- 1Faculty of Agriculture and Marine Science, Kochi University, Nankoku, Japan
- 2Forestry and Forest Products Research Institute, Tsukuba, Japan
- 3Forest Department Sarawak, Kuching, Malaysia
- 4Japan International Research Centre for Agricultural Sciences, Tsukuba, Japan
To predict the dynamics of tropical rainforest ecosystems in response to climate change, it is necessary to understand the drought tolerance and related mechanisms of trees in tropical rainforests. In this study, we assessed the ecophysiological responses of seedlings of six dipterocarp species (Dipterocarpus pachyphyllus, Dryobalanops aromatica, Shorea beccariana, S. curtisii, S. parvifolia, and S. smithiana) to experimental short-term drought conditions. The seedlings were initially grown in plastic pots with sufficient irrigation; irrigation was then stopped to induce drought. Throughout the soil-drying period, we measured various ecophysiological parameters, such as maximum photosynthetic and transpiration rates, stomatal conductance, water-use efficiency, predawn water potential, the maximum quantum yield of photosystem II (Fv/Fm), leaf water characteristics (using pressure-volume curves), leaf water content, and total sugar and starch contents. In all six dipterocarp species studied, the Fv/Fm values dropped sharply when the soil water content fell below 8%. However, there were interspecific differences in physiological responses to such a decrease in soil water content: S. parvifolia and S. beccariana actively controlled their stomata during drought to reduce water consumption via an isohydric response, but showed an increase (S. parvifolia) or no change (S. beccariana) in leaf drought tolerance; Di. pachyphyllus and Dry. aromatica maintained photosynthesis and transpiration close to the wilting point during drought without reducing water consumption via an anisohydric response, and also increased their leaf drought tolerance over the drying period; and S. curtisii and S. smithiana maintained their photosynthetic capacity without stomatal closure, but showed no change or a slight decrease in leaf drought tolerance. Our results indicate that extreme drought can cause the death of dipterocarp seedlings via various drought response, which could substantially impact the future distribution, population dynamics, and structure of tropical rainforests.
Introduction
Global climate change has affected precipitation patterns worldwide, leading to increased drought severity and frequency (Huntington, 2006; Dai, 2013; Chadwick et al., 2015; Cook et al., 2015; Donat et al., 2016). Even in tropical rainforests, which have important roles in global carbon and water cycling (e.g., Lean and Warrilow, 1989; Kanae et al., 2001; Beer et al., 2010; Pan et al., 2011; Spracklen et al., 2012), changes in rainfall patterns and increased drought frequency have led to increased tree mortality (e.g., Nakagawa et al., 2000; Williamson et al., 2000; Clark, 2004; Meir and Grace, 2005; van Nieuwstadt and Sheil, 2005), in turn reducing water cycling (Phillips et al., 2009; Kumagai and Porporato, 2012; Feldpausch et al., 2016). El Niño–Southern Oscillation (ENSO) is considered to be a major driver of worsening droughts in Southeast Asia (Malhi and Wright, 2004; Kumagai and Porporato, 2012), and climate change will likely increase the severity and frequency of ENSO phenomena in the future (Timmermann et al., 1999; Breshears et al., 2009; Allen et al., 2010; Chen et al., 2016; Thirumalai et al., 2017; Wang et al., 2017; Cai et al., 2018; Rifai et al., 2019). ENSO-induced droughts can result in substantial carbon losses due to the death of tropical forest trees, which in turn exacerbates global warming (Feng et al., 2017). There is growing evidence that ENSO-induced droughts can severely impact tropical forest dynamics, resulting in increased mortality and decreased growth of trees (Nakagawa et al., 2000; Phillips et al., 2009, 2010, Itoh et al., 2012; Bennett et al., 2015). However, there are few studies on the ecophysiological responses of tropical rainforest tree species to severe drought, particularly in Southeast Asia (Burslem et al., 1996; Tyree et al., 1998; Cao, 2000; Baltzer et al., 2008; O’Brien et al., 2014, 2015, 2017a,2017b,2020; Inoue et al., 2017; Bittencourt et al., 2022).
Dipterocarpaceae is the dominant family in the lowland tropical rainforests of Southeast Asia, especially in the canopy and emergent layers (Ashton, 2014), and is critical to the forest dynamics and carbon and water cycles in these areas (Kumagai et al., 2006; Ghazoul, 2016). A significant increase in dipterocarp mortality was reported during a severe ENSO-induced drought (Nakagawa et al., 2000; Slik, 2004; van Nieuwstadt and Sheil, 2005; Newbery and Lingenfelder, 2009; Itoh et al., 2012). Moreover, experiments on the responses of several dipterocarp species to controlled drought revealed that mature trees of Dryobalanops aromatica increased their leaf drought tolerance via altered osmotic regulation (Inoue et al., 2017) without a significant reduction in leaf stomatal conductance (Yoshifuji et al., 2020). This species had a lower mortality rate under ENSO-induced drought compared to other dipterocarps (Hiromi et al., 2012), suggesting that responses to drought might vary widely among this family. In addition, the total non-structural carbohydrate (TNC) concentrations during a prolonged artificial drought experiment varied among dipterocarp species and were positively correlated with the timing of seedling death (O’Brien et al., 2014).
A plant species can be classified as isohydric or anisohydric depending on its stomatal behavior and hydraulic status in response to drought stress (Tardieu and Simonneau, 1998; Martínez-Vilalta et al., 2014; Kannenberg et al., 2017); this concept can be applied in assessments of the mechanisms underlying the short-term drought resistance and mortality of plant species (Qiu et al., 2022). As drought severity increases, isohydric species maintain stable midday leaf water potential via strict stomatal regulation, whereas anisohydric species experience fluctuations in water availability, with no discernible threshold of minimum leaf water potential (Martínez-Vilalta and Garcia-Forner, 2017). Because of the regulatory role of stomata in leaf conductance to water vapor (i.e., transpiration) and plant water status, classification as isohydric or anisohydric usually depends on stomatal behavior: isohydric species maintain relatively stable leaf water potential via stricter stomatal control, whereas anisohydric species exhibit less strict transpiration regulation (Jones, 1998; Tardieu and Simonneau, 1998; Martínez-Vilalta and Garcia-Forner, 2017). As a consequence, isohydric species are tolerant of short-term drought but may experience TNC depletion due to limited photosynthesis under prolonged drought. In contrast, anisohydric species maintain photosynthesis despite the risk of dehydration due to less strict stomatal regulation (Tardieu and Simonneau, 1998; McDowell et al., 2008). Changes in water potential and transpiration during non-drought periods have been detected in mature trees of both types of dipterocarps (Hiromi et al., 2012); however, the response to drought and relationship with other ecophysiological traits, such as photosynthesis, remain unclear in dipterocarp species (O’Brien et al., 2014).
This study was conducted to measure the stomatal behavior and hydraulic status of seedlings under short-term controlled drought conditions using six dominant dipterocarp species of a primary tropical rainforest in Borneo. We hypothesized that these species would exhibit different responses to drought, i.e., isohydric or anisohydric.
Materials and methods
Study site
This study was conducted in Lambir Hills National Park in Sarawak, Malaysia (4°20′N, 113°50′E). The study area has a humid tropical climate with minimal seasonal changes in rainfall or temperature (Kato et al., 1995). The mean annual precipitation and air temperature are 2,600 mm and 25.8°C, respectively (Kume et al., 2011). Although this location lacks a regular dry season (Kume et al., 2011), it sometimes experiences reduced precipitation in association with supra-annual climatic events, such as ENSO (Nakagawa et al., 2000; Sakai et al., 2006; Gomyo and Kuraji, 2009).
Study species
We selected six dipterocarp species for this study; Dipterocarpus pachyphyllus Meijer (DP), Dryobalanops aromatica Gaertn.f (DrA), Shorea beccariana Burck (SB), Shorea curtisii Dyer ex King (SC), Shorea parvifolia Dyer (SP), and Shorea smithiana Symington (SS). All of these species are evergreen and dominate the canopy and emergent layers at the study site. DrA, SB, SC, and SS are among the most important tree species in Lambir Hills National Park in terms of appearance frequency or basal area (Davies et al., 2005), and DP and SP are locally abundant, especially on the lower slopes of the forest (Momose et al., 1997; Ashton, 2004). DrA, SB, and SC are mainly distributed in upper slope with sandy soil, while DP, SP, and SS are distributed in lower slope with clay soil (Itoh et al., 1995; Hirai et al., 1997; Lee et al., 2002; Ashton, 2004; Hiromi et al., 2012; Kenzo et al., 2016). However, the distribution of these species is unrelated to soil pH (Lee et al., 2002). All six species are used for enrichment planting in tropical rainforests in Southeast Asia (e.g., Appanah and Weinland, 1993; Vincent and Davies, 2003; Hattori et al., 2013; Tuck et al., 2016; Perumal et al., 2017; Widiyatno et al., 2020).
Seeds were collected from at least three mother trees per species in Lambir Hills National Park during the landscape-scale masting event in January 2014 (Iku et al., 2017). More than 300 seeds (50 per species) were collected, each of which was planted in a plastic pot (8 cm × 20 cm) filled with a 50:50 mixture of clayey soil (collected from the nearby Lambir Forest) and sand. The seedlings were grown in a nursery under two layers of 70% shade cloth, which reduced direct sunlight to about 5%, for 7 months. This is because dipterocarp species consume stored nutrients in their seeds for about 1 month after germination and then become autotrophic (Ichie et al., 2001).
Drought treatment
From August to October 2014, watering was stopped after all pots were moved to a concrete-paved area under the eaves, where light conditions were comparable to those inside the nursery (Supplementary Appendix 1, 2). Each pot was randomly moved throughout the experiment so that all individuals experienced similar light conditions over time. Volumetric soil moisture was measured to assess soil drying using a soil moisture sensor (SM-200; Delta-T Devices, Cambridge, UK). We measured soil water content (SWC) five times within 1 day per pot, and the mean was taken as the value for the day. This procedure was implemented periodically for all pots. Soil water potential was measured simultaneously with SWC using a soil moisture sensor (Watermark 200SS-15; IRROMETER, Riverside, CA, USA) equipped with a temperature sensor (Watermark 200TS; IRROMETER) to determine the relationship between the SWC and soil water potential. Soil drying reached a minimum of −2.5 MPa (Supplementary Appendix 2A). The decrease in SWC after the experiment showed similar patterns among the six dipterocarp species studied (Supplementary Appendix 2B).
