- 1MED–Mediterranean Institute for Agriculture, Environment and Development & CHANGE–Global Change and Sustainability Institute, Instituto de Investigação e Formação Avançada, Departamento de Engenharia Rural, Escola de Ciências e Tecnologia, Universidade de Évora, Évora, Portugal
- 2Department of Forestry Sciences and Landscape Architecture (CIFAP), University of Trás-os-Montes and Alto Douro, Vila Real, Portugal
- 3Forest Research Centre (CEF), School of Agriculture, University of Lisbon, Lisbon, Portugal
The renewal and sustainability of the forest stands are attained through regeneration. There are three basic methods to regenerate high forest stands: natural regeneration, direct seeding, and planting. This study reviews the factors affecting regeneration, the three methods of regeneration and the effect of stand structure on regeneration. Overall, regeneration success can be quite variable due to edaphic and climatic conditions, seed losses and/or seedling mortality. The silvicultural system is relevant to the choice of the regeneration method. Natural regeneration is used in selection and irregular shelterwood systems whereas direct seeding and planting are used in uniform shelterwood and clear-cut systems. In the former, stand stocking management is primordial to regeneration success while for the latter are species selection, seed predators and spontaneous vegetation control.
1. Introduction
Regeneration is pivotal in silviculture as it guarantees the renewal and sustainability of the forest stands and productions in managed stands (Boudru, 1989; Smith et al., 1997). It comprises a set of processes and phases that determine their success and the recruitment of seedlings/saplings to the main stand (Grossnickle, 2018; Bogdziewicz et al., 2020). The regeneration encompasses seed production (Vacchiano et al., 2018; Bogdziewicz et al., 2020), dispersal of seeds/fruits (Gómez et al., 2019), seed/fruit predation (Huth et al., 2017; Löf et al., 2019), seed germination and emergence (Löf et al., 2019), seedling survival (Vaz et al., 2019; Guignabert et al., 2020), and seedling establishment (Davis and Jonhson, 1987; Leonardsson et al., 2015). The aforementioned is interlinked with the species traits (Hupperts et al., 2020; Brüllhardt et al., 2022), stand structure and silvicultural system (Tinya et al., 2019; Guignabert et al., 2020), site (Pernot et al., 2019; Rosenvald, 2020), and biotic agents (Ibáñez and McCarthy-Neumann, 2016; Long et al., 2021).
Forest stands can be regenerated by two methods: natural or artificial regeneration. The latter can be further divided into direct seeding and plantation (Smith et al., 1997). The stand structure of the main stand and its influence on regeneration and recruitment has been analyzed. The overall goal of the studies was to understand regeneration patterns as well as the development of methods, techniques and tools to improve the success of the renewal of the stands. Many studies have analyzed regeneration. Yet, to the authors’ best knowledge no study revised the effect of management and disturbances on the forest stands’ regeneration. The aims of this study are to analyze seed origin regeneration of managed forest stands and its success. This review is divided into the following sections: factors affecting regeneration (Section “2. Factors affecting regeneration”), regeneration and silvicultural systems (Section “3. Regeneration and silvicultural systems”), stand renewal with natural regeneration (Section “4. Stand renewal with natural regeneration”) and stand renewal with artificial regeneration, including direct seeding and plantation (Section “5. Stand renewal with artificial regeneration”).
For this review a search of publications was made on online knowledge library (b-on, which provides access to most publishers, including those in web of science and science direct). The following keywords were used: natural regeneration, direct seeding, plantation, clear-cut system, shelterwood system, selection system, and disturbance. The results were restricted to high forest regime from 1980 onward. The number of resulting papers publications was 5,322, from which were selected the ones that had information about the regeneration method, silvicultural system, composition, structure and/or disturbances, resulting in 118 papers to which were added 7 textbooks of silviculture. Some of the publications were used to characterize the regeneration (circa 85) and others provide examples (circa 40).
2. Factors affecting regeneration
Regeneration encompasses a set of processes from flowering to seedling establishment and recruitment to the main stand (Figure 1). Seed production is influenced by flowering and fruiting, which are related to tree resources, pollen, genetics, hormones and tree social position in the stand (Vacchiano et al., 2018; Bogdziewicz et al., 2020). The strategy of allocation of resources by the trees is first to grow and second to reproduction. This results in the seed production interannual variability, which seems to be linked to the resource availability per tree. The higher the available resources the higher the flowering and the fruit yield (Bogdziewicz et al., 2020). Frequently good seed production years are followed by low or very low production ones. This can be explained, at least partially, by resource depletion, as reproduction consumes large amounts of resources. Trees need to store resources for a shorter or longer time before high fruit seed occurs again (Bogdziewicz et al., 2020; Garcia-Barreda et al., 2021). Pollen quantity can be constrained by several factors, including phenological synchrony, precipitation, temperature, light, wind, and drought, which may reduce fruit production (Misson et al., 2011; Koenig et al., 2015). Inversely, the regulation by hormones and genetics may induce the bud flowers, reducing the influence of resource allocation and the pollen quantity in seed yield (Bogdziewicz et al., 2020). Additionally, genetics are related to fruit development. While for some species fruit maturation is annual or biannual (e.g., Quercus spp.) for others takes three (e.g., Pinus pinaster) or four (e.g., Pinus pinea) years. Tree social position in the canopy affects crown dimension and light availability, which influences the potential number of flowers/fruits. Trees in the upper canopy layers with large crowns tend to have higher seed yields than those in the middle and lower layers (Kremer and Bauhus, 2020). Flowering and fruiting occur mainly in the outer crown. The larger the crown area and/or surface, potentially the higher the fruit/seed yield (Gonçalves and Pommerening, 2012; Gonçalves et al., 2017). Frequently, regeneration is linked to years with large amounts of seed/fruit (masting). This is related to the allocation of resources to reproduction and synchrony in the seed production of many trees in the stand (Vacchiano et al., 2018; Bogdziewicz et al., 2020). The frequency of masting differs among species, from an annual to a decade periodicity (Mencuccini et al., 1995; Owens and Fernando, 2007; Parker et al., 2013).
Depending on the regeneration method seed can be collected (artificial regeneration) or not (natural regeneration). The collected seeds used for artificial regeneration can be used directly (direct seeding) or to produce plants in the nursery (plantation). Different methods are used to collect and store seeds/fruits. The methods depend on the seed/fruit characteristics (for details see Boudru, 1992; Smith et al., 1997).
Seed/fruit production can also be affected by other factors. The most common are losses by fungi and invertebrates (e.g., arachnids, flies), predation (e.g., ants, rodents, birds), and/or grazing (e.g., for Quercus spp., acorns grazed by sheep, goats or cattle). Losses by insects and fauna either reduce the amount of available seed or their germination capacity (Branco et al., 2002; Gea-Izquierdo et al., 2006; Lucas-Borja et al., 2010). Weather can affect seed yield. Frost, drought, high temperatures and low precipitation, potentially reduce seed yield. This is related to the reduction of flower pollination, e.g., rain during pollination season can reduce pollen availability; and damages to flowers/fruits, e.g., frost, low temperatures, and drought, that can result in fruit abortion (Pulido and Díaz, 2005; Bogdziewicz et al., 2020).
In natural regeneration, after maturation seed dispersal occurs. The dispersal of seeds/fruits is dependent on their traits, predation and weather. The primordial seed traits are form and weight. Light seeds tend to have longer dispersion distances, as long as wind (direction and intensity) conditions are favorable (Gómez, 2003; Calama et al., 2017). For example, seeds of Pinus nigra, Pinus sylvestris, Pinus pinaster, Pinus halepensis, and Abies alba are light and wind-dispersed up to 60–150 m, depending on the species (Calama et al., 2017; Huth et al., 2022). Heavy seeds (dispersed by gravity) have shorter dispersal distances. For example, up to 10 m for Pinus pinea and Quercus ilex (Gómez, 2003; Ganatsas and Thanasis, 2010). Fauna can act as predators or disseminators. Seeds/fruits are a food source for a large variety of fauna, from insects to birds and mammals. Fauna, depending on its density, consumes larger or smaller amounts of seeds/fruits. Some seeds/fruits are partially digested and keep their germination capacity. Others are stored (many buried in the soil) and not consumed. These two factors contribute to the dispersal of seeds/fruits, especially important for heavy seeds. Furthermore, seeds can be transported on the animals’ fur and feathers (especially the small and light ones), which may increase the distance of dispersal and also influence their spatial distribution (Gómez et al., 2019).
The effects of seed predation are stronger in low-production years than in masting years (Lucas-Borja et al., 2012). In low-production years a larger proportion of seeds is used as a food source, whereas in masting years satiation is reached for a much smaller percentage of the seed yield (Löf et al., 2019). Animal population density control and/or protection of regeneration may potentially increase the success of regeneration (Dey et al., 2019; Oliet et al., 2019; Vaz et al., 2019; Muñoz-Rengifo et al., 2020; Bolibok et al., 2021; Borderieux et al., 2021). For some species (e.g., for most Pinus spp.) seed availability can be affected by serotinity (Fernandes and Rigolot, 2007). For most Pinus spp. cones do not open spontaneously after maturation. They open after being exposed to heat (high temperatures or fire). Seed release is closely linked to fire, e.g., Pinus pinaster (Ribeiro et al., 2022) or high temperatures in the summer (Wyse et al., 2019).
Seed germination is dependent on species traits, predation, seed banks, seed germination rate, seedbed conditions and site. Species traits are related to seed dormancy. Some seeds have no dormancy, germinating shortly after ripening, as long as the weather is favorable (e.g., Quercus spp., Castanea sativa, and Pinus pinaster). Other species may have different types of dormancy, which has to be broken before germination occurs (e.g., Eucalyptus spp., Acacia spp.) (Florence, 1996; Löf et al., 2019). Seed predation can also occur after ripening and its effects are similar to those prior to ripening. Site is determinant of germination. Seed banks (i.e., amount of seeds stored in the trees and soil, not germinated) can store seeds for shorter or longer periods (a few years to several decades), depending on the seed traits, site and stand conditions, and predation. Yet, in time seeds lose their germination capacity (Tiebel et al., 2018).
The seed germination rate is determined by genetics and seed storage. If the seeds are collected and stored according to their traits, it is possible to preserve their germination capacity. When mature seeds fall on the forest floor, conditions may not ideal for their conservation. Thus, germination shortly after ripening for seeds without dormancy, prevents the reduction of the germination rates. Seeds with dormancy can be stored on the forest floor and germinate after dormancy is broken without losing germination ability. The site is primordial to regeneration, namely a suitable seedbed (i.e., seeds should be immersed in the soil and soil water content, precipitation and temperature are appropriated).
In the northern hemisphere seed maturation, germination and emergence occurs for most species in autumn, when water is available and temperature is mild; or in the next spring when the temperature has overcome the inferior threshold for germination. Seeding in autumn is preferred in climates with summer drought whereas in spring is preferred in cold climates (for details see Boudru, 1992; Smith et al., 1997). The autumn germination and emergence enables the seedlings to develop the root system, allowing them to mitigate water stress in the summer and dry springs (Löf et al., 2019).
