- 1Guangxi Key Laboratory of Forest Ecology and Conservation, College of Forestry, Guangxi University, Nanning, China
- 2Guangxi Colleges and Universities Key Laboratory for Cultivation and Utilization of Subtropical Forest Plantation, College of Forestry, Guangxi University, Nanning, China
- 3Academy of Forest Inventory and Planning, State Forestry Administration, Beijing, China
As phenolic acids accumulated in monocropping plantations, their allelopathic effects caused the soil’s fertility to decline and productivity to decrease. The effects of stand age and generational turnover of phenolic acids in soil remain unclear. Therefore, we conducted an experiment to examine the changes in phenolic acid content and accumulation characteristics in soils from Eucalyptus plantations in terms of planting years and generations. Soils were collected from three Eucalyptus plantations: a 6-year-old first-generation plantation (6a1g), 9-year-old first-generation plantation (9a1g) and 6-year-old second-generation plantation (6a2g). Seven phenolic acids were identified from different soil samples by high-performance liquid chromatography. In addition, exogenous phenolic acids were added to the soil samples to study the kinetics as well as the adsorption and desorption characteristics of soils. The total of seven phenolic compounds in 6a1g (38.451 μg g–1) was higher than that of the 9a1g and 6a2g soils, and the total of 6a2g (27.257 μg g–1) was higher than that of the 9a1g (15.536 μg g–1). In the tested soil, the p-hydroxybenzoic acid accounted for 51.6% (for 6a1g), 51.1% (for 9a1g) and 33.5% (for 6a2g) of the total amount of the seven phenolic acids, the p-hydroxybenzoic may have had a higher allelopathic capacity in these Eucalyptus plantation soils. Soil bulk density showed a strong positive correlation with vanillic and ferulic acids, and SOM also had an extremely positive correlation with p-hydroxybenzoic and coumalic acids. The findings demonstrated that the adsorption and desorption rates and amounts of each phenolic acid in the soil of 6a1g were higher than those in the soil of 9a2g and 6a2g using both a kinetic adsorption experiment and isothermal adsorption and desorption experiments. But among the three soils, 9a1g soil had the lowest adsorption rate, which resulted in the lowest accumulation capacity. It is noticed that the Pseudo-second-order kinetic model can be used to describe the kinetic adsorption better than the Pseudo-first-order kinetic model and the Elovich model, and the Freundlich model could fit the desorption isotherms better than the adsorption isotherms. However, more study of this phenomenon is essential to better understanding its mechanisms and how they function in order to address issues with soil degradation in future intergenerational Eucalyptus plantings.
1. Introduction
Phenolic acids, organic acids with active carboxyl groups on aromatic rings, are not only secondary plant metabolites in plants, but also major allelopathic compounds, occurring widely in soils, decomposing plant residues and roots (Zhao et al., 2022). Litter leachate, root exudates, and the byproducts of lignin-rich plant debris are the main sources of phenolic acids in soil. The species diversity, vegetation, environmental factors, and soil characteristics all affect the composition of soil phenolics (Zwetsloot et al., 2020), with significant differences between deciduous and conifer forests (Buondonno et al., 2014). Most phenolic acids in soil normally promote the growth of their source plants by allelopathic inhibition of other plants (Lockwood et al., 2013). On the other hand, many studies have suggested that the accumulation of phenolic acids in soil is one of the important factors that hinder continuous mono-cropping (Qu and Wang, 2008; Bao et al., 2022), directly or indirectly alter the soil’s microbial population, encourages soil sickness (Wu and Lin, 2020) and excessive concentrations of phenolic acids can inhibit the growth of plants (Kato-Noguchi and Kurniadie, 2021).
This allelopathy also assumes a significant role in different managed forestry systems, and a number of trees have been reported to exhibit this phenomenon (Reigosa and Gonzalez, 2006). It was reported that allelopathy due to phenolics is a cause of natural regeneration deficiency in subalpine spruce (Picea abies L. Karst.) forests (Latif et al., 2017), and germination bioassays showed their autotoxicity involving spruce with inhibitory effects on root elongation and seedling growth (Yang et al., 2006). In recent years, with the decline of plantation productivity in monoculturing consecutive forest land, the phenolic acids in soil have attracted the attention of many scholars (Li et al., 2020; Zhao et al., 2022). Increasing evidence from those widely planted tree species in the form of successive monocultures seemed to support this “self-toxicity” caused by phenolic acids (Wu et al., 2016). In the majority of cases, one tree species planted continuously for a long time may suffer from allelochemicals like phenolic acids secreted by the root system and released by litter decomposition, thus resulting in negative allelopathy in replanted forest soil (Abbas et al., 2017; Kato-Noguchi and Kurniadie, 2021).
In China, some fast-growing timber tree species are planted continuously on the same forestlands, such as Chinese fir and Eucalyptus, which are representative species of successive mono-cropping for multiple generations. The accumulation of phenolic substances in soil may be one of the important reasons for the yield reduction of Chinese fir under continuous cultivation in southern China (Tan et al., 2008; Liu et al., 2020). With the consecutively planted generations in poplar plantations, the target phenolic acids and their total amount had an accumulation trend in soils, presenting as rhizosphere soil of generation II > rhizosphere soil of generation I > non-rhizosphere soil of generation II > non-rhizosphere soil of generation I (Tan et al., 2008; Wang Y. et al., 2016). Phenolic acids inhibit photosynthesis, biomass reduction, low organic matter accumulation, leaf cell damage, fine root growth, nutrient uptake efficiency, and nitrogen transformation (Li et al., 2020; Sengupta and Pal, 2021). The evidence found in Chinese fir plantations showed that the water extracts from soils, litters, and fresh tissues inhibited seed germination (Chen and Wang, 2013), organ development, root vigor, and chlorophyll content (Chen et al., 2014; Li et al., 2020) of the fir, linking to vanillin, p-coumaric, ferulic, and p-hydroxybenzoic acids (Lin et al., 2010; Wei, 2017).
Eucalyptus is a fast-growing, high-yielding, broad-leaved tree species that is widely cultivated in tropical and subtropical regions, contributing economically to the pulp and timber industries. However, the sustainability of eucalyptus plantations is a worry in many areas given the current state of global change, which includes an increase in the frequency of drought events, a rise in global temperature, allelopathy, and a rapid spread of pests and diseases (Laclau et al., 2018). In those regions where Eucalyptus plantations have expanded to cover more forest land in consecutive monoculture and regenerate by sprouting for multiple generations after clearing, the allelopathic substances secreted by Eucalyptus have become a hotspot of research, because of their potential effects on the growth of Eucalyptus or other plants (Zhou et al., 2020). In Eucalyptus stands, the allelochemicals enter the soil by means of rain washing leaves, decomposition of litter, and root secretion (Becerra et al., 2018), resulted an invasion of exotic plants occurred in the understory as a result of reductions in understory grasses, woody plants, and soil N and P cycling indices (Zhou et al., 2020). Previously, we analyzed the differences in phenolic acid accumulation and the effects on soil enzyme activities between the pure and mixed Eucalyptus plantations (He et al., 2017; Liao et al., 2019), but the changes in soil phenolic acid with the planting time of eucalyptus on forestland need to be investigated.
Furthermore, phenolic acid accumulation in Eucalyptus plantation soils should be dependent on phenolic acid adsorption and degradation abilities as a result of root exudates littering in different forest types (Wang, 2016). However, the relationship between the adsorption dynamics of phenolic acids and their environmental behavior in Eucalyptus plantations is still unclear. Therefore, adsorption and desorption isotherms provide valuable information related to the quantification of phenolic acids in soil, and these data can be used to assess their possible effects in environmental systems. The introduction of a mathematical model provides us with a useful tool to reveal the environmental behavior of phenolic acids (Sun et al., 2012; Mahmoudi et al., 2016). However, some basic research questions need to be addressed, e.g., what factors might be relevant to the accumulation of phenolic acids process? How do the different phenolic acids change during the processes of plantation and regeneration? To solve these acknowledged problems, it might be helpful to understand the productivity decline of Eucalyptus plantations from the perspective of phenolic acid allelopathy.
To identify the stand age and generation effects of Eucalyptus plantations on soil phenolic acid, we made a comparison at our study location in China’s subtropical region. In this case, we hypothesize that there are obvious differences in the soil phenolic acid, and the differences may be different in various stand ages. In order to clarify the performance of phenolic acids in the soils of Eucalyptus plantations with planting time, we collected the soils from three intergenerational Eucalyptus plantations with research objectives: (1) to investigate the changes of phenolic acids in forestland soils; (2) to study the adsorption or desorption characteristics; and (3) to assess the changes in phenolic acids by using the kinetics and isotherm curves.
2. Materials and methods
2.1. Study sites and soil sampling
This study was performed in mid-2016 at the Experimental Center of Tropical Forestry (21°57′47″–22°19′27″N, 106°39′50″–106°59′30″E; 1045.9 m), Chinese Academy of Forestry, Pingxiang, Guangxi, China. Three forest types of pure Eucalyptus plantations were studied: 6-year-old first-generation forest (6a1g), 9-year-old first-generation forest (9a1g) and 6-year-old second-generation forest (6a2g; Table 1). Although the content of phenolic acids in rhizosphere soil is generally higher than that in bulk soil, because the rhizosphere is limited to a few millimeters of space away from the root surface, the non-rhizosphere soil, which accounts for the majority of forest soil, is the key to controlling the category and content of phenolic acids and determining the allelopathic effect in forest land. Thus, in this study, non-rhizosphere soil was collected to describe the content distribution of different soil phenolic acids, and the adsorption dynamic mechanism was further discussed. 12 sample plots of equal size (20 m x 20 m = 400 m2) were randomly selected in each forest type, the number of stems in each plot was calculated, and divided by the plot size. Finally, the average stem number of selected plots was multiplied by 10,000, and the densities ha–1 were recorded. In each plot, 12 non-rhizospheric soil samples were collected randomly, but each sampling point was at least 1 m away from the base of the surrounding tree trunks to ensure that no roots were collected. The depth of the soil collection was 0–30 cm. If there were roots in the soil samples collected, the roots and the surrounding soil particles within at least 1 cm were removed. The soils from the same plot were mixed together for later analysis; each mixed sample is not less than 500 g. All samples were preserved in separate zip-lock bags, then divided into two parts; one was frozen in an ultralow temperature freezer until use, and the remainder was used to determine the soil density, pH, and soil organic matter (Table 1).