Measurement of leaf gas exchange rate
We measured the leaf gas exchange rate and water potential before irrigation was stopped, at 20, 15, 13, and 10% SWC, and finally every 2 days after the SWC fell below 10%. The leaf light-saturated photosynthetic rate (Amax), transpiration rate (Emax), and stomatal conductance (gsmax) at light saturation, and the dark respiration rate (Rd) in fully expanded and apparently non-senescent leaves were measured with a portable photosynthesis apparatus (LI-6400, Li-Cor, Lincoln, NE, USA). We randomly selected five to eight individuals per species and continued the measurements all day until the SWC of each reached the desired value. To avoid midday photosynthesis depression, we measured Amax, Emax, and gsmax from 08:00 to 11:30, and Rd from sunset to predawn (Kenzo et al., 2015). The light intensity was set to 1,500 μmol photon m–2 s–1 for the measurement of Amax, Emax, and gsmax and at 0 μmol photon m–2 s–1 for the measurement of Rd using an internal light-emitting diode (Li-640B; Li-Cor) as a light source. The CO2 concentration in the chamber was maintained at 400 ppm, and the air temperature and relative humidity were approximately 30°C and 60%, respectively. The water-use efficiency (WUE), i.e., the ratio of Amax to gsmax, was also calculated (Kitahashi et al., 2008; Ichie et al., 2016).
Chlorophyll fluorescence
We measured the maximum quantum yield of photosystem II (Fv/Fm) of each individual using a fluorescence meter (MINI-PAM; WALZ, Effeltrich, Germany) at predawn (around 05:00). To quantify Fv/Fm, dark-adapted leaves were exposed to a saturating pulse of 6,000 μmol m–2 s–1 with a wavelength of 650 nm, for 0.8 s under predawn conditions. In the leaves that maintained photosynthetic functions, the values of Fv/Fm show around 0.8, though less than 0.2 is the threshold corresponding to drought-induced mortality (Woo et al., 2008; García-Fernández et al., 2013). Three leaves were selected from each individual and the average value was taken.
Leaf water potential
Predawn leaf water potential (Ψpd), water potential at the turgor loss point (Ψtlp), and osmotic potential at full turgor (Ψ100) were determined using a pressure chamber (Model 1002; PMS instruments, Corvallis, OR, USA). Ψpd was measured from 05:00 to 06:00 in five individuals per species selected randomly on the day when the SWC reached the specified values. Ψtlp and Ψ100 were determined from pressure–volume curves (Tyree and Hammel, 1972). The base of each twig was cut off under water after the Ψpd measurement and rehydrated with tap water for at least 12 h under dark conditions (Tyree et al., 1974). The roughly 15-cm-long tip of each shoot bearing 5–10 leaves was cut from the twig for the measurement, and a pressure chamber was used to generate pressure–volume curves. We were unable to measure Ψpd, Ψtlp, and Ψ100 at 25 and 20% SWC in SP and at 20% in SC due to equipment trouble.
Individual size and dry weight
Four or five individuals per species were selected randomly before the irrigation was stopped and the tree height, ground diameter, and leaf area of each were measured. During the experiment, the seedlings used for leaf water potential measurement were dug out of the pots, washed to carefully remove soil, and divided into root, stem, leaf, and bud organs. After the fresh weight of leaves was measured, the leaf area was determined using a flatbed scanner and image analysis software (Lia32 freeware; http://www.agr.nagoya-u.ac.jp/~shinkan/LIA32/index.html). We measured the dry weight of each organ sample after drying at 60°C for 48 h. The leaf water content during the experiment was calculated as follows: (Fresh weight–Dry weight)/Fresh weight × 100 (%).
TNC analysis
Using the organ samples from the dry weight measurement, we determined the starch and total sugar contents of the leaves, stems, and roots in each species before the start of the experiment, and at 10 and 7% SWC. The total sugar content was determined following the phenol–sulfuric acid method of Dubois et al. (1956), as modified by Ashwell (1966). The dried samples were ground into a fine powder using a Hi-speed Vibration Sample Mill (TI-100; CMT, Fukushima, Japan), and about 100 mg of each sample was extracted at 90°C overnight using 80% ethanol. The fractional transmittance was read using an ultraviolet–visible light spectrophotometer (UV-1400; Shimadzu, Kyoto, Japan) at 490 nm. The starch content was determined following modified the methods of Lawrence et al. (1990) and Newell et al. (2002). The analytical procedure followed Ichie et al. (2005). The TNC content was calculated as the sum of the starch and total sugar contents.
Data analysis
To evaluate the response of each physiological trait to soil drought, we assessed the relationships between physiological parameters, including Amax, gsmax, Emax, Fv/Fm, and leaf water content, with SWC using a logistic regression model that estimated three parameters: the upper limit of each physiological trait under moist conditions before the experiment, the slope coefficient showing the rate of decline or rise, and the SWC at 50% of the upper limit. We also used a four-parameter logistic regression model to examine the relationships of Rd, WUE, and Ψpd with SWC. In addition to the parameters in the three-parameter model, this model estimated the lower limit of each physiological trait under dry conditions. Before conducting the analyses, Amax, gsmax, Emax, Rd, and WUE values were converted into values relative to those under well-irrigated conditions just before stopping irrigation to eliminate intraspecific error. Both analyses were carried out using the drm function in the drc package of R statistical software (version 4.2.1; R Development Core Team, Vienna, Austria) (Ritz and Streibig, 2005). A similar analysis was also performed at the individual level. Finally, we determined the SWC at which each physiological trait reached 90 and 50% of the upper limit, and the average was taken for interspecific comparison.
Linear regression was used to examine the relationships of Ψ100 and Ψtlp with SWC. Analysis of variance was used to assess the interspecific differences of all physiological and morphological characteristics before the experiment, changes in sugar, starch, and TNC concentrations in each organ throughout the experiment, and differences in SWC among the six species in the SWC at which each physiological trait reached 90 and 50% of the upper limit. When the result was significant at P < 0.05, Tukey’s HSD test was used to compare the differences and changes. Principal component analysis (PCA) was conducted to estimate the physiological responses of the six species to soil drought using the prcomp function. R software (version 4.2.1) was used for all analyses (R Core Team, 2022).
Results
Initial size and dry weight
The species studied differed widely in size immediately before the experiment (Supplementary Appendix 3). SB had much higher values for all parameters than the other species, except that SS was equivalent to SB in height and total leaf area; there were few differences among DP, SC, and SP.
The dry weight of each organ differed significantly among the species (Supplementary Appendix 3). The root dry weight was highest in SB, i.e., more than twice that of the other species. The species also differed considerably in their TR (shoot-to-root) ratio, with SS having a value significantly higher than the other five species.
Leaf gas exchange
Gas exchange rate parameters immediately before the experiment showed interspecific differences (Supplementary Appendix 4). Amax, Emax, and gsmax were significantly lower in DrA and SC than in the other four species. Rd was significantly lower in DP than SP. WUE was significantly higher in DrA than in the other five species.
During the experiment, interspecific differences were observed in Amax, Emax, and gsmax with decreasing SWC (Figures 1A–C and Table 1). SP showed a decrease in gsmax immediately after the irrigation was stopped, and Amax and Emax decreased accordingly. Meanwhile, in SB, gsmax was maintained for a short period after irrigation was stopped, and gsmax, Amax, and Emax decreased rapidly thereafter. In contrast, DP, DrA, SC, and SS responded to the decrease in SWC by maintaining gsmax values for a long period, but their stomata closed rapidly below 12% SWC; at 7–10% SWC, the gsmax, Amax and Emax values of these four species were half the pre-drought-values (Figures 1A–C and Table 1).
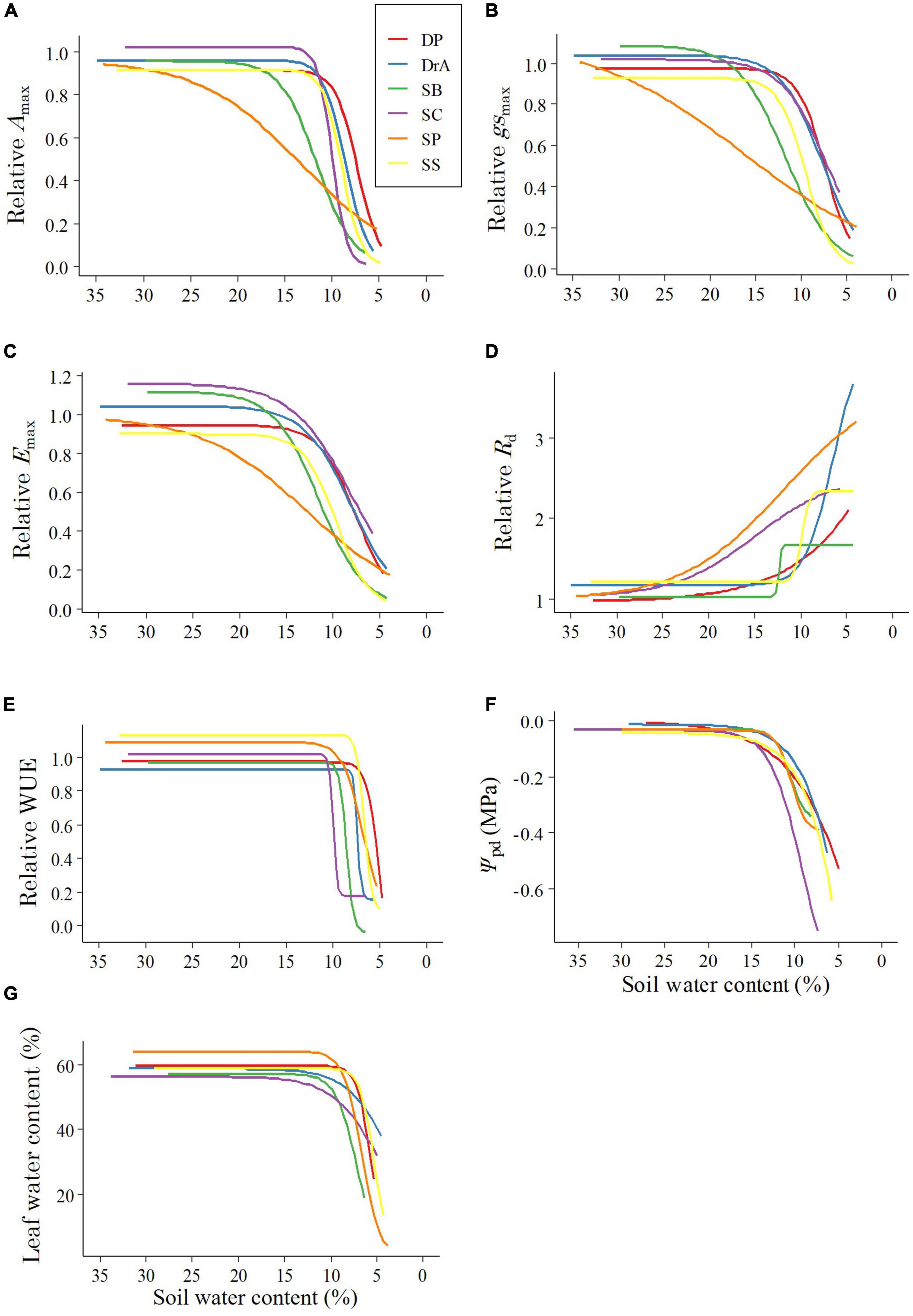
Figure 1. Changes in physiological parameters with decreasing soil water content: (A) Relative maximum photosynthetic rate (Amax), (B) relative stomatal conductance (gsmax), (C) relative transpiration rate (Emax), (D) relative dark respiration rate (Rd), (E) relative water-use efficiency (WUE), (F) predawn leaf water potential (Ψpd), (G) leaf water content. DP, Dipterocarpus pachyphyllus; DrA, Dryobalanops aromatica; SB, Shorea beccariana; SC, S. curtisii; SP, S. parvifolia; SS, S. smithiana.
