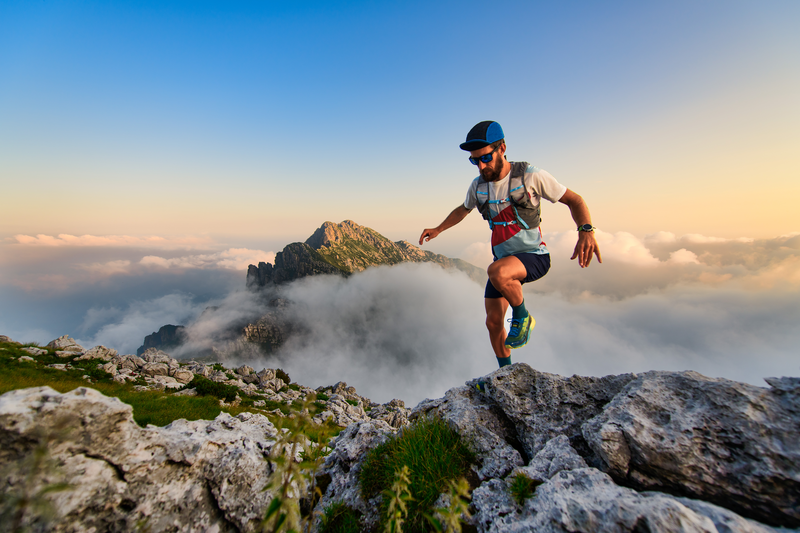
95% of researchers rate our articles as excellent or good
Learn more about the work of our research integrity team to safeguard the quality of each article we publish.
Find out more
ORIGINAL RESEARCH article
Front. For. Glob. Change , 25 April 2023
Sec. Forest Ecophysiology
Volume 6 - 2023 | https://doi.org/10.3389/ffgc.2023.1150413
This article is part of the Research Topic Understanding Forest Ecosystems: The Use of Stable Isotopes and Physiological Measurements View all 6 articles
The relationships among stand density and seasonal plant water source patterns in ponderosa pine (Pinus ponderosa) forests are important for informed management decisions in a bimodal climate with increasing variability in winter and monsoon precipitation inputs. Winter precipitation recharges soil moisture, yet it has declined over the past 20 years in the southwestern United States, and monsoon precipitation is becoming more variable in both magnitude and timing. Near Flagstaff, Arizona in August 2013 (monsoon), October 2013 (post-monsoon), and May 2014 (post winter snow melt), we measured soil moisture, soil water δD at five depths, and xylem water δD in Muhlenbergia montana (a perennial C4 grass), Festuca arizonica (a perennial C3 grass), and P. ponderosa seedlings (< 2 years old), saplings (2–5 cm basal diameter), and mature trees (> 60 cm diameter at breast height) in treated (thinned and burned) and untreated (no thinning, no burning) stands. We found that soil moisture was higher at all soil depths in treated stands in May, after snow melt, and this pattern persisted through August in the deepest soil (60 cm). We also found that, in all sampling months, δD in xylem water of grasses and pine seedlings indicated use of shallower soil water than for pine saplings and mature trees, presumably due to differences in rooting depths. Additionally, in August, δD in xylem water of pine saplings and mature trees indicated greater reliance on a deeper water source in untreated stands than in treated stands, likely due to greater competition for shallow water in untreated stands. Our isotopic data indicate that grasses and seedlings used predominantly monsoon water in August and October, while pine saplings and mature trees used predominantly winter water during all sampling months. Importantly, our data indicate that regenerating trees (seedlings and saplings) used both winter and monsoon seasonal water sources, suggesting an increasingly important role for monsoon precipitation if winter precipitation inputs continue to decline. Collectively, these findings demonstrate that management actions can benefit forests via increased soil moisture, that overstory trees rely predominantly on winter precipitation, and that monsoon precipitation is important for herbaceous species and younger, regenerating overstory trees.
Many coniferous forests in the western United States are overly dense due to past fire suppression and overgrazing, making them more vulnerable to the impacts of drought, pests, and wildfire (Covington et al., 1997). Management treatments including thinning and prescribed burning are therefore widespread across the region, as they can improve forest health, resilience, and productivity, as well as reduce fuel loads and fire risks (Fulé et al., 2001; van Mantgem et al., 2020). In terms of ecohydrology, thinning treatments can increase soil moisture via enhanced throughfall (Stogsdill et al., 1989; Simonin et al., 2007; Cardil et al., 2018; del Campo et al., 2022), thereby benefitting understory herbaceous species (Moore et al., 2006) as well as overstory tree water status, gas exchange, foliar nitrogen content (Kolb et al., 1998; Skov et al., 2004), and growth (Skov et al., 2005).
Precipitation in the southwestern U.S. (“Southwest”) is bimodal: winter storms originate from the Gulf of Alaska and bring cold air and moisture (as snow at higher elevations) to the region, while summer monsoon rains originate from the Gulf of Mexico and Gulf of California (Douglas et al., 1993; Adams and Comrie, 1997; Higgins et al., 1997; Leavitt et al., 2011). Because they occur sporadically during the summer (July through September) when evaporation rates and plant water use are high, monsoon rains have limited ability to penetrate to deep soil layers (Simpson et al., 1972), although an above-average monsoon can do so. Contrastingly, winter rain and snow fall steadily over a longer period (October through March) when evaporation rates are low and can recharge the soil profile to greater depth (Williams and Ehleringer, 2000). These two water sources have distinct isotopic compositions, with winter snows being relatively depleted of heavy isotopes (D and 18O) and monsoon rains being relatively enriched (Dutton et al., 2005). These precipitation differences can be used to determine plants’ relative reliance on winter and summer precipitation inputs (Ehleringer and Dawson, 1992).
