- 1Center for Ecological Research, Northeast Forestry University, Harbin, China
- 2Key Laboratory of Sustainable Forest Ecosystem Management-Ministry of Education, Northeast Forestry University, Harbin, China
- 3Northeast Asia Biodiversity Research Center, Northeast Forestry University, Harbin, China
The composition of non-structural carbohydrates (NSC), one of the photosynthetic products of plants, reflects the survival strategy of a plant. Although several studies have investigated variation of NSC content in woody plants over a set time scale, few studies have considered the dynamic variation of NSC over a continuous life history. In this study, the leaves, new twigs, and old branches of seven temperate broad-leaved tree species (diffuse-porous species: Betula platyphylla, Betula costata, Tilia amurensis, Acer pictum subsp. mono; ring-porous species: Ulmus davidiana var. japonica, Ulmus laciniata, Fraxinus mandshurica) were observed at three life history stages (seedling, sapling, and mature tree) to measure the dynamic changes of NSC and its influencing factors throughout the entire life cycles of these species. The results showed that life history, wood type, and environmental factors (soil nitrogen and phosphorus content, soil pH) significantly affected the NSC content in leaves and branches (including both new twigs and old branches). As plants grew, the NSC content in the leaves and branches generally showed an upward trend, meaning the total non-structural carbohydrate (TNC) content and soluble sugar (SS) content increased significantly, and the starch (ST) content was relatively stable. Lastly, there was no significant difference in NSC content between the canopy layers of mature trees. This indicates that the influence of life stage on NSC content in leaves and branches of plants may be dominated by genetics instead of being regulated by light factors.
1. Introduction
Forest vegetation, due to its role as a vital carbon sink in the global ecosystem (Yadav et al., 2022), is essential for the regulation of climate and carbon balance. To be a sink instead of a source, the net photosynthesis of forest vegetation must be positive (Beer et al., 2010), and thus photosynthetic products play a role in regulating forest productivity (Bonan, 2008). Non-structural carbohydrate (NSC), comprised of one of the photosynthetic products of plants (Zhang et al., 2013), is a mobile carbon pool (Wang and Wang, 2019). It participates in multiple physiological activities of plants, such as respiratory processes, metabolic activities, signal transduction, etc., and is of great significance for maintaining plant life activities. NSC mainly exists in the forms of soluble sugar (SS) and starch (ST) (Furze et al., 2021). SS, which includes oligosaccharides (sucrose, raffinose, and stachyose) and monosaccharides (glucose and fructose), provides direct energy materials for respiration, defense, plant stress signaling, phloem transport, and osmoregulation, while ST exists as a temporary or long-term energy store that plants can convert into SS when carbon demand exceeds supply (Piper and Paula, 2020; Signori-Müller et al., 2021a). The absorption and utilization of plant carbon can be judged based on variations in plant NSC content (Hoch et al., 2003), which reflect the adaptation strategies of plants when experiencing changes in the external environment (Myers and Kitajima, 2007). When the NSC content in plant tissues continues to decline, it means that the carbon demand is higher than the supply, and the carbon reserve is inadequate. When the NSC content is elevated or relatively stable, it means that the supply of carbon is greater than the demand, so carbon does not limit growth at all (Hoch et al., 2002). Thus, the growth status of forest vegetation can be characterized by the variation in a plant’s NSC content.
Studies on NSC in tree organs have mainly focused on the differences in intra- or interspecific concentration over various spatiotemporal scales, mostly in saplings and mature trees. However, there are few studies investigating the dynamic changes in NSC content over a continuous life history at the temporal scale. Some studies have shown that deciduous plants have evident seasonal fluctuations in the content of NSC and its components, with the lowest SS content in early summer and the highest content during dormancy, which is related to the germination and apoptosis of plant leaves (Piispanen and Saranpää, 2001; Regier et al., 2010). Similarly, at each developmental stage, woody plants differ in branch and leaf physiological and morphological characteristics (Mediavilla et al., 2013), and they vary in their adaptation strategies in response to a changing environment. This in turn leads to differences in carbon fixation, metabolism, allocation, and storage in vivo (Grulke and Retzlaff, 2001). In general, specific leaf weight, leaf nitrogen content, water use efficiency, stomatal conductance, and carbon assimilation all increase as diameter at breast height (DBH) increases (Thomas and Winner, 2002; Song et al., 2019). For example, in Pinus ponderosa, the concentration of mobile carbon pools increased significantly in the upper canopy tissues as tree height increased (Sala and Hoch, 2009); in Pinus koraiensis, the NSC content of current-year needles increased significantly at the leaf unfolding period as age increased, and the NSC content of current-year and perennial needles generally decreased significantly at the leaf fall period as age increased (Yan et al., 2022). Plants within the same community at different life history stages are subject to similar climatic and soil conditions (Zhang et al., 2018), but seedlings and saplings have relatively weaker light resources compared to mature trees (Liu et al., 2019). Further, plants at the seedling stage are more sensitive and vulnerable to changes in the external environment. In addition, organ age is one of the driving forces behind the shift in plant ecological strategies (Liu et al., 2021). It has been shown that tree size has a stronger effect on leaf traits than do environmental factors (Liu et al., 2020). Therefore, the patterns of change in NSC and the regulatory factors still need to be studied in depth at different life history stages (e.g., seedlings, saplings, and mature trees) (Hartmann et al., 2018; Signori-Müller et al., 2021b).
Non-structural carbohydrates (NSC) is distributed in most tree organs, such as the leaves, branches, trunks, and roots. Furze et al. (2019) showed that branches were the largest reservoir of total NSC and fluctuated seasonally, while total NSC in roots remained stable throughout the year. This is because the NSC content in branches were used to support springtime growth, and roots need relatively stable NSC content to cope with catastrophic situations. NSC is used for different purposes in different organs, and as a result, its distribution varies across organs. Similarly, species differ in their NSC distributions, which are one reason why NSC distributions are commonly associated with different biological characteristics of tree species (Hoch et al., 2003). Because most of the water absorbed by plant roots from the soil is dissipated by transpiration, and a small portion is transported to the leaves for photosynthesis, one wonders whether differences in water transport among plants lead to differences in NSC levels among species. Angiosperms can be divided into diffuse-porous species (relatively small diameter of the inner lumen of the duct) and ring-porous species (relatively large diameter of the inner lumen of the duct) (Taneda and Sperry, 2008). Studies have shown that the NSC storage of the entire tree overall is higher in ring-porous than in diffuse-porous species (Barbaroux and Bréda, 2002; Furze et al., 2019). In one example, the ST content of the whole tree was also higher in Quercus petraea, a ring-porous species, than in Fagus sylvatica, a diffuse-porous species (Barbaroux et al., 2003).
Numerous studies have confirmed that physiological ecological factors related to plant photosynthesis may affect the synthesis and distribution of NSC in plants to some extent, such as Nitrogen (N) and phosphorus (P) (Farrar and Jones, 2000; Millard et al., 2007). It has been shown that the response of TNC to soil N content varies. For example, TNC in plants increases with soil N content, but the increase is inhibited when the N content exceeds a certain threshold (Thomas et al., 2005). TNC content has a positive or insignificant response to the increase of environmental P content (Wang et al., 2014). However, few studies have explored the impact of multiple environmental factors along with plant size on NSC, simultaneously. Light intensity is another dominant factor in plant photosynthesis. Even in a single individual, different canopy positions can cause differences in plant light intensity and water availability (Yan et al., 2012). Therefore, canopy differences may lead to different NSC contents in different plants, even though several studies have found that canopy position had no significant effect on NSC (Yan et al., 2012, 2022; Li et al., 2017).