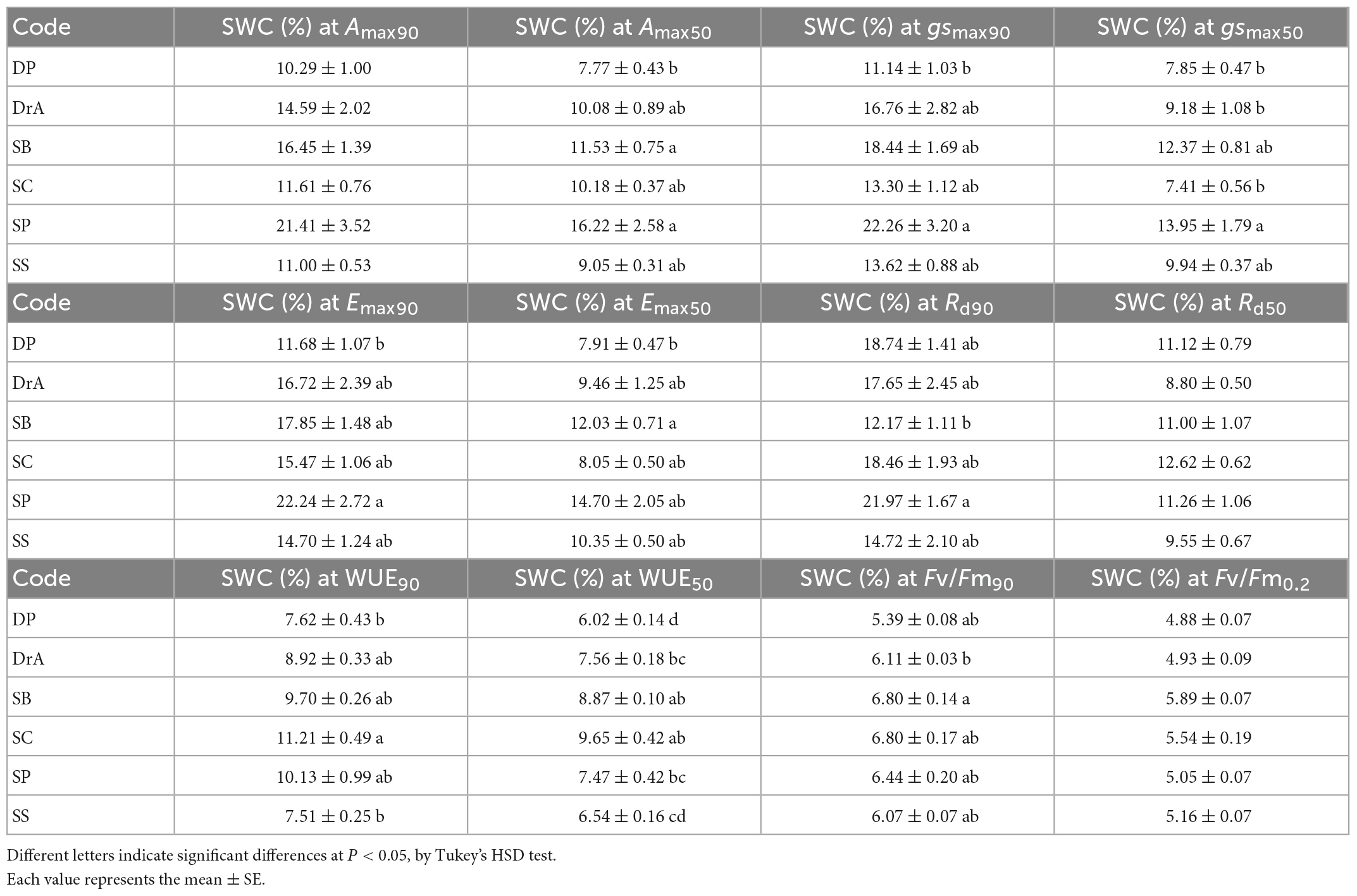
Table 1. Interspecific difference in soil water content at half (50%) and 90% of the initial physiological parameter.
After irrigation was stopped, the relative Rd began to increase under relatively high SWC in SP and SC, but only began to increase at low SWC for the other four species (Figure 1D and Table 1). Among the six species, DrA showed the lowest Rd with decreasing SWC. There was no interspecific difference in SWC at 50% of the difference between the initial and maximum Rd values (Table 1).
All species showed a sharp decline in WUE at 7–11% SWC (Figure 1E and Table 1). DP and SS reached 50% of the initial value at a significantly lower SWC compared to SB and SC (Table 1).
Leaf chlorophyll fluorescence
In all six species, Fv/Fm was about 0.8 before irrigation was stopped, and was maintained at approximately the same value after initially stopping irrigation (Figure 2 and Table 1). However, Fv/Fm decreased sharply when SWC fell below 8%. A significant interspecific difference was observed in the SWC at which Fv/Fm reached 90% of the initial value; however, there was no interspecific difference in the SWC at which Fv/Fm reached 0.2, i.e., the threshold corresponding to drought-induced death.
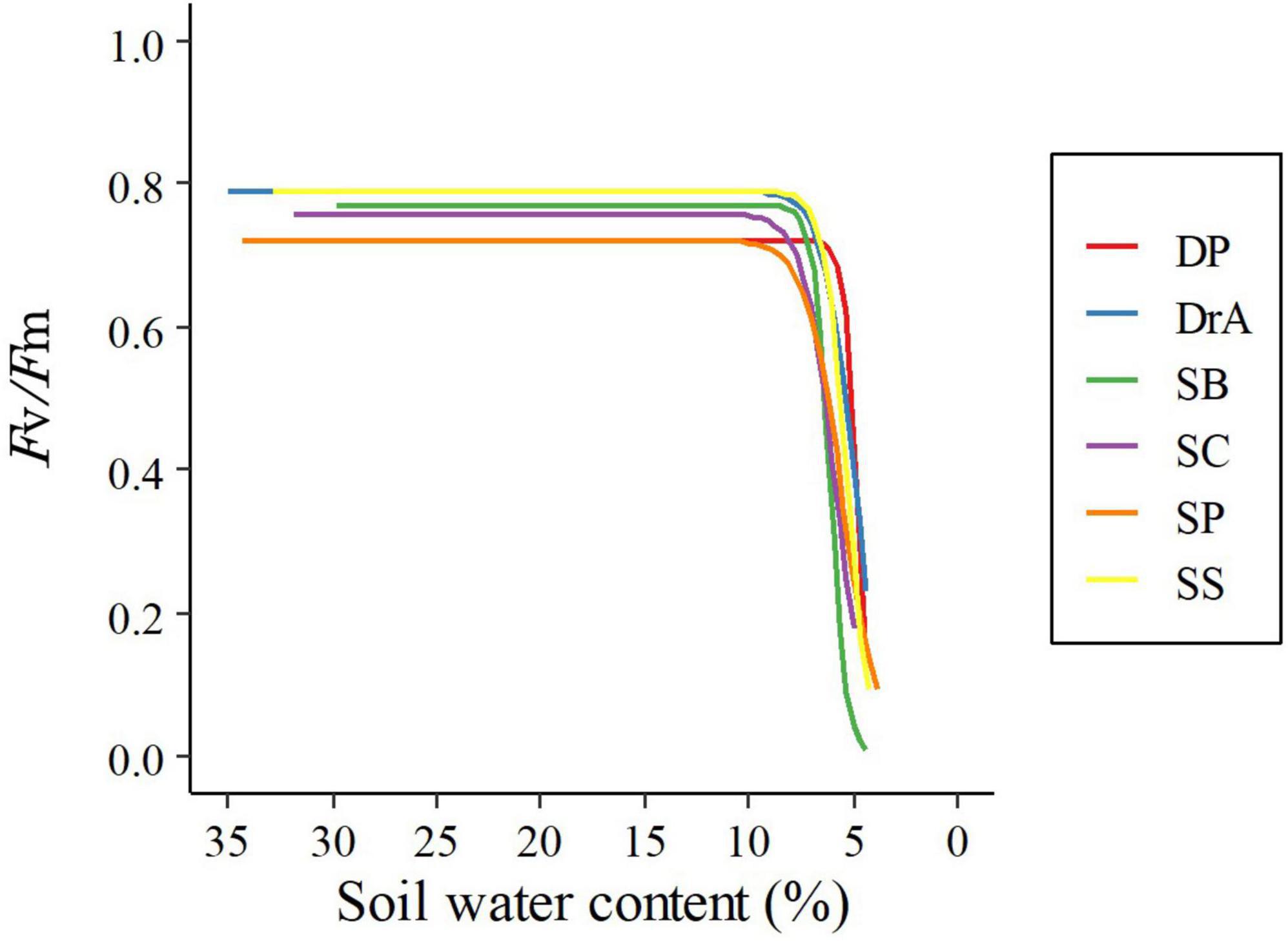
Figure 2. Relationship between the maximum quantum yield of photosystem II (Fv/Fm) and soil water content.