Seedling survival is determined by browsing, site, soil fungi, and plantation stress (in plantations). Seedling browsing can reduce leaf area and change seedling growth patterns. The former result in the reduction of photosynthesis and seedlings use seed reserves to survive. The seedlings of seeds with large reserves (e.g., Quercus spp.) can cope better with the reduction of leaf area than those with small reserves (e.g., Pinus spp.) (Löf et al., 2019; Vaz et al., 2019; Guignabert et al., 2020). Browsing may also affect seedling habit. Animals tend to browse mainly new branches and leaves. This originates in the development of one or several buds and seedlings may develop a shrubby habit (Vaz et al., 2019; Muñoz-Rengifo et al., 2020), especially for the species with weak epinastic control (Oliver and Larson, 1996; Cline, 1997). Site conditions, in particular weather, determine seedling survival, e.g., for Pinus nigra soil mobilization promoted germination and seedling density (Lucas-Borja et al., 2011).
Frosts, droughts and fires can result in the partial or total loss of the seedlings’ aerial part. Some species, e.g., Quercus spp., are well adapted to these disturbances (Pausas et al., 2009; Löf et al., 2019) and can sprout after frost events when temperature increases (frequently in the spring) whereas after drought or fire events it occurs with the increase of water availability in the soil (frequently in autumn after the first rainfalls). The sprouting results from the development of the root collar buds (Löf et al., 2019). Other species like Pinus spp., Abies spp., and Picea spp., are not able to sprout, not recovering their aerial part and usually die (Boudru, 1989; Smith et al., 1997). Fungal community in the soil can have positive, neutral and negative effects on seedling (Dalling et al., 2011; Ibáñez and McCarthy-Neumann, 2016; Long et al., 2021). This is related to seed traits, such as physical and chemical defenses and dormancy (Dalling et al., 2011). Moreover, while some fungi result in pathogenic interaction with the seedlings other fungi result in mutualistic interactions (Dalling et al., 2011; Long et al., 2021). Plantation stress is defined as the period between plantation and seedling establishment. This is the most critical phase in plantations, where most mortality occurs. It is related to the site and plant hardening (Grossnickle, 2018).
Seedling establishment depends on environmental (e.g., precipitation, temperature, and light), biological (e.g., species traits, competition, and browsing) and silvicultural (e.g., stand structure and silvicultural systems) factors (Davis and Jonhson, 1987; Smith et al., 1997; Leonardsson et al., 2015) as well as physiography and site quality (Ribeiro et al., 2022). Shade tolerance and main stand crown cover may constrain seedling development. The more shade-intolerant a specie is the lower should be the crown cover for a successful regeneration (Shelton and Cain, 2000). For these species, clear-cut and uniform shelterwood systems seem better suited (Bellefleur and LaRocque, 1983a,b; Lüpke, 1988). For shade-tolerant species irregular shelterwood and selection systems are preferred (Schütz, 1997; O’Hara, 2014). Tree age influences seed production, emergency, and growth of the seedlings. A study denoted that older trees of Quercus faginea produce larger acorns and seedlings while seeds from young trees have faster and higher germination rates and emergence. Thus, variability in structure may have a positive effect on the forest system’s sustainability (Alonso-Crespo et al., 2020). Spontaneous vegetation can have competitive interactions with regeneration, by using the available growing space and, due to its higher growth rates, outcompete regeneration; and facilitation interactions by the shelter provided, mainly shade and mitigating browsing (Smit et al., 2008; Caldeira et al., 2014; Leonardsson et al., 2015; Kolo et al., 2017; Muñoz-Rengifo et al., 2020). For example, shrub cover can promote the natural regeneration of Pinus pinaster and Quercus suber by decreasing temperature and solar radiation (Smit et al., 2008; Rodríguez-García et al., 2009).
3. Regeneration and silvicultural systems
Silvicultural systems can be grouped into three broad classes (Assmann, 1970; Matthews, 1989; Smith et al., 1997): clear-cut, shelterwood, and selection (Plenter).
In the clear-cut system when trees reach the end of the production cycle (rotation) all trees are cut. The regeneration is typically artificial by direct seeding or planting. Yet, for some species, well adapted and with frequent seed production, natural regeneration can be used (Huss, 2004). The choice of species is more restricted. Species shade-tolerant, sensitive to frosts and/or drought, and sensitive to competition with spontaneous vegetation can seldom be used. Natural regeneration is predominantly used with pioneer and shade-intolerant species (Boudru, 1989; Smith et al., 1997). Several types of clear-cut systems were developed to overcome its disadvantages, namely alternate strips, progressive strips, by patches and with seed trees. The subtypes differ from clear-cut in what concerns the cut area and regeneration type. Though in the subtypes all the area is cut within a short timeframe, with two or more cuts, these subtypes can mitigate the effects of cutting all the area. Both artificial and natural regeneration can be used. For a clear-cut system the primordial regeneration type is the artificial one (Boudru, 1989; Smith et al., 1997; Miina and Saksa, 2008).
The shelterwood system is characterized by at least four cutting interventions, and natural regeneration is the main renewal method. The cuts follow a sequence starting with the preparatory cut (if necessary), followed by seed cut, secondary cuts (in variable number) and final cut. In the latter the last trees of the main stand are removed, when regeneration does not need shelter. This system can be divided into two broad classes: uniform and irregular shelterwood. The former has been further divided, according to the spatial distribution of the cuts, in uniform shelterwood by strips, by groups or patches and by strips and patches (Assmann, 1970; Smith et al., 1997). The choice of the shelterwood variant is related to the structure of the stand. For even-aged stands, uniform shelterwood systems are better suited, but care should be taken so that regeneration develops in only one cohort. When the goal is to maintain or promote uneven-aged stands the irregular shelterwood systems are preferred. They enable to maintain or promote the heterogeneity of the structure (Smith et al., 1997; Raymond et al., 2009; O’Hara, 2014).
Clear-cut with seed tree and shelterwood systems are based on natural regeneration where the trees of the main stand provide seed and shelter. Their main differences are related to the number of seed trees per unit area (Hyppönen et al., 2005) and the number of cuts, both larger in the latter than in the former. Clear-cut with seed tree systems are based on one or a few years of seed production, and seed availability may be a limiting factor (Miina and Saksa, 2008). Shelterwood systems are based on several years of seed production which guarantees a higher number of seedlings (Béland et al., 2000; Dovčiak et al., 2003).
The selection system is characterized by more than one cohort and by the simultaneous removal of trees when they reach a target diameter and in tending. The main renewal method is natural regeneration. The selection system has been further divided in two subtypes according to the spatial distribution of the cuts, namely single tree and group (Schütz, 1997; Smith et al., 1997; O’Hara, 2014).
4. Stand renewal with natural regeneration
Natural regeneration can be defined as the establishment of trees from seeds produced and germinated in situ without or with human intervention (Assmann, 1970; Harmer, 1994a; Smith et al., 1997). Their advantages are linked to the seed produced in situ and a priori well-adapted to the site; regeneration with several years of seed production; different species regenerate in different niches, enabling mixed stands renewal or the conversion of pure in mixed stands if seed for all the desired species is available; and low costs (Davis and Jonhson, 1987; Löf et al., 2019; Petersson et al., 2019; Pommerening and Grabarnik, 2019). Its disadvantages are associated to stand structure, in particular trees with large crowns to produce a sufficient amount of seeds and the presence of the species to be regenerated; seed production variability; seedling/sapling losses; competition between regeneration and the main stand and/or spontaneous vegetation; suitability of seedbed (requiring sometimes soil mobilization); and weather conditions (e.g., wind, frost, and drought damages) (Harmer, 1994a,b, 1995; Klopčič et al., 2015; Tinya et al., 2019; Hupperts et al., 2020; Kremer and Bauhus, 2020; Scherrer et al., 2021).
Stand renewal by natural regeneration is primordially used with selection and shelterwood systems, thought to a lesser extent is also used with clear-cut systems (Figure 2). It is used in pure, mixed, even-aged and uneven-aged stands.
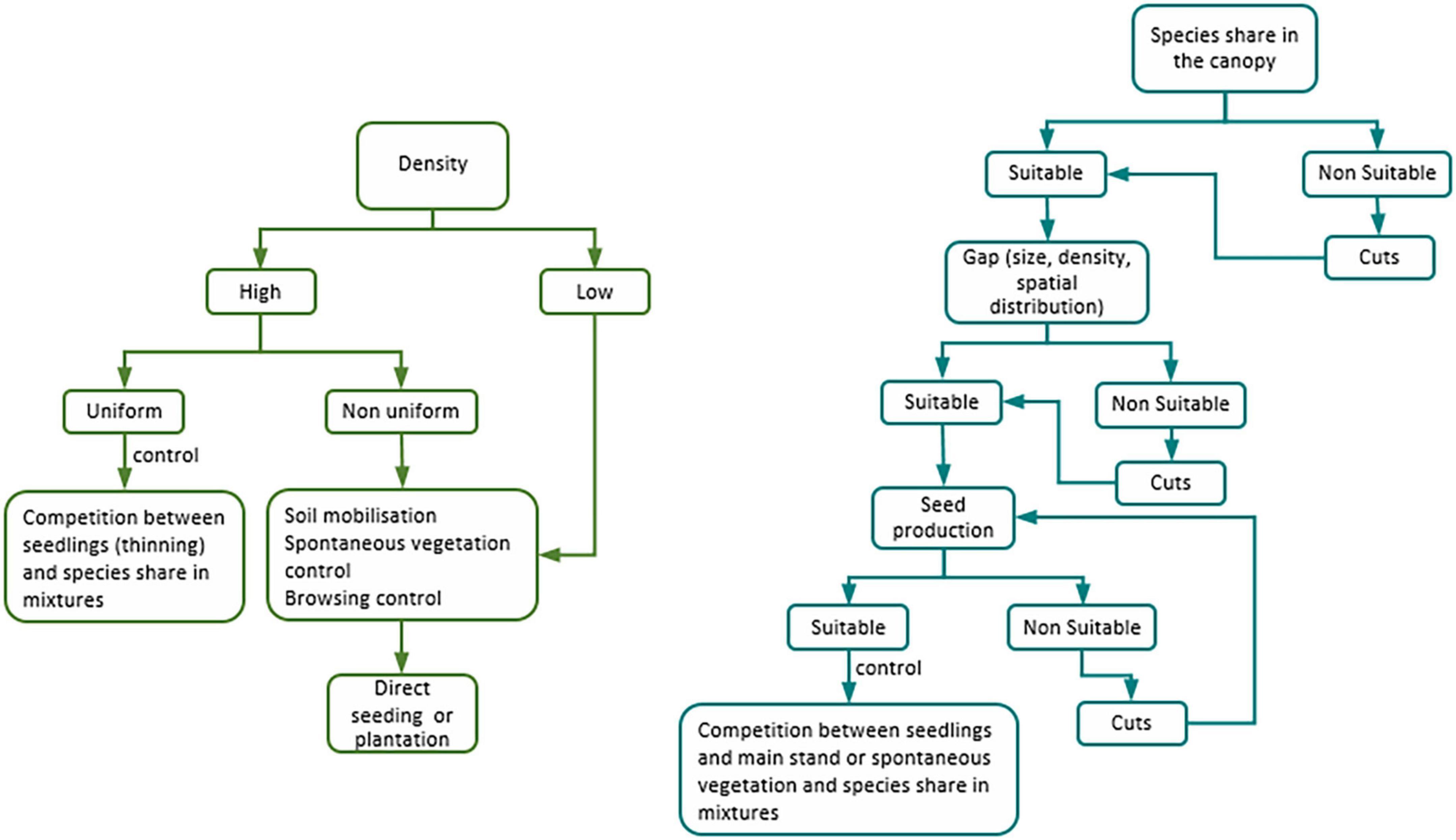
Figure 2. Factors influencing natural regeneration in clear-cut/uniform shelterwood (left) and irregular shelterwood and selection (right) systems.