In the Southwest, shallowly rooted plants generally use summer precipitation while deeply rooted plants rely on deep, winter-derived soil moisture (Ehleringer et al., 1991). Climate change has influenced both of these seasonal water inputs: winter precipitation and snowpack in the western United States have decreased in recent decades (Mote et al., 2018), while monsoon rains have become increasingly irregular (Prein et al., 2022) and are likely to weaken (Hoell et al., 2022) in response to future global warming (Pascale et al., 2017). Additionally, the region is predicted to become increasingly arid as temperatures rise (Seager et al., 2007; Seager and Vecchi, 2010; Jones and Gutzler, 2016; Prein et al., 2016; Seager and Ting, 2017; Mankin et al., 2021). This decrease in winter precipitation coupled with warming temperatures will likely increase plant water stress and forest susceptibility to fire, particularly in ponderosa pine (Pinus ponderosa) forests dominating much of the region.
While past work indicates that, in northern Arizona, mature P. ponderosa trees predominantly rely on winter precipitation from deeper soil layers (∼40 cm) throughout the year (Kerhoulas et al., 2013, 2017), little is known about the reliance of herbaceous understory plants or regenerating P. ponderosa seedlings and saplings on winter and monsoon moisture and if and how thinning treatments can influence these trends. For example, tree regeneration following widespread forest mortality related to drought, pests, and/or fire can be limited by hot, dry climate and also by altered stand structures with limited seed trees (Rodman et al., 2020, 2022; May et al., 2022). It seems likely that although overstory trees may rely on winter precipitation inputs in deeper soils, germination and establishment of shallowly-rooted regenerating seedlings and saplings may depend on monsoon precipitation inputs to surface soils. To test this, we investigated seasonal water use patterns in two common, native perennial grass species, Muhlenbergia montana (C4 grass) and Festuca arizonica (C3 grass), as well as P. ponderosa seedlings, saplings, and mature trees in thinned and unthinned forests in northern Arizona. We posed two questions: (1) To what extent is thinning able to increase forest soil moisture and how does this effect vary across the year? (2) To what extent do grasses, seedlings, saplings, and mature trees rely on winter and monsoon precipitation inputs and how do these trends vary across the year? For the first question, we hypothesized that thinning would increase soil moisture due increased snowpack accumulation and fewer trees using soil water and that this effect would be least pronounced during the monsoon season due to increased solar radiation and soil water evaporation in treated stands. For the second question, we hypothesized that during the spring after snowmelt, all plants would use winter water, while during the monsoon season, due to differences in rooting depths, grasses and seedlings would use shallow monsoon-derived water, saplings would use a mix of monsoon and winter waters, and trees would use deep winter-derived water.
Study trees are located in the Fort Valley Experimental Forest roughly 10 km northwest of Flagstaff, Arizona (N35°15′58″,W111°42′1″) at 2200 m ASL. In 1998, the study site was experimentally thinned to four average basal area densities: control 38 m2 ha–1, light thinning 22 m2 ha–1, moderate thinning 18 m2 ha–1, and heavy thinning 16 m2 ha–1, with each treatment replicated in three 17 ha units (Skov et al., 2005). We used three heavily thinned and three control plots, hereafter “thinned” and “unthinned,” respectively. Soils at this site are classified as basaltic, fine montmorillonitic complex of frigid Typic Argiborolls and Mollic Eutroboralfs (Mast et al., 1999); all plots were in close proximity to one another such that soils were considered comparable among plots. Sampling occurred in 2013 and 2014. Notably, 2013 had a record-setting high amount of monsoon precipitation, with 399 mm compared to the 2011–2021 mean of 220 ± 1 mm1 coupled with only 102 mm of winter precipitation, far below the 1991–2020 mean of 203 mm (PRISM Climate Group, Oregon State University)2.
All soil and xylem sampling occurred in August 2013 (during the monsoon), October 2013 (after the monsoon), and May 2014 (after winter snow melt). In each plot, five mature P. ponderosa trees were randomly selected for a total of 15 trees per treatment (thinned and unthinned). Soil sampling occurred within 30 feet of each study tree, where a 60 cm hole was dug (as possible, we could only dig to 60 cm at approximately 33% of the study trees due to encountering a rock layer or hardpan) and soil was sampled at five depths: 0–5, 9–11, 19–21, 39–41, and 59–61 cm; hereafter 5, 10, 20, 40, and 60 cm depths, respectively. As such, the 60 cm soil findings are based on a reduced sample size. Soil samples were double-bagged in ziplock plastic bags. In each sampling month, xylem samples were collected from C4 grass M. montana, C3 grass F. arizonica, and P. ponderosa seedlings (< 2 years old, as confirmed by tree-rings), saplings (2–5 cm basal diameter), and trees [diameter at breast height (DBH, 1.37 m) > 60 cm]; hereafter “grasses,” “seedlings,” “saplings,” and “trees”, respectively. Within a 5 m radius around each study tree, grasses, seedlings, and saplings were sampled, as present. For grasses, the clump of M. montana and of F. arizonica nearest to each study tree was sampled. To avoid evaporative enrichment, from each grass sample all photosynthetic material was removed and the belowground corm and root mass were placed in a glass screw top vial and sealed with wax film. Surrounding each study tree, the closest seedling was removed from the soil and the stem (without leaves) and root mass (to ensure a sufficient water sample, given the small stem size) were placed in a glass screw top vial and sealed with wax film. For saplings, stems were cut, placed in glass screw top vials, and sealed with wax film. For trees, a 5-mm increment borer was used to collect one breast height core from each study tree; cores were immediately placed in glass screw top vials and sealed with wax film. All soil and xylem samples were placed in a cooler while in the field and then transported to a 0°C freezer at the Arizona Climate and Ecosystems (ACE) Isotope Laboratory (formerly Colorado Plateau Stable Isotope Lab) at Northern Arizona University (NAU) until water extraction.
