- Guizhou Provincial Key Laboratory for Information Systems of Mountainous Areas and Protection of Ecological Environment, Guizhou Normal University, Guiyang, China
Litter decomposition is an essential process in nutrient cycling in terrestrial ecosystems. Phenolic acids have an allelopathic effect on the natural regeneration of rhododendron forests, which was recently observed in rhododendron forests in Southwest China. We investigated the distribution of phenolic acids and their relationship with soil nutrients during litter decomposition to provide a reference for the subsequent artificial management of rhododendron forests. High-performance liquid chromatography (HPLC) was used to analyze the contents of six phenolic acids in the litter layer, humus layer, and soil layer during litter decomposition. During the first 3 three months, the rapid decomposition of the litter from two early flowering rhododendron species released large amounts of phenolic acids, and the lower litter layer decomposed rapidly. In addition, the total phenolic acid content in the litter decreased by 257.60 μg/g (Rhododendron siderophyllum) and 53.12 μg/g (Rhododendron annae) in the first three 3 months. During subsequent litter decomposition, the protocatechuic acid content in the litters of Rhododendron siderophyllum ranged from 20.15 μg/g to 39.12 μg/g, and the ferulic acid content was 10.70 μg/g to –33.79 μg/g. The protocatechuic acid and ferulic acid contents in the litter of Rhododendron annae were in the ranges of 10.88—19.68 μg/g and 10.75—18.00 μg/g, respectively. The contents of these two phenolic acids and the trend of decomposition were different from those of gallic acid, chlorogenic acid, caffeic acid and syringic acid. The distribution of phenolic acids was influenced by soil organic matter (SOM), soil ammonium nitrogen (NH4+), soil nitrate nitrogen (NO3–) and soil available phosphorus (AP). The results indicate seasonal variations in phenolic acid release during litter decomposition. The amount of phenolic acid in the litter decreased after 18 months of decomposition, but it returned to the previous level in the soil and the humus after different trends. More research into the metabolism of phenolic acids is needed.
1. Introduction
Litter is a broad term for organic matter produced by aboveground plant components and returned to the ground to provide material and energy for decomposing agents while also maintaining ecosystem functions (Hättenschwiler et al., 2005). Litter decomposition in forest ecosystems provides nutrients to soil microorganisms and plant roots, representing an essential link in the biogeochemical cycle and energy flow of the ecosystem (Zechmeister-Boltenstern et al., 2015). Litter decomposition and transformation into soil occurs by leaching, crushing, and biodegradation. The three decomposition processes are tightly coupled to achieve nutrient release from litter (Wardle et al., 2004; Ristok et al., 2019). Research has shown that litter decomposition releases large amounts of phenolic acids, which are important secondary metabolites associated with plant growth (Zhang et al., 2019). In the natural environment, phenolic acids enter the environment through various mechanisms, such as plant root secretion (Wang et al., 2016), litter degradation (Chen H. et al., 2020), and microbial action (Pradisty et al., 2021). Litter decomposition is the primary method of phenolic acid release. In recent years, many phenolic acids have been isolated and identified as having allelopathic effects, such as ferulic acid, protocatechuic acid, chlorogenic acid, and gallic acid (Kalinova et al., 2007; Wang et al., 2017; Parmar et al., 2019). These phenolic acids have crucial ecological effects, influencing soil activity (Kobayashi, 2004; Hassan et al., 2021), causing plant autotoxicity (Liu et al., 2017), and changing plant competitiveness (Kato-Noguchi and Kurniadie, 2022). Studies have shown that soil factors directly affect phytotoxicity by controlling the content of secondary metabolites (Bosco et al., 2016; Scavo et al., 2019). These findings show that soil factors influence the ecological effects of plant secondary metabolites via pH changes, ion exchange, and microbial decomposition (Einhellig, 1987) and that secondary metabolites can influence soil conditions and nutrient cycling, affecting plant growth and development (Pal et al., 2015; Chauhan et al., 2021).
In the Baili Rhododendron National Forest Nature Reserve, Rhododendron annae Franch and Rhododendron siderophyllum Franch have an early flowering period and a high ornamental value. Phenolic acids released by litter decomposition are common secondary metabolites of plants and are an essential part of the forest ecosystem. The impact of phenolic acid accumulation in the environment and on soil fertility decline and plant growth renewal should not be overlooked (Ristok et al., 2017). Studies have shown large accumulations of understory rhododendron leaf litter and few seedlings in the Baili Rhododendron National Forest Nature Reserve. Researchers have carried out more studies from the perspective of aboveground plant ecology, such as stand structure and nutritional relationships (Fu et al., 2019; Tang et al., 2019). This study examines the contents and dynamic changes in six phenolic acids in the litter, humus, and soil layers during litter decomposition in Rhododendron annae Franch and Rhododendron siderophyllum Franch forests. We analyzed the relationship between the phenolic acid contents and soil factors and focused on belowground plant components. The objectives are to determine the effects of phenolic acids released into the soil during litter decomposition on rhododendron seedling growth and litter nutrient cycling.
2. Materials and methods
2.1. Study sites and plant material
The experimental site is the Baili Rhododendron National Forest Nature Reserve, located at the boundary between Dafang County and Qianxi County, Guizhou Province, China (105°45′00′′–106°04′45′′ E and 27°08′30′′–27°20′00′′ N). The altitude of this subtropical evergreen broad-leaved forest ranges from 1060 to 2121 m. The area has a typical subtropical warm and humid monsoon climate, with cloudy and wet conditions in winter and dry conditions in spring. The average annual temperature is 11.8°C, the average annual relative humidity is 84%, the annual precipitation is 1000∼1100 mm, and the average annual sunshine duration is 1335.5 h. The rainy season is from May to October, during which 70% of the annual precipitation falls. There are 43 species of rhododendrons, making this area the largest and most densely growing alpine rhododendron forest in the world. Two locations with early flowering rhododendrons, Rhododendron annae (105°53′E, 27°14′N) and Rhododendron siderophyllum (105°53′E, 27°15′N), were chosen as experimental sites. Information on the sample sites is listed in Table 1.
2.2. Litter decomposition bags and experimental design
Withered leaves of Rhododendron annae and Rhododendron siderophyllum were collected on January 11, 2020. After screening and drying, 15 g was quantitatively weighed, and the material was placed into a nylon mesh decomposition bag (15 cm × 20 cm) with a mesh diameter of 1 mm and sealed with nylon thread. On July 19, 2020, three duplicate plots of 5 m × 5 m were chosen in the two areas with the two rhododendron species. Litter bags were placed in the plots and covered with natural litter (Fujii and Takeda, 2010). Subsequently, the litter bags were retrieved in batches every 3–5 months (October 11, 2020, March 16, 2021, June 5, 2021, September 5, 2021, and January 8, 2022). Samples of the humus layer (0–20 cm) and the soil layer (depth 30–40 cm) were collected near the litter bags using the five-point sampling method. The litter samples were crushed in the laboratory with a pulverizer and passed through a 40-mesh screen for natural drying, while the humus and soil samples were directly passed through a 40-mesh screen. The sieved samples were bagged and stored in a −20°C refrigerator.
2.3. Identifying and quantifying phenolics in the samples
High-performance liquid chromatography (HPLC) with modifications was used to determine the phenolic acid content (Dhanani et al., 2017; Rezaei and Abedi, 2017; Manzano Durán et al., 2020). Briefly, 0.1 g of the sifted sample was mixed with 1 mL of methanol solution and oscillated for 1 h in an ultrasonic instrument at 30°C. The extract was centrifuged for 3 min at 5000 r/min. The supernatant was filtered through a 0.22 μM organic nylon filter with a multilayer membrane. Previous research and preliminary tests have confirmed the presence of gallic acid, protocatechuic acid, chlorogenic acid, caffeic acid, syringic acid, and ferulic acid in this sample (Kivrak and Kivrak, 2017; Fu et al., 2019). All concentrations were within the detection range of the HPLC column. The benchmark product was purchased from Solarbio (China), and a 2 mg/mL standard stock solution was prepared. It was gradient diluted to 50, 100, and 200 μg/mL. A Shimadzu InertSustain C18 analysis column (Japan) (4.6 mm × 150 mm, 5 μm) was used for the test. The flow rate of mobile phase A (0.1% phosphoric acid aqueous solution) and mobile phase B (100% acetonitrile) was fixed at 1 mL/min, and the operation time was 30 min. The gradient elution procedure was as follows: 0–5 min, 5: 95 (A: B) to 8: 92 (A: B); 5–10 min, 8: 92 (A: B) to 10: 90 (A: B); 10–30 min, 10: 90 (A: B) to 40: 60 (A: B). Each injection volume was 15 μL, and the sample was injected again at 10-min intervals after reaching equilibrium. Each sample was tested three times. The residence time of the reference sample served as a qualitative indicator of the sample’s phenolic acid collection, and the peak area at the detection wavelength of 275 nm served as a quantitative indicator.
