- Department of Geography, University of Zurich, Zurich, Switzerland
Peatlands store one-third of the world’s soil carbon, and their climate change response is a key unknown in the global carbon cycle-climate change feedback. In particular, peatland fine root responses to varied environmental changes are poorly constrained. Here, we synthesized fine root responses to warming and water level drawdown by performing a meta-analysis of existing data from boreal forested peatlands. We found seven studies and evaluated root responses from 65 observations. Overall, both warming (from 0 to 9.0°C) and water level drawdown (from 4.0 to 62.5 cm) increased fine root growth by over an order of magnitude, with plant functional type (PFT; graminoid, shrub, and tree) better predicting fine root biomass than treatment magnitude. We observed stronger responses for trees (+374.5% for warming and +868.6% for water level drawdown) than for shrubs (+44.0% for warming and +11.5% for water level drawdown) and graminoids (+59.5% for warming and −59.8% for water level drawdown). Among PFTs, tree fine roots increased significantly and non-linearly with increasing warming treatment, while graminoid fine roots responded significantly to lowering water level, decreasing 1.7% for every 1 cm decrease in water level. Fine roots in hollows, especially of shrubs, increased more strongly than those in hummocks, suggesting a possible flattening of peatland topography with sustained hollow growth from extended warming. Our synthesis highlights the important role of PFT’s in modulating fine root responses and the need for additional belowground data from these carbon-rich and globally relevant peatland soils. The altered fine root growth documented here, implies possible shifts in plant nutrient and water uptake as well as root inputs to soil carbon stocks, which in turn could strongly moderate and shape boreal peatland responses to future climate change.
1. Introduction
Peatlands cover only about 3% of the earth’s land surface but store up to one-third of global terrestrial soil carbon (C) in deep peat deposits (Gorham, 1991; Bridgham et al., 2006; Yu et al., 2010). But these ecosystems are facing increasing temperatures that are likely to exceed 5°C by the end of the century (Soong et al., 2020). Warming and associated soil drying could destabilize the large C pools, formed by the net C uptake over thousands of years, leading to an accelerated emission of greenhouse gases into the atmosphere (Gruber et al., 2004; IPCC, 2007; Limpens et al., 2008; Munir et al., 2014). Besides accelerated C loss, rising temperatures are expected to alter plant growth and functioning with implications on the C storage (Buttler et al., 2015; Zhong et al., 2020). Specifically, warming and associated hydrological changes may alter plant dynamics such as fine root production, which in turn can further influence ecosystem functions such as nutrient and water uptake, ecosystem respiration, and ultimately, soil C storage (Wan et al., 2004; Iversen et al., 2008, 2012; Jackson et al., 2017). Furthermore, fine roots are an important component of peatland carbon cycling, contributing between 25 and 75% of the annual net primary production (reviewed in Iversen et al., 2018) and providing C for peat formation (Laiho et al., 2003). Thus, changes in fine root growth could influence plant resource acquisition and primary productivity, which could influence the amount and quality of soil organic matter entering the soil (Laiho et al., 2003; Iversen et al., 2018). Fine root growth could further influence the decomposition of this soil organic matter if root exudation and rhizosphere priming also change (Jackson et al., 2017). To be able to understand these ecosystem functional responses, we first need to understand how environmental change influences fine root growth. However, mechanisms behind belowground plant responses to climate-driven changes are poorly understood in peatlands and thus, not well accounted for in our future estimates of peatland C balance.
Climate-driven changes are predominant environmental stress factors expected to affect belowground plant growth in northern peatlands (Murphy et al., 2009b; Iversen et al., 2018; Malhotra et al., 2020). Notably, key factors associated with climate change in peatlands, such as soil warming and associated lower water tables can alter belowground root production directly or indirectly through altered nutrient mineralization, water availability, and plant carbon fixation and allocation (Weltzin et al., 2003; Buttler et al., 2015; Hartmann et al., 2020; Zhong et al., 2020). However, the effect of warming on belowground root production is not consistent across plant functional types (PFTs) or microtopographical features. This is because as peatlands become warmer and drier, the relative abundance and productivity of dominant PFTs and belowground plant productivity and properties can change depending on PFTs sensitivity to environmental stressors (Weltzin et al., 2000; Camill, 2011; Lohila et al., 2011; Malhotra et al., 2020). For example, fine root biomass production can increase in warmer and drier peatlands (Bhuiyan et al., 2017; Malhotra et al., 2020; Iversen et al., 2022), but primarily for PFTs that are well-adapted to dry conditions, such as woody shrubs or trees (Munir et al., 2014). Due to these PFT differences in belowground responses, warmer and drier conditions are predicted to increase peatland shrubification i.e., a shift from a Sphagnum-dominated toward a woody shrub and tree dominated system (Weltzin et al., 2000; Camill, 2011; Lohila et al., 2011; McPartland et al., 2019; Malhotra et al., 2020). Shrub, graminoid and tree fine root response may also vary because the PFTs have different resource acquisition strategies given that they fall on the opposite ends of the “root economic spectrum” (RES; Bergmann et al., 2020). Thinly rooted shrubs and graminoids, with high specific root length (SRL), based on the RES, are expected to increase their resource acquisition by increasing fine root investment since roots are not as “costly” (“do-it-yourself approach”; Bergmann et al., 2020). On the other hand, thicker and lower SRL roots of trees, where investment to roots is costly, are expected to increase resource acquisition by increasing investment into mycorrhizal partners (“outsourcing approach”; Bergmann et al., 2020). Besides PFTs, microtopographical features in peatlands (such as hummocks and hollows) provide a moisture gradient to which plants respond differentially (Murphy et al., 2009b; Malhotra et al., 2016). Given that different species have different optimal growth conditions across microtopographical features (Bubier et al., 2006), changes in moisture conditions at these features will result in variable responses (Malhotra et al., 2020). For example, a lowering of the water table may make hummocks too dry for some PFTs such as graminoids but may make hollow moisture conditions optimal for shrub growth (Bubier et al., 2006). Other trajectories of root response to warming and drying are also possible, for example, a lower allocation to fine roots if nutrients are abundant and water is not limiting, for example, in a high precipitation site, or in a hollow relative to a hummock (Hartmann et al., 2020; Zhong et al., 2020). A synthetic quantification of fine-root responses to temperature and water table changes change is therefore necessary.
We focus here on boreal forested peatlands because boreal forests comprise one-third of the forested areas globally and carbon-rich peatland soils underlay 24% of the global boreal forests (Wieder and Vitt, 2006). Our knowledge of fine root dynamics in boreal peatlands has primarily been based on data from relative aboveground growth rates, sequential soil coring, root colonization of in-growth cores, or minirhizotrons (Reader and Stewart, 1972; Backeus, 1990; Laiho and Finér, 1996; Weltzin et al., 2000; Bond-Lamberty et al., 2004; Sullivan and Welker, 2005; Wieder, 2006; Murphy et al., 2009a; Murphy and Moore, 2010; Munir et al., 2014; D’Imperio et al., 2018; Iversen et al., 2018; Malhotra et al., 2020). However, fine-root responses to warming and drying, and interactions with PFTs or microtopography have not been synthesized across boreal peatland studies. Thus, our aims were to (1) quantify the observed range of fine root biomass responses to warming and water level drawdown in different peatland PFTs and micro topographic features and (2) evaluate the drivers of these fine-root changes. We conducted a meta-analysis of existing peer-reviewed literature on how fine root biomass responds to warming and water level drawdown in boreal peatlands (seven studies). We hypothesized that fine root biomass will increase linearly with both warming and water level drawdown due to a deeper aerobic layer as well as increased nutrient availability under warmer and drier conditions (Murphy et al., 2009a; Munir et al., 2017; Iversen et al., 2018, 2022). We also expected major peatland PFTs (trees, ericaceous shrubs, and graminoids) to vary in their fine-root responses. Under warmer and drier conditions, primarily driven by moisture limitation since nutrients are expected to increase with warming (Iversen et al., 2022), tree roots would increase their water acquisition by increasing “outsourcing” to mycorrhizal fungal partners (Defrenne et al., 2021), while thinly-rooted shrubs and graminoids would take a “do-it-yourself” approach instead by increasing production of new fine roots for foraging (Bergmann et al., 2020). Thus, we expected stronger fine root biomass responses in shrubs and graminoids than in trees to both warming and drying. Graminoid species are particularly sensitive to changes in moisture availability and thus should have the highest responses to water level drawdown (Bubier et al., 2006; Malhotra et al., 2016). We also expected microtopographical features to vary in their fine root response to warming and water level drawdown wherein fine root biomass would decrease through exacerbated moisture limitation in hummocks; but fine root biomass would increase in hollows where moisture conditions would become suitable for new PFTs such as shrubs. Lastly, non-linear responses of roots may be possible due to limiting moisture or nutrients in very high warming or water table treatments. For example, while some warming may stimulate growth, resulting in increased root growth, very high warming may push the system into a moisture limitation such that the plant may no longer be able to perform basic physiological functions, thus leading to decreased root growth.