In this study, four diffuse-porous species (Betula platyphylla, Betula costata, Tilia amurensis, Acer pictum subsp. mono) and three ring-porous species (Ulmus davidiana var. japonica, Ulmus laciniata, Fraxinus mandshurica) in a broad-leaved Korean pine forest in Northeast China were selected as the research subjects. The NSC content was determined in leaves, new twigs, and old branches in different life history stages (seedling, sapling, and mature trees) to explore whether life history, wood type, and soil factors (soil water content, soil N and P content, soil pH) would affect the NSC content in branches (new twigs, old branches) and leaves, and to analyze the dynamics of NSC in leaves, new twigs, and old branches throughout the duration of a continuous life history. In this regard, the following hypotheses were made: (1) life history, wood type, and soil factors all affect the NSC content of leaves, new twigs, and old branches; (2) the total non-structural carbohydrate (TNC) content in leaves, new twigs, and old branches tends to increase with tree growth, according to one of the following three conjectures (Figure 1): SS and ST both tend to increase; one of SS and ST tends to increase and the other is in a stable state; one of SS and ST shows an increasing trend and the other shows a decreasing trend, but the increase will be greater than the decrease; (3) The NSC content in leaves, new twigs, and old branches will not differ significantly among canopy layers.
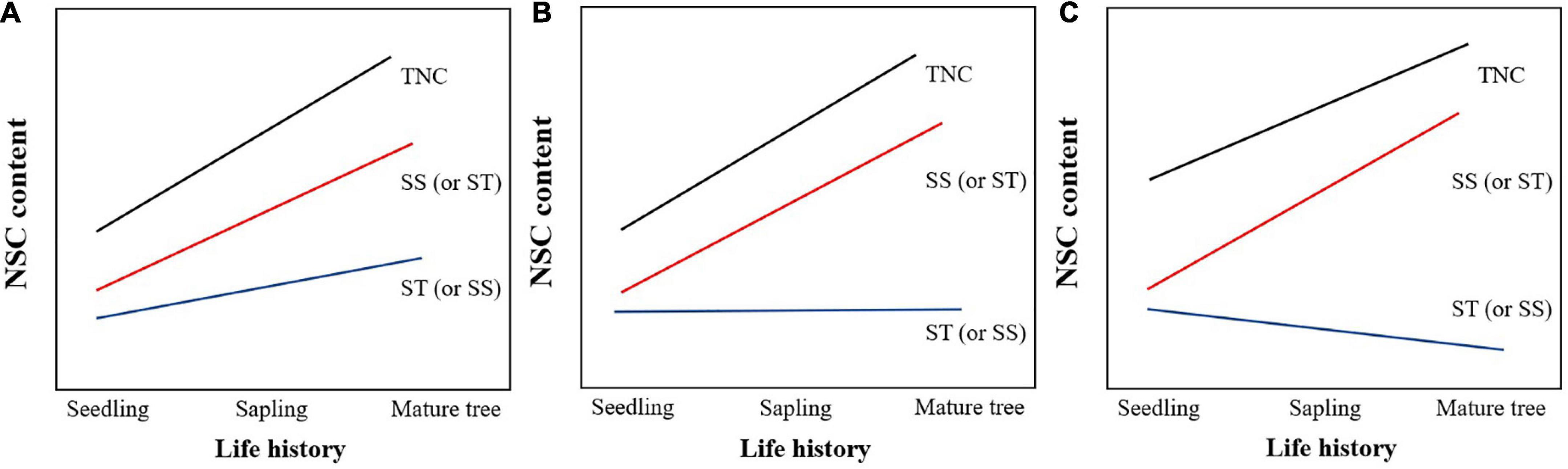
Figure 1. Hypothetical diagram of the changing trend of non-structural carbohydrates (NSC) content with life history. (A) SS and ST both tend to increase; (B) one of SS and ST tends to increase and the other is in a stable state; (C) one of SS and ST shows an increasing trend and the other shows a decreasing trend, but the increase will be greater than the decrease.
2. Materials and methods
2.1. Study region
The field survey was conducted in August 2020 in the Heilongjiang Liangshui National Nature Reserve (47°10’50”N, 128°53’20”E), located on the southern slope of the Xiao Hinggan Mountains. The reserve has an altitude range of 300–780 m and an average slope of 10°–15°, which classifies the region as having a temperate continental monsoon climate with four distinct seasons. The winter is lengthy and cold, and the summer is short and warm, with an average annual precipitation of about 676 mm. The average annual maximum temperature is 7.5° and minimum temperature is −6.6°, with a large annual temperature difference. The reserve is the most typical and intact coniferous and broad-leaved mixed forest in the northern temperate zone of China. P. koraiensis is the main established species, but other broad-leaved species are also abundant.
2.2. Experimental design
Seven broad-leaved tree species, which include B. platyphylla, B. albosinensis, T. amurensis, A. pictum subsp. mono, U. davidiana var. japonica, U. laciniata, and F. mandshurica were selected for study and classified into two major wood types, the first four of which were diffuse-porous species and the latter three of which were ring-porous species. To reduce the effect of light conditions on tree NSC, 30 individuals of each tree species were selected under full light conditions as far away from each other as possible and classified into different life history stages, according to the difference in DBH (Thomas, 2010; Masaki et al., 2021). And each of the three life stages, 10 individuals growing on the approximate south slope and well into their life history stage were selected. The categories were seedlings (DBH = 0∼3 cm), saplings (DBH = 18∼23 cm), and mature trees (DBH = 35∼40 cm) (Table 1). Leaves (morphologically intact and in great growth), new twigs (current year), and old branches (perennial) were sampled within each life history stage. To distinguish between new twigs and old branches, the nodal rings, which are formed by bud scale scars on the branches, were checked. Subsequently, the collected branches and leaves were marked and placed in a cryogenic foam box. After being brought back to the laboratory, they were placed in the oven at 105°C for 20 min and then dried at 65°C to a constant weight. Finally, the sample was crushed for the NSC determination.
Seedlings and saplings were measured twice. Mature trees were divided into three canopy layers—upper, middle, and lower, and measurements on each canopy layer were also repeated twice. In order to avoid the influence of spatial autocorrelation among the sample trees on the experimental results, the distance between any two sample trees had to be greater than 5 m.
2.3. NSC measurements
In this paper, NSC is defined as the free, low molecular weight sugars SS and ST, the sum of which is denoted as TNC (Liu et al., 2019; Signori-Müller et al., 2021a). NSC incorporates TNC, SS, and ST. These contents were measured using an improved phenol-sulfuric acid method (Buysse and Merckx, 1993), and their concentrations are expressed here as percentage dry weight (%DM).
First, 40 mg of the ground dry sample was inserted into a 10 mL centrifuge tube and soaked overnight in 10 mL of 80% ethanol. The soaked sample was centrifuged twice at 4,000 rpm in a DT5-4B low-speed benchtop centrifuge (Beijing Era Beili Centrifuge Co., Ltd., Beijing, China), and the supernatant was poured into a volumetric flask for constant volume, which was used to determine the SS. Then, the residue remaining after ethanol extraction was dried. Next 10 mL of deionized water was added, and the solution was mixed well. After gelatinization in a boiling water bath, the solution was cooled to below 60°C. One mL of 0.5% α-amylase solution was added, and the water bath was kept at 60°C for 1 h. The enzymatic solution was centrifuged at 2,000 rpm and filtered to maintain a constant volume with the deionized water for the determination of the ST content. Finally, SS and ST were determined. One mL of the sugar solution was taken into the glass tube, and then one mL of 28% phenol solution (dissolved in 80% ethanol) was added. Next, concentrated sulfuric acid was added immediately, and the glass tube was shaken for 1 min. The absorption value was measured by a spectrophotometer (722 N, Shanghai Yidi Analytical Instrument Co., Ltd., Shanghai, China) at 490 nm after 15 min.