2.4. Soil property analysis
The soil samples were air-dried and screened before analyzing their properties. The soil water content (SWC) was determined using the drying method (Ma et al., 2016), and the soil pH was determined using an acidity meter. The phenol disulfonic acid colorimetric method was used to determine soil nitrate nitrogen (NO3–), and the indigo blue colorimetric method was used to determine soil ammonium nitrogen (NH4+) (Sattolo et al., 2016). The additive potassium dichromate method was used to measure soil organic matter (SOM) (Bao, 2007). The soil available potassium (AK) content was measured by NH4OAc extraction flame spectrophotometry, and soil available phosphorus (AP) was determined using molybdenum-antimony resistance spectrophotometry (Grzebisz et al., 2020).
2.5. Data analysis
Statistical analysis was conducted using SPSS version 24 (IBM Corp.). Principal component analysis (PCA) and redundancy analysis (RDA) were used to evaluate the effects of the phenolic acid content on soil environmental factors. The stats, ggplot2 and vegan packages in R software, version 4.1.1 were used to draw the graphs (R Core Team, 2021). One-way ANOVA was used for analysis after a normal distribution test. The charts were produced using the software Origin 2021 (OriginLab Corp).
3. Results
3.1. Phenolic acid variation in the litters, humus, and soil
The samples obtained from the litter of Rhododendron annae and Rhododendron siderophyllum at different time points contained six phenolic acids: gallic acid, protocatechuic acid, chlorogenic acid, caffeic acid, syringic acid, and ferulic acid. The content analysis revealed that the concentrations of the six phenolic acids in the undecomposed litters (control group) of Rhododendron siderophyllum were significantly higher than the concentrations of phenolic acids in litters from different decomposition periods (P < 0.05) (Figure 1). Except for syringic acid and chlorogenic acid, the concentrations of the other four acids in undecomposed litters (control group) of Rhododendron annae were also significantly (P < 0.05) higher than those in the litters at other decomposition stages (Figures 2D, E). Additionally, the total content of phenolic acids in undecomposed samples of Rhododendron siderophyllum (315.64 μg/g) was higher than that of Rhododendron annae (97.58 μg/g).
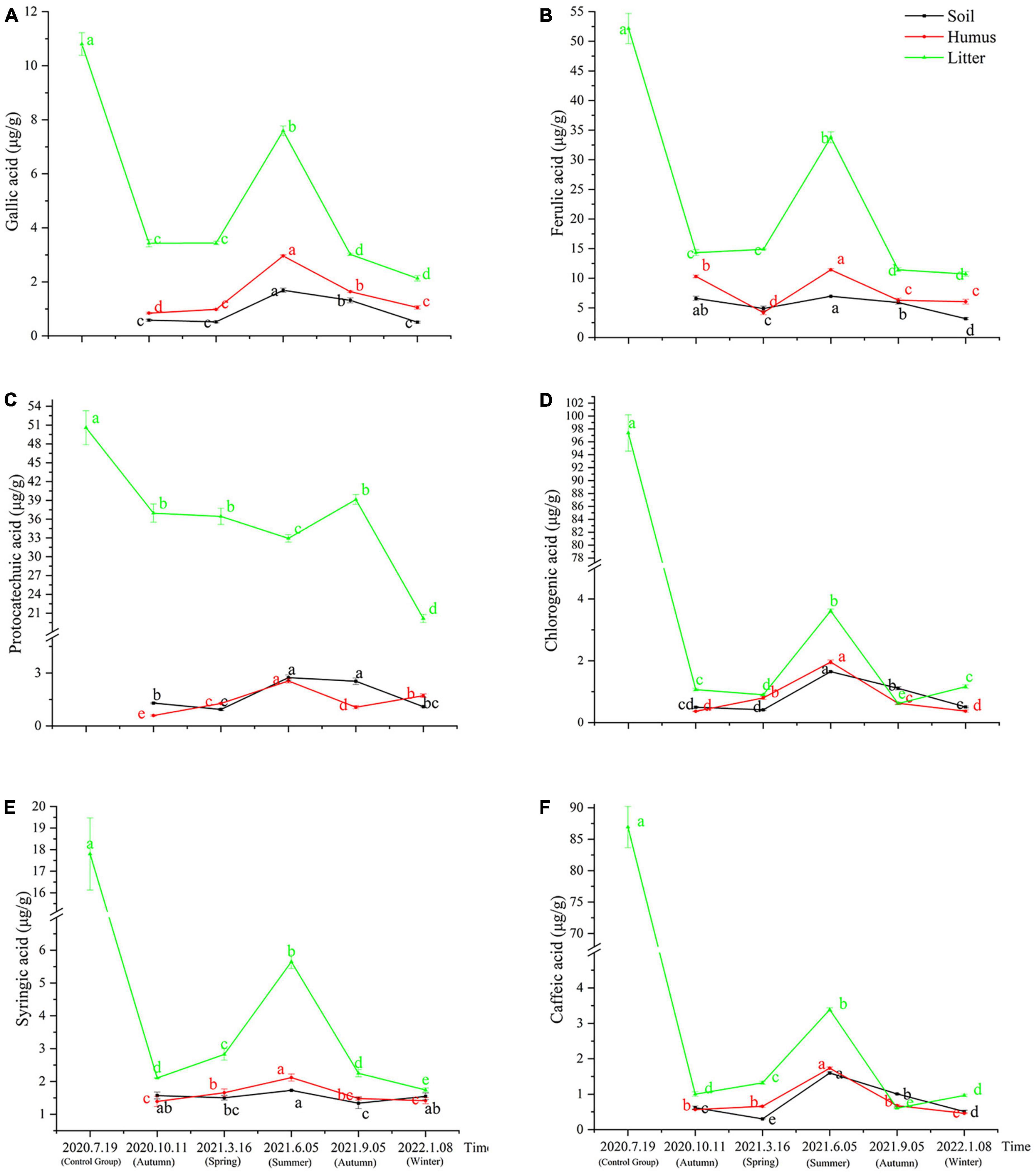
Figure 1. Changes of gallic acid (A), ferulic acid (B), protocatechuic acid (C), chlorogenic acid (D), syringic acid (E), and caffeic acid (F) content in soil, humus, and litter during various stages of litter decomposition in Rhododendron siderophyllum forest. The error bars represent the standard deviation of the mean (n = 3), and the lowercase letters on the column indicate significant differences (P < 0.05).