2. Materials and methods
2.1. Data compilation
For the meta-analysis, we searched peer-reviewed publications using Google Scholar, Web of Science, Scopus, Northern Research Station, and swisscovery with the keywords “roots” or “root biomass” and “peatland” or “wetland” or “bog” or “mire” or “fen” or “histosol” and “boreal” with the additional specification “warming,” “elevated temperature,” “water level drawdown,” “drainage,” or “lower water table” to narrow down the search. The search was conducted in January 2022 and included all articles published from January 1996 until December 2021. The following conditions had to be met for the study to be included:
(1) The study reported fine root biomass (< 2 mm or as reported by the study) responses to climate change factors in a boreal peatland.
(2) Studies had to have examined at least one of the two climate change factors, namely, warming and water level drawdown (WLD).
(3) Studies had to quantitatively provide data on the magnitude of warming or WLD to compare data points.
(4) Only in situ studies were considered. However, one exception was the Ge et al. (2012) study that sampled in situ but then moved their samples to controlled environmental chambers.
In total, we found seven peer-reviewed papers (Supplementary Table 1). From these studies, we collected data on the experimental site, treatment magnitude, experimental duration, experimental method, plant functional type (PFT) examined, topography, soil depth to which roots were analyzed, and the difference in fine root biomass between the control and treatment groups. In addition, we considered different treatments within a study (i.e., different sites, treatment magnitudes, etc.) as different observations. As a result, this current study consists of 65 data points including, 35 observations from warming experiments and 30 from water level drawdown experiments.
If the difference between treatments in fine root biomass was not explicitly stated, it was acquired from the graphs using the trial version of DigitizeIt version 2.5 (Bormann, 2015). Therefore, these data points are subject to a small, systematic error. When a treatment lasted for several years but only values for the individual years were provided, the result for the entire treatment period was calculated as the mean of all individual values. Missing data on climatic conditions of the study sites were complemented based on information from other studies conducted on the same study site [for Malhotra et al. (2020) from Sebestyen et al. (2020) and for Laiho and Finér (1996) from Murphy et al. (2009a)]. Topography (hummock or hollow) could not be determined conclusively for all data points. Data points were aggregated into three plant functional types: graminoid, shrub, and tree using categorizations based on species names provided in the papers. However, seven data points from Laiho and Finér (1996) were not categorizable as combined data for shrub and tree (apart from pine) fine root biomass combined was reported. Therefore, a fourth group called “shrub + tree” was used for these data points.
2.2. Data analyses
The difference in fine root biomass between the control and treatment groups was evaluated using the following equation. To better display the values, the results were converted from decimals to percentages by multiplying by 100.
The dataset was then analyzed and visualized primarily using JMP version 15.2 (SAS Institute Inc, 2014). Due to non-normality of data, analyses were run on log transformed differences to controls rather than on untransformed values. First, to determine whether trends are linear or non-linear, either a simple linear model (y = a⋅x + b) or a simple quadratic model (y = a⋅x2 + b⋅x + c) with the method of least squares was used to visualize and qualitatively analyze potential trends in the data. Once establishing linearity or making necessary data transformations to linearity, mixed-effects models were used to evaluate predictors of warming or water table responses. Random effects included data source (seven studies) while fixed effects were magnitude of treatment, duration of treatment, PFT, topography, soil depth to which roots were analyzed, MAT, MAP, Latitude, and Longitude. Interactions among PFT, topography and treatment were also checked but were not significant. Models were checked for variance inflation factors (VIFs) and any predictor with VIF > 2 was removed from the model.
3. Results
3.1. Highly variable fine root responses to warming and water level drawdown
The synthesized studies encompassed applied warming ranging from 0 to 9.0°C and water level drawdown ranging from a lowering of 4.0 to 62.5 cm. Fine root biomass responses to warming and water level drawdown were highly variable across the synthesized studies (Figure 1), and across plant functional types (Figure 2). Responses to warming and water level drawdown were highest for trees ranging from 105.5 to 708.5% with a median of 374.5% and from 165.5 to 1830.5% with a median of 782.5%, respectively (Figure 2). Shrub fine root biomass also increased for warming and water level drawdown, ranging from −76.5 to 244.5%, with a median of 44.0%, and from −3.0 to 26.0%, with a median of 11.5%, respectively (Figure 2). Graminoid fine root biomass increased for warming, with a median of 60.5% (range: −34.5 to 3950.0%), but decreased for water level drawdown, with a median of −77.8% (range: −96.0 to 1.5%). Non-assignable data points reported in the category “shrub + tree” increased with water level drawdown, with a median of 37.5% and ranging from −47.0 to 127.0%, (Figure 2). In the next two sections, we discuss the best predictors of these fine root responses to warming and water level drawdown.
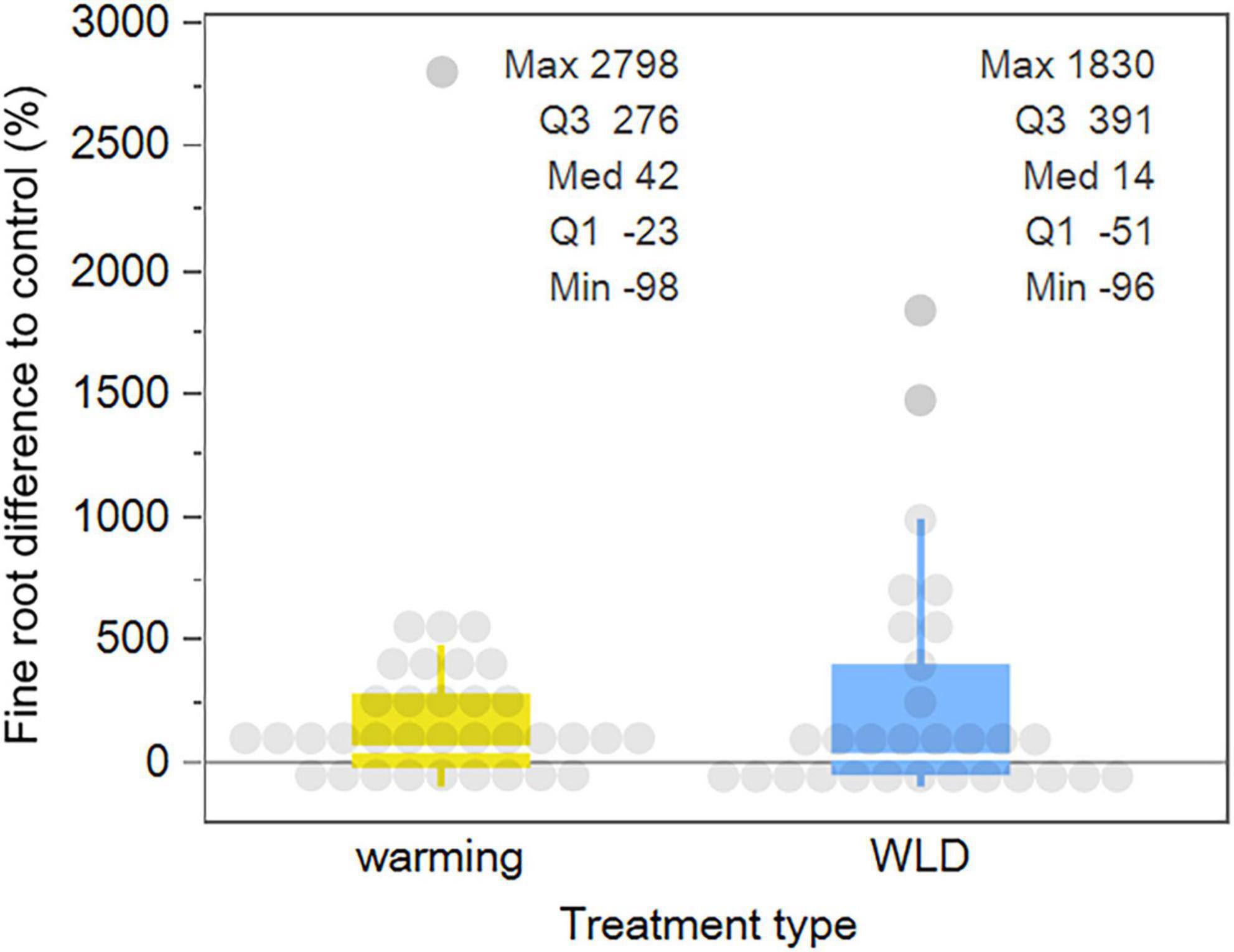
Figure 1. Distributions of fine root responses to warming and water level drawdown (WLD) across the synthesized seven studies. Box plots and reported values indicate minimum, maximum, and median values along with first and third quartiles (all units are % difference of treatment to control). Raw data points are also shown in gray. Positive values indicate that treatment fine root biomass increased while negative values indicate that fine root biomass decreased relative to control.