After a fire, in even-aged Eucalyptus regnans stands, the natural regeneration density was two-fold higher than after clear-cutting. Yet, the latter had a uniform distribution pattern whereas the former had a less uniform spatial arrangement and was in patches. Thus, in time, might promote structure heterogeneity. A concern with clear-cutting systems in Australia is the maintenance of habitats for flora and fauna. This can be achieved by retaining habitat trees and increasing stand structure heterogeneity to create (or maintain) different niches and habitats (Trouvé et al., 2021). In the Mediterranean region, Pinus pinaster stands, 3 years after a fire had from 17,000 to more than 100,000 seedlings/ha (Enes et al., 2019). Its development was mainly constrained by interspecific competition due to limited resources and ruled by self-thinning (Sales-Luis and Fonseca, 2004; Enes et al., 2019).
Regeneration density and height in even-aged conifer stands (e.g., Pinus sylvestris) under uniform and group shelterwood systems increased with the decrease of the main stand stocking. The poorer the site the lower should be the main stand stocking to decrease the aerial and the root competition between the main stand and the regeneration (Barbeito et al., 2011; Rosenvald, 2020; Huth et al., 2022). Regeneration distribution patterns tended to be homogenous in uniform shelterwood systems and clustered in group shelterwood ones. The distribution patterns were related to the spatial arrangement of the main stand trees (Barbeito et al., 2011). Shelterwood systems tended to increase seedlings’ age variability with the increase of the main stand stocking. This was likely related to the longer regeneration periods, the enhanced heterogeneity of the structure of the stand and the growth patterns of the recruited individuals (Huth et al., 2022). Soil mobilization increased regeneration density and promoted its growth due to the removal of spontaneous vegetation and/or organic layers (Barbeito et al., 2011; Huth et al., 2022), more noticeable in sites of poor quality than in good ones (Rosenvald, 2020). Soil broadcast mobilization resulted in the uniform distribution of the regeneration while patch mobilization tended to a clustered regeneration (Huth et al., 2022).
Crown cover and browsing are two determinant factors to regeneration success. In mature mixed even-aged stands of Picea abies, Abies alba, Fagus sylvatica, and Acer pseudoplatanus under shelterwood and clear-cut systems, regeneration density increased with the decrease of the crown cover. This was explained by the amount of water reaching the soil. After a cut, there was a short-lasting seed yield reduction. The main stand crown closure resulted in a sharp decrease in shade-intolerant species density. In the clear-cut system, seed production was scarce and competition between seedlings and spontaneous vegetation was strong. Furthermore, certain species needed higher seed quantities (Picea abies) than others (Abies alba, Fagus sylvatica, and Acer pseudoplatanus) to attain the same seedling density (Burschel et al., 1992). In pure and mixed stands of Fagus sylvatica and other broadleaved and conifer species, regeneration density decreased with the increase of crown cover, basal area and Fagus sylvatica in the upper canopy. Regeneration density showed a large variability from null to more than 10,000 seedlings/ha and was mostly of Fagus sylvatica. The density of other species either intolerant or semi-tolerant to shade (e.g., Picea abies, Abies alba, Carpinus betulus, and Acer pseudoplatanus) was lower (Žemaitis et al., 2019). Similarly, Quercus petraea regeneration density in pure and mixed stands was directly related to the species share in the canopy (the higher the share, the higher the regeneration density). For mixed stands, none of the eighteen secondary species affected negatively the regeneration of Quercus petraea. Several conifers (Pinus nigra, Pinus sylvestris, Pinus pinaster, and Pseudotsuga menziesii) and one broadleaved (Castanea sativa) species affected it positively. This was related to the direct sunlight reaching the understory, and the Quercus petraea’s competitive advantages over light-demanding conifers (Borderieux et al., 2021). The increase of browsing and crown cover in mixed stands of Quercus robur and Quercus petraea resulted in a sharp decrease in regeneration. This was due to the increase in the stand’s crown cover and deer population. The regeneration of the oaks was related to disturbances that increased light and better-suited environments for oak seedlings’ development (Petersson et al., 2019). Seedlings’ growth was promoted by reducing browsing pressure (Petersson et al., 2019; Knapp et al., 2021) and by species traits, e.g., tolerance to browsing of Fraxinus Americana and low palatability of Ostrya virginiana (Knapp et al., 2021).
The regeneration (density, composition, and structure) of uneven-aged (pure or mixed) stands was dependent on the dimension and spatial distribution of the canopy gaps under shelterwood and selection systems. The heterogeneity of the stand structure was promoted by cuts that resulted in canopy gaps. These gaps were characterized by size (small vs. large), dimension (equal vs. different sizes) and spatial arrangement (regular vs. irregular). The aforementioned was related to the species regeneration (Kern et al., 2017).
Other factors that correlate with regeneration density and composition are species traits, seed source and yield patterns, microsite conditions, competition (with the main stand and/or spontaneous vegetation), soil mobilization and browsing. For irregular shelterwood or selection systems, no relation was found between the regeneration density of shade-tolerant species and gap size (Knapp et al., 2019, 2021; Hupperts et al., 2020; VanderMolen et al., 2021). Inversely, semi-tolerant and intolerant species regeneration was higher in larger gaps (Schütz, 2006; Hessenmöller et al., 2018; Knapp et al., 2019, 2021; Brüllhardt et al., 2022). Shade-tolerant species were able to grow in small gaps where the light was low whereas less shade-tolerant species needed larger gaps that enabled light to reach the understory layers (Schütz, 2006; Hessenmöller et al., 2018; Knapp et al., 2019, 2021; Reuling et al., 2019; VanderMolen et al., 2021; Brüllhardt et al., 2022). Large gaps had higher composition diversity, as more species found suitable conditions to regenerate (Schütz, 2006; Klopčič et al., 2015; Tinya et al., 2019; Hupperts et al., 2020). Maintaining trees in the main stand tended to increase regeneration density due to seed availability increase (Hupperts et al., 2020; VanderMolen et al., 2021) and diversity if more than one specie was retained (Knapp et al., 2019). Yet, a lack of seed due to low or irregular seed production might reduce the regeneration density of all or some species (Knapp et al., 2021).
The natural regeneration spatial distribution pattern is dependent on microsite conditions, e.g., light, environment, soil water content, litter layer and fungi in the soil. The regeneration distribution patterns tended to be random when the conditions in the gaps were well-suited for a certain species (VanderMolen et al., 2021) and clustered when species traits required specific niches (Hupperts et al., 2020), e.g., direct sunlight (Lundqvist et al., 2019), soil water content (Hupperts et al., 2020), and litter depth (Knapp et al., 2021).
In irregular shelterwood and selection systems, the presence of shrubs reduced the density and the development of seedlings/saplings (cf. 2.). Shrub species used available growing space and frequently outcompeted the seedlings/saplings (Knapp et al., 2019; Reuling et al., 2019). Additionally, the competition between the main stand and the regeneration was dependent on the availability of growing space, in particular, light. The individuals of shade-tolerant species developed under low light environments (Klopčič et al., 2015; Petrovska et al., 2022) while shade-intolerant ones required lower stocking (Schütz, 2006; Lundqvist et al., 2019; Hupperts et al., 2020). For example, for the regeneration of pure uneven-aged stands of Pinus sylvestris a stocking ≤13 m2ha–1 was recommended (Lundqvist et al., 2019) whereas for light-demanding species in mixed uneven-aged stands was 21–25 m2ha–1 (Schütz, 2006; Hessenmöller et al., 2018; Tinya et al., 2019; Brüllhardt et al., 2022). Group selection systems are better suited to regenerate shade-intolerant species than single-tree ones (Klopčič et al., 2015; Tinya et al., 2019).
Soil mobilization increased the regeneration density by removing the competing vegetation and reducing the litter layer. In an irregular shelterwood system, Betula alleghaniensis density was higher in the gaps with site mobilization and with the removal of advanced regeneration (Reuling et al., 2019). Likewise, soil treatments promoted Betula alleghaniensis regeneration, as it was more affected by litter depth than Acer saccharum (Hupperts et al., 2020).
Regeneration development was dynamic over time, and species proportions and growth varied. This may influence the future stand structure (Knapp et al., 2019). A trend was found toward the reduction of the number of species and growth over time. The regeneration individuals from the main stand species were near the edge of the gaps. Thus, small gaps tended to maintain composition. To change composition stronger disturbances (silvicultural interventions) were needed (Scherrer et al., 2021). In mixed uneven-aged stands, in time, there was a trend toward a steep decrease in regeneration density, especially for the shade-intolerant species. This might affect the future composition, in particular of the shade-intolerant species (Klopčič et al., 2015). Irregular shelterwood and selection systems maintained or promoted the stand heterogeneity, but the latter was better suited due to the different gradients of light and niches (Tinya et al., 2019).
5. Stand renewal with artificial regeneration
5.1. Direct seeding
Direct seeding has been used for a long time, with several forest species (Huth et al., 2017). It encompasses a set of steps from the promotion of flowering/fruiting (cf. 2) to seed/fruit collection and storage (Boudru, 1989). It is a regeneration method in which the seeds are sown directly in the soil. Seeding can be done in the entire area or strips or patches. Direct seeding may be done manually, mechanically, or aerially (Grossnickle and Ivetić, 2017; Huth et al., 2017). The use of unmanned aerial vehicles (UAVs) has been explored and promoted in specific circumstances (e.g., Mohan et al., 2021). Direct seeding successful regeneration includes a set of factors (Figure 3). The selection of tree species suited to the site and seed quality are of primordial importance, to assure that germination and establishment rates are high. The more suitable season for seeding is the one that promotes its germination and growth when there is water availability in the soil and temperatures are mild. Seeding in the autumn, in temperate regions, has advantages as no seed storage is needed and the seedbed has suitable conditions. The disadvantages are related to the short time to prepare the seedbed and seeding. Seeding in the spring advantages are the higher germination rates and the disadvantages are the need to store the seeds, and adverse weather conditions, such as snow, frost and drought (Grossnickle and Ivetić, 2017; Huth et al., 2017).