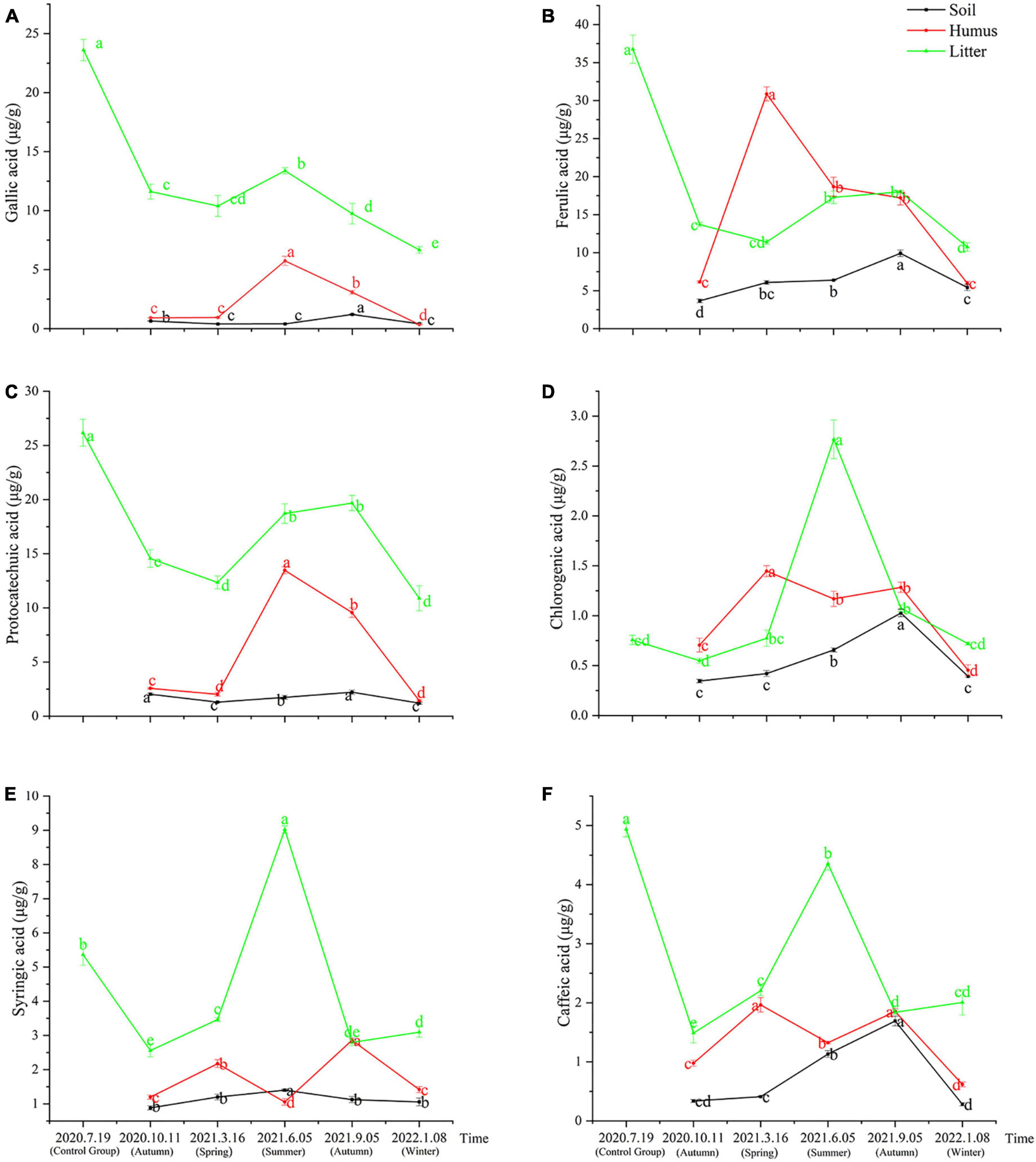
Figure 2. The variations of gallic acid (A), ferulic acid (B), protocatechuic acid (C), chlorogenic acid (D), syringic acid (E), and caffeic acid (F) content in soil, humus, and litter under Rhododendron annae forest at various stages of litter decomposition. The error bars represent the standard deviation of the mean (n = 3), and the lowercase letters on the column indicate significant differences (P < 0.05).
After 3 months of decomposition, the total content of phenolic acids in the litter of Rhododendron siderophyllum and Rhododendron annae decreased by 257.60 and 53.12 μg/g, respectively, and after further decomposition for 15 months, they decreased by 22.88 and 10.30 μg/g, respectively. The results also revealed that the decomposition rate of the litter was faster from July 2020, when the litter was buried without decomposition, to October 2020 (Figures 1, 2).
During Rhododendron siderophyllum litter decomposition, the distribution of phenolic acids except protocatechuic acid in the soil, humus and litter increased first and then decreased, with the highest value in June 2021. However, the content of ferulic acid in the soil was higher than that in the humus in March 2021 (Figure 1B). The contents of ferulic acid, chlorogenic acid and caffeic acid were also higher in the soil than in the humus in September 2021 (Figures 1B–D, F). The content of ferulic acid and protocatechuic acid in the litter was generally high, especially in the decomposition process of litters. The protocatechuic acid ranged from 20.15 to 39.12 μg/g, and the ferulic acid ranged from 10.70 to 33.79 μg/g (Figures 1B, C). In addition, protocatechuic acid also had a different changing trend from that of the other acids. That is, the content of protocatechuic acid decreased during the decomposition process, and it only increased from June to September 2021. After September 2021, the content of protocatechuic acid in the litters decreased significantly (P < 0.05) (Figure 1C).
During Rhododendron annae litter decomposition, the content of syringic acid in soil was significantly higher in June 2021 than in other decomposition periods (Figure 2E). In September 2021, the contents of chlorogenic acid, ferulic acid, protocatechuic acid, gallic acid and caffeic acid in the soil increased significantly (P < 0.05) (Figures 2A–D, F). The gallic acid and protocatechuic acid contents in the humus increased and decreased during decomposition, reaching a maximum in June 2021 (Figures 2A, C). From March to June 2021, the contents of ferulic acid, chlorogenic acid, syringic acid and caffeic acid in humus decreased significantly (P < 0.05). In addition, the ferulic acid content reached its maximum in March 2021 and was higher than that in the litter and soil (Figure 2B). The contents of protocatechuic acid and ferulic acid in the litter were in the range of 10.88–19.68 and 10.75–18.00 μg/g during litter decomposition. With the exception of ferulic acid and protocatechuic acid, the other acids in the litter of Rhododendron annae increased in summer and decreased in winter, reaching a maximum in June 2021 (Figure 2).
3.2. Soil chemical properties of the Rhododendron forests
The SOM and AP levels in the soil samples of the Rhododendron siderophyllum forest were the highest in June 2021, while the NH4+, NO3– and AK levels were the highest in March 2021. The contents of NO3–, NH4+ and AK in September 2021 were lower than those in other decomposition periods (Table 2). The physical and chemical parameters of the soil in the Rhododendron annae forest are listed in Table 3. Similar to the Rhododendron siderophyllum soil indices, NH4+, NO3– and AK were lower in September 2021 than in other periods. The SOM content in the Rhododendron annae soil was the lowest in June 2021, while the NO3–, NH4+, AP, AK, and SWC reached maximum values in March 2021.
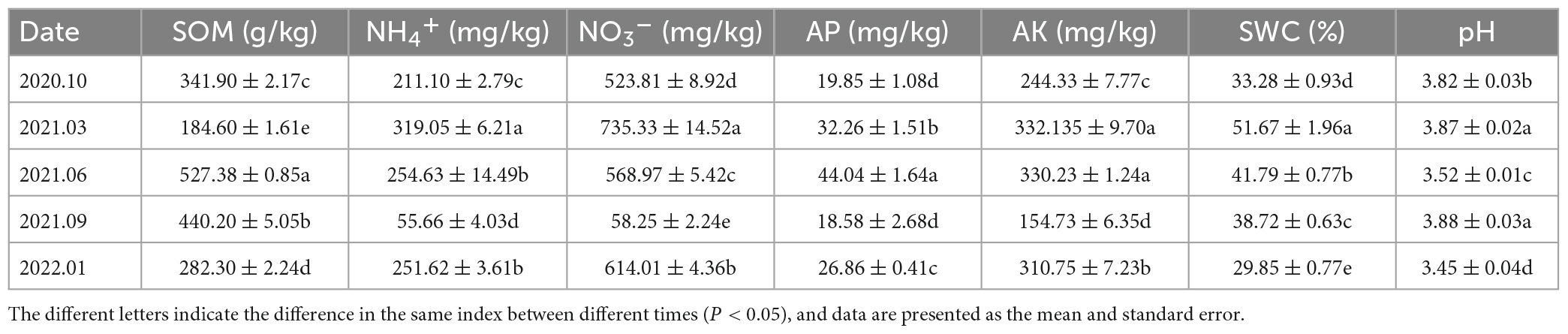
Table 2. The organic matter (SOM), ammonium nitrogen (NH4+), nitrate nitrogen (NO3–), available phosphorus (AP), available potassium (AK), water content (SWC), and pH (pH) contents of soil in the Rhododendron siderophyllum forest.