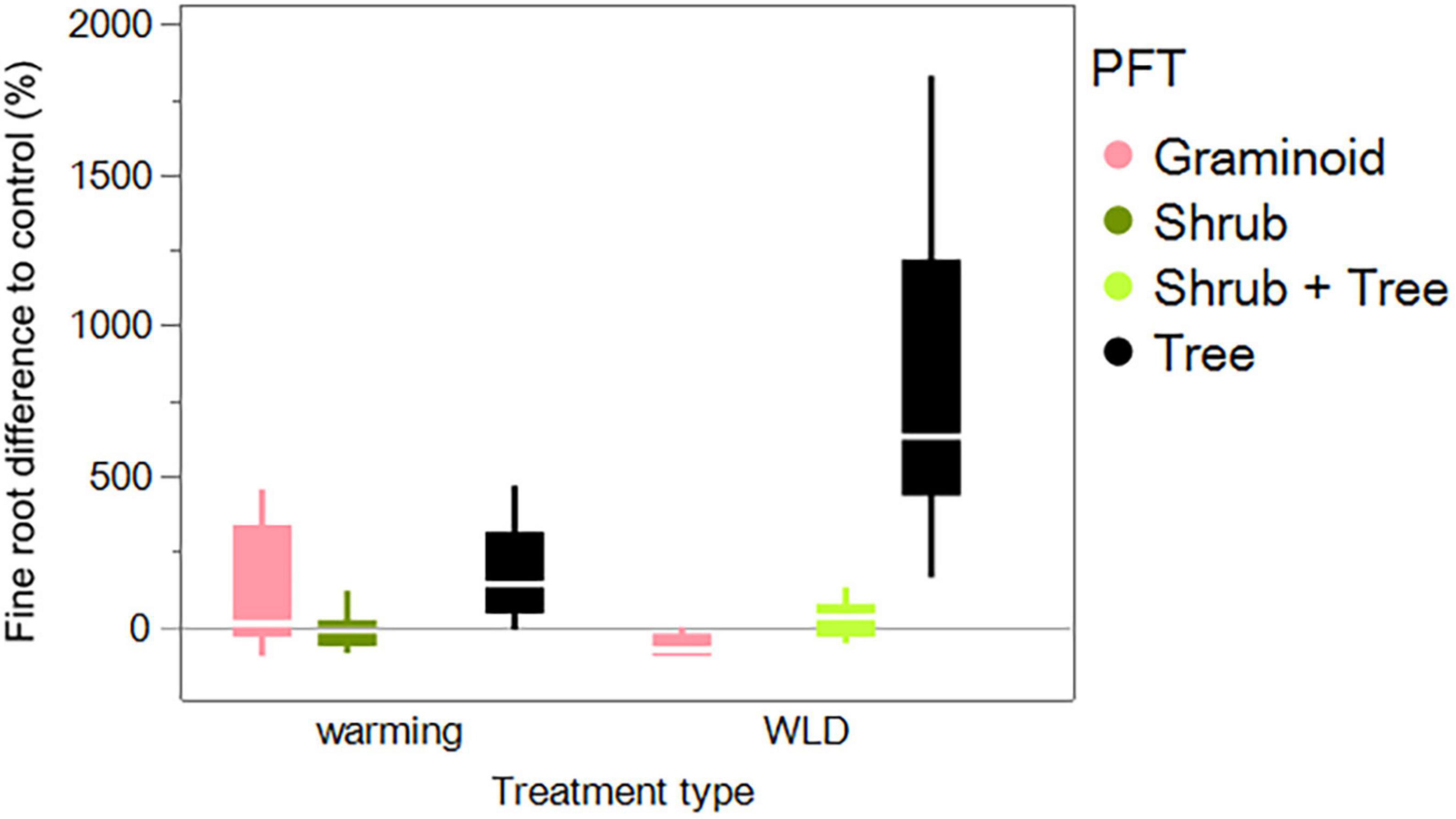
Figure 2. Overview of fine root biomass responses among plant functional types from seven studies. Data points on warming include 21 on graminoids, eight on shrubs, and eight on trees, with a mean of 377.3, 50.3, and 400.0% and a standard deviation of 499.2, 81.6, and 148.4%, respectively. Data points on water level drawdown (WLD) include 12 on graminoids, two on shrub, seven on “shrub + tree”, and nine on trees, with a mean of –59.8, 11.5, 38.6, and 868.6% and a standard deviation of 35.6, 22.0, 47.0, and 436.5%, respectively. Warming treatments range from 0.8 to 9.0°C, while WLD treatments range from 4.0 to 62.5 cm (Supplementary Table 2). For aesthetic reasons, the range of the y-axis has been adjusted so that one outlier is not visible (warming, graminoid: 3950.0%). In the warming treatment, there are no significant differences between the PFTs. In the WLD treatment, there are significant differences among PFTS [Based on an ANOVA where F(3,26) = 22.6, p < 0.0001]. Furthermore, a Tukey’s HSD test suggests that each of the PFTs in WLD treatment are significantly different from each other.
3.2. Plant type and topography drive fine root responses to warming
Fine root biomass responses to warming were primarily explained by topography and PFT (Table 1). The best fit model explained 40% [F(5,24) = 4.99, p = 0.0028] of the variance in fine root biomass warming responses and suggested that fine roots in hollows increased more than fine roots in hummocks (Table 1). Only trees and hollow shrubs showed significant fine root warming responses when analyzing each PFT and topography individually. In trees, 43% [F(2,9) = 6.09, p = 0.0210; quadratic regression] of the variation in the fine root response was explained by the magnitude of warming and in hollow shrubs, the 93% [F(1,2) = 46.7, p = 0.0208; positive linear regression] of the variation was explained albeit with a limited sample size of 4 (Figure 3).