2.4. Soil water and nutrient availability
Around the sample tree, a soil corer was used to collect about 10 cm of soil at the base of the trunk at three sampling points (the angle between any two sampling points was about 120°). The three soil samples were mixed evenly after removing obvious impurities, and then the soil water content (g/g) was determined by the oven-drying method (Liu et al., 2020). Soil total N content (mg/g) and total P content (mg/g) were measured using the AQ 400 automatic intermittent chemical analyzer (SEAL Analytical, Mequon, WI, USA) (Jing et al., 2017). Soil pH was measured by pH meter (HANNA pH211, Italy).
2.5. Data analysis
In this study, data was statistically analyzed in SPSS 22.0 and R-4.1.0 (R Core Team, 2021) and plotted in GraphPad Prism 8.0. Generalized linear models of DBH, wood type and environmental factors (soil water content, soil N and P content, soil pH) with NSC components were constructed using the “glm” function in R. One-way analysis of variance (ANOVA) and the least significant difference (LSD) was used to compare the differences in organ and life history of tree species under different wood types. The same method was used to test for differences between the different canopy layers of mature tree’s branches and leaves. To compare organs at different stages of life history in the two wood types, an independent sample T-test was performed. A linear regression was also run with NSC components against life history stages. All tests were performed at a significance level of α = 0.05.
3. Result
3.1. Factors influencing NSC concentration
Life history (DBH) had a significant effect on SS and TNC in leaves, new twigs, and old branches (p < 0.05), but only on ST in old branches (p < 0.05). The effect of wood type on TNC was significant in leaves, new twigs, and old branches (p < 0.05) (Table 2). The effects of environmental factors on NSC varied by organ (Table 2): soil water content only had a significant effect on SS and TNC content in leaves; soil N content had significant effects on TNC of leaves and new twigs, soil P content only had significant effects on TNC of new twigs, and soil pH had significant effects on TNC of leaves, new twigs, and old branches. Compared with soil P content, the effect of soil N content on leaves and branches (new twigs and old branches) was more significant and tended to be negatively correlated (Table 2).
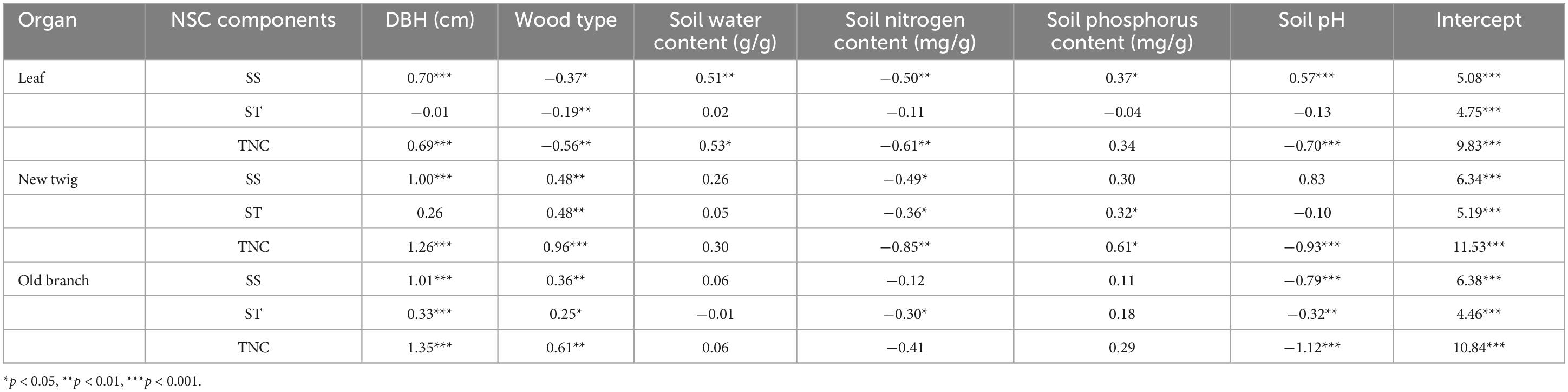
Table 2. The influence of wood type, life history [diameter at breast height (DBH)], and environmental factors (soil water content, soil nitrogen content, soil phosphorus content, soil pH) on non-structural carbohydrates (NSC) content.
3.2. Variations in NSC concentration with life history and organ
For the seven tree species, there was an overall increasing trend in the NSC content of leaves, new twigs, and old branches as the plant grew. For the diffuse-porous species, the SS content of leaves, new twigs, and old branches was significantly higher in the mature tree stage than in the other two life history stages, and the TNC content was significantly higher than in the seedling stage, while the ST content was stable in all life history stages of leaves and old branches, without significant differences (Figure 2). For the ring-porous species, the SS and TNC contents of leaves, new twigs, and old branches were significantly lower in the seedling stage than in the other two life history stages, while ST contents in leaves and new twigs did not differ significantly among the life history stages (Figure 2).
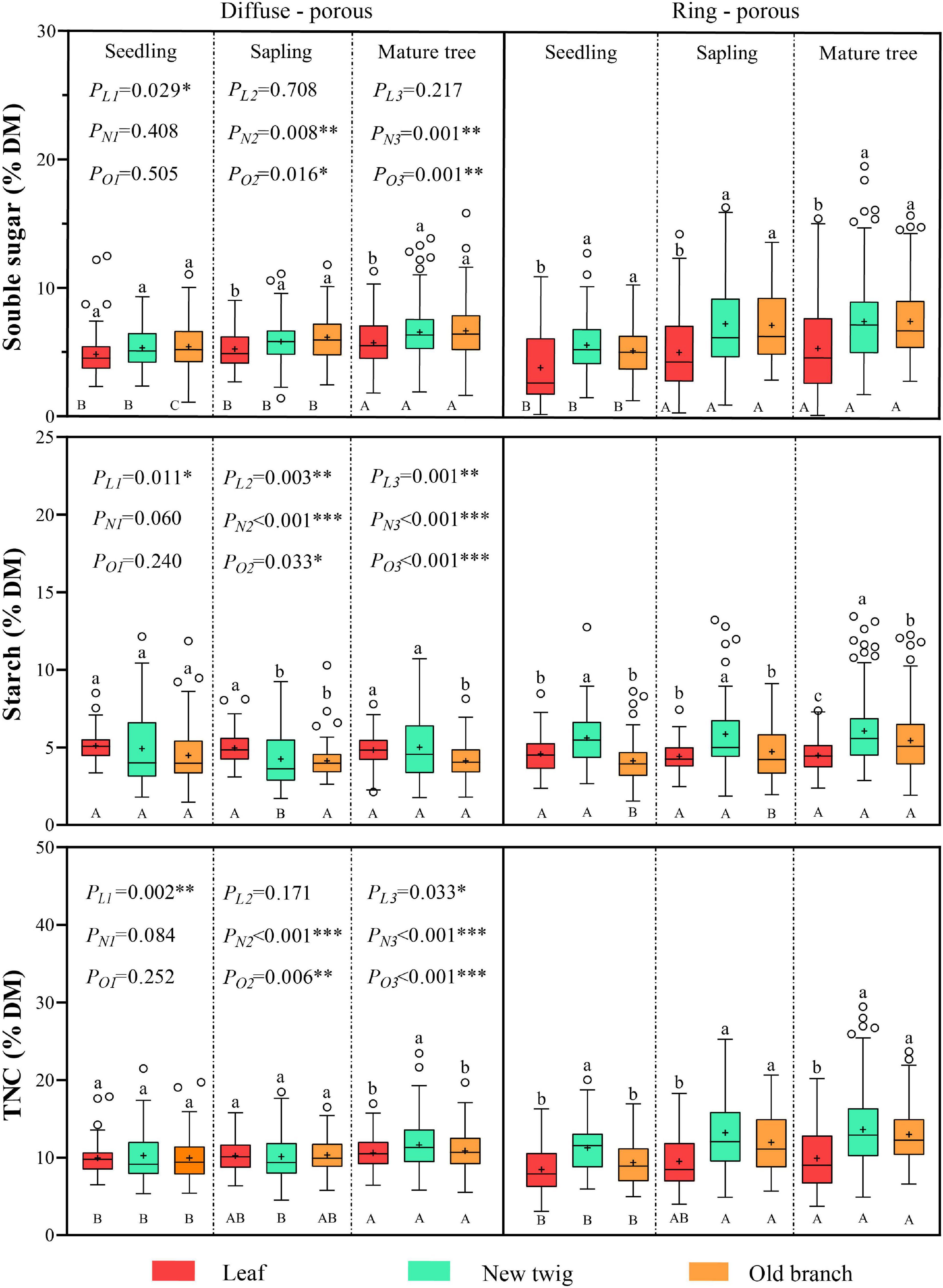
Figure 2. Non-structural carbohydrates (NSC) content in different organs of diffuse-porous and ring-porous species at each life history stage. Capital and lowercase letters indicate the differences between life history stages and organs under same wood types, respectively; P-values represent the comparison of leaves, new twigs and old branches in two species of wood types at the same life history stage, in which L, N, and O represent leaves, new twigs and old branches respectively; 1, 2, and 3 represent seedlings, sapling, and mature trees, respectively. Dots indicate outliers and “+” indicates mean values. *p < 0.05, **p < 0.01, ***p < 0.001.