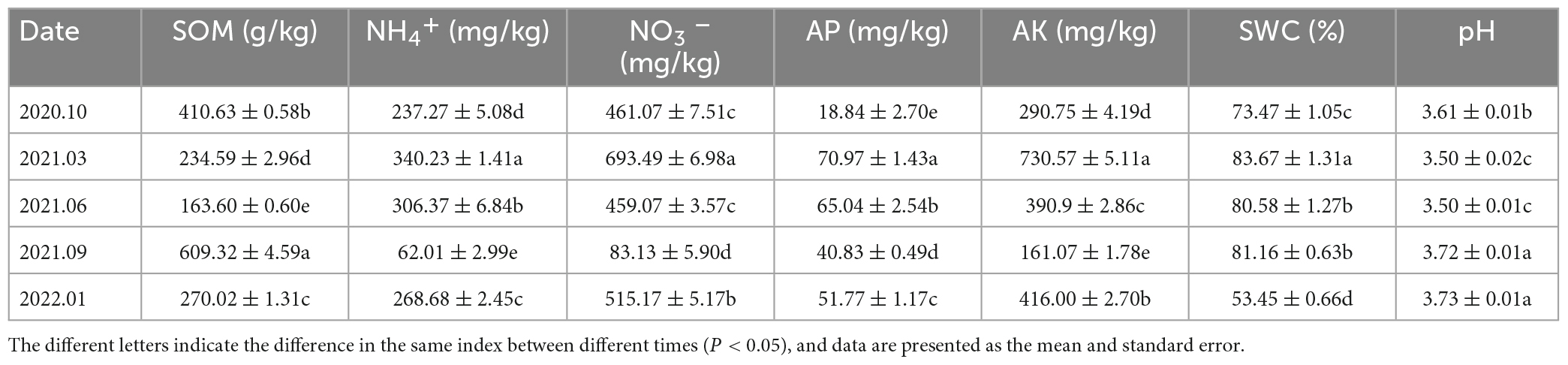
Table 3. The concentrations of soil organic matter (SOM), ammonium nitrogen (NH4+), nitrate nitrogen (NO3–), available phosphorus (AP), available potassium (AK), soil water content (SWC), and pH in the Rhododendron annae forest.
3.3. PCA of phenolic acids in different samples
The PCA results of the total phenolic acid concentration showed some overlap in the samples of Rhododendron annae and Rhododendron siderophyllum litter (confidence interval 95%) (Figure 3).
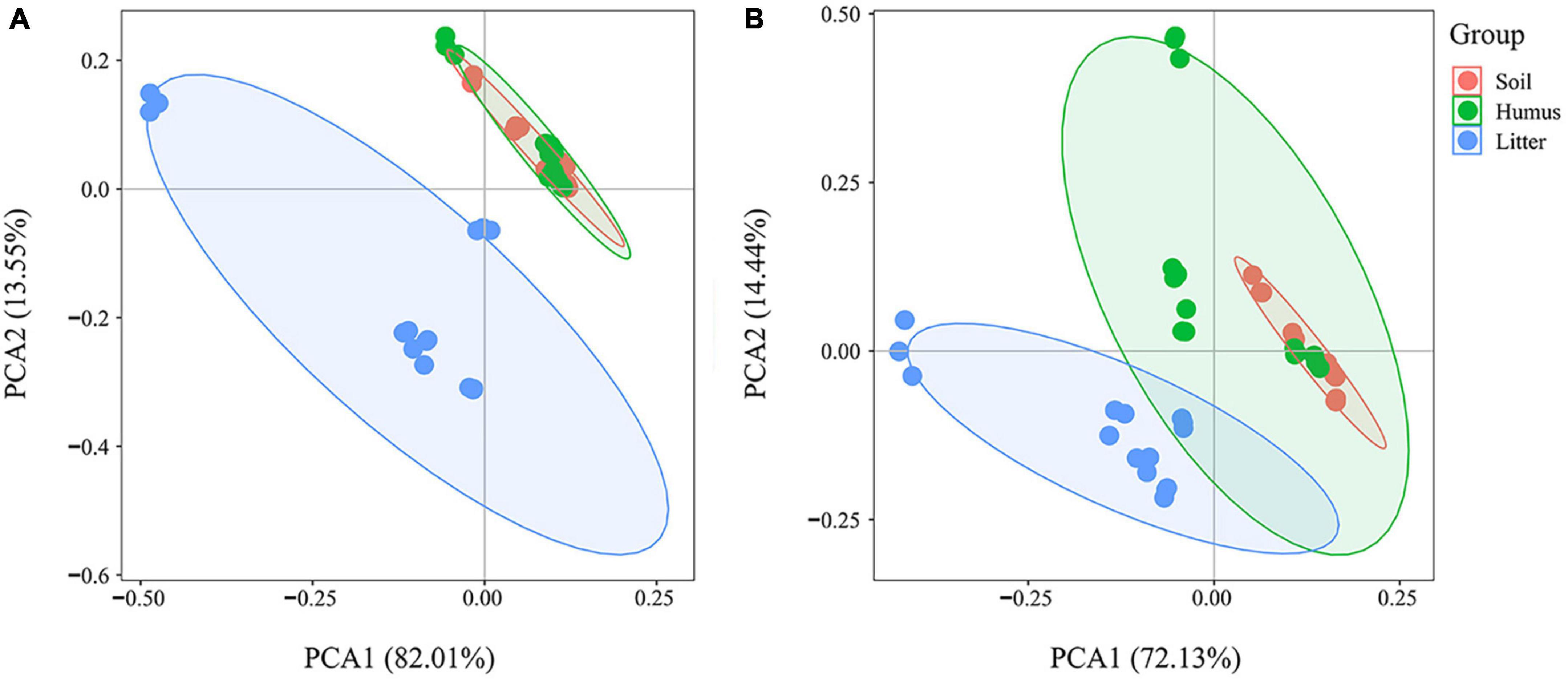
Figure 3. Principal component analysis of total phenolic acid concentration in the soil, humus, and litter samples of Rhododendron siderophyllum (A) and Rhododendron annae (B) during litter decomposition.
There were some differences in the contents of the litter, humus and soil layer. The results showed that the phenolic acid content of the litter was significantly separated from the humus and soil groups during the decomposition of Rhododendron siderophyllum (Figure 3A). The phenolic acid content in the soil layer overlapped with that in the humus. In the decomposition process of Rhododendron annae, the litter group was significantly separated from the soil group, and there was a certain overlap with the humus group (Figure 3B). The phenolic acid contents in the humus layer and the soil layer were similar.
3.4. Relationship between phenolic acid content and soil environmental factors
Redundancy analysis was used to investigate the relationship between the phenolic acid content and soil physical and chemical properties during the litter decomposition of Rhododendron siderophyllum (Figure 4A). The results showed that the cumulative explained variances of the first and second axes were 73.52 and 12.25%, respectively, and the total explained variance was 85.77%. The dominant environmental factors influencing the soil phenolic acid content were SOM (R2 = 0.91) and NO3– (R2 = 0.67). The SOM content was positively correlated with the contents of all phenolic acids and highly correlated with the ferulic acid content. AK, pH, NH4+, and NO3– were strongly negatively correlated with the contents of all phenolic acids except syringic acid.