2.2. Determination of soil bulk density, pH and SOM
Soil bulk density (ρB) values were determined using the core method (Mualem and Assouline, 1986). Undisturbed soil core samples were collected using a stainless steel coring ring from each plot at 0–30 cm depths. Soil cores were oven-dried at 105°C for 24 h. Bulk density was calculated by dividing the mass of oven dried soil by the core volume accordingly.
The pH of soil samples was measured using a digital pH meter (Starter 2100 pH Bench, OHAUS) after the soil was shaken with distilled water at a solid-to-water ratio of 1:2.5 (w/v) for an hour. SOC was computed using the oxidation method (Bao, 2000). Soil samples (0.5 g) were digested with 5 ml of 1 mol K2Cr2O7 and 5 ml of concentrated H2SO4 and titrated with FeSO4 after boiling at 175°C for 5 minutes. Soil organic matter calculated by multiplying SOC with 1.72.
2.3. Extraction of phenolic compounds
The soil samples (25 g) needed to stand in 25 mL of 1 M NaOH overnight. NaOH was chosen as the extractant mainly because it is commonly used in soil particle dispersant and organic material solubilization (Siqueira et al., 1991), which is conducive to improving the extraction of phenolic acid. The samples were shaken for 30 min, then the supernatants were filtered through 0.22 μm filter membranes after centrifugation (4,000 rpm, 10 min, 25°C). The filtrates were soured with 12 M HCl until the pH was reduced to 2.5. After standing for 2 hours, the precipitates of humic acid appeared, and then the mixtures were centrifuged for 10 min again. We used 1 mL of the supernatants for high-performance liquid chromatography.
2.4. High-performance liquid chromatography
Seven phenolic standard compounds were purchased from Sigma-Aldrich, including hydroxybenzoic, benzoic, vanilloid, ferulic, coumarate, salicylic, and cinnamic acids. Standard mixtures of these compounds were prepared. For each phenolic standard compound, 1, 5, 10, 50, and 100 mg were weighted, and dissolved in 2 mL of chromatographic grade methanol, then diluted with ultra-pure water (Millipore, Bedford, MA, USA). After methanol volatilization, the soil pH was adjusted by 0.5 M HCl or NaOH and then supplemented by ultra-pure water, resulting in concentrations of 1, 5, 10, 50, and 100 μg⋅mL–1. The standard curve was obtained by linear regression of the peak area and concentration of these seven phenolic acids.
Standards and sample analysis were carried out using a Waters-e2695 high-performance liquid chromatograph system (Milford, MA, USA). The phenolic acids were separated on a Luna 5u C18(2) column (250 × 4.6 mm; 100A; particle size, 5 microns; Phenomenex Inc., Torrance, CA, USA), with elution using 0.1% phosphoric acid (solvent A) and acetonitrile (solvent B) at a flow rate of 1.5 mL min–1. The gradient profile was as follows: 0-1 min, isocratic, 5% B in A; 1–20 min, 5–46 % B in A; 20–21 min, 46-5 % B in A. The eluent was monitored at 230 nm with a Waters 996PAD detector.
2.5. Accumulation mechanism experiment
The accumulation mechanism experiment was mainly divided into two parts: a kinetic adsorption experiment as well as isothermal adsorption and desorption experiments. The kinetic adsorption experiments were carried out on a constant shaking incubator at 180 rpm after 50 mL of phenolic acid mixed standard solutions with a specific concentration (100 μg/mL) were added to 5 g of ultraviolet-sterilized soil. After the start of the experiment, under sterile conditions, and at 1 h, 4 h, 8 h, 14 h, 24 h, 36 h, 48 h, 60 h, and 72 h, respectively, mixtures were centrifuged (4,000 × g, 10 min, 25°C), and then 2 mL of each supernatant was analyzed by high-performance liquid chromatography. To further evaluate the kinetics of the adsorption process (Qin et al., 2015), three of the most widely applied kinetic models were used to fit the experimental data, a kinetic model suitable for describing the phenolic acid adsorption process was explored. A pseudo-first-order model is expressed as (Tseng et al., 2003; Tan and Hameed, 2017):
where qe and qt (μg⋅g–1) are the amounts of phenolic acids adsorbed at equilibrium and at time t (h), respectively. k1 is the pseudo-first-order rate constant. Pseudo-first-order kinetic model is based on Lagergren’s first-order rate equation of solid adsorption capacity. It is mainly applied to the adsorption kinetic equation of the liquid phase and is suitable for the initial stage of adsorption. Based on the hypotheses, one is that adsorption is controlled by the pore diffusion step, and the other is that the adsorption rate is proportional to the difference between equilibrium adsorption capacity and t-moment adsorption capacity. If the adsorption process conforms to a pseudo-first-order kinetic model, the curve drawn by lg (qe-qt) and t should be a straight line. The model considers that the mass transfer resistance of particles is the limiting factor of adsorption.
A pseudo-second-order equation (Wang and Guo, 2020) has been widely used to predict kinetic adsorption. It was expressed as:
where k2 is the rate constant of second-order adsorption. The pseudo second-order kinetic model is based on an assumption that adsorption rate is controlled by chemical adsorption mechanisms, which involve electron sharing or electron transfer between adsorbent and adsorbate. It is consistent across the entire adsorption equilibrium time range and can generally explain the adsorption mechanism. The main idea of the pseudo-second order kinetic model is chemical adsorption, whole rate depends on chemical adsorption, and the adsorption capacity of the adsorbent mainly depends on active sites on the surface of the adsorbent. If the model is satisfied, a straight line should be obtained for mapping to t/qt and t. It is considered that the limiting factor of adsorption is the adsorption mechanism, not mass transfer.
The Elovich equation has the effect of characterizing this type of chemisorption and can be given in the following equation (Kajjumba et al., 2018).
where a is the initial adsorption rate and b is an indication of the number of sites available for adsorption. The phenolic acid uptake at any time, qt, was calculated using Eq. (4):
Whether, W (g) represents the mass of the soil samples and V (mL) is the volume of the drawing solution. C0 and Ce (μg⋅mL–1) are the initial and final (after adsorption) concentration of phenolic acid solution, respectively.
The isothermal adsorption experiments were conducted at 25°C. Five g of soil samples was added into 50 mL of phenolic acid mixed as standard solutions of different initial concentrations (100–400 μg⋅mL–1) in 100 mL Erlenmeyer flasks until the system reached adsorption equilibrium (shaking 24 h, then standing for 30 min). The concentrations of phenolic acid left in the supernatants (2 mL removed) were determined to fit the Freundlich (Eq. (5)) isotherm equation:
where Kd is the constant of adsorption equilibrium and n shows the intensity of sorption. The equation tends to the axiomatic linear form only for n = 1 (Hartley, 1976).
After removing 8 mL of supernatants from the excess solutions of isothermal adsorption experiment, the above amount of solution that remained was 50 mL by adding 10 mL Milli-Q water. The desorption solutions were determined until the system reached equilibrium (like the adsorption experiment). The amount (Cs’) and rate (Rd) of desorption were calculated according to Eqs. (6) and (7), respectively:
where Rd is the desorption rate and Ce′ (μg⋅mL–1) is the concentration of phenolic acid solution after desorption equilibrium.
2.6. Statistical analysis
The obtained data were collated in MS Excel and analyzed in SPSS 19.0. The content of phenolic acid is expressed by Mean ± SD. The differences of phenolic acid content among different forest types were detected with one-way ANOVA and LSD multiple comparisons. Because soil clay, organic matter, and pH are related to the amount of phenolic acid adsorbed by soil, R (3.2) software was used to conduct the correlation analysis between soil bulk density (instead of clay content, because of a significant positive correlation between them (R2 > 0.9), organic matter, pH, and phenolic acid accumulation in different soils.
3. Results
3.1. Quantitative analysis of soil phenolic acids
Seven phenolic acids, including p-hydroxybenzoic, benzoic, vanillic, ferulic, coumarate, salicylic, and cinnamic acids, were identified and quantified in the three types of Eucalyptus soils (Figure 1). The total of seven phenolic compounds in 6a1g (38.451 μg⋅g–1) was higher than that of the 9a1g and 6a2g soils, whereas in 6a2g (27.257 μg g–1) was found higher than the 9a1g (15.536 μg g–1). The percentages of p-hydroxybenzoic, vanillic, ferulic and coumarate acid varied among the different types of forest: 6a1g (51.6, 17.7, 7.8, and 19.7%), 9a1g (51.1, 7.1, 26.4, and 11.8%) and 6a2g (33.5, 26.6, 22.8, and 9.6%), although the other phenolic compounds made up less than 5% of the total. Benzoic, and cinnamic acids in 6a2g were significantly higher than in 9a1g, while coumalic acid in 6a2g and 9a1g was significantly lower than in 6a1g. In addition, no significant differences were observed for the vanillic, benzoic and salicyclic acids between 6a1g and 6a2g.
