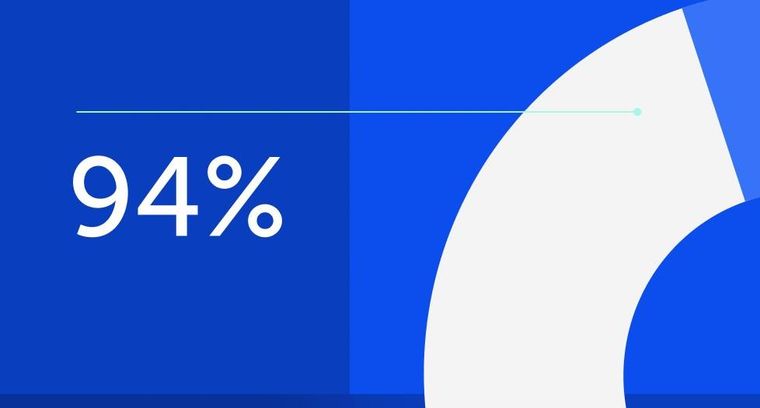
94% of researchers rate our articles as excellent or good
Learn more about the work of our research integrity team to safeguard the quality of each article we publish.
Find out more
ORIGINAL RESEARCH article
Front. For. Glob. Change, 13 July 2023
Sec. Tropical Forests
Volume 6 - 2023 | https://doi.org/10.3389/ffgc.2023.1131328
This article is part of the Research TopicClimate-Smart Solutions for Tropical Mountain EnvironmentsView all 6 articles
Maize (Zea mays) stover produced in the drier lowland areas on the volcanic foot slopes of Mount Kilimanjaro serves as an important additional fodder for smallholder zero-grazed dairy cattle farming in the humid highland areas of the region. The obtained cattle manure is used to replenish nutrients and manage soil fertility in banana-based agroforestry homegardens of the highlands, which contributes significantly to sustaining crop production, thus food security and the livelihoods of the community. The study aims to provide a preliminary evaluation of the amounts of stover removed from smallholder maize-based fields in the lowlands and the subsequent nutrient flows to the banana-based agroforestry homegardens of the highlands. In 54 smallholder maize fields selected at random from 11 villages in the Hai and Siha districts of the Kilimanjaro region, we conducted an agronomic survey to record the types and amounts of fertilizer used, plant spacing at harvest, and maize stover biomass yield. Most of the surveyed maize-based fields received inadequate nutrient inputs, which were limited to mineral nitrogen alone if any. Of the surveyed fields, 16.7% received recommended amounts of 74 kg N ha–1 by the Ministry of Agriculture, 46.3% sub-optimal amounts and 37.0% did not receive fertilizer at all. Plant density was only 50% of the recommended population density. While this was only a preliminary study on nutrient flows between maize and banana-based agroecosystems, our findings demonstrated that potassium exports via harvested banana bunches exceeded the inputs in the homegardens. We conclude that (i) soil fertility management techniques, preferably integrated soil fertility management, suitable for small-scale maize production in the lowlands should be formulated while optimizing plant density to increase production, (ii) maize-based agroforestry farming system adapted to drier conditions to deal with windstorm and soil erosion problems in the lowlands is urgently needed, and (iii) wider and more detailed research on nutrient flows between maize and banana-based agroecosystems should be conducted to document their respective soil nutrient balances and the sustainability of such practice.
Banana (Musa sp.) production in the humid upper slopes between 1,000 and 1,900 meters above sea level (m a.s.l) of Mount Kilimanjaro contributes significantly to maintaining food security for the Chagga people in the Kilimanjaro region for more than 2,000 years (Odner, 1971; Lejju et al., 2006). Bananas on the slopes of Mount Kilimanjaro are grown in smallholder organic agrosystems (Promar Consulting, 2011) in the backyards in a very unique land use type: dense “banana forest” with a scattered upper tree layer (Raeymaekers and Stevens, 2015; Vandekerckhove and Young, 2016), nicknamed as the Chagga homegardens. Banana, in its original ecosystem, is a plant that grows well in forest soils with high organic material where it acts as a pioneer crop, to close the forest gaps.
The existing banana/coffee/agroforestry systems (referred to herein as banana-based agroforestry systems) in the Chagga homegardens mimic the native forest banana ecosystem, which seems to explain well why smallholder organic banana ecosystems have survived for centuries on the slopes of Mount Kilimanjaro. First, there is a continuation application of cattle manure produced within the farmstead (Promar Consulting, 2011; Raeymaekers and Stevens, 2015; Vandekerckhove and Young, 2016) which replenishes soil nutrients, while at the same time improves soil organic matter suitable for banana plant growth. Second, farmers consistently implement fairly effective soil erosion control measures in the homegardens by establishing perennial pasture grasses in areas prone to erosion, thus making a closed system. Third, compatible multi-species trees in the Chagga homegardens serve as an important component in climate change adaptation, and most importantly they provide leaf litter materials, useful in recycling essential plant nutrients that have possibly leached below the rooting zone (Vourlitis et al., 2013) thus, improves the nutrient use efficiency of the system.