For diffuse-porous species, at the sapling stage, SS content was significantly lower in leaves than in branches, while ST content was significantly higher. At the mature tree stage, SS content was also significantly lower in leaves than in branches, while ST content was significantly lower in old branches than in leaves and new twigs, and TNC content was significantly higher in new twigs than in leaves and old branches (Figure 2). However, there was no significant difference in NSC content between leaves and branches at the seedling stage, and the fluctuation was slight among the three organs (Supplementary Table 1). For the ring-porous species, the differences in NSC content in leaves and branches were similar among the three life history stages, with the overall performance being higher in SS and TNC content for branches than for leaves, and significantly higher in ST content for new twigs than for leaves and old branches (Figure 2).
Diffuse-porous species had a higher NSC content in their leaves and a lower content in their new twigs than ring-porous species. The NSC content of the old branches was higher than that of the ring-porous species at the seedling stage only. In contrast, NSC content was lower than that of the ring-porous species at the other two life history stages (Figure 2). Comparing the two wood types, leaves were the only organ in which NSC content differed significantly at the seedling stage, but at the sapling and mature tree stages, the ST content in leaves differed significantly, and the NSC content in branches showed significant differences too (Figure 2).
Across both wood types, SS and TNC contents in leaves and branches increased significantly as life history stages progressed (Figure 3). For the diffuse-porous species, ST content decreased significantly only in leaves at a later life history stage (Figure 3A), while ST content in branches did not vary significantly with life history (Figures 3C, E). For the ring-porous species, ST content varied significantly only in old branches, and ST content in leaves and new twigs did not vary significantly with life history (Figures 3B, D, F).
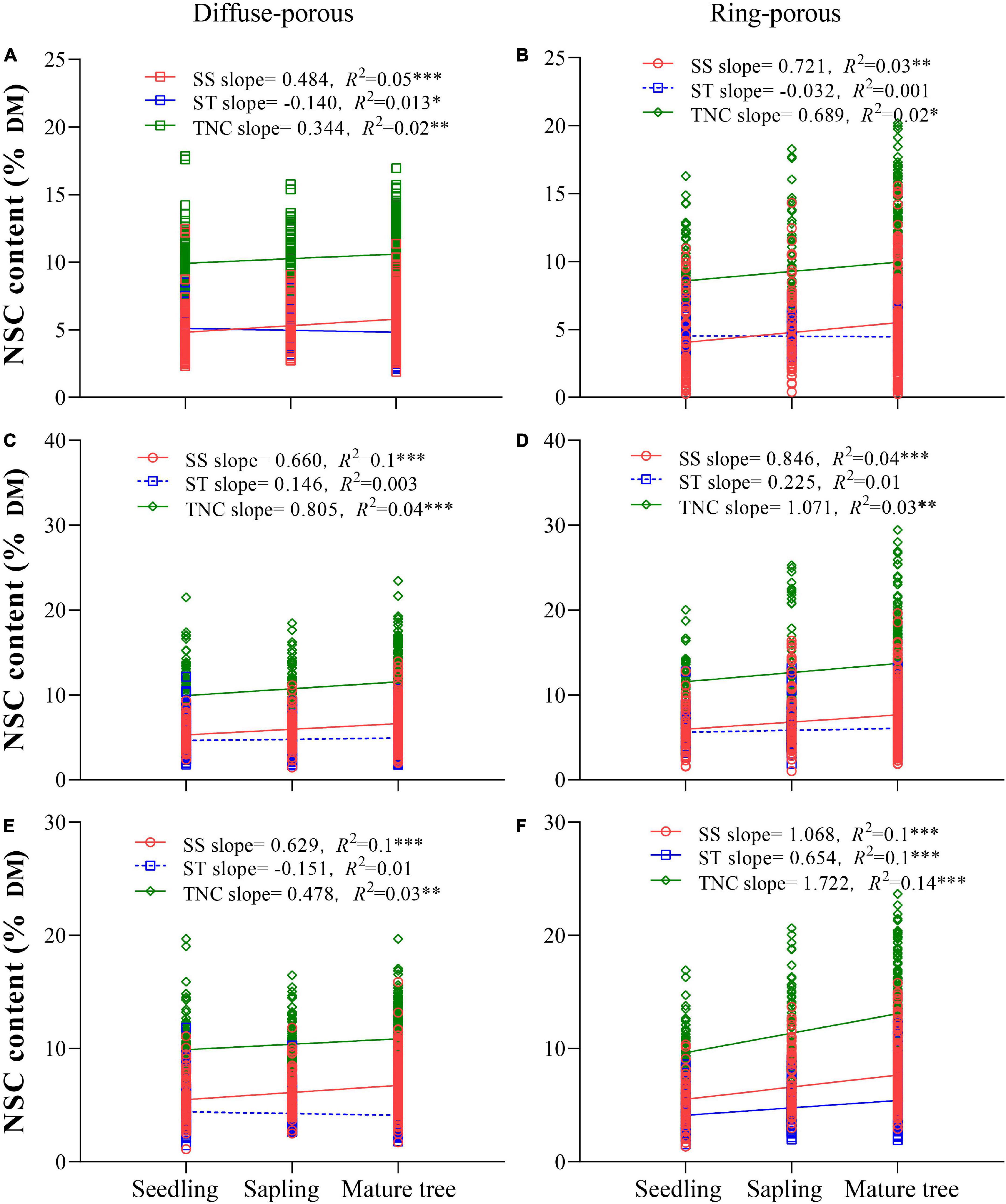
Figure 3. Variations of non-structural carbohydrates (NSC) components with life history in different organs of diffuse-porous and ring-porous species. (A,B) Shows leaf composition; (C,D) shows new twig composition; (E,F) shows old branch composition. The dashed lines indicate non-significant variation. *p < 0.05, **p < 0.01, ***p < 0.001.
3.3. Variation in NSC concentration with canopy
Among species of the same wood type, there was no significant difference in NSC content between different canopy layers in leaves and branches of the mature trees (Figure 4). The CV of NSC content in all three canopy layers of diffuse-porous species were: leaf TNC, 0.152∼0.178; new twig TNC, 0.236∼0.253; old branch TNC, 0.166∼0.194 (Supplementary Table 3). The CV of NSC content in leaves and branches of ring-porous species, in all three canopy layers, were: leaf TNC, 0.375∼0.425; new twig TNC, 0.332∼0.381; old branch TNC, 0.262∼0.299 (Supplementary Table 3). The CV of NSC content was similar among the three canopy layers of all species, regardless of wood type. The CV of NSC content in the canopy layers were smaller in the diffuse-porous than in the ring-porous species (Supplementary Table 3).