All field samples were stored, extracted, and isotopically analyzed at the NAU ACE Isotope Lab. Water was extracted from all samples on a cryogenic vacuum extraction line for 1 h (West et al., 2006). Although some recent work (e.g., Chen et al., 2020) has identified potential biases in plant source water extractions using a cryogenic vacuum line, this method has been used for decades for ecohydrological research and methodological fractionation seems to be negligible, particularly when working with natural abundance isotopes (Allen and Kirchner, 2022; Diao et al., 2022). Unfortunately, some of our August samples were lost due to complications with the cryogenic vacuum extraction process: 5 cm soil, 10 cm soil, and seedlings. For soils, glass wool was used during extraction to prevent particles from moving into the vacuum line and samples were weighed before and after water extraction to calculate % moisture using the following equation:
All extracted water samples were immediately placed in 2 ml screw top glass vials and stored in a refrigerator until isotopic analysis. Isotopic measurements were conducted using ring down spectroscopy on an off-axis cavity output spectrometer [Los Gatos Research Inc. (LGR), Mountain View, CA, USA] with a δD precision of 0.10‰. Although extracted stem water of some species can contain compounds that interfere with accurate laser-based δD measurements, this is a minor issue for Pinus and the effect is small for this genus (0.4‰) relative to the differences in δD that we report. Further, δD of neither grass, pine, nor soil water samples differed by more than 1‰ when analyzed by LGR versus isotope ratio mass spectrometry (IRMA) in a methodological comparison (West et al., 2010). All δD stable isotope values are reported in “delta” notation, which expresses the isotopic composition of a material relative to that of an accepted standard (Vienna Standard Mean Ocean Water, VSMOW) on a per mil (‰) basis:
where δ is the isotope ratio and Rsample and Rstandard are the molar ratios of heavy to light isotopes in the sample and the standard, respectively.
We used natural log regressions to estimate plant source water depths, a mixing model to calculate the % of monsoon water in soil and xylem waters, and 2-Way Analysis of Variance (ANOVA) to evaluate the significance of different effects on soil and xylem waters. To estimate plant source water depths, we used the isotopic signatures of soil water from different depths (20 to 60 cm for August due to loss of 5 and 10 cm samples during extraction; 0 to 60 cm for October and May) around each study tree to create a tree-specific logarithmic regression relating soil water δD to soil depth. Using this regression with xylem water isotopic signatures, we estimated, for each sampling month, the source water depth for each study tree and the two grasses, seedling, and sapling sampled by the study tree. To estimate the % of monsoon water in soil and xylem water samples, a two-source mixing model (Dawson et al., 2002) was used:
where δsample is the isotopic signature of soil or xylem, δwinter and δmonsoon are the mean δD signatures for winter (−91‰) and monsoon (−31‰) precipitation (Kerhoulas et al., 2013), respectively, and fmonsoon is the proportion of soil/xylem water contributed by monsoon precipitation. Importantly, while this mixing model is helpful to identify relative reliance on seasonal water inputs, we note that in this system, plant source waters within the soil profile are also influenced by rooting depths and evaporative enrichment at shallow depths; model results therefore require careful interpretation. For example, relatively enriched isotopic soil water δD values in May might not indicate greater monsoon water but rather greater evaporative enrichment. Seasonal precipitation δD values used for our analyses were based on precipitation samples collected in Flagstaff, Arizona in 2009 and 2010: monsoon (July through August) = −31 ± 6‰ and winter (October through March) = −91 ± 8‰ (Kerhoulas et al., 2013). For each sampling month, 2-Way ANOVAs were performed to evaluate the significance of treatment, depth, and treatment depth interactions on soil water and of treatment, sample (two grasses, seedlings, saplings, and trees), and treatment sample interactions on xylem water. To test the normality assumption, the Shapiro-Wilk goodness-of-fit test was used; when this assumption was violated, the Wilcoxon / Kruskal-Wallis test was used to evaluate effect significance. To test the equal variance assumption, Levene and Bartlett tests were used; when this assumption was violated, the Welch test was used to evaluate effect significance. All statistical analyses were conducted using JMP (SAS Institute, Cary, NC, USA). If an effect was found to be significant (p < 0.05), Tukey’s Honestly Significant Difference (HSD) test was used to identify differences among groups.
Mean soil moisture ranged from 9 to 25% and varied significantly with treatment and depth (Figure 1 and Table 1). In August, there was a significant interaction between treatment and depth (p = 0.03), with soil moisture at the 60 cm depth being significantly higher in thinned stands compared to unthinned stands. Notably, in unthinned stands, August soil moisture at 60 cm was lower than at 20 and 40 cm depths. In October, depth was a significant effect on soil moisture (p = 0.01), with 40 cm having the highest water content compared to 5 and 10 cm depths. In May, treatment (p < 0.0001) and depth (p < 0.0001) were both significant effects on soil moisture, with a significant interaction between the two effects (p = 0.01). As such, soil moisture was higher in thinned stands compared to unthinned stands at all depths except for 20 cm and increased with depth.