Leaf water potential and Ψtlp
Ψpd began to decrease at 12–16% SWC in all six species. Ψpd decreased more rapidly in SC than the other species, and showed the lowest values throughout the soil-drying period (Figure 1F). The Ψpd of DP and SS began to decrease at 15% SWC, and decreased slowly as the SWC dropped. Meanwhile, the Ψpd of SB and SP declined rapidly beginning around 12% SWC, but remained higher compared to the other four species. Finally, the Ψpd of DrA decreased slowly beginning around 12% SWC (Figure 1F). Overall, the values of Ψ100 and Ψtlp decreased with decreasing SWC in DrA, DP, and SP, but remained relatively constant, or even increased, in SB, SC, and SS (Figure 3).
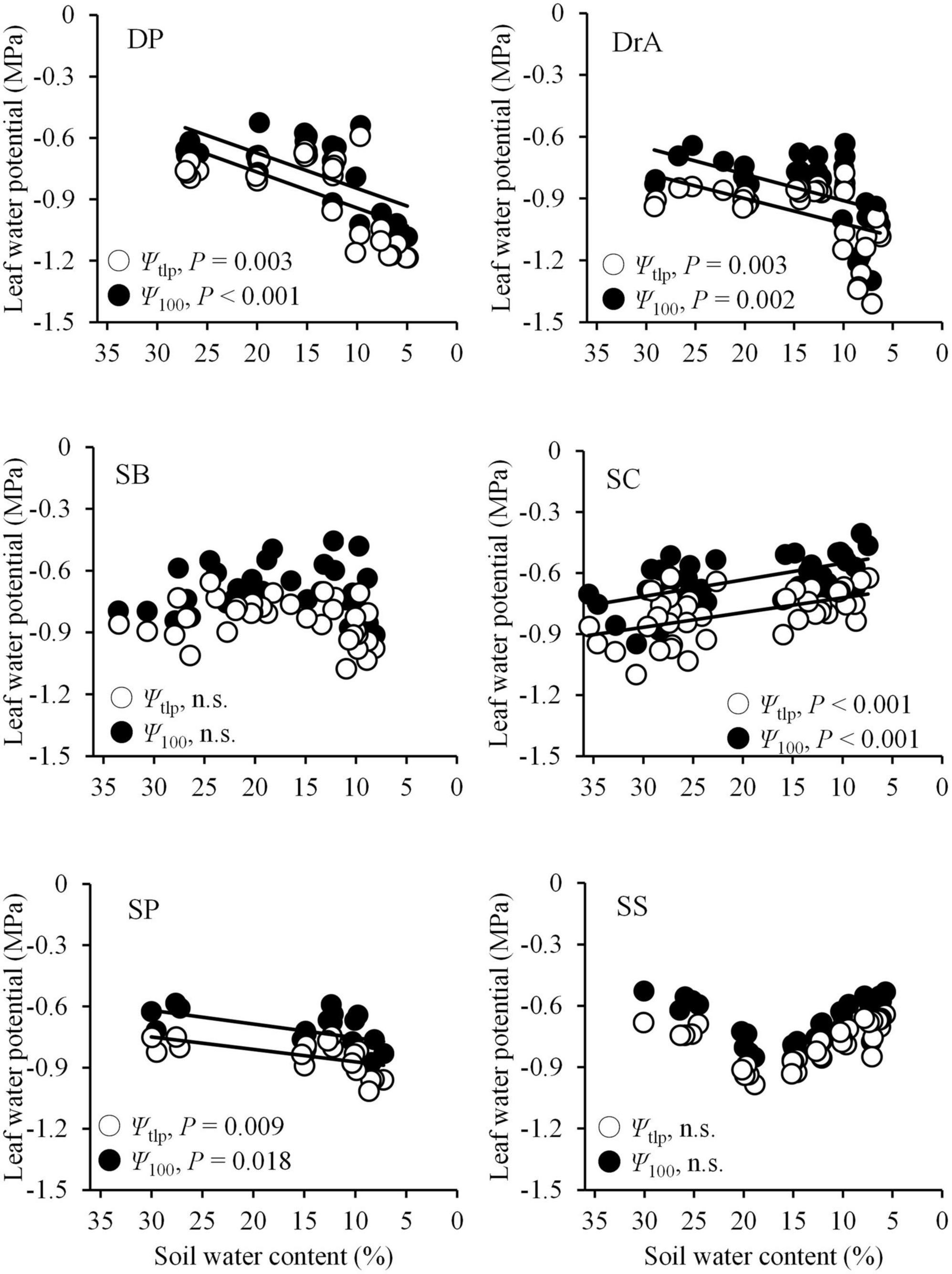
Figure 3. Changes in leaf water potential at the turgor loss point (Ψtlp: white circles) and osmotic potential at full turgor (Ψ100: black circles) with decreasing soil water content. The solid line indicates a significant relationship. n.s., not significant.
Leaf water content
The leaf water content immediately before the experiment was significantly differed among the six species; DP and SP showed the highest values (Supplementary Appendix 3). The leaf water content was positively related to Amax (P < 0.05, r = 0.84), Emax (P < 0.05, r = 0.83), and gsmax (P < 0.05, r = 0.87), while other leaf traits, such as LMA and stomatal size, were not significantly related to gas exchange traits (data not shown).
After stopping irrigation, the leaf water content of all species remained stable for a while, but varied with increasing drought intensity according to species (Figure 1G). In DrA and SC, the leaf water content started to decrease at a relatively fast rate at 15% SWC, but decreased slowly at lower SWC. SP and SB showed a rapid decline in leaf water content beginning around 10% SWC and reached below 40% the fastest. Finally, DP and SS maintained steady leaf water content until 7% SWC, below which they decreased rapidly.
TNC contents
TNC contents in leaves, stems, and roots tended to increase in all six species after stopping irrigation (Figure 4). Total sugar and starch contents did not change, or tended to increase in all the species; they did not decrease for any species.
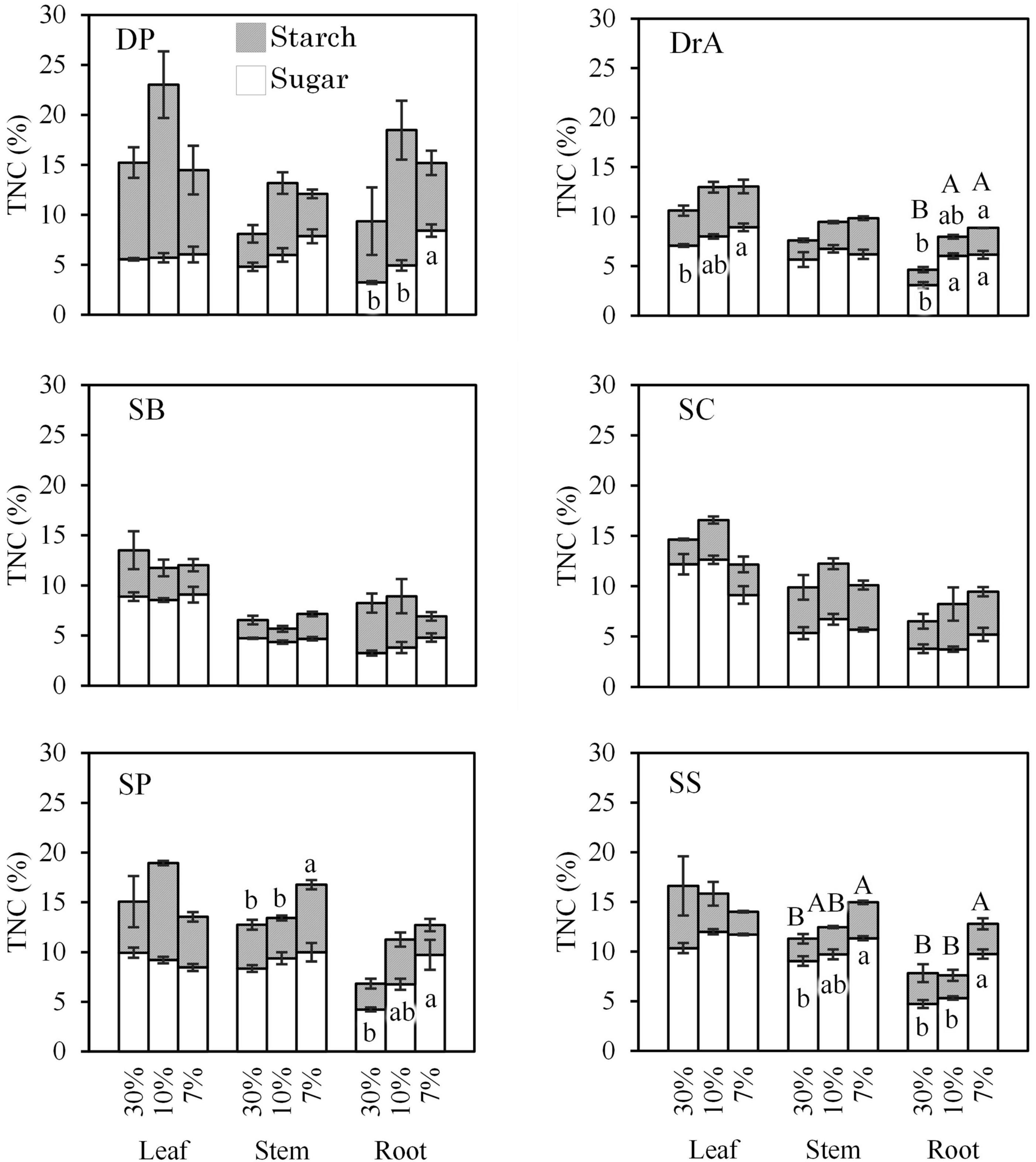
Figure 4. Total non-structural carbon (TNC) content in leaves, stems, and roots according to soil water content. Different uppercase (for TNC) and lowercase (for sugar and starch) letters indicate significant differences at P < 0.05. Error bars represent standard error.
Relationships among physiological parameters during drought
The PCA revealed different response patterns to soil drought among the six dipterocarp species studied. The first principal component (PC1) explained 48.0% of the variance; this dimension was related to the gas exchange response to drought (e.g., SWC at 50% of the initial gsmax, Emax, and Amax). The second principal component (PC2) explained 34.2% of the variance; this dimension was related to drought tolerance and death (e.g., SWC at 50% of the initial Rd and at a Fv/Fm of 0.2), and to variations in Ψtlp when SWC changed from 25 to 10%, (Figure 5 and Supplementary Appendix 5).