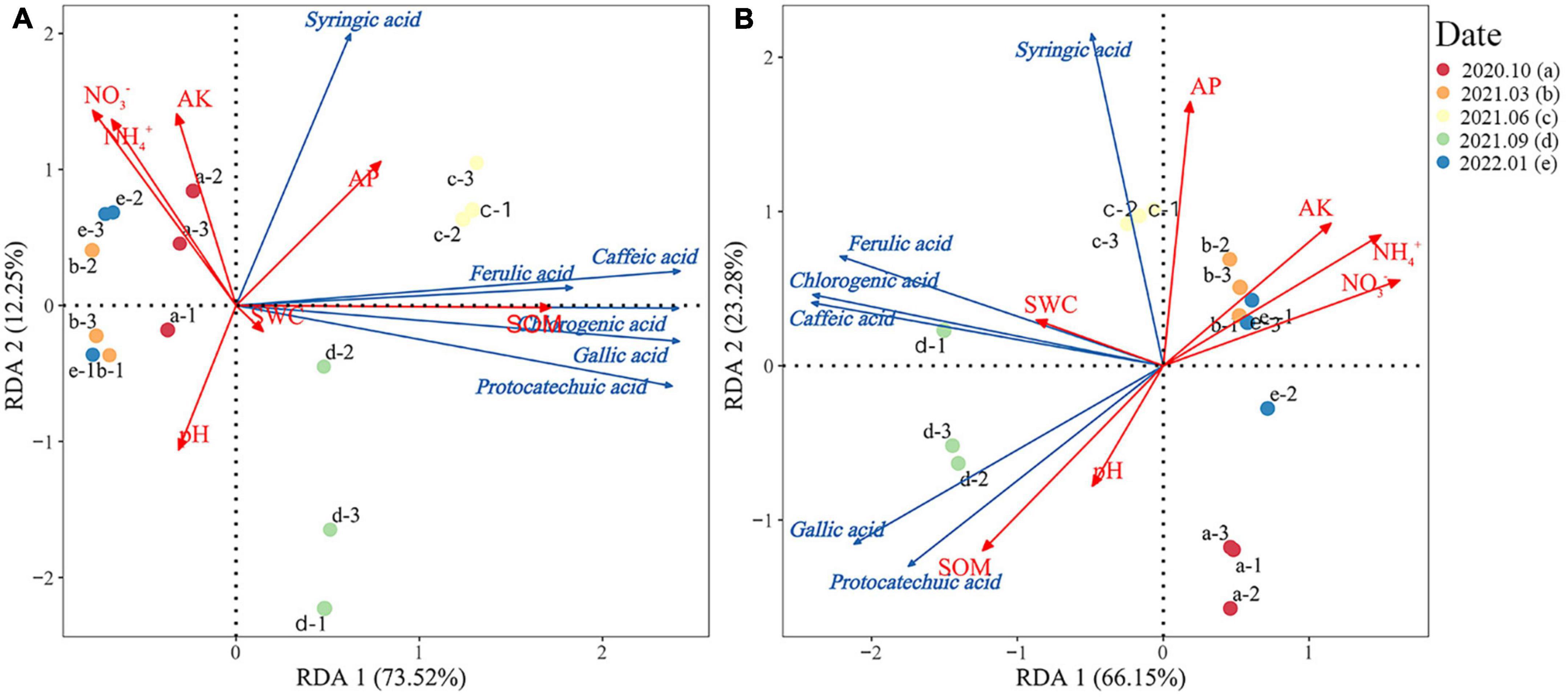
Figure 4. Redundancy analysis of the relationship between the phenolic acid content and soil physical and chemical properties during litter decomposition of Rhododendron siderophyllum (A) and Rhododendron annae (B). SOM, soil organic matter; NH4+, soil ammonium nitrogen; NO3−, soil nitrate nitrogen; AP, soil available phosphorus. Soil water content (SWC), available soil potassium content (AK), and pH indicate soil acidity and alkalinity.
The RDA of Rhododendron annae revealed that the cumulative explained variances of the first and second axes were 63.15 and 23.28%, respectively, with a total explained variance of 86.43% (Figure 4B). The dominant environmental factors influencing the phenolic acid content in Rhododendron annae soil were NO3– (R2 = 0.90), NH4+ (R2 = 0.89), SOM (R2 = 0.88), and AP (R2 = 0.84). NO3– and NH4+ were positively correlated with the syringic acid content and negatively correlated with the contents of the other phenolic acids. NH4+ was strongly negatively correlated with the gallic acid and protocatechuic acid contents. The gallic acid and protocatechuic acid contents were negatively correlated with AP, whereas the contents of the other phenolic acids were positively correlated. The SOM content was positively correlated with all phenolic acids except syringic acid.
3.5. Relationship between phenolic acid content and soil environmental factors at various levels
The RDA results of Rhododendron siderophyllum showed that the cumulative explained variance was 83.98% and that of the first and second was 69.93 and 14.05%, respectively (Figure 5A). The dominant factors influencing the phenolic acid contents in different layers were SOM (R2 = 0.75) and AP (R2 = 0.85). Syringic acid, gallic acid, and protocatechuic acid showed a strong positive correlation with AP in the humus layer.
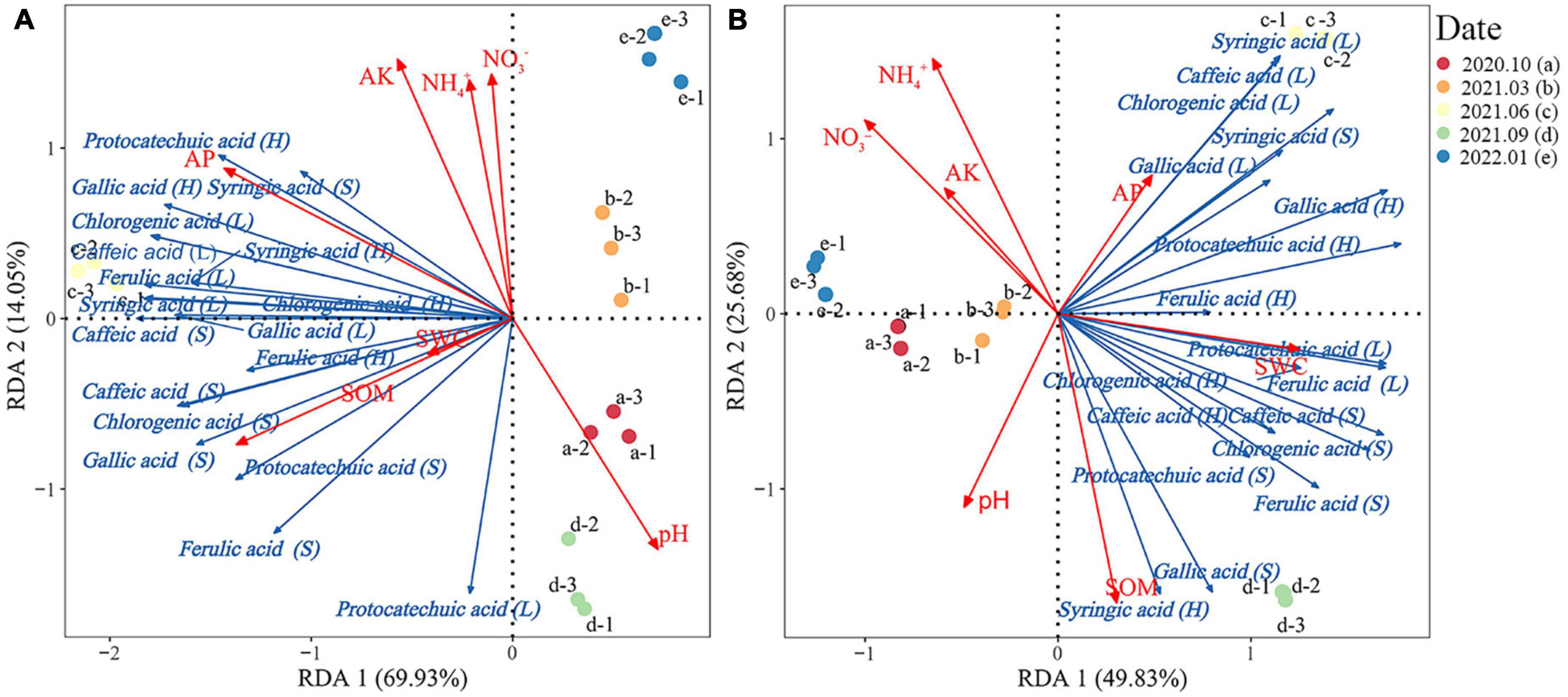
Figure 5. Redundancy analysis of the relationship between the phenolic acid content and soil physical and chemical properties in the soil, humus, and litter layers of Rhododendron siderophyllum (A) and Rhododendron annae (B). SOM, soil organic matter; NH4+, soil ammonium nitrogen; NO3−, soil nitrate nitrogen; AP, soil available phosphorus. Soil water content (SWC), available potassium content of the soil (AK), and pH indicate soil acidity and alkalinity.
The cumulative explained variance for Rhododendron annae was 75.51%, and that of the first and second axes was 49.83 and 25.68%, respectively (Figure 5B). The dominant factors influencing the phenolic acid contents in different layers were SOM (R2 = 0.87) and NH4+ (R2 = 0.78). Gallic acid, syringic acid, caffeic acid and chlorogenic acid in the litters were positively correlated with AP and negatively correlated with SOM.
4. Discussion
Forest litter decomposition provides nutrients for understory plant growth and releases large numbers of secondary metabolites into the environment (Chomel et al., 2016). Research has shown that different types of organic compounds dominate litter decomposition at different times, which can be divided into early decomposition controlled by their own nutrients and late decomposition controlled by lignin (Berg, 2014). In the early stage of litter decomposition, litter quality has a great influence (Heim and Frey, 2004). Intensive lignin degradation occurs during the first 200 d of litter decomposition, forming various phenolic derivatives, such as vanillin, vanillic acid, and ferulic acid (Klotzbücher et al., 2011). In this study, large amounts of phenolic acid were released from the litter of two early flowering rhododendrons during the 3-month decomposition period, indicating that the rapid decomposition resulted from the nutrients in the soil and litter layers. Since the leathery leaves of rhododendron are not easily decomposed by fungi, rhododendron litter decomposition depends on the physical traits in the early stages. As a result, multiple driving factors, such as the content of litter and soil nutrients, leaf structure, and microbial activity, influence the decomposition of early flowering rhododendron litter and the production of phenolic acids (Hoeber et al., 2020; Ramos et al., 2021). It has also been discovered that the effects of chemical and physical differences between leaf litter species may persist into the later stages of decomposition (Wickings et al., 2012).