Figure 1. Mean (± SE) soil moisture (left column), soil water δD (middle column), and the monsoon contribution to soil water (right column) at the Fort Valley Experimental Forest near Flagstaff, AZ, USA. Sampling occurred in August 2013 (top row of panels), October 2013 (middle row of panels), and May 2014 (bottom row of panels). Within each panel, asterisks denote a significant difference between thinned and unthinned treatments, and depths not sharing the same letter are significantly different. In the soil water δD panels (middle column), local monsoon (July through September; δD = –31 ± 6) and winter (October through March; δD = –91 ± 8) precipitation δD values are shown in orange and blue bars, respectively (Kerhoulas et al., 2013). Data are missing from 5 and 10 cm depths in August due to complications with the cryogenic vacuum extraction process.
Table 1. Mean (± SE) soil moisture, isotopic composition, and monsoon proportions in August 2013, October 2013, and May 2014.
Mean soil water δD signature ranged from −91 to −64‰ and varied significantly with treatment and depth (Figure 1 and Table 1). In August, treatment (p = 0.005) and depth (p < 0.0001) were significant effects on soil water δD; δD was significantly more enriched in unthinned stands compared to thinned stands at the 20 and 40 cm depths and was significantly more enriched at the 20 cm depth compared to the 40 and 60 cm depths. In October, δD was essentially invariable between treatments (p = 0.32) and among depths (p = 0.13). In May, treatment (p < 0.0001) and depth (p < 0.0001) were significant effects on soil water δD, with water being significantly more enriched in unthinned stands compared to thinned stands and δD depleting with depth.
Evaluating soil moisture in the context of percent monsoon water, trends closely mirrored the above δD soil water trends, with mean monsoon precipitation contribution to soil water ranging from 2 to 45% and treatment and depth both acting as significant effects (Figure 1 and Table 1). In August, treatment (p = 0.002) and depth (p < 0.0001) were significant effects on the proportion of monsoon rain in soil water; unthinned stands had significantly higher monsoon inputs in soil water compared to thinned stands at the 20 and 40 cm depths and the proportion of monsoon rain decreased with depth. In October, monsoon precipitation accounted for approximately 10–20% of soil water and did not significantly vary between treatments (p = 0.30) or among depths (p = 0.11). In May, treatment (p < 0.0001) and depth (p < 0.0001) both significantly influenced the proportion of monsoon rain in soil water, with monsoon inputs being higher in unthinned stands compared to thinned stands and decreasing with depth.
Mean xylem water δD ranged from −85 to −36‰ and varied significantly among plant samples (two grass species and P. ponderosa seedlings, saplings, and mature trees) but was not significantly influenced by treatment (Figure 2 and Table 2). In August, sample significantly (p < 0.0001) affected xylem water δD, with the two grass species having more enriched signatures compared to saplings and mature trees, and mature trees having more depleted signatures than saplings. In October, sample significantly (p < 0.0001) affected xylem water δD, with the two grasses and seedlings having enriched signatures compared to saplings and mature trees. In May, sample significantly (p < 0.0001) affected xylem water δD, with M. montana (C4 grass) and seedlings having the most enriched signatures, followed closely by F. arizonica (C3 grass), and saplings and mature trees having the most depleted signatures.
Figure 2. Mean (± SE) source water depth, xylem water δD, and the monsoon contribution to xylem water at the Fort Valley Experimental Forest near Flagstaff, AZ, USA. Sampling occurred in August 2013 (top row of panels), October 2013 (middle row of panels), and May 2014 (bottom row of panels). Within each panel, asterisks denote a significant difference between thinned and unthinned treatments, and samples not sharing the same letter are significantly different. Samples include: Muhlenbergia montana (MUMO), Festuca arizonica (FEAZ), Pinus ponderosa seedlings, P. ponderosa saplings, and P. ponderosa mature trees (PIPO). In the xylem water δD panels (middle column), local monsoon (July through September; δD = –31 ± 6) and winter (October through March; δD = –91 ± 8) precipitation δD values are shown in orange and blue bars, respectively (Kerhoulas et al., 2013); mean soil water δD values are also shown as different horizontal lines for the 5, 10, 20, 40, and 60 cm soil depths (thinned and unthinned stands pooled). Data are missing from seedlings and the 5 and 10 cm soil depths in August due to complications with the cryogenic vacuum extraction process.
Table 2. Mean (± SE) xylem water data from the Fort Valley Experimental Forest near Flagstaff, AZ, USA.
Our modeled source water depths based on xylem and soil water δD signatures ranged from 0 to 60 cm (on average) and found that sample and treatment had significant effects on source water depth (Figure 2 and Table 2). In August, treatment (p < 0.0001) and sample (p < 0.0001) were significant effects on source water depth, with a significant interaction between the two effects (p = 0.0001). During the monsoon, August source water depths were at the soil surface for the two grass species and were significantly deeper for saplings and mature trees; saplings and mature trees in unthinned stands used water from deeper depths (58 ± 13 cm and 60 ± 14 cm, respectively) compared to saplings and mature trees in thinned stands (12 ± 2 cm and 35 ± 3 cm, respectively). In October, source water depths varied significantly among samples (p < 0.0001), with grasses and seedlings using surface soil waters, saplings using water from 17 ± 5 cm depth, and mature trees using water from 31 ± 9 cm depth. In May, source water depths significantly varied among samples (p < 0.0001), with grasses and seedlings using surface soil waters, saplings using water from 24 ± 4 cm depth, and mature trees using water from 35 ± 4 cm depth.