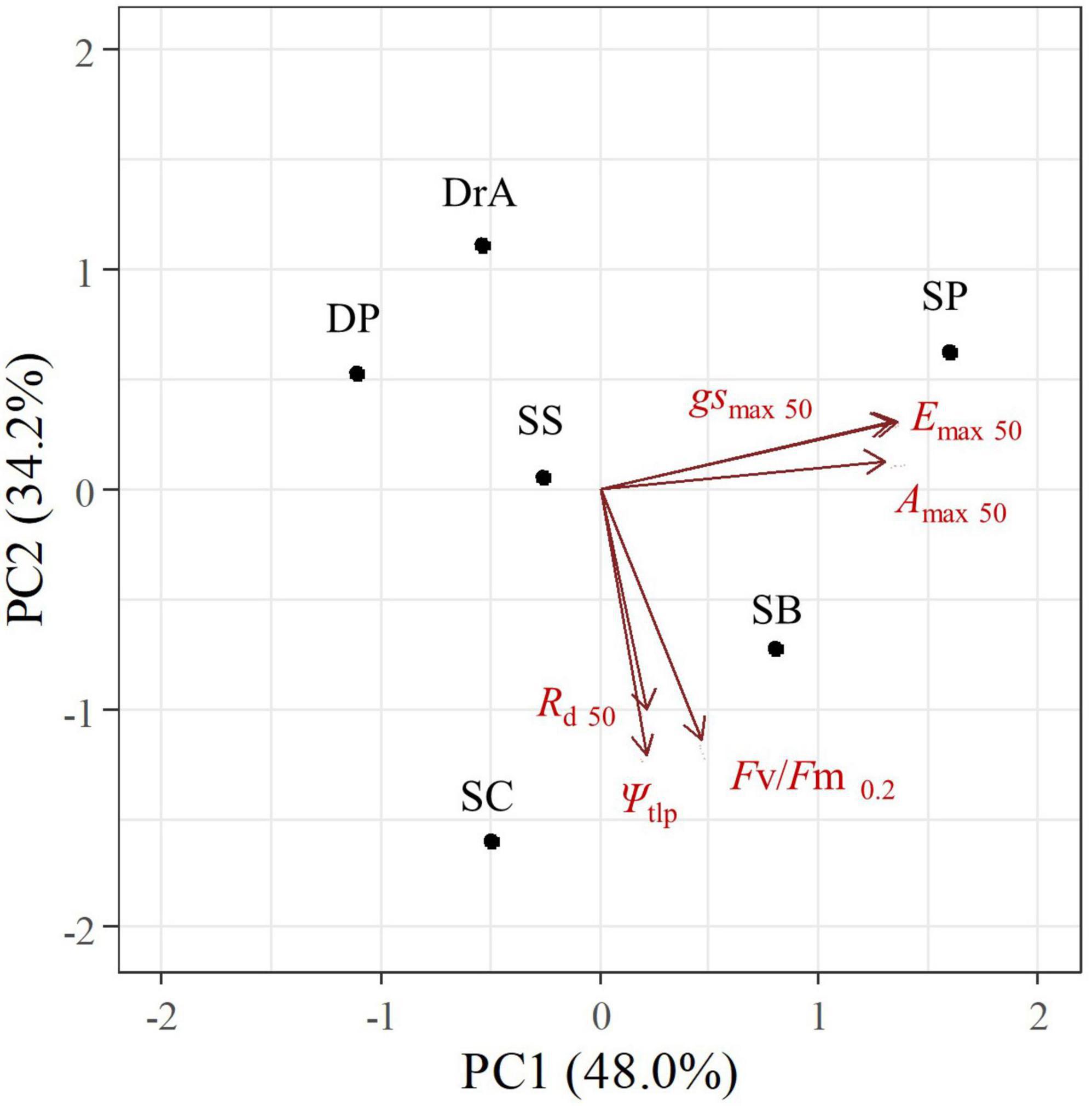
Figure 5. Results of principal component analysis of changes in physiological parameters in six dipterocarp species after stopping irrigation.
SP and SB exhibited an isohydric response, as illustrated by a rapid decline in gsmax and Emax, whereas DP, DrA, SS, and SC exhibited an anisohydric response, as evidenced by the late decline in PC1. Interestingly, DP, DrA, and SP showed increased drought resistance during the experiment, whereas SB, SC, and SS showed lower drought resistance in PC2. No significant correlations were found between PC1 or PC2 and the initial morphological and physiological characteristics of the six species (Supplementary Appendix 6).
Discussion
The six tropical dipterocarp species studied herein showed different stomatal and gas exchange behaviors toward soil drought, even within the same family and genus. SP and SB exhibited an isohydric response, with relatively early stomatal closure and a greater reduction in photosynthesis and transpiration with decreasing SWC. Both of these species reached 90 and 50% of their Amax, Emax, and gsmax values at higher SWC compared to the other species (Figures 1A–C and Table 1). Conversely, DP, DrA, SC, and SS maintained relatively higher gsmax values at lower SWC, and maintained Amax and Emax until the SWC dropped to around 8%, which was followed by a rapid decline (Figures 1A–C and Table 1); these trends are typical of an anisohydric response (Kumagai and Porporato, 2012; Roman et al., 2015). On the other hand, leaf water content may be a useful indicator of gas exchange parameters because the six species showed positive relations between leaf water content and Amax, Emax, and gsmax. A previous study also reported that leaf water content was associated with various physiological functions (Kramer, 1983). In addition, there were similar changes in leaf water content and gas exchange response during the soil drying. For example, the values of leaf water content, Amax and gsmax decreased rapidly at relatively high values of SWC in SP and SB (isohydric).
Although water stress under soil drying was greater in the anisohydric than isohydric species (Figure 1F), plasticity in drought tolerance did not coincide with stomatal behavior or gas exchange during the drought experiment in some species. Ψtlp did not change significantly, or even increased, with decreasing SWC in SB, SC, and SS; however, for DP, DrA, and SP, Ψtlp showed a marked decrease during the soil-drying period (Figure 3). Although a recent global synthesis revealed relatively low plasticity in Ψtlp in most species (Bartlett et al., 2014), high plasticity in Ψtlp has been reported in some anisohydric species (Meinzer et al., 1986, 2014; Martínez-Vilalta and Garcia-Forner, 2017). Our findings suggest that there are two types of responses by tree species to soil drought: some species show high plasticity in Ψtlp with increasing tolerance to drought, such as SP (isohydric) and DP and DrA (anisohydric), whereas others show low plasticity without increasing drought resistance, such as SB (isohydric) and SC and SS (anisohydric). Thus, despite anisohydric stomatal control, SC experienced substantial drought stress with poor plasticity in drought tolerance during the experiment; it showed the earliest decrease in Ψpd and lowest final Ψpd during the soil-drying period among the six species (Figure 1F).
Kumagai et al. (2004, 2005) observed an anisohydric response in the tropical rainforest in Lambir Hills National Park during an ENSO-induced severe drought (Kumagai and Porporato, 2012), possibly because many of the dominant dipterocarp species exhibited anisohydric responses to soil drying. In this study, four of the six studied dipterocarp species (DP, DrA, SS, and SC) exhibited an anisohydric-like response to drought in terms of stomatal regulation (Figures 1A–C and Figure 5). The response of forests to drought may be closely related to the behavior of the tree species forming the canopy and emergent layers, which generally make a large contribution to total carbon storage and water use in tropical rainforests (Kira, 1978; Kumagai et al., 2006; Rowland et al., 2015; McDowell et al., 2018). Although the present study focused on seedlings of dipterocarp species, if mature dipterocarp trees show a similar response to drought, the large number of tree species exhibiting anisohydric responses in this study may influence the forest-wide response to ENSO-induced drought. Supporting our findings, in a rainfall excursion experiment with mature DrA trees conducted in Lambir Hills National Park, stomatal conductance did not decrease significantly in response to soil drought (Yoshifuji et al., 2020); instead, leaf drought tolerance was enhanced via a decrease in Ψtlp (Inoue et al., 2017). The similar response by mature trees and seedlings of DrA suggests a close relationship between seedlings and mature trees with regard to drought response.
There was little difference in SWC-induced mortality among the six dipterocarp species studied, regardless of whether the response to drought was isohydric or anisohydric. Even though the response to drought varied widely among species, the timing of mortality was similar (Figure 2). O’Brien et al. (2014) showed that tropical tree seedlings, including dipterocarp species, depend on the accumulation of stored carbohydrates for survival under drought conditions. However, our findings suggest that, under short-term severe drought, dipterocarp species might experience poor osmoregulation with stored carbohydrates, leading to a similar timing of mortality. Higher plants accumulate osmolytes, such as proline and malondialdehyde, after exposure to water stress (Kavi Kishor et al., 2005); future studies should further clarify this response in dipterocarp species.
Although the soil conditions of the pots used in this study differed from the actual forest soil environment, which was less than 8% SWC in this study, drought events comparable to the SWC in this study have been recorded about every 10 years, including ENSO-induced strong droughts in Lambir Hills National Park (Ishizuka et al., 1998; Kumagai et al., 2009; Kumagai and Porporato, 2012; Ohashi et al., 2015). Such extreme soil drought conditions causing synchronous mortalities of seedlings of the six dipterocarp species at around 5% SWC in this study have not been recorded in Lambir Forest, but further climate change is likely to increase the severity and frequency of ENSO-induced droughts in Southeast Asian rainforests (Timmermann et al., 1999; Breshears et al., 2009; Allen et al., 2010; Chen et al., 2016; Corlett, 2016; Thirumalai et al., 2017; Wang et al., 2017; Cai et al., 2018; Rifai et al., 2019), which may alter rainforest structure. During shorter, less-severe droughts, differences in soil conditions may impact interspecific seedling survival. For example, SB, which has deep roots (Supplementary Appendix 2), may survive longer than the other species because it has access to deeper soil with higher SWC (Slot and Poorter, 2007; Poorter and Markesteijn, 2008; Markesteijn and Poorter, 2009). In addition, a lower Ψtlp may allow dipterocarp species to survive longer and recover after rainfall. The importance of seedling root depth to survival and recovery was reported in Bornean tropical rainforests subjected to severe drought events (Cao, 2000).
Conclusion
We conducted drought experiments on the seedlings of six dipterocarp species and observed different stomatal responses to drought, i.e., isohydric and anisohydric responses, which varied even within the same family. Interestingly, both isohydric and anisohydric species showed increased drought tolerance, while other isohydric and anisohydric species did not, indicating differences in the plasticity of drought tolerance regardless of whether there was a isohydric or anisohydric response. These findings indicate that an increase in the intensity and frequency of soil drought, as expected in tropical rainforests under climate change, may significantly alter forest dynamics and carbon and water cycles. Further research is needed to better predict the dynamics of seedling regeneration and drought-induced mortality in tropical rain forests under future climate change.