The integrity of the banana-based agroforestry systems on the humid upper slopes of Mount Kilimanjaro, on the other hand, has been negatively impacted by the high population pressure of up to 650 people per km2 (Zongolo et al., 2000) on the available land, forcing farmers to cut down trees to open more space for banana plants and other food crops. The average size of the farmstead in the humid highlands of Mount Kilimanjaro is 0.5 ha (Raeymaekers and Stevens, 2015; Vandekerckhove and Young, 2016) too small to produce adequate fodder to meet the all-year-round smallholder dairy cattle feed demand (Ikegami, 1994; Bill and Melinda Gates Foundation [BMGF], 2014; Ichinose et al., 2020). Supplementing the forage produced in the farmstead with feed concentrates could be an option but is too expensive for poorly resource-endowed smallholder farmers who are the major banana growers in the region. In response, smallholder farmers from the mixed banana/dairy cattle/agroforestry system of the uplands have established maize-based fields in the drier lowlands mainly to produce maize stover and common bean (Phaseolus vulgaris L.) haulms, which are transferred to the highlands as additional fodder to produce animal protein and manure.
Subsequently, the maize/common bean/agro-pastoral system has become a dominant land use type of the drier areas on the foot slopes of Mount Kilimanjaro (Kaihura et al., 2001). However, earlier studies e.g., Mizota et al. (1988), Kaihura et al. (1998), Pabst et al. (2013), and Maro et al. (2014) have indicated insufficient amounts of soil total carbon (C), nitrogen (N) and available phosphorus (P) as the most important crop production constraints in the drier lowland areas in the Kilimanjaro region. Low levels of soil total C in maize fields are attributed to the removal of crop residues (Figure 1A) as additional fodder for the zero-grazed smallholder dairy cattle farming (Figure 1B) in the highlands (Ikegami, 1994; Kaihura et al., 2001; Vandekerckhove and Young, 2016), while the leftovers are freely grazed by nomadic cattle herds roaming the lowlands (Figure 1C).
Figure 1. Stover transfers from maize-based fields in the lowlands (A) to feed zero-grazed smallholder dairy cattle in the uplands (B), subsequently, remains are freely grazed by cattle herds roaming on the land (C).
Maize stover and common bean haulms are the main sources of SOM in maize-based fields in the drier foothills of Mount Kilimanjaro. Since the obtained manure is used in the banana-based agroforestry homegardens of the uplands, a significant transfer across the Mount Kilimanjaro landscape occurs at the expense of nutrients from the maize-based smallholder fields of the lowlands. Maize stover transfers from maize-based fields in the lowlands to feed zero-grazed smallholder dairy cattle in the upland areas of Mount Kilimanjaro obviously aggravates the degradation of soil natural resources in the former areas. The close link between maize-based farming systems of the lowlands, the smallholder stable-fed dairy cattle, and the associated banana-based agroforestry homegardens in the uplands of Mount Kilimanjaro illustrate the complexity of the constraints farmers face in the maize growing zone. The objective of this study is to quantify as a preliminary assessment the amounts of maize stover removed from maize-based fields in the foot slopes of Mount Kilimanjaro, and the resulting nutrient flows to the banana-based agroforestry homegardens of the highlands.
The study site, which is situated between 800 and 1,381 m.a.s.l was selected to represent areas under maize-based farming systems in the Hai and Siha districts located on the southeast slopes of Mount Kilimanjaro in the Kilimanjaro region, Tanzania (Figure 2), with the major objective to produce maize stover as additional fodder for zero-grazed smallholder dairy cattle farming of the uplands. These lowland areas are characterized by a semi-arid climate with annual rainfall varying between 500 and 800 mm (Hamis, 2013). Normally, the rainfall pattern in the Upper zone above 1,200 m.a.s.l is bimodal, which allows farmers to grow common bean and maize sequentially between November and January, and March and June, respectively. In contrast, precipitation is much less and unimodal in the Lower zone below 1,000 m.a.s.l, where maize is grown in association with common bean between mid-March and June. Land preparation is done mechanically, mainly by tractors, while other field operations (planting, weeding, and harvesting) are done manually. Common bean is harvested by uprooting the whole plant and heaping in one point, where it can dry before threshing. On the other hand, maize cobs are picked by hand when thoroughly dry and collected in one point for threshing by simple motorized threshers. Subsequently, maize stover is harvested by cutting 2/3 of the stalk and transported by trucks to the highlands, together with common bean haulms as additional fodder for zero-grazed smallholder dairy cattle. The remaining 1/3 of the maize stover is lost to freely grazing cattle herds roaming the lowlands.