Evaluating xylem water in the context of percent monsoon water, monsoon contribution trends closely mirrored the above δD xylem water trends, with mean contribution ranging from 10 to 90% and significantly varying among samples (Figure 2 and Table 2). In August, the monsoon contribution to xylem water varied significantly among samples (p < 0.0001), with monsoon inputs representing 87 ± 2% of grass xylem water, 27 ± 2% of sapling xylem water, and 11 ± 1% of mature tree water. In October, the monsoon contribution to xylem water varied significantly among samples (p < 0.0001), with monsoon inputs representing 68 ± 2% of grass xylem water, 67 ± 4% of seedling xylem water, 20 ± 2% of sapling xylem water, and 14 ± 2% of mature tree water. In May, the monsoon contribution to xylem water varied significantly among samples (p < 0.0001), with monsoon inputs representing 63 ± 3% of grass xylem water, 73 ± 4% of seedling xylem water, 13 ± 3% of sapling xylem water, and 10 ± 1% of mature tree water.
Our findings indicate that while mature P. ponderosa trees rely primarily on winter precipitation inputs, understory grasses and seedlings use substantial amounts of monsoon rain water when it is available. Thus, to perpetuate P. ponderosa forests, both seasonal water inputs are critical. This study also demonstrates that thinning can increase soil moisture throughout the year, particularly in deeper soil layers that recharge via winter snows and from which mature trees appear to draw the bulk of their water. Moving into the future, as winter snow will likely continue to decrease (Seager and Vecchi, 2010; Mote et al., 2018) and the monsoon system will likely weaken (Pascale et al., 2017; Prein et al., 2022), improved understanding about the timing and extent of forest reliance on these seasonal precipitation inputs and if/how management efforts can maximize water availability is important for effective land stewardship.
A major finding of our work is that thinned stands had higher soil moisture than unthinned stands, presumably due to reduced canopy interception of snow coupled with reduced overstory water use. More specifically, in August, deep soil water (60 cm) was greater in thinned compared to unthinned stands. In unthinned stands, the low August 60 cm soil moisture (compared to 20 and 40 cm depths) suggests that winter water was depleted more quickly in these dense stands than in more open, thinned stands where 60 cm soil moisture was greatest.
Interestingly, in October, neither soil moisture nor monsoon contribution varied significantly among soil depths or between treatments, possibly indicating that by the end of the growing season, differences in soil water recharge, overstory water use, understory water use, and evaporation had balanced out to yield a uniform water availability throughout the soil, regardless of stand structure. Notably, 2013 was an above-average monsoon season following a winter with below-average precipitation. The fact that post-monsoon (October) deep (40–60 cm) soil moisture ranged from 21 to 25%, comparable to May 2014 soil moisture (19–29%) immediately after snowmelt, indicates that an above-average monsoon season can replenish deep soil water and that thinning treatments can increase this recharge.
Similar to August, in May, thinned stands had higher soil moisture at all soil depths compared to unthinned stands, soil moisture increased with depth, and unthinned stands had more enriched isotopic signatures throughout the soil compared to thinned stands. Although the soil water isotopic data and mixing model indicate greater monsoon water in unthinned than thinned stands in August and May, these patterns are likely driven by reduced winter snowpack in unthinned, dense stands yielding a relatively enriched soil water signature (Kerhoulas et al., 2013). For example, the relatively enriched May soil water δD in unthinned stands is likely driven by reduced snowpack accumulation under the dense canopy rather than a relatively high amount of monsoon rain input, as residual monsoon water in the soil from the previous summer is likely negligible after winter snow melt. Thus, these trends in soil moisture and isotopic composition collectively can be interpreted to indicate that thinned stands receive greater winter snowpack accumulation, which results in greater, more long-lasting recharge of deep soil water.
Another major finding of our work is that grasses and P. ponderosa seedlings used almost exclusively shallow soil water with a high proportion of monsoon input, while P. ponderosa saplings and trees used almost exclusively deep soil water with a high proportion of winter precipitation input. Stem water δD in understory grasses M. montana (C4) and F. arizonica (C3) and pine seedlings was largely reflective of monsoon rain use, particularly in August (during the monsoon) and October (immediately after the monsoon). Because germinants often suffer high mortality due to desiccation in the first summer (Cui and Smith, 1991), this is a notably vulnerable stage of life. Further, seedlings have limited space for water storage and also lack well established root systems and/or mycorrhizal partnerships for maximizing water uptake, making them particularly vulnerable to desiccation. Thus, our isotope-based finding that seedlings predominantly use shallow water highlights the importance of summer rains for the successful establishment of new overstory cohorts.