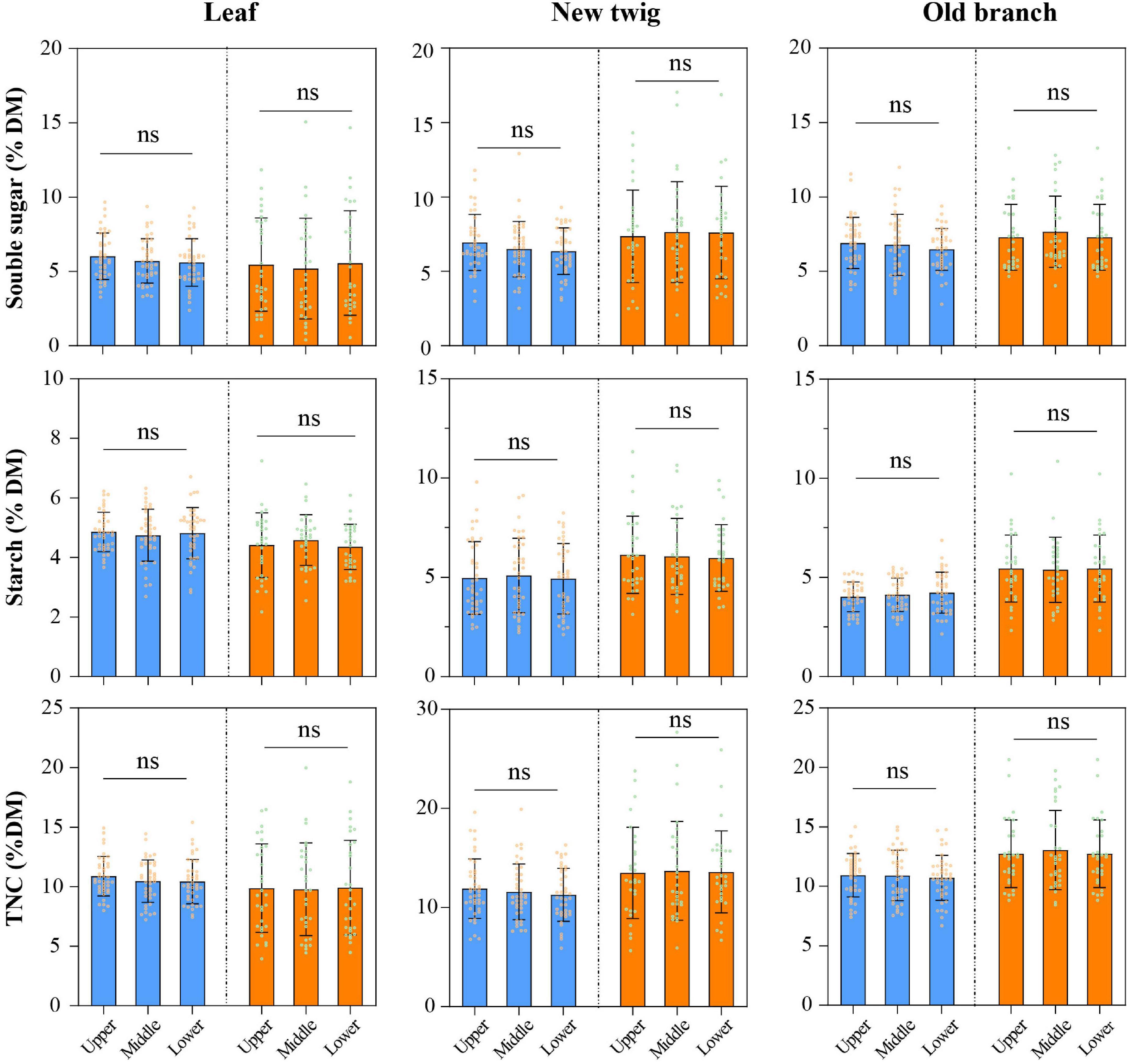
Figure 4. Differences among mature tree canopy layers of species by different wood types. Blue represents diffuse-porous species, orange represents ring-porous species. ns, no significant difference.
4. Discussion
4.1. Factors influencing NSC concentration
The functional traits of plants vary throughout the life cycle, from the time it is a seed, to when it becomes a full-grown individual, and so do the trade-offs between different stages of branches and leaves (Wright et al., 2010; Liu et al., 2019, 2021). Compared to mature trees, seedlings experience poor light conditions, have large, thin leaves (Augspurger and Bartlett, 2003; He and Yan, 2018), and have a relatively weak ability to intercept light and synthesize carbon (Niklas et al., 2007). Thus seedlings tend to compensate for the harsh environment with a relatively high specific leaf area (He and Yan, 2018). As trees grow, the plant exerts more energy into the branches to enhance their functions of mechanical support and water supply capacity so as to accommodate the increased leaf area and transpiration rate (Niinemets et al., 2004; Fan et al., 2017). Seedlings experience a stronger trade-off between growth rate and mortality compared to mature trees (Wright et al., 2010), and thus at the seedling stage, plants are resource acquisition-oriented. This means that seedlings prioritize nutrients for growth and development. In contrast, mature trees are relatively conservative in their resource use, and they only use increased resources when resisting adverse environments (He and Yan, 2018). From this, it has been inferred that carbohydrates, as essential energy substances for the growth and development processes of plants, are equally affected by the stages of life and the environmental factors in their distribution. This inference was verified in this study—-life history has a significant effect on NSC (Table 2).
It was confirmed that the diffuse- and ring-porous species differ in their TNC content (Barbaroux and Bréda, 2002; Barbaroux et al., 2003). Our results also showed that wood type had a significant effect on TNC content in leaves and branches (p < 0.05) (Table 2), which was consistent with previous studies. The synthesis of TNC is inseparable from the operation of the hydraulic system of various plant organs, and the water conductivity of the hydraulic system is also different due to the differences in xylem vessels in the two wood types (Taneda and Sperry, 2008; McCulloh et al., 2010). This may be one of the reasons for the significant effect of materiality on NSC.
Soil water content only had a significant effect on leaf TNC content (Table 2), which opposed Hypothesis 1. However, it is consistent with the findings of Lintunen et al. (2016). Soil N content had significant effects on the TNC of leaves and branches, and it was negatively correlated with NSC (Table 2). The N element is related to the synthesis of plant photosynthetic pigments, which in a certain range can promote the photosynthesis of plants (Nakaji et al., 2001). That a high N content inhibits photosynthetic pigment synthesis may be the reason for the negative correlation between soil N content and NSC. On the other hand, it is possible that plants need to absorb large amounts of N from the soil during their growing season, and this leads to higher carbon input for their morphogenesis (Eissenstat and Yanai, 1997; Wang et al., 2017). Soil pH affects the photosynthetic and osmoregulatory systems of plants (Yang et al., 2022), so it is significantly correlated with leaf and branch NSC components (Table 2).
4.2. Variation of NSC concentration with life history and organ
The differences in NSC across different life histories and organs characterize the differences in physiological regulation and adaptive strategies to the external environment (Augspurger and Bartlett, 2003). Overall, the NSC content in leaves and branches increased with maturing life stages, which is consistent with hypothesis 2. Sala and Hoch (2009) and Zhang et al. (2018) also showed that TNC storage in trees increases from the seedling to the mature tree stage. During these three growth stages, the photosynthetic capacity and water use efficiency of the plant gradually increases (Cavender-Bares and Bazzaz, 2000; Thomas, 2010), and hence the TNC synthesis content also showed an increasing trend. With the growth of trees, the tension of the xylem increases, and cavitation and embolism are more likely to occur (Sala and Hoch, 2009). The repair process may require a large amount of SS. The ST content of mature trees is higher than in saplings and seedlings, which is related to the survival strategy where big trees tend to reserve more energy in the event they must resist various environmental stresses. The results of this study further showed that the SS and TNC contents in leaves and branches of species of both wood types increased significantly with life history, and the ST content did not change significantly throughout the life history stages in either leaves or branches (Figure 3). This result confirmed conjecture 2 in hypothesis 2, that the variation in TNC was mainly caused by SS. This is consistent with the results of Zhang et al. (2013), where the changes in TNC were caused by NSC components in branches. Zhang et al. (2013) and Yan et al. (2022) also found that the increase of TNC in needles was more closely related to the increase of SS during the leaf unfolding period for P. koraiensis. This may be related to the season of the plant. Plants consume a large amount of ST in winter in response to cold stress and germination in spring (Piispanen and Saranpää, 2001; Lintunen et al., 2016), so a part of SS produced in the growing season was converted into ST to make up for the consumption, which may result in the change of TNC in summer more closely related to SS. However, Hoch et al. (2003) and Schadel et al. (2009) showed that the changes in branch TNC were more closely related to ST. It may be due to differences in sampling seasons and tree species.