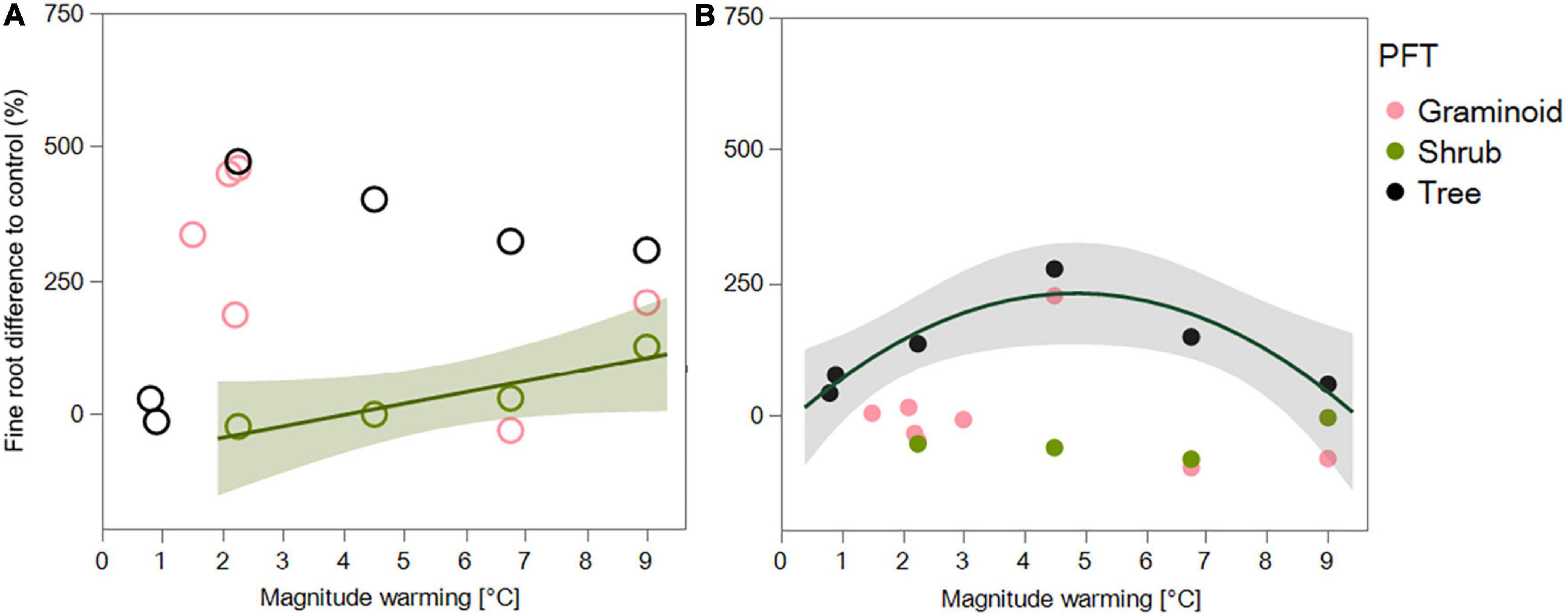
Figure 3. Fine root biomass responses to warming from four studies for hollows [(A); hollow circles] and hummocks [(B); solid circles]. Among plant functional types (PFTs), shrub fine roots had a significant response but only in hollows [shown as green line of fit and confidence interval; adjusted R2 = 0.93, F(1,2) = 46.7; p = 0.0208; In (difference to control) = –0.67–0.16*Magnitude warming]. Tree fine roots also had a significant quadratic response [shown as black line of fit and gray confidence interval; adjusted R2 = 0.43, F(2,9) = 6.09; p = 0.0210; In (difference to control) = 0.79 + 0.15 *Magnitude warming – 0.05*(Magnitude warming – 4.03)2].
3.3. Degree of drawdown and plant type regulate fine root responses to water level drawdown
Fine root biomass response to water level drawdown was significantly related to the magnitude of the drawdown and PFT (Table 2). Topography was not a significant driver of fine root response to water level drawdown. When analyzing PFTs individually, only graminoids responded significantly to water level drawdown [R2 = 0.87, F(1, 10) = 78.72, p < 0.0001; Figure 4]. The linear regression suggested a 1.74 ± 0.25% decrease in graminoid fine root biomass for every 1 cm decrease in water level (Figure 4).
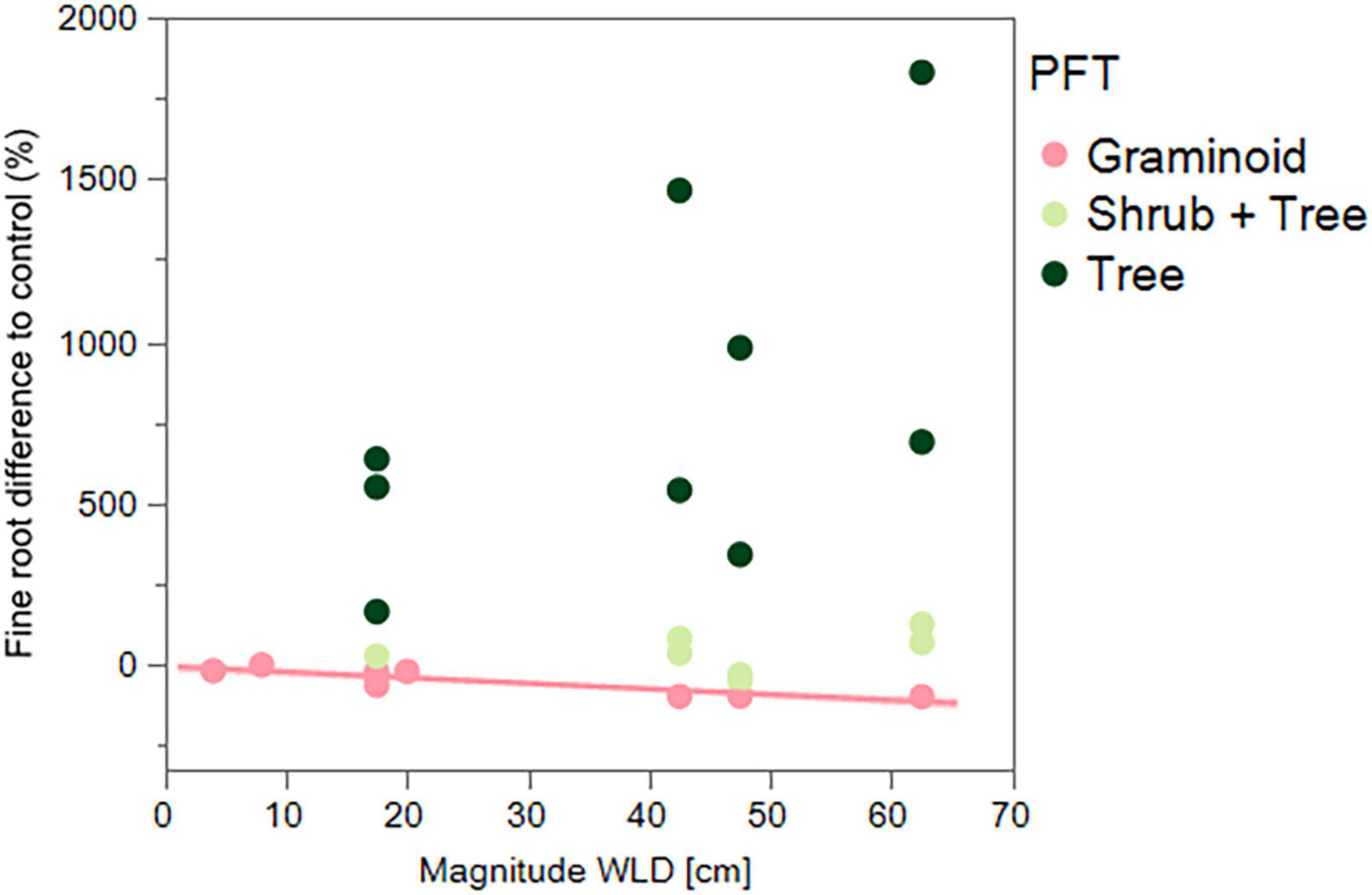
Figure 4. Fine root biomass responses to water level drawdown from four studies. When analyzing PFTs individually, only graminoids responded significantly to water level drawdown [shown as pink line of fit and confidence interval; adjusted R2 = 0.87, p < 0.0001; F(1, 10) = 78.7; In (difference to control) = 0.45–0.07*Magnitude WLD]. Despite a visual trend of increase, tree fine root biomass did not respond significantly to WLD [adjusted R2 = 0.21, p = 0.12; F(1,7) = 3.18; In (difference to control) = 1.30 + 0.19*Magnitude WLD].