The larger the number of seeds used, potentially, the higher the density of seedlings. Yet, other factors have to be considered: seed size (the larger the seed, potentially, the higher the success); and site microclimate (suitable conditions of water, temperature, and shade). Seedbed suitability through soil mobilization is short lasting but enables the seeds to be in contact with mineral soil and reduce competition with spontaneous vegetation, promoting the seedlings’ germination, survival, and growth (Huth et al., 2017). Seed covering depth depends on the seed dimensions and tends toward lower predation rates, though not valid for all predators. Germination rates vary for both buried and unburied seeds. Seeds unburied and protected by spontaneous vegetation may have success rates similar to those buried (Boudru, 1989; Grossnickle and Ivetić, 2017). Site conditions are influenced by vegetation (trees and spontaneous vegetation) which in turn constrain microclimate, including soil water availability and temperature, that influence positively germination and establishment rates (Grossnickle and Ivetić, 2017). Seedling survival and growth are dependent on a balance between the pathogenic and mutualistic fungi, and the latter mitigate the effects of the former. The effects of the fungi are dependent on the density of the adult trees in the stand (Long et al., 2021). The colonization by mycorrhiza fungi does not seem to be correlated with the size of the seed or the tolerance to shade of the species, but is dependent on the resources in the soil. The growth of the seedlings under high light levels increases with the increase of fungi colonization whereas under low light levels the opposite effect is observed. Additionally, light levels may originate a shift from positive to neutral or negative effects of the fungi colonization on the growth of seedlings (Ibáñez and McCarthy-Neumann, 2016). Stand structure and overstorey species are linked to the success of direct seeding. For example, shade-tolerant species regeneration is promoted by the main stand that provides shelter to seedlings/saplings and enhances their recruitment. But overstorey should provide simultaneously enough light to promote the growth rates, so seedlings are not maintained in the lower storeys in latency. Seedbed preparation is needed when the litter layer is thick and does not enable seeds to reach the mineral soil and to control spontaneous vegetation (Huth et al., 2017) either mechanically or chemically (Löf et al., 2019). Competition for the available growing space between seedlings and the main stand and/or spontaneous vegetation plays a key role in seedlings/saplings’ establishment and growth. It has a twofold effect, i.e., it can protect seedlings from extreme weather conditions (e.g., drought and frost) and browsing, and can compete for the available growing space. Silvicultural practices should aim for low competition between seedlings/saplings and other vegetation (Huth et al., 2017).
Predation of seeds and seedling browsing are two other major constraints to direct seeding success. The effects of the latter are those described for natural regeneration (cf. 2., 3.). The former varies per species and influences seedling density. The seeds of some species are vulnerable to predation (e.g., acorns, chestnuts, and beech nuts) whereas for other species essential oils and resins (e.g., Abies spp. seeds) act as a protection against biotic agents (Huth et al., 2017). Several alternatives have been used to minimize predation: control of the seed predators’ populations, balancing the rodents with their predators; involving the seeds in chemicals that repel seed predators (may have the disadvantage of reducing germination rates and seedlings establishment); using physical measures, either per area (fencing) or individually (seed and tree shelters, that might delay frost hardening); selection of sites with low seed predators; and seedling seasons and sowing depth that minimize the predation damages. However, the deeper the seed is sown the longer it takes to emerge and develop. Furthermore, seedlings may be damaged by herbicides. Inversely, seedling growth is promoted by site preparation (Löf et al., 2019).
Several advantages of direct seeding when compared to plantations can be pointed out: density is higher in direct seeding; the root systems develop naturally, particularly important for the species that develop long tap roots in the early stages of development (promoting the mitigation of drought and increasing seedling/sapling stability); lower risk of spread of rood diseases; the impact of seeding in the soil is lower; seeding can be done manually, mechanically or aerially; broadcast, strip, and/or patch seeding can be done, taking into account the advanced regeneration, terrain and/or stand variability; stand diversity can be promoted by seeding a mixture of species or seeding different species in different patches; regeneration of large areas in short timeframes is possible; there is no plantation shock; and the costs are lower, linked to the reduction of costs of seedling production in nurseries, transport and labor (Grossnickle and Ivetić, 2017; Huth et al., 2017; Löf et al., 2019; Pretzsch, 2020; Villalobos et al., 2020). The disadvantages are related to the amount of seed of good quality available and its price; the variability of germination rates; and the establishment rates, frequently lower than in plantations, due to weather, suitability of the seedbed, seed predation, and competition with spontaneous vegetation and/or trees of the main stand. The growth rates of seeded seedlings are lower than the planted ones, especially during establishment. After establishment, direct seeding seedlings have similar or higher growth rates than planted ones. It is more difficult to control spontaneous vegetation due to the smaller size of the direct seeding seedlings when compared to planted ones (Grossnickle and Ivetić, 2017; Huth et al., 2017; Löf et al., 2019).
The major advantage of strip and patch seeding, when compared with broadcast seeding, is related to the smaller amount of seed needed (Grossnickle and Ivetić, 2017; Huth et al., 2017). Mechanical seeding is restricted by the site (e.g., slope and soil, especially thin, and rocky soils), by a high density of the stands (particularly those with irregular distribution patterns), and when trees have shallow root systems or woody debris is large. Manual or aerial seeding can be used when mechanical is impossible. When regulations, laws, and certification schemes exclude the use of heavy machinery, lighter machinery, or devices with animal traction can be used. These two approaches are considered more efficient than manual seeding because are flexible in spatial terms and have better quality. The costs of manual, lighter machinery or animal traction seeding are higher than those of heavy machinery (Huth et al., 2017).
The effects of direct and no (natural regeneration) seeding under clear-cut and uniform shelterwood systems in Pinus pinaster stands were studied for sites where, historically, regeneration was successful (two sites) and unsuccessful (two sites) (Guignabert et al., 2020). Seed quantity enabled to regenerate the stands, though with higher density for direct seeding. The germination rates were dependent on the depth of the litter/debris layer, and seed predation, storage and conservation in the soil until site conditions were suitable for germination. The latter was also linked to Pinus pinaster provenances, e.g., Atlantic provenance germination rates decreased under drought. Germination and survival were lower in clear-cutting than in a uniform shelterwood system, which was related to the seeds’ viability in the soil (1 year). The regeneration in the clear-cut system resulted from the seed of the year before harvest. Inversely, in the uniform shelterwood system was from multiple seed productions. Direct seeding in the clear-cut system resulted in germination rates four times higher than those without seeding. The seeds sown had higher germination capacity and less time in the soil prior to germination. In general, the shelterwood system resulted in higher germination and survival rates, though variability was high, and increased with the increase of the stand crown cover (between 10–40%). This was related to the more suitable microclimate, i.e., lower soil temperature, high soil water content and lower transpiration. The main cause of regeneration mortality was the summer drought, but it was mitigated in the shelterwood systems (Guignabert et al., 2020).
Acorn predation, germination and development of direct seeding seedlings were studied for Quercus robur with soil scarification in patches and repellent treatments to acorns, in two clear-cut stands (Villalobos et al., 2020). The acorns were predominantly predated by rodents (ungulates were excluded by fencing). Acorn predation was rather variable and increased in the areas with spontaneous vegetation when piles of slash were nearby. The repellent treatments did not affect predation. The predation rate was higher for large acorns than for small ones. The size of the acorn did not influence seedling density, but large acorns originated larger seedlings and higher growth (Villalobos et al., 2020).
The direct seeding of a mixture of Betula pubescens, Sorbus aucuparia, and Alnus glutinosa was used to evaluate the viability of the restoration of native mixed-species stands (Willoughby et al., 2019). The regeneration density was higher in the direct seeded areas that in those unsown. After 5 years the latter had no regeneration. The differences were due to the amount of seed and the chemical removal of spontaneous vegetation in direct seeding, which enhanced the germination and development of the seedlings. The regeneration density was higher for Betula pubescens and Sorbus aucuparia than for Alnus glutinosa, suggesting that for the latter the amount of seed was insufficient or there were too few suitable niches. The growth rates of Betula pubescens and Sorbus aucuparia were similar to planted seedlings. Fertilization at 5 years of age maintained the growth rates of the seedlings. The direct seeding of the mixture of species promoted structure variability, both horizontally, and vertically. It also included individuals of natural regeneration (Willoughby et al., 2019).
5.2. Plantation
The plantation is an artificial regeneration method where seedlings produced in nurseries are planted on the site (Smith et al., 1997). It has been used for production, protection, conservation, and ecosystem restoration resulting in even-aged pure or mixed (frequently of two species) stands (FAO, 2020). For plantations’ establishment success, a set of factors should be considered: seed quality (cf. 2.), seedling production, site preparation, seedling development, and post-planting (Figure 4).
Seedling production in nurseries can be broadly grouped into bare-root and container-seedlings. The bare-root seedlings grow for 1–2 years in a soil bed. After, they are transplanted to another soil bed at a lower density until they reach the desired development. Then are collected without soil and planted on the site. The container-seedlings, frequently produced in greenhouses, can be grown in rigid-walled containers which are removed before planting; or soft-walled containers and planted with the container as it is degradable. The bare-foot seedlings have larger dimensions and are produced under field conditions. Its root system is less developed but more extensive than that of container-seedlings, due to the restricted volume of the containers (Pernot et al., 2019).
The choice between bare-root or container-seedlings is related to the site. In sites with low water availability and/or thin soils, container-seedlings tend to have higher survival rates due to their greater resistance to water stress. Inversely, bare-foot seedlings are better suited for sites where competition with other vegetation is expected due to their large size (Pernot et al., 2019 and references therein), and in deep and cooler soils (Paiva et al., 2010). Container-seedlings have the advantage of maintaining root system architecture, protection against dissection and injuries until planting (Argillier et al., 1991). Its disadvantages are related to the cost (cf. Grossnickle and El-Kassaby, 2016). The container dimension does not affect the plant quality of some species (e.g., Pinus ponderosa) (Pinto et al., 2011). For others might constrain root system development (e.g., Quercus spp. that develop a strong pivotal root) (Paiva et al., 2010). Compared with direct seeding, container-seedlings show better environmental adaptability and stress resistance because of their protected root systems (Duan and Abduwali, 2021).
A complementary strategy in seedling production is the inoculation with ectomycorrhizal fungi (e.g., Argillier et al., 1991; Rudawska et al., 2017), which improves the physiology and growth of the seedlings (Griebeler et al., 2021). In the transportation of seedlings from nurseries to plantation sites care should be taken. Damages by wind and heat (Zhigunov et al., 2014) or mechanical (Stjernberg, 1996) should be avoided, because they may reduce the survival and the growth of seedlings, especially the bare-foot ones (Stjernberg, 1996; Zhigunov et al., 2014).