Phenolic acids are secondary metabolites closely related to plant growth and are abundant in plants and soil (Blum et al., 1999). Litter decomposition is a significant source of phenolic acids (de Las Heras et al., 2020). A previous study found that the phenolic acid content was significantly higher in the litter layer than in the humus and soil layers (Li et al., 2019). The results of this study are basically consistent with these findings. The content of phenolic acids in the litter fluctuated seasonally after the rapid decomposition stage. With the exception of ferulic acid and protocatechuic acid, the content of phenolic acids in the litter was significantly higher in the summer than in the winter. The factors affecting litter decomposition and soil nutrient changes should be considered in combination with the specific plot conditions (Cou^teaux et al., 1995). Affected by geographical factors such as topography and elevation, the study area is accompanied by freezing and icing in winter (Gao, 2016). The alternation of dry and wet conditions not only affects the decomposition of litter but also affects the transformation and migration of soil nutrients (Dodd et al., 2015; Schmidt et al., 2016). Therefore, as the temperature rebounded, the soil nutrient content changed significantly. With changes in soil temperature and moisture, microbial activity increases, which promotes litter decomposition and the release of phenolic acids (Migliorini and Romero, 2020). Therefore, the content of phenolic acids in the soil, humus and litter of Rhododendron siderophyllum and Rhododendron annae began to increase gradually. In September 2021, the rainfall of Rhododendron siderophyllum and Rhododendron annae was as high as 125 and 154 mm, respectively. The increase in rainfall combined with karst geology affected the changes in phenolic acids released by litter decomposition and soil nutrient content (Wang et al., 2022). Furthermore, litter mass decreases after the rapid decomposition stage, and climate change increases the decomposition rate of temperature-sensitive low-mass litter (Canessa et al., 2021). As a result, litter quality, microbial activity, and climate change affect the content of some phenolic acids produced by litter decomposition in a rhododendron forest, and seasonal variations occur.
Secondary metabolites released into the environment by plants may promote or inhibit plant growth and development (Scavo et al., 2019). Previous research found that at certain concentrations, protocatechuic acid and ferulic acid could significantly reduce the early growth parameters of seeds and seedlings (Fu et al., 2019; Šćepanović et al., 2022). We found high levels of protocatechuic acid and ferulic acid, confirming the results of a previous study on the phenolic acid content of litter (Quan et al., 2022). However, since litter decomposes over time, the chemical composition and quantity of produced allelochemicals change to varying degrees due to physical and chemical changes in the environment (Blum et al., 1999). Target microorganisms breakdown ferulic acid to produce protocatechuic acid using the ketoadipate pathway, and other microorganisms continue to degrade protocatechuic acid (Harwood and Parales, 1996; Xie and Dai, 2015). As a result, the synthetic conversion pathway between these phenolic acids affects the content, influencing the allelochemical effect. Therefore, it is necessary to learn more about how these two phenolic acids are metabolized by microbes during the breakdown of rhododendron litter.
The content of phenolic acids in soil is affected by environmental factors such as soil geology, fertility, microbial quantity and composition (Inderjit and Cahill, 2015). Rhododendron grows primarily in acidic soils (Zhou et al., 2020). The content of some phenolic acids in the humus layer changed significantly during the decomposition period in this experiment, suggesting that the humus hydrolyzes rapidly under acidic conditions to produce various phenolic substances, such as protocatechuic acid and vanillin. Research has shown that certain phenolic acids restrict the activity of microbial populations to varying degrees, influencing SOM decomposition (Zwetsloot et al., 2020). SOM was found to be positively correlated with the phenolic acid content in the soil (except for syringic acid in Rhododendron annaeh) to varying degrees in this study. The likely reason is that diverse site conditions and litter with high levels of phenolic acids can influence SOM cycling (Prescott and Vesterdal, 2021). Phenolic acids can influence nitrification in soil, specifically the transformation of NH4+ to NO3– (Chen W. B. et al., 2020). This study found that the NH4+ and NO3– contents were negatively correlated with the phenolic acid content (except for syringic acid) in soil. Studies have shown that phenolic acids affect nitrogen fixation and conversion processes by affecting microorganisms, soil enzymes, plant roots, etc. Therefore, there was a negative correlation between the phenolic acid and nitrogen contents (Xue et al., 2006; Shen et al., 2021). In contrast, the explained variance between the phenolic acid content and the NH4+ content was high in different layers of the Rhododendron annae forest, indicating that phenolic acids affected the nutrients returned to the soil by litter decomposition, affecting the nitrogen content (Li et al., 2018). The gallic acid content in different layers was positively correlated with the soil AP content, which was consistent with the findings in studies of ancient tea gardens (Yang et al., 2022) and showed that a higher AP content promoted the accumulation of gallic acid in tea plants. However, there was a negative correlation between the contents of gallic acid and protocatechuic acid and AP in Rhododendron annae Franch in the soil, indicating that the content of these two phenolic acids decreased under high phosphorus conditions, which differs from the findings of other studies (Yang et al., 2022). Therefore, the effect of soil phenolic acids on soil P stress should be investigated in relation to specific plants and habitats.
5. Conclusion
The phenolic acid content of rhododendron litter decreased substantially under natural conditions during the first 3 months of decomposition, indicating a period of rapid decomposition of the rhododendron litter. Seasonal changes had a significant impact on the phenolic acid content in the litter during the subsequent decomposition process, with an increasing trend in summer. The contents of protocatechuic acid and ferulic acid were high in the three layers (soil, humus, and litter), and their distribution differed from those of other phenolic acids. The redundancy analysis results indicated that the dominant environmental factors affecting the distribution of the six phenolic acids were SOM, NH4+, NO3–, and AP. Our findings provide a preliminary understanding of the distribution and variation in phenolic acids in the litter of rhododendron forests. Since microbial degradation is the critical link between litter decomposition and nutrient return, more research on rhododendron phenolic acid allelochemicals is required to understand the metabolic mechanisms.
Data availability statement
The original contributions presented in this study are included in the article/supplementary material, further inquiries can be directed to the corresponding author.
Author contributions
CL conceived the structure of the manuscript and revised and approved the manuscript. YZ, JL, and WQ collected the materials and data. YZ and JL wrote the manuscript. All authors contributed to the article and approved the submitted version.
Funding
This work was supported by the National Natural Science Foundation of China (31960312) and the Guizhou Provincial Characteristic Key Laboratory [QJHKY (2021)002].
Conflict of interest
The authors declare that the research was conducted in the absence of any commercial or financial relationships that could be construed as a potential conflict of interest.
Publisher’s note
All claims expressed in this article are solely those of the authors and do not necessarily represent those of their affiliated organizations, or those of the publisher, the editors and the reviewers. Any product that may be evaluated in this article, or claim that may be made by its manufacturer, is not guaranteed or endorsed by the publisher.
References
Bao, S. D. (2007). Soil Agricultural Chemistry Analysis, 3rd Edn. Beijing: China Agriculture Press, 20–65.