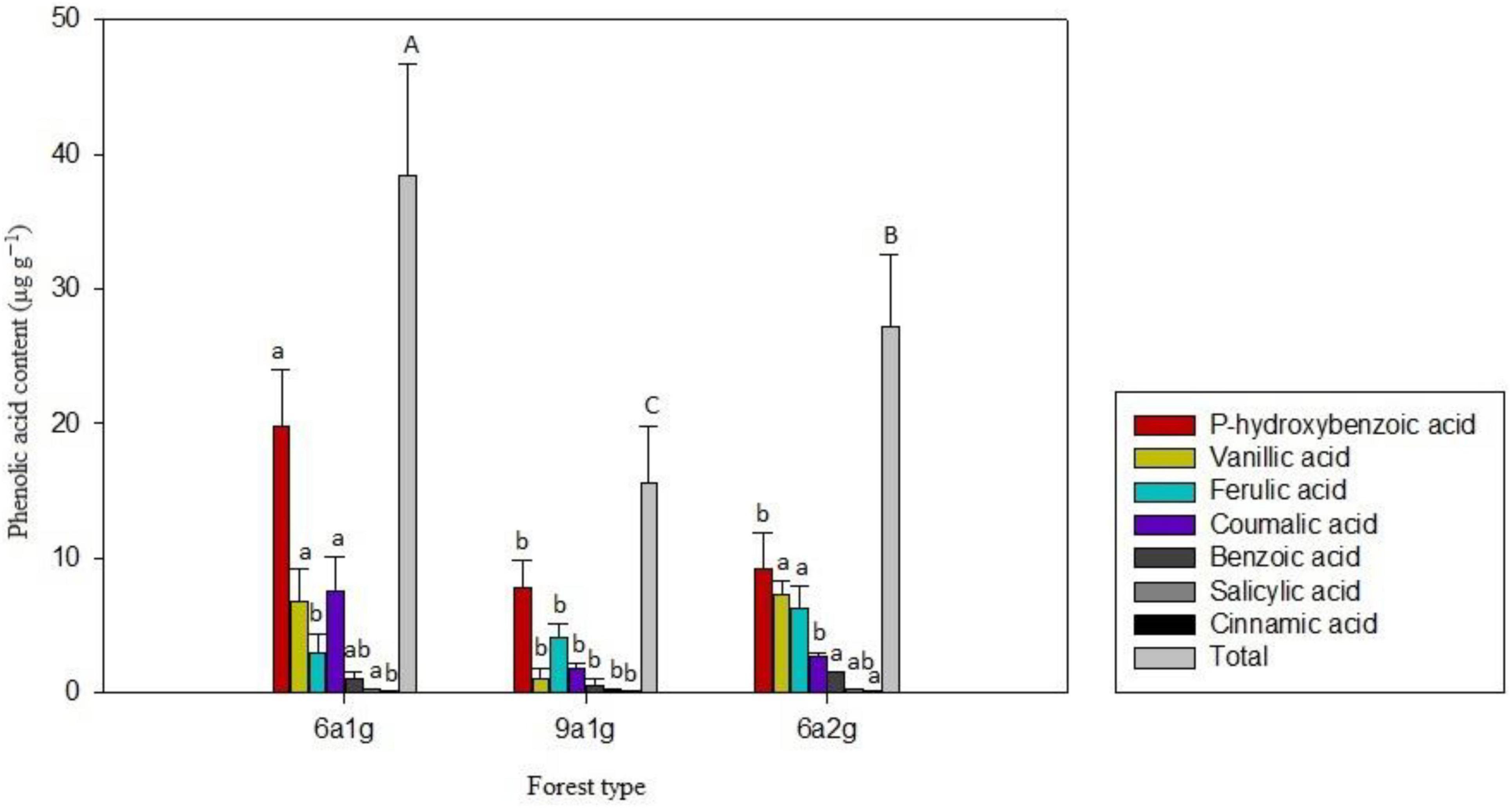
Figure 1. Phenolic acid content of the three Eucalyptus plantation soils. 6a1g, 6-year-old first-generation forest; 9a1g, 9-year-old first-generation forest; 6a2g, 6-year-old second-generation forest.
3.2. Relationship between phenolic acid content and soil characteristics
Soil bulk density, pH and soil organic matter were chosen to explore the relationship between them and phenolic acids in non-rhizosphere soil. Clay is usually considered to be positively correlated with soil phenolic acid content, mainly because of its strong adsorption capacity. We used the bulk density to replace the clay content because there is a positive correlation between them in general. Except for benzoic acid, the correlation analysis showed that pH had little effect on other phenolic acids. Soil bulk density showed a strong or an extremely strong positive correlation with vanillic, and ferulic acid, and SOM also had a strong or extremely strong positive correlation with p-hydroxybenzoic and coumalic acid (Figure 2).
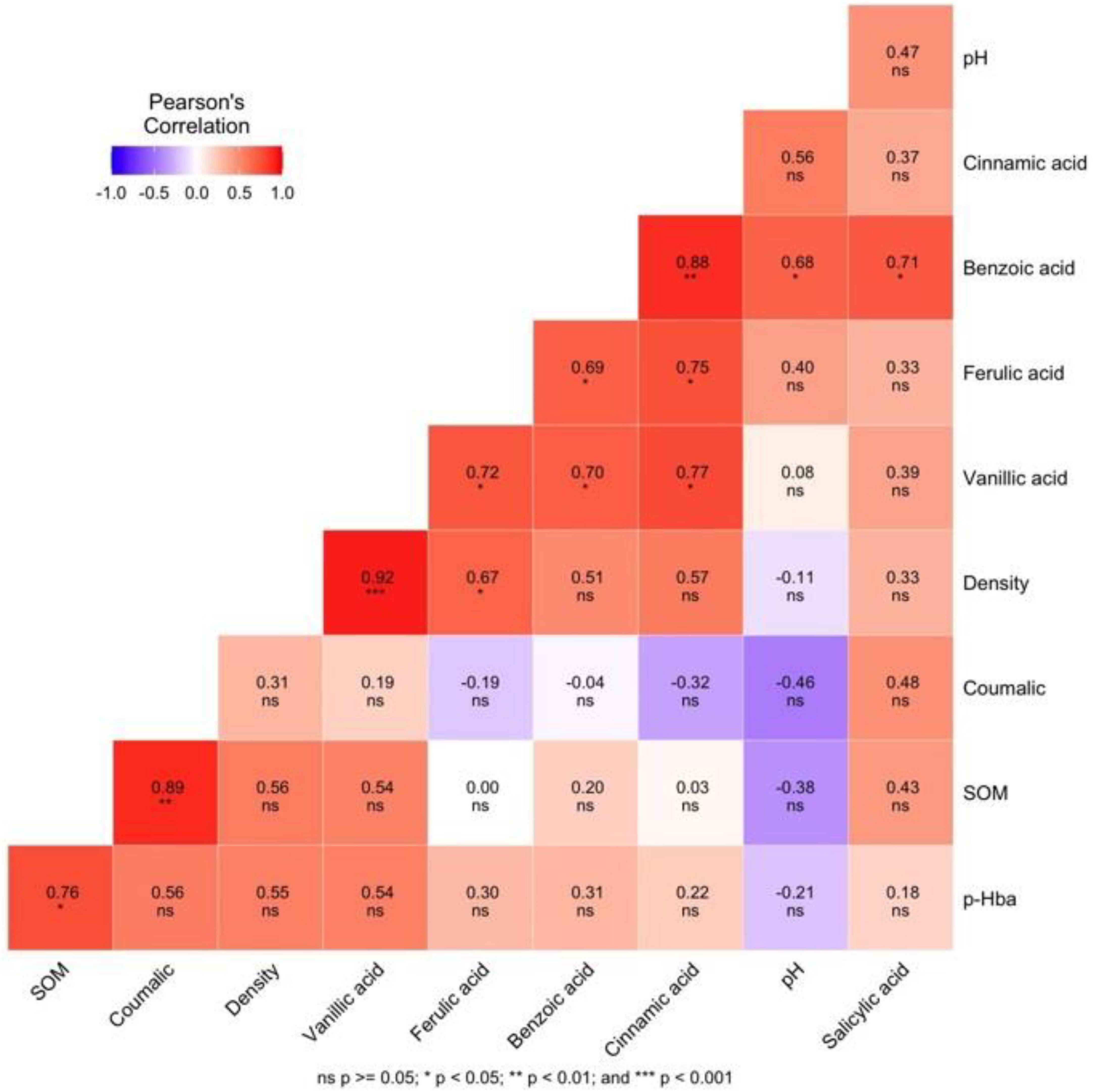
Figure 2. Correlation heat map of soil bulk density; pH and soil organic matter with phenolic acid contents. Red colors indicate positive relationships, and blue colors indicate negative relationships. Color, carries from white to dark red or dark blue trend to positive or negative correlations, respectively. SOM, soil organic matter; Density, soil bulk density; pHba, Phydroxybenzoic acid.
3.3. Kinetic adsorption study
Figures 3A, B shows the results of kinetic adsorption experiments conducted to determine the equilibrium time and adsorption quantity required for the uptake of different phenolic acid substances on the various soils of Eucalyptus. The equilibrium times in different types of Eucalyptus plantations were basically identical but varied for the different phenolic acids. The equilibrium times of p-hydroxybenzoic, vanilloid, ferulic, and benzoic acids were all 8 h in 6a1g, 9a1g, and 6a2g, while salicylic and cinnamic acids lasted nearly 36 h. Coumarate acid had the longest equilibrium time, lasting 60 hours. In terms of the adsorption quantity, in addition to coumarate acid, the other acids showed that the content in 6a1g was higher than in 9a1g or 6a2g. No obvious difference was observed for coumarate acid among the three plantation types. In the three different types of Eucalyptus plantations, cinnamic acid had the highest equilibrium adsorption quantity (about 700-1,000 μg⋅g–1) and p-hydroxybenzoic and benzoic acids were the lowest (100-200 μg⋅g–1) among the seven phenolic acids. Table 2 shows the kinetic adsorption parameters of three models. Based on R2, qe,exp and qe,cal values, the pseudo-second-order model (R2 > 0.95, qe,exp and qe,cal are similar) can be used to describe this kinetic adsorption better than the pseudo-first-order model (R2 < 0.9, qe,exp and qe,cal show differences). In addition to p-hydroxybenzoic in 6a2g, the Elovich model may also fit these data well (R2 > 0.8).