Silvicultural practices related to plantation are site preparation, planting time, seedling density, and pest and disease control (Masaba and Etemesi, 2021). Soil mobilization should be suited to the site, species traits and seedlings’ production type. For container-seedlings the soil clod should not be damaged (Paiva et al., 2010). Planting time should be coincident with suitable temperature and moisture levels (Masaba and Etemesi, 2021) to minimize plantation stress (Grossnickle, 2005). For example, in the Nordic countries, the plantation is recommended from May to early June. From summer to September there is a high risk of frost damage in autumn (Luoranen et al., 2018). One advantage of container versus bare-foot seedlings is that the former enables extending the plantation period in both the spring and winter seasons. The latter may be especially interesting for species less vulnerable to low temperatures (Zhigunov et al., 2014).
Plantation density depends on site conditions, species traits and management goals. For timber-oriented purposes, stands densities are frequently higher for good-quality sites and timber of smaller dimensions. For example, Zhigunov et al. (2014) referred to Picea abies 3,000–4,000 seedlings/ha for Russia and 1,800–2,000 seedlings/ha for southern Finland. Inversely, Mutke et al. (2012) for Pinus pinea mentioned 278 seedlings/ha for stands oriented for fruit production under annual drought. Planting can be done manually or mechanically. The latter is not frequently used due to its low cost-efficiency (Laine et al., 2016; Ersson et al., 2018). Besides, in plantations, a single seedling or a cluster of seedlings can be planted per hole (Duan and Abduwali, 2021). For multi-seedling planting, the design differs depending on the original growing space per seedling. The seedling plantation may follow a group or cluster-like spatial structure for plants spaced, e.g., about 1 to 2 m apart, or be closer to a nest-like structure when a high number of plants, e.g., 20–30 seedling per square meter is used (for a review on nest planting, see Saha et al. (2017), and the references cited there). Figure 5 shows regular patterns of a plantation in a square (a) and triangular (b) spacing and the nest design (c).
After plantation seedlings may undergo stress (plantation stress) when temperature and soil water content are less suitable for the development of the roots (for details see Grossnickle, 2005). It does not occur or is slight when soil and weather conditions promote the fast development of the root system. Inversely, it can last several years under harsh site conditions (i.e., the time needed for the seedling to develop the root system) and thus delaying the aerial part growth (Pernot et al., 2019). Good quality seedlings with an adequate balance between the aerial and root system are better suited. Moreover, suitable site preparation and fertilization at the plantation may improve the seedlings’ survival rates. Site conditions and seedlings’ quality are particularly important if stress is to fear, such as drought and competition with spontaneous vegetation (Grossnickle, 2018). At juvenile stages, growth is more dependent on soil nutrients, and fertilizer quantities are dependent on soil characteristics. Nitrogen and boron deficits are frequent, resulting in the seedlings’ growth delay (e.g., Zhigunov et al., 2014; Turner et al., 2021). Fertilization (at 3 years of age with nitrogen, phosphorous, and potassium) of plantations of Castanea sativa for timber attained higher height growth rate, diameter, and volume when compared with non-fertilized plantations. The promotion of the growth of the seedlings at early development stages by increasing survival rates and growth will be reflected in medium and long-term seedlings’ development (Ribeiro et al., 2019). The fertilization of a Picea abies plantation with boron on sites poor on this nutrient increased its availability. It improved seedling quality and growth and had longer-lasting effects on seedlings’ boron status (Riikonen et al., 2013). In a conifer plantation, seedling mortality increased with the decrease in intensity and frequency of the herbicide application (Sternberg et al., 2001). Inversely, in broadleaved plantations, the seedlings’ survival was higher with pre-planting herbicide application (Jacobs et al., 2004).
The spatial and temporal variation of biotic and abiotic disturbances on plantations was studied for 2 years of high and low precipitation in Mediterranean oaks [Quercus lobata (deciduous) and Quercus agrifolia (evergreen)] in the USA (López-Sánchez et al., 2019). Nearly all seedlings were affected by disturbances. The higher mortality was due to summer drought, followed by browsing and insect damage. Damages differed spatially and temporally. In the year with low precipitation insect damage and browsing were the primordial factors influencing regeneration. At the beginning of spring, the main cause of damage was the rodents, while from late spring to early summer was drought and browsing. Quercus agrifolia had a higher probability of survival than Quercus lobata. Cover of both trees and shrubs decreased drought damages but increased those of browsing. The aforesaid highlighted the influence not only of different disturbances but also of their interactions on regeneration (López-Sánchez et al., 2019).
The plantations of Pinus ponderosa, Pinus edulis, Pinus strobiformis, and Pseudotsuga menziesii post-fire highlighted the importance of landscape heterogeneity and topographical position on microclimate (Marsh et al., 2022). Areas with less solar radiation and higher moisture had higher seedling survival probability. Thus, microtopography knowledge can be a tool to select planting sites, enhancing the effectiveness of future plantations (Marsh et al., 2022). Inversely, the failure of a plantation of Pinus cembra (0.15% of survival rate) was related to the lack of knowledge of the site’s suitability in relation to the specie ecological traits (Fragnière et al., 2022).
Browsing in Quercus robur plantations in Poland was evaluated for three planting designs (single row, double row, and group planting) with three pre-commercial thinnings (Bolibok et al., 2021). The browsing damages on saplings were the lowest in group planting when compared with single or double-row planting, and diminished with the increase of the oaks’ height. The browsing damages were the lowest when pre-commercial thinning was carried out in summer for single row and in spring for double row and group planting. The methods developed promoted regeneration, saving the costs of fencing in areas under high browsing pressure (Bolibok et al., 2021).
The optimal densities for a given stand are species-specific and vary with age, which means that the initial tree spacing of a plantation only sometimes stays appropriate (criteria also applies to stands regenerated naturally). Adjustments through thinning may be necessary during the stand management of a plantation over a planning horizon. Thinning either based on the number of trees, basal area or stocking can be assessed through the ecological-based self-thinning law concept (e.g., Reineke, 1933). Examples are presented in Figure 6 for Pinus sylvestris, Pinus pinaster, and Quercus suber calculated as 60% of the self-thinning line, according to del Rìo et al. (2001), Sales-Luis and Fonseca (2004), and Fonseca et al. (2017), respectively. It is highlighted the different patterns of the decrease of tree number with average tree size of pure monospecific even-aged stands. In the example, Pinus spp. show a higher tolerance than Quercus suber meaning that, for a certain average tree size, the Quercus suber will reach the mortality threshold associated with the inter-tree competition with a smaller number of trees.
6. Discussion
Several factors that influence the success of regeneration for different silvicultural systems have been identified (Dey et al., 2019; Kremer and Bauhus, 2020). They are related to seed availability (Bogdziewicz et al., 2020; Garcia-Barreda et al., 2021), seed dispersal (Gómez et al., 2019; Huth et al., 2022), germination (Tiebel et al., 2018; Löf et al., 2019), and seedling survival (Grossnickle, 2018; Löf et al., 2019; Guignabert et al., 2020).
Natural regeneration is more frequently used with selection, shelterwood and clear-cut with seed tree systems. The main bottleneck for the regeneration development of shade-intolerant species is light. Higher success was attained in group selection and irregular shelterwood systems than in single tree selection systems and for stands with lower stocking (Schütz, 2006; Lundqvist, 2017; Hessenmöller et al., 2018; Brüllhardt et al., 2022). Inversely, in the clear-cut with seed trees and uniform shelterwood systems the main constraints are related to the number of seed years and the amount of seed (one year in the former and several years in the latter) and the site (Barbeito et al., 2011; Guignabert et al., 2020; Rosenvald, 2020).
Direct seeding advantages are related to the development of seedlings in situ, with high regeneration densities. It can be used in all silvicultural systems (Löf et al., 2019; Willoughby et al., 2019; Guignabert et al., 2020; Huth et al., 2022). Its main limitation is the availability of seeds of the desired species (Grossnickle and Ivetić, 2017; Huth et al., 2017).
Plantations are frequently linked to clear-cut systems, but can also be used in the shelterwood and selection (to a lesser extent) systems. Plantations can be used to regenerate stands maintaining the composition and structure, or to shift their composition and/or structure, for example to convert pure stands in mixed stands or to change from one specie to another. Its main advantages are related to planting seedlings that have the aerial and root systems developed. This is especially helpful for sites with less suited microclimatic and edaphic conditions. Moreover, planted seedlings have higher growth rates than seeded ones (Marsh et al., 2022). The main constraints are related to container dimension, damages on seedlings prior to planting and plantation stress (Zhigunov et al., 2014; Grossnickle and El-Kassaby, 2016; Andivia et al., 2019; Pernot et al., 2019).
Common to all regeneration methods’ success (establishment and growth) are the suitable site conditions, including soil, weather and topography (Dey et al., 2019; Kremer and Bauhus, 2020), browsing (Petersson et al., 2019; Vaz et al., 2019; Knapp et al., 2021), and the control of spontaneous vegetation and thus its competition with seedlings/saplings (Smit et al., 2008; Knapp et al., 2019; Reuling et al., 2019). Specific to selection and shelterwood systems are the competition between the main stand and regeneration, and the spatial distribution of regeneration, especially in mixed stands (Schütz, 2006; VanderMolen et al., 2021; Brüllhardt et al., 2022).
Conclusions
Overall, the primordial goal of regeneration is achieving the best-suited density and seedlings/saplings traits (vigor, form, size, and spatial arrangement) of the desired species and promoting their recruitment to the main stand. This can be achieved by natural or artificial (direct seeding or planting) regeneration. Yet, each silvicultural system has specificities that should be considered and future needs accounted for Table 1, especially under climatic changes. Moreover, regeneration can be promoted by the diversification of forest products that provide income. These products may support silvicultural practices, in particular those related to natural regeneration (Dey et al., 2019). Direct seeding and plantation can be used to complement natural regeneration or in afforestation and reforestation.
Author contributions
AG and TF contributed to the conception and design of the study, wrote the first draft of the manuscript, and wrote sections of the manuscript. Both authors contributed to the manuscript revision and read and approved the submitted version.
Funding
This work was funded by National Funds through FCT–Foundation for Science and Technology under the Project UIDB/05183/2020 for AG and UIDB/00239/2020 for TF, through the Forest Research Centre.
Acknowledgments
We would like to acknowledge COST CA19128: PEN-CaFoRR–Pan-European Network for Climate Adaptive Forest Restoration and Reforestation (http://www.pen-caforr.org/) and IUFRO Division 1-Silviculture for the fruitful discussions about the silviculture and regeneration of forest stands. We would also like to thank two reviewers that whose comments contributed to the manuscript improvement.