By comparing the NSC contents in leaves, new twigs, and old branches of species of the two wood types in each life history stage, it was found that the SS content was higher in branches than in leaves, and the ST content was higher in leaves than in branches (Figure 2). However, TNC content did not change consistently in the life stages of the diffuse-porous species, and for the ring-porous species, the contents were higher in the branches than in the leaves (Figure 2). Furze et al. (2019) suggested that high SS concentrations in branches helps trees resist low temperatures in the canopy. The carbon limitation hypothesis indicates that the stress of low temperatures weakens the photosynthetic capacity of plants and reduces their carbon assimilation capacity, which results in insufficient carbon supply, reduced NSC content, and reduced growth rate (Wardle, 1993; Wang and Wang, 2019). Therefore, increasing SS content in branches may be a conservative survival strategy for plants that need to resist external low-temperature environments. Ring-porous species are not only less resistant to low temperatures and drought (Yuan et al., 2021), but they also tend to have a higher risk of embolism and cavitation (Hack et al., 2006; Taneda and Sperry, 2008), which may result in higher SS content than in diffuse-porous species (Figure 2). Signori-Müller et al. (2021b) studied two types of plants with different growth rates in the Amazon forest. The results showed that the NSC content was higher in branches than in leaves for fast-growing species under sufficient light conditions, while the opposite was true for slow-growing species. Thus, the difference in NSC content in leaves and branches of woody plants is the embodiment of different survival strategies. Further discussion on the dynamic changes of NSC content in new twigs and old branches showed that the NSC content was higher in new twigs than in old branches, at all three life history stages (Figure 2). The results were similar to those of Zhang et al. (2013), regarding the NSC of new twigs and old branches of 12 tree species in temperate zones. According to the principle of near distribution of carbon sources, branches are the closest organ to the carbon source of leaves, and thus they have priority for claiming photosynthetic products. Further, because of their proximity to buds, old branches may be the most vital carbon source for tree germination and growth in the spring (Wong et al., 2003; Spann et al., 2008). The new twigs in the current growing season are old relative to the sprouting branches of the coming year, thus it is inferred that new twigs store more NSC than old branches during the growing season. This is both to maintain the growth and development of the current year, and to provide a source of carbon for the sprouting of leaves and branches in the coming year.
4.3. Variation of NSC concentration with canopy
To test hypothesis 3, this study analyzed the NSC content of mature tree canopies, and the results were consistent with our hypothesis (Figure 4). Zhang et al. (2015) studied the NSC concentration in temperate canopy branches and leaves and found that the canopy had no significant effect on the NSC concentration in leaves and twigs, but had a significant effect on coarse branches, which was relatively consistent with the results of this study. In the study on the effect of the canopy on the NSC of fast-growing and slow-growing trees in the Amazon forest, no significant difference was found in NSC concentration between different canopy branches and leaves (Signori-Müller et al., 2021b). The result suggests that variability in plant NSC may be more so determined by genetic characteristics than by variations in the vertical gradient of light environmental factors. However, Woodruff and Meinzer (2011) showed that the NSC content of branches above the canopy differed significantly among Douglas-fir (Pseudotsuga menziesii Mirb. Franco) individuals of different tree heights. Heights can indicate life history stages to a certain extent (Liu et al., 2020; Masaki et al., 2021), so the source of this difference may not only be attributed to changes in light gradients. Studies on evergreen conifer species have shown that the canopy layer influences needle NSC concentrations (Gholz and Cropper, 1991; Niinemets, 1997), which may be related to the selection of tree species types, their different habitats and phenological periods.
5. Conclusion
According to the dynamic NSC contents in leaves and branches of seven broad-leaved tree species in different life history stages, it was found that the NSC content of woody plants was not only affected by external environmental factors (soil N and P content, soil pH), but also correlated with their genetic characteristics (life history, wood type, etc.). As the trees aged and grew, the NSC content in leaves and branches also increased, while the ST content was relatively stable throughout the life history stages. Therefore, the increase of NSC content in plants during the growing season was likely caused by SS. The NSC content differed between the species of different wood types. Overall, the NSC content was higher in the leaves of the diffuse-porous than of the ring-porous species, while the NSC content was lower in the branches. However, this study found no significant difference in NSC content between the canopy layers of mature trees. Although the light environment was associated with NSC content in plants, the dynamic changes of NSC throughout the continuous life history of these seven broad-leaved species were not driven mainly by light factors, and the content fluctuations may be more closely related to their genetic characteristics.
Data availability statement
The raw data supporting the conclusions of this article will be made available by the authors, without undue reservation.
Author contributions
ZL and KW planned and designed the research. KW performed experiments, analyzed the data, and wrote the manuscript with substantial contributions from ZL and GJ. ZL provided helpful comments in the draft. All authors contributed to the article and approved the submitted version.
Funding
This work was supported by the National Key R&D Program of China (2022YFD2201100), the National Natural Science Foundation of China (31971636), and the Fundamental Research Funds for the Central Universities (2572022DS13).
Acknowledgments
We thank Dr. Annalise Elliot at the University of Kansas for her assistance with English language and grammatical editing of the manuscript. We also thank the responsible editor and two reviewers for their constructive and helpful comments that helped to improve the manuscript.
Conflict of interest
The authors declare that the research was conducted in the absence of any commercial or financial relationships that could be construed as a potential conflict of interest.
Publisher’s note
All claims expressed in this article are solely those of the authors and do not necessarily represent those of their affiliated organizations, or those of the publisher, the editors and the reviewers. Any product that may be evaluated in this article, or claim that may be made by its manufacturer, is not guaranteed or endorsed by the publisher.
Supplementary material
The Supplementary Material for this article can be found online at: https://www.frontiersin.org/articles/10.3389/ffgc.2023.1130604/full#supplementary-material
References
Augspurger, C. K., and Bartlett, E. A. (2003). Differences in leaf phenology between juvenile and adult trees in a temperate deciduous forest. Tree Physiol. 23, 517–525. doi: 10.1093/treephys/23.8.517
Barbaroux, C., and Bréda, N. (2002). Contrasting distribution and seasonal dynamics of carbohydrate reserves in stem wood of adult ring-porous sessile oak and diffuse-porous beech trees. Tree Physiol. 22, 1201–1210. doi: 10.1093/treephys/22.17.1201
Barbaroux, C., Bréda, N., and Dufrêne, E. (2003). Distribution of above-ground and below-ground carbohydrate reserves in adult trees of two contrasting broad-leaved species (Quercus petraea and Fagus sylvatica). New Phytol. 157, 605–615. doi: 10.1046/j.1469-8137.2003.00681.x
Beer, C., Reichstein, M., Tomelleri, E., Ciais, P., Jung, M., Carvalhais, N., et al. (2010). Terrestrial gross carbon dioxide uptake: Global distribution and covariation with climate. Science 329, 834–838.