Data availability statement
The original contributions presented in this study are included in the article/Supplementary material, further inquiries can be directed to the corresponding author.
Author contributions
TI, TT, YI, and TK conducted the drought experiment and measured physiological parameters. TI, SI, and TK interpreted results and wrote the manuscript. MM made site preparations. All authors contributed to the article and approved the submitted version.
Funding
This research was partly supported by a Grant-in-Aid for Scientific Research (Nos. 16K07795, 17H04623, 18H04149, and 21H05316) from the Ministry of Education, Science and Culture and by a JST/JICA-SATREPS (PUBS).
Acknowledgments
We are grateful to the Forest Department, Sarawak and Sarawak Forestry Corporation for their kind support for this study.
Conflict of interest
The authors declare that the research was conducted in the absence of any commercial or financial relationships that could be construed as a potential conflict of interest.
Publisher’s note
All claims expressed in this article are solely those of the authors and do not necessarily represent those of their affiliated organizations, or those of the publisher, the editors and the reviewers. Any product that may be evaluated in this article, or claim that may be made by its manufacturer, is not guaranteed or endorsed by the publisher.
Supplementary material
The Supplementary Material for this article can be found online at: https://www.frontiersin.org/articles/10.3389/ffgc.2023.1112852/full#supplementary-material
References
Allen, C. D., Macalady, A. K., Chenchouni, H., Bachelet, D., McDowell, N., Vennetier, M., et al. (2010). A global overview of drought and heat-induced tree mortality reveals emerging climate change risks for forests. For. Ecol. Manag. 259, 660–684. doi: 10.1016/j.foreco.2009.09.001
Appanah, S., and Weinland, G. (1993). Planting quality timber trees in Peninsular Malaysia: A review. Kuala Lumpur: Forest Research Institute Malaysia.
Ashton, P. (2014). On the forests of tropical Asia: Lest the memory fade. Kew: Royal Botanic Gardens.
Ashton, P. S. (2004). “Dipterocarpaceae,” in Tree flora of Sabah and Sarawak, Vol. 5, eds E. Soepadmo, L. G. Saw, and R. C. K. Chung (Kuala Lumpur: Forest Research Institute of Malaysia), 63–388.
Ashwell, G. (1966). New colorimetric methods of sugar analysis. VII. The phenol-sulfuric acid reaction for carbohydrates. Methods Enzymol. 8, 93–95.
Baltzer, J. L., Davies, S. J., Bunyavejchewin, S., and Noor, N. S. M. (2008). The role of desiccation tolerance in determining tree species distribution along the Malay-Thai Peninsula. Funct. Ecol. 22, 221–231. doi: 10.1111/j.1365-2435.2007.01374.x
Bartlett, M. K., Zhang, Y., Kreidler, N., Sun, S., Ardy, R., Cao, K., et al. (2014). Global analysis of plasticity in turgor loss point, a key drought tolerance trait. Ecol. Lett. 17, 1580–1590. doi: 10.1111/ele.12374
Beer, C., Reichstein, M., Tomelleri, E., Ciais, P., Jung, M., Carvalhais, N., et al. (2010). Terrestrial gross carbon dioxide uptake: Global distribution and covariation with climate. Science 329, 834–838. doi: 10.1126/science.1184984
Bennett, A. C., McDowell, N. G., Allen, C. D., and Anderson-Teixeira, K. J. (2015). Larger trees suffer most during drought in forests worldwide. Nat. Plants 1, 1–5. doi: 10.1038/NPLANTS.2015.139
Bittencourt, P. R. D. L., Bartholomew, D. C., Banin, L. F., Bin Suis, M. A. F., Nilus, R., Burslem, D. F., et al. (2022). Divergence of hydraulic traits among tropical forest trees across topographic and vertical environment gradients in Borneo. New Phytol. 235, 2183–2198. doi: 10.1111/nph.18280
Breshears, D. D., Myers, O. B., Meyer, C. W., Barnes, F. J., Zou, C. B., Allen, C. D., et al. (2009). Tree die-off in response to global change-type drought: Mortality insights from a decade of plant water potential measurements. Front. Ecol. Environ. 7:185–189. doi: 10.1890/080016
Burslem, D. F. R. P., Grabb, P. J., and Turner, I. M. (1996). Responses to simulated drought and elevated nutrient supply among shade-tolerant tree seedlings of lowland tropical forest in Singapore. Biotropica 28, 636–648. doi: 10.2307/2389050
Cai, W., Wang, G., Dewitte, B., Wu, L., Santoso, A., Takahashi, K., et al. (2018). Increased variability of eastern Pacific El Niño under greenhouse warming. Nature 564, 201–206. doi: 10.1038/s41586-018-0776-9
Cao, K. (2000). Water relations and gas exchange of tropical saplings during a prolonged drought in a Bornean heath forest, with reference to root architecture. J. Trop. Ecol. 16, 101–116. doi: 10.1017/S0266467400001292
Chadwick, R., Good, P., Martin, G., and Rowell, D. P. (2015). Large rainfall changes consistently projected over substantial areas of tropical land. Nat. Clim. Change 6, 177–181. doi: 10.1038/nclimate2805
Chen, C.-C., Lin, H.-W., Yu, J.-Y., and Lo, M.-H. (2016). The 2015 Borneo fires: What have we learned from the 1997 and 2006 El Niños? Environ. Res. Lett. 11:104003. doi: 10.1088/1748-9326/11/10/104003
Clark, D. A. (2004). Sources or sinks? The responses of tropical forests to current and future climate and atmospheric composition. Philos. Trans. R. Soc. Lond. B Biol. Sci. 359, 477–491. doi: 10.1098/rstb.2003.1426
Cook, B. I., Ault, T. R., and Smerdon, J. E. (2015). Unprecedented 21st century drought risk in the American Southwest and central plains. Sci. Adv. 1:e1400082. doi: 10.1126/sciadv.1400082
Corlett, R. T. (2016). The impacts of droughts in tropical forests. Trends Plant Sci. 21, 584–593. doi: 10.1016/j.tplants.2016.02.003
Dai, A. G. (2013). Increasing drought under global warming in observations and models. Nat. Clim. Change 3, 52–58. doi: 10.1038/nclimate1633
Davies, S. J., Tan, S., LaFrankie, J. V., and Potts, M. D. (2005). “Soil-related floristic variation in a hyperdiverse dipterocarp forest,” in Pollination ecology and the rain forest: Sarawak studies, eds D. W. Roubik, S. Sakai, and A. A. H. Karim (Berlin: Springer), 22–34. doi: 10.1007/b138701
Donat, M. G., Lowry, A. L., Alexander, L. V., O’Gorman, P. A., and Maher, N. (2016). More extreme precipitation in the world’s dry and wet regions. Nat. Clim. Change 6, 508–513. doi: 10.1038/nclimate2941
Dubois, M., Gilles, K. A., Hamilton, J. K., Rebers, P. A., and Smith, F. (1956). Colorimetric method for determination of sugars and related substances. Anal. Chem. 28, 350–356.
Feldpausch, T. R., Phillips, O. L., Brienen, R. J., Gloor, E., Lloyd, J., Lopez-Gonzalez, G., et al. (2016). Amazon forest response to repeated droughts. Glob. Biogeochem. Cycles 30, 964–982. doi: 10.1002/2015GB005133
Feng, X. H., Uriarte, M., Gonzalez, G., Reed, S., Thompson, J., Zimmerman, J. K., et al. (2017). Improving predictions of tropical forest response to climate change through integration of field studies and ecosystem modeling. Glob. Change Biol. 24, E213–E232. doi: 10.1111/gcb.13863
García-Fernández, A., Iriondo, J. M., Bartels, D., and Escudero, A. (2013). Response to artificial drying until drought-induced death in different elevation populations of a high-mountain plant. Plant Biol. 15, 93–100. doi: 10.1111/j.1438-8677.2012.00638.x
Ghazoul, J. (2016). Dipterocarp biology, ecology, and conservation. Oxford: Oxford University Press. doi: 10.1093/acprof:oso/9780199639656.001.0001
Gomyo, M., and Kuraji, K. (2009). Spatial and temporal variations in rainfall and the ENSO-rainfall relationship over Sarawak, Malaysian Borneo. SOLA 5, 41?44.