Figure 2. Map of the study site showing locations (green dots) of the surveyed maize-based fields in selected villages of Hai and Siha districts located on the southeast volcanic slopes of Mount Kilimanjaro in the Kilimanjaro region, central-northern Tanzania.
The study was conducted in August 2017 in 54 randomly selected smallholder maize-based fields covering the maize-growing areas of the study site. Field size and location (coordinates and elevation) were determined with a Garmin Etrex 30 Global Positioning System (GPS) with an accuracy of +/−4 m. In each field, four quadrants measuring 2 m by 4 m (referred to as sampling unit (SU) were demarcated in areas with uniform plant density and crop stand using a tape measure. Maize stover harvesting in the SU was done according to the farmers’ practice and weighed. Subsequently, dry matter yield (t ha–1 and field–1) was determined using Equations 1 and 2, respectively. Twelve randomly selected maize stalks from each surveyed field (three stalks per SU) were chopped into small pieces, and homogenized, then a subsample of about 50 g was taken for laboratory analyses. During the survey, the information on the type and quantity of fertilizer used was documented.
Where: SW stover weight (kg), SU sampling unit, SUA sampling unit area (8 m2), and FS field size (ha).
Maize stover samples were thoroughly washed with distilled water to remove dust, and oven dried at 70°C until constant weight. Then, the samples were ground with an agate ball mill to less than 2 mm, digested by 2 mL of concentrated nitric acid-analytical grade, and analyzed for phosphorus (P), potassium (K), magnesium (Mg), calcium (Ca), sulfur (S), boron (B), copper (Cu), iron (Fe), manganese (Mn), nickel (Ni), and zinc (Zn) concentrations by Inductively Coupled Plasma Optical Emission Spectrometry (ICP-OES; iCAP 7000 series; Thermo Scientific Inc.). Total N was determined by Dumas combustion using a Carlo Erba EA1108 elemental analyzer by subjecting samples between 0.5 and 1 mg, weighed in Sn-capsules, to oxidative digestion under a controlled oxygen supply at around 900°C. Mineral nutrient uptake by stover (MNUS) in kg ha–1 was calculated as the product of stover biomass (SB) and nutrient concentration in the sample (NCS) using Equation 3. This information was then used to quantify mineral nutrient uptake by stover per surveyed field (MNUSF) using Equation 4.
Where: MNUS mineral nutrient uptake by stover (kg ha–1), NCS nutrient concentration in the sample (kg nutrient t–1), SB stover biomass (t ha–1) in the respective field.
Where: MNUSF mineral nutrient uptake by stover in (kg field–1) and FS field size (ha).
The investigated variables, which included field size (ha), stover biomass (t ha–1 and t field–1), amount of fertilizer used (kg ha–1 and kg field–1), and mineral nutrient uptake by stover were subjected to one-way Analysis of Variance (ANOVA) using STATISTICA software to compare their differences among the surveyed maize fields.
The results indicate that the size of surveyed maize-based fields in the selected villages of Hai and Siha districts on the southeast volcanic slopes of Mount Kilimanjaro varied widely, and appeared to range between 0.2 and 9.6 ha, with an overall mean of 1.2 ha (Figure 3A). If fertilizer was used, it was usually only N fertilizer derived from urea. The results indicate further that farmers applied, on average, 39.7 kg N ha–1, which is below the recommended application rate of 74 Kg N ha–1 in the northern highlands of Tanzania (Marandu et al., 2014). Conversely, only 16.7% of the visited farmers were found to apply optimum amounts of N fertilizer in their maize-based fields, while 37.0% applied sub-optimal quantities of N and the remaining 46.3% did not apply fertilizer at all.
Figure 3. Distribution and comparison of the size of the surveyed maize-based fields (A) and maize stover biomass yield ha–1 (B) attained in the 2017 cropping season in the selected villages of Hai and Siha districts located on the southeast volcanic slopes of Mount Kilimanjaro in the Kilimanjaro region, central-northern Tanzania. * Are anomalous data points, caused by field size variations within the village. The lines dividing the groups are quartiles, the groups are quartile groups and lines perpendicular to the upper and lower quartiles are whiskers.