Conflict of interest
The authors declare that the research was conducted in the absence of any commercial or financial relationships that could be construed as a potential conflict of interest.
Publisher’s note
All claims expressed in this article are solely those of the authors and do not necessarily represent those of their affiliated organizations, or those of the publisher, the editors and the reviewers. Any product that may be evaluated in this article, or claim that may be made by its manufacturer, is not guaranteed or endorsed by the publisher.
References
Alonso-Crespo, I. M., Silla, F., Jiménez del Nogal, P., Fernández, M. J., Martínez-Ruiz, C., and Fernández-Santos, B. (2020). Effect of the mother tree age and acorn weight in the regenerative characteristics of Quercus faginea. Eur. J. For. Res. 139, 513–523. doi: 10.1007/s10342-020-01266-8
Andivia, E., Zuccarini, P., Grau, B., de Herralde, F., Villar-Salvador, P., and Savé, R. (2019). Rooting big and deep rapidly: The ecological roots of pine species distribution in southern Europe. Trees 33, 293–303. doi: 10.1007/s00468-018-1777-x
Argillier, C., Falconnet, G., and Greuz, J. (1991). “Production de plants forestiers,” in Guide technique du forestier méditerranéen français (Aix-en-Provence: CEMAGREF), 39.
Barbeito, I., LeMay, V., Calama, R., and Cañellas, I. (2011). Regeneration of Mediterranean Pinus sylvestris under two alternative shelterwood systems within a multiscale framework. Can. J. For. Res. 41, 341–351. doi: 10.1139/X10-214
Béland, M., Agestam, E., Ekö, P. M., Gemmel, P., and Nilsson, U. (2000). Scarification and seedfall affects natural regeneration of scots pine under two Shelterwood densities and a clear-cut in Southern Sweden. Scand. J. For. Res. 15, 247–255. doi: 10.1080/028275800750015064
Bellefleur, P., and LaRocque, G. (1983b). Compétition pour le rayonnement solaire en début de sucession secondaire dans une érablière à bouleau jaune et hêtre. Can. J. For. Res. 13, 514–521.
Bellefleur, P., and LaRocque, G. (1983a). Comparasion de la croissance d’espècesligneuses en milieu ouvert et sous couvert forestier. Can. J. For. Res. 13, 508–513.
Bogdziewicz, M., Ascoli, D., Hacket-Pain, A., Koenig, W. D., Pearse, I., Pesendorfer, M., et al. (2020). From theory to experiments for testing the proximate mechanisms of mast seeding: An agenda for an experimental ecology. Ecol. Lett. 23, 210–220. doi: 10.1111/ele.13442
Bolibok, L., Andrzejczyk, T., Szeligowski, H., and Liziniewicz, M. (2021). New methods of oak planting require modification of tending prescriptions under high browsing pressure – A case study from north-eastern Poland. For. Ecol. Manag. 497:119449. doi: 10.1016/j.foreco.2021.119449
Borderieux, J., Paillet, Y., Dalmasso, M., Mårell, A., Perot, T., and Vallet, P. (2021). The presence of shade-intolerant conifers facilitates the regeneration of Quercus petraea in mixed stands. For. Ecol. Manag. 491:119189. doi: 10.1016/j.foreco.2021.119189
Boudru, M. (1989). Forêt et sylviculture. Le traitement des forêts. Gembloux: Presses Agronomiques de Gembloux.
Boudru, M. (1992). Forêt et sylviculture. Boisements te reboisements artificieles. Gembloux: Presses Agronomiques de Gembloux.
Branco, M., Branco, C., Merouani, H., and Almeida, M. H. (2002). Germination success, survival and seedling vigour of Quercus suber acorns in relation to insect damage. For. Ecol. Manag. 166, 159–164. doi: 10.1016/S0378-1127(01)00669-7
Brüllhardt, M., Rotach, P., Forrester, D. I., and Bugmann, H. (2022). Sustainable regeneration in uneven-aged mixed deciduous forests managed by selection silviculture: The role of demographic structure. Forestry 95, 201–214. doi: 10.1093/forestry/cpab041
Burschel, P., El Kateb, H., and Monsandl, R. (1992). “Experiments in mixed mountain forests in Bavaria,” in The ecology and silviculture of mixed-species forests, eds M. J. Kelty, B. C. Larson, and C. D. Oliver (Dordrecht: Kluwer Academic Publishers), 183–215.
Calama, R., Manso, R., Lucas-Borja, M. E., Espelta, J. M., Piqué, M., Bravo, F., et al. (2017). Natural regeneration in Iberian pines: A review of dynamic processes and proposals for management. For. Syst. 26:eR02S. doi: 10.5424/fs/2017262-11255
Caldeira, M. C., Ibáñez, I., Nogueira, C., Bugalho, M. N., Lecomte, X., Moreira, A., et al. (2014). Direct and indirect effects of tree canopy facilitation in the recruitment of Mediterranean oaks. J. Appl. Ecol. 51, 349–358. doi: 10.1111/1365-2664.12189
Cline, M. G. (1997). Concepts and terminology of apical dominance. Am. J. Bot. 84, 1064–1069. doi: 10.2307/2446149
Dalling, J. W., Davis, A. S., Schutte, B. J., and Elizabeth Arnold, A. (2011). Seed survival in soil: Interacting effects of predation, dormancy and the soil microbial community: Seed survival in soil. J. Ecol. 99, 89–95. doi: 10.1111/j.1365-2745.2010.01739.x
del Rìo, M., Montero, G., and Bravo, F. (2001). Analysis of diameter–density relationships and self-thinning in non-thinned even-aged Scots pine stands. For. Ecol. Manag. 142, 79–87. doi: 10.1016/S0378-1127(00)00341-8
Dey, D. C., Knapp, B. O., Battaglia, M. A., Deal, R. L., Hart, J. L., O’Hara, K. L., et al. (2019). Barriers to natural regeneration in temperate forests across the USA. New For. 50, 11–40. doi: 10.1007/s11056-018-09694-6
Dovčiak, M., Reich, P. B., and Frelich, L. E. (2003). Seed rain, safe sites, competing vegetation, and soil resources spatially structure white pine regeneration and recruitment. Can. J. For. Res. 33, 1892–1904. doi: 10.1139/x03-115
Duan, J., and Abduwali, D. (2021). “Theory and methods of afforestation,” in Silviculture, ed. A. C. Gonçalves (London: IntechOpen), 77–99.
Enes, T., Lousada, J., Aranha, J., Cerveira, A., Alegria, C., and Fonseca, T. (2019). Size–density trajectory in regenerated maritime pine stands after fire. Forests 10:1057. doi: 10.3390/f10121057
Ersson, B., Laine, T., and Saksa, T. (2018). Mechanized tree planting in Sweden and Finland: Current state and key factors for future growth. Forests 9:370. doi: 10.3390/f9070370
Fernandes, P. M., and Rigolot, E. (2007). The fire ecology and management of maritime pine (Pinus pinaster Ait.). For. Ecol. Manag. 241, 1–13. doi: 10.1016/j.foreco.2007.01.010
Florence, R. G. (1996). Ecology and silviculture of eucalyptus forests. Clayton, VIC: Csiro Publishing.
Fonseca, T., Monteiro, L., Enes, T., and Cerveira, A. (2017). Self-thinning dynamics in cork oak woodlands: Providing a baseline for managing density. For. Syst. 26:e006. doi: 10.5424/fs/2017261-10105
Fragnière, Y., Sonnenwyl, V., Clément, B., and Kozlowski, G. (2022). Large-scale historical afforestation failure with Pinus cembra in the Swiss Prealps. New For. 53, 533–553. doi: 10.1007/s11056-021-09871-0
Ganatsas, P., and Thanasis, G. (2010). Pinus halepensis invasion in Pinus pinea habitat in Strofylia forest (site of NATURA 2000 network), southern Greece. J. Nat. Conserv. 18, 106–117. doi: 10.1016/j.jnc.2009.04.006
Garcia-Barreda, S., Sangüesa-Barreda, G., Madrigal-González, J., Seijo, F., González de Andrés, E., and Camarero, J. J. (2021). Reproductive phenology determines the linkages between radial growth, fruit production and climate in four Mediterranean tree species. Agric. For. Meteorol. 307:108493. doi: 10.1016/j.agrformet.2021.108493
Gea-Izquierdo, G., Cañellas, I., and Montero, G. (2006). Acorn production in Spanish holm oak woodlands. Invest. Agrar. Sist. Recur. For. 15, 339–354. doi: 10.5424/srf/2006153-00976
Gómez, J. M. (2003). Spatial patterns in long-distance dispersal of Quercus ilex acorns by jays in a heterogeneous landscape. Ecography 26, 573–584. doi: 10.1034/j.1600-0587.2003.03586.x
Gómez, J. M., Schupp, E. W., and Jordano, P. (2019). Synzoochory: The ecological and evolutionary relevance of a dual interaction. Biol. Rev. 94, 874–902. doi: 10.1111/brv.12481
Gonçalves, A. C., Afonso, A., Pereira, D. G., and Pinheiro, A. (2017). Influence of umbrella pine (Pinus pinea L.) stand type and tree characteristics on cone production. Agrofor. Syst. 91, 1019–1030. doi: 10.1007/s10457-016-9975-2
Gonçalves, A. C., and Pommerening, A. (2012). Spatial dynamics of cone production in Mediterranean climates: A case study of Pinus pinea L. in Portugal. For. Ecol. Manag. 266, 83–93. doi: 10.1016/j.foreco.2011.11.007
Griebeler, A. M., Araujo, M. M., Tabaldi, L. A., Steffen, G. P. K., Turchetto, F., Rorato, D. G., et al. (2021). Type of container and Trichoderma spp. inoculation enhance the performance of tree species in enrichment planting. Ecol. Eng. 169:106317. doi: 10.1016/j.ecoleng.2021.106317
Grossnickle, S. (2018). Seedling establishment on a forest restoration site – An ecophysiological perspective. Refor 6, 110–139. doi: 10.21750/REFOR.6.09.62
Grossnickle, S. C. (2005). Importance of root growth in overcoming planting stress. New For. 30, 273–294. doi: 10.1007/s11056-004-8303-2
Grossnickle, S. C., and El-Kassaby, Y. A. (2016). Bareroot versus container stocktypes: A performance comparison. New For. 47, 1–51. doi: 10.1007/s11056-015-9476-6
Grossnickle, S., and Ivetić, V. (2017). Direct seeding in reforestation – A field performance review. REFOR. 4, 94–142. doi: 10.21750/REFOR.4.07.46
Guignabert, A., Augusto, L., Delerue, F., Maugard, F., Gire, C., Magnin, C., et al. (2020). Combining partial cutting and direct seeding to overcome regeneration failures in dune forests. For. Ecol. Manag. 476:118466. doi: 10.1016/j.foreco.2020.118466
Harmer, R. (1994a). Natural regeneration of broadleaved trees in Britain: I. Historical aspects. Forestry 67, 179–188.