Berg, B. (2014). Decomposition patterns for foliar litter–a theory for influencing factors. Soil Biol. Biochem. 78, 222–232. doi: 10.1016/j.soilbio.2014.08.005
Blum, U., Shafer, S. R., and Lehman, M. E. (1999). Evidence for inhibitory allelopathic interactions involving phenolic acids in field soils: concepts vs. an experimental model. Crit. Rev. Plant Sci. 18, 673–693. doi: 10.1080/07352689991309441
Bosco, T., Bertiller, M. B., and Carrera, A. L. (2016). Combined effects of litter features, UV radiation, and soil water on litter decomposition in denuded areas of the arid Patagonian Monte. Plant Soil 406, 71–82. doi: 10.1007/s11104-016-2864-7
Canessa, R., van den Brink, L., Saldaña, A., Rios, R. S., Hättenschwiler, S., Mueller, C. W., et al. (2021). Relative effects of climate and litter traits on decomposition change with time, climate and trait variability. J. Ecol. 109, 447–458. doi: 10.1111/1365-2745.13516
Chauhan, A., Saini, R., and Sharma, J. C. (2021). Plant growth promoting rhizobacteria and their biological properties for soil enrichment and growth promotion. J. Plant Nutr. 45, 273–299. doi: 10.1080/01904167.2021.1952221
Chomel, M., Guittonny-Larcheveque, M., Fernandez, C., Gallet, C., DesRochers, A., Pare, D., et al. (2016). Plant secondary metabolites: A key driver of litter decomposition and soil nutrient cycling. J. Ecol. 104, 1527–1541. doi: 10.1111/1365-2745.12644
Chen, H., Liu, X., Blosser, G. D., Rücker, A. M., Conner, W. H., and Chow, A. T. (2020). Molecular dynamics of foliar litter and dissolved organic matter during the decomposition process. Biogeochemistry 150, 17–30. doi: 10.1007/s10533-020-00684-5
Chen, W. B., Chen, B. M., Liao, H. X., Su, J. Q., and Peng, S. L. (2020). Leaf leachates have the potential to influence soil nitrification via changes in ammonia-oxidizing archaea and bacteria populations. Eur. J. Soil Sci. 71, 119–131. doi: 10.1111/ejss.12844
Cou^teaux, M., Bottner, P., and Berg, B. (1995). Litter decomposition, climate and liter quality. Trends Ecol. Evol. 10, 63–66. doi: 10.1016/S0169-5347(00)88978-8
de Las Heras, P., Medina-Villar, S., Perez-Corona, M. E., and Vazquez-de-Aldana, B. R. (2020). Leaf litter age regulates the effect of native and exotic tree species on understory herbaceous vegetation of riparian forests. Basic Appl. Ecol. 48, 11–25. doi: 10.1016/j.baae.2020.07.005
Dhanani, T., Singh, R., and Kumar, S. (2017). Extraction optimization of gallic acid,(+)-catechin, procyanidin-B2,(–)-epicatechin,(–)-epigallocatechin gallate, and (–)-epicatechin gallate: their simultaneous identification and quantification in Saraca asoca. J. Food Drug Anal. 25, 691–698. doi: 10.1016/j.jfda.2016.08.004
Dodd, I. C., Puértolas, J., Huber, K., Pérez-Pérez, J. G., Wright, H. R., and Blackwell, M. S. (2015). The importance of soil drying and rewetting in crop phytohormonal and nutritional responses to deficit irrigation. J. Exp. Bot. 66, 2239–2252. doi: 10.1093/jxb/eru532
Einhellig, F. A. (1987). “Interactions among allelochemicals and other stress factors of the plant environment,” in Allelochemicals: Role of Agriculture and Forestry, ACS Symposium Series, Vol. 330, ed. G. R. Waller (Washington DC: American Chemical Society), doi: 10.1021/bk-1987-0330.ch032
Fu, Y. H., Quan, W. X., Li, C. C., Qian, C. Y., Tang, F. H., and Chen, X. J. (2019). Allelopathic effects of phenolic acids on seedling growth and photosynthesis in Rhododendron delavayi Franch. Photosynthetica 57, 377–387. doi: 10.32615/ps.2019.045
Fujii, S., and Takeda, H. (2010). Dominant effects of litter substrate quality on the difference between leaf and root decomposition process above-and belowground. Soil Biol. Biochem. 42, 2224–2230. doi: 10.1016/j.soilbio.2010.08.022
Gao, J. (2016). Analysis and assessment of the risk of snow and freezing disaster in China. Int. J. Disaster Risk Reduct. 19, 334–340. doi: 10.1016/j.ijdrr.2016.09.007
Grzebisz, W., Łukowiak, R., and Kotnis, K. (2020). Evaluation of nitrogen fertilization systems based on the in-season variability in the nitrogenous growth factor and soil fertility factors–a case of winter oilseed rape (Brassica napus L.). Agronomy 10:1701. doi: 10.3390/agronomy10111701
Harwood, C. S., and Parales, R. E. (1996). The beta-ketoadipate pathway and the biology of self-identity. Annu. Rev. Microbiol. 50, 553–591. doi: 10.1146/annurev.micro.50.1.553
Hassan, M. O., Mohamed, H. Y., and Aboellil, A. H. (2021). Allelopathic potential of Ficus retusa L. Leaf litter on understory vegetation in urban gardens. Acta Bot. Croat. 80, 131–139. doi: 10.37427/BOTCRO-2021-013
Hättenschwiler, S., Tiunov, A. V., and Scheu, S. (2005). Biodiversity and litter decomposition in terrestrial ecosystems. Annu. Rev. Ecol. Evol. Syst. 36, 191–218. doi: 10.1146/annurev.ecolsys.36.112904.151932
Heim, A., and Frey, B. (2004). Early stage litter decomposition rates for Swiss forests. Biogeochemistry 70, 299–313. doi: 10.1007/s10533-003-0844-5
Hoeber, S., Fransson, P., Weih, M., and Manzoni, S. (2020). Leaf litter quality coupled to Salix variety drives litter decomposition more than stand diversity or climate. Plant Soil 453, 313–328. doi: 10.1007/s11104-020-04606-0
Inderjit, I., and Cahill, J. F. (2015). Linkages of plant–soil feedbacks and underlying invasion mechanisms. AoB Plants 7:lv022. doi: 10.1093/aobpla/plv022
Kalinova, J., Vrchotova, N., and Triska, J. (2007). Exudation of allelopathic substances in buckwheat (Fagopyrum esculentum Moench). J. Agric. Food Chem. 55, 6453–6459. doi: 10.1021/JF070795U
Kato-Noguchi, H., and Kurniadie, D. (2022). Allelopathy and allelochemicals of Leucaena leucocephala as an invasive plant species. Plants 11:1672. doi: 10.3390/PLANTS11131672
Kivrak, S., and Kivrak, I. (2017). Assessment of phenolic profile of Turkish honeys. Int. J. Food Prop. 20, 864–876. doi: 10.1080/10942912.2016.1188307
Klotzbücher, T., Kaiser, K., Guggenberger, G., Gatzek, C., and Kalbitz, K. (2011). A new conceptual model for the fate of lignin in decomposing plant litter. Ecology 92, 1052–1062. doi: 10.1890/10-1307.1
Kobayashi, K. (2004). Factors affecting phytotoxic activity of allelochemicals in soil. Weed Biol. Manag. 4, 1–7. doi: 10.1002/chin.200435273
Li, C., Quan, W., Qian, C., and Wu, Y. (2019). Distribution of chemical compounds in different soil layers of rhododendron forest. Allelopathy J. 48, 191–202. doi: 10.26651/allelo.j/2019-48-2-1254
Li, H., Xie, H. C., Du, Z. L., Xing, X. S., Zhao, J., Guo, J., et al. (2018). The effects of phenolic acid on nitrogen metabolism in Populus euramericana ‘Neva’. J. For. Res. 29, 925–931. doi: 10.1007/s11676-017-0526-0
Liu, J., Li, D., Wang, D., Liu, Y., and Song, H. (2017). Allelopathic effects, physiological responses and phenolic compounds in litter extracts of Juniperus rigida Sieb. Et zucc. Chem. Biodivers. 14:e1700088. doi: 10.1002/cbdv.201700088
Ma, Y., Qu, L., Wang, W., Yang, X., and Lei, T. (2016). Measuring soil water content through volume/mass replacement using a constant volume container. Geoderma 271, 42–49. doi: 10.1016/j.geoderma.2016.02.003
Manzano Durán, R., Sánchez, J. E. F., Velardo-Micharet, B., and Gómez, M. J. R. (2020). Multivariate optimization of ultrasound-assisted extraction for the determination of phenolic compounds in plums (Prunus salicina Lindl.) by high-performance liquid chromatography (HPLC). Instrum. Sci. Technol. 48, 113–127. doi: 10.1080/10739149.2019.1662438
Migliorini, G. H., and Romero, G. Q. (2020). Warming and leaf litter functional diversity, not litter quality, drive decomposition in a freshwater ecosystem. Sci. Rep. 10:20333. doi: 10.1038/s41598-020-77382-7
Pal, P. K., Kumar, R., Guleria, V., Mahajan, M., Prasad, R., Pathania, V., et al. (2015). Crop-ecology and nutritional variability influence growth and secondary metabolites of Stevia rebaudiana Bertoni. BMC Plant Biol. 15:67. doi: 10.1186/s12870-015-0457-x
Parmar, A. G., Thakur, N. S., and Gunaga, R. P. (2019). Melia dubia Cav. Leaf litter allelochemicals have ephemeral allelopathic proclivity. Agroforest. Syst. 93, 1347–1360. doi: 10.1007/s10457-018-0243-5
Pradisty, N. A., Amir, A. A., and Zimmer, M. (2021). Plant species- and stage-specific differences in microbial decay of mangrove leaf litter: the older the better? Oecologia 195, 843–858. doi: 10.1007/S00442-021-04865-3
Prescott, C. E., and Vesterdal, L. (2021). Decomposition and transformations along the continuum from litter to soil organic matter in forest soils. For. Ecol. Manag. 498:119522. doi: 10.1016/j.foreco.2021.119522
Quan, W., Wang, A., Li, C., and Xie, L. (2022). Allelopathic potential and allelochemical composition in different soil layers of Rhododendron delavayi forest, southwest China. Front. Ecol. Evol. 10:963116. doi: 10.3389/FEVO.2022.963116
R Core Team. (2021). R: A language and environment for statistical computing (4.0.4). Vienna: R Foundation for Statistical Computing.