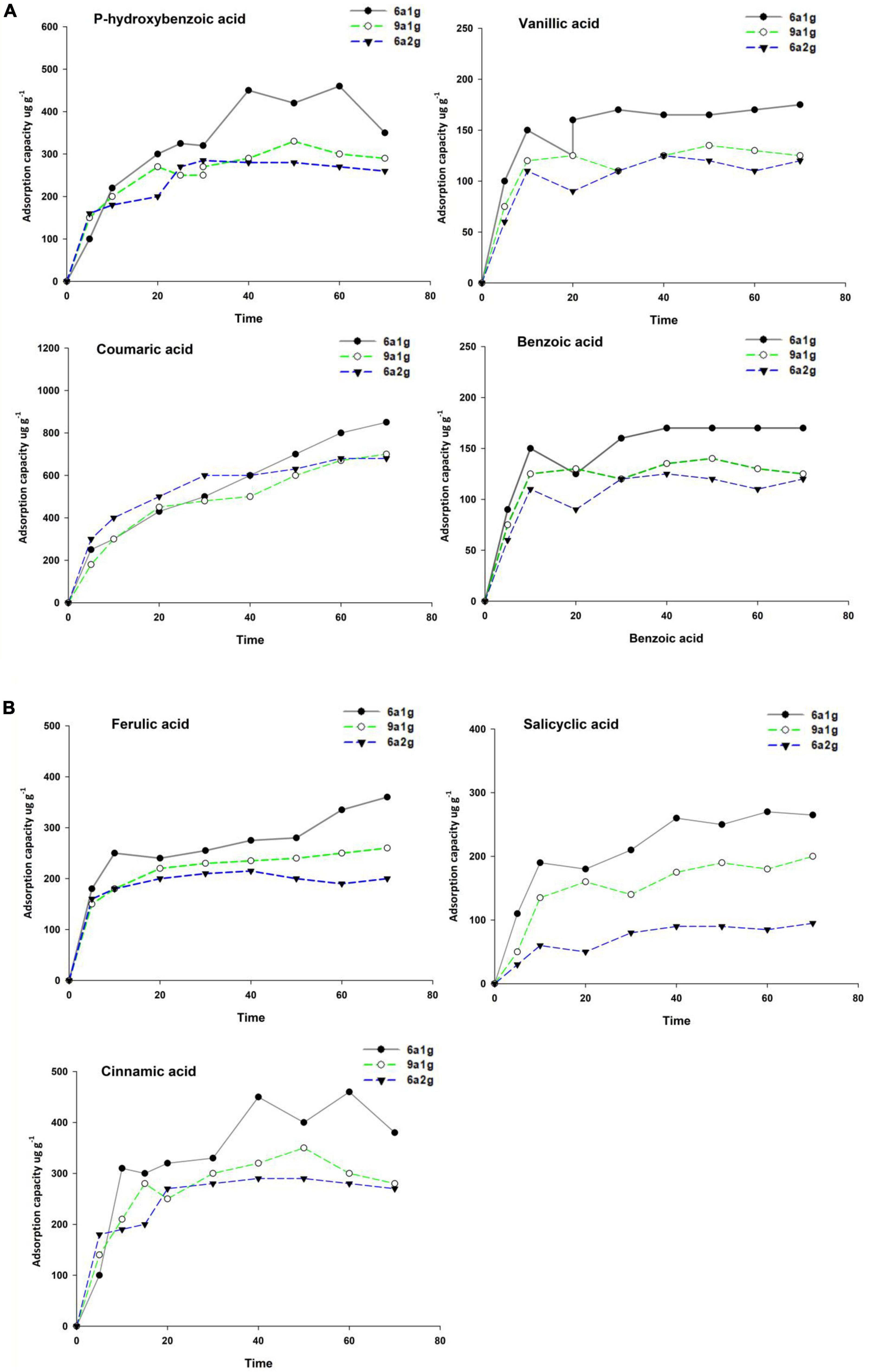
Figure 3. (A,B) Kinetic adsorption of seven phenolic acids in non-rhizospheric soils of different Eucalyptus plantations. 6a1g, 6-year-old first-generation forest; 9a1g, 9-year-old first-generation forest; 6a2g, 6-year-old second-generation forest.
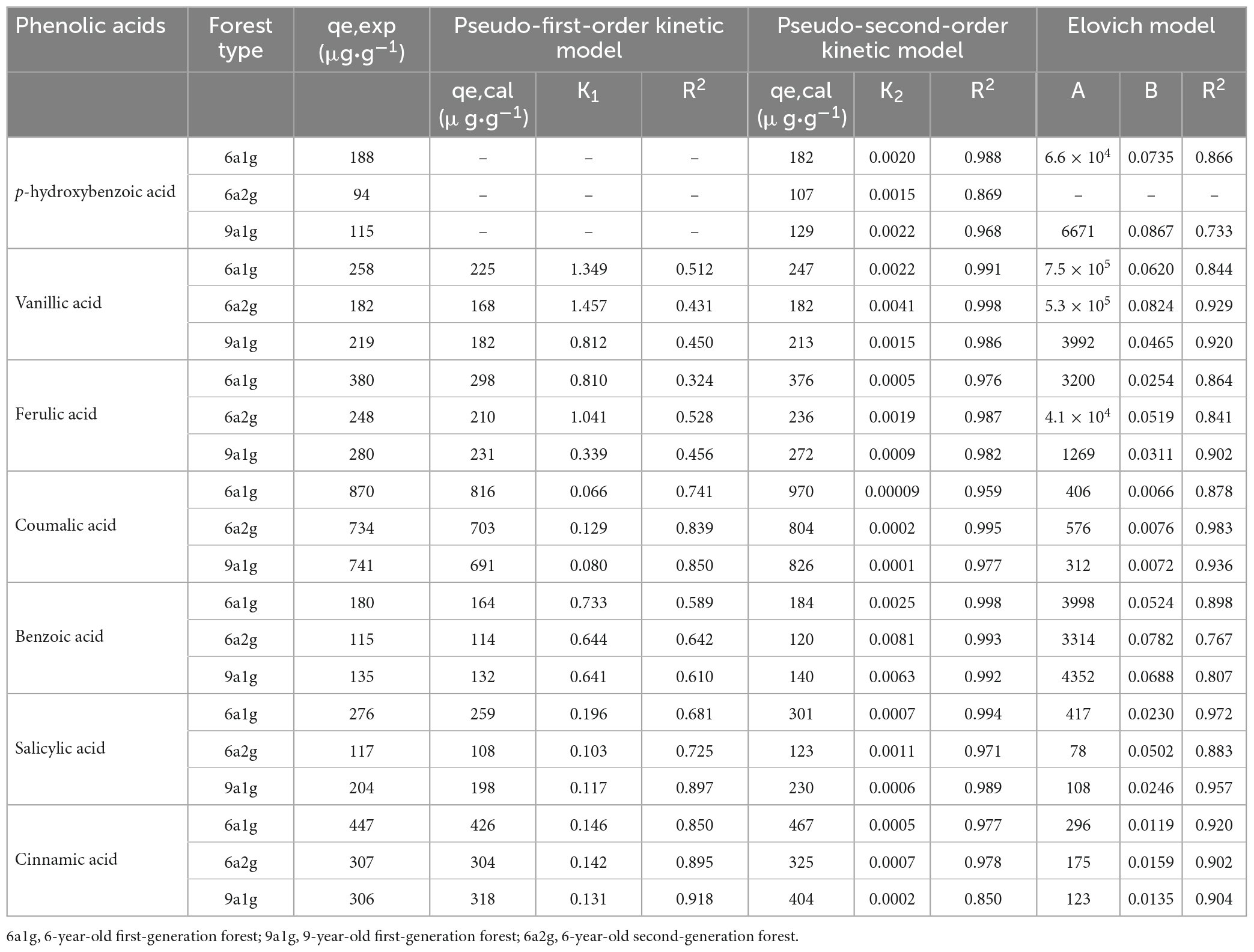
Table 2. Kinetic adsorption rate model equation constants and correlation coefficients for the adsorption of phenolic acids of three Eucalyptus plantation soils.
3.4. Adsorption and desorption experiments of three Eucalyptus plantation soils
The characteristics of the adsorption of seven phenolic acids were studied in three different soils and characterized through sorption isotherms. Sorption isotherms were described by the Freundlich equation with regression coefficients R2 > 0.9, except for the sorption isotherms of ferulic, coumarate, and benzoic acids in 9a1g and p-hydroxybenzoic, vanilloid, and salicylic acids in three soils (Table 3). The values of Kd were higher in 6a1g (0.645-4.364) than in 6a2g (0.046-1.493) for all of the phenolic acids, while the values of 1/n were the opposite. In addition to cinnamic acid, the Freundlich equation was unsatisfactory for the other phenolic acids in 9a1g. The Kd for cinnamic acid in the soils could be ranked in decreasing order: 6a1g > 6a2g > 9a1g. However, 6a1g soil had the highest average adsorption rate (12.313-22.807%) among the three soils for all seven phenolic acids.
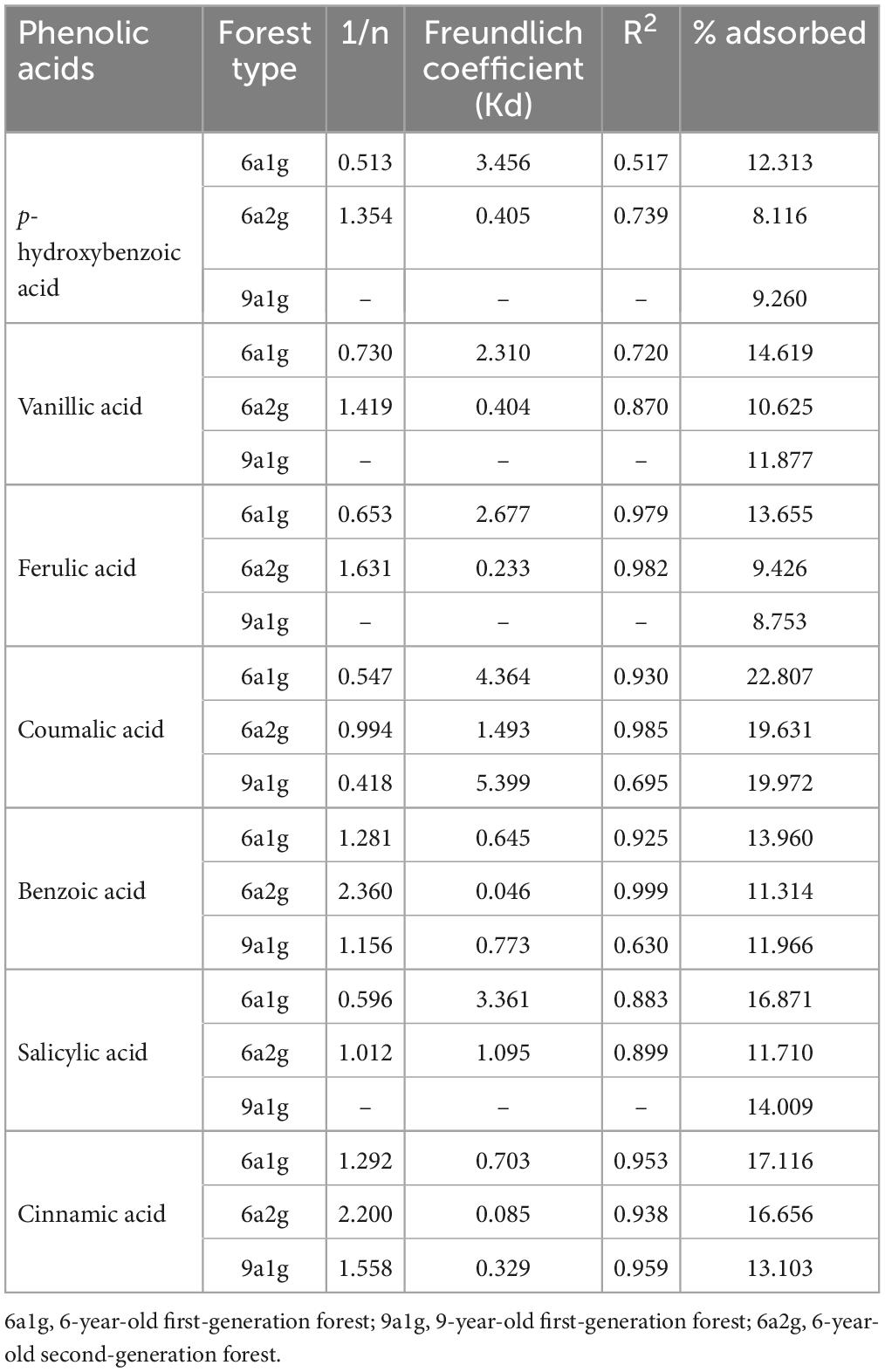
Table 3. Phenolic acid adsorption isotherm parameters for the Freundlich model of three Eucalyptus plantation soils.