4. Discussion
This meta-analysis quantifies the effects of climate change factors on belowground plant dynamics and provides evidence that warming, and water level drawdown influence fine root biomass production in boreal peatlands. We found strong non-linear and linear fine root biomass responses to warming and water level drawdown, respectively, with PFTs and topography varying significantly. Notably, we found that fine root biomass in hollows may increase more from warming than fine root biomass in hummocks and that tree fine roots have the strongest (and non-linear) warming increases across PFTs. Under lowered water tables, only graminoid fine roots showed a significant linear decrease in biomass. Since studies on the response of boreal peatland fine roots to global change drivers are limited and available data show inconsistent responses, our findings offer insights on the climate change drivers of boreal peatland plant roots. Moreover, our synthesis highlights the importance of investigating individual PFTs and topographic responses to climate change factors such as warming and water level drawdown. Lastly, the significantly altered fine root biomass responses to climate change (ranging between −96 and +1830% across treatments and PFTs) documented here imply that altered root inputs to soil carbon stocks, may not only affect overall ecosystem C balance but also peatland structure via plant community changes and resilience to environmental change via topographic changes.
4.1. Non-linear fine root responses to warming
We hypothesized a linear increase in fine-root biomass with increased warming and generally observed this. However, in trees we observed a non-linear relationship between warming and fine root biomass. Our analysis suggests a maximum tree fine root biomass growth at +5.4°C (relative to ambient), comparing well to a previously suggested value of +4.8°C (Lin et al., 2010; Figure 3). A possible reason for this quadratic relationship could be that, at first, warming stimulates increased plant growth both above and belowground both from direct temperature effects as well as from indirect increases in nutrients due to increased mineralization of organic matter (Munir et al., 2017; Iversen et al., 2022). However, continued warming may lead to a moisture or nutrient limitation (Poorter et al., 2012). For example, while plants are expected to allocate more belowground when nutrients are limiting, with sustained limitation and given the high nitrogen cost of fine roots, fine root growth increases may halt in the longer term (Iversen et al., 2008; Norby et al., 2010). Another reason, perhaps more likely in peatlands, could be warming-induced drying combined with increased evapotranspiration; wherein moisture becomes too limiting for physiological activity at a certain temperature threshold, and fine root increase stops (Lin et al., 2010; Malhotra et al., 2020).
Despite being highly variable, the overall warming-induced stimulation of fine root biomass in this study (on average +159.9%, with a 95% confidence interval of 107.1–212.8%, for warming treatments ranging from 0.8 to 9.0°C) is the first synthesized value of its kind. It is also worth noting that our reported fine-root responses are much larger than those reported in global meta-analyses for other ecosystems where fine roots increased by 8.7 (Wang et al., 2021) or 13% (Lin et al., 2010); highlighting that peatland fine root parameterization in models should be different than that of mineral soils. Nevertheless, our results are consistent with the few individual peatland studies using a response ratio approach, all of which were examined in this study; Sullivan and Welker (2005) on average +157.8%, with a 95% confidence interval of 27.9–287.7%, with warming treatments ranging from 0.8 to 0.9°C; Malhotra et al. (2020) on average +207.2%, with a 95% confidence interval of 131.9–282.5%, with warming treatments ranging from 2.25 to 9.0°C). Lastly, it is worth noting that our observed fine-root biomass changes could be a result of either an increase in fine-root production, a lengthening of the belowground growing season or decreases in fine-root mortality, or a combination of these different mechanisms (Malhotra et al., 2020; Defrenne et al., 2021). The exact mechanisms of fine root biomass change are important to test in future studies.
4.2. Water level drawdown responses strongest in graminoid fine roots
While water level drawdown generally increased fine root biomass (Figure 2), our predictive model suggests that this variability was mostly driven by PFT differences. As per our hypothesis we saw the strongest fine-root responses to water level drawdown in graminoids, wherein graminoid fine root biomass decreased significantly with water level drawdown.
While not significantly related to the degree of water level drawdown, in accordance with our hypothesis, we did see overall water level drawdown-induced stimulation of fine root biomass in this study (on average +246.4%, with a 95% confidence interval of 110.9–381.9%, for water level drawdown treatments ranging from 4.0 to 62.5 cm). To our knowledge, these are the first synthesized values for fine root water table response in boreal peatlands, and they are much higher than individual site studies reporting, for example, an average 12% increase in fine root biomass for a 17.5 cm drawdown (Murphy et al., 2009a). However, our high average response of fine roots to water level drawdown could be due to the large range of treatment magnitudes across our studies (a water level lowering of 4–62.5 cm) that represent not just likely climate change-driven water level changes but also changes from more drastic peatland drainage for human uses such as agriculture. It is also worth noting that if averaging across PFT and topography, the results of this meta-analysis indicate a linear increase in fine root biomass production of 7.2% per cm water level drawdown (Supplementary Figure 1). While the importance of PFT and microtopography are clear across our results, this value could serve as an ecosystem-scale reference for further investigations since, so far, the few individual studies discussing the nature of this relation did not report the slope of the linear regression, if any was performed at all (Laiho and Finér, 1996; Murphy et al., 2009a; Ge et al., 2012; Munir et al., 2014). This ecosystem-scale reference is particularly important for many boreal forested peatlands where graminoids may be a small component of the total ecosystem-scale fine root biomass (e.g., Iversen et al., 2018).
Water availability is an essential driver of fine root growth in boreal peatlands. Previous boreal peatland warming experiments have reported that it is not warming that determines fine root biomass responses, but drying (caused by warming), and that fine-root biomass production increases linearly with decreasing soil moisture (Malhotra et al., 2020). However, when moisture becomes too scarce, fine root production decreases after an initial increase (Lin et al., 2010; Malhotra et al., 2020). While our results suggest a linear fine root biomass response to water level drawdown (Figure 4), we expect non-linear responses as water level drawdown continues and moisture availability becomes a limiting factor for plant growth (Cagampan and Waddington, 2008; Macrae et al., 2013). Our compiled data only had either warming or water table drawdown as treatments and thus we could not explicitly test for interactions among the two factors. Future data collection and synthesis efforts could prioritize moisture x warming crossed designs to tease apart these interactions.
4.3. Warming and water table responses of fine roots are modulated by PFT and topography
Across both warming and water level drawdown treatments, our meta-analysis suggests that PFT and topography are more important predictors of fine root responses than the degree of treatment applied. Warming and water level drawdown seems to support a shift to woody PFTs (via increasing shrub and tree fine roots and decreasing graminoid fine roots). Sustained increases in woody PFT fine root growth may eventually drive peatland shrubification by outcompeting other PFTs (Malhotra et al., 2020; McPartland et al., 2019). We observed that both trees and shrubs may benefit from the warmer and drier improved growing conditions. Based on our limited data reflecting only fine roots and not mycorrhizae, we did not see strong support of our hypothesis that shrubs would increase their foraging capacity by increasing fine root biomass more than trees (that would take an approach of outsourcing to fungal symbionts; Bergmann et al., 2020). We had also expected that thinly-rooted graminoids would increase fine root biomass under warmer conditions but instead we only saw evidence for graminoid sensitivity to moisture (with decreasing graminoid fine root biomass under lower water tables). Either way, as a result of a shift toward woodier PFTs, increased shading could limit Sphagnum moss growth (Laine et al., 1995; Minkkinen et al., 1999; Mäkiranta et al., 2017; Norby et al., 2019; Malhotra et al., 2020). Sphagnum is a key ecosystem engineer in boreal peatlands and such changes in the plant community composition (and in the C source) would directly affect C cycling in peatlands by altering nutrients (Salmon et al., 2021), the amount and quality of organic matter inputs, and decomposition parameters that dictate peatland C storage (Ofiti et al., 2022).