Maize stover biomass ranged between 1.7 and 2.8 t ha–1 (Figure 3B), with an overall mean of 2.3 t ha–1. Surprisingly, there was no correlation (F = 0.236, p ≤ 0.05) between maize stover biomass and N fertilization (Figure 4), proving that N fertilization alone cannot considerably increase crop yields. Maize in most of the surveyed fields was grown in association with the common bean as an intercrop. However, the legume intercrop was already harvested during the survey period. The inter and intra-row spacing of maize at harvest ranged, respectively, between 0.75 and 0.95 m, and 0.45 and 0.65 m (data not shown), thus plant density ranged between 18,735 and 30,156 with an overall mean of 21,944 plants ha–1. Comparing the observed plant density with the optimal recommended population density of 44,444 plants ha–1 in the maize/common bean intercropping systems in northern Tanzania (Kihara et al., 2014), all surveyed maize fields in Hai and Siha districts had sub-optimal plant density.
Figure 4. Relationship between N fertilization and maize stover biomass ha–1 in the drier lowland zone of Hai and Siha districts located on the southeast volcanic slopes of Mount Kilimanjaro in the Kilimanjaro region, central-northern Tanzania.
The results in Figure 5 indicate the overall nutrient concentrations kg–1 dry-weight maize stover harvested from maize-based fields in the selected villages of Hai and Siha districts located on the southeast volcanic slopes of Mount Kilimanjaro in the Kilimanjaro region, central-northern Tanzania. The order for nutrient removal by the harvested maize stover in the study area was K > N > Ca > Mg > P > S > B > Zn > Cu > Mo > Ni (Figure 5), which was similar to research findings reported in the USA by Riedell and Osborne (2017) and Malaysia by Mubarak et al. (2002). The overall average nutrient exports from the lowland maize-based field by maize stover alone would amount to the values shown in Figure 6 if we take into account a model farm of 1 ha, which is a standard unit area. The results in Figure 6 further indicate that the values of nutrient export by the harvested maize stover from maize-based fields established in the drier areas of Hai and Siha districts in the Kilimanjaro region are much higher than nutrient inputs derived from external fertilizer inputs.
Figure 5. Distribution and comparison of macro-nutrients (A) and micro-nutrients (B) concentrations kg–1 dry-weight maize stover as grown under smallholder farmer conditions in the drier lowland areas of Hai and Siha districts located on the southeast volcanic slopes of Mount Kilimanjaro in the Kilimanjaro region, central-northern Tanzania. * Are anomalous data points, possibly caused by soil fertility management gradients among the surveyed fields. The lines dividing the groups are quartiles, the groups are quartile groups and lines perpendicular to the upper and lower quartiles are whiskers.
Figure 6. Distribution and comparison of macro-nutrients (A) and micro-nutrients (B) removal by maize stover ha–1 as grown under smallholder farmer conditions in the drier lowland areas of Hai and Siha districts located on the southeast volcanic slopes of Mount Kilimanjaro in the Kilimanjaro region, central-northern Tanzania. * Are anomalous data points, possibly caused by soil fertility management gradients among the surveyed fields. The lines dividing the groups are quartiles, the groups are quartile groups and lines perpendicular to the upper and lower quartiles are whiskers.
The small field sizes and low fertilizer use in the foothills of Mount Kilimanjaro as reported above indicate that maize production in these areas is constrained by land shortage and less-resource endowment, just like the banana production in the uplands (Ikegami, 1994; Soini, 2005; Ichinose et al., 2020). Likewise, the findings of this study further revealed that smallholder maize producers used mineral N only derived from urea as a top dress fertilizer. However, the fertilization rate was below the recommended rate of 74 kg N ha–1 (Marandu et al., 2014), indicating that maize production on the drier foot slopes of Mount Kilimanjaro operates under inadequate and imbalanced nutrient fertilizer inputs.
Maize stover biomass production ranged between 1.7 and 2.8 t ha–1, which is similar to the figures reported in previous studies e.g., Hellin et al. (2013) in Mexico, Jaleta et al. (2015) in Ethiopia, and Khanna and Paulson (2016) in the USA. Traditionally, farmers in the study area harvest 67% of the maize stover, similar to that published by Nunes et al. (2020) and Galindo et al. (2021) in the USA. While the remaining 23% of the maize stover (the lower part of the maize stalk) could sustain soil organic carbon (OC) essential for soil protection and ecosystem functions, these materials are lost to grazing cattle herds that roam the lowlands after crop harvest.