Through the Freundlich model (Table 4) the desorption isotherms fit very well. The regression coefficients were all greater than 0.9, except for the desorption isotherms of hydroxybenzoic, vanilloid, and salicylic acids in 9a1g soil and benzoic acid in 6a2g soil. The Freundlich coefficient (Kd) showed the order was 9a1g > 6a2g > 6a1g for p-hydroxybenzoic, vanillic, ferulic, coumalic, and salicylic acids, while for benzoic and cinnamic acids the highest ones were 6a2g and 6a1g, respectively. However, 1/n was the opposite of Kd.
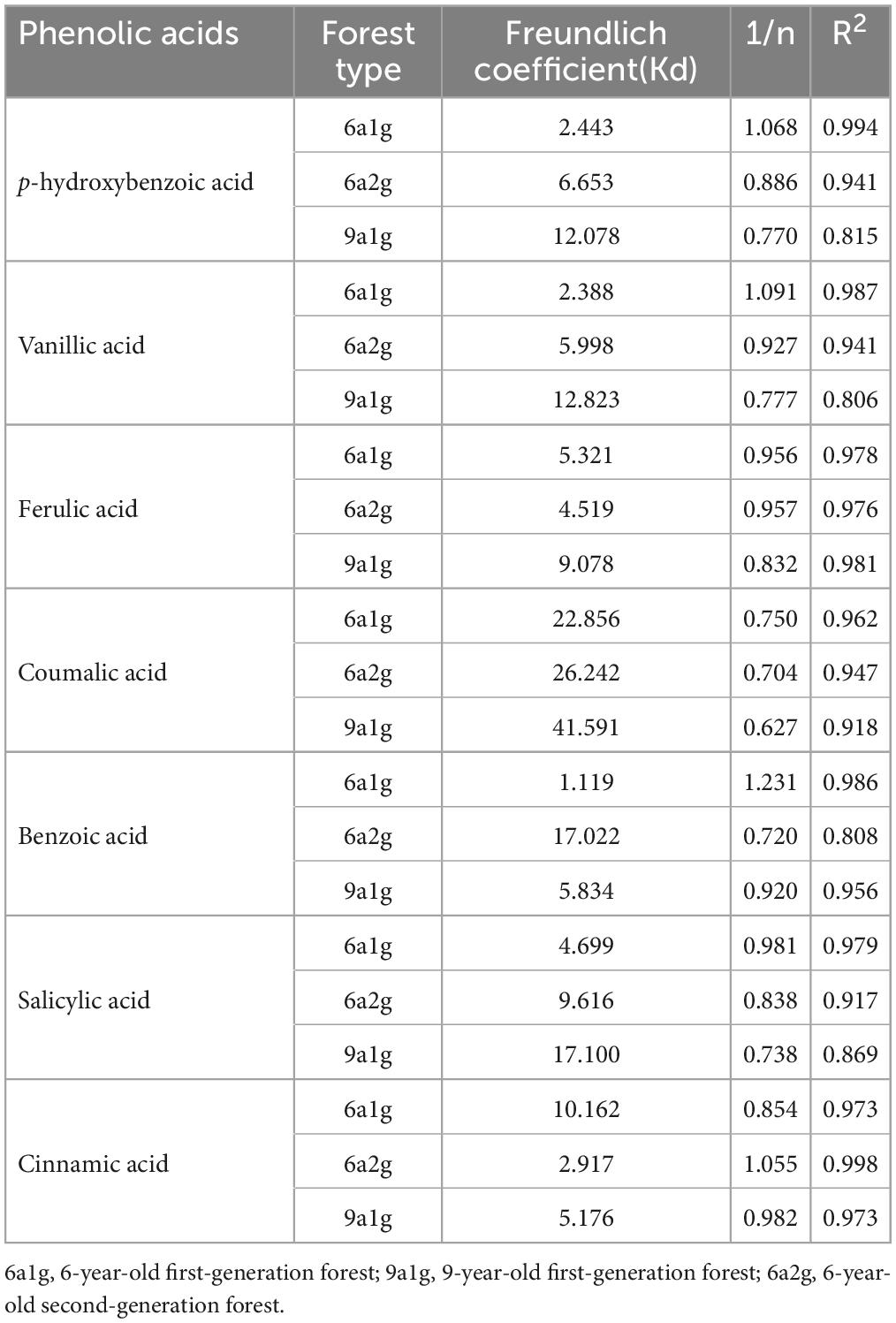
Table 4. Phenolic acid desorption isotherm parameters for the Freundlich model of three Eucalyptus plantation soils.
4. Discussion
4.1. Quantitative analysis of soil phenolic acids
We can usually separate and identify a variety of allelopathic phenolic acids in soil. For example, Chou and Muller (1972) identified p-hydroxybenzoic, p-coumaric, ferulic, syringic, and o-coumaric acids during research related to the allelopathic mechanisms of Arctostaphylos glandulosa var. zacaensis. Li et al. (1992) found that p-coumaric, ferulic, vanillic, and p-hydroxybenzoic acids, as well as p-hydroxybenzaldehyde, were the main allelochemicals in Sasa soil. Huang et al. (2013) also identified caffeic, ferulic, p-coumaric, chlorogenic, and p-hydroxybenzoic acids as the main phytotoxins. These phenolic acids can be divided into volatile, water-soluble, and compound phenolic acids. Volatile phenolic acids are difficult to accumulate in the soil. Several common phenolic acids are water-soluble phenolic acids, including the tested ones here. However, due to the adsorption, the content in the soil is very low, generally 0.1-30 μg g–1. When the content of water-soluble phenolic acids in soil is high, they can be adsorbed by soil humus substances and mineral colloids to form compound phenolic acids (Muscolo et al., 2020). As a result of being adsorbed by soil colloids, compound phenolic acids are insoluble in water and cannot be absorbed by plants, it is generally harmless to plants. When the content of water-soluble phenolic acids in soil decreases, the compound phenol acids are released from soil colloids and transformed into water-soluble phenolic acids, which is a dynamic balance. Li et al. (2002) reported that the content of water-soluble phenolic acids in the soils of Chinese fir forest and broad-leaved forest was between 6.58 and 35.88 μg⋅g–1, and the content of compound phenolic acids was between 420.10 and 1,907.47 μg g–1.
All of the seven target phenolic acids in this study were extracted and identified from soils of different types of Eucalyptus plantations, their mean total amounts were 15.28-38.37 μgg–1. The amplitude range was greater than that reported by Wang Y. et al. (2016), who reported that phenolic acid concentrations in the bulk soil of Poplar plantation forest soils ranged from 14.22 to 15.03 g g1. However, Djurdjevic et al. (2004) reported that the soil of Allium ursinum L. contained 160 μg g–1 free and 1,610 μg g–1 total bound phenolic acids. In our study, p-hydroxybenzoic acid from the soil of 6a1g was highest (19.81 μg g–1). In the soils, the total of seven phenolic acids in 6a1g soil was obviously higher than in 9a1g and 6a2g soils. Therefore, it was anticipated that the p-hydroxybenzoic acid could be a key source for phenolic acids to access soils and cause allelopathy. It is generally believed that the continuous tree planting will increase the phenolic acids in the soil year by year, and the reduction in forest production is caused by this accumulation of phenolic acids (Bai et al., 2019). But the result of our study shows that the phenolic acids were not accumulating with replanting and inter-generation in Eucalyptus plantations. The results of this study supported the hypothesis that the content of phenolic acids in soil usually varies with soil pH and nutrient status. Tan et al. (2008) identified five soil phenolic acids from the first, second, and third generations of P. nigra plantations and found that the total content of phenolic acids in the soils of the second and third generation forests was 137.87 and 64.18%, respectively. Therefore, continuous planting does not necessarily lead to the accumulation of soil phenolic acids. Phenolic acid accumulation is dynamically controlled by biotic and abiotic factors in the soil. Despite the lack of microbial evidence here, previous studies have confirmed that fungal growth and reproduction can be stimulated by phenolic acids as available C sources. According to He et al. (2001), some fungi, such as Endomycopsis sp. F2, Asperigillus orazge F3, Penicillium sp. F4, Oznyun sp. F7, and Papulaspora sp. F15, were good at degrading ferulic and p-hydroxybenzoic acids. Decarboxylase, hydroxylase, and oxygenase degrading phenolic acids and their coding genes have been isolated and identified (Wang W. B. et al., 2016). These gains partly explain why soil phenolic acids from Eucalyptus plantations failed to accumulate with continuous planting.