The decrease of graminoid fine roots with lower water table was expected as graminoids are better suited to wetter conditions than shrubs and trees. Unlike woody plants, graminoids have aerenchyma that transports O2 from the surface to the roots, allowing them to function under anaerobic conditions below the water table (Iversen et al., 2018). Therefore, graminoids are more likely to be negatively affected by warming-induced drying and, as a result, less able to compete with trees and shrubs under warming (Murphy et al., 2009a). However, one important caveat is that the data in our meta-analysis do not allow us to fully evaluate plant adaptation. In addition to missing diameter and specific root length data that could be more reflective of belowground adaptation strategies, rooting depth changes would also be needed to fully assess if graminoids are indeed not adapting to dry conditions as well as trees and shrubs. Nevertheless, we know from peatland survey studies (e.g., Bubier et al., 2006; Malhotra et al., 2016) that the aboveground biomass of graminoids and woody PFT abundances decreases and increase with a lower water table, respectively. Until we have better belowground niche tolerances of these PFTs, we can only make inferences about plant adaptation from aboveground surveys. We also expected that water level drawdown should increase the surface aerobic soil layer and improve conditions for growth of woody plants that do not form aerenchymatous roots (Murphy et al., 2009a; Iversen et al., 2018). However, while we did not see statistical evidence of shrub and tree fine roots increasing with lower water levels, we do see trends pointing toward increased fine roots although with a lot of variability (Figure 4). Furthermore, we observed a statistically significant increase in PFT-averaged water table response that is likely driven by the large tree fine root biomass responses (Figure 4 and Supplementary Figure 1). With continued drying, graminoids likely cannot compete with trees and shrubs under drier conditions (Laine et al., 1995) and these responses should have implications for various ecosystem processes, notably a potential reduction in methane emissions due to a decrease in plant-mediated methane transport through graminoids (Lai, 2009).
Topography was also an important predictor with higher fine root biomass responses to warming for sunken hollows than for raised hummocks, consistent with previous studies (Strack et al., 2006; Malhotra et al., 2020). Our results indicate that peatland microtopography predicts fine root growth more significantly than warming magnitude (Table 1). Continued higher fine root biomass in hollows with warming could raise them to hummocks and flatten the existing microtopography, influencing peatland resilience to environmental change and its C balance (Belyea and Malmer, 2004; Rietkerk and van de Koppel, 2008; Malhotra et al., 2016, 2020).
4.4. Limitations
This study is subject to several potential limitations that could be addressed in future research. First, there has been little research on boreal peatland fine root biomass responses to warming or water level drawdown (only seven studies synthesized here). Because of this limitation, our study had small sample sizes for some plant functional types and no observations for PFTs such as forbs. Despite great efforts developing manipulation experiments to assess climate change responses, there are still only very few in situ experiments in boreal peatlands, highlighting the need for further experimental development in this ecosystem. Our dataset only has one study site each from Canada, USA, and Greenland, as well as three sites from Finland; leaving large swaths of the boreal forest unrepresented in our study. For example, we did not have representative data from Scandinavia, Eastern Europe, Asia, Eastern Canada, USA, and Alaska. Thus, we require a lot more sites to be able to draw conclusive trends regarding fine root response to climate change in boreal forested peatlands. It is also important to note that even though, during our analyses, we always confirmed that our results were robust with and without study sources that were the most data rich, our data were dominated by two study sources and this bias should be noted while interpreting our results (Supplementary Figures 2, 3 and Supplementary Table 1). Another important limitation is that while we base our hypotheses on the root economic spectrum framework (Bergmann et al., 2020), we acknowledge that a more direct test of these hypotheses would require data on fine root diameter, specific root length and mycorrhizal colonization intensity. Since these data are rarely reported, we had to use fine root biomass response as a simple indicator of whether plants are increasing investment into roots (as opposed to into mycorrhizae). Thus, we recommend future peatland climate change experiments to quantify additional belowground traits. Lastly, the duration of most warming treatment studies examined was very short (max. 3.3 years). Therefore, ecosystem studies with longer durations should be assessed to verify that the trends in our study reflect long-term responses of peatland ecosystems.
5. Conclusion
Peatland plants are known to shape peat properties through their above and belowground functional traits, given the tight ecohydrological feedbacks in these ecosystems (Waddington et al., 2014; Malhotra et al., 2016). Our study showed that warming and water level drawdown can increase the environmental sensitivity of belowground plant components in boreal forested peatlands and the effects of lowered water level can be further modulated by plant functional type and peatland topography. Although we show that climate warming and associated water level drawdown will benefit fine root growth in shrub and tree PFTs, warming could still cause lasting impacts on species composition, which in turn could alter belowground C cycling. Indeed, we observed a decline in fine root biomass in the graminoid PFT and given that peatland plant communities are already projected to shift from bryophytes to vascular plants in response to future climate change (Dieleman et al., 2015; McPartland et al., 2019; Norby et al., 2019; Malhotra et al., 2020), shifts toward shrub/tree-dominated peatlands could decrease peat C storage (Hanson et al., 2020; Ofiti et al., 2022). Our study reports highly variable fine root biomass responses to warming and water table drawdown in boreal forested peatlands; and our synthesized PFT- and microtopography-dependent response functions could help improve predictions of peatland ecosystem structure and C sink function responses to climate change.
Data availability statement
All data presented in the manuscript are included in the Supplementary material (Supplementary Table 2), further inquiries can be directed to the corresponding author.
Author contributions
AM and MB conceived and designed the study and conducted the analyses, with input from NO. MB collected and combined the data and wrote the first manuscript draft. AM and NO wrote the different sections and refined the manuscript. All authors contributed to manuscript revision, read, and approved the submitted version.
Funding
We acknowledge funding support from the University of Zurich Stiftung für Wissenschaftliche Forschung (STWF-22-028) to AM and the Swiss National Science Foundation (Post-doc mobility grant P500PN_206708) to NO.
Conflict of interest
The authors declare that the research was conducted in the absence of any commercial or financial relationships that could be construed as a potential conflict of interest.
Publisher’s note
All claims expressed in this article are solely those of the authors and do not necessarily represent those of their affiliated organizations, or those of the publisher, the editors and the reviewers. Any product that may be evaluated in this article, or claim that may be made by its manufacturer, is not guaranteed or endorsed by the publisher.
Supplementary material
The Supplementary Material for this article can be found online at: https://www.frontiersin.org/articles/10.3389/ffgc.2023.1170252/full#supplementary-material
References
Backeus, I. (1990). Production and depth distribution of fine roots in a boreal open bog. Ann. Bot. Fenn. 27, 261–265.
Belyea, L. R., and Malmer, N. (2004). Carbon sequestration in peatland: Patterns and mechanisms of response to climate change. Glob. Chang. Biol. 10, 1043–1052. doi: 10.1111/j.1365-2486.2004.00783.x
Bergmann, J., Weigelt, A., Van Der Plas, F., Laughlin, D. C., Kuyper, T. W., Guerrero-Ramirez, N., et al. (2020). The fungal collaboration gradient dominates the root economics space in plants. Sci. Adv. 6:aba3756. doi: 10.1126/sciadv.aba3756
Bhuiyan, R., Minkkinen, K., Helmisaari, H. S., Ojanen, P., Penttilä, T., and Laiho, R. (2017). Estimating fine-root production by tree species and understorey functional groups in two contrasting peatland forests. Plant Soil 412, 299–316. doi: 10.1007/s11104-016-3070-3
Bond-Lamberty, B., Wang, C., and Gower, S. T. (2004). Net primary production and net ecosystem production of a boreal black spruce wildfire chronosequence. Glob. Chang. Biol. 10, 473–487. doi: 10.1111/j.1529-8817.2003.0742.x
Bridgham, S. D., Megonigal, J. P., Keller, J. K., Bliss, N. B., and Trettin, C. (2006). The carbon balance of North American wetlands. Wetlands 26, 889–916.