Total removal of maize stover in the current situation where maize is grown purely in non-agroforestry systems has a negative impact on the soil quality because it reduces organic materials, thus the amounts of soil OC accumulation in the cropland while exposing soils to water, and wind erosion. Therefore, as a short-term strategy, the development and strict enforcement of bylaws to protect the remaining 23% of maize stover after harvest from losses to the freely grazing nomadic cattle herds roaming the lowlands need special consideration if one wants to: (1) recycle essential plant nutrients following decomposition more efficiently, and (2) build up soil OC stocks. In addition, devising suitable/compatible, site-specific maize-based agroforestry systems adapted to smallholder farmers’ settings is urgently needed, with trees functioning as an important component of climate change adaptation but, most importantly trees shall act as (1) wind breaker, thus protecting wind erosion, and (2) an important source of organic materials (leaf litter), thus contributing to the increased accumulation of soil OC, important for improving soil aggregation and structure, increasing infiltration and water retention and sustaining ecosystem services (Blanco-Canqui and Lal, 2009).
We did not analyze mineral nutrient concentration in maize grains due to financial constraints, instead, values published by Codling et al. (2007), Arce and Caballero (2015), and Duarte et al. (2019) were used to estimate the average nutrient removal by maize cobs per field (Table 1). With total reliance on mineral N derived from urea (46% N) in replenishing soil fertility, the removal of each of the investigated nutrient elements by the above-ground maize biomass was found to be larger than the external inputs (Table 1). This indicates that the current maize-based farming systems of the lowlands in Hai and Siha districts mine the natural soil fertility. Apparently, the figures on nutrient removal would have doubled if the planting density would have been at 44,444 plants ha–1 as recommended for the maize/common bean intercropping system in central-northern Tanzania (Kihara et al., 2014).
Table 1. Nutrient flows and the corresponding field-level soil nutrient balance of smallholder maize-based fields in the drier lowlands of Hai and Siha districts located on the southeast slopes of Mount Kilimanjaro in the Kilimanjaro region, central-northern Tanzania.
Maize-based farming in the lowlands started way back in the early 1900s in an effort to produce maize grain for family consumption during lean banana production and additional fodder for zero-grazed smallholder dairy cattle of the highlands. Regrettably, the obtained manure from zero-grazed dairy cattle in the highlands is not brought back to replenish soil nutrients removed from maize-based fields in the lowlands by maize stover. This seems to threaten the sustainability of the existing maize-based farming of the lowlands if appropriate measures to rectify the situation are not implemented. Therefore, this study recommends the following in an attempt to close these nutrient cycles: (i) returning back nutrients removed from the fields by maize stover by bringing the obtained cattle manure from zero-grazing dairy cattle to the maize-based fields, (ii) device an appropriate crop fertilization package that will supplement soil nutrients that cannot be addressed by strategy (i) above, and (iii) imposing bylaws that restrict nomadic cattle herds from grazing in the maize-based fields during the off-season.
Merely for an indicative calculation exercise, we assumed that a typical surveyed household has an average banana-based agroforestry homegarden in the highlands of 0.5 ha (Raeymaekers and Stevens, 2015; Vandekerckhove and Young, 2016). This allowed us to draw a rough, average nutrient balance, keeping in mind that the range in surface area for banana-based agroforestry homegarden can vary substantially. From Table 1 we retain that large quantities of mineral nutrients can be transported uphill by this typical household to produce animal protein (meat and milk) each year. The obtained manure from zero-grazing smallholder dairy cattle is used to replenish nutrients and manage soil fertility in banana-based agroforestry homegardens of the uplands. With an average number of 3 crossbred dairy cattle per household (Raeymaekers and Stevens, 2015; Vandekerckhove and Young, 2016), and an average production of 4.5 kg dry manure per day (Fernandez-Rivera et al., 1995; Raussen, 1997), a resource-endowed household can produce 5 t of dry cattle manure year–1. Based on the nutrient contents of 5-3-12 g kg–1 N-P-K dry cattle manure (Baijukya et al., 2005), the obtained manure can add 25-15-60 kg N-P-K in a banana-based agroforestry homegarden each year (Figure 7).
Figure 7. Schematic relationship between a typical maize-based field in the drier foothill and 0.5 ha banana-based agroforestry homegarden in the more humid uplands of Mount Kilimanjaro located in the Kilimanjaro region in central-northern Tanzania, and the subsequent nutrient flows between these two agro-ecosystems each year.
Maize stover as explained above is an integral constituent of fodder in a zero-grazing smallholder dairy cattle farming of the highlands, contributing 25% of the annual forage budget. On the other hand, fresh fodder collected from forest reserve areas, common bean haulms transported from maize-based fields in the lowlands and, banana residues (pseudostem and leaves) each contributes about 25% of the total annual fodder requirements (Bariki Ulomi personal communication, 2022). In this light, 6.3, 3.8, and 15 kg of the ingested N, P and K, respectively, via maize stover is actually ending up in the manure, while other fodder (grasses, bean haulms, and banana residues) contributes to the remaining 18.7-11.2-45 kg N-P-K (Table 2).