Unlike our soil water findings, thinning treatments had little effect on understory and overstory water source patterns, except in August when saplings and trees in unthinned stands used significantly deeper water than counterparts in thinned stands, suggesting that high competition stimulated deeper rooting or greater reliance on water accessed by deep roots. Pairing this finding of relatively deep August water use by saplings and trees in unthinned stands with our finding of relatively high deep (60 cm) August soil moisture in thinned stands suggests that greater snowpack and reduced overstory water use after thinning extended the persistence of deep, winter-derived soil water throughout the growing season in thinned stands. In other words, saplings and trees in dense, unthinned stands likely had less snow-derived water input and greater competition for soil water such that by August, they were having to seek water at deeper depths compared to saplings and trees in more open, thinned stands with greater winter water input and less competition for this water. For saplings, while pre- (May) and post-monsoon (October) xylem water δD did not significantly differ from mature trees, during the monsoon, saplings had enriched August xylem water δD with greater monsoon contribution than mature trees, suggesting that these regenerating overstory trees use monsoon water inputs when available. Additionally, by using shallower water in August, saplings in thinned stands used (insignificantly) more monsoon water than saplings in unthinned stands, possibly indicating that treatments can enhance the use of summer water inputs (although our soil water δD data did not measure increased monsoon inputs in thinned stands). We note that further study is needed to improve understanding about how treatment influences source water depth, as a significant treatment effect was detected on sapling and tree source water depths in August but not on their corresponding xylem water δD values (Figure 2).
Our study highlights the importance of winter and monsoon precipitation for P. ponderosa forests and how management can possibly enhance forest resilience. As hypothesized, we found that forest thinning can benefit understory and overstory plants by increasing soil water availability and that this effect was most pronounced in the spring following snowmelt. More specifically, we found that decreased stand density fostered greater soil moisture at deep (60 cm) soil depths during the summer monsoon (August) and at all soil depths immediately after snowmelt in the spring (May). This management-facilitated increase in soil moisture could have a profound effect on forest resilience as the Southwest becomes warmer and drier. Our investigation of seasonal water source patterns among grasses, seedlings, saplings, and mature trees offers new insights on the importance of winter snows and monsoon rains. Broadly, our data illustrate that grasses and seedlings use very shallow soil water while saplings and mature trees use much deeper soil waters. In support of our hypothesis, we demonstrate that both seasonal precipitation inputs are important for regenerating P. ponderosa, with seedlings primarily relying on shallow soil waters largely derived from monsoon rains and saplings primarily relying on deep soil waters primarily derived from winter snowmelt but also using some shallow soil water derived from monsoon rains in August. This finding of seedling and sapling monsoon water use highlights the importance of this seasonal water source for new overstory recruitment, especially if winter snowpack continues to decrease. Remarkably, and somewhat surprisingly, our results indicate that even in years with low winter precipitation and high monsoon precipitation, mature trees at this site primarily rely on deep, winter-derived water throughout the year, even during the monsoon season. In summary, this study demonstrates that thinning forests can increase soil water availability for understory and overstory plants, conifer seedlings predominantly use monsoon water, and mature conifers predominantly use winter water; these findings are important as we strive to effectively manage and perpetuate resilient forests in the face of changing climate and fire regimes.
The raw data supporting the conclusions of this article will be made available by the authors, without undue reservation.
GK and NU designed the study. NU led the field and lab work. NU and LK analyzed the data and wrote the first draft of the manuscript. All authors contributed to the article and approved the final draft before submission.
We thank Jamie Brown and the Arizona Climate and Ecosystems (ACE) Isotope Laboratory at Northern Arizona University for assistance with lab work. We also thank two reviewers for their thoughtful suggestions that greatly improved this manuscript.
The authors declare that the research was conducted in the absence of any commercial or financial relationships that could be construed as a potential conflict of interest.
All claims expressed in this article are solely those of the authors and do not necessarily represent those of their affiliated organizations, or those of the publisher, the editors and the reviewers. Any product that may be evaluated in this article, or claim that may be made by its manufacturer, is not guaranteed or endorsed by the publisher.
Adams, D., and Comrie, A. (1997). The North American monsoon. Bull. Am. Meteorol. Soc. 78, 2197–2213. doi: 10.1175/1520-0477(1997)078<2197:TNAM>2.0.CO;2
Allen, S. T., and Kirchner, J. W. (2022). Potential effects of cryogenic extraction biases on plant water source partitioning inferred from xlyem-water isotope ratios. Hydrol. Process 36:e14483. doi: 10.1002/hyp.14483
Cardil, A., Imbert, J., Camarero, J., Primicia, I., and Castillo, F. (2018). Temporal interactions among throughfall, type of canopy and thinning drive radial growth in an Iberian mixed pine-beech forest. Agric. For. Meteorol. 252, 62–74. doi: 10.1016/j.agrformet.2018.01.004
Chen, Y., Helliker, B., Tang, X., Li, F., Zhou, Y., and Song, X. (2020). Stem water cryogenic extraction biases estimation in deuterium isotope composition of plant source water. Proc. Natl. Acad. Sci. 117, 33345–33350. doi: 10.1073/pnas.2014422117
Covington, W., Fulé, P., Moore, M., Hart, S., Kolb, T., Mast, J., et al. (1997). Restoring ecosystem health in ponderosa pine forests of the Southwest. J. For. 95, 23–29.