Harmer, R. (1994b). Natural regeneration of broadleaved trees in Britain: II. Seed Production and predation. Forestry 67, 275–286.
Harmer, R. (1995). Natural regeneration of broadleaved trees in Britain: III. Germination and establishment. Forestry 68, 1–9.
Hessenmöller, D., Bouriaud, O., Fritzlar, D., Elsenhans, A. S., and Schulze, E. D. (2018). A silvicultural strategy for managing uneven-aged beech-dominated forests in Thuringia, Germany: A new approach to an old problem. Scand. J. For. Res. 33, 668–680. doi: 10.1080/02827581.2018.1453081
Hupperts, S. F., Webster, C. R., Froese, R. E., and Dickinson, Y. L. (2020). Seedling and sapling recruitment following novel silvicultural treatments in Great Lakes northern hardwoods. For. Ecol. Manag. 462:117983. doi: 10.1016/j.foreco.2020.117983
Huss, J. (2004). “AFFORESTATION/stand establishment, treatment and promotion – European experience,” in Encyclopedia of forest sciences (Amsterdam: Elsevier), 14–27. doi: 10.1016/B0-12-145160-7/00224-6
Huth, F., Wehnert, A., Tiebel, K., and Wagner, S. (2017). Direct seeding of silver fir (Abies alba Mill.) to convert Norway spruce (Picea abies L.) forests in Europe: A review. For. Ecol. Manag. 403, 61–78. doi: 10.1016/j.foreco.2017.08.017
Huth, F., Wehnert, A., and Wagner, S. (2022). Natural regeneration of scots pine requires the application of silvicultural treatments such as overstorey density regulation and soil preparation. Forests 13:817. doi: 10.3390/f13060817
Hyppönen, M., Alenius, V., and Valkonen, S. (2005). Models for the establishment and height development of naturally regenerated Pinus sylvestris in Finnish Lapland. Scand. J. For. Res. 20, 347–357. doi: 10.1080/02827580510036391
Ibáñez, I., and McCarthy-Neumann, S. (2016). Effects of mycorrhizal fungi on tree seedling growth: Quantifying the parasitism–mutualism transition along a light gradient. Can. J. For. Res. 46, 48–57. doi: 10.1139/cjfr-2015-0327
Jacobs, D. F., Ross-Davis, A. L., and Davis, A. S. (2004). Establishment success of conservation tree plantations in relation to silvicultural practices in Indiana, USA. New For. 28, 23–36. doi: 10.1023/B:NEFO.0000031329.70631.d0
Kern, C. C., Burton, J. I., Raymond, P., D’Amato, A. W., Keeton, W. S., Royo, A. A., et al. (2017). Challenges facing gap-based silviculture and possible solutions for mesic northern forests in North America. Forestry 90, 4–17. doi: 10.1093/forestry/cpw024
Klopčič, M., Simončič, T., and Bončina, A. (2015). Comparison of regeneration and recruitment of shade-tolerant and light-demanding tree species in mixed uneven-aged forests: Experiences from the Dinaric region. Forestry 88, 552–563. doi: 10.1093/forestry/cpv021
Knapp, S. P., Kern, C. C., and Webster, C. R. (2021). Harvested opening size affects cohort development and failures in a second-growth northern hardwood forest. For. Ecol. Manag. 482:118804. doi: 10.1016/j.foreco.2020.118804
Knapp, S. P., Webster, C. R., and Kern, C. C. (2019). Can group selection with legacy retention change compositional trajectories in conventionally managed hardwoods? For. Ecol. Manag. 448, 174–186. doi: 10.1016/j.foreco.2019.06.005
Koenig, W. D., Knops, J. M. H., Carmen, W. J., and Pearse, I. S. (2015). What drives masting? The phenological synchrony hypothesis. Ecology 96, 184–192. doi: 10.1890/14-0819.1
Kolo, H., Ankerst, D., and Knoke, T. (2017). Predicting natural forest regeneration: A statistical model based on inventory data. Eur. J. For. Res. 136, 923–938. doi: 10.1007/s10342-017-1080-1
Kremer, K. N., and Bauhus, J. (2020). Drivers of native species regeneration in the process of restoring natural forests from mono-specific, even-aged tree plantations: A quantitative review. Restor. Ecol. 28, 1074–1086. doi: 10.1111/rec.13247
Laine, T., Kärhä, K., and Hynönen, A. (2016). A survey of the Finnish mechanized tree-planting industry in 2013 and its success factors. Silva Fenn. 50:14. doi: 10.14214/sf.1323
Leonardsson, J., Löf, M., and Götmark, F. (2015). Exclosures can favour natural regeneration of oak after conservation-oriented thinning in mixed forests in Sweden: A 10-year study. For. Ecol. Manag. 354, 1–9. doi: 10.1016/j.foreco.2015.07.004
Löf, M., Castro, J., Engman, M., Leverkus, A. B., Madsen, P., Reque, J. A., et al. (2019). Tamm review: Direct seeding to restore oak (Quercus spp.) forests and woodlands. For. Ecol. Manag. 448, 474–489. doi: 10.1016/j.foreco.2019.06.032
Long, Y., Yang, X., Cao, Y., Lv, G., Li, Y., Pan, Y., et al. (2021). Relationship between soil fungi and seedling density in the vicinity of adult conspecifics in an arid desert forest. Forests 12:92. doi: 10.3390/f12010092
López-Sánchez, A., Peláez, M., Dirzo, R., Fernandes, G. W., Seminatore, M., and Perea, R. (2019). Spatio-temporal variation of biotic and abiotic stress agents determines seedling survival in assisted oak regeneration. J. Appl. Ecol. 56, 2663–2674. doi: 10.1111/1365-2664.13500
Lucas-Borja, M. E., Fonseca, T. F., Lousada, J. L., Silva-Santos, P., Garcia, E. M., and Abellán, M. A. (2012). Natural regeneration of Spanish black pine [Pinus nigra Arn. ssp. salzmannii (Dunal) Franco] at contrasting altitudes in a Mediterranean mountain area. Ecol. Res. 27, 913–921. doi: 10.1007/s11284-012-0969-x
Lucas-Borja, M. E., Fonseca, T., Parresol, B. R., Silva-Santos, P., García-Morote, F. A., and Tíscar-Oliver, P. A. (2011). Modelling Spanish black pine seedling emergence: Establishing management strategies for endangered forest areas. For. Ecol. Manag. 262, 195–202. doi: 10.1016/j.foreco.2011.03.023
Lucas-Borja, M. E., Silva-Santos, P., Fonseca, T., López-Serrano, F. R., Tiscar, P. A., Martínez-García, E., et al. (2010). Modelling Spanish black pine postdispersal seed predation in Central-eastern Spain. For. Syst. 19, 393. doi: 10.5424/fs/2010193-9104
Lundqvist, L. (2017). Tamm review: Selection system reduces long-term volume growth in Fennoscandic uneven-aged Norway spruce forests. For. Ecol. Manag. 391, 362–375. doi: 10.1016/j.foreco.2017.02.011
Lundqvist, L., Ahlström, M. A., Axelsson, E. P., Mörling, T., and Valinger, E. (2019). Multi-layered Scots pine forests in boreal Sweden result from mass regeneration and size stratification. For. Ecol. Manag. 441, 176–181. doi: 10.1016/j.foreco.2019.03.044
Luoranen, J., Saksa, T., and Lappi, J. (2018). Seedling, planting site and weather factors affecting the success of autumn plantings in Norway spruce and Scots pine seedlings. For. Ecol. Manag. 41, 79–90. doi: 10.1016/j.foreco.2018.03.040
Lüpke, B. (1988). Silvicultural methods of oak regeneration with special respect to shade tolerant mixed species. For. Ecol. Manag. 106, 19–26.
Marsh, C., Crockett, J. L., Krofcheck, D., Keyser, A., Allen, C. D., Litvak, M., et al. (2022). Planted seedling survival in a post-wildfire landscape: From experimental planting to predictive probabilistic surfaces. For. Ecol. Manag. 525:120524. doi: 10.1016/j.foreco.2022.120524
Masaba, P. W., and Etemesi, I. N. (2021). Adaptation and Growth performance evaluation of nitrogen fixing tree/shrub species in Dello-menna district of Bale zone, Southeast Ethiopia. East Afr. For. Agrofor. 3, 54–61. doi: 10.37284/eajfa.3.1.336
Mencuccini, M., Piussi, P., and Zanzi Sulli, A. (1995). Thirty years of seed production in a subalpine Norway spruce forest: Patterns of temporal and spatial variation. For. Ecol. Manag. 76, 109–125. doi: 10.1016/0378-1127(95)03555-O
Miina, J., and Saksa, T. (2008). Predicting establishment of tree seedlings for evaluating methods of regeneration for Pinus sylvestris. Scand. J. For. Res. 23, 12–27. doi: 10.1080/02827580701779595
Misson, L., Degueldre, D., Collin, C., Rodriguez, R., Rocheteau, A., Ourcival, J.-M., et al. (2011). Phenological responses to extreme droughts in a Mediterranean forest. Glob. Change Biol. 17, 1036–1048. doi: 10.1111/j.1365-2486.2010.02348.x
Mohan, M., Richardson, G., Gopan, G., Aghai, M. M., Bajaj, S., Galgamuwa, G. A. P., et al. (2021). UAV-supported forest regeneration: Current trends, challenges and implications. Remote Sens. 13:2596. doi: 10.3390/rs13132596
Muñoz-Rengifo, J., Chirino, E., Cerdán, V., Martínez, J., Fosado, O., and Vilagrosa, A. (2020). Using field and nursery treatments to establish Quercus suber seedlings in Mediterranean degraded shrubland. iForest 13, 114–123. doi: 10.3832/ifor3095-013
Mutke, S., Calama, R., Gonza, S. C., Montero, G., Gordo, F. J., Bono, D., et al. (2012). “Mediterranean stone pine: Botany and horticulture,” in Horticultural reviews, ed. J. Janick (Hoboken, NJ: Wiley-Blackwell), 153–201.
O’Hara, K. L. (2014). Multiaged silviculture managing for complex forest stand structures. Oxford: Oxford University Press.
Oliet, J. A., Blasco, R., Valenzuela, P., Melero de Blas, M., and Puértolas, J. (2019). Should we use meshes or solid tube shelters when planting in Mediterranean semiarid environments? New For. 50, 267–282. doi: 10.1007/s11056-018-9659-z
Oliver, C. D., and Larson, B. C. (1996). Forest stand dynamics. New York, NY: John Wiley & Sons, Inc.
Owens, J. N., and Fernando, D. D. (2007). Pollination and seed production in western white pine. Can. J. For. Res. 37, 260–275. doi: 10.1139/x06-220
Paiva, V., Correia, C., and Silva, J. S. (2010). “A regeneração artificial em acções de reabilitação pós-incêndio,” in Ecologia do Fogo e Gestão de Áreas Ardidas, eds F. Moreira, F. X. Catry, J. S. Silva, and F. Rego (Lisboa: ISA Press), 253–288.