Table 2. Nutrient flows and the corresponding field-level nutrient balance of a typical 0.5 ha banana-based agroforestry homegarden in the highlands of Mount Kilimanjaro located in the Kilimanjaro region in central-northern Tanzania.
Banana bunches can export up to 88-12–318 kg N-P-K ha–1 year–1 (Meya et al., 2020). Translating these figures to the typical average 0.5 ha (average banana-based agroforestry homegarden in central-northern Tanzania), banana bunches alone can export up to 22-3-79 kg N-P-K year–1 (Table 2). With total dependence on cattle manure in addressing banana nutrient requirements, it was found that K removal by harvested banana bunches exceeded the external inputs, with an average balance of −19 kg from a 0.5 ha banana-based agroforestry homegarden year–1, inferring a fast soil K mining in banana-based agroforestry systems. These findings further explain the K shortage reported earlier by Raeymaekers and Stevens (2015) and Vandekerckhove and Young (2016) in banana plants grown on the southeast slopes of Mount Kilimanjaro and Mount Meru in central-northern Tanzania. Logically, the traditional soil fertility management practice that relies on the applications of cattle manure only does not supply sufficient amounts of K to offset the exports by harvested banana bunches. These findings reveal that it is challenging to fulfill nutritional K requirements for the bananas via cattle manure only in the study area. Therefore, supplementation of the accessible quantities of cattle manure with poultry manure and kitchen wastes (vegetable refusal and wood ash) should be given special concern to ensure the sustainability of the existing banana-based agroforestry systems, thus the livelihoods of the community.
Maize stover removal from maize-based fields as additional fodder for zero-grazed smallholder dairy cattle farming of the uplands together with free-grazing nomadic cattle herds roaming the lowlands during the off-season gradually mine the soils. This threatens the sustainability of the existing maize-based farming in the drier lowland areas in the absence of appropriate measures to rectify the situation. Therefore, future research should focus on: (1) developing innovative solutions to manage the remaining 23% of the maize stover, thus recycling nutrients whilst increasing the amounts of soil OC accumulation in the fields, important in sustaining ecosystem services and (2) devising agroforestry system adapted to drier areas, with trees component being crucial in (i) climate change adaptation and, (ii) most importantly blocking strong windstorms, thus reducing wind erosion, while at the same time (iii) adding leaf litter useful in augmenting soil nutrients and sustaining ecosystem services. Similarly, K removal from banana-based agroforestry homegardens exceeded addition rates, implying that farmers use inadequate external inputs because of the shortage of organic fertilizer resources. Therefore, a more detailed, longer-term, and broader study on nutrient flows in maize-based smallholder fields of the lowlands and banana-based agroforestry system of the uplands and the corresponding field-level soil nutrient balance is urgent and critically needed to design more sustainable nutrient management techniques that will conserve soils, and improve land productivity and profitability sustainably.
The raw data supporting the conclusions of this article will be made available by the authors, without undue reservation.
RM contributed to the conception and design of the study. AM conducted the field survey, analyzed the samples in the laboratory, performed the statistical data analysis, and wrote the first draft of the manuscript. KM reviewed and edited the first draft of the manuscript. PN, RS, and RM reviewed and edited the final draft of the manuscript, and approved the submitted version. All authors contributed to the article and approved the submitted version.
We thank indebted to the farmers of Hai and Siha districts in the Kilimanjaro region on whose fields the survey was conducted. In addition, we thank the agricultural extension staff of Hai and Siha districts for their support during the course of the field survey. We also thank Jan Diels and Sepper Deckers for suggestions and technical guidance with nutrient budget aspects of this manuscript.
The authors declare that the research was conducted in the absence of any commercial or financial relationships that could be construed as a potential conflict of interest.
All claims expressed in this article are solely those of the authors and do not necessarily represent those of their affiliated organizations, or those of the publisher, the editors and the reviewers. Any product that may be evaluated in this article, or claim that may be made by its manufacturer, is not guaranteed or endorsed by the publisher.
Arce, C. E., and Caballero, J. (2015). Agricultural sector risk assessment: Tanzania (p. 94883). World Bank Group Report. Tanzania: World Bank Group.
Baijukya, F. P., de Ridder, N., Masuki, K. F., and Giller, K. E. (2005). Dynamics of banana-based farming systems in Bukoba District, Tanzania: changes in land use, cropping and cattle keeping. Agric. Ecosyst. Environ. 106, 395–406. doi: 10.1016/j.agee.2004.08.010
Bill and Melinda Gates Foundation [BMGF] (2014). Multi crop value chain phase II Tanzania/Uganda cooking banana (Revisions). Seattle, WA: Bill and Melinda Gates Foundation, 90.
Blanco-Canqui, H., and Lal, R. (2009). Crop residue removal impacts on soil productivity and environmental quality. Crit. Rev. Plant Sci. 28, 139–163.