Adsorption behavior of phenolic acids can be regulated by soil physical and chemical characteristics, such as soil organic matter, clay, and pH, thereby acting on their accumulation in soil. Soil organic matter is colloidal with absorbability, mainly covered by negative charges, but if amino groups (–NH2) absorb H+, they become –NH3+ to adsorb phenolic acids. In addition, Zwetsloot et al. (2020) believed that compared with water extracts, partially solubilizing soil organic material by NaOH solutions should contribute to higher phenolic acid concentrations in NaOH extracts, suggesting that phenolic acids in soils exist predominantly in chemically bound rather than free forms. Cecchi et al. (2004) and Wang et al. (2010) studied the adsorption characteristics of phenolic acids and suggested that the adsorption capacity of benzoic acid strongly and positively depended on the content of soil organic matter and clay particles, thus affecting its retention in soil. Therefore, it is not surprising that there is a positive correlation between phenolic acids and organic matter and bulk density, while non-significant relationship of SOM and bulk density in the present study. According to research (Chaudhari et al., 2013), bulk density is a critical feature that may be used to estimate the concentration and transport of nutrients in the soil. Bulk density decreases inversely with soil organic matter concentration, meaning that the more organic matter there is, the lower the bulk density will be (Leifeld and Kögel-Knabner, 2005). Interestingly, with continuous planting time and generations, the variation in phenolic acid content may be related to the gradual accumulation of organic matter derived from first to second generation forests over time. This might be due to humic acid, fulvic acid, and humin are three components of soil organic matter that each have unique functional groups that are crucial to the cation exchange properties of soil. It affects the maintenance of the soil’s structure, moisture retention, nutrient turnover, cation exchange capability, and pollutant degradation (Min et al., 2015). Cecchi et al. (2004) showed that phenolic acid content was also regulated by soil pH; the higher the soil pH, the faster the decomposition rate of phenolic acids (Li et al., 2010). There is evidence that the adsorption capacity of phenolic acids is negatively correlated with soil pH (Diao et al., 2005). The adsorption of variable charges on organic acids, including phenolic compounds, is proportional to the soil acidity (Mitra et al., 2020). With the increase in soil pH, anionic organic acids preferentially adsorb on the surface of Fe and Al oxide minerals. As a result, the ability of soil to adsorb phenolic acids decreases. Therefore, more phenolic acids will enter the soil solution and be degraded. However, the correlation between pH and phenolic acids was not observed in Pearson’s analysis in the current study (Figure 2). In addition, metal ion content, molecular structure, and properties of phenolic acids are closely related to the content dynamics of different phenolic acids in soil. For example, the adsorption of cinnamic acid was stronger than that of benzoic acid in soil, and their adsorption could be enhanced by a methyl oxygen group or an acrylic acid side chain on the cyclic structure.
It should be noted that although p-hydroxybenzoic, vanilloid, ferulic, and coumarate acids were rich in these soils, we are not sure that they were able to lead to self-poisoning. Just listed as the allelopathic candidates at present, there are three reasons as follows: (1) Did the content of these soil phenolic acids reach or even exceed the threshold of toxicity to Eucalyptus? We cannot give a positive response. (2) There is no effective evidence to distinguish allelopathy from other pathological or environmental stresses on phenotypic, physiological, and biochemical levels. (3) If different phenolic acids are similar in their action sites and modes, antagonism or synergism probably occurs between them, showing their separate effects. In soils, phenolic chemicals can exist in three different forms: dissolved, sorbed, and polymerized. Despite the numerous studies on phenol, there is still considerable disagreement, for instance regarding phenol transformations in soil and the impact on the rate at which soil organic matter decomposes. It’s interesting to note that some authors claim phenols’ abundance in soils might vary depending on their forms, rather than their chemical makeup (Conant et al., 2011). By altering soil pH, nutrient availability, and enzyme activity, physically and chemically protected phenolics can survive longer than dissolved forms and provide feedback to SOM degrading microbes (Dungait et al., 2012). But the action site and mode of various phenolic acids still need to be further revealed. In an instant, we need to point out that the soil phenolic acid content has an intimate association with soil adsorption mechanisms and microbial decomposition. Adsorption is a complex process involving multiple factors, to be clear, the dynamic mechanism for adsorption of soil phenolic acids is conducive to how they accumulate in the soil.
4.2. Adsorption of different Eucalyptus plantation soils
Allelopathy must be based on a certain mass concentration of allelochemicals, which has been confirmed in many controlled experiments. The process of allelopathy is not only related to the intensity of allelochemicals secreted by plant roots but is also strongly influenced by microbial activities in soil and the adsorption capacity of soil colloids as well as allelochemicals. The study of the environmental behavior characteristics of allelochemicals in soils has become an important content to reveal the accumulation mechanism of allelochemicals. Among them, the study on the adsorption and desorption behavior of allelochemicals in soil can provide an important theoretical basis for solving this problem. In the prediction of the adsorption process, a rate-limiting step is an important factor. Kinetic studies help to identify the adsorption process and predict the required design of an accumulation mechanism (Hameed et al., 2008; Subramanyam and Das, 2009). For a solid-liquid adsorption, the solute transfer during the adsorption process can be either an external mass transfer for a nonporous medium or intra-particle diffusion for a porous medium, or both combined (Ncibi et al., 2008). Results of kinetic adsorption measurements in our study showed that the equilibrium timeframes in various Eucalyptus plantations were essentially the same; however, they differed for the various phenolic acids. Along with coumarate acid, the other acids demonstrated that the content in 6a1g was larger than that in 9a1g or 6a2g in terms of adsorption quantity (Table 2).
In this study, the second-order model could be used to very well describe the kinetic data for the adsorption of phenolic acids in different types of soil. The qe,cal values of the second-order model were very close to the experimental qe,exp (Fu et al., 2015), and the correlation coefficients (R2) were all greater than 0.95, with the exception of p-hydroxybenzoic in 6 a2g soil and cinnamic acid in 9 a1g soil (Table 2). Previous, studies also found that the adsorption of pesticides from aqueous solutions in soil and highly specific area-activated materials follows the pseudo-second-order kinetics model (Salman and Hameed, 2010; Long et al., 2015). Based on these data, the pseudo-second-order kinetic model was found to be the rate-limiting step (Subramanyam and Das, 2009). In this study, the adsorption of phenolic acids in different forests was also well described by the Elovich equation, indicating that the rate-limiting step involves chemisorption (Li et al., 2015). Phenolic concentrations in alkaline extracts are several-fold higher than in water extracts for most soils, because alkaline solutions partially solubilize organic material in soil; this indicates that phenolics in soils exist predominantly in chemically bound rather than free forms (Zwetsloot et al., 2020). The amount of phenolic acids adsorbed at equilibrium, qe, was highest in 6a1g than in the other forest types (Figures 3A, B). This result showed that 6a1g soil had the highest maximum adsorption capacity for the seven phenolic acids among the three types of soil analyzed here.
The adsorption and desorption isotherms were interpreted by the Freundlich model. The high values derived for the Freundlich coefficient, Kd, are an indication of strong adsorption or weak desorption of phenolic acids by soil particles (Cecchi et al., 2004; Mahmoudi et al., 2016). In terms of an adsorption isotherm, Kd, which was greater in 6a1g than in 6a2g, indicated that 6a1g soil had a higher sorptive capacity than 6a2g soil for all seven phenolic acids found here. Nevertheless, the Freundlich equation was not applicable to 9a1g soil. This might occur because it leads to some changes in the characteristics of the soil with the extension of continued planting years in 9a1g, while the stand was felled and regenerated by sprouting in 6a2g. Just, like Cecchi et al. (2004) reported, the sorption of the phenolic acids was dependent on a variety of soil characteristics. More follow-up studies are needed to verify this conjecture. In addition, from the adsorption rate of phenolic acids, it could be determined that the adsorption amounts of phenolic acids were very low and 6a1g soil had the highest adsorption rate among the three types of soil. In addition, the desorption capacities of benzoic and cinnamic acids were weakest in 6a2g soil and 6a1g soil, respectively. Meanwhile, 9a1g soil had the lowest desorption potential for the five other phenolic acids. When the 1/n value is between 0.1 and 1, the process is favorable (Vazquez et al., 2007). A 1/n value of 1.068, 1.091, and 1.231 clearly indicated the desorption processes of p-hydroxybenzoic, vanillic, and benzoic acids in 6a1g were worse than in 6a2g (0.886, 0.927, 0.720) and 9a1g (0.815, 0.777, 0.920).
This could be due to its accumulation and tendency to gather in the soils of first-generation plants, though how they accumulate in soils is not fully understood. Drought and nutrient-poor soils are two environmental factors that may encourage the metabolism and release of phenolic acids from live plants (Scavo et al., 2018). The results of the other study revealed that several phenolic acids were rapidly absorbed in the continuous cropping poplar plantation’s rhizosphere soils, whose rate of accumulation was higher than that of intergenerational planting soils (Tan et al., 2008). According to Narwal’s (2012) investigation, nutrient stress caused an increase in the total phenolic content. Such data suggested that plants under stress released more, which was probably related to the buildup of phenolic acids in soils, particularly in the rhizosphere. On the other hand, the distribution and degradation of soil were strongly affected by the environmental behavior of allelochemicals, namely their adsorption and retention. Therefore, the environment’s behavior may determine the fate of phenolic acid (Wang W. B. et al., 2016).
5. Conclusion
The results showed that the 6a1g soil had 38.451 μg g–1 of the seven phenolic acids, a high proportion of the total. P-hydroxybenzoic accounted for the highest percentage of the seven phenolic acids (benzoic, vanilloid, ferulic, coumarate, salicylic, and cinnamic acids), followed by vanillic, ferulic, and coumalic acids. The allelopathic potential of p-hydroxybenzoic may be greater in these Eucalyptus plantation soils. Furthermore, compared to second generation (6a2g) and first generation (9a1g) planting in Eucalyptus plantations, it was established that the total phenolic acids were highest in first generation (6a1g) forest types. Vanillic and ferulic acids positively correlated with soil bulk density, but p-hydroxybenzoic and coumalic acids positively correlated with SOM. Using both a kinetic adsorption experiment and isothermal adsorption and desorption experiments to describe this phenomenon, the results showed that the adsorption and desorption rates and amounts of each phenolic acid in 6a1g soil were higher compared with the other forest types. Therefore, the adsorption capacity might be an important factor causing 6a1g soil to have a high accumulation capacity for phenolic acids. In contrast, 9a1g soil had the lowest adsorption rate among the three soils; as a result, its accumulation capacity was also the lowest. Interestingly, the soil phenolic acid accumulation in the second-generation Eucalyptus plantation was not the highest. In order to address issues with soil deterioration in successive multi-generational Eucalyptus plantings, further research into this phenomenon will help to better understand its causes and how they function.