Parker, W. C., Noland, T. L., and Morneault, A. E. (2013). Comparative mast seed production in unmanaged and shelterwood white pine (Pinus strobus L.) stands in central Ontario. New For. 44, 613–628. doi: 10.1007/s11056-013-9366-8
Pausas, J. G., Marañon, T., Caldeira, M., and Pons, J. (2009). “Natural regeneration,” in Cork oak woodlands on the edge. Ecology, adaptive management, and restoration, eds J. Aronson, J. S. Pereira, and J. G. Pausas (Washington, DC: Island Press), 115–124.
Pernot, C., Thiffault, N., and DesRochers, A. (2019). Root system origin and structure influence planting shock of black spruce seedlings in boreal microsites. For. Ecol. Manag. 433, 594–605. doi: 10.1016/j.foreco.2018.11.043
Petersson, L. K., Milberg, P., Bergstedt, J., Dahlgren, J., Felton, A. M., Götmark, F., et al. (2019). Changing land use and increasing abundance of deer cause natural regeneration failure of oaks: Six decades of landscape-scale evidence. For. Ecol. Manag. 444, 299–307. doi: 10.1016/j.foreco.2019.04.037
Petrovska, R., Bugmann, H., Hobi, M. L., Ghosh, S., and Brang, P. (2022). Survival time and mortality rate of regeneration in the deep shade of a primeval beech forest. Eur. J. For. Res. 141, 43–58. doi: 10.1007/s10342-021-01427-3
Pinto, J. R., Marshall, J. D., Dumroese, R. K., Davis, A. S., and Cobos, D. R. (2011). Establishment and growth of container seedlings for reforestation: A function of stocktype and edaphic conditions. For. Ecol. Manag. 261, 1876–1884. doi: 10.1016/j.foreco.2011.02.010
Pommerening, A., and Grabarnik, P. (2019). Individual-based methods in forest ecology and management. Cham: Springer Nature.
Pretzsch, H. (2020). Density and growth of forest stands revisited. Effect of the temporal scale of observation, site quality, and thinning. For. Ecol. Manag. 460:117879. doi: 10.1016/j.foreco.2020.117879
Pulido, F. J., and Díaz, M. (2005). Regeneration of a Mediterranean oak: A whole-cycle approach. Écoscience 12, 92–102. doi: 10.2980/i1195-6860-12-1-92.1
Raymond, P., Bedard, S., Roy, V., Larouche, C., and Tremblay, S. (2009). The irregular shelterwood system: Review, classification, and potential application to forests affected by partial disturbances. J. For. 107, 405–413. doi: 10.1093/jof/107.8.405
Reineke, L. H. (1933). Perfecting a stand-density index for even-aged forests. J. Agric. Res. 46, 627–638.
Reuling, L. F., D’Amato, A. W., Palik, B. J., Martin, K. J., and Fassnacht, D. S. A. (2019). Initial tree regeneration response to natural-disturbance-based silviculture in second-growth northern hardwood forests. Can. J. For. Res. 49, 628–639. doi: 10.1139/cjfr-2018-0406
Ribeiro, S., Cerveira, A., Soares, P., and Fonseca, T. (2022). Natural regeneration of maritime pine: A review of the influencing factors and proposals for management. Forests 13:386. doi: 10.3390/f13030386
Ribeiro, S. L., Fonseca, T. F., and Pires, A. L. (2019). Influenece of fertilization on growth of young chestnut trees (Castanea sativa MILL.) managed for wood production. CERNE 25, 357–364. doi: 10.1590/01047760201925042660
Riikonen, J., Lehto, T., and Rikala, R. (2013). Effects of boron fertilization in the nursery or after planting on the performance of Norway spruce seedlings on boron-poor sites. New For. 44, 671–685. doi: 10.1007/s11056-013-9372-x
Rodríguez-García, E., Spies, T. A., and Bravo, F. (2009). “El matorral como herramienta para la regeneración natural de Pinus pinaster Ait. en ambientes mediterráneos,” in Proceedings of the 5o congreso forestal español—montes y sociedad: Saber qué hacer, Ávila, 1–11.
Rosenvald, R. (2020). Effects of stand parameters on conifer regeneration success in pine shelterwood stands in Estonia. Eur. J. For. Res. 139, 29–40.
Rudawska, M., Leski, T., Aučina, A., Karliński, L., Skridaila, A., and Ryliškis, D. (2017). Forest litter amendment during nursery stage influence field performance and ectomycorrhizal community of Scots pine (Pinus sylvestris L.) seedlings outplanted on four different sites. For. Ecol. Manag. 395, 104–114. doi: 10.1016/j.foreco.2017.04.002
Saha, S., Kuehne, C., and Bauhus, J. (2017). Lessons learned from oak cluster planting trials in central Europe. Can. J. For. Res. 47, 139–148. doi: 10.1139/cjfr-2016-0265
Sales-Luis, J. F., and Fonseca, T. F. (2004). The allometric model in the stand density management of Pinus pinaster Ait. in Portugal. Ann. For. Sci. 61, 807–814. doi: 10.1051/forest:2004077
Scherrer, D., Hiltebrand, F., Dengler, J., and Wohlgemuth, T. (2021). Mind the gaps: Comparison of representative vs opportunistic assessment of tree regeneration in central European beech forests. For. Ecol. Manag. 491:119179. doi: 10.1016/j.foreco.2021.119179
Schütz, J. (1997). Sylviculture 2. La gestion des forêts irrégulières et mélangées. Lausanne: Presses Polytechniques et Universitaires Romandes.
Schütz, J.-P. (2006). Modelling the demographic sustainability of pure beech plenter forests in Eastern Germany. Ann. For. Sci. 63, 93–100. doi: 10.1051/forest:2005101
Shelton, M. G., and Cain, M. D. (2000). Regenerating uneven-aged stands of loblolly and shortleaf pines: The current state of knowledge. For. Ecol. Manag. 129, 177–193.
Smit, C., Ouden, J., and Díaz, M. (2008). Facilitation of Quercus ilex recruitment by shrubs in Mediterranean open woodlands. J. Veg. Sci. 19, 193–200. doi: 10.3170/2007-8-18352
Smith, D. M., Larson, B. C., Kelty, M. J., and Ashton, P. M. S. (1997). The practice of silviculture. Applied forest ecology, 9th Edn. New York, NY: John Wiley & Sons, Inc.
Sternberg, M., Danin, A., and Noy-Meir, I. (2001). Effects of clearing and herbicide treatments on coniferous seedling establishment and growth in newly planted Mediterranean forests. For. Ecol. Manag. 148, 179–184. doi: 10.1016/S0378-1127(00)00528-4
Stjernberg, E. I. (1996). Mechanical shock during transportation: Effects on seedling performance. New For. 13, 395–414.
Tiebel, K., Huth, F., and Wagner, S. (2018). Soil seed banks of pioneer tree species in European temperate forests: A review. iForest 11, 48–57. doi: 10.3832/ifor2400-011
Tinya, F., Márialigeti, S., Bidló, A., and Ódor, P. (2019). Environmental drivers of the forest regeneration in temperate mixed forests. For. Ecol. Manag. 433, 720–728. doi: 10.1016/j.foreco.2018.11.051
Trouvé, R., Sherriff, R. M., Holt, L. M., and Baker, P. J. (2021). Differing regeneration patterns after catastrophic fire and clearfelling: Implications for future stand dynamics and forest management. For. Ecol. Manag. 498:119555. doi: 10.1016/j.foreco.2021.119555
Turner, J., Knott, J., Green, P., and Turner, S. (2021). Boron nutritional management in Australian forest plantations. Trees For. People 5:100120. doi: 10.1016/j.tfp.2021.100120
Vacchiano, G., Ascoli, D., Berzaghi, F., Lucas-Borja, M. E., Caignard, T., Collalti, A., et al. (2018). Reproducing reproduction: How to simulate mast seeding in forest models. Ecol. Modell. 376, 40–53. doi: 10.1016/j.ecolmodel.2018.03.004
VanderMolen, M. S., Knapp, S. P., Webster, C. R., Kern, C. C., and Dickinson, Y. L. (2021). Spatial patterning of regeneration failure in experimental canopy gaps 15–24 years post-harvest. For. Ecol. Manag. 499:119577. doi: 10.1016/j.foreco.2021.119577
Vaz, P. G., Bugalho, M. N., Fedriani, J. M., Branco, M., Lecomte, X., Nogueira, C., et al. (2019). Unravelling associations between tree-seedling performance, herbivory, competition, and facilitation in high nature value farmlands. J. Environ. Manag. 232, 1066–1074. doi: 10.1016/j.jenvman.2018.11.082
Villalobos, A., Schlyter, F., Olsson, G., Witzell, J., and Löf, M. (2020). Direct seeding for restoration of mixed oak forests: Influence of distance to forest edge, predator-derived repellent and acorn size on seed removal by granivorous rodents. For. Ecol. Manag. 477:118484. doi: 10.1016/j.foreco.2020.118484
Willoughby, I. H., Jinks, R. L., and Forster, J. (2019). Direct seeding of birch, rowan and alder can be a viable technique for the restoration of upland native woodland in the UK. Forestry 92, 324–338.
Wyse, S. V., Brown, J. E., and Hulme, P. E. (2019). Seed release by a serotinous pine in the absence of fire: Implications for invasion into temperate regions. AoB Plants 11:lz077. doi: 10.1093/aobpla/plz077
Žemaitis, P., Gil, W., and Borowski, Z. (2019). Importance of stand structure and neighborhood in European beech regeneration. For. Ecol. Manag. 448, 57–66. doi: 10.1016/j.foreco.2019.05.066
Keywords: natural regeneration, seeding, planting, silvicultural systems, success
Citation: Gonçalves AC and Fonseca TF (2023) Influence management and disturbances on the regeneration of forest stands. Front. For. Glob. Change 6:1123215. doi: 10.3389/ffgc.2023.1123215
Received: 13 December 2022; Accepted: 20 March 2023;
Published: 03 April 2023.
Edited by:
Thomas J. Dean, Louisiana State University, United StatesReviewed by:
Maciej Pach, University of Agriculture in Krakow, PolandManuel Esteban Lucas-Borja, University of Castilla-La Mancha, Spain
Copyright © 2023 Gonçalves and Fonseca. This is an open-access article distributed under the terms of the Creative Commons Attribution License (CC BY). The use, distribution or reproduction in other forums is permitted, provided the original author(s) and the copyright owner(s) are credited and that the original publication in this journal is cited, in accordance with accepted academic practice. No use, distribution or reproduction is permitted which does not comply with these terms.
*Correspondence: Ana Cristina Gonçalves, YWNhZ0B1ZXZvcmEucHQ=