Hattori, D., Kenzo, T., Kendawang, J. J., Ninomiya, I., and Sakurai, K. (2013). Rehabilitation of degraded tropical rainforest using dipterocarp trees in Sarawak Malaysia. Int. J. For. Res. 2013:83017. doi: 10.1155/2013/683017
Hirai, H., Matsumura, H., Hirotani, H., Sakurai, K., and Ogino, K. (1997). Soils and distribution of Dryobalanops aromatica and Dryobalanops lanceolata in mixed dipterocarp forest–a case study at Lambir Hills National Park, Sarawak, Malaysia. Tropics 7, 21–33. doi: 10.3759/tropics.7.21
Hiromi, T., Ichie, T., Kenzo, T., and Ninomiya, I. (2012). Interspecific variation in leaf water use associated with drought tolerance in four emergent dipterocarp species of a tropical rain forest in Borneo. J. For. Res. 17, 369–377. doi: 10.1007/s10310-011-0303-4
Huntington, T. G. (2006). Evidence for intensification of the global water cycle: Review and synthesis. J. Hydrol. 319, 83–95. doi: 10.1016/j.jhydrol.2005.07.003
Ichie, T., Inoue, Y., Takahashi, N., Kamiya, K., and Kenzo, T. (2016). Ecological distribution of leaf stomata and trichomes among tree species in a Malaysian lowland tropical rain forest. J. Plant Res. 129, 625–635. doi: 10.1007/s10265-016-0795-2
Ichie, T., Kenzo, T., Kitahashi, Y., Koike, T., and Nakashizuka, T. (2005). How does Dryobalanops aromatica supply carbohydrate resources for reproduction in a masting year? Trees 19, 703–710. doi: 10.1007/s00468-005-0434-3
Ichie, T., Ninomiya, I., and Ogino, K. (2001). Utilization of seed reserves during germination and early seedling growth by Dryobalanops lanceolata (Dipterocarpaceae). J. Trop. Ecol. 17, 371–378. doi: 10.1017/S0266467401001250
Iku, A., Itioka, T., Kishimoto-Yamada, K., Shimizu-Kaya, U., Mohammad, F. B., Hossman, M. Y., et al. (2017). Increased seed predation in the second fruiting event during an exceptionally long period of community-level masting in Borneo. Ecol. Res. 32, 537–545. doi: 10.1007/s11284-017-1465-0
Inoue, Y., Ichie, T., Kenzo, T., Yoneyama, A., Kumagai, T., and Nakashizuka, T. (2017). Effects of rainfall exclusion on leaf gas exchange traits and osmotic adjustment in mature canopy trees of Dryobalanops aromatica (Dipterocarpaceae) in a Malaysian tropical rain forest. Tree Physiol. 37, 1301–1311. doi: 10.1093/treephys/tpx053
Ishizuka, S., Tanaka, S., Sakurai, K., Hirai, H., Hirotani, H., Ogino, K., et al. (1998). Chracterization and distribution of soils at Lambir Hills National Park in Sarawak, Malaysia, with special reference to soil hardness and soil texture. Tropics 8, 31–44. doi: 10.3759/tropics.8.31
Itoh, A., Nanami, S., Harata, T., Ohkubo, T., Tan, S., Chong, L., et al. (2012). The effect of habitat association and edaphic conditions on tree mortality during El Niño-induced drought in a Bornean dipterocarp forest. Biotropica 44, 606–617. doi: 10.2307/23273031
Itoh, A., Yamakura, T., Ogino, K., and Lee, H. S. (1995). Survivorship and growth of seedlings of four dipterocarp species in a tropical rainforest of Sarawak, East Malaysia. Ecol. Res. 10, 327–338. doi: 10.1007/BF02347859
Jones, H. G. (1998). Stomatal control of photosynthesis and transpiration. J. Exp. Bot. 49, 387–398. doi: 10.1093/jexbot/49.suppl_1.387
Kanae, S., Oki, T., and Musiake, K. (2001). Impact of deforestation on regional precipitation over the Indochina Peninsula. J. Hydrometeorol. 2, 51–70. doi: 10.1175/1525-7541(2001)002<0051:IODORP>2.0.CO;2
Kannenberg, S. A., Novick, K. A., and Phillips, R. P. (2017). Coarse roots prevent declines in whole-tree non-structural carbohydrate pools during drought in an isohydric and an anisohydric species. Tree Physiol. 38, 1–9. doi: 10.1093/treephys/tpx119
Kato, M., Inoue, T., Hamid, A. A., Nagamitsu, T., Merdek, M. B., Nona, A. R., et al. (1995). Seasonality and vertical structure of light–attracted insect communities in a dipterocarp forest in Sarawak. Popul. Ecol. 37, 59–79. doi: 10.1007/BF02515762
Kavi Kishor, P. B., Sangam, S., Amrutha, R. N., Sri Laxmi, P., Naidu, K. R., Rao, K. R. S. S., et al. (2005). Regulation of proline biosynthesis, degradation, uptake and transport in higher plants: Its implications in plant growth and abiotic stress tolerance. Curr. Sci. 88, 424–438.
Kenzo, T., Ichie, T., Norichika, Y., Kamiya, K., Nanami, S., Igarashi, S., et al. (2016). Growth and survival of hybrid dipterocarp seedlings in a tropical rain forest fragment in Singapore. Plant Ecol. Divers. 9, 447–457. doi: 10.1080/17550874.2016.1265606
Kenzo, T., Inoue, Y., Yoshimura, M., Yamashita, M., Tanaka-Oda, A., and Ichie, T. (2015). Height-related changes in leaf photosynthetic traits in diverse Bornean tropical rain forest trees. Oecologia 177, 191–202. doi: 10.1007/s00442-014-3126-0
Kira, T. (1978). “Community architecture and organic matter dynamics in tropical rainforests of Southeast Asia with special reference to Pasoh forest, West Malaysia,” in Tropical trees as living systems, eds P. B. Tomlinson and M. H. Zimmerman (Cambridge: Cambridge University Press), 561–590.
Kitahashi, Y., Ichie, T., Maruyama, Y., Kenzo, T., Kitaoka, S., Matsuki, S., et al. (2008). Photosynthetic water use efficiency in tree crowns of Shorea beccariana and Dryobalanops aromatica in a tropical rain forest in Sarawak, East Malaysia. Photosynthetica 46, 151–155. doi: 10.1007/s11099-008-0025-9
Kumagai, T., and Porporato, A. (2012). Drought-induced mortality of a Bornean tropical rain forest amplified by climate change. J. Geophys. Res. 117:G02032. doi: 10.1029/2011JG001835
Kumagai, T., Ichie, T., Yoshimura, M., Yamashita, M., Kenzo, T., Saitoh, T. M., et al. (2006). Modeling CO2 exchange over a Bornean tropical rain forest using measured vertical and horizontal variations in leaf-level physiological parameters and leaf area densities. J. Geophys. Res. 111:D10107. doi: 10.1029/2005JD006676
Kumagai, T., Saitoh, T. M., Sato, Y., Morooka, T., Manfroi, O. J., Kuraji, K., et al. (2004). Transpiration, canopy conductance and the decoupling coefficient of a lowland mixed dipterocarp forest in Sarawak, Borneo: Dry spell effects. J. Hydrol. 287, 237–251. doi: 10.1016/j.jhydrol.2003.10.002
Kumagai, T., Saitoh, T. M., Sato, Y., Takahashi, H., Manfroi, O. J., Morooka, T., et al. (2005). Annual water balance and seasonality of evapotranspiration in a Bornean tropical rainforest. Agric. For. Meteorol. 128, 81–92. doi: 10.1016/j.agrformet.2004.08.006
Kumagai, T., Yoshifuji, N., Tanaka, N., Suzuki, M., and Kume, T. (2009). Comparison of soil moisture dynamics between a tropical rainforest and a tropical seasonal forest in Southeast Asia: Impact of seasonal and year-to-year variations in rainfall. Water Resour. Res. 45:W04413. doi: 10.1029/2008WR007307
Kume, T., Tanaka, N., Kuraji, K., Komatsu, H., Yoshifuji, N., Saitoh, T. M., et al. (2011). Ten-year evapotranspiration estimates in a Bornean tropical rainforest. Agric. For. Meteorol. 151, 1183–1192. doi: 10.1016/j.agrformet.2011.04.005
Lawrence, D. M., Halmer, P., and Bowles, D. J. (1990). Mobilisation of storage reserves during germination and early seedling growth of sugar beet. Physiol. Plant. 78, 421–429. doi: 10.1111/j.1399-3054.1990.tb09058.x
Lean, J., and Warrilow, D. (1989). Simulation of the regional climatic impact of Amazon deforestation. Nature 342, 411–413. doi: 10.1038/342411a0
Lee, H. S., Davies, S. J., LaFrankie, J. V., Tan, S., Yamakura, T., Itoh, A., et al. (2002). Floristic and structural diversity of mixed dipterocarp forest in Lambir Hills National Park, Sarawak, Malaysia. J. Trop. For. Sci. 14, 379–400.
Malhi, Y., and Wright, J. (2004). Spatial patterns and recent trends in the climate of tropical rainforest regions. Philos. Trans. R. Soc. Lond. B Biol. Sci. 359, 311–329. doi: 10.1098/rstb.2003.1433
Markesteijn, L., and Poorter, L. (2009). Seedling root morphology and biomass allocation of 62 tropical tree species in relation to drought–and shade–tolerance. J. Ecol. 97, 311–325. doi: 10.1111/j.1365-2745.2008.01466.x
Martínez-Vilalta, J., and Garcia-Forner, N. (2017). Water potential regulation, stomatal behaviour and hydraulic transport under drought: Deconstructing the iso/anisohydric concept. Plant Cell Environ. 40, 962–976. doi: 10.1111/pce.12846
Martínez-Vilalta, J., Poyatos, R., Aguade, D., Retana, J., and Mencuccini, M. (2014). A new look at water transport regulation in plants. New Phytol. 204, 105–115. doi: 10.1111/nph.12912
McDowell, N., Allen, C. D., Anderson-Teixeira, K., Brando, P., Brienen, R., Chambers, J., et al. (2018). Drivers and mechanisms of tree mortality in moist tropical forests. New Phytol. 219, 851–869. doi: 10.1111/nph.15027
McDowell, N., Pockman, W. T., Allen, C. D., Breshears, D. D., Cobb, N., Kolb, T. E., et al. (2008). Mechanisms of plant survival and mortality during drought: Why do some plants survive while others succumb to drought? New Phytol. 178, 719–739. doi: 10.1111/j.1469-8137.2008.02436.x
Meinzer, F. C., Rundel, P. W., Sharifi, M. R., and Nilsen, E. T. (1986). Turgor and osmotic relations of the desert shrub Larrea tridentata. Plant Cell Environ. 9, 467–476. doi: 10.1111/j.1365-3040.1986.tb01762.x
Meinzer, F. C., Woodruff, D. R., Marias, D. E., McCulloh, K. A., and Sevanto, S. (2014). Dynamics of leaf water relations components in co-occurring iso- and anisohydric conifer species. Plant Cell Environ. 37, 2577–2586. doi: 10.1111/pce.12327
Meir, P., and Grace, J. (2005). “The effects of drought on tropical forest ecosystems,” in Tropical forests & global atmospheric change, eds Y. Malhi and O. L. Phillips (Oxford: Oxford University Press), 75–84. doi: 10.1098/rstb.2003.1449
Momose, K., Yamauchi, M., Nagamitsu, T., Nagamasu, H., Hamid, A. A., and Inoue, T. (1997). “The canopy biology plot in a lowland dipterocarp forest in Sarawak,” in General flowering of tropical rainforests in Sarawak, eds T. Inoue and A. A. Hamid (Otsu: Center for Ecological Research, Kyoto University), 11–19.