Cui, M., and Smith, W. (1991). Photosynthesis, water relations and mortality in Abies lasiocarpa seedlings during natural establishment. Tree Physiol. 8, 37–46. doi: 10.1093/treephys/8.1.37
Dawson, T., Mambelli, S., Plamboeck, A., Templer, P., and Tu, K. (2002). Stable isotopes in plant ecology. Annu. Rev. Ecol. Syst. 33, 507–559. doi: 10.1146/annurev.ecolsys.33.020602.095451
del Campo, A., Otsuki, K., Serengil, Y., Blanco, J., Yousefpour, R., and Wei, X. (2022). A global synthesis on the effects of thinning on hydrological processes: implications for forest management. For. Ecol. Manag. 519:120324. doi: 10.1016/j.foreco.2022.120324
Diao, H., Schuler, P., Goldsmith, G., Siegwolf, R., Saurer, M., and Lehmann, M. (2022). Technical note: on uncertainties in plant water isotopic composition following extraction by cryogenic vacuum distillation. Hydrol. Earth Syst. Sci. 26, 5835–5847. doi: 10.5194/hess-26-5835-2022
Douglas, M., Maddox, R., Howard, K., and Reyes, S. (1993). The Mexican monsoon. J. Clim. 6, 1665–1677. doi: 10.1175/1520-0442(1993)006<1665:TMM>2.0.CO;2
Dutton, A., Wilkinson, B., Welker, J., Bowen, G., and Lohmann, K. (2005). Spatial distribution and seasonal variation in 18O/16O of modern precipitation and river water across the conterminous USA. Hydrol. Process. 19, 4121–4146. doi: 10.1002/hyp.5876
Ehleringer, J., and Dawson, T. E. (1992). Water uptake by plants: perspectives from stable isotope compmosition. Plant Cell Environ. 15, 1073–1082. doi: 10.1111/j.1365-3040.1992.tb01657.x
Ehleringer, J., Phillips, S., Schuster, W., and Sandquist, D. (1991). Differential utilization of summer rains by desert plants. Oecologia 88, 430–434. doi: 10.1007/BF00317589
Fulé, P., Waltz, A., Covington, W., and Heinlein, T. (2001). Measuring forest restoration effectiveness in reducing hazardous fuels. J. For. 99, 24–29.
Higgins, R., Yao, Y., and Wang, X. (1997). Influence of the North American monsoon system on the U.S. summer precipitation regime. J. Clim. 10, 2600–2622. doi: 10.1175/1520-0442(1997)010<2600:IOTNAM>2.0.CO;2
Hoell, A., Quan, X.-W., Hoerling, M., Fu, R., Mankin, J., Simpson, I., et al. (2022). Record low North American monsoon in 2020 reignites drought over the American Southwest. Bull. Am. Meteorol. Soc. 103, S26–S32. doi: 10.1175/BAMS-D-21-0129.1
Jones, S., and Gutzler, D. (2016). Spatial and seasonal variations in aridification across the Southwest North America. J. Clim. 29, 3639–4637. doi: 10.1175/JCLI-D-14-00852.1
Kerhoulas, L., Kolb, T., and Koch, G. (2013). Tree size, stand density, and the source of water used across seasons by ponderosa pine in northern Arizona. For. Ecol. Manag. 289, 425–433. doi: 10.1016/j.foreco.2012.10.036
Kerhoulas, L., Kolb, T., and Koch, G. (2017). The influence of monsoon climate on latewood growth of Southwestern ponderosa pine. Forests 8:140. doi: 10.3390/f8050140
Kolb, T., Holmberg, K., Wagner, M., and Stone, J. (1998). Regulation of ponderosa pine foliar physiology and insect resistance mechanisms by basal area treatments. Tree Physiol. 18, 375–381. doi: 10.1093/treephys/18.6.375
Leavitt, S., Woodhouse, C., Castro, C., Write, W., Meko, D., Touchan, R., et al. (2011). The North American monsoon in the U.S. Southwest: potential for investigation with tree-ring carbon isotopes. Quat. Int. 235, 101–107. doi: 10.1016/j.quaint.2010.05.006
Mankin, J., Simpson, I., Hoell, A., and Barlow, M. (2021). NOAA Drought Task Force report on the 2020-2021 Southwestern U.S. Drought. Available online at: https://www.drought.gov/sites/default/files/2021-09/NOAA-Drought-Task-Force-IV-Southwest-Drought-Report-9-23-21.pdf (accessed September 21, 2021).