Bubier, J. L., Moore, T. R., and Crosby, G. (2006). Fine-scale vegetation distribution in a cool temperate peatland. Can. J. Bot. 84, 910–923. doi: 10.1139/B06-044
Buttler, A., Robroek, B. J. M., Laggoun-Défarge, F., Jassey, V. E. J., Pochelon, C., Bernard, G., et al. (2015). Experimental warming interacts with soil moisture to discriminate plant responses in an ombrotrophic peatland. J. Veg. Sci. 26, 964–974. doi: 10.1111/jvs.12296
Cagampan, J. P., and Waddington, J. M. (2008). Moisture dynamics and hydrophysical properties of a transplanted acrotelm on a cutover peatland. Hydrol. Process. 1787, 1776–1787. doi: 10.1002/hyp
Camill, P. (2011). Patterns of boreal permafrost peatland vegetation across environmental gradients sensitive to climate warming. Can. J. Bot. 77, 721–733. doi: 10.1139/b99-008
Defrenne, C. E., Childs, J., Fernandez, C. W., Taggart, M., Nettles, W. R., Allen, M. F., et al. (2021). High-resolution minirhizotrons advance our understanding of root-fungal dynamics in an experimentally warmed peatland. Plants People Planet 3, 640–652. doi: 10.1002/ppp3.10172
Dieleman, C. M., Branfireun, B. A., Mclaughlin, J. W., and Lindo, Z. (2015). Climate change drives a shift in peatland ecosystem plant community: Implications for ecosystem function and stability. Glob. Chang. Biol. 21, 388–395. doi: 10.1111/gcb.12643
D’Imperio, L., Arndal, M. F., Nielsen, C. S., Elberling, B., and Schmidt, I. K. (2018). Fast responses of root dynamics to increased snow deposition and summer air temperature in an arctic wetland. Front. Plant Sci. 9:1258. doi: 10.3389/fpls.2018.01258
Ge, Z. M., Kellomäki, S., Zhou, X., Peltola, H., Wang, K. Y., and Martikainen, P. J. (2012). Seasonal physiological responses and biomass growth in a bioenergy crop (Phalaris arundinacea L.) under elevated temperature and CO2, subjected to different water regimes in boreal conditions. Bioenergy Res. 5, 637–648. doi: 10.1007/s12155-011-9170-2
Gorham, E. (1991). Northern peatlands: Role in the carbon cycle and probable responses to climatic warming. Ecol. Appl. 1, 182–195. doi: 10.2307/1941811
Gruber, N., Friedlingstein, P., Field, C. B., Valentini, R., Heimann, M., Richey, J. E., et al. (2004). “The vulnerability of the carbon cycle in the 21st century: an assessment of carbon-climate-human interactions,” in The global carbon cycle: integrating humans, climate and the natural world, eds C. B. Field and M. R. Raupach (Washington, DC: Island Press), 45–76.
Hanson, P. J., Griffiths, N. A., Iversen, C. M., Norby, R. J., Sebestyen, S. D., Phillips, J. R., et al. (2020). Rapid net carbon loss from a whole-ecosystem warmed Peatland. AGU Adv. 1:av000163. doi: 10.1029/2020av000163
Hartmann, H., Bahn, M., Carbone, M., and Richardson, A. D. (2020). Plant carbon allocation in a changing world – challenges and progress: Introduction to a Virtual Issue on carbon allocation: Introduction to a virtual issue on carbon allocation. New Phytol. 227, 981–988. doi: 10.1111/nph.16757
IPCC (2007). “Contribution of working group II to the fourth assessment report of the intergovernmental panel on climate change,” in Climate Change 2007 – Impacts, Adaptation and Vulnerability, eds M. L. Parry, O. F. Canziani, J. P. Palutikof, P. J. van der Linden, and C. E. Hanson (Cambridge: Cambridge University Press).
Iversen, C. M., Childs, J., Norby, R. J., Ontl, T. A., Kolka, R. K., Brice, D. J., et al. (2018). Fine-root growth in a forested bog is seasonally dynamic, but shallowly distributed in nutrient-poor peat. Plant Soil 424, 123–143. doi: 10.1007/s11104-017-3231-z
Iversen, C. M., Keller, J. K., Garten, C. T., and Norby, R. J. (2012). Soil carbon and nitrogen cycling and storage throughout the soil profile in a sweetgum plantation after 11 years of CO 2-enrichment. Glob. Chang. Biol. 18, 1684–1697. doi: 10.1111/j.1365-2486.2012.02643.x
Iversen, C. M., Latimer, J., Brice, D. J., Childs, J., Vander Stel, H. M., Defrenne, C. E., et al. (2022). Whole-Ecosystem Warming Increases Plant-Available Nitrogen and Phosphorus in an Ombrotrophic Bog. Ecosystems 26, 86–113. doi: 10.1007/s10021-022-00744-x
Iversen, C. M., Ledford, J., and Norby, R. J. (2008). CO2 enrichment increases carbon and nitrogen input from fine roots in a deciduous forest. New Phytol. 179, 837–847. doi: 10.1111/j.1469-8137.2008.02516.x
Jackson, R. B., Lajtha, K., Crow, S. E., Hugelius, G., Kramer, M. G., and Piñeiro, G. (2017). The ecology of soil carbon: Pools, vulnerabilities, and biotic and abiotic controls. Annu. Rev. Ecol. Evol. Syst. 48, 419–445. doi: 10.1146/annurev-ecolsys-112414-054234
Lai, D. Y. F. (2009). Methane dynamics in Northern Peatlands: A review. Pedosphere 19, 409–421. doi: 10.1016/S1002-0160(09)00003-4
Laiho, R., and Finér, L. (1996). Changes in root biomass after water-level drawdown on pine mires in southern Finland. Scand. J. For. Res. 11, 251–260. doi: 10.1080/02827589609382934
Laiho, R., Vasander, H., Penttilä, T., and Laine, J. (2003). Dynamics of plant-mediated organic matter and nutrient cycling following water-level drawdown in boreal peatlands. Glob. Biogeochem. Cycles 17:1053. doi: 10.1029/2002GB002015
Laine, J., Vasander, H., and Laiho, R. (1995). Long-Term Effects of Water Level Drawdown on the Vegetation of Drained Pine Mires in Southern Finland Author. J. Appl. Ecol. 32, 785–802.
