007). Plant invasion across space and time: Factors affecting nonindigenous species success during four stages of invasion. New Phytol. 176, 256–273. doi: 10.1111/j.1469-8137.2007.02207.x
Van der Maarel, E. (1979). Transformation of cover-abundance values in phytosociology and its effects on community similarity. Vegetation 39, 97–114. doi: 10.1007/BF00052021
Van Kleunen, M., Weber, E., and Fischer, M. (2010). A meta-analysis of trait differences between invasive and non-invasive plant species. Ecol. Lett. 13, 235–245. doi: 10.1111/j.1461-0248.2009.01418.x
Vítková, M., Müllerová, J., Sádlo, J., Pergl, J., and Pyšek, P. (2017). Black locust (Robinia pseudoacacia) beloved and despised: A story of an invasive tree in Central Europe. For. Ecol. Manage. 384, 287–302. doi: 10.1016/j.foreco.2016.10.057
Vítková, M., Sádlo, J., Roleček, J., Petřík, P., Sitzia, T., Müllerová, J., et al. (2020). Robinia pseudoacacia-dominated vegetation types of Southern Europe: Species composition, history, distribution and management. Sci. Total Environ. 707, 134857. doi: 10.1016/j.scitotenv.2019.134857
Von Holle, B., Neill, C., Largay, E. F., Budreski, K. A., Ozimec, B., Clark, S. A., et al. (2013). Ecosystem legacy of the introduced N2-fixing tree Robinia pseudoacacia in a coastal forest. Oecologia 172, 915–924. doi: 10.1007/s00442-012-2543-1
Walker, L. R., and Smith, S. D. (1997). “Impacts of Invasive Plants on Community and Ecosystem Properties,” in Assessment and Management of Plant Invasions, eds J. O. Luken and J. W. Thieret (New York, NY: Springer), doi: 10.1007/978-1-4612-1926-2_7
Warne, A. (2016). Black locust (Robinia pseudoacacia L.). Best Management Practices in Ontario. Peterborough, ON: Ontario Invasive Plant Council.
Wolkovich, E. M., and Cleland, E. E. (2011). The phenology of plant invasions: A community ecology perspective. Front. Ecol. Environ. 9:287–294. doi: 10.1890/100033
Wolkovich, E. M., and Cleland, E. E. (2014). Phenological niches and the future of invaded ecosystems with climate change. AoB Plants 6:plu013. doi: 10.1093/aobpla/plu013
Zhang, H. K., Roy, D. P., Yan, L., Li, Z., Huang, H., Vermote, E., et al. (2018). Characterization of Sentinel-2A and Landsat-8 top of atmosphere, surface, and nadir BRDF adjusted reflectance and NDVI differences. Remote Sens. Environ. 215, 482–494. doi: 10.1016/j.rse.2018.04.031
Zhang, X. Q., Liu, J., Welham, C. V. J., Liu, C. C., Li, D. N., Chen, L., et al. (2006). The effects of clonal integration on morphological plasticity and placement of daughter ramets in black locust (Robinia pseudoacacia). Flora Morphol. Distrib. Funct. Ecol. Plants 201, 547–554. doi: 10.1016/j.flora.2005.12.002
Keywords: chestnut coppice, invasive plant traits, vegetative regeneration, spring leaf phenology, remote sensing, root suckers
Citation: Saulino L, Rita A, Stinca A, Liuzzi G, Silvestro R, Rossi S and Saracino A (2023) Wildfire promotes the invasion of Robinia pseudoacacia in the unmanaged Mediterranean Castanea sativa coppice forests. Front. For. Glob. Change 6:1177551. doi: 10.3389/ffgc.2023.1177551
Received: 01 March 2023; Accepted: 26 April 2023;
Published: 10 May 2023.
Edited by:
Margarita Arianoutsou, National and Kapodistrian University of Athens, GreeceReviewed by:
Piermaria Corona, Council for Agricultural Research and Economics (CREA), ItalySlobodan Milanović, University of Belgrade, Serbia
Copyright © 2023 Saulino, Rita, Stinca, Liuzzi, Silvestro, Rossi and Saracino. This is an open-access article distributed under the terms of the Creative Commons Attribution License (CC BY). The use, distribution or reproduction in other forums is permitted, provided the original author(s) and the copyright owner(s) are credited and that the original publication in this journal is cited, in accordance with accepted academic practice. No use, distribution or reproduction is permitted which does not comply with these terms.
*Correspondence: Luigi Saulino, luigi.saulino@unina.it