Data availability statement
The original contributions presented in this study are included in the article/supplementary material, further inquiries can be directed to the corresponding author.
Author contributions
SU, YX, CL, and MY conceived the main idea of research and wrote the manuscript. SU, YX, SY, and MY revised the manuscript and provided suggestions. FC, SU, CL, and YX analyzed the data. CL and WL assessed data and performed data collection. All authors read and agreed to the published version of the manuscript.
Funding
This work was supported by Key Projects of Guangxi Natural Science Foundation (2021GXNSFDA196003), Guangxi Specific Grant for Innovation-driven Development Projects (AA17204087-6), and the National Natural Science Foundation of China (No. 31260176).
Acknowledgments
The authors thank all of the staff of the silviculture and tree physiological laboratories for their technical assistance and for providing access to their facilities.
Conflict of interest
The authors declare that the research was conducted in the absence of any commercial or financial relationships that could be construed as a potential conflict of interest.
Publisher’s note
All claims expressed in this article are solely those of the authors and do not necessarily represent those of their affiliated organizations, or those of the publisher, the editors and the reviewers. Any product that may be evaluated in this article, or claim that may be made by its manufacturer, is not guaranteed or endorsed by the publisher.
References
Abbas, T., Nadeem, M. A., Tanveer, A., Syed, S., Zohaib, A., Farooq, N., et al. (2017). Allelopathic influence of aquatic weeds on agro-ecosystems: A review. Planta Daninha 35:e017163146. doi: 10.1590/s0100-83582017350100020
Bai, Y., Wang, G., Cheng, Y., Shi, P., Yang, C., Yang, H., et al. (2019). Soil acidification in continuously cropped tobacco alters bacterial community structure and diversity via the accumulation of phenolic acids. Sci. Rep. 9, 1–18. doi: 10.1038/s41598-019-48611-5
Bao, L., Liu, Y., Ding, Y., Shang, J., Wei, Y., Tan, Y., et al. (2022). Interactions between phenolic acids and microorganisms in rhizospheric soil from continuous cropping of Panax notoginseng. Front. Microbial. 13:791603. doi: 10.3389/fmicb.2022.791603
Becerra, P. I., Catford, J. A., Luce McLeod, M., Andonian, K., Aschehoug, E. T., Montesinos, D., et al. (2018). Inhibitory effects of Eucalyptus globulus on understorey plant growth and species richness are greater in non-native regions. Glob. Ecol. Biogeogr. 27, 68–76. doi: 10.1111/geb.12676
Buondonno, A., Capra, G. F., Coppola, E., Dazzi, C., Grilli, E., Odierna, P., et al. (2014). Aspects of soil phenolic matter (SPM): An explorative investigation in agricultural, agroforestry, and wood ecosystems. Geoderma 213, 235–244. doi: 10.1016/j.geoderma.2013.08.026
Cecchi, A. M., Koskinen, W. C., Cheng, H. H., and Haider, K. (2004). Sorption–desorption of phenolic acids as affected by soil properties. Biol. Fertil. Soils 39, 235–242. doi: 10.1007/s00374-003-0710-6
Chaudhari, P. R., Ahire, D. V., Ahire, V. D., Chkravarty, M., and Maity, S. (2013). Soil bulk density as related to soil texture, organic matter content and available total nutrients of Coimbatore soil. Int. J. Sci. Res. Publ. 3, 1–8.
Chen, L. C., and Wang, S. L. (2013). Allelopathic behaviour of Chinese fir from plantations of different ages. Forestry 86, 225–230. doi: 10.1093/forestry/cps078
Chen, L. C., Wang, S. L., Wang, P., and Kong, C. H. (2014). Autoinhibition and soil allelochemical (cyclic dipeptide) levels in replanted Chinese fir (Cunninghamia lanceolata) plantations. Plant Soil 374, 793–801. doi: 10.1007/s11104-013-1914-7
Chou, C. H., and Muller, C. H. (1972). Allelopathic mechanisms of Arctostaphylos glandulosa var. Zacaensis. Am. Midl. Nat. 88, 324–347. doi: 10.2307/2424358
Conant, R. T., Ryan, M. G., Ågren, G. I., Birge, H. E., Davidson, E. A., Eliasson, P. E., et al. (2011). Temperature and soil organic matter decomposition rates–synthesis of current knowledge and a way forward. Glob. Chang. Biol. 17, 3392–3404. doi: 10.1111/j.1365-2486.2011.02496.x
Diao, G. Y., Wu, D. Q., and Yuan, P. (2005). Pentachlorophenol sorption in soils, south china: Experiments and controlling factors analysis. Ecol. Environ. 14, 640–644.
Djurdjevic, L., Dinic, A., Pavlovic, P., Mitrovic, M., Karadzic, B., and Tesevic, V. (2004). Allelopathic potential of Allium ursinum L. Biochem. Syst. Ecol. 32, 533–544. doi: 10.1016/j.bse.2003.10.001
Dungait, J. A., Hopkins, D. W., Gregory, A. S., and Whitmore, A. P. (2012). Soil organic matter turnover is governed by accessibility not recalcitrance. Glob. Chang. Biol. 18, 1781–1796. doi: 10.1111/j.1365-2486.2012.02665.x
Fu, J. W., Chen, Z. H., Wang, M. H., Liu, S. J., Zhang, J. H., Zhang, J. N., et al. (2015). Adsorption of methylene blue by a high-efficiency adsorbent (polydopamine microspheres): Kinetics, isotherm, thermodynamics and mechanism analysis. Chem. Eng. J. 259, 53–61. doi: 10.1016/j.cej.2014.07.101
Hameed, B. H., Tan, I. A. W., and Ahmad, A. (2008). Adsorption isotherm, kinetic modeling and mechanism of 2, 4, 6-trichlorophenol on coconut husk-based activated carbon. Chem. Eng. J. 144, 235–244. doi: 10.1016/j.cej.2008.01.028
Hartley, G. S. (1976). “Physical behaviour in the soil,” in Herbicides: Physiology, biochemistry, ecology, ed. L. Audus (London: Academic Press).
He, C., Li, Z. Y., Fang, H. X., Huang, X. L., Qin, Y. Y., and Yang, M. (2017). Dynamic of soil phenolic acids and enzyme activities in pure and mixed stands of Eucalyptus grandis×E.urophylla and Acacia mangium plantation. J. West China For. Sci. 46, 103–108.
He, S. J., Mao, X. G., and Li, C. H. (2001). Phenolic acid degradation fungi screened from successive plantation site of Chinese fir. Chin. J. Appl. Ecol. 12, 344–346.
Huang, L. F., Song, L. X., Xia, X. J., Mao, W. H., Shi, K., Zhou, Y. H., et al. (2013). Plant-soil feedbacks and soil sickness: From mechanisms to application in agriculture. J. Chem. Ecol. 39, 232–242. doi: 10.1007/s10886-013-0244-9
Kajjumba, G. W., Emik, S., Öngen, A., Özcan, H. K., and Aydın, S. (2018). “Modelling of adsorption kinetic processes—errors, theory and application,” in Advanced sorption process applications, ed. S. Edebali (Rijeka: IntechOpen), 1–19.
Kato-Noguchi, H., and Kurniadie, D. (2021). Allelopathy of Lantana camara as an invasive plant. Plants 10:1028. doi: 10.3390/plants10051028
Laclau, J. P., Mignard, E., Bouvet, J. M., and Mareschal, L. (2018). Managing eucalyptus plantations under global changes. Paris: Cirad, 17–18.
Latif, S., Chiapusio, G., and Weston, L. A. (2017). Allelopathy and the role of allelochemicals in plant defence. Adv. Bot. Res. 82, 19–54. doi: 10.1016/bs.abr.2016.12.001
Leifeld, J., and Kögel-Knabner, I. (2005). Soil organic matter fractions as early indicators for carbon stock changes under different land-use? Geoderma 124, 143–155. doi: 10.1016/j.geoderma.2004.04.009
Li, C. H., Li, M. H., He, S. J., and Chen, X. H. (2002). Studies on phenolic content and variation in soils of chinese-fir and broad-leaved stands. Sci. Silvae. Sin. 38, 9–14.
Li, H., Nishimura, H., Hasegawa, K., and Mizutani, J. (1992). Allelopathy of Sasa cernua. J. Chem. Ecol. 18, 1785–1796. doi: 10.1007/BF02751103
Li, Z. H., Wang, Q., Ruan, X., Pan, C. D., and Jiang, D. A. (2010). Phenolics and plant allelopathy. Molecules 15, 8933–8952.
Li, K., Zhang, T., Li, H., Zhang, L. D., and Li, F. (2020). Phenolic acids inhibit the photosynthetic productivity of poplar. Photosynthetica 58, 1210–1216. doi: 10.32615/ps.2020.071
Li, X., Wang, G. Z., Li, W. G., Wang, P., and Su, C. Y. (2015). Adsorption of acid and basic dyes by sludge-based activated carbon: Isotherm and kinetic studies. J. Cent. South Univ. 22, 103–113. doi: 10.1007/s11771-015-2500-3
Liao, K. B., Gao, H. D., Yang, M., and Liao, C. R. (2019). Species mixture changes the content of phenolic acids in the soil of Eucalyptus plantations. Austrian J. For. Sci. 136, 331–350.