Nakagawa, M., Tanaka, K., Nakashizuka, T., Ohkubo, T., Kato, T., Maeda, T., et al. (2000). Impact of severe drought associated with the 1997–1998 El Niño in a tropical forest in Sarawak. J. Trop. Ecol. 16, 355–367. doi: 10.1017/S0266467400001450
Newbery, D. M., and Lingenfelder, M. (2009). Plurality of tree species responses to drought perturbation in Bornean tropical rain forest. Plant Ecol. 201, 147–167. doi: 10.1007/978-90-481-2795-5_12
Newell, E. A., Mulkey, S. S., and Wright, S. J. (2002). Seasonal patterns of carbohydrate storage in four tropical tree species. Oecologia 131, 333–342. doi: 10.1007/s00442-002-0888-6
O’Brien, M. J., Burslem, D. F. R. P., Caduff, A., Tay, J., and Hector, A. (2015). Contrasting nonstructural carbohydrate dynamics of tropical tree seedlings under water deficit and variability. New Phytol. 205, 1083–1094. doi: 10.1111/nph.13134
O’Brien, M. J., Leuzinger, S., Philipson, C. D., Tay, J., and Hector, A. (2014). Drought survival of tropical tree seedlings enhanced by non-structural carbohydrate levels. Nature Clim. Change 4, 710–714. doi: 10.1038/nclimate2281
O’Brien, M. J., Ong, R., and Reynolds, G. (2017a). Intra-annual plasticity of growth mediates droughts resilience over multiple years in tropical seedling communities. Glob. Change Biol. 23, 4235–4244. doi: 10.1111/gcb.13658
O’Brien, M. J., Reynolds, G., Ong, R., and Hector, A. (2017b). Resistance of tropical seedlings to drought is mediated by neighbourhood diversity. Nat. Ecol. Evol. 1, 1643–1648. doi: 10.1038/s41559-017-0326-0
O’Brien, M. J., Valtat, A., Abiven, S., Studer, M. S., Ong, R., and Schmid, B. (2020). The role of soluble sugars during drought in tropical tree seedlings with contrasting tolerances. J. Plant Ecol. 13, 389–397. doi: 10.1093/jpe/rtaa017
Ohashi, M., Kume, T., Yoshifuji, N., Kho, L. K., Nakagawa, M., and Nakashizuka, T. (2015). The effects of an induced short-term drought period on the spatial variations in soil respiration measured around emergent trees in a typical Bornean tropical forest, Malaysia. Plant Soil 387, 337–349. doi: 10.1007/s11104-014-2303-6
Pan, Y., Birdsey, R. A., Fang, J., Houghton, R., Kauppi, P. E., Kurz, W. A., et al. (2011). A large and persistent carbon sink in the world’s forests. Science 333, 988–993. doi: 10.1126/science.1201609
Perumal, M., Wasli, M. E., Ying, H. S., Lat, J., and Sani, H. (2017). Association between soil fertility and growth performance of planted Shorea macrophylla (de Vriese) after enrichment planting at rehabilitation sites of Sampadi forest reserve, Sarawak, Malaysia. Int. J. For. Res. 2017:6721354. doi: 10.1155/2017/6721354
Phillips, O. L., Aragao, L., Lewis, S. L., Fisher, J. B., Lloyd, J., Lopez-Gonzalez, G., et al. (2009). Drought sensitivity of the Amazon rainforest. Science 323, 1344–1347. doi: 10.1126/science.1164033
Phillips, O. L., van der Heijden, G., Lewis, S. L., López-González, G., Aragão, L. E. O. C., Lloyd, J., et al. (2010). Drought–mortality relationships for tropical forests. New Phytol. 187, 631–646. doi: 10.1111/j.1469-8137.2010.03359.x
Poorter, L., and Markesteijn, L. (2008). Seedling traits determine drought tolerance of tropical tree species. Biotropica 40, 321–331. doi: 10.1111/j.1744-7429.2007.00380.x
Qiu, J., Crow, W. T., Wang, S., Dong, J., Li, Y., Garcia, M., et al. (2022). Microwave-based soil moisture improves estimates of vegetation response to drought in China. Sci. Total Environ. 849:157535. doi: 10.1016/j.scitotenv.2022.157535
R Core Team (2022). R: A language and environment for statistical computing. Vienna: R Foundation for Statistical Computing.
Rifai, S. W., Li, S., and Malhi, Y. (2019). Coupling of El Niño events and long-term warming leads to pervasive climate extremes in the terrestrial tropics. Environ. Res. Lett. 14:105002. doi: 10.1088/1748-9326/ab402f
Ritz, C., and Streibig, J. C. (2005). Bioassay analysis using R. J. Stat. Softw. 12:i05. doi: 10.18637/jss.v012.i05
Roman, D. T., Novick, K. A., Brzostek, E. R., Dragoni, D., Rahman, F., and Phillips, R. P. (2015). The role of isohydric and anisohydric species in determining ecosystem-scale response to severe drought. Oecologia 179, 641–654. doi: 10.1007/s00442-015-3380-9
Rowland, L., da Costa, A. C., Galbraith, D. R., Oliveira, R. S., Binks, O. J., Oliveira, A. A., et al. (2015). Death from drought in tropical forests is triggered by hydraulics not carbon starvation. Nature 528, 119–122. doi: 10.1038/nature15539
Sakai, S., Harrison, R. D., Momose, K., Kuraji, K., Nagamasu, H., Yasunari, T., et al. (2006). Irregular droughts trigger mass flowering in aseasonal tropical forests in Asia. Am. J. Bot. 93, 1134–1139. doi: 10.3732/ajb.93.8.1134
Slik, J. W. F. (2004). El Niño droughts and their effects on tree species composition and diversity in tropical rain forests. Oecologia 141, 114–120. doi: 10.1007/s00442-004-1635-y
Slot, M., and Poorter, L. (2007). Diversity of tropical tree seedling responses to drought. Biotropica 39, 683–690. doi: 10.1111/j.1744-7429.2007.00328.x
Spracklen, D. V., Arnold, S. R., and Taylor, C. M. (2012). Observations of increased tropical rainfall preceded by air passage over forests. Nature 489, 282–285. doi: 10.1038/nature11390
Tardieu, F., and Simonneau, T. (1998). Variability among species of stomatal control under fluctuating soil water status and evaporative demand: Modelling isohydric and anisohydric behaviours. J. Exp. Bot. 49, 419–432. doi: 10.1093/jexbot/49.suppl_1.419
Thirumalai, K., Dinezio, P. N., Okumura, Y., and Deser, C. (2017). Extreme temperatures in Southeast Asia caused by El Niño and worsened by global warming. Nat. Commun. 8, 15531–15531. doi: 10.1038/ncomms15531
Timmermann, A., Oberhuber, J., Bacher, A., Esch, M., Latif, M., and Roeckner, E. (1999). Increased El Niño frequency in a climate model forced by future greenhouse warming. Nature 398, 694–697. doi: 10.1038/19505
Tuck, S. L., O’Brien, M. J., Philipson, C. D., Saner, P., Tanadini, M., Dzulkifli, D., et al. (2016). The value of biodiversity for the functioning of tropical forests: Insurance effects during the first decade of the Sabah biodiversity experiment. Philos. Trans. R. Soc. Lond. B Biol. Sci. 283:20161451. doi: 10.1098/rspb.2016.1451
Tyree, M. T., and Hammel, H. T. (1972). The measurement of the turgor pressure and the water relations of plants by the pressure-bomb technique. J. Exp. Bot. 23, 267–282. doi: 10.1093/jxb/23.1.267
Tyree, M. T., Dainty, J., and Hunter, D. M. (1974). The water relations of hemlock (Tsuga canadensis). IV. The dependence of the balance pressure on temperature as measured by the pressure-bomb technique. Can. J. Bot. 52, 973–978. doi: 10.1139/b73-186
Tyree, M., Patiño, S., and Becker, P. (1998). Vulnerability to drought-induced embolism of Bornean heath and dipterocarp forest trees. Tree Physiol. 18, 583–588. doi: 10.1093/treephys/18.8-9.583
van Nieuwstadt, M. G. L., and Sheil, D. (2005). Drought, fire and tree survival in a Borneo rain forest, East Kalimantan, Indonesia. J. Ecol. 93, 191–201. doi: 10.1111/j.1365-2745.2004.00954.x
Vincent, A., and Davies, S. (2003). Effects of nutrient addition, mulching and planting-hole size on early performance of Dryobalanops aromatica and Shorea parvifolia planted in secondary forest in Sarawak, Malaysia. For. Ecol. Manag. 180, 261–271. doi: 10.1016/S0378-1127(02)00562-5
Wang, G., Cai, W., Gan, B., Wu, L., Santoso, A., Lin, X., et al. (2017). Continued increase of extreme El Niño frequency long after 1.5 °C warming stabilization. Nature Clim. Change 7:568. doi: 10.1038/nclimate3351
Widiyatno Hidayati, F., Hardiwinoto, S., Indrioko, S., Purnomo, S., and Jatmoko, et al. (2020). Selection of dipterocarp species for enrichment planting in a secondary tropical rainforest. For. Sci. Technol. 16, 206–215. doi: 10.1080/21580103.2020.1831620
Williamson, G. B., Laurance, W. F., Oliveira, A. A., Delamônica, P., Gascon, C., Lovejoy, T. E., et al. (2000). Amazonian tree mortality during the 1997 El Niño drought. Conserv. Biol. 14, 1538–1542. doi: 10.1046/j.1523-1739.2000.99298.x
Woo, N. S., Badger, M. R., and Pogson, B. J. (2008). A rapid, non-invasive procedure for quantitative assessment of drought survival using chlorophyll fluorescence. Plant Methods 4:27. doi: 10.1186/1746-4811-4-27
Yoshifuji, N., Kumagai, T., Ichie, T., Kume, T., Tateishi, M., Inoue, Y., et al. (2020). Limited stomatal regulation of the largest-size class of Dryobalanops aromatica in a Bornean tropical rainforest in response to artificial soil moisture reduction. J. Plant Res. 133, 175–191. doi: 10.1007/s10265-019-01161-3
Keywords: drought stress, isohydric/anisohydric behavior, leaf water potential, stomatal regulation, tropical rainforest
Citation: Ichie T, Igarashi S, Tanimoto T, Inoue Y, Mohizah M and Kenzo T (2023) Ecophysiological responses of seedlings of six dipterocarp species to short-term drought in Borneo. Front. For. Glob. Change 6:1112852. doi: 10.3389/ffgc.2023.1112852
Received: 30 November 2022; Accepted: 17 January 2023;
Published: 03 February 2023.
Edited by:
Narkis Morales, Pontificia Universidad Católica de Chile, ChileReviewed by:
Dapao Yu, Institute of Applied Ecology (CAS), ChinaTianyuan Yang, Anhui Agricultural University, China
Satoshi Kitaoka, Hokkaido University, Japan
Mai Kamakura, Kyoto University, Japan
Copyright © 2023 Ichie, Igarashi, Tanimoto, Inoue, Mohizah and Kenzo. This is an open-access article distributed under the terms of the Creative Commons Attribution License (CC BY). The use, distribution or reproduction in other forums is permitted, provided the original author(s) and the copyright owner(s) are credited and that the original publication in this journal is cited, in accordance with accepted academic practice. No use, distribution or reproduction is permitted which does not comply with these terms.
*Correspondence: Tomoaki Ichie, ichie@kochi-u.ac.jp