Codling, E. E., Mulchi, C. L., and Chaney, R. L. (2007). Grain yield and mineral element composition of maize grown on high phosphorus soils amended with water treatment residual. J. Plant Nutr. 30, 225–240. doi: 10.1080/01904160601117937
Duarte, A. P., Ferreira de Abreu, M., Francisco, E. A. B., Gitti, D. C., Gabriel, B. G., and Kappes, C. (2019). Reference values of grain nutrient content and removal for corn. Rev. Brasil. Cienc. 43:e0180102. doi: 10.1590/18069657rbcs20180102
Fernandez-Rivera, S., Willium, T. O., Hiernax, P., and Powell, M. (1995). “Faecal excretion by ruminant and manure availability for crop production in semi-arid West Africa,” in Livestock and sustainable nutrient cycling in mixed framing systems of Sub-Saharan Africa. Volume II: technical papers. Proceeding of an international conference held in Addis Ababa, Ethiopia, 22-26 November 1993 ILCA (International Livestock Centre for Africa Addis Ababa), Ethiopia, eds J. M. Powell, S. Fernandez-Rivera, T. O. Williams, and C. Renard (Addis Ababa: International Livestock Centre for Africa), 149–169.
Galindo, F. S., Strock, J. S., and Pagliari, P. H. (2021). Nutrient accumulation affected by corn stover management associated with nitrogen and phosphorus fertilization. Agriculture 11:1118. doi: 10.3390/agriculture11111118
Hamis, J. (2013). Study of rainfall trends and variability over Tanzania. Postgraduate diploma report. Kenya: University of Nairobi, 55.
Hellin, J., Erenstein, O., Beuchelt, T., Camacho, C., and Flores, D. (2013). Maize stover use and sustainable crop production in mixed crop–livestock systems in Mexico. Field Crops Res. 153, 12–21. doi: 10.1016/j.fcr.2013.05.014
Ichinose, Y., Higuchi, H., Kubo, R., Nishigaki, T., Kilasara, M., Shinjo, H., et al. (2020). Adaptation of farmland management strategies to maintain livelihood by the Chagga people in the Kilimanjaro highlands. Agric. Syst. 181:102829. doi: 10.1016/j.agsy.2020.102829
Ikegami, K. (1994). The traditional agrosilvipastoral complex system in the Kilimanjaro region, and its implications for the Japanese-assisted lower irrigation project. Afr. Study Monogr. 15, 189–210. doi: 10.14989/68126
Jaleta, M., Kassie, M., and Erenstein, O. (2015). Determinants of maize stover utilization as feed, fuel, and soil amendment in mixed crop-livestock systems, Ethiopia. Agric. Syst. 134, 17–23. doi: 10.1016/j.agsy.2014.08.010
Kaihura, F. B. S., Kully, I. K., Kilasara, M., Aune, J. B., Singh, B. R., and Lal, R. (1998). Impact of soil erosion on crop productivity and crop yield in Tanzania. Adv. Geoecol. 31, 375–381.
Kaihura, F. B. S., Stocking, M., and Kahembe, E. (2001). “Soil management and agrodiversity: a case study from Armumeru, Arusha, Tanzania,” in Paper presented by the symposium on: managing biodiversity in agricultural systems, (Montreal), 14.
Khanna, M., and Paulson, N. (2016). ”To harvest stover or not: is it worth it?” farmdoc daily (6):32. Urbana, IL: Department of Agricultural and Consumer Economics, University of Illinois at Urbana-Champaign.
Kihara, J., Tamene, L. D., Massawe, P., and Bekunda, M. (2014). Agronomic survey to assess crop yield, controlling factors and management implications: a case-study of Babati in northern Tanzania. Nutr. Cycl. Agroecosyst. 102, 5–16. doi: 10.1007/s10705-014-9648-3
Lejju, B. J., Robertshaw, P., and Taylor, D. (2006). Africa’s earliest bananas? J. Archaeol. Sci. 33, 102–113.
Marandu, A. E. T., Mbogoni, J. D. J., and Ley, G. J. (eds) (2014). Revised fertilizer recommendations for maize and rice in the Eastern, Southern Highlands and Lake Zones of Tanzania. Dares-Salaam: Ministry of Agriculture, Food Security and Cooperatives, Department of Research and Development.