Lin, K. M., Ye, F. M., Lin, Y., and Li, Q. S. (2010). Research advances of phenolic functional mechanisms in soils and plants. Chin. J. Eco Agric. 18, 1130–1137. doi: 10.3724/SP.J.1011.2010.01130
Liu, X., Wang, Y., Liu, Y., Chen, H., and Hu, Y. (2020). Response of bacterial and fungal soil communities to Chinese fir (Cunninghamia lanceolate) long-term monoculture plantations. Front. Microbiol. 11:181. doi: 10.3389/fmicb.2020.00181
Lockwood, J. L., Hoopes, M. F., and Marchetti, M. P. (2013). Invasion ecology, 2nd Edn. Hoboken, NJ: John Wiley & Sons.
Long, F., Zhu, A. N., Shi, H. C., Sheng, J. W., and Zhao, Z. (2015). Adsorption kinetics of pesticide in soil assessed by optofluidics-based biosensing platform. Chemosphere 120, 615–620. doi: 10.1016/j.chemosphere.2014.09.072
Mahmoudi, M., Rahnemaie, R., Es-haghi, A. J., and Malakouti, M. (2016). Kinetics of degradation and adsorption–desorption isotherms of thiobencarb and oxadiargyl in calcareous paddy fields. Chemosphere 91, 1009–1017. doi: 10.1016/j.chemosphere.2013.01.077
Min, K., Freeman, C., Kang, H., and Choi, S. U. (2015). The regulation by phenolic compounds of soil organic matter dynamics under a changing environment. Biomed Res. Int. 2015:825098. doi: 10.1155/2015/825098
Mitra, R., Singh, S. B., and Singh, B. (2020). Radiochemical evidence validates the involvement of root released organic acid and phytosiderphore in regulating the uptake of phosphorus and certain metal micronutrients in wheat under phosphorus and iron deficiency. J. Radioanal. Nucl. Chem. 326, 893–910. doi: 10.1007/s10967-020-07383-3
Mualem, Y., and Assouline, S. (1986). Mathematical model for rain drop distribution and rainfall kinetic energy. Trans. ASAE 29, 494–500. doi: 10.13031/2013.30179
Muscolo, A., Pizzeghello, D., Francioso, O., Sanchez Cortes, S., and Nardi, S. (2020). Effectiveness of humic substances and phenolic compounds in regulating plant-biological functionality. Agronomy 10:1553. doi: 10.3390/agronomy10101553
Ncibi, M. C., Mahjoub, B., and Seffen, M. (2008). Investigation of the sorption mechanisms of metal-complexed dye onto Posidonia oceanica (L.) fibres through kinetic modelling analysis. Bioresour. Technol. 99, 5582–5589. doi: 10.1016/j.biortech.2007.10.040
Qin, D. W., Niu, X., Qiao, M., Liu, G., Li, H. X., and Meng, Z. X. (2015). Adsorption of ferrous ions onto montmorillonites. Appl. Surf. Sci. 333, 170–177. doi: 10.1016/j.apsusc.2015.02.019
Qu, X. H., and Wang, J. G. (2008). Effect of amendments with different phenolic acids on soil microbial biomass, activity, and community diversity. Appl. Soil Ecol. 39, 172–179. doi: 10.1016/j.apsoil.2007.12.007
Reigosa, M. J., and Gonzalez, L. (2006). “Forest ecosystems and allelopathy,” in Allelopathy, eds M. Reigosa, N. Pedrol, and L. González (Dordrecht: Springer). doi: 10.1007/1-4020-4280-9
Salman, J. M., and Hameed, B. H. (2010). Adsorption of 2,4-dichlorophenoxyacetic acid and carbofuran pesticides onto granular activated carbon. Desalination 256, 129–135. doi: 10.1016/j.desal.2010.02.002
Scavo, A., Restuccia, A., and Mauromicale, G. (2018). Allelopathy: Principles and basic aspects for agroecosystem control. Sustain. Agric. Res. 28, 47–101. doi: 10.1007/978-3-319-90309-5_2
Sengupta, K., and Pal, S. (2021). “Rhizospheric plant–microbe interactions releasing antioxidants and phytostimulating compounds in polluted agroecosystems,” in Antioxidants in plant-microbe interaction, eds H. B. Singh, A. Vaishnav, and R. Sayyed (Singapore: Springer), 157–179. doi: 10.1007/978-981-16-1350-0_8
Siqueira, J. O., Nair, M. G., Hammerschmidt, R., Safir, G. R., and Putnam, A. R. (1991). Significance of phenolic compounds in plant-soil-microbial systems. Crit. Rev. Plant. Sci. 10, 63–121.
Subramanyam, B., and Das, A. (2009). Study of the adsorption of phenol by two soils based on kinetic and isotherm modeling analyses. Desalination 249, 914–921. doi: 10.1016/j.desal.2009.05.020
Sun, K., Gao, B., Ro, K. S., Novak, J. M., Wang, Z. Y., Herbert, S., et al. (2012). Assessment of herbicide sorption by biochars and organic matter associated with soil and sediment. Environ. Pollut. 163, 167–173. doi: 10.1016/j.envpol.2011.12.015
Tan, K. L., and Hameed, B. H. (2017). Insight into the adsorption kinetics models for the removal of contaminants from aqueous solutions. J. Taiwan Inst. Chem. Eng. 74, 25–48. doi: 10.1016/j.jtice.2017.01.024
Tan, X. M., Wang, H. T., Kong, L. G., and Wang, Y. P. (2008). Accumulation of phenolic acids in soil of a continuous cropping poplar plantation and their effects on soil microbes. J. Shandong Univ. 43, 14–19.
Tseng, R. L., Wu, F. C., and Juang, R. S. (2003). Liquid-phase adsorption of dyes and phenols using pinewood-based activated carbons. Carbon 41, 487–495. doi: 10.1016/S0008-6223(02)00367-6
Vazquez, I., Rodriguez-Iglesias, J., Maranon, E., Castrillon, L., and Alvarez, M. (2007). Removal of residual phenols from coke wastewater by adsorption. J. Hazard. Mater. 147, 395–400. doi: 10.1016/j.jhazmat.2007.01.019
Wang, S. Y. (2016). Analysis of the soil enzyme activity and microbial functional diversity of Cunninghamia lanceolata clones in continous planting obstacles soil of Cunninghamia lanceolata. Ph.D. thesis. Fuzhou: Fujian Agriculture and Forestry University.
Wang, J., and Guo, X. (2020). Adsorption kinetic models: Physical meanings, applications, and solving methods. J. Hazard. Mater. 390:122156.
Wang, W. B., Ma, X. S., Dong, Y. F., Wang, H. T., Wang, Y. P., and Yi, W. H. (2016). Community characteristics and degradation metabolism regulation of soil phenolic acid degrading bacteria in poplar plantations under continuous cropping and crop rotation. Chin. J. Appl. Environ. Biol. 22, 815–822.
Wang, Y. P., Yang, Y., Wang, H. T., Jung, Y. Z., and Wang, Z. Q. (2010). Sorption-desorption of two phenolic acids in poplar rhizosphere soil in continuous cropping plantation. Sci. Silvae Sin. 46, 48–55.
Wang, Y., Li, C., Wang, Q., Wang, H., Duan, B., and Zhang, G. (2016). Environmental behaviors of phenolic acids dominated their rhizodeposition in boreal poplar plantation forest soils. J. Soils Sediments 16, 1858–1870. doi: 10.1007/s11368-016-1375-8
Wei, X. X. (2017). Soil characteristics analysis and identification of phenolic acids under the continuous cropping obstacle of Chinese fir superior clones. Ph.D. thesis. Fuzhou: Fujian Agriculture and Forestry University.
Wu, H., and Lin, W. (2020). A commentary and development perspective on the consecutive monoculture problems of medicinal plants. Chin. J. Eco Agric. 28, 775–793.
Wu, H., Wu, L., Wang, J., Zhu, Q., Lin, S., Xu, J., et al. (2016). Mixed phenolic acids mediated proliferation of pathogens Talaromyces helicus and Kosakonia sacchari in continuously monocultured Radix pseudostellariae rhizosphere soil. Front. Microbiol. 7:335. doi: 10.3389/fmicb.2016.00335
Yang, M., Lin, S. Z., Huang, Y. H., and Cao, G. Q. (2006). Allelopathic effects of salicylic acid stress on membrane lipid peroxidation and osmosis-regulating substances in different Chinese fir clones. Acta Bot. Boreal. Occid Sin. 26, 2088–2093.
Zhao, Y., Xin, D., Lu, W., Zong, X., Niu, Y., Guo, X., et al. (2022). PeMPK7 is induced in an ROS-dependent manner and confers poplar para-hydroxybenzoic acid stress resistance through the removal of ROS. Ind. Crops Prod. 182:114861. doi: 10.1016/j.indcrop.2022.114861
Zhou, X., Zhu, H., Wen, Y., Goodale, U. M., Zhu, Y., Yu, S., et al. (2020). Intensive management and declines in soil nutrients lead to serious exotic plant invasion in Eucalyptus plantations under successive short-rotation regimes. Land Degrad. Dev. 31, 297–310. doi: 10.1002/ldr.3449
Keywords: phenolic acids, Eucalyptus plantations, adsorption isotherm, desorption isotherm, kinetic of adsorption
Citation: Ullah S, Xu Y, Liao C, Li W, Cheng F, Ye S and Yang M (2023) Continuous planting Eucalyptus plantations in subtropical China: Soil phenolic acid accumulation and adsorption physiognomies. Front. For. Glob. Change 6:1135029. doi: 10.3389/ffgc.2023.1135029
Received: 31 December 2022; Accepted: 13 February 2023;
Published: 02 March 2023.
Edited by:
Huma Qureshi, University of Chakwal, PakistanReviewed by:
Muhammad Shoaib Amjad, Women University of Azad Jammu and Kashmir Bagh, PakistanNasrullah Khan, Swiss Federal Institute for Forest, Snow and Landscape Research (WSL), Switzerland
Copyright © 2023 Ullah, Xu, Liao, Li, Cheng, Ye and Yang. This is an open-access article distributed under the terms of the Creative Commons Attribution License (CC BY). The use, distribution or reproduction in other forums is permitted, provided the original author(s) and the copyright owner(s) are credited and that the original publication in this journal is cited, in accordance with accepted academic practice. No use, distribution or reproduction is permitted which does not comply with these terms.
*Correspondence: Mei Yang, fjyangmei@126.com
†These authors have contributed equally to this work and share first authorship