Ramos, S. M., Graça, M. A., and Ferreira, V. (2021). A comparison of decomposition rates and biological colonization of leaf litter from tropical and temperate origins. Aquat. Ecol. 55, 925–940. doi: 10.1007/S10452-021-09872-3
Rezaei, E., and Abedi, M. (2017). Efficient Ultrasound-assisted extraction of cichoric acid from Echinacea purpurea root. Pharm. Chem. J. 51, 471–475. doi: 10.1007/s11094-017-1635-y
Ristok, C., Leppert, K. N., Franke, K., Scherer-Lorenzen, M., Niklaus, P. A., Wessjohann, L. A., et al. (2017). Leaf litter diversity positively affects the decomposition of plant polyphenols. Plant Soil 419, 305–317. doi: 10.1007/s11104-017-3340-8
Ristok, C., Leppert, K. N., Scherer-Lorenzen, M., Niklaus, P. A., and Bruelheide, H. (2019). Soil macrofauna and leaf functional traits drive the decomposition of secondary metabolites in leaf litter. Soil Biol. Biochem. 135, 429–437. doi: 10.1016/j.soilbio.2019.06.007
Sattolo, T., Otto, R., Mariano, E., and Kamogawa, M. Y. (2016). Adaptation and validation of colorimetric methods in determining ammonium and nitrate on tropical soils. Commun. Soil Sci. Plan. 47, 2547–2557. doi: 10.1080/00103624.2016.1243710
Scavo, A., Abbate, C., and Mauromicale, G. (2019). Plant allelochemicals: agronomic, nutritional and ecological relevance in the soil system. Plant Soil 442, 23–48. doi: 10.1007/s11104-019-04190-y
Šćepanović, M., Košćak, L., Šoštarčić, V., Pismarović, L., Milanović-Litre, A., and Kljak, K. (2022). Selected phenolic acids inhibit the initial growth of Ambrosia artemisiifolia L. Biology 11:482. doi: 10.3390/BIOLOGY11040482
Schmidt, A., John, K., Auge, H., Brandl, R., Horgan, F. G., Settele, J., et al. (2016). Compensatory mechanisms of litter decomposition under alternating moisture regimes in tropical rice fields. Appl. Soil Ecol. 107, 79–90. doi: 10.1016/j.apsoil.2016.05.014
Shen, Y., Tian, D., Hou, J., Wang, J., Zhang, R., Li, Z., et al. (2021). Forest soil acidification consistently reduces litter decomposition irrespective of nutrient availability and litter type. Funct. Ecol. 35, 2753–2762. doi: 10.1111/1365-2435.13925
Tang, F. H., Quan, W. X., Li, C. C., Huang, X. F., Wu, X. L., Yang, Q. A., et al. (2019). Effects of small gaps on the relationship among soil properties, topography, and plant species in subtropical rhododendron secondary forest, southwest China. Int. J. Environ. Res. Public Health 16:1919. doi: 10.3390/ijerph16111919
Wang, R. L., Liu, S. W., Xin, X. W., Chen, S., Peng, G. X., Su, Y. J., et al. (2017). Phenolic acids contents and allelopathic potential of 10 cultivars of alfalfa and their bioactivity. Allelopathy J. 40, 63–70. doi: 10.26651/2017-40-1-1066
Wang, Y., Dai, Q., Ding, P., Li, K., Yi, X., He, J., et al. (2022). Rapid response of runoff carrying nitrogen loss to extreme rainfall in gentle slope farmland in the karst area of SW China. Water Sui. 14:3341. doi: 10.3390/w14203341
Wang, Y., Li, C., Wang, Q., Wang, H., Duan, B., and Zhang, G. (2016). Environmental behaviors of phenolic acids dominated their rhizodeposition in boreal poplar plantation forest soils. J. Soil. Sediment. 16, 1858–1870. doi: 10.1007/s11368-016-1375-8
Wardle, D. A., Bardgett, R. D., Klironomos, J. N., Setala, H., Van Der Putten, W. H., and Wall, D. H. (2004). Ecological linkages between aboveground and belowground biota. Science 304, 1629–1633. doi: 10.1126/science.1094875
Wickings, K., Grandy, A. S., Reed, S. C., and Cleveland, C. C. (2012). The origin of litter chemical complexity during decomposition. Ecol. Lett. 15, 1180–1188. doi: 10.1111/j.1461-0248.2012.01837.x
Xie, X., and Dai, C. (2015). Degradation of a model pollutant ferulic acid by the endophytic fungus Phomopsis liquidambari. Bioresour. Technol. 179, 35–42. doi: 10.1016/j.biortech.2014.11.112
Xue, D., Yao, H., and Huang, C. (2006). Microbial biomass, N mineralization and nitrification, enzyme activities, and microbial community diversity in tea orchard soils. Plant Soil. 288, 319–331. doi: 10.1007/s11104-006-9123-2
Yang, G., Zhou, D., Wan, R., Wang, C., Xie, J., Ma, C., et al. (2022). HPLC and high-throughput sequencing revealed higher tea-leaves quality, soil fertility and microbial community diversity in ancient tea plantations: compared with modern tea plantations. BMC Plant Biol. 22:239. doi: 10.1186/s12870-022-03633-6
Zechmeister-Boltenstern, S., Keiblinger, K. M., Mooshammer, M., Peñuelas, J., Richter, A., Sardans, J., et al. (2015). The application of ecological stoichiometry to plant–microbial–soil organic matter transformations. Ecol. Monogr. 85, 133–155. doi: 10.1890/14-0777.1
Zhang, X., Wang, B., and Liu, Z. (2019). Impacts of plant secondary metabolites from conifer litter on the decomposition of Populus purdomii litter. J. For. Res. 30, 2237–2245. doi: 10.1007/s11676-018-0766-7
Zhou, X., Wang, Y., and Shen, S. (2020). Transcriptomic comparison reveals modifications in gene expression, photosynthesis, and cell wall in woody plant as responses to external pH changes. Ecotox. Environ. Safe. 203:111007. doi: 10.1016/j.ecoenv.2020.111007
Keywords: phenolic acids, Rhododendron forest, litter decomposition, nutrient content, metabolism
Citation: Zhao Y, Lin J, Quan W and Li C (2023) Distribution of six phenolic acids and soil nutrient relationships during litter decomposition in Rhododendron forests. Front. For. Glob. Change 6:1142510. doi: 10.3389/ffgc.2023.1142510
Received: 11 January 2023; Accepted: 28 March 2023;
Published: 12 April 2023.
Edited by:
Chao Fang, Nanjing University of Information Science and Technology, ChinaCopyright © 2023 Zhao, Lin, Quan and Li. This is an open-access article distributed under the terms of the Creative Commons Attribution License (CC BY). The use, distribution or reproduction in other forums is permitted, provided the original author(s) and the copyright owner(s) are credited and that the original publication in this journal is cited, in accordance with accepted academic practice. No use, distribution or reproduction is permitted which does not comply with these terms.
*Correspondence: Chaochan Li, Y2hhb2NoYW5sQGd6bnUuZWR1LmNu
†These authors have contributed equally to this work and share first authorship