Maro, G., Msanya, B., and Mrema, J. (2014). Soil fertility evaluation for coffee (Coffea arabica) in Hai and Lushoto districts, northern Tanzania. Int. J. Plant Soil Sci. 3, 934–947. doi: 10.9734/IJPSS/2014/8203
Meya, A. I., Ndakidemi, P. A., Mtei, K. M., Swennen, R., and Merckx, R. (2020). Optimizing soil fertility management strategies to enhance banana production in volcanic soils of the Northern Highlands, Tanzania. Agronomy 10:289. doi: 10.3390/agronomy10020289
Mizota, C., Kawasaki, I., and Wakatsuki, T. (1988). Clay mineralogy and chemistry of seven pedons formed in Volcanic ash, Tanzania. Geoderma 43, 131–141. doi: 10.1016/0016-7061(88)90039-0
Mubarak, A. R., Rosenani, A. B., Anuar, A. R., and Zauyah, S. (2002). Decomposition and nutrient release of maize stover and groundnut haulm under tropical field conditions of Malaysia. Commun. Soil Sci. Plant Anal. 33, 609–622.
Nunes, M. R., De, M., McDaniel, M. D., Kovar, J. L., Birrell, S., and Karlen, D. L. (2020). Science-based maize stover removal can be sustainable. Agron. J. 113, 3178–3192.
Odner, K. (1971). A preliminary report on an archaeological survey on the slopes of Kilimanjaro. Azania 6, 131–150.
Pabst, H., Kühnel, A., and Kuzyakov, Y. (2013). Effect of land use and elevation on microbial biomass and water extractable carbon in soils of Mount Kilimanjaro ecosystems. Appl. Soil Ecol. 67, 10–19. doi: 10.1016/j.apsoil.2013.02.006
Promar Consulting (2011). Fact-finding survey for the support aid to developing countries: agriculture, forestry and fisheries of Tanzania. Shinkawa Chuo-Ku: Promar consulting, 151.
Raeymaekers, S., and Stevens, B. (2015). Characterization of Banana-Based farming systems in the Upper-Pangani basin, Tanzania. MSc Thesis. Leuven: KU Leuven, 217.
Raussen, T. (1997). Integrated soil fertility management on small-scale farms in eastern province of Zambia. Nairobi: English press Ltd, 87.
Riedell, W. E., and Osborne, S. L. (2017). Row and forage crop rotation effects on maize nutrition and yield. Can. J. Plant Sci. 97, 645–653. doi: 10.1139/cjps-2017-0006
Soini, E. (2005). Land use change patterns and livelihood dynamics on the slopes of Mt. Kilimanjaro, Tanzania. Agric. Syst. 85, 306–323. doi: 10.1016/j.agsy.2005.06.013
Vandekerckhove, E., and Young, M. (2016). Characterization of banana-based farming systems in the Upper-Pangani basin, Tanzania. Master’s Thesis. Belgium: KU Leuven, 188.
Vourlitis, G. L., Lobo, F. A., Lawrence, S., Lucena, I. C., Borges, O. P. Jr., Dalmagro, H. J., et al. (2013). Variations in stand structure and diversity along a soil fertility gradient in a Brazilian savanna (Cerrado) in southern Mato Grosso. Soil Sci. Soc. Am. J. 77, 1370–1379. doi: 10.2136/sssaj2012.0336
Keywords: sustainability, maize stover, banana homegardens, nutrient flows, agroforestry, nutrient balance, cattle manure, agroecosystems
Citation: Meya AI, Swennen R, Ndakidemi PA, Mtei KM and Merckx R (2023) Maize stover transfers from maize fields to banana-based agroforestry homegardens and the corresponding nutrient flows in central-northern Tanzania. Front. For. Glob. Change 6:1131328. doi: 10.3389/ffgc.2023.1131328
Received: 24 December 2022; Accepted: 22 June 2023;
Published: 13 July 2023.
Edited by:
Didas Kimaro, Mwenge Catholic University (MWECAU), TanzaniaReviewed by:
Gopal Shankar Singh, Banaras Hindu University, IndiaCopyright © 2023 Meya, Swennen, Ndakidemi, Mtei and Merckx. This is an open-access article distributed under the terms of the Creative Commons Attribution License (CC BY). The use, distribution or reproduction in other forums is permitted, provided the original author(s) and the copyright owner(s) are credited and that the original publication in this journal is cited, in accordance with accepted academic practice. No use, distribution or reproduction is permitted which does not comply with these terms.
*Correspondence: Akida I. Meya, YWtpZGFpZ25hc0BnbWFpbC5jb20=, YWtpZGEubWV5YUBubS1haXN0LmFjLnR6
Disclaimer: All claims expressed in this article are solely those of the authors and do not necessarily represent those of their affiliated organizations, or those of the publisher, the editors and the reviewers. Any product that may be evaluated in this article or claim that may be made by its manufacturer is not guaranteed or endorsed by the publisher.
Research integrity at Frontiers
Learn more about the work of our research integrity team to safeguard the quality of each article we publish.