Bonan, G. B. (2008). Forests and climate change: Forcings, feedbacks, and the climate benefits of forests. Science 320, 1444–1449. doi: 10.1126/science.1155121
Buysse, J., and Merckx, R. (1993). An improved colorimetric method to quantify sugar content of plant tissue. J. Exp. Bot. 44, 1627–1629.
Cavender-Bares, J., and Bazzaz, F. A. (2000). Changes in drought response strategies with ontogeny in Quercus mibra: Implications for scaling from seedlings to mature trees. Oecologia 124, 8–18. doi: 10.1007/PL00008865
Fan, Z. X., Sterck, F., Zhang, S. B., Fu, P. L., and Hao, G. Y. (2017). Tradeoff between stem hydraulic efficiency and mechanical strength affects leaf-stem allometry in 28 Ficus tree species. Front. Plant Sci. 8:1619. doi: 10.3389/fpls.2017.01619
Farrar, J. F., and Jones, D. L. (2000). The control of carbon acquisition by roots. New Phytol. 147, 43–53.
Furze, M. E., Huggett, B. A., Aubrecht, D. M., Stolz, C. D., Carbone, M. S., and Richardson, A. D. (2019). Whole-tree nonstructural carbohydrate storage and seasonal dynamics in five temperate species. New Phytol. 221, 1466–1477. doi: 10.1111/nph.15462
Furze, M. E., Wainwright, D. K., Huggett, B. A., Knipfer, T., McElrone, A. J., and Brodersen, C. R. (2021). Ecologically driven selection of nonstructural carbohydrate storage in oak trees. New Phytol. 232, 567–578. doi: 10.1111/nph.17605
Gholz, H. L., and Cropper, W. P. Jr. (1991). Carbohydrate dynamics in mature Pinus elliottii var. elliottii trees. Can. J. For. Res. 21, 1742–1747.
Grulke, N. E., and Retzlaff, W. A. (2001). Changes in physiological attributes of ponderosa pine from seedling to mature tree. Tree Physiol. 21, 275–286. doi: 10.1093/treephys/21.5.275
Hack, U. G., Sperry, J. S., Wheeler, J. K., and Castro, L. (2006). Scaling of angiosperm xylem structure with safety and efficiency. Tree Physiol. 26, 689–701.
Hartmann, H., Adams, H. D., Hammond, W. M., Hoch, G., Landhäusser, S. M., Wiley, E., et al. (2018). Identifying differences in carbohydrate dynamics of seedlings and mature trees to improve carbon allocation in models for trees and forests. Environ. Exp. Bot. 152, 7–18.
He, D., and Yan, E. R. (2018). Size-dependent variations in individual traits and trait scaling relationships within a shade-tolerant evergreen tree species. Am. J. Bot. 105, 1165–1174. doi: 10.1002/ajb2.1132
Hoch, G., Marianne, P., and Christian, K. (2002). Altitudinal increase of mobile carbon pools in Pinus cembra suggests sink limitation of growth at the Swiss treeline. Oikos 98, 361–374.
Hoch, G., Richter, A., and Körner, C. (2003). Non-structural carbon compounds in temperate forest trees. Plant Cell Environ. 26, 1067–1081.
Jing, H., Zhou, H. X., Wang, G. L., Xue, S., Liu, G. B., and Duan, M. C. (2017). Nitrogen addition changes the stoichiometry and growth rate of different organs in Pinus tabuliformis seedlings. Front. Plant Sci. 8:1922. doi: 10.3389/fpls.2017.01922
Li, M. C., Kong, G. Q., and Zhu, J. J. (2017). Vertical and leaf-age-related variations of nonstructural carbohydrates in two alpine timberline species, southeastern Tibetan Plateau. J. For. Res. 14, 229–235.
Lintunen, A., Paljakka, T., Jyske, T., Peltoniemi, M., Sterck, F., von Arx, G., et al. (2016). Osmolality and non-structural carbohydrate composition in the secondary phloem of trees across a latitudinal gradient in Europe. Front. Plant Sci. 7:726. doi: 10.3389/fpls.2016.00726
Liu, C., Jin, G. Z., and Liu, Z. Z. (2021). Importance of organ age in driving intraspecific trait variation and coordination for three evergreen coniferous species. Ecol. Indic. 121:107099.
Liu, H. Y., Shangguan, H. L., Zhou, M., Airebule, P., Zhao, P. W., He, W. Q., et al. (2019). Differentiated responses of nonstructural carbohydrate allocation to climatic dryness and drought events in the Inner Asian arid timberline. Agric. For. Meteorol. 271, 355–361.
Liu, Z. L., Hikosaka, K., Li, F. R., and Jin, G. Z. (2020). Variations in leaf economics spectrum traits for an evergreen coniferous species: Tree size dominates over environment factors. Funct. Ecol. 34, 458–467.
Liu, Z. L., Jiang, F., Li, F. R., and Jin, G. Z. (2019). Coordination of intra and inter-species leaf traits according to leaf phenology and plant age for three temperate broadleaf species with different shade tolerances. For. Ecol. Manag. 434, 63–75.
Masaki, T., Kitagawa, R., Nakashizuka, T., Shibata, M., and Tanaka, H. (2021). Interspecific variation in mortality and growth and changes in their relationship with size class in an old-growth temperate forest. Ecol. Evol. 11, 8869–8881. doi: 10.1002/ece3.7720
McCulloh, K., Sperry, J. S., Lachenbruch, B., Meinzer, F. C., Reich, P. B., and Voelker, S. (2010). Moving water well: Comparing hydraulic efficiency in twigs and trunks of coniferous, ring-porous, and diffuse-porous saplings from temperate and tropical forests. New Phytol. 186, 439–450. doi: 10.1111/j.1469-8137.2010.03181.x
Mediavilla, S., Herranz, M., Gonzalez-Zurdo, P., and Escudero, A. (2013). Ontogenetic transition in leaf traits: A new cost associated with the increase in leaf longevity. J. Plant Ecol. 7, 567–575.
Millard, P., Sommerkorn, M., and Grelet, G.-A. (2007). Environmental change and carbon limitation in trees: A biochemical, ecophysiological and ecosystem appraisal. New Phytol. 175, 11–28. doi: 10.1111/j.1469-8137.2007.02079.x
Myers, J. A., and Kitajima, K. (2007). Carbohydrate storage enhances seedling shade and stress tolerance in a neotropical forest. J. Ecol. 95, 383–395.
Nakaji, T., Fukami, M., Dokiya, Y., and Izuta, T. (2001). Effects of high nitrogen load on growth, photosynthesis and nutrient status of Cryptomeria japonica and Pinus densiflora seedlings. Trees 15, 453–461.
Niinemets, Ü. (1997). Distribution patterns of foliar carbon and nitrogen as affected by tree dimensions and relative light conditions in the canopy of Picea abies. Trees 11, 144–154. doi: 10.1007/PL00009663
Niinemets, Ü., Sparrow, A., and Cescatti, A. (2004). Light capture efficiency decreases with increasing tree age and size in the southern hemisphere gymnosperm Agathis australis. Trees 19, 177–190. doi: 10.1007/s00468-004-0379-y
Niklas, K. J., Cobb, E. D., Niinemets, Ü., Reich, P. B., Sellin, A., Shipley, B., et al. (2007). “Diminishing returns” in the scaling of functional leaf traits across and within species groups. Proc. Natl. Acad. Sci. U.S.A. 104, 8891–8896. doi: 10.1073/pnas.0701135104
Piispanen, R., and Saranpää, P. (2001). Variation of non-structural carbohydrates in silver birch (Betula pendula Roth) wood. Trees 15, 444–451. doi: 10.1007/s004680100125
Piper, F. I., and Paula, S. (2020). The role of nonstructural carbohydrates storage in forest resilience under climate change. Curr. For. Rep. 6, 1–13.
R Core Team (2021). R: A language and environment for statistical computing. Vienna: R Foundation for Statistical Computing.