Limpens, J., Berendse, F., Blodau, C., Canadell, J. G., Freeman, C., Holden, J., et al. (2008). Peatlands and the carbon cycle: From local processes to global implications – a synthesis. Biogeosciences 5, 1475–1491. doi: 10.5194/bg-5-1475-2008
Lin, D., Xia, J., and Wan, S. (2010). Climate warming and biomass accumulation of terrestrial plants: A meta-analysis. New Phytol. 188, 187–198. doi: 10.1111/j.1469-8137.2010.03347.x
Lohila, A., Minkkinen, K., Aurela, M., Tuovinen, J. P., Penttilä, T., Ojanen, P., et al. (2011). Greenhouse gas flux measurements in a forestry-drained peatland indicate a large carbon sink. Biogeosciences 8, 3203–3218. doi: 10.5194/bg-8-3203-2011
Macrae, M. L., Devito, K. J., Strack, M., and Waddington, J. M. (2013). Effect of water table drawdown on peatland nutrient dynamics?: Implications for climate change. Biogeochemistry 112, 661–676. doi: 10.1007/s10533-012-9730-3
Mäkiranta, P., Laiho, R., Mehtätalo, L., Straková, P., Sormunen, J., Minkkinen, K., et al. (2017). Responses of phenology and biomass production of boreal fens to climate warming under different water-table level regimes. Glob. Chang. Biol. 24, 944–956. doi: 10.1111/gcb.13934
Malhotra, A., Brice, D. J., Childs, J., Graham, J. D., Hobbie, E. A., Vander Stel, H., et al. (2020). Peatland warming strongly increases fine-root growth. Proc. Natl. Acad. Sci. U.S.A. 117, 17627–17634. doi: 10.1073/pnas.2003361117
Malhotra, A., Roulet, N. T., Wilson, P., Giroux-Bougard, X., and Harris, L. I. (2016). Ecohydrological feedbacks in peatlands: An empirical test of the relationship among vegetation, microtopography and water table. Ecohydrology 9, 1346–1357. doi: 10.1002/eco.1731
McPartland, M. Y., Kane, E. S., Falkowski, M. J., Kolka, R., Turetsky, M. R., Palik, B., et al. (2019). The response of boreal peatland community composition and NDVI to hydrologic change, warming, and elevated carbon dioxide. Glob. Chang. Biol. 25, 93–107. doi: 10.1111/gcb.14465
Minkkinen, K., Vasander, H., Jauhiainen, S., Karsisto, M., and Laine, J. (1999). Post-drainage changes in vegetation composition and carbon balance in Lakkasuo mire, central Finland. Plant Soil 207, 107–120. doi: 10.1023/A:1004466330076
Munir, T. M., Xu, B., Perkins, M., and Strack, M. (2014). Responses of carbon dioxide flux and plant biomass to water table drawdown in a treed peatland in northern Alberta: A climate change perspective. Biogeosciences 11, 807–820. doi: 10.5194/bg-11-807-2014
Munir, T. M., Xu, B., and Strack, M. (2017). Mineral nitrogen and phosphorus pools affected by water table lowering and warming in a boreal forested peatland. Ecohydrology 10, e1893. doi: 10.1002/eco.1893
Murphy, M. T., McKinley, A., and Moore, T. R. (2009b). Variations in above- and below-ground vascular plant biomass and water table on a temperate ombrotrophic peatland. Botany 87, 845–853. doi: 10.1139/B09-052
Murphy, M., Laiho, R., and Moore, T. R. (2009a). Effects of water table drawdown on root production and aboveground biomass in a boreal Bog. Ecosystems 12, 1268–1282. doi: 10.1007/s10021-009-9283-z
Murphy, M. T., and Moore, T. R. (2010). Linking root production to aboveground plant characteristics and water table in a temperate bog. Plant Soil 336, 219–231. doi: 10.1007/s11104-010-0468-1
Norby, R. J., Childs, J., Hanson, P. J., and Warren, J. M. (2019). Rapid loss of an ecosystem engineer: Sphagnum decline in an experimentally warmed bog. Ecol. Evol. 9, 12571–12585. doi: 10.1002/ece3.5722
Norby, R. J., Warren, J. M., Iversen, C. M., Medlyn, B. E., and Mcmurtrie, R. E. (2010). CO 2 enhancement of forest productivity constrained by limited nitrogen availability. PNAS 107, 19368–19373. doi: 10.1073/pnas.1006463107
Ofiti, N. O. E., Solly, E. F., Hanson, P. J., Malhotra, A., Wiesenberg, G. L. B., and Schmidt, M. W. I. (2022). Warming and elevated CO2 promote rapid incorporation and degradation of plant-derived organic matter in an ombrotrophic peatland. Glob. Chang. Biol. 28, 883–898. doi: 10.1111/gcb.15955
Poorter, H., Niklas, K. J., Reich, P. B., Oleksyn, J., Poot, P., and Mommer, L. (2012). Biomass allocation to leaves, stems and roots: Meta-analyses of interspecific variation and environmental control. New Phytol. 193, 30–50. doi: 10.1111/j.1469-8137.2011.03952.x
Reader, R. J., and Stewart, J. M. (1972). The relationship between net primary production and accumulation for a Peatland in Southeastern Manitoba. Ecology 53, 1024–1037. doi: 10.2307/1935415
Rietkerk, M., and van de Koppel, J. (2008). Regular pattern formation in real ecosystems. Trends Ecol. Evol. 23, 169–175. doi: 10.1016/j.tree.2007.10.013
Salmon, V. G., Brice, D. J., Bridgham, S., Childs, J., Graham, J., Griffiths, N. A., et al. (2021). Nitrogen and phosphorus cycling in an ombrotrophic peatland: A benchmark for assessing change. Plant Soil 466, 649–674. doi: 10.1007/s11104-021-05065-x
Sebestyen, S. D., Verry, E. S., Elling, A. E., Kyllander, R. L., Roman, D. T., Burdick, J. M., et al. (2020). Marcell Experimental Forest daily maximum and minimum air temperature, 1961 - ongoing. Fort Collins, CO: Forest Service Research Data Archive, doi: 10.2737/RDS-2020-0063
Soong, J. L., Phillips, C. L., Ledna, C., Koven, C. D., and Torn, M. S. (2020). CMIP5 Models Predict Rapid and Deep Soil Warming Over the 21st Century. J. Geophys. Res. Biogeosci. 125, e2019JG005266. doi: 10.1029/2019JG005266
Strack, M., Waddington, J. M., Rochefort, L., and Tuittila, E.-S. (2006). Response of vegetation and net ecosystem carbon dioxide exchange at different peatland microforms following water table drawdown. J. Geophys. Res. 111, 1–10. doi: 10.1029/2005JG000145
Sullivan, P. F., and Welker, J. M. (2005). Warming chambers stimulate early season growth of an arctic sedge: Results of a minirhizotron field study. Oecologia 142, 616–626. doi: 10.1007/s00442-004-1764-3
Waddington, J. M., Morris, P. J., Kettridge, N., Granath, G., Thompson, D. K., and Moore, P. A. (2014). Hydrological feedbacks in northern peatlands. Ecohydrology 8, 113–127. doi: 10.1002/eco.1493
Wan, S., Norby, R. J., Pregitzer, K. S., Ledford, J., and O’Neill, E. G. (2004). CO2 enrichment and warming of the atmosphere enhance both productivity and mortality of maple tree fine roots. New Phytol. 162, 437–446. doi: 10.1111/j.1469-8137.2004.01034.x
Wang, J., Defrenne, C., McCormack, M. L., Yang, L., Tian, D., Luo, Y., et al. (2021). Fine-root functional trait responses to experimental warming: A global meta-analysis. New Phytol. 230, 1856–1867. doi: 10.1111/nph.17279
Weltzin, J. F., Bridgham, S. D., Pastor, J., Chen, J., and Harth, C. (2003). Potential effects of warming and drying on peatland plant community composition. Glob. Chang. Biol. 9, 141–151. doi: 10.1046/j.1365-2486.2003.00571.x
Weltzin, J. F., Pastor, J., Harth, C., Bridgham, S. D., Updegraff, K., and Chapin, C. T. (2000). Response of bog and fen plant communities to warming and water-table manipulations. Ecology 81, 3464–3478.
Wieder, R. K. (2006). “Primary Production in Boreal Peatlands,” in Boreal Peatland Ecosystems, eds R. K. Wieder and D. H. Vitt (Berlin: Springer Berlin Heidelberg), 145–164.
Yu, Z., Loisel, J., Brosseau, D. P., Beilman, D. W., and Hunt, S. J. (2010). Global peatland dynamics since the last glacial maximum. Geophys. Res. Lett. 37:L13402. doi: 10.1029/2010GL043584
Keywords: fine root, peatland, boreal, warming, water level drawdown, plant functional type (PFT), microtopography
Citation: Bucher M, Ofiti NOE and Malhotra A (2023) Plant functional types and microtopography mediate climate change responses of fine roots in forested boreal peatlands. Front. For. Glob. Change 6:1170252. doi: 10.3389/ffgc.2023.1170252
Received: 20 February 2023; Accepted: 03 April 2023;
Published: 05 May 2023.
Edited by:
Daniela Yaffar, University of Hamburg, GermanyReviewed by:
Lilli Zeh, Technical University Dresden, GermanyMonique Weemstra, Florida International University, United States
Copyright © 2023 Bucher, Ofiti and Malhotra. This is an open-access article distributed under the terms of the Creative Commons Attribution License (CC BY). The use, distribution or reproduction in other forums is permitted, provided the original author(s) and the copyright owner(s) are credited and that the original publication in this journal is cited, in accordance with accepted academic practice. No use, distribution or reproduction is permitted which does not comply with these terms.
*Correspondence: Avni Malhotra, YXZuaS5tYWxob3RyYUBnZW8udXpoLmNo