Mast, J., Fulé, P., Moore, M., Covington, W., and Waltz, A. (1999). Restoration of presettlement age structure of an Arizona ponderosa pine forest. Ecol. Appl. 9, 228–239. doi: 10.1890/1051-0761(1999)009[0228:ROPASO]2.0.CO;2
May, C., Zald, H., North, M., Gray, A., and Hurteau, M. (2022). Repeated burns fail to restore pine regeneration to the natural range of variability in a Sierra Nevada mixed-conifer forest, USA. Restorat. Ecol. e13863. doi: 10.1111/rec.13863
Moore, M., Casey, C., Bakker, J., Springer, J., Fulé, P., Covington, W., et al. (2006). Herbaceous vegetation responses (1992-2004) to restoration treatments in a ponderosa pine forest. Rangeland Ecol. Manag. 59, 135–144. doi: 10.2111/05-051R2.1
Mote, P., Li, S., Lettenmaier, D., Xiao, M., and Engel, R. (2018). Dramatic declines in snowpack in the western US. Npj Clim. Atmos. Sci 1, 1–6. doi: 10.1038/s41612-018-0012-1
Pascale, S., Boos, W., Bordoni, S., Llewellyn, D., Baker, S., Tighi, S., et al. (2017). Weakening of the North American monsoon with global warming. Nat. Clim. Change 7, 806–812. doi: 10.1038/nclimate3412
Prein, A., Holland, G., Rasmussen, R. M., Clark, M. P., and Tye, M. R. (2016). Running dry: the U.S. Southwest’s drift into a drier climate state. Geophys. Res. Lett. 43, 1–8. doi: 10.1002/2015GL066727
Prein, A., Towler, E., Ge, M., Llewellyn, D., Baker, S., Tighi, S., et al. (2022). Sub-seasonal predictability of North American Monsoon Precipitation. Geophys. Res. Lett. 49:e2021GL095602. doi: 10.1029/2021GL095602
Rodman, K., Andrus, R., Carlson, A. R., Carter, T. A., Chapman, T. B., Coop, J. D., et al. (2022). Rocky Mountain forests are poised to recover following bark beetle outbreaks but with altered composition. J. Ecol. 110, 2929–2949. doi: 10.1111/1365-2745.13999
Rodman, K., Veblen, T., Battaglia, M., Chamber, M., Fornwalt, P., Holden, Z., et al. (2020). A changing climate is snuffing out post-fire recovery in montane forests. Glob. Ecol. Biogeogr. 29, 2039–2051. doi: 10.1111/geb.13174
Seager, R., and Ting, M. (2017). Decadal drought vulnerability over North America: mechanisms and predictability. Curr. Clim. Change Rep. 3, 141–149. doi: 10.1007/s40641-017-0062-1
Seager, R., Ting, M., Held, I., Kushnir, Y., Lu, J., Vecchi, G., et al. (2007). Model projections of an imminent transition to a more arid climate in southwestern North America. Science 316, 1181–1184. doi: 10.1126/science.1139601
Seager, R., and Vecchi, G. (2010). Greenhouse warming and the 21st century hydroclimate of southwestern North America. Proc. Natl. Acad. Sci. U. S. A. 107, 21277–21282. doi: 10.1073/pnas.0910856107
Simonin, K., Kolb, T., Montes-Helu, M., and Koch, G. (2007). The influence of thinning on components of stand water balance in a ponderosa pine forest stand during and after extreme drought. Agric. For. Meteorol. 143, 266–276. doi: 10.1016/j.agrformet.2007.01.003
Simpson, E., Thorud, D., and Friedman, I. (1972). Distinguishing seasonal recharge to groundwater by deuterium analysis in southern Arizona. World Water Bal. 3, 623–633.
Skov, K., Kolb, T., and Wallin, K. (2004). Tree size and drought affect ponderosa pine physiological response to thinning and burning treatments. For. Sci. 50, 81–91.
Skov, K., Kolb, T., and Wallin, K. (2005). Difference in radial growth response to restoration thinning and burning treatments between young and old ponderosa pine in Arizona. Western J. Appl. For. 20, 36–43. doi: 10.1093/wjaf/20.1.36
Stogsdill, W., Wittwer, R., Hennessey, T., and Dougherty, P. (1989). Relationship between throughfall and stand density in a Pinus taeda plantation. For. Ecol. Manag. 29, 105–113. doi: 10.1016/0378-1127(89)90059-5
van Mantgem, P. J., Kerhoulas, L. P., Sherriff, R. L., and Wenderott, Z. J. (2020). Tree-ring evidence of forest management moderating drought responses: Implications for dry coniferous forests in the southwestern United States. Front. For. Glob. Change 3:41. doi: 10.3389/ffgc.2020.00041
West, A., Goldsmith, G., Brooks, P., and Dawson, T. (2010). Discrepancies between isotope ratio infrared spectroscopy and isotope ratio mass spectrometry for the stable isotope analysis of plant and soil waters. Rapid Commun. Mass Spectrom. 24, 1948–1954. doi: 10.1002/rcm.4597
West, A., Patrickson, S., and Ehleringer, J. (2006). Water extraction times for plant and soil materials used in stable isotope analysis. Rapid Commun. Mass Spectrom. 20, 1317–1321. doi: 10.1002/rcm.2456
Keywords: grasses, monsoon rain, precipitation, saplings, seedlings, stable isotopes, trees, winter snow
Citation: Kerhoulas LP, Umstattd N and Koch GW (2023) Seasonal water source patterns in a northern Arizona pine forest. Front. For. Glob. Change 6:1150413. doi: 10.3389/ffgc.2023.1150413
Received: 24 January 2023; Accepted: 29 March 2023;
Published: 25 April 2023.
Edited by:
Patrick F. Sullivan, University of Alaska Anchorage, United StatesReviewed by:
Jessica Young-Robertson, University of Alaska Fairbanks, United StatesCopyright © 2023 Kerhoulas, Umstattd and Koch. This is an open-access article distributed under the terms of the Creative Commons Attribution License (CC BY). The use, distribution or reproduction in other forums is permitted, provided the original author(s) and the copyright owner(s) are credited and that the original publication in this journal is cited, in accordance with accepted academic practice. No use, distribution or reproduction is permitted which does not comply with these terms.
*Correspondence: Lucy P. Kerhoulas, bHVjeS5rZXJob3VsYXNAaHVtYm9sZHQuZWR1
Disclaimer: All claims expressed in this article are solely those of the authors and do not necessarily represent those of their affiliated organizations, or those of the publisher, the editors and the reviewers. Any product that may be evaluated in this article or claim that may be made by its manufacturer is not guaranteed or endorsed by the publisher.
Research integrity at Frontiers
Learn more about the work of our research integrity team to safeguard the quality of each article we publish.