Regier, N., Streb, S., Zeeman, S. C., and Frey, B. (2010). Seasonal changes in starch and sugar content of poplar (Populus deltoides x nigra cv. Dorskamp) and the impact of stem girdling on carbohydrate allocation to roots. Tree Physiol. 30, 979–987.
Sala, A., and Hoch, G. (2009). Height-related growth declines in ponderosa pine are not due to carbon limitation. Plant Cell Environ. 32, 22–30. doi: 10.1111/j.1365-3040.2008.01896.x
Schadel, C., Blochl, A., Richter, A., and Hoch, G. (2009). Short-term dynamics of nonstructural carbohydrates and hemicelluloses in young branches of temperate forest trees during bud break. Tree Physiol. 29, 901–911. doi: 10.1093/treephys/tpp034
Signori-Müller, C., Oliveira, R. S., Barros, F., Tavares, J. V., and Galbraith, D. (2021a). Non-structural carbohydrates mediate seasonal water stress across Amazon forests. Nat. Commun. 12:2310. doi: 10.1038/s41467-021-22378-8
Signori-Müller, C., Oliveira, R. S., Valentim Tavares, J., Carvalho Diniz, F., Gilpin, M., Barros, V. F., et al. (2021b). Variation of non-structural carbohydrates across the fast–slow continuum in Amazon forest canopy trees. Funct. Ecol. 36, 341–355.
Song, Z. P., Liu, Y. H., Su, H. X., and Hou, J. H. (2019). N-P utilization of Acer mono leaves at different life history stages across altitudinal gradients. Ecol. Evol. 10, 851–862. doi: 10.1002/ece3.5945
Spann, T. M., Beede, R. H., and DeJong, T. M. (2008). Seasonal carbohydrate storage and mobilization in bearing and non-bearing pistachio (Pistacia vera) trees. Tree Physiol. 28, 207–213.
Taneda, H., and Sperry, J. S. (2008). A case-study of water transport in co-occurring ring- versus diffuse-porous trees: Contrasts in water-status, conducting capacity, cavitation and vessel refilling. Tree Physiol. 28, 1641–1651. doi: 10.1093/treephys/28.11.1641
Thomas, S. C. (2010). Photosynthetic capacity peaks at intermediate size in temperate deciduous trees. Tree Physiol. 30, 555–573. doi: 10.1093/treephys/tpq005
Thomas, S. C., and Winner, W. E. (2002). Photosynthetic differences between saplings and adult trees: An integration of field results by meta-analysis. Tree Physiol. 22, 117–127. doi: 10.1093/treephys/22.2-3.117
Thomas, V. F. D., Braun, S., and Flückiger, W. (2005). Effects of simultaneous ozone exposure and nitrogen loads on carbohydrate concentrations, biomass, and growth of young spruce trees (Picea abies). Environ. Pollut. 137, 507–516. doi: 10.1016/j.envpol.2005.02.002
Wang, K., Lei, H., Xia, Y., and Yu, G. Q. (2017). Responses of non-structural carbohydrates of poplar seedlings to increased precipitation and nitrogen addition. Chin. J. Appl. Ecol. 28, 399–407. doi: 10.13287/j.1001-9332.201702.012
Wang, X., Luo, W. T., Yu, Q., Yan, C. F., Xu, Z. W., Li, M. H., et al. (2014). Effects of nutrient addition on nitrogen, phosphorus and non-structural carbohydrates concentrations in leaves of dominant plant species in a semiarid steppe. Chin. J. Ecol. 33, 1795–1802.
Wang, Z. G., and Wang, C. K. (2019). Mechanisms of carbon source-sink limitations to tree growth. Chin. J. Plant Ecol. 43, 1036–1047. doi: 10.17521/cjpe.2019.0104
Wardle, P. (1993). “Causes of alpine timberline: A review of the hypotheses,” in Forest development in cold climates, eds J. N. Alden, J. L. Mastrantonio, and S. Ødum (Berlin: Springer), 89–103. doi: 10.1007/s004420050540
Wong, B. L., Baggett, K. L., and Rye, A. H. (2003). Seasonal patterns of reserve and soluble carbohydrates in mature sugar maple (Acer saccharum). Can. J. Bot. 81, 780–788. doi: 10.1139/b03-079
Woodruff, D. R., and Meinzer, F. C. (2011). Water stress, shoot growth and storage of non-structural carbohydrates along a tree height gradient in a tall conifer. Plant Cell Environ. 34, 1920–1930. doi: 10.1111/j.1365-3040.2011.02388.x
Wright, S. J., Kitajima, K., Kraft, N. J. B., Reich, P. B., Wright, I. J., Bunker, D. E., et al. (2010). Functional traits and the growth–mortality trade-off in tropical trees. Ecology 91, 3664–3674.
Yadav, V. S., Yadav, S. S., Gupta, S. R., Meena, R. S., Lal, R., Sheoran, N. S., et al. (2022). Carbon sequestration potential and CO2 fluxes in a tropical forest ecosystem. Ecol. Eng. 176:106541. doi: 10.1016/j.ecoleng.2022.106541
Yan, C. F., Han, S. J., Zhou, Y. M., Wang, C. G., Dai, G. H., Xiao, W. F., et al. (2012). Needle-age related variability in nitrogen, mobile carbohydrates, and δ13C within Pinus koraiensis tree crowns. PLoS One 7:e35076. doi: 10.1371/journal.pone.0035076
Yan, L. N., Zhang, Z. Y., Jin, G. Z., and Liu, Z. L. (2022). Variations of leaf nonstructural carbohydrates in an evergreen coniferous species: Needle age and phenology dominate over life history. Ecol. Indic. 136:108685. doi: 10.1016/j.ecolind.2022.108685
Yang, H., Wu, Y., Zhang, C., Wu, W., Lyu, L., and Li, W. (2022). Comprehensive resistance evaluation of 15 blueberry cultivars under high soil pH stress based on growth phenotype and physiological traits. Front. Plant Sci. 13:1072621. doi: 10.3389/fpls.2022.1072621
Yuan, D. Y., Zhu, L. J., Cherubini, P., Li, Z. S., Zhang, Y. D., and Wang, X. C. (2021). Species-specific indication of 13 tree species growth on climate warming in temperate forest community of northeast China. Ecol. Indic. 133:108389.
Zhang, H. Y., Wang, C. K., and Wang, X. C. (2013). Comparison of concentrations of non-structural carbohydrates between new twigs and old branches for 12 temperate species. Acta Ecol. Sin. 33, 5675–5685.
Zhang, H. Y., Wang, C. K., and Wang, X. C. (2015). Within-crown variation in concentrations of non-structural carbohydrates of five temperate tree species. Acta Ecol. Sin. 35, 6496–6506.
Keywords: broad-leaved tree species, leaves and branches, non-structural carbohydrates, survival strategies, life history
Citation: Wang K, Jin G and Liu Z (2023) Dynamic variation of non-structural carbohydrates in branches and leaves of temperate broad-leaved tree species over a complete life history. Front. For. Glob. Change 6:1130604. doi: 10.3389/ffgc.2023.1130604
Received: 23 December 2022; Accepted: 20 February 2023;
Published: 06 March 2023.
Edited by:
Ling Zhang, Jiangxi Agricultural University, ChinaReviewed by:
Bangliang Deng, Nanchang Institute of Technology, ChinaKnut Asbjørn Solhaug, Norwegian University of Life Sciences, Norway
Copyright © 2023 Wang, Jin and Liu. This is an open-access article distributed under the terms of the Creative Commons Attribution License (CC BY). The use, distribution or reproduction in other forums is permitted, provided the original author(s) and the copyright owner(s) are credited and that the original publication in this journal is cited, in accordance with accepted academic practice. No use, distribution or reproduction is permitted which does not comply with these terms.
*Correspondence: Zhili Liu, liuzl2093@126.com